Thank you for visiting nature.com. You are using a browser version with limited support for CSS. To obtain the best experience, we recommend you use a more up to date browser (or turn off compatibility mode in Internet Explorer). In the meantime, to ensure continued support, we are displaying the site without styles and JavaScript.
- View all journals
- My Account Login
- Explore content
- About the journal
- Publish with us
- Sign up for alerts
- Review Article
- Open access
- Published: 09 February 2024
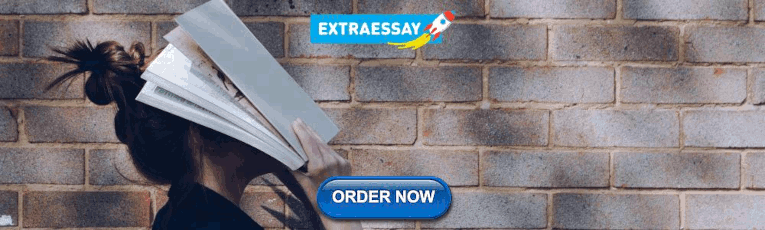
Major depressive disorder: hypothesis, mechanism, prevention and treatment
- Lulu Cui 1 , 2 , 3 ,
- Shu Li 1 , 2 , 3 ,
- Siman Wang 1 , 2 , 3 ,
- Xiafang Wu 1 , 2 , 3 ,
- Yingyu Liu 1 , 2 , 3 ,
- Weiyang Yu 1 , 2 , 3 ,
- Yijun Wang 1 , 2 , 3 ,
- Yong Tang ORCID: orcid.org/0000-0002-2543-066X 4 ,
- Maosheng Xia ORCID: orcid.org/0000-0003-4829-0812 5 &
- Baoman Li ORCID: orcid.org/0000-0002-3959-9570 1 , 2 , 3
Signal Transduction and Targeted Therapy volume 9 , Article number: 30 ( 2024 ) Cite this article
9861 Accesses
4 Altmetric
Metrics details
- Cellular neuroscience
- Diseases of the nervous system
Worldwide, the incidence of major depressive disorder (MDD) is increasing annually, resulting in greater economic and social burdens. Moreover, the pathological mechanisms of MDD and the mechanisms underlying the effects of pharmacological treatments for MDD are complex and unclear, and additional diagnostic and therapeutic strategies for MDD still are needed. The currently widely accepted theories of MDD pathogenesis include the neurotransmitter and receptor hypothesis, hypothalamic-pituitary-adrenal (HPA) axis hypothesis, cytokine hypothesis, neuroplasticity hypothesis and systemic influence hypothesis, but these hypothesis cannot completely explain the pathological mechanism of MDD. Even it is still hard to adopt only one hypothesis to completely reveal the pathogenesis of MDD, thus in recent years, great progress has been made in elucidating the roles of multiple organ interactions in the pathogenesis MDD and identifying novel therapeutic approaches and multitarget modulatory strategies, further revealing the disease features of MDD. Furthermore, some newly discovered potential pharmacological targets and newly studied antidepressants have attracted widespread attention, some reagents have even been approved for clinical treatment and some novel therapeutic methods such as phototherapy and acupuncture have been discovered to have effective improvement for the depressive symptoms. In this work, we comprehensively summarize the latest research on the pathogenesis and diagnosis of MDD, preventive approaches and therapeutic medicines, as well as the related clinical trials.
Similar content being viewed by others
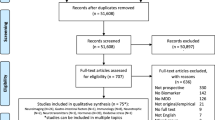
Prospective biomarkers of major depressive disorder: a systematic review and meta-analysis
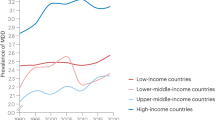
Major depressive disorder
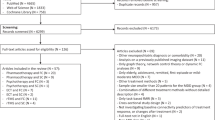
Brain connectivity in major depressive disorder: a precision component of treatment modalities?
Introduction.
Major depressive disorder (MDD), a main cause of disability worldwide, is characterized by physical changes such as tiredness, weight loss, and appetite loss. Anhedonia is a classic feature of MDD, and MDD is also accompanied by a lack of drive, sleep issues, cognitive challenges, and emotional symptoms such as guilt. 1 The prevalence of depression is increasing yearly. About 300 million people in the world are affected by MDD, which has become one of the main causes of disability. 2 In 2018, MDD ranked third in terms of disease burden according to the WHO, and it is predicted to rank first by 2030. 3 Pregnant women, elderly people, children, and others have a higher incidence rate of MDD, which may be related to genetic, psychological, and social factors. 4 Depression can be accompanied by recurrent seizures, which may occur even during remission or persist for longer than the disease itself. 5 Pharmacological therapies for MDD can effectively control symptoms; thus, patients may experience recurrence within a short time after discontinuing medication. 6 During recurrence, the patient experiences symptoms of low mood, loss of interest in life, fatigue, delayed thinking, and repeated fluctuations in mental state. 7
There is a certain correlation between the occurrence of MDD and social development. 8 A survey reported that with the development of the economy and increased life pressure, MDD has begun to emerge at a younger age, and the incidence of MDD in women is approximately twice that in men. 9 Specifically, women are more likely to develop depressive symptoms when they encounter social emergencies or are under significant stress. 8 Additionally, autumn and winter have been reported to be associated with a high incidence of MDD, namely, seasonal depression. 10
The clinical symptoms of MDD include a depressed mood, loss of interest, changes in weight or appetite, and increased likelihood of committing suicide. 11 These symptoms are also listed as the criteria for MDD in the Diagnostic and Statistical Manual of Mental Disorders (DSM-5). 12 In addition to the criteria listed in the DSM-5, the criteria reported in the International Classification of Diseases (ICD-10) are also used to guide clinical diagnosis. 13 However, due to the lack of characteristic symptoms and objective diagnostic evidence for MDD, identification and early prevention are difficult in the clinic. 14
Due to the complexity of the pathological mechanism of MDD, accurate diagnostic approaches and pharmacological therapeutic strategies are relatively limited. Several hypothesis were developed to explain MDD pathogenesis pathogenic including (i) the hypothalamic‒pituitary‒adrenal (HPA) axis dysfunction hypothesis, (ii) the monoamine hypothesis, (iii) the inflammatory hypothesis, (iv) the genetic and epigenetic anomaly hypothesis, (v) the structural and functional brain remodeling hypothesis, and (vi) the social psychological hypothesis 3 , 15 , 16 (Fig. 1 ). However, none of these hypotheses alone can fully explain the pathological basis of MDD, while many mechanisms proposed by these hypotheses interact with each other. In recent years, great progress has been made in identifying novel pharmacological therapies, diagnostic criteria, and nonpharmacological preventive measures for MDD, initiating related clinical trials. Specifically, increasing evidence suggests that astrocytic dysfunction plays a substantial role in MDD. 17 Pharmacological ablation of astrocytes in the medial prefrontal cortex (mPFC) causes depressive-like symptoms in experimental animals, 18 and postmortem studies of patients with MDD have shown reduced densities of glial cells in the prefrontal cortex (PFC), hippocampus and amygdala. 19 In addition, glial fibrillary acidic protein (GFAP), one of the markers of astrocytes, is expressed at various levels, 20 and the levels of connexins, 21 glutamine synthase (GS), glutamate transporter-1 (GLT-1), 21 , 22 and aquaporin-4 (AQP4) 23 are reduced in patients with MDD.
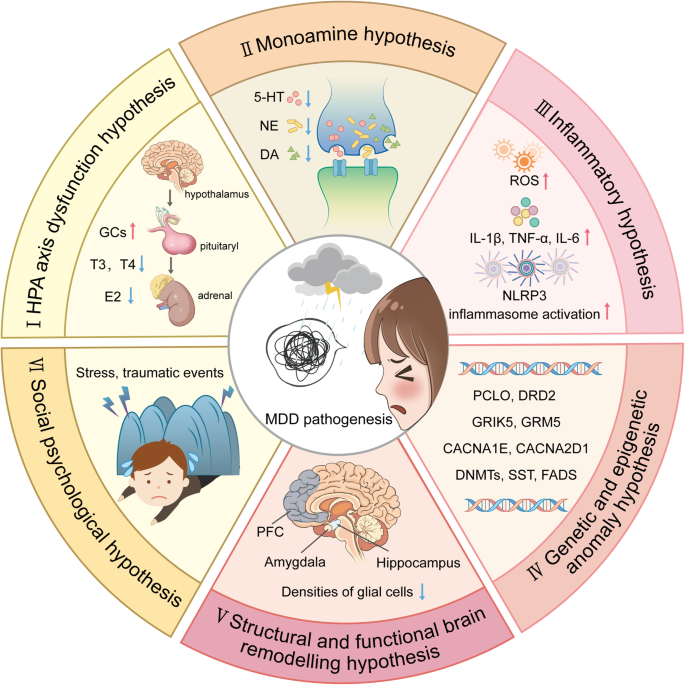
An outline map of the hypotheses to explain MDD pathogenesis. (I) HPA axis dysfunction hypothesis: high levels of glucocorticoids (GCs) play a core role in the pathogenesis of MDD, and thyroid hormone (TH) and estrogen are also involved in functions of the HPA axis; (II) the monoamine hypothesis: the functional deficiency of serotonin (5-HT), dopamine (DA) and norepinephrine (NE) are the main pathogenesis of MDD; (III) the inflammatory hypothesis: the neuro-inflammation induced by reactive oxygen species (ROS), inflammatory cytokines and inflammasomes activation is suggested to promote the occurrence of MDD; (IV) the genetic and epigenetic anomaly hypothesis: some genes are susceptible in the patients with MDD, including presynaptic vesicle trafficking (PCLO), D2 subtype of the dopamine receptor (DRD2), glutamate ionotropic receptor kainate type subunit 5 (GRIK5), metabotropic glutamate receptor 5 (GRM5), calcium voltage-gated channel subunit alpha1 E (CACNA1E), calcium voltage-gated channel auxiliary subunit alpha2 delta1(CACNA2D1), DNA methyltransferases (DNMTs), transcription levels of somatostatin (SST), fatty acid desaturase (FADS); (V) the structural and functional brain remodeling hypothesis: the postmortem results of patients with MDD are mostly associated with the reduced densities of glial cells in the prefrontal cortex (PFC), hippocampus, and amygdala; (VI) the social psychological hypothesis: the traumatic or stressful life events are the high risks of the occurrence of MDD. Adobe Illustrator was used to generate this figure
In this review, we summarize the latest research on the etiology, pathogenesis, diagnosis, prevention, mechanism, and pharmacological and nonpharmacological treatment of MDD as well as related clinical experiments.
Potential etiologies and pathogenic hypotheses
The common pathogenic factors.
Although the etiology of MDD is still unclear, it is widely accepted that MDD is associated with multiple pathogenic factor. In addition to well-known mental factors, MDD is also related to genetic factors, social stress, and even other common chronic diseases. Therefore, the etiology of MDD cannot be described from the perspective of a single factor.
Genetic factors
Although the etiology of MDD is still unclear, numerous studies have been performed and various models have been employed to explore the genetic factors, environmental factors and gene-environment interactions related to the disease. 24 Recent family, twin, and adoption studies suggests that genetic factors play a crucial role in the occurrence of MDD. 25 As a genetically diverse illness, MDD has a heritability of 30–50%. 26 Over 100 gene loci, including those associated with presynaptic vesicle trafficking (PCLO), dopaminergic neurotransmission (a primary target of antipsychotics), glutamate ionotropic receptor kainate type subunit 5 (GRIK5), and metabotropic glutamate receptor 5 (GRM5), and neuronal calcium signaling such as calcium voltage-gated channel subunit alpha1 E (CACNA1E) and calcium voltage-gated channel auxiliary subunit alpha2 delta1 (CACNA2D1), are found to be associated with an increased risk of MDD by genome-wide association studies. 19 , 27 , 28 In addition, rare copy number variants are also identified to be related to MDD risk, there may be three copy number variants (CNV) loci associated with Prader-Willis syndrome: 1q21.1 duplication, 15q11-13, and 16p11.2. However, no single genetic variation has been found to increase the risk of MDD thus far. 26 Genome Wide Association Studies (GWAS) identified 178 genetic risk loci and proposed over 200 candidate gene, using of biobank data, novel imputation methods, combined with clinical cases improved the ability to identify MDD specific pathways. 29 In the study of human MDD transcriptome, there are defects in the transcription levels of somatostatin (SST) in the subgenus anterior cingulate cortex and amygdala of MDD patients, 30 , 31 and SST levels are directly involved in the cellular processes that affect the synaptic output of intermediate neuronal circuits. 32 Recent studies revealed that gender specific genomic differences in MDD patients, the downregulation of the MDD-related gene Dusp6 in females leads to an increased susceptibility to stress, but this expression is not present in male mice. 33 In addition, studies of drug gene interactions, transcriptional genes associated with the risk of MDD are also reported, such as D2 subtype of the dopamine receptor (DRD2) and fatty acid desaturase (FADS), 34 which may serve as promising new targets for therapeutic intervention points. Thus, genetic variants are expected to have only minor effects on the overall risk of disease, and various hereditary factors combined with environmental factors such as stress are likely more essential for the development of MDD. 35
Stress factors
In addition to heritable factors, environmental influences such as stress also significantly contribute to the development of MDD, both independently and in conjunction with genetic factors. 26 Numerous studies have suggested that adverse life events can lead to the development of MDD. 18 A major depressive episode always follows a traumatic or stressful life event. In particular, severe events such as job loss, extramarital affairs and divorce are known to provoke the onset of the disease. 36 The exact pathological mechanism by which social stress results in the development of MDD is still not known, mainly due to the difficulty of separating social factors from genetic factors in patients and the impracticality of exposing disease model animals to relevant environmental factors. It has been proved that the changes in the structure and function of neurons may occur under the chronic stress and lead to the occurrence of MDD. 37 , 38 In some MDD patients, stress leads to long-term elevated glucocorticoids, resulting in synaptic structural changes and remodeling, and the stress-induced hyperactivity of the HPA axis leads to negative feedback imbalance of the HPA axis, which is also related to depression. 39 Studies on damage to microglia and astrocytes suggest the significance of glial cells in the development of environmental factor-induced depression-like behaviors in mice. 40 In addition, our previous studies proved that chronic environmental stress-induced depressive-like behaviors in mice can be dependent on purinergic ligand-gated ion channel 7 receptor (P2X 7 R) activation in astrocytes. 41
Comorbidity factors
The existence of various physiological and psychological comorbidities in patients with depression reveals a clear link between physical and mental health, which has given us a better understanding of MDD. The presence of MDD is a risk factor for a variety of complications, including neurodegenerative diseases (such as dementia, Alzheimer’s disease, and Parkinson’s disease), cardiovascular diseases (such as ischemic coronary artery disease and myocardial infarction), metabolic and endocrine diseases (such as obesity in females and diabetes in males), and some autoimmune diseases. 42 , 43 The relationship between the onset of MDD and several diseases is complex and potentially bidirectional in nature. 44 The impact of depression on society and the economy is increased by the existence of comorbidities. 45 Specifically, in 2018, comorbid disorders rather than MDD itself were responsible for 63% of all costs related to MDD in the United States. 46 , 47 Furthermore, compared to people without depression, patients with MDD have been demonstrated to have a shorter life expectancy. 48 Additionally, the worsening of comorbidities could be a factor in the premature mortality of MDD patients. 44
Neurotransmitter and receptor hypothesis
The traditional monoamine theory contends that in addition to common pathogenic factors, deficiencies in monoamine neurotransmitters, such as serotonin (5-HT), dopamine (DA) and norepinephrine (NE), are the root cause of clinical depression. 49 Selective serotonin reuptake inhibitors (SSRIs), a class of antidepressants that have been proven to successfully treat clinical depression, were developed in response to this hypothesis, which was derived primarily on the basis of the pharmacological mechanism of drug that were accidentally discovered to act as antidepressants. It is also crucial to note that astrocytes express NE transporter (NETT) and 5-HT transporter (SERT), which are the targets of some traditional antidepressants. 50 A previous study suggested that the function of astrocytes can be directly regulated by SSRIs. 51 Monoamine oxidase (MAO) activates the metabolism of adrenaline and triggers calcium signaling in astrocytes, 52 which suggests that antidepressants may directly affect astrocytes by preventing them from reabsorbing monoamines.
Serotonin (5-HT)
An essential neuromodulatory transmitter with specific neuroplastic properties is serotonin. Numerous investigations have demonstrated that 5-HT is intimately related to the pathophysiological process of major depression. The 5-HT hypothesis primarily asserts that a decrease in the 5-HT level is a risk factor for depression. 53 In addition, low levels of 5-HT and L-tryptophan, which is a precursor of 5-HT, 54 in blood platelets are also found in depressed people. Additionally, long-term treatment with fluoxetine, a typical SSRIs, reverses the stress-induced reduction in the quantity of astrocytic cells in the hippocampus in a tree shrew model of depression. 55
5-HT receptors, which are mostly found on the bodies and dendrites of neurons, play a role in the pathogenesis of MDD. 56 To date, 5-HT receptor subfamilies comprising 14 different receptor subunits expressed in various brain regions, namely, 5-HT 1A , 5-HT 1B , 5-HT 1D , 5-HT 1E , 5-HT 1F , 5-HT 2A , 5-HT 2B , 5-HT 2C , 5-HT 3 , 5-HT 4 , 5-HT 5A , 5-HT 5B , 5-HT 6 and 5-HT 7 , have been reported. Among these 5-HT receptor subtypes, the 5-HT 1 , 5-HT 2 , 5-HT 6 , and 5-HT 7 subtypes are expressed on brain and spinal astrocytes in humans and rodents. Numerous 5-HT receptors expressed on astrocytes are G-coupled proteins that are associated with changes in the concentration of free cytosolic calcium ([Ca 2+ ] i ). These changes may trigger the release of a variety of astrocyte-derived signaling modulators, which may control neuronal activity. 57 In astrocytes, 5-HT has a strong effect on the 5-HT 2B receptor. 58 5-HT receptors have been extensively studied to determine the pharmacological mechanism of antidepressants, and many novel pharmaceutical preparations are being investigated. For example, some novel antidepressants function as agonists of the 5-HT 1A , 5-HT 2B , or 5-HT 4 receptor or antagonists of the 5-HT 1B , 5-HT 2A , 5-HT 2C , 5-HT 3 , 5-HT 6 , or 5-HT 7 receptor. 59
Administration of fluoxetine in different concentrations to astrocytes expressing the 5-HT 2B receptor may activate distinct signaling pathways to control gene expression. Fluoxetine reduces the mRNA expression of c-Fos through the PI3K/AKT signaling pathway after acute application at concentrations below 1 μM, while the treatments with the higher doses (above 5 μM), it increases the gene expression of c-Fos via the MAPK/ERK signaling pathway in astrocytes. 60 Then, in the nucleus, the altered transcription factor c-Fos can further biphasic change the expression of caveoline under the chronic treatments, thus the alteration levels of caveoline on cellular membrane can finally affect the downstream activation of PTEN/PI3K/AKT/GSK3β 60 . The GSK3β polymorphisms are associated with the high risk of MDD in Chinese Han Population. 61 In our recent reports, the activation of GSK3β is also increased in the sorted astrocytes from the MDD-related stress-treated mice model and MDD clinic patients’ plasma. 62 In addition, after fluoxetine-mediated stimulation of the 5-HT 2B receptor in astrocytes, epidermal growth factor receptor (EGFR) is transactivated and subsequently activates the MAPK/ERK and PI3K/AKT signaling cascades, which control the expression of mRNA or proteins that may be linked to mood disorders, such as SERT. Ca 2+ -dependent phospholipase A2 (cPLA 2 ), adenosine deaminase acting on RNA 2 (ADAR2), and kainate receptor subtype 2 (GluK2) are all involved in kainate receptor signaling. 63 , 64 These discoveries promise astrocytic 5-HT 2B receptors can be the potential pharmacological target of SSRIs (Fig. 2 ).
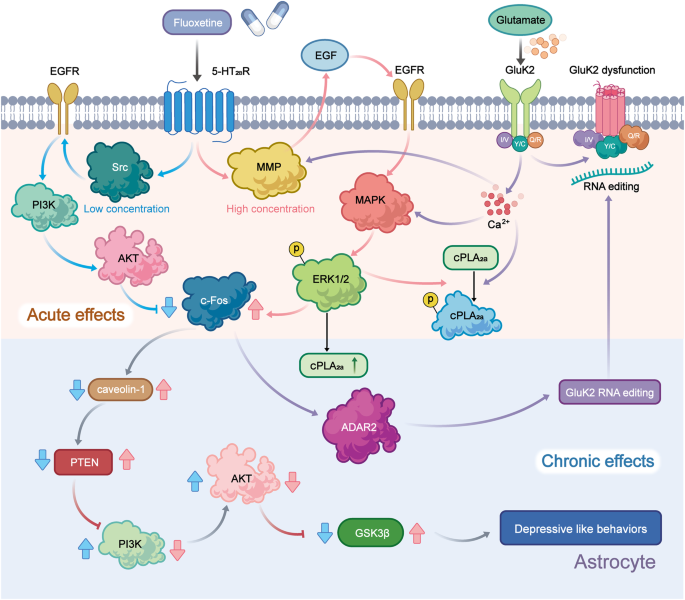
Schematic illustration of the pharmacological mechanism of fluoxetine in astrocytes. Acute treatment with fluoxetine at low concentrations (green arrows) stimulates Src, which phosphorylates EGF receptors by activating 5-HT 2B receptors (5-HT 2B R) and activates the PI3K/AKT signaling pathway. AKT phosphorylation induced by fluoxetine at low concentrations inhibits the expression of cFos and subsequently decreases the expression of caveolin-1 expression (chronic effects), which in turn decreases the membrane content of PTEN, induces phosphorylation and stimulation of PI3K and increases the phosphorylation of GSK3β, thus suppressing its activity. At higher concentrations, fluoxetine (red arrows) stimulates metalloproteinases (MMP) by activating 5-HT 2B R and induces the release of growth factors, which stimulates EGF receptors and activates the mitogen-activated protein kinases (MAPK)/ERK 1/2 signaling pathway. ERK 1/2 phosphorylation induced by fluoxetine at high concentrations stimulates the expression of cFos and subsequently increases the expression of caveolin-1 (chronic effects), which inhibits PTEN/PI3K/AKT/GSK3β, 60 ultimately leading to MDD like behavior. At high concentration, fluoxetine can also stimulate the activation of cPLA 2a by the transactivation of EGFR/MAPK/ERK 1/2 pathway, and the activated ERK 1/2 can also increases the expression of cPLA 2a at chronic treatments. 61 In addition, the increased expression of cFos induced by fluoxetine can further increases the RNA editing of GluK2 by increasing the expression of ADAR2 at the chronic treatments, the function of the edited GluK2 by fluoxetine is down-regulated, which causes the acute glutamated induced Ca 2+ -dependent ERK phosphorylation is suppressed. 63 Adobe Illustrator was used to generate this figure
Norepinephrine (NE)
NE released by the locus coeruleus (LC) can participate in regulating various neural functions, such as smell, movement, and sensation. 65 It is significant to note that after being released, noradrenaline (NA) is not restricted to the area around the synaptic cleft and can reach nearby glial cells. 66 Atomoxetine is a norepinephrine reuptake inhibitor (NRI) clinically used for the treatment of MDD. After systemic inflammatory attack with bacterial lipopolysaccharide (LPS), atomoxetine can decrease neuroinflammation in the rat cerebral cortex. 67
The bioavailability of 5-HT and NE are increased by antidepressants called serotonin/norepinephrine reuptake inhibitors (SNRIs), which belong to antidepressants. Currently, new SNRIs, including duloxetine (DXT), 68 desvenlafaxine (DVS), 69 and venlafaxine, 70 are widely used in MDD patients resistant to other treatments. Chronic treatment with DXT increases the expression of connexin 43 (Cx43), a crucial component of astrocyte gap junctions, in the rat PFC, preventing chronic unpredictable stress-induced dysfunction of astrocyte gap junctions and reversing the depressive-like behaviors caused by gap junction inhibition. 71 A novel therapeutic target for MDD is transforming growth factor β1 (TGF-β1), the expression of which is controlled by antidepressants. Venlafaxine has also been found to exert neuroprotection by boosting the production of type 2 fibroblast growth factor (FGF-2) and transforming growth factor 1 TGF-β1 in astrocytes following stroke. 72 However, the expression of protein markers of astrocytes and neurons is unaffected by DVS, and the chronic unpredictable mild stress (CUMS)-induced reduction in the levels of myelin- and oligodendrocyte-related proteins can be prevented by DVS. 69 DVS may reduce oligodendrocyte dysfunction in the CUMS mouse model by altering cholesterol production and reducing depression-like phenotypes. 69
Dopamine (DA)
There is increasing evidence that people with depression have reduced dopamine neurotransmission. 73 Astrocytes in the lateral habeula are involved in regulating depressive-like behavior, 74 whereas the reward circuit is mediated by the striatum. 75 The dorsolateral part of the striatum is linked to the drug-seeking behavior and drug addiction associated with psychiatric disorders. As the major input to the basal ganglia, the striatum and related nuclei are linked to psychiatric morbidity, while the chronic stress reduces dopamine levels in areas such as the striatum and hippocampus. 76 Due to processes involving dopamine D2 receptor signaling, 77 the glutamine level increases in the presence of dopaminergic lesions and decreases in the presence of a high DA level. 78 DA signaling is considered to play a key role in astrocyte-neuron crosstalk in the striatum. 79 Sulpiride is an antidepressant that blocks the ability of the GLT-1 inhibitor TFB-TBOA to induce synaptic depression 80 and partly attenuates the impact of fluorocitrate (a metabolic uncoupler that blocks aconitase in the tricarboxylic acid (TCA) cycle) on synaptic output. According to these results, astrocyte dysfunction results in an increase in DA levels, which decreases neuronal activity resulting from the binding of DA to dopamine D2 receptors, 80 which generates neuronal depolarization, reducing DA selectivity at dopamine D1-like receptors and promoting DA inhibition through dopamine D2 receptors, which may contribute to increasing extracellular glutamate levels. 81 An increase in DA signaling brought on by compromised astrocyte activity may induce a long-lasting change in striatal neurotransmission 80 since DA signaling is crucial for both structural and synaptic plasticity. 82
Glutamate is the main excitatory neurotransmitter in the central nervous system (CNS) 83 and can be released by neurons through exocytosis, which in turn activates extracellular N-methyl-D-aspartate receptors (eNMDARs) in neurons, leading to synaptic loss. 84 Exosynaptic glutamate also contributes to metabolism in neurons and astrocytes. When exosynaptic glutamate is taken up by astrocytes, it can become a substrate for glutamine synthesis or be metabolized by astrocytes and neurons. 85 In addition, extracellular glutamate can also promote glucose uptake by astrocytes and inhibit glucose uptake by neurons. Therefore, glutamate is an important signal that mediates the interaction between central neurons and astrocytes, and its normal release and transport are the result of the functional cooperation between neurons and astrocytes. Glutamate homeostasis and neurotransmission play a major role in the onset of depression and anxiety. Studies have shown that glutamate levels in frontal cortex samples from autopsied patients with severe depression are increased, and antidepressants can restore normal glutamate levels. 86 It has been observed in animal models that sustained glucocorticoid stimulation can increase the excitability of glutamatergic neurons and simultaneously decrease the number and plasticity of astrocytes, in addition to decreasing neuronal dendrite connectivity in the hippocampus and frontal cortex, leading to depression. 87
It is well-documented that astrocytes have a wide range of modulatory functions that may either increase or decrease the release of many different neurotransmitters. Specifically, astrocytes are essential regulators of glutamatergic neurotransmission, and reuptake of glutamate by astrocytes regulates excitatory synaptic activity. 85 When a large amount of glutamate is released from neuronal vesicles, glutamate clearance is mainly achieved by glutamate transporters (EAATs) on the membrane of astrocytes, which transport excess glutamate into astrocytes, where it is converted to glutamylamine through the action of glutamine synthase, reducing damage to neurons. 88 , 89 In the classic glutamate-glutamine cycle, astrocytes and neurons convert glutamate to the nonexcitatory amino acid glutamine, which is then released back into the extracellular space and absorbed by neurons. Alterations in astrocytic glutamate clearance are known to occur in schizophrenia and other psychiatric illnesses, and mice with glutamate/aspartate transporter (GLAST) deletion show phenotypic abnormalities such as mental and behavioral deficits. 90 , 91
Adenosine triphosphate (ATP)
Ectonucleotidases that are found in synapses can catabolize extracellular ATP to produce adenosine, and synapses also contain bidirectional nucleoside transporters that can release adenosine. 92 Adenosine primarily stimulates inhibitory A1 and facilitatory adenosine receptors (A 2A R) to play function. 93 Notably, depressive behavior is linked to purinergic signaling. Depressive-like symptoms are exacerbated by activation of P2X 7 R in glial cells. 94 Polimorphisms at P2X 7 R increase vulnerability to mood disorders whereas P2X 2 R-mediated neuronal activity is decreased in mice exposed to chronic stress due to insufficient ATP release from astrocytes. 95 According to our earlier studies, chronic sleep deprivation (SD) can cause depressive-like behaviors by increasing extracellular ATP levels in vivo. 41 Acting through P2X 7 R and FoxO3a cascade ATP inhibits expression of the 5-HT 2B receptor, the decrease in extracellular ATP levels caused by chronic stress and an increase in ATP levels caused by SD are both linked to depressive-like behaviors. 41 In detail, the elevated extracellular ATP induced by SD stress stimulates P2× 7 R and down-regulates the expression of 5-HT 2B R by suppressing the activation of AKT, which inhibits the phosphorylation of FoxO3a and promotes its transportation into the nucleus, the reduced 5-HT 2B R alleviates the inhibition of STAT3 to cPLA 2 , the activated cPLA 2 further increases the release of AA and PGE2, these indicators have high relationship with the depressive-like behaviors, because in P2X 7 R knockout mice, the above changes of these indicators and behavioral performance are all eliminated. 41 This increased activation of cPLA 2 and the elevated levels of AA and PGE2 in astrocytes are supported by our discoveries in MDD patients’ plasma. 62
After building a stress injury model in rats through maternal separation (MS), it is found that MS obviously reduces the total length of apical dendrites, however, the use of A 2A R antagonists could prevent synaptic loss 96 and reverse behavioral, electrophysiological, and morphological damage caused by MS, 97 this is related to the activity reconstruction of the HPA axis. In another study, the abnormally increased A 2A R in the lateral septum(LS) is a key factor in recurrent stress for leading to depressive-like behaviors. This function is mainly achieved by the increased activity of A 2A R-positive neurons and the inhibited activity of ambient neurons, associating with the neural circuits of dorsomedial hypothalamus(DMH) and lateral habenular(LHb). 98
Caffeine is an adenosine receptor antagonist, and epidemiological studies have shown that the intake of caffeine is closely related to the occurrence of suicide 99 and depression. 100 Since A 2A R polymorphisms are associated with emotional problems, adenosine A 2A R overexpression leads to emotional dysfunction, and A 2A R blockade protects against the persistent emotional disturbance brought on by stress. 101 Moreover, animal experiments have demonstrated that A 2A R are upregulated in chronic stress animal models. 102 Additionally, neuronal A1 receptors exhibit hypofunction caused by a decrease in astrocyte-derived adenosine levels; 103 this decrease, as well as depressive-like behavior, can be reversed by certain antidepressants. 104 , 105
HPA axis hypothesis
Stress and MDD are closely related, and stressful life events can often lead to depressive episodes. The activation of the HPA axis by stress can cause cognitive and emotional changes. 106 An increase in HPA activity is one of the most common neurobiological alterations in depressed people. Studies have shown that the main factor contributing to the elevation of hypothalamic-pituitary activity is the increased production of corticotropin-releasing hormone (CRH). In addition, pituitary adrenal corticotropic hormone (ACTH) is released in response to CRH, which in turn triggers the adrenal cortex to release glucocorticoids (GCs).
Glucocorticoids
The HPA axis, a component of the neuroendocrine system, is commonly associated with the stress response. Hyperactivity of the HPA axis is thought to be an important pathophysiological mechanism underlying depression. High HPA activity is among the most typical neurobiological alterations in depressed individuals. The HPA axis is the primary stress response system that produces GCs, which are a class of steroid hormones. There is evidence that GCs, which are released in response to stress, are harmful to neurons in various brain regions. The hypothalamic paraventricular nucleus (PVN) rapidly secretes CRH and arginine vasopressin (AVP) 107 when the HPA axis is activated by stress. The anterior pituitary is stimulated by CRH and AVP to produce ACTH, which in turn increases the release of GCs into the bloodstream. 108
The GC and mineralocorticoid (MC) receptors GR and MR are members of the nuclear receptor (NR) superfamily. Both NRs can be triggered by binding to either MCs (such as aldosterone) or GCs (such as cortisol). However, the affinity of MR for its ligands is 10 times higher than that of GR for its ligands. 109 , 110 GRs are expressesd at higher levels and particularly concentrated in the pituitary and hypothalamus, as well as a variety of regions of the limbic system (including the amygdala, hippocampus, and PFC), which are important for cognitive and psychological functions.
To prevent loss of control over the HPA axis, GCs exert negative feedback on the axis in all regions involved (the limbic system, hypothalamus, and pituitary). Some data suggest that HPA axis imbalance and high levels of GCs play a core role in the pathogenesis of MDD and suggest that GR may serve as an important target for treating depression. 111
Thyroid hormone
Thyroxine (T4) and triiodothyronine (T3) are the two primary Thyroid hormones (THs) that regulate metabolism, protein synthesis, the growth of bones, and nervous system development. Thyrotropin-releasing hormone (TRH), which regulates the synthesis of thyroid-stimulating hormone (TSH) by the anterior pituitary gland, is mostly produced by neurons in the PVN. TSH stimulates the thyroid gland to produce T3 and T4. The levels of serum-free T4 and free T3 are regulated by negative feedback from pituitary TSH release. Tissue deiodinase mostly transforms T4 into the less physiologically active metabolite reverse T3 and the more biologically active metabolite T4. 112
Overactivity of the HPA axis may be caused by damaged astrocytes and aberrant GR function. The HPA and hypothalamic-pituitary-thyroid (HPT) axes are inextricably linked. The most important related finding is that cortisol directly affects TRH secretion (which regulates TSH release), potentially through the response of GCs to TRH mRNA expression in neurons. According to research, hypercortisolemia may result in a reduction in TRH mRNA levels in the mid-caudal PVN. 113 TRH expression in the PVN is lower in nonpsychiatric patients treated with corticosteroids, and the mRNA levels of TRH are lower in the PVN of depressed patients who have recurrent suicidal thoughts. This suggests that the effect of hypothalamic TRH is weaker in these individuals.
THs are required for neuronal growth and function not only in the periphery but also in the CNS, 114 where they promote the formation of microglia, astrocytes, including radial glial cells, and oligodendrocytes. The role of THs in glial cells is becoming clear because of new discoveries in the field of glial cell biology. THs affect the shape and proliferation of astrocytes, as well as the organization and expression of GFAP/vimentin, and boost GS activity. 115 T3 has an effect on glial morphology and hence on glial function in the adult brain; therefore, it also has an effect on neuron-glia interactions. 115 , 116 It has been shown that T3 induces astrocyte proliferation by autocrine production of growth factors such as epidermal growth factor (EGF) and FGF-2. Apart from their proliferation-promoting impact, these growth factors increase and modify the pattern of deposition of the extracellular matrix components laminin and fibronectin, therefore boosting cell adherence and attachment to the substratum. Together with the discovery that animals with hypothyroidism and mice with TH receptor mutations display significant defects in glial development, these findings indicate that astrocytes are TH targets and that TH can protect neurons and astrocytes from glutamate toxicity. 115
The hippocampus is closely related to memory and learning, and estrogen plays an important role in these processes. Estrogen increases the proliferation, migration, and differentiation of neurons in the dentate gyrus to maintain hippocampal function and is also important for controlling the HPA axis. 117
Estrone (E1), estradiol (E2), and estriol (E3) are the three physiological estrogens; among these estrogens, E2 is the most active, and its level quickly decreases throughout menopause. 118 E2 has been demonstrated in numerous studies to alter systems involved in the pathophysiology of depression, including the serotonin and norepinephrine systems, and to considerably alleviate depressive symptoms in animal models. Estrogen therapy can decrease the quantity of 5-HT 1 and β-adrenergic receptors while increasing the quantity of 5-HT 1 receptors. 119 In addition, estradiol may influence the pathogenesis of male MDD patients. 120 In animal models, E2 has been shown to alleviate depressive-like behavior. 121 , 122 Estrogen receptor 1 (ER1) and estrogen receptor 2 (ER2) are transcription factors that are members of the NR family. Activating ER2 with a range of ER2 agonists has been reported to reduce stress-induced HPA activity and anxiety-like behaviors. 123 , 124
Astrocytes are estrogen targets, 125 as both ER1 and ER2 receptors are present on the astrocyte membrane or intracellularly in astrocytes. The transmembrane receptors ER and GPR30 have been shown to facilitate nongenomic and fast estrogen signaling in astrocytes, contributing to the neuroprotective effects of E2. In mature astrocytes differentiated from human induced pluripotent stem cells (iPSC)-derived astrocyte progenitors, ketamine can exert rapid antidepressant effects through the activation of amino-3-hydroxy-5-methyl-4-isoxazole propionic acid (AMPA) glutamate receptors, and estrogen enhances this effect of ketamine by increasing the gene expression of AMPA receptor subunits. 126
The obese gene (OB) encodes the hormone leptin, which is derived from adipocytes and the stomach and exerts its function through a specific receptor (OB-R). Leptin controls the function of the HPA axis 127 via its receptor in the hypothalamus. The cerebral cortex, hippocampus, hypothalamus, dorsal raphe (DR) nucleus, arcuate nucleus, and solitary tract nucleus are some regions of the brain that can express leptin receptors. Increasing experimental data have recently shown that leptin is linked to the pathological and physiological processes of numerous mental illnesses and plays a vital regulatory role in the CNS. 128 , 129 According to our previous reports, leptin can enhance the pharmacological effects of fluoxetine in astrocytes sorting from GFAP-GFP transgenic mice. 130 Leptin selectively increases the expression of the astrocytic 5-HT 2B receptor by activating the JAK2/STAT3 pathway, and fluoxetine in turn stimulates the 5-HT 2B receptor and increases the secretion of brain-derived neurotrophic factor (BDNF) from astrocytes in vivo, thus ameliorating depressive-like behaviors. 130 All of these findings indicate leptin’s potential to boost protein expression and functionally stimulate SERT.
Cytokine hypothesis
MDD is accompanied by changes in the levels of proinflammatory cytokines and trophic factors, including BDNF, interleukins (IL-1β, IL-6), and tumor necrosis factor alpha (TNF-α). Increasing data suggest that the production of certain cytokines by brain astrocytes plays a significant role in the pathogenesis of MDD.
Oxidative stress
Oxidative stress (OS), which is caused by an imbalance between antioxidants and reactive oxygen species (ROS), can harm proteins, lipids, or DNA. The activity of monoamine oxidase, the enzymes that break down monoamines such as DA, 5-HT and NE, is influenced by ROS and in turn can increase ROS production in mitochondria. The brain is more vulnerable to OS than other organs. In depression, OS plays a crucial role. 131 , 132 The brain is particularly sensitive to OS due to numerous variables, including rapid oxidative energy metabolism (a process through which ROS, which are harmful molecules, are constantly produced), high levels of unsaturated fatty acids (which are vulnerable to lipid peroxidation), and relatively low intrinsic antioxidant capability. 133 Adults with MDD exhibit ROS-mediated reductions in nitric oxide (NO)-dependent dilation. 134
Thioredoxin reductase, heme-oxygenase 1, glutathione, and glutathione peroxidase are only a few of the ROS-detoxifying enzymes that are abundant in astrocytes. 135 Astrocytes are the major producers of glutathione in the brain because they express a system xc-cyttine/glutamate antiporter, which does not exist in neurons; hence, neurons cannot synthesize glutathione. Notably, astrocytes can protect nearby neurons against toxic dosages of NO, H 2 O 2 , and superoxide anion in combination with NO, iron, or 6-hydroxydopamine in coculture systems, 135 indicating that neurons rely on the strong antioxidant capacity of astrocytes for protection against OS. Nuclear factor erythroid 2 (Nrf2), a redox-sensitive transcription factor required for coordinating the cellular antioxidant response, can be activated by astrocytes. In our recent study, lithium salt (Li + ) was found to effectively alleviate ischemia-induced anhedonia in mice by suppressing the production of mitochondrial ROS in glial cells. 136
Recent investigations have indicated that MDD is caused by increased ROS production and promotes inflammation. 137 The brain has weak antioxidative defenses and a high oxygen consumption rate, making it particularly susceptible to OS. Inflammasomes in microglia can be activated by ROS, which causes inflammatory cytokines, including TNF-α, IL-1β, and IFN-γ, to be produced. 138 Neuroendocrine-immune activities can be compromised by inflammation, which can also result in numerous disorders, such as MDD. Proinflammatory cytokines have become pathological indicators of MDD, and using the right antioxidants to combat ROS may be a useful method for treating MDD.
Proinflammatory cytokines
Higher levels of inflammation increase the chance of developing new-onset depression. 138 , 139 Although depression can cause inflammation, its cause is still unclear and may be influenced and regulated by immune cells, inflammatory cytokines, and the nervous system. In addition to contributing to the etiology of depression, activation of proinflammatory signaling pathways occurs as a result of elevated OS. 140 Evidence suggests that MDD is associated with the immune response, as shown by increased levels of IL-1β, TNF-α, and IL-6. 141 LPS-induced astrocyte activation also contributes to the symptoms of MDD. Systemic treatment with LPS induces depressive-like behaviors and increases the production of inducible nitric oxide synthase (iNOS), IL-1β, TNF-α, and GFAP in the hippocampus and cortex. Inhibition of activated astrocytes reduces neuroinflammation. These alterations are followed by amelioration of LPS-induced depressive-like behaviors. 142
Neurotrophic factors
In the vast majority of patients with severe depression, antidepressants affect the levels of neurotrophic factors. For example, the primary regultaory factor of neuronal survival, growth, and differentiation during development is BDNF. For the treatment of depression, targeting signaling transduction by BDNF and its receptor, tropomycin receptor kinase B (TrkB), is essential. 143 , 144 Recent research has shown a link between decreased hippocampal neurogenesis and low levels of BDNF and glial-derived neurotrophic factor (GDNF) in the brains of depressed individuals. 145 Under normal conditions, astrocytes release various nutrients and cytokines. After cell reactivation, the secretion of these factors is further increased. 146 According to previous studies, fluoxetine stimulates c-Fos expression and ERK 1/2 phosphorylation, which in turn promotes BDNF production in astrocytes sorting from GFAP-GFP transgenic mice. 147 Imipramine acts as an antidepressant by increasing the mRNA expression of BDNF in astrocytes. Fluoxetine also induces BDNF expression by activating cAMP-response element binding protein(CREB) through the PKA and/or ERK pathways. 148
BDNF is an essential molecule for neural plasticity and development and is related to several CNS diseases. Currently, it is known that BDNF can regulate the activity of neurons and that it is produced not only by neurons but also by astrocytes. 149 SSRIs and tricyclic antidepressants increase BDNF expression in cultured primary astrocytes, and BDNF overexpression in mouse hippocampal astrocytes is sufficient to promote neurogenesis and causes anxiolytic behavior. 149 By promoting neurotransmitter release, facilitating vesicle docking, and upregulating the expression of synaptic vesicle proteins, BDNF, which is released by astrocytes in response to long-term antidepressant therapy, may assist in increasing synaptic plasticity at presynaptic terminals. 150 In addition, astrocyte-secreted BDNF can stimulate adult hippocampal neurogenesis and may contribute to synaptic and structural plasticity that underlies the long-lasting behavioral effects of antidepressants. 150 Astrocytes can secrete numerous nerve growth factors. Vascular endothelial growth factor (VEGF) is a member of the vasoactive growth factor family. It exerts its unique molecular effects by binding and activating endothelial cell tyrosine kinase receptors. VEGF is traditionally associated with angiogenesis and its stimulation. Recent evidence indicates, however, that it also influences nerve cells and plays a crucial role in hippocampal neurogenesis and neuroprotection. 151
Inflammasomes
Neuroinflammation is a central pathophysiological mechanism and defining characteristic of MDD. Numerous elements in the periphery and CNS interact to generate neuroinflammation, thereby stimulating astrocytes. The nucleotide-binding domain and leucine-rich repeat protein-3 (NLRP3) inflammasome is one of the largest typical inflammasomes discovered thus far. It is composed of pro-Casp-1 protein, NLRP3, and apoptosis-associated speck-like protein (ASC). 152 The sensitization of the NLRP3 inflammasome and the suppression of BDNF synthesis result in MDD. 153 In our research, SD is found to reduce BDNF levels and induce depressive-like behaviors in the sorted astrocytes from GFAP-GFP transgenic mice by activating the NLRP3 inflammasome. 130 NLRP3 inflammasome activation causes astrocytes to produce more IL-1β and IL-18. 154 , 155
The release of proinflammatory cytokines is the primary consequence of the activation of caspase-1, a component of the NLRP3 inflammasome. In addition, it has been observed that stimulating NLRP3 inflammasome assembly can induce depression-like behaviors in rodents exposed to LPS or CUMS. 156 , 157 Research on the effect of astrocyte-specific NLRP3 knockout suggests that the astrocytic NLRP3 inflammasome exerts a significant effect on astrocytic pyroptosis via the Casp-1/GSDMD pathway in depression. 156 , 157 Therefore, efficient NLRP3 inflammasome inhibitors are novel therapeutic agents for MDD. As we previously reported, chronic SD can specifically activate the NLRP3 inflammasome and decrease the level of BDNF in astrocytes to ameliorate depressive-like behaviors. Fluoxetine can suppress the effects of SD on astrocytes by stimulating astrocytic 5-HT 2B receptors directly. 147 Additionally, in the middle cerebral artery occlusion (MCAO) stroke model of mice, Li + can significantly attenuate GSDMD-mediated glial pyroptosis by regulating the AKT/GSK3β/TCF4/β-catenin signaling pathway, in which, the activation of AKT induced by Li + can also increase the phosphorylation of FoxO3a and promote the transportation of FoxO3a from nucleus into cytoplasm, the reduced FoxO3a in nucleus dissolves its competition with TCF4 in order to confirm more β-catenin/TCF4 complex. The increased latter complex further up-regulates the expression and activation of STAT3 in nucleus, the latter further inhibits the activation of the NLRP3 inflammasome by increase UCP2 which can decrease the production of ROS from mitochondrion. 136 This neuroprotective mechanism of Li + after ischemia-reperfusion injuries contributes to the improved depressive-like behaviors, besides of motor and cognitive capacities. 136
In conclusion, there have been so many hypothesis to explain the pathogenesis of MDD associating with many booming researches (Fig. 3 ). However, it is still hard to adopt only one above hypothesis to completely reveal pathophysiology of MDD. The main problem may contribute to the limitations of the theoretical perspective and the limitations of detection methods. Some key scientific problems in the neurobiology of neurological and psychiatric disorders are still unclear, such as how to identify the pathological characteristic changes for mood disorders, how to metabolize the cerebral metabolic waste under the pathological condition,how to observe the instant interactions of neural cells and the real-time changes of intracellular organelles in the patients of MDD? In the pathological conditions, conducting research from the perspective of comprehensive collaboration of the whole body and increasing the proportion of new technological applications in research will open up the new paths to reveal the pathogenesis of MDD in the future.
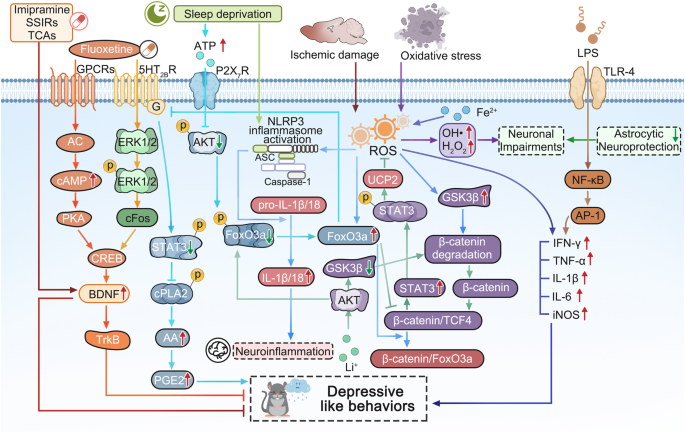
The molecular signaling schematic of cytokine hypothesis in the pathogenesis of MDD. The rodent performed the depressive like behaviors are impaired by some widely accepted risk factors, such as long-term sleep deprivation (SD), oxidative stress, lipopolysaccharide (LPS), ischemic damage and so on. Long-term SD can increase the extracellular ATP level, the latter inhibits the activation of AKT and the followed phosphorylation of FoxO3a by stimulating P2X7 receptors (P2X7R), the dephosphorylated FoxO3a translocates into the astrocytic nucleus, then the increased FoxO3a decreases the expression of 5-HT 2B R expression, which results the reduced phosphorylation of STAT3 which increases the activation of cPLA2 and the followed release of arachidonic acid (AA) and prostaglandin E2 (PGE2), finally causing the depressive-like behaviors. 41 Thus, antidepressant fluoxetine activates ERK 1/2 /cFos pathway by stimulating 5-HT 2B R and AC/cAMP/PKA pathway by activating GPCRs in order to increase the activation of CREB and the level of BDNF and TrkB, which can alleviate the depressive like behaviors induced by long-term SD. 147 , 148 As well as, imipramine, other SSIRs, and TCAs can also play antidepressive roles by increasing BDNF mRNA expression in astrocytes. 148 Ischemic stroke can trigger the increase of reactive oxygen species (ROS) which can induce the activation of NLRP3 inflammasome and the release of IL-1β/18, resulting in the neuroinflammation, however, Li + salt inhibits the activation of GSK3β and increases the phosphorylation of FoxO3a by activating AKT, which promotes the more FoxO3a transportation from nucleus into cytoplasm, and the reduced FoxO3a in nucleus lacks the competition with TCF4, the increased complex level of β-catenin and TCF4 further stimulates the expression and the phosphorylation of STAT3, which further induce the mRNA and protein expression of UCP2, then in mitochondrion, the increased UCP2 suppresses the production of ROS and results in the deactivation of NLRP3 inflammasomeincreases. 136 Superoxidation of Fe 2+ stimulates an increase in ROS, resulting in the production of inflammatory cytokines (including IFN-γ, TNF-α, IL-1β, IL-6) and inducible nitric oxide synthase (iNOS). 138 While, the treatments of oxidative stress (OS) can produce a large number of ROS, such as OH• and H 2 O 2 , resulting in neuronal impairments, while astrocytes can play their neuroprotective role by antioxidation. 135 Additionally, LPS can also increase TNF-α, IL-1β, and IL-6 by TLR-4/NFkB/AP-1 pathway and cause depressive-like behavior. 142 Adobe Illustrator was used to generate this figure
Interactions of multi-cells and multi-organs
Recently, increasing evidence has shown that pathological changes in a single cell type or brain region limited are insufficient explain the pathogenesis of MDD. This section mainly introduces the latest research on the pathogenesis of MDD, discussing the multiple interactions among neural cells and the multiple regulatory mechanisms between the brain and peripheral organs in detail.
The interaction between neuron and glial cell
Over the past few decades, studies on MDD have identified decreased PFC activity and excitatory/inhibitory (E/I) imbalance as probable mechanisms underlying depression. 158 Astrocytes are recognized to be essential for controlling neural network activity and to take part in higher brain activities. 159 To explore efficient treatments for MDD, it is important to focus on how to regulate the E/I balance and neuronal remodeling. 160
MDD-related marker proteins in neural cells
Astrocytes in the CNS form the neurovascular unit with neurons and blood vessels. The neurovascular unit mediates the exchange of nutrients and other functional substances between its components. 161 The blood-brain barrier (BBB) consists of endothelial cells tight junctions, a continuous basement membrane and astrocytic end-feet. Two proteins expressed on astrocytes, connexin 30 (Cx30) and Cx43, have been linked to the pathogenesis of depression. 162 Gap junctions that enable communication between astrocytes are formed by the membrane proteins Cx30 and Cx43. 163 Chronic unpredictable stress (CUS) and acute stress both specifically reduce the expression of the gap junction-forming proteins Cx30 and Cx43, 164 and the integrity of the BBB is weakened in mice lacking Cx30 and Cx43. 165
In addition to being an essential component of the developing astrocyte cytoskeleton, GFAP serves as the main intermediate filament protein in adult astrocytes. Although increased expression of GFAP is commonly observed in reactive astrogliosis, postmortem results suggest that the frequency and intensity of reactive astrogliosis are decreased in the brains of patients with MDD. 166 Accompanied by a decreased astrocyte density, the levels of GFAP and the GFAP intermediate filament domain are also reduced in brain samples from patients with MDD. 167 Researchers have even proposed that the GFAP content in serum can be used to determine the severity of MDD, 168 but this point is controversial.
AQP4, a kind of water channel, is mainly expressed on astrocytic end-feet in contact with blood vessels. The water channel AQP4 regulates the equilibrium of ions and water in the brain and is an essential part of the neurovascular unit. The vascular coverage of AQP4-immunopositive astrocytes in the orbitofrontal cortex (OFC) is lower in people with clinically significant depression than in psychiatrically healthy control patients. 169 In another postmortem study, it was found that the coverage of blood vessels by AQP4-positive astrocyte terminals was reduced in the OFC of MDD patients. 170 In addition, the K + -buffering capacity and presumably synaptic transmission are impaired in mice lacking AQP4, and impairment of these processes is associated with depressive-like behaviors. 171 In our previous study, we reported that the expression of AQP4 was decreased by exposure to CUMS, which contributed to dysfunction of glymphatic circulation and depressive-like behaviors in mice. 172 Additionally, the coverage of blood vessels by AQP4-positive astrocytic endfeet is decreased by 50% in MDD patients, indicating that decreased levels or mislocalization of AQP4 may contribute to the pathogenesis of MDD. 169 , 173
S100B is produced and secreted by astrocytes in the gray matter, 174 and changes in the levels of S100B in the blood and cerebrospinal fluid (CSF) of patients with MDD can cause glial cell dysfunction and damage. 175 , 176 In individuals with MDD, the number of S100B-immunopositive astrocytes in the pyramidal layer of the bilateral hippocampal CA1 region is decreased. 177 S100B secreted by damaged astrocytes can enter the extracellular space and CSF, 178 and the level of S100B is increased in the dorsolateral prefrontal cortex (dlPFC) of patients with MDD. 179 S100B levels are elevated in the CSF or serum of patients with MDD, 180 which suggests that S100B is a potential diagnostic biomarker for depressive episodes associated with MDD.
Communication between neurons and microglia plays an important role in the pathogenesis of depression. C-X3-C Motif Chemokine Ligand 1 (CX3CL1)- C-X3-C Motif Chemokine Ligand 1 receptor (CX3CR1) and OX-2 membrane glycoprotein (CD200)-OX-2 membrane glycoprotein receptor (CD200R) form ligand-receptor pairs, and these molecules are the most important chemokines and clusters of differentiation in maintaining CNS homeostasis. 181 CX3CL1 and CD200 are mainly expressed in neurons, and their receptors CX3CR1 and CD200R are expressed on microglia. 182 Activated microglia and decreased expression of CX3CL1 in the hippocampus were observed in an LPS-induced depression model. 183 CX3CR1-deficient mice show a temporary decrease in the number of microglia and a resulting deficiency of synaptic pruning, which may be related to neurodevelopmental and neuropsychiatric disorders. 184 However, CX3CR1-deficient mice show significant resistance to stress-induced depressive-like behaviors. 185 The level of CX3CL1 in the serum is increased in patients with moderate-severe depression compared with healthy subjects; thus, CX3CL1 could be used as a target for depression treatment. 186 Patients diagnosed with MDD with comorbid cocaine addiction show higher serum levels of CX3CL1. 187 Additionally, in a rat early-life social isolation (ESI) model, the expression of CD200 receptors in microglia is significantly reduced. 188 Exposure to unavoidable tail shock causes a decrease in CD200R expression in the hippocampus and amygdala, 189 and stress was also discovered to suppress CD200R expression in the hippocampus of rats. 190
Synaptic plasticity
Long-term potentiation (LTP) serves as the physiological basis for learning and conditioned responses. 191 Ketamine has a quick antidepressant effect, as it is a noncompetitive channel blocker of N-methyl-D-aspartate receptors (NMDARs). 192 Excessive glutamate in the synaptic cleft activates synaptic metabotropic glutamate receptors (mGluRs), which lead to neural excitotoxicity. 193 In a mouse model of chronic social defeat stress (CSDS), which causes depression, mGluR5 was shown to induce long-term depression (LTD). The major process responsible for synaptic plasticity is the mGluR-mediated LTD, which likely plays a significant role in the pathophysiological changes underlying depressive-like behaviors in the CSDS-induced depression paradigm. 194
ATP can mediate the activity of the astrocyte-neuron network, and ATP is a signaling molecule that also controls synaptic plasticity. 195 ATP can increase the expression of amino-3-hydroxy-5-methyl-4-isoxazole propionic acid receptors (AMPARs) by stimulating P2X 7 R and increasing the amplitude of miniature excitatory postsynaptic currents. 196 Stress exposure is a major pathogenic factor in disease models and can increase Ca 2+ -dependent release of ATP from neurons, which causes excitotoxicity. 197 , 198
Regulated in development and DNA damage response-1 (REDD1) is a stress response gene that can regulate development and the response to DNA damage. Virus-mediated overexpression of REDD1 in the rat PFC is sufficient to cause anxiety- and depressive-like behaviors and neuronal atrophy. 199 According to postmortem studies, the volume of the dlPFC is smaller and the density of neurons in the dlPFC is lower in MDD. 200 BDNF can modulate synaptic plasticity in the brain. TrkB is a functional receptor of BDNF. 201 BDNF produces antidepressant-like effects by increasing synaptic plasticity in a mouse model of CUMS. 202
Neuron-glia integrity
The term “tripartite synapse” was initially used to describe the intimate relationship between astrocytes and neurons at glutamatergic synapses, similar to the glutamate-glutamine cycle described above. 203 Moreover, glutamic acid decarboxylase, an enzyme that transforms glutamate into γ-aminobutyric acid (GABA), also exists in inhibitory GABAergic neurons. Increased inhibitory neurotransmission, glutamatergic/GABAergic E/I imbalance, and chronic stress-related emotional dysfunction reduce PFC activity. 204 , 205 In local circuits, various glutamatergic and GABAergic neurons interact in complicated ways to achieve E/I balance. 206 A meta-regression analysis indicated that glutamine and glutamate levels are decreased in the PFC, which is correlated with the therapies to MDD. 207 Global topological E/I imbalance in MDD is discovered through gene and protein expression of molecules related to inhibitory GABAergic and excitatory glutamatergic signaling in the postmortem MDD brains. 22 , 208 , 209 It shows the imbalance in cortical-subcortical limbic regions with decreased GABAergic signaling and increased glutamatergic signaling. 210 , 211 Meanwhile, GABAergic signaling is decreased in regions comprising the default mode network (DMN), while it is increased in the lateral prefrontal cortex (LPFC). 212 , 213 Stimulating P2X 7 R in neocortical nerve terminals can block the reuptake of GABA and glutamate by the presynaptic membrane and promote the release of these two neurotransmitters in the cerebral cortex of rats and humans, 214 , 215 and activation of P2X 7 R reduces the expression of GLAST. 216 This results in neuronal damage, a reduced number of synapses, decreased neurogenesis, and even impairment of key cerebral circuits that regulate mood.
Astrocytes are fundamental elements in synapses, participate in synaptogenesis and maturation, and maintain synaptic homeostasis. Ionic homeostasis in the extracellular space is critical for central nervous system function. 217 Astrocytes play an important role in maintaining extracellular K + homeostasis in the CNS, as well as H + , Cl - , and Ca 2+ homeostassis. 218 In addition, it also plays an important role in maintaining transmitter homeostasis, in which glutamate and GABA play particularly important roles. 219
In addition to the tripartite synapse, the more recent concepts of the four-part extracellular matrix and the microglial five-part synapse 220 also support the idea that glial dysfunction plays key roles in the early pathological features common to psychiatric disorders. 221 , 222 Under physiological conditions, microglia can play a neuroprotective role by producing cytokines. However, under pathological conditions, microglia can also affect the balance between excitatory and inhibitory synapses by phagocytosing synapses 223 and activating inflammatory factors in microglia. 224 In addition, the extracellular matrix (ECM) plays a significant role in maintaining normal communication in mature neural networks, which can limit the synaptic restriction of glutamate. 225 The components of the ECM are mainly produced by neurons and astrocytes, and microglia can also regulate the remodeling of the ECM. 226
Interaction mechanism in multi-organs
Abnormalities in cytokine levels in the brain and peripheral organs, disruption of the brain/immune system balance, and dysfunction of communication between the peripheral organs and the brain can cause neuroinflammation and depressive symptoms. For instance, cirrhosis and depression have been linked to intestinal dysbiosis, which results in intestinal barrier disruption, increasing bacterial translocation. Increased bacterial translocation then activates circulating immune cells, which produce cytokines and induce systemic inflammation. 227 In comparison with the healthy population, MDD patients have a much higher incidence and prevalence of chronic liver disease. 228 Inflammatory bowel disease (IBD) and irritable bowel syndrome (IBS) with increased intestinal permeability, which may have both inflammatory and autoimmune sources, are common comorbidities of MDD and anxiety. 229 , 230
Neuroendocrine-immune axis
Microglia secrete chemokines that disrupt the integrity of the BBB and increase the ability of immune cells to enter the brain parenchyma. 231 The stress response is a complex array of behavioral, neuroendocrine, autonomic, and immunological responses that enable adaptation to unpleasant psychological and physiological stimuli. 232 The HPA axis is a crucial endocrine system that orchestrates this response. 233 Stress can activate microglia, which are considered important immunocytes of the CNS. Mediators released by activated microglia can stimulate the HPA axis and induce GC production. 39 Similarly, high levels of GCs can also activate microglia, creating a vicious cycle. 234
Tryptophan (TRP) can be converted into a variety of biologically active molecules, and more than 95% of TRP is metabolized to kynurenine (KYN) and its breakdown products, with only a small portion of TRP being converted to 5-HT. 235 Indoleamine 2,3 dioxygenase (IDO) is an immune inducible enzyme that metabolizes TRP through the KYN pathway and plays an important role in the immune response. 236 In the brain, KYN is metabolized to the neurotoxic substance quinolinic acid (QUIN). 237
The primary GC in the HPA axis, corticosterone, plays a role in regulating the stress response in rodents. Stress, high GC levels, and serious depression are all linked. Analysis of transcriptomic changes associated with corticosterone-induced cytotoxicity revealed an association of neurite outgrowth-related genes with depression. Therapies for MDD may target the expression of genes involved in neurite formation, such as calpain 2 (Capn2), vesicle-associated membrane protein (Vamp7), and c-type natriuretic peptide (Cnp). 238
Consumption of a high-fat diet (HFD; for approximately 16 weeks) results in anxiety and anhedonic behaviors, and 4 months of HFD consumption results in increased levels of corticosterone and blood glucose, which also activate the innate immune system, increasing the release of inflammatory cytokines (i.e., IL-6, IL-1β, TNF-α). The behavioral abnormalities that arise from long-term consumption of a HFD are quickly reversed by ketamine. Additionally, giving HFD-fed rats a P2X 7 R antagonist greatly alleviates their anxiety. 239
Microbiota-gut–brain axis
In recent years, the microbiota-gut-brain axis has been reported to be disrupted in MDD. Stress stimulation can affect the gut microbiota, which in turn induces the production of inflammatory mediators (mainly IL-6 and IFN-γ) and a reduction in short-chain fatty acid levels. 240 The increased level of inflammatory cytokines may be caused by disturbance of the gut microbiota, which may also disrupt the gut barrier. 241 Alterations in the gut microbiota and inflammatory agents have an impact on the KYN pathway, metabolism, and toxin metabolism in the periphery. 242 Proinflammatory cytokines or toxic byproducts resulting from microbiota alterations may pass through the BBB and enter the brain. 243 This increases the levels of cytokines such as IL-1β and IL-6 and NLRP3 inflammasome activation in brain-resident cells. 244 In particular, microglia and astrocytes are activated and undergo atrophy, respectively. These glial cell changes, which affect the brain networks involved in learning and memory, mood regulation, and emotional regulation, may cause depressive symptoms or anxiety episodes. 245
According to clinical research, TRP and tryptophan catabolites (TRYCATs) may play a crucial role in psychiatric illnesses, including MDD. Peripheral and central inflammation can both stimulate the KYN pathway and trigger TRP metabolism and subsequent synthesis of various TRYCATs, including the toxic NMDAR activator QUIN, 246 which influences glutamate transmission, has a variety of immunomodulatory effects and has both neurotoxic and neuroprotective effects on the CNS. 141 Studies have proven that peripherally injected LPS increases the central and peripheral metabolism of TRP via the KYN pathway by exerting neurotoxic effects, inducing reactivation of microglia and astrocytes in the CNS. 247 Excessive production of QUIN, an NMDAR agonist, stimulates the release of glutamate and inhibits reuptake, leading to neuronal excitotoxicity. 248
Liver-brain axis
Patients with liver diseases often struggle with depression. According to one study on the frequency of liver disease and major depression in the United States, liver disease is linked to both major depression and suicidal thoughts. 249 A further population-based cohort study discovered that patients with MDD had much higher prevalence and incidence rates of chronic liver disease than the general population. 228 The incidence of depression is high in cirrhosis patients; moreover, depression is an independent predictor of mortality from cirrhosis. 250
An internal metabolic mechanism regulated by the liver can control depressive-like behavior. A crucial enzyme in epoxyeicosatrienoic acid (EET) signaling in the liver is epoxide hydrolase (sEH). Chronic stress selectively exacerbates sEH-induced depression-related changes in the liver while dramatically lowering the plasma levels of 14,15-EET. Deletion of hepatic epoxide hydrolase 2 (Ephx2) (which encodes sEH) rescues the chronic mild stress (CMS)-induced decrease in 14,15-EET plasma levels. 251 In a rat model of CUMS, electroacupuncture (EA) was found to downregulate P2X 7 R, NLRP3, and IL-1β expression in the prefrontal cortex and liver and relieved depression-like behavior. 252
In summary, as shown in Fig. 4 , although the etiology of MDD is still unclear, it is widely accepted that the common pathogenic factors of MDD are genetic, stress, and comorbidity. 3 The levels of monoamine neurotransmitters (5-HT, NE, and DA) are insufficient in the synaptic cleft of MDD patients, correspondingly, the explored antidepressants such as tricyclic antidepressants(TCAs), SSRIs and SNRIs almostly act on the channels responsible for inhibiting reuptake of these neurotransmitters. 51 Thus, according to these traditional pharmacological theories, these antidepressants always have the delayed clinical efficacy, this promises the potential new pharmacological mechanism still requires further study. As the well-known glutamate-glutamine cycle, astrocytes play key roles in resolving neuronal glutamate toxicity. However, under the MDD pathological condition, due to the decreased expression of EAATs in astrocytes, excessive glutamate in the synaptic cleft activates synaptic mGluRs, which leads to neuronal excitotoxicity. 194 In addition, the overdose glutamate can also be decarboxylated by glutamate decarboxylase (GAD) to GABA and activates the GABA receptors on the postsynaptic membranes. 206 In our previous studies, the expression of 5-HT 2B is selectively decreased in the sorting astrocytes from MDD model mice. 64 The antidepressants SSRIs and leptin can increase the expression of the astrocytic 5-HT 2B receptor. 147 Furthermore, OS plays a crucial role in the emergence of depression, including by elevating the levels of ROS and NO in the mitochondrion of astrocytes. 253 Proinflammatory signaling pathways are activated as a result of elevated OS, the mitochondrial dysfunction results in an increased generation of ROS and NO. 137 As well as, the pathogenesis of MDD are associated with the inflammatory-immune response, as shown by elevated levels of proinflammatory cytokines, mainly IL-1β, TNF-α, and IL-6. 141 The expression of neural cell marker proteins in neural cells, including Cx30/43, 162 GFAP, 167 AQP4, 172 and S100B, 177 are all decreased under MDD pathological conditions. In brain, KYN is metabolized by microglia to the neurotoxic metabolite QUIN and by astrocytes to the beneficial metabolite kynurenic acid (KynA), thus, QUIN is increased and KynA is decreased in MDD patients’ brain. 141 , 254 , 255 Recently, growing evidence support that the occurrence of MDD are the results of the correlational disorders from multiple systems or organs, not only limiting in brain. 227 , 228 The comorbidities of MDD have attracted widespread attention, the intestinal gut microbial dysbiosis, liver dysfunction, immune system disorders all play important roles in the pathogenesis of MDD. Stressful conditions can affect the gut microbiota, which in turn induces the production of inflammatory mediators (mainly IL-6 and IFN-γ). 256 Proinflammatory cytokines or toxic QUIN resulting from alterations in the microbiota may pass through the BBB and activate NMDARs. 243 Under the dysfunction of liver, the level of ammonia is increased in the brain. 257 The pathogenic factors of various organs at the body level and the pathological changes of glial cells at the cellular level should attract more attention to explain the pathogenesis of MDD.
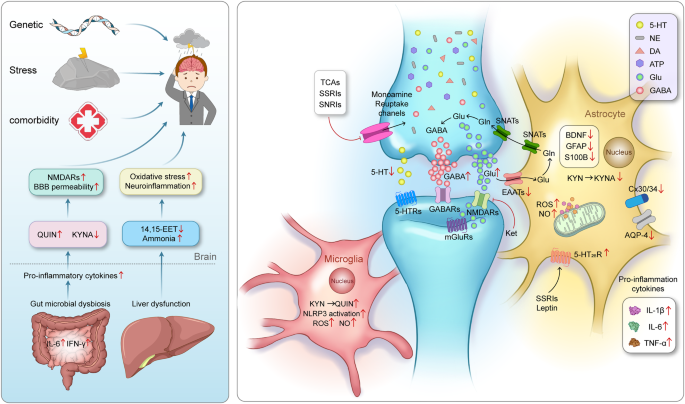
The pathogenesis of MDD is closely related to synapses, astrocytes, microglia, and their interactions as well as interactions among organ. Genetic factors, stress and comorbidities are considered the most common pathogenic factors of MDD 3 . The traditional monoamine theory contends that MDD may cause by the deficits in monoamine neurotransmitters. 49 Moreover, the other abnormal increase of neurotransmitters in the synaptic cleft, such as glutamate, GABA and ATP, has the high relationship with the pathogenesis of MDD. 41 , 496 The interaction between neurons and glial cells can induce the oxidative stress, pro-inflammatory cytokines released, the reduction of neurotrophic factors. The microbiota-gut-brain axis is clearly disrupted in MDD. 243 , 248 When liver dysfunction occurs and causes OS and neuroinflammation in the brain, which also contribute to the pathophysiology of MDD. 497 Adobe Illustrator was used to generate this figure
New diagnostic approaches
MDD is a prevalent psychiatric disorder worldwide and is expected to become one of top disease in terms of burden by 2030. 258 However, the current clinical diagnostic criteria for MDD are subjective, and diagnoses are mainly based on clinical symptoms, leading to high rates of missed and incorrect diagnoses. This section summarizes the newest research on diagnostic approaches for MDD, including serum indicators, neuroimaging indicators and multimodality scales. Research on new diagnostic approaches for MDD has the potential to improve our understanding of MDD pathogenesis and the accuracy of clinical diagnosis.
Potential serum indicators
The pathological mechanism of MDD can be studied in two ways: by exploring the pathophysiology of the disease and by identifying MDD-related neurobiological indicators4. Hence, identifying potential biomarkers for MDD could allow accurate diagnosis, faster treatment and effective monitoring of the disease. Recently, an increasing number of studies have confirmed the involvement of OS and neuroinflammation in MDD pathology. 259 , 260 Two novel biomarkers, serum nicotinamide adenine dinucleotide phosphate oxidase 1 (NOX1) and Raftlin, are reported to have good diagnostic value in MDD patients. The effectiveness of elevated NOX1 and Raftlin levels in diagnosing MDD has been evaluated in clinical trials; the related mechanism is that NOX1 can regulate the ROS-antioxidant balance in patients with MDD through OS and the inflammatory repsonse. 261 The serum level of the chemokine-like protein TAFA-5 (FAM19A5) has also been reported to be increased in patients with MDD, and increased serum FAM19A5 levels are associated with reactive astrogliosis, neuroinflammation, and neurodegeneration. 262 In addition, the level of serum FAM19A5 was shown to have a negative correlation with cortical thickness in specific brain regions. These findings suggest that serum FAM19A5 could be a potential biomarker for neurodegenerative changes in MDD.
Functional magnetic resonance imaging indicators
In addition to serum indicators, neuroimaging metrics are potential objective tools for improving the accuracy of MDD diagnosis and must be studied in death. In recent years, many researchers have tried to diagnose MDD using MRI by identifying disease-specific functional and/or structural abnormalities in patients with MDD compared with healthy subjects. 263 Structural MRI techniques, such as voxel-based morphometry (VBM), can be used to detect volume changes in gray matter. 264 It has been reported that abnormal gray matter volume (GMV) in several brain regions is positively correlated with MDD. 265 , 266 Regarding functional MRI, recent studies have revealed that cerebral functional abnormalities are not limited to specific brain regions in patients with MDD. These differences are also associated with hypoconnectivity within the frontoparietal network (FN), the DMN, and midline cortical regions. 267 , 268 Furthermore, resting-state functional magnetic resonance imaging (R-fMRI) is an emerging neuroimaging technique used to study functional connectivity in the brain and holds great potential in aiding clinical diagnosis. 269 It has the benefits of being noninvasive and easy to perform and offering high temporal and spatial resolution. 270 As a result, it has played a significant role in MDD research and is a superior technique for researching MDD pathogenesis and identifying neuroimaging markers for MDD. 271 Thus, indicators such as amplitude of low-frequency fluctuation (ALFF), fractional amplitude of low-frequency fluctuation (fALFF), regional homogeneity and functional connectivity (FC) have shown promise as neuroimaging markers for MDD. Recently, a study reported that increased average values of ALFF and fALFF in the right caudate and corpus callosum may serve as potential markers for diagnosing MDD. 272 Another study based on the largest R-fMRI database of MDD patients confirmed that the DMN plays a crucial role in MDD diagnosis, as DMN FC is reduced in patients with recurrent MDD. 273 These findings also suggest that the DMN should continue to be a prominent focus of MDD research.
New multi-modal evaluation scales
Given that structural and functional abnormalities are associated with MDD, 274 using multimodal approaches is more appropriate than relying on a single feature for the diagnosis of MDD. However, research results related to the effectiveness of neuroimaging techniques in diagnosing MDD remain inconsistent. 275 This may be attributed to variations in the types of structural and functional features examined; however, more importantly, very few studies have used multimodal approaches to diagnose MDD. 276 Recently, in a study utilizing multimodal MRI data, patients with MDD were successfully distinguished from healthy controls by radiomics analysis. 276 Radiomics is a rapidly developing field involving the extraction of quantitative information from diagnostic images, and it can be mainly divided into three steps: image acquisition, analysis and model building. 277 Additionally, omics and neuroimaging techniques can be combined to construct models for diagnosing MDD; specifically, 5-hydroxytryptamine receptor 1 A/1B methylation data can be integrated with resting-state functional connectivity (rsFC) data. It was shown that this combination could be used to more accurately distinguish patients with MDD from healthy subjects than R-fMRI data or DNA methylation data alone. 278
By now, the widely accepted objective diagnostic indicators or methods for MDD are still deficient. In addition to the unclear pathogenesis of MDD, insufficient sensitivity and accuracy of detection instruments are also the main reasons, especially the correlation between imaging characterization and disease-specific changes that need to be discussed.
Preventing the occurrence and recurrence of MDD
MDD is a disease with a high prevalence worldwide, 279 and preventing its occurrence and recurrence is crucial. Lifestyle medicine is an evolving medical specialty that aims to prevent chronic, noncommunicable diseases through lifestyle interventions. The goal of lifestyle medicine is to prevent the occurrence and recurrence of disease by improving sleep hygiene and diet, increasing physical exercise, avoiding sedentary behavior, increasing social support, and improving mood. In recent years, an increasing number of studies have demonstrated that the occurrence and recurrence of MDD can be prevented by means of lifestyle medicine; 280 we summarize these reports in this section.
Sleep improvements
Improving sleep is an important strategy to prevent the occurrence of depression. Insomnia is included in the diagnostic criteria for MDD. 281 However, few studies have examined whether treating insomnia can prevent the exacerbation of depressive symptoms. Treating insomnia can prevent the worsening of depressive symptoms, and cognitive behavioral therapy for insomnia (CBT-I) is a recommended intervention for treating insomnia to improve sleep and mood. 282 , 283 , 284 As a first-line treatment for insomnia, CBT-I includes cognitive therapy, stimulus management, sleep restriction, improved sleep hygiene, and relaxation. 282 , 285 CBT-I can also lead to sustained remission of insomnia-related disorders, and continuous treatment of insomnia with CBT-I can also reduce the occurrence and recurrence of MDD. 286 Circadian rhythm support (CRS) can strengthen the circadian rhythm by means of scheduled bright light exposure, physical activity, and body warming. 287 Although CRS has been reported to have only an indirect effect in alleviating sleep disturbance and depressive symptoms, 288 treatment with CRS may help maintain the beneficial effects of CBT-I. 288 , 289 In one study, 44% of untreated patients but 38%, 28% and 9% of patients treated with CRS, CBT-I, and CBT-I + CRS, respectively, experienced clinically significant worsening of depressive symptoms during a 1-year follow-up period. Between-group comparisons showed that the percentage of patients who experienced worsening of depressive symptoms was significantly different between the CBT-I + CRS group and the nontreated and CRS groups. 289 In a randomized controlled trial, exacerbation of depressive symptoms over one year was decreased in insomia patients with an increased risk of depression and insomnia patients treated by therapist-guided CBT-I combined with CRS; however, untreated insomnia patients with a high risk of depression experienced clinically significant worsening of depressive symptoms. 288 , 289
Disrupted sleep is a common symptom of depressive episodes and increases the risk of MDD, 290 but the correlation between the onset of sleep disturbance and MDD is still unclear. Additionally, patients with symptoms of sleep disturbance have a greater risk of MDD occurrence and recurrence. 290 , 291 One study suggests that disrupted sleep may affect monoamine function and the HPA axis, 292 even causing hyperarousal and inflammation. 293 Additional studies on the pathological mechanism of depression have suggested that the HPA axis is hyperactive in MDD patients and that sensitivity to negative feedback is decreased. 15 Additionally, one prospective cohort study reported that a history of sleep disorders can increase the risk of depression later in life and that subjective sleep problems are associated with clinically significant depressive symptoms. 294
Dietary adjustment
Dietary adjustment is an effective, safe, and widely applicable method for preventing MDD, especially by inhibiting MDD-related pathological inflammation. 295 Various nutrients can possess different anti-inflammatory properties; in contrast, there are many proinflammatory foods, such as those high in refined starch, sugar, and saturated fat and low in fiber and omega-3 fatty acids, 296 which can promote the occurrence of inflammation to increase the risk of MDD. 297 One study reported that the chance of being diagnosed with depression is higher among individuals who consume a proinflammtory diet than among those who consume an anti-inflammatory diet. 295 Stimulation of the innate immune system by proinflammatory foods can result in mild inflammation and chronic illness, which may contribute to an increased risk of MDD. 298 Furthermore, an increasing number of studies suggest that at the molecular and cellular levels, dietary factors have effects on neuronal function and synaptic plasticity, which may be implicated in the etiology of MDD. 299 , 300 Therefore, adherence to a healthier diet can reduce the incidence of MDD, which is of great significance for the clinical treatment and prevention of depression. 295
In addition, an increasing number of studies have identified the importance of the interaction among the microbiota, gut permeability, and immune-inflammatory processes in the pathophysiology of MDD. 301 Because the interaction of bacteria of some taxa in the gut with peripheral inflammation with the brain may be related to depression pathophysiology, 302 , 303 regulating the gut-microbe-brain axis may be a therapeutic and preventive strategy for psychiatric disorders. 304 Restoration of the gut eubiosis can prevent the occurrence of MDD, and probiotics can normalize the gut ecosystem. Additionally, by altering the microbiota and regulating gut permeability, a gluten-free diet can alter the activity of the gut-microbe-brain axis, which has been discovered to be related to the pathogenesis of MDD. 305 , 306 , 307 Other studies report that consuming a gluten-free diet and probiotic supplements together may inhibit the immune-inflammatory cascade in MDD patients, and decreased inflammation can improve the integrity of the gut barrier and alleviate depressive symptoms. 307 Similarly, dietary fiber can also improve immune function by regulating the gut microbiota to prevent the occurrence of MDD, 308 which is attributed to the inhibition of OS and inflammation.
Increasing evidence suggests that physical exercise can prevent some mental disorders in addition to cardiovascular disease. 280 , 309 This finding suggests that physical exercise may be able to prevent MDD. As reported in some studies, physical exercise can effectively prevent depression by affecting many molecular and cellular pathways; for instance, physical exercise can stimulate VEGF expression, 310 , 311 leading to cellular level changes, such as stimulation of angiogenesis, increased delivery of neurotrophic factors and oxygen by the vascular system, 312 an increase in the neurogenesis rate and induction of synaptogenesis. 312 , 313 Ultimately, VEGF improves function in the hippocampus, which is one of the brain regions related to depression and stress regulation. 314 , 315 , 316 Exercise also reduces the levels of proinflammatory factors (e.g., IL-6) and increases the levels of anti-inflammatory factors (e.g., IL-10), which is beneficial for preventing the occurrence of MDD. 317 , 318 , 319 Furthermore, physical exercise for approximately 45 minutes per day can significantly reduce the risk of MDD. 320 , 321 High-intensity activity, such as aerobic exercise, dancing, and the usage of exercise machines, and low-intensity exercises, including yoga and stretching, can all reduce the occurrence of MDD. 322 Specifically, the combination of aerobic exercise and stretching as a multimodal therapeutic strategies has a significant antidepressant effect in depressed inpatients. 323
Patients with MDD have significantly more sedentary than ordinary people, and they engage in less physical activity than what is recommended, i.e., an average of 150 min of moderate- to high-intensity physical activity weekly. 324 This finding suggests that decreasing sedentary behavior or increasing physical activity levels should be a priority to prevent the occurrence of disease. In psychiatric centers, aerobic exercise has received increasing attention as a valuable method of prevention. 324 Studies report that reduced depressive symptoms in MDD patients can be observed after increasing aerobic exercise and stretching exercise, with more significant alleviation of depressive symptoms after 8 weeks of aerobic exercise. 325 Reward positivity (RewP) and error-related negativity (ERN) were identified as potential biomarkers of the exercise treatment response in depression. 325 In individuals with MDD, aerobic exercise was found to be beneficial in ameliorating depressive symptoms, particularly in those with more severe depressive symptoms and a higher baseline RewP. 325 , 326 RewP may be useful for identifying those who will benefit from exercise as a treatment for depression. 325
Social intervention
Social support refers to the help provided by social relations and transactions. 327 Social support may be obtained from a variety of individuals, including family members, friends, coworkers, and community members. 328 Furthermore, a variety of factors, including the quantity and quality of support as well as subjectively perceived social support by individuals, impact the level of social support. 329 It has been reported that MDD patients often lack social support, and receiving adequate social support can confer greater resistance to stress and prevent the occurrence and recurrence of MDD. 330 , 331 Low-functioning social support or self-perceived poor social support causes worse symptoms and treatment outcomes in depressed patients. 332 , 333 , 334 A previous study also reported that patients who lack adequate social support are more likely to experience MDD. 335 Social support may have an influence on depression through neuroendocrine pathways, 336 , 337 and social support can improve a person’s psychological wellbeing and make the individual more resistant to stress. 337
Studies on structural social support, social network size, and mental health disorders have shown that less social contact and loneliness can cause more severe depressive symptoms. 338 For individuals with MDD, it is necessary not only to increase the frequency of social contact but also to improve self-awareness and foster close functional supporttive relationships. 335 , 339 Studies have reported that when controlling for all other variables, each aspect of social support is clearly associated with MDD, and to some extent, the occurrence of panic disorder in patients with MDD is more strongly associated with poor functional support. This finding suggests that functional support may be an important protective factor against MDD. 331 , 335 Social support itself, especially emotional support, 340 may alleviate and prevent depressive symptoms, and support from family members or friends can replace formal health care. 341
In general, the pathological development of MDD is a gradual transition from subclinical state to clinical pathological changes. It is crucial to identify the core targets that lead to pathological changes from quantitative to qualitative changes during this process, and the above preventive interventions, sleep improvement, physical exercise, dietary regulation, and social intervention, may prolong or reverse the subclinical pathological stage (Fig. 5 ).
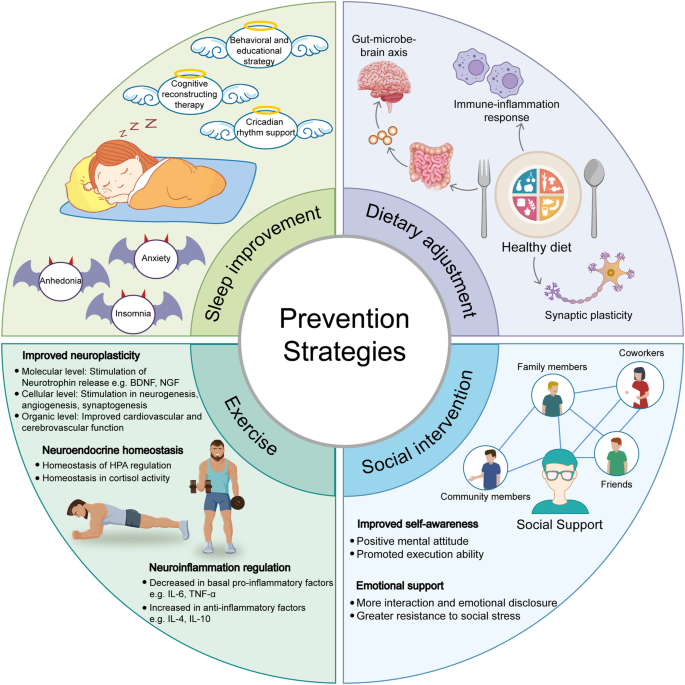
Schematic of prevention strategies for the occurrence and reoccurrence of MDD. An outline of various prevention strategies for MDD includes sleep improvement, dietary adjustment, exercise, and social intervention. Sleep disturbances have the high relationship with the occurrence of MDD, the anhedonia, anxiety and insomnia are the main symptoms of patients with MDD. The behavioral and educational strategies, cognitive reconstructing therapy and circadian rhythm support can be applied to improve sleep quality. 281 , 289 Dietary adjustments are also suggested to have the potential effects to prevent the occurrence or re-occurrence of MDD, the improvement mechanism of diet may involve in the regulated immune-inflammatory responses, the improved gut-microbe-brain axis and synaptic plasticity. 295 , 299 , 304 In addition, xxercise is an effective way to improve neuroplasticity, to maintain neuroendocrine homeostasis, and to regulate neuroinflammation, in order to effectively prevent the occurrence or re-occurrence of MDD. 280 , 309 Importantly, getting social support from family members, friends, coworkers and community members can be helpful for the MDD patients’ recovery, these social interventions can let patients get emotional support and improve their self-awareness. 328 , 340 Adobe Illustrator was used to generate this figure
Therapeutic drugs and strategies
This section summarizes new advances in research on the pharmacological mechanisms of common antidepressants and novel therapeutic strategies. Moreover, as laboratory animal models of MDD and other mental diseases are lacking, hindering the development of strategies for evaluating pharmacological effects and studying pathological mechanisms, we also discuss recent research on animal models.
The molecular mechanism of antidepressants
Tricyclic antidepressants.
In the late 1950s, the first TCAs were approved and used for the treatment of depression. 342 TCAs have a common three-ring chemical structure, and the main TCAs are imipramine, amitriptyline, clomipramine, desipramine and doxepin. The pharmacological mechanism of TCAs mainly involves its interaction with neurotransmitters in the brain, resulting in changes in neurotransmitter levels and an antidepressant effect. First, TCAs can inhibit the reuptake of neurotransmitters, leading to antidepressant effects. For example, they can influence the levels of 5-HT, NE, and to a lesser degree, DA, causing an increase in neurotransmitter concentrations in the synaptic gap and increasing neurotransmitter signaling to exert pharmacological effects. 343 However, different TCAs inhibit 5-HT and NE reuptake to varying degrees. For instance, amitriptyline, imipramine, and desipramine strongly inhibit 5-HT reuptake, 344 clomipramine specifically inhibits NE reuptake, and nortriptyline can inhibit both NE and 5-HT reuptake while also exerting central anticholinergic effects. 345 , 346 , 347 Additionally, TCAs can antagonize 5-HT 2A and 5-HT 2C , thereby increasing the release of NE and DA in cortical areas. 348 , 349 , 350 TCAs can bind to histamine receptors, especially H1 receptors, as well. 351 By blocking H1 receptors, they can induce sedation and drowsiness, which may benefit depressed patients with sleep disorders. 352 Furthermore, TCAs can also block muscarinic acetylcholine receptors, exerting anticholinergic effects and resulting in side effects such as dry mouth and constipation. 353
In addition to the above-known pharmacological mechanisms, some recent studies have reported that amitriptyline can induce the activation of fibroblast growth factor receptor (FGFR), leading to the production of GDNF. 354 In addition, amitriptyline can increase the expression of Cx43 to promote gap junction intercellular communication (GJIC) between astrocytes, thereby relieving depressive symptoms. 355 This suggests that TCAs may also ameliorate severe depression through additional mechanisms involving astrocytes that are independent of the monoamine system to some extent. Further exploration is needed to fully understand the specific mechanism. Another study demonstrated that FKBP51, a crucial modulator of the glucocorticoid receptor (GR) pathway, can bind to clomipramine and impede its interaction with PIAS4. Inhibition of this interaction subsequently hinders sumoylation; this alteration represents a newly discovered mechanism by which the antidepressant drug exerts its effect. 356
Selective serotonin reuptake inhibitor
According to a study, most severe depression patients are still advised to consider SSRIs as the initial choice for treatment. 350 The main representative SSRIs drugs include fluoxetine, sertraline, paroxetine, and escitalopram. The mechanisms of action of SSRIs are commonly known as follows: first, SSRIs can selectively inhibit SERT, inhibiting the reuptake of 5-HT in the synaptic cleft and thereby exerting pharmacological effects. 357 Second, SSRIs can impact the 5-HT signaling pathway, activating 5-HT 1A. 358 , 359 In addition, studies have shown that antagonism of 5-HT 2A/2C receptors can enhance the effects of SSRIs such as fluoxetine. 360 , 361 Third, long-term use of SSRIs can increase 5-HT transmission in the LC, 362 thereby increasing the release of GABA to exert inhibitory effects on NA neurons. 363 Fourth, long-term use of SSRIs is associated with neuroplasticity and neurogenesis in certain brain regions. 364 SSRIs have been found to increase the expression of BDNF, a protein crucial for neuronal growth and survival, by acting on TrkB, 365 which may contribute to the long-term therapeutic effects of SSRIs. Thus, our previous reports and others researches all suggested that astrocytic 5-HT 2B receptors may be the potential pharmacological target of SSIRs. 59 , 60 , 366 , 367 , 368
According to previous studies by our group, in the absence of SERT, SSRIs such as fluoxetine can act as direct agonists of astrocytic 5-HT 2B receptors to exert antidepressant-like effects. 60 , 64 , 179 , 366 , 369 In astroglia isolated from mice exposed to CUMS, fluoxetine activates the 5-HT 2B receptor, promoting ERK 1/2 phosphorylation. This increases downstream c-Fos expression, which in turn boosts BDNF synthesis. 147 Furthermore, administration of fluoxetine effectively inhibits SD-induced stimulation of the NLRP3 inflammasome by the AKT/STAT3 and ERK/STAT3 pathways in vivo, and SD dramatically triggers depressive-like behaviors by stimulating astrocytic P2X 7 Rs. 41 , 155 As previously mentioned, leptin may increase the expression of the 5-HT 2B receptor in astrocytes via the LepR/JAK2/STAT3 pathway, and fluoxetine may be more effective in increasing BDNF levels and alleviating depressive-like behaviors due to the leptin-mediated increase in 5-HT 2B receptor expression. 130 Both in vivo and in vitro, fluoxetine’s inhibitory actions on A1 reactive astrocytes depend on astrocytic 5-HT 2B R. 55 Recently, fluoxetine was shown to act as a 5-HT 2B agonist, and this finding is also supported by research by other groups. Fluoxetine has been reported to suppress the activation of A1 reactive astrocytes and decrease unusual behaviors in CMS-exposed mice. In vitro, Gq protein and b-arrestin1 are not necessary for fluoxetine’s effects on A1 astrocyte activation, and downstream signaling through astrocytic 5-HT 2B R is responsible for fluoxetine’s inhibitory effects on A1 astrocyte activation in primary culture. 55
Serotonin/norepinephrine reuptake inhibitors
SNRIs are often recommended as the initial choice for the treatment of MDD. Representative SNRIs include milnacipran, DXT, DVS, and venlafaxine. The molecular mechanisms of SNRIs can be summarized as follows: First, SNRIs inhibit the norepinephrine transporter (NET), which prevents the reuptake of NE into presynaptic neurons, leading to an increased concentration of NE in the synaptic cleft. 370 Second, similar to SSRIs, SNRIs also inhibit SERT, resulting in an increased concentration of 5-HT in the synaptic cleft. 371 For example, paroxetine and venlafaxine can inhibit SERT and, to a lesser extent, NET. 372 Third, SNRIs inhibit the reuptake of both NE and 5-HT; thus, they have a dual mechanism of action. This dual inhibitory effect is believed to contribute to the broader therapeutic effects of SNRIs compared to SSRIs. 373 Chronic treatment with fluoxetine has been shown to increase the expression of Cx43 in the rat PFC, which further prevents the dysfunction of astrocytic gap junctions induced by CUS and reverses the depressive-like behaviors caused by gap junction blockade. 71
In a randomized controlled trial, MRI scan were taken after treatment with duloxetine and desvenlafaxine, and the results showed that the thalamo-cortico-periaqueductal network, which is associated with the experience of pain, may be an important target of action of antidepressant drugs. 374
New potential pharmacological targets
The abovementioned antidepressants have been utilized as clinical therapies for MDD, but it is difficult to elucidate the exact pharmacological mechanisms of every medicine due to delayed clinical efficacy, poor treatment response to some patients, and difficulty in effectively controlling the incidence of suicide. Recently, several pharmacological agents have been discovered as potential antidepressants.
Ketamine, a noncompetitive antagonist of the NMDAR, has been shown to induce rapid and significant antidepressant effects within a few hours. 375 Due to the rapid antidepressant effects of ketamine, unlike the delayed effects of traditional antidepressant drugs, 376 research on this drug has continued and has revealed its mechanisms of action and potential drug targets. Ketamine can increase the level of BDNF in the prefrontal cortex, especially in the hippocampus, to exert antidepressant-like effects. 377 Studies have suggested that ketamine can increase the synthesis of synaptic proteins through BDNF signaling dependent on the activate protein kinase B (Akt) and mammalian target of rapamycin complex 1 (mTORC1) signaling cascades. 378 , 379 Ketamine may induce the activation of mTOR by the upstream kinase Akt, regulate the phosphorylation of GSK-3β, and exert antidepressant effects. 380 Ketamine can block NMDARs in postsynaptic principal neurons in the PFC and hippocampus, increase synaptic function through homeostatic mechanisms, and reverse synaptic defects caused by chronic stress. 381 , 382 Furthermore, by inhibiting NMDARs, ketamine can reduce the excitation of specific cortical GABAergic interneurons, resulting in a temporary increase in glutamate release that stimulates postsynaptic AMPA glutamate receptors. This, in turn, leads to the release of BDNF, activation of the TrkB receptor, and subsequent activation of the Akt/mTORC1 signaling pathway. These molecular events ultimately contribute to an increase in the number and functionality of synapses, leading to amelioration of depressive symptoms. 383
Similar as ketamine, some other psychedelics can also produce fast and persistent antidepressant effects. 384 Psilocybin, a classical psychedelic, can play its antidepressant roles by activating 5-HT 2A receptors (5-HT 2A R). 385 Thus, to block the 5-HT 2A R can not produce the antidepressant effects of psilocybin, only induce the hallucinogenic-like behaviors in mice. 386 This proposes 5-HT 2A R may not be the real pharmacological target for its antidepressant effects. Another study reports that the combination of lysergic acid diethylamide (LSD) and psilocybin may exert long-term antidepressant effects by promoting neural plasticity, which dose not involve in the hallucinogenic effects. 384 Additionally, to target 5-HT 2A R, the combination of LSD and psilocybin can lead to biased activation of the mediated signaling pathway and produce antidepressant effects without the side effects of hallucinations. 387 Thus, the administration of psilocybin can rapidly and persistently induce neuronal dendritic remodeling in the medial frontal cortex of mice, and the psilocybin-induced newly formed dendritic spines can successfully transform functional synapses, suggesting that synaptic rewiring may also be one pharmacological mechanism of the rapid antidepressant effects of psilocybin. 388 To further dissociate the hallucinogens effects from the psychedelics can be beneficial to develop more specific antidepressants with better therapeutic capacities.
Additionally, some novel potential therapeutic targets for MDD have also been reported, such as TGF-β1 389 and growth-associated protein 43 (GAP-43). 390 Multiple studies have shown that antidepressants may cause changes in TGF-β1 expression. Fluoxetine, paroxetine, venlafaxine, and sertraline have been shown to have the potential to increase the levels of TGF-β1, which may contribute to their antidepressant effects. 391 , 392 Venlafaxine has also been reported to exert neuroprotection by increasing the production of FGF-2 and TGF-β1 in astrocytes following stroke. 72 Then, chronic administration of desipramine has been shown to upregulate the expression of GAP-43 in the hippocampus of rats, potentially influencing neuronal plasticity in the CNS. 390 GAP-43 has been suggested as a relevant target for the pharmacological effects of antidepressants. 393 , 394
The most of above antidepressants have been widely used for the MDD patients according to the respective potential pharmacological actions (Fig. 6 ). Thus, the exactly neuromolecular mechanisms require deep studied and the new potential therapeutic targets and strategies still need further exploration.
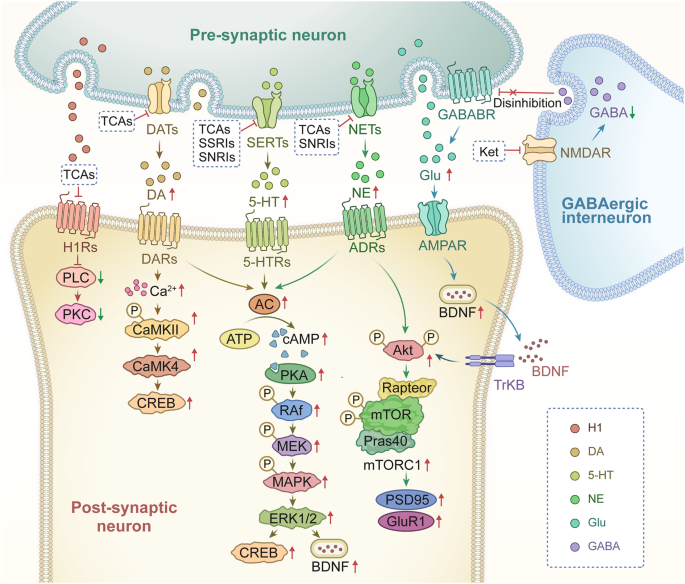
The molecular mechanisms of tricyclic antidepressants (TCAs), selective serotonin reuptake inhibitors (SSRIs), serotonin/norepinephrine reuptake inhibitors (SNRIs) and ketamine. TCAs can inhibit the protein kinase C (PKC) pathway by blocking the H1 receptors (H1Rs), 351 , 352 TCAs decreases the reuptake of dopamine (DA) by inhibiting dopamine transporters (DATs) in the presynaptic membrane, and increases the DA concentration in the synaptic gap, increase the effect of DA on dopamine receptors (DARs) of postsynaptic membrane. 343 The activated DARs increase Ca 2+ dependent CaMKII and CaMK4, as well as, the secretion of CREB. 498 , 499 In another way, the stimulated DARs by DA can also activate the cAMP-PKA pathway, which in turn activates the levels of CREB and BDNF by stimulating MAPK/ERK 1/2 pathway. 500 TCAs, SSRIs, and SNRIs can all inhibit the reuptake of 5-HT by SERTs, specially SSRIs have the selective inhibition on SERTs, which increase the concentration of 5-HT in the synaptic gap and play antidepressive roles by effecting on 5-HTRs in postsynaptic membrane, 343 , 344 which also activate the cAMP-PKA pathway. 49 , 501 Moreover, TCAs and SNRIs can also inhibit the reuptake of NE by NETs, which also increase the concentration of NE in the synaptic gap, and in turn activate the effect of NE on adrenoceptors (ADRs) and activate the cAMP-PKA pathway in postsynaptic membrane. 502 Besides of the AC/cAMP/PKA pathway, the effect of NE on ADRs can also activate protein kinase B (Akt) phosphorylation and mammalian target of rapamycin complex 1 (mTORC1) by stimulating TrkB, in order to promote the secretion of postsynaptic density 95 (PSD95) and glutamate receptor 1 (GluR1). 502 Ketamine works as the antagonist of NMDAR on GABAergic interneurons, it suppresses the excitation of subsets of GABAergic interneurons, which reduces the gamma aminobutyric acid (GABA) effects on gamma aminobutyric acid type B receptor (GABABR), and relieves the inhibition of GABAergic interneurons on the release of glutamate, the latter further stimulates AMPAR on postsynaptic membrane and increases the level of BDNF, even the release of BDNF stimulates the above TrkB/AKT/mTORC1 pathway. 503 , 504 Adobe Illustrator was used to generate this figure
Novel therapeutic strategies
New animal models.
Establishing animal models with pathological features representative of those seen in humans is key for advancing MDD research. Currently, the widely utilized animal models of MDD include CUMS, behavioral despair (BD), learned helplessness (LH), and CSDS, drug withdrawal, and transgenic animal models. 395 The CUMS model, one of the most commonly used animal models for MDD, 64 , 172 exhibits depressive-like behaviors. 396 , 397 According to a meta-analysis of 408 papers involving stress protocols, the most commonly used stressors for CUMS models are food and water deprivation, light cycle modification, wet bedding, cage tilting, social stress, and forced swimming. 398 Recently, we constructed an improved depression model named the chronic unpredictable mild restraint (CUMR) model by using environmental interference. 62 The stressors used to construct this CUMR mouse model included activity restriction, damp bedding, cage shaking, tail suspension, forced swimming, and 45° cage tilting. These stressors all restrict the activity of the mice; moreover, stressors that disturb physiological rhythms, chronic unpredictable rhythm disturbance (CURD), can cause manic-like behaviors in mice (Fig. 7 ). The disease-related pathological changes and serum indicators in the CUMR and CURD models are highly similar to those in patients in the clinic, and therapeutic medicines can effectively improve brain function and behavior in these models. 62
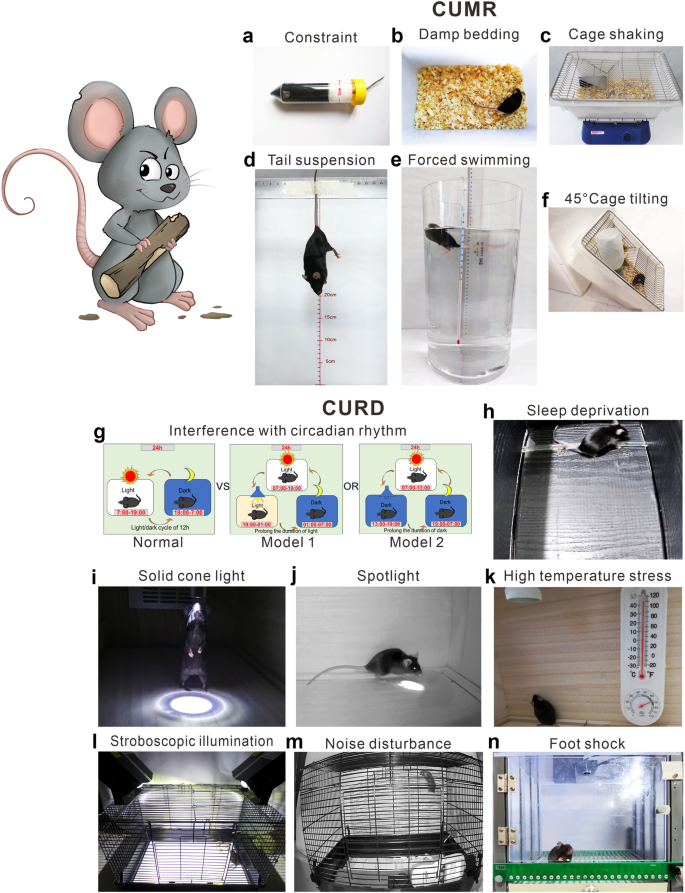
The protocol and stressors used for CURD and CUMR. In order to establish the CUMR model, a combination of various stressors includes interference of constraint ( a ), damp bedding ( b ), cage shaking ( c ), tail suspension ( d ), forced swimming ( e ), and cage tilting ( f ). Among these six stressors, two were randomly selected and administered daily for a duration of 3 weeks. On the other hand, to establish the CUMR model, a set of behavioral constraints includes circadian rhythm ( g ), sleep deprivation ( h ), interference of cone light ( i ), interference of followed spotlight ( j ), high temperature stress ( k ), stroboscopic illumination ( l ), noise disturbance ( m ), and foot shock ( n ). Similarly, two out of these eight constraints were randomly chosen and applied daily for a period of 3 weeks 62
Phototherapy
Phototherapy plays a significant role in regulating emotional behavior 399 and can have strong and rapid effects on mood and alertness. 400 , 401 , 402 There is increasing evidence for the therapeutic efficacy of phototherapy for MDD. 403 , 404 The combination of phototherapy and antidepressants has better effects than antidepressants alone. 402 , 405 Phototherapy utilizes bright light with a specific wavelength to stimulate the retina and affect the production of 5-HT and hormones in the brain. 406 Furthermore, phototherapy can alleviate depressive-like behavior by targeting the retinal-thalamic ventral lateral geniculate nucleus/intergeniculate leaflet-lateral habenula (retinal-vlGN/IGL-LHb) circuit; this mechanism may explain how phototherapy alleviates MDD. 407
Repetitive transcranial magnetic stimulation
Repetitive transcranial magnetic stimulation (rTMS) is an effective method used in clinical practice for treating patients with MDD. 408 Multiple evaluations and analyses have shown that rTMS can effectively treat MDD in patients from different age groups, including children and adolescents, 409 , 410 adults, 411 , 412 and elderly patients. 413 , 414 It is suggested that early use of rTMS in the treatment of depression in elderly patients may yield better results. 415 Furthermore, research has indicated that rTMS can effectively treat perinatal depression. 416 Increasing evidence suggests that rTMS of the anterior stimulation site of the left dlPFC can yield optimal treatment outcomes. 417 , 418 , 419 A randomized controlled trial demonstrated that the efficacy of rTMS in treating depression is linked to precise targeting of the dlPFC, the activity of which exhibits a negative correlation with subgenual cingulate cortex activity. 420 Identifying the optimal site for stimulation may further enhance the ability of rTMS to treat depression. 421 Recently, a retrospective study was conducted, which included 29 systematic evaluations and reanalyzed 15 meta-analyses to assess the effectiveness and safety of transcranial magnetic stimulation (TMS) for treating MDD in adults. 422 The results of the study indicated significant variations in the efficacy of TMS for MDD across different settings and revealed poor tolerability in certain populations, the further research is necessary to identify specific beneficiary populations for TMS in treating MDD and to personalize treatment based on comprehensive and detailed information. 422
Psychological intervention
MDD is characterized by a gradual onset and a high risk of relapse. 421 The American Medical Association recommends psychological interventions for individuals who are at a high risk of MDD. Some of the interventions commonly used for depression treatment include acceptance and commitment therapy, cognitive therapy, cognitive behavioral therapy (CBT), interpersonal therapy, and psychodynamic therapies. 423 Specifically, the combination of psychological interventions and antidepressants effectively decreases the risk of relapse in cases of MDD. 424 , 425 , 426
Acupuncture
Acupuncture, which mainly includes traditional body needling, moxibustion, EA, and laser acupuncture, is a traditional Chinese treatment modality used to treat various diseases. 427 Compared with pharmacological therapies, acupuncture is more cost-effective and has fewer side effects. 428 EA stimulation can effectively treat MDD; 429 , 430 , 431 however, the specific mechanism by which acupuncture treats depression remains unclear. In previous research, EA at the ST36 acupoint was shown to prevent shrinkage of the prefrontal cortical astrocytes and alleviate depressive-like behavior in mice exposed to CUMS. 432 The results of an 8-week clinical study involving 46 female patients with severe depression suggested that acupuncture may achieve therapeutic effects by modulating the corticostriatal reward/motivation circuit in patients with severe depression. 433 Additionally, studies indicate that EA may have the potential to promote neuronal regeneration and exert antidepressant effects by elevating the phosphorylation of cyclic adenosine monophosphate response element binding protein and the levels of BDNF. 434 Acupuncture at the GV20 and GV24 acupoints may alleviate depression symptoms by regulating the calmodulin-dependent protein kinase (CaMK) signaling pathway. 435 The antidepressant effect of EA may also be associated with increased synaptic transmission in the ventromedial prefrontal cortex (vmPFC). 436 A recent meta-analysis of 43 randomized controlled trials involving adult subjects with acupuncture for MDD demonstrated that acupuncture, either alone or in combination with antidepressants, significantly reduced the hamilton depression scal scores and had fewer adverse effects compared to antidepressants, however, further rigorous experiments are still required to determine the optimal frequency of acupuncture for MDD in order to achieve better efficacy. 437
In conclusion, the common antidepressants can improve some depressive symptoms in some patients with depression, but are always associated with the risk of adverse effects or recurrence. Although some new developed treatment methods can improve depression symptoms in a certain program, the compatibility between potential treatment mechanisms and pathological mechanisms still needs further research. In particular, the therapeutic principle of acupuncture still needs to be explored in depth, and the accompanied therapeutic mechanism and application potential of traditional Chinese medicine in depression deserve to be explored in depth.
Clinical research progress
In summary, the pathological features of MDD and pharmacological mechanism of antidepressants have been widely studied. Furthermore, there have been many clinical studies on MDD, and studies of human postmortem tissues and clinical medical images, multomics studies, and preclinical/clinical trials of new therapeutic drugs have improved our understanding of the disease mechanism.
Transcriptional studies of human postmortem tissue
A recent meta-analysis of eight transcriptome datasets identified 566 disease-related genes that are consistently up- or downregulated in patients with MDD. The brain regions in which these genes are expressed include the amygdala, subgenual anterior cingulate, and dorsolateral prefrontal cortex, and the associated molecular pathways include reduced neurotrophic support, neural signaling, and GABA function. 438 Through the discovery of nonoverlapping proteins that bind to calcium parvalbumin, calretinin, and the neural peptide somatostatin, subgroups of GABA interneurons that govern main pyramidal neurons differently were identified. 439 Decreased cortical levels of GABA and specific populations of GABA neurons have been reported in investigations of postmortem MDD patient tissues, 440 and the SST mRNA level is specifically decreased in patients with MDD. 213
The DR nucleus is the largest and most significant conduit of forebrain serotonergic input. 441 In postmortem samples of the human brain, several transcriptional regulators are dysregulated within the DR, including transcription-related elements (such as EGR1, TOB1, and CSDA), which bind to genes to stimulate their expression directly or in response to environmental cues, and NRs (NR4A2, NR4A3, THRA, and THRB), which are activated by ligands and regulate translation by targeting genes. 442 In addition, transporters for GRs generally regulate the activity of the HPA axis by negative feedback. 443 According to studies of postmortem brain tissues, hyperactivity of the HPA axis in MDD patients could be caused by methylation-mediated changes in GR transcription. 444 The expression of nerve growth factor-inducible protein A (NGFI-A), an enzyme that bindss exon 1 F of GR, is reduced in the hippocampus of patients with MDD, which may contribute to low methylation levels in the brain. 444 Moreover, in postmortem MDD patients, total GR levels are unchanged, while level of GRα in the amygdala and cingulate gyrus is decreased.
Sex-related molecular markers of MDD
Women are more likely than males to experience recurring MDD 445 and are twice as likely to experience MDD throughout their lifetimes. 446 Compared with male patients, female patients with MDD have symptoms that manifest sooner in the disease course, last longer, and are more severe; in addition, they experience hunger changes, weight fluctuations, and sleep difficulties more frequently. 447 , 448
In postmortem samples of patients who committed suicide due to MDD, the expression of DNA methyltransferases (DNMTs) in the frontopolar cortex was found to be more significantly increased in women than in men; elevated methylation is associated with decreased levels of the GABA A receptor alpha-1 subunit in men, which supports sex-related epigenetic alterations in transcription. 449 A gene array meta-analysis also revealed sex differences in MDD, with depressed females being more likely than depressed men to have lower production of somatostatin, a GABA neuron biomarker in corticolimbic brain regions according to postmortem analysis. 450 X-linked chromosomal polymorphisms affect the expression of the GABA-synthesizing enzyme and somatostatin. 450 Analyses of postmortem brain tissues showed an increase in the transcription of numerous glutamate-related genes in the prefrontal cortex in depressed women but not in depressed men; depressed women exhibited more alterations in glutamate receptor expression, while depressed men showed only GRM5 downregulation. 451
In postmortem brain specimens, there were no transcription differences between MDD men and controls, and the levels of 5-HT 1D receptors and the transcription factors NUDR and REST, which regulate 5-HT activity, in 5-HT-containing neurons in the ventral raphe nuclei were found to be higher in MDD females. 452 5-HT receptors and regulators were shown to exhibit sex-specific alterations in expression at the protein level, and postmortem investigations have largely focused on female subjects. The protein levels of 5-HT 1A R and NUDR, which regulate 5-HT signaling, in the prefrontal cortex were found to be lower in MDD women than in control subjects; however, this difference was not observed in MDD males compared with controls. 453 The NA/NE system, especially in the LC, is another monoaminergic system that exhibits sex-related variations and influences MDD risk. In fact, some researchers have found that the levels of microRNAs (miRNAs), short RNA molecules that control the expression of genes and play roles in psychological disorders, 454 are higher in the LC of suicidal female subjects than in the LC of suicidal male subjects. MiR-1179 is associated with GRIA3 and MAOA, which are involved in neuropsychiatric diseases. 455
OS is commonly linked to the onset of MDD. A study found that whereas cysteine and 1-methylinosine levels were much higher in males with MDD, they were significantly lower in females with MDD. 456 These metabolites are related to OS. Furthermore, several studies found a significant link between MDD and lipid metabolism; 457 for example, as 1-Oalkyl-2-acyl-PEs levels are decreased in MDD, showing a negative correlation with the extent of depression, lysophospholipid (LPC) and phospholipid (PC) levels are increased in MDD, exhibiting a substantial positive correlation with depression severity. 458 Similarly, a study found that men and women had different lipid concentrations. 456 These clinical data suggest that sex differences in MDD may result from differences in OS and lipid metabolism, but further research is required to make this connection.
Multiomics studies
Transcriptome studies, which explore relationships among the expression of genes and diseases, are regarded as an essential for investigating disease-causing mutations in genes, the mechanisms of disease development and progression, and disease-related target genes. 459 Dorsolateral prefrontal cortex tissues have been employed to identify genes and miRNAs that show changes in expression and biological processes that are altered in patients with MDD. 460 Serpin Family H Member 1 (SERPINH1), IL-8, humanin like-8 (MTRNRL8), and chemokine ligand 4 (CCL4) are among the genes whose expression is altered in MDD. 460 , 461 According to Gene Ontology (GO) enrichment analysis, MDD is related to decreased expression of genes related to oligodendrocyte development, glutamatergic neurotransmission modulation, and oxytocin receptor expression. These findings confirm that impairment of the blood-brain barrier and microglial, endothelial cell, ATPase, and astrocyte function exacerbate MDD; the involvement of these cells, molecules, and structures in MDD should be further investigated. 460
The field of study known as genomics focuses on the transcription of genes, the precise interactions among genes, and the control of gene activity. MDD has been linked to numerous biological processes, including energy metabolism. When the transcription of genes involved in glycolysis and glycogen synthesis was examined in the hippocampus of depressed rats, it was found that the mRNA expression of Slc2a3, which codes for GLUT3, is considerably increased. 462 Glyceraldehyde-3-phosphate dehydrogenase (GAPDH) and lactate dehydrogenase B (LDHB) mRNA levels were found to be substantially decreased in MDD. 462 The transcription of genes in the brain tissues of IL18 - / - mice was examined with the use of genome-wide microarrays, and the results revealed that urocortin 3 (Ucn3) expression was increased. 463 Ucn3 controls how the body processes glucose; 464 therefore, a change in Ucn3 expression will result in energy imbalance. Gene comethylation analysis was performed in the brains of individuals with MDD. The findings revealed that the methylation of genes associated with mitochondria was dramatically decreased, indicating impaired mitochondrial function. 465
Metabolomics has recently emerged as a useful technique for identifying markers and pathways associated with a wide range of diseases. 466 It is often used to analyze the mechanisms underlying disease occurrence and progression and the effects of small-molecule compounds. In one study, targeted metabolomic analysis of the CSF of 14 MDD patients who were not taking medication, 14 MDD patients in remission, and 18 healthy controls was performed. 467 An analysis of the tryptophan, tyrosine, purine pathways, and associated pathways revealed that in patients in remission, methionine levels were higher, while tryptophan and tyrosine levels were lower. The same group of patients also showed changes in the methionine-to-glutathione ratio, indicating alterations in OS and methylation. The levels of these same metabolites were altered in MDD patients who were not taking medication, although not to a significant degree. 467
Clinical medical imaging studies
MRI has been widely employed in research in recent years to pinpoint patterns of brain alterations linked to MDD. Many studies have demonstrated that structural and fMRI has outstanding potential as trustworthy imaging modalities for monitoring MDD treatment responses. A study indicated that MDD patients had large volume decreases in various frontal areas, such as the anterior cingulate cortex and OFC, which were linked to problems with stress management and emotional processing. 468 People with MDD also exhibited structural changes in their parietal lobes. 469 Alterations in the total gray matter volume and an increase in cortical thickness are the two findings that are most consistent. 470
The functional changes in the frontal lobe in MDD are hotly contested. A study discovered lower precuneus, supragenual anterior cingulate cortex, dorsomedial PFC, and dorsomedial thalamus lower activity when processing pleasant stimuli in MDD patients. 471 Another study found that during the processing of favorable self-indulgent information, individuals with MDD displayed higher activity in the medial PFC and anterior cingulate cortex. 472 The right hippocampus, parahippocampal gyrus, left amygdala, and the whole caudate nucleus all had functional changes in activity in MDD patients compared to healthy controls, indicating that the temporal lobe might be involved in the pathogenesis of MDD. 473
Although it is not feasible to evaluate synapse density directly in people in vivo, positron emission tomography (PET) can be utilized to gather useful information. It is thought that impairments of functional connections and synaptic atrophy are two factors that contribute to the symptoms of MDD. An indirect method of estimating synaptic density is to count the number of nerve terminals using synaptic vesicle glycoprotein 2 A (SV2A). The researchers examined synaptic density in MDD patients who were not taking any medication using positron emission PET with the SV2A radioligand [ 11 C] UCB-J. 474 The results revealed that reductions in the synapse density in areas connected with various processes, such as emotion control and thought (the dorsolateral prefrontal cortex, anterior cingulate cortex, and hippocampus), are related to to the severity of depressive disorders. Additionally, it was shown that compared with healthy subjects, subjects with MDD had reduced dlPFC resting-state connectivity throughout the brain. It was found that the dlPFC-posterior cingulate cortex connection was inversely negatively linked to the severity of depression symptoms and connected with synapse activity in the dlPFC, indicating that synaptic loss may impair antagonistism within the centers of both networks, which are typically at odds. 474
Preclinical and clinical trials of new therapeutic drugs
Esmethadone is a new, noncompetitive NMDAR antagonist 475 that exhibits fast antidepressant-like action by improving performance of rats in the forced swim test. 476 Esmethadone can also alleviate neural dysfunction linked to symptoms of depression by boosting the synapse and spine density and restoring spinogenesis, in addition to correcting depressive-like behaviors in animal models of depression. 378 , 477 Esmethadone was found to reduce cognitive symptoms in individuals with MDD in a stage II clinical study 478 and to increase the levels of circulating BDNF in normal individuals in a stage I clinical investigation. 479 In a phase II study involving patients who had received insufficient benefit from conventional antidepressants, esmethadone demonstrated immediate, strong, and long-lasting antidepressant benefits. 478
Ketamine is the most well-known rapid-acting antidepressant and an NMDAR antagonist. 383 GluN1, GluN2, and GluN3 are NMDAR subunits. 480 Ketamine exerts a quick and effective antidepressant effect by binding to the asparagine 616 residue of GluN1 and the leucine 642 residue of GluN2A. 192 In a clinical experiment, the effect of supplementary injection of subanesthetic doses of ketamine on thoughts of suicide in MDD patients was evaluated, and the results showed that the reduction in thoughts of suicide among MDD patients receiving ketamine was mostly sustained. 481 In several studies, a single dose of ketamine reduced immobility in the forced swim test immediately after injection and had effects similar to those of an antidepressant. 482 , 483
The S-enantiomer of ketamine, esketamine, has been approved by the U.S. Food and Drug Administration (FDA) for depression treatment. 383 Moreover, formulations of ketamine are also being developed, and intranasal esketamine spray has shown high efficacy in treating MDD. 484 Additionally, hydroxynorketamine (HNK), a metabolite of ketamine, can exert its anti-depressive effects by an NMDAR-independent mechanism. 377 One of these mechanisms involves increasing BDNF levels; an increasing number of studies have shown that BDNF signaling is an important target of antidepressants. 377 Thus, ketamine can also exert anti-inflammatory effects, a large amount of evidence suggests a tight relationship between neuroinflammation and the pathogenesis of MDD. 485 , 486 , 487 A summary of clinical trials related to new therapeutic drugs for MDD is shown in Table 1 .
The development of the present therapeutic medicines in clinic mainly targets the discovered pharmacological targets, mainly focusing on the key receptors or enzymes. However, at the organelle level of neural cells, the disturbed energy metabolism of mitochondria and the related RNA drugs, as well as the dysfunctions of lipid and glucose metabolism in psychopathological condition, still need deep exploration. Totally, the research on the mechanism of therapeutic drugs always requires the development of pathological mechanisms as support.
Conclusions and future perspectives
MDD is a heterogeneous disease, its pathological and pharmacological mechanisms are still unclear, and diagnostic and therapeutic methods for MDD are limited. SSRIs and SNRIs are the first-line treatments for MDD in the clinic; however, a sizable portion of MDD patients do not respond well to the currently available antidepressants. According to research on real-world sequential therapies, even after numerous treatment attempts, almost 30% of MDD patients do not experience remission. This suggests that the existing theories and hypotheses cannot completely explain the pathogenesis of MDD and that more research on the pharmacological mechanisms of currently available antidepressants is still needed. We mainly discussed the potential etiology and pathogenesis of MDD from the perspective of widely accepted theories, including the neurotransmitter and receptor hypothesis, HPA axis hypothesis, cytokine hypothesis, neuroplasticity hypothesis and systemic influence hypothesis. A more comprehensive understanding of the pathophysiological mechanisms of MDD might significantly improve our capacity to develop preventive and more effective therapeutic methods that can help reduce the burden of and pain caused by major depression. Knowledge of the cellular processes that drive these alterations and the symptoms they cause may offer crucial will provide insight for new treatments.
MDD is connected with several cellular and structural modifications in the nervous system. Nonetheless, in the majority of these alterations cannot be consistently observed in vivo. Therefore, several issues need to be considered in future research: (i) Studies of animal models have made important contributions to our understanding of the pathophysiology of major depression, and more representative animal models of MDD should be developed. (ii) Because of our incomplete understanding of the disease and the disease’s intrinsic intricacy, there is an urgent need to develop updated imaging technologies and imaging software to allow advances in our understanding of the disease. (iii) The therapeutic shortcomings of traditional antidepressants have prompted the need for further drug discovery and development. (iv) MDD is strongly associated with many systems, and it will be important to further elucidate the mechanisms associated with MDD and other pathological conditions.
Disease, G. B. D., Injury, I. & Prevalence, C. Global, regional, and national incidence, prevalence, and years lived with disability for 354 diseases and injuries for 195 countries and territories, 1990-2017: a systematic analysis for the Global Burden of Disease Study 2017. Lancet 392 , 1789–1858 (2018).
Article Google Scholar
Nagy, C. et al. Single-nucleus transcriptomics of the prefrontal cortex in major depressive disorder implicates oligodendrocyte precursor cells and excitatory neurons. Nat. Neurosci. 23 , 771–781 (2020).
Article CAS PubMed Google Scholar
Malhi, G. S. & Mann, J. J. Depression Lancet 392 , 2299–2312 (2018).
Article PubMed Google Scholar
Fellinger, M. et al. Seasonality in major depressive disorder: effect of sex and age. J. Affect Disord. 296 , 111–116 (2022).
Liu, C. H. et al. Role of inflammation in depression relapse. J. Neuroinflammation 16 , 90 (2019).
Article PubMed PubMed Central Google Scholar
Burcusa, S. L. & Iacono, W. G. Risk for recurrence in depression. Clin. Psychol. Rev. 27 , 959–985 (2007).
Monroe, S. M. & Harkness, K. L. Life stress, the “kindling” hypothesis, and the recurrence of depression: considerations from a life stress perspective. Psychol. Rev. 112 , 417–445 (2005).
Albert, K. M. & Newhouse, P. A. Estrogen, stress, and depression: cognitive and biological interactions. Annu. Rev. Clin. Psychol. 15 , 399–423 (2019).
Kessler, R. C., Chiu, W. T., Demler, O., Merikangas, K. R. & Walters, E. E. Prevalence, severity, and comorbidity of 12-month DSM-IV disorders in the National Comorbidity Survey Replication. Arch. Gen. Psychiatry 62 , 617–627 (2005).
Monteleone, P. & Maj, M. The circadian basis of mood disorders: recent developments and treatment implications. Eur. Neuropsychopharmacol. 18 , 701–711 (2008).
Rice, F. et al. Adolescent and adult differences in major depression symptom profiles. J. Affect Disord. 243 , 175–181 (2019).
Hasin, D. S. et al. Epidemiology of adult DSM-5 major depressive disorder and its specifiers in the United States. JAMA Psychiatry 75 , 336–346 (2018).
Howard, D. M. et al. Genome-wide association study of depression phenotypes in UK Biobank identifies variants in excitatory synaptic pathways. Nat. Commun. 9 , 1470 (2018).
Kovacs, M. & Lopez-Duran, N. Prodromal symptoms and atypical affectivity as predictors of major depression in juveniles: implications for prevention. J. Child Psychol. Psychiatry 51 , 472–496 (2010).
Stetler, C. & Miller, G. E. Depression and hypothalamic-pituitary-adrenal activation: a quantitative summary of four decades of research. Psychosom. Med. 73 , 114–126 (2011).
Milaneschi, Y., Lamers, F., Berk, M. & Penninx, B. Depression heterogeneity and its biological underpinnings: toward immunometabolic depression. Biol. Psychiatry 88 , 369–380 (2020).
Zhou, X. et al. Astrocyte, a promising target for mood disorder interventions. Front. Mol. Neurosci. 12 , 136 (2019).
Article CAS PubMed PubMed Central Google Scholar
Monroe, S. M. & Harkness, K. L. Major depression and its recurrences: life course matters. Annu. Rev. Clin. Psychol. 18 , 329–357 (2022).
Wray, N. R. et al. Genome-wide association analyses identify 44 risk variants and refine the genetic architecture of major depression. Nat. Genet. 50 , 668–681 (2018).
Gittins, R. A. & Harrison, P. J. A morphometric study of glia and neurons in the anterior cingulate cortex in mood disorder. J. Affect. Disord. 133 , 328–332 (2011).
Miguel-Hidalgo, J. J. et al. Glial and glutamatergic markers in depression, alcoholism, and their comorbidity. J. Affect. Disord. 127 , 230–240 (2010).
Sequeira, A. et al. Global brain gene expression analysis links glutamatergic and GABAergic alterations to suicide and major depression. PLoS ONE 4 , e6585 (2009).
Rajkowska, G. & Stockmeier, C. A. Astrocyte pathology in major depressive disorder: insights from human postmortem brain tissue. Curr. Drug Targets 14 , 1225–1236 (2013).
Tsuang, M. T., Taylor, L. & Faraone, S. V. An overview of the genetics of psychotic mood disorders. J. Psychiatr. Res 38 , 3–15 (2004).
Lohoff, F. W. Overview of the genetics of major depressive disorder. Curr. Psychiatry Rep. 12 , 539–546 (2010).
Kendall, K. M. et al. The genetic basis of major depression. Psychol. Med. 51 , 2217–2230 (2021).
Power, R. A. et al. Genome-wide association for major depression through age at onset stratification: Major Depressive Disorder Working Group of the psychiatric genomics consortium. Biol. Psychiatry 81 , 325–335 (2017).
Huang, C. et al. Proteomic analysis of olfactory bulb suggests CACNA1E as a promoter of CREB signaling in microbiota-induced depression. J. Proteom. 194 , 132–147 (2019).
Article CAS Google Scholar
Flint, J. The genetic basis of major depressive disorder. Mol. Psychiatry 28 , 2254–2265 (2023).
Tripp, A., Kota, R. S., Lewis, D. A. & Sibille, E. Reduced somatostatin in subgenual anterior cingulate cortex in major depression. Neurobiol. Dis. 42 , 116–124 (2011).
Seney, M. L., Tripp, A., McCune, S., Lewis, D. A. & Sibille, E. Laminar and cellular analyses of reduced somatostatin gene expression in the subgenual anterior cingulate cortex in major depression. Neurobiol. Dis. 73 , 213–219 (2015).
Hicks, E. M. et al. Integrating genetics and transcriptomics to study major depressive disorder: a conceptual framework, bioinformatic approaches, and recent findings. Transl. Psychiatry 13 , 129 (2023).
Labonte, B. et al. Sex-specific transcriptional signatures in human depression. Nat. Med. 23 , 1102–1111 (2017).
Levey, D. F. et al. Bi-ancestral depression GWAS in the Million Veteran Program and meta-analysis in >1.2 million individuals highlight new therapeutic directions. Nat. Neurosci. 24 , 954–963 (2021).
Cohen-Woods, S., Craig, I. W. & McGuffin, P. The current state of play on the molecular genetics of depression. Psychol. Med. 43 , 673–687 (2013).
Slavich, G. M. & Sacher, J. Stress, sex hormones, inflammation, and major depressive disorder: Extending Social Signal Transduction Theory of Depression to account for sex differences in mood disorders. Psychopharmacology 236 , 3063–3079 (2019).
McEwen, B. S., Nasca, C. & Gray, J. D. Stress effects on neuronal structure: hippocampus, amygdala, and prefrontal cortex. Neuropsychopharmacology 41 , 3–23 (2016).
Fries, G. R., Saldana, V. A., Finnstein, J. & Rein, T. Molecular pathways of major depressive disorder converge on the synapse. Mol. Psychiatry 28 , 284–297 (2023).
Keller, J. et al. HPA axis in major depression: cortisol, clinical symptomatology and genetic variation predict cognition. Mol. Psychiatry 22 , 527–536 (2017).
Dong, L., Li, B., Verkhratsky, A. & Peng, L. Cell type-specific in vivo expression of genes encoding signalling molecules in the brain in response to chronic mild stress and chronic treatment with fluoxetine. Psychopharmacology 232 , 2827–2835 (2015).
Xia, M. et al. Sleep deprivation selectively down-regulates astrocytic 5-HT(2B) receptors and triggers depressive-like behaviors via stimulating P2X(7) receptors in mice. Neurosci. Bull. 36 , 1259–1270 (2020).
Hare, D. L., Toukhsati, S. R., Johansson, P. & Jaarsma, T. Depression and cardiovascular disease: a clinical review. Eur. Heart J. 35 , 1365–1372 (2014).
Dunbar, J. A. et al. Depression: an important comorbidity with metabolic syndrome in a general population. Diabetes Care 31 , 2368–2373 (2008).
Arnaud, A. M. et al. Impact of major depressive disorder on comorbidities: a systematic literature review. J. Clin. Psychiatry 83 , 21r14328 (2022).
Sato, S. & Yeh, T. L. Challenges in treating patients with major depressive disorder: the impact of biological and social factors. CNS Drugs 27 , S5–10 (2013).
Greenberg, P. E., Fournier, A. A., Sisitsky, T., Pike, C. T. & Kessler, R. C. The economic burden of adults with major depressive disorder in the United States (2005 and 2010). J. Clin. Psychiatry 76 , 155–162 (2015).
Greenberg, P. E. et al. The economic burden of adults with major depressive disorder in the United States (2010 and 2018). Pharmacoeconomics 39 , 653–665 (2021).
Dean, J. & Keshavan, M. The neurobiology of depression: an integrated view. Asian J. Psychiatr. 27 , 101–111 (2017).
Bhatt, S., Devadoss, T., Manjula, S. N. & Rajangam, J. 5-HT(3) receptor antagonism a potential therapeutic approach for the treatment of depression and other disorders. Curr. Neuropharmacol. 19 , 1545–1559 (2021).
Inazu, M., Takeda, H. & Matsumiya, T. Functional expression of the norepinephrine transporter in cultured rat astrocytes. J. Neurochem. 84 , 136–144 (2003).
Schipke, C. G., Heuser, I. & Peters, O. Antidepressants act on glial cells: SSRIs and serotonin elicit astrocyte calcium signaling in the mouse prefrontal cortex. J. Psychiatr. Res. 45 , 242–248 (2011).
Novikova, I. N. et al. Adrenaline induces calcium signal in astrocytes and vasoconstriction via activation of monoamine oxidase. Free Radic. Biol. Med. 159 , 15–22 (2020).
Martin, H. et al. Insulin modulates emotional behavior through a serotonin-dependent mechanism. Mol. Psychiatry (2022). [Oline ahead of print].
Ogawa, S. et al. Plasma L-tryptophan concentration in major depressive disorder: new data and meta-analysis. J. Clin. Psychiatry 75 , e906–915 (2014).
Fang, Y. et al. Fluoxetine inhibited the activation of A1 reactive astrocyte in a mouse model of major depressive disorder through astrocytic 5-HT(2B)R/beta-arrestin2 pathway. J. Neuroinflammation 19 , 23 (2022).
Savitz, J., Lucki, I. & Drevets, W. C. 5-HT(1A) receptor function in major depressive disorder. Prog. Neurobiol. 88 , 17–31 (2009).
Verkhratsky, A., Rodriguez, J. J. & Parpura, V. Astroglia in neurological diseases. Future Neurol. 8 , 149–158 (2013).
Zhang, S. et al. 5-HT2B receptors are expressed on astrocytes from brain and in culture and are a chronic target for all five conventional ‘serotonin-specific reuptake inhibitors. Neuron Glia Biol. 6 , 113–125 (2010).
Verkhratsky, A., Parpura, V., Scuderi, C. & Li, B. Astroglial serotonin receptors as the central target of classic antidepressants. Adv. Neurobiol. 26 , 317–347 (2021).
Li, B. et al. Biphasic regulation of caveolin-1 gene expression by fluoxetine in astrocytes: opposite effects of PI3K/AKT and MAPK/ERK signaling pathways on c-fos. Front. Cell. Neurosci. 11 , 335 (2017).
Li, B., Zhang, S., Li, M., Hertz, L. & Peng, L. Chronic treatment of astrocytes with therapeutically relevant fluoxetine concentrations enhances cPLA2 expression secondary to 5-HT 2B -induced, transactivation-mediated ERK 1/2 phosphorylation. Psychopharmacology 207 , 1–12 (2009).
Li, X. et al. A novel murine model of mania. Mol. Psychiatry 28 , 3044–3054 (2023).
Li, B., Zhang, S., Zhang, H., Hertz, L. & Peng, L. Fluoxetine affects GluK2 editing, glutamate-evoked Ca(2+) influx and extracellular signal-regulated kinase phosphorylation in mouse astrocytes. J. Psychiatry Neurosci. 36 , 322–338 (2011).
Li, B. et al. Cell type-specific gene expression and editing responses to chronic fluoxetine treatment in the in vivo mouse brain and their relevance for stress-induced anhedonia. Neurochem. Res. 37 , 2480–2495 (2012).
Breton-Provencher, V., Drummond, G. T., Feng, J., Li, Y. & Sur, M. Spatiotemporal dynamics of noradrenaline during learned behaviour. Nature 606 , 732–738 (2022).
Mori, K. et al. Effects of norepinephrine on rat cultured microglial cells that express alpha1, alpha2, beta1 and beta2 adrenergic receptors. Neuropharmacology 43 , 1026–1034 (2002).
O’Sullivan, J. B., Ryan, K. M., Curtin, N. M., Harkin, A. & Connor, T. J. Noradrenaline reuptake inhibitors limit neuroinflammation in rat cortex following a systemic inflammatory challenge: implications for depression and neurodegeneration. Int. J. Neuropsychopharmacol. 12 , 687–699 (2009).
Choi, H. S. et al. The anti-inflammatory activity of duloxetine, a serotonin/norepinephrine reuptake inhibitor, prevents kainic acid-induced hippocampal neuronal death in mice. J. Neurol. Sci. 358 , 390–397 (2015).
Wang, J. et al. Desvenlafaxine prevents white matter injury and improves the decreased phosphorylation of the rate-limiting enzyme of cholesterol synthesis in a chronic mouse model of depression. J. Neurochem. 131 , 229–238 (2014).
Luo, Y., Kataoka, Y., Ostinelli, E. G., Cipriani, A. & Furukawa, T. A. National prescription patterns of antidepressants in the treatment of adults with major depression in the US Between 1996 and 2015: a population representative survey based analysis. Front. Psychiatry 11 , 35 (2020).
Sun, J. D., Liu, Y., Yuan, Y. H., Li, J. & Chen, N. H. Gap junction dysfunction in the prefrontal cortex induces depressive-like behaviors in rats. Neuropsychopharmacology 37 , 1305–1320 (2012).
Zepeda, R. et al. Venlafaxine treatment after endothelin-1-induced cortical stroke modulates growth factor expression and reduces tissue damage in rats. Neuropharmacology 107 , 131–145 (2016).
Bekhbat, M. et al. Correction to: functional connectivity in reward circuitry and symptoms of anhedonia as therapeutic targets in depression with high inflammation: evidence from a dopamine challenge study. Mol. Psychiatry 27 , 4122 (2022).
Cui, Y. et al. Astroglial Kir4.1 in the lateral habenula drives neuronal bursts in depression. Nature 554 , 323–327 (2018).
Skupio, U. et al. Astrocytes determine conditioned response to morphine via glucocorticoid receptor-dependent regulation of lactate release. Neuropsychopharmacology 45 , 404–415 (2020).
Rasheed, N. et al. Differential response of central dopaminergic system in acute and chronic unpredictable stress models in rats. Neurochem. Res. 35 , 22–32 (2010).
Morales, I., Fuentes, A., Ballaz, S., Obeso, J. A. & Rodriguez, M. Striatal interaction among dopamine, glutamate and ascorbate. Neuropharmacology 63 , 1308–1314 (2012).
Solis, O., Garcia-Sanz, P., Herranz, A. S., Asensio, M. J. & Moratalla, R. L-DOPA reverses the increased free amino acids tissue levels induced by dopamine depletion and rises GABA and tyrosine in the striatum. Neurotox. Res. 30 , 67–75 (2016).
Corkrum, M. et al. Dopamine-evoked synaptic regulation in the nucleus accumbens requires astrocyte activity. Neuron 105 , 1036–1047.e1035 (2020).
Adermark, L. et al. Astrocytes modulate extracellular neurotransmitter levels and excitatory neurotransmission in dorsolateral striatum via dopamine D2 receptor signaling. Neuropsychopharmacology 47 , 1493–1502 (2022).
Agren, R. & Sahlholm, K. Voltage-dependent dopamine potency at D(1)-like dopamine receptors. Front Pharmacol. 11 , 581151 (2020).
Fasano, C. et al. Dopamine facilitates dendritic spine formation by cultured striatal medium spiny neurons through both D1 and D2 dopamine receptors. Neuropharmacology 67 , 432–443 (2013).
Hao, Y. & Plested, A. J. R. Seeing glutamate at central synapses. J. Neurosci. Methods 375 , 109531 (2022).
Trudler, D. et al. alpha-synuclein oligomers induce glutamate release from astrocytes and excessive extrasynaptic NMDAR activity in neurons, thus contributing to synapse loss. J. Neurosci. 41 , 2264–2273 (2021).
Schmitz, F. et al. Methylphenidate decreases ATP levels and impairs glutamate uptake and Na(+),K(+)-ATPase activity in juvenile rat hippocampus. Mol. Neurobiol. 54 , 7796–7807 (2017).
Kucukibrahimoglu, E. et al. The change in plasma GABA, glutamine and glutamate levels in fluoxetine- or S-citalopram-treated female patients with major depression. Eur. J. Clin. Pharmacol. 65 , 571–577 (2009).
Chiba, S. et al. Chronic restraint stress causes anxiety- and depression-like behaviors, downregulates glucocorticoid receptor expression, and attenuates glutamate release induced by brain-derived neurotrophic factor in the prefrontal cortex. Prog. Neuropsychopharmacol. Biol. Psychiatry 39 , 112–119 (2012).
Sullivan, R. et al. Cloning, transport properties, and differential localization of two splice variants of GLT-1 in the rat CNS: implications for CNS glutamate homeostasis. Glia 45 , 155–169 (2004).
Zou, J. et al. Glutamine synthetase down-regulation reduces astrocyte protection against glutamate excitotoxicity to neurons. Neurochem. Int. 56 , 577–584 (2010).
Karlsson, R. M. et al. Assessment of glutamate transporter GLAST (EAAT1)-deficient mice for phenotypes relevant to the negative and executive/cognitive symptoms of schizophrenia. Neuropsychopharmacology 34 , 1578–1589 (2009).
Karlsson, R. M. et al. Reduced alcohol intake and reward associated with impaired endocannabinoid signaling in mice with a deletion of the glutamate transporter GLAST. Neuropharmacology 63 , 181–189 (2012).
Di Castro, M. A. et al. Local Ca2+ detection and modulation of synaptic release by astrocytes. Nat. Neurosci. 14 , 1276–1284 (2011).
Matos, M. et al. Deletion of adenosine A2A receptors from astrocytes disrupts glutamate homeostasis leading to psychomotor and cognitive impairment: relevance to schizophrenia. Biol. Psychiatry 78 , 763–774 (2015).
Yue, N. et al. Activation of P2X7 receptor and NLRP3 inflammasome assembly in hippocampal glial cells mediates chronic stress-induced depressive-like behaviors. J. Neuroinflammation 14 , 102 (2017).
Cao, X. et al. Astrocyte-derived ATP modulates depressive-like behaviors. Nat. Med. 19 , 773–777 (2013).
Cunha, G. M., Canas, P. M., Oliveira, C. R. & Cunha, R. A. Increased density and synapto-protective effect of adenosine A2A receptors upon sub-chronic restraint stress. Neuroscience 141 , 1775–1781 (2006).
Batalha, V. L. et al. Adenosine A(2A) receptor blockade reverts hippocampal stress-induced deficits and restores corticosterone circadian oscillation. Mol. Psychiatry 18 , 320–331 (2013).
Wang, M. et al. Lateral septum adenosine A(2A) receptors control stress-induced depressive-like behaviors via signaling to the hypothalamus and habenula. Nat. Commun. 14 , 1880 (2023).
Lucas, M. et al. Coffee, caffeine, and risk of completed suicide: results from three prospective cohorts of American adults. World J. Biol. Psychiatry 15 , 377–386 (2014).
Lucas, M. et al. Coffee, caffeine, and risk of depression among women. Arch. Intern. Med. 171 , 1571–1578 (2011).
Coelho, J. E. et al. Overexpression of adenosine A2A receptors in rats: effects on depression, locomotion, and anxiety. Front. Psychiatry 5 , 67 (2014).
Kaster, M. P. et al. Caffeine acts through neuronal adenosine A2A receptors to prevent mood and memory dysfunction triggered by chronic stress. Proc. Natl Acad. Sci. USA 112 , 7833–7838 (2015).
Hines, D. J., Schmitt, L. I., Hines, R. M., Moss, S. J. & Haydon, P. G. Antidepressant effects of sleep deprivation require astrocyte-dependent adenosine mediated signaling. Transl. Psychiatry 3 , e212 (2013).
Etievant, A. et al. Astroglial control of the antidepressant-like effects of prefrontal cortex deep brain stimulation. EBioMedicine 2 , 898–908 (2015).
Serchov, T. et al. Increased signaling via adenosine A1 receptors, sleep deprivation, imipramine, and ketamine inhibit depressive-like behavior via induction of Homer1a. Neuron 87 , 549–562 (2015).
Dostal, C. R., Carson Sulzer, M., Kelley, K. W., Freund, G. G. & McCusker, R. H. Glial and tissue-specific regulation of Kynurenine Pathway dioxygenases by acute stress of mice. Neurobiol. Stress 7 , 1–15 (2017).
Yang, W. et al. Dex modulates the balance of water-electrolyte metabolism by depressing the expression of AVP in PVN. Front. Pharmacol. 13 , 919032 (2022).
Jacobson, L. Forebrain glucocorticoid receptor gene deletion attenuates behavioral changes and antidepressant responsiveness during chronic stress. Brain Res. 1583 , 109–121 (2014).
Perez, P. Glucocorticoid receptors, epidermal homeostasis and hair follicle differentiation. Dermatoendocrinol 3 , 166–174 (2011).
Reichrath, J. Ancient friends, revisited: new aspects on the important role of nuclear receptor signalling for skin physiology and for the treatment of skin diseases. Dermatoendocrinol 3 , 121–124 (2011).
Herbert, J. & Lucassen, P. J. Depression as a risk factor for Alzheimer’s disease: genes, steroids, cytokines and neurogenesis - What do we need to know? Front. Neuroendocrinol. 41 , 153–171 (2016).
Rosenthal, L. J., Goldner, W. S. & O’Reardon, J. P. T3 augmentation in major depressive disorder: safety considerations. Am. J. Psychiatry 168 , 1035–1040 (2011).
Mokrani, M. C., Duval, F., Erb, A., Gonzalez Lopera, F. & Danila, V. Are the thyroid and adrenal system alterations linked in depression? Psychoneuroendocrinology 122 , 104831 (2020).
Stenzel, D. & Huttner, W. B. Role of maternal thyroid hormones in the developing neocortex and during human evolution. Front. Neuroanat. 7 , 19 (2013).
Mendes-de-Aguiar, C. B. et al. Thyroid hormone increases astrocytic glutamate uptake and protects astrocytes and neurons against glutamate toxicity. J. Neurosci. Res. 86 , 3117–3125 (2008).
Rastogi, L., Godbole, M. M., Sinha, R. A. & Pradhan, S. Reverse triiodothyronine (rT3) attenuates ischemia-reperfusion injury. Biochem. Biophys. Res. Commun. 506 , 597–603 (2018).
Isgor, C. & Watson, S. J. Estrogen receptor alpha and beta mRNA expressions by proliferating and differentiating cells in the adult rat dentate gyrus and subventricular zone. Neuroscience 134 , 847–856 (2005).
Russell, J. K., Jones, C. K. & Newhouse, P. A. The role of estrogen in brain and cognitive aging. Neurotherapeutics 16 , 649–665 (2019).
Biegon, A., Reches, A., Snyder, L. & McEwen, B. S. Serotonergic and noradrenergic receptors in the rat brain: modulation by chronic exposure to ovarian hormones. Life Sci. 32 , 2015–2021 (1983).
Arinami, H., Suzuki, Y., Tajiri, M., Tsuneyama, N. & Someya, T. Role of insulin-like growth factor 1, sex and corticosteroid hormones in male major depressive disorder. BMC Psychiatry 21 , 157 (2021).
Walf, A. A., Rhodes, M. E. & Frye, C. A. Antidepressant effects of ERbeta-selective estrogen receptor modulators in the forced swim test. Pharm. Biochem Behav. 78 , 523–529 (2004).
Frye, C. A. & Walf, A. A. Estrogen and/or progesterone administered systemically or to the amygdala can have anxiety-, fear-, and pain-reducing effects in ovariectomized rats. Behav. Neurosci. 118 , 306–313 (2004).
Hughes, Z. A. et al. WAY-200070, a selective agonist of estrogen receptor beta as a potential novel anxiolytic/antidepressant agent. Neuropharmacology 54 , 1136–1142 (2008).
Weiser, M. J., Wu, T. J. & Handa, R. J. Estrogen receptor-beta agonist diarylpropionitrile: biological activities of R- and S-enantiomers on behavior and hormonal response to stress. Endocrinology 150 , 1817–1825 (2009).
Irwin, R. W. et al. Selective oestrogen receptor modulators differentially potentiate brain mitochondrial function. J. Neuroendocrinol. 24 , 236–248 (2012).
Ho, M. F. et al. Ketamine and ketamine metabolites as novel estrogen receptor ligands: Induction of cytochrome P450 and AMPA glutamate receptor gene expression. Biochem. Pharmacol. 152 , 279–292 (2018).
Hochol, A. et al. Effects of neuropeptides B and W on the rat pituitary-adrenocortical axis: in vivo and in vitro studies. Int J. Mol. Med. 19 , 207–211 (2007).
CAS PubMed Google Scholar
Kurosawa, N., Shimizu, K. & Seki, K. The development of depression-like behavior is consolidated by IL-6-induced activation of locus coeruleus neurons and IL-1beta-induced elevated leptin levels in mice. Psychopharmacology 233 , 1725–1737 (2016).
Lee, J. Y. et al. The association between serum leptin levels and post-stroke depression: a retrospective clinical study. Ann. Rehabil. Med. 39 , 786–792 (2015).
Li, Z. et al. Fluoxetine improves behavioural deficits induced by chronic alcohol treatment by alleviating RNA editing of 5-HT(2C) receptors. Neurochem. Int. 134 , 104689 (2020).
de Morais, H. et al. Increased oxidative stress in prefrontal cortex and hippocampus is related to depressive-like behavior in streptozotocin-diabetic rats. Behav. Brain Res. 258 , 52–64 (2014).
Rawdin, B. J. et al. Dysregulated relationship of inflammation and oxidative stress in major depression. Brain Behav. Immun. 31 , 143–152 (2013).
Dringen, R. Metabolism and functions of glutathione in brain. Prog. Neurobiol. 62 , 649–671 (2000).
Greaney, J. L., Saunders, E. F. H. & Alexander, L. M. Short-term salicylate treatment improves microvascular endothelium-dependent dilation in young adults with major depressive disorder. Am. J. Physiol. Heart Circ. Physiol. 322 , H880–H889 (2022).
Belanger, M. & Magistretti, P. J. The role of astroglia in neuroprotection. Dialogues Clin. Neurosci. 11 , 281–295 (2009).
Chen, B. et al. The neuroprotective mechanism of lithium after ischaemic stroke. Commun. Biol. 5 , 105 (2022).
Maes, M. et al. Major differences in neurooxidative and neuronitrosative stress pathways between major depressive disorder and types I and II bipolar disorder. Mol. Neurobiol. 56 , 141–156 (2019).
Early, J. O. et al. Circadian clock protein BMAL1 regulates IL-1beta in macrophages via NRF2. Proc. Natl Acad. Sci. USA 115 , E8460–E8468 (2018).
Pasco, J. A. et al. Association of high-sensitivity C-reactive protein with de novo major depression. Br. J. Psychiatry 197 , 372–377 (2010).
Bakunina, N., Pariante, C. M. & Zunszain, P. A. Immune mechanisms linked to depression via oxidative stress and neuroprogression. Immunology 144 , 365–373 (2015).
Paul, E. R. et al. Peripheral and central kynurenine pathway abnormalities in major depression. Brain Behav. Immun. 101 , 136–145 (2022).
Wang, Y. et al. Inhibition of activated astrocyte ameliorates lipopolysaccharide- induced depressive-like behaviors. J. Affect Disord. 242 , 52–59 (2019).
Lenk, T., Rabet, S., Sprick, M., Raabe, G. & Schroder, U. Insight into the interaction of furfural with metallic surfaces in the electrochemical hydrogenation process. Chemphyschem 24 , e202200614 (2023).
Zhang, J. C., Yao, W. & Hashimoto, K. Brain-derived neurotrophic factor (BDNF)-TrkB signaling in inflammation-related depression and potential therapeutic targets. Curr. Neuropharmacol. 14 , 721–731 (2016).
Kennis, M. et al. Prospective biomarkers of major depressive disorder: a systematic review and meta-analysis. Mol. Psychiatry 25 , 321–338 (2020).
Norden, D. M., Trojanowski, P. J., Villanueva, E., Navarro, E. & Godbout, J. P. Sequential activation of microglia and astrocyte cytokine expression precedes increased Iba-1 or GFAP immunoreactivity following systemic immune challenge. Glia 64 , 300–316 (2016).
Li, X. et al. Leptin increases expression of 5-HT(2B) receptors in astrocytes thus enhancing action of fluoxetine on the depressive behavior induced by sleep deprivation. Front. Psychiatry 9 , 734 (2018).
Takano, K., Yamasaki, H., Kawabe, K., Moriyama, M. & Nakamura, Y. Imipramine induces brain-derived neurotrophic factor mRNA expression in cultured astrocytes. J. Pharmacol. Sci. 120 , 176–186 (2012).
Zhang, Y. et al. BDNF enhances electrophysiological activity and excitatory synaptic transmission of RA projection neurons in adult male zebra finches. Brain Res. 1801 , 148208 (2023).
Quesseveur, G. et al. BDNF overexpression in mouse hippocampal astrocytes promotes local neurogenesis and elicits anxiolytic-like activities. Transl. Psychiatry 3 , e253 (2013).
Zor, F. et al. Effect of VEGF gene therapy and hyaluronic acid film sheath on peripheral nerve regeneration. Microsurgery 34 , 209–216 (2014).
Sun, L. et al. Propofol directly induces caspase-1-dependent macrophage pyroptosis through the NLRP3-ASC inflammasome. Cell Death Dis. 10 , 542 (2019).
Alcocer-Gomez, E. & Cordero, M. D. NLRP3 inflammasome: a new target in major depressive disorder. CNS Neurosci. Ther. 20 , 294–295 (2014).
Beckwith, K. S. et al. Plasma membrane damage causes NLRP3 activation and pyroptosis during Mycobacterium tuberculosis infection. Nat. Commun. 11 , 2270 (2020).
Xia, M. et al. The ameliorative effect of fluoxetine on neuroinflammation induced by sleep deprivation. J. Neurochem. 146 , 63–75 (2018).
Jeon, S. A. et al. NLRP3 inflammasome contributes to lipopolysaccharide-induced depressive-like behaviors via indoleamine 2,3-dioxygenase induction. Int. J. Neuropsychopharmacol. 20 , 896–906 (2017).
Wong, M. L. et al. Inflammasome signaling affects anxiety- and depressive-like behavior and gut microbiome composition. Mol. Psychiatry 21 , 797–805 (2016).
Page, C. E. & Coutellier, L. Prefrontal excitatory/inhibitory balance in stress and emotional disorders: Evidence for over-inhibition. Neurosci. Biobehav. Rev. 105 , 39–51 (2019).
Santello, M., Toni, N. & Volterra, A. Astrocyte function from information processing to cognition and cognitive impairment. Nat. Neurosci. 22 , 154–166 (2019).
Castillo-Gomez, E., Perez-Rando, M., Vidueira, S. & Nacher, J. Polysialic acid acute depletion induces structural plasticity in interneurons and impairs the excitation/inhibition balance in medial prefrontal cortex organotypic cultures. Front. Cell. Neurosci. 10 , 170 (2016).
Haydon, P. G. & Carmignoto, G. Astrocyte control of synaptic transmission and neurovascular coupling. Physiol. Rev. 86 , 1009–1031 (2006).
Huang, D. et al. Dysfunction of astrocytic connexins 30 and 43 in the medial prefrontal cortex and hippocampus mediates depressive-like behaviours. Behav. Brain Res. 372 , 111950 (2019).
Nagy, C., Torres-Platas, S. G., Mechawar, N. & Turecki, G. Repression of astrocytic connexins in cortical and subcortical brain regions and prefrontal enrichment of H3K9me3 in depression and suicide. Int J. Neuropsychopharmacol. 20 , 50–57 (2017).
PubMed Google Scholar
Murphy-Royal, C. et al. Stress gates an astrocytic energy reservoir to impair synaptic plasticity. Nat. Commun. 11 , 2014 (2020).
Ezan, P. et al. Deletion of astroglial connexins weakens the blood-brain barrier. J. Cereb. Blood Flow Metab. 32 , 1457–1467 (2012).
Torres-Platas, S. G., Nagy, C., Wakid, M., Turecki, G. & Mechawar, N. Glial fibrillary acidic protein is differentially expressed across cortical and subcortical regions in healthy brains and downregulated in the thalamus and caudate nucleus of depressed suicides. Mol. Psychiatry 21 , 509–515 (2016).
Cobb, J. A. et al. Density of GFAP-immunoreactive astrocytes is decreased in left hippocampi in major depressive disorder. Neuroscience 316 , 209–220 (2016).
Steinacker, P. et al. Glial fibrillary acidic protein as blood biomarker for differential diagnosis and severity of major depressive disorder. J. Psychiatr. Res. 144 , 54–58 (2021).
Rajkowska, G., Hughes, J., Stockmeier, C. A., Javier Miguel-Hidalgo, J. & Maciag, D. Coverage of blood vessels by astrocytic endfeet is reduced in major depressive disorder. Biol. Psychiatry 73 , 613–621 (2013).
Rajkowska, G. & Miguel-Hidalgo, J. J. Glial pathology in major depressive disorder: an approach to investigate the coverage of blood vessels by astrocyte endfeet in human postmortem brain. Methods Mol. Biol. 1938 , 247–254 (2019).
Kong, H. et al. Aquaporin-4 knockout exacerbates corticosterone-induced depression by inhibiting astrocyte function and hippocampal neurogenesis. CNS Neurosci. Ther. 20 , 391–402 (2014).
Xia, M., Yang, L., Sun, G., Qi, S. & Li, B. Mechanism of depression as a risk factor in the development of Alzheimer’s disease: the function of AQP4 and the glymphatic system. Psychopharmacology 234 , 365–379 (2017).
Di Benedetto, B. et al. Fluoxetine requires the endfeet protein aquaporin-4 to enhance plasticity of astrocyte processes. Front. Cell. Neurosci. 10 , 8 (2016).
Du, J. et al. S100B is selectively expressed by gray matter protoplasmic astrocytes and myelinating oligodendrocytes in the developing CNS. Mol. Brain 14 , 154 (2021).
Schroeter, M. L., Abdul-Khaliq, H., Krebs, M., Diefenbacher, A. & Blasig, I. E. Serum markers support disease-specific glial pathology in major depression. J. Affect Disord. 111 , 271–280 (2008).
Michel, M. et al. Increased GFAP concentrations in the cerebrospinal fluid of patients with unipolar depression. Transl. Psychiatry 11 , 308 (2021).
Gos, T. et al. S100B-immunopositive astrocytes and oligodendrocytes in the hippocampus are differentially afflicted in unipolar and bipolar depression: a postmortem study. J. Psychiatr. Res. 47 , 1694–1699 (2013).
Michetti, F. et al. The S100B protein in biological fluids: more than a lifelong biomarker of brain distress. J. Neurochem. 120 , 644–659 (2012).
Zhang, L. et al. Changes in glial gene expression in the prefrontal cortex in relation to major depressive disorder, suicide and psychotic features. J. Affect Disord. 295 , 893–903 (2021).
Gules, E., Iosifescu, D. V. & Tural, U. Plasma neuronal and glial markers and anterior cingulate metabolite levels in major depressive disorder: a pilot study. Neuropsychobiology 79 , 214–221 (2020).
Chamera, K., Szuster-Gluszczak, M., Trojan, E. & Basta-Kaim, A. Maternal Immune Activation Sensitizes Male Offspring Rats to Lipopolysaccharide-Induced Microglial Deficits Involving the Dysfunction of CD200-CD200R and CX3CL1-CX3CR1 systems. Cells 9 , 1676 (2020).
Jiang, X. et al. Asperosaponin VI ameliorates the CMS-induced depressive-like behaviors by inducing a neuroprotective microglial phenotype in hippocampus via PPAR-gamma pathway. J. Neuroinflammation 19 , 115 (2022).
Tang, M. M., Lin, W. J., Pan, Y. Q. & Li, Y. C. Fibroblast growth factor 2 modulates hippocampal microglia activation in a neuroinflammation induced model of depression. Front. Cell. Neurosci. 12 , 255 (2018).
Zhan, Y. et al. Deficient neuron-microglia signaling results in impaired functional brain connectivity and social behavior. Nat. Neurosci. 17 , 400–406 (2014).
Hellwig, S. et al. Altered microglia morphology and higher resilience to stress-induced depression-like behavior in CX3CR1-deficient mice. Brain Behav. Immun. 55 , 126–137 (2016).
Merendino, R. A. et al. Involvement of fractalkine and macrophage inflammatory protein-1 alpha in moderate-severe depression. Mediat. Inflamm. 13 , 205–207 (2004).
Garcia-Marchena, N. et al. Inflammatory mediators and dual depression: Potential biomarkers in plasma of primary and substance-induced major depression in cocaine and alcohol use disorders. PLoS ONE 14 , e0213791 (2019).
Wang, H. T. et al. Early-Life Social Isolation-Induced Depressive-Like Behavior in Rats Results in Microglial Activation and Neuronal Histone Methylation that Are Mitigated by Minocycline. Neurotox. Res 31 , 505–520 (2017).
Frank, M. G., Fonken, L. K., Annis, J. L., Watkins, L. R. & Maier, S. F. Stress disinhibits microglia via down-regulation of CD200R: A mechanism of neuroinflammatory priming. Brain Behav. Immun. 69 , 62–73 (2018).
Fonken, L. K. et al. Neuroinflammatory priming to stress is differentially regulated in male and female rats. Brain Behav. Immun. 70 , 257–267 (2018).
Popov, A. et al. Astrocyte dystrophy in ageing brain parallels impaired synaptic plasticity. Aging Cell 20 , e13334 (2021).
Zhang, Y. et al. Structural basis of ketamine action on human NMDA receptors. Nature 596 , 301–305 (2021).
Wang, Q., Jie, W., Liu, J. H., Yang, J. M. & Gao, T. M. An astroglial basis of major depressive disorder? An overview. Glia 65 , 1227–1250 (2017).
Jiang, X., Lin, W., Cheng, Y. & Wang, D. mGluR5 facilitates long-term synaptic depression in a stress-induced depressive mouse model. Can. J. Psychiatry 65 , 347–355 (2020).
Guo, W. et al. Iptakalim alleviates synaptic damages via targeting mitochondrial ATP-sensitive potassium channel in depression. FASEB J. 35 , e21581 (2021).
Curro, D., Navarra, P., Samengo, I. & Martire, M. P2X7 receptors exert a permissive effect on the activation of presynaptic AMPA receptors in rat trigeminal caudal nucleus glutamatergic nerve terminals. J. Headache Pain 21 , 83 (2020).
Iwata, M. et al. Psychological stress activates the inflammasome via release of adenosine triphosphate and stimulation of the purinergic type 2X7 receptor. Biol. Psychiatry 80 , 12–22 (2016).
He, Y., Taylor, N., Fourgeaud, L. & Bhattacharya, A. The role of microglial P2X7: modulation of cell death and cytokine release. J. Neuroinflammation 14 , 135 (2017).
Ota, K. T. et al. REDD1 is essential for stress-induced synaptic loss and depressive behavior. Nat. Med 20 , 531–535 (2014).
Kang, H. J. et al. Decreased expression of synapse-related genes and loss of synapses in major depressive disorder. Nat. Med 18 , 1413–1417 (2012).
Tomassoni-Ardori, F. et al. Rbfox1 up-regulation impairs BDNF-dependent hippocampal LTP by dysregulating TrkB isoform expression levels. Elife 8 , e49673 (2019).
Wang, G. et al. Antidepressant-like effect of ginsenoside Rb1 on potentiating synaptic plasticity via the miR-134-mediated BDNF signaling pathway in a mouse model of chronic stress-induced depression. J. Ginseng Res. 46 , 376–386 (2022).
Lalo, U., Koh, W., Lee, C. J. & Pankratov, Y. The tripartite glutamatergic synapse. Neuropharmacology 199 , 108758 (2021).
Shepard, R., Page, C. E. & Coutellier, L. Sensitivity of the prefrontal GABAergic system to chronic stress in male and female mice: Relevance for sex differences in stress-related disorders. Neuroscience 332 , 1–12 (2016).
Shepard, R. & Coutellier, L. Changes in the prefrontal glutamatergic and parvalbumin systems of mice exposed to unpredictable chronic stress. Mol. Neurobiol. 55 , 2591–2602 (2018).
Bouamrane, L. et al. Reelin-haploinsufficiency disrupts the developmental trajectory of the E/I Balance in the Prefrontal Cortex. Front. Cell. Neurosci. 10 , 308 (2016).
Arnone, D., Mumuni, A. N., Jauhar, S., Condon, B. & Cavanagh, J. Indirect evidence of selective glial involvement in glutamate-based mechanisms of mood regulation in depression: meta-analysis of absolute prefrontal neuro-metabolic concentrations. Eur. Neuropsychopharmacol. 25 , 1109–1117 (2015).
Hu, Y. T., Tan, Z. L., Hirjak, D. & Northoff, G. Brain-wide changes in excitation-inhibition balance of major depressive disorder: a systematic review of topographic patterns of GABA- and glutamatergic alterations. Mol. Psychiatry 28 , 3257–3266 (2023).
Zhao, J. et al. Prefrontal alterations in GABAergic and glutamatergic gene expression in relation to depression and suicide. J. Psychiatr. Res. 102 , 261–274 (2018).
Douillard-Guilloux, G., Lewis, D., Seney, M. L. & Sibille, E. Decrease in somatostatin-positive cell density in the amygdala of females with major depression. Depress Anxiety 34 , 68–78 (2017).
Scifo, E. et al. Sustained molecular pathology across episodes and remission in major depressive disorder. Biol. Psychiatry 83 , 81–89 (2018).
Oh, D. H., Son, H., Hwang, S. & Kim, S. H. Neuropathological abnormalities of astrocytes, GABAergic neurons, and pyramidal neurons in the dorsolateral prefrontal cortices of patients with major depressive disorder. Eur. Neuropsychopharmacol. 22 , 330–338 (2012).
Sibille, E., Morris, H. M., Kota, R. S. & Lewis, D. A. GABA-related transcripts in the dorsolateral prefrontal cortex in mood disorders. Int J. Neuropsychopharmacol. 14 , 721–734 (2011).
Barros-Barbosa, A. R., Lobo, M. G., Ferreirinha, F., Correia-de-Sa, P. & Cordeiro, J. M. P2X7 receptor activation downmodulates Na(+)-dependent high-affinity GABA and glutamate transport into rat brain cortex synaptosomes. Neuroscience 306 , 74–90 (2015).
Barros-Barbosa, A. R. et al. Up-regulation of P2X7 receptor-mediated inhibition of GABA uptake by nerve terminals of the human epileptic neocortex. Epilepsia 57 , 99–110 (2016).
Liu, Y. P., Yang, C. S., Chen, M. C., Sun, S. H. & Tzeng, S. F. Ca(2+)-dependent reduction of glutamate aspartate transporter GLAST expression in astrocytes by P2X(7) receptor-mediated phosphoinositide 3-kinase signaling. J. Neurochem. 113 , 213–227 (2010).
Macaulay, N. & Zeuthen, T. Glial K(+) clearance and cell swelling: key roles for cotransporters and pumps. Neurochem. Res. 37 , 2299–2309 (2012).
Larsen, B. R. et al. Contributions of the Na(+)/K(+)-ATPase, NKCC1, and Kir4.1 to hippocampal K(+) clearance and volume responses. Glia 62 , 608–622 (2014).
Verkhratsky, A. & Nedergaard, M. Astroglial cradle in the life of the synapse. Philos. Trans. R. Soc. Lond. B Biol. Sci. 369 , 20130595 (2014).
Aramideh, J. A., Vidal-Itriago, A., Morsch, M. & Graeber, M. B. Cytokine signalling at the microglial penta-partite synapse. Int J. Mol. Sci. 22 , 13186 (2021).
Chung, W. S. et al. Astrocytes mediate synapse elimination through MEGF10 and MERTK pathways. Nature 504 , 394–400 (2013).
Yang, J. et al. Astrocytes contribute to synapse elimination via type 2 inositol 1,4,5-trisphosphate receptor-dependent release of ATP. Elife 5 , e15043 (2016).
Petrache, A. L. et al. Aberrant excitatory-inhibitory synaptic mechanisms in entorhinal cortex microcircuits during the pathogenesis of Alzheimer’s disease. Cereb. Cortex 29 , 1834–1850 (2019).
Arioz, B. I. et al. Melatonin attenuates LPS-Induced acute depressive-like behaviors and microglial NLRP3 inflammasome activation through the SIRT1/Nrf2 pathway. Front. Immunol. 10 , 1511 (2019).
Bikbaev, A., Frischknecht, R. & Heine, M. Brain extracellular matrix retains connectivity in neuronal networks. Sci. Rep. 5 , 14527 (2015).
Strackeljan, L. et al. Microglia depletion-induced remodeling of extracellular matrix and excitatory synapses in the hippocampus of adult mice. Cells 10 , 1862 (2021).
Leclercq, S., De Saeger, C., Delzenne, N., de Timary, P. & Starkel, P. Role of inflammatory pathways, blood mononuclear cells, and gut-derived bacterial products in alcohol dependence. Biol. Psychiatry 76 , 725–733 (2014).
Hsu, J. H., Chien, I. C. & Lin, C. H. Increased risk of chronic liver disease in patients with major depressive disorder: a population-based study. J. Affect Disord. 251 , 180–185 (2019).
Chen, D., Zhang, Y., Huang, T. & Jia, J. Depression and risk of gastrointestinal disorders: a comprehensive two-sample Mendelian randomization study of European ancestry. Psychol. Med . 53 , 7309–7321 (2023).
Facanali, C. B. G. et al. The relationship of major depressive disorder with Crohn’s disease activity. Clinics (Sao Paulo) 78 , 100188 (2023).
Ronaldson, P. T. & Davis, T. P. Regulation of blood-brain barrier integrity by microglia in health and disease: A therapeutic opportunity. J. Cereb. Blood Flow. Metab. 40 , S6–S24 (2020).
Qing, H. et al. Origin and Function of Stress-Induced IL-6 in Murine Models. Cell 182 , 1660 (2020).
Labad, J. et al. Hypothalamic-pituitary-adrenal axis function and exposure to stress factors and cannabis use in recent-onset psychosis. World J. Biol. Psychiatry 21 , 564–571 (2020).
Frank, M. G., Miguel, Z. D., Watkins, L. R. & Maier, S. F. Prior exposure to glucocorticoids sensitizes the neuroinflammatory and peripheral inflammatory responses to E. coli lipopolysaccharide. Brain Behav. Immun. 24 , 19–30 (2010).
Deng, Y. et al. Involvement of the microbiota-gut-brain axis in chronic restraint stress: disturbances of the kynurenine metabolic pathway in both the gut and brain. Gut Microbes 13 , 1–16 (2021).
Munn, D. H. & Mellor, A. L. Indoleamine 2,3 dioxygenase and metabolic control of immune responses. Trends Immunol. 34 , 137–143 (2013).
Gos, T. et al. Reduced microglial immunoreactivity for endogenous NMDA receptor agonist quinolinic acid in the hippocampus of schizophrenia patients. Brain Behav. Immun. 41 , 59–64 (2014).
Li, M. et al. Transcriptome profiles of corticosterone-induced cytotoxicity reveals the involvement of neurite growth-related genes in depression. Psychiatry Res. 276 , 79–86 (2019).
Dutheil, S., Ota, K. T., Wohleb, E. S., Rasmussen, K. & Duman, R. S. High-fat diet induced anxiety and anhedonia: impact on brain homeostasis and inflammation. Neuropsychopharmacology 41 , 1874–1887 (2016).
Skonieczna-Zydecka, K. et al. Faecal short chain fatty acids profile is changed in polish depressive women. Nutrients 10 , 1939 (2018).
Sampson, T. R. et al. Gut microbiota regulate motor deficits and neuroinflammation in a model of Parkinson’s disease. Cell 167 , 1469–1480.e1412 (2016).
Fan, L. et al. Total glycosides from stems of Cistanche tubulosa alleviate depression-like behaviors: bidirectional interaction of the phytochemicals and gut microbiota. Phytomedicine 83 , 153471 (2021).
Zhang, J. C. et al. Blockade of interleukin-6 receptor in the periphery promotes rapid and sustained antidepressant actions: a possible role of gut-microbiota-brain axis. Transl. Psychiatry 7 , e1138 (2017).
Pan, Y., Chen, X. Y., Zhang, Q. Y. & Kong, L. D. Microglial NLRP3 inflammasome activation mediates IL-1beta-related inflammation in prefrontal cortex of depressive rats. Brain Behav. Immun. 41 , 90–100 (2014).
Woodburn, S. C., Bollinger, J. L. & Wohleb, E. S. Synaptic and behavioral effects of chronic stress are linked to dynamic and sex-specific changes in microglia function and astrocyte dystrophy. Neurobiol. Stress 14 , 100312 (2021).
Zhang, K., Liu, R., Gao, Y., Ma, W. & Shen, W. Electroacupuncture relieves LPS-induced depression-like behaviour in rats through IDO-Mediated tryptophan-degrading pathway. Neuropsychiatr. Dis. Treat. 16 , 2257–2266 (2020).
Parrott, J. M., Redus, L. & O’Connor, J. C. Kynurenine metabolic balance is disrupted in the hippocampus following peripheral lipopolysaccharide challenge. J. Neuroinflammation 13 , 124 (2016).
Alzarea, S., Abbas, M., Ronan, P. J., Lutfy, K. & Rahman, S. The Effect of an alpha-7 nicotinic allosteric modulator PNU120596 and NMDA receptor antagonist memantine on depressive-like behavior induced by lps in mice: the involvement of brain microglia. Brain Sci. 12 , 1493 (2022).
Le Strat, Y., Le Foll, B. & Dubertret, C. Major depression and suicide attempts in patients with liver disease in the United States. Liver Int. 35 , 1910–1916 (2015).
Buganza-Torio, E. et al. Depression in cirrhosis - a prospective evaluation of the prevalence, predictors and development of a screening nomogram. Aliment. Pharmacol. Ther. 49 , 194–201 (2019).
Qin, X. H. et al. Liver soluble epoxide hydrolase regulates behavioral and cellular effects of chronic stress. Cell Rep. 29 , 3223–3234.e3226 (2019).
Pang, F. et al. Electroacupuncture alleviates depressive-like behavior by modulating the expression of P2X7/NLRP3/IL-1beta of prefrontal cortex and liver in rats exposed to chronic unpredictable mild stress. Brain Sci. 13 , 436 (2023).
Zhang, Y. et al. Effects of hydrogen-rich water on depressive-like behavior in mice. Sci. Rep. 6 , 23742 (2016).
Brown, S. J. et al. Sex- and suicide-specific alterations in the kynurenine pathway in the anterior cingulate cortex in major depression. Neuropsychopharmacology (2023). Online ahead of print.
Guillemin, G. J. et al. Characterisation of kynurenine pathway metabolism in human astrocytes and implications in neuropathogenesis. Redox Rep. 5 , 108–111 (2000).
Park, H. J., Kim, S. A., Kang, W. S. & Kim, J. W. Early-life stress modulates gut microbiota and peripheral and central inflammation in a sex-dependent manner. Int J. Mol. Sci. 22 , 1899 (2021).
Ninan, J. & Feldman, L. Ammonia levels and hepatic encephalopathy in patients with known chronic liver disease. J. Hosp. Med. 12 , 659–661 (2017).
Kautzky, A. et al. The influence of the rs6295 gene polymorphism on serotonin-1A receptor distribution investigated with PET in patients with major depression applying machine learning. Transl. Psychiatry 7 , e1150 (2017).
Das, R. et al. Higher levels of serum IL-1beta and TNF-alpha are associated with an increased probability of major depressive disorder. Psychiatry Res. 295 , 113568 (2021).
Lu, Z. et al. Oxidative stress and psychiatric disorders: evidence from the bidirectional mendelian randomization study. Antioxidants (Basel) 11 , 1386 (2022).
Hursitoglu, O. et al. Serum NOX1 and Raftlin as new potential biomarkers of Major Depressive Disorder: a study in treatment-naive first episode patients. Prog. Neuropsychopharmacol. Biol. Psychiatry 121 , 110670 (2023).
Han, K. M. et al. Serum FAM19A5 levels: A novel biomarker for neuroinflammation and neurodegeneration in major depressive disorder. Brain Behav. Immun. 87 , 852–859 (2020).
Winter, N. R. et al. Quantifying deviations of brain structure and function in major depressive disorder across neuroimaging modalities. JAMA Psychiatry 79 , 879–888 (2022).
Zhao, Y. et al. Gray matter abnormalities in non-comorbid medication-naive patients with major depressive disorder or social anxiety disorder. EBioMedicine 21 , 228–235 (2017).
Wise, T. et al. Common and distinct patterns of grey-matter volume alteration in major depression and bipolar disorder: evidence from voxel-based meta-analysis. Mol. Psychiatry 22 , 1455–1463 (2017).
Liu, Y. et al. Abnormal brain gray matter volume in patients with major depressive disorder: Associated with childhood trauma? J. Affect Disord. 308 , 562–568 (2022).
Kaiser, R. H., Andrews-Hanna, J. R., Wager, T. D. & Pizzagalli, D. A. Large-scale network dysfunction in major depressive disorder: a meta-analysis of resting-state functional connectivity. JAMA Psychiatry 72 , 603–611 (2015).
Wang, Y. et al. Topologically convergent and divergent functional connectivity patterns in unmedicated unipolar depression and bipolar disorder. Transl. Psychiatry 7 , e1165 (2017).
Kremneva, E. I., Sinitsyn, D. O., Dobrynina, L. A., Suslina, A. D. & Krotenkova, M. V. [Resting state functional MRI in neurology and psychiatry]. Zh. Nevrol. Psikhiatr Im. S S Korsakova 122 , 5–14 (2022).
Luo, Z. et al. Shared and specific dynamics of brain segregation and integration in bipolar disorder and major depressive disorder: A resting-state functional magnetic resonance imaging study. J. Affect. Disord. 280 , 279–286 (2021).
Drysdale, A. T. et al. Resting-state connectivity biomarkers define neurophysiological subtypes of depression. Nat. Med. 23 , 28–38 (2017).
Chen, Q. et al. Regional amplitude abnormities in the major depressive disorder: A resting-state fMRI study and support vector machine analysis. J. Affect. Disord. 308 , 1–9 (2022).
Yan, C. G. et al. Reduced default mode network functional connectivity in patients with recurrent major depressive disorder. Proc. Natl Acad. Sci. USA 116 , 9078–9083 (2019).
Pizzagalli, D. A. & Roberts, A. C. Prefrontal cortex and depression. Neuropsychopharmacology 47 , 225–246 (2022).
Muller, V. I. et al. Altered brain activity in unipolar depression revisited: meta-analyses of neuroimaging studies. JAMA Psychiatry 74 , 47–55 (2017).
Sun, K. et al. A two-center radiomic analysis for differentiating major depressive disorder using multi-modality MRI data under different parcellation methods. J. Affect. Disord. 300 , 1–9 (2022).
Mayerhoefer, M. E. et al. Introduction to radiomics. J. Nucl. Med. 61 , 488–495 (2020).
Xu, Z. et al. Combined HTR1A/1B methylation and human functional connectome to recognize patients with MDD. Psychiatry Res. 317 , 114842 (2022).
Verduijn, J. et al. Reconsidering the prognosis of major depressive disorder across diagnostic boundaries: full recovery is the exception rather than the rule. BMC Med. 15 , 215 (2017).
Blumenthal, J. A. & Rozanski, A. Exercise as a therapeutic modality for the prevention and treatment of depression. Prog. Cardiovasc. Dis. 77 , 50–58 (2023).
Baglioni, C., Spiegelhalder, K., Nissen, C. & Riemann, D. Clinical implications of the causal relationship between insomnia and depression: how individually tailored treatment of sleeping difficulties could prevent the onset of depression. EPMA J. 2 , 287–293 (2011).
Riemann, D. et al. European guideline for the diagnosis and treatment of insomnia. J. Sleep. Res. 26 , 675–700 (2017).
Soh, H. L., Ho, R. C., Ho, C. S. & Tam, W. W. Efficacy of digital cognitive behavioural therapy for insomnia: a meta-analysis of randomised controlled trials. Sleep. Med. 75 , 315–325 (2020).
Ho, F. Y., Chan, C. S., Lo, W. Y. & Leung, J. C. The effect of self-help cognitive behavioral therapy for insomnia on depressive symptoms: an updated meta-analysis of randomized controlled trials. J. Affect. Disord. 265 , 287–304 (2020).
Qaseem, A. et al. Management of chronic insomnia disorder in adults: a clinical practice guideline from the american college of physicians. Ann. Intern. Med. 165 , 125–133 (2016).
Irwin, M. R. et al. Prevention of incident and recurrent major depression in older adults with insomnia: a randomized clinical trial. JAMA Psychiatry 79 , 33–41 (2022).
Scheer, F. A., Pirovano, C., Van Someren, E. J. & Buijs, R. M. Environmental light and suprachiasmatic nucleus interact in the regulation of body temperature. Neuroscience 132 , 465–477 (2005).
Dekker, K. et al. Combined internet-based cognitive-behavioral and chronobiological intervention for insomnia: a randomized controlled trial. Psychother. Psychosom. 89 , 117–118 (2020).
Leerssen, J. et al. Treating insomnia with high risk of depression using therapist-guided digital cognitive, behavioral, and circadian rhythm support interventions to prevent worsening of depressive symptoms: a randomized controlled trial. Psychother. Psychosom. 91 , 168–179 (2022).
Zhai, L., Zhang, H. & Zhang, D. Sleep duration and depression among adults: a meta-analysis of prospective studies. Depress. Anxiety 32 , 664–670 (2015).
Baglioni, C. et al. Insomnia as a predictor of depression: a meta-analytic evaluation of longitudinal epidemiological studies. J. Affect. Disord. 135 , 10–19 (2011).
Utge, S. J. et al. Systematic analysis of circadian genes in a population-based sample reveals association of TIMELESS with depression and sleep disturbance. PLoS ONE 5 , e9259 (2010).
Irwin, M. R. Sleep and inflammation: partners in sickness and in health. Nat. Rev. Immunol. 19 , 702–715 (2019).
Hill Almeida, L. M. et al. Disrupted sleep and risk of depression in later life: A prospective cohort study with extended follow up and a systematic review and meta-analysis. J. Affect. Disord. 309 , 314–323 (2022).
Tolkien, K., Bradburn, S. & Murgatroyd, C. An anti-inflammatory diet as a potential intervention for depressive disorders: A systematic review and meta-analysis. Clin. Nutr. 38 , 2045–2052 (2019).
Galland, L. Diet and inflammation. Nutr. Clin. Pr. 25 , 634–640 (2010).
Oddy, W. H. et al. Dietary patterns, body mass index and inflammation: Pathways to depression and mental health problems in adolescents. Brain Behav. Immun. 69 , 428–439 (2018).
Bosma-den Boer, M. M., van Wetten, M. L. & Pruimboom, L. Chronic inflammatory diseases are stimulated by current lifestyle: how diet, stress levels and medication prevent our body from recovering. Nutr. Metab. (Lond.) 9 , 32 (2012).
Sanchez-Villegas, A. et al. The effect of the Mediterranean diet on plasma brain-derived neurotrophic factor (BDNF) levels: the PREDIMED-NAVARRA randomized trial. Nutr. Neurosci. 14 , 195–201 (2011).
Jiang, C. & Salton, S. R. The role of neurotrophins in major depressive disorder. Transl. Neurosci. 4 , 46–58 (2013).
Ogbonnaya, E. S. et al. Adult hippocampal neurogenesis is regulated by the microbiome. Biol. Psychiatry 78 , e7–9 (2015).
Zheng, P. et al. Gut microbiome remodeling induces depressive-like behaviors through a pathway mediated by the host’s metabolism. Mol. Psychiatry 21 , 786–796 (2016).
Jiang, H. et al. Altered fecal microbiota composition in patients with major depressive disorder. Brain Behav. Immun. 48 , 186–194 (2015).
Burokas, A. et al. Targeting the microbiota-gut-brain axis: prebiotics have anxiolytic and antidepressant-like effects and reverse the impact of chronic stress in mice. Biol. Psychiatry 82 , 472–487 (2017).
Bonder, M. J. et al. The influence of a short-term gluten-free diet on the human gut microbiome. Genome Med. 8 , 45 (2016).
Mohan, M. et al. Dietary gluten-induced gut dysbiosis is accompanied by selective upregulation of microRNAs with intestinal tight junction and bacteria-binding motifs in rhesus macaque model of celiac disease. Nutrients 8 , 684 (2016).
Karakula-Juchnowicz, H. et al. The study evaluating the effect of probiotic supplementation on the mental status, inflammation, and intestinal barrier in major depressive disorder patients using gluten-free or gluten-containing diet (SANGUT study): a 12-week, randomized, double-blind, and placebo-controlled clinical study protocol. Nutr. J. 18 , 50 (2019).
Khosravi, M. et al. The relationship between dietary patterns and depression mediated by serum levels of Folate and vitamin B12. BMC Psychiatry 20 , 63 (2020).
Schuch, F. B. et al. Exercise as a treatment for depression: a meta-analysis adjusting for publication bias. J. Psychiatr. Res. 77 , 42–51 (2016).
Morland, C. et al. Exercise induces cerebral VEGF and angiogenesis via the lactate receptor HCAR1. Nat. Commun. 8 , 15557 (2017).
Coelho, F. G. et al. Physical exercise modulates peripheral levels of brain-derived neurotrophic factor (BDNF): a systematic review of experimental studies in the elderly. Arch. Gerontol. Geriatr. 56 , 10–15 (2013).
Stimpson, N. J., Davison, G. & Javadi, A. H. Joggin’ the noggin: towards a physiological understanding of exercise-induced cognitive benefits. Neurosci. Biobehav Rev. 88 , 177–186 (2018).
De Rossi, P. et al. A critical role for VEGF and VEGFR2 in NMDA receptor synaptic function and fear-related behavior. Mol. Psychiatry 21 , 1768–1780 (2016).
Bugg, J. M. & Head, D. Exercise moderates age-related atrophy of the medial temporal lobe. Neurobiol. Aging 32 , 506–514 (2011).
Cole, R. C. et al. Cardiorespiratory fitness and hippocampal volume predict faster episodic associative learning in older adults. Hippocampus 30 , 143–155 (2020).
Zotcheva, E. et al. Associations of changes in cardiorespiratory fitness and symptoms of anxiety and depression with brain volumes: The HUNT Study. Front Behav. Neurosci. 13 , 53 (2019).
Dowlati, Y. et al. A meta-analysis of cytokines in major depression. Biol. Psychiatry 67 , 446–457 (2010).
Valkanova, V., Ebmeier, K. P. & Allan, C. L. CRP, IL-6 and depression: a systematic review and meta-analysis of longitudinal studies. J. Affect Disord. 150 , 736–744 (2013).
Kohler, C. A. et al. Peripheral cytokine and chemokine alterations in depression: a meta-analysis of 82 studies. Acta Psychiatr. Scand. 135 , 373–387 (2017).
Choi, K. W. et al. Assessment of bidirectional relationships between physical activity and depression among adults: a 2-sample mendelian randomization study. JAMA Psychiatry 76 , 399–408 (2019).
Schuch, F. B. et al. Physical activity and incident depression: a meta-analysis of prospective cohort studies. Am. J. Psychiatry 175 , 631–648 (2018).
Choi, K. W. et al. Physical activity offsets genetic risk for incident depression assessed via electronic health records in a biobank cohort study. Depress Anxiety 37 , 106–114 (2020).
Imboden, C. et al. Aerobic exercise or stretching as add-on to inpatient treatment of depression: Similar antidepressant effects on depressive symptoms and larger effects on working memory for aerobic exercise alone. J. Affect Disord. 276 , 866–876 (2020).
Vancampfort, D. et al. Sedentary behavior and physical activity levels in people with schizophrenia, bipolar disorder and major depressive disorder: a global systematic review and meta-analysis. World Psychiatry 16 , 308–315 (2017).
Brush, C. J. et al. A randomized trial of aerobic exercise for major depression: examining neural indicators of reward and cognitive control as predictors and treatment targets. Psychol. Med. 52 , 893–903 (2022).
Kujawa, A. et al. Reduced reward responsiveness predicts change in depressive symptoms in anxious children and adolescents following treatment. J. Child Adolesc. Psychopharmacol. 29 , 378–385 (2019).
Kandola, A., Ashdown-Franks, G., Hendrikse, J., Sabiston, C. M. & Stubbs, B. Physical activity and depression: towards understanding the antidepressant mechanisms of physical activity. Neurosci. Biobehav Rev. 107 , 525–539 (2019).
Yildirim, Y. & Kocabiyik, S. The relationship between social support and loneliness in Turkish patients with cancer. J. Clin. Nurs. 19 , 832–839 (2010).
Lin, J. et al. Perceived stressfulness mediates the effects of subjective social support and negative coping style on suicide risk in Chinese patients with major depressive disorder. J. Affect. Disord. 265 , 32–38 (2020).
Smith, L., Hill, N. & Kokanovic, R. Experiences of depression, the role of social support and its impact on health outcomes. J. Ment. Health 24 , 342–346 (2015).
Wang, X., Cai, L., Qian, J. & Peng, J. Social support moderates stress effects on depression. Int. J. Ment. Health Syst. 8 , 41 (2014).
Hybels, C. F., Pieper, C. F., Blazer, D. G. & Steffens, D. C. Heterogeneity in the three-year course of major depression among older adults. Int. J. Geriatr. Psychiatry 31 , 775–782 (2016).
Backs-Dermott, B. J., Dobson, K. S. & Jones, S. L. An evaluation of an integrated model of relapse in depression. J. Affect. Disord. 124 , 60–67 (2010).
Holma, I. A., Holma, K. M., Melartin, T. K., Rytsala, H. J. & Isometsa, E. T. A 5-year prospective study of predictors for disability pension among patients with major depressive disorder. Acta Psychiatr. Scand. 125 , 325–334 (2012).
Stewart, R. A., Patel, T. A., McDermott, K. A. & Cougle, J. R. Functional and structural social support in DSM-5 mood and anxiety disorders: a population-based study. J. Affect Disord. 308 , 528–534 (2022).
Ouakinin, S. R. S., Barreira, D. P. & Gois, C. J. Depression and obesity: integrating the role of stress, neuroendocrine dysfunction and inflammatory pathways. Front. Endocrinol. (Lausanne) 9 , 431 (2018).
Hallgren, M., Lundin, A., Tee, F. Y., Burstrom, B. & Forsell, Y. Somebody to lean on: Social relationships predict post-treatment depression severity in adults. Psychiatry Res. 249 , 261–267 (2017).
van Beljouw, I. M., Verhaak, P. F., Cuijpers, P., van Marwijk, H. W. & Penninx, B. W. The course of untreated anxiety and depression, and determinants of poor one-year outcome: a one-year cohort study. BMC Psychiatry 10 , 86 (2010).
Dour, H. J. et al. Perceived social support mediates anxiety and depressive symptom changes following primary care intervention. Depress Anxiety 31 , 436–442 (2014).
Teo, A. R., Choi, H. & Valenstein, M. Social relationships and depression: ten-year follow-up from a nationally representative study. PLoS ONE 8 , e62396 (2013).
Griffiths, K. M., Crisp, D. A., Barney, L. & Reid, R. Seeking help for depression from family and friends: a qualitative analysis of perceived advantages and disadvantages. BMC Psychiatry 11 , 196 (2011).
Hillhouse, T. M. & Porter, J. H. A brief history of the development of antidepressant drugs: from monoamines to glutamate. Exp. Clin. Psychopharmacol. 23 , 1–21 (2015).
Sulser, F., Vetulani, J. & Mobley, P. L. Mode of action of antidepressant drugs. Biochem. Pharmacol. 27 , 257–261 (1978).
Gillman, P. K. Tricyclic antidepressant pharmacology and therapeutic drug interactions updated. Br. J. Pharmacol. 151 , 737–748 (2007).
Taylor, C., Fricker, A. D., Devi, L. A. & Gomes, I. Mechanisms of action of antidepressants: from neurotransmitter systems to signaling pathways. Cell Signal. 17 , 549–557 (2005).
Jann, M. W. & Slade, J. H. Antidepressant agents for the treatment of chronic pain and depression. Pharmacotherapy 27 , 1571–1587 (2007).
Shultz, E. & Malone, D. A. Jr A practical approach to prescribing antidepressants. Cleve Clin. J. Med. 80 , 625–631 (2013).
Croom, K. F., Perry, C. M. & Plosker, G. L. Mirtazapine: a review of its use in major depression and other psychiatric disorders. CNS Drugs 23 , 427–452 (2009).
Benjamin, S. & Doraiswamy, P. M. Review of the use of mirtazapine in the treatment of depression. Expert Opin. Pharmacother. 12 , 1623–1632 (2011).
Cleare, A. et al. Evidence-based guidelines for treating depressive disorders with antidepressants: a revision of the 2008 British Association for Psychopharmacology guidelines. J. Psychopharmacol. 29 , 459–525 (2015).
Guloglu, C., Orak, M., Ustundag, M. & Altunci, Y. A. Analysis of amitriptyline overdose in emergency medicine. Emerg. Med. J. 28 , 296–299 (2011).
David, D. J. & Gourion, D. [Antidepressant and tolerance: determinants and management of major side effects]. Encephale 42 , 553–561 (2016).
Richelson, E. Antimuscarinic and other receptor-blocking properties of antidepressants. Mayo Clin. Proc. 58 , 40–46 (1983).
Hisaoka, K. et al. Tricyclic antidepressant amitriptyline activates fibroblast growth factor receptor signaling in glial cells: involvement in glial cell line-derived neurotrophic factor production. J. Biol. Chem. 286 , 21118–21128 (2011).
Morioka, N. et al. Amitriptyline up-regulates connexin43-gap junction in rat cultured cortical astrocytes via activation of the p38 and c-Fos/AP-1 signalling pathway. Br. J. Pharmacol. 171 , 2854–2867 (2014).
Budzinski, M. L. et al. Tricyclic antidepressants target FKBP51 SUMOylation to restore glucocorticoid receptor activity. Mol. Psychiatry 27 , 2533–2545 (2022).
Malison, R. T. et al. Reduced brain serotonin transporter availability in major depression as measured by [123I]-2 beta-carbomethoxy-3 beta-(4-iodophenyl)tropane and single photon emission computed tomography. Biol. Psychiatry 44 , 1090–1098 (1998).
Samuels, B. A. et al. 5-HT1A receptors on mature dentate gyrus granule cells are critical for the antidepressant response. Nat. Neurosci. 18 , 1606–1616 (2015).
Scorza, M. C. et al. Preclinical and clinical characterization of the selective 5-HT(1A) receptor antagonist DU-125530 for antidepressant treatment. Br. J. Pharmacol. 167 , 1021–1034 (2012).
Cremers, T. I. et al. Inactivation of 5-HT(2C) receptors potentiates consequences of serotonin reuptake blockade. Neuropsychopharmacology 29 , 1782–1789 (2004).
Boothman, L. J., Mitchell, S. N. & Sharp, T. Investigation of the SSRI augmentation properties of 5-HT(2) receptor antagonists using in vivo microdialysis. Neuropharmacology 50 , 726–732 (2006).
Haddjeri, N., de Montigny, C. & Blier, P. Modulation of the firing activity of noradrenergic neurones in the rat locus coeruleus by the 5-hydroxtryptamine system. Br. J. Pharmacol. 120 , 865–875 (1997).
Szabo, S. T., de Montigny, C. & Blier, P. Modulation of noradrenergic neuronal firing by selective serotonin reuptake blockers. Br. J. Pharmacol. 126 , 568–571 (1999).
Jang, S. W. et al. Amitriptyline is a TrkA and TrkB receptor agonist that promotes TrkA/TrkB heterodimerization and has potent neurotrophic activity. Chem. Biol. 16 , 644–656 (2009).
Casarotto, P. C. et al. Antidepressant drugs act by directly binding to TRKB neurotrophin receptors. Cell 184 , 1299–1313.e1219 (2021).
Li, B. et al. Down-regulation of GluK2 kainate receptor expression by chronic treatment with mood-stabilizing anti-convulsants or lithium in cultured astrocytes and brain, but not in neurons. Neuropharmacology 57 , 375–385 (2009).
Diaz, S. L., Narboux-Neme, N., Boutourlinsky, K., Doly, S. & Maroteaux, L. Mice lacking the serotonin 5-HT2B receptor as an animal model of resistance to selective serotonin reuptake inhibitors antidepressants. Eur. Neuropsychopharmacol. 26 , 265–279 (2016).
Sviridova, A. et al. The role of 5-HT(2B)-receptors in fluoxetine-mediated modulation of Th17- and Th1-cells in multiple sclerosis. J. Neuroimmunol. 356 , 577608 (2021).
Hertz, L. Isotope-based quantitation of uptake, release, and metabolism of glutamate and glucose in cultured astrocytes. Methods Mol. Biol. 814 , 305–323 (2012).
Arakawa, R. et al. Venlafaxine ER blocks the norepinephrine transporter in the brain of patients with major depressive disorder: a PET study using [18F]FMeNER-D2. Int J. Neuropsychopharmacol. 22 , 278–285 (2019).
Gould, G. G., Javors, M. A. & Frazer, A. Effect of chronic administration of duloxetine on serotonin and norepinephrine transporter binding sites in rat brain. Biol. Psychiatry 61 , 210–215 (2007).
Owens, M. J. et al. Estimates of serotonin and norepinephrine transporter inhibition in depressed patients treated with paroxetine or venlafaxine. Neuropsychopharmacology 33 , 3201–3212 (2008).
Sramek, J. J. et al. Exploratory biomarker study of the triple reuptake inhibitor sep-432 compared to the dual reuptake inhibitor duloxetine in healthy normal subjects. CNS Neurosci. Ther. 22 , 404–412 (2016).
Wang, Y. et al. The association between antidepressant treatment and brain connectivity in two double-blind, placebo-controlled clinical trials: a treatment mechanism study. Lancet Psychiatry 6 , 667–674 (2019).
Berman, R. M. et al. Antidepressant effects of ketamine in depressed patients. Biol. Psychiatry 47 , 351–354 (2000).
Murrough, J. W. et al. Antidepressant efficacy of ketamine in treatment-resistant major depression: a two-site randomized controlled trial. Am. J. Psychiatry 170 , 1134–1142 (2013).
Zanos, P. et al. NMDAR inhibition-independent antidepressant actions of ketamine metabolites. Nature 533 , 481–486 (2016).
Li, N. et al. mTOR-dependent synapse formation underlies the rapid antidepressant effects of NMDA antagonists. Science 329 , 959–964 (2010).
Li, N. et al. Glutamate N-methyl-D-aspartate receptor antagonists rapidly reverse behavioral and synaptic deficits caused by chronic stress exposure. Biol. Psychiatry 69 , 754–761 (2011).
Beurel, E., Grieco, S. F., Amadei, C., Downey, K. & Jope, R. S. Ketamine-induced inhibition of glycogen synthase kinase-3 contributes to the augmentation of alpha-amino-3-hydroxy-5-methylisoxazole-4-propionic acid (AMPA) receptor signaling. Bipolar Disord. 18 , 473–480 (2016).
Miller, O. H., Moran, J. T. & Hall, B. J. Two cellular hypotheses explaining the initiation of ketamine’s antidepressant actions: direct inhibition and disinhibition. Neuropharmacology 100 , 17–26 (2016).
Mingardi, J. et al. miR-9-5p is involved in the rescue of stress-dependent dendritic shortening of hippocampal pyramidal neurons induced by acute antidepressant treatment with ketamine. Neurobiol. Stress 15 , 100381 (2021).
Krystal, J. H., Charney, D. S. & Duman, R. S. A new rapid-acting antidepressant. Cell 181 , 7 (2020).
Moliner, R. et al. Psychedelics promote plasticity by directly binding to BDNF receptor TrkB. Nat. Neurosci. 26 , 1032–1041 (2023).
Halberstadt, A. L., Koedood, L., Powell, S. B. & Geyer, M. A. Differential contributions of serotonin receptors to the behavioral effects of indoleamine hallucinogens in mice. J. Psychopharmacol. 25 , 1548–1561 (2011).
Hesselgrave, N., Troppoli, T. A., Wulff, A. B., Cole, A. B. & Thompson, S. M. Harnessing psilocybin: antidepressant-like behavioral and synaptic actions of psilocybin are independent of 5-HT2R activation in mice. Proc. Natl Acad. Sci. USA 118 , e2022489118 (2021).
Cao, D. et al. Structure-based discovery of nonhallucinogenic psychedelic analogs. Science 375 , 403–411 (2022).
Shao, L. X. et al. Psilocybin induces rapid and persistent growth of dendritic spines in frontal cortex in vivo. Neuron 109 , 2535–2544 e2534 (2021).
Caraci, F. et al. Neurobiological links between depression and AD: The role of TGF-beta1 signaling as a new pharmacological target. Pharmacol. Res 130 , 374–384 (2018).
Chen, B., Wang, J. F., Sun, X. & Young, L. T. Regulation of GAP-43 expression by chronic desipramine treatment in rat cultured hippocampal cells. Biol. Psychiatry 53 , 530–537 (2003).
Lee, K. M. & Kim, Y. K. The role of IL-12 and TGF-beta1 in the pathophysiology of major depressive disorder. Int. Immunopharmacol. 6 , 1298–1304 (2006).
Sutcigil, L. et al. Pro- and anti-inflammatory cytokine balance in major depression: effect of sertraline therapy. Clin. Dev. Immunol. 2007 , 76396 (2007).
Zhao, Y. N. et al. Nelumbo nucifera gaertn stems (Hegeng) improved depression Behavior in CUMS mice by regulating NCAM and GAP-43 expression. Evid. Based Complement Altern. Med 2020 , 3056954 (2020).
Zavvari, F., Nahavandi, A. & Goudarzi, M. Fluoxetine attenuates stress-induced depressive-like behavior through modulation of hippocampal GAP43 and neurogenesis in male rats. J. Chem. Neuroanat. 103 , 101711 (2020).
Wang, Q., Timberlake, M. A. 2nd, Prall, K. & Dwivedi, Y. The recent progress in animal models of depression. Prog. Neuropsychopharmacol. Biol. Psychiatry 77 , 99–109 (2017).
Aten, S. et al. Chronic stress impairs the structure and function of astrocyte networks in an animal model of depression. Neurochem. Res. 48 , 1191–1210 (2023).
Liang, S. et al. Iron aggravates the depressive phenotype of stressed mice by compromising the glymphatic system. Neurosci. Bull. 36 , 1542–1546 (2020).
Antoniuk, S., Bijata, M., Ponimaskin, E. & Wlodarczyk, J. Chronic unpredictable mild stress for modeling depression in rodents: meta-analysis of model reliability. Neurosci. Biobehav Rev. 99 , 101–116 (2019).
Fernandez, D. C. et al. Light affects mood and learning through distinct retina-brain pathways. Cell 175 , 71–84.e18 (2018).
Smolders, K. C., de Kort, Y. A. & Cluitmans, P. J. A higher illuminance induces alertness even during office hours: findings on subjective measures, task performance and heart rate measures. Physiol. Behav. 107 , 7–16 (2012).
Liu, A. et al. Encoding of environmental illumination by primate melanopsin neurons. Science 379 , 376–381 (2023).
Lam, R. W. et al. Efficacy of bright light treatment, fluoxetine, and the combination in patients with nonseasonal major depressive disorder: a randomized clinical trial. JAMA Psychiatry 73 , 56–63 (2016).
Rutten, S. et al. Bright light therapy for depression in Parkinson disease: a randomized controlled trial. Neurology 92 , e1145–e1156 (2019).
Li, V. W. et al. Functional outcomes with bright light in monotherapy and combined with fluoxetine in patients with major depressive disorder: results from the LIFE-D trial. J. Affect. Disord. 297 , 396–400 (2022).
Guzel Ozdemir, P. et al. Comparison of venlafaxine alone versus venlafaxine plus bright light therapy combination for severe major depressive disorder. J. Clin. Psychiatry 76 , e645–654 (2015).
Lambert, G. W., Reid, C., Kaye, D. M., Jennings, G. L. & Esler, M. D. Effect of sunlight and season on serotonin turnover in the brain. Lancet 360 , 1840–1842 (2002).
Huang, L. et al. A visual circuit related to habenula underlies the antidepressive effects of light therapy. Neuron 102 , 128–142.e128 (2019).
McClintock, S. M. et al. Consensus recommendations for the clinical application of repetitive transcranial magnetic stimulation (rTMS) in the treatment of depression. J. Clin. Psychiatry 79 , 16cs10905 (2018).
Wall, C. A. et al. Adjunctive use of repetitive transcranial magnetic stimulation in depressed adolescents: a prospective, open pilot study. J. Clin. Psychiatry 72 , 1263–1269 (2011).
Qiu, H. et al. Efficacy and safety of repetitive transcranial magnetic stimulation in children and adolescents with depression: a systematic review and preliminary meta-analysis. J. Affect. Disord. 320 , 305–312 (2023).
Mutz, J. et al. Comparative efficacy and acceptability of non-surgical brain stimulation for the acute treatment of major depressive episodes in adults: systematic review and network meta-analysis. BMJ 364 , l1079 (2019).
Faller, J. et al. Daily prefrontal closed-loop repetitive transcranial magnetic stimulation (rTMS) produces progressive EEG quasi-alpha phase entrainment in depressed adults. Brain Stimul. 15 , 458–471 (2022).
Kaster, T. S. et al. Efficacy, tolerability, and cognitive effects of deep transcranial magnetic stimulation for late-life depression: a prospective randomized controlled trial. Neuropsychopharmacology 43 , 2231–2238 (2018).
Desbeaumes Jodoin, V., Miron, J. P. & Lesperance, P. Safety and efficacy of accelerated repetitive transcranial magnetic stimulation protocol in elderly depressed unipolar and bipolar patients. Am. J. Geriatr. Psychiatry 27 , 548–558 (2019).
Wathra, R. A. et al. Effect of prior pharmacotherapy on remission with sequential bilateral theta-burst versus standard bilateral repetitive transcranial magnetic stimulation in treatment-resistant late-life depression. Br. J. Psychiatry 223 , 504–506 (2023).
Cox, E. Q. et al. Repetitive transcranial magnetic stimulation for the treatment of postpartum depression. J. Affect. Disord. 264 , 193–200 (2020).
Pascual-Leone, A., Rubio, B., Pallardo, F. & Catala, M. D. Rapid-rate transcranial magnetic stimulation of left dorsolateral prefrontal cortex in drug-resistant depression. Lancet 348 , 233–237 (1996).
Zrenner, B. et al. Brain oscillation-synchronized stimulation of the left dorsolateral prefrontal cortex in depression using real-time EEG-triggered TMS. Brain Stimul. 13 , 197–205 (2020).
Fitzgerald, P. B. Targeting repetitive transcranial magnetic stimulation in depression: do we really know what we are stimulating and how best to do it? Brain Stimul. 14 , 730–736 (2021).
Rosen, A. C. et al. Targeting location relates to treatment response in active but not sham rTMS stimulation. Brain Stimul. 14 , 703–709 (2021).
Moffitt, T. E. et al. How common are common mental disorders? Evidence that lifetime prevalence rates are doubled by prospective versus retrospective ascertainment. Psychol. Med. 40 , 899–909 (2010).
Brini, S. et al. Efficacy and safety of transcranial magnetic stimulation for treating major depressive disorder: An umbrella review and re-analysis of published meta-analyses of randomised controlled trials. Clin. Psychol. Rev. 100 , 102236 (2023).
Qaseem, A., Barry, M. J. & Kansagara, D. Clinical Guidelines Committee of the American College of PhysiciansNonpharmacologic versus pharmacologic treatment of adult patients with major depressive disorder: a clinical practice guideline from the american college of physicians. Ann. Intern. Med. 164 , 350–359 (2016).
Biesheuvel-Leliefeld, K. E. et al. Effectiveness of psychological interventions in preventing recurrence of depressive disorder: meta-analysis and meta-regression. J. Affect. Disord. 174 , 400–410 (2015).
Breedvelt, J. J. F. et al. Psychological interventions as an alternative and add-on to antidepressant medication to prevent depressive relapse: systematic review and meta-analysis. Br. J. Psychiatry 219 , 538–545 (2021).
Guidi, J. & Fava, G. A. Sequential combination of pharmacotherapy and psychotherapy in major depressive disorder: a systematic review and meta-analysis. JAMA Psychiatry 78 , 261–269 (2021).
Tang, Y., Yin, H. Y., Rubini, P., Illes, P. & Acupuncture-Induced Analgesia: a neurobiological basis in purinergic signaling. Neuroscientist 22 , 563–578 (2016).
Chan, Y. Y., Lo, W. Y., Yang, S. N., Chen, Y. H. & Lin, J. G. The benefit of combined acupuncture and antidepressant medication for depression: a systematic review and meta-analysis. J. Affect Disord. 176 , 106–117 (2015).
Mischoulon, D., Brill, C. D., Ameral, V. E., Fava, M. & Yeung, A. S. A pilot study of acupuncture monotherapy in patients with major depressive disorder. J. Affect Disord. 141 , 469–473 (2012).
Yue, N. et al. Electro-acupuncture alleviates chronic unpredictable stress-induced depressive- and anxiety-like behavior and hippocampal neuroinflammation in rat model of depression. Front. Mol. Neurosci. 11 , 149 (2018).
Jung, J. et al. Lipidomics reveals that acupuncture modulates the lipid metabolism and inflammatory interaction in a mouse model of depression. Brain Behav. Immun. 94 , 424–436 (2021).
Lin, S. S. et al. Electroacupuncture prevents astrocyte atrophy to alleviate depression. Cell Death Dis. 14 , 343 (2023).
Wang, Z. et al. Acupuncture treatment modulates the corticostriatal reward circuitry in major depressive disorder. J. Psychiatr. Res. 84 , 18–26 (2017).
Duan, D. M., Tu, Y., Liu, P. & Jiao, S. Antidepressant effect of electroacupuncture regulates signal targeting in the brain and increases brain-derived neurotrophic factor levels. Neural Regen. Res. 11 , 1595–1602 (2016).
Bai, L. et al. Mechanisms underlying the antidepressant effect of acupuncture via the CaMK signaling pathway. Front Behav. Neurosci. 14 , 563698 (2020).
Cai, X. et al. Electroacupuncture alleviated depression-like behaviors in ventromedial prefrontal cortex of chronic unpredictable mild stress-induced rats: Increasing synaptic transmission and phosphorylating dopamine transporter. CNS Neurosci. Ther. 29 , 2608–2620 (2023).
Xu, G. et al. Clinical evidence for association of acupuncture with improved major depressive disorder: a systematic review and meta-analysis of randomized control trials. Neuropsychobiology 82 , 1–13 (2023).
Ding, Y. et al. Molecular and genetic characterization of depression: overlap with other psychiatric disorders and aging. Mol. Neuropsychiatry 1 , 1–12 (2015).
PubMed PubMed Central Google Scholar
Kay, R. B. & Brunjes, P. C. Diversity among principal and GABAergic neurons of the anterior olfactory nucleus. Front. Cell. Neurosci. 8 , 111 (2014).
Karolewicz, B. et al. Reduced level of glutamic acid decarboxylase-67 kDa in the prefrontal cortex in major depression. Int. J. Neuropsychopharmacol. 13 , 411–420 (2010).
Azmitia, E. C. Serotonin neurons, neuroplasticity, and homeostasis of neural tissue. Neuropsychopharmacology 21 , 33S–45S (1999).
Kerman, I. A. et al. Evidence for transcriptional factor dysregulation in the dorsal raphe nucleus of patients with major depressive disorder. Front. Neurosci. 6 , 135 (2012).
Keller-Wood, M. Hypothalamic-pituitary–adrenal axis-feedback control. Compr. Physiol. 5 , 1161–1182 (2015).
Alt, S. R. et al. Differential expression of glucocorticoid receptor transcripts in major depressive disorder is not epigenetically programmed. Psychoneuroendocrinology 35 , 544–556 (2010).
van Loo, H. M., Aggen, S. H., Gardner, C. O. & Kendler, K. S. Sex similarities and differences in risk factors for recurrence of major depression. Psychol. Med. 48 , 1685–1693 (2018).
Salk, R. H., Hyde, J. S. & Abramson, L. Y. Gender differences in depression in representative national samples: meta-analyses of diagnoses and symptoms. Psychol. Bull. 143 , 783–822 (2017).
Cavanagh, A., Wilson, C. J., Kavanagh, D. J. & Caputi, P. Differences in the expression of symptoms in men versus women with depression: a systematic review and meta-analysis. Harv. Rev. Psychiatry 25 , 29–38 (2017).
Marcus, S. M. et al. Gender differences in depression: findings from the STAR*D study. J. Affect Disord. 87 , 141–150 (2005).
Poulter, M. O. et al. GABAA receptor promoter hypermethylation in suicide brain: implications for the involvement of epigenetic processes. Biol. Psychiatry 64 , 645–652 (2008).
Seney, M. L. et al. The role of genetic sex in affect regulation and expression of GABA-related genes across species. Front. Psychiatry 4 , 104 (2013).
Gray, A. L., Hyde, T. M., Deep-Soboslay, A., Kleinman, J. E. & Sodhi, M. S. Sex differences in glutamate receptor gene expression in major depression and suicide. Mol. Psychiatry 20 , 1057–1068 (2015).
Goswami, D. B., May, W. L., Stockmeier, C. A. & Austin, M. C. Transcriptional expression of serotonergic regulators in laser-captured microdissected dorsal raphe neurons of subjects with major depressive disorder: sex-specific differences. J. Neurochem. 112 , 397–409 (2010).
Szewczyk, B. et al. Gender-specific decrease in NUDR and 5-HT1A receptor proteins in the prefrontal cortex of subjects with major depressive disorder. Int. J. Neuropsychopharmacol. 12 , 155–168 (2009).
Fiori, L. M. et al. miR-323a regulates ERBB4 and is involved in depression. Mol. Psychiatry 26 , 4191–4204 (2021).
Roy, B., Wang, Q., Palkovits, M., Faludi, G. & Dwivedi, Y. Altered miRNA expression network in locus coeruleus of depressed suicide subjects. Sci. Rep. 7 , 4387 (2017).
Zheng, P. et al. Identification of sex-specific urinary biomarkers for major depressive disorder by combined application of NMR- and GC-MS-based metabonomics. Transl. Psychiatry 6 , e955 (2016).
Liu, X. et al. Discovery and validation of plasma biomarkers for major depressive disorder classification based on liquid chromatography-mass spectrometry. J. Proteome Res. 14 , 2322–2330 (2015).
Liu, X. et al. Plasma lipidomics reveals potential lipid markers of major depressive disorder. Anal. Bioanal. Chem. 408 , 6497–6507 (2016).
Wang, Z., Gerstein, M. & Snyder, M. RNA-Seq: a revolutionary tool for transcriptomics. Nat. Rev. Genet 10 , 57–63 (2009).
Xu, Y. et al. Serum cytokines-based biomarkers in the diagnosis and monitoring of therapeutic response in patients with major depressive disorder. Int. Immunopharmacol. 118 , 110108 (2023).
Pantazatos, S. P. et al. Whole-transcriptome brain expression and exon-usage profiling in major depression and suicide: evidence for altered glial, endothelial and ATPase activity. Mol. Psychiatry 22 , 760–773 (2017).
Herbet, M. et al. Altered expression of genes involved in brain energy metabolism as adaptive responses in rats exposed to chronic variable stress; changes in cortical level of glucogenic and neuroactive amino acids. Mol. Med. Rep. 19 , 2386–2396 (2019).
Yamanishi, K. et al. Analysis of genes linked to depressive-like behaviors in interleukin-18-deficient mice: gene expression profiles in the brain. Biomed. Rep. 12 , 3–10 (2020).
Kuperman, Y. et al. Perifornical Urocortin-3 mediates the link between stress-induced anxiety and energy homeostasis. Proc. Natl Acad. Sci. USA 107 , 8393–8398 (2010).
Bustamante, A. C., Armstrong, D. L. & Uddin, M. Epigenetic profiles associated with major depression in the human brain. Psychiatry Res. 260 , 439–442 (2018).
Beger, R. D. et al. Metabolomics enables precision medicine: “A White Paper, Community Perspective”. Metabolomics 12 , 149 (2016).
Kaddurah-Daouk, R. et al. Cerebrospinal fluid metabolome in mood disorders-remission state has a unique metabolic profile. Sci. Rep. 2 , 667 (2012).
Schmaal, L. et al. Cortical abnormalities in adults and adolescents with major depression based on brain scans from 20 cohorts worldwide in the ENIGMA Major Depressive Disorder Working Group. Mol. Psychiatry 22 , 900–909 (2017).
Schmitgen, M. M. et al. Aberrant cortical neurodevelopment in major depressive disorder. J. Affect. Disord. 243 , 340–347 (2019).
Chen, Z. et al. High-field magnetic resonance imaging of structural alterations in first-episode, drug-naive patients with major depressive disorder. Transl. Psychiatry 6 , e942 (2016).
Grimm, S. et al. Increased self-focus in major depressive disorder is related to neural abnormalities in subcortical-cortical midline structures. Hum. Brain Mapp. 30 , 2617–2627 (2009).
Yoshimura, S. et al. Cognitive behavioral therapy for depression changes medial prefrontal and ventral anterior cingulate cortex activity associated with self-referential processing. Soc. Cogn. Affect. Neurosci. 9 , 487–493 (2014).
Yu, H. et al. Functional brain abnormalities in major depressive disorder using the Hilbert-Huang transform. Brain Imaging Behav. 12 , 1556–1568 (2018).
Holmes, S. E. et al. Lower synaptic density is associated with depression severity and network alterations. Nat. Commun. 10 , 1529 (2019).
Bettini, E. et al. Pharmacological comparative characterization of REL-1017 (Esmethadone-HCl) and Other NMDAR channel blockers in human heterodimeric N-methyl-D-aspartate receptors. Pharmaceuticals (Basel) 15 , 997 (2022).
Hanania, T., Manfredi, P., Inturrisi, C. & Vitolo, O. V. The N-methyl-D-aspartate receptor antagonist d-methadone acutely improves depressive-like behavior in the forced swim test performance of rats. Exp. Clin. Psychopharmacol. 28 , 196–201 (2020).
Fogaca, M. V. et al. N-Methyl-D-aspartate receptor antagonist d-methadone produces rapid, mTORC1-dependent antidepressant effects. Neuropsychopharmacology 44 , 2230–2238 (2019).
Fava, M. et al. REL-1017 (esmethadone) as adjunctive treatment in patients with major depressive disorder: a phase 2a randomized double-blind trial. Am. J. Psychiatry 179 , 122–131 (2022).
De Martin, S. et al. REL-1017 (Esmethadone) increases circulating BDNF levels in healthy subjects of a phase 1 clinical study. Front. Pharmacol. 12 , 671859 (2021).
Chou, T. H., Kang, H., Simorowski, N., Traynelis, S. F. & Furukawa, H. Structural insights into assembly and function of GluN1-2C, GluN1-2A-2C, and GluN1-2D NMDARs. Mol. Cell 82 , 4548–4563.e4544 (2022).
Hochschild, A. et al. Ketamine vs midazolam: Mood improvement reduces suicidal ideation in depression. J. Affect Disord. 300 , 10–16 (2022).
Burgdorf, J. et al. GLYX-13, a NMDA receptor glycine-site functional partial agonist, induces antidepressant-like effects without ketamine-like side effects. Neuropsychopharmacology 38 , 729–742 (2013).
Gigliucci, V. et al. Ketamine elicits sustained antidepressant-like activity via a serotonin-dependent mechanism. Psychopharmacology (Berl.) 228 , 157–166 (2013).
Daly, E. J. et al. Efficacy and safety of intranasal esketamine adjunctive to oral antidepressant therapy in treatment-resistant depression: a randomized clinical trial. JAMA Psychiatry 75 , 139–148 (2018).
Zhao, J. et al. Low-dose ketamine inhibits neuronal apoptosis and neuroinflammation in PC12 cells via alpha7nAChR mediated TLR4/MAPK/NF-kappaB signaling pathway. Int. Immunopharmacol. 117 , 109880 (2023).
Yang, Y. et al. Ketamine relieves depression-like behaviors induced by chronic postsurgical pain in rats through anti-inflammatory, anti-oxidant effects and regulating BDNF expression. Psychopharmacology (Berl.) 237 , 1657–1669 (2020).
Shibakawa, Y. S. et al. Effects of ketamine and propofol on inflammatory responses of primary glial cell cultures stimulated with lipopolysaccharide. Br. J. Anaesth. 95 , 803–810 (2005).
Abbasi, S., Hosseini, F., Modabbernia, A., Ashrafi, M. & Akhondzadeh, S. Effect of celecoxib add-on treatment on symptoms and serum IL-6 concentrations in patients with major depressive disorder: randomized double-blind placebo-controlled study. J. Affect. Disord. 141 , 308–314 (2012).
Akhondzadeh, S. et al. Clinical trial of adjunctive celecoxib treatment in patients with major depression: a double blind and placebo controlled trial. Depress Anxiety 26 , 607–611 (2009).
Nettis, M. A. et al. Augmentation therapy with minocycline in treatment-resistant depression patients with low-grade peripheral inflammation: results from a double-blind randomised clinical trial. Neuropsychopharmacology 46 , 939–948 (2021).
Hasebe, K. et al. Exploring interleukin-6, lipopolysaccharide-binding protein and brain-derived neurotrophic factor following 12 weeks of adjunctive minocycline treatment for depression. Acta Neuropsychiatr. 34 , 220–227 (2022).
Su, K. et al. Omega-3 fatty acids in the prevention of interferon-alpha-induced depression: results from arandomized, controlled trial. Biol. Psychiatry 76 , 559–566 (2014).
Berk, M. et al. Youth Depression Alleviation with Anti-inflammatory Agents (YoDA-A): a randomised clinical trial of rosuvastatin and aspirin. BMC Med . 18 , 16 (2020).
Meltzer-Brody, S. et al. Brexanolone injection in post-partum depression: two multicentre, double-blind, randomised, placebo-controlled, phase 3 trials. Lancet 392 , 1058–1070 (2018).
Leal, G. C. et al. Arketamine as adjunctive therapy for treatment-resistant depression: A placebo-controlled pilot study. J Affect. Disord. 330 , 7–15 (2023).
Kuga, N., Sasaki, T., Takahara, Y., Matsuki, N. & Ikegaya, Y. Large-scale calcium waves traveling through astrocytic networks in vivo. J. Neurosci. 31 , 2607–2614 (2011).
Shawcross, D. L. et al. Infection and systemic inflammation, not ammonia, are associated with Grade 3/4 hepatic encephalopathy, but not mortality in cirrhosis. J. Hepatol. 54 , 640–649 (2011).
Page, G. et al. The up-regulation of the striatal dopamine transporter’s activity by cAMP is PKA-, CaMK II- and phosphatase-dependent. Neurochem. Int. 45 , 627–632 (2004).
França, A. S. et al. D2 dopamine receptor regulation of learning, sleep and plasticity. Eur. Neuropsychopharmacol. 25 , 493–504 (2015).
Beaulieu, J. M. & Gainetdinov, R. R. The physiology, signaling, and pharmacology of dopamine receptors. Pharmacol. Rev. 63 , 182–217 (2011).
Opal, M. D. et al. Serotonin 2C receptor antagonists induce fast-onset antidepressant effects. Mol. Psychiatry 19 , 1106–1114 (2014).
Seki, K., Yoshida, S. & Jaiswal, M. K. Molecular mechanism of noradrenaline during the stress-induced major depressive disorder. Neural Regen. Res. 13 , 1159–1169 (2018).
Duman, R. S., Aghajanian, G. K., Sanacora, G. & Krystal, J. H. Synaptic plasticity and depression: new insights from stress and rapid-acting antidepressants. Nat. Med. 22 , 238–249 (2016).
Yang, B. et al. Comparison of R-ketamine and rapastinel antidepressant effects in the social defeat stress model of depression. Psychopharmacology 233 , 3647–3657 (2016).
Download references
Acknowledgements
This work was supported by the National Natural Science Foundation of China, MX [grant number 32271038] and BL [grant number 81871852]; Shenyang Science and Technology Innovation Talents Project, BL [grant number RC210251]; ‘ChunHui’ Program of Education Ministry, BL [grant number 2020703]; National Natural Science Foundation of China-Russian Science Foundation (NSFC-RSF), YT [grant number 82261138557]; Sichuan Provincial Administration of Traditional Chinese Medicine, YT [grant number 2023zd024].
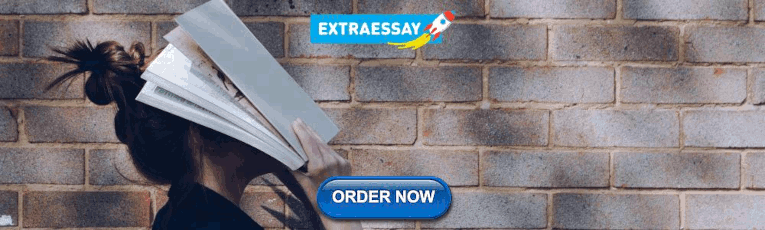
Author information
Authors and affiliations.
Department of Forensic Analytical Toxicology, School of Forensic Medicine, China Medical University, Shenyang, China
Lulu Cui, Shu Li, Siman Wang, Xiafang Wu, Yingyu Liu, Weiyang Yu, Yijun Wang & Baoman Li
Liaoning Province Key Laboratory of Forensic Bio-evidence Sciences, Shenyang, China
China Medical University Centre of Forensic Investigation, Shenyang, China
International Joint Research Centre on Purinergic Signalling/Key Laboratory of Acupuncture for Senile Disease (Chengdu University of TCM), Ministry of Education/School of Health and Rehabilitation, Chengdu University of Traditional Chinese Medicine/Acupuncture and Chronobiology Key Laboratory of Sichuan Province, Chengdu, China
Department of Orthopaedics, The First Hospital, China Medical University, Shenyang, China
Maosheng Xia
You can also search for this author in PubMed Google Scholar
Contributions
L.C., X.W., and B.L. provided direction and guidance throughout the preparation of this manuscript. L.C., X.W., and B.L. wrote and edited the manuscript. L.C., S.L., S.W., M.X., and B.L. reviewed and made significant revisions to the manuscript. L.C., S.L., S.W., X.W., Y.L., W.Y., Y.W., Y.T., M.X., and B.L. collected and prepared the related papers. All authors have read and approved the article.
Corresponding authors
Correspondence to Maosheng Xia or Baoman Li .
Ethics declarations
Competing interests.
The authors declare no competing interests.
Rights and permissions
Open Access This article is licensed under a Creative Commons Attribution 4.0 International License, which permits use, sharing, adaptation, distribution and reproduction in any medium or format, as long as you give appropriate credit to the original author(s) and the source, provide a link to the Creative Commons license, and indicate if changes were made. The images or other third party material in this article are included in the article’s Creative Commons license, unless indicated otherwise in a credit line to the material. If material is not included in the article’s Creative Commons license and your intended use is not permitted by statutory regulation or exceeds the permitted use, you will need to obtain permission directly from the copyright holder. To view a copy of this license, visit http://creativecommons.org/licenses/by/4.0/ .
Reprints and permissions
About this article
Cite this article.
Cui, L., Li, S., Wang, S. et al. Major depressive disorder: hypothesis, mechanism, prevention and treatment. Sig Transduct Target Ther 9 , 30 (2024). https://doi.org/10.1038/s41392-024-01738-y
Download citation
Received : 20 September 2023
Revised : 24 December 2023
Accepted : 28 December 2023
Published : 09 February 2024
DOI : https://doi.org/10.1038/s41392-024-01738-y
Share this article
Anyone you share the following link with will be able to read this content:
Sorry, a shareable link is not currently available for this article.
Provided by the Springer Nature SharedIt content-sharing initiative
Quick links
- Explore articles by subject
- Guide to authors
- Editorial policies


An official website of the United States government
The .gov means it’s official. Federal government websites often end in .gov or .mil. Before sharing sensitive information, make sure you’re on a federal government site.
The site is secure. The https:// ensures that you are connecting to the official website and that any information you provide is encrypted and transmitted securely.
- Publications
- Account settings
Preview improvements coming to the PMC website in October 2024. Learn More or Try it out now .
- Advanced Search
- Journal List
- Pharmaceuticals (Basel)

Monoamine Neurotransmitters Control Basic Emotions and Affect Major Depressive Disorders
1 Institute of Brain and Psychological Sciences, Sichuan Normal University, Chengdu 610066, China
2 Department of Psychology, Medical School, Jiangsu University, Zhenjiang 210023, China
3 Department of Neurology, Affiliated Lianyungang Hospital of Chinese Medicine, Nanjing University of Chinese Medicine, Nanjing 222000, China
4 School of Pharmacy, Chengdu University of Traditional Chinese Medicine, Chengdu 616000, China
Fushun Wang
Jason h. huang.
5 Department of Neurosurgery, Baylor Scott & White Health, Temple, TX 79409, USA
6 Department of Surgery, College of Medicine, Texas A&M University, Temple, TX 79409, USA
Associated Data
Data sharing not applicable.
Major depressive disorder (MDD) is a common and complex mental disorder, that adversely impacts an individual’s quality of life, but its diagnosis and treatment are not accurately executed and a symptom-based approach is utilized in most cases, due to the lack of precise knowledge regarding the pathophysiology. So far, the first-line treatments are still based on monoamine neurotransmitters. Even though there is a lot of progress in this field, the mechanisms seem to get more and more confusing, and the treatment is also getting more and more controversial. In this study, we try to review the broad advances of monoamine neurotransmitters in the field of MDD, and update its effects in many advanced neuroscience studies. We still propose the monoamine hypothesis but paid special attention to their effects on the new pathways for MDD, such as inflammation, oxidative stress, neurotrophins, and neurogenesis, especially in the glial cells, which have recently been found to play an important role in many neurodegenerative disorders, including MDD. In addition, we will extend the monoamine hypothesis to basic emotions; as suggested in our previous reports, the three monoamine neurotransmitters play different roles in emotions: dopamine—joy, norepinephrine—fear (anger), serotonins—disgust (sadness). Above all, this paper tries to give a full picture of the relationship between the MDD and the monoamine neurotransmitters such as DA, NE, and 5-HT, as well as their contributions to the Three Primary Color Model of Basic Emotions (joy, fear, and disgust). This is done by explaining the contribution of the monoamine from many sides for MDD, such the digestive tract, astrocytes, microglial, and others, and very briefly addressing the potential of monoamine neurotransmitters as a therapeutic approach for MDD patients and also the reasons for its limited clinical efficacy, side effects, and delayed onset of action. We hope this review might offer new pharmacological management of MDD.
1. Introduction
Major depressive disorder (MDD) is considered a serious public health issue and is one of the leading causes of disability, disease burden and suicide deaths worldwide [ 1 , 2 ]. Even though there has been a lot of progress in this field, its mechanisms seem to get more and more confusing, and the treatment is getting more and more controversial [ 3 ]. Currently, its diagnosis and treatment are not being accurately executed, and a symptom-based approach is utilized in most cases due to a lack of precise knowledge regarding the pathophysiology. So far, the first-line treatment is still based on monoamine neurotransmitters or most antidepressant drugs are still targeting the monoamine system (including serotonin, noradrenaline, and dopamine), which has been showed to be the substrate for emotions ever since the 1950s–1960s in the last century [ 4 , 5 , 6 , 7 ].
Rather than solely focusing on symptomology, it is imperative to understand the underlying causal mechanisms. Recent research has shown that the processing of information and regulation of emotions are altered in MDD [ 3 ]. Indeed, MDD is a kind of affective disorder characterized by a persistent depressive mood and a lack of interest or pleasure [ 8 ]. However, even though MDD is a kind of emotional disorder, we know almost nothing about the emotional mechanisms or psychological mechanisms of MDD. Thus, the current etiological aspects of MDD fail to explain many fundamental questions about MDD; for example, we do not know why depression tends to occur in some vulnerable populations, such as adolescents, women, and the elderly.
Currently, many depressed patients become insensitive to medication and even further exhibit refractory depression [ 9 , 10 ]. Because there is no systematic theoretical guidance, the diagnosis and treatment often face a dilemma [ 11 ]; antidepressant treatment options are limited and, in some cases, ineffective [ 12 ]. In our previous studies, we have proposed a hypothesis of mood alterations in depression from the basic emotional aspects [ 13 , 14 ]. In this paper, we reviewed recent advances for monoamine neurotransmitters in MDD and further explored their roles in the emotional mechanisms of MDD, hoping to provide a new perspective for the neural mechanisms of depression.
2. Monoamine Neurotransmitters Mediate Three Core Effects
Emotion plays a very important role in our daily lives, affecting our social interactions, with more than 80–90% of human psychological problems being emotional problems. However, emotion is considered to be one of the earliest studied but least known subjects among all life sciences. Recently, many papers have linked emotions to monoamine neurotransmitters, such as the “new three-dimensional model”, which suggested that emotions are mediated by three monoamine neurotransmitters, including (norepinephrine, dopamine, and serotonin). In addition, these three monoamines make up the three dimensions for the emotional cubic [ 6 ]. However, even though tons of studies support the role of monoamines in emotions, their effects are quite mixed. For examples, most psychological stimulants or antidepressants are targeting all three monoamines and are used for almost all of the psychological disorders such as depression and anxiety. Here, we tried to differentiate the roles of monoamines into basic emotions, and originally proposed the ‘ Three Primary Color Model of Basic Emotions ’, which hypothesized that human beings have three core affects, including reward (joy), punishment (disgust), and stress (fear and anger) [ 13 , 15 , 16 ]. The monoamines work together to make different emotions, like the three primary colors ( Figure 1 ). The dopamine (DA) system is involved in reward (joy), and the serotonin (5-HT) system is related to punishment (disgust or sadness), while noradrenaline (NE) is related to fear (anger) and the “fight or flight” behavior during stressful events. These three core affects might be called three prototypical emotions, which can be combined into a variety of compound emotions.

The primary color model of basic emotions. ( A ). Newton’s prismatic colors diagram, and Newton says there are seven primary colors. ( B ). The picture shows Ekman’s seven basic emotions (fear, anger, joy, surprise, contempt, sadness, and disguZ`st). ( C ). The picture shows our hypothesis of three primary emotions: joy, disgust (sadness), and fear (anger), which are linked to three monoamine neurotransmitters.
2.1. The Three Monoamines and Core Affects
The most important feature of depression is the absence of joyful emotions. Dopamine (DA) has become a synonym for joy and reward [ 17 ], thus increasing DA might be a good way for treating MDD [ 18 , 19 ], and, indeed, the US FDA (food and drug agency) recently approved ketamine as a drug capable of rapid antidepressant function in 2019 [ 20 , 21 ]. Ketamine itself is a drug and a stimulant, and one of its main functions is to increase release of dopamine. Even though many studies reported about the mechanisms of ketamine as antidepressants, ranging from effects on NMDA receptors to effects on GABA receptors [ 22 , 23 ], the major reason for ketamine to exert its antidepressant effect is related to DA release, possibly by activating astrocytic Ca 2+ signaling and changing the bursting of neurons in the Habula nucleus [ 24 ]. Thus, raising brain dopamine is certainly a quick way to change the depressed mood [ 25 ].
Norepinephrine (NE) has been known as the neurotransmitter for emotional arousal [ 26 ], and its major function is inducing “fight or flight” behavior [ 27 , 28 , 29 , 30 ], and the “fear and anger” emotion [ 31 ]. Epinephrine and NE are secreted by the LC (Locus Coeruleus) in the brain, while they are released to the blood by adrenal glands. It was in 1923 that Cannon suggested that the major function of the NE is inducing stress in the body, leading to ‘fight or flight’ behavior. We proposed for the first time that anger and fear might be twin emotions as they share the same neurotransmitter NE/ adrenaline [ 32 ]. Indeed, some previous reports also suggested that NE and adrenaline mediated fear and anger; for example, Kabitzke et al tried to distinguish fear and anger based on these two neurotransmitters, and suggested that NE mediates fear and adrenaline mediates anger [ 33 , 34 ]. Here we suggested that fear and anger are two faces of the same coin, and mediated by the same neurotransmitters, together with the sympathetic nervous system [ 35 ].
Disgust is the least studied basic emotions; recently, a growing number of studies suggest that disgust plays a significant role in our lives and in many psychological disorders [ 35 , 36 ]. Many experiments have confirmed the reward function of DA and the mood arousal function of NE, but the relationship between serotonin (5-HT) and depression is much more important, for most current antidepressants are targeting 5-HT [ 37 , 38 , 39 ]. However, the role of 5-HT in depression is complicated by a diversity of 5-HT receptors, and the prevalence of its distribution in the organism. 5-HT has a wide range of receptors, including 14 different receptor subtypes, and are distributed throughout the body, especially in the gastrointestinal tract [ 40 ]. More than 90% of the 5-HT in the body are released in the gastrointestinal tract, and many 5-HT receptors are also distributed in the intestine, such as 5-HT1 A [ 41 ]. 5-HT in the gut produces a possibility that gut alterations may be important in the pathophysiology of MDD [ 42 ]. This may be the reason that many studies have recently found that the brain–gut axis alternation is an important cause of depression, and indeed the greatest side effect of increased 5-HT is an increase in gastrointestinal nausea and vomiting response [ 43 ].
In the central nervous system, 5-HT is mainly released from the Raphe nucleus, whose major function is sleep, so the main side effect is to increase the patient’s sleep, instead of making the patient excited [ 44 ]. Even though many papers proposed the antidepressant function of 5-HT, the real function of central 5-HT in treating MDD might be inducing sleep, sedation, and inhibition of the compulsory thought [ 45 ]. Thus, the function of 5-HT might be suppressing the body’s excitability, instead of being an antidepressant. Indeed, the increase of 5-HT induces a sedative response in the body, which is a behavioral inhibition process [ 46 ], similar to the prolonged helpless state during depression [ 47 ]. Indeed, recent emphasis has been focused mainly on the effects of monoamines on behavioral inhibition [ 48 ]. In addition, recent studies found that the majority of psychological problems stem from the patient’s disgust with the people around them [ 47 ].
2.2. The Significance of Basic Emotion Theory
The hypothesis of monoamine transmitters as the neural basis for emotion was first proposed in the 1950s [ 5 ], and it is still used as the basis for developing antidepressants. However, it is not clear how monoamine transmitters affect emotions in MDD patients. Basic Emotion Theory (BET) is the most prevalent theory about emotion, which suggests that human emotions are composed of a limited number of basic emotions. The basic emotions are evolved to accomplish fundamental life tasks, and each primary emotion has a unique neural structure and physiological basis. Although most studies agreed upon the idea that emotions may consist of some discrete emotions, there is no consensus on the number of basic emotions. For example, Ekman proposed that there are seven basic emotions, including fear, anger, joy, sadness, disgust, and contempt [ 49 ]. The seven basic emotions proposed by Ekman is like the 7-color disk, or like Newton’s disk ( Figure 1 A,B), but there are only three primary colors. Recently, we redefined the basic emotion theory and made an analogy between emotion and colors [ 15 ]. We suggested that human beings may have only three basic emotions, and we originally proposed the ‘Three Primary Color Model of Basic Emotions’. To differentiate them with the definition of basic emotions in previous studies [ 50 , 51 , 52 ], we called them core affects, including reward (joy), punishment (disgust), and stress (fear and anger) ( Figure 1 C) [ 13 , 15 , 16 ]. These three core affects can be combined into a variety of compound emotions.
The Basic Emotion Theory (BET) dates back to Darwin’s book The Expressions of the Emotion in Man and Animals in 1872, in which he proposed that even insects have basic emotions which are similar to those of humans [ 15 , 53 ]. However, even though the brain structures of insects and humans are very different, both insects and humans have three monoamine neurotransmitters: DA, NE, and 5-HT [ 15 ]. Thus, we suggested that these three monoamine neurotransmitters are the neural basis for three core emotions [ 16 , 31 , 54 ]. The three primary color model of basic emotions fully supports the theory of monoamine transmitters for MDD ( Figure 1 ). Anyway, it is not clear what the role of these three core emotions and three monoamines is in the development of depression in addition to how depressed patients have developmental deficits in all three emotions, or if it is simply 5-HT dysfunction. This paper will review a large number of studies, including the genetic and epigenetic aspects of depression pathogenesis, various causative factors such as various developmental stages, and observed alterations in electrophysiological and functional magnetic resonance of the brain. In short, here we try to investigate the neural mechanisms of emotional alterations as well as monoamine neurotransmitters in MDD patients.
3. Monoamine in the Digestive System
The intestine, also known as the second brain, has a close connection with the brain [ 55 ]. Tremendous progress has been made in studying the bidirectional interactions between the gastrointestinal tract and the central nervous system [ 56 ], and understanding the interaction between the digestive system with the brain may provide novel approaches for prevention and treatment of MDD [ 57 , 58 ]. Indeed, the digestive system has also been found to play an important role in MDD, including liver [ 59 , 60 ] and intestine [ 61 ].
3.1. Monoamine and the Microorganisms in the Digestive System
In the digestive system, the increase of serotonin in the gastrointestinal tract leads to aversive nausea and vomiting reactions [ 62 ]. Recent studies found that gut microorganisms can activate enteroendocrine cells and produce corresponding hormones such as 5-hydroxytryptamine (5-HT), dopamine (DA), and norepinephrine (NE), which can affect the central nervous system [ 61 ]. The brain in turn can regulate gastrointestinal functions through the neuro-immune-endocrine system. At the same time, gut microbes and their metabolites can stimulate the enteric nervous system by regulating intestinal motility and participating in bidirectional gut–brain interactions [ 61 ]. During MDD, altered gut microbes modulate the brain mainly through the vagus nerve, the hypothalamic-pituitary-adrenal (HPA) axis, the hypothalamic-pituitary-gonadal (HPG) axis, the immune system, and metabolic pathways, thus causing the hippocampus to preside over mood, emotion, and cognitive functions, which may lead to MDD. Since gut microbes can regulate human emotions, learning, memory, social interaction, ingestion, and other behaviors, the related effects are called gut microbial regulation [ 61 ].
3.2. Brain–Gut Axis
The concept of the gut–brain axis emerged from the recent demonstration that the gut microbiota affect depressant behavior [ 63 ], and, recently, many scientists proposed that the brain–gut axis is a reason for MDD [ 64 , 65 , 66 , 67 ]. For example, Zhang et al. transplanted the gut microbiome of depressed patients into germ-free mice, and induced depression-like behaviors in the recipient mice, suggesting that alterations in the gut flora can induce depressive symptoms [ 68 ]. The human intestinal flora is a dynamic process in different stages of life, and adolescents are in the peak of growth and development, whose intestinal microorganisms are in an unstable state and more diverse. It is found that a surge of anaerobic bacteria in the gut flora of adolescents may be associated with the development of mood disorders such as anxiety and depression during adolescence [ 69 ].
However, no unifying hypothesis has been put forward to explain the physiologic significance of this remarkable phenomenon. Therefore, it is important to investigate the molecular mechanisms of the gut–brain axis interaction and seek new ways to regulate the function of the gut–brain axis for the treatment of adolescent depression. The intestines are closely connected with the brain and also in emotional regulation [ 70 ]. Disgust is a basic emotion induced by unpleasant and repulsive stimuli in the gut. Disgust emotion can induce behavior inhibition [ 49 ], warding off potential diseases and staying away from or avoiding spoiled food or other contaminants [ 14 , 71 ]. The brain–gut-axis induced disgust might be the reason for MDD; indeed, the brain–gut-axis affects disgust (sad) emotions via two main pathways [ 61 ]: (1) Metabolite pathways: Gut microbes can activate monoamines via stimulating the enteroendocrine cells to produce the 5-HT [ 61 ]. (2) Neuroendocrine pathway: The intestinal flora affect the monoamine system in the central nervous system by regulating the secretion of these neurotransmitters and hormones, such as endorphin, tryptophan, and 5-hydroxytryptamine. However, the brain–gut-axis also affects the vagal pathway: The enteric nerve forms synaptic connections with the vagus nerve, which composes the parasympathetic nervous system. The parasympathetic nervous system has been known to reduce the emotional arousal, known as the pleasure nerves [ 72 ].
3.3. Brain–Liver Axis
Chinese medicine has a long history related to believing that depression is due to inhibition of anger in the liver, and antidepressant medications in Chinese medicine often have a liver-regulating effect, such as the powder of bupleurum [ 73 ]. Recently, it has been found that patients with MDD had a significantly higher prevalence and incidence of chronic liver disease than the general population [ 74 ]; thus, the integrative activity of liver and brain might play a role in depression [ 59 , 60 ]. Part of the reason might be due to the metabolites, such as the bile acids [ 55 ]. Bile acids are steroid acids which are synthesized in the liver and further processed in the gut by digestive enzymes. Bile acids participate in a range of important host functions such as monoamine neurotransmitters, short-chain fatty acids, and indoles [ 75 ]. Non-alcoholic fatty liver disease has complex pathogenic mechanisms for MDD [ 76 ]. In addition, the liver is involved in the metabolism of many stress hormones, and neurotransmitters, for example, Cytochrome P450 (CYP), which is primarily expressed in the liver, are some of the main metabolizers of glucocorticoids and monoamines in the peripheral system [ 77 ]. Thus, the link between hepatic glucocorticoid metabolism and central monoamine transmission might be important in pathophysiology of stress-related disorders [ 77 ].
Previously, we proposed that NE and glucocorticoid and sympathetic system might be the neurotransmitter for anger and fear. Lazarus argues that a different expression of fear or anger in a given stressful situation is dependent on the cognitive appraisal of the situation [ 78 ]. He proposed that an individual will have the fear emotion, if his primary appraisal is having insufficient resources in a threatening situation; if he feels like he has sufficient resources, the individual will show the anger emotion [ 79 ]). However, this cognitive appraisal can be trained, such as for animals in CUMS (chronic unexpected mild stress) training. When the animals are trained at the early stage of CUMS training, they believe they are competent and show anger responses. However, with the training that has progressed for a long time, they are unsure about their competence, show no angry responses, and enter a stage of helplessness, which is a characteristic feature for MDD. Of note, this might be the reason why vulnerable groups, such as adolescents, the elderly, and women, are prone to depression because they can easily become helpless and are afraid to show angry emotions.
4. Monoamines and Astrocyte in the Brain
The monoamine hypothesis of MDD provides a robust theoretical framework, forming the core of a large jigsaw puzzle, around which we must look for the vital missing pieces [ 2 ]. Recent emphasis on astrocyte-centric cause of MDD has gained more attention during the past several decades [ 80 ], and a growing number of papers suggest that astrocytes play a key role in depression [ 2 ]. Astrocytes are the most abundant and versatile cells in the brain, and are involved in most brain functions acting as a passive housekeeper or as an active player [ 81 , 82 ]. Astrocytes were considered passive supporting cells, merely maintaining homeostasis by supplying nutrients and cleaning the metabolites [ 83 , 84 ]. Physiological studies during the past decades have found that astrocytes can actively work with these functions by monoamine induced Ca 2+ signaling [ 85 ]. It is found that astrocytes can actively work together in responses to monoamine neurotransmitters, glutamate, and purinergic bases [ 86 , 87 ].
Indeed, it is found that monoamine neurotransmitters and most antidepressants have been proved to work through astrocytes [ 82 ]. For example, we showed that the synchronized release of norepinephrine (NE) from locus coeruleus (LC) projections throughout the cerebral cortex mediate long-ranging Ca 2+ signals by activation of astrocytic α1-adrenergic receptors [ 88 ]. We also found that astrocytes appear to be the primary target for NE via triggering astrocytic Ca 2+ signaling through α1-adrenergic receptors; instead, astrocytes do not respond directly to glutamatergic signaling evoked by sensory stimulation; thus, astrocytes may coordinate the broad effects of neuromodulators on neuronal activity [ 88 , 89 ].
4.1. Astrocytic Loss Might Be a Reason for MDD
Unlike other diseases (e.g., brain injury, Alzheimer’s disease, Parkinson’s disease, etc.) where astrocytic activity is increased and GFAP (glial fibrillary acidic protein) proliferation (astrogliosis) is predominant, MDD patients exhibit reduced numbers and smaller size. Growing evidence reported astrocyte loss in key regions of the limbic system in depressed patients, which might account for the pathology of MDD [ 2 ]. Many studies in animal models have subsequently hinted at the possibility that astrocytic atrophy may play a causative role in the precipitation of depressive symptoms [ 82 ]. This reduction in cell number occurring first in astrocyte cells before that in neurons suggests that astrocyte lesions may be responsible for neuronal damage. This raises an intriguing possibility that the astrocytes may play a central role alongside neurons in the behavioral effects of antidepressant drugs.
4.2. Dysfunction of Buffering Ability in Astrocytes
The involvement of astrocytes in depression might also be due to its effects in buffering extracellular K + , as has been demonstrated, for example, in the study published in Nature by Hailan Hu’s laboratory (Cui et al, 2018). It was found that astrocytes in depressed mice have a reduced potassium buffering capacity and that astrocytes act as a ‘potassium reservoir’ in the perineuronal environment to maintain ion homeostasis of the perineuronal environment [ 90 ]. The most important function of astrocytes is to exchange nutrients and metabolites through the blood–brain barrier formed by the tight link between their terminal feet and the vascular epithelium, which can be called the glymphatic system [ 91 ]. In addition, antidepressants and monoamine neurotransmitters exert profound effects on the gene expression in astrocytes via releasing TGF-β. The inflammatory response induced by our previous experimental stress can trigger astrocytes to secrete some neuroactive substances such as TGF-β. We propose that TGF-β can lead to increased expression and depolarized distribution of NKCC1 and AQP4 in astrocytes, resulting in post-stress brain injury and depression [ 92 ].
4.3. Dysfunction of Glymphatic System
The glymphatic system is a recently defined system in the brain by our lab, to characterize the brain-wide paravascular pathway for cerebrospinal fluid (CSF) and interstitial fluid (ISF) exchange, which helps efficient clearance of solutes and waste from the brain [ 93 ]. Interstitial fluid was formed by cerebrospinal fluid that entered the brain along para-arterial channels, and the interstitial fluid was then cleaned from the brain via the para-venous pathways [ 94 ]. The normal function of glymphatic circulation depends on normal function of astrocytic activity, especially the polarized expression of aquaporin-4 (AQP4), which is also called the water channel. Many recent studies found that dysfunction of glymphatic transport, which can be induced by ageing or inflammation, is involved in MDD [ 95 ]. Liu et al reported that CUMS can induce depression-like symptoms by decreasing monoamines’ neurotransmitter concentration [ 96 ]. Moreover, CUMS decreased polarized expression of AQP4 and inhibited the glymphatic system. Some studies confirmed the "glymphatic dysfunction" hypothesis for MDD, which hypothesized that the stress induced dysfunctional glymphatic pathway serves as a bridge between monoamine disturbance and emotional disorders [ 94 , 97 ]. Indeed, it is found that NE can block the glymphatic system, which works more efficiently during sleep [ 98 ]. This is consistent with the idea that there are sleep problems for MDD patients; however, the role of basic emotion anger or NE is not clear.
5. Monoamines and Microglial in the Brain
Major depressive disorder (MDD) has been recently suggested to be related to inflammation [ 99 ]. In addition, many studies found that stress (fear and anger), which is an important core emotion as we previously suggested [ 31 ], really affects inflammation in MDD [ 100 , 101 ]. In addition, it is found that anger can induce cortisol while fear can induce inflammatory cytokine but a decrease in cortisol, which suggested that anger might mobilize energy and trigger adaptive processes, while fear promotes withdrawal behavior [ 102 ]. Thus, a lack in anger might be the reason for depression related inflammation, and indeed accumulating evidence started to reveal that stress related emotions might affect monoamine oxidase activity, and activate the primary immune cell microglia [ 95 ].
Many recent studies found that impairment of the microglia can lead to depression, and some forms of depression can be considered as a microglial disease (microgliopathy) [ 103 ]. Microglia activation induces releases of many inflammatory factors, such as IL-1β, tumor necrosis factor (TNF-α), and transforming growth factor (TGF-β). These inflammation factors are thought to be important factors for MDD [ 99 ]) by affecting the function of monoamine neurons. Studies suggested that stress related emotions, especially anger [ 104 ], can significantly elevate inflammatory factors, which can induce decreased monoaminergic neurotransmission via oxidative stress. Thus, abnormal function of astrocytes and microglia in the brain is also a major etiology of depression [ 105 ].
5.1. Microglia Cells
Microglia are the resident immune cells, and can induce release of proinflammatory cytokines to induce neuro-inflammation in the central nervous system. Microglia are not resting under physiological conditions; they play an important function in pruning synapses by actively monitoring the development and maturation of synapses. In stressful situations, they can be activated by ATP released from activated astrocytes, in order to induce neuroinflammation [ 106 ]. Microglia were activated by ATP to change its morphology with multiple protrusions, with highly branched or amoeboid morphology, and changed their function by releasing cytokines. Neuroinflammation and oxidative stress may provide the impetus for increased transcription of monoamine oxidase [ 107 ]; monoaminergic drugs can block both activation of microglia and reverse the depression in some way [ 108 ].
5.2. Early Life Stress Induces Microglia Vulnerability
Early life stress has been suggested to the major reason for MDD [ 109 ]. Early life stress induced inflammatory damage that can lead to abnormal neuronal plasticity via enhanced microglia activity and excessive synaptic pruning [ 110 ]. Many studies found that early life stress can induce microglia vulnerability in later lives [ 111 ], by altering phagocytic activity, gene expression, and morphology [ 109 ]. Consistently, it has been shown that chronic stress induces depressive symptoms by affecting microglia’s pruning ability and synaptic plasticity [ 112 ].
Microglia and neuronal interactions play important roles in synaptic plasticity and mediated behaviors during adolescence. Stressful lives during adolescence can induce rapid microglia changes in distribution, morphology, and function, significantly affecting neuronal and synaptic development [ 113 ]. Strong stress can induce inflammatory responses by releasing many cytokines, such as IL-6 and TNF-α, which are activated by enhanced expression of some proteins, such as the transcriptional fingerprint, especially the NF-κB pathway—together with reduced expression of other proteins, such as glucocorticoid receptor expression.
5.3. Acute Stress
Normal activation of microglia protects neurons by performing synaptic shearing and phagocytose excess proteins, but excessive neuroinflammatory response is neurotoxic and harmful. After acute stress, microglia are slowly activated by ATP (or excessive glutamate) via P2X7 receptors, which enhance intracellular Iba-1, CD68 expression, and enhanced expression of OX-42, RCA-21 etc., and resulted in activated inflammatory vesicles and the release of more inflammatory factors [ 114 ]. In addition, microglia release many miRNAs through exosomes [ 115 ]. Exosomes are structures to spread information, especially disease information like cancer and inflammation, by cellular excretion of proteins, mRNAs, and miRNAs in exosomes. It has been reported that stress responses (e.g., heat shock, and oxidative stress) can induce exosome secretion. miRNA expression in MDD patients is in an abnormally active state and can interfere with the normal function of glial cells and neurons [ 116 ]. Many miRNAs have been proven to play an important role in MDD pathogenesis and were suggested to be biomarkers for MDD, such as miR-1202. It follows that, if chronic stress is encountered, microglia undergo an inflammatory response and will also release many miRNAs through exosomes, thus interfering with the normal function of astrocytes and neurons [ 117 ]. In addition, inflammatory molecules can affect the developing brain by influencing the hypothalamus-pituitary-adrenal axis (HPA), glial cells, and monoamine metabolism [ 118 ].
6. Monoamines and Neuroendocrine
Inflammatory cytokines can modulate mood behavior and cognition by affecting brain monoamine levels, activating neuroendocrine responses, promoting excitotoxicity (increased glutamate levels), and impairing brain plasticity [ 119 ]. Monoaminergic modulation of emotion relies on both the nervous system and neuroendocrine. The major structure for neuroendocrine is the hypothalamus-pituitary-adrenal axis (HPA). HPA works as a very important complementary and executive system for the emotional function of the monoamine system. For example, cortisol and the adrenocorticotropin-releasing hormone (ACTH) are important neurotransmitters during angry responses, assisting in sympathetic regulation and emotion-related physiological functions [ 120 ]. In addition, the hypothalamic-pituitary-gonadal axis (HPG axis), which regulates estrogen and progesterone, has a very strong influence on aversive mood and may be the main reason for the high prevalence of depression in women [ 121 ]. In addition, oxytocin released by the hypothalamus is considered to be the neurotransmitter of love and attachment and plays a very important regulatory role in the recognition of facial expressions [ 122 , 123 ].
6.1. Hypothalamic-Pituitary-Adrenal (HPA)
The hypothalamic-pituitary-adrenal (HPA) axis is an important component of the neuroendocrine system and is involved in the regulation of the body’s stress response. Under physiological conditions, glucocorticoid (GC) negatively feeds back to the brain by regulating the release of monoamine neurotransmitters, inhibiting the activity of HPA, and weakening the stress response. However, when stressful stimuli persist, consistent high levels of glucocorticoids can lead to the abnormal release of monoamine neurotransmitters in the brain [ 124 ]. Glucocorticoid receptors (GR) and GR mRNA expression are reduced in peripheral blood and brain tissue of depressed patients, and antidepressant treatment ameliorates abnormalities in GR. Co-chaperone proteins of GR play important roles in normal folding, maturation, nuclear translocation, and binding of GR to DNA. Among them, FK506 binding protein 51 (FKBP51) is an important co-chaperone protein [ 125 ].
Enhanced FKBP5 transcription and translation reduce the sensitivity of GR. FKBP5 knockout mice had lower adrenal and thymus weights and corticosterone levels after chronic stress swimming for a longer time in the forced swimming test. In contrast, FKBP5 knockout mice showed an age-dependent antidepressant-like phenotype with enhanced stress resilience. Elevated basal FKBP5 levels in depressed patients can be a predictor of depressive disorders, while genetic polymorphisms in FKBP5 interact with early-life stress to increase the risk of developing depressive disorders. In terms of response to treatment, studies suggest that FKBP5 can be used as an independent factor to predict responsiveness to treatment in depressed patients [ 126 ]. It is found that hippocampal and prefrontal GR expression was reduced, and FKBP5 was elevated in adolescent chronic stress depression model animals. It is suggested that GR/FKBP5 might be a biomarker for MDD, for it is a key factor in the development and increased susceptibility to depression, and jointly mediate HPA axis function and stress response [ 127 ].
Regarding the regulation of FKBP5 expression, miR-511 was found to decrease the protein level of FKBP5 and downregulate the glucocorticoid-induced upregulation of FKBP5. In contrast, miR-511 overexpression significantly enhanced GR activity. Chronic stress experiences during adolescence lead to reduced miR-511 expression in the prefrontal lobe of adult animals. Functionally, FKBP5 might also be a biomarker because of its properties to regulate GR, such as regulating pathways related to immune function, autophagy, epigenetic remodeling, apoptosis, cell growth, cytoskeleton dynamics, and metabolism. In addition, miR-511 contributes to the age-dependent regulation of FKBP5 and neuronal development and differentiation [ 128 ].
6.2. Hypothalamic-Pituitary-Gonadal Axis (HPG)
Estrogen and several other sex hormones have been proved to affect emotions and play a role in MDD, which might be a major reason for high prevalence of MDD in women [ 129 ]. The pathogenesis of pre-perimenopausal depression is also suggested to be related to reduced levels of estrogen (estradiol, E2), and E2 replacement therapy has been used to treat perimenopausal depression [ 130 ]. However, recent studies have found that E2 has little effect on perimenopausal depression; instead, estrogen deficiency-induced female depression might be related to 5-hydroxytriptamine (5-HT) deficiency [ 36 ]. In addition, luteinizing hormone (LH) is significantly altered during the menstrual cycle, perinatal, and perimenopausal periods, which may be the main cause of depression [ 131 ]. Moreover, increased LH can enhance the release of cortisol and lead to Cushing’s syndrome and depression. In addition, depression is one of the typical symptoms of Cushing’s syndrome [ 132 ]. The main function of LH receptors is to promote glandular proliferation and hormone (transmitter) secretion. LH can activate the PI3K-Akt-mTOR pathway in ovarian epithelial cells and granulosa cells, increasing the phosphorylation of mTOR substrates S6K1 and EIF4EBP1 to promote hormone synthesis in the corpus luteum [ 133 ].
LH changes dramatically during the menstrual cycle, perinatal period, and perimenopause. LH secretion before ovulation can reach 3–8 times the basal level, and LH secretion increases to three times the previous level during perimenopause. During perimenopause, ovarian function gradually deteriorates and the ability to secrete E2 and progesterone decreases. The hypothalamic-pituitary-gonadal axis (HPG) is dysfunctional. Clinical studies suggested that persistent LH elevation can induce the clinical ACTH Independent Cushing’s Syndrome (ACTH) [ 134 ]. Recently, it has been reported that Cushing’s syndrome patients with increased cortisol secretion exhibit reduced mTOR function while inhibition of the mTOR pathway on the adrenal glands can reduce cortisol secretion. LH can activate the mTOR pathway on uterine membrane cells and granulosa cells, which leads to estrogen secretion. Studies also found a synchronous and persistent elevation of LH and cortisol in ovariectomized rats in a stressful environment, and we hypothesized that LH could induce cortisol secretion through the mTOR pathway [ 135 ]. Furthermore, the persistent increase in ACTH and cortisol secretion is an important predisposing factor for depression [ 136 ]. Therefore, increased peripheral LH secretion might be a reason for perimenopause MDD by inducing cortisol activity, which can lead to depression.
7. Neurotrophic, Nerve Regeneration, and Neuroplasticity
Major depressive disorder (MDD) is a common emotional disorder with etiological heterogeneity and complex molecular mechanisms which are not fully understood. Recent studies in postmortem or animals have shown that over-activated microglia can inhibit neurogenesis and induce MDD [ 137 ]. It is also found that neurotrophic/growth factors such as the brain-derived neurotrophic factor are decreased in postmortem brains from suicide victims, which suggests that altered trophic support might be a reason for the pathophysiology of MDD [ 138 ]. BDNF has recently been regarded as an interesting target for developing new antidepressant drugs [ 139 ].
In realizing the limitations of current antidepressant therapy, depression research has branched out to encompass other areas such as synaptic plasticity, neurogenesis, and brain structural remodeling as factors which influence mood and behavior. Monoamine neurons, their neural loops, and neural connections are in a lifelong process of reorganization and modification. Studies suggest that neuroplasticity is involved in the pathogenesis of depression. Synaptic plasticity includes structural plasticity and functional plasticity, which modulates the function of local neuronal networks or corresponding neural loops by altering normal neural firing, neurosecretion, and other activities by affecting the information transfer between neurons, and plasticity modulation targeting the corresponding neural loops will be the urgent need and main direction of research in these psychiatric disorders [ 140 ].
Reduced neuroplasticity is an important pathophysiological basis for psychiatric disorders including depression. Brain-derived neurotrophic factor (BDNF) is an important mediator involved in the regulation of normal brain development and neuroplasticity [ 141 ], and indeed BDNF abnormalities in signaling pathways play an important role in the development of depression [ 142 ]. BDNF gene polymorphisms may be risk factors for the development of depression and interact with the genetic environment to play a role in the development of depression. Based on the established database of comprehensive evaluation characteristics of adolescent bipolar demographics, genotyping, and behavior at the Institute of Psychology, Chinese Academy of Sciences, a bipolar design was used to isolate and identify the extent to which genetic, shared environmental, and nonshared factors influence adolescent mood by developing structural equation models [ 143 ]. The results revealed that the main effect of BDNF val66met on adolescent depression was not significant, which means that the allele present at the polymorphic locus did not predict depression. However, the BDNF val66met together with stressful life events interaction was significant, suggesting that adolescent individuals with different alleles at this polymorphic locus reacted differently after exposure to stressful life events [ 144 ]. In-depth analysis revealed that individuals with the Val allele were prone to depression after exposure to high levels of stressful life events relative to individuals who were met/met pure congenic. However, in the absence of stressful life events or at very low levels, Val allele carriers were again protected, which indicates that they were less prone to depression [ 145 ]. Furthermore, previous gene–environment interactions have failed to control well for confounding gene-environment correlations in study results. To better rule out such confusion, a bipartite design was used to isolate the part of the environment that is not genetically influenced as a pure environmental factor and observed the effect of the BDNF val66met polymorphic locus on depression by interacting with these pure environments. The findings confirmed previous findings that Val allele carriers are more sensitive to environmental factors in the Chinese population and are more prone to depression than met/met pure congeners at higher levels of stressful life events [ 146 ]; thus, BDNF and its related modulations might be a biomarker for MDD.
7.2. Epigenetics
Epigenetic mechanisms are important mechanisms for translating the effects of environmental factors into specific patterns of gene expression and can affect brain circuits over time [ 147 ]. The influences of early social environmental on animal and human behavior are associated with alterations in epigenetic modifications [ 148 ]. It is found that depressive chronic social stress continues to affect animals’ depressive behavior in adulthood, including deficits in social interest, impairments in executive function, and so on [ 149 ]. Epigenetic modifications in the medial prefrontal cortex BDNF gene are altered, primarily by elevated levels of histone H3K9 methylation (a transcriptional repressor of BDNF) in regions downstream from this promoter region, leading to an expression, mediating the long-term effects of adolescent depression on behavior and brain development [ 150 ].
8. Monoamine Neurotransmitter and Other Neurotransmitters
Psychological analyses have made a lot of progress in emotional studies, and these pioneering works have helped locate many important structures involved in basic emotions. However, the characteristic feature of monoamine neurotransmitters is their broad projection, which can affect the function of the whole brain and even the whole body [ 151 ]. Thus, monoamine neurotransmitters are also called neuromodulators, including dopamine, norepinephrine, and serotonin (DA, NE, and 5-HT). These monoamine neuromodulators might be the primary neural basis for emotions, and we suggested that emotions are nothing but neuromodulators [ 15 , 16 , 35 ].
The monoamine neuromodulators are the primary neural basis for emotions; however, there are many other chemicals in the brain that are related to emotions, such as corticotropin-releasing hormone (CRH) or cortisone, or oxytocin, or sex hormone. All these neurotransmitters, their mutual interaction and their influence on other processes, as stated before, might be different in individual patients; therefore, there might be many chemical dysfunctions that induce clinical heterogeneity among MDD patients. In this context, all chemical changes might play a role of biomarkers for the diagnosis of MDD. Overall, the monoamine neuromodulators might be the primary neural basis for emotions, and the other related chemicals, including the hormones, inflammation cytokines, neuro-tropical factors, or mRNA, LnRNA might work as biomarkers for MDD ( Figure 2 ).

The mechanisms of MDD. Pharmaceuticals have greatly supported the monoamine hypothesis by their efficient effects on the monoamine neurotransmitters. Their new effects should be investigated in many different systems, including neuroendocrine and digestive system, and also in many kinds of cells in the brain, including neurons and glia. Thus, the new mechanisms should be extended to neurotrophic, neuro-inflammation, metabolites as well as nerve regeneration.
There are many chemicals in the brain that are related to emotions; we cannot review them in this paper due to the paper’s length. We can use GABA as one example to introduce the interactions between monoamine and other chemicals. The specific neurotransmitters include glutamatergic excitatory neurons and GABAergic inhibitory interneurons, which are the main executive systems regulating neural circuit connections in emotional brain regions and between brain regions [ 152 ]. The structure and function of glutamatergic and GABAergic neurons in the prefrontal cortex and hippocampus of brain regions and the imbalance of their local loop activity are also the monoaminergic targets for the antidepressant drug treatments [ 153 ]. In addition, glutamatergic and GABAergic neurons can in turn counteract monoamine neurons; for example, direct or indirect excitatory neural projection from the prefrontal lobe can induce top-down emotional and cognitive regulation to the limbic system [ 154 ].
The interactions between glutamatergic and GABAergic systems can be affected by negative life events at early childhood, such as a mother–infant relationship and adolescent social stress, and results in emotional behavior [ 75 ]. GABAergic activates depending on ionotropic GABA A and metabotropic GABA B receptors. Some drugs targeting the GABA system, including GABA B receptor modulators, such as {"type":"entrez-protein","attrs":{"text":"CGP36742","term_id":"877561962"}} CGP36742 , CGP 51176, and {"type":"entrez-protein","attrs":{"text":"CGP56433","term_id":"876483748"}} CGP56433 , and GABAA receptor α2 and α5 subunit orthosteric agents, have been reported to have rapid antidepressant effects. On the other hand, NMDA receptors might also be a target for rapid antidepressant research, such as ketamine, which has been used as an antagonist for NMDA receptors. In addition, some other agonists or enhancers targeting the Glycine Binding Site (GBS) site on the NMDA receptor have also shown antidepressant effects [ 82 ]. However, their interaction with monoamine activity is not clear. Oxytocin has been recently found to be the neuromodulator for love and attachment [ 155 ]. Nasal application of oxytocin can induce positive emotions. In addition, the hormones from the hypothalamic-pituitary-adrenal (HPA) axis, such as CRH, ACTH (adrenocorticotropic hormone), and cortisol, have been proved to be involved in the stress process. Furthermore, the sex hormone progesterone has been proved to affect disgust [ 35 ]. Overall, many neurotransmitters have been suggested to be involved in the emotions.
9. Conclusions
The monoamine hypothesis has been largely supported by the pharmaceuticals that target monoamine neurotransmitters as a treatment for depression. Therefore, the first-line antidepressant drugs remain for raising monoamine neurotransmitters. However, these antidepressants have come under scrutiny due to their limited clinical efficacy, side effects, and delayed onset of action [ 156 ]. There is increasing evidence that glial cells play a role in the pathological mechanisms of mood disorders and the mode of action of antidepressant drugs. Thus, the new etiological hypotheses of MDD should extend the monoamine transmitter hypothesis to a broad area including many body systems and many kinds of brain cells, in order to clarify the main hypothesis for the pathogenesis of depression.
10. Future Perspectives:
This paper advanced the monoamine hypothesis for basic emotions and MDD; however, there are still some limitations for this review. MDD is quite a big field of study, and there are lots of publications every year; thus, we cannot give a full review of the literature. For example, we did not review the large amount of material about biomarkers for MDD, which is a very interesting approach for MMD diagnosis for precision medicine (especially for psychiatry). The interactions among the neurotransmitters involved in MMD, and their mutual interaction and their influence on other processes, as stated before, are very different in individual patients; therefore, it is critically important to screen the clinical heterogeneity among MDD patients, which will facilitate decision-making by introducing measurable biomarkers (i.e., indicators of normal biological processes, pathogenic processes, or responses to the exposure or intervention). This field of precision medicine will make tremendous progress in science and technology, enabling the acquisition and processing of enormous amounts of information and identification of associations between individual patient’s characteristics, as well as diagnosis, prognosis, and treatment methods for MDD.
Funding Statement
The authors are also supported by the National Nature Science Foundation in China (S.G., 82101602; F.W., 82171392).
Author Contributions
Conceptualization, writing-original draft, Y.J.; visualization, D.Z.; visualization, Y.L.; supervision, review and editing, S.G.; editing, J.D.; coneptualization, supervision, writing-original draft preparation, X.M.; reviewing and editing, S.X.; conceptualization, funding acquisition, supervision, writing-original draft preparation, F.W.; reviewing and editing, J.H.H. All authors have read and agreed to the published version of the manuscript.
Institutional Review Board Statement
Not applicable.
Informed Consent Statement
Data availability statement, conflicts of interest.
The authors declare that the research was conducted in the absence of any commercial or financial relationships that could be construed as a potential conflict of interest.
Publisher’s Note: MDPI stays neutral with regard to jurisdictional claims in published maps and institutional affiliations.
- Help & FAQ
History and evolution of the monoamine hypothesis of depression
Research output : Contribution to journal › Article › peer-review
The symptoms of depression can be improved by agents that act by various mechanisms to increase synaptic concentrations of monoamines. This finding led to the adoption of the monoamine hypothesis of depression, first put forward over 30 years ago, which proposes that the underlying biological or neuroanatomical basis for depression is a deficiency of central noradrenergic and/or serotonergic systems and that targeting this neuronal lesion with an antidepressant would tend to restore normal function in depressed patients. The hypothesis has enjoyed considerable support, since it attempts to provide a pathophysiologic explanation of the actions of antidepressants. However, in its original form it is clearly inadequate, as it does not provide a complete explanation for the actions of antidepressants, and the pathophysiology of depression itself remains unknown. The hypothesis has evolved over the years to include, for example, adaptive changes in receptors to explain why there should be only a gradual clinical response to antidepressant treatment when the increase in availability of monoamines is rapid. Still, the monoamine hypothesis does not address key issues such as why antidepressants are also effective in other disorders such as panic disorder, obsessive-compulsive disorder, and bulimia, or why all drugs that enhance serotonergic or noradrenergic transmission are not necessarily effective in depression. Despite these limitations, however, it is clear that the development of the monoamine hypothesis has been of great importance in understanding depression and in the development of safe and effective pharmacologic agents for its treatment.
ASJC Scopus subject areas
- Psychiatry and Mental health
Other files and links
- Link to publication in Scopus
- Link to the citations in Scopus
Fingerprint
- Antidepressive Agents Medicine & Life Sciences 100%
- History Medicine & Life Sciences 99%
- Depression Medicine & Life Sciences 87%
- Serotonin Agents Medicine & Life Sciences 30%
- Bulimia Medicine & Life Sciences 27%
- Panic Disorder Medicine & Life Sciences 25%
- Obsessive-Compulsive Disorder Medicine & Life Sciences 24%
- Therapeutics Medicine & Life Sciences 7%
T1 - History and evolution of the monoamine hypothesis of depression
AU - Hirschfeld, Robert M.A.
N2 - The symptoms of depression can be improved by agents that act by various mechanisms to increase synaptic concentrations of monoamines. This finding led to the adoption of the monoamine hypothesis of depression, first put forward over 30 years ago, which proposes that the underlying biological or neuroanatomical basis for depression is a deficiency of central noradrenergic and/or serotonergic systems and that targeting this neuronal lesion with an antidepressant would tend to restore normal function in depressed patients. The hypothesis has enjoyed considerable support, since it attempts to provide a pathophysiologic explanation of the actions of antidepressants. However, in its original form it is clearly inadequate, as it does not provide a complete explanation for the actions of antidepressants, and the pathophysiology of depression itself remains unknown. The hypothesis has evolved over the years to include, for example, adaptive changes in receptors to explain why there should be only a gradual clinical response to antidepressant treatment when the increase in availability of monoamines is rapid. Still, the monoamine hypothesis does not address key issues such as why antidepressants are also effective in other disorders such as panic disorder, obsessive-compulsive disorder, and bulimia, or why all drugs that enhance serotonergic or noradrenergic transmission are not necessarily effective in depression. Despite these limitations, however, it is clear that the development of the monoamine hypothesis has been of great importance in understanding depression and in the development of safe and effective pharmacologic agents for its treatment.
AB - The symptoms of depression can be improved by agents that act by various mechanisms to increase synaptic concentrations of monoamines. This finding led to the adoption of the monoamine hypothesis of depression, first put forward over 30 years ago, which proposes that the underlying biological or neuroanatomical basis for depression is a deficiency of central noradrenergic and/or serotonergic systems and that targeting this neuronal lesion with an antidepressant would tend to restore normal function in depressed patients. The hypothesis has enjoyed considerable support, since it attempts to provide a pathophysiologic explanation of the actions of antidepressants. However, in its original form it is clearly inadequate, as it does not provide a complete explanation for the actions of antidepressants, and the pathophysiology of depression itself remains unknown. The hypothesis has evolved over the years to include, for example, adaptive changes in receptors to explain why there should be only a gradual clinical response to antidepressant treatment when the increase in availability of monoamines is rapid. Still, the monoamine hypothesis does not address key issues such as why antidepressants are also effective in other disorders such as panic disorder, obsessive-compulsive disorder, and bulimia, or why all drugs that enhance serotonergic or noradrenergic transmission are not necessarily effective in depression. Despite these limitations, however, it is clear that the development of the monoamine hypothesis has been of great importance in understanding depression and in the development of safe and effective pharmacologic agents for its treatment.
UR - http://www.scopus.com/inward/record.url?scp=0034006802&partnerID=8YFLogxK
UR - http://www.scopus.com/inward/citedby.url?scp=0034006802&partnerID=8YFLogxK
M3 - Article
C2 - 10775017
AN - SCOPUS:0034006802
SN - 0160-6689
JO - Journal of Clinical Psychiatry
JF - Journal of Clinical Psychiatry
IS - SUPPL. 6
Advertisement
Natural Molecular Hydrogen Seepage Associated with Surficial, Rounded Depressions on the European Craton in Russia
- Published: 15 November 2014
- Volume 24 , pages 369–383, ( 2015 )
Cite this article
- Nikolay Larin 1 ,
- Viacheslav Zgonnik 2 ,
- Svetlana Rodina 1 ,
- Eric Deville 2 ,
- Alain Prinzhofer 2 nAff3 &
- Vladimir N. Larin 4
1726 Accesses
65 Citations
22 Altmetric
Explore all metrics
In the Russian part of the European craton, several thousands of subcircular structures ranging in size from a hundred meters to several kilometers in diameter have been identified throughout the region extending from Moscow to Kazakhstan. Generally, these structures correspond to minor morphological depressions. In cultivated areas, the periphery of these structures is often outlined by a ring of soil bleaching associated with growth anomalies of vegetation. The cores of the structures commonly correspond to marshes, sometimes with lakes. Subsoil gas composition of these structures was studied. For this purpose, portable gas detectors were used, and the results obtained were confirmed by gas chromatography analysis. Inside and around these structures, the concentration of molecular hydrogen in soil was much greater inside than outside, up to 1.25% at 1.2 m in soils. The hydrogen is associated with a small quantity of methane. We estimated a daily hydrogen flow seeping out at the surface is between 21,000 and 27,000 m 3 in one of these structures.
This is a preview of subscription content, log in via an institution to check access.
Access this article
Price includes VAT (Russian Federation)
Instant access to the full article PDF.
Rent this article via DeepDyve
Institutional subscriptions
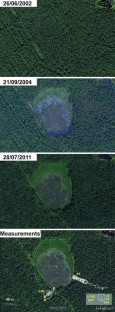
Similar content being viewed by others
Evidence for natural molecular hydrogen seepage associated with carolina bays (surficial, ovoid depressions on the atlantic coastal plain, province of the usa), integral assessment of the soil cover state in gas production area in the middle reaches of the angara river.
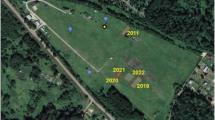
Influence of Spring Burns on the Properties of Humus Horizon of Chernozem in the Southeast of Western Siberia
Updates are published regularly on http://hydrogen-future.com/en/ .
Abrajano, T. A., Sturchio, N., Bohlke, J. K., Lyon, G., Poreda, R., & Stevens, C. (1988). Methane-hydrogen gas seeps, Zambales Ophiolite, Philippines: Deep or shallow origin? Chemical Geology, 71 , 211–222.
Article Google Scholar
Abrajano, T. A., Sturchio, N. C., Kennedy, B. M., Lyon, G. L., Muehlenbachs, K., & Bohlke, J. K. (1990). Geochemistry of reduced gas related to serpentinization of the Zambales ophiolite, Philippines. Applied Geochemistry, 5 , 625–630.
Angino, E. E., Coveney, R. M. J., Goebel, E. D., Zeller, E. J., & Dreschhoff, G. A. M. (1984). Hydrogen and nitrogen—origin, distribution, and abundance, a followup. Oil & Gas Journal, 82 , 142–146.
Google Scholar
Apps, J.A., Van De Kamp, P.C. (1993). Energy gases of abiogenic origin in the Earth’s crust. Future Energy Gases. United States Geological Survey Professional Paper, pp. 81–130.
Birina, L.M. (Ed.). (1962). Map of pre-quaternary formations: N-37-III. Scale 1:200000 . Geologic administration of Central regions (in Russian).
Charlou, J.-L. (2002). Geochemistry of high H 2 and CH 4 vent fluids issuing from ultramafic rocks at the Rainbow hydrothermal field (36°14′N, MAR). Chemical Geology, 191 , 345–359.
Charlou, J.-L., Fouquet, Y., Bougault, H., Donval, J. P., Etoubleau, J., Jean-Baptiste, P., et al. (1998). Intense CH 4 plumes generated by serpentinization of ultramafic rocks at the intersection of the 15°20′N fracture zone and the Mid-Atlantic Ridge. Geochimica et Cosmochimica Acta, 62 , 2323–2333.
Coveney, R. M. J., Goebel, E. D., Zeller, E. J., Dreschhoff, G. A. M., & Angino, E. E. (1987). Serpentinization and origin of hydrogen gas in Kansas. AAPG Bulletin, 71 , 39–48.
Cussler, E. L. (2009). Diffusion. Mass transfer in fluid systems. Cambridge University Press. Deville E., Guerlais S.-H. 2009. Cyclic activity of mud volcanoes: Evidences from Trinidad (SE Caribbean). Marine and Petroleum Geology, Special issue on mud volcanoes, 26 , 1681–1691.
Deville, E., & Guerlais, S.-H. (2009). Cyclic activity of mud volcanoes: Evidences from Trinidad (SE Caribbean). Marine and Petroleum Geology, 26 (9), 1681–1691.
Firstov, P. P., & Shirokov, V. A. (2005). Dynamics of molecular hydrogen and its relation to deformational processes at the Petropavlovsk–Kamchatskii geodynamic test site: Evidence from observations in 1999–2003. Geochemistry International, 43 , 1056–1064.
Freund, F., Dickinson, J. T., & Cash, M. (2002). Hydrogen in rocks: an energy source for deep microbial communities. Astrobiology, 2 (1), 83–92.
Iosifova, Yu. I., Gorbatkina, T. E., & Fadeeva, L. I. (1998). Geologic map of pre-quaternary sediments of Voronezh region. Scale 1:500 000. Ed. Gavryushova, E. A., Dashevskiy, V. V. Ministry of Natural Resources of Russian Federation (In Russian).
Gusev, A. (1997). Gas - geochemical effects of modern geodynamic activity of platform structures (on example of South - East of Belarus) . Schmidt Institute of Physics of the Earth of Russian Academy of Sciences. Dissertation (in Russian).
Hosgörmez, H., Etiope, G., & Yalçin, M. N. (2008). New evidence for a mixed inorganic and organic origin of the Olympic Chimaera fire (Turkey): A large onshore seepage of abiogenic gas. Geofluids, 4 , 263–273.
Ikorsky, S. V., Gigashvili, G. M., Lanyov, V. S., Narkotiev, V. D., & Petersilye, I. A. (1999). The investigation of gases during the Kola Superdeep Borehole Drilling (to 11.6 km Depth), in: Whiticar, M. J., Faber, E. (Eds.), Geologisches Jahrbuch Reihe , D. E. Schweizerbart Science Publishers, Hannover, pp. 145–152.
Isaev, E. I., Skorodumova, N. V., Ahuja, R., Vekilov, Y. K., & Johansson, B. (2007). Dynamical stability of Fe–H in the Earth’s mantle and core regions. PNAS of the USA, 104 , 9168–9171.
Jones, V.T., & Pirkle, R.J. (1981). Helium and hydrogen soil gas anomalies associated with deep or active faults, in: 181st ACS National Meeting . Atlanta.
Kotelnikova, S. (2002). Microbial production and oxidation of methane in deep subsurface. Earth-Science Reviews, 58 , 367–395.
Larin, V.N. (1993). Hydridic Earth: the New Geology of Our Primordially Hydrogen - rich Planet . Ed. C. W. Hunt. Polar publishing, Alberta.
Larin, N.V., Larin, V.N., & Gorbatikov A.V. (2010). Circular structures, caused by the deep seeping of hydrogen. Degassing of the Earth: Geotectonics, geodynamics, Deep Fluids, oil and gas; hydrocarbons and life . Russian Conference with International Participation Dedicated to the 100th Anniversary of Academician P.N. Kropotkin, p. 282 (in Russian).
Lin, L.-H., Hall, J., Lippmann-Pipke, J., Ward, J. A., Lollar, B. S., DeFlaun, M., et al. (2005). Radiolytic H 2 in continental crust: Nuclear power for deep subsurface microbial communities. Geochemistry, Geophysics, Geosystems, 6 (7), 1–13.
McCarthy, H., & McGuire, E. (1998). Soil gas studies along the Carlin trend, Eureka and Elko counties, Nevada. In: Tosdal, R.M. (Ed.), Contributions to the Gold Metallogeny of Northern Nevada. Open - File Report 98 - 338 1998 . U.S. Dept. of the Interior, U.S. Geological Survey, pp. 243–250.
Mukhin, Y. (1970). Main results of the deep hydrogeological studies in Central Russia sedimentation basin in connection with estimation of the prospects for oil and gas. Proceedings of VNIIGaz , (33/41), pp. 157–295 (in Russian).
Neal, C., & Stanger, G. (1983). Hydrogen generation from mantle source rocks in Oman. Earth and Planetary Science Letters, 66 , 315–320.
Newell, K. D., Doveton, J. H., Merriam, D. F., Lollar, B. S., Waggoner, W. M., & Magnuson, L. M. (2007). H 2 -rich and hydrocarbon gas recovered in a deep precambrian well in Northeastern Kansas. Natural Resources Research, 16 , 277–292.
Proskurowski, G., Lilley, M. D., Kelley, D. S., & Olson, E. J. (2006). Low temperature volatile production at the Lost City Hydrothermal Field, evidence from a hydrogen stable isotope geothermometer. Chemical Geology, 229 , 331–343.
Rogozhin, E. A., Gorbatikov, A. V., Larin, N. V., & Stepanova, M. Y. (2010). Deep structure of the Moscow Aulacogene in the western part of Moscow. Izvestiya, Atmospheric and Ocean. Physics, 46 , 973–981.
Sano, Y., Urabe, A., Wakita, H., & Wushiki, H. (1993). Origin of hydrogen–nitrogen gas seeps, Oman. Applied Geochemistry, 8 , 1–8.
Sato, M., Sutton, A. J., McGee, K. A., & Russell-Robinson, S. (1986). Monitoring of hydrogen along the San Andreas and Calaveras Faults in central California in 1980–1984. Journal of Geophysical Research, 91 , 12315–12326.
Semlitsch, R. D. (2000). Size does matter: The value of small isolated wetlands. National Wetlands Newsletter, 22 , 5–6.
Shcherbakov, A. V., & Kozlova, N. D. (1986). Occurrence of hydrogen in subsurface fluids and the relationship of anomalous concentrations to deep faults in the USSR. Geotectonics, 20 , 120–128.
Sherwood Lollar, B., Lacrampe-Couloume, G., Slater, G. F., Ward, J. A., Moser, D. P., Gihring, T. M., et al. (2006). Unravelling abiogenic and biogenic sources of methane in the Earth’s deep subsurface. Chemical Geology, 226 , 328–339.
Shestopalov, V. M., & Makarenko, A. N. (2013). On several results of research, developing the idea of V.I. Vernadskiy about “gas breathing” of the Earth. Geological Journal, 3 , 7–25. (in Russian).
Shik, S.M. (Ed.), (2000). Map of pre-quaternary formations: M-37, (38) (Voronezh). Scale 1:1000000 . FGUP “VSEGEI” (in Russian).
Shishov, S. I. (2010). Geography and soil geochemistry characteristics of delineated depressions in the Ryazan region. Bulletin of the Esenin Ryazan State University, 28 (3), 116–129. (in Russian).
Smith, N.J.P., Shepherd, T.J., Styles, M.T., & Williams, G.M., (2005). Hydrogen exploration: a review of global hydrogen accumulations and implications for prospective areas in NW Europe, in: Petroleum Geology: North-West Europe and Global Perspectives — Proceedings of the 6th Petroleum Geology Conference. Geological Society, London, pp. 349–358.
Sukhanova, N. I., Trofimov, S. Y., Polyanskaya, L. M., Larin, N. V., & Larin, V. N. (2013). Changes in the humus status and the structure of the microbial biomass in hydrogen exhalation places. Eurasian Soil Science, 46 , 135–144.
Syvorotkin, V. L. (2010). Hydrogen degassing of the earth: Natural disasters and the biosphere. Man and the Geosphere (pp. 307–347). New York: Nova Science Publishers.
Takai, K., Gamo, T., Tsunogai, U., Nakayama, N., Hirayama, H., Nealson, K.H., & Horikoshi, K. (2004). Geochemical and microbiological evidence for a hydrogen-based, hyperthermophilic subsurface lithoautotrophic microbial ecosystem (HyperSLiME) beneath an active deep-sea hydrothermal field. Extremophiles, 8, 269–82.
Toulhoat, H., Beaumont, V., Zgonnik, V., Larin, N. V., & Larin, V. N. (2012). Chemical differentiation of planets: a core issue. Submitted for publication. Available at: http://arxiv.org/abs/1208.2909 .
Urdukhanov, R. I., Nikolaev, I. N., Voytov, G. I., Daniyalov, M. G., Prutskaya, L. D., Parshikova, N. G., et al. (2002). Instability of the hydrogen field in atmosphere of soils and subsoils in response to the earthquake in Dagestan in 1998–2000. Proceedings of the Academy of Science, Section Geophysics, 385 (6), 818–822. (in Russian).
Vacquand, C., (2011). Genèse et mobilité de l’hydrogène dans les roches sédimentaires: source d’énergie naturelle ou vecteur énergétique stockable? PhD dissertation. IFP Energies Nouvelles and Institut de Physique du Globe de Paris. Available at: http://www.ipgp.fr/docs/publications/theses/20110318-vacquand.pdf .
Wakita, H., Nakamura, Y., Kita, I., Fujii, N., & Notsu, K. (1980). Hydrogen release: New indicator of fault activity. Science (New York, N.Y.), 210 , 188–190.
Ware, R. H., Roecken, C., & Wyss, M. (1985). The detection and interpretation of hydrogen in fault gases. Pure and Applied Geophysics PAGEOPH, 122 , 392–402.
Welhan, J. A., & Craig, H. (1979). Methane and hydrogen in East Pacific rise hydrothermal fluids. Geophysical Research Letters, 6 , 829–831.
Zgonnik, V., Beaumont, V., Deville, E., Larin, N., Pillot, D., & Farrell, K. Evidence for natural molecular hydrogen seepage associated with surficial, rounded depressions. The Atlantic Coastal Plain Province of the USA (Under review).
Download references
Acknowledgement
We thank Hervé Toulhoat and Armand Lattes for their support for this research topic, ZAO “NTK” for their assistance with funding for this research, Pavel Vodnev, Aleksandr Sysolin, Olga Veretennikova, Irina Katsura, Vladimir Larin (Jr.) for their assistance with the fieldwork, and the anonymous reviewers for their comments and critics.
Author information
Alain Prinzhofer
Present address: IPEXCO Rua Dezenove de Fevereiro, 69-71 Botafogo, Rio de Janeiro, RJ, CEP 22.280-030, Brazil
Authors and Affiliations
Russian Academy of Science, Schmidt Institute of Physics of the Earth, B. Gruzinskaya St. 10, 123995, Moscow, Russia
Nikolay Larin & Svetlana Rodina
IFP Energies Nouvelles, 1 & 4 Avenue de Bois Préau, 92852, Rueil-Malmaison Cedex, France
Viacheslav Zgonnik, Eric Deville & Alain Prinzhofer
Natural Hydrogen Energy Ltd, 24165 County Road 90, Ault, CO, 80610, USA
Vladimir N. Larin
You can also search for this author in PubMed Google Scholar
Corresponding author
Correspondence to Viacheslav Zgonnik .
Electronic supplementary material
Below is the link to the electronic supplementary material.
Supplementary material 1 (PDF 1520 kb)
Rights and permissions.
Reprints and permissions
About this article
Larin, N., Zgonnik, V., Rodina, S. et al. Natural Molecular Hydrogen Seepage Associated with Surficial, Rounded Depressions on the European Craton in Russia. Nat Resour Res 24 , 369–383 (2015). https://doi.org/10.1007/s11053-014-9257-5
Download citation
Received : 16 August 2014
Accepted : 03 November 2014
Published : 15 November 2014
Issue Date : September 2015
DOI : https://doi.org/10.1007/s11053-014-9257-5
Share this article
Anyone you share the following link with will be able to read this content:
Sorry, a shareable link is not currently available for this article.
Provided by the Springer Nature SharedIt content-sharing initiative
- Find a journal
- Publish with us
- Track your research
Academic Bibliography
- Download "2017 dpp is fs On two sid(...).pdf"
- See all downloads
- Search results
- On two-sided monogenic functions of a...

On two-sided monogenic functions of axial type
- Department of Mathematical analysis
- open access
Please use this url to cite or link to this publication: http://hdl.handle.net/1854/LU-8542068
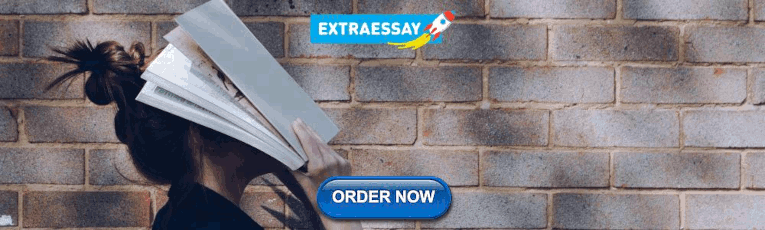
IMAGES
VIDEO
COMMENTS
The solutions were centrifuged for 15 minutes at 2,000 rpm, and 1 mL of the top layer of each tube was stored at −10 °C until ready for assay. The HPLC conditions were as follows: flow rate - 0.400 mL/minute, eluent - 80:20:0.1% (H 2 O:CH 3 CN:TFA), run time -5 minutes, detector - UV (205 nm), and temperature - 30 °C.
In the monoamine hypothesis, the functional deficiency of these neurotransmitters occurs through the degrading effects of the monoamine oxidases in the synaptic cleft [34, 35, 58, 59]. This is supported by experimental findings of increased monoamine oxidase enzyme activity in depressed individuals [34, 35].
Several hypothesis were developed to explain MDD pathogenesis pathogenic including (i) the hypothalamic‒pituitary‒adrenal (HPA) axis dysfunction hypothesis, (ii) the monoamine hypothesis, (iii ...
2.2. The Significance of Basic Emotion Theory. The hypothesis of monoamine transmitters as the neural basis for emotion was first proposed in the 1950s [ 5 ], and it is still used as the basis for developing antidepressants. However, it is not clear how monoamine transmitters affect emotions in MDD patients.
In the 1950s, the amine hypothesis of depression was proposed after it was observed that patients treated for hypertension with reserpine developed depression. 1 Since that time, pharmacologic therapy for treatment of depression has focused on increasing concentrations of brain monoamines, namely norepinephrine, serotonin, and dopamine.
Findings in patients with depression that support the monoamine-deficiency hypothesis include a relapse of depression with inhibition of tyrosine hydroxylase or depletion of dietary tryptophan, an ...
Abstract. Hypotheses about the pathophysiology of depression have evolved over time. This chapter covers the most important findings in this regard. First, the classical monoamine hypothesis posited that depression is caused by an alteration in levels of one or more of the monoamines: serotonin, norepinephrine, and dopamine.
The monoamine hypothesis of depression was first formulated over 30 years ago.1,2 The hypothesis pro-poses that there is an underlying biological basis for de-pression, namely a deficiency of the monoamine neuro-transmitters norepinephrine and/or serotonin in the brain. On the basis of this hypothesis, various classes of anti-depressant agents ...
The monoamine hypothesis has been accepted as the most common hypothesis of major depressive disorder (MDD) for a long period because of its simplicity and understandability. Actually, most currently used antidepressants have been considered to act based on the monoamine hypothesis. However, an important problem of the monoamine hypothesis has ...
European Journal of Neuroscience. Volume 55, Issue 9-10 p. 2895-2911. SPECIAL ISSUE REVIEW. ... including the monoamine hypothesis and the cytokine hypothesis. The monoamine hypothesis has been largely supported by the pharmaceuticals that target monoamine neurotransmitters as a treatment for depression. However, these antidepressants have come ...
The monoamine hypothesis of depression proposes that the underlying biological or neuroanatomical basis for depression is a deficiency of central noradrenergic and/or serotonergic systems and that targeting this neuronal lesion with an antidepressant would tend to restore normal function in depressed patients. The symptoms of depression can be improved by agents that act by various mechanisms ...
Monoamine hypothesis of major depression was formulated almost half a century ago, 21 which stated that deficiency of monoamine neurotransmitters, namely, norepinephrine and/or serotonin, underlies clinical depression. 22 Although this hypothesis mainly originated from the mechanism of action of serendipitously discovered antidepressant drugs ...
The Journal of Clinical Psychiatry, 61(Suppl6), 4-6. Abstract Argues that the monoamine hypothesis of depression does not address key issues such as why antidepressants are also effective in other disorders such as panic disorder, obsessive-compulsive disorder, and bulimia, and why all drugs that enhance serotonergic or noradrenergic ...
This ubiquitin incorporation into brain mitochondria observed only in the presence of ATP in the incubation medium increased sensitivity of monoamine oxidases (MAO) A and B to proteolytic inactivation by trypsin and papain, respectively. (Ubiquitin did not influence sensitivity of MAO B to trypsin and MAO A to papain).
The symptoms of depression can be improved by agents that act by various mechanisms to increase synaptic concentrations of monoamines. This finding led to the adoption of the monoamine hypothesis of depression, first put forward over 30 years ago, which proposes that the underlying biological or neuroanatomical basis for depression is a deficiency of central noradrenergic and/or serotonergic ...
Making the hypothesis that H 2 concentrations in entire structure are similar to our measurements, we can propose the flow for the entire surface of the structure. ... Oil & Gas Journal, 82, 142-146. Google Scholar Apps, J.A., Van De Kamp, P.C. (1993). Energy gases of abiogenic origin in the Earth's crust. Future Energy Gases.
In our research and publishing company you have the opportunity make an order for publication of textbooks, monographs and any other educational, fiction or scientific literature.. Also you can count on our full assistance in publishing in journals belonging to other publishers approved by High Qualification Committee of Russian Federation (HQC).
In this paper we study two-sided (left and right) axially symmetric solutions of a generalized Cauchy Riemann operator. We present three methods to obtain special solutions: via the Cauchy-Kowalevski extension theorem, via plane wave integrals and Funk Hecke's formula and via primitivation. Each of these methods is effective enough to generate all the polynomial solutions.