
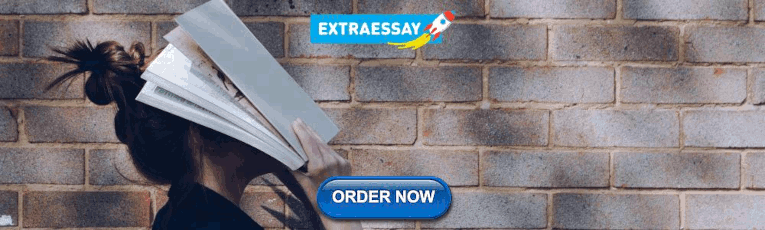
The Impact of Sleep on Learning and Memory
By Kelly Cappello, B.A.
For many students, staying awake all night to study is common practice. According to Medical News Today , around 20 percent of students pull all-nighters at least once a month, and about 35 percent stay up past three in the morning once or more weekly.
That being said, staying up all night to study is one of the worst things students can do for their grades. In October of 2019, two MIT professors found a correlation between sleep and test scores : The less students slept during the semester, the worse their scores.
So, why is it that sleep is so important for test scores? While the answer seems simple, that students simply perform better when they’re not mentally or physically tired, the truth may be far more complicated and interesting.
In the last 20 years, scientists have found that sleep impacts more than just students’ ability to perform well; it improves their ability to learn, memorize, retain, recall, and use their new knowledge to solve problems creatively. All of which contribute to better test scores.
Let’s take a look at some of the most interesting research regarding the impact of sleep on learning and memory.
How does sleep improve the ability to learn?
When learning facts and information, most of what we learn is temporarily stored in a region of the brain called the hippocampus. Some scientists hypothesize that , like most storage centers, the hippocampus has limited storage capacity. This means, if the hippocampus is full, and we try to learn more information, we won’t be able to.
Fortunately, many scientists also hypothesize that sleep, particularly Stages 2 and 3 sleep, plays a role in replenishing our ability to learn. In one study, a group of 44 participants underwent two rigorous sessions of learning, once at noon and again at 6:00 PM. Half of the group was allowed to nap between sessions, while the other half took part in standard activities. The researchers found that the group that napped between learning sessions learned just as easily at 6:00 PM as they did at noon. The group that didn’t nap, however, experienced a significant decrease in learning ability [1].
How does sleep improve the ability to recall information?
Humans have known about the benefits of sleep for memory recall for thousands of years. In fact, the first record of this revelation is from the first century AD. Rhetorician Quintilian stated, “It is a curious fact, of which the reason is not obvious, that the interval of a single night will greatly increase the strength of the memory.”
In the last century, scientists have tested this theory many times, often finding that sleep improves memory retention and recall by between 20 and 40 percent. Recent research has led scientists to hypothesize that Stage 3 (deep non-Rapid Eye Movement sleep, or Slow Wave Sleep) may be especially important for the improvement of memory retention and recall [2].
How does sleep improve long-term memory?
Scientists hypothesize that sleep also plays a major role in forming long-term memories. According to Matthew Walker, professor of neuroscience and psychology at UC Berkeley, MRI scans indicate that the slow brain waves of stage 3 sleep (deep NREM sleep) “serve as a courier service,” transporting memories from the hippocampus to other more permanent storage sites [3].
How does sleep improve the ability to solve problems creatively?
Many tests are designed to assess critical thinking and creative problem-solving skills. Recent research has led scientists to hypothesize that sleep, particularly REM sleep, plays a role in strengthening these skills. In one study, scientists tested the effect of REM sleep on the ability to solve anagram puzzles (word scrambles like “EOUSM” for “MOUSE”), an ability that requires strong creative thinking and problem-solving skills.
In the study, participants solved a couple of anagram puzzles before going to sleep in a sleep laboratory with electrodes placed on their heads. The subjects were woken up four times during the night to solve anagram puzzles, twice during NREM sleep and twice during REM sleep.
The researchers found that when participants were woken up during REM sleep, they could solve 15 to 35 percent more puzzles than they could when woken up from NREM sleep. They also performed 15 to 35 percent better than they did in the middle of the day [4]. It seems that REM sleep may play a major role in improving the ability to solve complex problems.
So, what’s the point?
Sleep research from the last 20 years indicates that sleep does more than simply give students the energy they need to study and perform well on tests. Sleep actually helps students learn, memorize, retain, recall, and use their new knowledge to come up with creative and innovative solutions.
It’s no surprise that the MIT study previously mentioned revealed no improvement in scores for those who only prioritized their sleep the night before a big test. In fact, the MIT researchers concluded that if students want to see an improvement in their test scores, they have to prioritize their sleep during the entire learning process. Staying up late to study just doesn’t pay off.
Interested in learning more about the impact of sleep on learning and memory? Check out this Student Sleep Guide .
Author Biography
Kelly Cappello graduated from East Stroudsburg University of Pennsylvania with a B.A. in Interdisciplinary Studies in 2015. She is now a writer, specialized in researching complex topics and writing about them in simple English. She currently writes for Recharge.Energy , a company dedicated to helping the public improve their sleep and improve their lives.
- Mander, Bryce A., et al. “Wake Deterioration and Sleep Restoration of Human Learning.” Current Biology, vol. 21, no. 5, 2011, doi:10.1016/j.cub.2011.01.019.
- Walker M. P. (2009). The role of slow wave sleep in memory processing. Journal of clinical sleep medicine : JCSM : official publication of the American Academy of Sleep Medicine, 5(2 Suppl), S20–S26.
- Walker, Matthew. Why We Sleep. Scribner, 2017.
- Walker, Matthew P, et al. “Cognitive Flexibility across the Sleep–Wake Cycle: REM-Sleep Enhancement of Anagram Problem Solving.” Cognitive Brain Research, vol. 14, no. 3, 2002, pp. 317–324., doi:10.1016/s0926-6410(02)00134-9.
Posted on Dec 21, 2020 | Tagged: learning and memory
© The Trustees of the University of Pennsylvania | Site best viewed in a supported browser . | Report Accessibility Issues and Get Help | Privacy Policy | Site Design: PMACS Web Team. | Sitemap
Featured Topics
Featured series.
A series of random questions answered by Harvard experts.
Explore the Gazette
Read the latest.
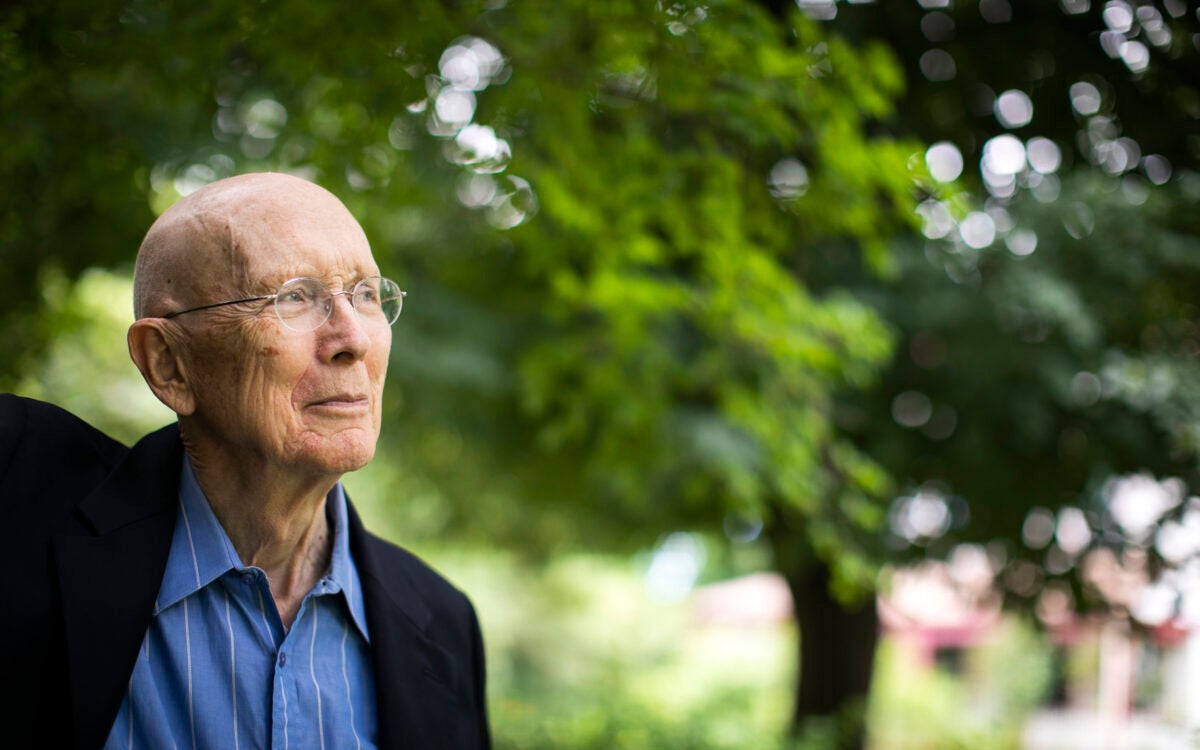
‘The scientist is not in the business of following instructions.’
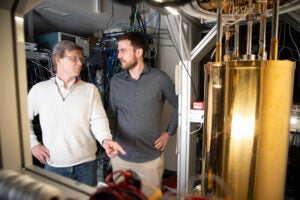
Glimpse of next-generation internet
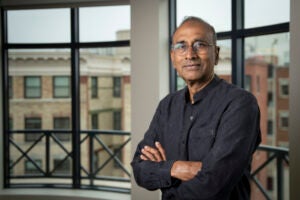
Science is making anti-aging progress. But do we want to live forever?
Brain practices new tasks while we sleep.
MGH News and Public Affairs
Scientists believe it plays a role in how we learn and form long-term memories
Why do we sleep? Scientists have debated this question for millennia, but a new study adds fresh clues for solving this mystery.
The findings, published in the Journal of Neuroscience, may help explain how humans form memories and learn, and could eventually aid the development of assistive tools for people affected by neurologic disease or injury. The study was conducted by Massachusetts General Hospital in collaboration with colleagues at Brown University, the Department of Veterans Affairs, and several other institutions.
Scientists studying laboratory animals long ago discovered a phenomenon known as “replay” that occurs during sleep, explains neurologist Daniel Rubin of the MGH Center for Neurotechnology and Neurorecovery, the lead author of the study. Replay is theorized to be a strategy the brain uses to remember new information. If a mouse is trained to find its way through a maze, monitoring devices can show that a specific pattern of brain cells, or neurons, will light up as it traverses the correct route. “Then, later on while the animal is sleeping, you can see that those neurons will fire again in that same order,” says Rubin.
Scientists believe that this replay of neuronal firing during sleep is how the brain practices newly learned information, which allows a memory to be consolidated — that is, converted from a short-term memory to a long-term one.
However, replay has only been convincingly shown in lab animals. “There’s been an open question in the neuroscience community: To what extent is this model for how we learn things true in humans? And is it true for different kinds of learning?” asks neurologist Sydney S. Cash, co-director of the Center for Neurotechnology and Neurorecovery at MGH and co-senior author of the study. Importantly, says Cash, understanding whether replay occurs with the learning of motor skills could help guide the development of new therapies and tools for people with neurologic diseases and injuries.
To study whether replay occurs in the human motor cortex — the brain region that governs movement — Rubin, Cash, and their colleagues enlisted a 36-year-old man with tetraplegia (also called quadriplegia), meaning he is unable to move his upper and lower limbs, in his case due to a spinal cord injury. The man, identified in the study as T11, is a participant in a clinical trial of a brain-computer interface device that allows him to use a computer cursor and keyboard on a screen. The investigational device is being developed by the BrainGate consortium, a collaborative effort involving clinicians, neuroscientists, and engineers at several institutions with the goal of creating technologies to restore communication, mobility, and independence for people with neurologic disease, injury, or limb loss. The consortium is directed by Leigh R. Hochberg of MGH, Brown University, and the Department of Veterans Affairs.
In the study, T11 was asked to perform a memory task similar to the electronic game Simon, in which a player observes a pattern of flashing colored lights, then has to recall and reproduce that sequence. He controlled the cursor on the computer screen simply by thinking about the movement of his own hand. Sensors implanted in T11’s motor cortex measured patterns of neuronal firing, which reflected his intended hand movement, allowing him to move the cursor around on the screen and click it at his desired locations. These brain signals were recorded and wirelessly transmitted to a computer.
That night, while T11 slept at home, activity in his motor cortex was recorded and wirelessly transmitted to a computer. “What we found was pretty incredible,” says Rubin. “He was basically playing the game overnight in his sleep.” On several occasions, says Rubin, T11’s patterns of neuronal firing during sleep exactly matched patterns that occurred while he performed the memory-matching game earlier that day.
“This is the most direct evidence of replay from motor cortex that’s ever been seen during sleep in humans,” says Rubin. Most of the replay detected in the study occurred during slow-wave sleep, a phase of deep slumber. Interestingly, replay was much less likely to be detected while T11 was in REM sleep, the phase most commonly associated with dreaming. Rubin and Cash see this work as a foundation for learning more about replay and its role in learning and memory in humans.
“Our hope is that we can leverage this information to help build better brain-computer interfaces and come up with paradigms that help people learn more quickly and efficiently in order to regain control after an injury,” says Cash, noting the significance of moving this line of inquiry from animals to human subjects. “This kind of research benefits enormously from the close interaction we have with our participants,” he adds, with gratitude to T11 and other participants in the BrainGate clinical trial.
Hochberg concurs. “Our incredible BrainGate participants provide not only helpful feedback toward the creation of a system to restore communication and mobility, but they also give us the rare opportunity to advance fundamental human neuroscience — to understand how the human brain works at the level of circuits of individual neurons,” he says, “and to use that information to build next-generation restorative neurotechnologies.”
Rubin is also an instructor in neurology at Harvard Medical School. Cash is an associate professor of neurology at HMS. Hochberg is a senior lecturer on neurology at HMS and professor of engineering at Brown University.
This work was supported by the Department of Veterans Affairs, the National Institute of Neurologic Disease and Stroke, the National Institute of Mental Health, Conquer Paralysis Now, the MGH-Deane Institute, the American Academy of Neurology, and the Howard Hughes Medical Institute at Stanford University.
Share this article
You might like.
George Whitesides became a giant of chemistry by keeping it simple
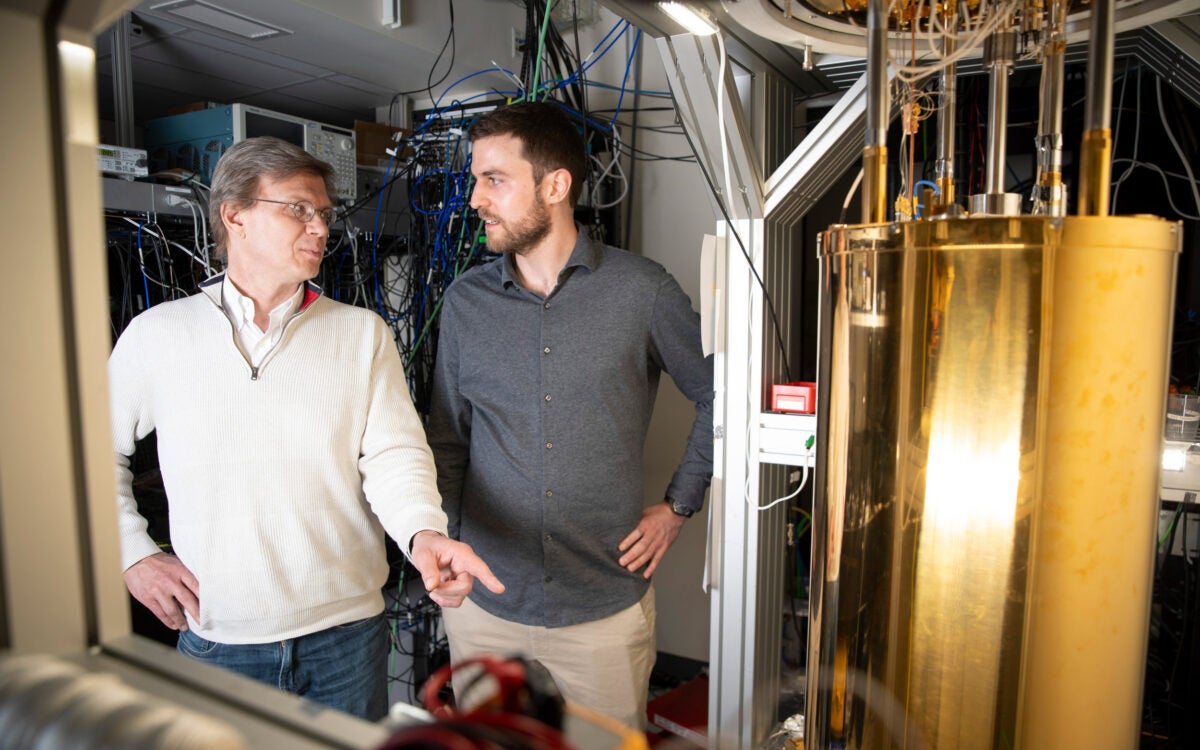
Physicists demo first metro-area quantum computer network in Boston
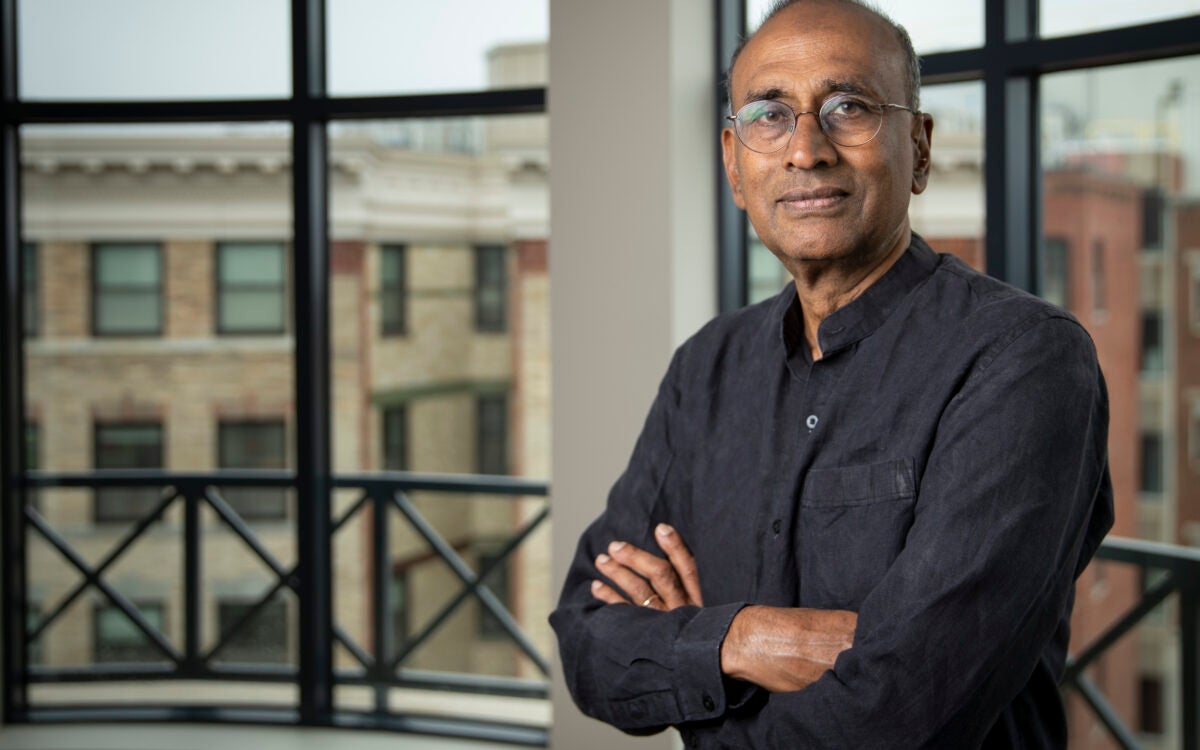
Nobel laureate details new book, which surveys research, touches on larger philosophical questions
Epic science inside a cubic millimeter of brain
Researchers publish largest-ever dataset of neural connections
Finding right mix on campus speech policies
Legal, political scholars discuss balancing personal safety, constitutional rights, academic freedom amid roiling protests, cultural shifts
Good genes are nice, but joy is better
Harvard study, almost 80 years old, has proved that embracing community helps us live longer, and be happier
- Frontiers in Sleep
- Sleep, Behavior and Mental Health
- Research Topics
The Role of Sleep in Learning and Memory
Total Downloads
Total Views and Downloads
About this Research Topic
Sleep is generally believed to prepare our brains for new learning and consolidate recently acquired memories. However, whether sleep is both necessary and sufficient for learning and memory remains a hotly debated empirical question. Advances in the basic, cognitive and computational neurosciences have ...
Keywords : Sleep, Learning, Memory, Consolidation, Polysomnography, EEG, fMRI
Important Note : All contributions to this Research Topic must be within the scope of the section and journal to which they are submitted, as defined in their mission statements. Frontiers reserves the right to guide an out-of-scope manuscript to a more suitable section or journal at any stage of peer review.
Topic Editors
Topic coordinators, recent articles, submission deadlines.
Submission closed.
Participating Journals
Total views.
- Demographics
No records found
total views article views downloads topic views
Top countries
Top referring sites, about frontiers research topics.
With their unique mixes of varied contributions from Original Research to Review Articles, Research Topics unify the most influential researchers, the latest key findings and historical advances in a hot research area! Find out more on how to host your own Frontiers Research Topic or contribute to one as an author.
Thank you for visiting nature.com. You are using a browser version with limited support for CSS. To obtain the best experience, we recommend you use a more up to date browser (or turn off compatibility mode in Internet Explorer). In the meantime, to ensure continued support, we are displaying the site without styles and JavaScript.
- View all journals
- Explore content
- About the journal
- Publish with us
- Sign up for alerts
- Perspective
- Published: 22 December 2023
Optimizing the methodology of human sleep and memory research
- Dezső Németh ORCID: orcid.org/0000-0002-9629-5856 1 , 2 , 3 na1 ,
- Emilie Gerbier 4 na1 ,
- Jan Born 5 ,
- Timothy Rickard 6 ,
- Susanne Diekelmann 5 , 7 ,
- Stuart Fogel ORCID: orcid.org/0000-0002-3227-5370 8 ,
- Lisa Genzel 9 ,
- Alexander Prehn-Kristensen 10 , 11 ,
- Jessica Payne 12 ,
- Martin Dresler ORCID: orcid.org/0000-0001-7441-3818 13 ,
- Peter Simor 2 , 14 ,
- Stephanie Mazza 15 ,
- Kerstin Hoedlmoser 16 ,
- Perrine Ruby 1 ,
- Rebecca M. C. Spencer 17 ,
- Genevieve Albouy 18 ,
- Teodóra Vékony 1 ,
- Manuel Schabus ORCID: orcid.org/0000-0001-5899-8772 16 &
- Karolina Janacsek 2 , 19 na1
Nature Reviews Psychology volume 3 , pages 123–137 ( 2024 ) Cite this article
1126 Accesses
15 Altmetric
Metrics details
- Human behaviour
- Learning and memory
- Long-term memory
Understanding the complex relationship between sleep and memory consolidation is a major challenge in cognitive neuroscience and psychology. Many studies suggest that sleep triggers off-line memory processes, resulting in less forgetting of declarative memory and performance stabilization in non-declarative memory. However, the role of sleep in human memory consolidation is still under considerable debate, and numerous contradictory and non-replicable findings have been reported. Methodological issues related to experimental designs, task characteristics and measurements, and data-analysis practices all influence the effects that are observed and their interpretation. In this Perspective, we review methodological issues in sleep and memory studies and suggest constructive solutions to address them. We believe that implementing these solutions in future sleep and memory research will substantially advance the field and improve understanding of the specific role of sleep in memory consolidation.
This is a preview of subscription content, access via your institution
Access options
Subscribe to this journal
Receive 12 digital issues and online access to articles
55,14 € per year
only 4,60 € per issue
Buy this article
- Purchase on Springer Link
- Instant access to full article PDF
Prices may be subject to local taxes which are calculated during checkout
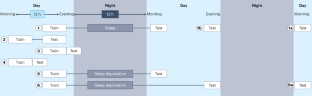
Similar content being viewed by others
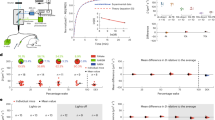
Brain clearance is reduced during sleep and anesthesia
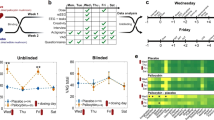
Microdosing with psilocybin mushrooms: a double-blind placebo-controlled study
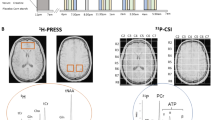
Single dose creatine improves cognitive performance and induces changes in cerebral high energy phosphates during sleep deprivation
Palagini, L. & Rosenlicht, N. Sleep, dreaming, and mental health: a review of historical and neurobiological perspectives. Sleep. Med. Rev. 15 , 179–186 (2011).
Article PubMed Google Scholar
Simor, P., Sifuentes-Ortega, R. & Peigneux, P. in Sleep Medicine Textbook (eds Bassetti, C., McNicholas, W., Paunio, T. & Peigneux, P.) Ch. A.5 (European Sleep Research Society, 2021).
Walker, M. P. The role of sleep in cognition and emotion. Ann. NY Acad. Sci. 1156 , 168–197 (2009).
Article PubMed ADS Google Scholar
Shochat, T. Impact of lifestyle and technology developments on sleep. Nat. Sci. Sleep 4 , 19–31 (2012).
Article PubMed PubMed Central Google Scholar
Bixler, E. Sleep and society: an epidemiological perspective. Sleep. Med. 10 , S3 (2009).
Matricciani, L. et al. Past, present, and future: trends in sleep duration and implications for public health. Sleep. Health 3 , 317–323 (2017).
Hale, L. & Guan, S. Screen time and sleep among school-aged children and adolescents: a systematic literature review. Sleep. Med. Rev. 21 , 50–58 (2015).
Ackermann, S. & Rasch, B. Differential effects of non-REM and REM sleep on memory consolidation? Curr. Neurol. Neurosci. Rep. 14 , 430 (2014).
Antony, J. W., Schönauer, M., Staresina, B. P. & Cairney, S. A. Sleep spindles and memory reprocessing. Trends Neurosci. 42 , 1–3 (2019).
Article CAS PubMed Google Scholar
Boyce, R., Williams, S. & Adamantidis, A. REM sleep and memory. Curr. Opin. Neurobiol. 44 , 167–177 (2017).
Diekelmann, S. & Born, J. The memory function of sleep. Nat. Rev. Neurosci. 11 , 114–126 (2010).
Diekelmann, S., Wilhelm, I. & Born, J. The whats and whens of sleep-dependent memory consolidation. Sleep. Med. Rev. 13 , 309–321 (2009).
Feld, G. B. & Born, J. Sculpting memory during sleep: concurrent consolidation and forgetting. Curr. Opin. Neurobiol. 44 , 20–27 (2017).
Lewis, P. A. & Durrant, S. J. Overlapping memory replay during sleep builds cognitive schemata. Trends Cogn. Sci. 15 , 343–351 (2011).
Mednick, S. C., Cai, D. J., Shuman, T., Anagnostaras, S. & Wixted, J. T. An opportunistic theory of cellular and systems consolidation. Trends Neurosci. 34 , 504–514 (2011).
Article CAS PubMed PubMed Central Google Scholar
Saletin, J. M. & Walker, M. P. Nocturnal mnemonics: sleep and hippocampal memory processing. Front. Neurol. 3 , 57 (2012).
Article Google Scholar
Siegel, D. J. Memory: an overview, with emphasis on developmental, interpersonal, and neurobiological aspects. J. Am. Acad. Child. Adolesc. Psychiat. 40 , 997–1011 (2001).
Article CAS Google Scholar
Stickgold, R. & Walker, M. P. Memory consolidation and reconsolidation: what is the role of sleep? Trends Neurosci. 28 , 408–415 (2005).
Stickgold, R. & Walker, M. P. Sleep-dependent memory triage: evolving generalization through selective processing. Nat. Neurosci. 16 , 139–145 (2013).
Tononi, G. & Cirelli, C. Sleep function and synaptic homeostasis. Sleep. Med. Rev. 10 , 49–62 (2006).
Tononi, G. & Cirelli, C. Sleep and the price of plasticity: from synaptic and cellular homeostasis to memory consolidation and integration. Neuron 81 , 12–34 (2014).
Walker, M. P. A refined model of sleep and the time course of memory formation. Behav. Brain Sci. 28 , 51–64 (2005).
Schönauer, M., Grätsch, M. & Gais, S. Evidence for two distinct sleep-related long-term memory consolidation processes. Cortex 63 , 68–78 (2015).
Plihal, W. & Born, J. Effects of early and late nocturnal sleep on declarative and procedural memory. J. Cogn. Neurosci. 9 , 534–547 (1997).
Feld, G. B., Weis, P. P. & Born, J. The limited capacity of sleep-dependent memory consolidation. Front. Psychol. 7 , 204960 (2016).
Diekelmann, S., Born, J. & Wagner, U. Sleep enhances false memories depending on general memory performance. Behav. Brain Res. 208 , 425–429 (2010).
Abel, M. & Bäuml, K. H. T. Retrieval-induced forgetting, delay, and sleep. Memory 20 , 420–428 (2012).
Payne, J. D. et al. Napping and the selective consolidation of negative aspects of scenes. Emotion 15 , 176–186 (2015).
Cairney, S. A., Durrant, S. J., Power, R. & Lewis, P. A. Complementary roles of slow-wave sleep and rapid eye movement sleep in emotional memory consolidation. Cereb. Cortex 25 , 1565–1575 (2015).
Rasch, B., Büchel, C., Gais, S. & Born, J. Odor cues during slow-wave sleep prompt declarative memory consolidation. Science 315 , 1426–1429 (2007).
Article CAS PubMed ADS Google Scholar
Rudoy, J. D., Voss, J. L., Westerberg, C. E. & Paller, K. A. Strengthening individual memories by reactivating them during sleep. Science 326 , 1079 (2009).
Article CAS PubMed PubMed Central ADS Google Scholar
Gais, S., Lucas, B. & Born, J. Sleep after learning aids memory recall. Learn. Mem. 13 , 259–262 (2006).
Walker, M. P., Brakefield, T., Hobson, J. A. & Stickgold, R. Dissociable stages of human memory consolidation and reconsolidation. Nature 425 , 616–620 (2003).
King, B. R., Hoedlmoser, K., Hirschauer, F., Dolfen, N. & Albouy, G. Sleeping on the motor engram: the multifaceted nature of sleep-related motor memory consolidation. Neurosci. Biobehav. Rev. 80 , 1–22 (2017).
Rasch, B. & Born, J. About sleep’s role in memory. Physiol. Rev. 93 , 681–766 (2013).
Fischer, S., Hallschmid, M., Elsner, A. L. & Born, J. Sleep forms memory for finger skills. Proc. Natl Acad. Sci. USA 99 , 11987–11991 (2002).
Born, J. & Wilhelm, I. System consolidation of memory during sleep. Psychol. Res. 76 , 192–203 (2012).
Vorster, A. P. & Born, J. Sleep and memory in mammals, birds and invertebrates. Neurosci. Biobehav. Rev. 50 , 103–119 (2015).
Pan, S. C. & Rickard, T. C. Sleep and motor learning: is there room for consolidation? Psychol. Bull. 141 , 812–834 (2015).
Mantua, J. Sleep physiology correlations and human memory consolidation: where do we go from here? Sleep 41 , zsx204 (2018).
Vertes, R. P. & Siegel, J. M. Time for the sleep community to take a critical look at the purported role of sleep in memory processing. Sleep 28 , 1228–1229 (2005).
Dastgheib, M., Kulanayagam, A. & Dringenberg, H. C. Is the role of sleep in memory consolidation overrated? Neurosci. Biobehav. Rev. 140 , 104799 (2022).
Cordi, M. J. & Rasch, B. How robust are sleep-mediated memory benefits? Curr. Opin. Neurobiol. 67 , 1–7 (2021).
Voderholzer, U. et al. Sleep restriction over several days does not affect long-term recall of declarative and procedural memories in adolescents. Sleep Med. 12 , 170–178 (2011).
Payne, J. D. et al. The role of sleep in false memory formation. Neurobiol. Learn. Mem. 92 , 327–334 (2009).
Ujma, P. P. Meta-analytic evidence suggests no correlation between sleep spindles and memory. Preprint at PsyArXiv https://doi.org/10.31234/osf.io/hjdex (2022).
Krakauer, J. W., Ghazanfar, A. A., Gomez-Marin, A., MacIver, M. A. & Poeppel, D. Neuroscience needs behavior: correcting a reductionist bias. Neuron 93 , 480–490 (2017).
Niv, Y. The primacy of behavioral research for understanding the brain. Behav. Neurosci. 135 , 601–609 (2021).
Peigneux, P., Fogel, S. & Smith, C. Memory processing in relation to sleep. Princip. Pract. Sleep Med . https://doi.org/10.1016/b978-0-323-24288-2.00022-2 (2017).
Schmidt, C., Collette, F., Cajochen, C. & Peigneux, P. A time to think: circadian rhythms in human cognition. Cogn. Neuropsychol. 24 , 755–789 (2007).
Barner, C., Schmid, S. R. & Diekelmann, S. Time-of-day effects on prospective memory. Behav. Brain Res. 376 , 112179 (2019).
Scullin, M. K. & McDaniel, M. A. Remembering to execute a goal: sleep on it! Psychol. Sci. 21 , 1028–1035 (2010).
Keisler, A., Ashe, J. & Willingham, D. T. Time of day accounts for overnight improvement in sequence learning. Learn. Mem. 14 , 669–672 (2007).
Taillard, J., Gronfier, C., Bioulac, S., Philip, P. & Sagaspe, P. Sleep in normal aging, homeostatic and circadian regulation and vulnerability to sleep deprivation. Brain Sci. 11 , 1003 (2021).
Tucker, M. A. et al. The relative impact of sleep and circadian drive on motor skill acquisition and memory consolidation. Sleep 40 , zsx096 (2017).
Payne, J. D., Ellenbogen, J. M., Walker, M. P. & Stickgold, R. in Learning and Memory: Comprehensive Reference 1st edn, Vol. 2 (eds Byrne, J. H.) 663–685 (Academic, 2008).
Dresler, M. et al. Neuroscience-driven discovery and development of sleep therapeutics. Pharmacol. Ther. 141 , 300–334 (2014).
Fenn, K. M., Nusbaum, H. C. & Margoliash, D. Consolidation during sleep of perceptual learning of spoken language. Nature 425 , 614–616 (2003).
Hallgató, E., Gyori-Dani, D., Pekár, J., Janacsek, K. & Nemeth, D. The differential consolidation of perceptual and motor learning in skill acquisition. Cortex 49 , 1073–1081 (2013).
Talamini, L. M., Nieuwenhuis, I. L. C., Takashima, A. & Jensen, O. Sleep directly following learning benefits consolidation of spatial associative memory. Learn. Mem. 15 , 233–237 (2008).
Tucker, M., McKinley, S. & Stickgold, R. Sleep optimizes motor skill in older adults. J. Am. Geriatr. Soc. 59 , 603–609 (2011).
Gais, S., Rasch, B., Dahmen, J. C., Sara, S. & Born, J. The memory function of noradrenergic activity in non-REM sleep. J. Cogn. Neurosci. 23 , 2582–2592 (2011).
Csabi, E., Varszegi-Schulz, M., Janacsek, K., Malecek, N. & Nemeth, D. The consolidation of implicit sequence memory in obstructive sleep apnea. PLoS One 9 , e109010 (2014).
Article PubMed PubMed Central ADS Google Scholar
Backhaus, J. et al. Impaired declarative memory consolidation during sleep in patients with primary insomnia: influence of sleep architecture and nocturnal cortisol release. Biol. Psychiat. 60 , 1324–1330 (2006).
Ahuja, S. et al. Role of normal sleep and sleep apnea in human memory processing. Nat. Sci. Sleep. 10 , 255–269 (2018).
Cellini, N. Memory consolidation in sleep disorders. Sleep. Med. Rev. 35 , 101–112 (2017).
Wallace, A. & Bucks, R. S. Memory and obstructive sleep apnea: a meta-analysis. Sleep 36 , 203–220 (2013).
PubMed PubMed Central Google Scholar
Csábi, E., Benedek, P., Janacsek, K., Katona, G. & Nemeth, D. Sleep disorder in childhood impairs declarative but not nondeclarative forms of learning. J. Clin. Exp. Neuropsychol. 35 , 677–685 (2013).
Rosenzweig, I. et al. Sleep apnoea and the brain: a complex relationship. Lancet Respir. Med. 3 , 404–414 (2015).
Wulff, K., Gatti, S., Wettstein, J. G. & Foster, R. G. Sleep and circadian rhythm disruption in psychiatric and neurodegenerative disease. Nat. Rev. Neurosci. 11 , 589–599 (2010).
Li, J., Vitiello, M. V. & Gooneratne, N. S. Sleep in normal aging. Sleep. Med. Clin. 13 , 1–11 (2018).
Bennett, I. J., Howard, J. H. & Howard, D. V. Age-related differences in implicit learning of subtle third-order sequential structure. J. Gerontol. Ser. B 62 , P98–P103 (2007).
Nissen, C. et al. Sleep-related memory consolidation in primary insomnia. J. Sleep. Res. 20 , 129–136 (2011).
Ellenbogen, J. M., Payne, J. D. & Stickgold, R. The role of sleep in declarative memory consolidation: passive, permissive, active or none? Curr. Opin. Neurobiol. 16 , 716–722 (2006).
Payne, J. D. et al. Memory for semantically related and unrelated declarative information: the benefit of sleep, the cost of wake. PLoS One 7 , e33079 (2012).
Gaskell, M. G., Cairney, S. A. & Rodd, J. M. Contextual priming of word meanings is stabilized over sleep. Cognition 182 , 109–126 (2019).
Schabus, M. et al. Sleep spindles and their significance for declarative memory consolidation. Sleep 27 , 1479–1485 (2004).
Himmer, L., Müller, E., Gais, S. & Schönauer, M. Sleep-mediated memory consolidation depends on the level of integration at encoding. Neurobiol. Learn. Mem. 137 , 101–106 (2017).
Jacoby, L. L. A process dissociation framework: separating automatic from intentional uses of memory. J. Mem. Lang. 30 , 513–541 (1991).
Sigman, M. & Dehaene, S. Parsing a cognitive task: a characterization of the mind’s bottleneck. PLoS Biol. 3 , 0334–0349 (2005).
Cohen, D. A., Pascual-Leone, A., Press, D. Z. & Robertson, E. M. Off-line learning of motor skill memory: a double dissociation of goal and movement. Proc. Natl Acad. Sci. USA 102 , 18237–18241 (2005).
Conte, F. & Ficca, G. Caveats on psychological models of sleep and memory: a compass in an overgrown scenario. Sleep. Med. Rev. 17 , 105–121 (2013).
Stickgold, R. Parsing the role of sleep in memory processing. Curr. Opin. Neurobiol. 23 , 847–853 (2013).
Ackermann, S., Hartmann, F., Papassotiropoulos, A., De Quervain, D. J. F. & Rasch, B. No associations between interindividual differences in sleep parameters and episodic memory consolidation. Sleep 38 , 951–959 (2015).
Schendan, H. E., Searl, M. M., Melrose, R. J. & Stern, C. E. An fMRI study of the role of the medial temporal lobe in implicit and explicit sequence learning. Neuron 37 , 1013–1025 (2003).
Fischer, S., Drosopoulos, S., Tsen, J. & Born, J. Implicit learning–explicit knowing: a role for sleep in memory system interaction. J. Cogn. Neurosci. 18 , 311–319 (2006).
Simor, P. et al. Deconstructing procedural memory: different learning trajectories and consolidation of sequence and statistical learning. Front. Psychol. 9 , 2708 (2019).
Freedberg, M., Toader, A. C., Wassermann, E. M. & Voss, J. L. Competitive and cooperative interactions between medial temporal and striatal learning systems. Neuropsychologia 136 , 107257 (2020).
Poldrack, R. A., & Packard, M. G. Competition among multiple memory systems: converging evidence from animal and human brain studies. Neuropsychologia 41 , 245–251 (2003).
Brown, R. M. & Robertson, E. M. Off-line processing: reciprocal interactions between declarative and procedural memories. J. Neurosci. 27 , 10468–10475 (2007).
Viczko, J., Sergeeva, V., Ray, L. B., Owen, A. M. & Fogel, S. M. Does sleep facilitate the consolidation of allocentric or egocentric representations of implicitly learned visual-motor sequence learning? Learn. Mem. 25 , 67–77 (2018).
Albouy, G. et al. Daytime sleep enhances consolidation of the spatial but not motoric representation of motor sequence memory. PLoS One 8 , e52805 (2013).
Song, S. & Cohen, L. G. Practice and sleep form different aspects of skill. Nat. Commun. 5 , 3407 (2014).
Chan, J. C. K. & McDermott, K. B. The testing effect in recognition memory: a dual process account. J. Exp. Psychol. Learn. Mem. Cogn. 33 , 431–437 (2007).
Soderstrom, N. C., Kerr, T. K. & Bjork, R. A. The critical importance of retrieval — and spacing — for learning. Psychol. Sci. 27 , 223–230 (2016).
Karpicke, J. D. & Roediger, H. L. The critical importance of retrieval for learning. Science 319 , 966–968 (2008).
Wiklund-Hörnqvist, C., Jonsson, B. & Nyberg, L. Strengthening concept learning by repeated testing. Scand. J. Psychol. 55 , 10–16 (2014).
Buschke, H. Selective reminding for analysis of memory and learning. J. Verbal Learn. Verbal Behav. 12 , 543–550 (1973).
Quan, S. F., Budhiraja, R. & Kushida, C. A. Associations between sleep quality, sleep architecture and sleep disordered breathing and memory after continuous positive airway pressure in patients with obstructive sleep apnea in the Apnea Positive Pressure Long-term Efficacy Study (APPLES). Sleep. Sci. 11 , 231–238 (2018).
Uguccioni, G. et al. Sleep-related declarative memory consolidation and verbal replay during sleep talking in patients with REM sleep behavior disorder. PLoS ONE 8 , e83352 (2013).
Mazza, S. et al. Relearn faster and retain longer: along with practice, sleep makes perfect. Psychol. Sci. 27 , 1321–1330 (2016).
Ellenbogen, J. M., Hulbert, J. C., Stickgold, R., Dinges, D. F. & Thompson-Schill, S. L. Interfering with theories of sleep and memory: sleep, declarative memory, and associative interference. Curr. Biol. 16 , 1290–1294 (2006).
Pöhlchen, D., Pawlizki, A., Gais, S. & Schönauer, M. Evidence against a large effect of sleep in protecting verbal memories from interference. J. Sleep. Res. 30 , e13042 (2021).
Klinzing, J. G., Rasch, B., Born, J. & Diekelmann, S. Sleep’s role in the reconsolidation of declarative memories. Neurobiol. Learn. Mem. 136 , 166–173 (2016).
Fowler, M. J., Sullivan, M. J. & Ekstrand, B. R. Sleep and memory. Science 179 , 302–304 (1973).
Cordi, M. J., Diekelmann, S., Born, J. & Rasch, B. No effect of odor-induced memory reactivation during REM sleep on declarative memory stability. Front. Syst. Neurosci. 8 , 100576 (2014).
Prehn-Kristensen, A. et al. Transcranial oscillatory direct current stimulation during sleep improves declarative memory consolidation in children with attention-deficit/hyperactivity disorder to a level comparable to healthy controls. Brain Stimul. 7 , 793–799 (2014).
Fenn, K. M. & Hambrick, D. Z. Individual differences in working memory capacity predict sleep-dependent memory consolidation. J. Exp. Psychol. Gen. 141 , 404–410 (2012).
Wilhelm, I., Diekelmann, S. & Born, J. Sleep in children improves memory performance on declarative but not procedural tasks. Learn. Mem. 15 , 373–377 (2008).
Marshall, L., Mölle, M., Hallschmid, M. & Born, J. Transcranial direct current stimulation during sleep improves declarative memory. J. Neurosci. 24 , 9985–9992 (2004).
Xue, G. et al. Greater neural pattern similarity across repetitions is associated with better memory. Science 330 , 97–101 (2010).
Young, D. R. & Bellezza, F. S. Encoding variability, memory organization, and the repetition effect. J. Exp. Psychol. Learn. Mem. Cogn. 8 , 545–559 (1982).
Ebbinghaus, H. Memory: a contribution to experimental psychology. Ann. Neurosci. 20 , 155 (2013).
Maski, K. et al. Sleep dependent memory consolidation in children with autism spectrum disorder. Sleep 38 , 1955–1963 (2015).
Baran, B. et al. Spared and impaired sleep-dependent memory consolidation in schizophrenia. Schizophr. Res. 199 , 83–89 (2018).
Nissen, M. J. & Bullemer, P. Attentional requirements of learning: evidence from performance measures. Cogn. Psychol. 19 , 1–32 (1987).
Brawn, T. P., Fenn, K. M., Nusbaum, H. C. & Margoliash, D. Consolidating the effects of waking and sleep on motor-sequence learning. J. Neurosci. 30 , 13977–13982 (2010).
Török, B., Janacsek, K., Nagy, D. G., Orbán, G. & Nemeth, D. Measuring and filtering reactive inhibition is essential for assessing serial decision making and learning. J. Exp. Psychol. Gen. 146 , 529–542 (2017).
Rickard, T. C., Cai, D. J., Rieth, C. A., Jones, J. & Ard, M. C. Sleep does not enhance motor sequence learning. J. Exp. Psychol. Learn. Mem. Cogn. 34 , 834–842 (2008).
Cai, D. J. & Rickard, T. C. Reconsidering the role of sleep for motor memory. Behav. Neurosci. 123 , 1153–1157 (2009).
Nettersheim, A., Hallschmid, M., Born, J. & Diekelmann, S. The role of sleep in motor sequence consolidation: stabilization rather than enhancement. J. Neurosci. 35 , 6696–6702 (2015).
Rieth, C. A., Cai, D. J., McDevitt, E. A. & Mednick, S. C. The role of sleep and practice in implicit and explicit motor learning. Behav. Brain Res. 214 , 470–474 (2010).
Rickard, T. C., Pan, S. C. & Gupta, M. W. Severe publication bias contributes to illusory sleep consolidation in the motor sequence learning literature. J. Exp. Psychol. Learn. Mem. Cogn. 48 , 1787–1796 (2022).
Ioannidis, J. P. A. Why most published research findings are false. PLoS Med. 2 , e124 (2005).
Maxwell, S. E., Lau, M. Y. & Howard, G. S. Is psychology suffering from a replication crisis?: What does ‘failure to replicate’ really mean? Am. Psychol. 70 , 487–498 (2015).
Aarts, A. A. et al. Estimating the reproducibility of psychological science. Science 349 , aac4716 (2015).
Wagner, U., Hallschmid, M., Rasch, B. & Born, J. Brief sleep after learning keeps emotional memories alive for years. Biol. Psychiat. 60 , 788–790 (2006).
Csábi, E. et al. Declarative and non-declarative memory consolidation in children with sleep disorder. Front. Hum. Neurosci. 9 , 709 (2016).
Newbury, C. R., Crowley, R., Rastle, K. & Tamminen, J. Sleep deprivation and memory: meta-analytic reviews of studies on sleep deprivation before and after learning. Psychol. Bull. 147 , 1215–1240 (2021).
Serdar, C. C., Cihan, M., Yücel, D. & Serdar, M. A. Sample size, power and effect size revisited: simplified and practical approachin pre-clinical, clinical and laboratory studies. Biochem. Med. 31 , 010502 (2021).
Berres, S. & Erdfelder, E. The sleep benefit in episodic memory: an integrative review and a meta-analysis. Psychol. Bull. 147 , 1309–1353 (2021).
Wagenmakers, E.-J., Lee, M., Lodewyckx, T. & Iverson, G. J. in Bayesian Evaluation of Informative Hypotheses 181–207 (Springer, 2008).
American Psychological Association. (2010). Publication manual of the American psychological association. American Psychological Association.
Dienes, Z. How Bayes factors change scientific practice. J. Math. Psychol. 72 , 78–89 (2016).
Article MathSciNet Google Scholar
Dienes, Z., Coulton, S. & Heather, N. Using Bayes factors to evaluate evidence for no effect: examples from the SIPS project. Addiction 113 , 240–246 (2018).
Dienes, Z. & Mclatchie, N. Four reasons to prefer Bayesian analyses over significance testing. Psychon. Bull. Rev. 25 , 207–218 (2018).
Wagenmakers, E. J. et al. Bayesian inference for psychology. Part I: Theoretical advantages and practical ramifications. Psychon. Bull. Rev. 25 , 35–57 (2018).
Rouder, J. N. Optional stopping: no problem for Bayesians. Psychon. Bull. Rev. 21 , 301–308 (2014).
Schönbrodt, F. D., Wagenmakers, E. J., Zehetleitner, M. & Perugini, M. Sequential hypothesis testing with Bayes factors: efficiently testing mean differences. Psychol. Meth. 22 , 322–339 (2017).
Brown, H. & Maylor, E. A. Memory consolidation effects on memory stabilization and item integration in older adults. Psychon. Bull. Rev. 24 , 1032–1039 (2017).
Van De Schoot, R., Winter, S. D., Ryan, O., Zondervan-Zwijnenburg, M. & Depaoli, S. Supplemental material for a systematic review of Bayesian articles in psychology: the last 25 years. Psychol. Meth. 22 , 217–239 (2017).
Ellis, P. D. The Essential Guide to Effect Sizes: Statistical Power, Meta-Analysis, and the Interpretation of Research Results (Cambridge Univ. Press, 2010).
Scullin, M. K. Sleep, memory, and aging: the link between slow-wave sleep and episodic memory changes from younger to older adults. Psychol. Aging 28 , 105–114 (2013).
Ujma, P. P. Sleep spindles and general cognitive ability — a meta-analysis. Sleep. Spindl. Cort. Up States 2 , 1–17 (2018).
Google Scholar
Sturman, M. C., Sturman, A. J. & Sturman, C. J. Uncontrolled control variables: the extent that a researcher’s degrees of freedom with control variables increases various types of statistical errors. J. Appl. Psychol. 107 , 9–22 (2022).
Lerner, I., Lupkin, S. M., Tsai, A., Khawaja, A. & Gluck, M. A. Sleep to remember, sleep to forget: rapid eye movement sleep can have inverse effects on recall and generalization of fear memories. Neurobiol. Learn. Mem. 180 , 107413 (2021).
Abdi, H. in Encyclopedia of Measurement and Statistics (ed. Salkind, N. J.) 103–107 https://doi.org/10.4135/9781412952644 (Sage, 2007).
Forstmeier, W., Wagenmakers, E. J. & Parker, T. H. Detecting and avoiding likely false-positive findings — a practical guide. Biol. Rev. 92 , 1941–1968 (2017).
Kruschke, J. K. Bayesian analysis reporting guidelines. Nat. Hum. Behav. 5 , 1282–1291 (2021).
Bódizs, R. et al. Prediction of general mental ability based on neural oscillation measures of sleep. J. Sleep. Res. 14 , 285–292 (2005).
Fang, Z. et al. Sleep spindles and intellectual ability: epiphenomenon or directly related? J. Cogn. Neurosci. 29 , 167–182 (2017).
Fang, Z., Ray, L. B., Owen, A. M. & Fogel, S. M. Brain activation time-locked to sleep spindles associated with human cognitive abilities. Front. Neurosci. 13 , 432131 (2019).
Fogel, S. M. & Smith, C. T. Learning-dependent changes in sleep spindles and stage 2 sleep. J. Sleep. Res. 15 , 250–255 (2006).
Daw, N. D., Niv, Y. & Dayan, P. Uncertainty-based competition between prefrontal and dorsolateral striatal systems for behavioral control. Nat. Neurosci. 8 , 1704–1711 (2005).
Fogel, S. M. & Smith, C. T. The function of the sleep spindle: a physiological index of intelligence and a mechanism for sleep-dependent memory consolidation. Neurosci. Biobehav. Rev. 35 , 1154–1165 (2011).
Lahl, O., Wispel, C., Willigens, B. & Pietrowsky, R. An ultra short episode of sleep is sufficient to promote declarative memory performance. J. Sleep. Res. 17 , 3–10 (2008).
Diekelmann, S., Biggel, S., Rasch, B. & Born, J. Offline consolidation of memory varies with time in slow wave sleep and can be accelerated by cuing memory reactivations. Neurobiol. Learn. Mem. 98 , 103–111 (2012).
Nishida, M. & Walker, M. P. Daytime naps, motor memory consolidation and regionally specific sleep spindles. PLoS One 2 , e341 (2007).
Schönauer, M., Geisler, T. & Gais, S. Strengthening procedural memories by reactivation in sleep. J. Cogn. Neurosci. 26 , 143–153 (2014).
Gais, S., Plihal, W., Wagner, U. & Born, J. Early sleep triggers memory for early visual discrimination skills. Nat. Neurosci. 3 , 1335–1339 (2000).
Mednick, S. C., Cai, D. J., Kanady, J. & Drummond, S. P. A. Comparing the benefits of caffeine, naps and placebo on verbal, motor and perceptual memory. Behav. Brain Res. 193 , 79–86 (2008).
Sopp, M. R., Michael, T. & Mecklinger, A. Effects of early morning nap sleep on associative memory for neutral and emotional stimuli. Brain Res. 1698 , 29–42 (2018).
Plihal, W., Weaver, S., Mölle, M., Fehm, H. L. & Born, J. Sensory processing during early and late nocturnal sleep. Electroencephalogr. Clin. Neurophysiol. 99 , 247–256 (1996).
Yaroush, R., Sullivan, M. J. & Ekstrand, B. R. Effect of sleep on memory: II. Differential effect of the first and second half of the night. J. Exp. Psychol. 88 , 361–366 (1971).
Mednick, S., Nakayama, K. & Stickgold, R. Sleep-dependent learning: a nap is as good as a night. Nat. Neurosci. 6 , 697–698 (2003).
Wamsley, E. J. Memory consolidation during waking rest. Trends Cogn. Sci. 23 , 171–173 (2019).
McDevitt, E. A., Duggan, K. A. & Mednick, S. C. REM sleep rescues learning from interference. Neurobiol. Learn. Mem. 122 , 51–62 (2015).
Cousins, J. N., Wong, K. F., Raghunath, B. L., Look, C. & Chee, M. W. L. The long-term memory benefits of a daytime nap compared with cramming. Sleep 42 , zsy207 (2019).
Piosczyk, H. et al. The effect of sleep-specific brain activity versus reduced stimulus interference on declarative memory consolidation. J. Sleep. Res. 22 , 406–413 (2013).
Schichl, M., Ziberi, M., Lahl, O. & Pietrowsky, R. The influence of midday naps and relaxation-hypnosis on declarative and procedural memory performance. Sleep. Hypn. 13 , 7–14 (2011).
Mednick, S. C., Makovski, T., Cai, D. J. & Jiang, Y. V. Sleep and rest facilitate implicit memory in a visual search task. Vis. Res. 49 , 2557–2565 (2009).
Schapiro, A. C., McDevitt, E. A., Rogers, T. T., Mednick, S. C. & Norman, K. A. Human hippocampal replay during rest prioritizes weakly learned information and predicts memory performance. Nat. Commun. 9 , 3920 (2018).
van Schalkwijk, F. J. et al. The effect of daytime napping and full‐night sleep on the consolidation of declarative and procedural information. J. Sleep Res. 28 , e12649 (2019).
Poldrack, R. A. & Gorgolewski, K. J. OpenfMRI: open sharing of task fMRI data. Neuroimage 144 , 259–261 (2017).
Gorgolewski, K., Esteban, O., Schaefer, G., Wandell, B. & Poldrack, R. OpenNeuro — a free online platform for sharing and analysis of neuroimaging data. F1000Res. 6 , 80190187 (2017).
Spitschan, M., Schmidt, M. H. & Blume, C. Principles of open, transparent and reproducible science in author guidelines of sleep research and chronobiology journals. Wellcome Open Res . https://wellcomeopenresearch.org/articles/5-172 (2021).
Prabhakar, J., Johnson, E. G., Nordahl, C. W. & Ghetti, S. Memory-related hippocampal activation in the sleeping toddler. Proc. Natl Acad. Sci. USA 115 , 6500–6505 (2018).
Schreiner, T., Petzka, M., Staudigl, T. & Staresina, B. P. Endogenous memory reactivation during sleep in humans is clocked by slow oscillation-spindle complexes. Nat. Commun. 12 , 3112 (2021).
Rakowska, M., Abdellahi, M. E. A., Bagrowska, P., Navarrete, M. & Lewis, P. A. Long term effects of cueing procedural memory reactivation during NREM sleep. Neuroimage 244 , 118573 (2021).
Nosek, B. A. et al. Promoting an open research culture. Science 348 , 1422–1425 (2015).
Simor, P., Gombos, F., Blaskovich, B. & Bódizs, R. Long-range alpha and beta and short-range gamma EEG synchronization distinguishes phasic and tonic REM periods. Sleep https://doi.org/10.1093/sleep/zsx210 (2018).
Simor, P., van Der Wijk, G., Gombos, F. & Kovács, I. The paradox of rapid eye movement sleep in the light of oscillatory activity and cortical synchronization during phasic and tonic microstates. Neuroimage 202 , 116066 (2019).
Foster, E. D. & Deardorff, A. Open science framework (OSF). J. Med. Libr. Assoc. 105 , 203–206 (2017).
Article PubMed Central Google Scholar
Nosek, B. A., Ebersole, C. R., DeHaven, A. C. & Mellor, D. T. The preregistration revolution. Proc. Natl Acad. Sci. USA 115 , 2600–2606 (2018).
Lindsay, D. S., Simons, D. J. & Lilienfeld, S. O. Research preregistration 101. APS https://www.psychologicalscience.org/observer/research-preregistration-101 (2016).
Hardwicke, T. E. & Wagenmakers, E. J. Reducing bias, increasing transparency and calibrating confidence with preregistration. Nat. Hum. Behav. 7 , 15–26 (2023).
Angelis, C. D. et al. Clinical trial registration: a statement from the International Committee of Medical Journal Editors. Lancet 364 , 911–912 (2004).
James, E., Gaskell, M. G. & Henderson, L. M. Offline consolidation supersedes prior knowledge benefits in children’s (but not adults’) word learning. Dev. Sci. 22 , e12776 (2019).
Soderberg, C. K. et al. Initial evidence of research quality of registered reports compared with the standard publishing model. Nat. Hum. Behav. 5 , 990–997 (2021).
Download references
Acknowledgements
The authors thank K. Schipper for her help and comments on the manuscript. This research was supported by the ANR grant awarded within the framework of the Inserm CPJ ANR-22-CPJ1-0042-01 (to D.N.); Hungary’s National Brain Research Program (project NAP2022-I-2/2022); NKFIH-OTKA PD 124148 (Principal Investigator K.J.); NKFI FK 142945 (Principal Investigator P.S.); Janos Bolyai Research Fellowship of the Hungarian Academy of Sciences (to K.J. and P.S.); and the French National Agency for Research (ANR, grant number ANR-15-CE33-0003, Principal Investigator S.M.).
Author information
These authors contributed equally: Dezső Németh, Emilie Gerbier, Karolina Janacsek.
Authors and Affiliations
Centre de Recherche en Neurosciences de Lyon CRNL U1028 UMR5292, INSERM, CNRS, Université Claude Bernard Lyon, Bron, France
Dezső Németh, Perrine Ruby & Teodóra Vékony
Institute of Psychology, ELTE Eötvös Lorand University, Budapest, Hungary
Dezső Németh, Peter Simor & Karolina Janacsek
Institute of Cognitive Neuroscience and Psychology, Hun-Ren Research Centre for Natural Sciences, Budapest, Hungary
Dezső Németh
Laboratoire Bases, Corpus, Langage (UMR 7320), CNRS, Université Côte d’Azur, Nice, France
Emilie Gerbier
Institute of Medical Psychology and Behavioral Neurobiology, University of Tübingen, Tübingen, Germany
Jan Born & Susanne Diekelmann
Department of Psychology, University of California San Diego, La Jolla, CA, USA
Timothy Rickard
Department of Psychiatry and Psychotherapy, University Hospital Tübingen, Tübingen, Germany
Susanne Diekelmann
School of Psychology, University of Ottawa, Ottawa, Canada
Stuart Fogel
Donders Institute for Brain Cognition and Behaviour, Radboud University, Nijmegen, Netherlands
Lisa Genzel
Institute for Child and Adolescent Psychiatry, Center for Integrative Psychiatry, University Hospital Schleswig-Holstein, Kiel, Germany
Alexander Prehn-Kristensen
Department of Psychology, Faculty of Human Sciences, MSH Medical School Hamburg, University of Applied Sciences and Medical University, Hamburg, Germany
Department of Psychology, University of Notre Dame, Notre Dame, IN, USA
Jessica Payne
Donders Institute for Brain, Cognition and Behavior, Radboud University Medical Center, Nijmegen, The Netherlands
Martin Dresler
Institute of Behavioural Sciences, Semmelweis University, Budapest, Hungary
Peter Simor
Forgetting Team, Centre de Recherche en Neurosciences de Lyon CRNL U1028 UMR5292, INSERM, CNRS, Université Claude Bernard Lyon, Bron, France
Stephanie Mazza
Department of Psychology, Centre for Cognitive Neuroscience, University of Salzburg, Salzburg, Austria
Kerstin Hoedlmoser & Manuel Schabus
Department of Psychological and Brain Sciences, University of Massachusetts, Amherst, MA, USA
Rebecca M. C. Spencer
Department of Movement Sciences, Katholieke Universiteit Leuven, Leuven, Belgium
Genevieve Albouy
Centre for Thinking and Learning, Institute for Lifecourse Development, School of Human Sciences, University of Greenwich, London, UK
Karolina Janacsek
You can also search for this author in PubMed Google Scholar
Contributions
D.N., E.G., J.B., T.R., S.D., S.F., L.G., A.P.-K., J.P., M.D., P.S., S.M., K.H., P.R., R.M.C.S., G.A., T.V., M.S. and K.J. contributed substantially to discussion of the content. All authors wrote the article. All authors reviewed and/or edited the manuscript before submission.
Corresponding author
Correspondence to Dezső Németh .
Ethics declarations
Competing interests.
The authors declare no competing interests.
Peer review
Peer review information.
Nature Reviews Psychology thanks M. Gareth Gaskell and the other, anonymous, reviewer(s) for their contribution to the peer review of this work.
Additional information
Publisher’s note Springer Nature remains neutral with regard to jurisdictional claims in published maps and institutional affiliations.
Rights and permissions
Springer Nature or its licensor (e.g. a society or other partner) holds exclusive rights to this article under a publishing agreement with the author(s) or other rightsholder(s); author self-archiving of the accepted manuscript version of this article is solely governed by the terms of such publishing agreement and applicable law.
Reprints and permissions
About this article
Cite this article.
Németh, D., Gerbier, E., Born, J. et al. Optimizing the methodology of human sleep and memory research. Nat Rev Psychol 3 , 123–137 (2024). https://doi.org/10.1038/s44159-023-00262-0
Download citation
Published : 22 December 2023
Issue Date : February 2024
DOI : https://doi.org/10.1038/s44159-023-00262-0
Share this article
Anyone you share the following link with will be able to read this content:
Sorry, a shareable link is not currently available for this article.
Provided by the Springer Nature SharedIt content-sharing initiative
Quick links
- Explore articles by subject
- Guide to authors
- Editorial policies
Sign up for the Nature Briefing newsletter — what matters in science, free to your inbox daily.

Sleep Loss and Emotion: A Systematic Review and Meta-Analysis
Saul Mcleod, PhD
Editor-in-Chief for Simply Psychology
BSc (Hons) Psychology, MRes, PhD, University of Manchester
Saul Mcleod, PhD., is a qualified psychology teacher with over 18 years of experience in further and higher education. He has been published in peer-reviewed journals, including the Journal of Clinical Psychology.
Learn about our Editorial Process
Olivia Guy-Evans, MSc
Associate Editor for Simply Psychology
BSc (Hons) Psychology, MSc Psychology of Education
Olivia Guy-Evans is a writer and associate editor for Simply Psychology. She has previously worked in healthcare and educational sectors.
Sleep and emotion are fundamentally intertwined, both being universal human experiences that profoundly shape our daily functioning and well-being. Our emotional states influence every facet of our lives, from our physical health and cognitive performance to our social relationships and overall life satisfaction. Similarly, sleep plays a vital role in regulating our emotional processing , reactivity, and mood.
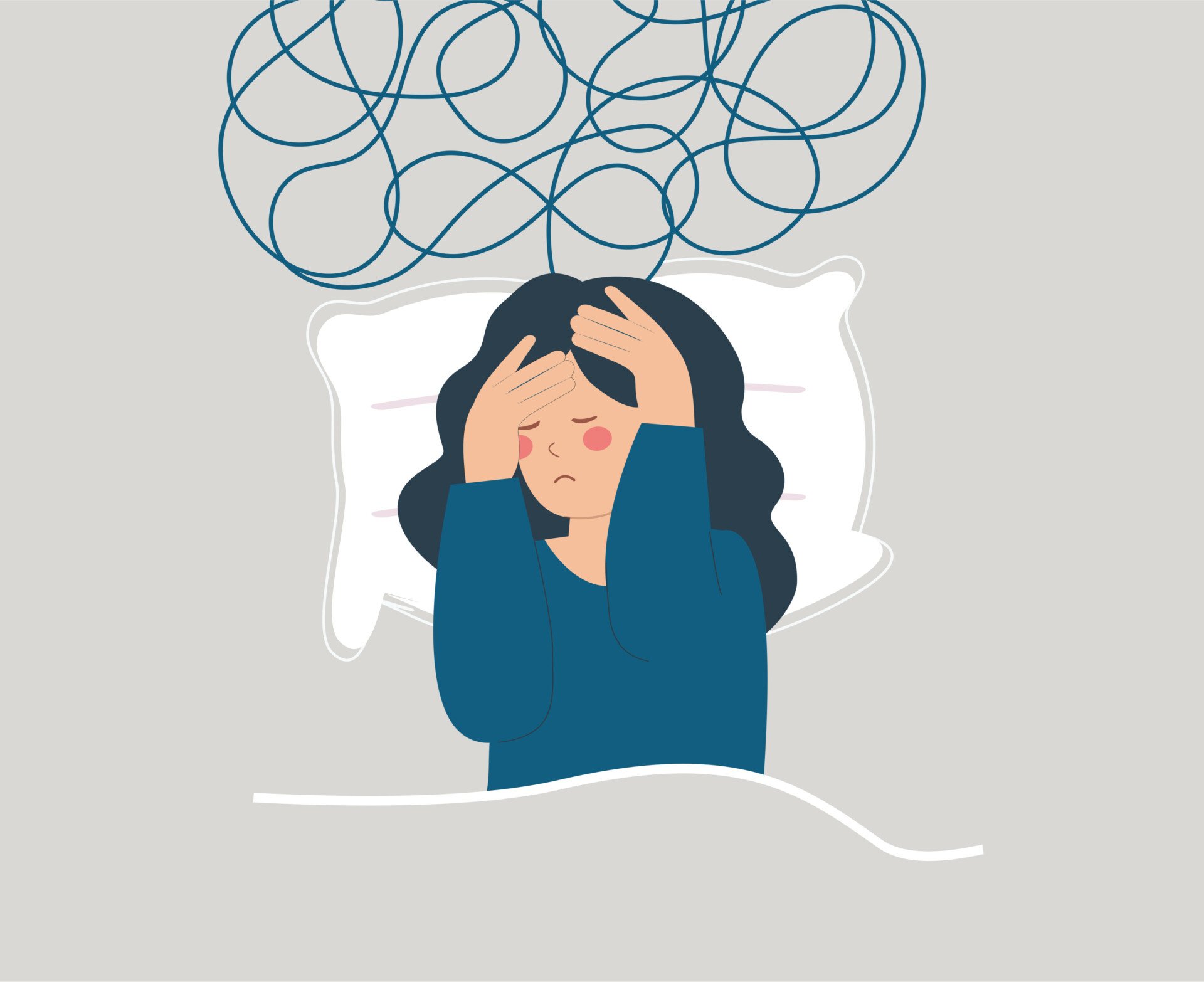
- This meta-analysis synthesized over 50 years of experimental research on sleep loss and emotion, examining the effects of sleep deprivation, sleep restriction, and sleep fragmentation on various emotional outcomes.
- Sleep loss resulted in reduced positive affect (SMD = -0.27 to -1.14), increased anxiety symptoms (SMD = 0.57-0.63), and blunted arousal in response to emotional stimuli (SMD = -0.20 to -0.53). Findings for negative affect, emotional valence, and depressive symptoms varied based on the type of sleep loss.
- Nonlinear dose-response effects were found for the amount of sleep loss on some emotional outcomes. Losing REM sleep had a stronger effect on unpleasant emotional reactivity compared to losing slow-wave sleep.
- While the research sheds light on the detrimental effects of sleep loss on emotions, it has some limitations such as relying predominantly on young adult samples and potential expectancy effects.
- The pervasiveness of insufficient sleep worldwide makes this an important public health issue with significant implications for emotional well-being and risk for psychiatric disorders.
Sleep loss is common in modern society, with large segments of the population regularly failing to obtain adequate sleep (Hafner et al., 2017).
Poor sleep is known to catalyze the development of emotional difficulties and affective disorders across the lifespan (Goldstein & Walker, 2014; Gregory et al., 2009).
While an increasing number of experimental studies have demonstrated the adverse effects of sleep loss on emotion in recent decades (Palmer & Alfano, 2017; Tempesta et al., 2018), findings have been mixed and a comprehensive quantitative synthesis was needed to integrate results across the heterogeneous research designs and consolidate conclusions.
This meta-analysis aimed to quantify the effects of various forms of experimental sleep loss (deprivation, restriction, fragmentation) on multiple aspects of emotional experience and elucidate factors that may influence these effects.
The researchers conducted a preregistered systematic review and meta-analysis following PRISMA guidelines.
They searched PubMed/MEDLINE, PsychINFO, and Web of Science databases for experimental studies examining the effects of sleep deprivation, sleep restriction, or sleep fragmentation on positive affect, negative affect, mood disturbance, emotional reactivity, anxiety symptoms and/or depressive symptoms in healthy populations.
Additional unpublished data was sought via listservs and contacting authors. Inclusion criteria required studies to have healthy samples, an experimental sleep loss manipulation, an adequate control/baseline condition, and an emotion-related dependent variable.
Two independent coders screened and extracted data from eligible studies.
Search strategy and terms
The search criteria included human studies mentioning experimental sleep manipulations and any emotion-related outcomes in the title/abstract. There were no restrictions on language, location or date.
Inclusion and exclusion criteria
Included studies had to 1) use healthy samples without psychiatric, sleep or medical disorders that impact sleep/emotions, 2) experimentally manipulate nighttime sleep deprivation, restriction or fragmentation, 3) have an adequate control/baseline condition, 4) assess an emotion-related dependent variable after the sleep manipulation.
Studies were excluded if they used specialized samples, nap/circadian protocols, or had an intervention prior to the emotional assessment that could affect outcomes.
Statistical measures
Standardized mean differences (Hedges’ g) were calculated for each eligible outcome. Multivariate multilevel random effects models were used to estimate overall effects for each sleep loss type and emotional outcome, accounting for non-independent effect sizes.
Mixed effects models examined potential moderators. Heterogeneity, outliers, and indices of publication bias were assessed.
The meta-analysis included 154 studies (N=5,717) yielding 1,338 effect sizes.
For sleep deprivation (k=599), significant effects were found for reduced positive affect (SMD=-0.86), increased negative affect (SMD=0.37), mood disturbance (SMD=0.71), blunted arousal (SMD=-0.53), and anxiety (SMD=0.63). Nonlinear dose-response effects showed negative affect, mood disturbance and anxiety peaking at 30-60 hours of wakefulness.
For sleep restriction (k=483), effects were significant for reduced positive affect (SMD=-0.56), increased negative affect (SMD=0.20), mood disturbance (SMD=0.56), greater unpleasantness (SMD=0.23), blunted arousal (SMD=-0.20), anxiety (SMD=0.57) and depression (SMD=0.46). Nonlinear dose-response effects found the largest deficits for positive affect and unpleasantness around 4 hours of sleep.
Sleep fragmentation (k=256) significantly reduced positive affect (SMD=-0.40) and blunted arousal (SMD=-0.36). There were no significant effects on negative affect, mood, or valence.
Some moderating effects of age, sex, and study characteristics emerged, though not consistently across outcomes. Importantly, losing REM sleep had a stronger effect than losing slow-wave sleep on ratings of unpleasantness to emotional stimuli.
This meta-analysis provides the most comprehensive picture to date of how sleep loss impacts human emotions.
It demonstrates that multiple aspects of emotional functioning are significantly altered by sleep deprivation, restriction, and fragmentation.
The most robust effects across all three types of sleep loss were found for reductions in positive affect, suggesting that inadequate sleep may be particularly detrimental for experiences and expressions of positive emotions.
This could have important mental health implications given the role of positive emotionality in psychological well-being and resilience (Fredrickson, 2001). The blunting of emotional arousal also seen after sleep loss may reflect impairments in top-down emotional processing.
In contrast, sleep loss effects on negative affect were smaller and less consistent across studies. The evolutionary importance of negative emotions for signaling threats and promoting survival could make negative affective responses more resistant to the effects of sleep loss compared to positive affect.
However, anxiety symptoms were consistently increased by sleep loss, indicating heightened feelings of apprehension and worry. The dose-response findings suggest these detrimental effects on negative affect and anxiety are exacerbated by more extreme sleep deprivation.
An intriguing finding was that losing REM sleep impacted ratings of emotional stimuli more than losing slow-wave sleep. This aligns with theories proposing a key role of REM sleep in emotional memory consolidation and maintaining emotional reactivity (Walker & van der Helm, 2009).
More targeted research comparing the effects of selective REM and slow-wave sleep deprivation on emotional reactivity and regulation is needed.
While only a small number of studies included children or older adults, some moderating effects of age did emerge, with sleep loss having stronger effects on mood disturbances in older individuals. Examining sleep-emotion dynamics in developmental and lifespan contexts is an important future direction.
Sex differences were also found for some outcomes, but not consistently, highlighting the need for more studies powered to detect potential gender differences in emotional vulnerability to sleep loss.
Overall, these findings underscore the consequences of insufficient sleep for affective experience and functioning. They provide an important foundation for further investigating the mechanisms linking sleep and emotion and developing interventions to mitigate the risks of sleep loss for emotional health.
- Preregistration of the study design and analytical plan
- Adherence to PRISMA guidelines for transparent reporting
- Comprehensive search strategy across multiple databases
- Inclusion of unpublished data to mitigate publication bias
- Rigorous coding procedures with two independent coders
- Examination of multiple types of sleep loss and emotional outcomes
- Advanced statistical methods accounting for non-independent effect sizes
- Assessment of heterogeneity, outliers, and publication bias indices
Limitations
- Most studies used young adult samples, limiting generalizability to other ages
- There was a lack of geographical diversity, with studies predominantly from Western countries
- Masking participants to sleep loss conditions is not possible, so expectancy effects may have influenced results
- Some analyses for specific emotional outcomes or sleep types had a small number of studies
- Variability in emotional assessments and sleep manipulation procedures across studies
Implications
The finding that even relatively modest amounts of sleep loss can have significant negative repercussions for emotional well-being has important real-world implications.
With up to one-third of the general adult population reporting insufficient sleep (Liu et al., 2016), a substantial proportion of people may be at heightened risk for emotional difficulties and affective disorders as a result of inadequate sleep.
This makes sleep a critical target for public health interventions aimed at promoting mental health.
The differential impacts of REM versus slow-wave sleep loss on emotional reactivity also have clinical relevance, suggesting sleep stage-specific interventions may be warranted for certain emotional issues.
The nonlinear dose-response effects for several outcomes indicate that sleeping less than 4-5 hours and/or being continuously awake for over 24 hours may represent particularly dangerous thresholds for emotional health.
Organizations and occupations where sleep loss is common (e.g., military, healthcare, shift work) need to be aware of the risks to emotional well-being and implement strategies to mitigate these effects.
Public policies regulating work hours and school start times should prioritize sleep to reduce adverse emotional consequences at the population level.
With the high rates of insufficient sleep among adolescents (Basch et al., 2014) and emerging mood disorders during this developmental period, optimizing sleep could be an important avenue for youth mental health promotion.
More broadly, initiatives to increase public awareness about the importance of sleep for emotional health, address barriers to adequate sleep, and promote evidence-based sleep hygiene practices have the potential to make a substantial and much-needed impact on psychological well-being and public health.
The current findings underscore the affective benefits of prioritizing sleep and the dangers of sacrificing it.
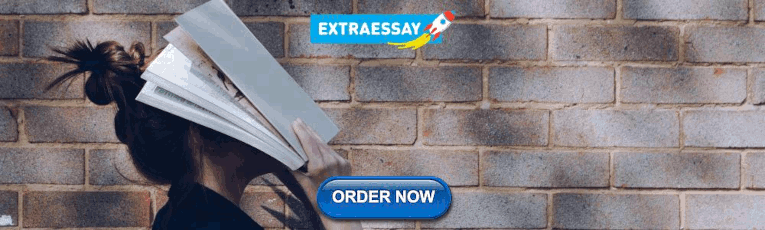
Primary reference
Palmer, C. A., Bower, J. L., Cho, K. W., Clementi, M. A., Lau, S., Oosterhoff, B., & Alfano, C. A. (2024). Sleep loss and emotion: A systematic review and meta-analysis of over 50 years of experimental research. Psychological Bulletin, 150 (4), 440–463. https://doi.org/10.1037/bul0000410
Other references
Basch, C. E., Basch, C. H., Ruggles, K. V., & Rajan, S. (2014). Prevalence of sleep duration on an average school night among 4 nationally representative successive samples of American high school students, 2007–2013. Preventing Chronic Disease, 11 , Article 140383. https://doi.org/10.5888/pcd11.140383
Fredrickson, B. L. (2001). The role of positive emotions in positive psychology. The broaden-and-build theory of positive emotions. American Psychologist, 56 (3), 218–226. https://doi.org/10.1037/0003-066X.56.3.218
Goldstein, A. N., & Walker, M. P. (2014). The role of sleep in emotional brain function. Annual Review of Clinical Psychology, 10, 679-708. https://doi.org/10.1146/annurev-clinpsy-032813-153716
Gregory, A. M., Rijsdijk, F. V., Lau, J. Y., Dahl, R. E., & Eley, T. C. (2009). The direction of longitudinal associations between sleep problems and depression symptoms: a study of twins aged 8 and 10 years. Sleep, 32 (2), 189-199. https://doi.org/10.1093/sleep/32.2.189
Hafner, M., Stepanek, M., Taylor, J., Troxel, W. M., & van Stolk, C. (2017). Why sleep matters—the economic costs of insufficient sleep: a cross-country comparative analysis. Rand Health Quarterly, 6 (4), 11. https://doi.org/10.7249/RB9962
Liu, Y., Wheaton, A. G., Chapman, D. P., Cunningham, T. J., Lu, H., & Croft, J. B. (2016). Prevalence of healthy sleep duration among adults — United States, 2014. MMWR. Morbidity and Mortality Weekly Report, 65 (6), 137–141. https://doi.org/10.15585/mmwr.mm6506a1
Palmer, C. A., & Alfano, C. A. (2017). Sleep and emotion regulation: An organizing, integrative review. Sleep Medicine Reviews, 31, 6-16. https://doi.org/10.1016/j.smrv.2015.12.006
Tempesta, D., Socci, V., De Gennaro, L., & Ferrara, M. (2018). Sleep and emotional processing. Sleep Medicine Reviews, 40, 183-195. https://doi.org/10.1016/j.smrv.2017.12.005
Walker, M. P., & van der Helm, E. (2009). Overnight therapy? The role of sleep in emotional brain processing. Psychological Bulletin, 135 (5), 731–748. https://doi.org/10.1037/a0016570
Keep Learning
- How does this meta-analysis advance our understanding of the relationship between sleep and emotion compared to previous individual studies? What are the advantages of synthesizing results across multiple studies?
- The strongest effects of sleep loss were found for positive affect. Why might inadequate sleep be particularly detrimental for positive emotions from an evolutionary or neurobiological perspective? What are the potential mental health implications of this finding?
- The study found some moderating effects of age on the sleep loss-emotion relationship. How might the effects of sleep loss on emotional functioning differ across the lifespan from childhood to older adulthood? What developmental factors could influence this?
- Losing REM sleep impacted emotional reactivity to unpleasant stimuli more than losing slow-wave sleep. What are the potential mechanisms that could explain this finding? How does it relate to theories about the role of REM sleep in emotional memory processing?
- Given the pervasiveness of insufficient sleep in the population, what public health strategies or policies could help mitigate the negative emotional consequences of sleep loss at a societal level? How could this research inform interventions for at-risk groups?
- What are some of the limitations of experimental sleep research for understanding real-world emotional functioning? How well do these controlled laboratory studies generalize to chronic partial sleep loss as it’s experienced in daily life?
- Where should sleep and emotion research go from here? What are the most pressing unanswered questions or promising future directions based on the current state of the science? What types of studies or methodologies are needed to advance the field?
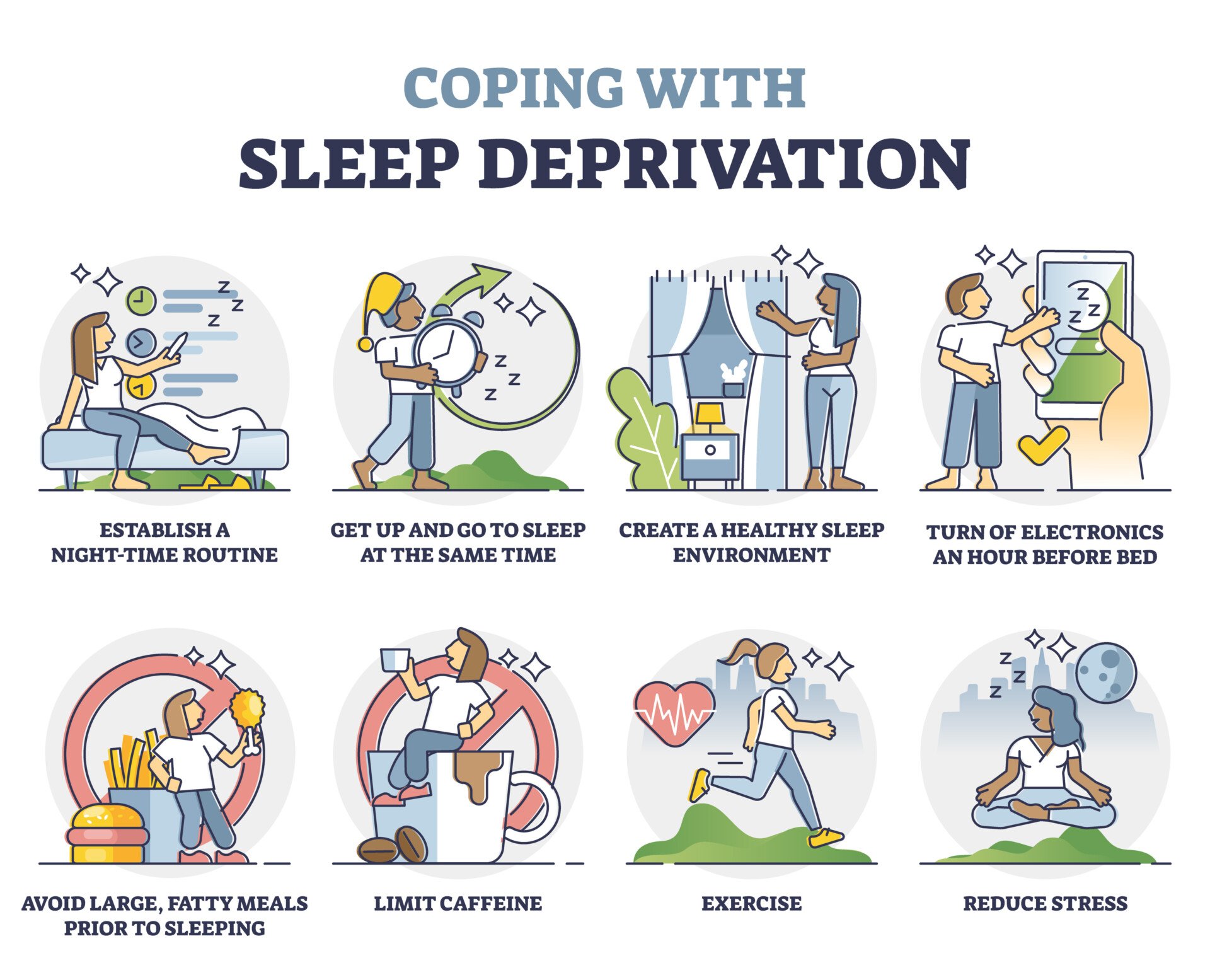
Related Articles
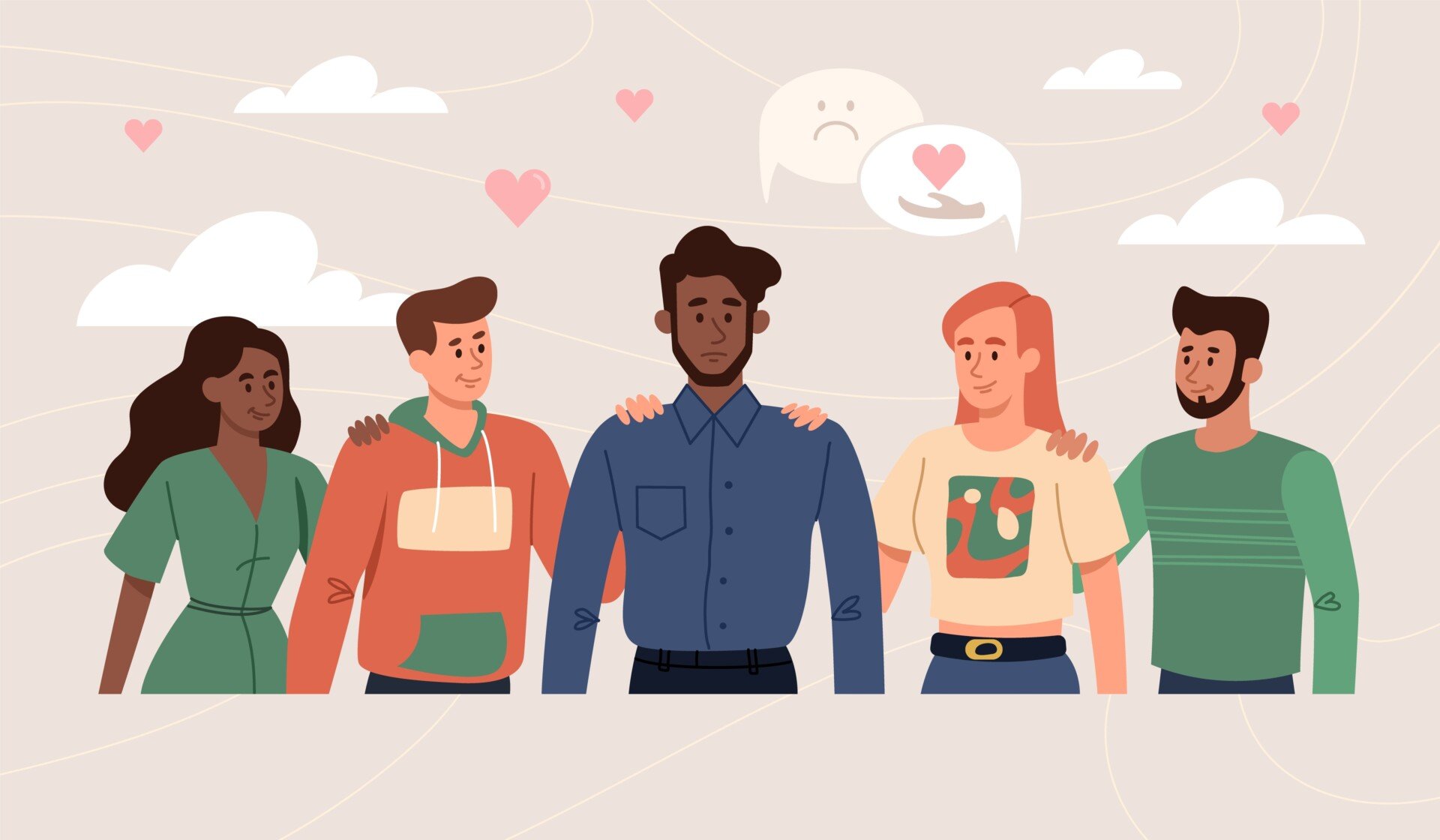
Clinical Psychology
A Study Of Social Anxiety And Perceived Social Support
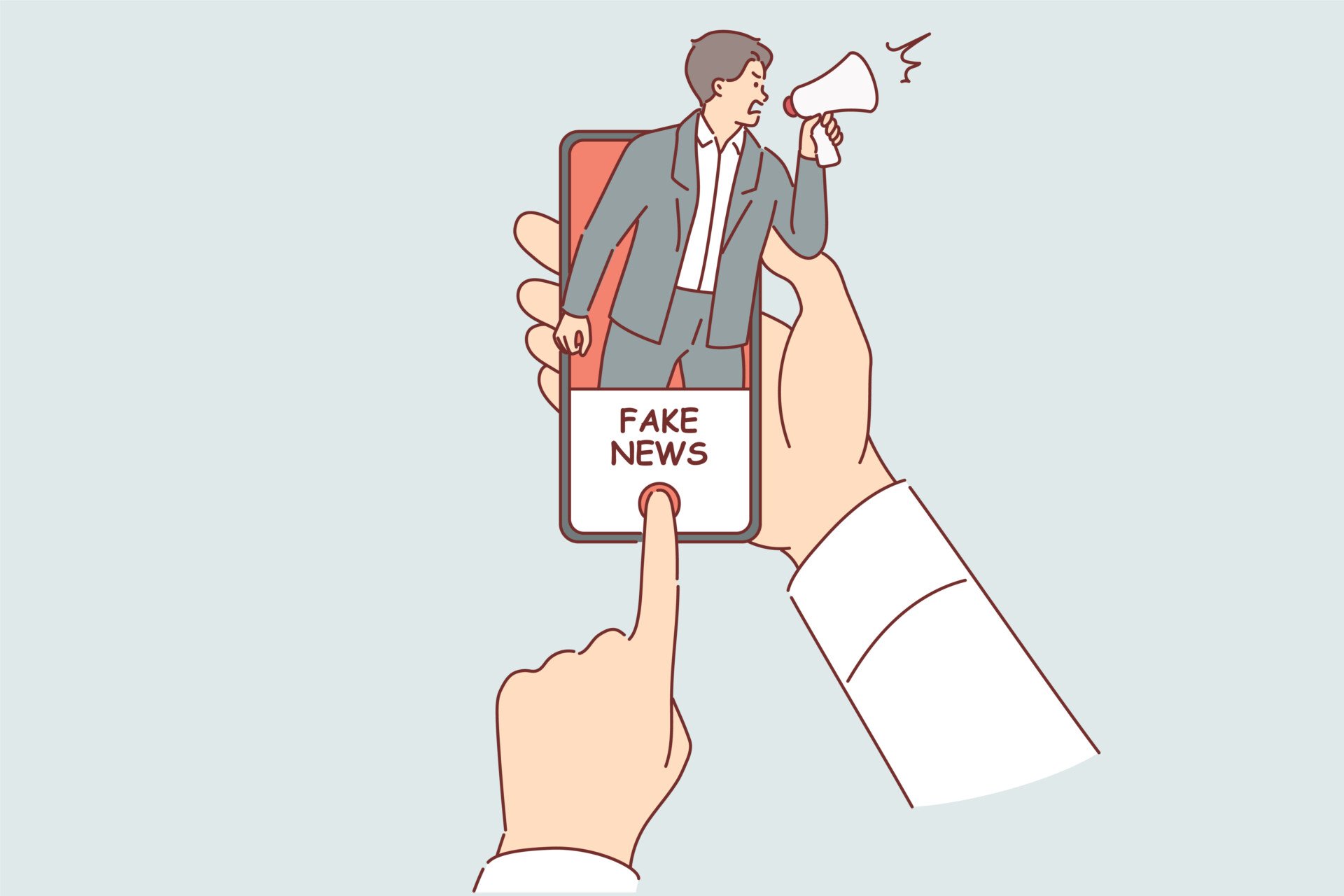
Psychological Impact Of Health Misinformation: A Systematic Review
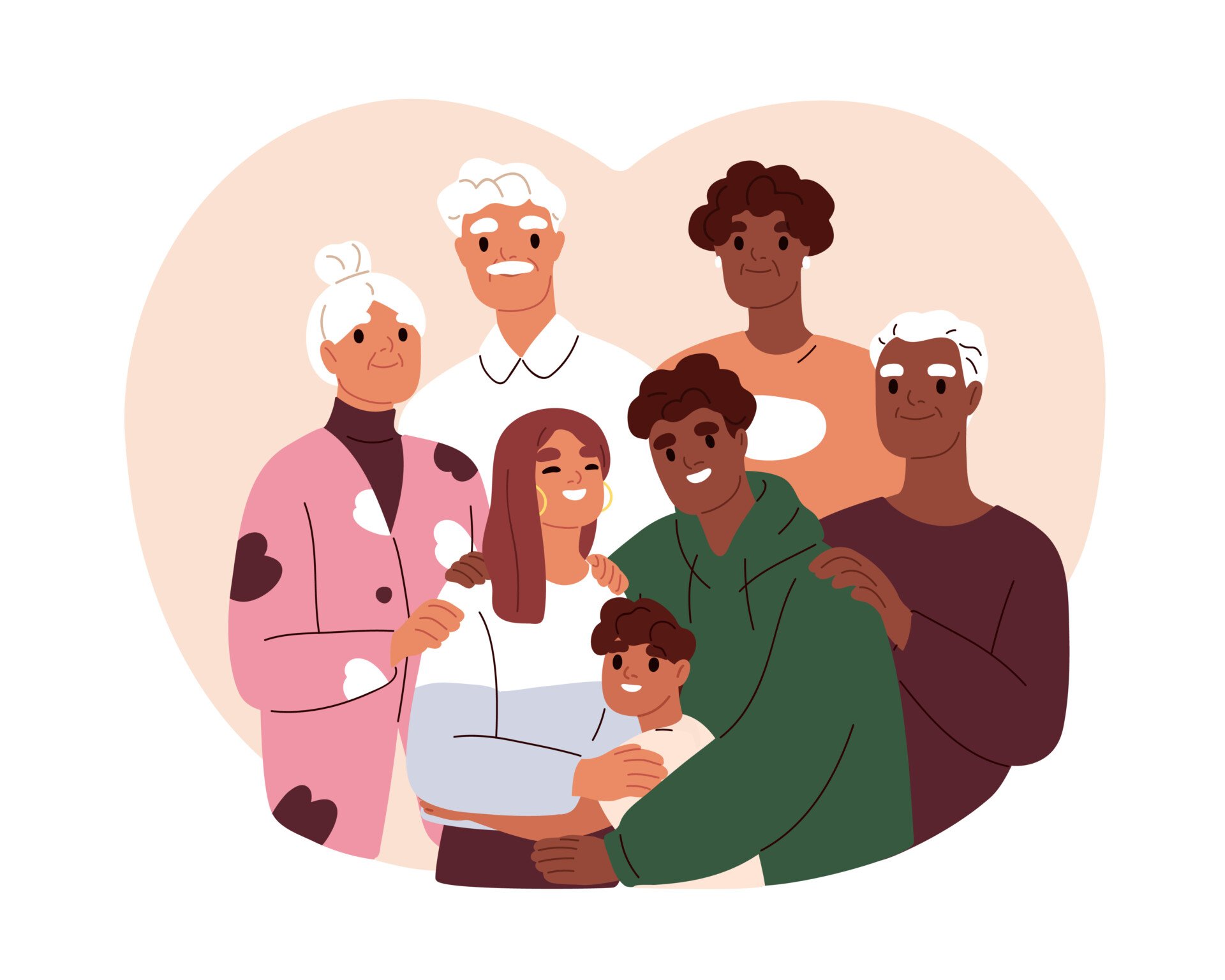
Family History Of Autism And ADHD Vary With Recruitment Approach And SES
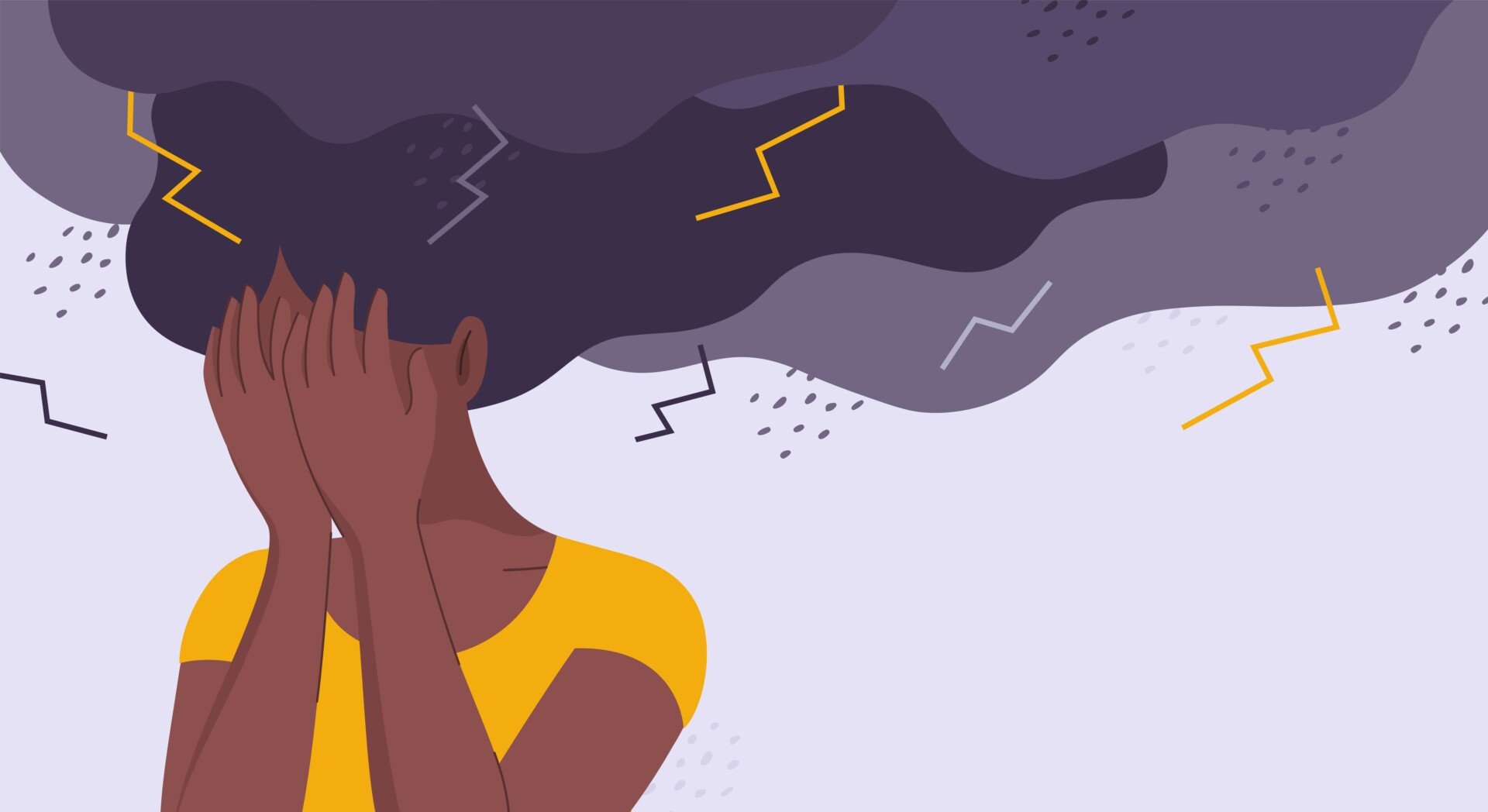
Measuring And Validating Autistic Burnout
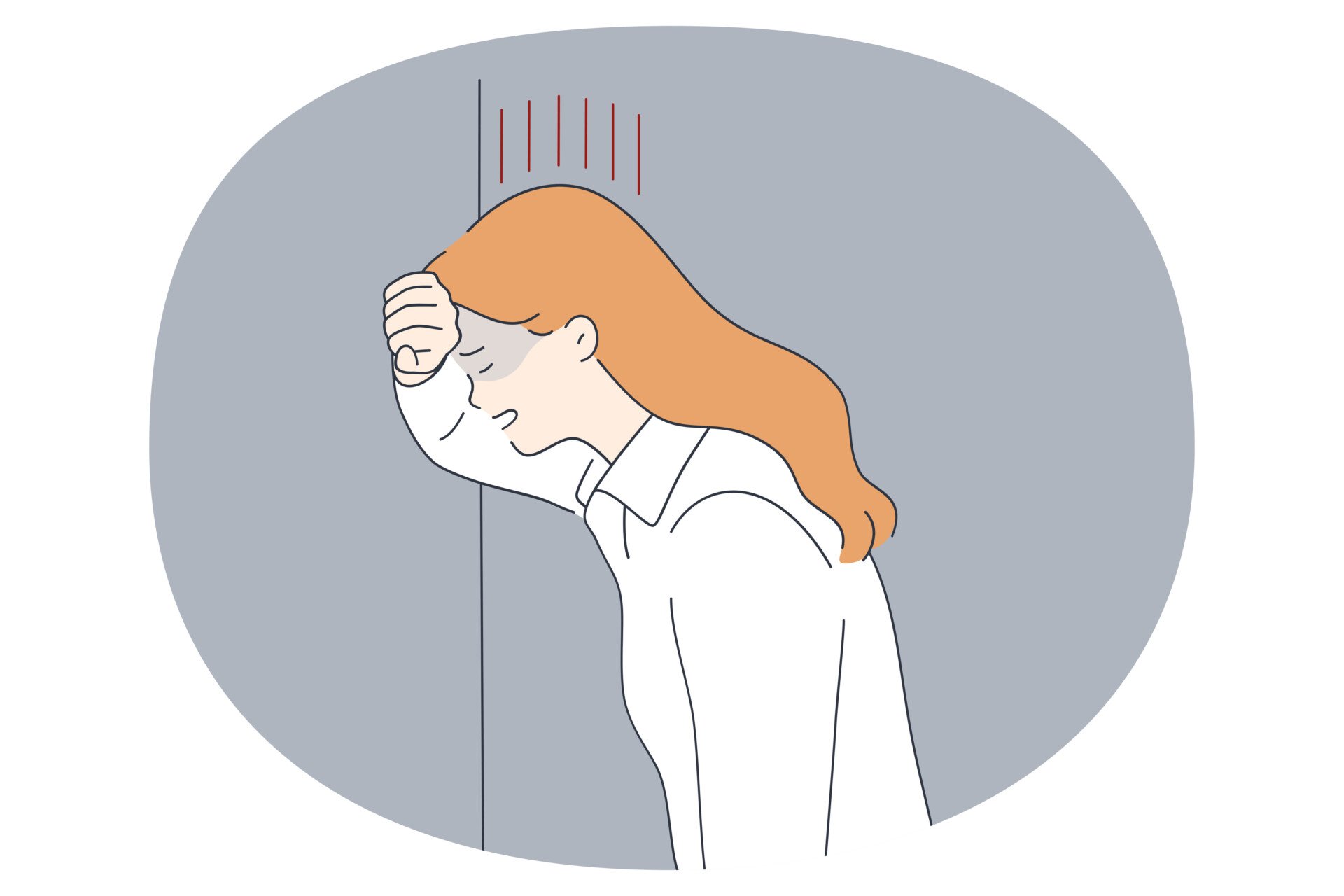
A Systematic Review of Grief and Depression in Adults

Anxiety in Early Adolescents During the Covid-19 Pandemic

A .gov website belongs to an official government organization in the United States.
A lock ( ) or https:// means you've safely connected to the .gov website. Share sensitive information only on official, secure websites.
- About Sleep
- Sleep Facts
- Sleep Resources
Sleep Facts and Stats
At a glance.
- The amount of sleep you need changes as you age.
- Several national surveys are used to study insufficient sleep (or short sleep duration) in U.S. children, high school students, and adults.
- These surveys show that not getting enough sleep has varied by age, location, racial/ethnic group, and over time in the United States.
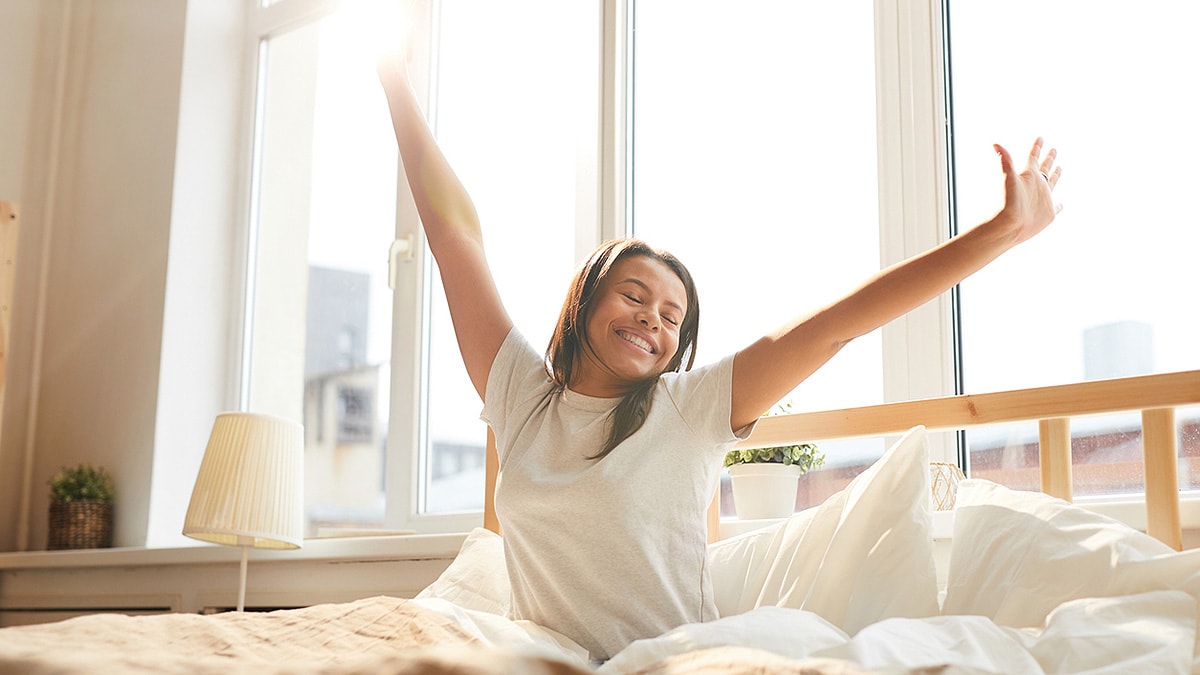
Populations
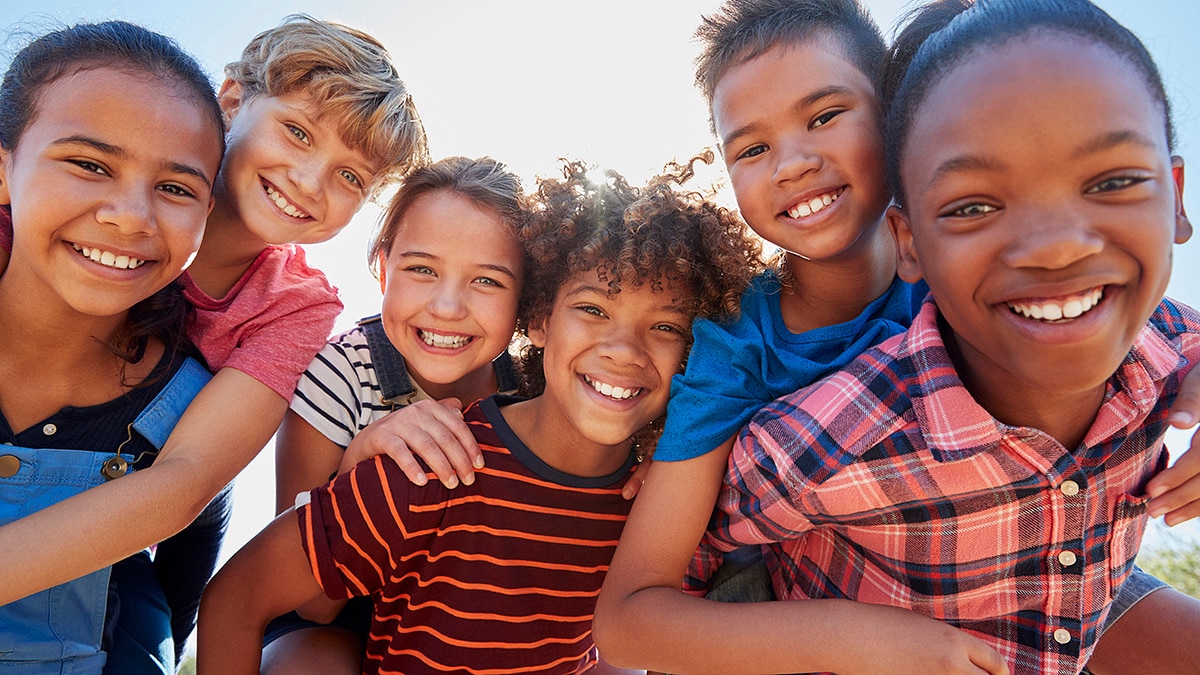
FastStats: Sleep in Children
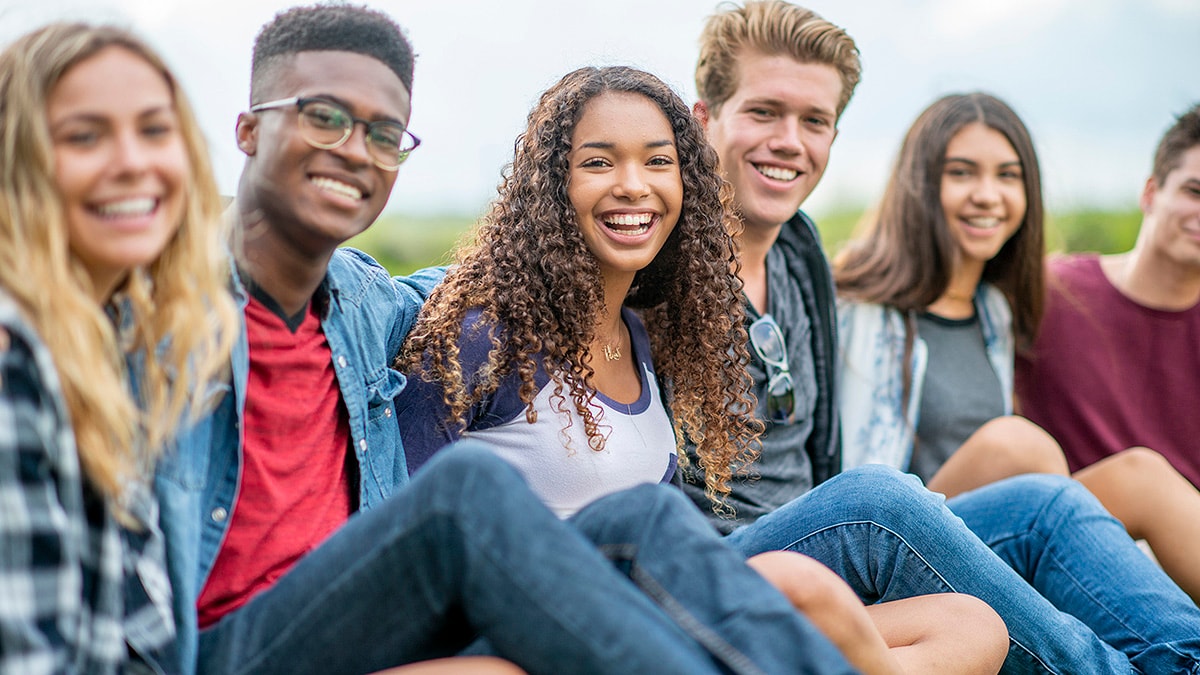
FastStats: Sleep in High School Students
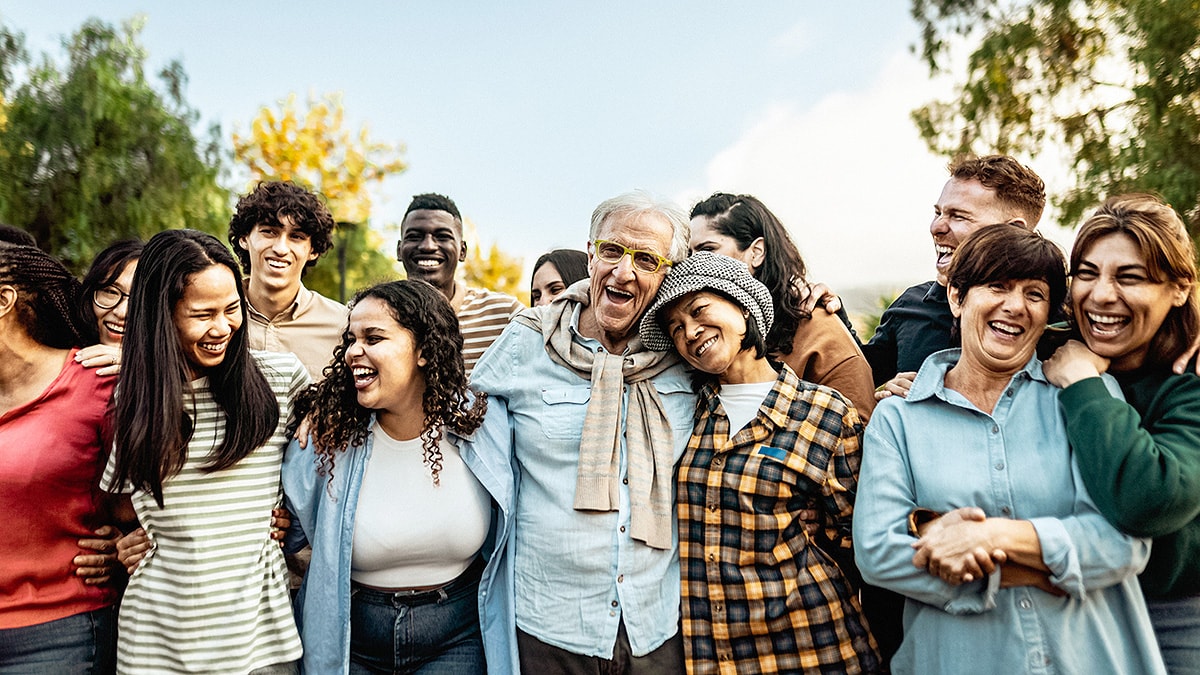
FastStats: Sleep in Adults
By sharing information and resources, CDC raises awareness about the importance of sleep health and its effect on public health.

An official website of the United States government
The .gov means it’s official. Federal government websites often end in .gov or .mil. Before sharing sensitive information, make sure you’re on a federal government site.
The site is secure. The https:// ensures that you are connecting to the official website and that any information you provide is encrypted and transmitted securely.
- Publications
- Account settings
Preview improvements coming to the PMC website in October 2024. Learn More or Try it out now .
- Advanced Search
- Journal List
- HHS Author Manuscripts

Sleep and Cognition
Maryann c. deak.
Department of Medicine, Division of Sleep Medicine, Brigham and Women’s Hospital and Harvard School of Medicine, 75 Francis Street, Boston, MA 02115, USA
Robert Stickgold
Center for Sleep and Cognition, Department of Psychiatry, Harvard Medical School, Beth Israel Deaconess Medical Center E/FD 861, 330 Brookline Ave. Boston, MA 02115, USA
Sleep is a complex physiologic state, the importance of which has long been recognized. Lack of sleep is detrimental to humans and animals. Over the past decade, an important link between sleep and cognitive processing has been established. Sleep plays an important role in consolidation of different types of memory and contributes to insightful, inferential thinking. While the mechanism by which memories are processed in sleep remains unknown, several experimental models have been proposed. This article explores the link between sleep and cognition by reviewing (1) the effects of sleep deprivation on cognition, (2) the influence of sleep on consolidation of declarative and non-declarative memory, and 3) some proposed models of how sleep facilitates memory consolidation in sleep.
The question of the function of sleep has fascinated people for thousands of years, and is now a subject of both basic and clinical research. One important facet of this question is how sleep contributes to cognitive processing and learning. A clue to the role of sleep in cognition is the detrimental effect of sleep deprivation on cognitive functioning. Over the past decade, growing interest in and experimentation on the role of sleep in learning and memory has led to an explosion of research, which has established a firm connection between sleep and memory. Although there are still many unknowns, recent studies have led to a better understanding of the contribution of sleep to cognition and of possible mechanisms underlying this relationship. This article offers an overview of current literature addressing the role of sleep in memory and cognitive processing.
Neurobiology of Sleep
Sleep is composed of physiologically and neurochemically distinct stages. Sleep stages are divided, first, into rapid eye movement sleep (REM) and non-rapid eye movement sleep (non-REM), which alternate throughout the night in a roughly 90-minute cycle ( Figure 1 ). Non-REM sleep is further divided into three stages. Stage N3, which is referred to as slow wave sleep (SWS), is prevalent during the first half of the night, while REM sleep is prevalent during the second half. †

Across the night, NREM and REM sleep cycle every 90 min in an ultradian manner, while the ratio of NREM to REM sleep shifts. During the first half of the night, NREM stage N3 (SWS) dominates, while N2 and REM sleep prevail in the latter half of the night. EEG patterns also differ significantly between sleep stages, with electrical oscillations such as K complexes and sleep spindles occurring during stage 2 NREM, slow (0.5–4Hz) delta waves developing in SWS, and theta waves seen during REM.
Transitions from wake to sleep and between the stages of sleep are accompanied by complex changes in pattern of neuronal firing and neurotransmitter release [ 1 ]. Wakefulness is a time when “all systems are go” from a neurophysiological perspective; the brainstem reticular formation is active; the pons releases norepinephrine, serotonin and acetylcholine; the posterior hypothalamus releases histamine. Orexin/hypocretin neurons in the lateral and posterior hypothalamus are also most active during wakefulness and play an important role in the stabilization of both wakefulness and sleep. Production of non-REM sleep is coordinated by the ventrolateral preoptic nucleus in the anterior hypothalamus. During non-REM sleep, norepinephrine, serotonin, acetylcholine and histamine release are decreased. The initiation of REM sleep is coordinated by communication between aminergic neurons, which produce norepinephrine, serotonin and histamine, and cholinergic neurons. During REM sleep, the aminergic neurons become nearly silent, while cholinergic neurons become highly active. These profound changes in neurophysiological state seen across the sleep cycle, with changes both in the activity of neuronal networks and in the neurochemical milieu of the brain, suggest that sleep evolved as a period of altered cognitive processing.
Effects of Sleep Deprivation
One element essential to understanding the role of sleep in cognition is observing the effects of lack of sleep on cognitive processing. This is particularly relevant in our society because of the common practices of acute sleep deprivation and chronic sleep restriction. While the impact of acute, total sleep deprivation is well established [ 2 ], the effects of chronic sleep restriction have only more recently been studied under carefully controlled conditions. In one seminal study, young adults were restricted to 4, 6, or 8 hours of time in bed per night for 14 days, under controlled laboratory conditions [ 3 ] ( Figure 2 ). Several times per day, subjects performed a psychomotor vigilance test (PVT) of attention, a digit-symbol substitution task (DSST) test of working memory, and a serial addition/subtraction test (SAST) of cognitive throughput. The performance of subjects restricted to 4 and 6 hours in bed per night worsened progressively over the 14 days on all cognitive tasks. After 14 days, their cognitive performance was similar to that of subjects who are totally sleep deprived for 24 to 48 hours. Similar findings of progressive worsening of performance on the PVT were reported in a second study in which time in bed was restricted to 3, 5, or 7 hours, over a shorter, 7 day period [ 4 ].

Three different neurobehavioral assays served to measure cognitive performance capability and subjective sleepiness. Each panel displays group averages for subjects in the 8 h (red lines), 6 h (green lines), and 4 h (lines) chronic sleep period conditions across 14 days, and in the 0 h (blue lines) sleep condition across 3 days. Upward corresponds to worse performance on the PVT (left), and to better performance on the DSST (center) and the SAST (right). The curves represent statistical non-linear model-based best-fitting profiles of the response to sleep deprivation. The mean ± s.e.m. ranges of performance for 1 and 2 days of total sleep deprivation are shown as light and dark gray bands, respectively, allowing comparison with the 14-day chronic sleep restriction conditions. For the DSST and SAST, these gray bands are curved parallel to the practice effect displayed by the subjects in the 8 h sleep period condition to compensate for different amounts of practice on these tasks. Adapted from Van Dongen et al. (2003) .
In addition to attention and memory tasks, sleep deprived patients have also shown deficits in executive function. In particular, several studies have demonstrated impairment on neuropsychological tests that depend on the prefrontal cortex [ 5 , 6 ]. Patients acutely sleep deprived for just 30 hours demonstrated deficits in verbal fluency, creative thinking and nonverbal planning.
Cognitive deficits in the setting of sleep deprivation have also been demonstrated in real-life circumstances demanding high level functioning. One such example is a series of studies performed on medical interns while “on the job”. In one of these studies, interns worked either a traditional schedule of 85 hours per week, or a modified schedule averaging 65 hours of work per week, which resulted in an additional 6 hours of sleep per week [ 7 ]. Interns working the traditional schedule had more than twice the rate of attention failures during work, as measured by the intrusion of slow rolling eye movements into wakefulness. Another study found that medical interns working in the intensive care unit on a traditional schedule consisting of extended shifts of greater than 24 hours made 36 percent more serious medical errors than interns on a modified schedule with shorter shifts and less total hours per week [ 8 ].
The question of what specific alterations in brain activation might underlie changes in performance in sleep-deprived individuals has been explored with functional magnetic resonance imaging (fMRI) [ 9 , 10 ]. Brain regions activated during performance of cognitive tasks in the sleep-deprived condition differ from those activated in the rested condition, in a manner that appears to be task-dependent. During performance of an arithmetic task, several brain regions that were activated in the rested condition, including prefrontal cortex, were less activated in the sleep-deprived condition [ 9 ]. But for a verbal memory task, not only were the areas activated in the rested condition maintained during sleep-deprivation, additional novel areas became activated in the sleep-deprived condition [ 10 ]. Increased activation in one of these areas, the parietal lobes, correlated with improved performance (even though performance overall was worse in the sleep-deprived condition), suggesting that this new area of activation can play a compensatory role. In summary, sleep deprivation results in dynamic changes in patterns of brain activation. These changes may result in either increased or depressed activity in brain regions typically required for a given task. In other instances, attempts to compensate for the negative effects of lack of sleep on cognition appear to recruit entirely new regions of the brain.
Taken as a whole, these and other studies of cognitive deficits associated with both acute total sleep deprivation and chronic sleep restriction paint a picture of dramatic impairment in attention, memory and executive function caused by lack of sleep, whether tested in the laboratory or under real-life workplace conditions. This impairment is accompanied by fundamental changes in brain activation patterns, which play an important role in determining how an individual performs when deprived of sleep.
Sleep-Dependent Memory Consolidation
Over the past 10 years, a growing body of literature has supported a role for sleep in the consolidation of several types of memory. This includes both declarative and non-declarative memory. By definition, non-declarative memory includes memories that cannot be recalled to conscious awareness. One type of non-declarative memory is procedural memory (for example, how to play tennis). Declarative memory is information that can be retrieved into conscious awareness, including memory for specific life events (episodic memory; e.g. , eating dinner last night) and memory for facts and general knowledge (semantic memory; e.g. , the capital of France).
Non-Declarative Memory
Sleep-dependent consolidation or enhancement has been demonstrated for several non-declarative procedural tasks, including visual and auditory discrimination tasks, motor sequence tasks and a motor adaptation task. The first task to show clear sleep-dependent enhancement was a visual texture discrimination task (VDT), developed by Karni et al. In this procedural task, subjects identify the orientation of an array of three diagonal bars embedded in a background of horizontal bars [ 11 ]. Having reported that task improvement could be seen 6 to 8 hours after training [ 12 ], they went on to demonstrate that performance improved over a night of sleep, but only when subjects were allowed REM sleep [ 13 ]. These results are confirmed by an experiment that compared performance on the VDT in subjects who were retested hours after training on the same day to subjects retested after a night of sleep [ 12 ]. The study found that overnight improvement was significantly better than daytime improvement [ 14 ] ( Figure 3a ) and correlated with the number of hours of post-training sleep [ 14 ]. While the initial study of Karni et al [ 12 ] suggested that the simple passage of time could lead to improvement, subsequent studies found improvement only when this time included sleep. When subjects’ sleep was recorded in the laboratory, overnight improvement correlated with both the amount of SWS early in the night and of REM sleep late in the night [ 14 ]. One to six additional nights of sleep after training resulted in significantly more improvement than seen after only one night [ 15 ]. In addition, subjects who were deprived of sleep the night following training did not demonstrate significant improvement on the VDT, even after two nights of recovery sleep [ 15 ]. Thus, sleep, and not the simple passage of time, appears to be required for post-training memory consolidation and improvement in performance.

Three different procedural tasks show sleep dependent improvements in performance. (A) Visual discrimination task: performance improvements were seen only in groups retested after a night of sleep (green circles), and not in groups retested after equivalent amounts of daytime wake (red circles). Adapted from Stickgold et al. 2000 . (B) Finger-tapping motor sequence task: performance improvements were seen only after a night of sleep (green bars). Left panel – subjects trained in the morning and retested that evening (PM) and again the next morning (AM); Center panel – the same as Left panel, except subjects wore mittens across the initial period of daytime wakefulness to eliminate interference as a possible cause of the lack of PM improvement; Right panel – subjects trained in the evening and retested the following morning and again that evening show improvement at both post-sleep tests. Adapted from Walker et al. 2002 . (C) Motor adaptation to a virtual sideways force shows improved accuracy in direction of movement after sleep, but not after an equivalent period of daytime wake. Adapted from Huber et al. 2004 .
As in the case of the VDT, the first hint that sleep was essential to the process of learning motor tasks came from the notion that the passage of time, in addition to practice alone, was required for improvement in motor skills [ 16 ]. In one experiment, subjects were asked to tap two five-element sequences of finger-to-thumb opposition [ 16 ]. Although improvement in speed and accuracy was seen during training, a marked further improvement was seen 24 hours later in the absence of additional training. When tested with a computerized version of this test, which involved typing the same numeric sequence (4-1-3-2-4), subjects demonstrated no further significant improvement when retested after 12 hours of daytime wake [ 17 ] ( Figure 3b ). However, when retested after 12 hours that contained a night of sleep, the subjects improved in speed by 20% ( Figure 3b ). Thus sleep, rather than the simple passage of time, appears to mediate this offline improvement in motor performance [ 18 , 19 ].
Another motor task where sleep dependence has been demonstrated is a motor adaptation task. In this computerized task, subjects move a cursor on the screen between a central starting point and one of eight targets locations, while overcoming an automatic rotation in the cursor at a fixed angle from hand position [ 20 ]. When tested 8 hours after training, mean directional error was significantly reduced in a group retested after an eight hour night of sleep compared to a group retested after 8 hours of daytime wakefulness ( Figure 3c ).
Declarative Memory
While the role of sleep in the consolidation of declarative memory was at one time controversial, recent evidence has increasingly supported such a function. Several studies have found a significant benefit of sleep on a declarative task that involves memorization of word pairs. Two studies suggested that subjects recalled word pairs better after sleeping during just the first half of a night of sleep than just the second half [ 21 ] [ 22 ] ( Figure 4a ). Additionally, subjects that were permitted to sleep, either early or late in the sleep interval, performed better than subjects that stayed awake. Since the first half of the night is rich in slow wave sleep, the authors concluded that slow wave sleep is likely playing an important role in the protection or enhancement of declarative memories [ 21 ] [ 22 ]. The benefits of sleep was also seen on the word pairs task in subjects who took daytime naps that contained only non-REM sleep when compared to subjects who stayed awake between training and retest [ 23 ]. Interestingly, sleep that occurs soon after a period of learning appears to be more beneficial than sleep that is delayed for several hours after learning [ 24 ].

Two different memory tasks show sleep-dependent improvements in performance. (A) Benefits of early and late night sleep on the paired associates list: performance improvement was significantly greater following early sleep (green bar left) compared to the early wake control (red bar left), and compared to late sleep (green bar right). There was no significant difference between the late sleep (green bar right) and late wake (red bar right) groups. * p< 0.05; **p<0.01. Adapted from Plihal and Born (1997) . (B) Paired associates task and effect of interference: there was a modest non-significant improvement in performance after sleep (green bar left compared to wake (red bar left). Performance improved significantly after sleep for the group that underwent interference training (green bar right) compared to the interference wake group (red bar right) and significantly more so than in the no interference condition (left). (*) 0.05 ≤ p ≤ 0.10, *p< 0.05, ***p<0.001. Adapted from Ellenbogen et al (2006) .
Sleep also benefits declarative memory by protecting such memories from interference. Ellenbogen et al. demonstrated this concept with an experiment during which subjects learn a list of unrelated word pair associates (A-B pairs), similar to previous word pair experiments [ 25 ]. After a 12-hour interval that contained either nocturnal sleep or daytime wakefulness, subjects in the interference group learned a new list of word pairs (A-C pairs), in which words from the original list (A) were paired with new words (C). All participants were then retested on the original list. Patients that did not undergo the interference testing demonstrated a modest, non-significant benefit from sleep ( Figure 4b ). However, for the group that underwent interference training, there was a large and significant benefit from sleep (76% correct recall versus 32% in the “wake” group) ( Figure 4b ). This study supports a role of sleep not only in rote memorization of words, but also in protection of memories that are threatened by subsequent associative interference.
A growing body of literature supports a special role of sleep in the consolidation of emotional declarative memories. During one experiment, subjects viewed both neutral and emotionally arousing pictures in the evening or in the morning [ 26 ]. Subjects again viewed the pictures intermixed with new pictures twelve hours later, after either sleeping or staying awake. The subjects were asked to identify which pictures they consciously remembered from the previous session, which pictures were only familiar, and which they thought were new. Subject performance improved overall after sleep. Most striking was the influence of sleep on recognition of emotional pictures, with recognition (identification of pictures as familiar by subjects) in the subjects who slept during the 12-hour interval between the first and second viewing being 42% greater than in the wake controls, while identification of neutral pictures did not differ significantly between the wake and sleep subjects. The study thus suggests that sleep may permit preferential retention of memories based on emotional content.
In another experiment, subjects were exposed to scenes involving either negative arousing objects or neutral objects, displayed on neutral background scenes [ 27 ]. After studying the pictures during baseline training, one group of subjects was retested 30 minutes later, while two additional groups were tested after a 12-hour period either of daytime wake or containing a night of sleep. Compared to performance at 30 minutes, subjects retested after a night of sleep were significantly better at recognizing negative images they had previously seen than the subjects tested after a period of wake ( Figure 5 ). Moreover, this improvement was not seen for the neutral images or for the neutral image backgrounds, suggesting that sleep permitted consolidation of memory for the emotional objects to the detriment of neutral memory. The emotional memory retention bias seen after periods of sleep may relate to an unconscious perception that emotional memories are most relevant to the individual; and, thus, sleep is playing a role in preserving that information which is deemed most significant.

Change in recognition rate of emotional or neutral images is demonstrated 12 hours from initial encoding compared to 30 minutes after encoding. Left: Neutral objects (striped bars) and their neutral backgrounds (solid bars) both show 6-11% deterioration across the 12 hr interval, whether across a period of daytime wake or across a night with sleep; Right: The same pattern is seen for emotional objects (striped bars) and neutral backgrounds (solid bars), except that emotional objects are recognized significantly better after a night of sleep than either objects or backgrounds in any other condition.
Sleep and Insight
In addition to playing a role in the stabilization and enhancement of different types of memory, sleep has also been implicated in the process of gaining creative insight and making broad connections among learned information. In an experiment by Wagner et al, subjects were presented with a task where discovery of a hidden rule greatly improves speed of performance [ 28 ]. After initial training, subjects were retested after an 8-hour period, which contained a night of sleep, a night of wake, or a day of wake. Sleep dramatically increased the likelihood of grasping the hidden rule, with 59% of the sleep group gaining insight compared with 23% of either group that stayed awake ( Figure 6a ). Similarly, Ellenbogen et al. looked at the concept of “relational memory”, which involves placing learned information in a context and making inferences by relating individual pieces of information [ 29 ]. Subjects were presented with a series of “premise pairs”, such as ”choose A over B”, “B over C”, “C over D”, and “D over E”. At retest 20 minutes, 12 hours, or 24 hours later, subjects were tested on their memory for these premise pairs, but also on their ability to integrate these premise pairs to infer, for example, that they should “choose B over D”. The ability to draw inferences was greatly improved after 12 or 24 hours compared to 20 minutes. Moreover, when a delay of 12 hours over a day of wake was compared to 12 hours over a night of sleep, subjects who slept between training and retest performed better with inference pairs that had a greater degree of separation, for example inferring that they should “choose B over E” ( Figure 6b ).

(A) Number reduction task: subjects were asked to determine the final digit in a series by processing digits from left to right according to two simple rules. However, subjects who gained insight into a hidden rule could determine the correct response after only the second digit presentation versus the sixth in those who did not gain insight. After initial training, subjects were retested after periods of wake or sleep – W/D: daytime wake; W/N: overnight sleep deprivation; S/N: nocturnal sleep. Subjects who slept had more than twice the chance of gaining insight compared to either of the wake groups. Adapted from Wagner et al (2004) . (B) Inference pair performance: After initial training, subjects were tested after 12 hours of daytime wake (red), 12 hours including a night of sleep (green), or a full 24-hr day (blue). 1°: first order association ( e.g. , A => C); 2°: second order association ( e.g. , A => D). In the wake group (red), there was no difference in performance between first and second order associations. Both sleep groups (green and blue) performed significantly better on second order associations compared to first order associations. * p<0.05. Adapted from Ellenbogen et al (2007) .
Sleep can also support the development and maintenance of a “big picture” view, enhancing the “gist” of a memory, at the expense of detail. This is evident in Payne et al.’s examination of the role of sleep in false memory formation [ 30 ]. Here, subjects were presented with a list of related words. However, a “critical word”, which connected all of the words in the list, was missing. For example, words like “ledge, sill, curtain and open” were present, but not “window”. Not only did subjects tested after 12 hours of sleep forget fewer of the studied words that did subjects tested after 12 hours of wake, but they also showed a numerical increase in recall of critical or gist words, which subjects falsely believe to be part of the original list, while those in the wake group showed a significant deterioration in the “recall”.
What aspect of sleep permits the incorporation of new information in these lists? Cai et al. studied the role of sleep in the development of associative networks [ 31 ]. The presence of REM sleep during a daytime nap, independent of total sleep time, enhanced performance in creative problem solving for items that subjects were exposed to prior to sleep. Another approach to understanding the processing of information in such associative networks has been to awaken subjects out of different sleep stages and quickly test their performance on various cognitive tasks [ 32 ] [ 33 ]. In one experiment, subjects were presented with a semantic priming task, which is designed to determine the strength of association between words [ 32 ]. Subjects were awakened out of REM and non-REM sleep for testing, as well as tested pre- and post-sleep. As expected, the associative strength of strongly related pairs (such as “hot – cold”) was markedly greater than that of weakly related pairs (such as “thief – wrong”) after awakenings from NREM sleep. In contrast, when subjects were awakened from REM sleep, the normally weakly related pairs showed greater associative strength than did the normally strongly related pairs. These results suggest that REM sleep may play an important role in identifying and strengthening previous weak cognitive associations. Another study compared performance on anagram word puzzles during wake and during awakenings from REM and non-REM sleep [ 33 ]. The number of anagrams solved following REM awakenings was 32% higher than those solved following non-REM awakenings, again suggesting that cognitive processing during REM may be a vital component in creative thinking and problem solving.
However, the role of REM sleep remains controversial. Individuals who have decreased amounts of REM sleep from medications or rare cases of brainstem damage appear to function normally [ 34 ]. Some studies involving suppression of REM sleep do not demonstrate impairments in learning, although creative insight has not specifically been tested [ 35 ]. The role of specific stages of sleep will be discussed in greater detail below.
Models of Memory Processing in Sleep
The mechanisms by which sleep facilitates memory consolidation are unclear. Several theoretical models have been proposed, not all of which are compatible. We review some of the major working theories here.
The first question is what processes occur in the brain during sleep that result in improved memory. One suggestion is the idea of “replay” of brain activity, i.e. reemergence of brain activity in sleep that occurred during learning in prior wakefulness. Wilson et al examined brain activity at the neuronal level in rats [ 36 ] [ 37 ]. They noted that hippocampal cells that fired together during a spatial behavioral task had an increased tendency to fire together during subsequent slow wave sleep, and tended to replicate sequences of neuronal firing representing movement along a spatial path. Peigneux et al. examined regional brain activity in humans by measuring cerebral blood flow with positron emission tomography (PET) [ 38 ]. They noted that hippocampal areas that were activated in human subjects while learning a route in a virtual town showed increased activation during subsequent slow wave sleep. Additionally, the amount of hippocampal activity seen during slow wave sleep correlated with task performance the next day. Maquet et al. also demonstrated regional activation using PET, this time with a serial reaction time task [ 39 ]. Multiple regions of the brain that were active during performance of the task were significantly more active during REM sleep in subjects who had recently executed the task than in those who had not trained on the task. These findings and those from other laboratories have led many researchers to conclude that recently encoded memories are reactivated and “replayed” during sleep, and that this replay mediates the memory processing seen to occur during sleep. In contrast to this notion that replay during sleep strengthens memories is the theory of “synaptic homeostasis”, which proposes that during sleep, particularly slow wave sleep, a process of synaptic downscaling occurs [ 40 ]. During this process total synaptic strength in the cortex is dramatically decreased, as a means of conserving energy and space within the brain. According to some formulations of this model, such downscaling could indirectly benefit learning and memory.
In addition to defining the processes, such as memory replay, that enhance specific memories during sleep, another important question is how sleep facilitates these processes. One approach to this question is to look at specific neurophysiologic features of sleep. One candidate is sleep spindles – short (~ 1 sec) bursts of rhythmic 12 to 16 Hertz (Hz) activity seen in the electroencephalogram (EEG) during non-REM sleep, which create feedback loops between the thalamus and the cortex [ 41 ]. These thalamo-cortical loops are believed to facilitate synapse formation and strengthening and thereby enhance memory [ 36 ]. Nishida and Walker observed differences in spindle activity between the “trained” and “untrained” hemisphere during a nap after subjects trained on a motor skill task [ 42 ]. The difference in spindle activity between the two hemispheres correlated with the amount of sleep-dependent improvement, with greater difference between the “learning” and “non-learning” hemispheres predicting greater improvement on the task after the nap. Other types of natural oscillation in the brain, including slow (<1 Hz) waves in the neocortex, theta (4 to 10 Hz) waves in the hippocampus, and gamma (40 to 100 Hz) waves in the cortex and thalamus, have also been implicated in sleep-dependent memory processing [ 43 ].
In addition to examining oscillatory patterns in the EEG, investigators have examined levels of hormones and neurotransmitters during sleep as possible contributors to memory consolidation. Levels of acetylcholine [ 44 ] and cortisol [ 45 ] both have been implicated in declarative memory processing during sleep.
Despite this research, the question of the mechanisms underlying sleep-dependent memory consolidation remain uncertain. It should be noted that these various models – memory replay, synaptic homeostasis, cortical oscillations, hormones and neuromodulators – are not mutually exclusive. Indeed, in the end, some or all of these processes may turn out to play a role in sleep-dependent memory processing.
Another approach to identifying mechanisms involved in sleep-dependent memory consolidation has been to investigate which stages of sleep mediate consolidation. Many studies that examine the role of sleep in memory consolidation have attempted to attribute consolidation of specific types of memory to particular stages of sleep. For example, non-declarative memory tasks have been linked to several stages of sleep, depending on the specific task in question. Consolidation of motor skills has been correlated with stage 2 sleep in the case of the motor sequence task (MST) [ 17 ] and SWS in the case of the motor adaptation task [ 20 ], while both REM sleep and SWS have been linked to performance on the visual discrimination task (VDT) [ 10 ] [ 11 ]. Declarative memory consolidation on the paired-associates word list has been correlated to non-REM sleep, specifically slow wave sleep [ 19 ] [ 20 ]. Sleep dependent improvements on other declarative tasks, where gist extraction or creative insight is required, have been correlated to REM sleep or a decrease in SWS [ 30 , 31 ].
However, many of the relationships between specific sleep stages and types of memory remain unclear and controversial. Researchers have yet to clarify which types of memory are consolidated in sleep, which components of memory consolidation occur during sleep, and how these relate to particular stages of sleep. For example, while specific sleep stages have generally been thought of as mediating consolidation for different types of memories, an alternate recently proposed model suggests that individual sleep stages mediate specific components of the consolidation process, independent of memory type [ 46 ]. According to this theory, slow wave sleep plays a role in stabilizing recently encoded memories at the synaptic level, while stage 2 and REM sleep play roles in integrating the memories into larger neuronal networks at the systems level. This systems-level consolidation links memories together and captures the essence of large amounts of information. Further studies are necessary to determine whether either or conceivably both of these models are correct.
Sleep is composed of a series of complex neurophysiological states that play important roles in learning, memory and cognitive processing. Studies that examine the effects of sleep deprivation have noted drastic deficits in cognitive processing. With careful experimentation involving various types of memory tasks, an important role for sleep in both non-declarative and declarative memory processing has been established. In addition to simply strengthening memories and improving their resistance to interference, sleep contributes to the process of gaining insight, making connections and integrating large amounts of information. Though our understanding of these processes has dramatically increased over the past 10 years, there remain many interesting, unanswered questions. The full range of specific types of memory that are consolidated during sleep remains to be clarified, as do the particular mechanisms and aspects of sleep physiology that underlie memory consolidation and cognitive processing during sleep.
† Prior to 2007, NREM sleep was classified into four stages, with NREM stages 3 and 4 collectively referred to as SWS.
Reviewer suggestions
Contributor Information
Maryann C. Deak, Department of Medicine, Division of Sleep Medicine, Brigham and Women’s Hospital and Harvard School of Medicine, 75 Francis Street, Boston, MA 02115, USA.
Robert Stickgold, Center for Sleep and Cognition, Department of Psychiatry, Harvard Medical School, Beth Israel Deaconess Medical Center E/FD 861, 330 Brookline Ave. Boston, MA 02115, USA.
Further Reading
- Stickgold R, Ellenbogen JM. Quiet! Sleeping brain at work. Scientific American Mind. 2008 Aug-Sep;:22–29. [ Google Scholar ]
- Diekelmann S, Wilhelm I, Born J. The whats and whens of sleep-dependent memory consolidation. Sleep Med Rev. 2009; 13 (5):309–321. [ PubMed ] [ Google Scholar ]
Transformer-Based Contrastive Learning Method for Automated Sleep Stages Classification
基于Transformer对比学习的自动睡眠分期方法
- Published: 10 May 2024
Cite this article
- Jin Ma ( 马进 ) 1 ,
- Ze Ren ( 任泽 ) 1 ,
- Tongtong Zhang ( 张彤彤 ) 1 ,
- Ying Ding ( 丁颍 ) 2 ,
- Yilei Lu ( 陆熠磊 ) 1 &
- Yinghong Peng ( 彭颖红 ) 3
24 Accesses
Explore all metrics
Automated sleep stages classification facilitates clinical experts in conducting treatment for sleep disorders, as it is more time-efficient concerning the analysis of whole-night polysomnography (PSG). However, most of the existing research only focused on public databases with channel systems incompatible with the current clinical measurements. To narrow the gap between theoretical models and real clinical practice, we propose a novel deep learning model, by combining the vision transformer with supervised contrastive learning, realizing the efficient sleep stages classification. Experimental results show that the model facilitates an easier classification of multi-channel PSG signals. The mean F1-scores of 79.2% and 76.5% on two public databases outperform the previous studies, showing the model’s great capability, and the performance of the proposed method on the children’s small database also presents a high mean accuracy of 88.6%. Our proposed model is validated not only on the public databases but the provided clinical database to strictly evaluate its clinical usage in practice.
自动睡眠分期由于其在分析整晚多导睡眠(PSG)信号方面具有高效性, 能够有效支持临床专家对睡眠障碍进行诊疗。然而, 现有的研究主要集中在与实际临床数据不相同的公共数据集上。为了缩小理论模型与实际临床实践之间的差距, 提出了一种新的深度学习模型, 将视觉Transformer与监督对比学习相结合, 实现有效的睡眠阶段分期。实验结果表明, 该模型能够更有效地对多通道PSG信号进行分期。在两个公开的睡眠数据库上该模型平均F1得分分别为79.2%和76.5%, 优于之前的研究, 表明了该模型强大的能力, 在儿童小数据库上的平均准确率也达到了88.6%。提出的模型不仅在公共数据库上进行了验证, 而且在提供的临床数据库上进行了验证, 以严格评估其在临床实践中的使用情况。
This is a preview of subscription content, log in via an institution to check access.
Access this article
Price includes VAT (Russian Federation)
Instant access to the full article PDF.
Rent this article via DeepDyve
Institutional subscriptions
Similar content being viewed by others
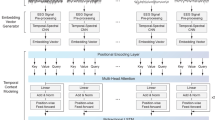
SleepExpertNet: high-performance and class-balanced deep learning approach inspired from the expert neurologists for sleep stage classification
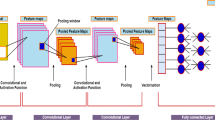
Automated classification of multi-class sleep stages classification using polysomnography signals: a nine- layer 1D-convolution neural network approach
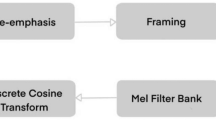
An automatic method using MFCC features for sleep stage classification
OHAYON M M. Epidemiological overview of sleep disorders in the general population [J]. Sleep Medicine Research , 2011, 2 (1): 1–9.
Article Google Scholar
TOBALDINI E, COSTANTINO G, SOLBIATI M, et al. Sleep, sleep deprivation, autonomic nervous system and cardiovascular diseases [J]. Neuroscience and Biobehavioral Reviews , 2017, 74 (Pt B): 321–329.
MAJDE J, KRUEGER J. Links between the innate immune system and sleep [J]. Journal of Allergy and Clinical Immunology , 2005, 116 (6): 1188–1198.
BARONE D A, CHOKROVERTY S. Neurologic diseases and sleep [J]. Sleep Medicine Clinics , 2017, 12 (1): 73–85.
ALI I ABOALAYON K, FAEZIPOUR M, AL-MUHAMMADI W S, et al. Sleep stage classification using EEG signal analysis: A comprehensive survey and new investigation [J]. Entropy , 2016, 18 (9): 272.
Berry R, QUAN S, Abreu A, et al. The AASM Manual for the Scoring of Sleep and Associated Events: Rules, Terminology and Technical Specifications, Version 2.6 [S]. Darien: American Academy of Sleep Medicine, 2020.
Google Scholar
TIAN P, HU J, QI J, et al. A hierarchical classification method for automatic sleep scoring using multi-scale entropy features and proportion information of sleep architecture [J]. Biocybernetics and Biomedical Engineering , 2017, 37 (2): 263–271.
HASSAN A R, BASHAR S K, BHUIYAN M I H. On the classification of sleep states by means of statistical and spectral features from single channel Electroencephalogram [C]// 2015 International Conference on Advances in Computing, Communications and Informatics . Kochi: IEEE, 2015: 2238–2243.
SEIFPOUR S, NIKNAZAR H, MIKAEILI M, et al. A new automatic sleep staging system based on statistical behavior of local extrema using single channel EEG signal [J]. Expert Systems with Applications , 2018, 104 : 277–293.
LECUN Y, BENGIO Y, HINTON G. Deep learning [J]. Nature , 2015, 521 (7553): 436–444.
MOUSAVI S, AFGHAH F, ACHARYA U R. SleepEEGNet: Automated sleep stage scoring with sequence to sequence deep learning approach [J]. PloS One , 2019, 14 (5): e0216456.
ZHANG H, WANG X, LI H, et al. Auto-annotating sleep stages based on polysomnographic data [J]. Patterns , 2022, 3 (1): 100371.
PERSLEV M, JENSEN M H, DARKNER S, et al. U-time: A fully convolutional network for time series segmentation applied to sleep staging [DB/OL]. (2019-10-24) [2023-05-16]. http://arxiv.org/abs/1910.11162
ZHONG Q H, LEI H B, CHEN Q R, et al. A sleep stage classification algorithm of wearable system based on multiscale residual convolutional neural network [J]. Journal of Sensors , 2021, 2021 : 8222721.
MICHIELLI N, ACHARYA U R, MOLINARI F. Cascaded LSTM recurrent neural network for automated sleep stage classification using single-channel EEG signals [J]. Computers in Biology and Medicine , 2019, 106 : 71–81.
PHAN H, CHEN O Y, TRAN M C, et al. XSleepNet: Multi-view sequential model for automatic sleep staging [J]. IEEE Transactions on Pattern Analysis and Machine Intelligence , 2022, 44 (9): 5903–5915.
JIN H H, YIN H B, HE L N. End-to-end single-channel automatic staging model for sleep EEG signal [J]. Computer Science , 2019, 46 (3): 242–247 (in Chinese).
JEON Y, KIM S, CHOI H S, et al. Pediatric sleep stage classification using multi-domain hybrid neural networks [J]. IEEE Access , 2019, 7 : 96495–96505.
SUPRATAK A, DONG H, WU C, et al. DeepSleepNet: A model for automatic sleep stage scoring based on raw single-channel EEG [J]. IEEE Transactions on Neural Systems and Rehabilitation Engineering , 2017, 25 (11): 1998–2008.
SUPRATAK A, GUO Y K. TinySleepNet: An efficient deep learning model for sleep stage scoring based on raw single-channel EEG [C]//2020 42nd Annual International Conference of the IEEE Engineering in Medicine & Biology Society . Montreal: IEEE, 2020: 641–644.
KEMP B, ZWINDERMAN A H, TUK B, et al. Analysis of a sleep-dependent neuronal feedback loop: The slow-wave microcontinuity of the EEG [J]. IEEE Transactions on Bio-Medical Engineering , 2000, 47 (9): 1185–1194.
GHASSEMI M M, MOODY B E, LEHMAN L W H, et al. You snooze, you win: The PhysioNet/computing in cardiology challenge 2018 [C]// 2018 Computing in Cardiology Conference . Maastricht: IEEE, 2018: 1–4.
WOLPERT E A. A manual of standardized terminology, techniques and scoring system for sleep stages of human subjects [J]. Archives of General Psychiatry , 1969, 20 (2): 246.
BOLSTAD B M, IRIZARRY R A, ASTRAND M, et al. A comparison of normalization methods for high density oligonucleotide array data based on variance and bias [J]. Bioinformatics , 2003, 19 (2): 185–193.
CHEN W X, MCDUFF D. DeepPhys: Video-based physiological measurement using convolutional attention networks [DB/OL]. (2018-05-21) [2023-05-16]. http://arxiv.org/abs/1805.07888
EOM H, LEE D, HAN S, et al. End-to-end deep learning architecture for continuous blood pressure estimation using attention mechanism [J]. Sensors , 2020, 20 (8): 2338.
GUILLOT A, THOREY V. RobustSleepNet: Transfer learning for automated sleep staging at scale [J]. IEEE Transactions on Neural Systems and Rehabilitation Engineering , 2021, 29 : 1441–1451.
VASWANI A, SHAZEER N, PARMAR N, et al. Attention is all you need [C]// 31st Conference on Neural Information Processing Systems . LongBeach: NIPS, 2017: 1–11.
DOSOVITSKIY A, BEYER L, KOLESNIKOV A, et al. An image is worth 16x16 words: Transformers for image recognition at scale [DB/OL]. (2020-10-22) [2023-05-16]. http://arxiv.org/abs/2010.11929
HE K, ZHANG X, REN S, et al. Deep residual learning for image recognition [C]// IEEE Conference on Computer Vision and Pattern Recognition . Las Vegas: IEEE, 2016: 770–778.
KRIZHEVSKY A, SUTSKEVER I, HINTON G E. ImageNet classification with deep convolutional neural networks [J]. Communications of the ACM , 2017, 60 (6): 84–90.
KRIZHEVSKY A. Learning multiple layers of features from tiny images [D]. Toronto: University of Toronto, 2009.
DEVLIN J, CHANG M W, LEE K, et al. BERT: Pre-training of deep bidirectional transformers for language understanding [DB/OL]. (2018-10-11) [2023-05-16]. http://arxiv.org/abs/1810.04805
BA J L,KIROS J R,HINTON G E. Layer normalization [DB/OL]. (2016-07-21) [2023-05-16]. http://arxiv.org/abs/1607.06450
BHATT D, PATEL C, TALSANIA H, et al. CNN variants for computer vision: History, architecture, application, challenges and future scope [J]. Electronics , 2021, 10 (20): 2470.
YIN W P, KANN K, YU M, et al. Comparative study of CNN and RNN for natural language processing [DB/OL]. (2017-02-07) [2023-05-16]. http://arxiv.org/abs/1702.01923
COHEN M X. Analyzing neural time series data: Theory and practice [M]. Cambridge: MIT Press, 2014
Book Google Scholar
SAUNSHI N, PLEVRAKIS O, ARORA S, et al. A theoretical analysis of contrastive unsupervised representation learning [C]// International Conference on Machine Learning . PMLR, 2019: 5628–5637.
KHOSLA P, TETERWAK P, WANG C, et al. Supervised contrastive learning [DB/OL]. (2020-04-23) [2023-05-16]. http://arxiv.org/abs/2004.11362
SOKOLOVSKY M, GUERRERO F, PAISARNSRISOMSUK S, et al. Deep learning for automated feature discovery and classification of sleep stages [J]. IEEE/ACM Transactions on Computational Biology and Bioinformatics , 2020, 17 (6): 1835–1845.
TSINALIS O, MATTHEWS P M, GUO Y K, et al. Automatic sleep stage scoring with single-channel EEG using convolutional neural networks [DB/OL]. (2016-10-05) [2023-05-16]. http://arxiv.org/abs/1610.01683
PHAN H, ANDREOTTI F, COORAY N, et al. DNN filter bank improves 1-max pooling CNN for single-channel EEG automatic sleep stage classification [C]//2018 40th Annual International Conference of the IEEE Engineering in Medicine and Biology Society . Honolulu: IEEE, 2018: 453–456.
YOU Y Y, ZHONG X Y, LIU G Z, et al. Automatic sleep stage classification: A light and efficient deep neural network model based on time, frequency and fractional Fourier transform domain features [J]. Artificial Intelligence in Medicine , 2022, 127 : 102279.
FIORILLO L, FAVARO P, FARACI F D. DeepSleepNet-lite: A simplified automatic sleep stage scoring model with uncertainty estimates [J]. IEEE Transactions on Neural Systems and Rehabilitation Engineering , 2021, 29 : 2076–2085.
KHALILI E, MOHAMMADZADEH ASL B. Automatic sleep stage classification using temporal convolutional neural network and new data augmentation technique from raw single-channel EEG [J]. Computer Methods and Programs in Biomedicine , 2021, 204 : 106063.
BAEK J, LEE C, YU H, et al. Automatic sleep scoring using intrinsic mode based on interpretable deep neural networks [J]. IEEE Access , 2022, 10 : 36895–36906.
ZHU T Q, LUO W, YU F. Convolution-and attention-based neural network for automated sleep stage classification [J]. International Journal of Environmental Research and Public Health , 2020, 17 (11): 4152.
TAUTAN A M, ROSSI A C, DE FRANCISCO R, et al. Automatic Sleep Stage Detection using a Single Channel Frontal EEG [C]// 2019 E-Health and Bioengineering Conference . Iasi: IEEE, 2019: 1–4.
Download references
Author information
Authors and affiliations.
School of Electronic, Information and Electrical Engineering, Shanghai Jiao Tong University, Shanghai, 200240, China
Jin Ma ( 马进 ), Ze Ren ( 任泽 ), Tongtong Zhang ( 张彤彤 ) & Yilei Lu ( 陆熠磊 )
Children’s Hospital of Shanghai, Shanghai, 200062, China
Ying Ding ( 丁颍 )
School of Mechanical Engineering, Shanghai Jiao Tong University, Shanghai, 200240, China
Yinghong Peng ( 彭颖红 )
You can also search for this author in PubMed Google Scholar
Corresponding author
Correspondence to Jin Ma ( 马进 ) .
Ethics declarations
Conflict of Interest The authors declare that they have no conflict of interest.
Additional information
Foundation item: the National Natural Science Foundation of China (No. 52375254), the Interdisciplinary Program of Shanghai Jiao Tong University (No. 21X010301670), the Open Project Program of SJTU-Pinghu Institute of Intelligent Optoelectronics (No. 2022SPIOE104)
Rights and permissions
Reprints and permissions
About this article
Ma, J., Ren, Z., Zhang, T. et al. Transformer-Based Contrastive Learning Method for Automated Sleep Stages Classification. J. Shanghai Jiaotong Univ. (Sci.) (2024). https://doi.org/10.1007/s12204-024-2734-z
Download citation
Received : 16 May 2023
Accepted : 27 June 2023
Published : 10 May 2024
DOI : https://doi.org/10.1007/s12204-024-2734-z
Share this article
Anyone you share the following link with will be able to read this content:
Sorry, a shareable link is not currently available for this article.
Provided by the Springer Nature SharedIt content-sharing initiative
- sleep stages classification
- vision transformer
- contrastive learning
- polysomnography (PSG) signal
- 视觉Transformer
Document code
- Find a journal
- Publish with us
- Track your research

Predicting Adverse Behavior in Individuals with Autism Spectrum Disorder Through Off-body Sleep Analysis
- Find this author on Google Scholar
- Find this author on PubMed
- Search for this author on this site
- ORCID record for Yashar Kiarashi
- For correspondence: [email protected]
- Info/History
- Preview PDF
Poor sleep quality in Autism Spectrum Disorder (ASD) individuals is linked to severe daytime behaviors. This study explores the relationship between a prior night's sleep structure and its predictive power for next-day behavior in ASD individuals. The motion was extracted using a low-cost near-infrared camera in a privacy-preserving way. Over two years, we recorded overnight data from 14 individuals, spanning over 2,000 nights, and tracked challenging daytime behaviors, including aggression, self-injury, and disruption. We developed an ensemble machine learning algorithm to predict next-day behavior in the morning and the afternoon. Our findings indicate that sleep quality is a more reliable predictor of morning behavior than afternoon behavior the next day. The proposed model attained an accuracy of 74% and a F1 score of 0.74 in target-sensitive tasks and 67% accuracy and 0.69 F1 score in target-insensitive tasks. For 7 of the 14, better-than-chance balanced accuracy was obtained (p-value<0.05), with 3 showing significant trends (p-value<0.1). These results suggest off-body, privacy-preserving sleep monitoring as a viable method for predicting next-day adverse behavior in ASD individuals, with the potential for behavioral intervention and enhanced care in social and learning settings.
Competing Interest Statement
The authors have declared no competing interest.
Funding Statement
This work was supported by were partially supported by the James M. Cox Foundation and Cox Enterprises Inc in support of Emorys Brain Health Center and Georgia Institute of Technology. GC is partially supported by the National Center for Advancing Translational Sciences of the National Institutes of Health under Award Number UL1TR002378.
Author Declarations
I confirm all relevant ethical guidelines have been followed, and any necessary IRB and/or ethics committee approvals have been obtained.
The details of the IRB/oversight body that provided approval or exemption for the research described are given below:
The study received approval from the Emory Institutional Review Board under the project title "Utilizing Technology to Accurately Track Sleep and Predict Adverse Behaviors in Autism" (IRB00003823).
I confirm that all necessary patient/participant consent has been obtained and the appropriate institutional forms have been archived, and that any patient/participant/sample identifiers included were not known to anyone (e.g., hospital staff, patients or participants themselves) outside the research group so cannot be used to identify individuals.
I understand that all clinical trials and any other prospective interventional studies must be registered with an ICMJE-approved registry, such as ClinicalTrials.gov. I confirm that any such study reported in the manuscript has been registered and the trial registration ID is provided (note: if posting a prospective study registered retrospectively, please provide a statement in the trial ID field explaining why the study was not registered in advance).
I have followed all appropriate research reporting guidelines, such as any relevant EQUATOR Network research reporting checklist(s) and other pertinent material, if applicable.
We have updated the size of the figures and margins.
Data Availability
All data produced in the present study are available upon reasonable request to the authors.
View the discussion thread.
Thank you for your interest in spreading the word about medRxiv.
NOTE: Your email address is requested solely to identify you as the sender of this article.

Citation Manager Formats
- EndNote (tagged)
- EndNote 8 (xml)
- RefWorks Tagged
- Ref Manager
- Tweet Widget
- Facebook Like
- Google Plus One
- Addiction Medicine (324)
- Allergy and Immunology (628)
- Anesthesia (165)
- Cardiovascular Medicine (2379)
- Dentistry and Oral Medicine (289)
- Dermatology (207)
- Emergency Medicine (379)
- Endocrinology (including Diabetes Mellitus and Metabolic Disease) (837)
- Epidemiology (11775)
- Forensic Medicine (10)
- Gastroenterology (703)
- Genetic and Genomic Medicine (3747)
- Geriatric Medicine (350)
- Health Economics (634)
- Health Informatics (2399)
- Health Policy (933)
- Health Systems and Quality Improvement (898)
- Hematology (341)
- HIV/AIDS (782)
- Infectious Diseases (except HIV/AIDS) (13318)
- Intensive Care and Critical Care Medicine (768)
- Medical Education (365)
- Medical Ethics (105)
- Nephrology (398)
- Neurology (3508)
- Nursing (198)
- Nutrition (527)
- Obstetrics and Gynecology (675)
- Occupational and Environmental Health (664)
- Oncology (1825)
- Ophthalmology (538)
- Orthopedics (219)
- Otolaryngology (287)
- Pain Medicine (233)
- Palliative Medicine (66)
- Pathology (446)
- Pediatrics (1035)
- Pharmacology and Therapeutics (426)
- Primary Care Research (420)
- Psychiatry and Clinical Psychology (3178)
- Public and Global Health (6145)
- Radiology and Imaging (1280)
- Rehabilitation Medicine and Physical Therapy (748)
- Respiratory Medicine (828)
- Rheumatology (379)
- Sexual and Reproductive Health (372)
- Sports Medicine (323)
- Surgery (402)
- Toxicology (50)
- Transplantation (172)
- Urology (146)
Appointments at Mayo Clinic
Kratom: unsafe and ineffective.
Users swear by kratom for mood enhancement and fatigue reduction, but safety issues and questions about its effectiveness abound.
If you read health news or visit vitamin stores, you may have heard about kratom, a supplement that is sold as an energy booster, mood enhancer, pain reliever and antidote for opioid withdrawal. However, the truth about kratom is more complicated, and the safety problems related to its use are concerning.
Kratom is an herbal extract that comes from the leaves of an evergreen tree (Mitragyna speciosa) grown in Southeast Asia. Kratom leaves can be chewed, and dry kratom can be swallowed or brewed. Kratom extract can be used to make a liquid product. The liquid form is often marketed as a treatment for muscle pain, or to suppress appetite and stop cramps and diarrhea. Kratom is also sold as a treatment for panic attacks.
Kratom is believed to act on opioid receptors. At low doses, kratom acts as a stimulant, making users feel more energetic. At higher doses, it reduces pain and may bring on euphoria. At very high doses, it acts as a sedative, making users quiet and perhaps sleepy. Some people who practice Asian traditional medicine consider kratom to be a substitute for opium.
Some people take kratom to avoid the symptoms of opioid withdrawal and because kratom may be bought more easily than prescription drugs.
Kratom is also used at music festivals and in other recreational settings. People who use kratom for relaxation report that because it is plant-based, it is natural and safe. However, the amount of active ingredient in kratom plants can vary greatly, making it difficult to gauge the effect of a given dose. Depending on what is in the plant and the health of the user, taking kratom may be very dangerous. Claims about the benefits of kratom can't be rated because reliable evidence is lacking.
Side effects and safety concerns
Although people who take kratom believe in its value, researchers who have studied kratom think its side effects and safety problems more than offset any potential benefits. Poison control centers in the United States received about 1,800 reports involving use of kratom from 2011 through 2017, including reports of death. About half of these exposures resulted in serious negative outcomes such as seizures and high blood pressure. Five of the seven infants who were reported to have been exposed to kratom went through withdrawal. Kratom has been classified as possibly unsafe when taken orally.
Kratom has a number of known side effects, including:
- Weight loss
- Chills, nausea and vomiting
- Changes in urine and constipation
- Liver damage
- Muscle pain
Kratom also affects the mind and nervous system:
- Hallucinations and delusion
- Depression and delusion
- Breathing suppression
- Seizure, coma and death
Kratom takes effect after five to 10 minutes, and its effects last two to five hours. The effects of kratom become stronger as the quantity taken increases. In animals, kratom appears to be more potent than morphine. Exposure to kratom has been reported in an infant who was breastfed by a mother taking kratom.
Many of the problems that occur with pain medications happen when these drugs are used at high doses or over a long period of time. It's not known exactly what level of kratom is toxic in people, but as with pain medications and recreational drugs, it is possible to overdose on kratom.
Research shows little promise
At one time, some researchers believed that kratom might be a safe alternative to opioids and other prescription pain medications. However, studies on the effects of kratom have identified many safety concerns and no clear benefits.
Kratom has been reported to cause abnormal brain function when taken with prescription medicines. When this happens, you may experience a severe headache, lose your ability to communicate or become confused.
In a study testing kratom as a treatment for symptoms of opioid withdrawal, people who took kratom for more than six months reported withdrawal symptoms similar to those that occur after opioid use. Too, people who use kratom may begin craving it and require treatments given for opioid addiction, such as naloxone (Narcan) and buprenorphine (Buprenex).
Kratom also adversely affects infant development. When kratom is used during pregnancy, the baby may be born with symptoms of withdrawal that require treatment.
In addition, substances that are made from kratom may be contaminated with salmonella bacteria. As of April 2018, more than 130 people in 38 states became ill with Salmonella after taking kratom. Salmonella poisoning may be fatal, and the U.S. Food and Drug Administration has linked more than 35 deaths to Salmonella-tainted kratom. Salmonella contamination has no obvious signs, so the best way to avoid becoming ill is to avoid products that may contain it.
Kratom is not currently regulated in the United States, and federal agencies are taking action to combat false claims about kratom. In the meantime, your safest option is to work with your doctor to find other treatment options.
There is a problem with information submitted for this request. Review/update the information highlighted below and resubmit the form.
From Mayo Clinic to your inbox
Sign up for free and stay up to date on research advancements, health tips, current health topics, and expertise on managing health. Click here for an email preview.
Error Email field is required
Error Include a valid email address
To provide you with the most relevant and helpful information, and understand which information is beneficial, we may combine your email and website usage information with other information we have about you. If you are a Mayo Clinic patient, this could include protected health information. If we combine this information with your protected health information, we will treat all of that information as protected health information and will only use or disclose that information as set forth in our notice of privacy practices. You may opt-out of email communications at any time by clicking on the unsubscribe link in the e-mail.
Thank you for subscribing!
You'll soon start receiving the latest Mayo Clinic health information you requested in your inbox.
Sorry something went wrong with your subscription
Please, try again in a couple of minutes
- Chien GCC, et al. Is kratom the new "legal high" on the block?: The case of an emerging opioid receptor agonist with substance abuse potential. Pain Physician. 2017;20:E195.
- Feng L, et al. New psychoactive substances of natural origin: A brief review. Journal of Food and Drug Analysis. 2017;25:461.
- Griffin III OH, et al. Do you get what you paid for? An examination of products advertised as kratom. Journal of Psychoactive Drugs. 2016;48:330.
- Drug Enforcement Administration. Kratom (Mitragyna speciosa korth). https://www.deadiversion.usdoj.gov/drug_chem_info/kratom.pdf. Accessed April 17, 2018.
- Yusoff NHM, et al. Opioid receptors mediate the acquisition, but not the expression of mitragynine-induced conditioned place preference in rats. Behavioural Brain Research. 2017;332:1.
- Diep J, et al. Kratom, an emerging drug of abuse: A case report of overdose and management of withdrawal. Anesthesia & Analgesia Case Reports. In press. Accessed May 2, 2018.
- Swogger MT, et al. Experiences of kratom users: A qualitative analysis. Journal of Psychoactive Drugs. 2015;47:360.
- Fox J, et al. Drugs of abuse and novel psychoactive substances at outdoor music festivals in Colorado. Substance Use & Misuse. In press. Accessed May 2, 2018.
- Kowalczuk AP, et al. Comprehensive methodology for identification of kratom in police laboratories. Forensic Science International. 2013;233:238.
- Fluyua D, et al. Biochemical benefits, diagnosis, and clinical risks of kratom. Frontiers in Psychiatry. 2017;8:62.
- Castillo A, et al. Posterior reversible leukoencephalopathy syndrome after kratom ingestion. Baylor University Medical Center Proceedings. 2017;30:355.
- Grundmann O. Patterns of kratom use and health impact in the US — Results from an online survey. Drug and Alcohol Dependence. 2017;176:63.
- Drago JD, et al. The harm in kratom. The Oncologist. 2017;22:1010.
- Pizarro-Osilla C. Introducing…kratom. In press. Accessed May 2, 2018.
- Kruegel AC, et al. The medicinal chemistry and neuropharmacology of kratom: A preliminary discussion of a promising medicinal plant and analysis of its potential for abuse. Neuropharmacology. In press. Accessed May 2, 2018.
- Ismail I, et al. Kratom and future treatment for the opioid addiction and chronic pain: Periculo beneficium? Current Drug Targets. In press. Accessed May 2, 2018.
- Singh D, et al. Kratom (Mitragyna speciosa) dependence, withdrawal symptoms and cravings in regular users. Drug and Alcohol Dependence. 2014;139:132.
- Swogger MT, et al. Kratom use and mental health: A systematic review. Drug and Alcohol Dependence. 2018;183:134.
- Food and Drug Administration. FDA investigates multistate outbreak of salmonella infections linked to products reported to contain kratom. https://www.fda.gov/Food/RecallsOutbreaksEmergencies/Outbreaks/ucm597265.htm. Accessed April 17, 2018.
- Food and Drug Administration. Statement from FDA Commissioner Scott Gottlieb, M.D., on FDA advisory about deadly risks associated with kratom. https://www.fda.gov/NewsEvents/Newsroom/PressAnnouncements/ucm584970.htm. Accessed April 17, 2018.
- Voelker R. Crackdown on false claims to ease opioid withdrawal symptoms. JAMA. 2018;319:857.
- Post S. Kratom exposures reported to United States poison control centers: 2011-2017. Clinical Toxicology. Published online February 20, 2019.
- Drug Enforcement Administration. Kratom—drug fact sheet. https://www.dea.gov/sites/default/files/2020-06/Kratom-2020.pdf. Accessed January 26, 2022.
- Therapeutic Research Center. Kratom. https://naturalmedicines.therapeuticresearch.com/databases/food,-herbs-supplements/professional.aspx?productid=1513. Accessed January 26, 2022.
- Umbehr G, et al. Acute liver injury following short-term use of the herbal supplement kratom. JAAPA. 2022;35:39.
- Medication-free hypertension control
- Alcohol: Does it affect blood pressure?
- Alpha blockers
- Ambien: Is dependence a concern?
- Angiotensin-converting enzyme (ACE) inhibitors
- Angiotensin II receptor blockers
- Antidepressant withdrawal: Is there such a thing?
- Antidepressants and alcohol: What's the concern?
- Antidepressants and weight gain: What causes it?
- Antidepressants: Can they stop working?
- Antidepressants for children and teens
- Antidepressants: Side effects
- Antidepressants: Selecting one that's right for you
- Antidepressants: Which cause the fewest sexual side effects?
- Anxiety: A cause of high blood pressure?
- Atypical antidepressants
- Automated external defibrillators: Do you need an AED?
- Beta blockers
- Beta blockers: Do they cause weight gain?
- Beta blockers: How do they affect exercise?
- Bipolar disorder
- Bipolar disorder and alcoholism: Are they related?
- Bipolar disorder in children: Is it possible?
- Bipolar medications and weight gain
- Bipolar treatment: I vs. II
- Blood pressure: Can it be higher in one arm?
- Blood pressure chart
- Blood pressure cuff: Does size matter?
- Blood pressure: Does it have a daily pattern?
- Blood pressure: Is it affected by cold weather?
- Blood pressure medication: Still necessary if I lose weight?
- Blood pressure medications: Can they raise my triglycerides?
- Blood pressure readings: Why higher at home?
- Blood pressure tip: Get more potassium
- Caffeine and hypertension
- Calcium channel blockers
- Calcium supplements: Do they interfere with blood pressure drugs?
- Can whole-grain foods lower blood pressure?
- Central-acting agents
- Choosing blood pressure medicines
- Clinical depression: What does that mean?
- Depression and anxiety: Can I have both?
- Depression, anxiety and exercise
- What is depression? A Mayo Clinic expert explains.
- Depression in women: Understanding the gender gap
- Depression (major depressive disorder)
- Depression: Supporting a family member or friend
- Diuretics: A cause of low potassium?
- High blood pressure and exercise
- Free blood pressure machines: Are they accurate?
- Home blood pressure monitoring
- Heart arrhythmia
- Heart Rhythm Conditions
- High blood pressure (hypertension)
- High blood pressure and cold remedies: Which are safe?
- High blood pressure and sex
- High blood pressure dangers
- How opioid use disorder occurs
- How to tell if a loved one is abusing opioids
- What is hypertension? A Mayo Clinic expert explains.
- Hypertension FAQs
- Hypertensive crisis: What are the symptoms?
- Insomnia: How do I stay asleep?
- Insomnia treatment: Cognitive behavioral therapy instead of sleeping pills
- Intervention: Help a loved one overcome addiction
- Isolated systolic hypertension: A health concern?
- Kratom for opioid withdrawal
- Lack of sleep: Can it make you sick?
- L-arginine: Does it lower blood pressure?
- Low blood pressure (hypotension)
- Male depression: Understanding the issues
- MAOIs and diet: Is it necessary to restrict tyramine?
- Marijuana and depression
- Medications and supplements that can raise your blood pressure
- Menopause and high blood pressure: What's the connection?
- Mental health: Overcoming the stigma of mental illness
- Mental health providers: Tips on finding one
- Mental illness
- Monoamine oxidase inhibitors (MAOIs)
- Natural remedies for depression: Are they effective?
- Nervous breakdown: What does it mean?
- Opioid stewardship: What is it?
- Pain and depression: Is there a link?
- Prescription drug abuse
- Prescription sleeping pills: What's right for you?
- Pulse pressure: An indicator of heart health?
- Reactive attachment disorder
- Resperate: Can it help reduce blood pressure?
- Selective serotonin reuptake inhibitors (SSRIs)
- Serotonin and norepinephrine reuptake inhibitors (SNRIs)
- Sleep deprivation: A cause of high blood pressure?
- Stress and high blood pressure
- Tapering off opioids: When and how
- Teen depression
- Teen drug abuse
- Nutrition and pain
- Pain rehabilitation
- Self-care approaches to treating pain
- Treatment-resistant depression
- Tricyclic antidepressants and tetracyclic antidepressants
- Unexplained weight loss
- Valerian: A safe and effective herbal sleep aid?
- Vasodilators
- How to measure blood pressure using a manual monitor
- How to measure blood pressure using an automatic monitor
- What is blood pressure?
- Vitamin B-12 and depression
- Can a lack of vitamin D cause high blood pressure?
- What are opioids and why are they dangerous?
- White coat hypertension
- Wrist blood pressure monitors: Are they accurate?
- Mayo Clinic Minute: Do not share pain medication
- Mayo Clinic Minute: Avoid opioids for chronic pain
- Mayo Clinic Minute: Be careful not to pop pain pills
Mayo Clinic does not endorse companies or products. Advertising revenue supports our not-for-profit mission.
- Opportunities
Mayo Clinic Press
Check out these best-sellers and special offers on books and newsletters from Mayo Clinic Press .
- Mayo Clinic on Incontinence - Mayo Clinic Press Mayo Clinic on Incontinence
- The Essential Diabetes Book - Mayo Clinic Press The Essential Diabetes Book
- Mayo Clinic on Hearing and Balance - Mayo Clinic Press Mayo Clinic on Hearing and Balance
- FREE Mayo Clinic Diet Assessment - Mayo Clinic Press FREE Mayo Clinic Diet Assessment
- Mayo Clinic Health Letter - FREE book - Mayo Clinic Press Mayo Clinic Health Letter - FREE book
- Kratom - unsafe and ineffective
Your gift holds great power – donate today!
Make your tax-deductible gift and be a part of the cutting-edge research and care that's changing medicine.
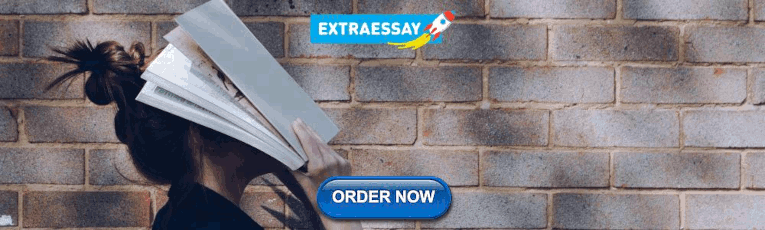
IMAGES
VIDEO
COMMENTS
Let's take a look at some of the most interesting research regarding the impact of sleep on learning and memory. ... Sleep research from the last 20 years indicates that sleep does more than simply give students the energy they need to study and perform well on tests. Sleep actually helps students learn, memorize, retain, recall, and use ...
Going beyond research strategies that focus on solidifying prior learning during sleep, a different approach considers the potential to learn new things during sleep. Such efforts have achieved limited success in the circumscribed territory of associative learning ( Arzi et al. 2012 ) and perceptual learning ( Andrillon et al. 2017 ).
Background. Sleep is an inseparable part of human health and life, and is pivotal to learning and practice as well as physical and mental health. 1 Studies have suggested that insufficient sleep, increased frequency of short-term sleep, and going to sleep late and getting up early affect the learning capacity, academic performance, and neurobehavioral functions. 2, 3 Previous studies have ...
Academic achievement and cognitive functions are influenced by sleep and mood/emotion. In addition, several other factors affect learning. A coherent overview of the resultant interrelationships ...
Scientists studying laboratory animals long ago discovered a phenomenon known as "replay" that occurs during sleep, explains neurologist Daniel Rubin of the MGH Center for Neurotechnology and Neurorecovery, the lead author of the study. Replay is theorized to be a strategy the brain uses to remember new information.
Well-controlled sleep studies conducted with healthy adults have shown that better sleep is associated with a myriad of superior cognitive functions, 1,2,3,4,5,6 including better learning and ...
Sleep across the Life Span. One pressing question about the sleep-memory link concerns how it manifests over one's lifetime. Spencer and Riggins examined this link at the younger end of the age spectrum.They review evidence that naps in early childhood are essential for memory consolidation, presenting a fascinating new hypothesis connecting the psychological, physiological, and ...
This work was supported by grants from the Swiss National Science Foundation (project number 320030-153387 and 320030-149561), the Clinical Research Priority Program 'Sleep and Health' of the ...
1. Introduction. Sleep is crucial for various human cognitive processes, including learning and memory (Diekelmann and Born, 2010, Fogel et al., 2012, Stickgold and Walker, 2013, Tamaki et al., 2020, Tononi and Cirelli, 2014).Our current understanding regarding the role of sleep in learning and memory has been built upon studies conducted both in animal models and human subjects.
Abstract. Converging evidence and new research methodologies from across the neurosciences permit the neuroscientific study of the role of sleep in off-line memory reprocessing, as well as the nature and function of dreaming. Evidence supports a role for sleep in the consolidation of an array of learning and memory tasks.
Research results on sleep and learning are presented and support different effects on the brain and learning, according to the age of the population. Sleep is a fundamental process for brain ...
About this Research Topic. Submission closed. Sleep is generally believed to prepare our brains for new learning and consolidate recently acquired memories. However, whether sleep is both necessary and sufficient for learning and memory remains a hotly debated empirical question. Advances in the basic, cognitive and computational neurosciences ...
Strategic opportunities in sleep and circadian research: report of the Joint Task Force of the Sleep Research Society and American Academy of Sleep Medicine. Sleep. 2014;37(2):219-227. Crossref Google Scholar; 74. Jackson CL, Walker JR, Brown MK, Das R, Jones NL. A workshop report on the causes and consequences of sleep health disparities. Sleep.
Building on these findings, Robertson et al., (2004) have recently demonstrated that explicit awareness of a specific motor sequence being learned modifies the subsequent development of a sleep-dependent learning correlated with NREM sleep. Visual Perceptual Learning. Karni et al. (1994) have demonstrated that learning on a visual texture discrimination task, which does not benefit from 4-12 ...
Study reveals how sleep boosts learning. Two distinct sleep stages appear to play vital, complementary roles in learning: one stage enhances overall performance, while the other stabilizes what we ...
There is a growing body of evidence suggesting a critical role of sleep in learning and memory (Diekelmann & Born, 2010).On the one hand, offline memory consolidation during sleep benefits both declarative and procedural memories acquired during preceding wake (Klinzing et al., 2019).On the other hand, memory encoding capacity has been argued to saturate gradually during wake, with sleep ...
2.3 Waking is for learning; sleep enables memory consolidation. Although waking is by far the best time for learning, sleep and REM dreams enhance memory consolidation. Memories are believed to be unstable and vulnerable to interference in the early hours after learning. Early memory is thought to depend upon active, resonating neural circuits ...
Sleep and learning. Multiple hypotheses explain the possible connections between sleep and learning in humans. Research indicates that sleep does more than allow the brain to rest; it may also aid the consolidation of long-term memories. REM sleep and slow-wave sleep play different roles in memory consolidation.
Most researchers investigating sleep-related consolidation of declarative memory choose a learning criterion between 40% and 80% 104,105, with a 60% criterion often used for word-pair learning or ...
Sleep's Role in Memory Consolidation . Sleep and memory Trusted Source National Institutes of Health (NIH) The NIH, a part of the U.S. Department of Health and Human Services, is the nation's medical research agency — making important discoveries that improve health and save lives. View Source share a complex relationship. Getting enough rest helps you process new information Trusted ...
This meta-analysis synthesized over 50 years of experimental research on sleep loss and emotion, examining the effects of sleep deprivation, sleep restriction, and sleep fragmentation on various emotional outcomes. ... Keep Learning. How does this meta-analysis advance our understanding of the relationship between sleep and emotion compared to ...
Background: Sleep problems associated with poor mental health and academic outcomes may have been exacerbated by the COVID-19 pandemic. Aims: To describe sleep in undergraduate students during the COVID-19 pandemic. Method: This longitudinal analysis included data from 9523 students over 4 years (2018-2022), associated with different pandemic phases. Students completed a biannual survey ...
At a glance. The amount of sleep you need changes as you age. Several national surveys are used to study insufficient sleep (or short sleep duration) in U.S. children, high school students, and adults. These surveys show that not getting enough sleep has varied by age, location, racial/ethnic group, and over time in the United States.
Objective . Sleep problems and executive dysfunction are associated with functional impairment in children with neurodevelopmental disorders. In this study, we aimed to investigate these aspects in children with Specific Learning Disorders (SLD) and SLD with comorbid Attention-Deficit Hyperactivity Disorder (ADHD), while also evaluating differences with typically developing (TD) children.
A clue to the role of sleep in cognition is the detrimental effect of sleep deprivation on cognitive functioning. Over the past decade, growing interest in and experimentation on the role of sleep in learning and memory has led to an explosion of research, which has established a firm connection between sleep and memory.
Sleep Disorder Analysis: Unveiling the Interplay Between Lifestyle Health and Sleep Quality. January 2024. January 2024. DOI: 10.1109/LT60077.2024.10468720. Conference: 2024 21st Learning and ...
Automated sleep stages classification facilitates clinical experts in conducting treatment for sleep disorders, as it is more time-efficient concerning the analysis of whole-night polysomnography (PSG). However, most of the existing research only focused on public databases with channel systems incompatible with the current clinical measurements. To narrow the gap between theoretical models ...
Poor sleep quality in Autism Spectrum Disorder (ASD) individuals is linked to severe daytime behaviors. This study explores the relationship between a prior night's sleep structure and its predictive power for next-day behavior in ASD individuals. The motion was extracted using a low-cost near-infrared camera in a privacy-preserving way. Over two years, we recorded overnight data from 14 ...
Join us for this afternoon's commencement exercises for our graduating class of 2024. #ForeverToThee24
Kratom is also sold as a treatment for panic attacks. Kratom is believed to act on opioid receptors. At low doses, kratom acts as a stimulant, making users feel more energetic. At higher doses, it reduces pain and may bring on euphoria. At very high doses, it acts as a sedative, making users quiet and perhaps sleepy.