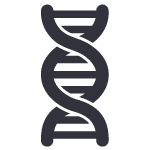
- DNA Replication
- Active Transport
- Cellular Receptors
- Endocytosis and Exocytosis
- Enzyme Inhibition
- Enzyme Kinetics
- Protein Structure
- Transcription of DNA
- Translation of DNA
- Anaerobic Respiration
- Electron Transport Chain
- Gluconeogenesis
- Calcium Regulation
- External Balance of Potassium
- Internal Balance of Potassium
- Sodium Regulation
- Cell Membrane
- Endoplasmic Reticulum
- Golgi Apparatus
- Mitochondria
- Blood Vessels
- Cellular Adaptations
- Epithelial Cells
- Muscle Histology
- Structure of Glands
- Control of Stroke Volume
- Control of Heart Rate
- Cardiac Cycle
- Cardiac Pacemaker Cells
- Conduction System
- Contraction of Cardiac Muscle
- Ventricular Action Potentials
- Blood Flow in Vessels
- Control of Blood Pressure
- Capillary Exchange
- Flow In Peripheral Circulation
- Venous Return
- Cardiac Muscle
- Hepatic Circulation
- Skeletal Muscle
- Airway Resistance
- Lung Volumes
- Mechanics of Breathing
- Gas Exchange
- Oxygen Transport in The Blood
- Transport of Carbon Dioxide in the Blood
- Ventilation-Perfusion Matching
- Chemoreceptors
- Cough Reflex
- Neural Control of Ventilation
- Respiratory Regulation of Acid-Base Balance
- Responses of The Respiratory System to Stress
- Regulation of Saliva
- Secretion of Saliva
- Gastric Acid Production
- Gastric Mucus Production
- Digestion and Absorption
- Histology and Cellular Function of the Small Intestine
- Absorption in the Large Intestine
- Large Intestinal Motility
- Bilirubin Metabolism
- Carbohydrate Metabolism in the Liver
- Lipid Metabolism in the Liver
- Protein and Ammonia Metabolism in the Liver
- Storage Functions of the Liver
- Bile Production
- Function of The Spleen
- Exocrine Pancreas
- Somatostatin
- Proximal Convoluted Tubule
- Loop of Henle
- Distal Convoluted Tubule and Collecting Duct
- Storage Phase of Micturition
- Voiding Phase of Micturition
- Antidiuretic Hormone
- Renin-Angiotensin-Aldosterone System
- Urinary Regulation of Acid-Base Balance
- Water Filtration and Reabsorption
- Development of the Reproductive System
- Gametogenesis
- Gonadotropins and the Hypothalamic Pituitary Axis
- Menstrual Cycle
- Placental Development
- Fetal Circulation
- Maternal Adaptations in Pregnancy
- Cells of the Nervous System
- Central Nervous System
- Cerebrospinal Fluid
- Neurotransmitters
- Peripheral Nervous System
- Action Potential
- Excitatory and Inhibitory Synaptic Signalling
- Resting Membrane Potential
- Synaptic Plasticity
- Synaptic Transmission
- Ascending Tracts
- Auditory Pathway
- Consciousness and Sleep
- Modalities of Sensation
- Pain Pathways
- Sensory Acuity
- Visual Pathway
- Descending Tracts
- Lower Motor Neurones
- Muscle Stretch Reflex
- Upper Motor Neurones
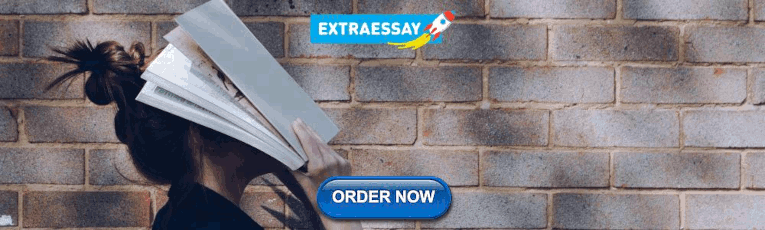
Aqueous Humour
- Ocular Accommodation
- Thyroid Gland
- Parathyroid Glands
- Adrenal Medulla
- Zona Glomerulosa
- Zona Fasciculata
- Zona Reticularis
- Endocrine Pancreas
- The Hypothalamus
- Anterior Pituitary
- Posterior Pituitary
- White Blood Cells – Summary
- Barriers to Infection
- Infection Recognition Molecules
- Phagocytosis
- The Complement System
- Antigen Processing and Presentation
- Primary and Secondary Immune Responses
- T Cell Memory
- Acute Inflammation
- Autoimmunity
- Chronic Inflammation
- Hypersensitivity Reactions
- Immunodeficiency
- Types of Immunity
- Antibiotics
- Viral Infection
- Blood Groups
- Coagulation
- Erythropoiesis
- Iron Metabolism
- Mononuclear Phagocyte System
Original Author(s): Teerajet Taechameekietichai Last updated: 20th October 2023 Revisions: 25
- 2 Composition
- 3 Movement of Aqueous Humour
- 4 Production of Aqueous Humour
- 5 Drainage of Aqeuous Humour
- 6.1 Assessing Glaucoma
- 6.2 Primary Open Angle Glaucoma
- 6.3 Primary Angle-closure Glaucoma
Aqueous humour is a transparent fluid located inside the eye, specifically within the anterior and posterior chambers. It has several key functions, and plays an important role in disease states such as glaucoma.
In this article, we will discuss the function, composition and production of aqueous humour, before considering some clinical applications.
The functions of aqueous humour are as follows:
- Maintaining the intraocular pressure and the shape of the globe.
- Providing nutrients and oxygen for ocular tissue including the posterior cornea, trabecular meshwork, and lens.
- Removal of metabolic by-products from intraocular cells.
- Facilitating the passage of light to the retina.
Composition
The composition of aqueous humour directly relates to its function within the eye.
Ultrafiltration of the aqueous humour within the ciliary body produces nearly protein-free liquid to provide an optically clear medium for vision.
The high level of lactate is due to anaerobic glycolysis by cells of the anterior eye segment e.g. lens epithelium. The high level of ascorbic acid protects against UV-radiation that can cause the formation of free radicals.
Table 1: Composition of aqueous humour relative to plasma
Movement of Aqueous Humour
The iris , ciliary body and choroid are part of the uvea. This is a layer of vascular tissue that delivers blood supply to the ocular tissues. The ciliary body , specifically the pars plicata (in the form of ciliary processes), is responsible for the production of aqueous humour.
The aqueous humour is constantly produced into the posterior chamber and then flows through the pupil into the anterior chamber (AC).
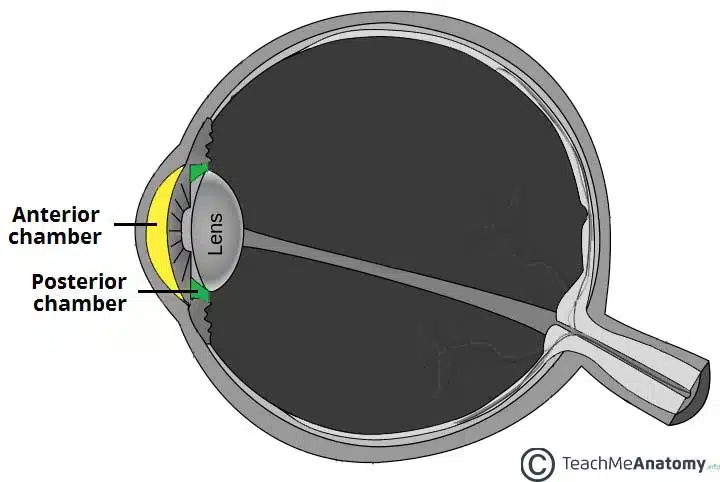
Fig 1 – Anatomy of the eye showing the anterior and posterior chambers
Production of Aqueous Humour
On average, the ciliary body produces around 2.5 μl of aqueous humour per minute. The 3 processes that play a part in this production include:
- Diffusion .
- Ultrafiltration.
- Active secretion.
While diffusion and ultrafiltration are passive processes, they help to collect ultrafiltrated plasma within the ciliary body’s stroma. The ciliary body epithelium then actively secretes the aqueous fluid into the posterior chamber. This active process involves an Na+/K+ ATPase that hydrolyses ATP for energy.
As the Na+/K+ ATPase actively transports Na+ ions into the posterior chamber, the water from the stroma of the ciliary body follows.
It is worth noting that the sympathetic nervous system affects the aqueous humour secretion. Beta-2 adrenoceptor and alpha-2 adrenoceptor activation increases and decreases the production of aqueous humour, respectively.
Drainage of Aqeuous Humour
From the AC, the aqueous fluid is drained through the trabecular meshwork into the canal of Schlemm , which then drains to episcleral veins. Schlemm’s canal (SC) is a circular structure, similar to a lymphatic vessel, that is located in the scleral sulcus right behind the corneoscleral junction. While the inner wall of SC communicates with the AC, the external portion communicates with the episcleral veins.
While the majority of aqueous humour is drained via the trabecular meshwork, approximately 10% exits through the uveoscleral route. The aqueous humour flows across the iris and anterior side of the ciliary muscle through the sclera into the suprachoroidal space, an area between the sclera and choroid.
Clinical Relevance
Assessing glaucoma.
Glaucoma is at term used to describe a group of diseases characterised by a loss of retinal ganglion cells and changes in the optic disc . As it has been shown that there is a link between increasing intraocular pressure (IOP) and worsening degeneration of retinal ganglion cells, most treatments aim to lower the IOP to slow or prevent the progression of glaucoma. To assess for glaucoma you must:
- Perform fundoscopy: Look at the optic nerve , assessing for an increased cup-to-disc ratio, deep or asymmetrical cupping, notching, or bayonetting of the blood vessels (kinking of vessels as they pass the cup).
- Measure intraocular pressure: Tonometry is used to estimate the pressure in the anterior chamber (normal range 10-21mmHg). This estimation must be corrected for with a measurement of the central corneal thickness ; a thin cornea leads to an underestimate of pressure which can lead to high pressures being missed
- Assess visual fields: An arcuate (arc-shaped) field loss may indicate early glaucoma. Treatment aims to preserve the visual fields so this is an important part of monitoring.
- Perform gonioscopy – Gonioscopy assesses the angle of drainage between the iris and cornea. This helps classify the type of glaucoma as well as informing the management plan.
Primary Open Angle Glaucoma
Primary Open Angle Glaucoma (POAG) is one type of glaucoma. It is characterised by a wide aqueous humour drainage angle , visual field loss and optic nerve changes in the absence of a primary cause. In this type of glaucoma, the IOP may be normal or elevated.
The aim of treatment is to reduce the pressure with topical drugs such as prostaglandin analogues or carbonic anhydrase inhibitors . In drug-resistant forms of the disease, surgery management may be indicated.
Primary Angle-closure Glaucoma
Primary Angle-closure Glaucoma (PACG) is another type of glaucoma where the drainage angle is so narrow that the iris is in contact with the trabecular meshwork, blocking the drainage of aqueous humour.
Relative pupillary block is one of the common mechanisms behind PACG. In relative pupillary block, the reduction of aqueous movement through the pupil contributes to a pressure difference between the anterior and posterior chambers. This causes anterior bowing of the iris, thus preventing the drainage of aqueous humour.
These patients may present more acutely with a red eye, headaches, nausea and seeing haloes around lights. On examination, it is classical to see a stony, firm red eye (due to the very high IOP), corneal oedema and a mid-dilated pupil.
These patients need emergency treatment with acetazolamide, pilocarpine and timolol before having surgery to drain aqueous humour between the anterior and posterior chambers by making a small break in the iris with a laser ( iridotomy ).
[start-clinical]
- Perform gonioscopy - Gonioscopy assesses the angle of drainage between the iris and cornea. This helps classify the type of glaucoma as well as informing the management plan.
[end-clinical]
Found an error? Is our article missing some key information? Make the changes yourself here!
Once you've finished editing, click 'Submit for Review', and your changes will be reviewed by our team before publishing on the site.
We use cookies to improve your experience on our site and to show you relevant advertising. To find out more, read our privacy policy .
Privacy Overview
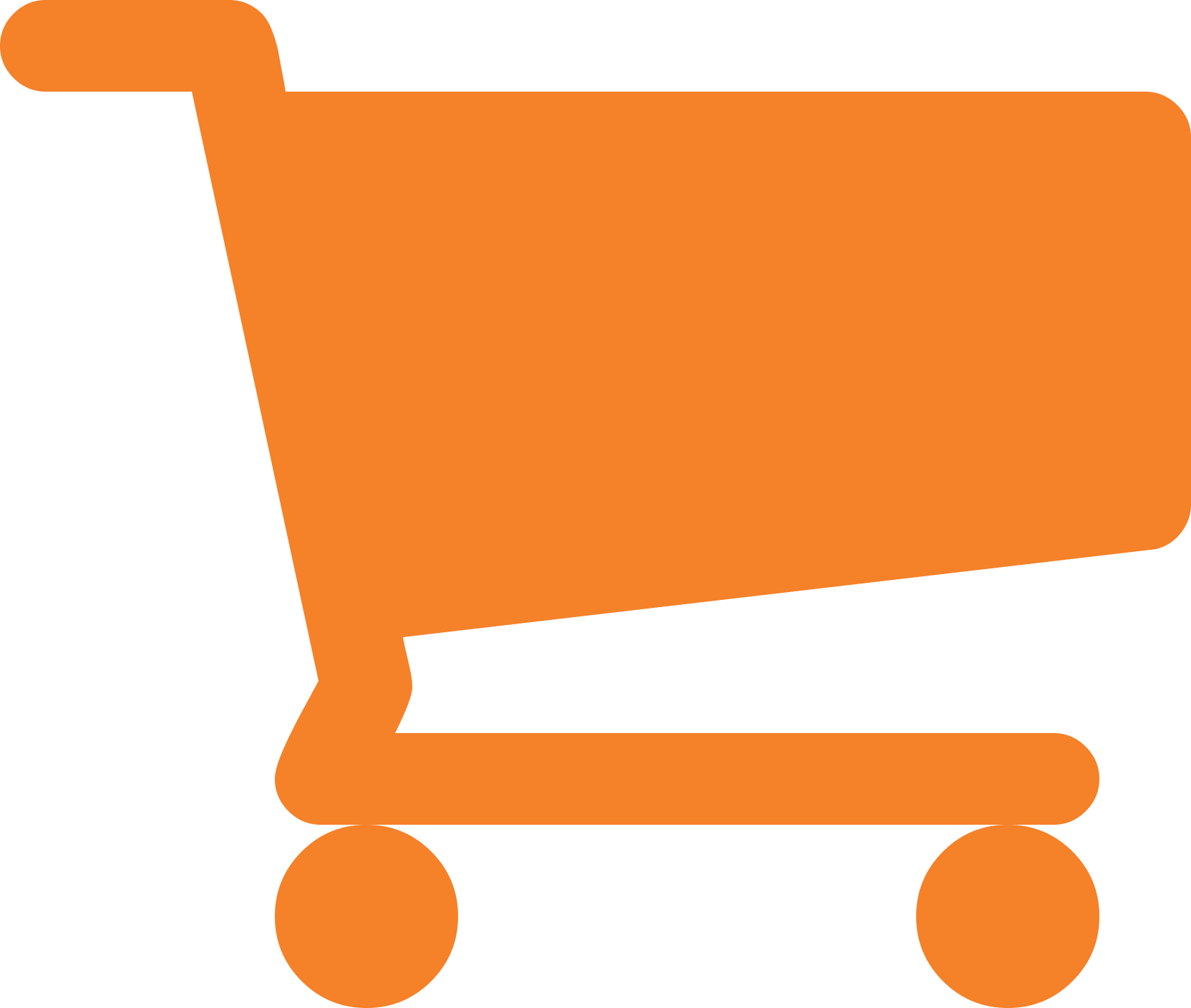
- For Ophthalmologists
- For Practice Management
- For Clinical Teams
- For Public & Patients
Museum of the Eye
- Eye Health A-Z
- Glasses & Contacts
- Tips & Prevention
- Ask an Ophthalmologist
- Patient Stories
- No-Cost Eye Exams
Aqueous Humor
Aqueous humor is the clear liquid inside the front part of the eye. It nourishes the eye and keeps it inflated. The eye constantly produces a small amount of aqueous humor while an equal amount flows out through the trabecular meshwork in the drainage angle .
Imbalances in the creation and drainage of aqueous humor can lead to high intraocular pressure (eye pressure or IOP) . High intraocular pressure is a major part of glaucoma and can damage vision .
Read an overview of general eye anatomy to learn how the parts of the eye work together .
- Find an Ophthalmologist Search Advanced Search
Free EyeSmart Newsletter
All content on the Academy’s website is protected by copyright law and the Terms of Service . This content may not be reproduced, copied, or put into any artificial intelligence program, including large language and generative AI models, without permission from the Academy.
- About the Academy
- Jobs at the Academy
- Financial Relationships with Industry
- Medical Disclaimer
- Privacy Policy
- Terms of Service
- Statement on Artificial Intelligence
- For Advertisers
- Ophthalmology Job Center
FOLLOW THE ACADEMY
Medical Professionals
Public & Patients
- Drainage Angle
- Ciliary Body
- Narrow Angles
Aqueous Humor
- Living reference work entry
- First Online: 01 January 2016
- Cite this living reference work entry
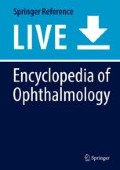
- Annette Giangiacomo 3
48 Accesses
This is a preview of subscription content, log in via an institution to check access.
Access this chapter
Institutional subscriptions
Further Reading
Allingham R et al (2005) Shields’ textbook of glaucoma. Lippincott Williams & Wilkins, Philadelphia
Google Scholar
Alward LM (2000) Glaucoma: the requisites in ophthalmology. Mosby, St. Louis
Download references
Author information
Authors and affiliations.
Emory University, 1365 Clifton Road, Atlanta, GA, 30322, USA
Annette Giangiacomo
You can also search for this author in PubMed Google Scholar
Corresponding author
Correspondence to Annette Giangiacomo .
Editor information
Editors and affiliations.
Dept of Ophthal & Optometry,AKH, Medical University of Wien Dept of Ophthal & Optometry,AKH, Wien, Austria
Ursula Schmidt-Erfurth
Department of Ophthalmology, Goethe University of Frankfurt am Main Department of Ophthalmology, Frankfurt am Main, Germany
Thomas Kohnen
Rights and permissions
Reprints and permissions
Copyright information
© 2012 Springer-Verlag Berlin Heidelberg
About this entry
Cite this entry.
Giangiacomo, A. (2012). Aqueous Humor. In: Schmidt-Erfurth, U., Kohnen, T. (eds) Encyclopedia of Ophthalmology. Springer, Berlin, Heidelberg. https://doi.org/10.1007/978-3-642-35951-4_65-4
Download citation
DOI : https://doi.org/10.1007/978-3-642-35951-4_65-4
Received : 24 September 2012
Accepted : 24 September 2012
Published : 16 June 2016
Publisher Name : Springer, Berlin, Heidelberg
Online ISBN : 978-3-642-35951-4
eBook Packages : Springer Reference Medicine Reference Module Medicine
- Publish with us
Policies and ethics
- Find a journal
- Track your research
Fastest Otolaryngology & Ophthalmology Insight Engine
- HEAD AND NECK SURGERY
- OPHTHALMOLOGY
- OTOLARYNGOLOGY
- Abdominal Key
- Anesthesia Key
- Basicmedical Key
- Otolaryngology & Ophthalmology
- Musculoskeletal Key
- Obstetric, Gynecology and Pediatric
- Oncology & Hematology
- Plastic Surgery & Dermatology
- Clinical Dentistry
- Radiology Key
- Thoracic Key
- Veterinary Medicine
- Gold Membership
Aqueous Humor: Secretion and Dynamics
Aqueous Humor: Secretion and Dynamics B’Ann True Gabelt Julie A. Kiland Baohe Tian Paul L. Kaufman The aqueous humor is a transparent, colorless solution continuously formed from plasma by the epithelial cells of the ciliary processes. It is secreted into the posterior chamber, passes from the posterior chamber through the pupil into the anterior chamber, and is drained at the anterior chamber angle. Most of the aqueous drains into the venous circulation via the trabecular meshwork, Schlemm’s canal, scleral collector channels, and aqueous and episcleral veins; the remainder drains into the orbit via the interstices of the ciliary muscle, the suprachoroidal space, and the sclera ( Fig. 1 ). The composition and formation of aqueous resembles that of cerebrospinal fluid. 1 Aqueous humor is thought to serve several functions: Fig. 1. Cross section through the anterior segment of the eye illustrating the chamber angle. Aqueous humor is formed by active secretion from the ciliary processes ( A ). Drainage occurs via the outflow pathways, principally the trabecular meshwork ( B ) and Schlemm’s canal ( C ) into the aqueous veins ( D ). A smaller proportion of the aqueous humor makes its way directly into the ciliary body (uveoscleral pathway) and is drained by way of the ciliary muscle, the suprachoroidal space, and the sclera ( E ). (From Karnezis TA, Murphy MB: Dopamine receptors and intraocular pressure. Trends Pharmacol Sci 9:389–390, 1988, with permission.) Aqueous delivers oxygen and nutrients to, and removes waste products, blood, macrophages, inflammatory products, or other debris from the posterior cornea, crystalline lens, and perhaps the anterior vitreous, structures that are necessarily avascular. Continuous formation and drainage of the aqueous helps maintain the intraocular pressure (IOP), necessary for maintaining the shape and internal alignment of the ocular structures and, consequently, optimal optical properties. The aqueous maintains a transparent and colorless medium of lower refractive index between the posterior cornea and the lens, and thus constitutes an important component of the eye’s optical system. Circulation of the aqueous in the anterior chamber occurs via hydrostatic phenomena, including mechanical forces caused by eyeball and head movements, thermal currents resulting from the temperature differential between the warmer vascular iris and the cooler avascular cornea, and the pressure gradients between the posterior chamber, anterior chamber, and episcleral veins. As the fluid bathes the anterior lens, iris, and corneal endothelium, its composition is altered as a result of the exchange of nutrients, cellular waste products, and other substances within these structures. The entire volume of the aqueous humor is replaced every 90 to 100 minutes. 2 Continuous formation and drainage of the aqueous is essential to the good health of the eye. In the absence of aqueous circulation, the cornea is thickened, the anterior chamber is absent, the iris is partly atrophic, and the lens is cataractous. 3 This chapter reviews the anatomy and physiology of aqueous humor circulation from formation to drainage. The ciliary body and its secretory mechanisms, the blood–aqueous barrier, the aqueous humor composition, the methods of measuring the aqueous flow rate and factors affecting it, the pathways of aqueous flow within the eye, and the aqueous outflow system are all discussed. ANATOMY OF THE CILIARY BODY The ciliary body forms a ring along the inner wall of the eyeball, and extends anteriorly from the scleral spur and iris to the ora serrata posteriorly. The greater part of the ciliary body mass is accounted for by the ciliary muscle, the bundles of which are arranged in three regional orientations: radial, circular, and longitudinal. When viewed in transverse section, the ciliary body appears as an isosceles triangle ( Fig. 2 ). The base of the triangle faces anteriorly, while one of its sides lies along the sclera, separated from it only by a potential space continuous with the suprachoroidal space. The other side, or inner portion of the ciliary body, is divided anatomically into two parts: the posterior portion (pars plana) and the anterior portion (pars plicata). Projecting inwardly from the pars plicata are approximately 70 villus-like structures: the ciliary processes. Viewed posteriorly, the ciliary processes appear as radial ridges, to which collectively the name corona ciliaris has been given. It is the ciliary processes that are responsible for aqueous formation. Fig. 2. Transverse section of the human ciliary body. (From Lütjen-Drecoll E, Rohen JW: Pathology of the trabecular meshwork in primary open angle glaucoma. In Kaufman PL, Mittag TW, eds.: Glaucoma. Textbook of Ophthalmology Series, Vol. 7. New York: CV Mosby, 1994:1.1–1.16, with permission.) The ciliary processes are long and slender in early life but become more blunt in later years. Their dimensions vary but average 2 mm in length anteroposteriorly, 0.5 mm in width, and 1 mm in height. 4 The processes are greatly convoluted. Structurally, they consist of capillaries surrounded by a loose connective tissue, encircled by a double epithelial layer. This structure provides a large surface area (in rabbits 5 approximately 5.7 cm 2 ) for capillaries to be in close proximity to the double layer of epithelium and for the epithelium to face the posterior chamber. This arrangement maximizes access of the ciliary body secretions into the small space of the posterior chamber. The blood supply of the ciliary body has a dual origin. 4 , 6 , 7 , 8 , 9 , 10 , 11 , 12 , 13 The two (medial and lateral) long posterior ciliary arteries, which penetrate the sclera posteriorly and travel anteriorly in the suprachoroid, give rise to the major arterial circle of the iris, the vascular structure that supplies the inner and anterior division of the ciliary muscle, ciliary processes and iris. 13 The seven anterior ciliary arteries, which penetrate the sclera anteriorly after supplying the extraocular rectus muscles, also contribute to the major iris arterial circle, and to the outer and posterior areas of the ciliary muscle, and the peripheral (anterior) area of the choroid. The microvasculature of the ciliary processes themselves, arising from the short radial ciliary arteries, which in turn arise from the major arterial circle of the iris, is arranged into three distinct vascular areas. The first of these is located at the anterior end of the major processes, with venous drainage achieved via venules passing the ciliary body. The second and third vascular areas supply the major and minor ciliary processes, and are drained posteriorly by venules located at the margin of the ciliary processes. The system of ciliary process venules in turn drains mainly through the vortex system of the choroid. Each short radial ciliary artery has many branches, providing an extensive capillary network ( Fig. 3 ). The capillaries of the ciliary processes are large, thin-walled, and highly fenestrated. Thus, the capillary network of the ciliary processes provides a large surface area of highly permeable vessels to initiate the process of aqueous formation. Fig. 3. A : Blood supply to the ciliary processes. LCM, longitudinal ciliary muscle; RCM, radial ciliary muscle; CCM, circular ciliary muscle. B : Vascular architecture in the human ciliary body. ( 1 ), Perforating branches of the anterior ciliary arteries; ( 2 ), major arterial circle of the iris; ( 3 ), first vascular territory. The second vascular territory is depicted in 4a , marginal route and 4b , capillary network in the center of this territory. ( 5 ), third vascular territory; ( 6 and 7 ), arterioles to the ciliary muscle; ( 8 ) recurrent choroidal arteries. Light circles, terminal arterioles; dark circle , efferent venous segment. ( A, From Caprioli J: The ciliary epithelia and aqueous humor. In Hart M, ed.: Adler’s Physiology of the Eye, 9th ed. St. Louis: Mosby Year-Book, 1992:228–247, with permission; B, From Funk R, Rohen JW: Scanning electron microscopic study on the vasculature of the human anterior eye segment, especially with respect to the ciliary processes. Exp Eye Res 51:651, 1990, with permission.) The capillaries are surrounded by the stroma, which consists mainly of loose connective tissue and collagen. A basement membrane, which is the thickened anterior continuation of Bruch’s membrane separating choroid and retinal pigment epithelium, separates the stroma from the epithelial layers. The ciliary epithelial cells have been subject to intensive study by light and electron microscopy. A striking feature is the interdigitation of the lateral surfaces of adjacent cells and the basal infoldings ( Fig. 4 ), which are characteristic features of secretory epithelia concerned with fluid transport. 14 The relation of the two epithelial cell layers is of importance because as the secreted aqueous is derived from an ultrafiltrate of blood in the stroma of the ciliary body, transport must occur across both layers. The double-layered ciliary epithelium itself is derived from anterior continuations of the retinal pigmented epithelium (forming the pigmented layer), and the neuroepithelium from which the retinal cells are derived (forming the nonpigmented layer). However, during embryogenesis, invagination of the neuroepithelium occurs, with the result that the apical surfaces of each cell layer in the ciliary epithelium face one another, while the basolateral surface of the nonpigmented layer faces directly into the posterior aqueous chamber. Conversely, the basolateral surface of the pigmented layer is tightly bound to the basement membrane. Cells of the pigmented epithelium contain many melanin granules. Gap junctions are present between the lateral interdigitations of the pigmented cells. Desmosomes also occur between the lateral interdigitations of the pigmented and nonpigmented epithelia and between their apical membranes. Fig. 4. Schematic diagram of nonpigmented and pigmented epithelial cells. Note apices of cells facing each other. Basal infoldings ( BI ); basement membrane ( BM ); ciliary channels ( CC ); desmosomes ( DES ); fenestrated capillary endothelium ( FE ); gap junction ( GJ ); melanosome ( MEL ); mitochondrion ( MIT ); red blood cell ( RBC ); rough endoplasmic reticulum ( RER ); tight junction ( TJ ). (From Caprioli J: The ciliary epithelia and aqueous humor. In Hart M (ed): Adler’s Physiology of The Eye, 9th ed, pp 228–247. St. Louis, Mosby Year-Book, 1992, with permission.) The cells of the inner nonpigmented epithelium possess numerous intermediate-sized mitochondria, and the rough endoplasmic reticulum is particularly well developed, indicative of active protein synthesis. Small vacuoles may be present in large numbers near the apex of these cells. Occasionally, a few pigmented granules may be seen. Tight junctions are present in the lateral interdigitations between the nonpigmented cells ( Fig. 4 ), thus forming a barrier for the passage of larger molecules between the cells. This important physiologic barrier, constituting part of the blood-aqueous barrier, is discussed further in a later section. The internal limiting membrane of the ciliary body is a more complex and thicker structure than its posterior counterpart on the retina, in part because it serves as the basement membrane for the inverted nonpigmented ciliary epithelium, and as the site of insertion of the zonular fibers, 4 to which the lens is attached. FORMATION AND SECRETION OF AQUEOUS HUMOR Early in the twentieth century, aqueous humor was regarded as a stagnant fluid. 15 However, this misconception was revoked after a number of experiments designed to investigate this were carried out, including Seidel’s procedure, 16 in which a cannula connected to a reservoir of indigo carmine dye was inserted into the anterior chamber of the rabbit eye. The reservoir was raised, thus creating a pressure of 15 mm Hg, and the dye was seen to enter the anterior chamber and subsequently the episcleral veins. From this, it was concluded that aqueous humor is continuously formed and drained, and it is to a large extent from this historic work that the modern study of aqueous humor dynamics has developed. Other aspects of the anatomy and physiology of aqueous drainage were discovered subsequently. Boerhaave first described the presence of the aqueous veins, 17 and Ascher 18 observed a clear fluid in veins of the episclera and demonstrated by means of external compression with a glass rod that these veins were interconnected with veins containing blood. Goldmann 19 demonstrated that these vessels contained aqueous humor by injecting fluorescein intravenously and observing the dye entering the anterior chamber and subsequently the aqueous veins. Ashton 20 identified an aqueous vein in a living human eye, and postmortem examination using a neoprene cast showed that there was a direct passage between the vessel and Schlemm’s canal. Three physiologic processes are known to contribute to the formation and chemical composition of the aqueous. These are diffusion, ultrafiltration (and the related dialysis), and active secretion. The first two are passive and, therefore, require no active cellular participation. Diffusion of solutes across cell membranes occurs down a concentration gradient. Substances with high lipid solubility coefficients that can easily penetrate biological membranes move readily in this way. Ultrafiltration is the term used to describe the bulk flow of blood plasma across the fenestrated ciliary capillary endothelia, into the ciliary stroma, which can be increased by augmentation of the hydrostatic driving force. This process is responsible for the formation of the reservoir of the plasma ultrafiltrate in the stroma, from which the posterior chamber aqueous is derived, via active secretion across the ciliary epithelium. Active secretion requires energy, normally provided via the hydrolysis of adenosine triphosphate (ATP). The energy is used to secrete substances against a concentration gradient. Of these three processes, active secretion is believed to contribute the most to the chemical composition and volume of the posterior chamber aqueous, accounting for 80% to 90% of total aqueous humor formation. 21 , 22 , 23 , 24 , 25 , 26 , 27 , 28 , 29 DIFFUSION Diffusion arises from the fact that the molecules in a fluid are in constant, random motion. The magnitude and rate of motion vary directly with the temperature. If the molecules in a liquid or gas are not evenly distributed, then simply by the laws of statistical probability the molecules will eventually reach a state of equilibrium whereby they are redistributed equally. For example, if there is initially a cloud of smoke particles on the right side of a closed room without air currents, more particles will move from the right side to the left, than from left to right. This process will continue until there is a relatively even distribution throughout the room, at which time the number of particles going from right to left at any moment will be equal to the number moving in the opposite direction. A similar process occurs in single solutions and in situations in which two solutions are separated by a membrane, as long as the membrane is permeable to at least some of the constituents of the solution (semipermeable membrane). Most capillary walls are permeable to water, dissolved gases, and many small molecules and ions. In a stagnant system, substances of higher concentration on one side of a semipermeable membrane show a net movement to the side of lower concentration until the concentrations are equal on both sides. When equilibrium is reached, movement across the membrane still occurs, but the number of particles going in one direction equals the number going the other way, thus yielding no net movement. It should be noted that water (or another solvent) participates in this process. Net movement of water is usually in the opposite direction of solute movement, since a higher concentration of solute means, in effect, a lower concentration of solvent. Fick’s law describes quantitatively the net movement of a substance across a semipermeable membrane where only diffusion is occurring: Rate of movement = K(C 1 – C 2 ) where C 1 = concentration on side with higher concentration C 2 = concentration on side with lower concentration K = constant, which depends on nature and permeability of membrane, nature of solute and solvent, and temperature. The less permeable the membrane to the solute or solvent, the lower the temperature, and the more viscous the fluid medium, then the longer will be the time required for equilibration. Therefore, diffusion tends to occur more rapidly through extracellular fluids than across cells. It should also be remembered that conditions for diffusion are markedly altered in a dynamic system such as the ciliary processes, in which blood is flowing rapidly, aqueous humor is constantly being formed and carried away, and other processes occur, such as those described below. DIALYSIS AND ULTRAFILTRATION In most biologic solutions, there exists a combination of salts, sugars, proteins, and other large molecules. Most biological membranes are permeable to water, salt, and some small organic molecules. However, these membranes are relatively impermeable to larger molecules, such as proteins. If a solution of protein and salt is separated from either water or a less concentrated salt solution by a membrane permeable to the salt and water but not to the protein, then there will be a net movement of water to the protein side by diffusion, and a movement of salt away from the protein side. The protein, of course, cannot move across the membrane. This process is called dialysis ( Fig. 5 ) and is utilized, for example, for removing unwanted salts and other toxic substances from the blood, using the peritoneum as the membrane (peritoneal dialysis), or by using a synthetic membrane such as that found in a dialysis machine (artificial kidney). Fig. 5. Dialysis. The presence of protein molecules ( large circles ) induces a net movement of water ( small dots ) across the semipermeable membrane to the protein side. There is also a net movement of salt molecules ( broken circles ) away from the protein side. With the exception of protein, movement of all molecules occurs in both directions, but net movement is in the direction as indicated by the solid arrows. (Courtesy of RL Stamper, MD.) By the addition of a hydrostatic pressure on the protein side of the system, the exchange of salt and water can be accelerated. This process is called ultrafiltration and differs from dialysis only because the hydrostatic pressure changes the rate of movement of ions and slightly changes their final respective concentrations. The fluid formed by the process of dialysis is called a dialysate, and that formed by ultrafiltration is called an ultrafiltrate. Ultrafiltration is the process that occurs across capillary walls due to the higher pressure and higher protein concentration in the plasma as compared with the extracellular space ( Fig. 6 ). Fig. 6. Ultrafiltration. This process is similar to dialysis, but with the addition of a hydrostatic pressure that increases the rate of net movement of water and salt molecules across the semipermeable membrane. The final equilibrium concentrations on either side of the membrane are the same as in dialysis. The hydrostatic pressure merely increases the rate at which equilibrium is achieved. (Courtesy of RL Stamper, MD.) GIBBS-DONNAN EQUILIBRIUM The salt does not distribute itself equally on both sides of the membrane. Since the protein carries an electrical charge (generally an overall negative charge at physiologic pH), the positive ions in solution tend to be bound to the negatively charged residues of the protein molecules. Thus, there is an excess of cations such as Na + or K + on the protein side of the membrane. This unequal distribution is called the Gibbs-Donnan effect, and the quantitative relationships between the various ions (at least in simple systems) is predictable ( Fig. 7 ). In order to maintain electrical balance, the total positive charges ( e.g., number of Na + and K + ions) on one side must equal the total negative charges ( e.g. , sum of negative protein charges, Cl – ions, and any other negative ions). Furthermore, it has been shown that the final equilibrium concentrations of Na + and Cl – on each side of the membrane are related according to the following equation 1 , 30 , 31 : Fig. 7. Gibbs-Donnan effect. Because protein molecules carry an overall electrical charge (usually negative) at physiological pH, the distribution of ions at equilibrium is altered slightly to reflect the tendency of the protein molecules to attract oppositely charged and repel like-charged ions. Therefore there is a higher concentration of cations on the protein side and a higher concentration of anions on the nonprotein side of the system. (Courtesy of RL Stamper, MD.) [Na + ] 1 /[Na + ] 2 = [CL – ] 2 /[CL – ] 1 Thus, if the aqueous humor were like extracellular fluid in most capillary beds of other parts of the body, we should expect to see a protein-free aqueous solution with ionic concentrations like those on side 2 of a Gibbs-Donnan ultrafiltrate of plasma. The Na + and K + concentrations should be less than plasma, and the Cl – and HCO 3 – concentrations should be slightly higher. Further, the actual values of the ratios of the concentrations of each respective ion in aqueous to plasma would conform to the values obtained experimentally when plasma is dialyzed against its own ultrafiltrate. In addition, no organic substance should be at higher concentration than in the plasma because diffusion and ultrafiltration can, at most, only equalize the concentrations of organic substances. These processes cannot promote an excess concentration on side 2 of the membrane. Although aqueous humor resembles a dialysate of plasma in many ways, as will be seen, the ionic concentrations do not quite fit the Gibbs-Donnan predictions, and some nonionic substances have higher concentrations in aqueous than in plasma ( Table 1 ). Such a condition can only occur in the presence of some active metabolic process. 1 , 22 Table 1. Aqueous Versus Dialysate Substance Concentration Ratio (aqueous/plasma) Concentration Ratio (dialysate/plasma) Na + 0.96 0.945 K + 0.955 0.96 Ca 2+ 0.58 0.65 Mg 2+ 0.78 0.80 Cl – 0.015 1.04 HCO 3 – 1.26 1.04 H 2 CO 3 1.29 1.00 Glucose 0.86 0.97 Urea 0.87 1.00 Values are for the rabbit eye. Although the chemical composition of aqueous humor bears some similarities to a plasma dialysate, the small differences are significant. These differences can only be accounted for theoretically by an active secretory mechanism. (Adapted from Davson H: The Aqueous Humor and the Intraocular Pressure. In: Physiology of the Eye, 5th ed, pp 3–95. New York, Pergamon Press, 1990.) SECRETION Secretion implies an active process that selectively transports some substances across the cell membrane. Because energy is consumed, substances can be moved across a concentration gradient in a direction opposite to that which would be expected by passive mechanisms alone. One example of this is the ability of the thyroid gland to accumulate iodide at up to 40 times the circulating plasma level. 27 , 32 One would expect the iodide concentration in the plasma and the thyroid gland to be similar if only diffusion and ultrafiltration were operating. Aqueous humor exhibits increased ascorbate, lactate, and certain amino acid concentrations as compared with plasma, as a consequence of active secretion. 1 Another way of testing for the presence of an active metabolic process is to apply specific metabolic inhibitors to the ciliary body and observe the effect on aqueous secretion. Ultrafiltration of fluid from the plasma to the posterior aqueous has been suggested to be responsible for approximately 70% of aqueous formation. 27 , 33 , 34 However, the results of experiments where metabolic inhibitors have been used have shown conclusively that this is not the case. For example, systemic 35 or intravitreal injection 36 of ouabain (an inhibitor of the enzyme sodium potassium-activated adenosine triphosphatase [ATPase]—Na + /K + ATPase) results in a decrease of up to 70% in aqueous formation, in a variety of species. The topical administration of vanadate (also a Na + /K + ATPase inhibitor) lowers aqueous secretion in rabbits 37 , 38 and monkeys. 39 Ouabain as well as a number of other ion transport and channel blocking drugs significantly reduce aqueous humor formation in arterially perfused bovine eyes. Bumetanide (a specific inhibitor of Na-K-2Cl cotransport) and furosemide (a nonspecific anion transport inhibitor) reduce aqueous humor formation by 35% and 45% in bovine eyes in vitro . Similarly, 4,4′-diisothiocyanatostilbene-2,2′-disulfonic acid (DIDS, a probable inhibitor of the Cl-HCO 3 exchanger, the Na-HCO 3 cotransporter, and chloride channel) and 5-nitro-2-(3-phenylpropylamino)-benzoic acid (NPPB, a chloride channel blocker in nonpigmented cells) reduce aqueous humor formation by 55% and 25%, respectively, in bovine eyes in vitro . 40 Also, catecholamines such as epinephrine, norepinephrine, isoproterenol and dopamine, that stimulate aqueous humor formation in humans also stimulate Na-K-Cl cotransport. 41 If the greatest proportion of aqueous secretion was attributable to ultrafiltration, then this would not occur. In addition, Bill 22 noted that the hydrostatic and oncotic forces that exist across the ciliary epithelium–posterior aqueous interface would favor resorption, not secretion, of aqueous humor. The ciliary process stroma has an oncotic pressure of approximately 14 mm Hg, because of its protein content. Because IOP in the healthy eye is maintained at approximately 15 mm Hg, a capillary hydrostatic pressure of greater than 29 mm Hg would be required to drive an ultrafiltrate. Capillary hydrostatic pressure in the ciliary stroma has been estimated to be 25 to 33 mm Hg. 14 It has been calculated 27 that a capillary pressure of greater than 50 mm Hg would be necessary to promote ultrafiltration as the major mechanism for the secretion of aqueous. The values of capillary hydrostatic pressure in the ciliary processes and a consideration of the hydrostatic and oncotic forces involved do not favor ultrafiltration as an important mechanism for aqueous humor secretion. Acetazolamide, and other specific inhibitors of the enzyme carbonic anhydrase, decrease the formation of aqueous by 40% to 60%. 42 , 43 , 44 A reduction in temperature, which inhibits most active metabolic processes, also results in a decrease in aqueous formation, to a greater extent than would be expected if only diffusion were operating. 1 , 45 Active transport systems usually exhibit a limit beyond which an increase in substrate concentration produces no further increase in transport. When this limit is reached, the system is said to be saturated. Thus, the fact that a transport mechanism for a substance can be saturated provides evidence of an active system. It has been demonstrated that by increasing the ascorbate concentration in plasma, a level of plasma ascorbate is reached above which no further increase in aqueous ascorbate concentration will occur. This provides evidence that the ascorbate transport system in the eye is saturable. Several membrane active transport systems have been identified in the ciliary epithelium that are known to pump various substances against a concentration gradient, including Na + /K + ATPase and amino acid membrane transporters ( Fig. 8 ). There are also passive transport proteins specific for HCO 3 – and Cl – . Membrane-bound Na + /K + ATPase is one important system involved in Na + and K + transport and is present mainly along the lateral cellular interdigitations of the nonpigmented ciliary epithelium. 35 , 46 , 47 , 48 , 49 , 50 , 51 The transmembrane Na + /K + transport protein is energized by the Gibbs free energy of hydrolysis of ATP, mediated by the ATPase enzyme intimately associated with it in the membrane. The ATP required for this process is derived predominantly from oxidative metabolism of glucose via the Krebs’ citric acid cycle. It is most likely that Na + is pumped across the cell membrane and Cl – is passively carried with it in order to maintain electrical neutrality, although the relative rate of transport of Cl – is unclear. 52 The Na + pump induces a potential difference across the ciliary body, ranging from 6 to 10 mV. Measurements of the potential difference across the ciliary epithelia indicate that the aqueous is positive with respect to the stroma. The magnitude of this potential difference is reduced after poisoning with ouabain. 53 These data are consistent with the hypothesis that Na + is the primary mover and that active transport of Cl – is probably small in comparison to that of Na + . 54 Interspecies differences in the aqueous-plasma ratios of Cl – ( e.g. , high in human, low in rabbits) might be explained by the relative proportions of Cl – actively transported. 55 Transepithelial electrical measurements in the isolated rabbit iris-ciliary body indicate that Na + /K + ATPase and HCO 3 – are required for active ion transport. 56 Fig. 8. Diagram of possible secretory pathways in the ciliary processes. AA , ascorbic acid; CA carbonic anhydrase. (From Wiederholt M, Helbig H, Korbmacher C. Ion transport across the ciliary epithelium: Lessons from cultured cells and proposed role of the carbonic anhydrase. In Carbonic Anhydrase. Basel: Verlag-Chemie, 1991:232–244, with permission.) However, recent studies suggest that active transport of Cl – ions plays an equally important role 40 , 41 , 57 providing the driving force for aqueous humor formation. 57 Shahidullah et al 40 found that in the isolated bovine eye, aqueous humor is formed mostly by processes involving active secretion and chloride transport. Recently, it has been discovered that water transport across many membranous barriers, including those in the eye, are facilitated by aquaporin (AQP) water channels. 58 The AQPs are a family of water channels expressed in animals, plants, and lower organisms. 58 There are at least 11 mammalian AQPs (AQP0 to AQP10) each of which is a small membrane protein approximately 30 kd in size. The eye expresses several AQPs at sites of fluid transport. AQP1 58 , 59 and AQP4 60 are expressed in the ciliary epithelium. AQP1-null mice have decreased IOP and aqueous humor production compared to normals. 58 Histochemical studies have demonstrated a number of active enzyme systems in the ciliary epithelia. These include nucleotide phosphatases (especially ATPase, as mentioned previously), adenylate cyclase, and carbonic anhydrase, the enzyme that forms HCO 3 – (the body’s alkaline ion) from CO 2 and H 2 O 61 , 62 , 63 , 64 , 65 by the following reaction: CO 2 +H 2 O→H 2 CO 3 →H + + HCO 3 – I II Carbonic anhydrase catalyzes step I of the reaction and step II is an almost instantaneous ionic dissociation. 66 , 67 The enzyme is localized in both the pigmented and the nonpigmented epithelial cells of the rabbit ciliary processes, 68 , 69 and monkey and human pigmented and nonpigmented epithelial cells, 70 most prominently in the basal and lateral membranes, but also in the cytoplasm. A variety of carbonic anhydrase inhibitors will reduce aqueous secretion, including acetazolamide, 71 methazolamide, methoxazolamide, ethoxzolamide, dichlorphenamide, 72 , 73 aminozolamide, 74 trifluormethazolamide, 75 6-hydroxyethoxzolamide, 76 dorzolamide, 77 , 78 , 79 brinzolamide, 80 , 81 , 82 and several biscarbonylamidothiadiazole compounds. 44 The site of action of these drugs is intraocular rather than systemic, because the unilateral injection of acetazolamide into the carotid artery lowers the IOP of only the ipsilateral feline eye. 83 Precisely how carbonic anhydrase helps mediate aqueous secretion has been a long-standing debate. However, in recent years many advances in understanding of this system have been achieved. In the past, it was suggested that acetazolamide caused constriction of afferent iris or ciliary arteries, thereby decreasing ultrafiltration and hence aqueous humor formation. 84 , 85 However, this view is no longer held. For example, Bill, 86 using radiolabeled microspheres, concluded that acetazolamide had no significant effect on the blood flow in the intraocular tissues in the rabbit eye, and that it was unlikely that the drug had any effect on blood flow in the anterior uvea. It has now been irrefutably demonstrated that acetazolamide exerts its ocular effects via a reduction in aqueous secretion, mediated by a direct effect on the ciliary epithelial cells. 26 The ciliary carbonic anhydrase system is now thought to function as described by Maren. 87 Inhibition of the enzyme decreases the rate of Na + and HCO 3 – transport into the posterior chamber by equimolar amounts, indicating a linkage of the accession of these two solutes into the posterior chamber of dogs and monkeys. 88 Furthermore, inhibition of carbonic anhydrase lowers the aqueous Cl – concentration in primates and the HCO 3 – concentration in rabbits. 24 , 89 The mechanism by which carbonic anhydrase activity is coupled to Na + and HCO 3 – movement into the posterior chamber is still subject to much study. However, it is now known that the primary reaction, which occurs within the cytosol of the nonpigmented ciliary epithelial cells, is the proteolysis of water to yield OH – and H + ions ( Fig. 9 ). The hydroxide ions so formed react with CO 2 catalytically (or noncatalytically when the enzyme is inhibited) to form HCO 3 – , which is passively transported to the aqueous humor, simultaneously with the active transport of Na + . The protons liberated from water pass into the blood circulation where they are buffered by proteins. The theoretical rates of flow of HCO 3 – , based on known constants and some assumptions regarding cell volume and pH of the secretory region, are shown at the bottom of Figure 9 . The catalytic rate ( i.e. , when active carbonic anhydrase is present) is 7 μmol/min, and the uncatalyzed is 0.07 μmol/min. Fig. 9. Model for HCO 3 – formation and its linkage to Na + transport in the ciliary processes. Observed rates are for monkey. Chemical rate constants (37°C) taken from the work of Maren. (From Maren TH: The kinetics of HCO 3 – synthesis related to fluid secretion, pH control, and CO 2 elimination. Annu Rev Physiol 50:695, 1988, with permission.) The uncatalyzed rate is found experimentally by inhibiting the enzyme in vivo by a large dose of acetazolamide or similar compound. Such inhibition, when complete, should yield the uncatalyzed rate of HCO 3 – formation. Measurements obtained experimentally indicate that the observed inhibited rate ( Fig. 9 ; 0.024 μmol/min) is not different from the calculated uncatalyzed rate of 0.07 μmol/min. Considering the assumptions about pH and cell volume, the difference between the calculated and experimentally measured inhibited rates of bicarbonate flow lies within acceptable limits of error. Inhibition of the flow of bicarbonate also leads to an inhibition of the flow of Na + . Several hypotheses have been put forward to explain this: (i) inhibition of carbonic anhydrase causes a decrease in HCO 3 – available for movement with Na + to the aqueous side to maintain electroneutrality; (ii) a reduction in intracellular pH may inhibit Na + -K + ATPase; and (iii) decreased availability of H + produced by the reaction catalyzed by carbonic anhydrase decreases H + /Na + exchange and reduces the availability of intracellular Na + for transport into the intercellular channel. A large excess of carbonic anhydrase has been found in the ciliary processes of all species studied. This has pharmacologic implications when one hopes to reduce aqueous secretion by inhibition of the enzyme. In order to achieve a clinically useful reduction in secretion and hence IOP, more than 99% of the enzyme must be inhibited. The sulfonamide carbonic anhydrase inhibitors have been in clinical use for glaucoma since 1955, when their action on the eye was discovered as an offshoot of their development as diuretics. 73 They have also been extremely useful in research on aqueous humor formation, because they are highly specific, having no other actions at concentrations below 1 μM. One major compound is acetazolamide but many ophthalmologists have used methazolamide or related compounds, which have a greater diffusibility than acetazolamide, and that act on the eye in lower doses, thereby achieving the desired ocular effect but having lesser action on other systems in the body where carbonic anhydrase is present, such as the secretory epithelia of the kidney. 42 Nonetheless, the systemic dose of sulfonamide carbonic anhydrase inhibitors necessary to significantly suppress aqueous secretion leads to undesirable side effects, even with methazolamide. 90 In recent years topically applied carbonic anhydrase inhibitors have been developed for clinical use. Dorzolamide has been used since 1995 80 , 91 , 92 and brinzolamide since 1998. 80 As a rule, the topically active carbonic anhydrase inhibitors have high lipid and water solubility, as well as high inhibitory potency and efficacy. Sufficient concentration is achieved in the ciliary processes via transcorneal, aqueous humor and iris absorption after instillation of a single drop. 43 , 92 , 93 This can result in essentially as large a decrease in IOP as with the sulfonamides 91 , 92 , 93 without the adverse side effects of oral acetazolamide. Dorzolamide has a high affinity for carbonic anhydrase and penetrates the eye well. However, some studies have found it to be a less effective at lowering IOP than oral acetazolamide. 94 Brinzolamide’s effectiveness equals that of dorzolamide. 80 , 81 , 95 , 96 However, both produce localized, albeit mild, side effects such as burning and stinging upon instillation and blurred vision. 95 , 96 Brinzolamide produces less ocular discomfort than dorzolamide 80 , 81 , 95 , 96 possibly due to the more physiologic pH of the preparation. 80 In summary, aqueous humor is mainly a product of active cellular secretion requiring metabolic energy. Na + and K + are transported from plasma to the posterior chamber, with HCO 3 – , Cl – and water following passively to maintain electrical and osmotic balance. Other substances such as certain amino acids are also actively transported. Some other small molecules probably appear in the aqueous via diffusion or ultrafiltration. The process begins with an ultrafiltrate of plasma in the extravascular spaces of the ciliary stroma. The ciliary epithelium (probably nonpigmented) then incorporates certain molecules and ions selectively for concentration and direct active secretion into the posterior chamber, while other substances are secreted passively. BLOOD–AQUEOUS BARRIER Large molecules such as proteins are present in the aqueous in only small quantities, even if their respective concentrations in the plasma are raised to high levels. In humans, normal plasma total protein levels are 6 g per 100 mL, compared to less than 20 mg per 100 mL in the aqueous, less than 0.5% that of plasma. 97 , 98 Thus, a restraint in the free passage of many solutes from the blood vessels of the ciliary stroma into the aqueous humor exists. This constitutes one component of the blood–aqueous barrier, 1 , 99 the anatomic correlate of which are the tight junctions between the interdigitating surfaces of the nonpigmented ciliary epithelial cells. Bill 100 , 101 demonstrated that albumin and other proteins pass through the ciliary capillary walls at a much faster rate than they enter the aqueous humor and concluded that a barrier to these substances existed at a site other than the vessel walls. The intravenous injection of horseradish peroxidase into the monkey leads to a rapid filling of the ciliary stroma by the enzyme. 102 The enzyme passes through the many fenestrations present in the blood capillaries of the ciliary body, since these have few tight junctions (similar to choroidal capillaries), and also fills the spaces between the pigmented cells. However, it is prevented from entering the aqueous by the tight junctions of the nonpigmented layer, between the cell apices. Comparable results have been reported in the mouse 103 , 104 and in the rabbit. 105 , 106 Numerous other studies have tested the blood–aqueous barrier using a number of different intravascular tracers designed to mimic the behavior of plasma-proteins. 2 The conclusion of these studies is that tight junctions between the apicolateral surfaces of the nonpigmented epithelium of the ciliary body, and between the endothelial cells of the iris vasculature, prevent the passage of plasma proteins into the aqueous humor. 102 , 103 , 104 , 105 , 107 , 108 , 109 , 110 , 111 The blood-aqueous barrier is not absolute. Water-soluble substances of medium molecular weight such as urea, creatinine, and certain sugars may penetrate at varying rates, but all are slower than their transit across capillary walls. Generally, the greater the lipid solubility coefficient of a substance, the greater its ability to penetrate the blood-aqueous barrier and pass to the posterior aqueous chamber. 1 , 112 , 113 , 114 In addition, regional differences of permeability in the ciliary body have been noted. For instance, the epithelia of the anterior portions of the ciliary processes of the rabbit are less permeable than the epithelia of the pars plana. 105 Substances such as mannitol are used clinically to reduce IOP. They function as hyperosmotic agents, by exploitation of the fact that they penetrate the blood–aqueous barrier only poorly but are distributed widely within the extracellular spaces of the body. Water is drawn from cells and the ocular fluids, balancing the high osmotic pressure induced in the extracellular space via the high concentrations of mannitol. The resulting loss of water from the eye leads to a reduction in the IOP. 1 The effect of hyperosmotic agents is most pronounced in eyes exhibiting pathologically elevated IOP. 115 Other examples of hyperosmotic agents include glycerin, urea, isosorbide, and ethanol. Urea was the first substance to be used for this purpose, however, it will slowly penetrate the blood–aqueous barrier, and hence the hyperosmotic effect of urea on the eye is shorter lived than that of mannitol. Ethanol is not used clinically because it penetrates the eye even more rapidly than urea and in the required doses has undesirable effects on the sensorium. Certain antibiotics ( e.g ., chloramphenicol, cephalothin, and ampicillin) are known to penetrate the blood-aqueous barrier well, whereas others do so only poorly ( e.g. , penicillin, methicillin, erythromycin, and gentamicin). A similar barrier to the passage of solutes exists in the retinal pigment epithelium, thus forming a blood–vitreous barrier between the vitreous and the blood capillaries of the choroid. Blood capillaries with tight junctions in their endothelia form further physiologic barriers (functionally similar to the blood–brain barrier) in the iris and retina. SECONDARY AQUEOUS The blood–aqueous barrier is fragile and may be disturbed by a variety of noxious stimuli. A corneal abrasion, paracentesis (withdrawal of a small volume of aqueous via a needle inserted into the anterior chamber), 116 , 117 , 118 , 119 , 120 intraocular infection, uveal inflammation, intraocular surgery, and certain drugs, applied topically (such as nitrogen mustard or an anticholinesterase, e.g. , echothiopate, diisopropyl flurophosphate [DFP], demecarium, neostigmine, or physostigmine), or delivered by intracarotid infusion, such as hyperosmotic agents (causing separation of the ciliary epithelial layers, opening of the blood–aqueous barrier, and severe, permanent damage to the pigmented epithelial cells 121 ), are all capable of breaking down the blood-aqueous barrier and inducing changes in the aqueous humor composition ( Table 2 ). Disruption of the blood–aqueous barrier has also been reported in the contralateral eyes of patients who have had cataract extraction and lens implantation surgery, 122 and after argon laser trabeculoplasty. 123 Severe damage to the blood-aqueous barrier occurs with cyclodestructive procedures used to treat advanced glaucoma and is evidenced by the prolonged or chronic presence of flare (the scattering of light upon slit-lamp examination by increased levels of protein in the anterior chamber). Table 2. Factors Interrupting the Blood-Aqueous Barrier I. Traumatic A. Mechanical 1. Paracentesis 2. Corneal abrasion 3. Blunt trauma 4. Intraocular surgery 5. Stroking of the iris B. Physical 1. X-ray 2. Nuclear radiation C. Chemical 1. Alkali 2. Irritants ( e.g., nitrogen mustard) II. Pathophysiologic A. Vasodilation 1. Histamine 2. Sympathectomy B. Corneal and intraocular infections C. Intraocular inflammation D. Prostaglandins E. Anterior segment ischemia III. Pharmacologic A. Melanocyte-stimulating hormone B. Nitrogen mustard C. Cholinergic drugs, especially cholinesterase inhibitors D. Plasma hyperosmolality (Courtesy of RL Stamper, MD) After breakdown of the blood–aqueous barrier, the resultant aqueous produced is known as secondary or plasmoid aqueous. 1 , 24 , 30 , 124 The most notable change is a marked increase in protein concentration. In this situation, the ionic composition of the aqueous approaches that of a simple dialysate of plasma, and substances that are normally barred from entering the aqueous now do so with ease. The unusually rapid rate of entry of substances such as fluorescein, Evan’s blue dye, albumin, or fibrinogen (which actually may allow the aqueous to coagulate) can be used as a diagnostic indicator of barrier breakdown. Many of the processes that disrupt the blood–aqueous barrier also lead to vasodilation. Vasodilation can occur by means of an axon reflex (as may be seen after abrasion of the cornea), a sudden drop in IOP, or inflammation. 125 , 126 In any case, vasodilation may be associated with loss of some of the tight junctions in the iridial vessels, which may help explain the breakdown of the barrier. 125 In addition, it has been suggested that plasma proteins and other constituents can leak in a retrograde manner through Schlemm’s canal, and, therefore, account for the breakdown of the barrier after paracentesis. 127 In this study, no changes in the permeability of the ciliary epithelium or iris to horseradish peroxidase were found after paracentesis, contradicting previous studies and conventional views of the mechanism of barrier breakdown. Release of prostaglandins causes vasodilation and many other findings associated with inflammation. Evidence points to the possible involvement of this class of compounds with breakdown of the blood-aqueous barrier. 124 , 128 , 129 , 130 , 131 , 132 , 133 , 134 Prostaglandins have been implicated in the irritative response after mechanical trauma to the eye, and cause miosis, vasodilation, release of protein into the aqueous, and elevated IOP. Prostaglandins applied topically to the eye in sufficiently high concentration cause breakdown of the tight junctions of the nonpigmented ciliary epithelium and increase the protein content of the aqueous humor, the highest levels of protein being found in the posterior chamber. 135 , 136 Pretreatment with inhibitors of prostaglandin synthesis such as indomethacin or aspirin will inhibit breakdown of the blood-aqueous barrier, as well as some of the other manifestations of inflammation ordinarily induced by the aforementioned process. 137 , 138 , 139 , 140 , 141 It may well be that prostaglandin release represents a final common pathway for the action of many different kinds of trauma and irritants. However, small doses of particular prostaglandins (especially prostaglandin F 2α [PGF 2α ] and certain congeners) applied topically to the eyes of cynomolgus monkeys and humans result in a large increase in uveoscleral outflow, with a concomitantly large decrease in IOP, without clinically evident ocular inflammation. 132 , 133 , 142 , 143 , 144 , 145 , 146 , 147 In the past decade, PGF 2α analogues such as latanoprost, 148 , 149 , 150 , 151 , 152 , 153 travoprost, and the prostamide, bimatoprost, have been approved for clinical use and are now some of the most commonly used drugs in the treatment of primary open-angle glaucoma (POAG) and ocular hypertension (see uveoscleral outflow section for greater detail). 154 , 155 AQUEOUS HUMOR COMPOSITION In the healthy eye, the aqueous humor has a refractive index taken to be constant at a value of 1.336. 156 Because this index of refraction is lower than that of the cornea, there is a slight divergence of light rays as they pass the cornea–aqueous interface. Both the viscosity and density of aqueous humor are slightly higher than that of pure water, while the osmolality is slightly higher than that of plasma. 30 , 157 , 158 , 159 , 160 The volume of the human anterior chamber is approximately 200 μL, 3 while that of the posterior chamber is approximately 60 μL. 1 This makes chemical analysis of the aqueous difficult because of the tiny volume of fluid as well as the relatively poor accessibility of the posterior chamber. Furthermore, it may account for the differences in values for the concentrations of many substances obtained by different investigators. The greatest difference between aqueous and plasma resides in the very low protein concentration in the aqueous ( Table 3 ), which is in the region of 0.5% that of plasma. 97 , 98 , 157 , 158 , 159 , 160 However, the composition of protein in the aqueous is also different from that in plasma. The ratio of the levels of the lower molecular weight plasma proteins (such as albumin and the γ-globulins) to the higher molecular weight proteins (such as the α-lipoproteins and the heavy immunoglobulins) is much higher in aqueous in the normal healthy eye than in plasma. 161 However, when the blood–aqueous barrier breaks down (as in uveitis), the composition and concentrations of protein in the aqueous are similar to that of plasma. 98 The levels of immunoglobulin in the aqueous have been determined, both in the normal eye and in eyes in which the barrier has broken down. 162 , 163 , 164 In the healthy eye, immunoglobulin (Ig) G is present at a concentration of approximately 3 mg per 100 mL, 164 while IgM, IgD, and IgA are absent, presumably because of their larger molecular structure. In eyes with uveitis, the concentration of IgG increases, and IgM and IgA appear also. In the normal aqueous, trace concentrations of active complement C2, C6, and C7 globulins are also present. 165 TABLE 3. Aqueous and Serum Protein Concentration Protein Human Rabbit Aqueous (g/100 mL) Serum (g/100 mL) Aqueous (g/100 mL) Serum (g/100 mL) Total protein 0.013 7.5 0.050 5.6 Globulin 0.003 2.5 0.025 2.5 Albumin 0.010 5 0.025 3.1 (Adapted from Davson H: The Eye. In: Vegetative Physiology and Biochemistry, Vol 1, 2nd ed, New York, Academic Press, 1969; and Duke-Elder S: The Aqueous Humour. The Physiology of the Eye and of Vision, Vol 4, pp 104–200. In Duke-Elder S (ed): System of Ophthalmology. St. Louis, CV Mosby, 1968) There are also trace quantities of several components of the fibrinolytic and coagulation system present in the aqueous, with the exception of plasminogen and plasminogen proactivator, which are present at more significant concentration. Only trace quantities of the inhibitors of plasminogen activation are present in the aqueous, 166 thereby ensuring that the aqueous outflow pathways remain free of fibrin. The aqueous humor of diseased eyes incorporates significant quantities of all of the major components of coagulation and fibrinolysis, giving rise to formation of intracameral clots; however, the composition of these clots is different from those that occur in blood vessels. 24 The α and γ lens crystallins are also present in only small amounts in the aqueous humor of healthy eyes, although the concentration of these proteins increases in cataract. 167 , 168 It has been calculated that in the normal healthy eye, the blood–aqueous barrier behaves as a semiporous membrane with a pore radius of approximately 10.4 nm. The protein concentration in the peripheral portion of the anterior chamber, close to the meshwork, may be much higher than in the more central region because of protein entry directly from the peripheral iris, as demonstrated in monkey and human eyes. 169 , 170 , 171 , 172 Inclusion of serum in the perfusand of monkey 173 or bovine 174 eyes decreases resistance washout. The reduction in washout may be the result of interactions of particular serum proteins and not due to the general level of serum proteins. 175 The concentration of amino acids, on the other hand, is frequently higher in the aqueous than in the plasma. 176 In the canine eye, however, there is a lower concentration of amino acids in the aqueous than in the plasma. 177 At least three transport systems for amino acids have been proposed in the eye, 55 one each for the acidic, basic, and neutral groups. A statistical study of the covariation of the concentration of amino acids and related compounds in human aqueous suggested the existence of six transport systems in the ciliary epithelia: three independent mechanisms for neutral amino acids, and independent mechanisms for basic amino acids, acidic amino acids, and urea. 178 The distribution of ions varies greatly amongst different species. For example, the monkey has a higher concentration of H + and Cl – and a lower concentration of HCO 3 – compared to plasma. On the other hand, rabbit aqueous has a lower concentration of Cl – and H + and a higher concentration of HCO 3 – compared to plasma. 179 Active transport of Cl – across the feline isolated ciliary epithelium has been reported. 52 The concentration of Na + in the aqueous is almost the same as in the plasma in many species. 1 , 30 , 180 However, the osmotic pressure of aqueous is slightly higher than that of plasma with respect to Na + , because of Gibbs-Donnan equilibrium. Most species tested have very high concentrations of ascorbate and lactate in the aqueous. Ascorbate is actively secreted into the posterior chamber, and the secretion mechanism will only function in the presence of ATP and a Na + gradient. The physiologic function of ascorbate remains to be elucidated; however, it is known to be concentrated by the lens epithelium 181 and has been shown to have a protective effect against UV-induced DNA damage to lens epithelium. 182 Ascorbate may function as an antioxidant, regulate the sol–gel balance of mucopolysaccharides in the trabecular meshwork, or partially absorb UV radiation 183 , 184 because diurnal mammals have approximately 35 times the concentration of aqueous ascorbate than nocturnal mammals. 185 The key oxidant in the aqueous humor, hydrogen peroxide, is normally present 186 as a result of reactions of ascorbic acid and trace metals. 187 Additional hydrogen peroxide and reactive oxygen species are generated by light-catalyzed reactions, metabolic pathways, and phagocytic or inflammatory processes. 186 , 187 Hydrogen peroxide affects aqueous outflow in the calf perfusion system. 188 Human trabecular meshwork cells exposed to 1 mmol of hydrogen peroxide show reduced adhesiveness to the extracellular matrix proteins fibronectin, laminin, and collagen types I and IV. 189 Extensive and repeated oxidative stress in vivo may result in reduced TM cell adhesion, leading to cell loss that is identified as one of the major culprits in glaucomatous conditions. 190 , 191 , 192 , 193 Lactate is produced as a result of the glycolytic degradation of glucose by both the ciliary body and the retina. 194 It diffuses into the posterior chamber but is present at only marginally higher concentration than in the plasma at this site. However, it accumulates in the anterior chamber at considerably higher concentration than in the plasma. Glucose, urea, and nonprotein nitrogen concentrations are slightly less than in plasma. 1 , 30 Glucose is thought to diffuse into the aqueous, where its concentration is approximately 80% that of plasma. Glucose also diffuses into the cornea. Its concentration within the corneal endothelium is approximately half that in the aqueous. In diabetes mellitus, the aqueous concentration of glucose is increased. High glucose levels in the aqueous humor may increase fibronectin synthesis and accumulation in the trabecular meshwork and accelerate the depletion of trabecular meshwork cells. In bovine trabecular meshwork cells grown in high-glucose medium, fibronectin mRNA is significantly upregulated, fibronectin immunofluorescence is more intense, and relative amounts of fibronectin protein are significantly increased. 195 Also the chemoattractant potential of fibronectin in aqueous humor is reported to play a role in trabecular meshwork cell loss in glaucoma. 196 Oxygen is also present in the aqueous humor, at a tension determined to lie between 13 to 80 mm Hg, depending upon the method of measurement. 197 , 198 , 199 , 200 , 201 The tension of oxygen in the aqueous can be decreased by topical epinephrine, possibly as a result of uveal vasoconstriction 198 or by the wearing of polymethylmethacrylate (perspex) contact lenses, which by restricting the normal passage of oxygen across the corneal epithelium, cause corneal hypoxia and thus an increase in movement of oxygen from the aqueous, across the corneal endothelium. 198 Berzelius 202 first demonstrated the presence of lipids in bovine aqueous. Since then, sphingomyelin, phosphatidyl choline, and lysophosphatidyl choline have all been shown to be present in the aqueous, 203 although at a concentration of less than 1 mg per 100 mL, because lipids are largely barred from entry to the aqueous by the blood–aqueous barrier. 204 Other substances, such as corticosteroids, 205 monoamine metabolites, 206 Cr 3+ ions, 207 vitamin B 12 , 208 sialic acid, 209 and hyaluronic acid 210 have all been found to exist in the aqueous of a number of different species. Hyaluronic acid covering the surfaces of the outflow pathways might prevent adherence of molecules to extracellular matrix components within the cribriform region and thereby prevent clogging of the outflow pathways. 211 Knepper et al 212 hypothesize that POAG is characterized by a decreased concentration of hyaluronic acid and increased turnover and downregulation of the hyaluronic acid receptor CD44 in the eye, which, in turn, may influence cell survival of TM and retinal ganglion cells. Corticosteroid regulation of IOP is proposed to occur via 11β-hydroxysteroid dehydrogenase (HSD)-1 expression that has been localized in the nonpigmented ciliary epithelium of human eyes. 213 , 214 , 215 This enzyme catalyzes the conversion of cortisone to cortisol which, in turn, induces sodium and concomitant water transport into the posterior chamber, through epithelial sodium channels, including Na+-K+-ATPase, 216 , 217 resulting in aqueous production. Levels of cortisol compared to cortisone in the aqueous humor are normally much greater than in the systemic circulation. 218 However, long-term interactions of cortisol in the aqueous with glucocorticoid receptors in the trabecular meshwork, could contribute to increasing outflow resistance in individuals susceptible to steroid induced glaucoma (see section on trabecular outflow ). Ingestion of the 11β-HSD antagonist carbenoxolone, decreased IOP in normal 213 and ocular hypertensive 214 human eyes. The role of the other compounds within the aqueous is not understood. Transforming growth factor (TGF) β2 is a component of normal aqueous humor detected in many mammalian eyes 219 , 220 , 221 , 222 and may play a role in glaucoma pathogenesis. The intrinsic activity of TGFβ2 is considered to be an important factor for the maintenance of the anterior chamber-associated immune deviation (ACAID). 219 , 223 (see section on Aqueous Outflow : Other Agents for more details). As the aqueous flows from the posterior chamber to the anterior chamber, changes occur. Nutrients diffuse into the lens, iris, and vitreous. 224 Diffusion from the posterior aqueous into the vitreous is a major contributor to the existence of concentration gradients of low molecular weight substances in the vitreous. 225 Waste products, such as lactate diffuse from the lens, iris, and corneal endothelium into the aqueous. Some exchanges of small molecules occur across the iridial vessels. Predictably, the chemical compositions of posterior chamber aqueous and anterior chamber aqueous are different. 30 To complicate the matter further, some substances appear to be actively transported out of the eye. Paraaminohippurate (PAH), diodrast, and penicillin are examples of large anions that are actively transported out of the eye. The system appears to be similar to that occurring in the renal tubules. This active transport system can be saturated and can also be inhibited by low temperatures and probenecid. 1 , 30 The nonpigmented ciliary epithelium has been implicated as the site of this active transport system within the anterior eye. Another independent transport system actively excretes injected iodide from the aqueous. This latter system resembles iodide transport mechanisms in the thyroid and salivary glands. 226 The significance to the normal physiology of the eye of these outward-directed transport systems has not been established. With the discovery that prostaglandins may be actively transported out of the eye, 227 , 228 some have suggested that such mechanisms may be useful to rid the eye of biologically active substances no longer needed, or which may even be detrimental. 45 Their removal from the eye to the blood facilitates their excretion via the hepatic route. There are other outwardly directed ion-uptake mechanisms present in the eye. The anterior uvea of the rabbit eye, for example, accumulates the anions cholate, glycocholate, deoxycholate, chenodeoxy-cholate, iodipamide, and o-iodohippurate. 229 , 230 At least one inwardly directed anion pump mechanism also operates—ascorbate is accumulated in the anterior chamber and lens, its concentration in the aqueous humor being approximately 20 times that in plasma. 181 This may help protect the anterior ocular structures from oxidative damage. Bárány, 231 making an analogy to the ion pump located at the renal peritubular cell border adjacent to the blood, which pumps simple cations from the blood to the kidney tubule, investigated whether there are any inwardly directed cation pump mechanisms from blood to aqueous. He concluded, however, that such mechanisms probably do not exist, at least within the rabbit eye. But outwardly directed cationic pump mechanisms have been reported. For example, iris–ciliary body preparations have been shown to accumulate the cation emepronium, 232 although a later report 233 questioned whether any other cations are actively eliminated from the eye. AQUEOUS FLOW The result of all of the biochemical processes previously discussed is the production of a quantity of fluid that circulates continuously. Many of the biochemical changes caused by diffusion occur as the fluid moves from the posterior chamber, through the pupil, around the anterior chamber, and into the outflow system. Superimposed on this bulk flow is the anterior chamber thermal circulation, which causes the aqueous closer to the cooler avascular posterior cornea (cooled additionally by evaporation of tears from the corneal epithelium) to move downward, whilst the aqueous closer to the warmer vascular anterior iris moves upward. In addition, movements of the eyes and head modify these flow parameters. In the human eye, the rate of aqueous formation is approximately 2.5 μL/min, while that in the rabbit is approximately 3 to 4 μL/min. Because of the formation rate and aqueous chamber volumes in the human eye, approximately 3% of the posterior chamber volume and 1% of the anterior chamber volume are replaced per minute 1 , 30 with the entire volume of the aqueous humor being replaced every 90 to 100 minutes. 2 The more rapidly the aqueous is formed, the less is the potential for diffusional exchange with the ciliary processes, lens, anterior iris, and posterior cornea. However, within physiologic limits, changes in the rate of formation probably do not significantly affect the diffusional exchange. Furthermore, the rate of aqueous formation contributes to the regulation of IOP. METHODS OF MEASURING RATES OF AQUEOUS FORMATION In 1951, Goldmann 234 first described a technique by which rate of flow of aqueous humor in the eye could be quantified. This technique was based on the measurement of the kinetics of unbound fluorescein in the plasma and concomitant fluorescence in the anterior chamber after intravenous injection. 3 Thereafter, other investigators devised techniques for the determination of aqueous flow, generally involving a cannulation of the anterior chamber with a needle, thus permitting drainage of aqueous or infusion of a fluid at a known rate. 235 , 236 , 237 , 238 , 239 , 240 , 241 For example, one can inject into the anterior chamber a known amount of dye, radioactively labeled substance, or large molecule, the concentration of which is easily measured. If the substance mixes rapidly with all of the aqueous, then repeated sampling of the fluid and measurement of the concentration provides an indirect measurement of bulk flow. The underlying assumption with this technique is that no change occurs in aqueous dynamics as a result of the injection or sampling technique. In practice such an assumption does not hold true, because the blood–aqueous barrier is breached by simple paracentesis. 242 Furthermore, this technique assumes that none of the substance leaves the eye by diffusion or any other way except by direct bulk flow (which includes outflow via the uveoscleral pathway). Later still, other techniques more applicable to the measurement of aqueous formation in the human eye were devised, which did not involve any invasive procedure. 243 , 244 , 245 , 246 , 247 , 248 , 249 These methods, discussed in the following section, fall essentially into one of two categories: (i) measurement of the rate of appearance or disappearance of a chemical substance from the aqueous or (ii) derivation of the aqueous formation rate from a mathematical formula (to be discussed) after obtaining measurements of the IOP, episcleral venous pressure, and resistance to aqueous outflow. 1 , 30 Each method incorporates intrinsic advantages and disadvantages, and specific sources of error. However, in spite of this, there exists good agreement for the values of aqueous formation obtained, and in several different species. MEASUREMENT OF FORMATION BY THE RATE OF APPEARANCE OR DISAPPEARANCE OF A CHEMICAL SUBSTANCE FROM THE AQUEOUS It is possible to inject a substance such as PAH or fluorescein into the bloodstream and maintain a high concentration there. Over a period of several hours, a small but measurable amount diffuses into the aqueous humor and reaches an equilibrated concentration. The injection is then stopped, and the level of the fluorescein or PAH in the blood subsequently declines rapidly, owing to renal clearance. The concentration of the substance in the aqueous, however, remains relatively constant over a short period of time, after which it starts to decrease, as fresh aqueous is formed not incorporating any PAH or fluorescein, because of low plasma levels. The rate of fresh aqueous formation can be calculated by measuring the declining concentration either by sampling the aqueous first in one eye, then later in the second eye, as in the case of PAH, or by optical means using fluorophotometry, as in the case of fluorescein ( Fig. 10 ). Bárány and Kinsey 250 developed this technique for PAH, and it was extended for use with fluorescein. 248 , 251 , 252 , 253 The technique using fluorescein has been modified by dropping the fluorescein topically onto the eye rather than by injecting it. 254 , 255 The dye in this case gains access to the anterior chamber via corneal absorption. There are, however, inherent problems with this approach, specifically, the problem of measurement of fluorescence in the anterior chamber and cornea, and the deduction of aqueous flow from the change in fluorescence over time. 3 The first problem was solved by the construction of a slit-lamp fluorometer. 256 , 257 The second was addressed by a number of investigators who devised several new experimental approaches. 55 , 250 , 258 Thus, despite the drawbacks of fluorophotometry using topically applied fluorescein, the technique has become the gold standard in studies involving the human eye. Jones and Maurice 248 realized that the corneal stroma could serve as a depot from which fluorescein could be introduced slowly into the anterior chamber. This method clarified the important role of the cornea in affecting the kinetics of topically applied drugs and tracers. Maurice’s technique is now used most frequently for the measurement of the rate of aqueous formation in the human eye. The method involves the application of fluorescein topically to the eye, which subsequently penetrates the corneal epithelium and enters the stroma. Fluorescein is not metabolized by the eye and thus can disappear in only three ways: (i) rediffusion through the corneal epithelium and loss with the tears, (ii) lateral diffusion into the limbal tissue, or (iii) penetration of the endothelium and entrance into the aqueous humor, from where it is washed away by flowing aqueous, or is lost by diffusion into the iris (approximately 10% of fluorescein in the aqueous is lost by this route in the human eye). 259 The third pathway of movement of fluorescein from the corneal stroma offers the least resistance, and thus is the major pathway of loss of fluorescein from the stroma. Fig. 10. Principles of measurement of aqueous flow by ocular fluorophotometry. A : Optical axis of eye is scanned for background fluorescence with a scanning ocular fluorophotometer. B : Topical application of drops of fluorophore (2% fluorescein) applied to cornea. C : After a suitable delay (approximately 15 hours), to allow fluorescein to diffuse from the corneal depot to the aqueous humor, the eye is scanned once again. D : Repeated scans at 30-minute to 1-hour intervals over a 3- to 6-hour period facilitate monitoring of decline in fluorescence of aqueous humor with time. This can be related mathematically to aqueous flow rate (a calculation often performed by computer) after subtraction of background fluorescence and derivation of anterior chamber volume from keratometry and pachymetry determinations. The graphs to the right of the diagrams indicate typical fluorescence patterns obtained along the optical axis at each stage in the procedure. C, cornea; AC, anterior chamber; L, lens; V, vitreous. The advantages of Maurice’s technique are that it is safe, repeatable, and objective. Studies of the human eye can span 18 to 24 hours after application of a single dose of fluorescein. The procedure disturbs the eye minimally, and the subject need not be constrained during the interval of measurement. Furthermore, the technique is reliable even when the rate of flow is not constant. 3 In one variation of this technique, fluorescein is introduced into the stroma simply by applying drops topically to the inferior fornix of the conjunctiva. 254 , 260 A waiting period of 6 hours or more allows the dye to become more uniformly distributed within the stroma. In another variation, fluorescein is applied by iontophoresis. 248 This procedure involves the introduction of fluorescein into the anterior chamber by forcing it through the cornea via the application of a small electric current. Fluorescence is measured in the stroma and in the anterior chamber at the beginning and end of an interval. Flow is the clearance of the dye during the interval minus the diffusional loss. The technique, however, is not applicable to eyes that lack an iridolenticular barrier between the anterior and posterior chambers. Furthermore, the technique measures only that portion of secreted aqueous that passes into the anterior chamber. 3 McLaren 261 developed a technique of measurement of aqueous flow based on flare. Flare (pathologic scattering of light resulting from the presence of protein in the aqueous resulting from inflammation and breakdown of the blood–aqueous barrier) was induced by argon laser photocoagulation of the iris in rabbits, and a scanning ocular spectrofluorophotometer was used to measure scattering in the anterior chamber. This method was used to study changes in aqueous flow over the diurnal cycle. A technique for the measurement of aqueous flow using corneal and vitreous depots of fluorescein in the rabbit eye has also been described. 262 , 263 It may be concluded that (i) movement of water into or out of the vitreous can cause large changes in the rate of movement of dye from the vitreous to the anterior chamber and can make interpretation of the vitreous method ambiguous and (ii) the vitreous method is probably superior for measuring sustained changes of the rate of aqueous flow over at least 10 hours, or perhaps several days, but it cannot be reliably used for measuring changes over shorter periods. Similar types of studies have been performed using iodide. 264 In all cases, it is assumed that the amount of substance leaving the eye by alternative routes is negligible compared with the dilution by fresh aqueous. By using the method of Maurice and introducing fluorescein by iontophoresis, a value of 2.48 ± 0.17 μL/min (mean ± standard error of the mean [SEM]) was calculated for aqueous flow in the human eye. 248 Holm 245 carried this method one step further. After forcing fluorescein into the anterior chamber, the fluorescein is carefully mixed by rapid eye movements. Then, as fresh aqueous humor not containing fluoresecein is produced, it forms a small, clear, growing bubble at the pupillary border. Multiple slit images are projected onto the bubble and photographed. The volume of the bubble can be estimated by measuring the deviation of each slit as it passes over the bubble and integrating the area of all the deviations. Two sets of photographs are taken 15 seconds apart in order to calculate the rate of change. However, the pupil must be miotic (constricted) for this technique to work, and a miosis is induced by topical application of pilocarpine, which may stimulate aqueous flow slightly. 265 Furthermore, the production and maintenance of the bubble of clear aqueous is technically difficult. For these reasons, Holm’s technique of iontophoresis has not gained widespread popularity in studies of aqueous humor secretion, either in the human or animal eye, although the results that have been obtained using this method agree closely with those obtained using Maurice’s technique of fluorophotometry. 3 In all the techniques whereby a fluorescent dye is used to measure aqueous flow, there are other potential confounding factors, such as binding of a proportion of the dye to ocular and/or plasma proteins, that must be considered. 266 However, under normal circumstances these factors do not compromise the measurements. MATHEMATICAL DERIVATION OF AQUEOUS FORMATION RATE The other major types of investigational tools used to estimate aqueous formation involve physical measurements. These methods depend on the following algebraic manipulation of the modified Goldmann equation describing IOP in terms of episcleral venous pressure (P e ), aqueous flow (F in ), trabecular outflow facility (C trab ), and uveoscleral outflow (F u ): IOP = P e + ((F in -F u )/C trab )) thus IOP-P e = ((F in -F u )/C trab )) and F in – F u = C trab (IOP – P e ) therefore F in = C trab (IOP – P e ) + F u In order to determine C trab and F u , the eye in a living anesthetized animal may be cannulated with a needle and perfused with a solution of mock aqueous humor 267 incorporating radiolabeled albumin (see later in this chapter). 268 , 269 A value for IOP can be obtained by tonometry, or (in experimental animals) cannulation of the anterior chamber with connection via tubing to a pressure transducer. An estimate of P e can be obtained in experimental animals by direct cannulation of an episcleral vein, or by measurement of the pressure necessary to completely collapse an episcleral vein. 270 (In practice these procedures would be repeated several times and a mean value calculated, because P e is not constant throughout the entire episcleral venous system). By substitution into the above equation, F in may be determined. F in can also be calculated by determination of the difference in radioactivity of a perfused radiolabeled solution before entering and after leaving the anterior chamber, the difference being related proportionally to total aqueous flow. These approaches, however, are applicable only to experimental animals; their invasive nature makes them unsuitable for use in the human eye. Another method for estimating the rate of aqueous flow involves the use of a perilimbal suction cup. 85 , 271 The perilimbal suction, theoretically, occludes the outflow channels of the eye, and thus IOP rises. The rate of increase in IOP depends not only on the rate of formation of aqueous humor but also on the elasticity or distensibility of the eye. Unfortunately, there is a question as to whether this method may also alter the blood flow to the ciliary body, thus possibly having an effect on aqueous formation. Furthermore, as the pressure rises in the eye, the rate of inflow of aqueous humor declines, a phenomenon known as pseudofacility. In addition, the suction cup may not totally close off the drainage of aqueous. Nevertheless, Galin, 271 using this method, calculated rates of aqueous formation that compare well with other methods. It is apparent that each of the foregoing methods has inherent errors and assumptions, yet despite this, the results indicate reasonable agreement among the various methods. The study of these methods has added much to the understanding of the factors that influence aqueous formation. In fact, it is not so much the absolute values themselves that are important but, rather the ability to compare changes in values for aqueous formation under different conditions that has been most informative. Of all of these methods, however, noninvasive fluorophotometry has become the method of choice for the determination of the rate of flow of aqueous. FACTORS AFFECTING AQUEOUS FORMATION The rate of formation of aqueous is not constant. Variations in the formation rate occur hourly and daily. 3 Knowledge concerning the cause(s) of this variation is particularly sparse. It is likely that many diverse physiologic systems, including the central nervous, endocrine, and cardiovascular systems, as well as changes in metabolic activity, all influence the production of aqueous. Aqueous secretion also exhibits a diurnal cycle. 1 , 24 , 272 It is hypothesized that the diurnal cycle of aqueous humor formation is regulated in part by a factors such as circulating catecholamines, epinephrine and norepinephrine, that have a circadian rhythm and partly by a factor that depends on the activity of the subject. 272 Further, the magnitude of the fluctuation in flow rate differs among different individuals. The ciliary body is innervated by nerves arising from the long posterior and short ciliary nerves, which run parallel to the long posterior and short ciliary arteries. These nerve fibers are of both the myelinated and nonmyelinated variety. Parasympathetic fibers originate in the Edinger-Westphal nucleus of the third cranial nerve, run with the inferior division of this nerve in the orbit, and synapse in the ciliary ganglion. 225 Sympathetic fibers synapse in the superior cervical ganglion and are distributed to the muscles and blood vessels of the ciliary body. Numerous unmyelinated nerve fibers surround the stromal vessels of the ciliary processes; these are most likely noradrenergic and subserve vasomotion. 24 Sensory fibers arise from the ophthalmic division of the trigeminal nerve and enter the ciliary body, but their distribution and function have not been well studied. However, despite the distribution of nerves to the ciliary body, little evidence for innervation of the ciliary epithelium itself has yet been found. 4 Wetzel and Eldred 273 found that some of the dendritic processes from peptidergic amacrine cells in the retina of the turtle formed a dense circumferentially oriented nerve fiber plexus and that collaterals from this plexus projected into and innervated the nonpigmented ciliary epithelium in the pars plana region of the ciliary body. The authors suggested that these peptidergic amacrine cells may be involved in the control of aqueous inflow. 273 It has also been found that stimulation of the ciliary ganglion or application of cholinergic agents, in nonprimates, will lead to an increase in aqueous formation, even in the isolated, perfused feline eye. 85 Additionally, in this model, sympathetic activity was reported to suppress aqueous secretion. Also, in the bovine perfused eye model, 274 delivery of the adrenergic agonist terbutaline via the intra-arterial route to the ciliary body was found to decrease the rate of aqueous secretion. 275 Results obtained with isolated perfused eyes of nonprimate animals, however, may bear little relationship to the in vivo physiologic situation reported in humans and subhuman primates. For example, adrenergic agonists are reported almost exclusively to increase aqueous secretion in primates. Topical epinephrine, norepinephrine, or isoproterenol stimulate secretion in the monkey eye, 276 , 277 and topical epinephrine or terbutaline stimulate aqueous secretion in the human eye. 278 , 279 Whether these processes work at the vascular level to change vascular tone or permeability or their effect is dependent on ciliary epithelial cell surface neurohumoral receptors associated with adenylate cyclase and/or guanylate cyclase activity has not yet been completely elucidated; however an amassing body of evidence points to the latter. 275 , 280 , 281 , 282 , 283 , 284 , 285 , 286 , 287 , 288 , 289 , 290 , 291 , 292 , 293 , 294 , 295 , 296 The α 2 -adrenoceptor agonists apraclonidine and brimonidine are reported to lower aqueous secretion and IOP in cats, rabbits, monkeys, 297 , 298 , 299 and humans. 300 The effect is thought to be mediated via a postjunctional or nonjunctional α 2 receptor present on the membrane of the nonpigmented ciliary epithelium 299 but may in part be centrally mediated via imidazoline receptors in some species. 301 The effect in monkeys is not abolished by superior cervical ganglionectomy, 299 indicating that intact sympathetic innervation is not required for the drugs to lower secretion and IOP. However, intact sympathetic innervation is required for a response to the α 2 -adrenoceptor agonists clonidine in rabbits 302 or brimonidine in cats and rabbits. 297 The lack of aqueous flow suppression by brimonidine in pentobarbital-anesthetized monkeys may reflect the suppression of neural and humoral catecholaminergic tone by this anesthetic, 303 especially relative to ketamine, which elevates such tone. 304 Timolol, a nonselective β-adrenergic antagonist, suppresses aqueous flow in both eyes of ketamine 305 but not pentobarbital-anesthetized 306 monkeys that have undergone unilateral superior cervical ganglionectomy, suggesting that the relevant β-receptors may be nonjunctional rather than postjunctional and that sympathetic neural tone to the ciliary body may play relatively little role in regulating aqueous humor formation in the monkey. It is thus not entirely clear to what extent aqueous secretion is under neuronal versus humoral adrenergic control. The effects of cholinergic drugs on aqueous humor formation and composition, and on the blood–aqueous barrier, are unclear, with conflicting results arising from various studies. In general, cholinergic drugs cause vasodilation 307 , 308 , 309 resulting in increased blood flow to the iris, ciliary processes and ciliary muscle. 309 , 310 The presence of flare (Tyndall effect, indicating increased protein concentration) and/or the detection of cells in the aqueous humor by biomicroscopy indicates that these agents can also cause breakdown in the blood-aqueous barrier. 311 Pilocarpine increases barrier permeability to iodide 264 and inulin. 312 Cholinergic drugs may alter the aqueous humor concentration of inorganic ions 313 and the movement of certain amino acids from the blood into the aqueous humor, and may also influence the outward-directed transport systems of the ciliary processes. 314 , 315 Under certain conditions, pilocarpine may increase pseudofacility. 316 , 317 Cholinergic agents or parasympathetic nerve stimulation have been reported to increase, decrease, or not alter aqueous humor formation rate and to slightly increase the episcleral venous pressure. 265 , 317 , 318 , 319 , 320 , 321 , 322 , 323 , 324 The minimal effect on the rate of aqueous humor formation and episcleral venous pressure are clearly not responsible for the drug-induced decrease in IOP that results from cholinergic therapy. Aqueous formation varies directly with the blood pressure in the internal carotid-ophthalmic arterial system in the primate 277 but only when blood pressure is altered artificially to a physiologically abnormal extent. For example, ligation of the internal carotid artery causes a profound drop in aqueous secretion. Formation is not significantly altered however by changes in blood pressure within the normal physiologic range for any given species. Aqueous formation rate diminishes slightly with age. After the age of approximately 10 years, formation declines by 3.2% to 3.5% per decade. 3 The mechanism underlying this decrease is unknown. An age-dependent loss of ciliary epithelial cells has not been described. Some authors have demonstrated a 5.8% loss of trabecular cells per decade in humans, 190 , 325 , 326 while others have reported a loss of corneal endothelial cells at the rate of 3.5% per decade. 327 Brubaker 3 suggested that the age dependency of the population of ciliary epithelial cells should be studied, because if it is found that aqueous formation parallels the number of secreting cells, it would suggest that although aqueous formation may depend on neuronal or hormonal stimulation, the normal rate of formation may depend on cell count. Alternatively, the decline in aqueous formation could be a result of the changes observed in the fine structure of aging ciliary epithelial cells. 328 Hypothermia leads to a decrease in aqueous formation, for example, a drop in body temperature of 7°C (about 19%) leads to approximately a 50% reduction in secretion, reflecting the deactivation of metabolic processes necessary to maintain active secretion. 45 The ultrafiltration component of aqueous humor formation is pressure-sensitive, decreasing with increasing IOP. This phenomenon is quantifiable and is termed pseudofacility, because a pressure-sensitive decrease in inflow appears as an increase in outflow facility when techniques such as tonography and constant pressure perfusion are used to measure outflow facility. 237 , 238 , 316 , 329 , 330 , 331 , 332 , 333 Although some sensory nerve endings exist in the ciliary body, they do not appear to be of the pressure-sensitive variety. 4 The initiating event of this pressure-induced response of the ciliary processes remains obscure. Bill and Bárány 237 reported that an artificially induced rise in IOP caused a reduction in secretion. This has been confirmed in the monkey. 334 An intracameral injection of erythrocytes yielded a partial blockade of the trabecular meshwork, and hence an elevation in IOP. This led to a suppression of aqueous secretion, corresponding to 0.06 μL/mm Hg per minute increase in pressure. Therefore with a normal secretion rate in the pentobarbital anesthetized cynomolgus monkey of approximately 1 μL/min, 335 an increase in IOP of 20 mm Hg should theoretically suppress aqueous secretion altogether. However, this does not in fact occur. It is well established that secretion continues, even against very high pressures. Increased IOP does decrease the blood flow in the ciliary body and may decrease secretion in this manner. 315 , 330 , 336 In addition to the acute effect of elevated IOP, some patients in the late stages of glaucoma may show hyposecretion and even have normal pressures despite almost totally occluded outflow channels. 337 It is not known if this is due to the same mechanism as the acute type of pressure-related hyposecretion. There is evidence, however, that much of the perceived reduction in aqueous secretion in response to increased IOP is caused by measurement artifact 237 , 238 , 268 , 315 , 334 , 338 and that the real magnitude of pseudofacility in the monkey is less than 0.02 μL/mm Hg per minute, or less than 5% of total facility. 238 , 339
Share this:
- Click to share on Twitter (Opens in new window)
- Click to share on Facebook (Opens in new window)
Related posts:
- A and V Patterns
- Theory and Practice of Spectacle Correction of Aniseikonia
- Plain Roentgenographic Evaluation of Orbital Disease
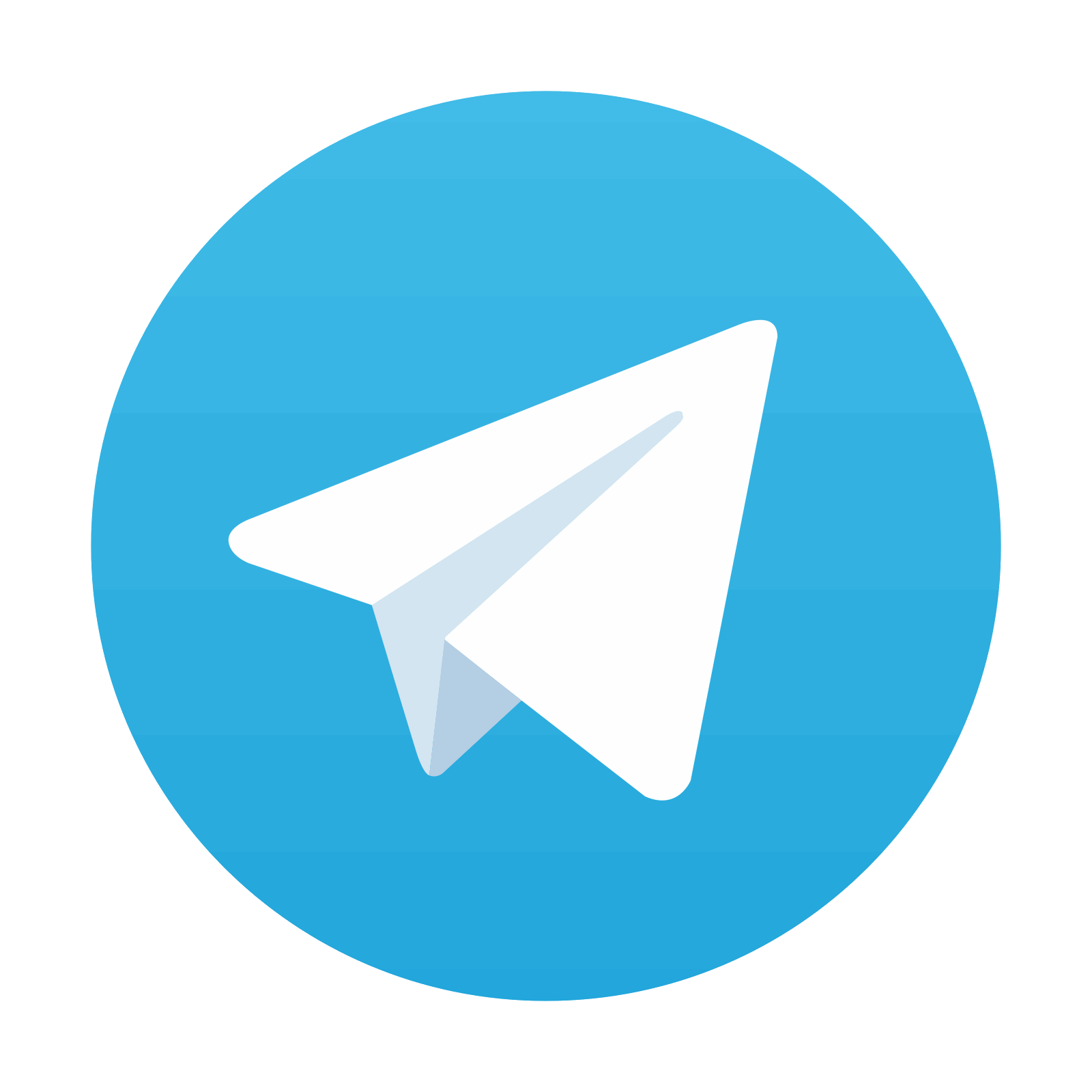
Stay updated, free articles. Join our Telegram channel
Comments are closed for this page.

Full access? Get Clinical Tree
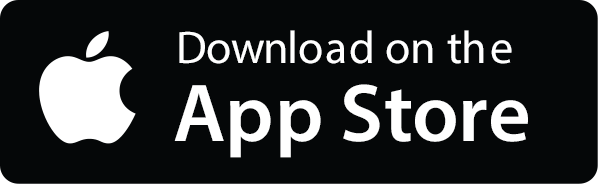

Open source ophthalmology education for students, residents, fellows, healthcare workers, and clinicians. Produced by the Moran Eye Center in partnership with the Eccles Library

Search Moran CORE
- Support CORE
- International
- About Moran
Aqueous Humor Production and Dynamics
Home / Glaucoma
Title: Aqueous Humor Production and Dynamics Author: Janine Yang MSIV, Susan Chortkoff MD Date: 10/4/2022 Keywords/Main Subject: Glaucoma, Aqueous Humor, Anatomy
The aqueous humor is a water-like fluid that fills the anterior chamber of the eye with continual production, secretion, and reabsorption. 1,2 The production, circulation and reabsorption of aqueous humor are vital processes maintaining homeostasis of the eye. Imbalances between the production and secretion of aqueous humor may lead to increased intraocular pressure and optic nerve damage such as in the setting of ocular hypertension or glaucoma. Therefore, understanding the physiology behind aqueous humor as well as changes observed in pathologic states is important in managing glaucomatous processes.
Chemistry and Function
Aqueous humor is produced by the ciliary body epithelium at a rate of 2-3 microliters per minute. 1-3 It is composed of organic and inorganic ions, carbon dioxide, amino acids, carbohydrates, glutathione, and water. 1,2,4 Important transport and enzymatic proteins involved in synthesis may be targeted in glaucoma drugs, discussed below. 1,2,5
Aqueous humor functions as a physical component allowing clear optics and filling the anterior chamber of the eye. 1-3 It is responsible for providing nourishment to the avascular components of the anterior chamber including the cornea and lens. 1-3 In addition, aqueous humor is responsible for removing waste products, blood, macrophages and other debris from the anterior chamber, including the trabecular meshwork. 1-3 The structure and function of the trabecular meshwork may become compromised by chronic oxidative stress from reactive oxygen species and insufficient antioxidant defense in the aqueous humor. 1-3,6,7 Decreased levels of antioxidants in aqueous humor are present in glaucomatous eyes versus normal eyes, consistent with the presence of increased oxidative stress and low-grade inflammation. 6,7
Production and Circulation
The primary anatomic structures vital to the homeostasis of aqueous humor include the ciliary body as the site of principle production, and the trabecular meshwork and uveoscleral pathway as the sites of primary outflow. 1,8 Aqueous humor is produced by the ciliary body via a multistep process closely correlating with systemic vascular blood flow. 1,9,10 Initially, blood enters the ciliary processes, which propels ultrafiltrate from the blood into the ciliary interstitial space via a pressure gradient. 1,9,10 Next, the ciliary epithelium transports plasma components from the basal to the apical surface in order to synthesize aqueous humor and transport it into the posterior chamber. 1,9,10 Passive diffusion and ultrafiltration are key in initial synthesis, and active secretion across a blood-aqueous barrier via aquaporins, Na-K-ATPase and carbonic anhydrase enzymes are necessary for final synthesis. 1,4,9,10 These active transport enzymes necessary for final synthesis are common pharmacologic targets in decreasing aqueous humor production. Although systemic blood flow via the ciliary artery is required for the initial production of ultrafiltrate, the production of aqueous humor is independent from systemic blood pressure due to a fixed rate of 4% filtration of plasma. 10 Therefore, there is minimal association between hypertension and elevated intraocular pressure. The estimated rate of aqueous humor production is approximately 2.4 microliters per minute, with diurnal variations leading to higher aqueous humor flow in the morning and lower flow in the evening. 1,9
While aqueous humor production is well documented, the mechanism of drainage is still poorly understood. There are two main drainage pathways – the conventional pathway (trabecular meshwork, Schlemm’s canal, collector channels, and the episcleral venous system), and the unconventional pathway (uveoscleral, uveovortex, uveolymphatic). 1,5,9
The conventional pathway involves passive drainage throughout the trabecular meshwork although the Schlemm’s canal has been documented with paracellular and intracellular pores. 1,5,9 The trabecular meshwork is a triangular porous structure composed of a layer of connective tissue and endothelium with sympathetic innervation from superior sympathetic ganglion, and parasympathetic innervation from the ciliary ganglion. 1,5,9 The meshwork may be divided into the uveal meshwork (iris root, ciliary body, peripheral cornea), corneoscleral meshwork (scleral spur), and juxtacanalicular meshwork (transition into Schlemm’s canal). 1,5,9 Schlemm’s canal is a structure with composition similar to venous vasculature, with fenestrated thin endothelium surrounded by connective tissue. 1,5,9 After drainage through the trabecular meshwork and the Schlemm’s canal, aqueous humor continues through collector channels into the episcleral venous system which deposits into the main venous system. 1,5,9
Resistance to outflow through the trabecular meshwork and Schlemm’s canal has been documented although it is poorly understood, yet resistance remains an important factor in regulating intraocular pressure and the pathogenesis of glaucomatous processes. In humans, up to 75% of aqueous outflow resistance is contributed by the trabecular meshwork while the remaining 25% is due to resistance beyond Schlemm’s canal. 1 The rate of outflow is directly influenced by iris and ciliary muscles which contract and relax based on cholinergic innervation and pharmacodynamics. 1,5,8,9,11 In ciliary contraction, the trabecular meshwork and Schlemm’s canal dilate, decreasing resistance and increasing outflow. 1,5,8,9,11 The rate of outflow is also influenced by intraocular pressure, with higher intraocular pressure altering the structure of endothelial lining in Schlemm’s canal to increase the number of porous vacuoles allowing increased outflow. 1,5,8,9,11 However, it is still debated if this finding substantially contributes to increasing outflow in glaucomatous eyes. 1,5,8,9,11 *
The unconventional pathway involves drainage into the orbital vasculature, vortex veins and ciliary lymphatics, contributing up to 25-40% of total aqueous outflow in cynomolgus and vervet monkey models. 2,5 The uveoscleral pathway involves diffusion into the sclera and episcleral through the orbital vasculature. 2,5 The uveovortex pathway involves osmotic absorption of fluid through the choroid, passing into the vortex veins. 2,5 Lastly, the uveolymphatic pathway involves drainage into lymphatic vessels within the ciliary body, although the extent of drainage under normal physiological conditions remains controversial. 2,5 In addition, the unconventional pathway also includes corneal, iridial and retinal routes, albeit less clinically significant. 12 Regardless of downflow pathway, all unconventional paths require drainage through the interstitial spaces of the ciliary muscle. 2,5,12 Resistance also exists within the unconventional pathway likely due to ciliary muscle tone, as seen with changes in outflow in the setting of pilocarpine, increasing ciliary tone and decreasing flow, and atropine, decreasing ciliary tone and increasing flow. 2,5,12 Therefore, the unconventional pathways are also clinically important in moderating intraocular pressure, and serve as a potential target in glaucoma therapy.
In the Setting of Glaucoma
Aqueous humor dynamics and circulation are vital in the pathogenesis of glaucomatous damage to the optic nerve. 1,9,10 One of the primary risk factors and causative factors in glaucoma is an elevated intraocular pressure above the normal physiologic range of 12 to 21 mmHg. 1,9,10 Extended periods of elevated intraocular pressure above 22 mmHg have been associated with development of glaucomatous damage. 1,9,10 Elevated intraocular pressure from increased resistance and decreased outflow of aqueous humor and/or increased production leads to compression of the optic nerve which limits oxygen and nutrition supply to the nerve. There are two main categories of glaucoma, each involving aqueous humor circulation and production in relation to resulting optic neuropathy.
Primary open angle glaucoma (POAG) causes optic neuropathy in the setting of an ‘open angle’, while primary angle closure glaucoma (PACG) in the setting of peripheral angle obstruction or narrowing. 1,9,10 The ‘angle’ refers to the intersection of the peripheral iris with the cornea, a key anatomical player in conventional aqueous humor drainage. 1,9,10 * as this is where the trabecular, Sclemm’s canal are located. In POAG where the iris-cornea angle remains open for drainage, the mechanism for glaucomatous damage includes overall increased resistance to outflow despite maintaining an open angle, and increased aqueous humor production. 1,9,10 In PACG, the iris-cornea angle is acutely or chronically obstructed which leads to significant decrease in aqueous humor outflow and an elevation in intraocular pressure. 1,9,10
Ocular hypertension (OHTN) is defined as elevated intraocular pressure without any signs of vision loss or optic neuropathy otherwise classified as glaucomatous changes. 2,3 Patients with OHTN have demonstrated reduced trabecular outflow and uveoscleral outflow with normal aqueous production which explains the mechanism for elevated intraocular pressure 2,3 This is a similar mechanism to patients with POAG. However, the understanding of elevated intraocular pressure in the setting of OHTN or POAG is limited, such as in situations with OHTN without POAG or normal tension glaucoma. 2,3
Current glaucoma pharmacologic and surgical therapies are targeted at decreasing aqueous humor production, such as carbonic anhydrase inhibitors or ciliary body ablative laser treatments, or increasing outflow, such as prostaglandin agonists, adrenergic agonists, and physical modifications to optimize or bypass the outflow pathways. 1,9

Taken from American Academy of Ophthalmology, Aqueous Humor Flow

Taken from article: Waly et al, 2010 (System Dynamic Model for Normal Intraocular Pressure)

Taken from: Toris CB, Koepsell SA, Yablonski ME, Camras CB. Aqueous humor dynamics in ocular hypertensive patients. J Glaucoma. Jun 2002;11(3):253-8. doi:10.1097/00061198-200206000-00015
- Goel M, Picciani RG, Lee RK, Bhattacharya SK. Aqueous humor dynamics: a review. Open Ophthalmol J . Sep 3 2010;4:52-9. doi:10.2174/1874364101004010052
- Sunderland DK SA. Physiology, Aqueous Humor Circulation.
- Toris CB, Koepsell SA, Yablonski ME, Camras CB. Aqueous humor dynamics in ocular hypertensive patients. J Glaucoma . Jun 2002;11(3):253-8. doi:10.1097/00061198-200206000-00015
- Macknight AD, McLaughlin CW, Peart D, Purves RD, Carre DA, Civan MM. Formation of the aqueous humor. Clin Exp Pharmacol Physiol . Jan-Feb 2000;27(1-2):100-6. doi:10.1046/j.1440-1681.2000.03208.x
- Johnson M, McLaren JW, Overby DR. Unconventional aqueous humor outflow: A review. Exp Eye Res . May 2017;158:94-111. doi:10.1016/j.exer.2016.01.017
- Ferreira SM, Lerner SF, Brunzini R, Evelson PA, Llesuy SF. Oxidative stress markers in aqueous humor of glaucoma patients. Am J Ophthalmol . Jan 2004;137(1):62-9. doi:10.1016/s0002-9394(03)00788-8
- Kaeslin MA, Killer HE, Fuhrer CA, Zeleny N, Huber AR, Neutzner A. Changes to the Aqueous Humor Proteome during Glaucoma. PLoS One . 2016;11(10):e0165314. doi:10.1371/journal.pone.0165314
- Brubaker RF. The flow of aqueous humor in the human eye. Trans Am Ophthalmol Soc . 1982;80:391-474.
- R TCYMT. Aqueous humor dynamics. In: DC CNL, ed. Atlas of Glaucoma . Second edition ed. Informa HK; 2007:13 – 28:chap 3.
- Kiel JW, Hollingsworth M, Rao R, Chen M, Reitsamer HA. Ciliary blood flow and aqueous humor production. Prog Retin Eye Res . Jan 2011;30(1):1-17. doi:10.1016/j.preteyeres.2010.08.001
- Roy Chowdhury U, Hann CR, Stamer WD, Fautsch MP. Aqueous humor outflow: dynamics and disease. Invest Ophthalmol Vis Sci . May 2015;56(5):2993-3003. doi:10.1167/iovs.15-16744
- Fautsch MP, Johnson DH. Aqueous humor outflow: what do we know? Where will it lead us? Invest Ophthalmol Vis Sci . Oct 2006;47(10):4181-7. doi:10.1167/iovs.06-0830
Faculty Approval by: Susan Chortkoff MD Copyright Statement: Copyright Janine Yang, ©2022. For further information regarding the rights to this collection, please visit: http://morancore.utah.edu/terms-of-use/ Identifier: Moran_CORE_126138
Filter by Specialty
- BASIC OPHTHALMOLOGY REVIEW
- OPHTHALMIC PATHOLOGY
- NEURO-OPHTHALMOLOGY
- PEDIATRIC OPHTHALMOLOGY AND STRABISMUS
- ORBIT, EYELIDS, AND LACRIMAL SYSTEM
- EXTERNAL DISEASE AND CORNEA
- INTRAOCULAR INFLAMMATION AND UVEITIS
- LENS AND CATARACT
- RETINA AND VITREOUS
- REFRACTIVE SURGERY
- OPHTHALMIC SURGERY
- ETHICS / RESEARCH / STATISTICS / GENETICS
- MORAN OPHTHALMOLOGY LEARNING EXPERIENCE
- ALLIED OPHTHALMIC TRAINING PROGRAM
- QUALITY IMPROVEMENT
Quick Links
Eccles Library NOVEL Webvision
Site Information
Guidelines for Authors Student Assignments Terms of Use Disclaimer
Social Media

- Download PDF
- Share X Facebook Email LinkedIn
- Permissions
THE AQUEOUS HUMOR : A CRITICAL REVIEW
NEW HAVEN, CONN. From the Section of Ophthalmology, Department of Surgery, Yale University School of Medicine.
The science of Galen prevailed until Andreas Vesaleus revealed by his dissection of the ocular tissue of man that the anterior humor of the eye did not resemble the white of an egg but was of a watery nature. Jacob Hovius (1702) some years later described the influx and efflux of this watery fluid in the anterior part of the eye. Since then a noteworthy amount of material has been written on the aqueous humor of man and animal, but a review of the literature discloses the fact that there is still little agreement as to the true nature and mode of formation of this intra-ocular fluid.
Several hypotheses have been presented to explain the mechanism of the formation of the aqueous humor. It is the consensus that the aqueous humor is derived from the circulating fluid—the blood and lymph—of the body. There is still some doubt as to
YUDKIN AM. THE AQUEOUS HUMOR : A CRITICAL REVIEW . Arch Ophthalmol. 1929;1(5):615–633. doi:10.1001/archopht.1929.00810010636011
Manage citations:
© 2024
Artificial Intelligence Resource Center
Ophthalmology in JAMA : Read the Latest
Browse and subscribe to JAMA Network podcasts!
Others Also Liked
Select your interests.
Customize your JAMA Network experience by selecting one or more topics from the list below.
- Academic Medicine
- Acid Base, Electrolytes, Fluids
- Allergy and Clinical Immunology
- American Indian or Alaska Natives
- Anesthesiology
- Anticoagulation
- Art and Images in Psychiatry
- Artificial Intelligence
- Assisted Reproduction
- Bleeding and Transfusion
- Caring for the Critically Ill Patient
- Challenges in Clinical Electrocardiography
- Climate and Health
- Climate Change
- Clinical Challenge
- Clinical Decision Support
- Clinical Implications of Basic Neuroscience
- Clinical Pharmacy and Pharmacology
- Complementary and Alternative Medicine
- Consensus Statements
- Coronavirus (COVID-19)
- Critical Care Medicine
- Cultural Competency
- Dental Medicine
- Dermatology
- Diabetes and Endocrinology
- Diagnostic Test Interpretation
- Drug Development
- Electronic Health Records
- Emergency Medicine
- End of Life, Hospice, Palliative Care
- Environmental Health
- Equity, Diversity, and Inclusion
- Facial Plastic Surgery
- Gastroenterology and Hepatology
- Genetics and Genomics
- Genomics and Precision Health
- Global Health
- Guide to Statistics and Methods
- Hair Disorders
- Health Care Delivery Models
- Health Care Economics, Insurance, Payment
- Health Care Quality
- Health Care Reform
- Health Care Safety
- Health Care Workforce
- Health Disparities
- Health Inequities
- Health Policy
- Health Systems Science
- History of Medicine
- Hypertension
- Images in Neurology
- Implementation Science
- Infectious Diseases
- Innovations in Health Care Delivery
- JAMA Infographic
- Law and Medicine
- Leading Change
- Less is More
- LGBTQIA Medicine
- Lifestyle Behaviors
- Medical Coding
- Medical Devices and Equipment
- Medical Education
- Medical Education and Training
- Medical Journals and Publishing
- Mobile Health and Telemedicine
- Narrative Medicine
- Neuroscience and Psychiatry
- Notable Notes
- Nutrition, Obesity, Exercise
- Obstetrics and Gynecology
- Occupational Health
- Ophthalmology
- Orthopedics
- Otolaryngology
- Pain Medicine
- Palliative Care
- Pathology and Laboratory Medicine
- Patient Care
- Patient Information
- Performance Improvement
- Performance Measures
- Perioperative Care and Consultation
- Pharmacoeconomics
- Pharmacoepidemiology
- Pharmacogenetics
- Pharmacy and Clinical Pharmacology
- Physical Medicine and Rehabilitation
- Physical Therapy
- Physician Leadership
- Population Health
- Primary Care
- Professional Well-being
- Professionalism
- Psychiatry and Behavioral Health
- Public Health
- Pulmonary Medicine
- Regulatory Agencies
- Reproductive Health
- Research, Methods, Statistics
- Resuscitation
- Rheumatology
- Risk Management
- Scientific Discovery and the Future of Medicine
- Shared Decision Making and Communication
- Sleep Medicine
- Sports Medicine
- Stem Cell Transplantation
- Substance Use and Addiction Medicine
- Surgical Innovation
- Surgical Pearls
- Teachable Moment
- Technology and Finance
- The Art of JAMA
- The Arts and Medicine
- The Rational Clinical Examination
- Tobacco and e-Cigarettes
- Translational Medicine
- Trauma and Injury
- Treatment Adherence
- Ultrasonography
- Users' Guide to the Medical Literature
- Vaccination
- Venous Thromboembolism
- Veterans Health
- Women's Health
- Workflow and Process
- Wound Care, Infection, Healing
- Register for email alerts with links to free full-text articles
- Access PDFs of free articles
- Manage your interests
- Save searches and receive search alerts

Enter a Search Term
Aqueous humor flow and function.
- Infographic

Aqueous humor is the fluid produced by the eye. It provides nutrition to the eye, as well as maintains the eye in a pressurized state.
Aqueous humor flows from the ciliary body into the anterior chamber, out through a spongy tissue at the front of the eye called the trabecular meshwork and into a drainage canal (dark blue region next to the trabecular meshwork). In open-angle glaucoma, fluid does not flow freely through the trabecular meshwork, causing an increase in intraocular pressure, damage to the optic nerve and vision loss.
Most, but not all, forms of glaucoma are characterized by high eye (intraocular) pressure. Intraocular pressure remains normal when some of the fluid (aqueous humor) produced by the eye's ciliary body flows out freely (follow blue arrow). This fluid provides nutrition to the eye and also maintains the eye in a pressurized state.
Glossary of Terms
Anterior chamber: The region of the eye between the cornea and the lens that contains aqueous humor.
Aqueous humor: The fluid produced in the eye.
Bruch's membrane: Located in the retina between the choroid and the retinal pigmented epithelium (RPE) layer; provides support to the retina and functions as the 'basement' membrane of the RPE layer.
Ciliary body: Part of the eye, above the lens, that produces the aqueous humor.
Choroid: Layer of the eye behind the retina, contains blood vessels that nourish the retina.
Cones: The photoreceptor nerve cells present in the macula and concentrated in the fovea (the very center of the macula); enable people to see fine detail and color.
Cornea: The outer, transparent structure at the front of the eye that covers the iris, pupil and anterior chamber.
Drusen: deposits of yellowish extra cellular waste products that accumulate within and beneath the retinal pigmented epithelium (RPE) layer.
Fovea: The pit or depression at the center of the macula that provides the greatest visual acuity.
Iris: The colored ring of tissue behind the cornea that regulates the amount of light entering the eye by adjusting the size of the pupil.
Lens: The transparent structure suspended behind the iris that helps to focus light on the retina.
Macula: The portion of the eye at the center of the retina that processes sharp, clear straight-ahead vision.
Optic nerve: The bundle of nerve fibers at the back of the eye that carry visual messages from the retina to the brain.
Photoreceptors: The light sensing nerve cells (rods and cones) located in the retina.
Pupil: The adjustable opening at the center of the iris through which light enters the eye.
Retina: The light sensitive layer of tissue that lines the back of the eye.
Retinal Pigmented Epithelium (RPE): A layer of cells that protects and nourishes the retina, removes waste products, prevents new blood vessel growth into the retinal layer and absorbs light not absorbed by the photoreceptor cells; these actions prevent the scattering of the light and enhance clarity of vision.
Rods: Photoreceptor nerve cells in the eyes that are sensitive to low light levels and are present in the retina, but outside the macula.
Sclera: The tough outer coat that protects the entire eyeball.
Trabecular meshwork: Spongy tissue located near the cornea through which aqueous humor flows out of the eye.
Vitreous: Clear jelly-like substance that fills the eye from the lens to the back of the eye.
- Glaucoma Toolkit (Information to Help You Understand and Manage Glaucoma)
- Expert Information on Glaucoma (Articles)
- Glaucoma Facts and Data (Fact Sheets)
Don't miss out.
Receive glaucoma breakthrough news, research updates, and inspiring stories.
Centers Near You
Selected Address
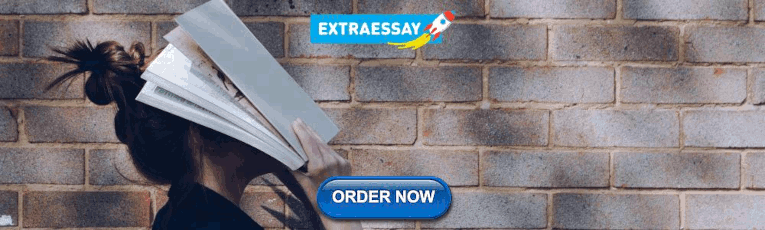
NVISION Procedures
Every NVISION patient is different. We look forward to working with you to determine the best eye surgery or nonsurgical treatment for your individual needs.
- LASIK Eye Surgery
- Contoura® Vision LASIK
- EVO ICL™ (Implantable Lens)
- SMILE™ Vision Correction
- Cataract Surgery
- Comprehensive Ophthalmology
- Lifestyle Lenses
- Vivity Extended Vision IOL
- Cross-Linking
- INTACS® Corneal Implants
- Oculoplastic
- Cosmetic Treatments
- Blepharoplasty
- Dry Eye Treatments
- Glaucoma Surgery
- PDT Eye Treatment
- Monovision LASIK
- Refractive Lens Exchange
- Pterygium Surgery
- Implantable Miniature Telescope Surgery
See Your Best This Winter!
Get $1000 off LASIK + Enjoy Easy and Fast LASIK Financing.**
Nvision conditions.
- Astigmatism
- Nearsightedness
- Farsightedness
- Keratoconus
- Macular Degeneration
- Coronavirus
NVISION Education
- ‣ LASIK Candidate Quiz ‣
- All About LASIK
- LASIK Safety & Success Rates
- LASIK Eye Surgery Cost
- LASIK for Astigmatism
- How Long Does LASIK Take?
- Glasses After Cataract Surgery
- Best Lens for Cataract Surgery
- When to Get Cataract Surgery
- What to Expect: Anesthesia
- Types of Cataracts
- Types of Lenses For Cataract Surgery
- PRK Surgery
- Guide to Laser Eye Surgery
- Types of Eye Surgery
- Corneal Cross-Linking
- Improving Eyesight
- Intraocular Lens
- Survey: America’s Bad Eye Care Habits
- Diet & Vision?
NVISION Locations
Start your journey to clearer vision now and book your appointment with us one of our many locations from our extensive network.
- Inland Empire
- Los Angeles
- Orange County
- Ventura County
- Fort Lauderdale
- Port St. Lucie
- West Palm Beach
- Indianapolis
- Kansas City
- Washington DC
- Minneapolis
- Lincoln City
- Philadelphia
- Corpus Christi
- South Texas
- Salt Lake City
- Virginia Beach
Find a nearby center
Medically Reviewed by Lee R. Katzman, M.D.
Last Updated Aug 30, 2023
Further Reading
- Is Eyebrow Tinting Safe?
- How Do Blind People Experience Their Dreams?
- Vision Care Guide for Veterans
- Young Adults & Vision Loss
- Botox Around the Eyes
- What You Should Know About LATISSE
- Stress & Vision
- The Different Types of Eye Doctor
- Eczema Around the Eyes
- Mental Health Effects of Vision Issues
- Pearle Vision vs. LensCrafters
- Remedies for Eye Bags
- Contrast Sensitivity Testing
- Eye Care Guide for Seniors
- Childhood Bullying
- Drugs That Cause Dilated Pupils
- Optic Nerve Cupping
- How to Test for Your Dominant Eye
- Guide to Bionic Eyes
- Vision Loss & Driving
- Types of Vision Tests
- Blindness From Staring at the Sun
- How Eye Patches Affect Your Good Eye
- Eye Damage From the Sun
- What Is Night Blindness?
- Eczema on the Eyelids
- Light Sensitivity
- Anatomy of the Eye
- Jaundice of the Eyes
- Visual Disturbances
- Eye Damage From Solar Eclipses
- Can Dilated Pupils Be Dangerous?
- Lupus and Eyes
- Microblading
- Vision Issues in Pregnancy
- Prosthetic Eyes
- Eye Disease Statistics
- How Often Should You Get Your Eyes Checked?
- 20/40 Vision
- Brown Eyes vs. Hazel Eyes
- 20/30 Vision
- Becoming an Ophthalmologist:
- Tea Bags for Eyes
- Cholesterol & Your Eyes
- Headache Caused By Eye Strain?
- Am I a Candidate for LASIK? Take our LASIK Candidate Quiz To Find Out!
- Current State of US Eye Health
- Facts, Stats & Myths - Blue Light
- Different Types of Eye Care Professionals
- Screen Time & Eyes
- Driving With Visual Impairments
- Learn & Compare Different Types of Vision Correction Procedures
- Salaries for Optometrists
- Career Options for the Visually Impaired
- Pros & Cons of Eye Colors
- Optometrists vs. Ophthalmologists
- Eye Refraction
- VR & Your Eyes
- Crow's Feet
- Guide to Enucleation
- Color Blindness Causes
Understanding Aqueous Humor and Vitreous Humor (The Differences)
Home / Vision Education / Understanding Aqueous Humor and Vitreous Humor (The Differences)
The human eye is perhaps the most evolved and relied-upon part of the body. From the moment you wake up, you use your eyesight to accomplish most, if not all, of your daily tasks.
Table of Contents
Eye anatomy, aqueous humor, maintaining the health of the aqueous humor, vitreous humor, maintaining the health of the vitreous humor, frequently asked questions.
Some parts of the eye are widely understood. These include the iris, pupil, lens and cornea. Other features of the eye, however, are unknown. As a result, caring for these lesser-known eye parts may prove difficult, especially if you don’t know where to start.
The vitreous humor and aqueous humor perhaps serve the best example of this. While they are important, few people understand these parts of the eye.
The aqueous humor is a clear fluid located at the front part of the eye. Because the eye doesn’t contain blood vessels, the aqueous humor is responsible for providing nutrients to the eye. The aqueous humor also drains out any excess material and waste from the eye.
The vitreous fluid, or vitreous humor, is a colorless, transparent, gel-like material. Vitreous humor is located between the retina and the lens. It is mainly composed of water with additional levels of protein, salts (electrolytes), sugar (glycosaminoglycan) and collagen.
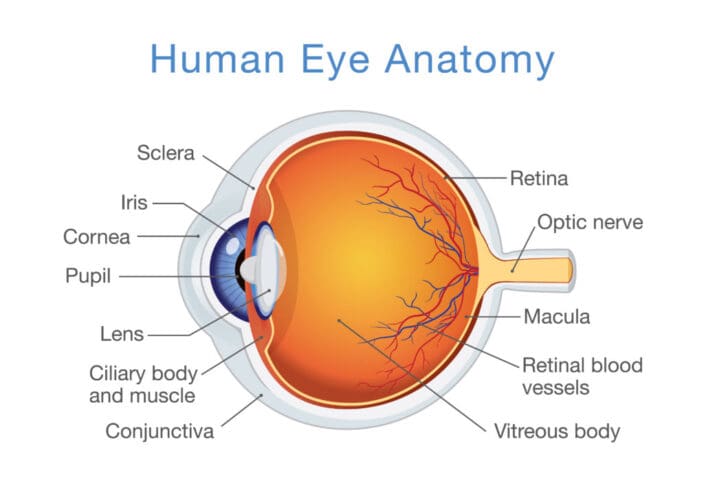
In simple terms, the eye is made up of two parts.
- Anterior chamber : This is between your iris and cornea; or the “front” part of the eye.
- Posterior chamber : This is everything behind the lens of the eye filled with a gel-like transparent fluid.
The aqueous humor is what fills up the eye’s anterior and posterior chamber. It is also responsible for your eye’s shape .
Formation of the aqueous humor is correlated with and sensitive to your body’s circadian rhythm. It is a water-like fluid between the cornea and iris.
The aqueous humor’s main job is to:
- Allow the cornea to expand, so it can protect the eye against dust, particles, and bacteria that can cause harm.
- Preserve ocular pressure.
- Transport nutrients, including vitamin C.
The aqueous humor is produced by a part of the eye called the ciliary body, located above the eye’s lens. The aqueous humor must enter and be drained from the eye at an equal rate, exiting the eye from a structure called the trabecular meshwork. This tissue lets fluid drain through it.
Consistent secretion of the aqueous humor is important to your eye’s health.
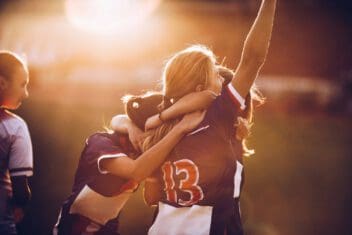
The aqueous humor needs to flow free continuously. Interference with the proper flow can lead to intraocular pressure, which can result in optic nerve damage and vision impairment. Regular eye check-ups can help your doctor detect any issues with the aqueous humor and curate an adequate remedy.
Various lifestyle choices can affect the aqueous humor’s health. The better your health, the more you can reduce the risk of intraocular pressure inside your eye. Some helpful tips:
- Always wear safety glasses if you’re working in a field that could affect the eye. For example, if you’re a carpenter, or work with a lot of dust, grains, or wind, invest in a good pair of safety glasses.
- Exercise regularly to relieve eye pressure naturally.
- Have regular (annual) eye checkups with an optometrist or ophthalmologist .
- Regulate caffeine intake.
- Try meditation and relaxation techniques.
Even if you take excellent care of your eye health , increased intraocular pressure is linked with age, conditions like diabetes, a family history of glaucoma, and certain ethnicities.
This is a type of liquid that is also clear. It mostly consists of sugar, salt, collagen, hyaluronic acid, and water.
The main difference between the vitreous humor and the aqueous humor is that there is a set amount of the vitreous humor in your eye, and it does not move freely about between the two chambers. It remains in the posterior chamber.
In children, the vitreous humor is milky and has a gel-like consistency. The gel-like liquid becomes clearer as you grow older, and in fact begins to liquefy.
People over the age of 50 may become affected by vitreous detachment as the vitreous humor dwindles. This causes the vitreous humor to change in consistency and become fibrous. Symptoms are:
- Increased floaters . These are small strands that look like cobwebs or small spots that look like shadows, but they go away once you try to focus on them.
- Light flashes . You may notice small light flashes in your side (peripheral) vision.
These floaters do not generally result in vision loss, but they can lead to a problems, such as:
- Retinal detachment . This happens when your retina becomes pulled or lifted from its regular position. It can lead to vision loss if not treated. When there are tears or smaller injuries, this is referred to as a retinal tear . Laser surgery can treat a tear, while traditional surgery may be needed for a retinal detachment.
- Macular hole . The macula is in the central part of the retina. It is the part of the eye that lets you see things in detail. If a part of your macula breaks, it could result in a hole that causes vision problems. Some macular holes heal on their own, while surgery is needed to repair others. Surgery involves removing the vitreous liquid and supplanting it with a mixture of gas and liquid. You will have to be face down for one to two days or even a few weeks after this surgery. You will usually not be allowed to fly for two months to prevent changes in air pressure from causing the bubble to increase in size, which could result in additional eye pressure.
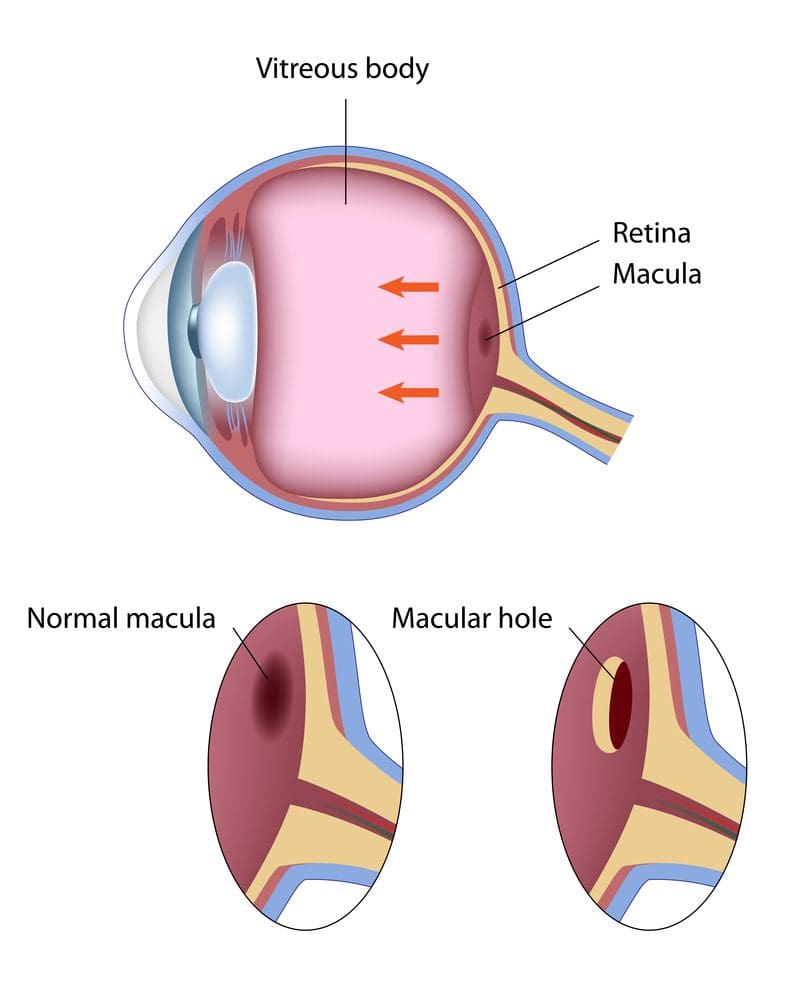
What does the aqueous humor do?
A clear fluid at the frontal part of the eye, the aqueous humor provides nutrients to parts of the eye that do not have blood supply. The clear aqueous humor also drains out at an equal rate to remove waste. It makes sure your eye is the right shape and maintains the right amount of pressure in the eye at all times.
Why is the vitreous humor important?
This clear fluid is located between the front part of the eye and the retina. It does not become replenished if some is lost. It plays a more important role in the early years of life, providing structure to the eye and protecting the retina.
Anything that gets stuck in the vitreous humor must be removed using surgical procedures.
What are some ways the aqueous humor and vitreous humor can be damaged?
The aqueous humor is constantly drained and replenished, but problems with the system that allows the aqueous humor to flow properly can increase intraocular pressure. Increased pressure in the eye can damage your optic nerve and lead to vision loss.
Glaucoma is often associated with imbalances in the aqueous humor. It means that your eye is either producing excess liquid or that your aqueous humor is not properly able to drain itself through the trabecular network.
The vitreous humor often becomes stringy as you get older. This may cause small parts to detach and float around your eye. Those who are age 50 or older may also suffer from retinal detachment or macular holes. These must be corrected using surgery.
- Aqueous Humor . (January 2019). American Academy of Ophthalmology.
- Facts About Vitreous Detachment . (August 2009). National Eye Institute.
- Aqueous Humor Dynamics: A Review . (September 2010). The Open Ophthalmology Journal .
- How Do We See? (July 2015). Arizona State University School of Life Sciences.
- Aqueous Humor Flow and Function . (July 2015). Bright Focus Foundation.
- Glaucoma . (November 2018). Mayo Clinic.
- Understanding Eye Anatomy – Function of the Vitreous Humor . (October 2017). Eye Pain Management.
- The Aqueous Humor . (August 2017). Vision Eye Institute.
- Facts About Retinal Detachment . (October 2009). National Eye Institute.
- Facts About Macular Hole . (April 2012). National Eye Institute.
The information provided on this page should not be used in place of information provided by a doctor or specialist. To learn more, read our Privacy Policy and Editorial Policy pages.

An official website of the United States government
The .gov means it’s official. Federal government websites often end in .gov or .mil. Before sharing sensitive information, make sure you’re on a federal government site.
The site is secure. The https:// ensures that you are connecting to the official website and that any information you provide is encrypted and transmitted securely.
- Publications
- Account settings
Preview improvements coming to the PMC website in October 2024. Learn More or Try it out now .
- Advanced Search
- Journal List
- HHS Author Manuscripts

Deconstructing aqueous humor outflow – the last 50 years
Associated data.
Herein partially summarizes one scientist-clinician’s wanderings through the jungles of primate aqueous humor outflow over the past ~45 years. Totally removing the iris has no effect on outflow facility or its response to pilocarpine, whereas disinserting the ciliary muscle (CM) from the scleral spur/trabecular meshwork (TM) completely abolishes pilocarpine’s effect. Epinephrine increases facility in CM disinserted eyes. Cytochalasins and latrunculins increase outflow facility, subthreshold doses of cytochalasins and epinephrine given together increase facility, and phalloidin, which has no effect on facility, partially blocks the effect of both cytochalasins and epinephrine. H-7, ML7, Y27632 and nitric oxide – donating compounds all increase facility, consistent with a mechanosensitive TM/SC. Adenosine A1 agonists increase and angiotensin II decrease facility. OCT and optical imaging techniques now permit visualization and digital recording of the distal outflow pathways in real time. Prostaglandin (PG) F2α analogues increase the synthesis and release of matrix metalloproteinases by the CM cells, causing remodeling and thinning of the interbundle extracellular matrix (ECM), thereby increasing uveoscleral outflow and reducing IOP. Combination molecules (one molecule, two or more effects) and fixed combination products (two molecules in one bottle) simplify drug regimens for patients. Gene and stem cell therapies to enhance aqueous outflow have been successful in laboratory models and may fill an unmet need in terms of patient compliance, taking the patient out of the delivery system. Functional transfer of genes inhibiting the rho cascade or decoupling actin from myosin increase facility, while genes preferentially expressed in the glaucomatous TM decrease facility. In live NHP, reporter genes are expressed for 2+ years in the TM after a single intracameral injection, with no adverse reaction. However, except for one recent report, injection of facility-effective genes in MOCAS have no effect in live NHP. While intracameral injection of an FIV.BOVPGFS-myc.GFP PGF synthase vector construct reproducibly induces an ~2mmHg reduction in IOP, the effect is much less than that of topical PGF 2⍺ analogue eyedrops, and dissipates after 5 months. The turnoff mechanism has yet to be defeated, although proteasome inhibition enhances reporter gene expression in monkey organ cultured anterior segments (MOCAS). Intracanalicular injection might minimize off-target effects that activate turn-off mechanisms. An AD-P21 vector injected sub-tenon is effective in ‘right-timing’ wound healing after trabeculectomy in live laser-induced glaucomatous monkeys. In human (H)OCAS, depletion of TM cells by saponification eliminates the aqueous flow response to pressure elevation, which can be restored by either cultured TM cells or by IPSC-derived TM cells.
There were many other steps along the way, but much was accomplished, biologically and therapeutically over the past half century of research and development focused on one very small but complex ocular apparatus. I am deeply grateful for this award, named for a giant in our field that none of us can live up to.
1. Introduction
I am honored and grateful to receive the 2018 Endré A. Balázs Prize, and to present the lecture named for him. Endré A. Balázs was a distinguished scientist and entrepreneur, whose outstanding contributions provided far-reaching progress in experimental eye research and in clinical ocular surgery ( “Endre Balazs,” 2015 ). Dr. Balazs was one of a group of scientists who came together in 1968 to establish an international organization to support vision research, which eventually became ISER. Dr. Balázs was the founding editor of Experimental Eye Research, which eventually became the official journal of ISER. He also co-founded the International Society for Hyaluronan Sciences. Dr. Balazs was a world leader in ophthalmic biochemistry and was instrumental in the development of products that revolutionized eye surgery, notably high-molecular weight hyaluronan, commercially known as Healon®. Importantly, he founded several companies and a philanthropic foundation dedicated to exploring and promoting the therapeutic potential of hyaluronic acid. Dr. Balazs’s complete biography is easily found ( “Endre-A-Balazs-doctor-who-found-acid-to-treat-arthritic-knees,” 2015 ) and is an inspiring story well worth reading.
2. Personal History
Mine is a 45-year story filled with amazing people and fascinating and often unexpected science, that redefined how we think about glaucoma, the world’s most common cause of irreversible vision loss. I always knew that I wanted to be both a physician and a scientist, but scientist-physician was a better descriptor for me than was physician-scientist. However, I approached my senior year of medical school with no idea of what my next step should be. Fortunately, the mandatory military service requirement in the US for physicians at that time provided two years of post-internship training and service at the NIH, where I learned a lot about biostatistics, epidemiology and clinical trials design, became engaged and married, and had time for my pin-the-tail-on-the-donkey tentative decision to do my residency training in ophthalmology to jell. By what I can only ascribe to a stroke of good fortune or the hand of a guardian angel, I landed at Washington University in St. Louis, under the tutelage of the great Bernard Becker and the terrific team he had assembled. Although the clinical demands of the residency did not allow much time for research, I was able to design and execute two small studies of the biochemical makeup of subretinal fluid as a predictor of surgical and visual outcome in patients with rhegmatogenous retinal detachments ( Kaufman, 1976 , 1975 ; Kaufman and Podos, 1973 ; Kaufman PL, 1974 , 1973 ). Small though they were, these studies taught me the joy of asking the “how” and “why” of pathophysiology and therapeutics.
Glaucoma and physiology/pharmacology seemed like the ideal intellectual setting for me. Dr. Becker; his protégé Steven Podos; Carl Kupfer, then Director of the National Eye Institute; and Ernst Bárány, the great ocular pharmacologist/physiologist and the world’s leading authority on aqueous humor dynamics, planned a two-year fellowship for me in Prof. Bárány’s lab at the University of Uppsala in Sweden, where he was the Chair of the Department of Medical Pharmacology. The NEI Study Section enthusiastically voted to support this, but then the application was “administratively withdrawn” when the Nixon Administration suspended all NIH training funds. I managed to secure a Seeing Eye Fellowship of $12,000, and another $2,000 from ALZA, the manufacturer of the pilocarpine Ocusert ( Lee et al., 1975 ) and the Alzet mini pumps ( Greenshaw, 1986 ).
In 1973, this princely sum of $14,000 did not go very far in Sweden. The 1973 Middle East War and oil embargo combined to drive down the value of the US dollar, but with a 6-month old child and minimal resources even for a 1-year fellowship, my wife and I made the final decision to go just three weeks before our scheduled departure shortly after the end of my residency training. Dr. Kupfer provided the security of a 2-year staff fellowship at the NEI when I returned if I had not secured an academic position by that time.
Life overall was good for us in Sweden. Wife Margaret, daughter Alison and I lived in a large if somewhat stark partially university-subsidized apartment. Laundry facilities were an outdoor quarter-mile walk away – lots of fun in the winter - with a drying room rather than automatic dryers, where everything was hung on drying racks and you then waited for magic to happen. We had old bicycles and a hand-me-down baby carriage, a rattletrap ancient car in our second year, and no discretionary money. Indeed, we dipped into savings to make ends meet. Despite the Spartan lifestyle, the apartment complex and the department were both collegial and we made friends - American, Canadian, German, Finnish and Swedish - that we have to this day, 45 years later.
3. Aqueous Humor Dynamics
3.1. physiology & pharmacology.
The work environment was off-the-charts phenomenal, after getting off to a slow start. It took about six months and a truly great laboratory instrument machine shop to work out the surgical techniques. We worked exclusively in non-human primates (and still do so), partnering with Prof. Anders Bill for certain boutique physiological experiments and Profs. Johannes Rohen and Elke Lütjen-Drecoll for light and electron microscopic studies.
3.1.a. Conventional (trabecular meshwork/Schlemm’s canal) outflow pathway
This pathway encompasses outflow from the anterior chamber through the various regions of the trabecular meshwork (uveal, trabecular, juxtacanalicular (cribriform), then across or through the inner wall endothelium of Schlemm’s canal into the canal lumen, then through an intrascleral vascular plexus (aqueous collector channels, intrascleral veins) that empty into the episcleral veins and from there eventually into the general venous circulation ( Fig. 1 ).

Aqueous humor outflow pathways. Aqueous humor, produced by the ciliary body, exits the eye through the conventional trabecular meshwork/ Schlemm’s canal pathway (plain arrows), and the unconventional uveoscleral pathway (crossed arrows). Aqueous humor production and outflow are always equal, and the resistance in the trabecular meshwork/Schlemm’s canal determinestheintraocularpressure(IOP). Increasedresistanceresultsinelevation in IOP, a causal risk factor for glaucoma. From Svedbergh, 1976 with permission.
3.1.a.1. Muscarinic cholinomimetics
We completely removed the iris via a small transcorneal incision in the live monkey eye ( Kaufman and Lütjen-Drecoll, 1975 ). After a short post-surgical recovery, the eyes were quiet and resting perfusion outflow facility and the facility response to intravenous pilocarpine were identical in the surgically aniridic and the contralateral iridic eyes, proving that the iris has no functional role in regulating trabecular meshwork outflow facility or its response to muscarinic cholinergic agonists ( Kaufman, 1979 ). Thus, to say that miotic drugs are a treatment for open angle glaucoma is somewhat a misnomer, as miosis has nothing to do with their facility-increasing effect. There is, however an intimate anatomic relationship between the ciliary muscle and the trabecular meshwork, with some of the muscle’s anterior tendons mingling with the elastic network of the trabecular meshwork, which in turn sends fibers that insert into special surface adaptations of the Schlemm’s canal inner wall endothelial cells ( Suppl Fig. 1 ) ( Rohen et al., 1981 , 1967 ).
When the ciliary muscle contracts as in response to muscarinic cholinergic agonist drugs, the meshwork is expanded, relaxed, and less stiff, and Schlemm’s canal dilates ( Lütjen-Drecoll, 1973 ), all contributing to increased outflow facility and reduced intraocular pressure (IOP). To prove that ciliary muscle contraction was mediating the facility increase, we used a transcameral goniotomy-type surgical approach to cut the ciliary muscle from the scleral spur over the entire 360-degree circumference at one sitting ( Kaufman and Bárány, 1976 ). This allowed the muscle’s elastic posterior attachments to retract posteriorly so that the posteriorly-pulled anterior tips reattached firmly to the inner scleral wall about 2 mm posterior to the scleral spur (Lütjen-Drecoll et al., 1977). In the non-inflamed eyes, now absent the iris and with the ciliary muscle disinserted from the scleral spur and trabecular meshwork/Schlemm’s canal (TM/SC) [ Fig. 2 ], resting outflow facility was modestly reduced ( Kaufman and Bárány, 1976 ), as expected if the meshwork became more compact and the canal narrowed absent the tonic pull of the ciliary muscle. Intravenous and intracameral pilocarpine had essentially no effect on outflow facility ( Kaufman and Bárány, 1976 ) [ Suppl Fig. 2 ]. This constellation of results proved that contraction of the ciliary muscle increased outflow facility, that pilocarpine’s facility-increasing effect was mediated solely by ciliary muscle contraction affecting TM and SC geometry with no primary drug effect on the TM/SC, and that the iris was completely uninvolved ( Kaufman, 1979 ; Kaufman and Bárány, 1976 ). It now became feasible to assess the effects of other drugs on outflow facility, free from potential confounding effects on ciliary muscle contraction/relaxation. There was still the hope that the miosis, accommodative and outflow facility effects of cholinomimetics could be decoupled, as the first two were hard-stop side effects in elderly folks with even early cataracts, and younger patients who still retained significant accommodative capacity, respectively. However, it turned out that all three functions were mediated by the same m3 muscarinic receptor subtype, and no way of pharmacologically separating them was found ( Gabelt and Kaufman, 1992 ).

A: Normal cynomolgus monkey anterior chamber angle. B: Gonioscopic appearance of surgically aniridic eye. C: Surgical aniridia and ciliary muscle disinsertion and retrodisplacement. Deep, fairly uniform retrodisplacement of ciliary body. Thinbandofmuscleremainsattachedtoscleralspur. D: Typical histologic appearance ofanterior chamber angle following ciliary muscle disinsertion; C = canal of Schlemm, filled with blood in lower half of the image; A = adhesion of retrodisplaced muscle to sclera; L = lens; N = naked sclera; SP=scleralspur;T=trabecularmeshwork.E:Light micrograph of the posterior portion of the trabecular meshwork/anterior portion of the ciliary muscle after perfusion with three sizes of latex spheres and thorotrast in gelatin. Streams of homogeneous gelatin, containing tracer particles (arrows), enter the ciliary body from the posterior portion of the trabecular meshwork and pass through the connective tissue between bundles of the ciliary muscle. CM: Ciliary muscle; SC: Schlemm’s canal (x 460). F: Monkey ciliary muscle intermuscular space showing latex spheres that had been injected into the anterior chamber. N: Nerve fiber (x 5500). G: Monkey suprachoroid at the posterior pole, showing 3 sizes of latex spheres that were injected into the anterior chamber. Th: Thorotrast. (X22,000). From: B - Kaufman PL, Lütjen-Drecoll 1975 ; C - Kaufman PL, Bárány EH 1976 ; D - Lütjen-Drecoll E, et al 1977 ; E, F, G - Inomata H, et al 1972; all with permission.
3.1.a.2. Adrenergics
Epinephrine, norepinephrine and 3’,5’-cyclic adenosine monophosphate (cAMP) (but not the inactive metabolite 5’AMP) increased facility similarly in iridic and aniridic eyes, and in CM-disinserted and non-CM-disinserted eyes. This proved that the TM/SC was not ‘dead’, and that epinephrine and its second messenger cAMP were acting directly on the TM/SC ( Kaufman, 1987a , 1986a , 1986b ; Kaufman et al., 1981 ; Kaufman and Rentzhog, 1981 ). We then defined other classes of compounds that provided significant insights into TM biology and regulation, and that might be ‘druggable’ for glaucoma therapy by upregulating or downregulating an outflow facility – related pathway ( Croft and Kaufman, 1995a , 1995b ; Hubbard et al., 1997 , 1996 ; Kaufman, 1987b ; Kaufman et al., 1982 , 1977 ; Kaufman and Erickson, 1982 ; Menage et al., 1995 ; Robinson and Kaufman, 1992 ; Svedbergh et al., 1978 ; Tian et al., 1997 ).
3.1.a.3. Actin cytoskeleton
3.1.a.3.a. cytochalasins.
Cytochalasins are fungal metabolites that disrupt actin microfilaments and the actin cytoskeleton. In essence they prevent the addition of actin monomers to one end of the microfilament without affecting the dissolution of actin monomers at the other end. ( Kaufman and Erickson, 1982 ) The process of monomer addition and deletion has been termed the “F-actin treadmill”, and keeps the filaments refreshed and stable. With cytochalasins present, the filaments lose but do not replenish monomers, and thus become shorter and weaker. This disrupts the actin microfilament system, impairs acto-myosin contractility, degrades and weakens cell-cell adherens attachments, as well as cell-ECM attachments (focal contacts), and relaxes the cells ( Kaufman et al., 1977 ; Kaufman and Erickson, 1982 ; Svedbergh et al., 1978 ; Takano et al., 1975 ). In cells with a robust actin cytoskeleton such as HTM and Schlemm’s canal inner wall cells this may have important functional consequences ( Inomata et al., 1972a ; Stamer et al., 2015 ). In live monkeys, the entire meshwork relaxes and expands and Schlemm’s canal dilates, allowing fluid to flow through the entire system more easily, i.e., outflow resistance decreases / outflow facility increases ( Gabelt and Kaufman, 2012 ). Unfortunately, given the two compartment – two pressure proximal conventional outflow system that comprises the anterior chamber, trabecular meshwork and Schlemm’s canal, the inner canal wall was ruptured and platelets attempted to plug the rupture as in blood vessels ( Fig. 3 ) ( Gabelt and Kaufman, 2012 ; Kaufman, 1987b ; Kaufman et al., 1977 ; Kaufman and Erickson, 1982 ; Robinson and Kaufman, 1991 ; Svedbergh et al., 1978 ; Tian et al., 1999 ).

Schlemm’s canal (SC) and cribriform meshwork approximately 30 min after intracameral infusion of 5 μg of cytochalasin B. Inner wall endothelium demonstrates ruptures (arrow) and abnormally large invaginations (I). Extracellular material (E) has been lost from some areas between the inner wall endothelium and the first subendothelial cell layer (and replaced by plasma (asterisk)) and is completely absent from most parts of the cribriform meshwork. The cribriform (juxtacanalicular) meshwork is greatly expanded. P, degranulated platelets; C, cellsof cribriform meshwork; M, swollen mitochondria; T, first corneoscleral trabeculum. From Svedbergh et al., 1978 , with permission.
While these findings were of great mechanistic interest, this was not a viable therapeutic approach. However, it did provide valuable therapeutic insights that utilized carefully developed epinephrine ( Kaufman, 1986a ) and cytochalasin B ( Kaufman and Erickson, 1982 ) dose – outflow facility response relationships. Sub-threshold doses of epinephrine and cytochalasin B given together intracamerally induced significant facility increases that were dose-dependent within the subthreshold range for either drug ( Robinson and Kaufman, 1991 ). Furthermore, addition of subthreshold doses of cytochalasin B to a maximal dose of epinephrine gave an additional response ( Robinson and Kaufman, 1991 ). Finally, phalloidin, which stabilizes actin microfilaments and protects them against the disruptive actions of cytochalasins, partially inhibited the facility-increasing effect of epinephrine ( Robinson and Kaufman, 1994 ). Thus, epinephrine was actually the first and the original cytoskeletal drug for increasing outflow facility, other claims notwithstanding. Indeed, epinephrine predated other cytoskeletal / actomyosin contractility-inhibiting drugs by well over half a century – even though its effector mechanism was completely unknown! Of course, and as is often the case, clues were there from other areas of science. Epinephrine acts as, among other things, a ß 2 -adrenergic receptor agonist, via the cAMP signaling cascade.( Bazrafkan et al., 2010 ; Neufeld and Sears, 1974 ). As noted above, in live monkeys, cAMP, but not its inactive metabolite 5’AMP, increases outflow facility, both in normal and in iridectomized and ciliary muscle inserted eyes ( Kaufman, 1986b ). Macrophage migration through blood vessel walls and lymphocyte adhesion to vessel walls are altered by epinephrine ( Petty and Martin, 1989 ) and those effects are inhibited by timolol, a non-selective ß 1 , ß 2 adrenergic receptor antagonist ( Petty and Martin, 1989 ). Thus, non-innervated white blood cells have ß-adrenergic receptors mediating cytoskeleton/actomyosin contractility-related functional effects. Welcome to the TM of the 21 st century!
3.1.a.3.b. Latrunculins
Recognizing that cytochalasin-based ‘war’ on the TM was not a viable therapeutic strategy, but believing that we had identified an important and potentially therapeutically useful control mechanism, we were drawn to work involving latrunculins, marine macrolides that are metabolites of Red Sea sponges. Latrunculins bind 1:1 with globular free actin monomers in cell cytoplasm, thus ‘drying up’ the pool of free actin available to add to the F-actin treadmill ( Spector et al., 1989 , 1983 ). Both latrunculin A and B, delivered intracamerally or topically to live monkey eyes, dramatically increased outflow facility by ~100–200% in normal ( Suppl Fig 3 ) and aniridic/ciliary muscle disinserted eyes ( Peterson et al., 2000 , 1999 ; Sabanay et al., 2006 ). Latrunculin B was carried forward into a phase 1 human clinical trial, which confirmed its safety and modest efficacy ( Rasmussen et al., 2014 ). It was not developed further because the company, Inspire Pharmaceuticals, was bought by Merck for other reasons, and Merck had no glaucoma drug development program.
3.1.a.4. Actomyosin contractility
3.1.a.4.a. kinase inhibitors.
Concurrently we approached the system from the actomyosin contractility aspect. Broad spectrum serine-threonine kinase inhibitors such as H-7, that can inhibit both the Rho kinase (RK) and myosin light-chain kinase (MLCK) pathways, prevent phosphorylation and activation of the myosin light chain and thereby inhibit actomyosin contractility ( Fig.4 ). This leads to degeneration of actin microfilaments and vinculin-rich cell-ECM junctions (focal contacts) ( Iizuka et al., 1999 ; Sabanay et al., 2004 ; Somlyo and Somlyo, 1994 ; Traube, 2006 ), and ultimately to expansion of the JCT and dilation of SC but without SCIWE breaks ( Fig 5 , Suppl Fig 4 ). In live monkeys, H-7, Y-27362 (selective RK inhibitor, Suppl Fig 5 ) and ML-7 (selective MLCK inhibitor) all increase outflow facility by 100–200% in live monkeys ( Honjo et al., 2001 ; Tian et al., 2004 , 2000 ; Tian and Kaufman, 2005 ; Vasantha Rao et al., 2001 ). RKIs were selected for development as glaucoma therapeutics perhaps because pharmaceutical companies were more familiar with them, and many companies had them in their libraries. At present, one such agent entered the commercial market in Japan in 2014 (ripasudil) ( Honjo and Tanihara, 2018 ; Inazaki et al., 2017 ; Inoue and Tanihara, 2017 , 2013 ) and another entered the USA market in 2018 (netarsudil) ( Kahook et al., 2019 ; Serle et al., 2018 ).

A: Focal adhesions (FAs) as a mechanosensors. FAs are multi-molecular complexes connectingthe extracellular matrix with the actin cytoskeleton. Heterodimeric trans membrane integrin receptors (pink) bind matrix proteins via their extracellular domains,while theircytoplasmicdomains are associated with a dense submembrane plaque containing more than 50 different proteins (“boxes” enclosed in the oval area) including structural elements as well as signal transduction proteins such as FAK, Src, ILK, etc. The plaque, in turn, is connected to the termini of actin filament bundles. The assembly and maintenance of FAs depend on local mechanical forces. These forces may be generated by myosin II-driven isometric contraction of the actin cytoskeleton, or by extracellular perturbations such as matrix stretching or fluid shear stress. Force-induced assembly of the adhesion plaque leads to the activation of a variety of signaling pathways that control cell proliferation, differentiation, and survival (e.g., MAP kinase and PI 3- kinase pathways) as well as the organization of the cytoskeleton (e.g., Rho family GTPase pathways). Rho, in particular, is an indispensable regulator of FA assembly affecting, via its immediate targets Dia1 and ROCK, actin polymerization and myosin II-driven contractility. Geiger and Bershadsky, 2002 with permission. B: Schematic drawing illustrating targets for agents known to disrupt the actin cytoskeleton to enhance outflow facility. C-3, Y-27632 and H-7 block the Rho cascade, inhibiting actomyosin contraction and disrupting actin stress fibers; H-7 and ML-7 block myosin light chain kinase phosphorylation of the myosin light chain to interfere with actinmyosin interactions; latrunculin sequesters monomeric G actin leading to microfilament disassembly; caldesmon negatively regulates actin-myosin interactions. Modified from original by Alexander Bershadsky, with permission.

Light micrographs of trabecular meshwork (TM) and Schlemm’s canal (SC) in monkey eyes treated with vehicle (a) or H-7 (1-[5-isoquinoline sulfonyl]-2-methyl piperazine), 300 μmol/L (b). The juxtacanalicular area (arrow in b) and intercellular spaces are extended. Inner wall endothelial cells in H-7–treated eyes were thinner than in controls, and cell-cell junctions were intact (not shown). Inner uveal TM were not significantly affected (b). From Sabanay et al., 2000 with permission.
3.1.a.4.b. Nitric oxide
Nitric oxide (NO) can be both a toxic free radical and an important signaling molecule ( Becquet et al., 1997 ). Acting through soluble guanylyl cyclase (cGMP) as the second messenger ( Suzuki et al., 2009 ), NO relaxes vascular endothelial cells and vascular smooth muscle by inhibiting various aspects of the rho cascade, ( Nathanson and McKee, 1995 ; Stamer et al., 2011 ) thereby dilating blood vessels. Compounds that contain a nitric oxide-donating moiety relax the TM and dilate SC and increase outflow facility after intracameral injection in non-human primates ( Gabelt et al., 2011 ; Heyne et al., 2013 ). cGMP itself injected into the anterior chamber also increases outflow facility ( Kee et al., 1994 ). Latanoprostene bunod, a single molecule with a PGF 2a backbone and an NO-donating moiety ( Garcia et al., 2016 ; Kaufman, 2017 ), lowers IOP in ocular hypertensive and POAG human subjects ( Kawase et al., 2016 ; Medeiros et al., 2016 ; Weinreb et al., 2015 ) by ~ 1 mmHg more than latanoprost ( Weinreb et al., 2015 ), and entered the US commercial market in 2018 ( Weinreb et al., 2018 ) after FDA approval in December 2017. Since NO inhibits the rho cascade, additivity between RKI and NO-donating compounds is uncertain.
3.1.a.4.c. TM/SC mechanosensitivity
Trabecular meshwork/ Schlemm’s canal inner wall (TM/SCIW) cells have an intrinsic actomyosin contractility mechanism that may be the efferent arm of an IOP-regulating mechano-sensitivity reflex. IOP, shear stress, various hormones and cytokines may be the afferent arms; endothelial nitric oxide synthase (eNOS) – NO may be a signaling arm ( Stamer et al., 2011 ); and cellular relaxation / contractility and cell-ECM / cell-cell adherens junction formation/degradation may be the efferent arms ( Kaufman and Rasmussen, 2012 ). Carreon and Johnstone have elegantly hypothesized and described the movements of the system at the macro level ( Carreon et al., 2017 ; Johnstone, 2014 ).
3.1.a.5. Adenosine agonists
Adenosine A 1 receptor agonists injected into the anterior chamber increase outflow facility in monkeys ( Tian et al., 1997 ), perhaps by upregulating the synthesis/release of various metalloproteinases affecting the TM / SCIW ( Crosson et al., 2005 ; Husain et al., 2006 ; Shearer and Crosson, 2002 ). A Phase III clinical trial of an adenosine A1R agonist yielded only modest IOP reduction, and its clinical future is uncertain ( Liebmann and Lee, 2017 ; Myers et al., 2016 ).
3.1.a.6. Conventional outflow facility-decreasing agents
There are several facility-decreasing molecular pathways in addition to glucocorticosteriods ( Montecchi-Palmer et al., 2017 ; Tripathi et al., 1994 ). For example, TGFß 2 , found in excess in the aqueous humor and TM of patients with POAG, decreases outflow facility in MOCAS ( Bhattacharya et al., 2009 ; Goel et al., 2012 ) while ergotamine and angiotensin II decrease facility in live monkeys ( Kaufman et al., 1981 ; Kaufman and Rentzhog, 1981 ) and angiotensin antagonists have been tested as potential anti-glaucoma agents ( Costagliola et al., 2000 ; Hirooka and Shiraga, 2007 ; Lotti and Pawlowski, 2009 ; Shah et al., 2000 ). These and other strategies discussed below may allow development of experimental molecular models for glaucoma in MOCAS or live monkeys.
3.1.a.7. ‘Distal’ conventional outflow pathway
Decades-old studies and mantra have attributed ~25% of conventional outflow resistance as residing beyond (i.e., downstream from) the inner wall endothelium of Schlemm’s canal, and likely beyond the canal itself ( Grant, 1963 , 1958 ). There was awareness of what is now called the distal conventional outflow pathway – the labrynth of intrascleral vessels connecting Schlemm’s canal and the episcleral veins ( Hamanaka et al., 1992 ), but their complex anatomy and physiology was poorly understood, and virtually nothing was known about their putative pathophysiology, if any. Recent studies have shown that the collector channels emanating from the outer wall of Schlemm’s canal may have contractile and perhaps sphincter-like properties ( Rohen and Rentsch, 1968 ), and even more distal intrascleral venules may have a contractile apparatus, so that resistance in this pathway under some conditions may be higher than previously thought ( de Kater et al., 1992 ; Gonzalez et al., 2017 ). Recent advances and future developments in optical coherence tomographic imaging e.g. OCT angiography ( Huang et al., 2018 ) may help unravel these mysteries.
3.1.b. Unconventional (uveoscleral) outflow pathway
This pathway encompasses the connective tissue-filled spaces between the sheaths surrounding the ciliary muscle bundles (~12 muscle fibers within each bundle) that communicate with the anterior chamber at the anterior face of the ciliary muscle, and with the supraciliary and suprachoroidal spaces all the way back to the macular and optic nerve head region ( Inomata et al., 1972b ), so that aqueous humor and ciliary process and choroidal stromal fluid and protein can be carried out of the eye (with debate as to how much is carried away trans-sclerally ( Anders Bill, 1967 ; Bill, 1971 , 1966 ; Inomata et al., 1972b , 1972a ), by the choroidal vasculature ( Pederson et al., 1977 ), and by recently described ciliary muscle/choroidal putative lymphatics ( Yücel et al., 2009 ). Intense ciliary muscle contraction by cholinimimetic drugs can constrict or even obliterate the spaces between the ciliary muscle bundles ( Rohen et al., 1967 ), impeding uveoscleral outflow dramatically ( A Bill, 1967 ) ( Suppl Fig 6 ), ( Crawford and Kaufman, 1987 ; Nilsson et al., 1989 ). This was utilized in the late 1980s to help delineate the mechanism by which PGF 2⍺ reduced IOP – see section 3.1.b.1 below.
3.1.b.1. Prostaglandin analogues
PGF 2α increases uveoscleral outflow ( Crawford et al., 1987 ; Crawford and Kaufman, 1987 ; Gabelt and Kaufman, 1989 ; Nilsson et al., 1989 )( Table 1 ) by increasing the synthesis and release of various matrix metalloproteinases and consequently decreasing and remodeling the collagenous extracellular matrix in the ciliary muscle ( Gaton et al., 2001 ). Increased uveoscleral outflow has been reported in monkey eyes with experimental autoimmune uveitis ( Mermoud et al., 1994 ; Toris and Pederson, 1987 ). Histologic studies have shown enlarged ciliary muscle inter-muscular and suprachoroidal spaces in eyes with experimental uveitis induced by various methods ( Liu et al., 1994 ; Toris and Pederson, 1987 ). The density of collagen type I in the extracellular matrix (ECM) of monkey ciliary muscle was reduced during anterior segment inflammation ( Sagara et al., 1999a ),indicating that reduction of ciliary muscle ECM may contribute to enhanced uveoscleral outflow during anterior segment inflammation. Twice daily topical treatment of cynomolgus monkey eyes with 2 μg PGF 2α -isopropyl ester increased matrix metalloproteinases 1, 2, and 3 in the monkey uveoscleral outflow pathway ( Sagara et al., 1999a ) and also reduced collagen types I, III and IV immunoreactivity in the ciliary muscle ( OCKLIND, 1998 ; Sagara et al., 1999a , 1999b ). PGF 2α analogs have become the most widely used anti-glaucoma medications worldwide ( Stein et al., 2015 , 2007 ).
Effect of topical PGF 2α on uveoscleral outflow in monkeys 5 th day of treatment bid with 2 μg PGF 2α – IE
Data are mean ± s.e.m. uveoscleral outflow (μl/min) for 9 animals, each contributing one treated and one control eye, following the ninth unilateral dose of PGF 2α on day 5.
3.1.c. Novel products: combinatorial molecules - one molecule, two effects; and combination products - two molecules, two effects, one or more effects for each molecule
The FDA recently approved two novel products. Latanoprostene bunod (Vyzulta®, Bausch & Lomb) is a combinatorial molecule; one molecule with two effects. It has a latanoprost backbone and an NO-donating esterified side chain ( Weinreb et al., 2018 ). Rocklatan®, (Aerie) is a fixed combination product; it contains two separate molecules, and conveys least two different effects, one or more for each molecule. It combines the rho kinase inhibitor Netarsudil (Rhopressa®, Aerie) with the prostaglandin PGF 2⍺ analogue latanoprost. ( Kahook et al., 2019 ; Serle et al., 2018 ).
4. Gene Therapy for Enhancing or Inhibiting Aqueous Outflow
4.1. background / unmet medical need.
Topical self-administered eye drops have become problematic for clinical glaucoma therapy, as they rely on the patient to be an accurate, reliable, reproducible part of the delivery system. Studies have shown that the latter is not the case ( Budenz, 2009 ; McKinnon et al., 2008 ; Nordstrom et al., 2005 ; Tsai, 2009 ). While various sustained release delivery systems are under development ( {"type":"clinical-trial","attrs":{"text":"NCT02371746","term_id":"NCT02371746"}} NCT02371746 , 2015 ; Vinod and Gedde, 2017 ; Walters et al., 2017 ), we have tried to develop a biological rather than a mechanical approach, namely gene transfer. This has nothing to do with hunting for and replacing a defective gene. Rather, the goal is to have relevant cells in the inflow or outflow pathways make more or less of something that affects an IOP–relevant physiological parameter so as to reduce IOP – whether or not an abnormality in that pathway was present. Thus, we have attempted to transfer genes that interfere with the rho pathway in the JCTM/SCIW to enhance conventional outflow facility, and genes that upregulate the PGF 2α – MMP pathway in the ciliary muscle to enhance uveoscleral outflow.
4.2. Reporter gene transfer to the TM and CM
We started with a self-complimentary AAV (scAAV) viral vector carrying the green fluorescent protein (GFP) gene injected into the anterior chamber of live monkeys, and achieved GFP expression in the TM easily visible gonioscopically at the slit lamp for over two years, with no clinically visible ocular inflammation ( Buie et al., 2010 ). A feline immunodeficient (FIV) viral vector carrying the GFP gene achieved the same outcome ( Barraza et al., 2009 ). Immunohistochemically, GFP was localized primarily to the TM and the most anterior part of longitudinal ciliary muscle ( Barraza et al., 2009 ; Buie et al., 2010 ) ( Fig. 6 ).

A, B, C: Monkey chamber angle after intracameral administration of scAAV.GFP vector, day 641 post injection, SLE - quiet, IOP normal. Panel A: A digital camera with a 175-W xenon nova light source and a 3-mm-6-cm 0° teleotoscope probe (Hopkins II; Karl Storz Endoscopy-America, Inc., Culver City, CA) captured the image. Panel B: A retinal camera (50EX; Topcon, Tokyo, Japan), fitted with a digital SLR color camera body (D1X (Nikon Instruments, Inc., Melville, NY); with standard clinical fluorescein exciter and barrier filters, and a gonioprism, captured the image. Panel C: A customized microscope (Nikon) with a 12-bit, monochromatic, cooled-CCD camera (Retiga, 2000RV; QImaging Burnaby, BC, Canada), a specific GFP exciter and barrier filter set and a gonioprism captured the image. D: Monkey anterior chamber angle after intracameral administration of FIV. GFP vector, day 515 post injection, SLE - quiet, IOP normal.
4.3. Functional gene transfer to the TM
4.3.a. mocas.
We then progressed to genes that, when overexpressed, would inhibit the rho cascade (the C3 exotoxin of Clostridium) or interfere with the actin/myosin interaction (caldesmon) in both cases inhibiting actomyosin contractility with consequent cellular relaxation and degradation of the actin cytoskeleton and cell-ECM attachments ( Gabelt et al., 2006 ; Grosheva et al., 2006 ; Liu X, Wang N, 2005 ; Liu et al., 2005 ; Slauson et al., 2015 ). An adenoviral vector carrying either of these transgenes, infused into our MOCAS system for freshly enucleated monkey eyes, induced a doubling of outflow facility ( Gabelt et al., 2006 ; Liu et al., 2005 ; Slauson et al., 2015 ) ( Table 2 ).
The effect of adenoviral vectors on outflow facility in monkey organ cultured anterior segment.
A: Caldesmon (1.5v10 7 plaque forming units, 20 μl); B: C3 (1.2×10 8 viral particles, 80 μl). Ad, Adenoviral vector; GFP, green fluorescent protein; Cald, caldesmon; Data are mean ± s.e.m. outflow facility; ratios are unitless. Ratio significantly different from 1.0 by the two-tailed paired t-test;
Several genes are known to drive outflow facility down in rodents and have also been implicated in the pathophysiology of human POAG. Examples among many are cochlin, SFRP1, TGF ß2 , and CTGF ( Fuchshofer and Tamm, 2012 ; Goel et al., 2012 ; Junglas et al., 2012 ; Lee et al., 2010 ; Montecchi-Palmer et al., 2017 ; Pang et al., 2015 ; Tamm et al., 2015 ; Webber et al., 2016 ). This opens the possibility of creating a local genetic molecular model of ocular hypertension/POAG in NHP, absent the outflow tissue disruption, inflammation and scarification that accompanies current laser ( Gaasterland and Kupfer, 1974 ) and trauma models, or the gross mechanical obstruction of microbead models ( Chan et al., 2018 ; Cone et al., 2012 ; Sappington et al., 2010 ). Indeed, it is possible that one or more of these genes may underlie human POAG pathophysiology. Locally overexpressing one of more of these genes in the NHP JCT/SCIWE may provide the long sought-after molecular model of NHP POAG ( Bhattacharya et al, 2009 ; ( Suppl Fig. 8 ), and a stable platform for testing new potential ocular hypertensive and glaucomatous optic neuroprotective therapies going forward.
4.3.b. Live monkeys
4.3.b.1. intracameral injection.
However, when FIV or scAAV vectors carrying either gene were injected intracamerally into live monkeys there was, with only one exception (high-titer scAAV.C3, that induced corneal endothelium dysfunction and consequent corneal edema, but no IOP or outflow changes (unpublished data), no physiological effect, no inflammation beyond that from the injection procedure itself, or any evidence at all that the eyes had been touched, save for the transcorneal needle tracks (unpublished data). One may hypothesize that in the live animal, where most of the aqueous humor drainage is through the TM/SC and the remainder is via the ciliary muscle, the ‘system’ shrugs its shoulders at GFP, which doesn’t really affect the cellular machinery. However, caldesmon and C3 exoenzyme, which significantly affect the TM cellular machinery, may elicit a strong turn-off response ( Aktas et al., 2014 ). Further, many cell types are exposed to the vector - corneal endothelium, scleral fibroblasts, TM, SC inner/outer wall, ciliary muscle, lens epithelium, iris pigment epithelium, etc. This might mount an ‘all hands-on deck’ turn-off response. Except for one recent report (Tan et al., 2019), injection of facility-effective genes in MOCAS have no effect in live NHP. While intracameral injection of an FIV.BOVPGFS-myc.GFP PGF synthase vector construct reproducibly induces an ~2mmHg reduction in IOP, the effect is much less than that of topical PGF 2⍺ analogue eyedrops, and dissipates after 5 months. The turnoff mechanism has yet to be defeated, although proteasome inhibition enhances reporter gene expression in monkey organ cultured anterior segments (MOCAS). In MOCAS, there is of course no vascular circulation and no connection to any systemic ‘assistance’, and there are also only a few cell types – corneal endothelium, scleral fibroblasts, TM and Schlemm’s canal cells - and a vastly reduced (and injured) number of ciliary muscle cells with no posterior attachments.
4.3.b.2. Intracanalicular injection
This difference, as well as a study showing that direct injection of adenoviral vectors into Schlemm’s canal was feasible in rat and human donor eyes and had potential in glaucoma treatment ( Hudde et al., 2005 ), suggested a live animal strategy of catheterizing Schlemm’s canal and passing the catheter 360 degrees around the entire circumference as in ABiC and GATT procedures ( Francis et al., 2017 ; Gallardo et al., 2018 ; Grover et al., 2018 ; Rahmatnejad et al., 2017 ). This has required modifications of the human ABiC catheter design, with generous collaboration from the engineers at Ellex iTrack. Both ab externo and ab interno cannulation of SC over 360 degrees has been achieved, with injection of trypan blue dye visible for up to four clock hours from the catheter tip during insertion ( Aktas et al., 2014 ) ( Fig. 7 ). The entire canal can be decorated blue by passing the catheter 360 degrees and then injecting slowly while withdrawing the catheter (unpublished data), with only a few small leaks/ruptures into the AC. We are currently administering cytoskeleton/contractility-modifying constructs via this route and are also looking at molecular modifications of both the vectors and the transgenes.

Drug delivery via catheterization of Schlemm’s Canal in monkeys A: Endoscopic camera image showing 6–0 prolene suture (solid arrow) and its end (dotted arrow) in Schlemm’s canal. B: Microcatheter in Schlemm’s canal (arrow). Note the blood in the canal blocking the gonioscopic view of thecatheterattherightofthepicture.C:LEDlightof the microcatheter in Schlemm’s canal. D: Endoscopic camera image of the temporal side of left eye at 3:00–4:00 o’clock showing trypan blue in Schlemm’s canalafterinjectionbycatheter(ostiumwasat12:00 o’clock, catheter tip at 1:00 o’clock). E: Infero-temporal site at 3:00–4:00 o’clock and the point where the visible dye column ended (arrow) at 5 o’clock.
4.3.b.3. Proteasome inhibition
We are also exploring proteasome inhibition. Short-term exposure of TM cells to the proteasome inhibitor MG132 increases the transduction efficiency of FIV-mediated reporter gene delivery to TM cells in culture and in the MOCAS model. A more even distribution and intensity of GFP transduction in the MOCAS TM was noted, but no transduction was seen in SCIW endothelium ( Aktas et al., 2018 ).
4.4. Prostaglandin F 2⍶ pathway gene transfer to the ciliary muscle
Currently, the most widely prescribed outflow drugs approved for clinical use are prostaglandin F 2α analogues. The delivery of genes for the prostaglandin F 2α and FP receptor biosynthetic pathways to the anterior chamber angle, and thence to the ciliary muscle, of live cats and monkeys has been achieved using lentiviral vectors, resulting in consistent low single digit IOP reduction, but far less than what is seen following topical administration of PGF 2⍺ analogue eyedrops. ( Barraza et al., 2010 ; Lee et al., 2014 ; Loewen et al., 2004 ). The IOP lowering effect in NHPs lasted for 5 months ( Suppl Fig. 9 ), ( Lee et al., 2014 ). In cats, the experiments were ended after 5 months, by design.
Although encouraging, these studies have identified a number of challenges to be overcome before prostaglandin gene therapy can be translated into the clinic: the IOP effect needs to be of greater magnitude, possibly by determining the mechanism whereby transgene expression is lost; engineering the vector to resist species-specific restriction factors or using proteasome inhibition to increase the transduction efficiency ( Aktas et al., 2018 ); identifying alternative promoters to better drive gene expression, including more ciliary muscle - and/or trabecular meshwork - specific promoters; and determining if species-specific PGF synthase or codon optimization is effective ( Aktas et al., 2018 ; Brandt et al., 2015 ; Lee et al., 2014 ; Slauson et al., 2015 ).
4.5. Post-glaucoma filtration surgery wound healing/antifibrosis
Glaucoma filtration surgery, commonly used for IOP lowering when medical and laser trabeculoplasty fail, uses a guarded sub conjunctival transscleral incision into the anterior chamber to allow the continuous long-term release of aqueous humor into the subconjunctival space, from whence it is resorbed. Antimetabolites, such as mitomycin C (MMC), are deployed subconjunctivally during surgery to block scarring of the ostomy during wound healing. Unfortunately, their use can result in undesirable side effects, such as tissue degeneration / cellular destruction leading to wound leaks, hypotony and ocular surface or intraocular infection. The human p21WAF-1/Cip-1 gene (rAd-p21), delivered via a recombinant adenovirus, was developed as a therapeutic alternative, ( Heatley et al., 2004 ; Perkins et al., 2002 ) causing cell cycle arrest rather than destruction of surrounding cells and invading fibroblasts. In vitro, treatment of Tenon fibroblasts with rAd.p21 resulted in a dose-dependent inhibition of DNA synthesis and cell growth ( Perkins et al., 2002 ). In vivo, in rabbits, rAd.p21 was comparable to mitomycin in inhibition of wound healing and fibroproliferation after filtration surgery without the complications of hypopyon and hyalitis that were associated with mitomycin ( Perkins et al., 2002 ). When delivered to live ocular hypertensive (following laser scarification of the TM) monkey eyes, sub-Tenon / episcleral rAd-p21 treatment resulted in open surgical ostomies by both functional (normalized IOP) and histological criteria for at least 9 months ( Heatley et al., 2004 ), without the tissue destruction seen in control animals treated with MMC ( Heatley et al., 2004 ).
5. Stem cell therapy
As with gene therapy, stem cell strategies have the potential to provide patients with one-time long term solutions. With age and glaucoma, trabecular meshwork and JCT cell counts decrease ( Alvarado et al., 1984 , 1981 ; Grierson and Howes, 1987 ). The goal with stem cell approaches is to replace or regenerate lost tissue in the outflow pathways, restoring TM function. TM stem cells have been identified and localized in the Schwalbe’s line / insert region of the TM in the primate eye. ( Braunger et al., 2014 ; Yun et al., 2016 ). Mesenchymal stem cells (MSC) and induced pluripotent stem cells (iPSC) have both been used in TM cell differentiation and regeneration applications. An advantage to these strategies is the ability to use autologous stem cells. Contractility and phagocytosis differ between TM cells and MSCs and can be used as a method to determine the extent to which a functional TM phenotype has been attained ( Snider et al., 2018 ). In a laser-damaged trabecular meshwork mouse model, intracamerally transplanted human trabecular meshwork stem cells (TMSCs) preferentially homed and integrated to the damaged TM region and expressed differentiated cell markers at 2 and 4 weeks. TMSC transplantation resulted in both ultrastructural and functional restoration. ( Yun et al., 2018 ) MSCs isolated from rat bone marrow, injected into the anterior chamber, significantly reduced IOP in rat eyes made hypertensive by episcleral vein cauterization. MSCs were localized in the ciliary processes and the TM ( Roubeix et al., 2015 ). Transplantation of induced pluripotent stem cell (iPSC) derived TM cells (iPSC-TM ) restored IOP and outflow facility in young ( Zhu et al., 2016 ) and aged (( Zhu et al., 2017 ) transgenic mice expressing a pathogenic form of human myocilin (Tg-MYOCY437H ) glaucoma. At 12 weeks after transplantation, IOP in iPSC-TM recipients was statistically lower and outflow facility was significantly improved compared to untreated controls ( Zhu et al., 2017 ). An ex vivo HOCAS system was developed to study outflow pathway cell loss effects on IOP homeostatic function. iPSCs were used to repopulate the cell depletion model. The differentiated cells (TM-like iPSCs) became similar to TM cells in both morphology and expression patterns and were able to restore IOP homeostatic function ( Abu-Hassan et al., 2015 ). Collectively, these studies show the potential for stem cell therapeutics targeted to the conventional TM outflow pathway.
6. Conclusion
We have come a long way in understanding and manipulating NHP and human aqueous humor outflow over the past half century. Yet, we still do not really understand the basic pathophysiology of the outflow dysfunction leading to ocular hypertension and POAG, nor even much of the normal physiology much less any putative pathophysiology of the distal conventional outflow pathway.
Finally, let me again thank ISER for bestowing this great honor upon me; my many friends, mentors, and close collaborators ‘in the business’ without whom this work would never have happened; and of course my intensely devoted, loyal and always supportive family who illuminate and inspire the long days and nights underpinning it.
- Cholinomimetics increase outflow facility solely via CM contraction
- Epinephrine, RKIs and NO ↑ facility by inhibiting TM contractility, relaxing TM and dilating SC
- PGF 2⍺ ↑ Fu by ↑ CM cell MMP synthesis/release, ↓ and remodeling CM ECM collagen
- Viral vectors (VV) transfer reporter genes expressing for > 2yrs in live monkey TM
- VV transfer genes inhibiting TM contractility and ↑ facility in MOCAS
- VV transfer genes that ↓ facility in MOCAS; molecular models for human POAG?
- iPSC cell-derived TM cells populated TM & rescued outflow in TM cell-depleted HOCAS
Supplementary Material
Suppl figure 4, suppl figure 1, suppl figure 2, suppl figure 3, suppl figure 5, suppl figure 6, suppl figure 7, suppl figure 8, suppl figure 9, acknowledgements:.
This work was supported in part by the Core Grant for Vision Research from the NIH to the University of Wisconsin-Madison (P30 EY016665), and by other grants from the NIH, the Glaucoma Research Foundation, and Research to Prevent Blindness.
Publisher's Disclaimer: This is a PDF file of an unedited manuscript that has been accepted for publication. As a service to our customers we are providing this early version of the manuscript. The manuscript will undergo copyediting, typesetting, and review of the resulting proof before it is published in its final form. Please note that during the production process errors may be discovered which could affect the content, and all legal disclaimers that apply to the journal pertain.
- Abu-Hassan DW, Li X, Ryan EI, Acott TS, Kelley MJ, 2015. Induced pluripotent stem cells restore function in a human cell loss model of open-angle glaucoma . Stem Cells 33 , 751–761. 10.1002/stem.1885 [ PMC free article ] [ PubMed ] [ CrossRef ] [ Google Scholar ]
- Aktas Z, Rao H, Slauson SR, Gabelt BT, Larsen IV, Sheridan RTC, Herrnberger L, Tamm ER, Kaufman PL, Brandt CR, 2018. Proteasome inhibition increases the efficiency of lentiviral vector-mediated transduction of trabecular meshwork . Investig. Ophthalmol. Vis. Sci 59 , 298–310. 10.1167/iovs.17-22074 [ PMC free article ] [ PubMed ] [ CrossRef ] [ Google Scholar ]
- Aktas Z, Tian B, McDonald J, Yamamato R, Larsen C, Kiland J, Kaufman PL, Rasmussen CA, 2014. Application of Canaloplasty in Glaucoma Gene Therapy: Where Are We? J. Ocul. Pharmacol. Ther 30 , 277–282. 10.1089/jop.2013.0203 [ PMC free article ] [ PubMed ] [ CrossRef ] [ Google Scholar ]
- Alvarado J, Murphy C, Juster R, 1984. Trabecular Meshwork Cellularity in Primary Open-angle Glaucoma and Nonglaucomatous Normals . Ophthalmology 91 , 564–579. 10.1016/S0161-6420(84)34248-8 [ PubMed ] [ CrossRef ] [ Google Scholar ]
- Alvarado J, Murphy C, Polansky J, Juster R, 1981. Age-related changes in trabecular meshwork cellularity . Investig. Ophthalmol. Vis. Sci 21 , 714–727. 10.1155/2013/295204 [ PubMed ] [ CrossRef ] [ Google Scholar ]
- Barraza RA, McLaren JW, Poeschla EM, 2010. Prostaglandin pathway gene therapy for sustained reduction of intraocular pressure . Mol. Ther 18 , 491–501. 10.1038/mt.2009.278 [ PMC free article ] [ PubMed ] [ CrossRef ] [ Google Scholar ]
- Barraza RA, Rasmussen CA, Loewen N, Cameron JD, Gabelt BT, Teo W-L, Kaufman PL, Poeschla EM, 2009. Prolonged Transgene Expression with Lentiviral Vectors in the Aqueous Humor Outflow Pathway of Nonhuman Primates . Hum. Gene Ther 20 , 191–200. 10.1089/hum.2008.086 [ PMC free article ] [ PubMed ] [ CrossRef ] [ Google Scholar ]
- Bazrafkan M, Panahi M, Saki G, Ahangarpour A, Zaeimzadeh N, 2010. Effect of aqueous extract of Ruta graveolens on spermatogenesis of adult rats . Int. J. Pharmacol 6 , 926–929. 10.3923/ijp.2010.926.929 [ CrossRef ] [ Google Scholar ]
- Becquet F, Courtois Y, Goureau O, 1997. Nitric oxide in the eye: Multifaceted roles and diverse outcomes . Surv. Ophthalmol 42 , 71–82. 10.1016/S0039-6257(97)84043-X [ PubMed ] [ CrossRef ] [ Google Scholar ]
- Bhattacharya SK, Gabelt BT, Ruiz J, Picciani R, Kaufman PL, 2009. Cochlin expression in anterior segment organ culture models after TGFβ2 treatment . Investig. Ophthalmol. Vis. Sci 50 , 551–559. 10.1167/iovs.08-2632 [ PMC free article ] [ PubMed ] [ CrossRef ] [ Google Scholar ]
- Bill A, 1971. Aqueous humor dynamics in monkeys (Macaca irus and Cercopithecus ethiops) . Exp. Eye Res 11 , 195–206. 10.1016/s0014-4835(71)80023-4 [ PubMed ] [ CrossRef ] [ Google Scholar ]
- Bill Anders, 1967. Effects of atropine and pilocarpine on aqueous humour dynamics in cynomolgus monkeys (Macaca irus) . Exp. Eye Res 6 , 120-IN3. 10.1016/S0014-4835(67)80062-9 [ PubMed ] [ CrossRef ] [ Google Scholar ]
- Bill A, 1967. Effects of atropine and pilocarpine on aqueous humour dynamics in cynomolgus monkeys (Macaca irus) . Exp. Eye Res 6 , 120–5. 10.1016/s00144-835(67)80062-9 [ PubMed ] [ CrossRef ] [ Google Scholar ]
- Bill A, 1966. Conventional and uveo-scleral drainage of aqueous humour in the cynomolgus monkey (Macaca irus) at normal and high intraocular pressures . Exp. Eye Res 5 , 45–54. 10.1016/s0014-4835(66)80019-2 [ PubMed ] [ CrossRef ] [ Google Scholar ]
- Brandt CR, Rao H, Gabelt BT, Aktas Z, Slauson SR, 2015. Genome deficient lentiviral particles improve FIV transduction efficiency of trabecular meshwork. Investig. Ophthalmol. Vis. Sci , 2015 Annual Meeting of the Association for Research in Vision and Ophthalmology, ARVO 2015. United States. 56, 3280. [ Google Scholar ]
- Braunger BM, Ademoglu B, Koschade SE, Fuchshofer R, Gabelt BT, Kiland JA, HennesBeann EA, Brunner KG, Kaufman PL, Tamm ER, 2014. Identification of adult stem cells in Schwalbe’s line region of the primate eye . Invest. Ophthalmol. Vis. Sci 55 , 7499–7507. 10.1167/iovs.14-14872 [ PMC free article ] [ PubMed ] [ CrossRef ] [ Google Scholar ]
- Budenz DL, 2009. A Clinician’s Guide to the Assessment and Management of Nonadherence in Glaucoma . Ophthalmology 116 , S43–S47. 10.1016/j.ophtha.2009.06.022 [ PubMed ] [ CrossRef ] [ Google Scholar ]
- Buie LKK, Rasmussen CA, Porterfield EC, Ramgolam VS, Choi VW, Markovic-Plese S, Samulski RJ, Kaufman PL, Borrás T, 2010. Self-complementary AAV virus (scAAV) safe and long-term gene transfer in the trabecular meshwork of living rats and monkeys . Investig. Ophthalmol. Vis. Sci 51 , 236–248. 10.1167/iovs.09-3847 [ PMC free article ] [ PubMed ] [ CrossRef ] [ Google Scholar ]
- Carreon T, van der Merwe E, Fellman RL, Johnstone M, Bhattacharya SK, 2017. Aqueous outflow - A continuum from trabecular meshwork to episcleral veins . Prog. Retin. Eye Res 57 , 108–133. 10.1016/j.preteyeres.2016.12.004 [ PMC free article ] [ PubMed ] [ CrossRef ] [ Google Scholar ]
- Chan A, Lynn MN, Tun SBB, Barathi VA, Aung T, 2018. Bilateral intraocular pressure (IOP) changes in a non human primate (NHP) microbead model of chronic IOP elevation: Can both eyes achieve similar elevations for therapeutics evalution? Invest. Ophthalmol. Vis. Sci [ Google Scholar ]
- Cone FE, Steinhart MR, Oglesby EN, Kalesnykas G, Pease ME, Quigley HA, 2012. The effects of anesthesia, mouse strain and age on intraocular pressure and an improved murine model of experimental glaucoma . Exp. Eye Res 99 , 27–35. 10.1016/j.exer.2012.04.006 [ PMC free article ] [ PubMed ] [ CrossRef ] [ Google Scholar ]
- Costagliola C, Verolino M, Leonarda De Rosa M, Iaccarino G, Ciancaglini M, Mastropasqua L, 2000. Effect of oral losartan potassium administration on intraocular pressure in normotensive and glaucomatous human subjects . Exp. Eye Res 71 , 167–171. 10.1006/exer.2000.0866 [ PubMed ] [ CrossRef ] [ Google Scholar ]
- Crawford K, Kaufman PL, 1987. Pilocarpine antagonizes prostaglandin f2α-induced ocular hypotension in monkeys: Evidence for enhancement of uveoscleral outflow by prostaglandin f2α . Arch. Ophthalmol 105 , 1112–1116. 10.1001/archopht.1987.01060080114039 [ PubMed ] [ CrossRef ] [ Google Scholar ]
- Crawford K, Kaufman PL, Gabelt BT, 1987. Effects of topical PGF2α on aqueous humor dynamics in cynomolgus monkeys . Curr. Eye Res 6 , 1035–1044. 10.3109/02713688709034874 [ PubMed ] [ CrossRef ] [ Google Scholar ]
- Croft MA, Kaufman PL, 1995a. Effect of daily topical ethacrynic acid on aqueous humor dynamics in monkeys . Curr. Eye Res 14 , 777–781. 10.3109/02713689508995799 [ PubMed ] [ CrossRef ] [ Google Scholar ]
- Croft MA, Kaufman PL, 1995b. Effect of daily topical ethacrynic acid on aqueous humor dynamics in monkeys . Curr. Eye Res 14 , 777–781. 10.3109/02713689508995799 [ PubMed ] [ CrossRef ] [ Google Scholar ]
- Crosson CE, Sloan CF, Yates PW, 2005. Modulation of conventional outflow facility by the adenosine A1 agonist N6-cyclohexyladenosine . Investig. Ophthalmol. Vis. Sci 46 , 3795–3799. 10.1167/iovs.05-0421 [ PubMed ] [ CrossRef ] [ Google Scholar ]
- de Kater AW, Shahsafaei A, Epstein DL, 1992. Localization of smooth muscle and nonmuscle actin isoforms in the human aqueous outflow pathway . Invest. Ophthalmol. Vis. Sci 33 , 424–9. [ PubMed ] [ Google Scholar ]
- Duan JC, Fulop A, 2015. Density-Tempered Marginalized Sequential Monte Carlo Samplers . J. Bus. Econ. Stat 33 , 192–202. 10.1080/07350015.2014.940081 [ CrossRef ] [ Google Scholar ]
- Endre-A-Balazs-doctor-who-found-acid-to-treat-arthritic-knees [WWW Document], 2015. . NY Times. [ Google Scholar ]
- Balazs Endre [WWW Document], 2015. . Harvard Eye . URL https://eye.hms.harvard.edu/endrebalazs (accessed 11.10.18). [ Google Scholar ]
- Francis BA, Akil H, Bert BB, 2017. Ab interno Schlemm’s Canal Surgery . Dev. Ophthalmol 59 , 127–146. 10.1159/000458492 [ PubMed ] [ CrossRef ] [ Google Scholar ]
- Fuchshofer R, Tamm ER, 2012. The role of TGF-β in the pathogenesis of primary open-angle glaucoma . Cell Tissue Res . 10.1007/s00441-011-1274-7 [ PubMed ] [ CrossRef ] [ Google Scholar ]
- Gaasterland D, Kupfer C, 1974. Experimental glaucoma in the rhesus . Invest. Ophthalmol. Vis. Sci 13 , 4208801. [ PubMed ] [ Google Scholar ]
- Gabelt BT, Hu Y, Vittitow JL, Rasmussen CR, Grosheva I, Bershadsky AD, Geiger B, Borrás T, Kaufman PL, 2006. Caldesmon transgene expression disrupts focal adhesions in HTM cells and increases outflow facility in organ-cultured human and monkey anterior segments . Exp. Eye Res 82 , 935–944. 10.1016/j.exer.2005.12.002 [ PubMed ] [ CrossRef ] [ Google Scholar ]
- Gabelt BT, Kaufman PL, 2012. Production and Flow of Aqueous Humor, in: (Eds: Kaufman PL, Alm A, Levin L, Nilsson S, VerHoeve J, W.S. (Ed.), Adler’s Physiology of the Eye . Saunders/Elsevier, pp. 274–307. 10.1016/b978-0-323-05714-1.00011-x [ CrossRef ] [ Google Scholar ]
- Gabelt BT, Kaufman PL, 1992. Inhibition of outflow facility and accommodative and miotic responses to pilocarpine in rhesus monkeys by muscarinic receptor subtype antagonists . J. Pharmacol. Exp. Ther 263 , 1133–9. [ PubMed ] [ Google Scholar ]
- Gabelt BT, Kaufman PL, 1989. Prostaglandin F2 alpha increases uveoscleral outflow in the cynomolgus monkey . Exp. Eye Res 49 , 389–402. [ PubMed ] [ Google Scholar ]
- Gabelt BT, Kaufman PL, Rasmussen CA, 2011. Effect of nitric oxide compounds on monkey ciliary muscle in vitro . Exp. Eye Res 93 , 321–327. 10.1016/j.exer.2010.12.003 [ PMC free article ] [ PubMed ] [ CrossRef ] [ Google Scholar ]
- Gallardo MJ, Supnet RA, Ahmed IIK, 2018. Circumferential viscodilation of Schlemm’s canal for open-angle glaucoma: Ab-interno vs ab-externo canaloplasty with tensioning suture . Clin. Ophthalmol 12 , 2493–2498. 10.2147/OPTH.S178962 [ PMC free article ] [ PubMed ] [ CrossRef ] [ Google Scholar ]
- Garcia GA, Ngai P, Mosaed S, Lin KY, 2016. Critical evaluation of latanoprostene bunod in the treatment of glaucoma . Clin. Ophthalmol 10.2147/OPTH.S103985 [ PMC free article ] [ PubMed ] [ CrossRef ] [ Google Scholar ]
- Gaton DD, Sagara T, Lindsey JD, Gabelt BT, Kaufman PL, Weinreb RN, 2001. Increased matrix metalloproteinases 1, 2, and 3 in the monkey uveoscleral outflow pathway after topical prostaglandin F2α-isopropyl ester treatment . Arch. Ophthalmol 119 , 1165–1170. 10.1001/archopht.119.8.1165 [ PubMed ] [ CrossRef ] [ Google Scholar ]
- Goel M, Sienkiewicz AE, Picciani R, Wang J, Lee RK, Bhattacharya SK, 2012. Cochlin, intraocular pressure regulation and mechanosensing . PLoS One 7 . 10.1371/journal.pone.0034309 [ PMC free article ] [ PubMed ] [ CrossRef ] [ Google Scholar ]
- Gonzalez JM, Ko MK, Hong Y-K, Weigert R, Tan JCH, 2017. Deep tissue analysis of distal aqueous drainage structures and contractile features . Sci. Rep 7 , 17071. 10.1038/s41598-017-16897-y [ PMC free article ] [ PubMed ] [ CrossRef ] [ Google Scholar ]
- Grant WM, 1963. Experimental Aqueous Perfusion in Enucleated Human Eyes . Arch. Ophthalmol 69 , 783–801. 10.1001/archopht.1963.00960040789022 [ PubMed ] [ CrossRef ] [ Google Scholar ]
- Grant WM, 1958. Further studies on facility of flow through the trabecular meshwork . AMA. Arch. Ophthalmol 60 , 523–33. [ PubMed ] [ Google Scholar ]
- Greenshaw AJ, 1986. Osmotic mini-pumps: A convenient program for weight-adjusted filling concentrations . Brain Res. Bull 16 , 759–761. 10.1016/0361-9230(86)90150-4 [ PubMed ] [ CrossRef ] [ Google Scholar ]
- Grierson I, Howes RC, 1987. Age-related depletion of the cell population in the human trabecular meshwork . Eye 1 , 204–210. 10.1038/eye.1987.38 [ PubMed ] [ CrossRef ] [ Google Scholar ]
- Grosheva I, Vittitow JL, Goichberg P, Gabelt BT, Kaufman PL, Borrás T, Geiger B, Bershadsky AD, 2006. Caldesmon effects on the actin cytoskeleton and cell adhesion in cultured HTM cells . Exp. Eye Res 82 , 945–958. 10.1016/j.exer.2006.01.006 [ PubMed ] [ CrossRef ] [ Google Scholar ]
- Grover DS, Smith O, Fellman RL, Godfrey DG, Gupta A, De Oca IM, Feuer WJ, 2018. Gonioscopy-Assisted transluminal trabeculotomy: An ab interno circumferential trabeculotomy: 24 months follow-up . J. Glaucoma 27 , 393–401. 10.1097/IJG.0000000000000956 [ PubMed ] [ CrossRef ] [ Google Scholar ]
- Hamanaka T, Bill A, Ichinohasama R, Ishida T, 1992. Aspects of the development of Schlemm’s canal . Exp. Eye Res 55 , 479–488. 10.1016/0014-4835(92)90121-8 [ PubMed ] [ CrossRef ] [ Google Scholar ]
- Heatley G, Kiland J, Faha B, Seeman J, Schlamp CL, Dawson DG, Gleiser J, Maneval D, Kaufman PL, Nickels RW, 2004. Gene therapy using p21 WAF-1/Cip-1 to modulate wound healing after glaucoma trabeculectomy surgery in a primate model of ocular hypertension . Gene Ther . 11 , 949–955. 10.1038/sj.gt.3302253 [ PubMed ] [ CrossRef ] [ Google Scholar ]
- Heyne GW, Kiland JA, Kaufman PL, Gabelt BT, 2013. Effect of nitric oxide on anterior segment physiology in monkeys . Investig. Ophthalmol. Vis. Sci 54 , 5103–5110. 10.1167/iovs.12-11491 [ PMC free article ] [ PubMed ] [ CrossRef ] [ Google Scholar ]
- Hirooka K, Shiraga F, 2007. Potential role for angiotensin-converting enzyme inhibitors in the treatment of glaucoma . Clin. Ophthalmol 1 , 217–23. [ PMC free article ] [ PubMed ] [ Google Scholar ]
- Honjo M, Inatani M, Kido N, Sawamura T, Yue BYJT, Honda Y, Tanihara H, 2001. Effects of protein kinase inhibitor, HA1077, on intraocular pressure and outflow facility in rabbit eyes . Arch. Ophthalmol 119 , 1171–1178. 10.1001/archopht.119.8.1171 [ PubMed ] [ CrossRef ] [ Google Scholar ]
- Honjo M, Tanihara H, 2018. Impact of the clinical use of ROCK inhibitor on the pathogenesis and treatment of glaucoma . Jpn. J. Ophthalmol 62 , 109–126. 10.1007/s10384-018-0566-9 [ PubMed ] [ CrossRef ] [ Google Scholar ]
- Huang AS, Francis BA, Weinreb RN, 2018. Structural and functional imaging of aqueous humour outflow: a review . Clin. Experiment. Ophthalmol 46 , 158–168. 10.1111/ceo.13064 [ PMC free article ] [ PubMed ] [ CrossRef ] [ Google Scholar ]
- Hubbard WC, Johnson M, Gong H, Gabelt BT, Peterson JA, Sawhney R, Freddo T, Kaufman PL, 1997. Intraocular pressure and outflow facility are unchanged following acute and chronic intracameral chondroitinase ABC and hyaluronidase in monkeys . Exp. Eye Res 65 , 177–190. 10.1006/exer.1997.0319 [ PubMed ] [ CrossRef ] [ Google Scholar ]
- Hubbard WC, Kee C, Kaufman PL, 1996. Aceclidine effects on outflow facility after ciliary muscle disinsertion . Ophthalmologica 210 , 303–307. 10.1159/000310729 [ PubMed ] [ CrossRef ] [ Google Scholar ]
- Hudde T, Apitz J, Bordes-Alonso R, Heise K, Johnson KTM, Steuhl KP, Geerling G, Pützer BM, 2005. Gene transfer to trabecular meshwork endothelium via direct injection into the Schlemm canal and in vivo toxicity study . Curr. Eye Res 30 , 1051–1059. 10.1080/02713680500323350 [ PubMed ] [ CrossRef ] [ Google Scholar ]
- Husain S, Shearer TW, Crosson CE, 2006. Mechanisms Linking Adenosine A1 Receptors and Extracellular Signal-Regulated Kinase 1/2 Activation in Human Trabecular Meshwork Cells . J. Pharmacol. Exp. Ther 320 , 258–265. 10.1124/jpet.106.110981 [ PubMed ] [ CrossRef ] [ Google Scholar ]
- Iizuka K, Yoshii A, Samizo K, Tsukagoshi H, Ishizuka T, Dobashi K, Nakazawa T, Mori M, 1999. A major role for the Rho-associated coiled coil forming protein kinase in G-protein-mediated Ca2+ sensitization through inhibition of myosin phosphatase in rabbit trachea . Br. J. Pharmacol 128 , 925–933. 10.1038/sj.bjp.0702864 [ PMC free article ] [ PubMed ] [ CrossRef ] [ Google Scholar ]
- Inazaki H, Kobayashi S, Anzai Y, Satoh H, Sato S, Inoue M, Yamane S, Kadonosono K, 2017. One-year efficacy of adjunctive use of Ripasudil, a rho-kinase inhibitor, in patients with glaucoma inadequately controlled with maximum medical therapy . Graefe’s Arch. Clin. Exp. Ophthalmol 255 , 2009–2015. 10.1007/s00417-017-3727-5 [ PubMed ] [ CrossRef ] [ Google Scholar ]
- Inomata H, Bill A, Smelser GK, 1972a. Aqueous humor pathways through the trabecular meshwork and into Schlemm’s canal in the cynomolgus monkey (Macaca irus). An electron microscopic study . Am. J. Ophthalmol 73 , 760–789. 10.1016/0002-9394(72)90394-7 [ PubMed ] [ CrossRef ] [ Google Scholar ]
- Inomata H, Bill A, Smelser GK, 1972b. Unconventional Routes of Aqueous Humor Outflow in Cynomolgus Monkey (Macaca Irus) . Am. J. Ophthalmol 73 , 893–907. 10.1016/0002-9394(72)90459-X [ PubMed ] [ CrossRef ] [ Google Scholar ]
- Inoue T, Tanihara H, 2017. Ripasudil hydrochloride hydrate: targeting Rho kinase in the treatment of glaucoma . Expert Opin. Pharmacother 18 , 1669–1673. 10.1080/14656566.2017.1378344 [ PubMed ] [ CrossRef ] [ Google Scholar ]
- Inoue T, Tanihara H, 2013. Rho-associated kinase inhibitors: A novel glaucoma therapy . Prog. Retin. Eye Res 10.1016/j.preteyeres.2013.05.002 [ PubMed ] [ CrossRef ] [ Google Scholar ]
- Johnstone MA, 2014. Intraocular Pressure Regulation: Findings of Pulse-Dependent Trabecular Meshwork Motion Lead to Unifying Concepts of Intraocular Pressure Homeostasis . J. Ocul. Pharmacol. Ther 30 , 88–93. 10.1089/jop.2013.0224 [ PMC free article ] [ PubMed ] [ CrossRef ] [ Google Scholar ]
- Junglas B, Kuespert S, Seleem AA, Struller T, Ullmann S, Bösl M, Bosserhoff A, Köstler J, Wagner R, Tamm ER, Fuchshofer R, 2012. Connective tissue growth factor causes glaucoma by modifying the actin cytoskeleton of the trabecular meshwork . Am. J. Pathol 180 , 2386–2403. 10.1016/j.ajpath.2012.02.030 [ PubMed ] [ CrossRef ] [ Google Scholar ]
- Kahook MY, Serle JB, Mah FS, Kim T, Raizman MB, Heah T, Ramirez-Davis N, Kopczynski CC, Usner DW, Novack GD, ROCKET-2 Study Group, 2019. Long-term Safety and Ocular Hypotensive Efficacy Evaluation of Netarsudil Ophthalmic Solution: Rho Kinase Elevated IOP Treatment Trial (ROCKET-2) . Am. J. Ophthalmol 200 , 130–137. 10.1016/j.ajo.2019.01.003 [ PubMed ] [ CrossRef ] [ Google Scholar ]
- Kaufman PL, 2017. Latanoprostene bunod ophthalmic solution 0.024% for IOP lowering in glaucoma and ocular hypertension . Expert Opin. Pharmacother 18 , 433–444. 10.1080/14656566.2017.1293654 [ PubMed ] [ CrossRef ] [ Google Scholar ]
- Kaufman PL, 1987a. Adenosine 3′,5′-cyclic-monophosphate and outflow facility in monkey eyes with intact and retrodisplaced ciliary muscle . Exp. Eye Res 44 , 415–423. 10.1016/S0014-4835(87)80175-6 [ PubMed ] [ CrossRef ] [ Google Scholar ]
- Kaufman PL, 1987b. Non-additivity of maximal pilocarpine and cytochalasin effects on outflow facility . Exp. Eye Res 44 , 283–291. 10.1016/S0014-4835(87)80012-X [ PubMed ] [ CrossRef ] [ Google Scholar ]
- Kaufman PL, 1986a. Epinephrine, norepinephrine, and isoproterenol dose-outflow facility response relationships in cynomolgus monkey eyes with and without ciliary muscle retrodisplacement . Acta Ophthalmol . 64 , 356–363. 10.1111/j.1755-3768.1986.tb06933.x [ PubMed ] [ CrossRef ] [ Google Scholar ]
- Kaufman PL, 1986b. Total iridectomy does not alter outflow facility responses to cyclic AMP in cynomolgus monkeys . Exp. Eye Res 43 , 441–447. 10.1016/S0014-4835(86)80079-3 [ PubMed ] [ CrossRef ] [ Google Scholar ]
- Kaufman PL, 1979. Aqueous humor dynamics following total iridectomy in the cynomolgus monkey . Investig. Ophthalmol. Vis. Sci 18 , 870–875. [ PubMed ] [ Google Scholar ]
- Kaufman PL, 1976. Prognosis of primary rhegmatogenous retinal detachments: 2. Accounting for and predicting final visual acuity in surgically reattached cases . Acta Ophthalmol . 54 , 61–74. 10.1111/j.1755-3768.1976.tb00419.x [ PubMed ] [ CrossRef ] [ Google Scholar ]
- Kaufman PL, 1975. Prognosis of primary rhegmatogenous retinal detachments: 1. Associations between clinical detachment characteristics, subretinal fluid butyrylcholinesterase and visual outcome following scleral buckling procedures . Acta Ophthalmol . 53 , 660–671. 10.1111/j.1755-3768.1975.tb01785.x [ PubMed ] [ CrossRef ] [ Google Scholar ]
- Kaufman PL, Bárány EH, 1976. Loss of acute pilocarpine effect on outflow facility following surgical disinsertion and retrodisplacement of the ciliary muscle from the scleral spur in the cynomolgus monkey . Invest. Ophthalmol. Vis. Sci 15 , 793–807. [ PubMed ] [ Google Scholar ]
- Kaufman PL, Barany EH, Bárány EH, 1981. Adrenergic drug effects on aqueous outflow facility following ciliary muscle retrodisplacement in the cynomolgus monkey . Investig. Ophthalmol. Vis. Sci 20 , 644–651. [ PubMed ] [ Google Scholar ]
- Kaufman PL, Bárány EH, Erickson KA, 1982. Effect of serotonin, histamine and bradykinin on outflow facility following ciliary muscle retrodisplacement in the cynomolgus monkey . Exp. Eye Res 35 , 191–199. 10.1016/S0014-4835(82)80066-3 [ PubMed ] [ CrossRef ] [ Google Scholar ]
- Kaufman PL, Bill A, Bárány EH, 1977. Effect of cytochalasin B on conventional drainage of aqueous humor in the cynomolgus monkey . Exp. Eye Res 25 , 411–414. 10.1016/S0014-4835(77)80037-7 [ PubMed ] [ CrossRef ] [ Google Scholar ]
- Kaufman PL, Erickson KA, 1982. Cytochalasin B and D dose - outflow facility response relationships in the cynomolgus monkey . Investig. Ophthalmol. Vis. Sci 23 , 646–650. [ PubMed ] [ Google Scholar ]
- Kaufman PL, Lütjen-Drecoll E, 1975. Total iridectomy in the primate in vivo: surgical technique and postoperative anatomy . Invest. Ophthalmol 14 , 766–71. [ PubMed ] [ Google Scholar ]
- Kaufman PL, Podos SM, 1973. Subretinal fluid butyrylcholinesterase. 1. Source of the enzyme and factors affecting its concentration in subretinal fluid from primary rhegmatogenous retinal detachments . Am. J. Ophthalmol 75 , 627–636. 10.1016/0002-9394(73)90814-3 [ PubMed ] [ CrossRef ] [ Google Scholar ]
- Kaufman PL, Rasmussen CA, 2012. Advances in glaucoma treatment and management: Outflow drugs . Investig. Ophthalmol. Vis. Sci 53 , 2495–2500. 10.1167/iovs.12-9483m [ PMC free article ] [ PubMed ] [ CrossRef ] [ Google Scholar ]
- Kaufman PL, Rentzhog L, 1981. Effect of total iridectomy on outflow facility responses to adrenergic drugs in cynomolgus monkeys . Exp. Eye Res 33 , 65–74. 10.1016/S0014-4835(81)80082-6 [ PubMed ] [ CrossRef ] [ Google Scholar ]
- Kaufman PL PS, 1974. Subretinal fluid butyrylcholinesterase. 2. Reoperated rhegmatogenous detachments and diabetic traction detachments . Am J Ophthalmol 19–24. [ PubMed ] [ Google Scholar ]
- Kaufman PL PS, 1973. The subretinal fluid in primary rhegmatogenous retinal detachment . Surv. Ophthalmol 100–116. [ PubMed ] [ Google Scholar ]
- Kawase K, Vittitow JL, Weinreb RN, Araie M, Hoshiai S, Hashida S, Iwasaki M, Kano K, Kato T, Kuwayama Y, Muramatsu T, Mitsuhashi M, Matsuzaki S, Nakajima T, Sato I, Yoshimura Y, 2016. Long-term Safety and Efficacy of Latanoprostene Bunod 0.024% in Japanese Subjects with Open-Angle Glaucoma or Ocular Hypertension : The JUPITER Study. Adv. Ther 33 , 1612–1627. 10.1007/s12325-016-0385-7 [ PMC free article ] [ PubMed ] [ CrossRef ] [ Google Scholar ]
- Kee C, Kaufman PL, Gabelt BT, 1994. Effect of 8-Br cGMP on aqueous humor dynamics in monkeys . Invest. Ophthalmol. Vis. Sci 35 , 2769–73. [ PubMed ] [ Google Scholar ]
- Lee ES, Gabelt BT, Faralli JA, Peters DM, Brandt CR, Kaufman PL, Bhattacharya SK, 2010. COCH transgene expression in cultured human trabecular meshwork cells and its effect on outflow facility in monkey organ cultured anterior segments . Investig. Ophthalmol. Vis. Sci 51 , 2060–2066. 10.1167/iovs.09-4521 [ PMC free article ] [ PubMed ] [ CrossRef ] [ Google Scholar ]
- Lee ES, Rasmussen CA, Filla MS, Slauson SR, Kolb AW, Peters DM, Kaufman PL, Gabelt BT, Brandt CR, 2014. Prospects for lentiviral vector mediated prostaglandin F synthase gene delivery in monkey eyes in vivo . Curr. Eye Res 10.3109/02713683.2014.884593 [ PMC free article ] [ PubMed ] [ CrossRef ] [ Google Scholar ]
- Lee P, Shen Y, Eberle M, 1975. The long-acting Ocusert-pilocarpine system in the management of glaucoma . Invest. Ophthalmol 14 , 43–6. [ PubMed ] [ Google Scholar ]
- Liebmann JM, Lee JK, 2017. Current therapeutic options and treatments in development for the management of primary open-angle glaucoma . Am. J. Manag. Care 23 , S279–S292. [ PubMed ] [ Google Scholar ]
- Liu GJ, Mizukawa A, Okisaka S, 1994. Mechanism of intraocular pressure decrease after contact transscleral continuous-wave Nd:YAG laser cyclophotocoagulation . Ophthalmic Res . 26 , 65–79. 10.1159/000267395 [ PubMed ] [ CrossRef ] [ Google Scholar ]
- Liu X, Wang N, K.P., 2005. Experimental Gene Therapy for Glaucoma with C3 Transferase . China Biotechnol . 25 , 109–117. [ Google Scholar ]
- Liu X, Hu Y, Filla MS, Gabelt BT, Peters DM, Brandt CR, Kaufman PL, 2005. The effect of C3 transgene expression on actin and cellular adhesions in cultured human trabecular meshwork cells and on outflow facility in organ cultured monkey eyes . Mol. Vis 11 , 1112–21. [ PubMed ] [ Google Scholar ]
- Loewen N, Fautsch MP, Teo WL, Bahler CK, Johnson DH, Poeschla EM, 2004. Longterm, targeted genetic modification of the aqueous humor outflow tract coupled with noninvasive imaging of gene expression in vivo . Investig. Ophthalmol. Vis. Sci 45 , 3091–3098. 10.1167/iovs.04-0366 [ PubMed ] [ CrossRef ] [ Google Scholar ]
- Lotti VJ, Pawlowski N, 2009. Prostaglandins Mediate the Ocular Hypotensive Action of the Angiotensin Converting Enzyme Inhibitor MK-422 (Enalaprilat) in African Green Monkeys . J. Ocul. Pharmacol. Ther 6 , 1–7. 10.1089/jop.1990.6.1 [ PubMed ] [ CrossRef ] [ Google Scholar ]
- Lütjen-Drecoll E, 1973. Structural Factors Influencing Outflow Facility and its Changeability Under Drugs A Study in Macaca Arctoides . Investig. Ophthalmol. Vis. Sci 12 , 280–294. [ PubMed ] [ Google Scholar ]
- Lutjen-Drecoll E, Kaufman PL, Barany EH, 1977. Light and electron microscopy of the anterior chamber angle structures following surgical disinsertion of the ciliary muscle in the cynomolgus monkey . Invest. Ophthalmol. Vis. Sci 16 , 218–225. [ PubMed ] [ Google Scholar ]
- McKinnon SJ, Goldberg LD, Peeples P, Walt JG, Bramley TJ, 2008. Current management of glaucoma and the need for complete therapy . Am. J. Manag. Care https://doi.org/10022 [pii] [ PubMed ] [ Google Scholar ]
- Medeiros FA, Martin KR, Peace J, Scassellati Sforzolini B, Vittitow JL, Weinreb RN, 2016. Comparison of Latanoprostene Bunod 0.024% and Timolol Maleate 0.5% in Open-Angle Glaucoma or Ocular Hypertension: The LUNAR Study . Am. J. Ophthalmol 168 , 250–259. 10.1016/j.ajo.2016.05.012 [ PubMed ] [ CrossRef ] [ Google Scholar ]
- Menage MJ, Croft MA, Gange SJ, Kaufman PL, 1995. ε-Aminocaproic acid does not inhibit outflow resistance washout in monkeys . Investig. Ophthalmol. Vis. Sci 36 , 1745–1749. [ PubMed ] [ Google Scholar ]
- Mermoud A, Baerveldt G, Mickler DS, Wu GS, Rao NA, 1994. Animal model for uveitic glaucoma . Graefe’s Arch. Clin. Exp. Ophthalmol 232 , 553–560. 10.1007/BF00181999 [ PubMed ] [ CrossRef ] [ Google Scholar ]
- Montecchi-Palmer M, Bermudez JY, Webber HC, Patel GC, Clark AF, Mao W, 2017. TGFβ2 induces the formation of cross-linked actin networks (CLANs) in human trabecular meshwork cells through the smad and non-smad dependent pathways . Investig. Ophthalmol. Vis. Sci 58 , 1288–1295. 10.1167/iovs.16-19672 [ PMC free article ] [ PubMed ] [ CrossRef ] [ Google Scholar ]
- Myers JS, Sall KN, DuBiner H, Slomowitz N, McVicar W, Rich CC, Baumgartner RA, 2016. A Dose-Escalation Study to Evaluate the Safety, Tolerability, Pharmacokinetics, and Efficacy of 2 and 4 Weeks of Twice-Daily Ocular Trabodenoson in Adults with Ocular Hypertension or Primary Open-Angle Glaucoma . J. Ocul. Pharmacol. Ther 32 , 555–562. 10.1089/jop.2015.0148 [ PMC free article ] [ PubMed ] [ CrossRef ] [ Google Scholar ]
- Nathanson JA, McKee M, 1995. Identification of an extensive system of nitric oxide-producing cells in the ciliary muscle and outflow pathway of the human eye . Invest. Ophthalmol. Vis. Sci 36 , 1765–73. [ PubMed ] [ Google Scholar ]
- {"type":"clinical-trial","attrs":{"text":"NCT02371746","term_id":"NCT02371746"}} NCT02371746 , 2015. Safety and Efficacy of ENV515 Travoprost Extended Release (XR) in Patients With Bilateral Ocular Hypertension or Primary Open Angle Glaucoma . Https://clinicaltrials.gov/show/nct02371746 . [ Google Scholar ]
- Neufeld AH, Sears ML, 1974. Cyclic-AMP in ocular tissues of the rabbit, monkey, and human . Invest. Ophthalmol 13 , 475–7. [ PubMed ] [ Google Scholar ]
- Nilsson SFE, Samuelsson M, Bill A, Stjernschantz J, 1989. Increased uveoscleral outflow as a possible mechanism of ocular hypotension caused by prostaglandin F2α−1-isopropylester in the cynomolgus monkey . Exp. Eye Res 48 , 707–716. 10.1016/0014-4835(89)90011-0 [ PubMed ] [ CrossRef ] [ Google Scholar ]
- Nordstrom BL, Friedman DS, Mozaffari E, Quigley HA, Walker AM, 2005. Persistence and adherence with topical glaucoma therapy . Am. J. Ophthalmol 140 , 598.e1–598.e11. 10.1016/j.ajo.2005.04.051 [ PubMed ] [ CrossRef ] [ Google Scholar ]
- Ocklind A, 1998. Effect of Latanoprost on the Extracellular Matrix of the Ciliary Muscle. A Study on Cultured Cells and Tissue Sections . Exp. Eye Res 67 , 179–191. 10.1006/exer.1998.0508 [ PubMed ] [ CrossRef ] [ Google Scholar ]
- Pang IH, Millar JC, Clark AF, 2015. Elevation of intraocular pressure in rodents using viral vectors targeting the trabecular meshwork . Exp. Eye Res 141 , 33–41. 10.1016/j.exer.2015.04.003 [ PMC free article ] [ PubMed ] [ CrossRef ] [ Google Scholar ]
- Pederson JE, Gaasterland DE, MacLellan HM, 1977. Uveoscleral aqueous outflow in the rhesus monkey: importance of uveal reabsorption . Invest. Ophthalmol. Vis. Sci 16 , 1008–7. [ PubMed ] [ Google Scholar ]
- Perkins TW, Faha B, Ni M, Kiland JA, Poulsen GL, Antelman D, Atencio I, Shinoda J, Sinha D, Brumback L, Maneval D, Kaufman PL, Nickells RW, 2002. Adenovirus-mediated gene therapy using human p21WAF-1/Cip-1 to prevent wound healing in a rabbit model of glaucoma filtration surgery . Arch. Ophthalmol 120 , 941–949. 10.1001/archopht.120.7.941 [ PubMed ] [ CrossRef ] [ Google Scholar ]
- Peterson JA, Tian B, Bershadsky AD, Volberg T, Gangnon RE, Spector I, Geiger B, Kaufman PL, 1999. Latrunculin-A increases outflow facility in the monkey . Investig. Ophthalmol. Vis. Sci 40 , 931–941. [ PubMed ] [ Google Scholar ]
- Peterson JA, Tian B, Geiger B, Kaufman PL, 2000. Effect of Latrunculin-B on outflow facility in monkeys . Exp. Eye Res 70 , 307–313. 10.1006/exer.1999.0797 [ PubMed ] [ CrossRef ] [ Google Scholar ]
- Petty HR, Martin SM, 1989. Combinative ligand-receptor interactions: Effects of CAMP, epinephrine, and met-enkephalin on RAW264 macrophage morphology, spreading, adherence, and microfilaments . J. Cell. Physiol 138 , 247–256. 10.1002/jcp.1041380205 [ PubMed ] [ CrossRef ] [ Google Scholar ]
- Rahmatnejad K, Pruzan NL, Amanullah S, Shaukat BA, Resende AF, Waisbourd M, Zhan T, Moster MR, 2017. Surgical Outcomes of Gonioscopy-assisted Transluminal Trabeculotomy (GATT) in Patients with Open-angle Glaucoma . J. Glaucoma 26 , 1137–1143. 10.1097/IJG.0000000000000802 [ PubMed ] [ CrossRef ] [ Google Scholar ]
- Rasmussen CA, Kaufman PL, Ritch R, Haque R, Brazzell RK, Vittitow JL, 2014. Latrunculin B Reduces Intraocular Pressure in Human Ocular Hypertension and Primary Open-Angle Glaucoma . Transl. Vis. Sci. Technol 3 , 1. 10.1167/tvst.3.5.1 [ PMC free article ] [ PubMed ] [ CrossRef ] [ Google Scholar ]
- Robinson JC, Kaufman PL, 1994. Phalloidin Inhibits Epinephrine’s and Cytochalasin B’s Facilitation of Aqueous Outflow . Arch. Ophthalmol 112 , 1610–1613. 10.1001/archopht.1994.01090240116035 [ PubMed ] [ CrossRef ] [ Google Scholar ]
- Robinson JC, Kaufman PL, 1992. The effect of 12(R)-hydroxyeicosatetraenoic acid on aqueous humor dynamics in the rabbit and cynomolgus monkey . Exp. Eye Res 54 , 813–5. [ PubMed ] [ Google Scholar ]
- Robinson JC, Kaufman PL, 1991. Cytochalasin B potentiates epinephrine’s outflow facility-increasing effect . Investig. Ophthalmol. Vis. Sci 32 , 1614–1618. [ PubMed ] [ Google Scholar ]
- Rohen JW, Futa R, Lütjen-Drecoll E, 1981. The fine structure of the cribriform meshwork in normal and glaucomatous eyes as seen in tangential sections . Invest. Ophthalmol. Vis. Sci 21 , 574–85. [ PubMed ] [ Google Scholar ]
- Rohen JW, Lütjen E, Bárány E, 1967. The relation between the ciliary muscle and the trabecular meshwork and its importance for the effect of miotics on aqueous outflow resistance . Albr. von Graefes Arch. für Klin. und Exp. Ophthalmol 172 , 23–47. 10.1007/bf00577152 [ PubMed ] [ CrossRef ] [ Google Scholar ]
- Rohen JW, Rentsch FJ, 1968. [ Morphology of Schlemm’s canal and related vessels in the human eye ]. Albrecht Von Graefes Arch. Klin. Exp. Ophthalmol 176 , 309–29. 10.1007/bf00421587 [ PubMed ] [ CrossRef ] [ Google Scholar ]
- Roubeix C, Godefroy D, Mias C, Sapienza A, Riancho L, Degardin J, Fradot V, Ivkovic I, Picaud S, Sennlaub F, Denoyer A, Rostene W, Sahel JA, Parsadaniantz SM, Brignole-Baudouin F, Baudouin C, 2015. Intraocular pressure reduction and neuroprotection conferred by bone marrow-derived mesenchymal stem cells in an animal model of glaucoma . Stem Cell Res. Ther 6 , 177. 10.1186/s13287-015-0168-0 [ PMC free article ] [ PubMed ] [ CrossRef ] [ Google Scholar ]
- Sabanay I, Tian B, Gabelt BT, Geiger B, Kaufman PL, 2006. Latrunculin B effects on trabecular meshwork and corneal endothelial morphology in monkeys . Exp. Eye Res 82 , 236–246. 10.1016/j.exer.2005.06.017 [ PubMed ] [ CrossRef ] [ Google Scholar ]
- Sabanay I, Tian B, Gabelt BT, Geiger B, Kaufman PL, 2004. Functional and structural reversibility of H-7 effects on the conventional aqueous outflow pathway in monkeys . Exp. Eye Res 78 , 137–150. 10.1016/j.exer.2003.09.007 [ PubMed ] [ CrossRef ] [ Google Scholar ]
- Sagara T, Gaton DD, Lindsey JD, Gabelt BT, Kaufman PL, Weinreb RN, 1999a. Reduction of collagen type I in the ciliary muscle of inflamed monkey eyes . Investig. Ophthalmol. Vis. Sci 40 , 2568–2576. [ PubMed ] [ Google Scholar ]
- Sagara T, Gaton DD, Lindsey JD, Gabelt BT, Kaufman PL, Weinreb RN, 1999b. Topical prostaglandin F(2α) treatment reduces collagen types I, III, and IV in the monkey uveoscleral outflow pathway . Arch. Ophthalmol 117 , 794–801. 10.1001/archopht.117.6.794 [ PubMed ] [ CrossRef ] [ Google Scholar ]
- Sappington RM, Carlson BJ, Crish SD, Calkins DJ, 2010. The microbead occlusion model: A paradigm for induced ocular hypertension in rats and mice . Investig. Ophthalmol. Vis. Sci 51 , 207–216. 10.1167/iovs.09-3947 [ PMC free article ] [ PubMed ] [ CrossRef ] [ Google Scholar ]
- Serle JB, Katz LJ, McLaurin E, Heah T, Ramirez-Davis N, Usner DW, Novack GD, Kopczynski CC, 2018. Two Phase 3 Clinical Trials Comparing the Safety and Efficacy of Netarsudil to Timolol in Patients With Elevated Intraocular Pressure: Rho Kinase Elevated IOP Treatment Trial 1 and 2 (ROCKET-1 and ROCKET-2) . Am. J. Ophthalmol 186 , 116–127. 10.1016/j.ajo.2017.11.019 [ PubMed ] [ CrossRef ] [ Google Scholar ]
- Shah GB, Sharma S, Mehta AA, Goyal RK, 2000. Oculohypotensive effect of angiotensin-converting enzyme inhibitors in acute and chronic models of glaucoma . J. Cardiovasc. Pharmacol 36 , 169–175. 10.1097/00005344-200036001-00075 [ PubMed ] [ CrossRef ] [ Google Scholar ]
- Shearer TW, Crosson CE, 2002. Adenosine A1receptor modulation of MMP-2 secretion by trabecular meshwork cells . Investig. Ophthalmol. Vis. Sci 43 , 3016–3020. [ PubMed ] [ Google Scholar ]
- Slauson SR, Peters DM, Schwinn MK, Kaufman PL, Gabelt BT, Brandt CR, 2015. Viral vector effects on exoenzyme C3 transferase-mediated actin disruption and on outflow facility . Investig. Ophthalmol. Vis. Sci 56 , 2431–2438. 10.1167/iovs.14-15909 [ PMC free article ] [ PubMed ] [ CrossRef ] [ Google Scholar ]
- Snider EJ, Vannatta RT, Schildmeyer L, Stamer WD, Ethier CR, 2018. Characterizing differences between MSCs and TM cells: Toward autologous stem cell therapies for the glaucomatous trabecular meshwork . J. Tissue Eng. Regen. Med 12 , 695–704. 10.1002/term.2488 [ PubMed ] [ CrossRef ] [ Google Scholar ]
- Somlyo AP, Somlyo AV, 1994. Signal transduction and regulation in smooth muscle . Nature . 10.1038/372231a0 [ PubMed ] [ CrossRef ] [ Google Scholar ]
- Spector I, Shochet NR, Kashman Y, Groweiss A, 1983. Latrunculins: Novel marine toxins that disrupt microfilament organization in cultured cells . Science (80-. ) . 219 , 493–495. 10.1126/science.6681676 [ PubMed ] [ CrossRef ] [ Google Scholar ]
- Spector I, Shorlet NR, Blasberger D, Kashman Y, 1989. Latrunculins - novel marine macrolides that disrupt microfilament organization and affert cell growth: I. Comparison with cytochalasin D . Cell Motil. Cytoskeleton 13 , 127–144. 10.1002/cm.970130302 [ PubMed ] [ CrossRef ] [ Google Scholar ]
- Stamer WD, Braakman ST, Zhou EH, Ethier CR, Fredberg JJ, Overby DR, Johnson M, 2015. Biomechanics of Schlemm’s canal endothelium and intraocular pressure reduction . Prog. Retin. Eye Res 10.1016/j.preteyeres.2014.08.002 [ PMC free article ] [ PubMed ] [ CrossRef ] [ Google Scholar ]
- Stamer WD, Lei Y, Boussommier-Calleja A, Overby DR, Ross Ethier C, 2011. eNOS, a pressure-dependent regulator of intraocular pressure . Investig. Ophthalmol. Vis. Sci 52 , 9438–9444. 10.1167/iovs.11-7839 [ PMC free article ] [ PubMed ] [ CrossRef ] [ Google Scholar ]
- Stein JD, Shekhawat N, Talwar N, Balkrishnan R, 2015. Impact of the introduction of generic latanoprost on glaucoma medication adherence . Ophthalmology 122 , 738–747. 10.1016/j.ophtha.2014.11.022 [ PMC free article ] [ PubMed ] [ CrossRef ] [ Google Scholar ]
- Stein JD, Sloan FA, Lee PP, 2007. Rates of Glaucoma Medication Utilization Among Older Adults with Suspected Glaucoma, 1992 to 2002 . Am. J. Ophthalmol 143 . 10.1016/j.ajo.2006.12.025 [ PMC free article ] [ PubMed ] [ CrossRef ] [ Google Scholar ]
- Suzuki H, Kimura K, Shirai H, Eguchi K, Higuchi S, Hinoki A, Ishimaru K, Brailoiu E, Dhanasekaran DN, Stemmle LN, Fields TA, Frank GD, Autieri MV, Eguchi S, 2009. Edothelial nitric oxide synthase inhibits G 12/13 and Rho-Kinase activated by the angiotensin II type-1 receptor implication in vascular migration . Arterioscler. Thromb. Vasc. Biol 29 , 217–224. 10.1161/ATVBAHA.108.181024 [ PMC free article ] [ PubMed ] [ CrossRef ] [ Google Scholar ]
- Svedbergh B, Lutjen-Drecoll E, Ober M, Kaufman PL, 1978. Cytochalasin B-induced structural changes in the anterior ocular segment of the cynomolgus monkey . Investig. Ophthalmol. Vis. Sci 17 , 718–734. [ PubMed ] [ Google Scholar ]
- Takano E, Kaufman PL, Bárány EH, 1975. [ Impression of the 1975 General Meeting of the Japan Nursing Association. Disappointing attitude of the Assembly toward the nursing system ]. Hokenfu. Zasshi 31 , 339–41. [ PubMed ] [ Google Scholar ]
- Tamm ER, Braunger BM, Fuchshofer R, 2015. Intraocular Pressure and the Mechanisms Involved in Resistance of the Aqueous Humor Flow in the Trabecular Meshwork Outflow Pathways , in: Progress in Molecular Biology and Translational Science. pp. 301–314. 10.1016/bs.pmbts.2015.06.007 [ PubMed ] [ CrossRef ] [ Google Scholar ]
- Tian B, Brumback LC, Kaufman PL, 2000. ML-7, chelerythrine and phorbol ester increase outflow facility in the monkey eye . Exp. Eye Res 71 , 551–566. 10.1006/exer.2000.0919 [ PubMed ] [ CrossRef ] [ Google Scholar ]
- Tian B, Gabelt BT, Crosson CE, Kaufman PL, 1997. Effects of adenosine agonists on intraocular pressure and aqueous humor dynamics in cynomolgus monkeys . Exp. Eye Res 64 , 979–989. 10.1006/exer.1997.0296 [ PubMed ] [ CrossRef ] [ Google Scholar ]
- Tian B, Gabelt BT, Geiger B, Kaufman PL, 1999. Combined effects of H-7 and cytochalasin B on outflow facility in monkeys . Exp. Eye Res 68 , 649–655. 10.1006/exer.1998.0647 [ PubMed ] [ CrossRef ] [ Google Scholar ]
- Tian B, Kaufman PL, 2005. Effects of the Rho kinase inhibitor Y-27632 and the phosphatase inhibitor calyculin A on outflow facility in monkeys . Exp. Eye Res 80 , 215–225. 10.1016/j.exer.2004.09.002 [ PubMed ] [ CrossRef ] [ Google Scholar ]
- Tian B, Wang RF, Podos SM, Kaufman PL, 2004. Effects of topical H-7 on outflow facility, intraocular pressure, and corneal thickness in monkeys . Arch. Ophthalmol 122 , 1171–1177. 10.1001/archopht.122.8.1171 [ PubMed ] [ CrossRef ] [ Google Scholar ]
- Toris CB, Pederson JE, 1987. Aqueous humor dynamics in experimental iridocyclitis . Investig. Ophthalmol. Vis. Sci 28 , 477–481. [ PubMed ] [ Google Scholar ]
- Traube M, 2006. Physiology of the Gastrointestinal Tract, in: Johnson L. (Ed.), Journal of Clinical Gastroenterology . Raven Press, p. 607. 10.1097/00004836-198910000-00040 [ CrossRef ] [ Google Scholar ]
- Tripathi RC, Li J, Chan WFA, Tripathi BJ, 1994. Aqueous humor in glaucomatous eyes contains an increased level of TGF-β2 . Exp. Eye Res 59 , 723–728. 10.1006/exer.1994.1158 [ PubMed ] [ CrossRef ] [ Google Scholar ]
- Tsai JC, 2009. A Comprehensive Perspective on Patient Adherence to Topical Glaucoma Therapy . Ophthalmology 116 , S30–S36. 10.1016/j.ophtha.2009.06.024 [ PubMed ] [ CrossRef ] [ Google Scholar ]
- Vasantha Rao P, Deng PF, Kumar J, Epstein DL, 2001. Modulation of aqueous humor outflow facility by the Rho kinase-specific inhibitor Y-27632 . Investig. Ophthalmol. Vis. Sci 42 , 1029–1037. [ PubMed ] [ Google Scholar ]
- Vinod K, Gedde SJ, 2017. Clinical investigation of new glaucoma procedures . Curr. Opin. Ophthalmol 10.1097/ICU.0000000000000336 [ PubMed ] [ CrossRef ] [ Google Scholar ]
- Walters TR, Lee SS, Goodkin ML, Whitcup SM, Robinson MR, 2017. Bimatoprost Sustained-Release Implants for Glaucoma Therapy: 6-Month Results From a Phase I/II Clinical Trial . Am. J. Ophthalmol 175 , 137–147. 10.1016/j.ajo.2016.11.020 [ PubMed ] [ CrossRef ] [ Google Scholar ]
- Webber HC, Bermudez JY, Sethi A, Clark AF, Mao W, 2016. Crosstalk between TGFβ and Wnt signaling pathways in the human trabecular meshwork . Exp. Eye Res 148 , 97–102. 10.1016/j.exer.2016.04.007 [ PMC free article ] [ PubMed ] [ CrossRef ] [ Google Scholar ]
- Weinreb RN, Liebmann JM, Martin KR, Kaufman PL, Vittitow JL, 2018. Latanoprostene Bunod 0.024% in Subjects with Open-angle Glaucoma or Ocular Hypertension: Pooled Phase 3 Study Findings . J. Glaucoma 27 , 7–15. 10.1097/IJG.0000000000000831 [ PMC free article ] [ PubMed ] [ CrossRef ] [ Google Scholar ]
- Weinreb RN, Ong T, Sforzolini BS, Vittitow JL, Singh K, Kaufman PL, Ackerman S, Branch J, Cottingham A, Day D, Depenbusch M, El-Hazari S, Firozvi A, Jorizzo P, Ou R, Peace J, Rotberg M, Schenker H, Smith S, Tyson F, Zaman F, Madzharova L, Toshev R, Vassilveva P, Misiuk-Hojło M, Kocięcki J, Liehneova I, Růžičková E, Scassellati Sforzolini B, Vittitow JL, Singh K, Kaufman PL, 2015. A randomised, controlled comparison of latanoprostene bunod and latanoprost 0.005% in the treatment of ocular hypertension and open angle glaucoma: The VOYAGER study . Br. J. Ophthalmol 99 , 738–745. 10.1136/bjophthalmol-2014-305908 [ PMC free article ] [ PubMed ] [ CrossRef ] [ Google Scholar ]
- Yücel YH, Johnston MG, Ly T, Patel M, Drake B, Gümüş E, Fraenkl SA, Moore S, Tobbia D, Armstrong D, Horvath E, Gupta N, 2009. Identification of lymphatics in the ciliary body of the human eye: A novel “uveolymphatic” outflow pathway . Exp. Eye Res 89 , 810–819. 10.1016/j.exer.2009.08.010 [ PubMed ] [ CrossRef ] [ Google Scholar ]
- Yun H, Wang Y, Zhou Y, Wang K, Sun M, Stolz DB, Xia X, Ethier CR, Du Y, 2018. Human stem cells home to and repair laser-damaged trabecular meshwork in a mouse model . Commun. Biol 1 , 216. 10.1038/s42003-018-0227-z [ PMC free article ] [ PubMed ] [ CrossRef ] [ Google Scholar ]
- Yun H, Zhou Y, Wills A, Du Y, 2016. Stem Cells in the Trabecular Meshwork for Regulating Intraocular Pressure . J. Ocul. Pharmacol. Ther 32 , 253–260. 10.1089/jop.2016.0005 [ PMC free article ] [ PubMed ] [ CrossRef ] [ Google Scholar ]
- Zhu W, Gramlich OW, Laboissonniere L, Jain A, Sheffield VC, Trimarchi JM, Tucker BA, Kuehn MH, 2016. Transplantation of iPSC-derived TM cells rescues glaucoma phenotypes in vivo . Proc. Natl. Acad. Sci 113 , E3492–E3500. 10.1073/pnas.1604153113 [ PMC free article ] [ PubMed ] [ CrossRef ] [ Google Scholar ]
- Zhu W, Jain A, Gramlich OW, Tucker BA, Sheffield VC, Kuehn MH, 2017. Restoration of aqueous humor outflow following transplantation of iPSC-derived trabecular meshwork cells in a transgenic mouse model of glaucoma . Investig. Ophthalmol. Vis. Sci 58 , 2054–2062. 10.1167/iovs.16-20672 [ PMC free article ] [ PubMed ] [ CrossRef ] [ Google Scholar ]
- DAILY PRACTICE
- IMAIOS DICOM Viewer
- vet-Anatomy
- Breast imaging learning tool
- Blog News, opinions & thoughts of anatomy, medical imaging
Articles talking about IMAIOS and its products
What our users say about us
Our commitments
Get help with your subscription, account and more
- Contact us Other questions?

- Aqueous humor
Humor aquosus
The aqueous humor fills the anterior and posterior chambers of the eyeball. It is small in quantity, has an alkaline reaction, and consists mainly of water, less than one-fiftieth of its weight being solid matter, chiefly chloride of sodium.
Aqueous humor is secreted into the posterior chamber by the ciliary body, specifically the non-pigmented epithelium of the ciliary body (pars plicata). It flows through the narrow cleft between the front of the lens and the back of the iris, to escape through the pupil into the anterior chamber, and then to drain out of the eye via the trabecular meshwork. From here, it drains into Schlemm's canal by one of two ways: directly, via aqueous vein to the episcleral vein, or indirectly, through collector channels to the episcleral vein by intrascleral plexus and eventually into the veins of the orbit.
Comparative anatomy in animals
Translations
Cookie preferences
IMAIOS and selected third parties, use cookies or similar technologies, in particular for audience measurement. Cookies allow us to analyze and store information such as the characteristics of your device as well as certain personal data (e.g., IP addresses, navigation, usage or geolocation data, unique identifiers). This data is processed for the following purposes: analysis and improvement of the user experience and/or our content offering, products and services, audience measurement and analysis, interaction with social networks, display of personalized content, performance measurement and content appeal. For more information, see our privacy policy .
You can freely give, refuse or withdraw your consent at any time by accessing our cookie settings tool. If you do not consent to the use of these technologies, we will consider that you also object to any cookie storage based on legitimate interest. You can consent to the use of these technologies by clicking "accept all cookies".
Cookie settings
When you visit IMAIOS, cookies are stored on your browser.
Some of them require your consent. Click on a category of cookies to activate or deactivate it. To benefit from all the features, it’s recommended to keep the different cookie categories activated.
These are cookies that ensure the proper functioning of the website and allow its optimization (detect browsing problems, connect to your IMAIOS account, online payments, debugging and website security). The website cannot function properly without these cookies, which is why they are not subject to your consent.
These cookies are used to measure audience: it allows to generate usage statistics useful for the improvement of the website.
- Google Analytics
We use cookies to enhance our website for you. Proceed if you agree to this policy or learn more about it.
- Essay Database >
- Essays Examples >
- Essay Topics
Essays on Aqueous Humor
1 sample on this topic
To many students, writing Aqueous Humor papers comes easy; others require the help of various kinds. The WowEssays.com collection includes professionally crafted sample essays on Aqueous Humor and related issues. Most definitely, among all those Aqueous Humor essay examples, you will find a piece that conforms with what you see as a worthy paper. You can be sure that virtually every Aqueous Humor paper showcased here can be used as a glowing example to follow in terms of overall structure and composing different chapters of a paper – introduction, main body, or conclusion.
If, however, you have a hard time coming up with a solid Aqueous Humor essay or don't have even a minute of extra time to explore our sample directory, our free essay writer service can still be of great aid to you. The thing is, our writers can craft a model Aqueous Humor paper to your personal needs and particular requirements within the pre-set timespan. Buy college essays today!
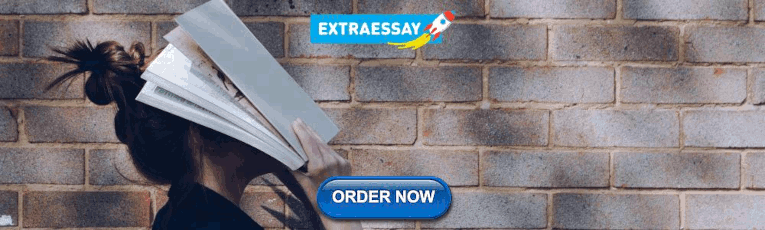
IMAGES
VIDEO
COMMENTS
Both are about 98% to 99% water. Vitreous humor also contains salts, sugars, proteins and collagen in addition to water. There are also phagocytes, or cells that help keep your eye clean. Along with water, aqueous humor also contains amino acids, electrolytes like sodium and potassium, ascorbic acid, glutathione and immunoglobulins.
The human eye is filled with two fluid-like substances, termed humors, which maintain the ocular pressure and shape of the eyeball. Aqueous humor is a water-like fluid that lies in front of the lens. Vitreous humor is a gel-like substance that lies behind the lens and in front of the retina. Aqueous humor is a low-viscosity fluid continuously being secreted and reabsorbed, with the balance ...
aqueous humour, optically clear, slightly alkaline liquid that occupies the anterior and posterior chambers of the eye (the space in front of the iris and lens and the ringlike space encircling the lens). The aqueous humour resembles blood plasma in composition but contains less protein and glucose and more lactic acid and ascorbic acid.It provides these nutrients (as well as oxygen) to eye ...
Aqueous humor also permits inflammatory cells and mediators to circulate in the eye in pathological conditions, as well as drugs to be distributed to different ocular structures . Aqueous humor provides a transparent and colorless medium between the cornea and the lens and constitutes an important component of the eye's optical system.
The aqueous humour is a transparent water-like fluid similar to blood plasma, but containing low protein concentrations.It is secreted from the ciliary body, a structure supporting the lens of the eyeball. It fills both the anterior and the posterior chambers of the eye, and is not to be confused with the vitreous humour, which is located in the space between the lens and the retina, also ...
Aqueous humour and Vitreous humour are substances that play a big role in maintaining the globe. They are gel like fluids inside the eye which help it maintain its shape, which plays an important role in overall eye health. AQUEOUS HUMOUR Aqueous humour is a watery fluid between the cornea and crystalline lens.
Aqueous humour is produced by non-pigmented epithelium cells of the ciliary body (ciliary processes). It is a transparent fluid located inside the eye, specifically within the anterior and posterior chambers. One of the factors that contribute to the change in the intraocular pressure (IOP) is the production and drainage of the aqueous humour. In this article, we will discuss aqueous humour ...
Abstract. Aqueous humor has numerous functions including optical transparency, structural integrity, and providing nutrition for the eye without the need for blood vessels that would be opaque. Ordinarily, no blood vessels are found in the cornea, lens, vitreous, or trabecular meshwork. Indeed, proportionately, the eye contains the largest ...
Aqueous humor is an optically clear, slightly alkaline ocular fluid that is continuously formed (~ 2.5 μL/min in humans) from plasma by epithelial cells of ciliary body [34].Three different processes - diffusion, ultrafiltration and active secretion - contribute to the chemical composition and formation of aqueous humor.
Aqueous humor is the clear liquid inside the front part of the eye. It nourishes the eye and keeps it inflated. The eye constantly produces a small amount of aqueous humor while an equal amount flows out through the trabecular meshwork in the drainage angle.. Imbalances in the creation and drainage of aqueous humor can lead to high intraocular pressure (eye pressure or IOP).
The aqueous humor is a small fraction of the transcellular fluid of the body in which, different from the rest, the highest value of hydrostatic tissue pressure is found: 10-15 mmHg; the same occurs with the cephalorachidian fluid. In Fig. 6.1, aqueous humor and cephalorachidian fluid occupy a tiny portion, and both are surrounded by arrows ...
Aqueous is an ultrafiltrate of plasma formed by the ciliary body; however, compared to plasma, aqueous is slightly hypertonic and acidic with a pH of 7.2 and contains much less protein and relatively less bicarbonate, calcium, and glucose. However, it contains much more ascorbate and relatively more hydrogen, chloride, and lactate.
The aqueous humor is a transparent, colorless solution continuously formed from plasma by the epithelial cells of the ciliary processes. It is secreted into the posterior chamber, passes from the posterior chamber through the pupil into the anterior chamber, and is drained at the anterior chamber angle. Most of the aqueous drains into the ...
Aqueous humor is produced by the ciliary body epithelium at a rate of 2-3 microliters per minute.1-3 It is composed of organic and inorganic ions, carbon dioxide, amino acids, carbohydrates, glutathione, and water.1,2,4 Important transport and enzymatic proteins involved in synthesis may be targeted in glaucoma drugs, discussed below.1,2,5.
Several hypotheses have been presented to explain the mechanism of the formation of the aqueous humor. It is the consensus that the aqueous humor is derived from the circulating fluid—the blood and lymph—of the body. There is still some doubt as to. References. 1.
Aqueous humor is the fluid produced by the eye. It provides nutrition to the eye, as well as maintains the eye in a pressurized state. Aqueous humor flows from the ciliary body into the anterior chamber, out through a spongy tissue at the front of the eye called the trabecular meshwork and into a drainage canal (dark blue region next to the trabecular meshwork).
Ciliary Blood Flow and its Role for Aqueous Humor Formation. J.W. Kiel, H.A. Reitsamer, in Encyclopedia of the Eye, 2010 Introduction. Aqueous humor is the clear liquid in the posterior and anterior chambers of the eye. It is produced by the ciliary processes, flows through the posterior chamber, then between the iris and lens through the pupil into the anterior chamber, and then leaves the ...
Results: The researchers found that high adaptive humor styles (affiliative humor and self-enhancing humor) were related to more positive impressions of another individual. A maladaptive style of humor (aggressive humor and self- defeating humor) correlated with more highly negative impressions of an individual.
The aqueous humor is a clear fluid located at the front part of the eye. Because the eye doesn't contain blood vessels, the aqueous humor is responsible for providing nutrients to the eye. The aqueous humor also drains out any excess material and waste from the eye. The vitreous fluid, or vitreous humor, is a colorless, transparent, gel-like ...
Aqueous humor outflow pathways. Aqueous humor, produced by the ciliary body, exits the eye through the conventional trabecular meshwork/ Schlemm's canal pathway (plain arrows), and the unconventional uveoscleral pathway (crossed arrows). Aqueous humor production and outflow are always equal, and the resistance in the trabecular meshwork ...
Definition: The aqueous humor is the watery fluid between the cornea and the crystalline lens. It maintains the pressure needed to inflate the eye and... Essays; Topics; ... More about Essay On Aqueous Humor. Cross Country Skiing Case Study 1584 Words | 7 Pages; Blurred Vision Nvq 108 Words | 1 Pages; A Son Of A Mob Humor Analysis
The aqueous humor fills the anterior and posterior chambers of the eyeball. It is small in quantity, has an alkaline reaction, and consists mainly of water, less than one-fiftieth of its weight being solid matter, chiefly chloride of sodium.Aqueous humor is secreted into the posterior chamber by the ciliary body, specifically the non-pigmented epithelium of the ciliary body (pars plicata).
Idiopathic uveitis (IU) and Vogt-Koyanagi-Harada (VKH) syndrome are common types of uveitis. However, the exact pathological mechanisms of IU and VKH remain unclear. Proteomic analysis of aqueous humor (AH), the most easily accessible intraocular fluid and a key site of uveitis development, may reveal potential biomarkers and elucidate uveitis pathogenesis. In this study, 44 AH samples ...
Having a sense of humor about yourself endears you to others. Satirical humor. Looking to the various faults of individuals, organizations, or society and mining them for comedic purposes. 2. Use the rule of three. The rule of three is a common rule in humor writing and one of the most common comedy writing secrets.
Get your free examples of research papers and essays on Aqueous Humor here. Only the A-papers by top-of-the-class students. Learn from the best! ... Blog Free Essay Writing Tools Quizzes and Tests Essay Topics Types of Essays Free Essay Examples Best Essay Writing Services. How It Works;