Lock-and-key model
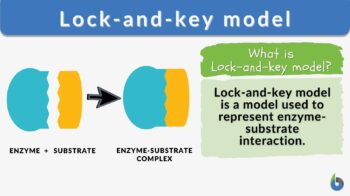
strong>Lock-and-key model n., [lɑk ænd ki ˈmɑdl̩] Definition: a model for enzyme-substrate interaction
Table of Contents
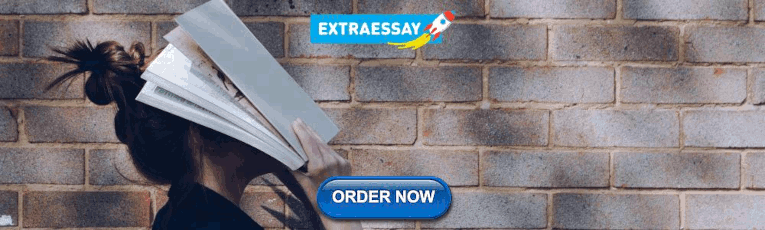
Lock-and-key model Definition
Lock-and-key model is a model for enzyme-substrate interaction suggesting that the enzyme and the substrate possess specific complementary geometric shapes that fit exactly into one another. In this model, enzymes are depicted as highly specific. They must bind to specific substrates before they catalyze chemical reactions . The term is a pivotal concept in enzymology to elucidate the intricate interaction between enzymes and substrates at the molecular level. In the lock-and-key model, the enzyme-substrate interaction suggests that the enzyme and the substrate possess specific complementary geometric shapes that fit exactly into one another. Like a key into a lock , only the correct size and shape of the substrate ( the key ) would fit into the active site ( the keyhole ) of the enzyme ( the lock ).
Compare: Induced fit model See also: enzyme , active site , substrate
Lock-and-key vs. Induced Fit Model
At present, two models attempt to explain enzyme-substrate specificity; one of which is the lock-and-key model , and the other is the Induced fit model . The lock and key model theory was first postulated by Emil Fischer in 1894. The lock-and-key enzyme action proposes the high specificity of enzymes. However, it does not explain the stabilization of the transition state that the enzymes achieve. The induced fit model (proposed by Daniel Koshland in 1958) suggests that the active site continues to change until the substrate is completely bound to the active site of the enzyme, at which point the final shape and charge are determined. Unlike the lock-and-key model, the induced fit model shows that enzymes are rather flexible structures. Nevertheless, Fischer’s Lock and Key theory laid an important foundation for subsequent research, such as during the refinement of the enzyme-substrate complex mechanism, as ascribed in the induced fit model. The lock-and-key hypothesis has opened ideas where enzyme action is not merely catalytic but incorporates a rather complex process in how they interact with the correct substrates with precision.
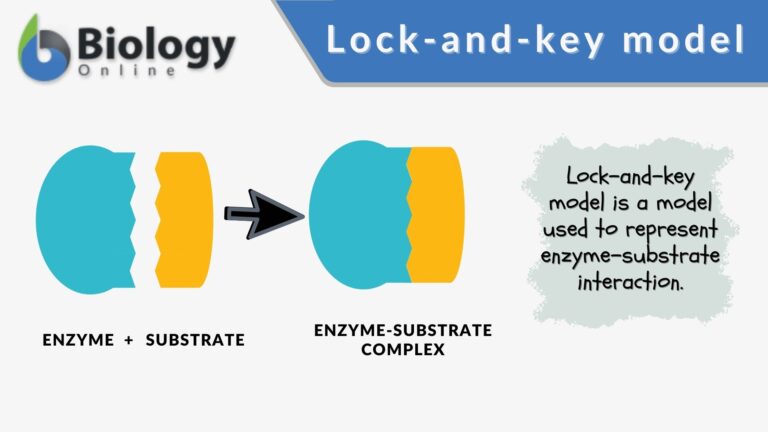
Key Components
Components of the lock and key model:
- Enzyme : the enzyme structure is a three-dimensional protein configuration, with an active site from where the substrate binds.
- Substrate : often an organic molecule, a substrate possesses a structural feature that complements the geometry of the enzyme’s active site.
In the lock and key model, both the enzymes and the substrates facilitate the formation of a complex that lowers the activation energy needed for a chemical transformation to occur. Such reduction in the activation energy allows the chemical reaction to proceed at a relatively faster rate, making enzymes crucial in various biological and molecular processes.
Lock-and-key Model Examples
Some of the common examples that are often discussed in the context of the Lock and Key Model are as follows:
- Enzyme lactate dehydrogenase with a specific active site for its substrates, pyruvate and lactate. The complex facilitates the interconversion of pyruvate and lactate during anaerobic respiration
- Enzyme carbonic anhydrase with a specific active site for the substrates carbon dioxide and water. The complex facilitates the hydration of carbon dioxide, forming bicarbonate
- Enzyme lysozyme binding with a bacterial cell wall peptidoglycan, which is a vital immune function
Choose the best answer.
Send Your Results (Optional)
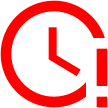
Time is Up!
- Aryal, S. and Karki, P. (2023). “Lock and Key Model- Mode of Action of Enzymes”. Microbenotes.com. https://microbenotes.com/lock-and-key-model-mode-of-action-of-enzymes/
- Farhana, A., & Lappin, S. L. (2023, May). Biochemistry, Lactate Dehydrogenase . Nih.gov; StatPearls Publishing. https://www.ncbi.nlm.nih.gov/books/NBK557536/
©BiologyOnline.com. Content provided and moderated by Biology Online Editors.
Last updated on January 11th, 2024
You will also like...
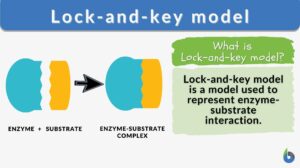
Freshwater Communities & Plankton
Planktons are microscopic organisms that live suspended in aquatic habitats. There are two groups: the phytoplanktons an..
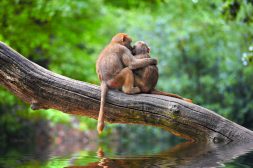
Population Regulation in an Ecosystem
With regard to the population size of a species and what factors may affect them, two factors have been defined. They ar..
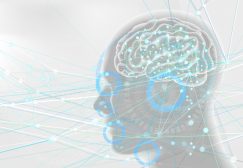
Sensory Systems
A sensory system is a part of the nervous system consisting of sensory receptors that receive stimuli from the internal ..
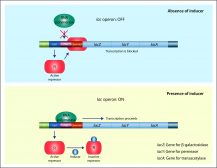
Gene Action – Operon Hypothesis
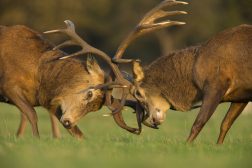
The Gene Pool and Population Genetics
According to Charles Darwin's theory of natural selection, preferable genes are favored by nature in the gene pool, and ..
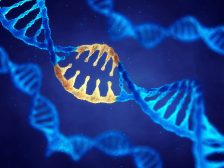
Genetic Information and Protein Synthesis
Genes are expressed through the process of protein synthesis. This elaborate tutorial provides an in-depth review of the..
Related Articles...
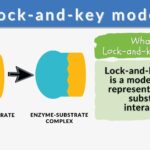
No related articles found
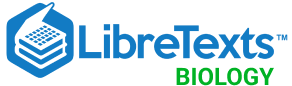
- school Campus Bookshelves
- menu_book Bookshelves
- perm_media Learning Objects
- login Login
- how_to_reg Request Instructor Account
- hub Instructor Commons
- Download Page (PDF)
- Download Full Book (PDF)
- Periodic Table
- Physics Constants
- Scientific Calculator
- Reference & Cite
- Tools expand_more
- Readability
selected template will load here
This action is not available.
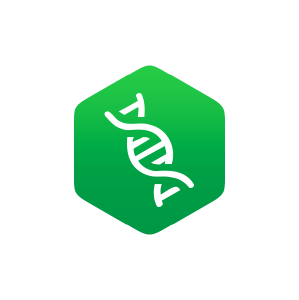
6.10: Enzymes - Active Site and Substrate Specificity
- Last updated
- Save as PDF
- Page ID 13122
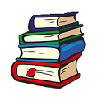
Learning Objectives
- Describe models of substrate binding to an enzyme’s active site.
Enzyme Active Site and Substrate Specificity
Enzymes bind with chemical reactants called substrates. There may be one or more substrates for each type of enzyme, depending on the particular chemical reaction. In some reactions, a single-reactant substrate is broken down into multiple products. In others, two substrates may come together to create one larger molecule. Two reactants might also enter a reaction, both become modified, and leave the reaction as two products.
The enzyme’s active site binds to the substrate. Since enzymes are proteins, this site is composed of a unique combination of amino acid residues (side chains or R groups). Each amino acid residue can be large or small; weakly acidic or basic; hydrophilic or hydrophobic; and positively-charged, negatively-charged, or neutral. The positions, sequences, structures, and properties of these residues create a very specific chemical environment within the active site. A specific chemical substrate matches this site like a jigsaw puzzle piece and makes the enzyme specific to its substrate.
Active Sites and Environmental Conditions
Environmental conditions can affect an enzyme’s active site and, therefore, the rate at which a chemical reaction can proceed. Increasing the environmental temperature generally increases reaction rates because the molecules are moving more quickly and are more likely to come into contact with each other.
However, increasing or decreasing the temperature outside of an optimal range can affect chemical bonds within the enzyme and change its shape. If the enzyme changes shape, the active site may no longer bind to the appropriate substrate and the rate of reaction will decrease. Dramatic changes to the temperature and pH will eventually cause enzymes to denature.
Induced Fit and Enzyme Function
For many years, scientists thought that enzyme-substrate binding took place in a simple “lock-and-key” fashion. This model asserted that the enzyme and substrate fit together perfectly in one instantaneous step. However, current research supports a more refined view called induced fit. As the enzyme and substrate come together, their interaction causes a mild shift in the enzyme’s structure that confirms an ideal binding arrangement between the enzyme and the substrate. This dynamic binding maximizes the enzyme’s ability to catalyze its reaction.
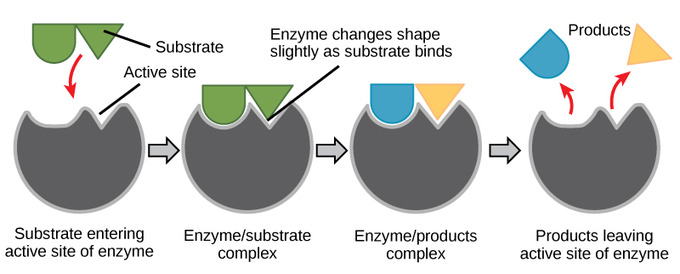
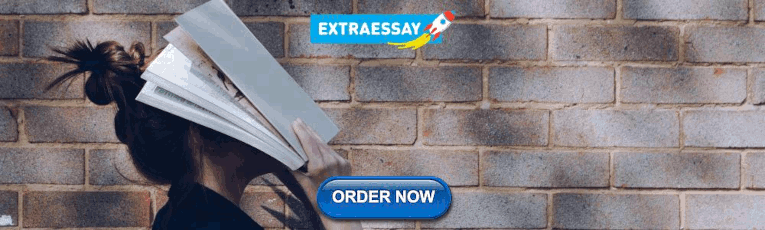
Enzyme-Substrate Complex
When an enzyme binds its substrate, it forms an enzyme-substrate complex. This complex lowers the activation energy of the reaction and promotes its rapid progression by providing certain ions or chemical groups that actually form covalent bonds with molecules as a necessary step of the reaction process. Enzymes also promote chemical reactions by bringing substrates together in an optimal orientation, lining up the atoms and bonds of one molecule with the atoms and bonds of the other molecule. This can contort the substrate molecules and facilitate bond-breaking. The active site of an enzyme also creates an ideal environment, such as a slightly acidic or non-polar environment, for the reaction to occur. The enzyme will always return to its original state at the completion of the reaction. One of the important properties of enzymes is that they remain ultimately unchanged by the reactions they catalyze. After an enzyme is done catalyzing a reaction, it releases its products (substrates).
- The enzyme ‘s active site binds to the substrate.
- Increasing the temperature generally increases the rate of a reaction, but dramatic changes in temperature and pH can denature an enzyme, thereby abolishing its action as a catalyst.
- The induced fit model states an substrate binds to an active site and both change shape slightly, creating an ideal fit for catalysis.
- When an enzyme binds its substrate it forms an enzyme-substrate complex.
- Enzymes promote chemical reactions by bringing substrates together in an optimal orientation, thus creating an ideal chemical environment for the reaction to occur.
- The enzyme will always return to its original state at the completion of the reaction.
- substrate : A reactant in a chemical reaction is called a substrate when acted upon by an enzyme.
- induced fit : Proposes that the initial interaction between enzyme and substrate is relatively weak, but that these weak interactions rapidly induce conformational changes in the enzyme that strengthen binding.
- active site : The active site is the part of an enzyme to which substrates bind and where a reaction is catalyzed.

An official website of the United States government
The .gov means it's official. Federal government websites often end in .gov or .mil. Before sharing sensitive information, make sure you're on a federal government site.
The site is secure. The https:// ensures that you are connecting to the official website and that any information you provide is encrypted and transmitted securely.
- Publications
- Account settings
- Browse Titles
NCBI Bookshelf. A service of the National Library of Medicine, National Institutes of Health.
Cooper GM. The Cell: A Molecular Approach. 2nd edition. Sunderland (MA): Sinauer Associates; 2000.
By agreement with the publisher, this book is accessible by the search feature, but cannot be browsed.

The Cell: A Molecular Approach. 2nd edition.
The central role of enzymes as biological catalysts.
A fundamental task of proteins is to act as enzymes —catalysts that increase the rate of virtually all the chemical reactions within cells. Although RNAs are capable of catalyzing some reactions, most biological reactions are catalyzed by proteins. In the absence of enzymatic catalysis, most biochemical reactions are so slow that they would not occur under the mild conditions of temperature and pressure that are compatible with life. Enzymes accelerate the rates of such reactions by well over a million-fold, so reactions that would take years in the absence of catalysis can occur in fractions of seconds if catalyzed by the appropriate enzyme. Cells contain thousands of different enzymes, and their activities determine which of the many possible chemical reactions actually take place within the cell.
- The Catalytic Activity of Enzymes
Like all other catalysts, enzymes are characterized by two fundamental properties. First, they increase the rate of chemical reactions without themselves being consumed or permanently altered by the reaction. Second, they increase reaction rates without altering the chemical equilibrium between reactants and products.
These principles of enzymatic catalysis are illustrated in the following example, in which a molecule acted upon by an enzyme (referred to as a substrate [ S ]) is converted to a product ( P ) as the result of the reaction. In the absence of the enzyme, the reaction can be written as follows:

The chemical equilibrium between S and P is determined by the laws of thermodynamics (as discussed further in the next section of this chapter) and is represented by the ratio of the forward and reverse reaction rates ( S → P and P → S , respectively). In the presence of the appropriate enzyme, the conversion of S to P is accelerated, but the equilibrium between S and P is unaltered. Therefore, the enzyme must accelerate both the forward and reverse reactions equally. The reaction can be written as follows:

Note that the enzyme ( E ) is not altered by the reaction, so the chemical equilibrium remains unchanged, determined solely by the thermodynamic properties of S and P .
The effect of the enzyme on such a reaction is best illustrated by the energy changes that must occur during the conversion of S to P ( Figure 2.22 ). The equilibrium of the reaction is determined by the final energy states of S and P , which are unaffected by enzymatic catalysis. In order for the reaction to proceed, however, the substrate must first be converted to a higher energy state, called the transition state . The energy required to reach the transition state (the activation energy ) constitutes a barrier to the progress of the reaction, limiting the rate of the reaction. Enzymes (and other catalysts) act by reducing the activation energy , thereby increasing the rate of reaction. The increased rate is the same in both the forward and reverse directions, since both must pass through the same transition state.
Figure 2.22
Energy diagrams for catalyzed and uncatalyzed reactions. The reaction illustrated is the simple conversion of a substrate S to a product P. Because the final energy state of P is lower than that of S , the reaction proceeds from left to right. For the (more...)
The catalytic activity of enzymes involves the binding of their substrates to form an enzyme- substrate complex ( ES ). The substrate binds to a specific region of the enzyme, called the active site . While bound to the active site , the substrate is converted into the product of the reaction, which is then released from the enzyme. The enzyme-catalyzed reaction can thus be written as follows:

Note that E appears unaltered on both sides of the equation, so the equilibrium is unaffected. However, the enzyme provides a surface upon which the reactions converting S to P can occur more readily. This is a result of interactions between the enzyme and substrate that lower the energy of activation and favor formation of the transition state.
- Mechanisms of Enzymatic Catalysis
The binding of a substrate to the active site of an enzyme is a very specific interaction. Active sites are clefts or grooves on the surface of an enzyme, usually composed of amino acids from different parts of the polypeptide chain that are brought together in the tertiary structure of the folded protein. Substrates initially bind to the active site by noncovalent interactions, including hydrogen bonds, ionic bonds, and hydrophobic interactions. Once a substrate is bound to the active site of an enzyme, multiple mechanisms can accelerate its conversion to the product of the reaction.
Although the simple example discussed in the previous section involved only a single substrate molecule, most biochemical reactions involve interactions between two or more different substrates. For example, the formation of a peptide bond involves the joining of two amino acids. For such reactions, the binding of two or more substrates to the active site in the proper position and orientation accelerates the reaction ( Figure 2.23 ). The enzyme provides a template upon which the reactants are brought together and properly oriented to favor the formation of the transition state in which they interact.
Figure 2.23
Enzymatic catalysis of a reaction between two substrates. The enzyme provides a template upon which the two substrates are brought together in the proper position and orientation to react with each other.
Enzymes accelerate reactions also by altering the conformation of their substrates to approach that of the transition state. The simplest model of enzyme- substrate interaction is the lock-and-key model , in which the substrate fits precisely into the active site ( Figure 2.24 ). In many cases, however, the configurations of both the enzyme and substrate are modified by substrate binding—a process called induced fit . In such cases the conformation of the substrate is altered so that it more closely resembles that of the transition state. The stress produced by such distortion of the substrate can further facilitate its conversion to the transition state by weakening critical bonds. Moreover, the transition state is stabilized by its tight binding to the enzyme, thereby lowering the required energy of activation.
Figure 2.24
Models of enzyme-substrate interaction. (A) In the lock-and-key model, the substrate fits precisely into the active site of the enzyme. (B) In the induced-fit model, substrate binding distorts the conformations of both substrate and enzyme. This distortion (more...)
In addition to bringing multiple substrates together and distorting the conformation of substrates to approach the transition state, many enzymes participate directly in the catalytic process. In such cases, specific amino acid side chains in the active site may react with the substrate and form bonds with reaction intermediates. The acidic and basic amino acids are often involved in these catalytic mechanisms, as illustrated in the following discussion of chymotrypsin as an example of enzymatic catalysis.
Chymotrypsin is a member of a family of enzymes (serine proteases) that digest proteins by catalyzing the hydrolysis of peptide bonds. The reaction can be written as follows:

The different members of the serine protease family (including chymotrypsin, trypsin, elastase, and thrombin) have distinct substrate specificities; they preferentially cleave peptide bonds adjacent to different amino acids. For example, whereas chymotrypsin digests bonds adjacent to hydrophobic amino acids, such as tryptophan and phenylalanine, trypsin digests bonds next to basic amino acids, such as lysine and arginine. All the serine proteases, however, are similar in structure and use the same mechanism of catalysis. The active sites of these enzymes contain three critical amino acids—serine, histidine, and aspartate—that drive hydrolysis of the peptide bond . Indeed, these enzymes are called serine proteases because of the central role of the serine residue.
Substrates bind to the serine proteases by insertion of the amino acid adjacent to the cleavage site into a pocket at the active site of the enzyme ( Figure 2.25 ). The nature of this pocket determines the substrate specificity of the different members of the serine protease family. For example, the binding pocket of chymotrypsin contains hydrophobic amino acids that interact with the hydrophobic side chains of its preferred substrates. In contrast, the binding pocket of trypsin contains a negatively charged acidic amino acid (aspartate), which is able to form an ionic bond with the lysine or arginine residues of its substrates.
Figure 2.25
Substrate binding by serine proteases. The amino acid adjacent to the peptide bond to be cleaved is inserted into a pocket at the active site of the enzyme. In chymotrypsin, the pocket binds hydrophobic amino acids; the binding pocket of trypsin contains (more...)
Substrate binding positions the peptide bond to be cleaved adjacent to the active site serine ( Figure 2.26 ). The proton of this serine is then transferred to the active site histidine. The conformation of the active site favors this proton transfer because the histidine interacts with the negatively charged aspartate residue. The serine reacts with the substrate , forming a tetrahedral transition state. The peptide bond is then cleaved, and the C-terminal portion of the substrate is released from the enzyme. However, the N-terminal peptide remains bound to serine. This situation is resolved when a water molecule (the second substrate) enters the active site and reverses the preceding reactions. The proton of the water molecule is transferred to histidine, and its hydroxyl group is transferred to the peptide, forming a second tetrahedral transition state. The proton is then transferred from histidine back to serine, and the peptide is released from the enzyme, completing the reaction.
Figure 2.26
Catalytic mechanism of chymotrypsin. Three amino acids at the active site (Ser-195, His-57, and Asp-102) play critical roles in catalysis.
This example illustrates several features of enzymatic catalysis; the specificity of enzyme- substrate interactions, the positioning of different substrate molecules in the active site , and the involvement of active-site residues in the formation and stabilization of the transition state. Although the thousands of enzymes in cells catalyze many different types of chemical reactions, the same basic principles apply to their operation.
In addition to binding their substrates, the active sites of many enzymes bind other small molecules that participate in catalysis. Prosthetic groups are small molecules bound to proteins in which they play critical functional roles. For example, the oxygen carried by myoglobin and hemoglobin is bound to heme, a prosthetic group of these proteins. In many cases metal ions (such as zinc or iron) are bound to enzymes and play central roles in the catalytic process. In addition, various low-molecular-weight organic molecules participate in specific types of enzymatic reactions. These molecules are called coenzymes because they work together with enzymes to enhance reaction rates. In contrast to substrates, coenzymes are not irreversibly altered by the reactions in which they are involved. Rather, they are recycled and can participate in multiple enzymatic reactions.
Coenzymes serve as carriers of several types of chemical groups. A prominent example of a coenzyme is nicotinamide adenine dinucleotide ( NAD + ), which functions as a carrier of electrons in oxidation-reduction reactions ( Figure 2.27 ). NAD + can accept a hydrogen ion (H + ) and two electrons (e - ) from one substrate , forming NADH. NADH can then donate these electrons to a second substrate, re-forming NAD + . Thus, NAD + transfers electrons from the first substrate (which becomes oxidized) to the second (which becomes reduced).
Figure 2.27
Role of NAD + in oxidation-reduction reactions. (A) Nicotinamide adenine dinucleotide (NAD + ) acts as a carrier of electrons in oxidation-reduction reactions by accepting electrons (e - ) to form NADH. (B) For example, NAD + can accept electrons from one substrate (more...)
Several other coenzymes also act as electron carriers, and still others are involved in the transfer of a variety of additional chemical groups (e.g., carboxyl groups and acyl groups; Table 2.1 ). The same coenzymes function together with a variety of different enzymes to catalyze the transfer of specific chemical groups between a wide range of substrates. Many coenzymes are closely related to vitamins, which contribute part or all of the structure of the coenzyme. Vitamins are not required by bacteria such as E. coli but are necessary components of the diets of human and other higher animals, which have lost the ability to synthesize these compounds.
Examples of Coenzymes and Vitamins.
- Regulation of Enzyme Activity
An important feature of most enzymes is that their activities are not constant but instead can be modulated. That is, the activities of enzymes can be regulated so that they function appropriately to meet the varied physiological needs that may arise during the life of the cell.
One common type of enzyme regulation is feedback inhibition , in which the product of a metabolic pathway inhibits the activity of an enzyme involved in its synthesis. For example, the amino acid isoleucine is synthesized by a series of reactions starting from the amino acid threonine ( Figure 2.28 ). The first step in the pathway is catalyzed by the enzyme threonine deaminase, which is inhibited by isoleucine, the end product of the pathway. Thus, an adequate amount of isoleucine in the cell inhibits threonine deaminase, blocking further synthesis of isoleucine. If the concentration of isoleucine decreases, feedback inhibition is relieved, threonine deaminase is no longer inhibited, and additional isoleucine is synthesized. By so regulating the activity of threonine deaminase, the cell synthesizes the necessary amount of isoleucine but avoids wasting energy on the synthesis of more isoleucine than is needed.
Figure 2.28
Feedback inhibition. The first step in the conversion of threonine to iso-leucine is catalyzed by the enzyme threonine deaminase. The activity of this enzyme is inhibited by isoleucine, the end product of the pathway.
Feedback inhibition is one example of allosteric regulation , in which enzyme activity is controlled by the binding of small molecules to regulatory sites on the enzyme ( Figure 2.29 ). The term “ allosteric regulation ” derives from the fact that the regulatory molecules bind not to the catalytic site, but to a distinct site on the protein ( allo = “other” and steric = “site”). Binding of the regulatory molecule changes the conformation of the protein, which in turn alters the shape of the active site and the catalytic activity of the enzyme. In the case of threonine deaminase, binding of the regulatory molecule (isoleucine) inhibits enzymatic activity. In other cases regulatory molecules serve as activators, stimulating rather than inhibiting their target enzymes .
Figure 2.29
Allosteric regulation. In this example, enzyme activity is inhibited by the binding of a regulatory molecule to an allosteric site. In the absence of inhibitor, the substrate binds to the active site of the enzyme and the reaction proceeds. The binding (more...)
The activities of enzymes can also be regulated by their interactions with other proteins and by covalent modifications, such as the addition of phosphate groups to serine, threonine, or tyrosine residues. Phosphorylation is a particularly common mechanism for regulating enzyme activity; the addition of phosphate groups either stimulates or inhibits the activities of many different enzymes ( Figure 2.30 ). For example, muscle cells respond to epinephrine (adrenaline) by breaking down glycogen into glucose, thereby providing a source of energy for increased muscular activity. The breakdown of glycogen is catalyzed by the enzyme glycogen phosphorylase, which is activated by phosphorylation in response to the binding of epinephrine to a receptor on the surface of the muscle cell. Protein phosphorylation plays a central role in controlling not only metabolic reactions but also many other cellular functions, including cell growth and differentiation.
Figure 2.30
Protein phosphorylation. Some enzymes are regulated by the addition of phosphate groups to the side-chain OH groups of serine (as shown here), threonine, or tyrosine residues. For example, the enzyme glycogen phosphorylase, which catalyzes the conversion (more...)
- Cite this Page Cooper GM. The Cell: A Molecular Approach. 2nd edition. Sunderland (MA): Sinauer Associates; 2000. The Central Role of Enzymes as Biological Catalysts.
- Disable Glossary Links
In this Page
Related items in bookshelf.
- All Textbooks
Recent Activity
- The Central Role of Enzymes as Biological Catalysts - The Cell The Central Role of Enzymes as Biological Catalysts - The Cell
Your browsing activity is empty.
Activity recording is turned off.
Turn recording back on
Connect with NLM
National Library of Medicine 8600 Rockville Pike Bethesda, MD 20894
Web Policies FOIA HHS Vulnerability Disclosure
Help Accessibility Careers
Complimentary 1-hour tutoring consultation Schedule Now
Induced-Fit Model of Enzymes
The induced-fit model proposes that the initial interaction between enzyme and substrate is relatively weak, but that these weak interactions rapidly induce conformational changes in the enzyme that strengthen binding.
For many years, scientists thought that enzyme-substrate binding took place in a simple “ lock-and-key ” fashion. This model asserted that the enzyme and substrate fit together perfectly in one instantaneous step. However, current research supports a more refined view called induced fit . As the enzyme and substrate come together, their interaction causes a mild shift in the enzyme’s structure that confirms an ideal binding arrangement between the enzyme and the substrate. This dynamic binding maximizes the enzyme’s ability to catalyze its reaction.

Practice Questions
Khan Academy
- Fighting the Flu with Oseltamivir
MCAT Official Prep (AAMC)
Section Bank C/P Section Question 47
• The enzyme‘s active site binds to the substrate
• The induced-fit model states a substrate binds to an active site and both change shape slightly, creating an ideal fit for catalysis.
• Enzymes promote chemical reactions by bringing substrates together in an optimal orientation, thus creating an ideal chemical environment for the reaction to occur.
lock-and-key: A model that asserts an enzyme and substrate fit together perfectly in one instantaneous step.
enzyme : A substance produced by a living organism which acts as a catalyst to bring about a specific biochemical reaction.
substrate : A reactant in a chemical reaction is called a substrate when acted upon by an enzyme.
induced fit : Proposes that the initial interaction between enzyme and substrate is relatively weak, but that these weak interactions rapidly induce conformational changes in the enzyme that strengthen binding.
catalyze : Cause or accelerate (a reaction) by acting as a catalyst.
Your Notifications Live Here
{{ notification.creator.name }} Spark
{{ announcement.creator.name }}
Trial Session Enrollment
Live trial session waiting list.

Next Trial Session:
{{ nextFTS.remaining.months }} {{ nextFTS.remaining.months > 1 ? 'months' : 'month' }} {{ nextFTS.remaining.days }} {{ nextFTS.remaining.days > 1 ? 'days' : 'day' }} {{ nextFTS.remaining.days === 0 ? 'Starts Today' : 'remaining' }} Starts Today
Recorded Trial Session
This is a recorded trial for students who missed the last live session.
Waiting List Details:
Due to high demand and limited spots there is a waiting list. You will be notified when your spot in the Trial Session is available.
- Learn Basic Strategy for CARS
- Full Jack Westin Experience
- Interactive Online Classroom
- Emphasis on Timing

Next Trial:
Free Trial Session Enrollment
Daily mcat cars practice.
New MCAT CARS passage every morning.
You are subscribed.
{{ nextFTS.remaining.months }} {{ nextFTS.remaining.months > 1 ? 'months' : 'month' }} {{ nextFTS.remaining.days }} {{ nextFTS.remaining.days > 1 ? 'days' : 'day' }} remaining Starts Today
Welcome Back!
Please sign in to continue..

Please sign up to continue.
{{ detailingplan.name }}.
- {{ feature }}
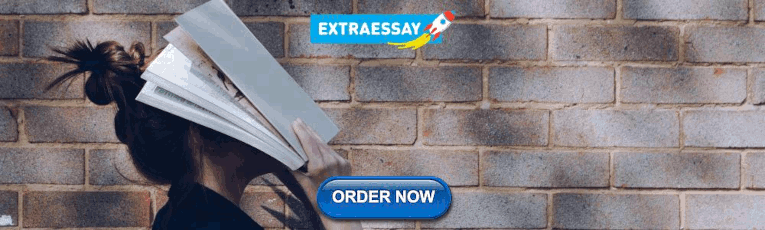
IMAGES
VIDEO
COMMENTS
A researcher proposes a model to explain how enzyme-substrate interactions determine enzyme specificity. The model is based on the idea that substrate molecules form favorable interactions with the amino acid side chains in an enzyme's active site. Based on the model, which of the following statements best explains an enzyme's specificity for a ...
A researcher proposes a model to explain how enzyme-substrate interactions determine enzyme specificity. The model is based on the idea that substrate molecules form favorable interactions with the amino acid side chains in an enzyme's active site. Based on the model, which of the following statements best explains an enzyme's specificity for a ...
A researcher proposes a model to explain how enzyme-substrate interactions determine enzyme specificity. The model is based on the idea that substrate molecules form favorable interactions with the amino acid side chains in an enzyme's active site. Based on the model, which of the following statements best explains an enzyme's specificity for a ...
At present, two models attempt to explain enzyme-substrate specificity; one of which is the lock-and-key model, and the other is the Induced fit model. The lock and key model theory was first postulated by Emil Fischer in 1894. The lock-and-key enzyme action proposes the high specificity of enzymes.
As the enzyme and substrate come together, their interaction causes a mild shift in the enzyme’s structure that confirms an ideal binding arrangement between the enzyme and the substrate. This dynamic binding maximizes the enzyme’s ability to catalyze its reaction. Figure 6.10.1 6.10. 1: Induced Fit: According to the induced fit model, both ...
Question: A researcher proposes a model to explain how enzyme-substrate interactions determine enzyme specificity. The model is based on the idea that substrate molecules form favorable interactions with the amino acid side chains in an enzymes active site.Based on the model, which of the following statements best explains an enzymes specificity for a particular
We created a dataset with experimentally confirmed enzyme-substrate pairs using the GO annotation database for UniProt IDs36 (Methods, “Creating a database with enzyme-substrate pairs”). We were able to extract 18351 enzyme-substrate pairs with experimental evidence for binding, containing 12156 unique enzymes and 1379 unique metabolites.
The simplest model of enzyme-substrate interaction is the lock-and-key model, in which the substrate fits precisely into the active site (Figure 2.24). In many cases, however, the configurations of both the enzyme and substrate are modified by substrate binding—a process called induced fit. In such cases the conformation of the substrate is ...
Because active sites are finely tuned to help a chemical reaction happen, they can be very sensitive to changes in the enzyme’s environment. Factors that may affect the active site and enzyme function include: Temperature. A higher temperature generally makes for higher rates of reaction, enzyme-catalyzed or otherwise.
The induced-fit model proposes that the initial interaction between enzyme and substrate is relatively weak, but that these weak interactions rapidly induce conformational changes in the enzyme that strengthen binding. For many years, scientists thought that enzyme-substrate binding took place in a simple “ lock-and-key ” fashion.