Academia.edu no longer supports Internet Explorer.
To browse Academia.edu and the wider internet faster and more securely, please take a few seconds to upgrade your browser .
Enter the email address you signed up with and we'll email you a reset link.
- We're Hiring!
- Help Center
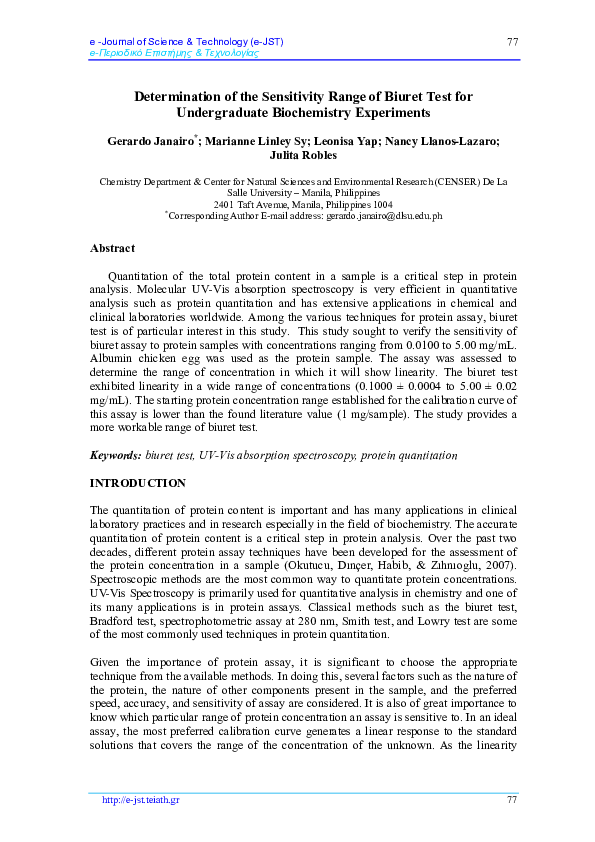
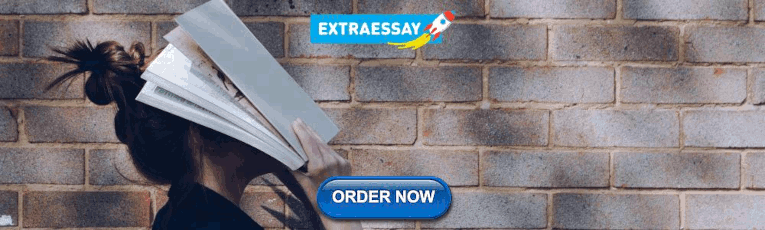
Determination of the Sensitivity Range of Biuret Test for Undergraduate Biochemistry Experiments

e-jst.teiath.gr
Related Papers
Chimica et Natura Acta
ahmad syauqi
Comparison between the references and the quantification of biuret standard curves can be performed using statistical methods. The objective of this study was to select a method of protein quantification of the biuret-spectrophotometric based on the similar standard curve of the compound. The study used experimental methods in the laboratory and references from a comparative scheme of mean that has categories of statistics called academic and practical terms. Two standard curves were tested with the reference data. The academic way was performed with a comparison of 11.779-12.401% confidence, and the quantification of the results of the protein mean of 8.211% and 10.17% showed no significant difference. The practical methods were carried out with the original reference data of 10.8-12.8%, and the result of the test confidence values of 9.163-11.180% and 7.596-8.826% showed different accuracy results. The quantifications of the protein biuret method displayed different results on how...
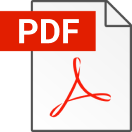
Indian Journal of Pharmaceutical Sciences
Sudha Gopinath
J. biol. Chem
Ravish Patel
Analytical Biochemistry
Rehana Parvin
A study of the applicability of a colorimetric biuret procedure for protein determination to a variety of biological preparation has revealed that the practice of using this procedure is undesirable because markedly apparent high values are obtained in this way. In some cases, this interference was mainly due to lipids. Use of suitable controls to account for the interfering opalescence in biuret color measurements considerably improved the method. Conditions affecting the reliability of the procedure are also described. For crude extracts the colorimetric phenol method was found to be more reliable. However, a prior separation of protein by TCA is often advantageous not alone for Kjeldahl analysis but also for colorimetric estimations. The actual chromogenicity of the protein mixtures of different biological extracts studied was found to be comparable to that of the commonly employed standard protein, bovine serum albumin.
Revista Do Instituto De Laticinios Cândido Tostes
Wesley Esteves
Joshua Caguioa
This study explores the potential of measuring protein concentration using spectrophotometry, unlike the standard protein quantification which uses Copper Sulfate (CuSO4) as biuret reagent, this study uses Magnesium Chloride (MgCl2) based biuret assay in determing the unknown concentration of Bovine Albumin Serum (BSA). Based from the result, MgCl2 based biuret reagent can yield accurate result when measuring the unknown concentration of BSA. Spectrophotometric data was treated through linear regression curve and revealed that protein concentration is directly proportional to the light absorbance of a material.Moreover, the determination acceptance of R=0.9719 obtained from 490 nm absorbance was utilized with linear equation (y=mx+b) to determine the unknown concentration of BSA. It was revealed that the unknown concentration computed was 19.73 mg/ml which is close to the actual value of 20.00 mg/ml.
Rehana Lebbe
Puspendu Karmakar
Miltiadis Pappas
Bicinchoninic acid, sodium salt, is a stable, water-soluble compound capable of forming an intense purple complex with cuprous ion (Cu1+) in an alkaline environment. This reagent forms the basis of an analytical method capable of monitoring cuprous ion produced in the reaction of protein with alkaline Cu2+ (biuret reaction). The color produced from this reaction is stable and increases in a proportional fashion over a broad range of increasing protein concentrations. When compared to the method of Lowry et al., the results reported here demonstrate a greater tolerance of the bicinchoninate reagent toward such commonly encountered interferences as nonionic detergents and simple buffer salts. The stability of the reagent and resulting chromophore also allows for a simplified, one-step analysis and an enhanced flexibility in protocol selection. This new method maintains the high sensitivity and low protein-to-protein variation associated with the Lowry technique.
RELATED PAPERS
Seismological Research Letters
Massimiliano Stucchi
S. Minutoli
Journal of PeriAnesthesia Nursing
Jan Odom-Forren
Lara Lázaro Touza
Eric Blanco
Medical Journal of Indonesia
Ihya Ridlo Nizomy
European Journal of Plant Pathology
Chrysi Vomvogianni
Bioscience Journal
Vitor Rosa de Oliveira
Revista Trilogia
Frank Vélez Penagos
Molecular Therapy
Antonio Vinciguerra
Juan David Troncoso Narvaez
Biochemical and Biophysical Research Communications
Jorge Capdevila
Jurnal Pendidikan Anak
Ledistar Sumin Naibaho ledistarsumin.2020
Journal of Engineering Advancements
Kazeem B . Adedeji
Perception & Psychophysics
Hilda Greenbaum
Canadian Journal of Neurological Sciences / Journal Canadien des Sciences Neurologiques
Jacob Hascalovici MD, PhD
Cognizance Journal of Multidisciplinary Studies
Sophomore T Vacalares
Arief Ardiansyah
Raximboy Yarmatov
Cristian Mosneanu
Vertex Revista Argentina de Psiquiatría
facundo ruffinatto
Matthieu Denoual
rtytrewer htytrer
Theoretical and Applied Genetics
Fang-Sheng Wu
See More Documents Like This
- We're Hiring!
- Help Center
- Find new research papers in:
- Health Sciences
- Earth Sciences
- Cognitive Science
- Mathematics
- Computer Science
- Academia ©2024
Identification Tests for Proteins (Casein and Albumin)
- First Online: 28 February 2020
Cite this protocol
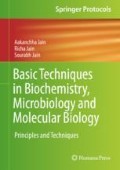
- Aakanchha Jain 4 ,
- Richa Jain 5 &
- Sourabh Jain 6
Part of the book series: Springer Protocols Handbooks ((SPH))
4068 Accesses
1 Citations
Casein, like proteins, is made up of many hundreds of individual amino acids. Each may have a positive or a negative charge, depending on the pH of the [milk] system. At some pH value, all the positive charges and all the negative charges on the [casein] protein will be in balance, so that the net charge on the protein will be zero. That pH value is known as the isoelectric point (IEP) of the protein and is generally the pH at which the protein is least soluble. For casein, the IEP is approximately 4.6, and it is the pH value at which acid casein is precipitated. In milk, which has a pH of about 6.6, the casein micelles have a net negative charge and are quite stable. During the addition of acid to milk, the negative charges on the outer surface of the micelle are neutralized (the phosphate groups are protonated), and the neutral protein precipitates.
This is a preview of subscription content, log in via an institution to check access.
Access this chapter
- Available as PDF
- Read on any device
- Instant download
- Own it forever
- Available as EPUB and PDF
- Compact, lightweight edition
- Dispatched in 3 to 5 business days
- Free shipping worldwide - see info
- Durable hardcover edition
Tax calculation will be finalised at checkout
Purchases are for personal use only
Institutional subscriptions
Author information
Authors and affiliations.
Bhagyoday Tirth Pharmacy College, Sagar, Madhya Pradesh, India
Aakanchha Jain
Centre for Scientific Research and Development, People’s University, Bhopal, Madhya Pradesh, India
Sagar Institute of Pharmaceutical Sciences, Sagar, Madhya Pradesh, India
Sourabh Jain
You can also search for this author in PubMed Google Scholar
Rights and permissions
Reprints and permissions
Copyright information
© 2020 Springer Science+Business Media, LLC, part of Springer Nature
About this protocol
Jain, A., Jain, R., Jain, S. (2020). Identification Tests for Proteins (Casein and Albumin). In: Basic Techniques in Biochemistry, Microbiology and Molecular Biology. Springer Protocols Handbooks. Humana, New York, NY. https://doi.org/10.1007/978-1-4939-9861-6_42
Download citation
DOI : https://doi.org/10.1007/978-1-4939-9861-6_42
Published : 28 February 2020
Publisher Name : Humana, New York, NY
Print ISBN : 978-1-4939-9860-9
Online ISBN : 978-1-4939-9861-6
eBook Packages : Springer Protocols
- Publish with us
Policies and ethics
- Find a journal
- Track your research
Direct analysis for urinary protein with biuret reagent, with use of urine ultrafiltrate blanking: comparison with a manual biuret method involving trichloroacetic acid precipitation
- PMID: 6697493
We describe a method for measuring urinary protein with a centrifugal analyzer. Biuret reagent is used, and blanking with an ultrafiltrate of urine eliminates interferences from the nonprotein, biuret-positive chromogens in urine. We compare results by this new method with those by a manual method in which trichloroacetic acid precipitation and biuret reagent are used. The new method shows good precision and excellent correlation (r = 0.997) with the manual method. The ease and convenience of this assay should make this a useful method for the routine clinical laboratory.
Publication types
- Comparative Study
- Anti-Bacterial Agents / urine
- Biuret Reaction
- Chemical Precipitation
- Colorimetry / methods*
- False Positive Reactions
- Proteins / analysis*
- Proteinuria / urine*
- Trichloroacetic Acid
- Ultrafiltration
- Anti-Bacterial Agents

An official website of the United States government
The .gov means it’s official. Federal government websites often end in .gov or .mil. Before sharing sensitive information, make sure you’re on a federal government site.
The site is secure. The https:// ensures that you are connecting to the official website and that any information you provide is encrypted and transmitted securely.
- Publications
- Account settings
Preview improvements coming to the PMC website in October 2024. Learn More or Try it out now .
- Advanced Search
- Journal List
- Elsevier - PMC COVID-19 Collection

Review: Detection and quantification of proteins in human urine
Sultan aitekenov.
a School of Sciences and Humanities, Department of Chemistry, Nazarbaev University, Nur-Sultan, Kazakhstan
Abduzhappar Gaipov
b School of Medicine, Department of Clinical Sciences, Nazarbaev University, Nur-Sultan, Kazakhstan
Rostislav Bukasov
Extensive medical research showed that patients, with high protein concentration in urine, have various kinds of kidney diseases, referred to as proteinuria. Urinary protein biomarkers are useful for diagnosis of many health conditions – kidney and cardio vascular diseases, cancers, diabetes, infections. This review focuses on the instrumental quantification (electrophoresis, chromatography, immunoassays, mass spectrometry, fluorescence spectroscopy, the infrared spectroscopy, and Raman spectroscopy) of proteins (the most of all albumin) in human urine matrix. Different techniques provide unique information on what constituents of the urine are. Due to complex nature of urine, a separation step by electrophoresis or chromatography are often used for proteomics study of urine. Mass spectrometry is a powerful tool for the discovery and the analysis of biomarkers in urine, however, costs of the analysis are high, especially for quantitative analysis. Immunoassays, which often come with fluorescence detection, are major qualitative and quantitative tools in clinical analysis. While Infrared and Raman spectroscopies do not give extensive information about urine, they could become important tools for the routine clinical diagnostics of kidney problems, due to rapidness and low-cost. Thus, it is important to review all the applicable techniques and methods related to urine analysis. In this review, a brief overview of each technique's principle is introduced. Where applicable, research papers about protein determination in urine are summarized with the main figures of merits, such as the limit of detection, the detectable range, recovery and accuracy, when available.
Graphical abstract

- • Urinary protein biomarkers are useful for diagnosis of many conditions: kidney and cardio vascular diseases, cancers.
- • Liquid chromatography – mass spectroscopy is a powerful tool for urine proteomics, but used mostly in science.
- • Immunoassays are widely used in both clinical and bio-analytical laboratories.
- • IR and Raman spectroscopies are promising tools for diagnostics of urine due to low-cost and rapidness.
1. Introduction
1.1. urine composition.
Urine is a readily available liquid for the medical diagnosis of patients. Urine tests are non-invasive procedures that involve no pain or discomfort for patients to determine problems with kidney function since extensive medical research showed that patients, with high protein concentration in urine, have various kinds of illnesses of the kidney, referred to as proteinuria [ 1 ]. Thus the precise and simple determination of urinary concentrations of total protein is important for diagnostic purposes. Since urine is a complex matrix, it poses challenges for the analytical determination of proteins and other constituents. Among the reasons that make urine challenging for researchers to analyze are: urine matrix is complex, it consists of various inorganic and organic compounds, from low-molar mass molecules to polymers; urine could contain cells, such as blood cells, or bacteria, which changes the composition of urine in time rapidly; an analytical method for diagnosis of proteinuria should cover protein presence in urine in a wide range from 0.01 mg/ml to 10 mg/ml. Also, it is important to note that the concentration of protein in urine taken from patients can vary widely depending on dieting, exercising, and time of the day a patient urinated. It is widely accepted that analysis of urea taken throughout a day is the best representation of any illnesses in the human body if any. The so-called – the urine 24-h volume test, that measures the amount of urine the human body produces in a day. Another option is to determine creatinine to protein ratio in urea since creatinine to protein ratio is positively correlated with 24-h volume test for quantifying proteinuria [ 2 , 3 ]. The noninvasive collection of samples and wide range of diagnostic targets found in urine makes urinalysis well suited for point-of-care (PoC) monitoring applications, in which testing is done at the time and place of patient care [ 4 ]. Table 1 shows constituents in urine taken from the urine 24-h volume test.
Composition of urine (averages of selected components in 24-h collection test) [ 5 ].
In healthy individuals, Tamm–Horsfall protein (also known as uromodulin) is the most abundant protein in urine (50%), followed by albumin (20%) and immunoglobulin (5%) [ 6 ]. Other constituents in the urine of either sick or healthy patients include, but not limited to: glucose; low abundant proteins, such as bilirubin, and urobilinogen; cells, such as Erythrocyte, Leukocyte, and other cells; bacteria [ 7 ].
1.2. Urine as a diagnostic tool
The urine of a healthy individual contains up to 150 mg of protein in total measured throughout a day, of which approximately 20 mg is albumin (human serum albumin) [ 1 ]. Albumin excretion of 30–300 mg a day, which is called microalbuminuria, is an early and sensitive marker of diabetic nephropathy [ 8 ], cardiovascular and renal disease [ 9 ]. The 15–30 mg/L albumin concentration is a critical value that could indicate kidney problems when it is repeatedly exceeded [ 10 ]. Though one of the main proteins in urine is albumin, there are thousands of other types of proteins. Furthermore, the removal of albumin from the urine helps to identify the low abundant proteins [ 11 ]. Marimuthu et al. by using high-resolution Fourier transform mass spectrometry were able to identify 1823 proteins in the urine of healthy subjects [ 12 ]. Using mass spectroscopy and sub-fractionating normal urine by successive steps (vesicle separation, CPLL, and solvent treatments) Santucci et al. were able to identify 3429 individual proteins [ 13 ]. All those discovered proteins could be potential biomarkers for diseases. In a review paper by Röthlisberger et al. it was stated that besides albumin, other proteins such as CD14, hh-FABP, BNP/NT-proBNP, NGAL, ORM1 are potential biomarkers of cardiovascular disease [ 14 ]. Another biomarker in human urine, Bence Jones protein (BJP), has an important diagnosis and prognosis value for multiple myeloma, cancer formed in a white blood cell called plasma cell [ 15 ]. Other types of chemical substances in urine can be important biomarkers as well, for example, urine microRNAs have the potential to be a valid marker for bladder cancer detection [ 16 ].
Proteinuria is the main clinical presentation of glomerular diseases (the glomerulus are complex capillary set that are located in the nephrons – renal cells) [ 17 ]. The glomerular diseases can be primarily when the disease caused by kidney diseases (such as glomerulonephritis), or secondary, when kidney glomerulus becomes target organ affected by different diseases such as diabetes and cardiovascular diseases, autoimmune and inflammatory disorders, amyloidosis and neoplasms, cancer and many other including genetic disorders [ 17 , 18 ]. The levels of proteinuria, which usually measures in clinical settings, may vary depending on severity of disease and glomerular injury, despite any cause of disease. Any detectable proteinuria in the urine between 30 and 300 mg/24h called albuminuria (or microalbuminuria), above the 300 mg/24h – proteinuria. Depending the amount of proteinuria they considered to named nephritic range proteinuria below 3.5 g/24h and nephrotic range proteinuria when protein loss excess the 3.5 g/24h (heavy proteinuria). The most of the cases, proteinuria caused by cardiovascular diseases limited with nephritic range proteinuria, whereas in renal diseases and cancer the proteinuria may reach nephrotic range proteinuria [ 19 , 20 ]. Since urine is in direct contact with kidneys, analysis of urine is important for diagnosis of renal diseases, such as chronic kidney disease. Several viral infections such as Epstein-Barr virus, hepatitis B and C viruses, herpes zoster, hantavirus, human immunodeficiency virus, dengue fever, COVID-19 and many others also may lead to proteinuria [ 21 , 22 ]. The potential mechanisms of proteinuria are related to primary affect to glomerulus and/or secondary to autoimmune response to infection [ 23 , 24 ]. Chronic kidney disease (CKD) is a major public health problem. Albuminuria, urinary sediment abnormality and other markers of kidney damage are criteria of CKD, according to international guidelines [ 25 ]. Fassett et al. summarized biomarkers for CKD in urine – cystatin C, β-trace protein, NGAL, KIM-1, NAG and many others [ 26 ]. A more recent article on this topic is discussed in Ref. [ 27 ].
In the United States, it was estimated in 2003 that 11% of the adult population (aged 20 or older) has chronic kidney disease [ 28 ]. In a review paper about the prevalence of chronic kidney disease (CKD) by Zhang et al., the authors studied relevant data across America, Europe, Asia, Australia [ 29 ]. They reported that the median prevalence of CKD was 7.2% in persons aged 30 years or older. In persons aged 64 years or older prevalence of CKD varied from 23.4% to 35.8%. The authors concluded that worldwide, CKD is becoming a common disease in the general population. In a more recent paper, Wei et al. studied data on kidney damage among elderly people in Wuhan, China [ 30 ]. The age-standardized prevalence of kidney damage decreased renal function and proteinuria was 17.2, 13.5, and 5.3%. In the US, patients aged 66 or older have a mortality rate of 111.2 per 1000 due to CKD, compared to 45.2 per 100 due to non-CKD reasons as of 2014 [ 31 ]. It remains among the few growing causes of mortality which made CKD the 13th leading cause of death in 2013 [ 32 ].
Other renal diseases include diabetic nephropathy, autosomal dominal polycystic kidney disease, paediatric renal disease, acute kidney injury, and renal transplant rejection [ 33 ]. Besides renal diseases, urine can also be a useful source of information related to cancers and non-renal diseases. Theodorescu et al. found biomarkers in urine related to urothelial carcinoma, also called transitional cell carcinoma, by the means of capillary electrophoresis coupled with mass spectroscopy [ 34 ]. It is the most common type of bladder cancer. Bhasin et al. developed a bioresistor device to detect bladder cancer marker DJ-1 in urine at concenctration of 10 pM in 1 min [ 35 ]. Zhang et al. in their review summarized information about cancer biomarkers in urine [ 36 ]. In another work related to non-renal disease, Zimmerli et al. investigated urine samples of patients with coronary artery disease [ 37 ]. Rossing et al. identified potential biomarkers for diabetes [ 38 ]. Raja et al. investigated into the potential of albuminuria as a biomarker of diabetic complications [ 39 ]. Moreover, researchers could diagnose patients with viruses from their urine samples. Yang et al. developed an immunoassay cassette with a handheld reader for HIV urine testing in point-of-care diagnostics [ 40 ]. Robles et al. analyzed viruses present in urine from patients with interstitial cystitis [ 41 ]. Other viruses in urine described in literature include – Zika virus [ 42 ], hepatitis C [ 43 ], human papiloma virus [ 44 ]. Niedrig et al. wrote a review about usefulness of saliva and urine for the diagnosis of emerging viruses [ 45 ]. They concluded that it is important to perform an investigation using non-invasive approaches, such as urine can provide, for the diagnostic of emerging viral diseases.
1.3. Clinical urine tests
Urinalysis is an abbreviation for clinical urine tests for diagnostic purposes. A urinalysis (UA) is one of the most common methods of medical diagnosis. There are three basic components to urinalysis [ 46 ]:
- • Gross/physical examination targets parameters that can be measured or quantified with the naked eye (or other senses), including volume, color, transparency, odor, and specific gravity.
- • Microscopic examination. The numbers and types of cells and/or material such as urinary casts can yield a great detail of information and may suggest a specific diagnosis.
- • Chemical examination of urine measures quantitatively and qualitatively for pH, blood, nitrite, protein, glucose, ketones, bilirubin, urobilinogen, ascorbic acid.
Conditions for storage of urine samples is an important consideration for chemical analysis, since undesired changes in unpreserved urine occur, such as a decrease of concentration of glucose due to consumption of it by cells or bacteria; a decrease of concentration of bilirubin due to photo-oxidation and etc [ 47 ]. The most common form of preservation of urine samples is refrigeration, also chemicals can be utilized too. Remer et al. suggest that high long-term stability and measurement validity for numerous clinical chemistry parameters stored at −22 °C without the addition of preservative for human urine can be achieved [ 48 ]. Specifically for free-light chains monoclonal immunoglobulin, Pieri et al. suggest to store urine sample at +4 °C, if analysis to be made within 72 h, otherwise for longer periods, the refrigeration should be done at −80 °C [ 49 ]. Moyle et al. found that collection and storage of canine urine samples in clean homopolymer polypropylene (HP), propylene copolymer (PC), or glass containers at 24 °C for 4 h, 4 °C for 12 h or −20 °C for 72 h is unlikely to result in clinically relevant decreases in measured protein to creatine values [ 50 ].
Historically, qualitative or semi-quantitative screening tests for urine protein relied on protein precipitation techniques. Proteins denature upon exposure to extremes of pH or temperature, and the most visible evidence of this is a decrease in solubility. In clinical laboratories, sulfosalicylic acid at room temperature may be used to detect urine protein [ 47 ]. This protein precipitation method detects all proteins—albumin and globulins. The analytical signal here is turbidity, which is the cloudiness or haziness of a fluid. This method can not detect protein below 0.05 mg/ml. Modern commercial reagent strips are available for routine protein screening use change in color due to acid-base reaction or formation of complexes between urine constituents and reagent strips material. Some of those reactions are described and compared in Ref. [ 51 ]: absorbance at 280 nm, which monitors tyrosine and tryptophan in protein (UV absorbance); the method of Lowry et al. based on complex formation between tyrosine, tryptophan or cysteine and heavy metals using Folin phenol reagent in the presence of copper ions under alkaline conditions (Lowry method) [ 52 ]; the Bradford method based on protein dye-binding using Coomassie brilliant blue (CBB method) [ 53 ]; the method of Smith et al. based on the formation of a purple complex between bicinchoninic acid and copper ions under alkaline conditions (BCA method) [ 54 ]; the method of Watanabe et al. based on protein-dye binding using pyrogallol redmolybdate reagent (PRM method) [ 55 ].
To date, different diagnostics methods in clinical settings can be used to ascertain urinary protein and albumin. Urine dipstick test, technique with acidic buffer precipitation and immuno-electrophoresis are commonly used diagnostic methods for urinary total protein quantification [ 56 ]. The protein-specific dipstick and immunochemical techniques are the fastest and cheapest way to determine proteins in the urine for diagnostic purposes. Modern automated urine analyzers are based on similar principles and are widely used in clinical routine tests [ 57 ]. A urine analyzer is a device used in the clinical setting to perform automatic urine testing. The units can detect and quantify a number of analytes including bilirubin, protein, glucose, and red blood cells. Many models contain urine strip readers, a type of reflectance photometer that can process several hundred strips per hour. Some analyzers can perform up to 240 tests an hour. Prices of urine analysis are relatively low, but they depend on a location/country and they are available from the most clinical laboratories The high-performance liquid chromatography mostly used for scientific purpose due to its relatively high cost, though relatively inexpensive versions of mass spectrometers are now available [ 58 ].
Prices of urine analysis are relatively low, but they depend on a location/country and they are available from the most clinical laboratories The high-performance liquid chromatography mostly used for scientific purpose due to its relatively high cost, though relatively inexpensive versions of mass spectrometers are now available [ 58 ].
1.4. Focus of this review
Several review papers are focused on urine proteomics. A review by Albalat et al. focuses on urinary proteins as potential biomarkers for mainly urine diseases, and capillary electrophoresis coupled mass spectroscopy as an instrumental method [ 33 ]. The review also gives information on biomarkers on non-renal diseases obtained from the urine. Kalantari et al. state that mass spectrometry as a detection technique is the most common [ 59 ]. The paper discusses technical aspects of urinary proteomics, proteomic technologies, and their advantage and disadvantages. As of 2015, several recent experiments are presented there which applied urinary proteome for biomarker discovery in renal diseases including diabetic nephropathy, immunoglobulin A (IgA) nephropathy, focal segmental glomerulosclerosis, lupus nephritis, membranous nephropathy, and acute kidney injury. Decramer et al. in their review paper focus on mass spectrometry-based urinary protein and peptide profiling [ 60 ]. The advantages and disadvantages of different mass spectroscopy methods are compared. Applications of urinary proteome analysis to urogenital and non-urogenital diseases are also discussed there.
This review, besides covering mass spectrometry, holds chapters discussing fluorescence spectroscopy, immunoassay, infrared, and Raman spectroscopy that was not given much attention compared from the reviews on urinalysis mentioned in the previous paragraph. This review also covers separation techniques – electrophoresis and chromatography. For each technique, a brief overview of the technique's principle is introduced. Where applicable, research papers about protein determination in urine are summarized with the main figures of merits, such as the limit of detection, the detectable range, recovery, and accuracy. Particularly, electrophoresis, chromatography, immunoassay, and fluorescence spectroscopy chapters are followed by summary tables with analytical parameters taken from individual research papers. It should be noted that not all analytical parameters are given in every paper, for example, sometimes recovery is unknown, and thus is not included in the following tables. Since the volumes used for all techniques are 100–1000 times smaller than a typical urine probe available from one patient for analysis, usually 50–100 mL, urine volume is not introduced in the tables. For instance for proteomics study, a typical sample size for high performance liquid chromatography (HPLC) ranges from few microliters to 0.1 mL [ 61 ]. A commercial immunoassay test kits developed by Valle et al. require 10 μL of urine [ 62 ]. This review ends with a paragraph about urinary protein biomarkers. Most of those biomarkers were identified by mass spectrometry or immunoassay methods. A summary table of experimental papers on urinary protein biomarkers with related diseases or health conditions is given.
2. Separation techniques
2.1. electrophoresis.
Electrophoresis is an electrokinetic process, with a long history of development, which separates charged particles (macro-molecules, such as proteins) in a fluid using a field of electrical charge [ 63 ]. Particles could be separated based on their charge or their mass. The electrophoretic method is a widely used method in a modern research laboratory, but not so much in a clinical laboratory. Electrophoresis is commonly used for proteins, peptides and nucleic acid analysis [ 64 ]. It could be applied as a qualitative or quantitative analytical technique on its own if a reference substance is given, or it could be used as a separation technique for a sample to be further examined by other techniques, such as mass spectroscopy, fluorescence spectroscopy and etc.
Aguzzie et al. successfully used the electrophoretic technique to detect Bence-Jones protein (BJP) in human urine with the limit of detection LOD of 1 mg/L to BJP, and LOD of 3 mg/L to other types of protein [ 65 ]. These results were obtained by immunofixation using Dako antisera on cellulose nitrate followed by further staining with gold. The authors claim that their technique does not require particular skill and is cheap. Such low levels of LOD allowed them to use unconcentrated urine samples, thereby eliminating the problem of the loss of low molecular weight proteins. Usually, though it is often necessary to concentrate the urine before electrophoresis because of the low urinary protein level [ 6 ]. This is a time-consuming process. Machii et al. used cellulose acetate membrane electrophoresis followed by colloidal silver staining on the urine of healthy subjects with success [ 6 ]. However, when they used silver staining on an agarose gel, urinary protein in healthy subjects was not detected. They estimated that urine has to be concentrated 10 times, furthermore, the pattern of the urinary fraction will change after the concentration procedure is conducted. Giovannoli et al. developed a noncompetitive capillary electrophoresis immunoassay with laser-induced fluorescence detection was used to determine human serum albumin (HSA) in buffer and urine [ 66 ]. The injection of an excess of labeled antibody off-line incubated with the analyte allows the surface capture of the free antibody and consequently the immunocomplex detection. In buffer, authors were able to achieve the limit of detection LOD of 0.60 mg/L, with the quantitative range up to 6.6 mg/L. Recovery in the urine matrix was dependent upon sample dilution and HSA added and was as low as 91 ± 8%.
One of the variations of the electrophoretic method is called gel electrophoresis, where a gel is used as an anticonvective medium. Particularly polyacrylamide gel PAGE is used for separating proteins due to the uniform pore size provided by the polyacrylamide. Jia et al. used PAGE gel electrophoresis with sodium dodecyl sulfate (SDS-PAGE) to show a good correlation (R 2 = 0.825) with the pyrogallol red-molybdate (PRM) assay technique used in clinical laboratories for urine samples [ 67 ]. Sodium dodecyl sulfate (SDS) coats proteins with a negative charge so that proteins are separated due to their size, not charge. The authors estimated that their method can detect protein as low as 5 mg/L. Tran et al. showed that the use of tube gel electrophoresis for protein separation was further expanded with the invention of gel-eluted liquid fraction entrapment electrophoresis (GELFrEE) [ 68 ]; shown in Fig. 1 .

Diagram of the GELFrEE device [ 68 ]. A gel column is utilized to achieve electrophoretic separation of proteins, analogous to SDS–PAGE, which are then eluted into the liquid-phase for manual collection. The fractionation can then be visualized by running a portion of the fractions on a SDS–PAGE gel. Reprinted from Refs. [ 95 ].
Bessonova et al. in their review paper compared capillary electrophoresis with five on-line preconcentration techniques, including field-amplified sample stacking (FASS), head-column field-amplified sample stacking (HC-FASS), stacking with a polymer solution, dynamic pH junction and large-volume sample stacking (LVSS) with reversed polarity for various analytes including protein in the urine [ 69 ]. The use of these preconcentration techniques makes it possible to increase sensitivity in the determination of analytes by a factor of 100 or higher. In another work by Bessonova et al., they found that the lowest LOD was obtained by the LVSS approach, and it was 15 mg/L with UV-detection [ 70 ].
Electrophoresis is often used to be followed by another method, such as mass spectroscopy. Wittke et al. used the on-line coupling of capillary electrophoresis (CE) with electrospray mass spectroscopy to distinguish the difference in polypeptide patterns in the urine of healthy individuals and patients with kidney disease [ 71 ]. Kiprijanovska et al. used two dimensional get electrophoresis coupled with mass spectroscopy (2D PAGE – MS) for mapping and identification purposes of the urine proteome [ 72 ]. For the same purposes, Nakayama et al. used cellulose acetate membrane electrophoresis coupled with liquid chromatography mass spectroscopy LC-MS [ 73 ].
2.2. Chromatography
Another widely used separation technique in chemistry is chromatography. In chromatography, a sample is dissolved in a fluid called the mobile phase, which carries it through a structure holding another material called the stationary phase. The various constituents of the mixture travel at different speeds, causing them to separate due to differential affinities (strength of adhesion, as an example) of the various components of the analyte towards the stationary and mobile phase results. Then resulting constituents can be further analyzed by various techniques, such as mass spectroscopy, UV-spectroscopy, fluorescence detection, etc. Depending on the mobile phase methods in chromatography could be classified as gas-chromatography (GC) [ 74 ], and liquid-chromatography (LC) [ 75 ]. Typically, proteomic sample size ranges from few microliters to 0.1 mL [61.
Ishida et al. quantitatively determined human serum albumin (HSA) in human plasma and urine by utilizing post-column high-performance liquid chromatography (HPLC) with fluorescence detection [ 76 ]. Particularly, they used 8-anilino-1-naphthalenesulfonic acid as a fluorophore to bind HSA. The sample size was 1 ml for urine, and the retention time was around 20 min. A linear relationship was observed between fluorescence peak and the amounts of HSA in both plasma and urine, as for the latter matrix, a linear relationship was observed up to 400 mg/L, with the correlation coefficient being 0.998. The LOD for HSA was 0.2 mg/L, while the recovery was 94.5 ± 3.8%. The same authors also compared their method with the immunological nephelometric method and obtained the correlation coefficient of 0.990 [ 77 ]. Often researchers compare their method with the immunoassay technique that is used in clinical practice. Brinkman et al. in their experiments using the immunonephelometric approach and HPLC determined that HPLC reveals higher values of HSA, especially in the lower concentration range, resulting in a higher prevalence of microalbuminuria [ 78 ]. Nephelometry only measures immunoreactive albumin, whereas HPLC measures both immunoreactive and immunoreactive intact albumin, as was found by Comper et al. with the HPLC method [ 79 ]. The authors also compared HPLC with radioimmunoassay (RIA), immunonephelometry (IN), and two different methods of immunoturbidimetry (IT).
Owen et al. used a new urinary albumin assay that uses size-exclusion high-performance liquid chromatography with UV-detection [ 80 ]. The limit of detection (LOD) of the assay based on a signal/noise ratio of 5 was 3.4 mg/L. The linearity of the HPLC assay was tested in a range of albumin concentrations from 4.3 to 240 mg/L. The maximum deviation from a mean recovery of 100% was 4.6% at a concentration of 66 mg/L. Total imprecision was less than 10% from 16 to 206 mg/L. They also conducted experiments to compare their approach with immunoturbidimetric (ITA) assay, which showed positive proportional bias, which decreased with increasing concentrations of albumin. Singh et al. used liquid chromatography with quadrupole time-of-flight mass spectrometry (Q-TOF, LC-MS) method for the quantification of urinary albumin using a 15 N-isotopically labeled bovine serum albumin as an internal standard in human urine matrix [ 81 ]. The limit of detection (LOD) was 4.84 mg/L, while the limit of quantification was 10.5 mg/L. Multiple calibration curves ranging from 4 to 625 mg/L were linear and were reproducible with the coefficient of determination (R 2 ) of 0.999.
Micellar electrokinetic capillary chromatography (MEKC) is a hybrid method that combines chromatographic and electrophoretic separation principles, extends the applicability of capillary electrophoretic methods to neutral analytes [ 82 ]. In micellar electrokinetic capillary chromatography, surfactants are added to the buffer solution in a concentration above their critical micellar concentrations, consequently, micelles are formed; micelles that undergo electrophoretic migration like any other charged particle. Glavac et al. utilized MEKC combined with UV-detection of urine samples of patients diagnosed with proteinuria without any sample pre-treatment before analysis [ 83 ]. They were able to simultaneously identify albumin (HSA), haemoglobin (HGB), myoglobin (MYO). For all three analytes, linear correlations were obtained with the correlation coefficient (R 2 ) being not less than 0.99. Particularly, for HSA, the limit of detection (LOD) was 0.115 mg/L, the limit of quantification (LOQ) was 0.383 mg/L, a linear range between 1 and 300 mg/L and the recovery was 101.9 ± 4.5%. In another work by Wu et al., a MEKC method combining field‐amplified sample injection (FASI) with UV-detection has been developed for the analysis of albumin (HSA) and transferrin (TRF) in human urine [ 84 ]. Field-amplified stacking or injection is an on-column (on-line) preconcentration technique which is based on the different conductivity between two solution plugs. For TRF, the authors obtained the following analytical parameters of the method: LOD 0.31 mg/L, linear range 1.00–100 mg/L with R 2 0.994. For HSA: LOD 0.14 mg/L, linear range 0.50–100 mg/L with R 2 0.999. The recoveries of TRF and HSA were 91.1–101.7%.
Jaffuel et al. performed Aquaporin-2 (AQP2) nephrotoxicity biomarker measurement by using liquid chromatography–multiple reaction monitoring cubed mass spectrometry assay (MRM mode in LC-MS/MS) [ 85 ]. AQPs regulate numerous downstream effector signaling molecules that promote cancer development and progression [ 86 ]. In numerous cancer types, AQP expression has shown a correlation with tumor stage and prognosis. Linearity is observed within the concentration range 0.5·10 −3 -50·10 −3 mg/L, intra and inter-assay precision ranged from 9 to 35% at the lower limit of quantification (LLOQ), and accuracy from 94 to 114%.
Overall, due to complex composition of biofluids, including the urine, liquid chromatog-raphy in particular HPLC, which is frequently coupled with MS for detection, remains a common method in bioanalytical laboratories to separate complex mixtures. A review by Novakova et al. states that in order to decrease time for an analysis without compromising resolution and separation efficiency, three main approaches in HPLC exist – the use of monolith columns, LC at high pressures and temperatures, and HPLC at ultra-high pressures [ 87 ]. By employing those approaches, time for analysis of biofluids could be done within few minutes up to half an hourhe Table 2 below shows a summary table of quantitative methods for application of electrophoresis and chromatography for protein determination in the human urine matrix.
Summary table of quantitative methods for application of electrophoresis and chromatography for protein determination in the human urine matrix (unless otherwise stated in the method section). CV (coefficient of variation) parameters are taken from maximum readings, for Recovery – the range is given.
3. Detection techniques
3.1. mass spectrometry.
Mass spectrometry is a powerful analytical technique that measures the mass-to-charge ratio of ions. Firstly, molecules in a sample must be ionized, which causes a large molecule to fragment into smaller ions; then those ions are separated by a magnetic field depending on their mass to charge ratio. The results are typically presented as a mass spectrum, a plot of intensity as a function of the mass-to-charge ratio. Usually, mass spectrometry is utilized after pre-treatment of a sample by electrophoresis or chromatography.
Mass spectrometry is a widely used technique for proteomics studies. The proteome is the entire set of proteins that is produced or modified by an organism. Research on urine proteomics is important for the identification purposes of reliable biomarkers that help the diagnosis of disease [ 90 ]. In a review by Kalantari et al. on urine proteomics is stated that common techniques for urinary proteome analysis are two-dimensional gel electrophoresis followed by mass spectrometry (2DE-MS), liquid chromatography coupled to mass spectrometry (LC-MS), surface-enhanced laser desorption/ionization coupled to mass spectrometry (SELDI-TOF), and capillary electrophoresis coupled to mass spectrometry (CE-MS) [ 59 ]. By using mass spectroscopy protein constituents of urine were identified. Also reviews by Albalat et al. and Decramer et al. focus on mass spectrometry-based urinary protein and peptide profiling [ 33 , 60 ]. The advantages and disadvantages of different mass spectrometry methods are compared. As was mentioned in the introductory part of this review, Marimuthu et al. by using high-resolution Fourier transform mass spectrometry were able to identify 1823 proteins in the urine of healthy subjects [ 12 ]. Santuccie et al. by using mass spectrometry and sub-fractionating normal urine by successive steps (vesicle separation, CPLL, and solvent treatments) were able to identify 3429 individual proteins [ 13 ].
Two ionization techniques in mass spectrometry are commonly used for protein studies: electrospray ionization (ESI) and matrix-assisted laser desorption/ionization (MALDI) [ 91 ]. In the ESI approach, ions are produced in an electrospray in which a high voltage is applied to a liquid to create an aerosol. ESI is different from other ionization processes (e.g. MALDI) since it may produce multiple-charged ions, effectively extending the mass range of the analyzer to accommodate the kDa-MDa orders of the magnitude observed in proteins and their associated polypeptide fragments [ 92 ]. In MALDI, a sample is dispersed in a certain solid matrix (e.g. aromatic compounds), which then irradiated by the laser that causes electronic excitation of molecules in the sample matrix [ 93 ]. Both methods ESI and MALDI produce relatively high molecular ions due to mild fragmentation of those approaches. It should be noted a specific method of MS applied to biomolecules, which is called tandem mass spectrometry, also known as MS/MS. In MS/MS two or more mass analyzers are coupled together using an additional reaction step to increase their abilities to analyze samples. In general, protein identification in mass spectroscopy is performed using either bottom-up or top-down approaches. Bottom-up protein identification methods rely on enzymatic digestion to break down proteins into smaller peptides that are easier to ionize and fragment, resulting in higher sequence coverage [ 94 ]. However, in top-down methods, intact proteins are injected into the mass spectrometer and subjected to fragmentation without pre-treatment [ 95 ]. Aside from better tracking of protein modifications, an advantage of top-down protein sequencing is that it complements imaging experiments by enabling mass measurement of the intact protein that relates more directly to the MALDI IMS generated signals [ 96 ].
Analysis of a mass-spectrum, a plot of intensity as a function of the mass-to-charge ratio, could be a challenge itself, especially for big molecules like proteins, since they can be divided in many fragments resulting in a complex spectrum. Usually, the mass-spectrum is compared by software with the existing database. Several computationally feasible approaches have emerged for performing protein inference from such data [ 97 ].
Mass spectrometry in itself does not allow for quantitative analysis due to the different physicochemical properties of different peptides and proteins [ [98] , [99] , [100] ]. However quantification in MS is possible if an internal standard is used, for such there are approaches based on labeling with stable isotopes (such as N-15, O-18), involving artificial labeling of peptides and proteins. On the other hand, there are label-free approaches, in which the samples retain their native isotope composition and are compared between separate measurements. In general, they could be classified into two categories: methods that involve comparing peptide signals at the level of LC-MS analysis; methods that involve counting the number of identified peptides or acquired fragment spectra. Also, it should be noted that label-based methods are relatively laborious while label-free methods are less accurate [ 98 ]. An example of quantitative protein determination, in this case – albumin, in the urine matrix by MS methods is described in a paper by Singh et al. [ 81 ]. They used N-15 isotopically labeled albumin as an internal standard. The authors achieved the limit of detection (LOD) of 4.84 mg/L, the limit of quantification of 10.5 mg/L with the linear range being from 4 to 625 mg/L, R 2 = 0.999. Chen et al. used isotope-labeled peptides as internal standards for quantification of 63 proteins in human urine by multiple reaction monitoring-based mass spectrometry for the discovery of potential bladder cancer biomarkers [ 101 ]. Bachmann et al. experimentally compared routine measurements of urinary albumin to isotope dilution tandem mass spectrometry (IDMS) [ 102 ]. They reported that median differences between the largest positive and negative biases versus IDMS were 45%, 37%, and 42% in the concentration intervals of 12–30 mg/L, 31–200 mg/L, and 201–1064 mg/L, respectively. Lieske et al. reported their current status on a developing reference method for quantification of urinary albumin by liquid chromatography coupled to tandem mass spectrometry (LC-MS/MS) [ 103 ]. They used three different labeled peptides as internal standards to cover the range of clinical values (5–1270 mg/L). Recovery was between 97% and 108% with an average of 103%. In conclusion, quantitative mass spectroscopy assays for peptides and proteins are difficult to implement, and the future will see standardization of MS validation and quality control requirements.
Overall, mass spectrometry coupled with liquid chromatography is a powerful tool for the discovery and the analysis of biomarkers of diseases from biofluid samples, which includes proteomics study of urine as well [ 104 ]. Many of urinary proteome biomarkers were discovered by this technique. However, quantitative protein determination represents a challenge by following this approach [ 105 ]. Thus, mass spectroscopy could be used for biomarker discovery but not for clinical applications [ 60 ]. Another consideration for an analytical technique is the cost of analysis. Practical usage of LC-MS is limited by factors such as instrument size, cost, ease of operation, though, low cost compact mass spectroscopy detectors coupled with cinematographic separation are being developed, their costs equal to around $50000 [ 58 ].
3.2. Immunoassay
Immunoassay is a widely used technique in clinical and research laboratories to determine qualitatively and quantitatively macromolecules (usually proteins) generated from organisms [ 106 ]. Immunoassay is based on a reaction between an antigen and an antibody, which is found in the adaptive immune system of vertebrates, that includes humans [ 107 ]. The reaction is carried out in-vitro (not in a living organism), and the result of it is the formation of a complex antibody-antigen formed by spatial complementary, like lock and key, thus such interactions are highly selective. Antibody-antigen complexes are bounded by weak forces such as electrostatic forces, hydrogen bonds, hydrophobic interactions, and van der Waals forces; which means that such bindings are reversible. The specific piece of the antigen to which an antibody binds is called the epitope. Usually, in analytical papers, the analyte is the antigen while the reagent is the antibody. Immunoassay methods have been widely used in many areas of pharmaceutical analysis such as diagnosis of diseases, therapeutic drug monitoring, clinical pharmacokinetics, and bioequivalence studies in drug discovery and pharmaceutical industries [ 108 ]. Conventional immuno-chemical based urinary albumin assays for diabetic patients as well as nondiabetic patients with renal disease, including those who may suffer hypertension and cardiovascular disease, now amount to 100 million assays per year worldwide as of 2004 [ 79 ]. The widespread immunoassay methods in the pharmaceutical analysis is attributed to their inherent specificity, high-throughput, and high sensitivity for the analysis of a wide range of analytes in biological samples as well the relatively low cost of the instruments, tools, or the reagents [ 109 ].
Based on signal detection immunoassays could be classified as follows:
Immunoassay experiments could be classified into types – competitive and non-competitive [ 106 , 109 ]. The competitive immunoassay relies on the competition between the antigen of interest (the analyte) and a constant amount of a similar but labeled antigen for a limited amount of specific antibody. When these immunoanalytical reagents are mixed and incubated, the analyte is bound to the antibody-forming an immune complex. This complex is separated from the unbound reagent fraction by physical or chemical separation technique, though sometimes separation is not required. Based on whether the separation step is or is not required, immunoassay methods can be classified into a heterogeneous or a homogeneous assay, respectively. The analysis is achieved by measuring the label activity in either of the bound or free fraction. On the other hand, the non-competitive assay, also called immunometric, uses an excess of labeled specific antibody toward the analyte of interest. It requires two antibodies that bind to non-overlapping epitopes on the analyte molecule. One of the two antibodies is bound to the solid phase, and the second one is labeled and used for detection. Labels commonly used in immunoassays include radioisotopes, fluorophores, and enzymes. For both types, the schematic diagrams are given below (for the competitive – Fig. 2 , for the non-competitive – Fig. 3 ), reprinted from Ref. [ 109 ].
- • Radioimmunoassay RIA. Detection is based on radioactive labeling of antigen with unstable isotopes such as I-125, C-14, H-3.
- • Enzyme immunoassay EIA with sub-categories: ELISA – Enzyme-linked immunosorbent assay; EMIT – Enzyme-multiplied immunoassay technique; MEIA – Microparticle enzyme immunoassay.
- • Fluoroimmunoassay FIA
- • Chemiluminescence immunoassay CLIA
- • Immunonephelometry IN. Detection is based on an antigen-antibody complex scattering light.
- • Immunoturbidimetry IT. Detection is based on an antigen-antibody complex blocking light thus increasing the turbidity of the sample.

Schematic diagram for the competitive immunoassays. Reprinted from Ref. [ 109 ].

Schematic diagram for the non-competitive immunoassay. Reprinted from Ref. [ 109 ].
As it was mentioned in the introductory part, the concentration of albumin higher than 2 mg/L could be indicative of potential kidney diseases [ 10 ]. Subsequently for many immunoassays methods for albumin determination in urine the limit of detection (LOD) of magnitude around 1 mg/L would be enough for clinical purposes, thus priority should be given to the parameters such as specificity, accuracy, costs, etc. However for low abundant proteins achieving lower LOD is important [ 110 ]. In 1980–1990 common immunoassays for urinary proteins for clinical purposes were developed. Comper et al. compared four immunoassays (RIA, IN, two IT) and high-performance liquid chromatography (HPLC) [ 79 ]. The correlation coefficients calculated were high (>0.85) which is indicative of a strong linear relationship between all assays studied. While the correlation was high, the slopes of the regression line were significantly different from 1, it means that certain methods tend to underestimate or overestimate. For example, the authors found that the Beckman immunonephelometry assay consistently gives values approximately threefold lower than the Dade-Behring immunoturbidimetry assay. There have been numerous other studies that have found similar levels of variation between different immunoassays and other techniques, such as in the paper by Shaikh et al. [ 111 ]. The authors claim that theirs LC-MS and HPLC assays both performed poorly at concentrations below 20 mg/L, while immunoturbidimetric assay underestimated albumin compared with LC-MS. Hoofnagle et al. state that immunoassays have four common problems of which a researcher should be aware [ 112 ]. Assay performed with one set of reagents does not measure the same concentration as another which results in patients having measurements performed on the same platform for continuity of care. The other three problems results in falsely low or high results depending on the analyte concentration, antibodies used. While immunoassays methods have their shortcomings they are still widely used in clinical practice. Modern immunoassays are actively being developed and some of their analytical parameters are given in the summary Table 3 .
Summary table of quantitative methods for application of immunoassay for protein determination in the human urine matrix (unless otherwise stated in the method section). CV (coefficient of variation) parameters are taken from maximum readings, for Recovery – the range is given.
3.3. Fluorescence spectroscopy
Fluorescence is a luminescence phenomenon in which a compound emits light after absorption of electromagnetic irradiation [ 133 ]. In most cases, the emitted light has a longer wavelength, and therefore lower energy, than the absorbed radiation. Often in chemical analysis, a sample is irradiated in UV or visible range, and as a result, fluorescence occurs in the visible and near-infrared region respectively. Fluorescent materials cease to glow nearly immediately when the radiation source stops, unlike phosphorescent materials, which continue to emit light for some time after. Fluorescence spectroscopy is one of the most common techniques with applications in geology, chemistry, medicine, and astronomy [ 133 ]. Because of the sensitivity that the method affords, fluorescent molecule concentrations as low as 1 part per trillion can be measured [ 134 ]. Fluorescence spectroscopy can be used on its own, and also it is often used in conjunction with high-performance liquid chromatography as a detector. Often fluorescence detection is used in immunoassays to either label antigen or antibody.
Usually, analyte molecules do not emit strong luminescence for their analytical detection. Hence an analyte usually modified with a fluorophore, or a fluorescent probe, in other words. The fluorophore (or a fluorescent probe) is a fluorescent chemical compound that can re-emit light upon light excitation. A suitable fluorescent probe should have the following characteristics: (i) is conveniently excitable, without simultaneous excitation of the biological matrix, and detectable with conventional instrumentation; (ii) is bright, that is, possesses a high molar absorption coefficient at the excitation wavelength and a high fluorescence quantum yield, (iii) is soluble in relevant buffers, cell culture media or body fluids, (iv) is sufficiently stable under relevant conditions, (v) has functional groups for site-specific labeling, (vi) has reported data about its photophysics, and (vi) is available in reproducible quality [ 135 ]. Most common fluorophores are usually organic molecules, that typically contain several combined aromatic groups or planar or cyclic molecules with several π bonds. Fluorophores are sometimes used alone, as a tracer in fluids, as a dye for staining of certain structures, as a substrate of enzymes, or as a probe or indicator (when its fluorescence is affected by environmental aspects such as polarity or ions). More generally they are covalently bonded to a macromolecule, serving as a marker (or dye, or tag, or reporter) for affine or bioactive reagents (antibodies, peptides, nucleic acids). Fluorophores are notably used to stain tissues, cells, or materials in a variety of analytical methods, i.e., fluorescent imaging and spectroscopy. Some examples of widely used fluorophores are fluorescein, and by its amine reactive isothiocyanate derivative fluorescein isothiocyanate, methylene blue. Over the last two decades, the role of semiconductor quantum dots (QD) and other types of nanoparticles have grown considerably in bionanalysis and bioimaging. Petryayeva et al. conclude that QDs as fluorophores and for other bioimaging purposes offer nontrivial advantages, such as the brightness needed for sensitive detection, the photostability needed for tracking dynamic processes, the multiplexing capability needed to elucidate complex systems or the nanoscale interface needed for biomolecular engineering of novel probes and biosensors [ 136 ]. The fluorescent labeling in immunoassays is the common practice (see page 20).
A method of fluorescence, where two light-sensitive molecules, that transfer energy between them, is called fluorescence resonance energy transfer. Fluorescence resonance energy transfer (FRET) involves the transfer of energy from a fluorescent donor molecule via a nonradiative dipole-dipole interaction to an acceptor molecule. The emission of the energy transfer-excited acceptor can be distinguished from donor emission by the use of a longer-wavelength filter (spatial resolution). The fluorescent lanthanide chelates of europium and terbium are attractive FRET donors. The use of a long-lifetime donor fluorophore and a short-lifetime acceptor fluorophore together with pulsed-laser excitation and time-resolved detection (temporal resolution) are effective in reducing the background signal. Qin et al. used time-resolved FRET for the determination of urinary albumin [ 118 ]. Particularly, they used, in the competitive homogeneous assay, an albumin-specific monoclonal antibody labeled with a stable fluorescent europium chelate as donor and an albumin labeled with cyanine 5 (Cy5) as acceptor. The limit of detection was 5.5 mg/L with the detectable range being 10–320 mg/L. Another interesting approach to fluorescence spectroscopy is the fluorescent quenching. Chawjiraphan et al. developed a method to determine HSA in urine and serum by the graphene oxide-mediated (GO) fluorescence quenching aptasensor [ 132 ]. When albumin was added to the complex GO with the fluorescence-labeled aptamer, the aptamer detached from the complex to bind albumin, which resulted by an increase in fluorescence intensity, as shown in Fig. 4 . The limit of detection was 0.05 mg/L and the detection range is 0.1–14.0 mg/L. Other quantitative works using the fluorescence spectroscopy are summarized in Table 4 .

Schematic of graphene oxide-mediated fluorescence quenching aptasensor for the detection of albuminuria in urine and HSA in human serum. When albumin was added to the complex GO with the fluorescence-labeled aptamer, the aptamer detached from the complex to bind albumin, which resulted by an increase in fluorescence intensity. Reprinted from Refs. [ 132 ].
Summary table of quantitative methods for application of fluorescence spectroscopy for protein determination in the human urine matrix (unless otherwise stated in the method section). CV(coefficient of variation) parameters are taken from maximum readings, for Recovery – the range is given.
3.4. The Infrared and Raman spectroscopies
The absorption of infrared radiation IR excites vibrational transitions of molecules [ 148 ]. The infrared spectral region covers wavelengths from 780 nm to 1000 μm which can be further subdivided into the near-infrared region from 780 nm to 2500 nm, the mid-infrared region from 2500 nm to 50 μm and the far-infrared region from 50 μm to 1000 μm. Since vibrational frequency and probability of absorption depend on the strength and polarity of the vibrating bonds, they are influenced by intra- and intermolecular effects [ 149 ]. The approximate position of an infrared absorption band is determined by the vibrating masses and the type of bond (single, double, triple), the exact position by electron-withdrawing or donating effects of the intra- and intermolecular environment and by coupling with other vibrations. Information that can be derived from the infrared spectrum:
- • Chemical structure of the vibrating group
- • Chemical properties of neighbouring groups in a molecule
- • Redox state
- • Bond parameters
- • Hydrogen bonding
- • Electric fields
- • Conformational freedom
Basically, all polar bonds contribute to the infrared absorption so there is no need to specifically label biomolecules to detect them. However, the infrared spectrum of biomolecules is challenging to analyze since they are complex with many overlapping bands. Certain mathematical and statistical procedures have to be utilized to analyze the resulting spectrum. The IR spectroscopy is often used to identify organic structures because functional groups give rise to characteristic bands both in terms of intensity and position (frequency). The IR spectra of organic molecules are often interpreted as having two regions: functional group region (>1500 cm −1 = 6.7 μm), and fingerprint region (<1500 cm −1 ). In the fingerprint region, there are many troughs that form an intricate pattern that can be used as a fingerprint to determine the compound. Modern infrared spectrometers are usually Fourier transform infrared (FTIR) spectrometers [ 149 ]. The FTIR spectrometer works differently than the dispersive spectrometer where a monochromatic light beam is absorbed by a sample. This technique shines a beam containing many frequencies of light at once and measures how much of that beam is absorbed by the sample. Next, the beam is modified to contain a different combination of frequencies, giving a second data point. This process is rapidly repeated many times over a short timespan. Afterward, a computer takes all this data and works backward to infer what the absorption is at each wavelength by a Fourier transform.
Another analytical technique that relies on molecular vibrations is Raman spectroscopy [ 150 ]. Raman spectroscopy is a powerful technique to determine vibrational modes of molecules to provide a structural fingerprint, like infrared spectroscopy, by which molecules can be identified. Infrared spectroscopy typically yields similar information because certain molecular vibrations can occur in both techniques but some only detectable in either, which makes them complimentary as well. Also, transitions that have large Raman intensities often have weak IR intensities and vice versa. Raman spectroscopy relies upon the inelastic scattering of photons. The laser light interacts with molecular vibrations, phonons or other excitations in the system, resulting in the energy of the laser photons being shifted up or down. The shift in energy gives information about the vibrational modes in the system. Raman spectra for biomolecules is hard to analyze due to overlapping bands, the same issue as in the IR spectroscopy. Certain mathematical procedures must be employed on Raman spectra to analyze a sample.
Despite difficulties in the analysis of large biomolecules such as proteins, the IR, and Raman spectroscopy make their advances in this regard. For the protein analysis with IR, the MIR region plays an important role since it includes bands that predominantly arise from three conformationally sensitive vibrations arising from the peptide backbone—namely, amide I, amide II, and amide III [ 151 ]. Amide group vibrations of the backbone have attracted much attention in protein IR spectroscopy, as they are native to all proteins and inform on secondary conformation and solvation. Such bands include the amide I region, between 1600 and 1700 cm-1, primarily resulting from C Created by potrace 1.16, written by Peter Selinger 2001-2019 O stretching vibration, the amide II band, related to CN stretch and NH in-plane bending, the amide III vibration, associated with CN stretching, NH bending, and CO in-plane bending, and, finally, the amide A (NH stretch) band. Conventional IR and Raman methods often lack enough sensitivity. Surface-Enhanced Infrared Absorption Spectroscopy (SEIRAS) [ 152 ], and Surface-Enhanced Raman spectroscopy SERS [ [153] , [154] , [155] ] improve sensitivity to overcome this problem. Both SEIRAS and SERS utilize the surface plasmon effect from the interaction of light with metallic nanoparticles. SERS has an enhancement factor (of the order of 10 6 –10 12 ) compared to the traditional Raman signal [ 156 ]. For SEIRA, the surface-enhancement is comparatively modest (~10 1 –10 3 ) [ 157 ]. Although the enhancement factor of SEIRAS is smaller than that of SERS, the cross-section for IR absorption is several orders of magnitude higher than the corresponding Raman cross-section. Despite the modest enhancement factor, the SEIRAS technique may be sufficient for many applications [ 152 ].
It should be emphasized again that the IR and Raman spectra are rich in data, and mathematical interpretations of those along with the right calibration procedure are important for analysis. Jessen et al. developed a method for simultaneous determination of glucose, triglycerides, urea, cholesterol, albumin and total protein in human plasma by Fourier transform infrared spectroscopy [ 158 ]. They put their samples of 40 μL without any reagent treatment or preconcentration into water-thermostated CaF cells to measure transmission. The spectra were recorded between 3100 and 950 cm −1 . In the analysis, the first derivative of the original spectra was used. The first derivative was computed by using the Savitzky–Golay algorithm with 9 smoothing points. For each sample, two IR spectra were recorded and the mean spectrum was subsequently corrected by the subtraction of a water blank spectrum. Since the IR spectra are complex, a calibration procedure is important. The authors, used between 144 and 169 patient samples for each analyte to cover a wide range of concentrations. Jessen et al. compared their results between the FTIR method by other reference methods, which were mainly based on immunoassays. For each analyte, the FTIR spectra are processed by a stepwise selection of wavenumbers giving the optimal least-squares correlation between the measured IR signal, and the analyte concentration measured by the reference method. The correlation coefficients between the FTIR and the reference method were 0.87<R 2 < 1.00, and specifically for albumin it was R 2 = 0.92. For albumin, the calibration range was 20–60 g/L. The authors concluded that the developed FTIR methods use a simple and robust technology to achieve stable and accurate results that meet international quality criteria for the measurement of glucose, triglycerides, urea, cholesterol, albumin, and total protein in human plasma.
Ma et al. synthesized thiourea-functionalized silica nanoparticles and used them for the preconcentration of albumin in urine [ 159 ]. The adsorbent with the analyte was then used for near-infrared diffuse reflectance spectroscopy measurement directly and the partial least squares model was established for quantitative prediction. Forty samples were taken as a calibration set for establishing the PLS model and 17 samples were used for validation of the method. The principle of the PLS method is the combination of different signal processing methods and wavenumber regions. The wavenumber regions that produced the best results in cross-validation were selected. The correlation coefficient and the root mean squared error of cross-validation is 0.9986 and 0.43, respectively. The residual predictive deviation value of the model is as high as 18.8. The recoveries of the 17 validation samples in the concentration range of 3.39–24.39 mg/L are between 95.9 and 113.1%. In another work by Hall et al., the authors performed multiple linear least-squares (MLR) and an enhanced partial least-squares (PLS) using this time the second-derivative of the absorbance data to determine analytes, including protein, in unmodified human serum [ 160 ]. Pezanniti et al. used a polynomial based spectral smoothing method to the urine matrix [ 161 ]. In general, all the mentioned works requires no specific reagents, they are fast and are able to quantify multiple analytes with one spectrum.
Premasiri et al. investigated urine by Raman spectroscopy [ 5 ]. The result was that since urea is in high concentration, normal Raman spectroscopy is sufficient for analysis of the analyte. However, all other components are in low concentrations requiring the use of SERS. Bispo et al. correlated the amount of urea, creatinine, and glucose in urine from patients with diabetes mellitus and hypertension with the risk of developing renal lesions by means of Raman spectroscopy and principal component analysis [ 162 ]. They took Raman spectra of control subjects and patients with illnesses, and then the spectra were submitted to principal component analysis (PCA) followed by discriminant analysis. The essence of PCA is to yield an orthogonal basis in which different individual dimensions of the data are uncorrelated. The discriminating model showed a better overall classification rate of 70%. The same approach was utilized by Almeida et al. to estimate concentrations of urea and creatinine in the human serum of normal and dialysis patients [ 163 ]. PCA showed high discrimination between dialysis and normality (95% accuracy). Senger et al. analyzed by the Raman spectroscopy three types of patients: patients receiving peritoneal dialysis (PD) therapy for end-stage kidney disease; patients receiving peritoneal dialysis (PD) therapy for end-stage kidney disease; urine from healthy human volunteers, as shown in Fig. 5 [ 164 ]. The authors used the set of mathematical procedures on spectra which included spectral processing (e.g., truncation, baselining, and vector normalization); principal component analysis (PCA); statistical analyses (ANOVA and pairwise comparisons); discriminant analysis of principal components (DAPC); and testing DAPC models using a leave-one-out build/test validation procedure. Their approach was able to identify “unknown” urine specimens as from PD patients or healthy human volunteers with better than 96% accuracy (with better than 97% sensitivity and 94% specificity).

Raman spectra of PD patient urine and spent dialysate.(A) Averaged, baselined, and vector normalized Raman spectra from 362 urine specimens obtained from patients receiving PD therapy for ESKD. (B) Averaged, baselined, and vector normalized Raman spectra from 395 spent PD dialysate specimens. Reprinted from Ref. [ 164 ].
Chamuah et al. used a blu-ray DVD as a SERS substrate for the detection of albumin, creatinine, and urea in urine [ 165 ]. The minimum concentration of albumin, creatinine, and urea which can be measured by Raman spectrometer is 0.1 mg/L, 0.2 mg/L, and 0.6 mg/L respectively. The authors investigated correlations between the SERS signal taken from their signature Raman peaks (albumin 1208 cm −1 , creatinine 1444 cm −1 , urea 1018 cm −1 ) and the concentration of the respective substance. The linear correlation coefficients (R 2 ) were 0.956 for albumin, 0.976 for creatinine, 0.982 for urea. Dou et al. determined quantitatively concentration of glucose, acetone, urea, and creatinine in the human urine [ 166 ]. Their detection limits were 410 mg/L, 400 mg/L, 49 mg/L, and 15 mg/L, respectively. Good correlations were obtained between the concentrations and the Raman intensities with the R values of 0.924 (glucose), 0.987 (acetone), 0.962 (urea), and 0.923 (creatinine), respectively. The authors showed that the excitation wavelength is an important factor to reduce fluorescence of urine, which reduces the signal to noise ratio. The laser with a wavelength of 750 nm performed better than 600 nm, 650 nm, 700 nm. Gaipov et al. have begun to study patients with elevated protein content in urine vs control group (normal protein content) using combination of hybrid Brillouin-Raman spectroscopy and SERS [ 167 ].
Chamuah et al. assigned the signature Raman peaks to the corresponding vibrations in analytes [ 165 ]:
- 1. For albumin – peaks at 1208 cm −1 and 1370 cm −1 are attributed to SO 2 symmetric stretch and CH bond deformation of albumin.
- 2. For creatinine – the Raman signature peaks are observed at 888 cm −1 , 958 cm −1 , and 1444 cm- 1 which could be due to C Created by potrace 1.16, written by Peter Selinger 2001-2019 O stretching, C–C stretching and CH3 anisometric deformation respectively.
- 3. For urea – a very strong Raman peak at 1018 cm −1 which corresponds to C–N stretching mode.
Throughout is important consideration for laboratory tests. Zhu et al. performed quantitative SERS detection of creatinine in urine, and then compared with a clinically validated enzymatic “creatinine kit” [ 168 ]. They wrote that SERS detection process could be completed within 2 min compared with 11 min for the creatinine kit, indicating the practicality of the quantitative SERS technique as high-throughput platform for relevant clinical and forensic analysis.
4. Urinary protein biomarkers
Human urine contains thousands of individual proteins [ 12 , 13 ]. Excessive presence of some of them are associated with certain diseases related not only to renal diseases, but also to cancer, diabetes, and infections. Those molecules that are associated with a certain biological condition are called biomarkers. Most of those biomarkers in human urine were identified by comparing patients with healthy individuals by the means of chromatography or electrophoretic separation followed by mass spectroscopy, or by the immunoassay. Literature review of urinary protein biomarkers with related diseases is summarized in Table 5 , corresponding diagnostic sensitivity and diagnostic specificity are given [ 169 ]. Some of the listed research papers used Western blot as a technique [ 170 ]. Due to the usage of antibodies, this technique was counted as the immunoassay in Table 5 . Most of the methods mentioned followed established procedures. To get a general idea of those experimental methods, refer to the previous paragraphs about separation and detection techniques, and Table 2 , Table 3 , Table 4
Protein biomarkers in urine. Sensitivity is the percentage of individuals who have a given disorder who are identified by the method as positive for the disorder. Specificity is the percentage of individuals who do not have a given condition who are identified by the method as negative for the condition.
Below are some observations and conclusions regarding Table 5 about the content of the listed research papers:
- • Urinary protein analysis are useful for not only renal diseases. Urine proteomics can be used for detection of at least 15 conditions, including 8 kinds of cancer; 5 kinds of renal complications; and at least 3 different infections. The list of health complications that can be diagnosed from urine analysis is bigger if non-protein substances are included, such as peptides, nucleic acids, low molar mass metabolites, and etc; that can be found in reviews [ 14 , 26 , 27 , 36 ].
- ◦ In total 34 references are included in Table 5 ; 12 of them utilized mass spectrometry, 24 utilized the immunoassay, and 1 paper – fluorescence spectroscopy.
- ◦ Authors of these research papers utilized the mass spectrometry methods with further validation by the immunoassay – [ [171] , [172] , [173] , [174] ].
- ◦ All of the mentioned work (except [ 129 ]) in the immunoassay followed manufacturer's protocol, or if a custom immunoassay was used, other established procedure from their own or previous works.
- ◦ ELISA-based immunoassay was the most popular method accounting for 13 references.
- • Diagnostic sensitivity and specificity increased if a panel of several biomarkers was considered simultaneously. Though, the panel should be carefully chosen as not all of the components provide increase in diagnostic sensitivity and specificity.
5. Conclusion
Urine is a readily available liquid for the medical diagnosis of patients. Urine tests are non-invasive procedures that involve no pain or discomfort for patients to determine problems with kidney function since extensive medical research showed that patients, with high protein concentration in urine, have various kinds of illnesses of the kidney, referred to as proteinuria [ 1 ]. Thus the precise and simple determination of urinary concentrations of total protein is important for diagnostic purposes. In this review, we focused on instrumental determination of abundant proteins in urine by electrophoresis, chromatography, mass spectrometry, immunoassay, fluorescence, IR, and Raman spectroscopy. The mentioned techniques are not mutually exclusive, for example, high performance liquid chromatography is usually a separation step followed by mass spectrometry, another example is labelling with fluorophores in immunoassay. Each technique has its own strength to analyze urine for specific information, hence, it is hard to compare them directly, and to find a common denominator for comparison. Overall, number of cited experimental papers that were covered by this review in Table 2 , Table 3 , Table 4 , Table 5 : electrophoresis and chromatography – 22 in total and 8 related to HSA detection; mass spectrometry – 17 in total and 5 related to HSA; immunoassay – 47 in total and 17 related to HSA; fluorescence spectroscopy – 22 in total and 19 related to HSA; IR and Raman spectroscopy – 10 in total; all topics – 101 papers. Thus, almost half of the cited literature by the means of immunoassays, which is in a good correlation that immunoassays are widespread in clinics and science.
The limit of detection is important parameter for evaluating an analytical method. In order to develop a method, the limit of detection should take into account which amount of analyte has clinical significance. The 15–30 mg/L albumin concentration is a critical value that could indicate kidney problems when it is repeatedly exceeded [ 10 ]. For the corresponding articles discussed above, the limit of detection for electrophoresis, chromatography, mass spectroscopy, immunoassay, fluorescence exceeded 2 mg/L for albumin, which is enough for the clinical purpose. For some methods, explained in Ref. [ 117 , 119 , 122 , 123 , 127 , 132 , 143 ], the limit of detection does not exceed 0.1 mg/L for albumin in the urine matrix. For low abundant proteins the limit of detection must be obviously much lower. For example, in the article for Aquaporin-2 determination by Jaffuel et al. the limit of detection was 5·10 −4 mg/L [ 85 ]. In another work by Zhao et al., the LOD for Nuclear matrix protein 22 was 1.7·10 −6 mg/L [ 110 ]. For the IR and Raman spectroscopy methods the LOD might not be so relevant factor. The IR and Raman methods is better characterized by how accurate a particular method can distinguish whether a spectra belongs to a healthy subject or not, as evidenced from works [ 158 , 164 ].
Electrophoresis and chromatography are powerful separation techniques which are accompanied by further detection, usually UV–vis spectrometry or fluorescence. Since the human urine matrix is very complex consisting of inorganic and organic compounds, those techniques are useful for urine analysis [ 7 ]. Though certain methods might require preconcentration techniques to enhance sensitivity [ 69 ]. High-performance liquid chromatography with mass spectroscopy detection is a particularly powerful tool for proteomics studies, as it was able to identify 1823 proteins in urine [ 12 ]. Mass spectroscopy in itself does not allow for quantitative analysis due to the different physicochemical properties of different peptides and proteins [ [98] , [99] , [100] ]. However quantification in MS is possible if an internal standard is used, for such there are approaches based on labeling with stable isotopes (such as N-15, O-18), involving artificial labeling of peptides and proteins, as in work by Singh et al., specifically in the urine matrix [ 81 ]. The immunoassay is widely used technique both in clinical practice and in research papers for urine analysis. Though different immunoassay methods can overestimate or underestimate an analyte concentration [ 79 ]. They can hurt patients due to inaccurate results [ 112 ]. The IR and Raman spectroscopy have advantage of easy preparation of samples to analyze. They require no specific reagents, or no reagents at all, no preconcentration or dilution, as evidenced from Refs. [ 158 , 162 , 165 ]. They are rapid. On the other hand, due to overlapping bands of large biomolecules, and the complexity of urine components – hard to analyze their spectra that require mathematical analysis.
Overall, there is no "silver bullet" modern quantification method for the analysis of proteins in urine that has been reported and validated so far. However, at least for the routine clinical diagnostics of kidney problems, applications of relatively direct methods (such as SERS or IR) spectroscopy may expand as instrumentation, substrates (at least SERS substrates), software and computing power for data analysis (peak deconvolution) become progressively less expensive.
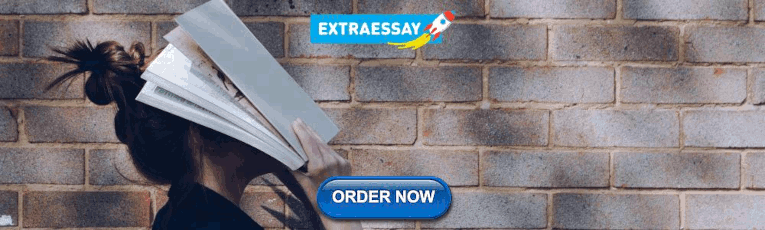
Funding Statement
The authors of this review acknowledge funding from the Nazarbayev University Collaborative Research Program (CRP) for 2020–2022 (Funder Project Reference: 091019CRP2105).
Declaration of competing interest
The authors declare that they have no known competing financial interests or personal relationships that could have appeared to influence the work reported in this paper.

Biuret Test: Principle, Procedure, and Uses
Protein analysis is done for various reasons; for example, in clinical laboratories, it is used for determining disease by analyzing serum proteins. The biuret test is one of the methods of protein analysis.
The biuret test is a colorimetric test that helps detect specific proteins or peptide bonds in given analytes. It is followed by spectrophotometry for quantification.
The test requires the use of a biuret reagent. This reagent is a solution that consists of hydrated copper (II) sulfate, sodium hydroxide, and potassium sodium tartrate.
The use of copper (II) ions present in the biuret reagent results in the formation of purple coloration if peptides are present. The intensity of the purple color is measured using a spectrophotometer.
Table of Contents
Principle of Biuret Test
Biuret test requires testing the analytes with biuret reagents. The reagent is a mixture of potassium sodium tartrate (KNaC 4 H 4 O 6 or C 4 H 4 KNaO 6 ), copper (II) sulfate or cupric sulfate (CuSO 4 ), and sodium hydroxide (NaOH).
Sodium hydroxide makes the solution alkaline, and potassium sodium tartrate is the chelating agent. The potassium sodium tartrate helps stabilize the cupric ions in the mixture and maintains the alkaline solution’s solubility.

The four nitrogen atoms present in the protein peptides bind to the reagent’s copper (II), resulting in a change of cupric ions to cuprous ions and displacement of peptide hydrogen under alkaline conditions.
Nitrogen binding to cupric ions also results in donating the lone pairs of an electron from nitrogen to the copper ions to form coordinated covalent bonds. The coordinate covalent bond with cupric ions forms a chelate complex that absorbs light with a wavelength of 540 nm, which imparts purple color. Hence, the formation of purple color indicates the presence of proteins in the analyte.
The test depends on the peptide bonds instead of the presence of amino acids in the sample, so it can help measure the protein concentration in whole tissue samples. Proteins purified using ammonium sulfate ((NH 4 ) 2 SO 4 ) may give false positive results due to nitrogen in ammonia.
Reagents and Materials Required
The reagents and materials required for the biuret test are as follows:
- The reagent required for performing a the test is biuret reagent . The biuret reagent is prepared by mixing 1% solution of CuSO 4 (1 gm CuSO 4 in 100 ml water) and 1.2 grams of potassium sodium tartrate. 10 ml 10% solution of NaOH (10 gm NaOH in 100 ml water) is added to the above mixture, known as a biuret reagent. The solution is blue in color due to the presence of the CuSO 4 .
- Other equipments required for the test are test tubes , a dropper or a pipette , a test tube holder, and a stand .
Procedure of Biuret Test
The following steps are followed to perform the biuret test:
- Take three clean and dry test tubes.
- In the first tube, add 1-2 ml test sample. Likewise, add 1-2 ml of egg albumin in the second one; in the third tube, add 1-2 ml of distilled water. The egg albumin is a positive control, whereas distilled water is a negative control for this test.
- Then, add 1-2 ml biuret reagent in all three tubes.
- After that, properly shake all the tubes to mix the reagent and samples/analytes. Then, let the mixture in the tubes stand for at least 5 minutes.
- Finally, observe the color change.
Precautionary measures
- Use test tube holders when holding the tubes with a solution.
- When preparing biuret reagent, handle NaOH carefully as it is a strong base that might cause corrosion when exposed to the skin.

Result Interpretation
The results of the biuret test are interpreted as follows:
Uses of Biuret Test
The biuret test is used mainly for diagnostic purposes, like determining serum proteins. Other applications of this test are as follows:
- The test helps in determining the type of proteins in unknown samples.
- It is used for quantification of protein by using a spectrophotometer alongside.
- It can help determine proteins in the urine, CSF, and other body fluids.
- The test helps determine the presence of specific proteins during food analysis.
Advantages of Biuret Test
The advantages of the biuret test are as follows:
- The test is simple and inexpensive.
- It can detect nitrogen from only peptide bonds.
- Very few components interfere with the test.
- The color is stable, so it causes less deviation.
- It is also a rapid test.
- It can detect proteins with at least four peptide bonds.
Disadvantages of Biuret Test
- The presence of amino acid histidine can give false positive results because there is a presence of nitrogen.
- If the buffer used to purify proteins has ammonium and magnesium salts, it can hinder the test.
- The presence of carbohydrates and fats can also hinder the test.
- This test alone cannot help in quantifying the protein in the sample; spectrophotometric analysis is required for quantification.
- Its sensitivity is lower than the Folin Lowry test.
- Only soluble proteins are helpful in this test, and different proteins give different colors, so standardization of colors is required for known proteins.
- A. Bianchi-Bosisio, PROTEINS | Physiological Samples, Editor(s): Paul Worsfold, Alan Townshend, Colin Poole, Encyclopedia of Analytical Science (Second Edition), Elsevier, 2005, Pages 357-375, ISBN 9780123693976, https://doi.org/10.1016/B0-12-369397-7/00494-5 . ( https://www.sciencedirect.com/science/article/pii/B0123693977004945 )
- Mohanlal Sukhadia University. (n.d.). Biuret test – Mohanlal Sukhadia University. https://www.mlsu.ac.in/econtents/2207_Biuret%20test.pdf .
Ashma Shrestha
Hello, I am Ashma Shrestha. I had recently completed my Masters degree in Medical Microbiology. Passionate about writing and blogging. Key interest in virology and molecular biology.
We love to get your feedback. Share your queries or comments Cancel reply
This site uses Akismet to reduce spam. Learn how your comment data is processed .
Recent Posts
Electrophoresis: Principles, Types, and Uses
Electrophoresis is a simple and sensitive separation technique in clinical and research laboratories. Since its discovery, it has been an essential tool used by biologists and chemists to separate...
Spectrophotometer: Principle, Parts, Types, and Uses
A spectrophotometer is a laboratory equipment that can measure the number of photons (the intensity of light) absorbed after passing through the solution of the sample. It can also detect the...
Talk to our experts
1800-120-456-456
- Biuret Test
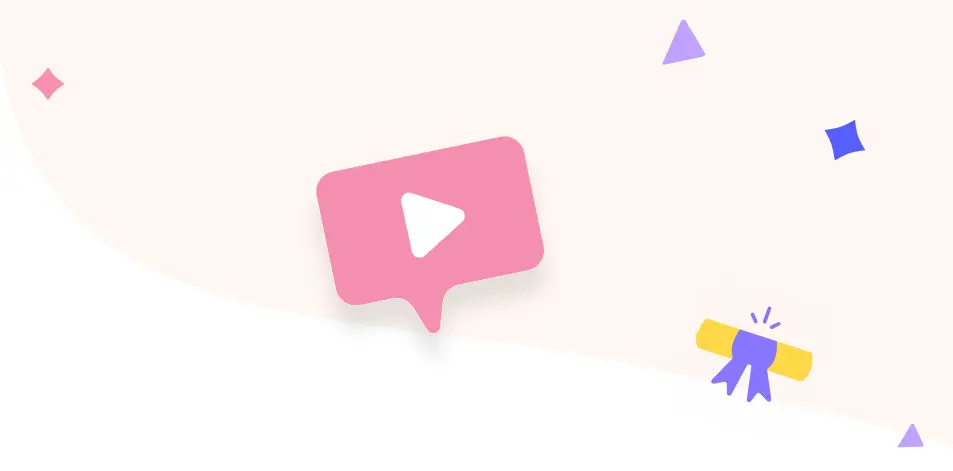
What is a Biuret Test?
The biuret test is a chemical test that can be used to see if an analyte has peptide bonds or not. As a result, the biuret test may be used to figure out how much protein is in the analyte. In this test, the presence of peptides induces the copper (II) ion to form pale purple (or mauve) coordination complexes (when the solution is sufficiently alkaline).
Overview of Biuret Test
Biuret is a compound produced by heating urea at 180 ℃. Biuret test is the name of a chemical test that utilises the Biuret reagents, which contains a 1% solution of Copper II sulphate (CuSO₄). It is the Cu₂⁺ in the Biuret reagent that forms a complex with the peptide bonds found in proteins. Hence, this test helps in determining peptide bonds in any substance.
When two acids are attached through carbonyl and amino groups, they are called peptide bonds. Moreover, the fundamental unit of protein is amino acids which are connected via peptide bonds. Notably, this experiment is a vital one and students about to appear on their boards in the near future should understand this chapter in detail.
Biuret Test Principle
This test is also known as Piotrowski’s test after the name of Gustaw Piotrowski, a polish physiologist who documented this test in 1857. Several other methods have been developed based on this method—for example, the modified Lowry test and BCA test.
However, the mechanism of this test works through a series of principles. They are discussed below.
In the presence of alkaline, when Biuret is reacted with dilute copper sulphate, a purple coloured substance is formed. The reason behind this colour is the formation of a chelate complex or the copper coordination complex.
Cu (II) or cupric ions create a chelate complex of violet colour, using oxygen of water and the unshared electron pairs of peptide nitrogen.
Since this complex absorbs light in 540 nm, it appears violet. In the presence of protein, it changes its colour from blue to violet.
(Image will be uploaded soon)
Naturally, the colour intensifies as the number of peptide bonds increases in protein.
Depending on this test’s principle, we can find the peptide bonds in any biological fluid.
This reaction takes place in a compound having at least two, H 2 N-CH 2 -, H 2 N-C, and H 2 N-CS- or similar groups attached directly or via a nitrogen or carbon atom .
One cupric ion is generally attached to six nearby peptide linkages through coordinate bonds.
Once you know about the principles of the Biuret test for protein, you must also concentrate on the detailed procedure of conducting. It has been discussed below in detail.
Biuret Test Manual
Materials Requirements
1% alanine and 5% albumin or egg white (as positive control)
Biuret reagents
Deionised water (as negative control)
Dry test tubes
Biuret Reagents
Biuret reagent is made of Copper sulphate (CuSO 4 ), sodium hydroxide (NaOH) and sodium-potassium tartrate (also known as Rochelle salt). Despite the name, this reagent does not contain Biuret ((H 2 N-CO-) 2 NH). It is a vital component of Biuret protein assay.
Biuret Reagent Preparation
It is formed by mixing NaOH in a solution of CuSO 4 , turning it alkaline. Following are the steps to yield 1000mL of Biuret reagent.
Take distilled water (500 ml) and dissolve pentavalent copper sulphate (1.5gm) and sodium-potassium tartrate (6gm).
Sodium potassium tartrate, a chelating substance that stabilises the copper ions.
Now take 2 molar hydroxide (375 ml)
Now take a volumetric flask and mix two solutions.
Finally, make it to 1000 ml by pouring distilled water.
Using the following steps, you can easily conduct this test.
First, take 3 dry and clean test tubes.
Now add 1 or 2 mL of the test solution, albumin and deionised water in the test tubes.
Add Biuret reagent (1-2 mL) in each test tube.
Now shake the solution well and let it stand for 5 minutes.
Finally, observe how the colour changes.
Often ammonium and magnesium ions hinder this test. However, using excess alkali, it can be removed.
Observation
(image will be uploaded soon)
Following is the Observation and Analysis of the Result for Each Sample Solution
Positive Result
The Colour turns purple.
All proteins and peptides give positive results.
Only amino acid, Histidine, gives a positive result.
Negative Result
No change in colour.
Also, to ensure that the test sample is alkaline, add a few drops of 5% sodium hydroxide solution to each test tube. Moreover, if you are using a Biuret reagent, ensure that you shake that well as it is prepared with a reagent of 1% Copper II sulphate and 5% sodium hydroxide.
Note: Do not forget to show your understanding of why 5% sodium hydroxide alkalises the sample and 1% Copper II sulphate forms a complex with peptide bonds with chemical reactions.
Increasing Biuret Test Sensitivity
Cu+ is a strong reducing agent that can react with Mo(VI) during Folin-Ciocalteu's test to produce molybdenum blue. Following this way, primarily proteins are detected in the concentrations between 0.005 and 2 mg/mL. On the other hand, Molybdenum blue can bind a few specific organic dyes such as malachite green and Auramine o. It results in the further amplification of the signal.
Cu + ions produce a deep purple complex with BCA or bicinchoninic acid. It allows detection of the proteins with a range of 0.0005 to 2 mg/mL. This is also referred to as "Pierce assay", honouring a reagent kit manufacturer.
Advantages
This method is the simplest and a rapid way to detect protein in a sample. Also, it is less expensive than the Kjeldahl test.
It provides a stable colour; hence, it does not cause deviations like other methods like UV absorption, Folin-Lowry, etc.
Apart from protein, very few compounds interfere in the test.
It only identifies N from protein or peptide bonds. It means it does not detect non-protein nitrogen.
Disadvantages
It is not as sensitive as the Folin Lowry test. Nonetheless, it is necessary for at least 2-4 mg protein to be detected.
A high concentration of ammonium salts and bile pigments influence the reaction.
It shows different colours for different proteins. For example, gelatine provides a pink-purple colour. Also, carbohydrates and lipids take away the clarity of the given solution.
The proteins have to be soluble for detection.
It is not an absolute test. The colours need to be standardised for known proteins such as BSA.
You can hasten the test simply by heating or by adding 30% isopropyl alcohol. It can reduce the reaction time from 35 to 10.
Significance of Biuret Test
It is widely used in determining the protein amount in urine.
Biuret test for protein is also useful to total protein's quantitative determination, using spectrophotometric analysis.
Biuret Test for Various Substances
The casein and pure protein content of skimmed milk, as well as whole milk's protein content, can be measured with the help of Biuret test solutions which consist of potassium hydroxide and a detergent. The Biuret test for protein is useful in the case of both cheese and meat as they get dissolved in potash lye or solutions of alkaline detergent. However, fat, lactose or turbidity disturbs this test. By extraction or additional measurements with a zinc-containing, copper-free, Biuret reagent, they are removed after mixing hydrogen peroxide.
Test Your Knowledge
1. Which colour do we get for a negative Biuret test?
Answer: (c)
2. What makes the Biuret reagent purple?
Peptide bonds
Hydrogen bond
Glycosidic bond
Answer: (a).
For further queries regarding the Biuret test for protein, visit our website. We have a wide range of notes on several topics of biochemistry and other subjects as well. You can also download model questions on each chapter and solve them to score excellent marks in every examination. Now you can also download our Vedantu app for better access to these materials.
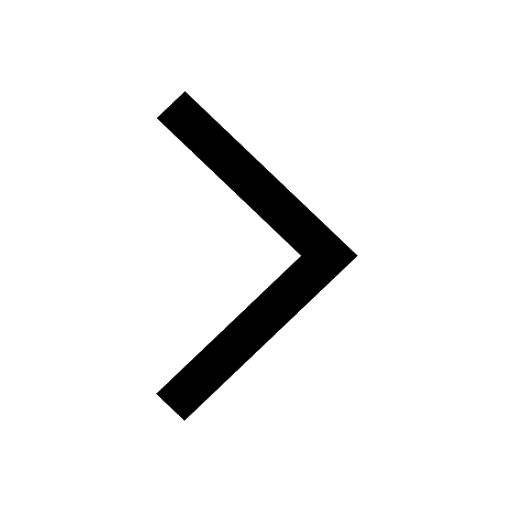
FAQs on Biuret Test
1. Which Free Amino Acids Give a Positive Biuret Test?
Histidine is the only amino acid that gives a positive result to the Biuret test. The reason behind it is that, in the absence of additional reducing agents, it acts itself as a reducing agent. However, if you add Histidine in large quantities, the reaction may alter to some degree.
2. What is the Use of CuSO 4 in Biuret Test for Protein?
CuSO 4 is used in the Biuret test because it is the main source of cupric II ions (Cu ++ ) in the solution.
3. Why is Biuret Solution Important?
Biuret solution is important primarily to test the presence of protein in any substance. Apart from that, it is also used to quantify the protein content in urine. As a matter of fact, the presence of excess protein in urine can result in kidney diseases and other complications like high pressure, diabetes mellitus, etc.
4. How Does Colour Change in Biuret Test?
The Biuret test is useful in finding out the protein in any substance, mainly through the changing of colours. For example, in the presence of protein, it turns pink-purple.
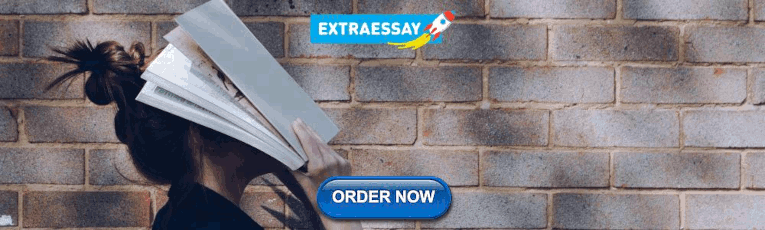
IMAGES
VIDEO
COMMENTS
Objective: To investigate the biuret reagent to detect proteins in the application, the impact of different test conditions for test results. Methods: The biuret method to select three different instruments, reagents, calibrators are arranged in combination to form 27 sets of detection systems, each detection system is a combination of 5 serum samples for testing, 5 measured values obtained ...
serves as the blank. 4. Now add 3 ml of Biuret reagent to all the test tubes including the test tubes labeled 'blank'. and 'unknown'. 5. Mix the contents of the tubes by vortexing / shaking the ...
The estimation of protein by the biuret and Greenberg methods - PMC. Journal List. Biochem J. v.36 (10-12); 1942 Dec. PMC1266873. As a library, NLM provides access to scientific literature. Inclusion in an NLM database does not imply endorsement of, or agreement with, the contents by NLM or the National Institutes of Health.
The biuret test exhibited linearity in a wide range of concentrations (0.1000 ± 0.0004 to 5.00 ± 0.02 mg/mL). ... Conference Paper. Nov 2022 ... in the detection of protein contaminants in drugs ...
Principle. Biuret test is a general test for compounds having a peptide bond. Biuret is a compound formed by heating urea to 180 °C. When biuret is treated with dilute copper sulfate in alkaline condition, a purple-colored compound is formed. This is the basis of biuret test widely used for identification of proteins and amino acids.
Edna Ogechi Nwachuku. Aim: This study evaluated the effects of chronic administration of some bouillon cubes on oxidative stress and liver function markers in female albino rats. Methodology: A ...
Additional key phrases: biuret; reference serum; bias; imprecision The main aim of this study was to evaluate the current methodology for the routine measurement of serum total protein with a view to identifying whether improvements were necessary and, if so, in what respect. The first part provides a brief
The modified Lowry method is a combination of the Biuret method, where copper ions react with the peptide bonds within the protein, and a reaction between Folin-Ciocalteu reagent and the ring structure on aromatic amino acids. The total reaction forms a stable, dark blue complex that can be read at 650-750 nm [ 3 ].
and real A1. Add concentrated biuret re-agent, and 10 min later measure absorbance A2 at 546 nm vs. reagent blank. Secondary wavelength was 700 nm. The test results were calculated against a one-point stan-dard. This biuret colorimetric method was relatively simple, fast, and accurate for the determination of protein in urine and cere-
The study makes the biuret test more useful to various applications like clinical laboratory practices and biochemical researches. Also, since the calibration curve for biuret test obtained in the study exhibit an R2 value of 0.9999, the study provides the best linear protein concentration range for biuret test.
Publish with us. Policies and ethics. The biuret reaction, used for the quantitative determination of proteins, is based upon the formation of a copper chelate with the peptide bonds of the protein at alkaline pH. In this complicated reaction one copper atom forms a complex with four peptide nitrogens.
The LOD of protein assays ranged from 0.3 mg/mL for BCA to 3.8 mg/mL for BCG. As the Biuret assay was less sensitive and had relatively higher standard deviation in its measurement, this assay was not quantifiable on paper platform. Similarly, the LOQs ranged from 1.2 mg/mL for BCA to 12.8 mg/mL for BCG. Based on the optimization parameters ...
Deionized water (negative control). Procedure. Take three clean and dry test tubes. Add 1-2 ml of the test solution, egg albumin, and deionized water in the respective test tubes. Add 1-2 ml of Biuret reagent to all the test tubes. Shake well and allow the mixtures to stand for 5 min. Observe for any color change.
Biuret Test is the test used to detect the presence of peptide bonds in the sample and to test for the presence of proteins or peptides. Proteins and peptides are polymers of amino acids. They are chains of amino acids as well as other biomolecules or ions or compounds. The amino acids are covalently bound to each other by a covalent bond ...
The biuret test exhibited linearity in a wide range of concentrations and the starting protein concentration range established for the calibration curve of this assay is lower than the found literature value (1 mg/sample). ... This research paper presents the DL and QL check done for four analytes viz Glucose, Urea, Creatinine and Calcium and ...
We describe a method for measuring urinary protein with a centrifugal analyzer. Biuret reagent is used, and blanking with an ultrafiltrate of urine eliminates interferences from the nonprotein, biuret-positive chromogens in urine. We compare results by this new method with those by a manual method i …
Response to the Biuret Test of Whey, Before and After Dialysis. These curves show that the response of the dialyzed whey agreed closely with ... This paper reports research undertaken in cooperation with the Quarter- master Food and Container Institute for the Armed Forces, and has been as- signed number 386 in the series of papers approved for ...
In the first half of the lab period, students carry out four macromolecule assays. Students add 20 drops (∼ 1 mL) of biuret reagent (0.25 mM CuSO 4 in 10 M NaOH) to 20 drops of each test substance and use the appearance of a purple color to confirm the presence of protein. To detect reducing sugars, students add 20 drops of Benedict's ...
Biuret test . 2mL filtrate + 1 drop of 2% copper sulphate sol. + 1mL of ... NaOH paper test . ... This can be explained by the fact that this research method is based on observing the appearance ...
1.1. Urine composition. Urine is a readily available liquid for the medical diagnosis of patients. Urine tests are non-invasive procedures that involve no pain or discomfort for patients to determine problems with kidney function since extensive medical research showed that patients, with high protein concentration in urine, have various kinds of illnesses of the kidney, referred to as ...
Procedure of Biuret Test. The following steps are followed to perform the biuret test: Take three clean and dry test tubes. In the first tube, add 1-2 ml test sample. Likewise, add 1-2 ml of egg albumin in the second one; in the third tube, add 1-2 ml of distilled water. The egg albumin is a positive control, whereas distilled water is a ...
Down in flames: The pyrolysis of urea and its decomposition products are studied in situ, for the first time.Biuret is a crucial intermediate for decomposition towards g-C 3 N 4.Extensive thermogravimetric studies of varying crucible geometry, heating rate and initial sample mass reveal that the concentration of reactive gases at the interface to the condensed sample residues is a crucial ...
Procedure. Using the following steps, you can easily conduct this test. First, take 3 dry and clean test tubes. Now add 1 or 2 mL of the test solution, albumin and deionised water in the test tubes. Add Biuret reagent (1-2 mL) in each test tube. Now shake the solution well and let it stand for 5 minutes.