- Reference Manager
- Simple TEXT file
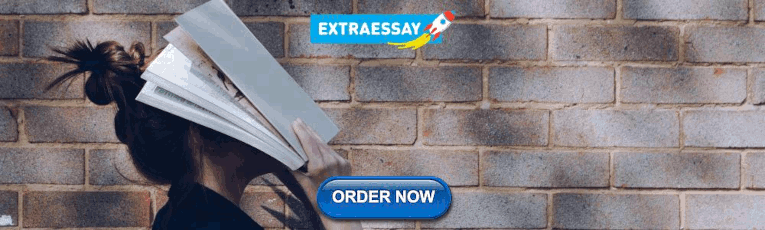
People also looked at
Review article, effects of water pollution on human health and disease heterogeneity: a review.

- 1 Research Center for Economy of Upper Reaches of the Yangtse River/School of Economics, Chongqing Technology and Business University, Chongqing, China
- 2 School of Economics and Management, Huzhou University, Huzhou, China
Background: More than 80% of sewage generated by human activities is discharged into rivers and oceans without any treatment, which results in environmental pollution and more than 50 diseases. 80% of diseases and 50% of child deaths worldwide are related to poor water quality.
Methods: This paper selected 85 relevant papers finally based on the keywords of water pollution, water quality, health, cancer, and so on.
Results: The impact of water pollution on human health is significant, although there may be regional, age, gender, and other differences in degree. The most common disease caused by water pollution is diarrhea, which is mainly transmitted by enteroviruses in the aquatic environment.
Discussion: Governments should strengthen water intervention management and carry out intervention measures to improve water quality and reduce water pollution’s impact on human health.
Introduction
Water is an essential resource for human survival. According to the 2021 World Water Development Report released by UNESCO, the global use of freshwater has increased six-fold in the past 100 years and has been growing by about 1% per year since the 1980s. With the increase of water consumption, water quality is facing severe challenges. Industrialization, agricultural production, and urban life have resulted in the degradation and pollution of the environment, adversely affecting the water bodies (rivers and oceans) necessary for life, ultimately affecting human health and sustainable social development ( Xu et al., 2022a ). Globally, an estimated 80% of industrial and municipal wastewater is discharged into the environment without any prior treatment, with adverse effects on human health and ecosystems. This proportion is higher in the least developed countries, where sanitation and wastewater treatment facilities are severely lacking.
Sources of Water Pollution
Water pollution are mainly concentrated in industrialization, agricultural activities, natural factors, and insufficient water supply and sewage treatment facilities. First, industry is the main cause of water pollution, these industries include distillery industry, tannery industry, pulp and paper industry, textile industry, food industry, iron and steel industry, nuclear industry and so on. Various toxic chemicals, organic and inorganic substances, toxic solvents and volatile organic chemicals may be released in industrial production. If these wastes are released into aquatic ecosystems without adequate treatment, they will cause water pollution ( Chowdhary et al., 2020 ). Arsenic, cadmium, and chromium are vital pollutants discharged in wastewater, and the industrial sector is a significant contributor to harmful pollutants ( Chen et al., 2019 ). With the acceleration of urbanization, wastewater from industrial production has gradually increased. ( Wu et al., 2020 ). In addition, water pollution caused by industrialization is also greatly affected by foreign direct investment. Industrial water pollution in less developed countries is positively correlated with foreign direct investment ( Jorgenson, 2009 ). Second, water pollution is closely related to agriculture. Pesticides, nitrogen fertilizers and organic farm wastes from agriculture are significant causes of water pollution (RCEP, 1979). Agricultural activities will contaminate the water with nitrates, phosphorus, pesticides, soil sediments, salts and pathogens ( Parris, 2011 ). Furthermore, agriculture has severely damaged all freshwater systems in their pristine state ( Moss, 2008 ). Untreated or partially treated wastewater is widely used for irrigation in water-scarce regions of developing countries, including China and India, and the presence of pollutants in sewage poses risks to the environment and health. Taking China as an example, the imbalance in the quantity and quality of surface water resources has led to the long-term use of wastewater irrigation in some areas in developing countries to meet the water demand of agricultural production, resulting in serious agricultural land and food pollution, pesticide residues and heavy metal pollution threatening food safety and Human Health ( Lu et al., 2015 ). Pesticides have an adverse impact on health through drinking water. Comparing pesticide use with health life Expectancy Longitudinal Survey data, it was found that a 10% increase in pesticide use resulted in a 1% increase in the medical disability index over 65 years of age ( Lai, 2017 ). The case of the Musi River in India shows a higher incidence of morbidity in wastewater-irrigated villages than normal-water households. Third, water pollution is related to natural factors. Taking Child Loess Plateau as an example, the concentration of trace elements in water quality is higher than the average world level, and trace elements come from natural weathering and manufacture causes. Poor river water quality is associated with high sodium and salinity hazards ( Xiao et al., 2019 ). The most typical water pollution in the middle part of the loess Plateau is hexavalent chromium pollution, which is caused by the natural environment and human activities. Loess and mudstone are the main sources, and groundwater with high concentrations of hexavalent chromium is also an important factor in surface water pollution (He et al., 2020). Finally, water supply and sewage treatment facilities are also important factors affecting drinking water quality, especially in developing countries. In parallel with China rapid economic growth, industrialization and urbanization, underinvestment in basic water supply and treatment facilities has led to water pollution, increased incidence of infectious and parasitic diseases, and increased exposure to industrial chemicals, heavy metals and algal toxins ( Wu et al., 1999 ). An econometric model predicts the impact of water purification equipment on water quality and therefore human health. When the proportion of household water treated with water purification equipment is reduced from 100% to 90%, the expected health benefits are reduced by up to 96%.. When the risk of pretreatment water quality is high, the decline is even more significant ( Brown and Clasen, 2012 ).
To sum up, water pollution results from both human and natural factors. Various human activities will directly affect water quality, including urbanization, population growth, industrial production, climate change, and other factors ( Halder and Islam, 2015 ) and religious activities ( Dwivedi et al., 2018 ). Improper disposal of solid waste, sand, and gravel is also one reason for decreasing water quality ( Ustaoğlua et al., 2020 ).
Impact of Water Pollution on Human Health
Unsafe water has severe implications for human health. According to UNESCO 2021 World Water Development Report , about 829,000 people die each year from diarrhea caused by unsafe drinking water, sanitation, and hand hygiene, including nearly 300,000 children under the age of five, representing 5.3 percent of all deaths in this age group. Data from Palestine suggest that people who drink municipal water directly are more likely to suffer from diseases such as diarrhea than those who use desalinated and household-filtered drinking water ( Yassin et al., 2006 ). In a comparative study of tap water, purified water, and bottled water, tap water was an essential source of gastrointestinal disease ( Payment et al., 1997 ). Lack of water and sanitation services also increases the incidence of diseases such as cholera, trachoma, schistosomiasis, and helminthiasis. Data from studies in developing countries show a clear relationship between cholera and contaminated water, and household water treatment and storage can reduce cholera ( Gundry et al., 2004 ). In addition to disease, unsafe drinking water, and poor environmental hygiene can lead to gastrointestinal illness, inhibiting nutrient absorption and malnutrition. These effects are especially pronounced for children.
Purpose of This Paper
More than two million people worldwide die each year from diarrhoeal diseases, with poor sanitation and unsafe drinking water being the leading cause of nearly 90% of deaths and affecting children the most (United Nations, 2016). More than 50 kinds of diseases are caused by poor drinking water quality, and 80% of diseases and 50% of child deaths are related to poor drinking water quality in the world. However, water pollution causes diarrhea, skin diseases, malnutrition, and even cancer and other diseases related to water pollution. Therefore, it is necessary to study the impact of water pollution on human health, especially disease heterogeneity, and clarify the importance of clean drinking water, which has important theoretical and practical significance for realizing sustainable development goals. Unfortunately, although many kinds of literature focus on water pollution and a particular disease, there is still a lack of research results that systematically analyze the impact of water pollution on human health and the heterogeneity of diseases. Based on the above background and discussion, this paper focuses on the effect of water pollution on human health and its disease heterogeneity.
Materials and Methods
Search process.
This article uses keywords such as “water,” “water pollution,” “water quality,” “health,” “diarrhea,” “skin disease,” “cancer” and “children” to search Web of Science and Google Scholar include SCI and SSCI indexed papers, research reports, and works from 1990 to 2021.
Inclusion-Exclusion Criteria and Data Extraction Process
The existing literature shows that water pollution and human health are important research topics in health economics, and scholars have conducted in-depth research. As of 30 December 2021, 104 related literatures were searched, including research papers, reviews and conference papers. Then, according to the content relevancy, 19 papers were eliminated, and 85 papers remained. The purpose of this review is to summarize the impact of water pollution on human health and its disease heterogeneity and to explore how to improve human health by improving water pollution control measures.
Information extracted from all included papers included: author, publication date, sample country, study methodology, study purpose, and key findings. All analysis results will be analyzed according to the process in Figure 1 .
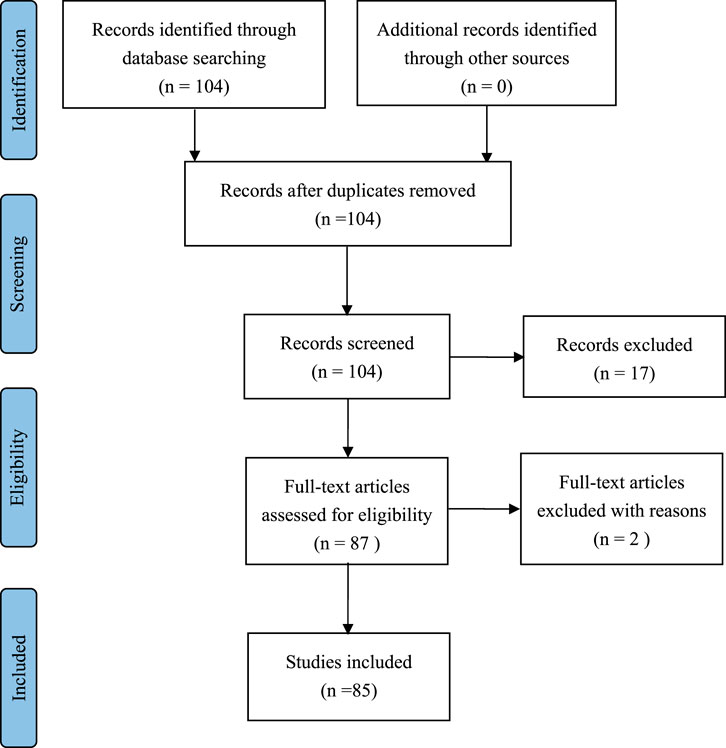
FIGURE 1 . Data extraction process (PRISMA).
The relevant information of the paper is exported to the Excel database through Endnote, and the duplicates are deleted. The results were initially extracted by one researcher and then cross-checked by another researcher to ensure that all data had been filtered and reviewed. If two researchers have different opinions, the two researchers will review together until a final agreement is reached.
Quality Assessment of the Literature
The JBI Critical Appraisal Checklist was used to evaluate the quality of each paper. The JBI (Joanna Briggs Institute) key assessment tool was developed by the JBI Scientific Committee after extensive peer review and is designed for system review. All features of the study that meet the following eight criteria are included in the final summary:1) clear purpose; 2) Complete information of sample variables; 3) Data basis; 4) the validity of data sorting; 5) ethical norms; (6); 7) Effective results; 8) Apply appropriate quantitative methods and state the results clearly. Method quality is evaluated by the Yes/No questions listed in the JBI Key Assessment List. Each analysis paper received 6 out of 8.
The quality of drinking water is an essential factor affecting human health. Poor drinking water quality has led to the occurrence of water-borne diseases. According to the World Health Organization (WHO) survey, 80% of the world’s diseases and 50% of the world’s child deaths are related to poor drinking water quality, and there are more than 50 diseases caused by poor drinking water quality. The quality of drinking water in developing countries is worrying. The negative health effects of water pollution remain the leading cause of morbidity and mortality in developing countries. Different from the existing literature review, this paper mainly studies the impact of water pollution on human health according to the heterogeneity of diseases. We focuses on diarrhea, skin diseases, cancer, child health, etc., and sorts out the main effects of water pollution on human health ( Table 1 ).
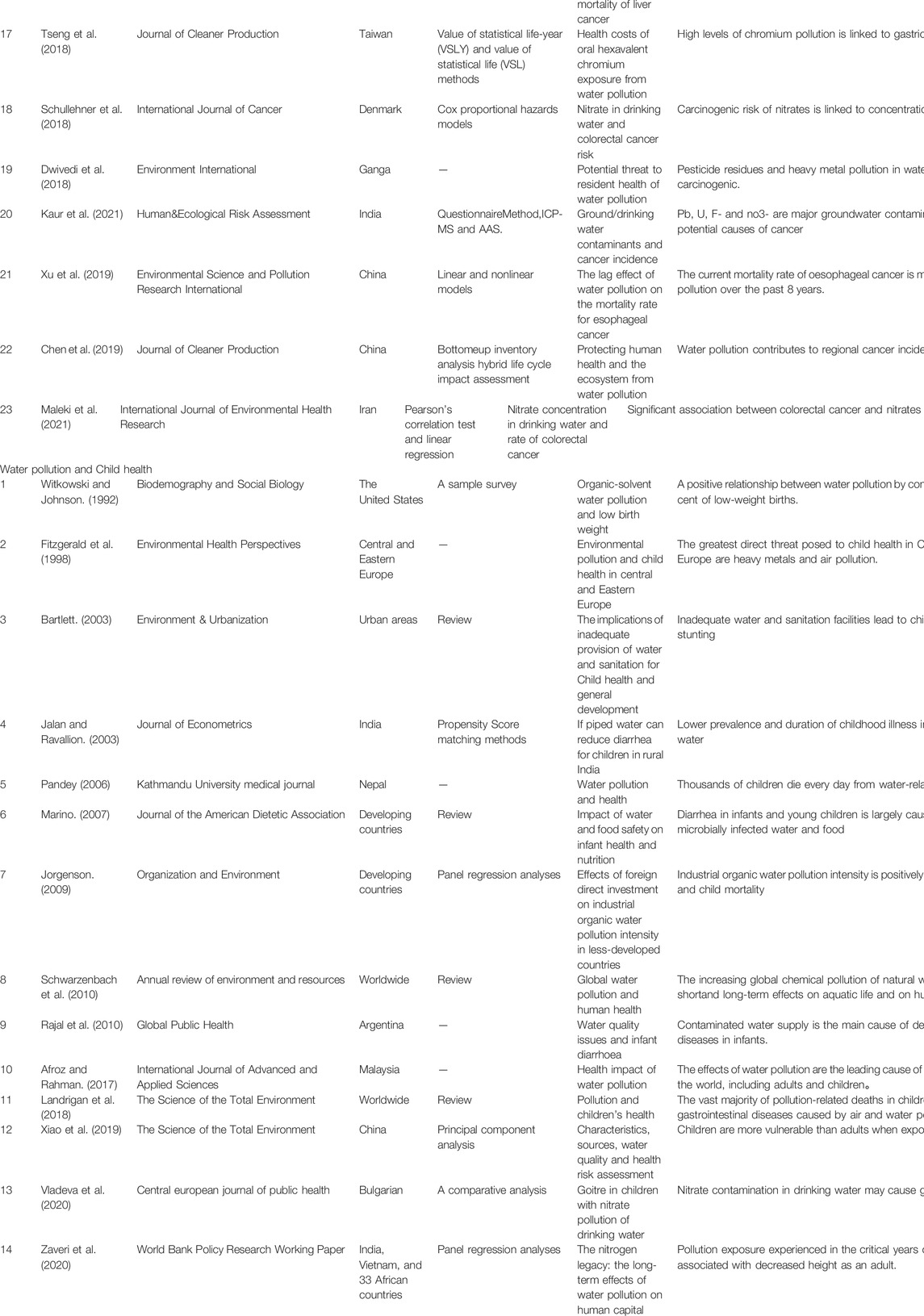
TABLE 1 . Major studies on the relationship between water pollution and health.
Water Pollution and Diarrhea
Diarrhea is a common symptom of gastrointestinal diseases and the most common disease caused by water pollution. Diarrhea is a leading cause of illness and death in young children in low-income countries. Diarrhoeal diseases account for 21% of annual deaths among children under 5 years of age in developing countries ( Waddington et al., 2009 ). Many infectious agents associated with diarrhea are directly related to contaminated water ( Ahmed and Ismail, 2018 ). Parasitic worms present in non-purifying drinking water when is consumed by human beings causes diseases ( Ansari and Akhmatov., 2020 ) . It was found that treated water from water treatment facilities was associated with a lower risk of diarrhea than untreated water for all ages ( Clasen et al., 2015 ). For example, in the southern region of Brazil, a study found that factors significantly associated with an increased risk of mortality from diarrhoea included lack of plumbed water, lack of flush toilets, poor housing conditions, and overcrowded households. Households without access to piped water had a 4.8 times higher risk of infant death from diarrhea than households with access to piped water ( Victora et al., 1988 )
Enteroviruses exist in the aquatic environment. More than 100 pathogenic viruses are excreted in human and animal excreta and spread in the environment through groundwater, estuarine water, seawater, rivers, sewage treatment plants, insufficiently treated water, drinking water, and private wells ( Fong and Lipp., 2005 ). A study in Pakistan showed that coliform contamination was found in some water sources. Improper disposal of sewage and solid waste, excessive use of pesticides and fertilizers, and deteriorating pipeline networks are the main causes of drinking water pollution. The main source of water-borne diseases such as gastroenteritis, dysentery, diarrhea, and viral hepatitis in this area is the water pollution of coliform bacteria ( Khan et al., 2013 ). Therefore, the most important role of water and sanitation health interventions is to hinder the transmission of diarrheal pathogens from the environment to humans ( Waddington et al., 2009 ).
Meta-analyses are the most commonly used method for water quality and diarrhea studies. It was found that improving water supply and sanitation reduced the overall incidence of diarrhea by 26%. Among Malaysian infants, having clean water and sanitation was associated with an 82% reduction in infant mortality, especially among infants who were not breastfed ( Esrey et al., 1991 ). All water quality and sanitation interventions significantly reduced the risk of diarrhoeal disease, and water quality interventions were found to be more effective than previously thought. Multiple interventions (including water, sanitation, and sanitation measures) were not more effective than single-focus interventions ( Fewtrell and Colford., 2005 ). Water quality interventions reduced the risk of diarrhoea in children and reduced the risk of E. coli contamination of stored water ( Arnold and Colford., 2007 ). Interventions to improve water quality are generally effective in preventing diarrhoea in children of all ages and under 5. However, some trials showed significant heterogeneity, which may be due to the research methods and their conditions ( Clasen et al., 2007 ).
Water Pollution and Skin Diseases
Contrary to common sense that swimming is good for health, studies as early as the 1950s found that the overall disease incidence in the swimming group was significantly higher than that in the non-swimming group. The survey shows that the incidence of the disease in people under the age of 10 is about 100% higher than that of people over 10 years old. Skin diseases account for a certain proportion ( Stevenson, 1953 ). A prospective epidemiological study of beach water pollution was conducted in Hong Kong in the summer of 1986–1987. The study found that swimmers on Hong Kong’s coastal beaches were more likely than non-swimmers to complain of systemic ailments such as skin and eyes. And swimming in more polluted beach waters has a much higher risk of contracting skin diseases and other diseases. Swimming-related disease symptom rates correlated with beach cleanliness ( Cheung et al., 1990 ).
A study of arsenic-affected villages in the southern Sindh province of Pakistan emphasized that skin diseases were caused by excessive water quality. By studying the relationship between excessive arsenic in drinking water caused by water pollution and skin diseases (mainly melanosis and keratosis), it was found that compared with people who consumed urban low-arsenic drinking water, the hair of people who consumed high-arsenic drinking water arsenic concentration increased significantly. The level of arsenic in drinking water directly affects the health of local residents, and skin disease is the most common clinical complication of arsenic poisoning. There is a correlation between arsenic concentrations in biological samples (hair and blood) from patients with skin diseases and intake of arsenic-contaminated drinking water ( Kazi et al., 2009 ). Another Bangladesh study showed that many people suffer from scabies due to river pollution ( Hanif et al., 2020 ). Not only that, but water pollution from industry can also cause skin cancer ( Arif et al., 2020 ).
Studies using meta-analysis have shown that exposure to polluted Marine recreational waters can have adverse consequences, including frequent skin discomfort (such as rash or itching). Skin diseases in swimmers may be caused by a variety of pathogenic microorganisms ( Yau et al., 2009 ). People (swimmers and non-swimmers) exposed to waters above threshold levels of bacteria had a higher relative risk of developing skin disease, and levels of bacteria in seawater were highly correlated with skin symptoms.
Studies have also suggested that swimmers are 3.5 times more likely to report skin diseases than non-swimmers. This difference may be a “risk perception bias” at work on swimmers, who are generally aware that such exposure may lead to health effects and are more likely to detect and report skin disorders. It is also possible that swimmers exaggerated their symptoms, reporting conditions that others would not classify as true skin disorders ( Fleisher and Kay. 2006 ).
Water Pollution and Cancer
According to WHO statistics, the number of cancer patients diagnosed in 2020 reached 19.3 million, while the number of deaths from cancer increased to 10 million. Currently, one-fifth of all global fevers will develop cancer during their lifetime. The types and amounts of carcinogens present in drinking water will vary depending on where they enter: contamination of the water source, water treatment processes, or when the water is delivered to users ( Morris, 1995 ).
From the perspective of water sources, arsenic, nitrate, chromium, etc. are highly associated with cancer. Ingestion of arsenic from drinking water can cause skin cancer and kidney and bladder cancer ( Marmot et al., 2007 ). The risk of cancer in the population from arsenic in the United States water supply may be comparable to the risk from tobacco smoke and radon in the home environment. However, individual susceptibility to the carcinogenic effects of arsenic varies ( Smith et al., 1992 ). A high association of arsenic in drinking water with lung cancer was demonstrated in a northern Chilean controlled study involving patients diagnosed with lung cancer and a frequency-matched hospital between 1994 and 1996. Studies have also shown a synergistic effect of smoking and arsenic intake in drinking water in causing lung cancer ( Ferreccio et al., 2000 ). Exposure to high arsenic levels in drinking water was also associated with the development of liver cancer, but this effect was not significant at exposure levels below 0.64 mg/L ( Lin et al., 2013 ).
Nitrates are a broader contaminant that is more closely associated with human cancers, especially colorectal cancer. A study in East Azerbaijan confirmed a significant association between colorectal cancer and nitrate in men, but not in women (Maleki et al., 2021). The carcinogenic risk of nitrates is concentration-dependent. The risk increases significantly when drinking water levels exceed 3.87 mg/L, well below the current drinking water standard of 50 mg/L. Drinking water with nitrate concentrations lower than current drinking water standards also increases the risk of colorectal cancer ( Schullehner et al., 2018 ).
Drinking water with high chromium content will bring high carcinogenicity caused by hexavalent chromium to residents. Drinking water intake of hexavalent chromium experiments showed that hexavalent chromium has the potential to cause human respiratory cancer. ( Zhitkovich, 2011 ). A case from Changhua County, Taiwan also showed that high levels of chromium pollution were associated with gastric cancer incidence ( Tseng et al., 2018 ).
There is a correlation between trihalomethane (THM) levels in drinking water and cancer mortality. Bladder and brain cancers in both men and women and non-Hodgkin’s lymphoma and kidney cancer in men were positively correlated with THM levels, and bladder cancer mortality had the strongest and most consistent association with THM exposure index ( Cantor et al., 1978 ).
From the perspective of water treatment process, carcinogens may be introduced during chlorine treatment, and drinking water is associated with all cancers, urinary cancers and gastrointestinal cancers ( Page et al., 1976 ). Chlorinated byproducts from the use of chlorine in water treatment are associated with an increased risk of bladder and rectal cancer, with perhaps 5,000 cases of bladder and 8,000 cases of rectal cancer occurring each year in the United States (Morris, 1995).
The impact of drinking water pollutants on cancer is complex. Epidemiological studies have shown that drinking water contaminants, such as chlorinated by-products, nitrates, arsenic, and radionuclides, are associated with cancer in humans ( Cantor, 1997 ). Pb, U, F- and no3- are the main groundwater pollutants and one of the potential causes of cancer ( Kaur et al., 2021 ). In addition, many other water pollutants are also considered carcinogenic, including herbicides and pesticides, and fertilizers that contain and release nitrates ( Marmot et al., 2007 ). A case from Hebei, China showed that the contamination of nitrogen compounds in well water was closely related to the use of nitrogen fertilizers in agriculture, and the levels of three nitrogen compounds in well water were significantly positively correlated with esophageal cancer mortality ( Zhang et al., 2003 ).
In addition, due to the time-lag effect, the impact of watershed water pollution on cancer is spatially heterogeneous. The mortality rate of esophageal cancer caused by water pollution is significantly higher downstream than in other regions due to the impact of historical water pollution ( Xu et al., 2019 ). A study based on changes in water quality in the watershed showed that a grade 6 deterioration in water quality resulted in a 9.3% increase in deaths from digestive cancer. ( Ebenstein, 2012 ).
Water Pollution and Child Health
Diarrhea is a common disease in children. Diarrhoeal diseases (including cholera) kill 1.8 million people each year, 90 per cent of them children under the age of five, mostly in developing countries. 88% of diarrhoeal diseases are caused by inadequate water supply, sanitation and hygiene (Team, 2004). A large proportion of these are caused by exposure to microbially infected water and food, and diarrhea in infants and young children can lead to malnutrition and reduced immune resistance, thereby increasing the likelihood of prolonged and recurrent diarrhea ( Marino, 2007 ). Pollution exposure experienced by children during critical periods of development is associated with height loss in adulthood ( Zaveri et al., 2020 ). Diseases directly related to water and sanitation, combined with malnutrition, also lead to other causes of death, such as measles and pneumonia. Child malnutrition and stunting due to inadequate water and sanitation will continue to affect more than one-third of children in the world ( Bartlett, 2003 ). A study from rural India showed that children living in households with tap water had significantly lower disease prevalence and duration ( Jalan and Ravallion, 2003 ).
In conclusion, water pollution is a significant cause of childhood diseases. Air, water, and soil pollution together killed 940,000 children worldwide in 2016, two-thirds of whom were under the age of 5, and the vast majority occurred in low- and middle-income countries ( Landrigan et al., 2018 ). The intensity of industrial organic water pollution is positively correlated with infant mortality and child mortality in less developed countries, and industrial water pollution is an important cause of infant and child mortality in less developed countries ( Jorgenson, 2009 ). In addition, arsenic in drinking water is a potential carcinogenic risk in children (García-Rico et al., 2018). Nitrate contamination in drinking water may cause goiter in children ( Vladeva et al.., 2000 ).
Discussions
This paper reviews the environmental science, health, and medical literature, with a particular focus on epidemiological studies linking water quality, water pollution, and human disease, as well as studies on water-related disease morbidity and mortality. At the same time, special attention is paid to publications from the United Nations and the World Health Organization on water and sanitation health research. The purpose of this paper is to clarify the relationship between water pollution and human health, including: The relationship between water pollution and diarrhea, the mechanism of action, and the research situation of meta-analysis; The relationship between water pollution and skin diseases, pathogenic factors, and meta-analysis research; The relationship between water pollution and cancer, carcinogenic factors, and types of cancer; The relationship between water pollution and Child health, and the major childhood diseases caused.
A study of more than 100 literatures found that although factors such as country, region, age, and gender may have different influences, in general, water pollution has a huge impact on human health. Water pollution is the cause of many human diseases, mainly diarrhoea, skin diseases, cancer and various childhood diseases. The impact of water pollution on different diseases is mainly reflected in the following aspects. Firstly, diarrhea is the most easily caused disease by water pollution, mainly transmitted by enterovirus existing in the aquatic environment. The transmission environment of enterovirus depends on includes groundwater, river, seawater, sewage, drinking water, etc. Therefore, it is necessary to prevent the transmission of enterovirus from the environment to people through drinking water intervention. Secondly, exposure to or use of heavily polluted water is associated with a risk of skin diseases. Excessive bacteria in seawater and heavy metals in drinking water are the main pathogenic factors of skin diseases. Thirdly, water pollution can pose health risks to humans through any of the three links: the source of water, the treatment of water, and the delivery of water. Arsenic, nitrate, chromium, and trihalomethane are major carcinogens in water sources. Carcinogens may be introduced during chlorine treatment from water treatment. The effects of drinking water pollution on cancer are complex, including chlorinated by-products, heavy metals, radionuclides, herbicides and pesticides left in water, etc., Finally, water pollution is an important cause of children’s diseases. Contact with microbiologically infected water can cause diarrhoeal disease in children. Malnutrition and weakened immunity from diarrhoeal diseases can lead to other diseases.
This study systematically analyzed the impact of water pollution on human health and the heterogeneity of diseases from the perspective of different diseases, focusing on a detailed review of the relationship, mechanism and influencing factors of water pollution and diseases. From the point of view of limitations, this paper mainly focuses on the research of environmental science and environmental management, and the research on pathology is less involved. Based on this, future research can strengthen research at medical and pathological levels.
In response to the above research conclusions, countries, especially developing countries, need to adopt corresponding water management policies to reduce the harm caused by water pollution to human health. Firstly, there is a focus on water quality at the point of use, with interventions to improve water quality, including chlorination and safe storage ( Gundry et al., 2004 ), and provision of treated and clean water ( Khan et al., 2013 ). Secondly, in order to reduce the impact of water pollution on skin diseases, countries should conduct epidemiological studies on their own in order to formulate health-friendly bathing water quality standards suitable for their specific conditions ( Cheung et al., 1990 ). Thirdly, in order to reduce the cancer caused by water pollution, the whole-process supervision of water quality should be strengthened, that is, the purity of water sources, the scientific nature of water treatment and the effectiveness of drinking water monitoring. Fourthly, each society should prevent and control source pollution from production, consumption, and transportation ( Landrigan et al., 2018 ). Fifthly, health education is widely carried out. Introduce environmental education, educate residents on sanitary water through newspapers, magazines, television, Internet and other media, and enhance public health awareness. Train farmers to avoid overuse of agricultural chemicals that contaminate drinking water.
Author Contributions
Conceptualization, XX|; methodology, LL; data curation, HY; writing and editing, LL; project administration, XX|.
This article is a phased achievement of The National Social Science Fund of China: Research on the blocking mechanism of the critical poor households returning to poverty due to illness, No: 20BJY057.
Conflict of Interest
The authors declare that the research was conducted in the absence of any commercial or financial relationships that could be construed as a potential conflict of interest.
Publisher’s Note
All claims expressed in this article are solely those of the authors and do not necessarily represent those of their affiliated organizations, or those of the publisher, the editors and the reviewers. Any product that may be evaluated in this article, or claim that may be made by its manufacturer, is not guaranteed or endorsed by the publisher.
Afroz, R., Rahman, A., and Rahman, A. (2017). Health Impact of River Water Pollution in Malaysia. Int. J. Adv. Appl. Sci. 4 (5), 78–85. doi:10.21833/ijaas.2017.05.014
CrossRef Full Text | Google Scholar
Ahmed, S., and Ismail, S. (2018). Water Pollution and its Sources, Effects and Management: a Case Study of Delhi. Int. J. Curr. Adv. Res. 7 (2), 10436–10442. doi:10.24327/ijcar.2018.10442.1768
Ansari, Z. Z., and Akhmatov, S. V. (2020). Impacts of Water Pollution on Human Health: A Case Study of Delhi .
Google Scholar
Arif, A., Malik, M. F., Liaqat, S., Aslam, A., Mumtaz, K., and Afzal, A. (2020). 3. Water Pollution and Industries. Pure Appl. Biol. (PAB) 9 (4), 2214–2224. doi:10.19045/bspab.2020.90237
Arnold, B. F., and Colford, J. M. (2007). Treating Water with Chlorine at Point-Of-Use to Improve Water Quality and Reduce Child Diarrhea in Developing Countries: a Systematic Review and Meta-Analysis. Am. J. Trop. Med. Hyg. 76 (2), 354–364. doi:10.4269/ajtmh.2007.76.354
PubMed Abstract | CrossRef Full Text | Google Scholar
Bartlett, S. (2003). Water, Sanitation and Urban Children: the Need to Go beyond “Improved” Provision. Environ. Urbanization 15 (2), 57–70. doi:10.1177/095624780301500220
Bessong, P. O., Odiyo, J. O., Musekene, J. N., and Tessema, A. (2009). Spatial Distribution of Diarrhoea and Microbial Quality of Domestic Water during an Outbreak of Diarrhoea in the Tshikuwi Community in Venda, South Africa. J. Health Popul. Nutr. 27 (5), 652–659. doi:10.3329/jhpn.v27i5.3642
Boldo, E., MartÍN-Olmedo, P., Medina, S., Pirard, P., Mouly, D., and Beaudeau, P. (2006). Towards the Quantification of Health Impacts Caused by Drinking-Water Pollution in European Countries. Epidemiology 17 (6), S447. doi:10.1097/00001648-200611001-01198
Brown, J., and Clasen, T. (2012). High Adherence Is Necessary to Realize Health Gains from Water Quality Interventions. PLoS ONE 7 (5), e36735–9. doi:10.1371/journal.pone.0036735
Cantor, K. P., Hoover, R., Mason, T. J., and McCabe, L. J. (1978). Associations of Cancer Mortality with Halomethanes in Drinking Water. J. Natl. Cancer Inst. 61 (4), 979
PubMed Abstract | Google Scholar
Cantor, K. P. (1997). Drinking Water and Cancer. Cancer Causes Control CCC 8 (3), 292–308. doi:10.1023/a:1018444902486
Chen, B., Wang, M., Duan, M., Ma, X., Hong, J., Xie, F., et al. (2019). In Search of Key: Protecting Human Health and the Ecosystem from Water Pollution in China. J. Clean. Prod. 228, 101–111. doi:10.1016/j.jclepro.2019.04.228
Cheung, W. H. S., Chang, K. C. K., Hung, R. P. S., and Kleevens, J. W. L. (1990). Health Effects of Beach Water Pollution in Hong Kong. Epidemiol. Infect. 105 (1), 139–162. doi:10.1017/s0950268800047737
Cheung, W. H. S., Hung, R. P. S., Chang, K. C. K., and Kleevens, J. W. L. (1991). Epidemiological Study of Beach Water Pollution and Health-Related Bathing Water Standards in Hong Kong. Water Sci. Technol. 23 (1-3), 243–252. doi:10.2166/wst.1991.0422
Chowdhary, P., Bharagava, R. N., Mishra, S., and Khan, N. (2020). Role of Industries in Water Scarcity and its Adverse Effects on Environment and Human Health. Environ. Concerns Sustain. Dev. , 235–256. doi:10.1007/978-981-13-5889-0_12
Clasen, T. F., Alexander, K. T., Sinclair, D., Boisson, S., Peletz, R., Chang, H. H., et al. (2015). Interventions to Improve Water Quality for Preventing Diarrhoea. Cochrane Database Syst. Rev. 10, CD004794. doi:10.1002/14651858.CD004794.pub3
Clasen, T., Schmidt, W.-P., Rabie, T., Roberts, I., and Cairncross, S. (2007). Interventions to Improve Water Quality for Preventing Diarrhoea: Systematic Review and Meta-Analysis. Bmj 334 (7597), 782. doi:10.1136/bmj.39118.489931.be
Conroy, R. M., Elmore-Meegan, M., Joyce, T., McGuigan, K. G., and Barnes, J. (1996). Solar Disinfection of Drinking Water and Diarrhoea in Maasai Children: a Controlled Field Trial. Lancet 348 (9043), 1695–1697. doi:10.1016/s0140-6736(96)02309-4
Dasgupta, P. (2004). Valuing Health Damages from Water Pollution in Urban Delhi, India: a Health Production Function Approach. Envir. Dev. Econ. 9 (1), 83–106. doi:10.1017/s1355770x03001098
Dwivedi, S., Mishra, S., and Tripathi, R. D. (2018). Ganga Water Pollution: A Potential Health Threat to Inhabitants of Ganga Basin. Environ. Int. 117, 327–338. doi:10.1016/j.envint.2018.05.015
Ebenstein, A. (2012). The Consequences of Industrialization: Evidence from Water Pollution and Digestive Cancers in China. Rev. Econ. Statistics 94 (1), 186–201. doi:10.1162/rest_a_00150
El-Kowrany, S. I., El- Zamarany, E. A., El-Nouby, K. A., El-Mehy, D. A., Abo Ali, E. A., and Othman, A. A. (2016). Water Pollution in the Middle Nile Delta, Egypt: an Environmental Study. J. Adv. Res. 7 (5), 781–794. doi:10.1016/j.jare.2015.11.005
Enrique Biagini, R. (1975). Chronic Arsenic Water Pollution in the Republic of Argentina. Med. Cutan. Ibero Lat. Am. 3 (6), 423
Esrey, S. A., Potash, J. B., Roberts, L., and Shiff, C. (1991). Effects of Improved Water Supply and Sanitation on Ascariasis, Diarrhoea, Dracunculiasis, Hookworm Infection, Schistosomiasis, and Trachoma. Bull. World Health Organ 69 (5), 609
Ferreccio, C., González, C., Milosavjlevic, V., Marshall, G., Sancha, A. M., and Smith, A. H. (2000). Lung Cancer and Arsenic Concentrations in Drinking Water in Chile. Epidemiology 11 (6), 673–679. doi:10.1097/00001648-200011000-00010
Fewtrell, L., and Colford, J. M. (2005). Water, Sanitation and Hygiene in Developing Countries: Interventions and Diarrhoea-A Review. Water Sci. Technol. A J. Int. Assoc. Water Pollut. Res. 52 (8), 133–142. doi:10.2166/wst.2005.0244
Fitzgerald, E. F., Schell, L. M., Marshall, E. G., Carpenter, D. O., Suk, W. A., and Zejda, J. E. (1998). Environmental Pollution and Child Health in Central and Eastern Europe. Environ. Health Perspect. 106 (6), 307–311. doi:10.1289/ehp.98106307
Fleisher, J. M., and Kay, D. (2006). Risk Perception Bias, Self-Reporting of Illness, and the Validity of Reported Results in an Epidemiologic Study of Recreational Water Associated Illnesses. Mar. Pollut. Bull. 52 (3), 264–268. doi:10.1016/j.marpolbul.2005.08.019
Fong, T.-T., and Lipp, E. K. (2005). Enteric Viruses of Humans and Animals in Aquatic Environments: Health Risks, Detection, and Potential Water Quality Assessment Tools. Microbiol. Mol. Biol. Rev. 69 (2), 357–371. doi:10.1128/mmbr.69.2.357-371.2005
Froom, P. (2009). Water Pollution and Cancer in Israeli Navy Divers. Int. J. Occup. Environ. Health 15 (3), 326–328. doi:10.1179/oeh.2009.15.3.326
Gundry, S., Wright, J., and Conroy, R. (2004). A Systematic Review of the Health Outcomes Related to Household Water Quality in Developing Countries. J. water health 2 (1), 1–13. doi:10.2166/wh.2004.0001
Halder, J., Islam, N., and Islam, N. (2015). Water Pollution and its Impact on the Human Health. Eh 2 (1), 36–46. doi:10.15764/eh.2015.01005
Hanif, M., Miah, R., Islam, M., and Marzia, S. (2020). Impact of Kapotaksha River Water Pollution on Human Health and Environment. Prog. Agric. 31 (1), 1–9. doi:10.3329/pa.v31i1.48300
Haseena, M., Malik, M. F., Javed, A., Arshad, S., Asif, N., Zulfiqar, S., et al. (2017). Water Pollution and Human Health. Environ. Risk Assess. Remediat. 1 (3), 20. doi:10.4066/2529-8046.100020
Henry, F. J., Huttly, S. R. A., Patwary, Y., and Aziz, K. M. A. (1990). Environmental Sanitation, Food and Water Contamination and Diarrhoea in Rural Bangladesh. Epidemiol. Infect. 104 (2), 253–259. doi:10.1017/s0950268800059422
Jalan, J., and Ravallion, M. (2003). Does Piped Water Reduce Diarrhea for Children in Rural India? J. Econ. 112 (1), 153–173. doi:10.1016/s0304-4076(02)00158-6
Jensen, P. K., Jayasinghe, G., Hoek, W., Cairncross, S., and Dalsgaard, A. (2004). Is There an Association between Bacteriological Drinking Water Quality and Childhood Diarrhoea in Developing Countries? Trop. Med. Int. Health 9 (11), 1210–1215. doi:10.1111/j.1365-3156.2004.01329.x
Jorgenson, A. K. (2009). Foreign Direct Investment and the Environment, the Mitigating Influence of Institutional and Civil Society Factors, and Relationships between Industrial Pollution and Human Health. Organ. Environ. 22 (2), 135–157. doi:10.1177/1086026609338163
Kaur, G., Kumar, R., Mittal, S., Sahoo, P. K., and Vaid, U. (2021). Ground/drinking Water Contaminants and Cancer Incidence: A Case Study of Rural Areas of South West Punjab, India. Hum. Ecol. Risk Assess. Int. J. 27 (1), 205–226. doi:10.1080/10807039.2019.1705145
Kazi, T. G., Arain, M. B., Baig, J. A., Jamali, M. K., Afridi, H. I., Jalbani, N., et al. (2009). The Correlation of Arsenic Levels in Drinking Water with the Biological Samples of Skin Disorders. Sci. Total Environ. 407 (3), 1019–1026. doi:10.1016/j.scitotenv.2008.10.013
Khan, S., Shahnaz, M., Jehan, N., Rehman, S., Shah, M. T., and Din, I. (2013). Drinking Water Quality and Human Health Risk in Charsadda District, Pakistan. J. Clean. Prod. 60, 93–101. doi:10.1016/j.jclepro.2012.02.016
Kochhar, N., Gill, G. S., Tuli, N., Dadwal, V., and Balaram, V. (2007). Chemical Quality of Ground Water in Relation to Incidence of Cancer in Parts of SW Punjab, India. Asian J. Water, Environ. Pollut. 4 (2), 107 doi:10.1086/114154
Kumar, S., Meena, H. M., and Verma, K. (2017). Water Pollution in India: its Impact on the Human Health: Causes and Remedies. Int. J. Appl. Environ. Sci. 12 (2), 275
Lai, W. (2017). Pesticide Use and Health Outcomes: Evidence from Agricultural Water Pollution in China. J. Environ. Econ. Manag. 86, 93–120. doi:10.1016/j.jeem.2017.05.006
Landrigan, P. J., Fuller, R., Fisher, S., Suk, W. A., Sly, P., Chiles, T. C., et al. (2018). Pollution and Children's Health. Sci. Total Environ. 650 (Pt 2), 2389–2394. doi:10.1016/j.scitotenv.2018.09.375
Lin, H.-J., Sung, T.-I., Chen, C.-Y., and Guo, H.-R. (2013). Arsenic Levels in Drinking Water and Mortality of Liver Cancer in Taiwan. J. Hazard. Mater. 262, 1132–1138. doi:10.1016/j.jhazmat.2012.12.049
Lu, Y., Song, S., Wang, R., Liu, Z., Meng, J., Sweetman, A. J., et al. (2015). Impacts of Soil and Water Pollution on Food Safety and Health Risks in China. Environ. Int. 77, 5–15. doi:10.1016/j.envint.2014.12.010
Marino, D. D. (2007). Water and Food Safety in the Developing World: Global Implications for Health and Nutrition of Infants and Young Children. J. Am. Dietetic Assoc. 107 (11), 1930–1934. doi:10.1016/j.jada.2007.08.013
Marmot, M., Atinmo, T., Byers, T., Chen, J., and Zeisel, S. H. (2007). Food, Nutrition, Physical Activity, and the Prevention of Cancer: a Global Perspective. Nutr. Bull.
Marr, A., and Dasgupta, N. (2009). Industrial Water Pollution in Dhaka, Bangladesh: Strategies and Incentives for Pollution Control in Small and Medium Enterprises. Int. J. Interdiscip. Soc. Sci. Annu. Rev. 3 (11), 97–108. doi:10.18848/1833-1882/cgp/v03i11/52752
Morris, R. D. (1995). Drinking Water and Cancer. Environ. Health Perspect. 103, 225. doi:10.2307/3432315
Moss, B. (2008). Water Pollution by Agriculture. Phil. Trans. R. Soc. B 363 (1491), 659–666. doi:10.1098/rstb.2007.2176
Page, T., Harris, R. H., and Epstein, S. S. (1976). Drinking Water and Cancer Mortality in Louisiana. Science 193 (4247), 55–57. doi:10.1126/science.935854
Pandey, S. (2006). Water Pollution and Health. Kathmandu Univ. Med. J. (KUMJ) 4 (1), 128. doi:10.1016/j.crvi.2013.04.013
Parris, K. (2011). Impact of Agriculture on Water Pollution in OECD Countries: Recent Trends and Future Prospects. Int. J. Water Resour. Dev. 27 (1), 33–52. doi:10.1080/07900627.2010.531898
Payment, P., Siemiatycki, J., Richardson, L., Renaud, G., Franco, E., and Prevost, M. (1997). A Prospective Epidemiological Study of Gastrointestinal Health Effects Due to the Consumption of Drinking Water. Int. J. Environ. Health Res. 7 (1), 5–31. doi:10.1080/09603129773977
Rabbani, M., Chowdhury, M., and Khan, N. A. (2010). Impacts of Industrial Pollution on Human Health: Empirical Evidences from an Industrial Hotspot (Kaliakoir) in Bangladesh. Asian J. Water, Environ. Pollut. 7 (1), 27
Rajal, V. B., Cruz, C., and Last, J. A. (2010). Water Quality Issues and Infant Diarrhoea in a South American Province. Glob. Public Health 5 (4), 348–363. doi:10.1080/17441690802447267
Rampen, F. H. J., Nelemans, P. J., and Verbeek, A. L. (1992). Is Water Pollution a Cause of Cutaneous Melanoma? Epidemiology 3, 263–265. doi:10.1097/00001648-199205000-00013
Royal Commission for Environmental Pollution 1979 Seventh Report. Agriculture and Pollution . London, UK: H.M.S.O .
Rusiñol, M., Fernandez-Cassi, X., Timoneda, N., Carratalà, A., and Abril, J. F. (2015). Evidence of Viral Dissemination and Seasonality in a Mediterranean River Catchment: Implications for Water Pollution Management. J. Environ. Manag. 159, 58–67. doi:10.1016/j.jenvman.2015.05.019
Schullehner, J., Hansen, B., Thygesen, M., Pedersen, C. B., and Sigsgaard, T. (2018). Nitrate in Drinking Water and Colorectal Cancer Risk: A Nationwide Population-Based Cohort Study. Int. J. Cancer 143 (1), 73–79. doi:10.1002/ijc.31306
Schwarzenbach, R. P., Egli, T., Hofstetter, T. B., Von Gunten, U., and Wehrli, B. (2010). Global Water Pollution and Human Health. Annu. Rev. Environ. Resour. 35, 109–136. doi:10.1146/annurev-environ-100809-125342
Sliman, N. A. (1978). Outbreak of Guillain-Barre Syndrome Associated with Water Pollution. Bmj 1 (6115), 751–752. doi:10.1136/bmj.1.6115.751
Smith, A. H., Hopenhayn-Rich, C., Bates, M. N., Goeden, H. M., Hertz-Picciotto, I., Duggan, H. M., et al. (1992). Cancer Risks from Arsenic in Drinking Water. Environ. Health Perspect. 97, 259–267. doi:10.1289/ehp.9297259
Stephens, J. K. (2002). Deterioration of Stored Domestic Water Quality and Diarrhoea in Zenu . University of Ghana .
Stevenson, A. H. (1953). Studies of Bathing Water Quality and Health. Am. J. Public Health Nations Health 43 (5 Pt 1), 529–538. doi:10.2105/ajph.43.5_pt_1.529
Team, S. H. (2004). Water, Sanitation and Hygiene Links to Health: Facts and Figures . World Health Organization .
Tondel, M., Rahman, M., Magnuson, A., Chowdhury, I. A., Faruquee, M. H., and Ahmad, S. A. (1999). The Relationship of Arsenic Levels in Drinking Water and the Prevalence Rate of Skin Lesions in Bangladesh. Environ. health Perspect. 107 (9), 727–729. doi:10.1289/ehp.99107727
Tseng, C.-H., Lei, C., and Chen, Y.-C. (2018). Evaluating the Health Costs of Oral Hexavalent Chromium Exposure from Water Pollution: A Case Study in Taiwan. J. Clean. Prod. 172, 819–826. doi:10.1016/j.jclepro.2017.10.177
Ustaoğlu, F., Tepe, Y., Taş, B., and Pag, N. (2020). Assessment of Stream Quality and Health Risk in a Subtropical Turkey River System: A Combined Approach Using Statistical Analysis and Water Quality Index. Ecol. Indic. , 113. doi:10.1016/j.ecolind.2019.105815
Vartiainen, T., Pukkala, E., Rienoja, T., Strandman, T., and Kaksonen, K. (1993). Population Exposure to Tri- and Tetrachloroethene and Cancer Risk: Two Cases of Drinking Water Pollution. Chemosphere 27 (7), 1171–1181. doi:10.1016/0045-6535(93)90165-2
Victora, C. G., Smith, P. G., Vaughan, J. P., Nobre, L. C., Lombard, C., Teixeira, A. M. B., et al. (1988). Water Supply, Sanitation and Housing in Relation to the Risk of Infant Mortality from Diarrhoea. Int. J. Epidemiol. 17 (3), 651–654. doi:10.1093/ije/17.3.651
Vladeva, S., Gatseva, P., and Gopina, G. (2000). Comparative Analysis of Results from Studies of Goitre in Children from Bulgarian Villages with Nitrate Pollution of Drinking Water in 1995 and 1998. Cent. Eur. J. Public Health 8 (3), 179
Waddington, H., Snilstveit, B., White, H., and Fewtrell, L. (2009). Water, Sanitation and Hygiene Interventions to Combat Childhood Diarrhoea in Developing Countries . New Delhi India Global Development Network International Initiative for Impact Evaluation Aug .
Witkowski, K. M., and Johnson, N. E. (1992). Organic-solvent Water Pollution and Low Birth Weight in Michigan. Soc. Biol. 39 (1-2), 45–54. doi:10.1080/19485565.1992.9988803
Wu, C., Maurer, C., Wang, Y., Xue, S., and Davis, D. L. (1999). Water Pollution and Human Health in China. Environ. Health Perspect. 107 (4), 251–256. doi:10.1289/ehp.99107251
Wu, H., Gai, Z., Guo, Y., Li, Y., Hao, Y., and Lu, Z. N. (2020). Does Environmental Pollution Inhibit Urbanization in China? A New Perspective through Residents' Medical and Health Costs. Environ. Res. 182 (Mar.), 109128–109128.9. doi:10.1016/j.envres.2020.109128
Xiao, J., Wang, L., Deng, L., and Jin, Z. (2019). Characteristics, Sources, Water Quality and Health Risk Assessment of Trace Elements in River Water and Well Water in the Chinese Loess Plateau. Sci. Total Environ. 650 (Pt 2), 2004–2012. doi:10.1016/j.scitotenv.2018.09.322
Xu, C., Xing, D., Wang, J., and Xiao, G. (2019). The Lag Effect of Water Pollution on the Mortality Rate for Esophageal Cancer in a Rapidly Industrialized Region in China. Environ. Sci. Pollut. Res. 26 (32), 32852–32858. doi:10.1007/s11356-019-06408-z
Xu, X., Wang, Q., and Li, C. (2022b). The Impact of Dependency Burden on Urban Household Health Expenditure and its Regional Heterogeneity in China: Based on Quantile Regression Method. Front. Public Health 10, 876088. doi:10.3389/fpubh.2022.876088
Xu, X., Yang, H., and Li, C. (2022a). Theoretical Model and Actual Characteristics of Air Pollution Affecting Health Cost: A Review. Ijerph 19, 3532. doi:10.3390/ijerph19063532
Yassin, M. M., Amr, S. S. A., and Al-Najar, H. M. (2006). Assessment of Microbiological Water Quality and its Relation to Human Health in Gaza Governorate, Gaza Strip. Public Health 120 (12), 1177. doi:10.1016/j.puhe.2006.07.026
Yau, V., Wade, T. J., de Wilde, C. K., and Colford, J. M. (2009). Skin-related Symptoms Following Exposure to Recreational Water: a Systematic Review and Meta-Analysis. Water Expo. Health 1 (2), 79–103. doi:10.1007/s12403-009-0012-9
Zaveri, E. D., Russ, J. D., Desbureaux, S. G., Damania, R., Rodella, A. S., and Ribeiro Paiva De Souza, G. (20203). The Nitrogen Legacy: The Long-Term Effects of Water Pollution on Human Capital . World Bank Policy Research Working Paper .
Zhang, X.-L., Bing, Z., Xing, Z., Chen, Z.-F., Zhang, J.-Z., Liang, S.-Y., et al. (2003). Research and Control of Well Water Pollution in High Esophageal Cancer Areas. Wjg 9 (6), 1187–1190. doi:10.3748/wjg.v9.i6.1187
Zhitkovich, A. (2011). Chromium in Drinking Water: Sources, Metabolism, and Cancer Risks. Chem. Res. Toxicol. 24 (10), 1617–1629. doi:10.1021/tx200251t
Keywords: water pollution, human health, disease heterogeneity, water intervention, health cost
Citation: Lin L, Yang H and Xu X (2022) Effects of Water Pollution on Human Health and Disease Heterogeneity: A Review. Front. Environ. Sci. 10:880246. doi: 10.3389/fenvs.2022.880246
Received: 21 February 2022; Accepted: 09 June 2022; Published: 30 June 2022.
Reviewed by:
Copyright © 2022 Lin, Yang and Xu. This is an open-access article distributed under the terms of the Creative Commons Attribution License (CC BY). The use, distribution or reproduction in other forums is permitted, provided the original author(s) and the copyright owner(s) are credited and that the original publication in this journal is cited, in accordance with accepted academic practice. No use, distribution or reproduction is permitted which does not comply with these terms.
*Correspondence: Xiaocang Xu, [email protected]
This article is part of the Research Topic
Bioaerosol Emission Characteristics and the Epidemiological, Occupational, and Public Health Risk Assessment of Waste and Wastewater Management
Human Impacts on the Environment
Humans impact the physical environment in many ways: overpopulation, pollution, burning fossil fuels, and deforestation. Changes like these have triggered climate change, soil erosion, poor air quality, and undrinkable water. These negative impacts can affect human behavior and can prompt mass migrations or battles over clean water.
Help your students understand the impact humans have on the physical environment with these classroom resources.
Earth Science, Geology, Geography, Physical Geography
Environmental Science and Pollution Research
Environmental Science and Pollution Research (ESPR) serves the international community in all broad areas of environmental science and related subjects with emphasis on chemical compounds.
- Covers all areas of Environmental Science and related subjects.
- Publishes on the natural sciences, but also includes the impacts of legislation, regulation, and the economy on pollution control.
- Safeguards international and interdisciplinary character through a global network of editorial board members.
- Official publication of the EuCheMS Division of Chemistry and the Environment.
- Authors from participating institutions can publish Open Choice at no cost.
- Philippe Garrigues
Societies and partnerships
Latest issue
Volume 31, Issue 14
Latest articles
Comments on: “spatio‑temporal evolution of groundwater quality and its health risk assessment in punjab (india) during 2000–2020” by praise shukla dericks, 10.1007/s11356-023–29200-6.
- Hardev Singh Virk
Removal of cadmium and cobalt from water by Slovak bentonites: efficiency, isotherms, and kinetic study
- Matej Šuránek
- Zuzana Melichová
- Maciej Thomas
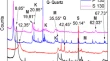
An ecological analysis of associations between ambient air pollution and cancer incidence rates in Taiwan
- Sheena Yi-Hsin Cheng
- Yi-Chiung Hsu
- Shih-Ping Cheng
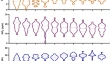
Molybdenum oxide-based catalyst towards better hydrogen production: effects of isothermal carburization
- Mohd Nor Latif
- Nur Syakirah Abdul Rahim
- Alinda Samsuri
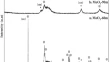
Natural magnetite as an effective and long-lasting catalyst for CWPO of azole pesticides in a continuous up-flow fixed-bed reactor
- Neus Lopez-Arago
- Macarena Munoz
- Jose A. Casas
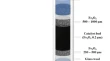
Journal updates
Meet the editors.
Learn more about the ESPR editors!
Special Issues
We invite you to browse through recently published special issues of Environmental Science and Pollution Research: Click here to get an overview of ESPR issues! All manuscripts will be peer reviewed as usual and following the journal's policies , with final decisions made by the Editor-in-Chief.
Special Issue Proposal Form
Call for Papers: Special Issue on PRR: Preventing, Removing and Recycling. Pollutant Toxic Ions and Molecules (PTIM2023)
Journal information.
- Astrophysics Data System (ADS)
- Biological Abstracts
- CAB Abstracts
- Chemical Abstracts Service (CAS)
- Current Contents/Agriculture, Biology & Environmental Sciences
- Engineering Village – GEOBASE
- Google Scholar
- INIS Atomindex
- Japanese Science and Technology Agency (JST)
- OCLC WorldCat Discovery Service
- Science Citation Index Expanded (SCIE)
- Semantic Scholar
- TD Net Discovery Service
- UGC-CARE List (India)
Rights and permissions
Springer policies
© Springer-Verlag GmbH Germany, part of Springer Nature
- Find a journal
- Publish with us
- Track your research
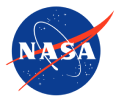
There is unequivocal evidence that Earth is warming at an unprecedented rate. Human activity is the principal cause.
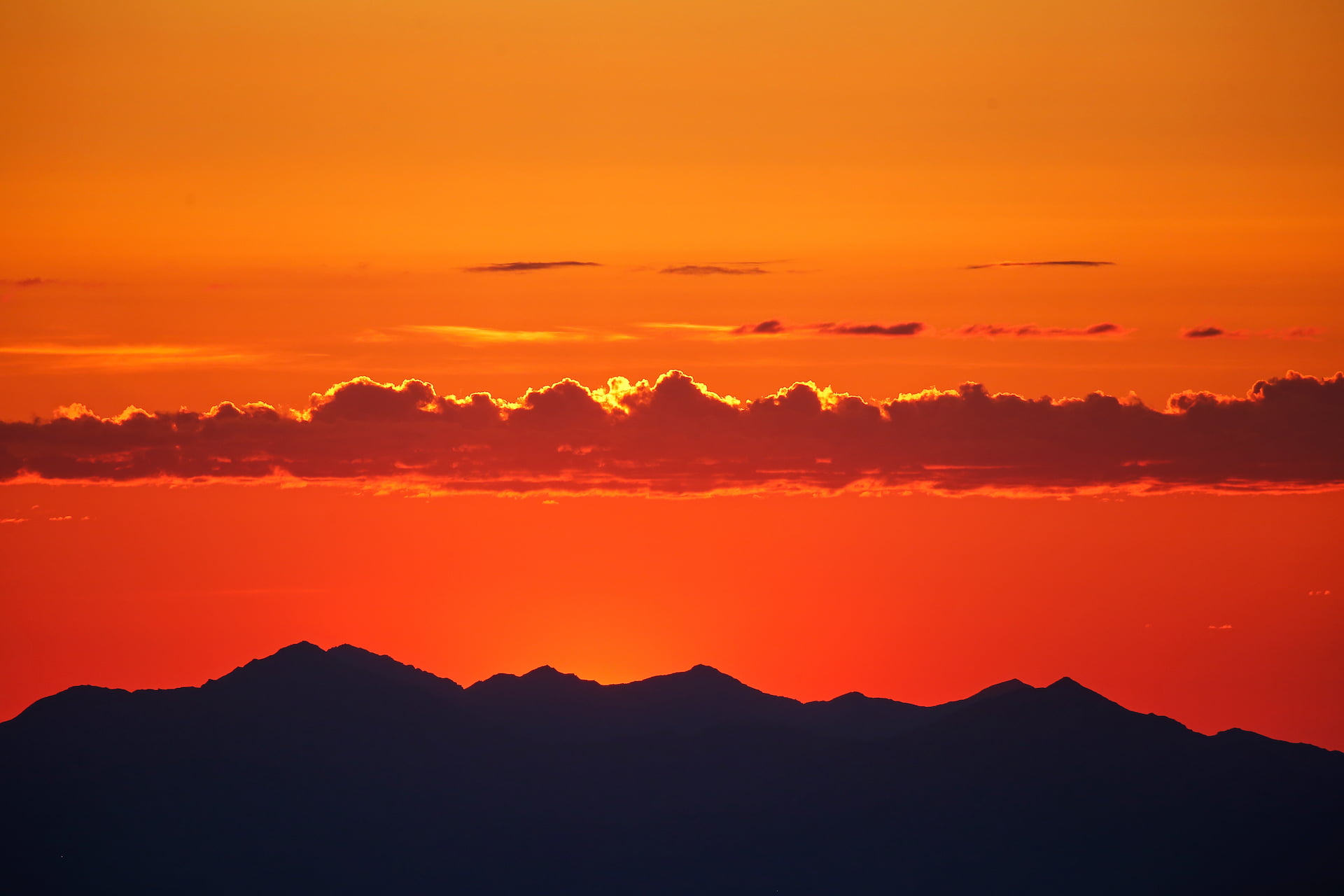
- While Earth’s climate has changed throughout its history , the current warming is happening at a rate not seen in the past 10,000 years.
- According to the Intergovernmental Panel on Climate Change ( IPCC ), "Since systematic scientific assessments began in the 1970s, the influence of human activity on the warming of the climate system has evolved from theory to established fact." 1
- Scientific information taken from natural sources (such as ice cores, rocks, and tree rings) and from modern equipment (like satellites and instruments) all show the signs of a changing climate.
- From global temperature rise to melting ice sheets, the evidence of a warming planet abounds.
The rate of change since the mid-20th century is unprecedented over millennia.
Earth's climate has changed throughout history. Just in the last 800,000 years, there have been eight cycles of ice ages and warmer periods, with the end of the last ice age about 11,700 years ago marking the beginning of the modern climate era — and of human civilization. Most of these climate changes are attributed to very small variations in Earth’s orbit that change the amount of solar energy our planet receives.
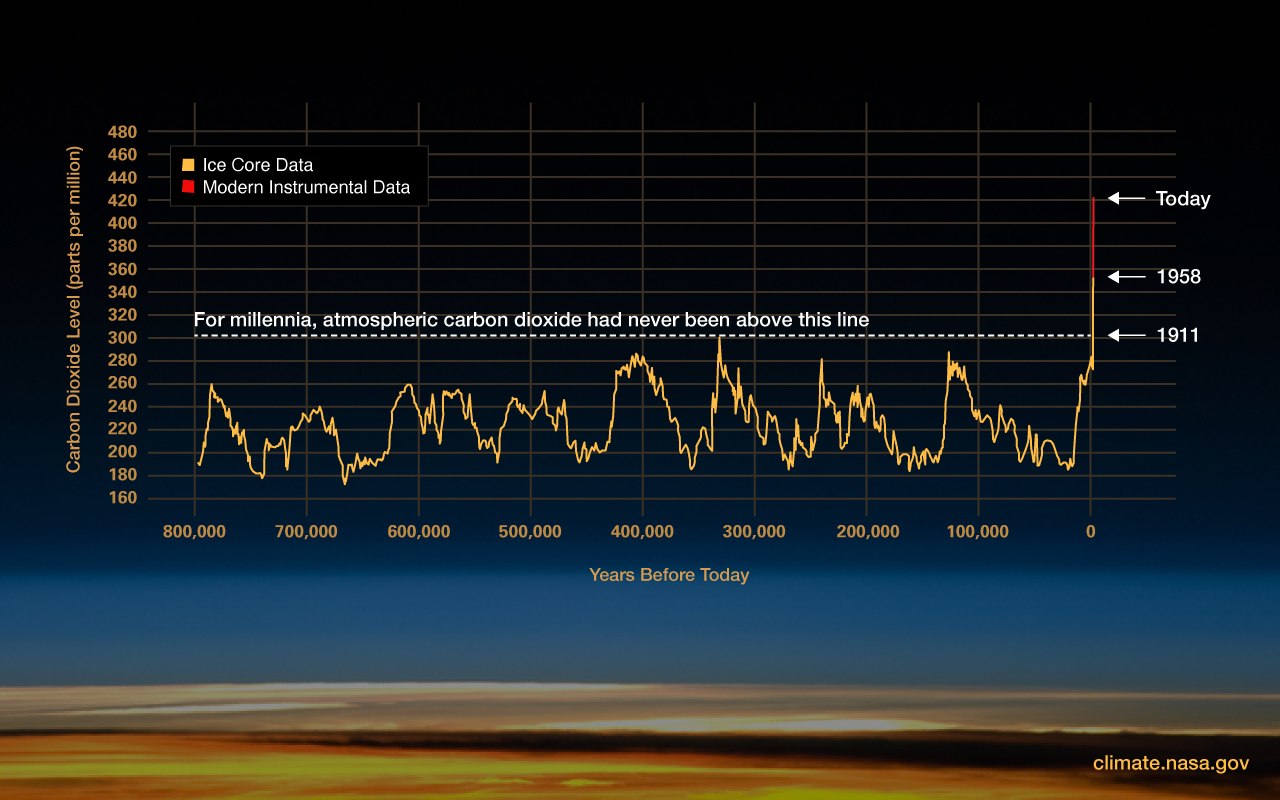
The current warming trend is different because it is clearly the result of human activities since the mid-1800s, and is proceeding at a rate not seen over many recent millennia. 1 It is undeniable that human activities have produced the atmospheric gases that have trapped more of the Sun’s energy in the Earth system. This extra energy has warmed the atmosphere, ocean, and land, and widespread and rapid changes in the atmosphere, ocean, cryosphere, and biosphere have occurred.
Earth-orbiting satellites and new technologies have helped scientists see the big picture, collecting many different types of information about our planet and its climate all over the world. These data, collected over many years, reveal the signs and patterns of a changing climate.
Scientists demonstrated the heat-trapping nature of carbon dioxide and other gases in the mid-19th century. 2 Many of the science instruments NASA uses to study our climate focus on how these gases affect the movement of infrared radiation through the atmosphere. From the measured impacts of increases in these gases, there is no question that increased greenhouse gas levels warm Earth in response.
Scientific evidence for warming of the climate system is unequivocal.
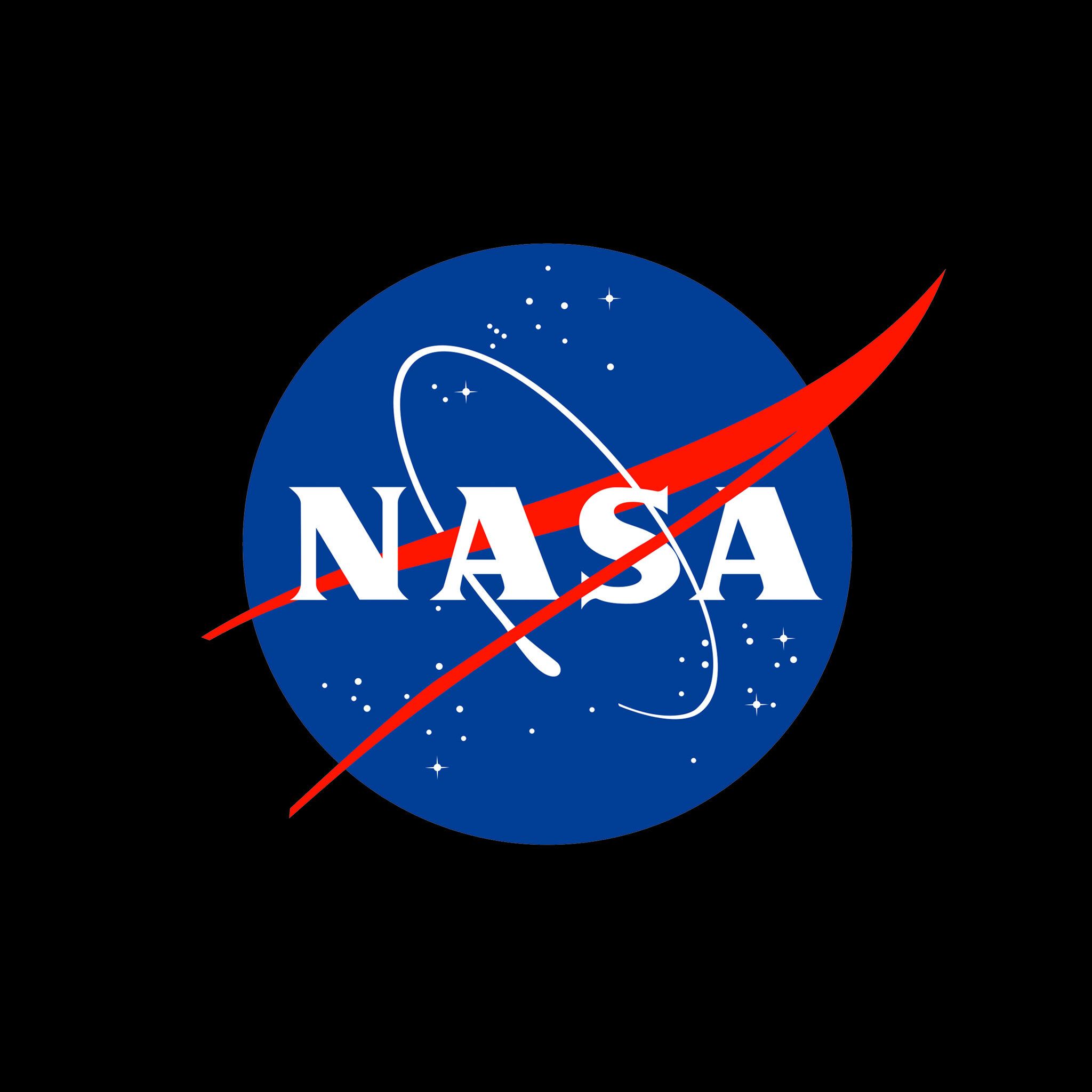
Intergovernmental Panel on Climate Change
Ice cores drawn from Greenland, Antarctica, and tropical mountain glaciers show that Earth’s climate responds to changes in greenhouse gas levels. Ancient evidence can also be found in tree rings, ocean sediments, coral reefs, and layers of sedimentary rocks. This ancient, or paleoclimate, evidence reveals that current warming is occurring roughly 10 times faster than the average rate of warming after an ice age. Carbon dioxide from human activities is increasing about 250 times faster than it did from natural sources after the last Ice Age. 3
The Evidence for Rapid Climate Change Is Compelling:
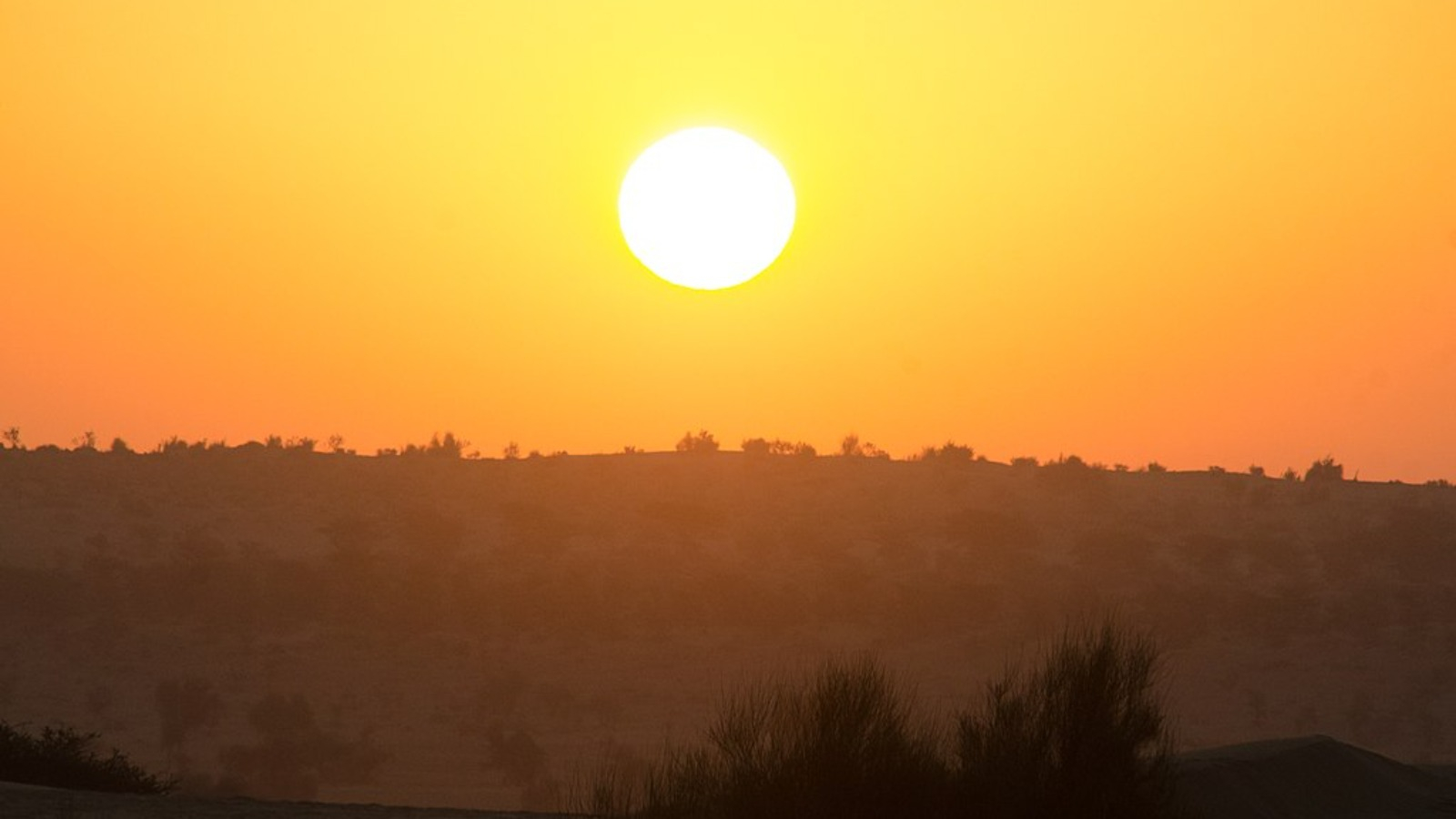
Global Temperature Is Rising
The planet's average surface temperature has risen about 2 degrees Fahrenheit (1 degrees Celsius) since the late 19th century, a change driven largely by increased carbon dioxide emissions into the atmosphere and other human activities. 4 Most of the warming occurred in the past 40 years, with the seven most recent years being the warmest. The years 2016 and 2020 are tied for the warmest year on record. 5 Image credit: Ashwin Kumar, Creative Commons Attribution-Share Alike 2.0 Generic.
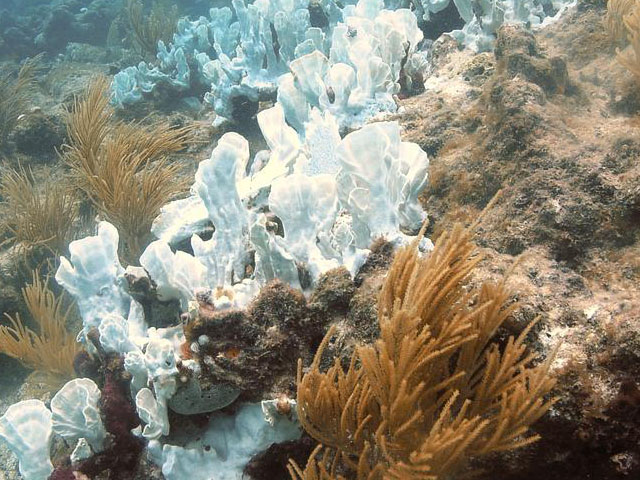
The Ocean Is Getting Warmer
The ocean has absorbed much of this increased heat, with the top 100 meters (about 328 feet) of ocean showing warming of 0.67 degrees Fahrenheit (0.33 degrees Celsius) since 1969. 6 Earth stores 90% of the extra energy in the ocean. Image credit: Kelsey Roberts/USGS
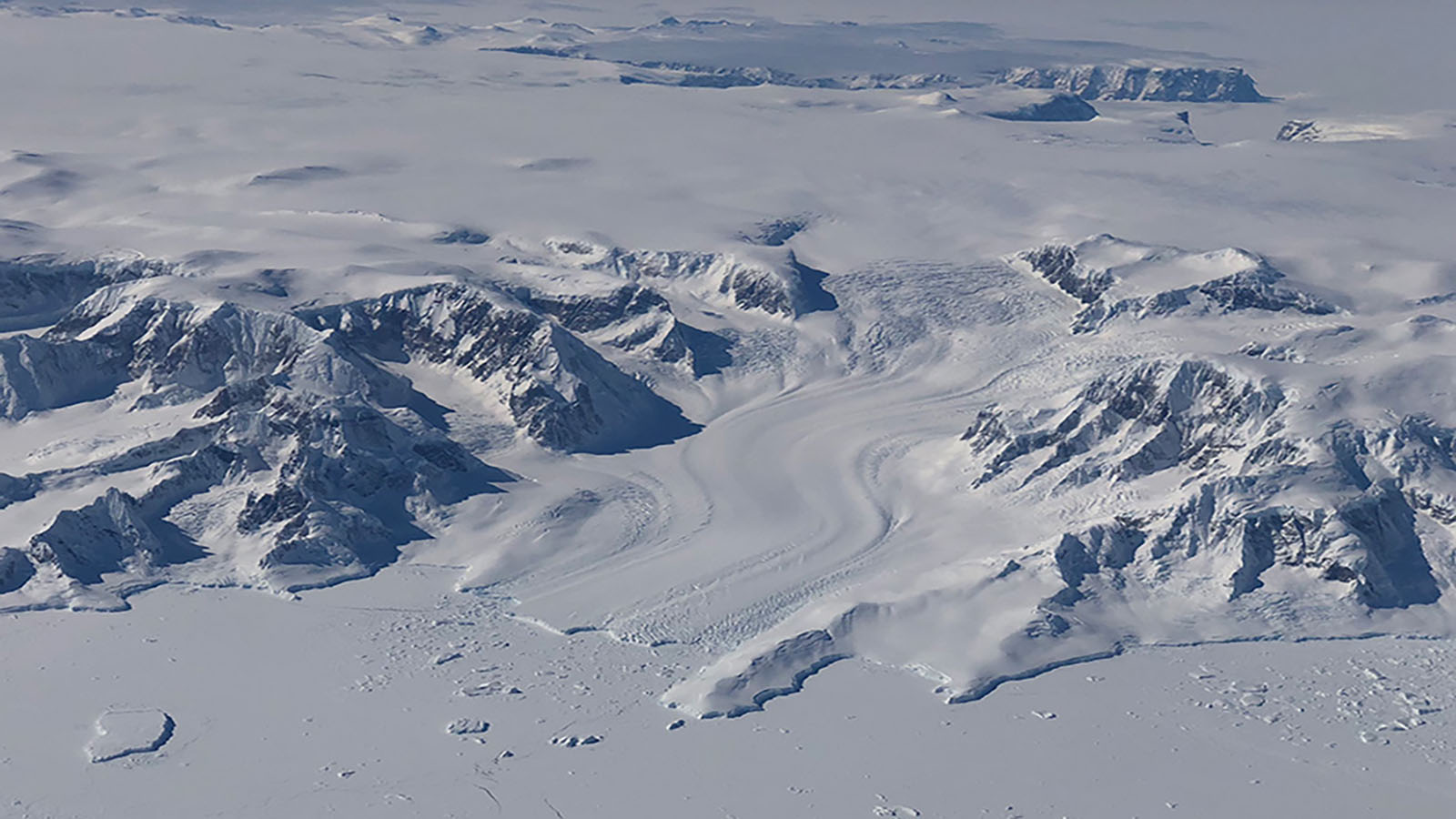
The Ice Sheets Are Shrinking
The Greenland and Antarctic ice sheets have decreased in mass. Data from NASA's Gravity Recovery and Climate Experiment show Greenland lost an average of 279 billion tons of ice per year between 1993 and 2019, while Antarctica lost about 148 billion tons of ice per year. 7 Image: The Antarctic Peninsula, Credit: NASA
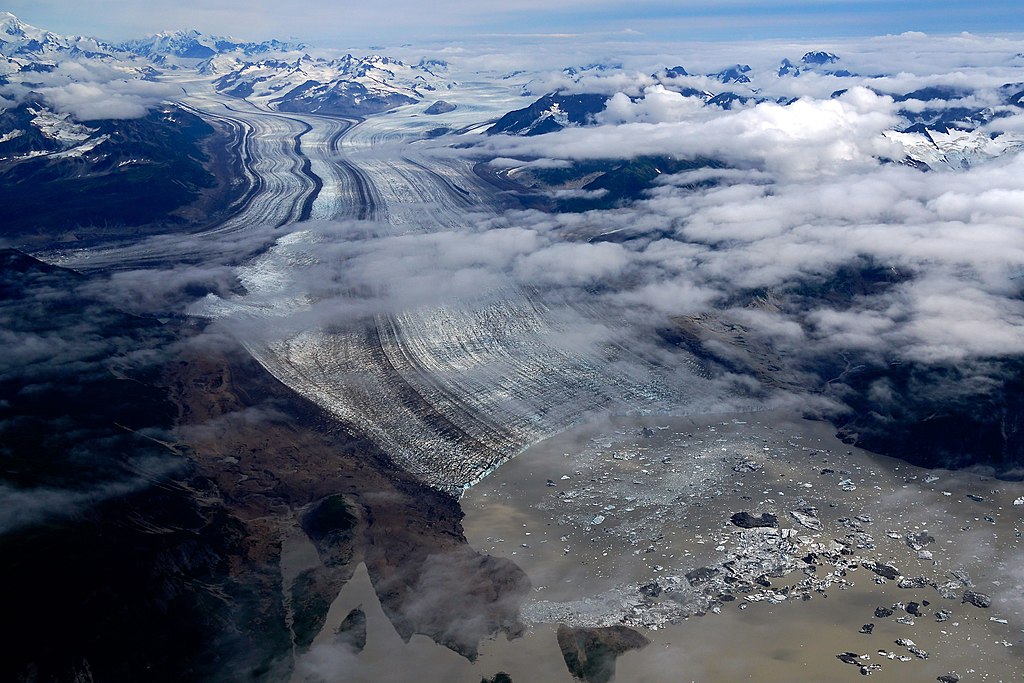
Glaciers Are Retreating
Glaciers are retreating almost everywhere around the world — including in the Alps, Himalayas, Andes, Rockies, Alaska, and Africa. 8 Image: Miles Glacier, Alaska Image credit: NASA
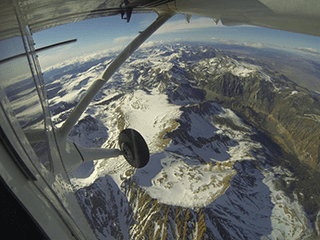
Snow Cover Is Decreasing
Satellite observations reveal that the amount of spring snow cover in the Northern Hemisphere has decreased over the past five decades and the snow is melting earlier. 9 Image credit: NASA/JPL-Caltech
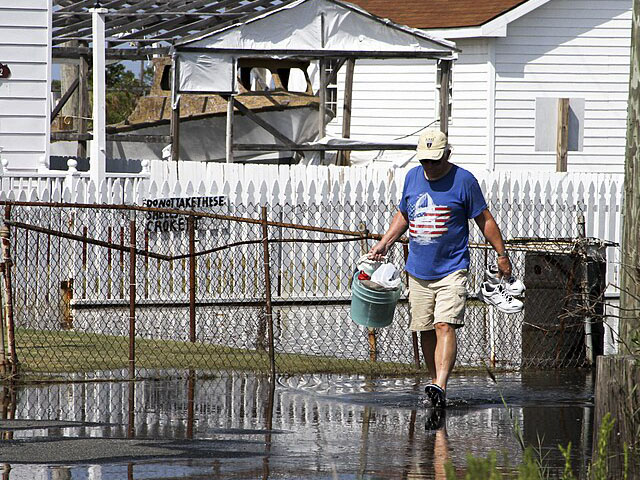
Sea Level Is Rising
Global sea level rose about 8 inches (20 centimeters) in the last century. The rate in the last two decades, however, is nearly double that of the last century and accelerating slightly every year. 10 Image credit: U.S. Army Corps of Engineers Norfolk District
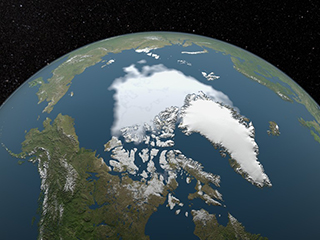
Arctic Sea Ice Is Declining
Both the extent and thickness of Arctic sea ice has declined rapidly over the last several decades. 11 Credit: NASA's Scientific Visualization Studio
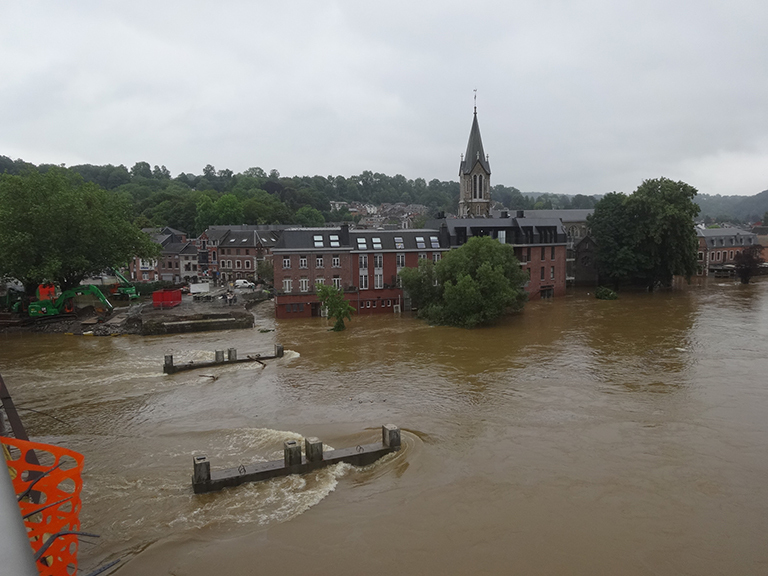
Extreme Events Are Increasing in Frequency
The number of record high temperature events in the United States has been increasing, while the number of record low temperature events has been decreasing, since 1950. The U.S. has also witnessed increasing numbers of intense rainfall events. 12 Image credit: Régine Fabri, CC BY-SA 4.0 , via Wikimedia Commons
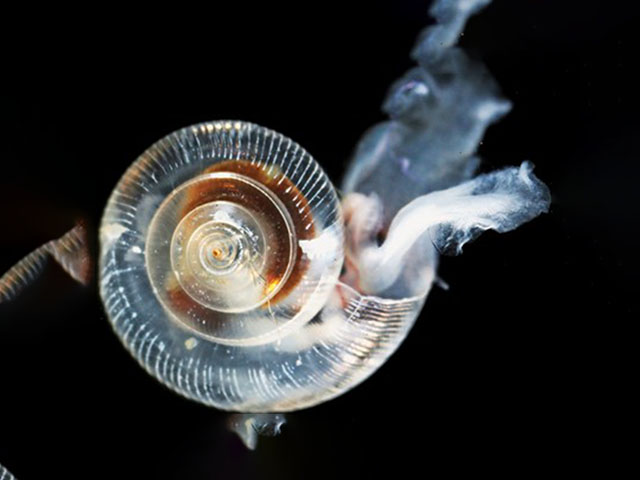
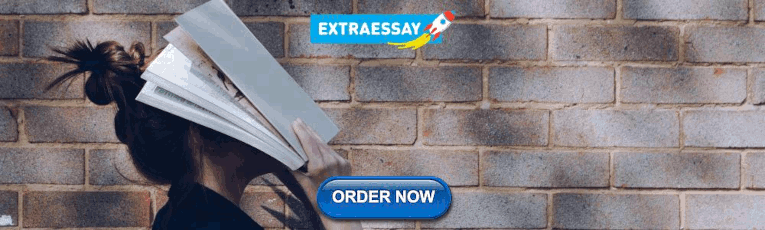
Ocean Acidification Is Increasing
Since the beginning of the Industrial Revolution, the acidity of surface ocean waters has increased by about 30%. 13 , 14 This increase is due to humans emitting more carbon dioxide into the atmosphere and hence more being absorbed into the ocean. The ocean has absorbed between 20% and 30% of total anthropogenic carbon dioxide emissions in recent decades (7.2 to 10.8 billion metric tons per year). 1 5 , 16 Image credit: NOAA
1. IPCC Sixth Assessment Report, WGI, Technical Summary . B.D. Santer et.al., “A search for human influences on the thermal structure of the atmosphere.” Nature 382 (04 July 1996): 39-46. https://doi.org/10.1038/382039a0. Gabriele C. Hegerl et al., “Detecting Greenhouse-Gas-Induced Climate Change with an Optimal Fingerprint Method.” Journal of Climate 9 (October 1996): 2281-2306. https://doi.org/10.1175/1520-0442(1996)009<2281:DGGICC>2.0.CO;2. V. Ramaswamy, et al., “Anthropogenic and Natural Influences in the Evolution of Lower Stratospheric Cooling.” Science 311 (24 February 2006): 1138-1141. https://doi.org/10.1126/science.1122587. B.D. Santer et al., “Contributions of Anthropogenic and Natural Forcing to Recent Tropopause Height Changes.” Science 301 (25 July 2003): 479-483. https://doi.org/10.1126/science.1084123. T. Westerhold et al., "An astronomically dated record of Earth’s climate and its predictability over the last 66 million years." Science 369 (11 Sept. 2020): 1383-1387. https://doi.org/10.1126/science.1094123
2. In 1824, Joseph Fourier calculated that an Earth-sized planet, at our distance from the Sun, ought to be much colder. He suggested something in the atmosphere must be acting like an insulating blanket. In 1856, Eunice Foote discovered that blanket, showing that carbon dioxide and water vapor in Earth's atmosphere trap escaping infrared (heat) radiation. In the 1860s, physicist John Tyndall recognized Earth's natural greenhouse effect and suggested that slight changes in the atmospheric composition could bring about climatic variations. In 1896, a seminal paper by Swedish scientist Svante Arrhenius first predicted that changes in atmospheric carbon dioxide levels could substantially alter the surface temperature through the greenhouse effect. In 1938, Guy Callendar connected carbon dioxide increases in Earth’s atmosphere to global warming. In 1941, Milutin Milankovic linked ice ages to Earth’s orbital characteristics. Gilbert Plass formulated the Carbon Dioxide Theory of Climate Change in 1956.
3. IPCC Sixth Assessment Report, WG1, Chapter 2 Vostok ice core data; NOAA Mauna Loa CO2 record O. Gaffney, W. Steffen, "The Anthropocene Equation." The Anthropocene Review 4, issue 1 (April 2017): 53-61. https://doi.org/abs/10.1177/2053019616688022.
4. https://www.ncei.noaa.gov/monitoring https://crudata.uea.ac.uk/cru/data/temperature/ http://data.giss.nasa.gov/gistemp
5. https://www.giss.nasa.gov/research/news/20170118/
6. S. Levitus, J. Antonov, T. Boyer, O Baranova, H. Garcia, R. Locarnini, A. Mishonov, J. Reagan, D. Seidov, E. Yarosh, M. Zweng, " NCEI ocean heat content, temperature anomalies, salinity anomalies, thermosteric sea level anomalies, halosteric sea level anomalies, and total steric sea level anomalies from 1955 to present calculated from in situ oceanographic subsurface profile data (NCEI Accession 0164586), Version 4.4. (2017) NOAA National Centers for Environmental Information. https://www.nodc.noaa.gov/OC5/3M_HEAT_CONTENT/index3.html K. von Schuckmann, L. Cheng, L,. D. Palmer, J. Hansen, C. Tassone, V. Aich, S. Adusumilli, H. Beltrami, H., T. Boyer, F. Cuesta-Valero, D. Desbruyeres, C. Domingues, A. Garcia-Garcia, P. Gentine, J. Gilson, M. Gorfer, L. Haimberger, M. Ishii, M., G. Johnson, R. Killick, B. King, G. Kirchengast, N. Kolodziejczyk, J. Lyman, B. Marzeion, M. Mayer, M. Monier, D. Monselesan, S. Purkey, D. Roemmich, A. Schweiger, S. Seneviratne, A. Shepherd, D. Slater, A. Steiner, F. Straneo, M.L. Timmermans, S. Wijffels. "Heat stored in the Earth system: where does the energy go?" Earth System Science Data 12, Issue 3 (07 September 2020): 2013-2041. https://doi.org/10.5194/essd-12-2013-2020.
7. I. Velicogna, Yara Mohajerani, A. Geruo, F. Landerer, J. Mouginot, B. Noel, E. Rignot, T. Sutterly, M. van den Broeke, M. Wessem, D. Wiese, "Continuity of Ice Sheet Mass Loss in Greenland and Antarctica From the GRACE and GRACE Follow-On Missions." Geophysical Research Letters 47, Issue 8 (28 April 2020): e2020GL087291. https://doi.org/10.1029/2020GL087291.
8. National Snow and Ice Data Center World Glacier Monitoring Service
9. National Snow and Ice Data Center D.A. Robinson, D. K. Hall, and T. L. Mote, "MEaSUREs Northern Hemisphere Terrestrial Snow Cover Extent Daily 25km EASE-Grid 2.0, Version 1 (2017). Boulder, Colorado USA. NASA National Snow and Ice Data Center Distributed Active Archive Center. doi: https://doi.org/10.5067/MEASURES/CRYOSPHERE/nsidc-0530.001 . http://nsidc.org/cryosphere/sotc/snow_extent.html Rutgers University Global Snow Lab. Data History
10. R.S. Nerem, B.D. Beckley, J. T. Fasullo, B.D. Hamlington, D. Masters, and G.T. Mitchum, "Climate-change–driven accelerated sea-level rise detected in the altimeter era." PNAS 15, no. 9 (12 Feb. 2018): 2022-2025. https://doi.org/10.1073/pnas.1717312115.
11. https://nsidc.org/cryosphere/sotc/sea_ice.html Pan-Arctic Ice Ocean Modeling and Assimilation System (PIOMAS, Zhang and Rothrock, 2003) http://psc.apl.washington.edu/research/projects/arctic-sea-ice-volume-anomaly/ http://psc.apl.uw.edu/research/projects/projections-of-an-ice-diminished-arctic-ocean/
12. USGCRP, 2017: Climate Science Special Report: Fourth National Climate Assessment, Volume I [Wuebbles, D.J., D.W. Fahey, K.A. Hibbard, D.J. Dokken, B.C. Stewart, and T.K. Maycock (eds.)]. U.S. Global Change Research Program, Washington, DC, USA, 470 pp, https://doi.org/10.7930/j0j964j6 .
13. http://www.pmel.noaa.gov/co2/story/What+is+Ocean+Acidification%3F
14. http://www.pmel.noaa.gov/co2/story/Ocean+Acidification
15. C.L. Sabine, et al., “The Oceanic Sink for Anthropogenic CO2.” Science 305 (16 July 2004): 367-371. https://doi.org/10.1126/science.1097403.
16. Special Report on the Ocean and Cryosphere in a Changing Climate , Technical Summary, Chapter TS.5, Changing Ocean, Marine Ecosystems, and Dependent Communities, Section 5.2.2.3. https://www.ipcc.ch/srocc/chapter/technical-summary/
Header image shows clouds imitating mountains as the sun sets after midnight as seen from Denali's backcountry Unit 13 on June 14, 2019. Credit: NPS/Emily Mesner Image credit in list of evidence: Ashwin Kumar, Creative Commons Attribution-Share Alike 2.0 Generic.
Discover More Topics From NASA
Explore Earth Science

Earth Science in Action
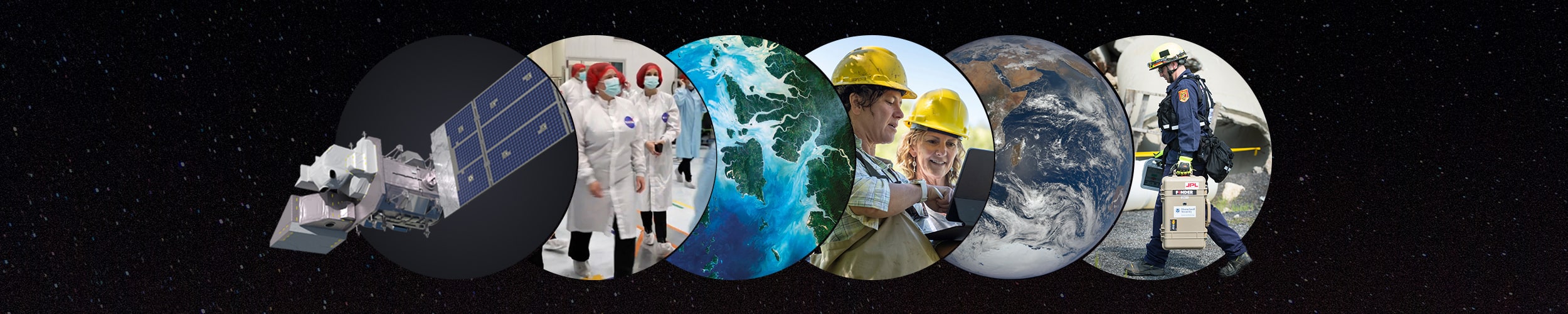
Earth Science Data
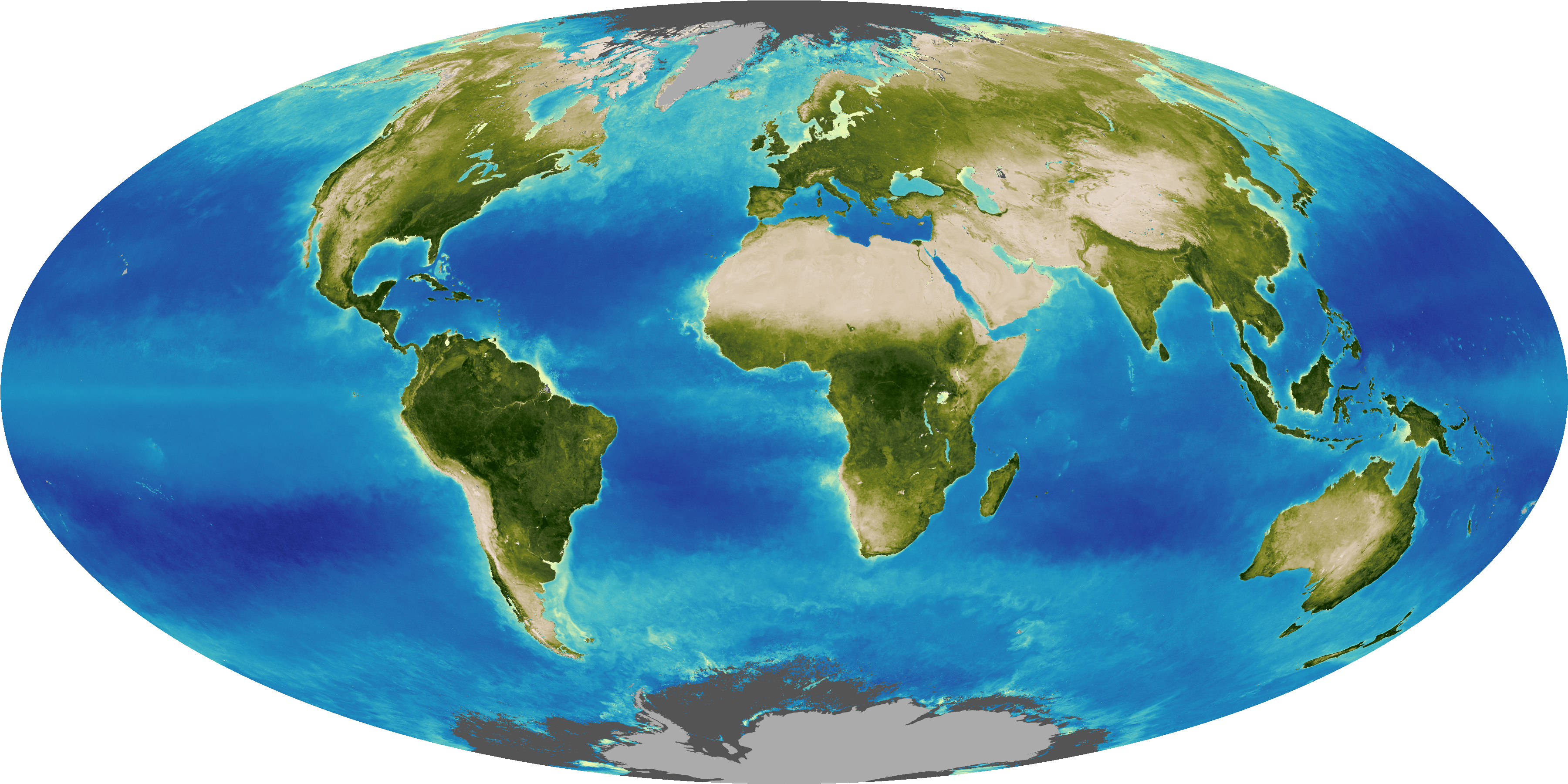
Facts About Earth
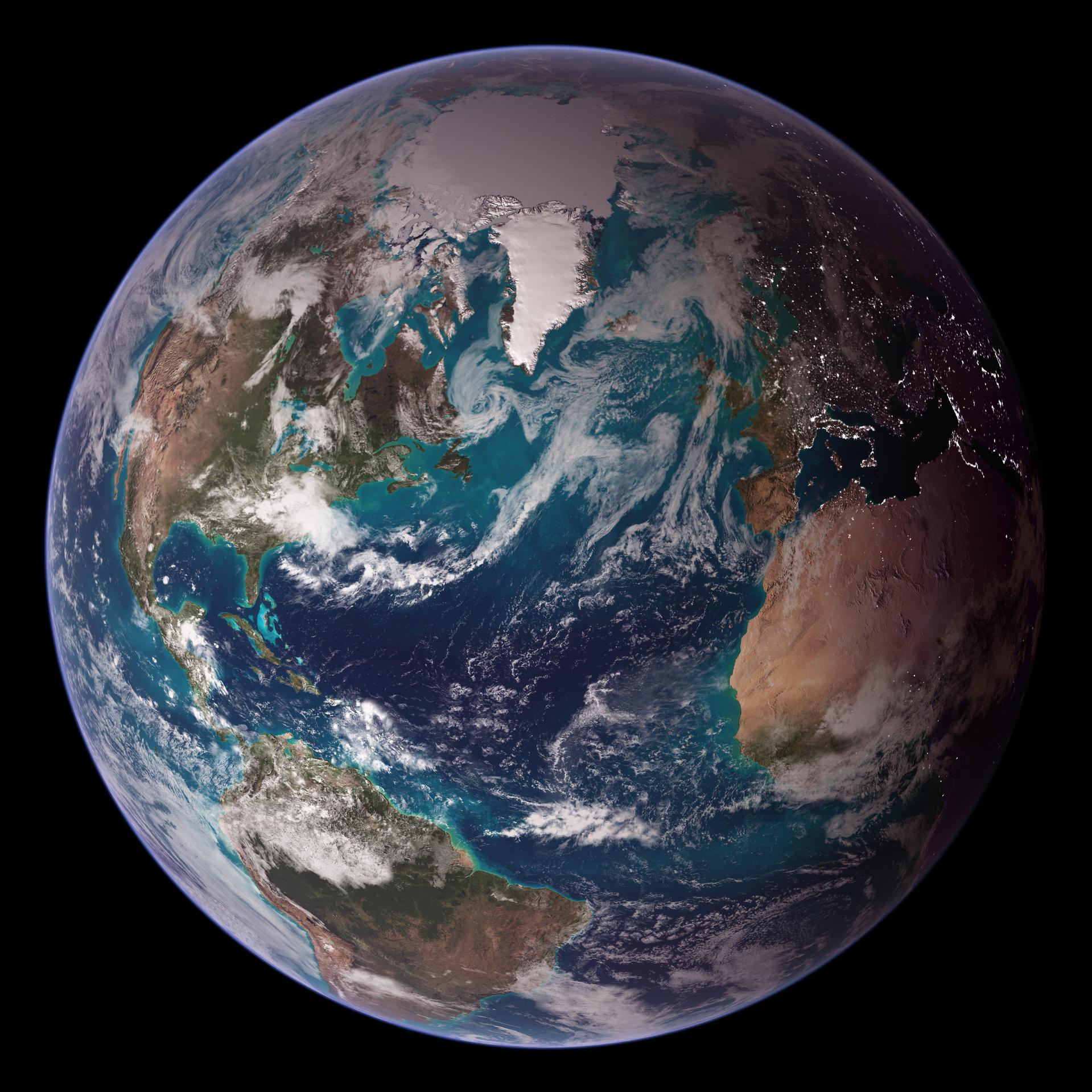

An official website of the United States government
The .gov means it’s official. Federal government websites often end in .gov or .mil. Before sharing sensitive information, make sure you’re on a federal government site.
The site is secure. The https:// ensures that you are connecting to the official website and that any information you provide is encrypted and transmitted securely.
- Publications
- Account settings
Preview improvements coming to the PMC website in October 2024. Learn More or Try it out now .
- Advanced Search
- Journal List
- Philos Trans R Soc Lond B Biol Sci
- v.364(1526); 2009 Jul 27
Plastics, the environment and human health: current consensus and future trends
Richard c. thompson.
1 Marine Biology and Ecology Research Centre, Marine Institute, University of Plymouth, Drake Circus, Plymouth PL4 8AA, UK
Charles J. Moore
2 Algalita Marine Research Foundation, Long Beach, CA 90803, USA
Frederick S. vom Saal
3 Division of Biological Sciences, University of Missouri, Columbia, MO 65201, USA
Shanna H. Swan
4 Department of Obstetrics and Gynecology, University of Rochester, Rochester, NY 14642, USA
Plastics have transformed everyday life; usage is increasing and annual production is likely to exceed 300 million tonnes by 2010. In this concluding paper to the Theme Issue on Plastics, the Environment and Human Health, we synthesize current understanding of the benefits and concerns surrounding the use of plastics and look to future priorities, challenges and opportunities. It is evident that plastics bring many societal benefits and offer future technological and medical advances. However, concerns about usage and disposal are diverse and include accumulation of waste in landfills and in natural habitats, physical problems for wildlife resulting from ingestion or entanglement in plastic, the leaching of chemicals from plastic products and the potential for plastics to transfer chemicals to wildlife and humans. However, perhaps the most important overriding concern, which is implicit throughout this volume, is that our current usage is not sustainable. Around 4 per cent of world oil production is used as a feedstock to make plastics and a similar amount is used as energy in the process. Yet over a third of current production is used to make items of packaging, which are then rapidly discarded. Given our declining reserves of fossil fuels, and finite capacity for disposal of waste to landfill, this linear use of hydrocarbons, via packaging and other short-lived applications of plastic, is simply not sustainable. There are solutions, including material reduction, design for end-of-life recyclability, increased recycling capacity, development of bio-based feedstocks, strategies to reduce littering, the application of green chemistry life-cycle analyses and revised risk assessment approaches. Such measures will be most effective through the combined actions of the public, industry, scientists and policymakers. There is some urgency, as the quantity of plastics produced in the first 10 years of the current century is likely to approach the quantity produced in the entire century that preceded.
1. Introduction
Many of the current applications and the predicted benefits of plastic follow those outlined by Yarsley and Couzens in the 1940s. Their account of the benefits that plastics would bring to a person born nearly 70 years ago, at the beginning of this ‘ plastic age ’, was told with much optimism:
It is a world free from moth and rust and full of colour, a world largely built up of synthetic materials made from the most universally distributed substances, a world in which nations are more and more independent of localised naturalised resources, a world in which man, like a magician, makes what he wants for almost every need out of what is beneath and around him ( Yarsley & Couzens 1945 , p. 152).
The durability of plastics and their potential for diverse applications, including widespread use as disposable items, were anticipated, but the problems associated with waste management and plastic debris were not. In fact the predictions were ‘ how much brighter and cleaner a world [it would be] than that which preceded this plastic age ’ ( Yarsley & Couzens 1945 , p. 152).
This paper synthesizes current understanding of the benefits and concerns surrounding the use of plastics and looks to challenges, opportunities and priorities for the future. The content draws upon papers submitted to this Theme Issue on Plastics, the Environment and Human Health together with other sources. While selected citations are given to original sources of information, we primarily refer the reader to the discussion of a particular topic, and the associated references, in the Theme Issue papers. Here, we consider the subject from seven perspectives: plastics as materials; accumulation of plastic waste in the natural environment; effects of plastic debris in the environment and on wildlife; effects on humans; production, usage, disposal and waste management solutions; biopolymers, degradable and biodegradable polymer solutions; and policy measures.
2. Plastics as materials: an overview
Plastics are inexpensive, lightweight, strong, durable, corrosion-resistant materials, with high thermal and electrical insulation properties. The diversity of polymers and the versatility of their properties are used to make a vast array of products that bring medical and technological advances, energy savings and numerous other societal benefits ( Andrady & Neal 2009 ). As a consequence, the production of plastics has increased substantially over the last 60 years from around 0.5 million tonnes in 1950 to over 260 million tonnes today. In Europe alone the plastics industry has a turnover in excess of 300 million euros and employs 1.6 million people ( Plastics Europe 2008 ). Almost all aspects of daily life involve plastics, in transport, telecommunications, clothing, footwear and as packaging materials that facilitate the transport of a wide range of food, drink and other goods. There is considerable potential for new applications of plastics that will bring benefits in the future, for example as novel medical applications, in the generation of renewable energy and by reducing energy used in transport ( Andrady & Neal 2009 ).
Virgin plastic polymers are rarely used by themselves and typically the polymer resins are mixed with various additives to improve performance. These additives include inorganic fillers such as carbon and silica that reinforce the material, plasticizers to render the material pliable, thermal and ultraviolet stabilizers, flame retardants and colourings. Many such additives are used in substantial quantities and in a wide range of products ( Meeker et al. 2009 ). Some additive chemicals are potentially toxic (for example lead and tributyl tin in polyvinyl chloride, PVC), but there is considerable controversy about the extent to which additives released from plastic products (such as phthalates and bisphenol A, BPA) have adverse effects in animal or human populations. The central issue here is relating the types and quantities of additives present in plastics to uptake and accumulation by living organisms ( Andrady & Neal 2009 ; Koch & Calafat 2009 ; Meeker et al. 2009 ; Oehlmann et al. 2009 ; Talsness et al. 2009 ; Wagner & Oehlmann 2009 ). Additives of particular concern are phthalate plasticizers, BPA, brominated flame retardants and anti-microbial agents. BPA and phthalates are found in many mass produced products including medical devices, food packaging, perfumes, cosmetics, toys, flooring materials, computers and CDs and can represent a significant content of the plastic. For instance, phthalates can constitute a substantial proportion, by weight, of PVC ( Oehlmann et al. 2009 ), while BPA is the monomer used for production of polycarbonate plastics as well as an additive used for production of PVC. Phthalates can leach out of products because they are not chemically bound to the plastic matrix, and they have attracted particular attention because of their high production volumes and wide usage ( Wagner & Oehlmann 2009 ; Talsness et al. 2009 ). Phthalates and BPA are detectable in aquatic environments, in dust and, because of their volatility, in air ( Rudel et al. 2001 , 2003 ). There is considerable concern about the adverse effects of these chemicals on wildlife and humans ( Meeker et al. 2009 ; Oehlmann et al. 2009 ). In addition to the reliance on finite resources for plastic production, and concerns about additive effects of different chemicals, current patterns of usage are generating global waste management problems. Barnes et al. (2009) show that plastic wastes, including packaging, electrical equipment and plastics from end-of-life vehicles, are major components of both household and industrial wastes; our capacity for disposal of waste to landfill is finite and in some locations landfills are at, or are rapidly approaching, capacity ( Defra et al. 2006 ). So from several perspectives it would seem that our current use and disposal of plastics is the cause for concern ( Barnes et al. 2009 ; Hopewell et al. 2009 ).
3. Accumulation of plastic waste in the natural environment
Substantial quantities of plastic have accumulated in the natural environment and in landfills. Around 10 per cent by weight of the municipal waste stream is plastic ( Barnes et al. 2009 ) and this will be considered later in §6 . Discarded plastic also contaminates a wide range of natural terrestrial, freshwater and marine habitats, with newspaper accounts of plastic debris on even some of the highest mountains. There are also some data on littering in the urban environment (for example compiled by EnCams in the UK; http://www.encams.org/home ); however, by comparison with the marine environment, there is a distinct lack of data on the accumulation of plastic debris in natural terrestrial and freshwater habitats. There are accounts of inadvertent contamination of soils with small plastic fragments as a consequence of spreading sewage sludge ( Zubris & Richards 2005 ), of fragments of plastic and glass contaminating compost prepared from municipal solid waste ( Brinton 2005 ) and of plastic being carried into streams, rivers and ultimately the sea with rain water and flood events ( Thompson et al. 2005 ). However, there is a clear need for more research on the quantities and effects of plastic debris in natural terrestrial habitats, on agricultural land and in freshwaters. Inevitably, therefore, much of the evidence presented here is from the marine environment. From the first accounts of plastic in the environment, which were reported from the carcasses of seabirds collected from shorelines in the early 1960s ( Harper & Fowler 1987 ), the extent of the problem soon became unmistakable with plastic debris contaminating oceans from the poles to the Equator and from shorelines to the deep sea. Most polymers are buoyant in water, and since items of plastic debris such as cartons and bottles often trap air, substantial quantities of plastic debris accumulate on the sea surface and may also be washed ashore. As a consequence, plastics represent a considerable proportion (50–80%) of shoreline debris ( Barnes et al. 2009 ). Quantities are highly variable in time and space, but there are reports of more than 100 000 items m –2 on some shorelines ( Gregory 1978 ) and up to 3 520 000 items km –2 at the ocean surface ( Yamashita & Tanimura 2007 ). Gyres and oceanic convergences appear to be particularly contaminated, as do enclosed seas such as the Mediterranean ( Barnes et al. 2009 ; Ryan et al. 2009 ). Despite their buoyant nature, plastics can become fouled with marine life and sediment causing items to sink to the seabed. For example, shallow seabeds in Brazil were more heavily contaminated than the neighbouring shorelines ( Oigman-Pszczol & Creed 2007 ), indicating that the seabed may be an ultimate sink even for initially buoyant marine debris ( Barnes et al. 2009 ). In some locations around Europe, it has been suggested that quantities on the seabed may exceed 10 000 items ha –1 , and debris has even been reported more than a 1000 m below the ocean surface, including accounts of inverted plastic bags passing a deep-sea submersible like an assembly of ghosts ( Gregory 2009 ). Quantitative data on the abundance of debris on the seabed are still very limited, but there are concerns that degradation rates in the deep sea will be especially slow because of darkness and cold ( Barnes et al. 2009 ; Ryan et al. 2009 ).
Monitoring the abundance of debris is important to establish rates of accumulation and the effectiveness of any remediation measures. Most studies assess the abundance of all types of anthropogenic debris including data on plastics and/or plastic items as a category. In general, the abundance of debris on shorelines has been extensively monitored, in comparison to surveys from the open oceans or the seabed. In addition to recording debris, there is a need to collect data on sources; for plastic debris this should include discharges from rivers and sewers together with littering behaviour. Here, the limited data we have suggest that storm water pulses provide a major pathway for debris from the land to the sea, with 81 g m –3 of plastic debris during high-flow events in the USA ( Ryan et al. 2009 ). Methods to monitor the abundance of anthropogenic debris (including plastics) often vary considerably between countries and organizations, adding to difficulties in interpreting trends. As a consequence, the United Nations Environment Programme and the OSPAR Commission are currently taking steps to introduce standardized protocols ( OSPAR 2007 ; Cheshire et al. 2009 ). Some trends are evident, however, typically with an increase in the abundance of debris and fragments between the 1960s and the 1990s ( Barnes et al. 2009 ). More recently, abundance at the sea surface in some regions and on some shorelines appears to be stabilizing, while in other areas such as the Pacific Gyre there are reports of considerable increases. On shorelines the quantities of debris, predominantly plastic, are greater in the Northern than in the Southern Hemisphere ( Barnes 2005 ). The abundance of debris is greater adjacent to urban centres and on more frequented beaches and there is evidence that plastics are accumulating and becoming buried in sediments ( Barnes et al. 2009 ; Ryan et al. 2009 ). Barnes et al. (2009) consider that contamination of remote habitats, such as the deep sea and the polar regions, is likely to increase as debris is carried there from more densely populated areas. Allowing for variability between habitats and locations, it seems inevitable, however, that the quantity of debris in the environment as a whole will continue to increase—unless we all change our practices. Even with such changes, plastic debris that is already in the environment will persist for a considerable time to come. The persistence of plastic debris and the associated environmental hazards are illustrated poignantly by Barnes et al. (2009) who describe debris that had originated from an aeroplane being ingested by an albatross some 60 years after the plane had crashed.
4. Effects of plastic debris in the environment and on wildlife
There are some accounts of effects of debris from terrestrial habitats, for example ingestion by the endangered California condor, Gymnogyps californianus ( Mee et al. 2007 ). However, the vast majority of work describing environmental consequences of plastic debris is from marine settings and more work on terrestrial and freshwater habitats is needed. Plastic debris causes aesthetic problems, and it also presents a hazard to maritime activities including fishing and tourism ( Moore 2008 ; Gregory 2009 ). Discarded fishing nets result in ghost fishing that may result in losses to commercial fisheries ( Moore 2008 ; Brown & Macfadyen 2007 ). Floating plastic debris can rapidly become colonized by marine organisms and since it can persist at the sea surface for substantial periods, it may subsequently facilitate the transport of non-native or ‘alien’ species ( Barnes 2002 ; Barnes et al. 2009 ; Gregory 2009 ). However, the problems attracting most public and media attention are those resulting in ingestion and entanglement by wildlife. Over 260 species, including invertebrates, turtles, fish, seabirds and mammals, have been reported to ingest or become entangled in plastic debris, resulting in impaired movement and feeding, reduced reproductive output, lacerations, ulcers and death ( Laist 1997 ; Derraik 2002 ; Gregory 2009 ). The limited monitoring data we have suggest rates of entanglement have increased over time ( Ryan et al. 2009 ). A wide range of species with different modes of feeding including filter feeders, deposit feeders and detritivores are known to ingest plastics. However, ingestion is likely to be particularly problematic for species that specifically select plastic items because they mistake them for their food. As a consequence, the incidence of ingestion can be extremely high in some populations. For example, 95 per cent of fulmars washed ashore dead in the North Sea have plastic in their guts, with substantial quantities of plastic being reported in the guts of other birds, including albatross and prions ( Gregory 2009 ). There are some very good data on the quantity of debris ingested by seabirds recorded from the carcasses of dead birds. This approach has been used to monitor temporal and spatial patterns in the abundance of sea-surface plastic debris on regional scales around Europe ( Van Franeker et al. 2005 ; Ryan et al. 2009 ).
An area of particular concern is the abundance of small plastic fragments or microplastics. Fragments as small as 1.6 µm have been identified in some marine habitats, and it seems likely there will be even smaller pieces below current levels of detection. A recent workshop convened in the USA by the National Oceanic and Atmospheric Administration concluded that microplastics be defined as pieces <5 mm with a suggested lower size boundary of 333 µm so as to focus on microplastics that will be captured using conventional sampling approaches ( Arthur et al. 2009 ). However, we consider it important that the abundance of even smaller fragments is not neglected. Plastic fragments appear to form by the mechanical and chemical deterioration of larger items. Alternative routes for microplastics to enter the environment include the direct release of small pieces of plastics that are used as abrasives in industrial and domestic cleaning applications (e.g. shot blasting or scrubbers used in proprietary hand cleansers) and spillage of plastic pellets and powders that are used as a feedstock for the manufacture of most plastic products. Data from shorelines, from the open ocean and from debris ingested by seabirds, all indicate that quantities of plastic fragments are increasing in the environment, and quantities on some shores are substantial (>10% by weight of strandline material; Barnes et al. 2009 ). Laboratory experiments have shown that small pieces such as these can be ingested by small marine invertebrates including filter feeders, deposit feeders and detritivores ( Thompson et al. 2004 ), while mussels were shown to retain plastic for over 48 days ( Browne et al. 2008 ). However, the extent and consequences of ingestion of microplastics by natural populations are not known.
In addition to the physical problems associated with plastic debris, there has been much speculation that, if ingested, plastic has the potential to transfer toxic substances to the food chain (see Teuten et al. 2009 ). In the marine environment, plastic debris such as pellets, fragments and microplastics have been shown to contain organic contaminants including polychlorinated biphenyls (PCBs), polycyclic aromatic hydrocarbons, petroleum hydrocarbons, organochlorine pesticides (2,2′-bis( p -chlorophenyl)-1,1,1 trichloroethane (DDT) and its metabolites; together with hexachlorinated hexane (HCH)), polybrominated diphenylethers (PBDEs), alkylphenols and BPA at concentrations ranging from ng g –1 to µg g –1 . Some of these compounds are added to plastics during manufacture while others adsorb to plastic debris from the environment. Work in Japan has shown that plastics can accumulate and concentrate persistent organic pollutants that have arisen in the environment from other sources. These contaminants can become orders of magnitude more concentrated on the surface of plastic debris than in the surrounding sea water ( Mato et al. 2001 ). Teuten et al. (2009) describe experiments to examine the transfer of these contaminants from plastics to seabirds and other animals. The potential for transport varies among contaminants, polymers and possibly also according to the state of environmental weathering of the debris. Recent mathematical modelling studies have shown that even very small quantities of plastics could facilitate transport of contaminants from plastic to organisms upon ingestion. This could present a direct and important route for the transport of chemicals to higher animals such as seabirds ( Teuten et al. 2007 , 2009 ), but will depend upon the nature of the habitat and the amount and type of plastics present. For instance, the extent to which the presence of plastic particles might contribute to the total burden of contaminants transferred from the environment to organisms will depend upon competitive sorption and transport by other particulates ( Arthur et al. 2009 ). The abundance of fragments of plastic is increasing in the environment; these particles, especially truly microscopic fragments less than the 333 µm proposed by NOAA (see earlier), have a relatively large surface area to volume ratio that is likely to facilitate the transport of contaminants, and because of their size such fragments can be ingested by a wide range of organisms. Hence, the potential for plastics to transport and release chemicals to wildlife is an emerging area of concern.
More work will be needed to establish the full environmental relevance of plastics in the transport of contaminants to organisms living in the natural environment, and the extent to which these chemicals could then be transported along food chains. However, there is already clear evidence that chemicals associated with plastic are potentially harmful to wildlife. Data that have principally been collected using laboratory exposures are summarized by Oehlmann et al. (2009) . These show that phthalates and BPA affect reproduction in all studied animal groups and impair development in crustaceans and amphibians. Molluscs and amphibians appear to be particularly sensitive to these compounds and biological effects have been observed in the low ng l –1 to µg l –1 range. In contrast, most effects in fish tend to occur at higher concentrations. Most plasticizers appear to act by interfering with hormone function, although they can do this by several mechanisms ( Hu et al. 2009 ). Effects observed in the laboratory coincide with measured environmental concentrations, thus there is a very real probability that these chemicals are affecting natural populations ( Oehlmann et al. 2009 ). BPA concentrations in aquatic environments vary considerably, but can reach 21 µg l –1 in freshwater systems and concentrations in sediments are generally several orders of magnitude higher than in the water column. For example, in the River Elbe, Germany, BPA was measured at 0.77 µg l –1 in water compared with 343 µg kg –1 in sediment (dry weight). These findings are in stark contrast with the European Union environmental risk assessment predicted environmental concentrations of 0.12 µg l –1 for water and 1.6 µg kg –1 (dry weight) for sediments.
Phthalates and BPA can bioaccumulate in organisms, but there is much variability between species and individuals according to the type of plasticizer and experimental protocol. However, concentration factors are generally higher for invertebrates than vertebrates, and can be especially high in some species of molluscs and crustaceans. While there is clear evidence that these chemicals have adverse effects at environmentally relevant concentrations in laboratory studies, there is a need for further research to establish population-level effects in the natural environment (see discussion in Oehlmann et al. 2009 ), to establish the long-term effects of exposures (particularly due to exposure of embryos), to determine effects of exposure to contaminant mixtures and to establish the role of plastics as sources (albeit not exclusive sources) of these contaminants (see Meeker et al. (2009) for discussion of sources and routes of exposure).
5. Effects on humans: epidemiological and experimental evidence
Turning to adverse effects of plastic on the human population, there is a growing body of literature on potential health risks. A range of chemicals that are used in the manufacture of plastics are known to be toxic. Biomonitoring (e.g. measuring concentration of environmental contaminants in human tissue) provides an integrated measure of an organism's exposure to contaminants from multiple sources. This approach has shown that chemicals used in the manufacture of plastics are present in the human population, and studies using laboratory animals as model organisms indicate potential adverse health effects of these chemicals ( Talsness et al. 2009 ). Body burdens of chemicals that are used in plastic manufacture have also been correlated with adverse effects in the human population, including reproductive abnormalities (e.g. Swan et al. 2005 ; Swan 2008 ; Lang et al. 2008 ).
Interpreting biomonitoring data is complex, and a key task is to set information into perspective with dose levels that are considered toxic on the basis of experimental studies in laboratory animals. The concept of ‘toxicity’ and thus the experimental methods for studying the health impacts of the chemicals in plastic, and other chemicals classified as endocrine disruptors, is currently undergoing a transformation (a paradigm inversion) since the disruption of endocrine regulatory systems requires approaches very different from the study of acute toxicants or poisons. There is thus extensive evidence that traditional toxicological approaches are inadequate for revealing outcomes such as ‘reprogramming’ of the molecular systems in cells as a result of exposure to very low doses during critical periods in development (e.g. Myers et al. 2009 ). Research on experimental animals informs epidemiologists about the potential for adverse effects in humans and thus plays a critical role in chemical risk assessments. A key conclusion from the paper by Talsness et al. (2009) is the need to modify our approach to chemical testing for risk assessment. As noted by these authors and others, there is a need to integrate concepts of endocrinology in the assumptions underlying chemical risk assessment. In particular, the assumptions that dose–response curves are monotonic and that there are threshold doses (safe levels) are not true for either endogenous hormones or for chemicals with hormonal activity (which includes many chemicals used in plastics) ( Talsness et al. 2009) .
The biomonitoring approach has demonstrated phthalates and BPA, as well as other additives in plastics and their metabolites, are present in the human population. It has also demonstrated that the most common human exposure scenario is to a large number of these chemicals simultaneously. These data indicate differences according to geographical location and age, with greater concentrations of some of these chemicals in young children. While exposure via house dust is extensive ( Rudel et al. 2008 ), it would appear that at least for some phthalates (e.g. diethylhexyl phthalate, DEHP), foodstuffs and to a lesser extent use of oral drugs probably present major uptake pathways ( Wormuth et al. 2006 ). Exposure data for BPA are similar but less extensive. While average concentrations of phthalates in selected populations worldwide appear quite similar, there is evidence of considerable variability in daily intake rates among individuals, and even within individuals ( Peck et al. 2009 ). Exposures through ingestion, inhalation and dermal contact are all considered important routes of exposure for the general population ( Adibi et al. 2003 ; Rudel et al. 2003 ). Koch & Calafat (2009 ) show that while mean/median exposures for the general population were below levels determined to be safe for daily exposure (USA, EPA reference dose, RfD; and European Union tolerable daily intake, TDI), the upper percentiles of di-butyl phthalate and DEHP urinary metabolite concentrations show that for some people daily intake might be substantially higher than previously assumed and could exceed estimated safe daily exposure levels. Current ‘safe’ exposure levels are typically based on the application of traditional toxicological assumptions regarding acute toxicants to calculate daily exposures for chemicals in a range of widely used plastic items. The toxicological consequences of such exposures, especially for susceptible subpopulations such as children and pregnant women, remain unclear and warrant further investigation. However, there is evidence of associations between urinary concentrations of some phthalate metabolites and biological outcomes ( Swan et al. 2005 ; Swan 2008 ). For example, an inverse relationship has been reported between the concentrations of DEHP metabolites in the mother's urine and anogenital distance, penile width and testicular decent in male offspring ( Swan et al. 2005 ; Swan 2008 ). In adults, there is some evidence of a negative association between phthalate metabolites and semen quality (Meeker & Sathyanarayana) and between high exposures to phthalates (workers producing PVC flooring) and free testosterone levels. Moreover, recent work ( Lang et al. 2008 ) has shown a significant relationship between urine levels of BPA and cardiovascular disease, type 2 diabetes and abnormalities in liver enzymes, and Stahlhut et al. (2009) have reported that exposure of adults in the USA to BPA is likely to occur from multiple sources and that the half-life of BPA is longer than previously estimated, and the very high exposure of premature infants in neonatal intensive-care units to both BPA and phthalates is of great concern ( Calafat et al. 2009 ). These data indicate detrimental effects in the general population may be caused by chronic low-dose exposures (separately or in combination) and acute exposure to higher doses, but the full extent to which chemicals are transported to the human population by plastics is yet to be confirmed.
Much has been learned about toxicological effects on humans from experiments using laboratory animals. This approach has been used to examine component chemicals used in plastic production. A summary of work on phthalates, BPA and tetrabromobisphenol A (TBBPA) is presented by Talsness et al. (2009) . The male reproductive tract is particularly sensitive to phthalate exposure. However, most reproductive effects are not exerted by phthalate diesters themselves, but by their monoester metabolites, which are formed in the liver. The majority of these studies have been done using rats as a model organism, with doses at least an order of magnitude higher than those to which humans are commonly exposed, but they have resulted in rapid, severe changes in the rat testis. Reproductive effects have also been described in mice and guinea pigs. Effects on pre- and early post-natal development are of particular concern, and recent animal studies have shown exposures to certain phthalates can result in severe disorders of the developing male reproductive system. It should be noted that most work on animals has used phthalate exposures much higher than estimated daily human exposures (see above), and researchers have only recently started to investigate possible biological effects within the range of median human phthalate exposure ( Talsness et al. 2009 ). This is of critical importance because epidemiological studies have reported associations between phthalate levels and a number of adverse health effects in humans ( Swan et al. 2005 ), suggesting that either humans are more sensitive to phthalates than experimental animals or that the testing paradigm used in traditional toxicological studies, which examines one phthalate at a time, has not served to accurately predict adverse effects from the mixture of phthalates to which humans are exposed ( Andrade et al. 2006 ; NAS 2008 ).
For BPA, there is an extensive published literature showing adverse effects of exposure at very low doses, based on administration during development and to adult experimental animals. In particular, unlike the case for experimental animal research on phthalates, there are now hundreds of experiments on laboratory animals using doses within the range of human exposures ( Vandenberg et al. 2007 ). The rate and extent to which BPA is metabolized affect the interpretation of these findings, but even very low doses of BPA have been shown to cause significant stimulation of insulin secretion followed by insulin resistance in mice, a significant decrease in sperm production by rats, a decrease in maternal behaviour in mice and disruption of hippocampal synapses, leading to the appearance of a brain typical of that seen in senility in both rats and monkeys. The greatest concerns with exposure to BPA are during development; BPA appears to affect brain development leading to loss of sex differentiation in brain structures and behaviour ( Talsness et al. 2009 ). A further important observation regarding adverse responses to developmental exposures of animals to very low doses of BPA is that many relate to disease trends in humans. Less has been published on effects of the flame retardant TBBPA, but there is evidence of effects on thyroid hormones, pituitary function and reproductive success in animals ( Talsness et al. 2009 ).
Despite the environmental concerns about some of the chemicals used in plastic manufacture, it is important to emphasize that evidence for effects in humans is still limited and there is a need for further research and in particular, for longitudinal studies to examine temporal relationships with chemicals that leach out of plastics ( Adibi et al. 2008 ). In addition, the traditional approach to studying the toxicity of chemicals has been to focus only on exposure to individual chemicals in relation to disease or abnormalities. However, because of the complex integrated nature of the endocrine system, it is critical that future studies involving endocrine-disrupting chemicals that leach from plastic products focus on mixtures of chemicals to which people are exposed when they use common household products. For example, in a study conducted in the USA, 80 per cent of babies were exposed to measurable levels of at least nine different phthalate metabolites ( Sathyanarayana et al. 2008 ), and the health impacts of the cumulative exposure to these chemicals need to be determined. An initial attempt at examining more than one phthalate as a contributor to abnormal genital development in babies has shown the importance of this approach ( Swan 2008 ). Studies of mixtures of chemicals therefore also need to extend beyond mixtures of the same class of chemical, such as mixtures of different phthalates or of different PCBs. For example, PVC (used in a wide range of products in the home including water pipes) may contain phthalates, BPA, flame retardants such as PBDEs or TBBPA, cadmium, lead and organotins, all of which have been shown in animal studies to result in obesity ( Heindel & vom Saal 2009 ). In addition, the monomer used to manufacture PVC plastic, vinyl chloride, is a known carcinogen and exposure can cause angiosarcoma of the liver among factory workers ( Bolt 2005 ; Gennaro et al. 2008 ). PVC in medical tubing has also been shown to be a source of high DEHP exposure among infants in neonatal intensive-care nurseries ( Green et al. 2005 ) and probably contributes to the high levels of BPA found in these babies since BPA is an additive in PVC plastic ( Calafat et al. 2009 ).
Examining the relationship between plastic additives and adverse human effects presents a number of challenges. In particular, the changing patterns of production and use of both plastics, and the additives they contain, as well as the confidential nature of industrial specifications makes exposure assessment particularly difficult. Evolving technology, methodology and statistical approaches should help disentangle the relationships between these chemicals and health effects. However, with most of the statistically significant hormone alternations that have been attributed to environmental and occupational exposures, the actual degree of hormone alteration has been considered subclinical. Hence, more information is required on the biological mechanisms that may be affected by plastic additives and in particular, low-dose chronic exposures. Meanwhile we should consider strategies to reduce the use of these chemicals in plastic manufacture and/or develop and test alternatives (for example citrates are being developed as substitute plasticizers). This is the goal of the new field of green chemistry, which is based on the premise that development of chemicals for use in commerce should involve an interaction between biologists and chemists. Had this approach been in place 50 years ago it would probably have prevented the development of chemicals that are recognized as likely endocrine disruptors ( Anastas & Beach 2007 ). There is also a need for industry and independent scientists to work more closely with, rather than against, each other in order to focus effectively on the best ways forward. For example, contrast comments on BPA by Bird (2005 ) with those of vom Saal (2005 ), and contrast comments in this volume on the safety of plastic additives by Andrady & Neal (2009 ) with those by Koch & Calafat (2009 ), Meeker et al. (2009 ), Oehlmann et al. (2009) and Talsness et al. (2009) .
6. Production, usage, disposal and waste management solutions
Accumulation of plastic debris in the environment and the associated consequences are largely avoidable. Considerable immediate reductions in the quantity of waste entering natural environments, as opposed to landfill, could be achieved by better waste disposal and material handling. Littering is a behavioural issue and some have suggested that it has increased in parallel with our use of disposable products and packaging. Perhaps increasing the capacity to recycle will help to reverse this trend such that we start to regard end-of-life materials as valuable feedstocks for new production rather than waste. To achieve this will require better education, engagement, enforcement and recycling capacity ( figure 1 a – f ). Unfortunately, we were unable to source a contribution on education and public engagement, but it is evident that social research on littering behaviour could be very informative. A recent report by EnCams in the UK examined attitudes towards littering in 2001 and then again in 2006. This indicated that despite greater awareness among the general public about the problems of littering, the propensity to litter had actually increased; five key attitudes and behaviours were noted and these offer valuable insight for future research ( EnCams 2006 ). There is evidence that appropriate education can influence behaviour. For example, pre-production plastic pellets (a feedstock for production of plastic products, also described as nurdles or mermaids tears) account for around 10 per cent, by number, of the plastic debris recorded on shorelines in Hawaii ( McDermid & McMullen 2004 ) and substantial quantities have been recorded on shorelines in New Zealand ( Gregory 1978 ). These pellets have entered the environment through spillage during transportation, handling and as cargo lost from ships. In the USA guidelines (Operation Clean-Sweep, figure 1 e ) on handling of resin pellets are reported to have reduced spillage during trials ( Moore et al . 2005 ). Conservation organizations such as the UK Marine Conservation Society play an important role in education, and the annual beach cleans they organize can be a good way to raise public awareness and to collect data on trends in the abundance of debris on shorelines (see www.mcsuk.org and Ocean Conservancy, International Coastal Cleanup www.oceanconservancy.org ). However, there is a pressing need for education to reduce littering at source ( figure 1 d and e ). This is especially important in urban settings where increased consumption of on-the-go/fast food coupled, in some locations, with a reduction in the availability of bins as a consequence of concerns about terrorism is likely to result in increased littering. Where plastic debris enters watercourses as a consequence of dumping or littering a range of strategies including catch basin inserts, booms and separators can be used to facilitate removal ( figure 1 f ).

Solutions include: ( a ) measures to reduce the production of new plastics from oil, here an example showing how small changes in product packing reduced the weight of packaging required by 70%, while ( b ) re-useable plastic packing crates have reduced the packaging consumption of the same retailer by an estimated 30,000 tonnes per annum; and ( c ) recycling; here, bales of used plastic bottles have been sorted prior to recycling into new items, such as plastic packaging or textiles. Measures to reduce the quantity of plastic debris in the natural environment include: ( d ) educational signage to reduce contamination via storm drains and ( e ) via industrial spillage, together with ( f ) booms to intercept and facilitate the removal of riverine debris. (Photographs ( a ) and ( b ), and associated usage statistics, courtesy of Marks and Spencer PLC; ( c ) courtesy of P. Davidson, WRAP; ( d , e , f ) courtesy of C. Moore, Algalita Marine Research Foundation.)
Substantial quantities of end-of-life plastics are disposed of to landfill. Waste generation statistics vary among countries and according to the rationale for data collection. For instance, plastics are a small component of waste by weight but a large component by volume. Temporal and spatial comparisons can thus be confounded, and data on quantities of waste recycled can be skewed according to categorization of various wastes. However, in many locations space in landfill is running out (e.g. Defra et al. 2006 ). It has also been suggested that because of the longevity of plastics, disposal to landfill may simply be storing problems for the future ( Barnes et al. 2009 ; Hopewell et al. 2009 ). For example, plasticizers and other additive chemicals have been shown to leach from landfills ( Teuten et al. (2009) and references therein). The extent of this varies according to conditions, particularly pH and organic content. There is evidence, however, that landfills can present a significant source of contaminants, such as BPA, to aquatic environments. Efficient treatment approaches are available and are in use in some countries ( Teuten et al. 2009 ).
From a waste management perspective, the three R's— reduce, reuse and recycle are widely advocated to reduce the quantities of plastic and especially plastics packaging the waste we generate ( figure 1 a – c ). Hopewell et al. (2009) outline the benefits and limitations of these strategies. They show that to be effective we need to consider the three R's in combination with each other and together with a fourth ‘R’, energy recovery . Indeed we also need to consider a 5th ‘R’, molecular redesign, as an emerging and potentially very important strategy. Hence, the three R's become five: ‘reduce, reuse, recycle, recover and redesign’. There are opportunities to ‘reduce’ usage of raw material by down gauging ( figure 1 a ) and there are also some opportunities to ‘reuse’ plastics, for example, in the transport of goods on an industrial (pallets, crates; figure 1 b ) and a domestic (carrier bags) scale. However, there is limited potential for wide-scale reuse of retail packaging because of the substantial back-haul distances and logistics involved in returning empty cartons to suppliers. Some of the energy content of plastics can be ‘recovered’ by incineration, and through approaches such as co-fuelling of kilns, reasonable energy efficiency can be achieved. These approaches have benefits compared with disposal to landfill since some of the energy content of plastics is recovered. However, energy recovery does not reduce the demand for raw material used in plastic production, hence it is considered less energy efficient than product recovery via recycling ( WRAP 2006 ; Defra 2007 ). In addition, concerns about emissions from incinerators ( Katami et al. 2002 ) can reduce the appeal of this waste disposal option. There is now strong evidence to indicate significant potential lies in increasing our ability to effectively recycle end-of-life plastic products ( WRAP 2006 , 2008 ; Defra 2007 ; fig 1 c ). Although thermoplastics have been recycled since the 1970s, the proportion of material recycled has increased substantially in recent years and represents one of the most dynamic areas of the plastic industry today ( WRAP 2006 , 2008 ).
The recycling message is simple; both industry and society need to regard end-of-life items, including plastics, as raw materials rather than waste. At present our consumption of fossil fuels for plastic production is linear, from oil to waste via plastics. It is essential to take a more cyclical approach to material usage, but achieving this goal is complex ( Hopewell et al. 2009 ). Greatest energy efficiency is achieved where recycling diverts the need for use of fossil fuels as raw materials ( figure 1 c ); good examples being the recycling of old polyethylene terephthalate (PET) bottles into new ones (closed-loop recycling) or where low-density polyethylene bottles are converted into waste bins (semi-closed loop). In addition to benefits as a consequence of more sustainable material usage, a recent life cycle analysis calculated that use of 100 per cent recycled PET rather than virgin PET to produce plastic bottles could give a 27 per cent reduction on CO 2 emissions ( WRAP 2008 ; Hopewell et al. 2009 ).
There are some very encouraging trends, with growth in mechanical recycling increasing at 7 per cent per annum in western Europe. However, there is considerable regional variation in recycling rates and globally only a small proportion of plastic waste is recycled (see Barnes et al. (2009) for US data; see Hopewell et al. (2009) for European data). Items made of a single polymer are easier and more efficient to recycle than composite items, films and mixed wastes. As a consequence, it is currently not possible to recycle a substantial proportion of the packaging in a typical shopping basket ( Hopewell et al. 2009 ). On reading the account by Hopewell et al. (2009) , the ingenuity of the separation procedures for recycling is evident (Fourier-transform near-infrared spectroscopy, optical colour separation, X-ray detection), but one cannot help but wonder why similar ingenuity has not been focused on designing products for better end-of-life recyclability. Historically, the main considerations for the design of plastic packaging have been getting goods safely to market and product marketing. There is an increasing urgency to also design products, especially packaging, in order to achieve material reduction and greater end-of-life recyclability. Public support for recycling is high in some countries (57% in the UK and 80% in Australia; Hopewell et al. 2009 ), and consumers are keen to recycle, but the small size and the diversity of different symbols to describe a product's potential recyclability, together with uncertainties as to whether a product will actually be recycled if it is offered for collection, can hinder engagement. In our opinion, what is needed is a simplification and streamlining of everyday packaging, to facilitate recyclability, together with clearer labelling to inform users. One option could be a traffic light system so that consumers can easily distinguish from printed product labelling between packages that use recycled content and have high end-of-life recyclability (marked with a green spot), those that have low end-of-life recyclability and are predominantly made of virgin polymer (red spot), and those which lie between these extremes (amber spot). With combined actions including waste reduction, design for end-of-life, better labelling for consumers, increased options for on-the-go disposal to recycling and improved recycling capability, Hopewell et al. (2009) consider it could be possible to divert the majority of plastic from landfill over the next few decades ( figure 1 a – c ). This will require consistency of policy measures and facilities among regions and will also require the cooperation of industry since ultimately there needs to be an acceptance of reduced usage and hence reduced income associated with the production of plastics from virgin polymer.
Molecular redesign of plastics (the 5th R) has become an emerging issue in green chemistry ( Anastas & Warner 1998 ; Anastas et al. 2000 ; Anastas & Crabtree 2009 ) that should be incorporated within the design and life cycle analysis of plastics. In this context, green chemists aspire to design chemical products that are fully effective, yet have little or no toxicity or endocrine-disrupting activity; that break down into innocuous substances if released into the environment after use; and/or that are based upon renewable feedstocks, such as agricultural wastes. One of the fundamental factors limiting progress on all other R's is that the design criteria used to develop new monomers have rarely included specifications to enhance reusability, recyclability or recovery of plastic once it has been used. Typically, such assessments have only been made after a product entered the marketplace and problems involving waste and/or adverse health effects have begun to appear. Had the guiding principles of Green Chemistry ( Anastas & Warner 1998 ) been available to inform the syntheses of polymers over the past century, perhaps some of the environmental and health concerns described in this Theme Issue would be more manageable. To date, the application of these design criteria to polymers has remained largely in the laboratory. Polylactic acid (PLA) ( Drumright et al. 2000 ), a biodegradable polymer sourced from corn and potatoes, has entered the marketplace and has the potential to make a valuable contribution among other strategies for waste management. However, life cycle analyses are required to help establish the most appropriate usage, disposal (e.g. Song et al. 2009 illustrate relatively slow degradability of PLA in home composting) and hence labelling, of biopolymers such as this ( WRAP 2009 ).
7. Biopolymers, degradable and biodegradable polymer solutions
Degradable polymers have been advocated as an alternative to conventional oil-based plastics and their production has increased considerably in recent decades. Materials with functionality comparable to conventional plastics can now be produced on an industrial scale; they are more expensive than conventional polymers and account for less than 1 per cent of plastics production ( Song et al. 2009 ). Biopolymers differ from conventional polymers in that their feedstock is from renewable biomass rather than being oil-based. They may be natural polymers (e.g. cellulose), or synthetic polymers made from biomass monomers (e.g. PLA) or synthetic polymers made from synthetic monomers derived from biomass (e.g. polythene derived from bioethanol) ( WRAP 2009 ). They are often described as renewable polymers since the original biomass, for example corn grown in agriculture, can be reproduced. The net carbon dioxide emission may be less than that with conventional polymers, but it is not zero since farming and pesticide production have carbon dioxide outputs ( WRAP 2009 ). In addition, as a consequence of our rapidly increasing human population, it seems unlikely that there will be sufficient land to grow crops for food, let alone for substantial quantities of packaging in which to wrap it. One solution is to recycle waste food into biopolymers; this has merit, but will ultimately be limited by the amount of waste food available.
Biopolymers that are designed to breakdown in an industrial composter are described as ‘biodegradables’ while those that are intended to degrade in a domestic composter are known as ‘compostable’. There are benefits of these biodegradable materials in specific applications, for example, with packaging of highly perishable goods where, regrettably, it can be necessary to dispose of perished unopened and unused product together with its wrapper. Song et al. (2009) show experimentally that degradation of biodegradable, as opposed to compostable, polymers can be very slow in home composters (typically less than 5% loss of biomass in 90 days). Degradation of these polymers in landfills is also likely to be slow and may create unwanted methane emissions. Hence, the benefits of biopolymers are only realized if they are disposed of to an appropriate waste management system that uses their biodegradable features. Typically, this is achieved via industrial composting at 50°C for around 12 weeks to produce compost as a useful product.
Some biopolymers, such as PLA, are biodegradable, but others such as polythene derived from bioethanol are not. A further complication is that degradable, as opposed to biodegradable, polymers (also called ‘oxo-biodegradable’, ‘oxy-degradable’ or ‘UV-degradable’) can also be made from oil-based sources but as a consequence are not biopolymers. These degradable materials are typically polyethylene together with additives to accelerate the degradation. They are used in a range of applications and are designed to break down under UV exposure and/or dry heat and mechanical stress, leaving small particles of plastic. They do not degrade effectively in landfills and little is known about the timescale, extent or consequences of their degradation in natural environments ( Barnes et al. 2009 ; Teuten et al. 2009 ). Degradable polymers could also compromise the quality of recycled plastics if they enter the recycling stream. As a consequence, use of degradable polymers is not advocated for primary retail packaging ( WRAP 2009 ).
There is a popular misconception that degradable and biodegradable polymers offer solutions to the problems of plastic debris and the associated environmental hazards that result from littering. However, most of these materials are unlikely to degrade quickly in natural habitats, and there is concern that degradable, oil-based polymers could merely disintegrate into small pieces that are not in themselves any more degradable than conventional plastic ( Barnes et al. 2009 ). So while biodegradable polymers offer some waste management solutions, there are limitations and considerable misunderstanding among the general public about their application ( WRAP 2007 ). To gain the maximum benefit from degradable, biodegradable and compostable materials, it is, therefore, essential to identify specific uses that offer clear advantages and to refine national and international standards (e.g. EN 13432, ASTM D6400-99) and associated product labelling to indicate appropriate usage and appropriate disposal.
8. Policy measures
Our intention when preparing this Theme Issue was to focus on the science surrounding all aspects pertinent to plastics, the environment and human health. There are some omissions from the volume, such as input from social scientists on how best to convey relevant information to influence littering behaviour, consumer choice and engagement with recycling. These omissions aside, to be of greatest value the science herein needs to be communicated beyond a purely scientific audience (see recommendations in table 1 ). This is in part the role of a Theme Issue such as this, and the final invited contribution to the volume examines the science–policy interface with particular reference to policy relating to plastics. Shaxson (2009) considers this interface from the perspectives of industry, the scientist and the policymaker. She emphasizes the need for policy relating to plastic to weigh societal and economic benefits against environmental and health concerns. This is a diverse subject area that will require a range of policies to focus at specific issues, including polymer safety, material reduction, reuse, recycling, biopolymers, biodegradable and compostable polymers, littering, dumping and industrial spillage. There are a range of appropriate measures ( National Research Council 2008 ) including information and recommendations (e.g. WRAP 2009 ), regulations (such as the Canadian Government restrictions on BPA in baby bottles), taxes (such as land fill tax, which incentivizes the diversion of waste from landfill to recycling), standards (such as EN 13432 covering compostable plastics) and allocation of funds for research, innovation and capacity building. However, the diversity of issues leads to an equally complex policy environment. In the UK, for example, there is not one, but many relevant policy interfaces and numerous policies. These activities are shared among several government departments, driven by national pressures, international obligations and European directives. In such a complex environment, even robust and clearly delivered information from the scientific community does not always have the most appropriate effects on the policy process.
Table 1.
Synthesis of current knowledge, uncertainty and recommended actions relevant to environmental and human health concerns arising from current production, use and disposal of plastics.
Shaxson presents evidence from case studies on policies relating to plastic litter in the marine environment and land-based plastic waste. She indicates that many plastic-related policy issues fall into what are defined as unstructured or badly structured problems—in essence, problems that lack consensus and clarity in the relevant policy question and in some cases lack clarity in the relevant knowledge base to inform any decision. Shaxson suggests such circumstances will require a reflexive approach to brokering knowledge between industry, scientists and policymakers, and that scientists will need to be prepared to make and facilitate value judgements on the basis of best evidence. From a UK perspective, she advocates using the science within this volume to help develop a ‘Plastics Road Map’, similar to the recently completed Milk and Dairy Road Map ( Defra 2008 ) to structure policy around plastics, the environment and human health and suggests that this be facilitated by appropriate and broad debate among relevant parties.
9. Plastics and the future
Looking ahead, we do not appear to be approaching the end of the ‘plastic age’ described by Yarsley and Couzens in the 1940s, and there is much that plastics can contribute to society. Andrady & Neal (2009) consider that the speed of technological change is increasing exponentially such that life in 2030 will be unrecognizable compared with life today; plastics will play a significant role in this change. Plastic materials have the potential to bring scientific and medical advances, to alleviate suffering and help reduce mankind's environmental footprint on the planet ( Andrady & Neal 2009 ). For instance, plastics are likely to play an increasing role in medical applications, including tissue and organ transplants; lightweight components, such as those in the new Boeing 787, will reduce fuel usage in transportation; components for generation of renewable energy and insulation will help reduce carbon emissions and smart plastic packaging will no doubt be able to monitor and indicate the quality of perishable goods.
In conclusion, plastics offer considerable benefits for the future, but it is evident that our current approaches to production, use and disposal are not sustainable and present concerns for wildlife and human health. We have considerable knowledge about many of the environmental hazards, and information on human health effects is growing, but many concerns and uncertainties remain. There are solutions, but these can only be achieved by combined actions (see summary table 1 ). There is a role for individuals, via appropriate use and disposal, particularly recycling; for industry by adopting green chemistry, material reduction and by designing products for reuse and/or end-of-life recyclability and for governments and policymakers by setting standards and targets, by defining appropriate product labelling to inform and incentivize change and by funding relevant academic research and technological developments. These measures must be considered within a framework of lifecycle analysis and this should incorporate all of the key stages in plastic production, including synthesis of the chemicals that are used in production, together with usage and disposal. Relevant examples of lifecycle analysis are provided by Thornton (2002) and WRAP (2006) and this topic is discussed, and advocated, in more detail in Shaxson (2009) . In our opinion, these actions are overdue and are now required with urgent effect; there are diverse environmental hazards associated with the accumulation of plastic waste and there are growing concerns about effects on human health, yet plastic production continues to grow at approximately 9 per cent per annum ( PlasticsEurope 2008 ). As a consequence, the quantity of plastics produced in the first 10 years of the current century will approach the total that was produced in the entire century that preceded.
Acknowledgements
We are indebted to James Joseph and Claire Rawlinson in the editorial office and Jessica Mnatzaganian in the journals production office at the Royal Society. Without their guidance and patience this volume would not have been possible. We also thank Dr J. P. Myers, Environmental Health Sciences, for his help in preparing text for the section on Green Chemistry.
One contribution of 15 to a Theme Issue ‘Plastics, the environment and human health’ .
- Adibi J. J., Perera F. P., Jedrychowski W., Camann D. E., Barr D., Jacek R., Whyatt R. M.2003 Prenatal exposures to phthalates among women in New York City and Krakow, Poland . Environ. Health Perspect. 111 , 1719–1722 [ PMC free article ] [ PubMed ] [ Google Scholar ]
- Adibi J. J., et al.2008 Characterization of phthalate exposure among pregnant women assessed by repeat air and urine samples . Environ. Health Perspect. 116 , 467–473 [ PMC free article ] [ PubMed ] [ Google Scholar ]
- Anastas P. T., Beach E. S.2007 Green chemistry: the emergence of a transformative framework . Green Chem. Lett. Rev. 1 , 9–24 ( doi:10.1080/17518250701882441 ) [ Google Scholar ]
- Anastas P. T., Crabtree R. H.(ed.)2009 Handbook of green chemistry—green catalysis. Vol I Homogenous catalysis . Handbook of Green Chemistry New York, NY: John Wiley & Sons [ Google Scholar ]
- Anastas P. T., Warner J. C.1998 Green chemistry: theory and practice Oxford, UK: Oxford University Press [ Google Scholar ]
- Anastas P. T., Bickart P. H., Kirchhoff M. M.2000 Designing safer polymers New York, NY: John Wiley and Sons, Wiley-Interscience [ Google Scholar ]
- Andrade A. J. M., Grande S. W., Talsness C. E., Grote K., Chahoud I.2006 A dose–response study following in utero and lactational exposure to di-(2-ethylhexyl)-phthalate (DEHP): non-monotonic dose–response and low dose effects on rat brain aromatase activity . Toxicology 227 , 185–192 ( doi:10.1016/j.tox.2006.07.022 ) [ PubMed ] [ Google Scholar ]
- Andrady A. L., Neal M. A.2009 Applications and societal benefits of plastics . Phil. Trans. R. Soc. B 364 , 1977–1984 ( doi:10.1098/rstb.2008.0304 ) [ PMC free article ] [ PubMed ] [ Google Scholar ]
- Arthur C., Baker J., Bamford H.2009. Proc. International Research Workshop on the occurrence, effects and fate of microplastic marine debris, 9–11 September 2008 NOAA Technical Memorandum NOS-OR&R30 [ Google Scholar ]
- Barnes D. K. A.2002 Biodiversity—invasions by marine life on plastic debris . Nature 416 , 808–809 ( doi:10.1038/416808a ) [ PubMed ] [ Google Scholar ]
- Barnes D. K. A.2005 Remote islands reveal rapid rise of southern hemisphere sea debris . Sci. World J. 5 , 915–921 [ PMC free article ] [ PubMed ] [ Google Scholar ]
- Barnes D. K. A., Galgani F., Thompson R. C., Barlaz M.2009 Accumulation and fragmentation of plastic debris in global environments . Phil. Trans. R. Soc. B 364 , 1985–1998 ( doi:10.1098/rstb.2008.0205 ) [ PMC free article ] [ PubMed ] [ Google Scholar ]
- Bird J.2005 Hyperbole or common sense . Chem. Ind. 5 , 14–15 [ Google Scholar ]
- Bolt H. M.2005 Vinyl chloride—a classical industrial toxicant of new interest . Crit. Rev. Toxicol. 35 , 307–323 ( doi:10.1080/10408440490915975 ) [ PubMed ] [ Google Scholar ]
- Brinton W. F.2005 Characterization of man-made foreign matter and its presence in multiple size fractions from mixed waste composting . Compost Sci. Utilizat. 13 , 274–280 [ Google Scholar ]
- Brown J., Macfadyen G.2007 Ghost fishing in European waters: impacts and management responses . Mar. Policy 31 , 488–504 ( doi:10.1016/j.marpol.2006.10.007 ) [ Google Scholar ]
- Browne M. A., Dissanayake A., Galloway T. S., Lowe D. M., Thompson R. C.2008 Ingested microscopic plastic translocates to the circulatory system of the mussel, Mytilus edulis (L.) . Environ. Sci. Technol. 42 , 5026–5031 ( doi:10.1021/es800249a ) [ PubMed ] [ Google Scholar ]
- Calafat A. M., Weuve J., Ye X. Y., Jia L. T., Hu H., Ringer S., Huttner K., Hauser R.2009 Exposure to bisphenol A and other phenols in neonatal intensive care unit premature infants . Environ. Health Perspec 117 , 639–644 ( doi:10.1289/ehp.0800265 ) [ PMC free article ] [ PubMed ] [ Google Scholar ]
- Cheshire A. C., et al.2009 UNEP/IOC Guidelines on Survey and Monitoring of Marine Litter UNEP Regional Seas Reports and Studies, No. 186; IOC Technical Series No. 83: xii+120 pp. [ Google Scholar ]
- Defra 2007 Waste strategy for England , p. 127 Norwich, UK: Department of Environment food and Rural Affairs, HMSO [ Google Scholar ]
- Defra 2008 The milk roadmap London, UK: Department of Environment, Food and Rural Affairs; See http://www.defra.gov.uk/environment/consumerprod/products/milk.htm#roadmap (accessed 10 July 2008). [ Google Scholar ]
- Defra Enviros, Wilson S., Hannan M.2006 Review of England's waste strategy . Environmental report under the ‘SEA’ directive , p. 96 London, UK: DEFRA [ Google Scholar ]
- Derraik J. G. B.2002 The pollution of the marine environment by plastic debris: a review . Mar. Pollut. Bull. 44 , 842–852 ( doi:10.1016/S0025-326X(02)00220-5 ) [ PubMed ] [ Google Scholar ]
- Drumright R. E., Gruber P. R., Henton D. E.2000 Polylactic acid technology . Adv. Mater. 12 , 1841–1846 ( doi:10.1002/1521-4095(200012)12:23<1841::AID-ADMA1841>3.0.CO;2-E ) [ Google Scholar ]
- EnCams 2006 Litter segmentation 2006 Wigan, UK: Environmental Campaigns Limited (ENCAMS) [ Google Scholar ]
- Gennaro V., Ceppi M., Crosignani P., Montanaro F.2008 Reanalysis of updated mortality among vinyl and polyvinyl chloride workers: confirmation of historical evidence and new findings . BMC Public Health 8 , article 21 ( doi:10.1186/1471-2458-8-21 ) [ PMC free article ] [ PubMed ] [ Google Scholar ]
- Green R., Hauser R., Calafat A. M., Weuve J., Schettler T., Ringer S., Huttner K., Hu H.2005 Use of di(2-ethylhexyl) phthalate-containing medical products and urinary levels of mono(2-ethylhexyl) phthalate in neonatal intensive care unit infants . Environ. Health Perspect. 113 , 1222–1225 [ PMC free article ] [ PubMed ] [ Google Scholar ]
- Gregory M. R.1978 Accumulation and distribution of virgin plastic granules on New Zealand beaches . N. Z. J. Mar. Freshwater Res. 12 , 339–414 [ Google Scholar ]
- Gregory M. R.2009 Environmental implications of plastic debris in marine settings—entanglement, ingestion, smothering, hangers-on, hitch-hiking and alien invasions . Phil. Trans. R. Soc. B 364 , 2013–2025 ( doi:10.1098/rstb.2008.0265 ) [ PMC free article ] [ PubMed ] [ Google Scholar ]
- Harper P. C., Fowler J. A.1987 Plastic pellets in New Zealand storm-killed prions ( Pachyptila spp.), 1958–1998 . Notornis 34 , 65–70 [ Google Scholar ]
- Heindel J. J., vom Saal F. S.2009 Overview of obesity and the role of developmental nutrition and environmental chemical exposures . Mol. Cell. Endocrinol ( doi:10.1016/j.mce.2009.02.025 ) [ PubMed ] [ Google Scholar ]
- Hopewell J., Dvorak R., Kosior E.2009 Plastics recycling: challenges and opportunities . Phil. Trans. R. Soc. B 364 , 2115–2126 ( doi:10.1098/rstb.2008.0311 ) [ PMC free article ] [ PubMed ] [ Google Scholar ]
- Hu G. X., Lian Q. Q., Ge R. S., Hardy D. O., Li X. K.2009 Phthalate-induced testicular dysgenesis syndrome: Leydig cell influence . Trends Endocrinol. Metab. 20 , 139–145 ( doi:10.1016/j.tem.2008.12.001 ) [ PMC free article ] [ PubMed ] [ Google Scholar ]
- Katami T., Yasuhara A., Okuda T., Shibamoto T.2002 Formation of PCDDs, PCDFs, and coplanar PCBs from polyvinyl chloride during combustion in an incinerator . Environ. Sci. Technol. 36 , 1320–1324 ( doi:10.1021/es0109904 ) [ PubMed ] [ Google Scholar ]
- Koch H. M., Calafat A. M.2009 Human body burdens of chemicals used in plastic manufacture . Phil. Trans. R. Soc. B 364 , 2063–2078 ( doi:10.1098/rstb.2008.0208 ) [ PMC free article ] [ PubMed ] [ Google Scholar ]
- Laist D. W.1997 Impacts of marine debris: entanglement of marine life in marine debris including a comprehensive list of species with entanglement and ingestion records . In Marine debris: sources, impacts and solutions (eds Coe J. M., Rogers B. D.), pp. 99–141 Berlin, Germany: Springer [ Google Scholar ]
- Lang I. A., Galloway T. S., Scarlett A., Henley W. E., Depledge M., Wallace R. B., Melzer D.2008 Association of urinary bisphenol A concentration with medical disorders and laboratory abnormalities in adults . J. Am. Med. Assoc. 300 , 1303–1310 ( doi:10.1001/jama.300.11.1303 ) [ PubMed ] [ Google Scholar ]
- Mato Y., Isobe T., Takada H., Kanehiro H., Ohtake C., Kaminuma T.2001 Plastic resin pellets as a transport medium for toxic chemicals in the marine environment . Environ. Sci. Technol. 35 , 318–324 ( doi:10.1021/es0010498 ) [ PubMed ] [ Google Scholar ]
- McDermid K. J., McMullen T. L.2004 Quantitative analysis of small-plastic debris on beaches in the Hawaiian archipelago . Mar. Pollut. Bull. 48 , 790–794 ( doi:10.1016/j.marpolbul.2003.10.017 ) [ PubMed ] [ Google Scholar ]
- Mee A., Rideout B. A., Hamber J. A., Todd J. N., Austin G., Clark M., Wallace M. P.2007 Junk ingestion and nestling mortality in a reintroduced population of California Condors Gymnogyps californianus . Bird Conserv. Int. 17 , 119–130 ( doi:10.1017/S095927090700069X ) [ Google Scholar ]
- Meeker J. D., Sathyanarayana S., Swan S. H.2009 Phthalates and other additives in plastics: human exposure and associated health outcomes . Phil. Trans. R. Soc. B 364 , 2097–2113 ( doi:10.1098/rstb.2008.0268 ) [ PMC free article ] [ PubMed ] [ Google Scholar ]
- Moore C. J.2008 Synthetic polymers in the marine environment: a rapidly increasing, long-term threat . Environ. Res 108 , 131–139 ( doi:10.1016/j.envres.2008.07.025 ) [ PubMed ] [ Google Scholar ]
- Moore C. J., Lattin G. L., Zellers A. F.2005 Working our way upstream: a snapshot of land based contributions of plastic and other trash to coastal waters and beaches of Southern California . In Proceedings of the Plastic Debris Rivers to Sea Conference, Algalita Marine Research Foundation, Long Beach, California [ Google Scholar ]
- Myers J. P., et al.2009 Why public health agencies cannot depend on good laboratory practices as a criterion for selecting data: the case of bisphenol A . Environ. Health Perspect. 117 , 309–315 [ PMC free article ] [ PubMed ] [ Google Scholar ]
- NAS. Phthalates and cumulative risk assessment: the tasks ahead. Washington, DC: National Academy of Sciences; 2008. [ PubMed ] [ Google Scholar ]
- National Research Council 2008 Tackling Marine Debris in the 21st century. Committee on the effectiveness of international and national measures to prevent and reduce marine debris and its impacts . Washington, DC: The National Academies Press [ Google Scholar ]
- Oehlmann J., et al.2009 A critical analysis of the biological impacts of plasticizers on wildlife . Phil. Trans. R. Soc. B 364 , 2047–2062 ( doi:10.1098/rstb.2008.0242 ) [ PMC free article ] [ PubMed ] [ Google Scholar ]
- Oigman-Pszczol S. S., Creed J. C.2007 Quantification and classification of marine litter on beaches along Armacao dos Buzios, Rio de Janeiro, Brazil . J. Coastal Res. 23 , 421–428 ( doi:10.2112/1551-5036(2007)23[421:QACOML]2.0.CO;2 ) [ Google Scholar ]
- OSPAR 2007 OSPAR pilot project on monitoring marine beach litter: monitoring of marine litter on beaches in the OSPAR region London, UK: OSPAR Commission [ Google Scholar ]
- Peck J. D., Sweeney A. M., Symanski E., Gardiner J., Silva M. J., Calafat A. M., Schantz S. L.2009 Intra- and inter-individual variability of urinary phthalate metabolite concentrations in Hmong women of reproductive age . J. Expo. Sci. Environ. Epidemiol ( doi:10.1038/jes.2009.4 ) [ PMC free article ] [ PubMed ] [ Google Scholar ]
- PlasticsEurope 2008 The compelling facts about plastics 2007: an analysis of plastics production, demanda and recovery in Europe , p. 24 Brussels, Australia: PlasticsEurope [ Google Scholar ]
- Rudel R. A., Brody J. G., Spengler J. C., Vallarino J., Geno P. W., Sun G., Yau A.2001 Identification of selected hormonally active agents and animal mammary carcinogens in commercial and residential air and dust samples . J. Air Waste Manag. Assoc. 51 , 499–513 [ PubMed ] [ Google Scholar ]
- Rudel R. A., Camann D. E., Spengler J. D., Korn L. R., Brody J. G.2003 Phthalates, alkylphenols, pesticides, polybrominated diphenyl ethers, and other endocrine-disrupting compounds in indoor air and dust . Environ. Sci. Technol. 37 , 4543–4553 ( doi:10.1021/es0264596 ) [ PubMed ] [ Google Scholar ]
- Rudel R. A., Dodson R. E., Newton E., Zota A. R., Brody J. G.2008 Correlations between urinary phthalate metabolites and phthalates, estrogenic compounds 4-butyl phenol and o -phenyl phenol, and some pesticides in home indoor air and house dust . Epidemiology 19 , S332 [ Google Scholar ]
- Ryan P. G., Moore C. J., van Franeker J. A., Moloney C. L.2009 Monitoring the abundance of plastic debris in the marine environment . Phil. Trans. R. Soc. B 364 , 1999–2012 ( doi:10.1098/rstb.2008.0207 ) [ PMC free article ] [ PubMed ] [ Google Scholar ]
- Sathyanarayana S., Karr C. J., Lozano P., Brown E., Calafat A. M., Liu F., Swan S. H.2008 Baby care products: possible sources of infant phthalate exposure . Pediatrics 121 , E260–E268 ( doi:10.1542/peds.2006-3766 ) [ PubMed ] [ Google Scholar ]
- Shaxson L.2009 Structuring policy problems for plastics, the environment and human health: reflections from the UK . Phil. Trans. R. Soc. B 364 , 2141–2151 ( doi:10.1098/rstb.2008.0283 ) [ PMC free article ] [ PubMed ] [ Google Scholar ]
- Song J. H., Murphy R. J., Narayan R., Davies G. B. H.2009 Biodegradable and compostable alternatives to conventional plastics . Phil. Trans. R. Soc. B 364 , 2127–2139 ( doi:10.1098/rstb.2008.0289 ) [ PMC free article ] [ PubMed ] [ Google Scholar ]
- Stahlhut R. W., Welshons W. V., Swan S. H.2009 Bisphenol A data in NHANES suggest longer than expected half-life, substantial non-food exposure, or both . Environ. Health Perspect 117 , 784–789 ( doi:10.1289/ehp.0800376 ) [ PMC free article ] [ PubMed ] [ Google Scholar ]
- Swan S. H.2008 Environmental phthalate exposure in relation to reproductive outcomes and other health endpoints in humans . Environ. Res 108 , 177–184 ( doi:10.1016/j.envres.2008.08.007 ) [ PMC free article ] [ PubMed ] [ Google Scholar ]
- Swan S. H., et al.2005 Decrease in anogenital distance among male infants with prenatal phthalate exposure . Environ. Health Perspect 113 , 1056–1061 [ PMC free article ] [ PubMed ] [ Google Scholar ]
- Talsness C. E., Andrade A. J. M., Kuriyama S. N., Taylor J. A., vom Saal F. S.2009 Components of plastic: experimental studies in animals and relevance for human health . Phil. Trans. R. Soc. B 364 , 2079–2096 ( doi:10.1098/rstb.2008.0281 ) [ PMC free article ] [ PubMed ] [ Google Scholar ]
- Teuten E. L., Rowland S. J., Galloway T. S., Thompson R. C.2007 Potential for plastics to transport hydrophobic contaminants . Environ. Sci. Technol. 41 , 7759–7764 ( doi:10.1021/es071737s ) [ PubMed ] [ Google Scholar ]
- Teuten E. L., et al.2009 Transport and release of chemicals from plastics to the environment and to wildlife . Phil. Trans. R. Soc. B 364 , 2027–2045 ( doi:10.1098/rstb.2008.0284 ) [ PMC free article ] [ PubMed ] [ Google Scholar ]
- Thompson R. C., Olsen Y., Mitchell R. P., Davis A., Rowland S. J., John A. W. G., McGonigle D., Russell A. E.2004 Lost at sea: where is all the plastic? Science 304 , 838–838 ( doi:10.1126/science.1094559 ) [ PubMed ] [ Google Scholar ]
- Thompson R., Moore C., Andrady A., Gregory M., Takada H., Weisberg S.2005 New directions in plastic debris . Science 310 , 1117. [ PubMed ] [ Google Scholar ]
- Thornton J.2002 Environmental Impacts of Polyvinyl Chloride Building Materials, A Healthy Building Network Report Washington, DC: Healthy Building Network [ Google Scholar ]
- Van Franeker J. A., et al.2005 Save the North Sea’ Fulmar Study 2002–2004: a regional pilot project for the Fulmar-Litter-EcoQO in the OSPAR area . In Alterra-rapport 1162 Wageningen: Alterra; See www.zeevogelgroep.nl . [ Google Scholar ]
- Vandenberg L. N., Hauser R., Marcus M., Olea N., Welshons W. V.2007 Human exposure to bisphenol A (BPA) . Reprod. Toxicol. 24 , 139–177 ( doi:10.1016/j.reprotox.2007.07.010 ) [ PubMed ] [ Google Scholar ]
- vom Saal F. S.2005 Low-dose BPA: confirmed by extensive literature . Chem. Ind. 7 , 14–15 [ Google Scholar ]
- Wagner M., Oehlmann J.2009 Endocrine disruptors in bottled mineral water: total estrogenic burden and migration from plastic bottles . Environ. Sci. Pollut. Res 16 , 278–286 [ PubMed ] [ Google Scholar ]
- Wormuth M., Scheringer M., Vollenweider M., Hungerbuhler K.2006 What are the sources of exposure to eight frequently used phthalic acid esters in Europeans? Risk Anal. 26 , 803–824 ( doi:10.1111/j.1539-6924.2006.00770.x ) [ PubMed ] [ Google Scholar ]
- WRAP 2006 Environmental benefits of recycling: an international review of life cycle comparisons for key materials in the UK recycling sector Banbury, UK: WRAP [ Google Scholar ]
- WRAP. Consumer attitudes to biopolymers. Banbury, UK: WRAP: 2007. [ Google Scholar ]
- WRAP 2008 The carbon impact of bottling Australian wine in the UK: PET and glass bottles , p. 34 Banbury, UK: WRAP [ Google Scholar ]
- WRAP 2009 Biopolymer packaging in UK grocery market , p. 4 Banbury, UK: WRAP [ Google Scholar ]
- Yamashita R., Tanimura A.2007 Floating plastic in the Kuroshio Current area, western North Pacific Ocean . Mar. Pollut. Bull. 54 , 485–488 ( doi:10.1016/j.marpolbul.2006.11.012 ) [ PubMed ] [ Google Scholar ]
- Yarsley V. E., Couzens E. G.1945 Plastics Middlesex: Penguin Books Limited [ Google Scholar ]
- Zubris K. A. V., Richards B. K.2005 Synthetic fibers as an indicator of land application of sludge . Environ. Pollut. 138 , 201–211 ( doi:10.1016/j.envpol.2005.04.013 ) [ PubMed ] [ Google Scholar ]
Thank you for visiting nature.com. You are using a browser version with limited support for CSS. To obtain the best experience, we recommend you use a more up to date browser (or turn off compatibility mode in Internet Explorer). In the meantime, to ensure continued support, we are displaying the site without styles and JavaScript.
- View all journals
- My Account Login
- Explore content
- About the journal
- Publish with us
- Sign up for alerts
- Open access
- Published: 28 March 2024
New water accounting reveals why the Colorado River no longer reaches the sea
- Brian D. Richter ORCID: orcid.org/0000-0001-7216-1397 1 , 2 ,
- Gambhir Lamsal ORCID: orcid.org/0000-0002-2593-8949 3 ,
- Landon Marston ORCID: orcid.org/0000-0001-9116-1691 3 ,
- Sameer Dhakal ORCID: orcid.org/0000-0003-4941-1559 3 ,
- Laljeet Singh Sangha ORCID: orcid.org/0000-0002-0986-1785 4 ,
- Richard R. Rushforth 4 ,
- Dongyang Wei ORCID: orcid.org/0000-0003-0384-4340 5 ,
- Benjamin L. Ruddell 4 ,
- Kyle Frankel Davis ORCID: orcid.org/0000-0003-4504-1407 5 , 6 ,
- Astrid Hernandez-Cruz ORCID: orcid.org/0000-0003-0776-5105 7 ,
- Samuel Sandoval-Solis 8 &
- John C. Schmidt 9
Communications Earth & Environment volume 5 , Article number: 134 ( 2024 ) Cite this article
5821 Accesses
660 Altmetric
Metrics details
- Water resources
Persistent overuse of water supplies from the Colorado River during recent decades has substantially depleted large storage reservoirs and triggered mandatory cutbacks in water use. The river holds critical importance to more than 40 million people and more than two million hectares of cropland. Therefore, a full accounting of where the river’s water goes en route to its delta is necessary. Detailed knowledge of how and where the river’s water is used can aid design of strategies and plans for bringing water use into balance with available supplies. Here we apply authoritative primary data sources and modeled crop and riparian/wetland evapotranspiration estimates to compile a water budget based on average consumptive water use during 2000–2019. Overall water consumption includes both direct human uses in the municipal, commercial, industrial, and agricultural sectors, as well as indirect water losses to reservoir evaporation and water consumed through riparian/wetland evapotranspiration. Irrigated agriculture is responsible for 74% of direct human uses and 52% of overall water consumption. Water consumed for agriculture amounts to three times all other direct uses combined. Cattle feed crops including alfalfa and other grass hays account for 46% of all direct water consumption.
Similar content being viewed by others
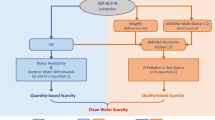
A triple increase in global river basins with water scarcity due to future pollution
Mengru Wang, Benjamin Leon Bodirsky, … Maryna Strokal
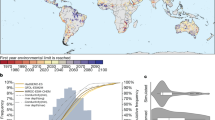
Environmental flow limits to global groundwater pumping
Inge E. M. de Graaf, Tom Gleeson, … Marc F. P. Bierkens
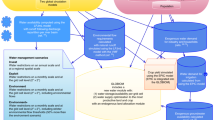
The global nexus of food–trade–water sustaining environmental flows by 2050
A. V. Pastor, A. Palazzo, … F. Ludwig
Introduction
Barely a trickle of water is left of the iconic Colorado River of the American Southwest as it approaches its outlet in the Gulf of California in Mexico after watering many cities and farms along its 2330-kilometer course. There were a few years in the 1980s in which enormous snowfall in the Rocky Mountains produced a deluge of spring snowmelt runoff capable of escaping full capture for human uses, but for most of the past 60 years the river’s water has been fully consumed before reaching its delta 1 , 2 . In fact, the river was overconsumed (i.e., total annual water consumption exceeding runoff supplies) in 16 of 21 years during 2000–2020 3 , requiring large withdrawals of water stored in Lake Mead and Lake Powell to accommodate the deficits. An average annual overdraft of 10% during this period 2 caused these reservoirs– the two largest in the US – to drop to three-quarters empty by the end of 2022 4 , triggering urgent policy decisions on where to cut consumption.
Despite the river’s importance to more than 40 million people and more than two million hectares (>5 million acres) of cropland—producing most of the vegetable produce for American and Canadian plates in wintertime and also feeding many additional people worldwide via exports—a full sectoral and crop-specific accounting of where all that water goes en route to its delta has never been attempted, until now. Detailed knowledge of how and where the river’s water is used can aid design of strategies and plans for bringing water use into balance with available supplies.
There are interesting historical reasons to explain why this full water budget accounting has not been accomplished previously, beginning a full century ago when the apportionment of rights to use the river’s water within the United States was inscribed into the Colorado River Compact of 1922 5 . That Compact was ambiguous and confusing in its allocation of water inflowing to the Colorado River from the Gila River basin in New Mexico and Arizona 6 , even though it accounts for 24% of the drainage area of the Colorado River Basin (Fig. 1 ). Because of intense disagreements over the rights to the Gila and other tributaries entering the Colorado River downstream of the Grand Canyon, the Compact negotiators decided to leave the allocation of those waters rights to a later time so that the Compact could proceed 6 . Arizona’s formal rights to the Gila and other Arizona tributaries were finally affirmed in a US Supreme Court decision in 1963 that also specified the volumes of Colorado River water allocated to California, Arizona, and Nevada 7 . Because the rights to the Gila’s waters lie outside of the Compact allocations, the Gila has not been included in formal accounting of the Colorado River Basin water budget to date 8 . Additionally, the Compact did not specify how much water Mexico—at the river’s downstream end—should receive. Mexico’s share of the river was not formalized until 22 years later, in the 1944 international treaty on “Utilization of the Waters of the Colorado and Tijuana Rivers and of the Rio Grande” (1944 Water Treaty) 9 . As a result of these political circumstances, full accounting for direct water consumption at the sectoral level—in which water use is accounted according to categories such as municipal, industrial, commercial, or agricultural uses—has not previously been compiled for the Gila River basin’s water, and sectoral accounting for Mexico was not published until 2023 10 .
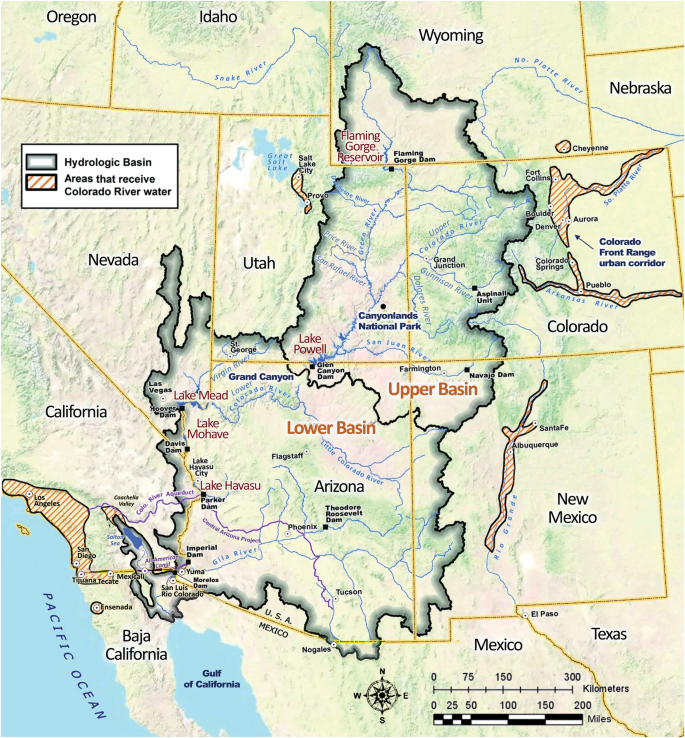
The physical boundary of the Colorado River Basin is outlined in black. Hatched areas outside of the basin boundary receive Colorado River water via inter-basin transfers (also known as ‘exports’). The Gila River basin is situated in the far southern portion of the CRB in Arizona, New Mexico, and Mexico. Map courtesy of Center for Colorado River Studies, Utah State University.
The US Bureau of Reclamation (“Reclamation”)—which owns and operates massive water infrastructure in the Colorado River Basin—has served as the primary accountant of Colorado River water. In 2012, the agency produced a “Colorado River Basin Water Supply and Demand Study” 8 that accounted for both the sectoral uses of water within the basin’s physical boundaries within the US as well as river water exported outside of the basin (Fig. 1 ). But Reclamation did not attempt to account for water generated from the Gila River basin because of that sub-basin’s exclusion from the Colorado River Compact, and it did not attempt to explain how water crossing the border into Mexico is used. The agency estimated riparian vegetation evapotranspiration for the lower Colorado River but not the remainder of the extensive river system. Richter et al. 11 published a water budget for the Colorado River that included sectoral and crop-specific water consumption but it too did not include water used in Mexico, nor reservoir evaporation or riparian evapotranspiration, and it did not account for water exported outside of the Colorado River Basin’s physical boundary as illustrated in Fig. 1 . Given that nearly one-fifth (19%) of the river’s water is exported from the basin or used in Mexico, and that the Gila is a major tributary to the Colorado, this incomplete accounting has led to inaccuracies and misinterpretations of “where the Colorado River’s water goes” and has created uncertainty in discussions based on the numbers. This paper provides fuller accounting of the fate of all river water during 2000–2019, including averaged annual consumption in each of the sub-basins including exports, consumption in major sectors of the economy, consumption in the production of specific types of crops, and water consumed by reservoir evaporation and riparian/wetland evapotranspiration.
Rising awareness of water overuse and prolonged drought has driven intensifying dialog among the seven US states sharing the basin’s waters as well as between the United States, Mexico, and 30 tribal nations within the US. Since 2000, six legal agreements affecting the US states and two international agreements with Mexico have had the effect of reducing water use from the Colorado River 7 :
In 2001, the US Secretary of the Interior issued a set of “Interim Surplus Guidelines” to reduce California’s water use by 14% to bring the state within its allocation as determined in the 1963 US Supreme Court case mentioned previously. A subsequent “Quantification Settlement Agreement” executed in 2003 spelled out details about how California was going to achieve the targeted reduction.
In 2007, the US Secretary of the Interior adopted a set of “Colorado River Interim Guidelines for Lower Basin Shortages and the Coordinated Operations for Lake Powell and Lake Mead” that reduced water deliveries to Arizona and Nevada when Lake Mead drops to specified levels, with increasing cutbacks as levels decline.
In 2012, the US and Mexican federal governments signed an addendum to the 1944 Water Treaty known as Minute 319 that reduced deliveries to Mexico as Lake Mead elevations fall.
In 2017, the US and Mexican federal governments established a “Binational Water Scarcity Contingency Plan” as part of Minute 323 that provides for deeper cuts in deliveries to Mexico under specified low reservoir elevations in Lake Mead.i
In 2019, the three Lower Basin states and the US Secretary of the Interior agreed to commitments under the “Lower Basin Drought Contingency Plan” that further reduced water deliveries beyond the levels set in 2007 and added specifications for deeper cuts as Lake Mead drops to levels lower than anticipated in the 2007 Guidelines.
In 2023, the states of California, Arizona and Nevada committed to further reductions in water use through the year 2026 12 .
With each of the above agreements, overall water consumption has been reduced but many scientists assert that these reductions still fall substantially short of balancing consumptive use with 21st century water supplies 2 , 13 . With all of these agreements—excepting the Interim Surplus Guidelines of 2001—set to expire in 2026, management of the Colorado River’s binational water supply is now at a crucial point, emphasizing the need for comprehensive water budget accounting.
Our tabulation of the Colorado River’s full water consumption budget (Table 1 ) provides accounting for all direct human uses of water as either agricultural or MCI (municipal, commercial, industrial), as well as indirect losses of water to reservoir evaporation and evapotranspiration from riparian or wetland vegetation including in the Salton Sea and in a wetland in Mexico (Cienega de Santa Clara) that receives agricultural return flows from irrigated areas in Arizona. We explicitly note that all estimates represent consumptive use , resulting from the subtraction of return flows from total water withdrawals. Table 2 provides a summary based only on direct human uses and does not include indirect consumption of water. We have provided Tables 1 and 2 in English units in our Supplementary Information as Tables SI-1 and SI-2 . We have lumped municipal, commercial, and industrial (MCI) uses together because these sub-categories of consumption are not consistently differentiated within official water delivery data for cities utilizing Colorado River water. More detail on urban water use by cities dependent on the river is available in Richter 14 , among other studies.
We differentiated water consumption geographically using the ‘accounting units’ mapped in Fig. 2 , which are based on the Colorado River Basin map as revised by Schmidt 15 ; importantly, these accounting units align spatially with Reclamation’s accounting systems for the Upper Basin and Lower Basin as described in our Methods, thereby enabling readers accustomed to Reclamation’s water-use reports to easily comprehend our accounting. We have also accounted for all water consumed within the Colorado River Basin boundaries as well as water exported via inter-basin transfers. Water exported outside of the basin includes 47 individual inter-basin transfer systems (i.e., canals, pipelines, pumps) that in aggregate export ~12% of the river’s water. We note that the Imperial Irrigation District of southern California is often counted as a recipient of exported water, but we have followed the rationale of Schmidt 15 by including it as an interior part of the Lower Basin even though it receives its Colorado River water via the All American Canal (Fig. 2 ).
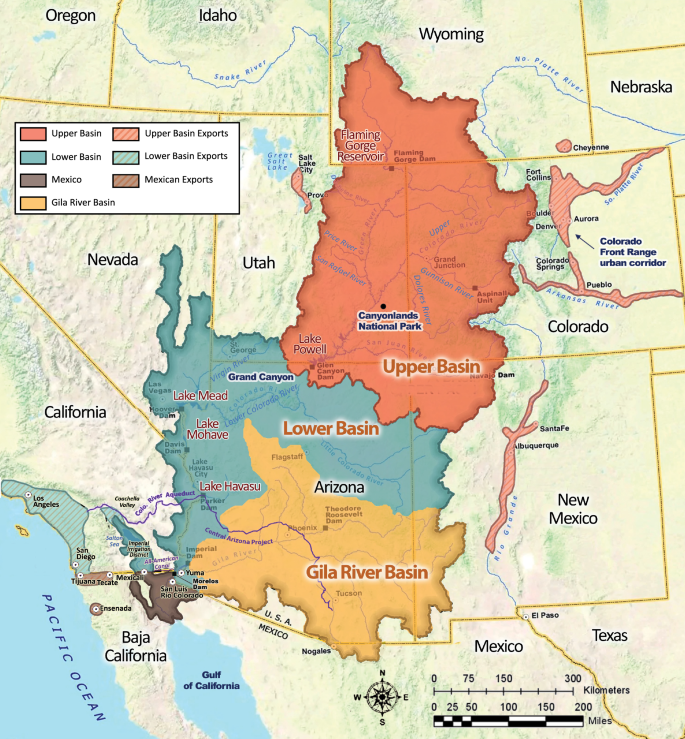
The water budget estimates presented in Tables 1 and 2 are summarized for each of the seven “accounting units” displayed here.
These results confirm previous findings that irrigated agriculture is the dominant consumer of Colorado River water. Irrigated agriculture accounts for 52% of overall consumption (Table 1 ; Figs. 3 and 4 ) and 74% of direct human consumption (Table 2 ) of water from the Colorado River Basin. As highlighted in Richter et al. 11 , cattle-feed crops (alfalfa and other hay) are the dominant water-consuming crops dependent upon irrigation water from the basin (Tables 1 and 2 ; Figs. 3 and 4 ). Those crops account for 32% of all water consumed from the basin, 46% of all direct water consumption, and 62% of all agricultural water consumed (Table 1 ; Fig. 3 ). The percentage of water consumed by irrigated crops is greatest in Mexico, where they account for 86% of all direct human uses (Table 2 ) and 80% of total water consumed (Table 1 ). Cattle-feed crops consume 90% of all water used by irrigated agriculture within the Upper Basin, where the consumed volume associated with these cattle-feed crops amounts to more than three times what is consumed for municipal, commercial, or industrial uses combined.
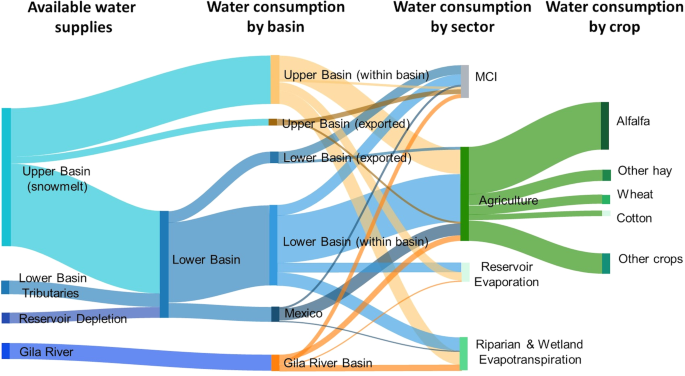
All estimates based on 2000–2019 averages. Both agriculture and MCI (municipal, commercial, and industrial) uses are herein referred to as “direct human uses.” “Indirect uses” include both reservoir evaporation as well as evapotranspiration by riparian/wetland vegetation.
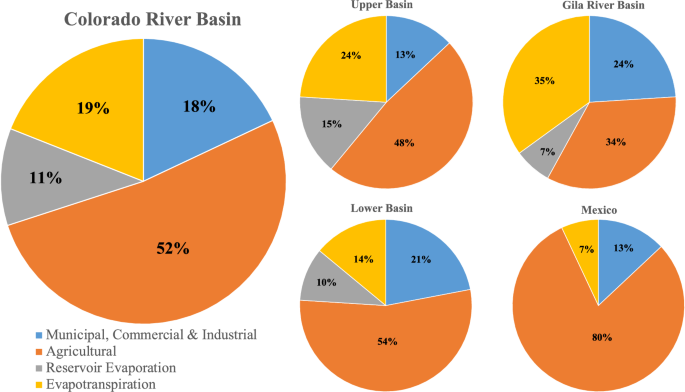
Water consumed by each sector in the Colorado River Basin and sub-basins (including exports), based on 2000–2019 averages.
Another important finding is that a substantial volume of water (19%) is consumed in supporting the natural environment through riparian and wetland vegetation evapotranspiration along river courses. This analysis—made possible because of recent mapping of riparian vegetation in the Colorado River Basin 16 —is an important addition to the water budget of the Colorado River Basin, given that the only previous accounting for riparian vegetation consumption has limited to the mainstem of the Colorado River below Hoover Dam and does not include vegetation upstream of Hoover Dam nor vegetation along tributary rivers 17 . Given that many of these habitats and associated species have been lost or became imperiled due to river flow depletion 18 —including the river’s vast delta ecosystem in Mexico—an ecologically sustainable approach to water management would need to allow more water to remain in the river system to support riparian and aquatic ecosystems. Additionally, 11% of all water consumed in the Colorado River Basin is lost through evaporation from reservoirs.
It is also important to note a fairly high degree of inter-annual variability in each sector of water use; for example, the range of values portrayed for the four water budget sectors shown in Fig. 5 equates to 24–47% of their 20-year averages. Also notable is a decrease in water consumed in the Lower Basin between the years 2000 and 2019 for both the MCI (−38%) and agricultural sectors (−15%), which can in part be attributed to the policy agreements summarized previously that have mandated water-use reductions.
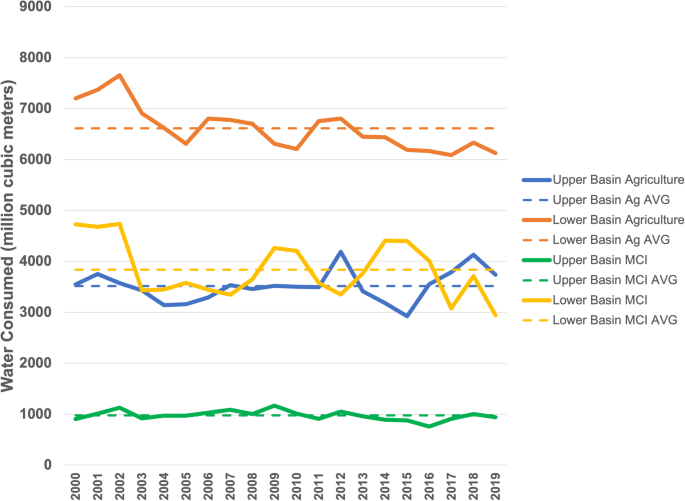
Inter-annual variability of water consumption within the Lower and Upper Basins, including water exported from these basins. The average (AVG) values shown are used in the water budgets detailed in Tables 1 and 2 .
The water accounting in Richter et al. 11 received a great deal of media attention including a front-page story in the New York Times 19 . These stories focused primarily on our conclusion that more than half (53%) of water consumed in the Colorado River Basin was attributable to cattle-feed crops (alfalfa and other hays) supporting beef and dairy production. However, that tabulation of the river’s water budget had notable shortcomings, as discussed previously. In this more complete accounting that includes Colorado River water exported outside of the basin’s physical boundary as well as indirect water consumption, we find that irrigated agriculture consumes half (52%) of all Colorado River Basin water, and the portion of direct consumption going to cattle-feed crops dropped from 53% as reported in Richter et al. 11 to 46% in this revised analysis.
These differences are explained by the fact that we now account for all exported water and also include indirect losses of water to reservoir evaporation and riparian/wetland evapotranspiration in our revised accounting, as well as improvements in our estimation of crop-water consumption. However, the punch line of our 2020 paper does not change fundamentally. Irrigated agriculture is the dominant consumer of water from the Colorado River, and 62% of agricultural water consumption goes to alfalfa and grass hay production.
Richter et al. 20 found that alfalfa and grass hay were the largest water consumers in 57% of all sub-basins across the western US, and their production is increasing in many western regions. Alfalfa is favored for its ability to tolerate variable climate conditions, especially its ability to persist under greatly reduced irrigation during droughts and its ability to recover production quickly after full irrigation is resumed, acting as a “shock absorber” for agricultural production under unpredictable drought conditions. The plant is also valued for fixing nitrogen in soils, reducing fertilizer costs. Perhaps most importantly, labor costs are comparatively low because alfalfa is mechanically harvested. Alfalfa is increasing in demand and price as a feed crop in the growing dairy industry of the region 21 . Any efforts to reduce water consumed by alfalfa—either through shifting to alternative lower-water crops or through compensated fallowing 20 —will need to compete with these attributes.
This new accounting provides a more comprehensive and complete understanding of how the Colorado River Basin’s water is consumed. During our study period of 2000–2019, an estimated average of 23.7 billion cubic meters (19.3 million acre-feet) of water was consumed each year before reaching its now-dry delta in Mexico. Schmidt et al. 2 have estimated that a reduction in consumptive use in the Upper and Lower Basins of 3–4 billion cubic meters (2.4–3.2 million acre-feet) per year—equivalent to 22–29% of direct use in those basins—will be necessary to stabilize reservoir levels, and an additional reduction of 1–3 billion cubic meters (~811,000–2.4 million acre-feet) per year will likely be needed by 2050 as climate warming continues to reduce runoff in the Colorado River Basin.
We hope that this new accounting will add clarity and a useful informational foundation to the public dialog and political negotiations over Colorado River Basin water allocations and cutbacks that are presently underway 2 . Because a persistent drought and intensifying aridification in the region has placed both people and river ecosystems in danger of water shortages in recent decades, knowledge of where the water goes will be essential in the design of policies for bringing the basin into a sustainable water supply-demand balance.
The data sources and analytical approaches used in this study are summarized below. Unless otherwise noted, all data were assembled for each year from 2000–2019 and then averaged. We acknowledge some inconsistency in the manner in which water consumption is measured or estimated across the various data sources and sectors used in this study, as discussed below, and each of these different approaches entail some degree of inaccuracy or uncertainty. We also note that technical measurement or estimation approaches change over time, and new approaches can yield differing results. For instance, the Upper Colorado River Commission is exploring new approaches for estimating crop evapotranspiration in the Upper Basin 22 . When new estimates become available we will update our water budget accordingly.
MCI and agricultural water consumption
The primary source of data on aggregate MCI (municipal, commercial, and industrial) and agricultural water consumption from the Upper and Lower Basins was the US Bureau of Reclamation. Water consumed from the Upper Basin is published in Reclamation’s five-year reports entitled “Colorado River—Upper Basin Consumptive Uses and Losses.” 23 These annual data have been compiled into a single spreadsheet used for this study 24 . Because measurements of agricultural diversions and return flows in the Upper Basin are not sufficiently complete to allow direct calculation of consumptive use, theoretical and indirect methods are used as described in the Consumptive Uses and Losses reports 25 . Reclamation performs these estimates for Colorado, Wyoming, and Utah, but the State of New Mexico provides its own estimates that are collaboratively reviewed with Reclamation staff. The consumptive use of water in thermoelectric power generation in the Upper Basin is provided to Reclamation by the power companies managing each generation facility. Reclamation derives estimates of consumptive use for municipal and industrial purposes from the US Geological Survey’s reporting series (published every 5 years) titled “Estimated Use of Water in the United States” at an 8-digit watershed scale 26 .
Use of shallow alluvial groundwater is included in the water accounting compiled by Reclamation but use of deeper groundwater sources—such as in Mexico and the Gila River Basin—is explicitly excluded in their accounting, and in ours. Reclamation staff involved with water accounting for the Upper and Lower Basins assume that groundwater use counted in their data reports is sourced from aquifers that are hydraulically connected to rivers and streams in the CRB (James Prairie, US Bureau of Reclamation, personal communication, 2023); because of this high connectivity, much of the groundwater being consumed is likely being sourced from river capture as discussed in Jasechko et al. 27 and Wiele et al. 28 and is soon recharged during higher river flows.
Water consumed from the Lower Basin (excluding water supplied by the Gila River Basin) is published in Reclamation’s annual reports entitled “Colorado River Accounting and Water Use Report: Arizona, California, and Nevada.” 3 These consumptive use data are based on measured deliveries and return flows for each individual water user. These data are either measured by Reclamation or provided to the agency by individual water users, tribes, states, and federal agencies 29 . When not explicitly stated in Reclamation reports, attribution of water volumes to MCI or agricultural uses was based on information obtained from each water user’s website, information provided directly by the water user, or information on export water use provided in Siddik et al. 30 . Water use by entities using less than 1.23 million cubic meters (1000 acre-feet) per year on average was allocated to MCI and agricultural uses according to the overall MCI-agricultural percentages calculated within each sub-basin indicated in Tables 1 and 2 for users of greater than 1.23 million cubic meters/year.
Disaggregation of water consumption by sector was particularly important and challenging for the Central Arizona Project given that this canal accounts for 21% of all direct water consumption in the Lower Basin. Reclamation accounts for the volumes of annual diversions into the Central Arizona Project canal but the structure serves 1071 water delivery subcontracts. We classified every unique Central Arizona Project subcontract delivery between 2000–2019 by its final water use to derive an estimated split between agricultural and MCI uses. Central Arizona Project subcontract delivery data were obtained from the current and archived versions of the project’s website summaries in addition to being directly obtained from the agency through a public information request. Subcontract deliveries were classified based on the final end use, including long-term and temporary leases of project water. This accounting also includes the storage of water in groundwater basins for later MCI or agricultural use. Additionally, water allocated to Native American agricultural uses that was subsequently leased to cities was classified as an MCI use.
Data for the Gila River basin was obtained from two sources. The Arizona Department of Water Resources has published data for surface water use in five “Active Management Areas” (AMAs) located in the Gila River basin: Prescott AMA, Phoenix AMA, Pinal AMA, Tucson AMA, and Santa Cruz AMA 31 . The water-use data for these AMAs is compiled from annual reports submitted by each water user (contractor) and then reviewed by the Arizona Department of Water Resources. The AMA water-use data are categorized by purpose of use, facilitating our separation into MCI and agricultural uses. These data are additionally categorized by water source; only surface water sourced from the Gila River hydrologic system was counted (deep groundwater use was not). The AMA data were supplemented with data for the upper Gila River basin provided by the University of Arizona 32 . We have assumed that all water supplied by the Gila River Basin is fully consumed, as the river is almost always completely dry in its lower reaches (less than 1% flows out of the basin into the Colorado River, on average 33 ).
Data for Mexico were obtained from Hernandez-Cruz et al. 10 based on estimates for 2008–2015. Agricultural demands were estimated from annual reports of irrigated area and water use published by the Ministry of Agriculture and the evapotranspiration estimates of the principal crops published by the National Institute for Forestry, Animal Husbandry, and Agricultural Research of Mexico 10 . The average annual volume of Colorado River water consumption in Mexico estimated by these researchers is within 1% of the cross-border delivery volume estimated by the Bureau of Reclamation for 2000–2019 in its Colorado River Accounting and Water Use Reports 3 .
Exported water consumption
Annual average inter-basin transfer volumes for each of 46 canals and pipelines exporting water outside of the Upper Basin were obtained from Reclamation’s Consumptive Uses and Losses spreadsheet 34 . Data for the Colorado River Aqueduct in the Lower Basin were obtained from Siddik et al. 30 Data for exported water in Mexico was available from Hernandez-Cruz et al. 10 . We assigned any seepage or evaporation losses from inter-basin transfers to their proportional end uses. All uses of exported water are considered to be consumptive uses with respect to the Colorado River, because none of the water exported out of the basin is returned to the Colorado River Basin.
We relied on data from Siddik et al. (2023) to identify whether the water exported out of the Colorado River Basin was for only MCI or agricultural use. When more than one water use purpose was identified, as well as for all major inter-basin transfers, we used government and inter-basin transfer project websites or information obtained directly from the project operator or water manager to determine the volume of water transferred and the end uses. Major recipients of exported water include the Coachella Valley Water District (California); Metropolitan Water District of Southern California (particularly for San Diego County, California); Northern Colorado Water Conservancy District; City of Denver (Colorado); the Central Utah Project; City of Albuquerque (New Mexico); and the Middle Rio Grande Conservancy District (New Mexico). We did not pursue sectoral water-use information for 17 of the 46 Upper Basin inter-basin transfers due to their relatively low volumes of water transferred by each system (<247,000 cubic meters or 2000 acre-feet), and instead assigned the average MCI or agricultural percentage (72% MCI, 28% agricultural) from all other inter-basin transfers in the Upper Basin. The export volume of these 17 inter-basin transfers sums to 9.76 million cubic meters (7910 acre-feet) per year, equivalent to 1% of the total volume exported from the Upper Basin.
Reservoir evaporation
Evaporation estimates for the Upper Basin and Lower Basin are based upon Reclamation’s HydroData repository 35 . Reclamation’s evaporation estimates are based on the standardized Penman-Monteith equation as described in the “Lower Colorado River Annual Summaries of Evapotranspiration and Evaporation” reports 17 . The Penman-Monteith estimates are based on pan evaporation measurements. Evaporation estimates for the Salt River Project reservoirs in the Gila River basin were provided by the Salt River Project in Arizona (Charlie Ester, personal communication, 2023).
Another consideration with reservoirs is the volume of water that seeps into the banks or sediments surrounding the reservoir when reservoir levels are high, but then drains back into the reservoir as water levels decline 36 . This has the effect of either exacerbating reservoir losses (consumptive use) or offsetting evaporation when bank seepage flows back into a reservoir. The flow of water into and out of reservoir banks is non-trivial; during 1999–2008, an estimated 247 million cubic meters (200,000 acre-feet) of water drained from the canyon walls surrounding Lake Powell into the reservoir each year, providing additional water supply 36 . However, the annual rate of alternating gains or losses has not been sufficiently measured at any of the basin’s reservoirs and therefore is not included in Tables 1 and 2 .
Riparian and wetland vegetation evapotranspiration
We exported the total annual evapotranspiration depth at a 30 meter resolution from OpenET 37 using Google Earth Engine from 2016 to 2019 to align with OpenET’s data availability starting in 2016. Total annual precipitation depths, sourced from gridMET 38 , were resampled to align with the evapotranspiration raster resolution. Subsequently, a conservative estimate of the annual water depth utilized by riparian vegetation from the river was derived by subtracting the annual precipitation raster from the evapotranspiration raster for each year. Positive differentials, indicative of river-derived evapotranspiration, were then multiplied by the riparian vegetation area as identified in the CO-RIP 16 dataset to estimate the total annual volumetric water consumption by riparian vegetation across the Upper, Lower, and Gila River Basins. The annual volumetric water consumption calculated over four years were finally averaged to get riparian vegetation evapotranspiration in the three basins. Because the entire flow of the Colorado River is diverted into the Canal Alimentador Central near the international border, very little riparian evapotranspiration occurs along the river south of the international border in the Mexico basin.
In addition to water consumed by riparian evapotranspiration within the Lower Basin, the Salton Sea receives agricultural drain water from both the Imperial Irrigation District and the Coachella Valley Irrigation District, stormwater drainage from the Coachella Valley, and inflows from the New and Alamo Rivers 39 . Combined inflows to the Sea during 2015–2019 were added to our estimates of riparian/wetland evapotranspiration in the Lower Basin.
Similarly, Mexico receives drainage water from the Wellton–Mohawk bypass drain originating in southern Arizona that empties into the Cienega de Santa Clara (a wetland); this drainage water is included as riparian/wetland evapotranspiration in the Mexico basin.
Crop-specific water consumption
The volumes of total agricultural consumption reported for each sub-basin in Tables 1 and 2 were obtained from the same data sources described above for MCI consumption and exported water. The portion (%) of those agricultural consumption volumes going to each individual crop was then allocated according to percentage estimates of each crop’s water consumption in each accounting unit using methods described in Richter et al. 20 and detailed here.
Monthly crop water requirements during 1981–2019 for 13 individual crops, representing 68.8% of total irrigated area in the US in 2019, were estimated using the AquaCrop-OS model (Table SI- 3 ) 40 . For 17 additional crops representing about 25.4% of the total irrigated area, we used a simple crop growth model following Marston et al. 41 as crop parameters needed to run AquaCrop-OS were not available. A list of the crops included in this study is shown in Table SI- 3 . The crop water requirements used in Richter et al. 11 were based on a simplistic crop growth model, often using seasonal crop coefficients whereas we use AquaCrop-OS 40 , a robust crop growth model, to produce more realistic crop growth and crop water estimates for major crops. AquaCrop-OS is an open-source version of the AquaCrop model 42 , a crop growth model capable of simulating herbaceous crops. Additionally, we leverage detailed local data unique to the US, including planting dates and subcounty irrigated crop areas, to produce estimates at a finer spatial resolution than the previous study. We obtained crop-specific planting dates from USDA 43 progress data at the state level. For crops that did not have USDA crop progress data, we used data from FAO 44 and CUP+ model 45 for planting dates. We used climate data (precipitation, minimum and maximum air temperature, reference ET) from gridMET 38 , soil texture data from ISRIC 46 database and crop parameters from AquaCrop-OS to run the model. The modeled crop water requirement was partitioned into blue and green components following the framework from Hoekestra et al. 47 , assuming that blue and green water consumed on a given day is proportional to the amount of green and blue water soil moisture available on that day. When applying a simple crop growth model, daily gridded (2.5 arc minutes) crop-specific evapotranspiration (ETc) was computed by taking the product of reference evapotranspiration (ETo) and crop coefficient (Kc), where ETo was obtained from gridMET. Crop coefficients were calculated using planting dates and crop coefficient curves from FAO and CUP+ model. Kc was set to zero outside of the growing season. We partitioned the daily ETc into blue and green components by following the methods from ref. 41 It is assumed that the crop water demands are met by irrigation whenever it exceeds effective precipitation (the latter calculated using the USDA Soil Conservation Service method (USDA, 1968 48 ). We obtained county level harvested area from USDA 43 and disaggregated to sub-county level using Cropland Data Layer (CDL) 49 and Landsat-based National Irrigation Dataset (LANID) 50 . The CDL is an annual raster layer that provides crop-specific land cover data, while the LANID provides irrigation status information. The CDL and LANID raster were multiplied and aggregated to 2.5 arc minutes to match the AquaCrop-OS output. We produced a gridded crop area map by using this resulting product as weights to disaggregate county level area. CDL is unavailable before 2008. Therefore, we used land use data from ref. 51 in combination with average CDL map and county level harvested area to produce gridded crop harvested area. We computed volumetric water consumption by multiplying the crop water requirement depth by the corresponding crop harvested area.
Data availability
All data compiled and analyzed in this study are publicly available as cited and linked in our Methods section. Our compilation of these data is also available from Hydroshare at: http://www.hydroshare.org/resource/2098ae29ae704d9aacfd08e030690392 .
Code availability
All model code and software used in this study have been accessed from sources cited in our Methods section. We used AquaCrop-OS (v5.0a), an open source version of AquaCrop crop growth model, to run crop simulations. This model is publicly available at http://www.aquacropos.com/ . For estimating riparian evapotranspiration, we used ArcGIS Pro 3.1.3 on the Google Earth Engine. Riparian vegetation distribution maps were sourced from Dryad at https://doi.org/10.5061/dryad.3g55sv8 .
Stromberg, J. C., Andersen, D. C. & Scott, M. L. Riparian floodplain wetlands of the arid and semiarid southwest In Wetland Habitats of North America: Ecology and Conservation Concern s , Chapter 24, pp. 343–356. (University of California Press, 2012). https://www.ucpress.edu/book/9780520271647/wetland-habitats-of-north-america .
Schmidt, J. C., Yackulic, C. B. & Kuhn, E. The Colorado River water crisis: Its origin and the future. WIREs Water https://doi.org/10.1002/wat2.1672 (2023).
Article Google Scholar
Colorado River Accounting and Water Use Report: Arizona, California, and Nevada. Interior Region 8: Lower Colorado Basin (US Bureau of Reclamation, 2023). Annual reports available under “Water Accounting Reports” at https://www.usbr.gov/lc/region/g4000/wtracct.html .
Water Operations: Historic Data (US Bureau of Reclamation, 2023). https://www.usbr.gov/rsvrWater/HistoricalApp.html .
Colorado River Compact , 1922 . US Bureau of Reclamation. https://www.usbr.gov/lc/region/pao/pdfiles/crcompct.pdf .
Kuhn, E. & Fleck, J. Science Be Dammed:How Ignoring Inconvenient Science Drained the Colorado River (The University of Arizona Press, 2019) https://uapress.arizona.edu/book/science-be-dammed .
Castle, A. & Fleck, J. The Risk of Curtailment under the Colorado River Compact ( https://doi.org/10.2139/ssrn.3483654 (2019).
US Bureau of Reclamation. Colorado River Basin Water Supply and Demand Study: Technical Report C – Water Demand Assessment https://www.usbr.gov/lc/region/programs/crbstudy/finalreport/Technical%20Report%20C%20-%20Water%20Demand%20Assessment/TR-C-Water_Demand_Assessmemt_FINAL.pdf (2012).
Utilization of the Waters of the Colorado and Tijuana Rivers and of the Rio Grande . International Treaty between the United States and Mexico, February 3, 1944. (International Boundary and Waters Commission, 1944). https://www.ibwc.gov/wp-content/uploads/2022/11/1944Treaty.pdf .
Hernández-Cruz, A. et al. Assessing water management strategies under water scarcity in the Mexican portion of the Colorado River Basin. J. Water Resour. Plan. Manag. 149 , 04023042 (2023).
Richter, B. D. et al. Water scarcity and fish imperilment driven by beef production. Nat. Sustain. 3 , 319–328 (2020).
Biden-Harris Administration announces historic Consensus System Conservation Proposal to protect the Colorado River Basin . US Department of the Interior, May 22, 2023. https://www.doi.gov/pressreleases/biden-harris-administration-announces-historic-consensus-system-conservation-proposal .
Wheeler, K. G. et al. What will it take to stabilize the Colorado River? Science 377 , 373–375 (2022).
Article ADS CAS PubMed Google Scholar
Richter, B. D. Decoupling urban water use from population growth in the Colorado River Basin. J. Water Plan. Manag. 149 , 2 (2023).
Google Scholar
Schmidt, J. C. Maps Matter: A few suggested changes to the Colorado River basin base map . Center for Colorado River Studies. (Utah State University, 2022).
Woodward, B. D. et al. Co-Rip: A riparian vegetation and corridor extent dataset for Colorado river basin streams and rivers. ISPRS Int. J. Geo Inform. 7 , 397 (2018).
Article ADS Google Scholar
Lower Colorado River Annual Summaries of Evapotranspiration and Evaporation . (US Bureau of Reclamation, Lower Colorado Region, 2023). https://www.usbr.gov/lc/region/g4000/wtracct.html .
Richter, B. D., Powell, E. M., Lystash, T. & Faggert, M. Protection and restoration of freshwater ecosystems. Chapter 5 in Miller, Kathleen A., Alan F. Hamlet, Douglas S. Kenney, and Kelly T. Redmond (Eds.) Water Policy and Planning in a Variable and Changing Climate . (CRC Press - Taylor & Francis Group, 2016).
Shao, Elena. “The Colorado River is shrinking. See what’s using all the water.” New York Times , May 22, 2023. https://www.nytimes.com/interactive/2023/05/22/climate/colorado-river-water.html .
Richter, B. D., et al. Alleviating water scarcity by optimizing crop mixes. Nat. Water . https://doi.org/10.1038/s44221-023-00155-9 .
Njuki, E. U.S. dairy productivity increased faster in large farms and across southwestern states . U.S. Economic Research Service, US Department of Agriculture, March 22, 2022. https://www.ers.usda.gov/amber-waves/2022/march/u-s-dairy-productivity-increased-faster-in-large-farms-and-across-southwestern-states/ .
Mefford, B. & Prairie J., eds. Assessing Agricultural Consumptive Use in the Upper Colorado River Basin - Phase III Report U.S. Bureau of Reclamation and the Upper Colorado River Commission. http://www.ucrcommission.com/reports-studies/ (2022).
Upper Basin Consumptive Uses and Losses (Bureau of Reclamation). Annual reports available at https://www.usbr.gov/uc/envdocs/plans.html .
Bureau of Reclamation. “Consumptive Uses and Losses spreadsheet 1971–2020” Colorado River Basin Natural Flow and Salt Data, Supporting data for consumptive uses and losses computation. https://www.usbr.gov/lc/region/g4000/NaturalFlow/documentation.html .
Upper Colorado River Basin Consumptive Uses and Losses Report 2016–2020 . US Department of Interior: Bureau of Reclamation. Five year reports available under “Colorado River-Consumptive Uses and Losses Reports” at https://www.usbr.gov/uc/envdocs/plans.html .
Estimated Use of Water in the United States . US Department of Interior: US Geological Survey. Reports available every five years at https://www.usgs.gov/mission-areas/water-resources/science/water-use-united-states .
Jasechko, S. et al. Widespread potential loss of streamflow into underlying aquifers across the USA. Nature 591 , 391–395 (2021).
Wiele, S. M., Leake, S. A., Owen-Joyce, S. J. & and McGuire, E. H. Update of the Accounting Surface Along the Lower Colorado River US Department of the Interior: US Geological Survey Scientific Investigations Report 2008–5113 (2008).
Bruce, B. W., et al. Comparison of U.S. Geological Survey and Bureau of Reclamation water-use reporting in the Colorado River Basin U.S. Geological Survey Scientific Investigations Report 2018–5021 . https://doi.org/10.3133/sir20185021 (2018).
Siddik, M. A. B., Dickson, K. E., Rising, J., Ruddell, B. L. & Marston, L. T. Interbasin water transfers in the United States and Canada. Sci. Data 10 , 27 (2023). Data spreadsheet provided by M.A.B. Siddik.
Article PubMed PubMed Central Google Scholar
Active Management Areas : AMA Annual Supply and Demand Dashboard (Arizona Department of Water Resources, 2023). https://azwater.gov/ama/ama-data .
Lacroix, K. M. et al. Wet water and paper water in the Upper Gila River Watershed https://extension.arizona.edu/sites/extension.arizona.edu/files/pubs/az1708-2016_0.pdf The University of Arizona Cooperative Extension, AZ1708. Data spreadsheet provided by A. Hullinger (2016).
Surface-Water Annual Statistics for the Nation: Gila River at Dome, Arizona . US Geological Survey. Available at https://waterdata.usgs.gov/nwis/annual/?referred_module=sw&site_no=09520500&por_09520500_5810=19975,00060,5810,1905,2024&year_type=C&format=html_table&date_format=YYYY-MM-DD&rdb_compression=file&submitted_form=parameter_selection_list .
Consumptive Uses and Losses spreadsheet 1971–2020 . Bureau of Reclamation, Colorado River Basin Natural Flow and Salt Data, Supporting data for consumptive uses and losses computation. https://www.usbr.gov/lc/region/g4000/NaturalFlow/documentation.html .
HydroData: Reservoir Data . US Bureau of Reclamation. https://www.usbr.gov/uc/water/ .
Myers, T. Loss rates from Lake Powell and their impact on management of the Colorado River. J. Am. Water Resour. Assoc. 49 , 1213–1224 (2013).
Melton, F. S. et al. OpenET: filling a critical data gap in water management for the western United States. J. Am. Water Resour. Assoc. 58 , 971–994 (2022).
Abatzoglou, J. T. Development of gridded surface meteorological data for ecological applications and modelling. Int. J. Climatol. 33 , 121–131 (2013).
Salton Sea Management Program: Long-Range Plan Public Draft (2022). California Natural Resources Agency. https://saltonsea.ca.gov/wp-content/uploads/2022/12/Salton-Sea-Long-Range-Plan-Public-Draft-Dec-2022.pdf .
Foster, T. et al. AquaCrop-OS: an open source version of FAO’s crop water productivity model. Agricul. Water Manag. 181 , 18–22 (2017).
Marston, L. T., et al. Reducing water scarcity by improving water productivity in the United States. Environ. Res. Lett. 15 https://doi.org/10.1088/1748-9326/ab9d39 (2020).
Steduto, P., Hsiao, T. C., Fereres, E. & Raes, D. Crop yield response to water (2012). 1028. Rome: Food and Agriculture Organization of the United Nations.
USDA, National Agricultural Statistics Service. “Quick Stats.” http://quickstats.nass.usda.gov .
Allen, R. G., Pereira, L. S., Raes, D. & Smith, M. FAO Irrigation and drainage paper No. 56 56, (e156. Food and Agriculture Organization of the United Nations, Rome, 1998).
Orange, M. N., Scott Matyac, J. & Snyder, R. L. Consumptive use program (CUP) model. IV Int. Symp. Irrig. Horticult. Crops 664 , 461–468 (2003).
Hengl, T. et al. SoilGrids250m: Global gridded soil information based on machine learning. PLoS One 12 , e0169748 (2017).
Hoekstra, A. Y. Green-blue water accounting in a soil water balance. Adv. Water Resour. 129 , 112–117 (2019).
USDA (US Department of Agriculture). A Method for Estimating Volume and Rate of Runoff in Small Watersheds . SCS-TP-149. Washington DC: Soil Conservation Service (1968).
Johnson, D. M., & Mueller, R. 2010. “Cropland Data Layer.” https://nassgeodata.gmu.edu/CropScape/ .
Xie, Y., Gibbs, H. K. & Lark, T. J. Landsat-based Irrigation Dataset (LANID): 30m resolution maps of irrigation distribution, frequency, and change for the US, 1997–2017. Earth Syst. Sci. Data 13 , 5689–5710 (2021).
Sohl, T. et al. Modeled historical land use and land cover for the conterminous United States. J. Land Use Sci. 11 , 476–499 (2016).
Download references
Acknowledgements
This paper is dedicated to our colleague Jack Schmidt in recognition of his retirement and enormous contributions to the science and management of the Colorado River. The authors thank James Prairie of the US Bureau of Reclamation, Luke Shawcross of the Northern Colorado Water Conservancy District, Charlie Ester of the Salt River Project, and Brian Woodward of the University of California Cooperative Extension for their assistance in accessing data used in this study. The authors also thank Rhett Larson at the Sandra Day O’Connor School of Law at Arizona State University for their review of Arizona water budget data, and the Central Arizona Project for providing delivery data by each subcontract. G.L., L.M., and K.F.D. acknowledge support by the United States Department of Agriculture National Institute of Food and Agriculture grant 2022-67019-37180. L.T.M. acknowledges the support the National Science Foundation grant CBET-2144169 and the Foundation for Food and Agriculture Research Grant No. FF-NIA19-0000000084. R.R.R. acknowledges the support the National Science Foundation grant CBET-2115169.
Author information
Authors and affiliations.
World Wildlife Fund, 1250 24th St NW, Washington, DC, 20037, USA
Brian D. Richter
Sustainable Waters, Crozet, Virginia, 22932, USA
The Charles E.Via, Jr, Department of Civil and Environmental Engineering, Virginia Tech, Blacksburg, VA, 24061, USA
Gambhir Lamsal, Landon Marston & Sameer Dhakal
Northern Arizona University, Flagstaff, AZ, 86011, USA
Laljeet Singh Sangha, Richard R. Rushforth & Benjamin L. Ruddell
Department of Geography and Spatial Sciences, University of Delaware, Newark, DE, 19716, USA
Dongyang Wei & Kyle Frankel Davis
Department of Plant and Soil Sciences, University of Delaware, Newark, DE, 19716, USA
Kyle Frankel Davis
Instituto de Investigaciones Oceanologicas, Universidad Autonoma de Baja California, Ensenada, Baja California, México
Astrid Hernandez-Cruz
Department of Land, Air and Water Resources, Univeristy of California at Davis, Davis, CA, 95616, USA
Samuel Sandoval-Solis
Center for Colorado River Studies, Utah State University, Logan, UT, 84322, USA
John C. Schmidt
You can also search for this author in PubMed Google Scholar
Contributions
B.D.R. designed the study, compiled and analyzed data, wrote the manuscript and supervised co-author contributions. G.L. compiled all crop data, estimated crop evapotranspiration, and prepared figures. S.D. compiled all riparian vegetation data and estimated riparian evapotranspiration. L.S.S. and R.R.R. accessed, compiled, and analyzed data from the Central Arizona Project. D.W. compiled data and prepared figures. A.H.-C. and S.S.-S. compiled and analyzed data for Mexico. J.C.S. compiled and analyzed reservoir evaporation data and edited the manuscript. L.M., B.L.R., and K.F.D. supervised data compilation and analysis and edited the manuscript.
Corresponding author
Correspondence to Brian D. Richter .
Ethics declarations
Competing interests.
The authors declare no competing interests.
Peer review
Peer review information.
Communications Earth & Environment thanks James Booker and Becky Bolinger for their contribution to the peer review of this work. Primary Handling Editors: Aliénor Lavergne and Carolina Ortiz Guerrero. A peer review file is available.
Additional information
Publisher’s note Springer Nature remains neutral with regard to jurisdictional claims in published maps and institutional affiliations.
Supplementary information
Peer review file, supplementary information, rights and permissions.
Open Access This article is licensed under a Creative Commons Attribution 4.0 International License, which permits use, sharing, adaptation, distribution and reproduction in any medium or format, as long as you give appropriate credit to the original author(s) and the source, provide a link to the Creative Commons licence, and indicate if changes were made. The images or other third party material in this article are included in the article’s Creative Commons licence, unless indicated otherwise in a credit line to the material. If material is not included in the article’s Creative Commons licence and your intended use is not permitted by statutory regulation or exceeds the permitted use, you will need to obtain permission directly from the copyright holder. To view a copy of this licence, visit http://creativecommons.org/licenses/by/4.0/ .
Reprints and permissions
About this article
Cite this article.
Richter, B.D., Lamsal, G., Marston, L. et al. New water accounting reveals why the Colorado River no longer reaches the sea. Commun Earth Environ 5 , 134 (2024). https://doi.org/10.1038/s43247-024-01291-0
Download citation
Received : 03 October 2023
Accepted : 27 February 2024
Published : 28 March 2024
DOI : https://doi.org/10.1038/s43247-024-01291-0
Share this article
Anyone you share the following link with will be able to read this content:
Sorry, a shareable link is not currently available for this article.
Provided by the Springer Nature SharedIt content-sharing initiative
By submitting a comment you agree to abide by our Terms and Community Guidelines . If you find something abusive or that does not comply with our terms or guidelines please flag it as inappropriate.
Quick links
- Explore articles by subject
- Guide to authors
- Editorial policies
Sign up for the Nature Briefing newsletter — what matters in science, free to your inbox daily.

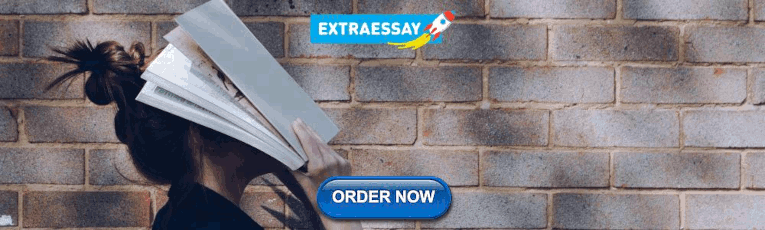
COMMENTS
Abstract. Humans have actually reduced yield from ecosystem services, owing to human-induced changes to components of the Earth's biodiversity and ecosystems along with economic development ...
Introduction. During the last century, research has been increasingly drawn toward understanding the human-nature relationship (1, 2) and has revealed the many ways humans are linked with the natural environment ().Some examples of these include humans' preference for scenes dominated by natural elements (), the sustainability of natural resources (5, 6), and the health benefits associated ...
Open. Human health and the environment are inextricably linked at local, national and global scales. Exposure to environmental issues, such as pollution, climate change, extreme heat events and ...
The deleterious effects of pollution manifest in elevated rates of cancer, cardiovascular disease, respiratory ailments, mental disorders, and diarrhea. Each year, approximately 7 million ...
Environmental hazards and their remediation. At the present time, environmental degradation; climate change; and natural calamities such as soil erosion, famine, floods, and rising sea level are the most common problems that restrain the path towards sustainable development (Li, 2020; Haseeb & Azam, 2021).The anthropogenic activities and climate change are catalysts for environmental hazards ...
Table 1: Major environmental pollutants, their sources, and impact on human health Pollutants and health effects are less studied because of the need for more information on exposure to pollutants in less developed countries, where waste management could be better, there is higher poverty, and the application of new technologies is limited The same situation is observed in many developed ...
We cannot solve this controversy in this paper. Instead, our research objective is to assess the total effect (i.e., direct and indirect effects) from population growth on the environment in Europe. ... Shortdridge A, Liu J. Exploring complexity in a human-environment system: An agent-based spatial model for multidisciplinary and multiscale ...
The direct interactions between people and nature are critically important in many ways, with growing attention particularly on their impacts on human health and wellbeing (both positive and negative), on people's attitudes and behaviour towards nature, and on the benefits and hazards to wildlife. A growing evidence base is accelerating the ...
Nature Climate Change (2023) Climate change and human-wildlife conflict are both pressing challenges for biodiversity conservation and human well-being in the Anthropocene. Climate change is a ...
The negative effects of rising temperatures on the environment, biodiversity and human health are becoming increasingly noticeable. The years 2020 and 2016 were among the hottest since the record ...
This paper examines three-level environmental impact risks associated with climate change on human mortality . It proposes a conceptual framework for developing an empirical event-based
Increasing demands on ecosystems, decreasing biodiversity, and climate change are among the most pressing environmental issues of our time. As changing weather conditions are leading to increased vector-borne diseases and heat- and flood-related deaths, it is entering collective consciousness: environmental issues are human health issues. In public health, the field addressing these issues is ...
Mercury has been shown to cause neurotoxicity, nephrotoxicity, and hepatotoxicity in humans. Cardiovascular toxicity has also been discovered in recent research. Levels of mercury in hair have been linked to oxidized LDL levels in atherosclerotic lesions, acute coronary failure and atherosclerosis (Yoshizawa et al., 2002).
Ecological and environmental pollution is a common important problem, and it is impossible to talk about the relationship between humans and nature without involving human behavior. The analysis of human behavior not only sheds light on understanding man but also represents the importance of ecological development and growth. This paper builds a framework of external and internal factors to ...
Background: More than 80% of sewage generated by human activities is discharged into rivers and oceans without any treatment, which results in environmental pollution and more than 50 diseases. 80% of diseases and 50% of child deaths worldwide are related to poor water quality.Methods: This paper selected 85 relevant papers finally based on the keywords of water pollution, water quality ...
An emphasis on human-environment interaction recognizes the complexity of historical and contemporary factors affecting society and environment at various scales; because of the value put on fieldwork, HEI research has an explicit concern with corroborating deductive and inductive perspectives. ... Malthus's An Essay on the Principle of ...
Article. Gregory Knapp. Human ecology can refer to a variety of disciplinary subfields or to the interdisciplinary project to systematically study human-environment relations; it may include ...
Grades. 5 - 8. Humans impact the physical environment in many ways: overpopulation, pollution, burning fossil fuels, and deforestation. Changes like these have triggered climate change, soil erosion, poor air quality, and undrinkable water. These negative impacts can affect human behavior and can prompt mass migrations or battles over clean water.
Environmental Science and Pollution Research is an online-only journal and its print ISSN (0944-1344) is ceased. Editor-in-Chief. Philippe Garrigues; Impact factor ... Call for Papers: Special Issue on PRR: Preventing, Removing and Recycling. Pollutant Toxic Ions and Molecules (PTIM2023) View all updates.
Human activities have an adverse effect on the environment by polluting the water we drink, the air we breathe, and the soil in which plants grow. Although the industrial revolution was a great success in terms of technology, society, and the provision of multiple services, it also introduced the production of huge quantities of pollutants ...
The authors review recent advances and current debates in epigenetics, including how epigenetic mechanisms interact with genetic variation, ageing, disease and the environment.
In this review article we have tried to explain the impacts and effects of human population's growth on the environment. Different factors which plays a vital role are been discussed in detail.
The current warming trend is different because it is clearly the result of human activities since the mid-1800s, and is proceeding at a rate not seen over many recent millennia. 1 It is undeniable that human activities have produced the atmospheric gases that have trapped more of the Sun's energy in the Earth system. This extra energy has warmed the atmosphere, ocean, and land, and ...
Today, environmental protection and sustainable development are two fundamental pillars of human existence. In this process, the major roles are played by governments and international organizations. Adherence to international obligations (Erga Omnes) and Jus Cogens are two important rudiments in environmental diplomacy towards sustainable ...
Plastics have transformed everyday life; usage is increasing and annual production is likely to exceed 300 million tonnes by 2010. In this concluding paper to the Theme Issue on Plastics, the Environment and Human Health, we synthesize current understanding of the benefits and concerns surrounding the use of plastics and look to future priorities, challenges and opportunities.
Postmodern, poststructuralist, and postcolonial approaches changed radically the basis of human-environment research. In this paper, we argue that geography needs to renew not only its ...
Persistent overuse of water supplies from the Colorado River during recent decades has substantially depleted large storage reservoirs and triggered mandatory cutbacks in water use. The river ...