
An official website of the United States government
The .gov means it’s official. Federal government websites often end in .gov or .mil. Before sharing sensitive information, make sure you’re on a federal government site.
The site is secure. The https:// ensures that you are connecting to the official website and that any information you provide is encrypted and transmitted securely.
- Publications
- Account settings
Preview improvements coming to the PMC website in October 2024. Learn More or Try it out now .
- Advanced Search
- Journal List
- Biomol Ther (Seoul)
- v.29(6); 2021 Nov 1
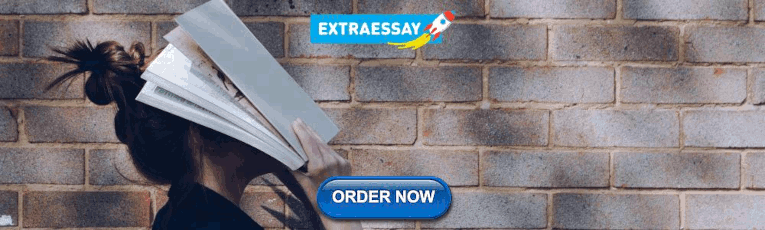
Lipid Metabolism, Disorders and Therapeutic Drugs – Review
Vijayakumar natesan.
1 Department of Biochemistry and Biotechnology, Faculty of Science, Annamalai University, Annamalainagar 608002, Tamilnadu, India
Sung-Jin Kim
2 Department of Pharmacology and Toxicology, Metabolic Diseases Research Laboratory, School of Dentistry, Kyung Hee University, Seoul 02447, Republic of Korea
Different lifestyles have an impact on useful metabolic functions, causing disorders. Different lipids are involved in the metabolic functions that play various vital roles in the body, such as structural components, storage of energy, in signaling, as biomarkers, in energy metabolism, and as hormones. Inter-related disorders are caused when these functions are affected, like diabetes, cancer, infections, and inflammatory and neurodegenerative conditions in humans. During the Covid-19 period, there has been a lot of focus on the effects of metabolic disorders all over the world. Hence, this review collectively reports on research concerning metabolic disorders, mainly cardiovascular and diabetes mellitus. In addition, drug research in lipid metabolism disorders have also been considered. This review explores lipids, metabolism, lipid metabolism disorders, and drugs used for these disorders.
INTRODUCTION
Lipids are organic compounds that are insoluble in water and soluble in organic solvents. They are esters of fatty acids, rarely containing alcohol or phosphate functional group molecules, and comprise triglycerides, phospholipids, and steroids. They are the energy reserves of animals and perform various functions, such as maintenance of body temperature, whilst being the key constituents of cell membranes and serving as chemical messengers ( Tocher, 2003 ; Ratnayake and Galli, 2009 ). The human body requires various types of useful lipid fat to maintain the healthy functions of its parts ( Ahmed et al ., 2020 ). Balancing lipid levels in the blood is an important part of staying healthy. Abnormal levels of blood lipids cause fat deposits in artery walls, which initiates complications inside the blood vessels. Causes for high lipid levels include diabetes, alcoholism, kidney disease, hypothyroidism, liver disease, and stress. Augmented lipids easily adhere to the blood’s circulating nerve walls, and the growing fatty scale causes a variety of atherosclerosis disorders, such as stroke or heart attack ( Nelson, 2013 ).
A lack of chemical reactions in our bodies causes metabolic diseases and lowers our quality of life. The enzymes needed to metabolize lipids may not work properly or are not produced enough ( Lattimer and Haub, 2010 ). Excessive lipids are stored, causes permanent cellular and tissue damage, predominantly in the brain and peripheral nervous system, resulting in metabolic disorders such as Gaucher’s disease, Tay-Sachs disease, Niemann-Pick disease (NPD), etc. ( Solomon and Muro, 2017 ). Obesity is now a common metabolic disorder, involving an excessive amount of body fat. It increases the risk of other diseases and health problems, such as heart disease, diabetes, high blood pressure, and certain cancers. Altered intestinal microbiota may stimulate hepatic fat deposition, also causing obesity and other metabolic disorders ( Arslan, 2014 ; Song et al ., 2019 ). Almost half of all cardiovascular disease-related fatalities occur as a result of a metabolic imbalance ( Knopp, 1999 ). Obesity is a major cause of cardio metabolic risk factors such as elevated plasma glucose levels, atherogenic dyslipidemia, elevated blood pressure, and so on ( Grundy, 2009 ).
According to the Mayo Clinic, while certain metabolic abnormalities can be discovered by continuous screening tests at birth, the majority are diagnosed after the onset of symptoms in adulthood. For example, the population of gut microbiota microorganisms in the human digestive system that are involved in beneficial metabolic action is high. ( Dibaise et al ., 2008 ). However, obese metabolic disease is caused by pathophysiological interactions that result in aberrant negative metabolic activity ( Hur and Lee, 2015 ). Based on the child’s viewpoint and progress, this condition can be detected as early as childhood. Because of deficiencies in the diagnostic and screening processes ( Denisenko et al ., 2020 ), physicians and drug researchers have yet to identify the optimal therapy for metabolic diseases (Metbd). Metbd caused by chemical reactions begins with obesity and progresses via different illnesses, such as infertility, hypothyroidism, hypoactive sexual desire disorder, nonalcoholic steatohepatitis, testosterone replacement, vaginal atrophy, cancer, type I diabetes, and type II diabetes ( Pischon et al ., 2008 ). In recent years, lipid metabolism disease insulin resistance has become a frequent worldwide concern, which necessitates more medication research and diagnosis ( Lark et al ., 2012 ; Monnerie et al ., 2020 ). Obesity-related illnesses are being caused by the excessive intake of saturated fat lipids ( Cena and Calder, 2020 ). The absence of certain lipids, such as polyunsaturated lipids and phospholipids, causes inflammation and disrupts the glucose-insulin balance ( Novgorodtseva et al ., 2011 ; Glass and Olefsky, 2012 ). Furthermore, several studies have indicated that the contribution of lipoxin A4 lipid levels has an influence on periodontal disease, kwonlic syndrome, and other chronic issues ( Doğan et al ., 2019 ). According to the National Institute of Neurological Disorders and Stroke, the impact of excessive fat accumulation (lipids) is the source of many health concerns, such as tissue damage, and liver, brain, bone marrow, peripheral nervous system, and spleen disorders. The data in this investigation reveals a variety of problems caused by alterations in lipid metabolism. As a result, this study summarizes the different Metbd and current medication development reports.
LIPID TYPES AND STRUCTURES
Aqueous insoluble lipids are molecules with complex structures as a result of several biochemical transformations ( Fahy et al ., 2011 ). Because of the participation of different enzymes and biological substances, the process of lipidomics is important to comprehend ( Nilsson et al ., 2019 ). Lipids contain hydrocarbons, a diverse and ubiquitous group of compounds that are non-polar soluble in organic solvents. They have significant structural variety, based on their variable chain length, and have a mass of oxidative, reductive, substitutional, and ring-forming capability, also with sugar residues and other functional groups ( Fahy et al ., 2011 ). Based on this, lipids are divided into several types, including saturated and unsaturated fatty acids, waxes, glycerol phospholipids, sphingo lipids, and glycosphingo lipids ( Fahy et al ., 2005 ). Lipids are liquids or non-crystalline solids with colorless, tasteless, and odorless qualities, and are energy-rich organic compounds with no ionic charge. The acetyl, propenyl, and isoprene functional groups of the building components of lipids also serve as hormones. Polyunsaturated fatty acids carry out a signaling function and are responsible for membrane structure ( Cani et al ., 2008 ; Bazinet and Layé , 2014 ).
LIPID OR FATTY ACID SYNTHESIS
Lipids or fatty acids are important components of the human body and have multiple functions in both health and diseases. Different lipids are synthesized by our body, based on the functional area, and are produced by lipogenic tissues in the presence of cytosol ( Tracey et al ., 2018 ). Lipids or fatty acids are synthesized from acetyl-CoA and NADPH through the action of enzymes called fatty acid synthases. Seven replications of four-cycle reactions were observed by Tracey et al . (2018) with various fatty synthesis mechanisms ( Nelson, 2013 ). Except for some essential fatty acids, the human body is able to synthesize most of the required fatty acids directly from precursors ( Nagy and Tiuca, 2017 ). Acetyl-CoA carboxylase beta (ACC2) is involved in the carboxylation of acetyl-CoA to malonyl-CoA. Malonyl-CoA is the substrate for fatty acid synthase complex and is also a key molecule regulator of both the biosynthesis and oxidation of fatty acids ( Leśniak et al ., 2015 ; Alves-Bezerra and Cohen, 2017 ). The Coronavirus host protein has ACC2 and a chief lipid complex, which are arranged on the mitochondrial membrane ( Castle et al ., 2009 ). Long chain fatty acid synthesis is found in all cells and organisms, serving as the universal building block of sphingolipids, glycerophospholipids, triacylglycerols, and wax-esters ( Uttaro, 2006 ). Similarly, three fatty acid biosynthetic pathways were observed in different parts of Toxoplasma ( Coppens et al ., 2014 ). From the results, this review observed the importance of lipid synthesis in various organisms and pathways.
LIPID FUNCTIONS AND ITS METABOLISM
Lipid metabolism is involved in different active functions of our body, such as energy storage, hormone regulation, nerve impulse transmission, and fat-soluble nutrient transportation. Lipids serves as an energy source with high caloric density, providing 9 kcal of energy when compared to protein and carbohydrates, which can also store 100,000 kcal of energy in our body functions without any intake of food for 30-40 days, only requiring sufficient water ( Ophardt, 2003 ). Biochemical lipids are stowed in cells all over the body, in specific varieties of connective tissue, named adipose. Lipids protect human organs, such as the spleen, liver, heart, and kidneys, from damage ( Church et al ., 2012 ).
Lipids that exist in the blood are absorbed through liver cells and provide the correct concentrations to various parts of the body. The liver plays a key and vital role in lipid metabolism ( Ophardt, 2003 ). The liver serves as a substitute reservoir for storing extensive quantities of excess fat. Through prolonged energy overload, the unspent excess energy is stored in adipose tissue and in hepatocytes in the form of triglycerides ( Huang et al ., 2011 ). The metabolism cycle is extended to the citric acid cycle, the urea cycle, and the citric cycle ( Arumugam and Natesan, 2017 ).
Fatty acids are degraded via oxidation, which releases large amounts of ATP and produces sensitive oxygen ( Rosca et al ., 2012 ). The glycerolipids biosynthesized through snglycerol-3-phosphate dominate in the liver and adipose tissue ( Athenstaedt and Daum, 2006 ). This review observed various useful metabolic functions of proteins enabling an understanding of metabolic disorders ( Huang and Freter, 2015 ; Trebatická et al ., 2017 ; Musso et al ., 2018 ; Yan and Horng, 2020 ).
LIPID METABOLISM DISORDERS
Increasing or decreasing levels of lipids cause various health effects in the human body, which are called disorders. These types of disorders usually increase triglyceride, LDL, or both lipid levels. The body requires the useful fatty acid HDL, which helps to transport bad cholesterol out of the body. Similarly, the accumulation of bad and unwanted lipids, such as fatty LDLs and triglyceride, damage the arteries and have serious consequences for cardiovascular health. Recently, Xiao et al . (2021) published an article on inherited complex lipid metabolism disorders, stating that over 80 diseases have been identified as complex lipid metabolism defects. They reviewed the physiological role of lipid metabolism in health disorders, which defines various metabolisms, such as nonlysosomal sphingolipids, acylceramides, etc. Lipid metabolism-based disorders were classified into five types by Fredrickson’s, based on the pathway and health effects ( Quispe et al ., 2019 ). When compared to a lower level of lipids, a higher amount of lipid accumulation in the body causes more health disorders, which is known as hyperlipidemia ( Natesan and Kim, 2021 ). Hyperlipidemia refers to a group of serious lipid disorders caused by an abnormally high level of unwanted lipids in the blood ( Verma, 2017 ). The Verma’s review classified hyperlipidemia based on the lipid type ( Fig. 1 ) ( Verma, 2017 ). Verma (2017) reviewed Fredrickson’s familial disorders classifications, symptoms, and treatments for each kind of disorder (Fredrickson and Lee, 1965).

Classifications of lipid metabolism disorders based on the nature of lipid and factors.
The discrete lipid metabolic disorders classification varies based on concentrations of classes of lipoproteins, and several disorders are now observable with structural defects in the presence or absence of apolipoproteins and lipid transfer proteins, respectively ( Schonfeld, 1990 ).
The peroxisome proliferator-activated receptors are a type of lipid, which are also called nuclear fatty acid receptors, that have been associated with playing a vital role in obesity connected to metabolic diseases like coronary artery disease, hyperlipidemia, and insulin resistance ( Azhar, 2010 ). The peroxisome proliferator-activated receptors involving regulated pathways that control various lipid disorders were also reported for medical treatment purposes ( Lee et al ., 2003 ). In addition, various lipid metabolism disorders, such as bone related disorders, osteoporosis, and atherosclerosis, are major worldwide health problems for postmenopausal females ( Bagger et al ., 2006 ). The hypothetical evidence proposes a relationship between lipid metabolism and bone, which are mutually regulated ( Tian and Yu, 2015 ); however, some conflicting results were observed, which require some Chinese human subjects. Myopathy and the severity of carnitine deficiency are caused by the excessive accumulation of lipid droplets on muscle fibers ( Di Mauro et al ., 1980 ). Metabolic systems of lipids or lipid abnormalities cause various disorders and diseases. Furthermore, excess lipid storage in the body causes a variety of disorders, including xanthoma, Bassen-Kornzweig syndrome, methylmalonic acid blood test, chylomicronemia syndrome, familial lipoprotein lipase deficiency, Niemann-Pick disease (NPD type-A and NPD type-B), methylmalonic academia, GM1 & GM2 gangliosidoses, Gaucher disease, Aside from these, the more serious consequences are cardiovascular disorders and diabetes, both of which had no symptoms at the time. Nowadays, these are the major health issues in the digital world. The most common causes of acquired hyperlipidemia are diabetes mellitus, alcohol consumption, hypothyroidism, renal failure, nephrotic syndrome, and continuous use of diuretics, estrogens, and β-blockers ( Stone, 1994 ; Reckless and Lawrence, 2003 ).
CLASSIFICATION OF LIPID METABOLISM DISORDERS
The best way of classifying lipid metabolism disorders is descriptively ( Table 1 ), based on the changes in concentration of the various types of lipids. LDL hypercholesterolemia is distinguished from mixed hyperlipoproteinemia, hypertriglyceridemia, and an isolated reduction in HDL cholesterol. All of these lipid metabolism disorders can be associated with elevated lipoprotein(a). The treatment of the individual lipid metabolism disorders is described below.
Descriptive classification of the dyslipoproteinemias
EXCLUSION OF SECONDARY LIPID METABOLISM DISORDERS
Secondary lipid metabolism abnormalities can cause a variety of illnesses. Diabetes mellitus, hypothyroidism (LDL hypercholesterolemia), renal illnesses (hypertriglyceridemia, mixed hyperlipoproteinemia, lipoprotein elevation), and cholestatic liver disorders are the most common clinically. Lipid metabolism disorders have also been found in the setting of other illnesses (e.g., lymphoma, Cushing syndrome, and porphyria). When the lipid metabolism problem is a secondary manifestation, the primary emphasis of treatment should be on the underlying illness. People with chronic diabetes or renal illness are frequently outliers to this rule, because adequate control or eradication of the underlying disease is not accomplished, and they exhibit symptoms of both primary and secondary lipid metabolism problems.
LIFESTYLE MODIFICATION
Lifestyle changes are important in the treatment of lipid metabolism problems. Regardless of the treatments used, the decrease in high LDL cholesterol concentrations seldom exceeds 10% ( Malhotra et al ., 2014 ). The biggest impact is obtained by reducing the consumption of saturated fatty acids, namely animal fats. Because the influence of orally ingested cholesterol is small, the current recommendations in the United States do not advise limiting cholesterol consumption at all. Lifestyle modifications, alone or in conjunction with changes in lipid concentrations, have a significantly larger effect on hypertriglyceridemia. Severe limits on alcohol consumption and a reduction in the intake of quickly absorbed carbohydrates can reduce triglyceride levels by more than 50% ( Hegele et al ., 2014 ). Regular exercise also increases the lipid profile. Even if the effect on lipid concentration is modest in certain situations, lifestyle changes might have a positive influence on the risk profile. In high-risk individuals, for example, a Mediterranean diet supplemented with extra olive oil or almonds results in a 30% reduction in relative risk ( Estruch et al ., 2013 ). Surprisingly, consuming nuts lowers LDL cholesterol; thus, it is debatable that at least some of the risk reduction is due to a beneficial impact on the lipid profile ( Wu et al ., 2014 ).
LDL HYPERCHOLESTEROLEMIA
According to European recommendations, the target concentration of LDL cholesterol should be determined by the total risk. If lifestyle changes alone are insufficient to achieve this aim, statin medication is the initial step in medical therapy. If the goal LDL cholesterol level is not reached after 4 to 6 weeks of therapy, the dose should be modified. In high-risk individuals, both lifestyle changes and statin therapy should begin at the same time ( Catapano et al ., 2011 ). According to the results of the IMPROVE-IT trial, ezetimibe should be administered if statin therapy alone fails to attain the target LDL cholesterol concentration. If the combination of a statin and ezetimibe is still ineffective, PCSK9 antibodies might be used. Patients with atherosclerosis and resistant LDL hypercholesterolemia might be treated with frequent lipid apheresis as a last option.
In Germany, other statins (lovastatin, fluvastatin, pravastatin, rosuvastatin, pitavastatin) play a limited role. Fluvastatin and pravastatin have lower side effect rates than atorvastatin and simvastatin; thus, they can be used in individuals who cannot take the latter ( Stroes et al ., 2015 ). Rosuvastatin has a very potent LDL cholesterol-lowering effect; however, patients in Germany must bear a portion of the expenditure. Acute coronary syndrome (ACS) is a unique condition. Initial research indicates that extremely early high-dose statin treatment improves the prognosis of ACS patients ( Cannon et al ., 2004 ). The most probable reason is that LDL cholesterol has no effect on endothelial function ( Sparrow et al ., 2001 ). In the meantime, however, these findings are being viewed with caution. Nonetheless, most recommendations suggest that patients with ACS begin therapy with a high-dose statin.
MIXED HYPERLIPOPROTEINEMIA
Because of its strong connection with metabolic syndrome, mixed hyperlipoproteinemia, characterized by elevated levels of both LDL cholesterol and triglycerides, is the most common lipid metabolism disease in diabetics ( Wu and Parhofer, 2014 ). In this case, too, the primary therapeutic objective is to keep LDL cholesterol levels in check. The essential measure in the treatment of hypertriglyceridemia is a change in the patient’s lifestyle. If the combination of lifestyle changes and statin medication does not achieve the desired concentrations, or at least normalize the triglyceride level, combined medical treatment may be explored ( Hegele et al ., 2014 ). In theory, statins can be used with omega-3 fatty acids or fibrates, although both of these combinations have performed poorly in endpoint trials ( Kromhout et al ., 2010 ). However, due to the poor design of these trials, no conclusive result can be drawn, as each of these two classes of drugs decreased cardiovascular risk in monotherapy studies ( Chaudhury et al ., 2017 ). In our facility, patients with very high risk and a combined lipid metabolic problem are treated with statin + fibrate or statin + omega-3 fatty acids after all other LDL cholesterol-lowering options have been exhausted. Without comparative research, neither of these two therapies can be favored over the other. It may be advisable to test both combinations and then stick with the one that is best tolerated and produces the greatest results.
HYPERTRIGLYCERIDEMIA
Triglyceride levels are frequently much higher than normal in isolated hypertriglyceridemia, whereas LDL cholesterol levels are modest. Total cholesterol levels might be high. Isolated hypertriglyceridemia, like mixed hyperlipoproteinemia, typically responds favorably to lifestyle changes. Moreover, there is no way to predict whether a specific patient will react well or poorly. Because no compelling studies have been published, there is no agreement on when medical therapy should begin ( Yuan et al ., 2007 ). However, the threshold is lower among people at high risk of atherosclerosis than when hypertriglyceridemia is detected accidentally in an otherwise healthy person. A fibrate can be administered if the triglyceride level remains above 400 mg/dL (4.6 mmol/L), after the application of lifestyle modification strategies. Fenofibrate and gemfibrozil appear to be the best alternatives. Alternatively, omega-3 fatty acids can be administered alone or in combination, as necessary ( Hegele et al ., 2014 ). Statins are typically ineffective in isolated hypertriglyceridemia since LDL cholesterol is frequently already extremely low at the start. Patients with known atherosclerosis should get a modest dosage of statin regardless of LDL cholesterol levels.
There is general agreement that if the triglyceride level stays above 400 mg/dL (4.6 mmol/L) despite implementation of lifestyle modification measures, a fibrate can be given. The best options seem to be fenofibrate or gemfibrozil (positive endpoint studies; should not be combined with statins). Alternatively, omega-3 fatty acids can be given, in combination if indicated ( Hegele et al ., 2014 ). Statins are generally of little use in isolated hypertriglyceridemia, because the LDL cholesterol is often already very low at the outset. Clients with known atherosclerosis should be given a modest dose of statins, regardless of LDL cholesterol levels.
SYMPTOMS OF HYPERLIPIDEMIA
In general, hyperlipidemia disorders do not have any noticeable symptoms, but they are regularly exposed by the health monitoring process or by routine examination and will cause a stroke or a heart attack if it reaches a dangerous stage. Patients with more than the maximum cholesterol level in the blood will be affected by xanthomas. In this disorder, cholesterol deposits itself under the skin and in the eyes ( Shattat, 2014 ). A raised level of triglycerides was reported at the same time, causing numerous pimples in diverse sites of the patients’ bodies. Familial hypercholesterolemia is a common autosomal-related disorder caused by elevated LDL cholesterol levels at birth. It also causes premature coronary artery disease and requires initial diagnosis to avoid expensive generic pharmacotherapy ( McGowan et al ., 2019 ).
CARDIOVASCULAR DISORDER OR DISEASE (CVD)
According to healthcare providers, the well-established stage of lipids in the body causes risk factors for CVD, and the analysis of lipid-screening test results plays a critical role in CVD risk assessment. The Framingham Risk Score is the most widely used authenticated lipid-screening technique ( Nelson, 2013 ). CVD, or coronary heart disease, causes serious health issues, such as heart attack, heart failure, and stroke ( Joynt et al ., 2003 ). CVD is caused by chronic inflammatory atherosclerosis, which develops gradually in the human body over several years. The CVD risk factor dyslipidemia is related to lipid metabolism and is affected by genes and proteins. Computed tomography investigations on various lipid molecules, such as cholesteryl ester transfer protein, lipoprotein lipase, polymorphisms of paraoxonase 1 and 2, and hepatic lipase, were used to measure the relationship between CVD and lipid metabolism. The Diabetes Heart Study included 620 European American volunteers, with 83% having type 2 diabetes mellitus. The results revealed that the Q192R variant of paraoxonase 1 and rs285 of lipoprotein lipase were linked with carotid artery calcium ( p =0.002 and 0.005, respectively) and paraoxonase-2 S311C was connected with coronary artery calcium ( p =0.037), which was proven by Burdon et al . (2005) . Goldberg et al . (2012) reported the lipid metabolism effect on the heart, observing that excess lipid accumulation causes severe chest pain by obstructing blood flow, reducing oxygen flow and resulting in a heart attack.
CVD kills one out of every three women. Sex-specific data concentrated on cardiovascular disease has been growing steadily. The average lifetime is reduced due to CVD in women who are at an age of approximately 50 years (≈40%). However, significant causes of CVD are depicted in Fig. 2 ( Garcia et al ., 2016 ).

Various reasons for cardiovascular disorders by lipids storage related metabolic actions.
DIABETES MELLITUS
Diabetes mellitus is another disorder caused by lipid metabolism that necessitates a continuous health monitoring strategy for long-term survival ( Amalan and Vijayakumar, 2015 ). The impact of insulin on lipid metabolism, which is influenced by diabetes, may be measured in four different ways ( Saudek and Eder, 1979 ). The proposed mechanism exposed the negative and positive effect on insulin in the regulation of triglyceride metabolism.
Lipid metabolism is altered and changes are observed during Gestational Diabetes Mellitus and the normal pregnancy period. Hepatic and adipose metabolism alters the concentrations of triacylglycerols, cholesterol, phospholipids, and fatty acids ( Amalan et al ., 2015 ). Then, in the first 8 weeks of pregnancy, there is a preliminary decrease, followed by a gradual increase in the majority of the fatty acid or lipid concentrations. At the same time, the higher concentrations of estrogen and insulin resistance are believed to be accountable for the hypertriglyceridemia of pregnancy ( Butte, 2000 ). Their research on pregnant women with GDM has revealed that diet control and exercise have managed their diabetes.
Diabetic dyslipidemia is a collection of many irregularities in fat, both LDL and HDL intolerance levels. This pattern of lipoprotein deviations is extremely atherogenic and is associated with a rise in plasma triglyceride levels. The clinical irregularities take place at a range of plasma triglyceride levels, which represent the upper normal range or mild hypertriglyceridemia (>1.5 mmol/L). In clinical practice, this means that triglyceride levels should be maintained as low as possible in non-insulin dependent diabetes mellitus patients ( Taskinen et al ., 1996 ).
An experimental report on the regulation of lipids with glucose metabolism in the post absorptive and postprandial conditions in six subjects (selective patients or volunteers) with insulin-treated diabetes mellitus, matched with eight non-diabetic volunteers or subjects, involved the investigation of blood or plasma concentrations of metabolites and fluxes across forearm and subcutaneous adipose tissue after an overnight fast and for 6 hours after a mixed meal (3.1 MJ, 41% from fat). The observation revealed that the wider spread of plasma (free) insulin concentrations in the diabetic group led to a wider range of plasma non-esterified fatty acid release from adipose tissue, plasma NEFA concentrations, and blood ketone body concentrations ( Frayn et al ., 1993 ). These studies and reviews have confirmed lipid metabolism and its impact on health issues in different ways.
CONTROL AND TREATMENT METHODS
These kinds of lipid-based disorders can be controlled by various methods, such as physical methods, a controlled food system, therapeutic lifestyle changes, drug therapy, and proper health checkups ( Fig. 3 ). Statins are the most potent class of medicine used for cardiovascular diseases. Being cholesterol-lowering drugs, statins are expected to ameliorate the cardiovascular problem, which lowers the acute-phase proteins ( Pahan, 2006 ). Table 2 shows some anti-lipidemia drugs, as well as their mechanisms and side effects ( Waller and Waller, 2014 ; Dias et al ., 2018 ).

Methods of controlling lipid disorder.
Anti-lipidemia drugs and their mechanism with side effects
This shows a list of anti-lipidemia drugs and their mechanism in fat or cholesterol control at different parts of body using different metabolic chemicals along with drugs side effects.
CONTROL OF SATURATED VS. UNSATURATED FATTY ACIDS (FAS)
The amounts of saturated FAs incorporated in cell membrane phospholipids change, depending on the source of FAs, de novo lipogenesis, or external lipid absorption. The lipogenic process raises the saturation level of cell membranes with saturated and monounsaturated fatty acids (MUFAs) ( Yue et al ., 2014 ; Fernandez et al ., 2020 ), which are less susceptible to lipid peroxidation than the polyunsaturated acyl chains (PUFAs) acquired mostly via food. In this approach, de novo lipogenesis helps to make cancer cells resilient to oxidative stress and chemotherapy ( Rysman et al ., 2010 ).
Nonetheless, an excess of saturated FAs in cell membranes might cause lipotoxicity. In this context, SCD1 inhibition promotes endoplasmic reticulum (ER) stress and death in cancer cells and reduces tumor development in colon and lung cancer xenograft models. The core regions of tumors are subjected to hypoxia and decreased nutritional availability during tumor development. Tumors have evolved several techniques for balancing the amounts of saturated vs. unsaturated FAs and anticipate lipotoxicity by increasing MUFA/PUFA absorption from plasma, which is then stored in lipid droplets (LDs) or integrated into phospholipids at the cell membranes. Because SCD1 activity requires oxygen, certain cancers rely on the activity of diglyceride acyltransferase (DGAT) during hypoxia to integrate MUFAs into triglyceride (TG), which is then deposited into LDs ( Fernandez et al ., 2020 ). Furthermore, cancers regulate the amounts of saturated vs. unsaturated FAs in phospholipids at the cell membranes via the Lands cycle. Recently, a mechanism known as ferroptosis has been identified, which is related with high amounts of MUFA/PUFAs in cell membrane phospholipids, causing cell death by oxidation via the Fenton pathway. Long-chain FA acyl CoA synthetases (ACSLs), which are involved in long chain FA activation, may regulate ferroptosis, because different isoforms employ different substrates. Conversely, although ACSL4’s major substrates are PUFAS, such as AA, ACSL3 may activate both MUFAs and PUFAs, allowing for better regulation of PUFA buildup in phospholipids ( Alwarawrah et al ., 2016 ). Furthermore, ACSL3 provides for better regulation of FA distribution between LD storage and fatty acid oxidation (FAO), allowing for better management of oxidative stress.
CURRENT TREATMENT MODALITIES
A variety of novel methods for the treatment of lipid metabolism diseases have been explored. Proprotein convertase subtilisin/kexin type 9 (PCSK9) antibodies are particularly significant ( Stein et al ., 2013 ). Even in individuals who have previously had combination statin and ezetimibe treatment, these medicines can result in a 50 to 60% decrease in LDL cholesterol ( Blom et al ., 2014 ). PCSK9 antibodies have just a little effect on triglyceride and HDL cholesterol concentrations. They do, however, reduce lipoprotein(a) levels by up to 30% ( Raal et al ., 2014 ; Parhofer, 2016 ). Until the endpoint comprehensive research is completed and published, PCSK9 antibodies can only be given to carefully selected patients, such as those with known atherosclerosis and prominent LDL hypercholesterolemia who cannot be treated by other means due to their levels being too high or who are unaware of statins.
Hyperlipidemia, a major cause of coronary heart disease, diabetes, and cancer, is prevalent throughout the world. Numerous studies and reviews have been reported on metabolism, the causes of lipid-based disorders, and the effects of fatty acids. Despite the fact that many drugs are available on the market, society continues to face problems as a result of CVD. To minimize the risk of cardiovascular related heart failure, heart attacks due to hyperlipidemia need novel drugs that can decrease lipids such as cholesterol and triglycerides in the blood. This review mainly focuses on compiling reports on lipids, metabolism, CVD, and diabetes related issues. Still, there is no complete report on lipid metabolism disorders and drug discovery. As a result, this review has been launched with only the most basic reports for further investigation.
- Ahmed S., Shah P., Ahmed O. StatPearls. StatPearls Publishing; Treasure Island: 2021. Biochemistry, lipids; pp. 11–19. [ Google Scholar ]
- Alves-Bezerra M., Cohen D. E. Triglyceride metabolism in the liver. Compr. Physiol. 2017; 8 :1–8. doi: 10.1002/cphy.c170012. [ PMC free article ] [ PubMed ] [ CrossRef ] [ Google Scholar ]
- Alwarawrah Y., Hughes P., Loiselle D., Carlson D. A., Darr D. B., Jordan J. L., Xiong J., Hunter L. M., Dubois L. G., Thompson J. W., Kulkarni M. M., Ratcliff A. N., Kwiek J. J., Haystead T. A. Fasnall, a selective FASN inhibitor, shows potent anti-tumor activity in the MMTV-Neu model of HER2(+) breast cancer. Cell Chem. Biol. 2016; 23 :678–688. doi: 10.1016/j.chembiol.2016.04.011. [ PMC free article ] [ PubMed ] [ CrossRef ] [ Google Scholar ]
- Amalan V., Vijayakumar N. Antihyperglycemic effect of p-coumaric acid on streptozotocin induced diabetic rats. Indian J. Appl. Res. 2015; 5 :10–13. [ Google Scholar ]
- Amalan V., Vijayakumar N., Ramakrishnan A. P-Coumaric acid regulates blood glucose and antioxidant levels in streptozotocin induced diabetic rats. J. Chem. Pharm. Res. 2015; 7 :831–839. [ Google Scholar ]
- Arslan N. Obesity, fatty liver disease and intestinal microbiota. World J. Gastroenterol. 2014; 44 :16452–16463. doi: 10.3748/wjg.v20.i44.16452. [ PMC free article ] [ PubMed ] [ CrossRef ] [ Google Scholar ]
- Arumugam R., Natesan V. Urea cycle pathway targeted therapeutic action of naringin against ammonium chloride induced hyperammonemic rats. Biomed. Pharmacother. 2017; 94 :1028–1037. doi: 10.1016/j.biopha.2017.08.028. [ PubMed ] [ CrossRef ] [ Google Scholar ]
- Athenstaedt K., Daum G. The life cycle of neutral lipids: synthesis, storage and degradation. Cell. Mol. Life Sci. 2006; 63 :1355–1369. doi: 10.1007/s00018-006-6016-8. [ PubMed ] [ CrossRef ] [ Google Scholar ]
- Azhar S. Peroxisome proliferator-activated receptors, metabolic syndrome and cardiovascular disease. Future Cardiol. 2010; 6 :657–691. doi: 10.2217/fca.10.86. [ PMC free article ] [ PubMed ] [ CrossRef ] [ Google Scholar ]
- Bagger Y. Z., Tankó L. B., Alexandersen P., Qin G., Christiansen C. Prospective epidemiological risk factors study group, author. Radiographic measure of aorta calcification is a site-specific predictor of bone loss and fracture risk at the hip. J. Intern. Med. 2006; 259 :598–605. doi: 10.1111/j.1365-2796.2006.01640.x. [ PubMed ] [ CrossRef ] [ Google Scholar ]
- Bazinet R. P., Layé S. Polyunsaturated fatty acids and their metabolites in brain function and disease. Nat. Rev. Neurosci. 2014; 15 :771–785. doi: 10.1038/nrn3820. [ PubMed ] [ CrossRef ] [ Google Scholar ]
- Blom D. J., Hala T., Bolognese M., Lillestol M. J., Toth P. D., Burgess L., Ceska R., Roth E., Koren M. J., Ballantyne C. M., Monsalvo M. L., Tsirtsonis K., Kim J. B., Scott R., Wasserman S. M., Stein E. A. DESCARTES Investigators, author. A 52-week placebo-controlled trial of evolocumab in hyperlipidemia. N. Engl J. Med. 2014; 370 :1809–1819. doi: 10.1056/NEJMoa1316222. [ PubMed ] [ CrossRef ] [ Google Scholar ]
- Burdon K. P., Langefeld C. D., Beck S. R., Wagenknecht L. E., Carr J. J., Freedman B. I., Herrington D., Bowden D. W. Association of genes of lipid metabolism with measures of subclinical cardiovascular disease in the Diabetes Heart Study. J. Med. Genet. 2005; 42 :720–724. doi: 10.1136/jmg.2004.029850. [ PMC free article ] [ PubMed ] [ CrossRef ] [ Google Scholar ]
- Butte N. F. Carbohydrate and lipid metabolism in pregnancy: normal compared with gestational diabetes mellitus. Am. J. Clin. Nutr. 2000; 71 :1256S–1261S. doi: 10.1093/ajcn/71.5.1256s. [ PubMed ] [ CrossRef ] [ Google Scholar ]
- Cani P. D., Delzenne N. M., Amar J., Burcelin R. Role of gut microflora in the development of obesity and insulin resistance following high-fat diet feeding. Pathol. Biol. 2008; 56 :305–309. doi: 10.1016/j.patbio.2007.09.008. [ PubMed ] [ CrossRef ] [ Google Scholar ]
- Cannon C. P., Braunwald E., McCabe C. H., Rader D. J., Rouleau J. L., Belder R., Joyal S. V., Hill K. A., Pfeffer M. A., Skene A. M. Pravastatin or Atorvastatin Evaluation, Infection Therapy-Thrombolysis in Myocardial Infarction 22 Investigators, author. Intensive versus moderate lipid lowering with statins after acute coronary syndromes. N. Engl. J. Med. 2004; 350 :1495–1504. doi: 10.1056/NEJMoa040583. [ PubMed ] [ CrossRef ] [ Google Scholar ]
- Castle J. C., Hara Y., Raymond C. K., Garrett-Engele P., Ohwaki K., Kan Z., Kusunoki J., Johnson J. M. ACC2 is expressed at high levels in human white adipose and has an isoform with a novel N-terminus. PLoS ONE. 2009; 4 :e4369. doi: 10.1371/journal.pone.0004369. [ PMC free article ] [ PubMed ] [ CrossRef ] [ Google Scholar ]
- Catapano A. L., Reiner Z., De Backer G., Graham I., Taskinen M. R., Wiklund O., Agewall S., Alegria E., Chapman M., Durrington P., Erdine S., Halcox J., Hobbs R., Kjekshus J., Filardi P. P., Riccardi G., Storey R. F., Wood D. European Society of Cardiology (ESC), author; European Atherosclerosis Society (EAS), author ESC/EAS guidelines for the management of dyslipidaemias. The Task Force for the management of dyslipidaemias of the European Society of Cardiology (ESC) and the European Atherosclerosis Society (EAS) Atherosclerosis. 2011; 217 :3–46. doi: 10.1016/j.atherosclerosis.2011.06.028. [ PubMed ] [ CrossRef ] [ Google Scholar ]
- Cena H., Calder P. C. Defining a healthy diet: evidence for the role of contemporary dietary patterns in health and disease. Nutrients. 2020; 12 :334. doi: 10.3390/nu12020334. [ PMC free article ] [ PubMed ] [ CrossRef ] [ Google Scholar ]
- Chaudhury A., Duvoor C., Reddy Dendi V. S., Kraleti S., Chada A., Ravilla R., Marco A., Shekhawat N. S., Montales M. T., Kuriakose K., Sasapu A., Beebe A., Patil N., Musham C. K., Lohani G. P., Mirza W. Clinical review of antidiabetic drugs: implications for type 2 diabetes mellitus management. Front. Endocrinol. 2017; 8 :6. doi: 10.3389/fendo.2017.00006. [ PMC free article ] [ PubMed ] [ CrossRef ] [ Google Scholar ]
- Church C., Horowitz M., Rodeheffer M. WAT is a functional adipocyte? Adipocyte. 2012; 1 :38–45. doi: 10.4161/adip.19132. [ PMC free article ] [ PubMed ] [ CrossRef ] [ Google Scholar ]
- Coppens I., Asai T., Tomavo S. Chapter 8 - Biochemistry and metabolism of Toxoplasma gondii: carbohydrates, lipids and nucleotides. In: Weiss L. M., Kim K., editors. Toxoplasma Gondii. 2nd ed. Academic Press; 2014. pp. 257–295. [ CrossRef ] [ Google Scholar ]
- Denisenko Y. K., Kytikova O. Y., Novgorodtseva T. P., Antonyuk M. V., Gvozdenko T. A., Kantur T. A. Lipid-induced mechanisms of metabolic syndrome. J. Obes. 2020; 2020 :5762395. doi: 10.1155/2020/5762395. [ PMC free article ] [ PubMed ] [ CrossRef ] [ Google Scholar ]
- Di Mauro S., Trevisan C., Hays A. Disorders of lipid metabolism in muscle. Muscle Nerve. 1980; 3 :369–388. doi: 10.1002/mus.880030502. [ PubMed ] [ CrossRef ] [ Google Scholar ]
- Dias S., Paredes S., Ribeiro L. Drugs involved in dyslipidemia and obesity treatment: focus on adipose tissue. Int. J. Endocrinol. 2018; 4 :2637418. doi: 10.1155/2018/2637418. [ PMC free article ] [ PubMed ] [ CrossRef ] [ Google Scholar ]
- Dibaise J. K., Zhang H., Crowell M. D., Krajmalnik-Brown R., Decker G. A., Rittmann B. E. Gut microbiota and its possible relationship with obesity. Mayo Clin. Proc. 2008; 4 :460–469. doi: 10.4065/83.4.460. [ PubMed ] [ CrossRef ] [ Google Scholar ]
- Doğan E. S. K., Doğan B., Fentoğlu O., Kırzıoğlu F. Y. The role of serum lipoxin A4 levels in the association between periodontal disease and metabolic syndrome. J. Periodontal Implant Sci. 2019; 49 :105–113. doi: 10.5051/jpis.2019.49.2.105. [ PMC free article ] [ PubMed ] [ CrossRef ] [ Google Scholar ]
- Estruch R., Ros E., Salas-Salvadó J., Covas M. I., Corella D., Arós F., Gómez-Gracia E., Ruiz-Gutiérrez V., Fiol M., Lapetra J., Lamuela-Raventos R. M., Serra-Majem L., Pintó X., Basora J., Muñoz M. A., Sorlí J. V., Martínez J. A., Martínez-González M. A. Primary prevention of cardiovascular disease with a mediterranean diet. N Engl J Med. 2013; 368 :1279–1290. doi: 10.1056/NEJMoa1200303. [ PubMed ] [ CrossRef ] [ Google Scholar ]
- Fahy E., Cotter D., Sud M., Subramaniam S. Lipid classification, structures and tools. Biochim. Biophy. Acta. 2011; 1811 :637–647. doi: 10.1016/j.bbalip.2011.06.009. [ PMC free article ] [ PubMed ] [ CrossRef ] [ Google Scholar ]
- Fahy E., Subramaniam S., Brown H. A., Glass C. K., Merrill A. H., Jr., Murphy R. C., Russell D. W., Seyama Y., Shaw W., Shimizu T., Spener F., van Meer G., VanNieuwenhze M. S., White S. H., Witztum J. L., Dennis E. A. A comprehensive classification system for lipids. J. Lipid Res. 2005; 46 :839–861. doi: 10.1194/jlr.E400004-JLR200. [ PubMed ] [ CrossRef ] [ Google Scholar ]
- Fernandez L. P., Gómez, de Cedrón M., Ramírez, de Molina A. Alterations of lipid metabolism in cancer: implications in prognosis and treatment. Front. Oncol. 2020; 10 :577420. doi: 10.3389/fonc.2020.577420. [ PMC free article ] [ PubMed ] [ CrossRef ] [ Google Scholar ]
- Frayn K. N., Coppack S. W., Humphreys S. M., Clark M. L., Evans R. D. Periprandial regulation of lipid metabolism in insulin-treated diabetes mellitus. Metabolism. 1993; 42 :504–510. doi: 10.1016/0026-0495(93)90110-A. [ PubMed ] [ CrossRef ] [ Google Scholar ]
- Fredrickson D. S., Lees R. S. Editorial: a system for phenotyping hyperlipoproteinemia. Circulation. 1965; 31 :321–327. doi: 10.1161/01.CIR.31.3.321. [ PubMed ] [ CrossRef ] [ Google Scholar ]
- Garcia M., Mulvagh S. L., Bairey Merz C. N., Buring J. E., Manson J. E. Cardiovascular disease in women: clinical perspectives. Circ. Res. 2016; 118 :1273–1293. doi: 10.1161/CIRCRESAHA.116.307547. [ PMC free article ] [ PubMed ] [ CrossRef ] [ Google Scholar ]
- Glass C. K., Olefsky J. M. Inflammation and lipid signaling in the etiology of insulin resistance. Cell Metab. 2012; 15 :635–645. doi: 10.1016/j.cmet.2012.04.001. [ PMC free article ] [ PubMed ] [ CrossRef ] [ Google Scholar ]
- Goldberg I. J., Trent C. M., Schulze P. C. Lipid metabolism and toxicity in the heart. Cell Metab. 2012; 15 :805–812. doi: 10.1016/j.cmet.2012.04.006. [ PMC free article ] [ PubMed ] [ CrossRef ] [ Google Scholar ]
- Grundy S. M. Advancing drug therapy of the metabolic syndrome. Nat. Rev. Drug Discov. 2009; 8 :341. doi: 10.1038/nrd2894. [ CrossRef ] [ Google Scholar ]
- Hegele R. A., Ginsberg H. N., Chapman M. J., Nordestgaard B. G., Kuivenhoven J. A., Averna M., Borén J., Bruckert E., Catapano A. L., Descamps O. S., Hovingh G. K., Humphries S. E., Kovanen P. T., Masana L., Pajukanta P., Parhofer K. G., Raal F. J., Ray K. K., Santos R. D., Stalenhoef A. F., Stroes E., Taskinen M. R., Tybjærg-Hansen A., Watts G. F., Wiklund O. European Atherosclerosis Society Consensus Panel, author. The polygenic nature of hypertriglyceridaemia: implications for definition, diagnosis, and management. Lancet Diabetes Endocrinol. 2014; 2 :655–666. doi: 10.1016/S2213-8587(13)70191-8. [ PMC free article ] [ PubMed ] [ CrossRef ] [ Google Scholar ]
- Huang C., Freter C. Lipid metabolism, apoptosis and cancer therapy. Int. J. Mol. Sci. 2015; 16 :924–949. doi: 10.3390/ijms16010924. [ PMC free article ] [ PubMed ] [ CrossRef ] [ Google Scholar ]
- Huang J., Borensztajn J., Reddy J. K. Hepatic lipid metabolism. In: Monga S., editor. Molecular Pathology of Liver Diseases. Molecular Pathology Library, Vol. 5. Springer; Boston: 2011. pp. 133–146. [ CrossRef ] [ Google Scholar ]
- Hur K. Y., Lee M. Gut microbiota and metabolic disorders. Diabetes Metab. J. 2015; 39 :198–203. doi: 10.4093/dmj.2015.39.3.198. [ PMC free article ] [ PubMed ] [ CrossRef ] [ Google Scholar ]
- Joynt K. E., Whellan D. J., Oconnor C. M. Depression and cardiovascular disease: mechanisms of interaction. Biol. Psychiatry. 2003; 54 :248–261. doi: 10.1016/S0006-3223(03)00568-7. [ PubMed ] [ CrossRef ] [ Google Scholar ]
- Knopp R. H. Drug treatment of lipid disorders. N. Engl. J. Med. 1999; 341 :498–511. doi: 10.1056/NEJM199908123410707. [ PubMed ] [ CrossRef ] [ Google Scholar ]
- Kromhout D., Giltay E. J., Geleijnse J. M. Alpha Omega Trial Group, author. n-3 fatty acids and cardiovascular events after myocardial infarction. N. Engl. J. Med. 2010; 363 :2015–2026. doi: 10.1056/NEJMoa1003603. [ PubMed ] [ CrossRef ] [ Google Scholar ]
- Lark D. S., Fisher-Wellman K. H., Neufer P. D. High-fat load: mechanism(s) of insulin resistance in skeletal muscle. Int. J. Obes. Suppl. 2012; 2 :S31–S36. doi: 10.1038/ijosup.2012.20. [ PMC free article ] [ PubMed ] [ CrossRef ] [ Google Scholar ]
- Lattimer J. M., Haub M. D. Effects of dietary fiber and its components on metabolic health. Nutrients. 2010; 2 :1266–1289. doi: 10.3390/nu2121266. [ PMC free article ] [ PubMed ] [ CrossRef ] [ Google Scholar ]
- Lee C. H., Olson P., Evans R. M. Minireview: lipid metabolism, metabolic diseases, and peroxisome proliferator-activated receptors. Endocrinology. 2003; 144 :2201–2207. doi: 10.1210/en.2003-0288. [ PubMed ] [ CrossRef ] [ Google Scholar ]
- Leśniak W., Bała M. M., Płaczkiewicz-Jankowska E., Topór-Mądry R., Jankowski M., Sieradzki J., Banasiak W. ARETAEUS2 Study Group, author. Cardiovascular risk management in type 2 diabetes of more than 10-year duration: Results of Polish ARETAEUS2-Grupa Study. Cardiol. J. 2015; 22 :150–159. doi: 10.5603/CJ.a2014.0067. [ PubMed ] [ CrossRef ] [ Google Scholar ]
- Malhotra A., Shafiq N., Arora A., Singh M., Kumar R., Malhotra S. Dietary interventions (plant sterols, stanols, omega-3 fatty acids, soy protein and dietary fibers) for familial hypercholesterolaemia. Cochrane Database Syst. Rev. 2014; 2014 :CD001918. doi: 10.1002/14651858.CD001918.pub3. [ PMC free article ] [ PubMed ] [ CrossRef ] [ Google Scholar ]
- McGowan M. P., Hosseini Dehkordi S. H., Moriarty P. M., Duell P. B. Diagnosis and treatment of heterozygous familial hypercholesterolemia. J. Am. Heart Assoc. 2019; 8 :e013225. doi: 10.1161/JAHA.119.013225. [ PMC free article ] [ PubMed ] [ CrossRef ] [ Google Scholar ]
- Monnerie S., Comte B., Ziegler D., Morais J. A., Pujos- Guillot E., Gaudreau P. Metabolomic and lipidomic signatures of metabolic syndrome and its physiological components in adults: a systematic review. Sci. Rep. 2020; 10 :669. doi: 10.1038/s41598-019-56909-7. [ PMC free article ] [ PubMed ] [ CrossRef ] [ Google Scholar ]
- Musso G., Cassader M., Paschetta E., Gambino R. Bioactive lipid species and metabolic pathways in progression and resolution of nonalcoholic steatohepatitis. Gastroenterology. 2018; 155 :282–302.e8. doi: 10.1053/j.gastro.2018.06.031. [ PubMed ] [ CrossRef ] [ Google Scholar ]
- Nagy K., Tiuca I.-D. Fatty Acids. IntechOpen; 2017. Importance of fatty acids in physiopathology of human body. doi: 10.5772/67407. [ CrossRef ] [ Google Scholar ]
- Natesan V., Kim S. J. Diabetic nephropathy - a review of risk factors, progression, mechanism, and dietary management. Biomol. Ther. (Seoul) 2021; 29 :365–372. doi: 10.4062/biomolther.2020.204. [ PMC free article ] [ PubMed ] [ CrossRef ] [ Google Scholar ]
- Nelson R. H. Hyperlipidemia as a risk factor for cardiovascular disease. Prim. Care. 2013; 40 :195–211. doi: 10.1016/j.pop.2012.11.003. [ PMC free article ] [ PubMed ] [ CrossRef ] [ Google Scholar ]
- Nilsson P. M., Tuomilehto J., Rydén L. The metabolic syndrome - what is it and how should it be managed? Eur. J. Prev. Cardiol. 2019; 26 :33–46. doi: 10.1177/2047487319886404. [ PubMed ] [ CrossRef ] [ Google Scholar ]
- Novgorodtseva T. P., Karaman Y. K., Zhukova N. V., Lobanova E. G., Antonyuk M. V., Kantur T. A. Composition of fatty acids in plasma and erythrocytes and eicosanoids level in patients with metabolic syndrome. Lipids Health Dis. 2011; 10 :82. doi: 10.1186/1476-511X-10-82. [ PMC free article ] [ PubMed ] [ CrossRef ] [ Google Scholar ]
- Ophardt C. E. Overview of lipid function. In Virtual ChemBook. Elmhurst College. 2003 Available from: http://chemistry.elmhurst.edu/vchembook/620fattyacid.html/
- Pahan K. Lipid-lowering drugs. Cell. Mol. Life Sci. 2006; 63 :1165–1178. doi: 10.1007/s00018-005-5406-7. [ PMC free article ] [ PubMed ] [ CrossRef ] [ Google Scholar ]
- Parhofer K. G. The treatment of disorders of lipid metabolism. Dtsch. Arztebl. Int. 2016; 113 :261–268. doi: 10.3238/arztebl.2016.0261. [ PMC free article ] [ PubMed ] [ CrossRef ] [ Google Scholar ]
- Pischon T., Nöthlings U., Boeing H. Obesity and cancer. Proc. Nutr. Soc. 2008; 67 :128–145. doi: 10.1017/S0029665108006976. [ PubMed ] [ CrossRef ] [ Google Scholar ]
- Quispe R., Hendrani A., Baradaran-Noveiry B., Martin S., Brown E., Kulkarni K., Banach M., Toth P. P., Brinton E. A., Jones S. R., Joshi P. Characterization of lipoprotein profiles in patients with hypertriglyceridemic Fredrickson-Levy and Lees dyslipidemia phenotypes: the Very Large Database of Lipids Studies 6 and 7. Arch. Med. Sci. 2019; 15 :1195–1202. doi: 10.5114/aoms.2019.87207. [ PMC free article ] [ PubMed ] [ CrossRef ] [ Google Scholar ]
- Raal F. J., Giugliano R. P., Sabatine M. S., Koren M. J., Langslet G., Bays H., Blom D., Eriksson M., Dent R., Wasserman S. M., Huang F., Xue A., Albizem M., Scott R., Stein E. A. Reduction in lipoprotein(a) with PCSK9 monoclonal antibody evolocumab (AMG 145): a pooled analysis of more than 1,300 patients in 4 phase II trials. J. Am. Coll. Cardiol. 2014; 63 :1278–1288. doi: 10.1016/j.jacc.2014.01.006. [ PubMed ] [ CrossRef ] [ Google Scholar ]
- Ratnayake W. M. N., Galli C. Fat and fatty acid terminology, methods of analysis and fat digestion and metabolism: a background review paper. Ann. Nutr. Metab. 2009; 55 :8–43. doi: 10.1159/000228994. [ PubMed ] [ CrossRef ] [ Google Scholar ]
- Reckless J. P. D., Lawrence J. M. Encyclopedia of Food Sciences and Nutrition. 2nd ed. Academic Press; 2003. Hyperlipidemia (hyperlipidaemia) pp. 3183–3192. [ CrossRef ] [ Google Scholar ]
- Rosca M. G., Vazquez E. J., Chen Q., Kerner J., Kern T. S., Hoppel C. L. Oxidation of fatty acids is the source of increased mitochondrial reactive oxygen species production in kidney cortical tubules in early diabetes. Diabetes. 2012; 61 :2074–2083. doi: 10.2337/db11-1437. [ PMC free article ] [ PubMed ] [ CrossRef ] [ Google Scholar ]
- Rysman E., Brusselmans K., Scheys K., Timmermans L., Derua R., Munck S., Van Veldhoven P. P., Waltregny D., Daniëls V. W., Machiels J., Vanderhoydonc F., Smans K., Waelkens E., Verhoeven G., Swinnen J. V. De novo lipogenesis protects cancer cells from free radicals and chemotherapeutics by promoting membrane lipid saturation. Cancer Res. 2010; 70 :8117–8126. doi: 10.1158/0008-5472.CAN-09-3871. [ PubMed ] [ CrossRef ] [ Google Scholar ]
- Saudek C. D., Eder H. A. Lipid metabolism in diabetes mellitus. Am. J. Med. 1979; 66 :843–852. doi: 10.1016/0002-9343(79)91126-4. [ PubMed ] [ CrossRef ] [ Google Scholar ]
- Schonfeld G. Inherited disorders of lipid transport. Endocrinol. Metab. Clin. North Am. 1990; 19 :229–257. doi: 10.1016/S0889-8529(18)30323-2. [ PubMed ] [ CrossRef ] [ Google Scholar ]
- Shattat G. F. A. Review article on hyperlipidemia: types, treatments and new drug targets. Biomed. Pharmacol. J. 2014; 7 :399–409. doi: 10.13005/bpj/504. [ CrossRef ] [ Google Scholar ]
- Solomon M., Muro S. Lysosomal enzyme replacement therapies: Historical development, clinical outcomes, and future perspectives. Adv. Drug Deliv. Rev. 2017; 118 :109–134. doi: 10.1016/j.addr.2017.05.004. [ PMC free article ] [ PubMed ] [ CrossRef ] [ Google Scholar ]
- Song X., Zhong L., Lyu N., Liu F., Li B., Hao Y., Xue Y., Li J., Feng Y., Ma Y., Hu Y., Zhu B. Inulin can alleviate metabolism disorders in ob/ob mice by partially restoring leptin-related pathways mediated by gut microbiota. Genomics Proteomics Bioinformatics. 2019; 17 :64–75. doi: 10.1016/j.gpb.2019.03.001. [ PMC free article ] [ PubMed ] [ CrossRef ] [ Google Scholar ]
- Sparrow C. P., Burton C. A., Hernandez M., Mundt S., Hassing H., Patel S., Rosa R., Hermanowski-Vosatka A., Wang P. R., Zhang D., Peterson L., Detmers P. A., Chao Y. S., Wright S. D. Simvastatin has anti-inflammatory and antiatherosclerotic activities independent of plasma cholesterol lowering. Arterioscler. Thromb. Vasc. Biol. 2001; 21 :115–121. doi: 10.1161/01.ATV.21.1.115. [ PubMed ] [ CrossRef ] [ Google Scholar ]
- Stein E. A., Honarpour N., Wasserman S. M., Xu F., Scott R., Raal F. J. Effect of the proprotein convertase subtilisin/kexin 9 monoclonal antibody, AMG 145, in homozygous familial hypercholesterolemia. Circulation. 2013; 128 :2113–2120. doi: 10.1161/CIRCULATIONAHA.113.004678. [ PubMed ] [ CrossRef ] [ Google Scholar ]
- Stone N. J. Secondary causes of hyperlipidemia. Med. Clin. North Am. 1994; 78 :117–141. doi: 10.1016/S0025-7125(16)30179-1. [ PubMed ] [ CrossRef ] [ Google Scholar ]
- Stroes E. S., Thompson P. D., Corsini A., Vladutiu G. D., Raal F. J., Ray K. K., Roden M., Stein E., Tokgözoğlu L., Nordestgaard B. G., Bruckert E., De Backer G., Krauss R. M., Laufs U., Santos R. D., Hegele R. A., Hovingh G. K., Leiter L. A., Mach F., März W., Newman C. B., Wiklund O., Jacobson T. A., Catapano A. L., Chapman M. J., Ginsberg H. N. European Atherosclerosis Society Consensus Panel, author. Statin-associated muscle symptoms: impact on statin therapy-European Atherosclerosis Society Consensus Panel Statement on Assessment, Aetiology and Management. Eur. Heart J. 2015; 36 :1012–1022. doi: 10.1093/eurheartj/ehv043. [ PMC free article ] [ PubMed ] [ CrossRef ] [ Google Scholar ]
- Taskinen M. R., Lahdenperä S., Syvänne M. New insights into lipid metabolism in non-insulin-dependent diabetes mellitus. Ann. Med. 1996; 28 :335–340. doi: 10.3109/07853899608999090. [ PubMed ] [ CrossRef ] [ Google Scholar ]
- Tian L., Yu X. Lipid metabolism disorders and bone dysfunction-interrelated and mutually regulated (review) Mol. Med. Rep. 2015; 12 :783–794. doi: 10.3892/mmr.2015.3472. [ PMC free article ] [ PubMed ] [ CrossRef ] [ Google Scholar ]
- Tocher D. R. Metabolism and functions of lipids and fatty acids in teleost fish. Rev. Fish. Sci. 2003; 11 :107–184. doi: 10.1080/713610925. [ CrossRef ] [ Google Scholar ]
- Tracey T. J., Steyn F. J., Wolvetang E. J., Ngo S. T. Neuronal lipid metabolism: multiple pathways driving functional outcomes in health and disease. Front. Mol. Neurosci. 2018; 11 :10. doi: 10.3389/fnmol.2018.00010. [ PMC free article ] [ PubMed ] [ CrossRef ] [ Google Scholar ]
- Trebatická J., Dukát A., Ďuračková Z., Muchová J. Cardiovascular diseases, depression disorders and potential effects of omega-3 fatty acids. Physiol. Res. 2017; 66 :363–382. doi: 10.33549/physiolres.933430. [ PubMed ] [ CrossRef ] [ Google Scholar ]
- Uttaro A. Biosynthesis of polyunsaturated fatty acids in lower eukaryotes. IUBMB Life. 2006; 58 :563–571. doi: 10.1080/15216540600920899. [ PubMed ] [ CrossRef ] [ Google Scholar ]
- Verma N. Introduction to hyperlipidemia and its treatment: a review. Int. J. Curr. Pharm. Res. 2017; 9 :6–14. doi: 10.22159/ijcpr.2017v9i1.16616. [ CrossRef ] [ Google Scholar ]
- Waller J. R., Waller D. G. Drugs for lipid disorders, antiplatelet drugs and fibrinolytics. Medicine. 2014; 42 :544–548. doi: 10.1016/j.mpmed.2014.06.015. [ CrossRef ] [ Google Scholar ]
- Wu L., Parhofer K. G. Diabetic dyslipidemia. Metabolism. 2014; 63 :1469–1479. doi: 10.1016/j.metabol.2014.08.010. [ PubMed ] [ CrossRef ] [ Google Scholar ]
- Wu L., Piotrowski K., Rau T., Waldmann E., Broedl U. C., Demmelmair H., Koletzko B., Stark R. G., Nagel J. M., Mantzoros C. S., Parhofer K. G. Walnut-enriched diet reduces fasting non-HDL-cholesterol and apolipoprotein B in healthy Caucasian subjects: a randomized controlled cross-over clinical trial. Metabolism. 2014; 63 :382–391. doi: 10.1016/j.metabol.2013.11.005. [ PubMed ] [ CrossRef ] [ Google Scholar ]
- Xiao C., Rossignol F., Vaz F. M., Ferreira C. R. Inherited disorders of complex lipid metabolism: a clinical review. J. Inherit. Metab. Dis. 2021; 44 :809–825. doi: 10.1002/jimd.12369. [ PubMed ] [ CrossRef ] [ Google Scholar ]
- Yan J., Horng T. Lipid metabolism in regulation of macrophage functions. Trends Cell Biol. 2020; 30 :979–989. doi: 10.1016/j.tcb.2020.09.006. [ PubMed ] [ CrossRef ] [ Google Scholar ]
- Yuan G., Al-Shali K. Z., Hegele R. A. Hypertriglyceridemia: its etiology, effects and treatment. Can. Med. Assoc. J. 2007; 176 :1113–1120. doi: 10.1503/cmaj.060963. [ PMC free article ] [ PubMed ] [ CrossRef ] [ Google Scholar ]
- Yue S., Li J., Lee S. Y., Lee H. J., Shao T., Song B., Cheng L., Masterson T. A., Liu X., Ratliff T. L., Cheng J. X. Cholesteryl ester accumulation induced by PTEN loss and PI3K/AKT activation underlies human prostate cancer aggressiveness. Cell Metab. 2014; 19 :393–406. doi: 10.1016/j.cmet.2014.01.019. [ PMC free article ] [ PubMed ] [ CrossRef ] [ Google Scholar ]
- Frontiers in Cell and Developmental Biology
- Membrane Traffic
- Research Topics
Novel Insights into the Modulation of Protein Function by Lipids and Membrane Organization
Total Downloads
Total Views and Downloads
About this Research Topic
A continuously growing amount of evidence underlines the active contribution of lipids and membrane organization to the regulation of the structure and function of transmembrane proteins. In general, lipids of the cell membrane can affect proteins through a mixture of direct and indirect mechanisms. While the ...
Keywords : protein function, lipids, membrane organization
Important Note : All contributions to this Research Topic must be within the scope of the section and journal to which they are submitted, as defined in their mission statements. Frontiers reserves the right to guide an out-of-scope manuscript to a more suitable section or journal at any stage of peer review.
Topic Editors
Topic coordinators, recent articles, submission deadlines, participating journals.
Manuscripts can be submitted to this Research Topic via the following journals:
total views
- Demographics
No records found
total views article views downloads topic views
Top countries
Top referring sites, about frontiers research topics.
With their unique mixes of varied contributions from Original Research to Review Articles, Research Topics unify the most influential researchers, the latest key findings and historical advances in a hot research area! Find out more on how to host your own Frontiers Research Topic or contribute to one as an author.
- Open access
- Published: 27 March 2024
The serum soluble ASGR1 concentration is elevated in patients with coronary artery disease and is associated with inflammatory markers
- Qin Luo 1 , 2 ,
- Jingfei Chen 3 ,
- Yanfeng Yi 1 , 2 ,
- Panyun Wu 1 , 2 ,
- Yingjie Su 4 ,
- Zhangling Chen 1 , 2 ,
- Hacı Ahmet Aydemir 5 ,
- Jianjun Tang 1 , 2 ,
- Zhenfei Fang 1 , 2 &
- Fei Luo 1 , 2
Lipids in Health and Disease volume 23 , Article number: 89 ( 2024 ) Cite this article
89 Accesses
Metrics details
Background and aims
Current research has suggested that asialoglycoprotein receptor 1 (ASGR1) is involved in cholesterol metabolism and is also related to systemic inflammation. This study aimed to assess the correlation between the serum soluble ASGR1 (sASGR1) concentration and inflammatory marker levels. Moreover, the second objective of the study was to assess the association between sASGR1 levels and the presence of coronary artery disease (CAD).
The study subjects included 160 patients who underwent coronary angiography. Ninety patients were diagnosed with CAD, while seventy age- and sex-matched non-CAD patients served as controls. We measured the serum sASGR1 levels using an ELISA kit after collecting clinical baseline characteristics.
Patients with CAD had higher serum sASGR1 levels than non-CAD patients did ( P < 0.0001). sASGR1 was independently correlated with the risk of CAD after adjusting for confounding variables (OR = 1.522, P = 0.012). The receiver operating characteristic (ROC) curve showed that sASGR1 had a larger area under the curve (AUC) than did the conventional biomarkers apolipoprotein B (APO-B) and low-density lipoprotein cholesterol (LDL-C). In addition, multivariate linear regression models revealed that sASGR1 is independently and positively correlated with high-sensitivity C-reactive protein (CRP) ( β = 0.86, P < 0.001) and WBC ( β = 0.13, P = 0.004) counts even after adjusting for lipid parameters. According to our subgroup analysis, this relationship existed only for CAD patients.
Our research demonstrated the link between CAD and sASGR1 levels, suggesting that sASGR1 may be an independent risk factor for CAD. In addition, this study provides a reference for revealing the potential role of sASGR1 in the inflammation of atherosclerosis.
Introduction
Atherosclerotic cardiovascular disease (ASCVD) is a fatal disease with a complex etiology worldwide. Over the last few decades, there has been constant updating and even subversion of the understanding of atherosclerosis [ 1 ]. In parallel, an increasing number of atherosclerotic markers have been discovered [ 2 , 3 ]. Disorders of lipid metabolism, especially hypercholesterolemia, are well-known pathogenic risk factors for ASCVD [ 4 ]. Lipoproteins are the initial points of interest. Oxidized low-density lipoprotein (ox-LDL) or small dense LDL enters the artery wall, which constitutes the classic early stage of atherosclerosis [ 2 , 5 ]. Of course, circulating adhesion molecules such as vascular cell adhesion molecule-1 (VCAM), intercellular adhesion molecule-1 (ICAM), and monocyte chemotactic protein-1 (MCP-1) play crucial roles in this stage [ 5 , 6 ]. High triglyceride (TG) and residual cholesterol levels are important pathogenic factors of ASCVD and were recognized when residual cardiovascular risk associated with statins was discovered [ 7 , 8 ]. Other lipoproteins, such as lipoprotein(a) [Lp(a)] and apolipoprotein B (APO-B), have also been found to be associated with the risk of atherosclerosis [ 5 ]. Evaluating the characteristics of coronary artery plaques using the latest invasive or noninvasive imaging methods can help predict the risk of cardiac events and guide personalized treatment strategies [ 9 , 10 , 11 ]. A higher plaque burden and high risk plaques are independent risk factors for myocardial ischemia [ 11 , 12 , 13 ]. In addition, recent studies have established that inflammatory markers participate in all stages of atherosclerosis, making them one of the most promising therapeutic targets for treating this disease [ 14 , 15 ].
Asialoglycoprotein receptor (ASGR) is a hepatic C-type lectin that is expressed mainly on the sinusoidal surface of hepatocytes [ 16 , 17 ]. The primary function of ASGR is to bind glycoproteins containing terminal galactose or GlcNAc residues (such as asialoglycoproteins) in circulation [ 18 , 19 ]. The complex is subsequently internalized under the coat of clathrin and transported to the lysosome for degradation [ 20 ]. As a hepatocyte membrane receptor, asialoglycoprotein receptor 1 (ASGR1) regulates hepatic cholesterol homeostasis by interacting with circulating asialoglycoproteins [ 21 ]. In animal studies, inhibiting hepatic ASGR1 or its binding to circulating asialoglycoproteins reduces serum cholesterol levels by promoting cholesterol efflux into the bile [ 21 ]. ASGR1 loss-of-function mutations are linked to a 0.4 mmol/l decrease in circulating non-high-density lipoprotein cholesterol (non-HDL-C) and a 34% reduction in the incidence of coronary artery disease (CAD) [ 22 ]. Notably, the effect of the ASGR1 mutation on the risk of CAD is significantly greater than that on non-HDL-C levels, suggesting that the significant reduction in the risk of CAD is not entirely explained by the effect of ASGR1 mutations on non-HDL-C levels [ 22 , 23 ]. Therefore, ASGR1 mutations may be involved in other protective mechanisms than the regulation of cholesterol homeostasis, such as inflammation. Indeed, some direct or indirect evidence has shown that ASGR1 is involved in biological processes related to systemic inflammation and vascular inflammation [ 24 , 25 , 26 , 27 ]. For example, proinflammatory cytokines upregulate the expression of ASGR1 [ 24 ]. The interaction of ASGR1 with epidermal growth factor receptor (EGFR) activates the extracellular signal-regulated kinase (ERK) pathway [ 25 ], which has been linked to inflammatory regulation and atherosclerosis [ 28 , 29 ]. However, the relationship between ASGR1 and inflammation in patients with CAD is unclear.
In contrast to ASGR1, which is located on the liver surface mentioned above, serum soluble ASGR1 (sASGR1), which is secreted by the liver, is another splicing variant of liver ASGR1 and is located in the circulation due to the absence of a transmembrane domain [ 30 ]. However, current research on sASGR1 is very limited. Although our previous study showed that sASGR1 is associated with LDL-C levels [ 31 ], the relationship between sASGR1 levels and the risk of CAD is still unclear.
C-reactive protein (CRP) levels and white blood cell (WBC) counts are the most basic and widely used indicators of systemic inflammation. In addition, CRP levels are positively correlated with the degree of coronary artery stenosis in patients with CAD [ 32 ]. Two recent studies have established that high-sensitivity C-reactive protein (hs-CRP) levels exhibit superior predictive power for future cardiovascular events and mortality risk when compared to circulating cholesterol levels in patients receiving statin therapy [ 33 , 34 ]. Similarly, WBC counts have been widely confirmed to be closely related to atherosclerosis and CAD [ 35 , 36 , 37 ]. Currently, there are no simple or effective methods for determining the level of ASGR1 expression on the hepatocyte membrane in clinical practice. Therefore, the purpose of this study was to assess the correlation between sASGR1 levels and inflammatory marker levels, including hs-CRP levels, WBC counts, and WBC subsets. Moreover, the second objective of the study was to assess the association between sASGR1 levels and the presence of CAD.
Materials and methods
Study population.
We consecutively included 160 patients admitted to the Second Xiangya Hospital of Central South University between September 2022 and September 2023 in this study. All patients underwent coronary angiography. Patients were categorized into two groups based on the results of coronary angiography: 90 patients with CAD and 70 patients without CAD. We matched the groups for age and sex. Four experienced angiographers performed coronary angiography, two of whom evaluated the vessels. To determine the severity of CAD, CAD patients were further subdivided into acute myocardial infarction (AMI) ( n = 26) and CAD without AMI ( n = 64) groups. The severity of coronary lesions was determined by the number of major coronary artery stenoses, which were classified as vessels ≤ 2 ( n = 51) or vessels > 2 ( n = 39). Furthermore, the Gensini score was also calculated to assess the severity of CAD. We excluded patients with infections, autoimmune diseases, liver diseases, renal failure, malignancies, or other serious diseases.
The diagnosis of CAD was based on angina pectoris manifestations, electrocardiogram changes, and coronary angiography, which indicated that the major vessel had a degree of stenosis greater than or equal to 50%. Elevated plasma troponin levels, together with evidence of acute myocardial ischemia, are diagnostic criteria for AMI [ 38 ]. Fasting blood glucose levels ≥ 126 mg/dl (7.0 mmol/L) or 2-hour postprandial blood glucose levels ≥ 200 mg/dL (11.1 mmol/L) were used to diagnose type 2 diabetes mellitus (T2DM). Repeated measures of blood pressure greater than or equal to 140/90 mmHg were used to diagnose hypertension.
The study was authorized by the ethics committee of Second Xiangya Hospital, which also found that it adhered to the ethical principles of the Declaration of Helsinki. Informed permission was obtained from all patients.
Sample size calculation
In the study assessing the association between sASGR1 levels and the presence of coronary artery disease (CAD), we used Power Analysis and Sample Size (PASS) software for sample size calculations. We set bilateral α = 0.05 and power = 0.9. The ratio of the sample size between the CAD group and the control group was 1.28:1. We set the mean and standard deviation of the sASGR1 levels for the two groups based on our preliminary results. The sample size calculation revealed that a sample of 55 CAD patients and 43 non-CAD patients achieved 90.31% power to reject the null hypothesis of equal means when the population mean difference was µ1- µ2 = 4–2.1 = 1.9 with standard deviations of 4 for the CAD group and 1.3 for the non-CAD group. Ultimately, 90 CAD patients and 70 non-CAD patients composed the sample.
Clinical characteristics and laboratory measurements
Basic information, including age, sex, body mass index (BMI), smoking status, and statin use, was collected and recorded. Peripheral blood samples were collected from patients through the elbow vein after they had fasted overnight. The serum was collected after centrifuging the blood sample for 10 min at 3,000 rpm and subsequently stored at -80 °C. Serum lipid parameters and hs-CRP levels were measured using a fully automated biochemical analyzer. WBCs, neutrophils, lymphocytes, and monocytes were counted using an automatic blood cell counter.
ELISA for determining the sASGR1 concentration
The serum concentration of sASGR1 was determined using sandwich enzyme-linked immunosorbent assay (ELISA) kits (JL41965; Jianglai Biology, Shanghai). Two measurements were repeated per sample to reduce random variations. Both the intraplate and interplate coefficients of variation (CVs) were less than 10%, suggesting that the assay has good repeatability. The recovery rate and linearity of this reagent kit were 95% and 91%, respectively (with a dilution ratio of 1:2). The minimum detectable concentration of serum sASGR1 was 0.156 ng/mL.
Statistical analyses
For the data analysis, we employed SPSS 25.0 statistical software. In addition, EmpowerStats statistical software (version 4.1) and R language (version 4.2.0) were used for subgroup analysis and interaction testing. P = 0.05 was used as the statistical criterion.
The continuous variables are displayed as the mean ± standard deviation or as medians and quartiles (Q1-Q3), and group comparisons were conducted using the independent-samples t test or Mann‒Whitney U test according to the type of data distribution. Chi-square tests were used to compare differences among categorical variables, which are presented as frequencies or percentages. The data were analyzed for a normal distribution by the D’Agostino–Pearson omnibus normality test. The Kruskal‒Wallis test was used to assess the association between sASGR1 levels and the severity of CAD. A multivariate logistic regression model was used to identify the factors that influence the presence of CAD. The diagnostic value of sASGR1 and traditional biomarkers in patients with CAD was assessed using receiver operating characteristic (ROC) curves. To further analyze the relationship between sASGR1 and inflammatory markers, we employed a stepwise multivariate regression model in which inflammatory markers were used as the dependent variables. For subgroup analysis, stratified linear regression models were employed, and likelihood ratio tests were applied to find any variations or interactions.
Baseline characteristics
The basic details and biochemical characteristics of the study subjects are listed in Table 1 . BMI, smoking status, statin use, history of hypertension, and T2DM status were greater in the CAD group than in the non-CAD group ( P < 0.05). The CAD group had higher serum TG, LDL-C, Lp(a), APO-B, and hs-CRP levels; WBC and neutrophil counts; and monocyte counts than did the non-CAD group ( P < 0.05). HDL-C levels were greater in non-CAD patients ( P < 0.05). There were no significant differences in age, sex, lymphocyte count, or other blood lipid parameters between the two patient groups.
Serum sASGR1 levels in the CAD and non-CAD groups
As shown in Fig. 1 , the serum level of sASGR1 in CAD patients was significantly greater than that in non-CAD patients [2.58 (1.8, 4.1) vs. 1.71 (1.3, 2.6) ng/ml, P < 0.0001]. Subsequently, we investigated the relationship between sASGR1 levels and the severity of CAD. As shown in Fig. 2 A, a lack of significant difference was observed between CAD patients with ≤ 2 diseased vessels and non-CAD patients [2.12 (1.5, 3.0) vs. 1.71 (1.3, 2.6) ng/ml, P = 0.078]. However, the level of sASGR1 in CAD patients with > 2 diseased vessels was significantly greater than that in non-CAD patients [2.76 (2.2, 4.8) vs. 1.71 (1.3, 2.6) ng/ml, P < 0.0001] or in CAD patients with ≤ 2 diseased vessels [2.76 (2.2, 4.8) vs. 2.12 (1.5, 3.0) ng/ml, P = 0.033]. Additionally, we separated patients into two groups according to the median (60) Gensini score to assess the association between sASGR1 level and CAD severity more precisely: Gensini score ≤ 60 ( n = 45) and Gensini score > 60 ( n = 45). However, there was no significant increase in the level of sASGR1 as the Gensini score increased (Fig. 2 B). In addition, we were unable to identify significant differences in the severity of CAD between individuals with and without AMI (Fig. 2 C).
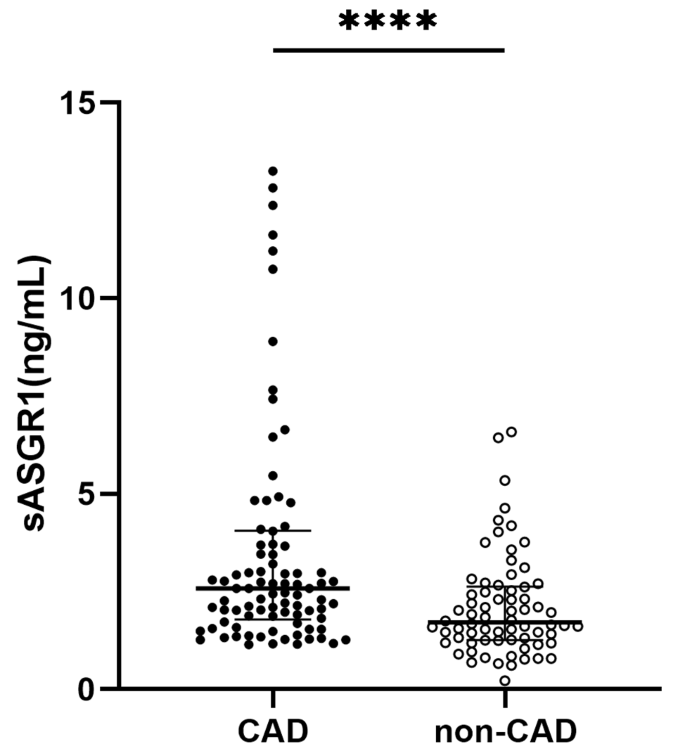
Comparison of serum sASGR1 levels between the CAD and non-CAD groups. The serum sASGR1 concentration in CAD patients ( n = 90) was significantly greater than that in non-CAD patients ( n = 70). [Mann‒Whitney U test, 2.58 (1.8, 4.1) vs. 1.71 (1.3, 2.6) ng/ml, P < 0.0001]. **** P < 0.0001
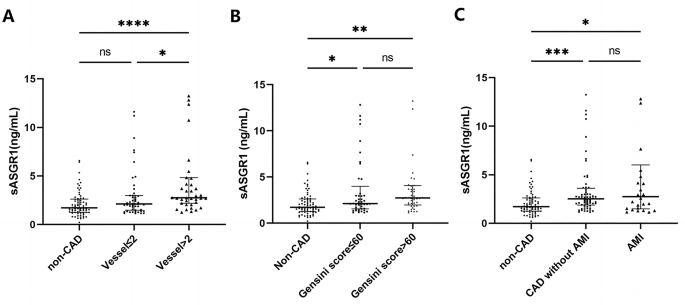
The relationship between sASGR1 levels and the severity of CAD. ( A ) sASGR1 levels in patients with different CAD statuses and numbers of involved vessels. Comparisons were evaluated by the Kruskal‒Wallis test. ns, not statistically significant. * P < 0.05. **** P < 0.0001. For vessels ≤ 2, the number of involved vessels was < 2; for vessels > 2, the number of involved vessels was more than 2. ( B ) Comparison of sASGR1 levels in patients with different Gensini scores. Comparisons were evaluated by the Kruskal‒Wallis test. ns, not statistically significant. * P < 0.05. ** P < 0.01. ( C ) sASGR1 levels in patients with different severities of CAD. Comparisons were evaluated by the Kruskal‒Wallis test. ns, not statistically significant. * P < 0.05. *** P < 0.001
Diagnostic value of sASGR1 and traditional biomarkers for CAD patients
We used receiver operating characteristic (ROC) curves to evaluate the diagnostic ability of sASGR1 levels for CAD (Fig. 3 ). The area under the curve (AUC) of sASGR1 was 0.691 (95% CI = 0.60–0.78, P < 0.001). Its diagnostic utility was better than that of the traditional biomarkers APO-B (AUC: 0.619, 95% CI: 0.53–0.71, P = 0.023) and LDL-C (AUC: 0.599, 95% CI: 0.50–0.69, P = 0.059), despite not being comparable to that of hs-CRP (AUC: 0.775, 95% CI: 0.70–0.85, P < 0.001), TG (AUC: 0.796, 95% CI: 0.72–0.87, P < 0.001), or total cholesterol (TC)/high-density lipoprotein cholesterol (HDL-C) (AUC: 0.723, 95% CI: 0.64–0.81, P < 0.001). sASGR1 has a cutoff value of 1.682 ng/ml for predicting the occurrence of CAD, with a sensitivity of 77.6% and a specificity of 54.2%. The sensitivity and specificity of the other biomarkers for predicting the presence of CAD were as follows: hs-CRP (sensitivity: 47.1%, specificity: 95.8%), APO-B (sensitivity: 41.2%, specificity: 87.5%), LDL-C (sensitivity: 35.3%, specificity: 91.7%), TC/HDL-C (sensitivity: 69.4%, specificity: 70.8%), and TG (sensitivity: 62.4%, specificity: 89.6%).
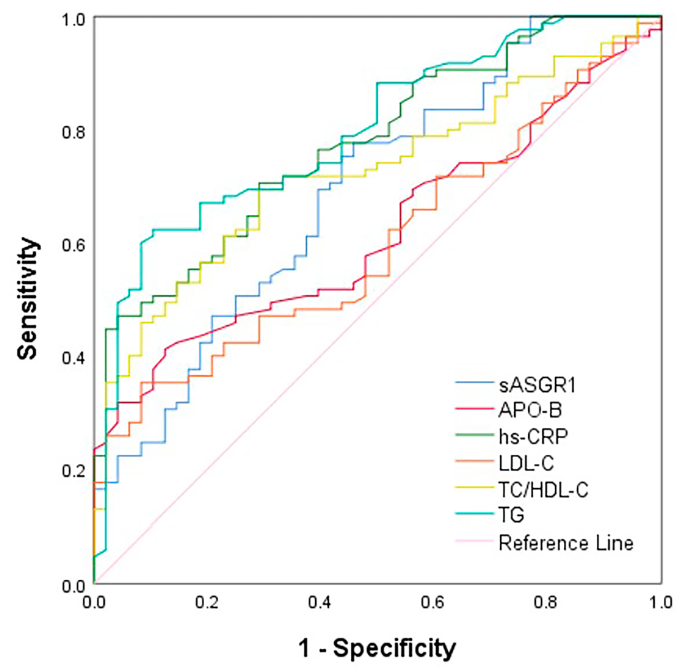
ROC curve analysis for the predictive value of serum sASGR1 and traditional biomarkers in the presence of CAD
sASGR1 is an independent influencing factor for the occurrence of CAD
A multivariate logistic regression model was used to identify the factors that influence the presence of CAD. We included independent variables based on the results of univariate analysis, clinical significance, sample size, and multicollinearity. The results showed that sASGR1, smoking, TG, hypertension, and T2DM were associated with an increased risk of CAD after controlling for BMI, smoking status, TG, LDL-C, hs-CRP, hypertension, and T2DM (Table 2 ). For every unit increase in the sASGR1 level, the risk of CAD increased by 0.52 times (OR = 1.522, 95% CI = 1.095, 2.115; P = 0.012).
Relationships between the serum sASGR1 concentration and inflammatory marker level
By setting hs-CRP levels, WBC counts, and WBC subsets as dependent variables, we used stepwise multivariate regression models to determine the correlation between sASGR1 levels and inflammatory marker levels (Table 3 ). After we adjusted for sex, age, BMI, smoking status, statin use, hypertension status, T2DM status, and CAD status (Model II), sASGR1 was positively associated with the levels of inflammatory markers [hs-CRP ( β = 1.00, p < 0.001), WBC count ( β = 0.12, P = 0.004), and neutrophil count ( β = 0.09, P = 0.011)]. Given that previous research has indicated a link between blood lipid levels and inflammation [ 39 ], we further adjusted for blood lipid parameters and found that the serum sASGR1 concentration was significantly positively correlated with the serum hs-CRP level ( β = 0.86, P < 0.001), WBC count ( β = 0.13, P = 0.004), and neutrophil count ( β = 0.10, P = 0.008). Additionally, a similar association was observed between sASGR1 concentration and monocyte count ( β = 0.009, P = 0.014).
Subgroup analysis of the correlation between sASGR1 and inflammatory marker levels
According to the interaction test (Table 4 ), the effect of sASGR1 on the hs-CRP level was significantly affected by age ( p for interaction = 0.015), sex ( p for interaction = 0.007), BMI ( p for interaction = 0.002), and hypertension status ( P for interaction = 0.019), indicating that the association between the sASGR1 concentration and the hs-CRP level differed according to these variables. Notably, even after we adjusted for factors such as age, sex, BMI, statin use, smoking status, and lipid parameters, the positive correlation between sASGR1 and hs-CRP levels was still significant in patients with CAD ( β = 0.8, P < 0.001). Among patients without CAD, this correlation was not observed ( P = 0.231).
Similarly, we also conducted subgroup analysis and interaction testing to determine the relationship between sASGR1 and WBC count. Age was the sole significant factor influencing the association between sASGR1 level and WBC count, in contrast to hs-CRP ( P for interaction < 0.001), as shown in Table 5 . Interestingly, similar to that of hs-CRP, a positive correlation between sASGR1 and WBC was observed only in CAD patients ( β = 0.1, P = 0.029).
According to our study, sASGR1 is independently and positively correlated with hs-CRP and WBC. According to our subgroup analysis, this relationship existed only for CAD patients. In addition, serum sASGR1 levels are elevated in CAD patients. sASGR1 is independently correlated with the risk of CAD after adjusting for confounding variables, and it has better diagnostic value than APO-B and LDL-C.
Since 2016, ASGR1 has received widespread attention from researchers due to its involvement in liver cholesterol metabolism [ 22 ]. Recent animal studies have shown that inhibiting ASGR1 on the hepatocyte membrane (named hASGR1) is expected to become a new strategy for reducing LDL-C levels [ 21 ]. Similarly, genetically mimicked ASGR1 inhibitors were associated with lower cholesterol levels and CAD risk [ 40 ]. In addition, an observational study showed that ASGR1 mRNA levels in peripheral blood mononuclear cells were lower in CAD patients than in non-CAD patients, but the underlying mechanism is still unknown [ 23 ]. However, there is currently no research on the role of serum sASGR1 in CAD patients. sASGR1 and LDL-C levels were found to be positively correlated in our recent study [ 31 ]. As expected, this study showed an increase in sASGR1 levels in CAD patients, which is consistent with the findings of a recent study [ 41 ]. In the present study, plasma proteomics analysis revealed ASGR1 to be a risk factor for ischemic heart disease. Thus, the results of our study lend support for sASGR1 as a potential biomarker for CAD. We also found that, after controlling for confounding variables, sASGR1 remained an independent risk factor for CAD (Table 2 ). As mentioned earlier, hASGR1 regulates liver cholesterol homeostasis by binding to circulating asialoglycoproteins, ultimately affecting plasma cholesterol levels and the risk of CAD [ 21 , 22 ]. Previous research revealed that while sASGR1 inhibits the binding of circulating asialoglycoproteins to hASGR1, it still binds to asialoglycoproteins and enters the liver as a complex [ 30 , 31 ]. Taken together, these findings imply that the entry of the sASGR1-asialoglycoprotein complex into hepatocytes may exert similar downstream biological effects as the binding of the asialoglycoprotein to hASGR1. Another hypothesis, however, is that the serum sASGR1 concentration might reflect the hASGR1 protein level. Given this, it will be intriguing to investigate whether blocking sASGR1 can lower plasma cholesterol and the risk of CAD in a manner similar to inhibiting hASGR1.
We evaluated the relationship between serum sASGR1 levels and the severity of CAD. Although the serum sASGR1 concentration was associated with the number of coronary artery lesions (Fig. 2 A), the level of sASGR1 did not significantly increase as the Gensini score increased (Fig. 2 B). The serum sASGR1 concentration has been reported to be positively correlated with LDL-C levels [ 31 ], but the correlation between LDL-C level and Gensini score is not significant [ 42 , 43 ]. The relationship between serum sASGR1 levels and the severity of CAD in this study remains uncertain, even though the Gensini score is a more accurate indicator of plaque burden and CAD severity than the number of vascular lesions. More precise methods, such as intravascular ultrasound and optical coherence tomography, may be needed to evaluate the relationship between sASGR1 and the severity of CAD. We failed to find that sASGR1 could be used to identify individuals who had an AMI (Fig. 2 C). Our previous study showed that there is no correlation between the serum troponin T concentration and sASGR1 level [ 31 ], which is consistent with the results of this study, indicating that the sASGR1 level is not related to the degree of myocardial injury.
We analyzed the diagnostic value of the sASGR1 level for CAD. Although it does not have the same diagnostic efficacy as hs-CRP, it is superior to LDL-C and APO-B. In addition, sASGR1 has advantages over hs-CRP (47.1%), APO-B (41.2%), TG (62.4%), and TC/HDL-C (69.4%) due to its relatively high diagnostic sensitivity (77.6%), indicating an advantage in the early screening of CAD.
In addition to its clear association with cholesterol metabolism, a small number of studies have shown that ASGR1 is associated with systemic inflammation. The most direct evidence shows that knockdown of Asgr1 in mouse liver and monocytes suppressed the expression of plasma inflammatory cytokines [interleukin-1 (IL-1), IL-6, and tumor necrosis factor (TNF-α)] [ 27 ]. However, these studies on the relationship between ASGR1 and systemic inflammation have focused mainly on the role of ASGR1 as a hepatocyte or monocyte membrane receptor. The relationships between serum sASGR1 levels and inflammatory marker levels in healthy individuals and patients with disease are unknown. Our findings showed that the serum sASGR1 concentration is positively correlated with inflammatory marker levels (hs-CRP and WBC), which supports and propels previous basic research. However, the causal link between sASGR1 and inflammatory markers warrants further exploration.
Inflammation is a key factor in the development of atherosclerosis and CAD. As a stable and reliable inflammatory marker, CRP has been shown to upregulate the expression of adhesion molecules and monocyte chemokines, inhibit the production of endothelial nitric oxide synthase (eNOS), and promote arterial thrombosis, indicating direct involvement in the occurrence of atherosclerosis [ 44 , 45 , 46 ]. Therefore, evaluating hs-CRP levels is highly important for CAD patients without hypercholesterolemia, as there is no urgent demand for lipid-lowering agents. The CANTOS study identified for the first time the ability of anti-inflammatory treatment to reduce cardiovascular events, which was independent of blood lipid levels [ 47 ]. An association between plasma CRP and LDL-C levels has been demonstrated in previous research [ 39 ]. Despite the fact that ASGR1 does affect circulating cholesterol levels, our subgroup analysis results showed that sASGR1 remains an independent influencing factor for hs-CRP and WBC count among individuals with CAD even after adjusting for lipid parameters (Table 3 ). Notably, patients without CAD did not exhibit this association (Tables 4 and 5 ). Thus, our findings suggest a potential connection between serum sASGR1 and inflammation in patients with CAD, independent of lipid metabolism disorders. This hypothesis has actually received indirect support from earlier studies. For instance, sialylation mediated by α2,3-sialyltransferases has been linked to the recruitment of circulating inflammatory myeloid cells to the atherosclerotic vascular endothelium [ 22 , 48 , 49 ]. Moreover, sASGR1 levels and monocyte counts were positively correlated according to the multivariate regression model (model III: β = 0.009, p = 0.014). ASGR1 is expressed in peripheral blood monocytes [ 50 ]. These findings suggest that, in addition to being secreted by hepatocytes, serum sASGR1 may also be secreted by monocytes. In summary, the positive correlation between the serum sASGR1 concentration and inflammatory marker (hs-CRP and WBC) levels in CAD patients supports the current view that ASGR1 is a risk factor for CAD. In addition, our research provides a reference for revealing the potential role of sASGR1 in the inflammation of atherosclerosis.
Study strengths and limitations
This is the first study in which we investigated the relationship between serum sASGR1 levels and CAD incidence, as well as inflammatory marker levels. This study has several limitations. First, this was a cross-sectional investigation, and the causal relationships between the serum sASGR1 concentration and CAD incidence or inflammatory marker levels could not be determined. However, further studies are needed to reveal the role of ASGR1 in the inflammation of atherosclerosis. Second, a relatively small sample size may hinder the ability to identify minute differences, even though the results of sample size calculations show that our sample size is appropriate for the main purpose of the study. These findings need to be confirmed in larger-sample studies. Third, the method of dividing the patients into groups with and without CAD may have resulted in selection bias. Fourth, we did not perform Western blot analysis of the serum sASGR1 concentration to confirm that this parameter is a biomarker for CAD, as suitable antibodies were not found. Finally, we did not investigate the correlation between sASGR1 levels and the levels of other inflammatory cytokines, such as ILs.
Conclusions
Our study suggested that serum sASGR1 levels are elevated in CAD patients and may be an independent risk factor for CAD. Moreover, sASGR1 was independently and positively correlated with inflammatory marker levels in CAD patients, even after controlling for lipid parameters. The potential role of sASGR1 in inflammation in atherosclerosis, independent of cholesterol metabolism, may need further study.
Data availability
The data that support the findings of this study are available from the corresponding author upon reasonable request.
Abbreviations
Atherosclerotic cardiovascular disease
Asialoglycoprotein receptor 1
- Coronary artery disease
Soluble ASGR1
Hepatic ASGR1
High-sensitivity CRP
- White blood cell count
Low-density lipoprotein cholesterol
High-density lipoprotein cholesterol
Vascular cell adhesion molecule-1
Intercellular adhesion molecule-1
Monocyte chemotactic protein-1
Apolipoprotein B
Apolipoprotein A1
Triglyceride
Total cholesterol
Lipoprotein (a)
Interleukin-1
Tumor necrosis factor
Epidermal growth factor receptor
Acute myocardial infarction
Type 2 Diabetes Mellitus
Body mass index
Receiver operating characteristic
Area under the curve
Enzyme-linked immunosorbent assay
Confidence interval
Libby P. The changing nature of atherosclerosis: what we thought we knew, what we think we know, and what we have to learn. Eur Heart J. 2021;42(47):4781–2.
Article PubMed PubMed Central Google Scholar
Tibaut M, Caprnda M, Kubatka P, Sinkovic A, Valentova V, Filipova S, et al. Markers of atherosclerosis: part 1 - serological markers. Heart Lung Circ. 2019;28(5):667–77.
Article PubMed Google Scholar
Tibaut M, Caprnda M, Kubatka P, Sinkovic A, Valentova V, Filipova S, et al. Markers of atherosclerosis: part 2 - genetic and imaging markers. Heart Lung Circ. 2019;28(5):678–89.
Ference BA, Ginsberg HN, Graham I, Ray KK, Packard CJ, Bruckert E, et al. Low-density lipoproteins cause atherosclerotic cardiovascular disease. 1. Evidence from genetic, epidemiologic, and clinical studies. A consensus statement from the European Atherosclerosis Society Consensus Panel. Eur Heart J. 2017;38(32):2459–72.
Article CAS PubMed PubMed Central Google Scholar
Della CV, Todaro F, Cataldi M, Tuttolomondo A. Atherosclerosis and its related laboratory biomarkers. Int J Mol Sci. 2023;24(21).
Libby P, Buring JE, Badimon L, Hansson GK, Deanfield J, Bittencourt MS, et al. Atherosclerosis Nat Rev Dis Primers. 2019;5(1):56.
Bhatt DL, Steg PG, Miller M, Brinton EA, Jacobson TA, Ketchum SB, et al. Cardiovascular Risk reduction with Icosapent Ethyl for Hypertriglyceridemia. N Engl J Med. 2019;380(1):11–22.
Article CAS PubMed Google Scholar
Nordestgaard BG. Triglyceride-Rich lipoproteins and Atherosclerotic Cardiovascular Disease: New insights from Epidemiology, Genetics, and Biology. Circ Res. 2016;118(4):547–63.
Stone PH, Libby P, Boden WE. Fundamental pathobiology of coronary atherosclerosis and clinical implications for chronic ischemic heart Disease Management-the Plaque hypothesis: a narrative review. Jama Cardiol. 2023;8(2):192–201.
Sarraju A, Nissen SE. Atherosclerotic plaque stabilization and regression: a review of clinical evidence. Nat Rev Cardiol. 2024.
Yang S, Koo BK, Hoshino M, Lee JM, Murai T, Park J, et al. CT angiographic and plaque predictors of functionally significant Coronary Disease and Outcome using machine learning. JACC Cardiovasc Imaging. 2021;14(3):629–41.
Lee JM, Choi KH, Koo BK, Park J, Kim J, Hwang D, et al. Prognostic implications of plaque characteristics and stenosis severity in patients with coronary artery disease. J Am Coll Cardiol. 2019;73(19):2413–24.
Severino P, D’Amato A, Pucci M, Infusino F, Adamo F, Birtolo LI et al. Ischemic heart Disease Pathophysiology paradigms overview: from plaque activation to Microvascular Dysfunction. Int J Mol Sci. 2020;21(21).
Libby P. Inflammation during the life cycle of the atherosclerotic plaque. Cardiovasc Res. 2021;117(13):2525–36.
CAS PubMed PubMed Central Google Scholar
Libby P. Inflammation in Atherosclerosis-No longer a theory. Clin Chem. 2021;67(1):131–42.
Ashwell G, Harford J. Carbohydrate-specific receptors of the liver. Annu Rev Biochem. 1982;51:531–54.
Ashwell G, Morell AG. The role of surface carbohydrates in the hepatic recognition and transport of circulating glycoproteins. Adv Enzymol Relat Areas Mol Biol. 1974;41(0):99–128.
Spiess M. The asialoglycoprotein receptor: a model for endocytic transport receptors. Biochemistry-Us. 1990;29(43):10009–18.
Article CAS Google Scholar
Stockert RJ. The asialoglycoprotein receptor: relationships between structure, function, and expression. Physiol Rev. 1995;75(3):591–609.
Trowbridge IS, Collawn JF, Hopkins CR. Signal-dependent membrane protein trafficking in the endocytic pathway. Annu Rev Cell Biol. 1993;9:129–61.
Wang JQ, Li LL, Hu A, Deng G, Wei J, Li YF, et al. Inhibition of ASGR1 decreases lipid levels by promoting cholesterol excretion. Nature. 2022;608(7922):413–20.
Nioi P, Sigurdsson A, Thorleifsson G, Helgason H, Agustsdottir AB, Norddahl GL, et al. Variant ASGR1 Associated with a reduced risk of coronary artery disease. N Engl J Med. 2016;374(22):2131–41.
Hamledari H, Sajjadi SF, Alikhah A, Boroumand MA, Behmanesh M. ASGR1 but not FOXM1 expression decreases in the peripheral blood mononuclear cells of diabetic atherosclerotic patients. J Diabetes Complications. 2019;33(8):539–46.
Nakaya R, Kohgo Y, Mogi Y, Nakajima M, Kato J, Niitsu Y. Regulation of asialoglycoprotein receptor synthesis by inflammation-related cytokines in HepG2 cells. J Gastroenterol. 1994;29(1):24–30.
Liu Y, Zhu L, Liang S, Yao S, Li R, Liu S, et al. Galactose protects hepatocytes against TNF-alpha-induced apoptosis by promoting activation of the NF-kappaB signaling pathway in acute liver failure. Lab Invest. 2015;95(5):504–14.
Lu Q, Liu J, Zhao S, Gomez CM, Laurent-Rolle M, Dong J, et al. SARS-CoV-2 exacerbates proinflammatory responses in myeloid cells through C-type lectin receptors and Tweety family member 2. Immunity. 2021;54(6):1304–19.
Shi R, Wang J, Zhang Z, Leng Y, Chen AF. ASGR1 promotes liver injury in sepsis by modulating monocyte-to-macrophage differentiation via NF-kappaB/ATF5 pathway. Life Sci. 2023;315:121339.
Qu WS, Tian DS, Guo ZB, Fang J, Zhang Q, Yu ZY, et al. Inhibition of EGFR/MAPK signaling reduces microglial inflammatory response and the associated secondary damage in rats after spinal cord injury. J Neuroinflammation. 2012;9:178.
Li N, McLaren JE, Michael DR, Clement M, Fielding CA, Ramji DP. ERK is integral to the IFN-gamma-mediated activation of STAT1, the expression of key genes implicated in atherosclerosis, and the uptake of modified lipoproteins by human macrophages. J Immunol. 2010;185(5):3041–8.
Liu J, Hu B, Yang Y, Ma Z, Yu Y, Liu S, et al. A new splice variant of the major subunit of human asialoglycoprotein receptor encodes a secreted form in hepatocytes. PLoS ONE. 2010;5(9):e12934.
Luo Q, Chen J, Su Y, Wu P, Wang J, Fang Z, et al. Correlation between serum soluble ASGR1 concentration and low-density lipoprotein cholesterol levels: a cross-sectional study. Lipids Health Dis. 2023;22(1):142.
Arroyo-Espliguero R, Avanzas P, Cosin-Sales J, Aldama G, Pizzi C, Kaski JC. C-reactive protein elevation and disease activity in patients with coronary artery disease. Eur Heart J. 2004;25(5):401–8.
Ridker PM, Bhatt DL, Pradhan AD, Glynn RJ, MacFadyen JG, Nissen SE. Inflammation and cholesterol as predictors of cardiovascular events among patients receiving statin therapy: a collaborative analysis of three randomized trials. Lancet. 2023;401(10384):1293–301.
Ridker PM, Lei L, Louie MJ, Haddad T, Nicholls SJ, Lincoff AM, et al. Inflammation and cholesterol as predictors of Cardiovascular events among 13970 contemporary high-risk patients with statin intolerance. Circulation. 2024;149(1):28–35.
Ortega E, Gilabert R, Nunez I, Cofan M, Sala-Vila A, de Groot E, et al. White blood cell count is associated with carotid and femoral atherosclerosis. Atherosclerosis. 2012;221(1):275–81.
Madjid M, Fatemi O. Components of the complete blood count as risk predictors for coronary heart disease: in-depth review and update. Tex Heart Inst J. 2013;40(1):17–29.
PubMed PubMed Central Google Scholar
Hasegawa T, Negishi T, Deguchi M. WBC count, atherosclerosis and coronary risk factors. J Atheroscler Thromb. 2002;9(5):219–23.
Thygesen K, Alpert JS, Jaffe AS, Chaitman BR, Bax JJ, Morrow DA, et al. Fourth Universal Definition of Myocardial Infarction (2018). Circulation. 2018;138(20):e618–51.
Varbo A, Benn M, Tybjaerg-Hansen A, Nordestgaard BG. Elevated remnant cholesterol causes both low-grade inflammation and ischemic heart disease, whereas elevated low-density lipoprotein cholesterol causes ischemic heart disease without inflammation. Circulation. 2013;128(12):1298–309.
Yang G, Schooling CM. Genetically mimicked effects of ASGR1 inhibitors on all-cause mortality and health outcomes: a drug-target mendelian randomization study and a phenome-wide association study. Bmc Med. 2023;21(1):235.
Mazidi M, Wright N, Yao P, Kartsonaki C, Millwood IY, Fry H, et al. Plasma proteomics to identify drug targets for ischemic heart disease. J Am Coll Cardiol. 2023;82(20):1906–20.
Koba S, Hirano T, Ito Y, Tsunoda F, Yokota Y, Ban Y, et al. Significance of small dense low-density lipoprotein-cholesterol concentrations in relation to the severity of coronary heart diseases. Atherosclerosis. 2006;189(1):206–14.
Li S, Guo YL, Zhao X, Zhang Y, Zhu CG, Wu NQ, et al. Novel and traditional lipid-related biomarkers and their combinations in predicting coronary severity. Sci Rep. 2017;7(1):360.
Pasceri V, Willerson JT, Yeh ET. Direct proinflammatory effect of C-reactive protein on human endothelial cells. Circulation. 2000;102(18):2165–8.
Verma S, Wang CH, Li SH, Dumont AS, Fedak PW, Badiwala MV, et al. A self-fulfilling prophecy: C-reactive protein attenuates nitric oxide production and inhibits angiogenesis. Circulation. 2002;106(8):913–9.
Danenberg HD, Szalai AJ, Swaminathan RV, Peng L, Chen Z, Seifert P, et al. Increased thrombosis after arterial injury in human C-reactive protein-transgenic mice. Circulation. 2003;108(5):512–5.
Ridker PM, Everett BM, Thuren T, MacFadyen JG, Chang WH, Ballantyne C, et al. Antiinflammatory therapy with Canakinumab for atherosclerotic disease. N Engl J Med. 2017;377(12):1119–31.
Frommhold D, Ludwig A, Bixel MG, Zarbock A, Babushkina I, Weissinger M, et al. Sialyltransferase ST3Gal-IV controls CXCR2-mediated firm leukocyte arrest during inflammation. J Exp Med. 2008;205(6):1435–46.
Doring Y, Noels H, Mandl M, Kramp B, Neideck C, Lievens D, et al. Deficiency of the sialyltransferase St3Gal4 reduces Ccl5-mediated myeloid cell recruitment and arrest: short communication. Circ Res. 2014;114(6):976–81.
Harris RL, van den Berg CW, Bowen DJ. ASGR1 and ASGR2, the genes that encode the Asialoglycoprotein receptor (Ashwell Receptor), are expressed in Peripheral Blood monocytes and Show Interindividual differences in transcript Profile. Mol Biol Int. 2012;2012:283974.
Download references
Acknowledgements
We would like to express our gratitude to all those who helped us during the writing of this manuscript.
This work was supported by the National Natural Science Foundation of China [grant number 82100495 to F. Luo, grant number 82201879 to J.F. Chen]; the Hunan Provincial Natural Science Foundation of China [grant number 2021JJ40852 to F. Luo, grant number 2022JJ40675 to J.F. Chen]; the Scientific Research Project of Hunan Provincial Health Commission [grant number 202203014009 to F. Luo, grant number B202305037231 to J.F. Chen]; the Scientific Research Launch Project for New Employees of the Second Xiangya Hospital of Central South University [to F. Luo and J.F. Chen]; the China Postdoctoral Science Foundation [grant number 331046 and 2023T160738 to F. Luo and J.F. Chen]; and the Key Research and Development Program of Hunan Province of China [grant number 2021SK2004 to Z.F. Fang].
Author information
Authors and affiliations.
Department of Cardiovascular Medicine, The Second Xiangya Hospital, Central South University, Changsha, 410011, Hunan, China
Qin Luo, Yanfeng Yi, Panyun Wu, Zhangling Chen, Jianjun Tang, Zhenfei Fang & Fei Luo
Research Institute of Blood Lipids and Atherosclerosis, the Second Xiangya Hospital, Central South University, Changsha, China
Reproductive Medicine Center, Department of Obstetrics and Gynecology, The Second Xiangya Hospital, Central South University, Changsha, Hunan, China
Jingfei Chen
Department of Emergency Medicine, Hengyang Medical School, The Affiliated Changsha Central Hospital, University of South China, Changsha, China
Kayakyolu Family Health Center, 33 Palandöken, Erzurum, Turkey
Hacı Ahmet Aydemir
You can also search for this author in PubMed Google Scholar
Contributions
FL, ZF and QL conceived the idea. QL wrote the manuscript; JC, YY and PW conducted the data collection and analysis. FL, ZC, YS, HAA, JT and ZF read through and corrected the manuscript. All authors read and approved the final manuscript.
Corresponding authors
Correspondence to Zhenfei Fang or Fei Luo .
Ethics declarations
Competing interests.
The authors declare no competing interests.
Ethics approval and consent to participate
The study conformed to the ethical guidelines of the Declaration of Helsinki and was approved by the ethics committee of the Second Xiangya Hospital. Informed consent was obtained from each patient.
Additional information
Publisher’s note.
Springer Nature remains neutral with regard to jurisdictional claims in published maps and institutional affiliations.
Rights and permissions
Open Access This article is licensed under a Creative Commons Attribution 4.0 International License, which permits use, sharing, adaptation, distribution and reproduction in any medium or format, as long as you give appropriate credit to the original author(s) and the source, provide a link to the Creative Commons licence, and indicate if changes were made. The images or other third party material in this article are included in the article’s Creative Commons licence, unless indicated otherwise in a credit line to the material. If material is not included in the article’s Creative Commons licence and your intended use is not permitted by statutory regulation or exceeds the permitted use, you will need to obtain permission directly from the copyright holder. To view a copy of this licence, visit http://creativecommons.org/licenses/by/4.0/ . The Creative Commons Public Domain Dedication waiver ( http://creativecommons.org/publicdomain/zero/1.0/ ) applies to the data made available in this article, unless otherwise stated in a credit line to the data.
Reprints and permissions
About this article
Cite this article.
Luo, Q., Chen, J., Yi, Y. et al. The serum soluble ASGR1 concentration is elevated in patients with coronary artery disease and is associated with inflammatory markers. Lipids Health Dis 23 , 89 (2024). https://doi.org/10.1186/s12944-024-02054-8
Download citation
Received : 28 December 2023
Accepted : 20 February 2024
Published : 27 March 2024
DOI : https://doi.org/10.1186/s12944-024-02054-8
Share this article
Anyone you share the following link with will be able to read this content:
Sorry, a shareable link is not currently available for this article.
Provided by the Springer Nature SharedIt content-sharing initiative
- Soluble asialoglycoprotein receptor 1
- Lipid metabolism
- High-sensitivity C-reactive protein
- Inflammation
Lipids in Health and Disease
ISSN: 1476-511X
- General enquiries: [email protected]
share this!
March 29, 2024
This article has been reviewed according to Science X's editorial process and policies . Editors have highlighted the following attributes while ensuring the content's credibility:
fact-checked
peer-reviewed publication
trusted source
Lipids with potential health benefits in herbal teas identified
by Hokkaido University
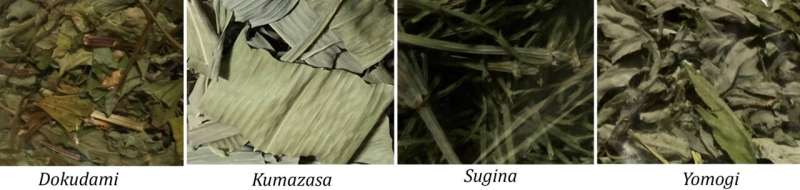
Herbal teas are enjoyed worldwide, not only for their taste and refreshment but also for a wide range of reputed health benefits. But the potential significance of a category of compounds called lipids in the teas has been relatively unexplored.
Researchers at Hokkaido University, led by Associate Professor Siddabasave Gowda and Professor Shu-Ping Hui of the Faculty of Health Sciences, have now identified 341 different molecular species from five categories of lipids in samples of four types of herbal tea. They published their results in the journal Food Chemistry .
Lipids are a diverse collection of natural substances that share the property of being insoluble in water. They include all of the fats and oils that are common constituents of many foods, but they have generally not been examined as significant components of teas.
The Hokkaido team selected four teas for their initial analysis : dokudami (Houttuynia cordata, fish mint), kumazasa (Sasa veitchii), sugina (Equisetum arvense, common horsetail) and yomogi (Artemisia princeps, Japanese mugwort).
"These herbs are native to Japan and have been widely consumed as tea from ancient times due to their medicinal properties," says Gowda. The medicinal benefits attributed to these and other herbal teas include antioxidant, antiglycation, anti-inflammatory, antibacterial, antiviral, anti-allergic, anticarcinogenic, antithrombotic, vasodilatory, antimutagenic, and anti-aging effects.
The lipids in the teas were separated and identified by combining two modern analytical techniques called high-performance liquid chromatography and linear ion trap-Orbitrap mass spectrometry.
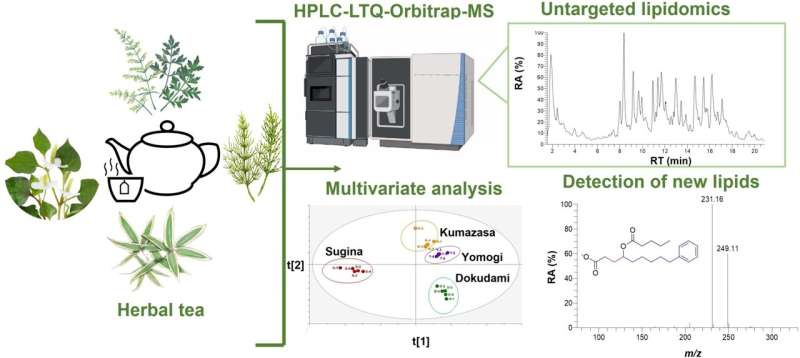
The analysis revealed significant variations in the lipids in the four types of tea, with each type containing some known bioactive lipids. These included a distinct category of lipids called short-chain fatty acid esters of hydroxy fatty acids (SFAHFAs), some of which had never previously been found in plants. SFAHFAs detected in tea could be a novel source of short-chain fatty acids, which are essential metabolites for maintaining gut health.
"The discovery of these novel SFAHFAs opens new avenues for research," says Hui, adding that the lipid concentrations found in the teas are at levels that could be expected to have significant nutritional and medical effects in consumers.
The lipids discovered also included α-linolenic acid, already known for its anti-inflammatory properties, and arachidonic acid which has been associated with a variety of health benefits . These two compounds are examples of a range of poly-unsaturated fatty acids found in the teas, a category of lipids that are well-known for their nutritional benefits.
"Our initial study paves the way for further exploration of the role of lipids in herbal teas and their broad implications for human health and nutrition," Gowda concludes. "We now want to expand our research to characterize the lipids in more than 40 types of herbal tea in the near future."
Journal information: Food Chemistry
Provided by Hokkaido University
Explore further
Feedback to editors

Newly hatched chicks can instantly recognize objects with vision, even if they've only ever experienced them by touch
9 minutes ago

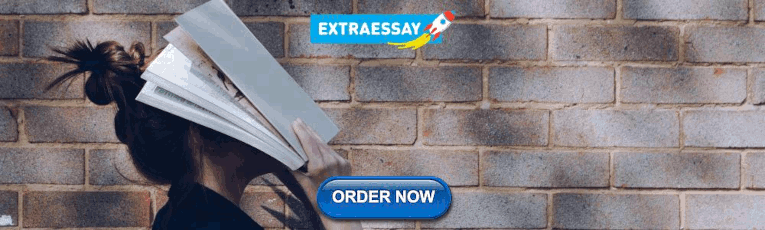
A return to roots: Lab builds its first stellarator in 50 years and opens the door for research into new plasma physics

A new estimate of US soil organic carbon to improve Earth system models

New research uses coaxial 'dish' antenna to scan for dark matter
2 hours ago

'Unheard of in structural biology': New enzyme models reveal disease insights

Transmitting entanglement between light and matter in the metropolitan network of Barcelona

These plants evolved in Florida millions of years ago. They may be gone in decades.

Focusing ultra-intense lasers to a single wavelength
3 hours ago

Classical optical neural network exhibits 'quantum speedup'
4 hours ago

How AI and deeper roots can help soil store more carbon
Relevant physicsforums posts, zirconium versus zirconium carbide for use with galinstan.
Mar 29, 2024
Electrolysis: Dark blue oxide from steel?
Mar 28, 2024
Identification of HOMO/LUMO in radicals
Mar 27, 2024
Quantum hybridized orbitals
New insight into the chemistry of solvents.
Mar 23, 2024
Is G10 material dangerous?
More from Chemistry
Related Stories

Discovery of 100 fatty acids opens new research paths
Jul 7, 2023

Acne bacteria trigger cells to produce fats, oils and other lipids essential to skin health, shows new research
Aug 23, 2023

Innovative microscopy technique reveals secrets of lipid synthesis inside cells
Jan 24, 2024

PFAS in blood are ubiquitous and are associated with an increased risk of cardiovascular diseases, finds study
Mar 11, 2024

LipidOz: New software enables identification of lipid double bond locations
Jan 23, 2024

Study reveals fatty acids hold clue to creating memories
Feb 6, 2024
Recommended for you

Researchers investigate the surface extraction of platinum catalysts in alkaline media
6 hours ago

Wound treatment hydrogel infused with amino acid kills bacteria naturally and promotes cell growth
8 hours ago

Engineers 'symphonize' cleaner ammonia production
Apr 1, 2024

Using a cellphone compass to measure tiny concentrations of compounds important for human health

New synthesis platform allows for rapid cancer drug synthesis and testing

Biochemists discover first new antibacterial class in decades
Let us know if there is a problem with our content.
Use this form if you have come across a typo, inaccuracy or would like to send an edit request for the content on this page. For general inquiries, please use our contact form . For general feedback, use the public comments section below (please adhere to guidelines ).
Please select the most appropriate category to facilitate processing of your request
Thank you for taking time to provide your feedback to the editors.
Your feedback is important to us. However, we do not guarantee individual replies due to the high volume of messages.
E-mail the story
Your email address is used only to let the recipient know who sent the email. Neither your address nor the recipient's address will be used for any other purpose. The information you enter will appear in your e-mail message and is not retained by Phys.org in any form.
Newsletter sign up
Get weekly and/or daily updates delivered to your inbox. You can unsubscribe at any time and we'll never share your details to third parties.
More information Privacy policy
Donate and enjoy an ad-free experience
We keep our content available to everyone. Consider supporting Science X's mission by getting a premium account.
E-mail newsletter
Thank you for visiting nature.com. You are using a browser version with limited support for CSS. To obtain the best experience, we recommend you use a more up to date browser (or turn off compatibility mode in Internet Explorer). In the meantime, to ensure continued support, we are displaying the site without styles and JavaScript.
- View all journals
- Explore content
- About the journal
- Publish with us
- Sign up for alerts
- News & Views
- Published: 07 April 2022
The emerging role of lipidomics in prediction of diseases
- Xianlin Han ORCID: orcid.org/0000-0002-8615-2413 1 , 2
Nature Reviews Endocrinology volume 18 , pages 335–336 ( 2022 ) Cite this article
1157 Accesses
6 Citations
22 Altmetric
Metrics details
- Risk factors
- Type 2 diabetes
A recent paper published in PLoS Biology reported the application of lipidomics in predicting the incidence of type 2 diabetes mellitus and cardiovascular diseases in a population cohort. The study demonstrates the role of lipidomics in prediction of diseases and translational research, which could herald the beginning of an era of quantitative lipidomics.
This is a preview of subscription content, access via your institution
Access options
Access Nature and 54 other Nature Portfolio journals
Get Nature+, our best-value online-access subscription
24,99 € / 30 days
cancel any time
Subscribe to this journal
Receive 12 print issues and online access
195,33 € per year
only 16,28 € per issue
Buy this article
Purchase on Springer Link
Instant access to full article PDF
Prices may be subject to local taxes which are calculated during checkout
Han, X. & Gross, R. W. The foundations and development of lipidomics. J. Lipid Res. 63 , 100164 (2022).
Article CAS Google Scholar
Yang, K. & Han, X. Lipidomics: Techniques, applications, and outcomes related to biomedical sciences. Trends Biochem. Sci. 41 , 954–969 (2016).
Lauber, C. et al. Lipidomic risk scores are independent of polygenic risk scores and can predict incidence of diabetes and cardiovascular disease in a large population cohort. PLoS Biol. 20 , e3001561 (2022).
Fernandez, C. et al. Plasma lipidome and prediction of type 2 diabetes in the population-based malmö diet and cancer cohort. Diabetes Care 43 , 366–373 (2020).
Ottosson, F. et al. A plasma lipid signature predicts incident coronary artery disease. Int. J. Cardiol. 331 , 249–254 (2021).
Article Google Scholar
Surma, M. A. et al. An automated shotgun lipidomics platform for high throughput, comprehensive, and quantitative analysis of blood plasma intact lipids. Eur. J. Lipid Sci. Technol. 117 , 1540–1549 (2015).
Khan, S. R. et al. The discovery of novel predictive biomarkers and early-stage pathophysiology for the transition from gestational diabetes to type 2 diabetes. Diabetologia 62 , 687–703 (2019).
Wang, M., Wang, C. & Han, X. Selection of internal standards for accurate quantification of complex lipid species in biological extracts by electrospray ionization mass spectrometry-What, how and why? Mass Spectrom. Rev. 36 , 693–714 (2017).
Köfeler, H. C. et al. Quality control requirements for the correct annotation of lipidomics data. Nat. Commun. 12 , 4771 (2021).
Köfeler, H. C. et al. Recommendations for good practice in MS-based lipidomics. J. Lipid Res. 62 , 100138 (2021).
Download references
Acknowledgements
The author acknowledges the support of by National Institutes of Health P30 AG013319, P30 AG044271, P30 AG066546 (Biomarker Core), U19 AG069701, and U54 NS110435.
Author information
Authors and affiliations.
Barshop Institute for Longevity and Aging Studies, University of Texas Health Science Center at San Antonio, San Antonio, TX, USA
Xianlin Han
Division of Diabetes, Department of Medicine, University of Texas Health Science Center at San Antonio, San Antonio, TX, USA
You can also search for this author in PubMed Google Scholar
Corresponding author
Correspondence to Xianlin Han .
Ethics declarations
Competing interests.
The author declares no competing interests.
Rights and permissions
Reprints and permissions
About this article
Cite this article.
Han, X. The emerging role of lipidomics in prediction of diseases. Nat Rev Endocrinol 18 , 335–336 (2022). https://doi.org/10.1038/s41574-022-00672-9
Download citation
Published : 07 April 2022
Issue Date : June 2022
DOI : https://doi.org/10.1038/s41574-022-00672-9
Share this article
Anyone you share the following link with will be able to read this content:
Sorry, a shareable link is not currently available for this article.
Provided by the Springer Nature SharedIt content-sharing initiative
This article is cited by
Diazobutanone-assisted isobaric labelling of phospholipids and sulfated glycolipids enables multiplexed quantitative lipidomics using tandem mass spectrometry.
- Ting-Jia Gu
- Peng-Kai Liu
Nature Chemistry (2024)
Prioritize biologically relevant ions for data-independent acquisition (BRI-DIA) in LC–MS/MS-based lipidomics analysis
- Grace Scheidemantle
- Xiaojing Liu
Metabolomics (2022)
Quick links
- Explore articles by subject
- Guide to authors
- Editorial policies
Sign up for the Nature Briefing newsletter — what matters in science, free to your inbox daily.

Scalable synthesis of lipid nanoparticles for nucleic acid drug delivery using an isometric channel-size enlarging strategy
- Research Article
- Published: 14 August 2023
- Volume 17 , pages 2899–2907, ( 2024 )
Cite this article
- Zesen Ma 1 , 2 na1 ,
- Haiyang Tong 3 , 4 na1 ,
- Sijin Lin 1 , 2 ,
- Li Zhou 5 ,
- Demeng Sun 5 ,
- Baoqing Li 1 , 2 , 6 ,
- Changlin Tian 4 , 5 , 6 &
- Jiaru Chu 1 , 2
279 Accesses
1 Altmetric
Explore all metrics
Lipid nanoparticles (LNPs) have emerged as highly effective delivery systems for nucleic acid-based therapeutics. However, the broad clinical translation of LNP-based drugs is hampered by the lack of robust and scalable synthesis techniques that can consistently produce formulations from early development to clinical application. In this work, we proposed a method to achieve scalable synthesis of LNPs by scaling inertial microfluidic mixers isometrically in three dimensions. Moreover, a theoretical predictive method, which controls the mixing time to be equal across different chips, is developed to ensure consistent particle size and size distribution of the synthesized LNPs. LNPs loaded with small interfering RNA (siRNA) were synthesized at different flow rates, exhibiting consistent physical properties, including particle size, size distribution and encapsulation efficiency. This work provides a practical approach for scalable synthesis of LNPs consistently, offering the potential to accelerate the transition of nucleic acid drug development into clinical application.
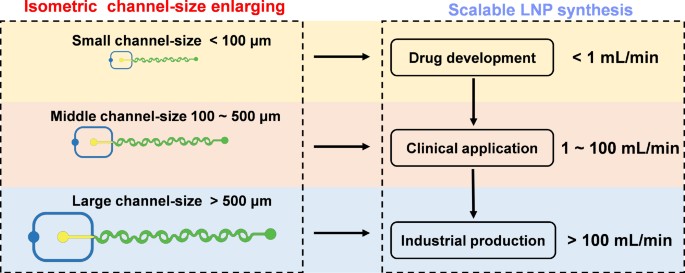
This is a preview of subscription content, log in via an institution to check access.
Access this article
Price includes VAT (Russian Federation)
Instant access to the full article PDF.
Rent this article via DeepDyve
Institutional subscriptions
Similar content being viewed by others
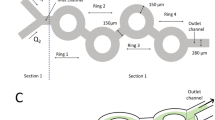
Optimal self-assembly of lipid nanoparticles (LNP) in a ring micromixer
Manon Ripoll, Elian Martin, … Pierre Wils
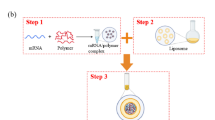
Continuous and size-control synthesis of lipopolyplex nanoparticles enabled by controlled micromixing performance for mRNA delivery
Shirong Song, Zhikai Liu, … Guangwen Chen
Microfluidic Production and Application of Lipid Nanoparticles for Nucleic Acid Transfection
Siavashy, S.; Soltani, M.; Ahmadi, M.; Landi, B.; Mehmanparast, H.; Ghorbani-Bidkorbeh, F. A comprehensive review of one decade of microfluidic platforms applications in synthesis of enhanced carriers utilized in controlled drug delivery. Adv. Mater. Technol. 2022 , 7 , 2101615.
Google Scholar
Shepherd, S. J.; Issadore, D.; Mitchell, M. J. Microfluidic formulation of nanoparticles for biomedical applications. Biomaterials 2021 , 274 , 120826.
CAS PubMed PubMed Central Google Scholar
Bendre, A.; Bhat, M. P.; Lee, K. H.; Altalhi, T.; Alruqi, M. A.; Kurkuri, M. Recent developments in microfluidic technology for synthesis and toxicity-efficiency studies of biomedical nanomaterials. Mater. Today Adv. 2022 , 13 , 100205.
CAS Google Scholar
Ferhan, A. R.; Park, S.; Park, H.; Tae, H.; Jackman, J. A.; Cho, N. J. Lipid nanoparticle technologies for nucleic acid delivery: A nanoarchitectonics perspective. Adv. Funct. Mater. 2022 , 32 , 2203669.
Evers, M. J. W.; Kulkarni, J. A.; Van Der Meel, R.; Cullis, P. R.; Vader, P.; Schiffelers, R. M. State-of-the-art design and rapid-mixing production techniques of lipid nanoparticles for nucleic acid delivery. Small Methods 2018 , 2 , 1700375.
Akinc, A.; Maier, M. A.; Manoharan, M.; Fitzgerald, K.; Jayaraman, M.; Barros, S.; Ansell, S.; Du, X. Y.; Hope, M. J.; Madden, T. D. et al. The Onpattro story and the clinical translation of nanomedicines containing nucleic acid-based drugs. Nat. Nanotechnol. 2019 , 14 , 1084–1087.
CAS PubMed ADS Google Scholar
Polack, F. P.; Thomas, S. J.; Kitchin, N.; Absalon, J.; Gurtman, A.; Lockhart, S.; Perez, J. L.; Pérez Marc, G.; Moreira, E. D.; Zerbini, C. et al. Safety and efficacy of the BNT162b2 mRNA covid-19 vaccine. N. Engl. J. Med. 2020 , 383 , 2603–2615.
CAS PubMed Google Scholar
Wei, Y. C.; Quan, L.; Zhou, C.; Zhan, Q. Q. Factors relating to the biodistribution & clearance of nanoparticles & their effects on in vivo application. Nanomedicine 2018 , 13 , 1495–1512.
Nakamura, T.; Kawai, M.; Sato, Y.; Maeki, M.; Tokeshi, M.; Harashima, H. The effect of size and charge of lipid nanoparticles prepared by microfluidic mixing on their lymph node transitivity and distribution. Mol. Pharmaceutics 2020 , 17 , 944–953.
Chen, S. M.; Tam, Y. Y. C.; Lin, P. J. C.; Sung, M. M. H.; Tam, Y. K.; Cullis, P. R. Influence of particle size on the in vivo potency of lipid nanoparticle formulations of siRNA. J Control Release 2016 , 235 , 236–244.
Prakash, G.; Shokr, A.; Willemen, N.; Bashir, S. M.; Shin, S. R.; Hassan, S. Microfluidic fabrication of lipid nanoparticles for the delivery of nucleic acids. Adv. Drug Delivery Rev. 2022 , 144 , 114197.
Hirsjärvi, S.; Sancey, L.; Dufort, S.; Belloche, C.; Vanpouille-Box, C.; Garcion, E.; Coll, J. L.; Hindré, F.; Benoît, J. P. Effect of particle size on the biodistribution of lipid nanocapsules: Comparison between nuclear and fluorescence imaging and counting. Int. J. Pharm. 2013 , 453 , 594–600.
PubMed Google Scholar
Qi, J. P.; Zhuang, J.; Lu, Y.; Dong, X. C.; Zhao, W. L.; Wu, W. In vivo fate of lipid-based nanoparticles. Drug Discovery Today 2017 , 22 , 166–172.
Piwowarczyk, L.; Mlynarczyk, D. T.; Krajka-Kuzniak, V.; Majchrzak-Celinska, A.; Budzianowska, A.; Tomczak, S.; Budzianowski, J.; Wozniak-Braszak, A.; Pietrzyk, R.; Baranowski, M. et al. Natural compounds in liposomal nanoformulations of potential clinical application in glioblastoma. Cancers 2022 , 14 , 6222.
Webb, C.; Forbes, N.; Roces, C. B.; Anderluzzi, G.; Lou, G.; Abraham, S.; Ingalls, L.; Marshall, K.; Leaver, T. J.; Watts, J. A. et al. Using microfluidics for scalable manufacturing of nanomedicines from bench to GMP: A case study using protein-loaded liposomes. Int. J. Pharm. 2020 , 582 , 119266.
Firmino, P. C. O. S.; Vianna, S. S. V.; Da Costa, O. M. M. M.; Malfatti-Gasperini, A. A.; Gobbi, A. L.; Lima, R. S.; De La Torre, L. G. 3D micromixer for nanoliposome synthesis: A promising advance in high mass productivity. Lab Chip 2021 , 21 , 2971–2985.
Erfle, P.; Riewe, J.; Cai, S. T.; Bunjes, H.; Dietzel, A. Horseshoe lamination mixer (HLM) sets new standards in the production of monodisperse lipid nanoparticles. Lab Chip 2022 , 22 , 3025–3044.
Huang, P. H.; Zhao, S. G.; Bachman, H.; Nama, N.; Li, Z. S.; Chen, C. Y.; Yang, S. J.; Wu, M. X.; Zhang, S. P.; Huang, T. J. Acoustofluidic synthesis of particulate nanomaterials. Adv. Sci. (Weinh.) 2019 , 6 , 1900913.
Zhao, S. G.; Huang, P. H.; Zhang, H. Y.; Rich, J.; Bachman, H.; Ye, J.; Zhang, W. F.; Chen, C. Y.; Xie, Z. M.; Tian, Z. H. et al. Fabrication of tunable, high-molecular-weight polymeric nanoparticles via ultrafast acoustofluidic micromixing. Lab Chip 2021 , 21 , 2453–2463.
Kimura, N.; Maeki, M.; Sato, Y.; Note, Y.; Ishida, A.; Tani, H.; Harashima, H.; Tokeshi, M. Development of the iLiNP device: Fine tuning the lipid nanoparticle size within 10 nm for drug delivery. ACS Omega 2018 , 3 , 5044–5051.
Cheung, C. C. L.; Al-Jamal, W. T. Sterically stabilized liposomes production using staggered herringbone micromixer: Effect of lipid composition and PEG-lipid content. Int. J. Pharm. 2019 , 566 , 687–696.
Khairnar, S. V.; Pagare, P.; Thakre, A.; Nambiar, A. R.; Junnuthula, V.; Abraham, M. C.; Kolimi, P.; Nyavanandi, D.; Dyawanapelly, S. Review on the scale-up methods for the preparation of solid lipid nanoparticles. Pharmaceutics 2022 , 14 , 1886.
Giorello, A.; Nicastro, A.; Berli, C. L. A. Microfluidic platforms for the production of nanoparticles at flow rates larger than one liter per hour. Adv. Mater. Technol. 2022 , 7 , 2101588.
Shepherd, S. J.; Warzecha, C. C.; Yadavali, S.; El-Mayta, R.; Alameh, M. G.; Wang, L. L.; Weissman, D.; Wilson, J. M.; Issadore, D.; Mitchell, M. J. Scalable mRNA and siRNA lipid nanoparticle production using a parallelized microfluidic device. Nano Lett 2021 , 21 , 5671–5680.
CAS PubMed PubMed Central ADS Google Scholar
Han, J. Y.; La Fiandra, J. N.; DeVoe, D. L. Microfluidic vortex focusing for high throughput synthesis of size-tunable liposomes. Nat. Commun. 2022 , 13 , 6997.
Lim, J. M.; Swami, A.; Gilson, L. M.; Chopra, S.; Choi, S.; Wu, J.; Langer, R.; Karnik, R.; Farokhzad, O. C. Ultra-high throughput synthesis of nanoparticles with homogeneous size distribution using a coaxial turbulent jet mixer. ACS Nano 2014 , 8 , 6056–6065.
Tomeh, M. A.; Mansor, M. H.; Hadianamrei, R.; Sun, W. Z.; Zhao, X. B. Optimization of large-scale manufacturing of biopolymeric and lipid nanoparticles using microfluidic swirl mixers. Int. J. Pharm. 2022 , 620 , 121762.
Xu, R. C.; Tomeh, M. A.; Ye, S. Y.; Zhang, P.; Lv, S. W.; You, R. R.; Wang, N.; Zhao, X. B. Novel microfluidic swirl mixers for scalable formulation of curcumin loaded liposomes for cancer therapy. Int. J. Pharm. 2022 , 622 , 121857.
Belliveau, N. M.; Huft, J.; Lin, P. J.; Chen, S. M.; Leung, A. K. K.; Leaver, T. J.; Wild, A. W.; Lee, J. B.; Taylor, R. J.; Tam, Y. K. et al. Microfluidic synthesis of highly potent limit-size lipid nanoparticles for in vivo delivery of siRNA. Mol. Ther. Nucl. Acids 2012 , 1 , e37.
Desai, D.; Guerrero, Y. A.; Balachandran, V.; Morton, A.; Lyon, L.; Larkin, B.; Solomon, D. E. Towards a microfluidics platform for the continuous manufacture of organic and inorganic nanoparticles. Nanomed. Nanotechnol. Biol. Med. 2021 , 35 , 102402.
Maeki, M.; Okada, Y.; Uno, S.; Sugiura, K.; Suzuki, Y.; Okuda, K.; Sato, Y.; Ando, M.; Yamazaki, H.; Takeuchi, M. et al. Mass production system for RNA-loaded lipid nanoparticles using piling up microfluidic devices. Appl. Mater. Today 2023 , 31 , 101754.
Toth, M. J.; Kim, T.; Kim, Y. Robust manufacturing of lipid-polymer nanoparticles through feedback control of parallelized swirling microvortices. Lab Chip 2017 , 17 , 2805–2813.
Zhang, J. S.; Wang, K.; Teixeira, A. R.; Jensen, K. F.; Luo, G. S. Design and scaling up of microchemical systems: A review. Annu. Rev. Chem. Biomol. Eng. 2017 , 8 , 285–305.
Elvira, K. S.; I Solvas, X. C.; Wootton, R. C. R.; deMello, A. J. The past, present and potential for microfluidic reactor technology in chemical synthesis. Nat. Chem. 2013 , 5 , 905–915.
Roces, C. B.; Lou, G.; Jain, N.; Abraham, S.; Thomas, A.; Halbert, G. W.; Perrie, Y. Manufacturing considerations for the development of lipid nanoparticles using microfluidics. Pharmaceutics 2020 , 12 , 1095.
Kulkarni, J. A.; Tam, Y. Y. C.; Chen, S.; Tam, Y. K.; Zaifman, J.; Cullis, P. R.; Biswas, S. Rapid synthesis of lipid nanoparticles containing hydrophobic inorganic nanoparticles. Nanoscale 2017 , 9 , 13600–13609.
Li, S. X.; Hu, Y. Z.; Li, A.; Lin, J. H.; Hsieh, K.; Schneiderman, Z.; Zhang, P. F.; Zhu, Y. N.; Qiu, C. H.; Kokkoli, E. et al. Payload distribution and capacity of mRNA lipid nanoparticles. Nat. Commun. 2022 , 13 , 5561.
Hamdallah, S. I.; Zoqlam, R.; Erfle, P.; Blyth, M.; Alkilany, A. M.; Dietzel, A.; Qi, S. Microfluidics for pharmaceutical nanoparticle fabrication: The truth and the myth. Int. J. Pharm. 2020 , 584 , 119408.
Layek, A.; Mishra, G.; Sharma, A.; Spasova, M.; Dhar, S.; Chowdhury, A.; Bandyopadhyaya, R. A Generalized three-stage mechanism of ZnO nanoparticle formation in homogeneous liquid medium. J. Phys. Chem. C 2012 , 116 , 24757–24769.
Buschmann, M. D.; Carrasco, M. J.; Alishetty, S.; Paige, M.; Alameh, M. G.; Weissman, D. Nanomaterial delivery systems for mRNA vaccines. Vaccines 2021 , 9 , 65.
Wei, T.; Cheng, Q.; Min, Y. L.; Olson, E. N.; Siegwart, D. J. Systemic nanoparticle delivery of CRISPR-Cas9 ribonucleoproteins for effective tissue specific genome editing. Nat. Commun. 2020 , 11 , 3232.
Chandler, M.; Panigaj, M.; Rolband, L. A.; Afonin, K. A. Challenges in optimizing RNA nanostructures for large-scale production and controlled therapeutic properties. Nonomedinine 2020 , 75 , 1331–1340.
Akinc, A.; Zumbuehl, A.; Goldberg, M.; Leshchiner, E. S.; Busini, V.; Hossain, N.; Bacallado, S. A.; Nguyen, D. N.; Fuller, J.; Alvarez, R. et al. A combinatorial library of lipid-like materials for delivery of RNAi therapeutics. Nat. Biotechnol. 2008 , 26 , 561–569.
Download references
Acknowledgements
This research work has been supported in part by Collaborative Innovation Program of Hefei Science Center, CAS (No. 2022HSC-CIP001), Anhui Province Key Laboratory of High Field Magnetic Resonance Imaging (No. KFKT-2022-0003), Joint Research Fund for Overseas Chinese, Hong Kong and Macao Young Scholars (No. 51929501), National Key R&D Program of China (No. 2022YFF0705002). The authors would like to acknowledge the USTC Experimental Center of Engineering and Material Sciences and the USTC center for Micro-and Nanoscale Research and Fabrication for technical support in microfabrication. The authors would like to acknowledge Wulin Zhu for the assistance in fabrication of microfluidic chip.
Author information
Zesen Ma and Haiyang Tong contributed equally to this work.
Authors and Affiliations
Department of Precision Machinery and Precision Instrumentation, University of Science and Technology of China, Hefei, 230027, China
Zesen Ma, Sijin Lin, Baoqing Li & Jiaru Chu
Key Laboratory of Precision Scientific Instrumentation of Anhui Higher Education Institutes, University of Science and Technology of China, Hefei, 230027, China
Science Island Branch, Graduate School, University of Science and Technology of China, Hefei, 230026, China
Haiyang Tong
Anhui Provincial Key Laboratory of High Field Magnetic Resonance Imaging, High Magnetic Field Laboratory, Hefei Institute of Physical Science, Chinese Academy of Sciences, Hefei, 230031, China
Haiyang Tong & Changlin Tian
Anhui Provincial Engineering Laboratory of Peptide Drugs, University of Science and Technology of China, Hefei, 230027, China
Li Zhou, Demeng Sun & Changlin Tian
School of Biomedical Engineering, Division of Life Sciences and Medicine, University of Science and Technology of China, Suzhou, 215127, China
Baoqing Li & Changlin Tian
You can also search for this author in PubMed Google Scholar
Corresponding authors
Correspondence to Baoqing Li or Changlin Tian .
Electronic Supplementary Material
12274_2023_6031_moesm1_esm.pdf, rights and permissions.
Reprints and permissions
About this article
Ma, Z., Tong, H., Lin, S. et al. Scalable synthesis of lipid nanoparticles for nucleic acid drug delivery using an isometric channel-size enlarging strategy. Nano Res. 17 , 2899–2907 (2024). https://doi.org/10.1007/s12274-023-6031-1
Download citation
Received : 21 June 2023
Revised : 20 July 2023
Accepted : 22 July 2023
Published : 14 August 2023
Issue Date : April 2024
DOI : https://doi.org/10.1007/s12274-023-6031-1
Share this article
Anyone you share the following link with will be able to read this content:
Sorry, a shareable link is not currently available for this article.
Provided by the Springer Nature SharedIt content-sharing initiative
- lipid nanoparticles
- nucleic acid delivery
- microfluidics
- scalable synthesis
- consistent particle size
- Find a journal
- Publish with us
- Track your research
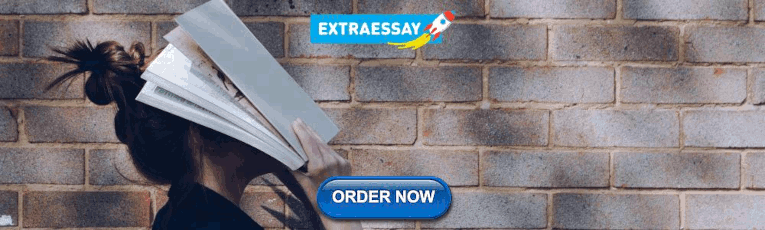
IMAGES
VIDEO
COMMENTS
LIPID TYPES AND STRUCTURES. Aqueous insoluble lipids are molecules with complex structures as a result of several biochemical transformations (Fahy et al., 2011).Because of the participation of different enzymes and biological substances, the process of lipidomics is important to comprehend (Nilsson et al., 2019).Lipids contain hydrocarbons, a diverse and ubiquitous group of compounds that are ...
Lipids articles from across Nature Portfolio. Lipids are hydrophobic or amphiphilic small molecules that include sterols, waxes, fatty acids and phospholipids, and are insoluble in water and ...
Abdominal aortic calcification (AAC) is a crucial indicator of cardiovascular health. This study aims investigates the associations between dietary fatty acid intake and AAC. Yan Xiao and Yingping Quan. Lipids in Health and Disease 2024 23 :73. Research Published on: 9 March 2024.
Lipid metabolism is a major regulator of T cell biology, and this Review Article highlights mechanisms by which diverse lipids modulate T cell signaling and opportunities for therapeutic ...
Research Article Collection: Lipid and Inflammation. Open Access. Zonal expression of StARD1 and oxidative stress in alcoholic-related liver disease. Journal of Lipid Research. Vol. 64 Issue 8 100413 Published online: July 18, 2023. ... Research Article Collection: Cholesterol Metabolism. Open Access.
About the journal. A journal of the American Society for Biochemistry and Molecular Biology . The Journal of Lipid Research focuses on the science of lipids in health and disease. The journal emphasizes lipid function and the biochemical and genetic regulation of lipid metabolism and aims to be on the forefront of …. View full aims & scope.
The Journal of Lipid Research focuses on the science of lipids in health and disease. The journal emphasizes lipid function and the biochemical and genetic regulation of lipid metabolism and aims to be on the forefront of the emerging areas of genomics, proteomics, metabolomics and lipidomics as they relate to lipid metabolism and function. More.
Lipidomics uses the principles of analytical chemistry to reveal the total lipid content and composition in a cell. In this Review, Xianlin Han provides an overview of lipidomics as applied to the ...
Research article Open access The lipid substrate preference of CETP controls the biochemical properties of HDL in fat/cholesterol-fed hamsters. Richard E. Morton, Daniel Mihna, Yan Liu. Article 100027 View PDF. Article preview.
Lipids. Lipids is a journal of the American Oil Chemists' Society (AOCS) covering the full range of lipid research, including chemistry, biochemistry, basic and clinical nutrition, and metabolism. We particularly welcome studies demonstrating novel mechanisms in lipid biochemistry or metabolism, as well as clinical or nutritional studies ...
Two C18 hydroxy-cyclohexenone fatty acids from mammalian epidermis: Potential relation to 12 R -lipoxygenase and covalent binding of ceramides. Journal of Biological Chemistry. Vol. 299Issue 6104739Published online: April 20, 2023.
Featured series: Lipids and Sleep Lipids in Health and Disease is calling for submissions to our new Collection on "Lipids and Sleep". This Collection is focusing on the link between sleep and lipid metabolism. We invite original research, topical and systematic review articles and meta-analyses.
Bringing lipid bilayers into shape. Lipid bilayers form the thin and floppy membranes that define the boundary of compartments such as cells. Now, a method to control the shape and size of ...
Furthermore, nonadherence to lipid‐lowering therapy could play a role in the association between lipid variability and the risk of CVD, as the beneficial effects of statins are quickly lost and often reversed when discontinued. 30, 31, 32 However, we found an association between variability in total cholesterol, LDL‐C, and HDL‐C, and risk ...
publishes research papers and review articles on chemical and physical aspects of lipids with primary emphasis on the relationship of these properties to biological functions and to biomedical applications. Accordingly, the journal covers: advances in synthetic and analytical lipid methodology; mass-spectrometry of lipids; chemical and physical ...
A continuously growing amount of evidence underlines the active contribution of lipids and membrane organization to the regulation of the structure and function of transmembrane proteins. In general, lipids of the cell membrane can affect proteins through a mixture of direct and indirect mechanisms. While the former comprise ligand-like binding of lipids at specific binding sites on proteins ...
Given that previous research has indicated a link between blood lipid levels and inflammation , we further adjusted for blood lipid parameters and found that the serum sASGR1 concentration was significantly positively correlated with the serum hs-CRP level (β = 0.86, P < 0.001), WBC count (β = 0.13, P = 0.004), and neutrophil count (β = 0.10 ...
Acne bacteria trigger cells to produce fats, oils and other lipids essential to skin health, shows new research Aug 23, 2023 Innovative microscopy technique reveals secrets of lipid synthesis ...
PLoS Biol. 20, e3001561 (2022). Lipidomics is an interdisciplinary research field, which emerged in the early 2000s, that relies on large scale analysis of lipid species 1. Lipidomics has become ...
Lipid nanoparticles (LNPs) have emerged as highly effective delivery systems for nucleic acid-based therapeutics. However, the broad clinical translation of LNP-based drugs is hampered by the lack of robust and scalable synthesis techniques that can consistently produce formulations from early development to clinical application. In this work, we proposed a method to achieve scalable synthesis ...
In this chapter, an overview of the major lipids in the diet with emphasis in nutritional aspects is provided. Triacylglycerols, i.e., glycerol esterified with three fatty acids, are the predominant constituents in dietary lipids. ... Progress in Lipid Research, 40 (4) (2001), pp. 231-268. View PDF View article View in Scopus Google Scholar ...
This study investigates the use of untapped mesopelagic species as a source of long-chain polyunsaturated omega-3 fatty acids (LC n-3 PUFAs) to meet the growing demand. The challenges faced by commercial fishing vessels, such as varying catch rates and species distribution affecting lipid levels, are addressed. Marine oils were produced post-catch using thermal separation and enzymatic ...
ACC 2024: Anticipating Advancements in Lipid Management. Erin D. Michos, MD, MHS, previews four late-breaking trials to be presented at ACC on novel therapies for lipid management. The first trial ...