Disclaimer » Advertising
- HealthyChildren.org
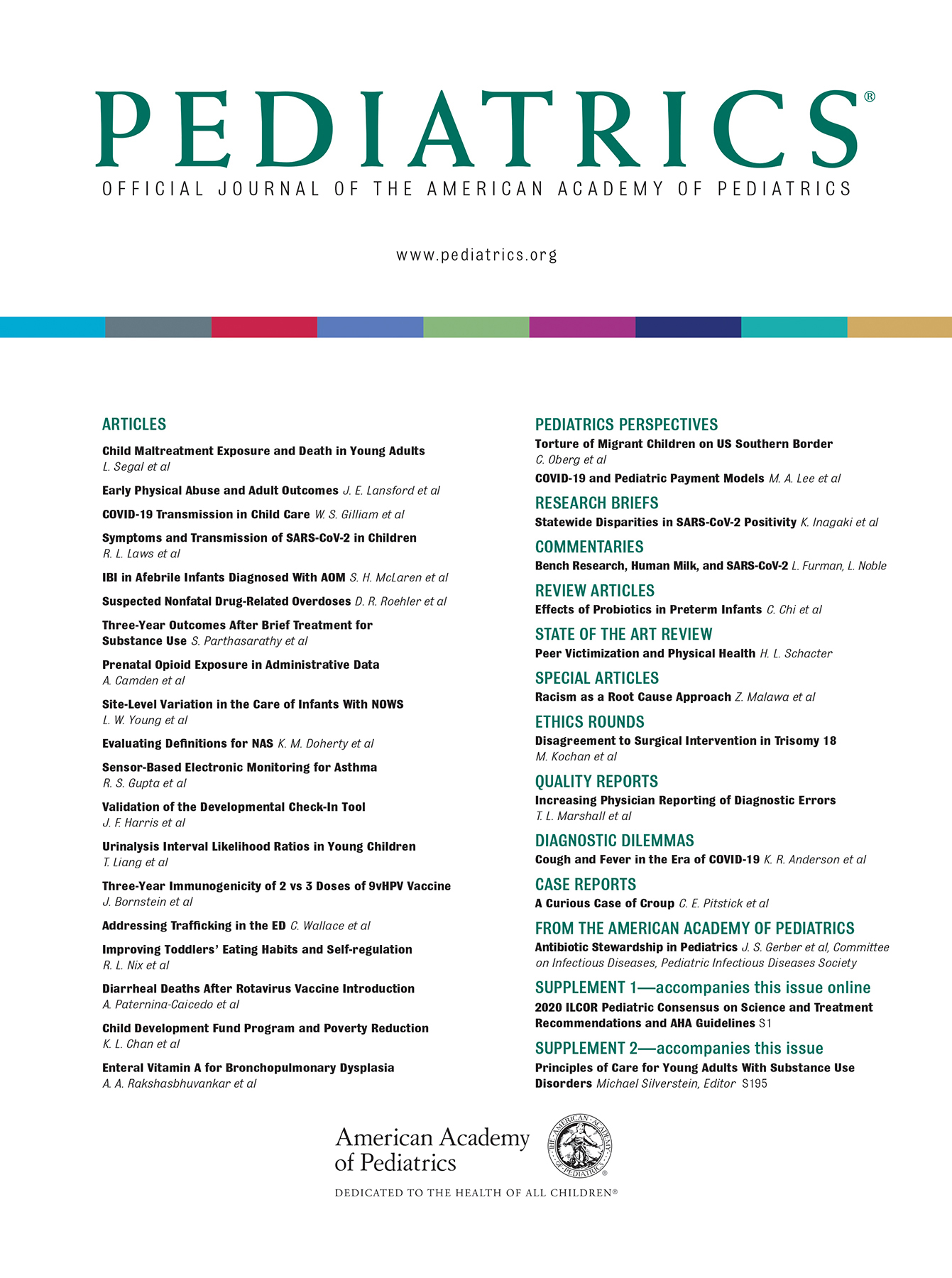
- Next Article
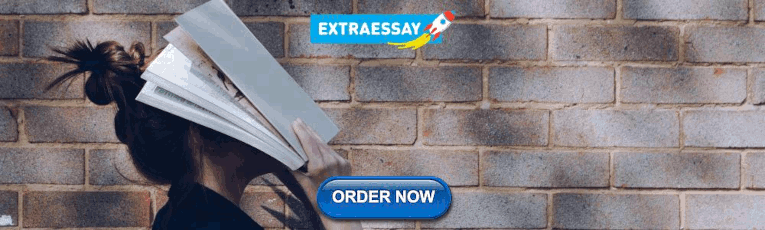
Prevaccine and Vaccine-Era Disease Estimates
Hepatitis a, hepatitis b, haemophilus influenzae type b, measles, mumps, and rubella, streptococcus pneumoniae, conclusions, acknowledgments, impact of routine childhood immunization in reducing vaccine-preventable diseases in the united states.
- Split-Screen
- Article contents
- Figures & tables
- Supplementary Data
- Peer Review
- CME Quiz Close Quiz
- Open the PDF for in another window
- Get Permissions
- Cite Icon Cite
- Search Site
Sandra E. Talbird , Justin Carrico , Elizabeth M. La , Cristina Carias , Gary S. Marshall , Craig S. Roberts , Ya-Ting Chen , Mawuli K. Nyaku; Impact of Routine Childhood Immunization in Reducing Vaccine-Preventable Diseases in the United States. Pediatrics August 2022; 150 (3): e2021056013. 10.1542/peds.2021-056013
Download citation file:
- Ris (Zotero)
- Reference Manager
Current routine immunizations for children aged ≤10 years in the United States in 2019 cover 14 vaccine-preventable diseases. We characterize the public-health impact of vaccination by providing updated estimates of disease incidence with and without universally recommended pediatric vaccines.
Prevaccine disease incidence was obtained from published data or calculated using annual case estimates from the prevaccine period and United States population estimates during the same period. Vaccine-era incidence was calculated as the average incidence over the most recent 5 years of available surveillance data or obtained from published estimates (if surveillance data were not available). We adjusted for underreporting and calculated the percent reduction in overall and age-specific incidence for each disease. We multiplied prevaccine and vaccine-era incidence rates by 2019 United States population estimates to calculate annual number of cases averted by vaccination.
Routine immunization reduced the incidence of all targeted diseases, leading to reductions in incidence ranging from 17% (influenza) to 100% (diphtheria, Haemophilus influenzae type b, measles, mumps, polio, and rubella). For the 2019 United States population of 328 million people, these reductions equate to >24 million cases of vaccine-preventable disease averted. Vaccine-era disease incidence estimates remained highest for influenza (13 412 per 100 000) and Streptococcus pneumoniae -related acute otitis media (2756 per 100 000).
Routine childhood immunization in the United States continues to yield considerable sustained reductions in incidence across all targeted diseases. Efforts to maintain and improve vaccination coverage are necessary to continue experiencing low incidence levels of vaccine-preventable diseases.
The United States childhood vaccination program has dramatically reduced morbidity, mortality, and disability for targeted diseases. Updated estimates of disease incidence and cases averted, reflecting changes in disease epidemiology, vaccine utilization, and vaccine recommendations (based on the 2017 to 2021 schedule), are needed.
The childhood vaccination program reduced the incidence of all targeted diseases—with reductions ranging from 17% (influenza) to 100% (diphtheria, Haemophilus influenzae type b, measles, mumps, polio, and rubella)—and averted >24 million disease cases for the 2019 United States population.
Childhood vaccination has dramatically reduced morbidity, mortality, and disability caused by vaccine-preventable diseases, with ∼21 million hospitalizations, 732 000 deaths, and 322 million cases of disease averted in the United States between 1994 and 2013. 1 Among diseases targeted by vaccines recommended before 1980, 3—polio, measles, and rubella—have achieved elimination status as defined by the World Health Organization 2 and 1—smallpox—has been eradicated. 3 Diphtheria and tetanus have declined markedly in incidence with routine immunization and are well controlled, 2 whereas the incidence of pertussis and mumps has declined when compared with prevaccine levels but still fluctuates given periodic outbreaks since vaccination was introduced. 3 The public health burden of diseases targeted in the childhood immunization program between 1980 and 2005, including hepatitis A, hepatitis B, invasive Haemophilus influenzae type b (Hib), varicella, and invasive pneumococcal disease (IPD), has decreased by more than 80% 3 ; reductions in related nontargeted diseases (eg, acute otitis media caused by Streptococcus pneumoniae ) have also been observed. 4 After 2005, the routine immunization schedule 5 for United States children ≤10 years of age targeted additional pathogens, such as rotavirus and further pneumococcal serotypes. 5
This study updates estimates of the reduction in overall and age-specific disease incidence associated with the routine childhood immunization program in the United States (based on the 2017 to 2021 vaccination schedule). This update incorporates changes in vaccine utilization rates and observed incidence of the targeted vaccine-preventable diseases since previous evaluations. 3 , 6 The present analysis will be of interest to policy makers, public health decision makers, and modelers concerned with public health interventions to minimize the burden of vaccine-preventable diseases. A companion study evaluated the value of the childhood immunization program for the 2017 United States birth cohort. 7
We estimated the epidemiologic impact of the United States routine childhood immunization program (ages ≤10 years) by calculating the percent reduction in overall and age-specific disease incidence rates for each disease targeted by the program. We multiplied the prevaccine and vaccine-era incidence rates (using age-specific data, where available) by 2019 United States population estimates, 8 accounting for underreporting where necessary, to calculate the 2019 clinical disease burden with and without childhood immunization and to estimate the cases averted by vaccination. As in previous studies, we assumed that the difference between incidence rates during these periods was entirely attributable to the childhood immunization program. 3 , 6
For the prevaccine period, we estimated disease incidence using published incidence estimates or calculated incidence using published annual case estimates and United States population data from the same period. For the vaccine era, we calculated incidence as the average incidence over the most recent 5 years of available surveillance data; we used published incidence estimates if surveillance data were not available. For both periods, we accounted for underreporting where necessary.
Table 1 summarizes the prevaccine and vaccine-era disease incidence sources. Age-specific incidence data were used for all diseases except diphtheria, polio, tetanus, and rotavirus. Incidence of Hib and rotavirus was limited to ages <5 years and diphtheria to ages ≤10 years, given lack of data in older age groups in the prevaccine period and the fact that clinical burden was largely limited to those age groups in both periods. Incidence of measles, mumps, and rubella was included only up to age 40 years, as prevaccine incidence data in ages ≥40 years was unavailable. For pneumococcal pneumonia, pneumococcal acute otitis media (AOM), and rotavirus, resource use estimates (ie, hospitalizations, emergency department [ED] visits, and outpatient visits) are reported instead of incidence and disease cases because of limitations in the source data.
Summary of Prevaccine and Vaccine-Era Disease Incidence Sources
ABC, Active Bacterial Core; AOM, acute otitis media; CDC, Centers for Disease Control and Prevention; ED, emergency department; IPD, invasive pneumococcal disease; NNDSS, National Notifiable Diseases Surveillance System.
Dates of immunization program initiation correspond to dates of vaccine licensure and/or routine recommended use. 3 , 96 For additional details on vaccines with multiple dates listed, please see Roush and Murphy 3 and Widdowson et al. 76
Prevaccine pertussis incidence estimates for ages >10 y were estimated from all cases reported by Roush and Murphy, 3 adjusted to account for the estimate from Cherry 95 that approximately 93% of pertussis infections in the first half of the 20th century were among ages <10 y.
An underreporting factor of 10 was taken from economic evaluations and burden-of-illness studies 53 – 55 and was multiplied by prevaccine pertussis incidence in ages >10 y and vaccine-era pertussis incidence for all ages; prevaccine incidence from birth to age 10 y (taken from Zhou et al) already accounted for underreporting. 9 This underreporting factor is conservative compared with previous studies that have tested underreporting of pertussis up to 100 to 200 times reported cases among adolescents and adults. 53 , 55 , 56
A prevaccine underreporting factor was calculated based on an estimated 48% of notifiable polio cases being paralytic in 1954. 74 This implied underreporting factor (1 of 0.48 = 2.1 cases per reported case) was used to calculate the estimated total number of notifiable polio cases (both paralytic and nonparalytic) based on the incidence of paralytic polio reported by Baicus. 75
The prevaccine underreporting factor (22.2) was calculated from the 1994 NNDSS report, 20 which reported that approximately 3.7 million cases of varicella occurred annually prevaccine, with 4% to 5% of cases reported. 11 – 15
Because cases of varicella were not reported by age in 2014 and 2015, the total cases were distributed by age using the same age distribution of cases from 2016 when calculating the age-specific 5-year incidence rate. The vaccine-era underreporting factor (10.4) was calculated based on the underreporting factor used by Roush and Murphy 3 (12.7 = 612 768 cases estimated of 48 445 cases reported by 33 states in 2006), adjusted for 40 states reporting varicella cases in 2015 versus 33 states in 2006 (12.7 × 33/40 = 10.4).
We obtained prevaccine diphtheria disease incidence for children aged ≤10 years from an economic evaluation by Zhou et al, 9 which estimated incidence from a 1916 to 1919 survey of childhood vaccine-preventable diseases in 31 353 United States children and physician-reported data. 10 We assumed the incidence reported by Zhou et al 9 for ages 5 to 9 years uniformly applied to all children ≤10 years. We calculated vaccine-era incidence among children aged ≤10 years as the average value over the most recent 5 years (2014 to 2018) of available data from the Centers for Disease Control and Prevention (CDC) National Notifiable Disease Surveillance System (NNDSS) reports. 11 – 15
We calculated prevaccine hepatitis A incidence using the average number of reported cases between 1990 and 1994 from the NNDSS 16 – 20 divided by the 1994 United States population for each respective age group. 21 We calculated vaccine-era incidence as the average value over the most recent 5 years (2014 to 2018) of available data from the NNDSS. 11 – 15 A systematic review and meta-analysis of underreporting of hepatitis A in nonendemic countries found that reported hepatitis A cases ranged from 4% to 97% of total estimated cases across 8 included studies, with a pooled proportion of 59%. 22 As a result, an underreporting factor of 1.7 (1/59% = 1.7) was applied for prevaccine and vaccine-era estimates, 22 which is similar to underreporting factors found in other studies. 23
We estimated prevaccine hepatitis B incidence as the average number of reported cases between 1976 and 1980 from the NNDSS 24 – 28 and calculated vaccine-era incidence as the average value over the most recent 5 years (2014 to 2018) of available data from the NNDSS. 11 – 15 The underreporting factor for hepatitis B (6.5) was obtained from a probabilistic model estimating underreporting of hepatitis A, B, and C. 23
We obtained prevaccine disease incidence for Hib for children aged <5 years for 1976 to 1984 from an economic analysis by Zhou et al. 29 We calculated overall incidence by summing the incidence values reported separately for Hib-related meningitis, epiglottitis, bacteremia, pneumonia, cellulitis, arthritis, and other invasive diseases reported in Zhou et al. 29 We calculated vaccine-era incidence among children aged <5 years as the average value over the most recent 5 years (2013–2017) of available data from CDC Active Bacterial Core (ABC) surveillance reports. 30 – 34
For influenza, instead of using data from the period before influenza vaccines were routinely recommended, we estimated prevaccine incidence among children aged ≤10 years by using the number of cases and averted cases estimated by the CDC, assuming all averted cases would have occurred without vaccination. 35 – 42 Specifically, we summed the number of reported cases to the cases averted by vaccination among children <5 years and children aged 5 to 10 years for 5 recent influenza seasons (2014–2015 to 2018–2019) and then divided the total number of cases by the number of children in the United States in each respective age group for the same period. 8 An average incidence across the 5 years was then calculated for both age groups. For vaccine-era incidence, we used the same source and calculated the average incidence over the same 5 recent seasons (2014–2015 to 2018–2019). Our analyses did not account for the impact of adolescent and adult influenza vaccination or herd immunity in older age groups; therefore, incidence of influenza was restricted to ages ≤10 years, and we attributed all changes in incidence to vaccination in this age cohort.
For measles, mumps, and rubella, we obtained prevaccine disease incidence from Zhou et al. 43 – 47 For the vaccine era, we calculated incidence as the average value over the most recent 5 years (2014 to 2018) of available data from the NNDSS. 11 – 15
We estimated prevaccine pertussis incidence for birth to 10 years from 2 economic evaluations of diphtheria, tetanus, and acellular pertussis vaccine, which derived age-specific risk of pertussis from United States data in the 1920s and from Sweden in the 1980s. 9 , 48 Prevaccine incidence for ages >10 years was calculated using the number of reported pertussis cases estimated by Roush and Murphy 3 for ages >10 years during 1934 to 1943 (before the start of routine pertussis vaccination in the late 1940s) divided by the size of the United States population >10 years old over the same period. 49 , 50 We calculated vaccine-era incidence as the average value over the most recent 5 years (2014 to 2018) of available data from the NNDSS. 11 – 15 An underreporting factor of 10 was applied in the prevaccine and vaccine eras ( Table 1 ). 51 – 56
For IPD, we calculated prevaccine disease incidence as the average value from the 1997 to 1999 ABC surveillance reports 57 – 59 and calculated vaccine-era incidence as the average value from the 2013 to 2017 ABC surveillance reports. 60 – 64
For pneumococcal pneumonia, we obtained prevaccine, age-specific, all-cause pneumonia hospitalization rates per 100 000 for the period 1997 to 1999 65 and all-cause outpatient visit rates per 100 000 for the period 1998 to 2000 66 , 67 ( Table 1 ). For the vaccine era, we used the incidence of all-cause pneumonia from 2014 based on an analysis of a large convenience insurance claims dataset (MarketScan) multiplied by the percentage hospitalized or treated in an outpatient or ED setting taken from the same study. 68 We multiplied the all-cause rates by the prevaccine 69 , 70 and postvaccine 71 , 72 percentage of all-cause pneumonia caused by pneumococcus ( Table 1 ).
For pneumococcal AOM, we used prevaccine, age-specific incidence from 1997 to 1999 and vaccine-era incidence from 2012 to 2014 from a retrospective analysis of the National Ambulatory Medical Care Survey comparing ambulatory visit rates before the introduction of 7-valent and following 13-valent pneumococcal conjugate vaccine. 4 We summed annual rates of physician office, hospital outpatient, and hospital ED visits to calculate a total annual ambulatory visit rate per 1000 children. To calculate pneumococcal AOM burden for each period, we multiplied all-cause rates by the percentage of AOM caused by pneumococcus in the prevaccine period (1995 to 2001) (44%) and vaccine era (2010 to 2016) (21%). 73
For polio, we obtained the average number of paralytic poliomyelitis cases for the period 1951 to 1954 (before the introduction of the first polio vaccine in 1955) from Roush and Murphy 3 . We divided the total number of cases by the average United States population size from 1951 to 1954 to estimate an overall incidence rate. 49 Age-specific data were not available in the prevaccine period; therefore, the same incidence rate was used for all ages. A prevaccine underreporting factor of 2.1 was applied ( Table 1 ). 74 , 75 We calculated vaccine-era incidence as the average value over the most recent 5 years (2014 to 2018) of available data from the NNDSS. 11 – 15
We calculated prevaccine estimates of rotavirus-related burden among children aged <5 years using 1993 to 2002 data on the cumulative individual risk of event by age 59 months for events including hospitalizations, ED visits, and hospital or ambulatory outpatient visits. 76 The median values were used to calculate annual probabilities of each type of rotavirus-related resource use. We further assumed rotavirus events were uniformly distributed from birth to age 5 years ( Supplemental Table 3 ). In the vaccine era, we calculated rotavirus-related burden by multiplying prevaccine event rates by the estimated reduction in hospitalizations 77 and reduction in ED and outpatient visits. 78
We calculated prevaccine tetanus incidence based on the number of cases reported during 1947 to 1949 (before routine vaccination began in the late 1940s 3 ) divided by the average size of the United States population during that same period. 49 Data were not available by age in the prevaccine period; therefore, the same incidence rate was used across all ages in the model. We calculated vaccine-era incidence as the average value over the most recent 5 years (2014 to 2018) of available data from the NNDSS. 11 – 15
We calculated prevaccine varicella incidence using the average number of reported varicella cases between 1990 and 1994 (before vaccine introduction in 1995) from the NNDSS 16 – 20 divided by the 1994 United States population for each respective age group. 20 , 21 We calculated vaccine-era incidence as the average value over the most recent 5 years (2014 to 2018) of available data from the NNDSS. 11 – 15 Underreporting factors of 22.2 and 10.4 were applied to prevaccine and vaccine-era incidence, respectively ( Table 1 ). 3 , 20
We report calculated incidence overall and by age for both the prevaccine and 2019 vaccine-era periods. We calculated the percent reduction in incidence overall and by age group for each disease by comparing the 2 periods. Using 2019 United States population estimates from the United States Census Bureau, we calculated the number of cases of each disease that would be expected in 2019 without and with the routine childhood immunization program and the number of cases of disease averted.
For infants (<1 year), prevaccine annual incidence per 100 000 was highest for pneumococcal AOM (49 324), influenza (18 903), measles (9200), and pertussis (4720) ( Supplemental Tables 3 – 5 ). For young children (ages 1 to 4 years), as for infants, incidence in the prevaccine period was highest for pneumococcal AOM (15 004–49 324), influenza (18 903), measles (10 641–11 503), and pertussis (4720), as well as for varicella (4519). For school-aged children (ages 5–18 years), prevaccine incidence varied by age group but was highest for influenza (14 066), varicella (389–6480), pneumococcal AOM (4840), and pertussis (131–4720). For adults, prevaccine incidence was highest for pneumococcal pneumonia (29–1553), rubella (300), mumps (99–256), and pertussis (131).
After vaccines were introduced, incidence decreased for all diseases evaluated ( Fig 1 ; Table 2 ). Incidence was reduced to less than 1 per 100 000 for 6 of the diseases: diphtheria, Hib, measles, polio, rubella, and tetanus. The incidence of mumps was reduced by >99% and varicella by 98%. The incidence of rotavirus-related hospitalizations among children aged <5 years was reduced by 91%; a lower reduction was observed for rotavirus-related ED visits (61%) and outpatient visits (45%). The incidence of pertussis was reduced by 91%, hepatitis A by 87%, hepatitis B by 86%, and IPD by 60%. Pneumococcal pneumonia hospitalization rates and outpatient visit rates decreased by 84% and 69%, respectively, and incidence of pneumococcal AOM decreased by 75%. The incidence of influenza among people aged <11 years was reduced by 17%.
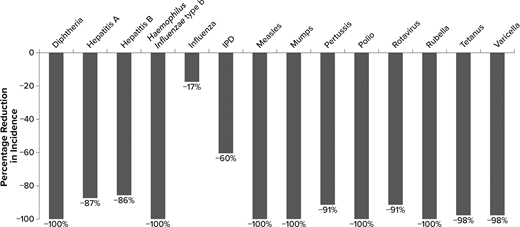
Percentage reduction in disease incidence in the vaccine era by disease. Percentage reduction for rotavirus is hospitalizations. IPD does not include pneumococcal pneumonia or acute otitis media. Percentage reductions in disease incidence round up to 100% for several diseases, although there are still some cases in the vaccine era ( Table 2 ). IPD, invasive pneumococcal disease.
Prevaccine and Vaccine-Era Disease Incidence Estimates, Annual Cases, and 2019 Cases Averted in the United States by Disease
Annual cases are rounded to the nearest thousand. AOM, acute otitis media; ED, emergency department; IPD, invasive pneumococcal disease.
Incidence estimates are adjusted by underreporting factors of 1.7 for hepatitis A, 6.5 for hepatitis B, 10.0 for pertussis (in ages 11 y and older prevaccine and all ages in the vaccine era), 2.1 for polio prevaccine (to capture paralytic and nonparalytic cases), 22.2 for varicella prevaccine, and 10.4 for varicella in the vaccine era (with all other diseases assumed fully reported and/or already adjusted to account for underreporting from the source data).
Prevaccine and vaccine-era case estimates are calculated using 2019 United States population estimates and are rounded to the nearest thousand. For Haemophilus influenzae type b and rotavirus, the population size for ages <5 y ( n = 19 576 683) was used to calculate annual cases. Annual cases for diphtheria and influenza were calculated using the population size for ages ≤10 y ( n = 43 833 518). The population size for ages <40 y ( n = 170 936 198) was used to calculate annual cases for measles, mumps, and rubella. For all other diseases, the total United States population size ( n = 328 239 523) was used to calculate annual prevaccine and vaccine-era cases.
Rotavirus and pneumococcal disease results are shown separately by healthcare resource use because of a lack of incidence data.
The calculated value for cases averted may not precisely equal the difference between the number of cases in the “with immunization” and “without immunization” period because of rounding.
For the 2019 United States population of 328 million people, the number of cases of each disease without and with the childhood immunization program and the estimated number of cases averted are shown in Table 2 . In the vaccine era with routine immunization, the annual number of cases of disease was 0 for polio, <10 cases per year for diphtheria and rubella, and <100 cases per year for Hib and tetanus. Pneumococcal AOM and influenza represented the largest clinical burden annually (>1 000 000 cases per year), followed by pertussis, pneumococcal pneumonia, outpatient rotavirus gastroenteritis, and outpatient varicella (between 100 000 and 1 million cases per year).
Routine immunization was estimated to avert over 24 million cases of vaccine-preventable disease in 2019 across all age groups, ranging from approximately 1000 cases of tetanus averted to more than 4.2 million varicella cases averted ( Table 2 ). Cases averted were greatest (>1 000 000) for influenza, measles, mumps, rubella, pertussis, varicella, and outpatient visits for pneumococcal AOM.
This analysis found that routine childhood immunization in the United States has continued to reduce the incidence of all targeted diseases. Landmark achievements have been the reduction in incidence of diphtheria, Hib, measles, polio, rubella, and tetanus to negligible levels (<1 case per 100 000 population annually); and >90% reduction in incidence for 10 diseases targeted by the routine childhood immunization program for children ≤10 years of age. These reductions equate to the prevention of over 24 million cases of disease for the 2019 US population.
Roush and Murphy 3 evaluated the impact of routine childhood immunization on vaccine-preventable diseases for which recommendations were in place before 2005, using 2006 disease data. Our estimates were generally consistent with the previous results and other published studies, 79 although we estimated a greater reduction in incidence of IPD (60% versus 34%) and of varicella (98% versus 85%). A potential explanation for these differences may be that our analysis used vaccine-era incidence from 2013 to 2017 for pneumococcal disease and from 2014 to 2018 for varicella, capturing the greater impact of the 13-valent pneumococcal conjugate vaccine (recommended in 2010 for infants) compared with the 7-valent pneumococcal conjugate vaccine and capturing the greater impact of 2-dose varicella vaccine compared with 1 dose (second dose added to recommendations in 2007). 80
With sustained vaccine coverage at levels greater than 80% for most pediatric vaccines (with the exception of hepatitis A, rotavirus, and annual influenza vaccine), many vaccine-preventable diseases are now controlled as a public health problem or eliminated in the United States. However, despite significant impact of vaccines, continued risk from these vaccine-preventable diseases remains. When whole-cell pertussis vaccine was withdrawn in Sweden in 1979 because of concerns about safety and efficacy, incidence rates of pertussis similar to those observed in the prevaccine era returned in Sweden within a few years; after introduction of the diphtheria, tetanus, and acellular pertussis vaccine in 1996, incidence rates decreased markedly compared with the 1986 to 1995 10-year period. 81 , 82 Similarly, despite elimination status being declared for measles in 2000, under-vaccination has led to continued measles outbreaks in the United States, jeopardizing elimination status for the disease. 83 – 85 Diphtheria outbreaks continue to occur where vaccination rates are low, particularly in areas of social disruption, and are often associated with high rates of mortality. 86 , 87 The most recent large outbreak occurred in Russia from 1990 to 1997, resulting in ∼115 000 cases and 3000 deaths across the population. 88 These experiences underscore the importance of continued immunization in sustaining reductions in incidence of infectious diseases.
This analysis includes some limitations. First, consistent with previous studies, 3 , 6 the analysis does not directly account for other public health measures (eg, better sanitation, healthcare access, and improved standards of care) that have been introduced over the past 70 years and likely contributed to the reduction in vaccine-preventable diseases. Furthermore, this analysis did not account for random error in the parameter estimates or account for the proportion of disease incidence reduction that may be attributed to adolescent and adult vaccines or to booster doses. As a result, the analysis may overestimate reductions in burden directly attributable to childhood immunization. Future analyses could address these methodological limitations using time-series analysis to identify and adjust for trends to explore the extent to which adolescent and adult vaccination programs, which have expanded since 2005, 80 , 89 , 90 contribute to reduction in disease incidence.
Second, owing to limited data on differences among racial and ethnic groups, this analysis did not account for racial or ethnic disparities in vaccine coverage and incidence of vaccine-preventable diseases. Evaluating the public health impact of routine immunization among racial and ethnic groups is an important direction for future research. Moreover, this analysis was limited in scope to vaccine-preventable disease for vaccines included in the United States routine childhood immunization program for children ages ≤10 years. Expansion of this analysis to include vaccine-preventable diseases, such as meningococcus and human papillomavirus targeted by routine adolescent vaccines, is another potential area of future research.
Third, because annual incidence varies substantially from year to year for many vaccine-preventable diseases, we have calculated prevaccine and vaccine-era incidence as averages across multiple years, where data allowed. Despite our efforts to estimate average incidence values in both periods, significant epidemics or outbreaks occurred for some diseases that may not be reflected in the annual averages used in this analysis. 91 For the vaccine era, data used to derive disease incidence were for years preceding the coronavirus disease 2019 (COVID-19) pandemic. There are multiple factors that may influence the impact of COVID-19 on the incidence of vaccine-preventable diseases. For example, behavior changes caused by nonpharmaceutical interventions, including lockdowns, face-covering use, and other social distancing measures may reduce the transmission of some diseases, while simultaneously causing disruptions to vaccine uptake and coverage for the pediatric population that may adversely impact the prevention of vaccine-preventable diseases. 92 – 94 Future surveillance and survey data will help to understand the impact of the COVID-19 pandemic and other potential “shocks” to the immunization program on the transmission of other vaccine-preventable diseases.
Routine childhood immunization in the United States has continued to reduce the incidence of all targeted vaccine-preventable diseases. In the vaccine era, the incidence of diphtheria, Hib, measles, polio, rubella, and tetanus has been reduced to <1 per 100 000; across all targeted diseases, ∼24 million cases have been averted because of vaccination for the 2019 United States population. Routine immunization remains an effective public health intervention to avert disease; maintenance of high rates of vaccination coverage is necessary for sustained impact.
We thank Kate Lothman of RTI Health Solutions, who provided medical writing support for the development of this manuscript and whose services were funded by Merck Sharp & Dohme LLC, a subsidiary of Merck & Co, Inc, Rahway, NJ, USA.
Ms Talbird, Mr Carrico, and Dr La conceptualized and designed the study, reviewed the literature, interpreted the results, and drafted the initial manuscript; Drs Chen, Nyaku, Carias, and Roberts conceptualized the study and provided input on the study design, secured funding, and interpreted the results; Dr Marshall interpreted the results; and all authors reviewed and revised the manuscript, approved the final manuscript as submitted, and agree to be accountable for all aspects of the work.
COMPANION PAPERS: companions to this article can be found online at http://www.pediatrics.org/cgi/doi/10.1542/peds.2021-056007 and http://www.pediatrics.org/cgi/doi/10.1542/peds.2022-057831 .
Dr La’s current affiliation is GSK, Philadelphia, Pennsylvania.
FUNDING: This study was funded by Merck Sharp & Dohme LLC, a subsidiary of Merck & Co, Inc, Rahway, NJ, USA.
CONFLICT OF INTEREST DISCLOSURES: Ms Talbird and Mr Carrico are employed by RTI Health Solutions, which received funding for the conduct of this study. Dr La was an employee of RTI Health Solutions when this study was conducted and is now an employee and shareholder in the GSK group of companies. Drs Chen, Carias, and Roberts are employees of Merck Sharp and Dohme LLC, a subsidiary of Merck & Co, Inc, Rahway, NJ, and are shareholders in Merck & Co, Inc. Rahway, NJ. Dr Nyaku was an employee of Merck Sharp & Dohme LLC, a subsidiary of Merck & Co, Inc, Rahway, NJ and a shareholder in Merck & Co, Inc, Rahway, NJ when this study was conducted. Dr Marshall has been an investigator on clinical trials funded by GlaxoSmithKline, Merck, Pfizer, Sanofi Pasteur, and Seqirus, and he has received honoraria from these companies for service on advisory boards and/or nonbranded presentations.
active bacterial core
acute otitis media
Centers for Disease Control and Prevention
coronavirus disease 2019
emergency department
Haemophilus influenzae type b
invasive pneumococcal disease
National Notifiable Disease Surveillance System

Supplementary data
Advertising Disclaimer »
Citing articles via
Email alerts.
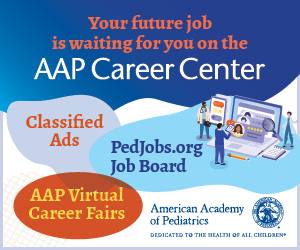
Affiliations
- Editorial Board
- Editorial Policies
- Journal Blogs
- Pediatrics On Call
- Online ISSN 1098-4275
- Print ISSN 0031-4005
- Pediatrics Open Science
- Hospital Pediatrics
- Pediatrics in Review
- AAP Grand Rounds
- Latest News
- Pediatric Care Online
- Red Book Online
- Pediatric Patient Education
- AAP Toolkits
- AAP Pediatric Coding Newsletter
First 1,000 Days Knowledge Center
Institutions/librarians, group practices, licensing/permissions, integrations, advertising.
- Privacy Statement | Accessibility Statement | Terms of Use | Support Center | Contact Us
- © Copyright American Academy of Pediatrics
This Feature Is Available To Subscribers Only
Sign In or Create an Account
We've detected unusual activity from your computer network
To continue, please click the box below to let us know you're not a robot.
Why did this happen?
Please make sure your browser supports JavaScript and cookies and that you are not blocking them from loading. For more information you can review our Terms of Service and Cookie Policy .
For inquiries related to this message please contact our support team and provide the reference ID below.
REVIEW article
The effect of covid-19 vaccine to the omicron variant in children and adolescents: a systematic review and meta-analysis.
- 1 Department of Outpatient, West China Hospital, Sichuan University, Chengdu, China
- 2 Department of Obstetrics and Gynecology, Peking University Third Hospital, Beijing, Beijing Municipality, China
- 3 National Clinical Research Center for Obstetrics and Gynecology, Peking University Third Hospital, Beijing, China
- 4 General Practice Ward/International Medical Center Ward, General Practice Medical Center, West China Hospital, Sichuan University, Chengdu, China
- 5 Department of Pediatrics, West China Second University Hospital, Sichuan University, Chengdu, Sichuan Province, China
- 6 Key Laboratory of Obstetric, Gynecologic and Pediatric Diseases and Birth Defects of Ministry of Education, West China Second University Hospital, Sichuan University, Chengdu, Sichuan Province, China
The final, formatted version of the article will be published soon.
Select one of your emails
You have multiple emails registered with Frontiers:
Notify me on publication
Please enter your email address:
If you already have an account, please login
You don't have a Frontiers account ? You can register here
Background: Omicron (B.1.1.529), a variant of SARS-CoV-2, has emerged as a dominant strain in COVID-19 pandemic. This development has raised concerns about the effectiveness of vaccination to Omicron, particularly in the context of children and adolescents. Our study evaluated the efficacy of different COVID-19 vaccination regimens in children and adolescents during the Omicron epidemic phase. Methods: We searched PubMed, Cochrane, Web of Science, and Embase electronic databases for studies published through March 2023 on the association between COVID-19 vaccination and vaccine effectiveness (VE) against SARS-CoV-2 infection in children and adolescents at the Omicron variant period. The effectiveness outcomes included mild COVID-19 and severe COVID-19. This study followed the Preferred Reporting Items for Systematic Reviews and Meta-Analyses guidelines and was prospectively registered in PROSPERO (CRD42023390481). Results: A total of 33 studies involving 16,532,536 children were included in the analysis. First, in children and adolescents aged 0-19 years, the overall VE of the COVID-19 vaccine is 45% (95% confidence interval [CI]: 40% to 50%). Subgroup analysis of VE during Omicron epidemic phase for different dosage regimens demonstrated that the VE was 50% (95% CI: 44% to 55%) for the 2-dose vaccination and 61% (95% CI: 45% to 73%) for the booster vaccination. Upon further analysis of different effectiveness outcomes during the 2-dose vaccination showed that the VE was 41% (95% CI: 35% to 47%) against mild COVID-19 and 71% (95% CI: 60% to 79%) against severe COVID-19. In addition, VE exhibited a gradual decrease over time, with the significant decline in the efficacy of Omicron for infection before and after 90 days following the 2-dose vaccination, registering 54% (95% CI: 48% to 59%) and 34% (95% CI: 21% to 56%), respectively. Conclusions: During the Omicron variant epidemic, the vaccine provided protection against SARS-CoV-2 infection in children and adolescents aged 0-19 years. Two doses of vaccination can provide effective protection severe COVID-19, with booster vaccination additionally enhancing VE.
Keywords: SARS-COV-2 variants, omicron, COVID-19 vaccines, Child, Adolescent
Received: 14 Nov 2023; Accepted: 27 Mar 2024.
Copyright: © 2024 Lu, Zeng, Yao, Luo and Ruan. This is an open-access article distributed under the terms of the Creative Commons Attribution License (CC BY) . The use, distribution or reproduction in other forums is permitted, provided the original author(s) or licensor are credited and that the original publication in this journal is cited, in accordance with accepted academic practice. No use, distribution or reproduction is permitted which does not comply with these terms.
* Correspondence: Tiechao Ruan, Department of Pediatrics, West China Second University Hospital, Sichuan University, Chengdu, 610041, Sichuan Province, China
Disclaimer: All claims expressed in this article are solely those of the authors and do not necessarily represent those of their affiliated organizations, or those of the publisher, the editors and the reviewers. Any product that may be evaluated in this article or claim that may be made by its manufacturer is not guaranteed or endorsed by the publisher.
Log in using your username and password
- Search More Search for this keyword Advanced search
- Latest content
- Current issue
- Browse by collection
- BMJ Journals More You are viewing from: Google Indexer
You are here
- Online First
- The role of COVID-19 vaccines in preventing post-COVID-19 thromboembolic and cardiovascular complications
- Article Text
- Article info
- Citation Tools
- Rapid Responses
- Article metrics

- Núria Mercadé-Besora 1 , 2 , 3 ,
- Xintong Li 1 ,
- Raivo Kolde 4 ,
- Nhung TH Trinh 5 ,
- Maria T Sanchez-Santos 1 ,
- Wai Yi Man 1 ,
- Elena Roel 3 ,
- Carlen Reyes 3 ,
- http://orcid.org/0000-0003-0388-3403 Antonella Delmestri 1 ,
- Hedvig M E Nordeng 6 , 7 ,
- http://orcid.org/0000-0002-4036-3856 Anneli Uusküla 8 ,
- http://orcid.org/0000-0002-8274-0357 Talita Duarte-Salles 3 , 9 ,
- Clara Prats 2 ,
- http://orcid.org/0000-0002-3950-6346 Daniel Prieto-Alhambra 1 , 9 ,
- http://orcid.org/0000-0002-0000-0110 Annika M Jödicke 1 ,
- Martí Català 1
- 1 Pharmaco- and Device Epidemiology Group, Health Data Sciences, Botnar Research Centre, NDORMS , University of Oxford , Oxford , UK
- 2 Department of Physics , Universitat Politècnica de Catalunya , Barcelona , Spain
- 3 Fundació Institut Universitari per a la recerca a l'Atenció Primària de Salut Jordi Gol i Gurina (IDIAPJGol) , IDIAP Jordi Gol , Barcelona , Catalunya , Spain
- 4 Institute of Computer Science , University of Tartu , Tartu , Estonia
- 5 Pharmacoepidemiology and Drug Safety Research Group, Department of Pharmacy, Faculty of Mathematics and Natural Sciences , University of Oslo , Oslo , Norway
- 6 School of Pharmacy , University of Oslo , Oslo , Norway
- 7 Division of Mental Health , Norwegian Institute of Public Health , Oslo , Norway
- 8 Department of Family Medicine and Public Health , University of Tartu , Tartu , Estonia
- 9 Department of Medical Informatics, Erasmus University Medical Center , Erasmus University Rotterdam , Rotterdam , Zuid-Holland , Netherlands
- Correspondence to Prof Daniel Prieto-Alhambra, Pharmaco- and Device Epidemiology Group, Health Data Sciences, Botnar Research Centre, NDORMS, University of Oxford, Oxford, UK; daniel.prietoalhambra{at}ndorms.ox.ac.uk
Objective To study the association between COVID-19 vaccination and the risk of post-COVID-19 cardiac and thromboembolic complications.
Methods We conducted a staggered cohort study based on national vaccination campaigns using electronic health records from the UK, Spain and Estonia. Vaccine rollout was grouped into four stages with predefined enrolment periods. Each stage included all individuals eligible for vaccination, with no previous SARS-CoV-2 infection or COVID-19 vaccine at the start date. Vaccination status was used as a time-varying exposure. Outcomes included heart failure (HF), venous thromboembolism (VTE) and arterial thrombosis/thromboembolism (ATE) recorded in four time windows after SARS-CoV-2 infection: 0–30, 31–90, 91–180 and 181–365 days. Propensity score overlap weighting and empirical calibration were used to minimise observed and unobserved confounding, respectively.
Fine-Gray models estimated subdistribution hazard ratios (sHR). Random effect meta-analyses were conducted across staggered cohorts and databases.
Results The study included 10.17 million vaccinated and 10.39 million unvaccinated people. Vaccination was associated with reduced risks of acute (30-day) and post-acute COVID-19 VTE, ATE and HF: for example, meta-analytic sHR of 0.22 (95% CI 0.17 to 0.29), 0.53 (0.44 to 0.63) and 0.45 (0.38 to 0.53), respectively, for 0–30 days after SARS-CoV-2 infection, while in the 91–180 days sHR were 0.53 (0.40 to 0.70), 0.72 (0.58 to 0.88) and 0.61 (0.51 to 0.73), respectively.
Conclusions COVID-19 vaccination reduced the risk of post-COVID-19 cardiac and thromboembolic outcomes. These effects were more pronounced for acute COVID-19 outcomes, consistent with known reductions in disease severity following breakthrough versus unvaccinated SARS-CoV-2 infection.
- Epidemiology
- PUBLIC HEALTH
- Electronic Health Records
Data availability statement
Data may be obtained from a third party and are not publicly available. CPRD: CPRD data were obtained under the CPRD multi-study license held by the University of Oxford after Research Data Governance (RDG) approval. Direct data sharing is not allowed. SIDIAP: In accordance with current European and national law, the data used in this study is only available for the researchers participating in this study. Thus, we are not allowed to distribute or make publicly available the data to other parties. However, researchers from public institutions can request data from SIDIAP if they comply with certain requirements. Further information is available online ( https://www.sidiap.org/index.php/menu-solicitudesen/application-proccedure ) or by contacting SIDIAP ([email protected]). CORIVA: CORIVA data were obtained under the approval of Research Ethics Committee of the University of Tartu and the patient level data sharing is not allowed. All analyses in this study were conducted in a federated manner, where analytical code and aggregated (anonymised) results were shared, but no patient-level data was transferred across the collaborating institutions.
This is an open access article distributed in accordance with the Creative Commons Attribution 4.0 Unported (CC BY 4.0) license, which permits others to copy, redistribute, remix, transform and build upon this work for any purpose, provided the original work is properly cited, a link to the licence is given, and indication of whether changes were made. See: https://creativecommons.org/licenses/by/4.0/ .
https://doi.org/10.1136/heartjnl-2023-323483
Statistics from Altmetric.com
Request permissions.
If you wish to reuse any or all of this article please use the link below which will take you to the Copyright Clearance Center’s RightsLink service. You will be able to get a quick price and instant permission to reuse the content in many different ways.
WHAT IS ALREADY KNOWN ON THIS TOPIC
COVID-19 vaccines proved to be highly effective in reducing the severity of acute SARS-CoV-2 infection.
While COVID-19 vaccines were associated with increased risk for cardiac and thromboembolic events, such as myocarditis and thrombosis, the risk of complications was substantially higher due to SARS-CoV-2 infection.
WHAT THIS STUDY ADDS
COVID-19 vaccination reduced the risk of heart failure, venous thromboembolism and arterial thrombosis/thromboembolism in the acute (30 days) and post-acute (31 to 365 days) phase following SARS-CoV-2 infection. This effect was stronger in the acute phase.
The overall additive effect of vaccination on the risk of post-vaccine and/or post-COVID thromboembolic and cardiac events needs further research.
HOW THIS STUDY MIGHT AFFECT RESEARCH, PRACTICE OR POLICY
COVID-19 vaccines proved to be highly effective in reducing the risk of post-COVID cardiovascular and thromboembolic complications.
Introduction
COVID-19 vaccines were approved under emergency authorisation in December 2020 and showed high effectiveness against SARS-CoV-2 infection, COVID-19-related hospitalisation and death. 1 2 However, concerns were raised after spontaneous reports of unusual thromboembolic events following adenovirus-based COVID-19 vaccines, an association that was further assessed in observational studies. 3 4 More recently, mRNA-based vaccines were found to be associated with a risk of rare myocarditis events. 5 6
On the other hand, SARS-CoV-2 infection can trigger cardiac and thromboembolic complications. 7 8 Previous studies showed that, while slowly decreasing over time, the risk for serious complications remain high for up to a year after infection. 9 10 Although acute and post-acute cardiac and thromboembolic complications following COVID-19 are rare, they present a substantial burden to the affected patients, and the absolute number of cases globally could become substantial.
Recent studies suggest that COVID-19 vaccination could protect against cardiac and thromboembolic complications attributable to COVID-19. 11 12 However, most studies did not include long-term complications and were conducted among specific populations.
Evidence is still scarce as to whether the combined effects of COVID-19 vaccines protecting against SARS-CoV-2 infection and reducing post-COVID-19 cardiac and thromboembolic outcomes, outweigh any risks of these complications potentially associated with vaccination.
We therefore used large, representative data sources from three European countries to assess the overall effect of COVID-19 vaccines on the risk of acute and post-acute COVID-19 complications including venous thromboembolism (VTE), arterial thrombosis/thromboembolism (ATE) and other cardiac events. Additionally, we studied the comparative effects of ChAdOx1 versus BNT162b2 on the risk of these same outcomes.
Data sources
We used four routinely collected population-based healthcare datasets from three European countries: the UK, Spain and Estonia.
For the UK, we used data from two primary care databases—namely, Clinical Practice Research Datalink, CPRD Aurum 13 and CPRD Gold. 14 CPRD Aurum currently covers 13 million people from predominantly English practices, while CPRD Gold comprises 3.1 million active participants mostly from GP practices in Wales and Scotland. Spanish data were provided by the Information System for the Development of Research in Primary Care (SIDIAP), 15 which encompasses primary care records from 6 million active patients (around 75% of the population in the region of Catalonia) linked to hospital admissions data (Conjunt Mínim Bàsic de Dades d’Alta Hospitalària). Finally, the CORIVA dataset based on national health claims data from Estonia was used. It contains all COVID-19 cases from the first year of the pandemic and ~440 000 randomly selected controls. CORIVA was linked to the death registry and all COVID-19 testing from the national health information system.
Databases included sociodemographic information, diagnoses, measurements, prescriptions and secondary care referrals and were linked to vaccine registries, including records of all administered vaccines from all healthcare settings. Data availability for CPRD Gold ended in December 2021, CPRD Aurum in January 2022, SIDIAP in June 2022 and CORIVA in December 2022.
All databases were mapped to the Observational Medical Outcomes Partnership Common Data Model (OMOP CDM) 16 to facilitate federated analytics.
Multinational network staggered cohort study: study design and participants
The study design has been published in detail elsewhere. 17 Briefly, we used a staggered cohort design considering vaccination as a time-varying exposure. Four staggered cohorts were designed with each cohort representing a country-specific vaccination rollout phase (eg, dates when people became eligible for vaccination, and eligibility criteria).
The source population comprised all adults registered in the respective database for at least 180 days at the start of the study (4 January 2021 for CPRD Gold and Aurum, 20 February 2021 for SIDIAP and 28 January 2021 for CORIVA). Subsequently, each staggered cohort corresponded to an enrolment period: all people eligible for vaccination during this time were included in the cohort and people with a history of SARS-CoV-2 infection or COVID-19 vaccination before the start of the enrolment period were excluded. Across countries, cohort 1 comprised older age groups, whereas cohort 2 comprised individuals at risk for severe COVID-19. Cohort 3 included people aged ≥40 and cohort 4 enrolled people aged ≥18.
In each cohort, people receiving a first vaccine dose during the enrolment period were allocated to the vaccinated group, with their index date being the date of vaccination. Individuals who did not receive a vaccine dose comprised the unvaccinated group and their index date was assigned within the enrolment period, based on the distribution of index dates in the vaccinated group. People with COVID-19 before the index date were excluded.
Follow-up started from the index date until the earliest of end of available data, death, change in exposure status (first vaccine dose for those unvaccinated) or outcome of interest.
COVID-19 vaccination
All vaccines approved within the study period from January 2021 to July 2021—namely, ChAdOx1 (Oxford/AstraZeneca), BNT162b2 (BioNTech/Pfizer]) Ad26.COV2.S (Janssen) and mRNA-1273 (Moderna), were included for this study.
Post-COVID-19 outcomes of interest
Outcomes of interest were defined as SARS-CoV-2 infection followed by a predefined thromboembolic or cardiac event of interest within a year after infection, and with no record of the same clinical event in the 6 months before COVID-19. Outcome date was set as the corresponding SARS-CoV-2 infection date.
COVID-19 was identified from either a positive SARS-CoV-2 test (polymerase chain reaction (PCR) or antigen), or a clinical COVID-19 diagnosis, with no record of COVID-19 in the previous 6 weeks. This wash-out period was imposed to exclude re-recordings of the same COVID-19 episode.
Post-COVID-19 outcome events were selected based on previous studies. 11–13 Events comprised ischaemic stroke (IS), haemorrhagic stroke (HS), transient ischaemic attack (TIA), ventricular arrhythmia/cardiac arrest (VACA), myocarditis/pericarditis (MP), myocardial infarction (MI), heart failure (HF), pulmonary embolism (PE) and deep vein thrombosis (DVT). We used two composite outcomes: (1) VTE, as an aggregate of PE and DVT and (2) ATE, as a composite of IS, TIA and MI. To avoid re-recording of the same complication we imposed a wash-out period of 90 days between records. Phenotypes for these complications were based on previously published studies. 3 4 8 18
All outcomes were ascertained in four different time periods following SARS-CoV-2 infection: the first period described the acute infection phase—that is, 0–30 days after COVID-19, whereas the later periods - which are 31–90 days, 91–180 days and 181–365 days, illustrate the post-acute phase ( figure 1 ).
- Download figure
- Open in new tab
- Download powerpoint
Study outcome design. Study outcomes of interest are defined as a COVID-19 infection followed by one of the complications in the figure, within a year after infection. Outcomes were ascertained in four different time windows after SARS-CoV-2 infection: 0–30 days (namely the acute phase), 31–90 days, 91–180 days and 181–365 days (these last three comprise the post-acute phase).
Negative control outcomes
Negative control outcomes (NCOs) were used to detect residual confounding. NCOs are outcomes which are not believed to be causally associated with the exposure, but share the same bias structure with the exposure and outcome of interest. Therefore, no significant association between exposure and NCO is to be expected. Our study used 43 different NCOs from previous work assessing vaccine effectiveness. 19
Statistical analysis
Federated network analyses.
A template for an analytical script was developed and subsequently tailored to include the country-specific aspects (eg, dates, priority groups) for the vaccination rollout. Analyses were conducted locally for each database. Only aggregated data were shared and person counts <5 were clouded.
Propensity score weighting
Large-scale propensity scores (PS) were calculated to estimate the likelihood of a person receiving the vaccine based on their demographic and health-related characteristics (eg, conditions, medications) prior to the index date. PS were then used to minimise observed confounding by creating a weighted population (overlap weighting 20 ), in which individuals contributed with a different weight based on their PS and vaccination status.
Prespecified key variables included in the PS comprised age, sex, location, index date, prior observation time in the database, number of previous outpatient visits and previous SARS-CoV-2 PCR/antigen tests. Regional vaccination, testing and COVID-19 incidence rates were also forced into the PS equation for the UK databases 21 and SIDIAP. 22 In addition, least absolute shrinkage and selection operator (LASSO) regression, a technique for variable selection, was used to identify additional variables from all recorded conditions and prescriptions within 0–30 days, 31–180 days and 181-any time (conditions only) before the index date that had a prevalence of >0.5% in the study population.
PS were then separately estimated for each staggered cohort and analysis. We considered covariate balance to be achieved if absolute standardised mean differences (ASMDs) were ≤0.1 after weighting. Baseline characteristics such as demographics and comorbidities were reported.
Effect estimation
To account for the competing risk of death associated with COVID-19, Fine-and-Grey models 23 were used to calculate subdistribution hazard ratios (sHRs). Subsequently, sHRs and confidence intervals were empirically calibrated from NCO estimates 24 to account for unmeasured confounding. To calibrate the estimates, the empirical null distribution was derived from NCO estimates and was used to compute calibrated confidence intervals. For each outcome, sHRs from the four staggered cohorts were pooled using random-effect meta-analysis, both separately for each database and across all four databases.
Sensitivity analysis
Sensitivity analyses comprised 1) censoring follow-up for vaccinated people at the time when they received their second vaccine dose and 2) considering only the first post-COVID-19 outcome within the year after infection ( online supplemental figure S1 ). In addition, comparative effectiveness analyses were conducted for BNT162b2 versus ChAdOx1.
Supplemental material
Data and code availability.
All analytic code for the study is available in GitHub ( https://github.com/oxford-pharmacoepi/vaccineEffectOnPostCovidCardiacThromboembolicEvents ), including code lists for vaccines, COVID-19 tests and diagnoses, cardiac and thromboembolic events, NCO and health conditions to prioritise patients for vaccination in each country. We used R version 4.2.3 and statistical packages survival (3.5–3), Empirical Calibration (3.1.1), glmnet (4.1-7), and Hmisc (5.0–1).
Patient and public involvement
Owing to the nature of the study and the limitations regarding data privacy, the study design, analysis, interpretation of data and revision of the manuscript did not involve any patients or members of the public.
All aggregated results are available in a web application ( https://dpa-pde-oxford.shinyapps.io/PostCovidComplications/ ).
We included over 10.17 million vaccinated individuals (1 618 395 from CPRD Gold; 5 729 800 from CPRD Aurum; 2 744 821 from SIDIAP and 77 603 from CORIVA) and 10.39 million unvaccinated individuals (1 640 371; 5 860 564; 2 588 518 and 302 267, respectively). Online supplemental figures S2-5 illustrate study inclusion for each database.
Adequate covariate balance was achieved after PS weighting in most studies: CORIVA (all cohorts) and SIDIAP (cohorts 1 and 4) did not contribute to ChAdOx1 subanalyses owing to sample size and covariate imbalance. ASMD results are accessible in the web application.
NCO analyses suggested residual bias after PS weighting, with a majority of NCOs associated positively with vaccination. Therefore, calibrated estimates are reported in this manuscript. Uncalibrated effect estimates and NCO analyses are available in the web interface.
Population characteristics
Table 1 presents baseline characteristics for the weighted populations in CPRD Aurum, for illustrative purposes. Online supplemental tables S1-25 summarise baseline characteristics for weighted and unweighted populations for each database and comparison. Across databases and cohorts, populations followed similar patterns: cohort 1 represented an older subpopulation (around 80 years old) with a high proportion of women (57%). Median age was lowest in cohort 4 ranging between 30 and 40 years.
- View inline
Characteristics of weighted populations in CPRD Aurum database, stratified by staggered cohort and exposure status. Exposure is any COVID-19 vaccine
COVID-19 vaccination and post-COVID-19 complications
Table 2 shows the incidence of post-COVID-19 VTE, ATE and HF, the three most common post-COVID-19 conditions among the studied outcomes. Outcome counts are presented separately for 0–30, 31–90, 91–180 and 181–365 days after SARS-CoV-2 infection. Online supplemental tables S26-36 include all studied complications, also for the sensitivity and subanalyses. Similar pattern for incidences were observed across all databases: higher outcome rates in the older populations (cohort 1) and decreasing frequency with increasing time after infection in all cohorts.
Number of records (and risk per 10 000 individuals) for acute and post-acute COVID-19 cardiac and thromboembolic complications, across cohorts and databases for any COVID-19 vaccination
Forest plots for the effect of COVID-19 vaccines on post-COVID-19 cardiac and thromboembolic complications; meta-analysis across cohorts and databases. Dashed line represents a level of heterogeneity I 2 >0.4. ATE, arterial thrombosis/thromboembolism; CD+HS, cardiac diseases and haemorrhagic stroke; VTE, venous thromboembolism.
Results from calibrated estimates pooled in meta-analysis across cohorts and databases are shown in figure 2 .
Reduced risk associated with vaccination is observed for acute and post-acute VTE, DVT, and PE: acute meta-analytic sHR are 0.22 (95% CI, 0.17–0.29); 0.36 (0.28–0.45); and 0.19 (0.15–0.25), respectively. For VTE in the post-acute phase, sHR estimates are 0.43 (0.34–0.53), 0.53 (0.40–0.70) and 0.50 (0.36–0.70) for 31–90, 91–180, and 181–365 days post COVID-19, respectively. Reduced risk of VTE outcomes was observed in vaccinated across databases and cohorts, see online supplemental figures S14–22 .
Similarly, the risk of ATE, IS and MI in the acute phase after infection was reduced for the vaccinated group, sHR of 0.53 (0.44–0.63), 0.55 (0.43–0.70) and 0.49 (0.38–0.62), respectively. Reduced risk associated with vaccination persisted for post-acute ATE, with sHR of 0.74 (0.60–0.92), 0.72 (0.58–0.88) and 0.62 (0.48–0.80) for 31–90, 91–180 and 181–365 days post-COVID-19, respectively. Risk of post-acute MI remained lower for vaccinated in the 31–90 and 91–180 days after COVID-19, with sHR of 0.64 (0.46–0.87) and 0.64 (0.45–0.90), respectively. Vaccination effect on post-COVID-19 TIA was seen only in the 181–365 days, with sHR of 0.51 (0.31–0.82). Online supplemental figures S23-31 show database-specific and cohort-specific estimates for ATE-related complications.
Risk of post-COVID-19 cardiac complications was reduced in vaccinated individuals. Meta-analytic estimates in the acute phase showed sHR of 0.45 (0.38–0.53) for HF, 0.41 (0.26–0.66) for MP and 0.41 (0.27–0.63) for VACA. Reduced risk persisted for post-acute COVID-19 HF: sHR 0.61 (0.51–0.73) for 31–90 days, 0.61 (0.51–0.73) for 91–180 days and 0.52 (0.43–0.63) for 181–365 days. For post-acute MP, risk was only lowered in the first post-acute window (31–90 days), with sHR of 0.43 (0.21–0.85). Vaccination showed no association with post-COVID-19 HS. Database-specific and cohort-specific results for these cardiac diseases are shown in online supplemental figures S32-40 .
Stratified analyses by vaccine showed similar associations, except for ChAdOx1 which was not associated with reduced VTE and ATE risk in the last post-acute window. Sensitivity analyses were consistent with main results ( online supplemental figures S6-13 ).
Figure 3 shows the results of comparative effects of BNT162b2 versus ChAdOx1, based on UK data. Meta-analytic estimates favoured BNT162b2 (sHR of 0.66 (0.46–0.93)) for VTE in the 0–30 days after infection, but no differences were seen for post-acute VTE or for any of the other outcomes. Results from sensitivity analyses, database-specific and cohort-specific estimates were in line with the main findings ( online supplemental figures S41-51 ).
Forest plots for comparative vaccine effect (BNT162b2 vs ChAdOx1); meta-analysis across cohorts and databases. ATE, arterial thrombosis/thromboembolism; CD+HS, cardiac diseases and haemorrhagic stroke; VTE, venous thromboembolism.
Key findings
Our analyses showed a substantial reduction of risk (45–81%) for thromboembolic and cardiac events in the acute phase of COVID-19 associated with vaccination. This finding was consistent across four databases and three different European countries. Risks for post-acute COVID-19 VTE, ATE and HF were reduced to a lesser extent (24–58%), whereas a reduced risk for post-COVID-19 MP and VACA in vaccinated people was seen only in the acute phase.
Results in context
The relationship between SARS-CoV-2 infection, COVID-19 vaccines and thromboembolic and/or cardiac complications is tangled. Some large studies report an increased risk of VTE and ATE following both ChAdOx1 and BNT162b2 vaccination, 7 whereas other studies have not identified such a risk. 25 Elevated risk of VTE has also been reported among patients with COVID-19 and its occurrence can lead to poor prognosis and mortality. 26 27 Similarly, several observational studies have found an association between COVID-19 mRNA vaccination and a short-term increased risk of myocarditis, particularly among younger male individuals. 5 6 For instance, a self-controlled case series study conducted in England revealed about 30% increased risk of hospital admission due to myocarditis within 28 days following both ChAdOx1 and BNT162b2 vaccines. However, this same study also found a ninefold higher risk for myocarditis following a positive SARS-CoV-2 test, clearly offsetting the observed post-vaccine risk.
COVID-19 vaccines have demonstrated high efficacy and effectiveness in preventing infection and reducing the severity of acute-phase infection. However, with the emergence of newer variants of the virus, such as omicron, and the waning protective effect of the vaccine over time, there is a growing interest in understanding whether the vaccine can also reduce the risk of complications after breakthrough infections. Recent studies suggested that COVID-19 vaccination could potentially protect against acute post-COVID-19 cardiac and thromboembolic events. 11 12 A large prospective cohort study 11 reports risk of VTE after SARS-CoV-2 infection to be substantially reduced in fully vaccinated ambulatory patients. Likewise, Al-Aly et al 12 suggest a reduced risk for post-acute COVID-19 conditions in breakthrough infection versus SARS-CoV-2 infection without prior vaccination. However, the populations were limited to SARS-CoV-2 infected individuals and estimates did not include the effect of the vaccine to prevent COVID-19 in the first place. Other studies on post-acute COVID-19 conditions and symptoms have been conducted, 28 29 but there has been limited reporting on the condition-specific risks associated with COVID-19, even though the prognosis for different complications can vary significantly.
In line with previous studies, our findings suggest a potential benefit of vaccination in reducing the risk of post-COVID-19 thromboembolic and cardiac complications. We included broader populations, estimated the risk in both acute and post-acute infection phases and replicated these using four large independent observational databases. By pooling results across different settings, we provided the most up-to-date and robust evidence on this topic.
Strengths and limitations
The study has several strengths. Our multinational study covering different healthcare systems and settings showed consistent results across all databases, which highlights the robustness and replicability of our findings. All databases had complete recordings of vaccination status (date and vaccine) and are representative of the respective general population. Algorithms to identify study outcomes were used in previous published network studies, including regulatory-funded research. 3 4 8 18 Other strengths are the staggered cohort design which minimises confounding by indication and immortal time bias. PS overlap weighting and NCO empirical calibration have been shown to adequately minimise bias in vaccine effectiveness studies. 19 Furthermore, our estimates include the vaccine effectiveness against COVID-19, which is crucial in the pathway to experience post-COVID-19 complications.
Our study has some limitations. The use of real-world data comes with inherent limitations including data quality concerns and risk of confounding. To deal with these limitations, we employed state-of-the-art methods, including large-scale propensity score weighting and calibration of effect estimates using NCO. 19 24 A recent study 30 has demonstrated that methodologically sound observational studies based on routinely collected data can produce results similar to those of clinical trials. We acknowledge that results from NCO were positively associated with vaccination, and estimates might still be influenced by residual bias despite using calibration. Another limitation is potential under-reporting of post-COVID-19 complications: some asymptomatic and mild COVID-19 infections might have not been recorded. Additionally, post-COVID-19 outcomes of interest might be under-recorded in primary care databases (CPRD Aurum and Gold) without hospital linkage, which represent a large proportion of the data in the study. However, results in SIDIAP and CORIVA, which include secondary care data, were similar. Also, our study included a small number of young men and male teenagers, who were the main population concerned with increased risks of myocarditis/pericarditis following vaccination.
Conclusions
Vaccination against SARS-CoV-2 substantially reduced the risk of acute post-COVID-19 thromboembolic and cardiac complications, probably through a reduction in the risk of SARS-CoV-2 infection and the severity of COVID-19 disease due to vaccine-induced immunity. Reduced risk in vaccinated people lasted for up to 1 year for post-COVID-19 VTE, ATE and HF, but not clearly for other complications. Findings from this study highlight yet another benefit of COVID-19 vaccination. However, further research is needed on the possible waning of the risk reduction over time and on the impact of booster vaccination.
Ethics statements
Patient consent for publication.
Not applicable.
Ethics approval
The study was approved by the CPRD’s Research Data Governance Process, Protocol No 21_000557 and the Clinical Research Ethics committee of Fundació Institut Universitari per a la recerca a l’Atenció Primària de Salut Jordi Gol i Gurina (IDIAPJGol) (approval number 4R22/133) and the Research Ethics Committee of the University of Tartu (approval No. 330/T-10).
Acknowledgments
This study is based in part on data from the Clinical Practice Research Datalink (CPRD) obtained under licence from the UK Medicines and Healthcare products Regulatory Agency. We thank the patients who provided these data, and the NHS who collected the data as part of their care and support. All interpretations, conclusions and views expressed in this publication are those of the authors alone and not necessarily those of CPRD. We would also like to thank the healthcare professionals in the Catalan healthcare system involved in the management of COVID-19 during these challenging times, from primary care to intensive care units; the Institut de Català de la Salut and the Program d’Analítica de Dades per a la Recerca i la Innovació en Salut for providing access to the different data sources accessible through The System for the Development of Research in Primary Care (SIDIAP).
- Pritchard E ,
- Matthews PC ,
- Stoesser N , et al
- Lauring AS ,
- Tenforde MW ,
- Chappell JD , et al
- Pistillo A , et al
- Duarte-Salles T , et al
- Hansen JV ,
- Fosbøl E , et al
- Chen A , et al
- Hippisley-Cox J ,
- Mei XW , et al
- Duarte-Salles T ,
- Fernandez-Bertolin S , et al
- Ip S , et al
- Bowe B , et al
- Prats-Uribe A ,
- Feng Q , et al
- Campbell J , et al
- Herrett E ,
- Gallagher AM ,
- Bhaskaran K , et al
- Raventós B ,
- Fernández-Bertolín S ,
- Aragón M , et al
- Makadia R ,
- Matcho A , et al
- Mercadé-Besora N ,
- Kolde R , et al
- Ostropolets A ,
- Makadia R , et al
- Rathod-Mistry T , et al
- Thomas LE ,
- ↵ Coronavirus (COVID-19) in the UK . 2022 . Available : https://coronavirus.data.gov.uk/
- Generalitat de Catalunya
- Schuemie MJ ,
- Hripcsak G ,
- Ryan PB , et al
- Houghton DE ,
- Wysokinski W ,
- Casanegra AI , et al
- Katsoularis I ,
- Fonseca-Rodríguez O ,
- Farrington P , et al
- Jehangir Q ,
- Li P , et al
- Byambasuren O ,
- Stehlik P ,
- Clark J , et al
- Brannock MD ,
- Preiss AJ , et al
- Schneeweiss S , RCT-DUPLICATE Initiative , et al
Supplementary materials
Supplementary data.
This web only file has been produced by the BMJ Publishing Group from an electronic file supplied by the author(s) and has not been edited for content.
- Data supplement 1
AMJ and MC are joint senior authors.
Contributors DPA and AMJ led the conceptualisation of the study with contributions from MC and NM-B. AMJ, TD-S, ER, AU and NTHT adapted the study design with respect to the local vaccine rollouts. AD and WYM mapped and curated CPRD data. MC and NM-B developed code with methodological contributions advice from MTS-S and CP. DPA, MC, NTHT, TD-S, HMEN, XL, CR and AMJ clinically interpreted the results. NM-B, XL, AMJ and DPA wrote the first draft of the manuscript, and all authors read, revised and approved the final version. DPA and AMJ obtained the funding for this research. DPA is responsible for the overall content as guarantor: he accepts full responsibility for the work and the conduct of the study, had access to the data, and controlled the decision to publish.
Funding The research was supported by the National Institute for Health and Care Research (NIHR) Oxford Biomedical Research Centre (BRC). DPA is funded through a NIHR Senior Research Fellowship (Grant number SRF-2018–11-ST2-004). Funding to perform the study in the SIDIAP database was provided by the Real World Epidemiology (RWEpi) research group at IDIAPJGol. Costs of databases mapping to OMOP CDM were covered by the European Health Data and Evidence Network (EHDEN).
Patient and public involvement Patients and/or the public were not involved in the design, or conduct, or reporting or dissemination plans of this research.
Provenance and peer review Not commissioned; externally peer reviewed.
Supplemental material This content has been supplied by the author(s). It has not been vetted by BMJ Publishing Group Limited (BMJ) and may not have been peer-reviewed. Any opinions or recommendations discussed are solely those of the author(s) and are not endorsed by BMJ. BMJ disclaims all liability and responsibility arising from any reliance placed on the content. Where the content includes any translated material, BMJ does not warrant the accuracy and reliability of the translations (including but not limited to local regulations, clinical guidelines, terminology, drug names and drug dosages), and is not responsible for any error and/or omissions arising from translation and adaptation or otherwise.
Read the full text or download the PDF:
Mpox Vaccine Protection Quickly Fades; Boosters Necessary: Study
By Dennis Thompson HealthDay Reporter

MONDAY, April 1, 2024 (HealthDay News) -- Vaccine protection against mpox fades quickly in the human immune system, even in people who’ve received the full two-dose regimen, a new real-world study shows.
Antibody levels fell to low or near zero within the first few months of getting the vaccine, unless the person had previously received a smallpox vaccine, scientists reported Saturday at the European Congress of Clinical Microbiology and Infectious Diseases in Barcelona.
In the study, less than half of men without prior smallpox vaccination had any detectible antibodies to mpox (formerly known as monkeypox) 28 days after getting their second jab, the findings showed.
“The results presented here indicate that long-term protective immunity might need a booster dose for its maintenance,” said researcher Dr. Klara Sonden , deputy state epidemiologist for the Public Health Agency of Sweden.
U.S. Cities With the Most Homelessness

The researchers noted that no clinical trials are underway to investigate a booster dose for the mpox vaccine, and that such trials are needed to inform public health guidelines.
Mpox cases have surged in the latter half of 2023 in European countries, following a sharp decline in the months after the vaccine was approved there in late 2022, researchers said.
A case study also presented at the meeting illustrates the point of the new study: A 35-year-old man had a breakthrough mpox infection despite having received two doses of Jynneos vaccine.
In January 2024, two years after getting the vaccine, the man attended a Vienna event at which he had multiple sexual partners, researchers said.
Five days later, the patient reported fever, chills, headache, discomfort urinating, bloody diarrhea and itching of his penis and anus, researchers said.
Tests revealed that the man had an active and severe mpox infection. It took 25 days for his scabs to fall off and a swab test to show the infection had ended.
“This was Austria's first monkeypox breakthrough case,” said Dr. Luigi Segagni-Lusignani , of the Public Health Authority in Vienna. “Despite no hospitalization, the clinical course was not less severe than in unvaccinated patients, with longer disease duration and higher scores on the monkeypox severity scale.”
For the antibody study, researchers followed up on 100 gay men who went to a sexual health clinic in Stockholm to receive the European mpox vaccine, Vaccinia Ankara–Bavarian Nordic.
Only men who’d been previously vaccinated against smallpox continued to carry potentially protective levels of mpox antibodies when retested at 28 days, researchers found.
Researchers believe that previous smallpox vaccination helped immune “memory” cells better adapt to the mpox virus, since the men already carried antibodies against smallpox.
“Monkeypox vaccination results in neutralizing antibodies only in a proportion of vaccinees, and a significant decline occurs already during the first month post-vaccination,” Sonden’s research team concluded.
The largest sexual health clinic in Sweden is planning to perform a randomized clinical trial of a booster dose, researchers said. However, there have been few mpox cases in Sweden.
Because the findings from the two studies were presented at a medical meeting, they should be considered preliminary until published in a peer-reviewed journal.
More information
The World Health Organization has more about mpox .
SOURCE: European Congress of Clinical Microbiology and Infectious Diseases, news release, March 30, 2024
Copyright © 2024 HealthDay . All rights reserved.
Join the Conversation
Tags: vaccines , sexually transmitted diseases
America 2024

Health News Bulletin
Stay informed on the latest news on health and COVID-19 from the editors at U.S. News & World Report.
Sign in to manage your newsletters »
Sign up to receive the latest updates from U.S News & World Report and our trusted partners and sponsors. By clicking submit, you are agreeing to our Terms and Conditions & Privacy Policy .
You May Also Like
The 10 worst presidents.
U.S. News Staff Feb. 23, 2024

Cartoons on President Donald Trump
Feb. 1, 2017, at 1:24 p.m.

Photos: Obama Behind the Scenes
April 8, 2022

Photos: Who Supports Joe Biden?
March 11, 2020
EXPLAINER: Rare Human Case of Bird Flu
Cecelia Smith-Schoenwalder April 2, 2024

The Worst Presidential Scandals
Seth Cline April 2, 2024

Trump Hits the Trail Amid Legal Dramas
Lauren Camera April 2, 2024

Labor Market Keeps on Chugging
Tim Smart April 2, 2024

Florida High Court OKs Abortion Vote
Lauren Camera April 1, 2024

The Week in Cartoons April 1-5
April 2, 2024, at 12:32 p.m.

Do you need a spring COVID-19 vaccine? Research backs extra round for high-risk groups
Recent studies suggest staying up-to-date on covid shots helps protect high-risk groups from severe illness.
Social Sharing
New guidelines suggest certain high-risk groups could benefit from having another dose of a COVID-19 vaccine this spring — and more frequent shots in general — while the broader population could be entering once-a-year territory, much like an annual flu shot.
Medical experts told CBC News that falling behind on the latest shots can come with health risks, particularly for individuals who are older or immunocompromised.
"Even when the risk of infection starts to increase, the vaccines still do a really good job at decreasing risk of severe disease," said McMaster University researcher and immunologist Matthew Miller.
Who needs another COVID shot?
Back in January, Canada's national vaccine advisory body set the stage for another round of spring vaccinations. In a statement , the National Advisory Committee on Immunization (NACI) stated that starting in spring 2024, individuals at an increased risk of severe COVID may get an extra dose of the latest XBB.1.5-based vaccines, which better protect against circulating virus variants.
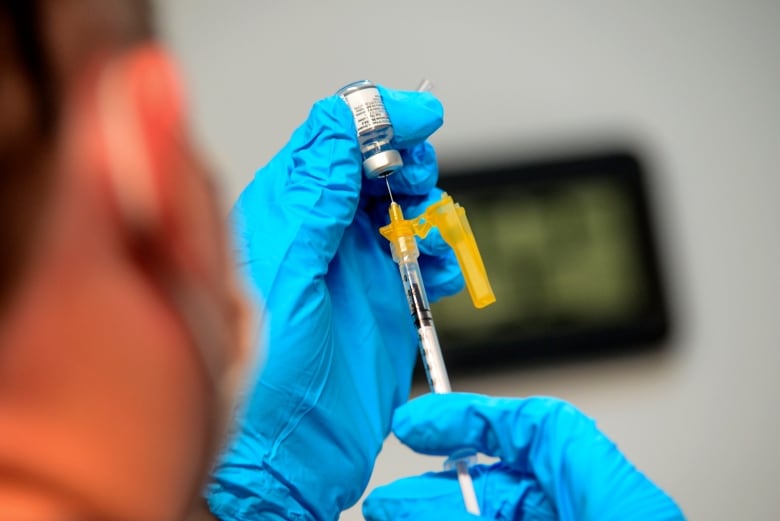
That means:
- Adults aged 65 and up.
- Adult residents of long-term care homes and other congregate living settings for seniors.
- Anyone six months of age or older who is moderately to severely immunocompromised.
The various spring recommendations don't focus on pregnancy, despite research showing clear links between a COVID infection while pregnant, and increased health risks. However, federal guidance does note that getting vaccinated during pregnancy can protect against serious outcomes.
"Vaccinated people can also pass antibodies to their baby through the placenta and through breastmilk," that guidance states .
What do the provinces now recommend?
Multiple provinces have started rolling out their own regional guidance based on those early recommendations — with a focus on allowing similar high-risk groups to get another round of vaccinations.
B.C. is set to announce guidance on spring COVID vaccines in early April, officials told CBC News, and those recommendations are expected to align with NACI's guidance.
In Manitoba , high-risk individuals are already eligible for another dose, provided it's been at least three months since their latest COVID vaccine.
- Older P.E.I. residents, others at risk, urged to get spring COVID vaccine booster
- High-risk groups can now book spring COVID-19 vaccination in Nova Scotia
Meanwhile Ontario's latest guidance , released on March 21, stresses that high-risk individuals may get an extra dose during a vaccine campaign set to run between April and June. Eligibility will involve waiting six months after someone's last dose or COVID infection.
Having a spring dose "is particularly important for individuals at increased risk of severe illness from COVID-19 who did not receive a dose during the Fall 2023 program," the guidance notes.
And in Nova Scotia , the spring campaign will run from March 25 to May 31, also allowing high-risk individuals to get another dose.
Specific eligibility criteria vary slightly from province-to-province, so Canadians should check with their primary care provider, pharmacist or local public health team for exact guidelines in each area.

Age still best determines when to get COVID vaccines, new research suggests
Why do the guidelines focus so much on age.
The rationale behind the latest spring guidelines, Miller said, is that someone's age remains one of the greatest risk factors associated with severe COVID outcomes, including hospitalization, intensive care admission and death.
"So that risk starts to shoot up at about 50, but really takes off in individuals over the age of 75," he noted.
Canadian data suggests the overwhelming majority of COVID deaths have been among older adults, with nearly 60 per cent of deaths among those aged 80 or older, and roughly 20 per cent among those aged 70 to 79.
People with compromised immune systems or serious medical conditions are also more vulnerable, Miller added.
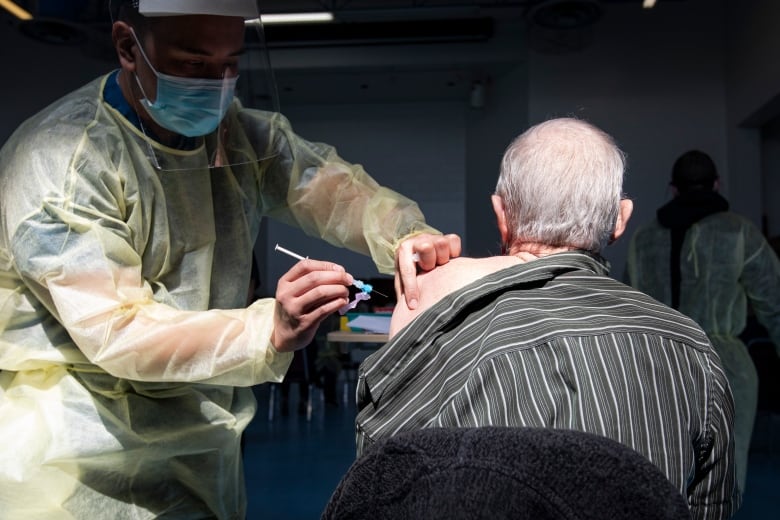
Will people always need regular COVID shots?
While the general population may not require shots as frequently as higher-risk groups, Miller said it's unlikely there will be recommendations any time soon to have a COVID shot less than once a year, given ongoing uncertainty about COVID's trajectory.
"Going forward, I suspect for pragmatic reasons, [COVID vaccinations] will dovetail with seasonal flu vaccine campaigns, just because it makes the implementation much more straightforward," Miller said.
- Just 15% of Canadians got updated COVID vaccines this fall, new figures show
- Spring COVID-19 vaccines available April 2 in N.B. for those at high-risk
"And although we haven't seen really strong seasonal trends with SARS-CoV-2 now, I suspect we'll get to a place where it's more seasonal than it has been."
In the meantime, the guidance around COVID shots remains simple at its core: Whenever you're eligible to get another dose — whether that's once or twice a year — you might as well do it.
What does research say?
One analysis, published in early March in the medical journal Lancet Infectious Diseases , studied more than 27,000 U.S. patients who tested positive for SARS-CoV-2, the virus behind COVID, between September and December 2023.
The team found individuals who had an updated vaccine reduced their risk of severe illness by close to a third — and the difference was more noticeable in older and immunocompromised individuals.
Another American research team from Stanford University recently shared the results from a modelling simulation looking at the ideal frequency for COVID vaccines.
- Elderly Canadians remain at higher risk of serious COVID from first infections, study suggests
- Spring vaccine dose suggested to protect seniors in Canada from severe COVID
The study in Nature Communications suggests that for individuals aged 75 and up, having an annual COVID shot could reduce severe infections from an estimated 1,400 cases per 100,000 people to around 1,200 cases — while bumping to twice a year could cut those cases even further, down to 1,000.
For younger, healthier populations, however, the benefit of regular shots against severe illness was more modest.
The outcome wasn't a surprise to Stanford researcher Dr. Nathan Lo, an infectious diseases specialist, since old age has consistently been a risk factor for severe COVID.
"It's almost the same pattern that's been present the entire pandemic," he said. "And I think that's quite striking."
More frequent vaccination won't prevent all serious infections, he added, or perhaps even a majority of those infections, which highlights the need for ongoing mitigation efforts.
ABOUT THE AUTHOR
Senior Health & Medical Reporter
Lauren Pelley covers health and medical science for CBC News, including the global spread of infectious diseases, Canadian health policy, pandemic preparedness, and the crucial intersection between human health and climate change. Two-time RNAO Media Award winner for in-depth health reporting in 2020 and 2022. Contact her at: [email protected]
- @LaurenPelley
Add some “good” to your morning and evening.
A vital dose of the week's news in health and medicine, from CBC Health. Delivered to your inbox every Saturday morning.
Thank you for visiting nature.com. You are using a browser version with limited support for CSS. To obtain the best experience, we recommend you use a more up to date browser (or turn off compatibility mode in Internet Explorer). In the meantime, to ensure continued support, we are displaying the site without styles and JavaScript.
- View all journals
- Explore content
- About the journal
- Publish with us
- Sign up for alerts
- Review Article
- Published: 12 April 2021
Vaccine development for emerging infectious diseases
- Jean-Louis Excler ORCID: orcid.org/0000-0002-6462-5101 1 ,
- Melanie Saville 2 ,
- Seth Berkley 3 &
- Jerome H. Kim ORCID: orcid.org/0000-0003-0461-6438 1
Nature Medicine volume 27 , pages 591–600 ( 2021 ) Cite this article
69k Accesses
190 Citations
92 Altmetric
Metrics details
- Viral infection
Examination of the vaccine strategies and technical platforms used for the COVID-19 pandemic in the context of those used for previous emerging and reemerging infectious diseases and pandemics may offer some mutually beneficial lessons. The unprecedented scale and rapidity of dissemination of recent emerging infectious diseases pose new challenges for vaccine developers, regulators, health authorities and political constituencies. Vaccine manufacturing and distribution are complex and challenging. While speed is essential, clinical development to emergency use authorization and licensure, pharmacovigilance of vaccine safety and surveillance of virus variants are also critical. Access to vaccines and vaccination needs to be prioritized in low- and middle-income countries. The combination of these factors will weigh heavily on the ultimate success of efforts to bring the current and any future emerging infectious disease pandemics to a close.
Similar content being viewed by others
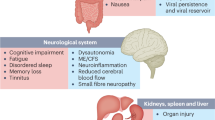
Long COVID: major findings, mechanisms and recommendations
Hannah E. Davis, Lisa McCorkell, … Eric J. Topol
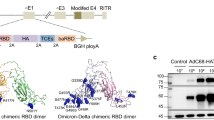
An intranasal combination vaccine induces systemic and mucosal immunity against COVID-19 and influenza
Man Xing, Gaowei Hu, … Dongming Zhou
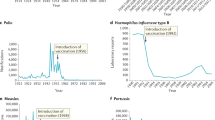
A guide to vaccinology: from basic principles to new developments
Andrew J. Pollard & Else M. Bijker
Newly emerging and reemerging infectious viral diseases have threatened humanity throughout history. Several interlaced and synergistic factors including demographic trends and high-density urbanization, modernization favoring high mobility of people by all modes of transportation, large gatherings, altered human behaviors, environmental changes with modification of ecosystems and inadequate global public health mechanisms have accelerated both the emergence and spread of animal viruses as existential human threats. In 1918, at the time of the ‘Spanish flu’, the world population was estimated at 1.8 billion. It is projected to reach 9.9 billion by 2050, an increase of more than 25% from the current 2020 population of 7.8 billion ( https://www.worldometers.info ). The novel severe acute respiratory syndrome coronavirus 2 (SARS-CoV-2) responsible for the coronavirus disease 2019 (COVID-19) pandemic 1 , 2 , 3 engulfed the entire world in less than 6 months, with high mortality in the elderly and those with associated comorbidities. The pandemic has severely disrupted the world economy. Short of lockdowns, the only means of control have been limited to series of mitigation measures such as self-distancing, wearing masks, travel restrictions and avoiding gatherings, all imperfect and constraining. Now with more than 100 million people infected and more than 2 million deaths, it seems that the addition of vaccine(s) to existing countermeasures holds the best hope for pandemic control. Taken together, these reasons compel researchers and policymakers to be vigilant, reexamine the approach to surveillance and management of emerging infectious disease threats, and revisit global mechanisms for the control of pandemic disease 4 , 5 .
Emerging and reemerging infectious diseases
The appearance of new infectious diseases has been recognized for millennia, well before the discovery of causative infectious agents. Despite advances in development of countermeasures (diagnostics, therapeutics and vaccines), world travel and increased global interdependence have added layers of complexity to containing these infectious diseases. Emerging infectious diseases (EIDs) are threats to human health and global stability 6 , 7 . A review of emerging pandemic diseases throughout history offers a perspective on the emergence and characteristics of coronavirus epidemics, with emphasis on the SARS-CoV-2 pandemic 8 , 9 . As human societies grow in size and complexity, an endless variety of opportunities is created for infectious agents to emerge into the unfilled ecologic niches we continue to create. To illustrate this constant vulnerability of populations to emerging and reemerging pathogens and their respective risks to rapidly evolve into devastating outbreaks and pandemics, a partial list of emerging viral infectious diseases that occurred between 1900 and 2020 is shown in Table 1 .
Although nonemerging infectious diseases (not listed in Table 1 ), two other major mosquito-borne viral infections are yellow fever and dengue. Yellow fever, known for centuries and an Aedes mosquito-borne disease, is endemic in more than 40 countries across Africa and South America. Since 2016, several yellow fever outbreaks have occurred in Angola, Democratic Republic of Congo, Nigeria and Brazil to cite a few 10 , raising major concerns about the adequacy of yellow fever vaccine supply. Four live attenuated vaccines derived from the live attenuated yellow fever strain (17D) 11 and prequalified by the WHO (World Health Organization) are available 12 .
Dengue is an increasing global public health threat with the four dengue virus types (DENV1–4) now cocirculating in most dengue endemic areas. Population growth, an expansion of areas hospitable for Aedes mosquito species and the ease of travel have all contributed to a steady rise in dengue infections and disease. Dengue is common in more than 100 countries around the world. Each year, up to 400 million people acquire dengue. Approximately 100 million people get sick from infection, and 22,000 die from severe dengue. Most seriously affected by outbreaks are the Americas, South/Southeast Asia and the Western Pacific; Asia represents ~70% of the global burden of disease ( https://www.cdc.gov/dengue ). Several vaccines have been developed 13 . A single dengue vaccine, Sanofi Pasteur’s Dengvaxia based on the yellow fever 17D backbone, has been licensed in 20 countries, but uptake has been poor. A safety signal in dengue-seronegative vaccine recipients stimulated an international review of the vaccine performance profile, new WHO recommendations for use and controversy in the Philippines involving the government, regulatory agencies, Sanofi Pasteur, clinicians responsible for testing and administering the vaccine, and the parents of vaccinated children 14 .
Two bacterial diseases, old scourges of humanity, are endemic and responsible for recurrent outbreaks and are increasingly antimicrobial resistant. Cholera, caused by pathogenic strains of Vibrio cholerae , is currently in its seventh global pandemic since 1817; notably, the seventh pandemic started in 1961 15 . Global mortality due to cholera infection remains high, mainly due to delay in rehydrating patients. The global burden of cholera is estimated to be between 1.4 and 4.3 million cases with about 21,000–143,000 deaths per year, mostly in Asia and Africa. Tragic outbreaks have occurred in Yemen and Haiti. Adding to rehydration therapy, antibiotics have been used in the treatment of cholera to shorten the duration of diarrhea and to limit bacterial spread. Over the years, antimicrobial resistance developed in Asia and Africa to many useful antibiotics including chloramphenicol, furazolidone, trimethoprim-sulfamethoxazole, nalidixic acid, tetracycline and fluoroquinolones. Several vaccines have been developed and WHO prequalified; these vaccines constitute a Gavi-supported global stockpile for rapid deployment during outbreaks 16 .
Typhoid fever is a severe disease caused by the Gram-negative bacterium Salmonella enterica subsp. enterica serovar Typhi ( S . Typhi). Antimicrobial-resistant S . Typhi strains have become increasingly common. The first large-scale emergence and spread of a novel extensively drug-resistant (XDR) S . Typhi clone was first reported in Sindh, Pakistan 17 , 18 , and has subsequently been reported in India, Bangladesh, Nepal, the Philippines, Iraq and Guatemala 19 , 20 . The world is in a critical period as XDR S . Typhi has appeared in densely populated areas. The successful development of improved typhoid vaccines (conjugation of the Vi polysaccharide with a carrier protein) with increased immunogenicity and efficacy including in children less than 2 years of age will facilitate the control of typhoid, in particular in XDR areas by decreasing the incidence of typhoid fever cases needing antibiotic treatment 21 , 22 .
A model of vaccine development for emerging infectious diseases
The understanding of emerging infectious diseases has evolved over the past two decades. A look back at the SARS-CoV outbreak in 2002 shows that—despite a small number of deaths and infections—its high mortality and transmissibility caused significant global disruption (see Table 1 ). The epidemic ended as work on vaccines was initiated. Since then, the disease has not reappeared—wet markets were closed and transmission to humans from civets ceased. Consequently, work on vaccines against SARS-CoV ended and its funding was cut. Only a whole inactivated vaccine 23 and a DNA vaccine 24 were tested in phase 1 clinical trials.
Following a traditional research and development pipeline, it takes between 5 and 10 years to develop a vaccine for an infectious agent. This approach is not well suited for the needs imposed by the emergence of a new pathogen during an epidemic. Figure 1 shows a comparison of the epidemic curves and vaccine development timelines between the 2014 West African Ebola outbreak and COVID-19. The 2014 Ebola epidemic lasted more than 24 months with 11,325 deaths and was sufficiently prolonged to enable the development and testing of vaccines for Ebola, with efficacy being shown for one vaccine (of several) toward the end of the epidemic 25 , 26 . What makes the COVID-19 pandemic remarkable is that the whole research and development pipeline, from the first SARS-CoV-2 viral sequenced to interim analyses of vaccine efficacy trials, was accomplished in just under 300 days 27 . Amid increasing concerns about unmitigated transmission during the 2013–2016 Western African Ebola outbreak in mid-2014, WHO urged acceleration of the development and evaluation of candidate vaccines 25 . To ensure that manufacturers would take the Ebola vaccine to full development and deployment, Gavi, the Vaccine Alliance, publicly announced support of up to US$300 million for vaccine purchase and followed that announcement with an advance purchase agreement. Ironically, there had been Ebola vaccines previously developed and tested for biodefense purposes in nonhuman primates, but this previous work was neither ‘ready’ for clinical trials during the epidemic nor considered commercially attractive enough to finish development 28 .
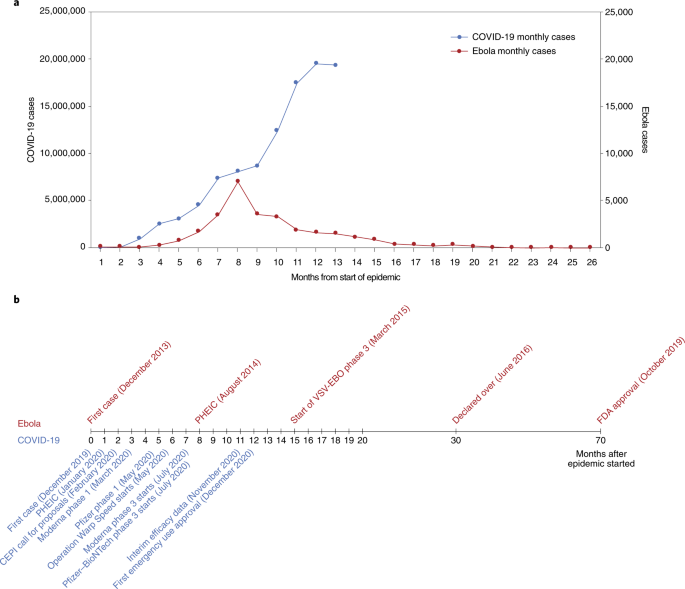
a , The number of months from the onset of the epidemic is shown against the number of reported cases per day. Note that the COVID-19 (left) and Ebola (right) axes are scaled differently. b , Vaccine development timelines for COVID-19 versus Ebola in the context of particular events during the respective outbreaks. PHEIC, public health emergency of international concern.
From these perceived shortcomings in vaccine development during public health emergencies arose the Coalition for Epidemic Preparedness Innovations (CEPI), a not-for-profit organization dedicated to timely vaccine development capabilities in anticipation of epidemics 29 , 30 . CEPI initially focused on diseases chosen from a list of WHO priority pathogens for EIDs—Middle East respiratory syndrome (MERS), Lassa fever, Nipah, Rift Valley fever (RVF) and chikungunya. The goal of CEPI was to advance candidate vaccines through phase 2 and to prepare stockpiles of vaccine against eventual use/testing under epidemic circumstances. CEPI had also prepared for ‘disease X’ by investing in innovative rapid response platforms that could move from sequence to clinical trials in weeks rather than months or years, such as mRNA and DNA technology, platforms that were useful when COVID-19 was declared a global health emergency in January 2020, and a pandemic in March 2020 31 , 32 .
CEPI has been able to fund several vaccine development efforts, among them product development by Moderna, Inovio, Oxford–AstraZeneca and Novavax. Providing upfront funding helped these groups to advance vaccine candidates to clinical trials and develop scaled manufacturing processes in parallel, minimizing financial risk to vaccine developers. The launch of the larger US-funded Operation Warp Speed 33 further provided companies with funding—reducing risks associated with rapid vaccine development and securing initial commitments in vaccine doses.
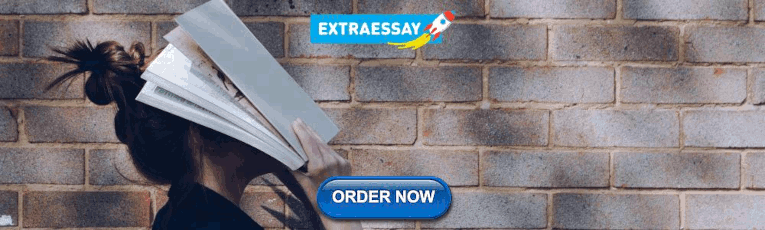
Vaccine platforms and vaccines for emerging infectious diseases
Vaccines are the cornerstone of the management of infectious disease outbreaks and are the surest means to defuse pandemic and epidemic risk. The faster a vaccine is deployed, the faster an outbreak can be controlled. As discussed in the previous section, the standard vaccine development cycle is not suited to the needs of explosive pandemics. New vaccine platform technologies however may shorten that cycle and make it possible for multiple vaccines to be more rapidly developed, tested and produced 34 . Table 2 provides examples of the most important technical vaccine platforms for vaccines developed or under development for emerging viral infectious diseases. Two COVID-19 vaccines were developed using mRNA technology (Pfizer–BioNTech 35 and Moderna 36 ), both showing safety and high efficacy, and now with US Food and Drug Administration (FDA) emergency use authorization (EUA) 37 , 38 and European Medicines Agency (EMA) conditional marketing authorization 39 , 40 . While innovative and encouraging for other EIDs, it is too early to assert that mRNA vaccines represent a universal vaccine approach that could be broadly applied to other EIDs (such as bacterial or enteric pathogens). While COVID-19 mRNA vaccines are a useful proof of concept, gathering lessons from their large-scale deployment and effectiveness studies still requires more work and time.
While several DNA vaccines are licensed for veterinary applications, and DNA vaccines have shown safety and immunogenicity in human clinical trials, no DNA vaccine has reached licensure for use in humans 41 . Recombinant proteins vary greatly in design for the same pathogen (for example, subunit, virus-like particles) and are often formulated with adjuvants but have longer development times. Virus-like particle-based vaccines used for hepatitis B and human papillomavirus are safe, highly immunogenic, efficacious and easy to manufacture in large quantity. The technology is also easily transferable. Whole inactivated pathogens (for example, SARS-CoV-2, polio, cholera) or live attenuated vaccines (for example, SARS-CoV-2, polio, chikungunya) are unique to each pathogen. Depending on the pathogen, these vaccines also may require biosafety level 3 manufacturing (at least for COVID-19 and polio), which may limit the possibility of technology transfer for increasing the global manufacturing capacity.
Other vaccines are based on recombinant vector platforms, subdivided into nonreplicating vectors (for example, adenovirus 5 (Ad5), Ad26, chimpanzee adenovirus-derived ChAdOx, highly attenuated vectors like modified vaccinia Ankara (MVA)) and live attenuated vectors such as the measles-based vector or the vesicular stomatitis virus (VSV) vector. Either each vector is designed with specific inserts for the pathogen targeted, or the same vector can be designed with different inserts for the same disease. The development of the Merck Ebola vaccine is an example. ERVEBO is a live attenuated, recombinant VSV-based, chimeric-vector vaccine, where the VSV envelope G protein was deleted and replaced by the envelope glycoprotein of Zaire ebolavirus . ERVEBO is safe and highly efficacious, now approved by the US FDA and the EMA, and WHO prequalified, making VSV an attractive ‘platform’ for COVID-19 and perhaps for other EID vaccines 26 although the −70 °C ultracold chain storage requirement still presents a challenge.
Other equally important considerations are speed of development, ease of manufacture and scale-up, ease of logistics (presentation, storage conditions and administration), technology transfer to other manufacturers to ensure worldwide supply, and cost of goods. Viral vectors such as Ad5, Ad26 and MVA have been used in HIV as well as in Ebola vaccines 42 . Finally, regulatory authorities do not approve platforms but vaccines. Each vaccine is different. However, with each use of a specific technology, regulatory agencies may, over time, become more comfortable with underlying technology and the overall safety and efficacy of the vaccine platform, allowing expedited review and approvals in the context of a pandemic 43 . With COVID-19, it meant that the regulatory authorities could permit expedited review of ‘platform’ technologies, such as RNA and DNA, that had been used (for other conditions) and had safety profiles in hundreds of people.
A heterologous prime–boost (HPB) vaccine approach has been extensively explored for HIV 44 and Ebola vaccines 42 . It is being investigated for COVID-19 vaccines with the Oxford–AstraZeneca AZD1222 and Gamaleya Sputnik V COVID-19 vaccines 45 or with the Pfizer–BioNTech vaccine ( https://www.comcovstudy.org.uk ). Other HPB combinations might be considered involving mRNA, DNA, viral vector-based and protein-based vaccines. This may offer the potential benefit of improving the immune response and avoiding mutlidose reactogenicity or anti-vector immune responses. Additionally, people previously vaccinated with the standard regimen (for example, single or two dose) could be offered a booster immunization with a different vaccine. This might mitigate current shortages in vaccines, particularly in low- and middle-income countries (LMICs). Such a matrix of HPB possibilities deserves further consideration by manufacturers, funders and regulators supported by clinical trial studies and assessment of implementation challenges.
Important improvements could speed up availability. Standardized labeling of vaccines so that they can be interchanged across countries and regions, date of production rather than expiration so that shelf life can be tracked, three-dimensional bar coding to allow critical information to be updated, standard indemnification and liability language that would allow agreement with all manufacturers, a no-fault compensation mechanism for serious adverse events related to vaccine administration, and regulatory harmonization are all critical and being worked on as part of the COVID-19 vaccine response and must be optimized for future outbreaks.
The pathway to EUA, licensure and beyond
Big pharmaceutical or biotechnology companies supported by organizations such as CEPI or efforts such as Operation Warp Speed have conducted efficacy trials in countries or regions with the highest SARS-CoV-2 incidence rates. The same groups have also committed funding for large-scale manufacturing at risk. With more than 60 vaccine candidates in clinical trials and another 170 in preclinical development (WHO COVID-19 vaccine landscape) 46 , it is uncertain whether vaccine candidates not in the first wave of testing/approvals will be able to progress to EUA and licensure based solely on results of randomized clinical efficacy trials with clinical endpoints. Regulators and ethics committees may decide that noninferiority clinical trials against comparator vaccines with proven clinical efficacy will be needed for further approvals. Would the demonstration of equivalence between immune responses generated by a new vaccine and those of a clinically proven efficacious vaccine (bridging studies) 47 be accepted by regulatory authorities and replace the need for noninferiority clinical endpoint studies? For that to occur there must be agreement on what are immune correlates of protection (ICP) to COVID-19, and these have yet to be identified. Moreover, it is not yet clear that ICP will translate equally between different vaccine platforms; for example, are immune responses generated by chimpanzee adenovirus the same as those generated by proteins or whole inactivated virus? As incidence rates of a disease decrease over time due to sustained mitigation measures and implementation of vaccination, larger sample sizes in multicountry trials, additional participant accrual time and complex logistics will likely be required for future approvals, compromising the speed of clinical development and increasing cost. Early deployment of scarce doses of still-investigational vaccines (under emergency use listing (EUL) or similar regulatory mechanisms) could bring additional public health benefits if accompanied by firm commitment to maintaining blinded follow-up of participants in ongoing or future placebo-controlled trials until a licensed vaccine is fully deployed in the population 48 .
Randomized controlled trials might underestimate the protective effect of vaccines at the population level. This would occur if the COVID-19 vaccine, in addition to conferring direct protection to individuals, reduces transmission of COVID-19 between individuals, providing protection to unvaccinated individuals and enhanced protection of vaccinated individuals in contact with vaccinated individuals. Vaccine-induced herd protection, which might be crucial to the public health value of a vaccine, will be missed when trials are individually randomized and analyses fail to take account of the geographical distribution of individuals in the population 49 . For these reasons, other clinical trial designs have been proposed once COVID-19 vaccines have achieved licensure via current phase 3 trials to assess how useful the vaccines will be in practice and addressing vaccine effectiveness, including the level of protection of both vaccinated and nonvaccinated individuals in targeted populations 50 .
In the particular context of the COVID-19 pandemic, whether regulatory authorities would require clinical endpoints in future efficacy trials or would consider ICP remains unclear. Clinical endpoints provide increased accuracy with regard to definitive clinical outcomes where outcome-related analyses using ICP are inferential. ICP will contribute to our understanding of viral pathogenesis and immunity, be useful for future approval of vaccines, and help in our understanding of waning of protective immunity following vaccination or infection. The paradox is that the higher the efficacy, the more difficult it will be to identify these correlates because there may not be enough infected vaccine recipients to compare with uninfected vaccine recipients. The analysis of ICP may be possible only in clinical trials showing a lower vaccine efficacy 50 . They would also not provide a rigorous evaluation of long-term safety and the potential for vaccine-associated enhanced respiratory disease 51 .
Pharmacovigilance and surveillance
In May 2020, the 42nd Global Advisory Committee on Vaccine Safety addressed pharmacovigilance preparedness for the launch of the future COVID-19 vaccines 52 . One of their recommendations was that infrastructure and capacity for surveillance of the safety of COVID-19 vaccines should be in place in all countries and engaged before a vaccine is introduced. The WHO’s COVID-19 vaccine safety surveillance manual develops the monitoring and reporting of adverse events following immunization and adverse events of special interest, data management systems and safety communication, and the need for postauthorization safety surveillance studies 53 . One critical element of this surveillance is the duration of the observation period. The implementation of this surveillance will require local, national, regional and global collaboration. While countries should include preparedness plans for COVID-19 vaccine safety in their overall plans for vaccine introduction, building on WHO guidance, it is imperative that the COVID-19 Vaccines Global Access (COVAX) initiative (coordinated by CEPI, Gavi, the Vaccine Alliance, and WHO) works with partners on capacity building and the practical aspects of implementation with technical and training support tailored to the settings.
In view of the public health urgency and the extensive vaccination campaigns foreseen worldwide, the EMA and the national competent authorities in EU member states have prepared themselves for the expected high data volume by putting pharmacovigilance plans specific for COVID-19 vaccines in place. Good pharmacovigilance practices include detailed requirements and guidance on the principles of a risk management plan (RMP) and requirements for vaccines. In addition, core RMP requirements for COVID-19 vaccines have been developed to facilitate and harmonize the preparation of RMPs by companies and their evaluation by assessors. The RMP preparation addresses the planning of the postauthorization safety follow-up of COVID-19 vaccines by marketing authorization holders, while acknowledging uncertainties in the pandemic setting and recommending ways to prepare for pharmacovigilance activities 54 . Similarly, the US Advisory Committee on Immunization Practices (ACIP) initially convened the COVID-19 Vaccine Safety Technical Working Group in June 2020 to advise the ACIP COVID-19 Vaccine Workgroup and the full ACIP on the safety monitoring of COVID-19 vaccines under development and pharmacovigilance postapproval 55 .
Key lessons could be learnt from past situations where new vaccines were introduced in response to pandemic and epidemic emergencies. For the 2009 H1N1 influenza pandemic, few countries had a pandemic preparedness plan that comprehensively addressed vaccine deployment and monitoring of adverse events. The African Vaccine Regulatory Forum, a regional network of regulators and ethics committees, working closely with regulators from other parts of the world, participated in the review of clinical trial protocols and results, the joint monitoring of trials and the joint authorization and deployment of vaccines 56 . Such models can be used to guide pharmacovigilance for the deployment of COVID-19 vaccines, particularly in LMICs with limited resources. The introduction of the first licensed dengue vaccine, while not in the context of an international public health emergency, illustrated a number of lessons for the pharmacovigilance of newly introduced vaccines, particularly the vaccine-associated enhanced disease that was observed 13 , 14 . Due to the significant sequence homology between SARS-CoV-2 and SARS-CoV, antibody-dependent enhancement (ADE) and vaccine-associated enhanced respiratory disease (VAERD) were raised as potential safety issues 57 , 58 . VAERD and ADE have not been described in current reports of SARS-CoV-2 vaccine phase 3 trials. Similarly, VAERD has not been reported in animal challenge studies with SARS-CoV-2 vaccines that conferred protection 50 . With ADE the effect of waning antibody titers after vaccination (or after infection) and potential safety signals are unknown, which emphasizes the importance of follow-up monitoring 57 .
Pregnant women seem to be disproportionately affected during pandemics and emerging pathogen outbreaks 59 , 60 . The Pregnancy Research Ethics for Vaccines, Epidemics, and New Technologies (PREVENT) Working Group has published a roadmap to guide the inclusion of the interests of pregnant women in the development and deployment of vaccines against emerging pathogens 61 , 62 .
Equally important is the surveillance on SARS-CoV-2 circulating strains as well as of other coronaviruses (MERS, seasonal) 63 . SARS-CoV-2 is evolving, with new lineages being reported all over the world. Amongst previous lineages, D614G was shown to have faster growth in vitro and enhanced transmission in small animals, and has subsequently become globally dominant 64 , 65 , 66 . Other variants of concern have been described in the UK (B.1.1.7) 67 and in Brazil (B.1.1.28.1/P1) 68 with higher capacity for transmission and, potentially, lethality. N501Y (B.1.351) isolated in South Africa has an increased affinity for the human ACE2 receptor, which together with the repeated and independent evolution of 501Y-containing lineages 69 strongly argues for enhanced transmissibility. The B.1.351 variant has nine spike alterations; it rapidly emerged in South Africa during the second half of 2020 and has shown resistance to neutralizing antibodies elicited by infection and vaccination with previously circulating lineages. The AstraZeneca COVID-19 vaccine rollout in South Africa was recently halted after the analysis showed minimal efficacy against mild and moderate cases due to B.1.351, which accounts for 90% of the cases in this country 70 . The Novavax vaccine efficacy is 86% against the variant identified in the UK and 60% against the variant identified in South Africa 71 . The efficacy of a single dose of Johnson & Johnson’s Ad26 was 57% against moderate to severe COVID-19 infection in South Africa 72 .
For the many people who have already been infected with SARS-CoV-2 globally and are presumed to have accumulated some level of immunity, new variants such as B.1.351 pose a significant reinfection risk, although vaccine-induced cell-mediated immune responses might mitigate this risk. Scientists do not know how variant lineages will evolve under vaccine-induced immune pressure during the vaccination rollout or whether choices that alter the schedule or dose may impact virus evolution. Whether vaccines efficacious against current circulating strains including the variants identified in the UK and Brazil will keep their efficacy against emerging variants is unknown and deserves enhanced global COVID-19 surveillance in both humans and animals, similar to those developed for influenza. Global influenza surveillance has been conducted through WHO’s Global Influenza Surveillance and Response System since 1952. The Global Influenza Surveillance and Response System is a global mechanism of surveillance, preparedness and response for seasonal, pandemic and zoonotic influenza, a global platform for monitoring influenza epidemiology and disease, and a global alert system for novel influenza viruses and other respiratory pathogens 73 . The Global Initiative on Sharing Avian Influenza Database ( https://www.gisaid.org ) promotes the rapid sharing of data from all influenza viruses and the coronavirus causing COVID-19. These include genetic sequence and related clinical and epidemiological data associated with human viruses, and geographical as well as species-specific data associated with avian and other animal viruses. This molecular epidemiology surveillance should be expanded to all EIDs, particularly the deadliest and most transmissible, as recently described for Ebola 25 . As with influenza, preparations for SARS-CoV-2 vaccine variants should be proactive, with a view that platforms such as mRNA could generate new vaccine strains very rapidly. A clear regulatory pathway for strain change needs discussion with the regulators.
Approval process for licensure and EUA and the risk of speed
Vaccines are classically approved by the country’s national regulatory authority such as the US FDA or by a centralized procedure through the EMA. Once approved for licensure by a stringent or functional national regulatory authority in the country of manufacture, the manufacturing company can submit a dossier for WHO prequalification. However, for SARS-CoV-2 vaccines intended for COVAX, WHO prequalification is not required for initial use if they have received WHO EUL. COVAX is one of three pillars of the Access to COVID-19 Tools Accelerator, which was launched in April 2020 by the WHO, the EC (European Commission) and France. Vaccines receiving WHO EUL can be purchased by UNICEF (United Nations International Children’s Emergency Fund), the largest purchaser of vaccines for Gavi, the Vaccine Alliance. Countries participating in COVAX can access the vaccines through the COVAX Facility either as 1 of the 98 self-financing countries or, for the 92 LMICs, funded through the Gavi COVAX advance market commitment (AMC; https://www.gavi.org ).
In the current pandemic situation, the US FDA is using the EUA process to allow initial use of the vaccines from Pfizer, Moderna and Johnson & Johnson 74 . EMA is taking the approach of conditional approval 75 . The WHO emergency use assessment and listing (EUAL) procedure was developed in the wake of the Ebola virus disease outbreak in Africa to expedite the availability of vaccines. The EUAL was intended as guidance for national regulatory authorities in circumstances when the “community may be more willing to tolerate less certainty about the efficacy and safety of products, given the morbidity and/or mortality of the disease and the shortfall of treatment and/or prevention options” 76 . In early 2020, the WHO issued a revised EUL procedure to assess whether submitted data demonstrate a reasonable likelihood that a vaccine’s quality, safety and performance are acceptable and that the benefits outweigh the foreseeable risks and uncertainties in the context of a public health emergency of international concern 77 . It is intended that vaccines approved through EUAL would eventually go to full review and receive prequalification. WHO member states have the prerogative through their national regulatory authority to use the EUL procedure to authorize the use of unlicensed vaccines.
Some countries have used their national regulatory authorities to secure approval of nationally produced vaccines. The Russian government approved the Ad26 and Ad5 combination-based COVID-19 vaccine, Sputnik V, produced by the Gamaleya Research Institute, for use by individuals aged 60 years and above 78 , 79 . China’s National Medical Products Agency has given conditional approval to the whole inactivated virus BBIBP-CorV COVID-19 vaccine developed by the Beijing Institute of Biological Products, a Sinopharm subsidiary 80 . The authorization allows the general public’s use of the inoculation and comes after the company announced that its vaccine proved 79.3% effective in phase 3 trials 81 . Although the interim results are not yet published, they must have been reviewed and approved by the Chinese Center for Disease Control and Prevention and National Medical Products Agency. The United Arab Emirates was first to approve the Sinopharm vaccine for EUA in early December 2020 based on interim analysis results 82 . The Sinovac CoronaVac vaccine was recently granted conditional approval on the basis of interim efficacy results 83 . The CanSinoBIO COVID-19 vaccine achieved 65.7% efficacy in preventing symptomatic cases in clinical trials (unpublished). The vaccine also showed a 90.98% success rate in stopping severe disease in one of its interim analysis. The vaccine was granted EUA in Mexico and Pakistan 84 .
Manufacturing—how to make more, faster
Production and distribution of hundreds of millions of doses of COVID-19 vaccine within a year of identification of the pandemic pathogen is unprecedented, and while the principles are straightforward, the manufacturing equation is complex and prone to delay. The technical platform utilized to make a vaccine (mRNA, whole inactivated virus, vector, protein with or without adjuvant), the dosage (low, mid, high), the schedule of vaccination (single or two dose) and the manufacturer capability, capacity and reputation are all important considerations for regulators and the WHO. The initial phase of manufacturing scale-up will be a key regulator of vaccine access initially. This could potentially be impacted by vaccine nationalism and the announced bilateral agreements between manufacturers and high-income countries. Companies such as Sinopharm, the Serum Institute of India or Bharat have a huge capacity for production but must supply the gigantic markets of China and India. Delays in the production of several western 85 and Chinese COVID-19 vaccines 86 have already been reported.
The Developing Countries Vaccine Manufacturers Network (DCVMN) was established in 2000 with the mission to increase the availability and affordability of quality vaccines to protect against known and emerging infectious diseases 87 . About 70% of the global EPI vaccine supplies and about 75% procured by UN (United Nations) agencies are produced by DCVMN members 88 . Several technology transfers to DCVMN members have occurred over the past decades to significantly contribute to global health. Following an initial collaboration on the oral cholera vaccine between Sweden and VABIOTECH in Vietnam, the International Vaccine Institute improved the vaccine and then transferred the technology back to VABIOTECH and to several DCVMN members, including Shantha Biotechnics (Shanchol), India; EuBiologics (Euvichol), Republic of Korea; and Incepta (Cholvax), Bangladesh. Shanchol, Euvichol and Euvichol Plus are WHO prequalified and the major contributors to the Gavi-supported global stockpile 16 while Cholvax is marketed in Bangladesh.
For COVID-19 vaccines, several companies have licensed or contracted vaccine production to other manufacturers—AstraZeneca and Novavax with the Serum Institute (India) and SK Bioscience (Korea); Moderna with Lonza (Switzerland), Johnson & Johnson with Biological E (India); and Chinese Sinovac with Butantan (Brazil) and BioFarma (Indonesia). Hopefully the license and contract manufacturing arrangements will allow the production of sufficient doses of vaccines to provide equitable access to at-risk populations globally 89 .
Under the pressures of the pandemic, and with the need for accelerated development of COVID-19 vaccines, optimization of more practical aspects of vaccine implementation, supply and dosing was secondary to the need for rapid proof of concept. COVID-19 mRNA vaccines and the VSV-EBO Ebola vaccine from Merck have a similar requirement for ultracold chain storage. While that might be overcome by relatively simple technology, the scalability of these technologies for universal vaccination is unknown. Additional development is needed to establish the stability of vaccines at higher temperatures (Pfizer mRNA). There is evidence to suggest the presence of some protection against COVID-19 after the first dose; this is critical information not only for COVID-19 but also to frame thinking around other EID vaccines.
Leave no one behind, or the unequal access to vaccines and treatments
The 2030 Agenda for Sustainable Development has the vision to leave no one behind, particularly low-income countries. COVID-19 has seen exceptionalism at either extreme. On the one hand, COVAX aims to provide at least 2 billion doses of WHO-approved vaccine to participating countries by the end of 2021—roughly 20% of each country’s vaccination needs. A total of 92 LMICs will receive vaccine largely through an AMC arranged by Gavi 90 . It now appears that the USA will join COVAX, which recently announced that it had secured agreements for sufficient doses to meet the 2021 target 50 .
Critically, vaccinating people in LMICs will require additional vaccine purchases, at a cost estimated in billions of dollars. In purely economic terms, it appears that such an investment could have substantial benefit for the global economy 91 . On the other hand, COVAX is on track to achieve its goals and poised to start delivering vaccines, and yet no AMC countries had yet been vaccinated when tens of millions of people were already being vaccinated in high-income countries. Among high-income countries, billions of doses have been preordered, several times more than justified by their populations. Can COVAX achieve its target of providing 2 billion doses by 2021, or will manufacturing bottlenecks lead to delay that will allow the coronavirus to continue to circulate in poorer countries and prolong the pandemic? If unable to access COVID-19 vaccines in a timely manner, the 2030 Agenda for Sustainable Development, especially Sustainable Development Goal 3 focusing on health, will be difficult to achieve, and low-income countries will be under extraordinary pressure as the COVID-19 pandemic forces them further into poverty and deeper inequality.
UN Secretary-General António Guterres has again stressed that COVID-19 vaccines must be a global public good, available to everyone, everywhere. “Vaccinationalism is self-defeating and would delay a global recovery” 92 . Modeling studies suggest that if high-income countries take the first 2 billion doses of available COVID-19 vaccines without regard to equity, global COVID-19 deaths will double 93 . Ensuring that all countries have rapid, fair and equitable access to COVID-19 vaccines is the promise of COVAX.
Final remarks
The lessons of the COVID-19 pandemic need to be compiled and applied to the development of future vaccines against emerging infectious diseases and novel pandemic pathogens. The permanent threat of emerging pathogens calls for vigilance, surveillance and preparedness for vaccine development and deployment, all crosscutting activities to be conducted flawlessly between epidemiologists, scientists, developers, human and veterinary health authorities, regulators and funders. Global health stakeholders have learned something about developing vaccines efficiently: they still have much to learn about making and using them with due regard to equity and access.
Zhu, N. et al. A novel coronavirus from patients with pneumonia in China, 2019. N. Engl. J. Med. 382 , 727–733 (2020).
CAS PubMed PubMed Central Google Scholar
Huang, C. et al. Clinical features of patients infected with 2019 novel coronavirus in Wuhan, China. Lancet 395 , 497–506 (2020).
Wang, D. et al. Clinical characteristics of 138 hospitalized patients with 2019 novel coronavirus-infected pneumonia in Wuhan, China. JAMA 323 , 1061–1069 (2020).
Friedler, A. Sociocultural, behavioural and political factors shaping the COVID-19 pandemic: the need for a biocultural approach to understanding pandemics and (re)emerging pathogens. Glob. Public Health 16 , 17–35 (2021).
Article PubMed Google Scholar
Gully, P. R. Pandemics, regional outbreaks, and sudden-onset disasters. Healthc. Manage. Forum 33 , 164–169 (2020).
Morens, D. M. & Fauci, A. S. Emerging infectious diseases: threats to human health and global stability. PLoS Pathog. 9 , e1003467 (2013).
Article CAS PubMed PubMed Central Google Scholar
Marston, H. D., Folkers, G. K., Morens, D. M. & Fauci, A. S. Emerging viral diseases: confronting threats with new technologies. Sci. Transl. Med. 6 , 253ps210 (2014).
Article Google Scholar
Morens, D. M. & Fauci, A. S. Emerging pandemic diseases: how we got to COVID-19. Cell 182 , 1077–1092 (2020).
Morens, D. M., Daszak, P. & Taubenberger, J. K. Escaping Pandora’s box—another novel coronavirus. N. Engl. J. Med. 382 , 1293–1295 (2020).
Article CAS PubMed Google Scholar
Sacchetto, L., Drumond, B. P., Han, B. A., Nogueira, M. L. & Vasilakis, N. Re-emergence of yellow fever in the neotropics—quo vadis? Emerg. Top. Life Sci. 4 , 399–410 (2020).
PubMed Google Scholar
World Health Organization. Yellow fever. https://www.who.int/biologicals/vaccines/yellow_fever/en/ (2015).
Juan-Giner, A. et al. Immunogenicity and safety of fractional doses of yellow fever vaccines: a randomised, double-blind, non-inferiority trial. Lancet 397 , 119–127 (2021).
Prompetchara, E., Ketloy, C., Thomas, S. J. & Ruxrungtham, K. Dengue vaccine: global development update. Asian Pac. J. Allergy Immunol. 38 , 178–185 (2020).
PubMed CAS Google Scholar
Thomas, S. J. & Yoon, I. K. A review of Dengvaxia ® : development to deployment. Hum. Vaccin. Immunother. 15 , 2295–2314 (2019).
Article PubMed PubMed Central Google Scholar
Hu, D. et al. Origins of the current seventh cholera pandemic. Proc. Natl Acad. Sci. USA 113 , E7730–E7739 (2016).
Shaikh, H., Lynch, J., Kim, J. & Excler, J. L. Current and future cholera vaccines. Vaccine 38 , A118–A126 (2020).
Klemm, E. J. et al. Emergence of an extensively drug-resistant Salmonella enterica serovar Typhi clone harboring a promiscuous plasmid encoding resistance to fluoroquinolones and third-generation cephalosporins. mBio 9 , e00105–18 (2018).
Yousafzai, M. T. et al. Ceftriaxone-resistant Salmonella Typhi outbreak in Hyderabad City of Sindh, Pakistan: high time for the introduction of typhoid conjugate vaccine. Clin. Infect. Dis. 68 , S16–S21 (2019).
Qamar, F. N. et al. Antimicrobial resistance in typhoidal salmonella: surveillance for enteric fever in Asia project, 2016–2019. Clin. Infect. Dis. 71 , S276–S284 (2020).
Marchello, C. S., Carr, S. D. & Crump, J. A. A systematic review on antimicrobial resistance among Salmonella Typhi worldwide. Am. J. Trop. Med. Hyg. 103 , 2518–2527 (2020).
Andrews, J. R. et al. Typhoid conjugate vaccines: a new tool in the fight against antimicrobial resistance. Lancet Infect. Dis. 19 , e26–e30 (2019).
D’Souza, M. P. & Frahm, N. Adenovirus 5 serotype vector-specific immunity and HIV-1 infection: a tale of T cells and antibodies. AIDS 24 , 803–809 (2010).
Lin, J. T. et al. Safety and immunogenicity from a phase I trial of inactivated severe acute respiratory syndrome coronavirus vaccine. Antivir. Ther. 12 , 1107–1113 (2007).
Martin, J. E. et al. A SARS DNA vaccine induces neutralizing antibody and cellular immune responses in healthy adults in a Phase I clinical trial. Vaccine 26 , 6338–6343 (2008).
Jacob, S. T. et al. Ebola virus disease. Nat. Rev. Dis. Primers 6 , 13 (2020).
Wolf, J. et al. Applying lessons from the Ebola vaccine experience for SARS-CoV-2 and other epidemic pathogens. NPJ Vaccines 5 , 51 (2020).
Ball, P. The lightning-fast quest for COVID vaccines—and what it means for other diseases. Nature 589 , 16–18 (2021).
Feldmann, H., Feldmann, F. & Marzi, A. Ebola: lessons on vaccine development. Annu. Rev. Microbiol. 72 , 423–446 (2018).
Gouglas, D., Christodoulou, M., Plotkin, S. A. & Hatchett, R. CEPI: driving progress toward epidemic preparedness and response. Epidemiol. Rev. 41 , 28–33 (2019).
Rottingen, J. A. et al. New vaccines against epidemic infectious diseases. N. Engl. J. Med. 376 , 610–613 (2017).
Sandbrink, J. B. & Shattock, R. J. RNA vaccines: a suitable platform for tackling emerging pandemics? Front. Immunol. 11 , 608460 (2020).
Jackson, N. A. C., Kester, K. E., Casimiro, D., Gurunathan, S. & DeRosa, F. The promise of mRNA vaccines: a biotech and industrial perspective. NPJ Vaccines 5 , 11 (2020).
US Department of Health and Human Health Services. Explaining Operation Warp Speed. https://health.mo.gov/living/healthcondiseases/communicable/novel-coronavirus-lpha/pdf/fact-sheet-operation-warp-speed.pdf 2020).
van Riel, D. & de Wit, E. Next-generation vaccine platforms for COVID-19. Nat. Mater. 19 , 810–812 (2020).
Article PubMed CAS Google Scholar
Polack, F. P. et al. Safety and efficacy of the BNT162b2 mRNA Covid-19 vaccine. N. Engl. J. Med. 383 , 2603–2615 (2020).
Baden, L. R. et al. Efficacy and safety of the mRNA-1273 SARS-CoV-2 vaccine. N. Engl. J. Med. 384 , 403–416 (2021).
US Food and Drug Administration. FDA takes key action in fight against COVID-19 by issuing emergency use authorization for first COVID-19 vaccine. https://www.fda.gov/news-events/press-announcements/fda-takes-key-action-fight-against-covid-19-issuing-emergency-use-authorization-first-covid-19 (2020).
US Food and Drug Administration. FDA takes additional action in fight against COVID-19 by issuing emergency use authorization for second COVID-19 vaccine. https://www.fda.gov/news-events/press-announcements/fda-takes-additional-action-fight-against-covid-19-issuing-emergency-use-authorization-second-covid (2020).
European Medicines Agency. EMA recommends first COVID-19 vaccine for authorisation in the EU. https://www.ema.europa.eu/en/news/ema-recommends-first-covid-19-vaccine-authorisation-eu (2020).
European Medicines Agency. EMA recommends COVID-19 Vaccine Moderna for authorisation in the EU. https://www.ema.europa.eu/en/news/ema-recommends-covid-19-vaccine-moderna-authorisation-eu (2021).
Liu, M. A. A Comparison of plasmid DNA and mRNA as vaccine technologies. Vaccines 7 , 37 (2019).
Article CAS PubMed Central Google Scholar
Pollard, A. J. et al. Safety and immunogenicity of a two-dose heterologous Ad26.ZEBOV and MVA-BN-Filo Ebola vaccine regimen in adults in Europe (EBOVAC2): a randomised, observer-blind, participant-blind, placebo-controlled, phase 2 trial. Lancet Infect. Dis . https://doi.org/10.1016/S1473-3099(20)30476-X (2020).
Adalja, A. A., Watson, M., Cicero, A. & Inglesby, T. Vaccine platform technologies: a potent tool for emerging infectious disease vaccine development. Health Secur. 18 , 59–60 (2020).
Excler, J. L. & Kim, J. H. Novel prime-boost vaccine strategies against HIV-1. Expert Rev. Vaccines 18 , 765–779 (2019).
European Pharmaceutical Review. AstraZeneca to test combination of AZD1222 and Sputnik V vaccines. https://www.europeanpharmaceuticalreview.com/news/136683/astrazeneca-to-test-combination-of-azd1222-and-sputnik-v-vaccines/ (2020).
World Health Organization. Draft landscape and tracker of COVID-19 candidate vaccines. https://www.who.int/publications/m/item/draft-landscape-of-covid-19-candidate-vaccines (2021).
Fritzell, B. Bridging studies. Dev. Biol. Stand. 95 , 181–188 (1998).
CAS PubMed Google Scholar
WHO Ad Hoc Expert Group on the Next Steps for Covid-19 Vaccine Evaluation. Placebo-controlled trials of Covid-19 vaccines—why we still need them. N. Engl. J. Med . 384 , e2 (2021).
Clemens, J., Brenner, R., Rao, M., Tafari, N. & Lowe, C. Evaluating new vaccines for developing countries. Efficacy or effectiveness? JAMA 275 , 390–397 (1996).
Kim, J. H., Marks, F. & Clemens, J. D. Looking beyond COVID-19 vaccine phase 3 trials. Nat. Med. 27 , 205–211 (2021).
Follmann, D. et al. Assessing durability of vaccine effect following blinded crossover in COVID-19 vaccine efficacy trials. Preprint at https://www.medrxiv.org/content/10.1101/2020.12.14.20248137v1 (2020).
World Health Organization. Global Advisory Committee on Vaccine Safety, 27–28 May 2020. https://www.who.int/vaccine_safety/committee/reports/May_2020/en/ (2020).
World Health Organization. COVID-19 vaccines: safety surveillance manual. https://apps.who.int/iris/handle/10665/338400 (2020).
European Medicines Agency. Pharmacovigilance plan of the EU Regulatory Network for COVID-19 Vaccines. https://www.ema.europa.eu/en/documents/other/pharmacovigilance-plan-eu-regulatory-network-covid-19-vaccines_en.pdf (2020).
Lee, G. M., Romero, J. R. & Bell, B. P. Postapproval vaccine safety surveillance for COVID-19 vaccines in the US. JAMA 324 , 1937–1938 (2020).
Kieny, M. P. & Rago, L. Regulatory policy for research and development of vaccines for public health emergencies. Expert Rev. Vaccines 15 , 1075–1077 (2016).
Lambert, P. H. et al. Consensus summary report for CEPI/BC March 12–13, 2020 meeting: assessment of risk of disease enhancement with COVID-19 vaccines. Vaccine 38 , 4783–4791 (2020).
Zellweger, R. M., Wartel, T. A., Marks, F., Song, M. & Kim, J. H. Vaccination against SARS-CoV-2 and disease enhancement - knowns and unknowns. Expert Rev. Vaccines 19 , 691–698 (2020).
Creanga, A. A. et al. Severity of 2009 pandemic influenza A (H1N1) virus infection in pregnant women. Obstet. Gynecol. 115 , 717–726 (2010).
Menendez, C., Lucas, A., Munguambe, K. & Langer, A. Ebola crisis: the unequal impact on women and children’s health. Lancet Glob. Health 3 , e130 (2015).
The PREVENT Working Group. Pregnant women and vaccines against emerging epidemic threats: ethics guidance on preparedness, research and response. http://vax.pregnancyethics.org/prevent-guidance (2018).
Krubiner, C. B. et al. Pregnant women and vaccines against emerging epidemic threats: ethics guidance for preparedness, research, and response. Vaccine 39 , 85–120 (2021).
Edridge, A. W. D. et al. Seasonal coronavirus protective immunity is short-lasting. Nat. Med. 26 , 1691–1693 (2020).
Plante, J. A. et al. Spike mutation D614G alters SARS-CoV-2 fitness. Nature https://doi.org/10.1038/s41586-020-2895-3 (2020).
Korber, B. et al. Tracking changes in SARS-CoV-2 spike: evidence that D614G increases infectivity of the COVID-19 virus. Cell 182 , 812–827 (2020).
Hou, Y. J. et al. SARS-CoV-2 D614G variant exhibits efficient replication ex vivo and transmission in vivo. Science 370 , 1464–1468 (2020).
Volz, E. et al. Evaluating the effects of SARS-CoV-2 spike mutation D614G on transmissibility and pathogenicity. Cell 184 , 64–75.e11 (2021).
Faria, N. R. Genomic characterisation of an emergent SARS-CoV-2 lineage in Manaus: preliminary findings. Virological https://virological.org/t/586 (2020).
Wibmer, C. K. et al. SARS-CoV-2 501Y.V2 escapes neutralization by South African COVID-19 donor plasma. Preprint at bioRxiv https://doi.org/10.1101/2021.01.18.427166 (2021).
BBC. Covid: South Africa halts AstraZeneca vaccine rollout over new variant. https://www.bbc.com/news/world-africa-55975052 (2021).
Mahase, E. Covid-19: Novavax vaccine efficacy is 86% against UK variant and 60% against South African variant. BMJ 372 , n296 (2021).
Johnson & Johnson. Johnson & Johnson announces single-shot Janssen COVID-19 vaccine candidate met primary endpoints in interim analysis of its phase 3 ENSEMBLE trial. https://www.jnj.com/johnson-johnson-announces-single-shot-janssen-covid-19-vaccine-candidate-met-primary-endpoints-in-interim-analysis-of-its-phase-3-ensemble-trial (2021).
World Health Organization. Global Influenza Surveillance and Response System (GISRS). https://www.who.int/influenza/gisrs_laboratory/en/
US Food and Drug Administration. Emergency use authorization. https://www.fda.gov/emergency-preparedness-and-response/mcm-legal-regulatory-and-policy-framework/emergency-use-authorization (2021).
European Medicines Agency. Conditional marketing authorization. https://www.ema.europa.eu/en/human-regulatory/marketing-authorisation/conditional-marketing-authorisation (2020).
Smith, M. J., Ujewe, S., Katz, R. & Upshur, R. E. G. Emergency use authorisation for COVID-19 vaccines: lessons from Ebola. Lancet 396 , 1707–1709 (2020).
World Health Organization. Emergency use listing procedure. https://www.who.int/publications/m/item/emergency-use-listing-procedure (2020).
Pharmaceutical Technology. Russia approves Sputnik V Covid-19 vaccine for senior citizens. https://www.pharmaceutical-technology.com/news/russia-sputnik-senior-citizens/ (2021).
Logunov, D. Y. et al. Safety and efficacy of an rAd26 and rAd5 vector-based heterologous prime-boost COVID-19 vaccine: an interim analysis of a randomised controlled phase 3 trial in Russia. Lancet https://doi.org/10.1016/S0140-6736(21)00234-8 (2021).
Xinhuanet. China approves first self-developed COVID-19 vaccine. http://www.xinhuanet.com/english/2020-12/31/c_139632402.htm (2020).
Sinopharm. China grants conditional market approval for Sinopharm CNBG’s COVID-19 vaccine. http://www.sinopharm.com/en/s/1395-4173-38862.html (2021).
CNBC. Dubai is offering the Pfizer vaccine to residents for free in addition to China’s Sinopharm shot. https://www.cnbc.com/2020/12/23/dubai-offering-pfizer-sinopharm-covid-vaccines-to-residents-for-free.html (2020).
Reuters. China approves Sinovac Biotech COVID-19 vaccine for general public use. https://www.reuters.com/article/us-health-coronavirus-vaccine-sinovac-idUSKBN2A60AY (2021).
Reuters. Pakistan approves Chinese CanSinoBIO COVID vaccine for emergency use. https://www.reuters.com/article/us-health-coronavirus-pakistan-vaccine/pakistan-approves-chinese-cansinobio-covid-vaccine-for-emergency-use-idUSKBN2AC1FG (2021).
Euronews. Coronavirus: 15m people in the UK have now had their first COVID jab—what about wider Europe? https://www.euronews.com/2021/02/14/coronavirus-15m-people-in-the-uk-have-now-had-their-first-covid-jab-what-about-wider-europ (2021).
Deng, C. & Malsin, J. China’s Covid-19 vaccine makers struggle to meet demand. The Wall Street Journal https://www.wsj.com/articles/chinas-covid-19-vaccine-makers-struggle-to-meet-demand-11612958560 (10 February 2021).
Pagliusi, S. et al. Developing countries vaccine manufacturers network: doing good by making high-quality vaccines affordable for all. Vaccine 31 , B176–B183 (2013).
Jadhav, S., Gautam, M. & Gairola, S. Role of vaccine manufacturers in developing countries towards global healthcare by providing quality vaccines at affordable prices. Clin. Microbiol. Infect. 20 , 37–44 (2014).
Kim, J. H. SARS-CoV-2 vaccine development, access, and equity. J. Exp. Med. 217 , e20201288 (2020).
Nhamo, G., Chikodzi, D., Kunene, H. P. & Mashula, N. COVID-19 vaccines and treatments nationalism: challenges for low-income countries and the attainment of the SDGs. Glob. Public Health 16 , 319–339 (2020).
World Bank. Global economy to expand by 4% in 2021; vaccine deployment and investment key to sustaining the recovery. https://www.worldbank.org/en/news/press-release/2021/01/05/global-economy-to-expand-by-4-percent-in-2021-vaccine-deployment-and-investment-key-to-sustaining-the-recovery (2021).
UN News. WHO chief warns against ‘catastrophic moral failure’ in COVID-19 vaccine access. https://news.un.org/en/story/2021/01/1082362 (18 January 2021).
Chinazzi, M. et al. The effect of travel restrictions on the spread of the 2019 novel coronavirus (COVID-19) outbreak. Science 368 , 395–400 (2020).
Download references
Author information
Authors and affiliations.
International Vaccine Institute, Seoul, Republic of Korea
Jean-Louis Excler & Jerome H. Kim
Coalition for Epidemic Preparedness Innovations (CEPI), London, UK
Melanie Saville
Gavi, the Vaccine Alliance, Geneva, Switzerland
Seth Berkley
You can also search for this author in PubMed Google Scholar
Contributions
J.-L.E., M.S., S.B. and J.H.K. equally contributed to the synopsis of the manuscript. J.-L.E. and J.H.K. wrote the text and tables of the manuscript. J.H.K. provided the figure. M.S. and S.B. edited the manuscript.
Corresponding authors
Correspondence to Jean-Louis Excler or Jerome H. Kim .
Ethics declarations
Competing interests.
J.-L.E. is a consultant for vaccine safety for the Brighton Collaboration, Johnson & Johnson and the US Military HIV Research Program. J.H.K. is a consultant to SK Bioscience. M.S. has a financial interest in Sanofi (shares). S.B. does not have any financial or nonfinancial conflicts of interest.
Additional information
Publisher’s note Springer Nature remains neutral with regard to jurisdictional claims in published maps and institutional affiliations.
Peer review information Nature Medicine thanks Robert Carnahan and David Morens for their contribution to the peer review of this work. Joao Monteiro was the primary editor on this article and managed its editorial process and peer review in collaboration with the rest of the editorial team.
Rights and permissions
Reprints and permissions
About this article
Cite this article.
Excler, JL., Saville, M., Berkley, S. et al. Vaccine development for emerging infectious diseases. Nat Med 27 , 591–600 (2021). https://doi.org/10.1038/s41591-021-01301-0
Download citation
Received : 27 January 2021
Accepted : 01 March 2021
Published : 12 April 2021
Issue Date : April 2021
DOI : https://doi.org/10.1038/s41591-021-01301-0
Share this article
Anyone you share the following link with will be able to read this content:
Sorry, a shareable link is not currently available for this article.
Provided by the Springer Nature SharedIt content-sharing initiative
This article is cited by
Nanotechnology’s frontier in combatting infectious and inflammatory diseases: prevention and treatment.
- Yujing Huang
- Xiaohan Guo
Signal Transduction and Targeted Therapy (2024)
BCG-booster vaccination with HSP90-ESAT-6-HspX-RipA multivalent subunit vaccine confers durable protection against hypervirulent Mtb in mice
- Kee Woong Kwon
- Han-Gyu Choi
- Sung Jae Shin
npj Vaccines (2024)
A novel epidemic network model incorporating infections induced by exposed individuals and environments
- Jingyuan Li
- Yijun Zhang
- Weiwei Xing
Nonlinear Dynamics (2024)
Potentiating humoral and cellular immunity using a novel hybrid polymer-lipid nanoparticle adjuvant for HBsAg-VLP vaccine
Journal of Nanobiotechnology (2023)
Attitudinal analysis of vaccination effects to lead endemic phases
- Donggyun Ku
- Seungjae Lee
Scientific Reports (2023)
Quick links
- Explore articles by subject
- Guide to authors
- Editorial policies
Sign up for the Nature Briefing newsletter — what matters in science, free to your inbox daily.

share this!
March 28, 2024
This article has been reviewed according to Science X's editorial process and policies . Editors have highlighted the following attributes while ensuring the content's credibility:
fact-checked
peer-reviewed publication
trusted source
Tuberculosis vaccine may enable elimination of the disease in cattle by reducing its spread
by University of Cambridge
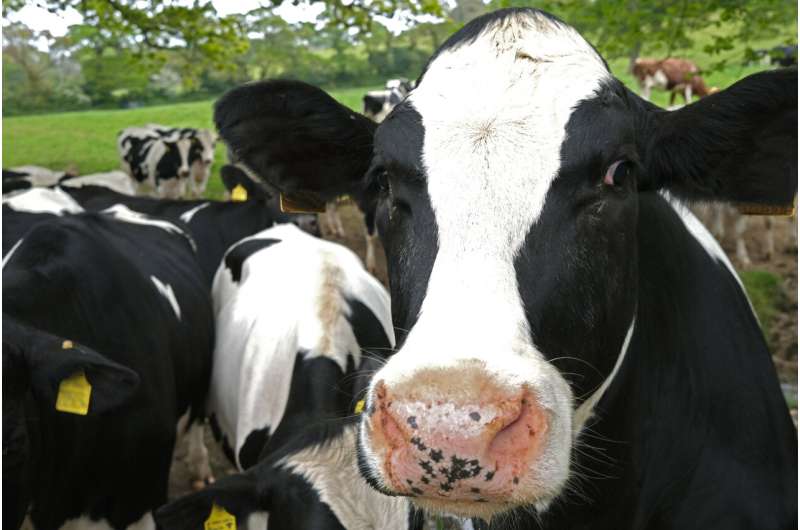
Vaccination not only reduces the severity of TB in infected cattle, but reduces its spread in dairy herds by 89%, research finds. The research, led by the University of Cambridge and Penn State University, improves prospects for the elimination and control of bovine tuberculosis (TB), an infectious disease of cattle that results in large economic costs and health impacts across the world. The study is published in the journal Science .
This is the first study to show that BCG-vaccinated cattle infected with TB are substantially less infectious to other cattle. This remarkable indirect effect of the vaccine beyond its direct protective effect has not been measured before.
The spillover of infection from livestock has been estimated to account for about 10% of human tuberculosis cases. While such zoonotic TB (zTB) infections are most commonly associated with gastrointestinal infections related to drinking contaminated milk, zTB can also cause chronic lung infections in humans. Lung disease caused by zTB can be indistinguishable from regular tuberculosis, but is more difficult to treat due to natural antibiotic resistance in the cattle bacteria.
TB remains endemic in many countries around the world, including in Europe and the Americas, where its control costs farmers and taxpayers hundreds of millions of dollars each year.
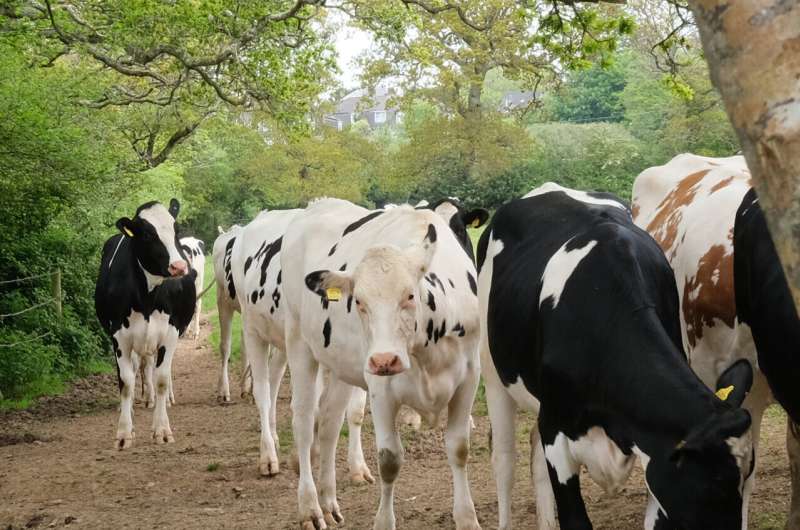
In the study, carried out in Ethiopia, researchers examined the ability of the vaccine, Bacillus Calmette-Guérin (BCG), to directly protect cattle that receive it, as well as to indirectly protect both vaccinated and unvaccinated cattle by reducing TB transmission. Vaccinated and unvaccinated animals were put into enclosures with naturally infected animals, in a novel crossover design performed over two years.
"Our study found that BCG vaccination reduces TB transmission in cattle by almost 90%. Vaccinated cows also developed significantly fewer visible signs of TB than unvaccinated ones. This suggests that the vaccination not only reduces the progression of the disease, but that if vaccinated animals become infected, they are substantially less infectious to others," said Andrew Conlan, Associate Professor of Epidemiology at the University of Cambridge's Department of Veterinary Medicine and a corresponding author of the study.
Using livestock census and movement data from Ethiopia, the team developed a transmission model to explore the potential for routine vaccination to control bovine tuberculosis.
"Results of the model suggest that vaccinating calves within the dairy sector of Ethiopia could reduce the reproduction number of the bacterium—the R 0 —to below 1, arresting the projected increase in the burden of disease and putting herds on a pathway towards elimination of TB," Conlan said.
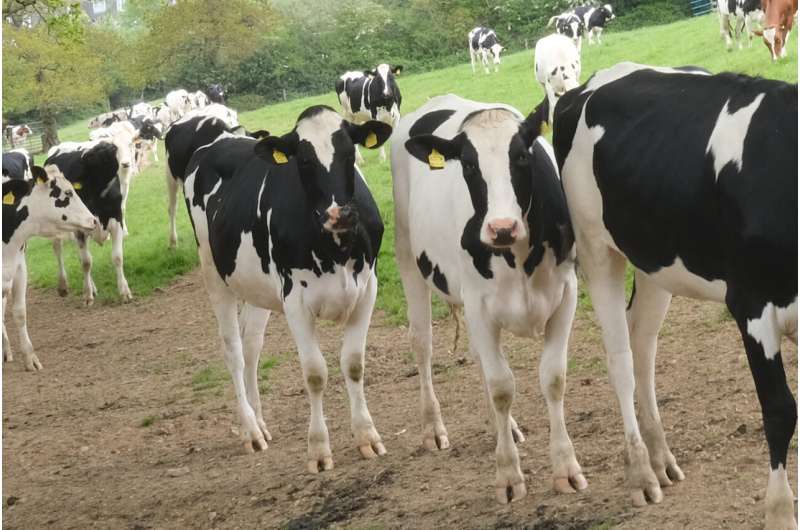
The team focused their studies in Ethiopia, a country with the largest cattle herd in Africa and a rapidly growing dairy sector that has a growing burden of bovine tuberculosis and no current control program, as a representative of similarly situated transitional economies.
"Bovine tuberculosis is largely uncontrolled in low- and middle-income countries , including Ethiopia," said Abebe Fromsa, associate professor of agriculture and veterinary medicine at Addis Ababa University in Ethiopia and the study's co-lead author. "Vaccination of cattle has the potential to provide significant benefits in these regions."
"For over a hundred years, programs to eliminate bovine tuberculosis have relied on intensive testing and slaughtering of infected animals," said Vivek Kapur, professor of microbiology and infectious diseases and Huck Distinguished Chair in Global Health at Penn State and a corresponding author of the study.
He added, "This approach is unimplementable in many parts of the world for economic and social reasons, resulting in considerable animal suffering and economic losses from lost productivity, alongside an increased risk of spillover of infection to humans. By vaccinating cattle, we hope to be able to protect both cattle and humans from the consequences of this devastating disease."
Professor James Wood, Alborada Professor of Equine and Farm Animal Science in the University of Cambridge's Department of Veterinary Medicine, noted that despite TB being more prevalent in lower-income countries, the United Kingdom, Ireland and New Zealand also experience considerable economic pressures from the disease which continues to persist despite intensive and costly control programs.
Wood said, "For over 20 years the UK government has pinned hopes on cattle vaccination for bovine tuberculosis as a solution to reduce the disease and the consequent costs of the controls. These results provide important support for the epidemiological benefit that cattle vaccination could have to reduce rates of transmission to and within herds."
Journal information: Science
Provided by University of Cambridge
Explore further
Feedback to editors

Study reports that age is the driving force in changing how stars move within galaxies
5 hours ago

Newly hatched chicks can instantly recognize objects with vision, even if they've only ever experienced them by touch
6 hours ago

A return to roots: Lab builds its first stellarator in 50 years and opens the door for research into new plasma physics
7 hours ago

A new estimate of US soil organic carbon to improve Earth system models

New research uses coaxial 'dish' antenna to scan for dark matter
8 hours ago

'Unheard of in structural biology': New enzyme models reveal disease insights

Transmitting entanglement between light and matter in the metropolitan network of Barcelona
9 hours ago

These plants evolved in Florida millions of years ago. They may be gone in decades.

Focusing ultra-intense lasers to a single wavelength

Classical optical neural network exhibits 'quantum speedup'
10 hours ago
Relevant PhysicsForums posts
What do large moles on the body indicate.
Mar 30, 2024
Avian flu - A new study led by a team from the University of Maryland
Mar 27, 2024
Are all biological catabolic reactions exergonic?
Mar 20, 2024
A First of Its Kind: A Calcium-based signal in the Human Brain
Mar 18, 2024
Biological culture and cultural biology
Mar 17, 2024
Potentially fatal dog parasite found in the Colorado River
Mar 15, 2024
More from Biology and Medical
Related Stories
Cost and scale of field trials for bovine tb vaccine may make them unfeasible, study suggests.
Jun 7, 2018

New vaccine will stop the spread of bovine tuberculosis
Nov 28, 2019

New tuberculosis tests pave way for cow vaccination programs
Jul 17, 2019

Clues of tuberculosis spread between cows and badgers
Dec 18, 2019

CDC says people can contract tuberculosis from deer
Oct 1, 2019

What's the best way to prevent tuberculosis transmission from wildlife to cattle?
Aug 23, 2023
Recommended for you

How AI and deeper roots can help soil store more carbon

Researchers discover corn reduces arsenic toxicity in soil
13 hours ago

Golfers' risk from pesticides used on turf grass is likely low, studies find
Apr 1, 2024

Scientist taps into lobsters' unusual habits to conquer the more than 120-year quest to farm them
Mar 29, 2024

Study highlights the potential of cyanobacteria as biofertilizers

Researchers discover key gene for toxic alkaloid in barley
Mar 28, 2024
Let us know if there is a problem with our content
Use this form if you have come across a typo, inaccuracy or would like to send an edit request for the content on this page. For general inquiries, please use our contact form . For general feedback, use the public comments section below (please adhere to guidelines ).
Please select the most appropriate category to facilitate processing of your request
Thank you for taking time to provide your feedback to the editors.
Your feedback is important to us. However, we do not guarantee individual replies due to the high volume of messages.
E-mail the story
Your email address is used only to let the recipient know who sent the email. Neither your address nor the recipient's address will be used for any other purpose. The information you enter will appear in your e-mail message and is not retained by Phys.org in any form.
Newsletter sign up
Get weekly and/or daily updates delivered to your inbox. You can unsubscribe at any time and we'll never share your details to third parties.
More information Privacy policy
Donate and enjoy an ad-free experience
We keep our content available to everyone. Consider supporting Science X's mission by getting a premium account.
E-mail newsletter

An official website of the United States government
The .gov means it’s official. Federal government websites often end in .gov or .mil. Before sharing sensitive information, make sure you’re on a federal government site.
The site is secure. The https:// ensures that you are connecting to the official website and that any information you provide is encrypted and transmitted securely.
- Publications
- Account settings
Preview improvements coming to the PMC website in October 2024. Learn More or Try it out now .
- Advanced Search
- Journal List
- Proc Natl Acad Sci U S A
- v.114(16); 2017 Apr 18

Simply put: Vaccination saves lives
Walter a. orenstein.
a Department of Medicine, Emory Vaccine Center, Emory University School of Medicine, Atlanta, GA, 30322;
b Department of Microbiology & Immunology, Emory Vaccine Center, Emory University School of Medicine, Atlanta, GA, 30322
Author contributions: W.A.O. and R.A. wrote the paper.
Few measures in public health can compare with the impact of vaccines. Vaccinations have reduced disease, disability, and death from a variety of infectious diseases. For example, in the United States, children are recommended to be vaccinated against 16 diseases ( 1 ). Table 1 highlights the impact in the United States of immunization against nine vaccine-preventable diseases, including smallpox and a complication of one of those diseases, congenital rubella syndrome, showing representative annual numbers of cases in the 20th century compared with 2016 reported cases ( 2 , 3 ). All of the diseases have been reduced by more than 90% and many have either been eliminated or reductions of 99% or more have been achieved. A recent analysis of vaccines to protect against 13 diseases estimated that for a single birth cohort nearly 20 million cases of diseases were prevented, including over 40,000 deaths ( 4 ). In addition to saving the lives of our children, vaccination has resulted in net economic benefits to society amounting to almost $69 billion in the United States alone. A recent economic analysis of 10 vaccines for 94 low- and middle-income countries estimated that an investment of $34 billion for the immunization programs resulted in savings of $586 billion in reducing costs of illness and $1.53 trillion when broader economic benefits were included ( 5 ). The only human disease ever eradicated, smallpox, was eradicated using a vaccine, and a second, polio, is near eradication, also using vaccines ( 6 , 7 ).
Comparison of 20th century annual morbidity and current estimates vaccine-preventable diseases
Vaccines not only provide individual protection for those persons who are vaccinated, they can also provide community protection by reducing the spread of disease within a population ( Fig. 1 ). Person-to-person infection is spread when a transmitting case comes in contact with a susceptible person. If the transmitting case only comes in contact with immune individuals, then the infection does not spread beyond the index case and is rapidly controlled within the population. Interestingly, this chain of human-to-human transmission can be interrupted, even if there is not 100% immunity, because transmitting cases do not have infinite contacts; this is referred to as “herd immunity” or “community protection,” and is an important benefit of vaccination.

( A ) A highly susceptible population in which a transmitting case is likely to come in contact with a susceptible person leading to a chain of person-to-person transmission. ( B ) A highly immune population in which a transmitting case is unlikely to come in contact with a susceptible person, thereby breaking the chain of transmission and achieving indirect protection of remaining susceptibles because they are not exposed.
Mathematical modelers can estimate on average how many persons the typical transmitting case is capable of infecting if all of the contacts were susceptible (i.e., a population of 100% susceptibility). This number is known as R 0 , or the basic reproductive number. The immunity threshold needed within the population for terminating transmission can be calculated in percent as ( R 0 − 1)/ R 0 × 100 and is a guide to setting immunity levels and vaccination coverage targets for various diseases ( 8 ). For example, measles is one of the most contagious of vaccine-preventable diseases, with an estimated immunity threshold of 92–94%. In contrast, the protection threshold for rubella is estimated at 83–85%. Thus, eliminating rubella transmission is easier than measles, and when there are gaps in immunization coverage leading to accumulation of susceptibles, measles is often the first vaccine-preventable disease identified. Because of community protection induced by vaccines, persons who cannot be vaccinated (e.g., have contraindications or are younger than the age for whom vaccines are recommended), as well as persons who fail to make an adequate immune response to the vaccine (although most vaccines are highly effective, they are not 100% effective), can be protected indirectly because they are not exposed ( Fig. 1 ). Thus, for most vaccines, achieving high levels of coverage is important not only for individual protection but in preventing disease in vulnerable populations that cannot be directly protected by vaccination. This provides the rationale for interventions to achieve high population immunity, such as removing barriers that may prevent access to vaccines (e.g., providing recommended vaccines without cost), as well as mandates for immunization requirements for attending school ( 9 ). There are many reasons why vaccinations may not be received as recommended. One extreme is outright opposition to vaccines. Probably even more common may be that making the effort to receive vaccines (e.g., making the healthcare visits at the appropriate time so vaccines can be administered) may be a low priority compared with other issues, so in the absence of having a mandate for vaccination, other things take priority. Thus, appropriate mandates could help in making vaccination a priority for all ( 10 ).
It’s often said that vaccines save lives, but this is not strictly true; it is vaccination that saves lives. A vaccine that remains in the vial is 0% effective even if it is the best vaccine in the world. Thus, it is imperative that we all work together to assure that a high level of coverage is obtained among populations for whom vaccines are recommended. In some sense, vaccines have become victims of their own success. Diseases that once induced fear and sparked desire for vaccines are now rare, and there is a false and dangerous sense of complacency among the public.
In addition, in recent years, growing numbers of persons have become hesitant about vaccines, fearing side effects and not appreciative of the enormous health and economic benefits that vaccines provide. A CDC report on 159 measles cases reported between January 4 and April 2, 2015, showed that 68 United States residents with measles were unvaccinated, and of these 29 (43%) cited philosophical or religious objections to vaccination ( 11 ). A 2014 national web-based poll of parents in the United States estimated that 90.8% (89.3–92.1%) reported accepting or planning to accept all recommended noninfluenza childhood vaccines, 5.6% (4.6–6.9%) reported intentionally delaying one or more, and 3.6% (2.8–4.5%) reported refusing one or more vaccines ( 12 ). A national survey of pediatricians in the United States reported that the proportion of pediatricians reporting parental vaccine refusals increased from 74.5% in 2006 to 87.0% in 2013 ( 13 ). A 67-country survey on the state of vaccine confidence reported an average of 5.8% of respondents globally were skeptical about the importance of vaccines, with that proportion rising to more than 15% in some countries ( 14 ). One of the major concerns in recent years has been the allegations that vaccines can cause autism. There are three major theories advanced on the role of vaccines in causing autism: ( i ) measles, mumps, rubella vaccine (MMR); ( ii ) thimerosal, an ethyl mercury containing preservative in many vaccines in the United States in the past, now mostly out of vaccines recommended for children; and ( iii ) too many vaccines ( 15 ). There have been multiple well-conducted studies and independent reviews of those studies by the Institute of Medicine (now the National Academy of Medicine) that do not support a role for vaccines in causing autism ( 16 ). Independent evaluation of the safety of the immunization schedule has found it to be extremely safe ( 17 ). However, translating the science into information capable of influencing vaccine skeptics has been difficult.
The National Vaccine Advisory Committee (NVAC) in the United States issued a report in 2015, with 23 recommendations to assure high levels of vaccine confidence ( 18 ). The recommendations have five focus areas: ( i ) measuring and tracking vaccine confidence, ( ii ) communication and community strategies to increase vaccine confidence, ( iii ) healthcare provider strategies to increase vaccine confidence, ( iv ) policy strategies to increase vaccine confidence, and ( v ) continued support and monitoring of the state of vaccine confidence. Critical to assuring confidence is evidence-based research to evaluate which interventions are most effective. The NVAC recommended that a repository of evidence-based best practices for informing, educating, and communicating with parents and others in ways that foster or increase vaccine confidence be created. And while we have focused on children, vaccine preventable diseases exact a substantial health burden in adults and immunization coverage rates for most recommended vaccines are substantially lower for adults than those achieved for recommended vaccines in children. Thus, there is need not only in enhancing immunization rates in children but also in adults.
In summary, vaccines are some of the most effective and also cost-effective prevention tools we have. But vaccines that are not administered to persons for whom they are recommended are not useful. It is incumbent upon all of us who work in the healthcare setting, as well as community leaders, to stress to our friends and colleagues the importance of vaccination both for the individual vaccinated as well as for the communities in which the individuals live. Also critically important, there remains an urgent need for greater emphasis on research to develop vaccines for global diseases for which vaccines either do not exist or need improvement.
Acknowledgments
The authors thank Dianne Miller, Ali Ellebedy, and Sandra Roush for their assistance in preparation of the manuscript.
See Perspective on page 4055 .
This paper is in the following e-collection/theme issue:
Published on 1.4.2024 in Vol 26 (2024)
Response of Unvaccinated US Adults to Official Information About the Pause in Use of the Johnson & Johnson–Janssen COVID-19 Vaccine: Cross-Sectional Survey Study
Response of unvaccinated us adults to official information about the pause in use of the johnson & johnson–janssen covid-19 vaccine: cross-sectional survey study.
Authors of this article:

Research Letter
- Vishala Mishra 1 * , MBBS, MMCi ;
- Joseph P Dexter 2, 3, 4 * , PhD
1 Department of Biostatistics and Bioinformatics, Duke University School of Medicine, Durham, NC, United States
2 Data Science Initiative, Harvard University, Allston, MA, United States
3 Department of Human Evolutionary Biology, Harvard University, Cambridge, MA, United States
4 Institute of Collaborative Innovation, University of Macau, Taipa, Macao
*all authors contributed equally
Corresponding Author:
Joseph P Dexter, PhD
Data Science Initiative
Harvard University
Science and Engineering Complex 1.312-10
150 Western Avenue
Allston, MA, 02134
United States
Phone: 1 8023381330
Email: [email protected]
Using a rapid response web-based survey, we identified gaps in public understanding of the Centers for Disease Control and Prevention’s messaging about the pause in use of the Johnson & Johnson–Janssen COVID-19 vaccine and estimated changes in vaccine hesitancy using counterfactual questions.
Introduction
On April 13, 2021, the Centers for Disease Control and Prevention (CDC) and Food and Drug Administration recommended a pause in use of the Johnson & Johnson (J&J)–Janssen COVID-19 vaccine due to 6 reports of cerebral venous sinus thrombosis in recently vaccinated individuals [ 1 ]. The announcement of the pause required development of a coordinated communication strategy under extreme time pressure and careful messaging by stakeholders to mitigate reduced public confidence in COVID-19 vaccines [ 2 ]. Moreover, official communication efforts had to consider the potential influence of already widespread misinformation about the vaccines on attitudes toward the pause [ 3 , 4 ]. In this survey study, we evaluated understanding and impressions of the CDC’s public web-based information about the J&J-Janssen pause among unvaccinated US adults.
Web-Based Survey About J&J-Janssen Pause
We administered the web-based survey to two cohorts of US adults recruited through Prolific between April 19-21, 2021 (cohort A), and April 21-23, 2021 (cohort B). Both cohorts were assembled using convenience sampling of unvaccinated adults. To obtain information about a population that especially needed targeted vaccine communication, the first cohort was restricted to individuals expressing neutral or negative sentiments about COVID-19 vaccines. The survey design and recruitment strategy are described in Multimedia Appendix 1 ; the survey questions are provided in Multimedia Appendices 2 and 3 .
Ethical Considerations
The study was approved by Harvard University’s Committee on the Use of Human Subjects (IRB20-2089), and participants agreed to a consent statement on the first page of the survey. Participants were paid US $2 for taking the survey. All study data were collected anonymously.
A total of 271 and 286 participants were included in cohorts A and B, respectively (demographic characteristics listed in Table 1 ). Across participants, the median number of correct responses to the comprehension questions was 6 in both cohort A (IQR 1.5; range 0-7) and cohort B (IQR 1.0; range 1-7). The total number of correct responses was negatively associated with intention not to seek vaccination in both cohort A (odds ratio 0.61, 95% CI 0.45-0.82; P =.001) and cohort B (odds ratio 0.48, 95% CI 0.31-0.74; P =.001; Multimedia Appendix 4 ). Although a majority of participants rated the passages as “clear and easy to read” (cohort A: n=229, 84.5%; cohort B: n=243, 85%), fewer indicated that they would be likely to share them on social media (cohort A: n=53, 19.6%; cohort B: n=75, 26.3%).
The web page mentioned “a small number of reports” of cerebral venous sinus thrombosis in individuals who received the J&J-Janssen vaccine. When asked to guess a specific number, 188 (69.4%) and 133 (46.5%) respondents in cohorts A and B, respectively, estimated 100 or more cases, at least an order of magnitude higher than the actual value; 176 (64.9%) and 128 (44.8%) respondents in cohorts A and B, respectively, estimated 10 or more deaths after vaccination ( Figure 1 ).
Responding to a counterfactual question, 127 (46.9%) and 139 (48.6%) participants in cohorts A and B, respectively, indicated that the pause reduced their confidence in the J&J-Janssen vaccine’s safety ( Figure 1 ). Most participants reported no change in their confidence in COVID-19 vaccines’ safety in general (cohort A: n=182, 67.2%; cohort B: n=194, 67.8%) or intention to receive the Pfizer-BioNTech or Moderna vaccine (cohort A: n=206, 76%; cohort B: n=211, 73.8%).
a Participants could select more than one option.
b Includes participants who selected “American Indian or Alaska Native,” “Native Hawaiian or Other Pacific Islander,” or “Another option not listed here.”
c Number of participants who gave the correct answer to each question.
d Number of participants who gave the indicated number of correct answers across all questions.
e Number of participants who answered “Strongly agree” or “Agree” about each description on a 6-point Likert scale.

In our web-based survey about the CDC’s messaging around the J&J-Janssen vaccine pause, many respondents overestimated the number of case reports that prompted the pause, often by several orders of magnitude. Since verbal descriptors are elastic concepts that can be misinterpreted, grounding them with numbers can reduce variability in risk perception and promote informed decision-making [ 5 ].
Respondents also expressed reduced confidence in the safety of the J&J-Janssen vaccine, highlighting the potential danger of conveying piecemeal information about risk during a pandemic response [ 3 ]. Encouragingly, the reduced confidence did not extend to mRNA COVID-19 vaccines, consistent with previous findings that overall vaccine hesitancy remained stable following the pause [ 6 ]. These results were obtained using the counterfactual format, which is less susceptible to overestimating shifts in beliefs than the change format ( Multimedia Appendix 1 ). The negative association between understanding of the passage and self-reported vaccine hesitancy suggests that more targeted messaging may have been useful to promote vaccine confidence [ 7 , 8 ].
Consistent with uncertainty management theory [ 9 ], individuals likely viewed the pause in different ways, leading to a spectrum of emotional responses and changes in behavior. Despite being a safety precaution, the pause introduced new uncertainties requiring effective management through clear and consistent messaging, highlighting the balance that must be maintained between fostering trust and preventing unnecessary alarm [ 10 ]. Given the limitations of the deficit model of scientific communication [ 11 ], just providing technically correct information is insufficient for effective communication during public health crises. Instead, attention should be given to the accessibility of information across diverse socioeconomic groups, in line with the knowledge gap hypothesis [ 12 ], and to countering misinformation by providing easy-to-use official guidance [ 6 , 7 ].
The study is limited by the convenience sampling strategy; the participants recruited were not representative of the US population as a whole, and the findings should not be generalized to other contexts. Since the study was conducted on the web, individuals with lower internet and health literacy may have been excluded.
Acknowledgments
We thank Vasudha Mishra, MBBS, for assistance with graphic design. This work was supported by a CoronaVirusFacts Alliance Grant from the Poynter Institute, a Harvard Data Science Fellowship, and the Institute of Collaborative Innovation at the University of Macau.
Data Availability
The data sets generated during this study are available from the corresponding author upon reasonable request.
Conflicts of Interest
None declared.
Additional information about survey methodology.
Survey administered to cohort A.
Survey administered to cohort B.
Supplemental tables about survey questions and ordinal logistic regression analysis.
- Karron RA, Key NS, Sharfstein JM. Assessing a rare and serious adverse event following administration of the Ad26.COV2.S vaccine. JAMA. Jul 22, 2021;325(24):2445-2447. [ CrossRef ] [ Medline ]
- Wood S, Schulman K. Beyond politics—promoting Covid-19 vaccination in the United States. N Engl J Med. Mar 18, 2021;384(7):e23. [ CrossRef ] [ Medline ]
- Lee JJ, Kang K, Wang MP, Zhao SZ, Wong JYH, O'Connor S, et al. Associations between COVID-19 misinformation exposure and belief with COVID-19 knowledge and preventive behaviors: cross-sectional online study. J Med Internet Res. Dec 13, 2020;22(11):e22205. [ FREE Full text ] [ CrossRef ] [ Medline ]
- Ginossar T, Cruickshank IJ, Zheleva E, Sulskis J, Berger-Wolf T. Cross-platform spread: vaccine-related content, sources, and conspiracy theories in YouTube videos shared in early Twitter COVID-19 conversations. Hum Vaccin Immunother. Dec 31, 2022;18(1):1-13. [ FREE Full text ] [ CrossRef ] [ Medline ]
- Edwards A, Elwyn G, Mulley A. Explaining risks: turning numerical data into meaningful pictures. BMJ. May 06, 2002;324(7341):827-830. [ FREE Full text ] [ CrossRef ] [ Medline ]
- Hsieh YL, Rak S, SteelFisher GK, Bauhoff S. Effect of the suspension of the J&J COVID-19 vaccine on vaccine hesitancy in the United States. Vaccine. Jan 24, 2022;40(3):424-427. [ FREE Full text ] [ CrossRef ] [ Medline ]
- Miller M, Castrucci BC. Changing the COVID-19 conversation: it's about language. JAMA Health Forum. Mar 01, 2021;2(2):e210020. [ FREE Full text ] [ CrossRef ] [ Medline ]
- Mishra V, Dexter JP. Comparison of readability of official public health information about COVID-19 on websites of international agencies and the governments of 15 countries. JAMA Netw Open. Aug 03, 2020;3(8):e2018033. [ FREE Full text ] [ CrossRef ] [ Medline ]
- Brashers D. Communication and uncertainty management. J Commun. 2001;51(3):477-497. [ CrossRef ]
- Petersen MB, Bor A, Jørgensen F, Lindholt MF. Transparent communication about negative features of COVID-19 vaccines decreases acceptance but increases trust. Proc Natl Acad Sci U S A. Jul 20, 2021;118(29):e2024597118. [ FREE Full text ] [ CrossRef ] [ Medline ]
- Reincke CM, Bredenoord AL, van Mil MH. From deficit to dialogue in science communication: the dialogue communication model requires additional roles from scientists. EMBO Rep. Oct 03, 2020;21(9):e51278. [ FREE Full text ] [ CrossRef ] [ Medline ]
- Lind F, Boomgaarden HG. What we do and don’t know: a meta-analysis of the knowledge gap hypothesis. Ann Int Commun Assoc. May 09, 2019;43(3):210-224. [ CrossRef ]
Abbreviations
Edited by A Mavragani; submitted 25.08.22; peer-reviewed by M Graham, T Ginossar, A Scherer; comments to author 25.01.23; revised version received 26.05.23; accepted 29.12.23; published 01.04.24.
©Vishala Mishra, Joseph P Dexter. Originally published in the Journal of Medical Internet Research (https://www.jmir.org), 01.04.2024.
This is an open-access article distributed under the terms of the Creative Commons Attribution License (https://creativecommons.org/licenses/by/4.0/), which permits unrestricted use, distribution, and reproduction in any medium, provided the original work, first published in the Journal of Medical Internet Research, is properly cited. The complete bibliographic information, a link to the original publication on https://www.jmir.org/, as well as this copyright and license information must be included.
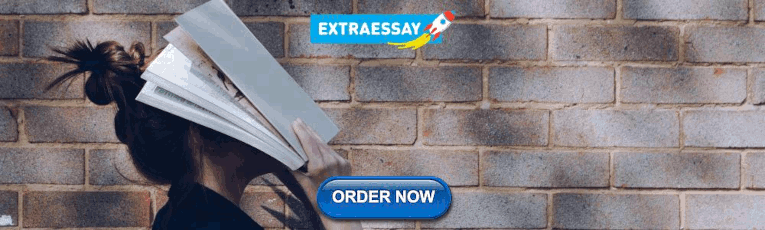
IMAGES
COMMENTS
Introduction "The impact of vaccination on the health of the world's peoples is hard to exaggerate. With the exception of safe water, no other modality has had such a major effect on mortality reduction and population growth" (Plotkin and Mortimer, 1988).The development of safe and efficacious vaccination against diseases that cause substantial morbidity and mortality has been one of the ...
Metrics. Vaccines have revolutionized modern medicine by preventing infectious diseases and safeguarding public health. This Collection showcases cutting-edge research on advancements in vaccine ...
A recent test-negative design study in Canada showed positive findings regarding vaccine effectiveness with the BNT162b2, mRNA-1273, and ChAdOx1 nCoV-19 vaccines against symptomatic disease with ...
The protective effects of vaccination and prior infection against severe Covid-19 are reviewed, with proposed directions for future research, including mucosal immunity and intermittent vaccine boo...
Vaccination is a powerful method of disease prevention that is relevant to people of all ages and in all countries, as the Covid-19 pandemic illustrates. Vaccination can improve people's chances ...
This Review, aimed at a broad scientific audience, provides an introductory guide to the history, development and immunological basis of vaccines, immunization and related issues to provide ...
Community‐based studies in five countries show consistent strong benefits from early rollouts of COVID‐19 vaccines. By the beginning of June 2021, almost 11% of the world's population had received at least one dose of a coronavirus disease 2019 (COVID‐19) vaccine. 1 This represents an extraordinary scientific and logistic achievement — in 18 months, researchers, manufacturers and ...
Vaccines articles from across Nature Portfolio. Vaccines are a clinical product that is composed of live or dead material from an infectious agent - bacterium, virus, fungus or parasite - that ...
Our analyses indicate that vaccine effectiveness generally decreases over time against SARS-CoV-2 infections, hospitalisations, and mortality. The baseline vaccine effectiveness levels for the omicron variant were notably lower than for other variants. Therefore, other preventive measures (eg, face-mask wearing and physical distancing) might be necessary to manage the pandemic in the long term.
The effectiveness of the mRNA vaccines in preventing COVID-19 disease progression in 2021 set new expectations about the role of prevention interventions for the disease. Efficacy observed in the trials was more than 90%.1,2 The efficacy of other vaccines evaluated in large randomised trials, such as the Oxford-AstraZeneca (70%) and Sputnik V (91%) vaccines, have been criticised for elements ...
Research and Development RBD Receptor binding domain NK (cells) Natural killer SARS-CoV-2 ... Since the mechanisms of action and undesirable effects of these vaccines are addressed in other articles of this special issue, we will focus on the efficacy of coronavirus disease 2019 (COVID-19) vaccines, more particularly on the four vaccines ...
Childhood vaccination has dramatically reduced morbidity, mortality, and disability caused by vaccine-preventable diseases, with ∼21 million hospitalizations, 732 000 deaths, and 322 million cases of disease averted in the United States between 1994 and 2013. 1 Among diseases targeted by vaccines recommended before 1980, 3—polio, measles, and rubella—have achieved elimination status as ...
There is no question that the current vaccines are effective and safe. The risk of severe reaction to a COVID-19 jab, say researchers, is outweighed by the protection it offers against the deadly ...
By the end of November 2021, scientists estimate that mRNA COVID-19 vaccines had prevented at least 1 million deaths, 10 million hospitalizations, and 36 million SARS-CoV-2 infections in the United States. Sometimes people who are fully vaccinated get a breakthrough infection, meaning that they test positive for SARS-CoV-2 or become ill with ...
Understanding Vaccine Safety and the Roles of the FDA and the CDC. Author: H. Cody Meissner, M.D. Author Info & Affiliations. Published April 27, 2022. N Engl J Med 2022;386: 1638 - 1645. DOI: 10. ...
Vaccines that protect against severe illness, death and lingering long Covid symptoms from a coronavirus infection were linked to small increases in neurological, blood, and heart-related ...
2. Vaccination strategies. Many efforts have been directed towards the development of the vaccines against COVID-19, to avert the pandemic and most of the developing vaccine candidates have been using the S-protein of SARS-CoV-2 (Dhama et al., 2020).As of July 2, 2020, the worldwide SARS-CoV-2 vaccine landscape includes 158 vaccine candidates, out of which 135 are in the preclinical or the ...
3 National Clinical Research Center for Obstetrics and Gynecology, Peking University Third Hospital, Beijing, ... Two doses of vaccination can provide effective protection severe COVID-19, with booster vaccination additionally enhancing VE. ... All claims expressed in this article are solely those of the authors and do not necessarily represent ...
Objective To study the association between COVID-19 vaccination and the risk of post-COVID-19 cardiac and thromboembolic complications. Methods We conducted a staggered cohort study based on national vaccination campaigns using electronic health records from the UK, Spain and Estonia. Vaccine rollout was grouped into four stages with predefined enrolment periods. Each stage included all ...
The current research is the most comprehensive network meta-analysis to compare the efficacy of nine new COVID-19 vaccines to prevent symptomatic and severe disease in the adult population ...
MONDAY, April 1, 2024 (HealthDay News) -- Vaccine protection against mpox fades quickly in the human immune system, even in people who've received the full two-dose regimen, a new real-world ...
Vaccines are needed to prevent coronavirus disease 2019 (Covid-19) and to protect persons who are at high risk for complications. The mRNA-1273 vaccine is a lipid nanoparticle-encapsulated mRNA ...
Development of a DNA-based lung cancer vaccine in the United Kingdom received funding for 2 years of laboratory research and initial manufacture of 3000 doses, according to a press release from ...
New guidelines suggest certain high-risk groups could benefit from having another dose of a COVID-19 vaccine this spring — and more frequent shots in general — while the broader population ...
Abstract. The coronavirus disease 2019 (COVID-19) pandemic is a global crisis, with devastating health, business and social impacts. Vaccination is a safe, simple, and effective way of protecting a person against COVID-19. By the end of August 2021, only 24.6% of the world population has received two doses of a COVID-19 vaccine.
Consequently, work on vaccines against SARS-CoV ended and its funding was cut. Only a whole inactivated vaccine 23 and a DNA vaccine 24 were tested in phase 1 clinical trials. Following a ...
N Engl J Med 2023;389:2233-2244. In a placebo-controlled, phase 2-3 trial, one dose of mRNA-1345 led to a lower incidence of RSV disease among adults 60 years of age or older. Solicited local ...
Vaccination not only reduces the severity of TB in infected cattle, but reduces its spread in dairy herds by 89%, research finds. The research, led by the University of Cambridge and Penn State ...
A recent economic analysis of 10 vaccines for 94 low- and middle-income countries estimated that an investment of $34 billion for the immunization programs resulted in savings of $586 billion in reducing costs of illness and $1.53 trillion when broader economic benefits were included ( 5 ). The only human disease ever eradicated, smallpox, was ...
Using a rapid response web-based survey, we identified gaps in public understanding of the Centers for Disease Control and Prevention's messaging about the pause in use of the Johnson & Johnson-Janssen COVID-19 vaccine and estimated changes in vaccine hesitancy using counterfactual questions.