Thank you for visiting nature.com. You are using a browser version with limited support for CSS. To obtain the best experience, we recommend you use a more up to date browser (or turn off compatibility mode in Internet Explorer). In the meantime, to ensure continued support, we are displaying the site without styles and JavaScript.
- View all journals
- Explore content
- About the journal
- Publish with us
- Sign up for alerts
- Published: 09 November 2020
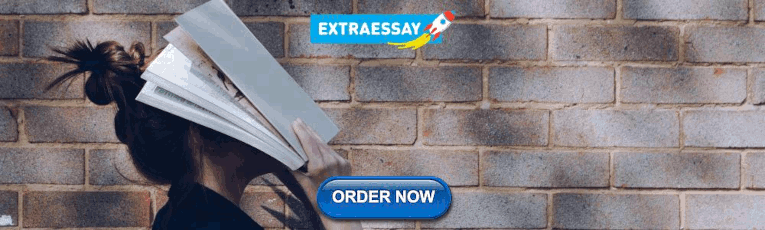
A global analysis of the social and environmental outcomes of community forests
- Reem Hajjar ORCID: orcid.org/0000-0003-0219-7313 1 , 2 na1 ,
- Johan A. Oldekop ORCID: orcid.org/0000-0003-0565-812X 2 , 3 na1 ,
- Peter Cronkleton 4 ,
- Peter Newton ORCID: orcid.org/0000-0002-3992-0483 2 , 5 ,
- Aaron J. M. Russell ORCID: orcid.org/0000-0002-0433-496X 6 , 7 &
- Wen Zhou 8
Nature Sustainability volume 4 , pages 216–224 ( 2021 ) Cite this article
3957 Accesses
76 Citations
121 Altmetric
Metrics details
- Developing world
- Environmental sciences
- Environmental social sciences
Community forest management (CFM) has been promoted for decades as a way to merge environmental conservation with economic development and natural resource rights agendas. Yet many of these initiatives have also led to substantial socioeconomic and environmental trade-offs. We present a comprehensive global analysis of environmental, income and natural resource rights outcomes of CFM, using data from 643 cases in 51 countries. We find that while the majority of cases reported positive environmental and income-related outcomes, forest access and resource rights were often negatively affected by policies to formalize CFM, countering one of CFM’s principal goals. Positive outcomes across all three dimensions were rare. We show that biophysical conditions, de facto tenure rights, national context, user-group characteristics and intervention types are key predictors of joint positive outcomes. These findings highlight key conducive conditions for CFM interventions, which can inform CFM design to ensure positive outcomes across multiple sustainability dimensions.
This is a preview of subscription content, access via your institution
Access options
Access Nature and 54 other Nature Portfolio journals
Get Nature+, our best-value online-access subscription
24,99 € / 30 days
cancel any time
Subscribe to this journal
Receive 12 digital issues and online access to articles
111,21 € per year
only 9,27 € per issue
Buy this article
- Purchase on Springer Link
- Instant access to full article PDF
Prices may be subject to local taxes which are calculated during checkout
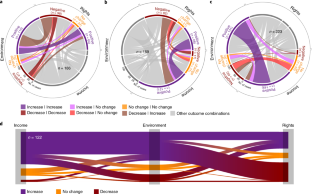
Similar content being viewed by others
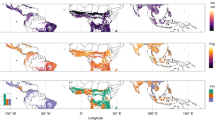
Global forest restoration and the importance of prioritizing local communities
J. T. Erbaugh, N. Pradhan, … A. Chhatre
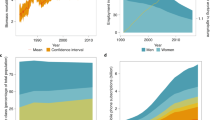
Forest-linked livelihoods in a globalized world
Johan A. Oldekop, Laura Vang Rasmussen, … Sarah J. Wilson
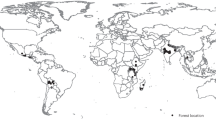
Community forest governance and synergies among carbon, biodiversity and livelihoods
Harry W. Fischer, Ashwini Chhatre, … Arun Agrawal
Data availability
The data used for this analysis is available at: http://www.forestlivelihoods.org/resources/ .
Code availability
All computer code used in this analysis is available from the authors upon reasonable request.
Houghton, R. A., Byers, B. & Nassikas, A. A. A role for tropical forests in stabilizing atmospheric CO 2 . Nat. Clim. Change 5 , 1022–1023 (2015).
Google Scholar
Newton, P., Oldekop, J. A., Brodnig, G., Karna, B. K. & Agrawal, A. Carbon, biodiversity, and livelihoods in forest commons: synergies, trade-offs, and implications for REDD+. Environ. Res. Lett. 11 , 044017 (2016).
Angelsen, A. et al. Environmental income and rural livelihoods: a global-comparative analysis. World Dev. 64 , S12–S28 (2014).
Lund, J. F., Rutt, R. L. & Ribot, J. Trends in research on forestry decentralization policies. Curr. Opin. Environ. Sustain. 32 , 17–22 (2018).
At a Crossroads: Consequential Trends in Recognition of Community-Based Forest Tenure (Rights and Resources Initiative, 2018).
Ribot, J. C., Agrawal, A. & Larson, A. M. Recentralizing while decentralizing: how national governments reappropriate forest resources. World Dev. 34 , 1864–1886 (2006).
Charnley, S. & Poe, M. R. Community forestry in theory and practice: where are we now? Annu. Rev. Anthropol. 36 , 301–337 (2007).
Cronkleton, P., Pulhin, J. M. & Saigal, S. Co-management in community forestry: how the partial devolution of management rights creates challenges for forest communities. Conserv. Soc. 10 , 91–102 (2012).
Gilmour, D. Forty Years of Community-Based Forestry: A Review of Its Extent and Effectiveness (FAO, 2016).
Baynes, J., Herbohn, J., Smith, C., Fisher, R. & Bray, D. Key factors which influence the success of community forestry in developing countries. Glob. Environ. Change 35 , 226–238 (2015).
Chhatre, A. & Agrawal, A. Trade-offs and synergies between carbon storage and livelihood benefits from forest commons. Proc. Natl Acad. Sci. USA 106 , 17667–17670 (2009).
CAS Google Scholar
Persha, L., Agrawal, A. & Chhatre, A. Social and ecological synergy: local rulemaking, forest livelihoods, and biodiversity conservation. Science 331 , 1606–1608 (2011).
Rasolofoson, R. A. et al. Impacts of community forest management on human economic well-being across Madagascar. Conserv. Lett. 10 , 346–353 (2017).
Oldekop, J. A., Sims, K. R. E., Karna, B. K., Whittingham, M. J. & Agrawal, A. Reductions in deforestation and poverty from decentralized forest management in Nepal. Nat. Sustain. 2 , 421–428 (2019).
Agrawal, A. & Benson, C. S. Common property theory and resource governance institutions: strengthening explanations of multiple outcomes. Environ. Conserv. 38 , 199–210 (2011).
Larson, A., Barry, D. & Dahal, G. R. New rights for forest-based communities? Understanding processes of forest tenure reform. Int. For. Rev. 12 , 78–96 (2010).
Hajjar, R., Kozak, R. A. & Innes, J. L. Is decentralization leading to ‘real’ decision-making power for forest-dependent communities? Case studies from Mexico and Brazil. Ecol. Soc. 17 , 12 (2012).
Newton, P. et al. What Are the Biophysical, Institutional, and Socioeconomic Contextual Factors Associated with Improvements in Livelihood and Environmental Outcomes in Forests Managed by Communities? A Systematic Review Protocol Working Paper 9 (CIFOR, 2015); https://doi.org/10.17528/cifor/005494
Hajjar, R. et al. The data not collected on community forestry. Conserv. Biol. 30 , 1357–1362 (2016).
Vyamana, V. Participatory forest management in the Eastern Arc Mountains of Tanzania: who benefits? Int. For. Rev. 11 , 239–253 (2009).
Mohammed, A. J. & Inoue, M. Forest-dependent communities’ livelihood in decentralized forest governance policy epoch: case study from West Shoa zone, Ethiopia. J. Nat. Resour. Policy Res. 5 , 49–66 (2013).
Nielsen, M. Improving the conservation status of the Udzungwa Mountains, Tanzania? The effect of joint forest management on bushmeat hunting in the Kilombero Nature Reserve. Conserv. Soc. 9 , 106–118 (2011).
Gobeze, T., Bekele, M., Lemenih, M. & Kassa, H. Participatory forest management and its impacts on livelihoods and forest status: the case of Bonga Forest in Ethiopia. Int. For. Rev. 11 , 346–358 (2009).
Mishra, T., Mandal, D. & Maiti, S. Evaluation of regeneration of Shorea robusta forests under joint forest management in West Bengal, India. Int. J. Environ. Sustain. Dev. 5 , 12–22 (2006).
Oyono, P., Samba, S. & Biyong, M. Beyond the decade of policy and community euphoria: the state of livelihoods under new local rights to forest in rural Cameroon. Conserv. Soc. 10 , 173–181 (2012).
Oldekop, J. A., Holmes, G., Harris, W. E. & Evans, K. L. A global assessment of the social and conservation outcomes of protected areas. Conserv. Biol. 30 , 133–141 (2016).
White, I., Royston, P. & Wood, A. Multiple imputation by chained equations: issues and guidance for practice. Stat. Med. 30 , 377–399 (2011).
Leuschner, C., Moser, G., Bertsch, C., Röderstein, M. & Hertel, D. Large altitudinal increase in tree root/shoot ratio in tropical mountain forests of Ecuador. Basic Appl. Ecol. 8 , 219–230 (2007).
Thanichanon, P., Schmidt-Vogt, D., Messerli, P., Heinimann, A. & Epprecht, M. Secondary forests and local livelihoods along a gradient of accessibility: a case study in northern Laos. Soc. Nat. Resour. 26 , 1283–1299 (2013).
Schlager, E. & Ostrom, E. Property-rights regimes and natural resources: a conceptual analysis. Land Econ. 68 , 249–262 (1992).
Place, F. & Hazell, P. Productivity effects of Indigenous land tenure systems in sub-Saharan Africa. Am. J. Agric. Econ. 75 , 10–19 (1993).
Gibson, C., Lehoucq, F. & Williams, J. Does privatization protect natural resources? Property rights and forests in Guatemala. Soc. Sci. Q. 83 , 206–225 (2002).
Bugri, J. T. The dynamics of tenure security, agricultural production and environmental degradation in Africa: evidence from stakeholders in north-east Ghana. Land Use Policy 25 , 271–285 (2008).
Cronkleton, P. & Larson, A. Formalization and collective appropriation of space on forest frontiers: comparing communal and individual property systems in the Peruvian and Ecuadoran Amazon. Soc. Nat. Resour. 28 , 496–512 (2015).
Nayak, P. K. & Berkes, F. Politics of co-optation: community forest management versus joint forest management in Orissa, India. Environ. Manage. 41 , 707–718 (2008).
Berkes, F., George, P. & Preston, R. J. Co-management: the evolution in theory and practice of the joint administration of living resources. Alternatives 18 , 12–18 (1991).
Humphries, S., Holmes, T., de Andrade, D. F. C., McGrath, D. & Dantas, J. B. Searching for win–win forest outcomes: learning-by-doing, financial viability, and income growth for a community-based forest management cooperative in the Brazilian Amazon. World Dev. https://doi.org/10.1016/j.worlddev.2018.06.005 (2018).
Bray, D. B. et al. Mexico’s community-managed forests as a global model for sustainable landscapes. Conserv. Biol. 17 , 672–677 (2003).
Balooni, K., Pulhin, J. & Inoue, M. When is Decentralization in Forest Management a Success and when is it a Failure? Case Studies from the Philippines (Indiana Univ., 2007).
Oldekop, J. A., Bebbington, A. J., Brockington, D. & Preziosi, R. F. Understanding the lessons and limitations of conservation and development. Conserv. Biol. 24 , 461–469 (2010).
Rudel, T. K. et al. Forest transitions: towards a global understanding of land use change. Glob. Environ. Change 15 , 23–31 (2005).
Erbaugh, J. T. & Oldekop, J. A. Forest landscape restoration for livelihoods and well-being. Curr. Opin. Environ. Sustain. 32 , 76–83 (2018).
Honey-Rosés, J., Maurer, M., Ramírez, M. I. & Corbera, E. Quantifying active and passive restoration in Central Mexico from 1986–2012: assessing the evidence of a forest transition. Restor. Ecol. 26 , 1180–1189 (2018).
Oldekop, J. A., Sims, K. R. E., Whittingham, M. J. & Agrawal, A. An upside to globalization: international outmigration drives reforestation in Nepal. Glob. Environ. Change 52 , 66–74 (2018).
Peluso, N. L. & Purwanto, A. B. The remittance forest: turning mobile labor into agrarian capital. Singap. J. Trop. Geogr. 39 , 6–36 (2018).
Sikor, T. & Nguyen, T. Q. Why may forest devolution not benefit the rural poor? Forest entitlements in Vietnam’s central highlands. World Dev. 35 , 2010–2025 (2007).
Pulhin, J. M. & Dressler, W. H. People, power and timber: the politics of community-based forest management. J. Environ. Manage. 91 , 206–214 (2009).
Jones, J. P. G. et al. Human migration to the forest frontier: implications for land use change and conservation management. Geo 5 , e00050 (2018).
Robson, J. P. & Berkes, F. Exploring some of the myths of land use change: can rural to urban migration drive declines in biodiversity? Glob. Environ. Change 21 , 844–854 (2011).
Brooks, J. S., Waylen, K. A. & Borgerhoff Mulder, M. How national context, project design, and local community characteristics influence success in community-based conservation projects. Proc. Natl Acad. Sci. USA 109 , 21265–21270 (2012).
Larson, A. M. & Soto, F. Decentralization of natural resource governance regimes. Annu. Rev. Environ. Resour. 33 , 213–239 (2008).
Anderson, J., Mehta, S., Epelu, E. & Cohen, B. Managing leftovers: does community forestry increase secure and equitable access to valuable resources for the rural poor? For. Policy Econ. 58 , 47–55 (2015).
Thanh, T. N. & Sikor, T. From legal acts to actual powers: devolution and property rights in the Central Highlands of Vietnam. For. Policy Econ. 8 , 397–408 (2006).
Larson, A. M., Cronkleton, P., Barry, D. & Pacheco, P. Tenure Rights and Beyond: Community Access to Forest Resources in Latin America (CIFOR, 2008).
Scheba, A. & Mustalahti, I. Rethinking ‘expert’ knowledge in community forest management in Tanzania. For. Policy Econ. 60 , 7–18 (2015).
Ameha, A., Nielsen, O. J. & Larsen, H. O. Impacts of access and benefit sharing on livelihoods and forest: case of participatory forest management in Ethiopia. Ecol. Econ. 97 , 162–171 (2014).
Lescuyer, G. Sustainable forest management at the local scale: a comparative analysis of community forests and domestic forests in Cameroon. Small-scale For. 12 , 51–66 (2013).
Guiang, E. S. & Castillo, G. Trends in forest ownership, forest resoures tenure and institutional arrangements in the Philippines: Are they contributing to better forest management and poverty reduction? (FAO, 2007).
Blackman, A., Corral, L., Lima, E. S. & Asner, G. P. Titling Indigenous communities protects forests in the Peruvian Amazon. Proc. Natl Acad. Sci. USA 114 , 4123–4128 (2017).
Sikor, T. et al. Community Forestry in Asia and the Pacific: Pathway to Inclusive Development (RECOFTC - The Center for People and Forests, 2013).
Wren-Lewis, L., Becerra-Valbuena, L. & Houngbedji, K. Formalizing land rights can reduce forest loss: experimental evidence from Benin. Sci. Adv. 6 , eabb6914 (2020).
Ostrom, E. Governing the Commons: The Evolution of Institutions for Collective Action (Cambridge Univ. Press, 1990).
Varughese, G. & Ostrom, E. The contested role of heterogeneity in collective action: some evidence from community forestry in Nepal. World Dev. 29 , 747–765 (2001).
Lambin, E. F. & Meyfroidt, P. Global land use change, economic globalization, and the looming land scarcity. Proc. Natl Acad. Sci. USA 108 , 3465–3472 (2011).
Scherr, S. J., White, A. & Kaimowitz, D. Making markets work for forest communities. Int. For. Rev. 5 , 67–73 (2003).
Agrawal, A. Forests, governance, and sustainability: common property theory and its contributions. Int. J. Commons 1 , 111–136 (2007).
Pagdee, A., Kim, Y. & Daugherty, P. J. What makes community forest management successful: a meta-study from community forests throughout the world. Soc. Nat. Resour. 19 , 33–52 (2006).
Hajjar, R. & Oldekop, J. A. Research frontiers in community forest management. Curr. Opin. Environ. Sustain. 32 , 119–125 (2018).
Methods (IFRI, 2013); http://ifri.forgov.org/resources/methods/
Persha, L., Fischer, H., Chhatre, A., Agrawal, A. & Benson, C. Biodiversity conservation and livelihoods in human-dominated landscapes: forest commons in South Asia. Biol. Conserv. 143 , 2918–2925 (2010).
Geist, H. J. & Lambin, E. F. Proximate causes and underlying driving forces of tropical deforestation. BioScience 52 , 143–150 (2002).
Rana, E., Thwaites, R. & Luck, G. Trade-offs and synergies between carbon, forest diversity and forest products in Nepal community forests. Environ. Conserv. 44 , 5–13 (2017).
Liaw, A. & Wiener, M. R Package ‘randomForest’ (The Comprehensive R Archive Network, 2018).
Breiman, L. Random forests. Mach. Learn. 45 , 5–32 (2001).
Genuer, R., Poggi, J. & Tuleau-Malot, C. Variable selection using random forests. Pattern Recognit. Lett. 31 , 2225–2236 (2010).
Harrell, F. Regression Modelling Strategies (Springer, 2015).
Download references
Acknowledgements
We thank the Evidence Based Forestry Initiative at the Centre for International Forestry Research (CIFOR) and the UK Department for International Development (DfID) for financing this research through its KNOWFOR programme grant. J.A.O. was supported through an EU FP7 Marie Curie Fellowship (FORCONEPAL). P.C. was supported through the CGIAR Research Program on Forest, Trees and Agroforestry (FTA), led by CIFOR. We also thank M. Vikas, M. Burbidge, A. Langeland and K. Gregory for their help in screening papers and extracting data and G. Steward, M. Grainger, M. Whittingham, R. Preziosi and E. W. Harris for their help with the statistical analysis.
Author information
These authors contributed equally: Reem Hajjar, Johan A. Oldekop.
Authors and Affiliations
Department of Forest Ecosystems and Society, Oregon State University, Corvallis, OR, USA
Reem Hajjar
Forests and Livelihoods: Assessment, Research, and Engagement (FLARE) network, School for Environment and Sustainability, University of Michigan, Ann Arbor, MI, USA
Reem Hajjar, Johan A. Oldekop & Peter Newton
Global Development Institute, The University of Manchester, Manchester, UK
Johan A. Oldekop
Center for International Forestry Research, La Molina, Lima, Peru
Peter Cronkleton
Environmental Studies Program, University of Colorado Boulder, Sustainability, Energy and Environment Community, Boulder, CO, USA
Peter Newton
Center for International Forestry Research, Jalan CIFOR, Situ Gede, Bogor Barat, Indonesia
Aaron J. M. Russell
Global Green Growth Institute, Naypyitaw, Myanmar
Yale School of the Environment and Department of Anthropology, Yale University, New Haven, CT, USA
You can also search for this author in PubMed Google Scholar
Contributions
R.H., J.A.O., P.N., A.J.M.R. and W.Z. conceived and designed the systematic review. R.H., J.A.O. and W.Z. conducted the review and data extraction. R.H. and J.A.O. conducted the analysis and drafted the manuscript. R.H., J.A.O., P.C., P.N., A.J.M.R. and W.Z. contributed to results interpretation and finalizing of the paper.
Corresponding authors
Correspondence to Reem Hajjar or Johan A. Oldekop .
Ethics declarations
Competing interests.
The authors declare no competing interests.
Additional information
Publisher’s note Springer Nature remains neutral with regard to jurisdictional claims in published maps and institutional affiliations.
Supplementary information
Supplementary information.
Supplementary Figs. 1–9 and Tables 1–3.
Reporting Summary
Rights and permissions.
Reprints and permissions
About this article
Cite this article.
Hajjar, R., Oldekop, J.A., Cronkleton, P. et al. A global analysis of the social and environmental outcomes of community forests. Nat Sustain 4 , 216–224 (2021). https://doi.org/10.1038/s41893-020-00633-y
Download citation
Received : 26 February 2020
Accepted : 01 October 2020
Published : 09 November 2020
Issue Date : March 2021
DOI : https://doi.org/10.1038/s41893-020-00633-y
Share this article
Anyone you share the following link with will be able to read this content:
Sorry, a shareable link is not currently available for this article.
Provided by the Springer Nature SharedIt content-sharing initiative
This article is cited by
The principles of natural climate solutions.
- Peter Woods Ellis
- Aaron Marr Page
- Susan C. Cook-Patton
Nature Communications (2024)
The effect of a political crisis on performance of community forests and protected areas in Madagascar
- Rachel A. Neugarten
- Ranaivo A. Rasolofoson
- Amanda D. Rodewald
The Property Rights Index (PRIF) can be used worldwide to compare different forest governance systems
- Richard Rimoli
- Liviu Nichiforel
- Laura Bouriaud
Scientific Reports (2023)
- Harry W. Fischer
- Ashwini Chhatre
- Arun Agrawal
Nature Climate Change (2023)
Towards epistemic diversity in sustainability transitions: an exploration of hybrid socio-technical systems
- Alejandro Balanzó-Guzmán
- Mónica Ramos-Mejía
Sustainability Science (2023)
Quick links
- Explore articles by subject
- Guide to authors
- Editorial policies
Sign up for the Nature Briefing: Anthropocene newsletter — what matters in anthropocene research, free to your inbox weekly.

- Review Paper
- Open access
- Published: 14 January 2015
Climate change impacts and adaptation in forest management: a review
- Rodney J. Keenan 1
Annals of Forest Science volume 72 , pages 145–167 ( 2015 ) Cite this article
52k Accesses
351 Citations
45 Altmetric
Metrics details
Key message
Adaptation of forest management to climate change requires an understanding of the effects of climate on forests, industries and communities; prediction of how these effects might change over time; and incorporation of this knowledge into management decisions. This requires multiple forms of knowledge and new approaches to forest management decisions. Partnerships that integrate researchers from multiple disciplines with forest managers and local actors can build a shared understanding of future challenges and facilitate improved decision making in the face of climate change.
Climate change presents significant potential risks to forests and challenges for forest managers. Adaptation to climate change involves monitoring and anticipating change and undertaking actions to avoid the negative consequences and to take advantage of potential benefits of those changes.
This paper aimed to review recent research on climate change impacts and management options for adaptation to climate change and to identify key themes for researchers and for forest managers.
The study is based on a review of literature on climate change impacts on forests and adaptation options for forest management identified in the Web of Science database, focusing on papers and reports published between 1945 and 2013.
One thousand one hundred seventy-two papers were identified in the search, with the vast majority of papers published from 1986 to 2013. Seventy-six percent of papers involved assessment of climate change impacts or the sensitivity or vulnerability of forests to climate change and 11 % (130) considered adaptation. Important themes from the analysis included (i) predicting species and ecosystem responses to future climate, (ii) adaptation actions in forest management, (iii) new approaches and tools for decision making under uncertainty and stronger partnerships between researchers and practitioners and (iv) policy arrangements for adaptation in forest management.
Conclusions
Research to support adaptation to climate change is still heavily focused on assessing impacts and vulnerability. However, more refined impact assessments are not necessarily leading to better management decisions. Multi-disciplinary research approaches are emerging that integrate traditional forest ecosystem sciences with social, economic and behavioural sciences to improve decision making. Implementing adaptation options is best achieved by building a shared understanding of future challenges among different institutions, agencies, forest owners and stakeholders. Research-policy-practice partnerships that recognise local management needs and indigenous knowledge and integrate these with climate and ecosystem science can facilitate improved decision making.
1 Introduction
Anthropogenic climate change presents potential risks to forests and future challenges for forest managers. Responding to climate change, through both mitigation and adaptation, may represent a paradigm shift for forest managers and researchers (Schoene and Bernier 2012 ). Climate change is resulting in increasing air temperature and changing precipitation regimes, including changes to snowfall and to the timing, amount and inter-annual variability of rainfall (IPCC 2013 ). Forests are widespread, long-lived ecosystems that are both intensively and extensively managed. They are potentially sensitive to these longer term climatic changes, as are the societies and economies that depend on them (Bernier and Schöne 2009 ). Climate change increases the potential consequences of many existing challenges associated with environmental, social or economic change.
Whilst forest ecosystems are resilient and many species and ecosystems have adapted historically to changing conditions, future changes are potentially of such magnitudes or will occur at rates that are beyond the natural adaptive capacity of forest species or ecosystems, leading to local extinctions and the loss of important functions and services, including reduced forest carbon stocks and sequestration capacity (Seppälä et al. 2009 ).
Recent global warming has already caused many changes in forests (Lucier et al. 2009 ). Aspects of climate change may be positive for some tree species in some locations. Tree growth is observed to be increasing in some locations under longer growing seasons, warmer temperatures and increased levels of CO 2 . However, many projected future changes in climate and their indirect effects are likely to have negative consequences for forests. Observed shifts in vegetation distribution (Kelly and Goulden 2008 ; Lenoir et al. 2010 ) or increased tree mortality due to drought and heat in forests worldwide (Allen et al. 2010 ) may not be due to human-induced climate change but demonstrate the potential impacts of rapid climate change. These impacts may be aggravated by other human-induced environmental changes such as increases in low elevation ozone concentrations, nitrogenous pollutant deposition, the introduction of exotic insect pests and pathogens, habitat fragmentation and increased disturbances such as fire (Bernier and Schöne 2009 ). Other effects of climate change may also be important for forests. Sea level rise is already impacting on tidal freshwater forests (Doyle et al. 2010 ) and tidal saltwater forests (mangroves) are expanding landward in sub-tropical coastal reaches taking over freshwater marsh and forest zones (Di Nitto et al. 2014 ).
With projected future change, species ranges will expand or contract, the geographic location of ecological zones will shift, forest ecosystem productivity will change and ecosystems could reorganise following disturbances into ecological systems with no current analogue (Campbell et al. 2009 ; Fischlin et al. 2009 ). Forests types differ in their sensitivity to climatic change. Bernier and Schöne ( 2009 ) considered boreal, mountain, Mediterranean, mangrove and tropical moist forests most vulnerable to climate change. However, there has been recent debate about the vulnerability of tropical moist forests (Corlett 2011 ; Huntingford et al. 2013 ; Feeley et al. 2012 ), and temperate forests in areas subject to drier climates may be more at risk (Choat et al. 2012 ).
Adapting to these changing and uncertain future conditions can be considered from a number of perspectives (McEvoy et al. 2013 ). Policy and management might be directed at avoiding or reducing the impact of climate-related events, reducing vulnerability to future climatic conditions, managing a broader suite of climate ‘risks’ or increasing resilience and capacity in forest ecological and production systems to recover from climate ‘shocks’.
Adapting forest management to climate change involves monitoring and anticipating change and undertaking actions to avoid the negative consequences or take advantage of potential benefits of those changes (Levina and Tirpak 2006 ). Adopting the principles and practices of sustainable forest management (SFM) can provide a sound basis for addressing the challenges of climate change. However, Innes et al. ( 2009 ) pointed out that our failure to implement the multi-faceted components of sustainable forest management in many forests around the world is likely to limit capacity to adapt to climate change. Forest managers will need to plan at multiple spatial and temporal scales and adopt more adaptive and collaborative management approaches to meet future challenges.
Whilst forest managers are accustomed to thinking in long time scales—considering the long-term implications of their decisions and factoring in uncertainty and unknowns into management—many are now responding to much shorter term social or economic imperatives. Local forestry practices are often based on an implicit assumption that local climate conditions will remain constant (Guariguata et al. 2008 ). Other social and economic changes will also continue to drive changes in forest management (Ince et al. 2011 ). For example, a growing global population, rapid economic development and increased wealth are driving demand for food and fibre crops and forest conversion to agriculture in many developing countries (Gibbs et al. 2010 ). Climate change mitigation objectives are increasing demands for wood-based bioenergy and the use of wood in construction and industrial systems. Increasing urbanisation is changing the nature of social demands on forests, and decreasing rural populations is limiting the availability of labour and capacity for intensive forest management interventions.
Ecosystem-based adaptation is being promoted as having the potential to incorporate sustainable management, conservation and restoration of ecosystems into adaptation to climate change (IUCN 2008 ). This can be achieved more effectively by integrating ecosystem management and adaptation into national development policies through education and outreach to raise societal awareness about the value of ecosystem services (Vignola et al. 2009 ).
Kimmins ( 2002 ) invoked the term ‘future shock’, first coined by Toffler ( 1970 ) to describe the situation where societal expectations from forests were changing faster than the institutional capacity for change in forest management organisations. The pace of climate change is likely to intensify this phenomenon. Empirically based management based on traditional ‘evidence-based’ approaches therefore will potentially not develop quickly enough for development of effective future management options. How can managers consider rapid change and incorporate the prospect of very different, but uncertain, future climatic conditions into their management decisions? What types of tools are needed to improve decision making capacity?
This study aimed to review the literature on studies to support forest management in a changing climate. It builds on the major review of Seppala ( 2009 ), in particular Chapter 6 of that report by Innes et al. ( 2009 ).
The study involved a systematic assessment of the literature based on the database Web of Science (Thomson-Reuters 2014 ), an online scientific citation indexing service that provides the capacity to search multiple databases, allowing in-depth exploration of the literature within an academic or scientific discipline.
The following search terms were used in the titles of publications:
(forest* or tree* or (terrestrial and ecosystem)) and climat* and (adapt* or impact* or effect* or respons*) and
(forest* or tree*) and climat* and vulnerabilit* or sensitivit*)
The search was restricted to publications between 1945 and 2013. References related solely to climate change mitigation were excluded, as were references where the word ‘climate’ simply referred to a study in a particular climatic zone. This left a database of 1172 publications for analyses (a spreadsheet of the papers revealed in the search can be obtained from the author). References were classified into various types of studies and different regions, again based on the titles. Not all papers identified in the search are referenced. The selection of themes for discussion and papers for citation was a subjective one, based on scanning abstracts and results from relevant individual papers. The focus was important themes from key papers and literature from the last 5 years. The review includes additional papers not revealed in the search relating to these themes including selected papers from the literature in the year 2014.
Of the published papers relating to climate impacts or adaptation selected for analysis, the vast majority of papers were published from 1986 onwards. The earliest paper dated from 1949 (Gentilli 1949 ) analysing the effects of trees on climate, water and soil. Most studies prior to 1986 (and even some published later) focused on the effects of trees on local or wider regional climate (Lal and Cummings 1979 ; Otterman et al. 1984 ; Bonan et al. 1992 ), the implications of climate variability (Hansenbristow et al. 1988 ; Ettl and Peterson 1995 ; Chen et al. 1999 ), studies of tree and forest responses across climatic gradients (Grubb and Whitmore 1966 ; Bongers et al. 1999 ; Davidar et al. 2007 ) or responses to historical climate (Macdonald et al. 1993 ; Huntley 1990 ; Graumlich 1993 ).
One thousand twenty-six papers specifically addressed future climate change (rather than historical climate or gradient analysis). Of these, 88 % studied impacts, effects, vulnerability or responses to climate change in tree species, forests, forest ecosystems or the forest sector (Fig. 1 ). The first study analysing the potential impacts of future climate change on terrestrial ecosystems was published in 1985 (Emanuel et al. 1985 ) with other highly cited papers soon after (Pastor and Post 1988 ; Cannell et al. 1989 ).
Publication numbers by publication year for publications relating to climate change and forests from a search of the Web of Science database to the end of 2013 (1025 in total, 896 publications studied climate change impacts, responses or vulnerability, 129 studied adaptation)
Twelve percent of papers (129) considered adaptation options, including 10 papers on adaptation in the forest sector. The first papers to focus on adaptation in the context of climate change were in 1996 with a number of papers published in that year (Kienast et al. 1996 ; Kobak et al. 1996 ; Dixon et al. 1996 ). Publications were then relatively few each year until the late 2000s with numbers increasing to 11 in 2009, 22 in 2010 and 27 in 2011. Publications on adaptation dropped to 14 papers in 2013. The ratio of adaptation-related papers has increased more recently, with 19 % of total publications on adaptation in the last 5 years. Most papers considering adaptation since the early 2000s have related to the integration of adaptation and forest management (e.g. Lindner 2000 ; Spittlehouse 2005 ; Kellomaki et al. 2008 ; Guariguata 2009 ; Bolte et al. 2009 ; Keskitalo 2011 ; Keenan 2012 ; Temperli et al. 2012 ).
Analyses of the implications of climate change for the forest sector have focused heavily on North America: Canada (Ohlson et al. 2005 ; Van Damme 2008 ; Rayner et al. 2013 ; Johnston et al. 2012 ) and the USA (Joyce et al. 1995 ; Blate et al. 2009 ; Kerhoulas et al. 2013 ); and Europe (Karjalainen et al. 2003 ; von Detten and Faber 2013 ). There has been a stronger consideration in recent years of social, institutional and policy issues (Ogden and Innes 2007b ; Kalame et al. 2011 ; Nkem et al. 2010 ; Spies et al. 2010 ; Somorin et al. 2012 ) and the assessment of adaptive capacity in forest management organisations and in society more generally (Keskitalo 2008 ; Lindner et al. 2010 ; Bele et al. 2013a ).
Regionally, there have been relatively few published journal articles on impacts or adaptation in forests in the Southern Hemisphere (Hughes et al. 1996 ; Williams 2000 ; Pinkard et al. 2010 ; Gonzalez et al. 2011 ; Mok et al. 2012 ; Breed et al. 2013 ), although there have been more studies in the grey literature for Australian forests (Battaglia et al. 2009 ; Cockfield et al. 2011 ; Medlyn et al. 2011 ; Stephens et al. 2012 ). There have been some valuable analyses for the tropics (Guariguata et al. 2008 , 2012 ; Somorin et al. 2012 ; Feeley et al. 2012 ).
Analysis of the publications identified the following key themes: (i) predicting species and ecosystem responses to future climate, (ii) adaptation actions in forest management, (iii) new approaches and tools for decision making under uncertainty and stronger partnerships between researchers and practitioners and (iv) policy arrangements for adaptation in forest management. These are discussed in more detail below.
3.1 Predicting species and ecosystem responses to future climate
Forest managers have long used climatic information in a range of ways in planning and decision making. Climate information has been used extensively to define and map vegetation types and ecological zones and for modelling habitat distributions of vertebrates and invertebrates (Daubenmire 1978 ; Pojar et al. 1987 ; Thackway and Cresswell 1992 ), for species and provenance selection (Booth et al. 1988 ; Booth 1990 ) and seed zone identification (Johnson et al. 2004 ), for forest fire weather risk assessment and fire behaviour modelling (Carvalho et al. 2008 ), for modelling forest productivity (Battaglia et al. 2004 ) and analysing the dynamics of a range of ecological processes (Anderson 1991 ; Breymeyer and Melillo 1991 ). Predicting species responses to future climate change presents a different set of challenges, involving consideration of predictions of future climate that are often outside the historical range of variability of many species. These challenges are discussed in the next section.
3.1.1 Species responses to climate
Aitken et al. ( 2008 ) argued that there were three possible fates for forest tree populations in rapidly changing climatic conditions: persistence through spatial migration to track their ecological niches, persistence through adaptation to new conditions in current locations or the extirpation of the species. Predicting the potential fate of populations in these conditions requires the integration of knowledge across biological scales from individual genes to ecosystems, across spatial scales (for example, seed and pollen dispersal distances or breadth of species ranges) and across temporal scales from the phenology of annual developmental cycle traits to glacial and interglacial cycles.
Whilst there has been widespread use of climatic information to predict future distributions in species distribution models (SDMs, Pearson and Dawson 2003 ; Attorre et al. 2008 ; Wang et al. 2012 ; Ruiz-Labourdette et al. 2013 ), understanding of the range of climatic and non-climatic factors that will determine the future range of a particular species remains limited. Many now feel that SDMs are of limited value in adaptation decision making or species conservation strategies. Some of these limitations are summarised in Table 1 .
For example, models indicate significant shifts in patterns of tree species distribution over the next century but usually without any intrinsic consideration of the biological capacity of populations to move, internal population dynamics, the extent and role of local adaptation or the effects of climate and land use (Aitken et al. 2008 ; Thuiller et al. 2008 ). In a recent study, Dobrowski et al. ( 2013 ) found that the predicted speed of movement of species to match the predicted rate of climate change appears to be well beyond the historical rates of migration. Whilst modelled outputs suggest that migration rates of 1000 m per year or higher will be necessary to track changing habitat conditions (Malcolm et al. 2002 ), actual migration rates in response to past change are generally considered to have been less than 100 m per year. This was reinforced by model predictions that incorporate species dispersal characteristics for five tree species in the eastern USA indicated very low probabilities of dispersal beyond 10–20 km from current species boundaries by 2100 (Iverson et al. 2004 ). Corlett and Westcott ( 2013 ) also argued that plant movements are not realistically represented in models used to predict future vegetation or carbon-cycle feedbacks and that fragmentation of natural systems is likely to slow migration rates.
However, these estimates do not account for the role of humans in influencing tree species distributions, which they have done for thousands of years (Clark 2007 ), and managed translocation may be an option for conserving many tree species, but there are significant unresolved technical and social questions about implementing translocation at a larger scale (Corlett and Westcott 2013 ).
Most early SDMs relied primarily on temperature envelopes to model future distribution, but factors such as precipitation and soil moisture are potentially more limiting and more important in determining distribution patterns (Dobrowski et al. 2013 ). Aitken et al. ( 2008 ) found that the degree to which variation in precipitation explains phenotypic variation among populations is greater in general for populations from continental than from maritime climates and greater for lower latitude than higher latitude populations. However, precipitation alone is often not a good predictor of variation and there is often a strong interaction with temperature (Andalo et al. 2005 ). Heat to moisture index or aridity is probably more important in determining future distribution or productivity than precipitation alone (Aitken et al. 2008 ; Harper et al. 2009 ; Wang et al. 2012 ). Soil properties (depth, texture and organic matter content) have a major influence on plant-available water, but few SDMs incorporate these.
Future precipitation is proving more difficult to model than temperature, due to the complex effects of topography, and there are more widely varying estimates between global circulation models (GCMs) of future change in precipitation (IPCC 2013 ). As such, there is more uncertainty around the extent to which moisture stress will change with warming and the extent to which natural selection pressures will change as a result. Even without changes in precipitation, increased temperatures will increase the length of growing season and potential evapotranspiration (PET) resulting in more water use over the year and greater risk plant water shortage and drought death.
Changes in the intervals of extreme events (extreme heat, cold, precipitation, humidity, wind) may also matter more than changes in the mean. Current forecasting approaches that produce future climate averages may make it difficult to detect non-linear ecosystem dynamics, or threshold effects, that could trigger abrupt ecosystem change (Campbell et al. 2009 ). Zimmermann et al. ( 2009 ) found that predictions of spatial patterns of tree species in Switzerland were improved by incorporating measures of extremes in addition to means in SDMs.
The risks of future climate will also depend on the management goal. If the aim is simply to conserve genetic diversity, risks of extinction or reduction in genetic diversity may be overstated by SDMs because much of the genetic variation within tree species is found within rather than among their populations, and the extinction of a relatively large proportion of a population is generally likely to result in relatively little overall loss of genetic diversity (Hamrick 2004 ). Local habitat heterogeneity (elevation, slope aspect, moisture, etc.) can preserve adaptive genetic variation that, when recombined and exposed to selection in newly colonised habitats, can provide for local adaptation. The longevity of individual trees can also retard population extinction and allow individuals and populations to survive until habitat recovery or because animal and wind pollination can provide levels of pollen flow that are sufficient to counteract the effects of genetic drift in fragmented populations. Consequently, widespread species with large populations, high fecundity and higher levels of phenotypic plasticity are likely to persist and adapt and have an overall greater tolerance to changing climates than predicted by SDMs (Alberto et al. 2013 ).
Tree species distributions have always been dynamic, responding to changing environmental conditions, and populations are likely to be sub-optimal for their current environments (Namkoong 2001 ; Wu and Ying 2004 ). These lag effects are important in predicting species responses to climate change. In a modelling study of Scots pine and silver birch, Kuparinen et al. ( 2010 ) predicted that after 100 years of climate change, the genotypic growth period length of both species will lag more than 50 % behind the climatically determined optimum. This lag is reduced by increased mortality of established trees, whereas earlier maturation and higher dispersal ability had comparatively minor effects. Thuiller et al. ( 2008 ) suggest that mechanisms for incorporating these ‘trailing edge’ effects into SDMs are a major area of research potential.
Trees are also capable of long-distance gene flow, which can have both adaptive evolution benefits and disadvantages. Kremer et al. ( 2012 ) found that there may be greater positive effects of gene flow for adaptation but that the balance of positive to negative consequences of gene flow differs for leading edge, core and rear sections of forest distributions.
Epigenetics—heritable changes that are not caused by changes in genetic sequences but by differences in the way DNA methylation controls the degree of gene expression—is another complicating factor in determining evolutionary response to climate change (Brautigam et al. 2013 ). For example, a recent study in Norway spruce ( Picea abies ) showed that the temperature during embryo development can dramatically affect cold hardiness and bud phenology in the offspring. In some cases, the offspring’s phenotype varied by the equivalent of 6° of latitude from what was expected given the geographic origin of the parents. It remains uncertain whether these traits are persistent, both within an individual’s lifetime and in its offspring and subsequent generations (Aitken et al. 2008 ). It is suggested that analysis of the epigenetic processes in an ecological context, or ‘ecological epigenetics’, is set to transform our understanding of the way in which organisms function in the landscape. Increased understanding of these processes can inform efforts to manage and breed tree species to help them cope with environmental stresses (Brautigam et al. 2013 ). Others argue that whilst investigating this evolutionary capacity to adapt is important, understanding responses of species to their changing biotic community is imperative (Anderson et al. 2012 ) and ‘landscape genomics’ may offer a better approach for informing management of tree populations under climate change (Sork et al. 2013 ).
These recent results indicate the importance of accounting for evolutionary processes in forecasts of the future dynamics and productivity of forests. Species experiencing high mortality rates or populations that are subject to regular disturbances such as storms or fires might actually be the quickest to adapt to a warming climate.
Species life history characteristics are also not usually well represented in most climate-based distribution models. Important factors include age to sexual maturity, fecundity, seed dispersal, competition or chilling or dormancy requirements (Nitschke and Innes 2008b ).
Competitive relationships within and between species are likely to be altered by climate change. Most models also assume open site growth conditions, rather than those within a forest, where the growth environment will be quite different. However, increased disturbance associated with climate change may create stand reinitiation conditions more often than has occurred in the past, altering competitive interactions.
Process-based models of species range shifts and ecosystem change may capture more of the life history variables and competition effects that will be important in determining responses to climate change (Kimmins 2008 ; Nitschke and Innes 2008a , b ). These can provide the basis for a more robust assessment framework that integrates biological characteristics (e.g. shade tolerance and seedling establishment) and disturbance characteristics (e.g. insect pests, drought and fire topkill). Matthews et al. ( 2011 ) integrated these factors into a decision support system that communicates uncertainty inherent in GCM outputs, emissions scenarios and species responses. This demonstrated a greater diversity among species to adapt to climate change and provides a more practical assessment of future species projections.
In summary, whilst SDMs and other climate-based modelling approaches can provide a guide to potential species responses, the extent to which future climate conditions will result in major range shifts or extinction of species is unclear and the value of this approach in adaptation and decision making is limited. The evidence from genetic studies seems to suggest that many species are reasonably robust to potential future climate change. Those with a wide geographic range, large populations and high fecundity may suffer local population extinction but are likely to persist and adapt whilst suffering adaptational lag for a few generations. For example, Booth ( 2013 ) considered that many eucalyptus species, some of which are widely planted around the world, had a high adaptive capacity even though their natural ranges are quite small.
However, large contractions or shifts in distribution could have significant consequences for different forest values and species with small populations, fragmented ranges, low fecundity or suffering declines due to introduced insects or diseases may have a higher sensitivity and are at greater risk in a changing climate (Aitken et al. 2008 ).
3.1.2 Ecosystem responses to climate
Projecting the fate of forest ecosystems under a changing climate is more challenging than for species. It has been well understood for some time that species will respond individualistically to climate change, rather than moving in concert, and that this is likely to result in ‘novel’ ecosystems, or groups of species, that are not represented in current classifications (Davis 1986 ). Forecasts need to consider the importance of these new species interactions and the confounding effects of future human activities.
Climate change affects a wide range of ecosystem functions and processes (Table 2 ). These include direct effects of temperature and precipitation on physiological and reproductive processes such as photosynthesis, water use, flowering, fruiting and regeneration, growth and mortality and litter decomposition. Changes in these processes will have effects on species attributes such as wood density or foliar nutrient status. Indirect effects will be exhibited through changing fire and other climate-driven disturbances. These will ultimately have impacts on stand composition, habitat structure, timber supply capacity, soil erosion and water yield.
Most early studies of forest ecosystem responses to climate change were built around ecosystem process models at various scales (Graham et al. 1990 ; Running and Nemani 1991 ; Rastetter et al. 1991 ). A number of recent studies have investigated the effects of past and current climate change on forest processes, often with surprising effects (Groffman et al. 2012 ).
Observed forest growth has increased recently in a number of regions, for example over the last 100 years in Europe (Pretzsch et al. 2014 ; Kint et al. 2012 ), and for more recent observations in Amazon forests (Phillips et al. 2008 ). In a major review, Boisvenue and Running ( 2006 ) found that at finer spatial scales, a trend is difficult to decipher, but globally, based on both satellite and ground-based data, climatic changes seemed to have a generally positive impact on forest productivity when water was not limiting. However, there can be a strong difference between species, complicating ecosystem level assessments (Michelot et al. 2012 ), and there are areas with little observed change (Schwartz et al. 2013 ). Generally, there are significant challenges in detecting the response of forests to climate change. For example, in the tropics, the lack of historical context, long-term growth records and access to data are real barriers (Clark 2007 ) and temperate regions also have challenges, even with well-designed, long-term experiments (Leites et al. 2012 ).
Projections of net primary productivity (NPP) under climate change indicate that there is likely to be a high level of regional variation (Zhao et al. 2013 ). Using a process model and climate scenario projections, Peters et al. ( 2013 ) predicted that average regional productivity in forests in the Great Lakes region of North America could increase from 67 to 142 %, runoff could potentially increase from 2 to 22 % and net N mineralization from 10 to 12 %. Increased productivity was almost entirely driven by potential CO 2 fertilization effects, rather than by increased temperature or changing precipitation. Productivity in these forests could shift from temperature limited to water limited by the end of the century. Reyer et al. ( 2014 ) also found strong regional differences in future NPP in European forests, with potential growth increases in the north but reduced growth in southern Europe, where forests are likely to be more water limited in the future. Again, assumptions about the impact of increasing CO 2 were a significant factor in this study.
In a different type of study using analysis of over 2400 long-term measurement plots, Bowman et al. ( 2014 ) found that there was a peaked response to temperature in temperate and sub-tropical eucalypt forests, with maximum growth occurring at a mean annual temperature of 11 °C and maximum temperature of the warmest month of 25–27 °C. Lower temperatures directly constrain growth, whilst high temperatures primarily reduced growth by reducing water availability but they also appeared to exert a direct negative effect. Overall, the productivity of Australia’s temperate eucalypt forests could decline substantially as the climate warms, given that 87 % of these forests currently experience a mean annual temperature above the ‘optimal’ temperature.
Incorporating the effects of rising CO 2 in models of future tree growth continues to be a major challenge. The sensitivity of projected productivity to assumptions regarding increased CO 2 was high in modelling studies of climate change impacts in commercial timber plantations in the Southern Hemisphere (Kirschbaum et al. 2012 ; Battaglia et al. 2009 ), and a recent analysis indicated a general convergence of different model predictions for future tree species distribution in Europe, with most of the difference between models due to the way in which this effect is incorporated (Cheaib et al. 2012 ). Increased CO 2 has been shown to increase the water-use efficiency of trees, but this is unlikely to entirely offset the effects of increased water stress on tree growth in drying climates (Leuzinger et al. 2011 ; Booth 2013 ). In general, despite studies extending over decades and improved understanding of biochemical processes (Franks et al. 2013 ), the impacts of increased CO 2 on tree and stand growth are still unresolved (Kallarackal and Roby 2012 ).
Integrating process model outputs with spatially explicit landscape models can improve understanding and projection of responses and landscape planning and this could provide for simulations of changes in ecological processes (e.g. tree growth, succession, disturbance cycles, dispersal) with other human-induced changes to landscapes (Campbell et al. 2009 ).
Investigation of current species responses to changing climate conditions may also guide improved prediction of patterns of future change in ecosystem distribution. For example, Allen et al. ( 2010 ) suggest that spatially explicit documentation of environmental conditions in areas of forest die-off is necessary to link mortality to causal climate drivers, including precipitation, temperature and vapour pressure deficit. Better prediction of climate responses will also require improved knowledge of belowground processes and soil moisture conditions. Assessments of future productivity will depend on accurate measurements of rates (net ecosystem exchange and NPP), changes in ecosystem level storage (net ecosystem production) and quantification of disturbances effects to determine net biome production (Boisvenue and Running 2006 ).
Hydrological conditions, runoff and stream flow are of critical importance for humans and aquatic organisms, and many studies have focused on the implications of climate change for these ecosystem processes. However, most of these have been undertaken at small catchment scale (Mahat and Anderson 2013 ; Neukum and Azzam 2012 ; Zhou et al. 2011 ) with few basin-scale assessments (van Dijk and Keenan 2007 ). However, the effects of climate and forest cover change on hydrology are complicated. Loss of tree cover may increase stream flow but can also increase evaporation and water loss (Guardiola-Claramonte et al. 2011 ). The extent of increasing wildfire will also be a major factor determining hydrological responses to climate change (Versini et al. 2013 ; Feikema et al. 2013 ).
Changing forest composition will also affect the habitat of vertebrate and invertebrate species. The implications of climate change for biodiversity conservation have been subject to extensive analysis (Garcia et al. 2014 ; Vihervaara et al. 2013 ; Schaich and Milad 2013 ; Clark et al. 2011 ; Heller and Zavaleta 2009 ; Miles et al. 2004 ). An integrated analytical approach, considering both impacts on species and habitat is important. For example, in a study of climate change impacts on bird habitat in the north-eastern USA, the combination of changes in tree distribution and habitat for birds resulted in significant impacts for 60 % of the species. However, the strong association of birds with certain vegetation tempers their response to climate change because localised areas of suitable habitat may persist even after the redistribution of tree species (Matthews et al. 2011 ).
Understanding thresholds in changing climate conditions that are likely to result in a switch to a different ecosystem state, and the mechanisms that underlie ecosystem responses, will be critical for forest managers (Campbell et al. 2009 ). Identifying these thresholds of change is challenging. Detailed process-based ecosystem research that identifies and studies critical species interactions and feedback loops, coupled with scenario modelling of future conditions, could provide valuable insights (Kimmins et al. 1999 , 2008 ; Walker and Meyers 2004 ). Also, rather than pushing systems across thresholds into alternative states, climate change may create a stepwise progression to unknown transitional states that track changing climate conditions, requiring a more graduated approach in management decisions (Lin and Petersen 2013 ).
Ultimately, management decisions may not be driven by whether we can determine future thresholds of change, but by observing the stressors that determine physiological limits of species distributions. These thresholds will depend on species physiology and local site conditions, with recent research demonstrating already observed ecosystem responses to climate change, including die-back of some species (Allen et al. 2010 ; Rigling et al. 2013 ).
3.1.3 Fire, pests, invasive species and disturbance risks
Many of the impacts of a changing future climate are likely to be felt through changing disturbance regimes, in particular fire. Forest fire weather risk and fire behaviour prediction have been two areas where there has been strong historical interaction between climate science and forest management and where we may see major tipping points driving change in ecosystem composition (Adams 2013 ). Fire weather is fundamentally under the control of large-scale climate conditions with antecedent moisture anomalies and large-scale atmospheric circulation patterns, further exacerbated by configuration of local winds, driving fire weather (Brotak and Reifsnyder 1977 ; Westerling et al. 2002 , 2006 ). It is therefore important to improve understanding of both short- and long-term atmospheric conditions in determining meteorological fire risk (Amraoui et al. 2013 ).
Increased fuel loads and changes to forest structure due to long periods of fire exclusion and suppression are increasing fire intensity and limiting capacity to control fires under severe conditions (Williams 2004 , 2013 ). Increasing urbanisation is increasing the interface between urban populations and forests in high fire risk regions, resulting in greater impacts of wildfire on human populations, infrastructure and assets (Williams 2004 ). Deforestation and burning of debris and other types of human activities are also introducing fire in areas where it was historically relatively rare (Tacconi et al. 2007 ).
In a recent study, Chuvieco et al. ( 2014 ) assessed ecosystem vulnerability to fire using an index based on ecological richness and fragility, provision of ecosystem services and value of houses in the wildland–urban interface. The most vulnerable areas were found to be the rainforests of the Amazon Basin, Central Africa and Southeast Asia; the temperate forest of Europe, South America and north-east America; and the ecological corridors of Central America and Southeast Asia.
In general, fire management policies in many parts of the world will need to cope with longer and more severe fire seasons, increasing fire frequency, and larger areas exposed to fire risk. This will especially be the case in the Mediterranean region of Europe (Kolström et al. 2011 ) and other fire-prone parts of the world such as South Eastern Australia (Hennessy et al. 2005 ). This will require improved approaches to fire weather modelling and behaviour prediction that integrate a more sophisticated understanding of the climate system with local knowledge of topography, vegetation and wind patterns. It will also require the development of fire management capacity where it had previously not been necessary. Increased fire weather severity could push current suppression capacity beyond a tipping point, resulting in a substantial increase in large fires (de Groot et al. 2013 ; Liu et al. 2010 ) and increased investment in resources and management efforts for disaster prevention and recovery.
Biotic factors may be more important than direct climate effects on tree populations in a changing climate. For example, insects and diseases have much shorter generation length and are able to adapt to new climatic conditions more rapidly than trees. However, if insects move more rapidly to a new environment whilst tree species lag, some parts of the tree population may be impacted less in the future (Regniere 2009 ).
The interaction of pests, diseases and fire will also be important. For example, this interaction will potentially determine the vulnerability of western white pine ( Pinus monticola ) ecosystems in Montana in the USA. Loehman et al. ( 2011 ) found that warmer temperatures will favour western white pine over existing climax and shade tolerant species, mainly because warmer conditions will lead to increased frequency and extent of wildfires that facilitates regeneration of this species.
3.2 Adaptation actions in forest management
The large majority of published studies relating to forests and climate change have been on vulnerability and impacts. These have increased understanding of the various relationships between forest ecosystems and climate and improved capacity to predict and assess ecosystem responses. However, managers need greater guidance in anticipating and responding to potential impacts of climate change and methods to determine the efficiency and efficacy of different management responses because they are generally not responding sufficiently to potential climate risks.
3.2.1 Adaptation actions at different management levels
A number of recent reviews have described adaptation actions and their potential application in different forest ecosystems being managed for different types of goods or services (Bernier and Schöne 2009 ; Innes et al. 2009 ; Lindner et al. 2010 ; Kolström et al. 2011 ), and adaptation guides and manuals have been developed (Peterson et al. 2011 ; Stephens et al. 2012 ) for different types of forest and jurisdictions. Adaptation actions can be primarily aimed at reducing vulnerability to increasing threats or shocks from natural disasters or extreme events, or increasing resilience and capacity to respond to progressive change or climate extremes. Adaptation actions can be reactive to changing conditions or planned interventions that anticipate future change. They may involve incremental changes to existing management systems or longer term transformational changes (Stafford Smith et al. 2011 ). Adaptation actions can also be applied at the stand level or at ownership, estate or national scales (Keskitalo 2011 ).
Recent research at the stand level in forests in the SE USA showed that forest thinning, often recommended in systems that are likely to experience increased temperature and decreased precipitation as a result of climate change, will need to be more aggressive than traditionally practised to stimulate growth of large residual trees, improve drought resistance and provide greater resilience to future climate-related stress (Kerhoulas et al. 2013 ).
An analysis of three multi-aged stand-level options in Nova Scotia, Canada, Steenberg et al. ( 2011 ) found that leaving sexually immature trees to build stand complexity had the most benefit for timber supply but was least effective in promoting resistance to climate change at the prescribed harvest intensity. Varying the species composition of harvested trees proved the most effective treatment for maximising forest age and old-growth area and for promoting stands composed of climatically suited target species. The combination of all three treatments resulted in an adequate representation of target species and old forest without overly diminishing the timber supply and was considered most effective in minimising the trade-offs between management values and objectives.
An estate level analysis of Austrian Federal Forests indicated that management to promote mixed stands of species that are likely to be well adapted to emerging environmental conditions, silvicultural techniques fostering complexity and increased management intensity might successfully reduce vulnerability, with the timing of adaptation measures important to sustain supply of forest goods and services (Seidl et al. 2011 ).
Whilst researchers are analysing different management options, the extent to which they are being implemented in practice is generally limited. For example, in four regions in Germany, strategies for adapting forest management to climate change are in the early stages of development or simply supplement existing strategies relating to general risk reduction or to introduce more ‘nature-orientated’ forest management (Milad et al. 2013 ). Guariguata et al. ( 2012 ) found that forest managers across the tropics perceived that natural and planted forests are at risk from climate change but were ambivalent about the value of investing in adaptation measures, with climate-related threats to forests ranked below others such as clearing for commercial agriculture and unplanned logging.
Community-based management approaches are often argued to be the most successful approach for adaptation. An analysis of 38 community forestry organisations in British Columbia found that 45 % were researching adaptation and 32 % were integrating adaptation techniques into their work (Furness and Nelson 2012 ). Whilst these community forest managers appreciated support and advice from government for adaptation, balancing this advice with autonomy for communities to make their own decisions was considered challenging.
In a study of communities impacted by drought in the forest zone of Cameroon, Bele et al. ( 2013b ) identified adaptive strategies such as community-created firebreaks to protect their forests and farms from forest fires, the culture of maize and other vegetables in dried swamps, diversifying income activities or changing food regimes. However, these coping strategies were considered to be incommensurate with the rate and magnitude of change being experienced and therefore no longer seen as useful. Some adaptive actions, whilst effective, were resource inefficient and potentially translate pressure from one sector to another or generated other secondary effects that made them undesirable.
3.2.2 Integrating adaptation and mitigation
In considering responses to climate change, forest managers will generally be looking for solutions that address both mitigation objectives and adaptation. To maintain or increase forest carbon stocks over the long term, the two are obviously inextricably linked (Innes et al. 2009 ). Whilst there are potentially strong synergies, Locatelli et al. ( 2011 ) identified potential trade-offs between actions to address mitigation and the provision of local ecosystem services and those for adaptation. They argued that mitigation projects can facilitate or hinder the adaptation of local people to climate change, whereas adaptation projects can affect ecosystems and their potential to sequester carbon.
Broadly, there has been little integration to date of mitigation and adaptation objectives in climate policy. For example, there is little connection between policies supporting the reducing emissions from deforestation and forest degradation plus (REDD+) initiatives and adaptation. Integrating adaptation into REDD+ can advance climate change mitigation goals and objectives for sustainable forest management (Long 2013 ). Kant and Wu ( 2012 ) considered that adaptation actions in tropical forests (protection against fire and disease, ensuring adequate regeneration and protecting against coastal impacts and desertification) will improve future forest resilience and have significant climate change mitigation value.
3.2.3 Sector-level adaptation
Analyses of climate change impacts and vulnerability at the sector level have been undertaken for some time (Lindner et al. 2002 ; Johnston and Williamson 2007 ; Joyce 2007 ). However, it has recently been argued (Wellstead et al. 2014 ) that these assessments, which focus on macro system-level variables and relationships, fail to account for the multi-level or polycentric nature of governance and the possibility that policy processes may result in the non-performance of critical tasks required for adaptation.
Joyce et al. ( 2009 ) considered that a toolbox of management options for the US National Forests would include the following: practices focused on reducing future climate change effects by building resistance and resilience into current ecosystems and on managing for change by enabling plants, animals and ecosystems to adapt to climate change. Sample et al. ( 2014 ) demonstrated the utility of this approach in a coniferous forest management unit in northwestern USA. It provided an effective means for guiding management decisions and an empirical basis for setting budgetary and management priorities. In general, more widespread implementation of already known practices that reduce the impact of existing stressors represents an important ‘no regrets’ strategy.
Johnston and Hesseln ( 2012 ) found that barriers to implementing adaptation across forest sector managers in Canada included inflexible tenure arrangements and regulatory environments which do not support innovation. Echoing calls for wider implementation of SFM as a key adaptation strategy (Innes et al. 2009 ), they argued that forest certification systems, participating in the Canadian model forest programme, and adopting criteria and indicators of SFM can support sectoral level adaptation.
Decentralised management approaches are considered to be a more appropriate governance arrangement for forest management, but Rayner et al. ( 2013 ) argued that a decentralised forest policy sector in Canada has resulted in limitations where policy, such as adaptation, requires a coherent national response. Climate change adaptation has led to an expansion of departmental mandates that is not being addressed by better coordination of the available policy capacity. Relevant federal agencies are not well represented in information networks, and forest policy workers report lower levels of internal and external networking than workers in related policy subsectors.
Economic diversification can be a valuable strategy to improve resilience to climate-related shocks. This can take a range of forms: developing new industries or different types of forest-based industries based on different goods or services. For the timber sector, the value of diversification as a risk management strategy for communities is open to question. Ince et al. ( 2011 ) pointed out that the forest sector operates in an international market and is susceptible to changes in the structure of this market. In the US forest sector, globalization has accelerated structural change, favouring larger and more capital-intensive enterprises and altering historical patterns of resource use. They suggest that future markets for timber will be driven by developments in these larger scale enterprises and may not lead to expansion of opportunities for smaller scale forest enterprises because development of niche markets or customised products is likely to be pursued aggressively by larger globally oriented enterprises to develop branding, product identity and product value. How to best diversify for adaptation therefore remains an open question.
Consequently, whilst policies that support economic diversification will be important, this may involve diversification well beyond traditional sectors. For example, in areas where there is a high probability that forests will be lost in favour of other ecosystems, such as grasslands, managers should recognise early on that their efforts and resources may best be focused outside forests (Innes et al. 2009 ). These adjustments will involve taking into account the perceptions of climate risk by various stakeholders, including individuals, communities, governments, private institutions and organisations (Adger et al. 2007 ). Vulnerability assessments and adaptation measures also need to be developed in a framework that takes into account the vulnerabilities and actions in other sectors that are linked to the forest sector, such as food, energy, health and water (Sonwa et al. 2012 ).
3.3 New approaches to decision making
Climate change presents new challenges for forest managers. Change is likely to happen faster than traditional, empirical approaches can provide evidence to support changes in management. Uncertainties in a range of aspects of future climate may also not be reduced through investment in research. Given that management for activities such as timber production can no longer be based solely on empirically derived growth and yield trajectories and management plans must incorporate uncertainty and the increased probability of extreme events, what types of tools are available to support these approaches? This section presents key points from the literature on decision making under uncertainty, adaptive management and resilience as a guide to future decision making in forest management.
3.3.1 Decision making under uncertainty
The future conditions for forest managers are subject to a high degree of uncertainty, and the future prospects for reducing these large uncertainties are limited. There is uncertainty regarding the trajectory of future increases in atmospheric greenhouse gases, what kind of effects these might have on the climate system and the effects of climatic changes on ecological and social systems and their capacity to adapt (see Fig. 2 ) (Wilby and Dessai 2010 ).
The cascade of uncertainty (Wilby and Dessai 2010 )
Consequently, many forest managers consider that the future situation is too uncertain to support long-term and potentially costly decisions that may be difficult to reverse. Dessai and Hulme ( 2004 ) argued that uncertainty per se should not be a reason for inaction. However, the critical issue for managers is deciding the types of actions to take and the timing and conditions under which they should be taken (Ogden and Innes 2007a ). A more reactive ‘wait and see’ approach (or ‘purposeful procrastination’) might be justified if uncertainty or costs are high relative to the expected impacts and risks, or if it is cheaper to implement interventions by waiting until after a significant disturbance (e.g. replanting an area with more fire- or drought-resistant tree species after a wildfire or drought-induced insect outbreak).
Effective adaptation requires setting clear objectives. Managers and policy makers need to decide whether they are trying to facilitate ecosystem adaptation through changing species composition or forest structure or trying to engineer resistance to change through proactive management strategies (Joyce et al. 2008 ). Establishing objectives often depends on the integration of the preferences of different stakeholders (Prato 2008 ), but changing social preferences presents another source of potential uncertainty.
Risk assessment and management provide a foundation for decision making in considering climate change in natural resource management. This approach provides both a qualitative and quantitative framework for evaluating climate change effects and adaptation options. Incorporating risk management approaches into forest management plans can provide a basis for managers to continue to provide forest conditions that meet a range of important values (Day and Perez 2013 ).
However, risk approaches generally requiring assigning probabilities to future events. In a comprehensive review, Yousefpour et al. ( 2011 ) identified a growing body of research literature on decision making under uncertainty, much of which has focused on price uncertainty and variation in timber production but is extending to multiple forest management objectives and other types of risk. They argue that we are actually in a stochastic transition from one known stable (but variable) climate state to a new but largely unknown and likely more rapidly changing set of future conditions.
Decision makers themselves may also not be the rational actors assumed by these models, with their decisions taken according to quite different assumptions, preferences and beliefs (Ananda and Herath 2009 ; Couture and Reynaud 2008 ). Therefore, the communication approach will be important in determining whether the information is acted on. In a recent study, Yousefpour et al. ( 2014 ) considered that the speed with which decision makers will form firm beliefs about future climate depends on the divergence among climate trajectories, the speed of change and short-term climate variability. Using a Bayesian modelling approach, they found that if a large change in climate occurs, the value of investing in knowledge and taking an adaptive approach would be positive and higher than a non-adaptive approach. In communicating about uncertainty, it may be better to focus discussion on the varying time in the future when things will happen, rather than on whether they will happen at all (Lindner et al. 2014 ).
Increased investment in climate science and projections or species distribution modelling may not necessarily decrease uncertainty in climate projections or impacts. Climate models are best viewed as heuristic tools rather than as accurate forecasts of the future (Innes et al. 2009 ). Trajectories of change in many other drivers of forest management (social, political or economic) are also highly uncertain (Keskitalo 2008 ) and the effects of these on the projected performance of management can be the same order of magnitude, requiring an integrated social-ecological perspective to adaptation (Seidl and Lexer 2013 ).
In a more ‘decision-centred’ approach, plausible scenarios of the potential range of future conditions are required. These can be derived from climate models but do not need to be accurate and precise ‘predictions’ of future climate states (Wilby and Dessai 2010 ). To support this type of approach, research needs to focus on improved understanding of tree and ecosystem responses and identifying those aspects of climate to which different forest types are most sensitive.
Devising strategies that are able to meet management objectives under a range of future scenarios is likely to be the most robust approach, recognising that these strategies are unlikely to be optimal under all future conditions. In some cases, the effect of different scenarios on forest growth may not be that great and differences in the present value of different management options are relatively small. For example, Eriksson et al. ( 2011 ) found that there was limited benefit in attempting to optimise management plans in accordance with future temperature scenarios.
Integration of climate change science and adaptation in forest management planning is considered important for building resilience in forest social and ecological systems (Keskitalo 2011 ; D’Amato et al. 2011 ; Chmura et al. 2011 ; Parks and Bernier 2010 ; Lindner et al. 2014 ). Forest restoration is becoming a more prominent aspect of forest management in many parts of the world and restoration approaches will also need to integrate understanding of future climate change to be successful (Stanturf et al. 2014 ).
3.3.2 Adaptive management, resilience and decisions
Adaptive management provides a mechanism to move forward when faced with future uncertainty (Innes et al. 2009 ). It can be viewed as a systematic process for continually improving management policies and practices by monitoring and then learning from the outcomes of operational programmes as a basis for incorporating adaptation actions into forest management. Whilst many management initiatives purport to implement these principles, they often lack essential characteristics of the approach (Innes et al. 2009 ).
However, effective adaptation to changing climate cannot simply involve adaptive management as it is currently understood. The pace of climate change is not likely to allow for the use of management as a tool to learn about the system by implementing methodologies to test hypotheses concerning known uncertainties (Holling 1978 ). Future climatic conditions may result in system states and dynamics that have never previously existed (Stainforth et al. 2007 ), so observation of past experience may be a poor guide for future action. Management will need to be more ‘forward-looking’, considering the range of possible future conditions and planning actions that consider that full range.
How does this translate into the practical guidance forest managers are seeking on how to adapt their current practices and, if necessary, their goals (Blate et al. 2009 )? Managers will need to consider trade-offs between different objectives under different conditions. For example, Seidl et al. ( 2011 ) showed that, to keep climate vulnerability in an Austrian forest low, Norway spruce will have to be replaced almost entirely by better adapted species. However, indicator weights that favoured timber production over C storage or biodiversity exerted a strong influence on the results. Wider social implications of imposing such drastic changes in forest landscapes will also deserve stronger consideration in decision making.
Ecosystem management will need to be reframed to accommodate the risks of a changing climate. Adaptive strategies, even without specific information on the future climate conditions of a target ecosystem, would enhance social and ecological resilience to address the uncertainties due to changing climate (Mori et al. 2013 ). These are likely to be more subject to change over the short to medium term, in response to more rapidly changing conditions.
Analysis of ecosystem resilience can provide a framework for these assessments. Resilience can be defined as ‘the capacity of ecosystems to absorb disturbance and reorganise so as to retain essentially the same function, structure and feedbacks – to have the same identity’ (Walker and Salt 2012 ). It is a function of the capacity of an ecosystem to resist change, the extent and pace of change and the ability of an ecosystem to reorganise following disturbance. The concept of resilience holds promise for informing future forest management, but Rist and Moen ( 2013 ) argue that its contributions are, so far, largely conceptual and offer more in terms of being a problem-framing approach than analytical or practical tools. There may also be trade-offs involved with focusing on resilience through retention of current species composition or using a more adaptation-oriented management approach after disturbances (Buma and Wessman 2013 ). Complexity theory and concepts can provide an appropriate framework for managing resilience (Messier et al. 2013 ).
Management decisions will ultimately depend on the costs and benefits of different options, but there are few examples of decision making frameworks that compare the costs of future impacts with the costs of different actions and the efficacy of those actions in reducing impacts. Ogden and Innes ( 2009 ) used a structured decision making process to identify and assess 24 adaptation options that managers considered important to achieve their regional goals and objectives of sustainable forest management in light of climate change. In the analysis of options for biodiversity conservation, Wintle et al. ( 2011 ) found that the amount of funding available for adaptation was a critical factor in deciding options aimed at minimising species extinctions in the mega-diverse fynbos biome of South Africa. When the available budget is small, fire management was the best strategy. If the budget is increased to an intermediate level, the marginal returns from more fire management were limited and the best strategy was added habitat protection. Above another budget threshold, increased investment should go into more fire management. By integrating ecological predictions in an economic decision framework, they found that making the choice of how much to invest is as important as determining what actions to take.
3.3.3 Adaptation as a social learning process
Whilst adaptation has been defined as ‘adjustment in natural or human systems in response to actual or expected climatic stimuli or their effects’ (Levina and Tirpak 2006 ), adaptation is essentially about meeting future human needs (Spittlehouse and Stewart 2003 ; Hahn and Knoke 2010 ). Consequently, it is inherently a social process. Forest landscapes are social-ecological systems that involve both nature and society (Innes et al. 2009 ), and resolving trade-offs between different management objectives to meet the different needs in society is an important element of sustainable forest management. As Kolström et al. ( 2011 ) pointed out, some proposed adaptation measures may change the balance between current objectives and stakeholder interests, and it will be important to consider the relative balance of different measures at the stand, management unit and landscape scales.
Those investigating adaptive management also recognise that it goes beyond the focus on scientific methods, statistical designs or analytical rigour favoured by its early proponents and that there is now an expectation of much greater stakeholder involvement, with the concept being renamed by some as adaptive, collaborative management (Innes et al. 2009 ). SFM and adaptation are as much about those who inhabit, work in or utilise forests as it is about managing the forest ecosystems themselves (White et al. 2010 ; Pramova et al. 2012 ; Fischer et al. 2013 ).
The choice of adaptation options will thus likely be relatively complex, even in cases where information and policy have been developed, and communication measures for forest management have been well formulated. Making such choices may require considerable knowledge, competence and commitment for implementation at the local level (Keskitalo 2011 ). Effective adaptation will require much greater cooperation between stakeholders, more flexibility for management actions and commitment of time to develop the social license for action in the absence of conclusive evidence or understanding. This will require venues for sharing perspectives on the nature of the problem (Fig. 3 ).
Adaptation as a social learning process. There is a need to provide situations to share different viewpoints on the nature of the problem as a basis for developing shared solutions (image source: John Rowley, http://ch301.cm.utexas.edu/learn/ )
3.3.4 Local and indigenous knowledge
The promotion of community-based forest management may increase local adaptive capacity by putting decisions in the hands of those people who first feel the effects of climate change (Gyampoh et al. 2009 ). In this context, local knowledge systems based on long-term observation and experience are likely to be of increasing importance in decision making. Adaptation strategies can benefit from combining scientific and indigenous knowledge, especially in developing countries (Gyampoh et al. 2009 ), with the translation of local forest knowledge into the language of formal forest science being considered an important step towards adaptation (Roberts et al. 2009 ). However, conservation and natural resource managers in government agencies have often discounted traditional local management systems (Scott 2005 ), although Spathelf et al. ( 2014 ) provided a useful approach for capturing local expert knowledge. Linking this type of knowledge with broader scientific understanding of ecosystem functioning and the global climate system will be a major challenge, requiring consideration of both technical and cultural issues (Caverley 2013 ), including intellectual property concerns of indigenous people (Lynch et al. 2010 ).
3.4 Policy arrangements for adaptation
Increasingly, many are arguing that effectively responding to climate change will require polycentric and multi-level governance arrangements (Peel et al. 2012 ). However, Nilsson et al. ( 2012 ) found that institutionalising of knowledge and knowledge exchange regarding climate change adaptation in Sweden was weak and that improved mechanisms are required for feedback from the local to the national level. Recent studies have described stronger relationships between scientific research and forest management to assess trade-offs and synergies, for participatory decision making and for shared learning (Blate et al. 2009 ; Littell et al. 2012 ; Klenk et al. 2011 ).
Many papers emphasised the need for greater flexibility in the policies, cultures and structures of forest management organisations (Brown 2009 ; von Detten and Faber 2013 ; Rayner et al. 2013 ). Because no single community or agency can prepare on their own for future impacts, inter-sectoral policy coordination will be required to ensure that policy developments in related policy sectors are not contradictory or counterproductive. Greater integration of information, knowledge and experience and collaborative projects involving scientists, practitioners and policy makers from multiple policy communities could increase focus on resilience, identify regions of large-scale vulnerability and provide a more rigorous framework for the analysis of vulnerability and adaptation actions (Thomalla et al. 2006 ).
There is also likely to be a greater need for cross-border implementation of different forest management options, requiring greater coordination between nation states and sub-national governments (Keenan 2012 ). Policy is the product of both ‘top-down’ and ‘bottom-up’ processes and these might sometimes be in conflict. Simply having ‘good policy’ in place is unlikely to be sufficient, as a great deal of what takes place at ‘street level’ is not determined by formal aims of central policy (Urwin and Jordan 2008 ). Having the right policies can send a strong political signal that adaptation needs to be considered seriously but flexibility in policy systems will be required to facilitate adaptive planning.
4 Discussion and conclusions
This broad survey of the literature indicated that, whilst there has been considerable development in research and thinking about adaptation in forest management over the last 10 years, research is still strongly focused on assessment of future impacts, responses and vulnerability of species and ecosystems (and in some cases communities and forest industries) to climate change. There has been some movement from a static view of climate based on long-term averages to a more detailed understanding of the drivers of different climate systems and how these affect the factors of greatest influence on different forest ecosystems processes, such as variability and extremes in temperature or precipitation or fire disturbance. For example, Guan et al. ( 2012 ) demonstrated that quasi-periodic climate variation on an inter-annual (ENSO) to inter-decadal (PDO) time scale can significantly influence tree growth and should be taken into account when assessing the impact of climate changes on forest productivity.
Adaptation is, in essence, about making good decisions for the future, taking into account the implications of climate change. It involves recognising and understanding potential future climate impacts and planning and managing for their consequences, whilst also considering the broader social, economic or other environmental changes that may impact on us, individually or collectively. To effectively provide a role in mitigation, delivering associated ecosystem services and benefits in poverty reduction (Eliasch 2008 ) forest management will have to adapt to a changing and highly variable climate. In achieving this, the roles and responsibilities of different levels of government, the private sector and different parts of the community are still being defined.
The broader literature emphasises that adaptation is a continuous process, involving a process of ‘adapting well’ to continuously changing conditions (Tompkins et al. 2010 ). This requires organisational learning based on past experience, new knowledge and a comprehensive analysis of future options. This can take place through ‘learning by doing’ or through a process of search and planned modification of routines (Berkhout et al. 2006 ). However, interpreting climate signals is not easy for organisations, the evidence of change is ambiguous and the stimuli are not often experienced directly within the organisation. For example, many forest managers in Australia currently feel little need to change practices to adapt to climate change, given both weak policy signals and limited perceived immediate evidence of increasing climate impacts (Cockfield et al. 2011 ). To explain and predict adaptation to climate change, the combination of personal experience and beliefs must be considered (Blennow et al. 2012 ). ‘Climate smart’ forest management frameworks can provide an improved basis for managing forested landscapes and maintaining ecosystem health and vitality based on an understanding of landscape vulnerability to future climatic change (Fig. 4 ) (Nitschke and Innes 2008a ).
Components of climate smart forest management (after Nitschke and Innes 2008a , b )
Many are now asking, do we really need more research to start adapting forest management to climate change? Whilst adaptation is often considered ‘knowledge deficit’ problem—where scientists provide more information and forest managers will automatically make better decisions—the reality is that the way in which this information is presented and how it is interpreted and received, will play major roles in determining potential responses. Successful adaptation will require dissemination of knowledge of potential climate impacts and suitable adaptation measures to decision makers at both practice and policy levels (Kolström et al. 2011 ) but it needs to go well beyond that.
Adaptation is, above all, a social learning process. It requires an understanding of sense of place, a capacity for individuals and society to consider potential future changes and what they mean for their circumstances. Leaders in forest management organisations will need to support a greater diversity of inputs into decision making, avoid creating rigid organisational hierarchies that deter innovation, and be inclusive, open and questioning (Konkin and Hopkins 2009 ). They will need to create more opportunities for interaction between researchers, managers and the community and space for reflection on the implications and the outcomes of management actions and unplanned events. Researchers will need to develop new modes of communication, providing knowledge in forms that are appropriate to the management decision and suitable for digestion by a range of different audiences.
From this analysis, key gaps in knowledge for adaptation may not be improved climate scenarios or better understanding of the biophysical responses of individual tree species or forest ecosystems to future climate. Knowledge gaps lie more in understanding the social and community attitudes and values that drive forest management and the decision making processes of forest managers, in order to work out how ‘climate intelligence’ can be built in to these processes.
The impacts of changing climate will vary locally. Consequently, managers must be given the flexibility to respond in ways that meet their particular needs and capacity to choose management options that are applicable to the local situation (Innes et al. 2009 ). This may not be consistent with rigid indicator-driven management assessment processes like forest certification. Whilst policy to support climate change mitigation is primarily a task for national governments and international agreements and processes, responsibility for supporting adaptation will fall more to sub-national and local governments, communities and the private sector. More active management will be required if specific values are to be maintained, particularly for forests in conservation reserves. This will require additional investment, but there has been little analysis to support the business case for investment in adaptation or to determine who should pay, particularly in developing countries.
We need to strengthen the relationship between climate science, forest research, forest managers and the community. Key challenges will include the setting of objectives for desired future conditions and accepting that we may not be able to maintain everything that forests have traditionally provided. It is important to discuss and agree on common goals in order to cope with, or benefit from, the challenges of future climates. Actively managing our forest ecosystems effectively and intelligently, using the best available knowledge and foresight capacity, can make those goals a reality.
Adams MA (2013) Mega-fires, tipping points and ecosystem services: managing forests and woodlands in an uncertain future. For Ecol Manag 294:250–261. doi: 10.1016/j.foreco.2012.11.039
Google Scholar
Adger WN, Agrawala S, Mirza MMQ, Conde C, O’Brien K, Pulhin J, Pulwarty R, Smit B, Takahashi K (2007) Assessment of adaptation practices, options, constraints and capacity. In: Parry ML, Canziani OF, Palutikof JP, van der Linden PJ, Hanson CE (eds) Climate change 2007: impacts, adaptation and vulnerability. Contribution of Working Group II to the Fourth Assessment Report of the Intergovernmental Panel of Climate Change (IPCC). Cambridge University Press, Cambridge, pp 717–743
Aitken SN, Yeaman S, Holliday JA, Wang T, Curtis-McLane S (2008) Adaptation, migration or extirpation: climate change outcomes for tree populations. Evol Appl 1:95–111. doi: 10.1111/j.1752-4571.2007.00013.x
PubMed Central PubMed Google Scholar
Alberto FJ, Aitken SN, Alia R, Gonzalez-Martinez SC, Hanninen H, Kremer A, Lefevre F, Lenormand T, Yeaman S, Whetten R, Savolainen O (2013) Potential for evolutionary responses to climate change evidence from tree populations. Glob Chang Biol 19:1645–1661. doi: 10.1111/gcb.12181
Allen CD, Macalady AK, Chenchouni H, Bachelet D, McDowell N, Vennetier M, Kitzberger T, Rigling A, Breshears DD, Hogg EH, Gonzalez P, Fensham R, Zhang Z, Castro J, Demidova N, Lim J-H, Allard G, Running SW, Semerci A, Cobb N (2010) A global overview of drought and heat-induced tree mortality reveals emerging climate change risks for forests. For Ecol Manag 259:660–684. doi: 10.1016/j.foreco.2009.09.001
Amraoui M, Liberato MLR, Calado TJ, DaCamara CC, Coelho LP, Trigo RM, Gouveia CM (2013) Fire activity over Mediterranean Europe based on information from Meteosat-8. For Ecol Manag 294:62–75. doi: 10.1016/j.foreco.2012.08.032
Ananda J, Herath G (2009) A critical review of multi-criteria decision making methods with special reference to forest management and planning. Ecol Econ 68:2535–2548. doi: 10.1016/j.ecolecon.2009.05.010
Andalo C, Beaulieu J, Bousquet J (2005) The impact of climate change on growth of local white spruce populations in Québec, Canada. For Ecol Manag 205:169–182. doi: 10.1016/j.foreco.2004.10.045
Anderson JM (1991) The effects of climate change on decomposition processes in grassland and coniferous forests. Ecol Appl 1:326–347. doi: 10.2307/1941761
Anderson JT, Panetta AM, Mitchell-Olds T (2012) Evolutionary and ecological responses to anthropogenic climate change. Plant Physiol 160:1728–1740. doi: 10.1104/pp. 112.206219
PubMed Central CAS PubMed Google Scholar
Attorre F, Francesconi F, Scarnati L, De Sanctis M, Alfo M, Bruno F (2008) Predicting the effect of climate change on tree species abundance and distribution at a regional scale. For Biogeosci For 1:132–139. doi: 10.3832/ifor0467-0010132
Battaglia M, Bruce J, Brack C, Baker T (2009) Climate change and Australia’s plantation estate: analysis of vulnerability and preliminary investigation of adaptation options.
Battaglia M, Sands P, White D, Mummery D (2004) CABALA: a linked carbon, water and nitrogen model of forest growth for silvicultural decision support. For Ecol Manag 193:251–282
Bele MY, Sonwa DJ, Tiani AM (2013a) Supporting local adaptive capacity to climate change in the Congo basin forest of Cameroon a participatory action research approach. Int J Clim Chang Strateg Manag 5:181–197. doi: 10.1108/17568691311327587
Bele MY, Tiani AM, Somorin OA, Sonwa DJ (2013b) Exploring vulnerability and adaptation to climate change of communities in the forest zone of Cameroon. Clim Chang 119:875–889. doi: 10.1007/s10584-013-0738-z
Berkhout F, Hertin J, Gann D (2006) Learning to adapt: organisational adaptation to climate change impacts. Clim Chang 78:135–156. doi: 10.1007/s10584-006-9089-3
Bernier P, Schöne D (2009) Adapting forests and their management to climate change: an overview. Unasylva 60:5–11
Blate GM, Joyce LA, Littell JS, McNulty SG, Millar CI, Moser SC, Neilson RP, O’Halloran K, Peterson DL (2009) Adapting to climate change in United States national forests. Unasylva 60:57–62
Blennow K, Persson J, Tome M, Hanewinkel M (2012) Climate change: believing and seeing implies adapting. PLoS ONE 7:e50182. doi: 10.1371/journal.pone.0050182
Boisvenue C, Running SW (2006) Impacts of climate change on natural forest productivity—evidence since the middle of the 20th century. Glob Chang Biol 12:862–882. doi: 10.1111/j.1365-2486.2006.01134.x
Bolte A, Eisenhauer DR, Ehrhart HP, Gross J, Hanewinkel M, Kolling C, Profft I, Rohde M, Rohe P, Amereller K (2009) Climate change and forest management—accordances and differences between the German states regarding assessments for needs and strategies towards forest adaptation. Landbauforschung Volkenrode 59:269–278
Bonan GB, Pollard D, Thompson SL (1992) Effects of boreal forest vegetation on global climate. Nature 359:716–718. doi: 10.1038/359716a0
Bongers F, Poorter L, Van Rompaey R, Parren MPE (1999) Distribution of twelve moist forest canopy tree species in Liberia and Cote d’Ivoire: response curves to a climatic gradient. J Veg Sci 10:371–382. doi: 10.2307/3237066
Booth TH (1990) Mapping regions climatically suitable for particular tree species at the global scale. For Ecol Manag 36:47–60
Booth TH (2013) Eucalypt plantations and climate change. For Ecol Manag 301:28–34. doi: 10.1016/j.foreco.2012.04.004
Booth TH, Nix HA, Hutchinson MF, Jovanovic T (1988) Niche analysis and tree species introduction. For Ecol Manag 23:47–59
Bowman DMJS, Williamson GJ, Keenan RJ, Prior LD (2014) A warmer world will reduce tree growth in evergreen broadleaf forests: evidence from Australian temperate and subtropical eucalypt forests. Glob Ecol Biogeogr 23:925–934. doi: 10.1111/geb.12171
Brautigam K, Vining KJ, Lafon-Placette C, Fossdal CG, Mirouze M, Marcos JG, Fluch S, Fraga MF, Guevara MA, Abarca D, Johnsen O, Maury S, Strauss SH, Campbell MM, Rohde A, Diaz-Sala C, Cervera MT (2013) Epigenetic regulation of adaptive responses of forest tree species to the environment. Ecol Evol 3:399–415. doi: 10.1002/ece3.461
Breed MF, Stead MG, Ottewell KM, Gardner MG, Lowe AJ (2013) Which provenance and where? Seed sourcing strategies for revegetation in a changing environment. Conserv Genet 14:1–10. doi: 10.1007/s10592-012-0425-z
Breymeyer A, Melillo JM (1991) Global climate change—the effects of climate change on production and decomposition in coniferous forests and grasslands. Ecol Appl 1:111–111. doi: 10.2307/1941804
Brooker RW, Travis JMJ, Clark EJ, Dytham C (2007) Modelling species’ range shifts in a changing climate: the impacts of biotic interactions, dispersal distance and the rate of climate change. J Theor Biol 245:59–65. doi: 10.1016/j.jtbi.2006.09.033
PubMed Google Scholar
Brotak EA, Reifsnyder WE (1977) Predicting major wildland fire occurrence. Fire Manag Notes 38:5–8
Brown HCP (2009) Climate change and Ontario forests: prospects for building institutional adaptive capacity. Mitig Adapt Strateg Glob Chang 14:513–536. doi: 10.1007/s11027-009-9183-8
Buma B, Wessman CA (2013) Forest resilience, climate change, and opportunities for adaptation: a specific case of a general problem. For Ecol Manag 306:216–225. doi: 10.1016/j.foreco.2013.06.044
Campbell EM, Saunders SC, Coates KD, Meidinger DV, MacKinnon A, O’Neil GA, MacKillop DJ, DeLong SC, Morgan. DG (2009) Ecological resilience and complexity: a theoretical framework for understanding and managing British Columbia’s forest ecosystems in a changing climate. B.C. Min. For. Range, For. Sci. Prog., Victoria, B.C.
Cannell MGR, Grace J, Booth A (1989) Possible impacts of climatic warming on trees and forests in the United Kingdom—a review. Forestry 62:337–364. doi: 10.1093/forestry/62.4.337
Carvalho A, Flannigan MD, Logan K, Miranda AI, Borrego C (2008) Fire activity in Portugal and its relationship to weather and the Canadian Fire Weather Index System. Int J Wildl Fire 17:328–338. doi: 10.1071/WF07014
Castagneri D, Motta R (2010) A research gap in the interactive effects of climate and competition on trees growth. In: Karam WP (ed) Tree growth: influences, layers and types. Nova Science, Hauppauge, pp 93–102
Caverley N (2013) The guardians of Mother Earth: a qualitative study of aboriginal knowledge keepers and their views on climate change adaptation in the South Selkirks region. Nativ Stud Rev 21:125–150
Cheaib A, Badeau V, Boe J, Chuine I, Delire C, Dufrêne E, François C, Gritti ES, Legay M, Pagé C, Thuiller W, Viovy N, Leadley P (2012) Climate change impacts on tree ranges: model intercomparison facilitates understanding and quantification of uncertainty. Ecol Lett 15:533–544. doi: 10.1111/j.1461-0248.2012.01764.x
Chen WJ, Black TA, Yang PC, Barr AG, Neumann HH, Nesic Z, Blanken PD, Novak MD, Eley J, Ketler RJ, Cuenca A (1999) Effects of climatic variability on the annual carbon sequestration by a boreal aspen forest. Glob Chang Biol 5:41–53. doi: 10.1046/j.1365-2486.1998.00201.x
CAS Google Scholar
Chmura DJ, Anderson PD, Howe GT, Harrington CA, Halofsky JE, Peterson DL, Shaw DC, St. Brad Clair J (2011) Forest responses to climate change in the northwestern United States: ecophysiological foundations for adaptive management. For Ecol Manag 261:1121–1142. doi: 10.1016/j.foreco.2010.12.040
Choat B, Jansen S, Brodribb TJ, Cochard H, Delzon S, Bhaskar R, Bucci SJ, Feild TS, Gleason SM, Hacke UG, Jacobsen AL, Lens F, Maherali H, Martinez-Vilalta J, Mayr S, Mencuccini M, Mitchell PJ, Nardini A, Pittermann J, Pratt RB, Sperry JS, Westoby M, Wright IJ, Zanne AE (2012) Global convergence in the vulnerability of forests to drought. Nature 491:752–755
CAS PubMed Google Scholar
Chuvieco E, Martinez S, Roman MV, Hantson S, Pettinari ML (2014) Integration of ecological and socio-economic factors to assess global vulnerability to wildfire. Glob Ecol Biogeogr 23:245–258. doi: 10.1111/geb.12095
Clark DA (2007) Detecting tropical forests’ responses to global climatic and atmospheric change: current challenges and a way forward. Biotropica 39:4–19. doi: 10.1111/j.1744-7429.2006.00227.x
Clark JS, Bell DM, Hersh MH, Nichols L (2011) Climate change vulnerability of forest biodiversity: climate and competition tracking of demographic rates. Glob Chang Biol 17:1834–1849. doi: 10.1111/j.1365-2486.2010.02380.x
Cockfield G, Maraseni T, Buys L, Sommerfeld J, Wilson C, Athukorala W (2011) Socioeconomic implications of climate change with regard to forests and forest management. Contribution of Work Package 3 to the Forest Vulnerability Assessment. National Climate Change Adaptation Research Facility, Gold Coast
Corlett RT (2011) Impacts of warming on tropical lowland rainforests. Trends Ecol Evol 26:606–613
Corlett RT, Westcott DA (2013) Will plant movements keep up with climate change? Trends Ecol Evol 28:482–488. doi: 10.1016/j.tree.2013.04.003
Couture S, Reynaud A (2008) Multi-stand forest management under a climatic risk: do time and risk preferences matter? Environ Model Assess 13:181–193
D’Amato AW, Bradford JB, Fraver S, Palik BJ (2011) Forest management for mitigation and adaptation to climate change: insights from long-term silviculture experiments. For Ecol Manag 262:803–816. doi: 10.1016/j.foreco.2011.05.014
Daubenmire RF (1978) Plant geography. Academic, New York
Davidar P, Rajagopal B, Mohandass D, Puyravaud JP, Condit R, Wright SJ, Leigh EG (2007) The effect of climatic gradients, topographic variation and species traits on the beta diversity of rain forest trees. Glob Ecol Biogeogr 16:510–518. doi: 10.1111/j.1466-8238.2007.00307.x
Davis MB (1986) Climatic instability, time lags and community disequilibrium. In: Diamond J, Case TJ (eds) Community ecology. Harper and Row, New York, pp 269–284
Day JK, Perez DM (2013) Reducing uncertainty and risk through forest management planning in British Columbia. For Ecol Manag 300:117–124. doi: 10.1016/j.foreco.2012.11.035
de Groot WJ, Flannigan MD, Cantin AS (2013) Climate change impacts on future boreal fire regimes. For Ecol Manag 294:35–44. doi: 10.1016/j.foreco.2012.09.027
Dessai S, Hulme M (2004) Does climate adaptation policy need probabilities? Clim Pol 4:107–128. doi: 10.1080/14693062.2004.9685515
Di Nitto D, Neukermans G, Koedam N, Defever H, Pattyn F, Kairo JG, Dahdouh-Guebas F (2014) Mangroves facing climate change: landward migration potential in response to projected scenarios of sea level rise. Biogeosciences 11:857–871. doi: 10.5194/bg-11-857-2014
Dixon RK, Krankina ON, Kobak KI (1996) Global climate change adaptation: examples from Russian boreal forests. Adapting to climate change: an international perspective.
Dobrowski SZ, Abatzoglou J, Swanson AK, Greenberg JA, Mynsberge AR, Holden ZA, Schwartz MK (2013) The climate velocity of the contiguous United States during the 20th century. Glob Chang Biol 19:241–251. doi: 10.1111/gcb.12026
Doyle TW, Krauss KW, Conner WH, From AS (2010) Predicting the retreat and migration of tidal forests along the northern Gulf of Mexico under sea-level rise. For Ecol Manag 259:770–777. doi: 10.1016/j.foreco.2009.10.023
Eliasch J (2008) Climate change: financing global forests. The Eliasch Review. HMSO
Emanuel WR, Shugart HH, Stevenson MP (1985) Climatic-change and the broad-scale distribution of terrestrial ecosystem complexes. Clim Chang 7:29–43. doi: 10.1007/bf00139439
Eriksson LO, Backéus S, Garcia F (2011) Implications of growth uncertainties associated with climate change for stand management. Eur J For Res 131:1199–1209. doi: 10.1007/s10342-011-0591-4
Ettl GJ, Peterson DL (1995) Extreme climate and variation in tree growth—individualistic response in sub-alpine fir ( Abies-lasiocarpa ). Glob Chang Biol 1:231–241. doi: 10.1111/j.1365-2486.1995.tb00024.x
Feeley KJ, Rehm EM, Machovina B (2012) Perspective: the responses of tropical forest species to global climate change: acclimate, adapt, migrate, or go extinct? Frontiers of Biogeography 4 (2)
Feikema PM, Sherwin CB, Lane PNJ (2013) Influence of climate, fire severity and forest mortality on predictions of long term streamflow: potential effect of the 2009 wildfire on Melbourne’s water supply catchments. J Hydrol 488:1–16. doi: 10.1016/j.jhydrol.2013.02.001
Fischer AP, Paveglio T, Carroll M, Murphy D, Brenkert-Smith H (2013) Assessing social vulnerability to climate change in human communities near public forests and grasslands: a framework for resource managers and planners. J For 111:357–365. doi: 10.5849/jof. 12-091
Fischlin A, Ayres M, Karnosky D, Kellomäki S, Louman B, Ong C, Plattner G-K, Santoso H, Thompson I, Booth TH, Marcar N, Scholes B, Swanston C, Zamolodchikov D (2009) Future environmental impacts and vulnerabilities. In: Seppälä R, Buck A, Katila P (eds) Adaptation of forests and people to climate change: a global assessment report, vol 22. IUFRO World Series, Helsinki, pp 53–100
Franks PJ, Adams MA, Amthor JS, Barbour MM, Berry JA, Ellsworth DS, Farquhar GD, Ghannoum O, Lloyd J, McDowell N, Norby RJ, Tissue DT, von Caemmerer S (2013) Sensitivity of plants to changing atmospheric CO2 concentration: from the geological past to the next century. New Phytol 197:1077–1094. doi: 10.1111/nph.12104
Furness E, Nelson H (2012) Community forest organizations and adaptation to climate change in British Columbia. For Chron 88:519–524
Garcia RA, Cabeza M, Rahbek C, Araújo MB (2014) Multiple dimensions of climate change and their implications for biodiversity. Science 344 (6183). doi:10.1126/science.1247579
Gentilli J (1949) Forest influences: the effects of woody vegetation on climatic water, and soil, with applications to the conservation of water and the control of floods and erosion. Geogr Rev 39:164–164. doi: 10.2307/211169
Gibbs HK, Ruesch AS, Achard F, Clayton MK, Holmgren P, Ramankutty N, Foley JA (2010) Tropical forests were the primary sources of new agricultural land in the 1980s and 1990s. Proc Natl Acad Sci 107:16732–16737. doi: 10.1073/pnas.0910275107
Gilman RT, Fabina NS, Abbott KC, Rafferty NE (2012) Evolution of plant–pollinator mutualisms in response to climate change. Evol Appl 5:2–16. doi: 10.1111/j.1752-4571.2011.00202.x
Gonzalez ME, Lara A, Urrutia R, Bosnich J (2011) Climatic change and its potential impact on forest fire occurrence in south-central Chile (33 degrees-42 degrees S). Bosque 32:215–219. doi: 10.4067/s0717-92002011000300002
Graham RL, Turner MG, Dale VH (1990) How increasing CO2 and climate change affect forests—at many spatial and temporal scales, there will be forest responses that will be affected by human activities. Bioscience 40:575–587. doi: 10.2307/1311298
Graumlich LJ (1993) Response of tree growth to climatic variation in the mixed conifer and deciduous forests of the upper Great-Lakes region. Can J For Res 23:133–143. doi: 10.1139/x93-020
Groffman PM, Rustad LE, Templer PH, Campbell JL, Christenson LM, Lany NK, Socci AM, Vadeboncoeur MA, Schaberg PG, Wilson GF, Driscoll CT, Fahey TJ, Fisk MC, Goodale CL, Green MB, Hamburg SP, Johnson CE, Mitchell MJ, Morse JL, Pardo LH, Rodenhouse NL (2012) Long-term integrated studies show complex and surprising effects of climate change in the northern hardwood forest. Bioscience 62:1056–1066. doi: 10.1525/bio.2012.62.12.7
Grubb PJ, Whitmore TC (1966) A comparison of montane and lowland rain forest in Ecuador. 2. Climate and its effects on distribution and physiognomy of forests. J Ecol 54:303. doi: 10.2307/2257951
Guan BT, Wright WE, Chung C-H, Chang S-T (2012) ENSO and PDO strongly influence Taiwan spruce height growth. For Ecol Manag 267:50–57. doi: 10.1016/j.foreco.2011.11.028
Guardiola-Claramonte M, Troch PA, Breshears DD, Huxman TE, Switanek MB, Durcik M, Cobb NS (2011) Decreased streamflow in semi-arid basins following drought-induced tree die-off: a counter-intuitive and indirect climate impact on hydrology. J Hydrol 406:225–233. doi: 10.1016/j.jhydrol.2011.06.017
Guariguata MR (2009) Tropical forest management and climate change adaptation. Rev Estud Soc 32:98–112
Guariguata MR, Cornelius JP, Locatelli B, Forner C, Sánchez-Azofeifa GA (2008) Mitigation needs adaptation: tropical forestry and climate change. Mitig Adapt Strateg Glob Chang 13:793–808. doi: 10.1007/s11027-007-9141-2
Guariguata MR, Locatelli B, Haupt F (2012) Adapting tropical production forests to global climate change: risk perceptions and actions. Int For Rev 14:27–38
Gyampoh BA, Amisah S, Idinoba M, Nkem J (2009) Using traditional knowledge to cope with climate change in rural Ghana. Unasylva 60:70–74
Hahn WA, Knoke T (2010) Sustainable development and sustainable forestry: analogies, differences, and the role of flexibility. Eur J For Res 129:787–801. doi: 10.1007/s10342-010-0385-0
Hamrick JL (2004) Response of forest trees to global environmental changes. For Ecol Manag 197:323–335. doi: 10.1016/j.foreco.2004.05.023
Hansenbristow KJ, Ives JD, Wilson JP (1988) Climatic variability and tree response within the forest alpine tundra ecotone. Ann Assoc Am Geogr 78:505–519. doi: 10.1111/j.1467-8306.1988.tb00221.x
Harper RJ, Smettem KRJ, Carter JO, McGrath JF (2009) Drought deaths in Eucalyptus globulus (Labill.) plantations in relation to soils, geomorphology and climate. Plant Soil 324:199–207. doi: 10.1007/s11104-009-9944-x
Heller NE, Zavaleta ES (2009) Biodiversity management in the face of climate change: a review of 22 years of recommendations. Biol Conserv 142:14–32. doi: 10.1016/j.biocon.2008.10.006
Hennessy K, Lucas C, Nicholls N, Bathols J, Suppiah R, Ricketts J (2005) Climate change impacts on fire-weather in south-east Australia. CSIRO Division of Marine and Atmospheric Research, Aspendale
Holling CS (1978) Adaptive environmental assessment and management. Wiley, Chichester
Hughes L, Cawsey EM, Westoby M (1996) Climatic range sizes of Eucalyptus species in relation to future climate change. Glob Ecol Biogeogr Lett 5:23–29. doi: 10.2307/2997467
Huntingford C, Zelazowski P, Galbraith D, Mercado LM, Sitch S, Fisher R, Lomas M, Walker AP, Jones CD, Booth BBB, Malhi Y, Hemming D, Kay G, Good P, Lewis SL, Phillips OL, Atkin OK, Lloyd J, Gloor E, Zaragoza-Castells J, Meir P, Betts R, Harris PP, Nobre C, Marengo J, Cox PM (2013) Simulated resilience of tropical rainforests to CO2-induced climate change. Nat Geosci 6:268–273
Huntley B (1990) European postglacial forests—compositional changes in response to climatic-change. J Veg Sci 1:507–518. doi: 10.2307/3235785
Ince PJ, Kramp AD, Skog KE, Yoo DI, Sample VA (2011) Modeling future U.S. forest sector market and trade impacts of expansion in wood energy consumption. J For Econ 17:142–156. doi: 10.1016/j.jfe.2011.02.007
Innes J, Joyce LA, Kellomäki S, Louman B, Ogden A, Parrotta J, Thompson I, Ayres M, Ong C, Santoso H, Sohngen B, Wreford A (2009) Management for adaptation. In: Seppälä R, Buck A, Katila P (eds) Adaptation of forests and people to climate change: a global assessment report, vol World Series Volume 22. IUFRO Helsinki, pp 135–186
IPCC (2013) Climate Change 2013: The physical science basis. Contribution of Working Group I to the Fifth Assessment Report of the Intergovernmental Panel on Climate Change [Stocker, T.F., D. Qin, G.-K. Plattner, M. Tignor, S.K. Allen, J. Boschung, A. Nauels, Y. Xia, V. Bex and P.M. Midgley (eds.)]. Cambridge, United Kingdom and New York, NY, USA
IUCN (2008) Ecosystem-based adaptation: an approach for building resilience and reducing risk for local communities and ecosystems. Submission to the Chair of the AWG-LCA with respect to the Shared Vision and Enhanced Action on Adaptation. International Union for the Conservation of Nature,
Iverson LR, Schwartz MW, Prasad AM (2004) How fast and far might tree species migrate in the eastern United States due to climate change? Glob Ecol Biogeogr 13:209–219
Johnson G, Sorensen FC, St Clair JB, Cronn RC (2004) Pacific Northwest forest tree seed zones: a template for native plants? Nativ Plants J 5:131–140
Johnston M, Hesseln H (2012) Climate change adaptive capacity of the Canadian forest sector. For Policy Econ 24:29–34. doi: 10.1016/j.forpol.2012.06.001
Johnston M, Lindner M, Parrotta J, Giessen L (2012) Adaptation and mitigation options for forests and forest management in a changing climate. For Policy Econ 24:1–2. doi: 10.1016/j.forpol.2012.09.007
Johnston M, Williamson T (2007) A framework for assessing climate change vulnerability of the Canadian forest sector. For Chron 83:358–361
Joyce LA (2007) The impacts of climate change on forestry. In: Adams DM, Haynes RW (eds) Resource and market projections for forest policy development: twenty-five years of experience with the US RPA Timber Assessment, vol 14. Managing Forest Ecosystems. pp 449–488
Joyce LA, Blate GM, Littell JS, McNulty SG, Millar CI, Moser SC, Neilson RP, O’Halloran K, Peterson DL (2008) National forests. Preliminary review of adaptation options for climate-sensitive ecosystems and resources. A report by the U.S. Climate Change Science Program and the Subcommittee on Global Change Research. US Environmental Protection Agency, Washington, DC
Joyce LA, Blate GM, McNulty SG, Millar CI, Moser S, Neilson RP, Peterson DL (2009) Managing for multiple resources under climate change: national forests. Environ Manag 44:1022–1032. doi: 10.1007/s00267-009-9324-6
Joyce LA, Mills JR, Heath LS, McGuire AD, Haynes RW, Birdsey RA (1995) Forest sector impacts from changes in forest productivity under climate change. J Biogeogr 22:703–713. doi: 10.2307/2845973
Kalame FB, Luukkanen O, Kanninen M (2011) Making the National Adaptation Programme of Action (NAPA) more responsive to the livelihood needs of tree planting farmers, drawing on previous experience in dryland Sudan. Forests 2:948–960. doi: 10.3390/f2040948
Kallarackal J, Roby TJ (2012) Responses of trees to elevated carbon dioxide and climate change. Biodivers Conserv 21:1327–1342. doi: 10.1007/s10531-012-0254-x
Kant P, Wu S (2012) Should adaptation to climate change be given priority over mitigation in tropical forests? Carbon Manag 3:303–311. doi: 10.4155/cmt.12.29
Karjalainen T, Pussinen A, Liski J, Nabuurs GJ, Eggers T, Lapvetelainen T, Kaipainen T (2003) Scenario analysis of the impacts of forest management and climate change on the European forest sector carbon budget. For Policy Econ 5:141–155. doi: 10.1016/s1389-9341(03)00021-2
Keenan RJ (2012) Adaptation of forests and forest management to climate change: an editorial. Forests 3:75–82. doi: 10.3390/f3010075
Kellomaki S, Peltola H, Nuutinen T, Korhonen KT, Strandman H (2008) Sensitivity of managed boreal forests in Finland to climate change, with implications for adaptive management. Phil Trans R Soc B 363:2341–2351. doi: 10.1098/rstb.2007.2204
Kelly AE, Goulden ML (2008) Rapid shifts in plant distribution with recent climate change. Proc Natl Acad Sci 105:11823–11826. doi: 10.1073/pnas.0802891105
Kerhoulas LP, Kolb TE, Hurteau MD, Koch GW (2013) Managing climate change adaptation in forests: a case study from the US Southwest. J Appl Ecol 50:1311–1320. doi: 10.1111/1365-2664.12139
Keskitalo EC (2008) Vulnerability and adaptive capacity in forestry in northern Europe: a Swedish case study. Clim Chang 87:219–234. doi: 10.1007/s10584-007-9337-1
Keskitalo ECH (2011) How can forest management adapt to climate change? Possibilities in different forestry systems. Forests 2:415–430. doi: 10.3390/f2010415
Kienast F, Brzeziecki B, Wildi O (1996) Long-term adaptation potential of Central European mountain forests to climate change: a GIS-assisted sensitivity assessment. For Ecol Manag 80:133–153. doi: 10.1016/0378-1127(95)03633-4
Kimmins JP (2002) Future shock in forestry—where have we come from; where are we going; is there a “right way” to manage forests? Lessons from Thoreau, Leopold, Toffler, Botkin and Nature. For Chron 78:263–271
Kimmins JP (2008) From science to stewardship: harnessing forest ecology in the service of society. For Ecol Manag 256:1625–1635. doi: 10.1016/j.foreco.2008.02.057
Kimmins JP, Blanco JA, Seely B, Welham C, Scoullar K (2008) Complexity in modelling forest ecosystems: how much is enough? For Ecol Manag 256:1646–1658. doi: 10.1016/j.foreco.2008.03.011
Kimmins JP, Mailly D, Seely B (1999) Modelling forest ecosystem net primary production: the hybrid simulation approach used in forecast. Ecol Model 122:195–224
Kint V, Aertsen W, Campioli M, Vansteenkiste D, Delcloo A, Muys B (2012) Radial growth change of temperate tree species in response to altered regional climate and air quality in the period 1901–2008. Clim Chang 115:343–363. doi: 10.1007/s10584-012-0465-x
Kirschbaum MUF, Watt MS, Tait A, Ausseil A-GE (2012) Future wood productivity of Pinus radiata in New Zealand under expected climatic changes. Glob Chang Biol 18:1342–1356. doi: 10.1111/j.1365-2486.2011.02625.x
Klenk NL, Adams BW, Bull GQ, Innes JL, Cohen SJ, Larson BC (2011) Climate change adaptation and sustainable forest management: a proposed reflexive research agenda. For Chron 87:351–357
Kobak KI, Turchinovich IY, Kondrasheva NY, Schulze ED, Schulze W, Koch H, Vygodskaya NN (1996) Vulnerability and adaptation of the larch forest in eastern Siberia to climate change. Water Air Soil Pollut 92:119–127
Kolström M, Lindner M, Vilén T, Maroschek M, Seidl R, Lexer MJ, Netherer S, Kremer A, Delzon S, Barbati A, Marchetti M, Corona P (2011) Reviewing the science and Implementation of climate change adaptation measures in European forestry. Forests 2:961–982. doi: 10.3390/f2040961
Konkin D, Hopkins K (2009) Learning to deal with climate change and catastrophic forest disturbances. Unasylva 60:17–23
Kremer A, Ronce O, Robledo-Arnuncio JJ, Guillaume F, Bohrer G, Nathan R, Bridle JR, Gomulkiewicz R, Klein EK, Ritland K, Kuparinen A, Gerber S, Schueler S (2012) Long-distance gene flow and adaptation of forest trees to rapid climate change. Ecol Lett 15:378–392. doi: 10.1111/j.1461-0248.2012.01746.x
PubMed Central Google Scholar
Kuparinen A, Savolainen O, Schurr FM (2010) Increased mortality can promote evolutionary adaptation of forest trees to climate change. For Ecol Manag 259:1003–1008. doi: 10.1016/j.foreco.2009.12.006
Lal R, Cummings DJ (1979) Clearing a tropical forest. 1. Effects on soil and micro-climate. Field Crop Res 2:91–107. doi: 10.1016/0378-4290(79)90012-1
Leites LP, Rehfeldt GE, Robinson AP, Crookston NL, Jaquish B (2012) Possibilities and limitations of using historic provenance tests to infer forest species growth responses to climate change. Nat Resour Model 25:409–433. doi: 10.1111/j.1939-7445.2012.00129.x
Lenoir J, Gegout JC, Dupouey JL, Bert D, Svenning JC (2010) Forest plant community changes during 1989–2007 in response to climate warming in the Jura Mountains (France and Switzerland). J Veg Sci 21:949–964. doi: 10.1111/j.1654-1103.2010.01201.x
Leuzinger S, Luo Y, Beier C, Dieleman W, Vicca S, Körner C (2011) Do global change experiments overestimate impacts on terrestrial ecosystems? Trends Ecol Evol 26:236–241
Levina E, Tirpak D (2006) Adaptation to climate change: key terms. OECD/IEA, Paris
Lin BB, Petersen B (2013) Resilience, regime shifts, and guided transition under climate change: examining the practical difficulties of managing continually changing systems. Ecology and Society 18 (1). doi:10.5751/es-05128-180128
Lindner M (2000) Developing adaptive forest management strategies to cope with climate change. Tree Physiol 20:299–307
Lindner M, Fitzgerald JB, Zimmermann NE, Reyer C, Delzon S, van der Maaten E, Schelhaas MJ, Lasch P, Eggers J, van der Maaten-Theunissen M, Suckow F, Psomas A, Poulter B, Hanewinkel M (2014) Climate change and European forests: what do we know, what are the uncertainties, and what are the implications for forest management? J Environ Manag 146:69–83. doi: 10.1016/j.jenvman.2014.07.030
Lindner M, Maroschek M, Netherer S, Kremer A, Barbati A, Garcia-Gonzalo J, Seidl R, Delzon S, Corona P, Kolström M, Lexer MJ, Marchetti M (2010) Climate change impacts, adaptive capacity, and vulnerability of European forest ecosystems. For Ecol Manag 259:698–709. doi: 10.1016/j.foreco.2009.09.023
Lindner M, Sohngen B, Joyce LA, Price DT, Bernier PY, Karjalainen T (2002) Integrated forestry assessments for climate change impacts. For Ecol Manag 162:117–136. doi: 10.1016/S0378-1127(02)00054-3
Littell JS, Peterson DL, Millar CI, O’Halloran KA (2012) U.S. national forests adapt to climate change through science-management partnerships. Clim Chang 110:269–296. doi: 10.1007/s10584-011-0066-0
Liu Y, Stanturf J, Goodrick S (2010) Trends in global wildfire potential in a changing climate. For Ecol Manag 259:685–697. doi: 10.1016/j.foreco.2009.09.002
Locatelli B, Evans V, Wardell A, Andrade A, Vignola R (2011) Forests and climate change in Latin America: linking adaptation and mitigation. Forests 2:431–450. doi: 10.3390/f2010431
Loehman RA, Clark JA, Keane RE (2011) Modeling effects of climate change and fire management on Western White Pine (Pinus monticola) in the Northern Rocky Mountains, USA. Forests 2:832–860. doi: 10.3390/f2040832
Long A (2013) REDD plus, adaptation, and sustainable forest management: toward effective polycentric global forest governance. Trop Conserv Sci 6:384–408
Lucier A, Ayres M, Karnosky D, Thompson I, Loehle C, Percy K, Sohngen B (2009) Forest responses and vulnerabilities to recent climate change. In: Seppälä R, Buck A, Katila P (eds) Adaptation of forests and people to climate change: a global assessment report, vol World Series Volume 22. IUFRO Helsinki, pp 29–52
Lynch AJJ, Fell DG, McIntyre-Tamwoy S (2010) Incorporating Indigenous values with ‘Western’ conservation values in sustainable biodiversity management. Aust J Environ Manag 17:244–255
Macdonald GM, Edwards TWD, Moser KA, Pienitz R, Smol JP (1993) Rapid response of treeline vegetation and lakes to past climate warming. Nature 361:243–246. doi: 10.1038/361243a0
Mahat V, Anderson A (2013) Impacts of climate and catastrophic forest changes on streamflow and water balance in a mountainous headwater stream in Southern Alberta. Hydrol Earth Syst Sci 17:4941–4956. doi: 10.5194/hess-17-4941-2013
Malcolm JR, Markham A, Neilson RP, Garaci M (2002) Estimated migration rates under scenarios of global climate change. J Biogeogr 29:835–849. doi: 10.1046/j.1365-2699.2002.00702.x
Matthews SN, Iverson LR, Prasad AM, Peters MP (2011) Changes in potential habitat of 147 North American breeding bird species in response to redistribution of trees and climate following predicted climate change. Ecography 34:933–945. doi: 10.1111/j.1600-0587.2011.06803.x
McEvoy D, Fünfgeld H, Bosomworth K (2013) Resilience and climate change adaptation: the importance of framing. Plan Pract Res 28:280–293. doi: 10.1080/02697459.2013.787710
Medlyn B, Zeppel M, Brouwers N, Howard K, O’Gara E, Hardy G, Lyons T, Li L, Evans B (2011) Biophysical impacts of climate change on Australia’s forests. Contribution of Work Package 2 to the Forest Vulnerability Assessment. National Climate Change Adaptation Research Facility, Gold Coast
Messier C, Puettmann K, Coates DJ (2013) Managing forests as complex adaptive systems: building resilience to the challenge of global change. Earthscan, London
Michelot A, Breda N, Damesin C, Dufrene E (2012) Differing growth responses to climatic variations and soil water deficits of Fagus sylvatica, Quercus petraea and Pinus sylvestris in a temperate forest. For Ecol Manag 265:161–171. doi: 10.1016/j.foreco.2011.10.024
Milad M, Schaich H, Konold W (2013) How is adaptation to climate change reflected in current practice of forest management and conservation? A case study from Germany. Biodivers Conserv 22:1181–1202. doi: 10.1007/s10531-012-0337-8
Miles L, Grainger A, Phillips O (2004) The impact of global climate change on tropical forest biodiversity in Amazonia. Glob Ecol Biogeogr 13:553–565. doi: 10.1111/j.1466-822X.2004.00105.x
Mok H-F, Arndt SK, Nitschke CR (2012) Modelling the potential impact of climate variability and change on species regeneration potential in the temperate forests of South-Eastern Australia. Glob Chang Biol 18:1053–1072. doi: 10.1111/j.1365-2486.2011.02591.x
Mori AS, Spies TA, Sudmeier-Rieux K, Andrade A (2013) Reframing ecosystem management in the era of climate change: issues and knowledge from forests. Biol Conserv 165:115–127. doi: 10.1016/j.biocon.2013.05.020
Namkoong G (2001) Forest genetics: pattern and complexity. Can J For Res 31:623–632. doi: 10.1139/cjfr-31-4-623
Neukum C, Azzam R (2012) Impact of climate change on groundwater recharge in a small catchment in the Black Forest, Germany. Hydrogeol J 20:547–560. doi: 10.1007/s10040-011-0827-x
Nilsson AE, Gerger Swartling Å, Eckerberg K (2012) Knowledge for local climate change adaptation in Sweden: challenges of multilevel governance. Local Environ 17:751–767. doi: 10.1080/13549839.2012.678316
Nitschke CR, Innes JL (2008a) Integrating climate change into forest management in South-Central British Columbia: an assessment of landscape vulnerability and development of a climate-smart framework. For Ecol Manag 256:313–327. doi: 10.1016/j.foreco.2008.04.026
Nitschke CR, Innes JL (2008b) A tree and climate assessment tool for modelling ecosystem response to climate change. Ecol Model 210:263–277. doi: 10.1016/j.ecolmodel.2007.07.026
Nkem J, Kalame FB, Idinoba M, Somorin OA, Ndoye O, Awono A (2010) Shaping forest safety nets with markets: adaptation to climate change under changing roles of tropical forests in Congo Basin. Environ Sci Pol 13:498–508. doi: 10.1016/j.envsci.2010.06.004
Ogden AE, Innes J (2007a) Incorporating climate change adaptation considerations into forest management planning in the boreal forest. Int For Rev 9:713–733. doi: 10.1505/ifor.9.3.713
Ogden AE, Innes JL (2007b) Perspectives of forest practitioners on climate change adaptation in the Yukon and Northwest Territories of Canada. For Chron 83:557–569
Ogden AE, Innes JL (2009) Application of structured decision making to an assessment of climate change vulnerabilities and adaptation options for sustainable forest management. Ecology and Society 14 (1). doi:11
Ohlson DW, McKinnon GA, Hirsch KG (2005) A structured decision-making approach to climate change adaptation in the forest sector. For Chron 81:97–103
Otterman J, Chou MD, Arking A (1984) Effects of nontropical forest cover on climate. J Clim Appl Meteorol 23:762–767. doi: 10.1175/1520-0450(1984)023<0762:eonfco>2.0.co;2
Parks CG, Bernier P (2010) Adaptation of forests and forest management to changing climate with emphasis on forest health: a review of science, policies and practices. For Ecol Manag 259:657–659. doi: 10.1016/s0378-1127(09)00903-7
Pastor J, Post WM (1988) Response of northern forests to CO2-induced climate change. Nature 334:55–58. doi: 10.1038/334055a0
Pearson RG, Dawson TP (2003) Predicting the impacts of climate change on the distribution of species: are bioclimate envelope models useful? Glob Ecol Biogeogr 12:361–371. doi: 10.1046/j.1466-822X.2003.00042.x
Peel J, Godden L, Keenan RJ (2012) Climate change law in an era of multi-level governance. Transl Environ Law 1:245–280. doi: 10.1017/S2047102512000052
Peters EB, Wythers KR, Zhang SX, Bradford JB, Reich PB (2013) Potential climate change impacts on temperate forest ecosystem processes. Can J For Res 43:939–950. doi: 10.1139/cjfr-2013-0013
Peterson DL, Millar CI, Joyce LA, Furniss MJ, Halofsky JE, Neilson RP, Morelli TL (2011) Responding to climate change in national forests: a guidebook for developing adaptation options. General Technical Report. USDA Forest Service, Pacific Northwest Research Station
Phillips OL, Lewis SL, Baker TR, Chao K-J, Higuchi N (2008) The changing Amazon forest. Phil Trans R Soc B 363:1819–1827. doi: 10.1098/rstb.2007.0033
Pinkard EA, Battaglia M, Bruce J, Leriche A, Kriticos DJ (2010) Process-based modelling of the severity and impact of foliar pest attack on eucalypt plantation productivity under current and future climates. For Ecol Manag 259:839–847. doi: 10.1016/j.foreco.2009.06.027
Pojar J, Klinka K, Meidinger DV (1987) Biogeoclimatic ecosystem classification in British Columbia. For Ecol Manag 22:119–154
Pramova E, Locatelli B, Djoudi H, Somorin OA (2012) Forests and trees for social adaptation to climate variability and change. Wiley Interdiscip Rev Clim Chang 3:581–596. doi: 10.1002/wcc.195
Prato T (2008) Conceptual framework for assessment and management of ecosystem impacts of climate change. Ecol Complex 5:329–338
Pretzsch H, Biber P, Schütze G, Uhl E, Rötzer T (2014) Forest stand growth dynamics in Central Europe have accelerated since 1870. Nat Commun 5. doi:10.1038/ncomms5967
Rastetter EB, Ryan MG, Shaver GR, Melillo JM, Nadelhoffer KJ, Hobbie JE, Aber JD (1991) A general biogeochemical model describing the responses of the C and N cycles in terrestrial ecosystems to changes in CO2, climate, and N deposition. Tree Physiol 9:101–126
Rayner J, McNutt K, Wellstead A (2013) Dispersed capacity and weak coordination: the challenge of climate change adaptation in Canada’s forest policy sector. Rev Policy Res 30:66–90. doi: 10.1111/ropr.12003
Regniere J (2009) Predicting insect continental distributions from species physiology. Unasylva 60:37–42
Reyer C, Lasch-Born P, Suckow F, Gutsch M, Murawski A, Pilz T (2014) Projections of regional changes in forest net primary productivity for different tree species in Europe driven by climate change and carbon dioxide. Ann For Sci 71:211–225. doi: 10.1007/s13595-013-0306-8
Rigling A, Bigler C, Eilmann B, Feldmeyer-Christe E, Gimmi U, Ginzler C, Graf U, Mayer P, Vacchiano G, Weber P, Wohlgemuth T, Zweifel R, Dobbertin M (2013) Driving factors of a vegetation shift from Scots pine to pubescent oak in dry Alpine forests. Glob Chang Biol 19:229–240. doi: 10.1111/gcb.12038
Rist L, Moen J (2013) Sustainability in forest management and a new role for resilience thinking. For Ecol Manag 310:416–427. doi: 10.1016/j.foreco.2013.08.033
Roberts G, Parrotta J, Wreford A (2009) Current adaptation measures and policies. In: Seppälä R, Buck A, Katila P (eds) Adaptation of forests and people to climate change: a global assessment report, vol World Series Volume 22. IUFRO Helsinki, pp 123–134
Ruiz-Labourdette D, Schmitz MF, Pineda FD (2013) Changes in tree species composition in Mediterranean mountains under climate change: indicators for conservation planning. Ecol Indic 24:310–323. doi: 10.1016/j.ecolind.2012.06.021
Running SW, Nemani RR (1991) Regional hydrologic and carbon balance responses of forests resulting from potential climate change. Clim Chang 19:349–368. doi: 10.1007/bf00151173
Sample VA, Halofsky JE, Peterson DL (2014) US strategy for forest management adaptation to climate change: building a framework for decision making. Ann For Sci 71:125–130. doi: 10.1007/s13595-013-0288-6
Schaich H, Milad M (2013) Forest biodiversity in a changing climate: which logic for conservation strategies? Biodivers Conserv 22:1107–1114. doi: 10.1007/s10531-013-0491-7
Schoene DHF, Bernier PY (2012) Adapting forestry and forests to climate change: a challenge to change the paradigm. For Policy Econ 24:12–19. doi: 10.1016/j.forpol.2011.04.007
Schwartz MW, Dolanc CR, Gao H, Strauss SY, Schwartz AC, Williams JN, Tang Y (2013) Forest structure, stand composition, and climate-growth response in montane forests of Jiuzhaigou National Nature Reserve, China. Plos One 8 (8). doi:10.1371/journal.pone.0071559
Scott D (2005) Integrating climate change into Canada’s National Parks System. In: Lovejoy T, Hannah L (eds) Climate change and biodiversity. Yale University Press, New Haven, pp 343–345
Seidl R, Lexer MJ (2013) Forest management under climatic and social uncertainty: trade-offs between reducing climate change impacts and fostering adaptive capacity. J Environ Manag 114:461–469. doi: 10.1016/j.jenvman.2012.09.028
Seidl R, Rammer W, Lexer MJ (2011) Adaptation options to reduce climate change vulnerability of sustainable forest management in the Austrian Alps. Can J For Res 41:694–706. doi: 10.1139/x10-235
Seppala R (2009) A global assessment on adaptation of forests to climate change. Scand J For Res 24:469–472. doi: 10.1080/02827580903378626
Seppälä R, Buck A, Katila P (2009) Adaptation of forests and people to climate change: a global assessment report, vol World Series Volume 22. Helsinki, IUFRO
Six DL (2009) Climate change and mutualism. Nat Rev Microbiol 7:686–686
Somorin OA, Brown HCP, Visseren-Hamakers IJ, Sonwa DJ, Arts B, Nkem J (2012) The Congo Basin forests in a changing climate: Policy discourses on adaptation and mitigation (REDD+). Glob Environ Chang 22:288–298. doi: 10.1016/j.gloenvcha.2011.08.001
Sonwa DJ, Somorin OA, Jum C, Bele MY, Nkem JN (2012) Vulnerability, forest-related sectors and climate change adaptation: the case of Cameroon. For Policy Econ 23:1–9. doi: 10.1016/j.forpol.2012.06.009
Sork VL, Aitken SN, Dyer RJ, Eckert AJ, Legendre P, Neale DB (2013) Putting the landscape into the genomics of trees: approaches for understanding local adaptation and population responses to changing climate. Tree Genet Genome 9:901–911. doi: 10.1007/s11295-013-0596-x
Spathelf P, van der Maaten E, van der Maaten-Theunissen M, Campioli M, Dobrowolska D (2014) Climate change impacts in European forests: the expert views of local observers. Ann For Sci 71:131–137. doi: 10.1007/s13595-013-0280-1
Spies TA, Giesen TW, Swanson FJ, Franklin JF, Lach D, Johnson KN (2010) Climate change adaptation strategies for federal forests of the Pacific Northwest, USA: ecological, policy, and socio-economic perspectives. Landsc Ecol 25:1185–1199. doi: 10.1007/s10980-010-9483-0
Spittlehouse DL (2005) Integrating climate change adaptation into forest management. For Chron 81:691–695
Spittlehouse DL, Stewart RB (2003) Adaption to climate change in forest management. BCJ Ecosyst Manag 4:1–11
Stafford Smith M, Horrocks L, Harvey A, Hamilton C (2011) Rethinking adaptation for a 4 degrees C world. Philos Transact A Math Phys Eng Sci 369:196–216
Stainforth DA, Allen MR, Tredger ER, Smith LA (2007) Confidence, uncertainty and decision-support relevance in climate predictions. Philos Trans R Soc A Math Phys Eng Sci 365:2145–2161. doi: 10.1098/rsta.2007.2074
Stanturf JA, Palik BJ, Dumroese RK (2014) Contemporary forest restoration: a review emphasizing function. For Ecol Manag 331:292–323
Steenberg JWN, Duinker PN, Bush PG (2011) Exploring adaptation to climate change in the forests of central Nova Scotia, Canada. For Ecol Manag 262:2316–2327. doi: 10.1016/j.foreco.2011.08.027
Stephens M, Pinkard L, Keenan RJ (2012) Plantation forest industry climate change adaptation handbook. Australian Forest Products Association, Canberra
Tacconi L, Moore PF, Kaimowitz D (2007) Fires in tropical forests—what is really the problem? Lessons from Indonesia. Mitig Adapt Strateg Glob Chang 12:55–66. doi: 10.1007/s11027-006-9040-y
Temperli C, Bugmann H, Elkin C (2012) Adaptive management for competing forest goods and services under climate change. Ecol Appl 22:2065–2077
Thackway R, Cresswell ID (1992) Environmental regionalisations of Australia: a user-oriented approach. Environmental Resources Information Network, Canberra
Thomalla F, Downing T, Spanger-Siegfried E, Han GY, Rockstrom J (2006) Reducing hazard vulnerability: towards a common approach between disaster risk reduction and climate adaptation. Disasters 30:39–48. doi: 10.1111/j.1467-9523.2006.00305.x
Thomson-Reuters (2014) Web of Science. http://thomsonreuters.com/thomson-reuters-web-of-science/ . Accessed 21 Aug 2014
Thuiller W, Albert C, Araújo MB, Berry PM, Cabeza M, Guisan A, Hickler T, Midgley GF, Paterson J, Schurr FM, Sykes MT, Zimmermann NE (2008) Predicting global change impacts on plant species’ distributions: future challenges. Perspect Plant Ecol Evol Syst 9:137–152. doi: 10.1016/j.ppees.2007.09.004
Toffler A (1970) Future shock. Bantam, Toronto
Tompkins EL, Adger WN, Boyd E, Nicholson-Cole S, Weatherhead K, Arnell N (2010) Observed adaptation to climate change: UK evidence of transition to a well-adapting society. Glob Environ Chang Hum Policy Dimens 20:627–635. doi: 10.1016/j.gloenvcha.2010.05.001
Urwin K, Jordan A (2008) Does public policy support or undermine climate change adaptation? Exploring policy interplay across different scales of governance. Glob Environ Chang Hum Policy Dimens 18:180–191. doi: 10.1016/j.gloenvcha.2007.08.002
Van Damme L (2008) Can the forest sector adapt to climate change? For Chron 84:633–634
van Dijk AIJM, Keenan RJ (2007) Planted forests and water in perspective. For Ecol Manag 251:1–9. doi: 10.1016/j.foreco.2007.06.010
Versini PA, Velasco M, Cabello A, Sempere-Torres D (2013) Hydrological impact of forest fires and climate change in a Mediterranean basin. Nat Hazards 66:609–628. doi: 10.1007/s11069-012-0503-z
Vignola R, Locatelli B, Martinez C, Imbach P (2009) Ecosystem-based adaptation to climate change: what role for policy-makers, society and scientists? Mitig Adapt Strateg Glob Chang 14:691–696. doi: 10.1007/s11027-009-9193-6
Vihervaara P, D’Amato D, Forsius M, Angelstam P, Baessler C, Balvanera P, Boldgiv B, Bourgeron P, Dick J, Kanka R, Klotz S, Maass M, Melecis V, Petrik P, Shibata H, Tang JW, Thompson J, Zacharias S (2013) Using long-term ecosystem service and biodiversity data to study the impacts and adaptation options in response to climate change: insights from the global ILTER sites network. Curr Opin Environ Sustain 5:53–66. doi: 10.1016/j.cosust.2012.11.002
von Detten R, Faber F (2013) Organizational decision-making by German state-owned forest companies concerning climate change adaptation measures. For Policy Econ 35:57–65. doi: 10.1016/j.forpol.2013.06.009
Walker B, Meyers JA (2004) Thresholds in ecological and social-ecological systems: a developing database. Ecology and Society 9 (2)
Walker B, Salt D (2012) Resilience practice: engaging the sources of our sustainability. Island Press, Washington, DC
Wang T, Campbell EM, O’Neill GA, Aitken SN (2012) Projecting future distributions of ecosystem climate niches: uncertainties and management applications. For Ecol Manag 279:128–140. doi: 10.1016/j.foreco.2012.05.034
Wellstead A, Rayner J, Howlett M (2014) Beyond the black box: forest sector vulnerability assessments and adaptation to climate change in North America. Environ Sci Pol 35:109–116. doi: 10.1016/j.envsci.2013.04.002
Westerling AL, Gershunov A, Cayan DR, Barnett TP (2002) Long lead statistical forecasts of area burned in western US wildfires by ecosystem province. Int J Wildl Fire 11:257–266. doi: 10.1071/wf02009
Westerling AL, Hidalgo HG, Cayan DR, Swetnam TW (2006) Warming and earlier spring increase western U.S. forest wildfire activity. Science 313:940–943. doi: 10.1126/science.1128834
White A, Hatcher J, Khare A, Liddle M, Molnar A, Sunderlin WD (2010) Seeing people through the trees and the carbon: mitigating and adapting to climate change without undermining rights and livelihoods. Social dimensions of climate change: equity and vulnerability in a warming world: 277–301
Wilby RL, Dessai S (2010) Robust adaptation to climate change. Weather 65:180–185. doi: 10.1002/wea.543
Williams J (2013) Exploring the onset of high-impact mega-fires through a forest land management prism. For Ecol Manag 294:4–10. doi: 10.1016/j.foreco.2012.06.030
Williams JE (2000) The biodiversity crisis and adaptation to climate change: a case study from Australia’s forests. Environ Monit Assess 61:65–74. doi: 10.1023/a:1006361917359
Williams JT (2004) Managing fire-dependent ecosystems: we need a public lands policy debate. Fire Manag Today 64:6–11
Wintle BA, Bekessy SA, Keith DA, van Wilgen BW, Cabeza M, Schroder B, Carvalho SB, Falcucci A, Maiorano L, Regan TJ, Rondinini C, Boitani L, Possingham HP (2011) Ecological-economic optimization of biodiversity conservation under climate change. Nat Clim Chang 1:355–359. doi: 10.1038/nclimate1227
Wu HX, Ying CC (2004) Geographic pattern of local optimality in natural populations of lodgepole pine. For Ecol Manag 194:177–198. doi: 10.1016/j.foreco.2004.02.017
Yousefpour R, Jacobsen JB, Meilby H, Thorsen BJ (2014) Knowledge update in adaptive management of forest resources under climate change: a Bayesian simulation approach. Ann For Sci 71:301–312. doi: 10.1007/s13595-013-0320-x
Yousefpour R, Jacobsen JB, Thorsen BJ, Meilby H, Hanewinkel M, Oehler K (2011) A review of decision-making approaches to handle uncertainty and risk in adaptive forest management under climate change. Ann For Sci 69:1–15. doi: 10.1007/s13595-011-0153-4
Zhao D, Wu S, Yin Y (2013) Responses of terrestrial ecosystems’ net primary productivity to future regional climate change in China. PLoS ONE 8:e60849. doi: 10.1371/journal.pone.0060849
Zhou GY, Wei XH, Wu YP, Liu SG, Huang YH, Yan JH, Zhang DQ, Zhang QM, Liu JX, Meng Z, Wang CL, Chu GW, Liu SZ, Tang XL, Liu XD (2011) Quantifying the hydrological responses to climate change in an intact forested small watershed in Southern China. Glob Chang Biol 17:3736–3746. doi: 10.1111/j.1365-2486.2011.02499.x
Zimmermann NE, Yoccoz NG, Edwards TC, Meier ES, Thuiller W, Guisan A, Schmatz DR, Pearman PB (2009) Climatic extremes improve predictions of spatial patterns of tree species. Proc Natl Acad Sci 106:19723–19728. doi: 10.1073/pnas.0901643106
Download references
Acknowledgments
Thanks to Linda Joyce for her comments on an earlier draft of this paper, to a number of anonymous reviewers for their thoughtful suggestions and to many colleagues that I have discussed these ideas with over the past five years.
Author information
Authors and affiliations.
Department of Forest and Ecosystem Science, The University of Melbourne, 221 Bouverie St., Carlton, VIC, 3010, Australia
Rodney J. Keenan
You can also search for this author in PubMed Google Scholar
Corresponding author
Correspondence to Rodney J. Keenan .
Additional information
Handling Editor: Erwin Dreyer
This research was partly undertaken during the period the author was Director of the Victorian Centre for Climate Change Adaptation Research and part-funded by the Victorian Government. It was completed with support from the University of Melbourne under a Special Studies Program grant.
Rights and permissions
Open Access This article is distributed under the terms of the Creative Commons Attribution License which permits any use, distribution, and reproduction in any medium, provided the original author(s) and the source are credited.
Reprints and permissions
About this article
Cite this article.
Keenan, R.J. Climate change impacts and adaptation in forest management: a review. Annals of Forest Science 72 , 145–167 (2015). https://doi.org/10.1007/s13595-014-0446-5
Download citation
Received : 01 September 2014
Accepted : 10 December 2014
Published : 14 January 2015
Issue Date : March 2015
DOI : https://doi.org/10.1007/s13595-014-0446-5
Share this article
Anyone you share the following link with will be able to read this content:
Sorry, a shareable link is not currently available for this article.
Provided by the Springer Nature SharedIt content-sharing initiative
- Climate change
- Vulnerability
- Forest management
Annals of Forest Science
ISSN: 1297-966X
- Submission enquiries: [email protected]
- General enquiries: [email protected]

You are here
Forest management and climate change: a literature review.
- Food and Agriculture Organization of the United Nations (FAO) www.fao.org
Focus themes
- Climate change agriculture and food security
- Climate change
- International climate change negotiations
- Climate change governance
- Climate change mitigation
- Climate change Forestry
- Environment
- Climate Change Adaptation
- Environment and Forestry
- forestry assessment
In collaboration with
- Service Climate and Development Knowledge Network (CDKN) www.cdkn.org/
- Content Partner Climate and Development Knowledge Network (CDKN) www.cdkn.org/
This literature review assesses current and potential future changes occurring within the forestry sector. It identifies challenges posed to forests and analyses the relationship between forests and climate change. While it is relatively safe to assume that temperature increase is a threat to the survival of many ecosystems other challenges, such as extreme weather and precipitation levels, are harder to predict. Other challenges identified include the management of climate change related human migration and maintaining an equitable balance between adapting to new market conditions and retaining ecological integrity. The review explores forest management options for mitigation and adaptation strategies with an emphasis on monitoring, capacity building, market influences and risk reduction. The review also identifies gaps in enabling conditions that require further research and action, including knowledge of potential climate change impacts, the capacity of forest managers to adapt, technology and the institutional environment.
Mitigation strategies highlighted in the review include:
- clearer definitions of land, forest and carbon rights
- raising awareness of climate change amongst forest managers
- documentation and sharing of best practice and lessons learned
- establishment of stakeholder platforms to ensure transparency and equity.
The document includes the following suggestions for strengthening adaptation measures.
- More research is required in monitoring systems, the adaptability and flexibility of different species to climate change, optimum management cycles, impact modelling and incentive mechanisms.
- Training in sustainable forest management should be improved.
- Improvements are needed in the institutional framework to clarify rights, ensure greater equity, provide a multi-sectoral approach, improve local governance and provide financial and technical assistance (particularly for carbon markets).
How good is this research?

Assessing the quality of research can be a tricky business. This blog from our editor offers some tools and tips.
Related website content

Climate change and rural sanitation
We need to move fast to mainstream climate change into planning for sanitation and hygiene access. In a challenging landscape, where do we start?
- Sanitation and hygiene
Register for webinar: Rural sanitation and climate change

New IDS research project seeks to re-imagine ‘shit’ as ‘brown gold’
Related content, impact of climate hazards on rural sanitation and hygiene practices in burkina faso, are livestock always bad for the planet, climate change 2021: the physical science bias, challenges for community‐based adaptation: discovering the potential for transformation, climate vulnerability and capacity analysis handbook,.

An official website of the United States government
The .gov means it’s official. Federal government websites often end in .gov or .mil. Before sharing sensitive information, make sure you’re on a federal government site.
The site is secure. The https:// ensures that you are connecting to the official website and that any information you provide is encrypted and transmitted securely.
- Publications
- Account settings
Preview improvements coming to the PMC website in October 2024. Learn More or Try it out now .
- Advanced Search
- Journal List
- Springer Nature - PMC COVID-19 Collection

A global systematic review of forest management institutions: towards a new research agenda
Jude ndzifon kimengsi.
1 Forest Institutions and International Development (FIID) Research Group, Chair of Tropical and International Forestry, Faculty of Environmental Sciences, Technische Universität Dresden, Dresden, Germany
2 Department of Geography, The University of Bamenda, Bamenda, Cameroon
Raphael Owusu
Shambhu charmakar, gordon manu.
3 Food and Agricultural Organization (FAO), Rome, Italy
Lukas Giessen
4 Chair of Tropical and International Forestry, Faculty of Environmental Sciences, Technische Universität Dresden, Dresden, Germany
Associated Data
Globally, forest landscapes are rapidly transforming, with the role of institutions as mediators in their use and management constantly appearing in the literature. However, global comparative reviews to enhance comprehension of how forest management institutions (FMIs) are conceptualized, and the varying determinants of compliance, are lacking. And so too, is there knowledge fragmentation on the methodological approaches which have and should be prioritized in the new research agenda on FMIs.
We review the regional variations in the conceptualization of FMIs, analyze the determinants of compliance with FMIs, and assess the methodological gaps applied in the study of FMIs.
A systematic review of 197 empirically conducted studies (491 cases) on FMIs was performed, including a directed content analysis.
First, FMIs literature is growing; multi-case and multi-country studies characterize Europe/North America, Africa and Latin America, over Asia. Second , the structure-process conceptualization of FMIs predominates in Asia and Africa. Third , global south regions report high cases of compliance with informal FMIs, while non-compliance was registered for Europe/North America in the formal domain. Finally, m ixed-methods approaches have been least employed in the studies so far; while the use of only qualitative methods increased over time, the adoption of only quantitative approaches witnessed a decrease.
Future research should empirically ground informality in the institutional set-up of Australia while also valorizing mixed-methods research globally. Crucially, future research should consider multidisciplinary and transdisciplinary approaches to explore the actor and power dimensions of forest management institutions.
Supplementary Information
The online version contains supplementary material available at 10.1007/s10980-022-01577-8.
Introduction
Globally, forests are at a crossroads—characterized by rapid transformation (Garcia et al. 2020 ). For instance, Global Forest Watch estimated that forest loss around the globe reached 29.7 million hectares as of 2016, indicating a 51% increase since 2015. For tropical forests, the loss was estimated at 12 million hectares (the size of Belgium) in 2018 (Weisse and Goldman 2017 ; Garcia et al. 2020 ). While such changes are linked to natural (e.g., climate change) and human-induced drivers such as land-use change (Rounsevell et al. 2006 ; Meyfroidt and Lambin 2011 ; Aguiar et al. 2016 ; Houghton and Nassikas 2018 ), they form part of a complex transformation system—mediated by socio-economic, political, and institutional forces (Malhi et al. 2014 ). This validates the role of institutions as a key enhancing or constraining factor in determining forest resources access, use and management (Cleaver 2017 ). Institutions are viewed as highly abstract and invisible conditions in the political environment. They constitute cognitive, normative, and regulatory structures which provide stability and meaning to social behaviour. Institutions are carried across multiple vehicles, including cultures, structures and routines, operating at multiple levels of jurisdiction (Scott 1995 ). The transformation of forest landscapes at various timescales is characterized by net tropical forest loss (Geist and Lambin 2002 ; Kissinger et al. 2012 ; Song et al. 2018 ). Furthermore, regional variations in the drivers (Curtis et al. 2018 ) exist: in Latin America, transformations are largely rooted in ranching and soybean expansion (Rudel et al. 2009 ; Verburg et al. 2014 ; Tyukavina et al. 2017 ), while subsistence agriculture drives the transformation process in Africa (Hosonuma et al. 2012 ; Tyukavina et al. 2018 ). Transformations in Asia are significantly linked to industrial processes and small-holder farming (Rudel et al. 2009 ; Turubanova et al. 2018 ).
While forests are declining (Weisse and Goldman 2017 ), their roles in the resolution of global socio-ecological challenges (e.g., climate change mitigation and poverty reduction) remain unrivalled (Oldekop et al. 2020 ; Nerfa et al. 2020 ). Scholars submit that governance mechanisms, especially the role of institutions, remain primordial in shaping forests access, use, and management. For this reason, institutions—the rules of the game—continually gain relevance (Agrawal and Gupta 2005 ; Dixon and Wood 2007 ; Kimengsi et al. 2021 ). Variations exist in the way institutions are conceptualized. For instance, following the structure process dichotomy (Fleetwood 2008a , b ), institutions relate to tissues of social relations linking groups and communities (structures) and a set of rules, conventions and values, among others (processes) (Fleetwood 2008a ; Bernardi et al. 2007 ). It is, however, difficult to provide a dividing line between the processes and structures; processes (rules) guide the formation of structures, while structures, on the other hand, oversee and enforce rules (Fleetwood 2008a ; Ntuli et al. 2021 ). However, structures differ from processes in terms of their functioning; structures could represent forest management organizations as an entity, and not the rules (processes) which they produce (Ntuli et al. 2021 ). Both structures and processes are subjected to a categorization as either formal (written and codified laws, largely state driven) and informal (unwritten or uncodified rules that transcend generations) (Osei-Tutu et al . 2014 ; Yeboah-Assiamah et al. 2017 ). Furthermore, and on the basis of source, institutions could be categorized following the endogenous—exogenous dichotomy; the former relates to community-specific complex and embedded rules, while the latter denotes institutions introduced by the state and international agencies (Yeboah-Assiamah et al. 2017 ; Kimengsi et al. 2022a , b , c ). By and large, these categories of institutions exist to provide order in the midst of ‘chaos’, with regards to the sustainable management of forest resources (Beunen and Patterson 2019 ).
While forest landscapes are transforming, institutions have also been subjected to several dimensions of change. Their evolution over time manifests through formation, reformation, disintegration, and modification in several contexts, including Africa (Haller et al. 2016 ; Friman 2020 ; Kimengsi et al. 2022a , b , c ), Asia (Haapal and White 2018 ; Steenbergen and Warren 2018 ), and Latin America (Faggin and Behagel 2018 ; Gebara 2019 ). This brings to fore the notion of ephemeral, intermittent and perennial institutions (Kimengsi et al. 2021 )—borrowed from the geographic classification of streams (Gomes et al. 2020 ). Ephemeral refers to short-term stream movements (institutional arrangements), intermittent is analogous to medium-term/seasonal streams (medium-term institutional arrangements), and perennial relates to streams that flow all through—analogous to more long-term, enduring institutions (Kimengsi et al. 2021 ). Therefore, the search for perennial (enduring) institutions is top on the scientific and policy agenda (Ostrom 1990 ; Kimengsi et al. 2021 ). This is important to support the attainment of objectives such as halting forest loss and improving forest cover and species diversity (Bare et al. 2015 ; Assa 2018 ), sustaining livelihoods and economic welfare (Buchenrieder and Balgah 2013 ; Foundjem-Tita et al. 2018 ), and engendering equity and fairness in the distribution of proceeds from forest systems (Faye et al. 2017 ). However, studies on institutions and institutional change are seemingly at an impasse; it seems difficult to proceed with the framing of forward-looking research questions linked to forest management institutions (FMIs). The impasse is rooted in the largely fragmented and unstructured institutional analysis around forest settings that harbor conflicts linked to emerging and persistent resource use inequalities (Gautam et al. 2004 ; Soliev et al. 2021 ). Additionally, the multiplicity of institutional variables and the lack of a consensus on which of the methods—qualitative or quantitative—is best suited for analyzing institutions and institutional change (Kimengsi et al. 2022a , b , c ) further validate the need to surmount this impasse. In this regard, a systematic review of the global knowledge base on FMIs is imminent. Furthermore, details on the methods to prioritize in future studies further validates the need for a review. Consequently, we seek answers to the following questions: (1) How have FMIs been conceptualized and analyzed globally? (2) How varied are the (non)compliance determinants and outcomes of FMIs? (3) How can we conceptually and methodologically advance research on FMIs? To provide answers to these interrogations, we undertake a review of FMIs. The study is inspired by an earlier review conducted in the context of sub-Saharan Africa (Kimengsi et al. 2022b ).
Materials and methods
Analytical framework.
In this review, we make use of the socio-ecological co-evolution framework (Pretzsch et al. 2014 ). The framework serves as a useful theoretical fundament to enhance understanding of the dynamics around forests and rural development. While allowing for the differentiation between humans and ecological subsystems, the framework also outlines the dynamic interactions between these two systems (Berkes et al. 1998 ; Pretzsch et al. 2014 ). The socio-ecological co-evolution framework is designed to enhance comprehension of the interactions between the social system (e.g., the community of forest users), the institutions that shape them, and the ecological system—forests. These interactions occur at the interface (management segment) of the framework. Besides providing a useful analytical lens to appreciate current levels of engagement in decision-making and the enforcement of institutional provisions, it also serves as a useful framework to understand how institutional change triggers the co-evolution of both ecological and social systems. The socio-ecological co-evolution framework is informed by the earlier works of Berkes et al. ( 1998 ), which bridged the hitherto divide between social research (centred around institutions), and ecological research, which emphasized cross-scale ecosystem dynamics. Worthy of note is the fact that other frameworks exist; for instance, the socio-ecological systems (SES) framework (Ostrom 2009 ) was proposed to explain complex systems involving resource systems (forests in this case), their resource units (e.g., timber), appropriators (e.g., timber exploiters), and governance systems (e.g., forest management rules) that continually interact to produce differential outcomes (Ostrom 2009 ). It explains that socio-ecological systems are constantly subjected to change. Some of these changes are rooted in institutions and institutional change processes (Rammel et al. 2007 ; Pretzsch et al. 2014 ). The socio-ecological co-evolution framework is employed for the following reasons: (1) with rapid transformations experienced in forest landscapes across the globe, scientific and policy circles need to extend their breadth of knowledge on how to further ‘marry’ social and ecological systems in forest management. (2) Institutional change is reflected through the decisions and actions of resource users at the interface of the framework. Therefore, understanding how these changes and their determinants precipitate (non)compliance is helpful in today’s dispensation, where forests are seen as crucial in stemming the upsurge of environmental crises. (3) The outcomes associated with the myriads of institutions need to be further appreciated to inform policy actors on the orientation of future FMIs. The socio-ecological co-evolution framework (Fig. 1 ) explains how changing societal demands and choices, influenced by the institutions in place, shape the type and magnitude of societal intervention in socio-ecological systems (e.g., forests).

Analytical framework for the systematic review.
Source: Based on Cleaver ( 2017 ), Haller et al. ( 2016 ), North ( 1990 ), Ostrom ( 1990 , 2005), and Pretzsch et al. ( 2014 ). NTFPs Non-Timber Forest Product
The review, guided by the research questions, focuses on the management phase and the social segment of the socio-ecological co-evolution framework. The management phase represents an interface—a point where management decisions under different forest categories such as plantation forests, forest reserves, community forests and landscapes in want of restoration, are implemented. Institutions and institutional change processes drive such decisions. The management operations are construed as forest-linked activities which are informed by institutions regulating timber and NTFPs exploitation, ecotourism, medicinal plants’ extraction and forest conservation. Institutional arrangements in this socio-ecological system culminate in the derivation of different management approaches, such as co-management and community-based forestry with a focus on livelihoods and conservation. The social segment of the framework focuses on the conceptualization of forest management institutions (for instance, structures vs processes, formal vs informal, and endogenous vs exogenous). This segment also captured forest management institutional compliance with an emphasis on the variations and determinants. The segment on outcomes explored the ecological, economic, socio-cultural, and political outcomes of FMIs. The framework also has a segment which explores methodological approaches employed in the study of FMIs.
Methodology
Data collection.
The systematic review approach (Nightingale 2009a , b ; Mengist et al. 2019 ) was employed in this study. Systematic reviews follow an established and standardized protocol for the search, appraisal and inclusion (or exclusion) of literature for subsequent analysis (Boell and Cecez-Kecmanovic 2015 ). This is different from the general review of literature which consists of a non-structured and highly subjective method of literature search and analysis (Kraus et al. 2020 ). The procedure was employed as follows: First a list of search terms (Appendix) was developed and used in the article search process. We targeted the following databases: Scopus, Science Direct, Google Scholar and Web of Science. Search terms such as forest management, forest governance, institutions, rules, norms, norms, laws, policies, community-based organizations, NGOs, associations, compliance, determinants, and outcomes were repeatedly employed in the search. The terms were combined with the respective regions (Africa, Asia, Australia, Europe/North America, Latin America), over a 15-year period (2006–2021). It should be noted that Europe and North America were clustered due to the observed similarity in their societal fabric and culture. We considered this timespan good enough to mirror contemporary evidence on the question of forest management institutions (FMIs). The search led to the initial identification of 920 articles. Four hundred thirty articles were identified from Web of Science, 104 from Google Scholar, 348 from Scopus and 38 from the Science Direct database. The search on Google Scholar did not produce a lot of articles. This is because grey literature was not considered during the search. Our emphasis was to derive literature which were published in internationally recognized databases. We then proceeded to deduplicate the articles—the deduplication process led to a reduction to 680 articles. Furthermore, article screening was performed with emphasis on the abstracts. This informed the decision to include or exclude the paper. In the selection, we targeted journal articles that were published in English and were empirically grounded. In cases where the abstract could not provide these details, we proceeded to review the methods and conclusions to inform inclusion (or exclusion). We excluded all grey literature during the article selection. This reduced the number of manuscripts to 197 (see Supplementary Excel Sheet), from which we derived 491 case studies; the cases were derived by considering the number of study areas that were included for analysis. We use ArcMap 10.5 to generate the map of the globe and the regions and/or countries where most of the case studies in this review paper were concentrated.
Data analysis
The articles retained were further read, and following the analytical framework (Fig. 1 ), a directed content analysis was performed (Hsieh and Shannon 2005 ). The directed content analysis began with a relevant theoretical framework—in this case, the socio-ecological coevolution framework. This framework provided a clear focus for the research questions under review. The key variables which were outlined in the framework (Fig. 1 ) informed the clustering of the data generated from the selected articles. For the selected articles, we read the abstract, methods and conclusion sections to generate data. The dataset was compiled in an excel sheet and further read; key texts which contained variables of interest were highlighted. These variables were then clustered following the established questions and themes for further analysis (Mayring 2000 ). Therefore, the highlighted texts, which contained data corresponding to the four thematic sections, were extracted from each article and organized under the main themes: conceptualization of institutions, institutional compliance, outcomes of forest management institutions and methodological approaches . We approached the conceptualization of institutions following the structure-process dimension (Fleetwood 2008a ), the formal and informal dichotomy (North 1990 ), the endogenous vs exogenous institutional lens (Kimengsi et al. 2021 ) and the state vs community-based institutional dichotomy (Ntuli et al. 2021 ). Compliance denotes the extent to which forest users adhere to the institutional provisions in their communities. This translates to forest management outcomes which could be ecological, socio-economic and even political (Haller et al. 2016 ).
These were recorded in a Microsoft Excel sheet (Artmann and Sartison 2018 ). We considered this approach appropriate, considering that software extraction might ignore salient details owing to the complex nature of institutional variables. Besides narratives and content analysis, we used descriptive statistics to report the variations across the five regions. The descriptive analysis further aided in establishing institutional compliance and its determinants, the ecological, socio-cultural, economic, and political outcomes linked to forest management institutions, and the variations in methodological approaches employed.
Attributes of reviewed papers and case studies
The review indicated that most of the articles emanate from Africa and Latin America—home to two of the world’s major forest ecosystems. This was followed by Asia. Case-wise, the study captured a total of 491 cases drawn from 99 countries across the globe (Fig. 2 ). The highest number of cases emanate from Europe/North America and Africa. This suggests that multi-case and multi-country studies have been significantly prioritized in these regions compared to single case/country studies for Asia and Australia.

Spatial distribution of case studies on forest management institutions (2006–2021)
In parts of Central Europe, studies to explore shifts towards new governance established that recent changes in institutional arrangements result from macro-political trends and the geopolitical strategy of some states (Sergent et al. 2018 ). In the United States and Canada, forest certification led to substantial changes in practices as enterprises embraced changes in forestry, environmental, social, and economic/system practices in the realm of forest certification (Moore et al. 2012 ). In the case of Africa, a comparative study of 38 countries reported that the activities of multinational corporations are associated with differential losses in forest cover—linked to weak governance (institutions) (Assa 2018 ). The review clearly shows that while political, geostrategic and religious forces defined the institutional change process in Europe/North America, economic interests through multinational companies shaped institutional change in Africa. The review established that some of the significant countries with regards to cases include Australia, Ecuador, and Germany (21 + cases). In addition, Bolivia, Brazil, Cameroon, Ghana, and Canada, India registered between 8 and 14 cases, while the remaining countries registered between 1 and 7 cases (Fig. 2 ).
Temporal evolution of papers and cases on forest management institutions
On the whole, the literature on institutions has grown over the last 15 years(Fig. 3 ). This could be linked to renewed interests to understand governance mishaps and to engage in getting institutions (including FMIs) right . In all, while the number of publications increased from 2006, the review shows that it witnessed a decline in 2009 and 2012. The growth in the literature is possibly explained by the interest to uncover institutional ‘relicts’ (in Africa) and rising environmental challenges in Latin America (bushfires and migration). Formal forest management institutions (structure and process) for forest products have received much attention in the 2000s literature. However, significant growth was observed in the literature of the 2010s, which included both formal (international) and informal (traditional and local) institutions and concepts such as ecosystem services, sustainable forest management, farm forestry, biodiversity, REDD + , and forest certification. This classification increasingly accommodated the use of endogenous and exogenous institutions, as well as state and community-based institutions. However, both classifications have been (mis)construed to represent formal and informal institutions.

Temporal evolution of papers on forest institutions across the globe for the past 15 years
Conceptualization of forest management institutions
From a structural dimension, institutions have been most conceptualized as structures in Asia and Africa. These predominate the informal structures where Asia and Africa account for 35% and 28%, respectively, of the review’s literature reporting on informal institutional structures. Latin America closely follows them with 22%. Literature from Asia and Africa further dominates in the classification of formal institutional structures with 28% and 25%, respectively. This is closely followed by Europe/North America (23%). Asia and Africa are ‘pace-setters” in the implementation of new forest management paradigms such as community-based forest management (Kimengsi and Bhusal, 2022 ). The introduction of these models saw the multiplication of management structures to oversee them. This explains why the literature significantly captures the structural dimension of institutions. Process-wise, literature from Asia and Africa accounts for 48% and 30%, respectively, of the literature on informal institutions, while Australia surprisingly reports none. In the formal domain, Asia, Europe/North America, and Latin America account for over 60% of the literature reporting the formal conceptualization of institutions (Table (Table1 1 ).
Total number of papers (studies) = 197
Literature from Africa and Asia showed similarities in the conceptualization of institutions (Table (Table2); 2 ); informal structures, for instance, chieftaincy and women groups, define and enforce processes (rules) which are conceived, for example, as taboos, beliefs, traditions, and customary rules. Studies in Cameroon and Burkina Faso in Africa report on bricolage manifestations involving formal and informal institutions (Kimengsi and Balgah 2021 ; Friman 2020 ), and the spatial variations in traditional institutions (Kimengsi et al. 2021 , 2022b ). In Europe/North America, the literature shows that informal structural institutions are conceptualized sparingly to include community leadership and inter-community forestry associations. Formally, they are reported as forest owners’ associations, political parties, protected area management, timber industry associations, resident associations, and state forest management. Informally, processes are conceived as local rules, while formally, they represent forest management policy, regulations, legal framework, local community forest governance, forest management strategies and forest marketing strategies. In Latin America, forest user groups, indigenous organizations and community management committees are frequently used in informal characterization, while labour unions, national services of protected areas, REDD + working group and Community general assemblies are used formally.
Global conceptualization of forest management institutions
Local land management rules constitute the key informal process in Latin America, while conservation laws, community-based forest policy, forest codes, forest laws, forest tenure agreements, and decentralized environmental policy appear in the formal conception of institutions. On the whole, a more diverse conceptualization of institutions (structures and processes) appear in the literature from Africa and Asia, followed by Latin America. The diversity is rooted in the diverse ethnic arrangements which characterize these regions. Africa, is the most ethnically diverse region in the world (Fearon 2003 ). This diversity accounts for the diversity in the nomenclature employed for forest management institutions—leading to the diversity in their conceptualization. With more empirical cases emanating from these settings, it is plausible to suggest that more in-depth and varied analysis about forest management institutions has been explored in these settings. Furthermore, the plethora of governance challenges in the management of natural resources (forest in this case) which is associated with such settings further explains the multifarious typification of institutions. In another dimension, structures and processes are surprisingly only conceptualized formally in the context of Australia—suggesting a significant drift away from informality to the pursuit of more formal, state-sanctioned institutions.
Compliance with forest management institutions
From the review, Africa, Asia, and Latin America report the highest cases of compliance in the informal institutional set-up. These settings have had a history linked to traditional institutions which were made to interact with colonially shaped institutions during their history. However, some degree of closeness to cultural institutions could be reported for these regions. The existence of compliance in the literature for Africa, Asia and Latin America is enough pointer to the multiplicity of institutional structures and processes which require monitoring against (non)compliance. Additionally, the interaction between formal and informal institutions, including the fallouts of colonial influence, led to the multiplicity of institutions. This possibly explains why compliance predominates the literature in the three regions. In Africa, for instance, pre-colonial types of resource use included the royal hunting preserves of the amaZulu and amaSwati people, and the kgotla system of land management practiced by the Batswana people (Ghai 1992 ; Fabricius 2004 ). Further, the making of access and use rules for natural resources in Mali (Moorehead 1989 ) and Botswana (Ostrom 1990 ), all indicate how endogenous cultural institutions shaped forest use. Likewise, Khasi, Garo and Jaintia tribes in Meghalaya of India, and traditional customary organization “Lembaga Adat” in Indonesia have not only conserved forest resources but also ensured its capacity to deliver ecosystem goods and services in sustainable manner (Mehring et al. 2011 ; Tiwari et al. 2013 ). Europe/North America registered few studies on informal institutional compliance, while this was non-existent for Australia—apparently due to the non-reported case of informal arrangements. Regarding non-compliance, articles from Latin America reported the highest case of non-compliance. This could be explained by the progressive decline in the informal institutions due to globalization and market forces which seemed to have permeated communities around the Amazon (Blundo-Canto et al. 2020 ). In the formal domain, non-compliance was significantly registered for Europe/North America, Africa, and Latin America (Fig. 4 ).

Global statistics of institutional compliance and non-compliance by regions. Left chart—papers ( N = 197, n for compliance = 133, n for non-compliance = 64, Right chart—Cases ( N = 491, n for compliance = 362, n for non-compliance = 129). Note Some papers reported compliance/compliance to formal and informal institutions simultaneously
It is important to note that some of the evoked reasons behind (non)compliance still require further investigation. For example, significant contextual variations in peoples’ attitudes and adherence to forest-sector institutions and governance in Asia, Africa, Latin America, and North America are directly linked to the disparities of key underlying and broader factors such as institutional models and policy frameworks for decentralization (Shackleton et al. 2002 ; Ribot 2003 ; Larson 2012 ; Mustalahti et al. 2020 ). Thus, a broader focus on institutional factors—rather than isolated reasons—is important to sufficiently explain (non-)compliance dynamics.
Forest management Institutional compliance determinants
From the analysis, political and economic factors were recurrent in the literature as key forces that influence institutional compliance. For instance, in Europe/North America, Latin America and Australia, political factors significantly influenced compliance (Table (Table3). 3 ). Some of these key determinants include conflicts, policy enforcement, power relations and governance structure (Latin America) and actor network, policy development, and governance structure (Europe/North America). Politics and geostrategy contributed to defining natural resource (forest in this case) institutions in Europe and North America. However, in Latin America, the rise in challenges linked to migration and bush fires also stand as key determinants of forest management institutional compliance. Economic factors in Europe/North America and Africa determined compliance. In Africa, economic factors linked to private enterprises and market-based mechanisms significantly featured in the literature as determinants of institutional compliance. For instance, aspects linked to donor income/investment and material aid, community forest expenditure and benefits, poverty were common. In Europe/North America, the economic viability of forest land use, forest certification, amongst others, were reported in the articles. In Nigeria, economic incentives (incomes) from farming activities, NTFPs use and non-traditional employment shaped compliance (Ezebilo 2011 ). On the whole, ecological, socio-cultural and demographic factors did not significantly explain compliance with forest management institutions. The case of ecological determinants in surprising given the litany of ecological campaigns which have been introduced in Africa (e.g., Leventon et al. 2014 ; Senganimalunje et al. 2016 ), Asia (Gilani et al. 2017 ) and Latin America (Entenmann and Schmitt 2013 ; Kowler et al. 2020 ) for instance, to foster conservation. Furthermore, socio-cultural diversity as viewed in Africa warrants some diversity in the way people adhere to institutions—both formal and informal. In Tanzania, trust in institutions was a significant predictor of participation intensity of the households in forest management (Luswaga and Nuppenau 2020 ). However, in Europe, differences in the attitudes of actors with regard to pursuing sustainable development significantly shaped compliance with forest management institutions (Jankovska et al. 2010 ).
Forest management institutional compliance determinants to by regions
Forest management institutional outcomes
The review indicates that political outcomes were the most significant for Europe/North America, followed by Latin America and Australia (Table (Table4). 4 ). Some key political outcomes included policy fragmentation, market formation failures, and reduced legitimacy of FSC certification (Europe/North America). This is understandable, considering that political and geostrategic forces were key determinants of institutional compliance. In Latin America,the setting up of provincial regulations which undermine enforcement of forest regime, rule breaking, challenges with the day-to-day operational institutions, inequitable benefit-sharing mechanism; the absence of law enforcement on sustainability of and access to non-wood forest products were common. Rule breaking is potentially triggered by increasing in-migration and the upsurge of bushfires. Bottazzi et al. ( 2014 ) showed how incentive-based systems of institutions facilitated the allocation and use of funds in REDD + programmes. In all these, deforestation persisted in the midst of lost and/or bypassed institutions (Carvalho et al. 2019 ). In Australia, divergent views characterized the seeking of solutions to enhance inter-departmental and inter-municipal coordination (Ordóñez et al. 2020 ). Positive ecological outcomes were significantly reported for Africa (forest or biodiversity protection/conservation, improved forest condition and surface water quality, sustainable forest or ecosystem management, planting of timber and fruit trees) and Latin America (fostering forest conservation, stabilization and/or decrease of deforestation, sustainable forest management). Furthermore, Europe/North America, Africa and Asia respectively reported positive economic outcomes linked to the generation of net monetary gains from parks, and from the wood harvesting and marketing (Europe/North America), higher incomes derived from certification, profits derived under community forestry, and the augmentation of household cash income (Africa). In Asia, studies report the positive outcomes linked to the forests’ substantial contribution to local livelihoods and income (Muhammed et al. 2008 ; Harada and Wiyono 2014 ; Barnes and van Laerhoven 2015 ).
Forest management institutional outcomes by regions
Some case studies have multiple institutional outcomes
Australia witnessed the most negative ecological outcomes. For instance, regional forest agreements were characterized by poor governance, leading to failures in biodiversity protection and ecosystem maintenance. This further precipitated the over-commitment of forest resources to wood production (Lindenmayer 2018 ). In Latin America, significant deforestation was observed for the Guarayos Indigenous Territory from 2000 to 2017—primarily driven by agricultural commodity production (He et al. 2019 ), while in Africa, Garekae et al. ( 2020 ) reported forest and wildlife decline in Botswana, linked to sectoral bias. Furthermore, the articles reported significant negative economic outcomes for Latin America, Asia, and Africa. Some of the reported outcomes include financial resource decline (Latin America), the timber-centric and market-oriented nature of community forests (Asia) (Bhusal et al. 2020 ), and the manifestations of elite capture in Africa. In Malawi, for instance, co-management programmes did not lead to positive outcomes, i.e., community organization, forest access, forest product availability and commercialization of forest products (Senganimalunje et al. 2016 ). On the whole socio-cultural outcomes were prevalent in Australia, Europe/North America, and Asia. Here, reported issues were linked to public acceptance of plantation policy, the improvement in communication of forest owners' associations and increased reliance on informal relationships. A case in point is linked to forest policy in Australia which led to several negative social impacts, including uncertainty, perceived injustice, and financial stress (Loxton et al. 2014 ). In the case of Asia, it was linked to inequalities among local actors, demographic changes and transformations in local social structures, gender inequality, successful collaboration between NGOs and community-based organizations , conflicts between communities and state forest enterprises (Adhikari and Lovett 2006 ; Barnes and van Laerhoven 2015 ).
Methodological approaches
The analysis reveals that globally mixed methods approaches have been least prioritized in the study of forest management institutions. For instance, between 2006 and 2021, we observed a growing trend in the application of qualitative methods; only 2 articles were reported in 2007, while this peaked to 99 in 2021. However, the use of quantitative and mixed methods approaches was significantly lower (Fig. 5 ). Considering the intricacies linked to the study of institutions, the prioritization of qualitative approaches is understandable. However, with growing interest in employing more robust data collection and analysis methods, it is only germane to report that studies have not prioritized mixed methods approaches so far (Malina et al. 2011 ; Karolina et al. 2021 ).

Cumulative distribution of paper and adoption of methods
A slight increase in the application of mixed methods approaches is observed (Table (Table5) 5 ) from 22% between 2006 and 2010 to 26% between 2016 and 2021, while there was a progressive decline in the sole application of quantitative methods from 37 to 23% within this time period. A slight decrease is also observed for qualitative methods, from 54 to 51% between 2011 and 2021.
Methods employed over time in the study of forest management institutions (% in parenthesis)
On the whole, while the use of only qualitative methods in the study of forest management institutions increased over time, the adoption of only quantitative approaches witnessed a decrease (Table (Table5). 5 ). Studies in Africa have largely prioritized the sole application of quantitative methods, as 42% of the papers reported this approach (Table (Table6), 6 ), while mixed methods (25%) were least prioritized. This could be linked to the growing ‘quantification revolution’ in research across the region. While quantitative analysis provides some pointers to institutional questions, they hide significant intricacies which could be revealed by solely qualitative or, better still, mixed methods analytical approaches. In Latin America, however, a significant proportion of the studies (40%) employed solely qualitative methods, followed by mixed methods (35%) and then quantitative methods (26%). Likewise, the highest proportion (58%) of studies draw from qualitative methods in Asia and Australia, whereas mixed-methods were prioritized as the second highest (37%) in Asia and the least (10%) in Australia (Table (Table6 6 ).
Methods used by different continents (percentage in parenthesis)
On the whole, while studies in Africa employed more of only quantitative methods over qualitative ones, research on forest management institutions in Europe and North America prioritized only qualitative methods over only quantitative ones. In North America/Europe, 73% of the studies employed the qualitative approach, followed by quantitative (19%). The least employed approach is mixed-methods, as only 8% used this approach (Table (Table6). 6 ). Overall, qualitative methods have been significantly employed globally except in Africa, while mixed methods were the least adopted in all regions except Asia and Latin America.
Perspectives on the conceptual and methodological advancement of research on FMIs
The literature so far presents a fragmented conceptualization of forest management institutions. For instance, institutions are broadly categorized as formal or informal on the one hand and as exogenous and endogenous on the other hand. This is based on the premise that not all endogenous institutions are informal institutions. A more detailed conceptualization which captures the formal and informal dimension, including the endogenous and exogenous categorization, is helpful to advance theoretical developments in the field of institutions in relation to forest management. Additionally, institutions seem to exhibit stream-like attributes; an approach which further conceptualizes them as ephemeral (very short-term arrangements made by forest actors to facilitate forest resource use conflict minimization), partially enduring ( arrangements that temporarily become a norm but fizzle out as new actors take over (Kimengsi et al. 2022b ); and enduring ( institutions are either codified (formal) and/or take the status of customs and values which transcend several generations (Ostrom 1990 ). In both cases, empirical studies geared towards establishing these proposed conceptual approaches are needed. Future research also needs to advance the “marriage” between actors and institutions.
From a methodological standpoint, further studies should prioritize methods based reviews (Palmatier et al. 2018 ) to enable researchers synthesize in detail, the design and instruments used so far, the approaches employed in data collection approaches and pros and cons linked to the methods employed. This will further inform the application of methodological approaches and instruments for future empirical studies on forest management institutions. Also, multi-country studies, employing mixed-methods approaches are needed to analyze institutions in forest use and management.
Review Limitations
This review provides an initial synthesis of the literature on forest management institutions from a global perspective. It is helpful in the identification of region-specific research needs in the ever-evolving field of institutions. A couple of limitations could be raised: Firstly, the conceptual analysis of institutions does not incorporate the exogenous versus endogenous dichotomy. With growing interest to further explore the typology and source of institutions, including whether management outcomes are a function of more endogenous or exogenous institutional arrangements (Kimengsi et al. 2022b ), future reviews and empirical studies should incorporate this dimension. Secondly, the regional clustering of institutions might shade details linked to how institutions are conceptualized and the outcomes they effectively produce. Although case studies are used, it is not possible in a single review to derive all these conceptual details, which might vary even within regions. Taking Africa, for instance, diversity in the region’s culture requires country-specific analysis of institutions. Latin America’s diversity precipitates ‘institutional shopping’ (Wartmann et al. 2016 Thirdly, institutions do not operate in isolation—therefore an actor-centred/power dimension is required to better appreciate institutional arrangements (Giessen et al. 2014 ; Ongolo et al. 2021 ). Therefore, a review of the actor and power dimensions of institutions is required to inform subsequent empirical studies. Fourthly, while the paper reports on compliance, the level of compliance is not reported, and the factors which militate for or against compliance. Fifthly, we selected articles which were exclusively published in English language and indexed in certain data bases. In doing so, we ignored papers which might have been published in French, Spanish, Amharic, Kiswahili, Nepali and other languages; such articles might have provided further compelling details on the region-specific dynamics of forest management institutions. We call for subsequent reviews to aim at valorizing such studies.
The current socio-ecological outcomes linked to the upsurge of pandemics (e.g. COVID-19) further justify the need to pay more attention to the management of forests and forest resources (Tollefson 2020 ; Saxena et al. 2021). These details, which vary over space and time, and may potentially assume a different dimension under the current COVID-19 scenario (Saxena et al. 2021), require extensive review and further empirical grounding. When pandemic prevention hinges on forest management to some extent, it is imperative to further explore the role of FMIs. Further reviews could emphasize the extent of compliance and the conditions under which (non)compliance prevails in the context of pandemics. Additionally, institutional change which is triggered by health crises (e.g., pandemics) still needs to be further established.
Finally, our review of the methods focused on providing a snapshot of the approaches, following the broad categorization of qualitative, quantitative and methods. This does not provide details on the specific qualitative methods employed (e.g., key informant interviews, participant observations, vignettes, focus group discussions). Future methods-based reviews should consider these.
To define conceptual and methodological pathways for future studies on forest management institutions (FMIs), this study undertakes a systematic review of the literature on FMIs using 197 papers (491 cases). From the study, the following conclusions are plausible: Firstly, while forest management institutions literature has witnessed a growth, this is most significant in Africa and Latin America. Secondly, the structure-process conceptualization of institutions (formal and informal) predominates in Asia and Africa. Process-wise, studies from Australia surprisingly did not report on a single process-linked institution. This merits further studies which pays attention to the identification of such institutions. The literature also reports on the drift away from informality to the pursuit of more formal, state-sanctioned institutional arrangements in Australia. Thirdly , global south regions—Africa, Asia, and Latin America—report the highest cases of compliance in the informal institutional set-up, while non-compliance was significantly registered for Europe/North America in the formal domain. Fourthly , politico-economic factors significantly influence institutional compliance in Europe/North America, while economic factors shape compliance in Africa. On the whole, ecological, socio-cultural, and demographic factors were reported to less significantly explain compliance with forest management institutions (FMIs). Fifthly , while forest management institutions in Europe/North America significantly contributed to determining politico-economic outcomes, those in Africa and Latin America contributed to positive ecological and negative economic outcomes. Finally, mixed methods approaches have been least prioritized in the study of forest management institutions; in Africa, the sole application of quantitative methods was prioritized. Future research needs to (1) extend the conceptualization of institutions, (2) increase multi-case and multi-country studies on FMIs especially for Asia and Australia, (3) empirically ground informality in the institutional set up of forest management in Australia, (4) establish in detail, the extent of (non)compliance, their spatio-temporal variations, and determinants, and (5) valorize the application of mixed-methods approaches in the study of FMIs across the globe.
Below is the link to the electronic supplementary material.
Open Access funding enabled and organized by Projekt DEAL. This research was funded by the Deutsche Forschungsgemeinschaft (DFG)—Projektnummer (437116427), Grant ID: F-010300-541-000-1170701.
Publisher's Note
Springer Nature remains neutral with regard to jurisdictional claims in published maps and institutional affiliations.
Contributor Information
Jude Ndzifon Kimengsi, Email: [email protected]_eduj , Email: [email protected] .
Raphael Owusu, Email: moc.oohay@usuwo60leahpar .
Shambhu Charmakar, Email: [email protected] .
Gordon Manu, Email: [email protected] .
Lukas Giessen, Email: [email protected] .
- Agrawal A, Gupta K. Decentralization and participation: the governance of common pool resources in Nepal’s Terai. World Dev. 2005; 33 (7):1101–1114. doi: 10.1016/j.worlddev.2005.04.009. [ CrossRef ] [ Google Scholar ]
- Aguiar APD, Vieira ICG, Assis TO, Dalla-Nora EL, Toledo PM, Santos-Junior RA, Batistella M, Coelho AS, Savaget EK, Aragaõ LEOC, et al. Land use change emission scenarios: anticipating a forest transition process in the Brazilian Amazon. Glob Change Biol. 2016; 22 :1821–1840. doi: 10.1111/gcb.13134. [ PubMed ] [ CrossRef ] [ Google Scholar ]
- Adhikari B, Lovett JC. Institutions and collective action: does heterogeneity matter in community-based resource management? J Dev Stud. 2006; 42 (3):426–445. doi: 10.1080/00220380600576201. [ CrossRef ] [ Google Scholar ]
- Artmann M, Sartison K. The role of urban agriculture as a nature-based solution: a review for developing a systemic assessment framework. Sustainability. 2018; 10 (6):1937. doi: 10.3390/su10061937. [ CrossRef ] [ Google Scholar ]
- Assa BSK. Foreign direct investment, bad governance and forest resources degradation: evidence in Sub-Saharan Africa. Economia Politica. 2018; 35 (1):107–125. doi: 10.1007/s40888-017-0086-y. [ CrossRef ] [ Google Scholar ]
- Bare M, Kauffman C, Miller DC. Assessing the impact of international conservation aid on deforestation in sub-Saharan Africa. Environ Res Lett. 2015; 10 (12):125010. doi: 10.1088/1748-9326/10/12/125010. [ CrossRef ] [ Google Scholar ]
- Barnes C, van Laerhoven F. Making it last? Analysing the role of NGO interventions in the development of institutions for durable collective action in Indian community forestry. Environ Sci Policy. 2015; 53 :192–205. doi: 10.1016/j.envsci.2014.06.008. [ CrossRef ] [ Google Scholar ]
- Berkes F, Colding J, Folke C, editors. Linking social and ecological systems. Management practices and social mechanisms for building resilience. Cambridge: Cambridge University Press; 1998. [ Google Scholar ]
- Bernardi F, Gonzalez JJ, Requena M. The sociology of social structure. In: Bryant B, Peck D, editors. 21st Century sociology: a reference handbook. Newbury: Sage; 2007. pp. 162–170. [ Google Scholar ]
- Beunen R, Patterson JJ. Analysing institutional change in environmental governance: exploring the concept of ‘institutional work’ J Environ Plan Manage. 2019; 62 (1):12–29. doi: 10.1080/09640568.2016.1257423. [ CrossRef ] [ Google Scholar ]
- Bhusal P, Karki P, Kimengsi JN. Timber distribution dynamics in scientifically managed community forests: learning from Nepal. Forests. 2020; 11 (10):1032. doi: 10.3390/f11101032. [ CrossRef ] [ Google Scholar ]
- Blundo-Canto G, Cruz-Garcia GS, Talsma EF, Francesconi W, Labarta R, Sanchez-Choy J, et al. Changes in food access by mestizo communities associated with deforestation and agrobiodiversity loss in Ucayali, Peruvian Amazon. Food Secur. 2020; 12 (3):637–658. doi: 10.1007/s12571-020-01022-1. [ CrossRef ] [ Google Scholar ]
- Boell SK, Cecez-Kecmanovic D. On being ‘systematic’ in literature reviews. In: Willcocks LP, Sauer C, Lacity MC, editors. Formulating Research Methods for Information Systems. London: Palgrave Macmillan; 2015. [ Google Scholar ]
- Bottazzi P, Crespo D, Soria H, Dao H, Serrudo M, Benavides JP, et al. Carbon sequestration in community forests: trade-offs, multiple outcomes and institutional diversity in the Bolivian Amazon. Dev Chang. 2014; 45 (1):105–131. doi: 10.1111/dech.12076. [ CrossRef ] [ Google Scholar ]
- Buchenrieder G, Balgah RA. Sustaining livelihoods around community forests: What is the potential contribution of wildlife domestication? J Modern Afr Stud. 2013; 51 (1):57–84. doi: 10.1017/S0022278X12000596. [ CrossRef ] [ Google Scholar ]
- Carvalho WD, Mustin K, Hilário RR, Vasconcelos IM, Eilers V, Fearnside PM. Deforestation control in the Brazilian Amazon: a conservation struggle being lost as agreements and regulations are subverted and bypassed. Perspect Ecol Conserv. 2019; 17 (3):122–130. [ Google Scholar ]
- Cleaver F. Development through bricolage: rethinking institutions for natural resource management. London: Routledge; 2017. [ Google Scholar ]
- Curtis PG, Slay CM, Harris NL, Tyukavina A, Hansen MC. Classifying drivers of global forest loss. Science. 2018; 361 :1108–1111. doi: 10.1126/science.aau3445. [ PubMed ] [ CrossRef ] [ Google Scholar ]
- Dixon AB, Wood AP. Local institutions for wetland management in Ethiopia: sustainability and state intervention. In: van Koppen B, Giordano M, Butterworth J, editors. Community-based water law and water resource management reform in developing countries. Wallingford: CABI International; 2007. pp. 130–145. [ Google Scholar ]
- Entenmann SK, Schmitt CB. Actors’ perceptions of forest biodiversity values and policy issues related to REDD+ implementation in Peru. Biodivers Conserv. 2013; 22 (5):1229–1254. doi: 10.1007/s10531-013-0477-5. [ CrossRef ] [ Google Scholar ]
- Ezebilo EE. Local participation in forest and biodiversity conservation in a Nigerian rain forest. Int J Sust Dev World. 2011; 18 (1):42–47. doi: 10.1080/13504509.2011.544389. [ CrossRef ] [ Google Scholar ]
- Fabricius C. Historical background to community-based natural resource management. In: Fabricius C, Koch E, editors. Rights, Resources and Rural Development Community-based Natural Resource Management in Southern Africa. Oxfordshire, New York: Earthscan; 2004. [ Google Scholar ]
- Faggin J, Behagel J. Institutional bricolage of sustainable forest management implementation in rural settlements in Caatinga biome. Brazil. International Journal of the Commons. 2018; 12 (2):275–299. doi: 10.18352/ijc.872. [ CrossRef ] [ Google Scholar ]
- Faye P, Haller T, Ribot R. Shaping rules and practice for more justice? Local conventions and local resistance in eastern Senegal. Hum Ecol. 2017; 8 (2017):1–11. [ Google Scholar ]
- Fearon JD. Ethnic structure and cultural diversity by country. J Econ Growth. 2003; 8 (June):195–222. doi: 10.1023/A:1024419522867. [ CrossRef ] [ Google Scholar ]
- Fleetwood S. Institutions and social structures. J Theory Soc Behav. 2008; 38 :30021–38308. doi: 10.1111/j.1468-5914.2008.00370.x. [ CrossRef ] [ Google Scholar ]
- Fleetwood S. Structure, institution, agency, habit and reflexive deliberation. J Inst Econ. 2008; 4 (2):183–203. [ Google Scholar ]
- Foundjem-Tita D, Duguma LA, Speelman S, Piabuo SM. Viability of community forests as social enterprises. Ecol Soc. 2018 doi: 10.5751/ES-10651-230450. [ CrossRef ] [ Google Scholar ]
- Friman J. Gendered woodcutting practices and institutional bricolage processes: the case of woodcutting permits in Burkina Faso. For Policy Econ. 2020; 111 :102045. doi: 10.1016/j.forpol.2019.102045. [ CrossRef ] [ Google Scholar ]
- Garcia CA, Savilaakso S, Verburg RW, Gutierrez V, Wilson SJ, Krug CB, Sassen M, Robinson BE, Moersberger H, Naimi B, Rhemtulla JM, Dessard H, Gond V, Vermeulen C, Trolliet F, Oszwald J, Quétier F, Pietsch SA, Bastin JF, Dray A, Araújo MB, Ghazoul J, Waeber PO. The global forest transition as a human affair. One Earth. 2020; 2 (5):417–428. doi: 10.1016/j.oneear.2020.05.002. [ CrossRef ] [ Google Scholar ]
- Gautam AP, Shivakoti GP, Webb EL. A review of forest policies, institutions, and changes in the resource condition in Nepal. Int for Rev. 2004; 6 (2):136–148. [ Google Scholar ]
- Gebara MF (2019) Understanding institutional bricolage: what drives behavior change towards sustainable land use in the Eastern Amazon? Int J Commons 13(1)
- Geist HJ, Lambin EF. Proximate causes and underlying driving forces of tropical deforestation. Bioscience. 2002; 52 :143–150. doi: 10.1641/0006-3568(2002)052[0143:PCAUDF]2.0.CO;2. [ CrossRef ] [ Google Scholar ]
- Ghai D (1992) Conservation, livelihood and democracy: social dynamics of environmental changes in Africa. Discussion Paper 33, United Nations Research Institute for Social Development. http://www.unrisd.org/unrisd/website/document.nsf/(httpPublications)/ . Accessed 10 Sept 2021
- Giessen L, Krott M, Möllmann T. Increasing representation of states by utilitarian as compared to environmental bureaucracies in international forest and forest-environmental policy negotiations. Forest Policy Econ. 2014; 38 :97–104. doi: 10.1016/j.forpol.2013.08.008. [ CrossRef ] [ Google Scholar ]
- Gilani HR, Yoshida T, Innes JL. A collaborative forest management user group's perceptions and expectations on REDD+ in Nepal. Forest Policy Econ. 2017; 80 :27–33. doi: 10.1016/j.forpol.2017.03.004. [ CrossRef ] [ Google Scholar ]
- Gomes PIA, Wai OWH, Dehini GK. Vegetation dynamics of ephemeral and perennial streams in mountainous headwater catchments. J Mater Sci. 2020; 17 :1684–1695. [ Google Scholar ]
- Haapal J, White P. Development through bricoleurs: portraying local personnel’s role in implementation of water resources development in rural Nepal. Water Alternat. 2018; 11 (3):979–998. [ Google Scholar ]
- Harada K, Wiyono (2014) Certification of a community-based forest enterprise for improving institutional management and household income: a case from Southeast Sulawesi, Indonesia. Small-Scale For 13(1):47–64
- Haller T, Acciaioli G, Rist S. Constitutionality: conditions for crafting local ownership of institution-building processes. Soc Nat Resour. 2016; 29 (1):68–87. doi: 10.1080/08941920.2015.1041661. [ CrossRef ] [ Google Scholar ]
- He Y, Baldiviezo JP, Agrawal A, Candaguira V, Perfecto I. Guardians of the forests: how should an indigenous community in eastern bolivia defend their land and forests under increasing political and economic pressures? Case Stud Environ. 2019; 3 :1–14. doi: 10.1525/cse.2019.sc.946307. [ CrossRef ] [ Google Scholar ]
- Hosonuma N, Herold M, De Sy V, De Fries RS, Brockhaus M, Verchot L, Angelsen A, Romijn E. An assessment of deforestation and forest degradation drivers in developing countries. Environ Res Lett. 2012; 7 :044009. doi: 10.1088/1748-9326/7/4/044009. [ CrossRef ] [ Google Scholar ]
- Houghton RA, Nassikas AA. Negative emissions from stopping deforestation and forest degradation, globally. Glob Change Biol. 2018; 24 :350–359. doi: 10.1111/gcb.13876. [ PubMed ] [ CrossRef ] [ Google Scholar ]
- Hsieh HF, Shannon SE. Three approaches to qualitative content analysis. Qual Health Res. 2005; 15 (9):1277–1288. doi: 10.1177/1049732305276687. [ PubMed ] [ CrossRef ] [ Google Scholar ]
- Jankovska ILZE, Straupe INGA, Panagopoulos THOMAS. Professionals awareness in promotion of conservation and management of urban forests as green infrastructure of Riga, Latvia. WSEAS Trans Environ Dev. 2010; 6 (8):614–623. [ Google Scholar ]
- Karolina V, Alif M, Sudharni S. The advantages and disadvantages of quantitative and qualitative approach for investigating washback in English language testing. EDUKATIF. 2021; 3 (5):2299–2310. [ Google Scholar ]
- Kimengsi JN, Abam CE. Forje GW (2021) Spatio-temporal analysis of the ‘last vestiges’ of endogenous cultural institutions: implications for Cameroon’s protected areas. GeoJournal. 2021 doi: 10.1007/s10708-021-10517-z. [ CrossRef ] [ Google Scholar ]
- Kimengsi JN, Balgah RA. Colonial hangover and institutional bricolage processes in forest use practices in Cameroon. Forest Policy Econ. 2021; 125 :102406. doi: 10.1016/j.forpol.2021.102406. [ CrossRef ] [ Google Scholar ]
- Kimengsi JN, Mairomi HW. COVID-19 and natural resource use practices in Cameroon. In: Akumbu PW, Nzweundji JG, editors. Responding to disease outbreak in Cameroon: lessons from COVID-19. Köln: Rüdiger Köppe; 2021. [ Google Scholar ]
- Kimengsi JN and Bhusal P (2022) Community forestry governance: lessons for Cameroon and Nepal. Soc Nat Resour 35(4):447–464
- Kimengsi JN, Grabek J, Giessen L, Balgah RA, Buchenrieder G (2022a) Forest management institutions and actor-centered conflicts in sub-Saharan Africa: contemporary realities and future avenues for research and policy. Forest Policy Econ 144:102846
- Kimengsi JN, Mukong AK, Giessen L, Pretzsch J. Institutional dynamics and forest use practices in the Santchou Landscape of Cameroon. Environ Sci Policy. 2022; 128 (2022):68–80. doi: 10.1016/j.envsci.2021.11.010. [ CrossRef ] [ Google Scholar ]
- Kimengsi JN, Owusu R, Djenontin INS, Pretzsch J, Giessen L, Buchenrieder G, Pouliot M, Acosta AN. What do we (not) know on forest management institutions in sub-Saharan Africa? A regional comparative review. Land Use Policy. 2022; 114 :105931. doi: 10.1016/j.landusepol.2021.105931. [ CrossRef ] [ Google Scholar ]
- Kissinger G, Herold M, De Sy V (2012) Drivers of deforestation and forest degradation: a synthesis report for REDD+ policymakers (Lexeme Consulting). https://www.cifor.org/knowledge/publication/5167/ . Accessed 18 Sept 2021
- Kowler L, Kumar Pratihast A, Ojeda P, del Arco A, Larson AM, Braun C, Herold M. Aiming for sustainability and scalability: community engagement in forest payment schemes. Forests. 2020; 11 (4):444. doi: 10.3390/f11040444. [ CrossRef ] [ Google Scholar ]
- Kraus S, Breier M, Dasí-Rodríguez S. The art of crafting a systematic literature review in entrepreneurship research. Int Entrep Manag J. 2020; 16 :1023–1042. doi: 10.1007/s11365-020-00635-4. [ CrossRef ] [ Google Scholar ]
- Larson AM (2012) Democratic decentralization in the forestry sector: lessons learned from Africa, Asia and Latin America. In: The politics of decentralization. Routledge, London, pp 46–76
- Leventon J, Kalaba FK, Dyer JC, Stringer LC, Dougill AJ. Delivering community benefits through REDD+: Lessons from joint forest management in Zambia. Forest Policy Econ. 2014; 44 :10–17. doi: 10.1016/j.forpol.2014.03.005. [ CrossRef ] [ Google Scholar ]
- Lindenmayer DB. Flawed forest policy: flawed regional forest agreements. Aust J Enviro Manag. 2018; 25 (3):258–266. doi: 10.1080/14486563.2018.1466372. [ CrossRef ] [ Google Scholar ]
- Loxton E, Schirmer J, Kanowski P. Social impacts of forest policy changes in Western Australia on members of the natural forest industry: implications for policy goals and decision-making processes. Forestry. 2014; 87 (3):363–376. doi: 10.1093/forestry/cpu011. [ CrossRef ] [ Google Scholar ]
- Luswaga H, Nuppenau EA. Participatory forest management in West Usambara Tanzania: what is the community perception on success? Sustainability. 2020; 12 (3):921. doi: 10.3390/su12030921. [ CrossRef ] [ Google Scholar ]
- Malhi Y, Gardner TA, Goldsmith GR, Silman MR, Zelazowski P. Tropical forests in the Anthropocene. Annu Rev Environ Resour. 2014; 39 :125–159. doi: 10.1146/annurev-environ-030713-155141. [ CrossRef ] [ Google Scholar ]
- Malina MA, Nørreklit HSO, Selto FH. Lessons learned: advantages and disadvantages of mixed method research. Qual Res Account Manag. 2011; 8 :59–71. doi: 10.1108/11766091111124702. [ CrossRef ] [ Google Scholar ]
- Mayring P (2000) Qualitative content analysis. Forum 1(2)
- Mehring M, et al. Local institutions: regulation and valuation of forest use-evidence from Central Sulawesi, Indonesia. Land Use Policy. 2011; 28 (4):736–747. doi: 10.1016/j.landusepol.2011.01.001. [ CrossRef ] [ Google Scholar ]
- Mengist W, Soromessa T, Legese G. Method for conducting systematic literature review and meta-analysis for environmental science research. MethodsX. 2019; 7 :100777. doi: 10.1016/j.mex.2019.100777(accessedon19.09.2021). [ PMC free article ] [ PubMed ] [ CrossRef ] [ Google Scholar ]
- Meyfroidt P, Lambin EF. Global forest transition: prospects for an end to deforestation. Annu Rev Environ Resour. 2011; 36 :343–371. doi: 10.1146/annurev-environ-090710-143732. [ CrossRef ] [ Google Scholar ]
- Moore SE, Cubbage F, Eicheldinger C. Impacts of forest stewardship council (FSC) and sustainable forestry initiative (SFI) forest certification in North America. J Forest. 2012; 110 (2):79–88. doi: 10.5849/jof.10-050. [ CrossRef ] [ Google Scholar ]
- Moorehead R. Changes taking place in common-property resource management in the Inland Niger Delta of Mali. In: Berkes F, editor. Common property resources. London: Belhaven; 1989. pp. 256–272. [ Google Scholar ]
- Muhammed N, Koike M, Haque F. Forest policy and sustainable forest management in Bangladesh: an analysis from national and international perspectives. New for. 2008; 36 (2):201–216. doi: 10.1007/s11056-008-9093-8. [ CrossRef ] [ Google Scholar ]
- Mustalahti I, Gutiérrez-Zamora V, Hyle M, Devkota BP, Tokola N. Responsibilization in natural resources governance: a romantic doxa? Forest Policy Econ. 2020; 111 :102033. doi: 10.1016/j.forpol.2019.102033. [ CrossRef ] [ Google Scholar ]
- Nerfa L, Rhemtulla JM, Zerriffi H. Forest dependence is more than forest income: Development of a new index of forest product collection and livelihood resources. World Dev. 2020; 125 (2020):104689. doi: 10.1016/j.worlddev.2019.104689. [ CrossRef ] [ Google Scholar ]
- Nightingale A. A guide to systematic literature reviews. Surg Infect (larchmt) 2009; 27 (9):381–384. [ Google Scholar ]
- Nightingale A. A guide to systematic literature reviews. Surgery. 2009; 27 (9):381–384. [ Google Scholar ]
- North D. Institutions, institutional change and economic performance. Cambridge: Cambridge University Press; 1990. [ Google Scholar ]
- Ntuli H, Mukong AK, Kimengsi JN. Institutions and environmental resource extraction within local communities in Mozambique. Forest Policy Econ. 2021; 139 :102724. doi: 10.1016/j.forpol.2022.102724. [ CrossRef ] [ Google Scholar ]
- Ordóñez C, Kendal D, Threlfall CG, Hochuli DF, Davern M, Fuller RA, et al. How urban forest managers evaluate management and governance challenges in their decision-making. Forests. 2020; 11 (9):963. doi: 10.3390/f11090963. [ CrossRef ] [ Google Scholar ]
- Oldekop JA, Rasmussen LV, Agrawal A, et al. Forest-linked livelihoods in a globalized world. Nat Plants. 2020; 6 :1400–1407. doi: 10.1038/s41477-020-00814-9. [ PubMed ] [ CrossRef ] [ Google Scholar ]
- Ongolo S, Giessen L, Karsenty A, Tchamba M, Krott M. Forestland policies and politics in Africa: recent evidence and new challenges. For Policy Econ. 2021; 127 (2021):102438. doi: 10.1016/j.forpol.2021.102438. [ CrossRef ] [ Google Scholar ]
- Osei-Tutu P, Pregernig M, Pokorny B. Legitimacy of informal institutions in contemporary local forest management: insights from Ghana. Biodivers Conserv. 2014; 23 (14):3587–3605. doi: 10.1007/s10531-014-0801-8. [ CrossRef ] [ Google Scholar ]
- Ostrom E. Governing the commons: the evolution of institutions for collective action. New York: Cambridge University Press; 1990. [ Google Scholar ]
- Ostrom E. A general framework for analyzing sustainability of social-ecological systems. Science. 2009; 2009 (325):419–422. doi: 10.1126/science.1172133. [ PubMed ] [ CrossRef ] [ Google Scholar ]
- Palmatier RW, Houston MB, Hulland J. Review articles: Purpose, process, and structure. J Acad Market Sci. 2018; 46 (1):1–5. doi: 10.1007/s11747-017-0563-4. [ CrossRef ] [ Google Scholar ]
- Pretzsch J, Darr D, Uibrig H, Auch E, editors. Forests and rural development. Berlin Heidelberg: Springer-Verlage; 2014. [ Google Scholar ]
- Rammel C, Stagl S, Wilfing H. Managing complex adaptive systems: a co-evolutionary perspective on natural resource management. Ecol Econ. 2007; 63 :9–21. doi: 10.1016/j.ecolecon.2006.12.014. [ CrossRef ] [ Google Scholar ]
- Ribot JC. Democratic decentralization of natural resources: institutional choice and discretionary power transfers in Sub-Saharan Africa. Public Admin Develop. 2003; 23 (1):53–65. doi: 10.1002/pad.259. [ CrossRef ] [ Google Scholar ]
- Rounsevell MDA, Reginster I, Araújo MB, Carter TR, Dendoncker N, Ewert F, House JI, Kankaapãã S, Leemans R, Metzger MJ, et al. A coherent set of future land-use change scenarios for Europe. Agric Ecosyst Environ. 2006; 114 :57–68. doi: 10.1016/j.agee.2005.11.027. [ CrossRef ] [ Google Scholar ]
- Rudel TK, Defries R, Asner GP, Laurance WF. Changing drivers of deforestation and new opportunities for conservation. Conserv Biol. 2009; 23 :1396–1405. doi: 10.1111/j.1523-1739.2009.01332.x. [ PubMed ] [ CrossRef ] [ Google Scholar ]
- Scott WR. Organizations, rational, natural and open systems. 4. New Jersey: Prentice Hall; 1995. [ Google Scholar ]
- Senganimalunje TC, Chirwa PW, Babalola FD, Graham MA. Does participatory forest management program lead to efficient forest resource use and improved rural livelihoods? Experiences from Mua-Livulezi Forest Reserve, Malawi. Agroforest Syst. 2016; 90 (4):691–710. doi: 10.1007/s10457-015-9826-6. [ CrossRef ] [ Google Scholar ]
- Sergent A, Arts B, Edwards P. Governance arrangements in the European forest sector: Shifts towards ‘new governance’or maintenance of state authority? Land Use Policy. 2018; 79 :968–976. doi: 10.1016/j.landusepol.2016.08.036. [ CrossRef ] [ Google Scholar ]
- Shackleton S, Campbell B, Wollenberg E, Edmunds D. Devolution and community-based natural resource management: creating space for local people to participate and benefit. Nat Resour Perspect. 2002; 76 (1):1–6. [ Google Scholar ]
- Soliev I, Theesfeld I, Abert E, Schramm W. Benefit sharing and conflict transformation: Insights for and from REDD+ forest governance in sub-Saharan Africa. For Policy Econ. 2021; 133 :102623. doi: 10.1016/j.forpol.2021.102623. [ CrossRef ] [ Google Scholar ]
- Song XP, Hansen MC, Stehman SV, Potapov PV, Tyukavina A, Vermote EF, Townshend JR. Global land change from 1982 to 2016. Nature. 2018; 560 :639–643. doi: 10.1038/s41586-018-0411-9. [ PMC free article ] [ PubMed ] [ CrossRef ] [ Google Scholar ]
- Steenbergen DJ, Warren C. Implementing strategies to overcome social-ecological traps. Ecol Soc. 2018 doi: 10.5751/ES-10256-230310. [ CrossRef ] [ Google Scholar ]
- Tiwari BK, et al. Institutional arrangement and typology of community forests of Meghalaya, Mizoram and Nagaland of North-East India. J for Res. 2013; 24 (1):179–186. doi: 10.1007/s11676-013-0337-x. [ CrossRef ] [ Google Scholar ]
- Tollefson J. Why deforestation and extinctions make pandemics more likely. Nature. 2020; 584 (13):175–176. doi: 10.1038/d41586-020-02341-1. [ PubMed ] [ CrossRef ] [ Google Scholar ]
- Turubanova S, Potapov PV, Tyukavina A, Hansen MC. Ongoing primary forest loss in Brazil, Democratic Republic of the Congo, and Indonesia. Environ Res Lett. 2018; 13 :074028. doi: 10.1088/1748-9326/aacd1c. [ CrossRef ] [ Google Scholar ]
- Tyukavina A, Hansen MC, Potapov PV, Stehman SV, Smith-Rodriguez K, Okpa C, Aguilar R. Types and rates of forest disturbance in Brazilian Legal Amazon, 2000–2013. Sci Adv. 2017; 3 :e1601047. doi: 10.1126/sciadv.1601047. [ PMC free article ] [ PubMed ] [ CrossRef ] [ Google Scholar ]
- Tyukavina A, Hansen MC, Potapov P, Parker D, Okpa C, Stehman SV, Kommareddy I, Turubanova S. Congo Basin forest loss dominated by increasing smallholder clearing. Sci Adv. 2018; 4 :t2993. doi: 10.1126/sciadv.aat2993. [ PMC free article ] [ PubMed ] [ CrossRef ] [ Google Scholar ]
- Verburg R, Rodrigues Filho S, Lindoso D, Debortoli N, Litre G, Bursztyn M. The impact of commodity price and conservation policy scenarios on deforestation and agricultural land use in a frontier area within the Amazon. Land Use Policy. 2014; 37 :14–16. doi: 10.1016/j.landusepol.2012.10.003. [ CrossRef ] [ Google Scholar ]
- Wartmann FM, Haller T, Backhaus N. “ Institutional shopping” for natural resource management in a protected area and indigenous territory in the Bolivian Amazon. Hum Organ. 2016; 75 (3):218–229. doi: 10.17730/1938-3525-75.3.218. [ CrossRef ] [ Google Scholar ]
- Weisse M, Goldman ED (2017). Global tree cover loss rose 51 percent in 2016, World Resources Institute blog, October 23, 2017. http://www.wri.org/blog/2017/10/global-tree-cover-loss-rose-51-percent-2016 . Accessed 15 Sept 2021.
- Yeboah-Assiamah E, Muller K, Domfeh KA. Institutional assessment in natural resource governance: a conceptual overview. Forest Policy Econ. 2017; 74 :1–12. doi: 10.1016/j.forpol.2016.10.006. [ CrossRef ] [ Google Scholar ]
- Open supplemental data
- Reference Manager
- Simple TEXT file
People also looked at
Systematic review article, a systematic literature review on the forest health biomonitoring technique: a decade of practice, progress, and challenge.
- Centre for Research in Development, Social and Environment (SEEDS), Faculty of Social Sciences and Humanities, Universiti Kebangsaan, Bangi, Malaysia
The approach for monitoring forest health such as canopy layer, air quality, soil texture has evolved in tandem with the advancement of new technology such as lab analysis, remote sensing etc. The application of biomonitoring techniques for example species diversity and morphological observation, on the other hand, has been positive and has made its own contribution to forest management. Many studies have been conducted in the last decade (2011–2021), which use the biomonitoring techniques in assessing the forest health status. Therefore, this study aims to systematically review the forest health biomonitoring techniques in the last decade. This study used the PRISMA guidelines as the protocol to search and analyze all the papers. This study selected 72 out of 538 papers for a thematic analysis which eventually identified four main biomonitoring techniques, namely: 1) diversity distribution, 2) morphological observations, 3) trace elements, minerals and physiological measurements, and 4) behavioral observations. The biomonitoring techniques applied to monitor forest health has evolved with numerous ways that can support existing technologies, as well as help educate people on the necessity of protecting and safeguarding the natural forest environment. This also will give more options to the authority in monitoring the forest health and not only focusing on technology.
1 Introduction
Forest health has been defined by the production of forest conditions which directly satisfy human needs and by resilience, recurrence, persistence, and biophysical processes which lead to sustainable ecological conditions ( Anderegg et al., 2020 ). The status of forest health has been declining globally, where global forest health has dropped nearly 60% from 1960 to 2019, mostly in the tropical region, due to urbanization and expansion of agricultural area ( Williams et al., 2021 ). This is the same reason that brings forth climate change, which also affects forest health. The decline of forest health status signifies that forest ecosystems cannot provide the services or functions they normally supply to humans ( Tebbett et al., 2021 ). The deterioration of forest ecosystem services implies a reduction in the forest’s ability to sustain local human populations through ecosystem services such as soil stability, water control, and local economic growth, as well as to offer recreational and cultural amenity value ( Delgado and Marín 2020 ). Hence, this will eventually affect people’s quality of life and wellbeing. According to the Millennium Economic Assessment (MEA) (2005) , human livelihoods are dependent on ecological services (particularly forest ecosystems), which help to support global employment and economic activity (food and timber production, marine fisheries and aquaculture, and recreation). Biodiversity loss, ecosystem degradation, and the resulting changes in ecosystem services have resulted in a fall in human wellbeing in some groups by intensifying poverty and increasing inequalities and inequities ( Tallis et al., 2018 ). Therefore, it is important and critical for humans to be able to monitor, measure and determine the status of forest health. On the other hand, the techniques used to monitor and measure the forest health status are also important things to be considered. The monitoring techniques used must be holistic, practical, and able to act as an early alarm of any significant changes happening to the forest health ( Abas 2021 ).
Forests are the most common terrestrial ecosystem on Earth and are found all across the world. Forests account for 75% of the biosphere’s gross primary output and comprise 80% of the planet’s plant biomass ( Duncanson et al., 2019 ). Net primary output in tropical forests is predicted to be 21.9 gigatonnes of biomass per year, 8.1 gigatonnes in temperate forests, and 2.6 gigatonnes in boreal forests ( Thakur et al., 2019 ). According to Newton et al. (2020) , over 2 billion people rely on forests. Forests provide people with shelter, livelihoods, water, food, and fuel security. All these activities, directly or indirectly, involve forests. Some are easy to figure out—fruit, paper, and wood from trees, and so on. Others are less obvious, such as by-products that go into everyday items like medicines, cosmetics, and detergents. Therefore, it is important to monitor the forest health status so that the forest can always provide the service it has usually given and human livelihoods and wellbeing will always be protected ( Diansyah et al., 2021 ). Forest health is usually defined as the production of forest conditions which directly satisfy human needs and have the resilience, recurrence, persistence, and biophysical processes which lead to sustainable ecological conditions. Numerous methods and parameters for monitoring biotic and abiotic forest components have been developed for example, the use of remote sensing ( Lausch et al., 2018 ), measuring SO 2 and NO 2 concentrations ( Davidson et al., 2020 ), satellite imaging ( Woodward et al., 2018 ), and vegetative index ( Rogers 2002 ). Biological indicators such as insects ( Allen et al., 2019 ), fungi ( Warwell et al., 2019 ), and plants ( Finger et al., 2021 ) have also been used widely to monitor and assess the health of forests.
Although there are many ways to monitor forest health, the use of biological indicators is important due to several advantages such as: 1) reflecting the overall environmental integrity comprising physical, chemical, and biological monitoring; 2) imparting an integrated and holistic measure of ecological conditions by uniting stresses over a period of time; and 3) providing a better understanding of a healthy environment for the public than other methods ( Medhi et al., 2020 ). Despite these advantages, efforts to review biomonitoring techniques systematically and constructively are still lacking, especially regarding forest health ecosystems ( Swislowski et al., 2020 ). Rather than inferring exposure from chemical concentrations in air, water, or soil, biomonitoring analyses personal exposure to dangerous chemicals by detecting the substances or their metabolites in human specimens such as blood or urine ( Bocca et al., 2019 ).
Bioaccumulation, biochemical modifications, morphological and behavioural observation, population- and community-level methods, and modelling are the most prevalent biomonitoring techniques ( Abas 2021 ). The significance of collecting, searching, and reviewing the biomonitoring techniques used to monitor forest health will help to create a better understanding and facilitate the identification of the research gaps that exist in this particular area ( Muhammad et al., 2018 ; Zulaini et al., 2019 ). Therefore, this study aims to systematically review the forest health biomonitoring techniques. In order to achieve this aim, three research objectives have been outlined which are: 1) to explore the spatial and temporal patterns of the studies; 2) to analyze the contextual issues discussed in the forest health biomonitoring techniques; and 3) to discover the biomonitoring techniques used to monitor forest health in the last decade between 2011–2021.
2 Research methodology
2.1 prisma statement (preferred reporting items for systematic reviews and meta-analyses).
The PRISMA statement ( Moher et al., 2009 ) served as the primary guideline for this systematic review investigation. PRISMA is a minimal set of monitoring components based on evidence for systematic analyses and meta-analysis. The example of relevant studies that used PRISMA statement are; Abas et al. (2022) that analyzed the local wisdom of the indigenous people in nature conservation, Owen (2020) that explored the climate change adaptation globally, and Malek et al. (2021) that determined the social indicator for building a smart city. The PRISMA statement was chosen for this study because of its strict procedure emphasizing the reporting of randomized trial assessment reviews, which may also be used as a basis for publishing comprehensive reviews of other types of studies, including intervention evaluations ( PRISMA 2021 ). PRISMA statement has been used to report the results of a systematic review evaluating the effects of an intervention, whether the review is limited to randomized controlled trials or includes other types of research.
2.2 Formulation of the research question
The study questions were developed using PICo. PICo is a key component of a question that developed to address knowledge gaps in a study. Caón and Buitrago-Gómez (2018) describe PICo as a tool that supports writers in developing appropriate research questions for review. PICo was often used in the field of health sciences and medical to develop an evidence-based clinical practice. PICo is built around three key concepts: population or problem, interest, and context. This study has incorporated three major features in the review based on these concepts: forest health monitoring (issue), forest health (interest), and biomonitoring approaches (context). Hence, this study asks the following questions: 1) What is the distribution pattern of biomonitoring techniques for forest health spatially and temporally? 2) What are the contextual issues discussed in this study? and 3) What is the theme that can be developed for the biomonitoring techniques used to assess and monitor forest health?
2.3 Systematic searching strategies
As shown in Figure 1 , the systematic searching strategies included three main strategies: identification, screening, and eligibility.
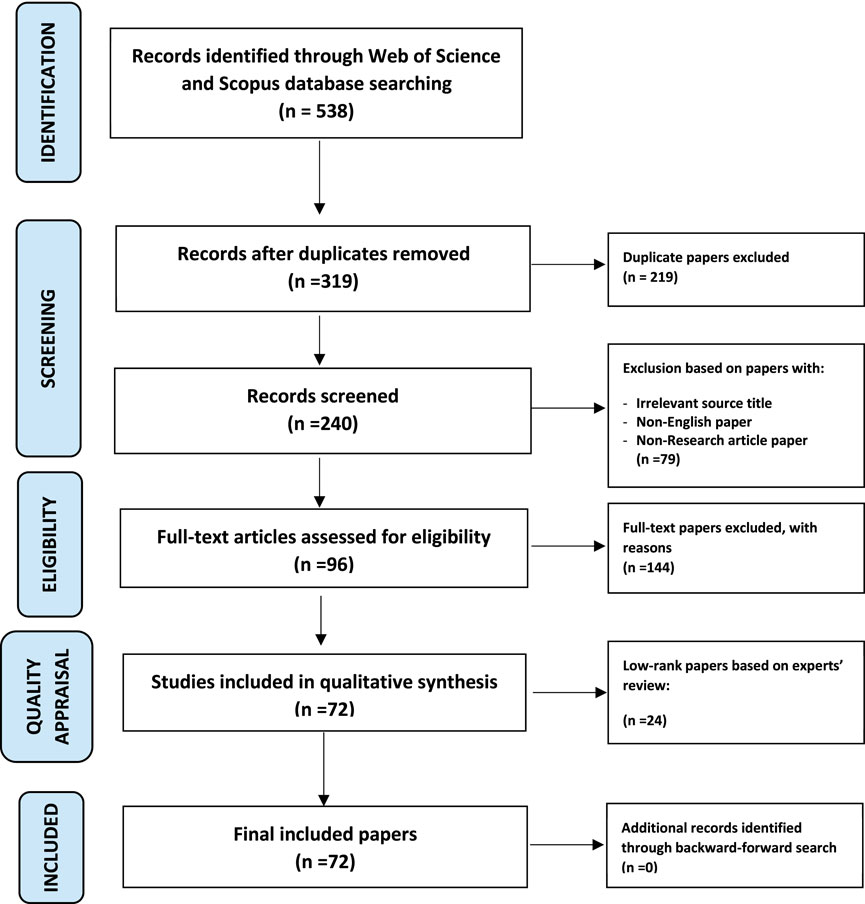
FIGURE 1 . Flow diagram of the process.
2.3.1 Identification
Identification is the process of searching for the appropriate keywords depending on the research questions. Three keywords, as well as their synonyms, related phrases, and variants, were employed in this investigation. The buzzwords were <forest health> and <biomonitoring>. The keywords were produced based on Okoli’s (2015) research question, and the identification method relied on online thesauruses, keywords from previous studies, keywords recommended by Web of Science (WoS), and keywords suggested by experts. As demonstrated in Table 1 , this study was able to enhance a series of keyword strings by searching for relevant publications in the WoS database and Scopus. WoS and Scopus are websites that offer subscription-based access to a variety of databases as well as detailed citation data for a wide range of academic subjects. The present researchers picked WoS and Scopus over other search engines since all journals in these two have gone through a rigorous editing procedure, assuring article quality before publication and preserving the publications’ impact factor rankings (Scopus 2021; WoS 2021). The search technique employing both databases yielded 538 articles that were relevant to the study’s research goals.
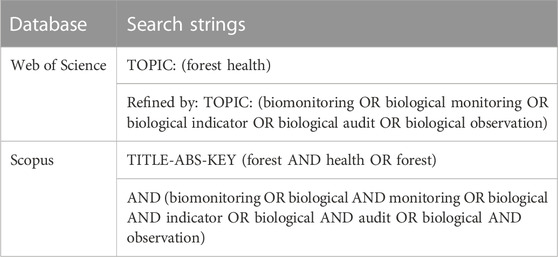
TABLE 1 . The search strings.
2.3.2 Screening
To eliminate duplicate records, all 538 articles were initially checked. Due to duplication, a total of 219 papers were removed from the database, leaving 319 articles. The remaining 319 papers were rescreened to confirm the review’s quality; only publications having empirical data and published in a journal were considered. Furthermore, only papers published in English were included in the review to minimize misunderstanding. This procedure eliminated 79 articles because they did not meet the inclusion requirements. The remaining 240 articles were employed in the third procedure, which was to determine eligibility.
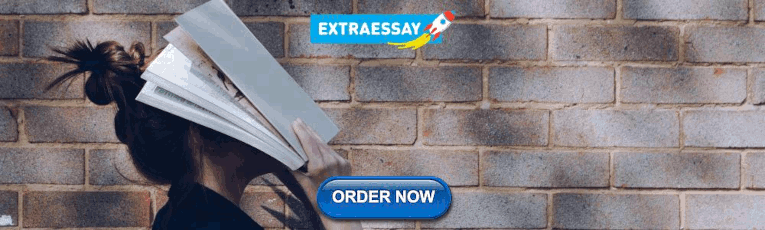
2.3.3 Eligibility
The third procedure is eligibility, in which the authors personally monitored the retrieved articles to ensure that all of the remaining articles (after the screening process) met the requirements. This was accomplished by reading the titles and abstracts of the papers. A total of 144 articles were excluded because they focused on ecological processes, social monitoring, forest ecological studies, forest conservation outreach, social-ecological systems, forest ecosystem monitoring, ecosystem services valuation and assessment, assessment of sustainable forest management, non-forest biomonitoring, focus on review, did not use empirical data, or were published as a chapter in a book. Hence, only 96 articles were through for the next stage.
2.4 Quality appraisal
The remaining articles were provided to two specialists for quality assessment in order to ensure the quality of the material. These two specialists are academics who have undertaken several studies on forest health. According to Petticrew and Roberts (2006) , the remaining papers were divided into three quality categories: good, moderate, and low. Only papers classified as high and moderate were examined. The specialists concentrated on the methodology of the articles in order to calculate the quality rating. Both experts had to concur that the paper’s quality had to be at least moderate in order for it to be included in the research. Before deciding whether or not to include or exclude articles from the review, they discussed any differences. Following this process, 50 articles were rated as high, 22 as moderate, and 24 as low. As a result, only 72 articles were eligible for review (refer to Annex 1).
2.5 Data abstraction and analysis
Thematic analysis was utilized in this study to create themes and sub-themes. According to Braun and Clarke (2006) , thematic analysis is used to find the themes and sub-themes that exist within the abstracted data by noticing patterns and motifs, grouping, counting, and highlighting parallels and correlations. Any abstracted data that was comparable or related was grouped together. Following a thorough analysis, four topics emerged in this study: 1) diversity distribution, 2) morphological observation, 3) trace elements, minerals, and physiological measurements, and 4) behavioral observations.
3.1 Spatial and temporal distribution of selected articles
A total of 72 articles were analyzed through a detailed screening procedure. Based on Figure 2A , the distribution of studies on the forest health biomonitoring techniques in the last decade (2011–2021) has been fluctuated but slowly increasing temporally. Most papers were published in 2016 and 2018 with nine papers respectively. Based on Figure 2B , all of the 72 papers were distributed among 29 countries, of which the United States of America (United States) had the most with 23 papers, followed by Brazil, China and Spain each with four papers. Figure 2C shows the distribution of the selected papers based on their continent of origin. The most papers come from North America with 26 papers and followed by Europe with 20 papers.
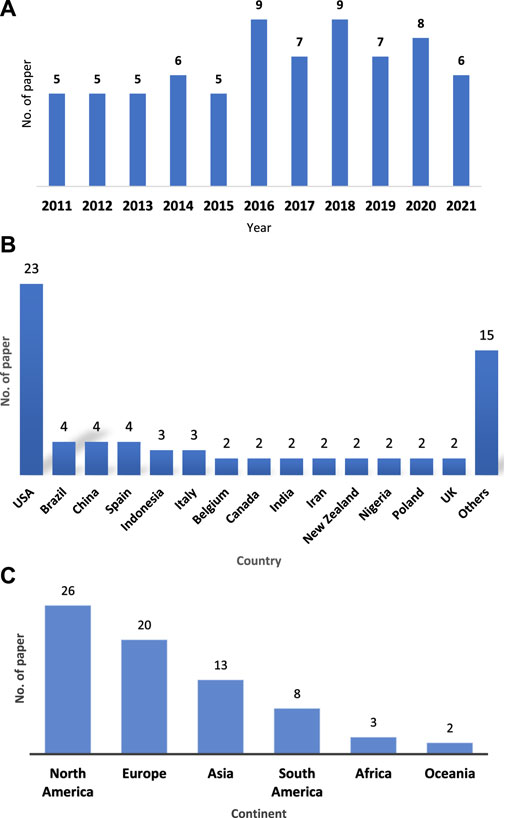
FIGURE 2 . (A) Temporal distribution of the selected papers. (B) Country distribution of the selected papers. (C) Continent distribution of the selected articles.
3.2 The forest health biomonitoring technique—Contextual issues
This study discovered four main contextual issues which were: 1) method used from the selected articles, 2) type of forest, 3) sub-type of forest, and 4) type of biological indicators. Based on Figure 3A , most of the selected articles used experimental design-type methods (67%) to study the biomonitoring techniques used for forest health assessment. In terms of type of forest, based on Figure 3B , 74% of the selected articles were studies on temperate forests, 18% on tropical forests. For the sub-type of forest ( Figure 3C ), most of the selected articles (23%) focused on coniferous forests, followed by mixed forests with 18%, lowland evergreen rainforests and montane forests both with 11%. Based on Figure 3D , 16 out of the 72 articles used vascular plants as biological indicators to monitor forest health, followed by insects with 13 articles, mammals with nine articles.
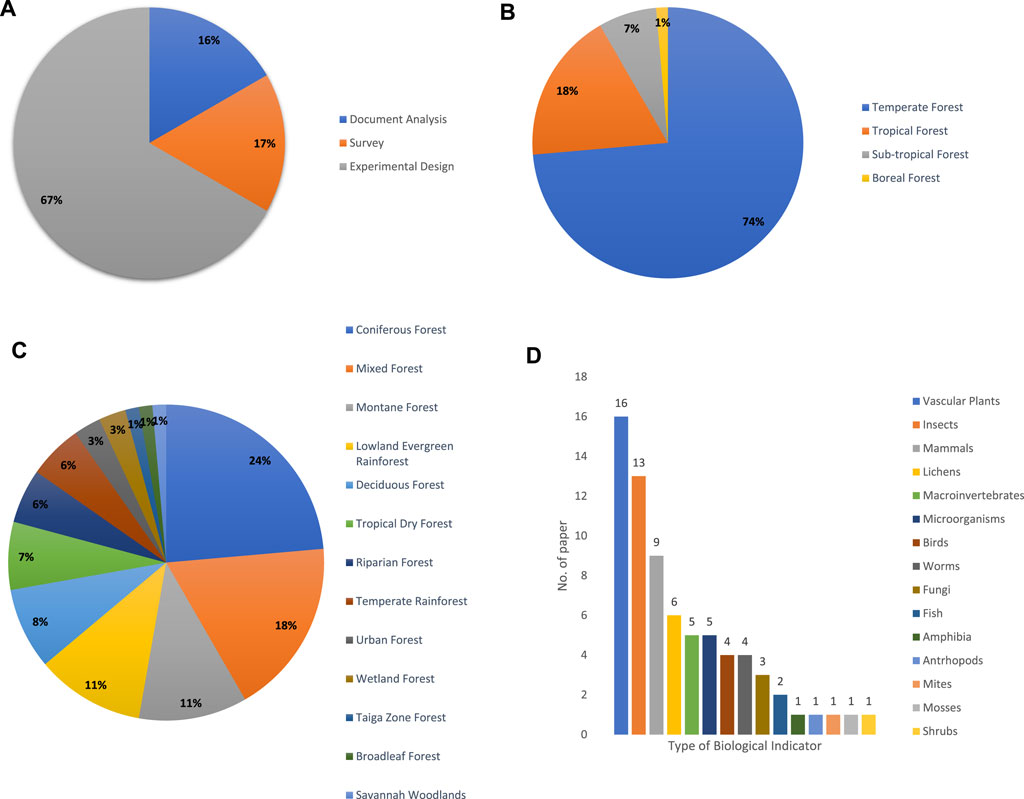
FIGURE 3 . (A) Methods used from the selected articles. (B) Type of forest. (C) Sub-type of forest. (D) Type of biological indicator.
3.3 The forest health biomonitoring technique—Thematic analysis
This study discovered four main themes for the biomonitoring techniques used to assess forest health which were: 1) diversity distribution, 2) morphological observation, 3) trace elements and physiological measurement and 4) behavioral observation. Based on Figure 4 , 56.94% of the selected articles studied the diversity distribution techniques, while 19.44% focused on morphological observation.
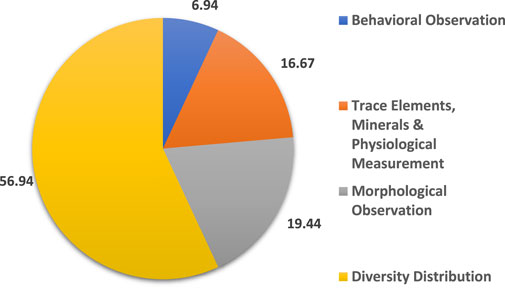
FIGURE 4 . Type of biomonitoring techniques.
4 Discussions
4.1 biomonitoring technique of the forest health—substantive issue, 4.1.1 diversity distribution.
The diversity distribution monitoring techniques is used to assess and measure forest health by calculating the frequency of individuals of certain species, identifying species diversity, analyzing by using the existing indices, etc. Vascular plants have been used in several countries, such as Indonesia ( Wulffraat and Morrison 2013 ; Fujiki et al., 2016 ), the United States ( Shearman et al., 2015 ; Guyon and Battaglia 2018 ) and Brazil ( Poorter et al., 2015 ) to monitor forest health through the forest communities stand index, canopy cover analysis through satellite imagery and forest inventory and analysis (FIA). Besides that, the diversity of insects has also been used to monitor forest health. For example, the diversity of dung beetles ( Sullivan et al., 2018 ; Somay et al., 2021 ) and bark beetles ( Rassati et al., 2016 ; Morris et al., 2018 ) have been analyzed and monitored to determine the soil moisture in the forest. Other than that, orchid bees ( Suni, 2017 ; Allen et al., 2019 ), moths ( Highland et al., 2013 ) and dragonflies ( Dolný et al., 2013 ) were used to observe the forest fertility and humidity. The individual frequency of small mammal species such as rodents ( Ahumada et al., 2011 ) and squirrels ( De La Sancha 2014 ) have been used to monitor the forest humidity and temperature. A specific index (the Living Planet Index) using the diversity of mammals such as deer ( Fisichelli and Miller, 2018 ) and forest specialists ( Green et al., 2020 ) has been developed to monitor forest health. On the other hand, a study by López-Baucells et al. (2017) used fruit bats as an indicator of good forest condition due to their need for fruit to survive. The diversity distribution of lichens and fungi were also used to monitor the forest health status. A study by Coyle and Hurlbert (2016) used macrolichen richness to measure the environmental heterogeneity and a study by Song et al. (2012) used the epiphytic lichen species distribution to determine the impact of climate change on forest health. In terms of the use of fungi, the existence of fungi usually brings negative signals to the forest health status. Numerous studies, such as Xu et al. (2012) , Warwell et al. (2019) and Zgrablić et al. (2016) , have shown that the existence of fungi such as Armillaria spp., Sphaeropsis sapinea, etc. indicate that the forest health status is in a very poor condition. Macroinvertebrates are one of the most notable biological indicators for freshwater quality, especially for river ecosystems. However, there are a few studies such as Deborde et al. (2016) , Kim et al. (2016) , Brogna et al. (2018) , Foomani et al. (2020) , and Edegbene et al. (2021) which have shown a significant relationship between forest health and river water quality. There are also studies that use other biological indicator distributions such as birds ( Laiolo et al., 2011 ; Wade et al., 2013 ; Gnass Giese et al., 2015 ), nematodes ( D’Errico et al., 2014 ; Nisa et al., 2021 ), amphibians ( Jongsma et al., 2014 ), microorganisms ( Hermans et al., 2020 ), fish ( Gottesman et al., 2020 ) and mites ( Meehan et al., 2019 ).
Diversity distribution has been the most notable method used to monitor forest health through biological indicators because of the dynamics of living things that always strive in order to survive. Living things always seek to evolve and adapt to their surroundings which will lead to variations of species. This process has been happening since the beginning of living things on Earth. Vascular plants such as gymnosperms, angiosperms, ferns, etc. have the highest numbers in forests, which means vascular plants are the most efficient and practical biological indicator to use for diversity distribution ( Moradi et al., 2019 ). Other than vascular plants, fungi and lichens are also favorites to be used as biological indicators to monitor forest health. Lichens are very dynamic in terms of species diversity distribution; they have a vast range in terms of sensitive species and tolerant species. Based on which species dominate the forest, indications of forest health can be determined ( Abas et al., 2019 ). Forests are also known as the home of megafauna such as mammals, insects, birds, amphibians, etc. The range of diversity of animals in the forest is often used to determine its condition. Mammals, insects, birds, and amphibians always respond to the forest conditions such as forest humidity, soil moisture, and light intensity ( Mohd-Taib et al., 2020 ).
However, organisms such as non-vascular plants, fish, and amphibians are still lacking in terms of studies for biomonitoring of forest health. According to Norhazrina et al. (2016) , non-vascular plant diversity distribution, such as mosses, liverworts, and hornworts, have a significant relationship with the forest humidity and the light intensity under the forest canopy. Therefore, they can act as good biological indicators for forest health. Other than that, fish and amphibian diversity distribution are highly dependent on the river water quality, which significantly correlates with the soil fertility of the forest ( Faruk et al., 2013 ). Fish and amphibians usually respond to the dissolved oxygen (DO) and pH values of the river water, where certain species such as toads and salmon can only be found in the upstream river due to its richness in terms of DO and neutral pH value ( Faudzi et al., 2021 ).
4.1.2 Morphological observation
The monitoring techniques using morphological observation of the biological component in the forest ecosystem includes observing physical changes such as color, size, and texture. Numerous studies have observed physical changes in vascular plants, such as the decolorization of leaves due to the lack of water and low humidity ( Kleinman et al., 2012 ; Kamoske et al., 2019 ; Finger et al., 2021 ) or disease and infection ( Smith 2012 ; Feldpausch et al., 2016 ; Donald et al., 2020 ). Besides that, a study by Sánchez-Salguero et al. (2017) found a significant correlation between the radial growth of trees and the soil moisture in the forest ecosystem. Mulvey and Bisbing (2016) also found a significant relationship between leaf size and CO2 concentration in the forest, where excessive CO2 would increase the size of tree leaves. On the other hand, there are several studies that used the physical changes in insects such as ants ( Krapfl et al., 2011 ; Skaldina and Sorvari 2017 ), elongate hemlock scale ( Gómez et al., 2015 ), and termites ( Kashian et al., 2018 ) to determine the soil moisture, soil pH and tree health. A study by Bal et al. (2018) observed nematode body moisture to determine the soil fertility in forests. Peterson et al. (2014) observed oomycete body color to assess the water quality of the river in the forest ecosystem.
The change of morphology of organisms such as color, texture, or size of a certain structure usually happens when there are sudden changes in the surroundings. This is similar to when the human skin turns red after sunbathing. According to Osman et al. (2021) , due to excessive CO2, vascular plants such as angiosperms usually adapt their structure by increasing the leaf size and wingless seeds. Besides that, due to decreasing forest humidity, some vascular plants will change the color of their leaves or bark as a sign of lack of water ( Nizam et al., 2009 ). Animals also have the ability to change morphological characteristics such as their skin color, body parts size, etc. However, according to Li et al. (2021) , changes in morphological characteristics of animals take a long time to happen and, in terms of monitoring forest health, this is only practical for long term monitoring.
4.1.3 Trace elements, minerals, and physiological measurement
Biological components can be monitored by analyzing trace elements, minerals, heavy metals, PAH, etc. , and also by assessing physiological changes such as the integrity of the cell membrane, the efficiency of chlorophyll, etc. Through these techniques, lichens have been widely used as the biological indicator to determine forest health. Lichens from forests were collected and analyzed to measure the concentrations of heavy metals such as Cr, Ni, Cu, Zn, Cd, and Pb ( Kłos et al., 2011 ), concentrations of S and N ( Cleavitt et al., 2015 ), the efficiency of the chlorophyll ( Loppi and Baragatti 2011 ) and the integrity of the cell membrane in the lichen ( Will-Wolf et al., 2018 ). Also, monitoring has been carried out on heavy metals such as K, Fe, Mg, Ca, Zn, Pb, Cd, and Cu from the internal organs of mammals, such as the liver of wild boar ( Kasprzyk et al., 2020 ), the colon of rats ( Naderi et al., 2017 ) and the blood of livestock ( Amadi et al., 2020 ). Physiological changes of microorganisms have been observed to understand and assess forest health using the integrity of their cell membrane ( Dhyani et al., 2019 ) and their genetic sequences ( Shi et al., 2019 ). Besides that, other studies analyzed the content of heavy metals in mosses ( Berisha et al., 2017 ), used fish blood to evaluate the genotoxicity ( Bühler et al., 2014 ), and vascular plants to determine the iodine concentration ( Roulier et al., 2018 ).
According to Abas et al. (2020) , trace elements can be found in some biological indicators, such as lichens. Lichens have unique features, such as the absence of a waxy cuticle on the thallus, allowing elements to enter the thallus. This makes lichens very good biological indicators that can accumulate air borne particles from their surroundings. Trace elements can also be found in animal’s internal organs such as the liver, brain, lungs, intestines, etc. ( Rahman et al., 2020 ). Besides that, according to Becklin et al. (2016) , a plant’s physiological features such as chlorophyll and cell membrane usually have reduced capacity under the stress of pollutants which can be one way to assess forest health.
4.1.4 Behavioral observation
The change in the behavior of certain organisms or biological components, such as reproductive rate, mortality rate, fatality rate, etc. , can determine the current status of forest health. The fecundity rate of vascular plants has been observed to aid understanding of how forest health status has changed ( de la Cruz et al., 2014 ; McMahon et al., 2019 ). On the other hand, the fatality rate of birds has been monitored to assess the temperature and humidity of a forest ( Bianchi et al., 2016 ). The growth pattern of shrubs has been mapped and analyzed to determine the soil fertility in forests, which also correlates with forest health ( Myron et al., 2021 ). Besides that, the zigzag sawfly eating pattern has been studied to analyze forest fertility and health ( Zúbrik et al., 2017 ).
The relationship between a change in behavior of an organism and the status of forest health has been found to be significant. According to Xu et al. (2021) , living things such as large mammals, reptiles and birds usually react and respond to sudden changes in their surroundings. A rise in temperature in an area, especially a forest, typically will significantly change the mortality and fatality rate of the vascular plants due to their dependency on soil moisture and air humidity to grow and survive ( Osman et al., 2021 ). Other than that, animals such as insects are usually less active in reproduction in rain and wet seasons but become more active when it is bright and in the warm season ( Alston et al., 2020 ). However, when it comes to large animals, it is quite difficult to monitor their behavior because there are no significant changes over short periods, and longer periods of monitoring are needed to observe any significant changes in their behavior ( Rahman et al., 2020 ).
4.2 Biomonitoring technique of the forest health—Current progress and practices
The present trend in biomonitoring technology has demonstrated a major influence as an alternate tool for measuring forest health status. The species richness of flora and wildlife in the forest itself is the most commonly used biomonitoring approach. According to Nisa et al. (2021) , the composition of the forest’s flora and wildlife may simply monitor its health state. For example, a forest with a large diversity of plants would have more fertile soil and will attract a greater range of animals ( Naderi et al., 2017 ). Aside from that, a large diversity of species in a forest indicates that the ecosystem service and function are optimum. According to Abas (2021) , biodiversity is commonly acknowledged as the primary driving factor in ecosystem function. Numerous of studies have been conducted to investigate the impact of tree species variety on several forest ecosystem services, including primary production ( Moradi et al., 2019 ). Furthermore, according to Finger et al. (2021) , a large diversity of fungi and lichens in the forest indicates that the forest is in a very active and healthy state. According to Hermans et al. (2020) , animal and fungal diversity play an important role in forest health, where the diversity of animal and fungal species affects many important processes, such as the availability of nutrients for tree growth, promoting timber growth, preventing soil erosion, recycling nutrients, and controlling pest populations.
4.3 The challenge for the biomonitoring technique of the forest health
The biomonitoring technology is still relatively new in comparison to other existing monitoring techniques for detecting forest health ( Faruk et al., 2013 ). The biomonitoring approach has a lot of promise, and it is not just limited to the utilisation of species diversity in the forest ecosystem. Several studies use changes in behaviour and appearance of organisms to measure forest health status ( Shearman et al., 2015 ). In addition, the trace element concentration inside the organism and its physiological changes may be tracked to evaluate how the forest health has altered ( Berisha et al., 2017 ). However, these techniques are currently insufficient for use in biomonitoring research, with the majority of studies focusing on species variety in the forest to establish the health status ( Kashian et al., 2018 ). This might be due to a shortage of botanists or zoologists who utilise the research topic to establish the forest health condition, as well as the observation process taking a very long time to conduct and finish, so the study will exhaust their money budget, time, and people resources ( Brogna et al., 2018 ).
4.4 Strength of the studied articles
This study has found three main strengths from the studied articles which firstly, the studied articles were coming from all over the continents. This show that the results that this study has shown are inclusive and cover all type of ecosystem around the globe. Secondly, the studied articles have covered and explored every type of forest that exist on the Earth biosphere. This means that every possible type of forest ecosystem has been represented in this study. This is very important to ensure that the themes that have been developed can be applied onto every type of forest that exist on Earth. Lastly, the type biological indicator that has been used to monitor the forest health is well-diverse and has explored every possible biological indicator that can used to determine the environmental quality. This means that the themes that have been developed are relevant, precise and inclusive. Determining the type of biological indicator that used to monitor the forest health is the main aim of this study, therefore it is important for it to be inclusive and well-represented.
4.5 Limitation of the studied articles
The study on the biomonitoring technique for forest health has shown several strengths and advantages. However, it also has several limitations where it can be improved in the future study. Firstly, the studied articles were mostly coming from the United States. The domination of article from United States proved that the study from other countries especially countries from the Asia region, South America and Oceania are still lacking and scientist from those countries are encouraged to explore the biomonitoring technique for the forest health in their particular locality. Secondly, all of the studied articles were written and published in English language and also been searched and screened from the Scopus and Web of Science database. Some of the articles that study on the biomonitoring technique of their local forest health may written in their native language and published on the local publisher database only. This is due to the language boundary and also the requirement from PRISMA statement which the article that selected for the study must published in the open access database with rigorous reviewing process.
4.6 Future research direction in forest health biomonitoring technique
As a result, for the limitation of the studied articles, also acknowledging the strength of it, this study found several future research directions in forest health biomonitoring technique that can explored by scientist and researcher in this field. Firstly, researcher and scientist from Asia, North America and Oceania should explore and do more study on this particular field where there are still lacking in term of number of the study. Especially for the country that have the tropical rainforest where the biological component is provenly much diverse and rich. Secondly, the method uses in assessing forest health through biomonitoring technique can be diversified where research method such as observation can be used also though it will consume much more energy and time. Lastly, the effort on translating the biomonitoring technique into the standard forest health monitoring guidelines should be taken seriously by the authorities and researcher.
5 Conclusion
This study confirms that there are several limitations and challenges to maximizing the use of biomonitoring to determine the forest health status. Firstly, information on the biomonitoring techniques used for the boreal forest type is lacking. Secondly, the biomonitoring techniques are dominated by plants, fungi, or small animals such as insects. However, studies of biomonitoring using large animals such as reptiles or mammals are still lacking due to the long period it takes to achieve significant results. Lastly, the decline of biological indicators in the forest due to anthropogenic activities and climate change has limited the choice of biological indicator for biomonitoring techniques to assess forest health status. We recommend that scientists and researchers explore boreal forest biological indicators for future studies. Plus, an established method for long term biomonitoring needs to be explored that can use large animals such as reptiles and mammals to assess forest health status. Also, there is an urgent need to conserve and preserve forest ecosystems to ensure the sustainability of biological indicators in the forest. Lastly, it is critical to have a standard parameter using biological indicators to determine the forest health quality globally. All these discoveries show that this study has succeeded in filling the gap and answering the questions raised earlier. It also opens a new dimension for research and discovery in the study of biomonitoring techniques for forest health.
Data availability statement
The original contributions presented in the study are included in the article/ Supplementary Material , further inquiries can be directed to the corresponding author.
Author contributions
AA is the only author for this article and all the works in this article is done by AA. AA also approved and read the final manuscript.
This study has been supported by Universiti Kebangsaan Malaysia through research grant (SK-2021-025).
Acknowledgments
This study acknowledges the contribution by Universiti Kebangsaan Malaysia through providing online database in searching for all the articles. Also, Rose Norman for her generous assistance in conducting the proofreading for this article.
Conflict of interest
The author declares that the research was conducted in the absence of any commercial or financial relationships that could be construed as a potential conflict of interest.
Publisher’s note
All claims expressed in this article are solely those of the authors and do not necessarily represent those of their affiliated organizations, or those of the publisher, the editors and the reviewers. Any product that may be evaluated in this article, or claim that may be made by its manufacturer, is not guaranteed or endorsed by the publisher.
Supplementary material
The Supplementary Material for this article can be found online at: https://www.frontiersin.org/articles/10.3389/fenvs.2023.970730/full#supplementary-material
Abas, A. (2021). A systematic review on biomonitoring using lichen as the biological indicator: A decade of practices, progress and challenges. Ecol. Indic. 121, 107197. doi:10.1016/j.ecolind.2020.107197
CrossRef Full Text | Google Scholar
Abas, A., Awang, A., and Aiyub, K. (2020). Analysis of heavy metal concentration using transplanted lichen Usnea misaminensis at Kota Kinabalu, Sabah (Malaysia). Appl. Ecol. Environ. Res. 18 (1), 1175–1182. doi:10.15666/aeer/1801_11751182
Abas, A., Aziz, A., and Awang, A. (2022). A systematic review on the local wisdom of indigenous people in nature conservation. Sustainability 14 (6), 3415. doi:10.3390/su14063415
Abas, A., Khalid, R. M., Rosandy, A. R., and Sulaiman, N. (2019). Lichens of pulau pangkor, perak, Malaysia. Malays. For. 82 (1), 59–66.
Google Scholar
Ahumada, J. A., Silva, C. E. F., Gajapersad, K., Hallam, C., Hurtado, J., Martin, E., et al. (2011). Community structure and diversity of tropical forest mammals: Data from a global camera trap network. Philosophical Trans. R. Soc. B Biol. Sci. 366 (1578), 2703–2711. doi:10.1098/rstb.2011.0115
PubMed Abstract | CrossRef Full Text | Google Scholar
Allen, L., Reeve, R., Nousek-McGregor, A., Villacampa, J., and MacLeod, R. (2019). Are orchid bees useful indicators of the impacts of human disturbance? Ecol. Indic. 103, 745–755. doi:10.1016/j.ecolind.2019.02.046
Alston, J. M., Joyce, M. J., Merkle, J. A., and Moen, R. A. (2020). Temperature shapes movement and habitat selection by a heat-sensitive ungulate. Landsc. Ecol. 35 (9), 1961–1973. doi:10.1007/s10980-020-01072-y
Amadi, C. N., Frazzoli, C., and Orisakwe, O. E. (2020). Sentinel species for biomonitoring and biosurveillance of environmental heavy metals in Nigeria. J. Environ. Sci. Health, Part C Toxicol. Carcinog. 38 (1), 21–60. doi:10.1080/26896583.2020.1714370
Anderegg, W. R., Trugman, A. T., Badgley, G., Konings, A. G., and Shaw, J. (2020). Divergent forest sensitivity to repeated extreme droughts. Nat. Clim. Change 10 (12), 1091–1095. doi:10.1038/s41558-020-00919-1
Bal, T. L., Storer, A. J., and Jurgensen, M. F. (2018). Evidence of damage from exotic invasive earthworm activity was highly correlated to sugar maple dieback in the Upper Great Lakes region. Biol. Invasions 20 (1), 151–164. doi:10.1007/s10530-017-1523-0
Becklin, K. M., Anderson, J. T., Gerhart, L. M., Wadgymar, S. M., Wessinger, C. A., and Ward, J. K. (2016). Examining plant physiological responses to climate change through an evolutionary lens. Plant Physiol. 172 (2), 635–649. doi:10.1104/pp.16.00793
Berisha, S., Skudnik, M., Vilhar, U., Sabovljević, M., Zavadlav, S., and Jeran, Z. (2017). Trace elements and nitrogen content in naturally growing moss Hypnum cupressiforme in urban and peri-urban forests of the Municipality of Ljubljana (Slovenia). Environ. Sci. Pollut. Res. 24 (5), 4517–4527. doi:10.1007/s11356-016-8201-0
Bianchi, M. A. F., Mello, R. H., Bianchi, H., Bermond, J. L., and Ibrahim, P. A. F. (2016). Restrain of birds with bottles of polyethylene terephthalate, tested in red-browed from the Atlantic Forest. Arq. Bras. Med. Veterinaria Zootec. 68 (1), 136–140. doi:10.1590/1678-4162-8604
Bocca, B., Ruggieri, F., Pino, A., Rovira, J., Calamandrei, G., Martínez, M. Á., et al. (2019). Human biomonitoring to evaluate exposure to toxic and essential trace elements during pregnancy. Part A. concentrations in maternal blood, urine and cord blood. Environ. Res. 177, 108599. doi:10.1016/j.envres.2019.108599
Braun, V., and Clarke, V. (2006). Using thematic analysis in psychology. Qual. Res. Psychol. 3 (2), 77–101. doi:10.1191/1478088706qp063oa
Brogna, D., Dufrêne, M., Michez, A., Latli, A., Jacobs, S., Vincke, C., et al. (2018). Forest cover correlates with good biological water quality. Insights from a regional study (Wallonia, Belgium). J. Environ. Manag. 211, 9–21. doi:10.1016/j.jenvman.2018.01.017
Bühler, D., Marinowic, D. R., De Barros, M. P., and Da Silva, L. B. (2014). Genetic damage induced by water pollutants in the freshwater fish Hyphessobrycon luetkenii (Characidae) in a reservoir of the Canela National Forest, Brazil. J. Freshw. Ecol. 29 (2), 295–299. doi:10.1080/02705060.2013.879539
Cañón, M., and Buitrago-Gómez, Q. (2018). The research question in clinical practice: A guideline for its formulation. Rev. Colomb. Psiquiatr. 47 (3), 193–200. doi:10.1016/j.rcp.2016.06.004
Cleavitt, N. L., Hinds, J. W., Poirot, R. L., Geiser, L. H., Dibble, A. C., Leon, B., et al. (2015). Epiphytic macrolichen communities correspond to patterns of sulfur and nitrogen deposition in the northeastern United States. Bryologist 118 (3), 304–324. doi:10.1639/0007-2745-118.3.304
Coyle, J. R., and Hurlbert, A. H. (2016). Environmental optimality, not heterogeneity, drives regional and local species richness in lichen epiphytes. Glob. Ecol. Biogeogr. 25 (4), 406–417. doi:10.1111/geb.12420
Davidson, C. J., Foster, K. R., and Tanna, R. N. (2020). Forest health effects due to atmospheric deposition: Findings from long-term forest health monitoring in the Athabasca Oil Sands Region. Sci. Total Environ. 699, 134277. doi:10.1016/j.scitotenv.2019.134277
de la Cruz, A. C., Gil, P. M., Fernández-Cancio, Á., Minaya, M., Navarro-Cerrillo, R. M., Sánchez-Salguero, R., et al. (2014). Defoliation triggered by climate induced effects in Spanish ICP Forests monitoring plots. For. Ecol. Manag. 331, 245–255. doi:10.1016/j.foreco.2014.08.010
De La Sancha, N. U. (2014). Patterns of small mammal diversity in fragments of subtropical Interior Atlantic Forest in eastern Paraguay. Mammalia 78 (4), 1–13. doi:10.1515/mammalia-2013-0100
Deborde, D. D. D., Hernandez, M. B. M., and Magbanua, F. S. (2016). Benthic macroinvertebrate community as an indicator of stream health: The effects of land use on stream benthic macroinvertebrates. Sci. Diliman 28 (2), 5–26.
Delgado, L. E., and Marín, V. H. (2020). Ecosystem services and ecosystem degradation: Environmentalist’s expectation? Ecosyst. Serv. 45, 101177. doi:10.1016/j.ecoser.2020.101177
D’Errico, G., Carletti, B., Schroder, T., Mota, M., Vieira, P., and Roversi, P. F. (2014). An update on the occurrence of nematodes belonging to the genus Bursaphelenchus in the Mediterranean area. Forestry 88 (5), 509–520. doi:10.1093/forestry/cpv028
Dhyani, A., Jain, R., and Pandey, A. (2019). Contribution of root-associated microbial communities on soil quality of Oak and Pine forests in the Himalayan ecosystem. Trop. Ecol. 60 (2), 271–280. doi:10.1007/s42965-019-00031-2
Diansyah, W., Abas, A., and Sakawi, Z. (2021). A systematic review on community forest management in southeast Asia: Current practices and impacts on biodiversity conservation and livelihood quality of local communities. Hum. Ecol. Rev. 27 (1), 3–21. doi:10.22459/her.27.01.2021.01
Dolný, A., Harabiš, F., Bártaa, D., Lhota, S., and Drozd, P. (2013). Aquatic insects indicate terrestrial habitat degradation: Changes in taxonomical structure and functional diversity of dragonflies in tropical rainforest of East Kalimantan. Trop. Zool. 25 (3), 141–157. doi:10.1080/03946975.2012.717480
Donald, F., Green, S., Searle, K., Cunniffe, N. J., and Purse, B. V. (2020). Small scale variability in soil moisture drives infection of vulnerable juniper populations by invasive forest pathogen. For. Ecol. Manag. 473, 118324. doi:10.1016/j.foreco.2020.118324
Duncanson, L., Armston, J., Disney, M., Avitabile, V., Barbier, N., Calders, K., et al. (2019). The importance of consistent global forest aboveground biomass product validation. Surv. Geophys. 40 (4), 979–999. doi:10.1007/s10712-019-09538-8
Edegbene, A. O., Arimoro, F. O., Odume, O. N., Ogidiaka, E., and Keke, U. N. (2021). Can macroinvertebrate traits Be explored and applied in biomonitoring riverine systems draining forested catchments? Front. Water 3. doi:10.3389/frwa.2021.607556
Faruk, A., Belabut, D., Ahmad, N., Knell, R. J., and Garner, T. W. J. (2013). Effects of oil-palm plantations on diversity of tropical Anurans. Conserv. Biol. 27 (3), 615–624. doi:10.1111/cobi.12062
Faudzi, R., Abas, A., Othman, N. W., and Mazlan, S. M. (2021). Effect of water quality on the abundance of firefly populations at Cherating River, Pahang, Malaysia. Environ. Asia 14 (1), 69–79. doi:10.14456/ea.2021.8
Feldpausch, T. R., Phillips, O. L., Brienen, R. J. W., Gloor, E., Lloyd, J., Lopez-Gonzalez, G., et al. (2016). Amazon forest response to repeated droughts. Glob. Biogeochem. Cycles 30 (7), 964–982. doi:10.1002/2015GB005133
Finger, D. J. I., McPherson, M. L., Houskeeper, H. F., and Kudela, R. M. (2021). Mapping bull kelp canopy in northern California using Landsat to enable long-term monitoring. Remote Sens. Environ. 254, 112243. doi:10.1016/j.rse.2020.112243
Fisichelli, N. A., and Miller, K. M. (2018). Weeds, worms, and deer: Positive relationships among common forest understory stressors. Biol. Invasions 20 (5), 1337–1348. doi:10.1007/s10530-017-1630-y
Foomani, A., Gholizadeh, M., Harsij, M., and Salavatian, S. M. (2020). River health assessment using macroinvertebrates and water quality parameters: A case of the shanbeh-bazar river, anzali wetland, Iran. Iran. J. Fish. Sci. 19 (5), 2274–2292. doi:10.22092/ijfs.2020.122380
Fujiki, S., Aoyagi, R., Tanaka, A., Imai, N., Kusma, A. D., Kurniawan, Y., et al. (2016). Large-scale mapping of tree-community composition as a surrogate of forest degradation in Bornean tropical rain forests. Land 5 (4), 45. doi:10.3390/land5040045
Gnass Giese, E. E., Howe, R. W., Wolf, A. T., Miller, N. A., and Walton, N. G. (2015). Sensitivity of breeding birds to the “human footprint” in weWesternreat Lakes forest landscapes. Ecosphere 6 (6), art90. doi:10.1890/ES14-00414.1
Gómez, S., Gonda-King, L., Orians, C. M., Orwig, D. A., Panko, R., Radville, L., et al. (2015). Interactions between invasive herbivores and their long-term impact on New England hemlock forests. Biol. Invasions 17 (2), 661–673. doi:10.1007/s10530-014-0757-3
Gottesman, B. L., Sprague, J., Kushner, D. J., Bellisario, K., Savage, D., McKenna, M. F., et al. (2020). Soundscapes indicate kelp forest condition. Mar. Ecol. Prog. Ser. 654, 35–52. doi:10.3354/meps13512
Green, E. J., McRae, L., Freeman, R., Harfoot, M. B. J., Hill, S. L. L., Baldwin-Cantello, W., et al. (2020). Below the canopy: Global trends in forest vertebrate populations and their drivers. Proc. R. Soc. B Biol. Sci. 287, 20200533. doi:10.1098/rspb.2020.0533
Guyon, L. J., and Battaglia, L. L. (2018). Ecological characteristics of floodplain forest reference sites in the Upper Mississippi River System. For. Ecol. Manag. 427, 208–216. doi:10.1016/j.foreco.2018.06.007
Hermans, S. M., Buckley, H. L., Case, B. S., Curran-Cournane, F., Taylor, M., and Lear, G. (2020). Using soil bacterial communities to predict physico-chemical variables and soil quality. Microbiome 8 (1), 79. doi:10.1186/s40168-020-00858-1
Highland, S. A., Miller, J. C., and Jones, J. A. (2013). Determinants of moth diversity and community in a temperate mountain landscape: Vegetation, topography, and seasonality. Ecosphere 4 (10), art129. doi:10.1890/ES12-00384.1
Jongsma, G. F. M., Hedley, R. W., Durães, R., and Karubian, J. (2014). Amphibian diversity and species composition in relation to habitat type and alteration in the mache-chindul reserve, northwest Ecuador. Herpetologica 70 (1), 34–46. doi:10.1655/HERPETOLOGICA-D-12-00068
Kamoske, A. G., Dahlin, K. M., Stark, S. C., and Serbin, S. P. (2019). Leaf area density from airborne LiDAR: Comparing sensors and resolutions in a temperate broadleaf forest ecosystem. For. Ecol. Manag. 433, 364–375. doi:10.1016/j.foreco.2018.11.017
Kashian, D. M., Bauer, L. S., Spei, B. A., Duan, J. J., and Gould, J. R. (2018). Potential impacts of emerald ash borer biocontrol on ash health and recovery in southern Michigan. Forests 9 (6), 296. doi:10.3390/f9060296
Kasprzyk, A., Kilar, J., Chwil, S., and Rudaś, M. (2020). Content of selected macro-and microelements in the liver of free-living wild boars ( Sus scrofa l.) from agricultural areas and health risks associated with consumption of liver. Animals 10 (9), 1–15. doi:10.3390/ani10091519
Kim, D. H., Chon, T. S., Kwak, G. S., Lee, S. B., and Park, Y. S. (2016). Effects of land use types on community structure patterns of benthic macroinvertebrates in streams of urban areas in the South of the Korea Peninsula. WaterSwitzerl. 8 (5), 187. doi:10.3390/w8050187
Kleinman, S. J., De Gomez, T. E., Snider, G. B., and Williams, K. E. (2012). Large-scale pinyon ips (<I>Ips confusus</I>) outbreak in southwestern United States tied with elevation and land cover. J. For. 110 (4), 194–200. doi:10.5849/jof.11-060
Kłos, A., Rajfur, M., Šrámek, I., and Wacławek, M. (2011). Use of lichen and moss in assessment of forest contamination with heavy metals in Praded and Glacensis Euroregions (Poland and Czech Republic). Water, Air, Soil Pollut. 222 (1–4), 367–376. doi:10.1007/s11270-011-0830-9
Krapfl, K. J., Holzmueller, E. J., and Jenkins, M. A. (2011). Early impacts of hemlock woolly adelgid in Tsuga canadensis forest communities of the southern Appalachian Mountains. J. Torrey Botanical Soc. 138 (1), 93–106. doi:10.3159/10-RA-031.1
Laiolo, P., Bañuelos, M. J., Blanco-Fontao, B., García, M., and Gutiérrez, G. (2011). Mechanisms underlying the bioindicator notion: Spatial association between individual sexual performance and community diversity. PLoS ONE 6 (7), e22724. doi:10.1371/journal.pone.0022724
Lausch, A., Borg, E., Bumberger, J., Dietrich, P., Heurich, M., Huth, A., et al. (2018). Understanding forest health with remote sensing, Part III: Requirements for a scalable multi-source forest health monitoring network based on data science approaches. Remote Sens. 10, 1120. doi:10.3390/rs10071120
Li, J., Dirzo, R., Wang, Y., Zeng, D., Liu, J., Ren, P., et al. (2021). Rapid morphological change in a small mammal species after habitat fragmentation over the past half-century. Divers. Distributions 27 (12), 2615–2628. doi:10.1111/ddi.13437
López-Baucells, A., Casanova, L., Puig-Montserrat, X., Espinal, A., Páramo, F., and Flaquer, C. (2017). Evaluating the use of Myotis daubentonii as an ecological indicator in Mediterranean riparian habitats. Ecol. Indic. 74, 19–27. doi:10.1016/j.ecolind.2016.11.012
Loppi, S., and Baragatti, E. (2011). Influence of the health status of pine trees on the diversity of epiphytic lichens. Nova Hedwig. 93 (1–2), 201–210. doi:10.1127/0029-5035/2011/0093-0201
Malek, J. A., Lim, S. B., and Yigitcanlar, T. (2021). Social inclusion indicators for building citizen-centric smart cities: A systematic literature review. Sustainability 13 (1), 376. doi:10.3390/su13010376
McMahon, S. M., Arellano, G., and Davies, S. J. (2019). The importance and challenges of detecting changes in forest mortality rates. Ecosphere 10 (2). doi:10.1002/ecs2.2615
Medhi, J., Dutta, J., and Chandra Kalita, M. (2020). Biomonitoring ecosystem: Modelling relationship with arthropods. Arthropods . doi:10.5772/intechopen.94313
Meehan, M. L., Song, Z., Lumley, L. M., Cobb, T. P., and Proctor, H. (2019). Soil mites as bioindicators of disturbance in the boreal forest in northern Alberta, Canada: Testing taxonomic sufficiency at multiple taxonomic levels. Ecol. Indic. 102, 349–365. doi:10.1016/j.ecolind.2019.02.043
Millennium Ecosystem Assessment (MEA) (2005). Ecosystems and human wellbeing: Synthesis report . Washington, DC: Island Press .
Mohd-Taib, F. S., Mohd-Saleh, W., Asyikha, R., Mansor, M. S., Ahmad-Mustapha, M., Mustafa-Bakray, N. A., et al. (2020). Effects of anthropogenic disturbance on the species assemblages of birds in the back mangrove forests. Wetl. Ecol. Manag. 28 (3), 479–494. doi:10.1007/s11273-020-09726-z
Moher, D., Liberati, A., Tetzlaff, J., and Altman, D. G.The PRISMA Group (2009). Preferred reporting items for systematic reviews and meta-analyses: The PRISMA statement. PLoS Med. 6 (7), e1000097. doi:10.1371/journal.pmed1000097
Moradi, S., Sheykhi Ilanloo, S., Kafash, A., and Yousefi, M. (2019). Identifying high-priority conservation areas for avian biodiversity using species distribution modeling. Ecol. Indic. 97, 159–164. doi:10.1016/j.ecolind.2018.10.003
Morris, J. L., Cottrell, S., Fettig, C. J., DeRose, R. J., Mattor, K. M., Carter, V. A., et al. (2018). Bark beetles as agents of change in social–ecological systems. Front. Ecol. Environ. 16, S34–S43. doi:10.1002/fee.1754
Muhammad, N., Hashim, N. H., Khairuddin, N. A., Yusof, H., Jusoh, S., Abas, A., et al. (2018). Identification of most tolerant lichen species to vehicular traffic’s pollutants: A case study at batu pahat. J. Adv. Res. Fluid Mech. Therm. Sci. 42 (1), 57–64.
Mulvey, R. L., and Bisbing, S. M. (2016). Complex interactions among agents affect shore pine health in southeast Alaska. Northwest Sci. 90 (2), 176–194. doi:10.3955/046.090.0209
Myron, K. J., Clarkson, B. D., and Gemmill, C. E. C. (2021). Biological flora of New Zealand 16: Pittosporum kirkii Hook.f. ex kirk, kirk’s kōhūhū, thick-leaved kohukohu. N. Z. J. Bot. 59 (1), 112–136. doi:10.1080/0028825X.2020.1770303
Naderi, M., Farashi, A., and Markov, G. (2017). Exploring contents of lead and cadmium in tissues of fat dormouse Glis glis (Linnaeus, 1766) (Rodentia: Gliridae) for use in monitoring of environmental pollutants in the Southern Caspian Coast Forests, Iran. Acta Zool. Bulg. 69 (1), 61–64.
Newton, P., Kinzer, A. T., Miller, D. C., Oldekop, J. A., and Agrawal, A. (2020). The number and spatial distribution of forest-proximate people globally. One Earth 3 (3), 363–370. doi:10.1016/j.oneear.2020.08.016
Nisa, R. U., Tantray, A. Y., Kouser, N., Allie, K. A., Wani, S. M., Alamri, S. A., et al. (2021). Influence of ecological and edaphic factors on biodiversity of soil nematodes. Saudi J. Biol. Sci. 28 (5), 3049–3059. doi:10.1016/j.sjbs.2021.02.046
Nizam, M. S., Ismail, P., Latiff, A., Shamsudin, I., and Faridah-Hanum, I. (2009). Diversity of tree communities and its relationships with soil properties in a peat swamp forest in Pahang, Peninsular Malaysia. Ecol. Environ. Conservation 15 (2), 307–318.
Norhazrina, N., Vanderpoorten, A., Hedenäs, L., and Patiño, J. (2016). What are the evolutionary mechanisms explaining the similar species richness patterns in tropical mosses? Insights from the phylogeny of the pantropical genus pelekium. Mol. Phylogenetics Evol. 105, 139–145. doi:10.1016/j.ympev.2016.08.008
Okoli, C. (2015). A guide to conducting a standalone systematic literature review. Commun. Assoc. Inf. Syst. 37, 43. doi:10.17705/1cais.03743
Osman, M. F., Lee, S. Y., Sarbini, S. R., Faudzi, S. M. M., Khamis, S., Zainudin, B. H., et al. (2021). Metabolomics-driven discovery of an introduced species and two Malaysian Piper betle L. Variants. Plants 10 (11), 2510. doi:10.3390/plants10112510
Peterson, E., Hansen, E., and Hulbert, J. (2014). Source or sink? The role of soil and water borne inoculum in the dispersal of phytophthora ramorum in Oregon tanoak forests. For. Ecol. Manag. 322, 48–57. doi:10.1016/j.foreco.2014.02.031
Petticrew, M., and Roberts, H. (2006). Systematic reviews in the social sciences: A practical guide . Malden, MA: Blackwell Publishing Ltd .
Poorter, L., van der Sande, M. T., Thompson, J., Arets, E. J. M. M., Alarcón, A., Álvarez-Sánchez, J., et al. (2015). Diversity enhances carbon storage in tropical forests. Glob. Ecol. Biogeogr. 24 (11), 1314–1328. doi:10.1111/geb.12364
PRISMA (2021). PRISMA. [online] Prisma-statement.org. Available at: < http://www.prisma-statement.org/ > (Accessed January 31, 2022).
Rahman, A., Talukdar, N. R., and Choudhury, P. (2020). Assessing some essential trace elements concentration in micro chiropteran bat (Megaderma lyra): A study in barak valley of Assam, India. Environ. Chem. Ecotoxicol. 2, 56–63. doi:10.1016/j.enceco.2020.02.002
Rassati, D., Faccoli, M., Battisti, A., and Marini, L. (2016). Habitat and climatic preferences drive invasions of non-native ambrosia beetles in deciduous temperate forests. Biol. Invasions 18 (10), 2809–2821. doi:10.1007/s10530-016-1172-8
Rogers, P. (2002). Using Forest Health Monitoring to assess aspen forest cover change in the southern Rockies ecoregion. For. Ecol. Manag. 155 (1–3), 223–236. doi:10.1016/S0378-1127(01)00560-6
Roulier, M., Bueno, M., Thiry, Y., Coppin, F., Redon, P. O., Le Hécho, I., et al. (2018). Iodine distribution and cycling in a beech (Fagus sylvatica) temperate forest. Sci. Total Environ. 645, 431–440. doi:10.1016/j.scitotenv.2018.07.039
Sánchez-Salguero, R., Camarero, J. J., Grau, J. M., de la Cruz, A. C., Gil, P. M., Minaya, M., et al. (2017). Analysing atmospheric processes and climatic drivers of tree defoliation to determine forest vulnerability to climate warming. Forests 8 (1), 13. doi:10.3390/f8010013
Shearman, T. M., Wang, G. G., and Bridges, W. C. (2015). Population dynamics of redbay (persea borbonia) after laurel wilt disease: An assessment based on forest inventory and analysis data. Biol. Invasions 17 (5), 1371–1382. doi:10.1007/s10530-014-0799-6
Shi, L., Dossa, G. G. O., Paudel, E., Zang, H., Xu, J., and Harrison, R. D. (2019). Changes in fungal communities across a forest disturbance gradient. Appl. Environ. Microbiol. 85 (12), 1–11. doi:10.1128/AEM.00080-19
Skaldina, O., and Sorvari, J. (2017). Wood ant colouration as an ecological indicator for the level of disturbance in managed coniferous forests. Ecol. Indic. 72, 444–451. doi:10.1016/j.ecolind.2016.08.039
Smith, G. (2012). Ambient ozone injury to forest plants in northeast and North central USA: 16 years of biomonitoring. Environ. Monit. Assess. 184 (7), 4049–4065. doi:10.1007/s10661-011-2243-z
Somay, L., Szigeti, V., Boros, G., Ádám, R., and Báldi, A. (2021). Wood pastures: A transitional habitat between forests and pastures for dung beetle assemblages. Forests 12 (1), 25–16. doi:10.3390/f12010025
Song, L., Liu, W. Y., and Nadkarni, N. M. (2012). Response of non-vascular epiphytes to simulated climate change in a montane moist evergreen broad-leaved forest in southwest China. Biol. Conserv. 152, 127–135. doi:10.1016/j.biocon.2012.04.002
Sullivan, C. D., Slade, E. M., Bai, M., Shi, K., and Riordan, P. (2018). Evidence of forest restoration success and the conservation value of community-owned forests in Southwest China using dung beetles as indicators. PLoS ONE 13 (11), e0204764. doi:10.1371/journal.pone.0204764
Suni, S. S. (2017). Dispersal of the orchid bee Euglossa imperialis over degraded habitat and intact forest. Conserv. Genet. 18 (3), 621–630. doi:10.1007/s10592-016-0902-x
Swislowski, P., Kríž, J., and Rajfur, M. (2020). The use of bark in biomonitoring heavy metal pollution of forest areas on the example of selected areas in Poland. Ecol. Chem. Eng. S 27, 195–210. doi:10.2478/eces-2020-0013
Tallis, H. M., Hawthorne, P. L., Polasky, S., Reid, J., Beck, M. W., Brauman, K., et al. (2018). An attainable global vision for conservation and human well-being. Front. Ecol. Environ. 16 (10), 563–570. doi:10.1002/fee.1965
Tebbett, S. B., Morais, R. A., Goatley, C. H., and Bellwood, D. R. (2021). Collapsing ecosystem functions on an inshore coral reef. J. Environ. Manag. 289, 112471. doi:10.1016/j.jenvman.2021.112471
Thakur, T. K., Swamy, S. L., Bijalwan, A., and Dobriyal, M. J. (2019). Assessment of biomass and net primary productivity of a dry tropical forest using geospatial technology. J. For. Res. 30 (1), 157–170. doi:10.1007/s11676-018-0607-8
Wade, A. S. I., Barov, B., Burfield, I. J., Gregory, R. D., Norris, K., and Butler, S. J. (2013). Quantifying the detrimental impacts of land-use and management change on European forest bird populations. PLoS ONE 8 (5), e64552. doi:10.1371/journal.pone.0064552
Warwell, M. V., McDonald, G. I., Hanna, J. W., Kim, M. S., Lalande, B. M., Stewart, J. E., et al. (2019). Armillaria altimontana Is associated with healthy Western white pine (Pinus monticola): Potential in situ biological control of the Armillaria root disease pathogen, A. solidipes. Forests 10 (4), 294. doi:10.3390/f10040294
Will-Wolf, S., Jovan, S., Nelsen, M. P., Trest, M. T., Rolih, K. M., and Reis, A. H. (2018). Lichen indices assess local climate and air quality status in the Mid-Atlantic Region, USA. Bryologist 121 (4), 461–479. doi:10.1639/0007-2745-121.4.461
Williams, D. R., Clark, M., Buchanan, G. M., Ficetola, G. F., Rondinini, C., and Tilman, D. (2021). Proactive conservation to prevent habitat losses to agricultural expansion. Nat. Sustain. 4 (4), 314–322. doi:10.1038/s41893-020-00656-5
Woodward, B. D., Evangelista, P. H., and Vorster, A. G. (2018). Mapping progression and severity of a Southern Colorado spruce beetle outbreak using calibrated image composites. Forests 9 (6), 336. doi:10.3390/f9060336
Wulffraat, S., and Morrison, J. (2013). Measuring biological indicators for status assessment of the heart of Borneo. Environ. Conserv. 40 (3), 277–286. doi:10.1017/S0376892913000064
Xu, A., Liu, C., Wan, Y., Bai, Y., and Li, Z. (2021). Monkeys fight more in polluted air. Sci. Rep. 11 (1), 654. doi:10.1038/s41598-020-80002-z
Xu, L., Ravnskov, S., Larsen, J., and Nicolaisen, M. (2012). Linking fungal communities in roots, rhizosphere, and soil to the health status of Pisum sativum. FEMS Microbiol. Ecol. 82 (3), 736–745. doi:10.1111/j.1574-6941.2012.01445.x
Zgrablić, Ž., Marjanović, H., and Diminić, D. (2016). Can we predict Sphaeropsis sapinea outbreak by monitoring fungal diversity in Austrian pine plantations? Sumar. List. 140 (1–2), 7–15. doi:10.31298/sl.140.1-2.1
Zúbrik, M., Galko, J., Gubka, A., Rell, S., Kunca, A., Nikolov, C., et al. (2017). Dispersal and larval hosts of the zigzag sawfly Aproceros leucopoda (Hymenoptera) in Slovakia, central Europe. Period. Biol. 119 (1), 55–62. doi:10.18054/pb.v119i1.4204
Zulaini, A. A. M., Muhammad, N., Asman, S., Hashim, N. H., Jusoh, S., Abas, A., et al. (2019). Evaluation 411 of transplanted lichens, Parmotrema Tinctorum and Usnea diffracta as bioindicator on heavy metals accumulation 412 in southern Peninsular Malaysia. J. Sustain. Sci. Manag. 14 (4), 1–13.
Keywords: forest ecosystem, ecological indicator, environmental monitoring, forest health, biomonitoring
Citation: Abas A (2023) A systematic literature review on the forest health biomonitoring technique: A decade of practice, progress, and challenge. Front. Environ. Sci. 11:970730. doi: 10.3389/fenvs.2023.970730
Received: 16 June 2022; Accepted: 12 January 2023; Published: 20 January 2023.
Reviewed by:
Copyright © 2023 Abas. This is an open-access article distributed under the terms of the Creative Commons Attribution License (CC BY). The use, distribution or reproduction in other forums is permitted, provided the original author(s) and the copyright owner(s) are credited and that the original publication in this journal is cited, in accordance with accepted academic practice. No use, distribution or reproduction is permitted which does not comply with these terms.
*Correspondence: Azlan Abas, [email protected]
- FAQ for users
- FAQ for providers
- Guidance to search function
- Tutorial Videos
- Share your information
- About Climate-ADAPT
- Dissemination materials
- Tutorial videos
- Inspiring Climate-ADAPT use cases
- Climate-ADAPT performance reporting
- Climate-ADAPT events and webinars
- Country specific promotions
- EU Adaptation Strategy
- EU Mission on Adaptation
- EU Reporting on Adaptation
- EU Covenant of Mayors
- EU Sustainable Finance Strategy
- EU funding of adaptation
- Agriculture
- Biodiversity
- Business and Industry
- Coastal areas
- Cultural heritage
- Disaster risk reduction
- Land use planning
- Marine and fisheries
- Mountain areas
- Water management
- EU REGIONAL POLICY
- European Climate Risk Assessment
- Just resilience
- Nature-based solutions
- Country Profiles
- Cities and towns
- Adriatic-Ionian
- Alpine Space
- Atlantic Area
- Central Europe
- Danube Area
- Mediterranean (Euro-Med)
- North West Europe
- Northern Periphery and Arctic
- South West Europe
- Outermost Regions
- Black Sea Basin (NEXT)
- Mediterranean Sea Basin (NEXT)
- Impacts, risks and vulnerabilities
- Adaptation options
- Uncertainty guidance
- Monitoring, Reporting and Evaluation
- Europe's vulnerability to climate change impacts occurring outside Europe
- Climate Services
- Indicators in Climate-ADAPT
- RESEARCH AND INNOVATION PROJECTS
- EUROPEAN CLIMATE DATA EXPLORER
- Adaptation Support Tool
- Urban Adaptation Support Tool
- Urban Adaptation Map Viewer
- Economic losses and fatalities
- Economic tools
- Case study explorer
- LIFE projects
- INTERREG projects
- Search the database
- EUROPEAN Climate and Health Observatory
- ADAPTATION IN THE FORESTRY SECTOR
Language preference detected
Do you want to see the page translated into ? Yes, reload the page using my language settings.
Forest Management and Climate Change: a literature review.
Description.
The document summarizes knowledge and experiences in forest management as a response to climate change, based on a literature review and a survey of forest managers. This is part of the publications series produced by the Forest and Climate Change Programme of FAO. The programme seeks to provide timely information and tools to a wide range of stakeholders, with the ultimate objective of assisting countries’ efforts to mitigate and adapt to climate change through actions consistent with sustainable forest management, with the aim to determine if and how forest management is changing or could change in order to respond effectively to climate change challenges and mitigation opportunities. It examines climate change impacts on forests and forest managers throughout the world and identifies what is needed to create an enabling policy, legal and institutional environment that would support forest managers’ efforts in mitigation and adaptation.
Reference information
Published in Climate-ADAPT Jun 07 2016 - Last Modified in Climate-ADAPT Dec 12 2023
Reviewed by:
Date of publication:, climate impacts:, adaptation elements:, geographic characterisation:.
Document Actions
Advertisement
Impact of Intensive Forest Management Practices on Wood Quality from Conifers: Literature Review and Reflection on Future Challenges
- Wood Structure and Function (A Koubaa, Section Editor)
- Open access
- Published: 08 March 2023
- Volume 9 , pages 101–130, ( 2023 )
Cite this article
You have full access to this open access article
- Julie Barrette 1 , 2 ,
- Alexis Achim 2 &
- David Auty 3
4733 Accesses
3 Citations
5 Altmetric
Explore all metrics
Purpose of Review
Intensive forest management practices are being implemented worldwide to meet future global demand for wood and wood products while facilitating the protection of natural forest ecosystems. A potential decline in wood properties associated with rapid tree growth makes it essential to quantify the potential impact of intensive management on the process of wood formation and, in turn, on its suitability for various end-uses.
Recent Findings
Wood produced over short rotations is generally of lower quality because wood properties tend to improve with cambial age (i.e. the number of annual growth rings from the pith). The intensification of silvicultural practices can thus have measurable consequences for the forest products value chain. The use of new planting material from tree improvement programs could offset such effects, but questions arise as to the effects of a changing climate on wood produced from these plantations and the best silvicultural approaches to manage them.
Based on these recent findings, we provide reflections on the need for a modelling framework that uses the effects of cambial age, ring width and position along the stem to summarise the effects of tree growth scenarios on wood properties. We then present challenges related to our limited understanding of the effects of several drivers of wood properties, such as climate variation, genetic material, and forest disturbances, among others, and highlight the need for further data collection efforts to better anticipate the quality attributes of the future wood fibre resource. We conclude by providing examples of promising new tools and technologies that will help move wood quality research forward by allowing (1) fast, efficient characterisation of wood properties, and (2) up-scaling predictions at the landscape level to inform forest management decisions.
Similar content being viewed by others
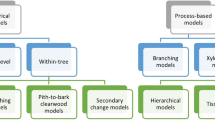
A Review of Progress and Applications in Wood Quality Modelling
David M. Drew, Geoffrey M. Downes, … Alexis Achim
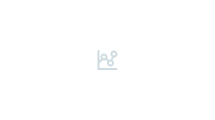
Wood formation and tree adaptation to climate
Cyrille B. K. Rathgeber, Patrick Fonti, … Philippe Rozenberg
Variation in wood basic density within and between tree species and site conditions of exclosures in Tigray, northern Ethiopia
Mengesteab Hailu Ubuy, Tron Eid & Ole Martin Bollandsås
Avoid common mistakes on your manuscript.
Introduction
Commonly understood definitions of intensive forest management practices have been in use for over 70 years [ 1 ], with adjustments made over subsequent decades by specialists in various sub-disciplines. However, most definitions in use today [ 2 – 7 ] converge around the principle that silvicultural treatments can be used to maximise wood and timber production. These silvicultural treatments include plantations of hybrid or fast-growing tree species (e.g. [ 8 ]), respacing (e.g. [ 9 ]), thinning (e.g. [ 10 ]), pruning (e.g. [ 11 ]), fertilisation (e.g. [ 12 ]), and vegetation control (e.g. [ 13 ])—with or without herbicides, insecticides or biocontrol of pests (e.g. [ 14 ]).
Currently, the most commonly mentioned solution in the scientific literature to meet future global demand for wood while allowing the protection of natural forest ecosystems relates to increasing wood supply from plantations [ 2 , 7 ]. Several jurisdictions around the world integrate the use of planted forests into their strategy for forest conservation [ 15 – 20 ]. As the adoption of intensive forest management practices increases, it is essential to consider their potential impact on the process of wood formation and, in turn, on wood quality. Indeed, silvicultural practices often result in important changes in growth patterns and tree form, which can lead to variability in wood properties, thereby influencing suitability for various end-uses [ 21 , 22 ] .
Pioneering work from Philip R. Larson in the 1960s [ 23 ] described the physiological processes underlying the deleterious effects of rapid growth on wood properties and emphasised that the concept of wood quality must consider specific end-uses. According to this principle—highlighted by many authors in recent years—the definition of wood quality may vary according to the desired end-product [ 24 – 28 ]. For the timber industry, for example, criteria such as wood density, relative proportions of heartwood and sapwood, proportion of juvenile wood (also referred to as corewood) and reaction wood, knots (size, status, frequency, etc.) and grain orientation are the most important to consider, while for the pulp and paper industries, fibre length, wood cell properties and chemical composition are the most relevant [ 29 ].
To better anticipate the consequences of silvicultural choices on future wood supplies, there is a need to understand the drivers of variation in wood properties [ 2 , 25 ]. In turn, such knowledge can help increase supply chain productivity and improve economic performance.
The intensification of silvicultural practices has measurable consequences for the forest products value chain. In the USA, for example, serious concerns were raised in 2010 regarding the bending design values for southern pine lumber from planted forests, which were found to be significantly lower than those obtained from natural forests. Consequently, the Southern Pine Inspection Bureau issued new reduced design values for some grades of southern pine dimension lumber [ 30 ]. This allowed the construction industry to use lumber from planted forests under revised building codes [ 31 ]. Such adjustments further highlight the relative nature of the wood quality concept; not only does it vary according to different end-uses, but it can also evolve over time for a specific product.
With this reality in mind, we aimed to assemble current knowledge on the effects of intensive silvicultural practices on important wood properties. This way, readers can interpret the information provided based on their own understanding of wood quality. Our review builds upon previous review papers on the effect of silvicultural treatments on wood properties [ 25 – 29 , 32 – 34 ]. Here, we focus more specifically on the effects of intensive silviculture on wood properties with an emphasis on coniferous species from around the world.
In line with current definitions of intensive forest management, papers considered in this review investigated the effects of ‘initial spacing’ thinning, respacing, pruning, fertilisation, site fertility, or genetics on wood properties. We used Google Scholar as our chosen search engine, as a preliminary comparison had shown it was the most inclusive among similar tools. The search terms included the following keywords: ‘the effect(s) of’, plus ‘initial spacing’, ‘thinning’, ‘respacing’, or ‘pruning’, ‘fertilization’ or ‘site fertility’ or ‘genetic(s)’ plus ‘wood properties’, ‘mechanical properties’, ‘wood density’, ‘lumber’, or ‘microfibril angle’, plus ‘plantation’. Once the search was completed, titles and abstracts of highlighted papers were sifted, and only relevant studies were included in the review. Main results from the retained papers were then summarised in the form of tables or graphs to facilitate the analysis. Figure 1 shows the location of each study included in this review.
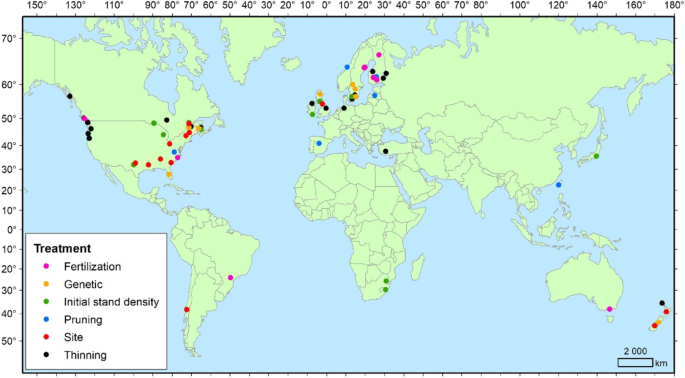
Geographic location of the research articles used in this review. If provided, the geographic coordinates of the study location were extracted; otherwise, we used the names of the location provided in the paper and extracted the coordinates for the studied area
The review is divided into four main sections. The first section summarises current knowledge on the effects of intensive forest management practices on wood properties. It is based on the main research findings extracted from the relevant empirical studies retained from our literature search. The subsequent sections provide reflections on (i) current knowledge gaps and future challenges, (ii) the need for a modelling framework to summarise the effects of intensive management practices on future wood properties under a changing climate and (iii) the emerging technologies in wood characterisation and utilisation that will help move wood quality research forward. We conclude by highlighting the detrimental effects of a decreasing age at harvest on wood quality and its implications for future production and processing.
Current Knowledge on the Effects of Intensive Forest Management Practices on Wood Properties
Initial spacing.
The choice of initial spacing at planting has an important effect on the secondary growth of trees, wood properties and volume production. Traditionally, the most common spacing used for conifer species was set at approximately 2 m in an orthogonal grid, which is equivalent to ~ 2500 seedlings per hectare [ 34 – 36 ]. However, in recent decades planting distances have tended to increase to encourage faster radial growth, leading to shorter rotations [ 34 , 37 – 40 ] and reduction in establishment costs [ 41 , 42 ]. In New Zealand, for example, typical stocking rates are now around 1,000 seedling per hectare, which represents an average square spacing of 3.2 m [ 43 ].
However, trees planted and grown at wider spacings generally have larger crowns that produce a larger juvenile core [ 32 , 34 , 39 , 44 , 45 ], which contains wood with lower density, higher MFA [ 46 , 47 ] (Fig. 2 , Table 1 ), larger knots [ 34 , 37 , 39 , 48 – 50 ], greater stem taper [ 34 , 51 ], increased stem curvature [ 52 ], and poor stem straightness in the bottom log [ 47 , 52 ]. The greater presence of sweep and leaning stems in a stand have also been associated with increased production of compression wood [ 34 ], which is associated with high longitudinal shrinkage and a greater propensity to sudden failure under loads than normal wood [ 53 ]. These changes in tree morphology and wood properties may eventually alter sawing patterns and thus affect sawmill recovery and efficiency [ 47 , 54 – 56 ].
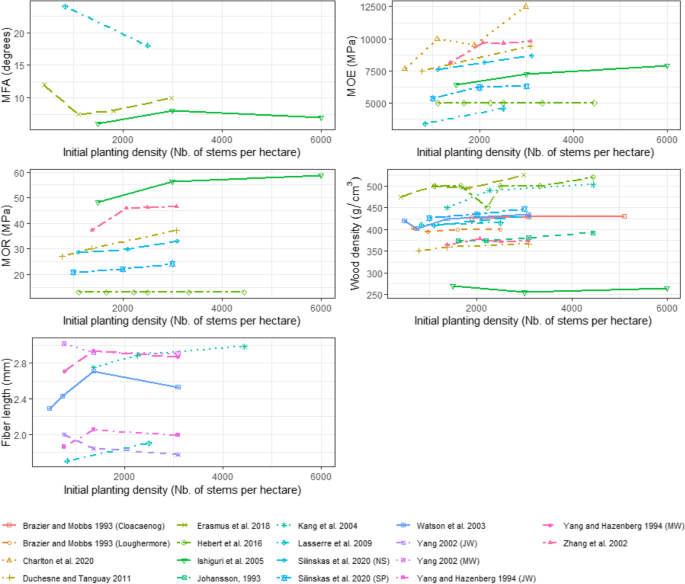
Variation of MFA (degrees), MOE (MPa), MOR (MPa), wood density (g cm −3 ) and fibre length (mm) along different initial planting densities for different published studies
Wider spacings are generally associated with lower modulus of elasticity (MOE) and modulus of rupture (MOR) in coniferous species [ 37 , 46 – 48 , 50 , 55 , 57 ] (Fig. 2 , Table 1 ). The effect of wide spacing on wood density, however, is often less severe and varies among species. For example, lower wood density was associated with wider spacings in jack pine ( Pinus banksiana Lamb.) [ 58 ], Scots pine ( Pinus sylvestris L . ), Norway spruce ( Picea abies (L.) Karst.) [ 57 ] and patula pine ( Pinus patula Schiede ex Schltdl. et Cham . ) [ 47 ]. Other studies revealed only slight reductions in wood density at wide spacings in Norway spruce [ 59 ], black spruce ( Picea mariana (Mill) B.S.P.) [ 50 ], and white spruce ( Picea glauca (Moench) Voss) [ 37 ]. Finally, wood density did not vary significantly with spacing in Sitka spruce ( Picea sitshensis (Bong.) Carr) [ 60 ], radiata pine ( Pinus radiata D. Don) [ 46 ], Japanese cedar ( Cryptomeria japonica ) [ 48 ], young jack pine [ 39 ] and western hemlock ( Tsuga heterophylla (Raf.) Sarg) [ 61 ] (Fig. 2 , Table 1 ). As can be seen from the jack pine studies [ 39 , 58 ], these effects also vary according to tree age.
Most research findings indicate a decrease in fibre length [ 44 , 46 , 58 , 61 ] with increased spacing (Fig. 2 , Table 1 ), with the exception of white spruce [ 45 ]. A reduction in cell wall thickness at wider spacings has also been reported in radiata pine [ 46 ].
While the adverse effects on wood quality attributes of planting at wider spacing are well documented, on some occasions this practice may also be beneficial. For example, on low fertility sites, or under serious drought conditions, wider spacing between trees can be advantageous, as more nutrients and water could be available to individual trees [ 62 ].
Thinning and Respacing
Thinning and respacing are intermediate treatments used to favour vigorous, healthy trees, which most likely will increase in size due to accelerated radial growth, thereby improving economic returns [ 34 , 63 ]. Respacing (or pre-commercial thinning) is applied to young stands before canopy closure and generally before individual stems have reached merchantable size. The effects of respacing on wood properties are comparable to those of initial spacing [ 9 , 64 – 67 ] (Table 2 ). Commercial thinning, in contrast, is usually applied to older stands after canopy closure, which corresponds, more or less, with the time of death of the lowest branches. Its effects on wood supplies are twofold. First, trees removed during thinning operations may include trees with low vigour and poor stem form, among other defects. Second, thinned trees are often of lower quality because they have not reached maturity and may thus contain a higher proportion of corewood [ 22 ]. However, little research has been dedicated to quantifying the specific properties of thinned stems. Instead, most work has focused on the effects of thinning on the properties of the crop at final harvest. Due to their older age and acceleration of radial growth at a later stage, commercial thinning may lead to a lower proportion of corewood in the remaining trees [ 68 ], while having little effect on knot size [ 34 , 63 ].
The timing and intensity of thinning or respacing treatments are thus critical, as implementing them too early or too aggressively may result in lower wood density [ 9 , 69 , 70 ], larger knots [ 65 , 67 , 71 ], inferior mechanical properties [ 65 , 72 ], shorter fibres [ 73 ] and lower grade lumber [ 10 , 65 ], all of which have been reported in trees grown at excessively wide initial spacing (> 2.5 m, Fig. 2 ).
When thinning treatments are applied optimally, the impact on structural wood quality or chemical composition can either be negligible [ 74 – 78 ], minor [ 69 , 79 ], or even beneficial [ 80 ]. Likewise, the production of compression wood has both been reported to increase [ 64 , 81 ] or decrease [ 75 ] after thinning (Table 2 ). Results of the latter study were interpreted as a potential response to phototropic stimuli, which might favour the inclination of stems as trees compete for space in unthinned stands. Compared with unthinned stands, trees in thinned stands may have the advantage to exhibit accelerated self-pruning of dead branches, because of the increased effects of wind, rain, snow, and solar radiation inside the stand [ 82 , 83 ]. In contrast, thinning may contribute to a faster lateral expansion of the tree crown [ 84 ]. Thinning has also been reported to stimulate the formation of epicormic branches in Sitka spruce, especially when combined with a high lift pruning treatment [ 85 ].
Artificial pruning is used to increase the length of the knot-free section of a log, which can increase both mechanical properties [ 34 , 86 , 87 ] and lumber value [ 33 ], especially in the most valuable lowest log of elite trees [ 32 , 88 ]. Knots cause local grain deviation in wood [ 89 ], which can cause reduced wood stiffness and strength [ 86 , 87 ]. A key effect of artificial pruning is to considerably shorten the time required to complete branch occlusion. This leads to fewer loose knots (i.e. knots formed after branch death). For example, the occlusion time for Norway spruce branch stubs can reach 50 years or more under self-pruning conditions [ 90 ], while it can occur after 4 to 12 years in pruned trees (2-cm-thick branch wounds) [ 89 ].
Usually, artificial pruning of branches is implemented on the lowest branches at an early age, i.e. when they are small, healthy and covered by a thin layer of bark [ 91 ]. Early intervention is recommended to minimise the problems of infections and to facilitate rapid occlusion [ 32 , 91 ]. However, pruned trees can be more susceptible to damage from browsing, or bark stripping and fraying, than unpruned trees [ 89 ]. Consequently, the density of ungulate populations should be considered before applying pruning treatments [ 89 , 92 ].
Other than damage from wildlife, several other factors may influence the response of trees to pruning. These include timing (i.e. seasonality), site fertility, soil moisture availability, climate, tree age, species, the tools used and pruning height [ 93 , 94 ]. According to Zobel [ 32 ], the optimum time for pruning is when the tree has reached about 12 cm in diameter at breast height. While Zobel [ 32 ] recommended removal of 33% of the live crown, more recent studies have shown it is possible to remove 50% of the live crown without a significant reduction in radial growth [ 11 , 95 , 96 ] or height growth [ 95 ]. However, lift pruning height has also been associated with the development of numerous epicormic sprouts, which can vary importantly according to species [ 85 ]. As highlighted in the same study, the relevance of using such a treatment should be considered carefully as pruning is a very expensive treatment that could end up costing two to three times the cost of thinning a stand. When applied, the frequency and severity of pruning treatments should be planned to allow for crown recovery and to limit the effects on growth, which can alter the dominance of pruned trees in the stand [ 93 , 94 ]. In some cases, tree pruning residues are considered as a useful feedstock for bioenergy, which can help mitigate dependence on fossil fuels [ 97 , 98 ].
Since pruning artificially increases crown base height, it is generally assumed that wood within the pruned area will reach mature characteristics earlier than in unpruned trees [ 11 , 32 , 64 ]. This implies that microfibril angle (MFA) would decrease while fibre length and wood density would slightly increase after pruning [ 64 ]. However, recent studies from Chiu et al. [ 77 ] in Taiwania ( Taiwania Cryptomerioides Hay) and in Douglas-fir ( Pseudotsuga menziesii (Mirb.) Franco) [ 28 , 99 ] did not find any effects of pruning on MFA, tracheid length, or wood density. In addition, some authors have suggested that heartwood formation can be regulated by pruning [ 100 , 101 ], although others disagree with this finding [ 102 ].
Pruning is often done in combination with thinning [ 71 , 77 , 89 , 96 ] or fertilising treatments [ 95 , 103 ]. Pruning can help limit the increase in stem taper generally caused by thinning [ 93 ], although whether this effect can be observed depends on stand density and age [ 94 ]. Pruning can also be applied to dead branches. Unlike pruning of live branches, pruning dead branches does not disrupt tree growth [ 94 ]. Its only effect would be to reduce the occurrence of loose knots.
Fertilisation
Fertilisation is often portrayed as a temporary increase in site fertility [ 104 ]. It can be used to help seedling establishment, especially on nutrient-poor sites. It can also accelerate growth and help tree development during the period of rapid juvenile growth (when nutrient demand is high), albeit to the detriment of wood quality. In the USA, mid-rotation fertilisation is a proven method for improving tree growth by counteracting nutrient limitations [ 12 , 105 ]. Even late-rotation fertilisation 5 to 10 years before harvesting has been practiced to improve tree growth of Douglas fir in Canada [ 106 ]. The area of forest treated with fertilisation has thus increased substantially in recent years [ 105 ]. Most often, the applied macronutrients are nitrogen, phosphorus and potassium (NPK). Their effects on wood quality depend on several factors, including the combination of nutrients applied, their concentration, the preexisting site conditions [ 107 ] and the species being treated [ 108 ].
Most studies measuring the effects of fertilisation treatments on wood properties have focused on wood density, with variable responses reported (Table 3 ). Some studies indicated a reduction in wood density in conifers after fertilisation [ 105 , 106 , 109 – 111 ], while others found either no decrease in density or only a slight decrease [ 74 , 112 , 113 ]. The effect on wood density also tends to last for a limited period of time, i.e. around 5 years following treatment (e.g. [ 109 , 111 ]). Generally, the reduction in wood density is more pronounced when fertilisation is applied at higher concentrations. For example, Antony et al. [ 105 ] showed a reduction in wood density of about 40 kg m −3 in a thinned loblolly pine ( Pinus taeda L.) plantation after a mid-rotation application of heavy nitrogen fertiliser treatment (336 kg ha −1 ), compared with the unfertilised control. The response of wood density to fertilisation treatments may also depend on the additional effects of stand density management operations.
Often, fertilisation is applied in combination with thinning treatments. For example, a recent study by Kantavichai et al. [ 114 ] reported that the decline in wood density following fertilisation was dependent on both initial stocking and the intensity of pre-commercial thinning treatments in Douglas-fir plantations in the US Pacific Northwest. According to their study, trees that produced the highest density and stiffest wood, came from stands that had been pre-commercially thinned to half their original stem density without further thinning and fertilisation treatments. Usually, when fertilisation and thinning are combined, the radial growth response is greater than when only fertilisation treatments are applied [ 112 , 113 ].
Aside from wood density, few studies have examined the effects of fertilisation on other wood properties. As for wood density, the effects on wood fibre characteristics seem to be more pronounced when heavy fertilisation treatments are applied. For example, Antony et al. [ 105 ] found a significant decrease in cell wall thickness and a significant increase in tracheid radial diameter under a heavy fertilisation treatment (336 kg ha −1 of N). At lower concentrations (e.g. 112 kg ha −1 or 224 kg ha −1 of N), the effects on wood fibre characteristics were either not significant or negligible. Mäkinen and Hynyen [ 112 ] found only small differences between the fertilisation treatments (control vs fertilised with 150 kg N + 75 kg P 2 O 5 + 75 kg K 2 O ha −1 ) in the earlywood: latewood ratio (3–9%), tracheid diameter (2–5%), tracheid length (4–5%) and cell wall thickness (0–10%) in Scots pine. Small increases in MFA after fertilisation have been reported, which led to a slight decrease in stiffness in loblolly pine and radiata pine [ 105 , 110 ]. To reduce the negative impacts of fertilisation on wood mechanical properties, Downes et al. [ 110 ] suggested applying mild fertilisation treatments more frequently. In terms of chemical properties, nitrogen fertilisation has been reported to increase lignin and extractive concentrations [ 107 , 115 ], which could affect pulp bleaching processes in pulp and paper manufacturing.
Silviculturists usually quantify site productivity using site index, which is typically defined as the average height of the dominant trees in even-aged stands at a given index age [ 116 ]. Higher values are therefore associated with higher overall site productivity. Since site conditions influences tree growth, it is generally assumed that wood properties will be affected too [ 24 ]. Studies looking at the link between site index and wood properties often show a decrease in wood properties with an increase in site index. For example, Antony et al. [ 117 ] observed that both MOE and MOR values decrease in loblolly pine with an increase in site index. Their observations were consistent with earlier ones made by Brazier and Mobbs [ 60 ] in Sitka spruce. Watson and Bradley [ 118 ] also reported a decrease in wood density with an increase in site index in several commercial conifer species, while fibre length showed the opposite trend.
Silviculturists can also use the concept of site quality to characterise a site. In this case, site quality is assessed using physical and biological variables—such as water and nutrient availability, temperature and precipitation, latitude or soil type—or using a classification system that stratifies the landscape into ecologically meaningful units [ 116 ]. Generally, most studies on the effects of site quality on wood properties have focused on wood density; however, recent efforts have been made to predict site level or regional variation in MOE and MOR for some coniferous species (e.g. [ 117 , 119 ]). For example, it has been reported that the MOE and MOR values of loblolly pine and radiata pine increased on warmer sites [ 117 , 119 ]. It is believed that air temperature measured in the autumn is the most important and consistent variable influencing MOE values as it influences the production of latewood [ 120 ]. As such, sites benefiting from an extending growing season were also found to result in lower MFA [ 121 ].
Temperature, rainfall and site fertility have also been recognised as environmental drivers of wood density (e.g. [ 122 ]). For example, in radiata pine growing in New Zealand, higher wood density was observed in warmer sites at lower elevations [ 122 ]. Moore et al. and Rossi et al. [ 65 , 123 ] also demonstrated that wood density decreased with increasing elevation and latitude in Sitka spruce and black spruce, respectively, while Giroud et al. [ 124 ] showed no effect of a latitudinal gradient on wood density in black spruce, jack pine and balsam fir. In black spruce, wood density was also found to be higher on either very dry (e.g. [ 125 ]) or wet sites (e.g. [ 126 ]).
Our review of literature has shown that studies based on site index tend to report contrasting results to those including site quality and climatic variables. It is thus difficult to summarise the effect of site productivity or site quality on wood properties. To better understand the variation in wood properties along a gradient of site conditions or site productivity, many different variables or combinations of variables should be considered and tested. Often, the influence of site productivity is studied in combination with climatic conditions and genetic factors (e.g. [ 127 ]). To meet the challenge of adapting forest management practices to climate change impacts, it will also be important for future studies to disentangle the effects of site from those of genotype on key determinants of wood quality [ 125 ].
Genetic improvement of forest trees, or tree breeding programs, have long been considered important for increasing growth rates and wood quality with respect to certain traits of interest, such as wood density. However, as plantation rotations were reduced over time, foresters and tree breeders realised that fast-grown trees contained a large proportion of corewood with unfavourable wood properties. This motivated forest geneticists to include more wood quality traits in tree improvement programs [ 128 – 131 ]. Traits such as wood density, microfibril angle, fibre properties, stiffness and dimensional stability are now commonly included in tree breeding programs. Among the traits tested, wood density, acoustic velocity (used to estimate wood stiffness), and microfibril angle appear to be the most heritable (Table 4 ). High narrow-sense heritability (i.e. the proportion of phenotypic variance due to additive genetic variation) values and their low standard errors suggest high genetic control for these traits [ 129 ]. However, estimates of individual narrow-sense heritability often vary with cambial age (i.e. the number of annual growth rings from the pith) and among species, which complicates the task of genetic selection [ 132 – 134 ].
Depending on the wood quality traits evaluated, heritability was found to increase with cambial age [ 133 – 135 ], while in some cases, it reached maximum values at a specific cambial age [ 133 , 136 ]. In addition, some traits of interest, such as wood stiffness, are highly correlated with other traits like microfibril angle and wood density, which themselves are not always well correlated (Table 5 ). Correlations between wood quality traits may also be complicated by environmental factors (genotype-by-environment interactions) [ 136 – 138 ]. To better control the often negative correlations between tree growth and wood quality characteristics, tree breeders stress the need for improving their understanding of the molecular genetics of wood traits [ 8 , 139 ].
Current Knowledge Gaps and Future Challenges
Over the past 40 years, the Earth’s temperature has risen by 0.18 °C per decade [ 140 ], which has resulted in an average increase in tree growing seasons of approximately 3.6 days per decade in Europe [ 141 ]. There are geographical variations in such effects, and in some regions, an extended growing season has already caused measurable phenological changes in trees [ 142 – 145 ], which could lead to changes in wood properties. Despite recent efforts, few studies have conducted in-depth investigations of the effects of climate warming on wood properties. According to a review on the wood anatomy of boreal species under a changing climate by Zhang et al. [ 26 ], the proportion of latewood in coniferous species appears to be negatively correlated with climate warming, while the proportion of earlywood and fibre length would likely increase with warming temperatures.
It is anticipated that water availability will be the most important limiting factor to tree growth in the twenty-first century, thus exceeding temperature across large portions of the boreal zone [ 146 •]. In areas, where acute drought stress is expected to increase, such as in Central Europe and western North America, changes in wood cell formation might be more pronounced [ 62 , 147 ]. This may affect conifers, as tracheid length is largely driven by the water availability during cell formation [ 148 , 149 ]. For example, Jyske et al. [ 69 ] observed an increase in the cell wall thickness of both earlywood and latewood tracheids of Norway spruce following induced drought. Despite MFA being considered to be under strong genetic control, a decrease in MFA has been observed under drought conditions [ 26 ]. Earlywood cell adaptations are believed to support hydraulic functional responses to climate, while latewood cells are believed to support mechanical functional responses to climate [ 150 ].
Drought-induced changes such as thicker cell walls and lower MFA may prove beneficial in terms of wood mechanical properties. However, drought can also cause significant reductions in annual radial growth and height increment [ 147 ], as well as drastically increasing the risk of tree mortality [ 151 ]. Such effects could largely overcome potential benefits in terms of wood properties. In comparison to broadleaved species, conifers have the tendency to maintain larger margins of safety from hydraulic failure, which may favour their chances of survival during drought periods [ 152 ]. However, this margin of safety varies between species according to their water potential regulation strategies (i.e. isohydric vs anisohydric) [ 151 ].
With the observed and predicted increases in global temperatures and changes in precipitation and radiation budgets, forests will also become more susceptible to a wider range of natural disturbances. While many uncertainties remain regarding changes in disturbance dynamics under climate change [ 153 – 155 ], warmer and drier conditions are expected to increase the frequency and severity of wildfire, drought and insect disturbances, while warmer and wetter conditions are likely to increase disturbances arising from wind and pathogens [ 154 ].
It remains uncertain how forest management practices should be adapted in response to climate change and new forest disturbance regimes [ 156 ]. Intensive forest management scenarios with short rotation lengths may bear the key advantage of reducing exposure time, thereby increasing the likelihood of a given crop reaching the final harvest prior to being disturbed. From a wood supply perspective, shorter rotations can confer an additional advantage of reduced risk of loss from catastrophic disturbances because younger stands tend to be less vulnerable to various hazards such as wind damage (e.g. [ 157 ]), insect attacks (e.g. [ 158 ]), drought stress (e.g. [ 159 ]) and, to some extent, wildfire (e.g. [ 160 ]). Following drought events, Bennett et al. [ 159 ] found that larger trees tend to suffer from higher mortality rates, which also suggests that shorter rotations can lead to a reduced risk. However, Büntgen et al. [ 161 ] reported that fast-grown trees may be more vulnerable to biotic stressors.
To reduce risk at the landscape level, one suggestion is to reduce stand density through more intensive thinning to limit growth restrictions caused by drought (e.g. [ 62 ]) or to increase resistance to insect attacks (e.g. [ 162 – 164 ]). In their systematic review of the effects of thinning, Moreau et al. [ 165 •] revealed a generally positive influence of thinning on forest resistance and resilience to stressors such as fire, drought, insects and pathogens. However, multiple factors may influence these processes, and the review, as well as other studies [ 157 , 166 ], also highlighted a tendency for windthrow resistance to decrease temporarily after thinning.
Despite uncertainties about future wind regimes, increases in wind exposure may also alter wood cell development. Under high wind exposure, conifers are more likely to develop compression wood [ 167 ], which is characterised by higher wood density, higher microfibril angle, shorter fibre or tracheid length, higher proportion of lignin, and thicker cell walls [ 22 ] than normal mature wood. The high exposure time needed to induce a compression wood response [ 167 ] suggests a significant increase in sustained wind speeds would be required to directly affect wood quality. However, if the occurrence of extreme wind events causes root systems to tilt rather than fully overturning (e.g. [ 168 ]), this will inevitably lead to the production of compression wood in conifers. This is a concern because the presence of this type of wood in solid wood products can lead to sudden failure under load stresses [ 34 , 53 , 169 ].
Genetic improvement programs have started to investigate the impact of different biotic and abiotic agents on wood quality traits, but several questions remain. For example, Nabais et al. [ 125 ] recently questioned the assumed link between wood density and drought tolerance and highlighted that, for some species, other variables, such as tree competition, soil fertility or resistance to pathogens, are important drivers of wood density variation that may undermine the assumed density-drought relationship. Therefore, they suggested that selection for drought tolerance should not systematically be based on wood density as a proxy. Other studies testing the relationships between tree resistance to insects and diseases on the one hand, and tree growth or wood quality traits on the other, are providing new knowledge that could guide tree improvement strategies. For example, Lenz et al. [ 170 ] recently showed that weevil resistance in Norway spruce is genetically positively correlated with other traits such as tree height, height-to-diameter ratio, and wood acoustic velocity. It is still unclear, however, how these relationships will vary among species and which wood quality traits might serve as reliable proxies of tree resistance to insects and diseases [ 171 ]. An important challenge to this work is the time required to confirm the efficacy and durability of the resistance of various genotypes to diseases or insects in different environments. A proposed way to overcome this challenge is to combine breeding with genetic engineering [ 172 ].
In summary, a key challenge of future wood quality research will be to predict the impact of intensive management practices under changing climatic conditions and a higher frequency and intensity of disturbances from biotic or abiotic stressors. Recent literature provides clear evidence that trees adapt their wood cell development in response to variations in temperature, water availability and CO 2 atmospheric concentration. However, linking environmental conditions to wood properties remains an emerging field of research. Further studies will be required before we can fully predict the effects of climate change—and its interactions with silvicultural treatments—on future wood quality attributes. An important limitation of the empirical approach generally used in wood quality studies is that the applicability of results is generally restricted to the range of tested conditions. It is practically impossible to test each potential combination of species, site, genetic material, silvicultural scenarios, and climatic conditions under which trees are managed. Also, the need to sample mature stands to evaluate the full impacts of silvicultural decisions implies that timely questions can only be answered several decades later (at least in temperate and boreal forests). In the meantime, the genetic material, silvicultural approach, species of interest, and even the wood properties of interest will have likely changed.
The Need for a Modelling Framework
Wood quality models can be used to anticipate wood properties in various conditions that may not have been empirically tested. A review by Drew et al. [ 173 ••] summarised recent progress in wood quality modelling and proposed a simple classification in two general groups, i.e. fully empirical and process-based. Here, we focus on the former and highlight how empirical knowledge such as that included in this review can be assembled into a statistical modelling framework that facilitates the extrapolation of results to untested conditions. This approach is based on the work of Larson [ 23 ], who proposed that the distinctive radial patterns of wood physico-mechanical properties were largely the result of crown processes, such that the type of xylem formed depends on both the proximity to the live crown and the age of the cambial initials, due to the differential distribution of growth regulators (i.e. auxin gradient) along the stem. Further understanding of the process of xylem formation in trees and of developmental constraints on cambial maturation led to the formulation of competing, but overlapping, hypotheses that the distinctive radial patterns of wood properties is driven by the need for optimising both mechanical stability and hydraulic efficiency in tree stems [ 174 ]. These concepts led to the development of a stem quality modelling approach whereby the systematic within-stem variation in wood properties is described as functions of cambial age, height in the stem and annual ring width, the latter being used as a proxy for crown vigour [ 175 – 179 ]. This modelling strategy allowed early assessments and comparisons of the impacts of any silvicultural scenarios on wood properties provided that their effects on tree growth could be simulated, i.e. predictions from growth models were used as input to the wood properties models [ 180 ].
Because this statistical modelling approach has the capacity to address the effects of multiple, potentially confounding factors [ 181 ], it has been widely used to detect and quantify the impact of drivers of wood properties variation, including site characteristics [ 126 ], silviculture [ 182 ] and genetics [ 183 ]. However, the use of annual ring width (or ring area) as a proxy for the effects of environmental conditions has important limitations, as both silvicultural treatments [ 47 , 182 ] and climatic conditions [ 184 , 185 ] have been shown to influence wood properties beyond the effects that could be anticipated from their effects on tree growth.
To improve model predictions, climatic variables are increasingly included as predictors of wood properties variation in such models (e.g. [ 70 , 186 – 188 ]). One approach is to develop correlations or response functions between seasonal or monthly climatic variables on the one hand, and annual ring-level wood properties on the other [ 186 ], but this may hinder the possibility of detecting the effects of acute climatic events such as frost and droughts that can alter wood properties [ 189 , 190 ]. Dendroclimatological approaches have been developed to quantify the effects of acute climatic events on tree growth [ 191 , 192 ], which could be applied to studies of wood properties. Babst et al. [ 193 ] provide a comprehensive synthesis of models developed to simulate radial growth as a function to climatic variables from dendrochronological measurements. Models driven by temperature, water balance and day length could offer a promising approach for simulating wood properties, which could be used in the described framework.
Key to informing silvicultural decision-making is the integration of wood properties models into growth modelling platforms. Ideally, these platforms should include risk assessment modules and allow simulations of changes in forest disturbance regimes under different climate projections [ 2 , 25 , 194 , 195 ]. Not only should platforms be user-friendly, but they should also follow the free and open source (FOSS) philosophy, so that the information is widely accessible, and the workflow is transparent and fully reproducible. CAPSIS is one notable example of such a platform that has been used extensively and has fostered international collaborations [ 196 ]. In addition to informing silvicultural decision-making before empirical tests can yield results, the developed platforms provide an identifiable locus for the integration of the most up-to-date knowledge on the links between growth conditions and wood properties. Such integration is key to meeting the challenges associated with ensuring that both wood supply and wood quality from intensively managed forests continues to meet demands associated with new product development.
Emerging Technologies in Wood Characterisation and Utilisation
New technologies offer fresh opportunities for fast and efficient characterisation of wood properties. For example, recent efforts have led to new algorithms or models for assessment of wood density and mechanical properties through high-resolution scanning technologies such as computed tomography (CT) (e.g. [ 197 , 198 ], micro-CT [ 199 ], and other methods using a combination of X-ray densitometry and ultrasonic measurements [ 200 ]. As they offer the possibility to characterise wood properties rapidly and at a fine scale, such technologies will facilitate greater knowledge acquisition on the effects of intensive forest management practices on wood quality.
A concomitant challenge is the need to expand wood properties assessments to scales that are relevant to the strategic decisions that must be made in forest management. Airborne laser scanning (ALS) data has been used to link statistical descriptors of forest inventory metrics to wood properties measured on sample plots, to produce wall-to-wall, landscape-level assessments of wood properties [ 201 – 203 ]. As they have yet to include explicit links between ALS metrics, stand structure and wood properties, such models require local parameterisation and validation. The fusion of terrestrial laser scanning (TLS) and ALS data may help include such explicit links between canopy structure, tree crown characteristics and wood properties, and thus improve model precision transferability across multiple scales [ 204 ]. Further development could include the use of spectral information from satellite imagery, which can complement the characterisation of forest structure given by ALS data by providing longitudinal estimates of photosynthetic activity or vegetation stress [ 205 , 206 ].
At the other end of the forest value chain, it will also be crucial to work closely with the engineered wood products industry to understand which wood quality traits most closely determine the suitability of wood for use in specific products [ 129 , 207 ]. The detrimental effects of intensive forest management practices on wood traits may be at least partially offset by innovations in wood processing technology. For example, corewood of fast-grown conifers can be used for the central portion of laminated beams or its fibre added to concrete or plastic composites [ 2 ]. Other examples include the production of oriented strand board (OSB), particleboard, and medium density fibreboard [ 208 ••]. Moore and Cown [ 208 ••] argued that corewood panels would have similar mechanical properties and internal bond strength to those from outerwood panels, although their dimensional stability could be compromised. Different processing options should be explored to develop wood products that provide the best possible alignment between market demand and the properties of the resource [ 209 ].
All things considered, the most important effects of intensive silvicultural practices on wood properties are related to the trend of decreasing age at harvest (i.e. rotation age) [ 25 , 32 , 34 , 174 , 208 ••, 210 ]. With advances in genetic improvement, trees can more rapidly reach a merchantable size, which leads to shorter rotations. This, in turn, leads to higher proportions of corewood, which is characterised by lower mechanical properties, poorer dimensional stability and overall lower quality of lumber for most end-uses [ 33 , 34 ].
As highlighted in this review, cambial age is a prime determinant of the within-stem variation in wood properties [ 174 ]. Many physico-mechanical properties, such as wood density, and strength and stiffness, are low near the pith, increase rapidly in the first few growth rings of the juvenile wood period and then attain more stable values at higher cambial ages [ 175 – 177 , 211 ]. Some properties, such as longitudinal shrinkage and microfibril angle (MFA), follow a decreasing trend with cambial age [ 211 – 213 ], and in these cases, lower values are generally more desirable. Some coniferous species have high density near the pith, but this is generally associated with a high microfibril angle, and thus has properties analogous to that of compression wood (also referred to as ‘flexure’ wood) [ 22 , 214 ].
Overall, the increasing demand for wood fibre combined with the needs to manage the risk of disturbance and protect natural forest ecosystems will imply that an increasing proportion of timber will come from intensively managed plantations at the global scale. Our review has highlighted that genetic selection can help mitigate the impact of a shift towards intensive forest management on future wood quality. However, the importance of cambial age as a driver of the variation of wood properties implies that for most species, sites, genetic material and end-uses, shorter rotations will come at the expense of overall wood quality.
In intensive silvicultural scenarios, the magnitude of this detrimental effect will obviously depend on the chosen rotation length, among other factors; however, it will also depend on the distribution of annual rings within the stem, which can be modified by the application of thinning, fertilising or pruning treatments. Delayed thinning, pruning and delayed harvesting can all help increase the proportion of mature wood in timber. Precise recommendations of silvicultural scenarios that can strike a balance between the needs to produce timber rapidly and maintain adequate wood properties will need to be devised for each species, genetic material and site of interest. Key to achieving this is the development of wood properties models and their integration into growth modelling simulators. Such simulation platforms exist but are only available for a limited number of species and sites. Although they offer an ideal framework to consider the effects of future climate on wood properties, this knowledge has rarely been included in wood quality simulators.
As several jurisdictions make the transition from visual grading of solid wood products to non-destructive mechanical grading [ 215 ], there is a growing concern that the lumber produced from intensively managed stands may not always meet the requirements and the grading specifications of the end-users. As highlighted in this review, the wood processing industry can adapt to some extent to the supply of wood of lesser quality, but some thresholds should not be exceeded. The minimum requirement of the C16 mechanical grade for timber frame construction in Europe provides an example of such threshold [ 65 , 216 ]. A better understanding of the links between silvicultural practices, visual grading, wood properties, and non-destructive mechanical grading is thus required [ 2 , 217 ]. This highlights the importance for silviculturists, wood scientists and tree breeders to work collaboratively to ensure that future wood quality will not deteriorate under rapidly changing conditions. A desired outcome of such collaborative efforts is that practitioners are given access to the knowledge and material that will allow them to select species and provenances with the best resistance and resilience to stressors without sacrificing either wood volume or quality.
Data Availability
No new data were generated or analysed in support of this research.
References
Papers of particular interest, published recently, have been highlighted as: • of importance •• of major importance.
Chapman HH. Forest management. Bristol, Connecticute: The Hildreth Press; 1950.
Google Scholar
Gardiner B, Moore J. Creating the wood supply of the future. In: Fenning T, editor. Challenges and opportunities for the world’s forests in the 21st century. Springer; 2014. pp. 677–704.
Tittler R, Filotas É, Kroese J, Messier C. Maximizing conservation and production with intensive forest management: it’s all about location. Environ Manage. 2015;56(5):1104–17. https://doi.org/10.1007/s00267-015-0556-3 .
Article Google Scholar
Paquette A, Messier C. The role of plantations in managing the world’s forests in the Anthropocene. Front Ecol Environ. 2010;8(1):27–34. https://doi.org/10.1890/080116 .
Bell FW, Pitt DG, Wester MC. Is intensive forest management a misnomer? An Ontario-based discussion of terminology and an alternative approac. For Chron. 2006;82(5):662–74. https://doi.org/10.5558/tfc82662-5 .
Bell FW, Parton J, Stocker N, Joyce D, Reid D, Wester M, et al. Developing a silvicultural framework and definitions for use in forest management planning and practice. For Chron. 2008;84(5):678–93. https://doi.org/10.5558/tfc84678-5 .
Park A, Wilson ER. Beautiful Plantations: can intensive silviculture help Canada to fulfill ecological and timber production objectives? For Chron. 2007;83(6):825–39. https://doi.org/10.5558/tfc83825-6 .
Wu H, Ivkovic M, Gapare W, Matheson A, Baltunis B, Powell M, et al. Breeding for wood quality and profit in Pinus radiata: a review of genetic parameter estimates and implications for breeding and deployment. NZ J Forest Sci. 2008;38(1):56–87.
Auty D, Moore J, Achim A, Lyon A, Mochan S, Gardiner B. Effects of early respacing on the density and microfibril angle of Sitka spruce wood. Forestry: An Int J Forest Res. 2017;91(3):307–19. https://doi.org/10.1093/forestry/cpx004 .
Krajnc L, Farrelly N, Harte AM. The effect of thinning on mechanical properties of Douglas fir, Norway spruce, and Sitka spruce. Ann For Sci. 2019;76(1):3.
Gartner BL, Robbins JM, Newton M. Effects of pruning on wood density and tracheid length in young Douglas-fir. Wood Fiber Sci. 2005;37(2):304–13.
CAS Google Scholar
Blazier MA, Scott DA, Coleman R. Mid-rotation silviculture timing influences nitrogen mineralization of loblolly pine plantations in the mid-south USA. Forests. 2015;6(4):1061–82.
Thiffault N, Roy V. Living without herbicides in Québec (Canada): historical context, current strategy, research and challenges in forest vegetation management. Eur J Forest Res. 2011;130(1):117–33.
Rolando C, Baillie B, Withers T, Bulman L, Garrett L. Pesticide use in planted forests in New Zealand. N Z J For. 2016;61(2):3–10.
Binkley CS. Preserving nature through intensive plantation forestry: the case for forestland allocation with illustrations from British Columbia. For Chron. 1997;73(5):553–9. https://doi.org/10.5558/tfc73553-5 .
Hartmann H, Daoust G, Bigué B. Negative or positive effects of plantation and intensive forestry on biodiversity: A matter of scale and perspective. For Chron. 2010;86(3):354–64. https://doi.org/10.5558/tfc86354-3 .
Ferraz SFB, Lima WdP, Rodrigues CB. Managing forest plantation landscapes for water conservation. Forest Ecol Manag. 2013;301:58–66. https://doi.org/10.1016/j.foreco.2012.10.015 .
Barrette M, Leblanc M, Thiffault N, Paquette A, Lavoie L, Bélanger L, et al. Issues and solutions for intensive plantation silviculture in a context of ecosystem management. For Chron. 2014;90(06):748–62. https://doi.org/10.5558/tfc2014-147 .
Sedjo RA, Botkin D. Using foret plantations to spare natural forests. Environ: Sci Policy Sustain Dev. 1997;39(10):14–30.
Gladstone WT, Thomas LF. Reducing pressure on natural forests through high-yield forestry. For Ecol Manage. 1990;35(1):69–78. https://doi.org/10.1016/0378-1127(90)90232-Z .
Zobel BJ, van Buijtenen JP. Wood variation and wood properties. In: Zobel BJ, van Buijtenen JP, editors. Wood variation: its causes and controls. Springer, Springer Berlin, Heidelberg; 1989. pp. 1–32.
Panshin AJ, De Zeeuw C. Textbook of wood technology: structure, identification, properties, and uses of the commercial woods of the United States and Canada. 4th ed. New York, NY, (USA): McGraw-Hill Book Company; 1980.
Larson PR. Wood formation and the concept of wood quality. Bulletin no 74 New Haven, CT: Yale University, School of Forestry; 1969. p. 1–54. https://elischolar.library.yale.edu/cgi/viewcontent.cgi?article=1085&context=yale_fes_bulletin .
Jozsa L, Middleton G. A discussion of wood quality attributes and their practical implications. Special Publication No. SP-34. Forintek Canada Corp. Vancouver, BC; 1994.
Moore J, Cown D. Wood quality variability—what is it, what are the consequences and what we can do about it. N Z J For. 2015;59:3–9.
Zhang S, Belien E, Ren H, Rossi S, Huang J. Wood anatomy of boreal species in a warming world: a review. iForest – Biogeosci Forestry. 2020;13(2):130–8. https://doi.org/10.3832/ifor3230-013 .
Haygreen JG, Bowyer JL. Forest products and wood science: an introduction. 3rd ed. Ames Iowa (USA); Iowa state university press; 1996.
Briggs DG, Smith R. Effects of silvicultural practices on wood properties of conifers: a review. In Oliver C, Hanley D, Johnson J editors. Douglas fir: stand management for the future. Seattle: University of Washington Press; 1986. pp. 108–117.
Listyanto T, Nichols JD. A review of relationships between wood quality and silvicultural practices. Jurnal Ilmu Kehutanan. 2009;3(2):116–26.
SPIB. Determination of design values for visually graded southern pine dimensional lumber. In: Bureau SPI, editor. Penascola, Fl. Lumber Grading Rules | SPIB | Southern; 2012. Pine Inspection Bureau. https://www.spib.org/wood-services/lumber-grading-rules .
Wood Design Focus. A Journal of contemporary wood engineering: 2012 Building Code Updates. Forest Products Society. 2012;22(4)
Zobel B. Silvicultural effects on wood properties. IPEF International. 1992;2(6):31–8.
Moore JR. Growing fit-for-purpose structural timber: what’s the target and how do we get there. N Z J For. 2012;57(3):17–24.
Macdonald E, Hubert J. A review of the effects of silviculture on timber quality of Sitka spruce. Forestry. 2002;75(2):107–38.
Liziniewicz M, Ekö PM, Agestam E. Effect of spacing on 23-year-old lodgepole pine (Pinus contorta Dougl. Var. latifolia) in southern Sweden. Scand J For Res. 2012;27(4):361–71.
Barrette J, Giroud G, Ward C, Serban L, Barrette M. Synthèse des connaissances sur la densité initiale de reboisement. Ministère des Forêts de la Faune et des Parcs: Direction de la recherche forestière. AT-SSRF-19. 2020. p. 25.
Duchesne I, Tanguay F. Effects of initial spacing on tree and wood attributes on lumber quality and value in white spruce - the stanley spacing trial case study: FPInnovations Report. 2011. p. 34.
Groot A, Cortini F. Effects of initial planting density on tree and stand development of planted black spruce up to age 30. For Chron. 2016;92(02):200–10. https://doi.org/10.5558/tfc2016-039 .
Hébert F, Krause C, Plourde P-Y, Achim A, Prégent G, Ménétrier J. Effect of tree spacing on tree level volume growth, morphology, and wood properties in a 25-year-old Pinus banksiana plantation in the boreal forest of Quebec. Forests. 2016;7(11):276.
McClain KM, Morris DM, Hills SC, Buse LJ. The effects of initial spacing on growth and crown development for planted northern conifers: 37-year results. For Chron. 1994;70(2):174–82. https://doi.org/10.5558/tfc70174-2 .
Huang C-H, Kronrad GD, Morton JD. The financially optimal loblolly pine planting density and management regime for nonindustrial private forestland in East Texas. South J Appl For. 2005;29(1):16–21.
Zhang S, Lei Y, Bowling C. Quantifying stem quality characteristics in relation to initial spacing and modeling their relationship with tree characteristics in black spruce (Picea mariana). North J Appl For. 2005;22(2):85–93.
NZFFA. NZFFA guide sheet no. 1: an introduction to growing radiata pine. 2007. https://www.nzffa.org.nz/farm-forestry-model/resource-centre/information-leaflets/nzffa-guide-sheets-2007/nzffa-guide-sheet-no-1/ . Accessed 2021.
Yang K, Hazenberg G. Impact of spacing on tracheid length, relative density, and growth rate of juvenile wood and mature wood in Picea mariana. Can J For Res. 1994;24(5):996–1007.
Yang K-C. Impact of spacing on juvenile wood and mature wood properties of white spruce ( Picea glauca ). Taiwan J For Sci. 2002;17(1):13–29.
Lasserre J-P, Mason EG, Watt MS, Moore JR. Influence of initial planting spacing and genotype on microfibril angle, wood density, fibre properties and modulus of elasticity in Pinus radiata D. Don corewood. For Ecol Manag. 2009;258(9):1924–31.
Erasmus J, Kunneke A, Drew DM, Wessels CB. The effect of planting spacing on Pinus patula stem straightness, microfibril angle and wood density. Forestry: Int J For Res. 2018;91(3):247–58. https://doi.org/10.1093/forestry/cpy005 .
Ishiguri F, Kasai S, Yokota S, Iizuka K, Yoshizawa N. Wood quality of sugi (Cryptomeria japonica) grown at four initial spacings. IAWA J. 2005;26(3):375–86.
Hein S, Weiskittel AR, Kohnle U. Effect of wide spacing on tree growth, branch and sapwood properties of young Douglas-fir [Pseudotsuga menziesii (Mirb.) Franco] in south-western Germany. Eur J For Res. 2008;127(6):481–93.
Zhang S, Chauret G, Ren H, Desjardins R. Impact of plantation black spruce initial spacing on lumber grade yield, bending properties and MSR yield. Wood Fiber Sci. 2002;34(3):460–75.
Smith JHG. Influences of spacing on radial growth and percentage latewood of Douglas-fir, western hemlock, and western redcedar. Can J For Res. 1980;10(2):169–75.
Belley D, Beaudoin M, Duchesne I, Vallerand S, Tong Q, Swift D. Assessment of white spruce and jack pine stem curvature from a Nelder spacing experiment. Wood Fiber Sci. 2013;45(3):237–49.
Sonderegger W, Mandallaz D, Niemz P. An investigation of the influence of selected factors on the properties of spruce wood. Wood Sci Technol. 2008;42(4):281–98.
Article CAS Google Scholar
Belley D, Duchesne I, Vallerand S, Barrette J, Beaudoin M. Computed tomography (CT) scanning of internal log attributes prior to sawing increases lumber value in white spruce (Picea glauca) and jack pine (Pinus banksiana). Can J For Res. 2019;49(12):1516–24. https://doi.org/10.1139/cjfr-2018-0409 .
Charlton RA, Naghizadeh Z, Ham C, Wessels CB. A value chain comparison of Pinus patula sawlog management regimes based on different initial planting densities and effect on wood quality. Forest Policy Econ. 2020;111:102067.
Yerbury M, Cooper R. Curve sawing spruce sawlogs containing sweep can reduce drying distortion when compared with conventional sawing. Forestry. 2010;83(4):443–50.
Šilinskas B, Varnagirytė-Kabašinskienė I, Aleinikovas M, Beniušienė L, Aleinikovienė J, Škėma M. Scots Pine and Norway Spruce Wood Properties at Sites with Different Stand Densities. Forests. 2020;11(5):587.
Kang K-Y, Zhang SY, Mansfield SD. The effects of initial spacing on wood density, fibre and pulp properties in jack pine (Pinus banksiana Lamb.). Holzforschung. 2004;58(5):455–63.
Johansson K. Effects of initial spacing on the stem and branch properties and graded quality of Picea abies (L.) Karst. Scand J For Res. 1992;7(1–4):503–14.
Brazier J, Mobbs I. The influence of planting distance on structural wood yields of unthinned Sitka spruce. Forestry: Int J For Res. 1993;66(4):333–52.
Watson P, Garner C, Robertson R, Reath S, Gee W, Hunt K. The effects of initial tree spacing on the fibre properties of plantation-grown coastal western hemlock. Can J For Res. 2003;33(12):2460–8. https://doi.org/10.1139/x03-171 .
Rais A, van de Kuilen J-WG, Pretzsch H. Growth reaction patterns of tree height, diameter, and volume of Douglas-fir (Pseudotsuga menziesii [Mirb] Franco) under acute drought stress in Southern Germany. Eur J For Res. 2014;133(6):1043–56.
Moore J. Wood properties and uses of Sitka spruce in Britain. Forestry Commission Research Report, Forestry Commission, Edinburgh. 2011; p. 1–48.
Barbour RJ, Marshall DD, Lowell EC. Managing for wood quality. In: Monserud RA, Haynes RW, Johnson AC, editors. Compatible forest management. Managing Forest Ecosystems, vol 8. Springer. Dordrecht; 2003. pp. 299–336.
Moore J, Achim A, Lyon A, Mochan S, Gardiner B. Effects of early re-spacing on the physical and mechanical properties of Sitka spruce structural timber. For Ecol Manage. 2009;258(7):1174–80.
Watt MS, Zoric B, Kimberley MO, Harrington J. Influence of stocking on radial and longitudinal variation in modulus of elasticity, microfibril angle, and density in a 24-year-old Pinus radiata thinning trial. Can J For Res. 2011;41(7):1422–31.
Auty D, Weiskittel AR, Achim A, Moore JR, Gardiner BA. Influence of early re-spacing on Sitka spruce branch structure. Ann For Sci. 2012;69(1):93–104. https://doi.org/10.1007/s13595-011-0141-8 .
Brüchert F, Becker G, Speck T. The mechanics of Norway spruce [Picea abies (L.) Karst]: mechanical properties of standing trees from different thinning regimes. For Ecol Manag. 2000;135(1):45–62. https://doi.org/10.1016/S0378-1127(00)00297-8 .
Jyske T, Kaakinen S, Nilsson U, Saranpää P, Vapaavuori E. Effects of timing and intensity of thinning on wood structure and chemistry in Norway spruce. Holzforschung. 2010;64(1):81–91.
Filipescu CN, Lowell EC, Koppenaal R, Mitchell AK. Modeling regional and climatic variation of wood density and ring width in intensively managed Douglas-fir. Can J For Res. 2014;44(3):220–9. https://doi.org/10.1139/cjfr-2013-0275 .
Wang S-Y, Lin C-J, Chiu C-M. Effects of thinning and pruning on knots and lumber recovery of Taiwania (Taiwania cryptomerioides) planted in the Lu-Kuei area. J Wood Sci. 2003;49(5):444–9.
Wang X, Ross RJ. Acoustic evaluation of Alaskan young-growth wood. In: Ross RJ, editors. Proceedings of the 15th International Symposium on Nondestructive Testing of Wood: Sept. 10-12, 2007. Madison: Forest Products Society; 2008. pp. 97–105.
Hussein A, Gee W, Watson P, Zhang S. Effect of precommercial thinning on residual sawmill chip kraft pulping and pulp quality in balsam fir. Wood Fiber Sci. 2007;38(1):179–86.
Jaakkola T, Mäkinen H, Saranpää P. Wood density in Norway spruce: changes with thinning intensity and tree age. Can J For Res. 2005;35(7):1767–78.
Cameron A, Thomas K. Effect of thinning on the development of compression wood in stems of Corsican pine. Eur J Forest Res. 2008;127(3):247–51.
Guller B. The effects of thinning treatments on density, MOE, MOR and maximum crushing strength of Pinus brutia Ten. wood. Annals For Sci. 2007;64(4):467–75.
Chiu C-M, Lin C-J, Wang S-Y. Tracheid length and microfibril angle of young Taiwania grown under different thinning and pruning treatments. Wood Fiber Sci. 2007;37(3):437–44.
Pfister O, Wallentin C, Nilsson U, Ekö P-M. Effects of wide spacing and thinning strategies on wood quality in Norway spruce (Picea abies) stands in southern Sweden. Scand J For Res. 2007;22(4):333–43. https://doi.org/10.1080/02827580701504951 .
Tong Q, Tanguay F, Zhang S. Impact of commercial thinning on annual radial growth and wood density in plantation-grown black spruce. Wood Fiber Sci. 2011;43(3):311–25.
Russo D, Marziliano PA, Macri G, Proto AR, Zimbalatti G, Lombardi F. Does thinning intensity affect wood quality? An analysis of Calabrian Pine in Southern Italy using a non-destructive acoustic method. Forests. 2019;10(4):303.
Barnett J, Jeronimidis G. editors. Wood quality and its biological basis. CRC Press; 2003.
Mäkinen H. Growth, suppression, death, and self-pruning of branches of Scots pine in southern and central Finland. Can J For Res. 1999;29(5):585–94.
Liziniewicz M, Ekö PM, Klang F. Effects of five tree-selection strategies when thinning spruce (Picea abies) stands: a case study in a field trail in southern Sweden. Scand J For Res. 2016;31(5):495–506.
Ganbaatar B, Jamsran T, Gradel A, Sukhbaatar G. Assessment of the effects of thinnings in scots pine plantations in Mongolia: a comparative analysis of tree growth and crown development based on dominant trees. For Sci Technol. 2021;17(3):135–43. https://doi.org/10.1080/21580103.2021.1963326 .
Deal RL, Barbour RJ, Mcclellan MH, Parry DL. Development of epicormic sprouts in Sitka spruce following thinning and pruning in south-east Alaska. Forestry: Int J For Res. 2003;76(4):401–12. https://doi.org/10.1093/forestry/76.4.401 .
As N, Goker Y, Dundar T. Effect of Knots on the physical and mechanical properties of scots pine. Wood Res. 2006;51(3):51–8.
Rocha MFV, Costa LR, Costa LJ, Araújo ACCd, Soares BCD, Hein PRG. Wood knots influence the modulus of elasticity and resistance to compression. Wood Science and Technology. Floresta Ambient. 2018;25(4). https://doi.org/10.1590/2179-8087.090617 .
Mederski PS, Szczawiński D, Giefing DF, Naparty K, Brunka M. Knot soundness and occlusion time after the artificial pruning of oak. For Res Pap. 2019;80(1):5–11.
Baders E, Donis J, Snepsts G, Adamovics A, Jansons A. Pruning effect on Norway spruce (Picea abies (L.) Karst.) growth and quality. For Stud. 2017;66(1):33–48.
Mäkinen H, Ojansuu R, Sairanen P, Yli-Kojola H. Predicting branch characteristics of Norway spruce (Picea abies (L.) Karst.) from simple stand and tree measurements. Forestry. 2003;76(5):525–46.
O’Hara KL. Pruning wounds and occlusion: a long-standing conundrum in forestry. J Forest. 2007;105(3):131–8.
Hörnberg S. Changes in population density of moose (Alces alces) and damage to forests in Sweden. For Ecol Manage. 2001;149(1–3):141–51.
O’Hara KL. Technical commentary: A biological justification for pruning in coastal Douglas-fir stands. West J Appl For. 1991;6(3):59–63.
Pinkard E, Beadle C. A physiological approach to pruning. Int For Rev. 2000;2(4):295–305.
Amateis RL, Burkhart HE. Growth of young loblolly pine trees following pruning. For Ecol Manage. 2011;262(12):2338–43.
Moreno-Fernández D, Sánchez-González M, Álvarez-González JG, Hevia A, Majada JP, Canellas I, et al. Response to the interaction of thinning and pruning of pine species in Mediterranean mountains. Eur J Forest Res. 2014;133(5):833–43.
Sagani A, Hagidimitriou M, Dedoussis V, editors. Techno-economic evaluation of tree pruning biomass fired power plants for electricity generation: the case of three prefectures in Greece. Proc. 22nd European Biomass Conference and Exhibition; 2014.
Laganière J, Paré D, Thiffault E, Bernier PY. Range and uncertainties in estimating delays in greenhouse gas mitigation potential of forest bioenergy sourced from Canadian forests. Gcb Bioenergy. 2017;9(2):358–69.
Gartner BL. Assessing wood characteristics and wood quality in intensively managed plantations. J Forest. 2005;103(2):75.
Taylor AM, Gartner BL, Morrell JJ. Heartwood formation and natural durability-a review. Wood Fiber Sci. 2002;34(4):587–611.
Margolis HA, Gagnon RR, Pothier D, Pineau M. The adjustment of growth, sapwood area, heartwood area, and sapwood saturated permeability of balsam fir after different intensities of pruning. Can J For Res. 1988;18(6):723–7.
Bergström B, Gref R, Ericsson A. Effects of pruning on heartwood formation in Scots pine trees. J For Sci. 2004;50(1):11–6.
Wiseman D, Smethurst P, Pinkard L, Wardlaw T, Beadle C, Hall M, et al. Pruning and fertiliser effects on branch size and decay in two Eucalyptus nitens plantations. For Ecol Manage. 2006;225(1–3):123–33.
Saarsalmi A, Mälkönen E. Forest fertilization research in Finland: a literature review. Scand J For Res. 2001;16(6):514–35.
Antony F, Jordan L, Schimleck LR, Daniels RF, Clark A III. The effect of mid-rotation fertilization on the wood properties of loblolly pine (Pinus taeda). IAWA J. 2009;30(1):49–58.
Filipescu CN, Trofymow JA, Koppenaal RS. Late-rotation nitrogen fertilization of Douglas-fir: growth response and fibre properties. Can J For Res. 2017;47(1):134–8. https://doi.org/10.1139/cjfr-2016-0306 .
Kaakinen S, Saranpää P, Vapaavuori E. Effects of growth differences due to geographic location and N-fertilisation on wood chemistry of Norway spruce. Trees. 2006;21(2):131. https://doi.org/10.1007/s00468-006-0103-1 .
Hart JF. A review of the effects of silviculture on wood quality. The University of British Columbia. Wood . 2010;493:27.
Jozsa L, Brix H. The effects of fertilization and thinning on wood quality of a 24-year-old Douglas-fir stand. Can J For Res. 1989;19(9):1137–45.
Downes GM, Nyakuengama JG, Evans R, Northway R, Blakemore P, Dickson RL, et al. Relationship between wood density, microfibril angle and stiffness in thinned and fertilized Pinus radiata. IAWA J. 2002;23(3):253–65.
Ortega Rodriguez DR, Tomazello-Filho M. Clues to wood quality and production from analyzing ring width and density variabilities of fertilized Pinus taeda trees. New Forest. 2019;50(5):821–43. https://doi.org/10.1007/s11056-018-09702-9 .
Mäkinen H, Hynynen J. Wood density and tracheid properties of Scots pine: responses to repeated fertilization and timing of the first commercial thinning. Forestry: Int J For Res. 2014;87(3):437–47.
Mörling T. Evaluation of annual ring width and ring density development following fertilisation and thinning of Scots pine. Ann For Sci. 2002;59(1):29–40.
Kantavichai R, Turnblom EC, Lowell EC. Effects of density control and fertilization on log wood quality from a douglas-fir stand in western oregon, USA. For Sci. 2020;66(2):191–201.
Anttonen S, Manninen A-M, Saranpää P, Kainulainen P, Linder S, Vapaavuori E. Effects of long-term nutrient optimisation on stem wood chemistry in Picea abies. Trees. 2002;16(6):386–94.
Skovsgaard JP, Vanclay JK. Forest site productivity: a review of the evolution of dendrometric concepts for even-aged stands. Forestry: Int J For Res. 2007;81(1):13–31. https://doi.org/10.1093/forestry/cpm041 .
Antony F, Jordan L, Schimleck LR, Clark A, Souter RA, Daniels RF. Regional variation in wood modulus of elasticity (stiffness) and modulus of rupture (strength) of planted loblolly pine in the United States. Can J For Res. 2011;41(7):1522–33. https://doi.org/10.1139/x11-064 .
Watson P, Bradley M. Canadian pulp fibre morphology: Superiority and considerations for end use potential. For Chron. 2009;85(3):401–8. https://doi.org/10.5558/tfc85401-3 .
Watt MS, Trincado G. Development of a generic model describing modulus of elasticity of Pinus radiata in Chile and New Zealand. For Ecol Manag. 2019;453:117583. https://doi.org/10.1016/j.foreco.2019.117583 .
Watt MS, Moore JR, Façon J-P, Downes GM, Clinton PW, Coker G, et al. Modelling the influence of stand structural, edaphic and climatic influences on juvenile Pinus radiata dynamic modulus of elasticity. For Ecol Manage. 2006;229(1–3):136–44.
Jordan L, He R, Hall DB, Clark AI, Daniels RF. Variation in loblolly pine ring microfibril angle in the southeastern United States. Wood Fiber Sci. 2007;39(2):352–63.
Palmer DJ, Kimberley MO, Cown DJ, McKinley RB. Assessing prediction accuracy in a regression kriging surface of Pinus radiata outerwood density across New Zealand. For Ecol Manage. 2013;308:9–16.
Rossi S, Cairo E, Krause C, Deslauriers A. Growth and basic wood properties of black spruce along an alti-latitudinal gradient in Quebec. Canada Annals of Forest Science. 2015;72(1):77–87.
Giroud G, Bégin J, Defo M, Ung C-H. Regional variation in wood density and modulus of elasticity of Quebec’s main boreal tree species. For Ecol Manage. 2017;400:289–99.
Nabais C, Hansen JK, David-Schwartz R, Klisz M, López R, Rozenberg P. The effect of climate on wood density: what provenance trials tell us? For Ecol Manage. 2018;408:148–56. https://doi.org/10.1016/j.foreco.2017.10.040 .
Pokharel B, Dech JP, Groot A, Pitt D. Ecosite-based predictive modeling of black spruce (Picea mariana) wood quality attributes in boreal Ontario. Can J For Res. 2014;44(5):465–75.
Carson SD, Cown DJ, McKinley RB, Moore JR. Effects of site, silviculture and seedlot on wood density and estimated wood stiffness in radiata pine at mid-rotation. NZ J Forest Sci. 2014;44(1):26. https://doi.org/10.1186/s40490-014-0026-3 .
Lenz P, Auty D, Achim A, Beaulieu J, Mackay J. Genetic improvement of white spruce mechanical wood traits—early screening by means of acoustic velocity. Forests. 2013;4(3):575–94.
Hassegawa M, Savard M, Lenz PR, Duchateau E, Gélinas N, Bousquet J, et al. White spruce wood quality for lumber products: priority traits and their enhancement through tree improvement. Forestry: Int J For Res. 2020;93(1):16–37.
Hannrup B, Cahalan C, Chantre G, Grabner M, Karlsson B, Bayon IL et al. Genetic parameters of growth and wood quality traits in Picea abies. Scand J For Res. 2004;19(1):14–29.
Kennedy SG, Cameron AD, Lee S. Genetic relationships between wood quality traits and diameter growth of juvenile core wood in Sitka spruce. Can J For Res. 2013;43(1):1–6.
Li X, Huber DAHA, Powell GLPL, White TLWL, Peter GFPF. Breeding for improved growth and juvenile corewood stiffness in slash pine. Can J For Res. 2007;37(10):1886–93. https://doi.org/10.1139/x07-043 .
Weng YH, Lu P, Adams GW, Fullarton MS, Tosh KJ. Genetic parameters of growth and stem quality traits for jack pine second-generation progeny tested in New Brunswick. Can J For Res. 2015;45(1):36–43. https://doi.org/10.1139/cjfr-2014-0106 .
Lenz P, Cloutier A, MacKay J, Beaulieu J. Genetic control of wood properties in Picea glauca—an analysis of trends with cambial age. Can J For Res. 2010;40(4):703–15.
Corriveau A, Beaulieu J, Daoust G. Heritability and genetic correlations of wood characters of Upper Ottawa Valley white spruce populations grown in Quebec. For Chron. 1991;67(6):698–705.
Chen Z-Q, Karlsson B, Mörling T, Olsson L, Mellerowicz EJ, Wu HX, et al. Genetic analysis of fiber dimensions and their correlation with stem diameter and solid-wood properties in Norway spruce. Tree Genet Genomes. 2016;12(6):1–12.
Desponts M, Perron M, DeBlois J. Rapid assessment of wood traits for large-scale breeding selection in Picea mariana [Mill.] BSP. Annals For Sci. 2017;74(3):53.
Jayawickrama K. Genetic parameter estimates for radiata pine in New Zealand and New South Wales: a synthesis of results. Silvae Genetica. 2001;50(2):45–53.
Beaulieu J, Doerksen T, Clément S, MacKay J, Bousquet J. Accuracy of genomic selection models in a large population of open-pollinated families in white spruce. Heredity. 2014;113(4):343–52.
Global_Climate_Report. 2021. https://www.ncdc.noaa.gov/sotc/global/202104 . Accessed 2021.
Menzel A, Fabian P. Growing season extended in Europe. Nature. 1999;397(6721):659. https://doi.org/10.1038/17709 .
Morin X, Lechowicz MJ, Augspurger C, O’keefe J, Viner D, Chuine I. Leaf phenology in 22 North American tree species during the 21st century. Glob Change Biol. 2009;15(4):961–75.
Deslauriers A, Morin H, Begin Y. Cellular phenology of annual ring formation of Abies balsamea in the Quebec boreal forest (Canada). Can J For Res. 2003;33(2):190–200.
Auty D, Achim A. The relationship between standing tree acoustic assessment and timber quality in Scots pine and the practical implications for assessing timber quality from naturally regenerated stands. Forestry. 2008;81(4):475–87.
Piao S, Liu Q, Chen A, Janssens IA, Fu Y, Dai J, et al. Plant phenology and global climate change: Current progresses and challenges. Glob Change Biol. 2019;25(6):1922–40.
Babst F, Bouriaud O, Poulter B, Trouet V, Girardin MP, Frank DC. Twentieth century redistribution in climatic drivers of global tree growth. Sci Adv. 2019;5(1):eaat4313. The paper highlights that water availability has replaced energy as the dominant limiting factor of tree growth across large portions of the boreal zone. This evidence suggests that drought is becoming the dominant limitation of tree growth worldwide .
Kurz-Besson CB, Lousada JL, Gaspar MJ, Correia IE, David TS, Soares PMM et al. Effects of recent minimum temperature and water deficit increases on Pinus pinaster radial growth and wood density in southern Portugal. Front Plant Sci. 2016;7(1170). https://doi.org/10.3389/fpls.2016.01170
Von Arx G, Archer SR, Hughes MK. Long-term functional plasticity in plant hydraulic architecture in response to supplemental moisture. Ann Bot. 2012;109(6):1091–100.
Primicia I, Camarero JJ, Imbert JB, Castillo FJ. Effects of thinning and canopy type on growth dynamics of Pinus sylvestris: inter-annual variations and intra-annual interactions with microclimate. Eur J Forest Res. 2013;132(1):121–35.
Björklund J, Seftigen K, Schweingruber F, Fonti P, von Arx G, Bryukhanova MV, et al. Cell size and wall dimensions drive distinct variability of earlywood and latewood density in Northern Hemisphere conifers. New Phytol. 2017;216(3):728–40.
McDowell N, Pockman WT, Allen CD, Breshears DD, Cobb N, Kolb T, et al. Mechanisms of plant survival and mortality during drought: why do some plants survive while others succumb to drought? New Phytol. 2008;178(4):719–39.
Pittermann J, Sperry JS, Hacke UG, Wheeler JK, Sikkema EH. Inter-tracheid pitting and the hydraulic efficiency of conifer wood: the role of tracheid allometry and cavitation protection. Am J Bot. 2006;93(9):1265–73.
Fleming RA. Climate change and insect disturbance regimes in Canada’s boreal forests. World Resour Rev. 2000;12(3):521–48.
Seidl R, Thom D, Kautz M, Martin-Benito D, Peltoniemi M, Vacchiano G, et al. Forest disturbances under climate change. Nat Clim Chang. 2017;7(6):395–402.
Jactel H, Koricheva J, Castagneyrol B. Responses of forest insect pests to climate change: not so simple. Curr Opin Insect Sci. 2019;35:103–8. https://doi.org/10.1016/j.cois.2019.07.010 .
Achim A, Moreau G, Coops NC, Axelson JN, Barrette J, Bédard S et al. The changing culture of silviculture. Forestry. 2022;95(2):143–52.
Gardiner B. Wind damage to forests and trees: a review with an emphasis on planted and managed forests. J For Res. 2021;26(4):248–66.
Bauce É, Crépin M, Carisey N. Spruce budworm growth, development and food utilization on young and old balsam fir trees. Oecologia. 1994;97(4):499–507.
Bennett AC, McDowell NG, Allen CD, Anderson-Teixeira KJ. Larger trees suffer most during drought in forests worldwide. Nature Plants. 2015;1(10):15139. https://doi.org/10.1038/nplants.2015.139 .
Kim D-W, Chung W, Lee B. Exploring tree crown spacing and slope interaction effects on fire behavior with a physics-based fire model. For Sci Technol. 2016;12(4):167–75. https://doi.org/10.1080/21580103.2016.1144541 .
Büntgen U, Krusic PJ, Piermattei A, Coomes DA, Esper J, Myglan VS, et al. Limited capacity of tree growth to mitigate the global greenhouse effect under predicted warming. Nat Commun. 2019;10(1):1–6.
Waring RH, Pitman GB. Modifying lodgepole pine stands to change susceptibility to mountain pine beetle attack. Ecology. 1985;66(3):889–97. https://doi.org/10.2307/1940551 .
Dodds KJ, Cooke RR, Hanavan RP. The Effects of Silvicultural Treatment on Sirex noctilio Attacks and Tree Health in Northeastern United States. Forests. 2014;5(11):2810–24.
Bauce É, Fuentealba A. Interactions between stand thinning, site quality and host tree species on spruce budworm biological performance and host tree resistance over a 6 year period after thinning. For Ecol Manage. 2013;304:212–23. https://doi.org/10.1016/j.foreco.2013.05.008 .
Moreau G, Chagnon C, Achim A, Caspersen J, D’Orangeville L, Sánchez-Pinillos M et al. Opportunities and limitations of thinning to increase resistance and resilience of trees and forests to global change. Forestry. 2022;95(5):595–615. The paper reviews recent literature to identify the positive and negative effects of thinning on both stand- and tree-level resistance and resilience to four stressors that are expected to increase in frequency and/or severity due to global change: drought, fire, insects and pathogens, and wind.
Peltola H, Gardiner B, Nicoll B. Mechanics of wind damage. Agric For Meteorol. 2013;151:328–44.
Gardiner B, Barnett J, Saranpää P, Gril J. The biology of reaction wood. Springer; 2014.
Book Google Scholar
Lindroth A, Lagergren F, Grelle A, Klemedtsson L, Langvall O, Weslien P, et al. Storms can cause Europe-wide reduction in forest carbon sink. Glob Change Biol. 2009;15(2):346–55.
Desch HE, Dinwoodie JM. Timber: structure, properties, conversion and use. Macmillan International Higher Education; 1996.
Lenz PRN, Nadeau S, Mottet M-J, Perron M, Isabel N, Beaulieu J, et al. Multi-trait genomic selection for weevil resistance, growth, and wood quality in Norway spruce. Evol Appl. 2020;13(1):76–94. https://doi.org/10.1111/eva.12823 .
Sniezko RA, Koch J. Breeding trees resistant to insects and diseases: putting theory into application. Biol Invasions. 2017;19(11):3377–400. https://doi.org/10.1007/s10530-017-1482-5 .
Naidoo S, Slippers B, Plett JM, Coles D, Oates CN. The road to resistance in forest trees. Front Plant Sci. 2019;10:273.
Drew DM, Downes GM, Seifert T, Eckes-Shepard A, Achim A. A Review of Progress and Applications in Wood Quality Modelling. Current Forestry Reports; 2022. p. 1–16. The paper reviews models that predict wood quality in standing trees. They propose a simple classification of wood quality models into two broad groupings: fully empirical and process-based. Comprehensive summaries of a wide range of published models in both categories are given.
Lachenbruch B, Moore JR, Evans R. Radial variation in wood structure and function in woody plants, and hypotheses for its occurrence. Size-and age-related changes in tree structure and function. Springer; 2011. pp. 121–64.
Auty D, Achim A, Macdonald E, Cameron AD, Gardiner BA. Models for predicting wood density variation in Scots pine. Forestry: Int J For Res. 2014;87(3):449–58.
Auty D, Achim A, Macdonald E, Cameron AD, Gardiner BA. Models for predicting clearwood mechanical properties of Scots pine. For Sci. 2016;62(4):403–13.
Gardiner B, Leban J-M, Auty D, Simpson H. Models for predicting wood density of British-grown Sitka spruce. Forestry. 2011;84(2):119–32.
Leban J-M, Haines DW. The modulus of elasticity of hybrid larch predicted by density, rings per centimeter, and age. Wood Fiber Sci. 1999;31(4):394–402.
Schimleck LR, Antony F, Mora C, Dahlen J. Mapping and modeling within-tree variation for loblolly pine pulp yield and lignin content. SN Appl Sci. 2021;3(4):468. https://doi.org/10.1007/s42452-021-04443-5 .
Houllier F, Leban J-M, Colin F. Linking growth modelling to timber quality assessment for Norway spruce. For Ecol Manage. 1995;74(1–3):91–102.
Bontemps J-D, Esper J. Statistical modelling and RCS detrending methods provide similar estimates of long-term trend in radial growth of common beech in north-eastern France. Dendrochronologia. 2011;29(2):99–107.
Auty D, Moore J, Achim A, Lyon A, Mochan S, Gardiner B. Effects of early respacing on the density and microfibril angle of Sitka spruce wood. Forestry: Int J For Res. 2018;91(3):307–19.
Soro A, Lenz P, Hassegawa M, Roussel J-R, Bousquet J, Achim A. Genetic influence on components of wood density variation in white spruce. Forestry: Int J For Res. 2021.
Drew DM, Allen K, Downes GM, Evans R, Battaglia M, Baker P. Wood properties in a long-lived conifer reveal strong climate signals where ring-width series do not. Tree Physiol. 2013;33(1):37–47.
Vaughan D, Auty D, Kolb T, Dahlen J, Sánchez Meador AJ, Mackes KH. Wood density variation in naturally regenerated stands of Pinus ponderosa in northern Arizona, USA. Can J For Res. 2021;51(4):583–94.
Franceschini T, Longuetaud F, Bontemps J-D, Bouriaud O, Caritey B-D, Leban J-M. Effect of ring width, cambial age, and climatic variables on the within-ring wood density profile of Norway spruce Picea abies (L.) Karst. Trees. 2013;27(4):913–25.
Wood LJ, Smith DJ, Hartley ID. Predicting softwood quality attributes from climate data in interior British Columbia, Canada. For Ecol Manag. 2016;361:81–9.
Xiang W, Auty D, Franceschini T, Leitch M, Achim A. Wood density-climate relationships are mediated by dominance class in black spruce (Picea mariana (Mill.) BSP). Forests. 2014;5(6):1163–84.
Candel-Pérez D, Lo Y-H, Blanco JA, Chiu C-M, Camarero JJ, González de Andrés E, et al. Drought-induced changes in wood density are not prevented by thinning in scots pine stands. Forests. 2018;9(1):4.
Benomar L, Bousquet J, Perron M, Beaulieu J, Lamara M. Tree maladaptation under mid-latitude early spring warming and late cold spell: implications for assisted migration. Front Plant Sci. 2022:2086.
Payette S, Fortin M-J, Morneau C. The recent sugar maple decline in southern Quebec: probable causes deduced from tree rings. Can J For Res. 1996;26(6):1069–78.
Moreau G, Chagnon C, Auty D, Caspersen J, Achim A. Impacts of climatic variation on the growth of black spruce across the forest-tundra ecotone: positive effects of warm growing seasons and heat waves are offset by late spring frosts. Front For Glob Change. 2020;3:613523.
Babst F, Bodesheim P, Charney N, Friend AD, Girardin MP, Klesse S, et al. When tree rings go global: Challenges and opportunities for retro-and prospective insight. Quatern Sci Rev. 2018;197:1–20.
West G, Moore J, Shula R, Harrington J, Snook J, Gordon J et al. Forest management DSS development in New Zealand. Implementation of DSS tools into the forestry practice. 2013:153–63.
Anderegg WRL, Hicke JA, Fisher RA, Allen CD, Aukema J, Bentz B, et al. Tree mortality from drought, insects, and their interactions in a changing climate. New Phytol. 2015;208(3):674–83. https://doi.org/10.1111/nph.13477 .
Dufour-Kowalski S, Courbaud B, Dreyfus P, Meredieu C, De Coligny F. Capsis: an open software framework and community for forest growth modelling. Ann For Sci. 2012;69(2):221–33.
Jacquin P, Mothe F, Longuetaud F, Billard A, Kerfriden B, Leban J-M. CarDen: a software for fast measurement of wood density on increment cores by CT scanning. Comput Electron Agric. 2019;156:606–17.
Freyburger C, Longuetaud F, Mothe F, Constant T, Leban J-M. Measuring wood density by means of X-ray computer tomography. Ann For Sci. 2009;66(8):804.
Dierick M, Van Loo D, Masschaele B, Van den Bulcke J, Van Acker J, Cnudde V, et al. Recent micro-CT scanner developments at UGCT. Nucl Instrum Methods Phys Res, Sect B. 2014;324:35–40.
Kharrat W, Koubaa A, Khlif M, Bradai C. Intra-ring wood density and dynamic modulus of elasticity profiles for black spruce and jack pine from X-ray densitometry and ultrasonic wave velocity measurement. Forests. 2019;10(7):569.
Luther JE, Skinner R, Fournier RA, van Lier OR, Bowers WW, Coté J-F, et al. Predicting wood quantity and quality attributes of balsam fir and black spruce using airborne laser scanner data. Forestry. 2014;87(2):313–26.
Hilker T, Frazer GW, Coops NC, Wulder MA, Newnham GJ, Stewart JD, et al. Prediction of wood fiber attributes from LiDAR-derived forest canopy indicators. Forest Sci. 2013;59(2):231–42.
Van Leeuwen M, Hilker T, Coops NC, Frazer G, Wulder MA, Newnham GJ, et al. Assessment of standing wood and fiber quality using ground and airborne laser scanning: a review. For Ecol Manage. 2011;261(9):1467–78.
Pyörälä J, Saarinen N, Kankare V, Coops NC, Liang X, Wang Y, et al. Variability of wood properties using airborne and terrestrial laser scanning. Remote Sens Environ. 2019;235:111474.
Barton CV. Advances in remote sensing of plant stress. Plant Soil. 2012;354(1):41–4.
Hilker T, Coops NC, Hall FG, Nichol CJ, Lyapustin A, Black TA et al. Inferring terrestrial photosynthetic light use efficiency of temperate ecosystems from space. J Geophys Res: Biogeosci. 2011;116(G3).
Dickinson Y, Wang X, Wiedenbeck J, Ross RJ. Effects of silviculture practices on engineering properties of northern hardwood species of the Great Lakes Region: a literature review. Gen Tech Rep FPL-GTR-269 Madison, WI: US Department of Agriculture, Forest Service, Forest Products Laboratory, 2019;17:269.
Moore JR, Cown DJ. Corewood (Juvenile Wood) and Its Impact on Wood Utilisation. Current Forestry Reports. 2017;3(2):107–18. https://doi.org/10.1007/s40725-017-0055-2 . This review focuses on describing the characteristics of corewood, the factors affecting its occurrence within a tree and the implications for wood utilisation .
Briggs D. Enhancing forest value productivity through fiber quality. J Forest. 2010;108(4):174–82.
Cave I, Walker J. Stiffness of wood in fast-grown plantation softwoods: the influence of microfibril angle. For Prod J. 1994;44(5):43.
Giroud G, Defo M, Bégin J. Determination of radial profiles of wood properties using a near infrared scanning system. J Near Infrared Spectrosc. 2021;29(1):24–32.
Auty D, Gardiner BA, Achim A, Moore JR, Cameron AD. Models for predicting microfibril angle variation in Scots pine. Ann For Sci. 2013;70(2):209–18.
Moore JR, Cown DJ, McKinley RB. Modelling microfibril angle variation in New Zealand-grown radiata pine. NZ J Forest Sci. 2014;44(1):1–11.
Telewski F. Structure and function of flexure wood in Abies fraseri. Tree Physiol. 1989;5(1):113–21.
Wang S-Y, Chen J-H, Tsai M-J, Lin C-J, Yang T-H. Grading of softwood lumber using non-destructive techniques. J Mater Process Technol. 2008;208(1):149–58. https://doi.org/10.1016/j.jmatprotec.2007.12.105 .
Moore JR, Lyon AJ, Searles GJ, Lehneke SA, Ridley-Ellis DJ. Within-and between-stand variation in selected properties of Sitka spruce sawn timber in the UK: implications for segregation and grade recovery. Ann For Sci. 2013;70(4):403–15.
Ridley-Ellis D, Gil-Moreno D, Harte AM. Strength grading of timber in the UK and Ireland in. Int Wood Prod J. 2021;2022:1–10.
Download references
Acknowledgements
This work was conducted as part of project number 142332173 led by Julie Barrette at the Direction de la Recherche Forestière (ministère des Ressources Naturelles et des Forêts, Québec, Canada) and took part of the network Matériaux Renouvelables Québec. We would like to thank Jean Noël for the production of Fig. 1 as well as two anonymous reviewers for providing insightful suggestions on a previous version of the manuscript.
Author information
Authors and affiliations.
Direction de La Recherche Forestière, Ministère Des Ressources Naturelles Et Des Forêts, Quebec, Canada
Julie Barrette
Centre de Recherche Sur Les Matériaux Renouvelables, Université Laval, Département Des Sciences du Bois Et de La Forêt, Quebec, Canada
Julie Barrette & Alexis Achim
School of Forestry, Northern Arizona University, 200 East Pine Knoll Drive, Flagstaff, AZ, 86011, USA
You can also search for this author in PubMed Google Scholar
Corresponding author
Correspondence to Julie Barrette .
Ethics declarations
Conflict of interest.
The authors declare that they have no conflict of interest.
Additional information
Publisher's note.
Springer Nature remains neutral with regard to jurisdictional claims in published maps and institutional affiliations.
This article is part of the Topical Collection on Wood Structure and Function
Rights and permissions
Open Access This article is licensed under a Creative Commons Attribution 4.0 International License, which permits use, sharing, adaptation, distribution and reproduction in any medium or format, as long as you give appropriate credit to the original author(s) and the source, provide a link to the Creative Commons licence, and indicate if changes were made. The images or other third party material in this article are included in the article's Creative Commons licence, unless indicated otherwise in a credit line to the material. If material is not included in the article's Creative Commons licence and your intended use is not permitted by statutory regulation or exceeds the permitted use, you will need to obtain permission directly from the copyright holder. To view a copy of this licence, visit http://creativecommons.org/licenses/by/4.0/ .
Reprints and permissions
About this article
Barrette, J., Achim, A. & Auty, D. Impact of Intensive Forest Management Practices on Wood Quality from Conifers: Literature Review and Reflection on Future Challenges. Curr Forestry Rep 9 , 101–130 (2023). https://doi.org/10.1007/s40725-023-00181-6
Download citation
Accepted : 30 January 2023
Published : 08 March 2023
Issue Date : April 2023
DOI : https://doi.org/10.1007/s40725-023-00181-6
Share this article
Anyone you share the following link with will be able to read this content:
Sorry, a shareable link is not currently available for this article.
Provided by the Springer Nature SharedIt content-sharing initiative
- Wood quality
- Intensive forest management
- Silvicultural treatments
- Future challenges
- Find a journal
- Publish with us
- Track your research
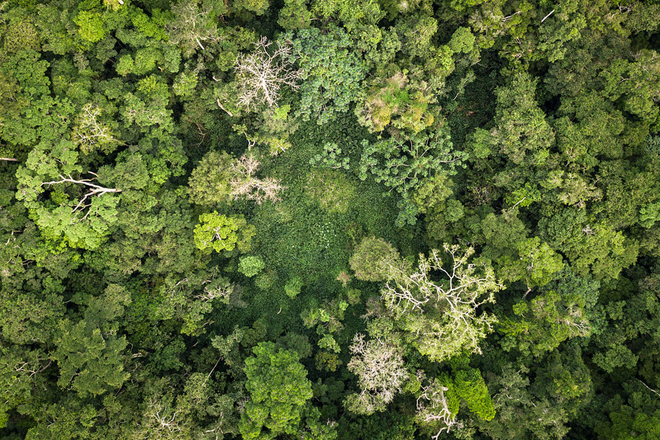
{{Global challenges}}
- {{Deforestation and biodiversity loss}}
- {{A climate in crisis}}
- {{Transforming food systems}}
- {{Unsustainable supply and value chains}}
- {{Extreme inequality}}
{{Governance}}
- {{Board of Trustees}}
- {{Leadership}}
{{CIFOR-ICRAF}}
- {{About CIFOR-ICRAF}}
- {{Partners}}
- {{Our network}}
- {{Work with us}}
- {{Contact us}}
{{Institutional Documents}}
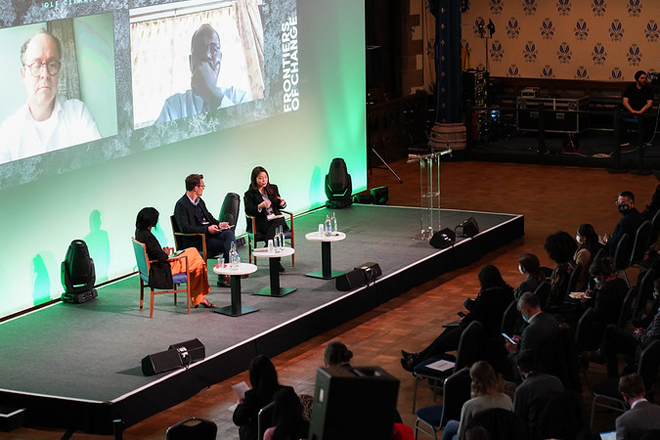
Explore eventos futuros e passados em todo o mundo e online, sejam hospedados pelo CIFOR-ICRAF ou com a participação de nossos pesquisadores.
UPCOMING EVENTS
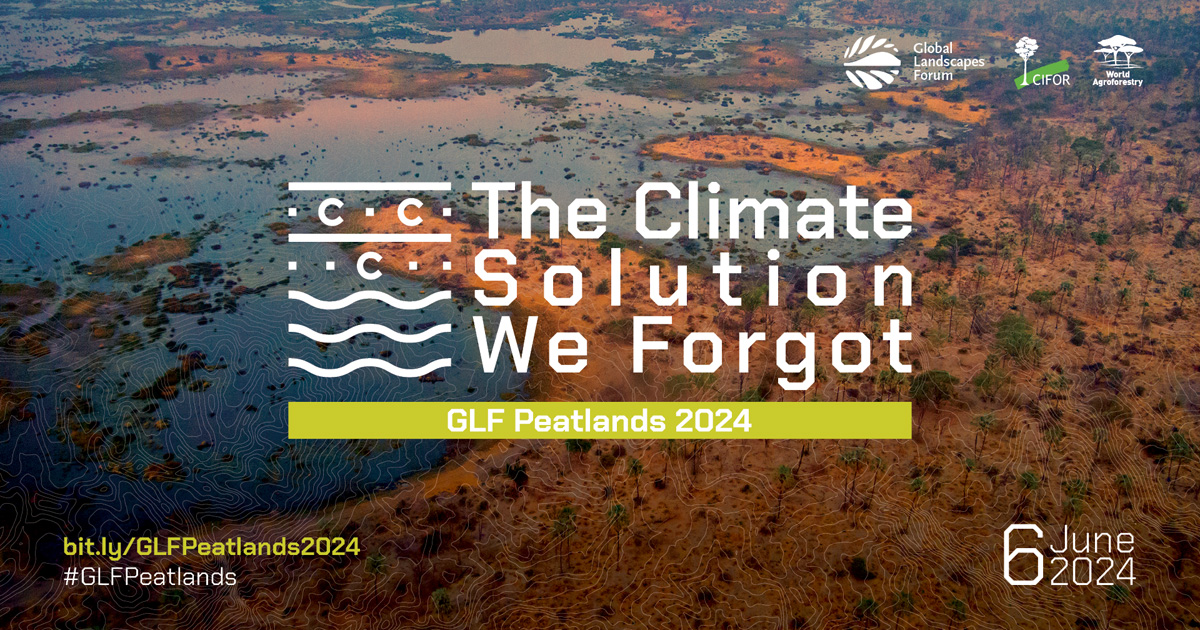
GLF Peatlands 2024: The Climate Solution We Forgot
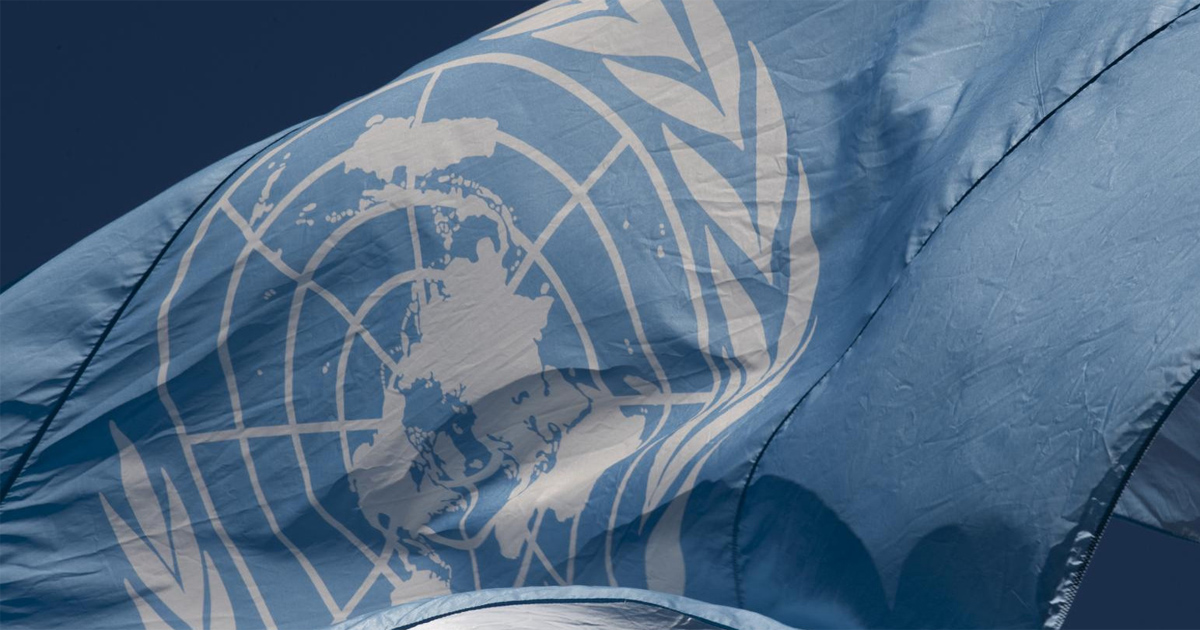
60th Sessions of the UNFCCC Subsidiary Bodies
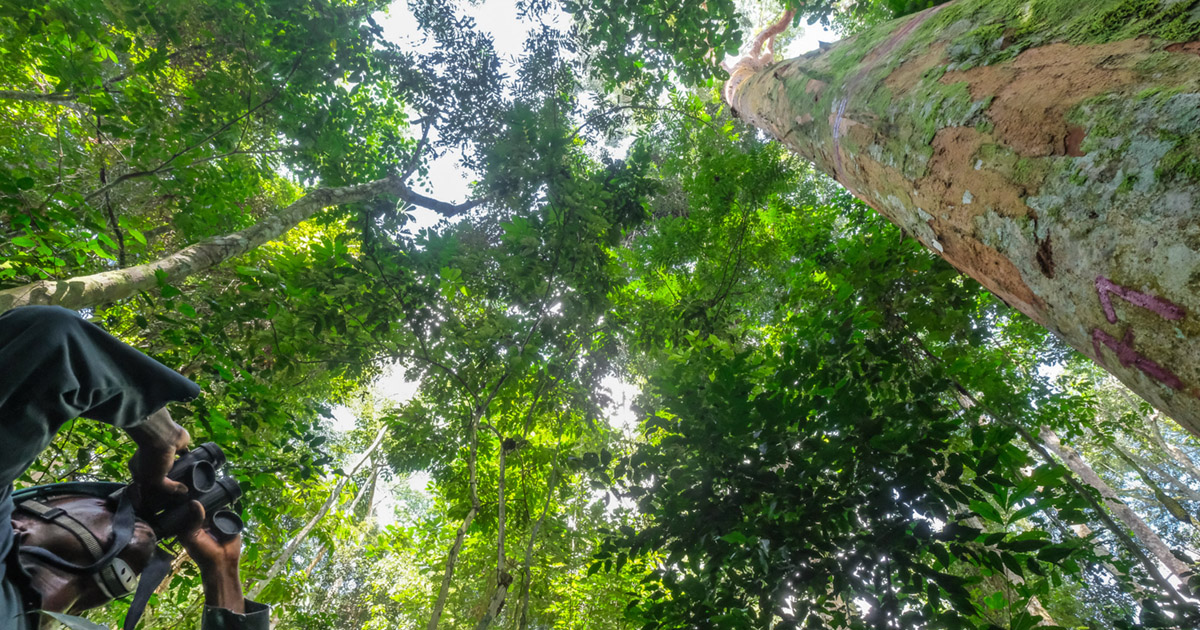
Forests & people: CIFOR-ICRAF at IUFRO 2024
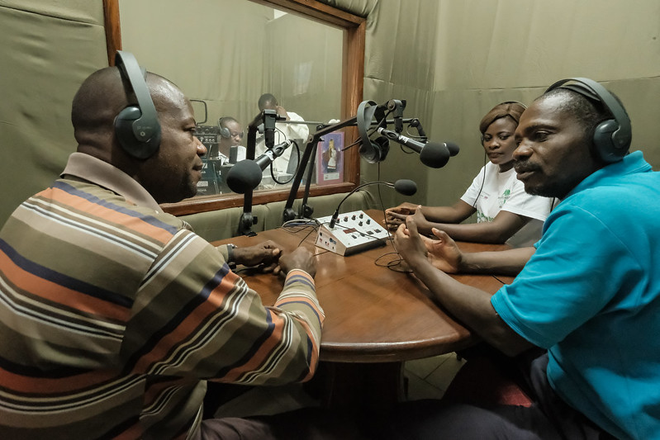
- Notícias sobre florestas
- Notícias do mundo agroflorestal
- Notícias de paisagem
- Mídia social
- Notícias corporativas
- Comunicados de imprensa
ÚLTIMAS NOTÍCIAS
A ciência precisa de canais de comunicação claros para cortar o ruído, para que a pesquisa tenha algum impacto. O CIFOR-ICRAF é tão apaixonado por compartilhar nosso conhecimento quanto por gerá-lo.
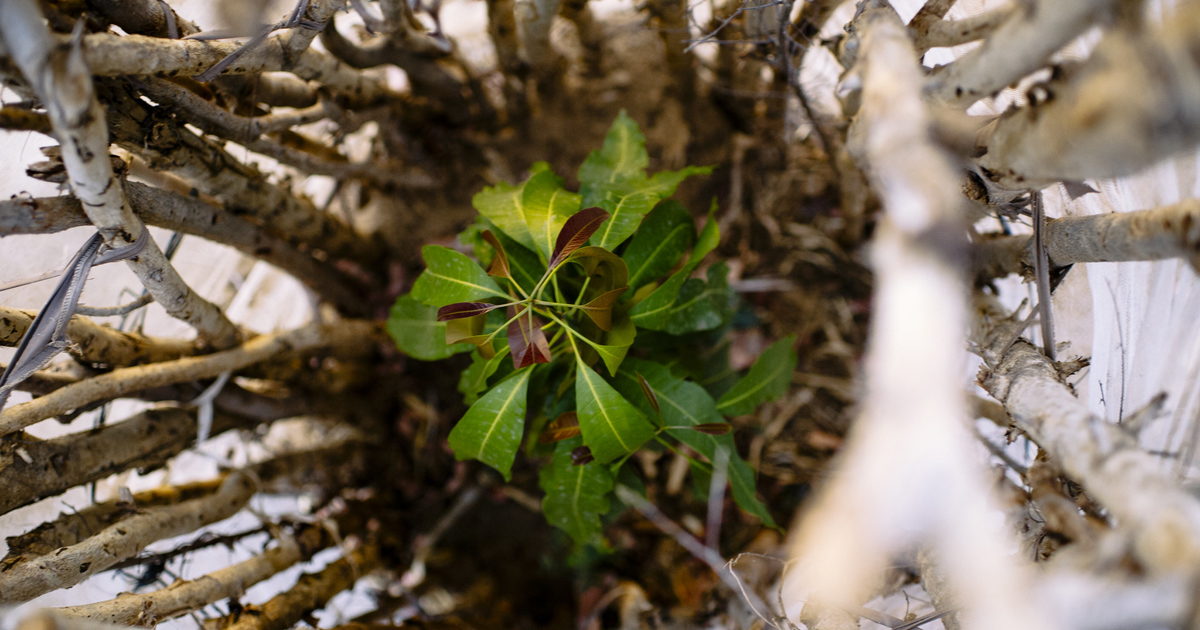
Research paper on Africa’s ‘forgotten’ foods wins prestigious Cozzarelli Prize in Washington, DC
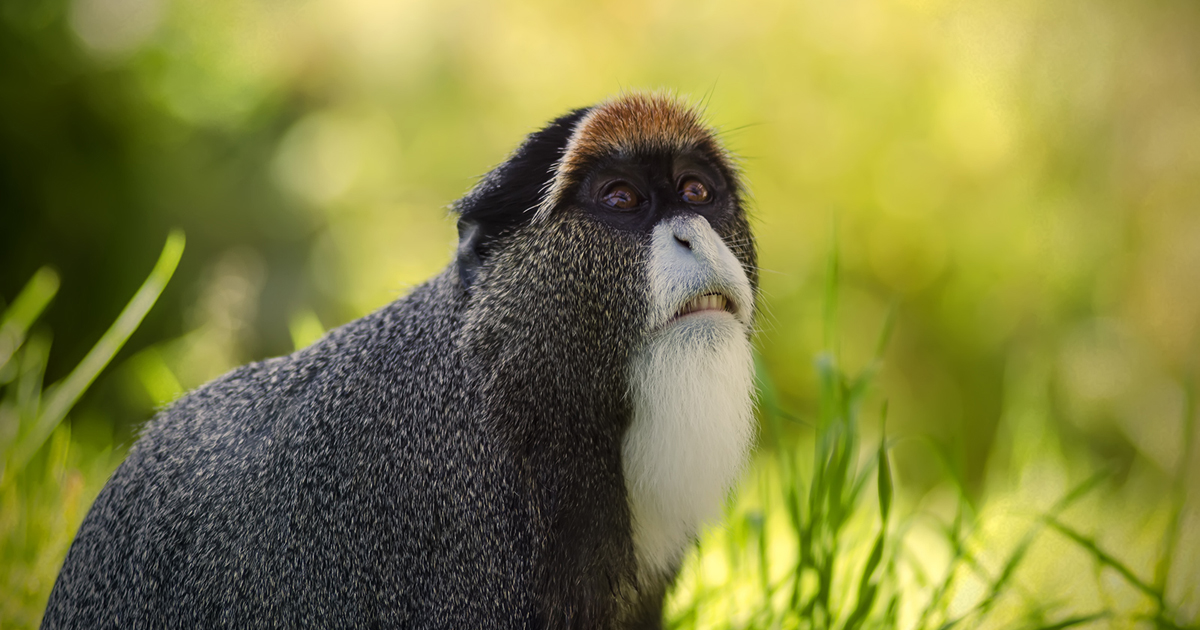
Lancement du Programme de gestion durable de la faune au Cameroun : La faune camerounaise sous les feux des projecteurs !
Découvrez les évènements passés et à venir dans le monde entier et en ligne, qu’ils soient organisés par le CIFOR-ICRAF ou auxquels participent nos chercheurs.
Jelajahi acara-acara mendatang dan yang telah lalu di lintas global dan daring, baik itu diselenggarakan oleh CIFOR-ICRAF atau dihadiri para peneliti kami.
- Actualités sur les forêts
- Actualités sur le monde de l’agroforesterie
- Actualités sur les paysages
- Réseaux sociaux
- Actualités des entreprises
- Communiqués de presse
Pour que la recherche ait un impact, la science a besoin de canaux de communication clairs pour aller droit au but. CIFOR-ICRAF est aussi passionné par le partage de ses connaissances que par leur production.
- Los Bosques en las Noticias
- Agroforestry World News
- Landscape News
- Redes sociales
- Noticias corporativas
- Notas de prensa
ÚLTIMAS NOTICIAS
Para que la investigación pueda generar algún impacto, los conocimientos científicos requieren de canales de comunicación claros. En CIFOR-ICRAF, compartir nuestros conocimientos nos apasiona tanto como generarlos.
Explore eventos futuros y pasados organizados por CIFOR-ICRAF o con la participación de nuestros investigadores.
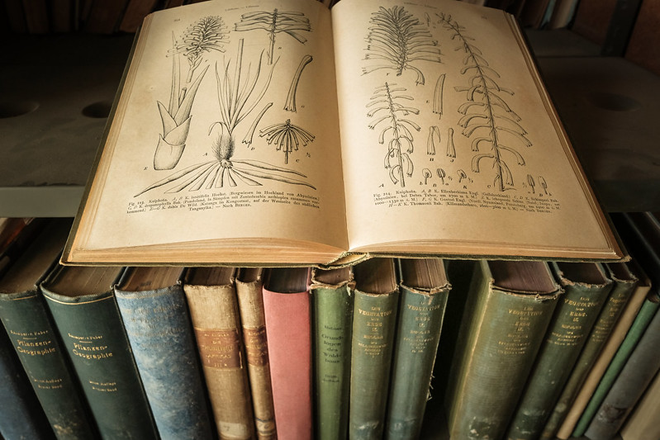
{{menu_nowledge_desc}}.
- {{Publications}}
- {{Presentations}}
- {{Datasets}}
- {{Podcasts}}
- Project database
{{menu_nowledge_tab_2}}
- {{Burkina Faso}}
- {{Cameroon}}
- {{Cote D’Ivoire}}
- {{Ethiopia}}
- {{Tanzania}}
- {{Bangladesh}}
- {{Indonesia}}
- {{Kyrgyzstan}}
- {{Philippines}}
- {{Sri Lanka}}
- {{Viet Nam}}
{{Latin America}}
- {{Germany}}
- Kabar Hutan
- World Agroforestry News
- Media sosial
- Kabar korporat
- Media rilis
Kabar terbaru
Ilmu pengetahuan membutuhkan saluran komunikasi yang jelas untuk mencapai tujuan, jika ingin dampaknya terlihat. CIFOR-ICRAF sangat bersemangat untuk berbagi pengetahuan sembari menghasilkan pengetahuan itu sendiri.
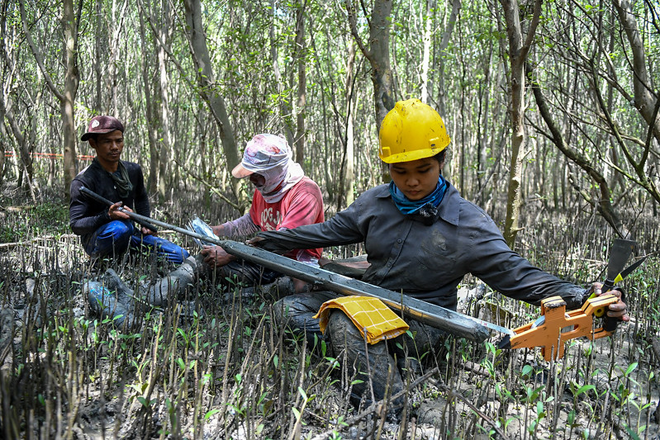
{{menu_research_desc}}
- {{Agroecology}}
- {{Agroforestry}}
- {{Biodiversity}}
- {{Bioenergy}}
- {{Climate change}}
- {{Finance, trade and investment}}
- {{Fire and haze}}
- {{Food security and nutrition}}
- {{Forest and landscape restoration}}
- {{Forest management}}
- {{Livelihoods}}
- {{Oil palm}}
- {{Soil health}}
- {{Tenure and tenure reform}}
- {{Tree genetic resources (TGR)}}
- {{Tree planting}}
- {{Wetlands and blue carbon}}
- {{Wild meat}}
- {{Trees and forest genetic resources, and biodiversity}}
- {{Sustainable value chains and investments}}
- {{Governance, equity and well-being}}
- {{Climate change, energy and low-carbon development}}
- {{Soil and land health}}
{{Innovations}}
- {{Transformative Partnership Platforms}}
- {{Engagement Landscapes}}
- {{Flagship Products}}
{{Flagship products}}
Our solutions to five global challenges.
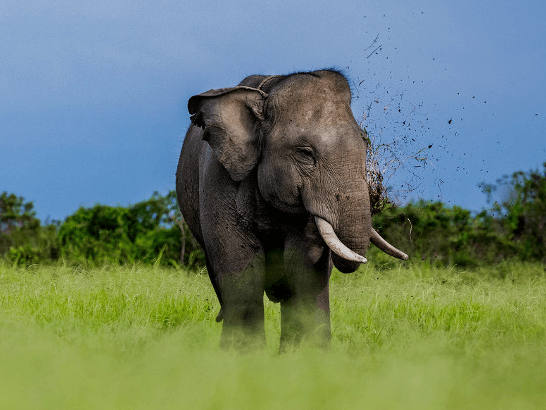
Biodiversity
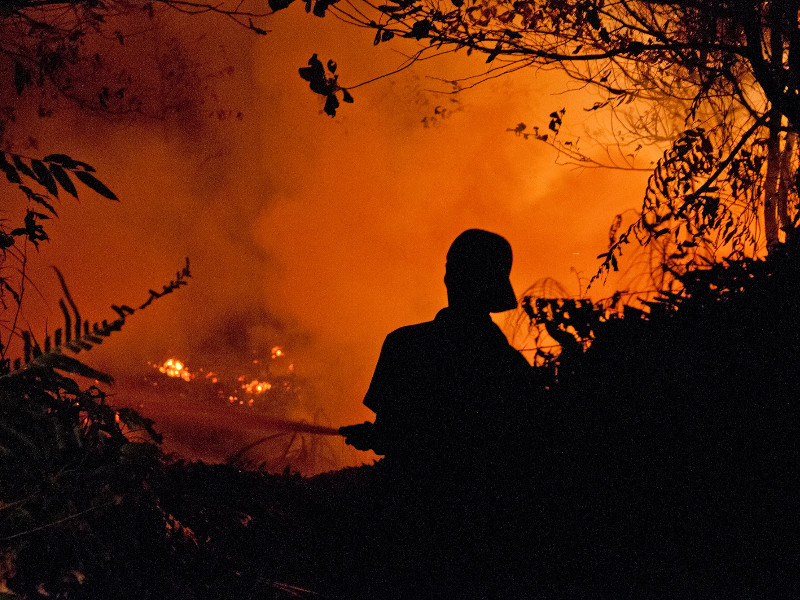
Value chains
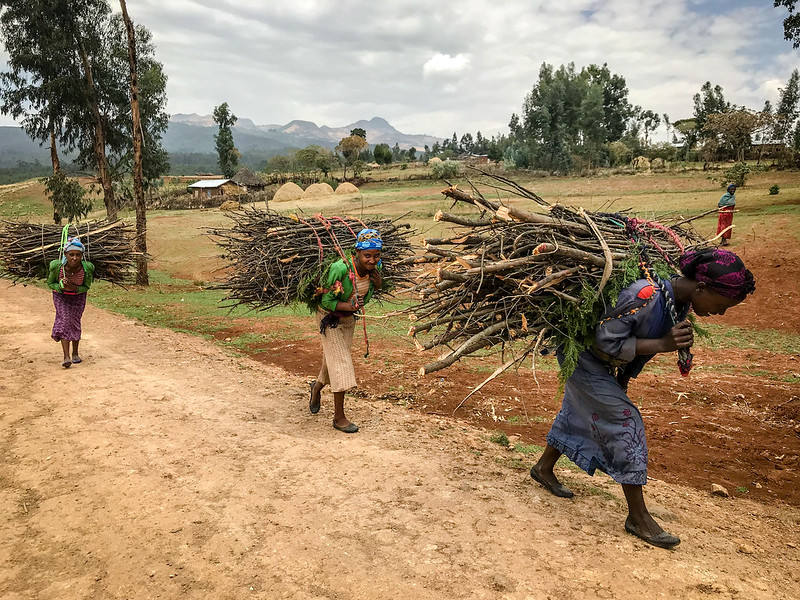
CIFOR–ICRAF achieves science-driven impact. We conduct innovative research, strengthen partners’ capacity and actively engage in dialogue with all stakeholders, bringing the latest insights on forests, trees, landscapes and people to global decision making.
- Agroecology
- Agroforestry
- Climate change
- Finance, trade and investment
- Fire and haze
- Food security and nutrition
- Forest and landscape restoration
- Forest management
- Livelihoods
- Multi-stakeholder platforms
- Soil health
- Tenure and tenure reform
- Tree genetic resources (TGR)
- Tree planting
- Wetlands and blue carbon
- Trees and forest genetic resources, and biodiversity
- Sustainable value chains and investments
- Governance, equity and well-being
- Climate change, energy and low-carbon development
- Soil and land health
- Innovations
- Transformative Partnership Platforms
- Engagement Landscapes
- Flagship Products
Flagship products
- Burkina Faso
- Cote D’Ivoire
- Philippines
Latin America
CIFOR–ICRAF publishes over 750 publications every year on agroforestry, forests and climate change, landscape restoration, rights, forest policy and much more – in multiple languages.
Explore our knowledge
Browse CIFOR–ICRAF’s published research in a wide range of formats, all of which are available for free online.
- Publications
- Presentations
This section includes knowledge products from CIFOR and ICRAF published since 2016. Please visit cifor.org or worldagroforestry.org for older publications, which will be added to this site over time.
Explore upcoming and past events across the globe and online, whether hosted by CIFOR-ICRAF or attended by our researchers.
- Forests News
- Social media
- Corporate news
- Press releases
- Media inquiries
Latest news
Science needs clear communication channels to cut through the noise, if research is to have any impact. CIFOR-ICRAF is as passionate about sharing our knowledge as we are in generating it.
CIFOR–ICRAF addresses local challenges and opportunities while providing solutions to global problems for forests, landscapes, people and the planet.
We deliver actionable evidence and solutions to transform how land is used and how food is produced: conserving and restoring ecosystems, responding to the global climate, malnutrition, biodiversity and desertification crises. In short, improving people’s lives.
Global challenges
- Deforestation and biodiversity loss
- A climate in crisis
- Transforming food systems
- Unsustainable supply and value chains
- Extreme inequality
- Board of Trustees
CIFOR-ICRAF
- About CIFOR-ICRAF
- Our network
- Work with us
Institutional Documents
Trees people planet, community forest management in the peruvian amazon: a literature review.

Export citation
Altmetric score:
Dimensions citation count:.
Publication year
Cossío, R. ; Menton, M. ; Cronkleton, P. ; Larson, A.M.
community forestry, government organizations
Related publications

Community forest management and the emergence of multi-scale governance institutions

Manejo forestal comunitario en la Amazonía peruana: Una revisión bibliográfica

Towards responsible and inclusive financing of the palm oil sector
Community forestry: current innovations and experiences
For general inquiries.
The Center for International Forestry Research and World Agroforestry (CIFOR-ICRAF) harnesses the power of trees, forests and agroforestry landscapes to address the most pressing global challenges of our time – biodiversity loss, climate change, food security, livelihoods and inequity. CIFOR and ICRAF are CGIAR Research Centers.
For general inquiries:
For media inquiries contact:.
- Five global challenges
GLOBAL OFFICES
Bangladesh, Benin, Brazil, Burkina Faso, Cameroon, China, Côte d’Ivoire, Democratic Republic of the Congo, Ethiopia, Germany, India, Indonesia, Kenya, Kyrgyzstan, Malawi, Mali, Nepal, Peru, Philippines, Rwanda, Sri Lanka, Tanzania, Uganda, Viet Nam, Zambia
Subscribe to the CIFOR-ICRAF news update
Privacy notice Term of use -->
© 2024 Center for International Forestry Research (CIFOR) and World Agroforestry (ICRAF) | CIFOR and ICRAF are CGIAR Research Centers | Privacy notice of CIFOR-ICRAF
This website uses cookies so that we can provide you with the best website experience. By clicking “I Accept” you acknowledge the use of cookies and to our Privacy Notice ( CIFOR-ICRAF ) and our Terms of Use ( CIFOR and ICRAF ).
Please use a modern browser for a better browsing experience. X
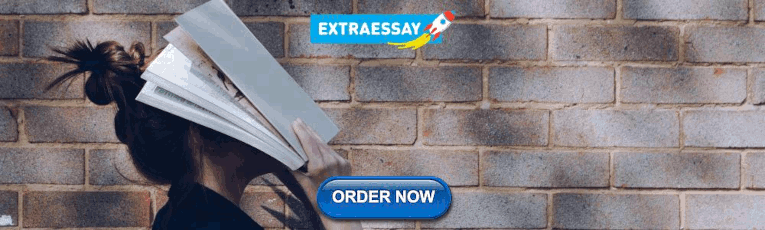
IMAGES
VIDEO
COMMENTS
Context Globally, forest landscapes are rapidly transforming, with the role of institutions as mediators in their use and management constantly appearing in the literature. However, global comparative reviews to enhance comprehension of how forest management institutions (FMIs) are conceptualized, and the varying determinants of compliance, are lacking. And so too, is there knowledge ...
Review and synthesis Effectiveness of forestry best management practices in the United States: Literature review Richard Cristana,⇑, W. Michael Austa, M. Chad Boldinga, Scott M. Barretta, John F. Munsellb, Erik Schillingc a Department of Forest Resources & Environmental Conservation (FREC), Virginia Tech, 228 Cheatham Hall, 310 West Campus Drive, VA 24061, USA
Explore the latest full-text research PDFs, articles, conference papers, preprints and more on FOREST MANAGEMENT. Find methods information, sources, references or conduct a literature review on ...
An analysis of 643 cases of community forest management shows that most led to improved environmental and income outcomes, but access and resource rights often deteriorate when formalizing such ...
The study is based on a review of literature on climate change impacts on forests and adaptation options for forest management identified in the Web of Science database, focusing on papers and reports published between 1945 and 2013. ... Adaptation of forest management to climate change requires an understanding of the effects of climate on ...
Introduction. Sustainable forest management is a traditional research field with an outstanding history. Since Von Carlowitz (Citation 1713) practically invented the term sustainability in the context of forest management, the general understanding of what sustainable forest management (SFM) is, has undertaken an evolutionary process.Between 1980 and 1990 research communities increasingly ...
1 INTRODUCTION. Land management strongly affects the ability of ecosystems to sequester and store carbon (C). Natural climate solutions (NCS), a set of land management, conservation and restoration practices aimed at mitigating climate change, have been introduced as cost-effective tools that increase C sequestration in terrestrial ecosystems (Fargione et al., 2018; Griscom et al., 2017 ...
The systemic literature review on the environmental impacts of FCs started with twelve records provided by the Forest and Nature Conservation Policy group (FNP) of Wageningen University and Research during the month of March 2018. ... certified forest management units succeed in providing better living conditions for workers and their family ...
Our goals are to inform forest management decision making to increase forest resilience, and to identify the most important evidence gaps for future research. ... We carried out a literature review using a systematic search method to identify published sources of empirical data on the relationship between forest management and resilience to ...
Species Distribution Modelling (SDM) techniques were originally developed in the mid-1980s. In this century they are gaining increasing attention in the literature and in practical use as a powerful tool to support forest management strategies especially under climate change. In this review paper we consider species occurrence datasets ...
Purpose of Review This review aims to discover the most common topics and trends in international scientific forest sector research between January 2000 and December 2019 and to test the suitability of a quantitative topic-modeling method to extract topics from the data. The results will be helpful for both researchers and policy decision-makers in identifying emerging research topics and ...
These previous literature reviews focus on broader categories of operations such as timber harvesting, site preparation, and biomass harvesting while this review focuses on more specific operational categories (timber harvesting, skid trails, forest roads, streamside management zones, site preparation, etc.) and seeks to comprehensively ...
It's not specifically from the point of information technology but from a general point of view. Thinking of the title of this conference, 'neural networks and artificial intelligence,' the talk follows this literature review on the topic of forest management by neural network and/or artificial intelligence.
Literature review on forest management and climate change. This literature review assesses current and potential future changes occurring within the forestry sector. It identifies challenges posed to forests and analyses the relationship between forests and climate change. While it is relatively safe to assume that temperature increase is a ...
Globally, forest landscapes are rapidly transforming, with the role of institutions as mediators in their use and management constantly appearing in the literature. However, global comparative reviews to enhance comprehension of how forest management institutions (FMIs) are conceptualized, and the varying determinants of compliance, are lacking.
This document summarizes knowledge and experiences in forest management as a response to climate change, based on a literature review and a survey of forest managers. This is part of an FAO-led process to prepare climate change guidelines for forest managers. It examines climate change impacts on forests and forest managers throughout the world.
Collaborative forest management: a review. R. John Petheram 1,2, Peter Stephen3 and Don Gilmour4. 1 School of Forest and Ecosystem Science, Creswick Campus, The University of Melbourne, Victoria ...
The approach for monitoring forest health such as canopy layer, air quality, soil texture has evolved in tandem with the advancement of new technology such as lab analysis, remote sensing etc. The application of biomonitoring techniques for example species diversity and morphological observation, on the other hand, has been positive and has made its own contribution to forest management. Many ...
Explore the latest full-text research PDFs, articles, conference papers, preprints and more on FOREST CONSERVATION. Find methods information, sources, references or conduct a literature review on ...
The document summarizes knowledge and experiences in forest management as a response to climate change, based on a literature review and a survey of forest managers. This is part of the publications series produced by the Forest and Climate Change Programme of FAO. The programme seeks to provide timely information and tools to a wide range of ...
Despite growing recognition of nature-based solutions (NBS), there remains a research gap in understanding their implementation in urban areas, which poses a significant challenge for urban forest development. Therefore, our paper aims to explore the intersection of NBS with urban forests (UF), identify current barriers, propose strategies to maximize the potential of urban forests as nature ...
Purpose of Review Intensive forest management practices are being implemented worldwide to meet future global demand for wood and wood products while facilitating the protection of natural forest ecosystems. A potential decline in wood properties associated with rapid tree growth makes it essential to quantify the potential impact of intensive management on the process of wood formation and ...
this literature review revealed that forest soils provide a predominantly differentiated soil structure—the basis for their high ecological functionality. The review also revealed that active management measures must be set in motion to preserve the vulnerable functional structures of forest soils under fast-changing environmental conditions ...
This review summarizes the published literature, as well as any available information provided by NGOs or project proponents, on the practice of community forest management (CFM) in the Peruvian Amazon. It provides an overview of literature related to land-use and forest management by rural populations in the Peruvian Amazon, placing this information in the broader context of the forestry ...