Advertisement
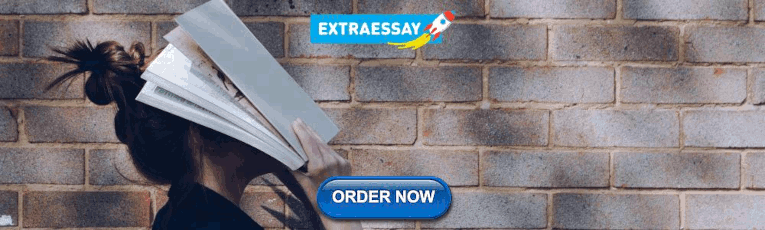
The role of disinfectants and sanitizers during COVID-19 pandemic: advantages and deleterious effects on humans and the environment
- Review Article
- Published: 15 May 2021
- Volume 28 , pages 34211–34228, ( 2021 )
Cite this article
- Kuldeep Dhama 1 ,
- Shailesh Kumar Patel 1 ,
- Rakesh Kumar 2 ,
- Rupali Masand 2 ,
- Jigyasa Rana 3 ,
- Mohd. Iqbal Yatoo 4 ,
- Ruchi Tiwari 5 ,
- Khan Sharun 6 ,
- Ranjan K. Mohapatra 7 ,
- Senthilkumar Natesan 8 ,
- Manish Dhawan 9 , 10 ,
- Tauseef Ahmad 11 , 12 ,
- Talha Bin Emran 13 ,
- Yashpal Singh Malik 14 &
- Harapan Harapan ORCID: orcid.org/0000-0001-7630-8413 15 , 16 , 17
33k Accesses
109 Citations
24 Altmetric
Explore all metrics
Disinfectants and sanitizers are essential preventive agents against the coronavirus disease 2019 (COVID-19) pandemic; however, the pandemic crisis was marred by undue hype, which led to the indiscriminate use of disinfectants and sanitizers. Despite demonstrating a beneficial role in the control and prevention of COVID-19, there are crucial concerns regarding the large-scale use of disinfectants and sanitizers, including the side effects on human and animal health along with harmful impacts exerted on the environment and ecological balance. This article discusses the roles of disinfectants and sanitizers in the control and prevention of the current pandemic and highlights updated disinfection techniques against severe acute respiratory syndrome coronavirus 2 (SARS-CoV-2). This article provides evidence of the deleterious effects of disinfectants and sanitizers exerted on humans, animals, and the environment as well as suggests mitigation strategies to reduce these effects. Additionally, potential technologies and approaches for the reduction of these effects and the development of safe, affordable, and effective disinfectants are discussed, particularly, eco-friendly technologies using nanotechnology and nanomedicine.
Similar content being viewed by others
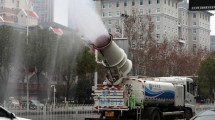
High concentration and high dose of disinfectants and antibiotics used during the COVID-19 pandemic threaten human health
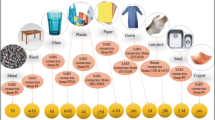
Environmental and health impacts of spraying COVID-19 disinfectants with associated challenges
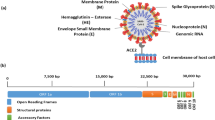
Environmental aspect and applications of nanotechnology to eliminate COVID-19 epidemiology risk
Avoid common mistakes on your manuscript.
Introduction
Intensive global research efforts have been engaged for the development of potential therapies and vaccines for coronavirus disease 2019 (COVID-19), caused by severe acute respiratory syndrome coronavirus 2 (SARS-CoV-2) (Dhama et al. 2020a , b ; WHO 2021 ), and the control of the COVID-19 pandemic remains the highest priority globally. Considering the current ineffectiveness of various strategies in the prevention of the spread of the virus, the lack of targeted treatments, and frequent increase in cases on a daily basis, disinfection is an important available measure to prevent COVID-19 spread and to combat SARS-CoV-2 directly.
Successful disinfection of SARS-CoV-2 is determined by the characteristics of the virus, properties of the disinfectants or sanitizers, and the environment where the virus is present or where disinfection is to be conducted. Disinfectants are chemical agents that are used to inactivate or destroy microorganisms, while sanitizers are available in the liquid, gel, or foam forms that are used to reduce the number of microorganisms present and to clean hands. SARS-CoV-2 is susceptible to disinfection (Rutala and Weber 2019 ; WHO 2020 ), exhibits stability at a broad range of pH values (pH 3–10) at room temperature (Chin et al. 2020 ), and is very much stable in a favorable environment (van Doremalen et al. 2020 ). SARS-CoV-2 persists for variable durations in different environments; for instance, it can reside on the outer layer of surgical masks for up to 7 days, and its presence on smooth surfaces (glass, plastic, banknotes, stainless steel) varies from 4 to 7 days (Chin et al. 2020 ). SARS-CoV-2 has not been detected on printing and tissue papers after 3 h, and treated wood and cloth show negative results for SARS-CoV-2 after 2 days (Chin et al. 2020 ). SARS-CoV-2 demonstrates increased stability on stainless steel and plastic as compared to copper and cardboard, and viable virus has been detected up to 72 h of application to such surfaces (van Doremalen et al. 2020 ). Furthermore, it has been detected in sewage and wastewater (Randazzo et al. 2020 ; Dhama et al. 2021 ).
SARS-CoV-2 is susceptible to a wide variety of disinfectants (Chin et al. 2020 ; United States Environmental Protection Agency (EPA), 2020). Lipid solvents, including ethanol (> 75%), formaldehyde (> 0.7%), isopropanol (> 70%), povidone-iodine (> 0.23%), sodium hypochlorite (> 0.21%), or hydrogen peroxide (H 2 O 2 ; > 0.5%), can also be used to inactivate SARS-CoV-2 (Duarte and de Santana 2020 ). Considering viral presence, persistence, stability, viability, and environmental influence on viral persistence, the disinfection of environments, such as offices, healthcare settings, public transportation, markets, restaurants, and auditoriums, is necessary to prevent the transmission and infection waves of COVID-19.
However, strategies and techniques of cleaning, sanitization, disinfection, and other methods to contain the devastating effects of the pandemic should be subjected to modification development over time according to their deleterious consequences on the environment and human health (Mukherjee et al. 2021 ). Hence, the present article highlights the beneficial usages, effectiveness, modes of antiviral action, and deleterious consequences of different disinfectants, along with a brief note on sanitizers, while also discussing the mitigation strategies with updated disinfection approaches to counter their harmful effects during the COVID-19 pandemic.
Disinfectants and their antiviral mechanisms
Disinfectants are chemical agents that are specifically formulated to inactivate or destroy microorganisms and include various classes, such as detergents, acids, oxidizing agents, alcohols, alkalis, aldehydes, biguanides, halogens, phenols, and quaternary ammonium compounds (QACs) (FDA 2020a ; Choi et al. 2021 ). Chemical disinfectants vary in their action mechanism, and the majority of disinfectants of a chemical nature target the outer lipid layer of coronaviruses (CoVs) and inactivate the viral particles (Choi et al. 2021 ). However, variations among the mechanisms of chemical disinfectants have been recorded. Detergents are a well-known category of chemical disinfectants that vary in their mechanism of action depending upon the class of disinfectant, the organism against which the disinfectant exhibits strong activity, the structure being affected, the surface or medium on which the disinfectant is applied, and the environment of application (Maris 1995 ; McDonnell and Russell 1999 ). Disinfectants may initiate three types of killing mechanisms that include cross-linking, coagulation, clumping, structural and functional disruption, and oxidation. These processes can occur through oxidation, hydrolysis, denaturation, or substitution (Ewart 2001 ). In case of viruses, they may affect the lipid membrane, cytoplasmic membrane, energy metabolism, cytoplasm, nucleus, enzymes, or proteins (Maris 1995 ). Non-ionic (uncharged) detergents are preferred over anionic detergents as they are good emulsifiers and exhibit better penetration and dispersion, decreased surface tension, lesser foaming property, and do not undergo complexation with hard water and result in microbial accumulation in the residue (Ewart 2001 ; CFSPH 2008 ).
Alcohol causes damage to microorganisms by denaturing proteins, leading to membrane damage and cell lysis (Ewart 2001 ; CFSPH 2008 ; Al-Sayah 2020 ). Ethanol shows appreciable activity on both living and non-living surfaces and evaporates quickly without leaving residue (CFSPH 2008 ). Ethanol at > 75% concentration acts as a potent virucidal agent that inactivates all lipophilic viruses (herpes, influenza, and vaccinia) and several hydrophilic viruses (adenovirus, rhinovirus, enterovirus, and rotaviruses). At a concentration of > 70%, ethanol and isopropanol inactivate CoVs within 30 s (Kampf et al. 2020a ). Isopropyl alcohol is extremely active against lipid viruses (CDC 2008 ). The primary mode of action is the coagulation and denaturation of proteins, apart from its lipid solvent properties.
Chlorine aids the oxidation of peptide links owing its electronegativity, and therefore, causes the oxidation of lipids and proteins and, in turn, inflicts damage on the membrane and cell wall of the microbes (McDonnell and Russell 1999 ). Moreover, hypochlorous acid is the most active compound, and it penetrates the cell layers even at a pH of 7. QACs damage the membrane permeability of microbes by irreversibly binding to phospholipids and proteins of the membrane (Gerba 2015 ). An alkaline pH (above 10.0) results in the disorganization of the peptidoglycan structure and leads to hydrolysis of the virus genome (Maris 1995 ). Phenolic compounds act specifically on the cell membrane and lead to the inactivation of the intracytoplasmic enzymes by forming unstable complexes (Sankar et al. 2016 ). Acids and alkalis mediate their antiviral action through H + and OH − ions that inflict damage on the amino acid bond in nucleic acids, modify the cytoplasmic pH, precipitate proteins, and saponify the lipids (Russell 1983 ; Maris 1995 ). H 2 O 2 catalyzes the oxidation and denaturation of proteins and lipids, causing membrane disorganization, resulting in swelling due to the saturation of H + ions (Russell 1983 ; Maris 1995 ; Al-Sayah 2020 ). Against SARS-CoV, H 2 O 2 exhibits virucidal activity at a 1–3% concentration and inactivates the virus within 1 min; however, the gaseous form is more efficient (Herzog et al. 2012 ; Goyal et al. 2014 ). H 2 O 2 -based non-touch disinfection techniques help reduce environmental contamination, particularly in hospital settings and intensive care units with infectious agents after routine cleaning (Huttner and Harbarth 2015 ; Blazejewski et al. 2015 ). Airborne H 2 O 2 in the form of vapor and dry mist has been used as an environmental disinfectant and to control infection in clinical settings (Falagas et al. 2011 ). Aldehydes demonstrate activity against CoVs at concentrations of 0.5–3% and result in viral deactivation within 2 min (Al-Sayah 2020 ). Povidone–iodine at a concentration of 1% or less triggers SARS-CoV inactivation within seconds (Kariwa et al. 2006 ; Eggers et al. 2018 ).
If the lipid envelope, glycoproteins, attachment (spike) proteins, and virion genome of CoVs, which impart infectivity to the virus, undergo damage or disruption, then the aftermath of such an occurrence is virus inactivation or exertion of effects on its infectivity (Al-Sayah 2020 ). Disinfectants have been evaluated and recommended against SARS-CoV-2 (Noorimotlagh et al. 2020 ). Among these, ethanol at a concentration > 70% and 2-propanol at a concentration of 70–100% have shown virucidal activity, while others have demonstrated either less effectivity or complete ineffectivity, with several agents posing a threat to human health and the environment (Kampf et al. 2020a ; Lai et al. 2020 ; Noorimotlagh et al. 2020 ; Schrank et al. 2020).
Necessity of disinfection during the COVID-19 pandemic
A recent study predicted that a considerable proportion of the global population would eventually be infected by SARS-CoV-2 (Giesecke 2020 ). The only available method of containing this pandemic is to prevent further transmission and to confer protection to individuals against exposure to the virus. Implementation of strict lockdowns, rampant testing, contact tracing, quarantine, isolation, and treatment approaches have decelerated the virus spread to a certain extent, and now it is imperative to adopt effective disinfection procedures to ensure the safety of populations after the lifting of lockdown and resumption of on-site work. Considering the persistence of SARS-CoV-2 on surfaces and the potential risk of infection through fomites, disinfection of the work environment is a priority before the resumption of regular working environments (ECDC 2020 ; van Doremalen et al. 2020 ). As SARS-CoV-2 survives in the environment with persistence ranging from hours (3 h in the air, 4 h on copper, and 24 h on cardboard) to days (2 to 3 days on both stainless steel and plastic), the disinfection of workplaces is imperative, especially where public visits or assemblies of crowds are inevitable (ECDC 2020 ; van Doremalen et al. 2020 ). Similarly, SARS-CoV-2 can persist for days on non-porous surfaces under 22°C and 65% relative humidity (Chin et al. 2020 ). Moreover, it has also been detected on desktops, printers, keyboards, doorknobs, gloves, and eye shields (ECDC 2020 ). A comparative study of SARS-CoV-1 and SARS-CoV-2 showed that the viability of the two CoVs is similar; however, SARS-CoV-2 spread is characterized by rapid dissemination and infection of more people (Gates 2020 ).
Although most SARS-CoV-2 transmissions occur in community settings, healthcare settings are also vulnerable to the establishment and spread of infections. In this context, hospitals engaged in treating patients with COVID-19 must be equipped with the most appropriate disinfection techniques and materials for the disinfection of healthcare personnel, hospital rooms, and medical equipment to avoid nosocomial transmission. The guidelines for the application of disinfectants in healthcare and non-healthcare settings issued by various agencies must be followed while using disinfectants in different environments (ECDC 2020 ; US EPA 2020 ).
Disinfection is a prerequisite for the control of infectious disease outbreaks, with SARS-CoV-2 containment being of utmost importance. It is vital to reduce the potential for virus contamination. Disinfection may also lessen the burden on other measures of pandemic control. Commonly, sodium hypochlorite, ethanol, and H 2 O 2 have been used and found to be more effective compared to benzalkonium chloride (BAC), chlorhexidine digluconate, povidoeyodine, and diluted ethyl alcohol, especially with reference to their application in hand hygiene, protective equipment sanitization, and in environmental disinfection (León Molina and Abad-Corpa 2021 ). Rowan and Laffey ( 2020 ) proposed the disinfection of personal protective equipment (PPE) for their reuse and the utilization of vaporized H 2 O 2 for the sterilization of filtering facepiece respirators and UV irradiation. Increased extent of liquid disinfection (Actichlor+) is being adopted in the USA and Ireland. This will help prevent a shortage of PPE. The European Centre for Disease Prevention and Control (ECDC) has suggested different cleaning options for different settings, which are described in Table 1 (ECDC 2020 ).
Moreover, several chemical disinfectants with high toxicity are being used for the decontamination of the surfaces of several environmental settings, such as clinical and surgical practices and water bodies, which is imperative to ensure well-being and safety. However, the development of new decontamination strategies, which neither leave residues nor induce toxicity, is vital. The following section emphasizes the updated and modified disinfection approaches to contain the overwhelming effects of the COVID-19 pandemic.
An overview of the important disinfectants and their antiviral mechanisms and the need for disinfection during the COVID-19 pandemic is illustrated in Fig. 1 .
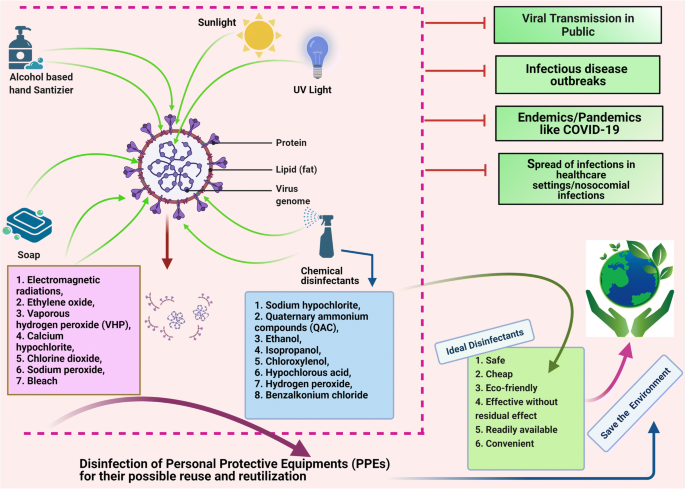
Disinfectants and their antiviral mechanisms (such as targeting lipid layer and spike proteins) and the need of disinfection during COVID-19 pandemic. The figure was created with BioRender.com
Updated disinfection approaches against SARS-CoV-2
Commonly used disinfectants against COVID-19 include detergents/soaps, alcohols, and chlorine. Chlorine is recommended as a disinfectant for indoor facilities (Yang et al. 2020 ). In healthcare settings, equipment, including imaging devices (e.g., endoscopes), scanners, bedding, and contact instruments, must be disinfected using appropriate disinfectants (Prochazka Zá Rate et al. 2020 ; Wan et al. 2020 ). To perform disinfection of the floor, environment, and table surfaces, application of 2 g/L of chlorine-containing disinfectant is recommended at least four times daily for at least 30 min, while application of UV irradiation and spraying techniques using 500 mg/L of chlorine-containing disinfectant for at least 30 min are the methods of choice for the disinfection of air (Barcelo 2020 ). Additionally, personal items, such as mobile phones, keys, credit cards, and writing pens, may be subjected to sanitization using 75% ethanol to ensure their disinfection (Yang et al. 2020 ). Virusend (TX-10) is a unique disinfectant that has already exhibited efficient activity against several microbial agents, including viruses. Virusend (TX-10) application was found to inactivate SARS-CoV-2 in an in vitro study based on surface and solution inactivation assays. This novel disinfectant rapidly decreased the SARS-CoV-2 viral titer by 4log 10 PFU/mL within a contact period of 1 min (Anderson et al. 2020 ).
The use of plasma-activated water is another alternative to conventional disinfectants that can efficiently be used to inactivate bacteria and bacteriophages via induction of direct damage to the biological macromolecules. Plasma-activated water can be used to inhibit SARS-CoV-2 pseudovirus infection via inactivation of the S protein (Guo et al. 2020 ). The alcohol-based hand sanitizers (ABHSs) that are commonly used on skin are not suitable for use on PPE. Therefore, new compounds or products should be developed that can be used to effectively disinfect PPE without causing detrimental effects to the surrounding skin tissue. Clyraguard copper iodine complex is a product that has exhibited potential efficacy in the decontamination of non-critical PPE (Mantlo et al. 2020 ).
As various environmental factors are considered to determine the transmission of SARS-CoV-2, disinfection of surfaces in work environments with diluted sodium hypochlorite (0.1%) is recommended (Eslami and Jalili 2020 ; Seymour et al. 2020 ). CoVs present on inanimate objects or surfaces may be inactivated by conducting treatment with biocidal agents, such as 0.5% H 2 O 2 , 62%–71% ethanol, and 0.1% sodium hypochlorite, for at least 1 min (Kampf et al. 2020a ). Presently, the disinfection of fabrics and carpets used in hospitals is nearly neglected, though such materials are highly prone to contamination by aerosols and dust. Standard procedures for the disinfection of surface coverings and linens include laundering, cleaning with water, and vacuuming, which, in many cases, are inadequate to remove infectious load (Malik et al. 2006a ). The carpets of shopping malls where a COVID-19-positive patient may have visited are disinfected by spraying 1% NaOCl, and the disinfection time is 30 min for spraying of disinfectants, as recommended by the WHO and the Central Pollution Control Board in India. Chlorine has been applied as a disinfectant to contain SARS-CoV-2 in indoor and outdoor spaces in China (China Ministry of Ecology and Environment 2020 ). Thermal methods involving temperatures of at least 50°C for conducting 30-min Uc radiation (100-μwcm −2 power) with a contact time of 15 to 20 min and H 2 O 2 vapor are being used for the disinfection of masks, especially N95 masks (Card et al. 2020 ; Seymour et al. 2020 ). Moreover, one study with the inclusion of original data based on 10 studies indicated that thermal disinfection at 80°C for 1 min, 65°C for 15 min, and 60°C for 30 min was highly effective in reducing CoV infectivity by at least 4 log 10 (Kampf et al. 2020b ). In this context, thermal aggregation of the membrane protein along with complete denaturation of nucleocapsid protein (55°C for 10 min) of SARS-CoV is suggested as a probable explanation of infectivity reduction (Wang et al. 2004 ; Lee et al. 2005 ).
The US Environmental Protection Agency (EPA) has published a list of effective disinfectants for use against SARS-CoV-2, including sodium hypochlorite, QAC, ethanol, isopropanol, hypochlorous acid, chloroxylenol, H 2 O 2 , BAC, and chlorine-based chemicals (US EPA 2020 ). Common disinfectants against SARS-CoV-2 are listed in Table 2 . BAC and related disinfectants are ubiquitously used and have been recommended by the FDA for use in soaps, hospital sanitation kits, and cleaning wipes; however, knowledge of their efficacy against SARS-CoV-2 is crucial, and thus, they must be evaluated. In addition to alcohols and ABHSs, QACs, such as BAC, have been evaluated against CoVs but have shown less activity; thus, similar to other disinfectants, including sodium hypochlorite, peroxides, aldehydes (formaldehyde and glutardialdehyde), and didecyldimethylammonium chloride, the above-mentioned agents should be subjected to proper analysis to avoid their improper application as disinfectants against SARS-CoV-2 (Kampf et al. 2020a ; Lai et al. 2020 ; Schrank et al. 2020 ). The CDC issued a warning regarding the use of products containing BAC for the prevention of COVID-19 (Schrank et al. 2020 ). Additionally, CoVs undergo destruction within 15 min of exposure to ultraviolet C (UVC) light (Darnell et al. 2004 ). The beta-CoVs, including SARS-CoV and Middle East respiratory syndrome (MERS) CoV, are effectively inactivated by germicidal ultraviolet (UV) irradiation; however, its efficacy for inactivating SARS-CoV-2 warrants further investigation (Leung and Ko 2020 ). Germicidal UV lamps for household disinfection should be used with extreme caution because their improper use can cause epidermal photo-toxicity and photo-keratitis (Leung and Ko 2020 ). UV radiation is more dangerous and may severely damage the eyes and skin; repeated exposure to such radiation can also cause skin cancer (ICNIRP 2004 ). The use of human disinfection chambers is an example of recent innovations developed in response to the COVID-19 pandemic (Wickramatillake and Kurukularatne 2020 ).
As the number of COVID-19 cases continues to increase worldwide, a considerable shortage of N95 respirators has emerged. Therefore, it is essential to note that N95 respirators can be reused after disinfection. In a recent study, N95 respirators were subjected to conditions of heat at temperatures ≤ 85°C and relative humidity ≤ 100%, which resulted in the inactivation of the virus without affecting the filtration properties of the masks (Liao et al. 2020 ). Furthermore, the use of H 2 O 2 and hot air is the most effective method for the industrial and home disinfection of face masks, respectively. In contrast, surgical masks and homemade or non-certified masks are slightly less and significantly less effective than PPE and face masks, respectively (Carlos Rubio-Romero et al. 2020 ). The disinfection of used masks is necessary for their safe reuse while there is an acute shortage; however, incorrect decontamination procedures can damage the filtering structure of the masks. Medical masks and N95 masks retain their blocking efficacy against over 99% of viruses in aerosols even after subjection to steam conditions in boiling water for 2 h, suggesting that they can be reused for several days with the application of steam decontamination between uses (Ma et al. 2020 ). Disinfection of the masks and PPE after use and prior disposal is imperative. Otherwise, they may become a source of environmental contamination of SARS-CoV-2. The disinfection of N95 respirators may be essential during pandemics, such as the present COVID-19 pandemic, to overcome the curtailment crisis. However, the decontamination method should not alter the efficiency of the filtration of the N95 respirators and surgical masks. The use of UV germicidal irradiation, microwave-generated steam, moist heat, and H 2 O 2 vapor techniques should be strictly followed for the efficient containment of SARS-CoV-2.
Considering the transmission of COVID-19 in public transport vehicles, such as aircraft, ships, trains, subways, and buses, public transportation staff and passengers are advised to adopt strict preventive measures. All surfaces in public transport vehicles must be appropriately disinfected and sanitized. For this purpose, surfaces can be sprayed or wiped with chlorine-containing disinfectants (COVID-19 Emergency Response Key Places Protection and Disinfection Technology Team 2020a ). A disinfectant should be used at an appropriate concentration and sufficient contact exposure time should be allowed with the surface to destroy the virus.
The precise timing, the location, and the mechanism of disinfection, the type of disinfectant to be used, and safety measures to be implemented for both public health and the environment must be determined (Iyiola et al. 2020 ; Nabi et al. 2020 ; Zhang et al. 2020 ). Determination of the minimum amount of disinfectant necessary, appropriate exposure levels, and appropriate level of contact is essential for optimum action (Sarada et al. 2020 ). Avoidance of indiscriminate sprays, minimization of public movement, and the development of low-risk or nontoxic but effective disinfectants for future safe applications should be an international priority (Iyiola et al. 2020 ; Nabi et al. 2020 ; Zhang et al. 2020 ). Additionally, efforts should be engaged for the development of disinfection systems, such as UVC-based disinfection trolleys, UVC germicidal lamp-based fogging chambers, dry heat sterilization, and HOCl-based chemical disinfectants (Sarada et al. 2020 ).
Disinfection and its deleterious effects on humans and the environment
Improper and inappropriate use of disinfectants can result in the exertion of adverse effects. Excessive use of disinfectants poses a potential threat to living beings and ecosystems (Chen et al. 2021 ; Ghafoor et al. 2021 ) as they present with a myriad side effect (Yari et al. 2020 ). Disinfectants can affect both the applicant and the environment and may have future deleterious consequences (Yari et al. 2020 ). Chemical agents used as highly concentrated, aerosolized, or atomized disinfectants can easily be inhaled or absorbed into the skin. For example, aerosolized particles can penetrate alveoli upon inhalation. The increased frequency and duration of exposure to disinfectants (e.g., in disinfection chambers) can cause harmful effects on human and animal health. Disinfectants may cause reactions in the mucosal lining, resulting in irritation, inflammation, swelling, and ulceration of the upper and lower respiratory tract. A few chemicals are absorbed quickly through the mucosa of various organs and organ systems (e.g., the central nervous system and gastrointestinal tract) into the bloodstream. A recent case study reported the infliction of severe corrosive damage to gastric, esophageal, and small intestinal mucosa after the intentional oral ingestion of 10 mL of ethanol-containing hand disinfectant for 3 weeks, as the patient aimed to perform self-disinfection against COVID-19 owing to a fear of infection by the virus (Binder et al. 2020 ). Direct contact of the cornea and skin with aerosols may cause severe irritation and irreversible damage (Wickramatillake and Kurukularatne 2020 ). In addition to dryness of the skin, ABHSs can lead to infection and poisoning, particularly in children, who are believed to be susceptible and thus are subject to a major health risk (Ghafoor et al. 2021 ). Bleach (diluted sodium hypochlorite), one of the most commonly used disinfectants, can be directly absorbed by the skin, leading to allergic reactions. Additionally, various harmful effects, such as acute cardiopulmonary arrest, gastrointestinal ailments (e.g., nausea, vomiting, and diarrhea), and renal problems, have occurred in individuals after the accidental inhalation and ingestion of bleach (Peck et al. 2011 ). QAC and bleach reportedly increase the risk of development of asthma, chronic obstructive pulmonary disease (COPD), infertility, and impaired brain development in children (Fair 2020 ). The potential impact of disinfectants on individuals with asthma remains to be investigated, especially in the case of disinfectants with strong odors. Such disinfectants can act as potential asthma triggers (Eldeirawi et al. 2020 ). Therefore, individuals with asthma should use safer disinfection alternatives. A recently published cohort study revealed that out of 55,000 health professionals who used QAC and bleach routinely, 663 developed COPD (European Lung Foundation 2017 ). A correlation between the concentration of sodium hypochlorite and microscopic/cellular alterations, including chromosomal aberrations, cell death (apoptotic and necrotic changes), and increased mitotic activity, has also been documented (Gul et al. 2009 ). Additionally, psychotic episodes associated with a fear of death from SARS-CoV-2 infection can result in the consumption of liquid disinfectants or inhalation of aerosol sprays containing chlorine in an effort to cleanse their body. This can result in the infliction of primary inhalational toxic lung injury, which can mimic the symptoms of clinical COVID-19 due to the development of acute respiratory distress syndrome (Willems et al. 2020 ).
Chemical compounds used as disinfectants are not only harmful to humans but also affect animals and aquatic ecosystems. Though the disinfection of wastewater originating from healthcare facilities, offices, public places, and other organizations, such as hotels and processing units, is essential for minimization of the likelihood of spreading infection and deleterious effects, application of these disinfectants can cause harm to both living organisms and the environment. Other disinfection practices, such as the washing of external floors, streets, and markets, also contribute to the discharge of disinfectants into sewage, rivers, and lakes (Subpiramaniyam 2021 ). Sodium hypochlorite is commonly used for the disinfection of hospital wastewater to prevent the spread of nosocomial infectious diseases. Therefore, such chemicals may gain entry into the sewage and cause pollution of drinking water resources (China Ministry of Ecology and Environment 2020 ). Furthermore, as both direct and indirect sewage effluents are discharged into rivers and lakes, aquatic ecosystems are at a risk of contamination with chemical disinfectants (Sedlak 2011 ; Subpiramaniyam 2021 ). Chlorine disinfectants threaten aquatic wildlife and plants as the agents catalyze the oxidation of their proteins and destruction of their cell walls (Sedlak 2011 ). Moreover, these chemicals may bind to other materials to form harmful compounds. For example, chlorine disinfectants undergo reactions with dissolved organic matter of surface water to produce disinfectant byproducts, such as haloacetic acids and trihalomethanes, which are highly toxic to aquatic flora and fauna (Sedlak 2011 ; Liu and Zhang 2014 ). Chlorine also undergoes reaction with organic matter in wastewater, thereby resulting in the generation of organic chlorine compounds that persist as environmental contaminants and may pose a considerable risk to aquatic ecosystems (Emmanuel et al. 2004 ). An effect of disinfectants may be exerted on microbial activity in wastewater treatment plants that may compromise the effective removal of pollutants (carbon, nitrose, and phosphorous). Extensive use of disinfectants against COVID-19 also poses potential risks to urban wildlife (Nabi et al. 2020 ). While humans can avoid the establishment of contact with disinfectants during the active disinfection of areas or localities, other organisms, including wild animals, are unable to do so, thus resulting in potential contact with corrosive or otherwise harmful substances (Nabi et al. 2020 ). The overuse of disinfectants has led to the death of animals, such as birds and weasels (You 2020 ). They exert toxicological effects on both terrestrial and aquatic animals (El-Nahhal and El-Nahhal 2020 ) and may have impacts on food and water sources (Zhang et al. 2020 ). Excessive use of disinfectants can lead to their enrichment, bioaccumulation, and biomagnification, resulting in toxicity, mutations, spread of antibiotic resistance genes, and the emergence of antibiotic-resistant bacteria (Chen et al. 2021 ).
The salient deleterious effects of disinfectants and sanitizers on humans and the environment during COVID-19 are depicted in Fig. 2 .
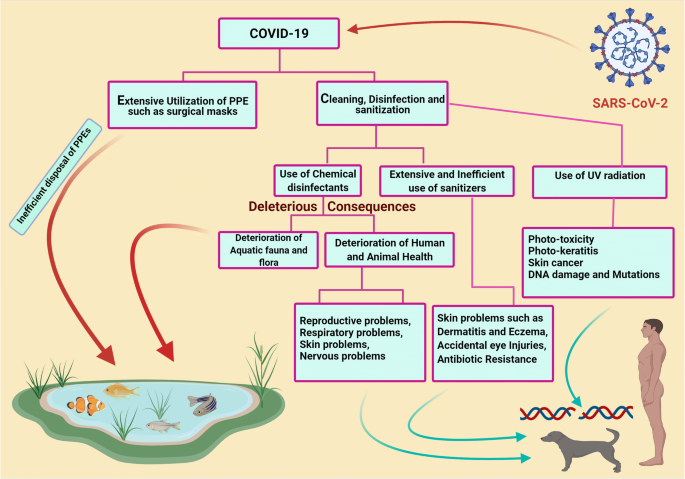
Deleterious effects of disinfectants and sanitizers in human and environment during COVID-19. The figure was created with BioRender.com
Disinfectants may also affect material surfaces (Bonin et al. 2020 ). Their corrosive nature may lead to the corrosion of important metal surfaces. Though four out of five disinfectants pose little or no risk to metals, further studies are warranted for the evaluation of the impact of disinfectants on surfaces subject to frequent and continuous use of various types of disinfectants, as these agents may exert adverse effects (Bonin et al. 2020 ). The increased use of disinfectants in response to the COVID-19 pandemic may lead to the occurrence of a secondary disaster in aquatic ecosystems worldwide. Therefore, sewage originating from medical institutions should be treated as per the guidelines provided by concerned authorities. This implies that sewage originating from healthcare facilities should be treated separately before combination with other sewage. Additionally, to remove remaining virus particles from the sewage originating from healthcare facilities, chemical agents, such as dibromo-dimethyl hydantoin, chlorine dioxide, and other chlorine-containing disinfectants, can be used (COVID-19 Emergency Response Key Places Protection and Disinfection Technology Team 2020b ). Analysis and re-evaluation of the current methods of sewage treatment are of utmost importance for preventing the transmission of COVID-19 via sewage; additionally, the substitution of conventional methods (chlorination and simple filtration) with advanced methods (centralized wastewater treatment, oxidation, filtration, and membrane technology) of sewage treatment is necessary to prevent the dissemination of SARS-CoV-2 throughout the environment (Núñez-Delgado 2020 ).
Mitigation strategies to reduce the deleterious consequences of disinfectants in humans and the environment
Safe and eco-friendly disinfectants should be used, and post-disinfection measures should be undertaken to avoid the occurrence of health hazards. In this context, light, including sunlight (Ratnesar-Shumate et al. 2020 ), UV light (Seyer and Sanlidag 2020 ; Zhao et al. 2020 ), and color light (Enwemeka et al. 2020 ), may demonstrate prospects and potential applications in managing the COVID-19 pandemic. However, further studies relating to this matter are warranted (Derraik et al. 2020 ; Ratnesar-Shumate et al. 2020 ; Seyer and Sanlidag 2020 ). Simulated sunlight can reportedly inactivate SARS-CoV-2 particles dried on stainless steel and suspended in simulated saliva or culture media (Ratnesar-Shumate et al. 2020 ). Moreover, 90% of infectious virus is inactivated at every 6.8 and 14.3 min of exposure in simulated saliva and culture media, respectively (Ratnesar-Shumate et al. 2020 ). This indicates that sunlight may be useful as a natural disinfectant for non-porous outdoor materials contaminated with SARS-CoV-2 (Ratnesar-Shumate et al. 2020 ). Special air-disinfecting machines are also presently being proposed (Zhao et al. 2020 ).
Hitherto, studies have shown the activity of alcohol-based disinfectants and sanitizers against several viral infections (Malik et al. 2006b ; Patnayak et al. 2008 ; Suman et al. 2020 ). The use of ethanol-based sanitizers is recommended for the prevention of the harmful effects of other chemical compounds on humans and animals. Direct spraying of bleach onto infected individuals or affected areas is discouraged. Disinfected surfaces must be subjected to drying and rinsing with water because disinfectants can persist for a long period on contaminated surfaces and may cause unintentional exposure to hazardous chemicals. Government agencies should, therefore, develop facilities for proper disinfectant drainage to minimize the harmful effects on aquatic flora and fauna. The use of eco-friendly technologies along with safe and effective disinfection methods is highly warranted, not only to combat the ongoing pandemic but also to protect the environment and living beings from hazardous chemicals.
Low-cost antibody-linked graphene sheets that function as environmental virus sensors have been synthesized; their application as coatings on face masks/PPEs represents a promising strategy to fight COVID-19 by minimizing the risk of transmission (Palmieri and Papi 2020 ). Moreover, magnetic nanomaterials or nanoparticles can be exploited as efficient alternatives for coating PPEs such as masks and eye-protecting glasses in order to produce reusable and environmentally friendly antiviral nanocoated PPEs (Tyagi et al. 2021 ). However, antiviral nanoparticles such as silver (Ag), copper (Cu), copper oxide (CuO), and zinc (Zn) have been incorporated on surfaces and PPE textiles, and can be a viable alternative to chemical disinfection processes (Valdez-Salas et al. 2021 ; Ruiz-Hitzky et al. 2020 ). In a recent study, the nanodisinfectant has been evaluated as a reliable technique for efficient disinfection, reusing, and even antimicrobial promotion of surgical masks for healthcare professionals (Valdez-Salas et al. 2021 ). In addition, modern nanotechnology and nanomedicine approaches have been harnessed to develop disinfection and treatment strategies to tackle increasing infection cases worldwide, especially challenges posed by pathogens of viral origin (Nikaeen et al. 2020 ). Recently, more sophisticated and modern strategies, such as the use of agriculture spraying drones and robotic machines, have been suggested to disinfect areas that pose a high risk of infection, such as stadiums and theaters, in a short timespan (Clay and Milk 2020 ; Khan et al. 2021 ). Various nanomaterials such as carbon nanotubes, graphene, or silver nanowires have been used to improve current physical disinfection methods (Kumar and Mohanty 2020 ; Palmieri and Papi 2020 ; Ruiz-Hitzky et al. 2020 ). Furthermore, nanomaterials have been proposed as possible disinfection candidates since they do not exhibit antiviral activities for single use but rather exhibit their action over a prolonged period of time (Campos et al. 2020 ; Ruiz-Hitzky et al. 2020 ), and this property can be used to produce sustainable and environmentally friendly disinfectants.
Furthermore, a recent study proposed that the use of a variety of physical techniques such as photolithography and laser surface modification, in conjunction with ion beam–assisted deposition, can be used to evolve biomaterial surfaces or self-cleaning surfaces with suitable topographical features and controlled cell adhesion (Kumari and Chatterjee 2021 ). In this sense, the antiviral behavior of aluminum surfaces with appropriately aligned ridges has been studied against SARS-CoV-2, and it was found that the self-disinfecting surfaces with coated nanoparticles are substantially effective against SARS-CoV-2 (Hasan et al. 2020a ; Hasan et al. 2020b ). Hence, the self-cleaning surfaces with minor deleterious consequences are incredibly effective at mitigating viral transmission by contact. Their ability should be explored further in the future to reduce the usage of chemical disinfectants.
Moreover, disinfectants are biocidal products; therefore, they are regulated by the Biocidal Products Regulation (BPR) (EU) in European Union countries and are appropriately evaluated before marketing (EPC 2020 ). However, considering the urgency of addressing the COVID-19 pandemic, a few agents may be provided for developing transitional measures without BPR for immediate use against SARS-CoV-2, such as 70–80% ethanol application for 1 min (Kampf et al. 2020a ). Most biocidal products with virucidal activity regulated under BPR are effective against SARS-CoV-2. This includes disinfectants used in hand hygiene and skin disinfection, albeit they may demonstrate limited biocidal activity against viruses or less remarkable activity against enveloped viruses (ECDC 2020 ). Hence, proper assessment before application and monitoring during use is of utmost importance for human health and environmental safety.
Appropriate use of disinfectants as recommended by various agencies should be practiced to counter SARS-CoV-2. The WHO ( 2020 ) has provided recommendations for the appropriate utilization of suitable disinfectants and at specified intervals. Similarly, cleaning and disinfection as per community facilities guidelines of the CDC (CDC 2020a ) and disinfection as per quarantine facility guidelines of the NCDC ( 2020 ) may be helpful. However, any disinfectant, regardless of its nature and properties, used for disinfection under a selective environment must meet local authority specifications and be used in an eco-friendly and efficient manner to prevent environmental contamination (WHO 2020 ).
Important mitigation strategies to reduce the deleterious consequences of disinfectants in humans and the environment during the COVID-19 pandemic are presented in Fig. 3 .
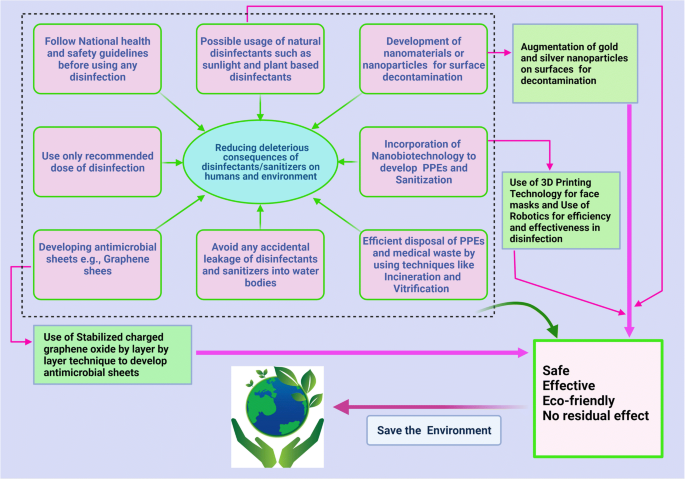
Mitigation strategies to reduce deleterious consequences of disinfectants in human and environment during COVID-19 pandemic. The figure was created with BioRender.com
Sanitizers during the COVID-19 pandemic
Sanitizer is an antimicrobial liquid, gel, or foam that is used to reduce the number of microorganisms present on a surface. Although alcohol-based hand rubs and washing with soap and water are effective against CoVs, due to ease of utilization, hand sanitizers have gained more popularity than other available options, including washing with soaps, chemical disinfection, exposure to sunlight, UV light, or heating. The increased frequency of sanitizer usage due to fear of developing COVID-19 has resulted in increased aerosol generation and, in certain cases, poses a potential hazard to exposed mucosal surfaces and skin. The adverse effects of alcohol used in hand sanitizers can be manifold and may lead to a condition called sanitizer aerosol-driven ocular surface disease owing to the increased sensitivity of eyes to the toxic effects of these sanitizers compared to that of skin (Ahn et al. 2010 ; Shetty et al. 2020 ). There has been a significant increase in the number of ocular injuries from 2020 to 2021 in the pediatric population due to inefficient use of ABHSs (Martin et al. 2021 ).
However, a few associated problems are caused by the market formulations of available hand sanitizers, and the capacity of chemists has increased manifold during the COVID-19 pandemic with emphasis on the use of hand sanitizers (Opatz et al. 2020 ). The use of hand sanitizers as a result of the COVID-19 pandemic has increased the number of cases of hand dermatitis in more than 90% of healthcare workers (HCWs) and hand eczema in nearly 14% of these cases (Guertler et al. 2020 ). Not all market formulations are effective for use. A screening of all WHO-recommended hand rub formulations (alcohol-based hand rubs) showed high virucidal activity with complete inactivation of SARS-CoV-2 (Kratzel et al. 2020 ). In this context, the Centers for Disease Control and Prevention (CDC) also recommended adopting practices for good hand hygiene, which includes proper handwashing with warm water and soap for a minimum period of 20 s and the use of ABHSs as the most effective approach to reduce COVID-19 infection (CDC 2020b ; Schrank et al. 2020 ).
Life-threatening clinical effects can be attributed to acute ethanol intoxication. A case study reported by Lim revealed that hand sanitizer application did not interfere with the course of treatment of infectious spondylitis or cause abnormal complications. However, during the current COVID-19 pandemic, it is expected that such intoxications will increase due to the increased use of hand sanitizers (Lim 2020 ).
Additionally, the toxicity and harmful effects of alcohol-based sanitizers on skin and their excessive use gradually lead to natural mutations in microbes and can contribute to the issue of antimicrobial resistance, which is already a significant threat to developing countries and continents, such as India, Pakistan, Africa, and Bangladesh (Mahmood et al. 2020 ).
To prevent the hazardous effects of ABHSs, the use of soap and water should be encouraged in susceptible individuals. The use of a face shield or protective goggles would be beneficial, wherein the frequent cleansing of hands is unavoidable. Moreover, the closure of eyes while pressing the nozzle of the sanitizer and maintenance of proper distance by ensuring that the sanitizer is below the eye level. Furthermore, keeping doors and windows open and avoiding sanitizer usage when air conditioning systems are activated may help reduce exposure to sanitizer droplets. Additionally, the most effective approach to confer protection to the eyes and mucosal surfaces from the harmful effects of sanitizers is by minimizing their use.
Steps to be followed while using disinfectants (National Pesticide Information Center 2020 ; COVID-19 Prevention: Enhanced Cleaning and Disinfection Protocols 2020 ):
Before using disinfectant, follow the precautionary statements on the accompanying label to prevent chemical exposure to self and surrounding individuals. Use appropriate recommended doses at appropriate intervals. Disinfectants containing 2 g/L chlorine need to be sprayed four times daily on highly infected areas (such as floors, tables, and beds of the contaminated/isolated areas and hospitals) for 30 min. A concentration of 0.5 g/L of chlorine is recommended for semi-contaminated areas. In case dilution of the disinfectant is required, it should not affect the final recommended concentration and should never be used in combination with other compounds.
While using disinfectants, wear appropriate PPE, such as goggles, gloves, long-sleeved shirts, long pants, and masks. The use of an appropriate mask for a specific purpose is essential rather than using any non-specific mask (Agrawal et al. 2020 ). For the community at large and for HCWs, surgical masks or three-layer cotton masks can be used. For HCWs during aerosol-generating procedures for a patient with COVID-19, respirator masks are recommended (Agrawal et al. 2020 ).
Ensure proper ventilation while conducting disinfection. Chlorine-based disinfectants with usual concentrations of 4% and 6% or glutaraldehyde-based disinfectants with higher vapor levels than the recommended 1.12–3.4% especially require well-ventilated rooms (Ghafoor et al. 2021 ; Kampf et al. 2020 ). There should be exhaust duct hoods, air systems with 7–15 air changes per hour, ductable fumigation hoods with disinfectant vapor absorbers, or straightener lids in dip baths (Foliente et al. 2001 ; Ghafoor et al. 2021 ).
Keep disinfectants away from the reach of children and pets. Children are particularly susceptible to poisoning by disinfectants (Ghafoor et al. 2021 ) with ingestion being the common exposure route (Rosenman et al. 2021 ).
Discard disposable protective items, such as gloves and masks, after using disinfectants, since they have limited efficacy (6–12 h) and are heat-sensitive, and thus, cannot tolerate the sterilization process (Rowan and Laffey 2020 ). Not only does the structure of PPE and masks change upon washing and drying, but changes are observed in also their quality and efficacy, such as through the deterioration of their filtration properties (Konda et al. 2020 ; Sharma et al. 2020 ).
Wash hands with soap and water after conducting disinfection, and apply the appropriate hand sanitizer.
Avoid spraying chloride- and hypochlorite-containing disinfectants to the most possible extent, as they are more harmful to surfaces, other organisms, and the environment than their alternatives (Lin et al. 2020 ); additionally, there are currently related concerns surrounding the enrichment, bioaccumulation, and biomagnification of disinfectants (Chen et al. 2021 ). Alcohol-based disinfectants, soaps, and detergents along with water and radiation can be comparatively beneficial; nevertheless, all specified options are detrimental when adopted in excess.
Conclusion and future prospects
The COVID-19 pandemic ushered in several challenges with its emergence and seemed to be unrestricted through current mitigation strategies. Considering the urgency of the situation caused by the global spread of SARS-CoV-2 with rising morbidity, alarming mortality, and global economic fallouts, the trend of efforts is shifting from strategies of lockdown, quarantine, testing, isolation, and treatment to the creation of an atmosphere of clean, healthy, and safe surroundings that provides a healthy working environment. Several prevention strategies, such as avoidance of close contact with sick people; avoiding touching eyes, nose, and mouth; staying home when sick; covering the mouth when coughing and sneezing; and undertaking the approaches for frequent disinfection and sanitization of hands and touched objects, fomites, and surfaces, are important to prevent virus transmission. In this context, a variety of chemicals and other virucidal agents have been used globally as disinfectants to render the environment free from SARS-CoV-2 to the highest extent possible to prevent further spread. Disinfectants are proving to be beneficial in this regard and have gained considerable attention recently as being effective, affordable, convenient, and readily available antimicrobial agents. Applications have been identified in every aspect of life, including at home, the office, healthcare facilities, other industries, and the surrounding environment. However, there remain concerns regarding the side effects on animal and human health, the environment, and ecological balance.
There is an urgent need for developing eco-friendly technologies that offer safer and more effective disinfection methods to combat the ongoing pandemic, along with conferring protection to the environment and living beings from the potentially hazardous effects of chemical disinfectants. Alternate and improved strategies are being devised for minimizing adverse effects. In this context, the use of graphene sheets as coatings for face masks offers a promising strategy for fighting COVID-19 by minimizing the risk of further transmission. The graphene coating of face masks is of particular interest because it can be reused as it is superhydrophobic, thereby reducing the likelihood of adherence of infectious drops, and its strong light-absorbing properties renders sterilization upon exposure to sunlight possible. Moreover, nanotechnology and nanomedicine approaches have been harnessed to develop novel disinfection and treatment strategies to tackle this pandemic more effectively.
The current pace of research and the evolution of numerous novel disinfectants against COVID-19 provides hope for the development of safe, effective, and convenient disinfectants that are affordable to all and accessible under diverse environments with minimum or no potential risk to health and surroundings. Meanwhile, during disinfectant use, precautionary and preventive measures should be adopted. An environmental impact assessment of the escalating use of disinfectants is urgently needed. Clear and comprehensive guidelines for disinfectant application are also necessary at regional, national, and international levels to reduce the deleterious consequences to both humans and the environment.
Data availability
Not applicable. This article does not produce new data.
Agrawal H, Singh S, Gupta N (2020) What all we should know about masks in COVID-19 pandemic. Indian J Surg Jun 9:1–2. https://doi.org/10.1007/s12262-020-02469-4
Article Google Scholar
Ahn J, Eum K, Kim Y, Oh SW, Kim YJ, Lee M (2010) Assessment of the dermal and ocular irritation potential of alcohol hand sanitizers containing aloe vera with in vitro and in vivo methods. Mol Cell Toxicol 6:397–404. https://doi.org/10.1007/s13273-010-0053-y
Article CAS Google Scholar
Al-Gheethi A, Al-Sahari M, Malek MA, Noman E, Al-Maqtari Q, Mohamed R, Talip BA, Alkhadher S, Hossain MS (2020) Disinfection methods and survival of SARS-CoV-2 in the environment and contaminated materials: a bibliometric analysis. Sustainability 12(18):7378. https://doi.org/10.3390/su12187378
Al-Sayah MH (2020) Chemical disinfectants of COVID-19: an overview. J Water Health 18(5):843–848. https://doi.org/10.2166/wh.2020.108
Anderson ER, Hughes GL, Patterson EI (2020) Inactivation of SARS-CoV-2 on surfaces and in solution with Virusend (TX-10), a novel disinfectant. bioRxiv [Preprint] 11.25.394288. https://doi.org/10.1101/2020.11.25.394288 .
Balagna C, Perero S, Percivalle E, Nepita EV, Ferraris M (2020) Virucidal effect against coronavirus SARS-CoV-2 of a silver nanocluster/silica composite sputtered coating. Open Ceramics 1:100006. https://doi.org/10.1016/j.oceram.2020.100006
Barcelo D (2020) An environmental and health perspective for COVID-19 outbreak: meteorology and air quality influence, sewage epidemiology indicator, hospitals disinfection, drug therapies and recommendations. J Environ Chem Eng 8(4):104006. https://doi.org/10.1016/j.jece.2020.104006
Binder L, Högenauer C, Langner C (2020) Gastrointestinal effects of an attempt to “disinfect” from COVID-19. Histopathology. 77:327–328. https://doi.org/10.1111/his.14137 (In press)
Blazejewski C, Wallet F, Rouzé A, Le Guern R, Ponthieux S, Salleron J, Nseir S (2015) Efficiency of hydrogen peroxide in improving disinfection of ICU rooms. Crit Care 19(1):30. https://doi.org/10.1186/s13054-015-0752-9
Bonin L, Vitry V, Olivier MG, Bertolucci-Coelho L (2020) Covid-19: effect of disinfection on corrosion of surfaces. Corros Eng Sci Technol 55(8):693–695. https://doi.org/10.1080/1478422X.2020.1777022
Campos EVR, Pereira AES, de Oliveira JL, Carvalho LB, Guilger-Casagrande M, de Lima R, Fraceto LF (2020) How can nanotechnology help to combat COVID-19? Opportunities and urgent need. J Nanobiotechnol 18(1):125. https://doi.org/10.1186/s12951-020-00685-4
Card KJ, Crozier D, Dhawan A, Dinh M, Dolson E, Farrokhian N, Gopalakrishnan V, Ho E, King ES, Krishnan N (2020) UV sterilization of personal protective equipment with idle laboratory biosafety cabinets during the Covid-19 pandemic. medRxiv. https://doi.org/10.1101/2020.03.25.20043489 .
Carlos Rubio-Romero J, Del Carmen P-FM, Antonio Torrecilla García J, Calero-Castro S (2020) Disposable masks: disinfection and sterilization for reuse, and non-certified manufacturing, in the face of shortages during the COVID-19 pandemic. Saf Sci 129:104830. https://doi.org/10.1016/j.ssci.2020.104830
CDC (2008) Chemical disinfectants (Guideline for disinfection and sterilization in healthcare facilities). https://www.cdc.gov/infectioncontrol/guidelines/disinfection/disinfection-methods/chemical.html #. Accessed 29 June 2020.
CDC (2020a) Cleaning and disinfection for community facilities. https://www.cdc.gov/coronavirus/2019-ncov/community/organizations/cleaning-disinfection.html . Accessed 13 June 2020
CDC (2020b) Hand hygiene recommendations. https://www.cdc.gov/coronavirus/2019-ncov/hcp/hand-hygiene.html . Accessed 17 May 2020.
CFSP (2008) Center for Food Security and Public Health (CFSPH). Disinfection101. http://www.cfsph.iastate.edu/Disinfection/Assets/Disinfection101.pdf . Accessed 17 May 2020.
Chen Z, Guo J, Jiang Y, Shao Y (2021) High concentration and high dose of disinfectants and antibiotics used during the COVID-19 pandemic threaten human health. Environ Sci Eur 33(1):11. https://doi.org/10.1186/s12302-021-00456-4
Chin A, Chu J, Perera M, Hui K, Yen HL, Chan M, Peiris M, Poon L (2020) Stability of SARS-CoV-2 in different environmental conditions. Lancet Microbe 1(1):E10. https://doi.org/10.1016/S2666-5247(20)30003-3
China Ministry of Ecology and Environment (2020) Will viruses and disinfection affect water quality? The Ministry of Ecology and Environment responded. www.mee.gov.cn/ywgz/ssthjbh/dbssthjgl/202003/t20200311_768408.shtml [in Chinese]. Accessed 29 June 2020.
Choi H, Chatterjee P, Lichtfouse E, Martel JA, Hwang M, Jinadatha C, Sharma VK (2021) Classical and alternative disinfection strategies to control the COVID-19 virus in healthcare facilities: a review. Environ Chem Lett:1–7. https://doi.org/10.1007/s10311-021-01180-4 (In press)
Clay and Milk (2020) Rantizo is using drone technology to sanitize stadiums. https://clayandmilk.com/2020/04/28/rantizo-is-using-drone-technology-to-sanitize-stadiums . Accessed 29 June 2020.
COVID-19 Emergency Response Key Places Protection and Disinfection Technology Team, Chinese Center for Disease Control and Prevention (2020a) Disinfection guideline of public transport during COVID-19 outbreak. Zhonghua Yu Fang Yi Xue Za Zhi 54:344–346. https://doi.org/10.3760/cma.j.cn112150-20200217-00129
COVID-19 Emergency Response Key Places Protection and Disinfection Technology Team, Chinese Center for Disease Control and Prevention (2020b) Technical guideline for disinfection of wastewater and wastes of medical organizations during COVID-19 outbreak. Zhonghua Yu Fang Yi Xue Za Zhi 54:353–356. https://doi.org/10.3760/cma.j.cn112150-20200217-00125
COVID-19 prevention: Enhanced cleaning and disinfection protocols (2020) Environment health and safety University of Washington. www.ehs.washington.edu . Accessed 29 June 2020.
Darnell ME, Subbarao K, Feinstone SM, Taylor DR (2004) Inactivation of the coronavirus that induces severe acute respiratory syndrome, SARS-CoV. J Virol Methods 121:85–91. https://doi.org/10.1016/j.jviromet.2004.06.006
Derraik JGB, Anderson WA, Connelly EA, Anderson Y(2020) Rapid evidence summary on SARS-CoV-2 survivorship and disinfection, and a reusable PPE protocol using a double-hit process. medRxiv. 2020. https://doi.org/10.1101/2020.04.02.20051409 .
Dhama K, Sharun K, Tiwari R, Dadar M, Malik YS, Singh KP, Chaicumpa W (2020a) COVID-19, an emerging coronavirus infection: advances and prospects in designing and developing vaccines, immunotherapeutics, and therapeutics. Hum Vaccin Immunother 16(6):1232–1238. https://doi.org/10.1080/21645515.2020.1735227
Dhama K, Khan S, Tiwari R, Sircar S, Bhat S, Malik YS, Singh KP, Chaicumpa W, Bonilla-Aldana DK, Rodriguez-Morales AJ (2020b) Coronavirus disease 2019-COVID-19. Clin Microbiol Rev 33(4):e00028–e00020. https://doi.org/10.1128/CMR.00028-20
Dhama K, Patel SK, Yatoo MI, Tiwari R, Sharun K, Dhama J, Natesan S, Malik YS, Singh KP, Harapan H (2021) SARS-CoV-2 existence in sewage and wastewater: A global public health concern? J Environ Manage 280:111825
Duarte PM, de Santana VTP (2020) Disinfection measures and control of SARS-COV-2 transmission. Global Biosecurity 1(3). https://doi.org/10.31646/gbio.64
ECDC (2020) European Centre for Disease Prevention and Control. Disinfection of environments in healthcare and nonhealthcare settings potentially contaminated with SARS-CoV-2. ECDC: Stockholm. https://www.ecdc.europa.eu/sites/default/files/documents/Environmental-persistence-of-SARS_CoV_2-virus-Optionsfor-cleaning2020-03-26_0.pdf . Accessed 9 Feb 2020.
Eggers M, Koburger-Janssen T, Ward LS, Newby C, Muller S (2018) Bactericidal and virucidal activity of povidone-iodine and chlorhexidine gluconate cleansers in an in vivo hand hygiene clinical simulation study. Infect Dis Ther 7(2):235–247. https://doi.org/10.1007/s40121-018-0202-5
Eldeirawi K, Huntington-Moskos L, Nyenhuis SM, Polivka B (2020) Increased disinfectant use among adults with asthma in the era of COVID-19. J Allergy Clin Immunol Pract. S2213-2198(20)31402-1. https://doi.org/10.1016/j.jaip.2020.12.038 .
El-Nahhal I, El-Nahhal Y (2020) Ecological consequences of COVID-19 outbreak. J Water Sci Eng 1:1–5. https://doi.org/10.13140/RG.2.2.24456.85769
Emmanuel E, Keck G, Blanchard JM, Vermande P, Perrodin Y (2004) Toxicological effects of disinfections using sodium hypochlorite on aquatic organisms and its contribution to AOX formation in hospital wastewater. Environ Int 30:891–900. https://doi.org/10.1016/j.envint.2004.02.004
Enwemeka CS, Bumah VV, Masson-Meyers DS (2020) Light as a potential treatment for pandemic coronavirus infections: a perspective. J Photochem Photobiol B 207:111891. https://doi.org/10.1016/j.jphotobiol.2020.111891
EPC (2020) European Parliament and Council. Regulation (EU) No 528/2012 of the European Parliament and of the Council of 22 May 2012 concerning the making available on the market and use of biocidal products 2012. https://eur-lex.europa.eu/legal-content/EN/TXT/?uri=CELEX%3A32012R0528 . Accessed 22 Mar 2020.
Eslami H, Jalili M (2020) The role of environmental factors to transmission of SARS-CoV-2 (COVID-19). AMB Express 10(1):92. https://doi.org/10.1186/s13568-020-01028-0
European Lung Foundation (2017) Nurses regular use of disinfectants is associated with developing COPD. ScienceDaily. https://www.sciencedaily.com/releases/2017/09/170910232514.htm . Accessed 9 May 2020.
Ewart SL (2001). Disinfectants and control of environmental contamination. In: Smith BL. editor. Large animal internal medicine: diseases of horses cattle, sheep and goats. 3rd edn. St. Louis: Mosby. pp. 1371-1380.
Fair D (2020) Issues of the environment: chemical impacts In fighting the spread of COVID-19. https://www.wemu.org/post/issues-environment-chemical-impacts-fighting-spread-covid-19 . Accessed 24 June 2020.
Falagas ME, Thomaidis PC, Kotsantis IK, Sgouros K, Samonis G, Karageorgopoulos DE (2011) Airborne hydrogen peroxide for disinfection of the hospital environment and infection control: a systematic review. J Hosp Infect 78(3):171–177. https://doi.org/10.1016/j.jhin.2010.12.006
FDA (2020a) Enforcement policy for sterilizers, disinfectant devices, and air purifiers during the coronavirus disease 2019 (COVID-19) public health emergency guidance for industry and Food and Drug Administration staff. https://www.fda.gov/media/136533/download . Accessed 17 May 2020.
FDA (2020b) UV lights and lamps: ultraviolet-C radiation, disinfection, and coronavirus. https://www.fda.gov/medical-devices/coronavirus-covid-19-and-medical-devices/uv-lights-and-lamps-ultraviolet-c-radiation-disinfection-and-coronavirus . Accessed 24 Jan 2021.
Foliente RL, Kovacs BJ, Aprecio RM, Bains HJ, Kettering JD, Chen YKJG (2001) Efficacy of high-level disinfectants for reprocessing GI endoscopes in simulated-use testing. Gastrointest Endosc 53(4):456–462. https://doi.org/10.1067/mge.2001.113380
Gates B (2020) Responding to Covid-19 - a once-in-a-century pandemic? N Engl J Med 382:1677–1679. https://doi.org/10.1056/NEJMp2003762
Gerba CP (2015) Quaternary ammonium biocides: efficacy in application. Appl Environ Microbiol 81(2):464–469. https://doi.org/10.1128/AEM.02633-14
Ghafoor D, Khan Z, Khan A, Ualiyeva D, Zaman N (2021) Excessive use of disinfectants against COVID-19 posing a potential threat to living beings. Curr Res Toxicol 2:159–168. https://doi.org/10.1016/j.crtox.2021.02.008
Giesecke J (2020) The invisible pandemic. Lancet. 395:e98. https://doi.org/10.1016/S0140-6736(20)31035-7
Goyal S. M. Chander Y. Yezli S. Otter J. A (2014) Evaluating the virucidal efficacy of hydrogen peroxide vapour. J Hosp Infect 86 (4), 255–259. https://doi.org/10.1016/j.jhin.2014.02.003
Guertler A, Moellhoff N, Schenck TL, Hagen CS, Kendziora B, Giunta RE, French LE, Reinholz M (2020) Onset of occupational hand eczema among healthcare workers during the SARS-CoV-2 pandemic - comparing a single surgical site with a COVID-19 intensive care unit. Contact Dermatitis 83:108–114. https://doi.org/10.1111/cod.13618
Gul S, Savsar A, Tayfa Z (2009) Cytotoxic and genotoxic effects of sodium hypochlorite on human peripheral lymphocytes in vitro. Cytotechnology. 59:113–119. https://doi.org/10.1007/s10616-009-9201-4
Guo L, Yao Z, Yang L, Zhang H, Qi Y, Gou L, Xi W, Liu D, Zhang L, Cheng Y, Wang X, Rong M, Chen H, Kong MG (2020) Plasma-activated water: an alternative disinfectant for S protein inactivation to prevent SARS-CoV-2 infection. Chem Eng J 20:127742. https://doi.org/10.1016/j.cej.2020.127742
Hasan J, Xu Y, Yarlagadda T, Schuetz M, Spann K, Yarlagadda PK (2020a) Antiviral and antibacterial nanostructured surfaces with excellent mechanical properties for hospital applications. ACS Biomater Sci Eng 6(6):3608–3618. https://doi.org/10.1021/acsbiomaterials.0c00348
Hasan J, Pyke A, Nair N, Yarlagadda T, Will G, Spann K, Yarlagadda PKDV (2020b) Antiviral nanostructured surfaces reduce the viability of SARS-CoV-2. ACS Biomater Sci Eng 6(9):4858–4861. https://doi.org/10.1021/acsbiomaterials.0c01091
Herzog A. B. Pandey A. K. Reyes-Gastelum D. Gerba C. P. Rose J. B. Hashsham S. A (2012) Evaluation of sample recovery efficiency for bacteriophage P22 on fomites. Appl Environ Microbiol 78 (22), 7915–7922. https://doi.org/10.1128/AEM.01370-12
Huttner BD, Harbarth S (2015) Hydrogen peroxide room disinfection--ready for prime time? Crit Care 19(1):216. https://doi.org/10.1186/s13054-015-0915-8
ICNIRP (2004) The International Commission on Non-Ionizing Radiation Protection guidelines on limits of exposure to ultraviolet radiation of wavelengths between 180 nm and 400. The International Commission on Non-Ionizing Radiation Protection. Health Phys. 2004; 87:171-186. https://www.icnirp.org/cms/upload/publications/ICNIRPUV2004.pdf . Accessed 10 Apr 2020.
Iyiola AO, Asiedu B, Fawole FJ (2020) Possible effects of COVID-19 on sustainability of aquatic ecosystems: an overview. Aquat Res 3:177–187. https://doi.org/10.3153/AR20016
Kampf G, Todt D, Pfaender S, Steinmann E (2020) Persistence of coronaviruses on inanimate surfaces and their inactivation with biocidal agents. J. Hosp Infect 104:246–251. https://doi.org/10.1016/j.jhin.2020.01.022
Kampf G, Todt D, Pfaender S, Steinmann E (2020a) Persistence of coronaviruses on inanimate surfaces and their inactivation with biocidal agents. J Hosp Infect 104:246–251. https://doi.org/10.1016/j.jhin.2020.01.022
Kampf G, Voss A, Scheithauer S (2020b) Inactivation of coronaviruses by heat. J Hosp Infect 105(2):348–349. https://doi.org/10.1016/j.jhin.2020.03.025
Kariwa H, Fujii N, Takashima I (2006) Inactivation of SARS coronavirus by means of povidone-iodine, physical conditions and chemical reagents. Dermatology 212(Suppl. 1):119–123. https://doi.org/10.1159/000089211
Khan H, Kushwah KK, Singh S, Urkude H, Maurya MR, Sadasivuni KK (2021) Smart technologies driven approaches to tackle COVID-19 pandemic: a review. 3. Biotech. 11(2):50. https://doi.org/10.1007/s13205-020-02581-y
Konda A, Prakash A, Moss GA, Schmoldt M, Grant GD, Guha S (2020) Aerosol filtration efficiency of common fabrics used in respiratory cloth masks. ACS Nano 14(5):6339–6347
Kratzel A, Todt D, V’kovski P, Steiner S, Gultom M, Thao TTN, Ebert N, Holwerda M, Steinmann J, Niemeyer D, Dijkman R, Kampf G, Drosten C, Steinmann E, Thiel V, Pfaender S (2020) Inactivation of severe acute respiratory syndrome coronavirus 2 by WHO-recommended hand rub formulations and alcohols. Emerg Infect Dis 26(7):1592–1595. https://doi.org/10.3201/eid2607.200915
Kumar RP, Mohanty S (2020) Are graphene and graphene-derived products capable of preventing COVID-19 infection? Med Hypotheses 144:110031. https://doi.org/10.1016/j.mehy.2020.110031
Kumar GD, Mishra A, Dunn L, Townsend A, Oguadinma IC, Bright KR, Gerba CP (2020) Biocides and novel antimicrobial agents for the mitigation of coronaviruses. Front Microbiol 11:1351. https://doi.org/10.3389/fmicb.2020.01351
Kumari S, Chatterjee K (2021 Feb 9) Biomaterials-based formulations and surfaces to combat viral infectious diseases. APL Bioeng 5(1):011503. https://doi.org/10.1063/5.0029486
Lai A, Bergna A, Acciarri C, Galli G, Zehender G (2020) Early phylogenetic estimate of the effective reproduction number of SARS-CoV-2. J Med Virol 92:675–679. https://doi.org/10.1002/jmv.25723
Lee YN, Chen LK, Ma HC, Yang HH, Li HP, Lo SY (2005) Thermal aggregation of SARS-CoV membrane protein. J Virol Methods 129(2):152–161. https://doi.org/10.1016/j.jviromet.2005.05.022
León Molina J, Abad-Corpa E (2021) Disinfectants and antiseptics facing coronavirus: synthesis of evidence and recommendations. Enferm Clin 31(Suppl 1):S84–S88. https://doi.org/10.1016/j.enfcli.2020.05.013
Leung KCP, Ko TCS (2020) Improper use of germicidal range ultraviolet lamp for household disinfection leading to phototoxicity in COVID-19 suspects. Cornea. 40:121–122. https://doi.org/10.1097/ICO.0000000000002397
Liao L, Xiao W, Zhao M, Yu X, Wang H, Wang Q, Chu S, Cui Y (2020) Can N95 respirators be reused after disinfection? How many times? ACS Nano 14:6348–6356. https://doi.org/10.1021/acsnano.0c03597
Lim DJ (2020) Intoxication by hand sanitizer due to delirium after infectious spondylitis surgery during the COVID-19 pandemic: a case report and literature review. Int J Surg Case Rep 77:76–79. https://doi.org/10.1016/j.ijscr.2020.10.086
Lin Q, Lim JYC, Xue K, Yew PYM, Owh C, Chee PL, Loh XJ (2020 May) Sanitizing agents for virus inactivation and disinfection. View. 24:e16. https://doi.org/10.1002/viw2.16
Lingayya H, Aayushi HZ, Alisha M, Jacqueline A, Ryna SS and Sriraksha BK (2020) Biocides for textiles against SARS-CoV 2. J Textile Sci Eng 10 10.37421/jtese.2020.10.424.
Liu J, Zhang X (2014) Comparative toxicity of new halophenolic DBPs in chlorinated saline wastewater effluents against a marine alga: halophenolic DBPs are generally more toxic than haloaliphatic ones. Water Res 65:64–72. https://doi.org/10.1016/j.watres.2014.07.024
Ma QX, Shan H, Zhang CM, Zhang HL, Li GM, Yang RM, Chen JM (2020) Decontamination of face masks with steam for mask reuse in fighting the pandemic COVID-19: experimental supports. J Med Virol 92:1971–1974. https://doi.org/10.1002/jmv.25921
Mahmood A, Eqan M, Pervez S, Alghamdi HA, Tabinda AB, Yasar A, Brindhadevi K, Pugazhendhi A (2020) COVID-19 and frequent use of hand sanitizers; human health and environmental hazards by exposure pathways. Sci Total Environ 742:140561. https://doi.org/10.1016/j.scitotenv.2020.140561
Malik YS, Allwood PB, Hedberg CW, Goyal SM (2006a) Disinfection of fabrics and carpets artificially contaminated with calicivirus: relevance in institutional and healthcare centres. J Hosp Infect 63:205–210. https://doi.org/10.1016/j.jhin.2006.01.013
Malik YS, Maherchandani S, Goyal SM (2006b) Comparative efficacy of ethanol and isopropanol against feline calicivirus, a norovirus surrogate. Am J Infect Control 34:31–35. https://doi.org/10.1016/j.ajic.2005.05.012
Malik YS, Sircar S, Bhat S, Sharun K, Dhama K, Dadar M, Tiwari R, Chaicumpa W (2020) Emerging novel coronavirus (2019-nCoV)-current scenario, evolutionary perspective based on genome analysis and recent developments. Vet Q 40(1):68–76. https://doi.org/10.1080/01652176.2020.1727993
Mantlo E, Rhodes T, Boutros J, Patterson-Fortin L, Evans A, Paessler S (2020) In vitro efficacy of a copper iodine complex PPE disinfectant for SARS-CoV-2 inactivation. F1000Res 9:674. https://doi.org/10.12688/f1000research.24651.2
Maris P (1995) Modes of action of disinfectants. Rev sci tech Off int Epiz 14(1):47–55. https://doi.org/10.20506/rst.14.1.829
Martin GC, Le Roux G, Guindolet D, Boulanger E, Hasle D, Morin E, Vodovar D, Vignal Gabison E, Descatha A; French PCC Research Group (2021) pediatric eye injuries by hydroalcoholic gel in the context of the coronavirus disease 2019 pandemic. JAMA Ophthalmol. e206346. 10.1001/jamaophthalmol.2020.6346.
McDonnell G, Russell AD (1999) Antiseptics and disinfectants: activity, action, and resistance. Clin Microbiol Rev 12(1):147–179. https://doi.org/10.1128/cmr.12.1.147
Moon J.M., Chun B.J., Min Y.I (2006) Hemorrhagic gastritis and gas emboli after ingesting 3% hydrogen peroxide. Int J Emerg Med 30(4):403–406. . https://doi.org/10.1016/j.jemermed.2005.05.036
Mukherjee S, Boral S, Siddiqi H, Mishra A, Meikap BC (2021) Present cum future of SARS-CoV-2 virus and its associated control of virus-laden air pollutants leading to potential environmental threat - a global review. J Environ Chem Eng 9(2):104973. https://doi.org/10.1016/j.jece.2020.104973
Nabi G, Wang Y, Hao Y, Khan S, Wu Y, Li D (2020) Massive use of disinfectants against COVID-19 poses potential risks to urban wildlife. Environ Res 188:109916. https://doi.org/10.1016/j.envres.2020.109916
National Pesticide Information Center (2020) Using disinfectants to control the COVID-19 virus. National Pesticide Information Center, Oregon State University Extension Services. http://npic.orst.edu/ingred/ptype/amicrob/covid19.html . Accessed 10 Apr 2020.
NCDC (2020) Guidelines for disinfection of quarantine facility (for COVID-19). https://ncdc.gov.in/WriteReadData/l892s/89168637271584172711.pdf . Accessed 13 June 2020.
Nikaeen G, Abbaszadeh S, Yousefinejad S (2020) Application of nanomaterials in treatment, anti-infection and detection of coronaviruses Nanomedicine (Lond). 15:1501–1512. https://doi.org/10.2217/nnm-2020-0117 (In press)
Noorimotlagh Z, Mirzaee SA, Jaafarzadeh N, Maleki M, Kalvandi G, Karami C (2020) A systematic review of emerging human coronavirus (SARS-CoV-2) outbreak: focus on disinfection methods, environmental survival, and control and prevention strategies. Environ Sci Pollut Res Int 28:1–15. https://doi.org/10.1007/s11356-020-11060-z
Núñez-Delgado A (2020) What do we know about the SARS-CoV-2 coronavirus in the environment? Sci Total Environ 727:138647. https://doi.org/10.1016/j.scitotenv.2020.138647
Opatz T, Senn-Bilfinger J, Richert C (2020) Thoughts on what chemists can contribute to fighting SARS-CoV-2 - a short note on hand sanitizers, drug candidates and outreach. Angew Chem Int Ed Eng 59:9236–9240. https://doi.org/10.1002/anie.202004721
Palmieri V, Papi M (2020) Can graphene take part in the fight against COVID-19? Nano Today 33:100883. https://doi.org/10.1016/j.nantod.2020.100883
Patnayak DP, Prasad AM, Malik YS, Ramakrishnan MA, Goyal SM (2008) Efficacy of disinfectants and hand sanitizers against avian respiratory viruses. Avian Dis 52:199–202. https://doi.org/10.1637/8097-082807-Reg.1
Peck B, Workeneh B, Kadikoy H, Patel SJ, Abdellatif A (2011) Spectrum of sodium hypochlorite toxicity in man-also a concern for nephrologists. NDT Plus 4:231–235. https://doi.org/10.1093/ndtplus/sfr053
Prochazka Zá Rate RA, Cabrera Cabrejos MC, Piscoya A, Vera Calderón AF (2020) Recomendaciones de la Sociedad de Gastroenterología del Perú para evitar la propagación del SARS-CoV-2 a través de procedimientos de endoscopía digestiva [Recommendations of the Society of Gastroenterology of Peru to avoid the spread of SARS-CoV-2 through digestive endoscopy procedures]. Rev Gastroenterol Peru 40:95–99
Google Scholar
Randazzo W, Truchado P, Cuevas-Ferrando E, Simón P, Allende A, Sánchez G (2020) SARS-CoV-2 RNA in wastewater anticipated COVID-19 occurrence in a low prevalence area. Water Res 181:115942. https://doi.org/10.1016/j.watres.2020.115942
Ratnesar-Shumate S, Williams G, Green B, Krause M, Holland B, Wood S, Bohannon J, Boydston J, Freeburger D, Hooper I, Beck K, Yeager J, Altamura LA, Biryukov J, Yolitz J, Schuit M, Wahl V, Hevey M, Dabisch P (2020) Simulated sunlight rapidly inactivates SARS-CoV-2 on surfaces. J Infect Dis 222 (2): 214-222. https://doi.org/10.1093/infdis/jiaa274 .
Rosenman KD, Reilly MJ, Wang L (2021) Calls to a state poison center concerning cleaners and disinfectants from the onset of the COVID-19 pandemic through April 2020. Public Health Rep 136(1):27–31. https://doi.org/10.1177/0033354920962437
Rowan NJ, Laffey JG (2020) Challenges and solutions for addressing critical shortage of supply chain for personal and protective equipment (PPE) arising from Coronavirus disease (COVID19) pandemic - case study from the Republic of Ireland. Sci Total Environ 725:138532. https://doi.org/10.1016/j.scitotenv.2020.138532
Ruiz-Hitzky E, Darder M, Wicklein B, Ruiz-Garcia C, Martin-Sampedro R, del Real G, Aranda P (2020) Nanotechnology responses to COVID-19. Advanced Healthcare Materials 9(19):2000979. https://doi.org/10.1002/adhm.202000979
Russell AD (1983) Principles of antimicrobial activity. In: Block SS (ed) Disinfection, sterilization and preservation, 3rd edn. Lea & Febiger, Philadelphia, pp 717–745
Rutala WA, Weber DJ (2019) Best practices for disinfection of noncritical environmental surfaces and equipment in health care facilities: a bundle approach. Am J Infect Control 47:A96–A105. https://doi.org/10.1016/j.ajic.2019.01.014
Sankar SA, Bhat KS, Anand J (2016) Sterilization and disinfection In: Essentials of medical microbiology. Jaypee Brothers, Medical Publishers Pvt. Limited, India. https://doi.org/10.5005/jp/books/12637_4 .
Sarada BV, Vijay R, Johnson R, Rao TN, Padmanabham G (2020) Fight against COVID-19: ARCI’s technologies for disinfection. Trans Indian Natl Acad Eng 5:349–354. https://doi.org/10.1007/s41403-020-00153-3
Schrank CL, Minbiole KPC, Wuest WM (2020) Are quaternary ammonium compounds, the workhorse disinfectants, effective against severe acute respiratory syndrome-coronavirus-2? ACS Infect Dis 6(7):1553–1557. https://doi.org/10.1021/acsinfecdis.0c00265
Sedlak DL (2011) von Gunten U (2011) Chemistry. The chlorine dilemma. Science. 331:42–43. https://doi.org/10.1126/science.1196397
Seyer A, Sanlidag T (2020) Solar ultraviolet radiation sensitivity of SARS-CoV-2. Lancet Microbe 1(1):e8–e9. https://doi.org/10.1016/S2666-5247(20)30013-6
Seymour N, Yavelak M, Christian C, Chapman B, Danyluk M (2020) COVID-19 FAQ for food service: cleaning and disinfection. EDIS. https://journals.flvc.org/edis/article/view/121172 . Accessed 10 April 2020.
Sharma SK, Mishra M, Mudgal SK (2020) Efficacy of cloth face mask in prevention of novel coronavirus infection transmission: a systematic review and meta-analysis. J Educ Health Promot 9:192. https://doi.org/10.4103/jehp.jehp_533_20
Shetty R, Jayadev C, Chabra A, Maheshwari S, D’Souza S, Khamar P, Sethu S, Honavar SG (2020) Sanitizer aerosol-driven ocular surface disease (SADOSD)-A COVID-19 repercussion? Indian J Ophthalmol 68(6):981–983. https://doi.org/10.4103/ijo.IJO_1308_20
Subpiramaniyam S (2021) Outdoor disinfectant sprays for the prevention of COVID-19: are they safe for the environment? Sci Total Environ 759:144289. https://doi.org/10.1016/j.scitotenv.2020.144289
Suman R, Javaid M, Haleem A, Vaishya R, Bahl S, Nandan D (2020) Sustainability of coronavirus on different surfaces. J Clin Exp Hepatol 10:386–390. https://doi.org/10.1016/j.jceh.2020.04.020
Talebian S, Wallace GG, Schroeder A, Stellacci F, Conde J (2020) Nanotechnology-based disinfectants and sensors for SARS-CoV-2. Nat Nanotechnol 15:618–621. https://doi.org/10.1038/s41565-020-0751-0
Tyagi PK, Tyagi S, Kumar A, Ahuja A, Gola D (2021) Contribution of nanotechnology in the fight against COVID-19. Biointerface Research In Applied Chemistry 2021 11(1):8233–8241. https://doi.org/10.33263/BRIAC111.82338241
US EPA O (2020) List N: disinfectants for use against SARSCoV-2. 2020. https://www.epa.gov/pesticide-registration/list-n-disinfectants-useagainst-sars-cov-2 . Accessed 7 Apr 2020.
Valdez-Salas B, Beltran-Partida E, Cheng N, Salvador-Carlos J, Valdez-Salas EA, Curiel-Alvarez M, Ibarra-Wiley R (2021) Promotion of surgical masks antimicrobial activity by disinfection and impregnation with disinfectant silver nanoparticles. Int J Nanomedicine 16:2689–2702. https://doi.org/10.2147/IJN.S301212
van Doremalen N, Bushmaker T, Morris DH, Holbrook MG, Gamble A, Williamson BN, Tamin A, Harcourt JL, Thornburg NJ, Gerber SI, Lloyd-Smith JO, de Wit E, Munster VJ (2020) Aerosol and surface stability of SARS-CoV-2 as compared with SARS-CoV-1. N Engl J Med 382(16):1564–1567. https://doi.org/10.1056/NEJMc2004973
Wan YL, Schoepf UJ, Wu CC, Giovagnoli DP, Wu MT, Hsu HH, Chang YC, Yang CT, Cherng WJ (2020) Preparedness and best practice in radiology department for COVID-19 and other future pandemics of severe acute respiratory infection. J Thorac Imaging 35(4):239–245. https://doi.org/10.1097/RTI.0000000000000529
Wang Y, Wu X, Wang Y, Li B, Zhou H, Yuan G, Fu Y, Luo Y (2004) Low stability of nucleocapsid protein in SARS virus. Biochemistry. 43(34):11103–11108. https://doi.org/10.1021/bi049194b
WHO (2020). Cleaning and disinfection of environmental surfaces in the context of COVID-19: interim guidance. https://www.who.int/publications/i/item/cleaning-and-disinfection-of-environmental-surfaces-inthe-context-of-covid-19 . Accessed 9 Feb 2021.
WHO (2021). DRAFT landscape of COVID-19 candidate vaccines https://www.who.int/publications/m/item/draft-landscape-of-covid-19-candidate-vaccines . Accessed 4 Mar 2021.
Wickramatillake A, Kurukularatne C (2020) SARS-CoV-2 human disinfection chambers: a critical analysis. Occup Med (Lond) 70(5):330–334. https://doi.org/10.1093/occmed/kqaa078
Willems LM, Samp PF, Pfeilschifter W (2020) Inhalation and ingestion of disinfectant as a mimic of COVID-19 infection. Dtsch Arztebl Int 117(29-30):499. https://doi.org/10.3238/arztebl.2020.0499
Yang Y, Wang H, Chen K, Zhou J, Deng S, Wang Y (2020) Shelter hospital mode: how to prevent novel coronavirus infection 2019 (COVID-19) hospital-acquired infection?. Infect Control Hosp Epidemiol. 1-4. https://doi.org/10.1017/ice.2020.97
Yao Y, Pan J, Liu Z, Meng X, Wang W, Kan H, Wang W (2020) No association of COVID-19 transmission with temperature or UV radiation in Chinese cities. Eur Respir J 55(5):2000517. https://doi.org/10.1183/13993003.00517-2020
Yari S, Moshammer H, Asadi AF, Mosavi Jarrahi A (2020) Side effects of using disinfectants to fight covid-19. Asian Pacific Journal of Environment and Cancer 3(1):9013. https://doi.org/10.31557/apjec.2020.3.1.9-13
You T (2020) More than 100 wild animals drop dead near coronavirus epicentre in china after workers ‘sprayed too much disinfectant’ to prevent coronavirus. https://www.dailymail.co.uk/news/article-8029271/100-wild-animals-drop-dead-near-coronavirus-epicentre.html . Accessed 29 June 2020.
Zaman F, Pervez A, Abreo K (2002) Isopropyl alcohol intoxication: a diagnostic challenge. Am J Kidney Dis 40(3):1–4. https://doi.org/10.1053/ajkd.2002.34938
Zhang YZ, Holmes EC (2020) A genomic perspective on the origin and emergence of SARS-CoV-2. Cell. 181:223–227. https://doi.org/10.1016/j.cell.2020.03.035
Zhang H, Tang W, Chen Y, Yin W (2020) Disinfection threatens aquatic ecosystems. Science. 368:146–147. https://doi.org/10.1126/science.abb8905
Zhao D, Zhang C, Chen JD (2020) Infection control in the medical imaging department during the cOVID-19 pandemic. J Med Imaging Radiat Sci 51(2):204–206. https://doi.org/10.1016/j.jmir.2020.03.005
Download references
Acknowledgements
All the authors acknowledge and thank their respective institutes and universities.
Author information
Authors and affiliations.
Division of Pathology, ICAR-Indian Veterinary Research Institute, Izatnagar, Bareilly, Uttar Pradesh, 243122, India
Kuldeep Dhama & Shailesh Kumar Patel
Department of Veterinary Pathology, Dr. G.C Negi College of Veterinary and Animal Sciences, CSK Himachal Pradesh Agricultural University, Palampur, Himachal Pradesh, 176062, India
Rakesh Kumar & Rupali Masand
Department of Veterinary Anatomy, Faculty of Veterinary and Animal Sciences, Rajeev Gandhi South Campus, Banaras Hindu University, Barkachha, Mirzapur, Uttar Pradesh, 231001, India
Jigyasa Rana
Division of Veterinary Clinical Complex, Faculty of Veterinary Sciences and Animal Husbandry, Shuhama, Alusteng Srinagar, Sher-E-Kashmir University of Agricultural Sciences and Technology of Kashmir, Shalimar, Srinagar, Jammu and Kashmir, 190006, India
Mohd. Iqbal Yatoo
Department of Veterinary Microbiology and Immunology, College of Veterinary Sciences, Uttar Pradesh Pandit Deen Dayal Upadhyaya Pashu Chikitsa Vigyan Vishwavidyalaya Evam Go Anusandhan Sansthan (DUVASU), Mathura, 281001, India
Ruchi Tiwari
Division of Surgery, ICAR-Indian Veterinary Research Institute, Izatnagar, Bareilly, Uttar Pradesh, 243122, India
Khan Sharun
Department of Chemistry, Government College of Engineering, Keonjhar, Odisha, 758002, India
Ranjan K. Mohapatra
Indian Institute of Public Health Gandhinagar, Lekawada, Gandhinagar, Gujarat, 382042, India
Senthilkumar Natesan
Department of Microbiology, Punjab Agricultural University, Ludhiana, 141004, India
Manish Dhawan
The Trafford Group of Colleges, Manchester, WA14 5PQ, UK
Department of Epidemiology and Health Statistics, School of Public Health, Southeast University, Nanjing, 210009, China
Tauseef Ahmad
Key Laboratory of Environmental Medicine Engineering, Ministry of Education, School of Public Health, Southeast University, Nanjing, 210009, China
Department of Pharmacy, BGC Trust University Bangladesh, Chittagong, 4381, Bangladesh
Talha Bin Emran
Division of Biological Standardization, ICAR-Indian Veterinary Research Institute, Izatnagar, Bareilly, Uttar Pradesh, 243122, India
Yashpal Singh Malik
Medical Research Unit, School of Medicine, Universitas Syiah Kuala, Banda Aceh, 23111, Indonesia
Harapan Harapan
Tropical Disease Centre, School of Medicine, Universitas Syiah Kuala, Banda Aceh, 23111, Indonesia
Department of Microbiology, School of Medicine, Universitas Syiah Kuala, Banda Aceh, 23111, Indonesia
You can also search for this author in PubMed Google Scholar
Contributions
KD and HH contributed to the review conception and design. SKP, RK, RM, JR, MIY, RT, KS, RKM, SN, MD, TA, TBE, and YSM performed the literature search and data analysis. KD, SKP, RK, RM, JR, MIY, RT, KS, RKM, SN, MD, TA, TBE, YSM, and HH drafted and/or critically revised the manuscript. All authors have read and approved the final version of the manuscript for publication.
Corresponding authors
Correspondence to Kuldeep Dhama or Harapan Harapan .
Ethics declarations
Ethics approval and consent to participate.
Not applicable
Consent for publication
Competing interests.
The authors declare no competing interests.
Additional information
Responsible Editor: Lotfi Aleya
Publisher’s note
Springer Nature remains neutral with regard to jurisdictional claims in published maps and institutional affiliations.
Rights and permissions
Reprints and permissions
About this article
Dhama, K., Patel, S.K., Kumar, R. et al. The role of disinfectants and sanitizers during COVID-19 pandemic: advantages and deleterious effects on humans and the environment. Environ Sci Pollut Res 28 , 34211–34228 (2021). https://doi.org/10.1007/s11356-021-14429-w
Download citation
Received : 10 March 2021
Accepted : 11 May 2021
Published : 15 May 2021
Issue Date : July 2021
DOI : https://doi.org/10.1007/s11356-021-14429-w
Share this article
Anyone you share the following link with will be able to read this content:
Sorry, a shareable link is not currently available for this article.
Provided by the Springer Nature SharedIt content-sharing initiative
- Disinfectants
- Environment
- Find a journal
- Publish with us
- Track your research
Introduction, Methods, Definition of Terms
Guideline for Disinfection and Sterilization in Healthcare Facilities (2008)
- Introduction
- Definition of Terms
In the United States, approximately 46.5 million surgical procedures and even more invasive medical procedures—including approximately 5 million gastrointestinal endoscopies—are performed each year. 2 Each procedure involves contact by a medical device or surgical instrument with a patient’s sterile tissue or mucous membranes. A major risk of all such procedures is the introduction of pathogens that can lead to infection. Failure to properly disinfect or sterilize equipment carries not only risk associated with breach of host barriers but also risk for person-to-person transmission (e.g., hepatitis B virus) and transmission of environmental pathogens (e.g., Pseudomonas aeruginosa ).
Disinfection and sterilization are essential for ensuring that medical and surgical instruments do not transmit infectious pathogens to patients. Because sterilization of all patient-care items is not necessary, health-care policies must identify, primarily on the basis of the items’ intended use, whether cleaning, disinfection, or sterilization is indicated.
Multiple studies in many countries have documented lack of compliance with established guidelines for disinfection and sterilization. 3-6 Failure to comply with scientifically-based guidelines has led to numerous outbreaks. 6-12 This guideline presents a pragmatic approach to the judicious selection and proper use of disinfection and sterilization processes; the approach is based on well-designed studies assessing the efficacy (through laboratory investigations) and effectiveness (through clinical studies) of disinfection and sterilization procedures.
This guideline resulted from a review of all MEDLINE articles in English listed under the MeSH headings of disinfection or sterilization (focusing on health-care equipment and supplies) from January 1980 through August 2006. References listed in these articles also were reviewed. Selected articles published before 1980 were reviewed and, if still relevant, included in the guideline. The three major peer-reviewed journals in infection control— American Journal of Infection Control, Infection Control and Hospital Epidemiology, and Journal of Hospital Infection —were searched for relevant articles published from January 1990 through August 2006. Abstracts presented at the annual meetings of the Society for Healthcare Epidemiology of America and Association for professionals in Infection Control and Epidemiology , Inc. during 1997–2006 also were reviewed; however, abstracts were not used to support the recommendations.
Sterilization describes a process that destroys or eliminates all forms of microbial life and is carried out in health-care facilities by physical or chemical methods. Steam under pressure, dry heat, EtO gas, hydrogen peroxide gas plasma, and liquid chemicals are the principal sterilizing agents used in health-care facilities. Sterilization is intended to convey an absolute meaning; unfortunately, however, some health professionals and the technical and commercial literature refer to “disinfection” as “sterilization” and items as “partially sterile.” When chemicals are used to destroy all forms of microbiologic life, they can be called chemical sterilants. These same germicides used for shorter exposure periods also can be part of the disinfection process (i.e., high-level disinfection).
Disinfection describes a process that eliminates many or all pathogenic microorganisms, except bacterial spores, on inanimate objects (Tables 1 and 2). In health-care settings, objects usually are disinfected by liquid chemicals or wet pasteurization. Each of the various factors that affect the efficacy of disinfection can nullify or limit the efficacy of the process.
Factors that affect the efficacy of both disinfection and sterilization include prior cleaning of the object; organic and inorganic load present; type and level of microbial contamination; concentration of and exposure time to the germicide; physical nature of the object (e.g., crevices, hinges, and lumens); presence of biofilms; temperature and pH of the disinfection process; and in some cases, relative humidity of the sterilization process (e.g., ethylene oxide).
Unlike sterilization, disinfection is not sporicidal. A few disinfectants will kill spores with prolonged exposure times (3–12 hours); these are called chemical sterilants . At similar concentrations but with shorter exposure periods (e.g., 20 minutes for 2% glutaraldehyde), these same disinfectants will kill all microorganisms except large numbers of bacterial spores; they are called high-level disinfectants . Low-level disinfectants can kill most vegetative bacteria, some fungi, and some viruses in a practical period of time (≤10 minutes). Intermediate-level disinfectants might be cidal for mycobacteria, vegetative bacteria, most viruses, and most fungi but do not necessarily kill bacterial spores. Germicides differ markedly, primarily in their antimicrobial spectrum and rapidity of action.
Cleaning is the removal of visible soil (e.g., organic and inorganic material) from objects and surfaces and normally is accomplished manually or mechanically using water with detergents or enzymatic products. Thorough cleaning is essential before high-level disinfection and sterilization because inorganic and organic materials that remain on the surfaces of instruments interfere with the effectiveness of these processes. Decontamination removes pathogenic microorganisms from objects so they are safe to handle, use, or discard.
Terms with the suffix cide or cidal for killing action also are commonly used. For example, a germicide is an agent that can kill microorganisms, particularly pathogenic organisms (“germs”). The term germicide includes both antiseptics and disinfectants. Antiseptics are germicides applied to living tissue and skin; disinfectants are antimicrobials applied only to inanimate objects. In general, antiseptics are used only on the skin and not for surface disinfection, and disinfectants are not used for skin antisepsis because they can injure skin and other tissues. Virucide, fungicide, bactericide, sporicide, and tuberculocide can kill the type of microorganism identified by the prefix. For example, a bactericide is an agent that kills bacteria. 13-18
To receive email updates about this page, enter your email address:
Exit Notification / Disclaimer Policy
- The Centers for Disease Control and Prevention (CDC) cannot attest to the accuracy of a non-federal website.
- Linking to a non-federal website does not constitute an endorsement by CDC or any of its employees of the sponsors or the information and products presented on the website.
- You will be subject to the destination website's privacy policy when you follow the link.
- CDC is not responsible for Section 508 compliance (accessibility) on other federal or private website.
Use of Household Disinfectants Research Paper
Populations which face the most risk, categories of hazardous elements, point 3: hazardous chemical groups in disinfectants.
The majority of household cleaning products such as disinfectants put up the warning “Keep out of Reach of Children” in heavy type on their markers. As buyers, we presume that if children are prevented from swallowing these products, they will be kept from harm. However, the most widespread means of exposure occur by means of the skin and respiratory tract. Children are habitually in contact with the deposits which housecleaning goods issue by crawling on the floor, lying down, and taking a seat on the sparkling floorboards. Children, in particular babies and toddlers, often stick their fingers on their orifice and in their noses, escalating the dangers of exposure. When a child eats his food, it is very likely that the foodstuff is put directly on a chair tray that has been freshly cleaned with a household cleaner or dish detergent. Thus toxic chemicals go into his body through his digestive route. Another issue here is that children’s exposure probability is superior to that of adults’ for the reason that even though the number of hazardous chemicals in any product is constant, the body of a child is smaller in size, which in effect increases the concentration of the toxic chemicals. Further, their immune systems are not completely developed. As a consequence, children, in all probability, face the gravest danger of chemical exposures by means of disinfecting products. In addition, populations with a definite threat are breast cancer sufferers, elderly people, those suffering from ailments such as asthma, people prone to allergies, and those with weak immune systems. (Scarfe, 2006)
Household disinfectants contain ingredients that can cause vulnerability in the central nervous system, reproductive systems, and various other critical human body systems. Consumers seldom have the spare time to be acquainted with or to seek vital knowledge relating to the products which they make use of. Further, the information is usually offered using a highly scientific lingo making extensive use of jargon that causes difficulties and proves to be quite tricky to interpret.
The three critical categories into which the majority of the harmful constituents in household disinfectant products fall are Carcinogens, Endocrine disruptors, and Neurotoxins.
Exposure to Carcinogens led to cancer and/or advances cancer’s intensification. Endocrine disruptors imitate human hormones, baffling the body with forged signals. Coming in contact with endocrine disruptors can bring about several health anxieties counting reproductive, developmental, and conduct tribulations. Endocrine disruptors are associated with abridged fertility, untimely puberty, miscarriage, menstrual troubles, weakened immune systems, anomalous prostate volume, ADHD, non-Hodgkin’s lymphoma, and a few cancers. Exposure to Neurotoxins modifies neurons, upsetting brain activity, which bring about various problems like headaches, challenged intellect, and other diseases linked with the nervous system. (Khan, 2006)
- Pesticides: Logical reasoning tells us that keeping off household microorganisms shields us from harm. Nevertheless, it should be remembered that these are fundamentally pesticides and thus harmful for living beings. They are fat-soluble, which makes it complicated to do away with them once they enter the body.
- Alkylphenol Ethoxylates (APEs): These function as surfactants, which mean that they reduce the surface tension of fluids and facilitate cleaning solutions to smear across the surface being uncontaminated and help in penetrating solids. They are proved a source of endocrine disruptors.
- Formaldehyde: Formaldehyde is generally recognized as a preservative. It is not common knowledge that it is also used as a germicide, bactericide, and fungicide, along with its other uses. Formaldehyde is a common ingredient in household cleaners and disinfectants. It is an established form of carcinogen. (Ozonoff, 2005)
- Organochlorines: Organochlorines are brought about by the blend of hydrogen and carbon. A number of Organochlorines are highly toxic. They are bio-accumulative and are used in the production of detergents and bleaches. These elements are generally referred to as disruptors of endocrine and carcinogens.
- Styrene: Styrene is a natural material extracted from the Styrax plant. Although Styrene is most frequently utilized in the production of numerous plastics, it also finds use in manufacturing floor waxes and other cleaners. Styrene is an identified carcinogen in addition to an endocrine disruptor. It is very harmful to the reproductive system, liver, and central nervous system.
- Phthalates: Phthalates are most often applied in the plastics industry. However, they are also used as haulers for perfumes and air fresheners. These compounds are categorized as inert chemicals, and thus, no product-labeling obligations are present for phthalates. They are established endocrine disruptors and alleged carcinogens. They are closely linked to hormonal aberrations, thyroid-related ailments, birth imperfections, and reproductive difficulties. (Bender, 2007)
- Volatile Organic Compounds (VOCs): These are discharged as gases, which remain suspended in the surrounding air. VOCs comprise an assortment of chemicals, and exposure to a number of VOCs leads to immediate and chronic undesirable health outcomes. They are commonly found in a number of perfumes, air fresheners, and disinfectants. VOCs usually consist of propane, butane, ethanol, phthalates, and/or formaldehyde. These compounds cause a range of human health vulnerabilities, and together they are perceived as reproductive contaminants, neurotoxins, liver contaminants, and carcinogens. (Tomes, 2005)
Bender, Herbert F. & Philipp Eisenbarth; 2007; Hazardous Chemicals: Control and Regulation in the European Market ; Wiley-VCH.
Khan, Faisal I. & Paul R. Amyotte; 2006; How to Make Inherent Safety Practice a Reality ; The Canadian Journal of Chemical Engineering; 81, 1, 2-16; Canadian Society for Chemical Engineering; Faculty of Engineering & Applied Science, Memorial University of Newfoundland, St. John’s, NF A1B 3X5, Canada; Department of Chemical Engineering, Faculty of Engineering, Dalhousie University, Halifax, NS B3J 2X4, Canada.
Ozonoff, David. Mary Ellen Colten, Adrienne Cupples, Timothy Heeren, Arthur Schatzkin, Thomas Mangione, Miriam Dresner, Theodore Colton; 2005; Health problems reported by residents of a neighborhood contaminated by a hazardous waste facility ; American Journal of Industrial Medicine; 11, 5, 581-597; Environmental Health Section, Boston University School of Public Health, Boston; Epidemiology and Biostatistics Section, Boston University School of Public Health, Boston; Center for Survey Research, University of Massachusetts, Boston
Scarfe, A. David. Cheng-Sheng Lee, Patricia J. O’Bryen; 2006; Aquaculture Biosecurity: Prevention, Control, and Eradication ; Blackwell Publishing.
Tomes, Nancy; 2005; The Gospel of Germs: Men, Women, and the Microbe in American Life ; Harvard University Press.
- Chicago (A-D)
- Chicago (N-B)
IvyPanda. (2021, December 2). Use of Household Disinfectants. https://ivypanda.com/essays/use-of-household-disinfectants/
"Use of Household Disinfectants." IvyPanda , 2 Dec. 2021, ivypanda.com/essays/use-of-household-disinfectants/.
IvyPanda . (2021) 'Use of Household Disinfectants'. 2 December.
IvyPanda . 2021. "Use of Household Disinfectants." December 2, 2021. https://ivypanda.com/essays/use-of-household-disinfectants/.
1. IvyPanda . "Use of Household Disinfectants." December 2, 2021. https://ivypanda.com/essays/use-of-household-disinfectants/.
Bibliography
IvyPanda . "Use of Household Disinfectants." December 2, 2021. https://ivypanda.com/essays/use-of-household-disinfectants/.
- Characteristics of Neurodegenerative Disorders
- Formaldehyde Exposure and Its Potential Health Risk
- Water Purification Process
- Medical Records, Informed Consent and HIPAA
- The Relationship Between the High Rate of Urbanization in Africa and AIDS Spread
- California’s Growing Healthcare Crisis: An Existing Reality
- Environmental Health Problems and Health Inequity
- Public Health: Pertussis Cases in Wisconsin
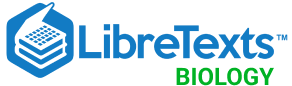
- school Campus Bookshelves
- menu_book Bookshelves
- perm_media Learning Objects
- login Login
- how_to_reg Request Instructor Account
- hub Instructor Commons
- Download Page (PDF)
- Download Full Book (PDF)
- Periodic Table
- Physics Constants
- Scientific Calculator
- Reference & Cite
- Tools expand_more
- Readability
selected template will load here
This action is not available.
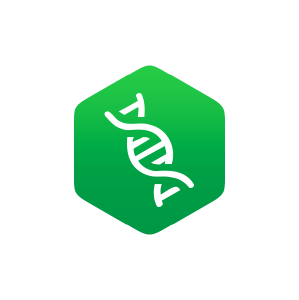
13.3: Using Chemicals to Control Microorganisms
- Last updated
- Save as PDF
- Page ID 5195
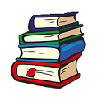
\( \newcommand{\vecs}[1]{\overset { \scriptstyle \rightharpoonup} {\mathbf{#1}} } \)
\( \newcommand{\vecd}[1]{\overset{-\!-\!\rightharpoonup}{\vphantom{a}\smash {#1}}} \)
\( \newcommand{\id}{\mathrm{id}}\) \( \newcommand{\Span}{\mathrm{span}}\)
( \newcommand{\kernel}{\mathrm{null}\,}\) \( \newcommand{\range}{\mathrm{range}\,}\)
\( \newcommand{\RealPart}{\mathrm{Re}}\) \( \newcommand{\ImaginaryPart}{\mathrm{Im}}\)
\( \newcommand{\Argument}{\mathrm{Arg}}\) \( \newcommand{\norm}[1]{\| #1 \|}\)
\( \newcommand{\inner}[2]{\langle #1, #2 \rangle}\)
\( \newcommand{\Span}{\mathrm{span}}\)
\( \newcommand{\id}{\mathrm{id}}\)
\( \newcommand{\kernel}{\mathrm{null}\,}\)
\( \newcommand{\range}{\mathrm{range}\,}\)
\( \newcommand{\RealPart}{\mathrm{Re}}\)
\( \newcommand{\ImaginaryPart}{\mathrm{Im}}\)
\( \newcommand{\Argument}{\mathrm{Arg}}\)
\( \newcommand{\norm}[1]{\| #1 \|}\)
\( \newcommand{\Span}{\mathrm{span}}\) \( \newcommand{\AA}{\unicode[.8,0]{x212B}}\)
\( \newcommand{\vectorA}[1]{\vec{#1}} % arrow\)
\( \newcommand{\vectorAt}[1]{\vec{\text{#1}}} % arrow\)
\( \newcommand{\vectorB}[1]{\overset { \scriptstyle \rightharpoonup} {\mathbf{#1}} } \)
\( \newcommand{\vectorC}[1]{\textbf{#1}} \)
\( \newcommand{\vectorD}[1]{\overrightarrow{#1}} \)
\( \newcommand{\vectorDt}[1]{\overrightarrow{\text{#1}}} \)
\( \newcommand{\vectE}[1]{\overset{-\!-\!\rightharpoonup}{\vphantom{a}\smash{\mathbf {#1}}}} \)
Learning Objectives
- Understand and compare various chemicals used to control microbial growth, including their uses, advantages and disadvantages, chemical structure, and mode of action
In addition to physical methods of microbial control, chemicals are also used to control microbial growth. A wide variety of chemicals can be used as disinfectants or antiseptics. When choosing which to use, it is important to consider the type of microbe targeted; how clean the item needs to be; the disinfectant’s effect on the item’s integrity; its safety to animals, humans, and the environment; its expense; and its ease of use. This section describes the variety of chemicals used as disinfectants and antiseptics, including their mechanisms of action and common uses.
In the 1800s, scientists began experimenting with a variety of chemicals for disinfection. In the 1860s, British surgeon Joseph Lister (1827–1912) began using carbolic acid, known as phenol, as a disinfectant for the treatment of surgical wounds (see Foundations of Modern Cell Theory ). In 1879, Lister’s work inspired the American chemist Joseph Lawrence (1836–1909) to develop Listerine, an alcohol-based mixture of several related compounds that is still used today as an oral antiseptic. Today, carbolic acid is no longer used as a surgical disinfectant because it is a skin irritant, but the chemical compounds found in antiseptic mouthwashes and throat lozenges are called phenolics.
Chemically, phenol consists of a benzene ring with an –OH group, and phenolics are compounds that have this group as part of their chemical structure (Figure \(\PageIndex{1}\)). Phenolics such as thymol and eucalyptol occur naturally in plants. Other phenolics can be derived from creosote, a component of coal tar. Phenolics tend to be stable, persistent on surfaces, and less toxic than phenol. They inhibit microbial growth by denaturing proteins and disrupting membranes.
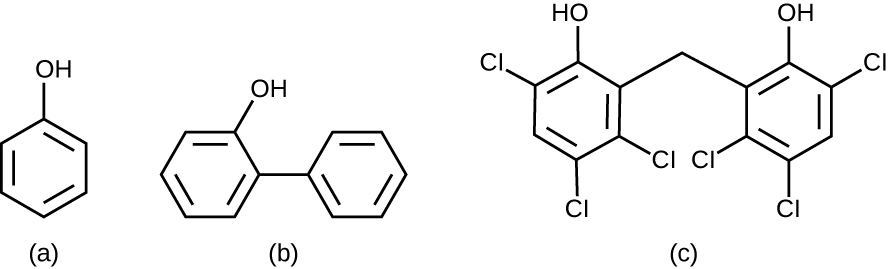
Since Lister’s time, several phenolic compounds have been used to control microbial growth. Phenolics like cresols(methylated phenols) and o-phenylphenol were active ingredients in various formulations of Lysol since its invention in 1889. o-Phenylphenol was also commonly used in agriculture to control bacterial and fungal growth on harvested crops, especially citrus fruits, but its use in the United States is now far more limited. The bisphenol hexachlorophene, a disinfectant, is the active ingredient in pHisoHex, a topical cleansing detergent widely used for handwashing in hospital settings. pHisoHex is particularly effective against gram-positive bacteria, including those causing staphylococcal and streptococcal skin infections. pHisoHex was formerly used for bathing infants, but this practice has been discontinued because it has been shown that exposure to hexachlorophene can lead to neurological problems.
Triclosan is another bisphenol compound that has seen widespread application in antibacterial products over the last several decades. Initially used in toothpastes, triclosan is now commonly used in hand soaps and is frequently impregnated into a wide variety of other products, including cutting boards, knives, shower curtains, clothing, and concrete, to make them antimicrobial. It is particularly effective against gram-positive bacteria on the skin, as well as certain gram-negative bacteria and yeasts. 1
Triclosan: Antibacterial Overkill?
Hand soaps and other cleaning products are often marketed as “antibacterial,” suggesting that they provide a level of cleanliness superior to that of conventional soaps and cleansers. But are the antibacterial ingredients in these products really safe and effective?
About 75% of antibacterial liquid hand soaps and 30% of bar soaps contain the chemical triclosan, a phenolic, (Figure \(\PageIndex{2}\)). 2 Triclosan blocks an enzyme in the bacterial fatty acid-biosynthesis pathway that is not found in the comparable human pathway. Although the use of triclosan in the home increased dramatically during the 1990s, more than 40 years of research by the FDA have turned up no conclusive evidence that washing with triclosan-containing products provides increased health benefits compared with washing with traditional soap. Although some studies indicate that fewer bacteria may remain on a person’s hands after washing with triclosan-based soap, compared with traditional soap, no evidence points to any reduction in the transmission of bacteria that cause respiratory and gastrointestinal illness. In short, soaps with triclosan may remove or kill a few more germs but not enough to reduce the spread of disease.
Perhaps more disturbing, some clear risks associated with triclosan-based soaps have come to light. The widespread use of triclosan has led to an increase in triclosan-resistant bacterial strains, including those of clinical importance, such as Salmonella enterica ; this resistance may render triclosan useless as an antibacterial in the long run. 3 4 Bacteria can easily gain resistance to triclosan through a change to a single gene encoding the targeted enzyme in the bacterial fatty acid-synthesis pathway. Other disinfectants with a less specific mode of action are much less prone to engendering resistance because it would take much more than a single genetic change.
Use of triclosan over the last several decades has also led to a buildup of the chemical in the environment. Triclosan in hand soap is directly introduced into wastewater and sewage systems as a result of the handwashing process. There, its antibacterial properties can inhibit or kill bacteria responsible for the decomposition of sewage, causing septic systems to clog and back up. Eventually, triclosan in wastewater finds its way into surface waters, streams, lakes, sediments, and soils, disrupting natural populations of bacteria that carry out important environmental functions, such as inhibiting algae. Triclosan also finds its way into the bodies of amphibians and fish, where it can act as an endocrine disruptor. Detectable levels of triclosan have also been found in various human bodily fluids, including breast milk, plasma, and urine. 5 In fact, a study conducted by the CDC found detectable levels of triclosan in the urine of 75% of 2,517 people tested in 2003–2004. 6 This finding is even more troubling given the evidence that triclosan may affect immune function in humans. 7
In December 2013, the FDA gave soap manufacturers until 2016 to prove that antibacterial soaps provide a significant benefit over traditional soaps; if unable to do so, manufacturers will be forced to remove these products from the market.
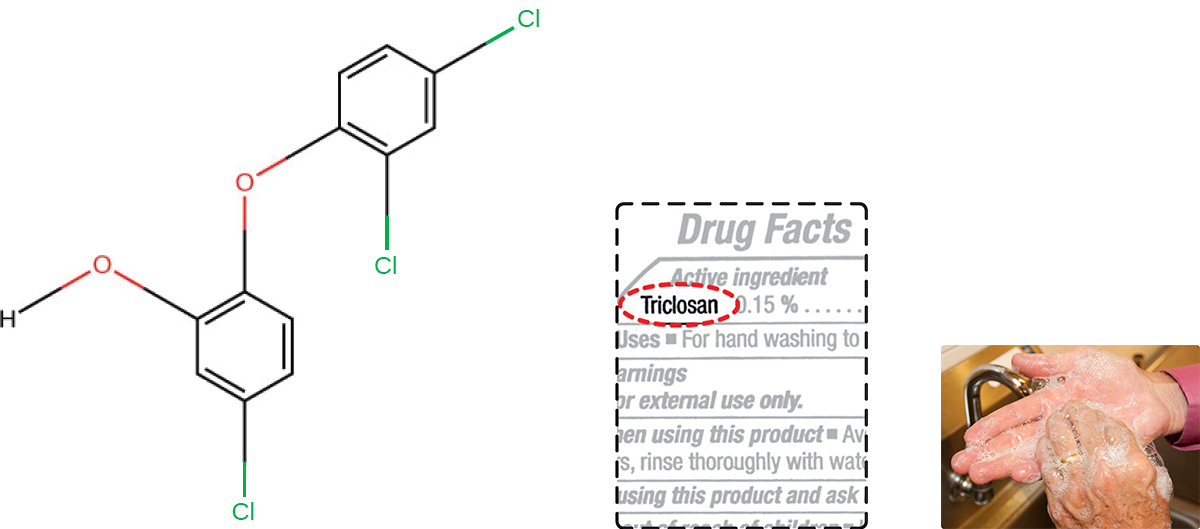
Exercise \(\PageIndex{1}\)
Why is triclosan more like an antibiotic than a traditional disinfectant?
Heavy Metals
Some of the first chemical disinfectants and antiseptics to be used were heavy metals. Heavy metals kill microbes by binding to proteins, thus inhibiting enzymatic activity (Figure \(\PageIndex{3}\)). Heavy metals are oligodynamic, meaning that very small concentrations show significant antimicrobial activity. Ions of heavy metals bind to sulfur-containing amino acids strongly and bioaccumulate within cells, allowing these metals to reach high localized concentrations. This causes proteins to denature.
Heavy metals are not selectively toxic to microbial cells. They may bioaccumulate in human or animal cells, as well, and excessive concentrations can have toxic effects on humans. If too much silver accumulates in the body, for example, it can result in a condition called argyria, in which the skin turns irreversibly blue-gray. One way to reduce the potential toxicity of heavy metals is by carefully controlling the duration of exposure and concentration of the heavy metal.
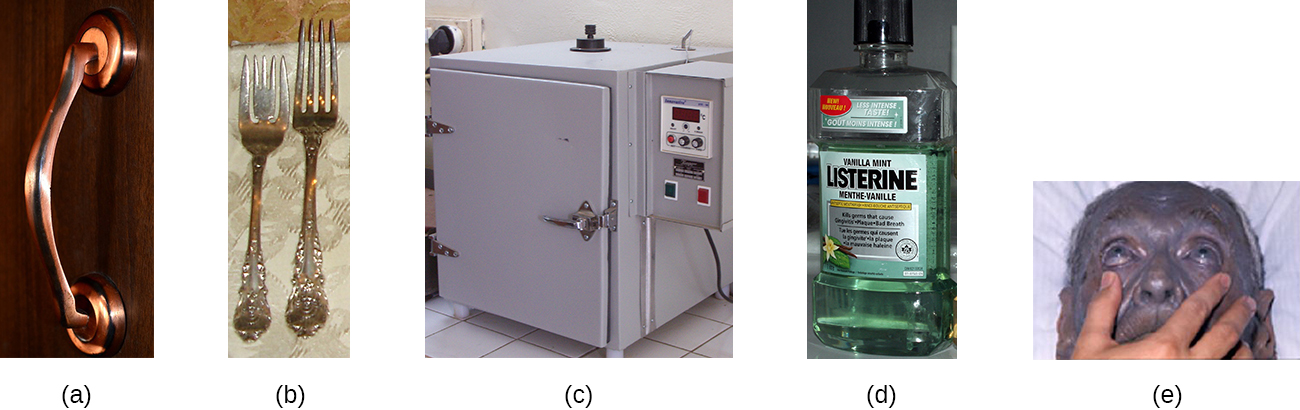
Mercury is an example of a heavy metal that has been used for many years to control microbial growth. It was used for many centuries to treat syphilis. Mercury compounds like mercuric chloride are mainly bacteriostatic and have a very broad spectrum of activity. Various forms of mercury bind to sulfur-containing amino acids within proteins, inhibiting their functions.
In recent decades, the use of such compounds has diminished because of mercury’s toxicity. It is toxic to the central nervous, digestive, and renal systems at high concentrations, and has negative environmental effects, including bioaccumulation in fish. Topical antiseptics such as mercurochrome, which contains mercury in low concentrations, and merthiolate, a tincture (a solution of mercury dissolved in alcohol) were once commonly used. However, because of concerns about using mercury compounds, these antiseptics are no longer sold in the United States.
Silver has long been used as an antiseptic. In ancient times, drinking water was stored in silver jugs. 8 Silvadene cream is commonly used to treat topical wounds and is particularly helpful in preventing infection in burn wounds. Silver nitrate drops were once routinely applied to the eyes of newborns to protect against ophthalmia neonatorum, eye infections that can occur due to exposure to pathogens in the birth canal, but antibiotic creams are more now commonly used. Silver is often combined with antibiotics, making the antibiotics thousands of times more effective. 9 Silver is also commonly incorporated into catheters and bandages, rendering them antimicrobial; however, there is evidence that heavy metals may also enhance selection for antibiotic resistance. 10
Copper, Nickel, and Zinc
Several other heavy metals also exhibit antimicrobial activity. Copper sulfate is a common algicide used to control algal growth in swimming pools and fish tanks. The use of metallic copper to minimize microbial growth is also becoming more widespread. Copper linings in incubators help reduce contamination of cell cultures. The use of copper pots for water storage in underdeveloped countries is being investigated as a way to combat diarrheal diseases. Copper coatings are also becoming popular for frequently handled objects such as doorknobs, cabinet hardware, and other fixtures in health-care facilities in an attempt to reduce the spread of microbes.
Nickel and zinc coatings are now being used in a similar way. Other forms of zinc, including zinc chloride and zinc oxide, are also used commercially. Zinc chloride is quite safe for humans and is commonly found in mouthwashes, substantially increasing their length of effectiveness. Zinc oxide is found in a variety of products, including topical antiseptic creams such as calamine lotion, diaper ointments, baby powder, and dandruff shampoos.
Exercise \(\PageIndex{2}\)
Why are many heavy metals both antimicrobial and toxic to humans?
Other chemicals commonly used for disinfection are the halogens iodine, chlorine, and fluorine. Iodine works by oxidizing cellular components, including sulfur-containing amino acids, nucleotides, and fatty acids, and destabilizing the macromolecules that contain these molecules. It is often used as a topical tincture, but it may cause staining or skin irritation. An iodophor is a compound of iodine complexed with an organic molecule, thereby increasing iodine’s stability and, in turn, its efficacy. One common iodophor is povidone-iodine, which includes a wetting agent that releases iodine relatively slowly. Betadine is a brand of povidone-iodine commonly used as a hand scrub by medical personnel before surgery and for topical antisepsis of a patient’s skin before incision (Figure \(\PageIndex{4}\)).
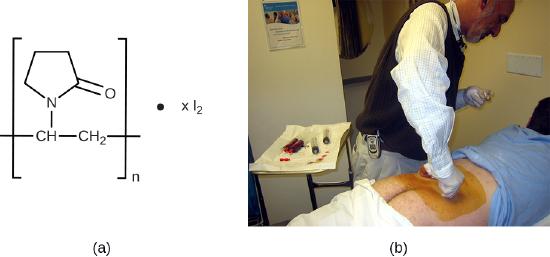
Chlorine is another halogen commonly used for disinfection. When chlorine gas is mixed with water, it produces a strong oxidant called hypochlorous acid, which is uncharged and enters cells easily. Chlorine gas is commonly used in municipal drinking water and wastewater treatment plants, with the resulting hypochlorous acid producing the actual antimicrobial effect. Those working at water treatment facilities need to take great care to minimize personal exposure to chlorine gas. Sodium hypochlorite is the chemical component of common household bleach, and it is also used for a wide variety of disinfecting purposes. Hypochlorite salts, including sodium and calcium hypochlorites, are used to disinfect swimming pools. Chlorine gas, sodium hypochlorite, and calcium hypochlorite are also commonly used disinfectants in the food processing and restaurant industries to reduce the spread of foodborne diseases. Workers in these industries also need to take care to use these products correctly to ensure their own safety as well as the safety of consumers. A recent joint statement published by the Food and Agriculture Organization (FAO) of the United Nations and WHO indicated that none of the many beneficial uses of chlorine products in food processing to reduce the spread of foodborne illness posed risks to consumers. 11
Another class of chlorinated compounds called chloramines are widely used as disinfectants. Chloramines are relatively stable, releasing chlorine over long periods time. Chloramines are derivatives of ammonia by substitution of one, two, or all three hydrogen atoms with chlorine atoms (Figure \(\PageIndex{5}\)).
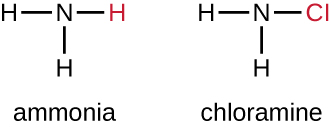
Chloramines and other cholorine compounds may be used for disinfection of drinking water, and chloramine tablets are frequently used by the military for this purpose. After a natural disaster or other event that compromises the public water supply, the CDC recommends disinfecting tap water by adding small amounts of regular household bleach. Recent research suggests that sodium dichloroisocyanurate (NaDCC) may also be a good alternative for drinking water disinfection. Currently, NaDCC tablets are available for general use and for use by the military, campers, or those with emergency needs; for these uses, NaDCC is preferable to chloramine tablets. Chlorine dioxide, a gaseous agent used for fumigation and sterilization of enclosed areas, is also commonly used for the disinfection of water.
Although chlorinated compounds are relatively effective disinfectants, they have their disadvantages. Some may irritate the skin, nose, or eyes of some individuals, and they may not completely eliminate certain hardy organisms from contaminated drinking water. The fungus Cryptosporidium , for example, has a protective outer shell that makes it resistant to chlorinated disinfectants. Thus, boiling of drinking water in emergency situations is recommended when possible.
The halogen fluorine is also known to have antimicrobial properties that contribute to the prevention of dental caries(cavities). 12 Fluoride is the main active ingredient of toothpaste and is also commonly added to tap water to help communities maintain oral health. Chemically, fluoride can become incorporated into the hydroxyapatite of tooth enamel, making it more resistant to corrosive acids produced by the fermentation of oral microbes. Fluoride also enhances the uptake of calcium and phosphate ions in tooth enamel, promoting remineralization. In addition to strengthening enamel, fluoride also seems to be bacteriostatic. It accumulates in plaque-forming bacteria, interfering with their metabolism and reducing their production of the acids that contribute to tooth decay.
Exercise \(\PageIndex{3}\)
What is a benefit of a chloramine over hypochlorite for disinfecting?
Alcohols make up another group of chemicals commonly used as disinfectants and antiseptics. They work by rapidly denaturing proteins, which inhibits cell metabolism, and by disrupting membranes, which leads to cell lysis. Once denatured, the proteins may potentially refold if enough water is present in the solution. Alcohols are typically used at concentrations of about 70% aqueous solution and, in fact, work better in aqueous solutions than 100% alcohol solutions. This is because alcohols coagulate proteins. In higher alcohol concentrations, rapid coagulation of surface proteins prevents effective penetration of cells. The most commonly used alcohols for disinfection are ethyl alcohol(ethanol) and isopropyl alcohol (isopropanol, rubbing alcohol) (Figure \(\PageIndex{6}\)).
Alcohols tend to be bactericidal and fungicidal, but may also be viricidal for enveloped viruses only. Although alcohols are not sporicidal, they do inhibit the processes of sporulation and germination. Alcohols are volatile and dry quickly, but they may also cause skin irritation because they dehydrate the skin at the site of application. One common clinical use of alcohols is swabbing the skin for degerming before needle injection. Alcohols also are the active ingredients in instant hand sanitizers, which have gained popularity in recent years. The alcohol in these hand sanitizers works both by denaturing proteins and by disrupting the microbial cell membrane, but will not work effectively in the presence of visible dirt.
Last, alcohols are used to make tinctures with other antiseptics, such as the iodine tinctures discussed previously in this chapter. All in all, alcohols are inexpensive and quite effective for the disinfection of a broad range of vegetative microbes. However, one disadvantage of alcohols is their high volatility, limiting their effectiveness to immediately after application.
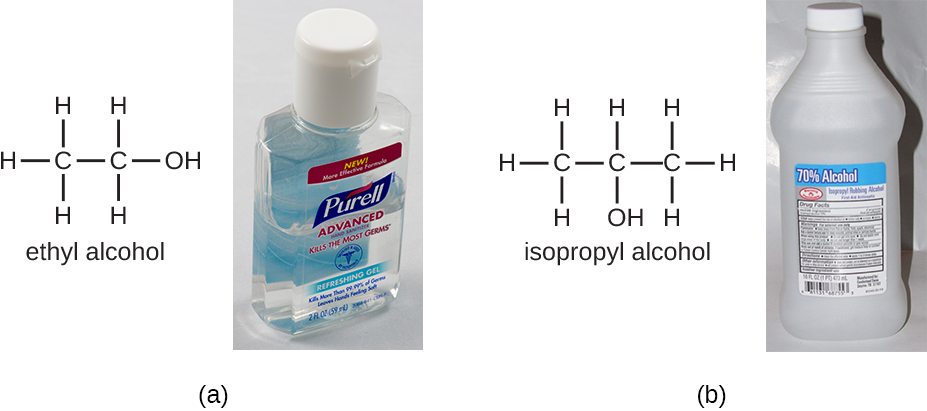
Exercise \(\PageIndex{4}\)
- Name at least three advantages of alcohols as disinfectants.
- Describe several specific applications of alcohols used in disinfectant products.
Surfactants
Surface-active agents, or surfactants, are a group of chemical compounds that lower the surface tension of water. Surfactants are the major ingredients in soaps and detergents. Soaps are salts of long-chain fatty acids and have both polar and nonpolar regions, allowing them to interact with polar and nonpolar regions in other molecules (Figure \(\PageIndex{7}\)). They can interact with nonpolar oils and grease to create emulsions in water, loosening and lifting away dirt and microbes from surfaces and skin. Soaps do not kill or inhibit microbial growth and so are not considered antiseptics or disinfectants. However, proper use of soaps mechanically carries away microorganisms, effectively degerming a surface. Some soaps contain added bacteriostatic agents such as triclocarban or cloflucarban, compounds structurally related to triclosan, that introduce antiseptic or disinfectant properties to the soaps.
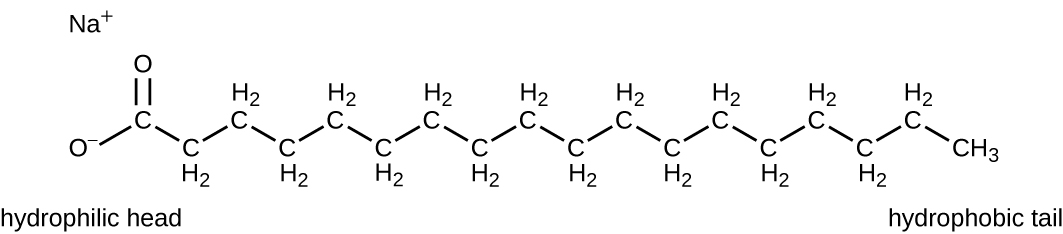
Soaps, however, often form films that are difficult to rinse away, especially in hard water, which contains high concentrations of calcium and magnesium mineral salts. Detergents contain synthetic surfactant molecules with both polar and nonpolar regions that have strong cleansing activity but are more soluble, even in hard water, and, therefore, leave behind no soapy deposits. Anionic detergents, such as those used for laundry, have a negatively charged anion at one end attached to a long hydrophobic chain, whereas cationic detergents have a positively charged cation instead. Cationic detergents include an important class of disinfectants and antiseptics called the quaternary ammonium salts (quats), named for the characteristic quaternary nitrogen atom that confers the positive charge (Figure \(\PageIndex{8}\)). Overall, quats have properties similar to phospholipids, having hydrophilic and hydrophobic ends. As such, quats have the ability to insert into the bacterial phospholipid bilayer and disrupt membrane integrity. The cationic charge of quats appears to confer their antimicrobial properties, which are diminished when neutralized. Quats have several useful properties. They are stable, nontoxic, inexpensive, colorless, odorless, and tasteless. They tend to be bactericidal by disrupting membranes. They are also active against fungi, protozoans, and enveloped viruses, but endospores are unaffected. In clinical settings, they may be used as antiseptics or to disinfect surfaces. Mixtures of quats are also commonly found in household cleaners and disinfectants, including many current formulations of Lysol brand products, which contain benzalkonium chlorides as the active ingredients. Benzalkonium chlorides, along with the quat cetylpyrimidine chloride, are also found in products such as skin antiseptics, oral rinses, and mouthwashes.
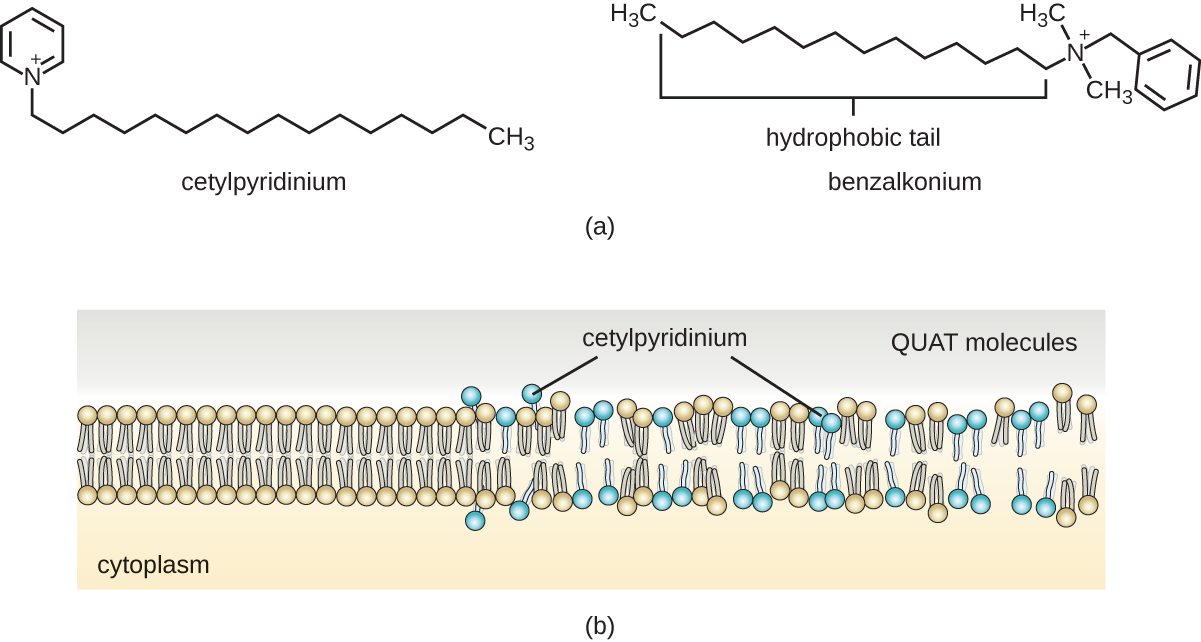
Exercise \(\PageIndex{5}\)
Why are soaps not considered disinfectants?
Handwashing the Right Way
Handwashing is critical for public health and should be emphasized in a clinical setting. For the general public, the CDC recommends handwashing before, during, and after food handling; before eating; before and after interacting with someone who is ill; before and after treating a wound; after using the toilet or changing diapers; after coughing, sneezing, or blowing the nose; after handling garbage; and after interacting with an animal, its feed, or its waste. Figure \(\PageIndex{9}\) illustrates the five steps of proper handwashing recommended by the CDC.
Handwashing is even more important for health-care workers, who should wash their hands thoroughly between every patient contact, after the removal of gloves, after contact with bodily fluids and potentially infectious fomites, and before and after assisting a surgeon with invasive procedures. Even with the use of proper surgical attire, including gloves, scrubbing for surgery is more involved than routine handwashing. The goal of surgical scrubbing is to reduce the normal microbiota on the skin’s surface to prevent the introduction of these microbes into a patient’s surgical wounds.
There is no single widely accepted protocol for surgical scrubbing. Protocols for length of time spent scrubbing may depend on the antimicrobial used; health-care workers should always check the manufacturer’s recommendations. According to the Association of Surgical Technologists (AST), surgical scrubs may be performed with or without the use of brushes (Figure \(\PageIndex{9}\)).
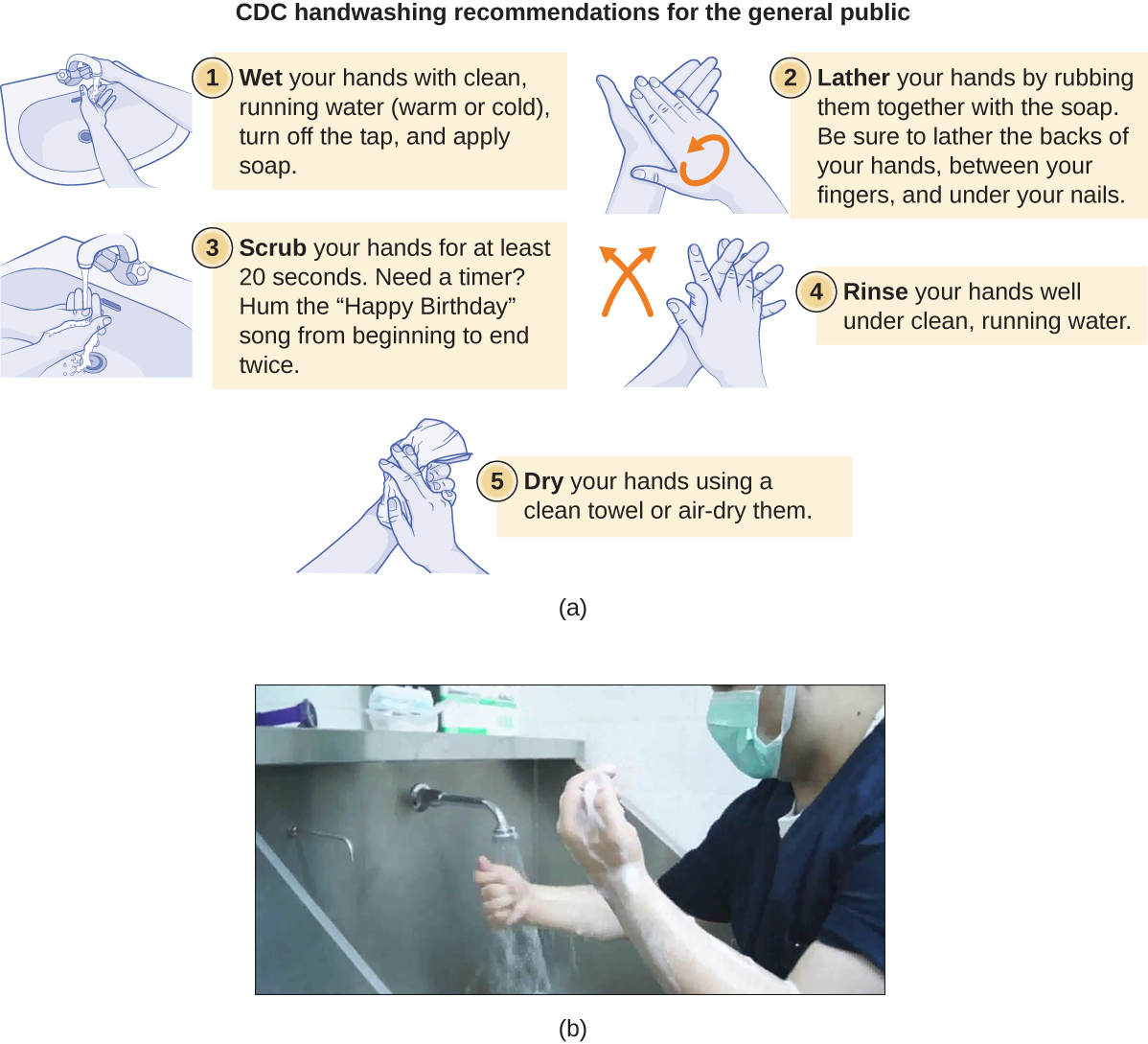
Link to Learning
To learn more about proper handwashing, visit the CDC’s website.
Bisbiguanides
Bisbiguanides were first synthesized in the 20th century and are cationic (positively charged) molecules known for their antiseptic properties (Figure \(\PageIndex{10}\)). One important bisbiguanide antiseptic is chlorhexidine. It has broad-spectrum activity against yeasts, gram-positive bacteria, and gram-negative bacteria, with the exception of Pseudomonas aeruginosa , which may develop resistance on repeated exposure. 13 Chlorhexidine disrupts cell membranes and is bacteriostatic at lower concentrations or bactericidal at higher concentrations, in which it actually causes the cells’ cytoplasmic contents to congeal. It also has activity against enveloped viruses. However, chlorhexidine is poorly effective against Mycobacterium tuberculosis and nonenveloped viruses, and it is not sporicidal. Chlorhexidine is typically used in the clinical setting as a surgical scrub and for other handwashing needs for medical personnel, as well as for topical antisepsis for patients before surgery or needle injection. It is more persistent than iodophors, providing long-lasting antimicrobial activity. Chlorhexidine solutions may also be used as oral rinses after oral procedures or to treat gingivitis. Another bisbiguanide, alexidine, is gaining popularity as a surgical scrub and an oral rinse because it acts faster than chlorhexidine.

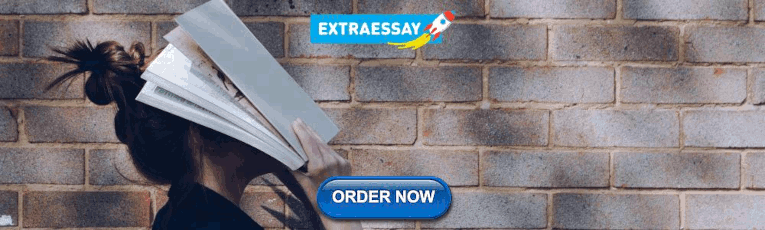
Exercise \(\PageIndex{6}\)
What two effects does chlorhexidine have on bacterial cells?
Alkylating Agents
The alkylating agents are a group of strong disinfecting chemicals that act by replacing a hydrogen atom within a molecule with an alkyl group (C n H 2n+1 ), thereby inactivating enzymes and nucleic acids (Figure \(\PageIndex{11}\)). The alkylating agent formaldehyde (CH 2 OH) is commonly used in solution at a concentration of 37% (known as formalin) or as a gaseous disinfectant and biocide. It is a strong, broad-spectrum disinfectant and biocide that has the ability to kill bacteria, viruses, fungi, and endospores, leading to sterilization at low temperatures, which is sometimes a convenient alternative to the more labor-intensive heat sterilization methods. It also cross-links proteins and has been widely used as a chemical fixative. Because of this, it is used for the storage of tissue specimens and as an embalming fluid. It also has been used to inactivate infectious agents in vaccine preparation. Formaldehyde is very irritating to living tissues and is also carcinogenic; therefore, it is not used as an antiseptic.
Glutaraldehyde is structurally similar to formaldehyde but has two reactive aldehyde groups, allowing it to act more quickly than formaldehyde. It is commonly used as a 2% solution for sterilization and is marketed under the brand name Cidex. It is used to disinfect a variety of surfaces and surgical and medical equipment. However, similar to formaldehyde, glutaraldehyde irritates the skin and is not used as an antiseptic.
A new type of disinfectant gaining popularity for the disinfection of medical equipment is o-phthalaldehyde (OPA), which is found in some newer formulations of Cidex and similar products, replacing glutaraldehyde. o-Phthalaldehyde also has two reactive aldehyde groups, but they are linked by an aromatic bridge. o-Phthalaldehyde is thought to work similarly to glutaraldehyde and formaldehyde, but is much less irritating to skin and nasal passages, produces a minimal odor, does not require processing before use, and is more effective against mycobacteria.
Ethylene oxide is a type of alkylating agent that is used for gaseous sterilization. It is highly penetrating and can sterilize items within plastic bags such as catheters, disposable items in laboratories and clinical settings (like packaged Petri dishes), and other pieces of equipment. Ethylene oxide exposure is a form of cold sterilization, making it useful for the sterilization of heat-sensitive items. Great care needs to be taken with the use of ethylene oxide, however; it is carcinogenic, like the other alkylating agents, and is also highly explosive. With careful use and proper aeration of the products after treatment, ethylene oxide is highly effective, and ethylene oxide sterilizers are commonly found in medical settings for sterilizing packaged materials.
β-Propionolactone is an alkylating agent with a different chemical structure than the others already discussed. Like other alkylating agents, β-propionolactone binds to DNA, thereby inactivating it (Figure \(\PageIndex{11}\)). It is a clear liquid with a strong odor and has the ability to kill endospores. As such, it has been used in either liquid form or as a vapor for the sterilization of medical instruments and tissue grafts, and it is a common component of vaccines, used to maintain their sterility. It has also been used for the sterilization of nutrient broth, as well as blood plasma, milk, and water. It is quickly metabolized by animals and humans to lactic acid. It is also an irritant, however, and may lead to permanent damage of the eyes, kidneys, or liver. Additionally, it has been shown to be carcinogenic in animals; thus, precautions are necessary to minimize human exposure to β-propionolactone. 14
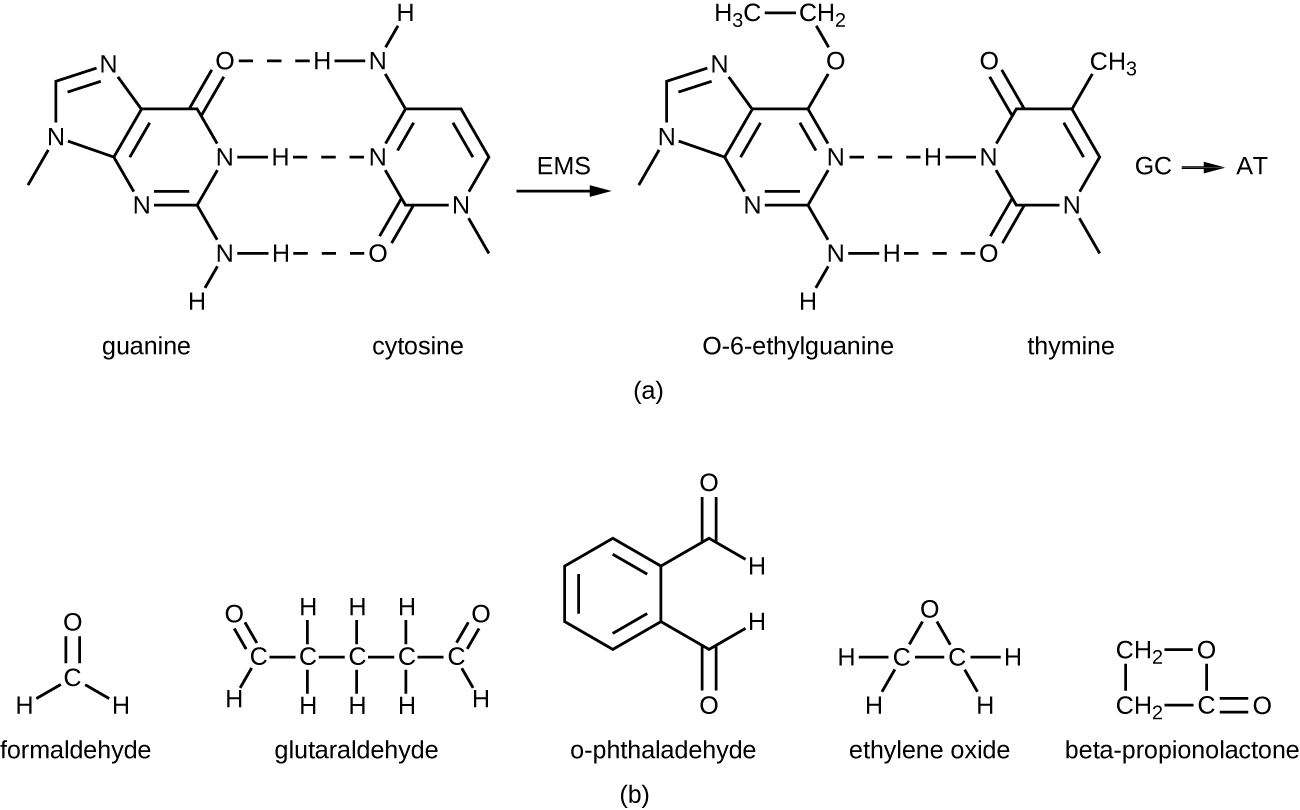
Exercise \(\PageIndex{7}\)
- What chemical reaction do alkylating agents participate in?
- Why are alkylating agents not used as antiseptics?
Diehard Prions
Prions , the acellular, misfolded proteins responsible for incurable and fatal diseases such as kuru and Creutzfeldt-Jakob disease (see Viroids, Virusoids, and Prions ), are notoriously difficult to destroy. Prions are extremely resistant to heat, chemicals, and radiation. They are also extremely infectious and deadly; thus, handling and disposing of prion-infected items requires extensive training and extreme caution.
Typical methods of disinfection can reduce but not eliminate the infectivity of prions. Autoclaving is not completely effective, nor are chemicals such as phenol, alcohols, formalin, and β-propiolactone. Even when fixed in formalin, affected brain and spinal cord tissues remain infectious.
Personnel who handle contaminated specimens or equipment or work with infected patients must wear a protective coat, face protection, and cut-resistant gloves. Any contact with skin must be immediately washed with detergent and warm water without scrubbing. The skin should then be washed with 1 N NaOH or a 1:10 dilution of bleach for 1 minute. Contaminated waste must be incinerated or autoclaved in a strong basic solution, and instruments must be cleaned and soaked in a strong basic solution.
For more information on the handling of animals and prion-contaminated materials, visit the guidelines published on the CDC and WHO websites.
Peroxygens are strong oxidizing agents that can be used as disinfectants or antiseptics. The most widely used peroxygen is hydrogen peroxide (H 2 O 2 ), which is often used in solution to disinfect surfaces and may also be used as a gaseous agent. Hydrogen peroxide solutions are inexpensive skin antiseptics that break down into water and oxygen gas, both of which are environmentally safe. This decomposition is accelerated in the presence of light, so hydrogen peroxide solutions typically are sold in brown or opaque bottles. One disadvantage of using hydrogen peroxide as an antiseptic is that it also causes damage to skin that may delay healing or lead to scarring. Contact lens cleaners often include hydrogen peroxide as a disinfectant.
Hydrogen peroxide works by producing free radicals that damage cellular macromolecules. Hydrogen peroxide has broad-spectrum activity, working against gram-positive and gram-negative bacteria (with slightly greater efficacy against gram-positive bacteria), fungi, viruses, and endospores. However, bacteria that produce the oxygen-detoxifying enzymes catalase or peroxidase may have inherent tolerance to low hydrogen peroxide concentrations (Figure \(\PageIndex{12}\)). To kill endospores, the length of exposure or concentration of solutions of hydrogen peroxide must be increased. Gaseous hydrogen peroxide has greater efficacy and can be used as a sterilant for rooms or equipment.
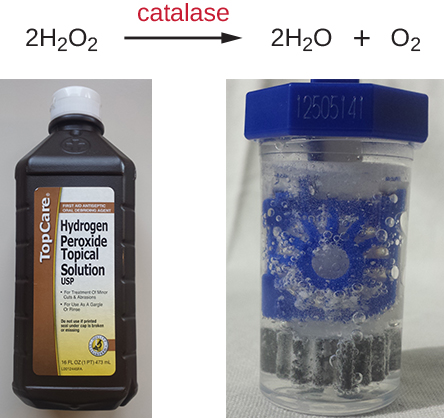
Plasma, a hot, ionized gas, described as the fourth state of matter, is useful for sterilizing equipment because it penetrates surfaces and kills vegetative cells and endospores. Hydrogen peroxide and peracetic acid, another commonly used peroxygen, each may be introduced as a plasma. Peracetic acid can be used as a liquid or plasma sterilant insofar as it readily kills endospores, is more effective than hydrogen peroxide even at rather low concentrations, and is immune to inactivation by catalases and peroxidases. It also breaks down to environmentally innocuous compounds; in this case, acetic acid and oxygen.
Other examples of peroxygens include benzoyl peroxide and carbamide peroxide. Benzoyl peroxide is a peroxygen that used in acne medication solutions. It kills the bacterium Propionibacterium acnes , which is associated with acne. Carbamide peroxide, an ingredient used in toothpaste, is a peroxygen that combats oral biofilms that cause tooth discoloration and halitosis (bad breath). 15 Last, ozone gas is a peroxygen with disinfectant qualities and is used to clean air or water supplies. Overall, peroxygens are highly effective and commonly used, with no associated environmental hazard.
Exercise \(\PageIndex{8}\)
How do peroxides kill cells?
Supercritical Fluids
Within the last 15 years, the use of supercritical fluids, especially supercritical carbon dioxide (scCO 2 ), has gained popularity for certain sterilizing applications. When carbon dioxide is brought to approximately 10 times atmospheric pressure, it reaches a supercritical state that has physical properties between those of liquids and gases. Materials put into a chamber in which carbon dioxide is pressurized in this way can be sterilized because of the ability of scCO 2 to penetrate surfaces.
Supercritical carbon dioxide works by penetrating cells and forming carbonic acid, thereby lowering the cell pH considerably. This technique is effective against vegetative cells and is also used in combination with peracetic acid to kill endospores. Its efficacy can also be augmented with increased temperature or by rapid cycles of pressurization and depressurization, which more likely produce cell lysis.
Benefits of scCO 2 include the nonreactive, nontoxic, and nonflammable properties of carbon dioxide, and this protocol is effective at low temperatures. Unlike other methods, such as heat and irradiation, that can degrade the object being sterilized, the use of scCO 2 preserves the object’s integrity and is commonly used for treating foods (including spices and juices) and medical devices such as endoscopes. It is also gaining popularity for disinfecting tissues such as skin, bones, tendons, and ligaments prior to transplantation. scCO 2 can also be used for pest control because it can kill insect eggs and larvae within products.
Exercise \(\PageIndex{9}\)
Why is the use of supercritical carbon dioxide gaining popularity for commercial and medical uses?
Chemical Food Preservatives
Chemical preservatives are used to inhibit microbial growth and minimize spoilage in some foods. Commonly used chemical preservatives include sorbic acid, benzoic acid, and propionic acid, and their more soluble salts potassium sorbate, sodium benzoate, and calcium propionate, all of which are used to control the growth of molds in acidic foods. Each of these preservatives is nontoxic and readily metabolized by humans. They are also flavorless, so they do not compromise the flavor of the foods they preserve.
Sorbic and benzoic acids exhibit increased efficacy as the pH decreases. Sorbic acid is thought to work by inhibiting various cellular enzymes, including those in the citric acid cycle, as well as catalases and peroxidases. It is added as a preservative in a wide variety of foods, including dairy, bread, fruit, and vegetable products. Benzoic acid is found naturally in many types of fruits and berries, spices, and fermented products. It is thought to work by decreasing intracellular pH, interfering with mechanisms such as oxidative phosphorylation and the uptake of molecules such as amino acids into cells. Foods preserved with benzoic acid or sodium benzoate include fruit juices, jams, ice creams, pastries, soft drinks, chewing gum, and pickles.
Propionic acid is thought to both inhibit enzymes and decrease intracellular pH, working similarly to benzoic acid. However, propionic acid is a more effective preservative at a higher pH than either sorbic acid or benzoic acid. Propionic acid is naturally produced by some cheeses during their ripening and is added to other types of cheese and baked goods to prevent mold contamination. It is also added to raw dough to prevent contamination by the bacterium Bacillus mesentericus , which causes bread to become ropy.
Other commonly used chemical preservatives include sulfur dioxide and nitrites. Sulfur dioxide prevents browning of foods and is used for the preservation of dried fruits; it has been used in winemaking since ancient times. Sulfur dioxide gas dissolves in water readily, forming sulfites. Although sulfites can be metabolized by the body, some people have sulfite allergies, including asthmatic reactions. Additionally, sulfites degrade thiamine, an important nutrient in some foods. The mode of action of sulfites is not entirely clear, but they may interfere with the disulfide bond (see Figure 7.4.5 ) formation in proteins, inhibiting enzymatic activity. Alternatively, they may reduce the intracellular pH of the cell, interfering with proton motive force-driven mechanisms.
Nitrites are added to processed meats to maintain color and stop the germination of Clostridium botulinum endospores. Nitrites are reduced to nitric oxide, which reacts with heme groups and iron-sulfur groups. When nitric oxide reacts with the heme group within the myoglobin of meats, a red product forms, giving meat its red color. Alternatively, it is thought that when nitric acid reacts with the iron-sulfur enzyme ferredoxin within bacteria, this electron transport-chain carrier is destroyed, preventing ATP synthesis. Nitrosamines, however, are carcinogenic and can be produced through exposure of nitrite-preserved meats (e.g., hot dogs, lunch meat, breakfast sausage, bacon, meat in canned soups) to heat during cooking.
Natural Chemical Food Preservatives
The discovery of natural antimicrobial substances produced by other microbes has added to the arsenal of preservatives used in food. Nisin is an antimicrobial peptide produced by the bacterium Lactococcus lactis and is particularly effective against gram-positive organisms. Nisin works by disrupting cell wall production, leaving cells more prone to lysis. It is used to preserve cheeses, meats, and beverages.
Natamycin is an antifungal macrolide antibiotic produced by the bacterium Streptomyces natalensis . It was approved by the FDA in 1982 and is used to prevent fungal growth in various types of dairy products, including cottage cheese, sliced cheese, and shredded cheese. Natamycin is also used for meat preservation in countries outside the United States.
Exercise \(\PageIndex{10}\)
What are the advantages and drawbacks of using sulfites and nitrites as food preservatives?
Key Concepts and Summary
- Heavy metals , including mercury, silver, copper, and zinc, have long been used for disinfection and preservation, although some have toxicity and environmental risks associated with them.
- Halogens , including chlorine, fluorine, and iodine, are also commonly used for disinfection. Chlorine compounds, including sodium hypochlorite , chloramines , and chlorine dioxide , are commonly used for water disinfection. Iodine, in both tincture and iodophor forms, is an effective antiseptic.
- Alcohols , including ethyl alcohol and isopropyl alcohol, are commonly used antiseptics that act by denaturing proteins and disrupting membranes.
- Phenolics are stable, long-acting disinfectants that denature proteins and disrupt membranes. They are commonly found in household cleaners, mouthwashes, and hospital disinfectants, and are also used to preserve harvested crops.
- The phenolic compound triclosan , found in antibacterial soaps, plastics, and textiles is technically an antibiotic because of its specific mode of action of inhibiting bacterial fatty-acid synthesis..
- Surfactants , including soaps and detergents, lower the surface tension of water to create emulsions that mechanically carry away microbes. Soaps are long-chain fatty acids, whereas detergents are synthetic surfactants.
- Quaternary ammonium compounds ( quats ) are cationic detergents that disrupt membranes. They are used in household cleaners, skin disinfectants, oral rinses, and mouthwashes.
- Bisbiguanides disrupt cell membranes, causing cell contents to gel. Chlorhexidine and alexidine are commonly used for surgical scrubs, for handwashing in clinical settings, and in prescription oral rinses.
- Alkylating agents effectively sterilize materials at low temperatures but are carcinogenic and may also irritate tissue. Glutaraldehyde and o-phthalaldehyde are used as hospital disinfectants but not as antiseptics. Formaldehyde is used for the storage of tissue specimens, as an embalming fluid, and in vaccine preparation to inactivate infectious agents. Ethylene oxide is a gas sterilant that can permeate heat-sensitive packaged materials, but it is also explosive and carcinogenic.
- Peroxygens , including hydrogen peroxide , peracetic acid , benzoyl peroxide , and ozone gas, are strong oxidizing agents that produce free radicals in cells, damaging their macromolecules. They are environmentally safe and are highly effective disinfectants and antiseptics.
- Pressurized carbon dioxide in the form of a supercritical fluid easily permeates packaged materials and cells, forming carbonic acid and lowering intracellular pH. Supercritical carbon dioxide is nonreactive, nontoxic, nonflammable, and effective at low temperatures for sterilization of medical devices, implants, and transplanted tissues.
- Chemical preservatives are added to a variety of foods. Sorbic acid , benzoic acid , propionic acid , and their more soluble salts inhibit enzymes or reduce intracellular pH.
- Sulfites are used in winemaking and food processing to prevent browning of foods.
- Nitrites are used to preserve meats and maintain color, but cooking nitrite-preserved meats may produce carcinogenic nitrosamines.
- Nisin and natamycin are naturally produced preservatives used in cheeses and meats. Nisin is effective against gram-positive bacteria and natamycin against fungi.
- 1 US Food and Drug Administration. “Triclosan: What Consumers Should Know.” 2015. www.fda.gov/ForConsumers/Cons.../ucm205999.htm. Accessed June 9, 2016.
- 2 J. Stromberg. “Five Reasons Why You Should Probably Stop Using Antibacterial Soap.” Smithsonian.com January 3, 2014. www.smithsonianmag.com/scienc...948078/?no-ist. Accessed June 9, 2016.
- 3 SP Yazdankhah et al. “Triclosan and Antimicrobial Resistance in Bacteria: An Overview.” Microbial Drug Resistance 12 no. 2 (2006):83–90.
- 4 L. Birošová, M. Mikulášová. “Development of Triclosan and Antibiotic Resistance in Salmonella enterica serovar Typhimurium.” Journal of Medical Microbiology 58 no. 4 (2009):436–441.
- 5 AB Dann, A. Hontela. “Triclosan: Environmental Exposure, Toxicity and Mechanisms of Action.” Journal of Applied Toxicology 31 no. 4 (2011):285–311.
- 6 US Centers for Disease Control and Prevention. “Triclosan Fact Sheet.” 2013. www.cdc.gov/biomonitoring/Tri...FactSheet.html. Accessed June 9, 2016.
- 7 EM Clayton et al. “The Impact of Bisphenol A and Triclosan on Immune Parameters in the US Population, NHANES 2003-2006.” Environmental Health Perspectives 119 no. 3 (2011):390.
- 8 N. Silvestry-Rodriguez et al. “Silver as a Disinfectant.” In Reviews of Environmental Contamination and Toxicology , pp. 23-45. Edited by GW Ware and DM Whitacre. New York: Springer, 2007.
- 9 B. Owens. “Silver Makes Antibiotics Thousands of Times More Effective.” Nature June 19 2013. http://www.nature.com/news/silver-ma...ective-1.13232
- 10 C. Seiler, TU Berendonk. “Heavy Metal Driven Co-Selection of Antibiotic Resistance in Soil and Water Bodies Impacted by Agriculture and Aquaculture.” Frontiers in Microbiology 3 (2012):399.
- 11 World Health Organization. “Benefits and Risks of the Use of Chlorine-Containing Disinfectants in Food Production and Food Processing: Report of a Joint FAO/WHO Expert Meeting.” Geneva, Switzerland: World Health Organization, 2009.
- 12 RE Marquis. “Antimicrobial Actions of Fluoride for Oral Bacteria.” Canadian Journal of Microbiology 41 no. 11 (1995):955–964.
- 13 L. Thomas et al. “Development of Resistance to Chlorhexidine Diacetate in Pseudomonas aeruginosa and the Effect of a ‘Residual’ Concentration.” Journal of Hospital Infection 46 no. 4 (2000):297–303.
- 14 Institute of Medicine. “Long-Term Health Effects of Participation in Project SHAD (Shipboard Hazard and Defense).” Washington, DC: The National Academies Press, 2007.
- 15 Yao, C.S. et al. “In vitro antibacterial effect of carbamide peroxide on oral biofilm.” Journal of Oral Microbiology Jun 12, 2013. http://www.ncbi.nlm.nih.gov/pmc/articles/PMC3682087/ . doi: 10.3402/jom.v5i0.20392.
13.4 Testing the Effectiveness of Antiseptics and Disinfectants
Learning objectives.
By the end of this section, you will be able to:
- Describe why the phenol coefficient is used
- Compare and contrast the disk-diffusion, use-dilution, and in-use methods for testing the effectiveness of antiseptics, disinfectants, and sterilants
The effectiveness of various chemical disinfectants is reflected in the terms used to describe them. Chemical disinfectants are grouped by the power of their activity, with each category reflecting the types of microbes and viruses its component disinfectants are effective against. High-level germicide s have the ability to kill vegetative cells, fungi, viruses, and endospores, leading to sterilization, with extended use. Intermediate-level germicides, as their name suggests, are less effective against endospores and certain viruses, and low-level germicides kill only vegetative cells and certain enveloped viruses, and are ineffective against endospores.
However, several environmental conditions influence the potency of an antimicrobial agent and its effectiveness. For example, length of exposure is particularly important, with longer exposure increasing efficacy. Similarly, the concentration of the chemical agent is also important, with higher concentrations being more effective than lower ones. Temperature, pH, and other factors can also affect the potency of a disinfecting agent.
One method to determine the effectiveness of a chemical agent includes swabbing surfaces before and after use to confirm whether a sterile field was maintained during use. Additional tests are described in the sections that follow. These tests allow for the maintenance of appropriate disinfection protocols in clinical settings, controlling microbial growth to protect patients, health-care workers, and the community.
Phenol Coefficient
The effectiveness of a disinfectant or antiseptic can be determined in a number of ways. Historically, a chemical agent’s effectiveness was often compared with that of phenol, the first chemical agent used by Joseph Lister . In 1903, British chemists Samuel Rideal (1863–1929) and J. T. Ainslie Walker (1868–1930) established a protocol to compare the effectiveness of a variety of chemicals with that of phenol, using as their test organisms Staphylococcus aureus (a gram-positive bacterium) and Salmonella enterica serovar Typhi (a gram-negative bacterium). They exposed the test bacteria to the antimicrobial chemical solutions diluted in water for 7.5 minutes. They then calculated a phenol coefficient for each chemical for each of the two bacteria tested. A phenol coefficient of 1.0 means that the chemical agent has about the same level of effectiveness as phenol. A chemical agent with a phenol coefficient of less than 1.0 is less effective than phenol. An example is formalin , with phenol coefficients of 0.3 ( S. aureus ) and 0.7 ( S. enterica serovar Typhi). A chemical agent with a phenol coefficient greater than 1.0 is more effective than phenol, such as chloramine, with phenol coefficients of 133 and 100, respectively. Although the phenol coefficient was once a useful measure of effectiveness, it is no longer commonly used because the conditions and organisms used were arbitrarily chosen.
Check Your Understanding
- What are the differences between the three levels of disinfectant effectiveness?
Disk-Diffusion Method
The disk-diffusion method involves applying different chemicals to separate, sterile filter paper disks ( Figure 13.31 ). The disks are then placed on an agar plate that has been inoculated with the targeted bacterium and the chemicals diffuse out of the disks into the agar where the bacteria have been inoculated. As the “lawn” of bacteria grows, zones of inhibition of microbial growth are observed as clear areas around the disks. Although there are other factors that contribute to the sizes of zones of inhibition (e.g., whether the agent is water soluble and able to diffuse in the agar), larger zones typically correlate to increased inhibition effectiveness of the chemical agent. The diameter across each zone is measured in millimeters.
- When comparing the activities of two disinfectants against the same microbe, using the disk-diffusion assay, and assuming both are water soluble and can easily diffuse in the agar, would a more effective disinfectant have a larger zone of inhibition or a smaller one?
Use-Dilution Test
Other methods are also used for measuring the effectiveness of a chemical agent in clinical settings. The use-dilution test is commonly used to determine a chemical’s disinfection effectiveness on an inanimate surface. For this test, a cylinder of stainless steel is dipped in a culture of the targeted microorganism and then dried. The cylinder is then dipped in solutions of disinfectant at various concentrations for a specified amount of time. Finally, the cylinder is transferred to a new test tube containing fresh sterile medium that does not contain disinfectant, and this test tube is incubated. Bacterial survival is demonstrated by the presence of turbidity in the medium, whereas killing of the target organism on the cylinder by the disinfectant will produce no turbidity.
The Association of Official Agricultural Chemists International (AOAC), a nonprofit group that establishes many protocol standards, has determined that a minimum of 59 of 60 replicates must show no growth in such a test to achieve a passing result, and the results must be repeatable from different batches of disinfectant and when performed on different days. Disinfectant manufacturers perform use-dilution tests to validate the efficacy claims for their products, as designated by the EPA.
- Is the use-dilution test performed in a clinical setting? Why?
In-Use Test
An in-use test can determine whether an actively used solution of disinfectant in a clinical setting is microbially contaminated ( Figure 13.32 ). A 1-mL sample of the used disinfectant is diluted into 9 mL of sterile broth medium that also contains a compound to inactivate the disinfectant. Ten drops, totaling approximately 0.2 mL of this mixture, are then inoculated onto each of two agar plates. One plate is incubated at 37 °C for 3 days and the other is incubated at room temperature for 7 days. The plates are monitored for growth of microbial colonies. Growth of five or more colonies on either plate suggests that viable microbial cells existed in the disinfectant solution and that it is contaminated. Such in-use tests monitor the effectiveness of disinfectants in the clinical setting.
- What does a positive in-use test indicate?
New Methods to Overcome Specific Challenges
Some pathogens have characteristics that make disinfectant efficacy difficult to prove. Human pappiloma virus (HPV), for example, cannot be cultured, so the previously described methods do not work. And since HPV's protein-only capsid enables it to survive on surfaces for an extended period of time, medical professionals rely on disinfectants to ensure the virus doesn't spread through facilities or equipment.
New Mexico State University's Michelle Ozbun , one of the foremost experts on HPV, developed a method to quantify (count) live virus on surfaces outside the body. The typical method for counting viruses like HPV is to grind up infected cells and measure the amount of viral DNA, but this method is imprecise and does not demonstrate the scope of the infection. To test the disinfectant's live virus was required. Instead of grinding up infected cells, Ozbun applied a stain that only attaches to viral RNA.
Using advanced microscopy, the quantities of infected cells can be determined with great accuracy. For the HVP disinfectant testing, Ozbun and her team applied various disinfectants to three HPV from three sources, and determined that the chemicals reduced the amount of virus by 10,000 times. This technique can be used for disinfectant testing for other protein-capsid viruses (like norovirus and poliovirus) and to produce accurate viral counting for other research or clinical applications.
Clinical Focus
Despite antibiotic treatment, Roberta’s symptoms worsened. She developed pyelonephritis , a severe kidney infection, and was rehospitalized in the intensive care unit (ICU). Her condition continued to deteriorate, and she developed symptoms of septic shock . At this point, her physician ordered a culture from her urine to determine the exact cause of her infection, as well as a drug sensitivity test to determine what antibiotics would be effective against the causative bacterium. The results of this test indicated resistance to a wide range of antibiotics, including the carbapenems, a class of antibiotics that are used as the last resort for many types of bacterial infections. This was an alarming outcome, suggesting that Roberta’s infection was caused by a so-called superbug : a bacterial strain that has developed resistance to the majority of commonly used antibiotics. In this case, the causative agent belonged to the carbapenem-resistant Enterobacteriaceae (CRE) , a drug-resistant family of bacteria normally found in the digestive system ( Figure 13.33 ). When CRE is introduced to other body systems, as might occur through improperly cleaned surgical instruments, catheters, or endoscopes, aggressive infections can occur.
CRE infections are notoriously difficult to treat, with a 40%–50% fatality rate. To treat her kidney infection and septic shock, Roberta was treated with dialysis, intravenous fluids, and medications to maintain blood pressure and prevent blood clotting. She was also started on aggressive treatment with intravenous administration of a new drug called tigecycline , which has been successful in treating infections caused by drug-resistant bacteria.
After several weeks in the ICU, Roberta recovered from her CRE infection. However, public health officials soon noticed that Roberta’s case was not isolated. Several patients who underwent similar procedures at the same hospital also developed CRE infections, some dying as a result. Ultimately, the source of the infection was traced to the duodenoscopes used in the procedures. Despite the hospital staff meticulously following manufacturer protocols for disinfection, bacteria, including CRE, remained within the instruments and were introduced to patients during procedures.
Go back to the previous Clinical Focus box.
Eye on Ethics
Who is responsible.
Carbapenem-resistant Enterobacteriaceae infections due to contaminated endoscopes have become a high-profile problem in recent years. Several CRE outbreaks have been traced to endoscopes, including a case at Ronald Reagan UCLA Medical Center in early 2015 in which 179 patients may have been exposed to a contaminated endoscope. Seven of the patients developed infections, and two later died. Several lawsuits have been filed against Olympus, the manufacturer of the endoscopes. Some claim that Olympus did not obtain FDA approval for design changes that may have led to contamination, and others claim that the manufacturer knowingly withheld information from hospitals concerning defects in the endoscopes.
Lawsuits like these raise difficult-to-answer questions about liability. Invasive procedures are inherently risky, but negative outcomes can be minimized by strict adherence to established protocols. Who is responsible, however, when negative outcomes occur due to flawed protocols or faulty equipment? Can hospitals or health-care workers be held liable if they have strictly followed a flawed procedure? Should manufacturers be held liable—and perhaps be driven out of business—if their lifesaving equipment fails or is found defective? What is the government’s role in ensuring that use and maintenance of medical equipment and protocols are fail-safe?
Protocols for cleaning or sterilizing medical equipment are often developed by government agencies like the FDA, and other groups, like the AOAC, a nonprofit scientific organization that establishes many protocols for standard use globally. These procedures and protocols are then adopted by medical device and equipment manufacturers. Ultimately, the end-users (hospitals and their staff) are responsible for following these procedures and can be held liable if a breach occurs and patients become ill from improperly cleaned equipment.
Unfortunately, protocols are not infallible, and sometimes it takes negative outcomes to reveal their flaws. In 2008, the FDA had approved a disinfection protocol for endoscopes, using glutaraldehyde (at a lower concentration when mixed with phenol), o-phthalaldehyde, hydrogen peroxide, peracetic acid, and a mix of hydrogen peroxide with peracetic acid. However, subsequent CRE outbreaks from endoscope use showed that this protocol alone was inadequate.
As a result of CRE outbreaks, hospitals, manufacturers, and the FDA are investigating solutions. Many hospitals are instituting more rigorous cleaning procedures than those mandated by the FDA. Manufacturers are looking for ways to redesign duodenoscopes to minimize hard-to-reach crevices where bacteria can escape disinfectants, and the FDA is updating its protocols. In February 2015, the FDA added new recommendations for careful hand cleaning of the duodenoscope elevator mechanism (the location where microbes are most likely to escape disinfection), and issued more careful documentation about quality control of disinfection protocols ( Figure 13.34 ).
There is no guarantee that new procedures, protocols, or equipment will completely eliminate the risk for infection associated with endoscopes. Yet these devices are used successfully in 500,000–650,000 procedures annually in the United States, many of them lifesaving. At what point do the risks outweigh the benefits of these devices, and who should be held responsible when negative outcomes occur?
As an Amazon Associate we earn from qualifying purchases.
This book may not be used in the training of large language models or otherwise be ingested into large language models or generative AI offerings without OpenStax's permission.
Want to cite, share, or modify this book? This book uses the Creative Commons Attribution License and you must attribute OpenStax.
Access for free at https://openstax.org/books/microbiology/pages/1-introduction
- Authors: Nina Parker, Mark Schneegurt, Anh-Hue Thi Tu, Philip Lister, Brian M. Forster
- Publisher/website: OpenStax
- Book title: Microbiology
- Publication date: Nov 1, 2016
- Location: Houston, Texas
- Book URL: https://openstax.org/books/microbiology/pages/1-introduction
- Section URL: https://openstax.org/books/microbiology/pages/13-4-testing-the-effectiveness-of-antiseptics-and-disinfectants
© Jan 10, 2024 OpenStax. Textbook content produced by OpenStax is licensed under a Creative Commons Attribution License . The OpenStax name, OpenStax logo, OpenStax book covers, OpenStax CNX name, and OpenStax CNX logo are not subject to the Creative Commons license and may not be reproduced without the prior and express written consent of Rice University.
An official website of the United States government
Here’s how you know
Official websites use .gov A .gov website belongs to an official government organization in the United States.
Secure .gov websites use HTTPS A lock ( Lock Locked padlock icon ) or https:// means you’ve safely connected to the .gov website. Share sensitive information only on official, secure websites.

- Health Topics
- Drugs & Supplements
- Medical Tests
- Medical Encyclopedia
- About MedlinePlus
- Customer Support
Cleaning, Disinfecting, and Sanitizing
Related issues, see, play and learn, videos and tutorials.
- No links available
Find an Expert
Patient handouts, where are germs found.
Germs are a part of everyday life. Some of them are helpful, but others are harmful and cause disease. They can be found everywhere - in our air, soil, and water. They are on our skin and in our bodies. Germs are also on the surfaces and objects that we touch.
Sometimes those germs can spread to you and make you sick. For example, there could be germs on a tv remote. You could get infected with the germs if you touch the remote and then rub your eyes or nose or eat with your hands.
How can I avoid getting germs from surfaces and objects?
To avoid becoming infected by germs from surfaces and objects, it is important to wash your hands often . But you can't wash your hands every time you touch something. So it's also important to regularly clean and disinfect surfaces and objects.
What is the difference between cleaning, sanitizing, and disinfecting?
Some people think that disinfecting is same thing as cleaning or sanitizing. But they are actually different:
- Cleaning removes dirt, dust, crumbs, and germs from surfaces or objects. When you clean, you will likely use soap (or detergent) and water to physically clean off the surfaces and objects. This may not necessarily kill the germs. But since you removed some of them, there are fewer germs that could spread infection to you.
- Disinfecting uses chemicals (disinfectants) to kill germs on surfaces and objects. Some common disinfectants are bleach and alcohol solutions. You usually need to leave the disinfectant on the surfaces and objects for a certain period of time to kill the germs. Disinfecting does not necessarily clean dirty surfaces or remove germs.
- Sanitizing could be done by either cleaning, disinfecting, or both. Sanitizing means that you are lowering the number of germs to a safe level. What is considered a safe level depends on public health standards or requirements at a workplace, school, etc. For example, there are sanitizing procedures for restaurants and other facilities that prepare food. What you do to sanitize will vary, depending on your needs. You might be mopping a floor using a mop, a chemical, and water. You might use a dishwasher to sanitize the dishes. Or you could be using an antibacterial wipe on a tv remote.
If you both clean and disinfect a surface or object, you can further lower the risk of spreading infection. There are products that clean and disinfect at the same time.
Which surfaces and objects do I need to clean and disinfect?
To prevent the spread of infection, you should regularly clean and disinfect surfaces and objects that are touched often. For example, in your house, this would include countertops, doorknobs, faucet and toilet handles, light switches, remotes, and toys.
How can I safely clean and disinfect?
It's important to be safe when using cleaning and disinfecting products:
- Store them in the containers they came in. Always follow the instructions and pay attention to the warnings on the label.
- Do not mix cleaners and disinfectants unless the labels say that it is safe to do so. Combining certain products (such as chlorine bleach and ammonia cleaners) can cause serious injury or even death.
- Check the label to see whether you need to use gloves to protect your hands and/or eye protection when using the products
- If you swallow, inhale, or get them on your skin , follow the directions on the label or get medical help
- Store them out of the reach of children
- Cleaners, Sanitizers, and Disinfectants (American Academy of Pediatrics)
- What's the Difference Between Products that Disinfect, Sanitize, and Clean Surfaces? (Environmental Protection Agency)
- When and How to Clean and Disinfect Your Home (Centers for Disease Control and Prevention) Also in Spanish
- How to Sanitize Your Phone and Other Devices (Federal Communications Commission) Also in Spanish
- Cleaning and Sanitizing with Bleach after an Emergency (Centers for Disease Control and Prevention) Also in Spanish
- COVID-19: Cleaning, Disinfecting, and Ventilation (Centers for Disease Control and Prevention) Also in Spanish
- How to Disinfect to Kill Germs (National Jewish Health)
- How to Disinfect your Workspace (Department of Homeland Security)
- Centers for Disease Control and Prevention Also in Spanish
- Environmental Protection Agency Also in Spanish

- Cleaning supplies and equipment (Medical Encyclopedia) Also in Spanish
- Cleaning to prevent the spread of germs (Medical Encyclopedia) Also in Spanish
The information on this site should not be used as a substitute for professional medical care or advice. Contact a health care provider if you have questions about your health.

Disinfection: Methods and Uses
Disinfection is a process whereby pathogenic organisms, but not necessarily all microorganisms or spores, are destroyed. Disinfection may be accomplished by physical or chemical means.
A number of factors influence the activity of disinfectants such as the following:
- Types of organisms present: Bacterial spores such as Bacillus spp., are the most resistant, followed by mycobacteria (acid-fast bacilli). Younger cells are usually more readily destroyed than mature organisms.
- Number of organisms present (microbial load): Time necessary for killing microorganisms increases in direct proportion with the number of organisms (microbial load). This is particularly true of instruments contaminated with organic material such as blood, pus, or mucus.
- Concentration of disinfectant : Generally, higher concentration of chemical disinfectants has higher killing power. Over a short-range, a small increase in concentration leads to an exponential rise in effectiveness but beyond a certain point, an increase in concentration may not raise the killing rate. Sometimes, an agent is more effective at lower concentrations. For example, 70% ethyl alcohol is more effective as a disinfectant than 95% ethyl alcohol.
- Amount of organics (blood, mucus, pus) present : The organic material should be mechanically removed before chemical sterilization to decrease the microbial load. This is analogous to removing dried food from utensils before placing them in a dishwasher and is important for cold sterilization of instruments such as bronchoscopes.
- Length of contact time : More is the exposure time to sterilant/disinfectant, the better is the efficacy.
- Type of water available (hard vs. soft): Hard water may reduce the rate of killing of microorganisms.
- Temperature and pH of the process: An increase in the temperature at which chemical acts often enhances its activity. A lower concentration of disinfectants can be used at a higher temperature. Heat kills more readily in acidic pH.
- Nature of surface to be disinfected (e.g., potential for corrosion; porous vs. nonporous surface)
Physical Methods of Disinfection
The three physical methods of disinfection are:
- Boiling at 100°C for 15 minutes kills vegetative bacteria.
- Pasteurizing at 63°C for 30 minutes or 72°C for 15 seconds kills food pathogens.
- Using nonionizing radiation s uch as ultraviolet (UV) light. UV rays are long wavelength and low energy. They do not penetrate well and organisms must have direct surface exposure, such as working surfaces of a biological safety cabinet (BSC) , for this form of disinfection to work.
Chemical Methods of Disinfection
When chemicals are used to destroy all life forms they are called chemical sterilants or biocides; however, these same chemicals used for shorter periods are disinfectants. Disinfectants are chemicals that kill microorganisms and are used on inanimate objects. Chemicals used on living tissue (skin) are called antiseptics.

Chemical disinfectants can be classified into four groups based on their microbicidal activity;
- Low-level disinfectants
- Intermediate-level disinfectants
- High-level disinfectants
- Chemical sterilant
Activity Level of Selected Chemical Disinfectants
Chemical disinfectants comprise many classes, including the following:
- Alcohols: They are among the most widely used disinfectants and antiseptics. Ethyl or isopropyl alcohol is non-sporicidal (does not kill spores) and evaporates quickly. Alcohols are best used on the skin as an antiseptic (surgical spirit). Clinical thermometers and small instruments can be disinfected by soaking in isopropyl alcohol for 10-15 minutes.
- Aldehydes: Formaldehyde, glutaraldehyde, and ortho-phthalaldehyde are commonly used aldehydes. They are sporicidal and can be used as chemical sterilants. Formaldehyde (formalin; i.e. formaldehyde in water) is used to preserve anatomical specimens and for fumigation of closed areas, such as operation theaters. They are generally not used as surface disinfectants because of their irritating fumes.
- Chlorine: It is the most commonly available disinfectant. Chlorine is most often used in the form of sodium hypochlorite (NaOCl), the compound known as household bleach. CDC recommends that tabletops be cleaned following blood spills with a 1:10 dilution of bleach . It is also used for municipal water supplies, swimming pools, dairy and food industries, etc.
- Iodine: Iodine compounds are widely employed antiseptics. Iodine is prepared either as tincture with alcohol or as an iodophor coupled with a neutral polymer, for example, povidone-iodine . “70% ethyl alcohol, followed by an iodophor, is the most common compound used for skin disinfection before drawing blood cultures or surgery”.
- Heavy metals: Salts of heavy metals, such as mercury, silver, zinc, and copper were widely used in the past as germicides but their use has been replaced by less toxic chemicals over the period. Eyedrop solution containing 1% silver nitrate is still instilled in the eyes of newborns to prevent ophthalmia neonatorum (an infection with Neisseria gonorrhoeae ). “Heavy metals containing mercury are no longer recommended because mercury is toxic to the environment”.
- Quaternary ammonium compounds (Quats): Quaternary ammonium compounds such as benzalkonium chloride, are used to disinfect bench-tops or other surfaces in the laboratory. However, organic materials, such as blood, may inactivate heavy metals or quaternary ammonium compounds, thus limiting their utility.
- Phenolics: Phenolics, such as the common laboratory disinfectant amphyl, are derivatives of carbolic acid (phenol). The addition of detergent results in a product that cleans and disinfects at the same time, and at a concentration between 2%-5% these products are widely used for cleaning benchtops.
References and further readings
- Madigan Michael T, Bender, Kelly S, Buckley, Daniel H, Sattley, W. Matthew, & Stahl, David A. (2018). Brock Biology of Microorganisms (15th Edition). Pearson.
- Procop, G. W., & Koneman, E. W. (2016). Koneman’s Color Atlas and Textbook of Diagnostic Microbiology (Seventh, International edition). Lippincott Williams and Wilkins.
- Tille, P. (2017). Bailey & Scott’s Diagnostic Microbiology (14 edition). Mosby.
- Willey, Joanne M, Sherwood, Linda M, & Woolverton, Christopher J. (2016). Prescott’s Microbiology (10 edition). McGraw-Hill Education.
Acharya Tankeshwar
Hello, thank you for visiting my blog. I am Tankeshwar Acharya. Blogging is my passion. As an asst. professor, I am teaching microbiology and immunology to medical and nursing students at PAHS, Nepal. I have been working as a microbiologist at Patan hospital for more than 10 years.
We love to get your feedback. Share your queries or comments Cancel reply
This site uses Akismet to reduce spam. Learn how your comment data is processed .
Recent Posts
Urea Cycle: Steps, End Products, and Functions
Amino nitrogen, a key component in the synthesis of amino acids or new nitrogenous products, can be toxic to the human body if not utilized to create new compounds. To prevent this, ureotelic...
Gluconeogenesis: Enzymes Involved, Steps, and Functions
During fasting, vigorous exercise, and hypoglycemic conditions, the body requires high glucose. Gluconeogenesis converts non-carbohydrate molecules like glycerol, pyruvate, lactate, glucogenic amino...

Lysol Disinfectant in Household
Microorganism control is critical for preventing disease transmission and infection, stopping deterioration and spoilage, and avoiding undesirable microbial contamination. There are numerous methods employed in countering the growth of microorganisms today. The techniques are divided into two categories: physical control and chemical control. Osmotic pressure, radiation, desiccation, high or low temperature, and filtration are physical control methods (Kaiser, 2021). At the same time, chemical control techniques include the use of antiseptics, chemotherapeutic antimicrobial chemicals, disinfectants, and antibiotics.
The difference between sterilization and disinfection is that sterilization is the procedure of killing all microorganisms, whereas disinfection is the act of removing or reducing hazardous microorganisms from inanimate items and surfaces. Sterilization also kills spores of numerous organisms that may be found on surfaces, in medication, liquids, or compounds like biological growth media. Decontamination of this severe kind is required during important periods, such as surgery or in places such as laboratories. Using disinfection in daily life is more practical. Antiseptics and disinfectants control infections and kill microorganisms like fungi, bacteria, and viruses (Brennan, 2021). Microorganisms on the skin are destroyed by antiseptics, while disinfectants kill germs on nonliving surfaces.
Lysol, Dettol, Ariel, Harpic, and bleach are the different types of disinfectants located in my household. The Lysol brand of disinfecting and cleaning is the product under investigation in this report. Lysol disinfectant kills germs, viruses, and dirt and soot that cause odors. The disinfectant has the duty to remove odor-causing microorganisms, the common cold virus, everyday dust, and challenging problems. The active ingredient in the disinfectant includes the benzalkonium chloride of 0.10% and acts as an antibacterial agent (“Lysol disinfectant spray,” n.d.). Furthermore, the active compound is effective in surface and hand sanitization. The Lysol brand has several inactive ingredients, including glycerin, citric acid, water, propylene glycol, cetrimonium chloride, and lauramine oxide (“Lysol disinfectant spray,” n.d.).
The benzalkonium chloride material is a quaternary ammonium compound that belongs to the chemical class of disinfectants and is extensively employed to control pathogenic fungi, bacteria, and other microorganisms on walls and floors (“Chemical disinfectants,” n.d.). In the medical field, quaternary ammonium compound is used to disinfect equipment. Furthermore, the compound acts as a sanitizing agent and plays a significant role in protecting individuals against contracting the viruses during viral pandemics.
Numerous disinfectants are employed for disinfection and sanitization in the biopharmaceutical field. They have various antimicrobial features based on their action style. Different modes of action from multiple disinfectants are crucial in preventing the growth of the resistance in bacteria. Lysol disinfectants work by attacking the cytoplasmic cell walls or the entire cytoplasm itself to kill germs (“Lysol touch of foam,” n.d.). An antimicrobial agent acts on a bacterial cell by first adhering to its surfaces and then penetrating the cellular membranes to reach its target areas.
The disinfectant is used to clean and sanitize hands and numerous surfaces such as door handles, tables, and walls in my household. The manufacturer’s instructions require that Lysol disinfectant spray cans be held in an upright manner of about six to eight inches from the surface. In addition, the spray should be applied to a pre-clean surface and sprayed for three to four seconds until the cleaning area is covered with mist. When sanitizing, a surface must be left wet for approximately ten seconds then allowed to air dry; during disinfection, however, the areas must remain wet for about three minutes and left to air dry (“Lysol disinfectant spray,” n.d.). According to the manufacturer’s directions on usage, it is evident that I underuse the product, thus denying it to be effective.
The disinfectant is effective, especially during the COVID-19 pandemic, as it protected my household against the deadly virus. I wash my hands thoroughly with Lysol each time I enter the house, and I believe it is because of this that I have not contracted the coronavirus. Moreover, I rarely contract flu and cold since the disinfectant kills the illness-causing germs; therefore, Lysol is an excellent product. Forms of fungi such as mildew and mold that grow in the bathroom are toxic and dangerous to health (Kerr, 2020). However, spraying Lysol on the bathroom walls kills molds and mildew, proving very effective.
The chemicals used in Lysol disinfectants can be treacherous to human health when utilized inaccurately. Mixing the Lysol with other cleaning products or spraying oneself or others with the product are a few scenarios of countless manners of misusing cleaning products. While using the disinfectant, the area should be well ventilated so that the harmful toxic fumes go out and you do not inhale them (Kerr, 2020). Lysol is designed for surfaces and should not be used on the body of humans or animals as its consequences might be detrimental. Once children’s toys are disinfected, they should be rinsed well with flowing water. Consuming Lysol is prohibited since it is poisonous and will have adverse effects on the body and death (Kerr, 2020).
In conclusion, I will follow the manufacturer’s instructions to ensure the maximum effectiveness of Lysol in the household. From my research, I have learned to let the surface remain wet for approximately three minutes and air dry. This is contrary to what I do: spray and wipe immediately, thereby denying the disinfectant to function successfully. When spraying, the Lysol bottle will be held about six inches to the surface to minimize the risk of the product splashing back to my face. I will change how I use the disinfectant for better results and reduce risk to my health.
Brennan, D. (2021). Difference between disinfectants and antiseptics . Web MD.
Chemical disinfectants. (n.d.). Infection control.
Kaiser, G. (2021). An overview to control of microorganisms. Libre Texts.
Kerr, J. (2020). Cleaning your home: Good. Accidentally poisoning yourself: Bad . The New York Times.
Lysol disinfectant spray. (n.d.). Lysol.
Lysol touch of foam. (n.d.). Daily med.
Cite this paper
- Chicago (N-B)
- Chicago (A-D)
StudyCorgi. (2023, March 16). Lysol Disinfectant in Household. https://studycorgi.com/lysol-disinfectant-in-household/
"Lysol Disinfectant in Household." StudyCorgi , 16 Mar. 2023, studycorgi.com/lysol-disinfectant-in-household/.
StudyCorgi . (2023) 'Lysol Disinfectant in Household'. 16 March.
1. StudyCorgi . "Lysol Disinfectant in Household." March 16, 2023. https://studycorgi.com/lysol-disinfectant-in-household/.
Bibliography
StudyCorgi . "Lysol Disinfectant in Household." March 16, 2023. https://studycorgi.com/lysol-disinfectant-in-household/.
StudyCorgi . 2023. "Lysol Disinfectant in Household." March 16, 2023. https://studycorgi.com/lysol-disinfectant-in-household/.
This paper, “Lysol Disinfectant in Household”, was written and voluntary submitted to our free essay database by a straight-A student. Please ensure you properly reference the paper if you're using it to write your assignment.
Before publication, the StudyCorgi editorial team proofread and checked the paper to make sure it meets the highest standards in terms of grammar, punctuation, style, fact accuracy, copyright issues, and inclusive language. Last updated: March 16, 2023 .
If you are the author of this paper and no longer wish to have it published on StudyCorgi, request the removal . Please use the “ Donate your paper ” form to submit an essay.
- Chemistry in Everyday Life
- Antiseptics And Disinfectants
Antiseptics and Disinfectants
What is disinfectant.
A disinfectant can be defined as an antimicrobial agent that can be applied on the surface of some objects in order to destroy the microorganisms residing on it.
What is an Antiseptic?
Antiseptics can be defined as antimicrobial agents which can be applied on the body of living organisms to inhibit the action of microbes. They are not injected into the body like the antibiotics, rather they are applied on the surface of the skin to heal the living tissues in case of wounds and cuts.
Dettol is the most commonly used antiseptic. It is a mixture of chloroxylenol and terpineol. Iodoform is also used as an antiseptic for wounds.
Table of Contents
How does it destroy the micro-organisms, types of disinfectants, types of antiseptics.
- Difference between Disinfectants and Antiseptics
- Frequently Asked Questions – FAQs
The simple answer to this is – antimicrobial agents.
A disinfectant is an antimicrobial agent. It is not necessary that a disinfectant will kill all the microorganisms. It is not as effective as sterilization. The process of sterilization kills all types of living forms but a disinfectant kills only certain types of microbes. It is generally applied to a floor or a drainage system. Most of the popularly used cleaning products used in homes and offices are nothing but disinfectants.
Some common types of disinfectants include:
- Air disinfectants: It is defined as the chemical substances which are used to kill the microorganisms that are suspended in the air. It can also be called as a disinfectant spray.
- Alcohol: It is seen that alcohols are used as disinfectants. Ethanol is the most common example in this case. Some other examples of disinfectants are, chlorine when it is in the concentration of 0.2 to 0.4 in aqueous solution and sulphur dioxide , which acts as a disinfectant in small concentrations.
Some antiseptics are germicidal in nature, implying that they have the ability to completely destroy microbes. These types of antiseptics are referred to as bacteriocidal antiseptics. Other antiseptics only inhibit the growth of microbes (or prevent the growth of microbes altogether). Such substances are commonly referred to as bacteriostatic antiseptics.
The Difference between Disinfectants and Antiseptics
Disinfectants and antiseptics are both used for killing the microbes but still, there is a difference between them.
- An antiseptic is used for killing the microbes on the living tissues whereas a disinfectant is applied on a non-living object.
- Secondly, the concentration of both differ. We can use the same chemical as a disinfectant and an antiseptic by varying its concentration.
- For example, phenol can be used as an antiseptic if its concentration is 0.2 % but to use it as a disinfectant the concentration should be 1%.
- We can broadly conclude that the cleaning products contain disinfectants and the healing products (for curing the living tissues) contain antiseptics.
- Both are similar in nature but vary in their concentration. Lysol is a disinfectant whereas Dettol is an antiseptic.
Frequently Asked Questions – FAQs
What are common antiseptics.
Chlorhexidine, povidone-iodine, chloroxylenol, isopropyl alcohol, hexachlorophene, benzalkonium chloride, and hydrogen peroxide are all often used as antiseptics in dermatologic surgery. They should be utilized for the majority, if not all, treatments that go into the dermis or deeper layers of the skin.
What is the difference between antiseptics and disinfectants?
In order to control infections, antiseptics and disinfectants are commonly employed. Biocides are chemicals that are used to kill microorganisms such as bacteria, viruses, and fungi. Germs on non living surfaces are killed with disinfectants. Microorganisms on your skin are killed by antiseptics.
Is bleach a disinfectant or antiseptic?
Bleach is a powerful disinfectant whose primary ingredient, sodium hypochlorite, kills bacteria, fungi, and viruses, including the influenza virus, although it is rapidly inactivated by organic matter. Within 10–60 minutes of contact time, diluted household bleach disinfects.
What is the use of disinfectant?
Disinfectants are chemical agents that are applied to non-living items to kill bacteria, viruses, fungi, mold, and mildews that are present.
What is an example of a disinfectant?
Chlorine, calcium and sodium hypochlorite, iodophor, phenol, ethanol, and quaternary ammonium compounds are some of the most often used chemical disinfectants. Disinfectants differ from sterilants in that they have a lower efficacy against dormant bacterial endospores.
For further details on this topic, download BYJU’S – The Learning App and experience the joy of learning with interactive video lessons.
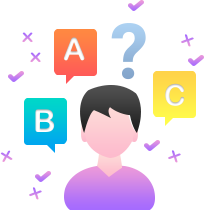
Put your understanding of this concept to test by answering a few MCQs. Click ‘Start Quiz’ to begin!
Select the correct answer and click on the “Finish” button Check your score and answers at the end of the quiz
Visit BYJU’S for all Chemistry related queries and study materials
Your result is as below
Request OTP on Voice Call
Leave a Comment Cancel reply
Your Mobile number and Email id will not be published. Required fields are marked *
Post My Comment

- Share Share
Register with BYJU'S & Download Free PDFs
Register with byju's & watch live videos.

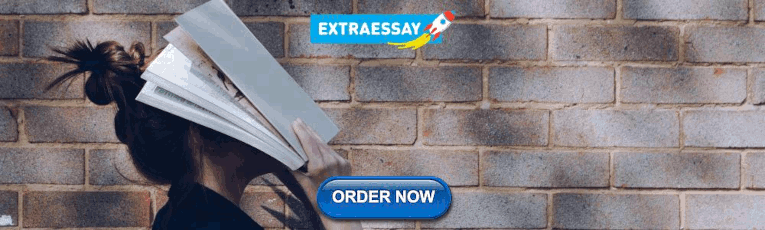
IMAGES
VIDEO
COMMENTS
Disinfectants are typically applied on non living objects in order to destroy microorganisms and this process are called disinfection. Order custom essay Sterilization and Disinfection Techniques for Microbial Control with free plagiarism report. 450+ experts on 30 subjects Starting from 3 hours delivery. Get Essay Help.
Disinfectants and sanitizers are essential preventive agents against the coronavirus disease 2019 (COVID-19) pandemic; however, the pandemic crisis was marred by undue hype, which led to the indiscriminate use of disinfectants and sanitizers. Despite demonstrating a beneficial role in the control and prevention of COVID-19, there are crucial concerns regarding the large-scale use of ...
Sterilization is intended to convey an absolute meaning; unfortunately, however, some health professionals and the technical and commercial literature refer to "disinfection" as "sterilization" and items as "partially sterile.". When chemicals are used to destroy all forms of microbiologic life, they can be called chemical sterilants.
Essay On Disinfectants. 1157 Words5 Pages. Disinfectants Are chemical substance, used to kill pathogenic and other harmful microorganisms on inanimate objects but are too toxic to be applied directly on living tissues. disinfection has a lower effect than sterilization . sterilization is an extreme chemical and physical operation that destroy ...
1. Introduction. Healthcare-associated infections (HAIs) contribute to patient morbidity and mortality with an estimated 687,000 infections and 72,000 deaths in the United States in 2015 [] and an additional 2.6 million annual infections in the European Union [].The burden of HAIs is higher in low- and middle-income countries [3,4,5].HAIs are often correlated with the presence of contaminated ...
Introduction. Multi drug resistant (MDR) microorganisms have become a major threat to both global health and economy. The World Health Organization (WHO) has declared that the microbial infections caused by antibiotic resistance bacteria will lead to massive preventable deaths (Collignon, 2017; Morrison and Zembower, 2020).They also estimated that the global economy will suffer over 100 ...
Introduction. Considerable progress has been made in understanding the mechanisms of the antibacterial action of antiseptics and disinfectants (215, 428, 437).By contrast, studies on their modes of action against fungi (426, 436), viruses (298, 307), and protozoa have been rather sparse.Furthermore, little is known about the means whereby these agents inactivate prions ().
Health care-associated infections are an important source of morbidity and mortality, with an estimated 1.7 million infections and 99,000 deaths annually in the United States.1 The major source of health care-associated pathogens is thought to be the patient's endogenous flora, but an estimated 20% are acquired via other transmission routes such as the environment, and 20%-40% are ...
Formaldehyde is a common ingredient in household cleaners and disinfectants. It is an established form of carcinogen. (Ozonoff, 2005) Organochlorines: Organochlorines are brought about by the blend of hydrogen and carbon. A number of Organochlorines are highly toxic. They are bio-accumulative and are used in the production of detergents and ...
There are a number of factors which influence the antimicrobial action of disinfectants and antiseptics, including: 1. The concentration of the chemical agent. 2. The temperature at which the agent is being used. Generally, the lower the temperature, the longer it takes to disinfect or decontaminate. 3.
Abstract and Figures. Control of microorganisms is vital to prevent infection. Prevention can be done in various ways, one of which is the use of disinfectants and antiseptics. The widespread use ...
Read our infographic on how to use surface disinfectant products. Six Steps for Safe & Effective Disinfectant Use (pdf) (423.8 KB) Contact Us to ask a question, provide feedback, or report a problem. Last updated on April 10, 2024. Six Steps for Safe & Effective Disinfectant use against SARS CoV 2 that causes CoVID 19.
In-Use Test. An in-use test can determine whether an actively used solution of disinfectant in a clinical setting is microbially contaminated (Figure 16.4.2 16.4. 2 ). A 1-mL sample of the used disinfectant is diluted into 9 mL of sterile broth medium that also contains a compound to inactivate the disinfectant.
According to a new science brief from the Centers for Disease Control and Prevention (CDC), the risk of catching COVID-19 by touching a contaminated surface is considered to be low. EPA's new factsheet has cleaning and disinfecting best practices and tips you can use during the COVID-19 pandemic. Cleaning and Disinfecting: Best Practices During the COVID-19 Pandemic (pdf) (76 KB)
Phenolics. In the 1800s, scientists began experimenting with a variety of chemicals for disinfection. In the 1860s, British surgeon Joseph Lister (1827-1912) began using carbolic acid, known as phenol, as a disinfectant for the treatment of surgical wounds (see Foundations of Modern Cell Theory).In 1879, Lister's work inspired the American chemist Joseph Lawrence (1836-1909) to develop ...
Additionally, personal items, such as mobile phones, keys, credit cards, and writing pens, may be subjected to sanitization using 75% ethanol to ensure their disinfection (Yang et al. 2020). Virusend (TX-10) is a unique disinfectant that has already exhibited efficient activity against several microbial agents, including viruses.
For the HVP disinfectant testing, Ozbun and her team applied various disinfectants to three HPV from three sources, and determined that the chemicals reduced the amount of virus by 10,000 times. This technique can be used for disinfectant testing for other protein-capsid viruses (like norovirus and poliovirus) and to produce accurate viral ...
Sanitizing: Composed of cleaning and disinfecting practices, sanitizing lowers the number of germs on surfaces and objects. In turn, this minimizes the spread of illnesses. Using a combination of these methods to sterilize the objects and surfaces we interact with decreases our health risk.
Disinfecting uses chemicals (disinfectants) to kill germs on surfaces and objects. Some common disinfectants are bleach and alcohol solutions. You usually need to leave the disinfectant on the surfaces and objects for a certain period of time to kill the germs. Disinfecting does not necessarily clean dirty surfaces or remove germs.
The three physical methods of disinfection are: Boiling at 100°C for 15 minutes kills vegetative bacteria. Pasteurizing at 63°C for 30 minutes or 72°C for 15 seconds kills food pathogens. Using nonionizing radiation s uch as ultraviolet (UV) light. UV rays are long wavelength and low energy. They do not penetrate well and organisms must have ...
Wear a new pair of gloves. Prepare your disinfectant solutions by numbering six small cups #1-6 using your permanent marker. Each numbered cup will match one section of your cutting board fomite. Fill each cup with a different disinfectant solution and write it in a data table. You should fill the first cup with water as a negative control.
Lysol disinfectant kills germs, viruses, and dirt and soot that cause odors. The disinfectant has the duty to remove odor-causing microorganisms, the common cold virus, everyday dust, and challenging problems. The active ingredient in the disinfectant includes the benzalkonium chloride of 0.10% and acts as an antibacterial agent ("Lysol ...
It can also be called as a disinfectant spray. Alcohol: It is seen that alcohols are used as disinfectants. Ethanol is the most common example in this case. Some other examples of disinfectants are, chlorine when it is in the concentration of 0.2 to 0.4 in aqueous solution and sulphur dioxide, which acts as a disinfectant in small concentrations.