- Open access
- Published: 07 March 2022
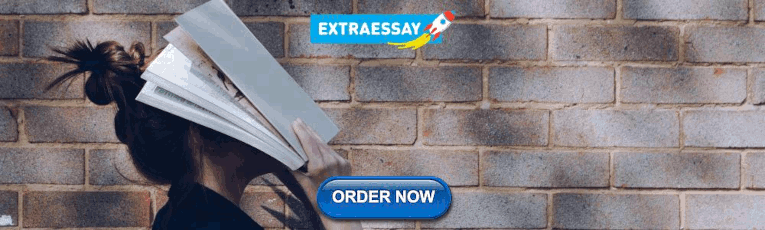
Supporting undergraduate students’ developing water literacy during a global pandemic: a longitudinal study
- Silvia-Jessica Mostacedo-Marasovic ORCID: orcid.org/0000-0001-6860-719X 1 ,
- Diane Lally 2 ,
- Destini N. Petitt 3 ,
- Holly White 4 &
- Cory Forbes 1
Disciplinary and Interdisciplinary Science Education Research volume 4 , Article number: 7 ( 2022 ) Cite this article
2308 Accesses
1 Citations
6 Altmetric
Metrics details
A Publisher Correction to this article was published on 22 March 2022
This article has been updated
To prepare students to address water-related challenges, undergraduate STEM education must provide them with opportunities to learn and reason about water issues. Water in Society is an introductory-level, innovative, and interdisciplinary undergraduate course offered annually at a large midwestern university. The course focuses on both disciplinary concepts and civic engagement, and is designed around a variety of interactive, research-based practices to support students’ learning, engagement with authentic data, scientific models and modeling, and collaboration and learning among peers. This study aims to evaluate, “ how have student outcomes and perceptions changed over five years of the course?”. The results are based on data from students ( n = 326) in five consecutive years of the course, during which time the course transitioned from a face-to-face model to fully asynchronous online model due, in part, to impacts of the COVID-19 pandemic. The particularly rapid and abrupt transition between 2020 and 2021 in response to COVID-19 led to many course changes, including modes of communication between instructors and students and opportunities for collaboration. Here, multiple measures are used to evaluate students’ learning about water concepts, model-based reasoning about socio-hydrologic systems, and perceptions of the course across all five years. By the end of each iteration of the course, students improved their knowledge of hydrologic concepts, independent of the course format or other student-level variables. However, results also show that students’ performance on complex socio-hydrologic systems modeling tasks, as well as their overall satisfaction with the course, decreased in Year 5 when the course was fully online. Results provide insight into efforts to move undergraduate STEM courses online and specific evidence of the COVID-19 pandemic’s impacts on undergraduate STEM teaching and learning about water.
Introduction
Water is a critical component supporting all life on Earth. It is interrelated with every human activity, such as agriculture, power generation, industrial production, mining, public and domestic supply, human health, education, and culture. Between 1900 and 2010, global water use increased by a six-fold factor; and, with population growth, economic development, and changes in consumption patterns, it continues to increase at a rate of 1% per year (Aquastat, n.d. in UNESCO & UN Water, 2020 ). In conjunction with climate change, this adds increasing pressure on both the quantity and quality of surface and groundwater resources (UNESCO & UN Water, 2020 ; IPCC, 2021 ). Furthermore, with human populations rapidly expanding, tensions around water resource access and use are likely to intensify. As a result, the world faces many socio-hydrologic issues (SHIs) that are complex in nature and involve both scientific and non-scientific dimensions. Efforts to conserve and effectively manage Earth’s water resources require decision-making based on scientific evidence, as well as social, economic, and policy considerations to ensure water security within complex socio-hydrological systems. Teaching and learning about water in an array of contexts, including undergraduate classrooms, is therefore pivotal to help future water researchers, practitioners, and global citizens develop water literacy, which involves hydrologic knowledge, skills, attitudes, and behaviors, to enable sustainable water management practices (King et al., 2012 ; McCarroll & Hamann, 2020 ; Su et al., 2011 ).
Despite evidence of the value of water and its importance across different STEM disciplines (King et al., 2012 ), research has found that there are many challenges to cultivating water literacy through education. At the undergraduate level, students may have inaccurate or incomplete understandings about water, the water cycle, or water systems (Arthurs & Elwonger, 2018 ; Attari et al., 2017 ; Cardak, 2009 ; Sibley et al., 2007 ), which can persist into adulthood (Duda et al., 2005 ; Williams et al., 2009 ) and need opportunities to develop understanding of environmental principles within a framework that foregrounds coupled human-natural systems (King et al., 2012 ). Many innovative educational strategies have been applied to improve or enhance undergraduate water education, including technological approaches (Habib et al., 2012 ; Li & Liu, 2003 ; Williams et al., 2009 ), developing and evaluating content material (White et al., 2017 ), and creating interdisciplinary courses (Willermet et al., 2013 ). While these studies highlight positive impacts of these approaches on undergraduate water education, they also illustrate persistent challenges in supporting undergraduate students’ learning about water and development of water literacy.
To contribute to these broader efforts, we have developed, offered, and evaluated the undergraduate Water in Society course at a large midwestern university since 2017. Water in Society is a 3-credit hour, introductory, interdisciplinary course offered to students from STEM and non-STEM majors that has served over n = 326 students in the past five years. The course has a dual focus on students 1) developing understanding of introductory-level core hydrologic concepts and 2) using that knowledge to analyze, reason, and make decisions about water-related issues. In an earlier publication, we described the origins and initial design of the course (Forbes et al., 2018 ). Since then, the course has maintained a consistent focus on a core set of innovative research-based practices to support students’ learning and engagement with authentic data, scientific models and modeling, and the use of evidence to make informed decisions about SHIs. However, there have also been changes to the course over time, particularly a shift from a more traditional, instructor-driven course to a hybrid, flipped, and more student-centered course design; and, most recently, to a fully online and asynchronous course in response to COVID-19. Since 2017, we have extensively studied these aspects of the course as it has evolved (Lally et al., 2020 ; Lally & Forbes., 2019 , 2020 ; Owens et al., 2020 ; White & Forbes, 2021 ), contributing to the broader body of research on undergraduate water education. Collectively, these studies have provided insight into the use of modeling and visualization tools to help students reason about hydrologic concepts and engage in decision-making about SHIs, as well as how the design of the course can support these outcomes. These studies build upon and contribute to insights from our broader body of research on undergraduate water education (Petitt & Forbes, 2019 ; Sabel et al., 2017 ).
Despite this multi-year discipline-based research effort, we have not yet conducted a comprehensive evaluation of the course outcomes. While prior studies have focused on specific course elements within one or two years, they have not sought to account for a broader range of outcomes across all five years as the course has evolved, or how these outcomes may have been impacted by the global COVID-19 pandemic. The present study aims to provide a systematized account of the five years of the course, in which we evaluate how students’ outcomes and perceptions have changed over five years, including the spring 2020 and 2021 semesters, in which the COVID-19 crisis necessitated a shift to virtual learning. It provides evidence about undergraduate water education from an interdisciplinary and holistic perspective, efforts to continue improving the course, and informs other education researchers and practitioners about strategies that could be used to improve the design of courses related with water resources. The research question guiding this study is, “ how have the students’ outcomes and perceptions changed over five years of the course?”.
Undergraduate water education
Teaching and learning about water remain an important priority within undergraduate education. Among hydrologists, for example, there has been a growing recognition of the need for innovative educational approaches to prepare the next generation of water scientists (Wagener et al., 2012 ). This has led to a growing body of work illustrating innovative approaches in undergraduate water science courses (Habib et al., 2012 ; Halvorson & Westcoat, 2002 ; Kingston et al., 2012 ; Li & Liu, 2003 ; Smith et al., 2006 ; Thompson et al., 2012 ). In addition, there is an emphasis on cultivating water literacy among the population to support science-based water-related decision-making (Attari et al., 2017 ; Covitt et al., 2009 ; Johnson & Courter, 2020 ; King et al., 2012 ; McCarroll & Hamann, 2020 ; Su et al., 2011 ). As King et al. ( 2012 ) observe, “Appreciating that the subject matter of hydrology is embedded in a larger context of causes and effects, which includes human decision-making and generates complex system behaviors, is a primary step in reframing hydrology education” (pg. 4025). In this sense, addressing the complexity of water-related challenges, requires that students from STEM and Non-STEM majors to develop an understanding about different natural and human dimensions of water. Within this context, some work has explored interdisciplinary courses for both STEM majors (including those in water sciences) and non-majors (Covitt et al., 2009 ; Forbes et al., 2018 ; Smith et al., 2006 ; Willermet et al., 2013 ; Williams et al., 2009 ). All of these efforts share a commitment to the idea that individuals must learn to apply disciplinary knowledge to the social, economic, legal, and political dimensions of water-related issues, or water literacy.
However, research has shown that water literacy in the United States remains underdeveloped. Students across the K-16 continuum, including undergraduate students, demonstrate an array of scientifically inaccurate conceptions about water and water systems (Arthurs & Elwonger, 2018 ; Attari et al., 2017 ; Cardak, 2009 ; Covitt et al., 2009 ; Halvorson & Westcoat, 2002 ; Sibley et al., 2007 ). For example, students may not fully grasp water vapor as a component of air (Cardak, 2009 ; Sibley et al., 2007 ), groundwater stocks and flows (Arthurs & Elwonger, 2018 ; Cardak, 2009 ), the relation between the built environment and water (Attari et al., 2017 ; Covitt et al., 2009 ), and interactions between natural and human dimensions of complex water systems (Halvorson & Westcoat, 2002 ). Prior research documents also observed that many commonly observed ideas students articulate (i.e., groundwater existing as underground reservoirs, evaporation occurring only from large bodies of surface water, the impact of Earth’s oceans on weather and climate, and the vast amount of Earth’s freshwater used for agricultural production), often underemphasize less-directly-observable components of water systems, such as soil moisture, groundwater, and water vapor, in their reasoning about hydrologic stocks and flows. Some evidence suggests these alternative ideas may carry over into adulthood (Duda et al., 2005 ). Collectively, while these findings highlight many characteristics of water and water systems that learners do grasp, they also identify specific areas in which water literacy can be enhanced, including through undergraduate education.
Reform-based, model-centric undergraduate teaching and learning
To support their learning about water and development of water literacy, undergraduate students should use computational, simulation-based models in classroom settings which, when combined with other pedagogical approaches, can support learners to develop a more comprehensive understanding of hydrology (AghaKouchak et al., 2013 ; Habib et al., 2012 ; Merwade & Ruddell, 2012 ). Models are an important tool with which to support students’ learning about complex systems, including water. Modeling helps students engage with otherwise inaccessible phenomena and develop skills, including explaining ideas, making connections between the real world and scientific concepts, evaluation of models and ideas, and metacognitive processes. While scientific models can take a variety of forms (visual representations, physical models, computer simulations, analogies, etc.) (Lally & Forbes, 2019 ; Merwade & Ruddell, 2012 ), here we focus on data-driven, computer-based computational models for water systems, tools commonly used by hydrologists. With these models at their disposal, students can explore multiple hypotheses, develop policies, and quickly run multiple scenarios involving hydrologic phenomena and socio-hydrologic systems (Gunn et al., 2002 ; Williams et al., 2009 ; Zigic & Lemckert, 2007 ).
Enhancing undergraduate water education using models, simulations, and visualizations harnesses the benefits of reform-based instruction, including active learning, to interact with authentic data for complex analysis and decision-making (AghaKouchak et al., 2013 ; Attari et al., 2017 ; Gunn et al., 2002 ; King et al., 2012 ; Lally & Forbes, 2019 , 2020 ; Merwade & Ruddell, 2012 ; Sibley et al., 2007 ; Zigic & Lemckert, 2007 ). Effective, model-centric instructional strategies align with best practices in undergraduate STEM instruction (Handelsman et al., 2004 ) and innovative teaching strategies to positively affect student outcomes (Gunn et al., 2002 ). Effective teaching and learning in STEM disciplines has evolved from traditional approaches that prioritize one-directional information transmission through instructor-led lectures and ‘canned’ activities with stringent, pre-determined outcomes. Instead, contemporary perspectives emphasize active learning, a process in which instructors and students co-participate in higher order thinking and co-create knowledge and meaning (Handelsman et al., 2004 ). Prior research has demonstrated the positive impact of reform-based pedagogy and active learning in undergraduate courses. Active learning involves an array of alternative classroom structures, including small and large group discussions, problem solving, practicing, questioning, and feedback, through which students, create, analyze, and evaluate evidence and knowledge claims for natural phenomena (Habib et al., 2012 ; Halvorson & Westcoat, 2002 ; Kingston et al., 2012 ; Li & Liu, 2003 ; Smith et al., 2006 ; Thompson et al., 2012 ; White et al., 2017 ; Willermet et al., 2013 ). This compelling evidence underlies current emphases on use of active learning strategies and principles of effective STEM instruction in undergraduate STEM courses.
Study design and context
This convergent mixed-methods evaluation study is based on student data from five consecutive years of the Water in Society course, offered annually in the spring semester at a large, R1 institution in the U.S. Midwest (Lally et al., 2020 ; Lally & Forbes, 2019 , 2020 ; Owens et al., 2020 ; White & Forbes, 2021 ). Over these five years, the course has gradually shifted from a lecture-based to a student-centered format, with varying types and degrees of active-learning opportunities. Furthermore, while the course content has remained largely the same, the modality of the course has shifted from traditional face-to-face (2017) to a flipped hybrid model (2018 and 2019), a split flipped and fully online modality (2020), to a fully asynchronous online model (2021). Students’ interactions with the content, instructors, and other students changed from a higher interaction between students and instructors and their peers in 2017 to a predominant degree of student-content interaction, followed by student-instructor interaction, and less student-student interaction in 2021 (Li et al., 2016 ) (Table 1 ).
Participants
At the beginning of the course, students were asked to sign a consent form approved by the university’s Institutional Review Board. Of the students who agreed to participate in research ( n = 326), the majority (85%) were STEM majors in programs offered through the institution’s primary colleges of science. Other majors represented were Arts (4.6%), Journalism and Mass Communication (2.1%), Education and Human Sciences (2.1%), Fine and Performing Arts (1.5%), Business Administration (1.2%), Agricultural Economics (1.2%), Architecture (0.9%), Public Affairs and Communication Services (0.3%), General studies (0.3%), and high school (0.6%). Over 2/3s of the students in the course were sophomore (30.1%) or juniors (36.8%), while 23.6% were seniors, and close to 10% were freshman (9.4%), post baccalaureates (0.3%) or high school students (0.6%) who joined the course as part of a state program. Students were relatively equal by gender, with 54% male, and 46% female (Appendix 1 ).
Data sources
This study is based on the data from three course assessments, assignments, and instruments. First, the Pre-/Post-test is an assessment consisting of 41 questions administered at the beginning and end of the course to evaluate the students’ understanding of hydrology-related concepts. Second, the Water Balance Model (WBM) is a data-driven, computer-based modeling tool designed to support student’s understanding of factors affecting the hydric balance. Students’ experience with the WBM is through a multi-week project where they engaged with a scenario where they had to evaluate the allocation of irrigation acreage considering climatic zones, the water table, rainfall, runoff, and the potential evapotranspiration. Lally & Forbes, 2019 developed a scoring rubric which evaluates students’ use and evaluation of the model. In the study, we used the score for model use which evaluates students’ use of the model to introduce evidence, provide a detailed and complex description and explanation, generalize, predict or hypothesize, organize their ideas, and generate new information in their analysis about the phenomenon. Third, the Mid-Semester Evaluation (MSE) is a course evaluation survey involving Likert scale and open-ended questions, administered at the mid-point of the semester. Except for the MSE, data is available for all five years. Each of these instruments are described more fully in prior publications (Forbes et al., 2018 ; Lally & Forbes 2019 , 2020 ). The number of students who responded to the instruments varied for each year and instrument (Table 2 ).
Data analysis
We calculated gain scores between the Pre and Post-tests to assess the differences between them. Then, we obtained descriptive statistics of all the quantitative scores using R program version 3.6.2. We performed ANOVAs to evaluate changes over time for the gain scores, Water Balance Model use scores, and the Mid-Semester Evaluation. In the cases in which we found statistically significant differences, we applied Tukey HSD Tests to identify the differences between years using R. Given the number of pairwise comparisons, we used the Bonferroni correction to establish the threshold for significance at p = .008 for post-hoc tests within each ANOVA.
To identify factors that would predict the gains or losses in students’ post-test scores, we performed an Analysis of Covariance (ANCOVA) where we included the categorical factors of gender, academic level and Major (STEM or Non-STEM), and all two-way interactions, and the covariate is the students’ pre-test scores. Pre-test scores have a negative linear relationship with gain scores setting a cap to students’ gain scores, where students who started the course with higher levels of knowledge about water concepts would be able to experience modest gains, whereas students with lower pre-test scores would be able to experience higher gains. In the cases in which we found statistically significant differences, we applied Tukey HSD Tests to identify the differences between the categorical variables. Furthermore, for the variables where we found differences, we ran ANOVAS to compare differences between years. These analyses were done using R program version 3.6.2. The full set of descriptive statistics, ANOVAS and corresponding Tukey HSD tests, and ANCOVA and corresponding Tukey HSD test are summarized in Appendix 2 ; 3 , 4 , 5 , 6 , 7 , 8 ; and 9 , respectively.
To analyze the qualitative data from the MSE, we evaluated, coded, and categorized each response to perform a Strengths, Weaknesses, Opportunities, and Threats (SWOT) analysis about students’ perceptions of the course. The SWOT analysis complemented the quantitative analysis from the MSE. First, we organized students’ comments by year, academic level, and Major using Word. Each comment received a number based on the order in which it was reviewed. We developed a code sheet using Excel with “strengths”, “weaknesses”, “opportunities”, and “threats” as main categories. We added columns representing the year, academic level, and Major to help keep track each response. As we read each response, we identified emergent sub-categories using representative words and assigned each comments’ number to a corresponding category and sub-category. When a comment represented two or more categories or sub-categories, it was added to the corresponding sections. As we read each comment, we also highlighted those that provided an ample description of the student’s opinions on the topic being discussed. With support of the code sheet, we developed a summary of strengths, weaknesses, opportunities, and threats for each question and year and re-organized the students’ responses accordingly using Word. The summary helped us identify students’ steady and changing perceptions of the course for each year. While developing the narrative, we traced back to the students’ responses and selected from those that were highlighted the most representative ones.
Students’ understanding of hydrologic concepts
Analysis of students’ Pre-tests, Post-tests, and gain scores show students’ Pre-test and gain scores varied across years while their Post-test scores were relatively consistent (Fig. 1 ). For the Pre-test, the Post-test, and the gain scores, the effect of year was statistically significant (Table 3 ). We observed that across all five years, students began the course each year with varying levels of knowledge of hydrologic concepts. Students’ knowledge of core hydrological concepts at the beginning of the semester was highest and equivalent in 2017 and 2020; while it was relatively lower in 2018, and particularly, 2019 and 2021. The 2019 group and 2021 had the lowest average score and the highest standard deviation between students whereas the 2017 and 2020 groups had the highest average scores and lowest standard deviations (Fig. 1 & Table 4 ). Differences between 2017 and 2020, and 2019 and 2021 Pre-test scores were not statistically significant at p = .008. However, all other pairwise comparisons across year were statistically significant at p = .008.
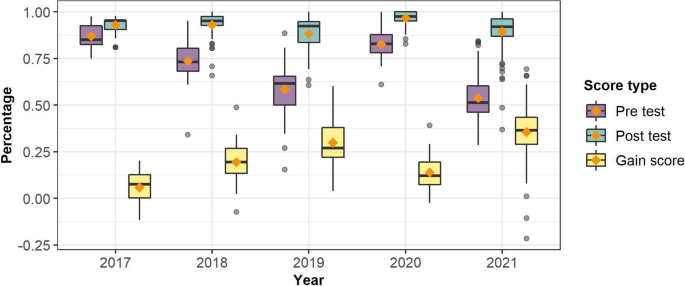
Pre-test, Post-test, and Gain scores (shown as percentages)
Compared to Pre-test scores, students’ scores on the Post-test were higher, more consistent, and relatively more similar to one another. By the end of the course, the students in the 2020, 2018 and 2017 had the least heterogeneity among students. The differences between the 2018 and 2019; 2019 and 2020; and 2020 and 2021 pairwise comparisons were statistically significant at p = .008. The 2019 and the 2021 groups exhibited the lowest Post-test scores (Table 5 ). These findings suggest that students in each year left the course with relatively similar levels of understanding of core hydrological concepts, except for 2019 and 2021 which exhibited Post-test scores that were approximately 5% lower than 2018, and 9% and 7% lower than 2020, respectively.
Analysis of the gain scores show that students’ knowledge of core hydrological concepts increased each year; however, the magnitude of these increases was different every year. We observed that between all possible pairs of years, except for 2018 and 2020, the mean differences were statistically-significant at p = .008. The 2021 ( M = 0.36, SD = 0.14) and 2019 groups ( M = 0.30, SD = 0.13) had the largest increase, but students’ individual gains were the most heterogeneous of all groups. It was followed by the 2018 group ( M = 0.19, SD = 0.10) and 2020 group ( M = 0.14, SD = 0.10) which were relatively similar. Gain scores for students in 2017 ( M = 0.06, SD = 0.08) were the lowest (Fig. 1 & Table 6 ).
Finally, results from the ANCOVA show that the effect of the Major (STEM or Non STEM) on gain scores was statistically significant at p < .01; whereas the effects of academic level, gender, and the interactions between Major and academic level were statistically significant at p < .05 (Table 7 ). The Tukey HSD tests showed differences in Major, but not in the other variables at p < .05. The ANOVA results show that the effect of year for the Major was statistically significant at p < .05 (Table 7 ). In 2018, STEM students ( M = 0.21, SD = 0.09) obtained larger gains than Non-STEM students ( M = 0.12, SD = 0.13) in the same cohort. To explain these differences, in 2018, Non-STEM students already had a high pre-test scores ( M = 0.79, SD = 0.10) compared to STEM students ( M = 0.73, SD = 0.10), thus limiting their gain scores. The post-test scores for Non-STEM students ( M = 0.91, SD = 0.11) suggest that they obtained modest improvements, and these were close to STEM students ( M = 0.93, SD = 0.06). To complement, in 2018, 7 out of 8 of Non-STEM students (87.5%) were Juniors or Seniors compared to a more diverse group of STEM students (60% were from Post-baccalaureate to Sophomore, and 40% were Juniors or Seniors). Results from the qualitative analysis indicate that their learning might have been already covered in a combination of previous courses in the past. As a Junior student indicated, “I think the activities are important, but they have been covered in other courses for me”. No other significant differences were observed. These findings suggest that students, regardless of their major, academic level, and gender, were able to improve their initial knowledge of hydrologic concepts.
Modeling assignment
Analysis of the modeling assignment show that students’ use scores for the Water Balance Model (WBM) between 2017, 2018, 2019 , and 2020 were relatively similar, as shown in Fig. 2 and Table 8 . However, the 2021 group, F (4,290) = 18.43, p = 0.00, exhibited statistically significant differences with the previous years at p= .008. Students in this group scored the lowest compared to other years and showed the largest heterogeneity among groups, with the first and third quartile ranging from 0.34 to 0.71 on a percentual scale. No other statistically significant differences were observed across years for the modeling assignment. These results suggest that while students’ model use in 2021 was the lowest, students’ model-based use was relatively similar across previous years.
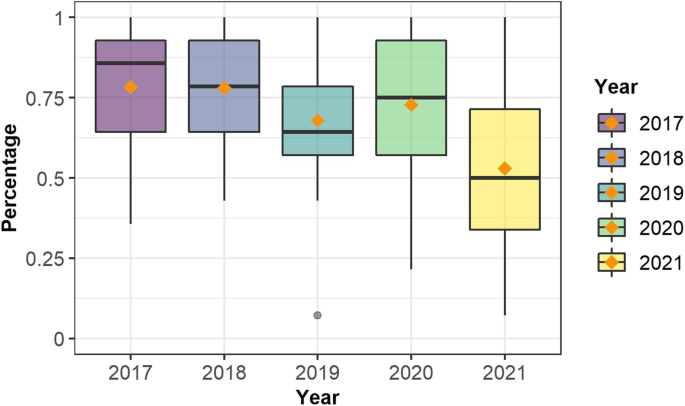
Water Balance Model’s use scores (shown as percentages)
Students’ overall perceptions of the course
In general, the analysis of the mid-semester course evaluation showed that the effect of year on student evaluation score was statistically significant, F (3,252) = 5.73, p = .000. The mean differences between 2020 and 2021 were statistically significant at p = .008. No other statistically significant differences were found. In this sense, it is possible to observe that students’ overall satisfaction with the course increased from 2017 to 2020. Considering a percentual scale, students evaluated the course the highest and most homogeneously in 2020, followed by 2018, and 2017. However, students’ evaluation dropped off in 2021 compared to 2020 (Fig. 3 & Table 9 ).
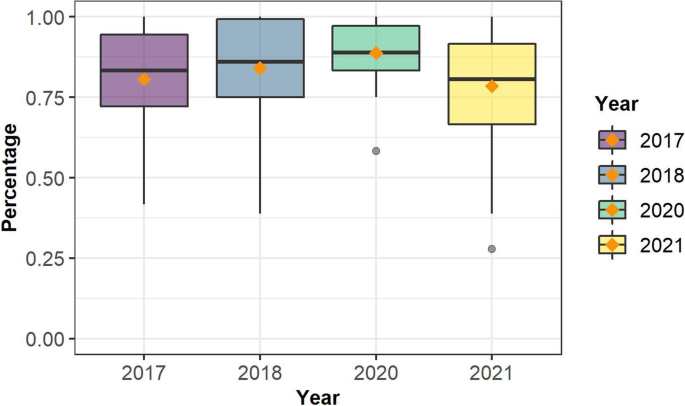
Mid Semester Total Evaluation scores (shown as percentages)
Results from the MSE suggest that over the years, students’ overall perception of the course was highly positive. As the course evolved, students' perceptions about the level of learning and opportunities to learn and provide different viewpoints improved all years (students’ agreement increased from 87% in 2017 to 93% in 2021, and from 85% in 2017 to 94% in 2021, respectively), and seem to have been the least affected by the transitions brought by COVID-19, as in 2021 students’ perception was less than 1% lower than the previous year. In this sense, the course has maintained an overarching value related to the importance of water for everyday life. In 2017 a student commented “I have learned a lot about how water contributes to more than just keeping humans hydrated, which is a good thing because I did not know how reliant we are on such a natural resource!”. The course also provided multiple views about water that helped students construct their own opinions and evaluate critically their own decisions. With regards to improvements, between 2017 to 2020, some students noted that the course could benefit from more in-depth content, especially regarding the social components of water, and the inclusion of cases addressing countries outside of the United States. In 2017, a student commented “I was expecting to go in depth on water policy and environmental justice issues throughout this course...”. Another student in 2017, who as an international student, commented that “it’s just hard to know where I would apply [the activities from the course] in my country” . In response, contents of the course were improved, and by 2021, students’ comments reflected how they valued the depth and breadth of water-related topics delivered through the videos and readings, and the introduction of global, national, and local cases. In that year, a student commented. “I am learning about water issues on the global level which I think is new to me”.
Other factors that seem to have been least affected by COVID-19 are the usefulness of the course to validate students’ current interests and even guide future career (students’ agreement increased from 75% in 2007 to 87% in 2021); the importance of course assessments as an important learning tool to review, clarify and demonstrate students’ learnings with a proper level of difficulty, application of decision-making skills to real-cases, and metacognition (students’ agreement increased from 78% in 2017 to 92% in 2021); and the learning outcomes (students’ agreement increased from 79% in 2017 to 93% in 2021) also improved over the years. These aspects showed a smaller than 2% difference in students’ agreement in 2021 compared to 2020. However, in 2020 and 2021, with the transition to a fully online and asynchronous mode, students experienced challenges with the assessments of the course. They expressed that, even with a similar workload compared to previous years, the daily assignments could be grouped together to reduce the workload and cognitive load. In 2020, a student said “... If I were to change something about the course, I would start with the duration of the assignments or the amount of work the students do”. Other improvements in the assessments identified by the students over the years include the need for more thorough explanations of the instructions, and time to develop them.
Students evaluated the team of instructors and learning assistants positively every year (students agreement increased from 89% in 2017 to 94% in 2020), and highlighted their expertise and pedagogy, support and guidance, engagement and delivery, feedback, and openness to address different opinions. However, these are areas where the impacts of COVID-19 are more visible in 2021, as there was a reduction in 4% to 5% of students’ perceptions compared to 2020. In 2021, as a result of the online and asynchronous mode, there was less teacher-student interaction, and less real-time opportunities to provide clarification which may have impacted students’ overall interaction with the course. In 2021 a student commented “This might be because of difficulties adjusting to COVID-19, but the online course is hard to be engaged and interested in”. Still, students valued their support and guidance through the feedback on assignments, and a Question-and-Answer module made accessible on Canvas to respond to students’ inquiries. With a higher degree of student-content interaction, the course videos were also important to support students’ engagement. In this sense, students recommended providing more emphasis to communication between instructors and students. Also, providing additional supports through a variety of course materials can help address some of the challenges of an asynchronous mode. As a student in 2021 indicated “I like how this class brings different types of material -- readings, videos, websites, etc. to students. This facilitates the assimilation and understanding of the key concepts being taught as the students view the learning process as dynamic”.
Working in a group environment was the area that seems most affected by the course transition resulting from COVID-19. Between 2017 and 2020 students’ perceptions about working in a group environment improved (students’ agreement increased from 80% in 2017 to 96% in 2020). However, there was a drop in 2021 compared to 2020 of 31%. In 2021, students emphasized the challenges reaching out to other students and coordinating group meetings online due to lack of responsiveness of other group members. A student shared “Group learning can be incredibly difficult in an online setting. I was lucky and got a really great group, but still online group work can be hard to organize from the student perspective”. Other challenges that were also reflected in previous years include the lack of cooperation and reliability of some group members; and the reduced opportunities for discussing ideas. A student in 2021 commented “... doing the infographic 100% asynchronously really allowed people to slack off. It was very difficult to maintain communication”. Still, over the years, including 2021, students highlighted that working in a functional group environment facilitated learning. Another affordance of the group environment is that it also enables students to learn and exchange different perspectives from various backgrounds. Students also highlighted that the group environment helped them develop personal and teamwork skills. Recommendations to support the group environment, particularly in an online setting, include providing more guidance, and follow-up to students. As a student in 2021 commented “[W] hen group projects are given the [re] should be more guidance given to allow for a better team-work” .
Water education is important to support students to develop water literacy, including knowledge, skills, attitudes, and behaviors to developing sustainable water management practices (Attari et al., 2017 ; King et al., 2012 ; McCarroll & Hamann, 2020 ; Su et al., 2011 ). This study builds upon prior research in the context of the Water in Society course (Forbes et al., 2018 ; Lally et al., 2020 ; Lally & Forbes, 2019 , 2020 ; Owens et al., 2020 ; White & Forbes, 2021 ), our broader undergraduate water education efforts (Petitt & Forbes, 2019 ; Sabel et al., 2017 ), as well as research in the field (AghaKouchak et al., 2013 ; Arthurs & Elwonger, 2018 ; Attari et al., 2017 ; Cardak, 2009 ; Covitt et al., 2009 ; Gunn et al., 2002 ; Habib et al., 2012 ; Johnson & Courter, 2020 ; King et al., 2012 ; Kingston et al., 2012 ; Li & Liu, 2003 ; Li et al., 2016 ; Merwade & Ruddell, 2012 ; Sibley et al., 2007 ; Smith et al., 2006 ; Su et al., 2011 ; Wagener et al., 2012 ; White et al., 2017 ; Willermet et al., 2013 ; Williams et al., 2009 ; Zigic & Lemckert, 2007 ). It provides important insight into efforts to cultivate undergraduate students’ water literacy through different course formats and the potential impacts of transitioning to a more student-content interaction (Li et al., 2016 ) as a result of the COVID-19 pandemic.
First, research has shown that students and adults may not hold scientifically accurate conceptions about water (Arthurs & Elwonger, 2018 ; Attari et al., 2017 ; Cardak, 2009 ; Covitt et al., 2009 ; Duda et al., 2005 ; Johnson & Courter, 2020 ; Sibley et al., 2007 ; Williams et al., 2009 ). Based on the results from the analysis of the Pre-/Post-tests, it is encouraging to see that each year the course has been effective in helping students develop introductory disciplinary knowledge, regardless of their initial understanding of hydrologic concepts, academic level, major, and gender, even with the transition to a fully online environment. Furthermore, the course has exhibited not only a high level of consistency in helping students attain what we believe a reasonable (88%) to high level (93% and above) of disciplinary understanding at the introductory level, but also engagement in data-driven modeling of real-world, water-related scenarios through the modeling assignment, for which students’ performance was similar across Years 1-4. While the relative consistency of these student outcomes can be interpreted as a positive, and we did observe some modest improvements in modeling sub-processes in a previous study (Lally & Forbes, 2019 ), it also suggests that changes to the course over time, particularly its increasingly hybrid nature and student-centeredness, did not substantially enhance student’s overall model-based reasoning as intended, at least as measured through the WBM task. We hypothesize that independent of the course format and structure, students may need various kinds of support learning to use and interpret the results from data-driven, computer-based models more effectively. Future research should continue to investigate what these supports are and how they can be employed to enhance undergraduate students’ learning about water across course formats.
Second, study findings show that students’ satisfaction with the course increased between 2017 to 2020. We interpret this result as affirmation of the more student-centered, research-based, and flipped nature of the course as it evolved over the five years. Prior research has shown that students respond favorably to research-based practices in undergraduate classrooms, including the use of class meetings for active learning, engagement with instructors, peers, and learning assistants, and collaborative problem-solving, all of which contrast with more traditional, one-directional, lecture-based classroom climates (Habib et al., 2012 ; Halvorson & Westcoat, 2002 ; Kingston et al., 2012 ; Li & Liu, 2003 ; Smith et al., 2006 ; Thompson et al., 2012 ; White et al., 2017 ; Willermet et al., 2013 ). In an engaging and learner-centric classroom, students are better positioned to internalize the importance of water resources management for their personal and professional future endeavors, recognize the relevance of the course to guide them in their selection of a water-related career, to develop decision-making skills, and to work with interdisciplinary teams. In general, this finding supports the idea that students generally respond favorably to student-centered undergraduate learning environments that foreground active learning and student engagement.
Third, study findings also yield insights into the impacts of the COVID-19 pandemic on undergraduate STEM education. As shown in the results, students’ satisfaction with the course decreased in 2021 after consistently increasing each year between 2017 to 2020. Additionally, in 2021, we observed a sizeable decrease in students’ performance on the WBM assignment, a critical opportunity for students to learn to analyze SHIs, after relatively stable outcomes across Years 1-4 (AghaKouchak et al., 2013 ; Attari et al., 2017 ; Handelsman et al., 2004 ; Gunn et al., 2002 ; King et al., 2012 ; Lally & Forbes, 2019 , 2020 ; Merwade & Ruddell, 2012 ; Sibley et al., 2007 ; Zigic & Lemckert, 2007 ). We believe these results to be attributable to disruptions brought about by COVID-19 for several specific reasons. Changes to the course in Year 5 (2021) fundamentally altered dynamics of collaboration between instructors and students, including peer interactions, especially as compared to the increasingly flipped and student-centered course model in Years 2-5. The decreases in students’ model-based reasoning and perceptions of the course in 2021 might have resulted from the transition to more participant-content interaction in which students mostly engaged with course materials and assignments and had less engagement with the instructor, teaching assistants, and peers in an asynchronous environment (Li et al., 2016 ). Although different opportunities for communication were provided, students may need additional encouragement to take advantage of these resources. Communication among peers is another area that may require additional support in an online and asynchronous setting. We also recognize the increasing levels of pandemic fatigue from which students were suffering in spring, 2021, by which time students had been experiencing a year or more of pandemic-related disruptions. The rapid and often uneven transition to a mostly or fully virtual undergraduate experience brought about by the pandemic was jarring and presented challenges for instructors and students alike. As such, findings from this research suggest that conditions brought about by COVID-19 may have impacted students’ experience with the course as compared to previous years.
Limitations and future research
While the present study illustrates longitudinal outcomes in undergraduate STEM education, including potential impacts of the COVID-19 pandemic, it is limited in a number of ways. First, it highlights only a subset of potential water-related student learning outcomes we may seek to cultivate. Subsequent research should explore additional outcomes related to undergraduate students’ learning about water systems and SHIs. Second, it is limited in scope to the specific population of students served by the course. Future research could replicate and elaborate upon the present study by focusing on larger and different student populations, which may provide insight into learning needs of particular groups of students. Such research may involve inclusion of additional student-level variables, such as access to technology, household socio-economic status, and affective orientations towards STEM, as well as course-related variables, such as communication practices with instructors, experience working in more independent environments, and engagement with other course elements, all of which may have contributed to challenges students experienced during the course amid the global pandemic. This work would help clarify how the course’s characteristics may have afforded and/or constrained students’ learning in the midst of COVID-19 and identify additional curricular and instructional supports for students.
Water-related issues remain one of the greatest challenges facing the global community. Education, and particularly undergraduate STEM education, has a critical role to play in adequately preparing individuals to problem-solve and address SHIs in varying domains of everyday life. Results presented here showcase one effort to prepare students to reason about real-world issues and how these efforts were impacted by another global challenge: the COVID-19 pandemic. As such, this research provides important contributions to the design and delivery of innovative undergraduate STEM educational interventions, including those foregrounding disciplinary concepts and model-based reasoning about socio-hydrologic systems, under unique and challenging educational circumstances brought about by the COVID-19 pandemic.
Availability of data and materials
The datasets used and/or analyzed during the current study are available from the corresponding author on reasonable request.
Change history
22 march 2022.
A Correction to this paper has been published: https://doi.org/10.1186/s43031-022-00050-5
AghaKouchak, A., Nakhjiri, N., & Habib, E. (2013). An educational model for ensemble streamflow simulation and uncertainty analysis. Hydrology and Earth System Sciences , 17 (2), 445–452. https://doi.org/10.5194/hess-17-445-2013 .
Article Google Scholar
Arthurs, L. A., & Elwonger, J. M. (2018). Mental models of groundwater residence: A deeper understanding of students’ preconceptions as a resource for teaching and learning about groundwater and aquifers. Journal of Astronomy & Earth Sciences Education , 5 (1), 53–56. https://doi.org/10.19030/jaese.v5i1.10192 .
Attari, S., Poinsatte-Jones, K., & Hinton, K. (2017). Perceptions of water systems. Judgement and Decision Making , 12 (3), 314–327 http://journal.sjdm.org/17/17124/jdm17124.html .
Google Scholar
Cardak, O. (2009). Science students’ misconceptions of the water cycle according to their drawings. Journal of Applied Sciences , 9 (5), 865–873 https://scialert.net/abstract/?doi=jas.2009.865.873 .
Covitt, B. A., Gunckel, K. L., & Anderson, C. W. (2009). Students’ developing understanding of water in environmental systems. The Journal of Environmental Education , 40 (3), 37–51. https://doi.org/10.3200/JOEE.40.3.37-51 .
Duda, M. D., De Michele, P. E., Jones, M., Criscione, A., Craun, C., & Winegord, & Herrick, J. B. (2005). Americans’ knowledge of and attitudes toward water and water-related issues . Harrisonburg: Responsive Management National Office.
Forbes, C. T., Brozović, N., Franz, T. E., Lally, D. E., & Petitt, D. N. (2018). Water in Society: An interdisciplinary course to support undergraduate students’ water literacy. Journal of College Science Teaching, 48 (1), 36–42. https://www.jstor.org/stable/26491338
Gunn, R. L., Mohtar, R. H., & Engel, B. A. (2002). World-Wide-Web--based soil and water quality modeling in undergraduate education. Journal of Natural Resources and Life Sciences Education , 31 , 141–147. https://doi.org/10.2134/jnrlse.2002.0141 .
Habib, E., Ma, Y., Williams, D., Sharif, H. O., & Hossain, F. (2012). HydroViz: Design and evaluation of a Web-based tool for improving hydrology education. Hydrology and Earth System Sciences , 16 , 3767–3781. https://doi.org/10.5194/hess-16-3767-2012 .
Halvorson, S. J., & Westcoat Jr., J. L. (2002). Problem-based inquiry on world water problems in large undergraduate classes. Journal of Geography , 101 (3), 91–102. https://doi.org/10.1080/00221340208978480 .
Handelsman, J., Ebert-May, D., Beichner, R., Bruns, P., Chang, A., DeHann, R., … Wood, W. (2004). Scientific teaching. Science , 304 (5670), 521–522. https://doi.org/10.1126/science.1096022 .
IPCC (2021). Summary for Policymakers. In V. Masson-Delmotte, P. Zhai, A. Pirani, S. L. Connors, C. Péan, S. Berger, et al. (Eds.), Climate Change 2021: The Physical Science Basis. Contribution of Working Group I to the Sixth Assessment Report of the Intergovernmental Panel on Climate Change . Cambridge University Press In Press.
Johnson, D., & Courter, J. (2020). Assessing water literacy at a primarily undergraduate university in Ohio. Natural Sciences Education , 49 (1), e20024. https://doi.org/10.1002/nse2.20024 .
King, E. G., O’Donnell, F. C., & Caylor, K. K. (2012). Reframing hydrology education to solve coupled human and environmental problems. Hydrology and Earth System Sciences , 16 , 4023–4031. https://doi.org/10.5194/hess-16-4023-2012 .
Kingston, D. G., Eastwood, W. J., Jones, P. I., Johnson, R., Marshall, S., & Hannah, D. M. (2012). Experiences of using mobile technologies and virtual field tours in physical geography: implications for hydrology education. Hydrology and Earth System Sciences , 16 , 1281–1286. https://doi.org/10.5194/hess-16-1281-2012 .
Lally, D., & Forbes, C. T. (2019). Modelling water systems in an introductory undergraduate course: Students’ use and evaluation of data-driven, computer-based models. International Journal of Science Education, 41 (14), 1999–2023. https://doi.org/10.1080/09500693.2019.1657252
Lally, D., & Forbes, C. T. (2020). Sociohydrologic Systems Thinking: An analysis of undergraduate students’ operationalization and modeling of coupled human-water systems. Water, 12 (1040). https://doi.org/10.3390/w12041040
Lally, D., Franz, T., & Forbes, C. (2020). Undergraduate education about water and climate change: Students’ use of a water balance model. The Journal of Sustainability Education.
Li, S.-G., & Liu, Q. (2003). Interactive Groundwater (IGW): An innovative digital laboratory for groundwater education and research. Computer Applications in Engineering Education , 11 (4), 179–202. https://doi.org/10.1002/cae.10052 .
Li, Y., Krasny, M., & Russ, A. (2016). Interactive learning in an urban environmental education online course. Environmental Education Research. , 22 (1), 111–128. https://doi.org/10.1080/13504622.2014.989961 .
McCarroll, M., & Hamann, H. (2020). What we know about water: A water literacy review. Water , 12 (10), 2803. https://doi.org/10.3390/w12102803 .
Merwade, V., & Ruddell, B. L. (2012). Moving university hydrology education forward with geoinformatics, data and modeling approaches. Hydrology & Earth System Sciences , 16 (8), 2393–2404. https://doi.org/10.5194/hess-16-2393-2012 .
Owens, D., Petitt, D., Lally, D., & Forbes, C. T. (2020). Cultivating water literacy in STEM education: Undergraduates’ socio-scientific reasoning about socio-hydrologic issues. Water, 12 (2857). https://doi.org/10.3390/w12102857
Petitt, D. N., & Forbes, C. T. (2019). Values use of undergraduate students in socio-hydrological reasoning: A comparative study. Natural Sciences Education, 48 (1), 1–12. https://doi.org/10.4195/nse2018.09.0016
Sabel, J. L., Vo, T., Alred, A., Dauer, J. M., & Forbes, C. T. (2017). Research and teaching: Undergraduate students’ scientifically informed decision making about socio-hydrological issues. Journal of College Science Teaching, 46 (6):71-79.
Sibley, D. F., Anderson, C. W., Heidemann, M., Merrill, J. E., Parker, J. M., & Szymanski, D. W. (2007). Box diagrams to assess students’ systems thinking about the rock, water and carbon cycles. Journal of Geoscience Education , 55 (2), 138–146. https://doi.org/10.5408/1089-9995-55.2.138 .
Smith, J. M., Edwards, P., & Raschke, J. (2006). Using technology and inquiry to improve student understanding of watershed concepts. Journal of Geography , 105 (6), 249–257. https://doi.org/10.1080/00221340608978694 .
Su, H.-J., Chen, M.-J., & Wang, J.-T. (2011). Developing a water literacy. Current Opinion in Environmental Sustainability , 3 (6), 571–519. https://doi.org/10.1016/j.cosust.2011.10.010 .
Thompson, S. E., Ngambeki, I., Troch, P. A., Sivapalan, M., & Evangelou, D. (2012). Incorporating student-centered approaches into catchment hydrology teaching: A review and synthesis. Hydrology and Earth System Sciences , 16 , 3263–3278. https://doi.org/10.5194/hess-16-3263-2012 .
UNESCO, & UN Water. (2020). The United Nations World Water Development Report 2020: Water and Climate Change. Accessed January 18, 2021, https://unesdoc.unesco.org/ark:/48223/pf0000372985.locale=en
Wagener, T., Kelleher, C., Weiler, M., McGlynn, B., Gooseff, M., Marshall, L., … Zappe, S. (2012). It takes a community to raise a hydrologist: The Modular Curriculum for Hydrologic Advancement (MOCHA). Hydrology and Earth System Sciences , 16 , 3405–3418. https://doi.org/10.5194/hess-16-3405-2012 .
White, H., & Forbes, C. T. (2021). An investigation of undergraduate students’ spatial thinking about groundwater. Journal of Geography in Higher Education. https://doi.org/10.1080/03098265.2021.2004582
White, T., Wymore, A., Dere, A., Hoffman, A., Washburne, J., & Conklin, M. (2017). Integrated interdisciplinary science of the critical zone as a foundational curriculum for addressing issues of environmental sustainability. Journal of Geoscience Education , 65 , 136–145. https://doi.org/10.5408/16-171.1 .
Willermet, C., Mueller, A., Juris, S. J., Drake, E., Upadhaya, S., & Chhetri, P. (2013). Water as life, death, and power: Building an integrated interdisciplinary course combining perspectives from anthropology, biology, and chemistry. Journal of the Scholarship of Teaching and Learning , 13 (5), 106–124 https://scholarworks.iu.edu/journals/index.php/josotl/article/view/3875 .
Williams, A., Lansey, K., & Washburne, J. (2009). A dynamic simulation-based water resources education tool. Journal of Environmental Management , 90 (1), 471–482. https://doi.org/10.1016/j.jenvman.2007.11.005 .
Zigic, S., & Lemckert, C. J. (2007). Development of an interactive computer-based learning strategy to assist in teaching water quality modelling. Computers & Education , 49 (4), 1246–1257. https://doi.org/10.1016/j.compedu.2006.01.011 .
Download references
Acknowledgements
We thank Nick Brozovic, Trenton Franz, staff from the UNL Statistical Cross Disciplinary Collaboration and Consulting Lab, and, most importantly, the students who have partnered with us to enhance understanding about water in the course.
This material is based upon work supported by the National Science Foundation under Grant No. DUE-1609598 and the Daugherty Water for Food Institute. Any opinions, findings, and conclusions or recommendations expressed in this material are those of the author(s) and do not necessarily reflect the views of the National Science Foundation.
Author information
Authors and affiliations.
University of Texas at Arlington, Texas, Arlington, USA
Silvia-Jessica Mostacedo-Marasovic & Cory Forbes
Industry, Lexington, Kentucky, USA
Diane Lally
University of North Carolina at Charlotte, Charlotte, North Carolina, USA
Destini N. Petitt
University of Maine, Orono, Maine, USA
Holly White
You can also search for this author in PubMed Google Scholar
Contributions
SJMM The author has made substantial contribution to the conception, and design of the work; the acquisition, analysis, and interpretation of data; the drafting and substantial revision of the work; and has approved the submitted version. The author has agreed both to be personally accountable for the author’s own contributions and to ensure that questions related to the accuracy or integrity of any part of the work, even ones in which the author was not personally involved are appropriately investigated, resolved, and the resolution documented in the literature. DL The author has made substantial contribution to the acquisition of data; the substantial revision of the work; and has approved the submitted version. The author has agreed both to be personally accountable for the author’s own contributions and to ensure that questions related to the accuracy or integrity of any part of the work, even ones in which the author was not personally involved are appropriately investigated, resolved, and the resolution documented in the literature. DP The author has made substantial contribution to the acquisition of data; the substantial revision of the work; and has approved the submitted version. The author has agreed both to be personally accountable for the author’s own contributions and to ensure that questions related to the accuracy or integrity of any part of the work, even ones in which the author was not personally involved are appropriately investigated, resolved, and the resolution documented in the literature. HW The author has made substantial contribution to the conception, and design of the work; the acquisition of data; the drafting and substantial revision of the work; and has approved the submitted version. The author has agreed both to be personally accountable for the author’s own contributions and to ensure that questions related to the accuracy or integrity of any part of the work, even ones in which the author was not personally involved are appropriately investigated, resolved, and the resolution documented in the literature. CF The author has made substantial contribution to the conception, and design of the work; the acquisition, analysis, and interpretation of data; the drafting and substantial revision of the work; and has approved the submitted version. The author has agreed both to be personally accountable for the author’s own contributions and to ensure that questions related to the accuracy or integrity of any part of the work, even ones in which the author was not personally involved are appropriately investigated, resolved, and the resolution documented in the literature.
Corresponding author
Correspondence to Silvia-Jessica Mostacedo-Marasovic .
Ethics declarations
Ethics approval and consent to participate.
This study has been approved by the University of Nebraska-Lincoln Institutional Review Board. All subjects provided written consent to participate in the research.
Consent for publication
All authors provide consent for publication.
Competing interests
The authors declare that they have no competing interests.
Additional information
Publisher’s note.
Springer Nature remains neutral with regard to jurisdictional claims in published maps and institutional affiliations.
This article has been updated to correct an incorrect author name.
Supplementary Information
Additional file 1: appendix 1..
Students’ demographics.
Additional file 2: Appendix 2.
Descriptive statistics.
Additional file 3: Appendix 3.
Pre-test scores: (a) ANOVAs and (b) Tukey HSD tests.
Additional file 4: Appendix 4.
Post-test scores: (a) ANOVAs and (b) Tukey HSD tests.
Additional file 5: Appendix 5.
Gain scores: (a) ANOVAs and (b) Tukey HSD tests.
Additional file 6: Appendix 6.
Water Balance Model: (a) ANOVAs and (b) Tukey HSD tests.
Additional file 7: Appendix 7.
Mid-Semester Evaluation: (a) ANOVAs and (b) Tukey HSD tests.
Additional file 8: Appendix 8.
Major: ANOVA.
Additional file 9: Appendix 9.
(a) ANCOVA: Type III Tests of Fixed effects and (b) Tukey HSD test.
Rights and permissions
Open Access This article is licensed under a Creative Commons Attribution 4.0 International License, which permits use, sharing, adaptation, distribution and reproduction in any medium or format, as long as you give appropriate credit to the original author(s) and the source, provide a link to the Creative Commons licence, and indicate if changes were made. The images or other third party material in this article are included in the article's Creative Commons licence, unless indicated otherwise in a credit line to the material. If material is not included in the article's Creative Commons licence and your intended use is not permitted by statutory regulation or exceeds the permitted use, you will need to obtain permission directly from the copyright holder. To view a copy of this licence, visit http://creativecommons.org/licenses/by/4.0/ .
Reprints and permissions
About this article
Cite this article.
Mostacedo-Marasovic, SJ., Lally, D., Petitt, D.N. et al. Supporting undergraduate students’ developing water literacy during a global pandemic: a longitudinal study. Discip Interdscip Sci Educ Res 4 , 7 (2022). https://doi.org/10.1186/s43031-022-00049-y
Download citation
Received : 15 October 2021
Accepted : 16 February 2022
Published : 07 March 2022
DOI : https://doi.org/10.1186/s43031-022-00049-y
Share this article
Anyone you share the following link with will be able to read this content:
Sorry, a shareable link is not currently available for this article.
Provided by the Springer Nature SharedIt content-sharing initiative
- Water literacy
- Water-based models
- Course effectiveness
Thank you for visiting nature.com. You are using a browser version with limited support for CSS. To obtain the best experience, we recommend you use a more up to date browser (or turn off compatibility mode in Internet Explorer). In the meantime, to ensure continued support, we are displaying the site without styles and JavaScript.
- View all journals
- Explore content
- About the journal
- Publish with us
- Sign up for alerts
- Review Article
- Published: 31 January 2023
Global water resources and the role of groundwater in a resilient water future
- Bridget R. Scanlon ORCID: orcid.org/0000-0002-1234-4199 1 ,
- Sarah Fakhreddine 1 , 2 ,
- Ashraf Rateb 1 ,
- Inge de Graaf ORCID: orcid.org/0000-0001-7748-868X 3 ,
- Jay Famiglietti 4 ,
- Tom Gleeson 5 ,
- R. Quentin Grafton 6 ,
- Esteban Jobbagy 7 ,
- Seifu Kebede 8 ,
- Seshagiri Rao Kolusu 9 ,
- Leonard F. Konikow 10 ,
- Di Long ORCID: orcid.org/0000-0001-9033-5039 11 ,
- Mesfin Mekonnen ORCID: orcid.org/0000-0002-3573-9759 12 ,
- Hannes Müller Schmied 13 , 14 ,
- Abhijit Mukherjee 15 ,
- Alan MacDonald ORCID: orcid.org/0000-0001-6636-1499 16 ,
- Robert C. Reedy 1 ,
- Mohammad Shamsudduha 17 ,
- Craig T. Simmons 18 ,
- Alex Sun 1 ,
- Richard G. Taylor 19 ,
- Karen G. Villholth 20 ,
- Charles J. Vörösmarty 21 &
- Chunmiao Zheng ORCID: orcid.org/0000-0001-5839-1305 22
Nature Reviews Earth & Environment volume 4 , pages 87–101 ( 2023 ) Cite this article
14k Accesses
117 Citations
290 Altmetric
Metrics details
- Hydrogeology
- Water resources
An Author Correction to this article was published on 29 March 2023
This article has been updated
Water is a critical resource, but ensuring its availability faces challenges from climate extremes and human intervention. In this Review, we evaluate the current and historical evolution of water resources, considering surface water and groundwater as a single, interconnected resource. Total water storage trends have varied across regions over the past century. Satellite data from the Gravity Recovery and Climate Experiment (GRACE) show declining, stable and rising trends in total water storage over the past two decades in various regions globally. Groundwater monitoring provides longer-term context over the past century, showing rising water storage in northwest India, central Pakistan and the northwest United States, and declining water storage in the US High Plains and Central Valley. Climate variability causes some changes in water storage, but human intervention, particularly irrigation, is a major driver. Water-resource resilience can be increased by diversifying management strategies. These approaches include green solutions, such as forest and wetland preservation, and grey solutions, such as increasing supplies (desalination, wastewater reuse), enhancing storage in surface reservoirs and depleted aquifers, and transporting water. A diverse portfolio of these solutions, in tandem with managing groundwater and surface water as a single resource, can address human and ecosystem needs while building a resilient water system.
Net trends in total water storage data from the GRACE satellite mission range from −310 km 3 to 260 km 3 total over a 19-year record in different regions globally, caused by climate and human intervention.
Groundwater and surface water are strongly linked, with 85% of groundwater withdrawals sourced from surface water capture and reduced evapotranspiration, and the remaining 15% derived from aquifer depletion.
Climate and human interventions caused loss of ~90,000 km 2 of surface water area between 1984 and 2015, while 184,000 km 2 of new surface water area developed elsewhere, primarily through filling reservoirs.
Human intervention affects water resources directly through water use, particularly irrigation, and indirectly through land-use change, such as agricultural expansion and urbanization.
Strategies for increasing water-resource resilience include preserving and restoring forests and wetlands, and conjunctive surface water and groundwater management.
This is a preview of subscription content, access via your institution
Access options
Access Nature and 54 other Nature Portfolio journals
Get Nature+, our best-value online-access subscription
$29.99 / 30 days
cancel any time
Subscribe to this journal
Receive 12 digital issues and online access to articles
$99.00 per year
only $8.25 per issue
Buy this article
Purchase on Springer Link
Instant access to full article PDF
Prices may be subject to local taxes which are calculated during checkout
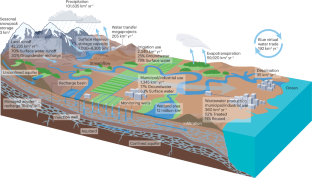
Similar content being viewed by others
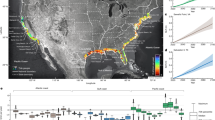
Disappearing cities on US coasts
Leonard O. Ohenhen, Manoochehr Shirzaei, … Robert J. Nicholls
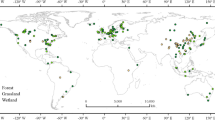
Meta-analysis shows the impacts of ecological restoration on greenhouse gas emissions
Tiehu He, Weixin Ding, … Quanfa Zhang
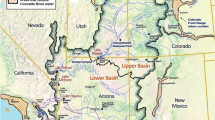
New water accounting reveals why the Colorado River no longer reaches the sea
Brian D. Richter, Gambhir Lamsal, … John C. Schmidt
Change history
29 march 2023.
A Correction to this paper has been published: https://doi.org/10.1038/s43017-023-00418-9
Vorosmarty, C. J. et al. Global threats to human water security and river biodiversity. Nature 467 , 555–561 (2010).
Article Google Scholar
Doell, P., Mueller Schmied, H., Schuh, C., Portmann, F. T. & Eicker, A. Global-scale assessment of groundwater depletion and related groundwater abstractions: combining hydrological modeling with information from well observations and GRACE satellites. Water Resour. Res. 50 , 5698–5720 (2014).
Wada, Y. et al. Global depletion of groundwater resources. Geophys. Res. Lett. 37 , L20402 (2010).
Douville, H. et al. in Climate Change 2021: The Physical Science Basis (eds Masson-Delmotte, V. et al.) 1055–1210 (IPCC, Cambridge Univ. Press, 2021).
Olivier, D. W. & Xu, Y. X. Making effective use of groundwater to avoid another water supply crisis in Cape Town, South Africa. Hydrogeol. J. 27 , 823–826 (2019).
Ozment, S. et al. Natural infrastructure in Sao Paulo’s water system. World Resources Institute Report 2013–2014: Interim Findings (2018).
Pascale, S., Kapnick, S. B., Delworth, T. L. & Cooke, W. F. Increasing risk of another Cape Town ‘Day Zero’ drought in the 21st century. Proc. Natl Acad. Sci. USA 117 , 29495 (2020).
Alley, W. M., Reilly, T. E. & Franke, O. L. Sustainability of ground-water resources. US Geological Survey Circular 1186 (1999).
Breslin, S. COP26 has 4 goals. Water is central to all of them. SIWI News https://siwi.org/latest/cop26-has-4-goals-water-is-central-to-all-of-them/ (2021).
Global Risks 2021 16th edition (World Economic Forum, 2021); https://www.weforum.org/reports/the-global-risks-report-2021/
The Water Challenge: The Roundtable on Water Financing (OECD, 2022); https://www.oecd.org/water/roundtable-on-financing-water.htm
The United Nations World Water Development Report 2018: Nature-Based Solutions for Water (United Nations World Water Assessment Program/UNESCO, 2018).
Browder, G., Ozment, S., Rehberger-Bescos, I., Gartner, T. & Lange, G. M. Integrating Green and Gray: Creating Next Generation Infrastructure (World Bank and World Resources Institute, 2019); https://openknowledge.worldbank.org/handle/10986/31430
Making Every Drop Count: Agenda for Water Action (High Level Panel on Water, United Nations and World Bank, 2018).
Lederer, E. M. Next UN assembly president warns world in dangerous crisis. Washington Post https://www.washingtonpost.com/world/next-un-assembly-president-warns-world-in-dangerous-crisis/2022/06/07/55075dce-e6b6-11ec-a422-11bbb91db30b_story.html (7 June 2022).
Tapley, B. D. et al. Contributions of GRACE to understanding climate change. Nat. Clim. Change 9 , 358–369 (2019).
Wada, Y. & Bierkens, M. F. P. Sustainability of global water use: past reconstruction and future projections. Environ. Res. Lett. https://doi.org/10.1088/1748-9326/9/10/104003 (2014).
Mekonnen, M. M. & Hoekstra, A. Y. Blue water footprint linked to national consumption and international trade is unsustainable. Nat. Food 1 , 792–800 (2020).
Rodell, M. et al. Emerging trends in global freshwater availability. Nature 557 , 651–659 (2018).
Save, H., Bettadpur, S. & Tapley, B. D. High-resolution CSR GRACE RL05 mascons. J. Geophys. Res. Solid Earth 121 , 7547–7569 (2016).
Tapley, B. D., Bettadpur, S., Watkins, M. & Reigber, C. The Gravity Recovery And Climate Experiment: mission overview and early results. Geophys. Res. Lett. https://doi.org/10.1029/2004gl019920 (2004).
Richey, A. S. et al. Quantifying renewable groundwater stress with GRACE. Water Resour. Res. 51 , 5217–5238 (2015).
Shamsudduha, M. & Taylor, R. G. Groundwater storage dynamics in the world’s large aquifer systems from GRACE: uncertainty and role of extreme precipitation. Earth Syst. Dyn. 11 , 755–774 (2020).
Vishwakarma, B. D., Bates, P., Sneeuw, N., Westaway, R. M. & Bamber, J. L. Re-assessing global water storage trends from GRACE time series. Environ. Res. Lett. 16 , 034005 (2021).
Pekel, J. F., Cottam, A., Gorelick, N. & Belward, A. S. High-resolution mapping of global surface water and its long-term changes. Nature 540 , 418–436 (2016).
Lehner, B. et al. High-resolution mapping of the world’s reservoirs and dams for sustainable river-flow management. Front. Ecol. Environ. 9 , 494–502 (2011).
Scanlon, B. R. et al. Global models underestimate large decadal declining and rising water storage trends relative to GRACE satellite data. Proc. Natl Acad. Sci. USA 115 , E1080–E1089 (2018).
Winter, T. C., Harvey, J. W., Franke, O. L. & Alley, W. M. Ground Water and Surface Water: A Single Resource . Circular 1139 (United States Geological Survey, 1998).
Konikow, L. F. Overestimated water storage. Nat. Geosci. 6 , 3 (2013).
Konikow, L. F. Contribution of global groundwater depletion since 1900 to sea-level rise. Geophys. Res. Lett. https://doi.org/10.1029/2011gl048604 (2011).
Pokhrel, Y. N. et al. Model estimates of sea-level change due to anthropogenic impacts on terrestrial water storage. Nat. Geosci. 5 , 389–392 (2012).
de Graaf, I. E. M. et al. A global-scale two-layer transient groundwater model: development and application to groundwater depletion. Adv. Water Resour. 102 , 53–67 (2017).
Rateb, A. et al. Comparison of groundwater storage changes from GRACE satellites with monitoring and modeling of major U.S. aquifers. Water Resour. Res. https://doi.org/10.1029/2020WR027556 (2020).
de Graaf, I. E. M., Gleeson, T., van Beek, L. P. H., Sutanudjaja, E. H. & Bierkens, M. F. P. Environmental flow limits to global groundwater pumping. Nature 574 , 90–94 (2019).
Sophocleous, M. From safe yield to sustainable development of water resources — the Kansas experience. J. Hydrol. 235 , 27–43 (2000).
Konikow, L. F. & Bredehoeft, J. D. Groundwater Resource Development: Effects and Sustainability (The Groundwater Project, 2020).
MacAllister, D. J., Krishan, G., Basharat, M., Cuba, D. & MacDonald, A. M. A century of groundwater accumulation in Pakistan and northwest India. Nat. Geosci. https://doi.org/10.1038/s41561-022-00926-1 (2022).
Scanlon, B. R. et al. Effects of climate and irrigation on GRACE-based estimates of water storage changes in major US aquifers. Environ. Res. Lett. https://doi.org/10.1088/1748-9326/ac16ff (2021).
McGuire, V. L. Water-Level and Recoverable Water In Storage Changes, High Plains Aquifer, Predevelopment to 2015 and 2013–15 . US Geological Survey Scientific Investigations Report 2017–5040 (2017); https://doi.org/10.3133/sir20175040
Faunt, C. C. Groundwater availability of the Central Valley Aquifer, California. US Geol. Surv. Prof. Pap . 1766 (2009).
Vorosmarty, C. J., Green, P., Salisbury, J. & Lammers, R. B. Global water resources: vulnerability from climate change and population growth. Science 289 , 284–288 (2000).
Mekonnen, M. M. & Hoekstra, A. Y. Four billion people facing severe water scarcity. Sci. Adv. https://doi.org/10.1126/sciadv.1500323 (2016).
Vorosmarty, C. J. & Sahagian, D. Anthropogenic disturbance of the terrestrial water cycle. Bioscience 50 , 753–765 (2000).
Gronwall, J. & Danert, K. Regarding groundwater and drinking water access through a human rights lens: self-supply as a norm. Water https://doi.org/10.3390/w12020419 (2020).
van Vliet, M. T. H. et al. Global water scarcity including surface water quality and expansions of clean water technologies. Environ. Res. Lett. https://doi.org/10.1088/1748-9326/abbfc3 (2021).
Podgorski, J. & Berg, M. Global threat of arsenic in groundwater. Science 368 , 845–850 (2020).
Yapiyev, V., Sagintayev, Z., Inglezakis, V. J., Samarkhanov, K. & Verhoef, A. Essentials of endorheic basins and lakes: a review in the context of current and future water resource management and mitigation activities in Central Asia. Water https://doi.org/10.3390/w9100798 (2017).
Pauloo, R. A., Fogg, G. E., Guo, Z. L. & Harter, T. Anthropogenic basin closure and groundwater salinization (ABCSAL). J. Hydrol. https://doi.org/10.1016/j.jhydrol.2020.125787 (2021).
Cao, T. Z., Han, D. M. & Song, X. F. Past, present, and future of global seawater intrusion research: a bibliometric analysis. J. Hydrol. https://doi.org/10.1016/j.jhydrol.2021.126844 (2021).
Werner, A. D. et al. Seawater intrusion processes, investigation and management: recent advances and future challenges. Adv. Water Resour. 51 , 3–26 (2013).
Held, I. M. & Soden, B. J. Robust responses of the hydrological cycle to global warming. J. Clim. 19 , 5686–5699 (2006).
Fan, X., Duan, Q. Y., Shen, C. P., Wu, Y. & Xing, C. Global surface air temperatures in CMIP6: historical performance and future changes. Environ. Res. Lett. 15 , 104056 (2020).
Tabari, H. Climate change impact on flood and extreme precipitation increases with water availability. Sci. Rep. https://doi.org/10.1038/s41598-020-70816-2 (2020).
Williams, A. P. et al. Large contribution from anthropogenic warming to an emerging North American megadrought. Science 368 , 314 (2020).
Arias, P. A. et al. in Climate Change 2021: The Physical Science Basis (eds Masson-Delmotte, V. et al.) 33−144 (IPCC, Cambridge Univ. Press, 2021).
van Dijk, A. et al. The Millennium Drought in southeast Australia (2001–2009): natural and human causes and implications for water resources, ecosystems, economy, and society. Water Resour. Res. 49 , 1040–1057 (2013).
Scanlon, B. R. et al. Hydrologic implications of GRACE satellite data in the Colorado River Basin. Water Resour. Res. 51 , 9891–9903 (2015).
Rateb, A., Scanlon, B. R. & Kuo, C. Y. Multi-decadal assessment of water budget and hydrological extremes in the Tigris-Euphrates Basin using satellites, modeling, and in-situ data. Sci. Total Environ. https://doi.org/10.1016/j.scitotenv.2020.144337 (2021).
Anyamba, A., Glennie, E. & Small, J. Teleconnections and interannual transitions as observed in African vegetation: 2015–2017. Remote Sens. https://doi.org/10.3390/rs10071038 (2018).
Scanlon, B. R. et al. Linkages between GRACE water storage, hydrologic extremes, and climate teleconnections in major African aquifers. Environ. Res. Lett. https://doi.org/10.1088/1748-9326/ac3bfc (2022).
Ul Hassan, W. & Nayak, M. A. Global teleconnections in droughts caused by oceanic and atmospheric circulation patterns. Environ. Res. Lett. https://doi.org/10.1088/1748-9326/abc9e2 (2021).
Shen, Z. X. et al. Drying in the low-latitude Atlantic Ocean contributed to terrestrial water storage depletion across Eurasia. Nat. Commun. 13 , 1849 (2022).
Dettinger, M. D. Atmospheric rivers as drought busters on the US West Coast. J. Hydrometeorol. 14 , 1721–1732 (2013).
Taylor, R. G. et al. Ground water and climate change. Nat. Clim. Change 3 , 322–329 (2013).
Cuthbert, M. O. et al. Observed controls on resilience of groundwater to climate variability in sub-Saharan Africa. Nature 572 , 230 (2019).
Hugonnet, R. et al. Accelerated global glacier mass loss in the early twenty-first century. Nature 592 , 726 (2021).
Zhao, F., Long, D., Li, X., Huang, Q. & Han, P. Rapid glacier mass loss in the Southeastern Tibetan Plateau since the year 2000 from satellite observations. Remote. Sens. Environ. 270 , 112853 (2022).
Li, X. Y. et al. Climate change threatens terrestrial water storage over the Tibetan Plateau. Nat. Clim. Change https://doi.org/10.1038/s41558-022-01443-0 (2022).
Yao, T. D. et al. The imbalance of the Asian water tower. Nat. Rev. Earth Environ. https://doi.org/10.1038/s43017-022-00299-4 (2022).
Immerzeel, W. W., van Beek, L. P. H. & Bierkens, M. F. P. Climate change will affect the Asian water towers. Science 328 , 1382–1385 (2010).
Immerzeel, W. W. et al. Importance and vulnerability of the world’s water towers. Nature 577 , 364 (2020).
Dery, S. J. et al. Detection of runoff timing changes in pluvial, nival, and glacial rivers of western Canada. Water Resour. Res. https://doi.org/10.1029/2008wr006975 (2009).
Siebert, S. et al. Groundwater use for irrigation – a global inventory. Hydrol. Earth Syst. Sci. 7 , 3977–4021 (2010).
Google Scholar
Scanlon, B. R. et al. Groundwater depletion and sustainability of irrigation in the US High Plains and Central Valley. Proc. Natl Acad. Sci. USA 109 , 9320–9325 (2012).
Dahlke, H. E. et al. in Advanced Tools for Integrated Water Resources Management Vol. 3 (eds Friesen, J. & Rodriguez-Sinobas, L.) 215–275 (Elsevier, 2018).
Reddy, V. R., Pavelic, P. & Hanjra, M. A. Underground taming of floods for irrigation (UTFI) in the river basins of South Asia: institutionalising approaches and policies for sustainable water management and livelihood enhancement. Water Policy 20 , 369–387 (2018).
McDonald, R. I., Weber, K. F., Padowski, J., Boucher, T. & Shemie, D. Estimating watershed degradation over the last century and its impact on water-treatment costs for the world’s large cities. Proc. Natl Acad. Sci. USA 113 , 9117–9122 (2016).
The State of the World’s Forests 2020. Forests, Biodiversity, and Peopl e (FAO/UNEP, 2020).
Convention on Wetlands. Global Wetland Outlook: Special Edition 2021 (Secretariat of the Convention on Wetlands, 2021).
Scanlon, B. R., Jolly, I., Sophocleous, M. & Zhang, L. Global impacts of conversions from natural to agricultural ecosystems on water resources: quantity versus quality. Water Resour. Res. https://doi.org/10.1029/2006WR005486 (2007).
Nosetto, M. D., Paez, R. A., Ballesteros, S. I. & Jobbagy, E. G. Higher water-table levels and flooding risk under grain vs. livestock production systems in the subhumid plains of the Pampas. Agric. Ecosyst. Environ. 206 , 60–70 (2015).
Favreau, G. et al. Land clearing, climate variability, and water resources increase in semiarid southwest Niger: a review. Water Resour. Res. https://doi.org/10.1029/2007wr006785 (2009).
Walker, C. D., Zhang, l, Ellis, T. W., Hatton, T. J. & Petheram, C. Estimating impacts of changed land use on recharge: review of modelling and other approaches appropriate for management of dryland salinity. Hydrogeol. J. 10 , 68–90 (2002).
Nosetto, M. D., Jobbagy, E. G., Jackson, R. B. & Sznaider, G. A. Reciprocal influence of crops and shallow ground water in sandy landscapes of the Inland Pampas. Field Crops Res. 113 , 138–148 (2009).
Gimenez, R., Mercau, J., Nosetto, M., Paez, R. & Jobbagy, E. The ecohydrological imprint of deforestation in the semiarid Chaco: insights from the last forest remnants of a highly cultivated landscape. Hydrol. Process. 30 , 2603–2616 (2016).
Eilers, R. G., Eilers, W. D. & Fitzgerald, M. M. A salinity risk index for soils of the Canadian prairies. Hydrogeol. J. 5 , 68–79 (1997).
Progress on Household Drinking Water, Sanitation and Hygiene 2000–2020: Five Years into the SDGs (WHO/UNICEF, 2021).
Cobbing, J. & Hiller, B. Waking a sleeping giant: realizing the potential of groundwater in sub-Saharan Africa. World Dev. 122 , 597–613 (2019).
Rockström, J. & Falkenmark, M. Agriculture: increase water harvesting in Africa. Nature 519 , 283–285 (2015).
MacAllister, D. J., MacDonald, A. M., Kebede, S., Godfrey, S. & Calow, R. Comparative performance of rural water supplies during drought. Nat. Commun. 11 , 1099 (2020).
Aboah, M. & Miyittah, M. K. Estimating global water, sanitation, and hygiene levels and related risks on human health, using global indicators data from 1990 to 2020. J. Water Health 20 , 1091–1101 (2022).
Abell, R. et al. Beyond the Source: The Environmental, Economic and Community Benefits of Source Water Protection (The Nature Conservancy, 2017).
Herrera-Garcia, G. et al. Mapping the global threat of land subsidence. Science 371 , 34–36 (2021).
Scanlon, B. R., Reedy, R. C., Faunt, C. C., Pool, D. & Uhlman, K. Enhancing drought resilience with conjunctive use and managed aquifer recharge in California and Arizona. Environ. Res. Lett. 11 , 035013 (2016).
Qadir, M. et al. Global and regional potential of wastewater as a water, nutrient and energy source. Nat. Resour. Forum 44 , 40–51 (2020).
Water Reuse within a Circular Economy Context . Global Water Security Issues Series 2 (UNESCO, 2020).
Jones, E. R., van Vliet, M. T. H., Qadir, M. & Bierkens, M. F. P. Country-level and gridded estimates of wastewater production, collection, treatment and reuse. Earth Syst. Sci. Data 13 , 237–254 (2021).
Jeuland, M. Challenges to wastewater reuse in the Middle East and North Africa. Middle East. Dev. J. 7 , 1–25 (2015).
Zhang, Y. & Shen, Y. Wastewater irrigation: past, present, and future. WIREs Water 6 , e1234 (2019).
Fito, J. & Van Hulle, S. W. H. Wastewater reclamation and reuse potentials in agriculture: towards environmental sustainability. Environ. Dev. Sust. 23 , 2949–2972 (2021).
Gao, L., Yoshikawa, S., Iseri, Y., Fujimori, S. & Kanae, S. An economic assessment of the global potential for seawater desalination to 2050. Water https://doi.org/10.3390/w9100763 (2017).
Ahdab, Y. D., Thiel, G. P., Bohlke, J. K., Stanton, J. & Lienhard, J. H. Minimum energy requirements for desalination of brackish groundwater in the United States with comparison to international datasets. Water Res. 141 , 387–404 (2018).
Jones, E., Qadir, M., van Vliet, M. T. H., Smakhtin, V. & Kang, S. M. The state of desalination and brine production: a global outlook. Sci. Total Environ. 657 , 1343–1356 (2019).
Lin, S. S. et al. Seawater desalination technology and engineering in China: a review. Desalination https://doi.org/10.1016/j.desal.2020.114728 (2021).
Martinez-Alvarez, V., Martin-Gorriz, B. & Soto-Garcia, M. Seawater desalination for crop irrigation — a review of current experiences and revealed key issues. Desalination 381 , 58–70 (2016).
Smith, K., Liu, S. M., Hu, H. Y., Dong, X. & Wen, X. H. Water and energy recovery: the future of wastewater in China. Sci. Total Environ. 637 , 1466–1470 (2018).
Pulido-Bosch, A. et al. Impacts of agricultural irrigation on groundwater salinity. Environ/ Earth Sci. https://doi.org/10.1007/s12665-018-7386-6 (2018).
Kurnik, J. The Next California: Phase 1: Investigating Potential in the Mid-Mississippi Delta River Region (The Markets Institute at WWF, 2020); https://www.worldwildlife.org/publications/the-next-california-phase-1-investigating-potential-in-the-mid-mississippi-delta-river-region
Senay, G. B., Schauer, M., Friedrichs, M., Velpuri, N. M. & Singh, R. K. Satellite-based water use dynamics using historical Landsat data (1984–2014) in the southwestern United States. Remote Sens. Environ. 202 , 98–112 (2017).
Gebremichael, M., Krishnamurthy, P. K., Ghebremichael, L. T. & Alam, S. What drives crop land use change during multi-year droughts in California’s Central Valley? Prices or concern for water? Remote Sens. https://doi.org/10.3390/rs13040650 (2021).
Brauman, K. A., Siebert, S. & Foley, J. A. Improvements in crop water productivity increase water sustainability and food security — a global analysis. Environ. Res. Lett. 8 , 024030 (2013).
Mekonnen, M. M., Hoekstra, A. Y., Neale, C. M. U., Ray, C. & Yang, H. S. Water productivity benchmarks: the case of maize and soybean in Nebraska. Agric. Water Manag. https://doi.org/10.1016/j.agwat.2020.106122 (2020).
Colaizzi, P. D., Gowda, P. H., Marek, T. H. & Porter, D. O. Irrigation in the Texas High Plains: a brief history and potential reductions in demand. Irrig. Drain. 58 , 257–274 (2008).
Scanlon, B. R., Gates, J. B., Reedy, R. C., Jackson, A. & Bordovsky, J. Effects of irrigated agroecosystems: (2). Quality of soil water and groundwater in the southern High Plains, Texas. Water Resour. Res. 46 , W09538 (2010).
Ward, F. A. & Pulido-Velazquez, M. Water conservation in irrigation can increase water use. Proc. Natl Acad. Sci. USA 105 , 18215–18220 (2008).
Grafton, R. Q. et al. The paradox of irrigation efficiency. Science 361 , 748–750 (2018).
Alcott, B. in The Jevons Paradox and the Myth of Resource Efficiency Improvements (eds Polimeni, J. M., Mayumi, K., & Giampetro, M.) 7–78 (Earthscan, 2008).
Aarnoudse, E. & Bluemling, B. Controlling Groundwater Through Smart Card Machines: The Case of Water Quotas and Pricing Mechanisms in Gansu Province, China . Groundwater Solutions Initiative for Policy and Practice (GRIPP) Case Profile Series 02 (International Water Management Institute, 2017); https://doi.org/10.5337/2016.224
Kinzelbach, W., Wang, H., Li, Y., Wang, L. & Li, N. Groundwater Overexploitation in the North China Plain: A Path to Sustainability (Springer, 2021).
McDougall, R., Kristiansen, P. & Rader, R. Small-scale urban agriculture results in high yields but requires judicious management of inputs to achieve sustainability. Proc. Natl Acad. Sci. USA 116 , 129–134 (2019).
Langemeyer, J., Madrid-Lopez, C., Mendoza Beltran, A. & Villalba Mendez, G. Urban agriculture — a necessary pathway towards urban resilience and global sustainability? Landsc. Urban Plan. 210 , 104055 (2021).
Palmer, L. Urban agriculture growth in US cities. Nat. Sust. 1 , 5–7 (2018).
Grafius, D. R. et al. Estimating food production in an urban landscape. Sci. Rep. 10 , 5141 (2020).
The State of Food Insecurity in the World 2015 (FAO/IFAD/WFP, 2015).
Kummu, M. et al. Lost food, wasted resources: global food supply chain losses and their impacts on freshwater, cropland, and fertiliser use. Sci. Total Environ. 438 , 477–489 (2012).
Gleick, P. H. Global freshwater resources: soft-path solutions for the 21st century. Science 302 , 1524–1528 (2003).
Miralles-Wilhelm, F. Nature-Based Solutions in Agriculture — Sustainable Management and Conservation of Land, Water, and Biodiversity (FAO/The Nature Conservancy, 2021).
McDonald, R. I. & Shemie, D. Urban Water Blueprint: Mapping Conservation Solutions to the Global Water Challenge (The Nature Conservancy, 2014); http://water.nature.org/waterblueprint
Kane, M. & Erickson, J. D. Urban metabolism and payment for ecosystem services: history and policy analysis of the New York city water supply. Adv. Econ. Environ. Resour. 7 , 307–328 (2007).
Greater Cape Town Water Fund: Business Case: Assessing the Return on Investment for Ecological Infrastructure Restoration (The Nature Conservancy, 2019).
Hu, J., Lu, Y. H., Fu, B. J., Comber, A. J. & Harris, P. Quantifying the effect of ecological restoration on runoff and sediment yields: a meta-analysis for the Loess Plateau of China. Prog. Phys. Geogr. Earth Environ. 41 , 753–774 (2017).
Liu, W. W. et al. Improving wetland ecosystem health in China. Ecol. Indic. https://doi.org/10.1016/j.ecolind.2020.106184 (2020).
Cities100: Chennai Is Restoring Waterbodies to Protect Against Flooding and Drought . C40 Knowledge Hub: Nordic Sustainability, South and West Asia, Chennai, Case Studies and Best Practice Examples https://www.c40knowledgehub.org/s/article/Cities100-Chennai-is-restoring-waterbodies-to-protect-against-flooding-and-drought?language=en_US (2019).
Chung, M. G., Frank, K. A., Pokhrel, Y., Dietz, T. & Liu, J. G. Natural infrastructure in sustaining global urban freshwater ecosystem services. Nat. Sust. 4 , 1068 (2021).
Qi, Y. F. et al. Addressing challenges of urban water management in Chinese sponge cities via nature-based solutions. Water https://doi.org/10.3390/w12102788 (2020).
Acreman, M. et al. Evidence for the effectiveness of nature-based solutions to water issues in Africa. Environ. Res. Lett. https://doi.org/10.1088/1748-9326/ac0210 (2021).
Livneh, B. & Badger, A. M. Drought less predictable under declining future snowpack. Nat. Clim. Change 10 , 452–458 (2020).
Mulligan, M., van Soesbergen, A. & Sáenz, L. GOODD, a global dataset of more than 38,000 georeferenced dams. Sci. Data 7 , 31 (2020).
International Commission on Large Dams https://www.icold-cigb.org/ (2022).
Yang, G., Guo, S., Liu, P. & Block, P. Integration and evaluation of forecast-informed multiobjective reservoir operations. J. Water Resour. Plan. Manag. 146 , 04020038 (2020).
Delaney, C. J. et al. Forecast informed reservoir operations using ensemble streamflow predictions for a multipurpose reservoir in northern California. Water Resour. Res . https://doi.org/10.1029/2019wr026604 (2020).
Amarasinghe, U. A., Muthuwatta, L., Surinaidu, L., Anand, S. & Jain, S. K. Reviving the Ganges water machine: potential. Hydrol. Earth Syst. Sci. 20 , 1085–1101 (2016).
Shamsudduha, M. et al. The Bengal water machine: quantified freshwater capture in Bangladesh. Science 377 , 1315–1319 (2022).
Chao, B. F., Wu, Y. H. & Li, Y. S. Impact of artificial reservoir water impoundment on global sea level. Science 320 , 212–214 (2008).
Zarfl, C., Lumsdon, A. E., Berlekamp, J., Tydecks, L. & Tockner, K. A global boom in hydropower dam construction. Aquat. Sci. 77 , 161–170 (2015).
Zarfl, C. et al. Future large hydropower dams impact global freshwater megafauna. Sci. Rep. https://doi.org/10.1038/s41598-019-54980-8 (2019).
Wheeler, K. G., Jeuland, M., Hall, J. W., Zagona, E. & Whittington, D. Understanding and managing new risks on the Nile with the Grand Ethiopian Renaissance Dam. Nat. Commun. https://doi.org/10.1038/s41467-020-19089-x (2020).
Di Baldassarre, G. et al. Water shortages worsened by reservoir effects. Nat. Sust. 1 , 617–622 (2018).
Dahlke, H. E., Brown, A. G., Orloff, S., Putnam, D. & O’Geen, T. Managed winter flooding of alfalfa recharges groundwater with minimal crop damage. Calif. Agric. 72 , 65–75 (2018).
Yang, Q. & Scanlon, B. R. How much water can be captured from flood flows to store in depleted aquifers for mitigating floods and droughts? A case study from Texas, US. Environ. Res. Lett. 14 , 054011 (2019).
Dillon, P. et al. Sixty years of global progress in managed aquifer recharge. Hydrogeol. J. https://doi.org/10.1007/s10040-018-1841-z. (2018).
Groundwater Replenishment System Technical Brochure, https://www.ocwd.com/media/10443/gwrs-technical-brochure-2021.pdf (2021).
Konikow, L. F. Groundwater Depletion in the United States (1900–2008) . US Geological Survey Scientific Investigation Report 2013–5079, http://pubs.usgs.gov/sir/2013/5079 (2013).
Hartog, N. & Stuyfzand, P. J. Water quality donsiderations on the rise as the use of managed aquifer recharge systems widens. Water 9 , 808 (2017).
Shumilova, O., Tockner, K., Thieme, M., Koska, A. & Zarfl, C. Global water transfer megaprojects: a potential solution for the water–food–energy nexus? Front. Environ. Sci. https://doi.org/10.3389/fenvs.2018.00150 (2018).
Long, D. et al. South-to-north water diversion stabilizing Beijing’s groundwater levels. Nat. Commun. https://doi.org/10.1038/s41467-020-17428-6 (2020).
Zhuang, W. Eco-environmental impact of inter-basin water transfer projects: a review. Environ. Sci. Pollut. Res. 23 , 12867–12879 (2016).
Hoekstra, A. Y. Virtual Water Trade : Proceedings of the International Expert Meeting on Virtual Water Trade (UNESCO-IHE, 2003).
Oki, T. & Kanae, S. Virtual water trade and world water resources. Water Sci. Technol. 49 , 203–209 (2004).
Dolan, F. et al. Evaluating the economic impact of water scarcity in a changing world. Nat. Commun. https://doi.org/10.1038/s41467-021-22194-0 (2021).
Hoekstra, A. Y. & Mekonnen, M. M. The water footprint of humanity. Proc. Natl Acad. Sci. USA 109 , 3232–3237 (2012).
Dalin, C., Wada, Y., Kastner, T. & Puma, M. J. Groundwater depletion embedded in international food trade. Nature 543 , 700–704 (2017).
Hanasaki, N., Inuzuka, T., Kanae, S. & Oki, T. An estimation of global virtual water flow and sources of water withdrawal for major crops and livestock products using a global hydrological model. J. Hydrol. 384 , 232–244 (2010).
Mekonnen, M. M. & Gerbens-Leenes, W. The water footprint of global food production. Water https://doi.org/10.3390/w12102696 (2020).
Australian Water Markets Report: 2019-20 Review and 2020-21 Outlook (Aither, 2020); https://aither.com.au/wp-content/uploads/2020/08/2020-Water-Markets-Report.pdf
Grafton, R. Q. & Wheeler, S. A. Economics of water recovery in the Murray–Darling Basin, Australia. Annu. Rev. Resour. Econ. 10 , 487–510 (2018).
Moench, M. Water and the potential for social instability: livelihoods, migration and the building of society. Nat. Resour. Forum 26 , 195–204 (2002).
Water Markets in Australia: A Short History (National Water Commission, 2011).
Kundzewicz, Z. W. & Döll, P. Will groundwater ease freshwater stress under climate change? Hydrol. Sci. J. 54 , 665–675 (2009).
A Snapshot of the World’s Water Quality: Towards a Global Assessment (UNEP, 2016).
Summary Progress Update 2021: SDG 6 — Water and Sanitation for All (UN-Water, 2021).
GEMStat: Global Environmental Monitoring System, https://gemstat.org/ (UNEP, 2022).
Akhmouch, A. & Correia, F. N. The 12 OECD principles on water governance — when science meets policy. Util. Policy 43 , 14–20 (2016).
Lankford, B., Bakker, K., Zeitoun, M. & Conway, B. D. Water Security: Principles, Perspectives, and Practices (Routledge, 2013).
Potapov, P. et al. Global maps of cropland extent and change show accelerated cropland expansion in the twenty-first century. Nat. Food 3 , 19 (2022).
Fan, Y., Li, H. & Miguez-Macho, G. Global patterns of groundwater table depth. Science 339 , 940–943 (2013).
Download references
Author information
Authors and affiliations.
Bureau of Economic Geology, Jackson School of Geosciences, University of Texas at Austin, Austin, TX, USA
Bridget R. Scanlon, Sarah Fakhreddine, Ashraf Rateb, Robert C. Reedy & Alex Sun
Department of Civil and Environmental Engineering, Carnegie Mellon University, Pittsburgh, PA, USA
Sarah Fakhreddine
Water Systems and Global Change, Wageningen University, Wageningen, The Netherlands
Inge de Graaf
Global Institute for Water Security, National Hydrology Research Center, University of Saskatchewan, Saskatoon, Canada
Jay Famiglietti
Department of Civil Engineering, University of Victoria, Victoria, British Columbia, Canada
Tom Gleeson
Crawford School of Public Policy, Australian National University, Canberra, ACT, Australia
R. Quentin Grafton
Grupo de Estudios Ambientales, IMASL, CONICET, Universidad Nacional de San Luis, San Luis, Argentina
Esteban Jobbagy
Center for Water Resources Research, School of Agricultural, Earth and Environmental Sciences, University of KwaZulu Natal, Durban, South Africa
Seifu Kebede
UK Meteorological Office, Exeter, UK
Seshagiri Rao Kolusu
Leonard Konikow Hydrogeologist, Reston, VA, USA
Leonard F. Konikow
Department of Hydraulic Engineering, Tsinghua University, Beijing, China
Department of Civil, Construction and Environmental Engineering, University of Alabama, Tuscaloosa, AL, USA
Mesfin Mekonnen
Institute of Physical Geography, Goethe University Frankfurt, Frankfurt am Main, Frankfurt, Germany
Hannes Müller Schmied
Senckenberg Leibniz Biodiversity and Climate Research Centre (SBiK-F), Frankfurt am Main, Frankfurt, Germany
School of Environmental Science and Engineering, Indian Institute of Technology Kharagpur, Kharagpur, India
Abhijit Mukherjee
British Geological Survey, Lyell Centre, Edinburgh, UK
Alan MacDonald
Institute for Risk and Disaster Reduction, University College London, London, UK
Mohammad Shamsudduha
National Centre for Groundwater Research and Training (NCGRT), College of Science and Engineering, Flinders University, Adelaide, South Australia, Australia
Craig T. Simmons
Department of Geography, University College London, London, UK
Richard G. Taylor
Water Cycle Innovation Ltd, Johannesburg, Gauten, South Africa
Karen G. Villholth
Environmental Sciences Initiative, Advanced Science Research Center at the CUNY Graduate Center, New York, NY, USA
Charles J. Vörösmarty
School of Environmental Science and Engineering, Southern University of Science and Technology, Shenzhen, China
Chunmiao Zheng
You can also search for this author in PubMed Google Scholar
Contributions
B.R.S. conceptualized the review and coordinated input. S.F. reviewed many of the topics and developed some of the figures. A.R. analysed GRACE satellite data and M.S. reviewed this output. Q.G. provided input on water economics. E.J. reviewed impacts of land-use change. S.R.K. provided data on future precipitation changes. L.F.K. provided detailed information on surface water/groundwater interactions. M.M. provided data on water trade. C.J.V. provided input on green and grey solutions. All authors reviewed the paper and provided edits.
Corresponding author
Correspondence to Bridget R. Scanlon .
Ethics declarations
Competing interests.
The authors declare no competing interests.
Peer review
Peer review information.
Nature Reviews Earth & Environment thanks Helen Dahlke, Diana Allen, who co-reviewed with Aspen Anderson, and the other, anonymous, reviewer(s) for their contribution to the peer review of this work.
Additional information
Publisher’s note Springer Nature remains neutral with regard to jurisdictional claims in published maps and institutional affiliations.
Supplementary information
Supplementary information, supplementary tables , rights and permissions.
Springer Nature or its licensor (e.g. a society or other partner) holds exclusive rights to this article under a publishing agreement with the author(s) or other rightsholder(s); author self-archiving of the accepted manuscript version of this article is solely governed by the terms of such publishing agreement and applicable law.
Reprints and permissions
About this article
Cite this article.
Scanlon, B.R., Fakhreddine, S., Rateb, A. et al. Global water resources and the role of groundwater in a resilient water future. Nat Rev Earth Environ 4 , 87–101 (2023). https://doi.org/10.1038/s43017-022-00378-6
Download citation
Accepted : 17 November 2022
Published : 31 January 2023
Issue Date : February 2023
DOI : https://doi.org/10.1038/s43017-022-00378-6
Share this article
Anyone you share the following link with will be able to read this content:
Sorry, a shareable link is not currently available for this article.
Provided by the Springer Nature SharedIt content-sharing initiative
This article is cited by
Rapid groundwater decline and some cases of recovery in aquifers globally.
- Scott Jasechko
- Hansjörg Seybold
- James W. Kirchner
Nature (2024)
Winter snow deficit was a harbinger of summer 2022 socio-hydrologic drought in the Po Basin, Italy
- Francesco Avanzi
- Francesca Munerol
- Luca Ferraris
Communications Earth & Environment (2024)
Development of Groundwater Levels Dataset for Chile since 1970
- Héctor Leopoldo Venegas-Quiñones
- Rodrigo Valdés-Pineda
- Ty P. A. Ferré
Scientific Data (2024)
Comparative life cycle assessment of environmental impacts and economic feasibility of tomato cultivation systems in northern plains of India
- Rohit Kumar
- Arvind Bhardwaj
- Kanhu Charan Pattnayak
Scientific Reports (2024)
Aquifer depletion exacerbates agricultural drought losses in the US High Plains
- Timothy Foster
- Nicholas Brozović
Nature Water (2024)
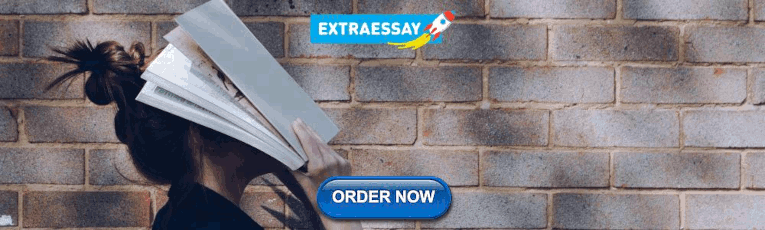
Quick links
- Explore articles by subject
- Guide to authors
- Editorial policies
Sign up for the Nature Briefing newsletter — what matters in science, free to your inbox daily.

- Skip to primary navigation
- Skip to main content
- Skip to primary sidebar
- Skip to footer
UCOWR home office supported by Southern Illinois University Carbondale
Universities Council on Water Resources
About JCWRE
JCWRE is listed in the Emerging Sources Citation Index (Web of Science) produced by Clarivate Analytics.
Aims and Scope The Journal of Contemporary Water Research and Education ( JCWRE ) disseminates original, peer-reviewed articles and comprehensive reviews on applied research, policy, education, and outreach/extension in water and watershed science and management, with international scope and an emphasis on transdisciplinary approaches and perspectives. Two themed issues a year focus on topics that emerge from conferences, water resources organizations, national training or education programs, and research and extension teams. One issue per year is reserved for general submissions. JCWRE is published by the Universities Council on Water Resources (UCOWR).
Publication The journal is published three times per year by UCOWR. It is available in electronic form on Wiley Online Library where it can be downloaded by researchers, educators, and policy-makers around the world. Issues are published in April, August, and December, unless unforeseen circumstances occur. The two themed issues are typically published in April and December. The issue reserved for general submissions is typically published in August.
Article Categories (New for 2021) JCWRE publishes articles in five categories: Original Research, Case Study, Review, Research Note, and Perspective Piece. Each category has specific author guidelines. View the Author Guidelines for more information. Your manuscript will not be considered for publication unless it follows the manuscript requirements. All categories, except Perspective Pieces, are peer-reviewed and subject to standard publication fees. Perspective Pieces are reviewed internally by our Editors and are not charged a publication fee.
Publication Fee The following publication charges apply per article: $300 for UCOWR members $500 for non-UCOWR members. These fees are subject to change.
Submission Deadlines JCWRE is currently accepting submissions for consideration. Manuscripts can be submitted at any time. Most general submissions are published in the August issue which is designated for open submissions. However, general submissions may be considered for themed issues if appropriate. If accepted before the issue is published, the article will be published as a sneak peek on our website ( https://ucowr.org/journal/jcwre-sneak-peek/ ). Manuscripts should be submitted by October 15 to be considered for the April issue; February 15 to be considered for the August issue; and June 15 to be considered for the December issue.
Questions? Contact the Editors: Jackie Gillespie (crimjac@siu.edu), Karl Williard (williard@siu.edu)
1231 Lincoln Drive, Mail Code 4526
Southern Illinois University Carbondale
Carbondale, IL 62901
UCOWR is grateful for the ongoing support from Southern Illinois University Carbondale!
For information, visit SIU online: www.siu.edu
Contact UCOWR:
- Email: ucowr@siu.edu
- Phone: 618.536.7571
- Fax: 618.453.7475
A comprehensive review of water quality indices (WQIs): history, models, attempts and perspectives
- Review paper
- Published: 11 March 2023
- Volume 22 , pages 349–395, ( 2023 )
Cite this article
- Sandra Chidiac ORCID: orcid.org/0000-0002-1822-119X 1 ,
- Paula El Najjar 1 , 2 ,
- Naim Ouaini 1 ,
- Youssef El Rayess 1 &
- Desiree El Azzi 1 , 3
16k Accesses
19 Citations
1 Altmetric
Explore all metrics
Water quality index (WQI) is one of the most used tools to describe water quality. It is based on physical, chemical, and biological factors that are combined into a single value that ranges from 0 to 100 and involves 4 processes: (1) parameter selection, (2) transformation of the raw data into common scale, (3) providing weights and (4) aggregation of sub-index values. The background of WQI is presented in this review study. the stages of development, the progression of the field of study, the various WQIs, the benefits and drawbacks of each approach, and the most recent attempts at WQI studies. In order to grow and elaborate the index in several ways, WQIs should be linked to scientific breakthroughs (example: ecologically). Consequently, a sophisticated WQI that takes into account statistical methods, interactions between parameters, and scientific and technological improvement should be created in order to be used in future investigations.
Similar content being viewed by others
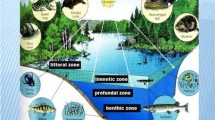
Water quality assessment of lake water: a review
Rachna Bhateria & Disha Jain
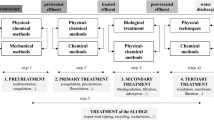
Advantages and disadvantages of techniques used for wastewater treatment
Grégorio Crini & Eric Lichtfouse
Irrigation Water Quality
Avoid common mistakes on your manuscript.
1 Introduction
Water is the vital natural resource with social and economic values for human beings (Kumar 2018 ). Without water, existence of man would be threatened (Zhang 2017 ). The most important drinking sources in the world are surface water and groundwater (Paun et al. 2016 ).
Currently, more than 1.1 billion people do not have access to clean drinking water and it is estimated that nearly two-thirds of all nations will experience water stress by the year 2025 (Kumar 2018 ).
With the extensive social and economic growth, such as human factors, climate and hydrology may lead to accumulation of pollutants in the surface water that may result in gradual change of the water source quality (Shan 2011 ).
The optimal quantity and acceptable quality of water is one of the essential needs to survive as mentioned earlier, but the maintenance of an acceptable quality of water is a challenge in the sector of water resources management (Mukate et al. 2019 ). Accordingly, the water quality of water bodies can be tested through changes in physical, chemical and biological characteristics related to anthropogenic or natural phenomena (Britto et al. 2018 ).
Therefore, water quality of any specific water body can be tested using physical, chemical and biological parameters also called variables, by collecting samples and obtaining data at specific locations (Britto et al. 2018 ; Tyagi et al. 2013 ).
To that end, the suitability of water sources for human consumption has been described in terms of Water Quality Index (WQI), which is one of the most effective ways to describe the quality of water, by reducing the bulk of information into a single value ranging between 0 and 100 (Tyagi et al. 2013 ).
Hence, the objective of the study is to review the WQI concept by listing some of the important water quality indices used worldwide for water quality assessment, listing the advantages and disadvantages of the selected indices and finally reviewing some water quality studies worldwide.
2 Water quality index
2.1 history of water quality concept.
In the last decade of the twentieth century, many organizations involved in water control, used the water quality indices for water quality assessment (Paun et al. 2016 ). In the 1960’s, the water quality indices was introduced to assess the water quality in rivers (Hamlat et al. 2017 ).
Horton ( 1965 ), initially developed a system for rating water quality through index numbers, offering a tool for water pollution abatement, since the terms “water quality” and “pollution” are related. The first step to develop an index is to select a list of 10 variables for the index’s construction, which are: sewage treatment, dissolved oxygen (DO), pH, coliforms, electroconductivity (EC), carbon chloroform extract (CCE), alkalinity, chloride, temperature and obvious pollution. The next step is to assign a scale value between zero and 100 for each variable depending on the quality or concentration. The last step, is to designate to each variable is a relative weighting factor to show their importance and influence on the quality index (the higher the assigned weight, the more impact it has on the water quality index, consequently it is more important) (Horton 1965 ).
Later on, Brown et al. ( 1970 ) established a new water quality index (WQI) with nine variables: DO, coliforms, pH, temperature, biochemical oxygen demand (BOD), total phosphate, nitrate concentrations, turbidity and solid content based on a basic arithmetic weighting using arithmetic mean to calculate the rating of each variable. These rates are then converted not temporary weights. Finally, each temporary weight is divided by the sum of all the temporary weights in order to get the final weight of each variable (Kachroud et al. 2019a ; Shah and Joshi 2017 ). In 1973, Brown et al., considered that a geometric aggregation (a way to aggregate variables, and being more sensitive when a variable exceeds the norm) is better than an arithmetic one. The National Sanitation Foundation (NSF) supported this effort (Kachroud et al. 2019a ; Shah and Joshi 2017 ).
Steinhart et al. ( 1982 ) developed a novel environmental quality index (EQI) for the Great Lakes ecosystem in North America. Nine variables were selected for this index: biological, physical, chemical and toxic. These variables were: specific conductance or electroconductivity, chloride, total phosphorus, fecal Coliforms, chlorophyll a , suspended solids, obvious pollution (aesthetic state), toxic inorganic contaminants, and toxic organic contaminants. Raw data were converted to subindex and each subindex was multiplied by a weighting factor (a value of 0.1 for chemical, physical and biological factors but 0.15 for toxic substances). The final score ranged between 0 (poor quality) and 100 (best quality) (Lumb et al. 2011a ; Tirkey et al. 2015 ).
Dinius ( 1987 ), developed a WQI based on multiplicative aggregation having a scale expressed with values as percentage, where 100% expressed a perfect water quality (Shah and Joshi 2017 ).
In the mid 90’s, a new WQI was introduced to Canada by the province of British Columbia, and used as an increasing index to evaluate water quality (Lumb et al. 2011b ; Shah and Joshi 2017 ). A while after, the Water Quality Guidelines Task Group of the Canadian Council of Ministers of the Environment (CCME) modified the original British Columbia Water Quality Index (BCWQI) and endorsed it as the CCME WQI in 2001(Bharti and Katyal 2011 ; Lumb et al. 2011b ).
In 1996, the Watershed Enhancement Program (WEPWQI) was established in Dayton Ohio, including water quality variables, flow measurements and water clarity or turbidity. Taking into consideration pesticide and Polycyclic Aromatic Hydrocarbon (PAH) contamination, is what distinguished this index from the NSFWQI (Kachroud et al. 2019a , b ).
Liou et al. (2003) established a WQI in Taiwan on the Keya River. The index employed thirteen variables: Fecal coliforms, DO, ammonia nitrogen, BOD, suspended solids, turbidity, temperature, pH, toxicity, cadmium (Cd), lead (Pb), copper (Cu) and zinc (Zn). These variables were downsized to nine based on environmental and health significance: Fecal coliforms, DO, ammonia nitrogen, BOD, suspended solids, turbidity, temperature, pH and toxicity. Each variable was converted into an actual value ranging on a scale from 0 to 100 (worst to highest). This index is based on the geometric means (an aggregation function that could eliminate the ambiguous caused from smaller weightings) of the standardized values (Akhtar et al. 2021 ; Liou et al. 2004 ; Uddin et al. 2021 ).
Said et al. ( 2004 ) implemented a new WQI using the logarithmic aggregation applied in streams waterbodies in Florida (USA), based on only 5 variables: DO, total phosphate, turbidity, fecal coliforms and specific conductance. The main idea was to decrease the number of variables and change the aggregation method using the logarithmic aggregation (this function does not require any sub-indices and any standardization of the variables). This index ranged from 0 to 3, the latter being the ideal value (Akhtar et al. 2021 ; Kachroud et al. 2019a , b ; Said et al. 2004 ; Uddin et al. 2021 ).
The Malaysian WQI (MWQI) was carried out in 2007, including six variables: DO, BOD, Chemical Oxygen Demand (COD), Ammonia Nitrogen, suspended solids and pH. For each variable, a curve was established to transform the actual value of the variable into a non-dimensional sub-index value.
The next step is to determine the weighting of the variables by considering the experts panel opinions. The final score is determined using the additive aggregation formula (where sub-indices values and their weightings are summed), extending from 0 (polluted) to 100 (clean) (Uddin et al. 2021 ).
The Hanh and Almeida indices were established respectively in 2010 on surface water in Vietnam and 2012 on the Potrero de los Funes in Argentina, based on 8 (color, suspended solids, DO, BOD, COD, chloride, total coliforms and orthophosphate) and 10 (color, pH, COD, fecal coliforms, total coliforms, total phosphate, nitrates, detergent, enterococci and Escherichia coli .) water quality variables. Both indices were based on rating curve- based sum-indexing system (Uddin et al. 2021 ).
The most recent developed WQI model in the literature was carried out in 2017. This index tried to reduce uncertainty present in other water quality indices. The West Java Water Quality Index (WJWQI) applied in the Java Sea in Indonesia was based on thirteen crucial water quality variables: temperature, suspended solids, COD, DO, nitrite, total phosphate, detergent, phenol, chloride, Zn, Pb, mercury (Hg) and fecal coliforms. Using two screening steps (based on statistical assessment), parameter (variable) redundancy was determined to only 9: temperature, suspended solids, COD, DO, nitrite, total phosphate, detergent, phenol and chloride. Sub-indices were obtained for those nine variables and weights were allocated based on expert opinions, using the same multiplicative aggregation as the NSFWQI. The WJWQI suggested 5 quality classes ranging from poor (5–25) to excellent (90–100) (Uddin et al. 2021 ).
2.2 Phases of WQI development
Mainly, WQI concept is based on many factors as displayed in Fig. 1 and described in the following steps:
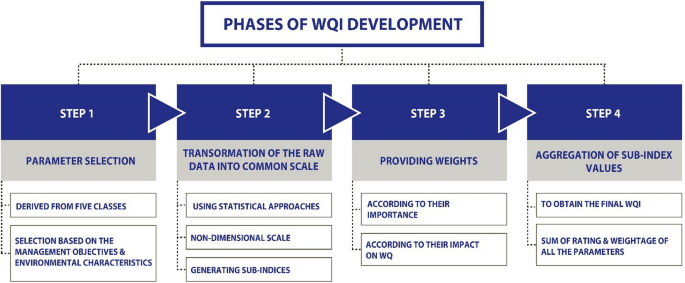
Phases of WQI development
Parameter selection for measurement of water quality (Shah and Joshi 2017 ):
The selection is carried out based on the management objectives and the environmental characteristics of the research area (Yan et al. 2015 ). Many variables are recommended, since they have a considerable impact on water quality and derive from 5 classes namely, oxygen level, eutrophication, health aspects, physical characteristics and dissolved substances (Tyagi et al. 2013 ).
Transformation of the raw data parameter into a common scale (Paun et al. 2016 ):
Different statistical approach can be used for transformation, all parameters are transformed from raw data that have different dimensions and units (ppm, saturation, percentage etc.) into a common scale, a non-dimensional scale and sub-indices are generated (Poonam et al. 2013 ; Tirkey et al. 2015 ).
Providing weights to the parameters (Tripathi and Singal 2019 ):
Weights are assigned to each parameter according to their importance and their impact on water quality, expert opinion is needed to assign weights (Tirkey et al. 2015 ). Weightage depends on the permissible limits assigned by International and National agencies in water drinking (Shah and Joshi 2017 ).
Aggregation of sub-index values to obtain the final WQI:
WQI is the sum of rating and weightage of all the parameters (Tripathi and Singal 2019 ).
It is important to note that in some indices, statistical approaches are commonly used such as factor analysis (FA), principal component analysis (PCA), discriminant analysis (DA) and cluster analysis (CA). Using these statistical approaches improves accuracy of the index and reduce subjective assumptions (Tirkey et al. 2015 ).
2.3 Evolution of WQI research
2.3.1 per year.
According to Scopus ( 2022 ), the yearly evolution of WQI's research is illustrated in Fig. 2 (from 1978 till 2022).
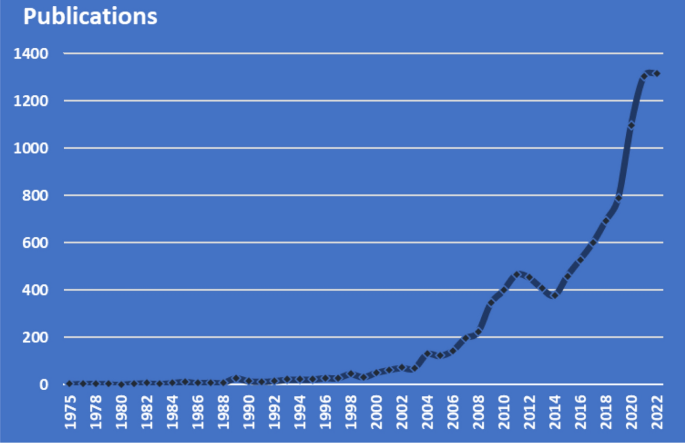
Evolution of WQI research per year (Scopus 2022 )
Overall, it is clear that the number of research has grown over time, especially in the most recent years. The number of studies remained shy between 1975 and 1988 (ranging from 1 to 13 research). In 1998, the number improved to 46 studies and increased gradually to 466 publications in 2011.The WQI's studies have grown significantly over the past decade, demonstrating that the WQI has become a significant research topic with the goal of reaching its maximum in 2022 (1316 studies) (Scopus, 2022 ).
2.3.2 Per country
In Fig. 3 , the development of WQI research is depicted visually per country from 1975 to 2022.
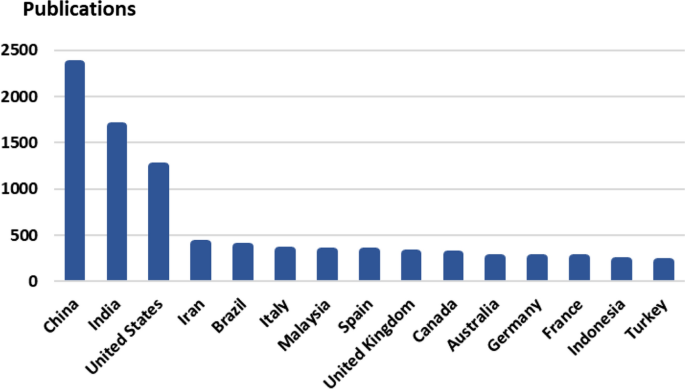
Evolution of WQI research per country (Scopus 2022 )
According to Scopus ( 2022 ), the top three countries were China, India and the United States, with 2356, 1678 and 1241 studies, respectively. Iran, Brazil, and Italy occupy the fourth, fifth, and sixth spots, respectively (409, 375 and 336 study). Malaysia and Spain have approximately the same number of studies, respectively 321 and 320 study. The studies in the remaining countries decrease gradually from 303 document in Spain to 210 documents in Turkey. This demonstrates that developing nations, like India, place a high value on the development of water quality protection even though they lack strong economic power, cutting-edge technology, and a top-notch scientific research team. This is because water quality is crucial to the long-term social and economic development of those nations (Zhang 2019 ).
2.4 Different methods for WQI determination
Water quality indices are tools to determine water quality. Those indices demand basic concepts and knowledge about water issues (Singh et al. 2013 ). There are many water quality indices such as the: National Sanitation Foundation Water Quality Index (NSFWQI), Canadian Council of Ministers of Environment Water Quality Index (CCMEWQI), Oregon Water Quality Index (OWQI), and Weight Arithmetic Water Quality Index (WAWQI) (Paun et al. 2016 ).
These water quality indices are applied in particular areas, based on many parameters compared to specific regional standards. Moreover, they are used to illustrate annual cycles, spatio-temporal variations and trends in water quality (Paun et al. 2016 ). That is to say that, these indices reflect the rank of water quality in lakes, streams, rivers, and reservoirs (Kizar 2018 ).
Accordingly, in this section a general review of available worldwide used indices is presented.
2.4.1 National sanitation foundation (NSFWQI)
The NSFWQI was developed in 1970 by the National Sanitation Foundation (NSF) of the United States (Hamlat et al. 2017 ; Samadi et al. 2015 ). This WQI has been widely field tested and is used to calculate and evaluate the WQI of many water bodies (Hamlat et al. 2017 ). However, this index belongs to the public indices group. It represents a general water quality and does not take into account the water’s use capacities, furthermore, it ignores all types of water consumption in the evaluation process (Bharti and Katyal 2011 ; Ewaid 2017 ).
The NSFWQI has been widely applied and accepted in Asian, African and European countries (Singh et al. 2013 ), and is based on the analysis of nine variables or parameters, such as, BOD, DO, Nitrate (NO 3 ), Total Phosphate (PO 4 ), Temperature, Turbidity, Total Solids(TS), pH, and Fecal Coliforms (FC).
Some of the index parameters have different importance, therefore, a weighted mean for each parameter is assigned, based on expert opinion which have grounded their opinions on the environmental significance, the recommended principles and uses of water body and the sum of these weights is equal to 1 (Table 1 ) (Ewaid 2017 ; Uddin et al. 2021 ).
Due to environmental issues, the NSFWQI has changed overtime. The TS parameter was substituted by the Total Dissolved Solids (TDS) or Total Suspended Solids (TSS), the Total Phosphate by orthophosphate, and the FC by E. coli (Oliveira et al. 2019 ).
The mathematical expression of the NSFWQI is given by the following Eq. ( 1 ) (Tyagi et al. 2013 ):
where, Qi is the sub-index for ith water quality parameter. Wi is the weight associated with ith water quality parameter. n is the number of water quality parameters.
This method ranges from 0 to 100, where 100 represents perfect water quality conditions, while zero indicates water that is not suitable for the use and needs further treatment (Samadi et al. 2015 ).
The ratings are defined in the following Table 2 .
In 1972, the Dinius index (DWQI) happened to be the second modified version of the NSF (USA). Expended in 1987 using the Delphi method, the DWQI included twelve parameters (with their assigned weights): Temperature (0.077), color (0.063), pH (0.077), DO (0.109), BOD (0.097), EC (0.079), alkalinity (0.063), chloride (0.074), coliform count (0.090), E. coli (0.116). total hardness (0.065) and nitrate (0.090). Without any conversion process, the DWQI used the measured variable concentrations directly as the sub-index values (Kachroud et al. 2019b ; Uddin et al. 2021 ).
Sukmawati and Rusni assessed in 2018 the water quality in Beratan lake (Bali), choosing five representative stations for water sampling representing each side of the lake, using the NSFWQI. NSFWQI’s nine parameters mentioned above were measured in each station. The findings indicated that the NSFWQI for the Beratan lake was seventy-eight suggesting a good water quality. Despite this, both pH and FC were below the required score (Sukmawati and Rusni 2019 ).
The NSFWQI indicated a good water quality while having an inadequate value for fecal coliforms and pH. For that reason, WQIs must be adapted and developed so that any minor change in the value of any parameter affects the total value of the water quality index.
A study conducted by Zhan et al. ( 2021 ) , concerning the monitoring of water quality and examining WQI trends of raw water in Macao (China) was established from 2002 to 2019 adopting the NSFWQI. NSFWQI's initial model included nine parameters (DO, FC, pH, BOD, temperature, total phosphates, and nitrates), each parameter was given a weight and the parameters used had a significant impact on the WQI calculation outcomes. Two sets of possible parameters were investigated in this study in order to determine the impact of various parameters. The first option was to keep the original 9-parameter model, however, in the second scenario, up to twenty-one parameters were chosen, selected by Principal Component Analysis (PCA).
The latter statistical method was used to learn more about the primary elements that contributed to water quality variations, and to calculate the impact of each attribute on the quality of raw water. Based on the PCA results, the 21-parameter model was chosen. The results showed that the quality of raw water in Macao has been relatively stable in the period of interest and appeared an upward trend overall. Furthermore, the outcome of environmental elements, such as natural events, the region's hydrology and meteorology, can have a significant impact on water quality. On the other hand, Macao's raw water quality met China's Class III water quality requirements and the raw water pollution was relatively low. Consequently, human activities didn’t have a significant impact on water quality due to effective treatment and protection measures (Zhan et al. 2021 ).
Tampo et al. ( 2022 ) undertook a recent study in Adjougba (Togo), in the valley of Zio River. Water samples were collected from the surface water (SW), ground water (GW) and treated wastewater (TWW), intending to compare the water quality of these resources for irrigation and domestic use.
Hence, WQIs, water suitability indicators for irrigation purposes (WSI-IPs) and raw water quality parameters were compared using statistical analysis (factor analysis and Spearman’s correlation).
Moreover, the results proposed that he water resources are suitable for irrigation and domestic use: TWW suitable for irrigation use, GW suitable for domestic use and SW suitable for irrigation use.
The NSFWQI and overall index of pollution (OPI) parameters were tested, and the results demonstrated that the sodium absorption ratio, EC, residual sodium carbonate, Chloride and FC are the most effective parameters for determining if water is suitable for irrigation.
On the other hand, EC, DO, pH, turbidity, COD, hardness, FC, nitrates, national sanitation foundation's water quality index (NSFWQI), and overall index of pollution (OPI) are the most reliable in the detection of water suitability for domestic use (Tampo et al. 2022 ).
Following these studies, it is worth examining the NSFWQI. This index can be used with other WQI models in studies on rivers, lakes etc., since one index can show different results than another index, in view of the fact that some indices might be affected by other variations such as seasonal variation.
Additionally, the NSFWQI should be developed and adapted to each river, so that any change in any value will affect the entire water quality. It is unhelpful to have a good water quality yet a low score of a parameter that can affect human health (case of FC).
2.4.2 Canadian council of ministers of the environment water quality index (CCMEWQI)
The Canadian Water Quality Index adopted the conceptual model of the British Colombia Water Quality Index (BCWQI), based on relative sub-indices (Kizar 2018 ).
The CCMEWQI provides a water quality assessment for the suitability of water bodies, to support aquatic life in specific monitoring sites in Canada (Paun et al. 2016 ). In addition, this index gives information about the water quality for both management and the public. It can furthermore be applied in many water agencies in various countries with slight modification (Tyagi et al. 2013 ).
The CCMEWQI method simplifies the complex and technical data. It tests the multi-variable water quality data and compares the data to benchmarks determined by the user (Tirkey et al. 2015 ). The sampling protocol requires at least four parameters sampled at least four times but does not indicate which ones should be used; the user must decide ( Uddin et al. 2021 ). Yet, the parameters may vary from one station to another (Tyagi et al. 2013 ).
After the water body, the objective and the period of time have been defined the three factors of the CWQI are calculated (Baghapour et al. 2013 ; Canadian Council of Ministers of the Environment 1999 ):
The scope (F1) represents the percentage of variables that failed to meet the objective (above or below the acceptable range of the selected parameter) at least once (failed variables), relative to the total number of variables.
The frequency (F2) represents the percentage of tests which do not meet the objectives (above or below the acceptable range of the selected parameter) (failed tests).
The amplitude represents the amount by which failed tests values did not meet their objectives (above or below the acceptable range of the selected parameter). It is calculated in three steps.
The excursion is termed each time the number of an individual parameter is further than (when the objective is a minimum, less than) the objective and is calculated by two Eqs. ( 4 , 5 ) referring to two cases. In case the test value must not exceed the objective:
For the cases in which the test value must not fall below the objective:
The normalized sum of excursions, or nse , is calculated by summing the excursions of individual tests from their objectives and diving by the total number of tests (both meetings and not meeting their objectives):
F3 is then calculated an asymptotic function that scales the normalized sum of the excursions from objectives (nse) to yield a range between 0 and 100:
Finally, the CMEWQI can be obtained from the following equation, where the index changes in direct proportion to changes in all three factors.
where 1.732 is a scaling factor and normalizes the resultant values to a range between 0 and 100, where 0 refers to the worst quality and one hundred represents the best water quality.
Once the CCME WQI value has been determined, water quality in ranked as shown in Table 3
Ramírez-Morales et al. ( 2021 ) investigated in their study the measuring of pesticides and water quality indices in three agriculturally impacted micro catchments in Costa Rica between 2012 and 2014. Surface water and sediment samples were obtained during the monitoring experiment.
The specifications of the water included: Pesticides, temperature, DO, oxygen saturation, BOD, TP, NO3, sulfate, ammonium, COD, conductivity, pH and TSS.
Sediment parameters included forty-two pesticides with different families including carbamate, triazine, organophosphate, phthalimide, pyrethroid, uracil, benzimidazole, substituted urea, organochlorine, imidazole, oxadiazole, diphenyl ether and bridged diphenyl.
WQIs are effective tools since they combine information from several variables into a broad picture of the water body's state. Two WQIs were calculated using the physicochemical parameters: The Canadian Council of Ministers of the Environment (CCME) WQI and the National Sanitation Foundation (NSF) WQI.
These were chosen since they are both extensively used and use different criteria to determine water quality: The NSF WQI has fixed parameters, weights, and threshold values, whereas the CCME has parameters and threshold values that are customizable.
The assessment of water quality using physico-chemical characteristics and the WQI revealed that the CCME WQI and the NSF WQI have distinct criteria. CCME WQI categorized sampling point as marginal/bad quality, while most sampling locations were categorized as good quality in the NSF WQI. Seemingly, the water quality classifications appeared to be affected by seasonal variations: during the wet season, the majority of the CCME WQI values deteriorated, implying that precipitation and runoff introduced debris into the riverbed. Thus, it’s crucial to compare WQIs because they use various factors, criteria, and threshold values, which might lead to different outcomes (Ramírez-Morales et al. 2021 ).
Yotova et al. ( 2021 ) directed an analysis on the Mesta River located between Greece and Bulgaria. The Bulgarian section of the Mesta River basin, which is under the supervision of the West-Aegean Region Basin Directorate, was being researched. The goal was to evaluate the surface water quality of ten points of the river using a novel approach that combines composite WQI developed by the CCME and Self organizing map (SOM) on the required monitoring data that include: DO, pH, EC, ammonium, nitrite, nitrate, total phosphate, BOD and TSS.
The use of WQI factors in SOM calculations allows for the identification of specific WQI profiles for various object groups and identifying groupings of river basin which have similar sampling conditions. The use of both could reveal and estimate the origin and magnitude of anthropogenic pressure. In addition, it might be determined that untreated residential wastewaters are to blame for deviations from high quality requirements in the Mesta River catchment.
Interestingly, this study reveals that WQI appear more accurate and specific when combined with a statistical test such as the SOM (Yotova et al. 2021 ).
2.4.3 Oregon water quality index (OWQI)
The Oregon Water Quality Index is a single number that creates a score to evaluate the water quality of Oregon’s stream and apply this method in other geographical region (Hamlat et al. 2017 ; Singh et al. 2013 ). The OWQI was widely accepted and applied in Oregon (USA) and Idaho (USA) (Sutadian et al. 2016 ).
Additionally, the OWQI is a variant of the NSFWQI, and is used to assess water quality for swimming and fishing, it is also used to manage major streams (Lumb et al. 2011b ). Since the introduction of the OWQI in 1970, the science of water quality has improved noticeably, and since 1978, index developers have benefited from increasing understanding of stream functionality (Bharti and Katyal 2011 ). The Oregon index belongs to the specific consumption indices group. It is a water classification based on the kind of consumption and application such as drinking, industrial, etc. (Shah and Joshi 2017 ).
The original OWQI dropped off in 1983, due to excessive resources required for calculating and reporting results. However, improvement in software and computer hardware availability, in addition to the desire for an accessible water quality information, renewed interest in the index (Cude 2001 ).
Simplicity, availability of required quality parameters, and the determination of sub-indexes by curve or analytical relations are some advantages of this approach (Darvishi et al. 2016a ). The process combines eight variables including temperature, dissolved oxygen (percent saturation and concentration), biochemical oxygen demand (BOD), pH, total solids, ammonia and nitrate nitrogen, total phosphorous and bacteria (Brown 2019 ). Equal weight parameters were used for this index and has the same effect on the final factor (Darvishi et al. 2016a ; Sutadian et al. 2016 ).
The Oregon index is calculated by the following Eq. 9 (Darvishi et al. 2016a ):
where,n is the number of parameters (n = 8) SI i is the value of parameter i.
Furthermore, the OWQI scores range from 10 for the worse case to 100 as the ideal water quality illustrated in the following Table 4 (Brown 2019 ).
Kareem et al. ( 2021 ) using three water quality indices, attempted to analyze the Euphrates River (Iraq) water quality for irrigation purposes in three different stations: WAWQI, CCMEWQI AND OWQI.
For fifteen parameters, the annual average value was calculated, which included: pH, BOD, Turbidity, orthophosphate, Total Hardness, Sulphate, Nitrate, Alkalinity, Potassium Sodium, Magnesium, Chloride, DO, Calcium and TDS.
The OWQI showed that the river is “very poor”, and since the sub-index of the OWQI does not rely on standard-parameter compliance, there are no differences between the two inclusion and exclusion scenarios, which is not the case in both WAWQI and CCMEWQI (Kareem et al. 2021 ).
Similarly, the OWQI showed a very bad quality category, and it is unfit for human consumption, compared to the NSFWQI and Wilcox indices who both showed a better quality of water in Darvishi et al., study conducted on the Talar River (Iran) (Darvishi et al. 2016b ).
2.4.4 Weighted arithmetic water quality index (WAWQI)
The weighted arithmetic index is used to calculate the treated water quality index, in other terms, this method classifies the water quality according to the degree of purity by using the most commonly measured water quality variables (Kizar 2018 ; Paun et al. 2016 ).This procedure has been widely used by scientists (Singh et al. 2013 ).
Three steps are essential in order to calculate the WAWQI:
Further quality rating or sub-index was calculated using the following equation (Jena et al. 2013 ):
Qn is the quality rating for the nth water quality parameter.
Vn is the observed value of the nth parameter at a given sampling station.
Vo is the ideal value of the nth parameter in a pure water.
Sn is the standard permissible value of the nth parameter.
The quality rating or sub index corresponding to nth parameter is a number reflecting the relative value of this parameter in polluted water with respect to its permissible standard value (Yogendra & Puttaiah 2008 ).
The unit weight was calculated by a value inversely proportional to the recommended standard values (Sn) of the corresponding parameters (Jena et al. 2013 ):
Wn is the unit weight for the nth parameter.
K is the constant of proportionality.
Sn is the standard value of the nth parameter.
The overall WQI is the aggregation of the quality rating (Qn) and the unit weight (Wn) linearly (Jena et al. 2013 ):
After calculating the WQI, the measurement scale classifies the water quality from “unsuitable water” to “excellent water quality” as given in the following Table 5 .
Sarwar et al. ( 2020 ) carried out a study in Chaugachcha and Manirampur Upazila of Jashore District (Bangladesh). The goal of this study was to determine the quality of groundwater and its appropriateness for drinking, using the WAWQI including nine parameters: turbidity, EC, pH, TDS, nitrate, ammonium, sodium, potassium and iron. Many samplings point was taken from Chaugachcha and Manirampur, and WQI differences were indicated (ranging from very poor to excellent). These variations in WQI were very certainly attributable to variances in geographical location. Another possibility could be variations in the parent materials from which the soil was created, which should be confirmed using experimental data. It is worth mentioning that every selected parameter was taken into consideration during calculation. Similarly, the water quality differed in Manirampur due to the elements contained in the water samples that had a big impact on the water quality (Sarwar et al. 2020 ).
In 2021, García-Ávila et al. undertook a comparative study between the CCMEWQI and WAWQI for the purpose of determining the water quality in the city of Azogues (Ecuador). Twelve parameters were analyzed: pH, turbidity, color, total dissolved solids, electrical conductivity, total hardness, alkalinity, nitrates, phosphates, sulfates, chlorides and residual chlorine over 6 months. The average WAWQI value was calculated suggesting that 16.67% of the distribution system was of 'excellent' quality and 83.33% was of 'good' quality, while the CCMEWQI indicated that 100% of the system was of ‘excellent’ quality.
This difference designated that the parameters having a low maximum allowable concentration have an impact on WAWQI and that WAWQI is a valuable tool to determine the quality of drinking water and have a better understanding of it (García-Ávila et al. 2022a , b ).
2.4.5 Additional water quality indices
The earliest WQI was based on a mathematical function that sums up all sub-indices, as detailed in the 2.1. History of water quality concept section (Aljanabi et al. 2021 ). The Dinius index (1972), the OWQI (1980), and the West Java index (2017) were later modified from the Horton index, which served as a paradigm for later WQI development (Banda and Kumarasamy 2020 ).
Based on eleven physical, chemical, organic, and microbiological factors, the Scottish Research Development Department (SRDDWQI) created in 1976 was based on the NSFWQI and Delphi methods used in Iran, Romania, and Portugal. Modified into the Bascaron index (1979) in Spain, which was based on 26 parameters that were unevenly weighted with a subjective representation that allowed an overestimation of the contamination level. The House index (1989) in the UK valued the parameters directly as sub-indices. The altered version was adopted as Croatia's Dalmatian index in 1999.
The Ross WQI (1977) was created in the USA using only 4 parameters and did not develop into any further indices.
In 1982, the Dalmatian and House WQI were used to create the Environmental Quality Index, which is detailed in Sect. 2.1 . This index continues to be difficult to understand and less powerful than other indices (Lumb et al. 2011a ; Uddin et al. 2021 ).
The Smith index (1990), is based on 7 factors and the Delphi technique in New Zealand, attempts to eliminate eclipsing difficulties and does not apply any weighting, raising concerns about the index's accuracy (Aljanabi et al. 2021 ; Banda and Kumarasamy 2020 ; Uddin et al. 2021 ).
The Dojildo index (1994) was based on 26 flexible, unweighted parameters and does not represent the water's total quality.
With the absence of essential parameters, the eclipse problem is a type of fixed-parameter selection. The Liou index (2004) was established in Taiwan to evaluate the Keya River based on 6 water characteristics that were immediately used into sub-index values. Additionally, because of the aggregation function, uncertainty is unrelated to the lowest sub-index ranking (Banda and Kumarasamy 2020 ; Uddin et al. 2021 ).
Said index (2004) assessed water quality using only 4 parameters, which is thought to be a deficient number for accuracy and a comprehensive picture of the water quality. Furthermore, a fixed parameter system prevents the addition of any new parameters.
Later, the Hanh index (2010), which used hybrid aggregation methods and gave an ambiguous final result, was developed from the Said index.
In addition to eliminating hazardous and biological indicators, the Malaysia River WQI (MRWQI developed in the 2.1 section) (2007) was an unfair and closed system that was relied on an expert's judgment, which is seen as being subjective and may produce ambiguous findings (Banda and Kumarasamy 2020 ; Uddin et al. 2021 ).
Table illustrated the main data of the studies published during 2020–2022 on water quality assessments and their major findings:
2.5 Advantages and disadvantages of the selected water quality indices
A comparison of the selected indices is done by listing the advantages and disadvantages of every index listed in the Table 7 below.
2.6 New attempts of WQI studies
Many studies were conducted to test the water quality of rivers, dams, groundwater, etc. using multiple water quality indices throughout the years. Various studies have been portrayed here in.
Massoud ( 2012 ) observed during a 5-year monitoring period, in order to classify the spatial and temporal variability and classify the water quality along a recreational section of the Damour river using a weighted WQI from nine physicochemical parameters measured during dry season. The WWQI scale ranged between “very bad” if the WQI falls in the range 0–25, to “excellent” if it falls in the range 91–100. The results revealed that the water quality of the Damour river if generally affected by the activities taking place along the watershed. The best quality was found in the upper sites and the worst at the estuary, due to recreational activities. If the Damour river is to be utilized it will require treatment prior any utilization (Massoud 2012 ).
Rubio-Arias et al. ( 2012 ) conducted a study in the Luis L. Leon dam located in Mexico. Monthly samples were collected at 10 random points of the dam at different depths, a total of 220 samples were collected and analyzed. Eleven parameters were considered for the WQI calculation, and WQI was calculated using the Weighted WQI equation and could be classified according to the following ranges: < 2.3 poor; from 2.3 to 2.8 good; and > 2.8 excellent. Rubio-Arias et al., remarked that the water could be categorized as good during the entire year. Nonetheless, some water points could be classified as poor due to some anthropogenic activities such as intensive farming, agricultural practices, dynamic urban growth, etc. This study confirms that water quality declined after the rainy season (Rubio-Arias et al. 2012 ).
In the same way, Haydar et al. ( 2014 ) evaluated the physical, chemical and microbiological characteristics of water in the upper and lower Litani basin, as well as in the lake of Qaraaoun. The samples were collected during the seasons of 2011–2012 from the determined sites and analyzed by PCA and the statistical computations of the physico-chemical parameters to extract correlation between variables. Thus, the statistical computations of the physico-chemical parameters showed a correlation between some parameters such as TDS, EC, Ammonium, Nitrate, Potassium and Phosphate. Different seasons revealed the presence of either mineral or anthropogenic or both sources of pollution caused by human interference from municipal wastewater and agricultural purposes discharged into the river. In addition, temporal effects were associated with seasonal variations of river flow, which caused the dilution if pollutants and, hence, variations in water quality (Haydar et al. 2014 ).
Another study conducted by Chaurasia et al., ( 2018 ), proposed a groundwater quality assessment in India using the WAWQI. Twenty-two parameters were taken into consideration for this assessment, however, only eight important parameters were chosen to calculate the WQI. The rating of water quality shows that the ground water in 20% of the study area is not suitable for drinking purpose and pollution load is comparatively high during rainy and summer seasons. Additionally, the study suggests that priority should be given to water quality monitoring and its management to protect the groundwater resource from contamination as well as provide technology to make the groundwater fit for domestic and drinking (Chaurasia et al. 2018 ).
Daou et al. ( 2018 ) evaluated the water quality of four major Lebanese rivers located in the four corners of Lebanon: Damour, Ibrahim, Kadisha and Orontes during the four seasons of the year 2010–2011. The assessment was done through the monitoring of a wide range of physical, chemical and microbiological parameters, these parameters were screened using PCA. PCA was able to discriminate each of the four rivers according to a different trophic state. The Ibrahim River polluted by mineral discharge from marble industries in its surroundings, as well as anthropogenic pollutants, and the Kadisha river polluted by anthropogenic wastes seemed to have the worst water quality. This large-scale evaluation of these four Lebanese rivers can serve as a water mass reference model (Daou et al. 2018 ).
Moreover, some studies compared many WQI methods. Kizar ( 2018 ), carried out a study on Shatt Al-Kufa in Iraq, nine locations and twelve parameters were selected. The water quality was calculated using two methods, the WAWQI and CWQI. The results revealed the same ranking of the river for both methods, in both methods the index decreased in winter and improved in other seasons (Kizar 2018 ).
On the other hand, Zotou et al. ( 2018 ), undertook a research on the Polyphytos Reservoir in Greece, taking into consideration thirteen water parameters and applying 5 WQIs: Prati’s Index of Pollution (developed in 1971, based on thirteen parameter and mathematical functions to convert the pollution concentration into new units. The results of PI classified water quality into medium classes (Gupta and Gupta 2021 ). Bhargava’s WQI (established in 1983, the BWQI categorize the parameters according to their type: bacterial indicators, heavy metals and toxins, physical parameters and organic and inorganic substances. The BWQI tends to classify the water quality into higher quality classes, which is the case in the mentioned study (Gupta and Gupta 2021 ). Oregon WQI, Dinius second index, Weighted Arithmetic WQI, in addition to the NSF and CCMEWQI. The results showed that Bhargava and NSF indices tend to classify the reservoir into superior quality classes, Prati’s and Dinius indices fall mainly into the middle classes of the quality ranking, while CCME and Oregon could be considered as “stricter” since they give results which range steadily between the lower quality classes (Zotou et al. 2018 ).
In their study, Ugochukwu et al. ( 2019 ) investigated the effects of acid mine drainage, waste discharge into the Ekulu River in Nigeria and other anthropogenic activities on the water quality of the river. The study was performed between two seasons, the rainy and dry season. Samples were collected in both seasons, furthermore, the physic-chemistry parameters and the heavy metals were analyzed. WQI procedure was estimated by assigning weights and relative weights to the parameters, ranking from “excellent water” (< 50) to “unsuitable for drinking” (> 300). The results showed the presence of heavy metals such as lead and cadmium deriving from acid mine drainage. In addition, the water quality index for all the locations in both seasons showed that the water ranked from “very poor” to “unsuitable for drinking”, therefore the water should be treated before any consumption, and that enough information to guide new implementations for river protection and public health was provided (Ugochukwu et al. 2019 ).
The latest study in Lebanon related to WQI was carried out by El Najjar et al. ( 2019 ), the purpose of the study was to evaluate the water quality of the Ibrahim River, one of the main Lebanese rivers. The samples were collected during fifteen months, and a total of twenty-eight physico-chemical and microbiological parameters were tested. The parameters were reduced to nine using the Principal Component Analysis (PCA) and Pearson Correlation. The Ibrahim WQI (IWQI) was finally calculated using these nine parameters and ranged between 0 and 25 referring to a “very bad” water quality, and between 91 and 100 referring to an “excellent” water quality. The IWQI showed a seasonal variation, with a medium quality during low -water periods and a good one during high-water periods (El Najjar et al. 2019 ).
3 Conclusion
WQI is a simple tool that gives a single value to water quality taking into consideration a specific number of physical, chemical, and biological parameters also called variables in order to represent water quality in an easy and understandable way. Water quality indices are used to assess water quality of different water bodies, and different sources. Each index is used according to the purpose of the assessment. The study reviewed the most important indices used in water quality, their mathematical forms and composition along with their advantages and disadvantages. These indices utilize parameters and are carried out by experts and government agencies globally. Nevertheless, there is no index so far that can be universally applied by water agencies, users and administrators from different countries, despite the efforts of researchers around the world (Paun et al. 2016 ). The study also reviewed some attempts on different water bodies utilizing different water quality indices, and the main studies performed in Lebanon on Lebanese rivers in order to determine the quality of the rivers (Table 6 ).
As mentioned in the article (Table 7 ); WQIs may undergo some limitations. Some indices could be biased, others are not specific, and they may not get affected by the value of an important parameter. Therefore, there is no interaction between the parameters.
Moreover, many studies exhibited a combination between WQIs and statistical techniques and analysis (such as the PCA, Pearson’s correlation etc.). with a view to obtain the relation between the parameters and which parameter might affect the water quality.
In other research, authors compared many WQIs to check the difference of water quality according to each index. Each index can provide different values depending on the sensitivity of the parameter. For that reason, WQIs should be connected to scientific advancements to develop and elaborate the index in many ways (example: ecologically). Therefore, an advanced WQI should be developed including first statistical techniques, such as Pearson correlation and multivariate statistical approach mainly Principal Component Analysis (PCA) and Cluster Analysis (CA), in order to determine secondly the interactions and correlations between the parameters such as TDS and EC, TDS and total alkalinity, total alkalinity and chloride, temperature and bacteriological parameters, consequently, a single parameter could be selected as representative of others. Finally, scientific and technological advancement for future studies such as GIS techniques, fuzzy logic technology to assess and enhance the water quality indices and cellphone-based sensors for water quality monitoring should be used.
Akhtar N, Ishak MIS, Ahmad MI, Umar K, Md Yusuff MS, Anees MT, Qadir A, Ali Almanasir YK (2021) Modification of the Water Quality Index (WQI) process for simple calculation using the Multi-Criteria Decision-Making (MCDM) Method: a review. Water 13:905. https://doi.org/10.3390/w13070905
Article CAS Google Scholar
Alexakis DE (2020) Meta-evaluation of water quality indices application into groundwater resources. Water 12:1890. https://doi.org/10.3390/w12071890
Article Google Scholar
Aljanabi ZZ, Jawad Al-Obaidy AHM, Hassan FM (2021) A brief review of water quality indices and their applications. IOP Conf Ser: Earth Environ Sci 779:012088. https://doi.org/10.1088/1755-1315/779/1/012088
Al-Kareem SA, ALKzwini RS (2022) Statistical analysis for water quality index for Shatt-Al-Hilla river in Babel city. Water Pract Technol 17:567–586. https://doi.org/10.2166/wpt.2022.004
Baghapour MA, Nasseri S, Djahed B (2013) Evaluation of Shiraz wastewater treatment plant effluent quality for agricultural irrigation by Canadian Water Quality Index (CWQI). Iran J Environ Health Sci Eng 10:27. https://doi.org/10.1186/1735-2746-10-27
Banda T, Kumarasamy M (2020) Development of a universal water quality index (UWQI) for South African River Catchments. Water 12:1534. https://doi.org/10.3390/w12061534
Betis H, St-Hilaire A, Fortin C, Duchesne S (2020) Development of a water quality index for watercourses downstream of harvested peatlands. Water Qual Res J 55:119–131. https://doi.org/10.2166/wqrj.2020.007
Bharti N, Katyal D (2011) Water quality indices used for surface water vulnerability assessment. Int J Environ Sci 2:154–173
CAS Google Scholar
Britto FB, do Vasco AN, Aguiar Netto ADO, Garcia CAB, Moraes GFO, Silva MGD (2018) Surface water quality assessment of the main tributaries in the lower São Francisco River, Sergipe. RBRH 23:6–23. https://doi.org/10.1590/2318-0331.231820170061
Brown D (2019) Oregon Water Quality Index: background, analysis and usage. State of Oregon Department of Environmental Quality, Laboratory and Environmental Assessment Program
Brown RM, McClelland NI, Deininger RA, Tozer RG (1970) A water quality index-do we dare. Water Sew Work 117:339–343
Calmuc M, Calmuc V, Arseni M, Topa C, Timofti M, Georgescu LP, Iticescu C (2020) A comparative approach to a series of physico-chemical quality indices used in assessing water quality in the lower Danube. Water 12:3239. https://doi.org/10.3390/w12113239
Canadian Council of Ministers of the Environment 2001 (1999) Canadian water quality guidelines for the protection of aquatic life: CCME Water Quality Index 1.0, Technical Report. Canadian environmental quality guidelines, Canadian Council of Ministers of the Environment, Winnipeg
Chaurasia AK, Pandey HK, Tiwari SK, Prakash R, Pandey P, Ram A (2018) Groundwater quality assessment using Water Quality Index (WQI) in parts of Varanasi District, Uttar Pradesh, India. J Geol Soc India 92:76–82. https://doi.org/10.1007/s12594-018-0955-1
Chen L, Tian Z, Zou K (2020) Water quality evaluation based on the water quality index method in Honghu Lake: one of the largest shallow lakes in the Yangtze River Economic Zone. Water Supp 20:2145–2155. https://doi.org/10.2166/ws.2020.111
Choi B, Choi SS (2021) Integrated hydraulic modelling, water quality modelling and habitat assessment for sustainable water management: a case study of the Anyang-Cheon stream. Korea Sustain 13:4330. https://doi.org/10.3390/su13084330
Choque-Quispe D, Froehner S, Palomino-Rincón H, Peralta-Guevara DE, Barboza-Palomino GI, Kari-Ferro A, Zamalloa-Puma LM, Mojo-Quisani A, Barboza-Palomino EE, Zamalloa-Puma MM, Martínez-Huamán EL, Calla-Florez M, Aronés-Medina EG, Solano-Reynoso AM, Choque-Quispe Y (2022) Proposal of a water-quality index for high Andean Basins: application to the Chumbao River, Andahuaylas. Peru Water 14:654. https://doi.org/10.3390/w14040654
Cong Thuan N (2022) Assessment of surface water quality in the Hau Giang province using geographical information system and statistical Aaproaches. J Ecol Eng 23:265–276. https://doi.org/10.12911/22998993/151927
Cristable RM, Nurdin E, Wardhana W (2020) Water quality analysis of Saluran Tarum Barat, West Java, based on National Sanitation Foundation-Water Quality Index (NSF-WQI). IOP Conf Ser: Earth Environ Sci 481:012068. https://doi.org/10.1088/1755-1315/481/1/012068
Cude CG (2001) Oregon Water Quality Index a tool for evaluating water quality management effectiveness. J Am Water Resour as 37:125–137. https://doi.org/10.1111/j.1752-1688.2001.tb05480.x
Da Silveira VR, Kunst Valentini MH, dos Santos GB, Nadaleti WC, Vieira BM (2021) Assessment of the water quality of the Mirim Lagoon and the São Gonçalo channel through qualitative indices and statistical methods. Water Air Soil Poll 232:217. https://doi.org/10.1007/s11270-021-05160-w
Daou C, Salloum M, Legube B, Kassouf A, Ouaini N (2018) Characterization of spatial and temporal patterns in surface water quality: a case study of four major Lebanese rivers. Environ Monit Assess 190:485. https://doi.org/10.1007/s10661-018-6843-8
Darvishi G, Kootenaei FG, Ramezani M, Lotfi E, Asgharnia H (2016a) Comparative investigation of river water quality by OWQI, NSFWQI and Wilcox indexes (Case study: The Talar River – IRAN). Arch Environ Prot 42:41–48. https://doi.org/10.1515/aep-2016-0005
Darvishi G, Kootenaei FG, Ramezani M, Lotfi E, Asgharnia H (2016b) Comparative investigation of river water quality by OWQI, NSFWQI and Wilcox indexes (Case study: The Talar River – IRAN). Arch Environ Prot. https://doi.org/10.1515/aep-2016-0005
De Oliveira MD, de Rezende OLT, de Fonseca JFR, Libânio M (2019) Evaluating the surface water quality index fuzzy and its influence on water treatment. J Water Process Eng 32:100890. https://doi.org/10.1016/j.jwpe.2019.100890
Deep A, Gupta V, Bisht L, Kumar R (2020) Application of WQI for water quality assessment of high-altitude snow-fed sacred Lake Hemkund. Garhwal Himal Sustain Water Resour Manag 6:89. https://doi.org/10.1007/s40899-020-00449-w
Deng L, Shahab A, Xiao H, Li J, Rad S, Jiang J, GuoYu Jiang P, Huang H, Li X, Ahmad B, Siddique J (2021) Spatial and temporal variation of dissolved heavy metals in the Lijiang River, China: implication of rainstorm on drinking water quality. Environ Sci Pollut R 28:68475–68486. https://doi.org/10.1007/s11356-021-15383-3
Dinius SH (1987) Design of an index of water quality. Water Resour Bull 23:833–843
Doderovic M, Mijanovic I, Buric D, Milenkovic M (2020) Assessment of the water quality in the Moraca River basin (Montenegro) using water quality index. Glas Srp Geogr Drus 100:67–81. https://doi.org/10.2298/GSGD2002067D
El Najjar P, Kassouf A, Probst A, Probst JL, Ouaini N, Daou C, El Azzi D (2019) High-frequency monitoring of surface water quality at the outlet of the Ibrahim River (Lebanon): a multivariate assessment. Ecol Indic 104:13–23. https://doi.org/10.1016/j.ecolind.2019.04.061
En-nkhili H, Najy M, Etebaai I, Talbi FZ, El Kharrim K, Belghyti D (2020) Application of water quality index for the assessment of Boudaroua lake in the Moroccan pre-rif. Conference GEOIT4W-2020: 1–5. https://doi.org/10.1145/3399205.3399248
Ewaid SH (2017) Water quality evaluation of Al-Gharraf river by two water quality indices. Appl Water Sci 7:3759–3765. https://doi.org/10.1007/s13201-016-0523-z
Fadel A, Kanj M, Slim K (2021) Water quality index variations in a Mediterranean reservoir: a multivariate statistical analysis relating it to different variables over 8 years. Environ Earth Sci 80:65. https://doi.org/10.1007/s12665-020-09364-x
Fraga MDS, da Silva DD, Reis GB, Guedes HAS, Elesbon AAA (2021) Temporal and spatial trend analysis of surface water quality in the Doce River basin, Minas Gerais, Brazil. Environ Dev Sustain 23:12124–12150. https://doi.org/10.1007/s10668-020-01160-8
Frîncu RM (2021) Long-term trends in water quality indices in the lower Danube and tributaries in Romania (1996–2017). Int J Environ Res Pub He 18:1665. https://doi.org/10.3390/ijerph18041665
Fu D, Chen S, Chen Y, Yi Z (2022) Development of modified integrated water quality index to assess the surface water quality: a case study of Tuo River. China Environ Monit Assess 194:333. https://doi.org/10.1007/s10661-022-09998-3
Galarza E, Cabrera M, Espinosa R, Espitia E, Moulatlet GM, Capparelli MV (2021) Assessing the quality of Amazon aquatic ecosystems with multiple lines of evidence: the case of the Northeast Andean foothills of Ecuador. B Environ Contam Tox 107:52–61. https://doi.org/10.1007/s00128-020-03089-0
Gamvroula DE, Alexakis DE (2022) Evaluating the performance of water quality indices: application in surface water of lake union, Washington State-USA. Hydrology 9:116. https://doi.org/10.3390/hydrology9070116
García-Ávila F, Jiménez-Ordóñez M, Torres-Sánchez J, Iglesias-Abad S, Cabello Torres R, Zhindón-Arévalo C (2022a) Evaluation of the impact of anthropogenic activities on surface water quality using a water quality index and environmental assessment. J Water Land Dev 53:58–67. https://doi.org/10.24425/JWLD.2022.140780
García-Ávila F, Zhindón-Arévalo C, Valdiviezo-Gonzales L, Cadme-Galabay M, Gutiérrez-Ortega H, del Pino LF (2022b) A comparative study of water quality using two quality indices and a risk index in a drinking water distribution network. Environ Technol Rev 11:49–61. https://doi.org/10.1080/21622515.2021.2013955
Ghani J, Ullah Z, Nawab J, Iqbal J, Waqas M, Ali A, Almutairi MH, Peluso I, Mohamed HRH, Shah M (2022) Hydrogeochemical characterization, and suitability assessment of drinking groundwater: application of geostatistical approach and geographic information system. Front Environ Sci 10:874464. https://doi.org/10.3389/fenvs.2022.874464
Giao NT, Nhien HTH, Anh PK, Van Ni D (2021) Classification of water quality in low-lying area in Vietnamese Mekong delta using set pair analysis method and Vietnamese water quality index. Environ Monit Assess 193:319. https://doi.org/10.1007/s10661-021-09102-1
Gomes FDG (2020) Climatic seasonality and water quality in watersheds: a study case in Limoeiro River watershed in the western region of São Paulo State, Brazil. Environ Sci Pollut Res 27:30034–30049. https://doi.org/10.1007/s11356-020-09180-7
Gruss L, Wiatkowski M, Pulikowski K, Kłos A (2021) Determination of changes in the quality of surface water in the river reservoir system. Sustainability 13:3457. https://doi.org/10.3390/su13063457
Gupta S, Gupta SK (2021) A critical review on water quality index tool: genesis, evolution and future directions. Ecol Inform 63:101299. https://doi.org/10.1016/j.ecoinf.2021.101299
Hachi T, Hachi M, Essabiri H, Boumalkha O, Doubi M, Khaffou M, Abba EH (2022) Statistical assessment of the water quality using water quality index and organic pollution index—Case study, Oued Tighza, Morocco. Mor J Chem 10:500–508. https://doi.org/10.48317/IMIST.PRSM/MORJCHEM-V10I3.33139
Hamlat A, Guidoum A, Koulala I (2017) Status and trends of water quality in the Tafna catchment: a comparative study using water quality indices. J Water Reuse Desal 7:228–245. https://doi.org/10.2166/wrd.2016.155
Haydar CM, Nehme N, Awad S, Koubaissy B, Fakih M, Yaacoub A, Toufaily J, Villeras F, Hamieh T (2014) Water quality of the upper Litani river Basin, Lebanon. Physcs Proc 55:279–284. https://doi.org/10.1016/j.phpro.2014.07.040
Horton RK (1965) An index-number system for rating water quality. J Water Pollut Con F 37:292–315
Google Scholar
Hu L, Chen L, Li Q, Zou K, Li J, Ye H (2022) Water quality analysis using the CCME-WQI method with time series analysis in a water supply reservoir. Water Supply 22:6281–6295. https://doi.org/10.2166/ws.2022.245
Jena V, Dixit S, Gupta S (2013) Assessment of water quality index of industrial area surface water samples. Int J Chemtech Res 5:278–283
Kachroud M, Trolard F, Kefi M, Jebari S, Bourrié G (2019a) Water quality indices: challenges and application limits in the literature. Water 11:361. https://doi.org/10.3390/w11020361
Kachroud M, Trolard F, Kefi M, Jebari S, Bourrié G (2019b) Water quality indices: challenges and application limits in the literature. Water. https://doi.org/10.3390/w11020361
Kareem SL, Jaber WS, Al-Maliki LA, Al-husseiny RA, Al-Mamoori SK, Alansari N (2021) Water quality assessment and phosphorus effect using water quality indices: Euphrates river- Iraq as a case study. Groundw Sustain Dev 14:100630. https://doi.org/10.1016/j.gsd.2021.100630
Khan I (2022) Hydrogeochemical and health risk assessment in and around a Ramsar-designated wetland, the Ganges River Basin, India: implications for natural and human interactions. Environ Monit and Asses 194:1–24. https://doi.org/10.1007/s10661-022-10154-0
Khan R, Saxena A, Shukla S, Sekar S, Goel P (2021) Effect of COVID-19 lockdown on the water quality index of river Gomti, India, with potential hazard of faecal-oral transmission. Environ Sci Pollut R 28:33021–33029. https://doi.org/10.1007/s11356-021-13096-1
Kizar FM (2018) A comparison between weighted arithmetic and Canadian methods for a drinking water quality index at selected locations in shatt al-kufa. IOP Conf Ser: Mater Sci Eng 433:012026. https://doi.org/10.1088/1757-899X/433/1/012026
Kothari V, Vij S, Sharma S, Gupta N (2021) Correlation of various water quality parameters and water quality index of districts of Uttarakhand. Environ Sustain Indic 9:100093. https://doi.org/10.1016/j.indic.2020.100093
Kulisz M, Kujawska J (2021) Application of artificial neural network (ANN) for water quality index (WQI) prediction for the river Warta. Poland. J Phys Conf Ser 2130:012028. https://doi.org/10.1088/1742-6596/2130/1/012028
Kumar A, Bojjagani S, Maurya A, Kisku GC (2022) Spatial distribution of physicochemical-bacteriological parametric quality and water quality index of Gomti river, India. Environ Monit Assess 194:159. https://doi.org/10.1007/s10661-022-09814-y
Kumar P (2018) Simulation of Gomti River (Lucknow City, India) future water quality under different mitigation strategies. Heliyon 4:e01074. https://doi.org/10.1016/j.heliyon.2018.e01074
Kunst Valentini MH, dos Santos GB, Duarte VH, Franz HS, Guedes HAS, Romani RF, Vieira BM (2021) Analysis of the influence of water quality parameters in the final WQI result through statistical correlation methods: Mirim lagoon, RS, Brazil, case study. Water Air Soil Pollut 232:363. https://doi.org/10.1007/s11270-021-05321-x
Lencha SM, Tränckner J, Dananto M (2021) Assessing the water quality of lake Hawassa Ethiopia—trophic state and suitability for anthropogenic uses—applying common water quality indices. Int J Environ Res Pub He 18:8904. https://doi.org/10.3390/ijerph18178904
Liou SM, Lo SL, Wang SH (2004) A generalized water quality index for Taiwan. Environ Monit Assess 96:35–52. https://doi.org/10.1023/B:EMAS.0000031715.83752.a1
Losa MS, González ARM, Hurtado DC (2022) Assessment of water quality with emphasis on trophic status in bathing areas from the central-southern coast of Cuba. Ocean Coast Res 70:e22019. https://doi.org/10.1590/2675-2824070.21096msl
Lumb A, Sharma TC, Bibeault JF (2011) A review of genesis and evolution of Water Quality Index (WQI) and some future directions. Water Qual Expos Hea. https://doi.org/10.1007/s12403-011-0040-0
Lumb LA, Sharma TC, Bibeault JF (2011) A review of genesis and evolution of Water Quality Index (WQI) and some future directions. Water Qual Expos Hea 3:11–24. https://doi.org/10.1007/s12403-011-0040-0
Luo P, Xu C, Kang S, Huo A, Lyu J, Zhou M, Nover D (2021) Heavy metals in water and surface sediments of the Fenghe river basin, China: assessment and source analysis. Water Sci Technol 84:3072–3090. https://doi.org/10.2166/wst.2021.335
Maity S, Maiti R, Senapati T (2022) Evaluation of spatio-temporal variation of water quality and source identification of conducive parameters in Damodar River, India. Environ Monit Assess 194:1–23. https://doi.org/10.1007/s10661-022-09955-0
Makubura R, Meddage DPP, Azamathulla H, Pandey M, Rathnayake U (2022) A simplified mathematical formulation for water quality index (WQI): a case study in the Kelani River Basin. Sri Lanka Fluids 7:147. https://doi.org/10.3390/fluids7050147
Massoud MA (2012) Assessment of water quality along a recreational section of the Damour River in Lebanon using the water quality index. Environ Monit Assess 184:4151–4160. https://doi.org/10.1007/s10661-011-2251-z
Hamdi KM, Lihan S, Hamdan N, Tay MG (2022) Water quality assessment and the prevalence of antibiotic- resistant bacteria from a recreational river in Kuching, Sarawak, Malaysia. J Sustain Sci Manag 17:37–59. https://doi.org/10.46754/jssm.2022.05.004
Moskovchenko DV, Babushkin AG, Yurtaev AA (2020) The impact of the Russian oil industry on surface water quality (a case study of the Agan River catchment, West Siberia). Environ Earth Sci 79:1–21. https://doi.org/10.1007/s12665-020-09097-x
Mukate S, Wagh V, Panaskar D, Jacobs JA, Sawant A (2019) Development of new integrated water quality index (IWQI) model to evaluate the drinking suitability of water. Ecol Indic 101:348–354. https://doi.org/10.1016/j.ecolind.2019.01.034
Muniz DHF, Malaquias JV, Lima JE, Oliveira-Filho EC (2020) Proposal of an irrigation water quality index (IWQI) for regional use in the Federal District, Brazil. Environ Monit Assess 192:1–15. https://doi.org/10.1007/s10661-020-08573-y
Murillo-Delgado JO, Jimenez-Torres HD, Alvarez-Bobadilla JI, Gutierrez-Ortega JA, Camacho JB, Valle PFZ, Barcelo-Quintal ID, Delgado ER, Gomez-Salazar S (2021) Chemical speciation of selected toxic metals and multivariate statistical techniques used to assess water quality of tropical Mexican Lake Chapala. Environ Monit Assess 193:1–25. https://doi.org/10.1007/s10661-021-09185-w
Muvundja FA, Walumona JR, Dusabe MC, Alunga GL, Kankonda AB, Albrecht C, Eisenberg J, Wüest A (2022) The land–water–energy nexus of Ruzizi River Dams (Lake Kivu outflow, African Great Lakes Region): status, challenges, and perspectives. Front Environ Sci 10:892591. https://doi.org/10.3389/fenvs.2022.892591
Nair HC, Joseph A, Padmakumari Gopinathan V (2020) Hydrochemistry of tropical springs using multivariate statistical analysis in Ithikkara and Kallada river basins, Kerala, India. Sustain Water Resour Manag 6:1–21. https://doi.org/10.1007/s40899-020-00363-1
Najah A, Teo FY, Chow MF, Huang YF, Latif SD, Abdullah S, Ismail M, El-Shafie A (2021) Surface water quality status and prediction during movement control operation order under COVID-19 pandemic: case studies in Malaysia. Int J Environ Sci Te 18:1009–1018. https://doi.org/10.1007/s13762-021-03139-y
Nong X, Shao D, Zhong H, Liang J (2020) Evaluation of water quality in the South-to-North Water diversion project of China using the water quality index (WQI) method. Water Res 178:115781. https://doi.org/10.1016/j.watres.2020.115781
Ortega-Samaniego QM, Romero I, Paches M, Dominici A, Fraíz A (2021) Assessment of physicochemical and bacteriological parameters in the surface water of the Juan Diaz River, Panama. WIT Trans Ecol Environ 251:95–104. https://doi.org/10.2495/WS210101
Othman F, Alaaeldin ME, Seyam M, Ahmed AN, Teo FY, Fai CM, Afan HA, Sherif M, Sefelnasr A, El-Shafie A (2020) Efficient River water quality index prediction considering minimal number of inputs variables. Eng Appl Comp Fluid Mech 14:751–763. https://doi.org/10.1080/19942060.2020.1760942
Panneerselvam B, Muniraj K, Duraisamy K, Pande C, Karuppannan S, Thomas M (2022) An integrated approach to explore the suitability of nitrate-contaminated groundwater for drinking purposes in a semiarid region of India. Environ Geochem Hlth 10:1–7. https://doi.org/10.1007/s10653-022-01237-5
Parween S, Siddique NA, Mahammad Diganta MT, Olbert AI, Uddin MG (2022) Assessment of urban river water quality using modified NSF water quality index model at Siliguri city, West Bengal, India. Environ Sustain Indic 16:100202. https://doi.org/10.1016/j.indic.2022.100202
Paun I, Cruceru L, Chiriac FL, Niculescu M, Vasile GG, Marin NM (2016) Water quality indices—methods for evaluating the quality of drinking water. In: Proceedings of the 19th INCD ECOIND International Symposium—SIMI 2016, “The Environment and the Industry”, Bucharest, Romania, 13–14 October 2016: 395–402. https://doi.org/10.21698/simi.2016.0055
Peluso J (2021) Comprehensive assessment of water quality through different approaches: physicochemical and ecotoxicological parameters. Sci Total Environ 800:149510. https://doi.org/10.1016/j.scitotenv.2021.149510
Peng H (2022) Hydrochemical characteristics and health risk assessment of groundwater in karst areas of southwest China: a case study of Bama, Guangxi. J Clean Prod 341:130872. https://doi.org/10.1016/j.jclepro.2022.130872
Phadatare SS, Gawande S (2016) Review paper on development of water quality index. Int Res J Eng Technol 5:765–767. https://doi.org/10.17577/IJERTV5IS050993
Poonam T, Tanushree B, Sukalyan C (2013) Water quality indices- important tools for water quality assessment: a review. Int J Adv Chem 1:15–28. https://doi.org/10.5121/ijac.2015.1102
Qu X, Chen Y, Liu H, Xia W, Lu Y, Gang DD, Lin LS (2020) A holistic assessment of water quality condition and spatiotemporal patterns in impounded lakes along the eastern route of China’s South-to-North water diversion project. Water Res 185:116275. https://doi.org/10.1016/j.watres.2020.116275
Radeva K, Seymenov K (2021) Surface water pollution with nutrient components, trace metals and metalloidsin agricultural and mining-affected river catchments: a case study for three tributaries of the Maritsa River, Southern Bulgaria. Geogr Pannonica 25:214–225. https://doi.org/10.5937/gp25-30811
Ramírez-Morales D, Pérez-Villanueva ME, Chin-Pampillo JS, Aguilar-Mora P, Arias-Mora V, Masís-Mora M (2021) Pesticide occurrence and water quality assessment from an agriculturally influenced Latin-American tropical region. Chemosphere 262:127851. https://doi.org/10.1016/j.chemosphere.2020.127851
Ristanto D, Ambariyanto A, Yulianto B (2021) Water quality assessment based on national sanitations foundation water quality index during rainy season in Sibelis and Kemiri estuaries Tegal City. IOP Conf Ser: Earth and Environ Sci 750:012013. https://doi.org/10.1088/1755-1315/750/1/012013
Rizani S, Feka F, Fetoshi O, Durmishi B, Shala S, Çadraku H, Bytyçi P (2022) Application of water quality index for the assessment the water quality in River Lepenci. Ecol Eng Environ Tech 23:189–201. https://doi.org/10.12912/27197050/150297
Roozbahani MM, Boldaji MN (2013) Water quality assessment of Karoun river using WQI. Int Res J Appl Basic Sci 5:628–632
Roșca OM (2020) Impact of anthropogenic activities on water quality parameters of glacial lakes from Rodnei mountains, Romania. Environ Res 182:109136. https://doi.org/10.1016/j.envres.2020.109136
Rubio-Arias H, Contreras-Caraveo M, Quintana RM, Saucedo-Teran RA, Pinales-Munguia A (2012) An overall water quality index (WQI) for a man-made aquatic reservoir in Mexico. Int J Env Res Pub He 9:1687–1698. https://doi.org/10.3390/ijerph9051687
Said A, Stevens DK, Sehlke G (2004) An innovative index for evaluating water quality in streams. Environ Manage 34:406–414. https://doi.org/10.1007/s00267-004-0210-y
Samadi MT, Sadeghi S, Rahmani A, Saghi MH (2015) Survey of water quality in Moradbeik river basis on WQI index by GIS. Environ Eng Manag J 2:7–11
Sarwar S, Ahmmed I, Mustari S, Shaibur MR (2020) Use of Weighted Arithmetic Water Quality Index (WAWQI) to determine the suitability of groundwater of Chaugachcha and Manirampur Upazila, Jashore, Bangladesh. Environ Biolog Res 2:22–30
Scopus (2022) Analyze search results Retrieved February 22, 2023, from https://www.scopus.com/term/analyzer.uri?sid=8eeff2944308f3417393fe6b0de5b7e1&origin=resultslist&src=s&s=TITLE-ABS-KEY%28water+quality+index%29&sort=cp-f&sdt=b&sot=b&sl=34&count=38419&analyzeResults=Analyze+results&txGid=68cf75652b70f07c51075648639736f3
Shah KA, Joshi GS (2017) Evaluation of water quality index for River Sabarmati, Gujarat. India Appl Water Sci 7:1349–1358. https://doi.org/10.1007/s13201-015-0318-7
Shan W (2011) Discussion on parameter choice for managing water quality of the drinking water source. Procedia Environ Sci 11:1465–1468. https://doi.org/10.1016/j.proenv.2011.12.220
Singh PK, Tiwari AK, Panigary BP, Mahato K (2013) Water quality indices used for water resources vulnerability assessment using GIS technique: a review. Int J Earth Sci Eng 6:1594–1600
Sofi MS, Hamid A, Bhat SU, Rashid I, Kuniyal JC (2022) Impact evaluation of the run-of-river hydropower projects on the water quality dynamics of the Sindh River in the Northwestern Himalayas. Environ Monit Assess 194:626. https://doi.org/10.1007/s10661-022-10303-5
Steinhart CE, Shcierow LJ, Sonzogni WC (1982) Environmental quality index for the great lakes. Water Resour Bull 18:1025–1031
Stričević L, Pavlović M, Filipović I, Radivojević A, Martić Bursać N, Gocić M (2021) Statistical analysis of water quality parameters in the basin of the Nišava River (Serbia) in the period 2009–2018. Geografie 126:55–73. https://doi.org/10.37040/geografie2021126010055
Sudhakaran S, Mahadevan H, Arun V, Krishnakumar AP, Krishnan KA (2020) A multivariate statistical approach in assessing the quality of potable and irrigation water environs of the Netravati River basin (India). Groundw Sustain Dev 11:100462. https://doi.org/10.1016/j.gsd.2020.100462
Sukmawati NMH, Rusni NW (2019) Assessment of Water Quality Index of Beratan lake using NSF WQI indicator. Warmadewa Med J 4:39–43
Sutadian AD, Muttil N, Yilmaz AG, Perera BJC (2016) Development of river water quality indice- A review. Environ Monit Assess 188:58. https://doi.org/10.1007/s10661-015-5050-0
Taloor AK, Pir RA, Adimalla N, Ali S, Manhas DS, Roy S, Singh AK (2020) Spring water quality and discharge assessment in the Basantar watershed of Jammu Himalaya using geographic information system (GIS) and water quality Index (WQI). G Groundw Sustain Dev 10:100364. https://doi.org/10.1016/j.gsd.2020.100364
Tampo L, Alfa-Sika Mande SL, Adekanmbi AO, Boguido G, Akpataku KV, Ayah M, Tchakala I, Gnazou MDT, Bawa LM, Djaneye-Boundjou G, Alhassan EH (2022) Treated wastewater suitability for reuse in comparison to groundwater and surface water in a peri-urban area: Implications for water quality management. Sci Total Environ 815:152780. https://doi.org/10.1016/j.scitotenv.2021.152780
Teodorof L, Ene A, Burada A, Despina C, Seceleanu-Odor D, Trifanov C, Ibram O, Bratfanof E, Tudor MI, Tudor M, Cernisencu I, Georgescu LP, Iticescu C (2021) Integrated assessment of surface water quality in Danube River Chilia branch. Appl Sci 11:9172. https://doi.org/10.3390/app11199172
Tirkey P, Bhattacharya T, Chakraborty S (2015) Water quality indices-important tools for water quality assessment: a review. Int J Adv Chem 1:15–28
Tripathi M, Singal SK (2019) Allocation of weights using factor analysis for development of a novel water quality index. Ecotox Environ Safe 183:109510. https://doi.org/10.1016/j.ecoenv.2019.109510
Tyagi S, Sharma B, Singh P, Dobhal R (2013) Water quality assessment in terms of water quality index. Am J Water Resour 1:34–38. https://doi.org/10.12691/ajwr-1-3-3
Uddin MG, Nash S, Rahman A, Olbert AI (2022) A comprehensive method for improvement of water quality index (WQI) models for coastal water quality assessment. Water Res 219:118532. https://doi.org/10.1016/j.watres.2022.118532
Uddin MG, Nash S, Olbert AI (2021) A review of water quality index models and their use for assessing surface water quality. Ecol Indic 122:107218. https://doi.org/10.1016/j.ecolind.2020.107218
Udeshani WAC, Dissanayake HMKP, Gunatilake SK, Chandrajith R (2020) Assessment of groundwater quality using water quality index (WQI): a case study of a hard rock terrain in Sri Lanka. Groundw Sustain Dev 11:100421. https://doi.org/10.1016/j.gsd.2020.100421
Ugochukwu U, Onuora O, Onuarah A (2019) Water quality evaluation of Ekulu river using water quality index (WQI). J Environ Stud 4:4. https://doi.org/10.13188/2471-4879.1000027
Uning R, Suratman S, Bedurus EA, Nasir FAM, Hock Seng T, Latif MT, Mostapa R (2021) The water quality and nutrients status in the Dungun River Basin, Terengganu. Am Soc Microbiol Sci J 16:1–14. https://doi.org/10.32802/asmscj.2021.837
Vaiphei SP, Kurakalva RM (2021) Hydrochemical characteristics and nitrate health risk assessment of groundwater through seasonal variations from an intensive agricultural region of upper Krishna River basin, Telangana. India. Ecotox Environ Safe 213:112073. https://doi.org/10.1016/j.ecoenv.2021.112073
Valentini M, dos Santos GB, Muller Vieira B (2021) Multiple linear regression analysis (MLR) applied for modeling a new WQI equation for monitoring the water quality of Mirim Lagoon, in the state of Rio Grande do Sul—Brazil. SN Appl Sci 3:70. https://doi.org/10.1007/s42452-020-04005-1
Varol S, Davraz A, Şener Ş, Şener E, Aksever F, Kırkan B, Tokgözlü A (2021) Assessment of groundwater quality and usability of Salda Lake Basin (Burdur/Turkey) and health risk related to arsenic pollution. J Environ Health Sci 19:681–706. https://doi.org/10.1007/s40201-021-00638-5
Vasistha P (2020) Assessment of spatio-temporal variations in lake water body using indexing method. Environ Sci Pollut R 27:41856–41875
Wang Q, Li Z, Xu Y, Li R, Zhang M (2022) Analysis of spatio-temporal variations of river water quality and construction of a novel cost-effective assessment model: a case study in Hong Kong. Environ Sci Pollut R 29:28241–28255. https://doi.org/10.1007/s11356-021-17885-6
Wong YJ, Shimizu Y, He K, Nik Sulaiman NM (2020) Comparison among different ASEAN water quality indices for the assessment of the spatial variation of surface water quality in the Selangor River basin. Malaysia Environ Monit Assess 192:644. https://doi.org/10.1007/s10661-020-08543-4
Xiao L, Zhang Q, Niu C, Wang H (2020) Spatiotemporal patterns in river water quality and pollution source apportionment in the Arid Beichuan River Basin of Northwestern China using positive matrix factorization receptor modeling techniques. Int J Env Res Pub He 17:5015. https://doi.org/10.3390/ijerph17145015
Xiong F, Chen Y, Zhang S, Xu Y, Lu Y, Qu X, Gao W, Wu X, Xin W, Gang DD, Lin LS (2022) Land use, hydrology, and climate influence water quality of China’s largest river. J Environ Manage 318:115581. https://doi.org/10.1016/j.jenvman.2022.115581
Yan F, Liu L, You Z, Zhang Y, Chen M, Xing X (2015) A dynamic water quality index model based on functional data analysis. Ecol Indic 57:249–258. https://doi.org/10.1016/j.ecolind.2015.05.005
Yang Z, Bai J, Zhang W (2021) Mapping and assessment of wetland conditions by using remote sensing images and POI data. Ecol Indic 127:107485. https://doi.org/10.1016/j.ecolind.2021.107485
Yılmaz E, Koç C, Gerasimov I (2020) A study on the evaluation of the water quality status for the Büyük Menderes River, Turkey. Sustain Water Resour Manag 6:100. https://doi.org/10.1007/s40899-020-00456-x
Yogendra K, Puttaiah ET (2008) Determination of water quality index and suitability of an urban waterbody in Shimoga Town, Karnataka. Proceedings of Taal 2007: The 12th world lake conference 342: 346
Yotova G, Varbanov M, Tcherkezova E, Tsakovski S (2021) Water quality assessment of a river catchment by the composite water quality index and self-organizing maps. Ecol Indic 120:106872. https://doi.org/10.1016/j.ecolind.2020.106872
Yuan H, Yang S, Wang B (2022) Hydrochemistry characteristics of groundwater with the influence of spatial variability and water flow in Hetao Irrigation District, China. Environ Sci Pollut R 20:1–5. https://doi.org/10.1007/s11356-022-20685-1
Zakir HM, Sharmin S, Akter A, Rahman MS (2020) Assessment of health risk of heavy metals and water quality indices for irrigation and drinking suitability of waters: a case study of Jamalpur Sadar area, Bangladesh. Environ Adv 2:100005. https://doi.org/10.1016/j.envadv.2020.100005
Zhan S, Zhou B, Li Z, Li Z, Zhang P (2021) Evaluation of source water quality and the influencing factors: a case study of Macao. Phys Chem Earth Parts a/b/c 123:103006. https://doi.org/10.1016/j.pce.2021.103006
Zhang L (2017) Different methods for the evaluation of surface water quality: the case of the Liao River, Liaoning Province, China. Int Rev Spat Plan Sustain Dev 5:4–18. https://doi.org/10.14246/irspsd.5.4_4
Zhang L (2019) Big data, knowledge mapping for sustainable development: a water quality index case study. Emerg Sci J 3:249–254. https://doi.org/10.28991/esj-2019-01187
Zhang ZM, Zhang F, Du JL, Chen DC (2022) Surface water quality assessment and contamination source identification using multivariate statistical techniques: a case study of the Nanxi River in the Taihu Watershed, China. Water 14:778. https://doi.org/10.3390/w14050778
Zhu X, Wang L, Zhang X, He M, Wang D, Ren Y, Yao H, Net Victoria Ngegla J, Pan H (2022) Effects of different types of anthropogenic disturbances and natural wetlands on water quality and microbial communities in a typical black-odor river. Ecol Indic 136:108613. https://doi.org/10.1016/j.ecolind.2022.108613
Zotou I, Tsihrintzis VA, Gikas GD (2018) Comparative assessment of various water quality indices (WQIs) in Polyphytos reservoir-Aliakmon River. Greece Proc 2:611. https://doi.org/10.3390/proceedings2110611
Download references
Author information
Authors and affiliations.
Department of Agricultural and Food Engineering, School of Engineering, Holy Spirit University of Kaslik, P.O.Box 446, Jounieh, Lebanon
Sandra Chidiac, Paula El Najjar, Naim Ouaini, Youssef El Rayess & Desiree El Azzi
FMPS HOLDING BIOTECKNO s.a.l. Research & Quality Solutions, Naccash, P.O. Box 60 247, Beirut, Lebanon
Paula El Najjar
Syngenta, Environmental Safety, Avenue des Près, 78286, Guyancourt, France
Desiree El Azzi
You can also search for this author in PubMed Google Scholar
Corresponding authors
Correspondence to Sandra Chidiac or Desiree El Azzi .
Ethics declarations
Conflict of interest.
All authors declare that they have no conflict of interest.
Additional information
Publisher's note.
Springer Nature remains neutral with regard to jurisdictional claims in published maps and institutional affiliations.
Rights and permissions
Springer Nature or its licensor (e.g. a society or other partner) holds exclusive rights to this article under a publishing agreement with the author(s) or other rightsholder(s); author self-archiving of the accepted manuscript version of this article is solely governed by the terms of such publishing agreement and applicable law.
Reprints and permissions
About this article
Chidiac, S., El Najjar, P., Ouaini, N. et al. A comprehensive review of water quality indices (WQIs): history, models, attempts and perspectives. Rev Environ Sci Biotechnol 22 , 349–395 (2023). https://doi.org/10.1007/s11157-023-09650-7
Download citation
Received : 07 December 2022
Accepted : 23 February 2023
Published : 11 March 2023
Issue Date : June 2023
DOI : https://doi.org/10.1007/s11157-023-09650-7
Share this article
Anyone you share the following link with will be able to read this content:
Sorry, a shareable link is not currently available for this article.
Provided by the Springer Nature SharedIt content-sharing initiative
- Water quality index (WQI)
- Water quality parameters
- Surface water
- Ground water
- Find a journal
- Publish with us
- Track your research
- Washington State University
- Go to wsu twitter
- Go to wsu facebook
- Go to wsu linkedin
CEREO and NSF Pathways Program takes students to Ecuador
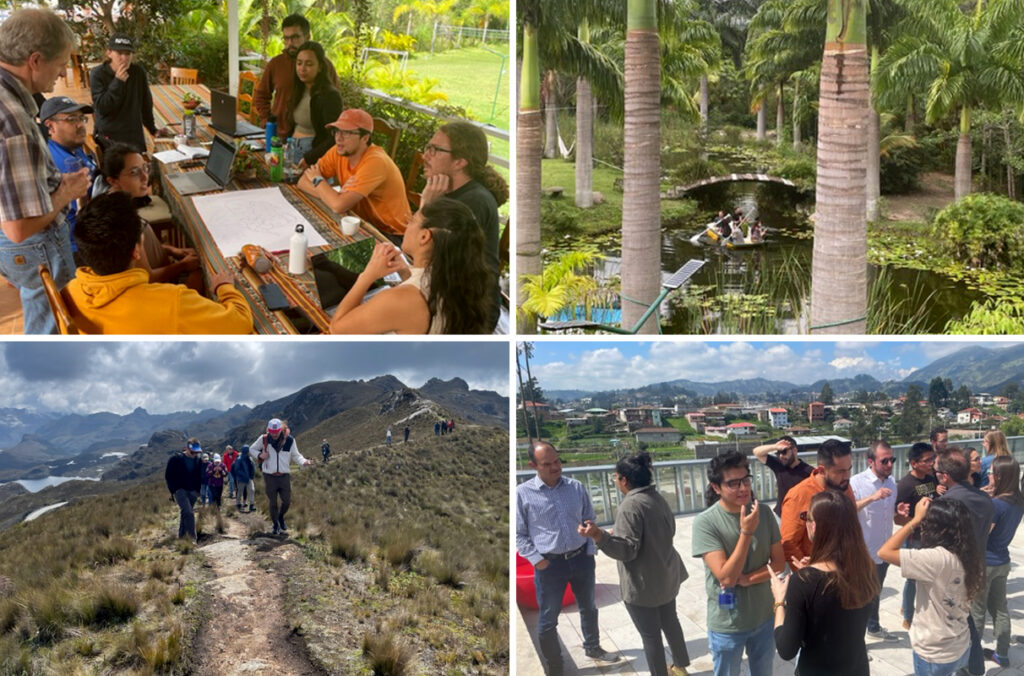
As part of a continuing global partnership, Washington State University’s Center for Environmental Research Education and Outreach took students to Ecuador early in 2024 to collaborate with their South American colleagues on water resource issues.
The event is a part of the Partnerships Along the Headwaters of the Americas for Young Scientists (Pathways) Program. Funded by the National Science Foundation’s International Research Experience for Students program, Pathways focuses on climate change effects on water storage and downstream uses that need to be managed.
In the first two years of the program, Pathways students went to Santiago and Frutillar in Chile, hosted by the University of Chile. This year, the Pathways Program traveled to Ecuador, hosted by the University of Cuenca, in the beautiful city of Cuenca.
Twelve Ecuadorian students and two Peruvian students joined 12 U.S.-based Pathways students for a two-week, intense program to understand issues related to Cuenca’s water supply.
WSU professors Alexander Fremier, Julie Padowski, and Jan Boll led the Ecuador summer school, along with Benjamin Warner from the University of New Mexico, and Rolando Célleri and Mario Cordova from the University of Cuenca.
“The interaction amongst U.S. and international students have been inspiring and long-lasting. This program has been one of the more rewarding projects in my career,” Boll said.
The interaction amongst U.S. and international students have been inspiring and long-lasting. This program has been one of the more rewarding projects in my career.” Jan Boll, professor WSU’s Center for Environmental Research Education and Outreach
Pathways is an outgrowth of the international Transect of the Americas project, which is also funded by the NSF. Boll, Fremier, and Padowski with more than 20 U.S. and international collaborators are studying the impacts of climate change on water storage systems (often called water towers) in headwater areas in mountain sites along the western Americas from Canada to Patagonia in Chile and Argentina.
Headwaters are linked to downstream areas where human activities depend on a reliable flow of quality water. The research team has adopted the term headwater dependent systems for these downstream areas. Pathways students go to sites in the area to conduct research on headwater dependent systems. This research gives the students time to interact with a variety of foreign mentors at universities and state organizations in Chile, Peru, Costa Rica, Ecuador, and Argentina.
The Pathways Program first prepared all students for the visit to Cuenca with a series of webinars in Fall 2023. During that time, students worked in groups to prepare white papers on water, climate, ecology, urban water supply, and water governance, so the entire team gained disciplinary adequacy. Once in Cuenca, the students reorganized into three interdisciplinary groups and took on topics such as “climate change effects and the 2023 drought”, “land use change effects on water quantity and quality in two headwater basins”, and “y ahora qué?” (And now what?”). In addition to practicing their interdisciplinary skills, students had an amazing cultural experience in Ecuador, and they learned about high mountain wetlands (called Páramo’s), the creativity involved with source water protection for water storage dams, and the history of water development in the region.
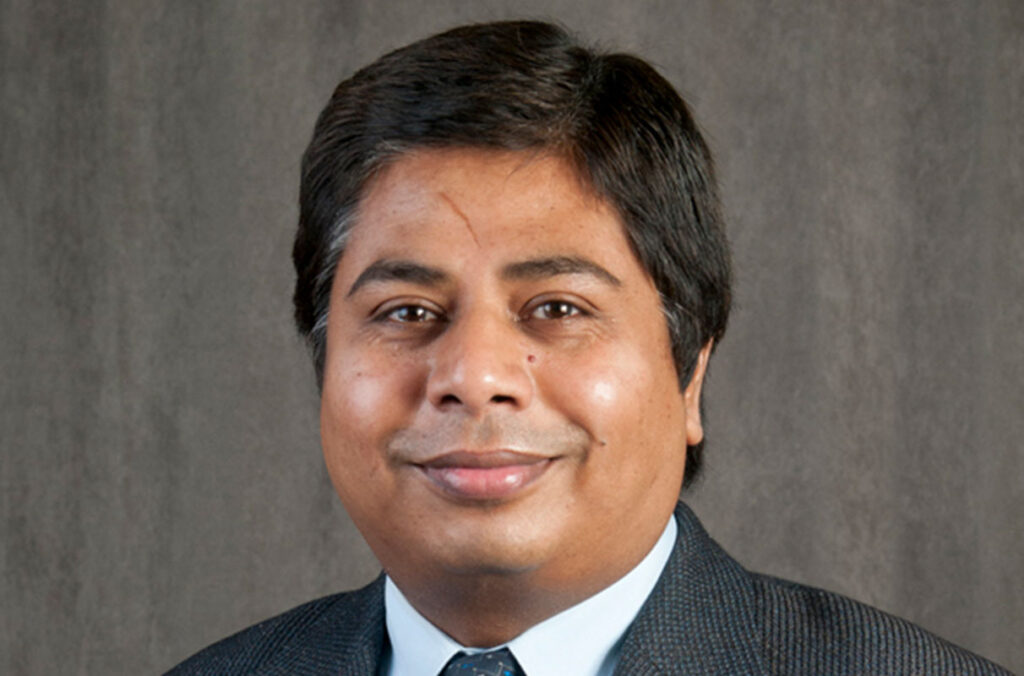
Mechanical engineering professor receives award for innovation in education
Recent news.
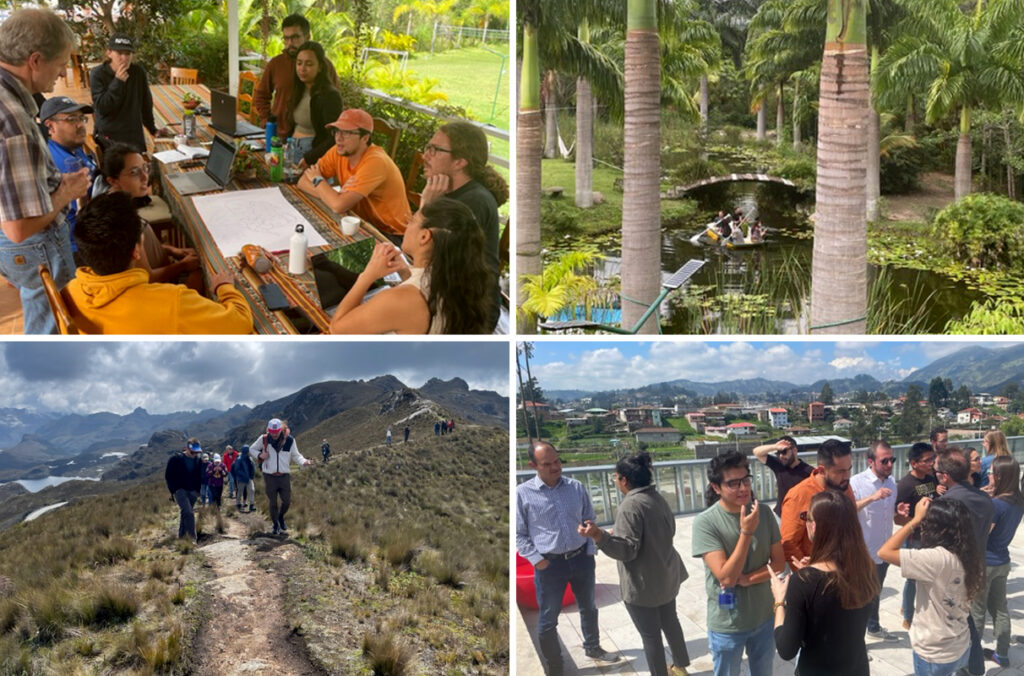
Exploring racial segregation, historical memory of Seattle
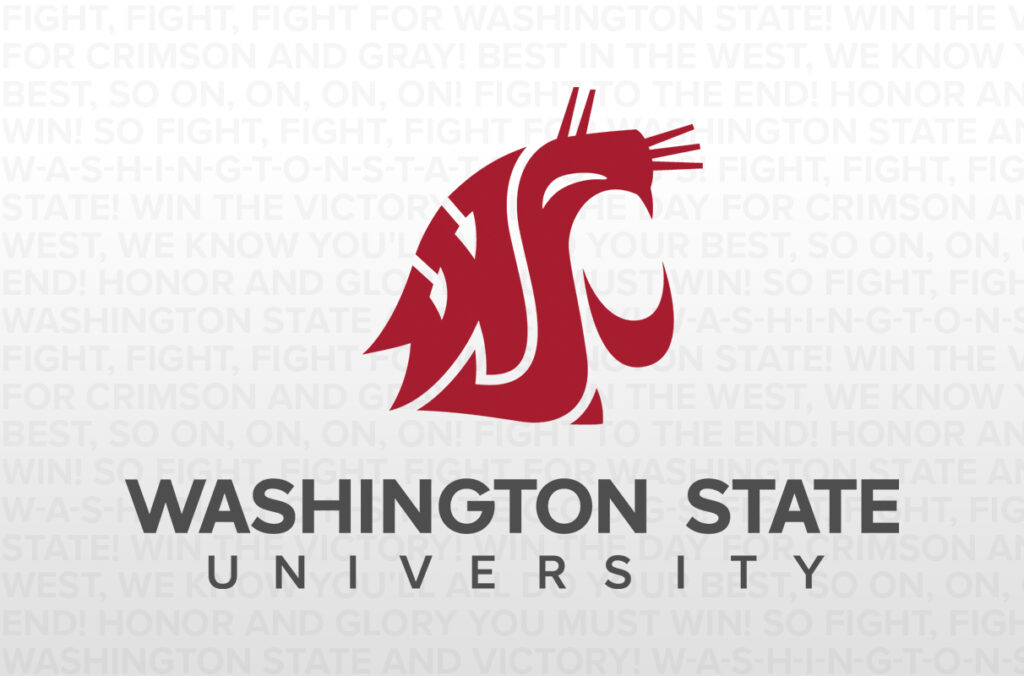
First provost candidate on campus Tuesday
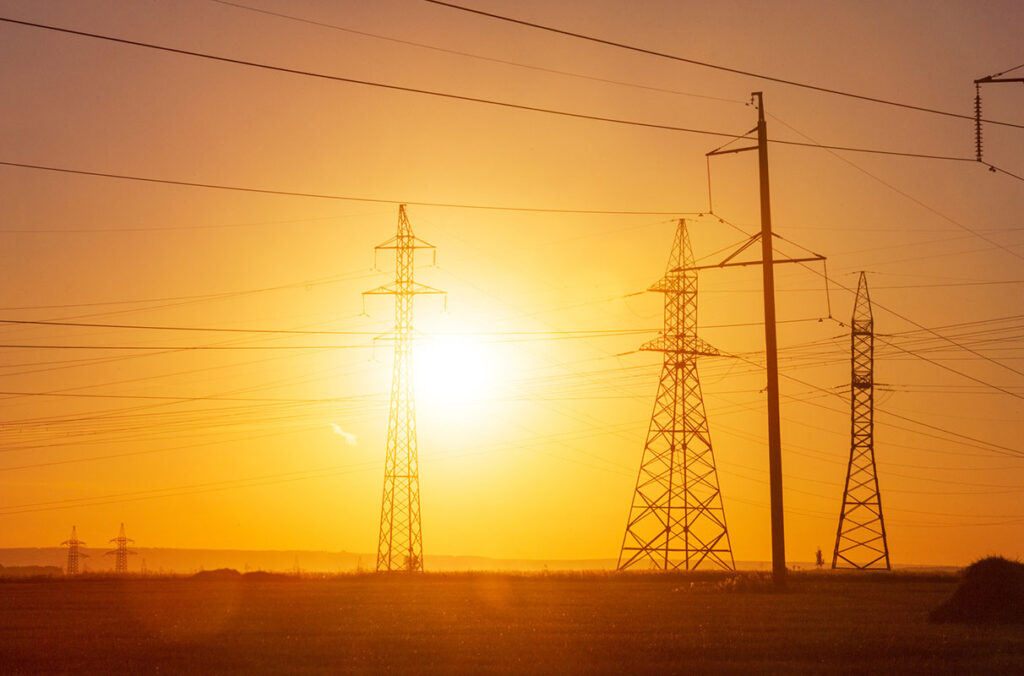
Planning tools aim to help prepare the power grid for future climate extremes
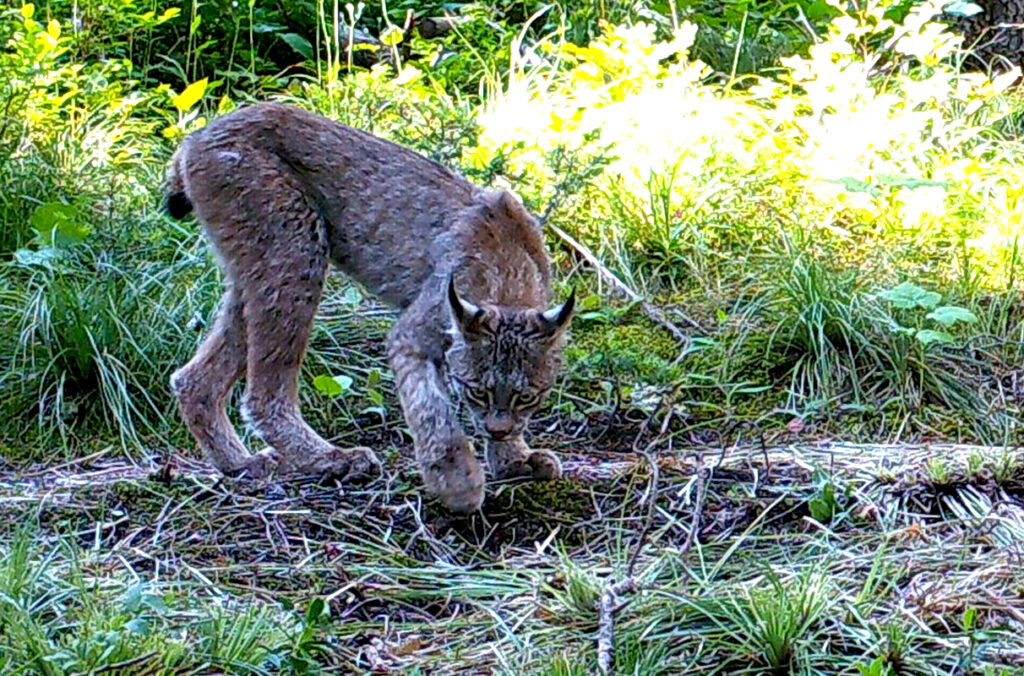
Canada lynx historic range in US likely wider than previously thought
Employee engagement survey feedback addressed in impact plans.
Generative Artificial Intelligence in education: Think piece by Stefania Giannini

Artificial Intelligence tools open new horizons for education, but we urgently need to take action to ensure we integrate them into learning systems on our terms. That is the core message of UNESCO’s new paper on generative AI and the future of education . In her think piece, UNESCO Assistant Director-General for Education, Stefania Giannini expresses her concerns that the checks and balances applied to teaching materials are not being used to the implementation of generative AI. While highlighting that AI tools create new prospects for learning, she underscores that regulations can only be built once the proper research has been conducted.
Readiness of schools to regulate the use of AI tools in education
In May, a UNESCO global survey of over 450 schools and universities found that fewer than 10% have developed institutional policies and/or formal guidance concerning the use of generative AI applications. The paper observes that in most countries, the time, steps and authorizations needed to validate a new textbook far surpass those required to move generative AI utilities into schools and classrooms. Textbooks are usually evaluated for accuracy of content, age-appropriateness, relevance of teaching and accuracy of content, cultural and social suitability which encompasses checks to protect against bias, before being used in the classroom.
Education systems must set own rules
The education sector cannot rely on the corporate creators of AI to regulate its own work. To vet and validate new and complex AI applications for formal use in school, UNESCO recommends that ministries of education build their capacities in coordination with other regulatory branches of government, in particular those regulating technologies.
Potential to undermine the status of teachers and the necessity of schools
The paper underscores that education should remain a deeply human act rooted in social interaction. It recalls that during the COVID-19 pandemic, when digital technology became the primary medium for education, students suffered both academically and socially. The paper warns us that generative AI in particular has the potential to both undermine the authority and status of teachers, and to strengthen calls for further automation of education: Teacher-less schools, and school-less education. It emphasizes that well-run schools, coupled with sufficient teacher numbers, training and salaries must be prioritized.
Education spending must focus on fundamental learning objectives
The paper argues that investment in schools and teachers, is the only way to solve the problem that today, at the dawn of the AI Era, 244 million children and youth are out of school and more than 770 million people are non-literate. Evidence shows that good schools and teachers can resolve this persistent educational challenge – yet the world continues to underfund them.
UNESCO’s response to generative AI in education
UNESCO is steering the global dialogue with policy-makers, EdTech partners, academia and civil society. The first global meeting of Ministers of Education took place in May 2023 and the Organization is developing policy guidelines on the use of generative AI in education and research, as well as frameworks of AI competencies for students and teachers for school education. These will be launched during the Digital Learning Week , which will take place at UNESCO Headquarters in Paris on 4-7 September 2023. The UNESCO Global Education Monitoring Report 2023 to be published on 26 July 2023 will focus on the use of technology in education.
UNESCO’s Recommendation on the Ethics of Artificial Intelligence
UNESCO produced the first-ever global standard on AI ethics – the ‘Recommendation on the Ethics of Artificial Intelligence’ in November 2021. This framework was adopted by all 193 Member States. The Recommendation stresses that governments must ensure that AI always adheres to the principles of safety, inclusion, diversity, transparency and quality.
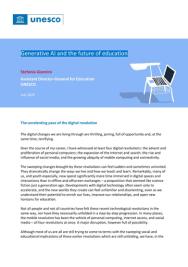
Related items
- Artificial intelligence
- Future of education
- Educational technology
- Digital learning week
- Educational trends
- Topics: Display
- See more add
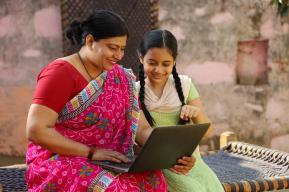
Other recent idea

Help | Advanced Search
Computer Science > Computers and Society
Title: genai detection tools, adversarial techniques and implications for inclusivity in higher education.
Abstract: This study investigates the efficacy of six major Generative AI (GenAI) text detectors when confronted with machine-generated content that has been modified using techniques designed to evade detection by these tools (n=805). The results demonstrate that the detectors' already low accuracy rates (39.5%) show major reductions in accuracy (17.4%) when faced with manipulated content, with some techniques proving more effective than others in evading detection. The accuracy limitations and the potential for false accusations demonstrate that these tools cannot currently be recommended for determining whether violations of academic integrity have occurred, underscoring the challenges educators face in maintaining inclusive and fair assessment practices. However, they may have a role in supporting student learning and maintaining academic integrity when used in a non-punitive manner. These results underscore the need for a combined approach to addressing the challenges posed by GenAI in academia to promote the responsible and equitable use of these emerging technologies. The study concludes that the current limitations of AI text detectors require a critical approach for any possible implementation in HE and highlight possible alternatives to AI assessment strategies.
Submission history
Access paper:.
- Other Formats

References & Citations
- Google Scholar
- Semantic Scholar
BibTeX formatted citation

Bibliographic and Citation Tools
Code, data and media associated with this article, recommenders and search tools.
- Institution
arXivLabs: experimental projects with community collaborators
arXivLabs is a framework that allows collaborators to develop and share new arXiv features directly on our website.
Both individuals and organizations that work with arXivLabs have embraced and accepted our values of openness, community, excellence, and user data privacy. arXiv is committed to these values and only works with partners that adhere to them.
Have an idea for a project that will add value for arXiv's community? Learn more about arXivLabs .
Colorado State University
Civil & environmental engineering walter scott, jr. college of engineering.
Walter Scott, Jr. College of Engineering
- Meet the Department Head
- Newsletters
- Admissions Information
- Curriculum & Advising
- What is Civil Engineering?
- What is Environmental Engineering?
- Bachelor-Master Accelerated Master’s Program (AMP)
- ABET Accreditation
- Internships
- Plan a Visit
- SUCCEED Program
- Academic Village
- Request Information
- Degree Information
- Graduate Application Information
- Non-Civil Engr BS Applicants
- Engineering BS Applicants
- Frequently Asked Questions
- Traditional Sub-Disciplines
- Grad Student Forms
- Grad Assistant Opportunities
- Graduate Distance Learning
- Degrees Conferred Last Five Years
- Course Descriptions
- Scholarships & Funding
- Student Associations & Clubs
- Available Student Jobs
- Research Focus Areas
- Research Centers and Institutes
- Research Laboratories
- International Programs
- Regular Faculty
- Administrative Staff
- Research Personnel
- Adjunct Faculty
- Joint Faculty
- Emeritus Faculty
- In Memoriam
- Faculty Awards
- Faculty Editorial Positions
- College Resources
- Faculty Resources
- 1930’s to 1940’s
- 1950’s to 1960’s
- 1970’s to 1980’s
- 1990’s to 2000
- 2001 to PRESENT
- Attend Engineering Events
- Nominate an Alum
- Join the Alumni Association
- Support Engineering
- Support CSU
- Search for:
Water Engineering and Science Seminar April 3: P. Andrew Jones, Esq.
“colorado water law for non-lawyers” p. andrew “andy” jones, esq. law office of p. andrew jones, wednesday, april 3, 2024 4:00pm in engineering 120.
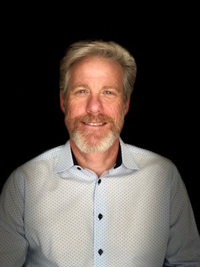
Andy will provide an overview of Colorado water law, relying on concepts first introduced in his book Colorado Water Law for Non-Lawyers, co-written with Tom Cech. This high-level summary will equip listeners with the basic tools to identify legal issues encountered in technical work involving Colorado water.
A Colorado native, P. Andrew (“Andy”) Jones graduated from Colorado State University in 1994 and Vermont Law School in 1997. His practice concentrates on water matters, and includes the representation of water districts, individuals, and corporate clients in water court litigation at trial and appellate levels. He is also active in legislative, administrative, and policy forums, where he represents clients’ interests in the development of water law and policy. He was appointed by the chief justice of the Colorado Supreme Court to serve on the Court’s standing Water Court Committee. His book, Colorado Water Rights for Non-Lawyers, written in cooperation with Thomas V. Cech, was published in 2009 by the University Press of Colorado. Learn more about Andy and his practice here: https://pandrewjones.com
Water Seminar Homepage
Winners of national penmanship contest crowned as handwriting is 'having a moment'
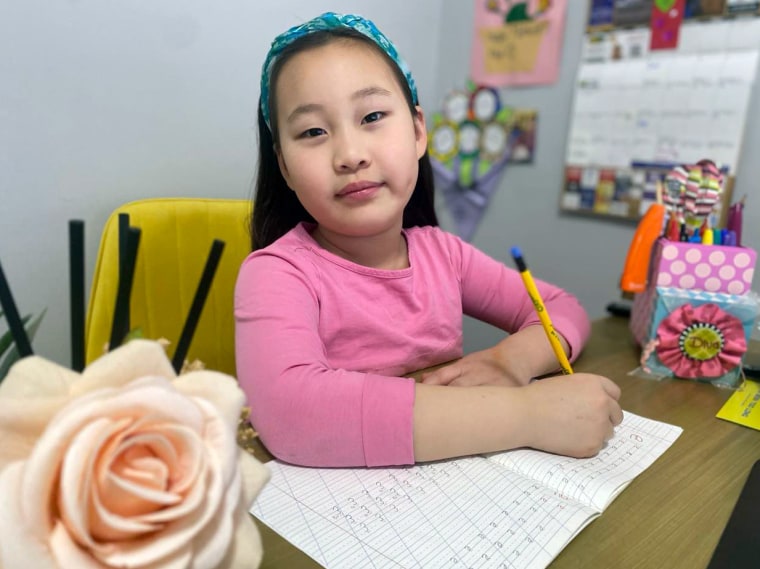
It’s regarded, hands down, as the Super Bowl of penmanship tournaments.
The Zaner-Bloser National Handwriting Contest, now in its 33rd year, crowned its 2024 grand champions on Monday, rewarding nine students from six states for their picture-perfect letters.
Ten-year old Zita Miller of White Bear Lake, Minnesota, took top honors in the fifth grade category. Her winning submission was one of the contest’s 80,000 entries.
“I like handwriting because it’s like art, drawing swirls and vines and curls,” Miller said, adding that she enjoys penning original mystery stories by hand.
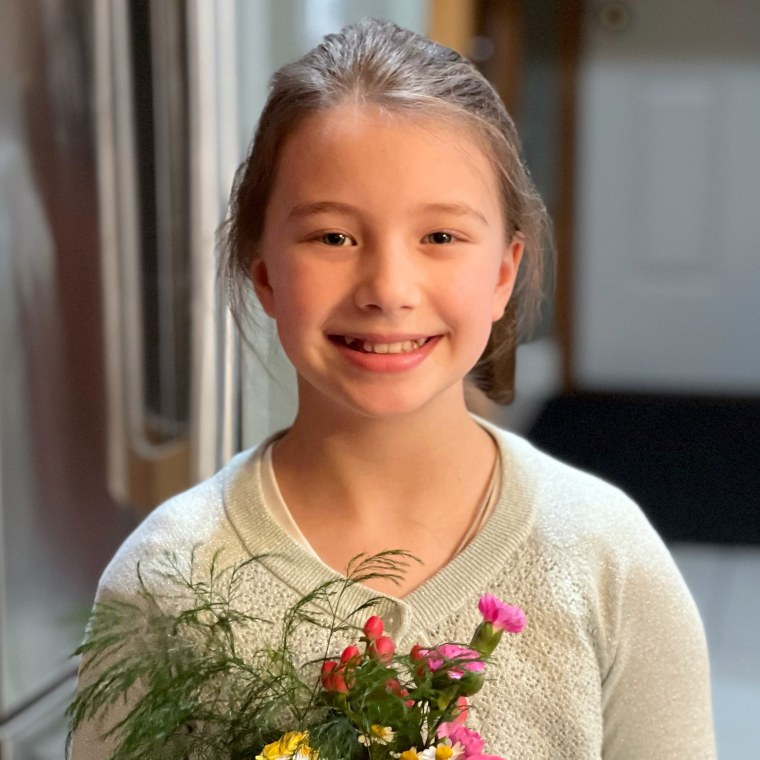
Namuun Baasanbold, from Carmel, Indiana, was named grand champion in the first grade category, and said she likes to give handwritten “love notes” to family and friends.
“Writing by hand makes me feel special,” she said.
The contest celebrates a centuries-old practice, but the victories come as handwriting is experiencing a kind of renaissance in the U.S. In January, California became the 22nd state to require cursive to be taught in schools — a significant jump from 2016, when just 12 states mandated it.
At the same time, various studies published over the past decade have detailed how writing with pencil and paper can benefit memory, cognitive development, reading comprehension and fine motor skills.
“Handwriting is definitely having a moment,” said Sharon Quirk-Silva, a member of the California state Assembly who sponsored the bill. She said she heard from people from all over the country who penned “beautifully handwritten notes” of support for the new law.
“We live in a very polarized nation. So many issues are contentious. But with this handwriting bill, we had full bipartisan support and goodwill. The importance of handwriting is something people seem to agree on,” she said.
Quirk-Silva said she backed the bill, in large part, because of her own experience — before becoming a lawmaker, she taught elementary school for 25 years.
“For years, technology has been taking over the curriculum in schools, with many kids being dormant in front of the screen, using two or three screens a day. Now, there’s a feeling of, ‘Let’s get pens and pencils back in kids’ hands,’” she said.
Although the California law mandates that first through sixth graders in the state receive cursive instruction, Quirk-Silva said she believes that writing by hand — in print or cursive — is an important language arts tool.
“It’s a way of slowing down a little bit, taking your thoughts from your brain to your hand and physically doing the writing,” she said.
Sophia Vinci-Booher, an assistant professor of psychology and human development at Vanderbilt University, said her research found that writing by hand enabled preschool students to form connections in the brain that likely support early letter recognition.
For that study , published in 2016, 20 children were asked to practice certain letters by writing them over and over, and practice others by pressing a button.
“Then we asked the children to go into an MRI scanner and look at those letters they’d been practicing,” Vinci-Booher explained. Her team analyzed the children’s brain activity to assess the functional connectivity between different areas of their brains.
“We found that the connection was stronger with letters they wrote by hand than those they tapped,” she said.
The research underscores the importance of the physical act of forming symbols, Vinci-Booher added.
“Writing by hand is a good thing for kids because it supports early reading development and it engages the fine motor system, which is developmentally important,” she said.
A 2021 study measured people’s brain activity during a memory task, this time finding that University of Tokyo students exhibited stronger activity and better recall after they had written information down on paper than when they did on a smartphone or even with a stylus on a tablet. The researchers suggested that the physical act of writing on paper provides the brain more details that trigger memory, and concluded that using paper notebooks can help students retain information in part because of their “tangible permanence.”
A similar study published in January compared the brain activity of students at the Norwegian University of Science and Technology who took notes by hand to the activity of those who typed their notes. The findings suggested that the students who wrote by hand had higher levels of electrical activity across a wide range of brain regions responsible for sensory processing and memory.
The results come as little surprise to many educators.
“I’ve seen firsthand that the kids learn more when they write by hand,” said Geeta Kadakia, who teaches second through fifth grade at the DAV Montessori School in Houston. “The lightbulb goes off through those achievements in handwriting, and handwriting leads to achievements in other areas, even math. When students make their numbers more neatly, their math scores improve.”
Laura Gajderowicz taught elementary school for 33 years in Indiana before retiring in 2022. She said she worried as she watched handwriting take a back seat to technology in U.S. classrooms in the early 2000s.
“Writing by hand does so much to help with the development of a student’s eye-hand coordination,” Gajderowicz said, adding: “I’m not against technology — I just think there’s a place at the table for both technology and handwriting when it comes to learning.”
This year, Gajderowicz served as a regional judge in the Zaner-Bloser contest.
“I was pleasantly surprised to see how many entries we had, especially from children in the upper grades,” she said.
Gajderowicz selected winners using criteria that analyzed the mechanics and precision of the letters students wrote, including their shapes, sizes, slant and spacing.
Contestants were asked to write the sentence, “The quick brown fox jumps over the lazy dog,” because it includes the entire alphabet, as well as a sentence explaining why handwriting makes them a better reader and writer.
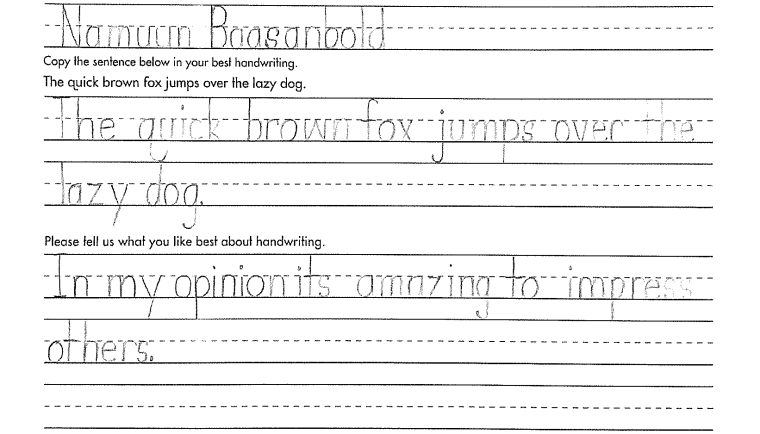
Baasanbold said she was “over the moon” to find out she won: “I screamed and celebrated with friends at a restaurant with pizza and an appetizer and a sundae for dessert,” she said.
Her prizes include a trophy and $500 — plus bragging rights.
“I like to use my handwriting to impress people,” she said.
Mary Pflum is a national field producer for NBC News, based in New York.
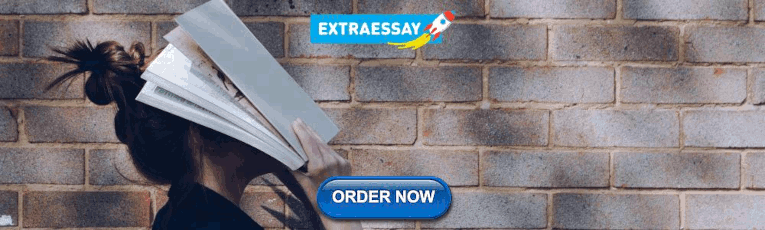
IMAGES
VIDEO
COMMENTS
Research Importance: The paper tackles and highlights the sustainable methods, techniques, ... Education: Water conservation is a crucial part of the increasingly important topic of sustainability, as it will help students to focus on social responsibility. Social responsibility is a key component of a sustainable development.
Water is critical to sustain human existence. Water literacy involves understanding the interactions within and between natural and human dimensions of water systems to support informed decision-making, an important outcome for learners of all ages. It is therefore critical to foster water literacy in today's global citizens, particularly through formal education. The purpose of this study ...
This paper seeks to synthesize and streamline the conception of water literacy. We conducted a systematic review of literature that defines or describes in detail either "water literacy" or ...
The papers presented here almost all come from reflecting on educational practice, rather than research as such. They have been developed out of reflection on the educational practice of university academics and out of professional desires to improve learning outcomes for our students, many of whom are practicing water professionals.
In their systematic review of climate change education, Monroe et al. (2017) described programs measuring knowledge, attitudes, and behavior. Thomas et al.'s (2018) review of 79 evaluations of conservation education programs reported cognitive, behavioral, social, and ecological outcomes. Thomas et al. (2018) also discussed a need for improved ...
The Integrative Graduate Education, Research, and Training (IGERT) program in the United States (1998-2015) was a forerunner model for graduate education and training in interdisciplinary environments. The last decade has seen a surge of new interdisciplinary doctoral programs in water that aim to implement the abovementioned principles.
Mostacedo-Marasovic et al. Disciplinary and Interdisciplinary Science Education Research (2022) 4:7 Page 5 of 12 except for 2019 and 2021 which exhibited Post-test scores that were approximately 5 ...
To prepare students to address water-related challenges, undergraduate STEM education must provide them with opportunities to learn and reason about water issues. Water in Society is an introductory-level, innovative, and interdisciplinary undergraduate course offered annually at a large midwestern university. The course focuses on both disciplinary concepts and civic engagement, and is ...
Omar Amahmid Department of Life and Earth Sciences, (Biology and Geology Research Units), Regional Centre for Careers in Education and Training CRMEF-Marrakech, Marrakesh40 000, ... Therefore water education topics should be incorporated in school curricula. The first objective of the present study was to explore the status of water education ...
Water-related challenges and environmental issues persist globally, including in Latin America, the Caribbean, and United States.An imperative step to improve the water management issues faced by many countries is to educate children on this important topic. Even though water conservation is not found in the basic (formal) education curricula of most the Americas (including the United States ...
Water Resources Research is an AGU hydrology journal publishing original research articles and ... Past investments and education tend to perpetuate a way of thinking among people, causing them to formulate and execute policies even if they are inferior to known alternatives. ... But clearly translating research results in ways that make them ...
In this Special Issue of Water, we invite contributions documenting research on the design, implementation, and impact of innovative water education programs that address these goals. Such programs might include K-12 or postsecondary curricula or courses, outreach programs with youth or adults, preservice teacher education, and/or professional ...
Water is a critical resource, but ensuring its availability faces challenges from climate extremes and human intervention. In this Review, we evaluate the current and historical evolution of water ...
JCWRE is listed in the Emerging Sources Citation Index (Web of Science) produced by Clarivate Analytics. Aims and Scope The Journal of Contemporary Water Research and Education (JCWRE) disseminates original, peer-reviewed articles and comprehensive reviews on applied research, policy, education, and outreach/extension in water and watershed science and management, with international scope and ...
A chain of 10 water molecules, linking the end of one a-helix (helix 9, 21 1-227) to the middle of another (helix 11, 272-285) is found from the X-ray diffraction data of glucoamylase-471, a ...
Our review focuses on primary data collection to understand how research on EJ and drinking water has captured the lived experiences of affected communities. Several EJ and drinking water papers represent the relationship between access and social vulnerability using secondary data (e.g., Balazs et al., 2011, 2012). Using secondary data such as ...
This study investigated the research trends, key areas, and directions of water rights (WRs) using bibliometric data based on the Science Citation Index Expanded (SCI-E) and Social Sciences Citation Index (SSCI). This paper presents a comprehensive review of annual output, research fields, cooperative networks, published journals, and hot spot evolution from 1971 to 2020. The results show that ...
Water Resources Research is an open access journal that publishes original research articles and commentaries on hydrology, water resources, and the social sciences of water that provide a broad understanding of the role of water in Earth's system.
Abstract— This paper presents water education issues and challenges in Timor-Leste and the way forward to in-troduce water education into school curricula. Subjects related to water education are not properly integrated into the cur- ... International Journal of Scientific & Engineering Research Volume 9, Issue 4, April-2018 ISSN 2229-5518 ...
1. Parameter selection for measurement of water quality (Shah and Joshi 2017):. The selection is carried out based on the management objectives and the environmental characteristics of the research area (Yan et al. 2015).Many variables are recommended, since they have a considerable impact on water quality and derive from 5 classes namely, oxygen level, eutrophication, health aspects, physical ...
Published since 1928, Water Environment Research (WER) is an international multidisciplinary water resource management journal for the dissemination of fundamental and applied research in all scientific and technical areas related to water quality and resource recovery. WER's goal is to foster communication and interdisciplinary research between water sciences and related fields such as ...
Abstract:. Colorado is fortunate to have a long-standing, reliable system called Prior Appropriation that is the basis for water rights administration in the state. That, coupled with a history of relying on science and engineering, has created a foundation for allocating the limited water resource in a state that relies on water for myriad uses including agricultural, municipal, commercial ...
Journal overview. Research Papers in Education has developed an international reputation for publishing significant research findings across the discipline of education. The distinguishing feature of the journal is that we publish longer articles than most other journals, to a limit of 12,000 words. We particularly focus on full accounts of ...
As part of a continuing global partnership, WSU's Center for Environmental Research Education and Outreach took students to Ecuador to collaborate with South American colleagues on water resource issues. ... students worked in groups to prepare white papers on water, climate, ecology, urban water supply, and water governance, so the entire team ...
Artificial Intelligence tools open new horizons for education, but we urgently need to take action to ensure we integrate them into learning systems on our terms. That is the core message of UNESCO's new paper on generative AI and the future of education. In her think piece, UNESCO Assistant Director-General for Education, Stefania Giannini ...
This study investigates the efficacy of six major Generative AI (GenAI) text detectors when confronted with machine-generated content that has been modified using techniques designed to evade detection by these tools (n=805). The results demonstrate that the detectors' already low accuracy rates (39.5%) show major reductions in accuracy (17.4%) when faced with manipulated content, with some ...
Engineering Building, Room A203. 1372 Campus Delivery. Fort Collins, CO 80523-1372 (970) 491-5048
A Feature Paper should be a substantial original Article that involves several techniques or approaches, provides an outlook for future research directions and describes possible research applications. Feature papers are submitted upon individual invitation or recommendation by the scientific editors and must receive positive feedback from the ...
Nine students won this year's national handwriting contest. A growing number of states are requiring cursive instruction, and research supports the benefits of writing on paper.