
An official website of the United States government
The .gov means it’s official. Federal government websites often end in .gov or .mil. Before sharing sensitive information, make sure you’re on a federal government site.
The site is secure. The https:// ensures that you are connecting to the official website and that any information you provide is encrypted and transmitted securely.
- Publications
- Account settings
Preview improvements coming to the PMC website in October 2024. Learn More or Try it out now .
- Advanced Search
- Journal List
- World J Diabetes
- v.6(6); 2015 Jun 25
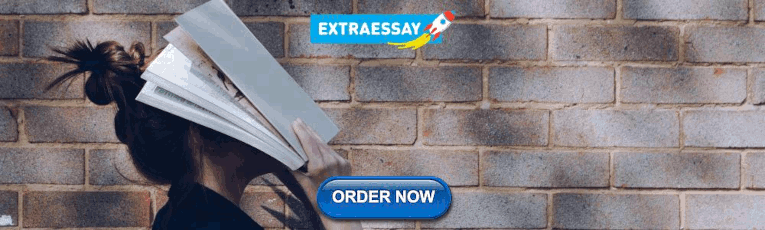
Diabetes mellitus: The epidemic of the century
Correspondence to: Akram T Kharroubi, PhD, Associate Professor of Biochemistry and Endocrinology, Dean of Faculty of Health Professions, Department of Medical Laboratory Sciences, Faculty of Health Professions, Al-Quds University, P.O. Box 51000, Abed Elhamaid Shoman Street, Beit Hanina-Jerusalem, Jerusalem 91000, Palestine. [email protected]
Telephone: +972-2-2791243 Fax: +972-2-2791243
The epidemic nature of diabetes mellitus in different regions is reviewed. The Middle East and North Africa region has the highest prevalence of diabetes in adults (10.9%) whereas, the Western Pacific region has the highest number of adults diagnosed with diabetes and has countries with the highest prevalence of diabetes (37.5%). Different classes of diabetes mellitus, type 1, type 2, gestational diabetes and other types of diabetes mellitus are compared in terms of diagnostic criteria, etiology and genetics. The molecular genetics of diabetes received extensive attention in recent years by many prominent investigators and research groups in the biomedical field. A large array of mutations and single nucleotide polymorphisms in genes that play a role in the various steps and pathways involved in glucose metabolism and the development, control and function of pancreatic cells at various levels are reviewed. The major advances in the molecular understanding of diabetes in relation to the different types of diabetes in comparison to the previous understanding in this field are briefly reviewed here. Despite the accumulation of extensive data at the molecular and cellular levels, the mechanism of diabetes development and complications are still not fully understood. Definitely, more extensive research is needed in this field that will eventually reflect on the ultimate objective to improve diagnoses, therapy and minimize the chance of chronic complications development.
Core tip: Diabetes mellitus is rising to an alarming epidemic level. Early diagnosis of diabetes and prediabetes is essential using recommended hemoglobin A1c criteria for different types except for gestational diabetes. Screening for diabetes especially in underdeveloped countries is essential to reduce late diagnosis. Diabetes development involves the interaction between genetic and non-genetic factors. Biomedical research continues to provide new insights in our understanding of the mechanism of diabetes development that is reviewed here. Recent studies may provide tools for the use of several genes as targets for risk assessment, therapeutic strategies and prediction of complications.
DEFINITION OF DIABETES MELLITUS
Diabetes mellitus is a group of metabolic diseases characterized by chronic hyperglycemia resulting from defects in insulin secretion, insulin action, or both. Metabolic abnormalities in carbohydrates, lipids, and proteins result from the importance of insulin as an anabolic hormone. Low levels of insulin to achieve adequate response and/or insulin resistance of target tissues, mainly skeletal muscles, adipose tissue, and to a lesser extent, liver, at the level of insulin receptors, signal transduction system, and/or effector enzymes or genes are responsible for these metabolic abnormalities. The severity of symptoms is due to the type and duration of diabetes. Some of the diabetes patients are asymptomatic especially those with type 2 diabetes during the early years of the disease, others with marked hyperglycemia and especially in children with absolute insulin deficiency may suffer from polyuria, polydipsia, polyphagia, weight loss, and blurred vision. Uncontrolled diabetes may lead to stupor, coma and if not treated death, due to ketoacidosis or rare from nonketotic hyperosmolar syndrome[ 1 - 3 ].
CLASSIFICATION OF DIABETES MELLITUS
Although classification of diabetes is important and has implications for the treatment strategies, this is not an easy task and many patients do not easily fit into a single class especially younger adults[ 1 , 4 - 6 ] and 10% of those initially classified may require revision[ 7 ]. The classical classification of diabetes as proposed by the American Diabetes Association (ADA) in 1997 as type 1, type 2, other types, and gestational diabetes mellitus (GDM) is still the most accepted classification and adopted by ADA[ 1 ]. Wilkin[ 8 ] proposed the accelerator hypothesis that argues “type 1 and type 2 diabetes are the same disorder of insulin resistance set against different genetic backgrounds”[ 9 ]. The difference between the two types relies on the tempo, the faster tempo reflecting the more susceptible genotype and earlier presentation in which obesity, and therefore, insulin resistance, is the center of the hypothesis. Other predictors of type 1 diabetes include increased height growth velocity[ 10 , 11 ] and impaired glucose sensitivity of β cells[ 12 ]. The implications of increased free radicals, oxidative stress, and many metabolic stressors in the development, pathogenesis and complications of diabetes mellitus[ 13 - 18 ] are very strong and well documented despite the inconsistency of the clinical trials using antioxidants in the treatment regimens of diabetes[ 19 - 21 ]. The female hormone 17-β estradiol acting through the estrogen receptor-α (ER-α) is essential for the development and preservation of pancreatic β cell function since it was clearly demonstrated that induced oxidative stress leads to β-cell destruction in ER-α knockout mouse. The ER-α receptor activity protects pancreatic islets against glucolipotoxicity and therefore prevents β-cell dysfunction[ 22 ].
TYPE 1 DIABETES MELLITUS
Autoimmune type 1 diabetes.
This type of diabetes constitutes 5%-10% of subjects diagnosed with diabetes[ 23 ] and is due to destruction of β cells of the pancreas[ 24 , 25 ]. Type 1 diabetes accounts for 80%-90% of diabetes in children and adolescents[ 2 , 26 ]. According to International Diabetes Federation (IDF), the number of youth (0-14 years) diagnosed with type 1 diabetes worldwide in 2013 was 497100 (Table (Table1) 1 ) and the number of newly diagnosed cases per year was 78900[ 27 ]. These figures do not represent the total number of type 1 diabetes patients because of the high prevalence of type 1 diabetes in adolescence and adults above 14 years of age. One reported estimate of type 1 diabetes in the United States in 2010 was 3 million[ 28 , 29 ]. The number of youth in the United States younger than 20 years with type 1 diabetes was estimated to be 166984 in the year 2009[ 30 ]. The prevalence of type 1 diabetes in the world is not known but in the United States in youth younger than 20 years was 1.93 per 1000 in 2009 (0.35-2.55 in different ethnic groups) with 2.6%-2.7% relative annual increase[ 26 , 31 ]. Type 1 diabetes is mainly due to an autoimmune destruction of the pancreatic β cells through T-cell mediated inflammatory response (insulitis) as well as a humoral (B cell) response[ 25 ]. The presence of autoantibodies against the pancreatic islet cells is the hallmark of type 1 diabetes, even though the role of these antibodies in the pathogenesis of the disease is not clear. These autoantibodies include islet cell autoantibodies, and autoantibodies to insulin (IAA), glutamic acid decarboxylase (GAD, GAD65), protein tyrosine phosphatase (IA2 and IA2β) and zinc transporter protein (ZnT8A)[ 32 ]. These pancreatic autoantibodies are characteristics of type 1 diabetes and could be detected in the serum of these patients months or years before the onset of the disease[ 33 ]. Autoimmune type 1 diabetes has strong HLA associations, with linkage to DR and DQ genes. HLA-DR/DQ alleles can be either predisposing or protective[ 1 ]. This autoimmune type 1 diabetes is characterized by the absence of insulin secretion and is more dominant in children and adolescents.
Number of subjects with type 1 diabetes in children (0-14 years), with diabetes in adults (20-79 years) and with hyperglycemia (type 2 or gestational diabetes) in pregnancy (20-49 years)
Data extracted from International Diabetes Federation Diabetes Atlas, 6th ed, 2013.
In addition to the importance of genetic predisposition in type 1 diabetes, several environmental factors have been implicated in the etiology of the disease[ 9 , 33 ]. Viral factors include congenital rubella[ 34 , 35 ], viral infection with enterovirus, rotavirus, herpes virus, cytomegalovirus, endogenous retrovirus[ 36 , 37 ] and Ljungan virus. Other factors include low vitamin D levels[ 38 ], prenatal exposure to pollutants, improved hygiene and living conditions decreased childhood infections in countries with high socioeconomic status leading to increased autoimmune diseases (hygiene hypothesis), early infant nutrition such as using cow’s milk formula instead of breast feeding[ 39 ] in addition to insulin resistance in early childhood due to obesity or increased height growth velocity. The role of environmental factors remains controversial[ 40 ]. Recent evidence supported the causative effect of viral infections in diabetes[ 41 - 43 ].
Type 1 diabetes often develops suddenly and can produce symptoms such as polydipsia, polyuria, enuresis, lack of energy, extreme tiredness, polyphagia, sudden weight loss, slow-healing wounds, recurrent infections and blurred vision[ 27 ] with severe dehydration and diabetic ketoacidosis in children and adolescents. The symptoms are more severe in children compared to adults. These autoimmune type 1 diabetes patients are also prone to other autoimmune disorders such as Graves’ disease, Hashimoto’s thyroiditis, Addison’s disease, vitiligo, celiac sprue, autoimmune hepatitis, myasthenia gravis, and pernicious anemia[ 1 ]. The complete dependence on insulin of type 1 diabetes patients may be interrupted by a honeymoon phase which lasts weeks to months or in some cases 2-3 years. In some children, the requirement for insulin therapy may drop to a point where insulin therapy could be withdrawn temporarily without detectable hyperglycemia[ 44 ].
Idiopathic type 1 diabetes
A rare form of type 1 diabetes of unknown origin (idiopathic), less severe than autoimmune type 1 diabetes and is not due to autoimmunity has been reported. Most patients with this type are of African or Asian descent and suffer from varying degrees of insulin deficiency and episodic ketoacidosis[ 45 ].
Fulminant type 1 diabetes
This is a distinct form of type 1 diabetes, first described in the year 2000, and has some common features with idiopathic type 1 diabetes being non-immune mediated[ 46 , 47 ]. It is characterized by ketoacidosis soon after the onset of hyperglycemia, high glucose levels (≥ 288 mg/dL) with undetectable levels of serum C-peptide, an indicator of endogenous insulin secretion[ 48 ]. It has been described mainly in East Asian countries and accounted for approximately 20% of acute-onset type 1 diabetes patients in Japan (5000-7000 cases) with an extremely rapid and almost complete beta-cell destruction resulting in nearly no residual insulin secretion[ 48 , 49 ]. Both genetic and environmental factors, especially viral infection, have been implicated in the disease. Anti-viral immune response may trigger the destruction of pancreatic beta cells through the accelerated immune reaction with no detectable autoantibodies against pancreatic beta cells[ 48 , 50 ]. Association of fulminant type 1 diabetes with pregnancy has also been reported[ 51 ].
TYPE 2 DIABETES MELLITUS
The global prevalence of diabetes in adults (20-79 years old) according to a report published in 2013 by the IDF was 8.3% (382 million people), with 14 million more men than women (198 million men vs 184 million women), the majority between the ages 40 and 59 years and the number is expected to rise beyond 592 million by 2035 with a 10.1% global prevalence. With 175 million cases still undiagnosed, the number of people currently suffering from diabetes exceeds half a billion. An additional 21 million women are diagnosed with hyperglycemia during pregnancy. The Middle East and North Africa region has the highest prevalence of diabetes (10.9%), however, Western Pacific region has the highest number of adults diagnosed with diabetes (138.2 millions) and has also countries with the highest prevalence (Figure (Figure1 1 )[ 27 ]. Low- and middle-income countries encompass 80% of the cases, “where the epidemic is gathering pace at alarming rates”[ 27 ]. Despite the fact that adult diabetes patients are mainly type 2 patients, it is not clear whether the reported 382 million adults diagnosed with diabetes also include type 1 diabetes patients.

Comparative prevalence of diabetes in adults (20-79 years) in countries with high prevalence (≥ 10%). Data extracted from International Diabetes Federation Diabetes Atlas, 6th ed, 2013.
More than 90%-95% of diabetes patients belong to this type and most of these patients are adults. The number of youth (less than 20 years) with type 2 diabetes in the United States in the year 2009 was 0.46 in 1000 and accounted for approximately 20% of type 2 diabetes in youth[ 26 ]. The increased incidence of type 2 diabetes in youth is mainly due to the change in the lifestyle of the children in terms of more sedentary life and less healthy food. Obesity is the major reason behind insulin resistance which is mainly responsible for type 2 diabetes[ 52 - 54 ]. The ADA recommends screening of overweight children and adolescence to detect type 2 diabetes[ 55 , 56 ]. The prevalence of obesity in children in on the rise[ 6 ] which is probably the main reason for the increased incidence of type 2 diabetes in the young (30.3% overall increase in type 2 diabetes in children and adolescence between 2001 and 2009)[ 26 ].
Insulin resistance in type 2 diabetes patients increases the demand for insulin in insulin-target tissues. In addition to insulin resistance, the increased demand for insulin could not be met by the pancreatic β cells due to defects in the function of these cells[ 18 ]. On the contrary, insulin secretion decreases with the increased demand for insulin by time due to the gradual destruction of β cells[ 57 ] that could transform some of type 2 diabetes patients from being independent to become dependent on insulin. Most type 2 diabetes patients are not dependent on insulin where insulin secretion continues and insulin depletion rarely occurs. Dependence on insulin is one of the major differences from type 1 diabetes. Other differences include the absence of ketoacidosis in most patients of type 2 diabetes and autoimmune destruction of β cells does not occur. Both type 1 and type 2 diabetes have genetic predisposition, however, it is stronger in type 2 but the genes are more characterized in type 1 (the TCF7L2 gene is strongly associated with type 2 diabetes)[ 58 ]. Due to the mild symptoms of type 2 diabetes in the beginning, its diagnosis is usually delayed for years especially in countries where regular checkup without symptoms is not part of the culture. This delay in diagnosis could increase the incidence of long-term complications in type 2 diabetes patients since hyperglycemia is not treated during this undiagnosed period.
In addition to diabetes, insulin resistance has many manifestations that include obesity, nephropathy, essential hypertension, dyslipidemia (hypertriglyceridemia, low HDL, decreased LDL particle diameter, enhanced postprandial lipemia and remnant lipoprotein accumulation), ovarian hyperandrogenism and premature adrenarche, non-alcoholic fatty liver disease and systemic inflammation[ 6 , 54 ]. The presence of type 2 diabetes in children and adolescence who are not obese[ 59 - 61 ], the occasional severe dehydration and the presence of ketoacidosis in some pediatric patients with type 2 diabetes[ 55 ] had led to the misclassification of type 2 to type 1 diabetes.
Some patients with many features of type 2 diabetes have some type 1 characteristics including the presence of islet cell autoantibodies or autoantibodies to GAD65 are classified as a distinct type of diabetes called latent autoimmune diabetes in adults (LADA)[ 62 ]. People diagnosed with LADA do not require insulin treatment. In a recent study, Hawa et al[ 63 ] reported 7.1% of European patients with type 2 diabetes with a mean age of 62 years, tested positive for GAD autoantibodies and the prevalence of LADA was higher in patients diagnosed with diabetes at a younger age. This classification of LADA as a distinct type of diabetes is still controversial[ 6 , 64 - 66 ].
Insulin resistance and signaling
Defects in the insulin-dependent substrate proteins IRS-1 and IRS-2 mediated signaling pathway are implicated in the development of metabolic disorders, mainly diabetes. This pathway mediates the cellular response to insulin and involves a large array of insulin-stimulated protein kinases including the serine/threonine kinase AKT and protein kinase C (PKC) that phosphorylate a large number of Ser/Thr residues in the insulin receptor substrate (IRS) proteins involved in the metabolic response to insulin[ 67 ]. In addition, other non-insulin dependent kinases including the AMP-activated protein kinase, c-Jun N-terminal protein kinase and G protein-coupled receptor kinase 2 that are activated under various conditions can phosphorylate the two insulin responsive substrates[ 67 - 71 ]. Disruption in the AKT and PKC kinases is central to the development of diabetes[ 72 ] and is associated with all major features of the disease including hyperinsulinemia, dyslipidemia and insulin resistance[ 73 ]. Replacing the wild type IRS-1 with a mutant version of the protein having alanine instead of tyrosine in three locations using genetic knock-in approach provided evidence to the central role of IRS-1 phosphorylation in the development of insulin resistance[ 74 ]. Using a similar approach to generate IRS-1 mutant with a single mutation involving a specific tyrosine residue, confirmed the role of IRS-1 phosphorylation in the development of insulin resistance pathogenesis[ 75 ]. The large cumulative evidence indicates a complex array of factors including environmental factors[ 76 ] and a wide range of cellular disturbances in glucose and lipid metabolism in various tissues[ 77 ] contribute to the development of insulin resistance. This condition generates complex cellular metabolic changes in a variety of tissues, mainly liver and muscles, that include the inability of the liver to transport and dispose glucose, control glucose production via gluconeogenesis, impaired storage of glucose as glycogen, de novo lipogenesis and hypertriglyceridemia[ 77 ]. Among the factors implicated in the development of insulin resistance, obesity is the most predominant risk factor leading to insulin insensitivity and diabetes which involves several mechanisms that participate in the pathogenesis of the disease[ 78 ]. Obesity-induced insulin resistance is directly linked to increased nutrient flux and energy accumulation in tissues that directly affect cell responsiveness to insulin[ 77 ]. However, it seems that other insulin-independent mechanisms are involved in the overall metabolic disturbances of glucose homeostasis and diabetes including activities in extra-hepatic tissues in addition to the central role of liver.
OTHER TYPES OF DIABETES MELLITUS
Monogenic diabetes.
Characterization of the genetic etiology of diabetes enables more appropriate treatment, better prognosis, and counseling[ 79 ]. Monogenic diabetes is due to a genetic defect in single genes in pancreatic β cells which results in disruption of β cell function or a reduction in the number of β cells. Conventionally, monogenic diabetes is classified according to the age of onset as neonatal diabetes before the age of six months or Maturity Onset Diabetes of the Young (MODY) before the age of 25 years. However, certain familial defects are manifested in neonatal diabetes, MODY or adult onset diabetes[ 2 , 9 , 80 ]. Others believe that classification of diabetes as MODY and neonatal diabetes is obsolete and monogenic diabetes is currently used relating specific genetic etiologies with their specific treatment implications[ 79 ]. Beta cell differentiation depends on the expression of the homeodomain transcription factor PDX1 where mutation in the gene results in early onset diabetes (MODY) and its expression decreases before the onset of diabetes[ 81 ]. The angiopoietin-like protein 8 (ANGPTL8) may represent a potential “betatrophin” that acts to promote the proliferation of beta cells, however, studies using mice lacking the ANGPTL8 active gene or overexpressed protein indicated that it did not seem to play a role in beta cells proliferation[ 82 ].
Mitochondrial diabetes is due to a point mutation in the mitochondrial DNA associated with deafness and maternal transmission of the mutant DNA can result in maternally-inherited diabetes[ 1 , 83 ].
Mutations that result in mutant insulin or the inability to convert proinsulin to insulin result in glucose intolerance in some of these cases. Genetic defects in the insulin receptor or in the signal transduction pathway of insulin have been demonstrated to result in hyperinsulinemia and modest hyperglycemia to severe diabetes[ 1 ].
Disease of the exocrine pancreas
Damage of the β cells of the pancreas due to diffused injury of the pancreas can cause diabetes. This damage could be due to pancreatic carcinoma, pancreatitis, infection, pancreatectomy, and trauma[ 1 ]. Atrophy of the exocrine pancreas leads to progressive loss of the β cells[ 84 ]. Accumulation of fat in the pancreas or pancreatic steatosis could lead to diabetes due to decreased insulin secretion but may require a long time before the damage to β cells occurs[ 85 ]. In most cases, extensive damage of the pancreas is required before diabetes occurs and the exocrine function of the pancreas is decreased in these patients[ 86 ]. Cirrhosis in cystic fibrosis may contribute to insulin resistance and diabetes[ 2 ].
Hormones and drugs
Diabetes has been found in patients with endocrine diseases that secrete excess hormones like growth hormone, glucocorticoids, glucagon and epinephrine in certain endocrinopathies like acromegaly, Cushing’s syndrome, glucagonoma, and pheochromocytoma, respectively[ 1 ]. Some of these hormones are used as drugs such as glucocorticoids to suppress the immune system and in chemotherapy and growth hormone to treat children with stunted growth.
Genetic syndromes
Diabetes has been detected in patients with various genetic syndromes such as Down syndrome, Klinefelter syndrome, Turner syndrome and Wolfram syndrome[ 1 ].
PREDIABETES
Individuals with prediabetes do not meet the criteria of having diabetes but are at high risk to develop type 2 diabetes in the future. According to the ADA Expert Committee, individuals are defined to have prediabetes if they have either impaired fasting plasma glucose (IFG) levels between 100-125 mg/dL (5.6-6.9 mmol/L) or impaired glucose tolerance test (IGT) with 2-h plasma glucose levels in the oral glucose tolerance test (OGTT) of 140-199 mg/dL (7.8-11.0 mmol/L). The World Health Organization (WHO) still adopts the range for IFG from 110-125 mg/dL (6.1-6.9 mmol/L). Prediabetes has been shown to correlate with increased cardiovascular mortality[ 87 , 88 ] and cancer[ 89 ]. The definition of prediabetes with the indicated cut off values is misleading since lower levels of glucose in the normal range are still correlated with cardiovascular disease in a continuous glycemic risk perspective[ 90 ]. In accordance with the recommendation of the ADA in 2009 to use hemoglobin A1c (HbA1c) to diagnose diabetes, ADA also recommended the use of an HbA1c (5.7%-6.4%) to diagnose prediabetes[ 91 ]. The number of people with IGT according to IDF was 316 million in 2013 (global prevalence 6.9% in adults) and is expected to rise to 471 million in 2030[ 27 ]. According to a report in 2014 by the Center for Disease Control and Prevention, 86 million Americans (1 out of 3) have prediabetes[ 92 ]. Four of the top ten countries with the highest prevalence of prediabetes are in the Middle East Arab States of the Gulf (Kuwait, Qatar, UAE and Bahrin with prevalence of 17.9%, 17.1%, 16.6% and 16.3%, respectively)[ 27 ]. The number of people diagnosed with prediabetes is different according to the method and criteria used to diagnose prediabetes. The number of people with prediabetes defined by IFG 100-125 mg/dL is 4-5 folds higher than those diagnosed using the WHO criteria of 110-125 mg/dL[ 93 ]. Diabetes and prediabetes diagnosed using an HbA1c criteria give different estimates compared to methods using FPG or OGTT. Higher percentages of prediabetes were diagnosed using HbA1c compared to FPG[ 94 - 96 ]. Prediabetes is associated with metabolic syndrome and obesity (especially abdominal or visceral obesity), dyslipidemia with high triglycerides and/or low HDL cholesterol, and hypertension[ 97 ]. Not all individuals with prediabetes develop diabetes in the future, exercise with a reduction of weight 5%-10% reduces the risk of developing diabetes considerably (40%-70%)[ 98 ]. Individuals with an HbA1c of 6.0%-6.5% have twice the risk of developing diabetes (25%-50%) in five years compared to those with an HbA1c of 5.5%-6.0%[ 99 ].
DIAGNOSTIC CRITERIA FOR DIABETES MELLITUS
Diabetes mellitus is diagnosed using either the estimation of plasma glucose (FPG or OGTT) or HbA1c. Estimation of the cut off values for glucose and HbA1c is based on the association of FPG or HbA1c with retinopathy. Fasting plasma glucose of ≥ 126 mg/dL (7.0 mmol/L), plasma glucose after 2-h OGTT ≥ 200 mg/dL (11.1 mmol/L), HbA1c ≥ 6.5% (48 mmol/mol) or a random plasma glucose ≥ 200 mg/dL (11.1 mmol/L) along with symptoms of hyperglycemia is diagnostic of diabetes mellitus. In addition to monitor the treatment of diabetes, HbA1c has been recommended to diagnose diabetes by the International Expert Committee in 2009[ 100 ] and endorsed by ADA[ 101 ], the Endocrine Society, the WHO[ 102 ] and many scientists and related organizations all over the world. The advantages and disadvantages of the different tests used to diagnose diabetes have been reviewed by Sacks et al[ 103 ]. The advantages of using HbA1c over FPG to diagnose diabetes include greater convenience and preanalytical stability, lower CV (3.6%) compared to FPG (5.7%) and 2h OGTT (16.6%), stronger correlation with microvascular complications especially retinopathy, and a marker for glycemic control and glycation of proteins which is the direct link between diagnosis of diabetes and its complications[ 104 - 109 ]. It is recommended to repeat the HbA1c test in asymptomatic patients within two weeks to reaffirm a single apparently diagnostic result[ 110 ].
A cut off value for HbA1c of ≥ 6.5% (48 mmol/mol) has been endorsed by many countries and different ethnic groups, yet ethnicity seems to affect the cut off values to diagnose diabetes[ 111 , 112 ]. Cut-off values of 5.5% (37 mmol/mol)[ 113 ] and 6.5% (48 mmol/mol)[ 114 ] have been reported in a Japanese study, 6.0% (42 mmol/mol) in the National Health and Nutrition Examination Survey (NHANES III), 6.2% (44 mmol/mol) in a Pima Indian study, 6.3% (45 mmol/mol) in an Egyptian study as reported by Davidson[ 105 ]; and three cut-off values for Chinese[ 112 ]. The Australians recommended the use of two cut-off values: ≤ 5.5% to “rule-out” and ≥ 7.0% to “rule-in” diabetes[ 115 ]. Variations in the prevalence of diabetes[ 94 , 116 - 119 ] and prediabetes[ 120 ] due to ethnicity have been documented. Most studies diagnosed less subjects with diabetes using HbA1c compared to FPG or OGTT[ 121 - 123 ]. Yet, other studies reported more subjects diagnosed with diabetes using HbA1c[ 96 , 124 - 126 ].
GESTATIONAL DIABETES
Hyperglycemia in pregnancy whether in the form of type 2 diabetes diagnosed before or during pregnancy or in the form gestational diabetes has an increased risk of adverse maternal, fetal and neonatal outcome. Mothers with gestational diabetes and babies born to such mothers have increased risk of developing diabetes later in life. Hyperglycemia in pregnancy is responsible for the increased risk for macrosomia (birth weight ≥ 4.5 kg), large for gestational age births, preeclampsia, preterm birth and cesarean delivery due to large babies[ 127 ]. Risk factors for gestational diabetes include obesity, personal history of gestational diabetes, family history of diabetes, maternal age, polycystic ovary syndrome, sedentary life, and exposure to toxic factors[ 3 ].
Diagnosis of type 2 diabetes before or during pregnancy is based on criteria mentioned before. Fasting plasma glucose ≥ 126 mg/dL (7.0 mmol/L) or 2-h plasma glucose ≥ 200 mg/dL (11.1 mmol/L) after a 75 g oral glucose load. However, gestational diabetes has been diagnosed at 24-28 wk of gestation in women not previously diagnosed with diabetes using two approaches: the first approach is based on the “one-step” International Association of the Diabetes and Pregnancy Study Groups (IADPSG) consensus[ 128 ] and recently adopted by WHO[ 129 ]. Gestational diabetes is diagnosed using this method by FPG ≥ 92 mg/dL (5.1 mmol/L), 1-h plasma glucose after a 75 g glucose load ≥ 180 mg/dL (10.0 mmol/L) or 2-h plasma glucose after a 75 g glucose load ≥ 153 mg/dL (8.5 mmol/L). This criteria is derived from the Hyperglycemia and Adverse Pregnancy Outcome (HAPO) study[ 127 ] even though the HAPO study showed a continuous relationship between hyperglycemia and adverse short-term pregnancy outcome with no threshold reported[ 130 ]. The second approach is used in the United States and is based on the “two-step” NIH consensus[ 131 ]. In the first step 1-h plasma glucose after a 50 g glucose load under nonfasting state ≥ 140 mg/dL (7.8 mmol/L) is followed by a second step under fasting conditions after a 100 g glucose load for those who screened abnormal in the first step. The diagnosis of gestational diabetes is made when at least two of the four plasma glucose levels are met. The four plasma glucose levels according to Carpenter/Coustan criteria are: FPG ≥ 95 mg/dL (5.3 mmol/L); 1-h ≥ 180 mg/dL (10.0 mmol/L); 2-h ≥ 155 mg/dL (8.6 mmol/L); and 3-h ≥ 140 mg/dL (7.8 mmol/L)[ 1 ].
The use IADPSC criteria in comparison with the Carpenter/Coustan criteria was associated with a 3.5-fold increase in GDM prevalence as well as significant improvements in pregnancy outcomes, and was cost-effective[ 132 ]. In another retrospective cohort study of women diagnosed with gestational diabetes, Ethridge et al[ 133 ] have shown that newborns of women diagnosed with gestational diabetes by IADPSG approach have greater measures of fetal overgrowth compared with Carpenter-Coustan “two-step” approach neonates. A strategy of using fasting plasma glucose as a screening test and to determine the need for OGTT is valid[ 134 , 135 ]. According to Sacks[ 136 ], correlation of glucose concentrations and the risk of subsequent complications will eventually lead to universal guidelines.
The use of ADA/WHO cut off value of HbA1c ≥ 6.5% (48 mmol/mol) to diagnose gestational diabetes is not recommended by the “one step” IADPSC criteria or the “two-step” NIH criteria. Further investigation is required in light of recent reports on HbA1c in combination with OGTT and its usefulness to predict adverse effect of gestational diabetes or obviate the use OGTT in all women with gestational diabetes[ 137 - 141 ].
DIABETES AND GENETICS
Diabetes is a complex disease that involves a wide range of genetic and environmental factors. Over the past several years, many studies have focused on the elucidation of the wide spectrum of genes that played a role in the molecular mechanism of diabetes development[ 142 - 144 ]. However, despite the vast flow of genetic information including the identification of many gene mutations and a large array of single nucleotide polymorphisms (SNPs) in many genes involved in the metabolic pathways that affect blood glucose levels, the exact genetic mechanism of diabetes remains elusive[ 145 , 146 ]. Evidently, a major complication is the fact that a single gene mutation or polymorphism will not impose the same effect among different individuals within a population or different populations. This variation is directly or indirectly affected by the overall genetic background at the individual, family or population levels that are potentially further complicated by interaction with highly variable environmental modifier factors[ 147 , 148 ].
Molecular genetics and type 2 diabetes
One of the major focuses of biomedical research is to delineate the collective and broad genetic variants in the human genome that are involved in the development of diabetes. This major effort will potentially provide the necessary information to understand the molecular genetics of the different forms of diabetes including type 1, type 2 and monogenic neonatal diabetes among individuals of all populations and ethnic groups. Despite the fact that linkage and association studies allowed the identification and characterization of many candidate genes that are associated with type 2 diabetes[ 144 , 149 , 150 ], however, not all of these genes showed consistent and reproducible association with the disease[ 151 ]. Genome wide association studies (GWAS) in various populations identified 70 loci associated with type 2 diabetes and revealed positive linkage of many mutations and SNPs that influence the expression and physiological impact of the related proteins and risk to develop type 2 diabetes. One study involved several thousand type 2 diabetes patients and control subjects from the United Kingdom allowed the identification of several diabetes putative loci positioned in and around the CDKAL1 , CDKN2A/B , HHEX/IDE and SLC30A8 genes in addition to the contribution of a large number of other genetic variants that are involved in the development of the disease[ 152 ]. Two similar studies from the Finns and Swedish populations and the United States resulted in the identification of similar single nucleotide variants[ 153 ] that are linked to the risk of acquiring type 2 diabetes[ 154 , 155 ]. The study in the United States population included in addition to type 2 diabetes, the association of the identified SNPs with the level of triglycerides in the tested subjects[ 155 ]. These SNPs are located near several candidate genes including IGFBP2 and CDKAL1 and other genes in addition to several other variants that are located near or in genes firmly associated with the risk of acquiring type 2 diabetes. Other GWAS analysis studies were performed in the Chinese, Malays, and Asian-Indian populations which are distinct from the European and United States populations in addition to meta-analysis of data from other populations in the region revealed relevant findings among patients with European ancestry[ 156 ]. The results of the combined analysis showed significant association of SNPs in the CDKAL1 , CDKN2A/B , HHEX , KCNQ1 and SLC30A8 genes after adjustment with gender and body mass index. More recently, meta-analysis of GWAS data involving African American type 2 diabetes patients identified similar loci to the previous studies with the addition of two novel loci, HLA-B and INS-IGF[ 157 ]. These results provide strong evidence of common genetic determinants including common specific genes that are linked to diabetes. A small list of specific genetic markers seem strongly associated with the risk of developing type 2 diabetes including the TCF7L2 [ 158 ] and CAPN10 [ 159 , 160 ] genes which also play a significant role in the risk and pathogenesis of the disease[ 158 , 159 ]. The association of TCF7L2 gene variants with type 2 diabetes and its mechanism of action received special attention by several investigators[ 161 , 162 ]. Over expression of the protein was shown to decrease the sensitivity of beta islet cells to secrete insulin[ 163 , 164 ] and was more precisely involved in the regulation of secretary granule fusion that constitute a late event in insulin secretion pathway[ 165 ]. The role of TCF7L2 in insulin secretion was partially clarified[ 166 ] that involves modifying the effect of incretins on insulin secretion by lowering the sensitivity of beta cells to incretins. Several other genes have been found to be significantly associated with the risk of developing type 2 diabetes including a specific SNP in a hematopoietically-expressed homeobox ( HHEX ) gene[ 167 ]. The islet zinc transporter protein (SLC30A8)[ 168 ] showed positive correlation with the risk of developing type 2 diabetes where variant mutations in this gene seem protective against the disease which provides a potential tool for therapy[ 169 ]. More recently, a low frequency variant of the HNF1A identified by whole exome sequencing was associated with the risk of developing type 2 diabetes among the Latino population and potentially may serve as a screening tool[ 170 ]. Genetic variants and specific combined polymorphisms in the interleukin and related genes including interlukin-6 ( IL-6 ), tumor necrosis factor-α and IL-10 genes were found to be associated with greater risk of developing type 2 diabetes[ 171 ], in addition to genetic variants in the genes for IL12B , IL23R and IL23A genes[ 172 ]. In a study involving the hormone sensitive lipase responsible for lipolysis in adipose tissues, a deletion null mutation, which resulted in the absence of the protein from adipocytes, was reported to be associated with diabetes[ 173 ]. Nine specific rare variants in the peroxisome proliferator-activated receptor gamma ( PPARG ) gene that resulted in loss of the function of the protein in adipocytes differentiation, were significantly associated with the risk of developing type 2 diabetes[ 174 ]. In addition, certain SNPs in the alpha 2A adrenergic receptor ( ADRA2A ) gene, involved in the sympathetic nervous system control of insulin secretion and lipolysis, were found to be associated with obesity and type 2 diabetes[ 175 ]. Link analysis between the melatonin MT2 receptor ( MTNR1B ) gene, a G-protein coupled receptor, identified 14 mutant variants from 40 known variants revealed by exome sequencing, to be positively linked with type 2 diabetes[ 176 ]. The authors suggested that mutations in the MT2 gene could provide a tool with other related genes in modifying therapy for type 2 diabetes patients based on their specific genetic background to formulate personalized therapies which potentially may ensures the optimum response. Interestingly, mutations in the clock[ 177 , 178 ] and Bmal1 [ 179 ] transcription factor genes which are involved in beta cells biological clock affecting growth, survival and synaptic vesicle assembly in these cells, resulted in reduced insulin secretion and diabetes. Evidently, prominent metabolic functions involve the production of specific reactive metabolites, leading to oxidative stress, which affect lipids, proteins and other biological compounds leading to serious damage in various tissues and organs. Mutations and SNPs in the antioxidant genes, including superoxide dismutase, catalase and glutathione peroxidase, that decrease their activity are implicated in the risk and pathogenesis of type 2 diabetes[ 180 ]. The metabolic syndrome was shown to be associated with the development of type 2 diabetes in a population that is described as highly endogenous especially in individuals over 45 years of age[ 181 ]. Since consanguinity marriages is high in this population, screening for this syndrome among families could provide an informative marker on the risk of developing type 2 diabetes[ 181 ].
Molecular genetics of type 1 diabetes
Even though type 1 diabetes is basically described as an autoimmune disease that results in the destruction of pancreatic beta cells, however, single gene mutations and SNPs have been found to be associated with the susceptibility to this type of diabetes. Initially, two gene mutations were linked to the development of type 1 diabetes including the autoimmune regulator ( AIRE ) gene which affect the immune tolerance to self antigens leading to autoimmunity[ 182 ] and the FOXP3 gene which results in defective regulatory T cells[ 183 ]. In addition, a mutation in the histone deacetylase SIRTI gene predominantly expressed in beta cells involved in the regulation of insulin secretion[ 184 ] and played a role in modulating the sensitivity of peripheral tissues to insulin[ 185 ] was detected in type 1 diabetes patients[ 186 ]. Recently, additional mutations and SNPs in the CTLA-4 +49A/G and HLA-DQB1 and INS gene VNTR alleles were found to be associated with type 1 diabetes, which have the advantage of differentiating between Latent autoimmune type 1 diabetes and type 2 diabetes[ 187 ]. The HLA-DQB1, in combination with HLA-DR alleles and a polymorphism in PTPN22 gene seem to be associated with the age onset of late type 1 diabetes[ 188 , 189 ]. Two specific polymorphisms in the promoter region of a transmembrane protein (DC-SIGN) gene expressed in macrophages and played an important role of T- cell activation and inflammation were found to be protective against type 1 diabetes[ 190 ]. An innovative non-parametric SNP enrichment tool using summary GWAS DATA allowed the identification of association between several transcription factors and type 1 diabetes and are located in a type 1 diabetes susceptibility region[ 191 ]. Nine SNP variants in several genes associated with type 1 diabetes, not including the major histocompatibility gene region, were identified using extensive GWAS analysis[ 192 ]. Furthermore, several novel SNPs in a region in chromosome 16 located in the CLEC16A gene were shown to be associated with type 1 diabetes and seem to function through the reduced expression of DEX1 in B lymphoblastoid cells[ 193 ]. Since more than 40 regions in the human genome were identified to be associated with the susceptibility to type 1 diabetes[ 194 - 196 ], a weighted risk model was developed utilizing selected genes SNPs could be used for testing infants for these genetic markers that could provide insights in the susceptibility to type 1 diabetes development or safe prevention of the disease among young children[ 197 ].
Molecular genetics of monogenic diabetes
A large array of genes were identified to be involved in the development of monogenic diabetes[ 80 ] which represent about 2%-5% of diabetes patients. Monogenic diabetes results primarily from gene defects that lead to a decrease in beta cell number or function. Monogenic diabetes genes were identified using linkage studies or code for proteins that directly affected glucose homeostasis. The majority of genes responsible for monogenetic diabetes code for either transcription factors that participate in the control of nuclear gene expression or proteins that are located on the cell membrane, cytoplasm and endoplasmic reticulum, proteins involved in insulin synthesis and secretion, exocrine pancreatic proteins and autoimmune diabetes proteins[ 80 ]. The collective function of these proteins is their participation in glucose metabolism at different levels. Evidently, the hierarchy of a specific gene in the overall glucose metabolism pathway determines the onset of diabetes in the patient and whether it is neonataly expressed or have late onset expression (adulthood). Consequently, molecular defects in the structure and function of these genes lead to the disturbance of plasma glucose level, the primary pathological sign of diabetes. The molecular mechanism of permanent neonatal diabetes mellitus (PNDP) in addition to MODY explains the observed phenotype of monogenetic diabetes that involves loss of function of the expressed mutant protein. The first gene implicated in monogenic diabetes was the glucokinase ( GCK ) gene[ 198 ] which functions as a pancreatic sensor for blood glucose where more than 70 mutations in the gene were identified that affected its activity[ 199 ]. A recent study on GCK gene mutations causing neonatal and childhood diabetes showed that the majority of mutations resulted in the loss of the enzyme function primarily due to protein instability[ 148 , 150 ]. Two hepatocytes nuclear factor genes that code for the HNF4A and HNF1A transcription factors were closely associated with MODY1 and MODY2[ 148 , 149 ]. Definitely, a whole list of other genes involved in monogenic diabetes are either overlooked or included in the genetic determinants of type 1 and type 2 diabetes which will be identified and clarified through more careful future studies.
MOLECULAR GENETICS OF DIABETES COMPLICATIONS
In addition to the genetic determinants of diabetes, several gene mutations and polymorphisms have been associated with the clinical complications of diabetes. The cumulative data on diabetes patients with a variety of micro- and macrovascular complications support the presence of strong genetic factors involved in the development of various complications[ 200 ]. A list of genes have been reported that are associated with diabetes complications including ACE and AKR1B1 in nephropathy, VEGF and AKRB1 in retinopathy and ADIPOQ and GLUL in cardiovascular diseases[ 200 ]. A study on Chinese patients revealed a single SNP in the promoter region of the smooth muscle actin ( ACTA2 ) gene correlates with the degree of coronary artery stenosis in type 2 diabetes patients[ 201 ]. Furthermore, the alpha kinase 1 gene ( ALPK1 ) identified as a susceptibility gene for chronic kidney disease by GWAS[ 202 ], was demonstrated in type 2 diabetes patients[ 203 ]. Three additional genes have been strongly correlated with this risk of diabetic retinopathy (DR) including the vascular endothelial growth receptor, aldose reductase and the receptor for advanced glycation products genes[ 204 ] where specific polymorphisms in these genes seem to increase the risk of DR development in diabetes patients[ 204 ]. A significant differential proteome (involving 56 out of 252 proteins) is evident that characterizes vitreous samples obtained from diabetes patients with the complication in comparison to diabetes patients without the complication and control individuals[ 205 ]. Interestingly, a large portion of these proteins (30 proteins) belong to the kallikrein-kinin, coagulation and complement systems including complement C3, complement factor 1, prothrombin, alpha-1-antitrypsin and antithrombin III that are elevated in diabetic patients with retinopathy[ 205 ]. In addition, 2 single nucleotides polymorphisms in the human related B7-I gene seem to mediate podocyte injury in diabetic nephropathy[ 206 ]. Furthermore, increased concentration of the ligand of B7-1 correlates with the progression of end-stage renal disease (ESRD) in diabetes patients[ 206 ]. These results indicate that B7-I inhibition may serve as a potential target for diabetes nephropathy prevention and/or treatment. Recently, it was shown that direct correlation is evident between circulating levels of tumor necrosis factors 1 and 2 and increased risk of ESRD in American Indian patients[ 207 ]. The link between diabetes and proper bone development and health is evident. Studies using animal models with major significant reduction in insulin receptor (IR) in osteoprogenitor cells resulted in thin and rod-like weak bones with high risk of fractures[ 208 ]. Similar findings were observed in animal models with bone-specific IR knockdown animals which points to the central role of IR in the proper development of bones[ 208 ]. Type 2 diabetes is also associated with mitochondrial dysfunction in adipose tissues. Using knockout animal models of specific mitochondrial genes led to significant reduction in key electron transport complexes expression and eventually adipocytes death[ 209 ]. These animals exhibited Insulin resistance in addition to other complications that can potentially lead to cardiovascular disease[ 209 ].
Diabetes mellitus is the epidemic of the century and without effective diagnostic methods at an early stage, diabetes will continue to rise. This review focuses on the types of diabetes and the effective diagnostic methods and criteria to be used for diagnosis of diabetes and prediabetes. Evidently, diabetes is a complex disease with a large pool of genes that are involved in its development. The precise identification of the genetic bases of diabetes potentially provides an essential tool to improve diagnoses, therapy (more towards individualized patient targeted therapy) and better effective genetic counseling. Furthermore, our advanced knowledge of the association between medical genetics and the chronic complications of diabetes, will provide an additional advantage to delay or eradicate these complications that impose an immense pressure on patient’s quality of life and the significantly rising cost of health-care services.
Conflict-of-interest: The authors declare that there is no conflict of interest associated with this manuscript.
Open-Access: This article is an open-access article which was selected by an in-house editor and fully peer-reviewed by external reviewers. It is distributed in accordance with the Creative Commons Attribution Non Commercial (CC BY-NC 4.0) license, which permits others to distribute, remix, adapt, build upon this work non-commercially, and license their derivative works on different terms, provided the original work is properly cited and the use is non-commercial. See: http://creativecommons.org/licenses/by-nc/4.0/
Peer-review started: November 23, 2014
First decision: February 7, 2015
Article in press: April 14, 2015
P- Reviewer: Hegardt FG, Surani S, Traub M S- Editor: Gong XM L- Editor: A E- Editor: Wang CH
- Reference Manager
- Simple TEXT file
People also looked at
Hypothesis and theory article, type 2 diabetes mellitus: a pathophysiologic perspective.
- Department of Medicine, Duke University, Durham, NC, United States
Type 2 Diabetes Mellitus (T2DM) is characterized by chronically elevated blood glucose (hyperglycemia) and elevated blood insulin (hyperinsulinemia). When the blood glucose concentration is 100 milligrams/deciliter the bloodstream of an average adult contains about 5–10 grams of glucose. Carbohydrate-restricted diets have been used effectively to treat obesity and T2DM for over 100 years, and their effectiveness may simply be due to lowering the dietary contribution to glucose and insulin levels, which then leads to improvements in hyperglycemia and hyperinsulinemia. Treatments for T2DM that lead to improvements in glycemic control and reductions in blood insulin levels are sensible based on this pathophysiologic perspective. In this article, a pathophysiological argument for using carbohydrate restriction to treat T2DM will be made.
Introduction
Type 2 Diabetes Mellitus (T2DM) is characterized by a persistently elevated blood glucose, or an elevation of blood glucose after a meal containing carbohydrate ( 1 ) ( Table 1 ). Unlike Type 1 Diabetes which is characterized by a deficiency of insulin, most individuals affected by T2DM have elevated insulin levels (fasting and/or post glucose ingestion), unless there has been beta cell failure ( 2 , 3 ). The term “insulin resistance” (IR) has been used to explain why the glucose levels remain elevated even though there is no deficiency of insulin ( 3 , 4 ). Attempts to determine the etiology of IR have involved detailed examinations of molecular and intracellular pathways, with attribution of cause to fatty acid flux, but the root cause has been elusive to experts ( 5 – 7 ).

Table 1 . Definition of type 2 diabetes mellitus.
How Much Glucose Is in the Blood?
Keeping in mind that T2DM involves an elevation of blood glucose, it is important to understand how much glucose is in the blood stream to begin with, and then the factors that influence the blood glucose—both exogenous and endogenous factors. The amount of glucose in the bloodstream is carefully controlled—approximately 5–10 grams in the bloodstream at any given moment, depending upon the size of the person. To calculate this, multiply 100 milligrams/deciliter × 1 gram/1,000 milligrams × 10 deciliters/1 liter × 5 liters of blood. The “zeros cancel” and you are left with 5 grams of glucose if the individual has 5 liters of blood. Since red blood cells represent about 40% of the blood volume, and the glucose is in equilibrium, there may be an extra 40% glucose because of the red blood cell reserve ( 8 ). Adding the glucose from the serum and red blood cells totals about 5–10 grams of glucose in the entire bloodstream.
Major Exogenous Factors That Raise the Blood Glucose
Dietary carbohydrate is the major exogenous factor that raises the blood glucose. When one considers that it is common for an American in 2021 to consume 200–300 grams of carbohydrate daily, and most of this carbohydrate is digested and absorbed as glucose, the body absorbs and delivers this glucose via the bloodstream to the cells while attempting to maintain a normal blood glucose level. Thinking of it in this way, if 200–300 grams of carbohydrates is consumed in a day, the bloodstream that holds 5–10 grams of glucose and has a concentration of 100 milligrams/deciliter, is the conduit through which 200,000–300,000 milligrams (200 grams = 200,000 milligrams) passes over the course of a day.
Major Endogenous Factors That Raise the Blood Glucose
There are many endogenous contributors that raise the blood glucose. There are at least 3 different hormones that increase glucose levels: glucagon, epinephrine, and cortisol. These hormones increase glucose levels by increasing glycogenolysis and gluconeogenesis ( 9 ). Without any dietary carbohydrate, the normal human body can generate sufficient glucose though the mechanism of glucagon secretion, gluconeogenesis, glycogen storage and glycogenolysis ( 10 ).
Major Exogenous Factors That Lower the Blood Glucose
A reduction in dietary carbohydrate intake can lower the blood glucose. An increase in activity or exercise usually lowers the blood glucose ( 11 ). There are many different medications, employing many mechanisms to lower the blood glucose. Medications can delay sucrose and starch absorption (alpha-glucosidase inhibitors), slow gastric emptying (GLP-1 agonists, DPP-4 inhibitors) enhance insulin secretion (sulfonylureas, meglitinides, GLP-1 agonists, DPP-4 inhibitors), reduce gluconeogenesis (biguanides), reduce insulin resistance (biguanides, thiazolidinediones), and increase urinary glucose excretion (SGLT-2 inhibitors). The use of medications will also have possible side effects.
Major Endogenous Factors That Lower the Blood Glucose
The major endogenous mechanism to lower the blood glucose is to deliver glucose into the cells (all cells can use glucose). If the blood glucose exceeds about 180 milligrams/deciliter, then loss of glucose into the urine can occur. The blood glucose is reduced by cellular uptake using glut transporters ( 12 ). Some cells have transporters that are responsive to the presence of insulin to activate (glut4), others have transporters that do not require insulin for activation. Insulin-responsive glucose transporters in muscle cells and adipose cells lead to a reduction in glucose levels—especially after carbohydrate-containing meals ( 13 ). Exercise can increase the glucose utilization in muscle, which then increases glucose cellular uptake and reduce the blood glucose levels. During exercise, when the metabolic demands of skeletal muscle can increase more than 100-fold, and during the absorptive period (after a meal), the insulin-responsive glut4 transporters facilitate the rapid entry of glucose into muscle and adipose tissue, thereby preventing large fluctuations in blood glucose levels ( 13 ).
Which Cells Use Glucose?
Glucose can used by all cells. A limited number of cells can only use glucose, and are “glucose-dependent.” It is generally accepted that the glucose-dependent cells include red blood cells, white blood cells, and cells of the renal papilla. Red blood cells have no mitochondria for beta-oxidation, so they are dependent upon glucose and glycolysis. White blood cells require glucose for the respiratory burst when fighting infections. The cells of the inner renal medulla (papilla) are under very low oxygen tension, so therefore must predominantly use glucose and glycolysis. The low oxygen tension is a result of the countercurrent mechanism of urinary concentration ( 14 ). These glucose-dependent cells have glut transporters that do not require insulin for activation—i.e., they do not need insulin to get glucose into the cells. Some cells can use glucose and ketones, but not fatty acids. The central nervous system is believed to be able to use glucose and ketones for fuel ( 15 ). Other cells can use glucose, ketones, and fatty acids for fuel. Muscle, even cardiac muscle, functions well on fatty acids and ketones ( 16 ). Muscle cells have both non-insulin-responsive and insulin-responsive (glut4) transporters ( 12 ).
Possible Dual Role of an Insulin-Dependent Glucose-Transporter (glut4)
A common metaphor is to think of the insulin/glut transporter system as a key/lock mechanism. Common wisdom states that the purpose of insulin-responsive glut4 transporters is to facilitate glucose uptake when blood insulin levels are elevated. But, a lock serves two purposes: to let someone in and/or to keep someone out . So, one of the consequences of the insulin-responsive glut4 transporter is to keep glucose out of the muscle and adipose cells, too, when insulin levels are low. The cells that require glucose (“glucose-dependent”) do not need insulin to facilitate glucose entry into the cell (non-insulin-responsive transporters). In a teleological way, it would “make no sense” for cells that require glucose to have insulin-responsive glut4 transporters. Cells that require glucose have glut1, glut2, glut3, glut5 transporters—none of which are insulin-responsive (Back to the key/lock metaphor, it makes no sense to have a lock on a door that you want people to go through). At basal (low insulin) conditions, most glucose is used by the brain and transported by non-insulin-responsive glut1 and glut3. So, perhaps one of the functions of the insulin-responsive glucose uptake in muscle and adipose to keep glucose OUT of the these cells at basal (low insulin) conditions, so that the glucose supply can be reserved for the tissue that is glucose-dependent (blood cells, renal medulla).
What Causes IR and T2DM?
The current commonly espoused view is that “Type 2 diabetes develops when beta-cells fail to secrete sufficient insulin to keep up with demand, usually in the context of increased insulin resistance.” ( 17 ). Somehow, the beta cells have failed in the face of insulin resistance. But what causes insulin resistance? When including the possibility that the environment may be part of the problem, is it possible that IR is an adaptive (protective) response to excess glucose availability? From the perspective that carbohydrate is not an essential nutrient and the change in foods in recent years has increased the consumption of refined sugar and flour, maybe hyperinsulinemia is the cause of IR and T2DM, as cells protect themselves from excessive glucose and insulin levels.
Insulin Is Already Elevated in IR and T2DM
Clinical experience of most physicians using insulin to treat T2DM over time informs us that an escalation of insulin dose is commonly needed to achieve glycemic control (when carbohydrate is consumed). When more insulin is given to someone with IR, the IR seems to get worse and higher levels of insulin are needed. I have the clinical experience of treating many individuals affected by T2DM and de-prescribing insulin as it is no longer needed after consuming a diet without carbohydrate ( 18 ).
Diets Without Carbohydrate Reverse IR and T2DM
When dietary manipulation was the only therapy for T2DM, before medications were available, a carbohydrate-restricted diet was used to treat T2DM ( 19 – 21 ). Clinical experience of obesity medicine physicians and a growing number of recent studies have demonstrated that carbohydrate-restricted diets reverse IR and T2DM ( 18 , 22 , 23 ). Other methods to achieve caloric restriction also have these effects, like calorie-restricted diets and bariatric surgery ( 24 , 25 ). There may be many mechanisms by which these approaches may work: a reduction in glucose, a reduction in insulin, nutritional ketosis, a reduction in metabolic syndrome, or a reduction in inflammation ( 26 ). Though there may be many possible mechanisms, let's focus on an obvious one: a reduction in blood glucose. Let's assume for a moment that the excessive glucose and insulin leads to hyperinsulinemia and this is the cause of IR. On a carbohydrate-restricted diet, the reduction in blood glucose leads to a reduction in insulin. The reduction in insulin leads to a reduction in insulin resistance. The reduction in insulin leads to lipolysis. The resulting lowering of blood glucose, insulin and body weight reverses IR, T2DM, AND obesity. These clinical observations strongly suggest that hyperinsulinemia is a cause of IR and T2DM—not the other way around.
What Causes Atherosclerosis?
For many years, the metabolic syndrome has been described as a possible cause of atherosclerosis, but there are no RCTs directly targeting metabolic syndrome, and the current drug treatment focuses on LDL reduction, so its importance remains controversial. A recent paper compared the relative importance of many risk factors in the prediction of the first cardiac event in women, and the most powerful predictors were diabetes, metabolic syndrome, smoking, hypertension and BMI ( 27 ). The connection between dietary carbohydrate and fatty liver is well-described ( 28 ). The connection between fatty liver and atherosclerosis is well-described ( 29 ). It is very possible that the transport of excess glucose to the adipose tissue via lipoproteins creates the particles that cause the atherosclerotic damage (small LDL) ( Figure 1 ) ( 30 – 32 ). This entire process of dietary carbohydrate leading to fatty liver, leading to small LDL, is reversed by a diet without carbohydrate ( 26 , 33 , 34 ).
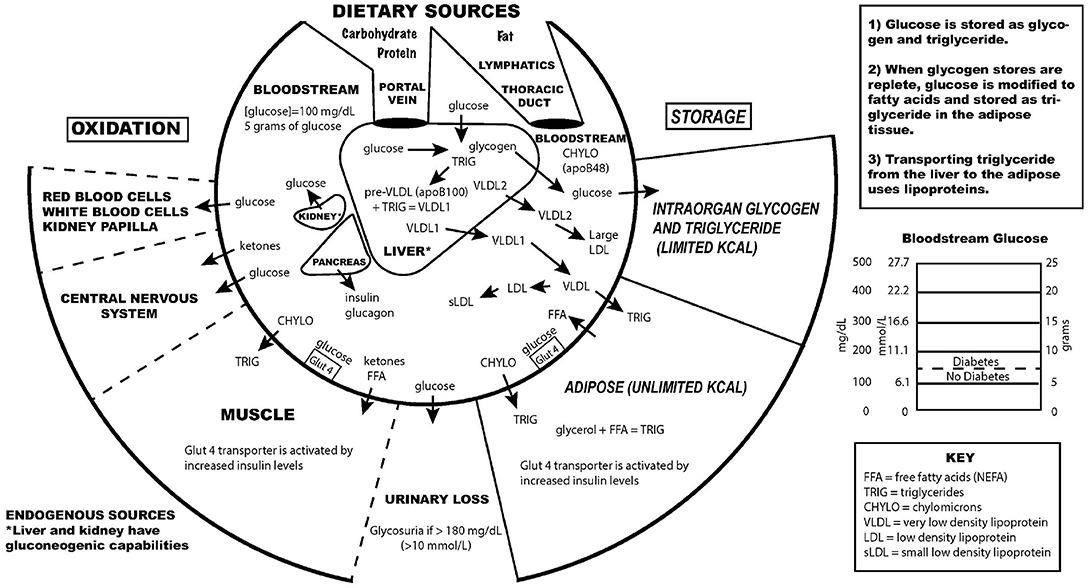
Figure 1 . Key aspects of the interconnection between glucose and lipoprotein metabolism.
Reducing dietary carbohydrate in the context of a low carbohydrate, ketogenic diet reduces hyperglycemia and hyperinsulinemia, IR and T2DM. In the evaluation of an individual for a glucose abnormality, measure the blood glucose and insulin levels. If the insulin level (fasting or after a glucose-containing meal) is high, do not give MORE insulin—instead, use an intervention to lower the insulin levels. Effective ways to reduce insulin resistance include lifestyle, medication, and surgical therapies ( 23 , 35 ).
The search for a single cause of a complex problem is fraught with difficulty and controversy. I am not hypothesizing that excessive dietary carbohydrate is the only cause of IR and T2DM, but that it is a cause, and quite possibly the major cause. How did such a simple explanation get overlooked? I believe it is very possible that the reductionistic search for intracellular molecular mechanisms of IR and T2DM, the emphasis on finding pharmaceutical (rather than lifestyle) treatments, the emphasis on the treatment of high total and LDL cholesterol, and the fear of eating saturated fat may have misguided a generation of researchers and clinicians from the simple answer that dietary carbohydrate, when consumed chronically in amounts that exceeds an individual's ability to metabolize them, is the most common cause of IR, T2DM and perhaps even atherosclerosis.
While there has historically been a concern about the role of saturated fat in the diet as a cause of heart disease, most nutritional experts now cite the lack of evidence implicating dietary saturated fat as the reason for lack of concern of it in the diet ( 36 ).
The concept of comparing medications that treat IR by insulin-sensitizers or by providing insulin itself was tested in the Bari-2D study ( 37 ). Presumably in the context of consuming a standard American diet, this study found no significant difference in death rates or major cardiovascular events between strategies of insulin sensitization or insulin provision.
While lifestyle modification may be ideal to prevent or cure IR and T2DM, for many people these changes are difficult to learn and/or maintain. Future research should be directed toward improving adherence to all effective lifestyle or medication treatments. Future research is also needed to assess the effect of carbohydrate restriction on primary or secondary prevention of outcomes of cardiovascular disease.
Data Availability Statement
The original contributions presented in the study are included in the article/supplementary material, further inquiries can be directed to the corresponding author/s.
Author Contributions
The author confirms being the sole contributor of this work and has approved it for publication.
Conflict of Interest
EW receives royalties from popular diet books and is founder of a company based on low-carbohydrate diet principles (Adapt Your Life, Inc.).
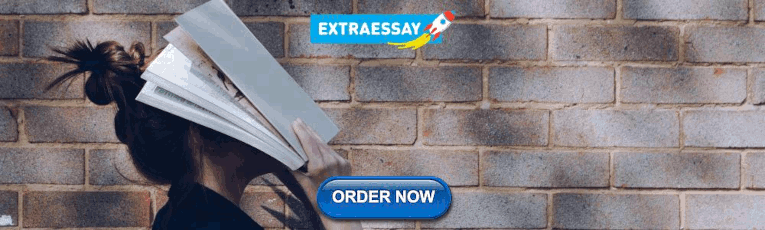
Publisher's Note
All claims expressed in this article are solely those of the authors and do not necessarily represent those of their affiliated organizations, or those of the publisher, the editors and the reviewers. Any product that may be evaluated in this article, or claim that may be made by its manufacturer, is not guaranteed or endorsed by the publisher.
1. American Diabetes Association. Classification and diagnosis of diabetes. Diabetes Care . (2016) 39 (Suppl. 1):S13–22. doi: 10.2337/dc16-S005
PubMed Abstract | CrossRef Full Text | Google Scholar
2. Bogardus C, Lillioja S, Howard BV, Reaven G, Mott D. Relationships between insulin secretion, insulin action, and fasting plasma glucose concentration in nondiabetic and noninsulin-dependent diabetic subjects. J Clin Invest. (1984) 74:1238–46. doi: 10.1172/JCI111533
3. Reaven GM. Compensatory hyperinsulinemia and the development of an atherogenic lipoprotein profile: the price paid to maintain glucose homeostasis in insulin-resistant individuals. Endocrinol Metab Clin North Am. (2005) 34:49–62. doi: 10.1016/j.ecl.2004.12.001
4. DeFronzo RA, Ferrannini E. Insulin resistance. A multifaceted syndrome responsible for NIDDM, obesity, hypertension, dyslipidemia, and atherosclerotic cardiovascular disease. Diabetes Care. (1991) 14:173–94. doi: 10.2337/diacare.14.3.173
5. Eckel RH, Grundy SM, Zimmet PZ. The metabolic syndrome. Lancet. (2005) 365:1415–28. doi: 10.1016/S0140-6736(05)66378-7
6. Yaribeygi H, Farrokhi FR, Butler AE, Sahebkar A. Insulin resistance: review of the underlying molecular mechanisms. J Cell Physiol. (2019) 234:8152–61. doi: 10.1002/jcp.27603
7. Shulman GI. Cellular mechanisms of insulin resistance. J Clin Invest. (2000) 106:171–6. doi: 10.1172/JCI10583
8. Guizouarn H, Allegrini B. Erythroid glucose transport in health and disease. Pflugers Arch. (2020) 472:1371–83. doi: 10.1007/s00424-020-02406-0
9. Petersen MC, Vatner DF, Shulman GI. Regulation of hepatic glucose metabolism in health and disease. Nat Rev Endocrinol. (2017) 13:572–87. doi: 10.1038/nrendo.2017.80
10. Tondt J, Yancy WS, Westman EC. Application of nutrient essentiality criteria to dietary carbohydrates. Nutr Res Rev. (2020) 33:260–70. doi: 10.1017/S0954422420000050
11. Colberg SR, Hernandez MJ, Shahzad F. Blood glucose responses to type, intensity, duration, and timing of exercise. Diabetes Care. (2013) 36:e177. doi: 10.2337/dc13-0965
12. Mueckler M, Thorens B. The SLC2 (GLUT) family of membrane transporters. Mol Aspects Med. (2013) 34:121–38. doi: 10.1016/j.mam.2012.07.001
13. Bryant NJ, Govers R, James DE. Regulated transport of the glucose transporter GLUT4. Nat Rev Mol Cell Biol. (2002) 3:267–77. doi: 10.1038/nrm782
14. Epstein FH. Oxygen and renal metabolism. Kidney Int. (1997) 51:381–5. doi: 10.1038/ki.1997.50
15. Cahill GF. Fuel metabolism in starvation. Annu Rev Nutr. (2006) 26:1–22. doi: 10.1146/annurev.nutr.26.061505.111258
16. Murashige D, Jang C, Neinast M, Edwards JJ, Cowan A, Hyman MC, et al. Comprehensive quantification of fuel use by the failing and nonfailing human heart. Science. (2020) 370:364–8. doi: 10.1126/science.abc8861
17. Skyler JS, Bakris GL, Bonifacio E, Darsow T, Eckel RH, Groop L, et al. Differentiation of diabetes by pathophysiology, natural history, and prognosis. Diabetes. (2017) 66:241–55. doi: 10.2337/db16-0806
18. Westman EC, Yancy WS, Mavropoulos JC, Marquart M, McDuffie JR. The effect of a low-carbohydrate, ketogenic diet versus a low-glycemic index diet on glycemic control in type 2 diabetes mellitus. Nutr Metab. (2008) 5:36. doi: 10.1186/1743-7075-5-36
CrossRef Full Text | Google Scholar
19. Allen F. The treatment of diabetes. Boston Med Surg J. (1915) 172:241–7. doi: 10.1056/NEJM191502181720702
20. Osler W, McCrae T. The Principles and Practice of Medicine . 9th ed. New York and London: Appleton & Company (1923).
21. Lennerz BS, Koutnik AP, Azova S, Wolfsdorf JI, Ludwig DS. Carbohydrate restriction for diabetes: rediscovering centuries-old wisdom. J Clin Invest. (2021) 131:e142246. doi: 10.1172/JCI142246
22. Steelman GM, Westman EC. Obesity: Evaluation and Treatment Essentials . 2nd ed. Boca Raton: CRC Press, Taylor & Francis Group (2016). 340 p.
23. Athinarayanan SJ, Adams RN, Hallberg SJ, McKenzie AL, Bhanpuri NH, Campbell WW, et al. Long-term effects of a novel continuous remote care intervention including nutritional ketosis for the management of type 2 diabetes: a 2-year non-randomized clinical trial. Front Endocrinol. (2019) 10:348. doi: 10.3389/fendo.2019.00348
24. Lim EL, Hollingsworth KG, Aribisala BS, Chen MJ, Mathers JC, Taylor R. Reversal of type 2 diabetes: normalisation of beta cell function in association with decreased pancreas and liver triacylglycerol. Diabetologia. (2011) 54:2506–14. doi: 10.1007/s00125-011-2204-7
25. Isbell JM, Tamboli RA, Hansen EN, Saliba J, Dunn JP, Phillips SE, et al. The importance of caloric restriction in the early improvements in insulin sensitivity after Roux-en-Y gastric bypass surgery. Diabetes Care. (2010) 33:1438–42. doi: 10.2337/dc09-2107
26. Bhanpuri NH, Hallberg SJ, Williams PT, McKenzie AL, Ballard KD, Campbell WW, et al. Cardiovascular disease risk factor responses to a type 2 diabetes care model including nutritional ketosis induced by sustained carbohydrate restriction at 1 year: an open label, non-randomized, controlled study. Cardiovasc Diabetol. (2018) 17:56. doi: 10.1186/s12933-018-0698-8
27. Dugani SB, Moorthy MV, Li C, Demler OV, Alsheikh-Ali AA, Ridker PM, et al. Association of lipid, inflammatory, and metabolic biomarkers with age at onset for incident coronary heart disease in women. JAMA Cardiol. (2021) 6:437–47. doi: 10.1001/jamacardio.2020.7073
28. Duwaerts CC, Maher JJ. Macronutrients and the adipose-liver axis in obesity and fatty liver. Cell Mol Gastroenterol Hepatol. (2019) 7:749–61. doi: 10.1016/j.jcmgh.2019.02.001
29. Zhang L, She Z-G, Li H, Zhang X-J. Non-alcoholic fatty liver disease: a metabolic burden promoting atherosclerosis. Clin Sci Lond Engl. (1979) 134:1775–99. doi: 10.1042/CS20200446
30. Horton TJ, Drougas H, Brachey A, Reed GW, Peters JC, Hill JO. Fat and carbohydrate overfeeding in humans: different effects on energy storage. Am J Clin Nutr. (1995) 62:19–29. doi: 10.1093/ajcn/62.1.19
31. Packard C, Caslake M, Shepherd J. The role of small, dense low density lipoprotein (LDL): a new look. Int J Cardiol. (2000) 74 (Suppl. 1):S17–22. doi: 10.1016/S0167-5273(99)00107-2
32. Borén J, Chapman MJ, Krauss RM, Packard CJ, Bentzon JF, Binder CJ, et al. Low-density lipoproteins cause atherosclerotic cardiovascular disease: pathophysiological, genetic, and therapeutic insights: a consensus statement from the European Atherosclerosis Society Consensus Panel. Eur Heart J. (2020) 41:2313–30. doi: 10.1093/eurheartj/ehz962
33. Yancy WS, Olsen MK, Guyton JR, Bakst RP, Westman EC. A low-carbohydrate, ketogenic diet versus a low-fat diet to treat obesity and hyperlipidemia: a randomized, controlled trial. Ann Intern Med. (2004) 140:769. doi: 10.7326/0003-4819-140-10-200405180-00006
34. Tendler D, Lin S, Yancy WS, Mavropoulos J, Sylvestre P, Rockey DC, et al. The effect of a low-carbohydrate, ketogenic diet on nonalcoholic fatty liver disease: a pilot study. Dig Dis Sci. (2007) 52:589–93. doi: 10.1007/s10620-006-9433-5
35. Pories WJ, Swanson MS, MacDonald KG, Long SB, Morris PG, Brown BM, et al. Who would have thought it? An operation proves to be the most effective therapy for adult-onset diabetes mellitus. Ann Surg. (1995) 222:339–50. doi: 10.1097/00000658-199509000-00011
36. Astrup A, Magkos F, Bier DM, Brenna JT, de Oliveira Otto MC, Hill JO, et al. Saturated fats and health: a reassessment and proposal for food-based recommendations: JACC state-of-the-art review. J Am Coll Cardiol. (2020) 76:844–57. doi: 10.1016/j.jacc.2020.05.077
37. A randomized trial of therapies for type 2 diabetes and coronary artery disease. N Engl J Med . (2009) 360:2503–15. doi: 10.1056/NEJMoa0805796
Keywords: type 2 diabetes, insulin resistance, pre-diabetes, carbohydrate-restricted diets, hyperinsulinemia, hyperglycemia
Citation: Westman EC (2021) Type 2 Diabetes Mellitus: A Pathophysiologic Perspective. Front. Nutr. 8:707371. doi: 10.3389/fnut.2021.707371
Received: 09 May 2021; Accepted: 20 July 2021; Published: 10 August 2021.
Reviewed by:
Copyright © 2021 Westman. This is an open-access article distributed under the terms of the Creative Commons Attribution License (CC BY) . The use, distribution or reproduction in other forums is permitted, provided the original author(s) and the copyright owner(s) are credited and that the original publication in this journal is cited, in accordance with accepted academic practice. No use, distribution or reproduction is permitted which does not comply with these terms.
*Correspondence: Eric C. Westman, ewestman@duke.edu
This article is part of the Research Topic
Carbohydrate-restricted Nutrition and Diabetes Mellitus
Thank you for visiting nature.com. You are using a browser version with limited support for CSS. To obtain the best experience, we recommend you use a more up to date browser (or turn off compatibility mode in Internet Explorer). In the meantime, to ensure continued support, we are displaying the site without styles and JavaScript.
- View all journals
- My Account Login
- Explore content
- About the journal
- Publish with us
- Sign up for alerts
- Open access
- Published: 18 April 2024
Assessing the predictive value of insulin resistance indices for metabolic syndrome risk in type 2 diabetes mellitus patients
- Hadi Bazyar 1 , 2 ,
- Ahmad Zare Javid 3 , 4 ,
- Mahmood Reza Masoudi 5 ,
- Fatemeh Haidari 6 ,
- Zeinab Heidari 7 ,
- Sohrab Hajializadeh 1 ,
- Vahideh Aghamohammadi 8 &
- Mehdi Vajdi 9
Scientific Reports volume 14 , Article number: 8917 ( 2024 ) Cite this article
145 Accesses
Metrics details
- Endocrinology
- Medical research
Limited research has explored the effectiveness of insulin resistance (IR) in forecasting metabolic syndrome (MetS) risk, especially within the Iranian population afflicted with type 2 diabetes mellitus (T2DM). The present investigation aimed to assess the efficacy of IR indices in predicting the risk of MetS among T2DM patients. Convenient sampling was utilized to select four hundred subjects with T2DM. Metabolic factors and IR indices, including the Waist Circumference-Triglyceride Index (WTI), Triglyceride and Glucose Index (TyG index), the product of TyG index and abdominal obesity indices, and the Metabolic Score for Insulin Resistance (METS-IR), were evaluated. Logistic regression, coupled with modeling, was employed to explore the risk of MetS. The predictive performance of the indices for MetS stratified by sex was evaluated via receiver operating characteristic (ROC) curve analysis and estimation of the area under the curve (AUC) values. The TyG-Waist Circumference (TyG-WC) index exhibited the largest AUCs in both males (0.91) and females (0.93), while the TyG-Body Mass Index (TyG-BMI) demonstrated the smallest AUCs (0.77 in males and 0.74 in females). All indices significantly predicted the risk of MetS in all subjects before and after adjustment (p < 0.001 for all). The TyG-WC index demonstrated the highest odds ratios for MetS (8.06, 95% CI 5.41–12.00). In conclusion, all IR indices assessed in this study effectively predicted the risk of MetS among Iranian patients with T2DM, with the TyG-WC index emerging as the most robust predictor across both genders.
Similar content being viewed by others
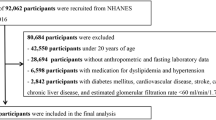
Lipid indices as simple and clinically useful surrogate markers for insulin resistance in the U.S. population
Juncheol Lee, Bongyoung Kim, … Shinje Moon
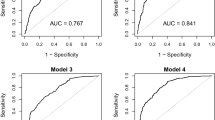
Waist-to-height ratio and metabolic phenotype compared to the Matsuda index for the prediction of insulin resistance
Katharina Lechner, Benjamin Lechner, … Helene von Bibra
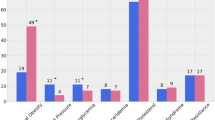
Validity assessment of the single-point insulin sensitivity estimator (spise) for diagnosis of cardiometabolic risk in post-pubertal hispanic adolescents
Paulina Correa-Burrows, Estela Blanco, … Raquel Burrows
Introduction
Insulin resistance (IR) arises from inadequate physiological responses due to reduced sensitivity of peripheral tissues to insulin, leading to elevated insulin levels through compensatory mechanisms involving pancreatic β-cell insulin production 1 . Predominantly affecting muscle, liver, and adipose tissue, IR onset in muscle tissue stems from immune-induced inflammatory changes and excess free fatty acids. With impaired glucose uptake by muscles, surplus glucose is redirected to the liver, triggering increased lipogenesis and release of free fatty acids, thereby promoting fat accumulation outside adipose tissue and exacerbating IR 2 , 3 . In individuals with compromised insulin signaling, such as those with type 2 diabetes mellitus (T2DM), insulin fails to suppress hepatic gluconeogenesis, even in the fed state, critically influencing blood glucose regulation 4 . Recognized as a major risk factor for metabolic syndrome (MetS), T2DM, and cardiovascular diseases (CVD), early detection of IR is vital for preventing these conditions 5 , 6 , 7 . While the glucose clamp technique serves as the gold standard for quantifying IR 8 , its complexity, cost, and invasiveness limit its routine use in laboratories 9 , 10 . Therefore, simpler methods like the homeostasis model assessment of insulin resistance (HOMA-IR) have been widely adopted since its proposal in 1985 11 . However, challenges with insulin measurement availability and standardization have prompted the exploration of alternative IR prediction approaches, including lipid ratios and visceral fat index (VAI) 9 , 12 , 13 . The triglyceride-glucose index (TyG index), derived from circulating triglyceride and glucose concentrations, has emerged as a promising tool for IR assessment, outperforming HOMA-IR in predictive accuracy 13 , 14 , 15 . Its strong correlation with IR, high diagnostic sensitivity and specificity, and ease of clinical application make it particularly valuable 9 , 10 , 12 , 13 , 14 . Additionally, obesity, prevalent among individuals with T2DM, is closely linked to IR. Anthropometric measures such as body mass index (BMI), waist circumference (WC), and waist-to-height ratio (WHtR) are commonly used due to their practicality. Combined TyG-related parameters, such as TyG-BMI and TyG-WC, exhibit superior performance compared to the standalone TyG index in IR evaluation 16 , 17 , 18 . Simultaneous consideration of WC and TG values, known as the waist circumference-triglyceride index (WTI), offers enhanced effectiveness in investigating MetS, T2DM, and CVD prevalence compared to individual parameters 19 , 20 , 21 . Moreover, the majority of individuals with T2DM are overweight or obese, and it is anticipated that a significant proportion of them will develop MetS 22 , 23 .
Given the scarcity of research on IR index effectiveness in predicting MetS risk among Iranian T2DM patients, this study seeks to evaluate the predictive capacity of IR indices, including WTI, TyG index, the product of TyG index and abdominal obesity indices, and METS-IR, in this population.
The prevalence of Metabolic Syndrome (MetS), as per the International Diabetes Federation (IDF) criteria, was found to be 63.3% in the study sample. The demographic and clinical characteristics of subjects across quartiles of the Triglyceride-Glucose (TyG) index scores are presented in Tables 1 and 2 . Compared to individuals in quartile 4, those in quartile 1 exhibited significantly lower values for weight, Body Mass Index (BMI), Waist Circumference (WC), Hip Circumference (HC), Fasting Blood Glucose (FBG), Hemoglobin A1C (HbA1C), Triglycerides (TG), Total Cholesterol (TC), Low-Density Lipoprotein Cholesterol (LDL-C), LDL/HDL Cholesterol ratio (LDL.HDL-c), Atherogenic Index of Plasma (AIP), Systolic Blood Pressure (SBP), Diastolic Blood Pressure (DBP), Mean Arterial Pressure (MAP), prevalence of MetS, TyG index score, TyG-BMI, TyG-WC, TyG-Waist-to-Hip Ratio (TyG-WHR), TyG-Waist-to-Height Ratio (TyG-WHtR), Waist Circumference-Triglyceride Index (WTI), and Metabolic Score for Insulin Resistance (METS-IR) (p < 0.001). Post-hoc pairwise comparisons revealed a significant reduction in FBG, HbA1C, TG, TC, LDL-C, AIP, TyG index score, TyG-BMI, TyG-WC, TyG-WHR, TyG-WHtR, and WTI in the third quartile compared to the fourth quartile (p < 0.001). Additionally, a significant reduction in mean weight, BMI, WC, HC, FBG, TG, LDL-C, LDL.HDL-c, AIP, TyG index score, TyG-BMI, TyG-WC, TyG-WHR, TyG-WHtR, WTI, and METS-IR were observed in the second quartile of TyG index score compared to the third and fourth quartiles (p < 0.001). Conversely, a significant increase in HDL-C was observed in the first quartile compared to the fourth quartile (p < 0.001).
Optimal cut-off values for IR indices in predicting MetS risk in patients with T2DM are presented in Table 3 . The predictive performance of anthropometric indices (TyG index, TyG-BMI, TyG-WC, TyG-WHR, TyG-WHtR, WTI, and METS-IR) for MetS, stratified by sex, was evaluated using receiver operating characteristic (ROC) curve analysis, and the corresponding area under the curve (AUC) values are depicted in Figs. 1 , 2 , 3 , 4 , 5 .
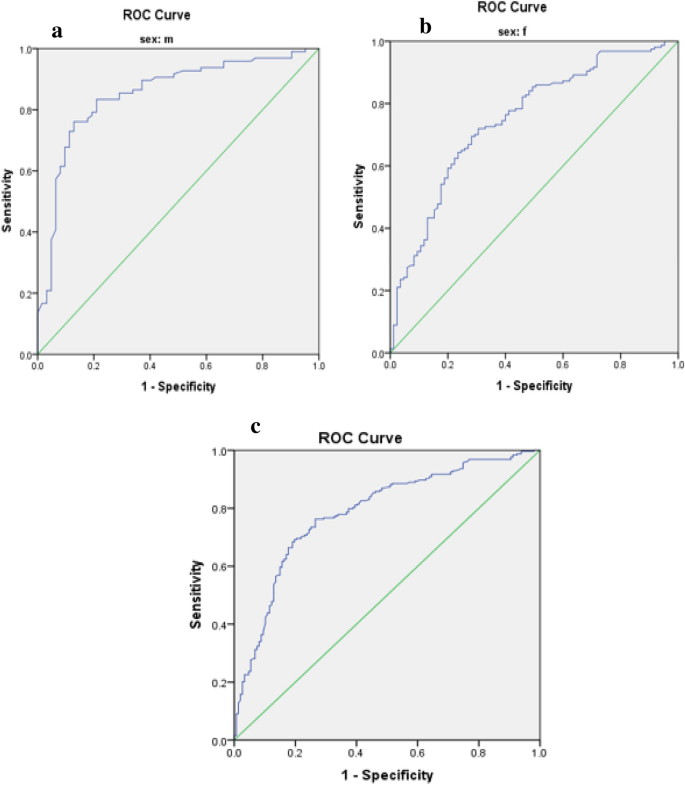
Roc Curve for TyG index ( a male, b female, c total).
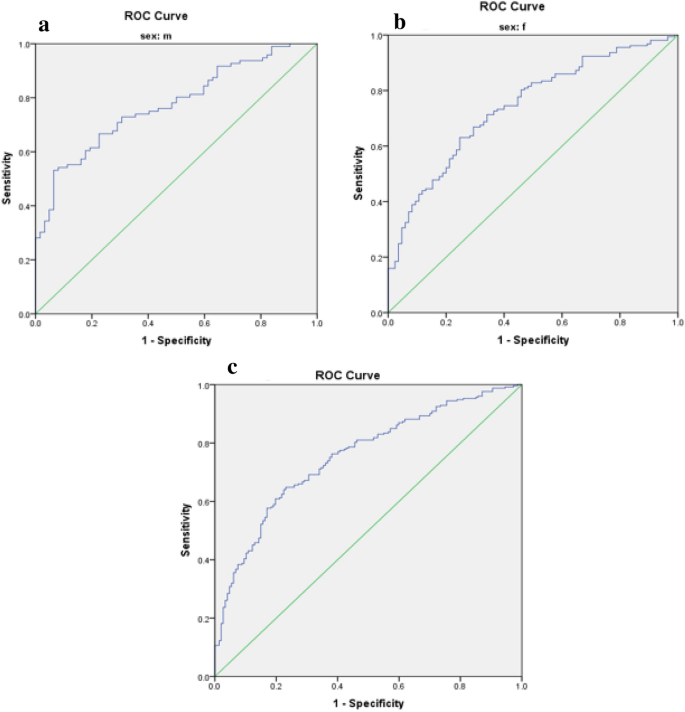
Roc Curve for TyG-BMI index ( a male, b female, c total).
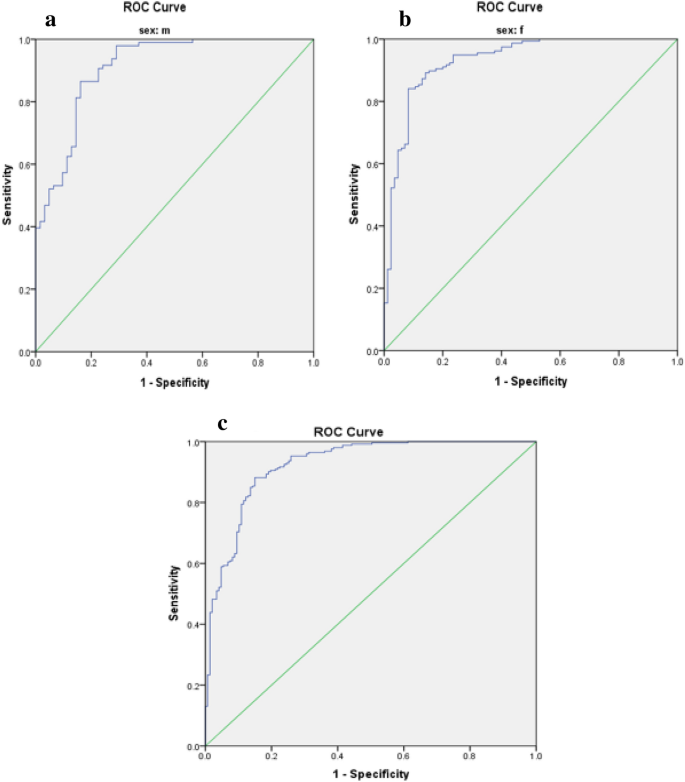
Roc Curve for TyG.WC index ( a male, b female, c total).
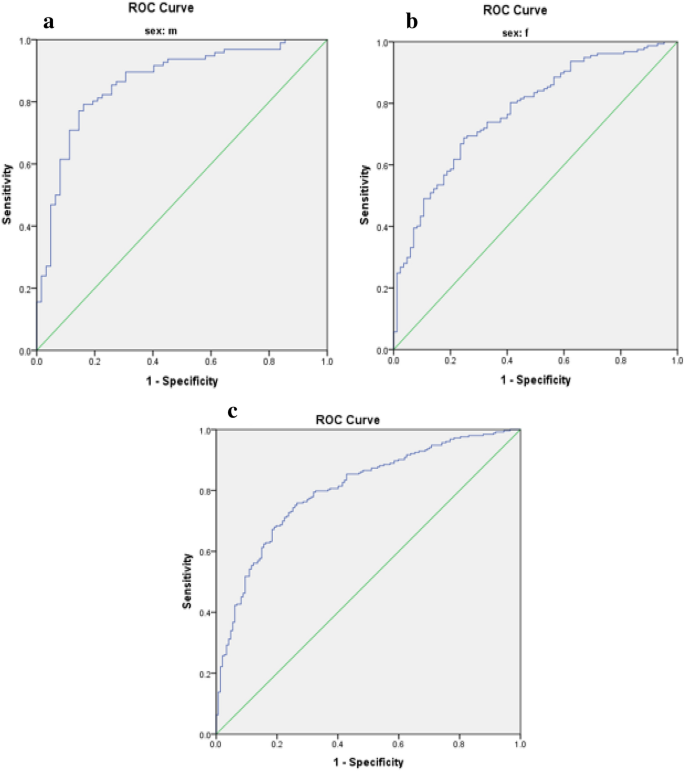
Roc Curve for total TyG-WHR index ( a male, b female, c total).
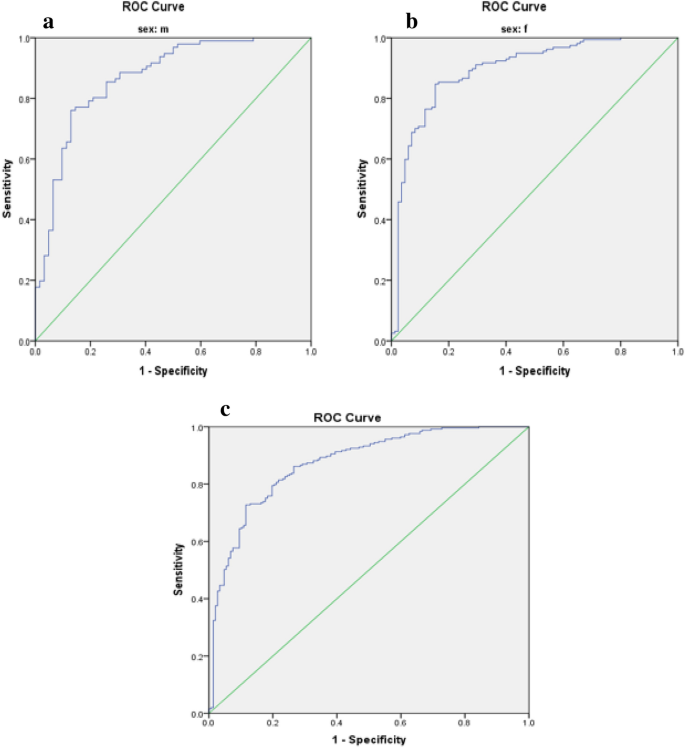
Roc Curve for total TyG-WHtR index ( a male, b female, c total).
The TyG-WC index exhibited the largest AUCs in both males and females (0.91 and 0.93, respectively) (Fig. 3 , Table 3 ), while the TyG-BMI demonstrated the smallest AUCs (0.77 in males and 0.74 in females) (Fig. 2 , Table 3 ).
Odds ratios (95% CI) for MetS, with IR indices as independent variables among participants, are presented in Table 4 . All indices significantly predicted the risk of MetS in all subjects before and after adjustment (p < 0.001). The TyG-WC index presented the highest odds ratios for MetS (8.06, 95% CI 5.41–12.00).
The coexistence of Metabolic Syndrome (MetS) in diabetic individuals is associated with the development of both microvascular and macrovascular complications, as evidenced by previous research 24 , 25 . Within our study sample, MetS was found to be prevalent in 63.3% of participants. Notably, all insulin resistance (IR) indices investigated demonstrated predictive potential for MetS risk. Among these indices, the TyG-WC index exhibited the most pronounced area under the curve (AUC) values and highest odds ratios for MetS among patients diagnosed with Type 2 Diabetes Mellitus (T2DM).
In line with our findings, prior investigations have also demonstrated the predictive capability of the TyG index for Metabolic Syndrome (MetS). The predictive capacity of the TyG index can be elucidated by mechanisms involving glucotoxicity and lipotoxicity, alongside the intimate associations of its constituent components (triglycerides and fasting plasma glucose) with insulin resistance, a pivotal factor in MetS pathogenesis 18 , 26 , 27 , 28 . However, combining the TyG index with measures of adiposity such as body mass index (BMI) and waist circumference (WC) may enhance predictive accuracy 29 . Indeed, in our study, the composite of the TyG index with abdominal obesity indices such as WC and waist-to-height ratio (WHtR) demonstrated higher odds ratios for MetS compared to the TyG index alone. Khan et al. revealed that the TyG index, with its robust area under the curve (AUC) of 0.764, outperforms other traditional markers such as fasting blood glucose, triglycerides, small dense LDL-c, non-HDL-c, and HOMA-IR in predicting MetS 26 . Similarly, Gui et al. demonstrated the predictive potential of various obesity- and lipid-related indices for MetS in middle-aged and older adults, with TyG-BMI and the Chinese visceral adiposity index (CVAI) emerging as the most effective markers for predicting MetS in men and women, respectively 30 . In a cross-sectional study, Raimi et al. assessed the utility of the TyG index in identifying MetS among Nigerians, concluding that it was effective in predicting MetS. Furthermore, combining anthropometric and TyG index indicators enhanced predictive accuracy, consistent with our findings 18 . Similarly, in our study, TyG-WC and TyG-WHtR exhibited the largest AUCs in both genders, with overall AUC values higher than those reported by Raimi et al. This suggests that TyG-WC and TyG-WHtR may possess greater predictive utility in our population. Both waist circumference (WC) and waist-to-height ratio (WHtR) serve as markers of visceral adiposity, which correlates more strongly with cardiovascular disease (CVD) risk than BMI, a measure of overall obesity 31 . Notably, WHtR, corrected for height, may offer superior predictive capability compared to WC alone. Indeed, previous studies have demonstrated that WHtR identifies individuals at early health risks more effectively than a composite index combining BMI and WC 18 , 32 , 33 . Moreover, in a study by Laurindo et al. conducted among the Brazilian population, the TyG-WC index exhibited the largest AUC (0.849) for detecting MetS using IR indices 34 . Differences in AUC values between studies may be attributed to differences in mean fasting plasma glucose and triglyceride levels, variation in study populations (diabetic versus non-diabetic individuals), and ethnic diversity.
Mao et al. conducted a study aiming to identify the optimal predictors and cut-off points for Metabolic Syndrome (MetS) among Chinese adults with Type 2 Diabetes Mellitus (T2DM). Their findings indicated that TyG-WC was the most effective predictor of MetS among women, while BMI emerged as the best predictor for both genders combined 35 . In contrast, our study revealed that TyG-WC was the superior predictor of MetS for both women and men. Another study utilizing data from the 2013–2016 US National Health and Nutrition Examination Survey found TyG-WC to be more robust in predicting MetS among the non-Hispanic population, though gender-specific analysis was not conducted 36 . Our findings demonstrated that TyG-WC outperformed TyG-BMI in MetS prediction, with TyG-WC exhibiting the largest area under the curve (AUC) and TyG-BMI the smallest. Body Mass Index (BMI) is commonly regarded as a general indicator of obesity, while waist circumference (WC) is considered a measure of central obesity 37 . However, the distribution of adipose tissue, particularly visceral fat, holds greater significance in metabolic dysfunction and insulin resistance. WC is closely associated with cardiometabolic risks 38 , highlighting its importance in predicting MetS. Moreover, in a study by Song et al., in addition to MetS, the product of the TyG index and anthropometric indices was also employed for predicting non-alcoholic fatty liver disease and Type 2 Diabetes Mellitus. TyG-WC exhibited superiority over TyG-BMI in predicting non-alcoholic fatty liver disease, further emphasizing the utility of WC as a predictor of metabolic disorders 39 .
In the present study, both the Waist-Triglyceride Index (WTI) and Metabolic Syndrome-Insulin Resistance (METS-IR) significantly predicted the risk of Metabolic Syndrome (MetS) in all participants, both before and after adjusting for relevant factors. Yang et al. highlighted the Waist-Triglyceride (WT) index, calculated as the product of waist circumference (WC) and triglyceride levels, as strongly associated with coronary heart disease risk 40 . Additionally, the WT index demonstrated effectiveness in screening for MetS in individuals with Type 2 Diabetes Mellitus (T2DM) 41 . Recently, Liu et al. introduced a modified form of the WT index, termed WTI, which exhibited a robust ability to identify MetS 42 . Similarly, Endukuru et al. demonstrated that WTI had the highest predictive ability for detecting low high-density lipoprotein cholesterol (HDL-C), elevated blood pressure, and high triglyceride levels in women compared to other indices 43 . Several studies have also demonstrated the high predictive capacity of WTI for discriminating MetS 44 , 45 . The METS-IR was developed by Chavolla et al. to evaluate insulin sensitivity, validated against the euglycemic–hyperinsulinemic clamp. Moreover, they found that METS-IR was associated with ectopic fat accumulation and could better predict incident T2DM than the triglyceride to high-density lipoprotein cholesterol ratio (TG/HDL-C) and TyG index in the Mexican population 46 . Han et al. investigated the association of various insulin resistance indicators, including METS-IR, TG/HDL-C, TyG-BMI, and TyG index, with serum uric acid levels in patients with T2DM, revealing significant associations between all indices and serum uric acid levels 47 . Furthermore, Zhang et al. demonstrated that METS-IR could predict the incidence of major adverse cardiovascular events in individuals with ischemic cardiomyopathy and T2DM 48 . It has been reported that METS-IR is strongly associated with hypertension even in individuals with normal weight 49 . Pathophysiological studies have elucidated that insulin resistance can perturb the lipid metabolism of the entire body, increase cardiac lipotoxicity, and induce oxidative stress and endothelial dysfunction, ultimately culminating in dyslipidemia, hypertension, and cardiovascular disease 50 .
Variations in the literature may stem from differences in chosen anthropometric indices, gender, ethnicity, underlying conditions, participant age, confounder variables, and criteria used to define Metabolic Syndrome (MetS), such as those by WHO, IDF, ATP III, and AHA/NHLBI. A limitation of our study is its cross-sectional design, which doesn't establish causality. Additionally, our focus on the Iranian population may limit generalizability. However, our study is the first to explore IR indices in predicting MetS risk among Iranian T2DM patients, and it includes both genders and employs multivariable logistic regression across three models.
All IR indices examined predicted MetS risk in our study, with the TyG-WC index emerging as the most effective predictor for both genders among Iranian T2DM patients.
Study design and participants
In this cross-sectional investigation, 400 Iranian patients diagnosed with Type 2 Diabetes Mellitus (T2DM) were prospectively enrolled from the Endocrine and Metabolism Clinic of Golestan Hospital, located in Ahvaz City, during the period spanning from March to May 2023. Patients were selected utilizing a convenient consecutive sampling method. Inclusion criteria comprised willingness to participate, age between 18 and 60 years, and a minimum of 2 years since the diagnosis of T2DM. Exclusion criteria consisted of insulin usage, pregnancy or lactation, smoking, alcohol consumption, incomplete demographic or anthropometric data, adherence to specialized diets, recent intake of antioxidant supplements within the last 3 months, and presence of comorbidities such as renal, hepatic, thyroidal, neoplastic, HIV, or infectious diseases.
A structured questionnaire was employed to collect demographic and baseline characteristics, encompassing sociodemographic factors such as gender, age, educational level, occupation, ethnicity, duration of diabetes, physical activity, and medication history. The study protocol adhered to the principles outlined in the Declaration of Helsinki and was approved by the Ethics Committee in Research of Sirjan University of Medical Sciences (Ethical code: IR.SIRUMS.REC.1401.017, Approval date: 18-03-2023). Written informed consent was obtained from all participants before their involvement. The sample size was determined based on the study conducted by Zhang et al. 51 and the utilization of the TyG-WHtR index, employing the formula (n = (z1 − a/2) 2 . SD 2 /d 2 ) with a precision (d) of 0.05, a standard deviation (SD) of 0.45, and a confidence level of 95%, resulting in a final sample size of 400 subjects.
Definition of MetS
Metabolic Syndrome (MetS) was defined according to the criteria established by the International Diabetes Federation (IDF), which includes the presence of central obesity, defined as a waist circumference (WC) equal to or greater than 95 cm for both genders based on guidelines provided by the Iranian National Obesity Committee 52 , in addition to meeting two or more of the following criteria: fasting blood glucose (FBG) levels equal to or greater than 100 mg/dL, or receiving medications for hyperglycemia; triglyceride (TG) levels equal to or greater than 150 mg/dL, or receiving medications for hypertriglyceridemia; low levels of high-density lipoprotein cholesterol (HDL-C), defined as less than 40 mg/dL in men and less than 50 mg/dL in women, or receiving drug treatment for low HDL-C; and elevated blood pressure, indicated by systolic blood pressure (SBP) equal to or greater than 130 mmHg or diastolic blood pressure (DBP) equal to or greater than 85 mmHg, or receiving drug treatment for hypertension 53 .
Blood pressure (BP) measurement
Blood pressure (BP) measurements were taken by a trained professional following a 20-min rest period for the patients, between 8:00 and 9:00 AM. This procedure was iterated thrice consecutively, and the average of the three successive readings was utilized for analysis. The mean arterial pressure (MAP) and pulse pressure (PP) were calculated employing the following formulas 54 :
where SBP represents systolic blood pressure and DBP represents diastolic blood pressure, both measured in millimeters of mercury (mmHg).
Biochemical assessment
Serum levels of fasting blood glucose (FBG) with a coefficient of variation (CV) interassay of 1.2% and lipid profile parameters, including triglycerides (TG) with a CV interassay of 1.6%, total cholesterol (TC) with a CV interassay of 2%, high-density lipoprotein cholesterol (HDL-C) with a CV interassay of 1.8%, low-density lipoprotein cholesterol (LDL-C) with a CV interassay of 1.29%, and very low-density lipoprotein (VLDL), were measured following a 12-h fasting period. Blood samples of 5 cc were drawn from each participant. FBG and the lipid profile were determined utilizing the enzymatic method with Pars Azmoon kits (Tehran, Iran) and analyzed on an auto analyzer (Hitachi 902, Japan). The Atherogenic Index of Plasma (AIP) was calculated using the logarithm of the TG to HDL-C ratio 55 . Hemoglobin A1c (HbA1c) levels in whole blood were quantified via automated high-performance liquid chromatography (HPLC) utilizing an exchange ion method with a DS5 set (DREW, United Kingdom).
Measurement of anthropometric indices and physical activity
All anthropometric assessments were conducted by a trained professional. Weight was measured using a digital scale manufactured in Japan with a precision of 0.1 kg, with participants asked to remove their shoes and wear minimal clothing. Height was determined using a tape measure with a precision of 0.5 cm. Body Mass Index (BMI) was calculated using the formula: weight in kilograms divided by the square of height in meters. Waist circumference (WC) was measured at the narrowest point of the torso with a precision of 0.5 cm, while hip circumference (HC) was assessed at the most prominent part of the hip area using a tape measure 56 , 57 , 58 . Waist-to-hip ratio (WHR) was computed by dividing WC by HC. Additionally, Waist-to-height ratio (WHtR) was obtained by dividing WC by height 59 .
Formulas for calculating novel indices of insulin resistance (IR) were applied as follows 18 , 36 :
Physical activity levels were assessed using the International Physical Activity Questionnaire (IPAQ), and results were reported as metabolic equivalent hours per week (METs hr/wk) 60 .
Statistical analysis
Data analysis was conducted using SPSS version 23 software. The normal distribution of the data was assessed using the Kolmogorov–Smirnov statistical test. Quantitative variables were compared between two groups using the independent t-test, while qualitative variables were compared using the chi-square test. Differences in variables across quartiles of the Triglyceride and glucose index (TyG index) were examined using One-way ANOVA with Post hoc (Least Significant Difference, LSD) analysis. To investigate the risk of Metabolic Syndrome (MetS), logistic regression was utilized, incorporating models with both crude and adjusted effects for potential confounding factors such as age, gender, ethnicity, educational level, occupation, duration of disease, physical activity, and medication usage. The predictive capacity of anthropometric indices (TyG index, TyG-BMI, TyG-WC, TyG-WHR, TyG-WHtR, Waist circumference-triglyceride index (WTI), and Metabolic Syndrome-Insulin Resistance (METS-IR)) for MetS stratified by sex was evaluated through receiver operating characteristic (ROC) curve analysis, with the area under the curve (AUC) values calculated. Figures 1 , 2 , 3 , 4 , 5 depict the results, highlighting the best predictors for both genders alongside their optimal threshold values. Quantitative data are presented as mean ± standard deviation (SD), while qualitative data are expressed as frequencies (percentages). A significance level of p < 0.05 was considered statistically significant.
Ethics declarations
The research protocol was in accordance with the guidelines of the Declaration of Helsinki. The Ethics Committee in Research of Sirjan University of Medical Sciences approved the study protocol (Ethical code: IR.SIRUMS.REC.1401.017, Approval date: 18-03-2023). The informed written consent form was acquired from all subjects at the starting of the study. For illiterate participants, informed consent was obtained from their guardian/legally authorized representative.
Data availability
All data and materials are fully presented in the manuscript.
Abbreviations
Area under the curve
Body mass index
Homeostasis model assessment of insulin resistance
Insulin resistance
Mean arterial pressure
- Metabolic syndrome
Metabolic score for insulin resistance
Pulse pressure
Receiver operating characteristic
- Type 2 diabetes mellitus
Triglyceride and glucose index
TyG-waist circumference
TyG-body mass index
Visceral fat index
Waist circumference
Waist to height ratio
Waist circumference-triglyceride index
Cerf, M. E. Beta cell dysfunction and insulin resistance. Front. Endocrinol. 4 , 43179 (2013).
Article Google Scholar
Bugianesi, E., McCullough, A. J. & Marchesini, G. Insulin resistance: A metabolic pathway to chronic liver disease. Hepatology 42 , 987–1000 (2005).
Article CAS PubMed Google Scholar
Freeman, A. M. & Pennings, N. Insulin resistance. (2018).
Hatting, M., Tavares, C. D., Sharabi, K., Rines, A. K. & Puigserver, P. Insulin regulation of gluconeogenesis. Ann. N. Y. Acad. Sci. 1411 , 21–35 (2018).
Article ADS CAS PubMed Google Scholar
Gast, K. B., Tjeerdema, N., Stijnen, T., Smit, J. W. & Dekkers, O. M. Insulin resistance and risk of incident cardiovascular events in adults without diabetes: Meta-analysis. PLoS ONE 7 , e52036 (2012).
Article ADS CAS PubMed PubMed Central Google Scholar
Nilsson, P. M., Tuomilehto, J. & Rydén, L. The metabolic syndrome–What is it and how should it be managed?. Eur. J. Prev. Cardiol. 26 , 33–46 (2019).
Article PubMed Google Scholar
Xia, C. et al. Lipid accumulation product is a powerful index for recognizing insulin resistance in non-diabetic individuals. Eur. J. Clin. Nutr. 66 , 1035–1038 (2012).
DeFronzo, R. A., Tobin, J. D. & Andres, R. Glucose clamp technique: A method for quantifying insulin secretion and resistance. Am. J. Physiol.-Endocrinol. Metab. 237 , E214 (1979).
Article CAS Google Scholar
Du, T. et al. Clinical usefulness of lipid ratios, visceral adiposity indicators, and the triglycerides and glucose index as risk markers of insulin resistance. Cardiovasc. Diabetol. 13 , 1–10 (2014).
Matthews, D. R. et al. Homeostasis model assessment: Insulin resistance and β-cell function from fasting plasma glucose and insulin concentrations in man. Diabetologia 28 , 412–419 (1985).
Miller, W. G. et al. Toward standardization of insulin immunoassays. Clin. Chem. 55 , 1011–1018 (2009).
Ren, X. et al. Association between triglyceride to HDL-C ratio (TG/HDL-C) and insulin resistance in Chinese patients with newly diagnosed type 2 diabetes mellitus. PLoS ONE 11 , e0154345 (2016).
Article PubMed PubMed Central Google Scholar
Kim-Dorner, S.-J., Deuster, P. A., Zeno, S. A., Remaley, A. T. & Poth, M. Should triglycerides and the triglycerides to high-density lipoprotein cholesterol ratio be used as surrogates for insulin resistance?. Metabolism 59 , 299–304 (2010).
Guerrero-Romero, F. et al. The product of triglycerides and glucose, a simple measure of insulin sensitivity. Comparison with the euglycemic-hyperinsulinemic clamp. J. Clin. Endocrinol. Metab. 95 , 3347–3351 (2010).
Simental-Mendía, L. E., Rodríguez-Morán, M. & Guerrero-Romero, F. The product of fasting glucose and triglycerides as surrogate for identifying insulin resistance in apparently healthy subjects. Metab. Syndr. Relat. Disord. 6 , 299–304 (2008).
Er, L.-K. et al. Triglyceride glucose-body mass index is a simple and clinically useful surrogate marker for insulin resistance in nondiabetic individuals. PLoS ONE 11 , e0149731 (2016).
Article MathSciNet PubMed PubMed Central Google Scholar
Zheng, S. et al. Triglyceride glucose-waist circumference, a novel and effective predictor of diabetes in first-degree relatives of type 2 diabetes patients: Cross-sectional and prospective cohort study. J. Transl. Med. 14 , 1–10 (2016).
Article ADS Google Scholar
Raimi, T. H. et al. Triglyceride-glucose index and related parameters predicted metabolic syndrome in Nigerians. Metab. Syndr. Relat. Disord. 19 , 76–82 (2021).
Article CAS PubMed PubMed Central Google Scholar
Zhao, K. et al. Association between the hypertriglyceridemic waist phenotype and prediabetes in Chinese adults aged 40 years and older. J. Diabetes Res. 2018 , 1–9 (2018).
Google Scholar
Sam, S. et al. Hypertriglyceridemic waist phenotype predicts increased visceral fat in subjects with type 2 diabetes. Diabetes Care 32 , 1916–1920 (2009).
Tanko, L. B. et al. Enlarged waist combined with elevated triglycerides is a strong predictor of accelerated atherogenesis and related cardiovascular mortality in postmenopausal women. Circulation 111 , 1883–1890 (2005).
Cardenas, V. et al. Depression is associated with increased risk for metabolic syndrome in latinos with type 2 diabetes. Am. J. Geriatr. Psychiatry 25 , 646–653 (2017).
Nsiah, K., Shang, V. O., Boateng, K. A. & Mensah, F. Prevalence of metabolic syndrome in type 2 diabetes mellitus patients. Int. J. Appl. Basic Med. Res. 5 , 133–138 (2015).
Katsiki, N., Anagnostis, P., Kotsa, K., Goulis, D. G. & Mikhailidis, D. P. Obesity, metabolic syndrome and the risk of microvascular complications in patients with diabetes mellitus. Curr. Pharm. Des. 25 , 2051–2059 (2019).
Lee, M.-Y. et al. Association between metabolic syndrome and microvascular and macrovascular disease in type 2 diabetic mellitus. Am. J. Med. Sci. 355 , 342–349 (2018).
Article ADS PubMed Google Scholar
Khan, S. H. et al. Metabolic clustering of risk factors: Evaluation of triglyceride-glucose index (TyG index) for evaluation of insulin resistance. Diabetol. Metab. Syndr. 10 , 1–8 (2018).
Mirr, M., Skrypnik, D., Bogdański, P. & Owecki, M. Newly proposed insulin resistance indexes called TyG-NC and TyG-NHtR show efficacy in diagnosing the metabolic syndrome. J. Endocrinol. Investig. 44 , 2831–2843 (2021).
Shin, K.-A. Triglyceride and glucose (TyG) index is a clinical surrogate marker for the diagnosis of metabolic syndrome. Biomed. Sci. Lett. 23 , 348–354 (2017).
Khamseh, M. E. et al. Triglyceride glucose index and related parameters (triglyceride glucose-body mass index and triglyceride glucose-waist circumference) identify nonalcoholic fatty liver and liver fibrosis in individuals with overweight/obesity. Metab. Syndr. Relat. Disord. 19 , 167–173 (2021).
Gui, J., Li, Y., Yuan, T. & Zhang, L. Obesity-and lipid-related indices as a predictor of obesity metabolic syndrome in a national cohort study. Front. Public Health 11 , 1073824 (2023).
Nalini, M. et al. Comparing anthropometric indicators of visceral and general adiposity as determinants of overall and cardiovascular mortality. Arch. Iran. Med. 22 , 301 (2019).
PubMed Google Scholar
Ashwell, M. & Gibson, S. Waist-to-height ratio as an indicator of ‘early health risk’: Simpler and more predictive than using a ‘matrix’based on BMI and waist circumference. BMJ Open 6 , e010159 (2016).
Gibson, S. & Ashwell, M. A simple cut-off for waist-to-height ratio (0· 5) can act as an indicator for cardiometabolic risk: Recent data from adults in the Health Survey for England. Br. J. Nutr. 123 , 681–690 (2020).
Fornari Laurindo, L. et al. Detection of metabolic syndrome using insulin resistance indexes: A cross-sectional observational cohort study. Endocrines 4 , 257–268 (2023).
Mao, J., Gan, S., Zhou, Q., Zhou, H. & Deng, Z. Optimal anthropometric indicators and cut points for predicting metabolic syndrome in Chinese patients with type 2 diabetes mellitus by gender. Diabetes Metab. Syndr. Obes. 16 , 505–514 (2023).
Okosun, I. S., Okosun, B., Lyn, R. & Airhihenbuwa, C. Surrogate indexes of insulin resistance and risk of metabolic syndrome in non-Hispanic White, non-Hispanic Black and Mexican American. Diabetes Metab. Syndr. Clin. Res. Rev. 14 , 3–9 (2020).
Kissebah, A. H. et al. Relation of body fat distribution to metabolic complications of obesity. J. Clin. Endocrinol. Metab. 54 , 254–260 (1982).
Pouliot, M.-C. et al. Waist circumference and abdominal sagittal diameter: best simple anthropometric indexes of abdominal visceral adipose tissue accumulation and related cardiovascular risk in men and women. Am. J. Cardiol. 73 , 460–468 (1994).
Song, S., Son, D.-H., Baik, S.-J., Cho, W.-J. & Lee, Y.-J. Triglyceride glucose-waist circumference (TyG-WC) is a reliable marker to predict non-alcoholic fatty liver disease. Biomedicines 10 , 2251 (2022).
Yang, R., Liu, X., Lin, Z. & Zhang, G. Correlation study on waist circumference-triglyceride (WT) index and coronary artery scores in patients with coronary heart disease. Eur. Rev. Med. Pharmacol. Sci. 19 , 113–118 (2015).
CAS PubMed Google Scholar
Ma, C.-M. et al. Three novel obese indicators perform better in monitoring management of metabolic syndrome in type 2 diabetes. Sci. Rep. 7 , 9843 (2017).
Article ADS PubMed PubMed Central Google Scholar
Liu, P. J., Lou, H. P. & Zhu, Y. N. Screening for metabolic syndrome using an integrated continuous index consisting of waist circumference and triglyceride: A preliminary cross-sectional study. Diabetes Metab. Syndr. Obes. 18 , 2899–2907 (2020).
Endukuru, C. K., Gaur, G. S., Dhanalakshmi, Y., Sahoo, J. & Vairappan, B. Cut-off values and clinical efficacy of body roundness index and other novel anthropometric indices in identifying metabolic syndrome and its components among Southern-Indian adults. Diabetol. Int. 13 , 188–200 (2022).
Yin, Q., Zheng, J., Cao, Y., Yan, X. & Zhang, H. Evaluation of novel obesity and lipid-related indices as indicators for the diagnosis of metabolic syndrome and Premetabolic syndrome in Chinese women with polycystic ovary syndrome. Int. J. Endocrinol. 2021 , 1–6 (2021).
Adejumo, E. N. et al. Anthropometric parameter that best predict metabolic syndrome in South west Nigeria. Diabetes Metab. Syndr. Clin. Res. Rev. 13 , 48–54 (2019).
Bello-Chavolla, O. Y. et al. METS-IR, a novel score to evaluate insulin sensitivity, is predictive of visceral adiposity and incident type 2 diabetes. Eur. J. Endocrinol. 178 , 533–544 (2018).
Han, R., Zhang, Y. & Jiang, X. Relationship between four non-insulin-based indexes of insulin resistance and serum uric acid in patients with type 2 diabetes: A cross-sectional study. Diabetes Metab. Syndr. Obes. Targets Ther. 9 , 1461–1471 (2022).
Zhang, X. et al. Metabolic score for insulin resistance (METS-IR) predicts adverse cardiovascular events in patients with type 2 diabetes and ischemic cardiomyopathy. Diabetes Metab. Syndr. Obes. 5 , 1283–1295 (2023).
Liu, X. Z., Fan, J. & Pan, S. J. METS-IR, a novel simple insulin resistance indexes, is associated with hypertension in normal-weight Chinese adults. J. Clin. Hypertens. 21 , 1075–1081 (2019).
Yang, W. et al. The metabolic score for insulin resistance (METS-IR) predicts cardiovascular disease and its subtypes in patients with hypertension and obstructive sleep apnea. Clin. Epidemiol. 15 , 177–189 (2023).
Zhang, X. et al. Association of metabolic syndrome with TyG index and TyG-related parameters in an urban Chinese population: A 15-year prospective study. Diabetol.Metab. Syndr. 14 , 1 (2022).
AZIZI, F. et al. Appropriate waist circumference cut-off points among Iranian adults: the first report of the Iranian National Committee of Obesity. (2010).
KG, A. International diabetes federation task force on epidemiology and prevention; hational heart, lung, and blood institute; American heart association; world heart federation; international atherosclerosis society; international association for the study of obesity: harmonizing the metabolic syndrome: a joint interim statement of the international diabetes federation task force on epidemiology and prevention; national heart, lung, and blood institute; American heart association; world heart federation; international atherosclerosis society; and international association for the study of obesity. Circulation 120 , 1640–1645 (2009).
Bazyar, H. et al. The effects of rutin supplement on blood pressure markers, some serum antioxidant enzymes, and quality of life in patients with type 2 diabetes mellitus compared with placebo. Front. Nutr. https://doi.org/10.3389/fnut.2023.1214420 (2023).
Dobiasˇova, M. Vol. 50 1113–1115 (Oxford University Press, 2004).
Haidari, F., Aghamohammadi, V., Mohammadshahi, M., Ahmadi-Angali, K. & Asghari-Jafarabadi, M. Whey protein supplementation reducing fasting levels of anandamide and 2-AG without weight loss in pre-menopausal women with obesity on a weight-loss diet. Trials 21 , 1–10 (2020).
Haidari, F., Aghamohammadi, V., Mohammadshahi, M. & Ahmadi-Angali, K. Effect of whey protein supplementation on levels of endocannabinoids and some of metabolic risk factors in obese women on a weight-loss diet: A study protocol for a randomized controlled trial. Nutr. J. 16 , 1–5 (2017).
Behbahani, H. B. et al. Glycemic index, glycemic load, dietary insulin index, and dietary insulin load in relation to cardiometabolic risk factors among participants with atherosclerosis: a cross-sectional study. BMC Nutr. 9 , 98 (2023).
Yoo, E.-G. Waist-to-height ratio as a screening tool for obesity and cardiometabolic risk. Korean J. Pediatr. 59 , 425 (2016).
Aadahl, M. & Jørgensen, T. Validation of a new self-report instrument for measuring physical activity. Med. Sci. Sports Exerc. 35 , 1196–1202 (2003).
Download references
Acknowledgements
The authors extend their appreciation to Endocrinology and Metabolism clinic employees of Golestan Hospital and also the laboratory staff of Golestan Hospital in Ahvaz.
This work has been financially supported by the Student Research Committee of Sirjan School of Medical Sciences (No: 401000024).
Author information
Authors and affiliations.
Student Research Committee, Sirjan School of Medical Sciences, Sirjan, Iran
Hadi Bazyar & Sohrab Hajializadeh
Department of Public Health, Sirjan School of Medical Sciences, Sirjan, Iran
Hadi Bazyar
Nutrition and Metabolic Diseases Research Center, Clinical Sciences Research Institute, Ahvaz Jundishapur University of Medical Sciences, Ahvaz, Iran
Ahmad Zare Javid
Department of Nutrition, School of Allied Medical Sciences, Ahvaz Jundishapur University of Medical Sciences, Ahvaz, Iran
Sirjan School of Medical Sciences, Sirjan, Iran
Mahmood Reza Masoudi
School of Health, Medical and Applied Sciences, Central Queensland University, Brisbane, Australia
Fatemeh Haidari
Student Research Committee, Ahvaz Jundishapur University of Medical Sciences, Ahvaz, Iran
Zeinab Heidari
Department of Nutrition, Khalkhal University of Medical Science, Khalkhal, Iran
Vahideh Aghamohammadi
Department of Community Nutrition, School of Nutrition and Food Science, Isfahan University of Medical Sciences, Isfahan, Iran
Mehdi Vajdi
You can also search for this author in PubMed Google Scholar
Contributions
HB: all steps of research and writing the manuscript, A-ZJ: drafting of the manuscript, MR-M: drafting of the manuscript, FH: revision of the manuscript, ZH: drafting and revision of the manuscript, SH: drafting of the manuscript, VA: corresponding author, supervision and all steps of research and writing the manuscript, MV: co-corresponding and all steps of research. All authors reviewed the manuscript. The final version of the manuscript was approved by all authors.
Corresponding authors
Correspondence to Vahideh Aghamohammadi or Mehdi Vajdi .
Competing interests
The authors declare no competing interests.
Additional information
Publisher's note.
Springer Nature remains neutral with regard to jurisdictional claims in published maps and institutional affiliations.
Rights and permissions
Open Access This article is licensed under a Creative Commons Attribution 4.0 International License, which permits use, sharing, adaptation, distribution and reproduction in any medium or format, as long as you give appropriate credit to the original author(s) and the source, provide a link to the Creative Commons licence, and indicate if changes were made. The images or other third party material in this article are included in the article's Creative Commons licence, unless indicated otherwise in a credit line to the material. If material is not included in the article's Creative Commons licence and your intended use is not permitted by statutory regulation or exceeds the permitted use, you will need to obtain permission directly from the copyright holder. To view a copy of this licence, visit http://creativecommons.org/licenses/by/4.0/ .
Reprints and permissions
About this article
Cite this article.
Bazyar, H., Zare Javid, A., Masoudi, M.R. et al. Assessing the predictive value of insulin resistance indices for metabolic syndrome risk in type 2 diabetes mellitus patients. Sci Rep 14 , 8917 (2024). https://doi.org/10.1038/s41598-024-59659-3
Download citation
Received : 31 October 2023
Accepted : 12 April 2024
Published : 18 April 2024
DOI : https://doi.org/10.1038/s41598-024-59659-3
Share this article
Anyone you share the following link with will be able to read this content:
Sorry, a shareable link is not currently available for this article.
Provided by the Springer Nature SharedIt content-sharing initiative
- Insulin resistance indices
By submitting a comment you agree to abide by our Terms and Community Guidelines . If you find something abusive or that does not comply with our terms or guidelines please flag it as inappropriate.
Quick links
- Explore articles by subject
- Guide to authors
- Editorial policies
Sign up for the Nature Briefing newsletter — what matters in science, free to your inbox daily.


Siblings with unique genetic change help scientists progress drug search for type 1 diabetes
Two siblings who have the only known mutations in a key gene anywhere in the world have helped scientists gain new insights that could help progress the search for new treatments in type 1 diabetes.
Type 1 diabetes (also known as autoimmune diabetes) is a devastating and life-long disease, in which the patient's immune cells wrongly destroy the insulin producing beta cells in the pancreas. People living with autoimmune diabetes need to test their blood sugar and inject insulin throughout their lives to control their blood sugars and prevent complications.
Autoimmune diabetes with clinical onset in very early childhood is rare and can result from a variety of genetic variants. However, there are many cases of early onset diabetes without known genetic explanation. In addition, some cancer patients treated with a category of immunotherapy known as immune checkpoint inhibitors -- which target the same pathway that the mutation was found in -- are prone to developing autoimmune diabetes. The reason why only this category of cancer immunotherapy can trigger autoimmune diabetes is not well understood. Like type 1 diabetes, genetic or immunotherapy-associated autoimmune diabetes requires life-long insulin replacement therapy -- there is currently no cure.
The new research, published in the Journal of Experimental Medicine , began when researchers studied two siblings who were diagnosed with a rare genetic form of autoimmune diabetes in the first weeks of life. The University of Exeter offers free genetic testing worldwide for babies diagnosed with diabetes before they are nine months old. For most of these babies, this service provides a genetic diagnosis and in around half of these babies, it allows for a change in treatment.
When researchers tested the two siblings in the study, no mutation in any of the known causes was identified. The Exeter team then performed whole genome sequencing to look for previously unknown causes of autoimmune diabetes. Through this sequencing, they found a mutation in the gene encoding PD-L1 in the siblings and realised it could be responsible for their very-early-onset autoimmune diabetes.
Study authorDr Matthew Johnson, from the University of Exeter, UK, said: "PD-L1 has been particularly well studied in animal models because of its crucial function in sending a stop signal to the immune system and its relevance to cancer immunotherapy. But, to our knowledge, nobody has ever found humans with a disease-causing mutation in the gene encoding PD-L1. We searched the globe, looking at all the large-scale datasets that we know of, and we haven't been able to find another family. These siblings therefore provide us with a unique and incredibly important opportunity to investigate what happens when this gene is disabled in humans."
The PD-L1 protein is expressed on many different cell types. Its receptor, PD-1, is expressed exclusively on immune cells. When the two proteins bind together it provides a stop signal to the immune system, preventing collateral damage to the bodies tissues and organs.
Researchers from the Rockefeller Institute in New York and King's College London joined forces with Exeter to study the siblings, with funding from Wellcome, The Leona M. and Harry B. Helmsley Charitable Trust, Diabetes UK, and the US National Institutes for Health. After contacting the family's clinician in Morocco, the Exeter team visited the siblings where they were living to collect samples and return them to King's College London, within the crucial ten-hour window for analysis while the immune cells were still alive. The London and New York teams then performed extensive analysis on the siblings' cells.
Study co-author Dr Masato Ogishi, from the Rockefeller University in New York, said: "We first showed that the mutation completely disabled the function of PD-L1 protein. We then studied the immune system of the siblings to look for immunological abnormalities that could account for their extremely early-onset diabetes. As we previously described another two siblings with PD-1 deficiency, both of whom had multi-organ autoimmunity including autoimmune diabetes and extensive dysregulation in their immune cells, we expected to find severe dysregulation of the immune system in the PD-L1-deficient siblings. To our great surprise, their immune systems looked pretty much normal in almost all aspects throughout the study. Therefore, PD-L1 is certainly indispensable for preventing autoimmune diabetes but is dispensable for many other aspects of human immune system. We think that PD-L2, another ligand of PD-1, albeit less well-studied than PD-L1, may be serving as a back-up system when PD-L1 is not available. This concept needs to be further investigated in the context of artificial blockade for PD-L1 as cancer immunotherapy."
Study co-author Professor Timothy Tree, from King's College London, said: "Through studying this one set of siblings -- unique in the world to our knowledge -- we have found that the PD-L1 gene is essential for avoiding autoimmune diabetes, but is not essential for 'everyday' immune function. This leads us to the grand question; 'what is the role of PD-L1 in our pancreas making it critical for preventing our immune cells destroying our beta cells?' We know that under certain conditions beta cells express PD-L1. However, certain types of immune cells in the pancreas also express PD-L1. We now need to work out the "communication" between different cell types that is critical for preventing autoimmune diabetes.
"This finding increases our knowledge of how autoimmune forms of diabetes such as type 1 diabetes develop. It opens up a new potential target for treatments that could prevent diabetes in the future. Simultaneously, it gives new knowledge to the cancer immunotherapy field by uniquely providing the results of completely disabling PD-L1 in a person, something you could never manipulate in studies. Reducing PD-L1 is already effective for cancer treatment, and boosting it is now being investigated as a type 1 diabetes treatment -- our findings will help accelerate the search for new and better drugs."
Dr Lucy Chambers, Head of Research Communications at Diabetes UK, said: "Pioneering treatments that alter the behaviour of the immune system to hold off its attack on the pancreas are already advancing type 1 diabetes treatment in the USA, and are awaiting approval here in the UK.
"By zeroing in on the precise role of an important player in the type 1 diabetes immune attack, this exciting discovery could pave the way for treatments that are more effective, more targeted and more transformational for people with or at risk of type 1 diabetes."
Helmsley Program Officer Ben Williams said: "New drugs often fail in development because scientific discoveries made in animal models don't translate into humans. As such, drug developers strongly prefer to pursue new drugs where human genetic evidence supports the drug's target. This study provides such compelling evidence that PD-L1 is a high-priority target to treat T1D, and should be pursued with the ambition of eventually reducing the burden of this difficult to manage disease."
The paper is entitled 'Human inherited PD-L1 deficiency is clinically and immunologically less severe than PD-1 deficiency' and is published in the Journal of Experimental Medicine. The research was supported by the National Institute of Health and Care Research (NIHR) Exeter Biomedical Research Centre and The NIHR Exeter Clinical Research Facility.
- Immune System
- Personalized Medicine
- Diseases and Conditions
- Hormone Disorders
- Medical Topics
- Diabetes mellitus type 1
- Diabetes mellitus type 2
- Erectile dysfunction
- Gene therapy
- Alzheimer's disease
Story Source:
Materials provided by University of Exeter . Original written by Louise Vennells. Note: Content may be edited for style and length.
Journal Reference :
- Matthew B. Johnson et al. Human inherited PD-L1 deficiency is clinically and immunologically less severe than PD-1 deficiency . JEM , 2024 DOI: 10.1084/jem.20231704
Cite This Page :
Explore More
- Two Species Interbreeding Created New Butterfly
- Warming Antarctic Deep-Sea and Sea Level Rise
- Octopus Inspires New Suction Mechanism for ...
- Cities Sinking: Urban Populations at Risk
- Puzzle Solved About Ancient Galaxy
- How 3D Printers Can Give Robots a Soft Touch
- Combo of Multiple Health Stressors Harming Bees
- Methane Emission On a Cold Brown Dwarf
- Remarkable Memories of Mountain Chickadees
- Predicting Future Marine Extinctions
Trending Topics
Strange & offbeat.
- Program Finder
- Admissions Services
- Course Directory
- Academic Calendar
- Hybrid Campus
- Lecture Series
- Convocation
- Strategy and Development
- Implementation and Impact
- Integrity and Oversight
- In the School
- In the Field
- In Baltimore
- Resources for Practitioners
- Articles & News Releases
- In The News
- Statements & Announcements
- At a Glance
- Student Life
- Strategic Priorities
- Inclusion, Diversity, Anti-Racism, and Equity (IDARE)
- What is Public Health?
Research Gaps Around Type 1 Diabetes
A large body of research on Type 2 diabetes has helped to develop guidance, informing how patients are diagnosed, treated, and manage their lifestyle. In contrast, Type 1 diabetes, often mistakenly associated only with childhood, has received less attention.
In this Q&A, adapted from the April 17 episode of Public Health On Call , Stephanie Desmon speaks to Johns Hopkins epidemiologists Elizabeth Selvin , PhD '04, MPH, and Michael Fang , PhD, professor and assistant professor, respectively, in the Department of Epidemiology, about recent findings that challenge common beliefs about type 1 diabetes. Their conversation touches on the misconception that it’s solely a childhood condition, the rise of adult-onset cases linked to obesity, and the necessity for tailored approaches to diagnosis and care. They also discuss insulin prices and why further research is needed on medications like Ozempic in treating Type 1 diabetes.
I want to hear about some of your research that challenges what we have long understood about Type 1 diabetes, which is no longer called childhood diabetes.
MF: Type 1 diabetes was called juvenile diabetes for the longest time, and it was thought to be a disease that had a childhood onset. When diabetes occurred in adulthood it would be type 2 diabetes. But it turns out that approximately half of the cases of Type 1 diabetes may occur during adulthood right past the age of 20 or past the age of 30.
The limitations of these initial studies are that they've been in small clinics or one health system. So, it's unclear whether it's just that particular clinic or whether it applies to the general population more broadly.
We were fortunate because the CDC has collected new data that explores Type 1 diabetes in the U.S. Some of the questions they included in their national data were, “Do you have diabetes? If you do, do you have Type 1 or Type 2? And, at what age were you diagnosed?”
With these pieces of information, we were able to characterize how the age of diagnosis of Type 1 diabetes differs in the entire U.S. population.
Are Type 1 and Type 2 diabetes different diseases?
ES: They are very different diseases and have a very different burden. My whole career I have been a Type 2 diabetes epidemiologist, and I’ve been very excited to expand work with Type 1 diabetes.
There are about 1.5 million adults with Type 1 diabetes in the U.S., compared to 21 million adults with Type 2 diabetes. In terms of the total cases of diabetes, only 5 to 10 percent have Type 1 diabetes. Even in our largest epidemiologic cohorts, only a small percentage of people have Type 1 diabetes. So, we just don't have the same national data, the same epidemiologic evidence for Type 1 diabetes that we have for Type 2. The focus of our research has been trying to understand and characterize the general epidemiology and the population burden of Type 1 diabetes.
What is it about Type 1 that makes it so hard to diagnose?
MF: The presentation of symptoms varies by age of diagnosis. When it occurs in children, it tends to have a very acute presentation and the diagnosis is easier to make. When it happens in adulthood, the symptoms are often milder and it’s often misconstrued as Type 2 diabetes.
Some studies have suggested that when Type 1 diabetes occurs in adulthood, about 40% of those cases are misdiagnosed initially as Type 2 cases. Understanding how often people get diagnosed later in life is important to correctly diagnose and treat patients.
Can you talk about the different treatments?
MF: Patients with Type 1 diabetes are going to require insulin. Type 2 diabetes patients can require insulin, but that often occurs later in the disease, as oral medications become less and less effective.
ES: Because of the epidemic of overweight and obese in the general population, we’re seeing a lot of people with Type 1 diabetes who are overweight and have obesity. This can contribute to issues around misdiagnosis because people with Type 1 diabetes will have signs and will present similarly to Type 2 diabetes. They'll have insulin resistance potentially as a result of weight gain metabolic syndrome. Some people call it double diabetes—I don't like that term—but it’s this idea that if you have Type 1 diabetes, you can also have characteristics of Type 2 diabetes as well.
I understand that Type 1 used to be considered a thin person's disease, but that’s not the case anymore. MF: In a separate paper, we also explored the issue of overweight and obesity in persons with Type 1 diabetes. We found that approximately 62% of adults with Type 1 diabetes were either overweight or obese, which is comparable to the general U.S. population.
But an important disclaimer is that weight management in this population [with Type 1 diabetes] is very different. They can't just decide to go on a diet, start jogging, or engage in rigorous exercise. It can be a very, very dangerous thing to do.
Everybody's talking about Ozempic and Mounjaro—the GLP-1 drugs—for diabetes or people who are overweight to lose weight and to solve their diabetes. Where does that fit in with this population?
ES: These medications are used to treat Type 2 diabetes in the setting of obesity. Ozempic and Mounjaro are incretin hormones. They mediate satiation, reduce appetite, slow gastric emptying, and lower energy intake. They're really powerful drugs that may be helpful in Type 1 diabetes, but they're not approved for the management of obesity and Type 1 diabetes. At the moment, there aren't data to help guide their use in people with Type 1 diabetes, but I suspect they're going to be increasingly used in people with Type 1 diabetes.
MF: The other piece of managing weight—and it's thought to be foundational for Type 1 or Type 2—is dieting and exercising. However, there isn’t good guidance on how to do this in persons with Type 1 diabetes, whereas there are large and rigorous trials in Type 2 patients. We’re really just starting to figure out how to safely and effectively manage weight with lifestyle changes for Type 1 diabetics, and I think that's an important area of research that should continue moving forward.
ES: Weight management in Type 1 diabetes is complicated by insulin use and the risk of hypoglycemia, or your glucose going too low, which can be an acute complication of exercise. In people with Type 2 diabetes, we have a strong evidence base for what works. We know modest weight loss can help prevent the progression and development of Type 2 diabetes, as well as weight gain. In Type 1, we just don't have that evidence base.
Is there a concern about misdiagnosis and mistreatment? Is it possible to think a patient has Type 2 but they actually have Type 1?
MF: I think so. Insulin is the overriding concern. In the obesity paper, we looked at the percentage of people who said their doctors recommended engaging in more exercise and dieting. We found that people with Type 1 diabetes were less likely to receive the same guidance from their doctor. I think providers may be hesitant to say, “Look, just go engage in an active lifestyle.”
This is why it's important to have those studies and have that guidance so that patients and providers can be comfortable in improving lifestyle management.
Where is this research going next?
ES: What's clear from these studies is that the burden of overweight and obesity is substantial in people with Type 1 diabetes and it's not adequately managed. Going forward, I think we're going to need clinical trials, clear clinical guidelines, and patient education that addresses how best to tackle obesity in the setting of Type 1 diabetes.
It must be confusing for people with Type 1 diabetes who are hearing about people losing all this weight on these drugs, but they go to their doctor who says, “Yeah, but that's not for you.”
ES: I hope it's being handled more sensitively. These drugs are being used by all sorts of people for whom they are not indicated, and I'm sure that people with Type 1 diabetes are accessing these drugs. I think the question is, are there real safety issues? We need thoughtful discussion about this and some real evidence to make sure that we're doing more good than harm.
MF: Dr. Selvin’s group has published a paper, estimating that about 15% of people with Type 1 diabetes are on a GLP-1. But we don't have great data on what potentially can happen to individuals.
The other big part of diabetes that we hear a lot about is insulin and its price. Can you talk about your research on this topic?
MF: There was a survey that asked, “Has there been a point during the year when you were not using insulin because you couldn’t afford it?” About 20% of adults under the age of 65 said that at some point during the year, they couldn't afford their insulin and that they did engage in what sometimes is called “cost-saving rationing” [of insulin].
Medicare is now covering cheaper insulin for those over 65, but there are a lot of people for whom affordability is an issue. Can you talk more about that?
MF: The fight is not over. Just because there are national and state policies, and now manufacturers have been implementing price caps, doesn't necessarily mean that the people who need insulin the most are now able to afford it.
A recent study in the Annals of Internal Medicine looked at states that adopted or implemented out-of-pocket cost caps for insulin versus those that didn't and how that affected insulin use over time. They found that people were paying less for insulin, but the use of insulin didn't change over time. The $35 cap is an improvement, but we need to do more.
ES: There are still a lot of formulations of insulin that are very expensive. $35 a month is not cheap for someone who is on insulin for the rest of their lives.
- Overweight and Obesity in People With Type 1 Diabetes Nearly Same as General Population
- The Impacts of COVID-19 on Diabetes and Insulin
- Why Eli Lilly’s Insulin Price Cap Announcement Matters
Related Content
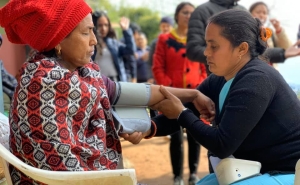
New Grant Enables Johns Hopkins Researchers to Implement Community Health Worker-Led Health Interventions for Noncommunicable Diseases in Nepal
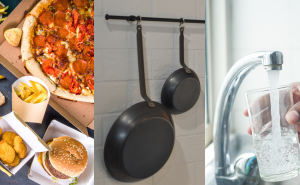
The Omnipresence of PFAS—and What We Can Do About Them
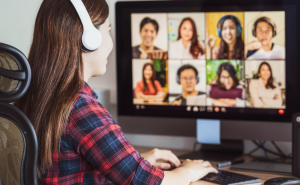
Summer Institute in Data to Policy
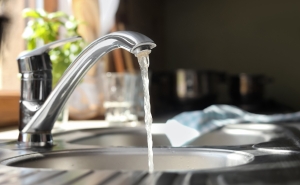
Filters and Digital Health Program Reduced Participants' Arsenic Levels by Nearly Half in American Indian Households Relying on Well Water
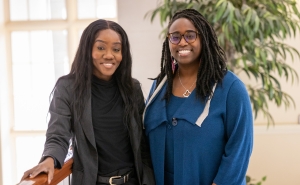
Student Spotlight: Glendedora Dolce
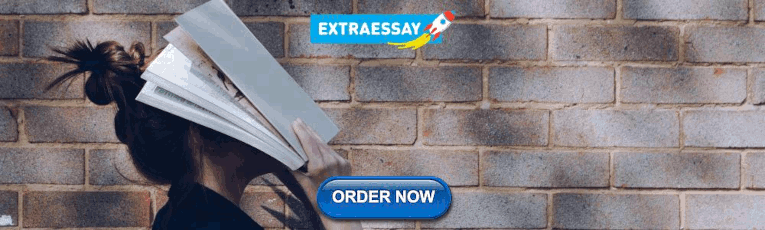
IMAGES
COMMENTS
Different classes of diabetes mellitus, type 1, type 2, gestational diabetes and other types of diabetes mellitus are compared in terms of diagnostic criteria, etiology and genetics. The molecular genetics of diabetes received extensive attention in recent years by many prominent investigators and research groups in the biomedical field.
Diabetes mellitus (DM) also known as simply diabetes, is a group of metabolic. diseases in which there are high blood sugar levels over a prolonged period This high. blood sugar produces the ...
Effects of intensive blood-pressure control in type 2 diabetes mellitus. N Engl J Med 2010;362:1575-1585. Crossref. ... Chronicle of Diabetes Research and Practice, 3, 1, ...
Diabetic keratopathy (DK) poses a significant challenge in diabetes mellitus, yet its molecular pathways and effective treatments remain elusive. The aim of our research was to explore the pyroptosis-related genes in the corneal epithelium of the streptozocin-induced diabetic rats. Methods. After sixteen weeks of streptozocin intraperitoneal ...
Diabetes mellitus. 1. Introduction. Diabetes is a long-term, persistent disease that occurs due to the body's inability to process and regulate blood glucose due to the over-secretion of insulin from the pancreas or the inability of the insulin to regulate the blood glucose levels [ 1 ]. Insulin is a polypeptide hormone secreted by the beta ...
The best evidence for a link between diabetes mellitus and breast cancer comes from a systematic review of six prospective cohort studies and more than 150,000 women, in which the hazard ratio (HR ...
Prevention of Cardiovascular Disease in Type 1 Diabetes. C. Manrique-Acevedo, I.B. Hirsch, and R.H. EckelN Engl J Med 2024;390:1207-1217. More than half of newly diagnosed cases of type 1 diabetes ...
Diabetes mellitus (DM) underscores a rising epidemic orchestrating critical socio-economic burden on countries globally. Different treatment options for the management of DM are evolving rapidly because the usual methods of treatment have not completely tackled the primary causes of the disease and are laden with critical adverse effects. Thus, this narrative review explores different ...
Diabetes drug slows development of Parkinson's disease. The drug, which is in the same family as blockbuster weight-loss drugs such as Wegovy, slowed development of symptoms by a small but ...
New estimates published this week in The Lancet indicate that more than 1·31 billion people could be living with diabetes by 2050 worldwide. That's 1·31 billion people living with a disease that causes life-altering morbidity, high rates of mortality, and interacts with and exacerbates many other diseases. The increase in prevalence (up from 529 million in 2021) is expected to be driven by ...
Diabetes remains a substantial public health issue. Type 2 diabetes, which makes up the bulk of diabetes cases, is largely preventable and, in some cases, potentially reversible if identified and managed early in the disease course. However, all evidence indicates that diabetes prevalence is increasing worldwide, primarily due to a rise in obesity caused by multiple factors. Preventing and ...
Type 2 Diabetes Mellitus (T2DM) is characterized by chronically elevated blood glucose (hyperglycemia) and elevated blood insulin (hyperinsulinemia). When the blood glucose concentration is 100 milligrams/deciliter the bloodstream of an average adult contains about 5-10 grams of glucose. Carbohydrate-restricted diets have been used effectively to treat obesity and T2DM for over 100 years ...
Diabetes mellitus (DM), o r simply diabetes, is a group of metabolic diseases in which a person has. high blood sugar, either because the body does not produce enough insulin, or because cells do ...
Type 1 diabetes cannot be prevented with current knowledge. Effective approaches are available to prevent type2 diabetes and to prevent the complications and premature death that can result from all types of diabetes. These include policies and practices across whole populations and within specific settings (school,
Type 2 diabetes mellitus (T2DM) is a metabolic disorder sparked by insulin resistance and dysfunction of the β cells. Monotherapy and combinatorial drug therapies represents the first line treatment choices for the said disorder. Nanotechnology based approaches offers promising potential in terms of therapeutic efficacy and improved quality of ...
Diabetes mellitus is a very common disorder in all over the world, often simply referred to as diabetes, is a group of metabolic diseases (Also known as metabolic syndrome and a slow poison) in ...
Limited research has explored the effectiveness of insulin resistance (IR) in forecasting metabolic syndrome (MetS) risk, especially within the Iranian population afflicted with type 2 diabetes ...
These findings indicate that LPE addresses type 2 diabetes mellitus by activating the AMPK energy metabolic pathway and regulating the Nrf2 oxidative stress and NF-κB inflammatory signaling pathways. ... Feature papers represent the most advanced research with significant potential for high impact in the field. A Feature Paper should be a ...
The paper is entitled 'Human inherited PD-L1 deficiency is ... Diabetes mellitus type 1; ... type 2 diabetes is on the rise. A research group has discovered a new gene that may hold the key to ...
There are about 1.5 million adults with Type 1 diabetes in the U.S., compared to 21 million adults with Type 2 diabetes. In terms of the total cases of diabetes, only 5 to 10 percent have Type 1 diabetes. Even in our largest epidemiologic cohorts, only a small percentage of people have Type 1 diabetes. So, we just don't have the same national ...
Please use one of the following formats to cite this article in your essay, paper or report: APA. Sai Lomte, Tarun. (2024, April 15). Exercise shown to curb appetite in diabetes and prediabetes ...
On the other hand, diabetes mellitus (DM) is a chronic disease that can develop due to a lack of insulin secretion or insulin sensitivity. Recently, CS-based nanocarriers have been extensively applied for DM therapy. Oral delivery of insulin is the most common use of CS nanoparticles in DM therapy, and they improve the pharmacological ...