
An official website of the United States government
The .gov means it’s official. Federal government websites often end in .gov or .mil. Before sharing sensitive information, make sure you’re on a federal government site.
The site is secure. The https:// ensures that you are connecting to the official website and that any information you provide is encrypted and transmitted securely.
- Publications
- Account settings
Preview improvements coming to the PMC website in October 2024. Learn More or Try it out now .
- Advanced Search
- Journal List
- Int J Environ Res Public Health

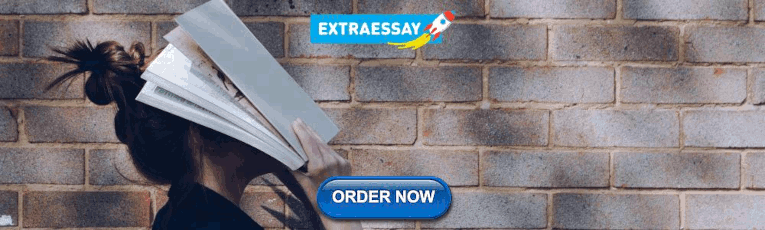
Environmental Sustainability Impacts of Solid Waste Management Practices in the Global South
Ismaila rimi abubakar.
1 College of Architecture and Planning, Imam Abdulrahman Bin Faisal University, Dammam 31441, Saudi Arabia
Khandoker M. Maniruzzaman
2 Department of Urban and Regional Planning, College of Architecture and Planning, Imam Abdulrahman Bin Faisal University, Dammam 31441, Saudi Arabia
Umar Lawal Dano
Faez s. alshihri, maher s. alshammari, sayed mohammed s. ahmed, wadee ahmed ghanem al-gehlani.
3 Department of Architecture, College of Architecture and Planning, Imam Abdulrahman Bin Faisal University, Dammam 32141, Saudi Arabia
Tareq I. Alrawaf
4 Department of Landscape Architecture, College of Architecture and Planning, Imam Abdulrahman Bin Faisal University, Dammam 31441, Saudi Arabia
Associated Data
No data were reported in this review article.
Solid waste management (SWM) is one of the key responsibilities of city administrators and one of the effective proxies for good governance. Effective SWM mitigates adverse health and environmental impacts, conserves resources, and improves the livability of cities. However, unsustainable SWM practices, exacerbated by rapid urbanization and financial and institutional limitations, negatively impact public health and environmental sustainability. This review article assesses the human and environmental health impacts of SWM practices in the Global South cities that are the future of global urbanization. The study employs desktop research methodology based on in-depth analysis of secondary data and literature, including official documents and published articles. It finds that the commonplace SWM practices include mixing household and commercial garbage with hazardous waste during storage and handling. While waste storage is largely in old or poorly managed facilities such as storage containers, the transportation system is often deficient and informal. The disposal methods are predominantly via uncontrolled dumping, open-air incinerators, and landfills. The negative impacts of such practices include air and water pollution, land degradation, emissions of methane and hazardous leachate, and climate change. These impacts impose significant environmental and public health costs on residents with marginalized social groups mostly affected. The paper concludes with recommendations for mitigating the public and environmental health risks associated with the existing SWM practices in the Global South.
1. Introduction
Solid waste management (SWM) continues to dominate as a major societal and governance challenge, especially in urban areas overwhelmed by the high rate of population growth and garbage generation. The role of SWM in achieving sustainable development is emphasized in several international development agendas, charters, and visions. For example, sustainable SWM can help meet several United Nations’ Sustainable Development Goals (SDG), such as ensuring clean water and sanitation (SDG6), creating sustainable cities and inclusive communities (SDG11), mitigating climate change (SDG13), protecting life on land (SDG15), and demonstrating sustainable consumption and production patterns (SDG12) ( https://sdgs.un.org/goals , accessed on 26 September 2022). It also fosters a circular urban economy that promotes reductions in the consumption of finite resources, materials reuse and recycling for waste elimination, pollution reduction, cost saving, and green growth
However, coupled with economic growth, improved lifestyle, and consumerism, cities across the globe will continue to face an overwhelming challenge of SWM as the world population is expected to rise to 8 billion by 2025 and to 9.3 billion by 2050, out of which around 70% will be living in urban areas [ 1 , 2 ]. In developing countries, most cities collect only 50–80% of generated waste after spending 20–50% of their budgets, of which 80–95% are spent on collecting and transporting waste [ 3 , 4 ]. Moreover, many low-income countries collect as low as 10% of the garbage generated in suburban areas, which contributes to public health and environmental risks, including higher incidents of diarrhea and acute respiratory infections among people, particularly children, living near garbage dumps [ 5 ]. Obstacles to effective municipal SWM include lack of awareness, technologies, finances, and good governance [ 6 , 7 , 8 ].
Removing garbage from homes and businesses without greater attention to what was then carried out with it has also been the priority of municipal SWM in several cities of developing countries [ 9 ]. In most developing countries, garbage collected from households is disposed of in landfills or dumpsites, the majority of which are projected to reach their capacities within a decade. The unsustainable approach of dumping or burning waste in an open space, usually near poor communities on the city edge, or throwing garbage into water bodies was an acceptable garbage disposal strategy. Similarly, several cities still use old-generation or poorly managed facilities and informal uncontrolled dumping or open-air waste burning. Often, these practices affect marginalized social groups near the disposal sites [ 10 ]. Moreover, this approach poses several sustainability problems, including resource depletion, environmental pollution, and public health problems, such as the spread of communicable diseases.
However, ever since the advent of the environmental movement in the 1960s, there has been a far-reaching appreciation of environmental and public health risks of unsustainable SWM practices. In the 1970s and onward, SWM was a technical issue to be resolved using technology; hence, the emphasis and investments were placed on garbage collection equipment [ 5 ]. Although modern technology can significantly reduce emissions of hazardous substances, by the 1990s, that viewpoint changed when municipalities become unable to evacuate and dispose of garbage effectively without the active involvement of service users and other stakeholders [ 5 ]. The inability of the public sector in the global South to deliver sufficient improvement of SWM, coupled with the pressure from the financial institutions and other donor agencies, led to privatization policies at the end of the decade. However, as privatization failed to provide municipal SWM services to the poor and marginalized communities, the current global thinking on addressing municipal SWM problems is changing.
A more sustainable waste management approach prioritizes practices such as reduced production, waste classifications, reuse, recycling, and energy recovery over the common practices of landfilling, open dumps, and open incineration [ 11 , 12 , 13 ]. This approach, which is still at an early stage but getting increased attention in the Global South, is more inclusive and environment-friendly and has less negative impact on human health and the environment than the common practices [ 14 , 15 , 16 ]. As such, there is a need to assess SWM practices in the Global South and their impacts on environmental and human health because 90% of the expected growth in the urban population by 2050 is expected to happen here. So far, there are a few studies on the impacts of SWM practices on human health and the environment in the global regions.
Therefore, this review article addresses this knowledge gap by assessing the negative impacts of the dominant SWM practices on human and environmental health. Section 2 presents the research methodology. Section 3 reviews the major SWM practices in the Global South and assesses the environmental and public health implications of SWM practices in the Global South cities. While Section 4 discusses the implications of the findings and proffers recommendations that could help authorities to deal with SWM challenges and mitigate public and environmental health risks associated with unsustainable SWM practices, Section 5 concludes the paper.
2. Materials and Methods
The present paper utilizes a desktop research method of collecting and analyzing relevant data from the existing literature, as utilized in some previous studies [ 17 , 18 ]. The method consists of three iterative stages shown in Figure 1 : (a) scoping, (b) collecting relevant literature, and (c) data analysis. Firstly, the scoping stage involves defining and understanding the research problem under investigation and setting the study scope and boundary. The scope of the paper is to explore human and environmental impacts of SWM practices toward policy and practical recommendations for a more sustainable SWM system, with the Global South as the study boundary. This stage also helped identify relevant keywords to search for during the literature review in the second stage.

The flow chart of the research method (Source: [ 18 ] (p. 4)).
The second stage involved identifying and collecting relevant literature from online sources. The researchers utilized Google Scholar and Scopus databases to identify peer-reviewed academic works (peer-reviewed articles, conference proceedings, and books) as well as the gray literature. The literature that satisfied the following three inclusion criteria was identified and downloaded: (1) It is related to the study’s objective; (2) it is in the English language; and (3) it was published within the last twenty years, although some old documents about established concepts and approaches were also accessed. The downloaded gray literature includes newspaper articles, statistics, technical reports, and website contents from international development organizations such as the World Health Organization (WHO), the United Nations, and the World Bank.
In the last stage, the authors organized, analyzed, and synthesized the data collected from the literature. The downloaded works were organized according to the similarity of topics, even though some fit in more than one category. Then, each document was thoroughly examined, and themes concerned with SWM practices and their human and environmental impacts were collated, synthesized, and harmonized. Finally, the themes were summarized in Table A1 , Table A2 and Table A3 (see Appendix A ) and discussed. Implications and recommendations of the findings are then highlighted.
3. Results and Discussion
3.1. solid waste management practices in the global south.
Global municipal solid waste (MSW) generation rose from 1.3 billion tons in 2012 to 2.1 billion tons (0.74 kg/capita/day) as of 2016, which by 2050 is expected to increase by 70% to reach a total of 3.40 billion tons or 1.42 kg/capita/day [ 19 ]. The per capita MSW generation varies among regions and countries. In the EU (European Union), it ranges from 0.3–1.4 kg/capita/day [ 20 ], and in some African cities, the average is 0.78 kg/capita/day [ 21 ]. In Asia, urban areas generate about 760,000 tons of MSW per day, which is expected to increase to 1.8 million tons per day or 26% of the world’s total by 2025, despite the continent housing 53% of the world’s population [ 22 , 23 ]. In China, the total MSW generation was around 212 million tons (0.98 kg/capita/day) in 2006, out of which 91.4%, 6.4%, and 2.2% were disposed of via landfilling, incineration, and composting [ 24 ]. In 2010, only 660 Chinese cities produced about 190 million tons of MSW, accounting for 29% of the world’s total, while the total amount of solid waste in China could reach at least 480 million tons in 2030 [ 25 ]. In China, industrial waste (more than one billion tons) was five times the amount of MSW generated in 2002, which is expected to generate approximately twice as much MSW as the USA, while India will overtake the USA in MSW generation by 2030 [ 26 ].
In Malaysia, while the average rate of MSW generation was about 0.5–0.8 kg/person/day, Kuala Lumpur’s daily per capita generation rate was 1.62 kg in 2008 [ 27 ], which is expected to reach 2.23 kg in 2024 [ 28 ]. About 64% of Malaysia’s waste consists of household and office waste, 25% industrial waste, 8% commercial waste, and 3% construction waste [ 29 ]. In Sri Lanka, the assessed mean waste generation in 1999 was 6500 tons/day or 0.89 kg/cap/day, which is estimated to reach 1.0 kg/cap/day by 2025 [ 30 ]. With a 1.2% population growth rate, the total MSW generation in 2009 was approximately 7250 tons/day [ 31 ]. In Ghana, the solid waste generation rate was 0.47 kg/person/day, or about 12,710 tons per annum, consisting of biodegradable waste (0.318), non-biodegradable (0.096), and inert and miscellaneous waste (0.055) kg/person/day, respectively [ 32 ].
Moreover, global SWM costs are anticipated to increase to about $375.5 billion in 2025, with more than four-fold increases in lower- to middle-income countries and five-fold increases in low-income countries [ 33 ]. Globally, garbage collection, transportation, and disposal pose a major cost component in SWM systems [ 19 ]. Inadequate funding militates against the optimization of MSW disposal services. Table 1 compares the everyday SWM practices in low-, middle- and high-income countries according to major waste management steps. The literature indicates that waste generation rates and practices depend on the culture, socioeconomic status, population density, and level of commercial and industrial activities of a city or region.
Common MSW management practices by country’s level of economic development (adapted from [ 34 ]).
3.2. Environmental and Public Health Impacts of SWM Practices in the Global South
- (a) Weak and Inadequate SWM System
Many problems in the cities of the global South are often associated with a weak or inadequate SWM system, which leads to severe direct and indirect environmental and public health issues at every stage of waste collection, handling, treatment, and disposal [ 30 , 31 , 32 , 33 , 34 ]. Inadequate and weak SWM results in indiscriminate dumping of waste on the streets, open spaces, and water bodies. Such practices were observed in, for example, Pakistan [ 35 , 36 ], India [ 37 ], Nepal [ 38 ], Peru [ 39 ], Guatemala [ 40 ], Brazil [ 41 ], Kenya [ 42 ], Rwanda [ 43 ], South Africa [ 44 , 45 ], Nigeria [ 46 ], Zimbabwe [ 47 ], etc.
The problems associated with such practices are GHG emissions [ 37 , 48 ], leachates [ 40 , 44 , 49 ], the spread of diseases such as malaria and dengue [ 36 ], odor [ 35 , 38 , 50 , 51 ], blocking of drains and sewers and subsequent flooding [ 52 ], suffocation of animals in plastic bags [ 52 ], and indiscriminate littering [ 38 , 39 , 53 ].
- (b) Irregular Waste Collection and Handling
Uncollected and untreated waste has socioeconomic and environmental costs extending beyond city boundaries. Environmental sustainability impacts of this practice include methane (CH 4 ) emissions, foul odor, air pollution, land and water contamination, and the breeding of rodents, insects, and flies that transmit diseases to humans. Decomposition of biodegradable waste under anaerobic conditions contributes to about 18% and 2.9% of global methane and GHG emissions, respectively [ 54 ], with the global warming effect of about 25 times higher than carbon dioxide (CO 2 ) emissions [ 55 ]. Methane also causes fires and explosions [ 56 ]. Emissions from SWM in developing countries are increasing due to rapid economic growth and improved living standards [ 57 ].
Irregular waste collection also contributes to marine pollution. In 2010, 192 coastal countries generated 275 million metric tons of plastic waste out of which up to 12.7 million metric tons (4.4%) entered ocean ecosystems [ 58 ]. Moreover, plastic waste collects and stagnates water, proving a mosquito breeding habitat and raising the risks of dengue, malaria, and West Nile fever [ 56 ]. In addition, uncollected waste creates serious safety, health, and environmental consequences such as promoting urban violence and supporting breeding and feeding grounds for flies, mosquitoes, rodents, dogs, and cats, which carry diseases to nearby homesteads [ 4 , 19 , 59 , 60 ].
In the global South, scavengers often throw the remaining unwanted garbage on the street. Waste collectors are rarely protected from direct contact and injury, thereby facing serious health threats. Because garbage trucks are often derelict and uncovered, exhaust fumes and dust stemming from waste collection and transportation contribute to environmental pollution and widespread health problems [ 61 ]. In India’s megacities, for example, irregular MSW management is one of the major problems affecting air and marine quality [ 62 ]. Thus, irregular waste collection and handling contribute to public health hazards and environmental degradation [ 63 ].
- (c) Landfilling and Open Dumping
Most municipal solid waste in the Global South goes into unsanitary landfills or open dumps. Even during the economic downturn during the COVID-19 pandemic, the amount of waste heading to landfill sites in Brazil, for example, increased due to lower recycling rates [ 64 ]. In Johor, Malaysia, landfilling destroys natural habitats and depletes the flora and fauna [ 65 ]. Moreover, landfilling with untreated, unsorted waste led to severe public health issues in South America [ 66 ]. Based on a study on 30 Brazilian cities, Urban and Nakada [ 64 ] report that 35% of medical waste was not properly treated before disposal, which poses a threat to public health, including the spread of COVID-19. Landfills and open dumps are also associated with high emissions of methane (CH 4 ), a major GHG [ 67 , 68 ]. Landfills and wastewater release 17% of the global methane emission [ 25 ]. About 29 metric tons of methane are emitted annually from landfills globally, accounting for about 8% of estimated global emissions, with 1.3 metric tons released from landfills in Africa [ 7 ]. The rate of landfill gas production steadily rises while MSW accumulates in the landfill emissions. Released methane and ammonia gases can cause health hazards such as respiratory diseases [ 37 , 69 , 70 , 71 ]. Since methane is highly combustible, it can cause fire and explosion hazards [ 72 ].
Open dumping sites with organic waste create the environment for the breeding of disease-carrying vectors, including rodents, flies, and mosquitoes [ 40 , 45 , 51 , 73 , 74 , 75 , 76 , 77 , 78 , 79 ]. Associated vector-borne diseases include zika virus, dengue, and malaria fever [ 70 , 71 , 72 , 73 , 74 , 75 , 76 , 77 , 78 , 79 , 80 ]. In addition, there are risks of water-borne illnesses such as leptospirosis, intestinal worms, diarrhea, and hepatitis A [ 80 , 81 ].
Odors from landfill sites, and their physical appearance, affect the lives of nearby residents by threatening their health and undermining their livelihoods, lowering their property values [ 37 , 38 , 68 , 82 , 83 , 84 ]. Moreover, the emission of ammonia (NH 3 ) from landfill sites can damage species’ composition and plant leaves [ 85 ]. In addition, the pollutants from landfill sites damage soil quality [ 73 , 84 ]. Landfill sites also generate dust and are sources of noise pollution [ 86 ].
Air and water pollution are intense in the hot and rainy seasons due to the emission of offensive odor, disease-carrying leachates, and runoff. Considerable amounts of methane and CO 2 from landfill sites produce adverse health effects such as skin, eyes, nose, and respiratory diseases [ 69 , 87 , 88 ]. The emission of ammonia can lead to similar problems and even blindness [ 85 , 89 ]. Other toxic gaseous pollutants from landfill sites include Sulphur oxides [ 89 ]. While less than 20% of methane is recovered from landfills in China, Western nations recover up to 60% [ 90 ].
Several studies report leachate from landfill sites contaminating water sources used for drinking and other household applications, which pose significant risks to public health [ 36 , 43 , 53 , 72 , 75 , 83 , 91 , 92 , 93 , 94 , 95 ]. For example, Hong et al. [ 95 ] estimated that, in 2006, the amount of leachates escaping from landfill sites in Pudong (China) was 160–180 m 3 per day. On the other hand, a properly engineered facility for waste disposal can protect public health, preserve important environmental resources, prevent clogging of drainages, and prevent the migration of leachates to contaminate ground and surface water, farmlands, animals, and air from which they enter the human body [ 61 , 96 ]. Moreover, heat in summer can speed up the rate of bacterial action on biodegradable organic material and produce a pungent odor [ 60 , 97 , 98 ]. In China, for example, leachates were not treated in 47% of landfills [ 99 ].
Co-mingled disposal of industrial and medical waste alongside municipal waste endangers people with chemical and radioactive hazards, Hepatitis B and C, tetanus, human immune deficiency, HIV infections, and other related diseases [ 59 , 60 , 100 ]. Moreover, indiscriminate disposal of solid waste can cause infectious diseases such as gastrointestinal, dermatological, respiratory, and genetic diseases, chest pains, diarrhea, cholera, psychological disorders, skin, eyes, and nose irritations, and allergies [ 10 , 36 , 60 , 61 ].
- (d) Open Burning and Incineration
Open burning of MSW is a main cause of smog and respiratory diseases, including nose, throat, chest infections and inflammation, breathing difficulty, anemia, low immunity, allergies, and asthma. Similar health effects were reported from Nepal [ 101 ], India [ 87 ], Mexico, [ 69 ], Pakistan [ 52 , 73 , 84 ], Indonesia [ 88 ], Liberia [ 50 ], and Chile [ 102 ]. In Mumbai, for example, open incineration emits about 22,000 tons of pollutants annually [ 56 ]. Mongkolchaiarunya [ 103 ] reported air pollution and odors from burning waste in Thailand. In addition, plastic waste incineration produces hydrochloric acid and dioxins in quantities that are detrimental to human health and may cause allergies, hemoglobin deficiency, and cancer [ 95 , 104 ]. In addition, smoke from open incineration and dumpsites is a significant contributor to air pollution even for persons staying far from dumpsites.
- (e) Composting
Composting is a biological method of waste disposal that entails the decomposing or breaking down of organic wastes into simpler forms by naturally occurring microorganisms, such as bacteria and fungi. However, despite its advantage of reducing organic waste by at least half and using compost in agriculture, the composting method has much higher CO 2 emissions than other disposal approaches. In Korea, for example, composting has the highest environmental impact than incineration and anaerobic digestion methods [ 105 ]. The authors found that the environmental impact of composting was found to be 2.4 times higher than that of incineration [ 105 ]. Some reviews linked composting with several health issues, including congested nose, sore throat and dry cough, bronchial asthma, allergic rhinitis, and extrinsic allergic alveolitis [ 36 , 106 ].
4. Implications and Recommendations
As discussed in the section above, there are many negative impacts of unsustainable SWM practices on the people and the environment. Although all waste treatment methods have their respective negative impacts, some have fewer debilitating impacts on people and the environment than others. The following is the summary of key implications of such unsustainable SWM practices.
- Uncollected organic waste from bins, containers and open dumps harbors rodents, insects, and reptiles that transmit diseases to humans. It also produces odor due to the decomposition of organic wastes, especially in the summer, and leachates that migrate and contaminate receiving underground and surface waters.
- Open dumps and non-engineered landfills release methane from decomposing biodegradable waste under anaerobiotic conditions. Methane is a key contributor to global warming, and it can cause fires and explosions.
- Non-biodegradable waste, such as discarded tires, plastics, bottles, and tins, pollutes the ground and collects water, thus creating breeding grounds for mosquitoes and increasing the risk of diseases such as malaria, dengue, and West Nile fever.
- Open burning of MSW emits pollutants into the atmosphere thereby increasing the incidences of nose and throat infections and inflammation, inhalation difficulties, bacterial infections, anemia, reduced immunity, allergies, and asthma.
- Uncontrolled incineration causes smog and releases fine particles, which are a major cause of respiratory disease. It also contributes to urban air pollution and GHG emissions significantly.
- Incineration and landfilling are associated with reproductive defects in women, developmental defects in children, cancer, hepatitis C, psychosocial impacts, poisoning, biomarkers, injuries, and mortality.
Therefore, measures toward more sustainable SWM that can mitigate such impacts must be worked out and followed. The growing complexity, costs, and coordination of SWM require multi-stakeholder involvement at each process stage [ 7 ]. Earmarking resources, providing technical assistance, good governance, and collaboration, and protecting environmental and human health are SWM critical success factors [ 47 , 79 ]. As such, local governments, the private sector, donor agencies, non-governmental organizations (NGOs), the residents, and informal garbage collectors and scavengers have their respective roles to play collaboratively in effective and sustainable SWM [ 40 , 103 , 107 , 108 ]. The following are key practical recommendations for mitigating the negative impacts of unsustainable SWM practices enumerated above.
First, cities should plan and implement an integrated SWM approach that emphasizes improving the operation of municipalities to manage all stages of SWM sustainably: generation, separation, transportation, transfer/sorting, treatment, and disposal [ 36 , 46 , 71 , 77 , 86 ]. The success of this approach requires the involvement of all stakeholders listed above [ 109 ] while recognizing the environmental, financial, legal, institutional, and technical aspects appropriate to each local setting [ 77 , 86 ]. Life Cycle Assessment (LCA) can likewise aid in selecting the method and preparing the waste management plan [ 88 , 110 ]. Thus, the SWM approach should be carefully selected to spare residents from negative health and environmental impacts [ 36 , 39 , 83 , 98 , 111 ].
Second, local governments should strictly enforce environmental regulations and better monitor civic responsibilities for sustainable waste storage, collection, and disposal, as well as health hazards of poor SWM, reflected in garbage littering observable throughout most cities of the Global South [ 64 , 84 ]. In addition, violations of waste regulations should be punished to discourage unsustainable behaviors [ 112 ]. Moreover, local governments must ensure that waste collection services have adequate geographical coverage, including poor and minority communities [ 113 ]. Local governments should also devise better SWM policies focusing on waste reduction, reuse, and recycling to achieve a circular economy and sustainable development [ 114 , 115 ].
Third, effective SWM requires promoting positive public attitudes toward sustainable waste management [ 97 , 116 , 117 , 118 ]. Therefore, public awareness campaigns through print, electronic, and social media are required to encourage people to desist from littering and follow proper waste dropping and sorting practices [ 36 , 64 , 77 , 79 , 80 , 82 , 91 , 92 , 119 ]. There is also the need for a particular focus on providing sorting bins and public awareness about waste sorting at the source, which can streamline and optimize subsequent SWM processes and mitigate their negative impacts [ 35 , 45 , 46 , 64 , 69 , 89 , 93 ]. Similarly, non-governmental and community-based organizations can help promote waste reduction, separation, and sorting at the source, and material reuse/recycling [ 103 , 120 , 121 , 122 ]. In Vietnam, for example, Tsai et al. [ 123 ] found that coordination among stakeholders and appropriate legal and policy frameworks are crucial in achieving sustainable SWM.
Fourth, there is the need to use environmentally friendly technologies or upgrade existing facilities. Some researchers prefer incineration over other methods, particularly for non-recyclable waste [ 44 , 65 ]. For example, Xin et al. [ 124 ] found that incineration, recycling, and composting resulted in a 70.82% reduction in GHG emissions from solid waste in Beijing. In Tehran city, Iran, Maghmoumi et al. [ 125 ] revealed that the best scenario for reducing GHG emissions is incinerating 50% of the waste, landfilling 30%, and recycling 20%. For organic waste, several studies indicate a preference for composting [ 45 , 51 , 75 ] and biogas generation [ 15 , 42 , 68 ]. Although some researchers have advocated a complete ban on landfilling [ 13 , 42 ], it should be controlled with improved techniques for leak detection and leachate and biogas collection [ 126 , 127 ]. Many researchers also suggested an integrated biological and mechanical treatment (BMT) of solid waste [ 66 , 74 , 95 , 119 ]. In Kenya, the waste-to-biogas scheme and ban on landfill and open burning initiatives are estimated to reduce the emissions of over 1.1 million tons of GHG and PM2.5 emissions from the waste by more than 30% by 2035 [ 42 ]. An appropriately designed waste disposal facility helps protect vital environmental resources, including flora, fauna, surface and underground water, air, and soil [ 128 , 129 ].
Fifth, extraction and reuse of materials, energy, and nutrients are essential to effective SWM, which provides livelihoods for many people, improves their health, and protects the environment [ 130 , 131 , 132 , 133 , 134 , 135 , 136 ]. For example, recycling 24% of MSW in Thailand lessened negative health, social, environmental, and economic impacts from landfill sites [ 89 ]. Waste pickers play a key role in waste circularity and should be integrated into the SWM system [ 65 , 89 , 101 , 137 ], even to the extent of taking part in decision-making [ 138 ]. In addition, workers involved in waste collection should be better trained and equipped to handle hazardous waste [ 87 , 128 ]. Moreover, green consumption, using bioplastics, can help reduce the negative impacts of solid waste on the environment [ 139 ].
Lastly, for effective SWM, local authorities should comprehensively address SWM challenges, such as lack of strategic SWM plans, inefficient waste collection/segregation and recycling, insufficient budgets, shortage of qualified waste management professionals, and weak governance, and then form a financial regulatory framework in an integrated manner [ 140 , 141 , 142 ]. Effective SWM system also depends on other factors such as the waste generation rate, population density, economic status, level of commercial activity, culture, and city/region [ 37 , 143 ]. A sustainable SWM strives to protect public health and the environment [ 144 , 145 ].
5. Conclusions
As global solid waste generation rates increase faster than urbanization, coupled with inadequate SWM systems, local governments and urban residents often resort to unsustainable SWM practices. These practices include mixing household and commercial garbage with hazardous waste during storage and handling, storing garbage in old or poorly managed facilities, deficient transportation practices, open-air incinerators, informal/uncontrolled dumping, and non-engineered landfills. The implications of such practices include air and water pollution, land degradation, climate change, and methane and hazardous leachate emissions. In addition, these impacts impose significant environmental and public health costs on residents with marginalized social groups affected mostly.
Inadequate SWM is associated with poor public health, and it is one of the major problems affecting environmental quality and cities’ sustainable development. Effective community involvement in the SWM requires promoting positive public attitudes. Public awareness campaigns through print, electronic, and social media are required to encourage people to desist from littering and follow proper waste-dropping practices. Improper SWM also resulted in water pollution and unhealthy air in cities. Future research is needed to investigate how the peculiarity of each Global South country can influence selecting the SWM approach, elements, aspects, technology, and legal/institutional frameworks appropriate to each locality.
Reviewed literature on the impacts of SWM practices in Asia (compiled by authors).
Reviewed literature on the impacts of SWM practices in South America (compiled by authors).
Reviewed literature on the impacts of SWM practices in Africa (compiled by authors).
Funding Statement
This research received no external funding.
Author Contributions
Conceptualization, I.R.A. and K.M.M.; methodology, I.R.A., K.M.M. and U.L.D.; validation, I.R.A., K.M.M. and U.L.D.; formal analysis, I.R.A. and K.M.M.; investigation, I.R.A., K.M.M., U.L.D., F.S.A., M.S.A., S.M.S.A. and W.A.G.A.-G.; resources, I.R.A., K.M.M., U.L.D., F.S.A., M.S.A., S.M.S.A., W.A.G.A.-G. and T.I.A.; data curation, U.L.D., F.S.A., M.S.A., S.M.S.A. and W.A.G.A.-G.; writing—original draft preparation, I.R.A., K.M.M., U.L.D., F.S.A., M.S.A., S.M.S.A. and W.A.G.A.-G.; writing—review and editing, I.R.A., K.M.M. and U.L.D.; supervision, F.S.A. and T.I.A.; project administration, I.R.A.; funding acquisition, I.R.A., K.M.M., U.L.D., F.S.A., M.S.A., S.M.S.A., W.A.G.A.-G. and T.I.A. All authors have read and agreed to the published version of the manuscript.
Institutional Review Board Statement
Not applicable.
Informed Consent Statement
Data availability statement, conflicts of interest.
The authors declare no conflict of interest in conducting this study.
Publisher’s Note: MDPI stays neutral with regard to jurisdictional claims in published maps and institutional affiliations.
Advertisement
Municipal solid waste management in Russia: potentials of climate change mitigation
- Original Paper
- Open access
- Published: 29 July 2021
- Volume 19 , pages 27–42, ( 2022 )
Cite this article
You have full access to this open access article
- C. Wünsch ORCID: orcid.org/0000-0002-3839-6982 1 , 2 &
- A. Tsybina 3
4523 Accesses
2 Citations
23 Altmetric
Explore all metrics
The goal of this study was to assess the impact of the introduction of various waste management methods on the amount of greenhouse gas emissions from these activities. The assessment was carried out on the example of the Russian waste management sector. For this purpose, three scenarios had been elaborated for the development of the Russian waste management sector: Basic scenario, Reactive scenario and Innovative scenario. For each of the scenarios, the amount of greenhouse gas emissions generated during waste management was calculated. The calculation was based on the 2006 Intergovernmental Panel on Climate Change Guidelines for National Greenhouse Gas Inventories. The results of the greenhouse gas net emissions calculation are as follows: 64 Mt CO 2 -eq./a for the basic scenario, 12.8 Mt CO 2 -eq./a for the reactive scenario, and 3.7 Mt CO 2 -eq./a for the innovative scenario. An assessment was made of the impact of the introduction of various waste treatment technologies on the amounts of greenhouse gas emissions generated in the waste management sector. An important factor influencing the reduction in greenhouse gas emissions from landfills is the recovery and thermal utilization of 60% of the generated landfill gas. The introduction of a separate collection system that allows to separately collect 20% of the total amount of generated municipal solid waste along with twofold increase in the share of incinerated waste leads to a more than threefold reduction in total greenhouse gas emissions from the waste management sector.
Similar content being viewed by others
Greenhouse gas emissions and cost assessments of municipal solid waste treatment and final disposal in maputo city.
Leticia Sarmento dos Muchangos, Akihiro Tokai & Atsuko Hanashima

Toward sustainable waste management: estimating emissions from alternative practices in Gulf Cooperation Council countries
A. Alsulaili, F. Alshamali & A. Aldabbous
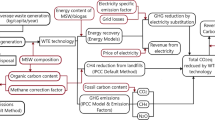
The analysis of electricity production and greenhouse-gas emission reduction from municipal solid waste sector in Oman
W. A. Qazi & M. F. M. Abushammala
Avoid common mistakes on your manuscript.
Introduction
Population growth, urbanization and changing life style have resulted in increased amounts of generated solid waste, which poses serious challenges for many cities and authorities around the world (Abu Qdais et al. 2019 ; Chen 2018 ; Dedinec et al. 2015 ). In 2011, world cities generated about 1.3 Gt of solid waste; this amount is expected to increase to 2.2 Gt by 2025 (Hoornweg and Bhada-Tata 2012 ). Unless properly managed on a national level, solid waste causes several environmental and public health problems, which is adversely reflected on the economic development of a country (Abu Qdais 2007 ; Kaza et al. 2018 ).
One of the important environmental impact of the waste management sector are the generated greenhouse gas (GHG) emissions. These emissions come mostly from the release of methane from organic waste decomposition in landfills (Wuensch and Kocina 2019 ). The waste management sector is responsible for 1.6 Gt carbon dioxide equivalents (CO 2 -eq.) of the global GHG direct anthropogenic emissions per year (Fischedick et al. 2014 ), which accounts for approx. 4% of the global GHG emissions (Papageorgiou et al. 2009 ; Vergara and Tchobanoglous 2012 ). The disposal of municipal solid waste (MSW) contributes to 0.67 Gt CO 2 -eq./a worldwide (Fischedick et al. 2014 ), which is approx. 1.4% of the global GHG emissions. Per capita emissions in developed countries are estimated to be about 500 kg CO 2 -eq./a (Wuensch and Kocina 2019 ), while in the developing and emerging countries, it is around 100 kg CO 2 -eq./a per person. This low contribution of waste management sector comparing to other sectors of the economy, such as energy and transportation, might be the reason for the small amount of research that aims to study GHG emissions from the waste management sector (Chung et al. 2018 ).
However, it is important to consider that the mitigation of GHG emissions from waste management sector is relatively simple and cost-effective as compared to other sectors of the economy. Several studies proved that separate waste collection and composting of biowaste as well as landfilling with landfill gas recovery is currently found to be one of the most effective and economically sound GHG emissions mitigation options (Chen 2018 ; EI-Fadel and Sbayti 2000 ; Yedla and Sindhu 2016 ; Yılmaz and Abdulvahitoğlu 2019 ). Metz et al. 2001 estimated that 75% of the savings of methane recovered from landfills can be achieved at net negative direct cost, and 25% at cost of about 20 US$/Mg CO 2 -eq./a. In any country of the world, the potential of the waste management sector is not yet fully utilized; the implementation of relatively simple and inexpensive waste treatment technologies might contribute to national GHG mitigation goals and convert the sector from a net emitter into a net reducer of GHG emissions (Crawford et al. 2009 ; Voigt et al. 2015 ; Wuensch and Simon 2017 ).
While there are many well-established solutions and technologies for the reduction in GHG emitted from the waste sector, there is no universal set of options that suits all the countries. When thinking to adapt certain solutions of GHG mitigation, it is important to take into account local circumstances such as waste quantities and composition, available infrastructure, economic resources and climate (Crawford et al. 2009 ).
It is expedient to assess how the introduction of modern waste management methods affects the amount of GHG emissions from the waste management process by the example of those countries in which the waste management sector is undergoing reform. These countries include the Russian Federation, where the values of targets for the waste management industry until 2030 are legally established (Government of the Russian Federation 2018 ). In addition, on February 8, 2021, Russia issued a Presidential Decree “On Measures to Implement State Scientific and Technical Policy in the Field of Ecology and Climate,” which prescribes the creation of a Federal Program for the Creation and Implementation of Science-Intensive Technologies to Reduce Greenhouse Gas Emissions (Decree of the President of the Russian Federation 2021 ).
The goal of this study was to quantify the impact of the introduction of various modern waste treatment methods on the volume of GHG emissions from the waste management sector using the example of Russia. To achieve this goal, the following objectives were set and solved:
Elaborate scenarios for the development of the waste management industry, based on the established Industry Development Strategy for the period up to 2030 (Government of the Russian Federation 2018 )
Determine the weighted average morphological composition of MSW;
Select emission factors for various waste treatment methods;
Calculate GHG emissions under each scenario and analyze the calculation results.
The study was conducted from November 2019 to May 2020; the text was updated in March 2021 in connection with the changed situation, as climate change issues began to play an important role on the agenda in Russia. The study and its calculations are theoretical in nature and did not involve experimental research. It was carried out by the authors at their place of work—in Germany (Technische Universität Dresden, Merseburg University of Applied Sciences) and in Russia (Perm National Research Polytechnic University).
Greenhouse gas emissions related to municipal solid waste management sector in Russia
According to the State Report on the Status of Environmental Protection of the Russian Federation of 2018 (Ministry of Natural Resources and Ecology of the Russian Federation 2019 ), the volume of generated MSW has increased by 17% from 235.4 to 275.4 m 3 (49.9 to 58.4 Mt) during the time period 2010 to 2018. With approx. 147 million inhabitants, the annual per capita generation rate is about 400 kg. Until now, MSW management in Russia has been disposal driven. More than 90% of MSW generated is transported to landfills and open dump sites; 30% of the landfills do not meet sanitary requirements (Korobova et al. 2014 ; Tulokhonova and Ulanova 2013 ). According to the State Register of the Waste Disposal Facilities in Russia, there were 1,038 MSW landfills and 2,275 unregistered dump sites at the end of 2018 (Rosprirodnadzor 2019 ). Such waste management practices are neither safe nor sustainable (Fedotkina et al. 2019 ), as they pose high public health and environmental risks and lead to the loss of valuable recyclable materials such as paper, glass, metals and plastics which account for an annual amount of about 15 Mt (Korobova et al. 2014 ).
According to the United Nations Framework Convention on Climate Change (UNFCCC) requirements, the signatory parties of the convention need to prepare and submit national communication reports that document GHG emissions and sinks in each country by conducting an inventory based on Intergovernmental Panel on Climate Change (IPCC) guidelines (UNFCCC 2006 ). Being the fourth biggest global emitter of GHG emissions, Russia submitted its latest National Inventory Report (NIR) to UNFCCC in April 2019. The report documents national GHG emissions by source and removals by sink (Russian Federation 2019 ). The total emissions had been decreased from 3.2 Gt in 1990 to about 2.2 Gt of CO 2 -eq. in 2017, which implies 30% reduction over a period of 27 years. At the same time, the emissions from the disposal of solid waste increased from 33 Mt in 1990 by more than 100% to 69 Mt CO 2 -eq. in 2017. In terms of methane emissions, Russian solid waste disposal sector is the second largest emitter in the country and accounts for 18.1% of the total emitted methane mostly in the form of landfill gas, while the energy sector is responsible for 61.2% of methane emissions (Russian Federation 2019 ).
Landfill gas recovery from MSW landfills is not widely practiced in the Russian Federation. According to the statistics of the Russian Ministry of Natural Resources and Ecology, the share of landfill gas energy in the total renewable energy produced in Russia was 8.61%, 5.43%, 2.77% and 2.59% in 2011, 2012, 2013 and 2014, respectively (Arkharov et al. 2016 ). Different studies show that the potential of recovering energy from landfill gas in the Russian Federation is high (Arkharov et al. 2016 ; Sliusar and Armisheva 2013 ; Starostina et al. 2018 ; Volynkina et al. 2009 ).
Waste-to-energy technology is still in its infancy in Russia; the country is lagging in this area (Tugov 2013 ). Despite that, there is a great interest among the public as well as the private sector in the possibilities of the recovery of energy from MSW. In April 2014, the State Program “Energy Efficiency and Energy Development” was approved, which includes a subprogram on the development of renewable energy sources in the Russian Federation (Government of the Russian Federation 2014 ). In this program, MSW was considered as a source of renewable energy. Until the year 2017, there were only four waste incineration plants in Moscow region processing 655,000 Mg MSW per year, with only one incinerator recovering energy in form of heat and electricity (Dashieva 2017 ). In the nearest future, the construction of four additional incinerators in Moscow region and one in the city of Kazan is planned. The annual total combined capacity of the four new plants in Moscow will be about 2.8 Mt (Bioenergy International 2019 ). In the Kazan incinerator, 0.55 Mt of MSW will be treated annually, which eventually will allow ceasing of landfilling of solid waste in the Republic of Tatarstan (Bioenergy International 2019 ; Regnum 2017 ). The construction of these five new incineration plants is part of the Comprehensive Municipal Solid Waste Strategy adopted by the Russian government in 2013 (Plastinina et al. 2019 ). The focus of this strategy is the reduction in the amount of landfilled waste by creating an integrated management system and industrial recycling of waste.
Separate collection of MSW and the recycling of different waste fractions at the moment plays only a negligible role in the Russian Federation.
Materials and methods
Scenarios of the development of municipal solid waste management system.
To assess the current situation and the potential for reducing GHG emissions from the MSW management industry, three scenarios of the development of the Russian waste management system had been elaborated. The developed scenarios are based on the official statistics data on the amount of waste generated and treated, and also on the adopted legislative acts that determine the development directions of the Russian waste management system and set targets in these areas (Council for Strategic Development and National Projects 2018 ). That is why the developed scenarios include such measures to improve the waste management system as elimination of unauthorized dump sites, introduction of landfill gas collection and utilization systems at the landfills, incineration of waste with energy recovery, separate collection of waste, and recycling of utilizable waste fractions, and do not include other waste-to-energy technologies and waste treatment strategies contributing to climate change mitigation. Separate collection and treatment of biowaste is not applied in the national waste management strategy of the Russian Federation (Government of the Russian Federation 2018 ) and therefore was beyond the scope of the elaborated scenarios. For the purpose of the current study, three scenarios had been developed.
Scenario 1: BASIC (business as usual)
This scenario is based on the current waste management practices, under which 90% of the generated mixed MSW is disposed of on landfills and dump sites. According to the 6th National Communication Report of the Russian Federation to UNFCCC, the total MSW generated that found its way to managed landfills Footnote 1 was 49.209 Mt in 2009, while the amount of MSW disposed in unmanaged disposal sites (dumps) was 5.067 Mt. In 2017, the amount of MSW generated was 58.4 Mt with 10% being diverted from landfills: 3% incinerated and 7% recycled (Ministry of Natural Resources and Ecology of the Russian Federation 2019 ). According to Russian Federation 2019 , landfill gas recovery is not taking place at Russian landfills. This scenario implies the closure of unorganized dump sites, with all the waste to be disposed of on managed dump sites or landfills only.
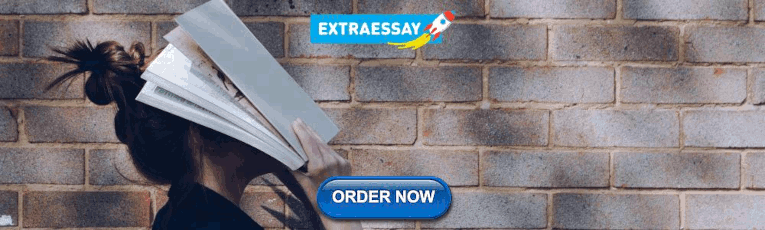
Scenario 2: REACTIVE (moderate development)
The reactive scenario implies a moderate development of the waste management sector, based on the construction of several large incinerators, a small increase in the share of waste to be recycled and the disposal of remaining waste at sanitary landfills, Footnote 2 with the closure of all the existing unorganized dump sites. In this scenario, all Russian regions were divided into two clusters: the first cluster included the city of Moscow and the Republic of Tatarstan, where new waste incinerators are being built, and the second cluster which includes — all the other cities and regions.
Moscow and the Republic of Tatarstan
In Moscow and Tatarstan together, 8.586 Mt of mixed MSW is generated annually (Cabinet of Ministers of the Republic of Tatarstan 2018 ; Department of Housing and Communal Services of the city of Moscow 2019 ). In an attempt to introduce the waste-to-energy technology in Russia, an international consortium that consists of Swiss, Japanese and Russian firms is currently involved in constructing five state-of-the-art incineration plants in these two areas. Four incinerators are to be built in the Moscow region and one in Kazan, the capital of the Republic of Tatarstan. The annual combined capacity of the four plants in Moscow will be about 2.8 Mt of MSW, and the one of Kazan 0.55 Mt (Bioenergy International 2019 ; Regnum 2017 ). In this scenario, it is assumed that compared to the basic scenario, the share of waste undergone recycling is increased to 10%, i.e., 0.859 Mt annually. Furthermore, these 10% would be transferred to recycling plants to recover secondary raw materials. The remaining 4.377 Mt of mixed MSW would be disposed of in sanitary landfills.
Other cities and regions
In the other cities and regions of Russia, in accordance with the Development Strategy of Waste Recycling Industry until 2030 (Government of the Russian Federation 2018 ), over two hundred new eco-techno parks (i.e., waste recycling complexes) will be built. These facilities will receive mixed MSW that will be sorted there for recycling purposes. Under this scenario, it is also assumed that compared to the basic scenario, the share of waste undergone recycling is increased to 10%, thus transferring 4.982 Mt annually of the mixed MSW to recycling plants. The remaining 44.932 Mt of MSW are disposed of in sanitary landfills.
Scenario 3: INNOVATIVE (active development)
This scenario is based on the legally established priority areas for the development of the industry (Council for Strategic Development and National Projects 2018 ; Government of the Russian Federation 2018 ). The scenario implies deep changes in the industry with the introduction of technologies for incineration, separate collection and recycling of waste. In this scenario, the regions of Russia are divided into three clusters, in accordance with the possibilities of improving the infrastructure for waste management and the need for secondary resources and energy received during the processing of waste. When determining the share of waste to which this or that treatment method is applied, federal targets (Council for Strategic Development and National Projects 2018 ; Government of the Russian Federation 2018 ) and estimates made by the World Bank (Korobova et al. 2014 ) were used.
The first cluster includes two huge, densely populated urban agglomerations in which large incineration plants are under construction: Moscow and Tatarstan. With the construction of new waste incinerators, 3.35 Mt of mixed MSW will be incinerated annually. It is assumed that some 10% of mixed MSW (0.859 Mt) generated in these two regions is to be transferred to eco-techno parks for secondary raw material recovery. Some 20% of the MSW (1.712 Mt) is to be recovered from separately collected waste, and the rest of 2.66 Mt (31%) to be disposed of in sanitary landfills.
Cities with more than 0.5 million inhabitants
This cluster includes large urban agglomerations with developed industry and high demand for materials and energy resources. In this cluster, approx. 28 Mt of MSW is generated annually (Korobova et al. 2014 ). Under this scenario, it is assumed that waste incineration plants are also built in some larger cities, besides Moscow and Kazan. However, the exact quantity and capacity of these plants is yet unknown; it was assumed that in comparison with the basic scenario, in this scenario, the share of incinerated waste increased to 10%, the share of recycled waste to 15%, and a separate waste collection system is partially implemented. Hereby, 10% of the generated mixed MSW (2.79 Mt) is undergoing incineration, 15% (4.185 Mt) is transferred to sorting facilities for secondary raw material recovery, some 20% of the MSW (5.58 Mt) is recovered from separately collected waste and the rest 55% (15.345 Mt) is disposed of in sanitary landfills.
Smaller cities with less than 0.5 million inhabitants and rural areas
This cluster includes smaller cities and towns with some industrial enterprises, as well as rural areas. The amount of waste generated annually in this group of settlements is 21.914 Mt. It is assumed that no waste is incinerated, 15% of the mixed MSW (3.287 Mt) is transferred to sorting facilities for secondary raw material recovery, 10% (2.191 Mt) is recovered from separately collected waste, and the rest 75% (16.435 Mt) is disposed of in sanitary landfills.
Waste flow diagrams corresponding to the three scenarios with their input and output flows are shown in Fig. 1 .
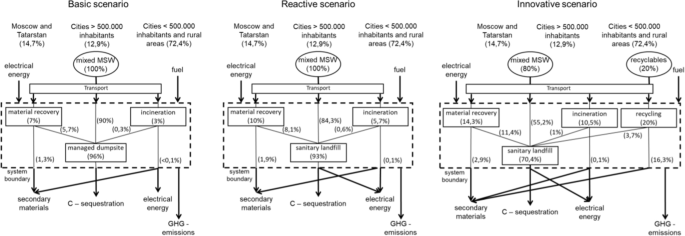
MSW management scenarios with model inputs and outputs
In all the three scenarios, mixed MSW is transferred to sorting facilities where the recovery of valuable materials by mostly hand sorting takes place. Detailed accounts of process efficiency for material recovery facilities, in terms of recovery rates and quality of recovered materials, are scarce in the published literature (Cimpan et al. 2015 ). In the study of Cimpan et al., 2015 , at least three data sets were evaluated with the result that 13–45% of paper, 3–49% of glass, 35–84% of metals and 1–73% of plastics were recovered from the plant input of these materials. Two other studies report similar recovery rates between 60 and 95% for paper, glass, plastic and aluminum for hand and automatic sorting test trials (CalRecovery, Inc and PEER Consultants 1993 ; Hryb 2015 ). Based on this data and the results of the authors’ own experimental studies on manual waste sorting in Russia, the recovery rates for the most valuable waste fractions, including paper/cardboard, glass, metals and plastics had been calculated (Table 1 ). In the Scenario 3, separate collection of paper/cardboard, glass and plastic is introduced. Recovery rates related to the input of the corresponding waste type into each waste management cluster (see Table 1 ) for Moscow and Tatarstan as well as for the cities with more than 0.5 million inhabitants are considered to be higher than for the settlements with less than 0.5 million inhabitants.
For the comparison of GHG emissions of the three elaborated scenarios, a specific assessment model was elaborated.
Model structure
The calculation of the amounts of released and avoided GHG emissions for the different considered waste treatment technologies are based on the 2006 IPCC Guidelines for National Greenhouse Gas Inventories. The IPCC methodology is scientifically widely recognized and used internationally, which makes the results easy comprehensible and easier to compare with other studies.
For the elaboration of the model that would allow calculating the GHG balance emissions, the upstream-operating-downstream (UOD) framework (Gentil et al. 2009 ) was used, where direct emissions from waste management procedures and indirect emissions from upstream and downstream activities are differentiated. On the upstream side, the indirect GHG emissions, like those related to fuel and material extraction, processing and transport as well as plant construction and commissioning, are excluded from the consideration. Indirect emissions from infrastructure construction on the downstream side are outside the system boundaries and not accounted for as they are relatively low (Boldrin et al. 2009 ; Mohareb et al. 2011 ). Direct GHG emissions from the waste transport are also excluded from the system boundaries since they are negligible comparing to the direct emissions from the waste processing/treatment (Weitz et al. 2002 ; Wuensch and Simon 2017 ). Since indirect GHG emissions avoided due to energy and material substitution, as well as carbon sequestration in the downstream processes is significant, they are included into the model. The conceptual framework of the model and its boundaries are shown in Fig. 2 .
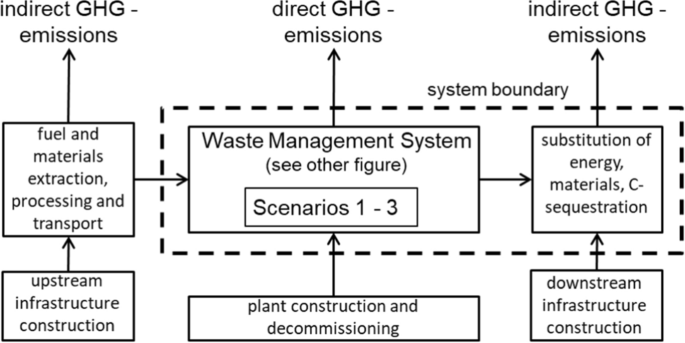
Conceptual framework of the model showing upstream and downstream processes along with the system boundaries [derived from Abu Qdais et al. ( 2019 )]
The inputs to the model are waste (its quantity, composition, carbon content fixed in biomass and no-biomass), as well as energy and fuel that are used in the waste treatment processes (see Table 2 and Figs. 1 , 2 and 3 ). The outputs include generated and delivered electricity, recovered secondary materials and sequestrated carbon.
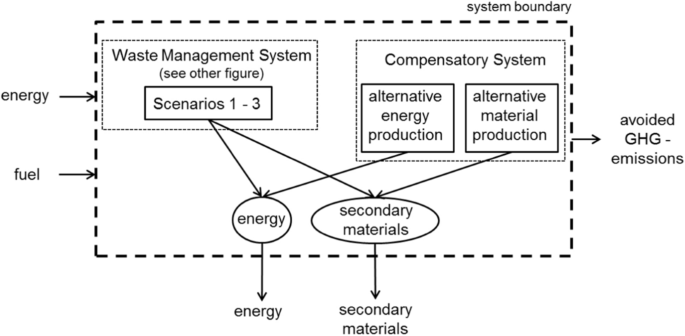
Compensatory system for the substitution of primary materials and energy [derived from Abu Qdais et al. ( 2019 )]
The analysis of MSW composition is not regularly done in Russia, and only a limited number of studies on this subject are published. Since waste composition is the basis for the determination of direct GHG emissions from waste management activities, accurate data is desirable. The Russian Federation is a huge country with both densely populated urban areas and sparsely populated rural areas. Due to the different settlement structures, the waste compositions also differ a lot. It is not expedient to assume an average composition for the entire country. Therefore, hereinafter three clusters had been considered to define waste compositions. The first cluster includes Moscow and the Republic of Tatarstan, since in these regions, a larger amount of mixed MSW is/will be incinerated in the nearest future. The second cluster includes the cities with the population of more than 0.5 million people, and the third cluster includes the settlements with the population of less than 0.5 million people. The waste compositions for these three clusters given in Table 2 are weighted averages of the results of a number of experimental studies of waste composition which were found in sources of the literature published after 2010 and further analyzed. Weighted average here means that the respective data on waste composition that was found for a city or region was included in the weighted average with the proportion that the amount of MSW generated in the city or region takes up as part of the total mass of MSW generated in the respective cluster.
To determine the avoidance of GHG emissions in the downstream processes by means of energy and material substitution as well as carbon sequestration, a compensatory system must be used. In Fig. 3 , the compensatory system for the substitution of energy and primary materials is shown.
Emission factors
Waste incineration.
It is necessary to know the emission factors when calculating GHG emissions from thermal treatment of waste, and also when compiling national emissions inventories (Larsen and Astrup 2011 ). Information on GHG emission factors of various solid waste treatment technologies for each country is of great importance for the assessment of GHGs emitted as a result of adopting a certain technology. However, such factors are not available for the Russian Federation, which implies using the data available in the literature for the countries with the conditions similar to the Russian ones, examining local circumstances of solid waste management system (Friedrich and Trois 2013 ; Larsen and Astrup 2011 ; Noya et al. 2018 ).
There are different factors affecting GHG emission levels from waste incineration. One of the most important factors in determining CO 2 emissions is the amount of fossil carbon in the waste stream meant for incineration. Non-CO 2 emissions are more dependent on the incineration technology and conditions, and for modern waste incinerators, the amounts of non-CO 2 emissions are negligible (Johnke 2001 ; Sabin Guendehou et al. 2006 ).
The amount of fossil carbon was calculated based on waste composition, carbon content and share of fossil carbon given in Table 2 ; the resulting fossil carbon content in wet waste was 0.117 kg C/kg. For the indirectly avoided GHG emissions, the recovery of electricity with a net efficiency of 24% for all the scenarios and for the Scenario 3 also from metals contained in the incinerator slag to substitute primary metals was considered. The recovery of heat in form of process steam or district heat was not considered in the scenarios (Dashieva 2017 ). Further parameters for the calculation of GHG emissions from waste incineration are given in Table 3 .
For the calculation of the impact of the methane released from landfills to climate change over a 100 years’ time horizon, the first-order decay kinetics model was used. Almost 80% of the Russian MSW landfills occupy an area larger than 10 ha (Volynkina and Zaytseva 2010 ). Here, it is assumed that all the MSW is highly compacted and disposed of in deep landfills under anaerobic conditions without the recovery of landfill gas (Govor 2017 ). Since no landfill gas is recovered, in Scenario 1, only the sequestrated non-biodegradable biogenic carbon in the landfill results in avoided GHG emissions. There is an intention in Russia to introduce the collection of landfill gas as the primary measure to reduce GHG emissions from the waste management sector (Government of the Russian Federation 2018 ; Ministry of Natural Resources and Ecology of the Russian Federation 2013 ) within the next years. In the literature, methane recovery rates between 9% (Scharff et al. 2003 ) and 90% (Spokas et al. 2006 ) are reported. For example, most US landfills are well-controlled and managed; in particular, in California, gas collection efficiencies are as high as 82.5% (Kong et al. 2012 ). Based on these values, for both Scenario 2 and Scenario 3, landfill gas recovery is introduced with a recovery rate of 60%. Under these two scenarios, in addition to carbon sequestration, the recovered landfill gas is used to produce electricity, which results in avoided indirect GHG emissions. Other parameters used for the calculation are mainly taken from the latest Russian National Inventory Report where IPCC default parameters were used (Pipatti et al. 2006 ; Russian Federation 2019 ). The parameters used for the calculation of GHG emissions from landfills for all the three scenarios are shown in Table 4 .
- Material recovery
In all the scenarios, some part of mixed MSW is treated in eco-techno parks, where valuable secondary raw materials like metals, paper, glass and plastics are recovered, and the sorting residues are forwarded to landfills. In addition, separate collection of some amounts of paper, glass, and plastics in the Scenario 3 is presumed. The corresponding recovery rates are already given in Table 1 . Each recovered secondary material substitutes a certain amount of primary material. Since the production of primary materials is usually connected with higher energy and raw material consumption than that of the secondary materials, more GHGs are released during the production of the former ones. Therefore, every unit of recovered secondary material obtained leads to a reduction in released GHGs.
GHG emission or substitution factors are developed for specific geographical areas and technologies, and their appropriateness to other circumstances may be questionable (Turner et al. 2015 ). The application of one specific emission factor for a recovered material in the whole Russian Federation would already be debatable due to the size of the country. Perhaps that is why emission factors for Russia cannot be found in the literature. For this study, the average values of GHG emission/substitution factors determined for other industrial countries from the study of (Turner et al. 2015 ) were used. The amounts of avoided GHG, i.e., the values of the emission factors in CO 2 equivalents for the recovered valuable waste fractions, including steel, aluminum, paper/cardboard, glass and plastic, are given in Table 5 .
In Table 5 , the used equivalent factor (Global Warming Potential over a time horizon of 100 years) of released methane versus carbon dioxide, the emission factor of the use of fuel oil in the waste incineration process and the substitution factor of delivered electrical power are shown. The emission factor of the generated electricity in the Russian Federation is relatively low, since approx. half (52%) of the electricity is produced by natural gas and approx. 13% by hydro- and nuclear power, while only 13% is produced by coal (British Petrolium 2019 ; U.S. Energy Information Administration 2017 ). The electricity mix factor is therefore only 0.358 Mg CO 2 -eq./MWh generated electricity (Gimadi et al. 2019 ).
Results and discussion
The population of the Russian Federation is expected to decrease in the next decades (United Nations 2019 ), but due to the economic growth, the amount of waste generated per capita is expected to increase in the same ratio; that is why the calculation of the GHG emissions for all the three scenarios was based on an assumed fixed annually amount of 58.4 Mt of MSW. Average waste compositions were calculated for this study on the basis of eleven waste analyses conducted in different Russian cities between 2010 and 2017 and grouped into three clusters (Moscow and Tatarstan, cities with more than 0.5 million inhabitants and cities/settlements with less than 0.5 million inhabitants). From the available literature data for the countries with conditions similar to Russian ones, emission factors were adopted to be further used in calculations of GHG emissions from waste disposal on managed and sanitary landfills, waste incineration and waste recycling with the recovery of secondary raw materials.
In Fig. 4 , the amounts of CO 2 -equivalent emissions per year that contribute to global warming for each of the three scenarios considered in the study are shown. Since the emissions related to the collection and transportation of waste, as well as energy consumption in the upstream side, are almost similar for all the treatment processes (Komakech et al. 2015 ), and as they are relatively small compared to the operational and downstream emissions (Boldrin et al. 2009 ; Friedrich and Trois 2011 ), they were not considered in the model. Avoided and sequestrated emissions were subtracted from the direct emissions to calculate GHG net emission values.
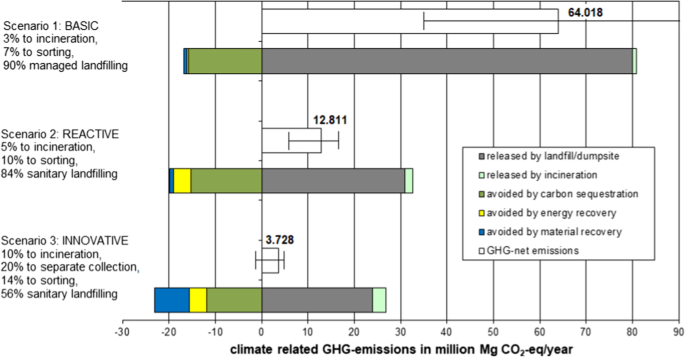
Global warming contribution of the three considered scenarios
The basic scenario (mostly managed landfilling without landfill gas recovery) gives the highest GHG net emissions among all the analyzed scenarios of approx. 64 Mt CO 2 -eq./a, followed by the reactive scenario (mostly sanitary landfilling with landfill gas recovery) with approx. 12.8 Mt CO 2 -eq./a of GHG net emissions. The innovative scenario (sanitary landfilling with landfill gas recovery and increased shares of MSW incineration, separate collection and material recovery) had shown an almost neutral GHG balance with approx. 3.7 Mt CO 2 -eq./a of GHG net emissions.
To assess the impact of the introduction of various waste treatment methods on the amount of GHG emissions from the waste management sector, the specific GHG emissions for each scenario as a whole was calculated, as well as “within” scenarios for each considered waste management process/method (Table 6 ).
The amount of specific total GHG emissions under Scenario 2 is five times less than under Scenario 1. Such a large difference is due to the modernization of existing managed dumpsites (Scenario 1), instead of which MSW is disposed of at sanitary landfills equipped with landfill gas and leachate collection systems, with intermediate insulating layers and top capping (Scenario 2). Such a transition from managed dumpsites to sanitary landfills leads not only to a decrease in the amount of specific released GHG emissions by approx. 1 Mg CO 2 -eq./Mg MSW, but also to a decrease in total emissions due to avoided emissions in the amount of 0.053 Mg CO 2 -eq./Mg MSW generated by energy recovery.
The amount of specific total GHG emissions under Scenario 3 is 3.4 times less than under Scenario 2. This reduction is mainly due to an almost twofold increase in the volume of waste incinerated, along with the introduction of a separate waste collection system (Scenario 3). At the same time, in Scenario 3, the share of plastic in the mixed waste stream sent to incineration is less than in Scenarios 1 and 2 (see Fig. 1 ). Climate-related GHG from waste incineration are generated mainly due to the plastic contained in the waste. Therefore, in Scenario 3, less GHG emissions are released during waste incineration. Reduction in GHG emissions from waste incineration is also facilitated by the recovery of metals from the bottom ash, which occurs only in Scenario 3.
In Scenario 3, the total amount of recycled material is larger than in Scenario 2, since not only part of the mixed waste is recycled, but also separately collected. According to the Scenario 3, metals are not included in the waste fractions collected separately. Metals have a comparably high GHG substitution factor (see Table 5 ); this explains the slight decrease in avoided GHG emissions due to material recovery in Scenario 3 compared to Scenario 2 because of a decreased share of metals in the total waste stream sent for recycling.
Many studies confirm GHG emissions reduction by the application of these waste treatment concepts. It is shown that the recovery of landfill gas from managed landfills has a high potential to reduce GHG emissions from landfills (EI-Fadel and Sbayti 2000 ; Friedrich and Trois 2016 ; Lee et al. 2017 ; Starostina et al. 2014 ). The transfer from the disposal of mixed MSW on landfills to the incineration on waste incineration or waste-to-energy plants leads to further reduction in GHG emissions (Bilitewski and Wuensch 2012 ; Chen 2018 ; Voigt et al. 2015 ). The recovery of secondary materials from MSW allows avoiding additional amounts of GHG emissions (Björklund and Finnveden 2005 ; Franchetti and Kilaru 2012 ; Turner et al. 2015 ; Wuensch and Simon 2017 ).
It should be noted that the calculated results of the direct GHG emissions from landfilling and waste incineration are subject to uncertainties. Waste composition (Table 2 ) and the parameters set/assumed for the landfills (Table 4 ) and waste incineration (Table 3 ) affect the level of the results. Indirect downstream emissions from recovered secondary materials and substituted energy cannot be provided with accuracy, as indicated by missing data for the substitution factors of recovered secondary materials in Russia and the variability of the scenarios for substituted electricity. To get an impression about the possible fluctuation range of the determined results, a sensitivity analysis was carried out. Therefore, all values shown in Tables 1 , 3 , 4 and 5 were ones decreased by 10% and once increased by 10%. The impact of the sensitivity analysis on the GHG net emissions is shown as error bars in Fig. 4 . The results of the sensitivity analysis show a range for the GHG net emissions of the basic scenario between 35.129 and 91.446 Mt CO 2 -eq./a, for the reactive scenario between 5.133 and 16.324 Mt CO 2 -eq./a and for the innovative scenario from − 1.516 to 4.871 Mt CO 2 -eq./a.
All the exact values of the final results shown in Fig. 4 as well as the graphical representation of the results of the sensitivity analysis can be checked in the provided supplementary materials.
The most recent data about global GHG emissions from solid waste disposal shows that direct emissions contribute with 0.67 Gt CO 2 -eq./a (Fischedick et al. 2014 ) to about 1.4% of the total anthropogenic GHG emissions of 49 Gt CO 2 -eq./a (Edenhofer et al. 2015 ). For the Russian Federation, the contribution of the direct emissions from the MSW management accounts for approx. 3.7% of the total GHG emissions of the country of around 2.2 Gt CO 2 -eq./a (Russian Federation 2019 ). In this study, the potential of different waste management methods in relation to climate change impact was assessed using the example of the Russian waste management industry. For this purpose, three scenarios had been developed and analyzed:
Basic scenario (business as usual), based on the existing waste management practices. The scenario implies that 90% of the generated mixed MSW is disposed of on managed dumpsites, 7% is undergone material recovery and 3% incinerated. All the unorganized dumpsites are closed; on managed dumpsites, there is no landfill gas recovery.
Reactive scenario (moderate development). This scenario implies construction of a number of large waste incineration plants and an increase in the share of waste to be recycled so that 84.3% of generated MSW is disposed of in sanitary landfills, 10% is sent to recycling plants for material recovery, and 5.7% is incinerated.
Innovative scenario (active development). This scenario assumes partial implementation of a separate waste collection system and broader introduction of waste processing technologies. As a result, 20% of the total generated MSW is collected separately and then recycled, 14.3% undergoes material recovery, 55.2% is disposed of in sanitary landfills, and 10.5% is incinerated.
For determining weighed average morphological composition of MSW, three clusters of human settlements had been considered, and the respective data on waste compositions had been analyzed. The first cluster includes Moscow and the Republic of Tatarstan, the second cluster includes the major cities (those with the population of more than 0.5 million people), and the third cluster includes the minor cities and rural areas.
For determining emission factors, both own calculation results and reference data from the National Inventory Report and other sources were used. Thus, the amount of fossil carbon, being one of the most important factors determining CO 2 emissions from waste incineration, was calculated based on the waste composition, carbon content and the share of fossil carbon in the waste. For the calculation of the amount of CH 4 released from MSW landfills, the first-order decay kinetics model was used. Avoided GHG emissions are the result of sequestrated non-biodegradable biogenic carbon in landfills (all the scenarios) and recovered landfill gas used to produce electricity (Scenarios 2 and 3). With the use of emission factors for material recovery included those for the recovered valuable waste fractions steel, aluminum, paper and cardboard, glass and plastic, GHG emissions were calculated under each scenario. As it was expected, the basic scenario gives the highest amount of total GHG net emissions of approx. 64 Mt CO 2 -eq./a (1.096 Mg CO 2 -eq./Mg MSW). Under the reactive scenario, the amount of total GHG net emissions is approx. 12.8 Mt CO 2 -eq./a (0.219 Mg CO 2 -eq./Mg MSW), and under the innovative scenario, it is about 3.7 Mt CO 2 -eq./a (0.064 Mg CO 2 -eq./Mg MSW).
The calculation of specific GHG emissions made it possible to assess the extent to which the introduction of various waste treatment methods makes it possible to reduce GHG emissions resulting from the respective waste treatment processes. Analysis of the results of these calculations showed that the transition from managed dumpsites to sanitary landfills can reduce total GHG emissions from the Russian waste management sector by up to 5 times. The introduction of a separate collection system (in which 20% of waste is collected separately) with a simultaneous twofold increase in the share of waste incinerated has led to a more than threefold reduction in total GHG emissions from the sector of Russian waste management. Another factor influencing the reduction in GHG emissions from waste incineration is the recovery of metals from the bottom ash.
Direct GHG emissions can be further reduced with a shift from landfilling to treatment of mixed MSW in material recovery facilities and waste incinerators or even to separate collection and treatment of MSW. In addition, indirect downstream emissions can be avoided by a significant amount via energy and material recovery. With a separate collection and treatment of biowaste and the recovery of district heat from waste incineration process, further GHG mitigation can be obtained. With these additional measures, the MSW industry of the Russian Federation could become a net avoider from a net emitter.
For this study, a number of parameters and emission factors from the literature where used, which does not precisely reflect the situation in Russia. Conducting further research for determining country specific, for a huge country like Russia, possibly even region-specific data and emission factors resulting in the development of a corresponding database would be useful to minimize these uncertainties.
Data availability
Not applicable.
Acknowledgements
The authors wish to thank all who assisted in conducting this work.
Open Access funding enabled and organized by Projekt DEAL.
Author information
Authors and affiliations.
Institute of Waste Management and Circular Economy, Department of Hydrosciences, Technische Universität Dresden, Pratzschwitzer Str. 15, 01796, Pirna, Germany
Department of Engineering and Natural Sciences, Merseburg University of Applied Sciences, 06217, Merseburg, Germany
Environmental Protection Department, Perm National Research Polytechnic University, Prof. Pozdeev Street 14, Perm, Russia, 614013
You can also search for this author in PubMed Google Scholar
Contributions
CW involved in conceptualization, data curation, methodology, validation, visualization, writing — original draft, and writing — review and editing. AT involved in conceptualization, data curation, investigation, and writing — original draft.
Corresponding author
Correspondence to C. Wünsch .
Ethics declarations
Conflict of interest.
The authors declare that they have no conflict of interest.
Additional information
Editorial responsibility: Maryam Shabani
Supplementary Information
Below is the link to the electronic supplementary material.
Supplementary file 1 (PDF 225 KB)
Supplementary file 2 (xlsx 37 kb), supplementary file 3 (xlsx 21 kb), rights and permissions.
Open Access This article is licensed under a Creative Commons Attribution 4.0 International License, which permits use, sharing, adaptation, distribution and reproduction in any medium or format, as long as you give appropriate credit to the original author(s) and the source, provide a link to the Creative Commons licence, and indicate if changes were made. The images or other third party material in this article are included in the article's Creative Commons licence, unless indicated otherwise in a credit line to the material. If material is not included in the article's Creative Commons licence and your intended use is not permitted by statutory regulation or exceeds the permitted use, you will need to obtain permission directly from the copyright holder. To view a copy of this licence, visit http://creativecommons.org/licenses/by/4.0/ .
Reprints and permissions
About this article
Wünsch, C., Tsybina, A. Municipal solid waste management in Russia: potentials of climate change mitigation. Int. J. Environ. Sci. Technol. 19 , 27–42 (2022). https://doi.org/10.1007/s13762-021-03542-5
Download citation
Received : 17 September 2020
Revised : 13 March 2021
Accepted : 09 July 2021
Published : 29 July 2021
Issue Date : January 2022
DOI : https://doi.org/10.1007/s13762-021-03542-5
Share this article
Anyone you share the following link with will be able to read this content:
Sorry, a shareable link is not currently available for this article.
Provided by the Springer Nature SharedIt content-sharing initiative
- Climate change
- Greenhouse gases
- Russian Federation
- Incineration
- Separate collection of waste
- Waste composition
- Find a journal
- Publish with us
- Track your research
City Council votes on a long-term garbage contract with Richard's Disposal. See what happened
Contract secured with one amendment added by the jackson city council. but if richard's disposal doesn't agree to the amendment, council will have to vote again.
Trash crisis averted, at least for the time being. Garbage will continue to be collected in Jackson.
In a 4-3 vote, and after 2 hours of discussion, the Jackson City Council approved a six-year contract with Richard's Disposal Inc., with an amendment calling for the removal of trash carts. The long-term contract will go into effect April 1. The current emergency contract with Richard's Disposal expires on March 31.
It was similar party lines as last year's vote. Ward 1 Councilman Ashby Foote, Ward 3 Councilman Kenneth Stokes and Ward 5 Councilman Vernon Hartley voted to reject Richard's. Ward 2 Councilwoman Angelique Lee, Ward 4 Councilman Brian Grizzell, Ward 6 Councilman and Council President Aaron Banks and Ward 7 Councilwoman Virgi Lindsay voted to approve.
Banks, who voted against Richard's last year, was the flipped vote.
But the vote almost failed due to the amendment approved by the council earlier Tuesday that would remove trash carts from the contract. The original contract presented to the council called for Richard's to purchase 45,000 carts. Initially, the amended Richard's contract was rejected, 4-3, on Tuesday, after Grizzell sided with Foote, Stokes and Hartley. Grizell said he wanted to keep the trash carts, which is why he sided with those council members historically opposed to Richard's.
Lee leaned back away from the mic as did Grizzell. Lee quickly called for a vote to reconsider, and Grizzell changed his mind, giving Richard's the needed four votes and thus avoiding another trash crisis.
Jackson's 2023 trash crisis: Mayor: 'Race has been all in this,' referring to heart of Jackson, MS, trash crisis
Trash crisis timeline: Council will vote on a new garbage vendor in the coming weeks. How did we get here? See more
Finally, residents have an answer to a question that has been looming over the city all year: Would Jacksonians have to face a trash crisis for the second year in a row? Or would city leaders be able to set aside their differences and agree on a long-term contract?
It wasn't without fireworks, as expected. Council members screamed at each other; council members screamed at Jackson Mayor Chokwe Antar Lumumba; the mayor fired back. Both Alvin Richard, the president and CEO of Richard's Disposal, and Lee Bush, president and CEO of National Collections United LLC., — the second and only other bidder — were in attendance.
Those against Richard's — Foote, Stokes and Hartley — complained that the city waited until the last minute to figure out the contract, therefore not giving the council enough time to review, that the RFP process wasn't done fairly and that there weren't enough vendors who bid on the RFP because trash companies were scared of the city's two ongoing legal disputes with Richard's Disposal.
Richard's Disposal's lawsuits: Richard's Disposal filed a lawsuit to block the latest RFP issued by the city. See more
"We had nine months to get this thing together," Hartley said. "We've been goaded into this situation."
Lumumba defended the timeline.
"We said that there was still a legal case and we had to protect the interest of residents," Lumumba said. "We did it (issued the RFP) in spite of the fact that we knew not only would it cost us more money, but there were potential risks to us ... It brought us to the point that Tori (former City Attorney Catoria Martin), at the time that she said we were issuing the RFP, that it would come right at this time."
The $64 million contract, at $891,000 per month, with Richard's Disposal is over a period of six years, with four options of one-year each which can be exercised by the city. Garbage will be collected twice a week Monday- Saturday.
The long-term contract is about a $83,000 monthly increase from the city's current emergency contract with Richard's, which cost $808,035 per month. Back in 2021, Richard's Disposal submitted a bid for a long-term contract worth $765,000 per month. Accepting the bid in 2021, taxpayers would have saved $43,035 per month, $516,420 per year and $1,549,260 over a three-year period compared to the emergency contract. That savings would be even greater compared to the new contract.
Grizzell and Lee made this point, that Richard's bid has increased every year, several times throughout the debate.
"I just wanted to say that if cost was an issue, we should have settled this then (last year) versus now," Grizzell said.
Much of the debate centered on carts
The initial contract stated that Richard's would provide roughly 45,000, 96-gallon trash carts for residents, though using them is optional. If a resident has a cart already, they can use that one. Trash bags on the street will also be picked up.
The council voted, 5-2, on an amendment that would remove the carts from the contract. Foote, Lee, Stokes, Banks and Hartley voted to remove the carts. Grizzell and Lindsay voted against.
Ward 6 town hall: Things got contentious at town hall meeting to discuss garbage pick up. See what happened
At the end of the day, the removal of the carts has to be negotiated by the city and Richard's Disposal. Gloria Greene, the attorney for Richard's Disposal told the council they would be willing to negotiate.
If Richard's Disposal doesn't agree to the removal of the carts, then the contract will have to be voted on again by the council, according to City Attorney Drew Martin.
If the company does agree to the removal of carts, it could potentially knock the price of the contract down more than $4 million. Right now, each cart costs $96. Doing the math, 45,000 carts cost the city around $4.3 million.
So, which company had the lowest bid?
Only two companies submitted bids in response to the city's 2023 RFP for solid waste collection: Richard's Disposal and National Collections United LLC.
Richard's Disposal submitted a bid on the contract for $891,000 per month for six years, according to the contract. National Collections United LLC submitted a bid for $874,000, according to Bush, who told the council members their bid during the meeting.
More details on Richard's contract: How much will new trash contract cost Jackson? Mayor releases deal with Richard's Disposal
Both companies were reviewed by an evaluation committee, with Richard's Disposal ultimately being selected. This raised concerns from Stokes and Hartley, who questioned why the company with the lowest bid wasn't chosen.
In response, Lumumba handed out a memo, dated Feb. 7, to council members, the press and members of the public. Although National Collections United LLC submitted the lowest bid, the memo states the company wasn't able to meet the minimum requirements the RFP required.
Those requirements included evidence of purchased garbage trucks, evidence of an insurance bond for the garbage trucks in the circumstance that an accident happens and evidence that the companies have a "minimum of five years of experience collecting and transporting solid waste for a governmental entity with a population at least as large" as Jackson.
To each of those requirements, according to the memo, National Collections United LLC responded: "We have not completed the information requested. We're sending the information we have on file that includes. Pictures of proposed truck and other certificates attached."
Richard Disposal responded, according to the memo, that they "currently have 21 leased" garbage trucks in possession, that the company "did submit a bid bond for this project," and the company "has had the City of New Orleans Residential Solid Waste Contract since 2007," as well as servicing the City of East Baton Rouge for the past two years. Richard's Disposal has also been servicing the City of Jackson for the past two years.
MDEQ fines for 2023 trash crisis: Jackson gets 4 MDEQ violations and is being fined for April's trash crisis. See how much
"I didn't disqualify anybody," Lumumba said. "It was the committee in its scoring, and it was the legal department in its review of the minimum requirements of the RFP, that said that those requirements didn't match."
Stokes' amendments
Stokes attempted to make four amendments to the contract. After making a motion for some of the amendments, the councilman could be seen laughing, while other council members shook their heads in annoyance. City Attorney Drew Martin told Stokes that he believes some of his amendments would be illegal due to state procurement laws.
Each amendment failed in a 4-3 vote. Foote, Stokes and Hartley voted in favor; Lee, Grizzell, Banks and Lindsay all voted against.
Stokes four failed amendments were as follows:
- An amendment to remove Richard's Disposal and give the contract to National Collections United, LLC. Martin advised that he believed this would be illegal under Mississippi's procurement laws.
- An amendment to make the contract last for only two years, until 2025. Martin said he believed the price of the contract would increase if this was supported.
- An amendment to change the price of the contract from $891,000 to $765,000, which was Richard's previous bid on the city's 2021 RFP.
- An amendment to give the contract to National Collections United LLC., and make Richard's Disposal a subcontractor. Again, Martin advised that he believes this would be illegal under Mississippi's procurement laws.
The Ward 3 councilman was vehemently opposed to the city doing any business with Richard's Disposal. Before the vote on the long-term contract and for the second meeting in a row, Stokes introduced an ordinance that would ban the city from doing any business at all with Richard's Disposal. That ordinance failed again in a 4-3 vote.
Banks continues push for local, minority subcontractor
One of the issues Banks has been fighting for was an amendment of the contract that required Richard's Disposal to use a local, minority subcontractor. He specifically fought for National Collections United LLC., to be that subcontractor during the meeting, as he did at a town hall he hosted last week.
It came to the point where he asked council members to take a straw poll, not an official vote, on who would be in favor of the amendment.
Mayor defends selection of Richard's: Lumumba addresses rumors on the selection of Richard's Disposal. See what he said
Grizzell, as well as Lindsay, refused to participate in the straw poll.
"The last time I checked we lived in the United States of America. A capitalistic democracy. There's no way as a business owner somebody is going to force me to do business with anybody else," Grizzell said. "That's between them to work out … that has nothing to do with the council."
Banks, who by this point was visibly worn out from all of the meeting's discussion, said he wasn't trying to force Richard's Disposal, but was only trying to see how the council feels about the amendment.
No vote or straw poll took place on Banks' proposed amendment.
Got a story idea? Want to talk about issues facing the City of Jackson? Contact Charlie Drape at [email protected].
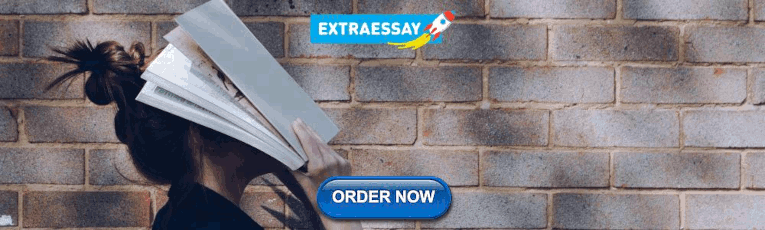
COMMENTS
Abstract. This study investigates landfill and incineration waste management strategies as potential and prevalent traditional methods of disposing of municipality-generated waste because of their cheapness and simplicity but requires a high level of management to mitigate their negative impacts. Interestingly, sustainability in municipal solid ...
iv URBAN DEVELOPMENT SERIES - KNOWLEDGE PAPERS Annexes A. Map of Regions 36 B. Map of Income Distribution 38 C. Availability of MSW Data by Country 40 D. Countries Excluded for Lack of Data 45 E. Estimated Solid Waste Management Costs 46 F. MSW Generation Data for Cities Over 100,000 47 G. MSW Collection Data for Cities Over 100,000 63 H. MSW Disposal Methods for Cities Over 100,000 71
Solid-waste management, the collecting, treating, and disposing of solid material that is discarded because it has served its purpose or is no longer useful. ... American refuse is usually lighter, for example, than European or Japanese refuse. In the United States paper and paperboard products make up close to 40 percent of the total weight of ...
The generation, recycling, composting, combustion with energy recovery, and landfilling of MSW have all changed dramatically over the last few decades [].From under 10% of produced MSW in 1980 to 35.0% in 2017, the combined recycling and composting rate have increased (Fig. 1.2).Recycling alone (without composting) increased from 14.5 million tons (9.6% of MSW) in 1980 to 69 million tons (23.6 ...
The concept of effective solid waste management is a long-term goal for municipalities to achieve. The composition of Indian waste is different as compared to other developed countries — so the adoption of the strategies could not be the same. ... Working Paper No. 356 Solid Waste Management in India An Assessment of Resource Recovery and ...
Solid Waste Management Practices in the Global South. Global municipal solid waste (MSW) generation rose from 1.3 billion tons in 2012 to 2.1 billion tons (0.74 kg/capita/day) as of 2016, which by 2050 is expected to increase by 70% to reach a total of 3.40 billion tons or 1.42 kg/capita/day [ 19 ].
Abstract. Solid waste management is a crucial process that involves controlling the generation, storage, collection, transfer, processing, and disposal of solid wastes. It encompasses a range of technologies aimed at harnessing energy from waste materials. The six functional components of solid waste management include waste identification, on ...
Solid waste management (SWM) has always been an integral feature of every human society and has become a growing global concern as urban populations continue to grow as well as consumption patterns change. ... (46%) among all types of waste, followed by paper (17%), plastic (10%), glass (5%), metal (4%), and other (18%) (see Fig. 1.2 and Table ...
Abstract. With the increase in dependence on plastic since the late 20th century, plastic pollution has also increased. A pollution that was measured at barely a few hundred tonnes in the 1950s is ...
3.1. Solid Waste Management Practices in the Global South. Global municipal solid waste (MSW) generation rose from 1.3 billion tons in 2012 to 2.1 billion tons (0.74 kg/capita/day) as of 2016, which by 2050 is expected to increase by 70% to reach a total of 3.40 billion tons or 1.42 kg/capita/day [19].
report, the current amount of waste produc ed in the. Philippine cities wi ll increase by approximately. 165% in 2025 - from abo ut 29,315 to 77, 776 tons. per day (Ng, 2012). The increasing ...
Solid waste management is a term that refers to the storage and disposal process for solid wastes. This also provides recycled options for things that don't belong to trash or waste. As long as humans have lived in villages and rural areas, the problem has been trash or solid waste. The solid waste management used in solid,
This study provides the first life cycle assessment (LCA) for municipal solid waste waste management system in one of the largest cities in Europe, Moscow. Its significance stems from recent important changes in the waste management system, the introduction of limited source separate collection in 2020, and the first examination of sorted municipal solid waste (MSW) composition.
The researchers used the LCA-IWM model to compare various alternatives of solid waste management. Recently, Vinitskaia et al. (2021) [39] used LCA to evaluate the existing and the proposed ...
This term paper, "Global Solid Waste Problem and Management" is published exclusively on IvyPanda's free essay examples database. You can use it for research and reference purposes to write your own paper.
and Satpal Singh in the paper, "Sustainable Municipal Solid Waste Management in India: A Policy Agenda" policy agenda for sustainable SWM needs to drive behavioral change amongst citizens, elected representatives and decision-makers to minimize waste and maximize reuse and recycling. As the J&K G25 strategy proposes
Norman Wade. Solid. Waste Management Term Paper: Solid waste management is the complex of the collection, transportation, recycling or utilization of wastes and the control over this process. Under the term of wastes we understand the solid results of the human activity. It is obvious that people produce too many wastes, which spoil and worsen ...
The analysis shows the average mean weight composition of household waste sortingat source ranges from 0.50kg to 0.63kg paper, 2.21kg to 3.29kg putrescibles, 0.85kg to 1.41kg glass, 0.52kg to 0.68 plastic and 0.89kg to 1.20kg cans. The average percentage composition of wastes ranges from 10% paper to 45% putrescibles.
Municipal solid waste accounts for approximately 1-2% of all waste generated, which is equivalent to 65 million tons in 2019 for Russia. More than 90% of all municipal solid waste is disposed into landfill sites, with only 5-7% being recycled. Food is the main source of waste composition in landfills, comprising over 34%.
Theoretical Study on Solid Waste Management. Shailesh Kumar Dewangan 1, Akshat Jaiswal 2, Ajeet jaiswal 3, Nishu Jaiswal 4, Mamta Chandra 5, Sourav Tirkey 6. 1 Asst. Professor & HOD Department of ...
Population growth, urbanization and changing life style have resulted in increased amounts of generated solid waste, which poses serious challenges for many cities and authorities around the world (Abu Qdais et al. 2019; Chen 2018; Dedinec et al. 2015).In 2011, world cities generated about 1.3 Gt of solid waste; this amount is expected to increase to 2.2 Gt by 2025 (Hoornweg and Bhada-Tata 2012).
LAHORE: The Lahore Waste Management Company (LWMC) claimed to have collected 130,000 tonnes of solid waste from different parts of the city and disposed it off at Mahmood Booti landfill at the ...
Today's Paper | March 30, 2024. Home Latest Pakistan Opinion Business World Culture Prism Sport Magazines Tech Popular Archive Flood Donations Home Latest Pakistan Opinion Business World Culture Prism Sport Magazines Tech Popular Archive Flood Donations Search. Search . Email Your Name * ...
The long-term contract will go into effect April 1. The current emergency contract with Richard's Disposal expires on March 31. It was similar party lines as last year's vote.
Later in Dec 2021, the Sindh Assembly passed an amended local government bill placing the Solid Waste Management Board under mayors of the metropolitan/municipal corporations in the province. The amended laws allowed mayors in all divisional metropolitan municipal corporations to look after their functions in a bid to improve the performance of ...
ABSTRACT: This research paper presents a comprehensive review on solid waste management. from an Indian perspective. It provides an overview of the current status, challenges, and. opportunities ...
KARACHI: The provincial government on Friday finally approved devolution of the Sindh Solid Waste Management Board (SSWMB) to division level by forming separate boards, to be headed by mayors, for ...
Today's Paper | March 31, 2024. ... BAHAWALPUR: A plan to outsource the solid waste management operations in Bahawalpur division has been finalised, said divisional Commissioner Nadir Chattha.
One of the main problems in Nepali municipalities that needs a long-term solution is the solid waste management [14]. According to the preliminary study and review, most municipalities' waste ...
You're watching live coverage of COMMITTEE: Audit & Risk - 27 March 2024. The full agenda for the meeting is available on our website -...