EURASIP Journal on Wireless Communications and Networking
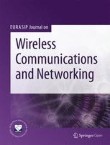
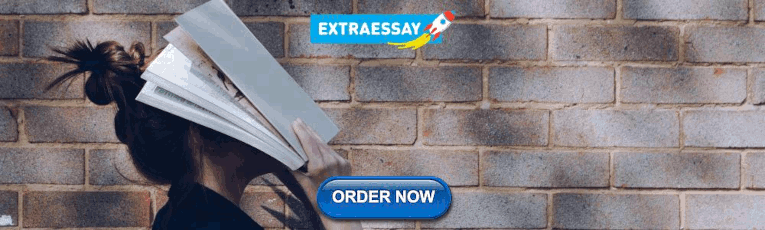
Featured Collection from the 2022 EuCNC & 6G Summit: "6G Connectivity for a Sustainable World"
Open special issues
- Holographic MIMO Systems and Applications
Deadline for submissions: 1 April 2024
EURASIP Journal on Wireless Communications and Networking welcomes proposals for Special Issues on timely topics relevant to the field of signal processing. If you are interested in publishing a collection with us, please read our guidelines here.
View our collection of published special issues
- Most accessed
A precise mathematical approach for analyzing the performance of MIMO space–time block code systems over Weibull fading channels
Authors: Abdelmajid Bessate, Youssef Miftah, Hussain Ben-Azza and Faissal El Bouanani
Performance evaluation of pair selection algorithms in device-to-device communication using relay-assisted techniques
Authors: Abdullahi Adan Omar, Kannan Pauliah Nadar, Chettiyar Vani Vivekanand, E. Anna Devi, A. Jasmine Xavier, T. M. Inbamalar, L. Magthelin Therase, J. Vanitha, C. Andrews Sonia and J. Binisha Rose
Design and analysis antennas of transverse polarization on the dielectric waveguide
Authors: Mohamed N. Shaaban, Aydar R. Nasybullin and Yuri E. Sedelnikov
An adaptive transmission strategy based on cloud computing in IoV architecture
Authors: Bin Li, Vivian Li, Miao Li, John Li, Jiaqi Yang and Bin Li
A modified LSTM with QoS aware hybrid AVO algorithm to enhance resource allocation in D2D communication
Authors: Shaik Ahmed Pasha and Noor Mohammed Vali Mohamad
Most recent articles RSS
View all articles
Handover management in high-dense femtocellular networks
Authors: Mostafa Zaman Chowdhury and Yeong Min Jang
A review of communication-oriented optical wireless systems
Authors: Deva K Borah, Anthony C Boucouvalas, Christopher C Davis, Steve Hranilovic and Konstantinos Yiannopoulos
Text feature extraction based on deep learning: a review
Authors: Hong Liang, Xiao Sun, Yunlei Sun and Yuan Gao
The Correction to this article has been published in EURASIP Journal on Wireless Communications and Networking 2018 2018 :42
LTE and IEEE 802.11p for vehicular networking: a performance evaluation
Authors: Zeeshan Hameed Mir and Fethi Filali
A simple block diagonal precoding for multi-user MIMO broadcast channels
Authors: Md Hashem Ali Khan, K M Cho, Moon Ho Lee and Jin-Gyun Chung
Most accessed articles RSS
Call for Special Issues
EURASIP Journal on Wireless Communications and Networking (JWCN) welcomes Special Issues on timely topics related to the field of signal processing. The objective of Special Issues is to bring together recent and high quality works in a research domain, to promote key advances in the science and applications of wireless communications and networking technologies with emphasis on original results relating to the theory and/or applications of wireless communications and networking, to provide overviews of the state-of-the-art in emerging domains.
Special issue proposals in the format of a single PDF document, are required to be submitted by e-mail to [email protected] . Please include in the subject line ‘JWCN Special Issue Proposal’.
Read more here
EURASIP Best paper awards 2022
We are pleased to announce that the following Research Article published in EURASIP Journal on Wireless Communications and Networking has been awarded the 2022 EURASIP best paper award!
Rate-splitting multiple access for downlink communication systems: bridging, generalizing, and outperforming SDMA and NOMA Authors : Yijie Mao, Bruno Clerckx, and Victor O.K. Li
The award ceremony will be presented at the upcoming edition of EUSIPCO to be held in September 2023.
Society affiliation
The European Association for Signal Processing (EURASIP) was founded on 1 September 1978 to improve communication between groups and individuals that work within the multidisciplinary, fast growing field of signal processing in Europe and elsewhere, and to exchange and disseminate information in this field all over the world. The association exists to further the efforts of researchers by providing a learned and professional platform for dissemination and discussion of all aspects of signal processing including continuous- and discrete-time signal theory, applications of signal processing, systems and technology, speech communication, and image processing and communication. EURASIP members are entitled to a 10% discount on the article-processing charge. To claim this discount, the corresponding author must enter the membership code when prompted. This can be requested from their EURASIP representative.
Your browser needs to have JavaScript enabled to view this video
Latest Tweets
Your browser needs to have JavaScript enabled to view this timeline
Editor's Quote
Eduard Jorswieck, PhD Technische Universität Braunschweig
- Aims and Scope
- Editorial Board
- Sign up for article alerts and news from this journal
- Follow us on Twitter
- Follow us on Facebook
Who reads the journal?
Learn more about the impact the EURASIP Journal on Wireless Communications and Networking has worldwide
Annual Journal Metrics
2022 Citation Impact 2.6 - 2-year Impact Factor 2.5 - 5-year Impact Factor 1.098 - SNIP (Source Normalized Impact per Paper) 0.985 - SJR (SCImago Journal Rank)
2023 Speed 23 days submission to first editorial decision for all manuscripts (Median) 157 days submission to accept (Median)
2023 Usage 1,226,841 downloads 99 Altmetric mentions
- More about our metrics
Affiliated with
Add a new code entry for this paper
Remove a code repository from this paper, mark the official implementation from paper authors, add a new evaluation result row, remove a task, add a method, remove a method, edit datasets, fractional delay alignment modulation for spatially sparse wireless communications.
29 Mar 2024 · Zhiwen Zhou , Zhiqiang Xiao , Yong Zeng · Edit social preview
Delay alignment modulation (DAM) is a novel transmission technique for wireless systems with high spatial resolution by leveraging delay compensation and path-based beamforming, to mitigate the inter-symbol interference (ISI) without resorting to complex channel equalization or multi-carrier transmission. However, most existing studies on DAM consider a simplified scenario by assuming that the channel multi-path delays are integer multiples of the signal sampling interval. This paper investigates DAM for the more general and practical scenarios with fractional multi-path delays. We first analyze the impact of fractional multi-path delays on the existing DAM design, termed integer DAM (iDAM), which can only achieve delay compensations that are integer multiples of the sampling interval. It is revealed that the existence of fractional multi-path delays renders iDAM no longer possible to achieve perfect delay alignment. To address this issue, we propose a more generic DAM design called fractional DAM (fDAM), which achieves fractional delay pre-compensation via upsampling and fractional delay filtering. By leveraging the Farrow filter structure, the proposed approach can eliminate ISI without real-time computation of filter coefficients, as typically required in traditional channel equalization techniques. Simulation results demonstrate that the proposed fDAM outperforms the existing iDAM and orthogonal frequency division multiplexing (OFDM) in terms of symbol error rate (SER) and spectral efficiency, while maintaining a comparable peak-to-average power ratio (PAPR) as iDAM, which is considerably lower than OFDM.
Code Edit Add Remove Mark official
Tasks edit add remove, datasets edit, results from the paper edit add remove, methods edit add remove.
Artificial Intelligence in Wireless Communications - Evolution Towards 6G Mobile Networks
Ieee account.
- Change Username/Password
- Update Address
Purchase Details
- Payment Options
- Order History
- View Purchased Documents
Profile Information
- Communications Preferences
- Profession and Education
- Technical Interests
- US & Canada: +1 800 678 4333
- Worldwide: +1 732 981 0060
- Contact & Support
- About IEEE Xplore
- Accessibility
- Terms of Use
- Nondiscrimination Policy
- Privacy & Opting Out of Cookies
A not-for-profit organization, IEEE is the world's largest technical professional organization dedicated to advancing technology for the benefit of humanity. © Copyright 2024 IEEE - All rights reserved. Use of this web site signifies your agreement to the terms and conditions.

An official website of the United States government
Here’s how you know
Official websites use .gov A .gov website belongs to an official government organization in the United States.
Secure .gov websites use HTTPS A lock ( Lock A locked padlock ) or https:// means you’ve safely connected to the .gov website. Share sensitive information only on official, secure websites.
https://www.nist.gov/publications/wireless-channel-characterization-uwb-communication-capsule-endoscopy
Wireless Channel Characterization for UWB Communication in Capsule Endoscopy
Download paper, additional citation formats.
- Google Scholar
Help | Advanced Search
Computer Science > Information Theory
Title: performance analysis of integrated sensing and communication networks with blockage effects.
Abstract: Communication-sensing integration represents an up-and-coming area of research, enabling wireless networks to simultaneously perform communication and sensing tasks. However, in urban cellular networks, the blockage of buildings results in a complex signal propagation environment, affecting the performance analysis of integrated sensing and communication (ISAC) networks. To overcome this obstacle, this paper constructs a comprehensive framework considering building blockage and employs a distance-correlated blockage model to analyze interference from line of sight (LoS), non-line of sight (NLoS), and target reflection cascading (TRC) links. Using stochastic geometric theory, expressions for signal-to-interference-plus-noise ratio (SINR) and coverage probability for communication and sensing in the presence of blockage are derived, allowing for a comprehensive comparison under the same parameters. The research findings indicate that blockage can positively impact coverage, especially in enhancing communication performance. The analysis also suggests that there exists an optimal base station (BS) density when blockage is of the same order of magnitude as the BS density, maximizing communication or sensing coverage probability.
Submission history
Access paper:.
- HTML (experimental)
- Other Formats
References & Citations
- Google Scholar
- Semantic Scholar
BibTeX formatted citation

Bibliographic and Citation Tools
Code, data and media associated with this article, recommenders and search tools.
- Institution
arXivLabs: experimental projects with community collaborators
arXivLabs is a framework that allows collaborators to develop and share new arXiv features directly on our website.
Both individuals and organizations that work with arXivLabs have embraced and accepted our values of openness, community, excellence, and user data privacy. arXiv is committed to these values and only works with partners that adhere to them.
Have an idea for a project that will add value for arXiv's community? Learn more about arXivLabs .
Wireless Personal Communications
An International Journal
- Aydin Sezgin,
- Ramjee Prasad
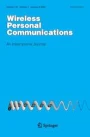
Latest issue
Volume 134, Issue 1
Latest articles
Research on uav-aided wsns node positioning task planning in field environment.
- Renwen Chen
- Junyi Zhang
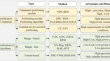
A New Discrete Learning-Based Logistic Regression Classifier for Bankruptcy Prediction
- Mehdi Khashei
- Sepideh Etemadi
- Negar Bakhtiarvand
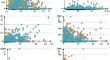
Dual Secret Key Virtualization Through TCC Based Wavelength Allocation and BB84 Protocol in QKD over Optical Networks
- Shravan Kumar Sehgal
- Rashmi Gupta
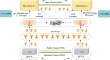
Detection of Epileptic Seizure from EEG Signals Using Majority Rule Based Local Binary Pattern
- S. Ramakrishnan
- R. Geetha Rajakumari
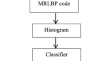
Smart-ESP System for Emotion Strength Prediction for Static Facial Images
- T. T. Mirnalinee
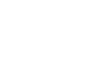
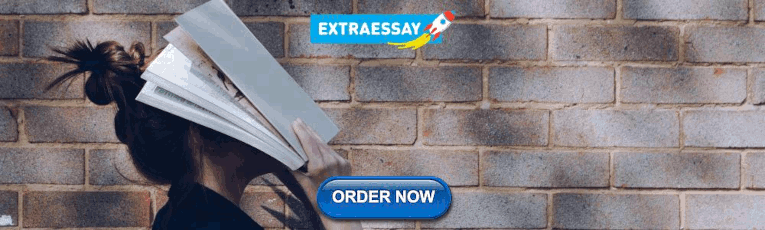
Journal updates
Covid-19 and impact on peer review.
As a result of the significant disruption that is being caused by the COVID-19 pandemic we are very aware that many researchers will have difficulty in meeting the timelines associated with our peer review process during normal times. Please do let us know if you need additional time. Our systems will continue to remind you of the original timelines but we intend to be highly flexible at this time.
Call for Papers
A full list of all current calls for papers and open special issues is available.
Journal information
- ACM Digital Library
- Current Contents/Electronics & Telecommunications Collection
- EI Compendex
- Google Scholar
- Japanese Science and Technology Agency (JST)
- OCLC WorldCat Discovery Service
- Science Citation Index Expanded (SCIE)
- TD Net Discovery Service
- UGC-CARE List (India)
Rights and permissions
Springer policies
© Springer Science+Business Media, LLC, part of Springer Nature
- Find a journal
- Publish with us
- Track your research
- Boise State News
- Share on Facebook
- Share on Twitter
- Share on LinkedIn
- Share through Email
- Alumni and Giving
- Faculty and Staff in Action
- Health, Science and Technology
Gluck wins research award for virtual reality paper
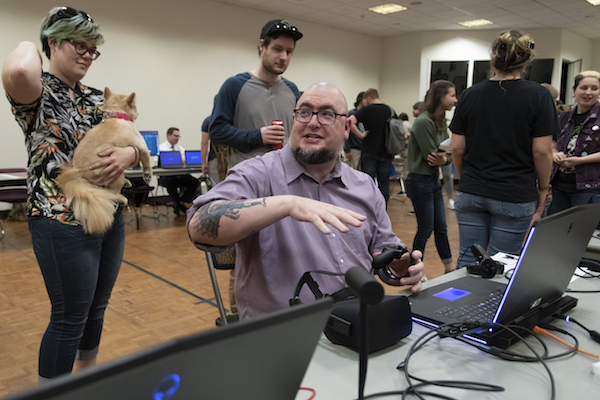
Aaron Gluck, clinical assistant professor in Games, Interactive Media, Mobile Technology (GIMM) and a Boise State alum, won the award for best paper at the IDEATExR Workshop. IDEATExR called for papers related to inclusion, diversity, equity, accessibility, transparency and ethics in extended reality.
Gluck’s winning paper, “Don’t Forget Our Presence: Exploring VR for Older Adults,” built on earlier research from his dissertation. Gluck and his coauthors noted that virtual reality (VR) had tremendous benefits for adults 65 and older—like socialization, rehabilitation and well-being. Despite these benefits, very few older adults use VR.
The research explored VR accessibility needs among the older population. Gluck’s work demonstrated the need for VR hardware and applications to support older users.
Gluck joined the GIMM faculty in 2023. Before earning his Ph.D., Gluck part of the first GIMM cohort and graduated in 2019.
Office of Communications and Marketing
Thank you for visiting nature.com. You are using a browser version with limited support for CSS. To obtain the best experience, we recommend you use a more up to date browser (or turn off compatibility mode in Internet Explorer). In the meantime, to ensure continued support, we are displaying the site without styles and JavaScript.
- View all journals
- My Account Login
- Explore content
- About the journal
- Publish with us
- Sign up for alerts
- Perspective
- Open access
- Published: 26 March 2024
Ecological countermeasures to prevent pathogen spillover and subsequent pandemics
- Raina K. Plowright ORCID: orcid.org/0000-0002-3338-6590 1 ,
- Aliyu N. Ahmed ORCID: orcid.org/0000-0001-6039-1101 2 ,
- Tim Coulson ORCID: orcid.org/0000-0001-9371-9003 3 ,
- Thomas W. Crowther ORCID: orcid.org/0000-0001-5674-8913 4 ,
- Imran Ejotre 5 ,
- Christina L. Faust ORCID: orcid.org/0000-0002-8824-7424 6 ,
- Winifred F. Frick ORCID: orcid.org/0000-0002-9469-1839 7 , 8 ,
- Peter J. Hudson ORCID: orcid.org/0000-0003-0468-3403 9 ,
- Tigga Kingston ORCID: orcid.org/0000-0003-3552-5352 10 ,
- P. O. Nameer ORCID: orcid.org/0000-0001-7110-6740 11 ,
- M. Teague O’Mara ORCID: orcid.org/0000-0002-6951-1648 7 ,
- Alison J. Peel ORCID: orcid.org/0000-0003-3538-3550 12 ,
- Hugh Possingham ORCID: orcid.org/0000-0001-7755-996X 13 ,
- Orly Razgour ORCID: orcid.org/0000-0003-3186-0313 14 ,
- DeeAnn M. Reeder ORCID: orcid.org/0000-0001-8651-2012 15 ,
- Manuel Ruiz-Aravena ORCID: orcid.org/0000-0001-8463-7858 1 , 12 nAff26 ,
- Nancy B. Simmons ORCID: orcid.org/0000-0001-8807-7499 16 ,
- Prashanth N. Srinivas ORCID: orcid.org/0000-0003-0968-0826 17 ,
- Gary M. Tabor ORCID: orcid.org/0000-0003-4711-1018 18 ,
- Iroro Tanshi 19 , 20 , 21 ,
- Ian G. Thompson ORCID: orcid.org/0000-0003-3445-8696 22 ,
- Abi T. Vanak ORCID: orcid.org/0000-0003-2435-4260 23 , 24 ,
- Neil M. Vora ORCID: orcid.org/0000-0002-4989-3108 25 ,
- Charley E. Willison ORCID: orcid.org/0000-0002-7272-1080 1 &
- Annika T. H. Keeley ORCID: orcid.org/0000-0001-7237-6259 18
Nature Communications volume 15 , Article number: 2577 ( 2024 ) Cite this article
7282 Accesses
751 Altmetric
Metrics details
- Epidemiology
- Policy and public health in microbiology
- Viral infection
Substantial global attention is focused on how to reduce the risk of future pandemics. Reducing this risk requires investment in prevention, preparedness, and response. Although preparedness and response have received significant focus, prevention, especially the prevention of zoonotic spillover, remains largely absent from global conversations. This oversight is due in part to the lack of a clear definition of prevention and lack of guidance on how to achieve it. To address this gap, we elucidate the mechanisms linking environmental change and zoonotic spillover using spillover of viruses from bats as a case study. We identify ecological interventions that can disrupt these spillover mechanisms and propose policy frameworks for their implementation. Recognizing that pandemics originate in ecological systems, we advocate for integrating ecological approaches alongside biomedical approaches in a comprehensive and balanced pandemic prevention strategy.
Similar content being viewed by others
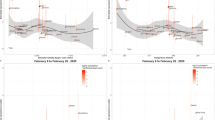
The role of environmental factors on transmission rates of the COVID-19 outbreak: an initial assessment in two spatial scales
Canelle Poirier, Wei Luo, … Mauricio Santillana
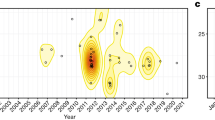
Pathogen spillover driven by rapid changes in bat ecology
Peggy Eby, Alison J. Peel, … Raina K. Plowright
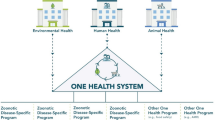
A generalizable one health framework for the control of zoonotic diseases
Ria R. Ghai, Ryan M. Wallace, … Casey Barton Behravesh
Introduction
Reducing the risk of future pandemics requires investment in prevention, preparedness, and response. At present, most attention and funding is allocated to mitigation after a pathogen is already circulating in humans, prioritizing outbreak detection and medical countermeasures such as vaccines and therapeutics 1 . By contrast, primary pandemic prevention—defined as reducing the likelihood a pathogen transmits from its animal host into humans (zoonotic spillover; Fig. 1 ) 2 —has received less attention in global conversations, policy guidance, and practice 1 , 2 . Given the time delays in identifying and responding to outbreaks, and the inequity in treatment distributions, investing in pandemic prevention is essential to achieve efficient, equitable, and cost-effective protection from disease.
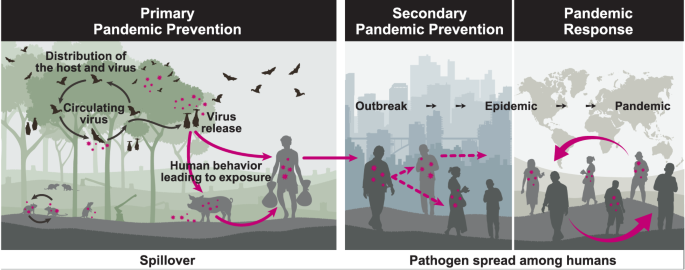
Primary pandemic prevention is the set of actions taken to reduce the risk of pathogen spillover from animals to humans, focusing on processes upstream of the spillover event (left panel). By contrast, secondary pandemic prevention (middle panel) focuses on limiting the spread of an outbreak to prevent its escalation into an epidemic or a pandemic. Pandemic response (right panel) involves actions taken to address a pandemic once one is underway. Although not illustrated here, pandemic preparedness involves developing capabilities to respond to a pandemic if one were to occur, and can be implemented concurrently with primary and secondary pandemic prevention. The nature of interventions varies across these phases: Primary pandemic prevention emphasizes ecological and behavioral interventions, but also encompasses biosafety practices in virological research 83 , whereas secondary pandemic prevention and response prioritize epidemiological and biomedical interventions. Definitions: an outbreak is “an increase, often sudden, in the number of cases of a disease in a particular area 84 ”; an epidemic is an outbreak extending over a wider geographic area 84 ; and a pandemic is “an epidemic occurring worldwide, or over a very wide area, crossing international boundaries and usually affecting a large number of people 84 ”.
To effectively prevent pandemics, we must recognize two key points: first that pandemics almost always start with a microbe infecting a wild animal in a natural environment and second that human-caused land-use change often triggers the events–whether through wildlife trade or other distal activities–that facilitate spillover of microbes from wild animals to humans 3 . As land-use change becomes more intense and extensive, the risk of zoonotic spillovers, and subsequent epidemics and pandemics, will increase. Designing land management and conservation strategies to explicitly limit spillover is central to meeting the challenge of pandemic prevention at a global scale.
Herein, we present a roadmap for reducing pathogen transmission from wildlife to humans and other animals. We show how strategic conservation and restoration of nature for reservoir hosts, and mitigation of risks for humans most at risk—what we define as ecological countermeasures—can prevent spillover and protect human and animal health, while also addressing key drivers of climate change and biodiversity loss.
Mechanisms of spillover
Despite hundreds of thousands of potentially zoonotic microbes circulating in nature 4 , pandemics are rare. Microbes, termed pathogens if they cause disease, must overcome a series of barriers, simplified and described below, to transmit from a wild animal to a human. Crossing those barriers requires the alignment of specific conditions—including ecological, epidemiological, immunological, and behavioral conditions—that are often complex and dynamic 5 .
First, the distribution of the species that maintains the zoonotic pathogen in nature (the reservoir host) and the species that is infected (the recipient host) must be connected, usually through overlapping distributions. Once wildlife reservoir hosts and humans overlap, the second barrier is the immune functions within wildlife hosts that keep potential zoonotic pathogens at low levels. Particular stressors (e.g., habitat loss, lack of food) can increase host viral infection and shedding 6 . A pathogen that passes through this second barrier and is shed by the animal host encounters a third barrier: humans must be exposed to a pathogen for spillover to occur. That exposure depends on specific interactions or behaviors of humans and the virus-shedding host. Exposure to the pathogen may be through direct contact, such as a bite, or indirect contact with the reservoir host’s excreta or a non-vertebrate vector (e.g., blood-feeding parasite). Often a bridging host species, such as commercially traded wildlife or a domestic animal, is infected by the reservoir host and subsequently amplifies and transmits the pathogen to humans. The fourth barrier is human susceptibility. The pathogen must be able to establish an infection within humans by overcoming structural and immunological barriers (e.g., binding to a human cell). Those barriers are substantial–one reason pandemics are rare–protecting humans from a continuous rain of microbes from soils, plants, and animals 5 . Fifth, after establishing an infection within a single human, the pathogen must be able to amplify within this new host, be excreted (e.g., through respiration), and then transmitted onward and exponentially 7 . If any of these barriers is not overcome, a pandemic cannot occur 5 .
Land use-induced spillover
Intact ecosystems provide the first line of defense against new pandemics because they strengthen the first three barriers to spillover (minimizing distribution overlap, host stress, and human exposure) and hence decrease the likelihood that the conditions for spillover occur or align 3 . Conversely, land-use changes and other environmental disturbances erode those first three barriers to spillover by changing the reservoir hosts’ spatial behavior and allostatic load (energy and stress budget), as well as altering human behavior. In this context, we identify targeted ecological countermeasures designed to decrease these risks (Fig. 2 ).
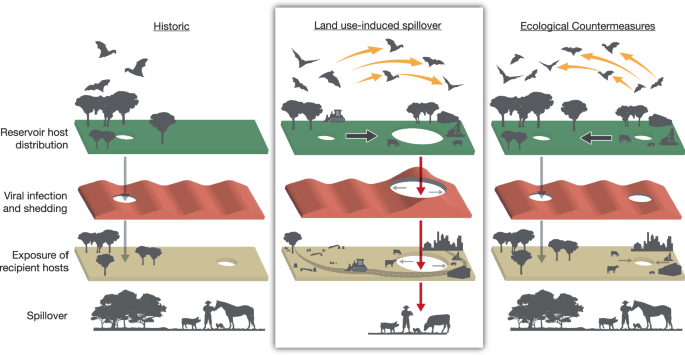
Historic (left panel): Historically, reservoir hosts and large human populations (and their domestic animals) were more separated, viruses circulated at low levels with seasonal fluctuations in prevalence, and the holes in the barriers to spillover were small and did not align 5 . Land use-induced spillover (middle panel): Land-use change increases the risk of spillover by driving two phenotypic changes in reservoir hosts: changes in behavior that alter how they use space, and changes in reservoir host energy and stress levels (allostatic load) that influence viral infection and shedding. Land-use change can also lead to emergent human behaviors that increase exposure to pathogens. Land-use change generally increases the overlap of reservoir, human, and bridging hosts; increases the probability that reservoir hosts are shedding pathogens; and increases the probability that humans are exposed to those pathogens. In sum, these changes increase the size and alignment of the holes in the barrier to spillover. Ecological countermeasures (right panel): Ecological countermeasures can address all three issues. Retaining natural resources reduces the overlap of humans and domestic recipient hosts in space and time, reduces the probability of allostatic overload and reduces the likelihood of emergent human behaviors that facilitate exposure.
We focus on ecological countermeasures in bats since several major epidemics and pandemics (e.g., those caused by SARS-CoV-2, Ebola virus, SARS-CoV-1, MERS-CoV, and Nipah virus) have an evolutionary origin in bats (but notably do not cause disease in their bat reservoir hosts) 8 . Certain bat species are also the hosts of four of the nine diseases prioritized by the World Health Organization as having the potential to generate epidemics that pose a great risk to public health, and for which there are insufficient countermeasures 9 . However, the ecological countermeasures we present also apply to other host taxa, particularly species that are susceptible to local resource depletion and can sustain the circulation of potential pathogens (e.g., species that aggregate in large numbers like colonial nesting birds, or in spatially structured but extensive aggregations, such as prairie dogs and other rodents). For species tied to permanent refuges (roosts, breeding grounds, burrow systems and warrens), loss of habitat may quickly push populations into allostatic overload or in more mobile species, prompt resource tracking and migration with attendant energetic costs and risks.
Reservoir host energy and stress (allostatic load)
Healthy animals maintain a positive energy balance, where energy inputs either from foraging or stored reserves of fat, balance or exceed energy expenditure required for survival and reproduction (Fig. 3 ). This balance of energy in physiological systems occurs through allostasis—a dynamic process that integrates the neuroendocrine, metabolic, cardiovascular, and immune systems to adapt to varying conditions. Animals regularly adapt to increased energy demands needed to migrate, hibernate, or reproduce. The total resources an animal requires at any given time is an animal’s “allostatic load” 10 , 11 . Allostatic load is frequently estimated with biomarkers such as cortisol, a glucocorticoid hormone indicative of stress 12 , or related energetic and immune metrics, such as total white-blood-cell count, the neutrophil-to-lymphocyte ratio, and immune regulatory markers. When in balance, glucocorticoid hormones help manage energy usage and have generally beneficial effects on immunity. For example, they mediate anti-inflammatory processes, support T cell maintenance, and enhance the functions of Th2, Th17 and B cells, which collectively bolster the body’s defense against infection and keep immune responses in check 13 , 14 . Across millennia, animals evolved the capacity to maintain allostasis under predictable variations in their environments, precisely aligning energetically expensive activities with periods of maximum food availability 15 (Fig. 3 ).
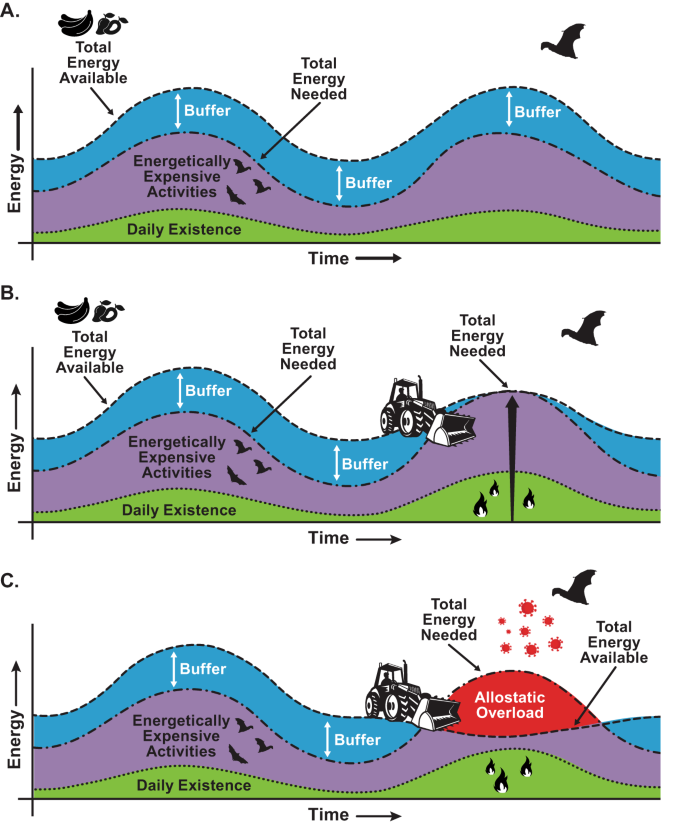
Bats have evolved mechanisms to meet their exceptionally high energy needs under prevailing environmental conditions. A Baseline levels of energy (green) are required for basic daily activities – to fuel cells, to move around, to find food and water, and to maintain the immune system. At any given time, a certain amount of food - or energy - is available (blue+purple+green), which varies seasonally. Bats optimize their energy intake and energy expenditure, timing expensive activities like migration and reproduction (purple) to periods in which more food is available. Under normal conditions, an energetic buffer (blue) exists providing energetic wiggle room for years with poor food availability. B Perturbations in the environment, whether natural (e.g., fire in some instances) or man-made (e.g., downstream effects of global climate change, habitat destruction, etc.) increase the amount of energy needed for survival and reproduction. For example, animals may be required to travel greater distances to locate food and resting sites. Such increased exertion diminishes the energetic buffer that enables them to withstand periods of resource scarcity. C At its worst, these perturbations result in a reversal of fortune; less energy is available than the bat needs. In these conditions, or with disturbance or harassment, animals experience allostatic overload (red). This leads to suppression of immune function, and increased susceptibility to viral infection and shedding. Figure adapted, in part, from concepts in 10 .
Animals are less able to manage the physiological and behavioral challenges that arise from unpredictable environmental changes, particularly those caused by human activities. Perhaps the most common consequence of environmental change is decreased food availability, leading to weight loss 16 . When food is limited, energy expenditure may exceed energy input and the animal shifts into a state of allostatic overload (Fig. 3 ).
Habitat destruction, degradation, and fragmentation profoundly increase the likelihood of allostatic overload. This risk is compounded when animals face repeated stressors, such as cave disturbance or harassment 17 . To survive, animals must divert energy from other systems, including their immune defenses 14 , 16 . The effects of allostatic overload are largely mediated by the chronically elevated glucocorticoid hormones, which can lead to immune system dysregulation, impaired resistance to infection, and a shift in the balance between pro-inflammatory and anti-inflammatory processes. This state, the effects of which accumulate over an animal’s lifetime, facilitates viral infection and shedding 13 , 18 , 19 , 20 . Consequently, animals experiencing allostatic overload may shed more pathogens for longer periods, increasing the risk of spillover. Empirical evidence underscores the link between stress, acute food deprivation, and low body weight with higher probability, magnitude, and duration of viral shedding, as observed in bats 21 , 22 , 23 , 24 , 25 and birds 26 , 27 .
Reservoir host spatial behavior
Changes in land use not only affect the energy needs of reservoir hosts but also alter how reservoir hosts use space, including how they encounter humans, livestock, or other bridging hosts. Typically, animals have home ranges sufficient for them to acquire the resources they need such as food, water, shelter, and mates. Some species, especially those dependent on unpredictable or briefly available food, may need to migrate or move regularly to find these resources. Land-use changes can limit the amount and accessibility of food resources. In response, and to avoid or mitigate allostatic overload, animals often need to expand their search area or modify their home ranges to find sufficient food 28 , 29 . For example, fruit-eating bats Dermanura watsoni were observed to have larger daily feeding ranges in degraded habitats 30 . Such adaptations may increase the likelihood of encounters and, consequently, pathogen transmission between reservoir hosts, humans, and livestock. This may be especially true if they must traverse resource-sparse areas to find food, increasing stress and mortality risk. A study in Uganda, for example, showed increased contact between humans and non-human primates with increasing forest fragmentation 31 .
Moreover, wildlife populations may adapt to areas where they historically did not occur, and some species that host zoonotic pathogens have proven more likely to thrive in disturbed landscapes than in undisturbed sites 32 . For example, in response to the loss of winter habitat, Australian Pteropus alecto bats, carriers of Hendra virus, are shifting to agricultural and urban areas. Here, they feed on suboptimal but reliable foods in proximity to livestock 33 .
Increased zoonotic risk, then, often coincides with stressful life stages or times and places of resource scarcity 21 , 33 , 34 . Understanding which animals are most likely to modify their distributions, or are at the highest risk of allostatic overload, helps target countermeasures to spillover. For example, the P. alecto bats that shifted to novel agricultural and urban habitats shed higher levels of Hendra virus than bats in traditional habitats, especially during winter and after periods of food scarcity 22 , 35 . This combination of factors breaches the barriers earlier noted and has led to a higher probability of spillover 22 .
Human behavior
Although human interaction with a pathogen is a fundamental component of pathogen spillover, mere spatial overlap between humans and virus-shedding reservoir hosts is not sufficient for spillover. Specific human behaviors (not always within one’s control) that provide a transmission route and sufficient dose for infection are usually required—for example, harvesting guano or date palm sap 36 , 37 , 38 , visiting a tourist cave 34 , or butchering wildlife with inadequate protection 39 . Such behaviors, which increase the frequency and intensity of contact with wildlife and wildlife excreta, can become more prevalent because of land-use change, frequently precipitated by the construction of new roads. While road construction, if designed well, can bring benefits such as employment, reduced transportation costs, and development 40 , roads also facilitate increased access to wildlife habitats. This access can enable activities such as the extraction of wild animals for food and trade, timber harvest, and livestock grazing, following deforestation 41 , 42 . New settlements that follow roads may also promote synanthropic responses of wildlife; for example, bats are commonly found roosting on roofs of rural homes 43 .
Road construction not only alters exposure opportunities but also introduces people into communities that lack immunity to local pathogens. By contrast, Indigenous Peoples and local communities (IPLCs) who have coexisted with these environments may have some protective immunity to local pathogens through repeated exposures. This is evident from the presence of antibodies to various outbreak-prone viruses in populations with frequent wildlife exposure. For example, antibodies to filoviruses were detected in bat harvesters in remote northeast India 44 and antibodies to SARS-related coronavirus have been identified in people residing near caves in Yunnan Province, China 45 . Such evidence suggests that while pandemics may be rare, local spillovers could be relatively common. Furthermore, the construction of roads not only increases the risk of exposure for those lacking immunity but also facilitates the rapid spread of novel pathogens once they have entered the human population, thereby increasing the likelihood of a pandemic.
Apart from the direct impact of road construction, there is a multitude of factors relating to deforestation and forest degradation that could affect human exposure to pathogens, including agricultural practices such as the cultivation of palm oil and extractive industries, notably mining 46 . Typically, such activities are either preceded by or necessitate the building of roads, further intertwining human exposure with infrastructural development. IPLCs living in and around forests, aren’t always the main beneficiaries of these activities and can be actively harmed by them 47 , 48 . For example, land-use change can result in decreased income and food security, incentivizing some individuals to increase hunting and bush travel. This underscores the need for development projects, including road construction, to take holistic approaches that optimize outcomes for people rather than focusing on single outcomes that can have unintended consequences. Such an approach could deliver much of the economic benefits to people while reducing environmental and social damage. Individual human behaviors that increase spillover risk must be considered in the context of such socio-ecological factors–including vulnerabilities and inequalities—as well as in a historical and cultural context 49 .
Ecological countermeasures defined
We define ecological countermeasures as actions that protect and restore wildlife habitat or mitigate wildlife-human interactions to reduce the risk of pathogen spillover. These measures are strategically designed to increase the resilience of reservoir host populations, reduce stress and likelihood of viral shedding, prevent distributional shifts, and protect vulnerable human communities. By addressing these factors, ecological countermeasures target the root causes of spillover. They effectively strengthen barriers to spillover and decrease the likelihood that the conditions for spillover align.
We propose a tiered approach that considers the land-use context surrounding the habitats of reservoir hosts (Fig. 4 ), focusing on enhancing habitat integrity, heterogeneity, and connectivity. In our view, the most effective strategy to reduce the probability of another pandemic is to preserve intact ecosystems and bolster their resilience through restoration and the creation of buffer zones. This priority is driven by the likelihood that the next pandemic will be triggered by an as-yet-unknown pathogen, referred to as “Disease X” by the World Health Organization 50 , that has had scarce opportunities for spillover or for evolutionary adaptation in bridging hosts. Our primary emphasis should be on maintaining and enhancing the integrity and resilience of still-intact landscapes to prevent new interfaces that could enable the emergence of Disease X.
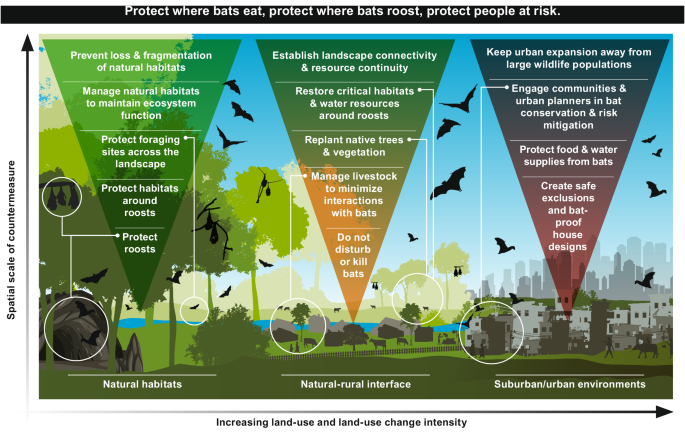
We propose a tiered approach that considers the land-use context surrounding the habitats of reservoir hosts. Because the next pandemic is most likely to be triggered by a pathogen that is currently limited in its exposure to human populations, the highest priority should be to preserve intact ecosystems and enhance their resilience through restoration and increasing connectivity. In regions where humans and reservoir hosts share landscapes, we prioritize the safeguarding of critical areas needed for reservoir hosts’ feeding, resting, and social aggregation. Simultaneously, we aim to protect human communities and livestock most at risk of exposure to zoonotic pathogens.
In regions where humans and reservoir hosts share landscapes, we prioritize the safeguarding of critical areas needed for reservoir hosts’ feeding, resting, and social aggregation. Simultaneously, we aim to protect human communities most at risk of exposure to zoonotic pathogens. In the following sections, we explain how these strategies target the fundamental drivers of pathogen spillover and promote the health of both wildlife and human populations. While we focus on bats as reservoir hosts, ecological countermeasures are relevant across diverse reservoir host species, as long as specific ecological contexts and local practices are considered 51 . We present these strategies with a simple policy-focused message as they would apply to bats: protect where bats forage (where bats eat), protect where bats roost (where bats sleep), and protect people at risk (Fig. 4 ).
Protect where bats forage
The quality of foraging areas determines the energetic buffer protecting individuals from allostatic overload in times of increased energetic costs or reduced resource availability (Fig. 2b ). If animals have enough nutritious food, they are less likely to become energetically or physiologically stressed, reducing the risk of allostatic overload and infection and shedding (Fig. 2c ). Moreover, the location of bat foraging areas relative to human activity determines the spatial overlap with potential recipient hosts. If enough food is available in relatively unmodified landscapes, or immediately around roosts, bats are also less likely to use areas with higher human population densities. Thus, protecting where bats eat not only ensures that they are healthy, but that they are spatially separated from people.
In natural landscapes (Fig. 4 , left panel), the overarching priority is to preserve or improve the integrity of ecosystems that animals inhabit, as previously outlined. This may entail securing extensive areas of unmodified habitats, and proactively managing these landscapes to prevent fragmentation and degradation.
In landscapes that have already been degraded (Fig. 4 , middle panel), the focus should shift to protecting, restoring, and connecting key food sources that sustain reservoir hosts during periods of resource scarcity (e.g., winter or the dry season) and through energy-demanding life stages (e.g., pregnancy and lactation). Additionally, in environments facing degradation from land-use and climate change, ecological countermeasures are crucial for mitigating food shortages caused by habitat deterioration across multiple scales.
The natural-rural interface often presents a heterogeneous landscape to bats, characterized by a mix of high-quality foraging habitats embedded in or interdigitating with degraded habitats or areas of human land use. These areas, while fragmented, can still offer valuable nutritional resources. It is crucial to protect key foraging sites, especially those outside of protected areas, and to preserve habitats surrounding roosts. A priority is to maintain or create connectivity among quality habitat patches to ensure a consistent flow of resources. Thereafter, efforts should be directed towards the restoration of critical habitats and water sources, particularly in the vicinity of roosts, coupled with strategic livestock management to reduce interactions with bats. Active management strategies should aim to maximize the benefits of human land-uses such as croplands and plantations, for both humans and bats 52 , 53 .
In suburban and urban settings (Fig. 4 , right panel), priority activities focus on the separation of bats and people through strategic planning and restricting human access. At the broadest scale, urban expansion plans should avoid encroaching on large wildlife habitats. Within urban areas, it is crucial to preserve bat foraging resources without inadvertently increasing contact with human populations. This necessitates a collaborative effort between local communities, urban planners and bat experts who understand the requirements of local species. For example, ornamental or landscaping trees used in city planning may attract fruit-eating bats (such as members of the Pteropodidae and Phyllostomidae families) in subtropical and tropical regions. This is also true for fruit trees in residential backyards 54 . A practical approach might include selecting alternative landscaping species and planting bat-attractive trees in areas that are less accessible to humans. Wildlife-safe protective netting around backyard fruit trees can also limit bats’ access to ripe fruits and minimize fruit loss 43 , 52 , 53 . Box 1 provides real-life examples of preserving or enhancing bat foraging habitat and Supplementary Table 1 provides more examples of ecological countermeasures.
Box 1 Real-life examples illustrate the importance of protecting or enhancing where bats forage
In subtropical Australia, no Hendra virus spillovers occurred when Pteropus species bats left agricultural areas to feed on pulses of nectar in winter-flowering forests 33 . In some areas of the subtropics, over 90% of these crucial habitats have been cleared and the remaining forest flowers on multi-year cycles. Consequently, the occurrence of abundant winter flowering has become increasingly rare 33 . Restoring these habitats would target animals’ needs during predictable periods of scarcity, decrease their allostatic load, and reduce their reliance on human-dominated areas for food. Replanting winter habitats would be a sustainable, scalable, and effective strategy to reduce the risk of spillover of not just Hendra virus, but other viruses carried by Pteropus species bats.
Great fruit-eating bats ( Artibeus lituratus ) captured in areas of Colombia that used agroforestry had higher body weights and body condition scores than those within conventional farming areas 85 . Thus, emphasizing agroforestry in agricultural landscapes can provide critical food and shelter for bats 86 , 87 , 88 , 89 , 90 . In turn, bat predation of agricultural insect pests provides economic and ecological benefits to agriculture by increasing crop yields and reducing pesticide applications 90 .
To improve the foraging efficiency of wild little brown bats ( Myotis lucifugus ), insect density was increased using UV light lures 91 . This approach aimed to reduce the bats’ allostatic load and their susceptibility to white-nose syndrome, a disease caused by a fungal pathogen that does not pose a risk of spillover to people. Increased fat reserves can improve a bat’s ability to survive this disease. Bats had reduced commuting costs and increasing foraging efficiency, demonstrating that bats behaviorally respond to increased prey availability during critical energetic periods. This work highlights the potential benefits of restoring and enhancing habitats near bat hibernacula to improve the resilience of reservoir host species.
Agave plants are being restored along bat migration corridors in the southwest United States and northeast Mexico to provide nectar for Mexican Long-nosed bats ( Leptonycteris nivali ) and Lesser Long-nosed bats ( Leptonycteris yerbabuenae ) during energetically expensive migration 92 . In the first five years, over 80,000 agaves were planted within 50 km of six key bat roosts, encompassing both migratory and maternity roosts. This restoration effort not only aids bats but also benefits farmers and rural communities in Mexico, as wild agaves are also harvested for food and beverages, livestock fodder, fencing materials, and other uses. Agaves hold significant cultural value and contribute to the livelihoods of rural Mexican communities 92 . Consequently, restoring bat foraging habitat is an example of how conservation efforts can simultaneously enhance human well-being when co-benefits are identified and integrated.
Protect where bats roost
Roosts are locations where bats sleep, shelter, mate, socialize, and raise their young. With few exceptions, bats cannot construct shelters and must roost in pre-existing natural (e.g., caves, rock crevices, tree cavities, and tree foliage) or human-made (e.g., buildings, bridges, mines) structures. Moreover, species are typically highly selective of their roost sites, seeking out particular microclimates, light conditions, ingress, and egress conditions. The number of bats using a roost can vary greatly, containing anywhere from a few bats to hundreds of thousands, depending on the species and nature of the roost.
Protecting the roost includes minimizing disturbance and persecution—conversely, often a first response to an outbreak of a bat-borne pathogen. Disturbance not only causes stress, impairing their immune responses but can also force bats into new areas. This increases their energy expenditure and likelihood of contact with humans 22 , 55 . Moreover, culling bats has been linked to increased active infection within bat populations (e.g., rabies in vampire bats [ Desmodus rotundus 56 ] and Marburg virus in Egyptian fruit bats [ Rousettus aegyptiacus 21 ], and a greater risk of spillover.
Roosts are typically small natural features, and protecting roost sites is a specific management action that can reduce the risk of pathogen spillover. This may require establishing protection buffers around roosts or installing physical barriers (Fig. 4 , and Supplementary Table 1 ). Such buffers are also vital for preserving the quality and quantity of foraging habitats surrounding the roost. Engaging local communities is another key strategy, especially if the roost holds cultural or use value, as is common with caves 57 . Local communities are less likely to harm bats if they are aware of bat natural history, and have previously engaged in environmental education 58 , and are aware of the benefits of bat presence 59 .
Protect people at risk
The third countermeasure, focused on the safety of humans and livestock in proximity to reservoir hosts, is less ecologically oriented but is crucial in mitigating pathogen exposure risk (Fig. 4 , Supplementary Table 1 ). Pathogen exposure can occur through contact with reservoir hosts, their body fluids, excreta, or through aerosols and droplets derived from these sources. Thus, identifying and modifying human behaviors that elevate the risk of such exposures is essential.
For communities reliant on bat-associated economic activities, such as guano harvesting, tourism, and wildlife consumption 45 , 56 , 60 , 61 , adopting safe practices is critical (Supplementary Table 1 ). Additional measures may include restricting and regulating the trade of bats 62 and preventing contact between bats and farmed wildlife 63 . When the specific mechanisms of pathogen spillover are understood, the implementation of preventative measures can be relatively straightforward. In Bangladesh, an effective measure to prevent Nipah virus transmission is covering the areas of date palm trees where sap is collected, which prevents bats from contaminating the sap and transmitting the Nipah virus to humans 64 . In Malaysia, a regulation requiring fruit trees to be planted at a distance from pig sties may explain the lack of subsequent Nipah virus spillovers 65 . Similarly, keeping horses away from trees frequented by bats at night may reduce the risk of Hendra virus transmission between bats and horses 66 .
Box 2 lists interventions in the context of the degree of human landscape modification. Future work must assess the relative effectiveness, feasibility, and prioritization of these countermeasures across different countries and regions since the underlying conditions and legal landscapes will vary. Additionally, given the dynamic nature of climate and land use-induced changes impacting natural and human environments, a flexible, iterative, and adaptive approach is essential for prioritization of these countermeasures 67 .
Box 2 Countermeasures in the context of degree of human landscape modification
Ecological countermeasures that protect where bats eat and roost, and protect people at risk, must consider the activities of bats and humans in the landscape. Countermeasures can be implemented at a range of geographic extents and within different contexts of degrees of human modification (Fig. 4 and Supplementary Table 1 )
In large wild areas, protect where bats forage and roost:
Maintain or increase the integrity of ecosystems by preventing the destruction and fragmentation of natural areas.
In shared landscapes dominated by natural areas interspersed with human land uses:
Protect where bats eat:
Connect protected areas.
Preserve and restore vegetation diversity and structural complexity in bat foraging habitats.
Protect and restore habitats that provide food during periods of resource scarcity and high energetic demand.
Maintain or restore landscape heterogeneity through, for example, wide buffers of natural vegetation along sensitive habitat like streams and wetlands.
Promote sustainable agriculture and forestry practices that support bat foraging and roosting.
Minimize disruption to water sources used by bats.
Protect natural areas when planning new developments.
Protect where bats roost:
Limit human access to roost sites to minimize disturbances.
Create buffers of foraging habitat around known roosts.
Protect a diversity of roosting options for bats, including large cavity-bearing trees, tree snags, and caves.
Provide alternative roosting options such as boxes and hollow trees.
Protect people at risk:
Manage livestock to reduce interactions with bats and bat excreta.
Provide information on risks and risk mitigation associated with certain activities.
Use personal protective equipment for individuals in contact with bats or their excreta.
Vaccinate at-risk populations for endemic bat-borne pathogens such as Ebola or rabies and potentially against pandemic potential pathogens in the future.
Empower communities as stewards of the local land and wildlife, including bats.
In heavily modified landscapes such as intensively farmed and urban areas:
Preserve where bats eat and roost:
Conserve remaining natural habitats that provide shelter or food.
Maintain and restore connectivity.
Restore foraging habitat near roosts.
Restore habitat buffers around roosts.
Increase the proportion of native plant species that provide food and shelter for bats in remnant natural areas away from people.
Exclude bats from human food (e.g. fruit trees) and water supplies.
Exclude humans from roosts in public buildings and structures (e.g. churches, bridges, culverts).
Humanely exclude bats from houses and construct bat-proof housing.
Actively involve communities in risk mitigation measures.
Policy outlook
Currently, multilateral policy discussions focus predominantly on enhancing pandemic preparedness (e.g., developing new vaccines, readying healthcare systems) 1 , 68 . While these capacities are undeniably important, integrating a more balanced approach that also prioritizes spillover prevention could reduce human suffering and negative economic impacts in the long term. Despite this, prioritizing prevention proves challenging and is overshadowed by reactive strategies that are activated only after a pathogen is already circulating among humans. This is evident in the current draft of the World Health Organization (WHO) Pandemic Agreement, which does not mention “primary pandemic prevention” and uses the word “prevention” only in the context of secondary prevention measures such as early detection and outbreak response 69 .
Although the importance of pandemic prevention is well-acknowledged, the concept of using ecological countermeasures—actions that protect and restore wildlife habitat or mitigate wildlife-human interactions—as a preventative strategy is only emerging. Ecological countermeasures offer multiple advantages: not only can they prevent spillover, but they engage multiple sectors in action beyond public health, and they contribute multiple co-benefits including climate change mitigation, biodiversity protection, and added ecosystem services (e.g., pest control and pollination by bats). Feedback among these sectors calls for integrated approaches. For example, both climate change and biodiversity loss can intensify processes that drive spillover. Excess heat, extreme climate events, and changing plant phenology are likely to increase allostatic load and alter wildlife (and human) spatial behavior 70 . The loss of biodiversity, including predator species, often leaves ecosystems dominated by species that are more competent hosts for zoonotic pathogens 32 . Together these processes escalate the need for ecological countermeasures.
Ecological countermeasures support, strengthen, and work in accord with existing and future policy frameworks, including those under the United Nations Framework Convention on Climate Change’s Paris Agreement, the Convention on Biological Diversity (CBD)’s Kunming-Montreal Global Biodiversity Framework, the UN Sustainable Development Goals, the UN Decade on Ecosystem Restoration, the new Pandemic Fund through the World Bank, and the WHO Pandemic Agreement. Such existing policy efforts offer opportunities for nations to invest in and incorporate primary pandemic prevention alongside preparedness efforts 1 .
Centrally, ecological countermeasures are fundamentally equitable because health benefits almost always accrue regardless of access to health systems. We’ve seen with COVID-19 and mpox that the most vulnerable populations, at greatest risk of infection and adverse outcomes, often had limited access to vaccines 71 . By contrast, spillover prevention benefits everyone globally, irrespective of individuals’ access to health systems 1 , 72 , 73 .
An Intergovernmental Panel for Pandemics
Many international entities have mandates that include enhancing pandemic prevention, preparedness, and response, including the One Health High-Level Expert Panel, the Global Preparedness Monitoring Board, and the Quadripartite. Such bodies all address unique and important issues, but none acts as an official scientific body that regularly assesses and synthesizes the full breadth of the latest data on pandemic prevention, preparedness, and response.
To address this, we strongly support the establishment of an Intergovernmental Panel for Pandemics, which could eventually come to fruition with the passage of the WHO Pandemic Agreement. This panel, if created, would provide regular scientific assessments to guide governments as they implement policies and programs related to pandemics. The scope of such a panel must include primary pandemic prevention alongside preparedness and response. The panel could be modeled after the Intergovernmental Panel on Climate Change or the Intergovernmental Platform on Biodiversity and Ecosystem Services 74 , 75 .
We recognize a risk of fragmentation with multiple different panels focused on climate, biodiversity, and pandemics. It is critical, therefore, to assure their coordination. By doing so, repeated efforts can be avoided, and, where applicable, intersectoral solutions can be implemented to harness co-benefits and synergies across sectors.
Moreover, there is a need to critically evaluate the evidence for the effectiveness of various pandemic prevention, preparedness, and response strategies. Although the global health community widely endorses strategies such as disease surveillance, perhaps largely due to their familiarity and experience with such methods, investments in primary prevention remain unprioritized. This raises a critical question: is there evidence that surveillance offers a greater reduction in pandemic risk compared to primary pandemic prevention (for example, is surveillance likely to activate response strategies in time to prevent spread of a pathogen with high transmissibility and pre-symptomatic spread)? To address these issues, an independent, broadly representative body could provide unbiased and politically neutral evaluation of the various strategies, encompassing prevention, preparedness, mitigation, and response 75 .
Metrics for pandemic prevention
Any program to mitigate pandemic risk through the conservation and restoration of nature must be evaluated to ensure it has the intended impact. Thus, we propose that the Intergovernmental Panel for Pandemics develop clear and robust metrics. These metrics should not only evaluate primary pandemic prevention efforts but also integrate them into existing biodiversity and climate change frameworks. Such metrics could monitor program performance, ensure accountability and transparency, and guide equitable wealth distribution to local communities based on program outcomes.
Numerous existing biodiversity assessment metrics could be shared with pandemic prevention metrics. Examples include the Ecological Integrity Index, STAR biodiversity index, and SEED biocomplexity metric, all in line with the CBD protocols. Additionally, there needs to be metrics specifically addressing spillover risk, including the guidance presented here (e.g., protect habitats where reservoir hosts forage and rest, especially during periods of resource scarcity; and reduce land-use changes that increase human-wildlife encounters).
The development of these metrics presents an opportunity to maximize the co-benefits of biodiversity preservation, climate change mitigation, and pandemic prevention. Such an integrated and synergistic approach should increase the success of program implementation globally 75 , 76 . For instance, restoration of koala ( Phascolarctos cinereus ) habitats in Australia, if strategically focused on trees that both support koalas and provide nectar for bats, could concurrently restore water catchments, sequester carbon, and reduce the risk of bat virus spillovers 33 .
Empowering local communities through One Health efforts
The One Health approach–popularized in recent years to optimize the health of people, animals, and ecosystems 77 –offers opportunities to implement ecological countermeasures for primary pandemic prevention. Currently, however, One Health efforts are overwhelmingly focused on disease surveillance in livestock and humans, rarely considering environmental drivers of emerging health threats 78 . One of the bottlenecks to advancing a more holistic One Health practice is the lack of practitioners across the animal-human-environment fields. To bridge this gap, we propose the creation of networks of ecosystem health workers to operationalize One Health and support local communities in implementing primary pandemic prevention. Those ecosystem health workers—who may include local forestry, wildlife, veterinary, medical, or public health officers–could be trained in, and help develop and implement, locally relevant ecological countermeasures, while embedded in larger governmental One Health teams. Their duties could include environmental education and ecological consultation (Supplementary Table 1 ), and information collection relevant to management actions (Box 3 ). They could also engage local universities and create pipelines for research on ecological countermeasure implementation and monitoring. They could ensure that local information is reported to national and international entities to inform effective, equitable decision-making 79 .
In parallel, it is essential to recognize the vital role of IPLCs in this framework. Integrating the perspectives and knowledge of IPLCs is not just a matter of cultural respect and justice; it is also a pragmatic strategy for designing and implementing appropriate, feasible and practical ecological countermeasures. Collaborating with IPLCs will help ensure that countermeasures align with local context and meaningfully incorporate local and Indigenous knowledge. IPLCs have managed natural ecosystems for thousands of years, and their involvement is increasingly seen as critical for reaching global climate and conservation goals 80 . Engaging IPLCs as equal partners in designing and implementing solutions to threats such as pandemics and climate change will increase the chances of successful outcomes 80 , 81 .
Box 3 Key questions for risk assessment and mitigation through ecological countermeasures, using bats as an example
Natural systems focus:
Which species of bats are present?
To what extent are local roost sites and foraging areas mapped?
Are local roost sites, and buffers around these sites, protected from disturbance?
What and where are the highest-quality habitats for these species in each season?
What resources are limited, either seasonally or consistently?
What habitat is required to ensure food is available during critical life stages?
How well are the local bat biology and movement patterns understood?
Human interactions focus:
Is land-use change likely to change the distribution and decrease the availability of bat foraging grounds, increase encounter rates with humans, or increase disturbance to roosts?
What is the nature of current bat-human interactions?
Are bat-human interactions increasing and, if so, why?
What are the attitudes of local communities toward bats, and why?
Who has regulatory authority to implement countermeasures?
Who are the key stakeholders needed to develop implementation mechanisms?
Is the available information sufficient to make informed decisions or actions?
Can areas critical to bats’ viability and health be protected or restored?
What steps can be taken to reduce contact between people and bats?
Expand the evidence base for ecological countermeasures
Our current understanding of pathogen spillover is characterized by vast knowledge inequalities. Biomedical aspects of spillover are extensively explored, while ecological components of spillover are under-represented. For example, thousands of publications detail the entry of bat-origin coronaviruses into human cells, but only a few studies explore their circulation in nature 82 . Moreover, studies on spillover are relatively rare but studies that examine the entire spillover process—from environmental drivers to reservoir hosts to human infections—are exceptionally rare. Therefore, our understanding of spillover is built on partial knowledge, such as studies demonstrating increased frequency of animal-human contact following habitat loss, or higher shedding in animals under stress (Supplementary Table 2 ). Although there is strong evidence for these component drivers of spillover, there is a critical need for studies that encompass the entire spectrum of spillover stages, including wildlife ecology, wildlife viral dynamics, human exposure, and human infection. Such studies need to be transdisciplinary, landscape-scale, with replication in space and time, shared data, and integration of local knowledge. Critically, these investigations must be grounded in the ecological systems where pandemics are likely to originate.
Pandemics have predominantly been addressed through a biomedical lens. While biomedical approaches are an essential part of the pandemic response toolbox, the genesis of a pandemic is rooted in ecological systems, necessitating ecological approaches for prevention. By aligning our research priorities with this understanding, we can build a comprehensive set of preemptive countermeasures that mitigate pandemic risk.
Conclusions
Spillover is an ecological process and, in the realm of human health, an ecological problem. While the human health issues arising from spillover events, such as outbreaks and pandemics, are addressed by epidemiological and biomedical countermeasures (e.g., testing, isolation, vaccines), the ecological aspects of spillover necessitate ecological solutions. In an ideal world, successful ecological countermeasures, which prevent spillover, would greatly reduce the need for biomedical countermeasures. We do not live in an ideal world; thus, we must move forward on both fronts.
To date, biomedical countermeasures to treat pandemics have received far more attention than ecological countermeasures. Our goal here has been to highlight the use of targeted ecological interventions as sensible, equitable, and efficient methods to prevent pandemics. While currently underutilized, ecological countermeasures have demonstrated potential in preventing spillover 33 , 76 . As challenges such as climate change, biodiversity loss, and a growing global population intensify, the relevance and necessity of ecological approaches for pandemic prevention are expected to increase.
Although we illustrate the science of ecological countermeasures using bats as a case study, the concepts are applicable across various wildlife reservoir host taxa, including ungulates, primates, and rodents. To reduce the likelihood of pandemics, we must protect where animals forage and rest so that we can keep wildlife healthy, minimize allostatic load, reduce the need for animals to alter their spatial behavior, and minimize risky human-wildlife encounters.
The current confluence of political will, resources, and scientific evidence for primary pandemic prevention provides an opportunity to incorporate ecological countermeasures into multiple policy frameworks. Such countermeasures can help prevent pandemics by, in part, protecting and restoring nature across the globe. Explicit consideration of such countermeasures within global land management and conservation strategies is key to simultaneously addressing the intertwined threats of biodiversity loss, climate change and global pandemics.
Vora, N. M. et al. Want to prevent pandemics? Stop spillovers. Nature 605 , 419–422 (2022).
Article ADS CAS PubMed Google Scholar
Markotter, W. et al. Prevention of zoonotic spillover: From relying on response to reducing the risk at source. PLoS Pathog. 19 , e1011504 (2023).
Article CAS PubMed PubMed Central Google Scholar
Plowright, R. K. et al. Land use-induced spillover: a call to action to safeguard environmental, animal, and human health. Lancet Planet Health 5 , e237–e245 (2021).
Article PubMed PubMed Central Google Scholar
Carroll, D. et al. The Global Virome Project. Science 359 , 872–874 (2018).
Plowright, R. K. et al. Pathways to zoonotic spillover. Nat. Rev. Microbiol. 15 , 502–510 (2017).
Plowright, R. K. et al. Transmission or within-host dynamics driving pulses of zoonotic viruses in reservoir–host populations. PLoS Negl. Trop. Dis. 10 , e0004796 (2016).
Wasik, B. R. et al. Onward transmission of viruses: how do viruses emerge to cause epidemics after spillover? Philos. Trans. R. Soc. Lond. B Biol. Sci. 374 , 20190017 (2019).
Banerjee, A. et al. Novel insights into immune systems of bats. Front. Immunol. 11 , 26 (2020).
World Health Organization. Prioritizing Diseases for Research and Development in Emergency Contexts https://www.who.int/activities/prioritizing-diseases-for-research-and-development-in-emergency-contexts (2022).
Wingfield, J. C. et al. How birds cope physiologically and behaviourally with extreme climatic events. Philos. Trans. R. Soc. Lond. B Biol. Sci. 372 , 20160140 (2017).
McEwen, B. S. & Wingfield, J. C. Allostasis and allostatic load*. In Encyclopedia of Stress 2nd edn (ed. Fink, G.) 135–141 (Academic Press, 2007).
Wingfield, J. C. The concept of allostasis: coping with a capricious environment. J. Mammal. 86 , 248–254 (2005).
Article Google Scholar
Shimba, A., Ejima, A. & Ikuta, K. Pleiotropic effects of glucocorticoids on the immune system in circadian rhythm and stress. Front. Immunol. 12 , 706951 (2021).
Shimba, A. & Ikuta, K. Immune-enhancing effects of glucocorticoids in response to day-night cycles and stress. Int. Immunol. 32 , 703–708 (2020).
Article CAS PubMed Google Scholar
Reeder, D. M. & Kramer, K. M. Stress in free-ranging mammals: integrating physiology, ecology, and natural history. J. Mammal. 86 , 225–235 (2005).
Acevedo-Whitehouse, K. & Duffus, A. L. J. Effects of environmental change on wildlife health. Philos. Trans. R. Soc. Lond. B Biol. Sci. 364 , 3429–3438 (2009).
Phelps, K. L. & Kingston, T. Environmental and biological context modulates the physiological stress response of bats to human disturbance. Oecologia 188 , 41–52 (2018).
Article ADS PubMed Google Scholar
Godbout, J. P. & Glaser, R. Stress-induced immune dysregulation: implications for wound healing, infectious disease and cancer. J. Neuroimmune Pharmacol. 1 , 421–427 (2006).
Article PubMed Google Scholar
Shimba, A. & Ikuta, K. Control of immunity by glucocorticoids in health and disease. Semin. Immunopathol. 42 , 669–680 (2020).
Guito, J.C. et al. Coordinated inflammatory responses dictate Marburg virus control by reservoir bats. Nat. Commun. 15 , 1826 (2024).
Amman, B. R. et al. Marburgvirus resurgence in Kitaka Mine bat population after extermination attempts, Uganda. Emerg. Infect. Dis. 20 , 1761–1764 (2014).
Becker, D. J., Eby, P., Madden, W., Peel, A. J. & Plowright, R. K. Ecological conditions predict the intensity of Hendra virus excretion over space and time from bat reservoir hosts. Ecol. Lett. 26 , 23–36 (2023).
Seltmann, A. et al. Seasonal fluctuations of astrovirus, but not coronavirus shedding in bats inhabiting human-modified tropical forests. Ecohealth 14 , 272–284 (2017).
Ruhs, E. C. et al. Applications of VirScan to broad serological profiling of bat reservoirs for emerging zoonoses. Front. Public Health 11 , 1212018 (2023).
Subudhi, S., Rapin, N. & Misra, V. Immune system modulation and viral persistence in bats: understanding viral spillover. Viruses 11 , 192 (2019).
Owen, J. C. et al. Reservoir hosts experiencing food stress alter transmission dynamics for a zoonotic pathogen. Proc. Biol. Sci. 288 , 20210881 (2021).
CAS PubMed PubMed Central Google Scholar
Gervasi, S. S., Burgan, S. C., Hofmeister, E., Unnasch, T. R. & Martin, L. B. Stress hormones predict a host superspreader phenotype in the West Nile virus system. Proc. Biol. Sci. 284 , 20171090 (2017).
PubMed PubMed Central Google Scholar
Mueller, T. et al. How landscape dynamics link individual- to population-level movement patterns: a multispecies comparison of ungulate relocation data. Glob. Ecol. Biogeogr. 20 , 683–694 (2011).
Tucker, M. A. et al. Moving in the Anthropocene: global reductions in terrestrial mammalian movements. Science 359 , 466–469 (2018).
Ripperger, S. P., Kalko, E. K. V., Rodríguez-Herrera, B., Mayer, F. & Tschapka, M. Frugivorous bats maintain functional habitat connectivity in agricultural landscapes but rely strongly on natural forest fragments. PLoS ONE 10 , e0120535 (2015).
Bloomfield, L. S. P., McIntosh, T. L. & Lambin, E. F. Habitat fragmentation, livelihood behaviors, and contact between people and nonhuman primates in Africa. Landsc. Ecol. 35 , 985–1000 (2020).
Gibb, R. et al. Zoonotic host diversity increases in human-dominated ecosystems. Nature 584 , 398–402 (2020).
Eby, P. et al. Pathogen spillover driven by rapid changes in bat ecology. Nature 613 , 340–344 (2023).
Schmidt, J. P. et al. Spatiotemporal fluctuations and triggers of ebola virus spillover. Emerg. Infect. Dis. 23 , 415–422 (2017).
Lunn, T. J. et al. Periodic shifts in viral load increase risk of spillover from bats. Preprint at bioRxiv https://doi.org/10.1101/2023.09.06.556454 (2023).
Luby, S. P. et al. Recurrent zoonotic transmission of Nipah virus into humans, Bangladesh, 2001–2007. Emerg. Infect. Dis. 15 , 1229–1235 (2009).
Arankalle, V. A. et al. Genomic characterization of Nipah virus, West Bengal, India. Emerg. Infect. Dis. 17 , 907–909 (2011).
Hegde, S. T. et al. Investigating rare risk factors for Nipah virus in Bangladesh: 2001–2012. Ecohealth 13 , 720–728 (2016).
Leroy, E. M. et al. Human Ebola outbreak resulting from direct exposure to fruit bats in Luebo, Democratic Republic of Congo, 2007. Vector Borne Zoonotic Dis. 9 , 723–728 (2009).
Vilela, T. et al. A better Amazon road network for people and the environment. Proc. Natl Acad. Sci. USA 117 , 7095–7102 (2020).
Article ADS CAS PubMed PubMed Central Google Scholar
Laurance, W. F., Goosem, M. & Laurance, S. G. W. Impacts of roads and linear clearings on tropical forests. Trends Ecol. Evol. 24 , 659–669 (2009).
Poulsen, J. R., Clark, C. J., Mavah, G. & Elkan, P. W. Bushmeat supply and consumption in a tropical logging concession in northern Congo. Conserv. Biol. 23 , 1597–1608 (2009).
Voigt, C. C. et al. Bats and buildings: the conservation of synanthropic bats. In Bats in the Anthropocene: Conservation of Bats in a Changing World (eds Voigt, C. C. & Kingston, T.) 427–462 (Springer International Publishing, 2016).
Dovih, P. et al. Filovirus-reactive antibodies in humans and bats in Northeast India imply zoonotic spillover. PLoS Negl. Trop. Dis. 13 , e0007733 (2019).
Wang, N. et al. Serological evidence of bat SARS-related coronavirus infection in humans, China. Virol. Sin. 33 , 104–107 (2018).
Pacheco, P. et al. Deforestation: A Threat to People and Nature https://www.worldwildlife.org/stories/deforestation-fronts (2021).
Nicas, J. & Moriyama, V. The Amazon’s largest isolated tribe is dying. N. Y. Times Illegal Gold Rush Poisons Indigenous People in Amazon Haven. Available online: https://www.nytimes.com/2023/03/25/world/americas/brazil-amazon-indigenous-tribe.html (2023).
Cornwall, W. Illegal gold mines flood Amazon forests with toxic mercury. Science https://doi.org/10.1126/science.ada0701 (2022).
Nakkeeran, N. et al. Beyond behaviour as individual choice: a call to expand understandings around social science in health research. Wellcome Open Res 6 , 212 (2021).
Honigsbaum, M. Disease X and other unknowns. Lancet 393 , 1496–1497 (2019).
Prist, P. R. et al. Promoting landscapes with a low zoonotic disease risk through forest restoration: the need for comprehensive guidelines. J. Appl. Ecol. 60 , 1510–1521 (2023).
Law, B., Park, K. J. & Lacki, M. J. Insectivorous bats and silviculture: balancing timber production and bat conservation. In Bats in the Anthropocene: Conservation of Bats in a Changing World (eds. Voigt, C. & Kingston, T.) 105–150 (Springer, Cham, 2016).
Williams-Guillén, K., Olimpi, E., Maas, B., Taylor, P. J. & Arlettaz, R. Bats in the anthropogenic matrix: challenges and opportunities for the conservation of Chiroptera and their ecosystem services in agricultural landscapes. In Bats in the Anthropocene: Conservation of bats in a Changing World (eds. Voigt, C. & Kingston, T.) 151–186 (Springer, Cham, 2016).
Amman, B. R., Schuh, A. J., Albariño, C. G. & Towner, J. S. Marburg virus persistence on fruit as a plausible route of bat to primate filovirus transmission. Viruses 13 , 2394 (2021).
Seltmann, A. et al. Habitat disturbance results in chronic stress and impaired health status in forest-dwelling paleotropical bats. Conserv Physiol. 5 , cox020 (2017).
Streicker, D. G. et al. Ecological and anthropogenic drivers of rabies exposure in vampire bats: implications for transmission and control. Proc. Biol. Sci. 279 , 3384–3392 (2012).
Furey, N. M. & Racey, P. A. Conservation ecology of cave bats. In Bats in the Anthropocene: Conservation of Bats in a Changing World 463–500 (2016).
Reid, J. L. Knowledge and experience predict indiscriminate bat‐killing intentions among Costa Rican men. Biotropica 48 , 394–404 (2016).
Deshpande, K., Vanak, A. T., Devy, M. S. & Krishnaswamy, J. Forbidden fruits? Ecosystem services from seed dispersal by fruit bats in the context of latent zoonotic risk. Oikos 2022 , (2022).
Huong, N. Q. et al. Coronavirus testing indicates transmission risk increases along wildlife supply chains for human consumption in Vietnam, 2013–2014. PLoS ONE 15 , e0237129 (2020).
Vora, N. M. et al. Bat and lyssavirus exposure among humans in area that celebrates bat festival, Nigeria, 2010 and 2013. Emerg. Infect. Dis. 26 , 1399–1408 (2020).
Osofsky, S. A., Lieberman, S., Walzer, C., Lee, H. L. & Neme, L. A. An immediate way to lower pandemic risk: (not) seizing the low-hanging fruit (bat). Lancet Planet Health 7 , e518–e526 (2023).
Biggs, D. et al. Governance principles for the wildlife trade to reduce spillover and pandemic risk. CABI One Health https://doi.org/10.1079/cabionehealth.2023.0013 (2023).
Khan, S. U. et al. A randomized controlled trial of interventions to impede date palm sap contamination by bats to prevent nipah virus transmission in Bangladesh. PLoS ONE 7 , e42689 (2012).
Pulliam, J. R. C. et al. Agricultural intensification, priming for persistence and the emergence of Nipah virus: a lethal bat-borne zoonosis. J. R. Soc. Interface 9 , 89–101 (2012).
Martin, G., Webb, R. J., Chen, C., Plowright, R. K. & Skerratt, L. F. Microclimates might limit indirect spillover of the bat borne zoonotic Hendra virus. Microb. Ecol. 74 , 106–115 (2017).
Article ADS PubMed PubMed Central Google Scholar
Plowright, R. K., Sokolow, S. H., Gorman, M. E., Daszak, P. & Foley, J. E. Causal inference in disease ecology: investigating ecological drivers of disease emergence. Front. Ecol. Environ. 6 , 420–429 (2008).
Kache, P. A., Cook, S., Sizer, N., Hannah, L. & Vora, N. M. Urgent need for integrated pandemic policies on pathogen spillover. Lancet Planet Health 5 , e668–e669 (2021).
Mettenleiter, T. C. et al. Draft of WHO Pandemic Agreement plays down primary prevention. Lancet 403 , 525–526 (2024).
Carlson, C. J. et al. Climate change increases cross-species viral transmission risk. Nature 607 , 555–562 (2022).
Asiedu, K. G. A year after outbreak, Africa waits for its share of mpox vaccines. Los Angel. Times (2023).
Adetifa, I., Muyembe, J.-J., Bausch, D. G. & Heymann, D. L. Mpox neglect and the smallpox niche: a problem for Africa, a problem for the world. Lancet 401 , 1822–1824 (2023).
Kozlov, M. WHO may soon end mpox emergency—but outbreaks rage in Africa. Nature 614 , 600–601 (2023).
ADS CAS PubMed Google Scholar
Phelan, A. L. How climate law can help to prevent the next pandemic. Nature 605 , 397 (2022).
Oppenheim, B., Brown, K. & Waldman, R. The world needs an intergovernmental panel on pandemic risk. Nat. Med. 27 , 934 (2021).
Hopkins, S. R. et al. How to identify win–win interventions that benefit human health and conservation. Nat. Sustain. 4 , 298–304 (2020).
World Health Organization News. Tripartite and UNEP support OHHLEP’s definition of ‘One Health’ (World Health Organization, 2021). https://www.who.int/news/item/01-12-2021-tripartite-and-unep-support-ohhlep-s-definition-of-one-health .
Ahmed, T., Tahir, M. F., Boden, L. & Kingston, T. Future directions for One Health research: regional and sectoral gaps. One Health 17 , 100584 (2023).
Greer, S. L., Bekker, M. P. M., Azzopardi-Muscat, N. & McKee, M. Political analysis in public health: middle-range concepts to make sense of the politics of health. Eur. J. Public Health 28 , 3–6 (2018).
Article PubMed Central Google Scholar
Walker, W. S. et al. The role of forest conversion, degradation, and disturbance in the carbon dynamics of Amazon indigenous territories and protected areas. Proc. Natl Acad. Sci. USA 117 , 3015–3025 (2020).
Jones, I. J. et al. Improving rural health care reduces illegal logging and conserves carbon in a tropical forest. Proc. Natl Acad. Sci. USA 117 , 28515–28524 (2020).
Ruiz-Aravena, M. et al. Ecology, evolution and spillover of coronaviruses from bats. Nat. Rev. Microbiol. 20 , 299–314 (2022).
Rasmussen, A. L. et al. Virology—the path forward. J. Virol. 98 , e0179123 (2024).
The World Bank. Establishment of a Financial Intermediary Fund for Pandemic Prevention, Preparedness and Response . http://documents.worldbank.org/curated/en/733191656685369495/Establishment-of-a-Financial-Intermediary-Fund-for-Pandemic-Prevention-Preparedness-and-Response (2022).
Chacón-Pacheco, J. J. & Ballesteros-Correa, J. MEJOR CONDICIÓN CORPORAL DE Artibeus lituratus EN FRAGMENTOS DE BOSQUE SECO ASOCIADOS A SISTEMAS SILVOPASTORILES QUE EN SISTEMAS CONVENCIONALES DE GANADERÍA EN CÓRDOBA, COLOMBIA. Oecol. Aust. 23 , 589–605 (2019).
Harvey, C. A. & González Villalobos, J. A. Agroforestry systems conserve species-rich but modified assemblages of tropical birds and bats. Biodivers. Conserv. 16 , 2257–2292 (2007).
Helbig-Bonitz, M. et al. Bats are not birds—different responses to human land‐use on a tropical mountain. Biotropica 47 , 497–508 (2015).
Williams-Guillén, K. & Perfecto, I. Effects of agricultural intensification on the assemblage of leaf-nosed bats (phyllostomidae) in a coffee landscape in Chiapas, Mexico. Biotropica 42 , 605–613 (2010).
Olimpi, E. M. & Philpott, S. M. Agroecological farming practices promote bats. Agric. Ecosyst. Environ. 265 , 282–291 (2018).
Tuneu-Corral, C. et al. Pest suppression by bats and management strategies to favour it: a global review. Biol. Rev. Camb. Philos. Soc. 98 , 1564–1582 (2023).
Frick, W. F. et al. Bats increased foraging activity at experimental prey patches near hibernacula. Ecol. Solut. Evid. 4 , e12217 (2023).
Frick, W. F., de Wit, L. A., Ibarra, A., Lear, K. & O’Mara, M. T. Conserving bats and their foraging habitats. In A Natural History of Bat Foraging (eds. Russo, D. & Fenton, B.) Ch. 16, 305–325 (Academic Press, 2024).
Download references
Acknowledgements
We are grateful to Sonia Altizer, Andrew Breed, Daphne Carlson-Bremer, Peggy Eby, Lee Hannah, Eric Moise Bakwo Fils, and Paul Webala for the discussions that helped shape this manuscript. Thank you to Mary Noel at Blu Skye Consulting for helping organize a workshop that generated ideas for this manuscript, Robyn Egloff for help with figures, and Scott Bischke, Erica Fleishman, and Brooklin Hunt for comments on a draft of the manuscript. Funding: Cornell Center for Pandemic Prevention, Preparedness, and Response (R.K.P., C.E.W.); National Science Foundation DEB-1716698, EF-2133763, EF-2231624 (R.K.P., P.J.H., A.J.P., M.R.A.); Defense Advanced Research Projects Agency PREEMPT program Cooperative Agreement D18AC00031 (R.K.P., P.J.H., A.J.P., M.R.A., A.T.H.K.); National Institute of Allergy and Infectious Diseases of the National Institutes of Health R01AI151144 (D.M.R. and I.E.); Montpellier Advanced Knowledge Institute On Transitions (R.K.P.); Natural Environment Research Council NE/V014730/1 (C.L.F.). The views, opinions, or findings expressed are those of the authors and should not be interpreted as representing the official views or policies of the Department of Defense or the U.S. Government.
Author information
Manuel Ruiz-Aravena
Present address: Department of Wildlife, Fisheries and Aquaculture, Mississippi State University, Starkville, USA
Authors and Affiliations
Department of Public and Ecosystem Health, Cornell University, Ithaca, NY, 14853, USA
Raina K. Plowright, Manuel Ruiz-Aravena & Charley E. Willison
Medical Research Council Unit The Gambia, London School of Hygiene and Tropical Medicine, London, WC1E 7HT, UK
Aliyu N. Ahmed
Department of Biology, University of Oxford, Oxford, OX1 3SZ, UK
Tim Coulson
Department of Environmental Systems Science, ETH Zürich, Zürich, 8092, Switzerland
Thomas W. Crowther
Department of Biology, Muni University, P.O. Box 725, Arua, Uganda
Imran Ejotre
School of Biodiversity, One Health and Veterinary Medicine, University of Glasgow, Glasgow, G12 8QQ, UK
Christina L. Faust
Bat Conservation International, Austin, TX, 78746, USA
Winifred F. Frick & M. Teague O’Mara
Department of Ecology and Evolutionary Biology, University of California, Santa Cruz, CA, 95064, USA
Winifred F. Frick
Centre for Infectious Disease Dynamics, Pennsylvania State University, State College, PA, 16801, USA
Peter J. Hudson
Department of Biological Sciences, Texas Tech University, Lubbock, TX, 79409-3131, USA
Tigga Kingston
College of Climate Change and Environmental Science, Kerala Agricultural University, Kerala, 680 656, India
P. O. Nameer
Centre for Planetary Health and Food Security, Griffith University, Nathan, QLD, 4111, Australia
Alison J. Peel & Manuel Ruiz-Aravena
School of Biological Sciences, University of Queensland, Brisbane, QLD, 4072, Australia
Hugh Possingham
Biosciences, University of Exeter, Exeter, EX4 4PS, UK
Orly Razgour
Department of Biology, Bucknell University, Lewisburg, PA, 17937, USA
DeeAnn M. Reeder
Department of Mammalogy, Division of Vertebrate Zoology, American Museum of Natural History, New York City, NY, 10024, USA
Nancy B. Simmons
Institute of Public Health, Bengaluru, Karnataka, 560070, India
Prashanth N. Srinivas
Center for Large Landscape Conservation, Bozeman, MT, 59771, USA
Gary M. Tabor & Annika T. H. Keeley
Department of Biology, University of Washington, Seattle, WA, 98195, USA
Iroro Tanshi
Small Mammal Conservation Organization, Benin City, 300251, Nigeria
Department of Animal and Environmental Biology, University of Benin, Benin City, 300000, Nigeria
Australian Capital Territory, Canberra, 2605, Australia
Ian G. Thompson
Centre for Policy Design, Ashoka Trust for Research in Ecology and the Environment, Bengaluru, Karnataka, 560064, India
Abi T. Vanak
School of Life Sciences, University of KwaZulu-Natal, Durban, 4041, South Africa
Conservation International, Arlington, VA, 22202, USA
Neil M. Vora
You can also search for this author in PubMed Google Scholar
Contributions
All authors contributed to idea generation, writing, and editing. R.K.P., A.T.H.K., I.G.T., T.C., O.R., C.L.F., and A.T.V. led working groups that facilitated the first draft of each section; R.K.P., D.M.R., and O.R. developed the figures.
Corresponding author
Correspondence to Raina K. Plowright .
Ethics declarations
Competing interests.
The authors declare no competing interests.
Peer review
Peer review information.
Nature Communications thanks the anonymous reviewer(s) for their contribution to the peer review of this work.
Additional information
Publisher’s note Springer Nature remains neutral with regard to jurisdictional claims in published maps and institutional affiliations.
Supplementary information
Supplementary information, rights and permissions.
Open Access This article is licensed under a Creative Commons Attribution 4.0 International License, which permits use, sharing, adaptation, distribution and reproduction in any medium or format, as long as you give appropriate credit to the original author(s) and the source, provide a link to the Creative Commons licence, and indicate if changes were made. The images or other third party material in this article are included in the article’s Creative Commons licence, unless indicated otherwise in a credit line to the material. If material is not included in the article’s Creative Commons licence and your intended use is not permitted by statutory regulation or exceeds the permitted use, you will need to obtain permission directly from the copyright holder. To view a copy of this licence, visit http://creativecommons.org/licenses/by/4.0/ .
Reprints and permissions
About this article
Cite this article.
Plowright, R.K., Ahmed, A.N., Coulson, T. et al. Ecological countermeasures to prevent pathogen spillover and subsequent pandemics. Nat Commun 15 , 2577 (2024). https://doi.org/10.1038/s41467-024-46151-9
Download citation
Received : 31 January 2024
Accepted : 16 February 2024
Published : 26 March 2024
DOI : https://doi.org/10.1038/s41467-024-46151-9
Share this article
Anyone you share the following link with will be able to read this content:
Sorry, a shareable link is not currently available for this article.
Provided by the Springer Nature SharedIt content-sharing initiative
By submitting a comment you agree to abide by our Terms and Community Guidelines . If you find something abusive or that does not comply with our terms or guidelines please flag it as inappropriate.
Quick links
- Explore articles by subject
- Guide to authors
- Editorial policies
Sign up for the Nature Briefing newsletter — what matters in science, free to your inbox daily.

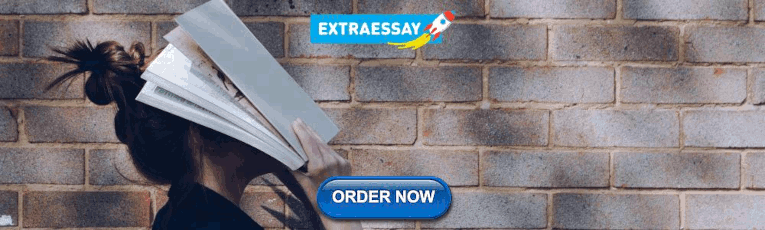
IMAGES
VIDEO
COMMENTS
The demand for wireless connectivity has grown exponentially over the last few decades. Fifth-generation (5G) communications, with far more features than fourth-generation communications, will soon be deployed worldwide. A new paradigm of wireless communication, the sixth-generation (6G) system, with the full support of artificial intelligence, is expected to be implemented between 2027 and ...
The Journal of Wireless Communications and Networking is riding on the 5th generation waves of the upcoming mobile communication systems with support of signal processing techniques and tools.Driven by the novel use cases for cyber physical systems, for the internet of things, and of the tactile internet, the journal will further grow and develop to take holistic and multi-disciplinary views ...
With the high demand for advanced services and the increase in the number of connected devices, current wireless communication systems are required to expand to meet the users' needs in terms of quality of service, throughput, latency, connectivity, and security. 5G, 6G, and Beyond (xG) aim at bringing new radical changes to shake the wireless communication networks where everything will be ...
2. WIRELESS NETWORKS EVOLUTION: 1G TO 6G. Starting from 1980, every 10 years a n ew w irel ess comm unicati on network generation appears [9], [10] t o date they are 5 generations. Figure 1 ...
The fifth generation (5G) organize is required to help essentially enormous measure of versatile information traffic and immense number of remote associations. To accomplish better spectrum, energy-efficiency, as a nature of quality of service (QoS) in terms of delay, security and reliability is a requirement for several wireless connectivity. Massive Multiple-input Multiple-output (mMIMO) is ...
The advent of a new generation of wireless communications has punctuated the dawn of every decade in recent times. Upgrades to mobile electronic systems represent faster and more robust capabilities of data transfer but bring with it a wide set of complementary changes as they are underpinned by harmonised specific spectrum bands, fresh international technical standards, new network operation ...
This work stands out for addressing a crucial problem in wideband wireless communications: the efficient distribution of signals across a wide spectrum. ... Tweeting your research paper boosts ...
A. Evolution of Wireless Communication Technologies and Systems: From 1G to Future 6G Wireless communication technology has progressed dramat-ically in the last several decades and wireless communications, which significantly impacted and changed our everyday lives in profound ways, has already become an integral part of our daily lives.
The field of terahertz wireless communication is growing rapidly. Here the authors discuss the challenges for the architectures of wireless platforms above 100 GHz and their potential applications.
6G W ireless Communications: Future T echnologies. and Research Challenges. Samar El meadawy 1and RaedM .S hubair 23. 1 Information Engineering and Technology Department, German University in ...
This paper proposes a novel wireless communication system to cater to a personalized service needs of both privacy-sensitive and privacy-insensitive users. We design the system based on based on multi-agent federated weighting deep reinforcement learning (MAFWDRL). The system, while fulfilling service requirements for users, facilitates real ...
Wireless communication is a rapidly expanding field that has completely changed the way people obtain information and communicate. Mobile telephony, wireless local area networks (WLANs), and ...
By freeing the user from the cord, personal communications networks, wireless LAN's, mobile radio networks and cellular systems, harbor the promise of fully distributed mobile computing and communications, any time, anywhere. Focusing on the networking and user aspects of the field, Wireless Networks provides a global forum for archival value ...
Near-field communication (NFC) is based on a simple idea. Two coils of conductors in close proximity can exchange electrical power over short distances (<5 cm) through wireless inductive coupling ...
The International Journal of Communication Systems is a communications journal publishing research papers on public and private communication ... of massive MIMO (m-MIMO) system in 2 GHz spectrum. The present generation of wireless networks must be rethought due to the rapidly rising user base, huge volume data transfer, wide coverage ...
Stay informed on the latest trending ML papers with code, research developments, libraries, methods, and datasets. ... Fractional Delay Alignment Modulation for Spatially Sparse Wireless Communications 29 Mar 2024 ... (DAM) is a novel transmission technique for wireless systems with high spatial resolution by leveraging delay compensation and ...
With the deployment of the 5G in wireless communications, the researchers' interest is focused on the sixth generation networks. This forthcoming generation is expected to replace the 5G network by the end of 2030. Artificial intelligence is one of the leading technologies in 5G, beyond 5G, and future 6G networks. Intelligence is endowing the tendency to throw open the capabilities of the 5G ...
The IEEE 802.11 standard for wireless local area networking (WLAN), commercially known as Wi-Fi, has become a necessity in our day-to-day life. Over a billion Wi-Fi access points connect close to hundred billion of IoT devices, smart phones, tablets, laptops, desktops, smart TVs, video cameras, monitors, printers, and other consumer devices to the Internet to enable millions of applications to ...
Abstract. Communication started with Telegraphy in the 1840s developing with Telephony some decade later and radio at the beginning of the century. The modern Telecommunication age is here. We ...
Our research also provides further information on the time domain characteristics and multipath propagation for the UWB communication channel with a capsule endoscope. We show that the time domain channel responses typically consist of very few noticeable delayed paths. We also conjecture that the more feasible frequency range within the ...
Communication-sensing integration represents an up-and-coming area of research, enabling wireless networks to simultaneously perform communication and sensing tasks. However, in urban cellular networks, the blockage of buildings results in a complex signal propagation environment, affecting the performance analysis of integrated sensing and communication (ISAC) networks. To overcome this ...
Wireless Personal Communications is an archival, peer reviewed, scientific and technical journal addressing mobile communications and computing. It investigates theoretical, engineering, and experimental aspects of radio communications, voice, data, images, and multimedia. A partial list of topics includes propagation, system models, speech and ...
Gluck's winning paper, "Don't Forget Our Presence: Exploring VR for Older Adults," built on earlier research from his dissertation. Gluck and his coauthors noted that virtual reality (VR) had tremendous benefits for adults 65 and older—like socialization, rehabilitation and well-being. Despite these benefits, very few older adults use VR.
This paper is focused on elements of Wireless Communication system, Types of Wireless Communication, Advantage & Disadvantage of it, Smart city, wireless network security. Discover the world's ...
In this Perspective, the authors discuss the importance of preventing zoonotic spillover to prevent pandemics. They highlight mechanisms by which environmental changes can enable spillover ...
The wireless technology have a series of benefits in relation to the wired networks and can be an alternative to the networks used traditionally in industry. This paper presents an overview on ...