
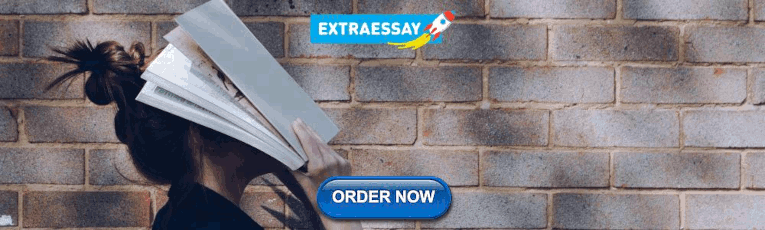
Materials Advances
Nanomaterials: a review of synthesis methods, properties, recent progress, and challenges.

* Corresponding authors
a Center of Research Excellence in Desalination & Water Treatment, King Fahd University of Petroleum and Minerals, Dhahran 31261, Saudi Arabia E-mail: [email protected] , [email protected]
b Center for Environment and Water, King Fahd University of Petroleum and Minerals, Dhahran 31261, Saudi Arabia
c Interdisciplinary Research Center for Membranes and Water Security, King Fahd University of Petroleum and Minerals, Dhahran 31261, Saudi Arabia
d Department of Chemical & Biological Engineering, University of Alabama, Tuscaloosa, Alabama 35487-0203, USA E-mail: [email protected] , [email protected]
e Department of Mechanical Engineering, King Fahd University of Petroleum and Minerals, Dhahran 31261, Saudi Arabia
Nanomaterials have emerged as an amazing class of materials that consists of a broad spectrum of examples with at least one dimension in the range of 1 to 100 nm. Exceptionally high surface areas can be achieved through the rational design of nanomaterials. Nanomaterials can be produced with outstanding magnetic, electrical, optical, mechanical, and catalytic properties that are substantially different from their bulk counterparts. The nanomaterial properties can be tuned as desired via precisely controlling the size, shape, synthesis conditions, and appropriate functionalization. This review discusses a brief history of nanomaterials and their use throughout history to trigger advances in nanotechnology development. In particular, we describe and define various terms relating to nanomaterials. Various nanomaterial synthesis methods, including top-down and bottom-up approaches, are discussed. The unique features of nanomaterials are highlighted throughout the review. This review describes advances in nanomaterials, specifically fullerenes, carbon nanotubes, graphene, carbon quantum dots, nanodiamonds, carbon nanohorns, nanoporous materials, core–shell nanoparticles, silicene, antimonene, MXenes, 2D MOF nanosheets, boron nitride nanosheets, layered double hydroxides, and metal-based nanomaterials. Finally, we conclude by discussing challenges and future perspectives relating to nanomaterials.
Article information

Download Citation
Permissions.

N. Baig, I. Kammakakam and W. Falath, Mater. Adv. , 2021, 2 , 1821 DOI: 10.1039/D0MA00807A
This article is licensed under a Creative Commons Attribution-NonCommercial 3.0 Unported Licence . You can use material from this article in other publications, without requesting further permission from the RSC, provided that the correct acknowledgement is given and it is not used for commercial purposes.
To request permission to reproduce material from this article in a commercial publication , please go to the Copyright Clearance Center request page .
If you are an author contributing to an RSC publication, you do not need to request permission provided correct acknowledgement is given.
If you are the author of this article, you do not need to request permission to reproduce figures and diagrams provided correct acknowledgement is given. If you want to reproduce the whole article in a third-party commercial publication (excluding your thesis/dissertation for which permission is not required) please go to the Copyright Clearance Center request page .
Read more about how to correctly acknowledge RSC content .
Social activity
Search articles by author, advertisements.
- Search Menu
- Advance Articles
- Author Guidelines
- Open Access Options
- Self-Archiving Policy
- About International Journal of Low-Carbon Technologies
- Editorial Board
- Advertising and Corporate Services
- Journals Career Network
- Dispatch Dates
- Journals on Oxford Academic
- Books on Oxford Academic

Article Contents
1. introduction, 2. systematic literature review: methods and materials, 3. results of the bibliographic analysis of data, 4. applications of nanotechnology in ev, 5. summary of key research findings, 6. conclusions, declaration of competing interest, acknowledgements, author contributions.
- < Previous
A systematic review of nanotechnology for electric vehicles battery

- Article contents
- Figures & tables
- Supplementary Data
Pulkit Kumar, Harpreet Kaur Channi, Atul Babbar, Raman Kumar, Javed Khan Bhutto, T M Yunus Khan, Abhijit Bhowmik, Abdul Razak, Anteneh Wogasso Wodajo, A systematic review of nanotechnology for electric vehicles battery, International Journal of Low-Carbon Technologies , Volume 19, 2024, Pages 747–765, https://doi.org/10.1093/ijlct/ctae029
- Permissions Icon Permissions
Nanotechnology has increased electric vehicle (EV) battery production, efficiency and use. Nanotechnology is explored in this electric car battery illustration. Nanoscale materials and topologies research has increased battery energy density, charge time and cycle life. Nanotubes, graphene and metal oxides improve energy storage, flow and charging/discharge. Solid-state and lithium-air high-energy batteries are safer, more energy dense and more stable using nanoscale catalysts. Nanotechnology improves battery parts. Nanostructured fluids reduce lithium dendrite, improving batteries. Nanocoating electrodes may reduce damage and extend battery life. Nanotechnology benefits the planet. Nanomaterials allow battery parts to employ ordinary, safe materials instead of rare, harmful ones. Nanotechnology promotes battery recycling, reducing waste. Change does not influence stable, cost-effective or scalable items. Business opportunities for nanotechnology-based EV batteries need more research. High-performance, robust and environmentally friendly batteries might make electric cars more popular and transportation more sustainable with research and development. An outline of EV battery nanotechnology researchexamines the publication patterns, notable articles, collaborators and contributions. This issue was researched extensively, indicating interest. Research focuses on anode materials, energy storage and battery performance. A research landscape assessment demonstrates EV battery nanotechnology’s growth and future. A comprehensive literature review examined nanosensors in EVs. Our study provides a solid foundation for understanding the current state of research, identifying major trends and discovering nanotechnology breakthroughs in EV sensors by carefully reviewing, characterizing and rating important papers.
The future of nanotechnology with electric vehicles (EVs) is uncertain. Researchers and engineers use nano-manipulating materials to boost EVs’ speed, efficiency and longevity [ 1 ]. Nanotechnology makes coatings for EVs, battery technology, energy harvesting, sensors, catalysis and lightweight materials possible [ 2 ]. By enhancing energy storage, charging speed, component weight and durability, nanotechnology makes electric automobiles more efficient and practical, which could help circumvent transportation system limitations. A cleaner, greener and more sustainable transportation future will be ushered in as nanotechnology improves the capabilities of electric automobiles and increases their widespread acceptability. Nanotechnology’s quick growth has generated exciting potential for the EV revolution [ 3 ]. The use of nanotechnology can improve the performance, efficiency and longevity of EVs. Battery technology, lightweight materials, energy harvesting, sensors, catalysis and coatings are all examples of applications where scientists and engineers have taken advantage of nanoparticles’ unique capabilities [ 4 ]. The batteries used in EVs are improving because of nanotechnology [ 5 ].
Graphene and carbon nanotubes are two examples of nanoscale materials that improve energy storage, electrode surface area and electrode conductivity. The lightweight materials made possible by nanotechnology may also impact EV design [ 6 ]. Nanomaterial composites, including carbon nanotubes and nanofibers, have superior strength-to-weight ratios. Lightening the load improves the vehicle’s mileage, range and handling. EVs use nanotechnology for energy collection. Nanogenerators convert the kinetic energy produced by the car’s motion and vibrations into electrical power. Nanostructured solar panels on the vehicle’s surface capture sun energy for EV charging [ 7 ].
Nanotechnology allows for the development sophisticated sensors that keep tabs on and control the operation of EVs. The susceptible and accurate nanosensors can measure temperature, pressure and gas concentrations, increase safety, give better battery management and enhance vehicle performance. Catalysis and fuel cell technologies, which provide alternatives to EV batteries, rely heavily on nanotechnology [ 8 ]. Using nanocatalysts, fuel cells can convert energy more cleanly. Fuel cells are an option for EVs because of their longer ranges and shorter recharging times [ 9 ]. Intelligent coatings and self-repairing materials can be produced via nanotechnology for EVs. Scratches, corrosion and weathering are all thwarted by nanocoating, and the coating’s self-healing properties repair minor damage [ 10 ]. These coatings’ durability, hydrophobicity and UV resistance increase EVs’ aesthetics, longevity and sustainability. As progress in nanotechnology advances, the outlook for EVs improves. Nanotechnology can make transportation systems more effective, practical and environmentally friendly [ 11 ]. Figure 1 shows the few primary ways nanotechnology can be applied to EVs. Cleaner, greener transportation may lie in the hands of EVs that utilize nanoparticles and nanoscale engineering.

Nanotechnology in EVs.
To further explore how EV batteries might be improved, it is vital to provide a more comprehensive explanation of the specific roles played by nanotubes, graphene, metal oxides and nanoscale catalysts. Nanomaterials are crucial in enhancing different performance characteristics of EV batteries [ 12 ]. The remarkable conductivity features of nanotubes and graphene enable efficient electron transit, hence increasing the overall conductivity of the battery. Metal oxides enhance batteries’ energy storage capacity, improving their efficiency in storing and releasing energy during charge and discharge processes [ 13 ]. In addition, catalysts at the nanoscale operate as promoters to enhance the speed of reaction kinetics, optimizing the charge and discharge efficiency. Furthermore, integrating these nanomaterials improves EV batteries’ security and durability by addressing concerns regarding excessive heat generation [ 14 ] and establishing a more substantial structural foundation [ 15 ]. An in-depth investigation into the unique effects of each nanomaterial on energy storage [ 16 ], conductivity, charge/discharge processes, safety and stability issues offers a detailed comprehension of their combined influence on the advancement of EV battery technology [ 17 ].
Nanotechnology has become a major force in improving the performance and economy of batteries for EVs. Materials like nanotubes, graphene, metal oxides and nanoscale catalysts play key roles in this. Nanotubes and graphene are great for storing energy because they are highly conductive and have a lot of surface area [ 15 ]. This makes them better electrode materials for lithium-ion batteries, which are widely used in EVs. These materials make it easier for electrons and ions to move faster, which leads to more energy and faster charging and discharging. On the other hand, metal oxides are great alternatives to standard cathode materials because they have higher capacity and better cycling stability. Their nanoscale size makes charge transfer even more efficient, which lowers the energy lost during cycles [ 18 ]. Graphene is a single layer of carbon atoms grouped in a hexagonal lattice. It is very good at conducting electricity and is also very strong. It makes the conductivity better when added to battery electrodes, which speeds up the charging and discharging processes. Nanotubes, like carbon nanotubes, have a lot of surface area and can carry electricity well. This has helped make electrode materials that are light and work well. Metal oxides, such as manganese oxide and titanium oxide, have better electrochemical qualities [ 19 ]. This meets the need for materials in EV batteries that have a higher energy density and last longer between cycles.
Moreover, tiny catalysts have completely changed the electrochemical processes that happen inside batteries. These helpers, which are usually made of precious metals like platinum and palladium, make the processes that reduce and release oxygen easier in fuel cells and metal–air batteries. This makes them work better overall. Nanostructuring these catalysts also makes them more active, which means they can convert and store energy more efficiently. When it comes to safety, nanomaterials help make improved battery systems possible [ 20 ]. Nanoscale coatings and additives can make lithium-ion batteries safer by making them more stable at high temperatures, lowering the risk of burning, and stopping dendrites from forming. Nanomaterials also make things more stable, which means batteries last longer. This means they don’t need to be replaced as often, and throwing away batteries has less of an effect on the world [ 21 ]. According to the researchers, adding nanotubes, graphene, metal oxides and tiny catalysts to the design and make-up of EV batteries is a big step forward for making them safer, more stable and better at storing energy and conducting electricity. As scientists continue to study and improve these nanotechnology uses, the chances of making electric transportation more efficient and environmentally friendly grow [ 22 ].
1.1. Importance of nanosensors
Sanguesa et al. (2021) reviewed EV battery technological trends, charging techniques and future research challenges and prospects. They discussed the pros and cons of lead-acid, nickel-metal hydride, lithium-ion and lithium-sulfur EV batteries. They also discussed EV charging standards and modes such as AC and DC, slow and rapid, wireless and bidirectional. They discussed nanosensors’ high sensitivity, low power consumption, miniaturization, fabrication, integration and calibration concerns for battery management [ 23 ]. A literature overview on EV technology and its various uses, including smart grid, vehicle to grid, and vehicle to home, was presented. Energy efficiency, emissions reduction, grid stability, battery cost, range anxiety and charging infrastructure were only some of the topics covered. Several global EV programs and projects were highlighted, including Tesla, Nissan Leaf and Dubai Smart City [ 24 ]. A systematic literature review (SLR) on EV consumer acceptance was published by Han et al. [ 25 ]. They synthesized the methodology, ideas and variables from 57 peer-reviewed publications published between 2015 and 2022 on EV purchase, behavior and usage intentions. They found that attitudes, norms, perceived behavioral control, awareness, knowledge, personal values, emotions and social influence affect EV adoption. They also highlighted literature gaps and suggested additional research [ 25 ]. The authors of Basu et al. (2018) provided a synopsis of EVs and EV sensors. The advantages and disadvantages of the various varieties of EVs, including fuel cell EVs (FCEVs), battery EVs (BEVs) and hybrid EVs (HEVs), were discussed. Additionally, they deliberated on the evolving landscape of micro-electro-mechanical system- (MEMS) based miniaturization for sensors and devices utilized in diverse EV applications and the various types of sensors associated with battery and position monitoring [ 26 ].
1.2. Literature review
The low-carbon mobility transition relies on plug-in EVs, including battery and hybrid EVs. Daramy-Williams et al. (2019) found many user experience topics, including driving and travel behaviors, vehicle interactions and subjective factors. EVs have increased the utilization of electric batteries. Many studies have developed and improved battery cell voltage equalization methods by adding noteworthy features [ 27 ]. These cell equalization techniques were the subject of a thorough and methodical review (Das et al., 2020). This SLR focuses on recent efforts that have built energy management storage system (EMSs) for HEVs [ 28 ]. The study conducted by Torreglosa et al. (2020) aimed to provide a quantitative analysis of the chosen works. Notwithstanding advancements in driving range and recharge alternatives, these and additional market impediments persist, rendering the present market share of BEVs inconsequential [ 29 ]. In light of the advancement of hydrogen fuel cell stacks, an emerging powertrain architecture concept for N1 class-type cars was described (Castillo et al., 2020). The fuel cell extended range electric vehicles (FC-EREV)concept combines a battery-electric arrangement with a hydrogen-powered fuel cell stack that acts as a range extender. The lithium-ion battery in EVs can sustain an operational temperature range of 15°C to 35°C employing a battery thermal management system (BTMS) [ 30 ].
Tete et al. (2021) conducted a comprehensive review of experimental and numerical analyses of BTMS utilized in electric and hybrid vehicles. This review encompassed approaches such as air, liquid, phase change material, heat pipe and refrigeration cooling for battery cooling systems. EVs need high-energy batteries. The maximum capacity of lithium-air battery theory using graphene under optimal electron conduction conditions and the experimental maximum obtained by optimizing the structure geometry, examples of structural engineering using carbon fiber and carbon nanotubes in cathode fabrication to perform the reaction properly while providing space for lithium oxide placement, are examined [ 31 ]. Suryatna et al. (2022) described the battery’s mechanism and analyze its constituent parts. As a result of the growth of green logistics and the support of new energy car development policies both domestically and globally, logistics and distribution have embraced EVs as an alternative to traditional fuel vehicles [ 32 ]. Ye et al. (2022) examined the most recent breakthroughs in EV routing models and solution algorithms in logistics and distribution. Passenger vehicles contribute significantly to glasshouse gas (GHG) emissions; thus, precise and current estimates of the comparative emissions of the key types of alternative power trains are essential to support evidence-based policy recommendations [ 33 ]. A systematic review and harmonization of the most recent scientific literature on this subject was presented in Raugei (2022). The findings show that battery BEVs are the most promising option for decarbonizing the passenger vehicle fleet across all global regions studied, with the potential for −70% reductions in GHG emissions compared to conventional gasoline-powered internal combustion engine vehicles. EVs are becoming increasingly prevalent as many nations set net-zero carbon targets for the foreseeable future [ 34 ].
The status, characteristics and application scope of global lithium ion battery (LIB) safety standards and regulations were examined by Lai et al. (2022). The rational test standard upgrade is reviewed in light of recent EV and energy storage power plant fires. Direct drive offers improved systematic economy, more flexible wheel control and better passenger comfort by eliminating the gearbox and transmission [ 35 ]. Cai et al. (2022) reviewed vehicle direct-drive methods and contemporary electrical machine improvements for direct-drive propulsion systems. FCEVs for long-haul applications can reduce road freight CO 2 emissions until long-distance battery-electric mobility matures, depending on the hydrogen fuel source [ 36 ]. Pardhi et al. (2022) examined FCEV powertrain topologies for long-distance HD applications, as well as their operating constraints, cooling requirements, waste heat recovery methods, cutting-edge powertrain control, energy and thermal management strategies, as well as over-the-air route data-based predictive powertrain management with V2X connectivity. Batteries powering EVs provide a promising way to reduce pollution and uncertainty.
In contrast to battery degeneration’s scalability and temporal constraints, machine learning (ML) approaches offer a non-invasive, accurate and low-processing solution [ 37 ]. Sharma and Bora (2023) evaluated these problems objectively and comprehensively. EVs are becoming mainstream as more governments set near-term net-zero carbon ambitions [ 38 ]. Senol et al. (2023) conducted a comprehensive literature analysis in their study, which focused on integrating power networks and Li-ion battery technologies, particularly emphasizing their performance under unfavorable weather circumstances [ 39 ]. Increasing multiscale modeling and design for battery efficiency and safety management was reviewed by Kiran MD et al. (2024). This article shows how machine learning-based data analysis in battery research has advanced, setting the groundwork for cloud and digital battery management to produce trustworthy onboard applications [ 40 ]. Electron and ion transport affect the battery’s energy production under application conditions and how much energy can be used [ 41 ]. The transport mechanisms of ions and electrons for active materials, in addition to positive and negative composite electrodes, were examined by Quilty et al. (2023). Simultaneously, contemporary EVs exhibit a confident capacity to reduce fossil fuel consumption [ 42 ]. Gevorkov et al. (2023) reviewed and analyzed multiport converters’ key characteristics, topologies, pros and cons and applications. Operando characterization is not new, but techniques that can track commercial battery properties under realistic conditions have unlocked a trove of chemical, thermal and mechanical data that could revolutionize lithium-ion device development and use [ 43 ]. The innovative dual-ion battery that is built on aluminum and has a cathode made of three-dimensional graphene possesses high-energy density, low cost and the ability to charge and discharge faster than other batteries, making it an ideal choice for grid storage and personal gadgets by Zhang et al. (2016) [ 44 ]. A novel calcium-ion battery with a high discharge voltage and 95% capacity retention can function consistently at room temperature. This battery has the potential to serve as an alternative to lithium-ion batteries by Wang et al. (2018) [ 45 ]. For sodium-based energy storage applications, this study conducted by Mu et al. (2020) successfully synthesizes high-performance anodes with high-fraction active materials. These anodes achieve good rate capability and cycling stability [ 46 ]. Zhang et al. (2023) examined the difficulties encountered by power systems that heavily rely on inverter-based resources (IBR), specifically emphasizing the inadequate capacity to support voltage and frequency. The authors suggest reorganizing the virtual synchronous generator (VSG’s) control blocks by aligning the control blocks of a VSG with the control perspective of conventional synchronous generators. The reorganization above streamlines and clarifies the control pathway governing the system’s active and reactive power output, enabling easier virtual inertia and attenuation parameters to be adjusted. The article presents a compact signal model for IBR controlled by VSG, including voltage and current loops modules, instantaneous power calculation and an LC (inductors (L) and capacitors (C)) filter [ 47 ]. A current discrepancy that occurs during charge and discharging in high-temperature superconducting (HTS) non-insulation closed-loop coils may result in novel phenomena, such as a rapid decrease or increase in magnetic field at particular positions, and may influence operational current judgment by Lu et al. (2022) [ 48 ]. Wu et al. (2023) centers on the advancements in rare earth-barium-copper oxides superconducting tapes, with a specific emphasis on the nanocomposite EuBa2Cu3O7-δ superconducting films. These films hold significant importance in the context of high-field magnet applications. The scientists utilize a pulsed laser deposition methodology with an exceedingly rapid growth rate of up to 100 nm/s—two orders of magnitude higher than traditional approaches. This enables them to accomplish both rapid growth and a substantial capacity to transport field current [ 49 ]. Shen et al. (2024) have presented an innovative energy management approach that seeks to optimize the functionality of energy storage systems in EVs, specifically focusing on reducing the aging of lithium-ion batteries caused by high-frequency power requirements. Fuzzy logic control and ensemble empirical mode decomposition are incorporated into the proposed method. At the outset, the power demand of EVs is decomposed into intrinsic mode function components. Subsequently, permutation entropy is utilized to reconstruct each component into low- or high-frequency components [ 50 ]. A proposal was made by Zhao et al. (2023) for the preview-based human-like trajectory planning model (PHTPM), which is subsequently evaluated and assessed through comparative and generalizability tests. The findings indicate that the implementation of the driver preview feature empowers PHTPM to precisely emulate the attributes of proficient drivers during left turns while surpassing them during right turns [ 51 ]. A critical scenario search technique for intelligent vehicle testing that is based on the social cognitive optimization algorithm has been suggested, and the findings show that the proposed method has the potential to increase both the search efficiency, and the coverage of important scenarios was figured out by Zhu et al. (2023) [ 52 ]. This research presents a novel model that has the potential to improve our understanding of the behavior of lithium deposition and pave the way for the development of stable and secure lithium metal anodes through the utilization of bimetallic metal organic framework- (MOF) derived materials in the construction of three-dimensional frameworks studied by Wei et al. (2023) [ 53 ]. Based on the findings of the analysis conducted by Yue et al. (2023), it can be concluded that the stability of the road system in the presence of incident effects is directly connected to the severity of the event, the signal control strategy, the penetration rate and the spatial distribution of autonomous vehicles. In conclusion, simulation results are carried out to demonstrate the efficacy of our suggested event management policy in enhancing the rate of recovery and the stability of road networks [ 54 ]. In order to solve the issue in which the response quality is decreased as a result of factors such as parameter mismatch and disturbance, an adaptive disturbance observer-based improved super-twisting sliding mode control (ISTSMC-ADOB) has been developed. With the purpose of achieving adaptive compensation, avoiding the usage of high-gain feedback, and expanding the applicability of the conventional disturbance observer, an adaptive disturbance observer, also known as an ADOB, was intentionally constructed by Lu et al. (2022) [ 55 ]. Yu et al. (2023) has discussed the difficulties that arise when attempting to implement aqueous zinc-ion batteries in practical applications. The paper focuses on the unstable electrode/electrolyte interface that is related to inhomogeneous zinc deposition and side reactions. The solution that has been offered involves the utilization of L-carnitine (L-CN) as an effective addition for the purpose of stabilizing electrodes and extending the lifespan of batteries. When L-CN is present in minute quantities, it exhibits a remarkable synergy between quaternary ammonium cations, COO− anions and hydroxyl groups. This synergy can influence the electrochemical deposition/insertion of Zn 2+ and the activity of water molecules [ 56 ]. Hou et al. (2023) have centered on developing a self-powered, lightweight biomimetic mouse whisker sensor (BMWS) that draws inspiration from the extraordinarily perceptive whisker detection exhibited by mice. The BMWS, unlike its predecessors that attempted to imitate animal whiskers, surmounts drawbacks, including a cumbersome design, dependence on external power and restricted application scenarios. By utilizing the triboelectric effect and a meticulously engineered framework, the BMWS exhibits exceptional capabilities in detecting collisions and maintaining signal stability. Intelligent early warning, direction identification, hole width discrimination and real-time distance sensing are among the many duties in which it excels [ 57 ]. A novel mechanism and technology for directed energy deposition-arc in the production of large parts, referred to as alternating-arc-based additive manufacturing and enabled by a polarity-switching self-adaptive shunt, are presented Yan et al. (2023). The experimental outcomes demonstrate that the proposed system facilitates the alternating passage of current through the welding wire and the substrate, generating electronegative arcs at the anode with the wire and electropositive arcs at the cathode with the substrate. By modulating the arcs, decoupling control between thermal force and mass transfer is accomplished. The electropositive arc is responsible for cathode cleaning and molten pool temperature, whereas the electronegative arc regulates wire melting, particle size and temperature [ 58 ]. A battery–supercapacitor hybrid energy storage system and an accompanying energy management strategy are proposed in the article by Wang et al. (2024) in a response to the growing need for compact motors with high output torque, specifically for use in mobile robotics. This method draws inspiration from automobiles’ high-capacity hybrid energy systems. In conditions of minimal torque, the motor operates on battery power, with any excess power being directed toward charging the supercapacitor. When faced with torque overload conditions, the motor is supplied with high current by swiftly discharging the supercapacitor, which guarantees an instantaneous increase in output power. The objective of the energy management strategy is to regulate the supercapacitor’s charging and discharging processes to prevent interference with the motor’s power supply from the battery and maintain current stability and control during discharge [ 59 ].

Process of SLR.

Flow chart of selection of data for bibliometric analysis.
The resources utilized in this SLR comprise bibliometric data extracted from various sources, such as journal articles, conference papers, scholarly surveys, books, essays and review articles. These sources are analyzed to determine author affiliations, country collaboration maps, citation patterns, keywords plus and the frequency of author keywords. The present study employs a systematic procedure to select research publications about nanomaterials in EVs. The literature search involved comprehensive exploration using databases like Scopus. Key search terms included ‘nanotechnology in EV batteries,’ ‘electrode nanocoating’ and related variations. Potential biases in article selection were mitigated by employing rigorous inclusion criteria, prioritizing peer-reviewed studies and avoiding undue influence from commercial interests. Figure 2 shows the process of SLR.
The Scopus article research selections were restricted in the first step, as shown in Figure 3 and Table 1 . From 1991 to July 2023, this analysis uncovered 2361 publications concerning EVs, nanotechnology, nanomaterials and sustainable development. We have 2322 articles remaining after eliminating concise surveys, notes, erratum editorials and letters. After publication status-based exclusions of research papers, 2309 remained. The final subjects that were excluded from consideration were the following: astronomy and physics, pharmacology, toxicology, pharmaceutics, earth and planetary science, health profession, agriculture and biological science, neuroscience, immunology, microbiology, business and management, accounting, economics, econometrics and finance, arts and humanities and dentistry. One thousand five hundred forty products were remaining.
Bibliometric analysis is a method that quantifies scientific publications by examining citation patterns. Citations, according to a bibliometric study, reveal the impact, influence and connections of academic journals [ 60 ]. The bibliometric analysis theory posits that scientific knowledge is transmitted through scholarly publications, wherein the number and caliber of citations gauge the scientific significance of an article or publication it garners [ 61 ]. Bibliometric researchers utilize citation data to quantify author output, journal influence, collaboration patterns and research trends. According to this theory, more people cite influential articles [ 62 ].
Additionally, intellectual networks between authors, institutions and fields may be reflected in citations [ 63 , 64 ]. By quantifying these citation patterns, bibliometric analysis evaluates research output, influence and collaboration [ 63 ]. Bibliometric research is regulated by mathematical and statistical models, including co-citation analysis, citation counts, h-index and bibliographic coupling. Academics can quantify and contrast scholastic output, discern notable works and authors and monitor the evolution of specific publications or research domains through these techniques. Citation patterns can disclose the structure and dynamics of scientific knowledge, aiding policymakers, institutions and researchers in making informed decisions regarding funding allocation, research evaluation and scholarly communication, according to bibliometric analysis [ 65 ].
By examining the bibliography, the most cited journals are determined. Energy and environmental science, nanoenergy, ACS Applied Materials and Interfaces and the Journal of Materials Chemistry , all of which have contributed to the study of nanomaterials in EVs, are utilized in the proposed research. Numerous scholarly periodicals indicate that nanomaterials are an emerging trend for energy storage and lightweight materials to reduce the weight of EVs, as demonstrated by this review [ 66 ]. Bibliometric analysis is an expert methodology that assists researchers in assessing the advancement of various approaches through the statistical distribution of data about citation analysis, keywords, author affiliation and country of contribution. The top journals are shown in Table 2 .
Detailed analysis of nanomaterials in EV data is presented in this section. In recent decades, the quantity of publications and literary sources is evaluated in Sections 3.1 and 3.2. Additionally, the country production analysis is presented in Section 3.3. The thematic evaluation of the keywords in the publications is presented in Section 3.4. Additionally, the collaboration map of the top 15 nations, most cited countries, country production over time and source dynamics is provided in Section 3.5. Section 3.6 provides a comprehensive analysis of the findings regarding author keywords, most common terms, a tree map of keywords and word clouds. Section 3.7 presents three field plots depicting the relationships between author keywords, author countries and keywords plus.
3.1. Literature analysis of publications
Figure 4 illustrates the annual distribution of publications from 1991 to 2023. However, upon examining the graph, we have identified a substantial fluctuation in the number of publications on nanomaterials in EVs. The number of publications in this field was ‘0’ before 2003 but rose after 2005. However, between 2012 and 2021, there has been a significant increase in the number of publications devoted to investigations. The calculated annual growth rate was approximately 12.79%.
3.2. Institutions
A visual representation of the collaborations between the five most prestigious institutions worldwide and their publications over the last 30 years is presented in Figure 5 . As stated, five major institutions contribute to the Scopus Journal, with Nanyang Technological University ranking first with 945 publications and Beijing University ranking second with 586 publications. Simultaneously, the University of Science and Technology of China, the School of Material Science and Engineering and Tsinghua University ranked third, fourth and fifth in the Scopus database from 1991 to 2023, with net publications of 528, 475 and 409, respectively.
Main information of data taken from Scopus.
Top 15 journals in the research area under the study, 3.3. analysis of countries.
Diverse nations have contributed to academic journals concerning the application of nanotechnology to EVs. The cumulative number of publications originates from the 10 countries in Figure 6 . Based on the analysis, China holds the highest position regarding the total number of articles. Following that, India and the United States occupied the second and third positions, respectively, with 1584 and 480 publications, while China produced 2370 articles within the specified period.
3.4. Thematic evaluation of keywords
Based on the evaluation of keywords from 1991 to 2023, as shown in Figure 7 , several authors have repeatedly utilized a small number of keywords. The following terms were examined following the analysis: self-powered sensors, batteries, triboelectric nanogenerator, nanotechnology, renewable energy, lithium-ion batteries, lithium-ion batteries, electrode material and electrochemical performance.

Annual distribution of the publications.

Top 5 productive institutions.

Top 15 countries with the maximum number of publications.

Thematic evaluation of keywords.
3.5. Country collaboration
The collaborative efforts of numerous nations are illustrated in Figure 8 , and Figure 9 shows the cloud-based structure of the various country collaborations. Based on the bibliometric survey’s analysis, the following countries have been identified as having the greatest number of collaborations in the field of nanotechnology for EVs: China, India, the United States, South Korea, Germany, Japan, the United Kingdom, the Philippines, Singapore, France, Australia, Canada, Italy, Spain and Malaysia.

Country collaboration map.

Cloud-based structure of the various country collaborations.
3.6. Keywords
Each node in Figure 10a corresponds to a distinct keyword. We opted to include only the top 50 out of 1000 keywords. The prevailing research topics identified were ‘nanotechnology,’ ‘lithium,’ ‘electrodes,’ ‘electric batteries,’ ‘nanostructured materials’ and ‘energy efficiency.’ A cloud structure of keywords is depicted in Figure 10b , which resembles the analysis in Figure 10a ; however, the distinction between the two figures lies in the quantity of keywords. The authors spent the most time gathering the following 50 keywords for the cloud of keywords: ‘nanotechnology,’ ‘lithium-ion batteries,’ ‘lithium,’ ‘electrodes,’ ‘electric batteries’ and lithium compounds. In contrast, Figure 10c illustrates the tree map structure of keywords as they appear in the publications of various authors.

a. Keyword co-occurrence network. b Keywords cloud of top 50 keywords. c. Tree map of keywords.
3.7. Three-field plot
The three field plots between author keywords, author countries and keywords plus were depicted in Figure 11 . The preceding 20-author keywords, utilized by most authors in their publications, are displayed on the left side of the plot. The author countries to which they belong are then indicated in the middle. The top 20 countries were analyzed, with China, the United States, Korea and India ranking the highest. The concluding segment of the narrative presents keywords plus, which includes the top 20 keywords and those used by the author. Concerning the keywords, this plot is structured to examine the research field and the authors’ collaborative network.

Three field plots between author keywords, author countries and keywords plus.
3.8. Bibliometric analysis challenges
Researchers may encounter several problems and restrictions when using R-Studio Biblioshiny for bibliometric analysis. One big problem is that bibliographic records are very different from one another. This is because different sources may use different formats and terminologies, which makes it hard to combine and standardize data. It might also be hard to ensure the bibliometric study’s accuracy if the data’s quality and completeness vary from database to database [ 67 ]. Another problem is that scientific literature is always changing; new papers are always being added, and databases might not be updated very often, which could mean the results are incomplete or outdated. Also, full-text articles may not always be available or easily accessible, which could affect the study’s completion. Researchers may also have trouble developing relevant search queries because the buzzwords and the criteria they use to include or leave out information can greatly affect the results [ 68 ]. Even with these problems, R-Studio Biblioshiny for bibliometric analysis is still a useful tool that helps researchers get around many of these issues and learn important things about the scholarly world.
Additionally, picking the right bibliometric indicators and measures presents different problems. To measure the effect, output and teamwork, researchers must be meticulous in picking the right factors. If you misinterpret metrics or only use a small group of indicators, you might come to the wrong conclusions about the importance of certain study topics or authors [ 69 ]. Also, because some fields are changing and combining different areas of study, it might be hard to correctly group papers into the right research domains. When doing bibliometric analysis, it is also very important to think about ethics, especially when there are disagreements about who wrote a paper or when someone cites themselves in a paper. To ensure the results are accurate, it is important to balance automated data extraction and human verification [ 70 ]. There may also be problems with how bibliometric analyses can be repeated since different researchers may get different results depending on how they read parameters or how the data sources are changed. Even with these problems and restrictions, R-Studio Biblioshiny has an easy-to-use design and many tools that can help with many of them. As software is constantly updated and made better, some problems may be solved. This makes bibliometric analysis a useful and ever-evolving way to understand the scholarly scene. As the scientific literature changes quickly, researchers should stay alert and deal with these problems to ensure their results are strong and correct [ 71 ].
Nanotechnology is currently leading the way in transforming numerous aspects of EV technology. Nanoscale materials, including carbon nanotubes and graphene, are important in battery technology because they improve electrode conductivity, surface area and energy storage. This leads to enhanced energy density, prolonged battery life and accelerated charging, effectively mitigating apprehensions regarding charging durations and range anxiety [ 72 ]. In addition to batteries, nanomaterials can generate lightweight and robust materials for EV construction. This is demonstrated through the integration of carbon nanotubes and nanofibers into composites. This phenomenon fortifies the structure and positively impacts the vehicle’s energy efficiency, range and overall performance [ 73 ]. Energy harvesting in EVs is further facilitated by nanotechnology, as nanostructured solar panels capture solar radiation, and nanogenerators convert mechanical energy into electrical power, thereby contributing to sustainable electricity generation [ 74 ]. Incorporating nanomaterials into supercapacitors and energy storage improves regenerative braking and power delivery, increasing energy storage and charge–discharge rates [ 75 ]. Nanosensors, renowned for their accuracy and sensitivity, provide advantages to EV control and monitoring systems by facilitating the observation of gas, pressure, temperature and concentrations for enhanced performance and safety.
Furthermore, progress in catalysis and fuel cell technologies is facilitated by nanotechnology, which has the potential to supplant conventional EV batteries and enhance the efficiency of energy conversion [ 76 ]. Intelligent coatings and self-healing materials, enabled by nanotechnology, safeguard EV surfaces against corrosion, weathering and abrasion by forming hydrophobic, UV-resistant and durable coatings that increase longevity. Fundamentally, nanotechnology manifests as a paradigm-shifting influence, initiating an era of enhanced EV performance, sustainability and innovation [ 77 ]. Figure 12 shows nanotechnology’s applications, challenges and future perspectives in EVs.

Nanotechnology for EVs.
4.1. Challenges of nanotechnology in EVs
Although nanotechnology can greatly enhance EVs, it presents considerable obstacles that must be thoughtfully assessed and resolved inventively. The significant challenge of scalability arises from the difficulties associated with mass-producing nanotechnology processes, which hinder the consistent and superior integration of nanomaterials into EV components. The complexities inherent in nanotechnology further complicate issues about scalability, thereby demanding progress in manufacturing methodologies to guarantee extensive implementation [ 78 , 79 ]. Nanotechnology materials and processes are more expensive to manufacture; thus, cost-effectiveness is essential. Nanomaterials in EV batteries and lightweight components may raise production costs, affecting EV affordability and consumer acceptability. Another major challenge is EV nanoparticle and nanostructure stability. Nanomaterials must be durable and reliable to maintain EV performance and safety, especially under harsh operational conditions [ 80 ].
These stability difficulties require continual research and development to improve nanomaterial robustness and compatibility with EV dynamics. In EV nanotechnology integration, environmental concerns are essential [ 81 ]. Nanomaterial manufacturing, use and disposal must meet environmental goals to address health and ecological problems throughout the life cycle of nanotechnology in EVs. Research and collaboration between the scientific and manufacturing sectors aim to overcome these limitations and advance nanotechnology’s use in EVs. Scalability, cost-effectiveness, long-term stability and environmental impact can be addressed to realize nanotechnology’s transformative promise in EVs, enabling sustainable, efficient and widely accessible electric mobility [ 82–84 ].
4.2. Commercial potential of nanotechnology in EV batteries
The discourse on the commercial viability of nanotechnology-enabled EV batteries involves a comprehensive examination of the promising breakthroughs and the obstacles and limitations that hinder their widespread use. Nanotechnology has become a robust and influential factor in energy storage, specifically EV batteries [ 85 ]. These technological developments are crucial for achieving higher performance metrics, including increased energy storage capacity, enhanced conductivity, efficient charge and discharge cycles and enhanced safety and stability. When considering the economic potential of nanotechnology in EV batteries, it is crucial to carefully examine the obstacles that could impede its smooth absorption into the market [ 86 ].
An essential component of this topic revolves around comprehending the market’s preparedness for EV batteries that incorporate nanotechnology. Although the potential advantages are significant, the market must be sufficiently prepared to adopt these technical advancements. Market preparation covers various elements, including establishing infrastructure for manufacturing and distribution, creating regulatory frameworks that can handle emerging technology and promoting consumer awareness and acceptance [ 87 ]. Evaluating the present level of preparedness offers valuable information regarding the timeframe and viability of implementing nanotechnology-enhanced EV batteries on a broader scope. Simultaneously, it is crucial to tackle the obstacles posed by incorporating nanotechnology in EV batteries. The issues encompass a wide spectrum, from intricate technological obstacles to financial constraints. Nanomaterials, including nanotubes, graphene and metal oxides, provide remarkable advancements at the molecular scale. However, their ability to be scaled up and their cost-effectiveness in industrial applications must be thoroughly evaluated. The complexities of production processes, potential environmental consequences and the requirement for specialized knowledge provide problems that require careful and thorough consideration [ 88 ].
Moreover, the conversation should include the possible obstacles to the adoption that could hinder the universal approval of nanotechnology-enabled EV batteries. Barriers can appear in different ways, such as economic, technological and societal issues. Economic factors encompass the aggregate expenses of production, prospective price increases for consumers and the economic viability for producers [ 89 ]. Technological impediments encompass challenges such as the requirement for standardized testing protocols, concerns regarding reliability and compatibility issues with current infrastructure. The success of nanotechnology-enabled EV batteries is heavily influenced by societal variables, including how the public perceives and accepts new technologies. The economic feasibility of EV batteries enhanced by nanotechnology also depends on effectively addressing safety issues and environmental consequences [ 90 ]. Although nanomaterials provide advancements in battery safety and stability, it is crucial to conduct a comprehensive assessment of the potential hazards related to the manufacturing and disposal of nanomaterials. Establishing or modifying regulatory frameworks is necessary to guarantee the secure and conscientious incorporation of nanotechnology into the electric car industry [ 91 ].
Strong competition in the EV sector complicates commercializing nanotechnology-enabled batteries. Given the ongoing advancements in traditional battery technologies and other energy storage solutions, it is crucial to consider the placement of nanotech-enabled batteries in this changing landscape [ 92 ]. An essential aspect of evaluating the commercial success of nanotechnology-enabled EV batteries is comprehending the competitive dynamics, developing distinctive selling factors and distinguishing them from existing technologies. Moreover, the influence of government regulations and incentives in defining the commercial path of nanotechnology-enabled EV batteries should not be underestimated [ 93 ]. Implementing supportive regulations, financial incentives and research funding can greatly expedite the implementation and acceptance of these advanced energy storage options. On the other hand, if there are no well-defined norms or obstacles in regulations, it could impede innovation and discourage investment in the emerging market of nanotechnology-enabled EV batteries [ 94 ].
4.3. Nanoscale breakthroughs boost battery performance
One of the most important factors in improving the overall performance of EV batteries is the adoption of nanoscale enhancements, which are advances on a smaller scale. The essential components of the battery, such as electrodes and catalysts, are subjected to transformations due to using materials such as nanotubes, graphene, metal oxides and nanoscale catalysts [ 95 ]. The nanoscale dimensions of these materials contribute to an increase in surface area, an improvement in conductivity and an enhancement in electrochemical characteristics. Due to these enhancements, the battery’s cycle life is extended, its charge and discharge rates are accelerated and its energy density is increased [ 96 ]. Furthermore, the improvements in nanotechnology also address safety concerns by reducing the dangers of overheating and limiting the creation of dendrites. As a result, the cumulative effect of these small-scale advancements dramatically raises the overall efficiency, reliability and sustainability of EV batteries, paving the way for a more optimistic future in electric transportation [ 86 ].
4.4. Future perspectives of nanotechnology
A comprehensive evaluation of the environmental consequences of incorporating nanomaterials and nanotechnology processes into EVs is imperative. This underscores the criticality of assessing health and environmental risks to ascertain nanotechnology’s feasibility and ecological sustainability in this domain. When considering the future, nanotechnology can fundamentally transform numerous facets of EV technology [ 97 ]. Nanomaterials possessing enhanced energy storage properties such as higher energy densities, faster charging capabilities and longer cycle lifetimes hold promise for substantially augmenting EV batteries’ range, efficiency and overall performance. Furthermore, continuous investigations into nanomaterials have the potential to yield sophisticated lightweight materials that integrate composites and nanoscale structures. Such materials would augment EV construction materials’ strength while decreasing their weight. Consequently, this could enhance maneuverability, range and energy efficiency [ 98 , 99 ].
Nanotechnology can create smart, functional surfaces with self-cleaning, anti-fogging and anti-icing qualities that improve EV visibility and efficiency while reducing maintenance. Nanosensors in EVs enable real-time battery health, temperature and performance monitoring, improving charging, safety and maintenance [ 100 ]. As research advances, sustainable nanomaterials that use abundant, non-toxic resources to make the EV sector more environmentally friendly become a priority. As EV nanomaterial recycling reduces resource depletion and waste throughout the lifecycle of EVs, it supports a circular economy. These advances demonstrate the continuous commitment to leverage nanotechnology’s transformative potential in EVs, balancing innovation with environmental responsibility for a sustainable electric mobility future [ 101 , 102 ].
Readers interested in delving more into nanotechnology in batteries will find that internet resources that clarify complicated topics are an excellent place to begin their exploration [ 103 ]. Websites like Nano.gov provide explanations of nanotechnology and its uses that are suitable for beginners. These explanations include the role that nanotechnology plays in battery technology. In addition, educational sites such as Khan Academy offer illuminating video lessons on nanoscience and its applications in the real world, which makes the process of learning more interesting and accessible [ 104 ]. Exploring articles on respected science websites such as Scientific American or National Geographic can provide in-depth yet understandable insights into how nanomaterials contribute to breakthroughs in battery efficiency. This is especially helpful for individuals who prefer a more hands-on approach [ 105 ]. The last point is that books such as Nanotechnology for Dummies by Earl Boysen and Nancy Boysen offer a straightforward guide to comprehending nanotechnology. This makes it an invaluable resource for readers anxious to dispel the mystery surrounding this fascinating field [ 106 ].
This study conducted a systematic literature analysis to analyze bibliometric data from various sources to gain insights into the changing research landscape of nanomaterials in EVs. The study primarily examined author affiliations, nation collaboration maps, citation patterns, keywords and the frequency of author keywords. The research publication selection process is depicted in Figure 2 , highlighting the meticulousness and thoroughness of the literature evaluation. Between 1991 and July 2023, the research yielded 2361 articles about EVs, nanotechnology, nanomaterials and sustainable development. By applying the exclusion criteria, the selection was narrowed down to 1540 articles that were deemed relevant, resulting in a strong dataset for bibliometric analysis. Prominent journals such as Energy and Environmental Science , Nano Energy , ACS Applied Materials and Interfaces and the Journal of Materials Chemistry emphasized the importance of nanoparticles in the context of EVs. The significance of bibliometric analysis in comprehending the impact, influence and interconnections among academic journals was underscored. Citation patterns were employed to assess the scientific value of articles, monitor research trends and evaluate collaboration networks. The study demonstrated the ever-changing nature of how scientific knowledge is spread and highlighted the importance of bibliometric research in guiding politicians, institutions and researchers when making decisions. Figure 3 depicts how the Scopus article research selections were reduced in the first step.
Section 4 included an in-depth examination of nanomaterials in EVs, including a study of publishing trends, country production, thematic evaluation of keywords, collaboration maps and field plots. Figure 4 depicts the yearly dissemination of publications from 1991 to 2023. It shows a notable increase in research efforts beginning in 2005, with an estimated annual growth rate of around 12.79%. The collaboration map depicted in Figure 5 prominently showcased the significant contributions made by prominent universities, with Nanyang Technological University and Beijing University taking the lead in research endeavors. Figure 6 provides an overview of the worldwide contributions to nanotechnology in EVs, with China, India and the United States as the primary countries leading in this field. The analysis of keywords ( Figure 7 ) revealed the consistent presence of terms such as self-powered sensors, batteries, triboelectric nanogenerator, nanotechnology, renewable energy, lithium-ion batteries, electrode material and electrochemical performance. These terms indicate the main themes of the research. Figures 8 and 9 depict the cooperative endeavors of several nations, with particular emphasis on China, India, the United States, South Korea, Germany, Japan, the United Kingdom, the Philippines, Singapore, France, Australia, Canada, Italy, Spain and Malaysia, which are recognized as significant contributors in this sector. Figures 10 and 11 visually depict keyword analysis, revealing the most common study topics and the network of writers who collaborated. A list of the 50 most significant keywords was compiled, which included terms such as ‘nanotechnology,’ ‘lithium,’ ‘electrodes,’ ‘electric batteries,’ ‘nanostructured materials,’ and ‘energy efficiency.’ The treemap structure ( Figure 10c ) displayed the distribution of keywords in publications by different authors, providing a detailed comprehension of the research landscape. To summarize, this SLR thoroughly examines nanomaterials in EVs, utilizing bibliometric methodologies to uncover patterns in research, collaborations and important theme areas. The results emphasize the increasing significance of nanotechnology in the progression of EV technologies. Research gaps may exist in comprehending the precise applications of nanomaterials, tackling issues related to scalability and cost-efficiency, and investigating potential environmental consequences. Potential areas for future research could prioritize interdisciplinary investigations, technological advancements and sustainable methodologies to facilitate the incorporation of nanomaterials into EV technologies. The findings obtained from this work provide valuable contributions to the wider discussion on the convergence of nanotechnology and electric cars, offering guidance for future research efforts in this rapidly changing and developing subject.
In conclusion, the bibliometric analysis conducted on nanotechnology in EVs provides valuable insights into this field’s research landscape and trends. The study highlights the growing interest and research output dedicated to nanotechnology’s applications in electric cars, showcasing its significance and potential impact. By examining publication trends, influential articles, collaborating institutions and key contributors, the analysis helps identify the key areas of research focus and the leading contributors in the field. This information can guide future research directions, collaboration opportunities and resource allocation. The analysis also highlights the challenges and opportunities associated with nanotechnology in EVs. It reveals the need to address scalability, cost-effectiveness, long-term stability and environmental impact to realize the potential of nanotechnology in this domain fully.
Moreover, the bibliometric analysis is a foundation for further exploration and understanding of nanotechnology in EVs. It provides a basis for researchers, policymakers and industry stakeholders to assess the progress made, identify knowledge gaps and develop strategies to accelerate the development and adoption of nanotechnology in EVs. Overall, the bibliometric analysis of nanotechnology in EVs is a valuable tool that enhances our understanding of the research landscape, highlights emerging trends and offers insights into the future perspectives of this exciting field. It paves the way for further research and collaboration, ultimately contributing to advancing and implementing nanotechnology in EVs for a greener and more sustainable transportation future.
The authors declare that there is no conflict of interest in this work.
The authors extend their appreciation to the Deanship of Scientific Research at King Khalid University, Saudi Arabia, for funding this work through the Research Group Program under grant no. RGP 2/88/44.
Pulkit Kumar: Conceptualization, Methodology, Software, Writing – Original Draft, Data Curation, Writing – Review & Editing; Harpreet Kaur Channi: Conceptualization, Methodology, Supervision, Writing – Review & Editing, Formal analysis; Atul Babbar: Investigation, Supervision, Resources, Formal analysis; Raman Kumar: Investigation, Supervision, Conceptualization, Methodology, Formal analysis, Writing – Review & Editing; Javed Khan Bhutto: Formal analysis, Investigation, Supervision T.M. Yunus Khan: Investigation, Visualization; Abhijit Bhowmik: Formal analysis, Investigation, Supervision; Abdul Razak: Formal analysis, Investigation, Supervision; Anteneh Wogasso Wodajo: Formal analysis, Visualization, Supervision
Thomas J , Patil RS , John J . et al. A comprehensive outlook of scope within exterior automotive plastic substrates and its coatings . Coatings (Basel) 2023 ; 13 :1569. https://doi.org/10.3390/coatings13091569 .
Google Scholar
Channi HK , Kumar R . The role of smart sensors in smart city . Smart Sensor Networks: Analytics, Sharing and Control 2022 ; 92 : 27 – 48 . https://doi.org/10.1007/978-3-030-77214-7_2 .
Parameswaran AK . et al. Recent progress of nanotechnology in the research framework of all-solid-state batteries . Nano Energy 2023 ; 105 :107994. https://doi.org/10.1016/j.nanoen.2022.107994 .
Kaur H , Kumar R , Kumar P . et al. 2024 . Efficient and cost-effective renewable energy integration of photovoltaic and hydro in rural India using homer pro: a case study of Chupki, Punjab. In Talpa Sai PHVS, Potnuru S, Avcar M, Ranjan Kar V (eds). Intelligent Manufacturing and Energy Sustainability: Proceedings of ICIMES 2023 . Springer . 281 – 91 .
Google Preview
Saraogi AK , Ibrahim M , Sangeethkumar E . et al. Battery materials for electric vehicle—a comprehensive review . Mater Today: Proc 2023 ; 72 : 2206 – 11 .
Naik N , Suresh P , Yadav S . et al. A review on composite materials for energy harvesting in electric vehicles . Energies 2023 ; 16 : 3348 . https://doi.org/10.3390/en16083348 .
Haghani M , Sprei F , Kazemzadeh K . et al. Trends in electric vehicles research . Transp Res Part D: Transp Environ 2023 ; 123 :103881. https://doi.org/10.1016/j.trd.2023.103881 .
Khan FNU , Rasul MG , Sayem A . et al. Design and optimization of lithium-ion battery as an efficient energy storage device for electric vehicles: a comprehensive review . J Energy Storage 2023 ; 71 :108033. https://doi.org/10.1016/j.est.2023.108033 .
Saha RK , Kumar R , Dev N . et al. Structural modeling and analysis of fuel cell: a graph-theoretic approach . Comput Sci 2023 ; 9 :e1510. https://doi.org/10.7717/peerj-cs.1510 .
Hameed MM , Mansor MB , Azau MAM . et al. Computational design and analysis of LiFePO4 battery thermal management system (BTMS) using thermoelectric cooling/thermoelectric generator (TEC–TEG) in electric vehicles (EVs) . J Energy Storage 2023 ; 72 :108394. https://doi.org/10.1016/j.est.2023.108394 .
Malik S , Muhammad K , Waheed Y . Nanotechnology: a revolution in modern industry . Molecules 2023 ; 28 : 661 . https://doi.org/10.3390/molecules28020661 .
Wali S . et al. Grid-connected lithium-ion battery energy storage system towards sustainable energy: a patent landscape analysis and technology updates . J Energy Storage 2024 ; 77 :109986. https://doi.org/10.1016/j.est.2023.109986 .
Shahzad K , Cheema II . Low-carbon technologies in automotive industry and decarbonizing transport . J Power Sources 2024 ; 591 :233888. https://doi.org/10.1016/j.jpowsour.2023.233888 .
Ahmad A , Prakash O , Sarangi SK . et al. Thermal and CFD analyses of sustainable heat storage-based passive greenhouse dryer operating in no-load condition . Sustain For 2023 ; 15 : 12067 . https://doi.org/10.3390/su151512067 .
Sharma YK , Kumar Y , Sharma S . et al. Nanotechnologies in the renewable energy sector . Renewable Energy Innovations: Biofuels, Solar, and Other Technologies 2024 ; 41 – 82 . https://doi.org/10.1002/9781119785712.ch2 .
Shu X . et al. Sustainability assessment of energy storage technologies based on commercialization viability: MCDM model . Sustain For 2023 ; 15 : 4707 . [Online]. Available: https://www.mdpi.com/2071–1050/15/6/4707 .
Nazir S , Zhang JM , Junaid M . et al. Metal-based nanoparticles: basics, types, fabrications and their electronic applications . Z Phys Chem 2024 ; 0 . https://doi.org/10.1515/zpch-2023-0375 .
Alemu MA , Worku AK , Getie MZ . Recent advances in electrically rechargeable transition metal-based-air batteries for electric mobility . Inorg Chem Commun 2024 ; 159 :111742. https://doi.org/10.1016/j.inoche.2023.111742 .
Cao J , Ji Y , Shao Z . Nanotechnologies in ceramic electrochemical cells . Chem Soc Rev 2024 ; 53 : 450 – 501 . https://doi.org/10.1039/D3CS00303E .
Aryanfar A , Elias F , Goddard WA . Enhancing the thermal dissipation in batteries via inclusion of central heat sink . J Electrochem Energy Convers Storage 2024 ; 21 . https://doi.org/10.1115/1.4062712 .
Mishra S , Mishra AK . 2024 . Supercapacitors: carbon-based nanostructures for supercapacitor application. In Sonkar P, Ganesan V (eds). Nanomaterials for Sustainable Energy Applications . CRC Press . 77 – 100 .
Zhao Y , Geng C , Wang L . et al. Design and modification of metal sulfide-based catalysts for lithium-sulfur batteries . Particuology 2024 ; 86 : 86 – 100 . https://doi.org/10.1016/j.partic.2023.04.010 .
Sanguesa JA , Torres-Sanz V , Garrido P . et al. A review on electric vehicles: technologies and challenges . Smart Cities 2021 ; 4 : 372 – 404 . [Online]. Available: https://doi.org/10.3390/smartcities4010022 . Accessed on (15 November, 2023) . https://www.mdpi.com/2624–6511/4/1/22 .
Ali MU , Zafar A , Nengroo SH . et al. Towards a smarter battery management system for electric vehicle applications: a critical review of lithium-ion battery state of charge estimation . Energies 2019 ; 12 : 446 . https://doi.org/10.3390/en12030446 .
Han D , Hosamo H , Ying C . et al. A comprehensive review and analysis of nanosensors for structural health monitoring in bridge maintenance: innovations, challenges, and future perspectives . Appl Sci 2023 ; 13 : 11149 . [Online]. Available: . https://www.mdpi.com/2076–3417/13/20/11149 . Accessed on (20 November, 2023) .
Basu AK , Tatiya S , Bhattacharya S . Overview of electric vehicles (EVs) and EV sensors . Sensors for Automotive and Aerospace Applications 2019 ; 107 – 22 . https://doi.org/10.1007/978-981-13-3290-6_7 .
Daramy-Williams E , Anable J , Grant-Muller S . A systematic review of the evidence on plug-in electric vehicle user experience . Transp Res Part D: Transp Environ 2019 ; 71 : 22 – 36 . https://doi.org/10.1016/j.trd.2019.01.008 .
Das UK . et al. Advancement of lithium-ion battery cells voltage equalization techniques: a review . Renew Sustain Energy Rev 2020 ; 134 :110227. https://doi.org/10.1016/j.rser.2020.110227 .
Torreglosa JP , Garcia-Triviño P , Vera D . et al. Analyzing the improvements of energy management systems for hybrid electric vehicles using a systematic literature review: how far are these controls from rule-based controls used in commercial vehicles? Appl Sci 2020 ; 10 : 8744 . https://doi.org/10.3390/app10238744 .
Castillo O , Álvarez R , Domingo R . Opportunities and barriers of hydrogen–electric hybrid powertrain vans: a systematic literature review . Processes 2020 ; 8 : 1261 . https://doi.org/10.3390/pr8101261 .
Tete PR , Gupta MM , Joshi SS . Developments in battery thermal management systems for electric vehicles: a technical review . J Energy Storage 2021 ; 35 :102255. https://doi.org/10.1016/j.est.2021.102255 .
Suryatna A , Raya I , Thangavelu L . et al. A review of high-energy density lithium-air battery technology: investigating the effect of oxides and nanocatalysts . J Chem 2022 ; 2022 : 1 – 32 . https://doi.org/10.1155/2022/2762647 .
Ye C , He W , Chen H . Electric vehicle routing models and solution algorithms in logistics distribution: a systematic review . Environ Sci Pollut Res 2022 ; 29 : 57067 – 90 . https://doi.org/10.1007/s11356-022-21559-2 .
Raugei M . Update on the life-cycle GHG emissions of passenger vehicles: literature review and harmonization . Energies 2022 ; 15 : 7163 . https://doi.org/10.3390/en15197163 .
Lai X , Yao J , Jin C . et al. A review of lithium-ion battery failure hazards: test standards, accident analysis, and safety suggestions . Batteries 2022 ; 8 : 248 . https://doi.org/10.3390/batteries8110248 .
Cai S , Kirtley JL , Lee CH . Critical review of direct-drive electrical machine systems for electric and hybrid electric vehicles . IEEE Trans Energy Convers 2022 ; 37 : 2657 – 68 . https://doi.org/10.1109/TEC.2022.3197351 .
Pardhi S , Chakraborty S , Tran D-D . et al. A review of fuel cell powertrains for long-haul heavy-duty vehicles: technology, hydrogen, energy and thermal management solutions . Energies 2022 ; 15 : 9557 . https://doi.org/10.3390/en15249557 .
Sharma P , Bora BJ . A review of modern machine learning techniques in the prediction of remaining useful life of lithium-ion batteries . Batteries 2023 ; 9 : 13 . https://doi.org/10.3390/batteries9010013 .
Senol M , Bayram IS , Naderi Y . et al. Electric vehicles under low temperatures: a review on battery performance, charging needs, and power grid impacts . IEEE Access 2023 ; 11 : 39879 – 912 . https://doi.org/10.1109/ACCESS.2023.3268615 .
Kiran MD , B R LY , Babbar A . et al. Tribological properties of CNT-filled epoxy-carbon fabric composites: optimization and modelling by machine learning . J Mater Res Technol 2024 ; 28 : 2582 – 601 . https://doi.org/10.1016/j.jmrt.2023.12.175 .
He R , Xie W , Wu B . et al. Towards interactional management for power batteries of electric vehicles . RSC Adv 2023 ; 13 : 2036 – 56 . https://doi.org/10.1039/D2RA06004C .
Quilty CD , Wu D , Li W . et al. Electron and ion transport in lithium and lithium-ion battery negative and positive composite electrodes . Chem Rev 2023 ; 123 : 1327 – 63 . https://doi.org/10.1021/acs.chemrev.2c00214 .
Gevorkov L , Domínguez-García JL , Romero LT . et al. Modern MultiPort converter technologies: a systematic review . Appl Sci 2023 ; 13 : 2579 . https://doi.org/10.3390/app13042579 .
Zhang X , Tang Y , Zhang F . et al. A novel aluminum–graphite dual-ion battery . Adv Energy Mater 2016 ; 6 : 1502588 . https://doi.org/10.1002/aenm.201502588 .
Wang M , Jiang C , Zhang S . et al. Reversible calcium alloying enables a practical room-temperature rechargeable calcium-ion battery with a high discharge voltage . Nat Chem 2018 ; 10 : 667 – 72 . https://doi.org/10.1038/s41557-018-0045-4 .
Mu S , Liu Q , Kidkhunthod P . et al. Molecular grafting towards high-fraction active nanodots implanted in N-doped carbon for sodium dual-ion batteries . Natl Sci Rev 2020 ; 8 : nwaa178 . https://doi.org/10.1093/nsr/nwaa178 .
Zhang X , Gong L , Zhao X . et al. Voltage and frequency stabilization control strategy of virtual synchronous generator based on small signal model . Energy Rep 2023 ; 9 : 583 – 90 . https://doi.org/10.1016/j.egyr.2023.03.071 .
Lu L , Wu W , Gao Y . et al. Study on current discrepancy and redistribution of HTS non-insulation closed-loop coils during charging/discharging and subsequent transient process toward steady-state operation . Supercond Sci Technol 2022 ; 35 :095001. https://doi.org/10.1088/1361-6668/ac7dfe .
Wu Y , Wu H , Zhao Y . et al. Metastable structures with composition fluctuation in cuprate superconducting films grown by transient liquid-phase assisted ultra-fast heteroepitaxy . Mater Today Nano 2023 ; 24 :100429. https://doi.org/10.1016/j.mtnano.2023.100429 .
Shen Y , Xie J , He T . et al. CEEMD-fuzzy control energy management of hybrid energy storage systems in electric vehicles . IEEE Trans Energy Convers 2024 ; 39 : 555 – 66 . https://doi.org/10.1109/TEC.2023.3306804 .
Zhao J , Song D , Zhu B . et al. A human-like trajectory planning method on a curve based on the driver preview mechanism . IEEE Trans Intell Transp Syst 2023 ; 24 : 11682 – 98 . https://doi.org/10.1109/TITS.2023.3285430 .
Zhu B , Sun Y , Zhao J . et al. A critical scenario search method for intelligent vehicle testing based on the social cognitive optimization algorithm . IEEE Trans Intell Transp Syst 2023 ; 24 : 7974 – 86 . https://doi.org/10.1109/TITS.2023.3268324 .
Wei T , Zhou Y , Sun C . et al. An intermittent lithium deposition model based on CuMn-bimetallic MOF derivatives for composite lithium anode with ultrahigh areal capacity and current densities . Nano Res 2023 ; 1 – 7 . https://doi.org/10.1007/s12274-023-6187-8 .
Yue W , Li C , Wang S . et al. Cooperative incident management in mixed traffic of CAVs and human-driven vehicles . IEEE Trans Intell Transp Syst 2023 ; 24 : 12462 – 76 . https://doi.org/10.1109/TITS.2023.3289983 .
Lu Y , Tan C , Ge W . et al. Adaptive disturbance observer-based improved super-twisting sliding mode control for electromagnetic direct-drive pump . Smart Mater Struct 2022 ; 32 :017001.
Yu H , Chen D , Ni X . et al. Reversible adsorption with oriented arrangement of a zwitterionic additive stabilizes electrodes for ultralong-life Zn-ion batteries . Energ Environ Sci 2023 ; 16 : 2684 – 95 . https://doi.org/10.1039/D3EE00982C .
Hou X , Xin L , Fu Y . et al. A self-powered biomimetic mouse whisker sensor (BMWS) aiming at terrestrial and space objects perception . Nano Energy 2023 ; 118 :109034. https://doi.org/10.1016/j.nanoen.2023.109034 .
Yan Z , Hu Q , Jiang F . et al. Mechanism and technology evaluation of a novel alternating-arc-based directed energy deposition method through polarity-switching self-adaptive shunt . Addit Manuf 2023 ; 67 :103504. https://doi.org/10.1016/j.addma.2023.103504 .
Wang Z , Li J , Hu C . et al. Hybrid energy storage system and management strategy for motor drive with high torque overload . J Energy Storage 2024 ; 75 :109432. https://doi.org/doi:10.1016/j.est.2023.109432 .
Sidhu AS , Singh S , Kumar R . Bibliometric analysis of entropy weights method for multi-objective optimization in machining operations . Mater Today: Proc 2022 ; 50 : 1248 – 55 . https://doi.org/10.1016/j.matpr.2021.08.132 .
Kumar R , Rani S , Awadh MA . Exploring the application sphere of the internet of things in industry 4.0: a review, bibliometric and content analysis . Sensor 2022 ; 22 : 4276 . https://doi.org/10.3390/s22114276 .
Kumar R , Goel P . Exploring the domain of interpretive structural modelling (ISM) for sustainable future panorama: a bibliometric and content analysis . Arch Comput Methods Eng 2022 ; 29 : 2781 – 810 . https://doi.org/10.1007/s11831-021-09675-7 .
Kaur S , Kumar R , Kaur R . et al. Piezoelectric materials in sensors: bibliometric and visualization analysis . Mater Today: Proc 2022 ; 65 : 3780 – 6 . https://doi.org/10.1016/j.matpr.2022.06.484 .
A. S. Sidhu , S. Singh , and R. Kumar , "Bibliometric analysis of entropy weights method for multi-objective optimization in machining operations," in 2nd International Conference on Functional Materials, Manufacturing and Performances, ICFMMP 2021 , Volume 50, Part 5 , 2021 , Elsevier Ltd , pp. 1248 – 1255 , [Online]. Available: https://www.scopus.com/inward/record.uri?eid=2-s2.0-85124946918&doi=10.1016%2fj.matpr.2021.08.132&partnerID=40&md5=06e988ed2dd8421efbcc139f893d1dcb . Accessed on (20 November, 2023) .
Kumar R , Singh S , Sidhu AS . et al. Bibliometric analysis of specific energy consumption (SEC) in machining operations: a sustainable response . Sustain For 2021 ; 13 :5617. [Online]. Available: https://doi.org/10.3390/su13105617 . https://www.mdpi.com/2071-1050/13/10/5617 .
Rani S , Kumar R . Bibliometric review of actuators: key automation technology in a smart city framework . Mater Today: Proc 2022 ; 60 : 1800 – 7 . https://doi.org/10.1016/j.matpr.2021.12.469 .
Paganin L , Borsato M . A critical review of design for reliability-a bibliometric analysis and identification of research opportunities . Procedia Manuf 2017 ; 11 : 1421 – 8 . https://doi.org/10.1016/j.promfg.2017.07.272 .
Donthu N , Kumar S , Mukherjee D . et al. How to conduct a bibliometric analysis: an overview and guidelines . J Bus Res 2021 ; 133 : 285 – 96 . https://doi.org/doi:10.1016/j.jbusres.2021.04.070 .
Agbodjan YS , Wang J , Cui Y . et al. Bibliometric analysis of zero energy building research, challenges and solutions . Sol Energy 2022 ; 244 : 414 – 33 . https://doi.org/10.1016/j.solener.2022.08.061 .
Xiong H , Wang Y , Guo X . et al. Current status and future challenges of groundwater vulnerability assessment: a bibliometric analysis . J Hydrol 2022 ; 615 :128694. https://doi.org/10.1016/j.jhydrol.2022.128694 .
Akhtar MN , Haleem A , Javaid M . Exploring the advent of Medical 4.0: a bibliometric analysis systematic review and technology adoption insights . Informatics Health 2024 ; 1 : 16 – 28 . https://doi.org/10.1016/j.infoh.2023.10.001 .
Wong K , Dia S . Nanotechnology in batteries . J Energy Resour Technol 2017 ; 139 :014001. https://doi.org/10.1115/1.4034860 .
Yang B , Ji J , Zhang X . et al. Passive cooling of lithium-ion batteries based on flexible phase change materials: molecular structure, interactions and mechanistic aspects . J Mol Liq 2023 ; 391 :123340. https://doi.org/10.1016/j.molliq.2023.123340 .
Kumar M , Farwaha HS , Kumar R . et al. Thermal performance evaluation of solar collector with rice husk graphene-PCM: bioengineering approach . Case Stud Therm Eng 2023 ; 52 :103773. https://doi.org/10.1016/j.csite.2023.103773 .
Chen Y , Fan Z , Liang L . et al. Nanotechnology in modern practical electric vehicles . Highl Sci Eng Technol 2023 ; 46 : 1 – 7 . https://doi.org/10.54097/hset.v46i.7656 .
Mahardhika SP , Putriani O . 2023 . A review of artificial intelligence-enabled electric vehicles in traffic congestion management. In ICSEDTI 2022: Proceedings of the 1st International Conference on Sustainable Engineering Development and Technological Innovation, ICSEDTI 2022, 11–13 October 2022 . Tanjungpinang, Indonesia : European Alliance for Innovation . 255 .
Sun L . Nanotechnology in the field of electric vehicles . Highl Sci Eng Technol 2023 ; 43 : 327 – 32 . https://doi.org/10.54097/hset.v43i.7436 .
Wang Y . Application of advanced nanotechnology in electric vehicles . Highl Sci Eng Technol 2023 ; 46 : 8 – 13 . https://doi.org/10.54097/hset.v46i.7657 .
Shukla S , Khan R , Saxena A . et al. Future of modern society: sustainability in green nanotechnology . Nanoremediation 2023 ; 393 – 410 . https://doi.org/10.1016/B978-0-12-823874-5.00001-2 .
Kurapati SK , Mahendar Reddy N , Sujithra R . et al. Nanomaterials and nanostructures in additive manufacturing: properties, applications, and technological challenges . Nanotechnology-Based Additive Manufacturing: Product Design, Properties and Applications 2023 ; 1 : 53 – 102 . https://doi.org/10.1002/9783527835478.ch3 .
Madheswaran DK , Vengatesan S , Varuvel EG . et al. Nanofluids as a coolant for polymer electrolyte membrane fuel cells: recent trends, challenges, and future perspectives . J Clean Prod 2023 ; 424 :138763. https://doi.org/10.1016/j.jclepro.2023.138763 .
Zero N . Nano-technology to achieve net zero goals . MBMST 2023 2023 ; 392 : 32 .
Bosu S , Rajamohan N . Recent advancements in hydrogen storage-comparative review on methods, operating conditions and challenges . Int J Hydrogen Energy 2024 ; 52 : 352 – 70 . https://doi.org/10.1016/j.ijhydene.2023.01.344 .
Shilkov VI , Anikin YV , Grishchenko YO . 2023 . Promising innovations for green economy and cleaner production in agriculture. In Trukhachev VI (ed). Unlocking Digital Transformation of Agricultural Enterprises: Technology Advances, Digital Ecosystems, and Innovative Firm Governance . Springer . 177 – 87 .
Soukupová G , Jindra M , Lapka T . et al. Novel silicon nanoparticles-based carbonized polypyrrole nanotube composites as anode materials for Li-ion batteries . J Power Sources 2024 ; 593 :233976. https://doi.org/10.1016/j.jpowsour.2023.233976 .
Poorshakoor E , Darab M . Advancements in the development of nanomaterials for lithium-ion batteries: a scientometric review . J Energy Storage 2024 ; 75 :109638. https://doi.org/10.1016/j.est.2023.109638 .
Hussain W , Sulaman M , Sandali Y . et al. An extensive exploration of non-conventional sources for preparing NiS and its potential applications in battery and photodegradation processes . Mater Sci Eng B 2024 ; 299 :116918. https://doi.org/10.1016/j.mseb.2023.116918 .
Gao S-L , Qin Z-X , Wang B-F . et al. Lithium recovery from the spent lithium-ion batteries by commercial acid-resistant nanofiltration membranes: a comparative study . Desalination 2024 ; 572 :117142. https://doi.org/10.1016/j.desal.2023.117142 .
Thomas F , Mahdi L , Lemaire J . et al. Technological advances and market developments of solid-state batteries: a review . Materials 2024 ; 17 : 239 . https://doi.org/10.3390/ma17010239 .
Schiavi PG , Marrani AG , Russina O . et al. Aqueous electrochemical delithiation of cathode materials as a strategy to selectively recover lithium from waste lithium-ion batteries . J Energy Chem 2024 ; 88 : 144 – 53 . https://doi.org/10.1016/j.jechem.2023.09.040 .
Kharabati S , Saedodin S . A systematic review of thermal management techniques for electric vehicle batteries . J Energy Storage 2024 ; 75 :109586. https://doi.org/10.1016/j.est.2023.109586 .
Divya N , Saju M , Roshna A . et al. 2024 . Smart nanomaterials in energy applications. In Tailored Functional Materials for Clean and Sustainable Development . Apple Academic Press . 263 – 80 .
Karjalainen P , Leinonen V , Olin M . et al. Real-world emissions of nanoparticles, particulate mass and black carbon from a plug-in hybrid vehicle compared to conventional gasoline vehicles . Environ Adv 2024 ; 15 :100454. https://doi.org/10.1016/j.envadv.2023.100454 .
Munonde TS , Raphulu MC . Review on titanium dioxide nanostructured electrode materials for high-performance lithium batteries . J Energy Storage 2024 ; 78 :110064. https://doi.org/10.1016/j.est.2023.110064 .
Lim JM , Jang YS , van T. Nguyen H . et al. Advances in high-voltage supercapacitors for energy storage systems: materials and electrolyte tailoring to implementation . Nanoscale Adv 2023 ; 5 : 615 – 26 . https://doi.org/10.1039/D2NA00863G .
Shi R , Shen Z , Yue Q . et al. Advances in functional organic material-based interfacial engineering on metal anodes for rechargeable secondary batteries . Nanoscale 2023 ; 15 : 9256 – 89 . https://doi.org/10.1039/D3NR01306E .
Edoziuno FO , Adediran AA , Nwaeju CC . et al. 2023 . Nanomaterials. In Verma C, Srivastava V, ... Ebenso EE (eds). Smart Anticorrosive Materials . Elsevier . 19 – 30 . https://doi.org/10.1016/B978-0-323-95158-6.00023-0 .
Huang Z , Li Y , Xu Y . Diverse nanotechnology applications for electric vehicles . Highl Sci Eng Technol 2023 ; 43 : 438 – 49 . https://doi.org/10.54097/hset.v43i.7462 .
Aravindan M . et al. Fuelling the future: a review of non-renewable hydrogen production and storage techniques . Renew Sustain Energy Rev 2023 ; 188 :113791.
Raj KS , Baskaran N , Nair PP . et al. Current scenario and future perspective of food waste into Li-ion based batteries—a critical review . J Hazard Mater Adv 2023 ; 10 :100317. https://doi.org/10.1016/j.hazadv.2023.100317 .
Yang Z . Nanomaterials as well their applications and effects in batteries . Highl Sci Eng Technol 2023 ; 32 : 73 – 82 . https://doi.org/10.54097/hset.v32i.4944 .
Mahdavi Dehkharghani F , Ghahremanlou M , Zandi Z . et al. Future energy and therapeutic perspectives of green nano-technology: recent advances and challenges . Nano Micro Biosyst 2023 ; 2 : 11 – 21 .
Shah SSA , Zafar HK , Javed MS . et al. Mxenes for Zn-based energy storage devices: nano-engineering and machine learning . Coord Chem Rev 2024 ; 501 :215565. https://doi.org/10.1016/j.ccr.2023.215565 .
Li Z , Zhang Y , Zhang S . et al. Phase change materials for lithium-ion battery thermal management systems: a review . J Energy Storage 2024 ; 80 :110259. https://doi.org/10.1016/j.est.2023.110259 .
Karthikeyan B , Velvizhi G . A state-of-the-art on the application of nanotechnology for enhanced biohydrogen production . Int J Hydrogen Energy 2024 ; 52 : 536 – 54 . https://doi.org/10.1016/j.ijhydene.2023.04.237 .
Xie H , Ahmad T , Zhang D . et al. Community-based virtual power plants’ technology and circular economy models in the energy sector: a techno-economy study . Renew Sustain Energy Rev 2024 ; 192 :114189. https://doi.org/10.1016/j.rser.2023.114189 .
Email alerts
Citing articles via, affiliations.
- Online ISSN 1748-1325
- Print ISSN 1748-1317
- Copyright © 2024 Oxford University Press
- About Oxford Academic
- Publish journals with us
- University press partners
- What we publish
- New features
- Open access
- Institutional account management
- Rights and permissions
- Get help with access
- Accessibility
- Advertising
- Media enquiries
- Oxford University Press
- Oxford Languages
- University of Oxford
Oxford University Press is a department of the University of Oxford. It furthers the University's objective of excellence in research, scholarship, and education by publishing worldwide
- Copyright © 2024 Oxford University Press
- Cookie settings
- Cookie policy
- Privacy policy
- Legal notice
This Feature Is Available To Subscribers Only
Sign In or Create an Account
This PDF is available to Subscribers Only
For full access to this pdf, sign in to an existing account, or purchase an annual subscription.
Thank you for visiting nature.com. You are using a browser version with limited support for CSS. To obtain the best experience, we recommend you use a more up to date browser (or turn off compatibility mode in Internet Explorer). In the meantime, to ensure continued support, we are displaying the site without styles and JavaScript.
- View all journals
- Explore content
- About the journal
- Publish with us
- Sign up for alerts
- Review Article
- Published: 06 December 2016
The role of nanotechnology in the development of battery materials for electric vehicles
- Zonghai Chen 1 ,
- Zifeng Ma 2 ,
- Feng Pan 3 ,
- Larry A. Curtiss 4 &
- Khalil Amine 1
Nature Nanotechnology volume 11 , pages 1031–1038 ( 2016 ) Cite this article
15k Accesses
528 Citations
29 Altmetric
Metrics details
- Nanoparticles
- Nanostructures
A Corrigendum to this article was published on 10 January 2017
This article has been updated
A significant amount of battery research and development is underway, both in academia and industry, to meet the demand for electric vehicle applications. When it comes to designing and fabricating electrode materials, nanotechnology-based approaches have demonstrated numerous benefits for improved energy and power density, cyclability and safety. In this Review, we offer an overview of nanostructured materials that are either already commercialized or close to commercialization for hybrid electric vehicle applications, as well as those under development with the potential to meet the requirements for long-range electric vehicles.
This is a preview of subscription content, access via your institution
Access options
Subscribe to this journal
Receive 12 print issues and online access
251,40 € per year
only 20,95 € per issue
Rent or buy this article
Prices vary by article type
Prices may be subject to local taxes which are calculated during checkout
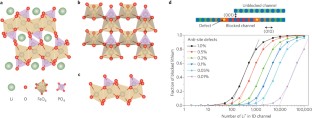
Similar content being viewed by others
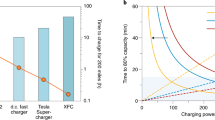
Challenges and opportunities towards fast-charging battery materials
Yayuan Liu, Yangying Zhu & Yi Cui
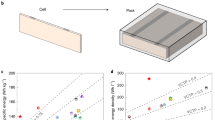
Thermally modulated lithium iron phosphate batteries for mass-market electric vehicles
Xiao-Guang Yang, Teng Liu & Chao-Yang Wang
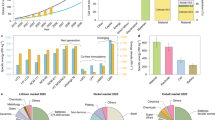
High-nickel layered oxide cathodes for lithium-based automotive batteries
Wangda Li, Evan M. Erickson & Arumugam Manthiram
Change history
14 december 2016.
In the original version of this Review Article Feng Pan's affiliation should have read: 'School of Advanced Materials, Peking University, Shenzhen Graduate School, Shenzhen 518055, PR China'. This has been updated in the online versions of the Review.
Dunn, B., Kamath, H. & Tarascon, J. M. Electrical energy storage for the grid: a battery of choices. Science 334 , 928–935 (2011).
Article CAS Google Scholar
Etacheri, V., Marom, R., Elazari, R., Salitra, G. & Aurbach, D. Challenges in the development of advanced Li-ion batteries: a review. Energy. Environ. Sci. 4 , 3243–3262 (2011).
CAS Google Scholar
Goodenough, J. B. & Park, K.-S. The Li-ion rechargeable battery: a perspective. J. Am. Chem. Soc. 135 , 1167–1176 (2013).
Bruce, P. G., Freunberger, S. A., Hardwick, L. J. & Tarascon, J.-M. Li–O2 and Li–S batteries with high energy storage. Nat. Mater. 11 , 19–29 (2012).
Lu, J. et al. Aprotic and aqueous Li–O2 batteries. Chem. Rev. 114 , 5611–5640 (2014).
Chen, Z., Lu, Z. & Dahn, J. R. Staging phase transitions in Li x CoO2 . J. Electrochem. Soc. 149 , A1604–A1609 (2002).
Padhi, A. K., Nanjundaswamy, K. S. & Goodenough, J. B. Phospho-olivines as positive-electrode materials for rechargeable lithium batteries. J. Electrochem. Soc. 144 , 1188–1194 (1997).
Chung, S. Y., Bloking, J. T. & Chiang, Y. M. Electronically conductive phospho-olivines as lithium storage electrodes. Nat. Mater. 1 , 123–128 (2002).
Malik, R., Zhou, F. & Ceder, G. Kinetics of non-equilibrium lithium incorporation in LiFePO4 . Nat. Mater. 10 , 587–590 (2011).
Zhang, K. et al. Conformal coating strategy comprising N-doped carbon and conventional graphene for achieving ultrahigh power and cyclability of LiFePO4 . Nano Lett. 15 , 6756–6763 (2015).
Lepage, D., Michot, C., Liang, G., Gauthier, M. & Schougaard, S. B. A soft chemistry approach to coating of LiFePO4 with a conducting polymer. Angew. Chem. Int. Ed. 50 , 6884–6887 (2011).
Hu, C. et al. Suppressing Li3PO4 impurity formation in LiFePO4/Fe2P by a nonstoichiometry synthesis and its effect on electrochemical properties. Mater. Lett. 65 , 1323–1326 (2011).
Herle, P. S., Ellis, B., Coombs, N. & Nazar, L. F. Nano-network electronic conduction in iron and nickel olivine phosphates. Nat. Mater. 3 , 147–152 (2004).
Zhang, P. X. et al. First-principles study on the electronic structure of a LiFePO4 (010) surface adsorbed with carbon. J. Alloys Compd. 540 , 121–126 (2012).
Nishimura, S.-i. et al. Experimental visualization of lithium diffusion in Li x FePO4 . Nat. Mater. 7 , 707–711 (2008).
Malik, R., Burch, D., Bazant, M. & Ceder, G. Particle size dependence of the ionic diffusivity. Nano Lett. 10 , 4123–4127 (2010).
Yuan, A., Tian, L., Xu, W. & Wang, Y. Al-doped spinel LiAl0.1Mn1.9O4 with improved high-rate cyclability in aqueous electrolyte. J. Power Sources 195 , 5032–5038 (2010).
Shkrob, I. A. et al. Manganese in graphite anode and capacity fade in Li ion batteries. J. Phys. Chem. C 118 , 24335–24348 (2014).
Kumagai, N., Komaba, S., Kataoka, Y. & Koyanagi, M. Electrochemical behavior of graphite electrode for lithium ion batteries in Mn and Co additive electrolytes. Chem. Lett. 29 , 1154–1155 (2000).
Google Scholar
Lin, Y.-M. et al. Enhanced high-rate cycling stability of LiMn2O4 cathode by ZrO2 coating for Li-ion battery. J. Electrochem. Soc. 152 , A1526–A1532 (2005).
Kim, J.-S. et al. The electrochemical stability of spinel electrodes coated with ZrO2, Al2O3, and SiO2 from colloidal suspensions. J. Electrochem. Soc. 151 , A1755–A1761 (2004).
Lu, J. et al. Effectively suppressing dissolution of manganese from spinel lithium manganate via a nanoscale surface-doping approach. Nat. Commun. 5 , 5693 (2014).
Yao, J., Shen, C., Zhang, P., Gregory, D. & Wang, L. Surface coating of LiMn2O4 spinel via in situ hydrolysis route: effect of the solution. Ionics 19 , 739–745 (2013).
Liu, Y., Lv, J., Fei, Y., Huo, X. & Zhu, Y. Improvement of storage performance of LiMn2O4/graphite battery with AlF3-coated LiMn2O4 . Ionics 19 , 1241–1246 (2013).
Komaba, S. et al. Impact of 2-vinylpyridine as electrolyte additive on surface and electrochemistry of graphite for C/LiMn2O4 Li-ion cells. J. Electrochem. Soc. 152 , A937–A946 (2005).
Zhan, C., Qiu, X., Lu, J. & Amine, K. Tuning the Mn deposition on the anode to improve the cycle performance of the Mn-based lithium ion battery. Adv. Mater. Interfaces 3 , 1500856 (2016).
Sun, Y.-K. et al. The role of AlF3 coatings in improving electrochemical cycling of Li-enriched nickel-manganese oxide electrodes for Li-ion batteries. Adv. Mater. 24 , 1192–1196 (2012).
Chebiam, R. V., Kannan, A. M., Prado, F. & Manthiram, A. Comparison of the chemical stability of the high energy density cathodes of lithium-ion batteries. Electrochem. Commun. 3 , 624–627 (2001).
Liu, L. & Chen, X. Titanium dioxide nanomaterials: self-structural modifications. Chem. Rev. 114 , 9890–9918 (2014).
Chen, Z., Qin, Y., Amine, K. & Sun, Y. K. Role of surface coating on cathode materials for lithium-ion batteries. J. Mater. Chem. 20 , 7606–7612 (2010).
Cho, J., Kim, Y. W., Kim, B., Lee, J. G. & Park, B. A breakthrough in the safety of lithium secondary batteries by coating the cathode material with AlPO4 nanoparticles. Angew. Chem. Int. Ed. 42 , 1618–1621 (2003).
Chen, Z. & Dahn, J. R. Methods to obtain excellent capacity retention in LiCoO2 cycled to 4.5 V. Electrochim. Acta 49 , 1079–1090 (2004).
Meng, X., Yang, X.-Q. & Sun, X. Emerging applications of atomic layer deposition for lithium-ion battery studies. Adv. Mater. 24 , 3589–3615 (2012).
Sun, Y.-K., Myung, S.-T., Kim, M.-H., Prakash, J. & Amine, K. Synthesis and characterization of Li[(Ni0.8Co0.1Mn0.1)0.8(Ni0.5Mn0.5)0.2]O2 with the microscale core–shell structure as the positive electrode material for lithium batteries. J. Am. Chem. Soc. 127 , 13411–13418 (2005).
Sun, Y. K. et al. Nanostructured high-energy cathode materials for advanced lithium batteries. Nat. Mater. 11 , 942–947 (2012).
Noh, H.-J. et al. Cathode material with nanorod structure — an application for advanced high-energy and safe lithium batteries. Chem. Mater. 25 , 2109–2115 (2013).
Xu, M. et al. Tris (pentafluorophenyl) phosphine: an electrolyte additive for high voltage Li-ion batteries. Electrochem. Commun. 18 , 123–126 (2012).
Abe, K., Ushigoe, Y., Yoshitake, H. & Yoshio, M. Functional electrolytes: novel type additives for cathode materials, providing high cycleability performance. J. Power Sources 153 , 328–335 (2006).
Tarnopolskiy, V. et al. Beneficial influence of succinic anhydride as electrolyte additive on the self-discharge of 5 V LiNi0.4Mn1.6O4 cathodes. J. Power Sources 236 , 39–46 (2013).
von Cresce, A. & Xu, K. Electrolyte additive in support of 5 V Li ion chemistry. J. Electrochem. Soc. 158 , A337–A342 (2011).
Winter, M., Besenhard, J. O., Spahr, M. E. & Novák, P. Insertion electrode materials for rechargeable lithium batteries. Adv. Mater. 10 , 725–763 (1998).
Dahn, J. et al. in Lithium Batteries: New Materials, Developments, and Perspectives (ed. Pistoria, G.) Ch. 1 (Elsevier, 1994).
Ohzuku, T., Ueda, A. & Yamamoto, N. Zero-strain insertion material of Li[Li1/3Ti5/3]O4 for rechargeable lithium cells. J. Electrochem. Soc. 142 , 1431–1435 (1995).
Park, C.-M., Kim, J.-H., Kim, H. & Sohn, H.-J. Li-alloy based anode materials for Li secondary batteries. Chem. Soc. Rev. 39 , 3115–3141 (2010).
Reddy, M., Subba Rao, G. & Chowdari, B. Metal oxides and oxysalts as anode materials for Li ion batteries. Chem. Rev. 113 , 5364–5457 (2013).
Endo, M., Kim, C., Nishimura, K., Fujino, T. & Miyashita, K. Recent development of carbon materials for Li ion batteries. Carbon 38 , 183–197 (2000).
Buqa, H., Goers, D., Holzapfel, M., Spahr, M. E. & Novák, P. High rate capability of graphite negative electrodes for lithium-ion batteries. J. Electrochem. Soc. 152 , A474–A481 (2005).
Aurbach, D., Markovsky, B., Weissman, I., Levi, E. & Ein-Eli, Y. On the correlation between surface chemistry and performance of graphite negative electrodes for Li ion batteries. Electrochim. Acta 45 , 67–86 (1999).
Smart, M. C. et al. Irreversible capacities of graphite in low-temperature electrolytes for lithium-ion batteries. J. Electrochem. Soc. 146 , 3963–3969 (1999).
Jeong, S.-K., Inaba, M., Abe, T. & Ogumi, Z. Surface film formation on graphite negative electrode in lithium-ion batteries: AFM study in an ethylene carbonate-based solution. J. Electrochem. Soc. 148 , A989–A993 (2001).
Kulova, T. L. et al. Electrochemical characteristics of negative electrodes made of ozone-treated graphite for lithium-ion batteries. Russ. J. Electrochem. 37 , 1017–1023 (2001).
Li, H. & Zhou, H. Enhancing the performances of Li-ion batteries by carbon-coating: present and future. Chem. Commun. 48 , 1201–1217 (2012).
Yoshio, M., Wang, H., Fukuda, K., Hara, Y. & Adachi, Y. Effect of carbon coating on electrochemical performance of treated natural graphite as lithium-ion battery anode material. J. Electrochem. Soc. 147 , 1245–1250 (2000).
Yoshio, M., Wang, H. & Fukuda, K. Spherical carbon-coated natural graphite as a lithium-ion battery-anode material. Angew. Chem. Int. Ed. 42 , 4203–4206 (2003).
Yoshio, M. et al. Improvement of natural graphite as a lithium-ion battery anode material, from raw flake to carbon-coated sphere. J. Mater. Chem. 14 , 1754–1758 (2004).
Gao, J. et al. Suppression of PC decomposition at the surface of graphitic carbon by Cu coating. Electrochem. Commun. 8 , 1726–1730 (2006).
Yang, L. C., Guo, W. L., Shi, Y. & Wu, Y. P. Graphite@MoO3 composite as anode material for lithium ion battery in propylene carbonate-based electrolyte. J. Alloys Compd. 501 , 218–220 (2010).
Guo, K., Pan, Q. & Fang, S. Poly(acrylonitrile) encapsulated graphite as anode materials for lithium ion batteries. J. Power Sources 111 , 350–356 (2002).
Zhang, H.-L. et al. Electrochemical performance of pyrolytic carbon-coated natural graphite spheres. Carbon 44 , 2212–2218 (2006).
Park, G., Gunawardhana, N., Nakamura, H., Lee, Y. & Yoshio, M. Suppression of Li deposition on surface of graphite using carbon coating by thermal vapor deposition process. J. Power Sources 196 , 9820–9824 (2011).
Nozaki, H., Nagaoka, K., Hoshi, K., Ohta, N. & Inagaki, M. Carbon-coated graphite for anode of lithium ion rechargeable batteries: carbon coating conditions and precursors. J. Power Sources 194 , 486–493 (2009).
Ding, Y.-S. et al. Characteristics of graphite anode modified by CVD carbon coating. Surf. Coat. Technol. 200 , 3041–3048 (2006).
Lee, M.-L. et al. Atomic layer deposition of TiO2 on negative electrode for lithium ion batteries. J. Power Sources 244 , 410–416 (2013).
Lu, W., Donepudi, V. S., Prakash, J., Liu, J. & Amine, K. Electrochemical and thermal behavior of copper coated type MAG-20 natural graphite. Electrochim. Acta 47 , 1601–1606 (2002).
Pan, Q., Guo, K., Wang, L. & Fang, S. Novel modified graphite as anode material for lithium-ion batteries. J. Electrochem. Soc. 149 , A1218–A1223 (2002).
Veeraraghavan, B., Paul, J., Haran, B. & Popov, B. Study of polypyrrole graphite composite as anode material for secondary lithium-ion batteries. J. Power Sources 109 , 377–387 (2002).
Zhang, H.-L., Li, F., Liu, C. & Cheng, H.-M. Poly(vinyl chloride) (PVC) coated idea revisited: influence of carbonization procedures on PVC-coated natural graphite as anode materials for lithium ion batteries. J. Phys. Chem. C 112 , 7767–7772 (2008).
Ouyang, C. Y., Zhong, Z. Y. & Lei, M. S. Ab initio studies of structural and electronic properties of Li4Ti5O12 spinel. Electrochem. Commun. 9 , 1107–1112 (2007).
Prakash, A. S. et al. Solution-combustion synthesized nanocrystalline Li4Ti5O12 as high-rate performance Li-ion battery anode. Chem. Mater. 22 , 2857–2863 (2010).
Rahman, M. M., Wang, J.-Z., Hassan, M. F., Wexler, D. & Liu, H. K. Amorphous carbon coated high grain boundary density dual phase Li4Ti5O12-TiO2: a nanocomposite anode material for Li-ion batteries. Adv. Energy Mater. 1 , 212–220 (2011).
Kim, H.-K., Bak, S.-M. & Kim, K.-B. Li4Ti5O12/reduced graphite oxide nano-hybrid material for high rate lithium-ion batteries. Electrochem. Commun. 12 , 1768–1771 (2010).
Shen, L., Uchaker, E., Zhang, X. & Cao, G. Hydrogenated Li4Ti5O12 nanowire arrays for high rate lithium ion batteries. Adv. Mater. 24 , 6502–6506 (2012).
Chiu, H.-c. & Demopoulos, G. P. A novel green approach to synthesis of nanostructured Li4Ti5O12 anode material. ECS Trans. 50 , 119–126 (2013).
Chen, J., Yang, L., Fang, S., Hirano, S.-i. & Tachibana, K. Synthesis of hierarchical mesoporous nest-like Li4Ti5O12 for high-rate lithium ion batteries. J. Power Sources 200 , 59–66 (2012).
Laumann, A. et al. Rapid green continuous flow supercritical synthesis of high performance Li4Ti5O12 nanocrystals for Li ion battery applications. J. Electrochem. Soc. 159 , A166–A171 (2011).
Bai, Y., Wang, F., Wu, F., Wu, C. & Bao, L.-y. Influence of composite LiCl–KCl molten salt on microstructure and electrochemical performance of spinel Li4Ti5O12 . Electrochim. Acta 54 , 322–327 (2008).
Li, J., Jin, Y.-L., Zhang, X.-G. & Yang, H. Microwave solid-state synthesis of spinel Li4Ti5O12 nanocrystallites as anode material for lithium-ion batteries. Solid State Ion. 178 , 1590–1594 (2007).
Shen, L. et al. Three-dimensional coherent titania–mesoporous carbon nanocomposite and its lithium-ion storage properties. ACS Appl. Mater. Interfaces 4 , 2985–2992 (2012).
Djenizian, T., Hanzu, I. & Knauth, P. Nanostructured negative electrodes based on titania for Li-ion microbatteries. J. Mater. Chem. 21 , 9925–9937 (2011).
Liu, H. et al. Mesoporous TiO2–B microspheres with superior rate performance for lithium ion batteries. Adv. Mater. 23 , 3450–3454 (2011).
Boukamp, B. A., Lesh, G. C. & Huggins, R. A. All-solid lithium electrodes with mixed-conductor matrix. J. Electrochem. Soc. 128 , 725–729 (1981).
Winter, M. & Besenhard, J. O. Electrochemical lithiation of tin and tin-based intermetallics and composites. Electrochim. Acta 45 , 31–50 (1999).
Wu, H. & Cui, Y. Designing nanostructured Si anodes for high energy lithium ion batteries. Nano Today 7 , 414–429 (2012).
Yoshio, M. et al. Carbon-coated Si as a lithium-ion battery anode material. J. Electrochem. Soc. 149 , A1598–A1603 (2002).
Johnson, C. S., Li, N., Lefief, C. & Thackeray, M. M. Anomalous capacity and cycling stability of x Li(2)MnO(3)center dot(1− x )LiMO2 electrodes (M = Mn, Ni, Co) in lithium batteries at 50 degrees C. Electrochem. Commun. 9 , 787–795 (2007).
Obrovac, M. N., Christensen, L., Le, D. B. & Dahn, J. R. Alloy design for lithium-ion battery anodes. J. Electrochem. Soc. 154 , A849–A855 (2007).
Sandu, I., Moreau, P., Guyomard, D., Brousse, T. & Roué, L. Synthesis of nanosized Si particles via a mechanochemical solid–liquid reaction and application in Li-ion batteries. Solid State Ion. 178 , 1297–1303 (2007).
Phan, V. P., Pecquenard, B. & Le Cras, F. High-performance all-solid-state cells fabricated with silicon electrodes. Adv. Funct. Mater. 22 , 2580–2584 (2012).
Szczech, J. R. & Jin, S. Nanostructured silicon for high capacity lithium battery anodes. Energy Environ. Sci. 4 , 56–72 (2011).
Liu, N. et al. a yolk-shell design for stabilized and scalable li-ion battery alloy anodes. Nano Lett. 12 , 3315–3321 (2012).
Chan, C. K. et al. High-performance lithium battery anodes using silicon nanowires. Nat. Nanotech. 3 , 31–35 (2008).
Cui, L.-F., Ruffo, R., Chan, C. K., Peng, H. & Cui, Y. Crystalline-amorphous core–shell silicon nanowires for high capacity and high current battery electrodes. Nano Lett. 9 , 491–495 (2009).
Hertzberg, B., Alexeev, A. & Yushin, G. Deformations in Si–Li anodes upon electrochemical alloying in nano-confined space. J. Am. Chem. Soc. 132 , 8548–8549 (2010).
Choi, N.-S., Yao, Y., Cui, Y. & Cho, J. One dimensional Si/Sn-based nanowires and nanotubes for lithium-ion energy storage materials. J. Mater. Chem. 21 , 9825–9840 (2011).
Ma, H. et al. Nest-like silicon nanospheres for high-capacity lithium storage. Adv. Mater. 19 , 4067–4070 (2007).
Magasinski, A. et al. High-performance lithium-ion anodes using a hierarchical bottom-up approach. Nat. Mater. 9 , 353–358 (2010).
Yin, J. et al. Micrometer-scale amorphous Si thin-film electrodes fabricated by electron-beam deposition for Li-ion batteries. J. Electrochem. Soc. 153 , A472–A477 (2006).
Mazouzi, D., Lestriez, B., Roué, L. & Guyomard, D. Silicon composite electrode with high capacity and long cycle life. Electrochem. Solid State Lett. 12 , A215–A218 (2009).
Chan, C. K., Patel, R. N., O'Connell, M. J., Korgel, B. A. & Cui, Y. Solution-grown silicon nanowires for lithium-ion battery anodes. ACS Nano 4 , 1443–1450 (2010).
Liu, N. et al. A pomegranate-inspired nanoscale design for large-volume-change lithium battery anodes. Nat. Nanotech. 9 , 187–192 (2014).
Xu, W. et al. Lithium metal anodes for rechargeable batteries. Energy. Environ. Sci. 7 , 513–537 (2014).
Cheng, X.-B. et al. A review of solid electrolyte interphases on lithium metal anode. Adv. Sci. 3 , 1500213 (2015).
Kim, J.-S., Kim, D. W., Jung, H. T. & Choi, J. W. Controlled lithium dendrite growth by a synergistic effect of multilayered graphene coating and an electrolyte additive. Chem. Mater. 27 , 2780–2787 (2015).
Van Noorden, R. A better battery. Nature 507 , 26–28 (2014).
Nazar, L. F., Cuisinier, M. & Pang, Q. Lithium-sulfur batteries. MRS Bull. 39 , 436–442 (2014).
Manthiram, A., Fu, Y. Z. & Su, Y. S. Challenges and prospects of lithium–sulfur batteries. Acc. Chem. Res. 46 , 1125–1134 (2013).
Yin, Y. X., Xin, S., Guo, Y. G. & Wan, L. J. Lithium–sulfur batteries: electrochemistry, materials, and prospects. Angew. Chem. Int. Ed. 52 , 13186–13200 (2013).
Zhang, B., Qin, X., Li, G. R. & Gao, X. P. Enhancement of long stability of sulfur cathode by encapsulating sulfur into micropores of carbon spheres. Energy. Environ. Sci. 3 , 1531–1537 (2010).
Liang, C. D., Dudney, N. J. & Howe, J. Y. Hierarchically structured sulfur/carbon nanocomposite material for high-energy lithium battery. Chem. Mater. 21 , 4724–4730 (2009).
Ji, X. L., Lee, K. T. & Nazar, L. F. A highly ordered nanostructured carbon-sulphur cathode for lithium-sulphur batteries. Nat. Mater. 8 , 500–506 (2009).
Seh, Z. W. et al. Sulphur–TiO2 yolk-shell nanoarchitecture with internal void space for long-cycle lithium-sulphur batteries. Nat. Commun. 4 , 1331 (2013).
Song, M. K., Zhang, Y. G. & Cairns, E. J. A long-life, high-rate lithium/sulfur cell: a multifaceted approach to enhancing cell performance. Nano Lett. 13 , 5891–5899 (2013).
Chen, R. J. et al. Graphene-based three-dimensional hierarchical sandwich-type architecture for high-performance Li/S batteries. Nano Lett. 13 , 4642–4649 (2013).
Lu, J. et al. A lithium–oxygen battery based on lithium superoxide. Nature 529 , 377–382 (2016).
Lu, J. et al. A nanostructured cathode architecture for low charge overpotential in lithium–oxygen batteries. Nat. Commun. 4 , 2383 (2013).
Download references
Acknowledgements
This work was supported by the US Department of Energy under Contract DE-AC0206CH11357 with the main support provided by the Vehicle Technologies Office, Department of Energy (DOE) Office of Energy Efficiency and Renewable Energy (EERE). We also acknowledge support from the Chinese Electric Power Research Institute (CEPRI).
Author information
Authors and affiliations.
Chemical Sciences and Engineering Division, Argonne National Laboratory, Argonne, 60439, Illinois, USA
Jun Lu, Zonghai Chen & Khalil Amine
Department of Chemical Engineering, Institute of Electrochemical and Energy Technology, Shanghai Jiao Tong University, 200240, Shanghai, China
School of Advanced Materials, Peking University, Shenzhen Graduate School, Shenzhen, 518055, PR China
Material Science Division, Argonne National Laboratory, Argonne, 60439, Illinois, USA
Larry A. Curtiss
You can also search for this author in PubMed Google Scholar
Corresponding authors
Correspondence to Feng Pan , Larry A. Curtiss or Khalil Amine .
Ethics declarations
Competing interests.
The authors declare no competing financial interests.
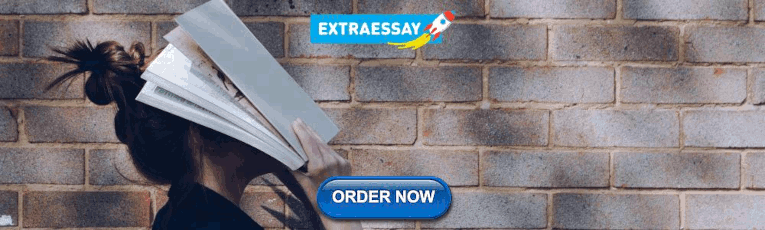
Rights and permissions
Reprints and permissions
About this article
Cite this article.
Lu, J., Chen, Z., Ma, Z. et al. The role of nanotechnology in the development of battery materials for electric vehicles. Nature Nanotech 11 , 1031–1038 (2016). https://doi.org/10.1038/nnano.2016.207
Download citation
Received : 27 January 2016
Accepted : 12 September 2016
Published : 06 December 2016
Issue Date : December 2016
DOI : https://doi.org/10.1038/nnano.2016.207
Share this article
Anyone you share the following link with will be able to read this content:
Sorry, a shareable link is not currently available for this article.
Provided by the Springer Nature SharedIt content-sharing initiative
This article is cited by
Sequencing polymers to enable solid-state lithium batteries.
- Shantao Han
Nature Materials (2023)
Preparation of double-shell Si@SnO2@C nanocomposite as anode for lithium-ion batteries by hydrothermal method
- Shao-Hua Wang
Rare Metals (2023)
Investigation of reduced lithium titanate spinel as insertion host for rechargeable batteries
- Minji Jeong
- Min Jin Kim
- Si Hyoung Oh
Korean Journal of Chemical Engineering (2023)
Electrical investigation and enhancement of optical, structural, and dielectric properties of flexible PVDF/LiZnVO4 nanocomposites
- I. S. Elashmawi
- A. M. Ismail
- A. Y. Yassin
Discover Materials (2023)
Conversion reaction lithium metal batteries
- Zhaopeng Li
- Lifang Jiao
Nano Research (2023)
Quick links
- Explore articles by subject
- Guide to authors
- Editorial policies
Sign up for the Nature Briefing newsletter — what matters in science, free to your inbox daily.


An official website of the United States government
The .gov means it’s official. Federal government websites often end in .gov or .mil. Before sharing sensitive information, make sure you’re on a federal government site.
The site is secure. The https:// ensures that you are connecting to the official website and that any information you provide is encrypted and transmitted securely.
- Publications
- Account settings
Preview improvements coming to the PMC website in October 2024. Learn More or Try it out now .
- Advanced Search
- Journal List
- Iran J Public Health
- v.48(10); 2019 Oct
Theoretical and Practical Principles on Nanoethics: A Narrative Review Article
Saeed biroudian.
1. Department of Medical Ethics, School of Traditional Medicine, Shahid Beheshti University of Medical Sciences, Tehran, Iran
Mahmoud ABBASI
2. Ethics and Law Research Center, Shahid Beheshti University of Medical Sciences, Tehran, Iran
Mehrzad KIANI
Background:.
Interests in nanotechnology and its application in medical research, diagnosis, and treatment of diseases continuously grow. The study identified the theoretical and practical principles of ethics in developed countries' nanomedical research to be used as the first step of development of a national nanoethics standard or guideline in Iran and developing countries.
The present study was done between 2012–2016 in Ethics and Law Research Center, Shahid Beheshti University of Medical Sciences, Tehran, Iran, which comprised a literature review and a comparative study to describe and compare the nanoethics situation and considerations of nanoethics in Australia, Canada, and USA.
The main ethical considerations in the three countries contain two major categories, including firstly, the nature of nanoparticles such as its diversity, rapid development of new and not well-defined nanoproducts and particles and unpredictable side effects of such nanoparticles; and secondly, the application of developed nanoparticles in areas such as justice, privacy protection, patient-physician relations, etc.
Conclusion:
It is controversial to develop an independent nanoethics standard or codes; however, national priorities and concerns, as well as specific nanoethics considerations, should be investigated before deciding to create such standards in each country. Overall, careful considerations have to take into account the justice, privacy protection, the inherent risks of nanomaterials and their possible side effects on patients and other study subjects, as well as considering characteristics of new developed nanoproducts and particles.
Introduction
Interest in nanotechnology and its application in medical research, diagnosis, and treatment of diseases are on a constant growth trend. Every day, a range of novel nano drugs, nano equipment and nanobiochips is introduced to the global market ( 1 , 2 ). Despite the benefits of such progress, nanomedical technology, like any other technology, is accompanied by a number of consequences ( 2 ). As the intersection of engineering, physics, biology, medicine, and chemistry, nanotechnology facilitates the application and empowerment of these sciences in the production of innovative products. Following the substantial progress of nanotechnology research over the past decade, increasing attention has been devoted novel drugs, drug delivery systems and treatment methods for diseases such as cancer to promote human health through nanotechnology ( 3 , 4 ).
The term “ nanotechnology ” is composed of the unit prefix “ nano ” (derived from a Greek word-meaning dwarf) which means one billionth and the word technology. Nanoscience deals with phenomena, properties, and interactions of various substances at the atomic, molecular, and macromolecular levels (sizes of 1–100 nm) where, especially at sizes smaller than 5 nm, materials manifest completely different characteristics compared to larger scales. Nanotechnology is hence the design, manipulation, and production of nanoscale (< 100 nm) equipment and systems through controlling the shape, size, properties, reactions, and performance of structures ( 5 ).
The European Science Foundation has defined nanomedicine as a branch of science involving the application of molecular knowledge and instruments to relieve pain and enhance human health by the prevention, diagnosis, and treatment of diseases and traumatic injuries ( 6 , 7 ).
Nanomedicine is the use of nanoscale approaches, theories, equipment, and structures to detect, prevent, or treat various illnesses by the identification and restoration of damaged tissues at the molecular level. In addition to its advantages in pharmaceutics, nanomedicine also seeks to provide advanced diagnostic pathology and treatment services at the molecular or even micro-molecular level. Attention to such consequences of nanomedicine is critical since this field of science deals directly with human health ( 8 ).
Ethics in Nanomedicine
Nanoethics is a set of ethical principles used in all nanotechnology-based research and diagnostic and therapeutic measures. It is a branch of bioethics, which is in turn categorized under professional ethics ( 9 , 10 ).
To describe better the concept of nanoethics in the context of medical ethics, it is necessary to know more about ethical concerns in nanomedicine.
Although nanomedicine has provided potential solutions to many long-standing unsolved medical problems, the ethical issues related to its emergence have been commonly neglected. Since ethical considerations have not developed at a pace similar to the science itself, investments are required to fill the gap between science and ethics ( 9 , 11 , 12 ). Raj Bawa and Summer Johnson (pioneers of discussions on social and ethical aspects of medicine) have highlighted investments on nanomedicine in pharmaceutical industry. Pharmaceutical companies' desire to maximize their profit and their consequent tendency toward investments in nanotechnology might prevent them from paying sufficient attention to social and ethical considerations ( 13 ).
While subjects such as human dignity, autonomy, and secrecy are indispensable to any biological study, they are even more prominent in nanomedicine research due to the nature of nanotechnology. As discussed earlier, nanomedicine is a branch of modern technology, which incorporates rapid developments and productions to facilitate the prevention, diagnosis, and treatment of diseases at cellular and biomolecular levels. Nevertheless, owing to the unclarity and unpredictability of long-term effects of nanomedicine, this field of science cannot be compared to any other branches of technology when ethical issues are concerned. In other words, despite the startling achievements of nanomedicine during the short period since its emergence, the obscurity of its long-term effects increases the significance of ethics in this field. Despite its undeniable benefits to modern medicine, nanomedicine (like any other field of research) has raised particular legal, social, and ethical issues whose neglect would lead to major crises in the future ( 11 , 12 ).
Nanotechnology in Iran
First sparkles of nanotechnology in Iran were lit in 2001, and two years after the beginning of the studies, “Iran Nanotechnology Initiative Council” was established in 2003 with direct support of parliament and presidency ( 14 ). Iran Nanotechnology Initiative Council created various workgroups for the development of nanotechnology such as Nanotechnology Development Workgroup, Human Resources Development Workgroup, Technology Infrastructures Workgroup, Public promotion and Culture-making Workgroup, etc. to perform a 10 yr strategic plan in Iran. After a decade of huge investment in nanotechnology, now, according 2016 statistics, Iran has more than 28000 researchers and 14000 Master and PhD students and graduates in this field, ranked 7th in the world in the number of Nano-articles in ISI and 9th in the citations, and first in Nanotechnology new method patents in USPTO ( 15 ).
Considering the dramatic development of nanotechnology in Iran, “Iran Nanotechnology Safety Network” was established in 2012. The aim of the network is to define national standards, regulations, and instructions on safety, environmental and ethical issues in nanotechnology.
The Ethics Committee of this network decided to provide a national standard for nanoethics to be considered in nanotechnology research and products ( 16 ).
During last two decades, some national guidelines of ethics in medical research have been developed ( 17 ); however, since nanomedicine is a novel technology in Iran's medical sector, no particular ethical considerations and codes have been developed to deal with the ethical issues arising from nanomedicine research. The identification and localization of the ethical policies adopted by pioneers of nanotechnology would hence minimize the adverse consequences of nanotechnology, especially nanomedicine, research and practice in Iran.
Therefore, the aim of this study was to investigate the status of nanoethics such as ethical considerations in nanomedicine in Australia, Canada, and the US as three pioneers in nanoethics as the first step to be able to define and develop a national standard for nanoethics in Iran. In fact, this comparative study is the first phase of this guideline/standard development in cooperation of “Iran Nanotechnology Safety Network”. Therefore, the present study sought to identify and summarize the theoretical and practical principles and considerations of ethics in nanomedical research in three pioneers of nanomedicine (Australia, Canada, and the US) in the light of a comparative study to be used later in development of a national standard/guideline.
This comparative study has described and compared situation and considerations of nanoethics in Australia, the US, and Canada as pioneers of nanoethics. Comparative research is a research methodology in which differences and similarities between phenomena are identified through comparisons between countries, cultures or other sectors. It is widely applied in macro sociology to answer questions at the intermediate and macro levels using internal and external analysis. ( 18 )
Therefore, the research comprised two distinct levels. At the first level, a literature review was conducted to describe and illuminate the existing status of nanoethics considerations. To this purpose, scientific databases, including PubMed, Scopus, and Web of Science, were searched using nanoethics, nanotechnology, nanomedicine, medical ethics, and ethics in nanotechnology as key terms. The next stage involved a comparative study of the status of nanoethics in, Australia, Canada, and USA. These three countries were selected due to their attention to medical ethics, as well as their keen interest and massive investments in nanomedicine. The available books, article, and online databases were again searched (with a number of key terms including applications of nanotechnology, nanomedicine, nanoproducts, medical ethics, and ethical codes in nanomedicine) to extract relevant information in each country.
An internal analysis was performed on the extracted information to determine the ethical standards governing nanomedicine studies, along with their strengths and weaknesses, in the target countries and to evaluate such standards based on the cultural context of each society. The ethical aspects of nanomedicine studies were first described in the target countries. The obtained data were further analyzed and summarized with the comparative approach. Finally, the findings of both levels and the ethical considerations in each country were meticulously analyzed.
The present study compared three countries in terms of ethical considerations in nanomedicine. The objective was to facilitate the establishment of ethical codes in nanomedicine research in Iran through the identification of the applied approached and developed ethical codes in the mentioned countries. The following sections will thus describe the findings of the comparative study.
Findings in Australia, Canada, and the US
Medical research and practice are deals directly with human health. As nanotechnology is a novel technology whose consequences cannot be easily predicted due to its nanoscale nature, the existing ethical codes do not seem to suffice. The subject of nanoethics is of particular significance in Iran, a country that is still a novice in this field despite its progress in the number of scientific articles and production of science. In order to facilitate the development of relevant ethical considerations and codes in the country, Table 1 summarizes the major ethical considerations in Australia, Canada, and the US.
Nanoethical considerations in Australia, Canada, and the US
Ethical considerations in these three countries are summarized in different codes. Each code is more described in literature related to each country. For example, Justice and nano-gap (in Australia and Canada) are described more as followed below. The other items of table are discussed in discussion section of this paper.
Justice as an ethical principle in bioresearch implies that all people should receive equal health facilities regardless of their personal characteristics such as ethnicity, gender, or age ( 32 ).
Nanotechnology is predicted to develop to more than two-trillion-dollar industry embracing advanced pharmaceutical products, improved medical imaging techniques, more precise surgical instruments, and unpredictable medical breakthroughs ( 33 ). Since such progress might be limited to countries with considerably high gross domestic products (at trillion-dollar levels), a wide gap, called a nano-gap, may be created between developed and developing countries ( 34 , 35 ). Developed countries would be more capable of producing and expanding nanotechnologies and benefiting from their production. Moreover, researchers may solely focus on introducing innovative nanotechnological solutions to the problems faced by developed countries. Developing countries would hence be forced to resolve their health and environmental issues through more sustainable, less technology-dependent strategies. In the absence of adequate resources to run their own research projects, these countries would have to rely on research findings of developed countries ( 34 , 36 ).
There are also raising concerns about intellectual property rights in nanotechnology, i.e. patents on nanotechnology might encompass large numbers of applications and thus restrict access to information and technology, especially in developing countries. The nano-gap might also broaden the gap between the rich and the poor in developed countries and make people access to different levels of novel technologies, particularly medical advances, based on their wealth ( 34 , 36 ).
The increasing application of nanotechnology and nanomedicine warrants attention to ethical issues in this field. Hence, the present study sought to redefine the theoretical and practical principles of ethics in nanomedicine in Iran and three pioneers of nanotechnology (Australia, Canada, and the US).
Ethics in nanomedicine refers to the ethical issues involved in the use of nanotechnology in medical research and development of diagnostic and therapeutic measures. It is a branch of bioethics, which can be categorized, in turn, under professional ethics. In the three studied countries, the main ethical considerations in nanomedicine can be classified into two major categories. The first group of concerns related to the nature, diversity, and unpredictable side effects of nanoparticles. The second group stemmed from the application of these materials can be summarized as:
- Uncertainty about the fair distribution of the benefits of nanotechnology: The benefits of nanotechnology might be exclusively available to developed countries (and increase the dependence of other nations on these countries) or even worse, to the upper classes of their societies.
- The risk of disclosure of confidential information: Nanotechnology might be able to access people's personal information without their consent. This would definitely jeopardize privacy protection (one of the most important ethical issues in medicine).
- Transformation of patient-physician relationships: The achieved progress may lead to the diagnosis of currently incurable diseases. Being diagnosed with an untreatable disease would lead to profound psychological consequences for the patients and their families. Furthermore, knowledge about the existence of incurable diseases (e.g. AIDS) causes a great level of public anxiety. On the other hand, patients may become able to diagnose and treat their own diseases. The possibility of self-diagnosis and self-treatment will result in disastrous social consequences. For instance, in case of diseases with overlapping symptoms, self-treatment may prevent timely and accurate diagnosis of the real disease and cause serious problems in the patient.
- Increased capabilities of humans: Nanotechnology may provide individuals with the chance to modify their appearance, performance, and even personality. Such possibilities would indubitably raise major ethical issues. This is particularly important when cloning and its consequent concerns, e.g. violation of human dignity, identify challenges, and interference in the laws of nature, are involved.
- Ethics in Biomedical Research: Since general research in nanotechnology involves human trials, issues related to privacy protection, autonomy, and human dignity are of critical significance in this field. In fact, the unclarity and unpredictability of the possible consequences of nanomaterials may affect the processes of information provision, obtaining informed consent, and privacy protection in nanomedicine research.
- Ethics in Diagnostic and Therapeutic Applications: Considering the increasing attention to the use of nanotechnology in the diagnosis and treatment of various diseases, the possible adverse effects of such measures on human health and the environment need to be thoroughly evaluated. Moreover, the ethical issues rising from the possible future applications of nanotechnology should be clearly described.
Due to the absence of a global standard or code of ethics in nanomedicine, developing a comprehensive ethical code would require an interdisciplinary study to identify the different aspects of ethical and social consequences of nanomedicine. This study provides an overview and summarized considerations of nanoethics in three studied countries. Our findings could be used as an overview as the first step to develop a national standard/guideline in Iran and also other countries more involved in nanomedicine.
Although some new ethical aspects and different considerations are mentioned in nanomedicine area, but there is a high overlap between common ethical principles of medical ethics and nanoethics; therefore, it is a controversy about development of a new independent field or codes of nanoethics according to previous studies and an independent ethics principles for nanoethics is needed. However, more studies are needed for each country to consider its own context, nanotechnology situation, national needs, concerns, and national medical ethics codes before deciding to develop any national nanoethics codes or standards.
Ethical considerations
Ethical issues (Including plagiarism, informed consent, misconduct, data fabrication and/or falsification, double publication and/or submission, redundancy, etc.) have been completely observed by the authors.
Acknowledgments
This paper derived from medical ethics Ph.D. thesis with number of 134 registered in Shahid Beheshti University of Medical Sciences, Tehran, Iran. Hereby, the authors would like to express their gratitude for the sincere cooperation and assistance of the professors and colleagues at the Research Center and Department of Medical Ethics of Shahid Beheshti University of Medical Sciences.
Conflict of interest
The authors declare that there is no conflict of interest.
Advertisement
A Review: Influence of Potential Nanomaterials for Civil Engineering Projects
- Review Paper
- Published: 12 October 2020
- Volume 45 , pages 2057–2068, ( 2021 )
Cite this article
- Ali Akbar Firoozi ORCID: orcid.org/0000-0002-5282-0575 1 ,
- Maryam Naji 2 ,
- Mahongo Dithinde 1 &
- Ali Asghar Firoozi 3
873 Accesses
8 Citations
Explore all metrics
The construction industry is increasingly turning to use of environmentally friendly materials in order to meet the sustainable aspect required by modern infrastructures. In the last two decades, the expansion of this concept and the increasing global warming have raised concerns on the extensive use of Portland cement and fly ash due to the high amount of carbon dioxide gas associated with their production. The development of nanotechnology and nanomaterials offers promising signs for a change in the way of construction and geotechnical projects. The aim of this research is to show potential of nanomaterials to replace traditional materials for civil engineering to optimize construction projects, i.e., to reduce global warming by using less amount of cement or fly ash, and finally drop cost of projects. The incorporation of variously manufactured nanomaterials into the matrices of conventional construction materials leads to drastic advancement in vital characteristics including mechanical strength, fatigue and damage resistance, durability, and lightness. However, due to the significance and serious disadvantages of using traditional materials like lime, cement, and fly ash as soil stabilizers, finding alternative additives is a matter of sustainability for construction projects.
This is a preview of subscription content, log in via an institution to check access.
Access this article
Price includes VAT (Russian Federation)
Instant access to the full article PDF.
Rent this article via DeepDyve
Institutional subscriptions
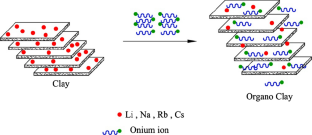
Similar content being viewed by others
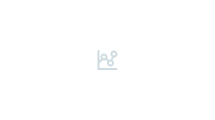
Smart and Functional Materials Based Nanomaterials in Construction Styles in Nano-Architecture
Ammar Sadik Dahlan
Enhancement in Shear Strength Characteristics of Soft Soil by Using Nanomaterials
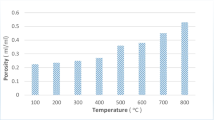
The effect of nanosilica incorporation on the mechanical properties of concrete exposed to elevated temperature: a review
Ibrahim Mohammed Nasser, Mohd Haziman Wan Ibrahim, … Abdullah Faisal Alshalif
Arabani M, Haghi A, Tanzadeh R (2015) Effects of nanoclay on mechanical properties of aged asphalt mixture. Chem Technol Key Dev Appl Chem Biochem Mater Sci 49:766–773
Google Scholar
Azzam WR (2012) Reduction of the shrinkage–swelling potential with polymer nanocomposite stabilization. J Appl Polym Sci 123(1):299–306
Article Google Scholar
Azzam WR (2014) Behavior of modified clay microstructure using polymer nanocomposites technique. Alex Eng J 53(1):143–150
Bashir R, Chisti H (2017) Nanotechnology for environmental control and remediation. In: Materials science and engineering: concepts, methodologies, tools, and applications. IGI Global, New York, pp 1217–1238
Burke DJ, Zhu S, Pablico-Lansigan MP, Hewins CR, Samia ACS (2014) Titanium oxide nanoparticle effects on composition of soil microbial communities and plant performance. Biol Fertil Soils 50(7):1169–1173
Chong KP, Garboczi EJ (2002) Smart and designer structural material systems. Prog Struct Mat Eng 4(4):417–430
Colvin VL (2003) The potential environmental impact of engineered nanomaterials. Nat Biotechnol 21(10):1166
Cui H, Yang S, Memon S (2015) Development of carbon nanotube modified cement paste with microencapsulated phase-change material for structural–functional integrated application. Int J Mol Sci 16(4):8027–8039
Drexler KE (1981) Molecular engineering: an approach to the development of general capabilities for molecular manipulation. Proc Natl Acad Sci 78(9):5275–5278
Faruk AN, Chen DH, Mushota C, Muya M, Walubita LF (2014) Application of nano-technology in pavement engineering: a literature review. In: Application of nanotechnology in pavements, geological disasters, and foundation settlement control technology, pp 9–16
Firoozi AA, Taha MR, Firoozi AA, Khan TA (2014a) Assessment of nano-zeolite on soil properties. Aust J Basic Appl Sci 8(19):292–295
Firoozi AA, Taha MR, Firoozi AA (2014b) Nanotechnology in civil engineering. EJGE 19:4673–4682
Firoozi AA, Taha MR, Firoozi AA, Khan TA (2015) Effect of ultrasonic treatment on clay microfabric evaluation by atomic force microscopy. Measurement 66:244–252
Firoozi AA, Taha MR, Khan TA, Hejazi F, Firoozi AA, Alsharef J (2019) A novel method for mixing nanomaterials with soil. In: Nano hybrids and composites, vol 25. Trans Tech Publications, New York, pp 46–68
Fulekar MH, Pathak B, Kale RK (2014) Nanotechnology: perspective for environmental sustainability. In: Fulekar M, Pathak B, Kale R (eds) Environment and sustainable development. Springer, New Delhi. https://doi.org/10.1007/978-81-322-1166-2_7
Chapter Google Scholar
Guo L (2014) Investigation of soil stabilization using biopolymers. Graduate Theses and Dissertations, 13909. https://lib.dr.iastate.edu/etd/13909
Hamzah MO, Kakar MR, Hainin MR (2015) An overview of moisture damage in asphalt mixtures. J Teknologi Sci Eng 73(4):125–131
Huang Y, Wang L (2016a) Experimental studies on nanomaterials for soil improvement: a review. Environ Earth Sci 75(6):497
Huang Y, Wang L (2016b) Laboratory investigation of liquefaction mitigation in silty sand using nanoparticles. Eng Geol 204:23–32
Huang Y, Wen Z, Wang L, Zhu C (2019) Centrifuge testing of liquefaction mitigation effectiveness on sand foundations treated with nanoparticles. Eng Geol 249:249–256
Kavussi A, Barghabani P (2014) The influence of nano materials on moisture resistance of asphalt mixes. Study Civ Eng Archit 3:36–40
Kiliaris P, Papaspyrides CD (2010) Polymer/layered silicate (clay) nanocomposites: an overview of flame retardancy. Prog Polym Sci 35(7):902–958
Lin PC, Lin S, Wang PC, Sridhar R (2014) Techniques for physicochemical characterization of nanomaterials. Biotechnol Adv 32(4):711–726
Naeini SA, Naderinia B, Izadi E (2012) Unconfined compressive strength of clayey soils stabilized with waterborne polymer. KSCE J Civ Eng 16(6):943–949
Onyejekwe S, Ghataora GS (2015) Stabilization of quarry fines using a polymeric additive and Portland cement. J Mater Civ Eng 28(1):04015070
Pacheco-Torgal F, Jalali S (2011) Nanotechnology: advantages and drawbacks in the field of construction and building materials. Constr Build Mater 25(2):582–590
Paul DR, Robeson LM (2008) Polymer nanotechnology: nanocomposites. Polymer 49(15):3187–3204
Peponi L, Puglia D, Torre L, Valentini L, Kenny JM (2014) Processing of nanostructured polymers and advanced polymeric based nanocomposites. Mater Sci Eng R Rep 85:1–46
Petrunin S, Vaganov V, Sobolev K (2015) The effect of functionalized carbon nanotubes on phase composition and strength of composites. In: Nanotechnology in construction. Springer, Cham, pp 245–251
Rana AK, Rana SB, Kumari A, Kiran V (2009) Significance of nanotechnology in construction engineering. Int J Recent Trends Eng 1(4):46
Roco MC, Bainbridge WS (2005) Societal implications of nanoscience and nanotechnology: maximizing human benefit. J Nanopart Res 7(1):1–13
Roco MC, Williams RS, Alivisatos P (1999) Nanotechnology research directions: IWGN workshop report. Vision for nanotechnology R&D in the next decade. National Science and Technology, Councilarlington
Book Google Scholar
Salvetat JP, Andrew G, Bnggs D, Bonard JM, Basca RR, Kulik AJ (1999) Review article polymer-matrix nanocomposites, processing, manufacturing, and application an overview. Phys Rev Lett 82:944
Santagata E, Baglieri O, Tsantilis L, Chiappinelli G, Aimonetto IB (2015) Effect of sonication on high temperature properties of bituminous binders reinforced with nano-additives. Constr Build Mater 75:395–403
Shah TK, Malecki HC, Adcock DJ (2014) US patent no. 8,664,573. US Patent and Trademark Office, Washington, DC
Shunmugasamy VC, Xiang C, Gupta N (2015) Clay/polymer nanocomposites: processing, properties, and applications. In: Hybrid and hierarchical composite materials. Springer, Cham, pp 161–200
Sobolev K, Shah SP (2015) Nanotechnology in construction. In: Proceedings of NICOM5. Springer, Berlin
Sparks S (2017) Nanotechnology: business applications and commercialization. CRC Press, New York
Taha MR, Taha OME (2012) Influence of nano-material on the expansive and shrinkage soil behavior. J Nanopart Res 14(10):1190
Walters R, Fini EH, Abu-Lebdeh T (2014) Introducing combination of nano-clay and bio-char to enhance asphalt binder. Int J Pavement Res Technol 7(6):451–455
Wang D, Pillai SC, Ho SH, Zeng J, Li Y, Dionysiou DD (2018) Plasmonic-based nanomaterials for environmental remediation. Appl Catal B 237:721–741
Wei Q, Yang D, Fan M, Harris HG (2013) Applications of nanomaterial-based membranes in pollution control. Cri Rev Environ Sci Technol 43(22):2389–2438
Wu Y, Pang H, Liu Y, Wang X, Yu S, Fu D, Wang X (2018) Environmental remediation of heavy metal ions by novel-nanomaterials: a review. Environ Pollut 246:608–620
Xu P, Zeng GM, Huang DL, Feng CL, Hu S, Zhao MH, Liu ZF (2012) Use of iron oxide nanomaterials in wastewater treatment: a review. Sci Total Environ 424:1–10
Yang J, Tighe S (2013) A review of advances of nanotechnology in asphalt mixtures. Proc Soc Behav Sci 96:1269–1276
Yang L, Yang L, Ding L, Deng F, Luo XB, Luo SL (2019) Principles for the application of nanomaterials in environmental pollution control and resource reutilization. In: Nanomaterials for the removal of pollutants and resource reutilization. Elsevier, Berlin, pp 1–23
Yusoff NIM, Breem AAS, Alattug HN, Hamim A, Ahmad J (2014) The effects of moisture susceptibility and ageing conditions on nano-silica/polymer-modified asphalt mixtures. Constr Build Mater 72:139–147
Zhu W, Bartos PJ, Porro A (2004) Application of nanotechnology in construction. Mater Struct 37(9):649–658
Download references
Acknowledgements
The authors gratefully acknowledge the support from Department of Civil Engineering, Universiti Kebangsaan Malaysia, which provided the facility for this research.
Author information
Authors and affiliations.
Department of Civil Engineering, Faculty of Engineering and Technology, University of Botswana, Gaborone, Botswana
Ali Akbar Firoozi & Mahongo Dithinde
Department of Civil Engineering, Higher Education Complex of Saravan, Saravan, Iran
Maryam Naji
Department of Petroleum Engineering, Shiraz University, Shiraz, Iran
Ali Asghar Firoozi
You can also search for this author in PubMed Google Scholar
Corresponding author
Correspondence to Ali Akbar Firoozi .
Ethics declarations
Competing interest.
The authors declare that they have no competing interest.
Ethics Approval and Consent to Participate
The presented work is part of research project entitled “Soil improvement using nanomaterials.” There is no any ethical conflict. Authors on behalf of an associated organization fully authorize to publish research.
Rights and permissions
Reprints and permissions
About this article
Firoozi, A.A., Naji, M., Dithinde, M. et al. A Review: Influence of Potential Nanomaterials for Civil Engineering Projects. Iran J Sci Technol Trans Civ Eng 45 , 2057–2068 (2021). https://doi.org/10.1007/s40996-020-00474-x
Download citation
Received : 04 September 2019
Accepted : 25 September 2020
Published : 12 October 2020
Issue Date : December 2021
DOI : https://doi.org/10.1007/s40996-020-00474-x
Share this article
Anyone you share the following link with will be able to read this content:
Sorry, a shareable link is not currently available for this article.
Provided by the Springer Nature SharedIt content-sharing initiative
- Nanomaterials
- Microscopy techniques
- Stabilization
- Construction projects
- Find a journal
- Publish with us
- Track your research
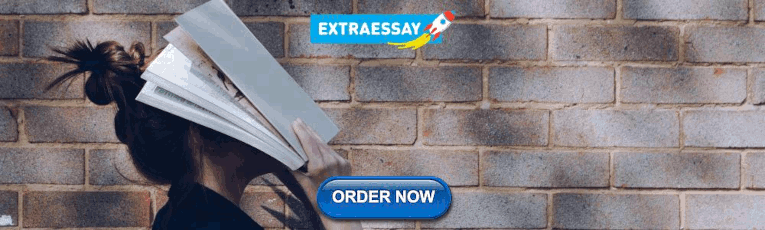
VIDEO
COMMENTS
Nanotechnology is a relatively new field of science and technology that studies tiny objects (0.1-100 nm). ... in this review, we present a thorough overview of NPs in general on their properties and therapeutic uses in different fields of science and technology, with specific emphasis on their therapeutic applications. ... The literature ...
Z. Javed Pakeeza Rubab Faiza Anum Ayesha Alam S. Raza. Agricultural and Food Sciences, Materials Science. Lahore Garrison University Journal of Life…. 2020. TLDR. This review aims to discuss the prospect of nanotechnology in the food sector and emphasize on the use of various nanomaterials for food industry. Expand.
Nanotechnology: A Review *1 Mandeep Kaur. Lecturer-ECE. 2 Gurjeevan Singh. DIC-ECE. 3 Kamal Khanna. ... Nanotechnology: A Brief Literature Review M.Ellin Doyle, Ph.D Food Research Institute ...
Nanotechnology: A Brief Literature Review M. Ellin Doyle, Ph.D. Food Research Institute, University of Wisconsin-Madison, Madison, WI 53706 [email protected] Contents
The application of nanotechnology in medicine and healthcare is referred to as nanomedicine, and it has been used to combat some of the most common diseases, including cardiovascular diseases and cancer. The present review provides an overview of the recent advances of nanotechnology in the aspects of imaging and drug delivery.
This review discusses a brief history of nanomaterials and their use throughout history to trigger advances in nanotechnology development. In particular, we describe and define various terms relating to nanomaterials. Various nanomaterial synthesis methods, including top-down and bottom-up approaches, are discussed.
Nanotechnology is a relatively new field of science and technology that studies tiny objects (0.1-100 nm). ... Nanotechnology as an alternative to improve the treatment of cutaneous leishmaniasis: A systematic review of the literature. Journal of Drug Delivery Science and Technology, Volume 75, 2022, Article 103622.
A comprehensive literature review examined nanosensors in EVs. Our study provides a solid foundation for understanding the current state of research, identifying major trends and discovering nanotechnology breakthroughs in EV sensors by carefully reviewing, characterizing and rating important papers.
History of nanotechnology. Human dreams and imagination often give rise to new science and technology. Nanotechnology, a 21st-century frontier, was born out of such dreams. Nanotechnology is defined as the understanding and control of matter at dimensions between 1 and 100 nm where unique phenomena enable novel applications. 1 Although human ...
A Literature Review of Nanotechnology. Divesh Garg. Published 2021. Materials Science, Environmental Science, Engineering. Many sectors, including information technology, electricity, environmental science, pharmacy, home security, food safety and transport allow nanotechnology to significantly improve and even revolutionise, among others.
Fig. 2 explores the various classes and categorisations of different nanotechnology-based materials having special applications and utilities in the domain of medicine. Several classes in the available literature have been reported, namely; metal nanoparticles, dendrimers, liposomes, biodegradable polymers, carbon-based nanomaterials, hydrogel nanocomposites, and many others too that support ...
Novel nano-organisms, called nanobes, are gaining interest among nanotechnology researchers as they are found during off-shore petroleum exploration on Triassic and Jurassic sandstones in Western Australia . These nanobes contains 20-150 nm diameter individual cells that are composed of a carbon, oxygen, nitrogen, DNA, membrane-bound ...
REVIEW OF LITERATURE 2.1. NANOTECHNOLOGY Nanotechnology is a multidisciplinary field, as it combines the knowledge from different disciplines: chemistry, physics, and biology amongst others ...
2.2. Nanotechnology and Computer Industry. Nanotechnology has taken its origins from microengineering concepts in physics and material sciences [].Nanoscaling is not a new concept in the computer industry, as technologists and technicians have been working for a long time to design such modified forms of computer-based technologies that require minimum space for the most efficient work.
We first review the critical role of nanotechnology in enabling cathode and anode materials of LIBs. ... several examples of Si nanostructures for anodes in LIBs have appeared in the literature 83 ...
Nevertheless, the remainder of this literature review describes in very general terms selected areas of application of nanotechnology products. In addition, Figure 2 collects the applications most frequently mentioned in the literature without distinguishing between those already on the market and those in the research phase.
Literature Review Yanlan Liu 2,3 Part of the book ... Nanotechnology is a new field of science that established on nowadays advanced scientific technology. The idea and hypothesis of nanotechnology was first suggested by a renowned American physicist Richard P. Feynman in 1959 in one of his report. As a pioneer of nanotechnology, he predicted ...
A review of the state-of-the-art of nano-technology in solar direct electricity generation systems. ... Before jumping into the literature on nano-enhanced PCMs, hereunder, some of the recent works on PVT systems equipped with PCMs and the use of nanofluids as the cooling medium are briefly reviewed. Generally, PCM is inserted under the solar ...
The application of nanotechnology for medical purposes has been termed nanomedicine and is defined as the use of nanomaterials for diagnosis, monitoring, control, prevention and treatment of diseases (Tinkle et al., 2014 ). However, the definition of nanomaterial has been controversial among the various scientific and international regulatory ...
Jeon, Nanotechnology, big things from a tiny world: a review, International Journal of u- and e-Service, Science and Technology 2(3) (2009), 29-37. [3] M. Ellin Doyle, Nanotechnology: A Brief Literature Review, FRI Briefings, 2006. [4] Mark T. Lusk and L. D. Carr, Nanoengineering defect structures on grapheme, Physical
The literature review is not claimed to be exhaustive, but the sample presents a broad range of published research papers giving ample insight into what scholars discuss when addressing the communication of nanotechnology to the public. The analysis was inspired by Carol Bacchi's (1999, 2012) approach to policy analysis. Her analytical and ...
The term "nanotechnology" is composed of the unit prefix ... At the first level, a literature review was conducted to describe and illuminate the existing status of nanoethics considerations. To this purpose, scientific databases, including PubMed, Scopus, and Web of Science, were searched using nanoethics, nanotechnology, nanomedicine ...
The development of nanotechnology and nanomaterials offers promising signs for a change in the way of construction and geotechnical projects. ... Walubita LF (2014) Application of nano-technology in pavement engineering: a literature review. In: Application of nanotechnology in pavements, geological disasters, and foundation settlement control ...