Click through the PLOS taxonomy to find articles in your field.
For more information about PLOS Subject Areas, click here .
Loading metrics
Open Access
Peer-reviewed
Research Article
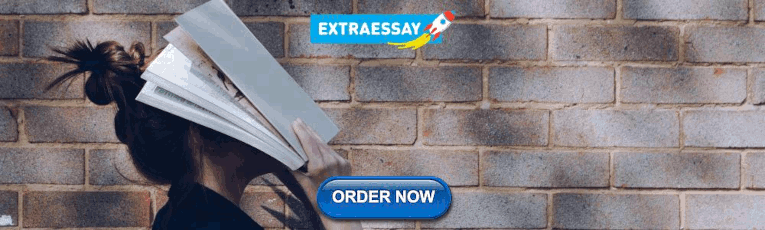
Research on drinking water purification technologies for household use by reducing total dissolved solids (TDS)
Roles Conceptualization, Data curation, Formal analysis, Investigation, Methodology, Project administration, Resources, Software, Supervision, Validation, Visualization, Writing – original draft, Writing – review & editing
* E-mail: [email protected]
Affiliation Redlands East Valley High School, Redlands, California, United States of America

- Bill B. Wang
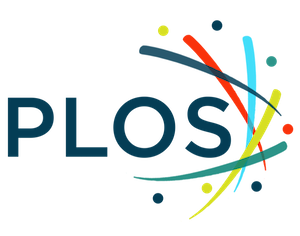
- Published: September 28, 2021
- https://doi.org/10.1371/journal.pone.0257865
- Reader Comments
This study, based in San Bernardino County, Southern California, collected and examined tap water samples within the area to explore the feasibility of adopting non-industrial equipment and methods to reduce water hardness and total dissolved solids(TDS). We investigated how water quality could be improved by utilizing water boiling, activated carbon and sodium bicarbonate additives, as well as electrolysis methods. The results show that heating is effective at lower temperatures rather than long boils, as none of the boiling tests were lower than the original value. Activated carbon is unable to lower TDS, because it is unable to bind to any impurities present in the water. This resulted in an overall TDS increase of 3.5%. However, adding small amounts of sodium bicarbonate(NaHCO 3 ) will further eliminate water hardness by reacting with magnesium ions and improve taste, while increasing the pH. When added to room temperature tap water, there is a continuous increase in TDS of 24.8% at the 30 mg/L mark. The new findings presented in this study showed that electrolysis was the most successful method in eliminating TDS, showing an inverse proportion where an increasing electrical current and duration of electrical lowers more amounts of solids. This method created a maximum decrease in TDS by a maximum of 22.7%, with 3 tests resulting in 15.3–16.6% decreases. Furthermore, when water is heated to a temperature around 50°C (122°F), a decrease in TDS of around 16% was also shown. The reduction of these solids will help lower water hardness and improve the taste of tap water. These results will help direct residents to drink more tap water rather than bottled water with similar taste and health benefits for a cheaper price as well as a reduction on plastic usage.
Citation: Wang BB (2021) Research on drinking water purification technologies for household use by reducing total dissolved solids (TDS). PLoS ONE 16(9): e0257865. https://doi.org/10.1371/journal.pone.0257865
Editor: Mahendra Singh Dhaka, Mohanlal Sukhadia University, INDIA
Received: June 22, 2021; Accepted: September 14, 2021; Published: September 28, 2021
Copyright: © 2021 Bill B. Wang. This is an open access article distributed under the terms of the Creative Commons Attribution License , which permits unrestricted use, distribution, and reproduction in any medium, provided the original author and source are credited.
Data Availability: All relevant data are within the manuscript and its Supporting Information files.
Funding: The author received no specific funding for this work.
Competing interests: The authors have declared that no competing interests exist.
Introduction
The concentration of total dissolved solids(TDS) present in water is one of the most significant factors in giving water taste and also provides important ions such as calcium, magnesium, potassium, and sodium [ 1 – 3 ]. However, water with high TDS measurements usually indicates contamination by human activities, such as soil and agricultural runoff caused by irrigation, unregulated animal grazing and wildlife impacts, environmentally damaging farming methods such as slash and burn agriculture, and the overuse of nitrate-based fertilizer [ 4 , 5 ], etc. Around tourist areas as well as state parks, these factors will slowly add up over time and influence the water sources nearby [ 5 ]. Water that flows through natural springs and waterways with high concentrations of organic salts within minerals and rocks, or groundwater that originates from wells with high salt concentration will also result in higher particle measurements [ 6 ].
Water sources can be contaminated by substances and ions such as nitrate, lead, arsenic, and copper [ 7 , 8 ] and may cause many health problems related to heavy metal consumption and poisoning. Water reservoirs and treatments plants that do not consider water contamination by motor vehicles, as well as locations that struggle to provide the necessary components required for water treatment will be more prone to indirect contamination [ 9 – 11 ]. Many plants are effective in ensuring the quality and reduction of these contaminants, but often leave out the secondary considerations, The United States Environmental Protection Agency(US EPA)’s secondary regulations recommend that TDS should be below 500 mg/L [ 2 ], which is also supported by the World Health Organization(WHO) recommendation of below 600 mg/L and an absolute maximum of less than 1,000 mg/L [ 3 ]. These substances also form calcium or magnesium scales within water boilers, heaters, and pipes, causing excess buildup and drain problems, and nitrate ions may pose a risk to human health by risking the formation of N -nitroso compounds(NOC) and less public knowledge about such substances [ 12 – 15 ]. Nitrates can pose a non-carcinogenic threat to different communities, but continue to slip past water treatment standards [ 15 ]. Furthermore, most people do not tolerate or prefer water with high hardness or chlorine additives [ 16 ], as the taste changes tremendously and becomes unpreferable. Even so, TDS levels are not accounted for in mandatory water regulations, because the essential removal of harmful toxins and heavy metals is what matters the most in water safety. Some companies indicate risks in certain ions and alkali metals, showing how water hardness is mostly disregarded and is not as well treated as commercial water bottling companies [ 17 , 18 ].
In Southern California, water quality is not as well maintained than the northern counties as most treatment plants in violation of a regulation or standard are located in Central-Southern California [ 19 ], with southern counties having the largest number of people affected [ 20 ]. This study is focused on the Redlands area, which has had no state code violations within the last decade [ 21 ]. A previous study has analyzed TDS concentrations throughout the Santa Ana Basin, and found concentrations ranging from 190–600 ppm as treated wastewater and samples obtained from mountain sites, taking into account the urban runoff and untreated groundwater as reasons for elevated levels of TDS but providing no solution in helping reduce TDS [ 22 ]. Also, samples have not been taken directly through home water supplies, where the consumer is most affected. Other water quality studies in this region have been focused on the elimination of perchlorates in soil and groundwater and distribution of nitrates, but such research on chemicals have ceased for the last decade, demonstrated by safe levels of perchlorates and nitrates in water reports [ 23 , 24 ]. In addition to these studies, despite the improving quality of the local water treatment process, people prefer bottled water instead of tap water because of the taste and hardness of tap water [ 25 ]. Although water quality tests are taken and documented regularly, the taste of the water is not a factor to be accounted for in city water supplies, and neither is the residue left behind after boiling water. The residue can build up over time and cause appliance damage or clogs in drainage pipes.
This study will build upon previous analyses of TDS studies and attempt to raise new solutions to help develop a more efficient method in reducing local TDS levels, as well as compare current measurements to previous analyses to determine the magnitude to which local treatment plants have improved and regulated its treatment processes.
Several methods that lower TDS are reviewed: boiling and heating tap water with and without NaHCO₃, absorption by food-grade activated carbon [ 26 , 27 ], and battery-powered electrolysis [ 28 – 30 ]. By obtaining water samples and determining the difference in TDS before and after the listed experiments, we can determine the effectiveness of lowering TDS. The results of this study will provide options for residents and water treatment plants to find ways to maintain the general taste of the tap water, but also preserve the lifespan of accessories and pipelines. By determining a better way to lower TDS and treat water hardness, water standards can be updated to include TDS levels as a mandatory measurement.
Materials and methods
All experiments utilized tap water sourced from Redlands homes. This water is partially supplied from the Mill Creek (Henry Tate) and Santa Ana (Hinckley) Water Sheds/Treatment Plants, as well as local groundwater pumps. Water sampling and sourcing were done at relatively stable temperatures of 26.9°C (80.42°F) through tap water supplies. The average TDS was measured at 159 ppm, which is slightly lower than the reported 175 ppm by the City of Redlands. Permission is obtained by the author from the San Bernardino Municipal Water Department website to permit the testing procedures and the usage of private water treatment devices for the purpose of lowering water hardness and improving taste and odor. The turbidity was reported as 0.03 Nephelometric Turbidity Units (NTU) post-treatment. Residual nitrate measured at 2.3mg/L in groundwater before treatment and 0.2 mg/L after treatment and perchlorate measured at 0.9 μg/L before treatment, barely staying below the standard of 1 μg/L; it was not detected within post-treatment water. Lead content was not detected at all, while copper was detected at 0.15 mg/L.
For each test, all procedures were done indoors under controlled temperatures, and 20 L of fresh water was retrieved before each test. Water samples were taken before each experimental set and measured for TDS and temperature, and all equipment were cleaned thoroughly with purified water before and after each measurement. TDS consists of inorganic salts and organic material present in solution, and consists mostly of calcium, magnesium, sodium, potassium, carbonate, chloride, nitrate, and sulfate ions. These ions can be drawn out by leaving the water to settle, or binding to added ions and purified by directly separating the water and ions. Equipment include a 50 L container, 1 L beakers for water, a graduated cylinder, a stir rod, a measuring spoon, tweezers, a scale, purified water, and a TDS meter. A standard TDS meter is used, operated by measuring the conductivity of the total amount of ionized solids in the water, and is also cleaned in the same manner as aforementioned equipment. The instrument is also calibrated by 3 pH solutions prior to testing.All results were recorded for and then compiled for graphing and analysis.
Heating/Boiling water for various lengths of time
The heating method was selected because heat is able to break down calcium bicarbonate into calcium carbonate ions that are able to settle to the bottom of the sample. Four flasks of 1 L of tap water were each heated to 40°C, 50°C, 60°C, and 80°C (104–176°F) and observed using a laser thermometer. The heated water was then left to cool and measurements were made using a TDS meter at the 5, 10, 20, 30, and 60-minute marks.
For the boiling experiments, five flasks of 1 L of tap water were heated to boil at 100°C (212°F). Each flask, which was labeled corresponding to its boiling duration, was marked with 2, 4, 6, 10, and 20 minutes. Each flask was boiled for its designated time, left to cool under open air, and measurements were made using a TDS meter at the 5, 10, 20, 30, 60, and 120-minute marks. The reason that the boiling experiment was extended to 120 minutes was to allow the water to cool down to room temperature.
Activated carbon as a water purification additive
This test was performed to see if food-grade, powdered activated carbon had any possibility of binding with and settling out residual particles. Activated carbon was measured using a milligram scale and separated into batches of 1, 2, 4, 5, 10, 30, and 50 mg. Each batch of the activated carbon were added to a separate flask of water and stirred for five minutes, and finally left to settle for another five minutes. TDS measurements were recorded after the water settled.
Baking soda as a water purification additive
To lower scale error and increase experimental accuracy, a concentration of 200 mg/L NaHCO₃ solution was made with purified water and pure NaHCO₃. For each part, an initial TDS measurement was taken before each experiment.
In separate flasks of 1 L tap water, each labeled 1, 2, 4, 5, 10, and 30 mg of NaHCO 3 , a batch was added to each flask appropriately and stirred for 5 minutes to ensure that everything dissolved. Measurements were taken after the water was left to settle for another 5 minutes for any TDS to settle.
Next, 6 flasks of 1 L tap water were labeled, with 5 mg (25 mL solution) of NaHCO₃ added to three flasks and 10 mg (50 mL solution) of NaHCO₃ added to the remaining three. One flask from each concentration of NaHCO₃ was boiled for 2 mins., 4 mins., or 6 mins., and then left to cool. A TDS measurement was taken at the 5, 10, 20, 30, 60, and 120-minute marks after removal from heat.
Electrolysis under low voltages
This test was performed because the ionization of the TDS could be manipulated with electricity to isolate an area of water with lower TDS. For this test, two 10cm long graphite pieces were connected via copper wiring to a group of batteries, with each end of the graphite pieces submerged in a beaker of tap water, ~3 cm apart.
Using groups of 1.5 V double-A batteries, 4 beakers with 40mL of tap water were each treated with either 7.5, 9.0, 10.5, and 12.0 V of current. Electrolysis was observed to be present by the bubbling of the water each test, and measurements were taken at the 3, 5, 7, and 10 minute marks.
Results/Discussion
Heating water to various temperatures until the boiling point.
The goal for this test was to use heat to reduce the amount of dissolved oxygen and carbon dioxide within the water, as shown by this chemical equation: Heat: Ca(HCO 3 ) 2 → CaCO 3 ↓ + H 2 O + CO 2 ↑.
This would decompose ions of calcium bicarbonate down into calcium carbonate and water and carbon dioxide byproducts.
Patterns and trends in decreasing temperatures.
The following trend lines are based on a dataset of changes in temperature obtained from the test results and graphed as Fig 1 .
- PPT PowerPoint slide
- PNG larger image
- TIFF original image
https://doi.org/10.1371/journal.pone.0257865.g001
To predict the precise temperature measurements of the tap water at 26.9°C, calculations were made based on Fig 1 . The fitting equations are in the format, y = a.e bx . The values for the fitting coefficients a and b, and correlation coefficient R 2 are listed in Table 1 as column a, b and R 2 . The calculated values and the target temperature are listed in Table 1 .
https://doi.org/10.1371/journal.pone.0257865.t001
Fig 2 was obtained by compiling TDS results with different temperatures and times.
https://doi.org/10.1371/journal.pone.0257865.g002
The fitting equations for Fig 2 are also in the format, y = a.e bx . The fitting coefficients a and b, and correlation coefficient R 2 values are listed in Table 2 . Based on the fitting curves in Fig 2 and the duration to the target temperature in Table 1 , We calculated the TDS at 26.9°C as listed in column calculated TDS in Table 2 based on the values we reported on Fig 2 .
https://doi.org/10.1371/journal.pone.0257865.t002
Based on the heating temperature and the calculated TDS with the same target water temperature, we obtained the following heating temperature vs TDS removal trend line and its corresponding fitting curve in Table 2 .
In Fig 1 , a trend in the rate of cooling is seen, where a higher heating temperature creates a steeper curve. During the first five minutes of cooling, the water cools quicker as the absorbed heat is quickly released into the surrounding environment. By the 10-minute mark, the water begins to cool in a linear rate of change. One detail to note is that the 100°C water cools quicker than the 80°C and eventually cools even faster than the 60°C graph. Table 1 supports this observation as the duration to target temperature begins to decrease from a maximum point of 94.8 mins to 80.95 mins after the 80°C mark.
As shown in Fig 2 , all TDS values decrease as the temperature starts to cool to room temperature, demonstrating a proportional relationship where a lower temperature shows lower TDS. This can partially be explained by the ions settling in the flasks. Visible particles can also be observed during experimentation as small white masses on the bottom, as well as a thin ring that forms where the edge of the water contacts the flask. When the water is heated to 40°C and cooled, a 3.8% decrease in TDS is observed. When 50°C is reached, the TDS drops at its fastest rate from an initial value of 202 ppm to 160 ppm after 60 minutes of settling and cooling. The TDS measurements in these experiements reach a maximum of 204 ppm at the 60°C mark. However, an interesting phenomenon to point out is that the water does not hit a new maximum at 100°C. meaning that TDS reaches a plateau at 60°C. Also, the rate of decrease begins to slow down after 20 minutes, showing that an unknown factor is affecting the rate of decrease. It is also hypothesized that the slight increase in TDS between the 5–20 minute range is caused by a disturbance in the settling of the water, where the temperature starts to decrease at a more gradual and constant rate. The unstable and easy formation of CaCO 3 scaling has also been the subject of a study of antiscaling methods, which also supports the result that temperature is a significant influence for scale formation [ 12 ].
In Table 2 , calculations for TDS and the time it takes for each test to cool were made. Using the data, it is determined that the test with 50°C water decreased the most by 16% from the initial measurement of 159ppm. This means that it is most effective when water is heated between temperatures of 40–60°C when it comes to lowering TDS, with a difference of ~7–16%. When water is heated to temperatures greater than 80°C, the water begins to evaporate, increasing the concentration of the ions, causing the TDS to increase substantially when cooled to room temperature.
Finally, in Fig 3 , a line of best fit of function f(x) = -0.0007x 3 + 0.1641x 2 –10.962x + 369.36 is used with R 2 = 0.9341. Using this function, the local minimum of the graph would be reached at 48.4°C.
https://doi.org/10.1371/journal.pone.0257865.g003
This data shows that heating water at low temperatures (i.e. 40–50°C) may be more beneficial than heating water to higher temperatures. This study segment has not been presented in any section within the United States EPA Report on water management for different residual particles/substances. However, warmer water temperatures are more prone to microorganism growth and algal blooms, requiring more intensive treatment in other areas such as chlorine, ozone, and ultraviolet disinfection.
Using the specific heat capacity equation, we can also determine the amount of energy and voltage needed to heat 1 L of water up to 50°C: Q = mcΔT, where c, the specific heat capacity of water, is 4.186 J/g°C, ΔT, the change in temperature from the experimental maximum to room temperature, is 30°C, and m, the mass of the water, is 1000 g. This means that the amount of energy required will be 125580 J, which is 0.035 kWh or 2.1 kW.
After taking all of the different measurements obtained during TDS testing, and compiling the data onto this plot, Fig 4 is created with a corresponding line of best fit:
https://doi.org/10.1371/journal.pone.0257865.g004
In Fig 4 , it can be observed that the relationship between the temperature of the water and its relative TDS value is a downwards facing parabolic graph. As the temperature increases, the TDS begins to decrease after the steep incline at 50–60°C. The line of best fit is represented by the function f(x) = -0.0142x 2 + 2.258x + 105.84. R 2 = 0.6781. Because the R 2 value is less than expected, factors such as the time spent settling and the reaction rate of the ions should be considered. To determine the specifics within this experiment, deeper research and prolonged studies with more highly accurate analyses must be utilized to solve this problem.
Boiling water for various amounts of time
Trend of boiling duration and rate of cooling..
Using the same methods to create the figures and tables for the previous section, Fig 5 depicts how the duration of time spent boiling water affects how fast the water cools.
https://doi.org/10.1371/journal.pone.0257865.g005
As seen in Fig 5 , within the first 10 minutes of the cooling time, the five different graphs are entwined with each other, with all lines following a similar pattern. However, the graph showing 20 minutes of boiling is much steeper than the other graphs, showing a faster rate of cooling. This data continues to support a previous claim in Fig 2 , as this is most likely represented by a relationship a longer the boil creates a faster cooling curve. This also shows that the first 5 minutes of cooling have the largest deviance compared to any other time frame.
The cooling pattern is hypothesized by possible changes in the orderly structure of the hydrogen bonds in the water molecules, or the decreased heat capacity of water due to the increasing concentration of TDS.
Effect on TDS as boiling duration increases.
In Fig 6 , all lines except for the 20-minute line are clustered in the bottom area of the graph. By excluding the last measurement temporarily due to it being an outlier, we have observed that the difference between the initial and final TDS value of each test decreases.
https://doi.org/10.1371/journal.pone.0257865.g006
Despite following a similar trend of an increase in TDS at the start of the tests and a slow decrease overtime, this experiment had an interesting result, with the final test measuring nearly twice the amount of particles compared to any previous tests at 310 ppm, as shown in Fig 6 . It is confirmed that the long boiling time caused a significant amount of water to evaporate, causing the minerals to be more concentrated, thus resulting in a 300 ppm reading. Fig 6 follows the same trend as Fig 2 , except the TDS reading veers away when the boiling duration reaches 20 minutes. Also, with the long duration of heating, the water has developed an unfavorable taste from intense concentrations of CaCO₃. This also causes a buildup of a thin crust of CaCO₃ and other impurities around the container that is difficult to remove entirely. This finding is in accordance with the introductory statement of hot boiling water causing mineral buildups within pipes and appliances [ 9 ]. A TDS reading of 300ppm is still well below federal secondary standards of TDS, and can still even be compared to bottled water, in which companies may fluctuate and contain 335ppm within their water [ 1 , 2 ].
This experiment continues to stupport that the cooling rate of the water increases as the time spent boiling increases. Based on this test, a prediction can be made in which an increased concentration of dissolved solids lowers the total specific heat capacity of the sample, as the total volume of water decreases. This means that a method can be derived to measure TDS using the heat capacity of a tap water mixture and volume, in addition to current methods of using the electrical conductivity of aqueous ions.
Adding food-grade activated carbon to untreated tap water
Fig 7 presents a line graph with little to no change in TDS, with an initial spike from 157 to 163 ppm. The insoluble carbon remains in the water and shows no benefit.
https://doi.org/10.1371/journal.pone.0257865.g007
The food-grade activated carbon proved no benefit to removing TDS from tap water, and instead added around 5–7 ppm extra, which settled down to around +4 ppm at 120 minutes. The carbon, which is not 100% pure from inorganic compounds and materials present in the carbon, can dissolve into the water, adding to the existing concentration of TDS. Furthermore, household tap water has already been treated in processing facilities using a variety of filters, including carbon, so household charcoal filters are not effective in further reducing dissolved solids [ 18 ].
Adding sodium bicarbonate solution to boiled tap water
As seen in Fig 8 , after adding 1 mg of NaHCO 3 in, the TDS rises to 161 ppm, showing a minuscule increase. When 4 mg was added, the TDS drops down to 158 ppm. Then, when 5 mg was added, a sudden spike to 172 ppm was observed. This means that NaHCO 3 is able to ionize some Ca 2+ and Mg 2+ ions, but also adds Na + back into the water. This also means that adding NaHCO 3 has little to no effect on TDS, with 4mg being the upper limit of effectiveness.
https://doi.org/10.1371/journal.pone.0257865.g008
To examine whether or not the temperature plays a role in the effectiveness in adding NaHCO 3 , a boiling experiment was performed, and the data is graphed in Fig 9 .
https://doi.org/10.1371/journal.pone.0257865.g009
Fig 9 presents the relationship between the amount of common baking soda(NaHCO₃) added, the boiling time involved, and the resulting TDS measurements. After boiling each flask for designated amounts of time, the results showed a downward trend line from a spike but does not reach a TDS value significantly lower than the initial sample. It is apparent that the NaHCO₃ has not lowered the TDS of the boiling water, but instead adds smaller quantities of ions, raising the final value. This additive does not contribute to the lowering of the hardness of the tap water. However, tests boiled with 5 mg/L of baking soda maintained a downward pattern as the water was boiled for an increasing amount of time, compared to the seemingly random graphs of boiling with 10 mg/L.
In some households, however, people often add NaHCO₃ to increase the pH for taste and health benefits. However, as shown in the test results, it is not an effective way of reducing TDS levels in the water [ 10 , 16 ], but instead raises the pH, determined by the concentration added. Even under boiling conditions, the water continues to follow the trend of high growth in TDS, of +25–43 ppm right after boiling and the slow drop in TDS (but maintaining a high concentration) as the particles settle to the bottom.
Utilizing the experimental results, we can summarize that after adding small batches of NaHCO3 and waiting up to 5 minutes will reduce water hardness making it less prone to crystallizing within household appliances such as water brewers. Also, this process raises the pH, which is used more within commercial water companies. However, the cost comes at increasing TDS.
Using electrolysis to treat TDS in tap water
Different voltages were passed through the water to observe the change in TDS overtime, with the data being compiled as Fig 10 .
https://doi.org/10.1371/journal.pone.0257865.g010
The process of electrolysis in this experiment was not to and directly remove the existing TDS, but to separate the water sample into three different areas: the anode, cathode, and an area of clean water between the two nodes [ 19 ]. The anions in the water such as OH - , SO 4 2- , HCO 3 - move to the anode, while the cations such as H + , Ca 2+ 、Mg 2+ 、Na + move to the cathode. The middle area would then be left as an area that is more deprived of such ions, with Fig 10 proving this.
As shown in Fig 10 , electrolysis is effective in lower the TDS within tap water. Despite the lines being extremely tangled and unpredictable, the general trend was a larger decrease with a longer duration of time. At 10 minutes, all lines except 10.5 V are approaching the same value, meaning that the deviation was most likely caused by disturbances to the water during measurement from the low volume of water. With each different voltage test, a decrease of 12.7% for 6.0 V, 14.9% for 9.0 V, 22.7% for 10.5 V. and 19.5% for 12.0 V respectfully were observed. In the treatment of wastewate leachate, a study has shown that with 90 minutes of electrical treatment, 34.58% of TDS content were removed, supporting the effectiveness of electricity and its usage in wastewater treatment [ 29 ].
This experiment concludes that electrolysis is effective in lowering TDS, with the possibility to improve this process by further experimentation, development of a water cleaning system utilizing this cathode-anode setup to process water. This system would be a more specific and limited version of a reverse osmosis system by taking away ions through attraction, rather than a filter.
The Southern Californian tap water supply maintains TDS values below the federal regulations. However, crystalline scale buildup in household appliances is a major issue as it is hard to clean and eliminate. To easily improve the taste and quality of tap water at home as well as eliminating the formation of scales, the following methods were demonstrated as viable:
- By heating water to around 50°C (122°F), TDS and water hardness will decrease the most. Also, the boiling process is effective in killing microorganisms and removing contaminants. This process cannot surpass 10 minutes, as the concentration of the ions in the water is too high, which poses human health risks if consumed. These, along with activated carbon and NaHCO₃ additives, are inefficient methods that have minimal effects for lowering TDS.
- Electrolysis is one of the most effective methods of eliminating TDS. Experiments have proven that increased current and duration of time helps lower TDS. However, this method has yet to be implemented into conventional commercial water filtration systems.
Also, some observations made in these experiments could not be explained, and require further research and experimentation to resolve these problems. The first observation is that TDS and increasing water temperature maintain a parabolic relationship, with a maximum being reached at 80°C, followed by a gradual decrease. The second observation is that when water is boiled for an increased duration of time, the rate of cooling also increases.
This experiment utilized non-professional scientific equipment which are prone to mistakes and less precise. These results may deviate from professionally derived data, and will require further study using more advanced equipment to support these findings.
Acknowledgments
The author thanks Tsinghua University Professor and PLOS ONE editor Dr. Huan Li for assisting in experimental setups as well as data processing and treatment. The author also thanks Redlands East Valley High School’s Dr. Melissa Cartagena for her experimental guidance, and Tsinghua University Professor Dr. Cheng Yang for proofreading the manuscript.
- View Article
- Google Scholar
- 2. United States Environmental Protection Agency. 2018 Edition of the Drinking Water Standards and Health Advisories Tables (EPA 822-F-18-001). US EPA, Washington D.C., USA, 2018; pp. 9–19.
- 3. World Health Organization. Guidelines for Drinking-Water Quality: Fourth Edition Incorporating the First Addendum. WHO, Geneva, Switzerland, 2017; pp. 7, 219–230, 423.
- PubMed/NCBI
- 6. Chloride, Salinity, and Dissolved Solids. 2019 Mar 1, [cited on 20 September 2020] Available from: https://www.usgs.gov/mission-areas/water-resources/science/chloride-salinity-and-dissolved-solids?qt-science_center_objects=0#qt-science_center_objects .
- 13. Shoukat, Ammara, Hussain, M., Shoukat, Asra. Effects of Temperature on Total dissolved Solid in water. Water Quality Study Conference, Mehran University Sindh, Pakistan, February 2020.
- 17. United States Environmental Protection Agency. Drinking Water Treatment Plant Residuals Management—Technical Report: Summary of Residuals Generation, Treatment, and Disposal at Large Community Water Systems. US EPA, Washington D.C., USA, 2011; pp. 177–182.
- 19. Exceedance and Compliance Status of Public Water Systems. 2012 Feb 1, [cited 22 September 2020] Available from: https://www.arcgis.com/apps/MapJournal/index.html?appid=143794cd74e344a29eb8b96190f4658b# .
- 20. 2019 Water Quality Status Report California. California Water Boards, 2019 July 1, [cited 22 September 2020] Available from: https://gispublic.waterboards.ca.gov/portal/apps/MapJournal/index.html?appid=6cde29ac0afc4d55b0fdaaae6bfc1aa4 .
- 21. City of Redlands—Water Quality Consumer Confidence Reports. City of Redlands, 2010–2020, [cited 23 September 2020] Available from: https://www.cityofredlands.org/post/water-quality .
- 25. Consumers’ Preference For Bottled Water Is Growing And They Want It Available Wherever Drinks Are Sold. 2019 Jan 8, [cited 23 September 2020] Available from: https://www.bottledwater.org/consumers’-preference-bottled-water-growing-and-they-want-it-available-wherever-drinks-are-sold .
- You are here:
- American Chemical Society
- Discover Chemistry
Recent advancements in water treatment
For immediate release, acs news service weekly presspac: january 19, 2022.
Generating clean, safe water is becoming increasingly difficult. Water sources themselves can be contaminated, but in addition, some purification methods can cause unintended harmful byproducts to form. And not all treatment processes are created equal with regard to their ability to remove impurities or pollutants. Below are some recent papers published in ACS journals that report insights into how well water treatment methods work and the quality of the resulting water. Reporters can request free access to these papers by emailing newsroom@acs.org .
“Drivers of Disinfection Byproduct Cytotoxicity in U.S. Drinking Water: Should Other DBPs Be Considered for Regulation?” Environmental Science & Technology Dec.15, 2021
In this paper, researchers surveyed both conventional and advanced disinfection processes in the U.S., testing the quality of their drinking waters. Treatment plants with advanced removal technologies, such as activated carbon, formed fewer types and lower levels of harmful disinfection byproducts (known as DBPs) in their water. Based on the prevalence and cytotoxicity of haloacetonitriles and iodoacetic acids within some of the treated waters, the researchers recommend that these two groups be considered when forming future water quality regulations.
“Complete System to Generate Clean Water from a Contaminated Water Body by a Handmade Flower-like Light Absorber” ACS Omega Dec. 9, 2021 As a step toward a low-cost water purification technology, researchers crocheted a coated black yarn into a flower-like pattern. When the flower was placed in dirty or salty water, the water wicked up the yarn. Sunlight caused the water to evaporate, leaving the contaminants in the yarn, and a clean vapor condensed and was collected. People in rural locations could easily make this material for desalination or cleaning polluted water, the researchers say.
“Data Analytics Determines Co-occurrence of Odorants in Raw Water and Evaluates Drinking Water Treatment Removal Strategies” Environmental Science & Technology Dec. 2, 2021
Sometimes drinking water smells foul or “off,” even after treatment. In this first-of-its-kind study, researchers identified the major odorants in raw water. They also report that treatment plants using a combination of ozonation and activated carbon remove more of the odor compounds responsible for the stink compared to a conventional process. However, both methods generated some odorants not originally present in the water.
“Self-Powered Water Flow-Triggered Piezocatalytic Generation of Reactive Oxygen Species for Water Purification in Simulated Water Drainage” ACS ES&T Engineering Nov. 23, 2021
Here, researchers harvested energy from the movement of water to break down chemical contaminants. As microscopic sheets of molybdenum disulfide (MoS2) swirled inside a spiral tube filled with dirty water, the MoS2 particles generated electric charges. The charges reacted with water and created reactive oxygen species, which decomposed pollutant compounds, including benzotriazole and antibiotics. The researchers say these self-powered catalysts are a “green” energy resource for water purification.
The American Chemical Society (ACS) is a nonprofit organization chartered by the U.S. Congress. ACS’ mission is to advance the broader chemistry enterprise and its practitioners for the benefit of Earth and all its people. The Society is a global leader in promoting excellence in science education and providing access to chemistry-related information and research through its multiple research solutions, peer-reviewed journals, scientific conferences, eBooks and weekly news periodical Chemical & Engineering News . ACS journals are among the most cited, most trusted and most read within the scientific literature; however, ACS itself does not conduct chemical research. As a leader in scientific information solutions, its CAS division partners with global innovators to accelerate breakthroughs by curating, connecting and analyzing the world’s scientific knowledge. ACS’ main offices are in Washington, D.C., and Columbus, Ohio.
To automatically receive press releases from the American Chemical Society, contact newsroom@acs.org .
Note: ACS does not conduct research, but publishes and publicizes peer-reviewed scientific studies.
Media Contact
ACS Newsroom newsroom@acs.org
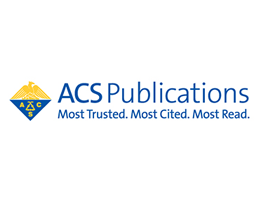
Discover Chemistry —Menu
- News Releases
- ACS in the News
Accept & Close The ACS takes your privacy seriously as it relates to cookies. We use cookies to remember users, better understand ways to serve them, improve our value proposition, and optimize their experience. Learn more about managing your cookies at Cookies Policy .
1155 Sixteenth Street, NW, Washington, DC 20036, USA | service@acs.org | 1-800-333-9511 (US and Canada) | 614-447-3776 (outside North America)
- Terms of Use
- Accessibility
Copyright © 2024 American Chemical Society
Accessibility Links
- Skip to content
- Skip to search IOPscience
- Skip to Journals list
- Accessibility help
- Accessibility Help
Click here to close this panel.
Purpose-led Publishing is a coalition of three not-for-profit publishers in the field of physical sciences: AIP Publishing, the American Physical Society and IOP Publishing.
Together, as publishers that will always put purpose above profit, we have defined a set of industry standards that underpin high-quality, ethical scholarly communications.
We are proudly declaring that science is our only shareholder.
Nanotechnology: an approach for water purification-review
Rama Sharma 1
Published under licence by IOP Publishing Ltd IOP Conference Series: Materials Science and Engineering , Volume 1116 , International Conference on Futuristic and Sustainable Aspects in Engineering and Technology (FSAET 2020) 18th-19th December 2020, Mathura, India Citation Rama Sharma 2021 IOP Conf. Ser.: Mater. Sci. Eng. 1116 012007 DOI 10.1088/1757-899X/1116/1/012007
Article metrics
1294 Total downloads
Share this article
Author e-mails.
Author affiliations
1 Department of Biotechnology, GLA University, Mathura
Buy this article in print
Clean water is the global need and need of life for all the human kinds. But the clean water resources are being contaminated in present time. Nanotechnology is an easy and practical approach to clean waste water by using different methods. Different types of bacteria, toxic chemicals like arsenic, mercury etc., and sediments can be removed by using nanotechnology. Nanomaterial based devices are being used for water purification. Nano filtration method has advantages over other conventional method as low pressure is required to pass the water through filters and these filters can be cleaned easily by back flushing. Smooth interior of carbon nanotubes make them convenient for the removal of almost all types of water contaminants. Because of larger surface area nanostructured materials have advantages over conventional micro structured materials.
Export citation and abstract BibTeX RIS
Content from this work may be used under the terms of the Creative Commons Attribution 3.0 licence . Any further distribution of this work must maintain attribution to the author(s) and the title of the work, journal citation and DOI.
Thank you for visiting nature.com. You are using a browser version with limited support for CSS. To obtain the best experience, we recommend you use a more up to date browser (or turn off compatibility mode in Internet Explorer). In the meantime, to ensure continued support, we are displaying the site without styles and JavaScript.
- View all journals
- Explore content
- About the journal
- Publish with us
- Sign up for alerts
- Review Article
- Published: 20 March 2008
Science and technology for water purification in the coming decades
- Mark A. Shannon 1 , 4 ,
- Paul W. Bohn 1 , 2 ,
- Menachem Elimelech 1 , 3 ,
- John G. Georgiadis 1 , 4 ,
- Benito J. Mariñas 1 , 5 &
- Anne M. Mayes 1 , 6
Nature volume 452 , pages 301–310 ( 2008 ) Cite this article
69k Accesses
6555 Citations
244 Altmetric
Metrics details
One of the most pervasive problems afflicting people throughout the world is inadequate access to clean water and sanitation. Problems with water are expected to grow worse in the coming decades, with water scarcity occurring globally, even in regions currently considered water-rich. Addressing these problems calls out for a tremendous amount of research to be conducted to identify robust new methods of purifying water at lower cost and with less energy, while at the same time minimizing the use of chemicals and impact on the environment. Here we highlight some of the science and technology being developed to improve the disinfection and decontamination of water, as well as efforts to increase water supplies through the safe re-use of wastewater and efficient desalination of sea and brackish water.
This is a preview of subscription content, access via your institution
Access options
Subscribe to this journal
Receive 51 print issues and online access
185,98 € per year
only 3,65 € per issue
Buy this article
- Purchase on Springer Link
- Instant access to full article PDF
Prices may be subject to local taxes which are calculated during checkout
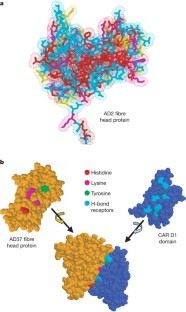
Similar content being viewed by others
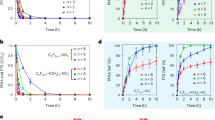
Near-complete destruction of PFAS in aqueous film-forming foam by integrated photo-electrochemical processes
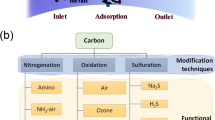
Removal of heavy metal ions from wastewater: a comprehensive and critical review
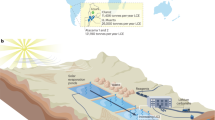
Environmental impact of direct lithium extraction from brines
Montgomery, M. A. & Elimelech, M. Water and sanitation in developing countries: including health in the equation. Environ. Sci. Technol. 41 , 17–24 (2007)
Article ADS PubMed Google Scholar
Lima, A. A. M. et al. Persistent diarrhea signals a critical period of increased diarrhea burdens and nutritional shortfalls: a prospective cohort study among children in northeastern brazil. J. Infect. Dis. 181 , 1643–1651 (2000)
Article CAS PubMed Google Scholar
Behrman, J. R., Alderman, H. & Hoddinott, J. Hunger and malnutrition. in Copenhagen Consensus—Challenges and Opportunities (London, 2004) OCLC 57489365 (London School of Hygiene and Tropical Medicine, 2004); 〈 http://www.copenhagenconsensus.com/Files/Filer/CC/Papers/ Hunger%5Fand%5FMalnutrition%5F070504.pdf 〉
Google Scholar
Singh, P. & Bengtson, L. The impact of warmer climate on melt and evaporation for the rainfed, snowfed and glacierfed basins in the Himalayan region. J. Hydrol. 300 , 140–154 (2005)
Article ADS Google Scholar
Shiyin, L., Wenxin, S., Shen, Y. & Li, G. Glacier changes since the Little Ice Age maximum in the western Qilian Shan, northwest China, and consequences of glacier runoff for water supply. J. Glaciol. 49 , 117–124 (2003)
Barnett, T. P., Adam, J. C. & Lettenmaier, D. P. Potential impacts of a warming climate on water availability in snow-dominated regions. Nature 438 , 303–309 (2005)
Article ADS CAS PubMed Google Scholar
Bradley, R. S., Vuille, M., Diaz, H. F. & Vergara, W. Threats to water supplies in the tropical Andes. Science 312 , 1755–1756 (2006)
van der Kooij, D. in Heterotrophic Plate Counts and Drinking-water Safety: The Significance of HPCs for Water Quality and Human Health (eds Bartram, J., Cotruvo, J., Exner, M., Fricker, C. & Glasmacher, A.) 199–232 (IWA Publishing, World Health Organization, Geneva, 2003)
Pitman, G. K. Bridging Troubled Waters—Assessing The World Bank Water Resources Strategy (World Bank Publications, Washington DC, 2002)
World Health Organization. Emerging Issues in Water and Infectious Disease 1–22 (World Health Organization, Geneva, 2003)
United States Environmental Protection Agency. 40 CFR parts 9, 141 & 142 National Primary Drinking Water Regulations: Long term 2 enhanced surface water treatment rule; final rule. Federal Register 71 , 653–702 (2006)
United States Environmental Protection Agency. 40 CFR parts 9, 141, & 142 National Primary Drinking Water Regulations: Stage 2 disinfectants and disinfection byproducts rule; final rule. Federal Register 71 , 388–493 (2006)
Krasner, S. W. et al. Occurrence of a new generation of disinfection byproducts. Environ. Sci. Technol. 40 , 7175–7185 (2006)
Muellner, M. G. et al. Haloacetonitriles vs. regulated haloacetic acids: are nitrogen-containing DBPs more toxic? Environ. Sci. Technol. 41 , 645–651 (2007)
Centers for Disease Control and Prevention. Safe Water Systems for the Developing World: A Handbook for Implementing Household-Based Water Treatment and Safe Storage Projects (CDC, Atlanta, 2000)
Simonet, J. & Gantzer, C. Inactivation of poliovirus 1 and f-specific RNA phages and degradation of their genomes by UV irradiation at 254 nanometers. Appl. Environ. Microbiol. 72 , 7671–7677 (2006)
Article CAS PubMed PubMed Central Google Scholar
Nuanualsuwan, S. & Cliver, D. O. Capsid functions of inactivated human picornaviruses and feline calicivirus. Appl. Environ. Microbiol. 69 , 350–357 (2003)
Coyne, C. B. & Bergelson, J. M. CAR: A virus receptor within the tight junction. Adv. Drug Deliv. Rev. 57 , 869–882 (2005)
Seiradake, E., Lortat-Jacob, H., Billet, O., Kremer, E. J. & Cusack, S. Structural and mutational analysis of human Ad37 and canine adenovirus 2 fiber heads in complex with the D1 domain of coxsackie and adenovirus receptor. J. Biol. Chem. 281 , 33704–33716 (2006)
Hawkins, C. L., Pattison, D. I. & Davies, M. J. Hypochlorite-induced oxidation of amino acids, peptides and proteins. Amino Acids 25 , 259–274 (2003)
Nightingdale, Z. D. et al. Relative reactivity of lysine and other peptides-bound amino acids to oxidation by hypochlorite. Free Radic. Biol. Med. 29 , 425–433 (2000)
Article Google Scholar
Bergt, C., Fu, X., Huq, N. P., Kao, J. & Heinecke, J. W. Lysine residues direct the chlorination of tyrosines in Y XX K motifs of apolipoprotein A-I when hypochlorous acid oxidizes high density lipoprotein. J. Biol. Chem. 279 , 7856–7866 (2004)
Pattison, D. I. & Davies, M. J. Kinetic analysis of the role of histidine chloramines in hypochlorous acid mediated protein oxidation. Biochemistry 44 , 7378–7387 (2005)
Medina-Kauwe, L. K. Endocytosis of adenovirus and adenovirus capsid proteins. Adv. Drug Deliv. Rev. 55 , 1485–1496 (2003)
Yates, M. V., Malley, J., Rochelle, P. & Hoffman, R. Effect of adenovirus resistance on UV disinfection requirements: A report on the state of adenovirus science. J. Am. Water Works Assoc. 98 , 93–106 (2006)
Article CAS Google Scholar
Li, Q., Liang, W. & Shang, J. K. Enhanced visible-light absorption from PdO nanoparticles in nitrogen-doped titanium oxide thin films. Appl. Phys. Lett. 90 , 063109 (2007)
Article ADS CAS Google Scholar
Fu, P., Luan, Y. & Dai, X. Preparation of activated carbon fibers supported TiO2 photocatalyst and evaluation of its photocatalytic reactivity. J. Mol. Catal. Chem. 221 , 81–88 (2004)
Medina-Valtierra, J., Garcia-Servin, J., Frausto-Reyes, C. & Calixto, S. The photocatalytic application and regeneration of anatase thin films with embedded commercial TiO2 particles deposited on glass microrods. Appl. Surf. Sci. 252 , 3600–3608 (2006)
Changrani, R. G. & Raupp, G. B. Two-dimensional heterogeneous model for a reticulated-foam photocatalytic reactor. Am. Inst. Chem. Eng. J. 46 , 829–842 (2000)
Molinari, R., Palmisano, L., Drioli, E. & Schiavello, M. Studies on various reactor configurations for coupling photocatalysis and membrane processes in water purification. J. Membr. Sci. 206 , 399–415 (2002)
Lin, H. & Valsaraj, K. T. Development of an optical fiber monolith reactor for photocatalytic wastewater treatment. J. Appl. Electrochem. 35 , 699–708 (2005)
Blanco-Galvez, J., Fernandez-Ibanez, P. & Malato-Rodriguez, S. Solar photocatalytic detoxification and disinfection of water: Recent overview. J. Solar Energy Eng. 129 , 4–15 (2007)
Gill, L. W. & McLoughlin, O. A. Solar disinfection kinetic design parameters for continuous flow reactors. J. Solar Energy Eng. 129 , 111–118 (2007)
Schwarzenbach, R. P. et al. The challenge of micropollutants in aquatic systems. Science 313 , 1072–1077 (2006)
Sarkar, S. et al. Well-head arsenic removal units in remote villages of Indian subcontinent: Field results and performance evaluation. Water Res. 39 , 2196–2206 (2005)
Khan, A. H. et al. Appraisal of a simple arsenic removal method for groundwater of Bangladesh. J. Environ. Sci. Health Part A 35 , 1021–1041 (2000)
Silliman, S. E., Boukari, M., Crane, P., Azonsi, F. & Neal, C. R. Observations on elemental concentrations of groundwater in central Benin. J. Hydrol. 335 , 374–388 (2007)
Rasul, S. B. et al. Electrochemical measurement and speciation of inorganic arsenic in groundwater of Bangladesh. Talanta 58 , 33–43 (2002)
Chen, Z. L., Akter, K. F., Rahman, M. M. & Naidu, R. Speciation of arsenic by ion chromatography inductively coupled plasma mass spectrometry using ammonium eluents. J. Sep. Sci. 29 , 2671–2676 (2006)
Sultan, J. & Gabryelski, W. Structural identification of highly polar nontarget contaminants in drinking water by ESI-FAIMS-Q-TOF-MS. Anal. Chem. 78 , 2905–2917 (2006)
Kuo, T.-C. et al. Gateable nanofluidic interconnects for multilayered microfluidic separational systems. Anal. Chem. 75 , 1861–1867 (2003)
Liu, J. W. et al. A catalytic beacon sensor for uranium with parts-per-trillion sensitivity and millionfold selectivity. Proc. Natl Acad. Sci. USA 104 , 2056–2061 (2007)
Article ADS CAS PubMed PubMed Central Google Scholar
Chang, I. H. et al. Miniaturized lead sensor based on lead-specific DNAzyme in a nanocapillary interconnected microfluidic device. Environ. Sci. Technol. 39 , 3756–3761 (2005)
Zhu, P. X. et al. Detection of water-borne E. coli O157 using the integrating waveguide biosensor. Biosens. Bioelectron. 21 , 678–683 (2005)
Snyder, S. A. et al. Role of membranes and activated carbon in the removal of endocrine disruptors and pharmaceuticals. Desalination 202 , 156–181 (2007)
Davis, A. P., Sheppard, D. N. & Smith, B. D. Development of synthetic membrane transporters for anions. Chem. Soc. Rev. 36 , 348–357 (2007)
Snyder, S. A., Vanderford, B. J. & Rexing, D. J. Trace analysis of bromate, chlorate, iodate, and perchlorate in natural and bottled waters. Environ. Sci. Technol. 39 , 4586–4593 (2005)
Garelick, H., Dybowska, A., Valsami-Jones, E. & Priest, N. D. Remediation technologies for arsenic contaminated drinking waters. J. Soils Sediments 5 , 182–190 (2005)
Schideman, L. C., Marinas, B. J., Snoeyink, V. L. & Campos, C. Three-component competitive adsorption model for fixed-bed and moving-bed granular activated carbon adsorbers. Part I. Model development. Environ. Sci. Technol. 40 , 6805–6811 (2006)
Magnuson, M. L. & Speth, T. F. Quantitative structure—Property relationships for enhancing predictions of synthetic organic chemical removal from drinking water by granular activated carbon. Environ. Sci. Technol. 39 , 7706–7711 (2005)
Yavuz, C. T. et al. Low-field magnetic separation of monodisperse Fe3O4 nanocrystals. Science 314 , 964–967 (2006)
Article PubMed Google Scholar
Fournier, D., Hawari, J., Streger, S. H., McClay, K. & Hatzinger, P. B. Biotransformation of N-nitrosodimethylamine by Pseudomonas mendocina KR1. Appl. Environ. Microbiol. 72 , 6693–6698 (2006)
Kraemer, S. M., Xu, J. D., Raymond, K. N. & Sposito, G. Adsorption of Pb(II) and Eu(III) by oxide minerals in the presence of natural and synthetic hydroxamate siderophores. Environ. Sci. Technol. 36 , 1287–1291 (2002)
Chaplin, B. P., Roundy, E., Guy, K. A., Shapley, J. R. & Werth, C. J. Effects of natural water ions and humic acid on catalytic nitrate reduction kinetics using an alumina supported Pd-Cu catalyst. Environ. Sci. Technol. 40 , 3075–3081 (2006)
Daiger, G. T., Rittmann, B. E., Adham, S. & Andreottola, G. Are membrane bioreactors ready for widespread application? Environ. Sci. Technol. 39 , 399A–406A (2005)
Yang, W. B., Cicek, N. & Ilg, J. State-of-the-art of membrane bioreactors: worldwide research and commercial applications in North America. J. Membr. Sci. 270 , 201–211 (2006)
Bixio, D. et al. Wastewater reuse in Europe. Desalination 189 , 89–101 (2006)
Kimura, K., Yamato, N., Yamamura, H. & Watanabe, Y. Membrane fouling in pilot-scale membrane bioreactors (MBRs) treating municipal wastewater. Environ. Sci. Technol. 39 , 6293–6299 (2005)
Ulbricht, M. & Belfort, G. Surface modification of ultrafiltration membranes by low temperature plasma.2. Graft polymerization onto polyacrylonitrile and polysulfone. J. Membr. Sci. 111 , 193–215 (1996)
Carroll, T., Booker, N. A. & Meier-Haack, J. Polyelectrolyte-grafted microfiltration membranes to control fouling by natural organic matter in drinking water. J. Membr. Sci. 203 , 3–13 (2002)
Deratani, A., Li, C. L., Wang, D. M. & Lai, J. Y. New trends in the preparation of polymeric membranes for liquid filtration. Ann. Chim.-Sci. Mater. 32 , 107–118 (2007)
Hester, J. F., Banerjee, P. & Mayes, A. M. Preparation of protein-resistant surfaces on poly(vinylidene fluoride) membranes via surface segregation. Macromolecules 32 , 1643–1650 (1999)
Hester, J. F. & Mayes, A. M. Design and performance of foul-resistant poly(vinylidene fluoride) membranes prepared in a single step by surface segregation. J. Membr. Sci. 202 , 119–135 (2002)
Wang, Y. Q. et al. Remarkable reduction of irreversible fouling and improvement of the permeation properties of poly(ether sulfone) ultrafiltration membranes by blending with pluronic F127. Langmuir 21 , 11856–11862 (2005)
Asatekin, A., Kang, S., Elimelech, M. & Mayes, A. M. Anti-fouling ultrafiltration membranes containing polyacrylonitrile- graft -poly(ethylene oxide) comb copolymer additives. J. Membr. Sci. 298 , 136–146 (2007)
Kang, S., Asatekin, A., Mayes, A. M. & Elimelech, M. Protein antifouling mechanisms of PAN UF membranes incorporating PAN-g-PEO additive. J. Membr. Sci. 298 , 42–50 (2007)
Ulbricht, M. Advanced functional polymer membranes. Polymer 47 , 2217–2262 (2006)
Akthakul, A., Salinaro, R. F. & Mayes, A. M. Antifouling polymer membranes with sub-nanometer size selectivity. Macromolecules 37 , 7663–7668 (2004)
Zhou, M., Kidd, T. J., Noble, R. D. & Gin, D. L. Supported lyotropic liquid crystal polymer membranes: promising materials for molecular-size-selective aqueous nanofiltration. Adv. Mater. 17 , 1850–1853 (2005)
Asatekin, A. et al. Antifouling nanofiltration membranes for membrane bioreactors from self-assembling graft copolymers. J. Membr. Sci. 285 , 81–89 (2006)
Revanur, R., McCloskey, B., Breitenkamp, K., Freeman, B. D. & Emrick, T. Reactive amphiphilic graft copolymer coatings applied to polyvinylidene fluoride ultrafiltration membranes. Macromolecules 40 , 3624–3630 (2007)
Yang, S. Y. et al. Nanoporous membranes with ultrahigh selectivity and flux for the filtration of viruses. Adv. Mater. 18 , 709–712 (2006)
Phillip, W. A., Rzayev, J., Hillmyer, M. A. & Cussler, E. L. Gas and water liquid transport through nanoporous block copolymer membranes. J. Membr. Sci. 286 , 144–152 (2006)
Nunes, S. P., Sforca, M. L. & Peinemann, K.-V. Dense hydrophilic composite membranes for ultrafiltration. J. Membr. Sci. 106 , 49–56 (1995)
Yoon, K. et al. High flux ultrafiltration membranes based on electrospun nanofibrous PAN scaffolds and chitosan coating. Polym. 47 , 2434–2441 (2006)
Lu, Y., Suzuki, T. & Zhang, W. Moore, J. S. &Mariñas, B. J. Nanofiltration membranes based on rigid star amphiphiles. Chem. Mater. 19 , 3194–3204 (2007)
Zhou, Y. & Tol, R. S. J. Evaluating the costs of desalination and water transport. Wat. Resour. Res. 41 , W03003,–1–10 (2005)
Veerapaneni, S., Long, B., Freeman, S. & Bond, R. Reducing energy consumption for seawater desalination. J. Am. Water Works Assoc. 99 , 95–106 (2007)
Morgan, L. A. et al. Solar distillation: a promising alternative for water provision with free energy, simple technology and a clean environment. Desalination 116 , 45–56 (1998)
Bourounia, K., Chaibib, M. T. & Tadrist, L. Water desalination by humidification and dehumidification of air: state of the art. Desalination 137 , 167–176 (2001)
McCutcheon, J. R., McGinnis, R. L. & Elimelech, M. A novel ammonia-carbon dioxide forward (direct) osmosis desalination process. Desalination 174 , 1–11 (2005)
Mathioulakis, E., Belessiotis, V. & Delyannis, E. Desalination by using alternative energy: review and state-of-the-art. Desalination 203 , 346–365 (2007)
Alonitis, S. A., Kouroumbas, K. & Vlachakis, N. Energy consumption and membrane replacement cost for seawater RO desalination plants. Desalination 157 , 151–158 (2003)
Seacord, T. F., Coker, S. D. & MacHarg, J. Affordable desalination collaboration 2005 results. In International Desalination And Water Reuse Quarterly (Green Global Publications, Anaheim, California, 2006)
Spiegler, K. S. & El-Sayed, Y. M. The energetics of desalination processes. Desalination 134 , 109–128 (2001)
Hummer, G., Rasaiah, J. C. & Nowotyta, J. P. Water conduction through the hydrophobic channel of a carbon nanotube. Nature 414 , 188–190 (2001)
Kalra, A., Garde, S. & Hummer, G. Osmotic water transport through carbon nanotube membranes. Proc. Natl Acad. Sci. USA 100 , 10175–10180 (2003)
Hinds, B. J. et al. Aligned multiwalled carbon nanotube membranes. Science 303 , 62–65 (2003)
Article ADS PubMed CAS Google Scholar
Holt, J. K. et al. Fast mass transport through sub-2-nanometer carbon nanotubes. Science 312 , 1034–1037 (2006)
Fornasiero, F. et al. Ion exclusion by sub 2-nm carbon nanotube pores. Proc. Natl. Acad. Sci. USA (in the press)
Walz, T., Smith, B. L., Zeidel, M. L., Engel, A. & Agre, P. Biologically-active 2-dimensional crystals of aquaporin chip. J. Biol. Chem. 269 , 1583–1586 (1994)
Qiao, R., Georgiadis, J. G. & Aluru, N. R. Differential ion transport induced electroosmosis and internal recirculation in heterogeneous osmosis membranes. Nano Lett. 6 , 995–999 (2006)
Ishida, H., Donowaki, K., Inoue, Y., Qi, Z. & Sokabe, M. Synthesis and ion channel formation of novel cyclic peptides containing a non-natural amino acid. Chem. Lett. Jpn 26 , 935–954 (1997)
Nednoor, P., Gavalas, V. G., Chopra, N., Hinds, B. J. & Bachas, L. G. Carbon nanotube based biomimetic membranes: mimicking protein channels regulated by phosphorylation. J. Mater. Chem. 17 , 1755–1757 (2007)
Parsegian, A. Energy of an ion crossing a low dielectric membrane: solutions to four relevant electrostatic problems. Nature 221 , 844–846 (1969)
Facciotti, M. T., Rouhani-Manshadi, S. & Glaeser, R. M. Energy transduction in transmembrane ion pumps. Trends Biochem. Sci. 29 , 445–451 (2004)
Martz, E. Protein explorer: easy yet powerful macromolecular visualization. Trends Biochem. Sci. 27 , 107–109 (2002)
van Raaij, M. J., Louis, N., Chroboczek, J. & Cusack, S. Structure of the human adenovirus serotype 2 fiber head domain at 1.5 Å resolution. Virology 262 , 333–343 (1999)
Download references
Acknowledgements
We acknowledge the US National Science Foundation Science and Technology Center, WaterCAMPWS , Center for Advanced Materials for the Purification of Water with Systems.
Author information
Authors and affiliations.
NSF STC WaterCAMPWS, University of Illinois at Urbana-Champaign, Urbana, Illinois 61801, USA,
Mark A. Shannon, Paul W. Bohn, Menachem Elimelech, John G. Georgiadis, Benito J. Mariñas & Anne M. Mayes
Department of Chemical and Biomolecular Engineering and Department of Chemistry, University of Notre Dame, Notre Dame, Indiana 46556, USA,
Paul W. Bohn
Department of Environmental and Chemical Engineering, Yale University, New Haven, Connecticut 06520, USA,
Menachem Elimelech
Department of Mechanical Science and Engineering, University of Illinois at Urbana-Champaign, Urbana, Illinois 61801, USA,
Mark A. Shannon & John G. Georgiadis
Department of Civil and Environmental Engineering, University of Illinois at Urbana-Champaign, Urbana, Illinois 61801, USA,
Benito J. Mariñas
Department of Materials Science and Engineering, Massachusetts Institute of Technology, Cambridge, Massachusetts 02139, USA,
Anne M. Mayes
You can also search for this author in PubMed Google Scholar
Corresponding author
Correspondence to Mark A. Shannon .
Rights and permissions
Reprints and permissions
About this article
Cite this article.
Shannon, M., Bohn, P., Elimelech, M. et al. Science and technology for water purification in the coming decades. Nature 452 , 301–310 (2008). https://doi.org/10.1038/nature06599
Download citation
Received : 14 July 2007
Accepted : 14 December 2007
Issue Date : 20 March 2008
DOI : https://doi.org/10.1038/nature06599
Share this article
Anyone you share the following link with will be able to read this content:
Sorry, a shareable link is not currently available for this article.
Provided by the Springer Nature SharedIt content-sharing initiative
This article is cited by
Bio-inspired design of next-generation ultrapermeable membrane systems.
- Mingheng Li
npj Clean Water (2024)
Fouling-resistant reverse osmosis membranes grafted with 2-aminoethanethiol having a low interaction energy with charged foulants
Wood-inspired metamaterial catalyst for robust and high-throughput water purification.
Nature Communications (2024)
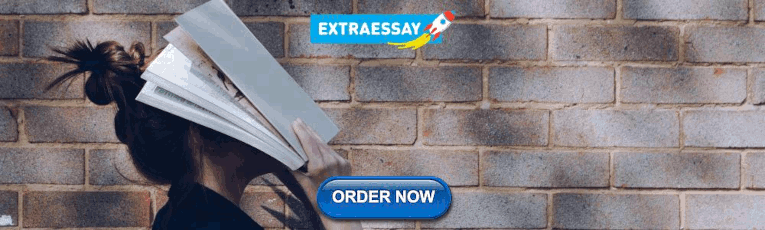
Walking-induced electrostatic charges enable in situ electroporated disinfection in portable water bottles
- Young-Jun Kim
- Zheng-Yang Huo
- Sang-Woo Kim
Nature Water (2024)
Bridging materials innovations to sorption-based atmospheric water harvesting devices
- Lenan Zhang
- Evelyn N. Wang
Nature Reviews Materials (2024)
By submitting a comment you agree to abide by our Terms and Community Guidelines . If you find something abusive or that does not comply with our terms or guidelines please flag it as inappropriate.
Quick links
- Explore articles by subject
- Guide to authors
- Editorial policies
Sign up for the Nature Briefing newsletter — what matters in science, free to your inbox daily.

A review of solar photovoltaic-powered water desalination technologies
- Original Article
- Published: 11 May 2024
- Volume 10 , article number 123 , ( 2024 )
Cite this article
- Albashir K. Elfaqih ORCID: orcid.org/0000-0001-6294-5868 1 ,
- Abdurazaq Elbaz 1 &
- Yousef M. Akash 1
13 Accesses
Explore all metrics
The availability of energy and water sources is basic and indispensable for the life of modernistic humans. Because of this importance, the interrelationship between energy derived from renewable energy sources and water desalination technologies has achieved great interest recently. So this paper reviews the photovoltaic (PV) system-powered desalination technologies as stand-alone systems or hybrid systems in the last decade, and this review includes the technologies of reverse osmosis (RO), electrodialysis (ED), reverse electrodialysis (RED), and membrane distillation (MD). This review aims to assess the extent of its development during this decade by evaluating the extent of the reduction in energy consumption and the costs of water production for each technology. Besides, this review reveals the significant developments in the use of photovoltaic system as a source of electric energy for various desalination technologies, especially their use with hybrid sources, such as wind turbines, thermal solar tubulars, and hydrogen fuel cells. These studies and research mainly focused on reverse osmosis technology. Also, most research indicates that PV-RO, PV-ED, and PV-RED systems are friendly to the environment, economical, and suitable for treating contaminated water, especially in remote areas. The review also shows that there is a development in other desalination technologies that can be powered by photovoltaic energy due to their lower energy consumption.
This is a preview of subscription content, log in via an institution to check access.
Access this article
Price includes VAT (Russian Federation)
Instant access to the full article PDF.
Rent this article via DeepDyve
Institutional subscriptions
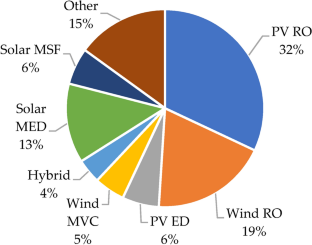
Data availability statement
Data sharing not applicable to this article as no datasets were generated or analyzed during the current study.
Abdalla AM et al (2022) Water desalination plant powered by solid oxide fuel cell technology in Egypt. J Clean Product 365:132570. https://doi.org/10.1016/j.jclepro.2022.132570
Article CAS Google Scholar
Abdulwahid AA et al (2022) Thermo-economic comparison of two models of combined transcritical CO2 refrigeration and multi-effect desalination system. Appl Energy 308:118320. https://doi.org/10.1016/j.apenergy.2021.118320
Abounahia N et al (2023) Desalination by the forward osmosis: advancement and challenges. Sci Total Environ 886:163901. https://doi.org/10.1016/j.scitotenv.2023.163901
Abu El-Maaty AE, Awad MM, Sultan GI, Hamed AM (2023) Innovative approaches to solar desalination: a comprehensive review of recent research. Energies 16(9):3957. https://doi.org/10.3390/en16093957
Article Google Scholar
Aghaei M, Kumar NM, Eskandari A, Ahmed H, de Oliveira AKV, Chopra SS (2020) Chapter 5 - Solar PV systems design and monitoring. In: Gorjian S, Shukla A (eds) Photovoltaic Solar Energy Conversion. Academic Press
Google Scholar
Ahdab JH, Yvana D (2021) Desalination of brackish groundwater to improve water quality and water supply. Global Groundwater. Elsevier, pp 559–575
Chapter Google Scholar
Ahmadi E, McLellan B, Mohammadi-Ivatloo B, Tezuka T (2020) The role of renewable energy resources in sustainability of water desalination as a potential fresh-water source: an updated review. Sustainability (switzerland). https://doi.org/10.3390/su12135233
Ahmed FE, Hashaikeh R, Hilal N (2020) Hybrid technologies: the future of energy efficient desalination – a review. Desalination 495:114659. https://doi.org/10.1016/j.desal.2020.114659
Ahmed FE, Khalil A, Hilal N (2021) Emerging desalination technologies: current status, challenges and future trends. Desalination 517:115183. https://doi.org/10.1016/j.desal.2021.115183
Al-Amshawee S, Yunus MYBM, Azoddein AAM, Hassell DG, Dakhil IH, Hasan HA (2019) Electrodialysis desalination for water and wastewater: a review. Chem Eng J 380:2020. https://doi.org/10.1016/j.cej.2019.122231
Alawad SM, Ben Mansour R, Al-Sulaiman FA, Rehman S (2023) Renewable energy systems for water desalination applications: a comprehensive review. Energy Convers Manage 286:117035. https://doi.org/10.1016/j.enconman.2023.117035
Alghoul MA et al (2016) Design and experimental performance of brackish water reverse osmosis desalination unit powered by 2 kW photovoltaic system. Renew Energy 93:101–114. https://doi.org/10.1016/j.renene.2016.02.015
Ali A, Tufa RA, Macedonio F, Curcio E, Drioli E (2018) Membrane technology in renewable-energy-driven desalination. Renew Sustain Energy Rev 81:1–21. https://doi.org/10.1016/j.rser.2017.07.047
Al-Karaghouli A, Kazmerski LL (2013) Energy consumption and water production cost of conventional and renewable-energy-powered desalination processes. Renew Sustain Energy Rev 24:343–356. https://doi.org/10.1016/j.rser.2012.12.064
Al-Obaidi MA, Zubo RHA, Rashid FL, Dakkama HJ, Abd-Alhameed R, Mujtaba IM (2022) Evaluation of solar energy powered seawater desalination processes: a review. Energies. https://doi.org/10.3390/en15186562
Alsebaeai MK, Ahmad AL (2020) Membrane distillation: progress in the improvement of dedicated membranes for enhanced hydrophobicity and desalination performance. J Ind Eng Chem 86:13–34. https://doi.org/10.1016/j.jiec.2020.03.006
Alsehli M et al (2022) Improving the performance of a hybrid solar desalination system under various operating conditions. Process Saf Environ Prot 162(June):706–720. https://doi.org/10.1016/j.psep.2022.04.044
Alsheghri A, Sharief SA, Rabbani S, Aitzhan NZ (2015) Design and cost analysis of a solar photovoltaic powered reverse Osmosis plant for Masdar institute. Energy Proced 75:319–324. https://doi.org/10.1016/j.egypro.2015.07.365
Alvarado L, Ramírez A, Rodríguez-Torres I (2009) Cr(VI) removal by continuous electrodeionization: study of its basic technologies. Desalination 249(1):423–428. https://doi.org/10.1016/j.desal.2009.06.051
Bahar R, Ng KC (2020) Fresh water production by membrane distillation (MD) using marine engine’s waste heat. Sustain Energy Technol Assess 42:100860. https://doi.org/10.1016/j.seta.2020.100860
Bait O (2020) 2019 ‘Direct and indirect solar–powered desalination processes loaded with nanoparticles: a review.’ Sustain Energy Technol Assess 37:100597. https://doi.org/10.1016/j.seta.2019.100597
Bamasag A et al (2023) Recent advances and future prospects in direct solar desalination systems using membrane distillation technology. J Clean Prod 385:135737. https://doi.org/10.1016/j.jclepro.2022.135737
Ben Abdallah S, Frikha N, Gabsi S (2013) Design of an autonomous solar desalination plant using vacuum membrane distillation, the MEDINA project. Chem Eng Res Design 91:2782–2788. https://doi.org/10.1016/j.cherd.2013.06.012
Bentamy A, Mbodji N, Hajji A, Design O, Performance F (2016 )‘Design and Cost Optimization of Small-Scale Pv-Powered Reverse Osmosis Desalination ( Case Study )’. https://doi.org/10.4229/EUPVSEC20162016-6AV.6.9
Bouguecha ST, Aly SE, Al-Beirutty MH, Hamdi MM, Boubakri A (2015) Solar driven DCMD: Performance evaluation and thermal energy efficiency. Chem Eng Res Des 100:331–340. https://doi.org/10.1016/j.cherd.2015.05.044
Bourouni K, Ben M’Barek T, Al Taee A (2011) Design and optimization of desalination reverse osmosis plants driven by renewable energies using genetic algorithms. Renew Energy 36(3):936–950. https://doi.org/10.1016/j.renene.2010.08.039
Bundschuh J, Ghaffour N, Mahmoudi H, Goosen M, Mushtaq S, Hoinkis J (2015) Low-cost low-enthalpy geothermal heat for freshwater production: Innovative applications using thermal desalination processes. Renew Sustain Energy Rev 43:196–206. https://doi.org/10.1016/j.rser.2014.10.102
Bundschuh J, Kaczmarczyk M, Ghaffour N, Tomaszewska B (2021) State-of-the-art of renewable energy sources used in water desalination: present and future prospects. Desalination 508:115035. https://doi.org/10.1016/j.desal.2021.115035
Busch M, Chu R, Kolbe U, Meng Q, Li S (2009) Ultrafiltration pretreatment to reverse osmosis for seawater desalination — three years field experience in the Wangtan Datang power plant. Desalin Water Treat 10(1–3):1–20. https://doi.org/10.5004/dwt.2009.702
Caldera U, Bogdanov D, Breyer C (2016) Local cost of seawater RO desalination based on solar PV and wind energy: a global estimate. Desalination 385:207–216. https://doi.org/10.1016/j.desal.2016.02.004
Campione A, Gurreri L, Ciofalo M, Micale G, Tamburini A, Cipollina A (2018) Electrodialysis for water desalination: a critical assessment of recent developments on process fundamentals, models and applications. Desalination 434:121–160. https://doi.org/10.1016/j.desal.2017.12.044
Cervantes-Rendón E et al (2022) Rural application of a low-pressure reverse osmosis desalination system powered by solar-photovoltaic energy for Mexican arid zones. Sustainability 14(17):10958. https://doi.org/10.3390/su141710958
Chafidz A, Rahma FN, Nurkhamidah S, Al-Zahrani S (2019) Portable solar-powered membrane distillation system to solve water and energy problems simultaneously. J Phys Conf Ser. https://doi.org/10.1088/1742-6596/1304/1/012018
Chen YH, Hung HG, Ho CD, Chang H (2021) Economic design of solar-driven membrane distillation systems for desalination. Membranes 11(1):1–20. https://doi.org/10.3390/membranes11010015
Christie KSS, Horseman T, Lin S (2020) Energy efficiency of membrane distillation: simplified analysis, heat recovery, and the use of waste-heat. Environ Int 138:105588. https://doi.org/10.1016/j.envint.2020.105588
Curto D, Franzitta V, Guercio A (2021) A review of the water desalination technologies. Appl Sci (switzerland) 11(2):1–36. https://doi.org/10.3390/app11020670
Del Rio-Gamero B, Rodríguez-López E, Schallenberg-Rodríguez J (2023) Desalination plant for irrigation purposes driven by an inland floating photovoltaic system. J Marine Sci Eng. https://doi.org/10.3390/jmse11050905
Domínguez EJM, Batista RP, de León Izquier JM (2022) Assessing the use of photovoltaic energy at a seawater reverse osmosis desalination plant: a case study of Porto Santo Desalination Plant (Madeira – Portugal). Desalin Water Treat 259:285–299. https://doi.org/10.5004/dwt.2022.28551
Easley JN, Talozi S, A. G. Winter V. (2023) Feasibility and design of solar-powered electrodialysis reversal desalination systems for agricultural applications in the Middle East and North Africa. Desalination 561:116628. https://doi.org/10.1016/j.desal.2023.116628
Eid M (2023) Using solar energy technology in sea water desalination in North Sinai according to the Egyptian sustainable development plan 2030. Suez Canal Engineering, Energy and Environmental Science 1(1):32–48. https://doi.org/10.21608/SCEEE.2023.279913
El Aimani S (2023) Modeling of reverse osmosis water desalination powered by photovoltaic solar energy. Green Energy Environ Technol. https://doi.org/10.5772/geet.15
El-Agouz SA et al (2022) Solar thermal feed preheating techniques integrated with membrane distillation for seawater desalination applications: Recent advances, retrofitting performance improvement strategies, and future perspectives. Process Saf Environ Prot 164:595–612. https://doi.org/10.1016/j.psep.2022.06.044
Elfaqih AK, Belhaj SO, Sawed AM (2022) Feasibility assessment of a BWRO desalination plant powered by renewable energy sources. J Appl Water Eng Res. https://doi.org/10.1080/23249676.2022.2144496
Elminshawy NAS, Gadalla MA, Bassyouni M, El-Nahhas K, Elminshawy A, Elhenawy Y (2020) A novel concentrated photovoltaic-driven membrane distillation hybrid system for the simultaneous production of electricity and potable water. Renew Energy 162:802–817. https://doi.org/10.1016/j.renene.2020.08.041
Elsaid K, Sayed ET, Abdelkareem MA, Mahmoud MS, Ramadan M, Olabi AG (2020) Environmental impact of emerging desalination technologies: a preliminary evaluation. J Environ Chem Eng 8(5):104099. https://doi.org/10.1016/j.jece.2020.104099
Engineering NA (2017) Frontiers of Engineering: Reports on Leading-Edge Engineering from the 2016 Symposium. National Academies Press
Essak L, Ghosh A (2022) Floating photovoltaics: a review. Clean Technol 4(3):752–769. https://doi.org/10.3390/cleantechnol4030046
Fardan A, Albuasa H, Sultan Aluminium Bahrain Philip Davies A (2022 )‘Design of an industrial Photovoltaic-Driven Reverse Osmosis (PV-RO) Plant in Bahrain’, pp. 1–22, [Online]. Available: https://doi.org/10.21203/rs.3.rs-1286052/v1
Fath HES et al (2008) PV and thermally driven small-scale, stand-alone solar desalination systems with very low maintenance needs. Desalination 225(1):58–69. https://doi.org/10.1016/j.desal.2006.11.029
Fernandez-Gonzalez C, Dominguez-Ramos A, Ibañez R, Irabien A (2015) Sustainability assessment of electrodialysis powered by photovoltaic solar energy for freshwater production. Renew Sustain Energy Rev 47:604–615. https://doi.org/10.1016/j.rser.2015.03.018
Fernandez-Gonzalez C, Dominguez-Ramos A, Ibañez R, Irabien A (2018) ‘Desalination by renewable energy-powered electrodialysis processes’, In: current trends and future developments on bio membranes: renewable energy integrated with membrane operations, Elsevier
Fodhil F, Bessenasse M, Cherrar I (2019) Feasibility study of grid-connected photovoltaic system for seawater desalination station in Algeria. Desalin Water Treat 165:35–44. https://doi.org/10.5004/dwt.2019.24350
Freire-Gormaly M, Bilton AM (2019) Design of photovoltaic powered reverse osmosis desalination systems considering membrane fouling caused by intermittent operation. Renew Energy 135:108–121. https://doi.org/10.1016/j.renene.2018.11.065
Fthenakis V, Atia AA, Morin O, Bkayrat R, Sinha P (2016) New prospects for PV powered water desalination plants: case studies in Saudi Arabia. Prog Photovolt Res Appl 24(4):543–550. https://doi.org/10.1002/pip.2572
G. G, A. G, and I. AF, (2019) Perspective of renewable desalination by using membrane distillation. Chem Eng Res Des 144:520–537. https://doi.org/10.1016/j.cherd.2019.02.036
Ghaffour N, Bundschuh J, Mahmoudi H, Goosen MFA (2015) Renewable energy-driven desalination technologies: a comprehensive review on challenges and potential applications of integrated systems. Desalination 356:94–114. https://doi.org/10.1016/j.desal.2014.10.024
Ghafoor A, Ahmed T, Munir A, Arslan C, Ahmad SA (2020) Techno-economic feasibility of solar based desalination through reverse osmosis’. Desalination 485:114464. https://doi.org/10.1016/j.desal.2020.114464
Ghazi ZM, Rizvi SWF, Shahid WM, Abdulhameed AM, Saleem H, Zaidi SJ (2022) An overview of water desalination systems integrated with renewable energy sources. Desalination 542:116063. https://doi.org/10.1016/j.desal.2022.116063
Ghoneim AA, Alabdulali HA (2020) Simulation and performance analysis of reverse osmosis water desalination system operated by a high concentrated photovoltaic system. Desalin Water Treat 177:29–39. https://doi.org/10.5004/dwt.2020.24895
Goh PS, Matsuura T, Ismail AF, Hilal N (2016) Recent trends in membranes and membrane processes for desalination. Desalination 391:43–60. https://doi.org/10.1016/j.desal.2015.12.016
Gökçekuş H, Kassem Y, Godwin MH, Babangida A (2022) Economic analysis of an off-grid solar PV for small scale desalination unit. Future Technol 1(3):26–43. https://doi.org/10.55670/fpll.futech.1.3.5
Gorjian S, Ghobadian B, Ebadi H, Ketabchi F, Khanmohammadi S (2020) In: applications of solar PV systems in desalination technologies. Elsevier Inc., UK
Hamiche AM, Stambouli AB, Flazi S, Tayeb A (2020) Desalination in algeria: Photovoltaic power plant for tmm (tahlyat myah magtaa) of oran as a case study. Handb Environ Chem 98:285–315. https://doi.org/10.1007/698_2020_538
He W, Wang Y, Shaheed MH (2015) Stand-alone seawater RO (reverse osmosis) desalination powered by PV (photovoltaic) and PRO (pressure retarded osmosis). Energy 86:423–435. https://doi.org/10.1016/j.energy.2015.04.046
He Q, Zheng H, Ma X, Wang L, Kong H, Zhu Z (2022) Artificial intelligence application in a renewable energy-driven desalination system: a critical review. Energy AI 7:100123. https://doi.org/10.1016/j.egyai.2021.100123
He H et al (2023a) Potential and economic viability of green hydrogen production from seawater electrolysis using renewable energy in remote Japanese islands. Renew Energy 202:1436–1447. https://doi.org/10.1016/j.renene.2022.12.046
He W, Huang G, Markides CN (2023b) Synergies and potential of hybrid solar photovoltaic-thermal desalination technologies. Desalination 552:116424. https://doi.org/10.1016/j.desal.2023.116424
He W, Wright NC, Amrose S, Buonassisi T, Peters IM, Winter AG (2019 )‘Preliminary field test results from a photovoltaic electrodialysis brackish water desalination system in Rural India’, 0–7. https://doi.org/10.1115/detc2018-86183
Herrando M et al (2023) A review of solar hybrid photovoltaic-thermal (PV-T) collectors and systems. Prog Energy Combust Sci 97:101072. https://doi.org/10.1016/j.pecs.2023.101072
Ibrahim SMA, Shabak AGM (2021) Design of a solar powered reverse osmosis system in Egypt. J Mech Energy Eng 5(2):125–140. https://doi.org/10.30464/10.30464/jmee.2021.5.2.125
Jafari A, Kebria MRS, Rahimpour A, Bakeri G (2018) Graphene quantum dots modified polyvinylidenefluride (PVDF) nanofibrous membranes with enhanced performance for air Gap membrane distillation. Chem Eng Process - Process Intensif 126:222–231. https://doi.org/10.1016/j.cep.2018.03.010
Jalihal P, Venkatesan R (2019) Advanced desalination technologies. Elsevier Inc
Janajreh I, Zhang H, El Kadi K, Ghaffour N (2023) Freeze desalination: current research development and future prospects. Water Res 229:119389. https://doi.org/10.1016/j.watres.2022.119389
Jia Y, Li F, Chen X, Wang M (2018) Model analysis on electrodialysis for inorganic acid recovery and its experimental validation. Sep Purif Technol 190:261–267. https://doi.org/10.1016/j.seppur.2017.08.067
Jones MA, Odeh I, Haddad M, Mohammad AH, Quinn JC (2016) Economic analysis of photovoltaic (PV) powered water pumping and desalination without energy storage for agriculture. Desalination 387:35–45. https://doi.org/10.1016/j.desal.2016.02.035
Jones E, Qadir M, van Vliet MTH, Smakhtin V, S. mu Kang, (2019) The state of desalination and brine production: a global outlook. Sci Total Environ 657:1343–1356. https://doi.org/10.1016/j.scitotenv.2018.12.076
Kadhim RA, Khudhair BH, Jaafar MS (2023) Comparative study of water desalination using reverse osmosis (RO) and electro-dialysis systems (ED): review. J Eng 29(4):61–77. https://doi.org/10.31026/j.eng.2023.04.04
Kalogirou SA (2005) Seawater desalination using renewable energy sources. Prog Energy Combust Sci 31(3):242–281. https://doi.org/10.1016/j.pecs.2005.03.001
Káňavová N, Machuča L (2014) A novel method for limiting current calculation in electrodialysis modules. Period Polytech, Chem Eng 58(2):125–130. https://doi.org/10.3311/PPch.7145
Karanikola V, Moore SE, Deshmukh A, Arnold RG, Elimelech M, Sáez AE (2019) Economic performance of membrane distillation configurations in optimal solar thermal desalination systems. Desalination 472:114164. https://doi.org/10.1016/j.desal.2019.114164
Karavas C-S, Arvanitis KG, Papadakis G (2019) Optimal technical and economic configuration of photovoltaic powered reverse osmosis desalination systems operating in autonomous mode. Desalination 466:97–106. https://doi.org/10.1016/j.desal.2019.05.007
Karimi L, Abkar L, Aghajani M, Ghassemi A (2015) Technical feasibility comparison of off-grid PV-EDR and PV-RO desalination systems via their energy consumption. Sep Purif Technol 151:82–94. https://doi.org/10.1016/j.seppur.2015.07.023
Kaya A, Evren Tok M, Koc M (2019) A levelized cost analysis for solar-energy-powered sea water desalination in the Emirate of Abu Dhabi. Sustainability (switzerland). https://doi.org/10.3390/su11061691
Kebria MRS, Rahimpour A (2020) Membrane Distillation: Basics, Advances, and Applications, vol. 11, no. tourism. IntechOpen
Khan ZU et al (2023) Electro-deionization (EDI) technology for enhanced water treatment and desalination: a review. Desalination 548:116254. https://doi.org/10.1016/j.desal.2022.116254
Korkmaz MS, Sahin AD (2023) Developing a micrositing methodology for floating photovoltaic power plants. Int J Environ Sci Technol 20(7):7621–7644. https://doi.org/10.1007/s13762-023-04961-2
Kumar S, Kumar M, Chowdhury S, Rajpurohit BS, Randhawa JK (2022) Environmental concerns and long-term solutions for solar-powered water desalination. J Clean Prod 345:131180. https://doi.org/10.1016/j.jclepro.2022.131180
Li G, Lu L (2020) Modeling and performance analysis of a fully solar-powered stand-alone sweeping gas membrane distillation desalination system for island and coastal households. Energy Convers Manage 205:112375. https://doi.org/10.1016/j.enconman.2019.112375
Li Q et al (2019a) An integrated, solar-driven membrane distillation system for water purification and energy generation. Appl Energy 237:534–548. https://doi.org/10.1016/j.apenergy.2018.12.069
Li S, Cai YH, Schäfer AI, Richards BS (2019b) Renewable energy powered membrane technology: a review of the reliability of photovoltaic-powered membrane system components for brackish water desalination. Appl Energy 253:113524. https://doi.org/10.1016/j.apenergy.2019.113524
Lopes MPC, Nogueira T, Santos AJL, Branco DC, Pouran H (2022) Technical potential of floating photovoltaic systems on artificial water bodies in Brazil. Renew Energy 181:1023–1033. https://doi.org/10.1016/j.renene.2021.09.104
Lotfy HR, Staš J, Roubík H (2022) Renewable energy powered membrane desalination — review of recent development. Environ Sci Pollut Res 29(31):46552–46568. https://doi.org/10.1007/s11356-022-20480-y
Ma C, Liu Z (2022) Water-surface photovoltaics: performance, utilization, and interactions with water eco-environment. Renew Sustain Energy Rev 167:112823. https://doi.org/10.1016/j.rser.2022.112823
Manju S, Sagar N (2017) Renewable energy integrated desalination: a sustainable solution to overcome future fresh-water scarcity in India. Renew Sustain Energy Rev 73:594–609. https://doi.org/10.1016/j.rser.2017.01.164
Manoj Kumar N, Chakraborty S, Kumar Yadav S, Singh J, Chopra SS (2022) Advancing simulation tools specific to floating solar photovoltaic systems – comparative analysis of field-measured and simulated energy performance. Sustain Energy Technol Assess 52:102168. https://doi.org/10.1016/j.seta.2022.102168
Marni Sandid A, Bassyouni M, Nehari D, Elhenawy Y (2021) Experimental and simulation study of multichannel air gap membrane distillation process with two types of solar collectors. Energy Convers Manage 243:114431. https://doi.org/10.1016/j.enconman.2021.114431
Mehmood A, Ren J (2021) Chapter 11 - Renewable energy-driven desalination for more water and less carbon. Renewable-Energy-Driven Future. Academic Press, pp 333–372
Mito MT, Ma X, Albuflasa H, Davies PA (2019) Reverse osmosis (RO) membrane desalination driven by wind and solar photovoltaic (PV) energy: State of the art and challenges for large-scale implementation. Renew Sustain Energy Rev 112:669–685. https://doi.org/10.1016/j.rser.2019.06.008
Mohamed ES, Papadakis G (2015) Advances of renewable energy powered desalination. Handbook of Clean Energy Systems. Wiley, pp 1–10
Mokheimer EMA, Sahin AZ, Al-Sharafi A, Ali AI (2013) Modeling and optimization of hybrid wind-solar-powered reverse osmosis water desalination system in Saudi Arabia. Energy Convers Manage 75:86–97. https://doi.org/10.1016/j.enconman.2013.06.002
Mostafaeipour A, Qolipour M, Rezaei M, Babaee-Tirkolaee E (2019) Investigation of off-grid photovoltaic systems for a reverse osmosis desalination system: a case study. Desalination 454:91–103. https://doi.org/10.1016/j.desal.2018.03.007
Moura Bernardes A, Rodrigues MAS (2014) Electrodialysis in water treatment. Springer, Berlin
Book Google Scholar
Mukherjee M et al (2022) Development of high performance pervaporation desalination membranes: a brief review. Process Saf Environ Prot 159:1092–1104. https://doi.org/10.1016/j.psep.2022.01.076
Muñoz-Cerón E, Osorio-Aravena JC, Rodríguez-Segura FJ, Frolova M, Ruano-Quesada A (2023) Floating photovoltaics systems on water irrigation ponds: technical potential and multi-benefits analysis. Energy 271:127039. https://doi.org/10.1016/j.energy.2023.127039
Nasr Aboelmaaty H (2022) A detailed review of solar desalination systems with different enhancement methods. SVU-Int J Eng Sci Appl 3(1):49–63. https://doi.org/10.21608/svusrc.2022.126110.1035
Ndadane SS, Inambao FL (2022) Consideration of design systems for solar powered reverse osmosis seawater desalination. Int J Mech Prod Eng Res Dev 11(6):183–203
Oré DG, Pretell VH, Pilco AW (2022) Design of an autonomous photovoltaic system for desalination of groundwater by electrodialysis. J Phys: Conf Ser 2180(1):12020. https://doi.org/10.1088/1742-6596/2180/1/012020
Pietrasanta AM, Mussati SF, Aguirre PA, Schmidhalter I, Morosuk T, Mussati MM (2023) Optimal sizing of seawater desalination systems using wind-solar hybrid renewable energy sources. Renew Energy. https://doi.org/10.1016/j.renene.2023.118970
Pimentel da Silva GD, Sharqawy MH (2020) Techno-economic analysis of low impact solar brackish water desalination system in the Brazilian Semiarid region. J Clean Prod 248:119255. https://doi.org/10.1016/j.jclepro.2019.119255
Qasim M, Badrelzaman M, Darwish NN, Darwish NA, Hilal N (2019) Reverse osmosis desalination: a state-of-the-art review. Desalination 459:59–104
Qasim M, Samad IU, Darwish NA, Hilal N (2021) Comprehensive review of membrane design and synthesis for membrane distillation. Desalination 518:115168. https://doi.org/10.1016/j.desal.2021.115168
Qtaishat MR, Banat F (2013) Desalination by solar powered membrane distillation systems. Desalination 308:186–197. https://doi.org/10.1016/j.desal.2012.01.021
Rashidi MM, Mahariq I, Murshid N, Wongwises S, Mahian O, Alhuyi Nazari M (2022) Applying wind energy as a clean source for reverse osmosis desalination: a comprehensive review. Alex Eng J 61(12):12977–12989. https://doi.org/10.1016/j.aej.2022.06.056
Saavedra A, Valdés H, Mahn A, Acosta O (2021) Comparative analysis of conventional and emerging technologies for seawater desalination: Northern chile as a case study. Membranes. https://doi.org/10.3390/membranes11030180
Sampedro T, Tristán C, Gómez-Coma L, Fallanza M, Ortiz I, Ibañez R (2023) Design of a reverse electrodialysis plant for salinity gradient energy extraction in a coastal wastewater treatment plant. Membranes 13(6):546. https://doi.org/10.3390/membranes13060546
Sarathe S, Baredar PV, Dwivedi G, Tapdiya S, Gaurav A (2022) Review of various types of renewable-powered desalination technologies with economic analysis. Mater Today: Proceed 56:326–335. https://doi.org/10.1016/j.matpr.2022.01.175
Sarbatly R, Chiam C-K (2013) Evaluation of geothermal energy in desalination by vacuum membrane distillation. Appl Energy 112:737–746. https://doi.org/10.1016/j.apenergy.2012.12.028
Schorr M (2011) Desalination: trends and technologies. IntechOpen
Sedighi M, Behvand Usefi MM, Ismail AF, Ghasemi M (2023) Environmental sustainability and ions removal through electrodialysis desalination: operating conditions and process parameters. Desalination 549:116319. https://doi.org/10.1016/j.desal.2022.116319
Selvi SR, Baskaran R (2015) Solar photovoltaic-powered membrane distillation as sustainable clean energy technology in desalination. Curr Sci 109(7):1247–1254. https://doi.org/10.18520/v109/i7/1247-1254
Shah KM et al (2022) Drivers, challenges, and emerging technologies for desalination of high-salinity brines: A critical review. Desalination 538:115827. https://doi.org/10.1016/j.desal.2022.115827
Shahid MK et al (2023) A review of membrane-based desalination systems powered by renewable energy sources. Water (switzerland). https://doi.org/10.3390/w15030534
Shalaby SM et al (2022) Membrane distillation driven by solar energy: a review. J Clean Prod 366:132949. https://doi.org/10.1016/j.jclepro.2022.132949
Sharbat A (2016) ‘Development of Photovoltaic electrodialysis desalination system’,In: Desalination and Water Purification Research and Development Program, no. 191, 15
Sharma VK et al (2022) Imperative role of photovoltaic and concentrating solar power technologies towards renewable energy generation. Int J Photoenergy 2022:3852484. https://doi.org/10.1155/2022/3852484
Sharon H, Reddy KS (2015) A review of solar energy driven desalination technologies. Renew Sustain Energy Rev 41:1080–1118. https://doi.org/10.1016/j.rser.2014.09.002
Shatat M, Riffat SB (2012) Water desalination technologies utilizing conventional and renewable energy sources. Int J Low-Carbon Technol 9(1):1–19. https://doi.org/10.1093/ijlct/cts025
Shatat M, Worall M, Riffat S (2013) Opportunities for solar water desalination worldwide: review. Sustain Cities Soc 9:67–80. https://doi.org/10.1016/j.scs.2013.03.004
Shokri A, Sanavi Fard M (2022) A sustainable approach in water desalination with the integration of renewable energy sources: environmental engineering challenges and perspectives. Environ Adv 9:100281. https://doi.org/10.1016/j.envadv.2022.100281
Soliman AM, Alharbi AG, Sharaf Eldean MA (2021) Techno-economic optimization of a solar-wind hybrid system to power a large-scale reverse osmosis desalination plant. Sustainability (switzerland). https://doi.org/10.3390/su132011508
Strathmann H (2010) Electrodialysis, a mature technology with a multitude of new applications. Desalination 264(3):268–288. https://doi.org/10.1016/j.desal.2010.04.069
Taft HL (2015) Water scarcity. In: Global Challenges for Agriculture. Elsevier Inc.,
Taha M, Al-Saed R (2018) Application potential of small-scale solar desalination for brackish water in the Jordan Valley, Palestine. Int J Environ Stud 75(1):214–225. https://doi.org/10.1080/00207233.2017.1403759
Taha Sayed E, Olabi AG, Elsaid K, Al Radi M, Alqadi R, Ali Abdelkareem M (2022) Recent progress in renewable energy based-desalination in the Middle East and North Africa MENA region. J Adv Res. https://doi.org/10.1016/j.jare.2022.08.016
Tarazona-Romero BE, Campos-Celador A, Maldonado-Muñoz YA (2022) ‘Can solar desalination be small and beautiful? A critical review of existing technology under the appropriate technology paradigm.’ Energy Res Soc Sci. https://doi.org/10.1016/j.erss.2022.102510
Tawalbeh M, Al-Othman A, Abdelwahab N, Alami AH, Olabi AG (2021) Recent developments in pressure retarded osmosis for desalination and power generation. Renew Sustain Energy Rev 138:110492. https://doi.org/10.1016/j.rser.2020.110492
Tedesco M, Hamelers HVM, Biesheuvel PM (2017a) Nernst-Planck transport theory for (reverse) electrodialysis: II. Effect of water transport through ion-exchange membranes. J Membr Sci 531:172–182. https://doi.org/10.1016/j.memsci.2017.02.031
Tedesco M, Cipollina A, Tamburini A, Micale G (2017b) Towards 1kW power production in a reverse electrodialysis pilot plant with saline waters and concentrated brines. J Membr Sci 522:226–236. https://doi.org/10.1016/j.memsci.2016.09.015
Thakkar H, Sankhala A, Ramana PV, Panchal H (2020) A detailed review on solar desalination techniques. Int J Ambient Energy 41(9):1066–1087. https://doi.org/10.1080/01430750.2018.1490351
Thi HTD, Pasztor T, Fozer D (2021) Comparison of desalination technologies using renewable energy sources with life cycle, PESTLE, and multi-criteria decision analyses. Water 13(21):3023
Vera E, Ruales J, Dornier M, Sandeaux J, Sandeaux R, Pourcelly G (2003) Deacidification of clarified passion fruit juice using different configurations of electrodialysis. J Chem Technol Biotechnol 78(8):918–925. https://doi.org/10.1002/jctb.827
Vidović V, Krajačić G, Matak N, Stunjek G, Mimica M (2023) Review of the potentials for implementation of floating solar panels on lakes and water reservoirs. Renew Sustain Energy Rev 178:113237. https://doi.org/10.1016/j.rser.2023.113237
Vo TTE, Ko H, Huh J-H, Park N (2021) Overview of solar energy for aquaculture: the potential and future trends. Energies. https://doi.org/10.3390/en14216923
Voutetaki A, Papadopoulos AI, Plakas K, Seferlis P (2022) Integration of an electrodialysis process for selective nitrate removal with renewable energy sources. Chem Eng Trans 94(3):721–726. https://doi.org/10.3303/CET2294120
Wright NC, de Zande G, Winter AG (2015) Justification, design, and analysis of a village-scale photovoltaic-powered electrodialysis reversal system for rural India. Int Des Eng Techn Conf Comput Inform Eng Conf 57076:043
Wu B, Maleki A, Pourfayaz F, Rosen MA (2018) Optimal design of stand-alone reverse osmosis desalination driven by a photovoltaic and diesel generator hybrid system. Sol Energy 163:91–103. https://doi.org/10.1016/j.solener.2018.01.016
Xu H, Jiang S, Xie MX, Jia T, Dai YJ (2022) Technical improvements and perspectives on humidification-dehumidification desalination — a review. Desalination 541:116029. https://doi.org/10.1016/j.desal.2022.116029
Yadav A, Labhasetwar PK, Shahi VK (2021) Membrane distillation using low-grade energy for desalination: a review. J Environ Chem Eng 9(5):105818. https://doi.org/10.1016/j.jece.2021.105818
Yadav A, Labhasetwar PK, Shahi VK (2022) Membrane distillation crystallization technology for zero liquid discharge and resource recovery: opportunities, challenges and futuristic perspectives. Sci Total Environ 806:150692. https://doi.org/10.1016/j.scitotenv.2021.150692
Zarzoum K, Zhani K, Ben Bacha H, Koschikowski J (2019) Experimental parametric study of membrane distillation unit using solar energy. Sol Energy 188:1274–1282. https://doi.org/10.1016/j.solener.2019.07.025
Zewdie TM, Habtu NG, Dutta A, Van der Bruggen B (2021) Solar-assisted membrane technology for water purification: a review. Water Reuse 11(1):1–32. https://doi.org/10.2166/wrd.2020.049
Zheng H (2017) Chapter 7 - solar desalination system combined with conventional technologies. In: Solar energy desalination technology. Elsevier, pp 537–622. https://doi.org/10.1016/B978-0-12-805411-6.00007-5
Download references
Author information
Authors and affiliations.
The Libyan Center for Solar Energy Research and Studies, Tripoli, Libya
Albashir K. Elfaqih, Abdurazaq Elbaz & Yousef M. Akash
You can also search for this author in PubMed Google Scholar
Corresponding author
Correspondence to Albashir K. Elfaqih .
Ethics declarations
Conflict of interest.
On behalf of all authors, the corresponding author states that there is no conflict of interest.
Additional information
Publisher's note.
Springer Nature remains neutral with regard to jurisdictional claims in published maps and institutional affiliations.
Rights and permissions
Springer Nature or its licensor (e.g. a society or other partner) holds exclusive rights to this article under a publishing agreement with the author(s) or other rightsholder(s); author self-archiving of the accepted manuscript version of this article is solely governed by the terms of such publishing agreement and applicable law.
Reprints and permissions
About this article
Elfaqih, A.K., Elbaz, A. & Akash, Y.M. A review of solar photovoltaic-powered water desalination technologies. Sustain. Water Resour. Manag. 10 , 123 (2024). https://doi.org/10.1007/s40899-024-01067-6
Download citation
Received : 29 July 2023
Accepted : 03 February 2024
Published : 11 May 2024
DOI : https://doi.org/10.1007/s40899-024-01067-6
Share this article
Anyone you share the following link with will be able to read this content:
Sorry, a shareable link is not currently available for this article.
Provided by the Springer Nature SharedIt content-sharing initiative
- Desalination
- Photovoltaic
- Reverse osmosis
- Electrodialysis
- Membrane distillation
- Find a journal
- Publish with us
- Track your research

An official website of the United States government
The .gov means it’s official. Federal government websites often end in .gov or .mil. Before sharing sensitive information, make sure you’re on a federal government site.
The site is secure. The https:// ensures that you are connecting to the official website and that any information you provide is encrypted and transmitted securely.
- Publications
- Account settings
Preview improvements coming to the PMC website in October 2024. Learn More or Try it out now .
- Advanced Search
- Journal List

Research on drinking water purification technologies for household use by reducing total dissolved solids (TDS)
Bill b. wang.
Redlands East Valley High School, Redlands, California, United States of America
Associated Data
All relevant data are within the manuscript and its Supporting Information files.
This study, based in San Bernardino County, Southern California, collected and examined tap water samples within the area to explore the feasibility of adopting non-industrial equipment and methods to reduce water hardness and total dissolved solids(TDS). We investigated how water quality could be improved by utilizing water boiling, activated carbon and sodium bicarbonate additives, as well as electrolysis methods. The results show that heating is effective at lower temperatures rather than long boils, as none of the boiling tests were lower than the original value. Activated carbon is unable to lower TDS, because it is unable to bind to any impurities present in the water. This resulted in an overall TDS increase of 3.5%. However, adding small amounts of sodium bicarbonate(NaHCO 3 ) will further eliminate water hardness by reacting with magnesium ions and improve taste, while increasing the pH. When added to room temperature tap water, there is a continuous increase in TDS of 24.8% at the 30 mg/L mark. The new findings presented in this study showed that electrolysis was the most successful method in eliminating TDS, showing an inverse proportion where an increasing electrical current and duration of electrical lowers more amounts of solids. This method created a maximum decrease in TDS by a maximum of 22.7%, with 3 tests resulting in 15.3–16.6% decreases. Furthermore, when water is heated to a temperature around 50°C (122°F), a decrease in TDS of around 16% was also shown. The reduction of these solids will help lower water hardness and improve the taste of tap water. These results will help direct residents to drink more tap water rather than bottled water with similar taste and health benefits for a cheaper price as well as a reduction on plastic usage.
Introduction
The concentration of total dissolved solids(TDS) present in water is one of the most significant factors in giving water taste and also provides important ions such as calcium, magnesium, potassium, and sodium [ 1 – 3 ]. However, water with high TDS measurements usually indicates contamination by human activities, such as soil and agricultural runoff caused by irrigation, unregulated animal grazing and wildlife impacts, environmentally damaging farming methods such as slash and burn agriculture, and the overuse of nitrate-based fertilizer [ 4 , 5 ], etc. Around tourist areas as well as state parks, these factors will slowly add up over time and influence the water sources nearby [ 5 ]. Water that flows through natural springs and waterways with high concentrations of organic salts within minerals and rocks, or groundwater that originates from wells with high salt concentration will also result in higher particle measurements [ 6 ].
Water sources can be contaminated by substances and ions such as nitrate, lead, arsenic, and copper [ 7 , 8 ] and may cause many health problems related to heavy metal consumption and poisoning. Water reservoirs and treatments plants that do not consider water contamination by motor vehicles, as well as locations that struggle to provide the necessary components required for water treatment will be more prone to indirect contamination [ 9 – 11 ]. Many plants are effective in ensuring the quality and reduction of these contaminants, but often leave out the secondary considerations, The United States Environmental Protection Agency(US EPA)’s secondary regulations recommend that TDS should be below 500 mg/L [ 2 ], which is also supported by the World Health Organization(WHO) recommendation of below 600 mg/L and an absolute maximum of less than 1,000 mg/L [ 3 ]. These substances also form calcium or magnesium scales within water boilers, heaters, and pipes, causing excess buildup and drain problems, and nitrate ions may pose a risk to human health by risking the formation of N -nitroso compounds(NOC) and less public knowledge about such substances [ 12 – 15 ]. Nitrates can pose a non-carcinogenic threat to different communities, but continue to slip past water treatment standards [ 15 ]. Furthermore, most people do not tolerate or prefer water with high hardness or chlorine additives [ 16 ], as the taste changes tremendously and becomes unpreferable. Even so, TDS levels are not accounted for in mandatory water regulations, because the essential removal of harmful toxins and heavy metals is what matters the most in water safety. Some companies indicate risks in certain ions and alkali metals, showing how water hardness is mostly disregarded and is not as well treated as commercial water bottling companies [ 17 , 18 ].
In Southern California, water quality is not as well maintained than the northern counties as most treatment plants in violation of a regulation or standard are located in Central-Southern California [ 19 ], with southern counties having the largest number of people affected [ 20 ]. This study is focused on the Redlands area, which has had no state code violations within the last decade [ 21 ]. A previous study has analyzed TDS concentrations throughout the Santa Ana Basin, and found concentrations ranging from 190–600 ppm as treated wastewater and samples obtained from mountain sites, taking into account the urban runoff and untreated groundwater as reasons for elevated levels of TDS but providing no solution in helping reduce TDS [ 22 ]. Also, samples have not been taken directly through home water supplies, where the consumer is most affected. Other water quality studies in this region have been focused on the elimination of perchlorates in soil and groundwater and distribution of nitrates, but such research on chemicals have ceased for the last decade, demonstrated by safe levels of perchlorates and nitrates in water reports [ 23 , 24 ]. In addition to these studies, despite the improving quality of the local water treatment process, people prefer bottled water instead of tap water because of the taste and hardness of tap water [ 25 ]. Although water quality tests are taken and documented regularly, the taste of the water is not a factor to be accounted for in city water supplies, and neither is the residue left behind after boiling water. The residue can build up over time and cause appliance damage or clogs in drainage pipes.
This study will build upon previous analyses of TDS studies and attempt to raise new solutions to help develop a more efficient method in reducing local TDS levels, as well as compare current measurements to previous analyses to determine the magnitude to which local treatment plants have improved and regulated its treatment processes.
Several methods that lower TDS are reviewed: boiling and heating tap water with and without NaHCO₃, absorption by food-grade activated carbon [ 26 , 27 ], and battery-powered electrolysis [ 28 – 30 ]. By obtaining water samples and determining the difference in TDS before and after the listed experiments, we can determine the effectiveness of lowering TDS. The results of this study will provide options for residents and water treatment plants to find ways to maintain the general taste of the tap water, but also preserve the lifespan of accessories and pipelines. By determining a better way to lower TDS and treat water hardness, water standards can be updated to include TDS levels as a mandatory measurement.
Materials and methods
All experiments utilized tap water sourced from Redlands homes. This water is partially supplied from the Mill Creek (Henry Tate) and Santa Ana (Hinckley) Water Sheds/Treatment Plants, as well as local groundwater pumps. Water sampling and sourcing were done at relatively stable temperatures of 26.9°C (80.42°F) through tap water supplies. The average TDS was measured at 159 ppm, which is slightly lower than the reported 175 ppm by the City of Redlands. Permission is obtained by the author from the San Bernardino Municipal Water Department website to permit the testing procedures and the usage of private water treatment devices for the purpose of lowering water hardness and improving taste and odor. The turbidity was reported as 0.03 Nephelometric Turbidity Units (NTU) post-treatment. Residual nitrate measured at 2.3mg/L in groundwater before treatment and 0.2 mg/L after treatment and perchlorate measured at 0.9 μg/L before treatment, barely staying below the standard of 1 μg/L; it was not detected within post-treatment water. Lead content was not detected at all, while copper was detected at 0.15 mg/L.
For each test, all procedures were done indoors under controlled temperatures, and 20 L of fresh water was retrieved before each test. Water samples were taken before each experimental set and measured for TDS and temperature, and all equipment were cleaned thoroughly with purified water before and after each measurement. TDS consists of inorganic salts and organic material present in solution, and consists mostly of calcium, magnesium, sodium, potassium, carbonate, chloride, nitrate, and sulfate ions. These ions can be drawn out by leaving the water to settle, or binding to added ions and purified by directly separating the water and ions. Equipment include a 50 L container, 1 L beakers for water, a graduated cylinder, a stir rod, a measuring spoon, tweezers, a scale, purified water, and a TDS meter. A standard TDS meter is used, operated by measuring the conductivity of the total amount of ionized solids in the water, and is also cleaned in the same manner as aforementioned equipment. The instrument is also calibrated by 3 pH solutions prior to testing.All results were recorded for and then compiled for graphing and analysis.
Heating/Boiling water for various lengths of time
The heating method was selected because heat is able to break down calcium bicarbonate into calcium carbonate ions that are able to settle to the bottom of the sample. Four flasks of 1 L of tap water were each heated to 40°C, 50°C, 60°C, and 80°C (104–176°F) and observed using a laser thermometer. The heated water was then left to cool and measurements were made using a TDS meter at the 5, 10, 20, 30, and 60-minute marks.
For the boiling experiments, five flasks of 1 L of tap water were heated to boil at 100°C (212°F). Each flask, which was labeled corresponding to its boiling duration, was marked with 2, 4, 6, 10, and 20 minutes. Each flask was boiled for its designated time, left to cool under open air, and measurements were made using a TDS meter at the 5, 10, 20, 30, 60, and 120-minute marks. The reason that the boiling experiment was extended to 120 minutes was to allow the water to cool down to room temperature.
Activated carbon as a water purification additive
This test was performed to see if food-grade, powdered activated carbon had any possibility of binding with and settling out residual particles. Activated carbon was measured using a milligram scale and separated into batches of 1, 2, 4, 5, 10, 30, and 50 mg. Each batch of the activated carbon were added to a separate flask of water and stirred for five minutes, and finally left to settle for another five minutes. TDS measurements were recorded after the water settled.
Baking soda as a water purification additive
To lower scale error and increase experimental accuracy, a concentration of 200 mg/L NaHCO₃ solution was made with purified water and pure NaHCO₃. For each part, an initial TDS measurement was taken before each experiment.
In separate flasks of 1 L tap water, each labeled 1, 2, 4, 5, 10, and 30 mg of NaHCO 3 , a batch was added to each flask appropriately and stirred for 5 minutes to ensure that everything dissolved. Measurements were taken after the water was left to settle for another 5 minutes for any TDS to settle.
Next, 6 flasks of 1 L tap water were labeled, with 5 mg (25 mL solution) of NaHCO₃ added to three flasks and 10 mg (50 mL solution) of NaHCO₃ added to the remaining three. One flask from each concentration of NaHCO₃ was boiled for 2 mins., 4 mins., or 6 mins., and then left to cool. A TDS measurement was taken at the 5, 10, 20, 30, 60, and 120-minute marks after removal from heat.
Electrolysis under low voltages
This test was performed because the ionization of the TDS could be manipulated with electricity to isolate an area of water with lower TDS. For this test, two 10cm long graphite pieces were connected via copper wiring to a group of batteries, with each end of the graphite pieces submerged in a beaker of tap water, ~3 cm apart.
Using groups of 1.5 V double-A batteries, 4 beakers with 40mL of tap water were each treated with either 7.5, 9.0, 10.5, and 12.0 V of current. Electrolysis was observed to be present by the bubbling of the water each test, and measurements were taken at the 3, 5, 7, and 10 minute marks.
Results/Discussion
Heating water to various temperatures until the boiling point.
The goal for this test was to use heat to reduce the amount of dissolved oxygen and carbon dioxide within the water, as shown by this chemical equation: Heat: Ca(HCO 3 ) 2 → CaCO 3 ↓ + H 2 O + CO 2 ↑.
This would decompose ions of calcium bicarbonate down into calcium carbonate and water and carbon dioxide byproducts.
Patterns and trends in decreasing temperatures
The following trend lines are based on a dataset of changes in temperature obtained from the test results and graphed as Fig 1 .

To predict the precise temperature measurements of the tap water at 26.9°C, calculations were made based on Fig 1 . The fitting equations are in the format, y = a.e bx . The values for the fitting coefficients a and b, and correlation coefficient R 2 are listed in Table 1 as column a, b and R 2 . The calculated values and the target temperature are listed in Table 1 .
Fig 2 was obtained by compiling TDS results with different temperatures and times.

The fitting equations for Fig 2 are also in the format, y = a.e bx . The fitting coefficients a and b, and correlation coefficient R 2 values are listed in Table 2 . Based on the fitting curves in Fig 2 and the duration to the target temperature in Table 1 , We calculated the TDS at 26.9°C as listed in column calculated TDS in Table 2 based on the values we reported on Fig 2 .
Based on the heating temperature and the calculated TDS with the same target water temperature, we obtained the following heating temperature vs TDS removal trend line and its corresponding fitting curve in Table 2 .
In Fig 1 , a trend in the rate of cooling is seen, where a higher heating temperature creates a steeper curve. During the first five minutes of cooling, the water cools quicker as the absorbed heat is quickly released into the surrounding environment. By the 10-minute mark, the water begins to cool in a linear rate of change. One detail to note is that the 100°C water cools quicker than the 80°C and eventually cools even faster than the 60°C graph. Table 1 supports this observation as the duration to target temperature begins to decrease from a maximum point of 94.8 mins to 80.95 mins after the 80°C mark.
As shown in Fig 2 , all TDS values decrease as the temperature starts to cool to room temperature, demonstrating a proportional relationship where a lower temperature shows lower TDS. This can partially be explained by the ions settling in the flasks. Visible particles can also be observed during experimentation as small white masses on the bottom, as well as a thin ring that forms where the edge of the water contacts the flask. When the water is heated to 40°C and cooled, a 3.8% decrease in TDS is observed. When 50°C is reached, the TDS drops at its fastest rate from an initial value of 202 ppm to 160 ppm after 60 minutes of settling and cooling. The TDS measurements in these experiements reach a maximum of 204 ppm at the 60°C mark. However, an interesting phenomenon to point out is that the water does not hit a new maximum at 100°C. meaning that TDS reaches a plateau at 60°C. Also, the rate of decrease begins to slow down after 20 minutes, showing that an unknown factor is affecting the rate of decrease. It is also hypothesized that the slight increase in TDS between the 5–20 minute range is caused by a disturbance in the settling of the water, where the temperature starts to decrease at a more gradual and constant rate. The unstable and easy formation of CaCO 3 scaling has also been the subject of a study of antiscaling methods, which also supports the result that temperature is a significant influence for scale formation [ 12 ].
In Table 2 , calculations for TDS and the time it takes for each test to cool were made. Using the data, it is determined that the test with 50°C water decreased the most by 16% from the initial measurement of 159ppm. This means that it is most effective when water is heated between temperatures of 40–60°C when it comes to lowering TDS, with a difference of ~7–16%. When water is heated to temperatures greater than 80°C, the water begins to evaporate, increasing the concentration of the ions, causing the TDS to increase substantially when cooled to room temperature.
Finally, in Fig 3 , a line of best fit of function f(x) = -0.0007x 3 + 0.1641x 2 –10.962x + 369.36 is used with R 2 = 0.9341. Using this function, the local minimum of the graph would be reached at 48.4°C.

This data shows that heating water at low temperatures (i.e. 40–50°C) may be more beneficial than heating water to higher temperatures. This study segment has not been presented in any section within the United States EPA Report on water management for different residual particles/substances. However, warmer water temperatures are more prone to microorganism growth and algal blooms, requiring more intensive treatment in other areas such as chlorine, ozone, and ultraviolet disinfection.
Using the specific heat capacity equation, we can also determine the amount of energy and voltage needed to heat 1 L of water up to 50°C: Q = mcΔT, where c, the specific heat capacity of water, is 4.186 J/g°C, ΔT, the change in temperature from the experimental maximum to room temperature, is 30°C, and m, the mass of the water, is 1000 g. This means that the amount of energy required will be 125580 J, which is 0.035 kWh or 2.1 kW.
After taking all of the different measurements obtained during TDS testing, and compiling the data onto this plot, Fig 4 is created with a corresponding line of best fit:

In Fig 4 , it can be observed that the relationship between the temperature of the water and its relative TDS value is a downwards facing parabolic graph. As the temperature increases, the TDS begins to decrease after the steep incline at 50–60°C. The line of best fit is represented by the function f(x) = -0.0142x 2 + 2.258x + 105.84. R 2 = 0.6781. Because the R 2 value is less than expected, factors such as the time spent settling and the reaction rate of the ions should be considered. To determine the specifics within this experiment, deeper research and prolonged studies with more highly accurate analyses must be utilized to solve this problem.
Boiling water for various amounts of time
Trend of boiling duration and rate of cooling.
Using the same methods to create the figures and tables for the previous section, Fig 5 depicts how the duration of time spent boiling water affects how fast the water cools.

As seen in Fig 5 , within the first 10 minutes of the cooling time, the five different graphs are entwined with each other, with all lines following a similar pattern. However, the graph showing 20 minutes of boiling is much steeper than the other graphs, showing a faster rate of cooling. This data continues to support a previous claim in Fig 2 , as this is most likely represented by a relationship a longer the boil creates a faster cooling curve. This also shows that the first 5 minutes of cooling have the largest deviance compared to any other time frame.
The cooling pattern is hypothesized by possible changes in the orderly structure of the hydrogen bonds in the water molecules, or the decreased heat capacity of water due to the increasing concentration of TDS.
Effect on TDS as boiling duration increases
In Fig 6 , all lines except for the 20-minute line are clustered in the bottom area of the graph. By excluding the last measurement temporarily due to it being an outlier, we have observed that the difference between the initial and final TDS value of each test decreases.

Despite following a similar trend of an increase in TDS at the start of the tests and a slow decrease overtime, this experiment had an interesting result, with the final test measuring nearly twice the amount of particles compared to any previous tests at 310 ppm, as shown in Fig 6 . It is confirmed that the long boiling time caused a significant amount of water to evaporate, causing the minerals to be more concentrated, thus resulting in a 300 ppm reading. Fig 6 follows the same trend as Fig 2 , except the TDS reading veers away when the boiling duration reaches 20 minutes. Also, with the long duration of heating, the water has developed an unfavorable taste from intense concentrations of CaCO₃. This also causes a buildup of a thin crust of CaCO₃ and other impurities around the container that is difficult to remove entirely. This finding is in accordance with the introductory statement of hot boiling water causing mineral buildups within pipes and appliances [ 9 ]. A TDS reading of 300ppm is still well below federal secondary standards of TDS, and can still even be compared to bottled water, in which companies may fluctuate and contain 335ppm within their water [ 1 , 2 ].
This experiment continues to stupport that the cooling rate of the water increases as the time spent boiling increases. Based on this test, a prediction can be made in which an increased concentration of dissolved solids lowers the total specific heat capacity of the sample, as the total volume of water decreases. This means that a method can be derived to measure TDS using the heat capacity of a tap water mixture and volume, in addition to current methods of using the electrical conductivity of aqueous ions.
Adding food-grade activated carbon to untreated tap water
Fig 7 presents a line graph with little to no change in TDS, with an initial spike from 157 to 163 ppm. The insoluble carbon remains in the water and shows no benefit.

The food-grade activated carbon proved no benefit to removing TDS from tap water, and instead added around 5–7 ppm extra, which settled down to around +4 ppm at 120 minutes. The carbon, which is not 100% pure from inorganic compounds and materials present in the carbon, can dissolve into the water, adding to the existing concentration of TDS. Furthermore, household tap water has already been treated in processing facilities using a variety of filters, including carbon, so household charcoal filters are not effective in further reducing dissolved solids [ 18 ].
Adding sodium bicarbonate solution to boiled tap water
As seen in Fig 8 , after adding 1 mg of NaHCO 3 in, the TDS rises to 161 ppm, showing a minuscule increase. When 4 mg was added, the TDS drops down to 158 ppm. Then, when 5 mg was added, a sudden spike to 172 ppm was observed. This means that NaHCO 3 is able to ionize some Ca 2+ and Mg 2+ ions, but also adds Na + back into the water. This also means that adding NaHCO 3 has little to no effect on TDS, with 4mg being the upper limit of effectiveness.

To examine whether or not the temperature plays a role in the effectiveness in adding NaHCO 3 , a boiling experiment was performed, and the data is graphed in Fig 9 .

Fig 9 presents the relationship between the amount of common baking soda(NaHCO₃) added, the boiling time involved, and the resulting TDS measurements. After boiling each flask for designated amounts of time, the results showed a downward trend line from a spike but does not reach a TDS value significantly lower than the initial sample. It is apparent that the NaHCO₃ has not lowered the TDS of the boiling water, but instead adds smaller quantities of ions, raising the final value. This additive does not contribute to the lowering of the hardness of the tap water. However, tests boiled with 5 mg/L of baking soda maintained a downward pattern as the water was boiled for an increasing amount of time, compared to the seemingly random graphs of boiling with 10 mg/L.
In some households, however, people often add NaHCO₃ to increase the pH for taste and health benefits. However, as shown in the test results, it is not an effective way of reducing TDS levels in the water [ 10 , 16 ], but instead raises the pH, determined by the concentration added. Even under boiling conditions, the water continues to follow the trend of high growth in TDS, of +25–43 ppm right after boiling and the slow drop in TDS (but maintaining a high concentration) as the particles settle to the bottom.
Utilizing the experimental results, we can summarize that after adding small batches of NaHCO3 and waiting up to 5 minutes will reduce water hardness making it less prone to crystallizing within household appliances such as water brewers. Also, this process raises the pH, which is used more within commercial water companies. However, the cost comes at increasing TDS.
Using electrolysis to treat TDS in tap water
Different voltages were passed through the water to observe the change in TDS overtime, with the data being compiled as Fig 10 .

The process of electrolysis in this experiment was not to and directly remove the existing TDS, but to separate the water sample into three different areas: the anode, cathode, and an area of clean water between the two nodes [ 19 ]. The anions in the water such as OH - , SO 4 2- , HCO 3 - move to the anode, while the cations such as H + , Ca 2+ 、Mg 2+ 、Na + move to the cathode. The middle area would then be left as an area that is more deprived of such ions, with Fig 10 proving this.
As shown in Fig 10 , electrolysis is effective in lower the TDS within tap water. Despite the lines being extremely tangled and unpredictable, the general trend was a larger decrease with a longer duration of time. At 10 minutes, all lines except 10.5 V are approaching the same value, meaning that the deviation was most likely caused by disturbances to the water during measurement from the low volume of water. With each different voltage test, a decrease of 12.7% for 6.0 V, 14.9% for 9.0 V, 22.7% for 10.5 V. and 19.5% for 12.0 V respectfully were observed. In the treatment of wastewate leachate, a study has shown that with 90 minutes of electrical treatment, 34.58% of TDS content were removed, supporting the effectiveness of electricity and its usage in wastewater treatment [ 29 ].
This experiment concludes that electrolysis is effective in lowering TDS, with the possibility to improve this process by further experimentation, development of a water cleaning system utilizing this cathode-anode setup to process water. This system would be a more specific and limited version of a reverse osmosis system by taking away ions through attraction, rather than a filter.
The Southern Californian tap water supply maintains TDS values below the federal regulations. However, crystalline scale buildup in household appliances is a major issue as it is hard to clean and eliminate. To easily improve the taste and quality of tap water at home as well as eliminating the formation of scales, the following methods were demonstrated as viable:
- By heating water to around 50°C (122°F), TDS and water hardness will decrease the most. Also, the boiling process is effective in killing microorganisms and removing contaminants. This process cannot surpass 10 minutes, as the concentration of the ions in the water is too high, which poses human health risks if consumed. These, along with activated carbon and NaHCO₃ additives, are inefficient methods that have minimal effects for lowering TDS.
- Electrolysis is one of the most effective methods of eliminating TDS. Experiments have proven that increased current and duration of time helps lower TDS. However, this method has yet to be implemented into conventional commercial water filtration systems.
Also, some observations made in these experiments could not be explained, and require further research and experimentation to resolve these problems. The first observation is that TDS and increasing water temperature maintain a parabolic relationship, with a maximum being reached at 80°C, followed by a gradual decrease. The second observation is that when water is boiled for an increased duration of time, the rate of cooling also increases.
This experiment utilized non-professional scientific equipment which are prone to mistakes and less precise. These results may deviate from professionally derived data, and will require further study using more advanced equipment to support these findings.
Acknowledgments
The author thanks Tsinghua University Professor and PLOS ONE editor Dr. Huan Li for assisting in experimental setups as well as data processing and treatment. The author also thanks Redlands East Valley High School’s Dr. Melissa Cartagena for her experimental guidance, and Tsinghua University Professor Dr. Cheng Yang for proofreading the manuscript.
Funding Statement
The author received no specific funding for this work.
Data Availability
- Share full article
Advertisement
Supported by
Is It Time to Stop Wasting Waste?
Circular sanitation systems could be lifelines on a water-stressed planet, but there are big barriers to overcome.

By Jane Withers
Jane Withers is a design writer and curator in London who specializes in water issues. She curated the exhibition “Water Pressure: Designing for the Future,” on view at MK&G, the museum of art and crafts in Hamburg, Germany, through Oct. 13.
This article is part of our Design special section about water as a source of creativity.
In the science fiction epic “Dune” (books and movies alike), the people who inhabit the brutally harsh desert planet Arrakis wear garments called stillsuits that allow them to drink their own recycled bodily waters.
These full-body survival suits, which look a bit like a techy version of an insect’s hard upper shell, absorb moisture from sweating and urination and filter out impurities to make potable water. This water is stored in pockets and sucked through a tube.
If this sounds like magical sci-fi thinking, the principle is similar to wastewater recycling systems already used in some water-stressed regions of the world.
And in space. Last year, NASA announced 98 percent of wastewater on the International Space Station was being recovered by a new system that distills sweat, urine and other moisture in the cabin into clean drinking water.
Waterborne infrastructure is largely taken for granted by those fortunate enough to have flushing toilets. But why does this technology persist? Does it make sense that vast quantities of fresh water are used to flush away human waste, while, according to UNICEF , about 1.5 billion people in the world still lack basic sanitation? Along with discarding a precious resource — in urban areas as much as 30 percent of fresh water is used to flush toilets — we are losing valuable nutrients that are needed in food production by not reclaiming them.
A number of recent initiatives, some more dreamy than others, propose to stanch the flow of flushed water. But the challenges are immense, given the cost of redesigning infrastructure for human wastewater disposal and implementing it on a global scale.
I recently visited Hamburg Water Cycle, in Germany, a pioneering wastewater reuse project in Hamburg’s new neighborhood of Jenfelder Au. Rather than following the convention of piping in water from outside the city and sending back waste to treatment plants, the new system separates wastewater into three streams for reuse locally.
Rainwater is collected for watering gardens. “Gray water” from kitchen and bathroom sinks is treated for irrigation and flushing. And “black water” from vacuum toilets, a bit like those on planes, is siphoned off to a local treatment plant where it is converted into biogas. The system reduces water use by 30 percent and generates electricity for 225 households and heat for 70.
This project demonstrates the pathways for water conservation in new buildings, but how we go about retrofitting existing homes and commercial buildings is much more complicated.
Dr. Upmanu Lall, the director of the Columbia Water Center at Columbia University, sees potential for alternative water systems in current attitudes toward other resources.
“It happened with lighting; most people have updated to LED technologies, and now the city gives incentives to phase out gas for cooking and heating,” he said, “People like to update their homes every 10 to 15 years; how can we leverage this cycle to transition to new water technologies? If we could get 10 percent of the population to update at a time, in 10 years we would have transitioned.”
The next question is how to reuse human “waste.” Agriculture depends on industrial fertilizers containing phosphorus from mined phosphate rock. The mineral is quickly depleted and often extracted in dangerous regions, but phosphorous can also be recovered from urine.
P-BANK is a demonstration public toilet inviting people to donate their urine for the recovery of phosphorous for reuse as garden fertilizer. According to P-BANK’s instigators, Bauhaus University Weimar in Germany, an average serving of urine (300 milliliters, or about 10 fluid ounces) contains about 200 milligrams of phosphorous, which is enough to grow three or four carrots. — at least theoretically. As of now, only Switzerland has approved the use of Aurin , a liquid fertilizer made from human urine.
In Kenya, the circular waste management systems provided by Sanivation, a nongovernmental organization, convert human waste to briquettes made from feces mixed with organic matter, called super logs, and used for fuel. Another nongovernmental organization, Washking, in Ghana, where the dearth of infrastructure means more people have a smartphone than a toilet, installs low-cost toilets fitted with systems that break down organic material to turn waste into compost.
Far from revolutionary, arguments about wet versus dry sanitation, the value of “waste” and the crisis of polluted waterways would have been familiar to sanitary reformers 150 years ago.
In the 19th century, according to Barbara Penner in her 2013 book “ Bathroom, ” urine harvesting and sewage farming were taken seriously.
“Government officials, sanitarians, scientists and entrepreneurs dreamed of finding a way to utilise human manure,” she writes. “Most were driven by a sincere horror of how water-closets polluted the rivers and wasted the ‘God-given’ nutrients in human excreta. Most also believed however that reusing sewage could be lucrative — not an unreasonable idea in an age when there was still a working organic economy.”
Fast forward to the 1970s. Environmentalists appalled like their predecessors by water pollution and intrigued by the potential of reclaiming nutrients from human waste added water and energy conservation to the agenda as the environmental cost of waterborne sewerage became apparent. Source books for alternative living like Whole Earth Catalog and Mother Earth News publicized a host of off-grid systems for autonomous lifestyles.
But if circular systems didn’t take off back then, what’s changed today? Will the urgencies of water scarcity and climate change overcome obstacles to allow water recycling to be a key part of future drinking water strategies? Although wastewater can be treated to make it safe for drinking, in many places regulations prohibit this because of safety concerns. Another formidable obstacle is public reluctance to drink recycled water. Or at least when people realize it is recycled.
Water-scarce Singapore already recycles all wastewater including sewage, which is served to the public under the innocuous-sounding brand name NEWater. Other water-tech marketeers are tackling the “yuck” factor straight on. Epic OneWater Brew is marketed as a beer made from gray water, a ploy to promote the environmental company Epic Cleantec’ s recycling strategies. But this is a gentle provocation compared to a Berlin brew made of treated sewage, an initiative both to support Xylem Water Solution’s technology and to demonstrate to the public that water reuse is a viable way to protect scarce resources.
There are signs the threshold might be shifting.
Franklin Antonio Hall Sparks Research Collaborations
The fruits of these team efforts are presented at the icra 2024 conference.
- Ioana Patringenaru - [email protected]
Published Date
Share this:, article content.
From improving tools for robot-assisted surgery to studying how humans prefer to interact with robots, collaborations across different departments at the Jacobs School of Engineering are in the spotlight in this year’s accepted papers for the 2024 IEEE International Conference on Robotics and Automation.
The interdisciplinary collaborations were partially ignited by the opening of Franklin Antonio Hall in 2022, which is home to various collaboratories bringing together research from across disciplines, including computer scientists, mechanical engineers and electrical engineers, into shared lab spaces.
ICRA brings together robotics researchers, students and industrial partners from around the world to discuss the latest innovations and breakthroughs, highlighting the role of robotics and automation in addressing global challenges.
Incidentally, the theme of this year’s ICRA conference is “CONNECT+”--reflecting the conference’s aim of helping to build relationships and potential collaborations with participants within and outside their field of study.
“This year’s papers accepted at ICRA from UC San Diego researchers show that the collaborative spaces housed in the new Franklin Antonio Hall are bearing fruit,” said Henrik Christensen, a professor in the Department of Computer Science and Engineering and Director of the Contextual Robotics Institute here at UC San Diego.
The following research will be presented at ICRA:
Robust Surgical Tool Tracking with Pixel-based Probabilities for Projected Geometric Primitives
A paper on improving tools for robot-assisted surgery would not have been possible without sharing the same building with electrical engineers, said Christopher D’Ambrosia, who earned his PhD in Christensen’s research group.
D’Ambrosia worked closely with the research group of Michael Yip, a professor in the UC San Diego Department of Electrical and Computer Engineering. Coauthors from Yip’s group on the paper were Florian Richter, Zih-Yun Ciu, Nikhil Shinde and Fei Liu.
Being in Franklin Antonio Hall allowed the researchers to hold spontaneous meetings, as well as scheduled group meetings and Q&A sessions. The PhD students could; share hardware, such as the DaVinci surgical robot platform; and work together at the various whiteboards available in the building.
“It 1000% would not have been possible to do this together without FAH,” D’Ambrosio said. “For example, one of us could easily pick up where someone else left off on a common workstation because we could easily shift our seats that day to the place where we were needed.
{/exp:typographee}
Robot Navigation in risky, crowded environments: understanding human preferences
Ph.D. students from the research groups of mechanical engineering professor Sonia Martinez and computer science professor Laurel Riek had been at work on a paper about how humans prefer to interact with robots in risky, crowded environments before their work shifted during the COVID 19 pandemic. Martinez and Riek both say the collaboration made them look forward to moving into Franklin Antonio Hall, which made it easier to work together.
Open X-Embodiment: Robotic Learning Datasets and RT-X Models (robotics-transformer-x.github.io)
Another collaboration stemming from being located in Franklin Antonio is the involvement of three UC San Diego engineering faculty members on a paper bringing together academic and industry researchers to develop a wide range of datasets for embodied AI and robotics. The three faculty members–Christensen, computer science professor Hao Su; and electrical and computer engineering Xiaolong Wang–used to be located in three different buildings. Moving into the same space at Franklin Antonio Hall allowed them to dramatically increase their collaborations, Christensen said.
Also of note, the lead author of the paper, Quan Vuong, is a former PhD student in Christensen’s research group here at UC San Diego.
In addition to these rich collaborations, Franklin Antonio Hall continues to be a center of innovative work in a wide range of robotics-related fields, as illustrated by these additional ICRA 2024 papers:
Nikolai Atanasov Optimal Scene Graph Planning with Large Language Model Guidance : Hamiltonian Dynamics Learning from Point Cloud Observations for Nonholonomic Mobile Robot Control website: https://altwaitan.github.io/DLFO/
Hao Su EasyHeC (ootts.github.io)
Tania Morimoto Hapstick: a soft flexible joystick for stiffness rendering via fiber jamming
Xiaolong Wang Robot Synesthesia: In-hand Manipulation with Visuotactile Sensing Sim2Real Manipulation on Unknown Objects with Tactile-based reinforcement learning
Michael Yip Tracking Snake-like Robots in the Wild Using Only a Single Camera
Learn more about research and education at UC San Diego in: Artificial Intelligence
You May Also Like
Metabolism of autism reveals developmental origins, a new guide to success, during peak of covid-19 some lacked access to safe water and lavatories, stay in the know.
Keep up with all the latest from UC San Diego. Subscribe to the newsletter today.
You have been successfully subscribed to the UC San Diego Today Newsletter.
Campus & Community
Arts & culture, visual storytelling.
- Media Resources & Contacts
Signup to get the latest UC San Diego newsletters delivered to your inbox.
Award-winning publication highlighting the distinction, prestige and global impact of UC San Diego.
Popular Searches: Covid-19 Ukraine Campus & Community Arts & Culture Voices
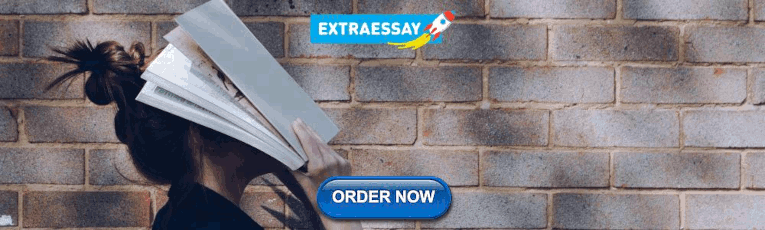
IMAGES
VIDEO
COMMENTS
This review paper focuses on the various types of Filtration techniques. available and whic h tec hnique is most suitable for which t ype of region. W e. will study the t ypes of natural ...
In a News & Views in June 2023 4 we highlighted a paper studying the fundamental aspects of water transport in reverse osmosis membranes 5. Among the papers we published, a clear example is the ...
This study, based in San Bernardino County, Southern California, collected and examined tap water samples within the area to explore the feasibility of adopting non-industrial equipment and methods to reduce water hardness and total dissolved solids(TDS). We investigated how water quality could be improved by utilizing water boiling, activated carbon and sodium bicarbonate additives, as well ...
In 2019, about 6% of public water utilities in the U.S. had a health-based violation. Due to the high risk of exposure to various contaminants in drinking water, point-of-use (POU) drinking water ...
The aim of this study was to find out whether domestic water purification systems could eliminate the essential materials such as fluoride besides filtrating the heavy ions and other unwanted particles out of water. Materials and Method. In this study, 6 frequently used commercial brands of water purifiers in Ahwaz were compared. ...
PRACTICAL APPLICATION VALUE OF SUSTAINABLE WATER PURIFICATION MATERIALS. The sustainability of water purification processes has long been an ongoing concern for researchers. However, the road to a large-scale application is long and challenging. Among currently available methods, membrane separation appears to have the greatest potential, as ...
This requires investment in water purification technologies. World Water Day offers an opportunity to discuss whether such investment will help achieve this laudable goal. ... Chemical Research in ...
Dec.15, 2021. In this paper, researchers surveyed both conventional and advanced disinfection processes in the U.S., testing the quality of their drinking waters. Treatment plants with advanced removal technologies, such as activated carbon, formed fewer types and lower levels of harmful disinfection byproducts (known as DBPs) in their water.
The area of water purification has been one of the most dynamic research fields in recent years with strong public policy implications with over 39,000 papers. Similarly, the areas of nanomaterials and nanoprocesses have been one of the most dynamic research fields in recent years with significant public policy implications with over 1,000,000 ...
Abstract. There are number of water purification techniques but the adsorption is one of the most simplest, effective and economical method for wastewater purification. In this article a large number of solid adsorbents such as Natural adsorbents, Agricultural Wastes, Industrial wastes, Biomass, Nanoadsorbents: Carbon based nanomaterials, Nobel ...
Sustainable nanotechnology has made substantial contributions in providing contaminant-free water to humanity. In this Review, we present the compelling need for providing access to clean water through nanotechnology-enabled solutions and the large disparities in ensuring their implementation. We also discuss the current nanotechnology frontiers in diverse areas of the clean water space with ...
Clean water scarcity has threatened the human health, and sustainability of the environment and ecosystems (Gleick and Cooley, 2021; Zhao et al., 2021).Recent studies showed that around four billion people forming two-thirds of the global population live under aggressive water scarcity conditions for at least one month of the year (Mekonnen and Hoekstra, 2016).
Nanotechnology is an easy and practical approach to clean waste water by using different methods. Different types of bacteria, toxic chemicals like arsenic, mercury etc., and sediments can be removed by using nanotechnology. Nanomaterial based devices are being used for water purification. Nano filtration method has advantages over other ...
In drinking water treatment, filtration plays an important role in the multi-barrier approach employed for the removal of pathogens. The presence of suspended solids and other particulate matter in water increases the resistance of most microbes to disinfection. Therefore, high performance in the removal of particles achieved by granular filtration can increase the disinfection efficiency ...
This paper critically reviews the growth and achievement in organic and inorganic membrane studies for RO and NF procedures. The review will start by introducing the synthesis method and structural properties of recent RO and NF membranes, followed by discussing and comparing water purification performance of representative RO and NF membranes made from organic and inorganic materials.
The need for research into water purification is pressing. In an extensive Review Article, Mark Shannon et al. highlight the developing technologies that — it is hoped — can provide our ...
Water sources such as lakes, rivers, and ground water supply much of the water for domestic use. Some of the water that reaches our household faucets has also been used for other purposes. Water from these various sources is treated to remove impurities and to make it suitable for human consumption. Several steps can form the treatment process. Large items and particles can be filtered out ...
Furthermore, considering the importance of drinking water at the regional and global level, there is a need for an updated study to identify new trends in water treatment research based on information provided by Scopus and WoS, two of the main multidisciplinary academic databases worldwide (Visser et al., Citation 2021>).
The first step in the process of wastewater treatment is usually screening, which removes the solid waste present in wastewater, such as pieces of cloth, hair, paper, wood, cork, kitchen solids, fecal matter, and the like. Screens are selected on the basis of the size of the solid waste present in the water. 7.5.1.2.
The availability of energy and water sources is basic and indispensable for the life of modernistic humans. Because of this importance, the interrelationship between energy derived from renewable energy sources and water desalination technologies has achieved great interest recently. So this paper reviews the photovoltaic (PV) system-powered desalination technologies as stand-alone systems or ...
International Journal of Research and Innovation in Social Science, 2024, vol. 8, issue 4, 1115-1120 Abstract: This research explores the development of a novel water filtration system. Using a mix of online and direct surveys, the study reveals strong support for addressing water supply challenges in specific areas of the campus.
Reusing reclaimed water is of paramount importance to achieve the 2030 Agenda for Sustainable Development Goals 6 and 13. In Europe, a recent Regulation set minimum requirements for water reuse in agriculture. However, some challenges remain considering microbial risks and their prevention. In this study, two urban wastewater treatment plants (WWTPs) were investigated from the perspective of ...
DOI: 10.1002/awwa.2142 Corpus ID: 261392376; How Data Gaps in Rulemaking Affect Water Affordability @article{Berahzer2023HowDG, title={How Data Gaps in Rulemaking Affect Water Affordability}, author={Stacey Isaac Berahzer and Janet Clements and Jonathan Betts and Claire Sheridan}, journal={Journal ‐ American Water Works Association}, year={2023}, volume={115}, url={https://api ...
The results of this study will provide options for residents and water treatment plants to find ways to maintain the general taste of the tap water, but also preserve the lifespan of accessories and pipelines. ... Water Research, 2002, 36 (14); pp. 3647-3653, doi: 10.1016/s0043-1354(02)00049- [Google Scholar] 25. Consumers ...
Drinking water treatment sludge (DWTS) is a growing problem worldwide that is typically buried in landfills. This study thus proposes the use of calcined WTS as a novel supplemental cementitious material, based on an analysis of the effects of adding it to Portland cement composites. Experimental research was done on using WTS to partially replace cement at 0%, 5%, 10%, and 15% by weight.
Water Research publishes refereed, original research papers on all aspects of the science and technology of the anthropogenic water cycle, water quality, and its management worldwide. A broad outline of the journal's scope includes: •Treatment processes for water and wastewaters (municipal, agricultural, industrial, and on-site treatment ...
Jane Withers is a design writer and curator in London who specializes in water issues. She curated the exhibition "Water Pressure: Designing for the Future," on view at MK&G, the museum of art ...
Robot Navigation in risky, crowded environments: understanding human preferences. Ph.D. students from the research groups of mechanical engineering professor Sonia Martinez and computer science professor Laurel Riek had been at work on a paper about how humans prefer to interact with robots in risky, crowded environments before their work shifted during the COVID 19 pandemic.