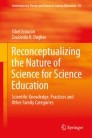
Reconceptualizing the Nature of Science for Science Education pp 41–65 Cite as
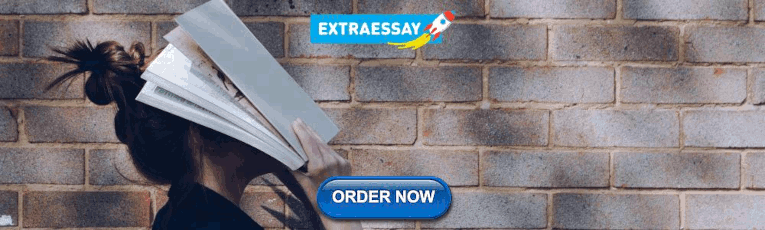
Aims and Values of Science
- Sibel Erduran 4 &
- Zoubeida R. Dagher 5
- First Online: 01 January 2014
1971 Accesses
Part of the book series: Contemporary Trends and Issues in Science Education ((CTISE,volume 43))
This chapter explores the role of aims and values in science. In particular, the epistemic and cognitive aims and values are emphasized, as the social, political and cultural aims and values, are revisited in Chap. 7 in the context of the discussion on social contexts of science. To guide the discussion in this chapter, the following example questions are posed: What are the aims and values of science, and how do values function? For instance, what values come into play when scientists choose between theories? Do values apply similarly across different functions in science? How do values limit or expand scientific knowledge? The components of scientific aims and values are discussed as originally described by various philosophers of science and the review is extended to draw some implications for science education. Examples are drawn to show how scientific aims and values can be promoted in science lessons particularly in relation to assessment of a range of values. The discussion is contextualized in a contemporary curriculum standards document.
- Science Education
- Soft Tissue Sarcoma
- Science Lesson
- Epistemic Attitude
- Argumentation Framework
These keywords were added by machine and not by the authors. This process is experimental and the keywords may be updated as the learning algorithm improves.
This is a preview of subscription content, log in via an institution .
Buying options
- Available as PDF
- Read on any device
- Instant download
- Own it forever
- Available as EPUB and PDF
- Compact, lightweight edition
- Dispatched in 3 to 5 business days
- Free shipping worldwide - see info
- Durable hardcover edition
Tax calculation will be finalised at checkout
Purchases are for personal use only
AAAS. (1989). Science for all Americans . Washington, DC: American Association for the Advancement of Science.
Google Scholar
Allchin, D. (1999). Values in science. Science & Education, 8 , 1–12.
Article Google Scholar
Carrier, M. (2013). Values and objectivity in science: Value-ladenness, pluralism and the epistemic attitude. Science & Education, 22 , 2547–2568.
Collins, J. J., Acquavella, J. F., & Friedlander, B. (1992). Reconciling old and new findings on dioxin. Epidemiology, 3 (1), 65–69.
Douglas, H. (2000). Inductive risk and values in science. Philosophy of Science, 67 (4), 559–579.
Erduran, S., & Jimenez-Aleixandre, M. P. (Eds.). (2008). Argumentation in science education: Perspectives from classroom-based research . Dordrecht, The Netherlands: Springer.
Fingerhut, M. A., Halperin, W. E., Marlow, D. A., Piacitelli, L. A., Honchar, P. A., Sweeney, M. H., et al. (1991). Cancer mortality in workers exposed to 2,3,7,8-Tetrachlorodibenzo-P-Dioxin. New England Journal of Medicine, 324 , 212–218. doi: 10.1056/NEJM199101243240402 .
Gluud, L. L. (2006). Bias in clinical intervention research. American Journal of Epidemiology, 163 (6), 493–501.
Harding, S. (1991). Whose science? Whose knowledge? Thinking from women’s lives . Ithaca, NY: Cornell University Press.
Harris, S. (2012). The moral landscape . London: Bantam Press.
Hempel, C. G. (1965). Aspects of scientific explanation and other essays in the philosophy of science . New York: Free Press.
Irzik, G., & Nola, R. (2011). A family resemblance approach to the nature of science. Science & Education, 20 , 591–607.
Irzik, G., & Nola, R. (2014). New directions for nature of science research. In M. Matthews (Ed.), International handbook of research in history, philosophy and science teaching (pp. 999–1021). Dordrecht, The Netherlands: Springer.
Chapter Google Scholar
Kolsto, S. D., & Ratcliffe, M. (2008). Social aspects of argumentation. In S. Erduran & M. P. Jimenez-Aleixandre (Eds.), Argumentation in science education: Perspectives from classroom-based research (pp. 117–136). Dordrecht, The Netherlands: Springer.
Kuhn, T. S. (1977). The essential tension . Chicago: University of Chicago Press.
Lee, M.-H., Wu, Y.-T., & Tsai, C.-C. (2009). Research trends in science education from 2003 to 2007: A content analysis of publications in selected journals. International Journal of Science Education, 31 (15), 1999–2020.
Longino, H. (1990). Science as social knowledge: Values and objectivity in scientific inquiry . Princeton, NJ: Princeton University Press.
Longino, H. E. (1993). Essential tensions—Phase two: Feminist, philosophical, and social studies of science. In L. M. Antony & C. Witt (Eds.), A mind of one’s own. Feminist essays on reason and objectivity (pp. 257–272). Boulder, CO: Westview Press.
Longino, H. (1995). Gender, politics and the theoretical virtues. Synthese, 104 , 383–397.
Longino, H. (1997). Feminist epistemology as a local epistemology. Aristotelian Society Supplementary Volume, 71 (1), 19–36.
Longino, H. E. (2002). The fate of knowledge . Princeton, NJ: Princeton University Press.
Machamer, P., & Douglas, H. (1999). Cognitive and social values. Science & Education, 8 , 45–54.
NGSS Lead States. (2013). Next generation science standards: For states by states . Appendix H. Retrieved from http://www.nextgenscience.org/next-generation-science-standards
Popper, K. R. (1957). Science: Conjectures and refutations. In Conjectures and refutations. The growth of scientific knowledge (pp. 43–78). London: Routledge, 1963, 2002.
Popper, K. R. (1963). Conjectures and refutations . London: Routledge and Kegan Paul.
Popper, K. R. (1975). Objective knowledge . Oxford, UK: Clarendon.
Resnick, D. (2007). The price of truth: How many affects the norms of science . Oxford, UK: Oxford University Press.
Book Google Scholar
van Frassen, B. (1980). The scientific image . Oxford, UK: Clarendon.
von Glasersfeld, E. (1989). Cognition, construction of knowledge and teaching. Synthese, 80 , 121–40.
Wilholt, T. (2009). Bias and values in scientific research. Studies in History and Philosophy of Science, 40 , 92–101.
Download references
Author information
Authors and affiliations.
Department of Education and Professional Studies, University of Limerick, Limerick, Ireland
Sibel Erduran
School of Education, University of Delaware, Newark, DE, USA
Zoubeida R. Dagher
You can also search for this author in PubMed Google Scholar
Rights and permissions
Reprints and permissions
Copyright information
© 2014 Springer Netherlands
About this chapter
Cite this chapter.
Erduran, S., Dagher, Z.R. (2014). Aims and Values of Science. In: Reconceptualizing the Nature of Science for Science Education. Contemporary Trends and Issues in Science Education, vol 43. Springer, Dordrecht. https://doi.org/10.1007/978-94-017-9057-4_3
Download citation
DOI : https://doi.org/10.1007/978-94-017-9057-4_3
Published : 11 July 2014
Publisher Name : Springer, Dordrecht
Print ISBN : 978-94-017-9056-7
Online ISBN : 978-94-017-9057-4
eBook Packages : Humanities, Social Sciences and Law Education (R0)
Share this chapter
Anyone you share the following link with will be able to read this content:
Sorry, a shareable link is not currently available for this article.
Provided by the Springer Nature SharedIt content-sharing initiative
- Publish with us
Policies and ethics
- Find a journal
- Track your research
- Search Menu
- Browse content in Arts and Humanities
- Browse content in Archaeology
- Anglo-Saxon and Medieval Archaeology
- Archaeological Methodology and Techniques
- Archaeology by Region
- Archaeology of Religion
- Archaeology of Trade and Exchange
- Biblical Archaeology
- Contemporary and Public Archaeology
- Environmental Archaeology
- Historical Archaeology
- History and Theory of Archaeology
- Industrial Archaeology
- Landscape Archaeology
- Mortuary Archaeology
- Prehistoric Archaeology
- Underwater Archaeology
- Urban Archaeology
- Zooarchaeology
- Browse content in Architecture
- Architectural Structure and Design
- History of Architecture
- Residential and Domestic Buildings
- Theory of Architecture
- Browse content in Art
- Art Subjects and Themes
- History of Art
- Industrial and Commercial Art
- Theory of Art
- Biographical Studies
- Byzantine Studies
- Browse content in Classical Studies
- Classical History
- Classical Philosophy
- Classical Mythology
- Classical Literature
- Classical Reception
- Classical Art and Architecture
- Classical Oratory and Rhetoric
- Greek and Roman Papyrology
- Greek and Roman Epigraphy
- Greek and Roman Law
- Greek and Roman Archaeology
- Late Antiquity
- Religion in the Ancient World
- Digital Humanities
- Browse content in History
- Colonialism and Imperialism
- Diplomatic History
- Environmental History
- Genealogy, Heraldry, Names, and Honours
- Genocide and Ethnic Cleansing
- Historical Geography
- History by Period
- History of Emotions
- History of Agriculture
- History of Education
- History of Gender and Sexuality
- Industrial History
- Intellectual History
- International History
- Labour History
- Legal and Constitutional History
- Local and Family History
- Maritime History
- Military History
- National Liberation and Post-Colonialism
- Oral History
- Political History
- Public History
- Regional and National History
- Revolutions and Rebellions
- Slavery and Abolition of Slavery
- Social and Cultural History
- Theory, Methods, and Historiography
- Urban History
- World History
- Browse content in Language Teaching and Learning
- Language Learning (Specific Skills)
- Language Teaching Theory and Methods
- Browse content in Linguistics
- Applied Linguistics
- Cognitive Linguistics
- Computational Linguistics
- Forensic Linguistics
- Grammar, Syntax and Morphology
- Historical and Diachronic Linguistics
- History of English
- Language Evolution
- Language Reference
- Language Acquisition
- Language Variation
- Language Families
- Lexicography
- Linguistic Anthropology
- Linguistic Theories
- Linguistic Typology
- Phonetics and Phonology
- Psycholinguistics
- Sociolinguistics
- Translation and Interpretation
- Writing Systems
- Browse content in Literature
- Bibliography
- Children's Literature Studies
- Literary Studies (Romanticism)
- Literary Studies (American)
- Literary Studies (Asian)
- Literary Studies (European)
- Literary Studies (Eco-criticism)
- Literary Studies (Modernism)
- Literary Studies - World
- Literary Studies (1500 to 1800)
- Literary Studies (19th Century)
- Literary Studies (20th Century onwards)
- Literary Studies (African American Literature)
- Literary Studies (British and Irish)
- Literary Studies (Early and Medieval)
- Literary Studies (Fiction, Novelists, and Prose Writers)
- Literary Studies (Gender Studies)
- Literary Studies (Graphic Novels)
- Literary Studies (History of the Book)
- Literary Studies (Plays and Playwrights)
- Literary Studies (Poetry and Poets)
- Literary Studies (Postcolonial Literature)
- Literary Studies (Queer Studies)
- Literary Studies (Science Fiction)
- Literary Studies (Travel Literature)
- Literary Studies (War Literature)
- Literary Studies (Women's Writing)
- Literary Theory and Cultural Studies
- Mythology and Folklore
- Shakespeare Studies and Criticism
- Browse content in Media Studies
- Browse content in Music
- Applied Music
- Dance and Music
- Ethics in Music
- Ethnomusicology
- Gender and Sexuality in Music
- Medicine and Music
- Music Cultures
- Music and Media
- Music and Religion
- Music and Culture
- Music Education and Pedagogy
- Music Theory and Analysis
- Musical Scores, Lyrics, and Libretti
- Musical Structures, Styles, and Techniques
- Musicology and Music History
- Performance Practice and Studies
- Race and Ethnicity in Music
- Sound Studies
- Browse content in Performing Arts
- Browse content in Philosophy
- Aesthetics and Philosophy of Art
- Epistemology
- Feminist Philosophy
- History of Western Philosophy
- Metaphysics
- Moral Philosophy
- Non-Western Philosophy
- Philosophy of Language
- Philosophy of Mind
- Philosophy of Perception
- Philosophy of Science
- Philosophy of Action
- Philosophy of Law
- Philosophy of Religion
- Philosophy of Mathematics and Logic
- Practical Ethics
- Social and Political Philosophy
- Browse content in Religion
- Biblical Studies
- Christianity
- East Asian Religions
- History of Religion
- Judaism and Jewish Studies
- Qumran Studies
- Religion and Education
- Religion and Health
- Religion and Politics
- Religion and Science
- Religion and Law
- Religion and Art, Literature, and Music
- Religious Studies
- Browse content in Society and Culture
- Cookery, Food, and Drink
- Cultural Studies
- Customs and Traditions
- Ethical Issues and Debates
- Hobbies, Games, Arts and Crafts
- Lifestyle, Home, and Garden
- Natural world, Country Life, and Pets
- Popular Beliefs and Controversial Knowledge
- Sports and Outdoor Recreation
- Technology and Society
- Travel and Holiday
- Visual Culture
- Browse content in Law
- Arbitration
- Browse content in Company and Commercial Law
- Commercial Law
- Company Law
- Browse content in Comparative Law
- Systems of Law
- Competition Law
- Browse content in Constitutional and Administrative Law
- Government Powers
- Judicial Review
- Local Government Law
- Military and Defence Law
- Parliamentary and Legislative Practice
- Construction Law
- Contract Law
- Browse content in Criminal Law
- Criminal Procedure
- Criminal Evidence Law
- Sentencing and Punishment
- Employment and Labour Law
- Environment and Energy Law
- Browse content in Financial Law
- Banking Law
- Insolvency Law
- History of Law
- Human Rights and Immigration
- Intellectual Property Law
- Browse content in International Law
- Private International Law and Conflict of Laws
- Public International Law
- IT and Communications Law
- Jurisprudence and Philosophy of Law
- Law and Politics
- Law and Society
- Browse content in Legal System and Practice
- Courts and Procedure
- Legal Skills and Practice
- Primary Sources of Law
- Regulation of Legal Profession
- Medical and Healthcare Law
- Browse content in Policing
- Criminal Investigation and Detection
- Police and Security Services
- Police Procedure and Law
- Police Regional Planning
- Browse content in Property Law
- Personal Property Law
- Study and Revision
- Terrorism and National Security Law
- Browse content in Trusts Law
- Wills and Probate or Succession
- Browse content in Medicine and Health
- Browse content in Allied Health Professions
- Arts Therapies
- Clinical Science
- Dietetics and Nutrition
- Occupational Therapy
- Operating Department Practice
- Physiotherapy
- Radiography
- Speech and Language Therapy
- Browse content in Anaesthetics
- General Anaesthesia
- Neuroanaesthesia
- Clinical Neuroscience
- Browse content in Clinical Medicine
- Acute Medicine
- Cardiovascular Medicine
- Clinical Genetics
- Clinical Pharmacology and Therapeutics
- Dermatology
- Endocrinology and Diabetes
- Gastroenterology
- Genito-urinary Medicine
- Geriatric Medicine
- Infectious Diseases
- Medical Toxicology
- Medical Oncology
- Pain Medicine
- Palliative Medicine
- Rehabilitation Medicine
- Respiratory Medicine and Pulmonology
- Rheumatology
- Sleep Medicine
- Sports and Exercise Medicine
- Community Medical Services
- Critical Care
- Emergency Medicine
- Forensic Medicine
- Haematology
- History of Medicine
- Browse content in Medical Skills
- Clinical Skills
- Communication Skills
- Nursing Skills
- Surgical Skills
- Browse content in Medical Dentistry
- Oral and Maxillofacial Surgery
- Paediatric Dentistry
- Restorative Dentistry and Orthodontics
- Surgical Dentistry
- Medical Ethics
- Medical Statistics and Methodology
- Browse content in Neurology
- Clinical Neurophysiology
- Neuropathology
- Nursing Studies
- Browse content in Obstetrics and Gynaecology
- Gynaecology
- Occupational Medicine
- Ophthalmology
- Otolaryngology (ENT)
- Browse content in Paediatrics
- Neonatology
- Browse content in Pathology
- Chemical Pathology
- Clinical Cytogenetics and Molecular Genetics
- Histopathology
- Medical Microbiology and Virology
- Patient Education and Information
- Browse content in Pharmacology
- Psychopharmacology
- Browse content in Popular Health
- Caring for Others
- Complementary and Alternative Medicine
- Self-help and Personal Development
- Browse content in Preclinical Medicine
- Cell Biology
- Molecular Biology and Genetics
- Reproduction, Growth and Development
- Primary Care
- Professional Development in Medicine
- Browse content in Psychiatry
- Addiction Medicine
- Child and Adolescent Psychiatry
- Forensic Psychiatry
- Learning Disabilities
- Old Age Psychiatry
- Psychotherapy
- Browse content in Public Health and Epidemiology
- Epidemiology
- Public Health
- Browse content in Radiology
- Clinical Radiology
- Interventional Radiology
- Nuclear Medicine
- Radiation Oncology
- Reproductive Medicine
- Browse content in Surgery
- Cardiothoracic Surgery
- Gastro-intestinal and Colorectal Surgery
- General Surgery
- Neurosurgery
- Paediatric Surgery
- Peri-operative Care
- Plastic and Reconstructive Surgery
- Surgical Oncology
- Transplant Surgery
- Trauma and Orthopaedic Surgery
- Vascular Surgery
- Browse content in Science and Mathematics
- Browse content in Biological Sciences
- Aquatic Biology
- Biochemistry
- Bioinformatics and Computational Biology
- Developmental Biology
- Ecology and Conservation
- Evolutionary Biology
- Genetics and Genomics
- Microbiology
- Molecular and Cell Biology
- Natural History
- Plant Sciences and Forestry
- Research Methods in Life Sciences
- Structural Biology
- Systems Biology
- Zoology and Animal Sciences
- Browse content in Chemistry
- Analytical Chemistry
- Computational Chemistry
- Crystallography
- Environmental Chemistry
- Industrial Chemistry
- Inorganic Chemistry
- Materials Chemistry
- Medicinal Chemistry
- Mineralogy and Gems
- Organic Chemistry
- Physical Chemistry
- Polymer Chemistry
- Study and Communication Skills in Chemistry
- Theoretical Chemistry
- Browse content in Computer Science
- Artificial Intelligence
- Computer Architecture and Logic Design
- Game Studies
- Human-Computer Interaction
- Mathematical Theory of Computation
- Programming Languages
- Software Engineering
- Systems Analysis and Design
- Virtual Reality
- Browse content in Computing
- Business Applications
- Computer Security
- Computer Games
- Computer Networking and Communications
- Digital Lifestyle
- Graphical and Digital Media Applications
- Operating Systems
- Browse content in Earth Sciences and Geography
- Atmospheric Sciences
- Environmental Geography
- Geology and the Lithosphere
- Maps and Map-making
- Meteorology and Climatology
- Oceanography and Hydrology
- Palaeontology
- Physical Geography and Topography
- Regional Geography
- Soil Science
- Urban Geography
- Browse content in Engineering and Technology
- Agriculture and Farming
- Biological Engineering
- Civil Engineering, Surveying, and Building
- Electronics and Communications Engineering
- Energy Technology
- Engineering (General)
- Environmental Science, Engineering, and Technology
- History of Engineering and Technology
- Mechanical Engineering and Materials
- Technology of Industrial Chemistry
- Transport Technology and Trades
- Browse content in Environmental Science
- Applied Ecology (Environmental Science)
- Conservation of the Environment (Environmental Science)
- Environmental Sustainability
- Environmentalist Thought and Ideology (Environmental Science)
- Management of Land and Natural Resources (Environmental Science)
- Natural Disasters (Environmental Science)
- Nuclear Issues (Environmental Science)
- Pollution and Threats to the Environment (Environmental Science)
- Social Impact of Environmental Issues (Environmental Science)
- History of Science and Technology
- Browse content in Materials Science
- Ceramics and Glasses
- Composite Materials
- Metals, Alloying, and Corrosion
- Nanotechnology
- Browse content in Mathematics
- Applied Mathematics
- Biomathematics and Statistics
- History of Mathematics
- Mathematical Education
- Mathematical Finance
- Mathematical Analysis
- Numerical and Computational Mathematics
- Probability and Statistics
- Pure Mathematics
- Browse content in Neuroscience
- Cognition and Behavioural Neuroscience
- Development of the Nervous System
- Disorders of the Nervous System
- History of Neuroscience
- Invertebrate Neurobiology
- Molecular and Cellular Systems
- Neuroendocrinology and Autonomic Nervous System
- Neuroscientific Techniques
- Sensory and Motor Systems
- Browse content in Physics
- Astronomy and Astrophysics
- Atomic, Molecular, and Optical Physics
- Biological and Medical Physics
- Classical Mechanics
- Computational Physics
- Condensed Matter Physics
- Electromagnetism, Optics, and Acoustics
- History of Physics
- Mathematical and Statistical Physics
- Measurement Science
- Nuclear Physics
- Particles and Fields
- Plasma Physics
- Quantum Physics
- Relativity and Gravitation
- Semiconductor and Mesoscopic Physics
- Browse content in Psychology
- Affective Sciences
- Clinical Psychology
- Cognitive Psychology
- Cognitive Neuroscience
- Criminal and Forensic Psychology
- Developmental Psychology
- Educational Psychology
- Evolutionary Psychology
- Health Psychology
- History and Systems in Psychology
- Music Psychology
- Neuropsychology
- Organizational Psychology
- Psychological Assessment and Testing
- Psychology of Human-Technology Interaction
- Psychology Professional Development and Training
- Research Methods in Psychology
- Social Psychology
- Browse content in Social Sciences
- Browse content in Anthropology
- Anthropology of Religion
- Human Evolution
- Medical Anthropology
- Physical Anthropology
- Regional Anthropology
- Social and Cultural Anthropology
- Theory and Practice of Anthropology
- Browse content in Business and Management
- Business Ethics
- Business Strategy
- Business History
- Business and Technology
- Business and Government
- Business and the Environment
- Comparative Management
- Corporate Governance
- Corporate Social Responsibility
- Entrepreneurship
- Health Management
- Human Resource Management
- Industrial and Employment Relations
- Industry Studies
- Information and Communication Technologies
- International Business
- Knowledge Management
- Management and Management Techniques
- Operations Management
- Organizational Theory and Behaviour
- Pensions and Pension Management
- Public and Nonprofit Management
- Strategic Management
- Supply Chain Management
- Browse content in Criminology and Criminal Justice
- Criminal Justice
- Criminology
- Forms of Crime
- International and Comparative Criminology
- Youth Violence and Juvenile Justice
- Development Studies
- Browse content in Economics
- Agricultural, Environmental, and Natural Resource Economics
- Asian Economics
- Behavioural Finance
- Behavioural Economics and Neuroeconomics
- Econometrics and Mathematical Economics
- Economic History
- Economic Systems
- Economic Methodology
- Economic Development and Growth
- Financial Markets
- Financial Institutions and Services
- General Economics and Teaching
- Health, Education, and Welfare
- History of Economic Thought
- International Economics
- Labour and Demographic Economics
- Law and Economics
- Macroeconomics and Monetary Economics
- Microeconomics
- Public Economics
- Urban, Rural, and Regional Economics
- Welfare Economics
- Browse content in Education
- Adult Education and Continuous Learning
- Care and Counselling of Students
- Early Childhood and Elementary Education
- Educational Equipment and Technology
- Educational Strategies and Policy
- Higher and Further Education
- Organization and Management of Education
- Philosophy and Theory of Education
- Schools Studies
- Secondary Education
- Teaching of a Specific Subject
- Teaching of Specific Groups and Special Educational Needs
- Teaching Skills and Techniques
- Browse content in Environment
- Applied Ecology (Social Science)
- Climate Change
- Conservation of the Environment (Social Science)
- Environmentalist Thought and Ideology (Social Science)
- Natural Disasters (Environment)
- Social Impact of Environmental Issues (Social Science)
- Browse content in Human Geography
- Cultural Geography
- Economic Geography
- Political Geography
- Browse content in Interdisciplinary Studies
- Communication Studies
- Museums, Libraries, and Information Sciences
- Browse content in Politics
- African Politics
- Asian Politics
- Chinese Politics
- Comparative Politics
- Conflict Politics
- Elections and Electoral Studies
- Environmental Politics
- European Union
- Foreign Policy
- Gender and Politics
- Human Rights and Politics
- Indian Politics
- International Relations
- International Organization (Politics)
- International Political Economy
- Irish Politics
- Latin American Politics
- Middle Eastern Politics
- Political Behaviour
- Political Economy
- Political Institutions
- Political Methodology
- Political Communication
- Political Philosophy
- Political Sociology
- Political Theory
- Politics and Law
- Public Policy
- Public Administration
- Quantitative Political Methodology
- Regional Political Studies
- Russian Politics
- Security Studies
- State and Local Government
- UK Politics
- US Politics
- Browse content in Regional and Area Studies
- African Studies
- Asian Studies
- East Asian Studies
- Japanese Studies
- Latin American Studies
- Middle Eastern Studies
- Native American Studies
- Scottish Studies
- Browse content in Research and Information
- Research Methods
- Browse content in Social Work
- Addictions and Substance Misuse
- Adoption and Fostering
- Care of the Elderly
- Child and Adolescent Social Work
- Couple and Family Social Work
- Developmental and Physical Disabilities Social Work
- Direct Practice and Clinical Social Work
- Emergency Services
- Human Behaviour and the Social Environment
- International and Global Issues in Social Work
- Mental and Behavioural Health
- Social Justice and Human Rights
- Social Policy and Advocacy
- Social Work and Crime and Justice
- Social Work Macro Practice
- Social Work Practice Settings
- Social Work Research and Evidence-based Practice
- Welfare and Benefit Systems
- Browse content in Sociology
- Childhood Studies
- Community Development
- Comparative and Historical Sociology
- Economic Sociology
- Gender and Sexuality
- Gerontology and Ageing
- Health, Illness, and Medicine
- Marriage and the Family
- Migration Studies
- Occupations, Professions, and Work
- Organizations
- Population and Demography
- Race and Ethnicity
- Social Theory
- Social Movements and Social Change
- Social Research and Statistics
- Social Stratification, Inequality, and Mobility
- Sociology of Religion
- Sociology of Education
- Sport and Leisure
- Urban and Rural Studies
- Browse content in Warfare and Defence
- Defence Strategy, Planning, and Research
- Land Forces and Warfare
- Military Administration
- Military Life and Institutions
- Naval Forces and Warfare
- Other Warfare and Defence Issues
- Peace Studies and Conflict Resolution
- Weapons and Equipment

- < Previous chapter
- Next chapter >
29 Values in Science
Heather Douglas, University of Waterloo
- Published: 10 December 2015
- Cite Icon Cite
- Permissions Icon Permissions
After describing the origins and nature of the value-free ideal for science, this chapter details three challenges to the ideal: the descriptive challenge (arising from feminist critiques of science, which led to deeper examinations of social structures in science), the boundary challenge (which questioned whether epistemic values can be distinguished from nonepistemic values), and the normative challenge (which questioned the ideal qua ideal on the basis of inductive risk and scientific responsibility). The chapter then discusses alternative ideals for values in science, including recent arguments regarding epistemic values, arguments distinguishing direct from indirect roles for values, and arguments calling for more attention to getting the values right. Finally, the chapter turns to the many ways in which values influence science and the importance of getting a richer understanding of the place of science within society in order to address the questions about the place of values in science.
1 Introduction
Science takes place in society. Although this might seem obvious, incorporating an understanding of this into philosophy of science has driven dramatic changes in the understanding of values in science in recent years. Seeing science as fully embedded in and responsible to the broader society that supports it, the scientific endeavor becomes fully value-laden. This change is not just for the description of science performed by all-too-human actors who inevitably bring their values with them into their science. The change also applies to science in the ideal. However, the distinctive nature of science must also be described and protected from abuse or the misuse of values in science. The issues for philosophers thus become (1) What are the implications of the embedding of science in society? (2) When and how are values legitimate? (3) Which values are legitimate? And (4), what is the ideal for science embedded in society?
The changes that this conceptual shift have wrought take place against the backdrop of the value-free ideal. This chapter will first describe the value-free ideal before moving on to the challenges philosophers have leveled against it. It will then describe the terrain of how to conceive of the role of values in science once the value-free ideal is rejected qua ideal. As we will see, challenging questions of the place and role of science in society come to the fore, with important implications for the philosophy of science.
2 The Value-Free Ideal
The value-free ideal has historical roots stretching back centuries ( Proctor 1991 ), but the contemporary formulation dates from the Cold War era ( Douglas 2009 : ch. 3). Precursors to the contemporary value-free ideal, including Max Weber, did not articulate the full-fledged value-free ideal, suggesting instead that values served to help focus scientists on what was significant and that science could not proceed without values ( Douglas 2011 ). That values shape what scientists chose to explore was, and remains, an uncontroversial way in which values influence science. That scientists’ interests and concerns help direct scientists’ attention in some directions and not others seemed unproblematic and was never contested by philosophers of science. But, by 1950, this was thought to be part of the “logic of discovery” in science rather than the “logic of justification.” If values played a role in the logic of justification, the worry was (and remains) that science could be corrupted.
It was not until a debate over the role of values in science erupted in the 1950s that the value-free ideal emerged in its fully articulated form. The debate began with the work of C. West Churchman (1948) and Richard Rudner (1953) and their acknowledgment that, in addition to values shaping the direction of research, values were required to set what was thought to be sufficient evidence. Rudner pointedly argued that because science was not a deductive process and there was always an inductive gap between the evidence and the scientific claims being made (“no scientific hypothesis is ever completely verified”), one had to decide “that the evidence is sufficiently strong or that the probability is sufficiently high to warrant the acceptance of the hypothesis.” ( Rudner 1953 , 2) Making this decision involved considering “the importance, in the typically ethical sense, of making a mistake in accepting or rejecting the hypothesis” ( Rudner 1953 , 2). This meant that social and ethical values had a legitimate role in deciding whether a scientific claim is sufficiently justified—the determination of sufficient justification can include social and ethical values weighing the importance of a mistake and whether we have enough evidence to consider such a risk worth worrying about. For example, if we think a false-positive type of error is more worrisome, we might demand more evidence. If we think a false-negative type of error is more worrisome, we might demand less before finding a claim “sufficiently warranted.” Whether one or the other is more worrisome is a value judgment, one that often depends on social and ethical valuations of the consequences of error. What counts as sufficient warrant thus depends on the claim and the context in which it is made and whether there are serious implications of error in that context.
Such a view brought values, however, into the heart of doing science. And, as such, there was substantial resistance to it. Philosophers like Richard Jeffrey and Isaac Levi resisted this line of argument, albeit in different ways. Jeffrey argued that scientists did not need to accept and reject hypotheses; instead, he thought they should just assign probabilities to them ( Jeffrey 1956 ). Rudner had anticipated this approach, however, and had argued that even in assigning probabilities, one needed to decide that the probability was sufficiently warranted. The problem of assessing sufficiency might be mitigated, but it was not eliminated.
Levi took a different tack, arguing that, as a normative matter, scientists should not consider the broader societal implications or the context of their work when assessing scientific claims. Levi suggested that certain “canons of inference” should be used when examining evidence and its relationship to hypotheses and that such canons were entirely sufficient for addressing the inferential gap that confronted scientists ( Levi 1960 ). For Levi, such canons demanded a uniform response from scientists when confronting judgments about the strength of evidence.
It was this line of argument that helped to formulate the value-free ideal for science. The canons of inference were to include such considerations as simplicity, scope, and explanatory power, aspects that were soon to be called “epistemic” or “cognitive” values. The value-free ideal was the idea that social and ethical values should influence only the external aspects of science—such as which projects were undertaken and which methodologies were thought to be ethically acceptable—but that in the heart of science, at the moment of inference, no social and ethical values were to have any role whatsoever. Rather, only the canons of inference (the epistemic values) should shape scientists’ judgments.
It was in Thomas Kuhn’s 1977 paper, “Objectivity, Value Judgment, and Theory Choice,” that such canons of inference came to be called “values.” Kuhn argued that one cannot generate an algorithm or set of rules for scientific theory choice and that the process of judgment regarding theories is better characterized as a value judgment, one informed by the characteristics desired of good scientific theories, characteristics that in Kuhn’s view included “accuracy, consistency, scope, simplicity, and fruitfulness” ( Kuhn 1977 , 322). By establishing a shared set of values used to inform theory choice, science could remain objective even if some values were essential to science. In addition, scientists could disagree about which theories to adopt (as historical work showed they did) and still be working within the canons of scientific inference because some scientists might emphasize particular values over others or have different interpretations of what instantiated the values properly. What made science objective, on this picture, was an adherence to excluding social and ethical values from the inferential aspects of science. It was this sense of which values should be allowed in (the epistemic values only) at the crucial point in science (when making inferences about which theories to accept) that constituted the value-free ideal (see also Laudan 1984 ; McMullin 1983 ).
3 Challenges to the Value-Free Ideal
The value-free ideal, a somewhat inaccurate label for the epistemic-values-only-in-scientific-inference ideal, has been challenged in a number of ways. I describe three main approaches to critiquing the ideal: the descriptive challenge, the boundary challenge, and the normative challenge. These are not meant to be fully distinct challenges, but the thrust of each of the three leads in different directions.
3.1 The Descriptive Challenge
The first challenge began in the 1980s, as feminist scholars of science noted that science did not appear to be value-free. Although some scientific claims under scrutiny could be shown to be due to poor methodology, others could not. Instead, they appeared to be the result of either a lack of sufficient alternative explanations or theories, or the result of insufficiently examined background assumptions. Feminist philosophers argued that even good science, science thought to be exemplary, appeared value-laden in important ways. ( Fausto-Sterling 1985 ; Harding 1986 , 1991 ; Keller and Longino 1996 ; Nelson 1990 ) Their work showed how fields that carefully gathered evidence, that followed the traditional practices of scientific inference, still managed to produce blatantly sexist results. Examples such as early primatology ( Haraway 1989 ), anthropological accounts of human development ( Longino 1990 ), and biological accounts of sperm–egg interactions ( Martin 1996 ) were shot through with sexist presuppositions that blinded scientists to alternative explanations of phenomena and thus led to acceptance of views later shown to be inadequate. The scientists who did such work were (generally) not committing fraud or allowing social values to shape their inferences in obvious ways, but, once the science was scrutinized, it seemed anything but value-free.
The problems unearthed by this work showed the pervasive difficulty of eliminating values from the scientific process and the results produced—you could only test whichever theories were available, and you could only test them using your background assumptions. What theories you had available and which background assumptions you held often reflected your values. Values could not be removed from science on this account.
This line of argument is also tied to the understanding of science as underdetermined by the available evidence (in the tradition of Duhem, Neurath, and Quine; see Brown 2013 a ), particularly transient underdetermination ( Anderson 2004 ; Biddle 2013 ) Although all of the evidence may one day be in and make clear what we should think, as actual epistemic actors, we are not in that position. The evidence does not clearly determine which claims are the right ones, nor does it indicate that we have all the plausible options on the table for consideration, nor even whether our background assumptions are adequate. If a surprising result does arise, we don’t know precisely where the problem lies. Against this backdrop of uncertainty, we still make judgments about what is scientifically supported. This has been called the “gap argument” for the role of values in science ( Brown 2013 a ; Elliott 2011 a ; Intemann 2005 ).
The recognition of this gap between theory and evidence, and the possibility that it is filled by values, has led many feminists to call for increased diversity in science and better scrutiny of theories within science. If background assumptions used by scientists often “encode social values” ( Longino 1990 , 216), we need better scientific practices to uncover such encoding and help us generate background assumptions with better values. Such work led philosophers to consider more carefully the social structure of the scientific community and scientific practices, to see if improving those could assist in both ferreting out these problematic values and ensuring better science. Feminist philosophers invigorated the field of social epistemology, which examines more closely the social conditions of knowledge production and what kinds of conditions we should consider good for reliable knowledge production ( Longino 1990 , 2002 ). I will call this the descriptive challenge to the value-free ideal: that science, even science done well, is not value-free, and so the ideal is irrelevant. This approach turns elsewhere, to social structures, for ideals for science.
Helen Longino, for example, has argued for a set of principles that should structure epistemic communities for robust knowledge production. An epistemic community should instantiate public forums for criticism, prima facie equality of intellectual authority, responsiveness to criticism, and shared values in order to be able to produce claims worth calling “knowledge” ( Longino 1990 , 2002 ). Miriam Solomon, in contrast, focuses on the range of “decision-vectors” (influences on decisions scientists make) and argues for two principles to guide the distribution of effort within a scientific community: efforts should be distributed equitably (i.e., proportionally) with respect to empirical successes of different theories, and effort should be distributed equally with respect to nonempirical factors (i.e., there should be no clustering of effort in science for purely social or political reasons) ( Solomon 2001 ). Both of these approaches value diversity in science for creating critical pressure on existing scientific approaches and for bringing new ideas into the fore, thus fostering debate and discussion (see also Intemann 2009 ).
This emphasis on the social structures of science produced by the descriptive challenge has illuminated much about science. By examining how scientific communities should be structured, and in particular how such structures would reveal the hidden values embedded in science, social epistemological approaches have much to teach us about values in science. But such approaches on their own are not enough to take down the value-free ideal qua ideal, nor enough to replace it. One could still argue that the goal of science should be the removal of values from science, and social approaches help us ferret out those values, thus leaving the ideal intact. If, on the other hand, one embraced rejecting the ideal, the social-level norms (about the structure of epistemic communities) do not tell individual actors what counts as a good argument within those communities. Some guidance on the legitimate (and illegitimate) grounds for inference in science is still needed so that actors can know what is (and what is not) appropriate argumentation. Section 4 on alternative ideals takes up this challenge.
3.2 The Boundary Challenge
In addition to the descriptive challenge, philosophers critiqued the value-free ideal on another front: on the plausibility that a clear distinction between epistemic and nonepistemic values could be made. The value-free ideal, in its post-Levi/Kuhn form, requires a clear distinction between the values that are allowed to influence theory choice (often called epistemic values) and those that are not (the nonepistemic social and ethical values). If this distinction cannot withstand scrutiny, then the value-free ideal collapses.
Several philosophers have challenged the boundary between epistemic and nonepistemic values. Phyllis Rooney, for example, argued that when we examine the epistemic values espoused by scientists at different historical moments, we can often recognize the nonepistemic values influencing what is thought to be purely epistemic ( Rooney 1992 ). In examining episodes in the history of physics, Rooney finds cultural or religious values shaping supposedly epistemic values as reasons for accepting or rejecting theories. Longino argued that the selection of epistemic values by Kuhn and others was arbitrary and, indeed, that other values could also be argued to be important criteria for theory selection, including ones that seemed opposite to the canonical ones ( Longino 1995 , 1996 ). She proposed that, in contrast to the traditional epistemic values of consistency, simplicity, scope, and fruitfulness, equally plausible are such values as novelty, applicability, and ontological heterogeneity, values that arise out of feminist criticisms of science, criticisms that are motivated by a concern for social justice. For example, feminist scientists value novelty in scientific theories because the traditional theories were so often laden with sexism. Feminist scientists also value ontological heterogeneity so that scientists are more likely to recognize the diversity among the individuals they are studying ( Longino 1996 , 45–47). How the value-free ideal is to be maintained as a coherent ideal when the boundary between epistemic and nonepistemic values appears to be porous is not clear.
Arguments about how to understand the epistemic values in science have become more complex in recent years. As will be discussed later, examinations of the traditional set of epistemic values within a context of rejecting the value-free ideal have opened up new possibilities and insights about epistemic values. It is possible to have differences in purpose and emphasis among values, including among epistemic and nonepistemic values, without having a clear distinction that can bear the weight of the value-free ideal.
3.3 The Normative Challenge
The third challenge to the value-free ideal confronts the ideal more directly. Rather than undermine its plausibility or feasibility, this approach argues that the ideal, qua ideal, is the wrong ideal for science. This argument draws from the insights of Churchman, and Rudner and from even earlier work by William James ( Magnus 2013 ), but provides a stronger articulation of and basis for those insights. I will call this the normative challenge. (This has been called the “error argument”; see Brown 2013 a ; Elliott 2011 a .) Because this approach challenges the ideal qua ideal, it must replace the value-free ideal with an alternative ideal by which to manage values in science.
There are several conceptual bases that combine in the normative challenge to the value-free ideal. First, as with the descriptive challenge and arguments from underdetermination, the endemic uncertainty in science is central. That we never have conclusive proof for our scientific theories or complete evidence for our hypotheses means that there is always some uncertainty regarding scientific knowledge. (This was also a key premise in Rudner’s argument.) It is because of this uncertainty that there is an inductive gap involved in every scientific claim and an “inductive risk” that accompanies every decision to make that claim—a risk that one makes an inaccurate claim or fails to make an accurate one ( Hempel 1965 ).
Second, the epistemic authority of science and of scientists in society is acknowledged. Scientists do not just do science for themselves, but also for the broader society that supports scientific research. Scientists are generally granted—and indeed we think they should be granted—a prima facie epistemic authority regarding their research areas. As the bearers of our most reliable source of knowledge, scientists should speak and (mostly) expect to be believed. They should also expect their claims about the world to serve as a further basis for decision-making. If one examines the history of science advising, scientists were brought into the heart of government decision-making increasingly throughout the twentieth century, across developed democratic countries, albeit through varied institutional mechanisms ( Douglas 2009 , ch. 2; Lentsch and Weingart 2009 , 2011 ). The rise of scientific epistemic authority is both descriptively manifested and to be normatively desired.
This baseline epistemic authority brings with it general responsibilities to be neither reckless nor negligent in one’s actions ( Douglas 2003 , 2009 , ch. 4). Although this is a responsibility every person has, scientists must consider in particular the impact of their authoritative statements and whether they think they have sufficient evidence for their claims. The inductive risk scientists bear when deciding on a claim must be confronted. To do so requires considering the context(s) in which scientific claims will likely be used and what the consequences of making an error would be (whether it is a false-positive or false-negative error). Evidential sufficiency is not set at a fixed level across all possible contexts ( Holter 2014 ; Miller 2014 a ; Wilholt 2013 ). Although good, solid evidence is generally needed to support a scientific claim (a coin toss will not do), how much evidence is enough, what constitutes sufficient evidence, will vary depending on what is at stake (see also Elliott and Resnik 2014 ).
For example, if one is testing a new treatment for a disease, the level of evidential sufficiency will vary depending on whether the disease has an alternative, mostly successful treatment or whether there is no existing treatment, as well as on how deadly or harmful the disease is. If the disease is highly fatal and there is no treatment, a lower threshold of evidential sufficiency is often warranted before we claim the treatment has enough evidence to support its implementation. If the disease is mild and/or we have successful treatments, we can legitimately demand a stricter standard of safety and efficacy before releasing it on the market. Even disciplines have different levels of sufficient evidence attached to them. High-energy physics, with theories that are far removed from practical application and thus have few impacts of withholding acceptance (low false-negative impacts), can demand very high evidential thresholds to avoid the embarrassment (and potential for wasted effort) of prematurely accepted (and later proved false) claims ( Staley 2014 ). Other areas of science, both because of the technical difficulties of achieving very high levels of statistical significance and because the costs of error are more evenly distributed, legitimately accept a lower threshold (often—but not universally—two standard deviations) for evidential sufficiency.
In addition to the flexibility in standards of evidential sufficiency found in scientific practice, the argument from inductive risk also demonstrates the importance of inductive risk at more stages in the research process than the decision of whether the evidence is sufficient to support the conclusions of a research project. Inductive risk also plays an important role earlier in the scientific process, when data must be characterized. Even here, scientists can be confronted with ambiguous events that they must decide what to do with ( Miller 2014 a ). Should they discard them (potentially lowering the power of their study)? Should they characterize them one way or another? Should they give up on the study until a more precise methodology can be found? Each of these choices poses inductive risks for the scientist, a chance that a decision could be the wrong one and thus will incur the consequences of error.
For example, Douglas (2000) describes a case of scientists attempting to characterize the changes in rat liver slides. The rats had been dosed with an important environmental contaminant (dioxin) for 2 years at various levels and then killed and autopsied. Changes to the livers, and whether the rats’ livers exhibited carcinogenic changes, were assessed visually. But some of the slides were interpreted differently by different toxicologists. ( Douglas [2000] discusses three different sets of evaluations taking place over the course of 12 years.) There were clear inductive risks to describing a liver slide as having a malignant versus a benign change or even no substantial change at all. Although one might balk at the need for interpretation of the slides and the expert disagreement regarding it, doing the study over again and culturing the liver tissues would be prohibitively expensive (costing millions of dollars). Weighing the consequences of error could help scientists decide how much evidence was enough—or how sure they needed to be—to assign any particular characterization.
The value-free ideal is thus too restrictive in excluding values from moments of inference in science. It is also too permissive in that the epistemic values the value-free ideal allows are given too much free rein. Consider, for example, the epistemic value of simplicity. Should scientists select a theory because it is simple or elegant? Only if the world actually were simple or elegant, which it only sometimes is. Other times it is complex and messy. To allow such a value to play the same role as evidence gives too much weight to such a value and moves science away from its core empiricism ( Douglas 2009 , ch. 5). Because scientists have responsibilities to be neither reckless nor negligent, and thus to consider and weigh the consequences of mistakes, and because scientists always confront inductive risk in doing studies and making claims, the value-free ideal is the wrong ideal. It is also the wrong ideal because it allows epistemic values too much ability to influence science. Taken together, these arguments seem destructive of the value-free ideal qua ideal.
Nevertheless, we cannot do without some ideal for values in science. The descriptive challenge and resultant emphasis on social structure in science are insufficient sources of alternative ideals. In addition to norms for social structures, we need norms to address the concern that values could be used in place of evidence. It would be deeply problematic for the epistemic authority of science if we were to decide that what best characterizes the rat liver slide is what we would like it to be or we were to decide that a drug is safe and effective (or not) because we would like it to be (or not). In order to understand what is or is not a good use of values in scientific reasoning, we need an alternative ideal for values in science.
4 Alternative Ideals for Values in Science
There are several contenders for a replacement ideal once the value-free ideal is set aside. As noted earlier, allowing any values to play any role whatsoever seems to open the door to wishful thinking and a complete undermining of the value of science to society. What to say in the face of these concerns has brought out a number of distinct arguments. Some of these ideals depend on rethinking the terrain of values that can influence science, particularly the nature of epistemic values.
4.1 Rethinking Epistemic Values in Science
As noted in section 3.2 , some philosophers have challenged the boundary between epistemic and nonepistemic values as being too porous to support the value-free ideal. But with the value-free ideal set aside, there has been renewed interest in a possible topography of values to articulate a new ideal.
One approach has been to redefine what is meant by epistemic and to acknowledge the context dependence of epistemic values. In his 2010 paper, Daniel Steel argues that epistemic values should be defined as those values that “promote the attainment of truth” ( Steel 2010 , 15). Some of these values Steel suggests are “intrinsic,” that is, “manifesting that value constitutes an attainment of or is necessary for truth” ( Steel 2010 , 15). Examples of such values include empirical adequacy and internal consistency. This is a rather small set of values, as others such as Laudan (2004) and Douglas (2009) (where they are called “epistemic criteria”) have noted. Extrinsic epistemic values, on the other hand, “promote the attainment of truth without themselves being indicators or requirements of truth” ( Steel 2010 , 18). Examples include simplicity, external consistency, testability, and open discourse in science. Whether such values actually promote the attainment of truth will depend on the context. For example, external consistency promotes truth only when the additional theories one is being consistent with are true. With this account of epistemic values in hand, Steel argues that nonepistemic values are allowable in science only so long as they do not “hinder or obstruct the attainment of truth” ( Steel 2010 , 25).
Douglas (2013) , on the other hand, has articulated a different terrain for the traditional epistemic values. Starting with a core set of values (similar to Steel’s intrinsic epistemic values), one can then make further distinctions among the remaining values from the Kuhnian set. For example, one can distinguish between values that are instantiated by the claims or theories on their own and values that are instantiated by a scientific claim and the evidence that supports it. Douglas (2013) notes that conflating these two different ways in which a value is instantiated in science has led to some confusion. For example, simplicity applied to and exemplified in a theory on its own is properly not epistemic, but instead at best a reflection of the fact that simplicity makes theories easier to work with ( Douglas 2009 , 107). On the other hand, simplicity instantiated by a theory in relationship to evidence (e.g., a best fit curve) is in fact a good reason to adopt a theory and is properly epistemic (i.e., indicative of truth) ( Douglas 2013 ; Forster and Sober 1994 ). With this distinction in hand, one can distinguish between epistemic values (reliable guides for inference) and cognitive values (attributes of theories that make them productive or easier to use). These complexities in the terrain for epistemic values are then reflected in replacement ideals.
4.2 Distinguishing Roles for Values in Science
Douglas (2009) both argues against the value-free ideal for science and proposes an alternative ideal to properly constrain values in science. The alternative ideal depends on a distinction between two kinds of roles for values in science: a direct role and an indirect role. In the direct role, the values serve as reasons in themselves for the choices being made and directly assess those choices. For example, when a scientist decides to pursue a particular project because she is interested in the subject, her interest in the subject is a value judgment that serves in a direct role for the decision to pursue it. She pursues a project because of her values. Similarly, when a scientist decides not to use a particular methodology because it would cause extreme suffering in the primates on which he is experimenting, his moral concern for suffering in primates is a value that serves in a direct role in his decision not to use that methodological approach. He decides not to use that method because of his values. Douglas (2009) argues that the direct role is acceptable (indeed, often laudable) in deciding which projects to pursue, in making methodological choices, and in deciding what to do with the science produced (i.e., in the “external” phases of science).
This direct role is to be distinguished from an indirect role for values in science. In the indirect role, the values help determine whether the evidence or reasons one has for one’s choice are sufficient (sufficiently strong or well supported) but do not contribute to the evidence or reasons themselves. In the indirect role, values assess whether evidence is sufficient by examining whether the uncertainty remaining is acceptable. A scientist would assess whether a level of uncertainty is acceptable by considering what the possible consequences of error would be (false positives or false negatives) by using values to weigh those consequences. In this way, the indirect role captures the concerns over inductive risk by using values to weigh the consequences of error in order to assess evidential sufficiency. The values do not contribute to the weight of evidence because that would be the values serving in a direct role. Douglas (2009) argues that in the “internal” phases of science, in evidence characterization and interpretation (i.e., when scientists decide what to make of the available evidence), (nonepistemic) values should play only an indirect role.
In short, the alternative to the value-free ideal is that (nonepistemic) values should be constrained to the indirect role when scientists are characterizing phenomena and assessing hypotheses with respect to available evidence. If values were allowed to play a direct role, the values would be reasons to accept a hypothesis or to characterize phenomena, and this would shift science away from empiricism. Most problematically, values in a direct role during evidential assessment would be equivalent to allowing wishful thinking into the heart of science. If values could play a direct role in the assessment of evidence, a preference for a particular outcome could act as a reason for that outcome or for the rejection of a disliked outcome. In the indirect role, acting to assess evidential sufficiency, values do not have such potency.
Kevin Elliott (2011 b , 2013 ) has raised a conceptual challenge for the direct versus indirect role distinction. He has argued that it is not clear what the distinction refers to, whether it is a distinction about the logical role for values or a distinction about which kinds of consequences are important: intended or unintended. Conceptually, the distinction is first and foremost about the logical role for values in science, in particular about assessing whether evidence is sufficient for a claim (or specific phenomena are sufficiently captured by a description). One relevant consideration for assessing evidential sufficiency is whether the uncertainty regarding the claim is acceptable. In assessing the acceptability of uncertainty, one considers the two directions of error, false positive or false negative, and the potential consequences of these errors. Values are used to weigh the seriousness of these consequences.
One may wonder, however, what the role is for the epistemic values (as described earlier—the genuinely epistemic values such as internal consistency, empirical adequacy, or explanatory power of a theory with respect to evidence). These values should be conceived of as part of the epistemic evaluation of science—they help us assess how strong the evidence is in relationship to the claim being made or whether the claim is even an adequate contender for our consideration ( Douglas 2009 , 2013 ). As such, they are central to the assessment of how much uncertainty we have (and less about the acceptability of such uncertainty, where inductive risk considerations arise). They have what Matthew Brown has called “lexical priority,” along with the evidence ( Brown 2013 a ). Once these values have been utilized to assess how much uncertainty we think there is, the other values (social, ethical, and cognitive ) must compete to help weigh whether the evidence (and its relationship to the theory) is enough. It is here that inductive risk is crucial, that the indirect role is central, and that a direct role is prohibited.
One can ask further, however, whether this distinction between the roles for values in science is useful in assessing what scientists do. Because the distinction between a direct role (the value serves as a reason for a choice) and an indirect role (values are used to assess the sufficiency of evidence) depends on the reasoning of the scientist, and the actual reasoning is not always transparent—even to the scientists themselves—the distinction may not be helpful in policing acceptable and unacceptable roles for values in science in practice ( Elliott 2011 b ; Hicks 2014 ; Steel and Whyte 2012 ). Daniel Steel and Kyle Powys Whyte have argued that their alternative ideal, that “non-epistemic values should not conflict with epistemic values [both intrinsic and extrinsic, i.e., any value that is truth-promoting] in the design, interpretation, or dissemination of research that is practically and ethically permissible,” does better than an ideal that is based on the roles that values play in the reasoning of scientists ( Steel and Whyte 2012 , 164). Their essay examines the methodological choices and interpretations of evidence in environmental justice research. (Unfortunately, the test is not as incisive as one could hope for because both direct and indirect roles for values are allowable for methodological choices in science—see later discussion.) But, in order to apply this ideal, we need to know which extrinsic epistemic values are in play in a given context, which means knowing which ones will promote the attainment of the truth. Depending on where the truth lies, this can change from context to context and be only apparent in hindsight, well after the dust has settled ( Elliott 2013 ).
One need not intuit, however, a scientist’s reasoning in each particular instance for the role-based ideal to be useful. (No ideal for reasoning can meet such a standard.) Instead, one can look at patterns of argumentation to see whether, for example, scientists respond in appropriate ways to new pieces of evidence ( Douglas 2006 ). If values serve in an appropriate indirect role, new evidence (particularly evidence that reduces uncertainty) should shift a scientist’s claims. If a scientist remains intransigent, either he must espouse particularly strong values (a strong concern for the impacts of a false positive, for example, with no concern for a false negative), or his values are playing an improper direct role. In either case, there are grounds for rejecting that particular scientist’s views. If we disagree with their weighing of inductive risk, we should not rely on them ( Douglas 2015 ). If they are using values in an improper role, we should not rely on them either. Finally, the purpose of an ideal is as much about guiding our own reasoning as for policing the behavior of others. We need some sense of when and how using values is appropriate in science, without the benefit of hindsight, before we know where the truth lies.
4.3 Arguing for the Right Values in Science
Another approach taken to the problem of a replacement ideal has been to argue for scientists adopting the right values. For example, in her book Philosophy of Science After Feminism , Janet Kourany argues for an ideal of socially responsible science ( Kourany 2010 ). In this ideal, science should meet jointly both epistemic and social standards. It is science instantiating the right values that is important to Kourany, whether those values influence the external or internal parts of science. As Kourany argues, the ideal of socially responsible science “maintains that sound social values as well as sound epistemic values must control every aspect of the research process” ( Kourany 2013 , 93–94). One can view Kourany’s position as requiring “the joint necessity of evidence and values” ( Brown 2013 b , 68). Getting the values right is thus central to having a responsible science. Kourany argues for more intensive discussion both within scientific specialties and with the groups affected by scientific communities for developing the codes that will guide responsible science.
Kevin Elliott (2013) has argued that rather than focus on the different roles that values can play in science, we should focus on the various goals scientists have in addition to epistemic goals and ensure those goals are both clearly articulated and that the values employed further them (see also Elliott and McKaughan 2014 ). As he says, “a particular value can appropriately influence a scientist’s reasoning in a particular context only to the extent that the value advances the goals that are prioritized in that context” ( Elliott 2013 , 381). If a scientist prefers a speedy test to an accurate test, then she should make that clear and use the faster test. Such an approach “allows non-epistemic values to count as reasons for accepting a model or hypothesis as long as they promote the goals of the assessment” ( Elliott 2013 , 381).
Daniel Hicks further argues that “philosophers of science should undertake a deeper engagement with ethics” in order to grapple with a fuller picture of the aims of science and the aims of other endeavors of which science is a part ( Hicks 2014 , 3; see also Anderson 2004 ). Hicks thinks we should analyze whether certain values, particularly nonepistemic values, should have priority in a particular context based on what the constitutive goals are for that context. Thus, in analyzing the machinations of the pharmaceutical industry, Hicks suggests that placing profit motive over good science only appears to make sense for the industry but is, in fact, self-undermining because the ultimate aim of the industry is not profit but good health ( Hicks 2014 ). Distorting science is wrong not because of the harm it does to science or our epistemic enterprise, but because doing so subverts the correct order of values, placing profits over health. It is this sort of analysis that Hicks thinks we can use to assess whether the influence of values is legitimate or not in science—analyzing whether we have the right values prioritized.
Matthew Brown has argued for a return to a more pragmatic image of inquiry (drawn from John Dewey), one that considers the incorporation of values in science a necessary and ongoing feature ( Brown 2012 ). Brown further urges us to be wary of accepting the “lexical priority of evidence,” both because evidence may in fact be suspect and because value judgments are often the result of careful inquiry and reflection, even grounded in empirical information, rather than mere subjective preferences ( Anderson 2004 ; Brown 2013 a ). Brown urges that we coordinate evidence, values, and theory in our inquiries and that all must fit together well in a way that “resolves the problem that spurred the inquiry” at the start of the process ( Brown 2013 a , 837). Success is defined as resolving the problem that spurred the inquiry, and it assures us that the parts of inquiry have ultimately worked properly.
One might wonder whether these approaches undermine the particular empirical character of science by suggesting that values can play an even broader array of roles in scientific inference as long as they are the right values. For example, even with the requirement of joint satisfaction of epistemic and ethical considerations for theory acceptance, we can be faced with situations where the evidence strongly supports a theory we find unwelcome because of our value commitments. Should we reject such a theory solely because it does not live up to our value judgments (even though the epistemic reasons for it are strong)? Whether such approaches can avoid the problems of wishful thinking (or excessively motivated reasoning) remains to be seen. Regardless, if one examines the broader set of contexts in which values influence science, getting the values right proves to be paramount.
5 More Locations for Values in Science
Thus far, we have discussed the value-free ideal, its challengers, and contenders for replacements, including further examinations of the nature of epistemic and cognitive values. This debate has focused primarily on the assessment of evidence and the crucial moments of inference in science. But values play a role in science at far more than these “internal” aspects of science. Values also help shape the direction of research, the methodologies pursued, and the ways in which research is disseminated and applied. Whether or not one judges the challenges to the value-free ideal as successful, the debate about values in inference only scratches the surface of the full range of ways in which values influence scientific practice. And this broader array has proved to crucially influence what evidence we have to examine and which theories or hypotheses get pursued. Regardless of the proper place of values in inference, the broader array of locations of values in science deeply shapes what we claim to know ( Okruhlik 1994 ). Depending on what research we do and how we collect evidence, our inferences will be substantially altered. Considering this broader array raises an interesting set of challenges for philosophers of science. Restricting roles for values across this array is inappropriate—values play both direct and indirect roles legitimately in the locations discussed herein. Whether the emphases on diversity arising from the social epistemic approaches to science are sufficient remains an open question.
5.1 Values in Research Direction
Values deeply shape which projects scientists pursue. From societal decisions of which projects to fund, to corporate decisions for which projects to pursue (and how to pursue them), to individual decisions for where to place effort, the evidence we eventually have to consider depends on scientists’, institutional, and societal values. The decisions here can be fraught. For example, decisions on whether (and under what conditions) to pursue potentially dangerous research must weigh the potential epistemic and societal benefits of pursuit against the potential societal risks of pursuit. (Such research is often called “dual-use” but sometimes the danger has little to do with intentional uses.) In examples such as “gain-of-function” research on pathogenic viruses, such weightings involve fraught and highly charged value-based debates ( Casadevall, Howard, and Imperiale 2014 ; Douglas 2014 a , 975–977; Evans 2014 ; Lipsitch 2014 ).
Additional examples of the importance of values (and institutional structures instantiating values) in shaping scientific research agendas are found in the work of Hugh Lacey and Philip Kitcher, among others. Although a staunch proponent of the value-free ideal for scientific inference (for whether to accept or reject scientific theories), Lacey has argued for greater attention to which research projects are taken up, noting that what looks like a good project can be deeply shaped by (potentially problematic and one-sided) social values ( Lacey 1999 , 2005 ). He has focused in particular on agricultural research, comparing the intensive efforts to create mass-marketed genetically modified organisms (GMOs) with more locally based agro-ecology research and finds the imbalance of efforts problematic. Kitcher has elucidated tensions regarding whether some research should be pursued at all, questioning whether democratic societies should support research that seeks to empirically undermine presumptions of shared equality central to democratic systems ( Kitcher 2001 ). These questions of which knowledge is worth pursuing (as evaluated from within liberal democracies) remain pressing.
5.2 Values and Methodological Choices
In addition to which projects to pursue, among the most important decisions scientists make concern the methodologies they use. These can be general questions, such as whether to utilize human subjects in a controlled setting or whether to observe humans and attempt to find a naturally occurring control cohort. These can be very specific, detailed questions, such as whether to utilize a particular source of information (e.g., death certificates versus medical records, or census tracts versus zip codes; see Steel and Whyte 2012 ) as being sufficiently appropriate or reliable for a study. At both the specific and general levels of questions, values play a vital and potent role.
The importance of values in methodological choices can be readily seen in the ethical prohibitions we have for some options ( Douglas 2014 b ). Despite the knowledge we might gain, we rightly do not think it acceptable to round people up involuntarily and submit them to various experimental conditions. Regardless of whether we think the conditions might harm or help the people subjected to them, to do so without their informed consent is deeply unethical. This reflects a strongly held (and well-justified) concern for human autonomy that the value of knowledge does not trump. Beyond human subject research, ethical considerations have the potential to restrict other areas of research as well, arising, for example, from ethical concern over inflicting unnecessary suffering on animals. What counts as an acceptable or necessary level of suffering is contentious.
The ability of social and ethical values (such as the concern over human autonomy or animal suffering) to restrict the avenues of research open to scientists speaks to the potency of values in methodological choices. Values can legitimately play a powerful and direct role in deciding which research projects can and cannot be pursued. But, at the same time, we do not want social and ethical values to determine the epistemic outcomes of research. We know there are techniques scientists can employ to “game” the research process, skewing results in a particular favor. How should the pervasive, potent, and potentially problematic influence of values here be managed?
This is not merely an academic question. As detailed by Michaels (2008) and McGarity and Wagner (2008) , scientists have perfected ways of structuring research projects so that they appear to be an open-ended process of inquiry to the casual observer but are, in fact, set up to generate a particular result. Torsten Wilholt, for example, describes a case where researchers were ostensibly testing for the estrogenic potential of particular synthetic substances (due to concern over hormone disruption) but ensured a negative outcome to the study by testing the substances on a mouse strain known for estrogen insensitivity ( Wilholt 2009 ). Other ways of ensuring particular outcomes include using an insufficient exposure or dosage level so that no difference between the dosed and control group appears, doing multiple trials and publishing only the successful ones (an approach trial registries are attempting to thwart), and using inappropriate testing conditions so that results are irrelevant to actual practice ( Wickson 2014 ).
So, although values can legitimately restrict the methodological choices of scientists, we don’t want such values to shape research so that genuine tests are avoided. For this decision context, discussing roles for values is not helpful. What is needed is a clear examination of the values employed and whether they are the right ones.
If researchers choose to use a noncoercive methodology when working with human subjects for ethical reasons, we should applaud them not because of the role the values are (or are not) playing, but because the researchers are displaying or using the right values. And if researchers deliberately select a research method so that particular desired results are produced (i.e., the results are practically guaranteed by the methods chosen so no genuine test is possible), we should excoriate them not for the role values are playing but for the values on display; in this case, an abuse of the authority of science while giving short shrift to the value of genuine discovery, for whatever other interest or gain being sought.
Yet there is still difficult conceptual terrain to be explored. As Steel and Whyte (2012) note, it is acceptable for ethical and moral considerations to trump epistemic ones in shaping methodological choices. How extensive are these considerations? How are we to weigh, in cases where the values are contested—for example, in potentially dangerous research (because the methods are risky to humans or to the environment) or in cases where animal suffering must be inflicted in order to pursue a particular research program—which is more important, the value of the knowledge to be gained or the ethical issues at stake? Values in methodological issues force us to confront these difficult problems.
5.3 Values Everywhere Else
Values in inference, in decisions about which projects to pursue, and in decisions about which methodologies to employ do not exhaust the places where values influence science. Consider these additional locations:
The language we choose to use can embed values in science. Sometimes these choices hinder or hamper the ability of scientists to do good work (as in the case of using the term “rape” to describe duck behavior, Dupré 2007 ), and sometimes the value-inflected language choice can make research epistemically and socially stronger (as in the decision to call a collection of behaviors “spousal abuse,” Root 2007 ). This issue does not belong to social science in particular; natural science domains can also have value-inflected language debates ( Elliott 2011 a ). An analysis of how values embedded in language, or in the “thick concepts” that are often central topics of investigation, is needed, including the ideals that should guide their use. ( Alexandrova 2014 ).
Values can play an important role in the construction and testing of models. As Biddle and Winsberg (2010) note, models, especially models of great complexity, often have a particular historical development that reflects certain priorities (e.g., scientists want to reflect temperature patterns more than precipitation patterns), which then become embedded in how the models work. These priorities are value judgments, and they are value judgments that subsequently affect how different modules in the models combine and how we estimate uncertainties regarding the models. Winsberg (2012) further argues that we cannot unpack all the value judgments embedded in models, nor can we capture them all in uncertainty estimates, nor can we expect scientists to be fully aware of what they are doing. What this means for the use of models—particularly complex computer models—in scientific assessments of public import remains unclear, except that more work needs to be done ( Parker 2014 ).
Values are crucial in deciding how to use and disseminate science. Even if potentially dangerous research is pursued, debates about whether to disseminate the knowledge gleaned are as fraught with value judgments as decisions about whether to pursue that research. Despite a baseline Mertonian norm of openness, some research should potentially be kept confidential ( Douglas 2014 a ). This can be true because of a desire to avoid weapons proliferation or because of a desire to protect the privacy of individuals involved in the research.
6 Implications for Science in Society
What is perhaps most interesting about the wide-ranging discussion concerning values in science is how important the conceptualization of science and society is for what one thinks the norms should be. The normative challenge to the value-free ideal—that what counts as sufficient evidence could vary by context and the implications of error—is perfectly rational. Indeed, it has been noted by epistemologists such as John Heil (1983) and the burgeoning interest in pragmatic encroachment among epistemologists ( Miller 2014 b ). If one is to object to a role for values in science or to the presence of particular values in science, one has to do so on the grounds that the role of science in society makes the presence of values problematic (e.g., Betz 2013 ). And this is where the debate is properly joined. Given the rationality of and need for values in science, how should we conceive of the relationship between science and society? Does the importance of science in society place additional constraints or requirements on the presence of values in science?
The inductive risk argument gains its traction because of the view of the responsibilities of scientists articulated in Douglas (2003 , 2009 ). Is this the right view? More specifically, do particular institutional contexts in which scientists operate (e.g., within advising structures, academia, private industry, government science) alter the nature of the role responsibilities scientists have, even in ways that alter their general responsibilities? If institutional demands clash with general moral responsibilities, what should scientists do?
The relationship between science and democratic societies also needs further exploration (following on the work of Brown [2009] and Kitcher [2001 , 2011] ). Should scientists’ judgments be free of social and ethical values to preserve democratic ideals (as Betz [2013] has argued), or should they be value explicit ( Douglas 2009 ; Elliott and Resnik 2014 ) or embedded in value-laden collaborations to preserve democratic ideals ( Douglas 2005 ; Elliott 2011 a )? Stephen John has argued that having varying standards for scientists’ assertions depending on the expected audience is an unworkable approach ( John 2015 ) and has argued instead for uniform high evidential standards for the public assertions of scientists. Is this the right standard, or should scientists’ assertions be more nuanced, carrying with them clear expressions of why scientists think the evidence is enough, so that audience members can interpret them properly? Would such an approach be more workable than the complex institutional structures John proposes (e.g., distinguishing between public and private assertions, generating different norms for each, and structuring institutions accordingly)? Furthermore, what is the correct basis for trust in science by the broader society, and what role should values play in science given that basis ( Elliott 2006 ; Wilholt 2013 )?
In short, what the values in science debate forces us to confront is the need to theorize—in a grounded way by assessing actual science policy interfaces, actual practices of science communication, and actual research decision contexts—what the proper role is of science in society. It is exactly where philosophy of science should be.
Acknowledgments
My thanks to Ty Branch, Matt Brown, Kevin Elliott, Dan Hicks, Paul Humphreys, and Ted Richards for their invaluable advice and assistance with this paper. Deficiencies remaining are mine alone.
Alexandrova, A. ( 2014 ). “ Well-being and Philosophy of Science. ” Philosophy Compass 10(3), 1–13.
Google Scholar
Anderson, E. ( 2004 ). “ Uses of Value Judgments in science: A General Argument, with Lessons from a Case Study of Feminist Research on Divorce. ” Hypatia 19(1), 1–24.
Betz, G. ( 2013 ). “ In Defence of the Value Free Ideal. ” European Journal for Philosophy of Science 3(2), 207–220.
Biddle, J. ( 2013 ). “ State of the Field: Transient Underdetermination and Values in Science. ” Studies in History and Philosophy of Science Part A 44(1), 124–133.
Biddle, J. , and Winsberg, E. ( 2010 ). “Value Judgements and the Estimation of Uncertainty in Climate Modeling.” In P. D. Magnus and J. Busch (eds.), New waves in philosophy of science (New York: Palgrave Macmillan), 172–197.
Google Preview
Brown, M. B. ( 2009 ). Science in Democracy: Expertise, Institutions, and Representation (Cambridge, MA: MIT Press).
Brown, M. J. ( 2012 ). “ John Dewey’s Logic of Science. ” HOPOS: The Journal of the International Society for the History of Philosophy of Science 2(2), 258–306.
Brown, M. J. ( 2013 a ). “ Values in Science Beyond Underdetermination and Inductive Risk. ” Philosophy of Science 80(5), 829–839.
Brown, M. J. ( 2013 b ). “ The Source and Status of Values for Socially Responsible Science. ” Philosophical Studies 163(1), 67–76.
Casadevall, A. , Howard, D. , and Imperiale, M. J. ( 2014 ). “ An Epistemological Perspective on the Value of Gain-of-Function Experiments Involving Pathogens With Pandemic Potential. ” mBio 5(5), e01875–e01914.
Churchman, C. W. ( 1948 ). “ Statistics, Pragmatics, Induction. ” Philosophy of Science 15(3), 249–268.
Douglas, H. ( 2000 ). “ Inductive Risk and Values in Science. ” Philosophy of Science 67(4), 559–579.
Douglas, H. ( 2003 ). “ The Moral Responsibilities of Scientists (Tensions Between Autonomy and Responsibility).” American Philosophical Quarterly 40(1), 59–68.
Douglas, H. ( 2005 ). “Inserting the Public into Science.” In S. Massen and P. Weingart (eds.), Democratization of Expertise? Exploring Novel Forms of Scientific Advice in Political Decision-Making (Dordrecht: Springer), 153–169.
Douglas, H. ( 2006 ). “Bullshit at the Interface of Science and Policy: Global Warming, Toxic Substances and Other Pesky Problems.” In G. L. Hardcastle and G. A. Reisch (eds.), Bullshit and Philosophy (Chicago: Open Court Press), 215–228.
Douglas, H. ( 2009 ). Science, Policy, and the Value-Free Ideal (Pittsburgh, PA: University of Pittsburgh Press).
Douglas, H. ( 2011 ). “Facts, Values, and Objectivity.” In I. C. Jarvie and J. Zamora-Bonilla (eds.), The Sage Handbook of the Philosophy of Social Science (London: Sage Publications), 513–529.
Douglas, H. ( 2013 ). “ The Value of Cognitive Values. ” Philosophy of Science 80(5), 796–806.
Douglas, H. ( 2014 a ). “ The Moral Terrain of Science. ” Erkenntnis 79(5), 961–979.
Douglas, H. ( 2014 b ). “Values in Social Science.” In N. Cartwright and E. Montuschi (eds.), Philosophy of Social Science: A New Introduction (Oxford: Oxford University Press), 162–182.
Douglas, H. ( 2015 ). “ Politics and Science Untangling Values, Ideologies, and Reasons. ” The Annals of the American Academy of Political and Social Science 658(1), 296–306.
Dupré, J. ( 2007 ). “Fact and Value.” In H. Kincaid , J. Dupré , and A. Wylie (eds.), Value-Free Science?: Ideals and Illusions (Oxford: Oxford University Press), 27–41.
Elliott, K. C. ( 2006 ). “ An Ethics of Expertise Based on Informed Consent. ” Science and Engineering Ethics 12(4), 637–661.
Elliott, K.C. ( 2011 a ). Is a Little Pollution Good for You? Incorporating Societal Values in Environmental Research (New York: Oxford University Press).
Elliott, K. C. ( 2011 b ). “ Direct and Indirect Roles for Values in Science. ” Philosophy of Science 78(2), 303–324.
Elliott, K. C. ( 2013 ). “ Douglas on Values: From Indirect Roles to Multiple Goals. ” Studies in History and Philosophy of Science Part A 44(3), 375–383.
Elliott, K. C. , and McKaughan, D. J. ( 2014 ). “ Nonepistemic Values and the Multiple Goals of Science. ” Philosophy of Science 81(1), 1–21.
Elliott, K. C. , and Resnik, D. B. ( 2014 ). “ Science, Policy, and the Transparency of Values. ” Environmental Health Perspectives 122(7), 647–650.
Evans, N. G. ( 2014 ). “ Valuing Knowledge: A Reply to the Epistemological Perspective on the Value of Gain-of-Function Experiments. ” mBio 5(5), e01993-14.
Fausto-Sterling, A. ( 1985 ). Myths of Gender: Biological Theories About Women and Men (New York: Basic Books).
Forster, M. , and Sober, E. ( 1994 ). “ How to Tell When Simpler, More Unified, or Less Ad Hoc Theories Will Provide More Accurate Predictions. ” British Journal for the Philosophy of Science 45(1), 1–35.
Haraway, D. J. ( 1989 ). Primate Visions: Gender, Race, and Nature in the World of Modern Science (New York/London: Routledge).
Harding, S. G. ( 1986 ). The Science Question in Feminism (Ithaca, NY: Cornell University Press).
Harding, S. G. ( 1991 ). Whose Science? Whose Knowledge? (Ithaca, NY: Cornell University Press).
Heil, J. ( 1983 ). “ Believing What One Ought. ” Journal of Philosophy 80(11), 752–765.
Hempel, C. G. ( 1965 ). “Science and Human Values.” In C.G. Hempel (ed.), Aspects of Scientific Explanation (New York: Free Press), 81–96.
Hicks, D. J. ( 2014 ). “ A New Direction for Science and Values. ” Synthese 191(14), 3271–3295.
Holter, B. (2014). “The Epistemic Significance of Values in Science.” University of Calgary: http://hdl.handle.net/11023/1317
Intemann, K. ( 2005 ). “ Feminism, Underdetermination, and Values in Science. ” Philosophy of Science 72(5), 1001–1012.
Intemann, K. ( 2009 ). “ Why Diversity Matters: Understanding and Applying the Diversity Component of the National Science Foundation’s Broader Impacts Criterion. ” Social Epistemology 23(3–4), 249–266.
Jeffrey, R. C. ( 1956 ). “ Valuation and Acceptance of Scientific Hypotheses. ” Philosophy of Science 23(3), 237–246.
John, S. ( 2015 ). “ Inductive Risk and the Contexts of Communication. ” Synthese 192(1), 79–96.
Keller, E. F. , and Longino, H. E. (eds.). ( 1996 ). Feminism and Science (Oxford: Oxford University Press).
Kitcher, P. ( 2001 ). Science, Truth, and Democracy (Oxford: Oxford University Press).
Kitcher, P. ( 2011 ). Science in a Democratic Society (Amherst, NY: Prometheus Books).
Kourany, J. ( 2010 ). Philosophy of Science After Feminism (Oxford: Oxford University Press).
Kourany, J. A. ( 2013 ). “ Meeting the Challenges to Socially Responsible Science: Reply to Brown, Lacey, and Potter. ” Philosophical Studies 163(1), 93–103.
Kuhn, T. S. ( 1977 ). “Objectivity, Value Judgment, and Theory Choice.” In T. Kuhn (ed.), The Essential Tension (Chicago: University of Chicago Press), 320–339.
Lacey, H. ( 1999 ). Is Science Value Free?: Values and Scientific Understanding (New York: Routledge).
Lacey, H. ( 2005 ). Values and Objectivity in Science: The Current Controversy About Transgenic Crops (Lanham, MD: Rowman and Littlefield).
Laudan, L. ( 1984 ). Science and Values: The Aims of Science and their Role in Scientific Debate (Oakland: University of California Press).
Laudan, L. ( 2004 ). “The Epistemic, the Cognitive, and the Social.” In P. Machamer and G. Wolters (eds.) Science, Values, and Objectivity (Pittsburgh, PA: University of Pittsburgh Press), 14–23.
Lentsch, J. , and Weingart, P. (eds.). ( 2009 ). Scientific Advice to Policy Making: International Comparison (Farmington Hills, MI: Barbara Budrich Publishers).
Lentsch, J. , and Weingart, P. (eds.). ( 2011 ). The Politics of Scientific Advice: Institutional Design for Quality Assurance (Cambridge: Cambridge University Press).
Levi, I. ( 1960 ). “ Must the Scientist Make Value Judgements? ” Journal of Philosophy 57(11), 345–357.
Lipsitch, M. ( 2014 ). “ Can Limited Scientific Value of Potential Pandemic Pathogen Experiments Justify the Risks? ” mBio 5(5), e02008-14.
Longino, H. E. ( 1990 ). Science as Social Knowledge: Values and Objectivity in Scientific Inquiry (Princeton, NJ: Princeton University Press).
Longino, H. E. ( 1995 ). “ Gender, Politics, and the Theoretical Virtues. ” Synthese 104(3), 383–397.
Longino, H. E. ( 1996 ). “Cognitive and Non-Cognitive Values in Science: Rethinking the Dichotomy.” In L. H. Nelson and H. Nelson (eds.), Feminism, Science, and the Philosophy of Science (Dordrecht: Kluwer), 39–58.
Longino, H. E. ( 2002 ). The Fate of Knowledge (Princeton, NJ: Princeton University Press).
Magnus, P. D. ( 2013 ). “ What Scientists Know Is Not a Function of What Scientists Know. ” Philosophy of Science 80(5), 840–849.
Martin, E. ( 1996 ). “The Egg and the Sperm: How Science Has Constructed a Romance Based on Stereotypical Male-Female Roles.” in E. F. Keller and H. Longino (eds.), Feminism and Science (Oxford: Oxford University Press), 103–117.
McGarity, T. O. , and Wagner, W. E. ( 2008 ). Bending Science: How Special Interests Corrupt Public Health Research (Cambridge, MA: Harvard University Press).
McMullin, E. ( 1983 ). “Values in Science.” In P. D. Asquith and T. Nickles (eds.), PSA: Proceedings of the Biennial Meeting of the 1982 Philosophy of Science Association vol. 1 (East Lansing, MI: Philosophy of Science Association), 3–28.
Michaels, D. ( 2008 ). Doubt Is Their Product: How Industry’s Assault on Science Threatens Your Health (Oxford: Oxford University Press).
Miller, B. ( 2014 a ). “ Catching the WAVE: The Weight-Adjusting Account of Values and Evidence. ” Studies in History and Philosophy of Science Part A 47, 69–80.
Miller, B. ( 2014 b ). “ Science, Values, and Pragmatic Encroachment on Knowledge. ” European Journal for Philosophy of Science 4(2), 253–270.
Nelson, L. H. ( 1990 ). Who Knows: From Quine to a Feminist Empiricism (Philadelphia, PA: Temple University Press).
Okruhlik, K. ( 1994 ). “ Gender and the Biological Sciences. ” Canadian Journal of Philosophy 24(supp1), 21–42.
Parker, W. ( 2014 ). “ Values and Uncertainties in Climate Prediction, Revisited. ” Studies in History and Philosophy of Science Part A 46, 24–30.
Proctor, R. ( 1991 ). Value-free Science?: Purity and Power in Modern Knowledge (Cambridge, MA: Harvard University Press).
Rooney, P. ( 1992 ). “On Values in Science: Is the Epistemic/Non-Epistemic Distinction Useful?” In K. Okruhlik , D. Hull and M. Forbes (eds.), PSA: Proceedings of the Biennial Meeting of the Philosophy of Science Association, Volume One (Chicago: Philosophy of Science Association), 13–22.
Root, M. ( 2007 ). “Social Problems.” In H. Kincaid , J. Dupré , and A. Wylie (eds.), Value-Free Science?: Ideals and Illusions (New York: Oxford University Press), 42–57.
Rudner, R. ( 1953 ). “The Scientist Qua Scientist Makes Value Judgments.” Philosophy of Science 20(1), 1–6.
Staley, K. (2014). “Inductive Risk and the Higgs Boson,” presented at Philosophy of Science Association Meetings, November 2014, Chicago Illinois.
Steel, D. ( 2010 ). “ Epistemic Values and the Argument from Inductive Risk. ” Philosophy of Science 77(1), 14–34.
Steel, D. , and Whyte, K. P. ( 2012 ). “ Environmental Justice, Values, and Scientific Expertise. ” Kennedy Institute of Ethics Journal 22(2), 163–182.
Solomon, M. ( 2001 ). Social Empiricism (Cambridge, MA: MIT Press).
Wickson, F. (2014). “Good Science, Bad Researchers and Ugly Politics: Experimenting with a Norwegian Model,” presented at the American Association for the Advancement of Science Annual Meeting, Feburary 2015, Chicago Illinois.
Wilholt, T. ( 2009 ). “ Bias and Values in Scientific Research. ” Studies in History and Philosophy of Science Part A 40(1), 92–101.
Wilholt, T. ( 2013 ). “ Epistemic Trust in Science. ” British Journal for the Philosophy of Science 64(2), 233–253.
Winsberg, E. ( 2012 ). “ Values and Uncertainties in the Predictions of Global Climate Models. ” Kennedy Institute of Ethics Journal 22(2), 111–137.
- About Oxford Academic
- Publish journals with us
- University press partners
- What we publish
- New features
- Open access
- Institutional account management
- Rights and permissions
- Get help with access
- Accessibility
- Advertising
- Media enquiries
- Oxford University Press
- Oxford Languages
- University of Oxford
Oxford University Press is a department of the University of Oxford. It furthers the University's objective of excellence in research, scholarship, and education by publishing worldwide
- Copyright © 2024 Oxford University Press
- Cookie settings
- Cookie policy
- Privacy policy
- Legal notice
This Feature Is Available To Subscribers Only
Sign In or Create an Account
This PDF is available to Subscribers Only
For full access to this pdf, sign in to an existing account, or purchase an annual subscription.

An official website of the United States government
The .gov means it's official. Federal government websites often end in .gov or .mil. Before sharing sensitive information, make sure you're on a federal government site.
The site is secure. The https:// ensures that you are connecting to the official website and that any information you provide is encrypted and transmitted securely.
- Publications
- Account settings
- Browse Titles
NCBI Bookshelf. A service of the National Library of Medicine, National Institutes of Health.
National Academy of Sciences (US), National Academy of Engineering (US), and Institute of Medicine (US) Committee on Science, Engineering, and Public Policy. On Being a Scientist: Responsible Conduct in Research. Washington (DC): National Academies Press (US); 1995.
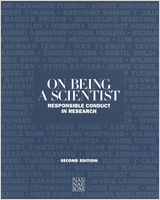
On Being a Scientist: Responsible Conduct in Research.
- Hardcopy Version at National Academies Press
Values in Science
Scientists bring more than just a toolbox of techniques to their work. Scientist must also make complex decisions about the interpretation of data, about which problems to pursue, and about when to conclude an experiment. They have to decide the best ways to work with others and exchange information. Taken together, these matters of judgment contribute greatly to the craft of science, and the character of a person's individual decisions helps determine that person's scientific style (as well as, on occasion, the impact of that person's work).
Much of the knowledge and skill needed to make good decisions in science is learned through personal experience and interactions with other scientists. But some of this ability is hard to teach or even describe. Many of the intangible influences on scientific discovery—curiosity, intuition, creativity—largely defy rational analysis, yet they are among the tools that scientists bring to their work.
When judgment is recognized as a scientific tool, it is easier to see how science can be influenced by values. Consider, for example, the way people judge between competing hypotheses. In a given area of science, several different explanations may account for the available facts equally well, with each suggesting an alternate route for further research. How do researchers pick among them?
Scientists and philosophers have proposed several criteria by which promising scientific hypotheses can be distinguished from less fruitful ones. Hypotheses should be internally consistent so that they do not generate contradictory conclusions. Their ability to provide accurate experimental predictions, sometimes in areas far removed from the original domain of the hypothesis, is viewed with great favor. With disciplines in which experimentation is less straightforward, such as geology, astronomy, or many of the social sciences, good hypotheses should be able to unify disparate observations. Also highly prized are simplicity and its more refined cousin, elegance.
Other kinds of values also come into play in science. Historians, sociologists, and other students of science have shown that social and personal beliefs—including philosophical, thematic, religious, cultural, political, and economic beliefs—can shape scientific judgment in fundamental ways. For example, Einstein's rejection of quantum mechanics as an irreducible description of nature—summarized in his insistence that "God does not play dice"—seems to have been based largely on an aesthetic conviction that the physical universe could not contain such an inherent component of randomness. The nineteenth-century geologist Charles Lyell, who championed the idea that geological change occurs incrementally rather than catastrophically, may have been influenced as much by his religious views as by his geological observations. He favored the notion of a God who is an unmoved mover and does not intervene in His creation. Such a God, thought Lyell, would produce a world in which the same causes and effects keep cycling eternally, producing a uniform geological history.
Does holding such values harm a person's science? In some cases the answer has to be "yes." The history of science offers a number of episodes in which social or personal beliefs distorted the work of researchers. The field of eugenics used the techniques of science to try to demonstrate the inferiority of certain races. The ideological rejection of Mendelian genetics in the Soviet Union beginning in the 1930s crippled Soviet biology for decades.
Despite such cautionary episodes, it is clear that values cannot—and should not—be separated from science. The desire to do good work is a human value. So is the conviction that standards of honesty and objectivity need to be maintained. The belief that the universe is simple and coherent has led to great advances in science. If researchers did not believe that the world can be described in terms of a relatively small number of fundamental principles, science would amount to no more than organized observation. Religious convictions about the nature of the universe have also led to important scientific insights, as in the case of Lyell discussed above.
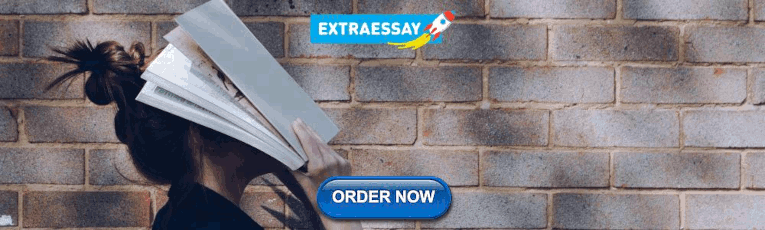
POLYWATER AND THE ROLE OF SKEPTICISM
The case of polywater demonstrates how the desire to believe in a new phenomenon can sometimes overpower the demand for solid, well-controlled evidence. In 1966 the Soviet scientist Boris Valdimirovich Derjaguin lectured in England on a new form of water that he claimed had been discovered by another Soviet scientist, N. N. Fedyakin. Formed by heating water and letting it condense in quartz capillaries, this "anomalous water," as it was originally called, had a density higher than normal water, a viscosity 15 times that of normal water, a boiling point higher than 100 degrees Centigrade, and a freezing point lower than zero degrees.
Over the next several years, hundreds of papers appeared in the scientific literature describing the properties of what soon came to be known as polywater. Theorists developed models, supported by some experimental measurements, in which strong hydrogen bonds were causing water to polymerize. Some even warned that if polywater escaped from the laboratory, it could autocatalytically polymerize all of the world's water.
Then the case for polywater began to crumble. Because polywater could only be formed in minuscule capillaries, very little was available for analysis. When small samples were analyzed, polywater proved to be contaminated with a variety of other substances, from silicon to phospholipids. Electron microscopy revealed that polywater actually consisted of finely divided particulate matter suspended in ordinary water.
Gradually, the scientists who had described the properties of polywater admitted that it did not exist. They had been misled by poorly controlled experiments and problems with experimental procedures. As the problems were resolved and experiments gained better controls, evidence for the existence of polywater disappeared.
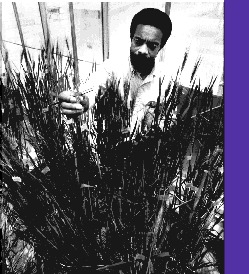
The empirical link between scientific knowledge and the physical, biological, and social world constrains the influence of values in science. Researchers are continually testing their theories about the world against observations. If hypotheses do not accord with observations, they will eventually fall from favor (though scientists may hold on to a hypothesis even in the face of some conflicting evidence since sometimes it is the evidence rather than the hypothesis that is mistaken).
The social mechanisms of science also help eliminate distorting effects that personal values might have. They subject scientific claims to the process of collective validation, applying different perspectives to the same body of observations and hypotheses.
The challenge for individual scientists is to acknowledge and try to understand the suppositions and beliefs that lie behind their own work so that they can use that self-knowledge to advance their work. Such self-examination can be informed by study in many areas outside of science, including history, philosophy, sociology, literature, art, religion, and ethics. If narrow specialization and a single-minded focus on a single activity keep a researcher from developing the perspective and fine sense of discrimination needed to apply values in science, that person's work can suffer.
- Cite this Page National Academy of Sciences (US), National Academy of Engineering (US), and Institute of Medicine (US) Committee on Science, Engineering, and Public Policy. On Being a Scientist: Responsible Conduct in Research. Washington (DC): National Academies Press (US); 1995. Values in Science.
- PDF version of this title (831K)
Recent Activity
- Values in Science - On Being a Scientist Values in Science - On Being a Scientist
Your browsing activity is empty.
Activity recording is turned off.
Turn recording back on
Connect with NLM
National Library of Medicine 8600 Rockville Pike Bethesda, MD 20894
Web Policies FOIA HHS Vulnerability Disclosure
Help Accessibility Careers

Science Literacy: Concepts, Contexts, and Consequences (2016)
Chapter: summary.
Science is a way of knowing about the world. At once a process, a product, and an institution, science enables people to both engage in the construction of new knowledge as well as use information to achieve desired ends. Access to science—whether using knowledge or creating it—necessitates some level of familiarity with the enterprise and practice of science: we refer to this as science literacy .
Science literacy is desirable not only for individuals, but also for the health and well-being of communities and society. More than just basic knowledge of science facts, contemporary definitions of science literacy have expanded to include understandings of scientific processes and practices, familiarity with how science and scientists work, a capacity to weigh and evaluate the products of science, and an ability to engage in civic decisions about the value of science. Although science literacy has traditionally been seen as the responsibility of individuals, individuals are nested within communities that are nested within societies—and, as a result, individual science literacy is limited or enhanced by the circumstances of that nesting.
In response to a request from the National Institutes of Health (NIH), the National Academies of Sciences, Engineering, and Medicine established an ad hoc committee to study the role of science literacy in public support of science. The study committee, composed of 12 experts across an array of research areas, was tasked with considering existing data about science literacy and health literacy and research on the association of science literacy with public support of science, health literacy, and behaviors related to health. The committee was asked to synthesize the available research literature on science literacy, make
recommendations on the need to improve the understanding of science and scientific research in the United States, and consider the relationship between science literacy and support for and use of science and research. In addition, the statement of task guiding this study asked the following questions:
- What is the consensus on metrics for science literacy in the United States?
- What is the evidence on how those measures have changed over time?
- How does this compare to other nations?
- Support for, attitudes on, and perception of scientific research?
- Use of scientific knowledge?
- Perception of U.S. international standing in science?
- Health literacy?
- Behaviors related to health?
- Is lack of science literacy associated with decreased support for science and/or research?
DEFINING AND MEASURING SCIENCE AND HEALTH LITERACY
Science literacy is often construed as knowing the basic facts established by science, but the concept entails much more. We identified three aspects of science literacy common to most applications of the term: content knowledge, understanding of scientific practices, and understanding of science as a social process. We also identified four additional aspects of science literacy that, while less common, provide some insight into how the term has been used: foundational literacy, epistemic knowledge, identifying and judging scientific expertise, and dispositions and habits of mind. Given this range of aspects, it is not surprising that there is no clear consensus about which aspects of science literacy are most salient or important. Different aspects may be more or less important depending on the context.
CONCLUSION 1 The committee identified many aspects of science literacy, each of which operates differently in different contexts. These aspects include (but may not be limited to): (1) the understanding of scientific practices (e.g., formulation and testing of hypotheses, probability/risk, causation versus correlation); (2) content knowledge (e.g., knowledge of basic facts, concepts, and vocabulary); and (3) understanding of science as a social process (e.g., the criteria for the assignment of expertise, the role of peer review, the accumulation of accepted findings, the existence of venues for discussion and critique, and the nature of funding and conflicts of interest).
Though science literacy has been defined in many ways, the aspects highlighted above are some of the most common ideas emerging in the literature, and they represent what some scholars expect would be useful or valuable for individuals using science in their lives, interacting with science information, and making decisions related to science. When considering why science literacy itself would be valuable, some scholars emphasize a personal rationale, defining the term in the context of how science knowledge and knowledge of science can be beneficial to people in their daily lives. Indicators developed to measure science literacy have focused on creating a marker for science knowledge and differentiating between individuals’ capabilities.
CONCLUSION 2 Historically, the predominant conception of science literacy has focused on individual competence.
We identify foundational literacy as one aspect of the definition of science literacy. For the purposes of this report, the committee includes numeracy as part of foundational literacy. As such, foundational literacy encompasses the skills and capacities necessary to process and be fluent in the use of words, language, numbers, and mathematics. Domain literacies, like science literacy and health literacy, emerge when a particular set of knowledge or competencies become socially important. The committee recognizes that all domain literacies depend on foundational literacy but may also encompass other skills and knowledge.
CONCLUSION 3 Foundational literacy (the ability to process information—oral and written, verbal and graphic—in ways that enable one to construct meaning) is a necessary but not sufficient condition for the development of science literacy.
Formal definitions of health literacy have developed independently of definitions of science literacy. Because the health literacy field has focused on health behaviors and outcomes, research has examined how health literacy operates in a wide variety of settings and media and has uncovered structural impediments in the health care system. Features of these new, more comprehensive definitions of health literacy, which include aspects such as (1) system demands and complexities as well as individual skills and abilities; (2) measurable inputs, processes, and outcomes; (3) potential for an analysis of change; and (4) linkages between informed decisions and action.
CONCLUSION 4 Concerns about the relationship of health literacy to health outcomes have led to a reconceptualization of health literacy as a property not just of the individual but also of the system, with attention
to how the literacy demands placed on individuals by that system might be mitigated.
This reconceptualization of health literacy informed the committee’s understanding of science literacy. As a result, the committee supports expanding contemporary perspectives on science literacy to encompass the ways that broader social structures can shape an individual’s science literacy. In addition, the committee questions the common understanding that science literacy is, or should be seen only as a property of individuals—something that only individual people develop, possess, and use. Research on individual-level science literacy provides invaluable insight, but it likely offers an incomplete account of the nature, development, distribution, and impacts of science literacy within and across societies. The committee asserts that societies and communities can possess science literacy in ways that may transcend the aggregation of individuals’ knowledge and accomplishments. The committee’s stance here is relatively new to the field of science literacy: it emerged as a direct result of the opportunity to examine science literacy in relationship to health literacy.
In light of this understanding, the committee organized its thinking about the questions posed in the charge by examining evidence at three levels of science literacy: the society, the community, and the individual. We chose this organization to contrast purposefully with the default understanding of literacy as an individual accomplishment. As a result, the committee chose to delve first into what science literacy looks like at its largest level of social organization—the society.
SCIENCE LITERACY AT THE LEVEL OF SOCIETY
There are four primary rationales for the importance of science literacy: personal, economic, democratic, and cultural. Each of them makes claims about the value of science literacy for nations and societies. Perhaps the most commonly heard claim is that a more science-literate population helps democratic societies make prudent and equitable decisions about policy issues that involve science. Currently, the available evidence does not provide enough information to draw conclusions on whether such claims are justified or not.
Research on science literacy at the level of a nation or whole society can be split into two perspectives. We refer to the first one as the aggregate perspective—empirical work that aggregates data about individuals, usually collected through large public opinion surveys or tests with samples representative of a population, and examines patterns in the whole or by groups. The vast majority of scholarly inquiry at the society level in the field of science literacy, as well as the public discourse, has focused on the aggregate perspective. We refer to the second one as the structural perspective—an alternative way to consider science literacy at the society level by examining the role of social structures. Social
structures could include (but would not be limited to) formal policies and institutions (e.g., schools and the scientific establishment) and emergent cultural properties, such as norms of political participation, social and economic stratification, and the presence of diverse groups and worldviews. There is very little research on science literacy from a structural perspective.
Currently, what is measured on science literacy at the society level comes from large public opinion surveys among adults and survey tests of adolescents in many countries. Indicators of adults’ knowledge of science are limited to a narrow range of measures on public surveys. It is difficult to draw strong conclusions on cross-national performance from these measures. However, survey responses over time have shown much stability in terms of average performance on knowledge questions, and no country for which there are data consistently outperforms other countries on all questions.
CONCLUSION 5 The population of adults in the United States performs comparably to adults in other economically developed countries on most current measures of science knowledge.
The large public opinion surveys in different countries also include measures of attitudes toward science. On these measures, there are many similarities among countries, and response trends have been stable across multiple survey years, particularly in the United States (for which there are more data). The percentage of respondents reporting positive attitudes has been (and remains) quite high, notably in regard to the perceived benefits created by science for societies and support for scientific research.
CONCLUSION 6 Current evidence, though limited, shows that populations around the world have positive attitudes toward science and support public funding for scientific research. These attitudes have been generally stable over time. In addition, the same evidence reveals an overall high level of trust in scientists and in scientific institutions.
In reviewing the literature and data from surveys on science literacy, as well as those on foundational literacy and health literacy, the committee found significant disparities in knowledge and access to knowledge. Much more is known about disparities in foundational literacy and health literacy than disparities in science literacy. The committee encourages new research in this area to examine the extent of disparities in science literacy and the social structures that contribute to them.
CONCLUSION 7 Within societies, evidence shows that severe disparities in both foundational literacy and health literacy exist and are associated with structural features such as distribution of income and access to high-
quality schooling. Though direct evidence for such structural disparities in science literacy is scarce, we conclude they too exist, in part because the possession of foundational literacy is so integral to the development of science literacy
SCIENCE LITERACY AT THE COMMUNITY LEVEL
Evidence from case studies suggests that science literacy can be expressed in a collective manner—i.e., resources are distributed and organized in such a way that the varying abilities of community members work in concert to contribute to their overall well-being. Science literacy in a community does not require that each individual attain a particular threshold of knowledge, skills, and abilities; rather, it is a matter of that community having sufficient shared capability necessary to address a science-related issue. Examples of such collective capability and action abound.
However, research does not yet show the extent to which communities are able to mobilize to respond to issues at a local level or what features of particular communities enable them to develop and deploy science literacy in effective ways. Evidence from case studies suggests that the success of communities is constrained by structural conditions and depends, at least in part, on the development of scientific knowledge throughout the community and the organization and composition of the community, including the strength and diversity of relationships with scientists and health professionals, scientific institutions, and health systems. The data show that particularly under-resourced communities are more susceptible to the types of environmental and health crises in which science literacy-informed community activism would be crucial, yet they often have the least access to resources that support development and use of science literacy. Additional research is needed to understand the various features and contexts that enable or prevent community science literacy and action.
CONCLUSION 8 There is evidence from numerous case studies that communities can develop and use science literacy to achieve their goals. Science literacy can be expressed in a collective manner when the knowledge and skills possessed by particular individuals are leveraged alongside the knowledge and skills of others in a given community.
The committee also finds that communities can and do contribute to new scientific knowledge in diverse and substantive ways, often in collaboration with scientists. Community involvement has helped to bring new questions to light, provide data that would otherwise be unavailable, encourage the integration of qualitative and observational data with experimental data, increase the robustness and public relevance of data collection strategies, garner political and community support for conclusions, produce new instruments and technologies,
and build community awareness and knowledge. Though the evidence describing this phenomenon is still case based, the committee finds that the creation of new scientific knowledge is a compelling demonstration of science literacy.
CONCLUSION 9 Based on evidence from a limited but expanding number of cases, communities can meaningfully contribute to science knowledge through engagement in community action, often in collaboration with scientists.
SCIENCE LITERACY AT THE INDIVIDUAL LEVEL
Research on science literacy at the individual level has largely assessed individuals’ knowledge using content knowledge assessments and measures of understanding of scientific principles administered through large public surveys. These widely used surveys have provided valuable insight into science knowledge, but constraints on length and demands for comparability over time and across nations mean that they may be limited in what they can capture about science literacy. The existing empirical evidence at the individual level on the value of science literacy is drawn largely from two separate research fields: science literacy and health literacy. Studies on the impact of health literacy have largely examined the relationship between knowledge and behaviors related to health. In contrast, most of the literature on science literacy assesses the relationship between science knowledge and attitudes toward, perceptions of, and support for science.
CONCLUSION 10 Research examining the application of science literacy and health literacy has focused on different things: studies on the impact of health literacy have looked for impact on health-related behaviors and actions (e.g., compliance with medical advice, shared decision making, etc.), whereas studies on the impact of science literacy have mostly examined its relationship to individual attitudes toward science and support for scientific research.
Attitudes have been measured by assessing the adult population’s evaluation of the social impact of science and technology. These attitudes have been further separated into two groups: a set of broad attitudes toward science and technology that reflect an individual’s assessment of the scientific research enterprise generally and a more focused set of attitudes toward specific scientific controversies, such as nuclear power, climate change, stem cell research, and genetically modified foods. Findings demonstrate that context matters when looking at the relationship between knowledge and perceptions of and support for science. Though science knowledge plays a role, many other factors influence an individual’s support for science and scientific research.
CONCLUSION 11 Available research does not support the claim that increasing science literacy will lead to appreciably greater support for science in general.
Though there appears to be a small, positive relationship between general science knowledge and general attitudes toward science, scholars have shown that this relationship becomes more complicated when assessing science knowledge and attitudes toward specific science issues. Knowledge impacts diverse sub-groups in the population differently depending on a host of factors, including levels of religiosity, political predispositions and worldviews, and scientific deference. These patterns seem to vary depending on the specific scientific issue being explored and the culture in which the data are collected. In fact, there is often an interaction between knowledge and worldviews such that enhanced knowledge has been associated, in cases of controversial issues, with increased polarization, affecting attitudes toward those specific science issues.
CONCLUSION 12 Measures of science literacy in adult populations have focused on a very limited set of content and procedural knowledge questions that have been asked within the constraints of large population surveys. Though available measures are limited in scope, evidence suggests they are reasonable indicators of one aspect of science literacy, science knowledge. Studies using these measures observe a small, positive relationship between science literacy and attitudes toward and support for science in general.
CONCLUSION 12a An individual’s general attitude toward science does not always predict that same individual’s attitude toward a specific science topic, such as genetic engineering or vaccines.
CONCLUSION 12b Some specific science issues evoke reactions based on worldviews (e.g., ideology, religion, deference to scientific authority) rather than on knowledge of the science alone.
Research examining the relationship between science literacy, health literacy, and behaviors related to health is limited, but the available examples highlight the weak correlation between science literacy, health literacy, and behaviors. Like the relationship between science knowledge and attitudes toward science, the causal pathway between science literacy, health literacy, and behaviors is complex and mediated by a number of personal and external factors.
These weak relationships suggest that efforts to simply promote knowledge and understanding to change behavior or attitudes may have limited results. Efforts should focus on increasing knowledge while also removing impediments to actions and lowering the literacy demands of particular situations.
CONCLUSION 13 The commonly used measures of science and health literacy, along with other measures of scientific knowledge, are only weakly correlated with action and behavior across a variety of contexts.
MOVING FORWARD THROUGH RESEARCH
The committee offers a conceptualization of science literacy at multiple levels of social organization that is relatively new to the field of science literacy. In order to demonstrate the value of this conception, it will be necessary to develop an evidence base that investigates science literacy in all its complexity.
Recommendation: The committee recommends that, in keeping with contemporary thinking, the scientific community, the research community, and other interested stakeholders continue to expand conceptions of science literacy to encompass (a) an understanding of how social structures might support or constrain an individual’s science literacy and (b) an understanding that societies and communities can demonstrate science literacy in ways that go beyond aggregating the science literacy of the individuals within them.
Recommendation: The committee recommends that the research community take on a research agenda that pursues new lines of inquiry around expanding conceptions of science literacy.
The committee notes many places where further research would inform thinking about science literacy. In Chapter 6 we outline a series of research questions as a way of thinking about creating new measures and expanding the information available to better understand. Our questions cover four broad topics: (1) the relationship between science knowledge and attitudes toward science; (2) the utility of science literacy; (3) the relationship of science literacy to other literacy skills; and (4) the role of science literacy for citizens as decision makers.
This page intentionally left blank.
Science is a way of knowing about the world. At once a process, a product, and an institution, science enables people to both engage in the construction of new knowledge as well as use information to achieve desired ends. Access to science—whether using knowledge or creating it—necessitates some level of familiarity with the enterprise and practice of science: we refer to this as science literacy.
Science literacy is desirable not only for individuals, but also for the health and well- being of communities and society. More than just basic knowledge of science facts, contemporary definitions of science literacy have expanded to include understandings of scientific processes and practices, familiarity with how science and scientists work, a capacity to weigh and evaluate the products of science, and an ability to engage in civic decisions about the value of science. Although science literacy has traditionally been seen as the responsibility of individuals, individuals are nested within communities that are nested within societies—and, as a result, individual science literacy is limited or enhanced by the circumstances of that nesting.
Science Literacy studies the role of science literacy in public support of science. This report synthesizes the available research literature on science literacy, makes recommendations on the need to improve the understanding of science and scientific research in the United States, and considers the relationship between scientific literacy and support for and use of science and research.
READ FREE ONLINE
Welcome to OpenBook!
You're looking at OpenBook, NAP.edu's online reading room since 1999. Based on feedback from you, our users, we've made some improvements that make it easier than ever to read thousands of publications on our website.
Do you want to take a quick tour of the OpenBook's features?
Show this book's table of contents , where you can jump to any chapter by name.
...or use these buttons to go back to the previous chapter or skip to the next one.
Jump up to the previous page or down to the next one. Also, you can type in a page number and press Enter to go directly to that page in the book.
Switch between the Original Pages , where you can read the report as it appeared in print, and Text Pages for the web version, where you can highlight and search the text.
To search the entire text of this book, type in your search term here and press Enter .
Share a link to this book page on your preferred social network or via email.
View our suggested citation for this chapter.
Ready to take your reading offline? Click here to buy this book in print or download it as a free PDF, if available.
Get Email Updates
Do you enjoy reading reports from the Academies online for free ? Sign up for email notifications and we'll let you know about new publications in your areas of interest when they're released.
- Advanced Search
- All new items
- Journal articles
- Manuscripts
- All Categories
- Metaphysics and Epistemology
- Epistemology
- Metaphilosophy
- Metaphysics
- Philosophy of Action
- Philosophy of Language
- Philosophy of Mind
- Philosophy of Religion
- Value Theory
- Applied Ethics
- Meta-Ethics
- Normative Ethics
- Philosophy of Gender, Race, and Sexuality
- Philosophy of Law
- Social and Political Philosophy
- Value Theory, Miscellaneous
- Science, Logic, and Mathematics
- Logic and Philosophy of Logic
- Philosophy of Biology
- Philosophy of Cognitive Science
- Philosophy of Computing and Information
- Philosophy of Mathematics
- Philosophy of Physical Science
- Philosophy of Social Science
- Philosophy of Probability
- General Philosophy of Science
- Philosophy of Science, Misc
- History of Western Philosophy
- Ancient Greek and Roman Philosophy
- Medieval and Renaissance Philosophy
- 17th/18th Century Philosophy
- 19th Century Philosophy
- 20th Century Philosophy
- History of Western Philosophy, Misc
- Philosophical Traditions
- African/Africana Philosophy
- Asian Philosophy
- Continental Philosophy
- European Philosophy
- Philosophy of the Americas
- Philosophical Traditions, Miscellaneous
- Philosophy, Misc
- Philosophy, Introductions and Anthologies
- Philosophy, General Works
- Teaching Philosophy
- Philosophy, Miscellaneous
- Other Academic Areas
- Natural Sciences
- Social Sciences
- Cognitive Sciences
- Formal Sciences
- Arts and Humanities
- Professional Areas
- Other Academic Areas, Misc
- Submit a book or article
- Upload a bibliography
- Personal page tracking
- Archives we track
- Information for publishers
- Introduction
- Submitting to PhilPapers
- Frequently Asked Questions
- Subscriptions
- Editor's Guide
- The Categorization Project
- For Publishers
- For Archive Admins
- PhilPapers Surveys
- Bargain Finder
- About PhilPapers
- Create an account
Science and Values
- Biomedical Ethics ( 91,907 | 42,675)
- Medical Ethics ( 20,513 | 12,993)
- Scientific Research Ethics ( 462 )
- Feminist Philosophy of Science ( 495 )
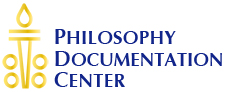

25,000+ students realised their study abroad dream with us. Take the first step today
Meet top uk universities from the comfort of your home, here’s your new year gift, one app for all your, study abroad needs, start your journey, track your progress, grow with the community and so much more.

Verification Code
An OTP has been sent to your registered mobile no. Please verify
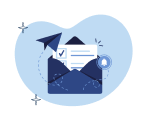
Thanks for your comment !
Our team will review it before it's shown to our readers.
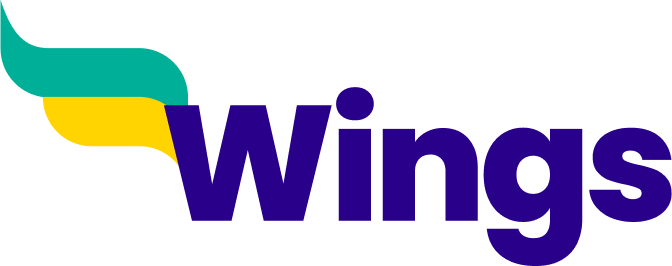
- School Education /
Essay on Science: Sample for Students in 100,200 Words
- Updated on
- Oct 28, 2023
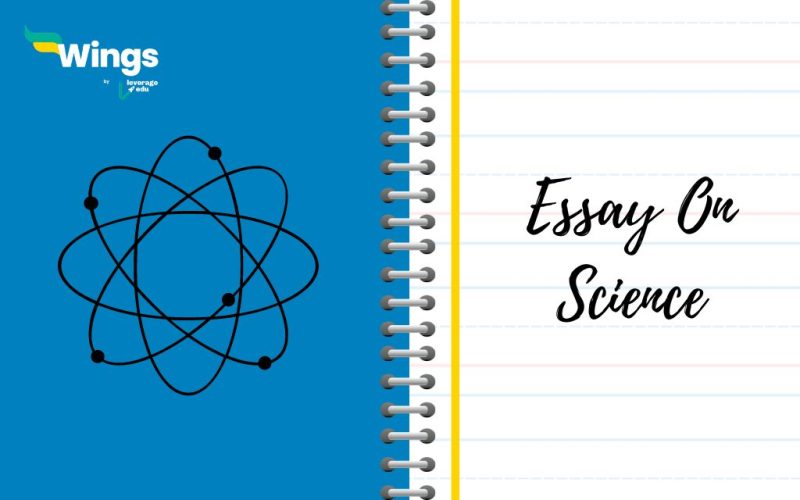
Science, the relentless pursuit of knowledge and understanding, has ignited the flames of human progress for centuries. It’s a beacon guiding us through the uncharted realms of the universe, unlocking secrets that shape our world. In this blog, we embark on an exhilarating journey through the wonders of science. We’ll explore the essence of science and its profound impact on our lives. With this we will also provide you with sample essay on science in 100 and 200 words.
Must Read: Essay On Internet
What Is Science?
Science is a systematic pursuit of knowledge about the natural world through observation, experimentation, and analysis. It aims to understand the underlying principles governing the universe, from the smallest particles to the vast cosmos. Science plays a crucial role in advancing technology, improving our understanding of life and the environment, and driving innovation for a better future.
Branches Of Science
The major branches of science can be categorized into the following:
- Physical Science: This includes physics and chemistry, which study the fundamental properties of matter and energy.
- Biological Science : Also known as life sciences, it encompasses biology, genetics, and ecology, focusing on living organisms and their interactions.
- Earth Science: Geology, meteorology, and oceanography fall under this category, investigating the Earth’s processes, climate, and natural resources.
- Astronomy : The study of celestial objects, space, and the universe, including astrophysics and cosmology.
- Environmental Science : Concentrating on environmental issues, it combines aspects of biology, chemistry, and Earth science to address concerns like climate change and conservation.
- Social Sciences : This diverse field covers anthropology, psychology, sociology, and economics, examining human behavior, society, and culture.
- Computer Science : Focused on algorithms, data structures, and computing technology, it drives advancements in information technology.
- Mathematics : A foundational discipline, it underpins all sciences, providing the language and tools for scientific analysis and modeling.
Wonders Of Science
Science has numerous applications that profoundly impact our lives and society: Major applications of science are stated below:
- Medicine: Scientific research leads to the development of vaccines, medicines, and medical technologies, improving healthcare and saving lives.
- Technology: Science drives technological innovations, from smartphones to space exploration.
- Energy: Advances in physics and chemistry enable the development of renewable energy sources, reducing reliance on fossil fuels.
- Agriculture: Biology and genetics improve crop yields, while chemistry produces fertilizers and pesticides.
- Environmental Conservation : Scientific understanding informs efforts to protect ecosystems and combat climate change.
- Transportation : Physics and engineering create efficient and sustainable transportation systems.
- Communication : Physics and computer science underpin global communication networks.
- Space Exploration : Astronomy and physics facilitate space missions, expanding our understanding of the cosmos.
Must Read: Essay On Scientific Discoveries
Sample Essay On Science in 100 words
Science, the bedrock of human progress, unveils the mysteries of our universe through empirical investigation and reason. Its profound impact permeates every facet of modern life. In medicine, it saves countless lives with breakthroughs in treatments and vaccines. Technology, a child of science, empowers communication and innovation. Agriculture evolves with scientific methods, ensuring food security. Environmental science guides conservation efforts, preserving our planet. Space exploration fuels dreams of interstellar travel.
Yet, science requires responsibility, as unchecked advancement can harm nature and society. Ethical dilemmas arise, necessitating careful consideration. Science, a double-edged sword, holds the potential for both salvation and destruction, making it imperative to harness its power wisely for the betterment of humanity.
Sample Essay On Science in 250 words
Science, often regarded as humanity’s greatest intellectual endeavor, plays an indispensable role in shaping our world and advancing our civilization.
At its core, science is a methodical pursuit of knowledge about the natural world. Through systematic observation, experimentation, and analysis, it seeks to uncover the underlying principles that govern our universe. This process has yielded profound insights into the workings of the cosmos, from the subatomic realm to the vastness of space.
One of the most remarkable contributions of science is to the field of medicine. Through relentless research and experimentation, scientists have discovered vaccines, antibiotics, and groundbreaking treatments for diseases that once claimed countless lives.
Furthermore, science has driven technological advancements that have reshaped society. The rapid progress in computing, for instance, has revolutionized communication, industry, and research. From the ubiquitous smartphones in our pockets to the complex algorithms that power our digital lives, science, and technology are inseparable partners in progress.
Environmental conservation is another critical arena where science is a guiding light. Climate change, a global challenge, is addressed through rigorous scientific study and the development of sustainable practices. Science empowers us to understand the impact of human activities on our planet and to make informed decisions to protect it.
In conclusion, science is not just a field of study; it is a driving force behind human progress. As we continue to explore the frontiers of knowledge, science will remain the beacon guiding us toward a brighter future.
Science is a boon due to innovations, medical advancements, and a deeper understanding of nature, improving human lives exponentially.
Galileo Galilei is known as the Father of Science.
Science can’t address questions about personal beliefs, emotions, ethics, or matters of subjective experience beyond empirical observation and measurement.
We hope this blog gave you an idea about how to write and present an essay on science that puts forth your opinions. The skill of writing an essay comes in handy when appearing for standardized language tests. Thinking of taking one soon? Leverage Edu provides the best online test prep for the same via Leverage Live . Register today to know more!
Amisha Khushara
With a heart full of passion for writing, I pour my emotions into every piece I create. I strive to connect with readers on a personal level, infusing my work with authenticity and relatability. Writing isn't just a skill; it's my heartfelt expression to touch hearts and minds.
Leave a Reply Cancel reply
Save my name, email, and website in this browser for the next time I comment.
Contact no. *

Connect With Us
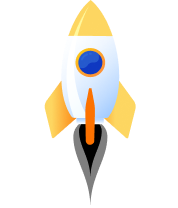
25,000+ students realised their study abroad dream with us. Take the first step today.

Resend OTP in

Need help with?
Study abroad.
UK, Canada, US & More
IELTS, GRE, GMAT & More
Scholarship, Loans & Forex
Country Preference
New Zealand
Which English test are you planning to take?
Which academic test are you planning to take.
Not Sure yet
When are you planning to take the exam?
Already booked my exam slot
Within 2 Months
Want to learn about the test
Which Degree do you wish to pursue?
When do you want to start studying abroad.
January 2024
September 2024
What is your budget to study abroad?
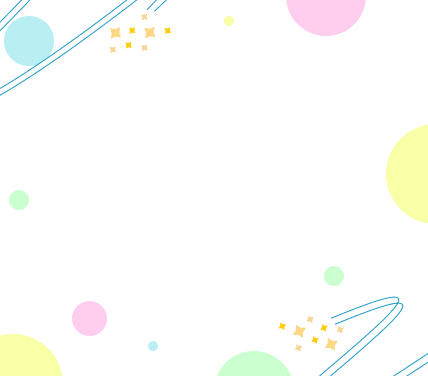
How would you describe this article ?
Please rate this article
We would like to hear more.
Have something on your mind?

Make your study abroad dream a reality in January 2022 with

India's Biggest Virtual University Fair

Essex Direct Admission Day
Why attend .

Don't Miss Out
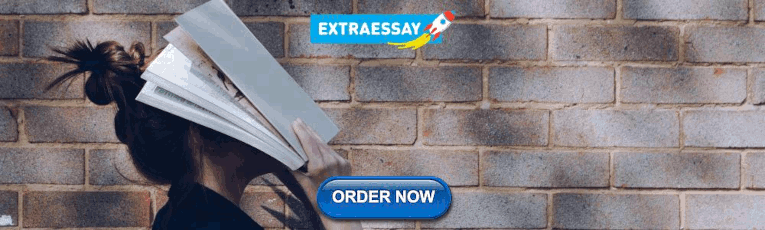
IMAGES
VIDEO
COMMENTS
This essay examines the important roles for values in science, from deciding which research projects are worth pursuing, to shaping good methodological approaches (including ethical concerns), to assessing the sufficiency of evidence for scientific claims. I highlight the necessity of social and ethical value judgements in science, particularly ...
Values in Science. Science is, of course, a human practice. And when we do science, we entangle values, including social and ethical values, in that science. The questions are how values interweave with science, whether it is legitimate and necessary, and ultimately what to do about it. Critics of the value-free ideal for science initially ...
Abstract. Protagonists in the so-called Science Wars differ most markedly in their views about the role of values in science and what makes science valuable. Scientists and philosophers of science have traditionally considered the principal aims of science to be explanation and application. Only cognitive values should influence what is taken ...
Indiana University. Protagonists in the so-called Science Wars differ most markedly in their views about the role of values in science and what makes science valuable. Scientists and philoso- phers of science have traditionally considered the principal aims of science to be expla- nation and application.
The Element explores a range of strategies for distinguishing between appropriate and inappropriate value influences. It concludes by proposing an approach for managing values in science that relies on justifying, prioritising, and implementing norms for scientific research practices and institutions. Element.
The Joy of Science by physicist and science popularizer, Jim al-Khalili, is among the most recent books to make this argument. The book is presented in the form of eight short lessons on how to lead 'a more rational' life. Each chapter draws on particular values that guide scientific practice, explains the benefits to be had from the wider adoption of these values, and gives advice on how ...
Values in relation to science can be considered from epistemic, cognitive, cultural, social, political, moral and ethical perspectives. Considering the vast amount of work within philosophy of science and sociology of science on the subject of values, and the ongoing debate about their distinction, the aim is to clarify and summarize a functional notion of values for science education purposes.
Does holding such values harm a person's science? In some cases the answer has to be "yes." The history of science offers a number of episodes in which social or personal beliefs distorted the work of researchers. The field of eugenics used the techniques of science to try to demonstrate the inferiority of certain races.
Abstract. After describing the origins and nature of the value-free ideal for science, this chapter details three challenges to the ideal: the descriptive challenge (arising from feminist critiques of science, which led to deeper examinations of social structures in science), the boundary challenge (which questioned whether epistemic values can be distinguished from nonepistemic values), and ...
This normal functioning of science is full of values: a scientist must be honest, modest in front of the experimental results, always critical, rejecting any dogmatism and any fraud, analyzing all the possible bias, but also creative, developing his / her work with imagination and rigor, and more and more collectively.
Science used to be valued as a means of getting to know the world; now, owing to the triumph of technique, it is conceived as showing how to change the world. The new point of view, which is adopted in practice throughout America and Russia, and in theory by many modern philosophers, was first proclaimed by Marx in 1845, in his Theses on ...
The Value Of Science∗ Richard P. Feynman From time to time people suggest to me that scientists ought to give more consideration to social problems - especially that they should be more re-sponsible in considering the impact of science on society. It seems to be generally believed that if the scientists would only look at these very dif-
Values in Science. Scientists bring more than just a toolbox of techniques to their work. Scientist must also make complex decisions about the interpretation of data, about which problems to pursue, and about when to conclude an experiment. They have to decide the best ways to work with others and exchange information.
III. Freedom to Doubt. Perhaps the greatest value of science is the freedom it provides us to doubt. Scientific knowledge is a body of statements of varying degrees of certainty — some most ...
The Element explores a range of strategies for distinguishing between appropriate and inappropriate value influences. It concludes by proposing an approach for managing values in science that relies on justifying, prioritising, and implementing norms for scientific research practices and institutions. Cambridge Core - Philosophy of Science ...
Summary. Science is a way of knowing about the world. At once a process, a product, and an institution, science enables people to both engage in the construction of new knowledge as well as use information to achieve desired ends. Access to science—whether using knowledge or creating it—necessitates some level of familiarity with the ...
Summary. Science and values is a multifaceted discussion in the philosophy of science, as there are a variety of ways the conjunction of the two can be understood. Two major theses in this area are (1) that scientific inquiry, rather than being a simple matter of evidence and logic or rule-governed inference, requires a variety of value ...
To do so leads to what he described as an "open channel.". "It is our responsibility as scientists… to proclaim the value of this freedom; to teach how doubt is not to be feared but ...
Science treats all humans equally. Scientists are concerned with the content of the scientific work, not with the person who produced it. Science is open to all, regardless of nationality, race, religion, or sex. These values of science are universal values worth defending, not just to promote the pursuit of science but to produce a better and ...
The Value of Science. R. Feynman. Published 1 December 1955. Philosophy. From time to time, people suggest to me that scientists ought to give more consideration to social problems -especially that they should be more responsible in considering the impact of science upon society. This same suggestion must be made to many other scientists, and ...
Science used to be valued as a means of getting to know the world; now, owing to the triumph of technique, it is conceived as showing how to change the world. The new point of view, which is adopted in practice throughout America and Russia, and in theory by many modem philosophers, was first proclaimed by Marx in 1845, in his Theses on ...
Sample Essay On Science in 100 words. Science, the bedrock of human progress, unveils the mysteries of our universe through empirical investigation and reason. Its profound impact permeates every facet of modern life. In medicine, it saves countless lives with breakthroughs in treatments and vaccines. Technology, a child of science, empowers ...
Last Name 2 so. In these ways, he believes that science offers value to society, and it is the responsibility of all scientists to ensure the values science offers are maintained. Response The essay offers important facts about science and how the values are intertwined with the rest of society. People must understand that science is a key to society's good and bad.