
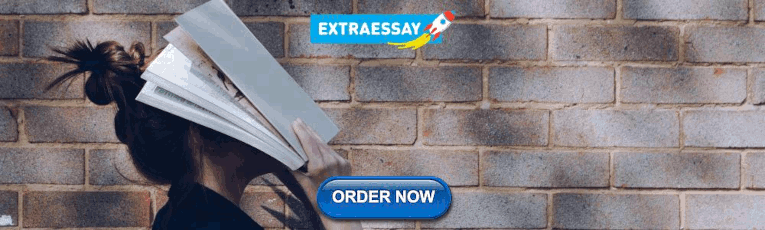
Essay On Disinfectants
Disinfectants Are chemical substance, used to kill pathogenic and other harmful microorganisms on inanimate objects but are too toxic to be applied directly on living tissues. disinfection has a lower effect than sterilization . sterilization is an extreme chemical and physical operation that destroy all kinds of life . Disinfectants have different properties than the other "antimicrobial" agents such as antiseptics which kill microorganisms only on living tissues . Disinfectants workes by destroying the cell wall of the microorganism or interfering with the metabolism . Disinfectants are used in dentalnsurgeries , hospitals to kill pathogenic organisms . Antisepsis Are chemical agents that may be applied topically to kill or stop the growth of the pathogenic microorganismss on living tissues such as the skin, epithelial …show more content…
The disks are then placed on an agar plate that has been cultivated with the targeted bacteria , the disinfectant diffuse out of the disks into the agar where the bacteria have been cultivated . As the of bacteria start to grows , zones of inhibition of microbial growth are showen as clear areas around the disks . larger zones typically correlate to increased inhibition effectiveness of the disinfectant agent. • Use-Dilution Test The dilution test is commonly used to detect the disinfection efficiency . first , a cylinder of stainless steel is put in a culture of a microorganism and then dried . The cylinder is then put in solutions of disinfectants that have various concentrations for a fixed time. Finally, the cylinder is transferred to a new test tube containing fresh sterile medium , and this test tube is incubated .if there is turbidity shown in the medium , Bacterial survival is presence , whereas killing of the target organism on the cylinder by the disinfectant will produce no
Unknown Culture Lab Report
After 5 days the plates were removed from the cold room and the gram-negative test for Colony A on the EMB agar showed pink fisheye colonies which lead to the conclusion that the gram-negative organism within Unknown #21 was Enterobacter aerogenes. Had the pigmentation been metallic green, the organism would have been identified as Escherichia coli, and had there been no pigmentation at all a Triple Sugar Iron agar (TSI) test among other tests would have been
Unknown Bacteria Lab Report
Differential media allows for the differentiation between two similar micro-organisms through how the bacteria may handle certain compounds found in the media or the different reactions that may take place when the bacteria is exposed to the medium (3). Selective media on the other hand allow only certain microbes to grow. This is due to the plate containing a limited amount of nutrients, compounds and chemicals that will deter the growth of certain bacteria (3). Dyes, antimicrobial substances, salts, certain growth inhibitors and, antibiotics are also found on this type of medium (3). The differential and selective media mentioned in this lab are as follows:
Lab Report Identifying Unknown Microorganisms
I expect to learn the biochemical differences in bacteria from this lab. Also, how to identify different species of bacteria. Material & Methods For the first day of the practical, an unknown specimen was provided
Explain Why Was America's Complacency Was Shattered In The Stock Market Crash
Many villages must use chemicals to purify their drinking water. 7. In medieval times an infected person was placed in isolation. 8. Dentists have special equipment to sterilize their instruments.
Unknown Lab Report
The tube was placed back in incubation for 96 more hours to observe any more positives. 2.10 Catalase Test A trypticase soy agar plate was used and after incubation, four drops of 3% Hydrogen Peroxide was added to the plate to flow over the bacterial growth. A presence of bubbling was observed. 2.11 Starch Hydrolysis
Antisepsis Dbq
Antisepsis wasn’t the only way to prevent illness. By the end of the 18th century people had found a way to try to prevent smallpox, a disease that had caused around 60 million deaths in Europe in that century alone. They had noticed that the survivors of smallpox never developed the disease again, so they began to scratch small pieces of smallpox sores into their skin, which would give them a mild case of smallpox, so they wouldn’t develop full-blown smallpox later. This was called variolation. They only problem was that sometimes it would cause a full-blown case instead of a mild one.
Pglo Transformation Lab Report
After the directed time, those solutions are immediately moved to a hot water bath for 50 seconds. Following this, the solutions are immediately moved back to the ice again. Heat shock “increases the permeability of the cell membrane to DNA” (Lab manual). The results for this experiment are significant because we want to see what plates will have growth. The bacteria with the gene GFP causes bacteria to have a green glow under UV light.
Unknown Microbiology Essay
Being able to identify unknown microbes from systematic testing is what makes the field of microbiology so important, especially in infectious disease control. Using the testing procedure laid out by the microbiology field we are able to identify unknown bacteria present in our everyday lives, and along the way learn a lot about their characteristics that separate them from other types of bacteria. Being able to do this is vital in order for us to understand why microbes are present in certain places, how they are able to grow and what restricts their growth, that way they can be combatted if necessary. These techniques for determining unknowns are also important for isolating and testing infectious disease microbes in order to prevent spreading. Another important aspect of being able to identify unknown microbes is the
Hand Sanitizer Case Study
This episode was about a man named Joshua who was very successful and pretty much had life figured out. He had a six-figure salary managing 14 different convenience stores. He also had a beautiful home, wife, and kids by the age of 21. But Joshua was overweight and at age 27 he decided to have gastric bypass surgery to help him with his weight loss. After the surgery, he wasn 't losing weight as quick as he wanted and he had back issues which prevented him from exercising.
Beta Vulgaris Lab Report
To prepare the solutions a 70% ethanol solution was used to make 40%. This was calculated using the C1V1=C2V2 formula. A photo spectrometer was used to measure, in arbitrary units, the change in membrane permeability of the B. Vulgaris cells. To begin, the B. Vulgaris samples were put into vials containing the distilled water, 40% and 70% Ethanol solutions. As soon as the B. Vulgaris samples were added to the vials a time zero sample was taken from the vials.
Essay On Yeast Infection
Yeast infections most often occur in the moist areas of the body particularly on the mouth and genital area in both men and women. These are caused by many factors including poor diet, hygiene and stress management while the symptoms include red, painful and itchy sores, lesions and rashes. The good news is that natural remedies for yeast infection are often the only methods that anybody with a yeast infection will need to treat the condition. And even when over-the-counter medications are necessary, the following natural remedies are still a must because of their preventive value. Yeast infections are less likely to occur with healthy lifestyle changes since the causes are directly addressed instead of just providing temporary relief for the symptoms.
Dog Saliva Hypothesis
Introduction It has long been said, even in biblical references (Luke 16:19-31) that dogs have somewhat of ‘special powers’ with regards to their healing abilities. (Patching, 2008) In some areas of the world dog saliva would be used as an antibacterial because it contains some similar properties to that of disinfectants, which would theoretically be able to kill harmful bacteria in wounds and aid in the process of the healing. If a dog has an open wound, the dog is likely to lick the wound in order to ensure that their saliva has direct contact with the open sore to prevent growth of bacteria that could lead to infection.
Picot Question
Hand washing or isolation of the sick persons with infections in the prevention of hospital acquired infections. 5. Does the use of hand washing, and antisepsis lower the rate of hospital acquired infections? The fifth PICOT question is selected because of the reported low compliance percentage among medical caregivers.
Kinzua Dam Case Study
An act of attacking with a Chemicl agent is typically from an aerosal type or disseminating the agent into other avenues of contact. However, for this particular scenario, a Chemical-Biological Agent will be used in the rare form of being part of an explosive. The particular chemical agent in reference will be petroleum-contaminated water.
Kirby Bauer Diffusion Test Lab Report
Aseptic technique was initiated at the beginning of this experiment by cleaning the work surface with disinfected wipes. Personal protectives equipment was also worn. The material utilized in this experiment was: S. epidermidis culture broth, sterile cotton swab, streak plate, forceps in 70% alcohol, a lit tea light, and the three antibiotic disks (novobiocin, gentamicin, penicillin). The first step, I divided a plate into three quadrants and labelled them with the different antibiotic names. Using the lit tea light, like a bursen burner, I flamed the mouth of the S. epidermidis culture.
More about Essay On Disinfectants
Related topics.
- Carbon dioxide
- Environmentalism
Sterilization and Disinfection Techniques for Microbial Control
The application of heat is used for sterilization the duration of time and appropriate temperature should be considered to guarantee destruction of all microorganisms. Wherein, disinfection is used to apply chemicals to objects like chlorination of water so that pathogens will be killed. The implementation of aseptic techniques using air filtration in susceptible areas, use of ultraviolet light in an area where microorganisms usually thrive like operating rooms.
The ingredients like quaternary ammonium salts, this is a component of Lysol which is used as a toilet bowl cleaner and disinfectant spray, these chemical can destroy cell membranes and can also disrupt the membrane of some viruses. The compound named phenol which can be derived from ordinary plant which is also a common ingredient of disinfectants that can disrupt cell membrane to control enzyme function. Disinfectants are typically applied on non living objects in order to destroy microorganisms and this process are called disinfection.
Order custom essay Sterilization and Disinfection Techniques for Microbial Control with free plagiarism report
The difference between disinfectants and antibiotics should be distinguished when disinfectants are improperly used it can harm even the person using it that is why household disinfectants should be properly used and kept away from children. Bitrex is a component of some disinfectants which is a special bitter substance that can discourage intake of food for human or animals and it should be used with extra care. In addition, the use of other cleaning products mixed with the indoor disinfectant should be avoided hence chemical reaction can occur that may cause more harm.
Conclusion The odor of the disinfectant like Lysol even if it has fragrance does not mean that it is safe to smell it is quite irritating to the nose due of its chemical component and I have learned that there should always be safety rules to follow before applying disinfectant in the house, its effect is tolerable for man the continuous use of it may soon give the human body a drastic affect that could damage cells that would lead to metabolic abnormality and impair the physiologic activity of the body that ends up to occurrence of diseases once the immune system shuts down.
The contact of various infectants to our skin especially to the food we ingest should be avoided again this is due to the fact that this chemical solutions serve its purpose but once the body inhaled or ingest too much of it and in instances that it already accumulated in the body but is not excreted by our organs thoroughly it will stay inside our body. Its damage will be observed once it develops antagonistic effect to our immune system and to the normal flora of microorganism inside our body.
In short, disinfectants should only be used frequently in places where too much types of microorganism exist like hospitals. In our household, proper hygiene like frequent washing of hands before and after eating, on handling of foods, especially after coming from the rest room because the stool is the carrier of almost all microorganisms that can infect man. The proper use of disinfectants should be observed carefully by reading the label before use because even if they control the growth of microorganisms it does not mean that it will not give harm to us humans.
Cite this Page
Sterilization and Disinfection Techniques for Microbial Control. (2016, Aug 22). Retrieved from https://phdessay.com/disinfectants/
Run a free check or have your essay done for you
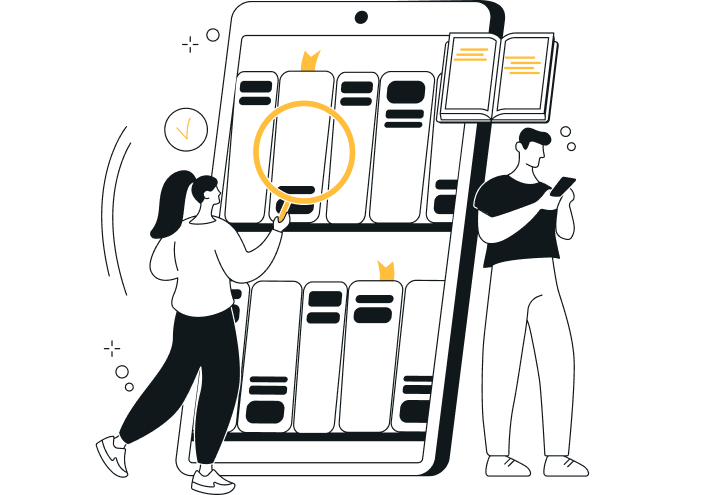
More related essays
Food is vital for humans to survive, the population of the world is immense as it approaches 6 billion and all these humans need to be fed on a continual.
Occupational Therapy provides a unique contribution to the cardiac rehabilitation setting. It plays a distinct role in the interdisciplinary practice as well as in client-centered care whilst addressing cardiac disease.
This assignment considers physical activity in four different special population groups. Each population group is identified as being 'special' because they have specific physiological and psychological needs that require physical.
The aim of Boo.com was to develop into the popular online international sports retail company. It was supposed to be a European trademark, but with a worldwide demand. At the.
This literature position is to supply a basic cognition about tools and techniques application for undertaking information direction in current building patterns. This paper will show a better understanding about.
A esteemed axiom from Winston Churchill goes that people shape edifices, and so edifices transform people. The affects from edifices, or more specifically, from the built environment to people is.
Net Present value The present value of an investments future net cash flows minus the initial investment. If positive, the investment should be considered (unless an even better investment exists),.
MICHELLE LEE IAT 343 – D104 #301097226 ANIMATION ESSAY The two three-dimensional animated films that are chosen are Pocoyo animation shorts and a music video by Genki Rockets. I am.
We use cookies to give you the best experience possible. By continuing we’ll assume you’re on board with our cookie policy
Save time and let our verified experts help you.
Thank you for visiting nature.com. You are using a browser version with limited support for CSS. To obtain the best experience, we recommend you use a more up to date browser (or turn off compatibility mode in Internet Explorer). In the meantime, to ensure continued support, we are displaying the site without styles and JavaScript.
- View all journals
- Explore content
- About the journal
- Publish with us
- Sign up for alerts
- Review Article
- Published: 30 August 2023
Disinfectants and antiseptics: mechanisms of action and resistance
- Jean-Yves Maillard ORCID: orcid.org/0000-0002-8617-9288 1 &
- Michael Pascoe ORCID: orcid.org/0000-0002-7794-8970 1
Nature Reviews Microbiology volume 22 , pages 4–17 ( 2024 ) Cite this article
5848 Accesses
7 Citations
19 Altmetric
Metrics details
- Antimicrobial resistance
- Clinical microbiology
- Infectious diseases
Chemical biocides are used for the prevention and control of infection in health care, targeted home hygiene or controlling microbial contamination for various industrial processes including but not limited to food, water and petroleum. However, their use has substantially increased since the implementation of programmes to control outbreaks of methicillin-resistant Staphylococcus aureus , Clostridioides difficile and severe acute respiratory syndrome coronavirus 2. Biocides interact with multiple targets on the bacterial cells. The number of targets affected and the severity of damage will result in an irreversible bactericidal effect or a reversible bacteriostatic one. Most biocides primarily target the cytoplasmic membrane and enzymes, although the specific bactericidal mechanisms vary among different biocide chemistries. Inappropriate usage or low concentrations of a biocide may act as a stressor while not killing bacterial pathogens, potentially leading to antimicrobial resistance. Biocides can also promote the transfer of antimicrobial resistance genes. In this Review, we explore our current understanding of the mechanisms of action of biocides, the bacterial resistance mechanisms encompassing both intrinsic and acquired resistance and the influence of bacterial biofilms on resistance. We also consider the impact of bacteria that survive biocide exposure in environmental and clinical contexts.
This is a preview of subscription content, access via your institution
Access options
Access Nature and 54 other Nature Portfolio journals
Get Nature+, our best-value online-access subscription
$29.99 / 30 days
cancel any time
Subscribe to this journal
Receive 12 print issues and online access
$209.00 per year
only $17.42 per issue
Rent or buy this article
Prices vary by article type
Prices may be subject to local taxes which are calculated during checkout
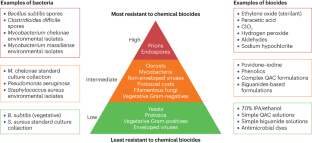
Similar content being viewed by others
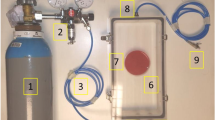
Exploration of oxygen-mediated disinfection of medical devices reveals a high sensitivity of Pseudomonas aeruginosa to elevated oxygen levels
Francis M. Cavallo, Richard Kommers, … Jan Maarten van Dijl
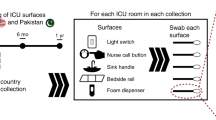
Spatiotemporal dynamics of multidrug resistant bacteria on intensive care unit surfaces
Alaric W. D’Souza, Robert F. Potter, … Gautam Dantas
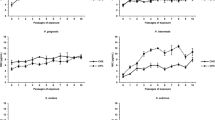
Development of antiseptic adaptation and cross-adapatation in selected oral pathogens in vitro
Tim Verspecht, Esteban Rodriguez Herrero, … Wim Teughels
Fraise, A. in Principles and Practice of Disinfection, Preservation and Sterilization 5th edn (eds Fraise, A. P., Maillard, J.-Y. & Sattar, S.) 1–4 (Wiley-Blackwell, 2013).
Pasteur, L. On the extension of the germ theory to the etiology of certain common diseases [French]. Comptes Rendus de l’Académie des Sciences XC, 1033–1044 (1880).
Walker, L., Levine, H. & Jucker, M. Koch’s postulates and infectious proteins. Acta Neuropathol. 112 , 1–4 (2006).
Article CAS PubMed PubMed Central Google Scholar
Carter, K. C. Ignaz Semmelweis, Carl Mayrhofer, and the rise of germ theory. Med. Hist. 29 , 33–53 (1985).
Maillard, J.-Y. et al. Does microbicide use in consumer products promote antimicrobial resistance? A critical review and recommendations for a cohesive approach to risk assessment. Microb. Drug. Res. 19 , 344–354 (2013). This opinion paper highlights the issues associated with a lack of definition of ‘biocide resistance’ and with a lack of consensus for measuring bacterial resistance to biocides.
Article Google Scholar
European Commission. Scientific Committee on Emerging and Newly Identified Health Risks (SCENIHR). Assessment of the Antibiotic Resistance Effects of Biocides. European Commission http://ec.europa.eu/health/ph_risk/committees/04_scenihr/docs/scenihr_o_021.pdf (2009).
Mueller, S. & Beraud, S. S. L. The Biocides Market in the Times of Coronavirus. S&P Global Commodity Insights https://www.spglobal.com/commodityinsights/en/ci/research-analysis/the-biocides-market-in-the-times-of-coronavirus.html (2020).
Maillard, J.-Y. Resistance of bacteria to biocides. Microbiol. Spectrum 6 , ARBA-0006-2017 (2018).
Ko, S., An, H. S., Bang, J. H. & Park, S. W. An outbreak of Burkholderia cepacia complex pseudobacteremia associated with intrinsically contaminated commercial 0.5% chlorhexidine solution. Am. J. Infect. Control 43 , 266–268 (2015).
Article CAS PubMed Google Scholar
Nakashima, A. K., McCarthy, M. A., Martone, W. J. & Anderson, R. L. Epidemic septic arthritis caused by Serratia marcescens and associated with benzalkonium chloride antiseptic. J. Clin. Microbiol. 25 , 1014–1018 (1987).
Hsueh, P.-R. et al. Nosocomial pseudoepidemic caused by Bacillus cereus traced to contaminated ethyl alcohol from a liquor factory. J. Clin. Microbiol. 37 , 2280–2284 (1999).
Poole, K. Mechanisms of bacterial biocide and antibiotic resistance. J. Appl. Microbiol. 92 , 55S–64S (2002).
Article PubMed Google Scholar
Griffiths, P. A., Babb, J. R., Bradley, C. R. & Fraise, A. P. Glutaraldehyde resistant Mycobacterium chelonae from endoscope washer disinfectors. J. Appl. Microbiol. 82 , 519–526 (1997).
Martin, D. J. H., Denyer, S. P., McDonnell, G. & Maillard, J.-Y. Resistance and cross-resistance to oxidising agents of bacterial isolates from endoscope washer disinfectors. J. Hosp. Infect. 69 , 377–383 (2008). This paper presents evidence of vegetative bacteria isolated from an endoscope washer disinfector (using chlorine dioxide high-level disinfection), resistant to in-use concentration of chlorine dioxide and other reactive biocides.
Martin, D. J. H., Wesgate, R., Denyer, S. P., McDonnell, G. & Maillard, J.-Y. Bacillus subtilis vegetative isolate surviving chlorine dioxide exposure: an elusive mechanism of resistance. J. Appl. Microbiol. 119 , 1541–1551 (2015).
Russell, A. D. Biocides — mechanisms of action and microbial resistance. World J. Microbiol. Biotechnol. 8 , 58–59 (1992).
McDonnell, G. & Russell, A. D. Antiseptics and disinfectants: activity, action, and resistance. Clin. Microbiol. Rev. 12 , 147–179 (1999). This review highlights the limitation of biocide efficacy depending on their chemistry and the propensity for microbial resistance resulting from exposure to a low concentration of a biocide.
Russell, A. D. Biocide use and antibiotic resistance: the relevance of laboratory findings to clinical and environmental situations. Lancet Infect. Dis. 3 , 794–803 (2003).
Maillard, J.-Y. Impact of benzalkonium chloride, benzethonium chloride and chloroxylenol on bacterial resistance and cross-resistance to antimicrobials. J. Appl. Microbiol. 133 , 3322–3346 (2022).
Wand, M. E. & Sutton, J. M. Efflux-mediated tolerance to cationic biocides, a cause for concern? Microbiology 168 , 1263 (2022).
Article CAS Google Scholar
Vijayakumar, R. & Sandle, T. A review on biocide reduced susceptibility due to plasmid-borne antiseptic-resistant genes — special notes ion pharmaceutical environmental isolates. J. Appl. Microbiol. 126 , 1011–1022 (2019).
Jones, I. A. & Joshi, L. Biocide use in the antimicrobial era: a review. Molecules 26 , 2276 (2021).
Al-Adham, I., Haddadin, R. & Collier, P. in Principles and Practice of Disinfection, Preservation and Sterilization 5th edn (eds Fraise, A. P., Maillard, J.-Y. & Sattar, S.) 5–70 (Wiley-Blackwell, 2013).
Singer, A. C., Shaw, H., Rhodes, V. & Hart, A. Review of antimicrobial resistance in the environment and its relevance to environmental regulators. Front. Microbiol. 7 , 1728 (2016).
Article PubMed PubMed Central Google Scholar
Leggett, M. J., Setlow, P., Sattar, S. A. & Maillard, J.-Y. Assessing the activity of microbicides against bacterial spores: knowledge and pitfalls. J. Appl. Microbiol. 120 , 1174–1180 (2016).
Forbes, S. et al. Formulation of biocides increases antimicrobial potency and mitigates the enrichment of nonsusceptible bacteria in multispecies. Appl. Environ. Microbiol. 83 , e3054-16 (2017).
Maillard, J.-Y. Bacterial target sites for biocide action. J. Appl. Microbiol. 92 , 16S–27S (2002).
Sani, M.-A. et al. Maculatin 1.1 disrupts Staphylococcus aureus lipid membranes via a pore mechanism. Antimicrob. Agents Chemother. 57 , 3593–3600 (2013).
Johnston, M. D., Hanlon, G. W., Denyer, S. P. & Lambert, R. J. W. Membrane damage to bacteria caused by single and combined biocides. J. Appl. Microbiol. 94 , 1015–1023 (2003).
Barros, A. C., Melo, L. F. & Pereira, A. A multi-purpose approach to the mechanisms of action of two biocides (benzalkonium chloride and dibromonitrilopropionamide): discussion of Pseudomonas fluorescens ’ viability and death. Front. Microbiol. 13 , 842414 (2022).
Linley, E., Denyer, S. P., McDonnell, G., Simons, C. & Maillard, J.-Y. Use of hydrogen peroxide as a biocide: new consideration of its mechanisms of biocidal action. J. Antimicrob. Chemother. 67 , 1589–1596 (2012).
Setlow, B., Atluri, S., Kitchel, R., Koziol-Dube, K. & Setlow, P. Role of dipicolinic acid in resistance and stability of spores of Bacillus subtilis with or without DNA-protective α/β-type small acid-soluble proteins. J. Bacteriol. 188 , 3740–3747 (2006).
Leggett, M. J. et al. Resistance to and killing by the sporicidal microbicide peracetic acid. J. Antimicrob. Chemother. 70 , 773–779 (2015).
Alkhalifa, S. et al. Analysis of the destabilization of bacterial membranes by quaternary ammonium compounds: a combined experimental and computational study. ChemBioChem 21 , 1510–1516 (2020).
Bore, E. et al. Adapted tolerance to benzalkonium chloride in Escherichia coli K-12 studied by transcriptome and proteome analyses. Microbiology 153 , 935–946 (2007).
Roth, M. et al. Transcriptomic analysis of E. coli after exposure to a sublethal concentration of hydrogen peroxide revealed a coordinated up-regulation of the cysteine biosynthesis pathway. Antioxidants 11 , 655 (2022).
Denyer, S. P. & Maillard, J.-Y. Cellular impermeability and uptake of biocides and antibiotics in Gram-negative bacteria. J. Appl. Microbiol. 92 , 35S–45S (2002).
Denyer, S. P. Mechanisms of action of biocides. Int. Biodeter. 26 , 89–100 (1990).
McMurry, L. M., Oethinger, M. & Levy, S. B. Triclosan targets lipid synthesis. Nature 394 , 531–532 (1998).
Simões, L. C. et al. Persister cells in a biofilm treated with a biocide. Biofouling 27 , 403–411 (2011).
Fernandes, S., Gomes, I. B., Sousa, S. F. & Simões, M. Antimicrobial susceptibility of persister biofilm cells of Bacillus cereus and Pseudomonas fluorescens . Microorganisms 10 , 160 (2022).
Maillard, J.-Y. Usage of antimicrobial biocides and products in the healthcare environment: efficacy, policies, management and perceived problems. Ther. Clin. Risk Manag. 1 , 340–370 (2005).
Google Scholar
Russell, A. D. & McDonnell, G. Concentration: a major factor in studying biocidal action. J. Hosp. Infect. 44 , 1–3 (2000).
Lambert, P. A. Cellular impermeability and uptake of biocides and antibiotics in Gram-positive bacteria and mycobacteria. J. Appl. Microbiol. 92 , 46S–54S (2002).
Lambert, R. J. W., Hanlon, G. W. & Denyer, S. P. The synergistic effect of EDTA/antimicrobial combinations on Pseudomonas aeruginosa . J. Appl. Microbiol. 96 , 244–253 (2004).
Leggett, M. J., McDonnell, G., Denyer, S. P., Setlow, P. & Maillard, J.-Y. Bacterial spore structures and their protective role in biocide resistance. J. Appl. Microbiol. 113 , 485–498 (2012).
Maillard, J.-Y. Innate resistance to sporicides and potential failure to decontaminate. J. Hosp. Infect. 77 , 204–209 (2011).
Vickery, K. et al. Presence of biofilm containing viable multiresistant organisms despite terminal cleaning on clinical surfaces in an intensive care unit. J. Hosp. Infect. 80 , 52–55 (2012).
Hu, H. et al. Intensive care unit environmental surfaces are contaminated by multidrug-resistant bacteria in biofilms: combined results of conventional culture, pyrosequencing, scanning electron microscopy, and confocal laser microscopy. J. Hosp. Infect. 91 , 35–44 (2015).
Ledwoch, K. et al. Beware biofilm! Dry biofilms containing bacterial pathogens on multiple healthcare surfaces; a multi-centre study. J. Hosp. Infect. 100 , E47–E56 (2018).
Ledwoch, K. et al. Is a reduction in viability enough to determine biofilm susceptibility to a biocide? Infect. Control. Hosp. Epidemiol. 42 , 1486–1492 (2021).
Bridier, A., Dubois-Brissonnet, F., Greub, G., Thomas, V. & Briandet, R. Dynamics of the action of biocides in Pseudomonas aeruginosa biofilms. Antimicrob. Agents Chemother. 55 , 2648–2654 (2011).
Stewart, P. S. Antimicrobial tolerance in biofilms. Microbiol. Spectr. https://doi.org/10.1128/microbiolspec.MB-0010-2014 (2015).
Bas, S., Kramer, M. & Stopar, D. Biofilm surface density determines biocide effectiveness. Front. Microbiol. 8 , 2443 (2017).
Araújo, P. A., Mergulhão, F., Melo, L. & Simões, M. The ability of an antimicrobial agent to penetrate a biofilm is not correlated with its killing or removal efficiency. Biofouling 30 , 673–683 (2014).
Wood, T. K., Knabel, S. J. & Kwana, B. W. Bacterial persister cell formation and dormancy. Appl. Environ. Microbiol. 79 , 7116–7121 (2013).
Podlesek, Z. & Bertok, D. Z. The DNA damage inducible SOS response is a key player in the generation of bacterial persister cells and population wide tolerance. Front. Microbiol. 4 , 1785 (2020).
Ciusa, M. L. et al. A novel resistance mechanism to triclosan that suggests horizontal gene transfer and demonstrates a potential selective pressure for reduced biocide susceptibility in clinical strains of Staphylococcus aureus . Int. J. Antimicrob. Agents 40 , 210–220 (2012).
Jia, Y., Lu, H. & Zhua, L. Molecular mechanism of antibiotic resistance induced by mono- and twin-chained quaternary ammonium compounds. Sci. Total Environ. 832 , 155090 (2022).
Schindler, B. D. & Kaatz, G. W. Multidrug efflux pumps of Gram-positive bacteria. Drug Res. Updates 27 , 1–13 (2016).
Poole, K. Outer membranes and efflux: the path to multidrug resistance in Gram-negative bacteria. Curr. Pharm. Biotechnol. 3 , 77–98 (2002).
Chitsaz, M. & Brown, M. H. The role played by drug efflux pumps in bacterial multidrug resistance. Essays Biochem. 61 , 127–139 (2017).
Rajamohan, G., Srinivasan, V. B. & Gebreyes, W. A. Novel role of Acinetobacter baumannii RND efflux transporters in mediating decreased susceptibility to biocides. J. Antimicrob. Chemother. 65 , 228–232 (2010).
LaBreck, P. T. et al. Systematic analysis of efflux pump-mediated antiseptic resistance in Staphylococcus aureus suggests a need for greater antiseptic stewardship. mSphere 5 , e00959-19 (2020).
Wand, M. E., Darby, E. M., Blair, J. M. A. & Sutton, J. M. Contribution of the efflux pump AcrAB-TolC to the tolerance of chlorhexidine and other biocides in Klebsiella spp. J. Med. Microbiol. 71 , 001496 (2022).
Fernández-Cuenca, F. et al. Reduced susceptibility to biocides in Acinetobacter baumannii : association with resistance to antimicrobials, epidemiological behaviour, biological cost and effect on the expression of genes encoding porins and efflux pumps. J. Antimicrob. Chemother. 70 , 3222–3229 (2015).
Kim, M. et al. Widely used benzalkonium chloride disinfectants can promote antibiotic resistance. Appl. Environ. Microbiol. 84 , 1201–1218 (2018).
Nordholt, N., Kanaris, O., Schmidt, S. B. I. & Schreiber, F. Persistence against benzalkonium chloride promotes rapid evolution of tolerance during periodic disinfection. Nat. Commun. 12 , 6792 (2021).
Amsalu, A. et al. Efflux pump-driven antibiotic and biocide cross-resistance in Pseudomonas aeruginosa isolated from different ecological niches: a case study in the development of multidrug resistance in environmental hotspots. Microorganisms 8 , 1647 (2020).
Sánchez, M. B. et al. Predictive studies suggest that the risk for the selection of antibiotic resistance by biocides is likely low in St enotrophomonas maltophilia . PLoS ONE 10 , e0132816 (2015).
Bay, D. C. & Turner, R. J. Diversity and evolution of the small multidrug resistance protein family. BMC Evol. Biol. 9 , 140 (2009).
Hansen, L. S., Jensen, L. B., Sørensen, H. I. & Sørensen, S. J. Substrate specificity of the OqxAB multidrug resistance pump in Escherichia coli and selected enteric bacteria. J. Antimicrob. Chemother. 60 , 145–147 (2007).
Kaatz, G. W. & Seo, S. M. Effect of substrate exposure and other growth condition manipulations on norA expression. J. Antimicrob. Chemother. 54 , 364–369 (2004).
Mima, T., Joshi, S., Gomez-Escalada, M. & Schweizer, H. P. Identification and characterization of TriABC-OpmH, a triclosan efflux pump of Pseudomonas aeruginosa requiring two membrane fusion proteins. J. Bacteriol. 189 , 7600–7609 (2007).
Buffet-Bataillon, S., Tattevin, P., Maillard, J.-Y., Bonnaure-Mallet, M. & Jolivet-Gougeon, A. Efflux pump induction by quaternary ammonium compounds and fluoroquinolone resistance in bacteria. Future Microbiol. 11 , 81–92 (2016).
Reza, A., Sutton, J. M. & Rahman, K. M. Effectiveness of efflux pump inhibitors as biofilm disruptors and resistance breakers in Gram-negative (ESKAPEE) bacteria. Antibiotics 8 , 229 (2019).
Kvist, M., Hancok, V. & Klemm, O. P. Inactivation if efflux pumps abolishes bacterial biofilm formation. Appl. Environ. Microbiol. 74 , 7376–7382 (2008).
Soto, S. M. Role of efflux pumps in the antibiotic resistance of bacteria embedded in a biofilm. Virulence 4 , 223–229 (2013).
Chevalier, S. et al. Structure function and regulation of Pseudomonas aeruginosa porins. FEMS Microbiol. Rev. 41 , 698–772 (2017).
Svetlíková, Z. et al. Role of porins in the susceptibility of Mycobacterium smegmatis and Mycobacterium chelonae to aldehyde-based disinfectants and drugs. Antimicrob. Agents Chemother. 53 , 4015–4018 (2009).
Stahl, C. et al. MspA provides the main hydrophilic pathway through the cell wall of Mycobacterium smegmatis . Mol. Microbiol. 40 , 451–464 (2001).
Pereira, B. M. P., Wang, X. K. & Tagkopoulos, I. Biocide-induced emergence of antibiotic resistance in Escherichia coli . Front. Microbiol. 12 , 640923 (2021).
Silver, S. Bacterial silver resistance: molecular biology and uses and misuse of silver compounds. FEMS Microbiol. Rev. 27 , 341–353 (2003).
Casado Muñoz, M. C. et al. Comparative proteomic analysis of a potentially probiotic Lactobacillus pentosus MP-10 for the identification of key proteins involved in antibiotic resistance and biocide tolerance. Int. J. Food Microbiol. 222 , 8–15 (2016).
Allen, M. J., White, G. F. & Morby, A. P. The response of Escherichia coli to exposure to the biocide polyhexamethylene biguanide. Microbiology 152 , 989–1000 (2006).
Motgatla, R. M., Gouws, P. A. & Brözel, V. S. Mechanisms contributing to hypochlorous acid resistance of a Salmonella isolate from a poultry-processing plant. J. Appl. Microbiol. 92 , 566–573 (2002).
Wu, C. H. A review of microbial injury and recovery methods in food. Food Microbiol. 25 , 735–744 (2008).
Yildiz, F. H. & Schoolnik, G. K. Vibrio cholerae O1 E1 Tor: identification of a gene cluster required for the rugose colony type, exopolysaccharide production, chlorine resistance and biofilm formation. Proc. Natl Acad. Sci. USA 96 , 4028–4033 (1999).
Koska, M. et al. Distinct long- and short-term adaptive mechanisms in Pseudomonas aeruginosa . Microbiol. Spectr. 10 , e0304322 (2022).
Keim, K. C., George, I. K., Reynolds, L. & Smith, A. C. The clinical significance of Staphylococcus aureus small colony variants. Lab. Med. 54 , 227–234 (2023).
Seaman, P. F., Ochs, D. & Day, M. J. Small-colony variants: a novel mechanism for triclosan resistance in methicillin-resistant Staphylococcus aureus . J. Antimicrob. Chemother. 59 , 43–450 (2007).
Pitton, M. et al. Mutation to ispA produces stable small-colony variants of Pseudomonas aeruginosa that have enhanced aminoglycoside resistance. Antimicrob. Agents Chemother. 66 , e0062122 (2022).
Zhou, S., Rao, Y., Li, J., Huang, Q. & Rao, X. Staphylococcus aureus small-colony variants: formation, infection, and treatment. Microbiol. Res. 260 , 127040 (2022).
Fischer, A. J. Small colonies, bigger problems? New evidence that Staphylococcus aureus small colony variants can worsen lung inflammation in cystic fibrosis rats. Infect. Immun. 90 , e0041322 (2022).
McNamara, P. J. & Proctor, R. A. Staphylococcus aureus small colony variants, electron transport and persistent infections. Int. J. Antimicrob. Agents 14 , 117–122 (2000).
Gilman, S. & Saunders, V. A. Accumulation of gentamicin by Staphylococcus aureus : the role of the transmembrane electrical potential. J. Antimicrob. Chemother. 17 , 37–44 (1986).
Guo, H. et al. Biofilm and small colony variants — an update on Staphylococcus aureus strategies toward drug resistance. Int. J. Mol. Sci. 23 , 1241 (2022).
Wesgate, R., Fanning, S., Hu, Y. & Maillard, J.-Y. The effect of exposure to microbicide residues at ‘during use’ concentrations on antimicrobial susceptibility profile, efflux, conjugative plasmid transfer and metabolism of Escherichia coli . Antimicrob. Agents Chemother. 64 , e01131-20 (2020).
Bischofberger, A. M., Baumgartner, M., Pfrunder-Cardozo, K. R., Allen, R. C. & Hall, A. R. Associations between sensitivity to antibiotics, disinfectants and heavy metals in natural, clinical and laboratory isolates of Escherichia coli . Environ. Microbiol. 22 , 2664–2679 (2020).
Webber, M. A., Coldham, N. G., Woodward, M. J. & Piddock, L. J. V. Proteomic analysis of triclosan resistance in Salmonella enterica serovar Typhimurium. J. Antimicrob. Chemother. 62 , 92–97 (2008).
Condell, O. et al. Comparative analysis of Salmonella susceptibility and tolerance to the biocide chlorhexidine identifies a complex cellular defense network. Front. Microbiol. 5 , 373 (2014). This paper identifies the expression of multiple mechanisms in response to biocide exposure, reporting for the first time, to our knowledge, a complex cellular defence network and emphasizing that bacterial response to biocide stress does rely on a combination of mechanisms.
Curiao, T. et al. Multiple adaptive routes of Salmonella enterica Typhimurium to biocide and antibiotic exposure. BMC Genomics 17 , 491 (2016).
Pi, B. R., Yu, D. L., Hua, X. T., Ruan, Z. & Yu, Y. S. Genomic and transcriptome analysis of triclosan response of a multidrug-resistant Acinetobacter baumannii strain, MDR-ZJ06. Arch. Microbiol. 199 , 223–230 (2017).
Curiao, T. et al. Polymorphic variation in susceptibility and metabolism of triclosan-resistant mutants of Escherichia coli and Klebsiella pneumoniae clinical strains obtained after exposure to biocides and antibiotics. Antimicrob. Agents Chemother. 59 , 3413–3423 (2015).
McMurry, L. M., Oethinger, M. & Levy, S. B. Overexpression of marA , soxS , or acrAB produces resistance to triclosan in laboratory and clinical strains of Escherichia coli . FEMS Microbiol. Lett. 166 , 305–309 (1998).
Bailey, A. M. et al. Exposure of Escherichia coli and serovar Typhimurium to triclosan induces a species-specific response, including drug detoxification. J. Antimicrob. Chemother. 64 , 973–985 (2009).
Dejoies, L., Le Neindre, K., Reissier, S., Felden, B. & Cattoir, V. Distinct expression profiles of regulatory RNAs in the response to biocides in Staphylococcus aureus and Enterococcus faecium . Sci. Rep. 11 , 6892 (2021). This paper documents the impact of biocide exposure at a subinhibitory concentration on the expression of small RNA (sRNA) in Staphylococcus aureus and Enterococcus faecium , demonstrating that sRNA-mediated responses were mostly repressed potentially leading to specific bacterial response and adaptation to biocides.
Demple, B. Redox signaling and gene control in the Escherichia coli soxRS oxidative stress regulon — a review. Gene 179 , 53–57 (1996).
Koutsolioutsou, A., Pena-Llopis, S. & Demple, B. Constitutive soxR mutations contribute to multiple-antibiotic resistance in clinical Escherichia coli isolates. Antimicrob. Agents Chemother. 49 , 2746–2752 (2005).
Wand, M. E., Bock, L. J., Bonney, L. C. & Sutton, J. M. Mechanisms of increased resistance to chlorhexidine and cross-resistance to colistin following exposure of Klebsiella pneumoniae clinical isolates to chlorhexidine. Antimicrob. Agents Chemother. 61 , e01162-16 (2016).
PubMed PubMed Central Google Scholar
Kastbjerg, V. G., Hein-Kristensen, L. & Gram, L. Triclosan-induced aminoglycoside-tolerant Listeria monocytogenes isolates can appear as small-colony variants. Antimicrob. Agents Chemother. 58 , 3124–3132 (2014).
McMurry, L. M., McDermott, P. F. & Levy, S. B. Genetic evidence that InhA of Mycobacterium smegmatis is a target for triclosan. Antimicrob. Agents Chemother. 43 , 711–713 (1999).
International Organization for Standardization. ISO: 20776-1. Clinical Laboratory Testing and In Vitro Diagnostic Test Systems: Susceptibility Testing of Infectious Agents and Evaluation of Performance of Antimicrobial Susceptibility Test Devices. Part 1. Reference Method for Testing the In Vitro Activity of Antimicrobial Agents Against Rapidly Growing Aerobic Bacteria Involved in Infectious Diseases (British Standard Institute, 2006).
European Committee on Antimicrobial Susceptibility Testing (EUCAST). Breakpoint Tables for Interpretation of MICs and Zone Diameters. Version 4.0 (EUCAST, 2014).
Andrews, J. M. BSAC Working Party on Susceptibility Testing. BSAC standardized disc susceptibility testing method (version 8). J. Antimicrob. Chemother. 64 , 454–489 (2009).
Bock, L. J., Hind, C. K., Sutton, J. M. & Wand, M. E. Growth media and assay plate material can impact on the effectiveness of cationic biocides and antibiotics against different bacterial species. Lett. Appl. Microbiol. 66 , 368–377 (2018).
Kampf, G. Suitability of methods to determine resistance to biocidal active substances and disinfectants — a systematic review. Hygiene 2 , 109–119 (2022).
Kahlmeter, G. et al. European harmonization of MIC breakpoints for antimicrobial susceptibility testing of bacteria. J. Antimicrob. Chemother. 52 , 145–148 (2003).
Coelho et al. The use of machine learning methodologies to analyse antibiotic and biocide susceptibility in Staphylococcus aureus . PLoS ONE 8 , e55582 (2013).
Walsh, S. E. et al. Development of bacterial resistance to several biocides and effects on antibiotic susceptibility. J. Hosp. Infect. 55 , 98–107 (2003).
Alonso-Calleja, C., Guerrero-Ramos, E., Alonso-Hernando, A. & Capita, R. Adaptation and cross-adaptation of Escherichia coli ATCC 12806 to several food-grade biocides. Food Control 56 , 86–94 (2015).
Cowley, N. L. et al. Effects of formulation on microbicide potency and mitigation of the development of bacterial insusceptibility. Appl. Environ. Microbiol. 81 , 7330–7338 (2015).
Wesgate, R., Grasha, P. & Maillard, J.-Y. Use of a predictive protocol to measure the antimicrobial resistance risks associated with biocidal product usage. Am. J. Infect. Control 44 , 458–464 (2016).
Randall, L. P. et al. Commonly used farm disinfectants can select for mutant Salmonella enterica serovar Typhimurium with decreased susceptibility to biocides and antibiotics without compromising virulence. J. Antimicrob. Chemother. 60 , 1273–1280 (2007).
Weber, D. J., Rutala, W. A. & Sickbert-Bennett, E. E. Outbreaks associated with contaminated antiseptics and disinfectants. Antimicrob. Agents Chemother. 51 , 4217–4224 (2007). This review presents evidence of bacterial contamination of biocidal products and highlights the reasons for product failure, including contamination with an intrinsically resistant bacterium or spore, or product misuse.
Maillard, J.-Y. in Blocks’ Disinfection, Sterilization and Preservation 6th edn (eds McDonnell, G. & Hansen, J.) 44–67 (Wolters Kluwer, 2020).
de Frutos, M. et al. Serratia marcescens outbreak due to contaminated 2% aqueous chlorhexidine. Enferm. Infecc. Microbiol. Clin. 35 , 624–629 (2016).
PubMed Google Scholar
Anyiwo, C. E., Coker, A. O. & Daniel, S. O. Pseudomonas aerugin osa in postoperative wounds from chlorhexidine solutions. J. Hosp. Infect. 3 , 189–191 (1982).
Wishart, M. M. & Riley, T. V. Infection with Pseudomonas maltophilia hospital outbreak due to contaminated disinfectant. Med. J. Aust. 2 , 710–712 (1976).
Georgia Division of Public Health. Abscesses in an allergy practice due to Mycobacterium chelonae . Georgia Epidemiol. Rep. 6 , 2 (1960).
Guinness, M. & Levey, J. Contamination of aqueous dilutions of Resiguard disinfectant with Pseudomonas. Med. J. Aust. 2 , 392 (1976).
Cason, J. S., Jackson, D. M., Lowbury, E. J. & Ricketts, C. R. Antiseptic and septic prophylaxis for burns: use of silver nitrate and of isolators. Br. Med. J. 2 , 1288–1294 (1966).
Duarte, R. S. et al. Epidemic of postsurgical infections caused by Mycobacterium massiliense . J. Clin. Microbiol. 47 , 2149–2155 (2009).
Ben Miloud, S., Ali, M. M., Boutiba, I., Van Houdt, R. & Chouchani, C. First report of cross resistance to silver and antibiotics in Klebsiella pneumoniae isolated from patients and polluted water in Tunisia. Water Environ. J. 35 , 730–739 (2021).
Molina-González, D., Alonso-Calleja, C., Alonso-Hernando, A. & Capita, R. Effect of sub-lethal concentrations of biocides on the susceptibility to antibiotics of multi-drug resistant Salmonella enterica strains. Food Control 40 , 329–334 (2014).
Amos, G. C. A. et al. The widespread dissemination of integrons throughout bacterial communities in a riverine system. ISME J. 12 , 681–691 (2018).
Randall, L. P. et al. Fitness and dissemination of disinfectant-selected multiple-antibiotic-resistant (MAR) strains of Salmonella enterica serovar Typhimurium in chickens. J. Antimicrob. Chemother. 61 , 156–162 (2008).
Cole, E. C. et al. Investigation of antibiotic and antibacterial agent cross-resistance in target bacteria from homes of antibacterial product users and nonusers. J. Appl. Microbiol. 95 , 664–676 (2003).
Carson, R. T., Larson, E., Levy, S. B., Marshall, B. M. & Aiello, A. E. Use of antibacterial consumer products containing quaternary ammonium compounds and drug resistance in the community. J. Antimicrob. Chemother. 62 , 1160–1162 (2008).
Short, F. L. et al. Benzalkonium chloride antagonises aminoglycoside antibiotics and promotes evolution of resistance. eBioMedicine 73 , 103653 (2021).
Liu, Q. et al. Frequency of biocide-resistant genes and susceptibility to chlorhexidine in high-level mupirocin-resistant, methicillin-resistant Staphylococcus aureus (MuH MRSA). Diagn. Microbiol. Infect. Dis. 82 , 278–283 (2015). This paper shows multiple efflux gene carriage in Staphylococcus aureus clinical isolates, where most of the isolates harbour two or more efflux pump gene determinants.
Hijazi, K. et al. Susceptibility to chlorhexidine amongst multidrug-resistant clinical isolates of Staphylococcus epidermidis from bloodstream infections. Int. J. Antimicrob. Agents 48 , 86–90 (2016).
Conceição, T., Coelho, C., de Lencastre, H. & Aires-de-Sousa, M. High prevalence of biocide resistance determinants in Staphylococcus aureus isolates from three African countries. Antimicrob. Agents Chemother. 60 , 678–681 (2015).
Wand, M. E. et al. Characterization of pre-antibiotic era Klebsiella pneumoniae isolates with respect to antibiotic/disinfectant susceptibility and virulence in Galleria mellonella . Antimicrob. Agents Chemother. 59 , 3966–3972 (2015).
Lin, F. et al. Molecular characterization of reduced susceptibility to biocides in clinical isolates of Acinetobacter baumannii . Front. Microbiol. 8 , 1836 (2017).
Elkhatib, W. F., KhaIiI, M. A. F. & Ashour, H. M. Integrons and antiseptic resistance genes mediate resistance of Acinetobacter baumannii and Pseudomonas aeruginosa isolates from intensive care unit patients with wound infections. Curr. Mol. Med. 19 , 286–293 (2019).
Goodarzi, R., Yousefimashouf, R., Taheri, M., Nouri, F. & Asghari, B. Susceptibility to biocides and the prevalence of biocides resistance genes in clinical multidrug-resistant Pseudomonas aeruginosa isolates from Hamadan, Iran. Mol. Biol. Rep. 48 , 5275–5281 (2021).
Namaki, M. et al. Prevalence of resistance genes to biocides in antibiotic-resistant Pseudomonas aeruginosa clinical isolates. Mol. Biol. Rep. 49 , 2149–2155 (2022).
Boutarfi, Z. et al. Biocide tolerance and antibiotic resistance of Enterobacter spp. isolated from an Algerian hospital environment. J. Glob. Antimicrob. Res. 18 , 291–297 (2019).
Medardus, J. J. et al. In-feed use of heavy metal micronutrients in U.S. swine production systems and its role in persistence of multidrug-resistant Salmonellae. Appl. Environ. Microbiol. 80 , 2317–2325 (2014).
Correa, J. E., De Paulis, A., Predari, S., Sordelli, D. O. & Jeric, P. E. First report of qacG , qacH and qacJ genes in Staphylococcus haemolyticus human clinical isolates. J. Antimicrob. Chemother. 62 , 956–960 (2008).
Jiang, X. et al. Examination of quaternary ammonium compound resistance in Proteus mirabilis isolated from cooked meat products in China. Front. Microbiol. 8 , 2417 (2017).
Jiang, X. et al. Characterization and horizontal transfer of qacH -associated class 1 integrons in Escherichia coli isolated from retail meats. Int. J. Food Microbiol. 258 , 12–17 (2017).
Wales, A. D. & Davies, R. H. Co-selection of resistance to antibiotics, biocides and heavy metals, and its relevance to foodborne pathogens. Antibiotics 4 , 567–604 (2015).
Pal, C. et al. Metal resistance and its association with antibiotic resistance. Adv. Microb. Physiol. 70 , 261–313 (2017).
Sidhu, M. S., Heir, E., Leegaard, T., Wiger, K. & Holck, A. Frequency of disinfectant resistance genes and genetic linkage with beta-lactamase transposon Tn552 among clinical staphylococci. Antimicrob. Agents Chemother. 46 , 2797–2803 (2002).
Harrison, K. R., Kappell, A. D. & McNamara, P. J. Benzalkonium chloride alters phenotypic and genotypic antibiotic resistance profiles in a source water used for drinking water treatment. Environ. Poll. 257 , 113472 (2020).
Siani, H. & Maillard, J.-Y. Best practice in healthcare environment decontamination. Eur. J. Infect. Control. Infect. Dis. 34 , 1–11 (2015).
CAS Google Scholar
Van Asselt, A.J. & te Giffel, M. C. in Handbook of Hygiene Control in the Food Industry (eds Lelieveld, H. L. M., Mostert, M. A. & Holah, J.) 69–92 (Woodhead Publishing, 2005).
Maillard, J.-Y. et al. Reducing antibiotic prescribing and addressing the global problem of antibiotic resistance by targeted hygiene in the home and everyday life settings: a position paper. Am. J. Infect. Control. 48 , 1090–1099 (2020).
Wellcome Trust. The Global Response to AMR. Momentum, Success, and Critical Gaps. Wellcome Trust https://cms.wellcome.org/sites/default/files/2020-11/wellcome-global-response-amr-report.pdf (2020).
O’Neil, J. Tackling Drug-Resistant Infections Globally; Final Report and Recommendations. Wellcome Trust and HM Government https://amr-review.org/sites/default/files/160518_Final%20paper_with%20cover.pdf (2016).
Zhang, M., Chen, L., Ye, C. & Yu, X. Co-selection of antibiotic resistance via copper sock loading on bacteria from drinking water bio-filter. Eviron. Poll. 233 , 132–141 (2018).
Fernando, D. M., Xu, W., Loewen, P. C., Zhanel, G. G. & Kumar, A. Triclosan can select for an AdeIJK-overexpressing mutant of Acinetobacter baumannii ATCC 17978 that displays reduced susceptibility to multiple antibiotics. Antimicrob. Agents Chemother. 58 , 6424–6431 (2014).
Mc Cay, P. H., Ocampo-Sosa, A. O. & Fleming, G. T. A. Effect of subinhibitory concentrations of benzalkonium chloride on the competitiveness of Pseudomonas aeruginosa grown in continuous culture. Microbiology 156 , 30–38 (2010).
Mavri, A. & Smole Možina, S. Development of antimicrobial resistance in Campylobacter jejuni and Campylobacter coli adapted to biocides. Int. J. Food Microbiol. 160 , 304–312 (2013).
Tong, C. et al. Chlorine disinfectants promote microbial resistance in Pseudomonas sp. Environ. Res. 199 , 111296 (2021).
Download references
Author information
Authors and affiliations.
School of Pharmacy and Pharmaceutical Sciences, Cardiff University, Wales, UK
Jean-Yves Maillard & Michael Pascoe
You can also search for this author in PubMed Google Scholar
Contributions
The authors contributed equally to all aspects of the manuscript.
Corresponding author
Correspondence to Jean-Yves Maillard .
Ethics declarations
Competing interests.
J.-Y.M. is the Director of Biocide Consult Ltd. M.P. declares no competing interests.
Peer review
Peer review information.
Nature Reviews Microbiology thanks Anabela Borges, Ilias Tagkopoulos, Manuel Simões and the other, anonymous, reviewer(s) for their contribution to the peer review of this work.
Additional information
Publisher’s note Springer Nature remains neutral with regard to jurisdictional claims in published maps and institutional affiliations.
Related links
ECHA: https://echa.europa.eu/information-on-chemicals/biocidal-active-substances
ECHA, Biocidal Product Regulation: https://echa.europa.eu/regulations/biocidal-products-regulation/legislation
Supplementary information
Supplementary information, rights and permissions.
Springer Nature or its licensor (e.g. a society or other partner) holds exclusive rights to this article under a publishing agreement with the author(s) or other rightsholder(s); author self-archiving of the accepted manuscript version of this article is solely governed by the terms of such publishing agreement and applicable law.
Reprints and permissions
About this article
Cite this article.
Maillard, JY., Pascoe, M. Disinfectants and antiseptics: mechanisms of action and resistance. Nat Rev Microbiol 22 , 4–17 (2024). https://doi.org/10.1038/s41579-023-00958-3
Download citation
Accepted : 28 July 2023
Published : 30 August 2023
Issue Date : January 2024
DOI : https://doi.org/10.1038/s41579-023-00958-3
Share this article
Anyone you share the following link with will be able to read this content:
Sorry, a shareable link is not currently available for this article.
Provided by the Springer Nature SharedIt content-sharing initiative
This article is cited by
Operando investigation of the synergistic effect of electric field treatment and copper for bacteria inactivation.
- Mourin Jarin
Nature Communications (2024)
Quick links
- Explore articles by subject
- Guide to authors
- Editorial policies
Sign up for the Nature Briefing newsletter — what matters in science, free to your inbox daily.

13.3 Using Chemicals to Control Microorganisms
Learning objectives.
By the end of this section, you will be able to:
- Understand and compare various chemicals used to control microbial growth, including their uses, advantages and disadvantages, chemical structure, and mode of action
In addition to physical methods of microbial control, chemicals are also used to control microbial growth. A wide variety of chemicals can be used as disinfectants or antiseptics. When choosing which to use, it is important to consider the type of microbe targeted; how clean the item needs to be; the disinfectant’s effect on the item’s integrity; its safety to animals, humans, and the environment; its expense; and its ease of use. This section describes the variety of chemicals used as disinfectants and antiseptics, including their mechanisms of action and common uses.
In the 1800s, scientists began experimenting with a variety of chemicals for disinfection. In the 1860s, British surgeon Joseph Lister (1827–1912) began using carbolic acid, known as phenol , as a disinfectant for the treatment of surgical wounds (see Foundations of Modern Cell Theory ). In 1879, Lister’s work inspired the American chemist Joseph Lawrence (1836–1909) to develop Listerine, an alcohol-based mixture of several related compounds that is still used today as an oral antiseptic. Today, carbolic acid is no longer used as a surgical disinfectant because it is a skin irritant, but the chemical compounds found in antiseptic mouthwashes and throat lozenges are called phenolics .
Chemically, phenol consists of a benzene ring with an –OH group, and phenolics are compounds that have this group as part of their chemical structure ( Figure 13.19 ). Phenolics such as thymol and eucalyptol occur naturally in plants. Other phenolics can be derived from creosote, a component of coal tar. Phenolics tend to be stable, persistent on surfaces, and less toxic than phenol. They inhibit microbial growth by denaturing proteins and disrupting membranes.
Since Lister’s time, several phenolic compounds have been used to control microbial growth. Phenolics like cresols (methylated phenols) and o-phenylphenol were active ingredients in various formulations of Lysol since its invention in 1889. o-Phenylphenol was also commonly used in agriculture to control bacterial and fungal growth on harvested crops, especially citrus fruits, but its use in the United States is now far more limited. The bisphenol hexachlorophene , a disinfectant, is the active ingredient in pHisoHex, a topical cleansing detergent widely used for handwashing in hospital settings. pHisoHex is particularly effective against gram-positive bacteria, including those causing staphylococcal and streptococcal skin infections. pHisoHex was formerly used for bathing infants, but this practice has been discontinued because it has been shown that exposure to hexachlorophene can lead to neurological problems.
Triclosan is another bisphenol compound that has seen widespread application in antibacterial products over the last several decades. Initially used in toothpastes, triclosan has also been used in hand soaps and impregnated into a wide variety of other products, including cutting boards, knives, shower curtains, clothing, and concrete, to make them antimicrobial. However, in 2016 the FDA banned the marketing of over-the-counter antiseptic products containing triclosan and 18 other chemicals. This ruling was based on the lack of evidence of safety or efficacy, as well as concerns about the health risks of long-term exposure (See Micro Connections below). In 2019 the FDA issued an updated ban ruling to included 28 chemicals. Rulings on benzalkonium chloride, ethyl alcohol, and isopropyl alcohol have been deferred to allow for the submission of additional safety and efficacy data. 8
Micro Connections
Triclosan: antibacterial overkill.
Hand soaps and other cleaning products are often marketed as “antibacterial,” suggesting that they provide a level of cleanliness superior to that of conventional soaps and cleansers. But are the antibacterial ingredients in these products really safe and effective?
About 75% of antibacterial liquid hand soaps and 30% of bar soaps contain the chemical triclosan, a phenolic, ( Figure 13.20 ). 9 Triclosan blocks an enzyme in the bacterial fatty acid-biosynthesis pathway that is not found in the comparable human pathway. Although the use of triclosan in the home increased dramatically during the 1990s, more than 40 years of research by the FDA have turned up no conclusive evidence that washing with triclosan-containing products provides increased health benefits compared with washing with traditional soap. Although some studies indicate that fewer bacteria may remain on a person’s hands after washing with triclosan-based soap, compared with traditional soap, no evidence points to any reduction in the transmission of bacteria that cause respiratory and gastrointestinal illness. In short, soaps with triclosan may remove or kill a few more germs but not enough to reduce the spread of disease.
Perhaps more disturbing, some clear risks associated with triclosan-based soaps have come to light. The widespread use of triclosan has led to an increase in triclosan-resistant bacterial strains, including those of clinical importance, such as Salmonella enterica ; this resistance may render triclosan useless as an antibacterial in the long run. 10 11 Bacteria can easily gain resistance to triclosan through a change to a single gene encoding the targeted enzyme in the bacterial fatty acid-synthesis pathway. Other disinfectants with a less specific mode of action are much less prone to engendering resistance because it would take much more than a single genetic change.
Use of triclosan over the last several decades has also led to a buildup of the chemical in the environment. Triclosan in hand soap is directly introduced into wastewater and sewage systems as a result of the handwashing process. There, its antibacterial properties can inhibit or kill bacteria responsible for the decomposition of sewage, causing septic systems to clog and back up. Eventually, triclosan in wastewater finds its way into surface waters, streams, lakes, sediments, and soils, disrupting natural populations of bacteria that carry out important environmental functions, such as inhibiting algae. Triclosan also finds its way into the bodies of amphibians and fish, where it can act as an endocrine disruptor. Detectable levels of triclosan have also been found in various human bodily fluids, including breast milk, plasma, and urine. 12 In fact, a study conducted by the CDC found detectable levels of triclosan in the urine of 75% of 2,517 people tested in 2003–2004. 13 This finding is even more troubling given the evidence that triclosan may affect immune function in humans. 14
In December 2013, the FDA gave soap manufacturers until 2016 to prove that antibacterial soaps provide a significant benefit over traditional soaps; if unable to do so, manufacturers will be forced to remove these products from the market.
Check Your Understanding
- Why is triclosan more like an antibiotic than a traditional disinfectant?
Heavy Metals
Some of the first chemical disinfectants and antiseptics to be used were heavy metals . Heavy metals kill microbes by binding to proteins, thus inhibiting enzymatic activity ( Figure 13.21 ). Heavy metals are oligodynamic, meaning that very small concentrations show significant antimicrobial activity. Ions of heavy metals bind to sulfur-containing amino acids strongly and bioaccumulate within cells, allowing these metals to reach high localized concentrations. This causes proteins to denature.
Heavy metals are not selectively toxic to microbial cells. They may bioaccumulate in human or animal cells, as well, and excessive concentrations can have toxic effects on humans. If too much silver accumulates in the body, for example, it can result in a condition called argyria , in which the skin turns irreversibly blue-gray. One way to reduce the potential toxicity of heavy metals is by carefully controlling the duration of exposure and concentration of the heavy metal.
Mercury is an example of a heavy metal that has been used for many years to control microbial growth. It was used for many centuries to treat syphilis. Mercury compounds like mercuric chloride are mainly bacteriostatic and have a very broad spectrum of activity. Various forms of mercury bind to sulfur-containing amino acids within proteins, inhibiting their functions.
In recent decades, the use of such compounds has diminished because of mercury’s toxicity. It is toxic to the central nervous, digestive, and renal systems at high concentrations, and has negative environmental effects, including bioaccumulation in fish. Topical antiseptics such as mercurochrome , which contains mercury in low concentrations, and merthiolate , a tincture (a solution of mercury dissolved in alcohol) were once commonly used. However, because of concerns about using mercury compounds, these antiseptics are no longer sold in the United States.
Silver has long been used as an antiseptic. In ancient times, drinking water was stored in silver jugs. 15 Silvadene cream is commonly used to treat topical wounds and is particularly helpful in preventing infection in burn wounds. Silver nitrate drops were once routinely applied to the eyes of newborns to protect against ophthalmia neonatorum , eye infections that can occur due to exposure to pathogens in the birth canal, but antibiotic creams are more now commonly used. Silver is often combined with antibiotics, making the antibiotics thousands of times more effective. 16 Silver is also commonly incorporated into catheters and bandages, rendering them antimicrobial; however, there is evidence that heavy metals may also enhance selection for antibiotic resistance. 17
Copper, Nickel, and Zinc
Several other heavy metals also exhibit antimicrobial activity. Copper sulfate is a common algicide used to control algal growth in swimming pools and fish tanks. The use of metallic copper to minimize microbial growth is also becoming more widespread. Copper linings in incubators help reduce contamination of cell cultures. The use of copper pots for water storage in underdeveloped countries is being investigated as a way to combat diarrheal diseases. Copper coatings are also becoming popular for frequently handled objects such as doorknobs, cabinet hardware, and other fixtures in health-care facilities in an attempt to reduce the spread of microbes.
Nickel and zinc coatings are now being used in a similar way. Other forms of zinc, including zinc chloride and zinc oxide , are also used commercially. Zinc chloride is quite safe for humans and is commonly found in mouthwashes, substantially increasing their length of effectiveness. Zinc oxide is found in a variety of products, including topical antiseptic creams such as calamine lotion, diaper ointments, baby powder, and dandruff shampoos.
- Why are many heavy metals both antimicrobial and toxic to humans?
Other chemicals commonly used for disinfection are the halogens iodine , chlorine , and fluorine . Iodine works by oxidizing cellular components, including sulfur-containing amino acids, nucleotides, and fatty acids, and destabilizing the macromolecules that contain these molecules. It is often used as a topical tincture, but it may cause staining or skin irritation. An iodophor is a compound of iodine complexed with an organic molecule, thereby increasing iodine’s stability and, in turn, its efficacy. One common iodophor is povidone-iodine , which includes a wetting agent that releases iodine relatively slowly. Betadine is a brand of povidone-iodine commonly used as a hand scrub by medical personnel before surgery and for topical antisepsis of a patient’s skin before incision ( Figure 13.22 ).
Chlorine is another halogen commonly used for disinfection. When chlorine gas is mixed with water, it produces a strong oxidant called hypochlorous acid, which is uncharged and enters cells easily. Chlorine gas is commonly used in municipal drinking water and wastewater treatment plants, with the resulting hypochlorous acid producing the actual antimicrobial effect. Those working at water treatment facilities need to take great care to minimize personal exposure to chlorine gas. Sodium hypochlorite is the chemical component of common household bleach , and it is also used for a wide variety of disinfecting purposes. Hypochlorite salts, including sodium and calcium hypochlorites, are used to disinfect swimming pools. Chlorine gas, sodium hypochlorite, and calcium hypochlorite are also commonly used disinfectants in the food processing and restaurant industries to reduce the spread of foodborne diseases. Workers in these industries also need to take care to use these products correctly to ensure their own safety as well as the safety of consumers. A recent joint statement published by the Food and Agriculture Organization (FAO) of the United Nations and WHO indicated that none of the many beneficial uses of chlorine products in food processing to reduce the spread of foodborne illness posed risks to consumers. 18
Another class of chlorinated compounds called chloramines are widely used as disinfectants. Chloramines are relatively stable, releasing chlorine over long periods time. Chloramines are derivatives of ammonia by substitution of one, two, or all three hydrogen atoms with chlorine atoms ( Figure 13.23 ).
Chloramines and other cholorine compounds may be used for disinfection of drinking water, and chloramine tablets are frequently used by the military for this purpose. After a natural disaster or other event that compromises the public water supply, the CDC recommends disinfecting tap water by adding small amounts of regular household bleach. Recent research suggests that sodium dichloroisocyanurate (NaDCC) may also be a good alternative for drinking water disinfection. Currently, NaDCC tablets are available for general use and for use by the military, campers, or those with emergency needs; for these uses, NaDCC is preferable to chloramine tablets. Chlorine dioxide, a gaseous agent used for fumigation and sterilization of enclosed areas, is also commonly used for the disinfection of water.
Although chlorinated compounds are relatively effective disinfectants, they have their disadvantages. Some may irritate the skin, nose, or eyes of some individuals, and they may not completely eliminate certain hardy organisms from contaminated drinking water. The protozoan parasite Cryptosporidium , for example, has a protective outer shell that makes it resistant to chlorinated disinfectants. Thus, boiling of drinking water in emergency situations is recommended when possible.
The halogen fluorine is also known to have antimicrobial properties that contribute to the prevention of dental caries (cavities). 19 Fluoride is the main active ingredient of toothpaste and is also commonly added to tap water to help communities maintain oral health. Chemically, fluoride can become incorporated into the hydroxyapatite of tooth enamel, making it more resistant to corrosive acids produced by the fermentation of oral microbes. Fluoride also enhances the uptake of calcium and phosphate ions in tooth enamel, promoting remineralization. In addition to strengthening enamel, fluoride also seems to be bacteriostatic. It accumulates in plaque-forming bacteria, interfering with their metabolism and reducing their production of the acids that contribute to tooth decay.
- What is a benefit of a chloramine over hypochlorite for disinfecting?
Alcohols make up another group of chemicals commonly used as disinfectants and antiseptics. They work by rapidly denaturing proteins, which inhibits cell metabolism, and by disrupting membranes, which leads to cell lysis. Once denatured, the proteins may potentially refold if enough water is present in the solution. Alcohols are typically used at concentrations of about 70% aqueous solution and, in fact, work better in aqueous solutions than 100% alcohol solutions. This is because alcohols coagulate proteins. In higher alcohol concentrations, rapid coagulation of surface proteins prevents effective penetration of cells. The most commonly used alcohols for disinfection are ethyl alcohol (ethanol) and isopropyl alcohol (isopropanol, rubbing alcohol) ( Figure 13.24 ).
Alcohols tend to be bactericidal and fungicidal, but may also be viricidal for enveloped viruses only. Although alcohols are not sporicidal, they do inhibit the processes of sporulation and germination. Alcohols are volatile and dry quickly, but they may also cause skin irritation because they dehydrate the skin at the site of application. One common clinical use of alcohols is swabbing the skin for degerming before needle injection. Alcohols also are the active ingredients in instant hand sanitizer s, which have gained popularity in recent years. The alcohol in these hand sanitizers works both by denaturing proteins and by disrupting the microbial cell membrane, but will not work effectively in the presence of visible dirt.
Last, alcohols are used to make tincture s with other antiseptics, such as the iodine tinctures discussed previously in this chapter. All in all, alcohols are inexpensive and quite effective for the disinfection of a broad range of vegetative microbes. However, one disadvantage of alcohols is their high volatility, limiting their effectiveness to immediately after application.
- Name at least three advantages of alcohols as disinfectants.
- Describe several specific applications of alcohols used in disinfectant products.
Surfactants
Surface-active agents, or surfactants , are a group of chemical compounds that lower the surface tension of water. Surfactants are the major ingredients in soaps and detergents . Soaps are salts of long-chain fatty acids and have both polar and nonpolar regions, allowing them to interact with polar and nonpolar regions in other molecules ( Figure 13.25 ). They can interact with nonpolar oils and grease to create emulsions in water, loosening and lifting away dirt and microbes from surfaces and skin. Soaps do not kill or inhibit microbial growth and so are not considered antiseptics or disinfectants. However, proper use of soaps mechanically carries away microorganisms, effectively degerming a surface. Some soaps contain added bacteriostatic agents such as triclocarban or cloflucarban , compounds structurally related to triclosan, that introduce antiseptic or disinfectant properties to the soaps.
Soaps, however, often form films that are difficult to rinse away, especially in hard water, which contains high concentrations of calcium and magnesium mineral salts. Detergents contain synthetic surfactant molecules with both polar and nonpolar regions that have strong cleansing activity but are more soluble, even in hard water, and, therefore, leave behind no soapy deposits. Anionic detergents , such as those used for laundry, have a negatively charged anion at one end attached to a long hydrophobic chain, whereas cationic detergents have a positively charged cation instead. Cationic detergents include an important class of disinfectants and antiseptics called the quaternary ammonium salts (quats) , named for the characteristic quaternary nitrogen atom that confers the positive charge ( Figure 13.26 ). Overall, quats have properties similar to phospholipids, having hydrophilic and hydrophobic ends. As such, quats have the ability to insert into the bacterial phospholipid bilayer and disrupt membrane integrity. The cationic charge of quats appears to confer their antimicrobial properties, which are diminished when neutralized. Quats have several useful properties. They are stable, nontoxic, inexpensive, colorless, odorless, and tasteless. They tend to be bactericidal by disrupting membranes. They are also active against fungi, protozoans, and enveloped viruses, but endospores are unaffected. In clinical settings, they may be used as antiseptics or to disinfect surfaces. Mixtures of quats are also commonly found in household cleaners and disinfectants, including many current formulations of Lysol brand products, which contain benzalkonium chlorides as the active ingredients. Benzalkonium chlorides, along with the quat cetylpyrimidine chloride , are also found in products such as skin antiseptics, oral rinses, and mouthwashes.
- Why are soaps not considered disinfectants?
Handwashing the Right Way
Handwashing is critical for public health and should be emphasized in a clinical setting. For the general public, the CDC recommends handwashing before, during, and after food handling; before eating; before and after interacting with someone who is ill; before and after treating a wound; after using the toilet or changing diapers; after coughing, sneezing, or blowing the nose; after handling garbage; and after interacting with an animal, its feed, or its waste. Figure 13.27 illustrates the five steps of proper handwashing recommended by the CDC.
Handwashing is even more important for health-care workers, who should wash their hands thoroughly between every patient contact, after the removal of gloves, after contact with bodily fluids and potentially infectious fomites, and before and after assisting a surgeon with invasive procedures. Even with the use of proper surgical attire, including gloves, scrubbing for surgery is more involved than routine handwashing. The goal of surgical scrubbing is to reduce the normal microbiota on the skin’s surface to prevent the introduction of these microbes into a patient’s surgical wounds.
There is no single widely accepted protocol for surgical scrubbing. Protocols for length of time spent scrubbing may depend on the antimicrobial used; health-care workers should always check the manufacturer’s recommendations. According to the Association of Surgical Technologists (AST), surgical scrubs may be performed with or without the use of brushes ( Figure 13.27 ).
Link to Learning
To learn more about proper handwashing, visit the CDC’s website.
Bisbiguanides
Bisbiguanides were first synthesized in the 20th century and are cationic (positively charged) molecules known for their antiseptic properties ( Figure 13.28 ). One important bisbiguanide antiseptic is chlorhexidine . It has broad-spectrum activity against yeasts, gram-positive bacteria, and gram-negative bacteria, with the exception of Pseudomonas aeruginosa , which may develop resistance on repeated exposure. 20 Chlorhexidine disrupts cell membranes and is bacteriostatic at lower concentrations or bactericidal at higher concentrations, in which it actually causes the cells’ cytoplasmic contents to congeal. It also has activity against enveloped viruses. However, chlorhexidine is poorly effective against Mycobacterium tuberculosis and nonenveloped viruses, and it is not sporicidal. Chlorhexidine is typically used in the clinical setting as a surgical scrub and for other handwashing needs for medical personnel, as well as for topical antisepsis for patients before surgery or needle injection. It is more persistent than iodophors, providing long-lasting antimicrobial activity. Chlorhexidine solutions may also be used as oral rinses after oral procedures or to treat gingivitis. Another bisbiguanide, alexidine , is gaining popularity as a surgical scrub and an oral rinse because it acts faster than chlorhexidine.
- What two effects does chlorhexidine have on bacterial cells?
Alkylating Agents
The alkylating agent s are a group of strong disinfecting chemicals that act by replacing a hydrogen atom within a molecule with an alkyl group (C n H 2n+1 ), thereby inactivating enzymes and nucleic acids ( Figure 13.29 ). The alkylating agent formaldehyde (CH 2 O) is commonly used in solution at a concentration of 37% (known as formalin ) or as a gaseous disinfectant and biocide. It is a strong, broad-spectrum disinfectant and biocide that has the ability to kill bacteria, viruses, fungi, and endospores, leading to sterilization at low temperatures, which is sometimes a convenient alternative to the more labor-intensive heat sterilization methods. It also cross-links proteins and has been widely used as a chemical fixative. Because of this, it is used for the storage of tissue specimens and as an embalming fluid. It also has been used to inactivate infectious agents in vaccine preparation. Formaldehyde is very irritating to living tissues and is also carcinogenic; therefore, it is not used as an antiseptic.
Glutaraldehyde is structurally similar to formaldehyde but has two reactive aldehyde groups, allowing it to act more quickly than formaldehyde. It is commonly used as a 2% solution for sterilization and is marketed under the brand name Cidex. It is used to disinfect a variety of surfaces and surgical and medical equipment. However, similar to formaldehyde, glutaraldehyde irritates the skin and is not used as an antiseptic.
A new type of disinfectant gaining popularity for the disinfection of medical equipment is o-phthalaldehyde (OPA), which is found in some newer formulations of Cidex and similar products, replacing glutaraldehyde. o-Phthalaldehyde also has two reactive aldehyde groups, but they are linked by an aromatic bridge. o-Phthalaldehyde is thought to work similarly to glutaraldehyde and formaldehyde, but is much less irritating to skin and nasal passages, produces a minimal odor, does not require processing before use, and is more effective against mycobacteria.
Ethylene oxide is a type of alkylating agent that is used for gaseous sterilization. It is highly penetrating and can sterilize items within plastic bags such as catheters, disposable items in laboratories and clinical settings (like packaged Petri dishes), and other pieces of equipment. Ethylene oxide exposure is a form of cold sterilization, making it useful for the sterilization of heat-sensitive items. Great care needs to be taken with the use of ethylene oxide, however; it is carcinogenic, like the other alkylating agents, and is also highly explosive. With careful use and proper aeration of the products after treatment, ethylene oxide is highly effective, and ethylene oxide sterilizers are commonly found in medical settings for sterilizing packaged materials.
β-Propionolactone is an alkylating agent with a different chemical structure than the others already discussed. Like other alkylating agents, β-propionolactone binds to DNA, thereby inactivating it ( Figure 13.29 ). It is a clear liquid with a strong odor and has the ability to kill endospores. As such, it has been used in either liquid form or as a vapor for the sterilization of medical instruments and tissue grafts, and it is a common component of vaccines, used to maintain their sterility. It has also been used for the sterilization of nutrient broth, as well as blood plasma, milk, and water. It is quickly metabolized by animals and humans to lactic acid. It is also an irritant, however, and may lead to permanent damage of the eyes, kidneys, or liver. Additionally, it has been shown to be carcinogenic in animals; thus, precautions are necessary to minimize human exposure to β-propionolactone. 21
- What chemical reaction do alkylating agents participate in?
- Why are alkylating agents not used as antiseptics?
Diehard Prions
Prions, the acellular, misfolded proteins responsible for incurable and fatal diseases such as kuru and Creutzfeldt-Jakob disease (see Viroids, Virusoids, and Prions ), are notoriously difficult to destroy. Prions are extremely resistant to heat, chemicals, and radiation. They are also extremely infectious and deadly; thus, handling and disposing of prion-infected items requires extensive training and extreme caution.
Typical methods of disinfection can reduce but not eliminate the infectivity of prions. Autoclaving is not completely effective, nor are chemicals such as phenol, alcohols, formalin, and β-propiolactone. Even when fixed in formalin, affected brain and spinal cord tissues remain infectious.
Personnel who handle contaminated specimens or equipment or work with infected patients must wear a protective coat, face protection, and cut-resistant gloves. Any contact with skin must be immediately washed with detergent and warm water without scrubbing. The skin should then be washed with 1 N NaOH or a 1:10 dilution of bleach for 1 minute. Contaminated waste must be incinerated or autoclaved in a strong basic solution, and instruments must be cleaned and soaked in a strong basic solution.
For more information on the handling of animals and prion-contaminated materials, visit the guidelines published on the WHO website.
Peroxygens are strong oxidizing agents that can be used as disinfectants or antiseptics. The most widely used peroxygen is hydrogen peroxide (H 2 O 2 ), which is often used in solution to disinfect surfaces and may also be used as a gaseous agent. Hydrogen peroxide solutions are inexpensive skin antiseptics that break down into water and oxygen gas, both of which are environmentally safe. This decomposition is accelerated in the presence of light, so hydrogen peroxide solutions typically are sold in brown or opaque bottles. One disadvantage of using hydrogen peroxide as an antiseptic is that it also causes damage to skin that may delay healing or lead to scarring. Contact lens cleaners often include hydrogen peroxide as a disinfectant.
Hydrogen peroxide works by producing free radicals that damage cellular macromolecules. Hydrogen peroxide has broad-spectrum activity, working against gram-positive and gram-negative bacteria (with slightly greater efficacy against gram-positive bacteria), fungi, viruses, and endospores. However, bacteria that produce the oxygen-detoxifying enzymes catalase or peroxidase may have inherent tolerance to low hydrogen peroxide concentrations ( Figure 13.30 ). To kill endospores, the length of exposure or concentration of solutions of hydrogen peroxide must be increased. Gaseous hydrogen peroxide has greater efficacy and can be used as a sterilant for rooms or equipment.
Plasma, a hot, ionized gas, described as the fourth state of matter, is useful for sterilizing equipment because it penetrates surfaces and kills vegetative cells and endospores. Hydrogen peroxide and peracetic acid , another commonly used peroxygen, each may be introduced as a plasma. Peracetic acid can be used as a liquid or plasma sterilant insofar as it readily kills endospores, is more effective than hydrogen peroxide even at rather low concentrations, and is immune to inactivation by catalases and peroxidases. It also breaks down to environmentally innocuous compounds; in this case, acetic acid and oxygen.
Other examples of peroxygens include benzoyl peroxide and carbamide peroxide . Benzoyl peroxide is a peroxygen that used in acne medication solutions. It kills the bacterium Propionibacterium acnes , which is associated with acne. Carbamide peroxide, an ingredient used in toothpaste, is a peroxygen that combats oral biofilms that cause tooth discoloration and halitosis (bad breath). 22 Last, ozone gas is a peroxygen with disinfectant qualities and is used to clean air or water supplies. Overall, peroxygens are highly effective and commonly used, with no associated environmental hazard.
- How do peroxides kill cells?
Supercritical Fluids
Within the last 15 years, the use of supercritical fluids , especially supercritical carbon dioxide (scCO 2 ), has gained popularity for certain sterilizing applications. When carbon dioxide is brought to approximately 10 times atmospheric pressure, it reaches a supercritical state that has physical properties between those of liquids and gases. Materials put into a chamber in which carbon dioxide is pressurized in this way can be sterilized because of the ability of scCO 2 to penetrate surfaces.
Supercritical carbon dioxide works by penetrating cells and forming carbonic acid , thereby lowering the cell pH considerably. This technique is effective against vegetative cells and is also used in combination with peracetic acid to kill endospores. Its efficacy can also be augmented with increased temperature or by rapid cycles of pressurization and depressurization, which more likely produce cell lysis.
Benefits of scCO 2 include the nonreactive, nontoxic, and nonflammable properties of carbon dioxide, and this protocol is effective at low temperatures. Unlike other methods, such as heat and irradiation, that can degrade the object being sterilized, the use of scCO 2 preserves the object’s integrity and is commonly used for treating foods (including spices and juices) and medical devices such as endoscopes. It is also gaining popularity for disinfecting tissues such as skin, bones, tendons, and ligaments prior to transplantation. scCO 2 can also be used for pest control because it can kill insect eggs and larvae within products.
- Why is the use of supercritical carbon dioxide gaining popularity for commercial and medical uses?
Chemical Food Preservatives
Chemical preservatives are used to inhibit microbial growth and minimize spoilage in some foods. Commonly used chemical preservatives include sorbic acid , benzoic acid , and propionic acid , and their more soluble salts potassium sorbate , sodium benzoate , and calcium propionate , all of which are used to control the growth of molds in acidic foods. Each of these preservatives is nontoxic and readily metabolized by humans. They are also flavorless, so they do not compromise the flavor of the foods they preserve.
Sorbic and benzoic acids exhibit increased efficacy as the pH decreases. Sorbic acid is thought to work by inhibiting various cellular enzymes, including those in the citric acid cycle, as well as catalases and peroxidases . It is added as a preservative in a wide variety of foods, including dairy, bread, fruit, and vegetable products. Benzoic acid is found naturally in many types of fruits and berries, spices, and fermented products. It is thought to work by decreasing intracellular pH, interfering with mechanisms such as oxidative phosphorylation and the uptake of molecules such as amino acids into cells. Foods preserved with benzoic acid or sodium benzoate include fruit juices, jams, ice creams, pastries, soft drinks, chewing gum, and pickles.
Propionic acid is thought to both inhibit enzymes and decrease intracellular pH, working similarly to benzoic acid. However, propionic acid is a more effective preservative at a higher pH than either sorbic acid or benzoic acid. Propionic acid is naturally produced by some cheeses during their ripening and is added to other types of cheese and baked goods to prevent mold contamination. It is also added to raw dough to prevent contamination by the bacterium Bacillus mesentericus , which causes bread to become ropy.
Other commonly used chemical preservatives include sulfur dioxide and nitrites . Sulfur dioxide prevents browning of foods and is used for the preservation of dried fruits; it has been used in winemaking since ancient times. Sulfur dioxide gas dissolves in water readily, forming sulfites . Although sulfites can be metabolized by the body, some people have sulfite allergies, including asthmatic reactions. Additionally, sulfites degrade thiamine, an important nutrient in some foods. The mode of action of sulfites is not entirely clear, but they may interfere with the disulfide bond (see Figure 7.21 ) formation in proteins, inhibiting enzymatic activity. Alternatively, they may reduce the intracellular pH of the cell, interfering with proton motive force-driven mechanisms.
Nitrites are added to processed meats to maintain color and stop the germination of Clostridium botulinum endospores. Nitrites are reduced to nitric oxide , which reacts with heme groups and iron-sulfur groups. When nitric oxide reacts with the heme group within the myoglobin of meats, a red product forms, giving meat its red color. Alternatively, it is thought that when nitric acid reacts with the iron-sulfur enzyme ferredoxin within bacteria, this electron transport-chain carrier is destroyed, preventing ATP synthesis. Nitrosamines, however, are carcinogenic and can be produced through exposure of nitrite-preserved meats (e.g., hot dogs, lunch meat, breakfast sausage, bacon, meat in canned soups) to heat during cooking.
Natural Chemical Food Preservatives
The discovery of natural antimicrobial substances produced by other microbes has added to the arsenal of preservatives used in food. Nisin is an antimicrobial peptide produced by the bacterium Lactococcus lactis and is particularly effective against gram-positive organisms. Nisin works by disrupting cell wall production, leaving cells more prone to lysis. It is used to preserve cheeses, meats, and beverages.
Natamycin is an antifungal macrolide antibiotic produced by the bacterium Streptomyces natalensis . It was approved by the FDA in 1982 and is used to prevent fungal growth in various types of dairy products, including cottage cheese, sliced cheese, and shredded cheese. Natamycin is also used for meat preservation in countries outside the United States.
- What are the advantages and drawbacks of using sulfites and nitrites as food preservatives?
- 8 US Food and Drug Administration. "FDA Issues Final Rule on Safety and Effectiveness of Antibacterial Soaps." 2016. https://www.fda.gov/news-events/press-announcements/fda-issues-final-rule-safety-and-effectiveness-antibacterial-soaps. Accessed October 29, 2020.
- 9 J. Stromberg. “Five Reasons Why You Should Probably Stop Using Antibacterial Soap.” Smithsonian.com January 3, 2014. http://www.smithsonianmag.com/science-nature/five-reasons-why-you-should-probably-stop-using-antibacterial-soap-180948078/?no-ist. Accessed June 9, 2016.
- 10 SP Yazdankhah et al. “Triclosan and Antimicrobial Resistance in Bacteria: An Overview.” Microbial Drug Resistance 12 no. 2 (2006):83–90.
- 11 L. Birošová, M. Mikulášová. “Development of Triclosan and Antibiotic Resistance in Salmonella enterica serovar Typhimurium.” Journal of Medical Microbiology 58 no. 4 (2009):436–441.
- 12 AB Dann, A. Hontela. “Triclosan: Environmental Exposure, Toxicity and Mechanisms of Action.” Journal of Applied Toxicology 31 no. 4 (2011):285–311.
- 13 US Centers for Disease Control and Prevention. “Triclosan Fact Sheet.” 2013. http://www.cdc.gov/biomonitoring/Triclosan_FactSheet.html. Accessed June 9, 2016.
- 14 EM Clayton et al. “The Impact of Bisphenol A and Triclosan on Immune Parameters in the US Population, NHANES 2003-2006.” Environmental Health Perspectives 119 no. 3 (2011):390.
- 15 N. Silvestry-Rodriguez et al. “Silver as a Disinfectant.” In Reviews of Environmental Contamination and Toxicology , pp. 23-45. Edited by GW Ware and DM Whitacre. New York: Springer, 2007.
- 16 B. Owens. “Silver Makes Antibiotics Thousands of Times More Effective.” Nature June 19 2013. http://www.nature.com/news/silver-makes-antibiotics-thousands-of-times-more-effective-1.13232
- 17 C. Seiler, TU Berendonk. “Heavy Metal Driven Co-Selection of Antibiotic Resistance in Soil and Water Bodies Impacted by Agriculture and Aquaculture.” Frontiers in Microbiology 3 (2012):399.
- 18 World Health Organization. “Benefits and Risks of the Use of Chlorine-Containing Disinfectants in Food Production and Food Processing: Report of a Joint FAO/WHO Expert Meeting.” Geneva, Switzerland: World Health Organization, 2009.
- 19 RE Marquis. “Antimicrobial Actions of Fluoride for Oral Bacteria.” Canadian Journal of Microbiology 41 no. 11 (1995):955–964.
- 20 L. Thomas et al. “Development of Resistance to Chlorhexidine Diacetate in Pseudomonas aeruginosa and the Effect of a ‘Residual’ Concentration.” Journal of Hospital Infection 46 no. 4 (2000):297–303.
- 21 Institute of Medicine. “Long-Term Health Effects of Participation in Project SHAD (Shipboard Hazard and Defense).” Washington, DC: The National Academies Press, 2007.
- 22 Yao, C.S. et al. “In vitro antibacterial effect of carbamide peroxide on oral biofilm.” Journal of Oral Microbiology Jun 12, 2013. http://www.ncbi.nlm.nih.gov/pmc/articles/PMC3682087/. doi: 10.3402/jom.v5i0.20392.
As an Amazon Associate we earn from qualifying purchases.
This book may not be used in the training of large language models or otherwise be ingested into large language models or generative AI offerings without OpenStax's permission.
Want to cite, share, or modify this book? This book uses the Creative Commons Attribution License and you must attribute OpenStax.
Access for free at https://openstax.org/books/microbiology/pages/1-introduction
- Authors: Nina Parker, Mark Schneegurt, Anh-Hue Thi Tu, Philip Lister, Brian M. Forster
- Publisher/website: OpenStax
- Book title: Microbiology
- Publication date: Nov 1, 2016
- Location: Houston, Texas
- Book URL: https://openstax.org/books/microbiology/pages/1-introduction
- Section URL: https://openstax.org/books/microbiology/pages/13-3-using-chemicals-to-control-microorganisms
© Jan 10, 2024 OpenStax. Textbook content produced by OpenStax is licensed under a Creative Commons Attribution License . The OpenStax name, OpenStax logo, OpenStax book covers, OpenStax CNX name, and OpenStax CNX logo are not subject to the Creative Commons license and may not be reproduced without the prior and express written consent of Rice University.
- Skip to Content
- Skip to Menu
- Increase Text Size
[1].png)
Why It's Important to Clean, Disinfect and Sanitize
- Category: General
- Posted On: Jan 13, 2021
While it’s easier to pinpoint our sickness's cause if exposed to someone who fell ill, we must also keep in mind that germs and bacteria easily spread onto objects and surfaces quickly and easily. From coughing or sneezing, droplets spread out into the air and land on anything underneath it. Even if you weren’t directly exposed to someone under the weather, their germs might have migrated by people touching things in one area and leaving a trail from one room to another. Therefore, we need to cover our noses and mouth to prevent droplets from spreading, even if you aren’t sick. Not only is it good practice, but it’s hygienic and keeps the areas you occupy free from possible buildup of dirt and bacteria.
But merely reducing our droplets spread isn’t the only measure we can take to prevent spreading germs. When we take the time to clean the spaces we use—our offices and homes, most prominently—we keep our health in check and the people around us. Cleaning, however, isn’t as simple as we make it out to be. The term ‘cleaning’ is typically used as a catch-all for reducing dirt, debris, grime, germs and pathogens, but there are different cleaning habits: cleaning, disinfecting and sanitizing.
What are the differences between the two? And more importantly, why is it important to understand them individually? Between cleaning, disinfecting, and sanitizing, each offers solutions to a much larger problem: stop germs from spreading across shared spaces and reducing the chances of getting sick. All are important and good practices to increase our chances of staying healthy, but one might be better than the other depending on the environment, cleaning solutions and people involved in the cleaning process.
The main differences between cleaning, disinfecting and sanitizing according to the CDC :
- Cleaning: Using soaps or detergent with water physically removes dirt, grime and germs from surfaces. Cleaning areas remove debris and bacteria but does not kill them. However, it does lower pathogens numbers and lowers the risk of infections spreading.
- Disinfecting: The best way to disinfect surfaces and objects is with chemical cleaning agents so germs and bacteria are killed. While this does not completely clean or remove dirt and germs, it lowers the risk of getting sick due to the killing of live pathogens.
- Sanitizing: Composed of cleaning and disinfecting practices, sanitizing lowers the number of germs on surfaces and objects. In turn, this minimizes the spread of illnesses.
Using a combination of these methods to sterilize the objects and surfaces we interact with decreases our health risk. Some pathogens and allergens wreak havoc on our immune system year-round despite illnesses seemingly more prominent during the winter months; when more people are indoors to stay out of the cold, the chances of getting sick skyrocket. The least we can do for ourselves is to find solutions to support our health.
By cleaning the spaces we use, it prevents us from getting sick from:
- Influenza: Flu shots are one of the most common ways to protect yourself against contracting influenza , and it’s essential to get one every year. These shots are updated every year based on the most prevalent strain of the virus each season. But the flu spreads easily, which is why it’s essential to clean the areas you interact with, cover your nose and mouth when coughing or sneezing and understand that the contagious peak is anywhere from three to four days. Disinfecting and sanitizing will kill and remove influenza germs and reduce the risk of sickness and possible emergency medical treatment from complications.
- Colds: Like the flu, colds spread through respiratory droplets because most germs are spread by hands. Anyone can catch it, and there isn’t a direct cure for it yet. However, when we’re diligent about reducing the germs that carry cold viruses, we eliminate our chances of getting it and spreading it to others. The first signs of a cold are sore throats and runny noses, so if you do get sick, stay home to protect others.
- Allergies: Fortunately, allergies and seasonal allergies do not spread from person to person like viral infections, but they can still cause problems for individuals who are allergic to dust, pollen, animal dandruff and more. Allergies spread similarly to germs because they are particles that float through the air or land on surfaces. When in contact with the body, irritation happens, leading to uncomfortable symptoms—from itchy eyes to runny noses, rhinitis or sinus allergies. However, not everyone is affected by allergens, but for those that are, it’s critical to keep up a regular procedure of dusting, cleaning and minimizing time outdoors in areas where pollen is high.
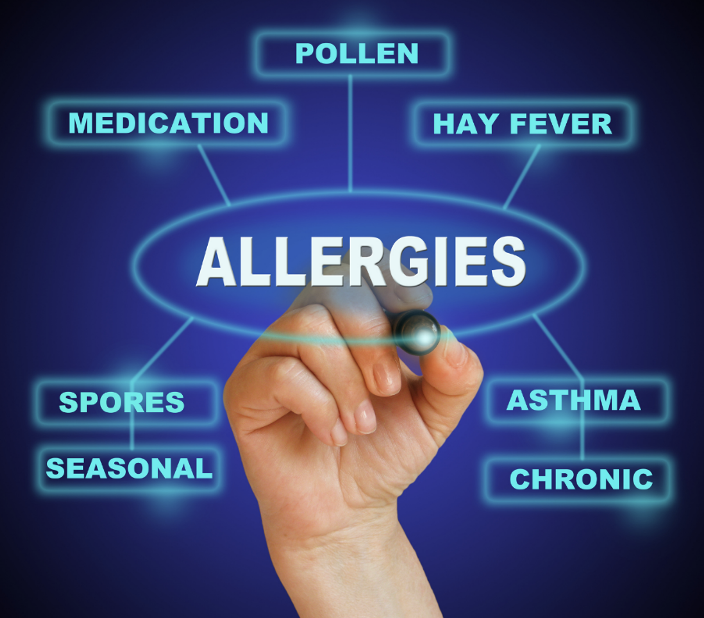
- Bad indoor air quality: While outdoor air is commonly associated with allergens, indoor air also holds irritants. In fact, poor indoor air is a significant problem for health issues, including irritation of the eyes, nose and throat. It can also induce headaches, dizziness and fatigue. Poor air quality is often linked to the HVAC unit's insufficient circulation within the building and results from the pollution that enters into the space and gets trapped inside. Asbestos, formaldehyde, radon, paints, fumes, pesticides, mold and mildew contribute to the air's health indoors. Minimizing these particles with regular upkeep and cleaning can detract from illnesses.
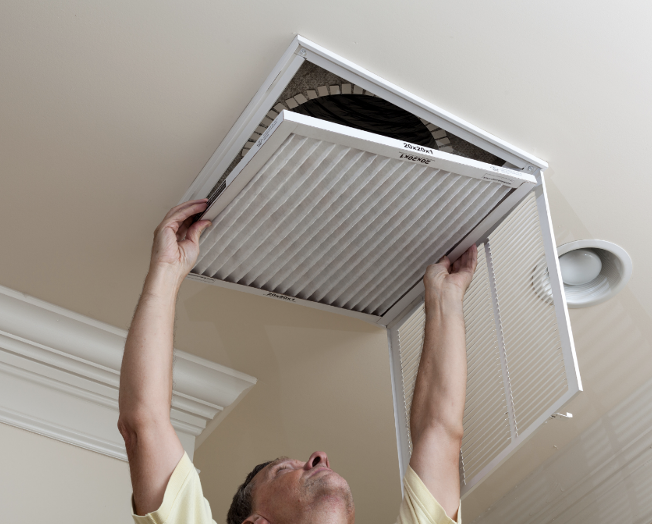
- Stomach flu: Unlike influenza, stomach flu is an entirely different type of illness that affects the intestines . It’s also different from food poisoning because the stomach flu is a contagious virus that manifests within one or two days of contraction; food poisoning usually hits within one to six hours of eating or drinking contaminated items. Most people fall ill from the stomach flu from being in contact with someone who is sick. Washing your hands and cleaning surfaces and objects can prevent the spread of the stomach flu.
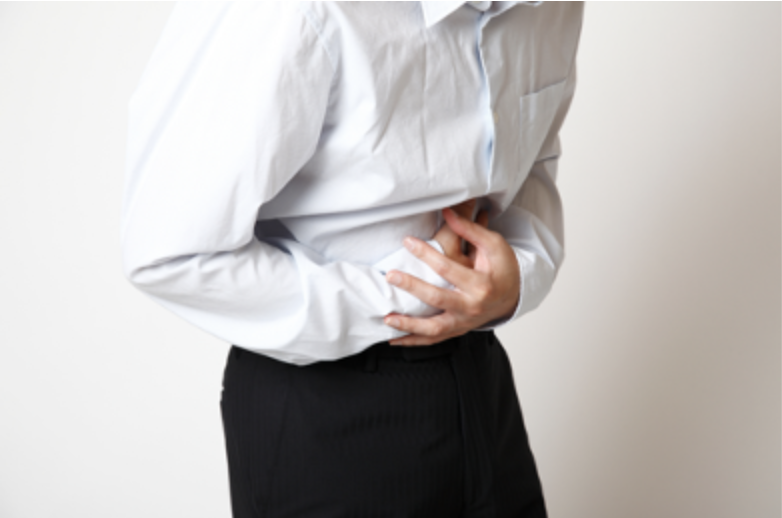
Additional cleaning habits can also include washing your hands frequently and wearing a mask, especially if you think you’re coming down with an illness. The main reason to be mindful of cleaning, disinfecting, and sanitizing is that we’re protecting ourselves and the health of others around us. Unfortunately, sickness affects us all, and when we do our part to minimize it, we create environments that remain happy and productive.
If you’re feeling unwell, think you’ve been exposed to an infection or have allergies that are causing you severe problems, consider stopping by Baptist Emergency Room & Urgent Care.
Baptist Emergency Room & Urgent Care's emergency room is open 24/7, and urgent care is available from 7 a.m. to 8 p.m. every day. Appointments are unnecessary - walk in, and our healthcare professionals are ready to help at both of our locations.
Navarre - 8888 Navarre Pkwy., Navarre, FL 32566. Call the center at 850-750-5698.
Nine Mile - 9400 University Parkway Suite 101A Pensacola, FL 32514. Call the center at 448-227-4600.
Baptist Emergency Room & Urgent Care is comprised of board-certified ER-trained physicians and professionally trained ER nurses who deliver quality medical care. Our urgent care and emergency room can effectively treat various medical conditions. From fever to fractures, allergies to abdominal pain, and colds to concussions, we have you covered with on-site labs, CT, X-rays, and ultrasound.
Disinfection
Guideline for Disinfection and Sterilization in Healthcare Facilities (2008)
- Methods of Disinfection
Many disinfectants are used alone or in combinations (e.g., hydrogen peroxide and peracetic acid) in the health-care setting. These include alcohols, chlorine and chlorine compounds, formaldehyde, glutaraldehyde, ortho -phthalaldehyde, hydrogen peroxide, iodophors, peracetic acid, phenolics, and quaternary ammonium compounds. Commercial formulations based on these chemicals are considered unique products and must be registered with EPA or cleared by FDA. In most instances, a given product is designed for a specific purpose and is to be used in a certain manner. Therefore, users should read labels carefully to ensure the correct product is selected for the intended use and applied efficiently.
Disinfectants are not interchangeable, and incorrect concentrations and inappropriate disinfectants can result in excessive costs. Because occupational diseases among cleaning personnel have been associated with use of several disinfectants (e.g., formaldehyde, glutaraldehyde, and chlorine), precautions (e.g., gloves and proper ventilation) should be used to minimize exposure 318, 480, 481 . Asthma and reactive airway disease can occur in sensitized persons exposed to any airborne chemical, including germicides. Clinically important asthma can occur at levels below ceiling levels regulated by OSHA or recommended by NIOSH. The preferred method of control is elimination of the chemical (through engineering controls or substitution) or relocation of the worker.
The following overview of the performance characteristics of each provides users with sufficient information to select an appropriate disinfectant for any item and use it in the most efficient way.
- Chlorine and chlorine compounds
- Formaldehyde
- Glutaraldehyde
- Hydrogen peroxide
- Ortho-phthalaldehyde (OPA)
- Peracetic acid
- Peracetic acid and hydrogen peroxide
- Quaternary ammonium compounds
- Other germicides
- Metals as microbicides
- Ultraviolet radiation
- Pasteurization
- Flushing- and washer-disinfectors
- Regulatory Framework for Disinfectants and Sterilants
To receive email updates about this page, enter your email address:
Exit Notification / Disclaimer Policy
- The Centers for Disease Control and Prevention (CDC) cannot attest to the accuracy of a non-federal website.
- Linking to a non-federal website does not constitute an endorsement by CDC or any of its employees of the sponsors or the information and products presented on the website.
- You will be subject to the destination website's privacy policy when you follow the link.
- CDC is not responsible for Section 508 compliance (accessibility) on other federal or private website.
Advertisement
The role of disinfectants and sanitizers during COVID-19 pandemic: advantages and deleterious effects on humans and the environment
- Review Article
- Published: 15 May 2021
- Volume 28 , pages 34211–34228, ( 2021 )
Cite this article
- Kuldeep Dhama 1 ,
- Shailesh Kumar Patel 1 ,
- Rakesh Kumar 2 ,
- Rupali Masand 2 ,
- Jigyasa Rana 3 ,
- Mohd. Iqbal Yatoo 4 ,
- Ruchi Tiwari 5 ,
- Khan Sharun 6 ,
- Ranjan K. Mohapatra 7 ,
- Senthilkumar Natesan 8 ,
- Manish Dhawan 9 , 10 ,
- Tauseef Ahmad 11 , 12 ,
- Talha Bin Emran 13 ,
- Yashpal Singh Malik 14 &
- Harapan Harapan ORCID: orcid.org/0000-0001-7630-8413 15 , 16 , 17
32k Accesses
100 Citations
23 Altmetric
Explore all metrics
Disinfectants and sanitizers are essential preventive agents against the coronavirus disease 2019 (COVID-19) pandemic; however, the pandemic crisis was marred by undue hype, which led to the indiscriminate use of disinfectants and sanitizers. Despite demonstrating a beneficial role in the control and prevention of COVID-19, there are crucial concerns regarding the large-scale use of disinfectants and sanitizers, including the side effects on human and animal health along with harmful impacts exerted on the environment and ecological balance. This article discusses the roles of disinfectants and sanitizers in the control and prevention of the current pandemic and highlights updated disinfection techniques against severe acute respiratory syndrome coronavirus 2 (SARS-CoV-2). This article provides evidence of the deleterious effects of disinfectants and sanitizers exerted on humans, animals, and the environment as well as suggests mitigation strategies to reduce these effects. Additionally, potential technologies and approaches for the reduction of these effects and the development of safe, affordable, and effective disinfectants are discussed, particularly, eco-friendly technologies using nanotechnology and nanomedicine.
Similar content being viewed by others
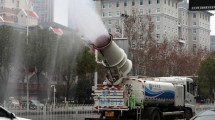
High concentration and high dose of disinfectants and antibiotics used during the COVID-19 pandemic threaten human health
Zhongli Chen, Jinsong Guo, … Ying Shao
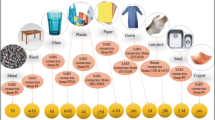
Environmental and health impacts of spraying COVID-19 disinfectants with associated challenges
Shakeel Ahmad Bhat, Farooq Sher, … Eder C. Lima
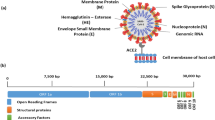
Environmental aspect and applications of nanotechnology to eliminate COVID-19 epidemiology risk
Eman Serag & Marwa El-Zeftawy
Avoid common mistakes on your manuscript.
Introduction
Intensive global research efforts have been engaged for the development of potential therapies and vaccines for coronavirus disease 2019 (COVID-19), caused by severe acute respiratory syndrome coronavirus 2 (SARS-CoV-2) (Dhama et al. 2020a , b ; WHO 2021 ), and the control of the COVID-19 pandemic remains the highest priority globally. Considering the current ineffectiveness of various strategies in the prevention of the spread of the virus, the lack of targeted treatments, and frequent increase in cases on a daily basis, disinfection is an important available measure to prevent COVID-19 spread and to combat SARS-CoV-2 directly.
Successful disinfection of SARS-CoV-2 is determined by the characteristics of the virus, properties of the disinfectants or sanitizers, and the environment where the virus is present or where disinfection is to be conducted. Disinfectants are chemical agents that are used to inactivate or destroy microorganisms, while sanitizers are available in the liquid, gel, or foam forms that are used to reduce the number of microorganisms present and to clean hands. SARS-CoV-2 is susceptible to disinfection (Rutala and Weber 2019 ; WHO 2020 ), exhibits stability at a broad range of pH values (pH 3–10) at room temperature (Chin et al. 2020 ), and is very much stable in a favorable environment (van Doremalen et al. 2020 ). SARS-CoV-2 persists for variable durations in different environments; for instance, it can reside on the outer layer of surgical masks for up to 7 days, and its presence on smooth surfaces (glass, plastic, banknotes, stainless steel) varies from 4 to 7 days (Chin et al. 2020 ). SARS-CoV-2 has not been detected on printing and tissue papers after 3 h, and treated wood and cloth show negative results for SARS-CoV-2 after 2 days (Chin et al. 2020 ). SARS-CoV-2 demonstrates increased stability on stainless steel and plastic as compared to copper and cardboard, and viable virus has been detected up to 72 h of application to such surfaces (van Doremalen et al. 2020 ). Furthermore, it has been detected in sewage and wastewater (Randazzo et al. 2020 ; Dhama et al. 2021 ).
SARS-CoV-2 is susceptible to a wide variety of disinfectants (Chin et al. 2020 ; United States Environmental Protection Agency (EPA), 2020). Lipid solvents, including ethanol (> 75%), formaldehyde (> 0.7%), isopropanol (> 70%), povidone-iodine (> 0.23%), sodium hypochlorite (> 0.21%), or hydrogen peroxide (H 2 O 2 ; > 0.5%), can also be used to inactivate SARS-CoV-2 (Duarte and de Santana 2020 ). Considering viral presence, persistence, stability, viability, and environmental influence on viral persistence, the disinfection of environments, such as offices, healthcare settings, public transportation, markets, restaurants, and auditoriums, is necessary to prevent the transmission and infection waves of COVID-19.
However, strategies and techniques of cleaning, sanitization, disinfection, and other methods to contain the devastating effects of the pandemic should be subjected to modification development over time according to their deleterious consequences on the environment and human health (Mukherjee et al. 2021 ). Hence, the present article highlights the beneficial usages, effectiveness, modes of antiviral action, and deleterious consequences of different disinfectants, along with a brief note on sanitizers, while also discussing the mitigation strategies with updated disinfection approaches to counter their harmful effects during the COVID-19 pandemic.
Disinfectants and their antiviral mechanisms
Disinfectants are chemical agents that are specifically formulated to inactivate or destroy microorganisms and include various classes, such as detergents, acids, oxidizing agents, alcohols, alkalis, aldehydes, biguanides, halogens, phenols, and quaternary ammonium compounds (QACs) (FDA 2020a ; Choi et al. 2021 ). Chemical disinfectants vary in their action mechanism, and the majority of disinfectants of a chemical nature target the outer lipid layer of coronaviruses (CoVs) and inactivate the viral particles (Choi et al. 2021 ). However, variations among the mechanisms of chemical disinfectants have been recorded. Detergents are a well-known category of chemical disinfectants that vary in their mechanism of action depending upon the class of disinfectant, the organism against which the disinfectant exhibits strong activity, the structure being affected, the surface or medium on which the disinfectant is applied, and the environment of application (Maris 1995 ; McDonnell and Russell 1999 ). Disinfectants may initiate three types of killing mechanisms that include cross-linking, coagulation, clumping, structural and functional disruption, and oxidation. These processes can occur through oxidation, hydrolysis, denaturation, or substitution (Ewart 2001 ). In case of viruses, they may affect the lipid membrane, cytoplasmic membrane, energy metabolism, cytoplasm, nucleus, enzymes, or proteins (Maris 1995 ). Non-ionic (uncharged) detergents are preferred over anionic detergents as they are good emulsifiers and exhibit better penetration and dispersion, decreased surface tension, lesser foaming property, and do not undergo complexation with hard water and result in microbial accumulation in the residue (Ewart 2001 ; CFSPH 2008 ).
Alcohol causes damage to microorganisms by denaturing proteins, leading to membrane damage and cell lysis (Ewart 2001 ; CFSPH 2008 ; Al-Sayah 2020 ). Ethanol shows appreciable activity on both living and non-living surfaces and evaporates quickly without leaving residue (CFSPH 2008 ). Ethanol at > 75% concentration acts as a potent virucidal agent that inactivates all lipophilic viruses (herpes, influenza, and vaccinia) and several hydrophilic viruses (adenovirus, rhinovirus, enterovirus, and rotaviruses). At a concentration of > 70%, ethanol and isopropanol inactivate CoVs within 30 s (Kampf et al. 2020a ). Isopropyl alcohol is extremely active against lipid viruses (CDC 2008 ). The primary mode of action is the coagulation and denaturation of proteins, apart from its lipid solvent properties.
Chlorine aids the oxidation of peptide links owing its electronegativity, and therefore, causes the oxidation of lipids and proteins and, in turn, inflicts damage on the membrane and cell wall of the microbes (McDonnell and Russell 1999 ). Moreover, hypochlorous acid is the most active compound, and it penetrates the cell layers even at a pH of 7. QACs damage the membrane permeability of microbes by irreversibly binding to phospholipids and proteins of the membrane (Gerba 2015 ). An alkaline pH (above 10.0) results in the disorganization of the peptidoglycan structure and leads to hydrolysis of the virus genome (Maris 1995 ). Phenolic compounds act specifically on the cell membrane and lead to the inactivation of the intracytoplasmic enzymes by forming unstable complexes (Sankar et al. 2016 ). Acids and alkalis mediate their antiviral action through H + and OH − ions that inflict damage on the amino acid bond in nucleic acids, modify the cytoplasmic pH, precipitate proteins, and saponify the lipids (Russell 1983 ; Maris 1995 ). H 2 O 2 catalyzes the oxidation and denaturation of proteins and lipids, causing membrane disorganization, resulting in swelling due to the saturation of H + ions (Russell 1983 ; Maris 1995 ; Al-Sayah 2020 ). Against SARS-CoV, H 2 O 2 exhibits virucidal activity at a 1–3% concentration and inactivates the virus within 1 min; however, the gaseous form is more efficient (Herzog et al. 2012 ; Goyal et al. 2014 ). H 2 O 2 -based non-touch disinfection techniques help reduce environmental contamination, particularly in hospital settings and intensive care units with infectious agents after routine cleaning (Huttner and Harbarth 2015 ; Blazejewski et al. 2015 ). Airborne H 2 O 2 in the form of vapor and dry mist has been used as an environmental disinfectant and to control infection in clinical settings (Falagas et al. 2011 ). Aldehydes demonstrate activity against CoVs at concentrations of 0.5–3% and result in viral deactivation within 2 min (Al-Sayah 2020 ). Povidone–iodine at a concentration of 1% or less triggers SARS-CoV inactivation within seconds (Kariwa et al. 2006 ; Eggers et al. 2018 ).
If the lipid envelope, glycoproteins, attachment (spike) proteins, and virion genome of CoVs, which impart infectivity to the virus, undergo damage or disruption, then the aftermath of such an occurrence is virus inactivation or exertion of effects on its infectivity (Al-Sayah 2020 ). Disinfectants have been evaluated and recommended against SARS-CoV-2 (Noorimotlagh et al. 2020 ). Among these, ethanol at a concentration > 70% and 2-propanol at a concentration of 70–100% have shown virucidal activity, while others have demonstrated either less effectivity or complete ineffectivity, with several agents posing a threat to human health and the environment (Kampf et al. 2020a ; Lai et al. 2020 ; Noorimotlagh et al. 2020 ; Schrank et al. 2020).
Necessity of disinfection during the COVID-19 pandemic
A recent study predicted that a considerable proportion of the global population would eventually be infected by SARS-CoV-2 (Giesecke 2020 ). The only available method of containing this pandemic is to prevent further transmission and to confer protection to individuals against exposure to the virus. Implementation of strict lockdowns, rampant testing, contact tracing, quarantine, isolation, and treatment approaches have decelerated the virus spread to a certain extent, and now it is imperative to adopt effective disinfection procedures to ensure the safety of populations after the lifting of lockdown and resumption of on-site work. Considering the persistence of SARS-CoV-2 on surfaces and the potential risk of infection through fomites, disinfection of the work environment is a priority before the resumption of regular working environments (ECDC 2020 ; van Doremalen et al. 2020 ). As SARS-CoV-2 survives in the environment with persistence ranging from hours (3 h in the air, 4 h on copper, and 24 h on cardboard) to days (2 to 3 days on both stainless steel and plastic), the disinfection of workplaces is imperative, especially where public visits or assemblies of crowds are inevitable (ECDC 2020 ; van Doremalen et al. 2020 ). Similarly, SARS-CoV-2 can persist for days on non-porous surfaces under 22°C and 65% relative humidity (Chin et al. 2020 ). Moreover, it has also been detected on desktops, printers, keyboards, doorknobs, gloves, and eye shields (ECDC 2020 ). A comparative study of SARS-CoV-1 and SARS-CoV-2 showed that the viability of the two CoVs is similar; however, SARS-CoV-2 spread is characterized by rapid dissemination and infection of more people (Gates 2020 ).
Although most SARS-CoV-2 transmissions occur in community settings, healthcare settings are also vulnerable to the establishment and spread of infections. In this context, hospitals engaged in treating patients with COVID-19 must be equipped with the most appropriate disinfection techniques and materials for the disinfection of healthcare personnel, hospital rooms, and medical equipment to avoid nosocomial transmission. The guidelines for the application of disinfectants in healthcare and non-healthcare settings issued by various agencies must be followed while using disinfectants in different environments (ECDC 2020 ; US EPA 2020 ).
Disinfection is a prerequisite for the control of infectious disease outbreaks, with SARS-CoV-2 containment being of utmost importance. It is vital to reduce the potential for virus contamination. Disinfection may also lessen the burden on other measures of pandemic control. Commonly, sodium hypochlorite, ethanol, and H 2 O 2 have been used and found to be more effective compared to benzalkonium chloride (BAC), chlorhexidine digluconate, povidoeyodine, and diluted ethyl alcohol, especially with reference to their application in hand hygiene, protective equipment sanitization, and in environmental disinfection (León Molina and Abad-Corpa 2021 ). Rowan and Laffey ( 2020 ) proposed the disinfection of personal protective equipment (PPE) for their reuse and the utilization of vaporized H 2 O 2 for the sterilization of filtering facepiece respirators and UV irradiation. Increased extent of liquid disinfection (Actichlor+) is being adopted in the USA and Ireland. This will help prevent a shortage of PPE. The European Centre for Disease Prevention and Control (ECDC) has suggested different cleaning options for different settings, which are described in Table 1 (ECDC 2020 ).
Moreover, several chemical disinfectants with high toxicity are being used for the decontamination of the surfaces of several environmental settings, such as clinical and surgical practices and water bodies, which is imperative to ensure well-being and safety. However, the development of new decontamination strategies, which neither leave residues nor induce toxicity, is vital. The following section emphasizes the updated and modified disinfection approaches to contain the overwhelming effects of the COVID-19 pandemic.
An overview of the important disinfectants and their antiviral mechanisms and the need for disinfection during the COVID-19 pandemic is illustrated in Fig. 1 .
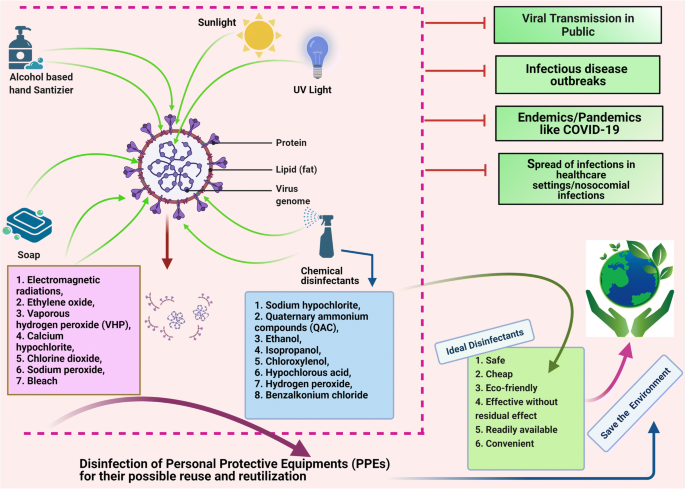
Disinfectants and their antiviral mechanisms (such as targeting lipid layer and spike proteins) and the need of disinfection during COVID-19 pandemic. The figure was created with BioRender.com
Updated disinfection approaches against SARS-CoV-2
Commonly used disinfectants against COVID-19 include detergents/soaps, alcohols, and chlorine. Chlorine is recommended as a disinfectant for indoor facilities (Yang et al. 2020 ). In healthcare settings, equipment, including imaging devices (e.g., endoscopes), scanners, bedding, and contact instruments, must be disinfected using appropriate disinfectants (Prochazka Zá Rate et al. 2020 ; Wan et al. 2020 ). To perform disinfection of the floor, environment, and table surfaces, application of 2 g/L of chlorine-containing disinfectant is recommended at least four times daily for at least 30 min, while application of UV irradiation and spraying techniques using 500 mg/L of chlorine-containing disinfectant for at least 30 min are the methods of choice for the disinfection of air (Barcelo 2020 ). Additionally, personal items, such as mobile phones, keys, credit cards, and writing pens, may be subjected to sanitization using 75% ethanol to ensure their disinfection (Yang et al. 2020 ). Virusend (TX-10) is a unique disinfectant that has already exhibited efficient activity against several microbial agents, including viruses. Virusend (TX-10) application was found to inactivate SARS-CoV-2 in an in vitro study based on surface and solution inactivation assays. This novel disinfectant rapidly decreased the SARS-CoV-2 viral titer by 4log 10 PFU/mL within a contact period of 1 min (Anderson et al. 2020 ).
The use of plasma-activated water is another alternative to conventional disinfectants that can efficiently be used to inactivate bacteria and bacteriophages via induction of direct damage to the biological macromolecules. Plasma-activated water can be used to inhibit SARS-CoV-2 pseudovirus infection via inactivation of the S protein (Guo et al. 2020 ). The alcohol-based hand sanitizers (ABHSs) that are commonly used on skin are not suitable for use on PPE. Therefore, new compounds or products should be developed that can be used to effectively disinfect PPE without causing detrimental effects to the surrounding skin tissue. Clyraguard copper iodine complex is a product that has exhibited potential efficacy in the decontamination of non-critical PPE (Mantlo et al. 2020 ).
As various environmental factors are considered to determine the transmission of SARS-CoV-2, disinfection of surfaces in work environments with diluted sodium hypochlorite (0.1%) is recommended (Eslami and Jalili 2020 ; Seymour et al. 2020 ). CoVs present on inanimate objects or surfaces may be inactivated by conducting treatment with biocidal agents, such as 0.5% H 2 O 2 , 62%–71% ethanol, and 0.1% sodium hypochlorite, for at least 1 min (Kampf et al. 2020a ). Presently, the disinfection of fabrics and carpets used in hospitals is nearly neglected, though such materials are highly prone to contamination by aerosols and dust. Standard procedures for the disinfection of surface coverings and linens include laundering, cleaning with water, and vacuuming, which, in many cases, are inadequate to remove infectious load (Malik et al. 2006a ). The carpets of shopping malls where a COVID-19-positive patient may have visited are disinfected by spraying 1% NaOCl, and the disinfection time is 30 min for spraying of disinfectants, as recommended by the WHO and the Central Pollution Control Board in India. Chlorine has been applied as a disinfectant to contain SARS-CoV-2 in indoor and outdoor spaces in China (China Ministry of Ecology and Environment 2020 ). Thermal methods involving temperatures of at least 50°C for conducting 30-min Uc radiation (100-μwcm −2 power) with a contact time of 15 to 20 min and H 2 O 2 vapor are being used for the disinfection of masks, especially N95 masks (Card et al. 2020 ; Seymour et al. 2020 ). Moreover, one study with the inclusion of original data based on 10 studies indicated that thermal disinfection at 80°C for 1 min, 65°C for 15 min, and 60°C for 30 min was highly effective in reducing CoV infectivity by at least 4 log 10 (Kampf et al. 2020b ). In this context, thermal aggregation of the membrane protein along with complete denaturation of nucleocapsid protein (55°C for 10 min) of SARS-CoV is suggested as a probable explanation of infectivity reduction (Wang et al. 2004 ; Lee et al. 2005 ).
The US Environmental Protection Agency (EPA) has published a list of effective disinfectants for use against SARS-CoV-2, including sodium hypochlorite, QAC, ethanol, isopropanol, hypochlorous acid, chloroxylenol, H 2 O 2 , BAC, and chlorine-based chemicals (US EPA 2020 ). Common disinfectants against SARS-CoV-2 are listed in Table 2 . BAC and related disinfectants are ubiquitously used and have been recommended by the FDA for use in soaps, hospital sanitation kits, and cleaning wipes; however, knowledge of their efficacy against SARS-CoV-2 is crucial, and thus, they must be evaluated. In addition to alcohols and ABHSs, QACs, such as BAC, have been evaluated against CoVs but have shown less activity; thus, similar to other disinfectants, including sodium hypochlorite, peroxides, aldehydes (formaldehyde and glutardialdehyde), and didecyldimethylammonium chloride, the above-mentioned agents should be subjected to proper analysis to avoid their improper application as disinfectants against SARS-CoV-2 (Kampf et al. 2020a ; Lai et al. 2020 ; Schrank et al. 2020 ). The CDC issued a warning regarding the use of products containing BAC for the prevention of COVID-19 (Schrank et al. 2020 ). Additionally, CoVs undergo destruction within 15 min of exposure to ultraviolet C (UVC) light (Darnell et al. 2004 ). The beta-CoVs, including SARS-CoV and Middle East respiratory syndrome (MERS) CoV, are effectively inactivated by germicidal ultraviolet (UV) irradiation; however, its efficacy for inactivating SARS-CoV-2 warrants further investigation (Leung and Ko 2020 ). Germicidal UV lamps for household disinfection should be used with extreme caution because their improper use can cause epidermal photo-toxicity and photo-keratitis (Leung and Ko 2020 ). UV radiation is more dangerous and may severely damage the eyes and skin; repeated exposure to such radiation can also cause skin cancer (ICNIRP 2004 ). The use of human disinfection chambers is an example of recent innovations developed in response to the COVID-19 pandemic (Wickramatillake and Kurukularatne 2020 ).
As the number of COVID-19 cases continues to increase worldwide, a considerable shortage of N95 respirators has emerged. Therefore, it is essential to note that N95 respirators can be reused after disinfection. In a recent study, N95 respirators were subjected to conditions of heat at temperatures ≤ 85°C and relative humidity ≤ 100%, which resulted in the inactivation of the virus without affecting the filtration properties of the masks (Liao et al. 2020 ). Furthermore, the use of H 2 O 2 and hot air is the most effective method for the industrial and home disinfection of face masks, respectively. In contrast, surgical masks and homemade or non-certified masks are slightly less and significantly less effective than PPE and face masks, respectively (Carlos Rubio-Romero et al. 2020 ). The disinfection of used masks is necessary for their safe reuse while there is an acute shortage; however, incorrect decontamination procedures can damage the filtering structure of the masks. Medical masks and N95 masks retain their blocking efficacy against over 99% of viruses in aerosols even after subjection to steam conditions in boiling water for 2 h, suggesting that they can be reused for several days with the application of steam decontamination between uses (Ma et al. 2020 ). Disinfection of the masks and PPE after use and prior disposal is imperative. Otherwise, they may become a source of environmental contamination of SARS-CoV-2. The disinfection of N95 respirators may be essential during pandemics, such as the present COVID-19 pandemic, to overcome the curtailment crisis. However, the decontamination method should not alter the efficiency of the filtration of the N95 respirators and surgical masks. The use of UV germicidal irradiation, microwave-generated steam, moist heat, and H 2 O 2 vapor techniques should be strictly followed for the efficient containment of SARS-CoV-2.
Considering the transmission of COVID-19 in public transport vehicles, such as aircraft, ships, trains, subways, and buses, public transportation staff and passengers are advised to adopt strict preventive measures. All surfaces in public transport vehicles must be appropriately disinfected and sanitized. For this purpose, surfaces can be sprayed or wiped with chlorine-containing disinfectants (COVID-19 Emergency Response Key Places Protection and Disinfection Technology Team 2020a ). A disinfectant should be used at an appropriate concentration and sufficient contact exposure time should be allowed with the surface to destroy the virus.
The precise timing, the location, and the mechanism of disinfection, the type of disinfectant to be used, and safety measures to be implemented for both public health and the environment must be determined (Iyiola et al. 2020 ; Nabi et al. 2020 ; Zhang et al. 2020 ). Determination of the minimum amount of disinfectant necessary, appropriate exposure levels, and appropriate level of contact is essential for optimum action (Sarada et al. 2020 ). Avoidance of indiscriminate sprays, minimization of public movement, and the development of low-risk or nontoxic but effective disinfectants for future safe applications should be an international priority (Iyiola et al. 2020 ; Nabi et al. 2020 ; Zhang et al. 2020 ). Additionally, efforts should be engaged for the development of disinfection systems, such as UVC-based disinfection trolleys, UVC germicidal lamp-based fogging chambers, dry heat sterilization, and HOCl-based chemical disinfectants (Sarada et al. 2020 ).
Disinfection and its deleterious effects on humans and the environment
Improper and inappropriate use of disinfectants can result in the exertion of adverse effects. Excessive use of disinfectants poses a potential threat to living beings and ecosystems (Chen et al. 2021 ; Ghafoor et al. 2021 ) as they present with a myriad side effect (Yari et al. 2020 ). Disinfectants can affect both the applicant and the environment and may have future deleterious consequences (Yari et al. 2020 ). Chemical agents used as highly concentrated, aerosolized, or atomized disinfectants can easily be inhaled or absorbed into the skin. For example, aerosolized particles can penetrate alveoli upon inhalation. The increased frequency and duration of exposure to disinfectants (e.g., in disinfection chambers) can cause harmful effects on human and animal health. Disinfectants may cause reactions in the mucosal lining, resulting in irritation, inflammation, swelling, and ulceration of the upper and lower respiratory tract. A few chemicals are absorbed quickly through the mucosa of various organs and organ systems (e.g., the central nervous system and gastrointestinal tract) into the bloodstream. A recent case study reported the infliction of severe corrosive damage to gastric, esophageal, and small intestinal mucosa after the intentional oral ingestion of 10 mL of ethanol-containing hand disinfectant for 3 weeks, as the patient aimed to perform self-disinfection against COVID-19 owing to a fear of infection by the virus (Binder et al. 2020 ). Direct contact of the cornea and skin with aerosols may cause severe irritation and irreversible damage (Wickramatillake and Kurukularatne 2020 ). In addition to dryness of the skin, ABHSs can lead to infection and poisoning, particularly in children, who are believed to be susceptible and thus are subject to a major health risk (Ghafoor et al. 2021 ). Bleach (diluted sodium hypochlorite), one of the most commonly used disinfectants, can be directly absorbed by the skin, leading to allergic reactions. Additionally, various harmful effects, such as acute cardiopulmonary arrest, gastrointestinal ailments (e.g., nausea, vomiting, and diarrhea), and renal problems, have occurred in individuals after the accidental inhalation and ingestion of bleach (Peck et al. 2011 ). QAC and bleach reportedly increase the risk of development of asthma, chronic obstructive pulmonary disease (COPD), infertility, and impaired brain development in children (Fair 2020 ). The potential impact of disinfectants on individuals with asthma remains to be investigated, especially in the case of disinfectants with strong odors. Such disinfectants can act as potential asthma triggers (Eldeirawi et al. 2020 ). Therefore, individuals with asthma should use safer disinfection alternatives. A recently published cohort study revealed that out of 55,000 health professionals who used QAC and bleach routinely, 663 developed COPD (European Lung Foundation 2017 ). A correlation between the concentration of sodium hypochlorite and microscopic/cellular alterations, including chromosomal aberrations, cell death (apoptotic and necrotic changes), and increased mitotic activity, has also been documented (Gul et al. 2009 ). Additionally, psychotic episodes associated with a fear of death from SARS-CoV-2 infection can result in the consumption of liquid disinfectants or inhalation of aerosol sprays containing chlorine in an effort to cleanse their body. This can result in the infliction of primary inhalational toxic lung injury, which can mimic the symptoms of clinical COVID-19 due to the development of acute respiratory distress syndrome (Willems et al. 2020 ).
Chemical compounds used as disinfectants are not only harmful to humans but also affect animals and aquatic ecosystems. Though the disinfection of wastewater originating from healthcare facilities, offices, public places, and other organizations, such as hotels and processing units, is essential for minimization of the likelihood of spreading infection and deleterious effects, application of these disinfectants can cause harm to both living organisms and the environment. Other disinfection practices, such as the washing of external floors, streets, and markets, also contribute to the discharge of disinfectants into sewage, rivers, and lakes (Subpiramaniyam 2021 ). Sodium hypochlorite is commonly used for the disinfection of hospital wastewater to prevent the spread of nosocomial infectious diseases. Therefore, such chemicals may gain entry into the sewage and cause pollution of drinking water resources (China Ministry of Ecology and Environment 2020 ). Furthermore, as both direct and indirect sewage effluents are discharged into rivers and lakes, aquatic ecosystems are at a risk of contamination with chemical disinfectants (Sedlak 2011 ; Subpiramaniyam 2021 ). Chlorine disinfectants threaten aquatic wildlife and plants as the agents catalyze the oxidation of their proteins and destruction of their cell walls (Sedlak 2011 ). Moreover, these chemicals may bind to other materials to form harmful compounds. For example, chlorine disinfectants undergo reactions with dissolved organic matter of surface water to produce disinfectant byproducts, such as haloacetic acids and trihalomethanes, which are highly toxic to aquatic flora and fauna (Sedlak 2011 ; Liu and Zhang 2014 ). Chlorine also undergoes reaction with organic matter in wastewater, thereby resulting in the generation of organic chlorine compounds that persist as environmental contaminants and may pose a considerable risk to aquatic ecosystems (Emmanuel et al. 2004 ). An effect of disinfectants may be exerted on microbial activity in wastewater treatment plants that may compromise the effective removal of pollutants (carbon, nitrose, and phosphorous). Extensive use of disinfectants against COVID-19 also poses potential risks to urban wildlife (Nabi et al. 2020 ). While humans can avoid the establishment of contact with disinfectants during the active disinfection of areas or localities, other organisms, including wild animals, are unable to do so, thus resulting in potential contact with corrosive or otherwise harmful substances (Nabi et al. 2020 ). The overuse of disinfectants has led to the death of animals, such as birds and weasels (You 2020 ). They exert toxicological effects on both terrestrial and aquatic animals (El-Nahhal and El-Nahhal 2020 ) and may have impacts on food and water sources (Zhang et al. 2020 ). Excessive use of disinfectants can lead to their enrichment, bioaccumulation, and biomagnification, resulting in toxicity, mutations, spread of antibiotic resistance genes, and the emergence of antibiotic-resistant bacteria (Chen et al. 2021 ).
The salient deleterious effects of disinfectants and sanitizers on humans and the environment during COVID-19 are depicted in Fig. 2 .
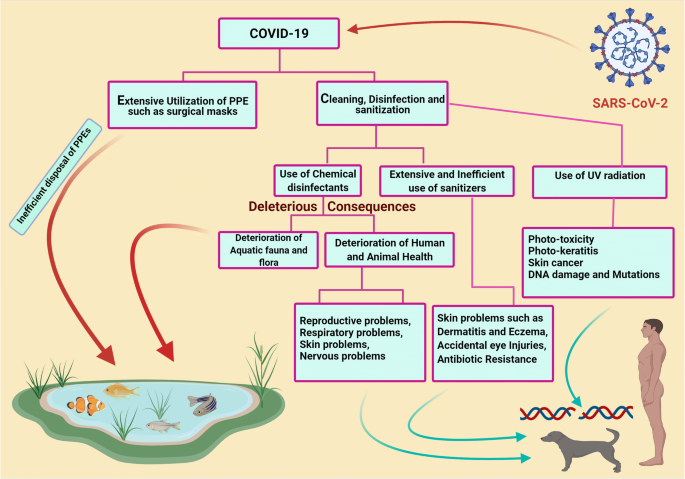
Deleterious effects of disinfectants and sanitizers in human and environment during COVID-19. The figure was created with BioRender.com
Disinfectants may also affect material surfaces (Bonin et al. 2020 ). Their corrosive nature may lead to the corrosion of important metal surfaces. Though four out of five disinfectants pose little or no risk to metals, further studies are warranted for the evaluation of the impact of disinfectants on surfaces subject to frequent and continuous use of various types of disinfectants, as these agents may exert adverse effects (Bonin et al. 2020 ). The increased use of disinfectants in response to the COVID-19 pandemic may lead to the occurrence of a secondary disaster in aquatic ecosystems worldwide. Therefore, sewage originating from medical institutions should be treated as per the guidelines provided by concerned authorities. This implies that sewage originating from healthcare facilities should be treated separately before combination with other sewage. Additionally, to remove remaining virus particles from the sewage originating from healthcare facilities, chemical agents, such as dibromo-dimethyl hydantoin, chlorine dioxide, and other chlorine-containing disinfectants, can be used (COVID-19 Emergency Response Key Places Protection and Disinfection Technology Team 2020b ). Analysis and re-evaluation of the current methods of sewage treatment are of utmost importance for preventing the transmission of COVID-19 via sewage; additionally, the substitution of conventional methods (chlorination and simple filtration) with advanced methods (centralized wastewater treatment, oxidation, filtration, and membrane technology) of sewage treatment is necessary to prevent the dissemination of SARS-CoV-2 throughout the environment (Núñez-Delgado 2020 ).
Mitigation strategies to reduce the deleterious consequences of disinfectants in humans and the environment
Safe and eco-friendly disinfectants should be used, and post-disinfection measures should be undertaken to avoid the occurrence of health hazards. In this context, light, including sunlight (Ratnesar-Shumate et al. 2020 ), UV light (Seyer and Sanlidag 2020 ; Zhao et al. 2020 ), and color light (Enwemeka et al. 2020 ), may demonstrate prospects and potential applications in managing the COVID-19 pandemic. However, further studies relating to this matter are warranted (Derraik et al. 2020 ; Ratnesar-Shumate et al. 2020 ; Seyer and Sanlidag 2020 ). Simulated sunlight can reportedly inactivate SARS-CoV-2 particles dried on stainless steel and suspended in simulated saliva or culture media (Ratnesar-Shumate et al. 2020 ). Moreover, 90% of infectious virus is inactivated at every 6.8 and 14.3 min of exposure in simulated saliva and culture media, respectively (Ratnesar-Shumate et al. 2020 ). This indicates that sunlight may be useful as a natural disinfectant for non-porous outdoor materials contaminated with SARS-CoV-2 (Ratnesar-Shumate et al. 2020 ). Special air-disinfecting machines are also presently being proposed (Zhao et al. 2020 ).
Hitherto, studies have shown the activity of alcohol-based disinfectants and sanitizers against several viral infections (Malik et al. 2006b ; Patnayak et al. 2008 ; Suman et al. 2020 ). The use of ethanol-based sanitizers is recommended for the prevention of the harmful effects of other chemical compounds on humans and animals. Direct spraying of bleach onto infected individuals or affected areas is discouraged. Disinfected surfaces must be subjected to drying and rinsing with water because disinfectants can persist for a long period on contaminated surfaces and may cause unintentional exposure to hazardous chemicals. Government agencies should, therefore, develop facilities for proper disinfectant drainage to minimize the harmful effects on aquatic flora and fauna. The use of eco-friendly technologies along with safe and effective disinfection methods is highly warranted, not only to combat the ongoing pandemic but also to protect the environment and living beings from hazardous chemicals.
Low-cost antibody-linked graphene sheets that function as environmental virus sensors have been synthesized; their application as coatings on face masks/PPEs represents a promising strategy to fight COVID-19 by minimizing the risk of transmission (Palmieri and Papi 2020 ). Moreover, magnetic nanomaterials or nanoparticles can be exploited as efficient alternatives for coating PPEs such as masks and eye-protecting glasses in order to produce reusable and environmentally friendly antiviral nanocoated PPEs (Tyagi et al. 2021 ). However, antiviral nanoparticles such as silver (Ag), copper (Cu), copper oxide (CuO), and zinc (Zn) have been incorporated on surfaces and PPE textiles, and can be a viable alternative to chemical disinfection processes (Valdez-Salas et al. 2021 ; Ruiz-Hitzky et al. 2020 ). In a recent study, the nanodisinfectant has been evaluated as a reliable technique for efficient disinfection, reusing, and even antimicrobial promotion of surgical masks for healthcare professionals (Valdez-Salas et al. 2021 ). In addition, modern nanotechnology and nanomedicine approaches have been harnessed to develop disinfection and treatment strategies to tackle increasing infection cases worldwide, especially challenges posed by pathogens of viral origin (Nikaeen et al. 2020 ). Recently, more sophisticated and modern strategies, such as the use of agriculture spraying drones and robotic machines, have been suggested to disinfect areas that pose a high risk of infection, such as stadiums and theaters, in a short timespan (Clay and Milk 2020 ; Khan et al. 2021 ). Various nanomaterials such as carbon nanotubes, graphene, or silver nanowires have been used to improve current physical disinfection methods (Kumar and Mohanty 2020 ; Palmieri and Papi 2020 ; Ruiz-Hitzky et al. 2020 ). Furthermore, nanomaterials have been proposed as possible disinfection candidates since they do not exhibit antiviral activities for single use but rather exhibit their action over a prolonged period of time (Campos et al. 2020 ; Ruiz-Hitzky et al. 2020 ), and this property can be used to produce sustainable and environmentally friendly disinfectants.
Furthermore, a recent study proposed that the use of a variety of physical techniques such as photolithography and laser surface modification, in conjunction with ion beam–assisted deposition, can be used to evolve biomaterial surfaces or self-cleaning surfaces with suitable topographical features and controlled cell adhesion (Kumari and Chatterjee 2021 ). In this sense, the antiviral behavior of aluminum surfaces with appropriately aligned ridges has been studied against SARS-CoV-2, and it was found that the self-disinfecting surfaces with coated nanoparticles are substantially effective against SARS-CoV-2 (Hasan et al. 2020a ; Hasan et al. 2020b ). Hence, the self-cleaning surfaces with minor deleterious consequences are incredibly effective at mitigating viral transmission by contact. Their ability should be explored further in the future to reduce the usage of chemical disinfectants.
Moreover, disinfectants are biocidal products; therefore, they are regulated by the Biocidal Products Regulation (BPR) (EU) in European Union countries and are appropriately evaluated before marketing (EPC 2020 ). However, considering the urgency of addressing the COVID-19 pandemic, a few agents may be provided for developing transitional measures without BPR for immediate use against SARS-CoV-2, such as 70–80% ethanol application for 1 min (Kampf et al. 2020a ). Most biocidal products with virucidal activity regulated under BPR are effective against SARS-CoV-2. This includes disinfectants used in hand hygiene and skin disinfection, albeit they may demonstrate limited biocidal activity against viruses or less remarkable activity against enveloped viruses (ECDC 2020 ). Hence, proper assessment before application and monitoring during use is of utmost importance for human health and environmental safety.
Appropriate use of disinfectants as recommended by various agencies should be practiced to counter SARS-CoV-2. The WHO ( 2020 ) has provided recommendations for the appropriate utilization of suitable disinfectants and at specified intervals. Similarly, cleaning and disinfection as per community facilities guidelines of the CDC (CDC 2020a ) and disinfection as per quarantine facility guidelines of the NCDC ( 2020 ) may be helpful. However, any disinfectant, regardless of its nature and properties, used for disinfection under a selective environment must meet local authority specifications and be used in an eco-friendly and efficient manner to prevent environmental contamination (WHO 2020 ).
Important mitigation strategies to reduce the deleterious consequences of disinfectants in humans and the environment during the COVID-19 pandemic are presented in Fig. 3 .
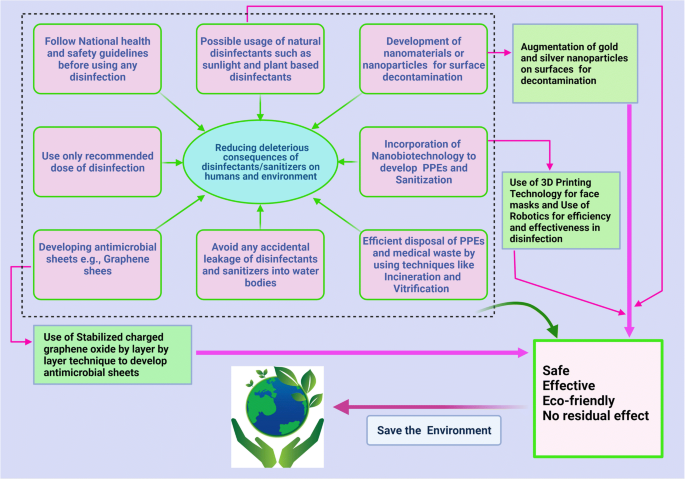
Mitigation strategies to reduce deleterious consequences of disinfectants in human and environment during COVID-19 pandemic. The figure was created with BioRender.com
Sanitizers during the COVID-19 pandemic
Sanitizer is an antimicrobial liquid, gel, or foam that is used to reduce the number of microorganisms present on a surface. Although alcohol-based hand rubs and washing with soap and water are effective against CoVs, due to ease of utilization, hand sanitizers have gained more popularity than other available options, including washing with soaps, chemical disinfection, exposure to sunlight, UV light, or heating. The increased frequency of sanitizer usage due to fear of developing COVID-19 has resulted in increased aerosol generation and, in certain cases, poses a potential hazard to exposed mucosal surfaces and skin. The adverse effects of alcohol used in hand sanitizers can be manifold and may lead to a condition called sanitizer aerosol-driven ocular surface disease owing to the increased sensitivity of eyes to the toxic effects of these sanitizers compared to that of skin (Ahn et al. 2010 ; Shetty et al. 2020 ). There has been a significant increase in the number of ocular injuries from 2020 to 2021 in the pediatric population due to inefficient use of ABHSs (Martin et al. 2021 ).
However, a few associated problems are caused by the market formulations of available hand sanitizers, and the capacity of chemists has increased manifold during the COVID-19 pandemic with emphasis on the use of hand sanitizers (Opatz et al. 2020 ). The use of hand sanitizers as a result of the COVID-19 pandemic has increased the number of cases of hand dermatitis in more than 90% of healthcare workers (HCWs) and hand eczema in nearly 14% of these cases (Guertler et al. 2020 ). Not all market formulations are effective for use. A screening of all WHO-recommended hand rub formulations (alcohol-based hand rubs) showed high virucidal activity with complete inactivation of SARS-CoV-2 (Kratzel et al. 2020 ). In this context, the Centers for Disease Control and Prevention (CDC) also recommended adopting practices for good hand hygiene, which includes proper handwashing with warm water and soap for a minimum period of 20 s and the use of ABHSs as the most effective approach to reduce COVID-19 infection (CDC 2020b ; Schrank et al. 2020 ).
Life-threatening clinical effects can be attributed to acute ethanol intoxication. A case study reported by Lim revealed that hand sanitizer application did not interfere with the course of treatment of infectious spondylitis or cause abnormal complications. However, during the current COVID-19 pandemic, it is expected that such intoxications will increase due to the increased use of hand sanitizers (Lim 2020 ).
Additionally, the toxicity and harmful effects of alcohol-based sanitizers on skin and their excessive use gradually lead to natural mutations in microbes and can contribute to the issue of antimicrobial resistance, which is already a significant threat to developing countries and continents, such as India, Pakistan, Africa, and Bangladesh (Mahmood et al. 2020 ).
To prevent the hazardous effects of ABHSs, the use of soap and water should be encouraged in susceptible individuals. The use of a face shield or protective goggles would be beneficial, wherein the frequent cleansing of hands is unavoidable. Moreover, the closure of eyes while pressing the nozzle of the sanitizer and maintenance of proper distance by ensuring that the sanitizer is below the eye level. Furthermore, keeping doors and windows open and avoiding sanitizer usage when air conditioning systems are activated may help reduce exposure to sanitizer droplets. Additionally, the most effective approach to confer protection to the eyes and mucosal surfaces from the harmful effects of sanitizers is by minimizing their use.
Steps to be followed while using disinfectants (National Pesticide Information Center 2020 ; COVID-19 Prevention: Enhanced Cleaning and Disinfection Protocols 2020 ):
Before using disinfectant, follow the precautionary statements on the accompanying label to prevent chemical exposure to self and surrounding individuals. Use appropriate recommended doses at appropriate intervals. Disinfectants containing 2 g/L chlorine need to be sprayed four times daily on highly infected areas (such as floors, tables, and beds of the contaminated/isolated areas and hospitals) for 30 min. A concentration of 0.5 g/L of chlorine is recommended for semi-contaminated areas. In case dilution of the disinfectant is required, it should not affect the final recommended concentration and should never be used in combination with other compounds.
While using disinfectants, wear appropriate PPE, such as goggles, gloves, long-sleeved shirts, long pants, and masks. The use of an appropriate mask for a specific purpose is essential rather than using any non-specific mask (Agrawal et al. 2020 ). For the community at large and for HCWs, surgical masks or three-layer cotton masks can be used. For HCWs during aerosol-generating procedures for a patient with COVID-19, respirator masks are recommended (Agrawal et al. 2020 ).
Ensure proper ventilation while conducting disinfection. Chlorine-based disinfectants with usual concentrations of 4% and 6% or glutaraldehyde-based disinfectants with higher vapor levels than the recommended 1.12–3.4% especially require well-ventilated rooms (Ghafoor et al. 2021 ; Kampf et al. 2020 ). There should be exhaust duct hoods, air systems with 7–15 air changes per hour, ductable fumigation hoods with disinfectant vapor absorbers, or straightener lids in dip baths (Foliente et al. 2001 ; Ghafoor et al. 2021 ).
Keep disinfectants away from the reach of children and pets. Children are particularly susceptible to poisoning by disinfectants (Ghafoor et al. 2021 ) with ingestion being the common exposure route (Rosenman et al. 2021 ).
Discard disposable protective items, such as gloves and masks, after using disinfectants, since they have limited efficacy (6–12 h) and are heat-sensitive, and thus, cannot tolerate the sterilization process (Rowan and Laffey 2020 ). Not only does the structure of PPE and masks change upon washing and drying, but changes are observed in also their quality and efficacy, such as through the deterioration of their filtration properties (Konda et al. 2020 ; Sharma et al. 2020 ).
Wash hands with soap and water after conducting disinfection, and apply the appropriate hand sanitizer.
Avoid spraying chloride- and hypochlorite-containing disinfectants to the most possible extent, as they are more harmful to surfaces, other organisms, and the environment than their alternatives (Lin et al. 2020 ); additionally, there are currently related concerns surrounding the enrichment, bioaccumulation, and biomagnification of disinfectants (Chen et al. 2021 ). Alcohol-based disinfectants, soaps, and detergents along with water and radiation can be comparatively beneficial; nevertheless, all specified options are detrimental when adopted in excess.
Conclusion and future prospects
The COVID-19 pandemic ushered in several challenges with its emergence and seemed to be unrestricted through current mitigation strategies. Considering the urgency of the situation caused by the global spread of SARS-CoV-2 with rising morbidity, alarming mortality, and global economic fallouts, the trend of efforts is shifting from strategies of lockdown, quarantine, testing, isolation, and treatment to the creation of an atmosphere of clean, healthy, and safe surroundings that provides a healthy working environment. Several prevention strategies, such as avoidance of close contact with sick people; avoiding touching eyes, nose, and mouth; staying home when sick; covering the mouth when coughing and sneezing; and undertaking the approaches for frequent disinfection and sanitization of hands and touched objects, fomites, and surfaces, are important to prevent virus transmission. In this context, a variety of chemicals and other virucidal agents have been used globally as disinfectants to render the environment free from SARS-CoV-2 to the highest extent possible to prevent further spread. Disinfectants are proving to be beneficial in this regard and have gained considerable attention recently as being effective, affordable, convenient, and readily available antimicrobial agents. Applications have been identified in every aspect of life, including at home, the office, healthcare facilities, other industries, and the surrounding environment. However, there remain concerns regarding the side effects on animal and human health, the environment, and ecological balance.
There is an urgent need for developing eco-friendly technologies that offer safer and more effective disinfection methods to combat the ongoing pandemic, along with conferring protection to the environment and living beings from the potentially hazardous effects of chemical disinfectants. Alternate and improved strategies are being devised for minimizing adverse effects. In this context, the use of graphene sheets as coatings for face masks offers a promising strategy for fighting COVID-19 by minimizing the risk of further transmission. The graphene coating of face masks is of particular interest because it can be reused as it is superhydrophobic, thereby reducing the likelihood of adherence of infectious drops, and its strong light-absorbing properties renders sterilization upon exposure to sunlight possible. Moreover, nanotechnology and nanomedicine approaches have been harnessed to develop novel disinfection and treatment strategies to tackle this pandemic more effectively.
The current pace of research and the evolution of numerous novel disinfectants against COVID-19 provides hope for the development of safe, effective, and convenient disinfectants that are affordable to all and accessible under diverse environments with minimum or no potential risk to health and surroundings. Meanwhile, during disinfectant use, precautionary and preventive measures should be adopted. An environmental impact assessment of the escalating use of disinfectants is urgently needed. Clear and comprehensive guidelines for disinfectant application are also necessary at regional, national, and international levels to reduce the deleterious consequences to both humans and the environment.
Data availability
Not applicable. This article does not produce new data.
Agrawal H, Singh S, Gupta N (2020) What all we should know about masks in COVID-19 pandemic. Indian J Surg Jun 9:1–2. https://doi.org/10.1007/s12262-020-02469-4
Article Google Scholar
Ahn J, Eum K, Kim Y, Oh SW, Kim YJ, Lee M (2010) Assessment of the dermal and ocular irritation potential of alcohol hand sanitizers containing aloe vera with in vitro and in vivo methods. Mol Cell Toxicol 6:397–404. https://doi.org/10.1007/s13273-010-0053-y
Article CAS Google Scholar
Al-Gheethi A, Al-Sahari M, Malek MA, Noman E, Al-Maqtari Q, Mohamed R, Talip BA, Alkhadher S, Hossain MS (2020) Disinfection methods and survival of SARS-CoV-2 in the environment and contaminated materials: a bibliometric analysis. Sustainability 12(18):7378. https://doi.org/10.3390/su12187378
Al-Sayah MH (2020) Chemical disinfectants of COVID-19: an overview. J Water Health 18(5):843–848. https://doi.org/10.2166/wh.2020.108
Anderson ER, Hughes GL, Patterson EI (2020) Inactivation of SARS-CoV-2 on surfaces and in solution with Virusend (TX-10), a novel disinfectant. bioRxiv [Preprint] 11.25.394288. https://doi.org/10.1101/2020.11.25.394288 .
Balagna C, Perero S, Percivalle E, Nepita EV, Ferraris M (2020) Virucidal effect against coronavirus SARS-CoV-2 of a silver nanocluster/silica composite sputtered coating. Open Ceramics 1:100006. https://doi.org/10.1016/j.oceram.2020.100006
Barcelo D (2020) An environmental and health perspective for COVID-19 outbreak: meteorology and air quality influence, sewage epidemiology indicator, hospitals disinfection, drug therapies and recommendations. J Environ Chem Eng 8(4):104006. https://doi.org/10.1016/j.jece.2020.104006
Binder L, Högenauer C, Langner C (2020) Gastrointestinal effects of an attempt to “disinfect” from COVID-19. Histopathology. 77:327–328. https://doi.org/10.1111/his.14137 (In press)
Blazejewski C, Wallet F, Rouzé A, Le Guern R, Ponthieux S, Salleron J, Nseir S (2015) Efficiency of hydrogen peroxide in improving disinfection of ICU rooms. Crit Care 19(1):30. https://doi.org/10.1186/s13054-015-0752-9
Bonin L, Vitry V, Olivier MG, Bertolucci-Coelho L (2020) Covid-19: effect of disinfection on corrosion of surfaces. Corros Eng Sci Technol 55(8):693–695. https://doi.org/10.1080/1478422X.2020.1777022
Campos EVR, Pereira AES, de Oliveira JL, Carvalho LB, Guilger-Casagrande M, de Lima R, Fraceto LF (2020) How can nanotechnology help to combat COVID-19? Opportunities and urgent need. J Nanobiotechnol 18(1):125. https://doi.org/10.1186/s12951-020-00685-4
Card KJ, Crozier D, Dhawan A, Dinh M, Dolson E, Farrokhian N, Gopalakrishnan V, Ho E, King ES, Krishnan N (2020) UV sterilization of personal protective equipment with idle laboratory biosafety cabinets during the Covid-19 pandemic. medRxiv. https://doi.org/10.1101/2020.03.25.20043489 .
Carlos Rubio-Romero J, Del Carmen P-FM, Antonio Torrecilla García J, Calero-Castro S (2020) Disposable masks: disinfection and sterilization for reuse, and non-certified manufacturing, in the face of shortages during the COVID-19 pandemic. Saf Sci 129:104830. https://doi.org/10.1016/j.ssci.2020.104830
CDC (2008) Chemical disinfectants (Guideline for disinfection and sterilization in healthcare facilities). https://www.cdc.gov/infectioncontrol/guidelines/disinfection/disinfection-methods/chemical.html #. Accessed 29 June 2020.
CDC (2020a) Cleaning and disinfection for community facilities. https://www.cdc.gov/coronavirus/2019-ncov/community/organizations/cleaning-disinfection.html . Accessed 13 June 2020
CDC (2020b) Hand hygiene recommendations. https://www.cdc.gov/coronavirus/2019-ncov/hcp/hand-hygiene.html . Accessed 17 May 2020.
CFSP (2008) Center for Food Security and Public Health (CFSPH). Disinfection101. http://www.cfsph.iastate.edu/Disinfection/Assets/Disinfection101.pdf . Accessed 17 May 2020.
Chen Z, Guo J, Jiang Y, Shao Y (2021) High concentration and high dose of disinfectants and antibiotics used during the COVID-19 pandemic threaten human health. Environ Sci Eur 33(1):11. https://doi.org/10.1186/s12302-021-00456-4
Chin A, Chu J, Perera M, Hui K, Yen HL, Chan M, Peiris M, Poon L (2020) Stability of SARS-CoV-2 in different environmental conditions. Lancet Microbe 1(1):E10. https://doi.org/10.1016/S2666-5247(20)30003-3
China Ministry of Ecology and Environment (2020) Will viruses and disinfection affect water quality? The Ministry of Ecology and Environment responded. www.mee.gov.cn/ywgz/ssthjbh/dbssthjgl/202003/t20200311_768408.shtml [in Chinese]. Accessed 29 June 2020.
Choi H, Chatterjee P, Lichtfouse E, Martel JA, Hwang M, Jinadatha C, Sharma VK (2021) Classical and alternative disinfection strategies to control the COVID-19 virus in healthcare facilities: a review. Environ Chem Lett:1–7. https://doi.org/10.1007/s10311-021-01180-4 (In press)
Clay and Milk (2020) Rantizo is using drone technology to sanitize stadiums. https://clayandmilk.com/2020/04/28/rantizo-is-using-drone-technology-to-sanitize-stadiums . Accessed 29 June 2020.
COVID-19 Emergency Response Key Places Protection and Disinfection Technology Team, Chinese Center for Disease Control and Prevention (2020a) Disinfection guideline of public transport during COVID-19 outbreak. Zhonghua Yu Fang Yi Xue Za Zhi 54:344–346. https://doi.org/10.3760/cma.j.cn112150-20200217-00129
COVID-19 Emergency Response Key Places Protection and Disinfection Technology Team, Chinese Center for Disease Control and Prevention (2020b) Technical guideline for disinfection of wastewater and wastes of medical organizations during COVID-19 outbreak. Zhonghua Yu Fang Yi Xue Za Zhi 54:353–356. https://doi.org/10.3760/cma.j.cn112150-20200217-00125
COVID-19 prevention: Enhanced cleaning and disinfection protocols (2020) Environment health and safety University of Washington. www.ehs.washington.edu . Accessed 29 June 2020.
Darnell ME, Subbarao K, Feinstone SM, Taylor DR (2004) Inactivation of the coronavirus that induces severe acute respiratory syndrome, SARS-CoV. J Virol Methods 121:85–91. https://doi.org/10.1016/j.jviromet.2004.06.006
Derraik JGB, Anderson WA, Connelly EA, Anderson Y(2020) Rapid evidence summary on SARS-CoV-2 survivorship and disinfection, and a reusable PPE protocol using a double-hit process. medRxiv. 2020. https://doi.org/10.1101/2020.04.02.20051409 .
Dhama K, Sharun K, Tiwari R, Dadar M, Malik YS, Singh KP, Chaicumpa W (2020a) COVID-19, an emerging coronavirus infection: advances and prospects in designing and developing vaccines, immunotherapeutics, and therapeutics. Hum Vaccin Immunother 16(6):1232–1238. https://doi.org/10.1080/21645515.2020.1735227
Dhama K, Khan S, Tiwari R, Sircar S, Bhat S, Malik YS, Singh KP, Chaicumpa W, Bonilla-Aldana DK, Rodriguez-Morales AJ (2020b) Coronavirus disease 2019-COVID-19. Clin Microbiol Rev 33(4):e00028–e00020. https://doi.org/10.1128/CMR.00028-20
Dhama K, Patel SK, Yatoo MI, Tiwari R, Sharun K, Dhama J, Natesan S, Malik YS, Singh KP, Harapan H (2021) SARS-CoV-2 existence in sewage and wastewater: A global public health concern? J Environ Manage 280:111825
Duarte PM, de Santana VTP (2020) Disinfection measures and control of SARS-COV-2 transmission. Global Biosecurity 1(3). https://doi.org/10.31646/gbio.64
ECDC (2020) European Centre for Disease Prevention and Control. Disinfection of environments in healthcare and nonhealthcare settings potentially contaminated with SARS-CoV-2. ECDC: Stockholm. https://www.ecdc.europa.eu/sites/default/files/documents/Environmental-persistence-of-SARS_CoV_2-virus-Optionsfor-cleaning2020-03-26_0.pdf . Accessed 9 Feb 2020.
Eggers M, Koburger-Janssen T, Ward LS, Newby C, Muller S (2018) Bactericidal and virucidal activity of povidone-iodine and chlorhexidine gluconate cleansers in an in vivo hand hygiene clinical simulation study. Infect Dis Ther 7(2):235–247. https://doi.org/10.1007/s40121-018-0202-5
Eldeirawi K, Huntington-Moskos L, Nyenhuis SM, Polivka B (2020) Increased disinfectant use among adults with asthma in the era of COVID-19. J Allergy Clin Immunol Pract. S2213-2198(20)31402-1. https://doi.org/10.1016/j.jaip.2020.12.038 .
El-Nahhal I, El-Nahhal Y (2020) Ecological consequences of COVID-19 outbreak. J Water Sci Eng 1:1–5. https://doi.org/10.13140/RG.2.2.24456.85769
Emmanuel E, Keck G, Blanchard JM, Vermande P, Perrodin Y (2004) Toxicological effects of disinfections using sodium hypochlorite on aquatic organisms and its contribution to AOX formation in hospital wastewater. Environ Int 30:891–900. https://doi.org/10.1016/j.envint.2004.02.004
Enwemeka CS, Bumah VV, Masson-Meyers DS (2020) Light as a potential treatment for pandemic coronavirus infections: a perspective. J Photochem Photobiol B 207:111891. https://doi.org/10.1016/j.jphotobiol.2020.111891
EPC (2020) European Parliament and Council. Regulation (EU) No 528/2012 of the European Parliament and of the Council of 22 May 2012 concerning the making available on the market and use of biocidal products 2012. https://eur-lex.europa.eu/legal-content/EN/TXT/?uri=CELEX%3A32012R0528 . Accessed 22 Mar 2020.
Eslami H, Jalili M (2020) The role of environmental factors to transmission of SARS-CoV-2 (COVID-19). AMB Express 10(1):92. https://doi.org/10.1186/s13568-020-01028-0
European Lung Foundation (2017) Nurses regular use of disinfectants is associated with developing COPD. ScienceDaily. https://www.sciencedaily.com/releases/2017/09/170910232514.htm . Accessed 9 May 2020.
Ewart SL (2001). Disinfectants and control of environmental contamination. In: Smith BL. editor. Large animal internal medicine: diseases of horses cattle, sheep and goats. 3rd edn. St. Louis: Mosby. pp. 1371-1380.
Fair D (2020) Issues of the environment: chemical impacts In fighting the spread of COVID-19. https://www.wemu.org/post/issues-environment-chemical-impacts-fighting-spread-covid-19 . Accessed 24 June 2020.
Falagas ME, Thomaidis PC, Kotsantis IK, Sgouros K, Samonis G, Karageorgopoulos DE (2011) Airborne hydrogen peroxide for disinfection of the hospital environment and infection control: a systematic review. J Hosp Infect 78(3):171–177. https://doi.org/10.1016/j.jhin.2010.12.006
FDA (2020a) Enforcement policy for sterilizers, disinfectant devices, and air purifiers during the coronavirus disease 2019 (COVID-19) public health emergency guidance for industry and Food and Drug Administration staff. https://www.fda.gov/media/136533/download . Accessed 17 May 2020.
FDA (2020b) UV lights and lamps: ultraviolet-C radiation, disinfection, and coronavirus. https://www.fda.gov/medical-devices/coronavirus-covid-19-and-medical-devices/uv-lights-and-lamps-ultraviolet-c-radiation-disinfection-and-coronavirus . Accessed 24 Jan 2021.
Foliente RL, Kovacs BJ, Aprecio RM, Bains HJ, Kettering JD, Chen YKJG (2001) Efficacy of high-level disinfectants for reprocessing GI endoscopes in simulated-use testing. Gastrointest Endosc 53(4):456–462. https://doi.org/10.1067/mge.2001.113380
Gates B (2020) Responding to Covid-19 - a once-in-a-century pandemic? N Engl J Med 382:1677–1679. https://doi.org/10.1056/NEJMp2003762
Gerba CP (2015) Quaternary ammonium biocides: efficacy in application. Appl Environ Microbiol 81(2):464–469. https://doi.org/10.1128/AEM.02633-14
Ghafoor D, Khan Z, Khan A, Ualiyeva D, Zaman N (2021) Excessive use of disinfectants against COVID-19 posing a potential threat to living beings. Curr Res Toxicol 2:159–168. https://doi.org/10.1016/j.crtox.2021.02.008
Giesecke J (2020) The invisible pandemic. Lancet. 395:e98. https://doi.org/10.1016/S0140-6736(20)31035-7
Goyal S. M. Chander Y. Yezli S. Otter J. A (2014) Evaluating the virucidal efficacy of hydrogen peroxide vapour. J Hosp Infect 86 (4), 255–259. https://doi.org/10.1016/j.jhin.2014.02.003
Guertler A, Moellhoff N, Schenck TL, Hagen CS, Kendziora B, Giunta RE, French LE, Reinholz M (2020) Onset of occupational hand eczema among healthcare workers during the SARS-CoV-2 pandemic - comparing a single surgical site with a COVID-19 intensive care unit. Contact Dermatitis 83:108–114. https://doi.org/10.1111/cod.13618
Gul S, Savsar A, Tayfa Z (2009) Cytotoxic and genotoxic effects of sodium hypochlorite on human peripheral lymphocytes in vitro. Cytotechnology. 59:113–119. https://doi.org/10.1007/s10616-009-9201-4
Guo L, Yao Z, Yang L, Zhang H, Qi Y, Gou L, Xi W, Liu D, Zhang L, Cheng Y, Wang X, Rong M, Chen H, Kong MG (2020) Plasma-activated water: an alternative disinfectant for S protein inactivation to prevent SARS-CoV-2 infection. Chem Eng J 20:127742. https://doi.org/10.1016/j.cej.2020.127742
Hasan J, Xu Y, Yarlagadda T, Schuetz M, Spann K, Yarlagadda PK (2020a) Antiviral and antibacterial nanostructured surfaces with excellent mechanical properties for hospital applications. ACS Biomater Sci Eng 6(6):3608–3618. https://doi.org/10.1021/acsbiomaterials.0c00348
Hasan J, Pyke A, Nair N, Yarlagadda T, Will G, Spann K, Yarlagadda PKDV (2020b) Antiviral nanostructured surfaces reduce the viability of SARS-CoV-2. ACS Biomater Sci Eng 6(9):4858–4861. https://doi.org/10.1021/acsbiomaterials.0c01091
Herzog A. B. Pandey A. K. Reyes-Gastelum D. Gerba C. P. Rose J. B. Hashsham S. A (2012) Evaluation of sample recovery efficiency for bacteriophage P22 on fomites. Appl Environ Microbiol 78 (22), 7915–7922. https://doi.org/10.1128/AEM.01370-12
Huttner BD, Harbarth S (2015) Hydrogen peroxide room disinfection--ready for prime time? Crit Care 19(1):216. https://doi.org/10.1186/s13054-015-0915-8
ICNIRP (2004) The International Commission on Non-Ionizing Radiation Protection guidelines on limits of exposure to ultraviolet radiation of wavelengths between 180 nm and 400. The International Commission on Non-Ionizing Radiation Protection. Health Phys. 2004; 87:171-186. https://www.icnirp.org/cms/upload/publications/ICNIRPUV2004.pdf . Accessed 10 Apr 2020.
Iyiola AO, Asiedu B, Fawole FJ (2020) Possible effects of COVID-19 on sustainability of aquatic ecosystems: an overview. Aquat Res 3:177–187. https://doi.org/10.3153/AR20016
Kampf G, Todt D, Pfaender S, Steinmann E (2020) Persistence of coronaviruses on inanimate surfaces and their inactivation with biocidal agents. J. Hosp Infect 104:246–251. https://doi.org/10.1016/j.jhin.2020.01.022
Kampf G, Todt D, Pfaender S, Steinmann E (2020a) Persistence of coronaviruses on inanimate surfaces and their inactivation with biocidal agents. J Hosp Infect 104:246–251. https://doi.org/10.1016/j.jhin.2020.01.022
Kampf G, Voss A, Scheithauer S (2020b) Inactivation of coronaviruses by heat. J Hosp Infect 105(2):348–349. https://doi.org/10.1016/j.jhin.2020.03.025
Kariwa H, Fujii N, Takashima I (2006) Inactivation of SARS coronavirus by means of povidone-iodine, physical conditions and chemical reagents. Dermatology 212(Suppl. 1):119–123. https://doi.org/10.1159/000089211
Khan H, Kushwah KK, Singh S, Urkude H, Maurya MR, Sadasivuni KK (2021) Smart technologies driven approaches to tackle COVID-19 pandemic: a review. 3. Biotech. 11(2):50. https://doi.org/10.1007/s13205-020-02581-y
Konda A, Prakash A, Moss GA, Schmoldt M, Grant GD, Guha S (2020) Aerosol filtration efficiency of common fabrics used in respiratory cloth masks. ACS Nano 14(5):6339–6347
Kratzel A, Todt D, V’kovski P, Steiner S, Gultom M, Thao TTN, Ebert N, Holwerda M, Steinmann J, Niemeyer D, Dijkman R, Kampf G, Drosten C, Steinmann E, Thiel V, Pfaender S (2020) Inactivation of severe acute respiratory syndrome coronavirus 2 by WHO-recommended hand rub formulations and alcohols. Emerg Infect Dis 26(7):1592–1595. https://doi.org/10.3201/eid2607.200915
Kumar RP, Mohanty S (2020) Are graphene and graphene-derived products capable of preventing COVID-19 infection? Med Hypotheses 144:110031. https://doi.org/10.1016/j.mehy.2020.110031
Kumar GD, Mishra A, Dunn L, Townsend A, Oguadinma IC, Bright KR, Gerba CP (2020) Biocides and novel antimicrobial agents for the mitigation of coronaviruses. Front Microbiol 11:1351. https://doi.org/10.3389/fmicb.2020.01351
Kumari S, Chatterjee K (2021 Feb 9) Biomaterials-based formulations and surfaces to combat viral infectious diseases. APL Bioeng 5(1):011503. https://doi.org/10.1063/5.0029486
Lai A, Bergna A, Acciarri C, Galli G, Zehender G (2020) Early phylogenetic estimate of the effective reproduction number of SARS-CoV-2. J Med Virol 92:675–679. https://doi.org/10.1002/jmv.25723
Lee YN, Chen LK, Ma HC, Yang HH, Li HP, Lo SY (2005) Thermal aggregation of SARS-CoV membrane protein. J Virol Methods 129(2):152–161. https://doi.org/10.1016/j.jviromet.2005.05.022
León Molina J, Abad-Corpa E (2021) Disinfectants and antiseptics facing coronavirus: synthesis of evidence and recommendations. Enferm Clin 31(Suppl 1):S84–S88. https://doi.org/10.1016/j.enfcli.2020.05.013
Leung KCP, Ko TCS (2020) Improper use of germicidal range ultraviolet lamp for household disinfection leading to phototoxicity in COVID-19 suspects. Cornea. 40:121–122. https://doi.org/10.1097/ICO.0000000000002397
Liao L, Xiao W, Zhao M, Yu X, Wang H, Wang Q, Chu S, Cui Y (2020) Can N95 respirators be reused after disinfection? How many times? ACS Nano 14:6348–6356. https://doi.org/10.1021/acsnano.0c03597
Lim DJ (2020) Intoxication by hand sanitizer due to delirium after infectious spondylitis surgery during the COVID-19 pandemic: a case report and literature review. Int J Surg Case Rep 77:76–79. https://doi.org/10.1016/j.ijscr.2020.10.086
Lin Q, Lim JYC, Xue K, Yew PYM, Owh C, Chee PL, Loh XJ (2020 May) Sanitizing agents for virus inactivation and disinfection. View. 24:e16. https://doi.org/10.1002/viw2.16
Lingayya H, Aayushi HZ, Alisha M, Jacqueline A, Ryna SS and Sriraksha BK (2020) Biocides for textiles against SARS-CoV 2. J Textile Sci Eng 10 10.37421/jtese.2020.10.424.
Liu J, Zhang X (2014) Comparative toxicity of new halophenolic DBPs in chlorinated saline wastewater effluents against a marine alga: halophenolic DBPs are generally more toxic than haloaliphatic ones. Water Res 65:64–72. https://doi.org/10.1016/j.watres.2014.07.024
Ma QX, Shan H, Zhang CM, Zhang HL, Li GM, Yang RM, Chen JM (2020) Decontamination of face masks with steam for mask reuse in fighting the pandemic COVID-19: experimental supports. J Med Virol 92:1971–1974. https://doi.org/10.1002/jmv.25921
Mahmood A, Eqan M, Pervez S, Alghamdi HA, Tabinda AB, Yasar A, Brindhadevi K, Pugazhendhi A (2020) COVID-19 and frequent use of hand sanitizers; human health and environmental hazards by exposure pathways. Sci Total Environ 742:140561. https://doi.org/10.1016/j.scitotenv.2020.140561
Malik YS, Allwood PB, Hedberg CW, Goyal SM (2006a) Disinfection of fabrics and carpets artificially contaminated with calicivirus: relevance in institutional and healthcare centres. J Hosp Infect 63:205–210. https://doi.org/10.1016/j.jhin.2006.01.013
Malik YS, Maherchandani S, Goyal SM (2006b) Comparative efficacy of ethanol and isopropanol against feline calicivirus, a norovirus surrogate. Am J Infect Control 34:31–35. https://doi.org/10.1016/j.ajic.2005.05.012
Malik YS, Sircar S, Bhat S, Sharun K, Dhama K, Dadar M, Tiwari R, Chaicumpa W (2020) Emerging novel coronavirus (2019-nCoV)-current scenario, evolutionary perspective based on genome analysis and recent developments. Vet Q 40(1):68–76. https://doi.org/10.1080/01652176.2020.1727993
Mantlo E, Rhodes T, Boutros J, Patterson-Fortin L, Evans A, Paessler S (2020) In vitro efficacy of a copper iodine complex PPE disinfectant for SARS-CoV-2 inactivation. F1000Res 9:674. https://doi.org/10.12688/f1000research.24651.2
Maris P (1995) Modes of action of disinfectants. Rev sci tech Off int Epiz 14(1):47–55. https://doi.org/10.20506/rst.14.1.829
Martin GC, Le Roux G, Guindolet D, Boulanger E, Hasle D, Morin E, Vodovar D, Vignal Gabison E, Descatha A; French PCC Research Group (2021) pediatric eye injuries by hydroalcoholic gel in the context of the coronavirus disease 2019 pandemic. JAMA Ophthalmol. e206346. 10.1001/jamaophthalmol.2020.6346.
McDonnell G, Russell AD (1999) Antiseptics and disinfectants: activity, action, and resistance. Clin Microbiol Rev 12(1):147–179. https://doi.org/10.1128/cmr.12.1.147
Moon J.M., Chun B.J., Min Y.I (2006) Hemorrhagic gastritis and gas emboli after ingesting 3% hydrogen peroxide. Int J Emerg Med 30(4):403–406. . https://doi.org/10.1016/j.jemermed.2005.05.036
Mukherjee S, Boral S, Siddiqi H, Mishra A, Meikap BC (2021) Present cum future of SARS-CoV-2 virus and its associated control of virus-laden air pollutants leading to potential environmental threat - a global review. J Environ Chem Eng 9(2):104973. https://doi.org/10.1016/j.jece.2020.104973
Nabi G, Wang Y, Hao Y, Khan S, Wu Y, Li D (2020) Massive use of disinfectants against COVID-19 poses potential risks to urban wildlife. Environ Res 188:109916. https://doi.org/10.1016/j.envres.2020.109916
National Pesticide Information Center (2020) Using disinfectants to control the COVID-19 virus. National Pesticide Information Center, Oregon State University Extension Services. http://npic.orst.edu/ingred/ptype/amicrob/covid19.html . Accessed 10 Apr 2020.
NCDC (2020) Guidelines for disinfection of quarantine facility (for COVID-19). https://ncdc.gov.in/WriteReadData/l892s/89168637271584172711.pdf . Accessed 13 June 2020.
Nikaeen G, Abbaszadeh S, Yousefinejad S (2020) Application of nanomaterials in treatment, anti-infection and detection of coronaviruses Nanomedicine (Lond). 15:1501–1512. https://doi.org/10.2217/nnm-2020-0117 (In press)
Noorimotlagh Z, Mirzaee SA, Jaafarzadeh N, Maleki M, Kalvandi G, Karami C (2020) A systematic review of emerging human coronavirus (SARS-CoV-2) outbreak: focus on disinfection methods, environmental survival, and control and prevention strategies. Environ Sci Pollut Res Int 28:1–15. https://doi.org/10.1007/s11356-020-11060-z
Núñez-Delgado A (2020) What do we know about the SARS-CoV-2 coronavirus in the environment? Sci Total Environ 727:138647. https://doi.org/10.1016/j.scitotenv.2020.138647
Opatz T, Senn-Bilfinger J, Richert C (2020) Thoughts on what chemists can contribute to fighting SARS-CoV-2 - a short note on hand sanitizers, drug candidates and outreach. Angew Chem Int Ed Eng 59:9236–9240. https://doi.org/10.1002/anie.202004721
Palmieri V, Papi M (2020) Can graphene take part in the fight against COVID-19? Nano Today 33:100883. https://doi.org/10.1016/j.nantod.2020.100883
Patnayak DP, Prasad AM, Malik YS, Ramakrishnan MA, Goyal SM (2008) Efficacy of disinfectants and hand sanitizers against avian respiratory viruses. Avian Dis 52:199–202. https://doi.org/10.1637/8097-082807-Reg.1
Peck B, Workeneh B, Kadikoy H, Patel SJ, Abdellatif A (2011) Spectrum of sodium hypochlorite toxicity in man-also a concern for nephrologists. NDT Plus 4:231–235. https://doi.org/10.1093/ndtplus/sfr053
Prochazka Zá Rate RA, Cabrera Cabrejos MC, Piscoya A, Vera Calderón AF (2020) Recomendaciones de la Sociedad de Gastroenterología del Perú para evitar la propagación del SARS-CoV-2 a través de procedimientos de endoscopía digestiva [Recommendations of the Society of Gastroenterology of Peru to avoid the spread of SARS-CoV-2 through digestive endoscopy procedures]. Rev Gastroenterol Peru 40:95–99
Google Scholar
Randazzo W, Truchado P, Cuevas-Ferrando E, Simón P, Allende A, Sánchez G (2020) SARS-CoV-2 RNA in wastewater anticipated COVID-19 occurrence in a low prevalence area. Water Res 181:115942. https://doi.org/10.1016/j.watres.2020.115942
Ratnesar-Shumate S, Williams G, Green B, Krause M, Holland B, Wood S, Bohannon J, Boydston J, Freeburger D, Hooper I, Beck K, Yeager J, Altamura LA, Biryukov J, Yolitz J, Schuit M, Wahl V, Hevey M, Dabisch P (2020) Simulated sunlight rapidly inactivates SARS-CoV-2 on surfaces. J Infect Dis 222 (2): 214-222. https://doi.org/10.1093/infdis/jiaa274 .
Rosenman KD, Reilly MJ, Wang L (2021) Calls to a state poison center concerning cleaners and disinfectants from the onset of the COVID-19 pandemic through April 2020. Public Health Rep 136(1):27–31. https://doi.org/10.1177/0033354920962437
Rowan NJ, Laffey JG (2020) Challenges and solutions for addressing critical shortage of supply chain for personal and protective equipment (PPE) arising from Coronavirus disease (COVID19) pandemic - case study from the Republic of Ireland. Sci Total Environ 725:138532. https://doi.org/10.1016/j.scitotenv.2020.138532
Ruiz-Hitzky E, Darder M, Wicklein B, Ruiz-Garcia C, Martin-Sampedro R, del Real G, Aranda P (2020) Nanotechnology responses to COVID-19. Advanced Healthcare Materials 9(19):2000979. https://doi.org/10.1002/adhm.202000979
Russell AD (1983) Principles of antimicrobial activity. In: Block SS (ed) Disinfection, sterilization and preservation, 3rd edn. Lea & Febiger, Philadelphia, pp 717–745
Rutala WA, Weber DJ (2019) Best practices for disinfection of noncritical environmental surfaces and equipment in health care facilities: a bundle approach. Am J Infect Control 47:A96–A105. https://doi.org/10.1016/j.ajic.2019.01.014
Sankar SA, Bhat KS, Anand J (2016) Sterilization and disinfection In: Essentials of medical microbiology. Jaypee Brothers, Medical Publishers Pvt. Limited, India. https://doi.org/10.5005/jp/books/12637_4 .
Sarada BV, Vijay R, Johnson R, Rao TN, Padmanabham G (2020) Fight against COVID-19: ARCI’s technologies for disinfection. Trans Indian Natl Acad Eng 5:349–354. https://doi.org/10.1007/s41403-020-00153-3
Schrank CL, Minbiole KPC, Wuest WM (2020) Are quaternary ammonium compounds, the workhorse disinfectants, effective against severe acute respiratory syndrome-coronavirus-2? ACS Infect Dis 6(7):1553–1557. https://doi.org/10.1021/acsinfecdis.0c00265
Sedlak DL (2011) von Gunten U (2011) Chemistry. The chlorine dilemma. Science. 331:42–43. https://doi.org/10.1126/science.1196397
Seyer A, Sanlidag T (2020) Solar ultraviolet radiation sensitivity of SARS-CoV-2. Lancet Microbe 1(1):e8–e9. https://doi.org/10.1016/S2666-5247(20)30013-6
Seymour N, Yavelak M, Christian C, Chapman B, Danyluk M (2020) COVID-19 FAQ for food service: cleaning and disinfection. EDIS. https://journals.flvc.org/edis/article/view/121172 . Accessed 10 April 2020.
Sharma SK, Mishra M, Mudgal SK (2020) Efficacy of cloth face mask in prevention of novel coronavirus infection transmission: a systematic review and meta-analysis. J Educ Health Promot 9:192. https://doi.org/10.4103/jehp.jehp_533_20
Shetty R, Jayadev C, Chabra A, Maheshwari S, D’Souza S, Khamar P, Sethu S, Honavar SG (2020) Sanitizer aerosol-driven ocular surface disease (SADOSD)-A COVID-19 repercussion? Indian J Ophthalmol 68(6):981–983. https://doi.org/10.4103/ijo.IJO_1308_20
Subpiramaniyam S (2021) Outdoor disinfectant sprays for the prevention of COVID-19: are they safe for the environment? Sci Total Environ 759:144289. https://doi.org/10.1016/j.scitotenv.2020.144289
Suman R, Javaid M, Haleem A, Vaishya R, Bahl S, Nandan D (2020) Sustainability of coronavirus on different surfaces. J Clin Exp Hepatol 10:386–390. https://doi.org/10.1016/j.jceh.2020.04.020
Talebian S, Wallace GG, Schroeder A, Stellacci F, Conde J (2020) Nanotechnology-based disinfectants and sensors for SARS-CoV-2. Nat Nanotechnol 15:618–621. https://doi.org/10.1038/s41565-020-0751-0
Tyagi PK, Tyagi S, Kumar A, Ahuja A, Gola D (2021) Contribution of nanotechnology in the fight against COVID-19. Biointerface Research In Applied Chemistry 2021 11(1):8233–8241. https://doi.org/10.33263/BRIAC111.82338241
US EPA O (2020) List N: disinfectants for use against SARSCoV-2. 2020. https://www.epa.gov/pesticide-registration/list-n-disinfectants-useagainst-sars-cov-2 . Accessed 7 Apr 2020.
Valdez-Salas B, Beltran-Partida E, Cheng N, Salvador-Carlos J, Valdez-Salas EA, Curiel-Alvarez M, Ibarra-Wiley R (2021) Promotion of surgical masks antimicrobial activity by disinfection and impregnation with disinfectant silver nanoparticles. Int J Nanomedicine 16:2689–2702. https://doi.org/10.2147/IJN.S301212
van Doremalen N, Bushmaker T, Morris DH, Holbrook MG, Gamble A, Williamson BN, Tamin A, Harcourt JL, Thornburg NJ, Gerber SI, Lloyd-Smith JO, de Wit E, Munster VJ (2020) Aerosol and surface stability of SARS-CoV-2 as compared with SARS-CoV-1. N Engl J Med 382(16):1564–1567. https://doi.org/10.1056/NEJMc2004973
Wan YL, Schoepf UJ, Wu CC, Giovagnoli DP, Wu MT, Hsu HH, Chang YC, Yang CT, Cherng WJ (2020) Preparedness and best practice in radiology department for COVID-19 and other future pandemics of severe acute respiratory infection. J Thorac Imaging 35(4):239–245. https://doi.org/10.1097/RTI.0000000000000529
Wang Y, Wu X, Wang Y, Li B, Zhou H, Yuan G, Fu Y, Luo Y (2004) Low stability of nucleocapsid protein in SARS virus. Biochemistry. 43(34):11103–11108. https://doi.org/10.1021/bi049194b
WHO (2020). Cleaning and disinfection of environmental surfaces in the context of COVID-19: interim guidance. https://www.who.int/publications/i/item/cleaning-and-disinfection-of-environmental-surfaces-inthe-context-of-covid-19 . Accessed 9 Feb 2021.
WHO (2021). DRAFT landscape of COVID-19 candidate vaccines https://www.who.int/publications/m/item/draft-landscape-of-covid-19-candidate-vaccines . Accessed 4 Mar 2021.
Wickramatillake A, Kurukularatne C (2020) SARS-CoV-2 human disinfection chambers: a critical analysis. Occup Med (Lond) 70(5):330–334. https://doi.org/10.1093/occmed/kqaa078
Willems LM, Samp PF, Pfeilschifter W (2020) Inhalation and ingestion of disinfectant as a mimic of COVID-19 infection. Dtsch Arztebl Int 117(29-30):499. https://doi.org/10.3238/arztebl.2020.0499
Yang Y, Wang H, Chen K, Zhou J, Deng S, Wang Y (2020) Shelter hospital mode: how to prevent novel coronavirus infection 2019 (COVID-19) hospital-acquired infection?. Infect Control Hosp Epidemiol. 1-4. https://doi.org/10.1017/ice.2020.97
Yao Y, Pan J, Liu Z, Meng X, Wang W, Kan H, Wang W (2020) No association of COVID-19 transmission with temperature or UV radiation in Chinese cities. Eur Respir J 55(5):2000517. https://doi.org/10.1183/13993003.00517-2020
Yari S, Moshammer H, Asadi AF, Mosavi Jarrahi A (2020) Side effects of using disinfectants to fight covid-19. Asian Pacific Journal of Environment and Cancer 3(1):9013. https://doi.org/10.31557/apjec.2020.3.1.9-13
You T (2020) More than 100 wild animals drop dead near coronavirus epicentre in china after workers ‘sprayed too much disinfectant’ to prevent coronavirus. https://www.dailymail.co.uk/news/article-8029271/100-wild-animals-drop-dead-near-coronavirus-epicentre.html . Accessed 29 June 2020.
Zaman F, Pervez A, Abreo K (2002) Isopropyl alcohol intoxication: a diagnostic challenge. Am J Kidney Dis 40(3):1–4. https://doi.org/10.1053/ajkd.2002.34938
Zhang YZ, Holmes EC (2020) A genomic perspective on the origin and emergence of SARS-CoV-2. Cell. 181:223–227. https://doi.org/10.1016/j.cell.2020.03.035
Zhang H, Tang W, Chen Y, Yin W (2020) Disinfection threatens aquatic ecosystems. Science. 368:146–147. https://doi.org/10.1126/science.abb8905
Zhao D, Zhang C, Chen JD (2020) Infection control in the medical imaging department during the cOVID-19 pandemic. J Med Imaging Radiat Sci 51(2):204–206. https://doi.org/10.1016/j.jmir.2020.03.005
Download references
Acknowledgements
All the authors acknowledge and thank their respective institutes and universities.
Author information
Authors and affiliations.
Division of Pathology, ICAR-Indian Veterinary Research Institute, Izatnagar, Bareilly, Uttar Pradesh, 243122, India
Kuldeep Dhama & Shailesh Kumar Patel
Department of Veterinary Pathology, Dr. G.C Negi College of Veterinary and Animal Sciences, CSK Himachal Pradesh Agricultural University, Palampur, Himachal Pradesh, 176062, India
Rakesh Kumar & Rupali Masand
Department of Veterinary Anatomy, Faculty of Veterinary and Animal Sciences, Rajeev Gandhi South Campus, Banaras Hindu University, Barkachha, Mirzapur, Uttar Pradesh, 231001, India
Jigyasa Rana
Division of Veterinary Clinical Complex, Faculty of Veterinary Sciences and Animal Husbandry, Shuhama, Alusteng Srinagar, Sher-E-Kashmir University of Agricultural Sciences and Technology of Kashmir, Shalimar, Srinagar, Jammu and Kashmir, 190006, India
Mohd. Iqbal Yatoo
Department of Veterinary Microbiology and Immunology, College of Veterinary Sciences, Uttar Pradesh Pandit Deen Dayal Upadhyaya Pashu Chikitsa Vigyan Vishwavidyalaya Evam Go Anusandhan Sansthan (DUVASU), Mathura, 281001, India
Ruchi Tiwari
Division of Surgery, ICAR-Indian Veterinary Research Institute, Izatnagar, Bareilly, Uttar Pradesh, 243122, India
Khan Sharun
Department of Chemistry, Government College of Engineering, Keonjhar, Odisha, 758002, India
Ranjan K. Mohapatra
Indian Institute of Public Health Gandhinagar, Lekawada, Gandhinagar, Gujarat, 382042, India
Senthilkumar Natesan
Department of Microbiology, Punjab Agricultural University, Ludhiana, 141004, India
Manish Dhawan
The Trafford Group of Colleges, Manchester, WA14 5PQ, UK
Department of Epidemiology and Health Statistics, School of Public Health, Southeast University, Nanjing, 210009, China
Tauseef Ahmad
Key Laboratory of Environmental Medicine Engineering, Ministry of Education, School of Public Health, Southeast University, Nanjing, 210009, China
Department of Pharmacy, BGC Trust University Bangladesh, Chittagong, 4381, Bangladesh
Talha Bin Emran
Division of Biological Standardization, ICAR-Indian Veterinary Research Institute, Izatnagar, Bareilly, Uttar Pradesh, 243122, India
Yashpal Singh Malik
Medical Research Unit, School of Medicine, Universitas Syiah Kuala, Banda Aceh, 23111, Indonesia
Harapan Harapan
Tropical Disease Centre, School of Medicine, Universitas Syiah Kuala, Banda Aceh, 23111, Indonesia
Department of Microbiology, School of Medicine, Universitas Syiah Kuala, Banda Aceh, 23111, Indonesia
You can also search for this author in PubMed Google Scholar
Contributions
KD and HH contributed to the review conception and design. SKP, RK, RM, JR, MIY, RT, KS, RKM, SN, MD, TA, TBE, and YSM performed the literature search and data analysis. KD, SKP, RK, RM, JR, MIY, RT, KS, RKM, SN, MD, TA, TBE, YSM, and HH drafted and/or critically revised the manuscript. All authors have read and approved the final version of the manuscript for publication.
Corresponding authors
Correspondence to Kuldeep Dhama or Harapan Harapan .
Ethics declarations
Ethics approval and consent to participate.
Not applicable
Consent for publication
Competing interests.
The authors declare no competing interests.
Additional information
Responsible Editor: Lotfi Aleya
Publisher’s note
Springer Nature remains neutral with regard to jurisdictional claims in published maps and institutional affiliations.
Rights and permissions
Reprints and permissions
About this article
Dhama, K., Patel, S.K., Kumar, R. et al. The role of disinfectants and sanitizers during COVID-19 pandemic: advantages and deleterious effects on humans and the environment. Environ Sci Pollut Res 28 , 34211–34228 (2021). https://doi.org/10.1007/s11356-021-14429-w
Download citation
Received : 10 March 2021
Accepted : 11 May 2021
Published : 15 May 2021
Issue Date : July 2021
DOI : https://doi.org/10.1007/s11356-021-14429-w
Share this article
Anyone you share the following link with will be able to read this content:
Sorry, a shareable link is not currently available for this article.
Provided by the Springer Nature SharedIt content-sharing initiative
- Disinfectants
- Environment
- Find a journal
- Publish with us
- Track your research

Long-lasting disinfectant promises to help fight pandemics
University of Central Florida researchers have developed a nanoparticle-based disinfectant that can continuously kill viruses on a surface for up to seven days -- a discovery that could be a powerful weapon against COVID-19 and other emerging pathogenic viruses.
The findings, by a multidisciplinary team of the university's virus and engineering experts and the leader of an Orlando technology firm, were published this week in ACS Nano , a journal of the American Chemical Society.
Christina Drake, a UCF alumna and founder of Kismet Technologies, was inspired to develop the disinfectant after making a trip to the grocery store in the early days of the pandemic. There she saw a worker spraying disinfectant on a refrigerator handle, then wiping off the spray immediately.
"Initially my thought was to develop a fast-acting disinfectant," she said, "but we spoke to consumers -- like doctors and dentists -- to find out what they really wanted from a disinfectant. What mattered the most to them was something long-lasting that would continue to disinfect high-touch areas like doorhandles and floors long after application."
Drake partnered with Dr. Sudipta Seal, a UCF materials engineer and nanosciences expert, and Dr. Griff Parks, a College of Medicine virologist who is also associate dean of research and director of the Burnett School of Biomedical Sciences. With funding from the National Science Foundation, Kismet Tech and the Florida High Tech Corridor, the researchers created a nanoparticle-engineered disinfectant.
Its active ingredient is an engineered nanostructure called cerium oxide, which is known for its regenerative antioxidant properties. The cerium oxide nanoparticles are modified with small amounts of silver to make them more potent against pathogens.
"It works both chemically and mechanically," explained Seal, a who has been studying nanotechnology for more than 20 years. "The nanoparticles emit electrons that oxidize the virus, rendering it inactive. Mechanically, they also attach themselves to the virus and rupture the surface almost like popping a balloon."
Most disinfecting wipes or sprays will disinfect a surface within three to six minutes of application but have no residual effects. This means surfaces need to be wiped down repeatedly to stay clean from a number of viruses like COVID-19. The nanoparticle formulation maintains its ability to inactivate microbes and continues to disinfect a surface for up to seven days after a single application.
"The disinfectant has shown tremendous antiviral activity against seven different viruses," explained Parks, whose lab was responsible for testing the formulation against "a dictionary" of viruses. "Not only did it show antiviral properties toward coronavirus and rhinovirus, but it also proved effective against a wide range of other viruses with different structures and complexities. We are hopeful that with this amazing range of killing capacity, this disinfectant will also be a highly effective tool against other new emerging viruses. "
The scientists are confident the solution will have a major impact in health care settings, in particular, reducing the rate of hospital acquired infections -- such as Methicillin-resistant Staphylococcus Aureus (MRSA), Pseudomonas aeruginosa and Clostridium difficile -- which cause infections that affect more than one in 30 patients admitted to U.S. hospitals.
And unlike many commercial disinfectants, the formulation has no harmful chemicals, which indicates it will be safe to use on any surface. Regulatory testing for irritancy on skin and eye cells, as required by the U.S. Environmental Protection Agency, showed no harmful effects.
"Many household disinfectants currently available contain chemicals that can be harmful to the body with repeated exposure," Drake said. "Our nanoparticle-based product will have a high safety rating will play a major role in reducing overall chemical exposure for humans."
More research is needed before the product can go to market, which is why the next phase of the study will look at how the disinfectant performs outside of the lab in real world applications. That work will look at how the disinfectant is affected by external factors such as temperature or sunlight. The team is in talks with a local hospital network to test the product in their facilities.
"We're also exploring developing a semi-permanent film to see if we can coat and seal a hospital floor or door handles, areas where you need things to be disinfected and even with aggressive and persistent contact," Drake added.
Seal joined UCF's Department of Materials Science and Engineering, which is part of UCF's College of Engineering and Computer Science, in 1997. He has an appointment at the College of Medicine and is a member of UCF's prosthetics Cluster Biionix. He is the former director of UCF's Nanoscience Technology Center and Advanced Materials Processing Analysis Center. He received his doctorate in materials engineering with a minor in biochemistry from the University of Wisconsin and was a postdoctoral fellow at the Lawrence Berkeley National Laboratory at the University of California Berkeley.
Parks came to UCF in 2014 after 20 years at the Wake Forest School of Medicine, where he was professor and chairman of the Department of Microbiology and Immunology. He earned his doctorate in biochemistry at the University of Wisconsin and was an American Cancer Society Fellow at Northwestern University.
The study was co-authored by post-doctoral researchers Candace Fox, from UCF's College of Medicine and Craig Neal from UCF's College of Engineering and Computer Sciences and graduate students, Tamil Sakthivel, Udit Kumar and Yifei Fu from UCf's College of Engineering and Computer Sciences.
- Infectious Diseases
- Medical Topics
- Personalized Medicine
- Engineering
- Civil Engineering
- Engineering and Construction
- Materials Science
- Human parainfluenza viruses
- Microorganism
- Foodborne illness
- Water purification
Story Source:
Materials provided by University of Central Florida . Original written by Christin Senior. Note: Content may be edited for style and length.
Journal Reference :
- Craig J. Neal, Candace R. Fox, Tamil Selvan Sakthivel, Udit Kumar, Yifei Fu, Christina Drake, Griffith D. Parks, Sudipta Seal. Metal-Mediated Nanoscale Cerium Oxide Inactivates Human Coronavirus and Rhinovirus by Surface Disruption . ACS Nano , 2021; DOI: 10.1021/acsnano.1c04142
Cite This Page :
Explore More
- Robot, Can You Say 'Cheese'?
- Researchers Turn Back the Clock On Cancer Cells
- Making Long-Term Memories: Nerve-Cell Damage
- A Solar Cell You Can Bend and Soak in Water
- Risk Factors for Faster Brain Aging
- Deep Space Objects Can Become 'Ice Bombs'
- Want to Feel Young? Protect Your Sleep
- Century-Old Powdered Milk in Antarctica
- New Artificial Reef Stands Up to Storms
- Persistent Hiccups in a Far-Off Galaxy
Trending Topics
Strange & offbeat.
We use cookies to enhance our website for you. Proceed if you agree to this policy or learn more about it.
- Essay Database >
- Essays Examples >
- Essay Topics
Essays on Disinfectant
7 samples on this topic
The variety of written assignments you might get while studying Disinfectant is stunning. If some are too difficult, an expertly crafted sample Disinfectant piece on a related topic might lead you out of a deadlock. This is when you will definitely acknowledge WowEssays.com ever-widening directory of Disinfectant essay samples meant to ignite your writing creativity.
Our directory of free college paper samples showcases the most vivid instances of top-notch writing on Disinfectant and related topics. Not only can they help you develop an interesting and fresh topic, but also exhibit the effective use of the best Disinfectant writing practices and content structuring techniques. Also, keep in mind that you can use them as a source of dependable sources and factual or statistical data processed by real masters of their craft with solid academic experience in the Disinfectant area.
Alternatively, you can take advantage of practical write my essay assistance, when our experts provide a unique example essay on Disinfectant tailored to your personal specifications!
Example Of Sanitizers Better Than Soap And Water Essay
Perfect model essay on hand washing of nursing students: an observational study.
Kimiesha Nichols
Good Essay About Water Disinfection
Learn to craft research papers on effects of chlorine with this example, the importance of water in everyday life essay examples, free essay on national patient safety goal 7.
275 words = 1 page double-spaced
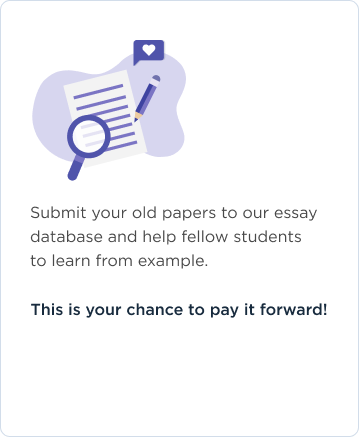
Password recovery email has been sent to [email protected]
Use your new password to log in
You are not register!
By clicking Register, you agree to our Terms of Service and that you have read our Privacy Policy .
Now you can download documents directly to your device!
Check your email! An email with your password has already been sent to you! Now you can download documents directly to your device.
or Use the QR code to Save this Paper to Your Phone
The sample is NOT original!
Short on a deadline?
Don't waste time. Get help with 11% off using code - GETWOWED
No, thanks! I'm fine with missing my deadline
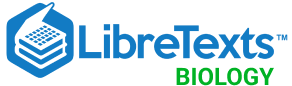
- school Campus Bookshelves
- menu_book Bookshelves
- perm_media Learning Objects
- login Login
- how_to_reg Request Instructor Account
- hub Instructor Commons
- Download Page (PDF)
- Download Full Book (PDF)
- Periodic Table
- Physics Constants
- Scientific Calculator
- Reference & Cite
- Tools expand_more
- Readability
selected template will load here
This action is not available.
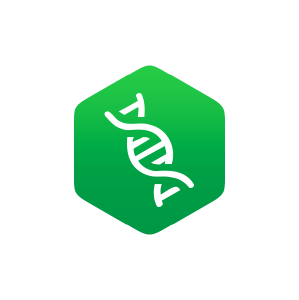
13.3: Using Chemicals to Control Microorganisms
- Last updated
- Save as PDF
- Page ID 5195
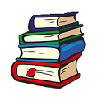
Learning Objectives
- Understand and compare various chemicals used to control microbial growth, including their uses, advantages and disadvantages, chemical structure, and mode of action
In addition to physical methods of microbial control, chemicals are also used to control microbial growth. A wide variety of chemicals can be used as disinfectants or antiseptics. When choosing which to use, it is important to consider the type of microbe targeted; how clean the item needs to be; the disinfectant’s effect on the item’s integrity; its safety to animals, humans, and the environment; its expense; and its ease of use. This section describes the variety of chemicals used as disinfectants and antiseptics, including their mechanisms of action and common uses.
In the 1800s, scientists began experimenting with a variety of chemicals for disinfection. In the 1860s, British surgeon Joseph Lister (1827–1912) began using carbolic acid, known as phenol, as a disinfectant for the treatment of surgical wounds (see Foundations of Modern Cell Theory ). In 1879, Lister’s work inspired the American chemist Joseph Lawrence (1836–1909) to develop Listerine, an alcohol-based mixture of several related compounds that is still used today as an oral antiseptic. Today, carbolic acid is no longer used as a surgical disinfectant because it is a skin irritant, but the chemical compounds found in antiseptic mouthwashes and throat lozenges are called phenolics.
Chemically, phenol consists of a benzene ring with an –OH group, and phenolics are compounds that have this group as part of their chemical structure (Figure \(\PageIndex{1}\)). Phenolics such as thymol and eucalyptol occur naturally in plants. Other phenolics can be derived from creosote, a component of coal tar. Phenolics tend to be stable, persistent on surfaces, and less toxic than phenol. They inhibit microbial growth by denaturing proteins and disrupting membranes.
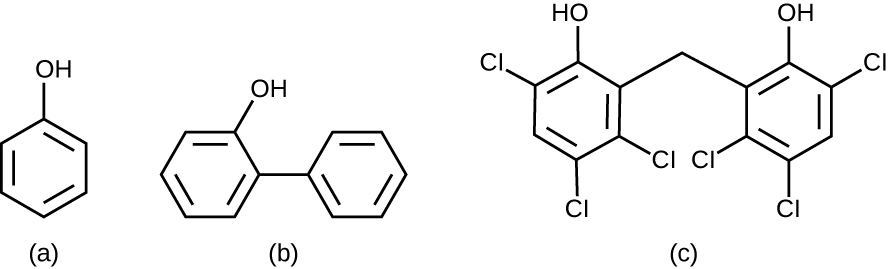
Since Lister’s time, several phenolic compounds have been used to control microbial growth. Phenolics like cresols(methylated phenols) and o-phenylphenol were active ingredients in various formulations of Lysol since its invention in 1889. o-Phenylphenol was also commonly used in agriculture to control bacterial and fungal growth on harvested crops, especially citrus fruits, but its use in the United States is now far more limited. The bisphenol hexachlorophene, a disinfectant, is the active ingredient in pHisoHex, a topical cleansing detergent widely used for handwashing in hospital settings. pHisoHex is particularly effective against gram-positive bacteria, including those causing staphylococcal and streptococcal skin infections. pHisoHex was formerly used for bathing infants, but this practice has been discontinued because it has been shown that exposure to hexachlorophene can lead to neurological problems.
Triclosan is another bisphenol compound that has seen widespread application in antibacterial products over the last several decades. Initially used in toothpastes, triclosan is now commonly used in hand soaps and is frequently impregnated into a wide variety of other products, including cutting boards, knives, shower curtains, clothing, and concrete, to make them antimicrobial. It is particularly effective against gram-positive bacteria on the skin, as well as certain gram-negative bacteria and yeasts. 1
Triclosan: Antibacterial Overkill?
Hand soaps and other cleaning products are often marketed as “antibacterial,” suggesting that they provide a level of cleanliness superior to that of conventional soaps and cleansers. But are the antibacterial ingredients in these products really safe and effective?
About 75% of antibacterial liquid hand soaps and 30% of bar soaps contain the chemical triclosan, a phenolic, (Figure \(\PageIndex{2}\)). 2 Triclosan blocks an enzyme in the bacterial fatty acid-biosynthesis pathway that is not found in the comparable human pathway. Although the use of triclosan in the home increased dramatically during the 1990s, more than 40 years of research by the FDA have turned up no conclusive evidence that washing with triclosan-containing products provides increased health benefits compared with washing with traditional soap. Although some studies indicate that fewer bacteria may remain on a person’s hands after washing with triclosan-based soap, compared with traditional soap, no evidence points to any reduction in the transmission of bacteria that cause respiratory and gastrointestinal illness. In short, soaps with triclosan may remove or kill a few more germs but not enough to reduce the spread of disease.
Perhaps more disturbing, some clear risks associated with triclosan-based soaps have come to light. The widespread use of triclosan has led to an increase in triclosan-resistant bacterial strains, including those of clinical importance, such as Salmonella enterica ; this resistance may render triclosan useless as an antibacterial in the long run. 3 4 Bacteria can easily gain resistance to triclosan through a change to a single gene encoding the targeted enzyme in the bacterial fatty acid-synthesis pathway. Other disinfectants with a less specific mode of action are much less prone to engendering resistance because it would take much more than a single genetic change.
Use of triclosan over the last several decades has also led to a buildup of the chemical in the environment. Triclosan in hand soap is directly introduced into wastewater and sewage systems as a result of the handwashing process. There, its antibacterial properties can inhibit or kill bacteria responsible for the decomposition of sewage, causing septic systems to clog and back up. Eventually, triclosan in wastewater finds its way into surface waters, streams, lakes, sediments, and soils, disrupting natural populations of bacteria that carry out important environmental functions, such as inhibiting algae. Triclosan also finds its way into the bodies of amphibians and fish, where it can act as an endocrine disruptor. Detectable levels of triclosan have also been found in various human bodily fluids, including breast milk, plasma, and urine. 5 In fact, a study conducted by the CDC found detectable levels of triclosan in the urine of 75% of 2,517 people tested in 2003–2004. 6 This finding is even more troubling given the evidence that triclosan may affect immune function in humans. 7
In December 2013, the FDA gave soap manufacturers until 2016 to prove that antibacterial soaps provide a significant benefit over traditional soaps; if unable to do so, manufacturers will be forced to remove these products from the market.
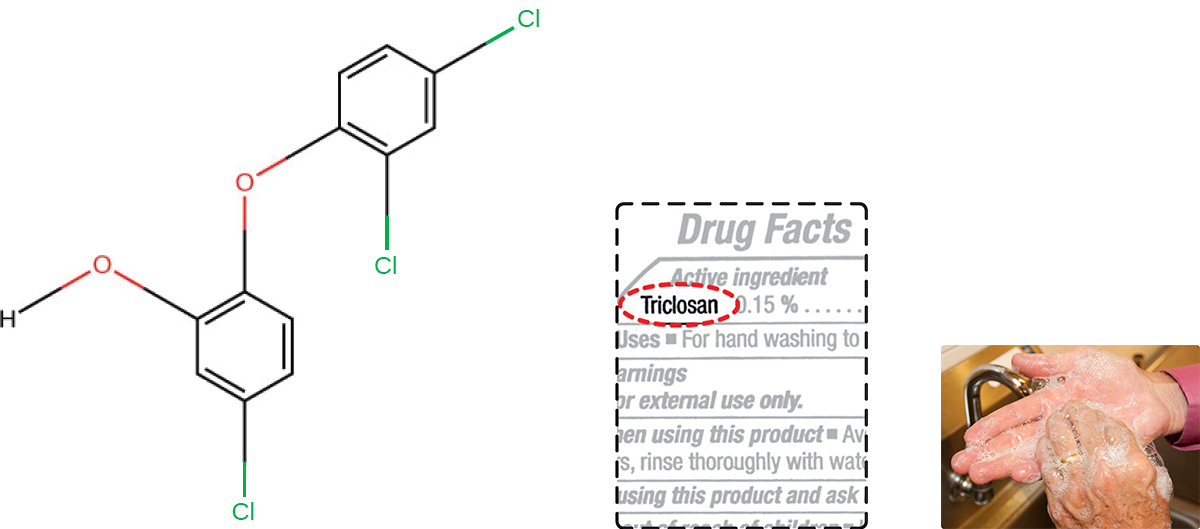
Exercise \(\PageIndex{1}\)
Why is triclosan more like an antibiotic than a traditional disinfectant?
Heavy Metals
Some of the first chemical disinfectants and antiseptics to be used were heavy metals. Heavy metals kill microbes by binding to proteins, thus inhibiting enzymatic activity (Figure \(\PageIndex{3}\)). Heavy metals are oligodynamic, meaning that very small concentrations show significant antimicrobial activity. Ions of heavy metals bind to sulfur-containing amino acids strongly and bioaccumulate within cells, allowing these metals to reach high localized concentrations. This causes proteins to denature.
Heavy metals are not selectively toxic to microbial cells. They may bioaccumulate in human or animal cells, as well, and excessive concentrations can have toxic effects on humans. If too much silver accumulates in the body, for example, it can result in a condition called argyria, in which the skin turns irreversibly blue-gray. One way to reduce the potential toxicity of heavy metals is by carefully controlling the duration of exposure and concentration of the heavy metal.
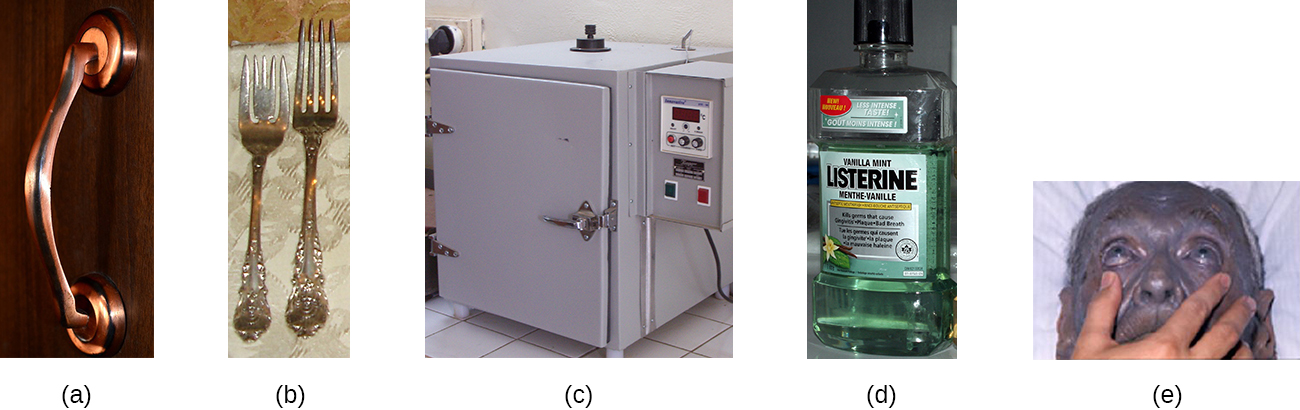
Mercury is an example of a heavy metal that has been used for many years to control microbial growth. It was used for many centuries to treat syphilis. Mercury compounds like mercuric chloride are mainly bacteriostatic and have a very broad spectrum of activity. Various forms of mercury bind to sulfur-containing amino acids within proteins, inhibiting their functions.
In recent decades, the use of such compounds has diminished because of mercury’s toxicity. It is toxic to the central nervous, digestive, and renal systems at high concentrations, and has negative environmental effects, including bioaccumulation in fish. Topical antiseptics such as mercurochrome, which contains mercury in low concentrations, and merthiolate, a tincture (a solution of mercury dissolved in alcohol) were once commonly used. However, because of concerns about using mercury compounds, these antiseptics are no longer sold in the United States.
Silver has long been used as an antiseptic. In ancient times, drinking water was stored in silver jugs. 8 Silvadene cream is commonly used to treat topical wounds and is particularly helpful in preventing infection in burn wounds. Silver nitrate drops were once routinely applied to the eyes of newborns to protect against ophthalmia neonatorum, eye infections that can occur due to exposure to pathogens in the birth canal, but antibiotic creams are more now commonly used. Silver is often combined with antibiotics, making the antibiotics thousands of times more effective. 9 Silver is also commonly incorporated into catheters and bandages, rendering them antimicrobial; however, there is evidence that heavy metals may also enhance selection for antibiotic resistance. 10
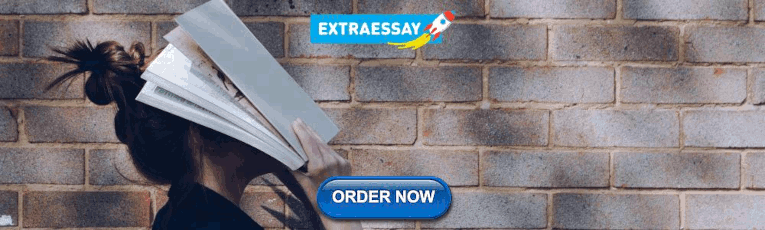
Copper, Nickel, and Zinc
Several other heavy metals also exhibit antimicrobial activity. Copper sulfate is a common algicide used to control algal growth in swimming pools and fish tanks. The use of metallic copper to minimize microbial growth is also becoming more widespread. Copper linings in incubators help reduce contamination of cell cultures. The use of copper pots for water storage in underdeveloped countries is being investigated as a way to combat diarrheal diseases. Copper coatings are also becoming popular for frequently handled objects such as doorknobs, cabinet hardware, and other fixtures in health-care facilities in an attempt to reduce the spread of microbes.
Nickel and zinc coatings are now being used in a similar way. Other forms of zinc, including zinc chloride and zinc oxide, are also used commercially. Zinc chloride is quite safe for humans and is commonly found in mouthwashes, substantially increasing their length of effectiveness. Zinc oxide is found in a variety of products, including topical antiseptic creams such as calamine lotion, diaper ointments, baby powder, and dandruff shampoos.
Exercise \(\PageIndex{2}\)
Why are many heavy metals both antimicrobial and toxic to humans?
Other chemicals commonly used for disinfection are the halogens iodine, chlorine, and fluorine. Iodine works by oxidizing cellular components, including sulfur-containing amino acids, nucleotides, and fatty acids, and destabilizing the macromolecules that contain these molecules. It is often used as a topical tincture, but it may cause staining or skin irritation. An iodophor is a compound of iodine complexed with an organic molecule, thereby increasing iodine’s stability and, in turn, its efficacy. One common iodophor is povidone-iodine, which includes a wetting agent that releases iodine relatively slowly. Betadine is a brand of povidone-iodine commonly used as a hand scrub by medical personnel before surgery and for topical antisepsis of a patient’s skin before incision (Figure \(\PageIndex{4}\)).
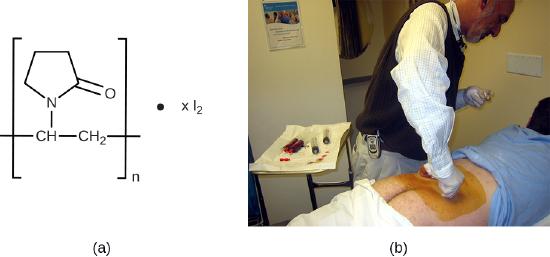
Chlorine is another halogen commonly used for disinfection. When chlorine gas is mixed with water, it produces a strong oxidant called hypochlorous acid, which is uncharged and enters cells easily. Chlorine gas is commonly used in municipal drinking water and wastewater treatment plants, with the resulting hypochlorous acid producing the actual antimicrobial effect. Those working at water treatment facilities need to take great care to minimize personal exposure to chlorine gas. Sodium hypochlorite is the chemical component of common household bleach, and it is also used for a wide variety of disinfecting purposes. Hypochlorite salts, including sodium and calcium hypochlorites, are used to disinfect swimming pools. Chlorine gas, sodium hypochlorite, and calcium hypochlorite are also commonly used disinfectants in the food processing and restaurant industries to reduce the spread of foodborne diseases. Workers in these industries also need to take care to use these products correctly to ensure their own safety as well as the safety of consumers. A recent joint statement published by the Food and Agriculture Organization (FAO) of the United Nations and WHO indicated that none of the many beneficial uses of chlorine products in food processing to reduce the spread of foodborne illness posed risks to consumers. 11
Another class of chlorinated compounds called chloramines are widely used as disinfectants. Chloramines are relatively stable, releasing chlorine over long periods time. Chloramines are derivatives of ammonia by substitution of one, two, or all three hydrogen atoms with chlorine atoms (Figure \(\PageIndex{5}\)).
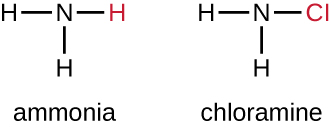
Chloramines and other cholorine compounds may be used for disinfection of drinking water, and chloramine tablets are frequently used by the military for this purpose. After a natural disaster or other event that compromises the public water supply, the CDC recommends disinfecting tap water by adding small amounts of regular household bleach. Recent research suggests that sodium dichloroisocyanurate (NaDCC) may also be a good alternative for drinking water disinfection. Currently, NaDCC tablets are available for general use and for use by the military, campers, or those with emergency needs; for these uses, NaDCC is preferable to chloramine tablets. Chlorine dioxide, a gaseous agent used for fumigation and sterilization of enclosed areas, is also commonly used for the disinfection of water.
Although chlorinated compounds are relatively effective disinfectants, they have their disadvantages. Some may irritate the skin, nose, or eyes of some individuals, and they may not completely eliminate certain hardy organisms from contaminated drinking water. The fungus Cryptosporidium , for example, has a protective outer shell that makes it resistant to chlorinated disinfectants. Thus, boiling of drinking water in emergency situations is recommended when possible.
The halogen fluorine is also known to have antimicrobial properties that contribute to the prevention of dental caries(cavities). 12 Fluoride is the main active ingredient of toothpaste and is also commonly added to tap water to help communities maintain oral health. Chemically, fluoride can become incorporated into the hydroxyapatite of tooth enamel, making it more resistant to corrosive acids produced by the fermentation of oral microbes. Fluoride also enhances the uptake of calcium and phosphate ions in tooth enamel, promoting remineralization. In addition to strengthening enamel, fluoride also seems to be bacteriostatic. It accumulates in plaque-forming bacteria, interfering with their metabolism and reducing their production of the acids that contribute to tooth decay.
Exercise \(\PageIndex{3}\)
What is a benefit of a chloramine over hypochlorite for disinfecting?
Alcohols make up another group of chemicals commonly used as disinfectants and antiseptics. They work by rapidly denaturing proteins, which inhibits cell metabolism, and by disrupting membranes, which leads to cell lysis. Once denatured, the proteins may potentially refold if enough water is present in the solution. Alcohols are typically used at concentrations of about 70% aqueous solution and, in fact, work better in aqueous solutions than 100% alcohol solutions. This is because alcohols coagulate proteins. In higher alcohol concentrations, rapid coagulation of surface proteins prevents effective penetration of cells. The most commonly used alcohols for disinfection are ethyl alcohol(ethanol) and isopropyl alcohol (isopropanol, rubbing alcohol) (Figure \(\PageIndex{6}\)).
Alcohols tend to be bactericidal and fungicidal, but may also be viricidal for enveloped viruses only. Although alcohols are not sporicidal, they do inhibit the processes of sporulation and germination. Alcohols are volatile and dry quickly, but they may also cause skin irritation because they dehydrate the skin at the site of application. One common clinical use of alcohols is swabbing the skin for degerming before needle injection. Alcohols also are the active ingredients in instant hand sanitizers, which have gained popularity in recent years. The alcohol in these hand sanitizers works both by denaturing proteins and by disrupting the microbial cell membrane, but will not work effectively in the presence of visible dirt.
Last, alcohols are used to make tinctures with other antiseptics, such as the iodine tinctures discussed previously in this chapter. All in all, alcohols are inexpensive and quite effective for the disinfection of a broad range of vegetative microbes. However, one disadvantage of alcohols is their high volatility, limiting their effectiveness to immediately after application.
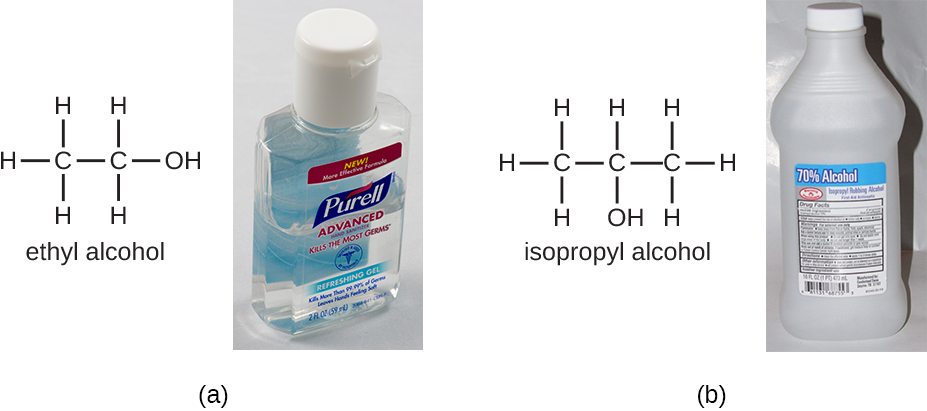
Exercise \(\PageIndex{4}\)
- Name at least three advantages of alcohols as disinfectants.
- Describe several specific applications of alcohols used in disinfectant products.
Surfactants
Surface-active agents, or surfactants, are a group of chemical compounds that lower the surface tension of water. Surfactants are the major ingredients in soaps and detergents. Soaps are salts of long-chain fatty acids and have both polar and nonpolar regions, allowing them to interact with polar and nonpolar regions in other molecules (Figure \(\PageIndex{7}\)). They can interact with nonpolar oils and grease to create emulsions in water, loosening and lifting away dirt and microbes from surfaces and skin. Soaps do not kill or inhibit microbial growth and so are not considered antiseptics or disinfectants. However, proper use of soaps mechanically carries away microorganisms, effectively degerming a surface. Some soaps contain added bacteriostatic agents such as triclocarban or cloflucarban, compounds structurally related to triclosan, that introduce antiseptic or disinfectant properties to the soaps.
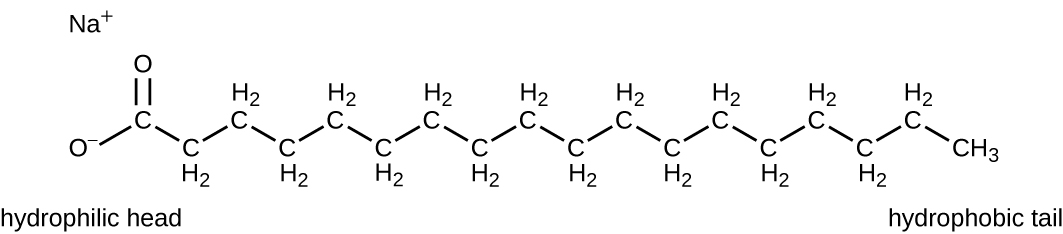
Soaps, however, often form films that are difficult to rinse away, especially in hard water, which contains high concentrations of calcium and magnesium mineral salts. Detergents contain synthetic surfactant molecules with both polar and nonpolar regions that have strong cleansing activity but are more soluble, even in hard water, and, therefore, leave behind no soapy deposits. Anionic detergents, such as those used for laundry, have a negatively charged anion at one end attached to a long hydrophobic chain, whereas cationic detergents have a positively charged cation instead. Cationic detergents include an important class of disinfectants and antiseptics called the quaternary ammonium salts (quats), named for the characteristic quaternary nitrogen atom that confers the positive charge (Figure \(\PageIndex{8}\)). Overall, quats have properties similar to phospholipids, having hydrophilic and hydrophobic ends. As such, quats have the ability to insert into the bacterial phospholipid bilayer and disrupt membrane integrity. The cationic charge of quats appears to confer their antimicrobial properties, which are diminished when neutralized. Quats have several useful properties. They are stable, nontoxic, inexpensive, colorless, odorless, and tasteless. They tend to be bactericidal by disrupting membranes. They are also active against fungi, protozoans, and enveloped viruses, but endospores are unaffected. In clinical settings, they may be used as antiseptics or to disinfect surfaces. Mixtures of quats are also commonly found in household cleaners and disinfectants, including many current formulations of Lysol brand products, which contain benzalkonium chlorides as the active ingredients. Benzalkonium chlorides, along with the quat cetylpyrimidine chloride, are also found in products such as skin antiseptics, oral rinses, and mouthwashes.
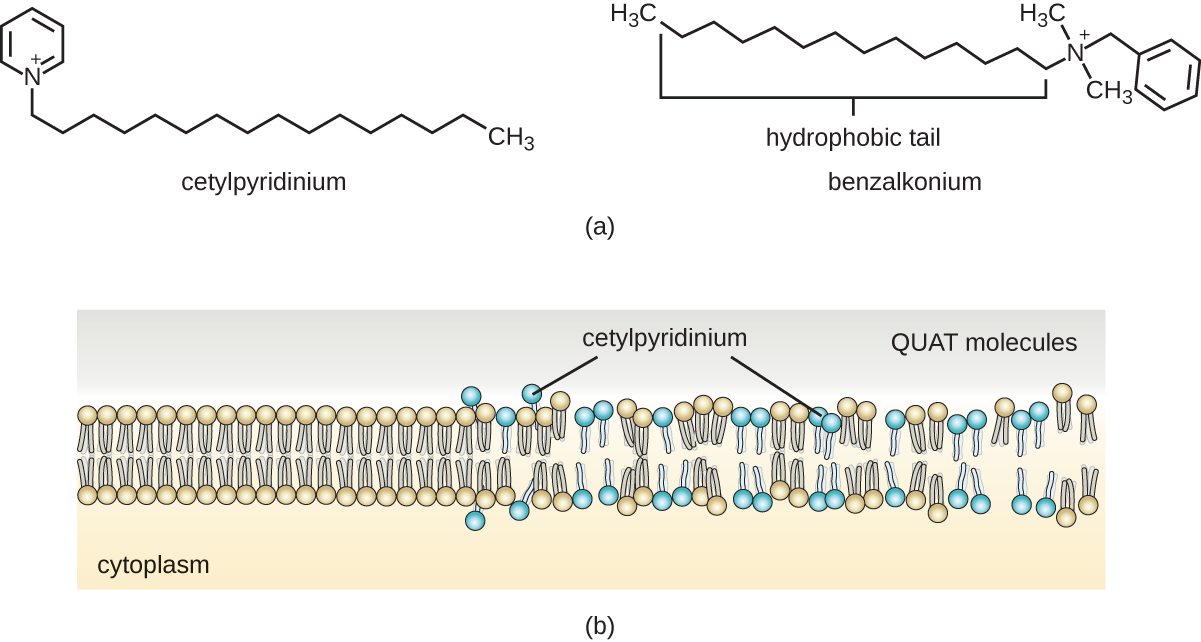
Exercise \(\PageIndex{5}\)
Why are soaps not considered disinfectants?
Handwashing the Right Way
Handwashing is critical for public health and should be emphasized in a clinical setting. For the general public, the CDC recommends handwashing before, during, and after food handling; before eating; before and after interacting with someone who is ill; before and after treating a wound; after using the toilet or changing diapers; after coughing, sneezing, or blowing the nose; after handling garbage; and after interacting with an animal, its feed, or its waste. Figure \(\PageIndex{9}\) illustrates the five steps of proper handwashing recommended by the CDC.
Handwashing is even more important for health-care workers, who should wash their hands thoroughly between every patient contact, after the removal of gloves, after contact with bodily fluids and potentially infectious fomites, and before and after assisting a surgeon with invasive procedures. Even with the use of proper surgical attire, including gloves, scrubbing for surgery is more involved than routine handwashing. The goal of surgical scrubbing is to reduce the normal microbiota on the skin’s surface to prevent the introduction of these microbes into a patient’s surgical wounds.
There is no single widely accepted protocol for surgical scrubbing. Protocols for length of time spent scrubbing may depend on the antimicrobial used; health-care workers should always check the manufacturer’s recommendations. According to the Association of Surgical Technologists (AST), surgical scrubs may be performed with or without the use of brushes (Figure \(\PageIndex{9}\)).
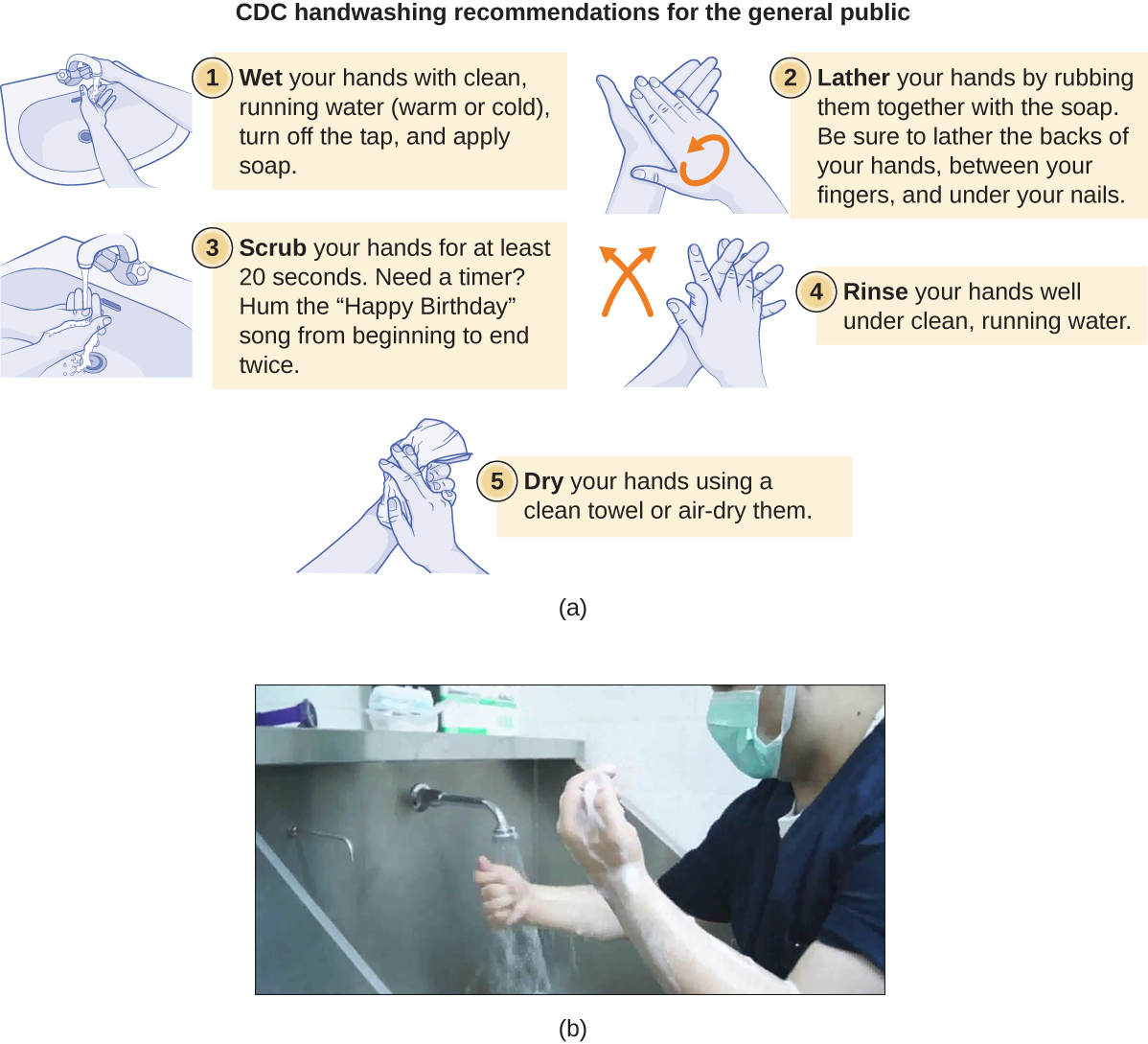
Link to Learning
To learn more about proper handwashing, visit the CDC’s website.
Bisbiguanides
Bisbiguanides were first synthesized in the 20th century and are cationic (positively charged) molecules known for their antiseptic properties (Figure \(\PageIndex{10}\)). One important bisbiguanide antiseptic is chlorhexidine. It has broad-spectrum activity against yeasts, gram-positive bacteria, and gram-negative bacteria, with the exception of Pseudomonas aeruginosa , which may develop resistance on repeated exposure. 13 Chlorhexidine disrupts cell membranes and is bacteriostatic at lower concentrations or bactericidal at higher concentrations, in which it actually causes the cells’ cytoplasmic contents to congeal. It also has activity against enveloped viruses. However, chlorhexidine is poorly effective against Mycobacterium tuberculosis and nonenveloped viruses, and it is not sporicidal. Chlorhexidine is typically used in the clinical setting as a surgical scrub and for other handwashing needs for medical personnel, as well as for topical antisepsis for patients before surgery or needle injection. It is more persistent than iodophors, providing long-lasting antimicrobial activity. Chlorhexidine solutions may also be used as oral rinses after oral procedures or to treat gingivitis. Another bisbiguanide, alexidine, is gaining popularity as a surgical scrub and an oral rinse because it acts faster than chlorhexidine.

Exercise \(\PageIndex{6}\)
What two effects does chlorhexidine have on bacterial cells?
Alkylating Agents
The alkylating agents are a group of strong disinfecting chemicals that act by replacing a hydrogen atom within a molecule with an alkyl group (C n H 2n+1 ), thereby inactivating enzymes and nucleic acids (Figure \(\PageIndex{11}\)). The alkylating agent formaldehyde (CH 2 OH) is commonly used in solution at a concentration of 37% (known as formalin) or as a gaseous disinfectant and biocide. It is a strong, broad-spectrum disinfectant and biocide that has the ability to kill bacteria, viruses, fungi, and endospores, leading to sterilization at low temperatures, which is sometimes a convenient alternative to the more labor-intensive heat sterilization methods. It also cross-links proteins and has been widely used as a chemical fixative. Because of this, it is used for the storage of tissue specimens and as an embalming fluid. It also has been used to inactivate infectious agents in vaccine preparation. Formaldehyde is very irritating to living tissues and is also carcinogenic; therefore, it is not used as an antiseptic.
Glutaraldehyde is structurally similar to formaldehyde but has two reactive aldehyde groups, allowing it to act more quickly than formaldehyde. It is commonly used as a 2% solution for sterilization and is marketed under the brand name Cidex. It is used to disinfect a variety of surfaces and surgical and medical equipment. However, similar to formaldehyde, glutaraldehyde irritates the skin and is not used as an antiseptic.
A new type of disinfectant gaining popularity for the disinfection of medical equipment is o-phthalaldehyde (OPA), which is found in some newer formulations of Cidex and similar products, replacing glutaraldehyde. o-Phthalaldehyde also has two reactive aldehyde groups, but they are linked by an aromatic bridge. o-Phthalaldehyde is thought to work similarly to glutaraldehyde and formaldehyde, but is much less irritating to skin and nasal passages, produces a minimal odor, does not require processing before use, and is more effective against mycobacteria.
Ethylene oxide is a type of alkylating agent that is used for gaseous sterilization. It is highly penetrating and can sterilize items within plastic bags such as catheters, disposable items in laboratories and clinical settings (like packaged Petri dishes), and other pieces of equipment. Ethylene oxide exposure is a form of cold sterilization, making it useful for the sterilization of heat-sensitive items. Great care needs to be taken with the use of ethylene oxide, however; it is carcinogenic, like the other alkylating agents, and is also highly explosive. With careful use and proper aeration of the products after treatment, ethylene oxide is highly effective, and ethylene oxide sterilizers are commonly found in medical settings for sterilizing packaged materials.
β-Propionolactone is an alkylating agent with a different chemical structure than the others already discussed. Like other alkylating agents, β-propionolactone binds to DNA, thereby inactivating it (Figure \(\PageIndex{11}\)). It is a clear liquid with a strong odor and has the ability to kill endospores. As such, it has been used in either liquid form or as a vapor for the sterilization of medical instruments and tissue grafts, and it is a common component of vaccines, used to maintain their sterility. It has also been used for the sterilization of nutrient broth, as well as blood plasma, milk, and water. It is quickly metabolized by animals and humans to lactic acid. It is also an irritant, however, and may lead to permanent damage of the eyes, kidneys, or liver. Additionally, it has been shown to be carcinogenic in animals; thus, precautions are necessary to minimize human exposure to β-propionolactone. 14
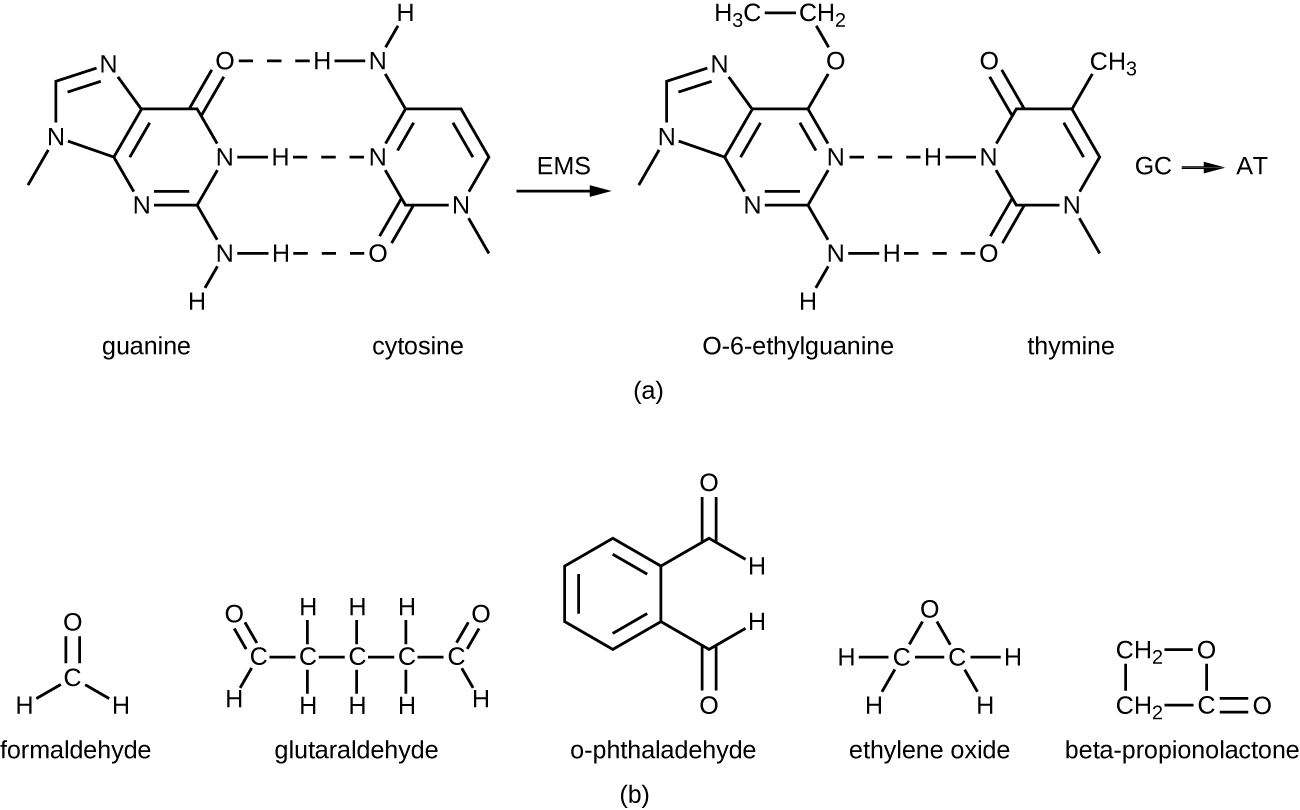
Exercise \(\PageIndex{7}\)
- What chemical reaction do alkylating agents participate in?
- Why are alkylating agents not used as antiseptics?
Diehard Prions
Prions , the acellular, misfolded proteins responsible for incurable and fatal diseases such as kuru and Creutzfeldt-Jakob disease (see Viroids, Virusoids, and Prions ), are notoriously difficult to destroy. Prions are extremely resistant to heat, chemicals, and radiation. They are also extremely infectious and deadly; thus, handling and disposing of prion-infected items requires extensive training and extreme caution.
Typical methods of disinfection can reduce but not eliminate the infectivity of prions. Autoclaving is not completely effective, nor are chemicals such as phenol, alcohols, formalin, and β-propiolactone. Even when fixed in formalin, affected brain and spinal cord tissues remain infectious.
Personnel who handle contaminated specimens or equipment or work with infected patients must wear a protective coat, face protection, and cut-resistant gloves. Any contact with skin must be immediately washed with detergent and warm water without scrubbing. The skin should then be washed with 1 N NaOH or a 1:10 dilution of bleach for 1 minute. Contaminated waste must be incinerated or autoclaved in a strong basic solution, and instruments must be cleaned and soaked in a strong basic solution.
For more information on the handling of animals and prion-contaminated materials, visit the guidelines published on the CDC and WHO websites.
Peroxygens are strong oxidizing agents that can be used as disinfectants or antiseptics. The most widely used peroxygen is hydrogen peroxide (H 2 O 2 ), which is often used in solution to disinfect surfaces and may also be used as a gaseous agent. Hydrogen peroxide solutions are inexpensive skin antiseptics that break down into water and oxygen gas, both of which are environmentally safe. This decomposition is accelerated in the presence of light, so hydrogen peroxide solutions typically are sold in brown or opaque bottles. One disadvantage of using hydrogen peroxide as an antiseptic is that it also causes damage to skin that may delay healing or lead to scarring. Contact lens cleaners often include hydrogen peroxide as a disinfectant.
Hydrogen peroxide works by producing free radicals that damage cellular macromolecules. Hydrogen peroxide has broad-spectrum activity, working against gram-positive and gram-negative bacteria (with slightly greater efficacy against gram-positive bacteria), fungi, viruses, and endospores. However, bacteria that produce the oxygen-detoxifying enzymes catalase or peroxidase may have inherent tolerance to low hydrogen peroxide concentrations (Figure \(\PageIndex{12}\)). To kill endospores, the length of exposure or concentration of solutions of hydrogen peroxide must be increased. Gaseous hydrogen peroxide has greater efficacy and can be used as a sterilant for rooms or equipment.
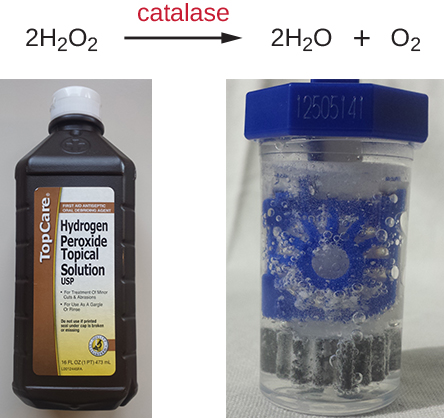
Plasma, a hot, ionized gas, described as the fourth state of matter, is useful for sterilizing equipment because it penetrates surfaces and kills vegetative cells and endospores. Hydrogen peroxide and peracetic acid, another commonly used peroxygen, each may be introduced as a plasma. Peracetic acid can be used as a liquid or plasma sterilant insofar as it readily kills endospores, is more effective than hydrogen peroxide even at rather low concentrations, and is immune to inactivation by catalases and peroxidases. It also breaks down to environmentally innocuous compounds; in this case, acetic acid and oxygen.
Other examples of peroxygens include benzoyl peroxide and carbamide peroxide. Benzoyl peroxide is a peroxygen that used in acne medication solutions. It kills the bacterium Propionibacterium acnes , which is associated with acne. Carbamide peroxide, an ingredient used in toothpaste, is a peroxygen that combats oral biofilms that cause tooth discoloration and halitosis (bad breath). 15 Last, ozone gas is a peroxygen with disinfectant qualities and is used to clean air or water supplies. Overall, peroxygens are highly effective and commonly used, with no associated environmental hazard.
Exercise \(\PageIndex{8}\)
How do peroxides kill cells?
Supercritical Fluids
Within the last 15 years, the use of supercritical fluids, especially supercritical carbon dioxide (scCO 2 ), has gained popularity for certain sterilizing applications. When carbon dioxide is brought to approximately 10 times atmospheric pressure, it reaches a supercritical state that has physical properties between those of liquids and gases. Materials put into a chamber in which carbon dioxide is pressurized in this way can be sterilized because of the ability of scCO 2 to penetrate surfaces.
Supercritical carbon dioxide works by penetrating cells and forming carbonic acid, thereby lowering the cell pH considerably. This technique is effective against vegetative cells and is also used in combination with peracetic acid to kill endospores. Its efficacy can also be augmented with increased temperature or by rapid cycles of pressurization and depressurization, which more likely produce cell lysis.
Benefits of scCO 2 include the nonreactive, nontoxic, and nonflammable properties of carbon dioxide, and this protocol is effective at low temperatures. Unlike other methods, such as heat and irradiation, that can degrade the object being sterilized, the use of scCO 2 preserves the object’s integrity and is commonly used for treating foods (including spices and juices) and medical devices such as endoscopes. It is also gaining popularity for disinfecting tissues such as skin, bones, tendons, and ligaments prior to transplantation. scCO 2 can also be used for pest control because it can kill insect eggs and larvae within products.
Exercise \(\PageIndex{9}\)
Why is the use of supercritical carbon dioxide gaining popularity for commercial and medical uses?
Chemical Food Preservatives
Chemical preservatives are used to inhibit microbial growth and minimize spoilage in some foods. Commonly used chemical preservatives include sorbic acid, benzoic acid, and propionic acid, and their more soluble salts potassium sorbate, sodium benzoate, and calcium propionate, all of which are used to control the growth of molds in acidic foods. Each of these preservatives is nontoxic and readily metabolized by humans. They are also flavorless, so they do not compromise the flavor of the foods they preserve.
Sorbic and benzoic acids exhibit increased efficacy as the pH decreases. Sorbic acid is thought to work by inhibiting various cellular enzymes, including those in the citric acid cycle, as well as catalases and peroxidases. It is added as a preservative in a wide variety of foods, including dairy, bread, fruit, and vegetable products. Benzoic acid is found naturally in many types of fruits and berries, spices, and fermented products. It is thought to work by decreasing intracellular pH, interfering with mechanisms such as oxidative phosphorylation and the uptake of molecules such as amino acids into cells. Foods preserved with benzoic acid or sodium benzoate include fruit juices, jams, ice creams, pastries, soft drinks, chewing gum, and pickles.
Propionic acid is thought to both inhibit enzymes and decrease intracellular pH, working similarly to benzoic acid. However, propionic acid is a more effective preservative at a higher pH than either sorbic acid or benzoic acid. Propionic acid is naturally produced by some cheeses during their ripening and is added to other types of cheese and baked goods to prevent mold contamination. It is also added to raw dough to prevent contamination by the bacterium Bacillus mesentericus , which causes bread to become ropy.
Other commonly used chemical preservatives include sulfur dioxide and nitrites. Sulfur dioxide prevents browning of foods and is used for the preservation of dried fruits; it has been used in winemaking since ancient times. Sulfur dioxide gas dissolves in water readily, forming sulfites. Although sulfites can be metabolized by the body, some people have sulfite allergies, including asthmatic reactions. Additionally, sulfites degrade thiamine, an important nutrient in some foods. The mode of action of sulfites is not entirely clear, but they may interfere with the disulfide bond (see Figure 7.4.5 ) formation in proteins, inhibiting enzymatic activity. Alternatively, they may reduce the intracellular pH of the cell, interfering with proton motive force-driven mechanisms.
Nitrites are added to processed meats to maintain color and stop the germination of Clostridium botulinum endospores. Nitrites are reduced to nitric oxide, which reacts with heme groups and iron-sulfur groups. When nitric oxide reacts with the heme group within the myoglobin of meats, a red product forms, giving meat its red color. Alternatively, it is thought that when nitric acid reacts with the iron-sulfur enzyme ferredoxin within bacteria, this electron transport-chain carrier is destroyed, preventing ATP synthesis. Nitrosamines, however, are carcinogenic and can be produced through exposure of nitrite-preserved meats (e.g., hot dogs, lunch meat, breakfast sausage, bacon, meat in canned soups) to heat during cooking.
Natural Chemical Food Preservatives
The discovery of natural antimicrobial substances produced by other microbes has added to the arsenal of preservatives used in food. Nisin is an antimicrobial peptide produced by the bacterium Lactococcus lactis and is particularly effective against gram-positive organisms. Nisin works by disrupting cell wall production, leaving cells more prone to lysis. It is used to preserve cheeses, meats, and beverages.
Natamycin is an antifungal macrolide antibiotic produced by the bacterium Streptomyces natalensis . It was approved by the FDA in 1982 and is used to prevent fungal growth in various types of dairy products, including cottage cheese, sliced cheese, and shredded cheese. Natamycin is also used for meat preservation in countries outside the United States.
Exercise \(\PageIndex{10}\)
What are the advantages and drawbacks of using sulfites and nitrites as food preservatives?
Key Concepts and Summary
- Heavy metals , including mercury, silver, copper, and zinc, have long been used for disinfection and preservation, although some have toxicity and environmental risks associated with them.
- Halogens , including chlorine, fluorine, and iodine, are also commonly used for disinfection. Chlorine compounds, including sodium hypochlorite , chloramines , and chlorine dioxide , are commonly used for water disinfection. Iodine, in both tincture and iodophor forms, is an effective antiseptic.
- Alcohols , including ethyl alcohol and isopropyl alcohol, are commonly used antiseptics that act by denaturing proteins and disrupting membranes.
- Phenolics are stable, long-acting disinfectants that denature proteins and disrupt membranes. They are commonly found in household cleaners, mouthwashes, and hospital disinfectants, and are also used to preserve harvested crops.
- The phenolic compound triclosan , found in antibacterial soaps, plastics, and textiles is technically an antibiotic because of its specific mode of action of inhibiting bacterial fatty-acid synthesis..
- Surfactants , including soaps and detergents, lower the surface tension of water to create emulsions that mechanically carry away microbes. Soaps are long-chain fatty acids, whereas detergents are synthetic surfactants.
- Quaternary ammonium compounds ( quats ) are cationic detergents that disrupt membranes. They are used in household cleaners, skin disinfectants, oral rinses, and mouthwashes.
- Bisbiguanides disrupt cell membranes, causing cell contents to gel. Chlorhexidine and alexidine are commonly used for surgical scrubs, for handwashing in clinical settings, and in prescription oral rinses.
- Alkylating agents effectively sterilize materials at low temperatures but are carcinogenic and may also irritate tissue. Glutaraldehyde and o-phthalaldehyde are used as hospital disinfectants but not as antiseptics. Formaldehyde is used for the storage of tissue specimens, as an embalming fluid, and in vaccine preparation to inactivate infectious agents. Ethylene oxide is a gas sterilant that can permeate heat-sensitive packaged materials, but it is also explosive and carcinogenic.
- Peroxygens , including hydrogen peroxide , peracetic acid , benzoyl peroxide , and ozone gas, are strong oxidizing agents that produce free radicals in cells, damaging their macromolecules. They are environmentally safe and are highly effective disinfectants and antiseptics.
- Pressurized carbon dioxide in the form of a supercritical fluid easily permeates packaged materials and cells, forming carbonic acid and lowering intracellular pH. Supercritical carbon dioxide is nonreactive, nontoxic, nonflammable, and effective at low temperatures for sterilization of medical devices, implants, and transplanted tissues.
- Chemical preservatives are added to a variety of foods. Sorbic acid , benzoic acid , propionic acid , and their more soluble salts inhibit enzymes or reduce intracellular pH.
- Sulfites are used in winemaking and food processing to prevent browning of foods.
- Nitrites are used to preserve meats and maintain color, but cooking nitrite-preserved meats may produce carcinogenic nitrosamines.
- Nisin and natamycin are naturally produced preservatives used in cheeses and meats. Nisin is effective against gram-positive bacteria and natamycin against fungi.
- 1 US Food and Drug Administration. “Triclosan: What Consumers Should Know.” 2015. www.fda.gov/ForConsumers/Cons.../ucm205999.htm. Accessed June 9, 2016.
- 2 J. Stromberg. “Five Reasons Why You Should Probably Stop Using Antibacterial Soap.” Smithsonian.com January 3, 2014. www.smithsonianmag.com/scienc...948078/?no-ist. Accessed June 9, 2016.
- 3 SP Yazdankhah et al. “Triclosan and Antimicrobial Resistance in Bacteria: An Overview.” Microbial Drug Resistance 12 no. 2 (2006):83–90.
- 4 L. Birošová, M. Mikulášová. “Development of Triclosan and Antibiotic Resistance in Salmonella enterica serovar Typhimurium.” Journal of Medical Microbiology 58 no. 4 (2009):436–441.
- 5 AB Dann, A. Hontela. “Triclosan: Environmental Exposure, Toxicity and Mechanisms of Action.” Journal of Applied Toxicology 31 no. 4 (2011):285–311.
- 6 US Centers for Disease Control and Prevention. “Triclosan Fact Sheet.” 2013. www.cdc.gov/biomonitoring/Tri...FactSheet.html. Accessed June 9, 2016.
- 7 EM Clayton et al. “The Impact of Bisphenol A and Triclosan on Immune Parameters in the US Population, NHANES 2003-2006.” Environmental Health Perspectives 119 no. 3 (2011):390.
- 8 N. Silvestry-Rodriguez et al. “Silver as a Disinfectant.” In Reviews of Environmental Contamination and Toxicology , pp. 23-45. Edited by GW Ware and DM Whitacre. New York: Springer, 2007.
- 9 B. Owens. “Silver Makes Antibiotics Thousands of Times More Effective.” Nature June 19 2013. http://www.nature.com/news/silver-ma...ective-1.13232
- 10 C. Seiler, TU Berendonk. “Heavy Metal Driven Co-Selection of Antibiotic Resistance in Soil and Water Bodies Impacted by Agriculture and Aquaculture.” Frontiers in Microbiology 3 (2012):399.
- 11 World Health Organization. “Benefits and Risks of the Use of Chlorine-Containing Disinfectants in Food Production and Food Processing: Report of a Joint FAO/WHO Expert Meeting.” Geneva, Switzerland: World Health Organization, 2009.
- 12 RE Marquis. “Antimicrobial Actions of Fluoride for Oral Bacteria.” Canadian Journal of Microbiology 41 no. 11 (1995):955–964.
- 13 L. Thomas et al. “Development of Resistance to Chlorhexidine Diacetate in Pseudomonas aeruginosa and the Effect of a ‘Residual’ Concentration.” Journal of Hospital Infection 46 no. 4 (2000):297–303.
- 14 Institute of Medicine. “Long-Term Health Effects of Participation in Project SHAD (Shipboard Hazard and Defense).” Washington, DC: The National Academies Press, 2007.
- 15 Yao, C.S. et al. “In vitro antibacterial effect of carbamide peroxide on oral biofilm.” Journal of Oral Microbiology Jun 12, 2013. http://www.ncbi.nlm.nih.gov/pmc/articles/PMC3682087/ . doi: 10.3402/jom.v5i0.20392.

An official website of the United States government
The .gov means it's official. Federal government websites often end in .gov or .mil. Before sharing sensitive information, make sure you're on a federal government site.
The site is secure. The https:// ensures that you are connecting to the official website and that any information you provide is encrypted and transmitted securely.
- Publications
- Account settings
- Browse Titles
NCBI Bookshelf. A service of the National Library of Medicine, National Institutes of Health.
National Research Council (US) Safe Drinking Water Committee. Drinking Water and Health: Volume 2. Washington (DC): National Academies Press (US); 1980.

Drinking Water and Health: Volume 2.
- Hardcopy Version at National Academies Press
II The Disinfection of Drinking Water
The goal of disinfection of public water supplies is the elimination of the pathogens that are responsible for waterborne diseases. The transmission of diseases such as typhoid and paratyphoid fevers, cholera, salmonellosis, and shigellosis can be controlled with treatments that substantially reduce the total number of viable microorganisms in the water.
While the concentration of organisms in drinking water after effective disinfection may be exceedingly small, sterilization (i.e., killing all the microbes present) is not attempted. Sterilization is not only impractical, it cannot be maintained in the distribution system. Assessment of the reduction in microbes that is sufficient to protect against the transmission of pathogens in water is discussed below.
Chlorination is the most widely used method for disinfecting water supplies in the United States. The near universal adoption of this method can be attributed to its convenience and to its highly satisfactory performance as a disinfectant, which has been established by decades of use. It has been so successful that freedom from epidemics of waterborne diseases is now virtually taken for granted. As stated in Drinking Water and Health (National Academy of Sciences, 1977), "chlorination is the standard of disinfection against which others are compared."
However, the discovery that chlorination can result in the formation of trihalomethanes (THM's) and other halogenated hydrocarbons has prompted the reexamination of available disinfection methodology to determine alternative agents or procedures (Morris, 1975).
The method of choice for disinfecting water for human consumption depends on a variety of factors (Symons et al. , 1977). These include:
- its efficacy against waterborne pathogens (bacteria, viruses, protozoa, and helminths);
- the accuracy with which the process can be monitored and controlled;
- its ability to produce a residual that provides an added measure of protection against possible posttreatment contamination resulting from faults in the distribution system;
- the aesthetic quality of the treated water; and
- the availability of the technology for the adoption of the method on the scale that is required for public water supplies.
Economic factors will also play a part in the final decision; however, this study is confined to a discussion of the five factors listed above as they apply to various disinfectants.
The propensity of various disinfection methods to produce by-products having effects on health (other than those relating to the control of infectious diseases) and the possibility of eliminating or avoiding these undesirable by-products are also important factors to be weighed when making the final decisions about overall suitability of methods to disinfect drinking water. The subcommittee has not attempted to deal with these problems since the chemistry of disinfectants in water and the toxicology of expected by-products have been studied by other subcommittees of the Safe Drinking Water Committee, whose reports appear in Chapter III of this volume (Chemistry) and Chapter IV (Toxicity) of Drinking Water and Health , Vol. 3.
- Organization of the Study
The general considerations noted in the immediately following material should be borne in mind when considering each method of disinfection. Available information on the obvious major candidates for drinking water disinfection—chlorine, ozone, chlorine dioxide, iodine, and bromine—is then evaluated for each method individually in the following sections. Other less obvious possibilities are also examined to see if they have been overlooked unjustly in previous studies or if it might be profitable to conduct further experimentation on them. Disinfection by chloramines is dealt with in parallel with that effected by chlorine because of the close relationship the former has to chlorine disinfection under conditions that might normally be encountered in drinking water treatment.
The evaluations in this report are not exhaustive literature reviews but, rather, are selections of the studies that, in the judgment of the committee, provide the most accurate and relevant information on the biocidal activities of each method of disinfection. The analytical methods that are described in this report are those that are most likely to be used by persons involved in disinfection research or water treatment. A review of all existing analytical methods, some of which may be more sophisticated than those described below, would be impractical within the constraints of time and space available and is not within the scope of this document.
After the methods of disinfection are examined individually, their major characteristics and biocidal efficacy are compared by means of summary tables and c · t (concentration, in milligrams per liter, times contact time, in minutes) values required for similar inactivations under identical conditions. The conclusions of the study are then recorded on the basis of this evidence.
- General Aspects of Disinfection
In any comparison of disinfection methods, certain considerations should be discussed at the outset since they are relevant to most, if not all, methods. The quality of the raw water (i.e., its content of solids and material that will react with the disinfectant), treatment of the water prior to disinfection, and the manner in which the disinfectant is applied to the water will directly affect the efficacy of all disinfectants. Equally applicable to all methods are appropriate standards for verifying the adequacy of disinfection, differences in response to disinfectants between organisms that were obtained directly from the field and those that have been acclimated to laboratory culture, and the maintenance of potability from treatment plant to the consumer's tap. The use of chlorination as presented in examples in the following pages does not imply that it is necessarily the method of choice. Rather, this method has been studied more thoroughly than other methods.
Raw Water Quality
In addition to potential pathogens, raw water may contain contaminants that may interfere with the disinfection process or may be undesirable in the finished product. These contaminants include inorganic and organic molecules, particulates, and other organisms, e.g., invertebrates. Variations among these contaminants arise from differences in regional geochemistry and between ground- and surface-water sources.
Disinfectant Demand
Many inorganic and organic molecules that occur in raw water exert a "demand," i.e., a capacity to react with and consume the disinfectant. Therefore, higher "demand" waters require a greater dose to achieve a specific concentration of the active species of disinfectant. This demand must be satisfied to ensure adequate biocidal treatment.
Ferrous ions, nitrites, hydrogen sulfide, and various organic molecules exert a demand for oxidizing disinfectants such as chlorine. The bulk of the nonparticulate organic material in raw water occurs as naturally derived humic substances, i.e., humic, fulvic, and hymatomelanic acids, which contribute to color in water. The structure of these molecules is not yet fully understood. However, they are known to be polymeric and to contain aromatic rings and carboxyl, phenolic, alcoholic hydroxyl, and methoxyl functional groups. Humic substances, when reacting with and consuming applied chlorine, produce chloroform (CHCl 3 ) and other THM's. Water, particularly surface waters, may also contain synthetic organic molecules whose demand for disinfectant will be determined by their structure. Ammonia and amines in raw water will react with chlorine to yield chloramines that do have some biocidal activity, unlike most products of these side reactions. If chlorination progresses to the breakpoint, i.e., to a free-chlorine residual, these chloramines will be oxidized causing more added chlorine to be consumed before a specific free-chlorine level is achieved. This phenomenon is discussed more fully below.
The nature of the demand reactions varies with the composition of the water and the disinfectant. Removal of the demand substances leaves a water with a lower requirement for a disinfectant to achieve an equivalent degree of protection against transmission of a waterborne disease.
Physical and Chemical Treatments
Various treatments applied to raw water to remedy undesirable characteristics, e.g., color, taste, odor, or turbidity, may affect the ultimate microbiological quality of the finished water. Microorganisms may be physically removed or the disinfectant demand of the water altered.
Presedimentation to remove suspended matter, coagulation with alum or other agents, and filtration reduce the organic material in the raw water and, thus, the disinfectant demand. Removal of ferrous iron similarly reduces the demand for oxidizing disinfectants as will aeration, which eliminates hydrogen sulfide. Prechlorination to a free chlorine residual is practiced early in the treatment sequence as one method to alter taste- and odor-producing compounds, to suppress growth of organisms in the treatment plant, to remove iron and manganese, and to reduce the interference of organic compounds in the coagulation process.
The necessity for these treatments or others is determined by the characteristics of the raw water. The selection of one of the various methods to achieve a particular result will be based upon cost-effectiveness in the particular situation. When chlorination is used, the application or point of application in the treatment sequence of some of the above-mentioned procedures can affect the undesirable THM content of the finished water.
Reduction of precursors in raw water by coagulation and settling prior to chlorination reduces final THM production (Hoehn et al. , 1977; Stevens et al. , 1975). The Louisville Water Company reduced THM concentrations leaving the plant by 40%-50% by shifting the point of chlorination from the presedimentation basin to the coagulation basin (Hubbs et al. , 1977). The available information on these variations is limited, and a universally applicable procedure cannot be recommended in view of the diverse treatments required for different raw waters.
Particulates and Aggregates
To inactivate organisms in water, the active chemical species must be able to reach the reactive site within the organism or on its surface. Inactivation will not result if this cannot occur. Microorganisms may acquire physical protection in water as a result of their being adsorbed to the enormous surfaces provided by clays, silt, and organic matter or to the surfaces of solids created during water treatment, e.g., aluminum or ferric hydrated oxides, calcium carbonate, and magnesium hydroxide. Viruses, bacteria, and protozoan cysts may be adsorbed to these surfaces. Such particles, with the adsorbed microorganisms, may aggregate to form clumps, affording additional protection. Organisms themselves may also aggregate or clump together so that organisms that are on the interior of the clump are shielded from the disinfectant and are not inactivated. Organisms may also be physically embedded within particles of fecal material, within larger organisms such as nematodes, or, in the case of viruses, within human body cells that have been discharged in fecal material.
To disinfect water adequately, the water must have been pretreated, when necessary, to reduce the concentration of solid materials to an acceptably low level. The primary drinking water turbidity standard of 1 nephelometric turbidity unit (NTU) is an attempt to assure that the concentration of particulates is compatible with current disinfection techniques. Where it is possible to obtain lower turbidities, this is desirable.
Disinfection studies in which the complications of adsorbed organisms, aggregation, or embedment were thought to occur were excluded from this study. The conclusions in this report should not be extrapolated to such situations as the disinfection of turbid or colored waters.
The Importance of Residuals
Water supplies are disinfected through the addition or dosage of a chemical or physical agent. With a chemical agent, such as a halogen, a given dosage should theoretically impart a predetermined concentration (residual) of the active agent in the water. From a practical point of view, most natural waters exert a "demand" for the disinfectant, as discussed above, so that the residual in the water is less than the calculated amount based on the dosage. The decrease in residual, which is caused by the demand, is rapid in most cases, but it may be prolonged until the residual eventually disappears. In addition, the chemical agent may decompose spontaneously, thereby yielding substances having little or no disinfection ability and exerting no measurable residual. For example, ozone not only reacts with substances in water that exert a demand, but it also decomposes rapidly. To achieve microbial inactivation with a chemical agent, a residual must be present for a specific time. Thus, the nature and level of the residual, together with time of exposure, are important in achieving disinfection or microbial inactivation. Because the nature of the dosage-residual relationship for natural waters has not been and possibly cannot be reliably defined, the efficacy of disinfection with a chemical agent must be based on a residual concentration/time of exposure relationship.
Residual measurements are important and useful in controlling the disinfection process. By knowing the residual-time relationship that is required to inactivate pathogenic or infectious agents, one can adjust the dosage of the disinfecting agent to achieve the residual that is required for effective disinfection with a given contact time. Thus, the effectiveness of the disinfection process can be controlled and/or judged by monitoring or measuring the residual.
Following disinfection of a water supply at a treatment plant, the water is distributed to the consumers. A persistent residual is important for continued protection of the water supply against subsequent contamination in the distribution system. Accidental or mechanical failures in the distribution system may result in the introduction of infectious agents into the water supply. In the presence of a residual, disinfection will continue and, as a result, offer continued protection to the users. Physical agents such as radiation may provide effective disinfection during application, but they do not impart any persistent residual to the water.
The dosage of a chemical agent that is used to effect microbial inactivation should not be so great that it imparts a health hazard to the water consumer. From another point of view, the aesthetic quality of the finished water should not be impaired by the dosage of the chemical agent or the residual that is required for effective disinfection. These qualities might include discoloration of water from potassium permanganate (KMnO 4 ) or iodine or problems of taste and odor from excessive chlorine.
Application of the Disinfectant
Optimum inactivation occurs when the disinfectant is distributed uniformly throughout the water. To disperse the chemical disinfectant when it is added to the water, it must be mixed effectively to assure that all of the water, however small the volume, receives its proportionate share of the chemical. Additions of a disinfectant at points in a flowing water stream, e.g., from submerged pipes, is seldom adequate to assure uniform concentration. In such cases, mechanical mixing devices are needed to disperse the disinfectant throughout the water. Disinfection by radiation treatment also requires good mixing to bring all of the water within the effective radiation distance.
Microbiological Considerations 1
Comparison of the biocidal efficacy of disinfectants is complicated by the need to control many variables, a need not realized in some early studies. Halogens in particular are significantly affected by the composition of the test menstruum and its pH, temperature, and halogen demand. For very low concentrations of halogen to be present over a testing period, halogen demand must be carefully eliminated. Different disinfectants may have different biocidal potential. In earlier work, analytical difficulties may have precluded defining exactly the species present, but new techniques allow the species to be defined for most disinfectants. Information on the species of disinfectant actually in the test system should be included in future reports on disinfection studies.
Investigators studying efficacy have usually adopted one of two extremes. Some have conducted carefully designed laboratory experiments with controls for as many variables as possible. Certain of these investigators have reduced the temperature to slow the inactivation reactions. Although these experiments yield good basic information and can be used to determine which variables are important, they often have little quantitative relationship to field situations. The other extreme, a field study or reconstruction of field conditions, is difficult to control. Moreover, their results are often not repeatable.
In addition to the variables noted above, prereaction of chemicals in the test system, the culture history of the organism being used, and the ''cleanup" procedures applied to it may also affect the observed results. Despite these problems, there have been some attempts to standardize efficacy testing.
Model Systems and Indicator Organisms
A major factor that influences the evaluation of the efficacy of a particular disinfectant is the test microorganism. There is a wide variation in susceptibility, not only among bacteria, viruses, and protozoa (cyst stage), but also among genera, species, and strains of the microorganism. It is impractical to obtain information on the inactivation by each disinfectant for each species and strain of pathogenic microorganism of importance in water. In addition, interpretation of the data would be confounded by the condition and source of the test microorganism (e.g., the degree of aggregation and whether the organisms were "naturally occurring" or laboratory preparations), the presence of solids and particulates, and the presence of materials that react with and consume the disinfectant.
The overwhelming majority of the literature on water disinfection concerns the inactivation of model microorganisms rather than the pathogens. These disinfectant model microorganisms have generally been nonpathogenic microorganisms that are as similar as possible to the pathogen and behave in a similar manner when exposed to the disinfectant. The disinfectant model systems are simpler, less fastidious, technically more workable systems that provide a way to obtain basic information concerning fundamental parameters and reactions. The information gained with the model systems can then be used to design key experiments in the more difficult systems. The disinfection model microorganism should be clearly distinguished from the indicator organism. The indicator microorganism, as defined in Drinking Water and Health (National Academy of Sciences, 1977), is a "microorganism whose presence is evidence that pollution (associated with fecal contamination from man or other warm-blooded animals) has occurred." Following are criteria for the indicator microorganism (Fair and Geyer, 1954):
The indicator should always be present when fecal material is present and absent in clean, uncontaminated water.
The indicator should die away in the natural aquatic environment and respond to treatment processes in a manner that is similar to that of the pathogens of interest.
The indicator should be more numerous than the pathogens.
The indicator should be easy to isolate, identify, and enumerate.
Only a restrictive application of the second criterion is necessary for a disinfection model. The response of the test microorganism to the disinfectant must be similar to that of the pathogen that it is intended to simulate. The disinfection model is not meant to function as an indicator microorganism.
During the latter part of the nineteenth century, investigators recognized the presence of a group of bacteria that occured in large numbers in feces and wastewater. The most significant member of this group (currently called the coliform group) is Escherichia coli. Since the late nineteenth century, this coliform group has served as an indicator of the degree of fecal contamination of water, and E. coli has been used routinely as a disinfection model for enteric pathogens. Butterfield and co-workers (Butterfield and Wattie, 1946; Butterfield et al. , 1943; Wattie and Butterfield, 1944) provided information on the inactivation of E. coli and other enteric bacterial pathogens with chlorine and chloramines. At pH values above 8.5, all strains of E. coli were more resistant to free chlorine than were Salmonella typhi strains. At pH values of 6.5 and 7.0, strains of S. typhi were more resistant. Only slight differences between the two genera were found when chloramines were used as the disinfectant. The bactericidal activity of chloramine was noticably less than that of free chlorine.
Bacteria of the coliform group, especially E. coli , have proved useful as an indicator and disinfection model for enteric bacterial pathogens but are poor indicators and disinfection models for nonbacterial pathogens. E. coli has been observed to be markedly more susceptible to chlorine than certain enteric viruses and cysts of pathogenic protozoa (Dahling et al. , 1972; Krusé, 1969).
The bacterial viruses of E. coli have received increased attention as possible disinfection models and indicators of enteric viruses in water and wastewater. At present, the data to justify the bacterial viruses as indicators for enteric viruses are limited and inconsistent. However, there is a growing body of knowledge on the utilization of bacterial viruses as disinfection models.
Hsu (1964) and Hsu et al. (1966) first reported the use of the f2 virus as a model for disinfection studies with iodine. They showed that inactivation of both the f2 virus and poliovirus 1 were inhibited by increasing concentrations of iodide ion and that both f2 RNA and poliovirus 1 RNA were resistant to iodination.
Dahling et al. (1972) compared the inactivation of two enteric viruses (poliovirus 1 and coxsackievirus A9), two DNA phages (T2 and T5), two RNA phages (f2 and MS2), and E. coli ATCC 11229 under demand-free conditions with free chlorine at pH 6.0. They found enteric viruses to be most resistant to free chlorine followed by RNA phages, E. coli, and the T phages.
Shah and McCamish (1972) compared the resistance of poliovirus 1 and the coliphages f2 and T2 to 4 mg/liter combined residual chlorine. The f2 virus was shown to be more resistant to this form of chlorine than poliovirus 1 and T2 coliphage.
Cramer et al. (1976) compared the inactivation of poliovirus 3 (Leon) and f2 with chlorine and iodine in buffered wastewater. Both viruses were treated together in the same reaction flask, thereby eliminating any inherent differences due to virus preparations and replicate systems. In wastewater effluent at pH 6.0 and 10.0 with a 30 mg/liter dosage of halogen under prereacted (halogen added to wastewater, allowed to react, viruses added at zero time) and dynamic (viruses added to wastewater, halogen added at zero time) conditions, f2 was, in each case, at least as or more resistant to chlorine and iodine than poliovirus 1. The f2 virus appears to be more sensitive to free chlorine but more resistant to combined chlorine than poliovirus 1 is.
Neefe et al. (1945) observed that the agent of infectious hepatitis was inactivated by breakpoint chlorination (free chlorine) but not completely inactivated by combined chlorine.
Engelbrecht et al. (1975) reported that the use of a yeast ( Candida parapsilosis ) and two acid-fast bacteria ( Mycobacterium fortuitum and Mycobacterium phlei ) may provide suitable disinfection models. They observed that the yeast was more resistant to free chlorine than were poliovirus 1 and the enteric bacteria under all conditions tested. The acid-fast bacilli were most resistant.
There is no generally accepted disinfection model for protozoan cysts. In disinfection studies for protozoan diseases, investigators have used the pathogen or its cysts. Work with such systems is, however, generally difficult.
The use of disinfection models provides useful information that is helpful to the comparison of the relative efficiencies of various disinfectants in the laboratory and in controlled field investigations. Strains of E. coli have been used extensively as models for enteric pathogenic bacteria. While not as widely accepted, the bacterial viruses of E. coli are used as disinfection models for enteric viruses. The difficulty of available methods has limited the number of disinfection studies with protozoan cysts.
Laboratory Cultures versus "Naturally Occurring" Organisms
The resistance or sensitivity to disinfectants of some bacteria (e.g., E. coli ) in the laboratory may bear very little resemblance to their responses in nature. This is true in spite of the fact that standardized procedures govern the conditions under which cells are grown, harvested, washed, etc., when they are used as inocula. Examples of such differences range from Gram-negative bacteria and their comparative resistance to disinfectants in general (Carson et al. , 1972; Favero et al. , 1971, 1975) to Gram-positive bacterial spores and heat resistance (Bond et al. , 1973) and to halogen resistance of Entamoeba histolytica cysts from simian hosts as opposed to those grown in in-vitro systems (Stringer et al. , 1975). Presumably, the mechanisms creating this phenomenon among these three groups vary widely.
The comparative resistance to disinfectants among Gram-negative bacteria varies greatly. A good example of this is the study of Favero and Drake (1966). They first applied the term "naturally occurring" to certain Gram-negative bacteria with the potential for rapid growth in water. They observed that Pseudomonas alcaligenes , a common bacterial contaminant in iodinated swimming pools, could grow well in swimming pool waters that had been sterilized by membrane filters and rendered free of iodine or chlorine. Starting with contaminated swimming pool water that contained a variety of bacteria, they isolated a pure culture of P. alcaligenes by an extinction-dilution technique in which filter-sterilized swimming pool water was used as the diluent and growth medium. Since these cells had been isolated in pure culture without exposure to conventional laboratory culture media, they were referred to as "naturally occurring" P. alcaligenes. Subsequent tests showed that these naturally occurring cells were significantly more resistant to free iodine than were cells of the same organism that had been subcultured one time on trypticase soy agar. In fact, standard disinfectant tests using the cells that had been subcultured on an enriched laboratory medium suggested that P. alcaligenes should never be found in pools that had been disinfected even minimally with iodine. This was obviously an erroneous assumption. The discovery that naturally occurring cells were extremely resistant to iodine explained the relatively high concentrations of P. alcaligenes that accumulated in pool water that had been iodinated for several weeks.
Subsequently, Favero et al. (1971, 1975) and Carson et al. (1972) published a series of papers showing that Pseudomonas aeruginosa could grow rapidly in distilled water, which they obtained from hospitals, and could reach high concentrations of cells that remained stable for a long time. Naturally occurring cells that were grown in distilled water reacted quite differently to chemical and physical stresses than did cells grown on standard laboratory culture media. For example, naturally occurring cells of P. aeruginosa were significantly more resistant to chlorine, quaternary ammonium compounds, and alkaline glutaraldehyde than were subcultured cells.
In halogen-disinfected waters, naturally occurring bacteria can be from one to two orders of magnitude more resistant to the disinfectant than cells of the same organism that had been subcultured on conventional laboratory culture media. Since standard disinfectant testing necessarily employs subcultured and washed bacterial cells, a false sense of confidence may be created if these data are used as an absolute criterion for the dilution of a disinfectant. These results could explain the frequent discrepancies between tests that are performed under laboratory conditions and those that are performed under field conditions.
If bacteria could be used in their naturally occurring state, one might explore the possibility of bridging the gaps between laboratory and field conditions by using this experimental system. The ability of some Gram-negative bacteria to grow in water makes it possible to produce and control large numbers of cells for such studies.
More difficult to answer is the more basic question of why naturally occurring cells of Gram-negative water bacteria become more sensitive to disinfectants when grown in a rich medium than the same strain when grown in water. One would expect the reverse to occur. Milbauer and Grossowicz (1959) showed that cells of E. coli were much more sensitive to chlorine when grown on a medium of glucose mineral salts than when grown on nutrient agar. Since Favero and Drake (1966) reported that filter-sterilized dehalogenated swimming pool water could be considered a minimal medium, one would expect that P. alcaligenes cells that were grown in this environment would be less resistant to iodine than those grown in trypticase soy broth. This phenomenon has not been explained. Evidently it is not primarily a genetic response since the extreme difference in iodine resistance occurs with one subpassage on trypticase soy agar.
Over the years various investigators have tried without success to "train" bacteria to become more resistant to chlorine and/or iodine (Favero, 1961; Favero et al. , 1964; Krusé, 1969). This failure is not surprising, because, if halogens are truly a general cytoplasmic poison that affects primarily the sulfhydryl groups of enzymes (see pp. 36-39), it would be very difficult for an organism to modify its physiology to the extent that it becomes resistant, very unlike the situation with antibiotics and bacteria. Consequently, the extreme resistance or differing resistances of naturally occurring bacteria can be attributed only to "environmental" factors and, perhaps, to the different compositions of cell walls and membranes. However, there have been no data to substantiate this hypothesis.
Despite the questions that have been raised by differences in the behavior of organisms under both laboratory and field conditions, valuable comparative information can be obtained from studies of disinfectants that are conducted in similar laboratory systems.
- Chlorine and Chloramines
Chlorine is a strong oxidizing disinfectant that has been used to treat drinking water supplies for more than 60 yr. The gas was named "chlorine" after the Greek word for green, "chloros," because of its characteristic color. About 1800, chlorine gas was used as a general disinfectant in both France and England. In the United States, electrolytically produced chlorine was first used directly for water disinfection for only a week or two in 1896 at the Louisville Experimental Station in Kentucky. The first continuous municipal application of chlorine (as sodium hypochlorite [NaOCl]) to water in the United States occurred in 1908 at Jersey City, New Jersey. This was followed in 1912 by the first full-scale use of liquid chlorine for water disinfection at Niagara Falls, New York, where solution-feed equipment was used. This use of chlorine successfully eliminated recurring outbreaks of typhoid fever. In 1913, improved solution-feed equipment was developed to measure chlorine gas, dissolve it in water, and apply the solution to the water supply. This equipment was first installed at Boonton, New Jersey, where it replaced the use of sodium hypochlorite. Other equally significant historical occurrences (Laubush, 1971; White, 1972) led to the eventual addition of chlorine to drinking water in most of the United States for disinfection to destroy or inactivate pathogenic microorganisms (see Drinking Water and Health , National Academy of Sciences, 1977).
Chemistry of Chlorine in Water

When chlorine is added to water, the following chemical reactions occur:

Extremely little molecular chlorine (Cl 2 ) is present at pH values greater than pH 3.0 and total chlorine concentrations of less than ~1,000 mg/liter (White, 1972). The hypochlorous acid (HOCl) that is produced further ionizes to form hypochlorite ion (OCl - ) and hydrogen ion (H + ) (Reaction 2). The dissociation of hypochlorous acid is dependent chiefly upon pH and, to a much lesser extent, temperature, with almost 100% hypochlorous acid present at pH 5 and almost 100% hypochlorite ion present at pH 10 ( Figure II-1 ). Free available chlorine refers to the concentration of hypochlorous acid and hypochlorite ion, as well as any molecular chlorine existing in a chlorinated water.
Figure II-1
Effect of pH on quantities of hypochlorous acid (HOCl) and hypochlorite ion (OCl - ) that are present in water. Data from Fair et al ., 1948.
Chloramine Formation: Inorganic Chloramines
During chlorination of a water supply for disinfection, chlorine will react with any ammonia (NH 3 ) in the water to form inorganic chloramines.
Furthermore, ammonia is sometimes deliberately added to chlorinated public water supplies to provide a combined available chlorine residual, i.e., inorganic chloramines. Chlorine will also react with organic amines. The organic chloramines that are produced (see below) are considered encompassed in the term "combined available chlorine."
Although inorganic chloramines are less effective oxidizing and disinfecting agents than hypochlorous acid and hypochlorite ion, they are more stable. Consequently, they will produce a residual in water that will persist for a longer time (Symons et al. , 1977).
Inorganic chloramines are formed when hypochlorous acid reacts with ammonia:

The chloramine that is formed in the reaction depends upon the ratio of ammonia to hypochlorous acid and the pH of the system. Dichloramine (NHCl 2 ) is the predominant form of chloramine at a 1:1 molar ratio of ammonia to chlorine at pH values of 5 and below, whereas at pH values of 9 and above, monochloramine (NH 2 Cl) predominates. Figure II-2 shows the proportions of monochloramine and dichloramine formed for pH values of 4 to 9 and temperatures of 0°C, 10°C, and 25°C (Morris, 1978, personal communication).
Figure II-2
Proportions of mono- and dichloramine (NH 2 Cl and NHCl 2 ) in water chlorination with equimolar concentrations of chlorine and ammonia. Data from J.C. Morris, personal communication.
Organic Chloramines
Chlorine is also known to combine slowly with organic or albumenoid nitrogen (amines) to form organic chloramines (Taras, 1953):

Although the reaction between organic amines and chlorine is generally considered to be slow, organic chloramines may be formed, thereby producing a stable combined available chlorine residual after many hours of contact. It is generally accepted that most organic chloramines have little disinfecting capability, i.e., less than the inorganic chloramines (Feng, 1966; Nusbaum, 1952).
Breakpoint Chlorination
Hypochlorous acid and other chlorine compounds having disinfecting ability by virtue of their being oxidizing agents will oxidize sulfites (SO 3 2- ), sulfides (S - ), and ferrous (Fe 2+ ) or manganous (Mn 2+ ) ions. The disinfecting species are reduced, and the products have no disinfecting activity. All of the interfering compounds that destroy the disinfecting ability of the added chlorine exert a "chlorine demand," which may be defined as the difference between the amount of chlorine applied and the quantity of free or combined available chlorine residual measured in the water at the end of a specified contact period. When chlorine is added to water with no chlorine demand, a linear relationship is established between the chlorine dosage and the free chlorine residual ( Figure II-3 ).
Figure II-3
Diagrammatic representation of completed breakpoint reaction. From Morris, 1978, personal communication.
However, when increasing amounts of chlorine are added to water containing reducing agents and ammonia, the so-called breakpoint phenomenon occurs. The breakpoint is that dosage of chlorine that produces the first detectable amount of free available chlorine residual.
When chlorine is added to water, it reacts with any reducing agents and ammonia that are present. It is believed that chlorine reacts first with the reducing agents. Since the chlorine is destroyed, no measurable residual is produced. Following the oxidation of these reducing agents, e.g., sulfides, sulfites, nitrites (NO 2 - ), and ferrous ions, the chlorine will react with ammonia to form inorganic chloramines. The quantity of monochloramine and dichloroamine that is formed is determined primarily by the pH of the water and the ratio of chlorine to ammonia. When the ratio by weight is less than 5:1, or the molar ratio is less than 1:1, and the pH is in the range of 6.5 to 8.5, the combined available chlorine residual is probably due primarily to monochloramine (Reaction 3). With additional chlorine, the ratio of chlorine to ammonia changes with the result that the monochloramines are converted to dichloramines (Reaction 4). When all of the ammonia has been reacted, a free available chlorine residual begins to develop. As the concentration increases, the previously formed chloramines are oxidized to nitrous oxide (N 2 O), nitrogen trichloride (NCl 3 ), and nitrogen (N 2 ). The reactions leading to the formation of these oxidized forms of nitrogen destroy the combined available chlorine residual so that the measurable residual in the water actually decreases. Upon completion of the oxidation of all the chloramines, the addition of more chlorine creates the breakpoint phenomenon. At the breakpoint dosage, some resistant chloramines may still be present, but at such small concentrations that they are unimportant.
As pointed out by Morris (1970), the occurrence of reactions giving rise to the ''breakpoint" is most rapid in the pH range 7.0 to 7.5. At greater and lesser pH values, it becomes slower and less distinct, e.g., at pH's < 6 or > 9 the concept of "breakpoint" is not significant. In the pH range 7.0 to 7.5 the "breakpoint" is about half developed within 10 min at 15°C to 20°C and is then substantially completed within about 2 hr.
Analytical Methods and Their Evaluation
Standard Methods (1976) lists six acceptable methods for the determination of chlorine residuals in natural and treated waters: iodometric methods, amperometric titration, the stabilized neutral orthotolidine (SNORT) method, the ferrous diethyl- p -phenylenediamine (DPD) method, the DPD colorimetric method, and the leuco crystal violet (LCV) method.
The amperometric, LCV, DPD, and SNORT methods are unaffected by dichloramine concentrations in the range of 0 to 9 mg/liter (as Cl 2 ) in the determination of free chlorine. If nitrogen trichloride is present, it reacts partially as free available chlorine in the amperometric, DPD, and SNORT methods. Nitrogen trichloride does not interfere with the LCV procedure for free chlorine. The sample color and turbidity may interfere with all colorimetric procedures. Thus, a compensation must be made. Also, organic contaminants in the sample may produce a false-free chlorine reading in most colorimetric methods.
Standard Methods (1976) contains data on the precision and accuracy of the methods used in the measurement of chlorine. These data were obtained from participating laboratories by the Analytical Reference Service (1969, 1971), which then operated in an agency that preceded the Environmental Protection Agency. However, as noted in Standard Methods (1976), these results are valuable only for comparison of the methods tested, and many factors, such as analytical skill, recognition of known interferences, and inherent limitations, determine the reliability of any given method. Moreover, some oxidizing agents, including free halogens other than chlorine, will appear quantitatively as free chlorine. This is also true of chlorine dioxide. Also, some nitrogen trichloride may be measured as free chlorine. The actions of interfering substances should be familiar to the analyst because they affect a particular method.
Although orthotolidine (i.e., orthotolidine and orthotolidine arsenite) methods have been widely used in many disinfection studies, they are omitted from the 14th edition of Standard Methods (1976) primarily because of their inaccuracy and high overall (average) total error in comparison with other available methods.
Research studies on disinfection are restricted by the limitations that are inherent in the methods themselves or by poor selection of methods by the investigator. The chemical conditions of the test water have not always been well-defined. The types of titratable chlorine, i.e., free (hypochlorous acid or hypochlorite ion) or combined (mono- or dichloramine) in the chlorinated water, have not always been differentiated, and the rates of microbial destruction or inactivation have not always been studied in experimental systems with little or no chlorine demand. In fact, reports prior to the 1940's have been especially difficult to interpret, because reliable test methods for distinguishing between free and combined chlorine, between hypochlorous acid and hypochlorite ion, and between mono- and dichloramine in solution were not developed until the 1950's. For example, many earlier researchers claimed to have tested mono- and dichloramine by controlling the pH and the ratio of chlorine to nitrogen. They used methods such as the orthotolidine or thiosulfate titrations to determine total chlorine residual. Much of this early work is now questionable, since it was not possible to detect free chlorine contamination in their chloramine solutions or the quantitative ratios between the mono- and dichloramine tested. in addition, these earlier studies had high chlorine demand in the test systems.
Some more contemporary studies have lacked quantitated information on chlorine residual and/or types of chlorine present in the test systems.
Biocidal Activity
In the absence of reducing agents, inorganic ammonia, and organic amines, the addition of chlorine to municipal water supplies will result in free available residual chlorine, represented by the hypochlorous acid or hypochlorite ion. The pH determines the relative amounts of each species. However, inorganic chloramines will be formed if the background level of ammonia in the water supply is significant or if ammonia is intentionally added during treatment. If such is the case, monochloramine would predominate due to the alkaline pH of most finished water (see Figure II-2 ).
In 1966, Feng proposed that the active forms of chlorine would exhibit disinfection properties in the following descending order:

Butterfield et al. (1943) published the first treatise on the use of chlorine demand-free water for studies of water disinfection. They proposed that to study the disinfectant capacity of any chlorine species, the test medium must meet certain exacting criteria. It must be nontoxic to bacteria except for the variables under study such as chlorine and pH, well buffered at the desired pH, free of all ammonia and organic matter capable of forming chlorine-addition products, free of background chlorine, and of such a nature that calculated additions of chlorine are recoverable after 5 min without a loss in residual and that free chlorine must still be present several hours after contact.
Most studies of combined chlorine have dealt with poorly defined mixtures of mono- and dichloramine. Also, test conditions have often been inadequately defined, poorly controlled, or both.
Efficacy against Bacteria
Free chlorine (hocl and ocl - ).
Butterfield et al. (1943) studied percentages of inactivation as functions of time for E. coli, Enterobacter aerogenes, Pseudomonas aeruginosa, Salmonella typhi, and Shigella dysenteriae. They used different levels of free chlorine at pH values ranging from 7.0 to 10.7 and two temperature ranges—2°C to 5°C and 20°C to 25°C. Their work is of great importance, since very few other studies have been conducted that dealt with the action of disinfectants on pathogens. Generally, they found that the primary factors governing the bactericidal efficacy of free available chlorine and combined available chlorine were:
- the time of contact between the bacteria and the bactericidal agent, i.e., the longer the time, the more effective the chlorine disinfection process;
- the temperature of the water in which contact is made, i.e., the lower the temperature, the less effective the chlorine disinfecting activity; and
- the pH of the water in which contact is made, i.e., the higher the pH, the less effective chlorination.
Thus, the test bacteria will be killed more rapidly at lower pH values and at higher temperatures. Since hypochlorous acid would predominate at lower pH's ( Figure II-1 ), the data of Butterfield et al. show that it is a better bactericide than the hypochlorite ion. For example, to produce a 100% inactivation of an initial inoculum of 8 × 10 5 E. coli in 400 ml of sterile chlorine demand-free water (2,000/ml) at 20°C-25°C with a chlorine level of 0.046 to 0.055 mg/liter, 1.0 min was required at pH 7.0, but at pH 8.5, 9.8, and 10.7, between 20 and 60 min of exposure were needed. At higher concentrations of chlorine, i.e., from 0.1 to 0.29 mg/liter, exposure of 1.0 min was required at pH 7.0, 10 min at pH 8.5, 20 min at 9.8, and 60 min at 10.7. A similar pH effect was noted for S. typhi.
Unfortunately, Butterfield et al. (1943) lifted their cells from agar slants but failed to wash them in demand-free water. The cells probably carried trace amounts of albumenoid nitrogen from the slants to the test flasks, thereby creating the small chlorine demand that the investigators had tried so carefully to avoid. The effect of such a trace amount of chlorine demand would be most apparent in test solutions with very low chlorine levels. In studies using approximately 0.1 mg/liter or less free chlorine at decreasing pH values, Butterfield et al. (1943) observed that the disinfection of the organisms required a very long time. This might indicate interference at the low levels due to the formation of combined chlorine.
Under very exact controlled test conditions of pH and temperature and using chlorine demand-free buffer systems, Scarpino et al. (1972) observed that at 5°C E. coli was 99% destroyed by 1.0 mg/liter hypochlorous acid at pH 6 in less than 10 s and at pH 10 by 1.0 mg/liter hypochlorite ion in about 50 s. Their studies, which totally eliminated any form of combined chlorine from the test solutions, indicated that hypochlorous acid was approximately 50 times more effective than the hypochlorite ion as a bactericide. Fair et al. (1948) and Berg (1966) analyzed the data of Butterfield et al. (1943) on the destruction of E. coli. They reported that hypochlorous acid was 70-80 times as bactericidal as hypochlorite ion.
Engelbrecht et al. (1975) investigated new microbial indicators of disinfection efficiency. In their chlorination studies, they noted the following decreasing order of resistance to free chlorine at pH values of 6, 7, and 10 and at 5°C and 20°C: acid-fast bacteria > yeasts > poliovirus > Salmonella typhimurium > E. coli.
Monochloramine (NH 2 Cl)
In 1948, Butterfield summarized previous results on the bactericidal properties of chloramines (and free chlorine) in water at pH values ranging from 6.5 to 10.7 and in two temperature ranges—2°C to 5°C and 20°C to 25°C. The test bacteria included strains of Escherichia coli, Enterobacter aerogenes, Pseudomonas aeruginosa, Salmonella typhi, and Shigella dysenteriae. Although he admitted that adequate tests for separate determination of free and combined chlorine forms were not used in these studies, the solutions were vigorously prepared to ensure the exclusions of free chlorine. Chloramines were determined using orthotolidine; readings made after 10 to 30 s at 20°C gave free chlorine levels, and those after standing for 10 min at 20°C were recorded as total residual chlorine. Since no free chlorine was reported (and should not have been found, according to the authors), the 10-min readings of total residual chlorine were also those of total chloramine levels. No distinction could be made between monochloramine and dichloramine. However, he estimated that at pH 6.5, 7.0, 7.8, 8.5, 9.5, and 10.5 the chloramines were present as monochloramine at 35%, 51%, 84%, 98%, 100%, and 100%, respectively. The balance was believed to be dichloramine.
Butterfield and his associates (Butterfield, 1948; Butterfield et al. , 1946) found that chloramine disinfection was always slower than that for free chlorine. For example, in order to achieve a 100% inactivation of the initial number of bacteria tested after 60 min of contact time at 20°C, 0.6 mg/liter chloramine was required at pH 7.0 and 1.2 mg/liter at pH 8.5. At 4°C, a 100% inactivation required 1.5 mg/liter chloramine at pH 7.0 and 1.8 mg/liter at pH 8.5. However, only 0.03 to 0.06 mg/liter free chlorine was needed at pH ranges of 7.0 and 8.5 at either 4°C or 22°C to achieve 100% inactivation in 20 min.
The bactericidal effects of monochloramine alone were confirmed by Siders et al. in 1973. They found that at 15°C E. coli was 99% destroyed in approximately 20 min using 1.0 ppm monochloramine in pH 9 borate buffer. Since E. coli was less resistant to monochloramine than were the animal viruses tested, Siders et al. questioned the validity of using E. coli as an indicator organism for measuring the viral quality of a chlorinated water supply. Chang (1971) had previously calculated that 4 mg/liter monochloramine would be needed to give 99.999% reduction of E. coli bacterium in 10 min at 25°C.
Dichloramine (NHCl 2 )
Chang (1971) calculated that 1.2 mg/liter dichloramine would be needed to give 99.999% reduction of enteric bacteria in 10 min at 25°C.
In carefully conceived studies, Esposito et al. (1974) examined the destruction rates of test organisms in contact with dichloramine in demand-free phthalate buffer at pH 4.5 and 15°C. Figure II-4 shows the comparisons that were made among enteroviruses (poliovirus 1 and coxsackievirus A9), the bacteriophage ΦX-174, and E. coli (ATCC 11229).
Figure II-4
Inactivation of various microorganisms with dichloramine (NHCl 2 ) at pH 4.5 and 15°C. From Esposito, 1974.
From a review of the literature and an analysis of the data, Chang (1971) calculated that the relative bactericidal efficiency of dichloramine to monochloramine was 3.3:1. However, Esposito (1974) and Esposito et al. (1974) showed experimentally that Chang's estimate was conservative. They found that dichloramine was 35 times more bactericidal than was monochloramine, not 3.3. At 15°C, poliovirus 1 was 17 times more resistant than coxsackievirus A9, 83 times more resistant than ΦX-174, and 1,700 times more resistant than E. coli to dichloramine ( Figure II-4 ). They observed that dichloramine was a better bactericide than monochloramine.
These chlorine derivatives exhibit some bactericidal activity, but markedly less than either free chlorine or the inorganic chloramines (Feng, 1966; Nusbaum, 1952).
In summary, the bactericidal efficiency of hypochlorous acid, the hypochlorite ion, monochloramine, and dichloramine have been accurately defined in recent years by investigators using rigidly controlled test conditions. The order of disinfection efficiency presented by Feng (1966) has been confirmed. Comparative c · t values are shown in Table II-3 at the end of the section on chlorine.
Dosages of Various Chlorine Species Required for 99% Inactivation of Escherichia Coli and Poliovirus 1.
Efficacy against Viruses
In reviewing disinfection of enteroviruses in water, Clarke and Chang (1959) excluded all studies on the inactivation of viruses by chlorine that were conducted before 1946. Their justification for this exclusion was the failure of these studies to differentiate between free and combined chlorine. Furthermore, they attributed the irregular virucidal results of some studies to the use of animal inoculation methods for assaying virus concentrations. For these same reasons, those studies have been omitted in this report. The advent of viral propogation techniques using tissue cultures (Enders et al. , 1949) enabled research of a more exacting nature to be performed, resulting in more precise virus inactivation data.
Generally, enteroviruses are more resistant to free chlorine than are the enteric bacteria (Chang, 1971; Clarke and Kabler, 1954; Scarpino et al. , 1972). For example, in what was probably the first well-defined study, Clarke and Kabler (1954) used purified coxsackievirus A2 to investigate viral inactivation in water by free chlorine. They carefully controlled their free chlorine residuals with a modified form of the orthotolidine test to determine total chlorine and an orthotolidine-arsenite method for free chlorine. (Combined chlorine was then calculated as the difference between "total" and "free" chlorine readings.) They measured virus recoveries by using suckling mice and the LD 50 quantitation procedure. Their results indicated that inactivation times for the virus increased with increasing pH (6.9 to 9.0), decreasing temperatures (27°C-29°C to 3°C-6°C), and decreasing total chlorine concentration. They estimated that approximately 7 to 46 times as much free chlorine was required to obtain comparable inactivation of coxsackievirus A2 as was required for a suspension of E. coli cells (Butterfield et al. , 1943). For instance, Butterfield et al. (1943) found that at pH 7.0 and at 2°C to 5°C, 99.9% of E. coli cells were inactivated in 5 min with 0.03 mg/liter of free chlorine. At approximately the same pH and temperature ranges, Clarke and Kabler (1954) observed 99.6% inactivation of coxsackievirus A2 in 5 min with 1.4 mg/liter of free chlorine, i.e., 46 times as much free chlorine as that required to inactivate E. coli cells. At a pH of 8.5 at 25°C, 99.9% of E. coli cells were inactivated in 3 min with 0.14 mg/liter of free chlorine (Butterfield et al. , 1943), while at a pH of 9.0 at 27°C to 29°C, 99.6% of the virus was inactivated in 3 min by 1.0 mg/liter of free chlorine. Thus, Clarke and Kabler's work showed that 7 times as much free chlorine was required to inactivate the test coxsackievirus compared to the time necessary to kill the bacterium E. coli. In a subsequent study, Clarke et al. (1956) found that adenovirus type 3, E. coli, and Salmonella typhi were all inactivated or destroyed at approximately the same concentration of free chlorine.
In 1958, Weidenkopf reported his studies on the rate of inactivation of poliovirus 1 as a function of free available chlorine (HOCl and OCl - ) and pH at 0°C. His results showed that 99% inactivation of poliovirus 1 was obtained with 0.10 mg/liter free chlorine in 10 min at pH 6.0 and of 0°C. At pH 6, most of the free chlorine should have been present as hypochlorous acid. Increasing the pH to 7.0 increased the time required for the same degree of inactivation by approximately 50%. At that pH, the free chlorine should have been a mixture containing predominantly hypochlorous acid and significant levels of hypochlorite ion. Both Weidenkopf (1958) and Clarke et al. (1956) indicated that an increase in pH (from 7.0 to 8.5, Weidenkopf; from 8.8 to 9.0, Clarke et al. ) increased the inactivation time about sixfold. At these pH's, the free chlorine should have been present as mixtures of both hypochlorous acid and hypochlorite ion, but predominantly as the ion.
After comparing these studies, Clarke et al. (1964) concluded that at 0°C to 6°C poliovirus 1 and coxsackievirus A2 were considerably more resistant to hypochlorous acid than was E. coli, while adenovirus type 3 was more sensitive ( Figure II-5 ). For 99% destruction of E. coli, a 99-s contact time was required when the system was dosed with 0.1 mg/liter free chlorine as hypochlorous acid. The same percentage of the adenovirus was inactivated in approximately one-third of that time by the same concentration of hypochlorous acid. Under the same conditions, 8.5 min was required to inactivate 99% of the poliovirus, i.e., approximately 5 times the contact time required for E. coli. Coxsackievirus required a contact time for 99% inactivation in excess of 40 min, more than 24 times that required for E. coli.
Figure II-5
Concentration-time relationship for 99% destruction of E. coli and several viruses by hypochlorous acid at 0°-6°C. From Clarke et al., 1964.
Clarke and Kabler (1954) and Clarke et al. (1956) reported that the time required for inactivation of coxsackievirus A2 and adenovirus increased with increasing pH, decreasing total chlorine concentrations, and decreasing temperatures. Clarke and Chang (1959) concluded from data in the literature that a 10°C increase in temperature increased the rate of virus inactivation by a factor of 2 to 3.
Kelly and Sanderson (1958) reported that each of six enteric viruses possessed a different sensitivity to chlorine. Their results suggested that the inactivation of enteric viruses in water at pH 7.0 and 25°C required a minimum free residual chlorine concentration of 0.3 mg/liter with a contact time of at least 30 min. With combined chlorine in water, a concentration of at least 9.0 mg/liter was necessary for a 99.7% inactivation of poliovirus at 25°C and a pH of 7.0. Poliovirus 1 (strain MK 500) was the most resistant strain tested and coxsackievirus B5 the most sensitive. Poliovirus 1 (Mahoney strain), poliovirus 2, coxsackievirus B1, and poliovirus 3 were intermediate in resistance. The virucidal efficiency of hypochlorous acid was more than 50 times greater than that of the chloramines (Kelley and Sanderson, 1958, 1960).
Liu et al. (1971) studied the manner in which 20 strains of human enteric viruses responded to free chlorine. They used Potomac River water that had been partially treated by coagulation with alum and filtration through sand. Chlorine was added to the water at one dosage, 0.5 mg/liter. The final pH was 7.8. They stored the sample at 2°C. There was a wide range of resistance to chlorine by the viruses. The most sensitive virus was reovirus type 1, which required 2.7 min for inactiving 4 logs (99.99%) of the virus with 0.5 mg/liter of free chlorine. The most resistant, as judged by extrapolating the experimental data, was poliovirus 2, which required 40 min for the same degree of inactivation. Using actual experimental data, the most resistant virus was echovirus 12, which required a contact time of greater than 60 min for 99.99% inactivation. Liu et al. (1971) concluded from their extrapolated values that the reoviruses were the least resistant to chlorine treatment, that both adenoviruses and echoviruses were less resistant, and that the polioviruses and coxsackieviruses were the most resistant. However, assuming a 20-min contact time, most of the viruses tested at pH 7.8 and 2°C would have been 99.99% inactivated with a free chlorine residual of 0.5 mg/liter.
Using six of the same virus strains studied by Liu et al. (1971), Engelbrecht et al. (1978) investigated the effect of pH on the kinetics of chlorine inactivation at 5.0 ± 0.2°C. The suspending medium was buffered, chlorine demand-free, distilled-deionized water. Each virus stock was also prepared so as to be chlorine demand-free. Table II-1 summarizes the results, giving the chlorine levels used and the time required for two logs (99%) inactivation of the viruses at pH 6.0 and 7.8 in phosphate buffer and at pH 10.0 in borate buffer. Because of the use of two different buffer systems, i.e., at pH 6.0 and 10.0, virus inactivation was determined at pH 7.8 with each of the two buffer systems. The kinetics of inactivation of poliovirus 1 at pH 7.8, using both the phosphate and borate buffer, were the same. The results shown in Table II-1 indicate that there is a significant difference in the time required for two logs inactivation for the various viruses at pH 6.0 and 10.0. In every case, the rate of inactivation at pH 10.0 was significantly less than at pH 6.0. The rank ordering in Table II-1 shows that there is also a wide range of sensitivity of related viruses to chlorine disinfection. For example, at pH 10.0, coxsackie B5 was 40 times more resistant than coxsackie A9. There are several cases in which the relative sensitivity to chlorine was altered (rank ordering) between pH 6.0 and 10.0, suggesting important effects of pH on the virion as well as on the chlorine species, i.e., hypochlorous acid versus. hypochlorite ion. This observation can be seen more clearly in Table II-2 in which the time required for two logs of inactivation of the various viruses and the ratio of inactivation times at pH 6.0 and 10.0 are compared. Even at pH 7.8, differences in relative sensitivity appear when ranked and compared to results at pH 6.0 or 10.0 ( Table II-1 ).
Time Required for 99% Inactivation by Free Residual Chlorine at 5.0°C ± 0.2°C.
Comparison of Virus Inactivation by Free Residual Chlorine at pH 6.0 and 10.0, at 5.0°C ± 0.2°C.
Combined Chlorine (NH 2 Cl and NHCl 2 )
Viral inactivation rates with chloramines have been found to be much slower than with free chlorine. For example, Kelly and Sanderson (1958) studied the effects of chlorine on several enteric viruses. They reported that at pH 7 at 25°C-28°C, 0.2-0.3 mg/liter free chlorine inactivated 99.9% of all test viruses in 8 min. At the same temperature and pH, combined chlorine at 0.7 mg/liter and at least 4 hr of contact time were needed to achieve 99.7% inactivation of the test viruses.
Although most viral inactivation studies with chloramines have not differentiated between mono- and dichloramine (Kelly and Sanderson, 1958; Lothrop and Sproul, 1969), Kelly and Sanderson (1958, 1960) noted that viral inactivation by chloramines proceeds more rapidly at pH 6-7 than at pH 8-10. This tendency indicates that dichloramine may be more virucidal than monochloramine since its proportion increases with increased hydrogen ion concentration.
In 1971, Chang proposed that 5.0 mg/liter dichloramine or 20 mg/liter monochloramine would be needed to inactivate enteroviruses by 99.99% in 10 min at 25°C. Subsequently, Siders et al. (1973) presented evidence of the first comparative studies on viral inactivation due solely to monochloramine. At pH 9 at 15°C, poliovirus 1 (Mahoney) was 10 times more resistant to monochloramine than was E. coli. Similarly, coxsackievirus A9 was approximately 4 times more resistant than E. coli. Siders' data can be compared to Chang's theory on the disinfectant capacity of monochloramine. Assume that poliovirus inactivation has a temperature coefficient for a 10°C rise (Q 10 ) of 3. Based on Chang's (1971) calculations that 20 mg/liter monochloramine at 25°C would be needed to achieve 99.999% reduction of enterovirus in 10 min, one would extrapolate that 3 × 20 or 60 mg/liter monochloramine would be needed at 15°C to achieve an equivalent reduction of poliovirus in 10 min. However, examination of the data of Siders et al. reveals that approximately 18 min were needed to achieve only 99% inactivation using 60 ppm monochloramine. Therefore, it appears that Chang slightly overestimated the virucidal capacity of monochloramine.
Efficacy against Parasites
Some studies, mostly in the field of wastewater treatment, have shown that ova and larvae of the helminth parasites that affect humans and that could occur in U.S. water supplies are resistant to current chlorination procedures. They can survive concentrations and exposure periods considerably in excess of those used in the treatment of municipal water supplies. In studies of various free-living nematodes, Chang et al. (1960) observed that 2.5 to 3.0 mg/liter of free chlorine for a 120-min contact period and 15 to 45 mg/liter of free chlorine for 1 min were not lethal. Free chlorine residuals as high as 95 to 100 mg/liter for 5 min killed only 40%-50% of the nematodes. Thus, it may be speculated that all the helminths, including their larvae, may approach the degree of resistance to chlorine that had been demonstrated by the free-living nematodes.
There have been a number of studies on the effectiveness of chlorine in destroying or inactivating cysts of the protozoan parasite, Entamoeba histolytica , in water, especially during the early 1940's (Brady et al. , 1943; Chang, 1944b; Chang and Fair, 1941). Varied results reflect primarily the different experimental conditions and techniques that were used. The presence of organic matter, pH, and temperature, as well as the concentration and form of chlorine and exposure period, have been shown to exert an influence on disinfection. However, the consensus is that, compared with bacteria, these cysts are rather resistant to current chlorination procedures, but are much less resistant than helminths.
Brady et al. (1943) conducted field-simulated studies with cysts in raw water that had been treated with calcium hypochlorite (CaOCl), resulting in pH levels ranging from approximately 7.5 to 8.0. They found that at temperatures of 23°C-26°C, exposures of 20 min and longer to residuals of 3 to 4 mg/liter were required to produce an estimated 99% cyst destruction as judged via a culture technique. Chang (1944b), also using a culture technique, studied the cysticidal effectiveness of calcium hypochlorite solution, chloramines, and gaseous chlorine in tap water as well as the effects of pH and organic matter on the biocidal activity. At contact periods of up to 30 min, gaseous chlorine was the most powerful, hypochlorite solution slightly less so, and chloramines the least. Increase in pH and organic matter reduced cysticidal efficacy. For comparison with Brady et al. (1943), the ''lethal" residual concentration in tap water at 18°C and pH 6.8-7.2 ranged from 2.8 to 3.2 mg/liter at 15 min, and from 1.8 to 2.2 mg/liter at 30 min (as estimated from a graph in Chang, 1944b).
Recently, Stringer et al. (1975) reported on comparative studies of the cysticidal efficacy of chlorine, bromine, and iodine as disinfectants. Using chlorine gas bubbled into buffered distilled water as stock, they obtained 99.9% cyst inactivation (as measured by excystment capability) after 15 min exposure to 2 mg/liter free chlorine in "clean water" at pH 6. However at pH 8 a contact time exceeding 60 min was required to achieve 99% mortality. In "secondary treated sewage effluent," Stringer et al. considered 13.7 mg/liter chlorine at pH 8 to be ineffectual as a cysticide.
In keeping with these findings, it is unlikely that the chlorine residuals generally maintained in distribution systems provide much protection against E. histolytica cysts in the event of contamination because of cross-connections, seepage, etc.
During the past 10 yr, a number of outbreaks of waterborne infections from Giardia lamblia (another intestinal protozoan) have been reported (National Academy of Sciences, 1977). Most incidents in the United States that were traced to municipal water supplies involved surface water sources where disinfection appeared to be the only treatment. The cysts of this parasite are thought to be as resistant to chlorine as those of E. hystolytica. However, there seem to be no studies of the resistance of this parasite to chlorine or other disinfectants.
Mechanism of Action
One of the earliest references to the mechanism of inactivation of microorganisms by chlorine resulted from the work of Chang (1944a,b). While studying the inactivation of E. histolytica cysts by chlorine, he observed greater uptake of chlorine and less survival at low pH than at high pH. This observation was associated with the increased inactivation efficiency of the undissociated hypochlorous acid. Supportive evidence for the hypothesis that permeability of the uncharged chlorine species is important in determining sensitivity to chlorine has been provided by Skvortsova and Lebedeva (1973), Kaminski et al. (1976), and Dennis (1977). Chang (1944a) also noted that the inactivation of amoebic cysts was accompanied by microscopic damage to the cell nucleus, which was dependent on chlorine penetration.
The importance of penetration and/or damage to the permeability barrier of the cell membrane as a result of exposure to chlorine has been observed by several investigators. In 1945, Rahn suggested that the inactivation of bacteria by chlorine was due to multiple injuries to the cell surface. From their work with bacterial spores, Kulikovsky et al. (1975) implicated permeability damage as a mechanism of chlorine inactivation. Studies with Escherichia coli have shown that chlorine causes leakage of cytoplasmic material, first protein, then RNA and DNA, into the suspending menstruum. It also inhibits the biochemical activities that are associated with the bacterial cell membrane (Venkobachar, 1975; Venkobachar et al. , 1977). Friberg (1957) observed that E. coli also loses nondialyzable phosphorus following exposure to chlorine. In a recent study, Haas (1978) demonstrated that chlorine caused certain bacteria and yeast to release organic matter or UV-absorbing material, presumably protein or nucleic acid or their precursors. This investigator also noted that chlorine affected the uptake and retention of potassium by these same microorganisms.
Green and Stumpf (1946) and Knox et al. (1948) indicated that destruction of bacteria by chlorine was caused by an inhibition of the mechanism of glucose oxidation. Specifically, they suggested that chlorine affected the aldolase enzyme of E. coli by oxidizing the sulfhydryl group that is associated with the enzyme. Venkobachar et al. (1977) recently reported that chlorine significantly inhibits both oxygen uptake and oxidative phosphorylation. The latter effect was attributed to inhibition of the respiratory enzyme rather than to a deficiency in phosphate uptake. However, it is unclear whether free or combined chlorine was used in these studies. Haas (1978) also observed chlorine to affect the respiration of bacteria as well as the rate of synthesis of protein and DNA. Others have also noted that chlorine affects the nucleic acids or physically damages DNA (Bocharov, 1970; Bocharov and Kulikovskii, 1971; Fetner, 1962; Rosenkranz, 1973; Shih and Lederberg, 1976a,b).
It appears that chlorine, having penetrated the cell wall, encounters the cell membrane and alters its permeability. Simultaneously or subsequently, the chlorine molecules may enter the cytoplasm and interfere with various enzymatic reactions. It should be noted that permeases and respiratory enzymes are associated with the cytoplasmic membrane of bacteria.
Chang (1971) supported the hypothesis that the rapid destruction of vegetative bacteria by chlorine was due to the extensive destruction of metabolic enzyme systems. He also addressed the subject of virus inactivation, commenting that viruses are generally more resistant to chlorine than bacteria. He associated this observation with the fact that viruses completely lack a metabolic enzyme system. He speculated that inactivation of viruses by chlorine probably result from the denaturation of the capsid protein. Furthermore, since protein denaturation is more difficult to achieve than destruction of enzymatic R—S—H bonds by oxidizing agents, it is understandable why greater levels of chlorine are required to inactivate viruses than bacteria. However, from their experimental work with the bacterial virus f2, Olivieri et al. (1975) concluded that chlorine caused initial lethal damage to the viral genome and that the capsid protein was affected after the virus was inactivated. Dennis (1977) reported that the incorporation of chlorine into the f2 bacterial virus is dependent on pH and that the higher rates of incorporation occur at lower pH values.
There is limited information in the literature on the mechanism of inactivation of microorganisms by chloramines. Nusbaum (1952) proposed that since low levels of inorganic chloramines were effective in inactivating bacteria, the mechanism of action must be essentially the same as that of hypochlorous acid on enzymes. Ingols et al. (1953) showed that monochloramine was not able to immediately and irreversibly oxidize sulfhydryl groups. Such oxidation would have resulted in the rapid inactivation of the bacteria. They hypothesized that since monochloramine required higher concentrations and longer contact times to destroy bacteria completely and could not readily and irreversibly oxidize the sulfhydryl groups of the glucose oxidation enzymes, its ability to inactivate microorganisms should be attributed to changes in enzymes that may not be involved in the inactivation of the organism by hypochlorous acid. Thus, while the sulfhydryl group may be the most vulnerable to a strong oxidant like hypochlorous acid, changes in other groups produced by the weaker oxidant, monochloramine, may lead also to microbial inactivation. More recent information indicates that the destructive effects of chloramine might be associated with the effects of chloramine on nucleic acids or DNA of cells (Fetner, 1962; Shih and Lederberg, 1976a,b).
Nusbaum (1952) suggested that the disinfective activity of dichloramine occurs by a mechanism similar to monochloramine, but there do not appear to be any data to support this contention.
Considering the mechanism of destruction or inactivation of microorganisms by chlorine and associated compounds, it is interesting to note that Fair et al. (1948) speculated that there might be three or four "targets" or points of attack and that perhaps all must be affected before there is death. This "multiple hit" concept supported the observation that monochloramine must alter groups other than the sulfhydryl group to be effective in the destruction of microorganisms.
Thus, the action of chlorine on microbes such as bacteria and amebic cysts may involve some or all of the steps in the following sequence: penetration of the disinfectant through the cell wall followed by attack on the cell membrane (the site of cellular respiration in bacteria) and disruption of permeability of the cell membrane, which leads to a loss of cell constituents, thereby disrupting metabolic functions within the cell including those involving nucleic acids. Changes in viability may result from this process. Experimental studies on virus (Olivieri et al. , 1975) demonstrated that chlorine caused initial damage on the viral nucleic acid while leaving the capsid protein unaffected until after the virus was inactivated.
Chlorine is the most widely used water supply disinfectant in the United States. Depending upon the predominant species of chlorine, hypochlorous acid, and/or hypochlorite ion, disinfection with chlorine can achieve greater than 99.9% destruction of bacteria. For example, a chlorine residual of 0.2 to 1.0 mg/liter and a contact time of 15 to 30 min will inactivate 99.9% of E. coli (Walton, 1969). According to Walton, a properly designed, constructed, and operated water treatment plant, consisting of chemical coagulation, sedimentation, filtration, and disinfection, can remove or destroy more than 99.999% of the coliform bacteria that are present. Although most investigations on the removal or destruction of bacteria have used E. coli , there is evidence that the bacterial pathogens, e.g., Salmonella typhi , respond somewhat similarly to E. coli.
Laboratory studies have demonstrated that that there is limited virus inactivation after the added chlorine has reacted with any ammonia that is in the water. Most inactivation probably occurs in the first few seconds before the chlorine has completed its reaction with ammonia (Olivieri et al. , 1971).
Table II-3 displays c · t values for E. coli and poliovirus inactivation for the various species of free and combined chlorine.
- Research Recommendations
Recent reports of enhanced chlorine resistance of certain viral and bacterial strains should be investigated and the mechanism of increased resistance elucidated, if the reports are corroborated.
Other recommendations, applicable to other agents as well as to chlorine, are included after the evaluations of the other methods of disinfection.
Chemistry of Ozone in Water
Ozone has the molecular formula O 3 , a molecular weight of 48 g/mol, and a density, as a gas, of 2.154 g/liter at 0°C and 1 atm. It is approximately 13 times more soluble in water than is oxygen. At saturation in water at 20°C, a 2% weight mixture of ozone and oxygen contains about 11 mg of ozone and 40 mg of oxygen per liter.
Ozone has a half-life in pure distilled water of approximately 40 min at pH 7.6, but this decreases to 10 min at pH 8.5 (Stumm, 1958) at 14.6°C. Rising temperatures increase the rate of decomposition. Because its half-life is so short, ozone must be generated on the site where it is to be used.
Ozone is a powerful oxidant that reacts rapidly with most organic and many inorganic compounds. It does not convert chloride to chlorine under test conditions (U.S. Environmental Protection Agency, 1976), nor does it react extensively with ammonia (NH 3 ) (Singer and Zilli, 1975). However, bromide and iodide are oxidized to bromine and iodine. Singer and Zilli (1975) reported that oxidation of ammonia was pH-dependent. At pH 7.0, 9% of a 29 mg/liter ammonia nitrogen (NH 3 —N) solution was oxidized in 30 min, but at pH 9.0 70% of a 24.4 mg/liter solution was oxidized in the same time. During disinfection, only minor amounts of ammonia are oxidized when ozone is used. Ozone's limited reaction with ammonia is desirable, but its fast reaction rate with most organic and many inorganic compounds further shortens its persistence in water.
Production and Application of Ozone
Ozone is produced on site from a stream of clean dry air or oxygen by passing an electrical discharge between electrodes that are separated by a dielectric. Approximately twice the percent of ozone by weight is obtained if oxygen, rather than air, is used as the feed stream. Power requirements are about 13 to 22 kWh/kg of ozone that is generated from air and approximately half that when oxygen is used (Rosen, 1972). Compressors and dryers may increase these requirements by 20% to 50%. Other factors affecting efficiency are the rate of gas flow, applied voltage, and the temperature of the gas. The heat that is produced during the process must be removed by cooling with either air or water.
The ozone gas stream must be fed into the water to effect the transfer of ozone. The usual methods are to inject the ozone gas stream through an orifice at the bottom of a co- or countercurrent contact chamber or to aspirate the gas into a contact chamber where it is mixed with the water mechanically. Successful design and operation of the contactor system is necessary to minimize costs of the operation.
Commercial equipment is available in a wide range of capacities—from a few grams of ozone per day to more than 40 kg/day. Larger capacities are obtained by adding additional units. Successful delivery of ozone to the water to be treated requires a dependable power supply and reasonably maintenance-free ozonization equipment.
Ozone has been used in a great number of water treatment plants throughout the world. However, in small institutions and private residences, its use appears limited, because it requires dependable power supplies and, usually, a second disinfectant to furnish a disinfecting residual in the system. The maintenance and repairs that are required for the specialized ozone generation equipment provide further barriers against the use of ozone by small institutions.
Analytical Methods
The disinfection process is usually controlled in one of two ways: by the dosage of a specified amount of ozone or by the maintenance of a specified minimum residual for a given time. Residual measurements in both the gas stream and water are sometimes required. Standard Methods (1976) contains descriptions of the measurement of ozone in water by the iodometric, orthotolidine-manganese sulfate, and orthotolidine-arsenite methods. Of these methods the iodometric method, which is subject to the fewest interferences, is the method of choice. Determinations must be made immediately since ozone decomposes rapidly.
In all three methods, the oxidant compounds that result from the reaction of ozone with contaminants in water may react with the test reagents, thereby indicating a higher concentration of ozone than is actually present. This is particularly true in the presence of organic matter, which results in the formation of organic peroxides. In the iodometric method this interference and others are minimized by stripping the ozone from the sample with nitrogen or air and absorbing it from this gas stream in an iodide solution. These and other methods are described in Standard Methods.
Schecter (1973) developed a UV spectrophotometric method to measure the triiodide that is formed by the oxidation of iodide by ozone. She reported a better sensitivity at low ozone concentrations (0.01 to 0.3 mg/liter) than achieved with the normal titration method. The effects of interferences on the direct measurement of ozone without sparging the ozone to a separate iodide solution were not indicated. These effects are noted in Standard Methods.
Analytical determination of ozone in water in the presence of other oxidants is poor. Considerable work in this area is needed.
As discussed above, ozone is unstable in water with a half-life of approximately 40 min at pH 7.6 and 14.6°C. Many regard the half-life in water supplies at higher ambient temperatures to be 10 to 20 min.
In a recent review of the literature, Peleg (1976) concluded that the possible species to be found in an ozone solution were ozone, hydroxyl radicals (·OH), hydroperoxyl radicals (HO 2 ·), oxide radicals (O·), ozonide radicals (O 3 ·), and, possibly, free oxygen atoms (·O·). Hydrogen peroxide (H 2 O 2 ) may also be present by dimerization of the hydroxyl radicals. There have been no studies on the disinfecting activities of these individual species except for those on hydrogen peroxide, which is a poor biocide (when compared to chlorine). Peleg concluded that evidence indicates that the dissociation species are better disinfectants than ozone.
Wuhrmann and Meyrath (1955) found that 99% of Escherchia coli were inactivated in 21 s with 0.0125 mg/liter residual ozone, in 62 s with 0.0023 mg/liter residual ozone, and in 100 s with 0.0006 mg/liter residual ozone at pH 7.0 and 12°C. Spores of a Bacillus species were much more resistant, requiring 2 min with 0.191 mg/liter residual ozone and 5 min with 0.049 mg/liter residual ozone for 99% inactivation. These data were obtained at pH 7.2 and 22°C. The observed ozone residuals were reported as being constant throughout the test periods.
Katzenelson et al. (1974) found that an initial residual ozone concentration of 0.04 mg/liter in demand-free water inactivated approximately 3 logs of E. coli in 50 s, while a concentration of 1.3 mg/liter achieved the same degree of inactivation in 5 s. They obtained their inactivation data from experiments in which the loss of initial ozone residuals did not exceed 20%. Their work was conducted at 1°C and pH 7.2. The ozone was determined by the method of Schecter (1973).
Using washed cells of E. coli, Bacillus megaterium, and Bacillus cereus, which were suspended in deionized water with 106 cells/ml, and different concentrations of ozone up to 0.71 mg/liter, Broadwater et al. (1973) demonstrated that the inactivation of all three test organisms gave an "all-or-none" response. They measured the ozone by stripping it into iodide at the end of the contact period. Consequently, any demand should have used up whatever ozone was needed. They did measure what they presumed to be ozone and not some breakdown product. Thus, they appear to have eliminated the ozone demand problem as much as is possible with present techniques. However, it is possible that their results reflect some effect or artifact not yet understood. With the constant contact time of 5 min, no inactivation of vegetative cells of E. coli or B. megaterium occurred until the initial residual concentration of ozone was 0.19 mg/liter, when the density of viable cells of both species decreased to "near zero." With the vegetative cells of B. cereus, an initial ozone residual of 0.12 mg/liter was required before any inactivation occurred, and then it was nearly complete. Spores of B. megaterium and B. cereus were not inactivated until the ozone residual reached approximately 2.29 mg/liter, when the spores of both organisms showed an "all-or-none" response. All of the inactivation experiments were performed at 28°C, but the pH was not reported.
Burleson et al. (1975) determined that E. coli, Staphylococcus aureus, Salmonella typhimurium, Shigella flexneri, Psuedomonas fluorescens, and Vibrio cholerae were all reduced by 7.5 logs in 15 s after exposure to approximately 0.5 mg/liter of ozone at 25°C in phosphate-buffered saline. The pH was not reported. In this study, the bacteria were placed in unozonized water (no initial residual), and the ozone was then sparged into the water. After 15 s the ozone concentration in solution reached approximately 0.5 mg/liter. This technique does not render quantitative data. Ozone was determined by spectrophotometric measurement of iodine that was released from iodide without stripping of the ozone.
The inactivation of E. coli with initial counts of about 5 ´ 10 5 /ml in buffered demand-free water at 11°C was measured by Ross et al. (1976). For 99% inactivation with 0.1 mg/liter of initial ozone residuals, contact times of 16.5 and 21 s were required at pH 6 and 10, respectively. Ozone was measured by the diethyl- p -phenylenediamine (DPD) method.
Farooq (1976) and Farooq et al. (1977a,b,c) studied the effects of pH and temperature in ozone inactivation studies with Mycobacterium fortuitum and Candida parapsilosis (a yeast). They concluded that if the ozone residual remains constant, the disinfection capability will not be affected by a change in pH. They also demonstrated that for a given dosage a rise in temperature increases the rate of inactivation, even though the ozone residual was decreased. (Ozone was less soluble at higher temperature). Vorchinskii (1963) concluded that whereas the bactericidal dose of ozone at 4°C to 6°C was unity, the corresponding dose at 18°C to 21°C was 1.6 and at 36°C to 38°C was 3.6.
The work of Farooq and his colleagues is in agreement with that of Morris (1976), who observed that the disinfection capability of ozone does not change significantly with pH, at least over the normal pH range (6 to 8.5) of water supplies.
Katzenelson et al. (1974) investigated the inactivation of poliovirus 1 at 5°C and pH 7.2. A 99% inactivation of the virus occurred in less than 8 s with 0.3 mg/liter of initial residual ozone. Their experimental methods were the same as those described above for bacteria. They also observed that inactivation resulted from two distinct stages (rates) of action. The first stage of inactivation, less than 8 s long, produced a virus inactivation of from 99% to 99.5%, depending upon the ozone residual. The second stage, which lasted from 1 to 5 min, still left some viruses infective. Additional work showed that the slower second stage inactivation apparently involved the inactivation of viruses that were clumped together. The single virus particles were inactivated during the first stage. After ultrasonic treatment, 99.5% of the virus was inactivated within 8 s and more than 99.99% within 3 min at an initial ozone residual of 0.1 mg/liter.
Coin et al. (1964) investigated the inactivation of poliovirus 1 (Mahoney) in a batch system in which a measured amount of ozone was introduced into a virus suspension in distilled and filtered river water. In distilled water, nearly 1 log of the virus was inactivated in 4 min at a 4-min ozone residual of approximately 0.23 mg/liter. In river water, the inactivation was in excess of 99.99% in 4 min when the ozone concentration, also measured at 4 min, exceeded 0.3 mg/liter. The ozone was measured by the iodide titration without stripping of the ozone. Rather large ozone demands existed in this system. An initial 5 mg/liter residual ozone concentration in the distilled water decreased to about 0.6 to 0.8 mg/liter in 4 min. The same initial residual in river water decreased to between 0.2 to 0.6 mg/liter. Temperature and pH were not reported.
Keller et al. (1974) studied ozone inactivation of viruses in a water supply by using both batch tests and a pilot plant with a 38 liter/min (10 gal/min) flow rate. Inactivation of poliovirus 2 and coxsackievirus B3 in 5 min was greater than 99.9% in the batch tests when the ozone residual was between 0.8 and 1.7 mg/liter at the end of the 5-min contact period. Initial ozone residuals varied from 1.6 to 2.8 mg/liter. At the pilot plant, greater than 99.999% inactivation of coxsackievirus B3 could be achieved with an ozone dosage of 1.45 mg/liter, which provided an ozone residual of 0.28 mg/liter after 1 min in lake water. Temperature and pH conditions were not reported. Ozone was measured by the iodide method without prior stripping of the ozone.
Burleson et al. (1975) obtained inactivations of > 99.9% for vesicular stomatitis virus, > 99.99% for encephalomyocarditis virus, and 99.99% for GD VII virus in 15 s with an ozone residual of approximately 0.5 mg/liter of ozone. The experiment was conducted in the same manner as that described above for their studies with bacteria. Evison (1977) reported data for the inactivation of a number of viruses in buffered water at pH 7.0 and 25°C ( Table II-4 ).
Ozone Required for Inactivation of Viruses in 10 Minutes at pH 7.0 and 25°C.
The reported ozone concentrations were evidently those measured initially and maintained by the addition of ozone during the experiment. Ozone was measured by a colorimetric version of Palin's DPD technique. The Evison data show that more ozone or a longer time are required for inactivation than do the data of other workers. This may have resulted from the virus purification used. Her viruses were purified by low-speed centrifugation and filtration through an 0.2-µm membrane filter. These cleanup procedures are neither as complete nor as thorough as those used by other investigators. The unremoved cell debris and organic matter offer protection to the virus. Either higher ozone residuals or longer contact times would be required to inactivate such preparations to the same extent as clean virus. Other data showed that the inactivation of coliphage 185 was relatively unaffected by pH's ranging from 6.0 to 8.0 at ozone concentrations exceeding 0.05 to 0.10 mg/liter. Evison (1977) also concluded that the rate of inactivation of the coliphage 185 by ozone was much less affected by temperature than was the inactivation by chlorine.
Farooq (1976) observed a 99% inactivation of 10 5 /ml poliovirus 1 in 30 s in distilled water at an initial ozone residual concentration between 0.23 and 0.26 mg/liter at 24°C and pH 7.0. In this study, ozone was measured by the Schecter (1973) method.
Sproul et al. (1978) reported total inactivation in 10 s of poliovirus 1 (Sabin), which had initial concentrations of 1.3 × 10 2 to 2.4 × 10 3 plaque-forming units (PFU)/ml. The initial ozone residual of 0.012 to 0.085 mg/liter decreased by approximately one-third in 40 s. The experiments were conducted with the Sharpe dynamic reactor. Ozone was measured by the Schecter (1973) method.
No studies on the efficacy of ozone as an antihelmintic agent appear to have been reported.
Ozone may have application as an antiparasitic agent in the treatment of water supplies but only limited information is available. Newton and Jones (1949) reported that ozone, with 5-min residuals as low as 0.3 mg/liter, inactivated from 98% to >99% of Entamoeba histolytica cysts that were suspended in water. Initial ozone residuals that were required to obtain 5-min residuals of 0.3 mg/liter varied from 0.7 mg/liter to 0.9 mg/liter. With the ozone concentrations used, the cysticidal action was not affected by temperatures from 10°C to 27°C nor by pH's between 6.5 to 8.0. Ozone was measured by titration of iodine, which was released from iodide directly in the reactors without removal of ozone by sparging.
Investigations of the inactivation of bacteria by ozone have centered on the action of ozone on the cell membrane. Scott and Lesher (1963) concluded from their work with E. coli that the primary attack of ozone occurred on the double bonds of the fatty acids in the cell wall and membrane and that there was a consequent change in the cell permeability. Cell contents then leaked into the water. This was confirmed by Smith (1967). Prat et al. (1968), examining extracts from ozonized E. coli, reported that thymine was more sensitive to ozone attack than were cytosine and uracil.
Riesser et al. (1977) showed that ozone attacked the protein capsid of poliovirus 2 in such a manner that the virus was not taken up into susceptible cells. An electrophoretic study showed complete loss of viral proteins in a poliovirus 2 sample that had showed an inactivation of 7 logs in 20 min.
Summary and Conclusions
Inactivation with ozone at specified ozone residuals is relatively insensitive to pH's between 6.0 and 8.5. Moreover, ozone does not react with ammonia over this same range when short detention times are used. The data on temperature are not sufficiently firm to permit conclusions concerning its effect on disinfection. Ozone must be generated on site, and the process is relatively energy intensive. To make economic comparisons of ozone with other disinfectants, the cost of local power must be ascertained.
Available kinetic data on ozone inactivation are presented in Table II-5 . The c · t product for 99% inactivation of E. coli appears to be approximately 0.006 at near-neutral pH values and at temperatures of ≤10°C. There was less consistency for the c · t products of poliovirus 1. These values varied from < 0.005 to 0.42 at pH 7 and at temperatures from 5°C to 25°C.
Concentration of Ozone and Contact Time Necessary for 99% Inactivation of Escherichia coli and Polio 1 Virus.
The c · t products vary over a broad range. These variations illustrate the difficulty of doing quantitative experimentation with ozone and microorganisms in water. Among other reasons, these difficulties are caused by undetected ozone demand in the water, poor analytical techniques for residual ozone, and nonuniformity of microorganisms from one laboratory to another. As an example of the latter, different strains of poliovirus with different inactivation rates are used, but the inactivation data are frequently not reported as strain-specific. Furthermore, viruses frequently exist in an undetected clumped state rather than in the presumed single discrete particle state. Higher c · t products are required for the clumped viruses than for the unclumped ones.
Because of ozone's relatively short half-life in water, another disinfectant must be added to maintain a disinfection capability in the distribution system. The most effective disinfectant, its optimum concentration, and method of addition must be determined. The disinfection process with ozone will probably be controlled by specifying the ozone residual at the beginning and end of a given contact time.
Future research studies with ozone should be conducted to:
- provide data on the inactivation of enteric pathogens;
- provide more data on the inactivation of protozoan cysts, especially those of Giardia lamblia ;
- provide analytical methods that are specific for ozone;
- provide additional definitive data on bacteria and viruses, and eliminate discrepancies; and
- provide operating data from full-scale drinking water plants to demonstrate reliability of operation, operating costs, ozone dosages, residuals, contact times, and disinfection results.
- Chlorine Dioxide
Chlorine dioxide (ClO 2 ) was first prepared in the early nineteenth century by Sir Humphrey Davey (1811). By combining potassium chlorate (KClO 3 ) and hydrochloric acid (HCl), he produced a greenish-yellow gas, which he named ''euchlorine." Later, this gas was found to be a mixture of chlorine dioxide and chlorine. The bleaching action of chlorine dioxide on wood pulp was recognized by Watt and Burgess (1854).
Large quantities of chlorine dioxide are produced each day in the United States. Although its primary application has been the bleaching of wood pulp, it is also used extensively for bleaching and dye stripping in the textile industry and for bleaching flour, fats, oils, and waxes (Gall, 1978).
In the United States, chlorine dioxide was first used in 1944 at the water treatment plant in Niagara Falls, New York, to control phenolic tastes and odors arising from the presence of industrial wastes, algae, and decaying vegetation (Synan et al. , 1945). Granstrom and Lee (1958) surveyed water treatment plants believed to be using chlorine dioxide.
The majority of respondents (956 plants) were using it for taste and odor control. Other uses reported were algal control (7 plants), iron and manganese removal (3 plants), and disinfection (15 plants).
Sussman (1978) compiled a partial listing of plants using chlorine dioxide. He reported that the compound is used primarily to control taste and odor in the United States. In England, Italy, and Switzerland, it is used for disinfection of water supplies.
The Chemistry of Chlorine Dioxide in Water
Chlorine dioxide reacts with a wide variety of organic and inorganic chemicals under conditions that are usually found in water treatment systems (Stevens et al. , 1978). However, two important reactions do not occur. Chlorine dioxide per se does not react to cause the formation of trihalomethanes (THM's) (Miltner, 1976). However, THM's will be formed if the chlorine dioxide is contaminated with chlorine. Such a situation may occur when chlorine is used in the preparation of chlorine dioxide.
Chlorine dioxide does not react with ammonia, but will react with other amines (Rosenblatt, 1978). The amine structure determines reactivity. Tertiary amines are more reactive with chlorine dioxide than secondary amines, which, in turn, are more reactive than primary amines.
Production and Application
Chlorine dioxide condenses to form an unstable liquid. Both the gas and liquid are sensitive to temperature, pressure, and light. At concentrations above 10% in air, chlorine dioxide may be explosive, and at 4% in air, it can be detonated by sparks (Gall, 1978; Sussman, 1978). As a result, the preparation and distribution of chlorine dioxide in bulk have not been deemed practical. It has been generated and used on site.
Sodium chlorate (NaClO 3 ) or sodium chlorite (NaClO 2 ) may be used to generate chlorine dioxide. The method of production will depend upon the amount of chlorine dioxide that is required. The reduction of sodium chlorate is the more efficient process and is generally used when large volumes and high concentrations of chlorine dioxide are needed. Commercial processes that are used in North America for large-scale production of chlorine dioxide are based on the three reactions listed below. To reduce the sodium chlorate, each process uses a different agent: sulfur dioxide (SO 2 ), methanol (CH 3 OH), and the chloride ion (Cl - ).
All of these processes are used in the pulp and paper industry. They can also be used to prepare chlorine dioxide for the large waterworks that might require several metric tons per day. Small quantities of chlorine are formed during the side reactions and intermediate reactions in these processes. A more detailed review of the chemistry that is involved in the production of chlorine dioxide from chlorate is given by Gall (1978) and Gordon et al. (1972).

Chlorine dioxide can be prepared from chlorine and sodium chlorite through the following reactions:

The theoretical weight ratio of sodium chlorite to chlorine is 1.00:0.39 (Dowling, 1974). With available sodium chlorite (80%), the weight ratio is 1:0.30. In practice, Gall (1978) recommended a chlorite to chlorine ratio of 1:1. The excess chlorine lowers the pH, thereby increasing the reaction rate and optimizing the yield of chlorine dioxide. Dowling (1974) reported that the maximum theoretical yield of chlorine dioxide was produced when the ratio was normally maintained at a minimum of 1.0:0.5.
Alternatively, chlorine dioxide may be prepared from sodium hypochlorite (NaOCl) and sodium chlorite. The sodium hypochlorite is acidified to yield hypochlorous acid (HOCl), and the chlorine dioxide is generated according to Reaction 10. Each of the methods produces a solution containing both chlorine and chlorine dioxide.
Chlorine dioxide may also be prepared by the addition of a strong acid, such as sulfuric acid (H 2 SO 4 ) or hydrochloric acid, to sodium chlorite as shown in the following reactions:

Although some investigators have claimed that this method produces chlorine-free chlorine dioxide, Feuss (1964) and Schilling (1956) reported that chlorine is also formed. Dowling (1974) indicated that chlorine was formed even when sulfuric acid was used.
Chlorine dioxide is one of the few stable nonmetallic inorganic free radicals (Rosenblatt, 1978). It does not contain available chlorine in the form of hypochlorous acid or hypochlorite ion (OCl - ). However, concentrations of chlorine dioxide are often reported in terms of available chlorine. The chlorine atom in chlorine dioxide has a valance of + 4. A reduction to chloride results in a gain of five electrons. In terms of available chlorine, chlorine dioxide has 263% or more than 2.5 times the oxidizing capacity of chlorine.

The weight ratio of chlorine dioxide to available chlorine is 67.45 to 35.45 or 1.9. However, in water treatment practices this increased oxidizing capacity is rarely realized. The reduction of chlorine dioxide depends heavily on pH and the nature of the reducing agent. At neutral or alkaline pH, chlorine dioxide is reduced to chlorite, a net gain of one electron. Thus, only one-fifth or 20% of its oxidizing capacity is utilized. At low pH, the chlorite (ClO 2 - ) is reduced to chloride (Cl - ) releasing the remaining four available electrons.
Chlorine dioxide may be determined iodometrically ( Standard Methods , 1976), amperometrically (Haller and Listek, 1948; Standard Methods , 1976), spectrophotometrically (Gordon et al. , 1972), and colorimetrically (Aston, 1950; Hodgen and Ingols, 1954; Masschelein, 1966; Palin, 1957, 1960, 1967, 1970, 1974, 1975; Post and Moore, 1959; Standard Methods , 1976).
Several studies contain comparisons of various analytical methods and procedures for the measurement of chlorine dioxide. Adams et al. (1966) compared the H-acid, tyrosine, amperometric, and diethyl- p -phenylenediamine (DPD) procedures for free chlorine, chlorine dioxide, and chlorite. They reported DPD to be the most reliable, as did Dowling (1974), Miltner (1976), and the U.S. Public Health Service (Miltner, 1976). Myhrstad and Samdal (1969) noted that the DPD method (Palin, 1957, 1960) yielded consistently higher residual measurements for chlorine dioxide than those that are produced with other analytical methods. After analysis with acid chrome violet K (Masschelein, 1966), chlorine dioxide was not observed in the water of the distribution system; however, chlorite was found. The residuals that were previously interpreted as chlorine dioxide were apparently due to chlorite.
Recently, more sophisticated procedures were suggested for the determination of chlorine dioxide. Moffa et al. (1975) reported the use of electron spin resonance, and Issacsson and Wettermark (1976) described a chemiluminescent method for active chlorine compounds. Stevenson et al. (1978) reviewed electrochemical methods and presented preliminary results for a membrane amperometric probe. Under development is a sensor that shows a linear response region from about 0.5 to 10 mg/liter. The response to hypochlorous acid and chloramines was low, and the sensor does not measure chlorite or other ionized species.
No one procedure appears to possess the necessary sensitivity, selectivity, and simplicity to permit reliable determinations in the treatment of water. Each of the titration methods are prone to error because of volatilization. They are time-consuming and particularly complex when differentiation of chlorine and oxychloro species are necessary. The colorimetric procedures require strict control of pH, temperature, and reaction times and will be affected by turbidity. In addition, the selectivity of the indicators for chlorine dioxide is questionable. The direct spectrophotometric determination of chlorine dioxide at 360 nm is selective and rapid but is not sufficiently sensitive for use in water. Limited experience with the more recent procedures (chemiluminescence and membrane amperometric probe) does not permit an evaluation.
In practice, the principal distinction that must be made is that between the active biocidal species (hypochlorous acid, the hypochloride ion, and chlorine dioxide), the moderately biocidal species (monochloramine [NH 2 Cl], dichloramine [NHCl 2 ], and nitrogen trichloride [NCl 3 ]), and the relatively nonbiocidal species (chlorite and chlorate [ClO 3 - ] ions). This is imperative when the primary purpose for the addition of chlorine dioxide is the inactivation of microorganisms. Furthermore, when the formation of THM's is to be considered, the distinction between free chlorine and chlorine dioxide becomes important.
Experimental data on the efficacy of chlorine dioxide as a disinfectant became available in the early 1940's. McCarthy (1944, 1945) reported that chlorine dioxide was an effective bactericide in water with a low organic content. When the levels of organic material in the water were high, chlorine dioxide was less effective.
Ridenour and Ingols (1947) reported that chlorine dioxide was at least as effective as chlorine against Escherichia coli after 30 min at similar residual concentrations. Both chlorine and chlorine dioxide residues were determined by the orthotolidine-arsenite (OTA) method. The bactericidal activity of chlorine dioxide was not affected by pH values from 6.0 to 10.0. Ridenour and Armbruster (1949) extended their observation to other enteric bacteria. The common waterborne pathogens were similarly inactivated with chlorine dioxide. They also reported that the efficiency of chlorine dioxide decreased as the temperature decreased from 20°C to 5°C.
Ridenour et al. (1949) found chlorine dioxide to be more effective than chlorine (based on OTA residuals) against bacterial endospores. They indicated that less weight of chlorine dioxide than chlorine is required to inactivate the spores of Bacillus mesentericus in either demand-free water or in waters containing ammonia. In the waters containing ammonia, chlorine had to be applied beyond breakpoint before efficient sporicidal activity was observed.
The work of Ridenour and colleagues is not discussed in depth because the small amounts of free chlorine that are produced during the generation of chlorine dioxide are not distinguished from the chlorine dioxide by the OTA method that they used for both stock solutions and residual determination. In their 1947 paper (Ridenour and Ingols, 1947), the survival measurements were ± and not quantitative. In their 1949 paper (Ridenour and Armbruster, 1949), the survival measurement was quantitative, but only one contact time was used, 5.0 min. Since chlorine dioxide is a rapidly acting disinfectant, c · t products may be misleading using this contact time.
Russian investigators (Bedulevich et al. , 1953; Trakhtman, 1946) found chlorine dioxide to be a more effective or at least as effective a bactericide as chlorine. Additional data were reported for Bacillus anthracis. They observed that the efficiency of chlorine dioxide decreased as the pH of the system containing the B. anthracis increased.
Early studies on disinfectant activity are difficult to interpret because the methods of preparing chlorine dioxide invariably included the addition or production of chlorine. Analytical procedures were not sufficiently advanced to differentiate between chlorine dioxide and other oxychloro species. Thus, the quantitative analyses of stock solutions and reports of dose and residual chlorine dioxide may be in error. This suggests that the initial and residual concentrations of chlorine dioxide were probably lower than reported values and that the comparative bactericidal efficiency would suffer accordingly. In addition, the older investigations did not take into account the volatility of chlorine dioxide. Depending upon concentration and length of exposure, losses of 7% to 30% can occur within an hour.
Many of the difficulties that were encountered during the early studies were overcome in a series of studies reported by Benarde and co-workers during the mid-1960's. Their work on disinfection was based heavily on the improved methods of preparing and analyzing chlorine dioxide, which were reported by Granstrom and Lee (1958). They prepared chlorine dioxide by oxidizing sodium chlorite with persulfate (S 2 O 8 2- ) under acid conditions. The resulting chlorine dioxide was swept to a collection vessel by high purity nitrogen gas. Chlorine dioxide was measured spectrophotometrically at 357 nm.
Bernarde et al. (1965) compared the bactericidal effectiveness of chlorine with that of chlorine dioxide at pH 6.5 and 8.5 in a demand-free buffered system. At pH 6.5, both chlorine and chlorine dioxide inactivated a freshly isolated strain of E. coli in less than 60 s. Chlorine was slightly more effective at the lower dosages at the lower pH. At pH 8.5, chlorine dioxide was dramatically more effective than chlorine. Greater than 99% inactivation of E. coli was observed in 15 s with 0.25 mg/liter chlorine dioxide, while, under the same conditions, chlorine required almost 5 min for similar inactivation. Chlorine dioxide was significantly more efficient than chlorine in the presence of high levels of organic and nitrogenous material. Bernarde et al. (1967a) also reported that temperature affects the rate of inactivation of bacteria with chlorine dioxide. A decrease in disinfectant activity was observed as temperature decreased from 30°C to 5°C. For 99% inactivation of E. coli with 0.25 mg/liter of chlorine dioxide, 190, 74, 41, and 16 s were required at 5°C, 10°C, 20°C, 30°C, respectively. More recent work by Cronier (1977) in a clean system also demonstrated the excellent bactericidal activity of chlorine dioxide. Results of both studies are shown in Table II-6 .
Concentrations of Chlorine Dioxide and Contact Times Necessary for 99% Inactivation of Escherichia coli .
In the mid-1940's, there were also investigations on the virucidal activity of chlorine dioxide. Ridenour and Ingols (1946) reported that chlorine dioxide was as effective as chlorine against a mouse-adapted strain of poliovirus (Lansing). Again, their comparison was based upon OTA-determined residual levels of each disinfectant. Hettche and Ehlbeck (1953) found chlorine dioxide to be more effective against poliovirus than either chlorine or ozone. In addition to the difficulties that are associated with the preparation and measurement of chlorine dioxide and chlorine, the early virus studies were also saddled with difficult and time-consuming virus test systems.
The first definitive work on the virucidal activity of chlorine dioxide was done in Göteborg, Sweden. Warriner (1967) showed that the rate of inactivation of poliovirus 3 increased with increasing pH at pH values of 5.6 to 8.5 (see Table II-7 ). Similar to the action on bacteria, the viral inactivation occurred rapidly. When chlorine and chlorine dioxide were combined, the inactivation was synergistic. The inactivation with the two chlorine species together was more efficient at lower pH, but the presence of chlorine dioxide enhanced inactivation by chlorine at the higher pH.
Concentrations of Chlorine Dioxide and Contact Times Necessary for 99% Inactivation of Viruses.
Cronier (1977) compared the inactivation of E. coli (ATCC 11229), poliovirus 1, and coxsackievirus A9. All of the microorganisms that were tested were sensitive to low concentrations (< 1 mg/liter) of chlorine dioxide. Poliovirus 1 and coxsackievirus A9 were more resistant than E. coli. Similar to its bactericidal activity, chlorine dioxide was more effective as a virucide at higher pH. Cronier also reported that on a weight basis, it was similar to hypochlorous acid and better than hypochlorite ion, monochloramine, and dichloramine.
The effect of bentonite (AlO 3 · 4SiO 2 · H 2 O) (added to the test as a model for turbidity) on disinfection with chlorine dioxide was studied by Scarpino et al. (1977). At turbidity levels below 2.29 nephelometric turbidity units (NTU) at 25°C, no protection was afforded to poliovirus 1 by the bentonite. However, at 3.22 and 14.1 NTU's, poliovirus inactivation was noticeably decreased. The bentonite at these levels appeared to offer protection to the virus.
The data that are available on the efficacy of chlorine dioxide on helminths or protozoan cysts do not appear to be suitable for comparison with the action of other disinfectants.
Comparative Biocidal Activity
The data presented in Tables II-6 and II-7 were collected for demand-free systems within the last 15 yr, when relatively reliable chemical methods and/or quantitative biocidal assay procedures were used. Table II-6 shows the concentration of chlorine dioxide necessary for 99% inactivation of E. coli strains. Even at 5°C and chlorine dioxide concentrations of 0.25 to 0.30 mg/liter, less than 2 min was required for 99% inactivation. Increases in chlorine dioxide concentration and/or temperature markedly reduced the contact time that was necessary. Table II-7 presents similar data for viruses. Increased chlorine dioxide concentration, temperature, and pH decreased the contact time that was required to produce 99% inactivation of the viruses. The amount of inactivation depended on the virus that was tested.
There is little information concerning the mode of action by which chlorine dioxide inactivates bacteria and viruses. Ingols and Ridenour (1948) suggested that the bactericidal effectiveness of chlorine dioxide is due to its adsorption on the cell wall with subsequent penetration into the cell where it reacts with enzymes containing sulfhydryl groups.
Benarde et al. (1967b) demonstrated that chlorine dioxide abruptly inhibited protein synthesis. The incorporation of 14 C-labeled amino acids into protein by whole cells stopped within a few seconds after the addition of chlorine dioxide. Subsequently, Olivieri (1968) reported a dose-response in the inhibition of protein synthesis in bacteria that had been treated with chlorine dioxide. The site of action was localized in the soluble portion (enzymes) of the cell extracts of treated cells without affecting the integrity of the ribosomes' function in protein synthesis.
Chlorine dioxide is an effective bactericide and virucide under the pH, temperature, and turbidity that are expected in the treatment of potable water. It should be noted that the U.S. Environmental Protection Agency (1978) has set an interim Maximum Contaminant Level of 1 mg/liter on chlorine dioxide (U.S. Environmental Protection Agency, 1978) because of the unresolved questions on its health effects (Symons et al. , 1977).
A simple, selective, and sensitive test for chlorine dioxide should be developed to monitor residual concentration.
Chlorine dioxide is currently used at several plants. A review of plant records and field studies on the stability and effectiveness of chlorine dioxide in the distribution system should be undertaken.
Studies should be directed toward evaluating the inactivation of protozoan cysts.
More information should be obtained on the mode of action by which chlorine dioxide inactivates bacteria, viruses, and cysts.
The use of iodine as a biocide has had a long history, primarily as an antiseptic for skin wounds and mucous surfaces of the body and, to a lesser degree, as a powerful sanitizing agent in hospitals and laboratories (Gershenfeld, 1977). The use of iodine as a disinfectant of drinking and swimming pool water has not been extensive, mainly because of the costs and problems that are involved in applying the dosage.
Aside from the emergency iodination of small volumes for field and emergency drinking water and limited experience with swimming pool disinfection (Black et al. , 1959), the only substantial experience with iodine disinfection of piped water system is that of Black et al. (1965, 1968). Two water systems serving three prisons in the state of Florida were disinfected satisfactorily with 1 mg/liter of iodine. A persistent residual was maintained throughout the distribution system despite a finished water pH of 8.0 to 9.5. No adverse effects on health were observed among those consuming the water.
Chemistry of Iodine in Water
Iodine is the only common halogen that is a solid at room temperature, and it possesses the highest atomic weight (126.91). Of the four common halogens, it is the least soluble in water, has the lowest standard oxidation potential for reduction to halide, and reacts least readily with organic compounds.

Diatomic iodine (I 2 ) reacts with water to form hypoiodous acid (HOI) and iodide ion (I - ). The effect of pH on this reaction is shown in Table II-8 .
Hydrolysis of Iodine at 25°C Showing the Percentage of Iodine Species at Different pH's.
The distribution of chemical species of iodine given in Table II-8 was taken from the calculations that were made by Chang (1958) from the equilibrium expression:

The value of K h , the hydrolysis constant, is given by Wyss and Strandskov (1945) as 3 × 10 -12 at 25°C. With iodine residuals at 0.5 mg/liter, which are expected in water systems, and a pH of 5, approximately 99% of the total iodine residual is present as iodine and only 1% as hypoiodous acid. At pH 7, the two forms are present in almost equal concentrations. At pH 8, only 12% is present as elemental iodine and 88% as hypoiodous acid, which can be converted to hypoiodite ion (OI - ).

The dissociation constant, K a , of hypoiodous acid (at 20°C) is 4.5 × 10 -13 . Consequently, the dissociation of hypoiodous acid, which occurs at high pH's, is not important for practical purposes. However, as confirmed by the field studies in Florida, hypoiodous acid can form iodate ion by autooxidation at pH values above 9.

The iodate ion possesses no disinfecting ability (Marks and Strandskov, 1950).
Iodine may be added to a municipal water supply by several procedures. One method is to employ nonhazardous solvents and solubilizing agents such as ethyl alcohol (C 2 H 5 OH) and potassium iodide (KI) to overcome the low concentration of aqueous iodine stock for solution feeders. Another method produces the required concentration of iodine by passing water through a bed of crystalline iodine (saturator). This has passing water through a bed of crystalline iodine (saturator). This has been used in many small, semipublic and private home water systems. Since the maximum concentration is limited by solubility to 200-300 mg/liter at the ambient temperatures that are expected for drinking water, some physical complications would accompany the introduction of this method into the large waterworks system in view of the large saturation beds required. The iodinated anion exchange resin bed and the vaporization technique are not sufficiently developed to be considered for use in public water supplies.
In certain circumstances, potassium iodide might be combined with an oxidation reaction to release iodine. The chemistry is simple, and the persistence of the iodine that is generated may be much better than chlorine. This method has been used in swimming pools and for dechlorination purposes where a chlorine residual may be exchanged for an iodine residual, or an iodine residual may be provided where ozone is the primary disinfectant.
Both amperometric titration and leuco crystal violet (LCV) colorimetric methods give acceptable results when used to measure free iodine in drinking water. Waters containing oxidized forms of manganese interfere with the LCV method. Also, when iodide ion exceeds 50 mg/liter and chloride ion exceeds 200 mg/liter, the amperometric method is preferred. Under unusual situations, where mixtures of chlorine, bromine, and ozone occur along with iodine, the problem of separation is difficult ( Standard Methods , 1976).
Table II-9 shows the relative resistance of bacteria, viruses, and cysts to inactivation by iodine.
Comparative Values from Confirmed Experiments on Disinfecting Water with Iodine at 23°C to 30°C, at pH 7.0.
Chang and Morris (1953), summarizing the development of a universal water disinfectant tablet for the military, concluded that iodine concentrations of 5 to 10 mg/liter were effective against bacterial pathogens. Iodine was less dependent than chlorine upon the pH, temperature, contact time, and secondary reactions with nitrogenous impurities in the water. As a cysticide, iodine was poor in water with a high pH. Consequently, the tablet that was formulated contained an acid buffer to lower the pH of the water. The tablet, called ''Globaline," released 8 mg/liter of iodine, which is extremely high compared to the amount that is possibly needed for public water supplies. The tablet is not widely accepted, since color, taste, and odor problems are fairly common (O'Connor and Kapoor, 1970).
Chambers et al. (1952) investigated the effect of iodine concentration, pH, exposure time, and temperature on 13 enteric bacteria in a clean system. Their results are not completely quantitative in that the reported iodine concentration is that required to inactivate all test organisms plated out after 1, 2, and 5 min of contact. This was equivalent to approximately 99.9% activation with the procedure that they followed. There was definitely an observed pH effect. At 2°C to 5°C, some bacterial species required 3 to 4 times as much iodine for similar inactivation at pH 9.0 as was required at pH 7.5. For 5 min of exposure, the required dosage for ≥99.9% bacterial inactivation in high pH water at 20°C-26°C was always less than 1 mg/liter. A summary of their work with E. coli is given in Table II-10 . This is the best available information on iodine as a bactericide.
TABLE II-10
Concentrations of Iodine and Contact Times Necessary for 99% Inactivation of Escherichia coli .
Studies on the efficacy of iodine on viruses have shown that viruses are more resistant to disinfection than are vegetative cells of bacteria. Krusé (1969) compared virus and bacterial inactivation. At an iodine dose of 10 mg/liter in 0.048 m M potassium iodide at room temperature and pH 7.0, E. coli was inactivated more rapidly than f2 virus but both were reduced by 4 logs in less than 1 min. However, when the potassium iodide concentration was raised to 0.5 M , bacterial inactivation was 4 logs in 1 min while virus inactivation was only 0.5 log in 1 hr ( Figure II-6 ).
Figure II-6
Survival of E. coli and f 2 bacteriophage reacted with 0.04 m M iodine containing (a) 0.048 m M potassium iodide (KI) at 37°C and (b) 0.5 m M potassium iodide at 0°C, and survival obtained with (c) 1 m M of a sulfhydryl reacting agent, p- (more...)
Berg et al. (1964) measured the dynamics of survival for poliovirus, coxsackievirus, and echovirus that were iodinated at pH 6.0 and at temperatures of 5°C, 15°C, and 25°C. The work of the Johns Hopkins group from 1962 to 1969 (Krusé, 1969) was primarily with the virus model f2, although some comparative poliovirus 1 work was done. Cramer et al. (1976) have shown that the mode of inactivation of these two viruses by iodine is similar. Data on the inactivation of virus by iodine are summarized in Table II-11 for both polio and f2. The results of inactivation studies by the various groups compare very favorably. At pH 6.0, iodine approaches the order of magnitude of virucidal activity of hypochlorous acid (HOCl). At the pH's likely to be maintained in the distribution system (pH 8.0), iodine is a vastly more effective virucide than combined chlorine and is not far removed from the activity range of free chlorine.
TABLE II-11
Concentrations of Iodine and Contact Times Necessary for 99% Inactivation of Polio and f 2 Viruses with Flash Mixing.
Many studies on cyst inactivation have been reported, but there are discrepancies in their results due mainly to differences in the test systems that were used. The dose, pH, and temperature that were used in many of the studies are in doubt as are the different sources, cleaning methods for cysts, number of cysts used per test, and determination of viability. Stringer (1970) compared the resistance to iodine disinfection of Entamoeba histolytica cysts obtained from in-vitro culturing with those harvested from a human carrier and mixed amoebic cysts from monkeys. In water at room temperature at pH 6.5, 3 times the iodine dose was required in 10 min of exposure for 99% inactivation with wild (naturally formed) cysts compared to the cultured cysts. Large numbers of amoebic cysts from simian hosts were more readily available than cysts from human stools. They served as a reliable model for human E. histolytica.
The most extensive and reliable data on the cysticidal properties of iodine are found in the work of Chang and Stringer and their co-workers (Change and Baxter, 1955; Chang et al. , 1955; Stringer, 1970; Stringer and Krusé, 1971; Stringer et al. , 1975). The only quantitative comparative study of the cysticidal properties of chlorine, bromine, and iodine believed to be published is that of Stringer, who reported a 99.9% inactivation. The earlier studies of others involved "total kills" and are quite dependent on the cyst density that was used.
All investigators more or less agree that iodine is an excellent cysticide in low pH waters (< pH 4.0), suggesting that molecular iodine (I 2 ), rather than hypoiodous acid, is the active agent. Chang (1958) observed that when the titratable iodine dosages exceed 20 mg/liter in the presence of iodide ion, the triiodide ion (I 3 - ) has a cysticidal efficiency that is equal to 1/11, 1/8, and 1/7 that of I 2 at 6°C, 25°C, and 35°C. He further calculated relative cysticidal efficiency of hypoiodous acid to be one-third that of 1 2 at 6°C and one-half that of I 2 at 20°C.
There may be the problem of ineffective iodine residual in the distribution system where cross-connection introduction of cyst contamination is a possibility. This is due to the practice (for corrosion control) of maintaining water in the distribution system at approximately pH 8.0. At the low halogen residual levels that are usually maintained, the most cysticidal form, I 2 , would be less prevalent than hypoiodous acid. Water at 25°C and pH 8.0 with a total iodine residual of 0.5, 1.0, and 2.0 mg/liter would contain only 0.06, 0.2, and 0.62 mg/liter of molecular iodine. Table II-12 shows the time in minutes required for 90% inactivation of simian cysts with residuals of bromine, chlorine, and iodine in water at pH 6.0, 7.0, and 8.0 and a temperature of 30°C. At pH 8.0, iodine is inferior to free bromine and chlorine. However, in the presence of excess ammonia, with which the halogens could react, bromine appears to be more effective at pH 8.0 than do similar dosages of iodine or chlorine. Residual iodine in water at pH 8.0 has the ability to persist.
TABLE II-12
Contact Times Necessary for Low Residual Bromine, Chlorine, and Iodine in Water at 30°C to Effect 99% Inactivation of Cysts from Simian Stools.
The kinetics of iodine as a cysticide in water at 20°C to 30°C have been assembled and compared. The cultured E. histolytica cyst data of Chang and co-workers (Chang and Baxter, 1955; Chang and Morris, 1953; Chang et al. , 1955) and the results of Stringer et al. (1975) present a consistent picture. For cultured cysts in water at pH 5 the c · t values for 99% inactivation calculated by Chang were 200 at 3°C, 130 at 10°C, and 65 at 23°C (Chang and Morris, 1953). Stringer et al. (1975) extended the cultured cyst data at 30°C for a range of pH given below:
These c · t values from Stringer et al. (1975) were approximately one-half the values they obtained with E. histolytica cysts from human stools and for mixed cysts from simian stools.
The failure of a strong commercial iodine disinfectant to inactivate poliovirus (Wallis et al. , 1963) led to interest in the responsible mechanism, especially the role of pH and iodide ion. Berg et al. (1964) claimed that iodine inactivation of coxsackievirus resulted from bio-molecular reaction with a single iodine molecule and that clumping of virions played a role in resistance to disinfection. Fraenkel-Conrat (1955) pointed out inconsistencies in the literature regarding the virucidal properties of iodine. Hsu (1964) and Hsu et al. (1965) clarified the mechanism of iodine on cells and virus. Hsu extracted fully active transforming DNA from iodine-inactivated Haemophilus parainfluenzae cells and just as much infectious RNA from f2 bacterial virus that had been inactivated (5 logs) by iodine as from noniodinated controls. Iodine inactivation, unlike the action of chlorine, appears to be attributable to a reaction with vital amino acids in proteins. Further experiments were conducted to determine whether sulfhydryl, tryptophanyl, histidyl, or tyrosyl groups were involved. With bacterial cells, there was a striking similarity between the kinetics of inactivation with iodine and the application of p-chloromercuribenzoic acid, a sulfhydryl reacting agent. While the active chemical species of iodine is not known, Hughes (1957), Allen and Keefer (1955), Bell and Gelles (1951), and Hsu (1964) hypothesized that the hydrated cationic iodine species (H 2 OI + ) attacks the base, first against the sulfhydroxyl groups, and is not materially affected by the presence of iodide ion as was tyrosine. When sulfhydroxyl groups are the site of inactivation, a low pH should favor the reaction. With the viruses, the sulfhydroxyl group is not involved. Evidence of tyrosine's involvement came from parallel experiments showing similar patterns of inactivation curves between iodination of f2 virus and L tyrosine. Li (1942, 1944) had shown that the presence of iodide ion and low pH iodine (I 2 ) inhibited the iodination of tyrosine. Both poliovirus and f2 virus inactivation with iodine was inhibited by the iodide ion (Cramer et al. , 1976). At pH 4.0, both polio and f2 viruses survived iodination whereas at pH 10 inactivation was complete. Although iodine decomposes rapidly to iodate (IO 3 - ) and iodide at pH 10, flash mixing of iodine (yielding hypoiodous acid) overcomes this difficulty effectively, and the virus inactivation is complete in less than 1 min (Longley, 1964). Therefore, there is evidence that iodine action, with little or no iodide present, is effected by the modification of protein without destroying DNA or RNA (Brammer, 1963; Hsu, 1964). The mode of action of iodine in cyst penetration and inactivation has not been studied.
Conclusions
Iodine has many features that are comparable to free chlorine and bromine as a water disinfectant, but iodamines are not formed. Free iodine is an effective bactericide over a relatively wide range of pH. Field studies on small public water systems have shown that low levels of 0.5 to 1 mg/liter of free iodine can be maintained in distribution systems and that the magnitude of residual is sufficient to produce safe drinking water with no adverse effects on human health. Like other halogens, the effectiveness of iodine against bacteria and cysts is significantly reduced by high pH, but unlike bromine and chlorine it is much more effective against viruses because of the enhanced iodination of tyrosine. Currently, its use is restricted primarily to emergency disinfection of field water supplies because of its high cost and because it is difficult to apply to large systems. The possible adverse health effects of increased iodide intake for susceptible individuals in the population must also be considered.
Studies should be conducted to determine the consequences for human health of the long-term consumption of iodine in drinking water with special regard for more susceptible subgroups of the population.
Chemistry of Bromine in Water
Bromine was first applied to water as a disinfectant in the form of liquid bromine (Br 2 ) (Henderson, 1935), but it can also be added as bromine chloride gas (BrCl) (Mills, 1975) or from a solid brominated ion exchange resin (Mills, 1969). Oxidation of bromide (Br - ) to bromine can also be accomplished either chemically or electrochemically. Oxidation with aqueous chlorine gives either bromine or hypobromous acid (HOBr), depending on the ratio of chlorine to bromide. Both bromine (Liebhafsky, 1934) and bromine chloride (Kanyaev and Shilov, 1940) hydrolyze to hypobromous acid:

Molecular bromine exists in water at moderately acid pH and high bromide concentrations since the equilibrium constant of Reaction 21 is 5.8 × 10 -9 at 25°C. Like chlorine, bromine chloride has a much higher hydrolysis constant than this, so it does not exist as the molecular form in appreciable concentrations under conditions of water treatment. The ratio of molecular bromine to hypobromous acid depends on both pH and bromide concentration. From the equilibrium expression for Reaction 21:

Thus, for a solution containing 10 mg/liter bromide and a pH of 6.3, 1% of the bromine is Br 2 , while at lower bromide concentrations or higher pH aqueous bromine occurs almost entirely as hypobromous acid, which is a very weak acid with a dissociation constant (for Reaction 24) of 2 × 10 -9 at 25°C (Farkas and Lewin, 1950).

The hypobromite ion (OBr - ) becomes the major form of bromine above pH 8.7 at 25°C. Lower temperature decreases both of the above equilibrium values, thereby increasing the pH range where hypobromous acid is the major chemical form of bromine in water.
Bromine and bromine chloride also react with basic nitrogen compounds to form combined bromine or bromamines (Galal-Gorchev and Morris, 1965; Johannesson, 1958; Johnson and Overby, 1971):

The observed breakpoint for ammonia (NH 3 ) solutions that have been treated with bromine is similar to that seen with chlorine. For bromine, this point corresponds to 17 mg/liter bromine for 1 mg/liter of ammonia nitrogen (NH 3 -N). At this point, a minimum of bromamine stability occurs (Inman et al., 1976) as ammonia nitrogen is oxidized to nitrogen gas:

At bromine-to-ammonia ratios higher than this and in the acid pH range, nitrogen tribromide (NBr 3 ) is stable. It is the most abundant bromamine in such aqueous solutions. At lower bromine concentrations, dibromamine (NHBr 2 ) can be present, but it is quite unstable. Only at alkaline pH values and very high ammonia concentrations such as those found in wastewater are significant quantities of monobromamine (NH 2 Br) formed. Organic bromamines are also formed, but there is little information on their forms or stability.
Bromine is produced by oxidation of bromide-rich brines (that contain between 0.05% and 0.6% bromide) with chlorine. Bromine is then stripped with steam or air and is collected as liquid Br 2 .
Bromine chloride is produced by mixing equal molar quantities of pure bromine and chlorine (Mills, 1975). It condenses to liquid bromine chloride below 5°C at I atm pressure or above 30 psig at 25°C. In the liquid phase, more than 80% of the liquid is bromine chloride, and the remainder is Br 2 and Cl 2 . In the gas phase, 40% of the bromine chloride dissociates to Br 2 and Cl 2 at 25°C.
Bromine has been applied to water as liquid Br 2 . The difficulties that are encountered when handling the liquid and its corrosive nature, especially when wet, have encouraged the use of bromine chloride. Liquid bromine chloride is removed from cylinders under moderate pressure. It is then vaporized, and the gas is metered in equipment that is similar to that used for chlorine. Like chlorine, bromine chloride is shipped as the dry liquid in steel containers. Gas feeders must be made of Teflon, Kynar, or Viton plastics because bromine chloride is more reactive than chlorine with polyvinylchloride plastics.
Bromine concentrations can be measured iodometrically by procedures that are identical to those used to measure total chlorine residuals ( Standard Methods , 1976). None of these procedures is capable of distinguishing free bromine (Br 2 , HOBr, OBr - ) from combined bromine (bromamines) or other oxidants that are capable of reacting with iodide under the slightly acid conditions used in these procedures. However, bromate does not interfere except at low pH (Kolthoff and Belcher, 1957). UV spectroscopy can be used to measure the bromamines selectively in the presence of one another and without interference from free bromine because none of the free bromine forms except Br 3 - has strong UV absorptivities (Johnson and Overby, 1971).
Since residual bromine was rarely measured in the studies cited here, c · t (concentration, mg/liter, times contact time, min) products have generally been calculated using the dosage of bromine added to the system.
The efficacy of bromine inactivation of bacteria has been summarized by Farkas-Hinsley (1966). Vegetative cells are readily inactivated by bromine, but reports often leave the type of bromine compound and its residual concentration as uncertain. Consequently, it is difficult to make quantitative comparisons with other disinfectants. Tanner and Pitner (1939) reported that the concentrations of hypobromous acid that are required to give ''complete kill" in 30 min are 0.15 mg/liter for Escherichia coli and 0.6 mg/liter for Salmonella typhi. Spores of Bacillus subtilis required more than 150 mg/liter of bromine. Krusé et al. (1970) found that 4 mg of bromine per liter as hypobromous acid gave 5 logs of disinfection of E. coli in 10 min at 0°C. At pH 4.5, where significant Br 2 was present, 2 mg/liter bromine at 0°C gave 4 logs of E. coli disinfection in 3 min. However, Krusé also reported that 0.1 M bromide decreased E. coli disinfection to 2 logs of inactivation from 4.5 logs at 0.001 M bromide, using 4 mg/liter bromine at pH 7.5 and 0°C in each case. This is in conflict with observations made at low pH, since high bromide should also produce bromine. The data at pH 4.5 showed 4 logs inactivation with a c · t of 6 compared to 5 logs inactivation from a c · t of 40 for hypobromous acid. Even these data, the best available, are based on the concentrations of bromine added to the solution. It is difficult to compare the various studies on disinfection by bromine, since the chemical species of bromine present in most instances have not been measured or reported.
The effect of the formation and decomposition of the bromamines on the efficacy of bacterial disinfection was first discussed by Johannesson (1958). He reported that 0.28 mg/liter of monobromamine expressed as bromine resulted in 99% inactivation in less than 1 min, while the same concentration of N -bromodimethylamine [N(CH 3 ) 2 Br] required 12 min for 99% inactivation of E. coli. The measurements of the halogen remaining after the experiments showed very little loss.
The efficacy of bromine inactivation of spores of Bacillus metiens and B. subtilis has been studied by Marks and Strandskov (1950) and Wyss and Stockton (1947). Both pairs of investigators found that the activity was markedly pH-dependent at low pH values where molecular bromine predominates. The activity increased rapidly from pH 4 to pH 3, the lowest that was tested. The c · t required for 99% inactivation was 20-25 at 25°C and pH 3.0. At pH 6 to 8, the c · t was 225 to 375 (Wyss and Stockton, 1947). At pH 9, where the hypobromite ion starts to predominate, the c · t increased to more than 500.
Marks and Strandskov (1950) also determined that at pH 7 and 25°C monobromamine was one-half as effective as hypobromous acid against B. metiens spores. N -bromosuccinimide was 1/17, and N -bromopiperidine was 1/800 as effective. Wyss and Stockton (1947) showed the effect of changing chemical form and concentration by increasing the concentration of ammonia that was added to a 20 mg/liter bromine solution at pH 7 containing B. subtilis spores. Table II-13 shows the measured residual and the time required for 99% inactivation. Before the ammonia is added to the solution, hypobromous acid is the major chemical form. When the ammonia nitrogen reaches 1 mg/liter, nitrogen tribromide predominates. At 2 mg/liter ammonia nitrogen, the breakpoint occurs with rapid loss of combined bromine residual. At 10 and 30 mg/liter ammonia nitrogen, dibromamine is present and is less stable and effective than hypobromous acid. At 1,000 mg/liter ammonia nitrogen, monobromamine has a c · t value for 99% inactivation that is as effective as nitrogen tribromide and hypobromous acid.
TABLE II-13
The Effect of Ammonia on Bromine Concentrations Required for 99% Inactivation of Bacillus subtilis Spores at 20 mg/liter, pH 7, 25°C.
The effect of temperature on the efficacy of hypobromous acid inactivation of B. subtilis spores is shown in Table II-14 (Wyss and Stockton, 1947). The activity increases approximately 2.3 times for each 10°C rise in temperature. After measuring washed vegetative cells of B. subtilis, Wyss and Stockton also found them to be 500 times less resistant. They reported no temperature data for their vegetative cell studies.
TABLE II-14
The Effect of Temperature on Bromine Concentrations Required for 99% Inactivation of Bacillus subtilis Spores with 25 mg/liter Bromine at pH 7.0.
Efficacy against Virus
Krusé et al. (1970) reported that 4 mg/liter of bromine as hypobromous acid at pH 7 and 0°C gave 3.7 logs of inactivation of f2 E. coli phage virus in 10 min. At higher bromide concentrations and lower pH, where Br 2 becomes the principal form of bromine, the rates of inactivation increased. A c · t of 40 was required for 3.7 logs inactivation at pH 7.0 and 0°C compared to a c · t of 1 for 4.5 logs inactivation at 0°C and pH 4.8. At pH 7.5 and with a concentration of 0.1 M bromide at 0°C, a c · t of 12 yielded 5.5 logs inactivation. In these studies the residual concentrations of bromine were probably not significantly different from those in solutions that did not contain added salts. In the presence of added bromide, amines were difficult to quantify. After 2 hr, Krusé and his colleagues found no viable virus in a solution of 4 mg/liter bromine, starting from an initial titer of 1.7 × 109 plaque-forming units (PFU)/ml at 0°C and pH 7.5, when excess ammonia was present. However, the addition of bromine to phage solutions that contained excess methylamine (CH 3 NH 2 ) and glycine (H 2 NCH 2 COOH) essentially stopped disinfection under these conditions. This may be due either to the formation of the N -bromo compounds or to the reduction of bromine.
Taylor and Johnson (1974) used Φx174 E. coli phage and measured concentrations of the disinfecting species in constant residual solutions. They reported that 0.32 mg/liter hypobromous acid required only 1.1 min or a c · t of 0.35 to inactivate 99% of this phage at 0°C. Compared to hypobromous acid, molecular bromine was approximately 3 times as fast, requiring Br 2 at c · t of 0.1. Nitrogen tribromide was less potent, requiring a c · t of 1.0 for 99% inactivation of this phage at 0°C.
The effect of temperature on Φx174 inactivation was measured for 0.16 mg/liter of hypobromous acid expressed as bromine at pH 7. The Arrhenius plot of In k against 1 /T (where T is temperature) from 273°K to 303°K gave a slope equivalent to 37 kcal/mol or an increase in rate of inactivation of 1.9 times for a rise in temperature from 15°C to 25°C.
Sharp's group (Floyd et al. , 1976, 1978; Sharp et al. , 1975, 1976) has made careful studies with measured, controlled residual concentrations. They demonstrated that even under these controlled chemical conditions the degree of aggregation or clumping had a marked effect on the apparent, observed inactivation rates with reovirus and type 1 poliovirus (Sharp et al. , 1975). Reoviruses, as single particles, required only 1 s for 3 logs of inactivation at pH 7 and 2°C with hypobromous acid at 0.46 mg/liter bromine. Aggregated samples gave much slower rates, especially at high levels of inactivation. At 3 logs of inactivation, the time required doubled for the same concentration conditions (Sharp et al. , 1976). Poliovirus 1 was also rapidly inactivated by hypobromous acid when single virus particles were studied (Floyd et al. , 1976). At 2°C and pH 7, only 3.5 mg/liter bromine or a c · t of 0.2 was required for 2 logs of inactivation. However, the rate of inactivation did not increase linearly with concentration at this temperature. Higher concentrations were much less efficient than longer exposures. At 1 mg/liter hypobromous acid, 7 s were required for 1 log of inactivation or a c · t of 0.23 for 2 logs of inactivation at 2°C and pH 7 with hypobromous acid. The inactivation rate increased only slightly with temperature at this concentration. At 10°C a c · t of 0.21 for 2 logs of inactivation and at 20°C a c · t of 0.06 was required for 99% inactivation with hypobromous acid.
The inactivation of single poliovirus particles in buffered, distilled water and constant residual concentrations for the other major bromine chemical forms have also been studied by Floyd et al. (1978). Table II-15 gives the calculated c · t required to yield 99% inactivation for these different forms near 1 mg/liter expressed as bromine. The values in Table II-15 depend on concentration except for Br 2 , for which time and concentration were inversely related as normally assumed from the Watson-Chick relationship (Morris, 1975). Floyd et al. (1978) also demonstrated that dibromamine, nitrogen tribromide, and hypobromous acid were less efficient at higher concentrations while the hypobromite ion became more effective as the concentration increased. The rate for the hypobromite ion is rapid compared to the other bromine compounds. This is interesting in light of the fact the hypochlorite ion (OCl - ) is generally considered to be a much poorer disinfectant than hypochlorous acid (HOCl).
TABLE II-15
Exposure (c·t) to Various Bromine Compounds Required for 99% Inactivation of Poliovirus 1, Mahoney.
There appears to be no information regarding the effectiveness of bromine as a disinfectant against eggs or larvae of helminth parasites in water.
With protozoa that are important to public health, attention appears to have been devoted primarily to the efficacy of bromine in destroying cysts of Entamoeba histolytica. Most relevant information has been provided by Stringer et al. (1975) as a result of their studies on the comparative cysticidal efficacies of various halogens (shown in Table II-12 in the section on iodine). They used bromine stock solutions that were prepared by bubbling nitrogen through bottles of elemental bromine. Concentrations were determined by a colorimetric bromocresol purple test. Cyst survival was evaluated by the excystation method that these investigators had developed.
In dose-response experiments in distilled water for exposures of 10 min and at pH levels from pH 4 to pH 10, bromine was found by Stringer et al. (1975) to be the most effective and fastest acting of the halogens tested over the widest pH range. At pH 4, 99.9% cyst mortality was obtained with 1.5 mg/liter of free bromine residual; whereas 2 mg/liter of chlorine and 5 mg/liter of iodine were required to attain the same mortality. Furthermore, increases in pH seemed to have less effect on the cysticidal efficacy of bromine as compared with the other halogens. At pH 10, 99.9% mortality was obtained with residuals of 4 mg/liter of bromine, 12 mg/liter of chlorine, and 20 mg/liter of iodine.
In studies more nearly simulating usual water treatment procedures, "flash mixing" of halogens at a dosage of 2 mg/liter with the cyst suspensions was used. In buffered distilled water, bromine again proved to be the most effective: 99.9% inactivation was obtained at pH 6 and pH 8 after 15 min of contact. Longer exposures at the lower pH were required for the other halogens in order to provide even less inactivation than this.
An interesting aspect of the study of Stringer et al. (1975) was that ammonia bromamines were nearly as cysticidal as free bromine except in waters of high pH ( Table II-12 ).
Thus, under conditions likely to be found during the treatment of natural water supplies, free and combined bromine appear to be a practical, effective cystide, at least as far as cysts of E. histolytica are concerned.
Mechanisms of Action
The pattern of bromine disinfection appears to be similar to that of chlorine. After comparing the activity of chlorine, bromine, and iodine against spores, Marks and Strandskov (1950) noted that Br 2 was 9 times more effective than hypobromous acid and that the hypobromite ion and tribromide ion (Br3 - ) had very low activity. They noted that a high degree of polarity contributed to the inactivity of the ionic forms and the reduced activity of the hypohalous acid compared to the free molecular halogen. They also found that "the killing rates of bacterial spores for the hypohalous acids and probably for the molecular halogens decrease in the [following] order: chlorine, bromine, iodine."
Many workers attribute this decrease to the greater oxidation potential of the halogens with lower molecular weight. However, the polarity and perhaps the sizes of the halogens may be important in getting the disinfectants to the vital site.
Olivieri et al. (1975) demonstrated that bromine was effective in inactivating both naked viral RNA and intact virus. The primary site of inactivation of f2 phage more likely involves the reaction of bromine with the protein coat of the virus, because inactivation of RNA that was prepared from bromine-treated virus lagged significantly behind the inactivation of intact virus. The mechanism of inactivation with bromine, as with the other halogens, involves moving the disinfectant to the vital site, mass transport, and the reactivity of the bromine with that site, oxidation. The effectiveness of bromamines as disinfectants may be explained by their relatively low polarity and high reactivity. The effectiveness of the hypobromite ion as a virucide may be due to the fact the high pH's of the solutions make the virus more sensitive to the ion. Or, it could be attributed to Olivieri's observation that bromine acts primarily on the protein coat of viruses.
Laboratory tests show that bromine is an effective bactericide and virucide. It is more effective than chlorine in the presence of ammonia.
As a cysticide, it is highly active. Bromine is active over a relatively wide range of pH, and it retains some of its effectiveness as hypobromous acid to above pH 9.
The major disadvantage of bromine as a disinfectant is its reactivity with ammonia or other amines that may seriously limit its effectiveness under conditions that are encountered in the treatment of drinking water. Data on the effectiveness of bromine against bacteria are complicated by this reactivity and the lack of characterization of the residual species in disinfection studies.
Further research is required to quantitate the effectiveness of the various species of bromine and the bromamines, both organic and inorganic, against bacteria, viruses, and cysts, particularly with bacteria.
The reactivity of bromine and the dosages that are required to maintain effective residual in natural water systems should be investigated.
The effectiveness of various technologies for application of bromine or bromine chloride to intended drinking water should be evaluated.
Ferrates are salts of ferric acid (H 2 FeO 4 ) in which iron is hexavalent. Fremy (1841) first synthesized potassium ferrate (K 2 FeO 4 ) in the mid-nineteenth century. Since then, a wide variety of metallic salts have been prepared. However, only a few of the preparations yield ferrates of sufficient purity and stability for use in the treatment of water. Ferrates are strong oxidizing agents that have a redox potential of-2.2 V or 0.7 V in acid and base, respectively (Wood, 1958).
The Chemistry of Ferrate in Water
Aqueous solutions of potassium ferrate are unstable and decompose to yield oxygen (O2), hydroxide (OH-), and insoluble hydrous iron oxide [FeO(OH)]:

The hydrous iron oxide is a coagulant that is commonly used in water treatment. Initial ferrate concentration, pH, temperature, and the surface character of the resulting hydrous iron oxide affect the rate of decomposition (Schreyer and Ockerman, 1951; Wagner et al. , 1952; Wood, 1958). Ferrate solutions are most stable in strong base (> 3 M or at pH 10 to 11). Schreyer and Ockerman (1951) reported that dilute aqueous solutions of ferrate are more stable than concentrated solutions. The presence of other inorganic ions also affects ferrate stability in aqueous solutions.
Ferrate reacts rapidly with reducing agents in solutions (Murmann, 1974). It will also oxidize ammonia (NH 3 ). The rates for this reaction increase with pH (optimum pH range 9.5-11.2), molar ratio of ferrate to ammonia, and temperature (Strong, 1973). The oxidation of ammonia by ferrate below pH 9 is markedly slower than that observed for chlorine. Some information is available on the reaction of ferrates with various organic compounds (Audette et al. , 1971; Becarud, 1966; Zhdanov and Pustovarova, 1967).
Preparation
Concentrated solutions of sodium ferrate (Na 2 FeO 4 ) may be prepared electrochemically from the more common iron forms. Scrap iron can be converted to ferrate iron with a 40% efficiency (Murmann, 1974). Potassium ferrate may be prepared by wet oxidation of Fe(III) with potassium permanganate (KMnO 4 ). Subsequent recrystallization yields a crystalline solid with greater than 90% purity (Schreyer et al. , 1950). Ferrate salts are not commercially available. Consequently, sufficient quantities for pilot or full-scale testing would have to be especially prepared.
Analytical Methods and Residuals
Aqueous ferrate solutions have a characteristic violet color that is similar to permanganate and a wavelength maximum at 505 nm in the visible portion of the electromagnetic spectrum. The molar extinction coefficient at 505 nm in 10 -4 M sodium hydroxide (NaOH) was 1,070 ± 30 1 M -7 cm -1 (Wood, 1958). Since ferrates are unstable, residual cannot be maintained.
Gilbert et al. (1976) reported the inactivation of a pure culture of Escherichia coli at pH 8.0. Increased ferrate concentrations from 1.2 to 6.0 mg/liter yielded increased rates of inactivation of E. coli. They observed 99% inactivation at 6.0 mg/liter, pH 8.0, and 27°C in approximately 8.5 min. The rates of inactivation of E. coli with ferrate appear to be of the same order of magnitude as monochloramine (NH 2 Cl). Waite (1978a,b) extended Gilbert's disinfection studies to include enteric pathogens and Gram-positive bacteria and evaluated the effects of pH and temperature. The rate of E. coli inactivation by ferrate increases as pH decreases from pH 8.0 to pH 6.0. At the lower pH value, the rate of ferrate decomposition is increased, and little inactivation was observed after 5 min. Several inconsistencies at low ferrate concentrations (0.12 mg/liter) were observed, but low-level inactivations (< 50% inactivation) are difficult to interpret. At lower temperatures, the biocidal activities of ferrate appear to increase as temperature increases. At higher temperatures, the ferrate decomposition becomes an important factor. Salmonella typhimurium and Shigella flexneri were inactivated in a manner similar to that of E. coli. However, considerably higher dosages of ferrate (> 12 mg/liter) were necessary for inactivation of Streptococcus faecalis. Ferrate concentrations of 12 mg/liter and 60 mg/liter required 5 min and 15 min, respectively, for 99% inactivation at pH 8.0. Other Gram-positive bacteria tested ( Bacillus cereus, Streptococcus bovis, and Staphylococcus aureus ) were noticeably more resistant to ferrates than were the enteric bacteria. Higher doses of ferrate (15-20 mg/liter) were also necessary to inactivate 99% to 99.9% of the bacteria in the presence of organic material in wastewater.
Waite (1978a,b) reported that the inactivation of the RNA bacterial f2 virus with ferrate appeared to be more dependent on pH than was bacterial inactivation. Considerably more viral inactivation was observed at pH 6.0 than at pH 8.0. At 1.2 mg/liter ferrate, just under 4 min were required for 99% inactivation, while for similar conditions 13 min were necessary at pH 8.0.
The disinfection efficacy of ferrate is summarized in Table II-16 . The ferrate dose is given in the table, since ferrate residuals were not reported. Ferrate decomposes rapidly in aqueous solution, and the dose represents an overestimate of ferrate concentration. Nevertheless, the c · t products (concentration, mg/liter, times contact time, min) indicate the difference in efficacy relative to the test microorganism and provide a crude idea of the c · t product for ferrate.
TABLE II-16
Concentrations of Ferrate (FeO 4 2 - ) and Contact Times Necessary for 99% Inactivation of Escherichia coli, Streptococcus faecalis, and f 2 Virus.
No studies have elucidated the mechanism of inactivation caused by ferrate.
While not as biocidal as the free halogens, chlorine dioxide, or ozone, ferrate appears to be similar to or slightly better than the chloramines as a bactericide and more active as a virucide than the chloramines. The combined application of ferrate as a disinfectant and a coagulant makes it an attractive alternate biocide. Far more information on the feasibility of large-scale preparation of ferrate and its biocidal activity (particularly under water treatment conditions) are necessary before ferrate can be given consideration as a water disinfectant for public systems.
- High pH Conditions
High pH values are obtained when drinking water is softened by the commonly used precipitation method, which removes calcium (Ca 2+ ) and magnesium (Mg 2+ ) ions that cause water hardness. In such cases, calcium hydroxide [Ca(OH) 2 ] is the usual source of hydroxide that is used to raise the pH. In this process, pH values as high as 10.5 are reached and maintained up to 6 hr. Low pH conditions are not used in water treatment processes. Therefore, while low pH's are lethal to most microorganisms, they are of less interest in this report than high pH conditions, which might have potential for disinfection through modification of current practices.
Attainment of Elevated pH
High pH values in water are obtained by using either calcium hydroxide or sodium hydroxide (NaOH). Sodium hydroxide is a by-product of the production of chlorine by the electrolysis method. Calcium hydroxide is prepared by reacting calcium oxide (CaO) with water. The calcium oxide is produced by heating calcium carbonate (CaCO 3 ) to drive off the carbon dioxide (CO 2 ). The calcium oxide is normally prepared off the site of the water treatment plant. However, it is often slaked on site at all but the smallest plants, which purchase calcium hydroxide and use it directly. A few, very large water treatment plants produce their own calcium hydroxide by calcining their water-softening sludge.
Hydroxide (OH - ) is added to water as a water slurry containing calcium hydroxide or as a water solution of sodium hydroxide. In the usual water-softening technique, the water is flocculated for 10-30 min to promote the precipitation reactions. The precipitates are then removed by sedimentation with retention times from 1 to 6 hr.
Disinfection with high pH is readily accomplished, but the required pH values are so high that the pH must be reduced before the water is consumed. In a full-scale water treatment plant, this is accomplished in the recarbonation stage, which is a normal component of the water-softening process. However, for small institutions or private residences the pH reduction requirement would mitigate against the use of this disinfection technique.
Analytical Methods and Process Control
The pH can be reliably measured with the glass electrode at pH values of approximately 7 and at room temperatures. Special care must be taken to obtain accurate values at other temperatures and at the elevated pH values that are necessary for disinfection with this process.
The hydroxide that is used to obtain the necessary pH values enters into few side reactions in natural water after the initial formation of metallic hydroxides and the reactions with carbon dioxide. The amounts of hydroxide for these reactions can be readily computed after the water has been chemically analyzed. There is a slow additional loss of hydroxide from the water by reaction with carbon dioxide entering from the atmosphere. However, this is not a serious problem.
The microbial inactivation under consideration in this chapter is that caused solely by high pH. When the pH of water is raised, the opportunity exists for precipitation of many compounds associated with carbonates, oxides, and hydroxides, among many others. Even in distilled water, calcium carbonate may precipitate if calcium hydroxide is used to raise the pH and if sufficient carbon dioxide enters the water during the experiment. These precipitates provide opportunities for adsorption and coagulation of microorganisms and will cause an increased removal over that obtained from the pH effect alone without the precipitate. The research cited below, unless otherwise noted, was conducted with procedures that obviated this problem or that made its effect insignificant.
Wattie and Chambers (1943) determined the times required to obtain 100% inactivation of several bacterial species at initial concentrations of approximately 1,500 organisms/ml in 20°C to 25°C dechlorinated tapwater, using calcium hydroxide for pH adjustment (see Table II-17 ). The inactivation was significantly less at each pH for each organism between 0°C to 1 C. At a pH of 11.5, the time for 100% inactivation of Escherichia coli was increased from 210 min between 20°C to 25°C to 355 min between 0°C to 1°C. The time for 100% inactivation of Salmonella typhi was increased from 75 min to 270 min for the same temperature change. No mention was made of difficulties from the formation of precipitates.
TABLE II-17
Contact Times Necessary for High pH Conditions to Effect 100% Inactivation of Initial Concentrations of 1,500 Organisms/ml.
Riehl et al. (1952) reported E. coli inactivations of 95% in 8 hr at pH 10.5 and 5°C, 100% in 2 hr at pH 10.5 and 15°C, and 100% in approximately 30 min at pH 10.5 and 25°C in distilled water. Calcium hydroxide was used for pH adjustment. Inactivations of 50% and 55% in 10 hr were noted for Salmonella montevideo and S. typhi, respectively, at 2°C and pH 10.6 in distilled water. Initial concentrations of organisms were approximately 1,000/ml. They also observed that higher temperatures gave more inactivation at the same pH and contact time and that the composition of the water did not markedly influence the bacterial survival.
Berg and Berman (1967) determined the inactivation of E. coli at 25°C in the dilution water medium that is described in the 11th edition of Standard Methods (1960) (see Table II-18 ). Sodium hydroxide was used to adjust the pH.
TABLE II-18
Inactivation of Escherichia coli by High pH Conditions at 25°C in a Dilution Medium.
Wentworth et al. (1968) reported that little or no poliovius 1 was inactivated in distilled water at pH 11.2 at room temperature in 90 min when calcium hydroxide or sodium hydroxide was used to increase the pH. For poliovirus 1 in distilled water without added salts, Sproul et al. (1970) showed that no inactivation occurred in 90 min at pH values of 10.5 or less when calcium hydroxide and sodium hydroxide furnished the hydroxide. Their work was done at 21°C to 22°C. Sproul (1975) obtained poliovirus 1 inactivations in 30 min of 7% at pH 11.5, 59% at pH 11.9 in 30 min, 94% at pH 12.1 in 20 min, and 99.83% at pH 12.5 in 5 min. He used distilled water with 100 mg/liter of sodium chloride (NaCl) at 22°C to 23°C.
Berg and Berman (1967) showed the inactivation of echovirus 7 AGKP8A1 at 25°C in a dilution water medium ( Standard Methods , 1960). They used sodium hydroxide to adjust pH (see Table II-19 ). From his work with the f2 bacteriophage, Donovan (1972) suggested that a calcium-virus complex was formed and that a large part of the observed decrease in titer at pH 11.5 was caused by an aggregation of the virus. Electron micrographs and chemical evidence supported the observation.
TABLE II-19
Inactivation of Echovirus 7 by High pH Conditions at 25°C in a Dilution Medium.
The susceptibility of protozoa or helminths to inactivation by high pH conditions does not appear to have been studied.
The inactivation mechanism of high pH on bacteria has not been examined. There is ample evidence to show that the poliovirus is inactivated by the disruption of the capsid and a loss of the RNA to the water (Boeye and Van Elsen, 1967; Maizel et al. , 1967; Van Elsen and Boeye, 1966). Boeye and Van Elsen (1967) suggested that, at pH 10 and at elevated temperatures (≥30°C), the RNA was released from the capsid in a degraded form or that it was quickly and extensively broken down after release. The applicability of this mechanism to enteroviruses other than poliovirus is unknown.
The utilization of high pH conditions for disinfection of water is feasible, but higher pH values than are normally used in water treatment and long contact times are required. At pH values of approximately 10.5, bacteria are inactivated in up to 600 min, but viruses are probably unaffected. A pH value of 12.0 to 12.5 and a contact time of approximately 30 min can probably yield 99.0% to 99.9% inactivation of most bacteria and of certain viruses. This process has a drawback: the pH that is necessary for effective disinfection must be reduced before the water can be consumed. Use of the high pH as the sole means of disinfection is not recommended. In many situations where water is softened by the precipitation process and where the water is of poor biological quality, the disinfection potential of this process could be used by increasing the pH above its present values.
Although this method has not been used deliberately for disinfection in the past, additional work on this method may be warranted since high pH conditions are attained in many treatment plants. Studies are needed to determine the pH values required for inactivation of protozoans, a broader and more representative group of viruses, and a larger group of enteric bacteria.
- Hydrogen Peroxide
Hydrogen peroxide (H 2 0 2 ) is a strong oxidizing agent that has been used for disinfection for more than a century. Its instability and the difficulty of preparing concentrated solutions have tended to limit its use. However, by 1950 electrochemical and other processes were developed to produce pure hydrogen peroxide in high concentration, which is known as stabilized hydrogen peroxide (Schumb et al. , 1955). This product has been subjected to increased study and application. Most recently it has been used to disinfect spacecraft (Wardle and Renninger, 1975), foods (Toledo, 1975), and contact lenses (Gasset et al. , 1975; Spaulding et al. , 1977). Although there has been some interest in using hydrogen peroxide as a disinfectant for wastewater (Taki and Hashimoto, 1977), it has been used more for control of bulking in the activated sludge waste treatment process (Sezgin et al. , 1978). Its use in drinking water disinfection appears minimal.
Analytical methods for hydrogen peroxide are based on oxidation or reduction with potassium permanganate (KMnO 4 ), potassium iodide (KI), or ceric sulfate [Ce(SO 4 ) 2 ] (Chadwick and Hoh, 1966). A colorimetric procedure based on the oxidation of titanium sulfate (TiOSO 4 ) (Snell and Snell, 1949) is sensitive to about 1 mg/liter in the absence of other oxidizing agents.
Commercially produced hydrogen peroxide is available in aqueous solution, usually ranging from 30% to 90%. It is stabilized during manufacture by addition of such compounds as sodium pyrophosphate (Na 4 P 2 O 7 ), acetophenetidin (CH 3 CONHC 6 H 4 OC 2 H 5 ), or acetanilide (CH 3 CONHC 6 H 5 ). As the concentration of hydrogen peroxide is decreased, the concentration of stabilizers is typically increased. Experience in the waterworks industry using hydrogen peroxide is nonexistent. However, hydrogen peroxide is widely used as a bleaching agent in making cotton textiles or in wood pulping (Chadwick and Hoh, 1966). Presumably, a concentrated solution would be diluted and applied with a chemical metering pump. Careful attention to safety in handling would be required because of the possibility of fire or explosion.
The few pre-1965 references on disinfection by hydrogen peroxide have been summarized by Yoshpe-Purer and Eylan (1968). Although its bactericidal activity was indicated, a need for catalytic Fe 2+ or Cu 2+ was reported. Yoshpe-Purer and Eylan (1968) worked with Escherichia coli, Salmonella typhi, and Staphylococcus aureus in pure culture and as a mixture of bacteria. They also studied the effect of hydrogen peroxide concentrations (from 30 to 60 mg/liter), contact time (10 to 420 min), and initial concentration of organisms. Without ever measuring residuals, they concluded that bacterial inactivations were relatively slow, that E. coli was more resistant to hydrogen peroxide than S. typhi or S. aureus, and that the required inactivation time was increased as the initial concentration of organisms was increased. Regardless of the hydrogen peroxide concentration or the type of organism used , all tests were claimed to be ''sterile" in 24 hr. Table II-20 summarizes some of these data.
TABLE II-20
Contact Times Necessary for Hydrogen Peroxide (H 2 O 2 ) to Effect 99% Inactivation of Various Concentrations of Escherichia coli, Salmonella typhi, and Staphylococcus aureus .
Toledo et al. (1973) studied inactivation of S. aureus and spores of several species of Bacillus and Clostridium with 25.8% (258,000 mg/liter) hydrogen peroxide. The time to reduce S. aureus by 6 logs was 1 min, whereas reduction of spores by 99% required from less than 1 min to approximately 17 min, depending on the species. Increasing the hydrogen peroxide concentration up to 41% (or 410,000 mg/liter) or increasing temperature from 24°C to 76°C significantly reduced the required inactivation time.
Several studies on virus inactivation by hydrogen peroxide have been reported. Lund (1963) obtained a 99% inactivation of poliovirus (Saukett strain) in about 6 hr with 0.3% (3,000 mg/liter) hydrogen peroxide. Mentel and Schmidt (1973) worked with rhinovirus (types 1A, 1B, and 7). They found that while a 1.5% (15,000 mg/liter) concentration required approximately 24 min for 99% inactivation, equivalent inactivation occurred in 4 min with a concentration of 3% (30,000 mg/liter).
Information is lacking on the effect of hydrogen peroxide on protozoa and helminths in water.
In none of the studies cited above is there any indication that the dosage of hydrogen peroxide was measured other than by the volumetric addition of hydrogen peroxide. No measurements of residual were reported, although Yoshpe-Purer and Eylan (1968) claimed that a residual was present for up to 13 days as indicated by inactivation of added doses of bacteria.
No studies have specifically identified the mechanism of action of hydrogen peroxide. Spaulding et al. (1977) believed that the hydrogen peroxide molecule itself was not responsible for the action but, rather, that the free hydroxyl radical (HO · ) that it produced was the specific inactivating agent. They claimed that the catalytic effect of iron or copper ions supported this theory. Yoshpe-Purer and Eylan (1968) also reported that free radicals were important but that sufficient catalyzing metal ions were available either from the tap water that they used or from the bacterial cells themselves.
Because of its relatively high cost and the high concentrations that are required to achieve disinfection in reasonable time, hydrogen peroxide is not a generally satisfactory disinfectant for drinking water.
If further research is to be conducted, the stability of hydrogen peroxide and its residual effect in the presence of organic material and other substances in water or distribution systems should be investigated. Parallel studies using chlorine and hydrogen peroxide, separately and in conjunction, should be conducted.
- Ionizing Radiation
Ionizing radiation may be electromagnetic or particulate. As used for disinfection or sterilization, electromagnetic radiation may be UV, gamma, or X rays, and the particles may be alpha or beta or neutrons, mesons, positrons, or neutrinos. This discussion is limited to gamma rays and beta particles (or electrons).
Although there is extensive literature on the use of ionizing radiation in the preservation of food and other materials (Silverman and Sinskey, 1977), little information is available on water treatment.
Application
Laboratory studies of destruction of microorganisms are generally conducted by exposing small containers holding the test suspensions to the shielded source of ionizing radiation. However, in a practical application, the shielded source must be designed to permit relatively thin sheets of flowing liquid to be exposed. This is particularly important for high-energy electrons that have a less penetrating power than gamma rays. The MIT study (Massachusetts Institute of Technology, 1977) included a field demonstration in which a plant treating approximately 380,000 liters/day (minimum dosage of 400,000 rads) was designed and operated. The energy source was a 50-kW electron accelerator operated at 850,000 V. Sludge was pumped under the electron beam through a drum system that produced a layer that was 1.2-m wide and 2-mm thick. The entire operation was conducted in a concrete vault to shield the operators against X rays, which are produced incidentally. Shielding of workers is a major requirement both for gamma ray and high-energy electron sources.
Analytical Methods and Residual
No residual is produced by ionizing radiation. Consequently, dosage has been measured exclusively. The MIT (1977) report and Silverman and Sinskey (1977) summarized analytical methods including calorimetry, photoluminescent dosimetry, colorimetry, and the use of ionization chamber instruments.
The first study of ionizing radiation in the treatment of water was reported by Dunn (1953). In addition to reviewing the literature and providing some discussion on ionizing radiation, he studied the use of a 1,000-Ci source of cobalt-60 (providing gamma rays) and a Van de Graaff generator (providing high-energy electrons). Working with natural waters and wastewaters that were not chemically or bacteriologically characterized or controlled, he exposed samples to a constant radiation flux (2,000 R/min from the cobalt-60 or electrons from 3-MV operation of the Van de Graaff generator). Varying only the time of exposure, Dunn found that it took 0.125 min (dosage 250,000 R) with the cobalt-60 source to reach 95.4% to 99.999% inactivation of total initial numbers of bacteria (as measured by a plate count at 37°C).
Ridenour and Armbruster (1956) studied the effect of a 10-kCi source of cobalt-60 (3,000 R/min) on a variety of natural waters, wastewaters, and pure cultures (approximately 2 × 107 organisms per milliliter) of 10 different organisms. They found that a dosage of 100,000 roentgen equivalent physical (rep) reduced the count of all species tested by > 99%. (In order of increasing resistance, the species were: Enterobacter aerogenes, Escherichia coli, Shigella flexneri, Salmonella typhi, S. sonnei, Salmonella sp., Staphylococcus aureus, S. paratyphi B, Streptococcus faecalis, and Bacillus subtilis. ) With river water, a dosage of from 50,000 to 100,000 rep reduced the total bacterial count and the coliform index by at least 99%, but 150,000 rep were required to reduce the streptococcus index by 99%. Varying the pH (5.0, 7.0, and 8.5) had no effect on the rate of inactivation.
Also using cobalt-60 (1,100 Ci), Lowe et al. (1956) exposed pure cultures of bacteria that were suspended in double distilled water and sterile settled sewage. The concentration of microorganisms was approximately 1 × 10 6 /ml. Although they did not report exposure time, they summarized their exposure data in rads (radiation absorbed dose). (See Table II-21 ).
TABLE II-21
Dosages of Cobalt-60 Irradiation Necessary for 99% Inactivation of Microorganisms in Distilled Water.
There have been more studies on the disinfection of wastewater than on disinfection of drinking water. Ballantine et al. (1969) and Compton et al. (1970) considered ionizing radiation not as good as other available methods but, with increased emphasis on water reclamation (Eliassen and Trump, 1973) and the use of high-energy electrons (Massachusetts Institute of Technology, 1977; Wright and Trump, 1956), the prospects for practical application seem improved. In the MIT (1977) study, which is the most systematic one conducted to date, washed cells or spores were suspended in 0.067- M phosphate buffer (1 × 10 8 cells/ml) and exposed to high-energy electrons from a Van de Graaff generator. The approximate dosages, in rads, required for a 99% inactivation of the organisms tested were: Escherichia coli (K12), 38,000; Salmonella typhimurium (LT2), 24,000; Micrococcus sp., 35,000; Aspergillus niger (spores), 78,000; S. typhimurium (24), 100,000; Streptococcus fecalis, 300,000; and Clostridium perfringens (spores), 400,000. The investigators found that the solids in the wastewater had no effect on disinfection.
Of the factors affecting disinfection, oxygen was most important. For example, for E. coli K12, the dosage required for 99% inactivation was more than doubled when an atmosphere of air was replaced by one of nitrogen, and almost halved when the air was replaced by an oxygen-rich atmosphere.
The inactivation of viruses does not appear to be influenced by the source of the ionizing radiation (Lea, 1955). It is affected by factors that are similar to those described above for bacterial inactivation (Ballentine et al. , 1969). Lowe et al. (1956) found that 32,000 rads were required for 99% inactivation of E. coli phage T3 (see Table II-21 ). In the MIT study (1977), from 300,000 to 420,000 rads were required for 99% inactivation of coxsackievirus B3, poliovirus 2, echovirus 7, reovirus 1, and adenovirus 5 (in order of increasing sensitivity), each suspended in 0.05 M glycine at pH 7.0.
The MIT study (1977) appears to be the only one in which the effect of ionizing radiation on both protozoa and helminths was investigated; however, no quantitative data were reported. Brannan et al. (1975) found that to attain a 90% reduction of embryonation of Ascaris lumbricoides eggs, a dosage of approximately 30,000 rads was required.
In a review of the literature, Silverman and Sinskey (1977) summarized the mode of action of disinfection by ionizing radiation. Two effects are recognized: the direct effect, in which the primary cellular target is DNA that is damaged by the energy released from the ionizing radiation, and the indirect effect, which is associated with the production of such substances in the cell menstruum as hydrogen peroxide (H 2 O 2 ), organic peroxides, and free radicals. Oxygen is important because it reacts with electrons and radicals, which in turn react to form hydrogen peroxide or ozone (O 3 ).
Ionizing radiation can disinfect water effectively; however, the large source, shielding, and relatively thin exposure layers that are required create difficult engineering and safety problems. The complex technology may limit application to large facilities that can provide for adequate safeguards. The absence of residual disinfection is also restrictive.
Any further research should address practical methods of delivering the ionizing radiation to the water mass. Basic engineering design, construction, and operation data should be developed.
- Potassium Permanganate
Potassium permanganate (KMnO 4 ) is a strong oxidizing agent, which was first used as a municipal water treatment chemical by Sir Alexander Houston of the London Metropolitan Water Board in 1913. In the United States, it was used in Rochester, New York, in 1927 and in Buffalo, New York, in 1928. Since 1948, it has been used more widely in waterworks as an algicide (Fitzgerald, 1964; Kemp et al. , 1966), as an oxidant to control tastes and odors (Spicher and Skrinde, 1963; Welch, 1963), to remove iron and manganese from solution (American Water Works Association, 1971; Shull, 1962), and, to a limited extent, as a disinfectant (Cleasby et al. , 1964).
The relatively limited information concerning disinfection with potassium permanganate is subject to criticism, because there have been no studies of the effects of organic constituents of the medium (test system) or destruction of a variety of organisms, especially pathogens.
Concentrations of potassium permanganate can be determined readily by iodometric titration using sodium thiosulfate (Na 2 S 2 O 3 ) as a titrant or by direct titration with ferrous sulfate. Manganese can be determined by atomic absorption spectrophotometry, or the permanganate ion (MnO 4 - ) can be measured colorimetrically ( Standard Methods , 1976). The minimum detectable concentration of manganese by the colorimetric procedures, using a 100-ml sample, is 50 µ g/liter. A concentration of 0.05 mg/liter or greater can be detected visually by the pink color that is imparted to the water (Welch, 1963).
Crystalline potassium permanganate is highly soluble in water (2.83 g/100 g at 0°C). In waterworks, it is prepared usually as a dilute solution (1% to 4%) and applied with a chemical metering pump (American Water Works Association, 1971). It also may be added as a solid using conventional dry-feed equipment.
Reduction of the permanganate ion produces insoluble manganese oxide (Mn 3 O 4 ) hydrates. To prevent distribution of turbid water or of water that will cause unsightly staining of plumbing fixtures, potassium permanganate most often is applied as a pretreatment that is followed by filtration. For example, addition of potassium permanganate to a finished water to maintain a residual in a distribution system is unacceptable because of the pink color of the compound itself or the brown color of the oxides.
Cleasby et al. (1964) and Kemp et al. (1966) summarized the few scientific references to disinfection by potassium permanganate that were published earlier than 1960. Although some bactericidal activity was indicated, no quantitative data were presented, and the early reports disagreed as to its value.
The most systematic study of disinfection by potassium permanganate has been made by Cleasby et al. (1964). They worked exclusively with Escherichia coli (prepared as a lactose broth culture) and studied the effect of potassium permanganate at doses of 1 to 16 mg/liter at pH values of 5.9, 7.4, and 9.2, temperatures of 0°C and 20°C, and contact times of 4 to 120 min. They concluded that bacterial inactivation was relatively ineffective, but slightly better at the higher temperature, and that increasing pH decreased disinfection rates. Table II-22 summarizes in tabular form their data, which were presented originally in graphic fashion.
TABLE II-22
Concentrations and Contact Times Necessary for Potassium Permanganate to Effect 99% Inactivation of a 48-Hr Escherichia coli Lactose Broth Culture.
At least two patent applications involve the use of potassium permanganate as a disinfectant for water in swimming pools (Heuston, 1972; Seidel, 1973). Seidel's patent covered use of a concentration of 0.1 to 0.2 mg/liter in a recirculating system including filtration through quartz gravel. She claimed removal of bacteria and algae, but the specific merit of potassium permanganate in her system cannot be determined from the patent application. Heuston (1972) proposed a tablet containing 0.001 g of potassium permanganate per 0.2 g tablet to give a dose of 1 mg/liter. Because the tablet includes potassium iodide (KI) and other oxidizing agents, it is impossible to assess the specific killing action of potassium permanganate.
A number of studies on inactivation by potassium permanganate have been reported. However, these dealt largely with the mode of action of potassium permanganate in viral inactivation (Lund, 1963, 1966) or at efforts to control human diseases (Peretts et al. , 1960; Schultz and Robinson, 1942; Wagner, 1951), animal diseases (Derbyshire and Arkell, 1971), or plant diseases (Eskarous and Habib, 1972; Hughes and Steindl, 1955). They provide few clear-cut quantitative data on biocidal activity of the compound.
Information is lacking on the effect of potassium permanganate on protozoans and helminths in water.
The mechanism of action of potassium permanganate has not been definitively identified. From the studies that have been conducted (Lund, 1963, 1966) it may be presumed that it exerts its disinfection activities by oxidizing compounds that are involved in essential cellular functions. However, Lund (1963) questioned the suggestion that the oxidative process was the mechanism for poliovirus inactivation, but was unable to exclude it as a possibility.
The relatively high cost, ineffective bactericidal action, and aesthetic unsuitability of maintaining a residual in the distribution system make potassium permanganate a generally unsatisfactory disinfectant for drinking water.
Additional studies on potassium permanganate are hardly warranted, but in the unlikely event that further research on its biocidal activities is conducted, it would be desirable to study the effect of organic material on its disinfection and the effect of the compound on organisms other than E. coli. To relate disinfection efficiency to more conventional practices, parallel studies using chlorine should be conducted.
Silver as a metal has been known for millennia, and its use as a water disinfectant dates back to the Persian king Cyrus. The term oligodynamic, which describes the killing effect of small concentrations, was coined by von Naegeli in 1893, but it is unscientific and its use should be rejected. The antibacterial action of silver and silver nitrate (AgNO 3 ) was noted first by Raulin in 1869. Since the late nineteenth century, but especially since World War II, there have been considerable efforts to exploit the use of silver as a disinfectant, particularly for individual (home) water systems and swimming pools. Silver has been used both as a salt, most commonly silver nitrate, or as metallic silver, either bound in filter beds, generated by electrolytic devices, or applied as a colloidal suspension.
The relatively limited information concerning disinfection with silver has been seriously criticized because accurate measurement of low concentrations, i.e., < 200 µ g/liter, has been difficult; a suitable neutralizing agent has not always been incorporated in test protocols; and adsorption of silver on surfaces of test vessels has confounded some studies. Despite these limitations, silver has been used in parts of Europe and Japan as a water disinfectant.
In water treatment, silver has been applied principally by dissolving the metal or by incorporating a silver compound in a filter medium, often an activated carbon filter. Romans (1954) has described older processes and patents. Davies (1976) reviewed processes for treating swimming pool water in which silver was added as a soluble salt and the silver ions were kept oxidized by the addition of persulfate; metallic silver was deposited on an activated carbon filter; silver was released from solid silver electrodes, which were alternately made anodic and cathodic; silver was released from an activated carbon filter containing silver and supplemented by passing the water through anodic silver screens; and silver was released from a pair of silver-copper alloy electrodes by applying a low reversing voltage.
During the past 6 yr, dozens of patents have been issued in Germany, Spain, South Africa, India, and the United States for devices or systems for adding silver to home drinking water systems or swimming pools. The home systems are most frequently combinations of activated carbon to remove tastes and odors and silver to prevent bacterial growth on the filter.
Because of the low solubility of silver, the dose is usually less than 50 µ g/liter. Presumably this is reduced rapidly because of the adsorption of silver to surfaces, but there is no information on the silver residual in treated water. However, the maximum contaminant level (MCL) is 0.05 mg/liter (U.S. Environmental Protection Agency, 1975).
Until recently there have been no satisfactory techniques for measuring silver at the µ g/liter level. Using a dithizone colorimetric method, the minimum detectable quantity of silver is 200 µ g/liter ( Standard Methods , 1976). Using an atomic absorption spectrophotometric method, the detection limit is 10 µ g/liter (U.S. Environmental Protection Agency, 1974), and, if a heated graphite furnace is used, the detection limit is reduced to 0.005 µ g/liter (Rattonetti, 1974).
One of the earliest studies was conducted by Just and Szniolis (1936), who found that 100 µ g of silver per liter disinfected water within 3 to 4 hr, that silver nitrate and metallic silver were equally effective, and that histopathological changes occurred in rats that were given water containing 400-1,000 µ g silver/liter for 100 days. Other systematic studies of silver disinfection have been conducted by Renn and Chesney (1953-1956), Wuhrmann and Zobrist (1958), and Chambers and Proctor (1960). These studies have been summarized by Woodward (1963). Table II-23 , adapted from Wuhrmann and Zobrist (1958), shows that in the concentrations used, silver acted slowly, but that there were increases in the rate of bacterial inactivation with increasing temperature and pH. Chambers and Proctor (1960) obtained similar results, but the inactivation rates of Renn and Chesney (1953-1956), who used comparable concentrations of silver, were faster.
TABLE II-23
Concentrations and Contact Times Necessary for Silver to Effect 99.9% Inactivation of Escherichia coli in 0.005 M Phosphate Buffer.
Both Wuhrmann and Zobrist (1958) and Chambers and Proctor (1960) found that the presence of phosphate (at 60 mg/liter) slowed bacterial inactivation. Because phosphate is typically found at much lower concentrations in drinking water, this observation has little practical significance, but it may account for disparate results in some laboratory studies.
Increasing water hardness slowed bacterial inactivation. According to Wuhrmann and Zobrist, an increase of 3 min was required to achieve 99.9% bacterial inactivation (at 20°C and pH 7.0) for each 10 mg/liter increase in hardness. Likewise, chlorides interfered with the action of silver. At 10 mg/liter, they increased the inactivation time by 25% and at 100 mg/liter, by 70%.
The source of silver seemed to be irrelevant to the inactivation rate because silver either added as silver nitrate or dissolved from metallic silver gave approximately equal results.
In most studies of the disinfection of water with silver, Escherichia coli has been the test organism. Wuhrmann and Zobrist also tested a Salmonella species and found it to be at least as sensitive as E. coli. Yasinskii and Kuznetsova (1973) observed that 90 µ g/liter inactivated Vibrio comma (1 × 10 6 cells/ml) in 30 min or 22.5 µ g/liter in 60 min.
Fair (1948) and Harrison (1947) suggested that silver salts were ineffective against cysts of Entamoeba histolytica, but the data provided in these reports were limited. By contrast, Newton and Jones (1949) observed that electrolytically produced silver in tap water gave greater than 99% cyst inactivation in 1 hr and highly variable residual concentrations that ranged between 17 and 33 mg/liter at pH's of 9.0 to 9.8. When silver nitrate in distilled water at 0.5 mg/liter was used, 4 to 6 hr were required for a 90% to 99% inactivation. At 5 mg/liter, a > 99% inactivation occurred in 3 hr, and at 30 mg/liter, a > 99% inactivation occurred in I to 2 hr.
Chang and Baxter (1955) used 150 mg/liter of silver nitrate (95 mg/liter Ag + ) with contact lines of 1 to 6 min. They concluded that cysticidal activity was only moderate compared to that of iodine. Apparently, there are no data on silver as a virucidal agent in water except for one study by Lund (1963), who decreased poliovirus activity by 2.5 logs in 4 hr with a concentration of approximately 68 mg/liter.
A good general review of silver and its compounds and a more thorough medical examination of their applications was published by Grier (1977), who concluded that there would be a ''significant place for silver compounds in the prevention and treatment of at least some bacterial diseases."
Romans (1954) summarized the mechanism of so-called oligodynamic activity: "However, there is much difference of opinion as to the form of the active principle, as to the mechanism of its action, and the value of the results obtained. Some authorities believe that the active form is a positively charged ion, some think it is a complex ion, others a salt and still others think it acts by formation of proteinates or merely as a catalyst. These are only a few of the ideas that have been expressed with formidable experimental support."
Chang (1970) attributed a direct action to silver in the nonreversible formation of silver-sulfhydryl complexes that could not function as hydrogen carriers:

He considered silver to be bacteriostatic as well as bactericidal. This would explain the relatively long contact times that are required for antibacterial activity at the concentrations that are normally used.
Zimmerman (1952) demonstrated that low concentrations of silver do not enter the cell but are adsorbed onto the bacterial surface just as silver tends to be adsorbed on other surfaces (Chambers and Proctor, 1960). According to Chang (1970), the adsorbed silver ions must immobilize the dehydrogenation process because bacterial respiration takes place at the cell surface membrane.
As a disinfectant, silver may be applicable to home treatment systems and swimming pools. These applications may be more effective in keeping filters free from bacteria than in actually inactivating organisms that are suspended in the water. This is because of the relatively low rate of solution of silver in water and its low biocidal activities.
Woodward (1963) ably summarized the situation: "The high cost of silver will limit it to specialty uses. The fact that silver does not impart taste, odor, or color to water makes it attractive for use. Its slow bactericidal action, although a disadvantage in some situations, may be an advantage in others, particularly where water is stored for long times before use, as on shipboard. Until some of the uncertainties regarding silver are resolved, it would be prudent to use it as a drinking water disinfectant only in situations where substantial factors of safety can be provided and where the bactericidal effectiveness of the procedure can be monitored."
Silver and its compounds are weak, costly disinfectants that are unsuitable for use in municipal drinking water supplies. To achieve acceptable disinfection in a reasonable time would require concentrations exceeding the MCL of 0.05 mg/liter.
Now that adequate chemical analytical techniques are available for measuring low concentrations of silver, it would be desirable to conduct disinfection studies in which both dose and residual are measured accurately. To relate disinfection efficiency to more conventional practices, parallel studies using chlorine should be conducted.
- Ultraviolet Light
Electromagnetic radiation, in wavelengths from 240 to 280 nm, is an effective agent for killing bacteria and other microorganisms in water (Luckiesh and Holladay, 1944). Conveniently, from a practical point of view, from 30% to more than 90% of the energy emitted by a low-pressure mercury arc, which is enclosed in special UV transmitting glass, is emitted at a wavelength of 253.7 nm (Anonymous, 1960; Childs, 1962; Luckiesh and Taylor, 1946).
Two basically different physical arrangements are commonly used for the application of UV light to water. In one, lamps are placed above the solution to be disinfected at the apex or focus of parabolic or elliptical reflectors. For this purpose, aluminum is preferred because of its high reflectance for the germicidal 253.7-nm wavelength (Luckiesh and Taylor, 1946). Although this is an efficient way of applying UV radiation to water, the open nature of the structure can permit contamination. Furthermore, it must operate at atmospheric pressure. Tubular reactors are more common in water treatment because they are sealed and operate under pressure.
To increase intensities and permit higher flow rates, multiple lamp reactors are being designed and put into use. These units generally contain lamps that are positioned parallel to the flow of water through them. A recent patent (Wood, 1974) describes an apparatus using a water film approximately 0.64 cm thick and a flow of liquid that is perpendicular to the lamp in a baffled system. This type of unit is designed for disinfection of fluids with low UV transmittance. For liquids with high transmittance like drinking water, such a design is inefficient, because insufficient depth is available for absorption of UV and a large fraction of the radiant energy is dissipated as heat when the UV is reflected on the walls of the contactor.
In units containing lamps that are surrounded by water, an insulation space must be provided to maintain their efficiency. The maximum efficiency of modern cold cathode lamps is near 40°C, dropping off to a 50% output at 24°C and 65°C. Lamps are normally placed in sleeves that are made of high-silica glass or quartz in order to maximize transmission at the 253.7-nm wavelength. Solarization or opacity can develop in the sleeve as it deteriorates with age. The sleeves must be cleaned regularly for efficient functioning.
Another consideration in the mechanical design of UV contactors is the degree of agitation that is provided and the plug flow characteristics of the contactor. In all disinfection systems that require several orders of magnitude of disinfection, short circuiting or nonplug flow characteristics of contactors, which bypass a part of the flow, limit the efficiency of the process. It was recognized by Cortelyou et al. in 1954 that the degree of agitation in the UV disinfection of water is important in bringing the target microorganism into close proximity to the UV source where the intensity is highest.
The dose, D , of electromagnetic radiation that is applied to a solution is commonly measured as the intensity of radiant energy input, I 0 , at the lamp surface or at some given distance from the lamp ( I 0 being expressed as µ W/cm 2 ), multiplied by the time of exposure, t, in seconds (Luckiesh and Holladay, 1944):

Another measurement of dose is chemical actinometry (Calvert and Pitts, 1966). In this method, a photochemical reaction with a known quantum yield is used to measure the intensity (quanta per second) of light that is absorbed by the actinometry solution. Knowing the time of exposure, the volume of solution, and the volume of sample analyzed, the moles of photochemical reaction per unit of time can be related through the quantum yield to the Einsteins or moles of photons per second that are absorbed by the fluid. From the wavelength of radiation, l, and the area of lamp surface, the average intensity at the lamp surface can be determined.

Neither of these measurements of energy input, I 0 , per unit area of lamp exposed considers the change in intensity through the depth of exposed fluid and the solid angle over which this energy interacts. Recently, attempts have been made to correct the decrease in intensity with depth in studies of wastewater disinfection where this problem is acute (Roeber and Hoot, 1975; Severin, 1978; Venosa et al., 1978). These studies have shown that the usual water quality indices, such as chemical oxygen demand (COD), color, and turbidity, do not adequately predict the loss of intensity through the solution. The direct measurement of UV transmittance has been successful. In spite of these difficulties, Huff et al. (1965) suggested that-color at a maximum level of 5 units, iron at 3.7 mg/liter, and turbidity up to 5 units did not decrease treatment efficiency below acceptable limits in a unit with a maximum water depth of approximately 7.5 cm.
Biocidal Dose
The biocidal dose of UV energy consists of the intensity of UV energy that is absorbed at the reactive site within the microbe over the time of interaction. The biocidal dose is then a function of the energy input from the UV source into the solution, dispersion of the energy as a function of distance from the source, the depth of the fluid between the organisms and the source as well as its absorptivity, and, finally, the losses and reflection of UV light within the contactor. All of these factors determine the actual intensity of radiation that is available to the microorganism at any one point within the contactor (Childs, 1962; Luckiesh and Holladay, 1944; Luckiesh et al. , 1944).
Quite early in the study of the batericidal action of UV light, Gates (1929) found that the relation between the intensity of incident energy and time required for bacterial destruction were not of equal importance in determining experimental disinfection. However, Hoather (1955) found that when one corrects for the transmitted intensity of radiation, taking into consideration the absorptivity of the water and dispersion as a function of distance from the source, the required time of exposure is inversely proportional to the calculated intensity of radiation penetrating the water for a given degree of inactivation. Finally, Oliver and Cosgrove (1975) used chemical actinometry and a pulsed laser to show that their coliform and streptococcal inactivation in wastewater depended only on the total dose that is delivered and not on the dose rate or intensity of light that impinged on the sample. Thus, the same number of µ W s/cm 2 provided the same disinfection regardless of the time and intensity that were used to produce that dosage.
Bacterial spores have also been studied with chemical actinometry. For certain stocks, the rate at which the energy was deposited affected the degree of response (Powers et al. , 1974). Powers and his colleagues observed that the effect of the dose rate was not due to geometric factors or intensity variations with depth and solution absorptivity. This explains many earlier results. They did find that dependence on dose rate was seen only for some of their stocks of spores, it was not as dramatic with monochromatic-filtered light of a wavelength of 253.7 nm, and it was observed only at very low doses. The explanations usually given for these low-dose effects are photochemical back reactions, enzymatic repair, and photoreactivation mechanisms (Deering and Setlow, 1963; Harm, 1968).
UV radiation produces no residual. Therefore, monitoring and control of disinfection efficiency are more difficult for UV than for chemical disinfectants.
However, this is not a major problem, because disinfection can be controlled by adjusting the contact time and the UV energy that is transmitted through the solution. The major disadvantage is the lack of a tracer for ensuring the integrity of the distribution system.
UV disinfection follows Chick's Law of kinetics (Luckiesh and Holladay, 1944):

where N is the number surviving at time t of an original population N 0 that has been exposed to an intensity I. Q is the dose for 1 log survival, one lethal unit exposure, or a lethe.
The variables discussed above interfere with the accurate determination of the average intensity and time of exposure in a practical UV contactor. Consequently, there is considerable disagreement in the literature concerning the absolute magnitude of Q for the microorganisms that have been studied.
Of the Gram-negative bacteria, Escherichia coli is consistently more resistant to disinfection by UV than are the Salmonella and Shigella species that have been studied (Cortelyou et al. , 1954; Kawabata and Harada, 1959). The vegetative forms of the Gram-positive bacteria were more resistant than E. coli. Kawabata and Harada found Streptococcus faecalis nearly 3 times more resistant than E. coli, while Bacillus subtilis was 4 times more resistant. The spores of B. subtilis were 6 times more resistant than E. coli.
Luckiesh and Holladay (1944) reported that a dosage of 2,400 µ W · s/cm 2 at a wavelength of 254 nm produced one lethe of disinfection of E. coli. Huff et al. (1965) studied a two-lamp shipboard disinfection unit with an approximately 75-cm long, 20-cm diam. unbaffled stainless steel cylinder. Their dosages were generally quite high, ranging from 3,000 to 11,000 µ W ··s/cm 2 at a maximum water depth of approximately 19 cm. At the lowest dosage, there was a 0.02% to 0.04% survival of E. coli, which, assuming Chick's Law, gives a dosage of less than 400 µ W · s/cm 2 /lethe. They also found approximately 2 logs less spore inactivation with the same dose as required for vegetative cells. They reported more than 4 logs of inactivation of polio-, echo-, and coxsackievirus with 4,000 µ W · s/cm 2 . Based on these results, the U.S. Department of Health, Education, and Welfare issued a policy statement on April 1, 1966, stating criteria for the acceptability of UV disinfecting units as a minimum dosage of 16,000 µ W · s/cm 2 with a maximum water depth of approximately 7.5 cm.
Morris (1972) has compared the dose of 254-nm UV energy required to inactivate a range of microorganisms suspended in droplets of buffer solution on an aluminum surface. Their results are shown in Table II-24 expressed as µ W · s/cm 2 /lethe.
TABLE II-24
Ultraviolet Energy Necessary to Inactivate Various Organisms.
Required dosages reported by Morris (1972) are similar in magnitude to those found by Huff et al. (1965). Both Huffs values and those of Morris (1972) are lower than those of Luckiesh and Holladay (1944), partly because of the additional dose of UV that was provided by reflections from the contactor and aluminum surface, which produce an estimated dosage approximately twice the values given. No studies of the action of UV light on parasitic helminths and protozoa in the treatment of drinking water appear to have been reported.
Inactivation by UV light is believed to act through the direct absorption of UV energy by the microorganism, causing a molecular rearrangement of one or more of the biochemical components that are essential to the organism's functioning. The major site of UV absorption in microorganisms is the purine and pyrimidine components of the nucleoproteins. Since the relative efficiency of disinfection by UV energy as a function of wavelength follows the absorption spectrum of these chromophore groups and because the first law of photochemistry states that the light that is absorbed by a molecule can produce photochemical change, the mechanism of action of UV probably occurs through the progressive biochemical change that is produced primarily in the nucleoproteins. Witkin (1976) has reviewed both UV mutagenesis and inducible DNA repair. The major specific mechanisms that have been suggested for UV damage involve the reversible formation of pyrimidine hydrates and pyrimidine dimers. Breaks in the bonding structure, known as nicking, also occur. The older literature has been reviewed by Reddish (1957).
The repair of damaged nucleoprotein with light of a wavelength longer than that of the damaging radiation is commonly referred to as photoreactivation (Witkin, 1976). This process, originally identified by Kelner (1949), has been studied extensively. It occurs in the visible wavelength range from 300 to 550 nm (Jagger, 1960; Kelner, 1949). In addition, repair of nucleoproteins that have been damaged by UV radiation can also occur in the dark. The mechanisms of repair of damage to DNA caused by UV radiation are generally thought to involve induced enzymes (Witkin, 1976), but repair has also been observed in inactive systems for which photochemical back reactions have been suggested (Powers et al. , 1974).
UV light produces no residual. Therefore, if a residual is desired, another disinfectant must be used. Current technology is limited to the use of UV light in small systems. Requirements for equipment maintenance limits widespread adoption of UV light for drinking water disinfection.
Tables II-25 and II-26 present significant characteristics of the methods that are considered for disinfection of drinking water.
TABLE II-25
Summary of Major Possible Disinfection Methods for Drinking Water.
TABLE II-26
Summary of Minor Possible Disinfection Methods for Drinking Water.
The biocidal activity of various disinfectants can be compared conveniently through the numerical value of the product (c · t) of the concentration of disinfectant (c) multiplied by the time of exposure (t) that is required to achieve a particular degree of inactivation (e.g., 99%) under similar conditions of pH, temperature, etc. The lower its c · t product, the more effective the disinfecting action of a particular agent.
Table II-27 displays c · t products for the methods that are regarded as the major possibilities for drinking water disinfection. The c · t products for similar inactivation under the same conditions obtained for other possible agents (ranked less promising by this or other criteria) are up to several orders of magnitude greater than those shown in Table II-27 , e.g., 33,000 and 10 6 for inactivation of E. coli and poliovirus 1 by hydrogen peroxide, respectively. Therefore, they were not included.
TABLE II-27
Comparative Efficacy of Disinfectants in the Production of 99% Inactivation of Microorganisms in Demand-Free Systems.
Table II-28 provides the current status of theoretically possible methods for disinfecting drinking water. To derive the conclusions in the table, the specific biocidal activity data summarized in Table II-27 were considered as well as information (or lack of it) on the practical application and reliability of the methods.
TABLE II-28
Status of Possible Methods for Drinking Water Disinfection.
Chlorination, ozonization, and the use of chlorine dioxide come closest to meeting the criteria desired. At this time none of the other possibilities considered can substitute for techniques presently used to disinfect drinking water.
The ultimate choice between methods will require weighing the characteristics detailed in this evaluation against the nature of byproducts to be expected from the use of the particular method and the potential toxicities of the by-products. Evaluations of these aspects of drinking water disinfection, also carried out by the Safe Drinking Water Committee, are reported in parallel to this study and should be consulted before final conclusions are drawn.
The lack of data, particularly data on pathogens or data that are amenable to comparison, on the biocidal efficacy of various disinfectants needs to be remedied. Particular emphasis should be placed on the methodology for determining biocidal activity and defining those parameters that need to be controlled for the study to yield data for comparative purposes. Disinfection efficacy studies should be conducted with actual pathogens (bacteria, viruses, and cysts of Giardia lamblia and Entamoeba histolytica ) as well as with model systems. Careful control of the character and concentration of the disinfecting species and of the degree of aggregation and other characteristics of the test organisms (or cysts) are required in these tests.
Studies should be conducted to define the mechanisms resulting in the different responses to disinfectants of laboratory-acclimated cultures and organisms that have been freshly isolated from natural conditions or that are resistant to specific disinfectants for other reasons. Techniques should be developed to facilitate accurate predictions from laboratory data to disinfectant susceptibilities under operating conditions in treatment plants.
There are no practical assays for many human viruses of known or suspected importance, e.g., hepatitis A virus and rotaviruses. Assay models should be developed for such viruses, and studies should be conducted on their susceptibilities to disinfection. Support is also needed for research that would result in better appraisals of the significance to human health of the presence of viruses in water supplies. Such studies should include investigation of the causative agents that are involved in the large number of illnesses presently characterized as ''gastroenteritis of unknown etiology" and of the doses of various enteroviruses that are required to establish infections.
In investigations of factors affecting biocidal activity in full-scale treatment plant operations, priority should be given to studying more thoroughly the effects of turbidity (both organically and inorganically caused) on disinfection.
It should be determined if any data are available from treatment plants on the effectiveness of ozone or chlorine dioxide in disease prevention, where these methods of disinfection are not used sequentially or, in the case of ozone, with subsequent chlorination. If such data, free of complications, are available, they should be compared with the records on the efficacy of chlorination disinfection.
- Bond, W.W., M.S. Favero, and M.R. Korber. 1973. Bacillus sp. ATCC 27380: a spore with extreme resistance to dry heat . Appl. Microbiol. 26:614-616. [ PMC free article : PMC379858 ] [ PubMed : 4751804 ]
- Buchanan, R.E., editor; , and N.E. Gibbons, editor. , eds. 1974. Bergey's Manual of Determinative Bacteriology , 8th ed. Williams & Wilkins, Inc., Baltimore, Md. 1246 pp.
- Butterfield, C.T., and E. Wattie. 1946. Influence of pH and temperature on the survival of coliforms and enteric pathogens when exposed to chloramine . Public Health Rep. 61:157-192. [ PubMed : 21012646 ]
- Butterfield, C.T., E. Wattie, S. Megregian, and C.W. Chambers. 1943. Influence of pH and temperature on the survival of coliforms and enteric pathogens when exposed to free chlorine . Public Health Rep. 58:1837-1866. [ PubMed : 21012646 ]
- Carson, L.A., M.S. Favero, W.W. Bond, and NJ. Petersen. 1972. Factors affecting comparative resistance of naturally occurring and subcultured Pseudomonas aeruginosa to disinfectants . Appl. Microbiol. 23:863-869. [ PMC free article : PMC380462 ] [ PubMed : 4624209 ]
- Cramer, W.N., K. Kawata, and C.W. Krusé. 1976. Chlorination and iodination of poliovirus and f 2 . J. Water Pollut. Control Fed. 48:61-76. [ PubMed : 176476 ]
- Dahling, D., P.V. Scarpino, M. Lucas, G. Berg, and S.L. Chang. 1972. Destruction of viruses and bacteria in water by chlorine . Abstr. Ann. Meet. Am. Soc. Microbiol. Washington, D.C. Abstr. No. E152, p. 26.
- Engelbrecht, R.S., B.F. Severin, M.T. Masarik, S. Farooq, and S.H. Lee. 1975. New Microbial Indicators of Disinfection Efficiency . U.S. National Technical Information Service, AD Report 1975, AD-A030547. 88 pp.
- Fair, G.M., and J.C. Geyer. 1954. Water Supply and Waste-Water Disposal . John Wiley & Sons, New York. 973 pp.
- Favero, M.S. 1961. Comparative chlorine resistance of some bacteria of public health significance . M.S. thesis. Washington State University, Pullman, Wash.
- Favero, MS., and C.H. Drake. 1966. Factors influencing the occurrence of high numbers of iodine-resistant bacteria in iodinated swimming pools . Appl. Microbiol. 14:627-635. [ PMC free article : PMC546799 ] [ PubMed : 5927040 ]
- Favero, M.S., C.H. Drake, and G.B. Randall. 1964. Use of staphylococci as indicators of swimming pool pollution . Public Health Rep. 79:61-70. [ PMC free article : PMC1915488 ] [ PubMed : 14105731 ]
- Favero, M.S., LA. Carson, W.W. Bond, and NJ. Petersen. 1971. Pseudomonas aeruginosa : growth in distilled water from hospitals . Science 173:836-838. [ PubMed : 4999114 ]
- Favero, M.S., NJ. Petersen, L.A. Carson, W.W. Bond, and S.H. Hindman. 1975. Gram-negative water bacteria in hemodialysis systems . Health Lab. Sci. 12:321-334. [ PubMed : 1236620 ]
- Hoehn, R.C., C.W. Randall, R.P. Good, and P.T.B. Shaffer. 1977. Chlorination and water treatment for minimizing trihalomethanes in drinking water . Pp. 519-535 in R.L. Jolley, editor; , H. Gorchev, editor; , and D.H. Hamilton, Jr., editor. , eds. Water Chlorination: Environmental Impact and Health Effects, Vol. 2 . Ann Arbor Science Publishers, Inc., Ann Arbor, Mich. 909 pp.
- Hsu, Y-C. 1964. Resistance of infectious RNA and transforming DNA to iodine which inactivates f 2 phage and cells . Nature 203:152-153.
- Hsu, Y-C., S. Nomura, and C.W. Krusé. 1966. Some bactericidal and virucidal properties of iodine not affecting infectious RNA and DNA . Am. J. Epidemiol. 82:317-328. [ PubMed : 4285343 ]
- Hubbs, S.A., J.S. Zogorski, D.A. Wilding, A.N. Arbuckle, G.D. Allgier, and R.L. Mullins. 1977. Trihalomethane reduction at the Louisville Water Company . Pp. 605-611 in R.L. Jolley, editor; , H. Gorchev, editor; , and D.H. Hamilton, Jr., editor. , eds. Water Chlorination: Environmental Impact and Health Effects, Vol. 2 . Ann Arbor Science Publishers, Inc., Ann Arbor, Mich. 909 pp.
- Krusé, C.W. 1969. Mode of action of halogens on bacteria and viruses and protozoa in water systems . Final report to the Commission on Environmental Hygiene of the Armed Forces Epidemiological Board, U.S. Army Med. Res. Dev. Command. Contract No. DA-49-193-MD-2314.
- Milbauer, R., and N. Grossowicz. 1959. Effect of growth conditions on chlorine sensitivity of Escherichia coli . Appl. Microbiol. 7:71-74. [ PMC free article : PMC1057470 ] [ PubMed : 13637886 ]
- Morris, J.C. 1975. Formation of halogenated organics by chlorination of water supplies . U.S. Environmental Protection Agency, Washington, D.C. Report No. 600/1-75-002. 54 pp.
- National Academy of Sciences. 1977. Drinking Water and Health . National Academy of Sciences, Washington, D.C. 939 pp.
- Neefe, J.R., J. Stokes, Jr., J.B. Baty, and J.G. Reinhold. 1945. Disinfection of water containing causative agent of infectious (epidemic) hepatitis . J. Am. Med. Assoc. 128:1076-1080.
- Shah, P.C., and J. McCamish. 1972. Relative chlorine resistance of poliovirus 1 and coliphages f 2 and T 2 in water . Appl. Microbiol. 24:658-659. [ PMC free article : PMC380631 ] [ PubMed : 4343866 ]
- Stevens, A.A., C.J. Slocum, D.R. Seeger, and G.R. Robeck. 1975. Chlorination of organics in drinking water . Pp. 77-104 in E.R. Jolley, editor. , ed. Water Chlorination: Environmental Impact and Health Effects, Vol. 1 . Ann Arbor Science Publishers, Inc., Ann Arbor, Mich. 439 pp.
- Stringer, R.P., W.N. Cramer, and C.W. Krusé. 1975. Comparison of bromine, chlorine and iodine as disinfectants for amoebic cysts . Pp. 193-209 in J.D. Johnson, editor. , ed. Disinfection: Water and Wastewater . Ann Arbor Science Publishers, Inc, Ann Arbor, Mich. 425 pp.
- Symons, J.M., J.K. Carswell, R.M. Clark, P. Dorsey, E.E Geldreich, W.P. Heffernam, J.C. Hoff, O.T. Love, L.J. McCabe, and A.A. Stevens. 1977. Ozone, Chlorine Dioxide, and Chloramines as Alternatives to Chlorine for Disinfection of Drinking Water: State of the Art . Water Supply Research Division, U.S. Environmental Protection Agency, Cincinnati, Ohio. 84 pp.
- Wattie, E., and C.T. Butterfield. 1944. Relative resistance of Escherichia coli and Eberthella typhosa to chlorine and chloramines . Public Health Rep. 59:1661-1671.
- Analytical Reference Service. 1969. Water Chlorine (Residual) No. 1 . Report No. 35. Environmental Control Administration, Cincinnati, Ohio. 126 pp.
- Analytical Reference Service. 1971. Water Chlorine (Residual) No. 1 . Report No. 40. Environmental Control Administration, Cincinnati, Ohio. 97 pp.
- Berg, A. 1966. Virus transmission by the water vehicle, III. Removal of viruses by water treatment procedures . Health Lab. Sci. 3:170-181. [ PubMed : 5942493 ]
- Bocharov, D.A. 1970. Content of nucleic acids in bacterial suspension after influence on it of chlorinated and alkaline solutions . Tr. Vses. Nauchno-Issled. Inst. Vet. Sanit. 34:242-252.
- Bocharov, D.A., and A.V. Kulikovskii. 1971. Structural and biochemical changes of bacteria after the action of some chlorine-containing preparations, Report 1 . Tr. Vses. Nauchno-Issled. Inst. Vet. Sanit. 38:165-170.
- Brady, F.J., M.F. Jones, and W.L Newton. 1943. Effect of chlorination of water on viability of cysts of Endamoeba histolytica . War Med. 3:409-419.
- Brigano, F.A.D., P.V. Scarpino, S. Cronier, and M.L. Zink. 1978. Effect of particulates on inactivation of enteroviruses in water . Presented at U.S. Environmental Protection Agency Symposium on Wastewater Disinfection. September 18-20, 1978. Cincinnati, Ohio.
- Butterfield, C.T. 1948. Bactericidal properties of chloramines and free chlorine in water . Public Health Rep. 63:934-940. [ PubMed : 18870921 ]
- Chang, S.L. 1944. a. Destruction of micro-organisms . J. Am. Water Works Assoc. 36:1192-1207.
- Chang, S.L. 1944. b. Studies on Endamoeba histolytica. III . Destruction of cysts of Endamoeba histolytica by a hypochlorite solution, chloramines in tap water and gaseous chlorine in tap water of varying degrees of pollution . War Med. 5:46-55.
- Chang, S.L. 1971. Modern concepts of disinfection . J. Sanit. Eng. Div. Am. Soc. Civ. Eng. 97:689-707.
- Chang, S.L., and G.M. Fair. 1941. Viability and destruction of the cysts of Endamoeba histolytica . J. Am. Water Works Assoc. 33:1705-1715.
- Chang, S.L., G. Berg, N.A. Clarke, and P.W. Kabler. 1960. Survival and protection against chlorination of human enteric pathogens in free living nematodes isolated from water supplies . Am. J. Trop. Med. Hyg. 9:136-142. [ PubMed : 13809161 ]
- Clarke, N.A., and S.L. Chang. 1959. Enteric viruses in water . J. Am. Water Works Assoc. 51:1299-1317.
- Clarke, N.A., and P.W. Kabler. 1954. Inactivation of purified coxsackie virus in water by chlorine . Am. J. Hyg. 59:119-127. [ PubMed : 13124330 ]
- Clarke, N.A., R.E. Stevenson, and P.W. Kabler. 1956. The inactivation of purified type 3 adenovirus in water by chlorine . Am. J. Hyg. 64:314-319. [ PubMed : 13372525 ]
- Clarke, N.A., G. Berg, P.W. Kabler, and S.L. Chang. 1964. Human enteric viruses in water source, survival and removal and removability . Pp. 523-541 in W.W. Eckenfelder, editor. , ed. Advances in Water Pollution Research, Vol. 2, Proceedings of the 1st International Conference on Water Pollution Research, London, September 1962 . Macmillan Co., New York.
- Dennis, W.H. 1977. The mode of action of chlorine on f 2 bacterial virus during disinfection . Sc.D. thesis. School of Hygiene and Public Health, Johns Hopkins University, Baltimore, Md.
- Dorn, J.M. 1974. A comparative study of disinfection of viruses and bacteria by monochloramine . MS. thesis. University of Cincinnati, Ohio. 67 pp.
- Enders, J.F., T.H. Weller, and F.C. Robbins. 1949. Cultivation of the Lansing strain of poliovirus in cultures of various human embryonic tissues . Science 109:85-87. [ PubMed : 17794160 ]
- Engelbrecht, R.S., M.J. Weber, C.A. Schmidt and B.L. Salter. 1978. Virus Sensitivity to Chlorine Disinfection of Water Supplies . EPA 600/2-78-123. U.S. Environmental Protection Agency, Washington, D.C. 44 pp.
- Esposito, P. 1974. The Inactivation of Viruses in Water by Dichloramine . M.S. thesis. University of Cincinnati, Ohio. 121 pp.
- Esposito, P., P.V. Scarpino, S.L. Chang, and G. Berg. 1974. Destruction by dichloramine of viruses and bacteria in water . Abstr. No. G99. Abstracts of the Annual Meeting, American Society for Microbiology, Washington, D.C.
- Fair, G.M., J.C. Morris, S.L. Chang, I. Weil, and R.J. Burden. 1948. The behavior of chlorine as a water disinfectant . J. Am. Water Works Assoc. 40:1051-1061.
- Feng, T.H. 1966. Behavior of organic chloramines in disinfection . J. Water Pollut. Control Fed. 38:614-628. [ PubMed : 5326132 ]
- Fetner, R.H. 1962. Chromosome breakage in Vicia faba by monochloramine . Nature 196:1122-1123.
- Friberg, L. 1957. Further quantitative studies on the reaction of chlorine with bacteria in water disinfection. 2. Experimental investigations with Cl 36 and P 32 . Acta Pathol. Microbiol. Scand. 40:67-80. [ PubMed : 13424278 ]
- Green, D.E., and P.K. Stumpf. 1946. The mode of action of chlorine . J. Am. Water Works Assoc. 38:1301-1305.
- Haas, C.N. 1978. Mechanisms of inactivation of new indicators of disinfection efficiency by free available chlorine . Ph.D. thesis. Department of Civil Engineering, University of Illinois at Urbana-Champaign. 158 pp.
- Ingols, R.S., H.A. Wyckoff, T.W. Kethley, H.W. Hodgen, E.L. Fincher, J.C. Hildebrand, and J.E. Mandel. 1953. Bactericidal studies of chlorine . Ind. Eng. Chem. 45:996-1000.
- Kaminski, J.J., M.M. Huycke, S.H. Selk, N. Bodor, and T. Higuchi. 1976. N-halo derivatives V: Comparative antimicrobial activity of soft N-chloramine systems . J. Pharm. Sci. 65:1737-1742. [ PubMed : 25954 ]
- Kelly, S., and W.W. Sanderson. 1958. The effect of chlorine in water on enteric viruses . Am. J. Public Health 48:1323-1334. [ PMC free article : PMC1551785 ] [ PubMed : 13583275 ]
- Kelly, S.M., and W.W. Sanderson. 1960. The effect of chlorine in water on enteric viruses. II. The effect of combined chlorine on poliomyelitis and coxsackie viruses . Am. J. Public Health 50:14-20. [ PMC free article : PMC1373209 ] [ PubMed : 14405317 ]
- Knox, W.E., P.K. Stumpf, D.E. Green, and V.H. Auerbach. 1948. The inhibition of sulfhydryl enzymes as the basis of the bactericidal action of chlorine . J. Bacteriol. 55:451-458. [ PMC free article : PMC518466 ] [ PubMed : 16561477 ]
- Kulikovsky, A., H.S. Pankratz, and H.L. Sadoff. 1975. Ultrastructural and chemical changes in spores of Bacillus ceres after action of disinfectants . J. Appl. Bacteriol. 38:39-46. [ PubMed : 803940 ]
- Laubush, E.J. 1971. Chlorination and other disinfection processes . Pp. 158-224 in Water Quality and Treatment, A Handbook of Public Water Supplies , 3rd ed. Prepared by the American Water Works Association, Inc., McGraw-Hill Book Company, New York.
- Liu, O.C., H.R. Seraichekas, E.W. Akin, D.A. Brashear, EL. Katz, and W.J. Hill, Jr. 1971. Relative resistance of twenty human enteric viruses to free chlorine in Potomac water . Pp. 171-195 in Virus and Water Quality: Occurrence and Control . Proceedings of the 13th Water Quality Conference conducted by the Department of Civil Engineering, University of Illinois at Urbana-Champaign, and the U.S. Environmental Protection Agency, Urbana.
- Lothrop, T.L., and O.J. Sproul. 1969. High-level inactivation of viruses in wastewater by chlorination . J. Water Pollut. Control Fed. 41:567-575. [ PubMed : 5772755 ]
- Morris, J.C. 1970. Modern Chemical Methods, Part I, International Courses in Hydraulic and Sanitary Engineering . International Institute of Hydraulics and Environmental Engineering, Delft, Netherlands.
- Nusbaum, I. 1952. Sewage chlorination mechanism. A survey of fundamental factors . Water and Sewage Works 99:295-297.
- Olivieri, V.P., T.K. Donovan, and K. Kawata. 1971. Inactivation of virus in sewage . J. Sanit. Eng. Div. Am. Soc. Civ. Eng. 97:661-673.
- Olivieri, V.P., C.W. Krusé, Y-C. Hsu, A.C. Griffiths, and K. Kawata. 1975. The comparative mode of action of chlorine, bromine and iodine on f 2 bacterial virus . Pp. 145-162 in J. D. Johnson, editor. , ed. Disinfection: Water and Wastewater . Ann Arbor Science Publishers, Ann Arbor, Mich. 425 pp.
- Rosenkranz, H.S. 1973. Sodium hypochlorite and sodium perborate: Preferential inhibitors of DNA polymerase-deficient bacteria . Mutat. Res. 21:171-174. [ PubMed : 4582988 ]
- Scarpino, P.V., G. Berg, S.L. Chang, D. Dahling, and M. Lucas. 1972. A comparative study of the inactivation of viruses in water by chlorine . Water Res. 6:959-965.
- Scarpino, P.V., M. Lucas, D.R. Dahliing, A. Berg, and S.L. Chang. 1974. Effectiveness of hypochlorous acid and hypochlorite ion in destruction of viruses and bacteria . Pp. 359-368 in A.J. Rubin, editor. , ed. Chemistry of Water Supply, Treatment and Distribution . Ann Arbor Science Publishers, Ann Arbor, Mich.
- Shih, K.L., and J. Lederberg. 1976. a. Chloramine mutagenesis in Bacillus subtilis . Science 192:1141-1143. [ PubMed : 818709 ]
- Shih, K.L, and J. Lederberg. 1976. b. Effects of chloramine on Bacillus subtilis deoxyribonucleic acid . J. Bacteriol. 125:934-945. [ PMC free article : PMC236169 ] [ PubMed : 815252 ]
- Siders, D.L, P.V. Scarpino, M. Lucas, G. Berg, and S.L. Chang. 1973. Destruction of viruses and bacteria in water by monochloramine . Abstr. No. E27. Abstracts of the Annual Meeting American Society for Microbiology, Washington, D.C.
- Skvortsova, E.R., and N.S. Lebedeva. Cited in M.N. Bekhtereva and O.A. Krainova 1973. Changes in the activity of enzyme systems in Bacillus anthracoides spores during germination and due to the action of calcium hypochlorite . Mikrobiologiya 44:791-795.
- Standard Methods for the Examination of Water and Wastewater , 14th ed. 1976. American Public Health Association, Washington, D.C. 1193 pp.
- Stringer, R.P., W.N. Cramer, and C.W. Krusé. 1975. Comparison of bromine, chlorine and iodine as disinfectants for amoebic cysts . Pp. 193-209 in J.D. Johnson, editor. , ed. Disinfection: Water and Wastewater . Ann Arbor Science Publishers, Ann Arbor, Mich. 425 pp.
- Symons, J.M., J.K. Carswell, R.M. Clarke, P. Dorsey, E.E. Geldreich, W.P. Heffernam, J.C. Hoff, O.T. Love, L.J. McCabe, and A.A. Stevens. 1977. Ozone, Chlorine Dioxide, and Chloramines as Alternatives to Chlorine for Disinfection of Drinking Water: State of the Art . Water Supply Research Division, U.S. Environmental Protection Agency, Cincinnati, Ohio. 84 pp.
- Taras, M.J. 1953. Effect of free residual chlorination on nitrogen compounds in water . J. Am. Water Works Assoc. 45:47-61.
- Venkobachar, C. 1975. Biochemical model for chlorine disinfection . Ph.D. thesis. Department of Civil Engineering, Indian Institute of Technology at Kanpur.
- Venkobachar, C., L. Iyengar, and A.V.S.P. Rao. 1977. Mechanism of disinfection: effect of chlorine on cell membrane functions . Water Res. 11:727-729.
- Walton, G. 1969. Water treatment: prevention of waterborne disease . Pp. 21-36 in U. Weise, editor. , ed. Influence of Raw Water Characteristics on Treatment, Proceedings 11th Sanitary Engineering Conference, University of Illinois, Urbana .
- Weidenkopf, S.J. 1958. Inactivation of Type I poliomyelitis virus with chlorine . Virology 5:56-67. [ PubMed : 13519749 ]
- White, G.C. 1972. Handbook of Chlorination . Von Nostrand Reinhold Company, New York. 744 pp.
- Broadwater, W.T., R.C. Hoehn, and P.H. King. 1973. Sensitivity of three selected bacterial species to ozone . Appl. Microbiol. 26:391-393. [ PMC free article : PMC379797 ] [ PubMed : 4201643 ]
- Burleson, G.R., T.M. Murray, and M. Pollard. 1975. Inactivation of viruses and bacteria by ozone, with and without sonication . Appl. Microbiol. 29:340-344. [ PMC free article : PMC186978 ] [ PubMed : 163616 ]
- Coin, L., C. Hannoun, and C. Gomella. 1964. Ozone inactivation of poliomyelitis virus present in water . (In French) La Presse Medicale 72(38):2153-2156. [ PubMed : 14186308 ]
- Evison, L.M. 1977. Disinfection of water using ozone: comparative studies with enteroviruses, phage and bacteria . Paper presented at the 3rd Congress of the International Ozone Institute, Paris.
- Farooq, S. 1976. Kinetics of inactivation of yeasts and acid-fast organisms with ozone . Ph.D. thesis. University of Illinois, Urbana. 134 pp. ( Diss. Abstr. Int. 37B:2406B, Nov.-Dec., 1976.)
- Farooq, S., E.S.K. Chian, and R.S. Engelbrecht. 1977. a. Basic concepts in disinfection with ozone . J. Water Pollut. Control Fed. 49:1818-1831. [ PubMed : 894833 ]
- Farooq, S., R.S Engelbrecht, and E.S.K. Chian. 1977. b. The effect of ozone bubbles on disinfection . Prog. Water Technol. 9:233-247.
- Farooq, S., R.S. Engelbrecht, and E.S.K. Chian. 1977. c. Influence of temperature and U.V. light on disinfection with ozone . Water Res. 11:737-741.
- Katzenelson, E., B. Kett, and H.R. Shuval. 1974. Inactivation kinetics of viruses and bacteria in water by use of ozone . J. Am. Water Works Assoc. 66:725-729.
- Keller, J.W., R.A. Morin, and T.J. Schaffernoth. 1974. Ozone disinfection pilot plant studies at Laconia, N.H . J. Am. Water Works Assoc. 66:730-733.
- Morris, J.C. 1976. The role of ozone in water treatment . Paper 29-26 in Proceedings of the 96th Annual Conference of the American Water Works Association, Vol. 2 . New Orleans, La.
- Newton, W.L., and M.F. Jones. 1949. Effect of ozone in water on cysts of Endamoeba histolytica . Am. J. Trop. Med. 29:669-681. [ PubMed : 18141385 ]
- Peleg, M. 1976. The chemistry of ozone in the treatment of water . Water Res. 10:361-365.
- Prat, R., C. Nofre, and A. Cier. 1968. Effects of sodium hyperchlorite, ozone, and ionizing radiation on the pyrimidine constituents of Escherichia coli . (In French) Annales de L'Institut Pasteur. 114:595-607. [ PubMed : 4938484 ]
- Riesser, V.N., J.R. Perrich, E.B. Silver, and J.R. McCammon. 1977. Possible mechanisms of poliovirus inactivation by ozone . Pp. 186-192 in E.G. Fochtman, editor; , R.G. Rice, editor; , and M.F. Browning, editor. , eds. Forum on Ozone Disinfection . International Ozone Institute, Cleveland, Ohio. 435 pp.
- Rosen, A.M. 1972. Ozone generation and its economical application wastewater treatment . Water and Sewage Works 119:114-120.
- Ross, W.R., J. van Leeuwen, and W.O.K. Grabow. 1976. Studies on disinfection and chemical oxidation with ozone and chlorine in water reclamation . Pp. 497-513 and discussion, pp. 509-510, in R.G. Rice, editor; , P. Pichet, editor; , and M.A. Vincent, editor. , eds. Proceedings of the Second International Symposium on Ozone Technology, Montreal, Canada, May 11-14, 1975 . Jamesville, N.Y. Ozone Press Internationale, Cleveland, Ohio. 725 pp.
- Schecter, H. 1973. Spectrophotometric method for determination of ozone in aqueous solutions . Water Res. 7:729-739.
- Scott, D.B. McN., and E.C. Lesher. 1963. Effect of ozone on survival and permeability of Escherichia coli . J. Bacteriol. 85:567-576. [ PMC free article : PMC278184 ] [ PubMed : 14174901 ]
- Singer, P.C., and W.B. Zilli. 1975. Ozonation of ammonia: application to wastewater treatment . Pp. 269-287 in R.G. Rice, editor; and M.E. Browning, editor. , eds. Proceedings First International Symposium on Ozone for Water and Wastewater Treatment . International Ozone Institute, Waterbury, Conn. 910 pp.
- Smith, D.K. 1967. Disinfection and sterilization of polluted water with ozone . P. 52 in Proceedings of 2nd Annual Symposium on Water Research . McMasters University, Hamilton, Ontario.
- Sproul, O.J., H.A. Emerson, H.A. Howser, D.M. Boyce, D.S. Walsh, and C.E. Buck. 1978. Effects of particulate matter on virus inactivation by ozone . Presented at the 98th Annual Conference of the American Water Works Association in Atlantic City, NJ. To be published by the American Water Works Association, Denver, Colo.
- Stumm, W. 1958. Ozone as a disinfectant for water and sewage . J. Boston Soc. Civ. Eng. 45:68-79.
- U.S. Environmental Protection Agency. 1976. Interim Treatment Guide for the Control of Chloroform and Other Trihalomethanes . Water Supply Research Division, Municipal Environmental Research Laboratory, U.S. Environmental Protection Agency, Cincinnati, Ohio. 251 pp.
- Vrochinskii, K.K. 1963. Decontaminating water with ozone . (In Russian) Gig. Sanita. 28(12):3-9. (Chemical Abstracts. 60:1039d. 1964.)
- Wuhrmann, K., and Meyrath. 1955. On the bactericidal effects of aqueous ozone solutions . (In German) Schweiz. Z. Path. Bakteriol. 18:1060-1069. [ PubMed : 13311469 ]
- Adams, D.B., D.H. Jackson, and J.W. Ogleby. 1966. Determination of trace quantities of chlorine, chlorine dioxide, chlorite and chloramines in water . Proc. Soc. Water Treat. Exam. 15(2): 117-150.
- Aston, R.N. 1950. Developments in the chlorine dioxide process . J. Am. Water Works Assoc. 42:151-154.
- Bedulevich, T.S., M.N. Svetlakova, and N.N. Trakhtman. 1953. Use of chlorine dioxide in purification of water . Gig. Sanit. 10:14-17. [ PubMed : 13128317 ]
- Benarde, M.A., B.M. Israel, V.P. Olivieri, and M.L. Granstrom. 1965. Efficiency of chlorine dioxide as a bactericide . Appl. Microbiol. 13:776-780. [ PMC free article : PMC1058342 ] [ PubMed : 5325940 ]
- Benarde, M.A., W.B. Snow, and V.P. Olivieri. 1967. a. Chlorine dioxide disinfection temperature effects . J. Appl. Bacteriol. 30:159-169. [ PubMed : 5343295 ]
- Benarde, M.A., W.B. Snow, V.P. Olivieri, and B. Davidson. 1967. b. Kinetics and mechanism of bacterial disinfection by chlorine dioxide . Appl. Microbiol. 15:257-265. [ PMC free article : PMC546889 ] [ PubMed : 5339839 ]
- Cronier, S.D. 1977. Destruction by chlorine dioxide of viruses and bacteria in water . M.Sc. thesis. University of Cincinnati, Ohio. 85 pp.
- Davy, H. 1811. On a combination of oxymuriatic gas and oxygene gas . Philos. Trans. R. Soc. London 101:155-162.
- Dowling, L.T. 1974. Chlorine dioxide in potable water treatment . Water Treat. Exam. 23:190-204.
- Feuss, J.X. 1964. Problems in determination of chlorine dioxide residuals . J. Am. Water Works Assoc. 56:607-615.
- Gall, R.J. 1978. Chlorine dioxide, an overview of its preparation and uses . Pp. 356-382 in R.G. Rice, editor; and J.A. Cotruvo, editor. , eds. Ozone/Chlorine Dioxide Oxidation Products of Organic Materials . Proceedings of a Conference held in Cincinnati, Ohio, November 17-18, 1976. Sponsored by the International Ozone Institute, Inc., and the U.S. Environmental Protection Agency. Ozone Press International, Cleveland, Ohio. 487 pp.
- Gordon, G., R.G. Kieffer, and D.H. Rosenblatt. 1972. The chemistry of chlorine dioxide . Pp. 201-286 in S.J. Lippard, editor. , ed. Progress in Inorganic Chemistry, Vol. 15 , Wiley Interscience, New York.
- Granstrom, M.L., and G.F. Lee. 1958. Generation and use of chlorine dioxide in water treatment . J. Am. Water Works Assoc. 50:1453-1466.
- Haller, J.F., and S.S. Listek. 1948. Determination of chlorine dioxide and other active chlorine compounds in water . Anal. Chem. 20:639-642.
- Hettche, O., and H.W.S. Ehlbeck. 1953. Epidemiology and prophylaxis of poliomyelitis in respect of the role of water in transfer . Arch. Hyg. Bakteriol. 137:440-449. [ PubMed : 13139474 ]
- Hodgen, H.W., and R.S. Ingols. 1954. Direct colorimetric method for the determination of chlorine dioxide in water . Anal. Chem. 26:1224-1226.
- Ingols, R.S., and G.M. Ridenour. 1948. Chemical properties of chlorine dioxide in water treatment . J. Am. Water Works Assoc. 40:1207-1227.
- Issacsson, U., and G. Wettermark. 1976. The determination of inorganic chlorine compounds by chemiluminescence reactions . Anal. Chim. Acta 83:227-239.
- Masschelein, W. 1966. Spectrophotometric determination of chlorine dioxide with acid chrome violet K . Anal. Chem. 38:1839-1841.
- Masschelein, W. 1967. Developments in the chemistry of chlorine dioxide and its applications . Chim. Ind. Genie Chim. 97:49-61.
- McCarthy, J.A. 1944. Bromine and chlorine dioxide as water disinfectants . J. N. Engl. Water Works Assoc. 58:55-68.
- McCarthy, J.A. 1945. Chlorine dioxide for the treatment of water supplies . J. N. Engl. Water Works Assoc. 59:252-264.
- Miltner, R.J. 1976. The Effect of Chlorine Dioxide on Trihalomethanes in Drinking Water . M.S. thesis. University of Cincinnati, Ohio. 88 pp.
- Moffa, P.E., E.C. Tifft, S.L. Richardson, and J.E. Smith. 1975. Bench-scale High Rate Disinfection of Combined Sewer Overflows with Chlorine and Chlorine Dioxide . U.S. Environmental Protection Agency Report No. EPA-670/2-75-021. Washington, D.C. 193 pp.
- Myhrstad, J.A., and J.E. Samdal. 1969. Behavior and determination of chlorine dioxide . J. Am. Water Works Assoc. 61:205-208.
- Olivieri, V.P. 1968. Chlorine dioxide and protein synthesis . M.S. thesis. West Virginia University, Morgantown. 63 pp.
- Palin, A.T. 1957. The determination of free and combined chlorine in water by the use of diethyl-p-phenylene diamine . J. Am. Water Works Assoc. 49:873.
- Palin, A.T. 1960. Colorimetric determination of chlorine dioxide in water . Water and Sewage Works 107:457-478.
- Palin, A.T. 1967. Methods for the determination in water of free and combined available chlorine, chlorine dioxide and chlorite, bromine, iodine and ozone using diethyl-p-phenylene diamine (DPD) . J. Inst. Water Eng. 21:537-547.
- Palin, A.T. 1970. Determining chlorine dioxide and chlorite . J. Am. Water Works A Palin, A.T. 1974. Analytical control of water disinfection with special reference to differential DPD methods for chlorine, chlorine dioxide, bromine, iodine, and ozone . J. Inst. Water Eng. 28:139-154.
- Palin, A.T. 1974. Analytical control of water disinfection with special reference to differential DPD methods for chlorine, chlorine dioxide, bromine, iodine, and ozone . J. Inst. Water Eng. 28:139-154.
- Palin, A.T. 1975. Current DPD methods for residual halogen compounds and ozone in water . J. Am. Water Works Assoc. 67:32-33.
- Post, M.A., and W.A. Moore. 1959. The determination of chlorine dioxide in treated surface waters . Anal. Chem. 31:1872-1874.
- Ridenour, G.M., and E.M. Armbruster. 1949. Bactericidal effects of chlorine dioxide . J. Am. Water Works Assoc. 41:537-550.
- Ridenour, G.M., and R.S. Ingols. 1946. Inactivation of poliomyelitis virus by "free" chlorine . Am. J. Public Health 36:639-44. [ PMC free article : PMC1625779 ] [ PubMed : 18016367 ]
- Ridenour, G.M., and R.S. Ingols. 1947. Bactericidal properties of chlorine dioxide . J. Am. Water Works Assoc. 39:561-567.
- Ridenour, G.M., R.S. Ingols, and E.H. Armbruster. 1949. Sporicidal properties of chlorine dioxide . Water and Sewage Works 96:279-283. [ PubMed : 18134396 ]
- Rosenblatt, D.H. 1978. Chlorine dioxide: chemical and physical properties . Pp. 332-343 in R.G. Rice, editor; and J.A. Cotruvo, editor. , eds. Ozone/Chlorine Dioxide Products of Organic Materials . Proceedings of a Conference held in Cincinnati, Ohio, November 17-19, 1976. Sponsored by the International Ozone Institute, Inc., and the U.S. Environmental Protection Agency. Ozone Press International, Cleveland, Ohio. 487 pp.
- Scarpino, P.V., S.D. Cronier, M.L. Zink, F.A.O. Brigano, and J.C. Hoff. 1977. Effect of particulates on disinfection of enterovirus and coliform bacteria in water by chlorine dioxide . Water Quality Technology Conference—Water Quality in the Distribution System. Kansas City, Mo. 19 pp.
- Schilling, K. 1956. Chlordioxyd in der Wasseraufbereitung . Wasser 23:95-101.
- Stevens, A.A., D.R. Seeger, and C.J. Slocum. 1978. Products of chlorine dioxide treatment of organic materials in water . Pp. 383-395 in R.G. Rice, editor; and J.A. Cotruvo, editor. , eds. Ozone/Chlorine Dioxide Products of Organic Materials . Proceedings of a Conference held in Cincinnati, Ohio, November 17-19, 1976. Sponsored by the International Ozone Institute, Inc., and the U.S. Environmental Protection Agency. Ozone Press International, Cleveland, Ohio. 487 pp.
- Stevenson, R.G., Jr., L.L. Dailey, and B.J. Ratigan. 1978. The continuous analysis of chlorine dioxide in process solutions . Pp. 23-24 in Preprints of Papers presented at the 175th National Meeting of the Environmental Chemistry Division, American Chemical Society, Anaheim, Calif., March 12-17, 1978.
- Sussman, S. 1978. Use of chlorine dioxide in water and wastewater treatment . Pp. 344-355 in R.G. Rice, editor; and J.A. Cotruvo, editor. , eds. Ozone/Chlorine Dioxide Oxidation Products of Organic Materials . Proceedings of a Conference held in Cincinnati, Ohio, November 1719, 1976. Sponsored by the International Ozone Institute, Inc., and the U.S. Environmental Protection Agency. Ozone Press International, Cleveland, Ohio. 487 pp.
- Symons, J.M., J.K. Carswell, R.M. Clark, P. Dorsey, E.E. Geldreich, W.P. Heffernam, J.C. Hoff, O.T. Love, L.J. McCabe, and A.A. Stevens. 1977. Ozone, Chlorine Dioxide and Chloramines as Alternatives to Chlorine for Disinfection of Drinking Water: State of the Art . Water Supply Research Division, U.S. Environmental Protection Agency, Cincinnati, Ohio. 84 pp.
- Synan, J.F., J.D. MacMahon, and G.P. Vincent. 1945. Chlorine dioxide in potable water treatment . Water Eng. 48:285-286.
- Trakhtman, N.N. 1946. Chlorine dioxide in water disinfection . Gig. Sanit. 11(10): 10-13.
- U.S. Environmental Protection Agency. 1978. Interim primary drinking water regulations: control of organic chemical contaminants in drinking water . Fed. Reg. 43(28):5756-5780.
- Warriner, T.R. 1967. Inaktwering av Poliovirus med Klordioxid . Institute for Vattenforsorgning och Avlopp, Publikation A67:284-290.
- Watt, C., and H. Burgess. 1854. Improvement in the manufacture of paper from wood . U.S. Patent No. 11,343.
- Allen, T.L., and R.M. Keefer. 1955. The formation of hypoiodous acid and hydrated iodine cation by the hydrolysis of iodine . J. Am. Chem. Soc. 77:2957-2960.
- Bell, R.P., and E. Gelles. 1951. The halogen cations in aqueous solution . J. Chem. Soc. Pt. III 2734-2740.
- Berg, G., S.L. Chang, and E.K. Harris. 1964. Devitalization of microorganisms by iodine. I. Dynamics of the devitalization of enteroviruses by elemental iodine . Virology 22:469-481. [ PubMed : 14166106 ]
- Black, A.P., J.B. Lackey, and E.W. Lackey. 1959. Effectiveness of iodine for the disinfection of swimming pool water . Am. J. Public Health 49:1060-1068. [ PMC free article : PMC1372877 ] [ PubMed : 13801138 ]
- Black, A.P., R.N. Kinman, W.C. Thomas, Jr., G. Freund, and E.D. Bird. 1965. Use of iodine for disinfection . J. Am. Water Works Assoc. 57:1401-1421.
- Black, A.P., W.C. Thomas, Jr., R.N. Kinman, W.P. Bonner, M.A. Keirn, J.J. Smith, Jr., and A.A. Jabero. 1968. Iodine for the disinfection of water . J. Am. Water Works Assoc. 60:69-83.
- Brammer, K.W. 1963. Chemical modification of viral ribonucleic acid. II. Bromination and iodination . Biochim. Biophys. Acta 72:217-229. [ PubMed : 14014915 ]
- Chambers, C.W., P.W. Kabler, G. Malaney, and A. Bryant. 1952. Iodine as a bactericide . Soap Sanit. Chem. 28(10): 149-151, 153,, 163,, 165.
- Chang, S.L. 1958. The use of active iodine as a water disinfectant . J. Am. Pharm. Assoc. 47:417-423. [ PubMed : 13549285 ]
- Chang, S.L., and J.C. Morris. 1953. Elemental iodine as a disinfectant for drinking water . Ind. Eng. Chem. 45:1009-1012.
- Chang, S.L., and M. Baxter. 1955. Studies on the destruction of cysts of Endamoeba histolytica. I. Establishment of the order of reaction in destruction of cysts of E. histolytica by elemental iodine and silver nitrate . Am. J. Hyg. 61:121-132. [ PubMed : 13228419 ]
- Chang, S.L., M. Baxter, and L. Eisner. 1955. Studies on the destruction of Endamoeba histolytica. II. Dynamics of destruction of cysts of E. histolytica in water by tri-iodine ion . Am. J. Hyg. 61:133-141. [ PubMed : 13228420 ]
- Fraenkel-Conrat, H. 1955. The reaction of tobacco mosaic virus with iodine . J. Biol. Chem. 217:373-381. [ PubMed : 13271401 ]
- Gershenfeld, L. 1977. Iodine . Pp. 196-218 in S.S. Block, editor. , ed. Disinfection, Sterilization, and Preservation , 2nd ed. Lea & Febiger, Philadelphia, Pa.
- Hsu, Y-C., S. Normura, and C.W. Krusé. 1965. Some bactericidal and viricidal properties of iodine not affecting infectious RNA and DNA . Am. J. Epidemiol. 82:317-328. [ PubMed : 4285343 ]
- Hughes, W.L. 1957. The chemistry of iodination . Ann. N.Y. Acad. Sci. 70:3-18. [ PubMed : 13488250 ]
- Krusé, C.W. 1969. Mode of action of halogens on bacteria and viruses and protozoa in water systems . Pp. 1-89 in Final report to the Commission on Environmental Hygiene of the Armed Forces Epidemiological Board, U.S. Army Med. Res. Dev. Command Contract No. DA-49-193-MD-2314.
- Li, C.H. 1942. Kinetics and mechanism of 2,6-di-iodotryosine formation . J. Am. Chem. Soc. 64:1147-1152.
- Li, C.H. 1944. Kinetics of reactions between iodine and histidine . J. Am. Chem. Soc. 66:225-227.
- Longley, K.E. 1964. Some aspects of iodination of bacteriophage f 2 . MSE essay. The Johns Hopkins University, Baltimore, Md.
- Marks, H.C., and F.B. Strandskov. 1950. Halogens and their mode of action . Ann. N.Y. Acad. Sci. 53:163-171. [ PubMed : 15433172 ]
- O'Connor, J.T., and S.K. Kapoor. 1970. Small quantity field disinfection . J. Am. Water Works Assoc. 62(2):80-84.
- Stringer, R.P. 1970. Amoebic cysticidal properties of halogens in water . Sc.D. thesis. Johns Hopkins University, Baltimore, Md.
- Stringer, R., and C.W. Krusé. 1971. Amoebic cysticidal properties of halogens in water . J. Sanit. Eng. Div. Am. Soc. Civ. Eng. 97:801-811.
- Stringer, R.P., W.N. Cramer, and C.W. Krusé. 1975. Comparison of bromine, chlorine and iodine as disinfectants for amoebic cysts . Pp. 193-209 in J.D. Johnson, editor. , ed. Disinfection: Water and Wastewater . Ann Arbor Science Publishers, Inc., Ann Arbor, Mich. 425 pp.
- Wallis, C., A.M. Behbehani, L.H. Lee, and M. Bianchi. 1963. The ineffectiveness of organic iodine (Wescodyne) as a viral disinfectant . Am. J. Hyg. 78:325-329. [ PubMed : 14071355 ]
- Wyss, O., and F.B. Strandskov. 1945. The germicidal action of iodine . Arch. Biochem. 6:261-268.
- Farkas, L, and M. Lewin. 1950. The dissociation constant of hypobromous acid . J. Am. Chem. Soc. 72:5766-5767.
- Farkas-Hinsley, H. 1966. Disinfection . Pp. 554-562 in Z.E. Jolles, editor. , ed. Bromine and Its Compounds . Academic Press, New York. 940 pp.
- Floyd, R., J.D. Johnson, and D.G. Sharp. 1976. Inactivation by bromine of single poliovirus particles in water . Appl. Environ. Microbiol. 31:298-303. [ PMC free article : PMC169763 ] [ PubMed : 11745 ]
- Floyd, R., D.G. Sharp, and J.D. Johnson. 1978. Inactivation of single poliovirus particles in water by hypobromite ion, molecular bromine, dibromamine, and tribromamine . Environ. Sci. Tech. 12:1031-1035.
- Galal-Gorchev, H., and J.C. Morris. 1965. Formation and stability of bromamide, bromimide, and nitrogen tribromide in aqueous solution . Inorg. Chem. 4:899-905.
- Henderson, C.T. 1935. Process of antisepticizing water . U.S. Patent No. 1995639.
- Inman, G.W., Jr., T.F. LaPointe, and J.D. Johnson. 1976. Kinetics of nitrogen tribromide decomposition in aqueous solution . Inorg. Chem. 15:3037-3042.
- Johannesson, J.K. 1958. Anomalous bactericidal action of bromamine . Nature 181:1799-1800. [ PubMed : 13566147 ]
- Johnson, J.D., and R. Overby. 1971. Bromine and bromamine disinfection chemistry . J. Sanit. Eng. Div. Am. Soc. Civ. Eng. 97:617-628.
- Kanyaev, N.P., and E.A. Shilov. 1940. Constants of some equilibrium reactions of hypobromous acid . Tr. Ivanov. Khim. Tekhnol. Inst. (USSR) 3:69-73.
- Kolthoff, I.M., and R. Belcher. 1957. Volumetric Analysis . Interscience Pub., New York. 714 pp.
- Krusé, C.W., Y. Hsu, A.C. Griffiths, and R Stringer. 1970. Halogen action on bacteria, viruses, and protozoa . Pp. 113-136 in Proceedings of the National Specialty Conference on Disinfection . American Society of Civil Engineers, New York.
- Liebhafsky, H.A. 1934. The equilibrium constant of the bromine hydrolysis and its variation with temperature . J. Am. Chem. Soc. 56:1500-1505.
- Mills, J.F. 1969. The control of microorganisms with polyhalide resins . U.S. Patent No. 3462363.
- Mills, J.F. 1975. Interhalogens and halogen mixtures as disinfectants . Pp. 113-143 in J.D. Johnson, editor. , ed. Disinfection: Water and Wastewater . Ann Arbor Science Publishers, Inc., Ann Arbor, Mich. 425 pp.
- Morris, J.C 1975. Aspects of the quantitative assessment of germicidal efficiency . Pp. 1-10 in J.D. Johnson, editor. , ed. Disinfection: Water and Wastewater . Ann Arbor Science Publishers, Inc., Ann Arbor, Mich. 425 pp.
- Olivieri, V.P., C.W. Krusé, Y.C. Hsu, A.C. Griffiths, and K. Kawata. 1975. The comparative mode of action of chlorine, bromine, and iodine on f 2 bacterial virus . Pp. 145-162 in J.D. Johnson, editor. , ed. Disinfection: Water and Wastewater . Ann Arbor Science Publishers, Inc., Ann Arbor, Mich. 425 pp.
- Sharp, D.G., R. Floyd, and J.D. Johnson. 1975. Nature of the surviving plaque forming unit of reovirus in water containing bromine . Appl. Microbiol. 29:94-101. [ PMC free article : PMC186917 ] [ PubMed : 1167388 ]
- Sharp, D.G., R. Floyd, and J.D. Johnson. 1976. Initial fast reaction of bromine on reovirus in turbulent flowing water . Appl. Environ. Microbiol. 31:173-181. [ PMC free article : PMC169744 ] [ PubMed : 11743 ]
- Stringer, R.P., W.N. Cramer, and C.W. Krusé. 1975. Comparison of bromine, chlorine, and iodine as disinfectants for amoebic cysts . Pp. 193-209 in J.D. Johnson, editor. , ed. Disinfection: Water and Wastewater . Ann Arbor Science Publishers, Inc., Ann Arbor, Mich. 425 pp.
- Tanner, F.W., and G. Pitner. 1939. Germicidal action of bromine . Proc. Soc. Exp. Biol. Med. 40:143-145.
- Taylor, D.G., and J.D. Johnson. 1974. Kinetics of viral inactivation by bromine . Pp. 369-408 in A.J. Rubin, editor. , ed. Chemistry of Water Supply, Treatment, and Distribution . Ann Arbor Science Publishers, Inc., Ann Arbor, Mich.
- Wyss, O., and J.R. Stockton. 1947. The germicidal action of bromine . Arch. Biochem. 12:267-271. [ PubMed : 20283578 ]
- Audette, R.J., R.J. Quail, and P.J. Smith. 1971. Ferrate (VI) ion, a novel oxidizing agent . Tetrahedron Lett. 3:279-282.
- Becarud, N. 1966. Analytical Study of Ferrates . Comm. Energie at France Rapt. No. 2895. 52 pp.
- Fremy, E.G. 1841. Studies of the action of alkaline peroxides on metal oxides . (In French) C.R. Acad. Sci. Ser. A 12:23-24.
- Gilbert, M.D., T.D. Waite, and C. Hare. 1976. An investigation of the applicability of ferrate ion for disinfection . J. Am. Water Works Assoc. 68:495-497.
- Murmann, R.K. 1974. The preparation and oxidative properties of ferrate (FeO 42 - ) . Studies directed towards its use as a water purifying agent. NTIS publication; PB Rept. 238-057. National Technical Information Service, Springfield, Va.
- Schreyer, J.M., and L.T. Ockerman. 1951. Stability of the ferrate (VI) ion in aqueous solution . Anal. Chem. 23:1312-1314.
- Schreyer, J.M., G.W. Thompson, and L.T. Ockerman. 1950. Ferrate oxidimetry. Oxidation of arsenite with potassium ferrate (VI) . Anal. Chem. 22:691-692.
- Strong, A.W. 1973. An Exploratory Work on the Oxidation of Ammonia by Potassium Ferrate (VI) . M.S thesis. Department of Chemical Engineering [Rept. OWRR-A-031-OHIO(2)] Ohio State University, Columbus. NTIS publication; PB-231 873. National Technical Information Service, Springfield, Va.
- Wagner, W.F., J.R. Gump, and E.N. Hart. 1952. Factors affecting the stability of aqueous potassium ferrate (VI) solutions . Anal. Chem. 24:1497-1498.
- Waite, T.D. 1978. a. Management of Wastewater Residuals with Iron (VI) Ferrate . First Annual Report Grant # ENV 76-83897. National Science Foundation: RANN, Washington, D.C. 207 pp.
- Waite, T.D. 1978. b. Inactivation of Salmonella sp., Shigella sp. , Streptococcus sp., and f2 virus by Iron (VI) Ferrate . Paper 33-4 presented at the Annual Meeting of the American Water Works Association, Atlantic City, NJ. 1978 Annual Conference Proceedings. American Water Works Association, Denver, Colo.
- Wood, R.H. 1958. The heat, free energy and entropy of the ferrate (VI) ion . J. Am. Chem. Soc. 80:2038-2041.
- Zhdanov, Y.A., and O.A. Pustovarova. 1967. Oxidation of alcohols and aldehydes by potassium ferrate . Zh. Onsch. Khim. 37(12):2780.
- Berg, G., and D. Berman. 1967. Final Report of Progress on Quarterly Contract AMXREC 66-51 and First Quarterly Report on Quartermaster Contract AMXRED 67-54 on Effectiveness of Military Phenolic Dry-Type Disinfectant (MIL-D-51061) Against Viruses . Federal Water Pollution Control Administration, Cincinnati, Ohio.
- Boeye, A., and A. Van Elsen. 1967. Alkaline disruption of poliovirus: kinetics and purification of RNA-free particles . Virology 33:335-343. [ PubMed : 4293235 ]
- Donovan, T.K. 1972. Virus inactivation associated with lime precipitation of phosphate from sewage . D.Sc. thesis. Johns Hopkins University, Baltimore, Md.
- Maizel, J.V., Jr., B.A. Phillips, and D.F. Summers. 1967. Composition of artificially produced and naturally occurring empty capsids of poliovirus type 1 . Virology 32:692-699. [ PubMed : 18614069 ]
- Riehl, M.L., H.H. Weiser, and B.T. Rheins. 1952. Effect of lime-treated water upon survival of bacteria . J. Am. Water Works Assoc. 44:466-470.
- Sproul, O.J. 1975. Investigation to Increase the Viricidal Capacity of Disinfectant, Germicidal and Fungicidal Phenolic, Dry Type . Technical Report TR 76-90 FSL, Department of Civil Engineering, University of Maine, Orono. 46 pp.
- Sproul, O.J., R.T. Thorup, D.F. Wentworth, and J.S. Atwell. 1970. Salt and virus inactivation by chlorine and high pH . Pp. 385-396 in Proceedings of the National Specialty Conference of Disinfection . American Society of Civil Engineers, New York.
- Standard Methods for the Examination of Water and Wastewater , 11th ed., p. 485. 1960. American Public Health Association, Inc., New York.
- Van Elsen, A., and A. Boeye. 1966. Disruption of type 1 poliovirus under alkaline conditions: role of pH, temperature and sodium dodecyl sulfate (SDS) . Virology 28:481-483. [ PubMed : 18611483 ]
- Wattie, E., and C.W. Chambers. 1943. Relative resistance of coliform organisms and certain enteric pathogens to excess-lime treatment . J. Am. Water Works Assoc. 35:709-720.
- Wentworth, D.F., R.T. Thorup, and O.J. Sproul. 1968. Poliovirus inactivation in water softening precipitation processes . J. Am. Water Works Assoc. 60:939-946.
- Chadwick, A.F., and G.L.K. Hoh. 1966. Hydrogen peroxide . Pp. 319-417 in Kirk-Othmer Encyclopedia of Chemical Technology, Vol. 11 , 2nd ed. Wiley Interscience Publishers, New York.
- Gasset, A.R., R.M. Ramer, and D. Katzin. 1975. Hydrogen peroxide sterilization of hydrophilic contact lenses . Arch. Ophthamol. 93:412-415. [ PubMed : 805580 ]
- Lund, E. 1963. Significance of oxidation in chemical inactivation of poliovirus . Arch. Gesamte Virusforsch. 12:648-660. [ PubMed : 13931660 ]
- Mentel, R., and J. Schmidt. 1973. Investigations on rhinovirus inactivation by hydrogen peroxide . Acta Virol. 17:351-354. [ PubMed : 4148216 ]
- Schumb, W.C., C.N. Satterfield, and R.L. Wentworth. 1955. Stabilization . Pp. 515-547 in Hydrogen Peroxide . Reinhold Publishing Corporation, New York.
- Sezgin, M., D. Jenkins, and D.S. Parker. 1978. A unified theory of filamentous activated sludge bulking . J. Water Pollut. Control Fed. 50:362-381.
- Snell, F.D., and C.T. Snell. 1949. Colorimetric Methods of Analysis: Including some Turbidimetric and Nephelometric Methods , 3rd ed. D. Van Nostrand Company, New York. 5 Vols.
- Spaulding, E.H., K.R. Cundy, and F.J. Turner. 1977. Chemical disinfection of medical and surgical materials . Pp. 654-684 in S.S. Block, editor. , ed. Disinfection, Sterilization, and Preservation , 2nd ed. Lea & Febiger, Philadelphia, Pa.
- Taki, M., and R. Hashimoto. 1977. Sterilization of biologically treated water. I. Sterilizing effects of chlorine and hydrogen peroxide . Mizu Shori Gijutsu (Jap.) 18(2):149-156.
- Toledo, R.T. 1975. Chemical sterilants for aseptic packaging . Food Technol. 29:102, 104,, 105,, 108,, 110,, 112.
- Toledo, R.T., F.E. Escher, and J.C. Ayres. 1973. Sporicidal properties of hydrogen peroxide against food spoilage organisms . Appl. Microbiol. 26:592-597. [ PMC free article : PMC379855 ] [ PubMed : 16349974 ]
- Wardle, M.D., and G.M. Renninger. 1975. Bactericidal effect of hydrogen peroxide on spacecraft isolates . Appl. Microbiol. 30:710-711. [ PMC free article : PMC187255 ] [ PubMed : 1190768 ]
- Yoshpe-Purer, Y., and E. Eylan. 1968. Disinfection of water by hydrogen peroxide . Health Lab. Sci. 5:233-238. [ PubMed : 4880129 ]
- Ballantine, D.S., L.A. Miller, D.F. Bishop, and F.A. Rohrman. 1969. The practicality of using atomic radiation for wastewater treatment J. Water Pollut. Control. Fed. 41:445-458.
- Brannan, J.P., D.M. Garst, and S. Langley. 1975. Inactivation of Ascaris lumbricoides eggs by heat, radiation, and thermoradiation . Sandia Laboratories Report Sand. 75-0163. Albuquerque, N.Mex. 26 pp.
- Compton, D.M.J., W.L. Whittemore, and S.J. Black. 1970. An evaluation of the applicability of ionizing radiation to the treatment of municipal waste waters and sewage sludge . Trans. Am. Nucl. Soc. 13:71-72.
- Dunn, C.G. 1953. Treatment of water and sewage by ionizing radiations . Sewage Ind. Wastes 25:1277-1281.
- Eliassen, R., and J.G. Trump. 1973. High Energy Electron Treatment of Wastewater and Sludge . Paper presented at 45th Annual Meeting of the California Water Pollution Control Association, San Diego.
- Lea, D.E. 1955. Actions of Radiations on Living Cells , 2nd ed. Cambridge University Press, London.
- Lowe, H.N., Jr., W.J. Lacy, B.F. Surkiewicz, and R.F. Jaeger. 1956. Destruction of microorganisms in water, sewage, and sewage sludge by ionizing radiations . J. Am. Water Works Assoc. 48:1363-1372.
- Massachusetts Institute of Technology. 1977. High Energy Electron Radiation of Wastewater Liquid Residuals . Report to US. National Science Foundation. NSF Grant ENV 74 13016, Dec. 31, 1977. National Science Foundation, Washington, D.C.
- Ridenour, G.M., and E.H. Armbruster. 1956. Effect of high-level gamma radiation on disinfection of water and sewage . J. Am. Water Works Assoc. 48:671-676.
- Silverman, G.F., and A.J. Sinskey. 1977. Sterilization by ionizing radiation . Pp. 542-567 in S.S. Block, editor. , ed. Disinfection, Sterilization, and Preservation , 2nd ed. Lea & Febiger, Philadelphia, Pa.
- Wright, K.A., and J.G. Trump. 1956. High energy electrons for the irradiation of blood derivatives . Pp. 230-239 in Proc. 6th Congress of the International Society of Blood Transfusion . Supplement to Acta Haematologica, 1956.
- American Water Works Association. 1971. Water Quality and Treatment: A Handbook of Public Water Supplies , 3rd ed. McGraw-Hill Book Company, New York. 654 pp.
- Cleasby, J.L., E.R. Baumann, and C.D. Black. 1964. Effectiveness of potassium permanganate for disinfection . J. Am. Water Works Assoc. 56:466-474.
- Derbyshire, J.B., and S. Arkell. 1971. Activity of some chemical disinfectants against Talfan virus and porcine adenovirus type 2 . Br. Vet. J. 127(3): 137-142. [ PubMed : 4323383 ]
- Eskarous, J.K., and H.M. Habib. 1972. Effect of potassium permanganate and toluquinone on mosaic symptoms and necrosis caused by tomato streak virus on tobacco . Adv. Front. Plant Sci. 29:125-169.
- Fitzgerald, G.P. 1964. Laboratory evaluation of potassium permanganate as an algicide for water reservoirs . Southwest Water Works J. 45(10): 16-17.
- Heuston, K.H. 1972. Tablets for the sterilization of water . Ger. Offen. 2:303, 364.
- Hughes, C.G., and D.R.L. Steindl. 1955. Ratoon stunting disease of sugar cane . Bur. Sugar Exp. Stn., Brisbane, Tech. Commun. No. 2:1-54.
- Kemp, H.T., R.G. Fuller, and R.S. Davidson. 1966. Potassium permanganate as an algicide . J. Am. Water Works Assoc. 58:255-263.
- Lund, E. 1963. Oxidative inactivation of poliovirus at different temperatures . Arch. Ges. Virusforsch. 13:375-386. [ PubMed : 14046083 ]
- Lund, E. 1966. Oxidative inactivation of adenovirus . Arch. Ges. Virusforsch. 19:32-37.
- Peretts, L.G., O.V. Bychkovskaia, M.A. Bazhedomova, N.S. Babina, and N.S. Semenova. 1960. Effect of potassium permanganate on poliomyelitus virus . Probl. Virol. 5:441-447.
- Schultz, E.W., and F. Robinson. 1942. The in vitro resistance of poliomyelitus virus to chemical agents . J. Infect. Dis. 70:193-200.
- Seidel, K. 1973. Purification of swimming pool water . Ger. Offen. 2:141, 620.
- Shull, K.E. 1962. Operating experiences at Philadelphia suburban treatment plants . J. Am. Water Works Assoc. 54:1232-1240.
- Spicher, R.G., and R.T. Skrinde. 1963. Potassium permanganate oxidation of organic contaminants in water supplies . J. Am. Water Works Assoc. 55:1174-1194.
- Wagner, R.R. 1951. Studies on the inactivation of influenza virus. Comparison of the effects of p-benzoquinone and various inorganic oxidizing agents . Yale J. Biol. Med. 23:288-298. [ PMC free article : PMC2599070 ] [ PubMed : 14818118 ]
- Welch, WA. 1963. Potassium permanganate in water treatment . J. Am. Water Works Assoc. 55:735-741.
- Chambers, C.W., and C.M. Proctor. 1960. The Bacteriological and Chemical Behavior of Silver in Low Concentrations . Robert A. Taft Sanitary Engineering Center, Tech. Rep. W604, U.S. Department of Health, Education, and Welfare. 18 pp.
- Chang, S.L., and M. Baxter. 1955. Studies on destruction of cysts of Entamoeba histolytica. I. Establishment of the order of reaction in destruction of cysts of E. histolytica by elemental iodine and silver nitrate . Am. J. Hyg. 61:121-132. [ PubMed : 13228419 ]
- Chang, S.L. 1970. Modern concept of disinfection . Pp. 635-681 in Proceedings of the National Specialty Conference on Disinfection . American Society of Civil Engineers, New York.
- Davies, R.L. 1976. Improved circulation systems use silver to maintain pool water . Swimming Pool Weekly and Swimming Pool Age, Data and Reference Annual 50 .
- Fair, G.M. 1948. Water disinfection and allied subjects . Pp. 520-531 in E.C. Andrus, editor; , D.W. Bronk, editor; , G.A. Garden, Jr., editor; , C.S. Keefer, editor; , J.S. Lockwood, editor; , J.T. Wearn, editor; , and M.C. Winternitz, editor. , eds. Advances in Military Medicine, Vol. 2 . Little, Brown and Company, Boston, Mass.
- Grier, N. 1977. Silver and its compounds . Pp. 395-407 in S.S. Block, editor. , ed. Disinfection, Sterilization, and Preservation , 2nd ed. Lea & Febiger, Philadelphia, Pa.
- Harrison, C.J. 1947. Purification of tea-estate water supplies . Indian Tea Association, Tockai Experimental Station, Memo 18. 10 pp.
- Just, J., and A. Szniolis. 1936. Germicidal properties of silver in water . J. Am. Water Works Assoc. 28:492-506.
- Lund, E. 1963. Significance of oxidation in chemical inactivation of poliovirus . Arch. Ges. Virusforsch. 12:648-660. [ PubMed : 13931660 ]
- Newton, W.L., and M.F. Jones. 1949. Effectiveness of silver ions against cysts of Entamoeba histolytica . J. Am. Water Works Assoc. 41:1027-1034.
- Rattonetti, A. 1974. Determination of soluble cadmium, lead, silver, and indium in rainwater and stream water with the use of flameless atomic absorption . Anal. Chem. 46:739-742. [ PubMed : 4825965 ]
- Renn, C.E., and W.E. Chesney. 1953-1956. Reports to Salem-Brosius, Inc., on Research on Hyla System of Water Disinfection.
- Romans, I.B. 1954. Oligodynamic metals . Pp. 388-428 in G.F. Reddish, editor. , ed. Antiseptics, Disinfectants, Germicides, and Chemical and Physical Sterilization . Lea & Febiger, Philadelphia, Pa.
- U.S. Environmental Protection Agency. 1974. Methods for Chemical Analysis of Water and Wastes . EPA-625/6-74-003. U.S. Environmental Protection Agency, Washington, D.C.
- U.S. Environmental Protection Agency. 1975. Interim primary drinking water regulations . Fed. Reg. 40(51): 11989-11998.
- Woodward, R.L. 1963. Review of the bactericidal effectiveness of silver . J. Am. Water Works Assoc. 55:881-886.
- Wuhrmann, K., and F. Zobrist. 1958. Bactericidal effect of silver in water . Schweiz. Z. Hydrol. 20:218-254.
- Yainskii, A.V., and V.F. Kuznetsova. 1973. Disinfection of water containing vibrios by silver ions . Aktual. Vopr. Sanit. Mikrobiol. 112-113.
- Zimmermann, W. 1952. Oligodynamic silver action. I. The action mechanism Z . Hyg. Infektionskr. 135:403-413. [ PubMed : 13039351 ]
- Anonymous. 1960. Germicidal Lamps and Applications . General Electric Bulletin LS-179. 15 pp.
- Calvert, J.G., and J.N. Pitts, Jr. 1966. Photochemistry . John Wiley, New York. 890 pp.
- Childs, C.B. 1962. Low-pressure mercury arc for ultraviolet calibration . Appl. Opt. 1:711-716.
- Cortelyou, J.R., M.A. McWhinnie, M.S. Riddiford, and J.E. Semrad. 1954. Effects of ultraviolet irradiation on large populations of certain water-borne bacteria in motion. I. The development of adequate agitation to provide an effective exposure period . Appl. Microbiol. 2:227-235. [ PMC free article : PMC1056998 ] [ PubMed : 13181406 ]
- Deering, R.A., and R.B. Setlow. 1963. Effects of ultraviolet light on thymidine dinucleotide and polynucleotide . Biochim. Biophys. Acta 68:526-534. [ PubMed : 14026389 ]
- Gates, F.L. 1929. A study of the bactericidal action of ultraviolet light II. The effect of various environmental factors and conditions . J. Gen. Physiol. 13:249-260. [ PMC free article : PMC2141035 ] [ PubMed : 19872522 ]
- Harm, W. 1968. Effects of dose fractionation on ultraviolet survival of Escherichia coli . Photochem. Photobiol. 7:73-86. [ PubMed : 4866689 ]
- Hoather, R.C. 1955. The penetration of ultra-violet radiation and its effects in waters . J. Inst. Water Eng. 9:191-207.
- Huff, C.B., H.F. Smith, W.D. Boring, and NA. Clarke. 1965. Study of ultraviolet disinfection of water and factors in treatment efficiency . Public Health Rep. 80:695-705. [ PMC free article : PMC1919663 ] [ PubMed : 14318944 ]
- Jagger, J. 1960. Photoreactivation . Radiat. Res. Suppl. 2:75-90. [ PubMed : 14406518 ]
- Kawabata, T., and T. Harada 1959. J. Illumination Society 36:89.
- Kelner, A. 1949. Photoreactivation of ultraviolet-irradiated Escherchia coli , with special reference to the dose-reduction principle and to ultraviolet-induced mutation . J. Bacteriol. 58:511-522. [ PMC free article : PMC385659 ] [ PubMed : 16561813 ]
- Luckiesh, M., and A.H. Taylor. 1946. Transmittance and reflectance of germicidal (2537) energy . J. Opt. Soc. Am. 36:227-234. [ PubMed : 21023098 ]
- Luckiesh, M., A.H. Taylor, and G.P. Kerr. 1944. Germicidal energy . Gen. Electr. Rev. 47(9):7-9.
- Luckiesh, M., and L.L Holladay. 1944. Disinfecting water by means of germicidal lamps . Gen. Electr. Rev. 47(4):45-50.
- Morris, E.J. 1972. The practical use of ultraviolet radiation for disinfection purposes . Med. Lab. Technol. 29:41-47. [ PubMed : 5038923 ]
- Oliver, B.G., and E.G. Cosgrove. 1975. The disinfection of sewage treatment plant effluents using ultraviolet light . Can. J. Chem. Eng. 53:170-174.
- Powers, E.L., M. Cross, and C.J. Varga. 1974. A dose-rate effect in the ultraviolet inactivation of bacterial spores . Photochem. Photobiol. 19:273-276. [ PubMed : 4207142 ]
- Reddish, G.G., editor. , ed. 1957. Antiseptics, Disinfectants, Fungicides and Chemical and Physical Sterilization , 2nd ed. Lea & Febiger, Philadelphia, Pa. 975 pp.
- Roeber, J.A., and F.M. Hoot. 1975. Ultraviolet Disinfection of Activated Sludge Effluent Discharging to Shellfish Waters . EPA 600/2-75/060 Municipal Environmental Research Laboratory Office of Research and Development, U.S. Environmental Protection Agency, Cincinnati, Ohio. 85 pp.
- Severin, B.F. 1978. Disinfection of Municipal Wastewater Effluents With Ultraviolet Light . Paper presented to Annual Meeting, Water Pollution Control Federation, Anaheim, Calif.
- Venosa, A.D., H.W. Wolf, and A.C. Petrasek. 1978. Ultraviolet disinfection of municipal effluents . Pp. 675-684 in R. Jolley, editor; , H. Gorchev, editor; , and D. H. Hamilton, Jr., editor. , eds. Water Chlorination: Environmental Impact and Health Effects, Vol. 2 . Ann Arbor Science Publishers, Ann Arbor, Mich. 909 pp.
- Witkin, E.M. 1976. Ultraviolet mutagenesis and inducible DNA repair in Escherichia coli . Bacteriol. Rev. 40:869-907. [ PMC free article : PMC413988 ] [ PubMed : 795416 ]
- Wood, M.D. 1974. Apparatus method for purifying fluids . U.S. Patent No. 3,837,800. September 24. (Chem. Abs. 85:4749v.)
Nomenclature in this report follows that recommended in the Eighth Edition of Bergey's Manual of Determinative Bacteriology (Buchanan and Gibbons, 1974). Thus, the name of an organism mentioned in the text may not be that used by the author of the work cited.
- Cite this Page National Research Council (US) Safe Drinking Water Committee. Drinking Water and Health: Volume 2. Washington (DC): National Academies Press (US); 1980. II, The Disinfection of Drinking Water.
- PDF version of this title (13M)
In this Page
Related information.
- PMC PubMed Central citations
- PubMed Links to PubMed
Recent Activity
- The Disinfection of Drinking Water - Drinking Water and Health The Disinfection of Drinking Water - Drinking Water and Health
Your browsing activity is empty.
Activity recording is turned off.
Turn recording back on
Connect with NLM
National Library of Medicine 8600 Rockville Pike Bethesda, MD 20894
Web Policies FOIA HHS Vulnerability Disclosure
Help Accessibility Careers
Have a language expert improve your writing
Run a free plagiarism check in 10 minutes, generate accurate citations for free.
- Knowledge Base
- Example of a great essay | Explanations, tips & tricks
Example of a Great Essay | Explanations, Tips & Tricks
Published on February 9, 2015 by Shane Bryson . Revised on July 23, 2023 by Shona McCombes.
This example guides you through the structure of an essay. It shows how to build an effective introduction , focused paragraphs , clear transitions between ideas, and a strong conclusion .
Each paragraph addresses a single central point, introduced by a topic sentence , and each point is directly related to the thesis statement .
As you read, hover over the highlighted parts to learn what they do and why they work.
Instantly correct all language mistakes in your text
Upload your document to correct all your mistakes in minutes
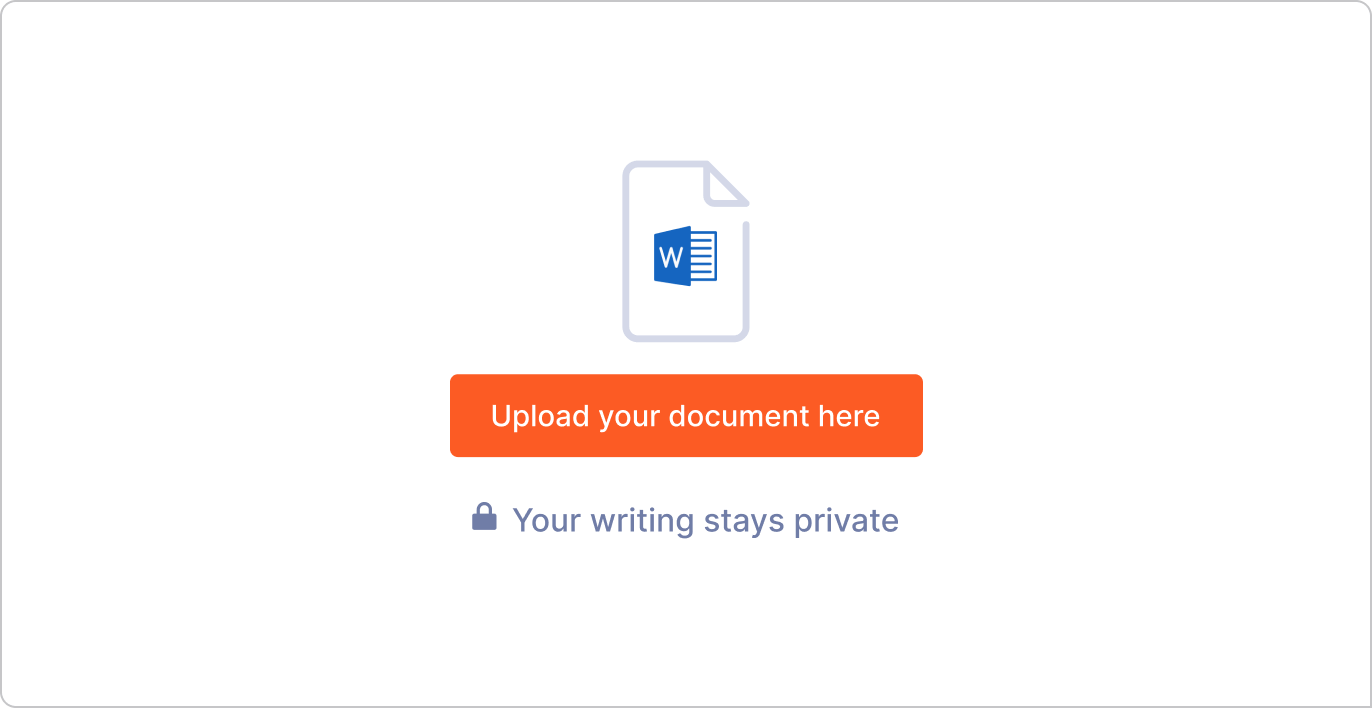
Table of contents
Other interesting articles, frequently asked questions about writing an essay, an appeal to the senses: the development of the braille system in nineteenth-century france.
The invention of Braille was a major turning point in the history of disability. The writing system of raised dots used by visually impaired people was developed by Louis Braille in nineteenth-century France. In a society that did not value disabled people in general, blindness was particularly stigmatized, and lack of access to reading and writing was a significant barrier to social participation. The idea of tactile reading was not entirely new, but existing methods based on sighted systems were difficult to learn and use. As the first writing system designed for blind people’s needs, Braille was a groundbreaking new accessibility tool. It not only provided practical benefits, but also helped change the cultural status of blindness. This essay begins by discussing the situation of blind people in nineteenth-century Europe. It then describes the invention of Braille and the gradual process of its acceptance within blind education. Subsequently, it explores the wide-ranging effects of this invention on blind people’s social and cultural lives.
Lack of access to reading and writing put blind people at a serious disadvantage in nineteenth-century society. Text was one of the primary methods through which people engaged with culture, communicated with others, and accessed information; without a well-developed reading system that did not rely on sight, blind people were excluded from social participation (Weygand, 2009). While disabled people in general suffered from discrimination, blindness was widely viewed as the worst disability, and it was commonly believed that blind people were incapable of pursuing a profession or improving themselves through culture (Weygand, 2009). This demonstrates the importance of reading and writing to social status at the time: without access to text, it was considered impossible to fully participate in society. Blind people were excluded from the sighted world, but also entirely dependent on sighted people for information and education.
In France, debates about how to deal with disability led to the adoption of different strategies over time. While people with temporary difficulties were able to access public welfare, the most common response to people with long-term disabilities, such as hearing or vision loss, was to group them together in institutions (Tombs, 1996). At first, a joint institute for the blind and deaf was created, and although the partnership was motivated more by financial considerations than by the well-being of the residents, the institute aimed to help people develop skills valuable to society (Weygand, 2009). Eventually blind institutions were separated from deaf institutions, and the focus shifted towards education of the blind, as was the case for the Royal Institute for Blind Youth, which Louis Braille attended (Jimenez et al, 2009). The growing acknowledgement of the uniqueness of different disabilities led to more targeted education strategies, fostering an environment in which the benefits of a specifically blind education could be more widely recognized.
Several different systems of tactile reading can be seen as forerunners to the method Louis Braille developed, but these systems were all developed based on the sighted system. The Royal Institute for Blind Youth in Paris taught the students to read embossed roman letters, a method created by the school’s founder, Valentin Hauy (Jimenez et al., 2009). Reading this way proved to be a rather arduous task, as the letters were difficult to distinguish by touch. The embossed letter method was based on the reading system of sighted people, with minimal adaptation for those with vision loss. As a result, this method did not gain significant success among blind students.
Louis Braille was bound to be influenced by his school’s founder, but the most influential pre-Braille tactile reading system was Charles Barbier’s night writing. A soldier in Napoleon’s army, Barbier developed a system in 1819 that used 12 dots with a five line musical staff (Kersten, 1997). His intention was to develop a system that would allow the military to communicate at night without the need for light (Herron, 2009). The code developed by Barbier was phonetic (Jimenez et al., 2009); in other words, the code was designed for sighted people and was based on the sounds of words, not on an actual alphabet. Barbier discovered that variants of raised dots within a square were the easiest method of reading by touch (Jimenez et al., 2009). This system proved effective for the transmission of short messages between military personnel, but the symbols were too large for the fingertip, greatly reducing the speed at which a message could be read (Herron, 2009). For this reason, it was unsuitable for daily use and was not widely adopted in the blind community.
Nevertheless, Barbier’s military dot system was more efficient than Hauy’s embossed letters, and it provided the framework within which Louis Braille developed his method. Barbier’s system, with its dashes and dots, could form over 4000 combinations (Jimenez et al., 2009). Compared to the 26 letters of the Latin alphabet, this was an absurdly high number. Braille kept the raised dot form, but developed a more manageable system that would reflect the sighted alphabet. He replaced Barbier’s dashes and dots with just six dots in a rectangular configuration (Jimenez et al., 2009). The result was that the blind population in France had a tactile reading system using dots (like Barbier’s) that was based on the structure of the sighted alphabet (like Hauy’s); crucially, this system was the first developed specifically for the purposes of the blind.
While the Braille system gained immediate popularity with the blind students at the Institute in Paris, it had to gain acceptance among the sighted before its adoption throughout France. This support was necessary because sighted teachers and leaders had ultimate control over the propagation of Braille resources. Many of the teachers at the Royal Institute for Blind Youth resisted learning Braille’s system because they found the tactile method of reading difficult to learn (Bullock & Galst, 2009). This resistance was symptomatic of the prevalent attitude that the blind population had to adapt to the sighted world rather than develop their own tools and methods. Over time, however, with the increasing impetus to make social contribution possible for all, teachers began to appreciate the usefulness of Braille’s system (Bullock & Galst, 2009), realizing that access to reading could help improve the productivity and integration of people with vision loss. It took approximately 30 years, but the French government eventually approved the Braille system, and it was established throughout the country (Bullock & Galst, 2009).
Although Blind people remained marginalized throughout the nineteenth century, the Braille system granted them growing opportunities for social participation. Most obviously, Braille allowed people with vision loss to read the same alphabet used by sighted people (Bullock & Galst, 2009), allowing them to participate in certain cultural experiences previously unavailable to them. Written works, such as books and poetry, had previously been inaccessible to the blind population without the aid of a reader, limiting their autonomy. As books began to be distributed in Braille, this barrier was reduced, enabling people with vision loss to access information autonomously. The closing of the gap between the abilities of blind and the sighted contributed to a gradual shift in blind people’s status, lessening the cultural perception of the blind as essentially different and facilitating greater social integration.
The Braille system also had important cultural effects beyond the sphere of written culture. Its invention later led to the development of a music notation system for the blind, although Louis Braille did not develop this system himself (Jimenez, et al., 2009). This development helped remove a cultural obstacle that had been introduced by the popularization of written musical notation in the early 1500s. While music had previously been an arena in which the blind could participate on equal footing, the transition from memory-based performance to notation-based performance meant that blind musicians were no longer able to compete with sighted musicians (Kersten, 1997). As a result, a tactile musical notation system became necessary for professional equality between blind and sighted musicians (Kersten, 1997).
Braille paved the way for dramatic cultural changes in the way blind people were treated and the opportunities available to them. Louis Braille’s innovation was to reimagine existing reading systems from a blind perspective, and the success of this invention required sighted teachers to adapt to their students’ reality instead of the other way around. In this sense, Braille helped drive broader social changes in the status of blindness. New accessibility tools provide practical advantages to those who need them, but they can also change the perspectives and attitudes of those who do not.
Bullock, J. D., & Galst, J. M. (2009). The Story of Louis Braille. Archives of Ophthalmology , 127(11), 1532. https://doi.org/10.1001/archophthalmol.2009.286.
Herron, M. (2009, May 6). Blind visionary. Retrieved from https://eandt.theiet.org/content/articles/2009/05/blind-visionary/.
Jiménez, J., Olea, J., Torres, J., Alonso, I., Harder, D., & Fischer, K. (2009). Biography of Louis Braille and Invention of the Braille Alphabet. Survey of Ophthalmology , 54(1), 142–149. https://doi.org/10.1016/j.survophthal.2008.10.006.
Kersten, F.G. (1997). The history and development of Braille music methodology. The Bulletin of Historical Research in Music Education , 18(2). Retrieved from https://www.jstor.org/stable/40214926.
Mellor, C.M. (2006). Louis Braille: A touch of genius . Boston: National Braille Press.
Tombs, R. (1996). France: 1814-1914 . London: Pearson Education Ltd.
Weygand, Z. (2009). The blind in French society from the Middle Ages to the century of Louis Braille . Stanford: Stanford University Press.
If you want to know more about AI tools , college essays , or fallacies make sure to check out some of our other articles with explanations and examples or go directly to our tools!
- Ad hominem fallacy
- Post hoc fallacy
- Appeal to authority fallacy
- False cause fallacy
- Sunk cost fallacy
College essays
- Choosing Essay Topic
- Write a College Essay
- Write a Diversity Essay
- College Essay Format & Structure
- Comparing and Contrasting in an Essay
(AI) Tools
- Grammar Checker
- Paraphrasing Tool
- Text Summarizer
- AI Detector
- Plagiarism Checker
- Citation Generator
Here's why students love Scribbr's proofreading services
Discover proofreading & editing
An essay is a focused piece of writing that explains, argues, describes, or narrates.
In high school, you may have to write many different types of essays to develop your writing skills.
Academic essays at college level are usually argumentative : you develop a clear thesis about your topic and make a case for your position using evidence, analysis and interpretation.
The structure of an essay is divided into an introduction that presents your topic and thesis statement , a body containing your in-depth analysis and arguments, and a conclusion wrapping up your ideas.
The structure of the body is flexible, but you should always spend some time thinking about how you can organize your essay to best serve your ideas.
Your essay introduction should include three main things, in this order:
- An opening hook to catch the reader’s attention.
- Relevant background information that the reader needs to know.
- A thesis statement that presents your main point or argument.
The length of each part depends on the length and complexity of your essay .
A thesis statement is a sentence that sums up the central point of your paper or essay . Everything else you write should relate to this key idea.
A topic sentence is a sentence that expresses the main point of a paragraph . Everything else in the paragraph should relate to the topic sentence.
At college level, you must properly cite your sources in all essays , research papers , and other academic texts (except exams and in-class exercises).
Add a citation whenever you quote , paraphrase , or summarize information or ideas from a source. You should also give full source details in a bibliography or reference list at the end of your text.
The exact format of your citations depends on which citation style you are instructed to use. The most common styles are APA , MLA , and Chicago .
Cite this Scribbr article
If you want to cite this source, you can copy and paste the citation or click the “Cite this Scribbr article” button to automatically add the citation to our free Citation Generator.
Bryson, S. (2023, July 23). Example of a Great Essay | Explanations, Tips & Tricks. Scribbr. Retrieved March 25, 2024, from https://www.scribbr.com/academic-essay/example-essay-structure/
Is this article helpful?
Shane Bryson
Shane finished his master's degree in English literature in 2013 and has been working as a writing tutor and editor since 2009. He began proofreading and editing essays with Scribbr in early summer, 2014.
Other students also liked
How to write an essay introduction | 4 steps & examples, academic paragraph structure | step-by-step guide & examples, how to write topic sentences | 4 steps, examples & purpose, unlimited academic ai-proofreading.
✔ Document error-free in 5minutes ✔ Unlimited document corrections ✔ Specialized in correcting academic texts
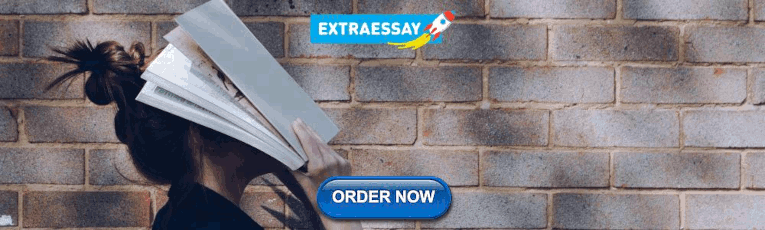
COMMENTS
Essay On Disinfectants. 1157 Words5 Pages. Disinfectants Are chemical substance, used to kill pathogenic and other harmful microorganisms on inanimate objects but are too toxic to be applied directly on living tissues. disinfection has a lower effect than sterilization . sterilization is an extreme chemical and physical operation that destroy ...
Disinfectants are typically applied on non living objects in order to destroy microorganisms and this process are called disinfection. Order custom essay Sterilization and Disinfection Techniques for Microbial Control with free plagiarism report. 450+ experts on 30 subjects Starting from 3 hours delivery. Get Essay Help.
In-depth knowledge of disinfection and sterilization is a key component of infection control. Sterilization completely removes a spore, whereas disinfection cannot. Disinfectants are classified as oxidants and non-oxidants. The decision regarding which method to apply is based on Spaulding's classification.
Introduction. Multi drug resistant (MDR) microorganisms have become a major threat to both global health and economy. The World Health Organization (WHO) has declared that the microbial infections caused by antibiotic resistance bacteria will lead to massive preventable deaths (Collignon, 2017; Morrison and Zembower, 2020).They also estimated that the global economy will suffer over 100 ...
Abstract and Figures. Control of microorganisms is vital to prevent infection. Prevention can be done in various ways, one of which is the use of disinfectants and antiseptics. The widespread use ...
The purpose of disinfectants and antiseptics is to kill target microorganisms, effectively reducing their number on skin, surfaces, materials or in water. Unlike chemotherapeutic antibiotics ...
Learning Objectives. Compare and contrast the disk-diffusion, use-dilution, and in-use methods for testing the effectiveness of antiseptics, disinfectants, and sterilants. The effectiveness of various chemical disinfectants is reflected in the terms used to describe them. Chemical disinfectants are grouped by the power of their activity, with ...
Level of germ reduction as a result of cleaning, sanitizing, disinfecting, and sterilizing. surfaces (Figure by Reza Ovissipour). Cleaning. Cleaning alone can reduce germs, and is also the first step before you can sanitize, disinfect, or sterilize. Cleaning removes the things you can see (for example, dirt, dust, or bits of food) and with-it ...
A rational approach to disinfection and sterilization. Almost 50 years ago, Earle H. Spaulding 15 devised a rational approach to disinfection and sterilization of patient-care items or equipment. This classification scheme is so clear and logical that it has been retained, refined, and successfully used by infection control professionals and others when planning methods for disinfection or ...
Phenolics tend to be stable, persistent on surfaces, and less toxic than phenol. They inhibit microbial growth by denaturing proteins and disrupting membranes. Figure 13.19 Phenol and phenolic compounds have been used to control microbial growth. (a) Chemical structure of phenol, also known as carbolic acid.
In-Use Test. An in-use test can determine whether an actively used solution of disinfectant in a clinical setting is microbially contaminated (Figure 16.4.2 16.4. 2 ). A 1-mL sample of the used disinfectant is diluted into 9 mL of sterile broth medium that also contains a compound to inactivate the disinfectant.
Sterilization is intended to convey an absolute meaning; unfortunately, however, some health professionals and the technical and commercial literature refer to "disinfection" as "sterilization" and items as "partially sterile.". When chemicals are used to destroy all forms of microbiologic life, they can be called chemical sterilants.
Sanitizing: Composed of cleaning and disinfecting practices, sanitizing lowers the number of germs on surfaces and objects. In turn, this minimizes the spread of illnesses. Using a combination of these methods to sterilize the objects and surfaces we interact with decreases our health risk.
Many disinfectants are used alone or in combinations (e.g., hydrogen peroxide and peracetic acid) in the health-care setting. These include alcohols, chlorine and chlorine compounds, formaldehyde, glutaraldehyde, ortho-phthalaldehyde, hydrogen peroxide, iodophors, peracetic acid, phenolics, and quaternary ammonium compounds.Commercial formulations based on these chemicals are considered unique ...
On the one hand, Disinfectants essays we present here evidently demonstrate how a really terrific academic paper should be developed. On the other hand, upon your demand and for a fair price, a pro essay helper with the relevant academic background can put together a top-notch paper model on Disinfectants from scratch.
Additionally, personal items, such as mobile phones, keys, credit cards, and writing pens, may be subjected to sanitization using 75% ethanol to ensure their disinfection (Yang et al. 2020). Virusend (TX-10) is a unique disinfectant that has already exhibited efficient activity against several microbial agents, including viruses.
Disinfectants and sanitizers are essential preventive agents against the coronavirus disease 2019 (COVID-19) pandemic; however, the pandemic crisis was marred by undue hype, which led to the indiscriminate use of disinfectants and sanitizers. Despite demonstrating a beneficial role in the control and prevention of COVID-19, there are crucial concerns regarding the large-scale use of ...
Long-lasting disinfectant promises to help fight pandemics. ScienceDaily . Retrieved March 24, 2024 from www.sciencedaily.com / releases / 2021 / 09 / 210901142713.htm
Get your free examples of research papers and essays on Disinfectant here. Only the A-papers by top-of-the-class students. Learn from the best! ... World of Writing Hub Blog Free Essay Writing Tools Quizzes and Tests Essay Topics Types of Essays Free Essay Examples. How It Works ...
Phenolics. In the 1800s, scientists began experimenting with a variety of chemicals for disinfection. In the 1860s, British surgeon Joseph Lister (1827-1912) began using carbolic acid, known as phenol, as a disinfectant for the treatment of surgical wounds (see Foundations of Modern Cell Theory).In 1879, Lister's work inspired the American chemist Joseph Lawrence (1836-1909) to develop ...
A pH value of 12.0 to 12.5 and a contact time of approximately 30 min can probably yield 99.0% to 99.9% inactivation of most bacteria and of certain viruses. This process has a drawback: the pH that is necessary for effective disinfection must be reduced before the water can be consumed.
An essay is a focused piece of writing that explains, argues, describes, or narrates. In high school, you may have to write many different types of essays to develop your writing skills. Academic essays at college level are usually argumentative : you develop a clear thesis about your topic and make a case for your position using evidence ...