
- HAIR & BODY
- HOME & TOOLS
- Derma Treatments
- Doctor Facelift
- Doctors Formula
- Dr Botanicals
- Dr Botanicals Apothecary
- Dr Botanicals Baby
- Dr Botanicals Baby Lux
- Feel Like Beauty
- Gold Serums
- Organic & Botanic
- Skin Research
- Skin Superfood
- skinChemists
- skinChemists London
- skinChemists Youth Series
- skinPharmacy
- Featured Outlet Best Sellers Shop All Accessories Skincare Kits
- Shop Categories Cleansers Exfoliators Eye Care Eye Pads Face Lift Facial Oils Facial Serums Lip Care Mascaras Masks Moisturisers Toners
- Shop Ingredients Bee Venom Collagen Green Caviar Hyaluronic Acid Probiotic Retinol Vitamin C Vitamin D
- Collections Biphase Brightening Cocoa Noir Japanese Orange Moroccan Rose Night Care Pomegranate Rose Quartz Sun Protection SPF Teeth Whitening Wrinkle Killer
- HAIR Conditioners Shampoos Hair Masks Hair Mists
- BODY Body Creams Body Oils Hand Creams Perfumes
- Aromatherapy Candles Diffusers Diffuser Oils
- Tools Beauty Electricals Facial Rollers Makeup Brushes Toothbrush
Your cart is empty
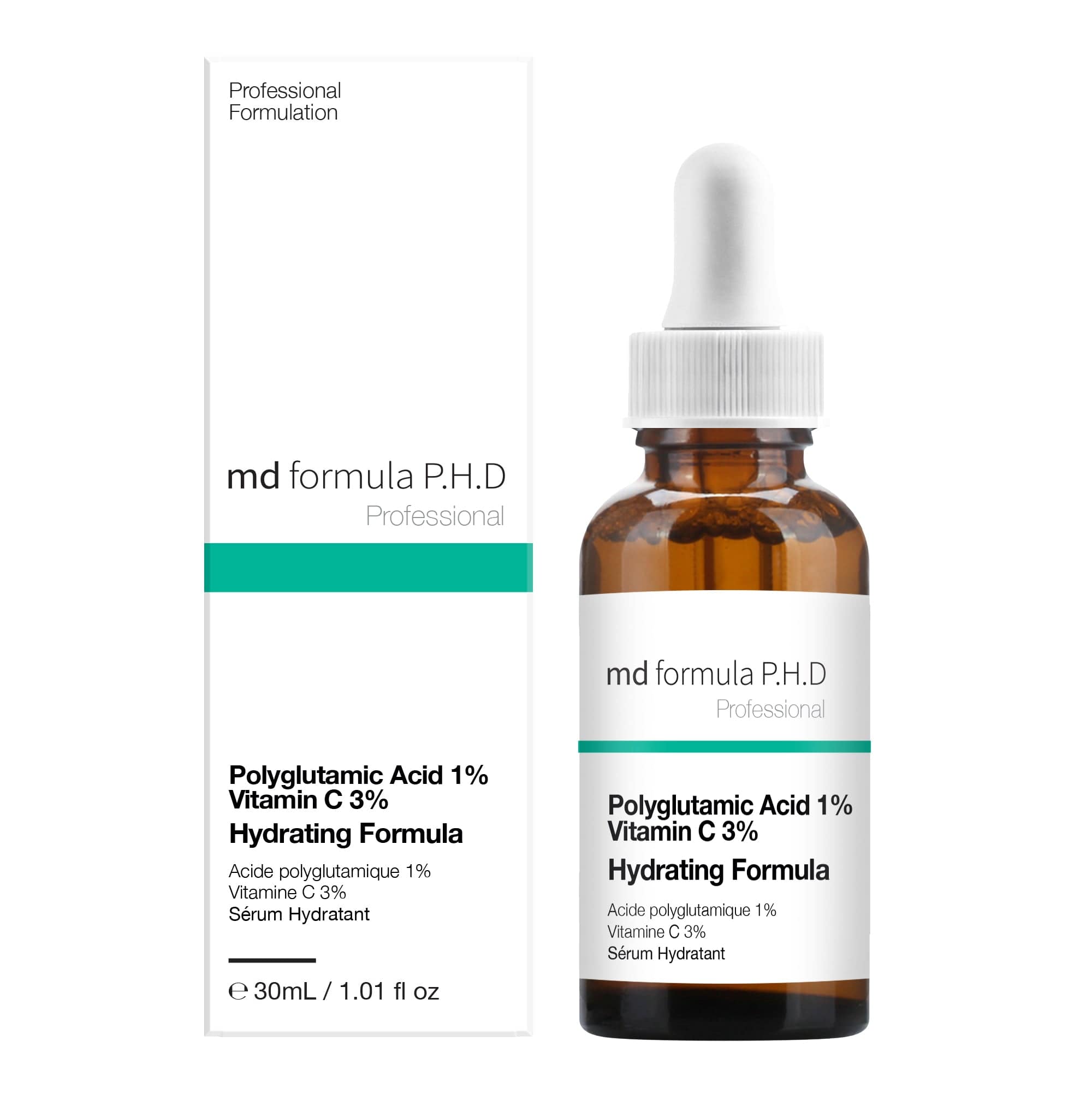
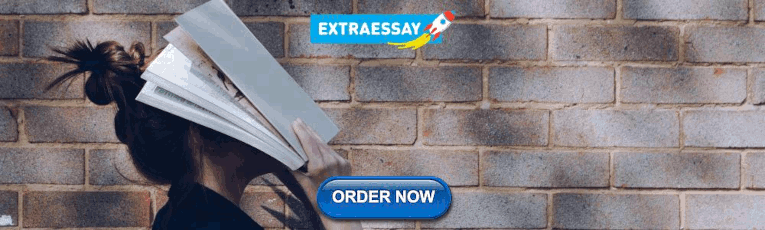
Hydrating Serum Polyglutamic Acid 1%, Vitamin C 3% 30ml
- Provides deep moisturization for hydrated skin
- Enhances complexion with temporary brightness
- Contains Polyglutamic Acid and Vitamin C for intense hydration and a radiant appearance
This formula is a skincare product that aims to provide deep moisturization and a temporary brighter complexion. It contains Polyglutamic Acid for intense hydration and Vitamin C for a radiant appearance. With its focus on hydration and brightening, this formula promotes healthy and hydrated skin.
This formula targets dry and dehydrated skin, promoting hydration and a brighter complexion. It is suitable for all skin types.
Choose options
Free delivery
On orders over £40
Extra 5% off
With code FLB
24/7 Customer service
Satisfied or Refunded
Secure payments
Information is processed securely
CONFIDENCE, COMMUNITY, AND JOY
- Skincare Ingredients A-Z
- Skin Concerns
- Hair Removal
- Moisturizers
- Tools and Techniques
- Hair Concerns
- Hair Styling
- Fashion Trends
- What to Wear
- Accessories
- Clothing and Apparel
- Celebrities
- Product and Brand News
- Trends and Innovation
- Amazon Picks
- Gift Guides
- Product Reviews
- Mental Health and Mood
- The Byrdie Team
- Editorial Guidelines
- Editorial Policy
- Terms of Use and Policies
- Privacy Policy
- Skincare Ingredients A–Z
Polyglutamic Acid Is the Hydrating Hero Ingredient Your Skincare Routine Needs
:max_bytes(150000):strip_icc():format(webp)/cdn.cliqueinc.com__cache__users__618__amy-lawrenson-1-618-1514897570-main_image-e162502b98da4adb8a4f2859c61c39b4.jpg)
Marcos Flores / Pexels
In This Article
About a month ago I heard the words polyglutamic acid (PGA) for the first time. I was listening to Charlotte Tilbury talk about her latest product launches at a virtual event (because that's the new normal). Tilbury was extolling the virtues of this little-known ingredient as a hydrating hero. In fact, she is so enamored with it, she factored it into her Magic Serum ($80).
Meet the Expert
- Charlotte Tilbury is a British makeup artist and the founder and chief creative officer of the makeup and skincare brand Charlotte Tilbury Beauty Ltd.
- Michelle Wong is a cosmetic scientist and the founder of Lab Muffin Beauty Science .
"Polyglutamic acid is a hydration powerhouse!" Tilbury tells Byrdie. "Polyglutamic acid is an incredible humectant that holds four times more moisture than hyaluronic acid (HA)—my skin just drinks it in!"
So why is an ingredient supposedly more moisture-banding than the hydration hero that is hyaluronic acid so little known? I called on Tilbury and scientist Michelle Wong of Lab Muffin Beauty Science to reveal more about the enigma that is polyglutamic acid. Plus, keep scrolling to shop the products that feature this buzzy skincare ingredient.
POLYGLUTAMIC ACID
TYPE OF INGREDIENT: Hydrator
MAIN BENEFITS: Moisturizes skin, reduces the appearance of wrinkles and pores, replenishes cell moisture.
WHO SHOULD USE IT: It is ideal for anyone with dry or dehydrated skin. Polyglutamic acid is safe for all skin types, however, if you have sensitive skin it is always best to patch test a new product.
WHEN YOU CAN USE IT: Polyglutamic acid can be used morning and night.
WORKS WELL WITH: It plays nicely with other skincare ingredients, especially hyaluronic acid and vitamin C.
DON’T USE WITH: There are no known negative reactions with polyglutamic acid.
What Is Polyglutamic Acid?
"Glutamic acid is an amino acid. Polyglutamic acid is created when lots of glutamic acid molecules are linked together," says Wong. "It's produced by bacterial fermentation and is found in Nattō." [Ed note: Nattō is a traditional Japanese food made from fermented soybean.]
Polyglutamic acid is very similar to another buzzy skincare hero, hyaluronic acid but, as experts note, it's perhaps even more moisturizing.
What Are the Skin Benefits?
"It's a very effective humectant that can grab onto water and keep skin hydrated," notes Wong.
"Polyglutamic acid helps seal the moisture in your skin, and enhances the skin’s elasticity," says Tilbury. "Part of the aging process involves the loss of HA in the skin, which is broken down by an enzyme called hyaluronidase. Products that contain PGA help to inhibit the action of hyaluronidase, resulting in more HA in the skin, and giving an overall moisturized, rejuvenated, and hydrated appearance to the skin."
Within her namesake brand's Magic Serum , Tilbury tells us that the PGA hydrates the skin, leading to a plumper-looking complexion and a diminished appearance of pores. "It makes makeup application so much easier too!" she adds.
How Does It Compare to Hyaluronic Acid?
"[Unlike HA], polyglutamic acid doesn't occur naturally in our bodies," Wong says. "Ingredient suppliers claim it can hold 10 times more water than hyaluronic acid, which is very impressive." Tilbury, on the other hand, claims it holds four times as much moisture because of its larger molecular size and can actually help the skin produce more hyaluronic acid. "Not only does it draw water into the cells, like HA, but it also forms a protective film on your skin that prevents water from evaporating," she claims. "I call it my plumper-looking skin filter!"
What both Wong and Tilbury do agree on is that polyglutamic acid is more effective at holding onto moisture than hyaluronic acid, however, don't be so quick to abandon HA in favor of PGA. "I see the two ingredients as friends rather than competitors," says Tilbury. "When used together, they can be seen as the ultimate recipe for hydrated skin."
Why Is It Under-the-Radar?
Polyglutamic acid is relatively new on the beauty market, Wong tells us. According to Tilbury, it also costs more than HA, which affects profit margins and could be one of the reasons we haven't seen it in that many products to date. That being said, as the popularity of this skincare powerhouse increases, we could eventually see more products, at lower price points, enter the market. Already, we see the acid as a key ingredient in at least one trendy skincare line, the Futurwise Slugging System .
Does Polyglutamic Acid Play Nice With All Skin Types?
Tilbury notes that "polyglutamic acid floods the skin with moisture—all skin types require hydration to function optimally but sensitive and dry skin types, in particular, will love the hydration boost this gives." Oily skin could also benefit from polyglutamic acid, but it's best to proceed with caution.
Is It Best Applied via a Serum?
Polyglutamic acid is mostly formulated into serum textures, and Wong tells us that "since it's a general humectant and effective at very low concentrations (as low as 0.1%), it can work well in lots of different formulations. Along with serums, it can be formulated into moisturizers, essences, toners, possibly even sunscreens though I don't know of any on the market yet." It also plays well with makeup, so you can layer it on the skin before adding in your other products .
"As more people learn about the benefits of PGA, it will gain the cult status of HA and we can expect to see it used in more products," predicts Tilbury. "I’m thrilled to have been one of the first to bottle this incredible, supercharged ingredient."
Interactions to Know About
There are no known ingredients that negatively interact with polyglutamic acid. In fact, according to Wong, polyglutamic acid "mixes well with other products, especially water-based ones." In other words, using it in conjunction with moisturizers , oils, etc. is kind of the point, as it can increase the absorption of other ingredients. That being said, if your skin does develop a rash or reacts negatively, it's always best to back off a new product and seek an expert's help.
Side Effects
As with pretty much any type of acid, it's possible to develop an allergic reaction to polyglutamic acid. Signs and symptoms would be red, tingling skin, hives, itchiness or swelling. If you do note any of the above—or find that your skin is dry and scaly rather than moist and well-hydrated—it's time to visit a dermatologist. Acids are sometimes best to introduce to your skin over time, so if you're worried about a negative reaction, try using a polyglutamic-containing product just once per week, and gradually working your way up to every day. This will allow your skin time to tolerate the acid.
Yes, you can use hyaluronic acid and polyglutamic acid together. When used in conjunction, both ingredients work to help moisturize the skin and repair the skin barrier.
Polyglutamic acid can be worn morning or night, and is best applied post-cleanse and prior to serums, moisturizers, sunscreens, or makeup.
Polyglutamic acid is thought to be safe for all skin types, but it works especially well on those with dry skin. As with any skincare ingredient, it's best to exercise caution when introducing a new product into your daily routine.
Related Stories
17 Spring Skincare Launches That Should Be On Your Radar
The 12 Best Foundations for Dry Skin That Offer Coverage and Hydration
The Benefits of Hyaluronic Acid Serums and How to Use Them
Marula Oil Is the Hydrating, Anti-Inflammatory Solution Derms Love—Here’s Why
The 15 Best Anti-Aging Serums to Address Fine Lines, Uneven Skin Texture, and Skin Firmness
The 16 Best Moisturizers for Dry Skin, Tested & Reviewed
How to Use Tranexamic Acid In Your Skincare Routine for a Brighter Complexion
The 12 Best Glycolic Acid Cleansers That Will Make Your Skin Glow
Propanediol: The Skincare Ingredient Derms Want You to Know
The 19 Best Face Moisturizers, Tested and Dermatologist Approved
Malachite Is the Ideal Stress-Reducing Skincare Ingredient for Crystal Lovers
Salicylic Acid Is the Acne-Fighting Ingredient Derms Want You to Use—Here's Why
The 23 Best Anti-Aging Eye Creams of 2024 for Wrinkles, Fine Lines, & Dark Circles
OLAY's New "Super Serum" Cocktails 5 Ingredients Without Irritation
The 12 Best Hyaluronic Acid Serums of 2024, Tested by Byrdie, Approved by Derms
The 15 Best Night Creams for Smooth, Hydrated Skin
Polyglutamic Acid Is Trending — Does It Deserve a Spot in Your Skin-Care Routine?
Updated on 5/5/2023 at 4:35 PM
:upscale()/2024/01/17/607/n/1922153/7ea189e265a7d7887df639.73618175_.jpg)
Thought hyaluronic acid was the best hydrator on the block? Turns out there's a new kid called polyglutamic acid coming for its crown. We know, we know: another day, another buzzy skin-care ingredient that promises to transform your skin. And this time, it's got a name that's not only difficult to say (try: poly-glue-ta-mick) but is also complicated enough to send your predictive text into a complete meltdown.
So, what exactly is polyglutamic acid (also known as PGA) — and is it worth the hype? Can it really rival good old hyaluronic acid, like so many people are claiming? We asked the experts for the lowdown. Keep reading for everything you need to know about polyglutamic acid.
What Is Polyglutamic Acid?
"Polyglutamic acid is a natural, biodegradable polymer of the amino acid glutamic acid produced by fermentation of Japanese soybeans with Bacillus subtilis," Marko Lens , MD, consultant plastic surgeon and founder of Zelens, tells POPSUGAR. That's a lot of science in one sentence, so let's break it down a little.
Polyglutamic acid is a type of ingredient called a peptide and is made up of a chain of a particular type of amino acid called glutamic acid. Still, despite being a peptide, it doesn't work in the same way as antiaging peptides you might have heard of but "acts as a moisturizing agent by locking moisture into the skin," explains Mark Curry , cofounder of The Inkey List.
It's a humectant, like hyaluronic acid , which means its primary role is to attract water like a magnet. Polyglutamic acid is made by fermenting Japanese soybeans with a particular strain of bacteria that can also be found in kimchi. Think of it a bit like the skin-care version of a sourdough starter, just with more bacteria and less yeast.
How Does Polyglutamic Acid Work?
So how does this little chain of amino acids work in our skin? "Polyglutamic acid is a powerhouse humectant that not only puts water into the skin but keeps it there as well," junior doctor and skin expert Kemi Fabusiwa says.
It holds onto this moisture by "creating a barrier over the skin to prevent hydration loss from the inside and prevent dehydration from the outside," says Ahmed El Muntasar , a general practitioner and aesthetician. "Polyglutamic acid can also potentially prevent something called hyaluronidase, which is the enzyme that breaks down hyaluronic acid in the skin." This last benefit is a biggie, as the natural levels of hyaluronic acid in our skin deplete as we age, meaning we're more prone to dehydration the older we get.
Who Is Polyglutamic Acid Good For?
Pretty much anyone can use the ingredient, but you'll see the biggest improvement if your skin is dehydrated or lacking in radiance. "By binding water to the skin, polyglutamic acid is a super, glow-inducing active for dewy skin," says Georgie Cleeve , founder of Oskia Skincare. "Due to its ability to attract water, it will help minimize fine lines and wrinkles and keep skin soft, supple, and healthy as well. PGA also has antioxidant properties, inhibits the production of melanin, and strengthens the barrier function."
"Ultimately, all skin types can benefit from polyglutamic acid as a hydrating active, but particularly mature and dry skin or those with a weakened barrier function," Cleeve says. The only caveat to this is if you have an oily skin type. "If you're someone that's naturally oily, then overhydrating your skin can actually break you out a little bit," Dr. Muntasar says. "I find that sometimes this is a bit of a fine balance." If this sounds like you, introduce polyglutamic acid into your routine slowly and sparingly to avoid blemishes.
As well as improving how your skin looks when you're bare-faced, polyglutamic acid can also improve the finish of your makeup. "By increasing the water content of the epidermis (the top layer of our skin), polyglutamic acid allows your liquid foundations to glide onto the skin better for a more dewy finish," Dr. Fabusiwa says. "Its ability to plump the skin and boost elasticity makes it the perfect partner to your foundation." Wear PGA beneath your base, or mix them together for maximum glow.
Polyglutamic Acid vs. Hyaluronic Acid
How does polyglutamic acid match up to the much-loved hero hydrator hyaluronic acid? "Polyglutamic acid has a large molecular size, while hyaluronic acid can have a much lower molecular weight, which allows significantly deeper penetration," Dr. Lens says. "That's why, in simple terms, I would say that PGA works above the surface and HA works below. Additionally, polyglutamic acid forms a soft, smooth film on the skin, which results in the protection of the outer layer. They are different molecules with different actions, but if we're talking about hydration efficacy, then polyglutamic acid will increase skin's moisture capacity more effectively than HA."
Hyaluronic acid is very good at providing deep-down hydration in the skin, but it is polyglutamic acid's protective film that allows this newer ingredient to deliver more visible, instant results. A nice way to visualize it is to imagine that hyaluronic acid is handing the skin a nice big glass of water. This is great, but unless you follow with moisturiser, it can escape — in the same way that water in an open glass can evaporate or be spilt. Polyglutamic acid is your skin-care equivalent of a sports drink. Not only does it have the extra benefit of electrolytes for more intense rehydration, but it also comes in a bottle with a lid so no liquid is lost.
PGA might be coming out on top in the battle of the humectants, but if you can, it's still more beneficial to use both ingredients in your routine rather than pick and choose. "It's not a competition, it's more of a logistic relationship," Dr. Muntasar says. "They work well together. Polyglutamic acid is a new ingredient that isn't very common or very popular because it is expensive and quite difficult to make, whereas hyaluronic acid is a lot more readily available. If you suffer from very dry skin, you can use both of these ingredients to really target those dry areas."
"Polyglutamic acid and hyaluronic acid are like scones and jam or butter and toast," Cleeve says. "They're the perfect combination, especially when cleverly combined using different molecular weights." For best results, use PGA during the day to create a silky canvas for makeup and HA for deep-down hydration as part of your evening routine.
How to Use Polyglutamic Acid in Your Routine
Polyglutamic acid is a friendly ingredient that plays nicely with other actives and is therefore easy to slot into your routine. "Polyglutamic is a great active to use both a.m. and p.m. and has a place in all products, from cleansers to serums and moisturizers," Cleeve says. "However, the best effect will be in serums and moisturizers." That's because these are left on the skin, rather than washed off, which gives them maximum opportunity to work their magic.
"Polyglutamic acid should always be applied directly against the skin in a water-based product and before anything that contains oils," Cleeve says. This sequence is important because oil is heavier than water. If you put your polyglutamic-acid product on over an oil, the oil will block its path, and the PGA won't be able to make its way into the skin.
Although it has acid in its name, polyglutamic acid doesn't exfoliate the skin, so there's no real risk of overdoing it. How often you use it will depend on your skin type and individual needs. "This is where trial and error really comes into play," Dr. Muntasar says. "Try the product, apply the recommended amounts, and if it works for you, then that's great. If not, you can always add a touch more."
Interested in adding this wonder ingredient to your routine? See some of our favorite polyglutamic-acid-infused skin-care products ahead.
:upscale()/2023/05/05/571/n/29590734/de22546bd8111801_Screenshot_2023-05-05_at_13.42.55.png)
Best Polyglutamic-Acid Sunscreen
The Inkey List Polyglutamic Acid Dewy Sunscreen ($15)
The best SPF is one you want to wear, which is why The Inkey List wanted to create a formula that felt and looked good on skin. Years in the making, this is the result: a nongreasy, super-hydrating formula that leaves skin glowing and protected.
:quality(70):extract_cover():upscale():fill(ffffff)/2023/05/05/571/n/29590734/de22546bd8111801_Screenshot_2023-05-05_at_13.42.55.png)
Best Polyglutamic-Acid Serum
Zelens Melatonin B12 Advanced Repair Serum ($73)
The key ingredients in this premium serum are melatonin and vitamin B12, chosen for their ability to renew and repair the skin. Polyglutamic acid is here in a supporting role, adding extra moisture into the mix so skin can function at its best.
:quality(70):extract_cover():upscale():fill(ffffff)/2023/05/05/876/n/44344577/shopr4oJ9O.jpg)
Best Soothing Polyglutamic Acid
Oskia Rest Day Barrier Repair Balm ($103)
If your skin is throwing a toddler-worthy tantrum, this is the product to get it off the floor and back into its happy place. Polyglutamic acid works alongside soothing colloidal oats, micro-algae, and ceramides to give stressed-out skin a hard reset.
:quality(70):extract_cover():upscale():fill(ffffff)/2023/05/05/876/n/44344577/shopJaNERT.jpg)
Best Plumping Polyglutamic Acid
Typology Plumping Serum With Polyglutamic Acid 3% + Red Seaweed Extract ($50)
Late nights taking their toll on your skin? Lock in moisture to refresh and smooth tired, lined complexions with this lightweight serum. It sells out fast, so add to cart if you see it in stock.
:quality(70):extract_cover():upscale():fill(ffffff)/2023/05/05/876/n/44344577/shopNjqjU5.jpg)
- Beauty Explainer
Move Over, Hyaluronic Acid—Polyglutamic Acid Retains 5 Times More Moisture
If you ever suffer from dry skin, chances are, you've reached for hyaluronic acid , and for good reason. This hydrating ingredient revives dry, tight, or flaky skin . As someone with extremely dry skin no matter what I throw at it, I often wish there was something even more hydrating than hyaluronic acid .
Enter polyglutamic acid. Consider polyglutamic acid hyaluronic acid's overachieving older sister. The two ingredients are cut from the same cloth and have many of the same benefits for skin, but with a few key differences. Keep reading to find out more about polyglutamic acid.
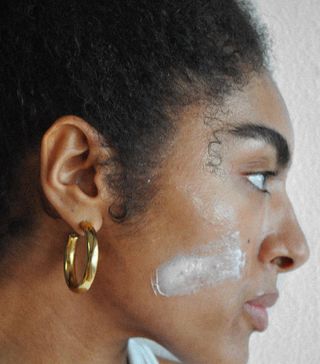
What are the benefits of polyglutamic acid?
Just like hyaluronic acid, Polyglutamic acid is a humectant. According to Elyse Love, MD, a board-certified dermatologist, that means the ingredient draws moisture into the skin when it is paired with a moisturizer. " This leads to increased skin hydration and improvement in the appearance of dullness and fine wrinkles," she explains. "It is a great ingredient for anyone who wants extra hydration to the skin, especially those who struggle with dull and/or dehydrated skin. It plays well with almost all other skin ingredients and works well for all skin types."
Hyaluronic Acid vs Polyglutamic Acid
The biggest difference between hyaluronic acid and polyglutamic acid is polyglutamic acid's ability to retain water. " It's been shown to retain moisture up to 5000 times its weight, which is more potent than hyaluronic acid," says David Kim, MD, MS, and board-certified dermatologist. "Hyaluronic acid can retain moisture up to 1000 times its weight."
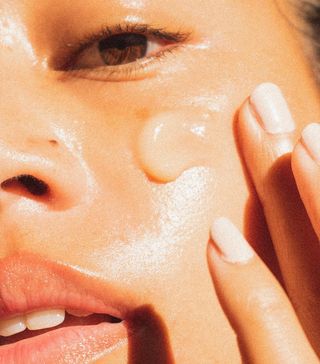
Our favorite polyglutamic acid products:
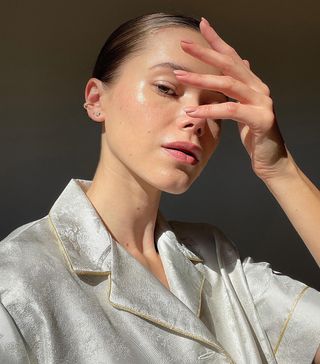
"Obagi Clinical Dark Spot Disruptor Discoloration Correcting Serum combines the hydrating effects of p olyglutamic a cid with the soothing and brightening effects of bakuchiol for a gentle, lightweight formula to improve the appearance of dark spots, dullness, and fine wrinkles," says Love.
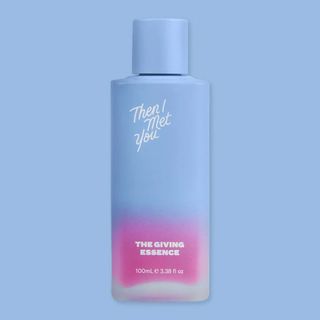
"It's a very light and gentle essence with polyglutamic acid and ferment filtrates that is good for all skin types, especially during the summer," says Kim.
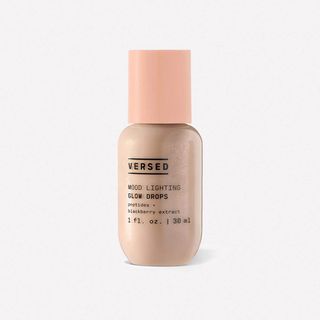
There's nothing better than a good makeup-skincare hybrid. Versed's Mood Lighting has all the skincare benefits of a serum with all the illuminating benefits of a highlighter. Polyglutamic acid is the star of the show, working to visibly plump skin.
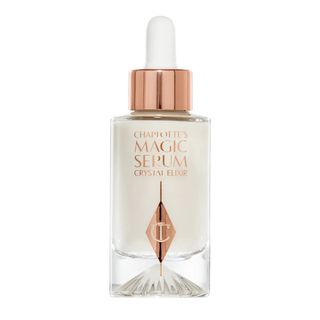
This supercharged serum is basically a one-stop shop for good skin in a bottle. Polyglutamic acid and vitamin C are blended together for a serum that plumps while brightening.
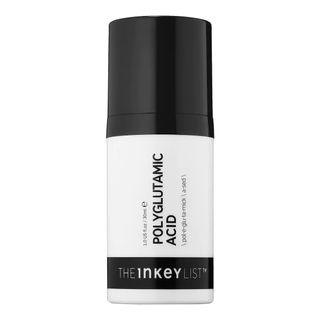
For just $15, this gel-like serum packs a major moisturizing punch. It helps lock in moisture while also priming skin for makeup application.
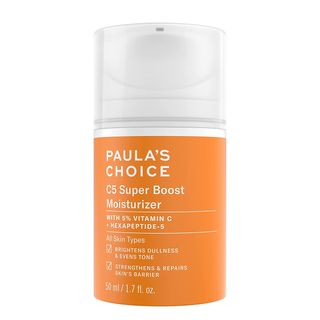
If you're after bright skin, try mixing in this vitamin C–rich moisturizer. A blend of peptides, polyglutamic acid, squalane, and a double-encapsulated blend of lipids help moisturize and strenghten skin.
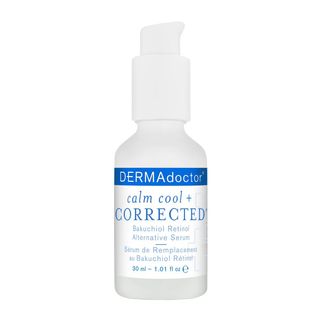
If you wants the benefits of retinol but have super sensitive skin, try reaching for this bakuchiol serum. Bakuchiol helps even texture and tone while polyglutamic acid plumps.
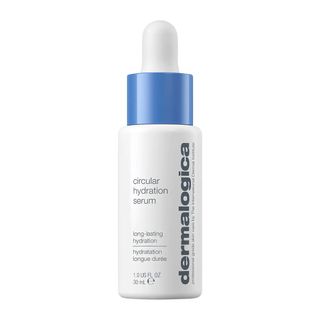
Consider this hydrating serum a triple threat. Mega moisturizers hyaluronic acid, algae extract, and polyglutamic acid are basically like a Gatorade for dehydrated skin.
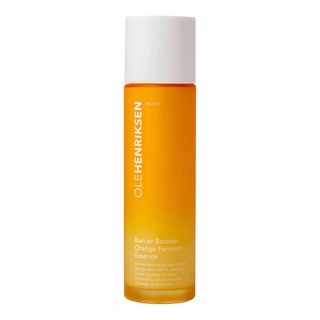
Using an essence is already a great way to add a blast of hydration to skin. This one has vitamin C and polyglutamic acid to brighten and moisturize.
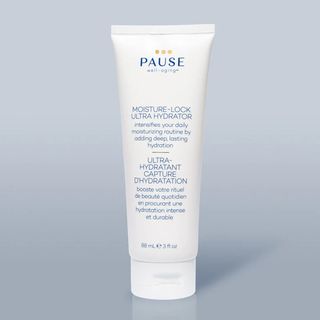
If you're after some serious hydration, this deeply moisturizing cream delivers. Polyglutamic acid and ceramides work in tandem with each other to hydrate and nourish the skin. Use it liberally anywhere you need a boost of moisture.
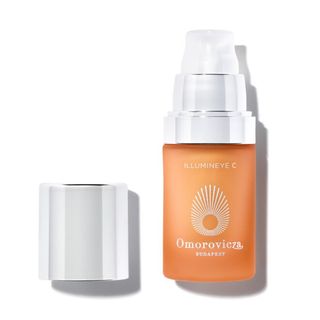
Puffy eyes and dark circles are no match for this brightening eye cream. The brand's essential moisture complex has a blend of polysaccharides, hyaluronic acid, polyglutamic acid for plump, hydrated under eyes.
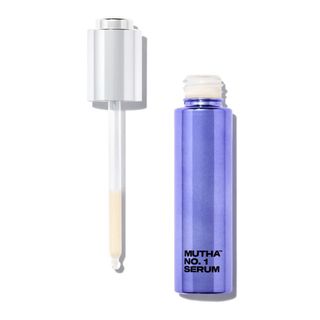
Mutha's multitasking serum has vitamin C, hyaluronic acid, polyglutamic acid, and camellia oil for supremely hydrated and radiant skin.
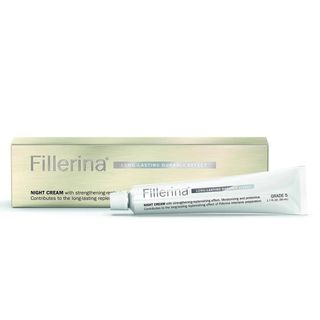
With 12 different weights of hyaluronic acid, this cream is about as hydrating as you can imagine. Polyglutamic acid also helps hydrate, while growth factors work to nourish and restore skin.
Other hydrating products we love:
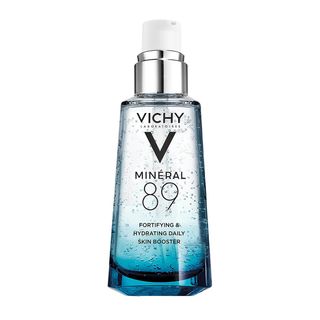
Next: Hyaluronic Acid Should Be Your Desert Island Skincare Ingredient—Here's Why
Katie Berohn is the associate beauty editor at Who What Wear. Previously, she worked as the beauty assistant for Good Housekeeping , Woman's Day, and Prevention magazines, all part of the Hearst Lifestyle Group. She graduated from the University of Colorado, Boulder, with a major in journalism and minor in technology, arts, and media, and earned her master's degree at NYU's graduate program for magazine journalism. In addition, Katie has held editorial internships at Denver Life magazine, Yoga Journal, and Cosmopolitan ; a digital editorial internship at New York magazine's The Cut ; a social good fellowship at Mashable ; and a freelance role at HelloGiggles . When she's not obsessing over the latest skincare launch or continuing her journey to smell every perfume on the planet, Katie can be found taking a hot yoga class, trying everything on the menu at New York's newest restaurant, or hanging out at a trendy wine bar with her friends.
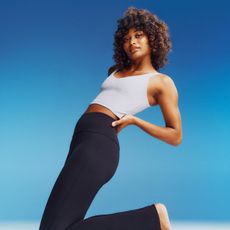
Sponsor content created with Lululemon.
By Humaa Hussain
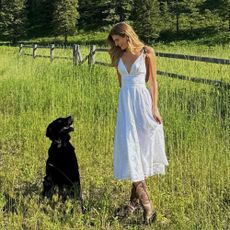
By Natalie Munro
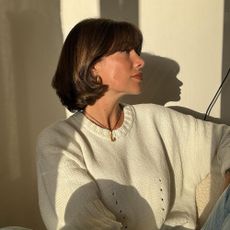
Could I achieve that Parisian glow?
By Grace Lindsay
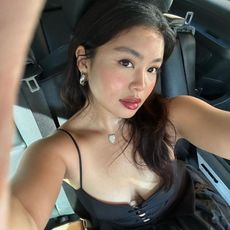
Take your pick.
By Grace Day
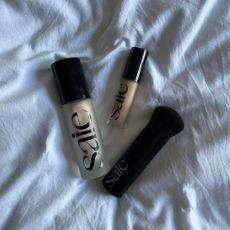
Glow in a bottle.
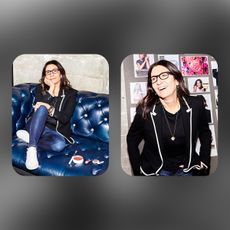
An industry icon.
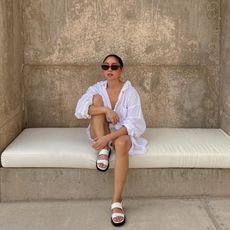
From head to toe.
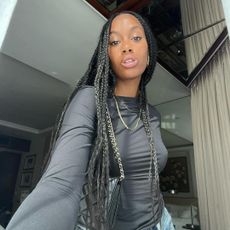
The underrated AHA you need to know about.
By Mica Ricketts
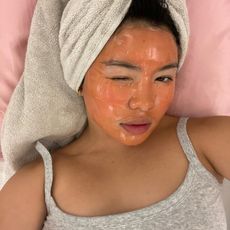
Take notes.
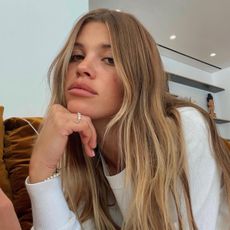
From £5 to £155.
- Contact Future's experts
- Terms and conditions
- Privacy policy
- Cookies policy
- Accessibility Statement
- Advertise with us
Who What Wear UK is part of Future plc, an international media group and leading digital publisher. Visit our corporate site . © Future Publishing Limited Quay House, The Ambury, Bath BA1 1UA. All rights reserved. England and Wales company registration number 2008885.
- Search Please fill out this field.
- Newsletters
- Sweepstakes
We independently evaluate all recommended products and services. If you click on links we provide, we may receive compensation. Learn more .
Dermatologists Say Polyglutamic Acid Is the Anti-Aging Ingredient 10 Times More Moisturizing Than Hyaluronic Acid
And these PGA-infused products will create an instant glass-skin finish.
Tamim is beauty commerce writer at InStyle, with nearly a decade of experience writing about beauty, fashion, wellness, and music.
:max_bytes(150000):strip_icc():format(webp)/tamim-alnuweiri-2000-725ca5d559e445eabd4c2f2718f11022.jpg)
In the skincare industry's current top 40, one ingredient reigns supreme — hyaluronic acid . It's a multitasking powerhouse of an ingredient you can find in any and every type of skincare product thanks to its multitude of benefits, like replenishing and retaining moisture, which helps reduce the appearance of fine lines and wrinkles, keeping the skin looking supple and smooth. But there is an under the radar anti-aging ingredient that a dermatologist refers to as a "close cousin" to HA — polyglutamic acid (PGA).
Put simply, "PGA keeps skin super dewy, hydrated and maintains the bounce or sponge-like quality of younger skin," Dr. Ava Shamban , MD, cosmetic dermatologist says. Like HA, it's a humectant but according to Dr. Shamban, "can hold 4,000 times its weight in water — 10 times more moisture than hyaluronic acid."
PGA has a relatively larger molecular size, which essentially means that it is useful for surface level, and not penetrative, hydration. Polyglutamic acid and hyaluronic acid, however, are not mutually exclusive ingredients in formulas. They are often used together because of their slightly differing properties, according to Dr. Shamban.
But the lesser-known PGA does have some specific advantages. First, PGA is an instant gratification ingredient. Dr. Shamban tells InStyle that it is more effective in diminishing the appearance of fine lines, wrinkles, and the appearance of pores. The acid's efficient hydration plumps the upper layers of your complexion , creating a quick-acting glass skin effect.
And while it doesn't penetrate the deep layers of the skin, it does prevent hyaluronidase — an enzyme responsible for breaking down the hyaluronic acid in your skin thereby accelerating aging. This is what makes it a great booster for HA skincare products.
To quickly summarize all of the science just thrown at you — PGA instantly plumps and hydrates your epidermis for a glass skin effect while also preventing the breakdown of pre-existing hyaluronic acid. It's a one-two punch that makes for a great anti-aging ingredient. If your interest is piqued, check out the products below.
Charlotte Tilbury Charlotte's Magic Serum
Part of Charlotte Tilbury's beloved Magic line, this serum combines PGA and vitamin C for an instantly plump and vibrant complexion.
Decorté Liposome Eye Cream
This eye cream instantly plumps and awakens your face thanks to the long lasting hydration effects of polyglutamic acid.
Glow Recipe Watermelon Glow Ultra-Fine Mist
Glow Recipe's Watermelon Mist is actually one of Dr. Shamban's PGA-infused products. "It combines both hyaluronic acid and polyglutamic acid for a throughout-the-day refresher to hydrate skin."
The Inkey List 3% Polyglutamic Acid
A budget-conscious choice that's perfect for dipping your toes in the hydrating waters of PGA, Dr. Shamban recommends this straightforward Inkey List serum.
Then I Met You The Giving Essence
Before you begin your skincare routine, start with this polyglutamic acid and red algae formulated essence. The combination of the two gently exfoliates dead, pore-clogging cells while hydrating the skin, creating an ideal canvas for the rest of your products.
Omorovicza Midnight Renewal Serum
Another Dr. Shamban recommendation, she says this Hungarian product is specifically for "more mature skin" thanks to ingredients such as retinol and oat lipid microalgae — the latter being protective against stress and optimizing the skin for overnight repair.
Shop More InStyle-Approved Picks
:max_bytes(150000):strip_icc():format(webp)/GettyImages-1779500227-d9e631dbe879428fb9d8448beb5eb59f.jpg)
Related Articles
- United Kingdom
Okay, What's Polyglutamic Acid?
Okay, what’s polyglutamic acid, what is polyglutamic acid , what does it do, how should i use it, what’s a good formula.
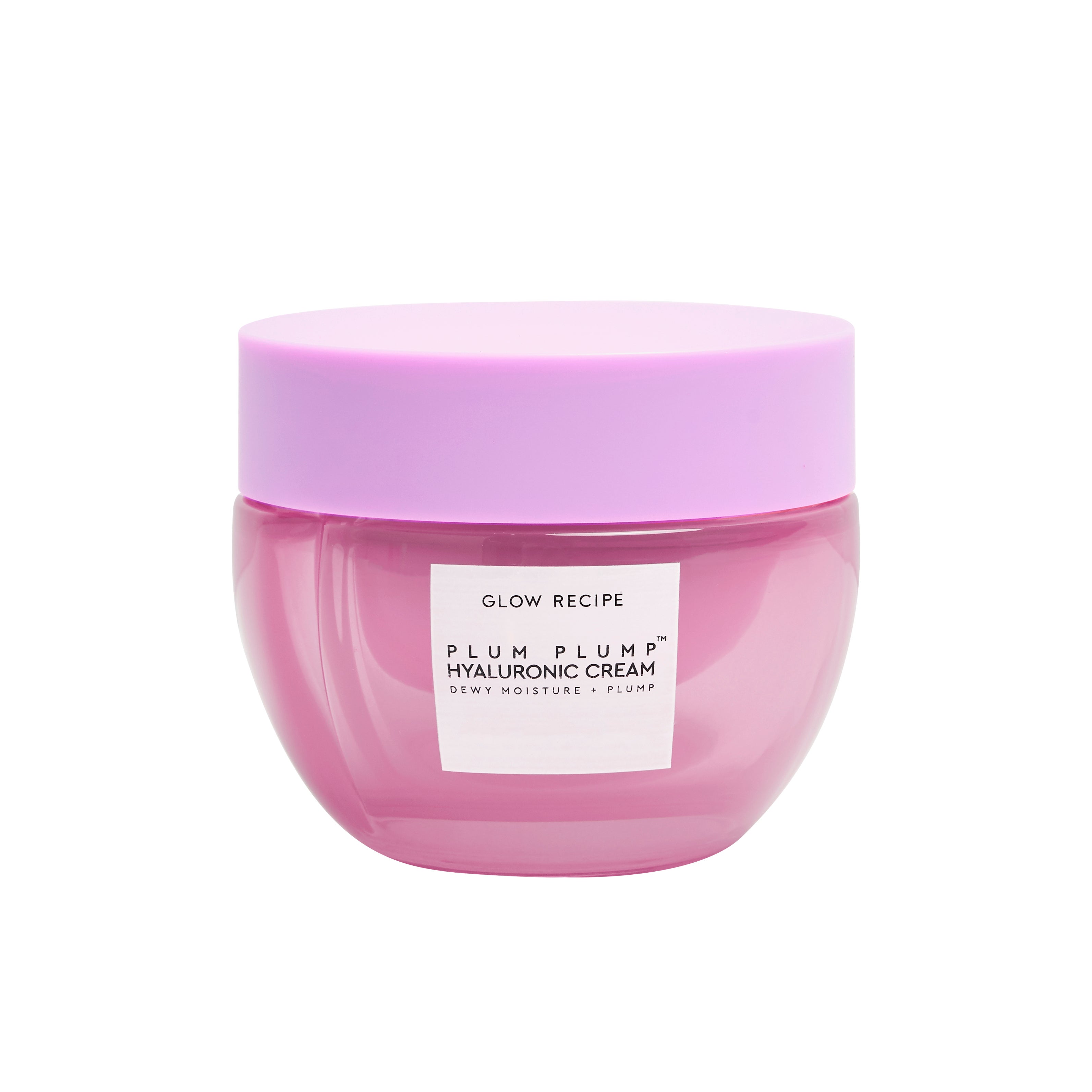
More from Skin Care
R29 original series.
I’ve Been Through 10 Bottles of This Ultra-Hydrating Serum That’s Got Me To Stop Wearing Makeup Almost Entirely—And It’s Back in Stock

Haven’t you heard? Polyglutamic acid is the buzzy hydrating skin-care hero in town that’s even more effective than hyaluronic acid. The potent active is finally getting the spotlight it deserves, and just so happens to be the key ingredient in the product I’ve been screaming about from the rooftops to anyone who will listen: FaceGym Hydrobound Daily Serum ($68). Trust me—this is one product (and ingredient) worth knowing about. Keep reading to find out more.
What is polyglutamic acid?
“Polyglutamic acid (PGA) within skincare is a biopolymer first discovered in jellyfish that prevents tissue dehydration in salt water. Nowadays it’s sourced via fermentation,” says Krupa Koestline, cosmetic chemist and founder of KKT Consultants . It’s actually found in natto, a sticky Japanese dish made from fermented soybeans (which is the same way it’s sourced for skin care).
The ingredient acts as a humectant, like hyaluronic acid, drawing in water to keep skin hydrated and maintain its moisture barrier over long periods of time. Cara Bondi , a cosmetic chemist who has worked with beauty brands like Tata Harper Skincare, Ursa Major, and Sol De Janeiro, notes that polyglutamic acid also smooths the appearance of wrinkles, increases moisture retention, softens skin, and evens skin tone.
The biggest difference between hyaluronic acid and polyglutamic acid is their molecular weight, which has an effect on their effectiveness to hydrate the skin. Polyglutamic acid molecules are larger than hyaluronic acid molecules, which means they stay on the surface of your skin to create a sort of film that locks in water. Of course, both ingredients are effective humectants (there’s a reason why HA is so beloved), but polyglutamic acid works just a little bit harder.
{{post.sponsorText}}
“Polyglutamic acid holds 4,000-5,000 times its weight in water. Hyaluronic acid is usually cited as 1,000 times its weight in water,” says Bondi. However, while polyglutamic acid is more effective, it’s a lot more expensive to source. According to derms, this is why we don’t often see the ingredient marketed. If you do, it will be more pricey than your trusted hyaluronic acid serum; around $60 - $70 dollars.
The polyglutamic acid serum that transformed my skin
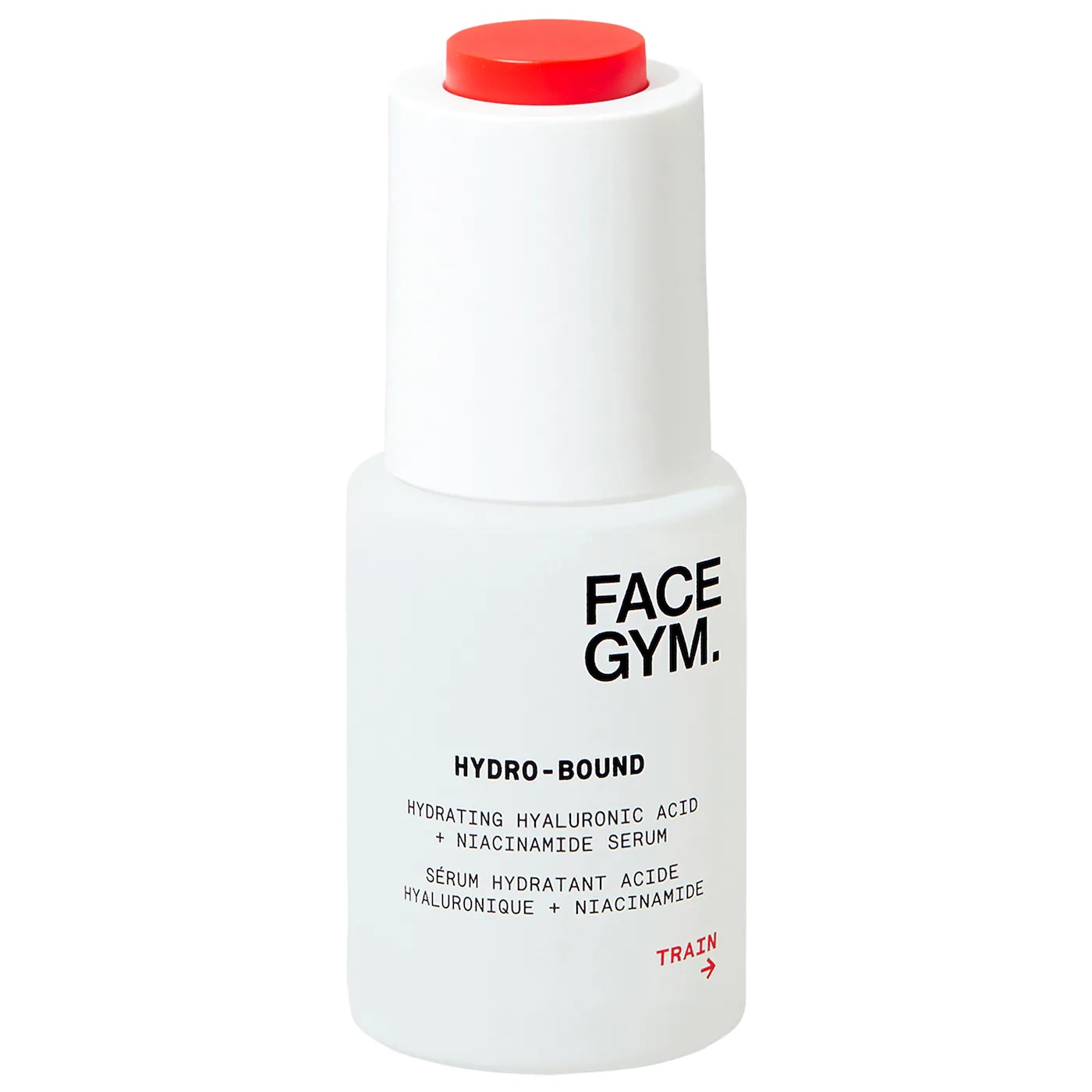
The larger, 30 mL bottle is back in stock for $77. The smaller, 15 mL bottle ($45) is currently on back-order. But trust me—go with the bigger bottle. You’ll need it.
Both of these powerhouse ingredients are present in Face Gym’s Hydrobound serum, which means you’re getting a double dose of hydration. Plus, it’s got niacinamide, which means the formula offers a trifecta of skin-perfecting, nourishing, and smoothing ingredients.
The combination of polyglutamic acid and multi-molecular Ha4 (hyaluronic acid) in Hydrobound delivers hydration and helps the skin naturally produce its own hyaluronic acid, improving skin elasticity and softening fine lines. “Polyglutamic acid is ideal for those with dry or dehydrated skin,” says Kim Nichols, MD , board-certified dermatologist and founder of NicholsMD in Greenwich, CT. Hydrobound also contains a bio-lifting complex that works with niacinamide to firm skin and rebuild damaged areas of the skin barrier.
It’s hard to play favorites when you’re a beauty editor but when you go through a bottle of a specific skin-care product every month, despite having endless choices, you tend to notice. Hydrobound is so good, it’s the only product that I religiously order and simply can’t live without.
Lifetime to date, I’ve been through about 10 bottles of this serum. The first few were gifted (thank you) but then I realized couldn’t be without this precious formula ever again, so I started buying multiple at a time. I love it because it’s lightweight, and almost feels like water on your face. In addition to the longterm hydration benefits it offers, I can instantly see it quenching my skin every time I apply. In the past year I’ve been using this serum, I’ve never received more compliments on how my complexion looks. I’ve gone makeup free almost every chance I get and my skin looks and feels healthy and clear–not at all dehydrated. I give this serum a 10 out of 10, and will keep buying it until they no longer stock the shelves with it.
Loading More Posts...
The Beach Is My Happy Place—and Here Are 3 Science-Backed Reasons It Should Be Yours, Too
Your official excuse to add "OOD" (ahem, out of doors) to your cal.
4 Mistakes That Are Causing You to Waste Money on Skin-Care Serums, According to an Esthetician
These Are the Best Anti-Chafing Denim Shorts—According to Some Very Happy Reviewers
{{ successMessage }}
Please wait a moment...
- Beauty Amplified
Get to Know Polyglutamic Acid, the Hydrating Ingredient Taking Over Right Now
When we think about hydrating ingredients, acids aren’t always the first thing to come to mind. Of course, the popularity of hyaluronic acid , with its ability to hold 1000 times its weight in water and all, changed all that. But a new ingredient, polyglutamic acid—PGA for short—has entered the scene. And it’s appearing in an increasing number of hydrating skincare products. We checked in with dermatologist Karan Lal, MD, to find out more about this popular ingredient—plus, we’re sharing five PGA-infused products worth getting your hands on now.
About the Expert :
Karan Lal, MD, is a board-certified dermatologist at Schweiger Dermatology Group in Hillsborough, New Jersey.
Quick question: what is polyglutamic acid.
Despite its long name, you’ll likely be hearing more and more about this powerhouse skincare ingredient. “Polyglutamic acid is the new humectant on the block,” says Dr. Lal. And like other humectants (i.e. hyaluronic acid, glycerin , and even sugar ), it works by attracting water molecules to the skin’s surface. The name itself also helps explain what it is—”poly” means many, denoting that it’s a compound of multiple glutamic acids. Therefore, says the derm, PGA “is a larger molecule [than similar ingredients], thus it sits on top of your skin and soaks up water.”
What Are the Benefits of Polyglutamic Acid?
Three words: hydration, hydration, hydration. “It’s a phenomenal moisturizer and it gives you dewy skin,” explains Dr. Lal. In addition to being great for dry and dehydrated skin, it’s also a great addition to anti-aging products. Look for it in everything from moisturizers to serums to eye creams, though it’s especially effective in water-soluble formulations, for obvious reasons. “Because it hydrates the top of the skin, it can help soften fine lines after you apply it,” says the derm, adding that “This means it’s great as a base before applying makeup.”
Even better, polyglutamic acid has no known side effects, and can be used by pretty much everyone. “PGA is great for all skin types and skin colors,” says the derm. “It’s especially great for patients with facial eczema who feel like they can’t get enough moisturizer on their face.” Plus, because PGA is non-irritating it plays well with other active ingredients. “Because it’s not an exfoliating acid it can be used with all of your other skincare products,” reassures Dr. Lal.
How Is Polyglutamic Acid Different From Hyaluronic Acid?
Hydrating? Check. Plumping? Check. Non-irritating? Check. If PGA sounds similar to hyaluronic acid, it’s because it is. “Similar to hyaluronic acid, it soaks up a lot of water which makes it a great hydrating agent,” explains Dr. Lal. The main differences are that hyaluronic acid is naturally found in our skin, whereas polyglutamic acid isn’t (it’s sourced from Japanese fermented soybeans called natto). Also, the molecule is much larger. “Polyglutamic acid retains four times more moisture than hyaluronic acid,” says the expert. That said, the two ingredients are often seen together on labels to double up on hydration benefits.
The Best Polyglutamic Products to Try Now
1. the inkey list polyglutamic acid.
This silky serum puts the focus on the PGA, featuring a 3% concentration of the ingredient. It can be applied both in the morning and at night for a boost of hydration, and creates a bouncy, smooth surface for makeup, or your next skincare steps.
2. CHARLOTTE TILBURY Magic Serum Crystal Elixir
Mixing the hydrating power of PGA with the brightening benefits of vitamin C? That’s the genius of Charlotte Tilbury. The formula boosts elasticity, and skin looks glowy and smoother with each use and over time.
3. FACE GYM Hydro-Bound Hydrating Face Serum
This hydrating serum blends the powers of PGA and multiple weights of hyaluronic acid, making it a great layer in any skincare routine, especially for dry skin. Plus, it has niacinamide to strengthen skin and leave it more even toned, too.
4. FARMACY Daily Greens Silicone-Free + Oil-Free Gel Moisturizer
Another great thing about polyglutamic acid? It’s great in oil-free formulas, such as this moisturizer. Also made with hyaluronic acid, as well as clarifying moringa, willowherb, and papaya, it’s ultra-hydrating yet lightweight and won’t clog pores.
5. VERSED Mood Lighting Lumizing Glow Drops
We love any product that offers a one-two punch, and this hydrating highlighting fluid both adds a subtle glow as it plumps skin with PGA and antioxidant-packed blackberry extract. Wear it alone or mixed with foundation, depending on how much radiance you’re going for.
Want hydrating skincare—and so much more—delivered each month with the IPSY Glam Bag? Take our Beauty Quiz now to get started. Already an Ipster? Refer your friends to earn points, which you can use toward products. Either way, don’t forget to check us out on Instagram and Twitter @IPSY.
Like this article? Share it with your friends by clicking the icons below!
More Stories
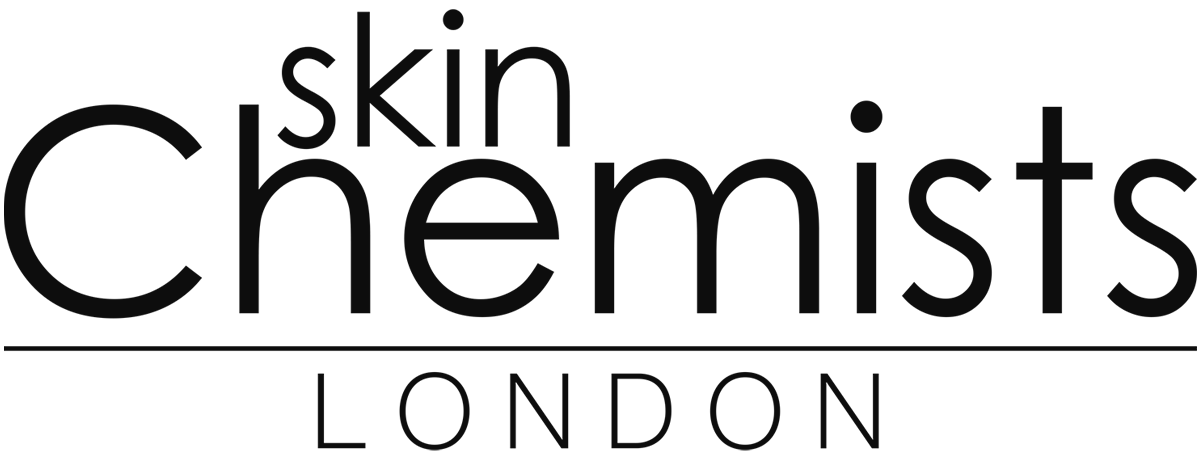
- BEST SELLERS
- DAILY DEALS
- SHOP BY PRODUCT Shop All Eyes Exfoliator Cleanser Mask Facial Serums Facial Oil Facial Moisturiser SPF Body Creams Shampoo Conditioner
- SHOP BY CONCERN Ageing, fine lines and wrinkles Dull Skin Dry Skin Loss Of Elasticity
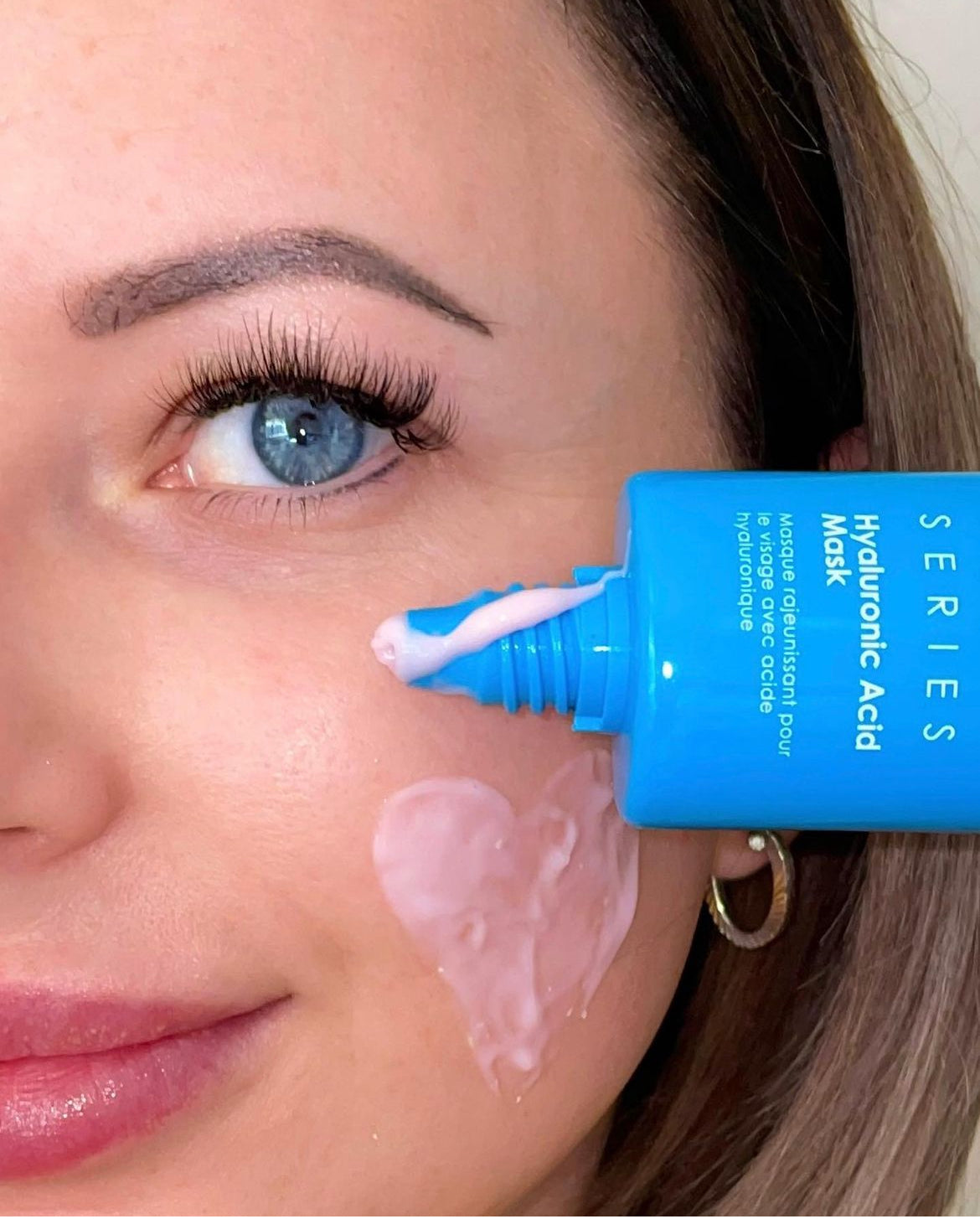
TAKE THE SKINCARE QUIZ
- Bundles & Gifts SHOP ALL Retinol Moisturise & Sooth Kit Caviar Anti-Aging Starter Kit Collagen Starter Kit Youth Series Green Caviar Hydrating Gift Set Youth Series Collagen Acne Prone Gift Set Youth Series Green Caviar Essentials Full Set Youth Series Collagen Tone & Flex Kit Youth Series Hyaluronic Acid Smooth & Condition Kit
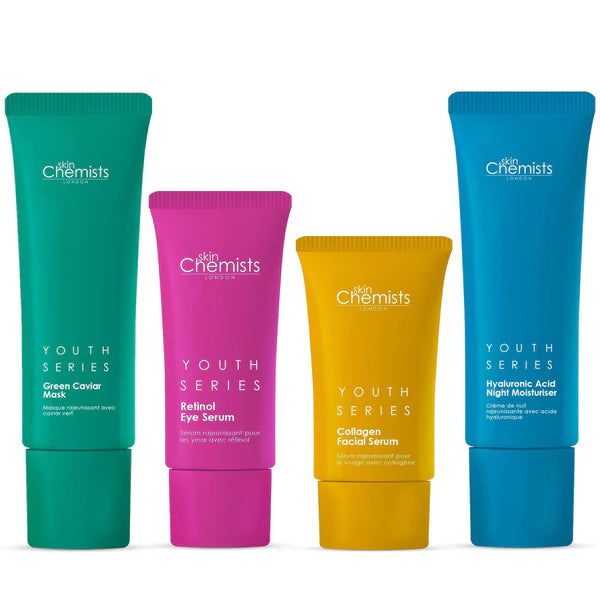
YOUTH SERIES WELCOME KIT
- Beauty Tips
- Daily Deals
- Discount Codes
Your cart is empty
20 products
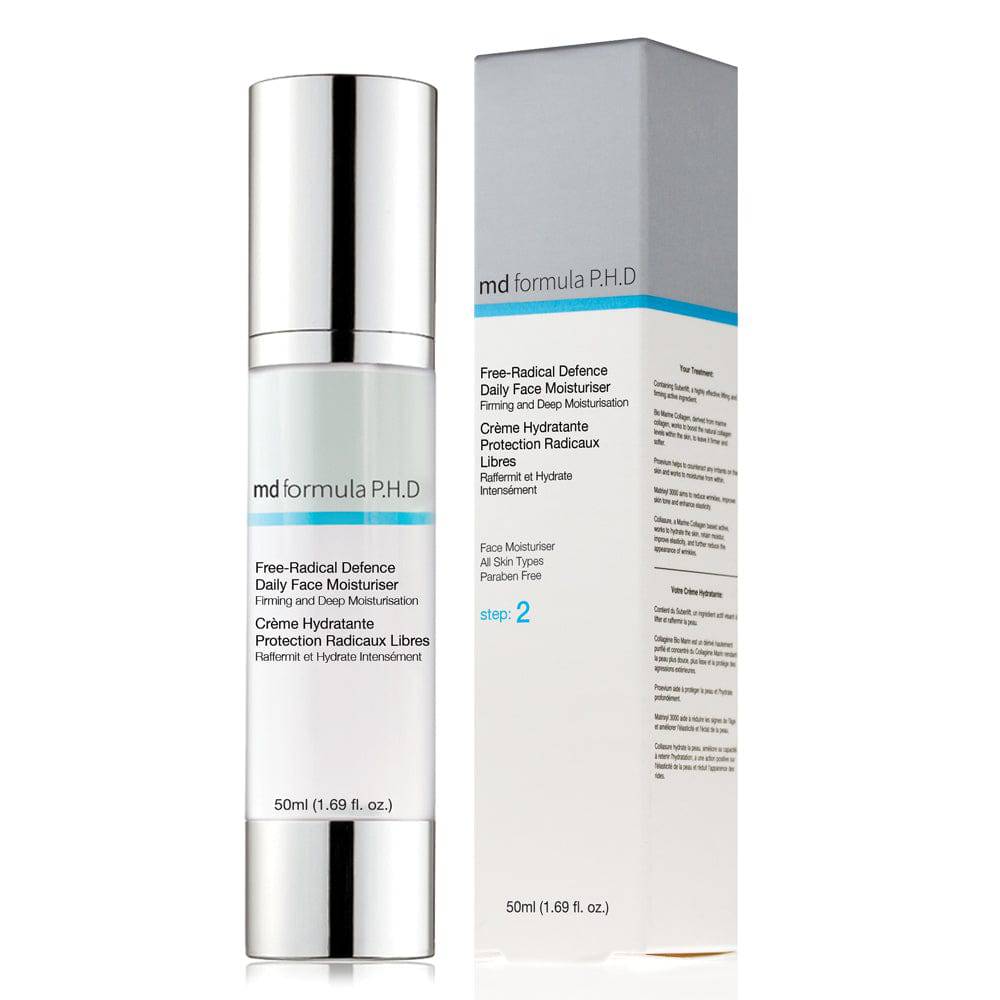
Molecular dynamics simulation and performance verification of γ-polyglutamic acid/cold water-soluble starch film formation and permeability
- ORIGINAL PAPER
- Open access
- Published: 26 March 2024
Cite this article
You have full access to this open access article
- Zuolong Yu 1 ,
- Yao Chen 2 ,
- Yongfei Chen 3 ,
- Yunxiao Wei 1 ,
- Changchun Fu 1 ,
- Dan Liu 1 ,
- Keshavan Niranjan 4 &
- Chao Han 1
431 Accesses
Explore all metrics
Six types of γ-polyglutamic acid (γ-PGA)/cold water-soluble starch (St) composite film models were constructed using molecular dynamics simulation, and their properties were investigated and compared with the corresponding experimental values. The compatibility between the composite film components was analyzed using the radial distribution function and mean square displacement (MSD). The hydrogen-bond number and bond energy were used to track the film-formation process. The mechanical property data of the films were extracted, and MSD was used to analyze the permeability of the film to carbon dioxide, oxygen, water vapor, and carbon-16 saturated fatty acids. Finally, the simulated values of mechanical properties and permeability were compared with the experimental values. The results demonstrated that γ-PGA is well compatible with St. The intramolecular and intermolecular hydrogen bonds of γ-PGA and St did not change considerably during the film-formation process. The simulated values of the mechanical properties exhibited a similar trend as the experimental values; however, in terms of permeability, a difference was observed between the initial values of the simulated design and actual material parameters, as well as the complexity of the experiment.
Similar content being viewed by others
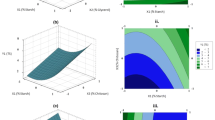
Analysis and Modeling of Mechanical and Barrier Properties of Arracacha Starch-Chitosan Composite Biodegradable Films
Omar R. Garcia, Magda I. Pinzón & Cristian C. Villa
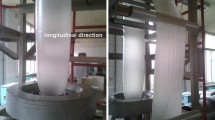
Effects of various nanomaterials on the properties of starch/poly(vinyl alcohol) composite films formed by blow extrusion process
Wentao Wang, Hui Zhang, … Haizhou Dong
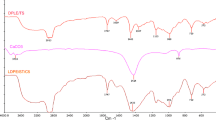
Preparation and Characterization of Hybrid Composite Film Based on Synthetic and Natural Materials
Waham Ashaier Laftah & Wan Aizan Wan Abdul Rahman
Avoid common mistakes on your manuscript.
Introduction
Starch, which is one of the most abundant polysaccharides on Earth, can be converted into different biobased materials through physical and chemical modifications, e.g., pharmaceutical excipients [ 1 , 2 ] and packaging plastics [ 3 , 4 ]. These materials can be applied in pharmaceutical [ 5 ] and environmental industries, and if the materials meet people’s dietary needs, they can be used in the food industry [ 6 , 7 ]. The physicochemical properties of starch differ according to its amylose and amylopectin content, and the solubility characteristics of starch in water differ according to the number of glucose units. Raw starch cannot be dissolved in water; it can only form a suspension or colloid. When the raw starch is processed and its molecular weight drops to thousands to tens or hundreds of thousands, it can be dissolved in hot water (referred to as soluble starch) [ 8 , 9 ] or cold water (referred to as cold water-soluble starch) [ 8 , 9 , 10 , 11 , 12 ]. Studies have shown that starch-based products obtained by different treatment methods have a wide application range [ 13 , 14 ].
Industrial γ-polyglutamic acid (γ-PGA) products can be obtained using the fermentation methods of different genetically engineered strains [ 15 , 16 ]. After separation and purification, γ-PGA can be used to prepare environmentally compatible products, e.g., hydrogels, nanoparticles, and packaging films [ 17 , 18 , 19 ]. γ-PGA with different molecular weights can be obtained by modifying genetically engineered bacteria and changing the fermentation conditions, which expand its applicability and enhance its application value [ 9 , 20 , 21 ].
Starch-based films are relatively inexpensive and environmentally friendly. Their poor mechanical and nonthermoplastic properties can be modified and improved via blending or copolymerization to enhance their application. In addition, the known antibacterial properties and intelligent manifestations of these films can broaden the application range of starch in the packaging field [ 22 , 23 , 24 , 25 ]. γ-PGA films are also receiving increased research interest in food packaging [ 19 ], medical materials [ 26 ], and heavy-metal adsorption [ 27 ] applications.
The molecular simulation technology developed in recent years can be used to investigate the interactions and mechanisms of various components at the molecular level, predict material properties, reduce experimental costs and time, and accelerate the development of new materials [ 28 , 29 ]. The compatibility [ 30 ], glass transition temperature [ 31 ], mechanical properties [ 32 ], and gas diffusion [ 33 ] of blend films can be effectively predicted using molecular dynamics (MD) techniques. Researchers have used MD for designing the preparation methods and determining the properties of biobased macromolecules, e.g., polysaccharides and proteins [ 34 , 35 ]. In this study, the complex starch molecule was simplified into cold water-soluble starch to prepare γ-PGA blends and obtain composite films with different mass ratios. The compatibility, mechanical properties, and permeability of the composite films were estimated via molecular simulation. The experimental results agreed with the simulated values, and comprehensive data were obtained from micro and macro perspectives. Meanwhile, it provided a reference for molecular dynamics simulation of biological macromolecules.
Methodology
Model and simulation methodology, modeling and relaxation.
In this study, first, molecular structures of γ-PGA, starch, and glycerol were constructed through the modeling function of Materials Studio 7.0 (Figs. 1 a, b, and d), and the degree of polymerization of γ-PGA and starch molecules was 10. Then, three types of molecular chains of the amorphous system were constructed using the Amorphous Cell module. The mass ratios of γ-PGA and starch in the amorphous system were 10:0, 8:2, 6:4, 4:6, 2:8, and 0:10, and the mass of glycerol was 10% of the total mass. (The six composite films are referred to as P10S0, P8S2, P6S4, P4S6, P2S8, and P0S10, respectively.) To preserve appropriate space between the molecules in the subsequent relaxation process of the system, the initial density of the model was set to 0.8 g/cm 3 , the summation method of electrostatic energy in nonbonding interaction energy was group-based, the summation method of van der Waals interaction energy was atom-based, and the truncation radius was 12.5 Å. Further, the COMPASSII force field was used, and the atomic charge was automatically allocated to the force field. After the model was constructed, the structure was optimized using the Forcite Plus module in Materials Studio. The optimization method was efficient enough to obtain a lower energy system.
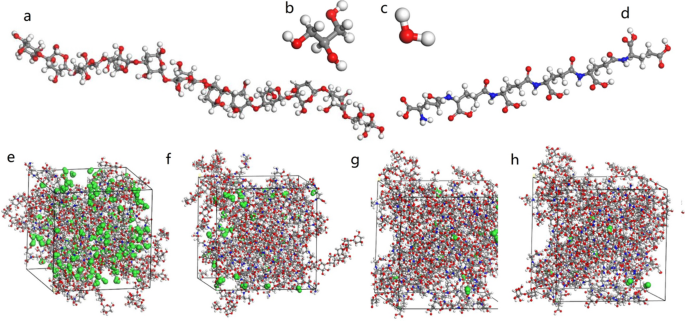
Simulation model of low energy monomer molecules and their blends with different water content: a starch; b glycerin; c water; d γ-polyglutamic acid; e , f , g , and h blend systems containing 100, 20, 10, and 1 g of water, respectively
Finally, the kinetic method was used to fully relax the model. To quickly eliminate the internal stress in the system, the total simulation time was set to 300 ps, and the system comprised a constant number of atoms at normal pressure and temperature (NPT). The pressure was 1 atm, and the temperature was 600 K. After relaxation, an NPT system was used in the model, where the temperature was 300 K, the pressure was 1 atm, the simulation time was 3 ns, and the time step of the two dynamics was 1 fs. A single frame was output every 1000 steps, the force field was COMPASSII, and the summation method of electrostatic energy was group-based. In addition, the summation method of van der Waals interaction energy was atom-based, and the truncation radius was 18.5 Å.
Film-formation mechanism
The P6S4 sample was selected as the simulation object. The Amorphous Cell tool in Materials Studio was used to set up four types of film liquids or film model structures with different water contents (100, 20, 10, and 1 g), from the initial film liquid to dry film-formation process. The MD parameters of the film-formation process were then analyzed. Note that the conditions of the MD simulation were the same as those described in Sect. " Modeling and relaxation ". Particularly, the model size ranges from 30 to 40 Å. The systems characterized by different water contents (obtained through experiments) are investigated from a simulation perspective. The simulation method indirectly represents the water content based on a specific number of water molecules and utilizes a dominant solution model. The temperature was changed from normal temperature to 333 K, the total simulation time was 1 ns, the time step was 1 fs, and the deterministic Nosé–Hoover Langevin algorithm was the temperature-control method. After the dynamics were completed, the trajectory file was analyzed to obtain the total number of hydrogen bonds in each system. Here, to further investigate the contribution of each component to the hydrogen bonds, the number of hydrogen bonds between each component was also extracted. In this study, the maximum length of the hydrogen bond was set to 2.5 Å and the minimum angle of the hydrogen bond was set to 90°.
Mechanical properties
The tensile mechanical properties of the systems were investigated. Equilibrium calculations for MD were conducted across all systems. Subsequently, 100 frames were selected from the model at the equilibrium stage to calculate the mechanical properties. The calculation method involved altering the strain of the model and calculating the stress of the system. Six strains, comprising three stretches and three compressions, were calculated for each structure, with a maximum deformation of 0.5%. In addition, the stress–strain curve was obtained by submitting the stress and strain data during the stretching process.
- Permeability
The permeation effect of different molecules in six composite film systems was investigated. During the investigation, oxygen, carbon dioxide (CO 2 ), water, and carbon-16 saturated fatty acid molecules were separately added to the model. The MD simulation process was the same as that described in Sect. " Modeling and relaxation ". In the diffusion–penetration–simulation process, the dynamics were integrated into microcanonical ensemble (NVT), the time step was 1 fs, and the total simulation time was 1000 ps.
Experimental verification
To validate the guiding significance of the simulation method, the corresponding simulation blending films with different proportions were prepared. The simulation and experimental results regarding rheological properties, mechanical properties, and permeability of the membrane toward four types of substances were compared.
Film preparation
The films were prepared using γ-PGA (70 KDa) (Nanjing Shineking Biotech, Co., Nanjing, China) and starch (96–100% solubility in water) (Shanghai Aladdin Biochemical Technology Co., Ltd., Shanghai, China) as the main substrates and glycerol as the additive, and the formula was as follows in Sect. " Modeling and relaxation ". In the specific experimental methods, the P6S4 sample was taken as an example. Here, 4 g of starch and a certain amount of water were stirred evenly and poured into a three-neck flask and then gelatinized by heating at 85 °C for 30 min. In a separate flask, 6 g of γ-PGA, 1 g of glycerol (Sinopharm Group Chemical Reagent Co. Ltd., Shanghai, China), and 100 mL of water were stirred and dissolved. This mixture was then added to the three-neck flask containing gelatinized starch and stirred for 10 min. After degassing, the resulting film liquid was cast on a glass plate and dried in an oven at 60 ℃ to form a film (with a moisture content of ~ 10% of the total mass). The mechanical properties and permeability values were tested after 48 h.
The storage modulus and shear rate curves of the film liquids were determined using a rheometer (MCR 102, Anton-Paar, Shanghai, China). The measurements were performed at a constant temperature of 80 °C using a conical plate with a diameter of 50 mm and an angle of 1° using the flow curve measurement mode. The shear rate was considered a variable with a range of 0.1–100 s −1 , the mode was linear scan, and 50 variable points were selected.
The sample was cut into strips with a length of 5 cm and a width of 0.5 cm. The tensile modulus (T) of the films was measured (TA.XT Plus, Stable Micro System, London, UK) along the vertical and horizontal directions using three strips. In total, six parallel samples were measured. The standard distance was 50 mm, and the sampling speed was 100 mm/min.
A strong alkali absorption method was used to determine CO 2 permeability [ 36 , 37 ]. A weighing bottle containing 200 mL of a 1-N KOH solution was sealed with the film to be tested and placed in a desiccator with a 10% relative humidity (RH) saturated salt solution. The system was balanced in a nitrogen (N 2 ) environment for 24 h, and then N 2 was replaced with CO 2 to maintain the partial pressure of CO 2 outside the film at 101 kPa. CO 2 was absorbed by the KOH solution through the film. The absorption time and weight gain of the weighing bottle were recorded, and the CO 2 transmittance was calculated as follows.
where \({\text{P}}_{{{\text{CO}}_{2} }}\) is the CO 2 permeation rate of the film (mg/(cm 2 ·d)), Δm is the mass of CO 2 absorbed by the KOH solution (mg), d is the number of days, and S is the effective area of the film (cm 2 ).
GB/T 16928–1997 and the ASTM material moisture permeability test method with slight modifications were used to determine water vapor permeability (WVP). In the test, 50 g of anhydrous calcium chloride (particle size = 2 mm) was placed in a 100-mL beaker at 25 °C. After measuring the thickness of the film, the mouth of the beaker was sealed with melted paraffin and placed in a desiccator at 100% RH. The sample bottles were removed from the desiccator and weighed every 24 h. Three parallel tests were conducted, and the bottles were measured continuously for one week. The results were calculated according to a process reported in the literature [ 38 ].
Unsaturated fat absorption method was used to determine oxygen permeability [ 36 , 37 ]. A 50-mL beaker containing 20 mL of oleic acid was sealed with the film to be tested and placed in a desiccator with a 10% RH saturated salt solution. The system was balanced under N 2 atmosphere for 24 h, and then, N 2 was replaced with O 2 to maintain the O 2 partial pressure outside the film at 101 kPa. O 2 was consumed via the oxidation of vegetable oil through the film. The weight gain of the beaker was recorded at intervals, and the O 2 penetration was calculated as follows:
where \({\text{P}}_{{{\text{O}}_{2} }}\) is the O 2 permeation rate of the film (mg/(cm 2 ·d)) and Δm is the mass of O 2 absorbed by oleic acid (mg).
To evaluate the vegetable oil permeability (P oil ) [ 39 ], 5 mL of vegetable oil was poured into a test tube and sealed with the film. The test tube was placed upside down on filter paper for one week. The filter paper was weighed every 24 h to calculate the P oil of the samples.
Statistical analysis
Note that the data from the experiments are expressed as the mean ± standard deviation. The statistical differences were analyzed according to variance and LSD analysis using the SPSS software (SPSS Inc., Chicago, Illinois, USA). In this evaluation, P < 0.05 was considered statistically significant.
Results and discussion
Equilibrium configuration.
Two criteria were used to distinguish the MD simulations to achieve equilibrium: (1) temperature fluctuation < 10 °C and (2) energy fluctuation < 100 fs. Note that these criteria must be constant or fluctuate (increase or decrease) slightly along a constant value. Generally, the system is considered to have reached equilibrium when the temperature and energy fluctuations are between 5 and 10% [ 40 ]. The temperature change is minor in the simulation of the composite film model (Fig. 2 ), and the energy change is shown in Fig. 3 . At the beginning of the MD simulation, the fluctuation gradually weakened within 10–15 ps (10000–15000 fs), and the energy increased gradually. Under the action of shear force, the molecular chain untangled, and the molecules broke free from the binding, resulting in an increase in the energy of the molecular system. Then, it stabilized quickly to a constant value. These results demonstrate that the system reached a thermodynamic equilibrium structure, and then, the energy and instantaneous temperature of the system fluctuated slowly around the equilibrium value, forming a relatively stable curve according to the equilibrium criterion. This indicates that all the mixed systems reached equilibrium via MD simulation.
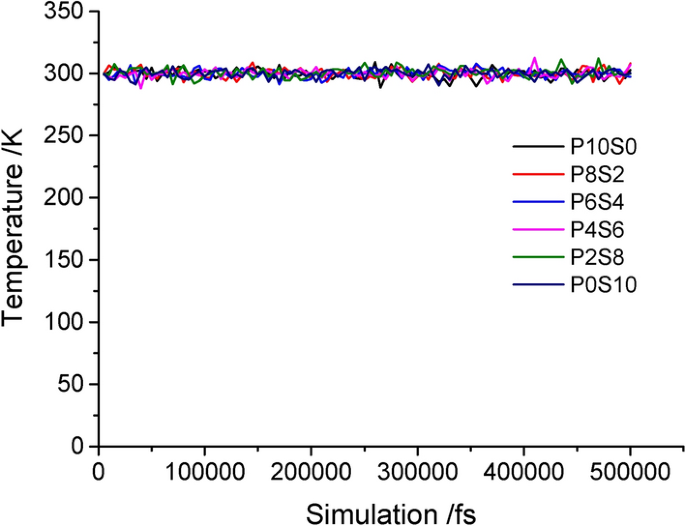
Temperature curves of composite films with simulation time
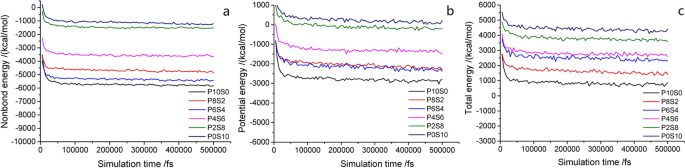
Energy curves of composite films with simulation time: a nonbond energy; b potential energy; and c total energy
Compatibility
The radial distribution function (g(r)) is performed on an atom as a characteristic physical quantity that reflects the microstructure of materials. It represents the ratio of the probability density of a molecule to the probability density of random distribution at distance r around another molecule. Thus, a larger g(r) value indicates better compatibility of the blend system. The intermolecular g(r) is used to reveal the interaction mode and nature between nonbonded atoms. Note that the hydrogen-bond interaction range is 2.6–3.1 Å, and the van der Waals interaction range is 3.1–5.0 Å [ 41 ].
Figure 4 (a) shows the g(r) values of the separate γ-PGA, St, and glycerol molecules. This figure shows that none of these three pure substances exhibits a strong peak at > 3 Å, verifying that the constructed molecular structure is amorphous [ 42 ]. However, no peak is observed, and the g(r) value approaches 1 at radial distances of > 4 Å, proving that the blend system has amorphous characteristics [ 43 ]. As shown in Figs. 4 b–g, the g(r) values of all the blends peak at ~ 5 Å. Thus, the main mode of interaction between the blends is considered as the van der Waals force. By comparing the g(r) values of the C–C bond between the γ-PGA/St molecules (Fig. 4 h), we found that the peak-height order of the blends was as follows: P6S4 > P4S6 > P2S8 > P8S2. This trend indicates that the blend system exhibits good compatibility, and when the quality of the two substances is equivalent, the compatibility is better. In addition, by comparing the nonbond energies of their respective systems (Fig. 3 a), the bonding energies of the blends are observed to follow the order of P6S4 > P8S2 > P4S6 > P2S8.
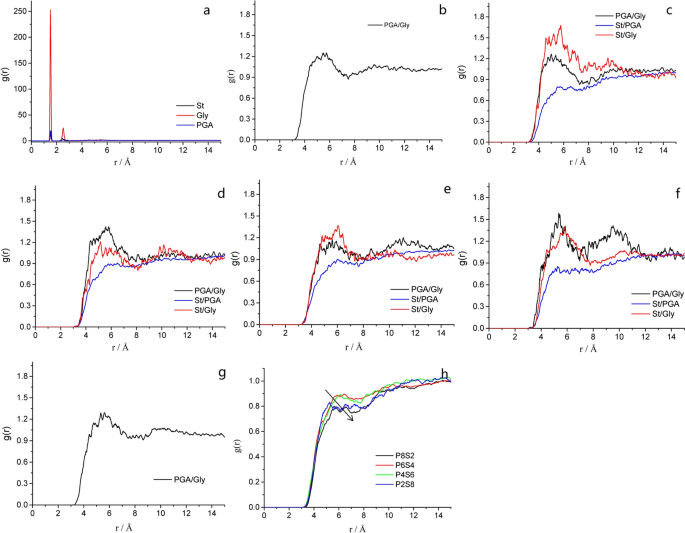
Radial distribution function (RDF) of C–C atoms in composite films and monomers: a RDF of γ-PGA, St, and Gly; b – g : RDF between molecules in P10S0, P8S2, P6S4, P4S6, P2S8, and P0S10, respectively; and h RDF comparison of γ-PGA/St in composite films
In this study, the mean square displacement (MSD) was considered to characterize the thermal motion displacement of the γ-PGA, St, and glycerol chains. Therefore, it was used to explore the mutual influence of motion ability in the films; a larger MSD value indicates a more intense thermal motion of molecules. The thermal motion of the glycerol molecules was the most intense, followed by γ-PGA and then St (Fig. 5 and Table 1 ). A comparison of different composite films indicates that the thermal motion of γ-PGA and St molecular chains was the most intense in P10S0 and P0S10. In the other composite films, the movements of the γ-PGA molecular chains were more intense than those of St, and the change trend of γ-PGA and St was opposite. These findings indicate that the movement ability of the molecular chains in the mixing system was affected by the change in the blending ratio. In the composite films with different mixing ratios, the force on the molecular chain of the system differed. The change in the mass ratio of γ-PGA/St can cause considerable changes in the secondary bond force, e.g., the hydrogen-bond force, affecting the spatial structure of the molecule and in turn affecting the movement activity of the molecule.
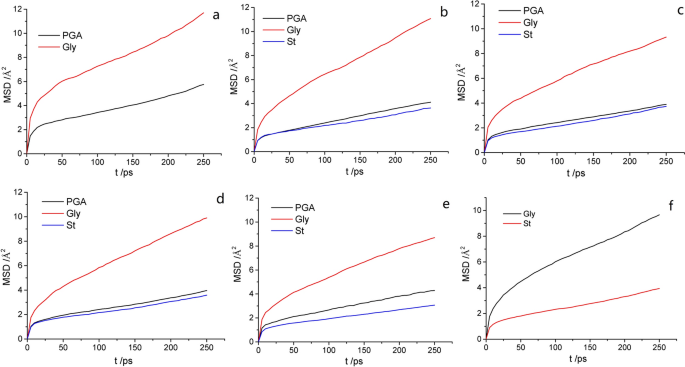
MSD of different molecules in composite films: a P10S0; b P8S2; c P6S4; d P4S6; e P2S8; and f P0S10
Film processing
Using P6S4 as an example, the hydrogen bonds within and between each molecule under the simulated conditions are shown in Fig. 6 . When various substances reached equilibrium after addition (Fig. 6 a), the numbers of hydrogen bonds between γ-PGA and water, St and water, and γ-PGA and St were the highest at approximately 250, 241, and 228, respectively. The numbers of intramolecular hydrogen bonds in St, γ-PGA, and water molecules were approximately 182, 171, and 123, respectively. In addition, the number of hydrogen bonds formed by glycerol was consistently the lowest. With the evaporation of water, the concentration of γ-PGA and St and the probability of their contact increased. The number of hydrogen bonds within and between the molecules increased gradually (Fig. 6 b), accounting for the majority of their total number of hydrogen bonds. The hydrogen bond between γ-PGA and St was the most evident, indicating that PGA and St can be mixed evenly and are well compatible in the film-formation process. The secondary bonds represented by hydrogen bonds between PGA and St exhibit microscopic changes. Table 2 shows that the changes in bond energy (i.e., the bond stretching energy (E bond ), bond angle bending energy (E agle ), dihedral angle torsion energy (E torsion ), and inversion energy (E inversion )) of the four composite films during the film-formation process were not significant because these processes were unaffected by external forces. Considerable changes occurred in the nonbonding energy (i.e., the van der Waals interaction energy (E vdw ), electrostatic energy (E coulomb ), and hydrogen bonding energy (E hbond )). Although the reduction of water mass was one factor, the increased interaction between γ-PGA and St decreased the reduction rate of the nonbond energy.
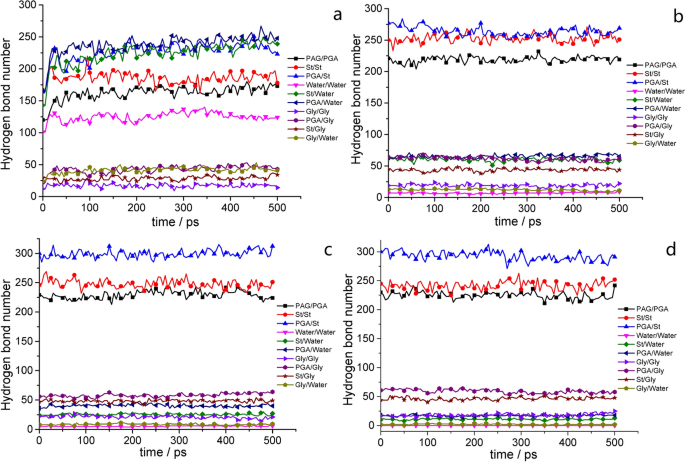
Number of intermolecular and intramolecular hydrogen bonds in film liquids or films with different water content: a 100; b 20; c 10; and d 1 g
The elastic modulus (E), shear modulus (G), and bulk modulus (K) are used to indicate the rigidity of the material under different force conditions. Note that the elastoplastic properties of a material are associated with E, and the hardness and tensile strength (T) are proportional to G. A positive Cauchy pressure value indicates good ductility of the material, whereas a higher value indicates better ductility of the system [ 44 ].
The mechanical properties of γ-PGA, St, and their composite films at four proportions obtained through MD simulation calculations are listed in Table 3 , including the E, K, G, and Poisson’s ratio (ν; the ratio of the transverse strain to the longitudinal strain) values. The values of the elastic constants of the C 11 , C 22 , C 33 , C 44 , C 55 , and C 66 groups, as well as the C 12 , C 13 , and C 23 groups, were similar, indicating that γ-PGA and St had good solubility. The ν value of the composite films was ~ 0.28, and the ν value of plastics is typically between 0.2 and 0.4, indicating that the mixed system exhibits some properties of plastics [ 45 ]. The Cauchy pressure values (C 12 –C 44 ) of various films were all positive, indicating that the composite films exhibit toughness. The Cauchy pressure values of the P0S10 and P10S0 samples were lower than those of the blend films, indicating that the ductility of the single principal component was poorer than that of the composite principal component and the brittleness was stronger. In addition, depending on the proportion of the components, the number of hydrogen bonds formed by the composite film varied. We found that the P4S6 sample exhibited the highest K value, the P0S10 sample showed the highest G value, and the P2S8 sample demonstrated the highest Young’s modulus (Y) value.
According to the MSD slope, the ability of gas to escape from the interface can be determined [ 46 ]. Figures 7 a–d shows that the diffusion of CO 2 , water vapor, O 2 , and vegetable oil in the composite films did not exhibit regular changes. The MSD of gas diffusion can also be converted into the diffusion coefficient to illustrate the molecular permeability (Fig. 8 ) [ 47 ]. The P10S0 sample exhibited the highest \({\text{P}}_{{{\text{CO}}_{2} }}\) . The P2S8 sample exhibited the highest WVP, and the P2S8 sample demonstrated the highest P oil . The P10S0 sample demonstrated the lowest \({\text{P}}_{{{\text{O}}_{2} }}\) . Note that the small molecules exhibited jumping diffusion in the polymer films, which was affected by the intermolecular force of the film. The small molecules were confined in the polymer film holes and caused small local vibrations most of the time. In addition, with the continuous movement of the polymer chain end, small molecules could jump from one hole to another [ 48 , 49 ]. The difference in the energy barrier, electrostatic interaction, and free volume fraction on the molecular chains of the membranes resulted in the difference in the diffusion coefficient of the gas through the films [ 50 ].
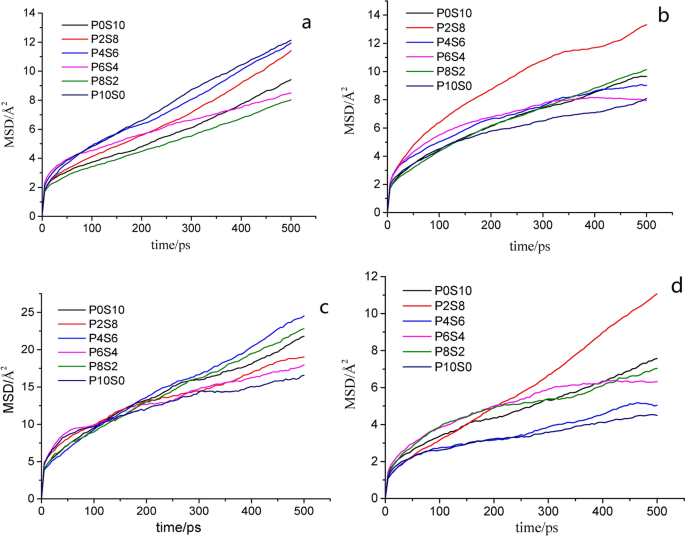
Permeability of composite films to four substances: a CO 2 , b water vapor, c O 2 , and d oil
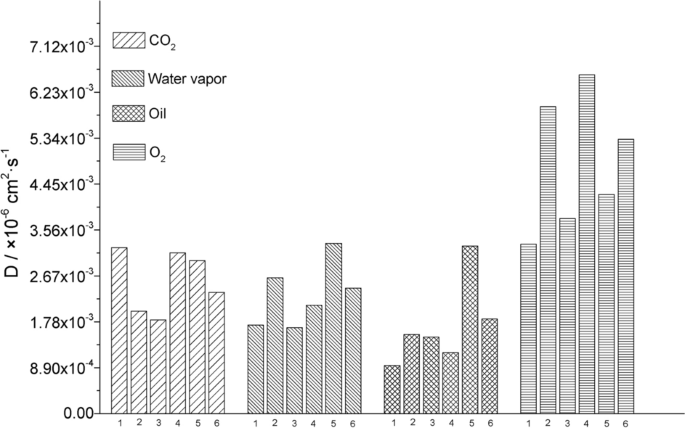
Diffusion coefficients of composite films to four substances: (1) P10S0, (2) P8S2, (3) P6S4, (4) P4S6, (5) P2S8, and (6) P0S10
Experimental data
The P0S10 sample exhibited the highest storage modulus (G′) (Fig. 9 ), and with an increasing mass ratio of γ-PGA, the G′ of the composite film liquid decreased gradually, and shear thinning occurred. We found that the G′ values of the P8S2 and P10S0 samples were similar. The variation trend of the G′ values was similar to that observed for the E values. Owing to the proportional relationship between G and G′, they exhibited the same variation pattern as the simulated G values (Table 3 ). As the mass ratio of St increased, the T initially decreased and then increased, which is closely related to the molecular interaction between γ-PGA and St, as well as their molecular weights.
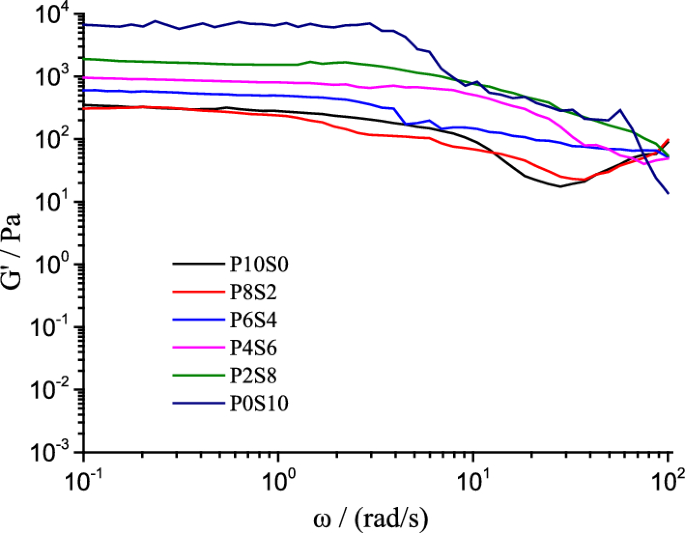
Storage modulus of composite film liquids
The experimental data validation for permeability (Table 4 ) showed that as the mass ratio of St increased, P2S8 exhibited the highest \({\text{P}}_{{{\text{CO}}_{2} }}\) , which was equivalent to that of P4S6 and consistent with the simulation results. However, the WVP of P0S10 was much lower than that of the other composite films; similarly, the data for \({\text{P}}_{{{\text{O}}_{2} }}\) and P oil were not consistent with the simulated values. In the simulation design, the molecular weights of the two macromolecular substances were considered to be close to the weight of only 10 monomers, while in the experiment, the molecular weight of γ-PGA was 70 KDa and the molecular weight of St was 100–200 glucose units. Thus, the difference between the simulated and experimental values was significant.
In this study, six mass ratios of γ-PGA/St composite films were simulated using MD. First, the energy-difference relationship of each composite film was compared when they reached their steady states. Owing to the secondary bond interaction between γ-PGA and St, their energy states changed in different ways. The solubility of γ-PGA and St was investigated based on their g(r) and MSD values, and the results demonstrated good compatibility. According to the film-formation process of the P6S4 sample, in the absence of an external force, the hydrogen-bond interaction between γ-PGA and St in the composite system was always the maximum with decreasing water mass. A comparison of the simulated and experimental values corresponding to the mechanical properties revealed similar variation trends between these values, indicating that the simulation method can be used for reference. However, when comparing the simulation and experimental values of permeability, the experimental settings for real substances could not be achieved due to the limitations of the simulation software conditions; therefore, the simulation values can only serve as guidance to a certain extent.
Data Availability
The data used in this study are confidential.
Labelle MA, Ispas-Szabo P, Tajer S, Xiao Y, Barbeau B, Mateescu MA (2023) Anionic and ampholytic high-amylose starch derivatives as excipients for pharmaceutical and biopharmaceutical applications: structure-properties correlations. Pharmaceutics 15:834
Article CAS PubMed PubMed Central Google Scholar
Kalita P, Ahmed AB, Sen S, Chakraborty R (2023) Citric acid esterified Glutinous Assam bora rice starch enhances disintegration and dissolution efficiency of model drug. Int J Biol Macromol 227:424–436
Article CAS PubMed Google Scholar
Rahardiyan D, Moko EM, Tan JS, Lee CK (2023) Thermoplastic starch (TPS) bioplastic, the green solution for single-use petroleum plastic food packaging—A review. Enzyme Microb Tech 168:110260
Article CAS Google Scholar
Siqueira LDV, Arias CILF, Maniglia BC, Tadini CC (2021) Starch-based biodegradable plastics: methods of production, challenges and future perspectives. Curr Opin Food Sci 38:122–130
Article Google Scholar
Saraf P, Movaghar MA, Montazer M, Rad MM (2023) Bio and photoactive starch/MnO 2 and starch/MnO 2 /cotton hydrogel nanocomposite. Int J Biol Macromol 193:681–692
Asharuddin SM, Othman N, Altowayti WAH, Bakar NA, Hassan A (2021) Recent advancement in starch modification and its application as water treatment agent. Environ Technol Inno 23:101637
Wang JP, Yuan SJ, Wang Y, Yu HQ (2013) Synthesis, characterization and application of a novel starch-based flocculant with high flocculation and dewatering properties. Water Res 47:2643–2648
Kusunoki K, Kawakami K, Shiraishi F, Kato K, Kai M (1982) A kinetic expression for hydrolysis of soluble starch by glucoamylase. Biotechnol Bioeng 24:347–354
Aboubacar A, Hamaker BR (2000) Low molecular weight soluble starch and its relationship with sorghum couscous stickiness. J Cereal Sci 31:119–126
Ma WP, Robyt JF (1987) Preparation and characterization of soluble starches having different molecular sizes and composition, by acid hydrolysis in different alcohols. Carbohyd Res 166:283–297
Jivan MJ, Yarmand M, Madadlou A (2004) Preparation of cold water-soluble potato starch and its characterization. J Food Sci Tech Mys 51:601–605
Choi YJ, Baik MY, Kim BY (2017) Characteristics of granular cold-water-soluble potato starch treated with alcohol and alkali. Food Sci Biotechnol 26:1263–1270
Anwar M, Birch EJ, Ding Y, Bekhit AED (2022) Water-soluble non-starch polysaccharides of root and tuber crops: extraction, characteristics, properties, bioactivities, and applications. Crit Rev Food Sci 62:2309–2341
Pascu B, Negrea A, Ciopec M, Duteanu N, Negrea P, Nemeş NS, Seiman C, Marian E, Micle O (2021) A green, simple and facile way to synthesize silver nanoparticles using soluble starch. ph studies and antimicrobial applications. Materials 14:4765
Yokoigawa K, Sato M, Soda K (2006) Simple improvement in freeze-tolerance of bakers’ yeast with poly-gamma-glutamate. J Biosci Bioeng 102:215–219
Xu J, Chen SW, Yu ZN (2005) Optimization of process parameters for poly gamma-glutamate production under solid state fermentation from Bacillus subtilis CCTCC202048. Process Biochem 40:3075–3081
Kim MH, Lee JN, Lee J, Lee H, Park WH (2020) Enzymatically cross-linked poly (gamma-glutamic acid) hydrogel with enhanced tissue adhesive property. ACS Biomater Sci Eng 6:3103–3113
Ikeda M, Akagi T, Yasuoka T, Nagao M, Akashi M (2008) Characterization and analytical development for amphiphilic poly (gamma-glutamic acid) as raw material of nanoparticle adjuvants. J Pharmaceut Biomed 150:460–468
Yu ZL, Wei YX, Fu CC, Sablani SS, Huang ZE, Han C, Li DN, Sun ZJ, Qin HZ (2023) Antimicrobial activity of gamma-poly (glutamic acid), a preservative coating for cherries. Colloid Surfaces B 225:113272
Sha Y, Zhang Y, Qiu Y, Xu Z, Li S, Feng X, Wang M, Xu H (2019) Efficient biosynthesis of low-molecular-weight poly-gamma-glutamic acid by stable overexpression of pgds hydrolase in bacillus amyloliquefaciens nb. J Agric Food Chem 67:282–290
Poo H, Park C, Kwak MS, Choi DY, Hong SP, Lee IH, Lim YT, Choi YK, Bae SR, Uyama H, Kim CJ, Sung MH (2010) New biological functions and applications of high-molecular-mass poly-gamma-glutamic acid. Chem Biodivers 7:1555–1562
He Y, Kong W, Wang W, Liu T, Yu L, Gong Q, Gao J (2012) Modified natural halloysite/potato starch composite films. Carbohyd Polym 87:2706–2711
Zhou P, Luo Y, Lv Z, Sun X, Zhang X (2021) Melt-processed poly (vinyl alcohol)/corn starch/nanocellulose composites with improved mechanical properties. Int J Biol Macromol 183:1903–1910
Yuan YL, Chen HY (2021) Preparation and characterization of a biodegradable starch-based antibacterial film containing nanocellulose and polyhexamethylene biguanide. Food Packaging Shelf 30:100718
Cui C, Ji N, Wang Y, Xiong L, Sun Q (2021) Bioactive and intelligent starch-based films: a review. Trends Food Sci Tech 116:854–869
Tong Z, Yang J, Lin L, Wang R, Cheng B, Chen Y, Tang L, Chen J, Ma X (2019) In situ synthesis of poly (gamma- glutamic acid)/alginate/AgNP composite microspheres with antibacterial and hemostatic properties. Carbohyd Polym 221:21–28
Bhattacharyya D, Hestekin JA, Brushaber P, Cullen L, Sikdar SK (1998) Novel poly-glutamic acid functionalized microfiltration membranes for sorption of heavy metals at high capacity. J Membrane Sci 141:121–135
Arenaza IMD, Meaurio E, Coto B, Sarasua JR (2010) Molecular dynamics modelling for the analysis and prediction of miscibility in polylactide/polyvinilphenol blends. Polymer 51:4431–4438
Rakkapao N, Vao-soongnern V (2014) Molecular simulation and experimental studies of the miscibility of chitosan/poly (ethylene oxide) blends. J Polym Res 21:606
Amini M, Mobli M, Khalili M, Ebadi-Dehaghani H (2018) Assessment of compatibility in Polypropylene/Poly (lactic acid)/Ethylene vinyl alcohol ternary blends: relating experiments and molecular dynamics simulation results. J Macromol Sci B 57:287–304
Bouzid L, Hiadsi S, Bensaid MO, Foudad FZ (2018) Molecular dynamics simulation studies of the miscibility and thermal properties of PMMA/PS polymer blend. Chinese J Phys 56:3012–3019
Wang YC, Ju SP, Chen CC, Chen HT, Hsieh JY (2014) Mechanical property prediction of starch/polymer composites by molecular dynamics simulation. RSC Adv 4:11475–11480
Liao LQ, Fu YZ, Liang XY, Mei LY, Liu YQ (2013) Diffusion of CO 2 molecules in polyethylene terephthalate/polylactide blends estimated by molecular dynamics simulations. B Korean Chem Soc 34:753–758
Meng Y, Shi X, Cai L, Zhang S, Ding K, Nie S, Luo C, Xu X, Zhang L (2018) Triple-helix conformation of a polysaccharide determined with light scattering, AFM, and molecular dynamics simulation. Macromolecules 51:10150–10159
Hoof BV, Markvoort AJ, Santen RGAV (2014) Molecular simulation of protein encapsulation in vesicle formation. J Phys Chem B 118:3346–3354
Article PubMed Google Scholar
Zhang HJ, Chi YJ, Sun B, Wang XB, Ning X (2010) Preparation of soy protein isolate-based food packaging film. Food Science 31:280–285
CAS Google Scholar
Guan R, Zhao X (2016) Preparation and characteristics of hordein/nano-TiO 2 edible film. Journal of the Chinese Cereals and Oils Association 31:66–70
Google Scholar
Kaya S, Kaya A (2000) Microwave drying effects on properties of whey protein isolate edible films. J Food Eng 43:91–96
Tang LY, Zhao H, Chen J, Gan J, Cai J (2003) Study on edible film’s forming property made of lac and starch and its application results. Food Science 1:23–27
Yang PY, Ju SP, Huang SM (2018) Predicted structural and mechanical properties of activated carbon by molecular simulation. Comp Mater Sci 143:43–54
Li M, Li F, Shen R, Guo X (2011) Molecular dynamics study of the structures and properties of RDX/GAP propellant. J Hazard Mater 186:2031–2036
Abou-Rachid H, Lussier LS, Ringuette S, Lafleur-Lambert X, Jaidann M, Brisson J (2008) On the correlation between miscibility and solubility properties of energetic plasticizers/polymer blends: modeling and simulation studies. Propell Explos Pyrot 33:301–310
Kang JW, Choia K, Jo WH, Hsu SL (1998) Structure-property relationships of polyimides: a molecular simulation approach. Polymer 39:7079–7087
Senkov ON, Miracle DB (2021) Generalization of intrinsic ductile-to-brittle criteria by Pugh and Pettifor for materials with a cubic crystal structure. Sci Rep 11:4531
Yang JQ, Zhang XL, Gao P, Gong XD, Wang GX (2014) Molecular dynamics and dissipative particle dynamics simulations of the miscibility and mechanical properties of GAP/DIANP blending systems. RSC Adv 4:41934–41941
Bullerjahn JT, Bülow SV, Hummer G (2020) Optimal estimates of self-diffusion coefficients from molecular dynamics simulations. J Chem Phys 153:024116
Venkatanarayanan RI, Lebga-Nebane JL, Wu L, Krishnan S (2021) Lithium coordination and diffusion coefficients of PEGylated ionic liquid and lithium salt blends: a molecular dynamics simulation study. J Mol Liq 331:115694
Tocci E, Bellacchio E, Russo N, Drioli E (2002) Diffusion of gases in PEEKs membranes: molecular dynamics simulations. J Membrane Sci 206:389–398
Lim SY, Tsotsis TT, Sahimi M (2003) Molecular simulation of diffusion and sorption of gases in an amorphous polymer. J. Chem. Phys. 119:496–504
Boyd RH, Pant PVK (1991) Molecular packing and diffusion in polyisobutylene. Macromolecules 24:6325–6331
Download references
Acknowledgements
This study was supported by the Basic Public Welfare Research Program of Zhejiang Province (LGN21C200019). Zuolong Yu was supported by a scholarship from the China Scholarship Council (202108330314).
Author information
Authors and affiliations.
Key Laboratory of Pollution Exposure and Health Intervention of Zhejiang Province, Biology and Environmental Engineering College, Zhejiang Shuren University, Hangzhou, 310015, China
Zuolong Yu, Yunxiao Wei, Changchun Fu, Dan Liu & Chao Han
Comprehensive Technical Service Center of Wenzhou Customs, Wenzhou, 325027, China
Hangzhou Yongzhan Environmental Technology Co., Ltd, Hangzhou, 310015, China
Yongfei Chen
Department of Food and Nutritional Sciences, University of Reading, Reading, RG6 6DZ, UK
Keshavan Niranjan
You can also search for this author in PubMed Google Scholar
Contributions
Zuolong Yu: Conceptualization, Methodology, Software, Data Curation, Writing - Original Draft, Project Administration. Yao Chen: Methodology, Software. Yongfei Chen:Resources, Validation. Yunxiao Wei: Data curation, Writing - Original draft preparation. ChangChun Fu: Visualization, Investigation, Formal analysis. Dan Liu: Investigation, Data Curation, Validation. Keshavan Niranjan: Writing - Review & Editing. Chao Han: Supervision.
Corresponding authors
Correspondence to Keshavan Niranjan or Chao Han .
Ethics declarations
Conflict of interest.
The authors declare that they have no known competing financial interests or personal relationships that could have appeared to influence the work reported in this paper.
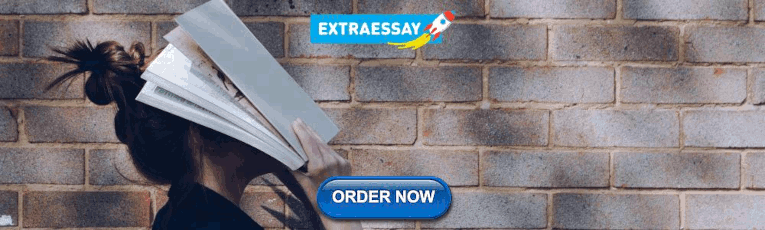
Additional information
Publisher's note.
Springer Nature remains neutral with regard to jurisdictional claims in published maps and institutional affiliations.
Rights and permissions
Open Access This article is licensed under a Creative Commons Attribution 4.0 International License, which permits use, sharing, adaptation, distribution and reproduction in any medium or format, as long as you give appropriate credit to the original author(s) and the source, provide a link to the Creative Commons licence, and indicate if changes were made. The images or other third party material in this article are included in the article's Creative Commons licence, unless indicated otherwise in a credit line to the material. If material is not included in the article's Creative Commons licence and your intended use is not permitted by statutory regulation or exceeds the permitted use, you will need to obtain permission directly from the copyright holder. To view a copy of this licence, visit http://creativecommons.org/licenses/by/4.0/ .
Reprints and permissions
About this article
Yu, Z., Chen, Y., Chen, Y. et al. Molecular dynamics simulation and performance verification of γ-polyglutamic acid/cold water-soluble starch film formation and permeability. Polym. Bull. (2024). https://doi.org/10.1007/s00289-024-05231-y
Download citation
Received : 25 January 2024
Revised : 20 February 2024
Accepted : 03 March 2024
Published : 26 March 2024
DOI : https://doi.org/10.1007/s00289-024-05231-y
Share this article
Anyone you share the following link with will be able to read this content:
Sorry, a shareable link is not currently available for this article.
Provided by the Springer Nature SharedIt content-sharing initiative
- γ-Polyglutamic acid
- Cold water-soluble starch
- Molecular simulation
Advertisement
- Find a journal
- Publish with us
- Track your research
Thank you for visiting nature.com. You are using a browser version with limited support for CSS. To obtain the best experience, we recommend you use a more up to date browser (or turn off compatibility mode in Internet Explorer). In the meantime, to ensure continued support, we are displaying the site without styles and JavaScript.
- View all journals
- My Account Login
- Explore content
- About the journal
- Publish with us
- Sign up for alerts
- Open access
- Published: 06 October 2017
Conformations and molecular interactions of poly-γ-glutamic acid as a soluble microbial product in aqueous solutions
- Ling-Ling Wang 1 ,
- Jian-Tao Chen 2 ,
- Long-Fei Wang 3 ,
- Guang-zhao Zhang 2 ,
- Han-Qing Yu ORCID: orcid.org/0000-0001-5247-6244 3 ,
- Xiao-dong Ye 4 &
- Qing-Shan Shi ORCID: orcid.org/0000-0003-4228-3080 1
Scientific Reports volume 7 , Article number: 12787 ( 2017 ) Cite this article
7059 Accesses
33 Citations
1 Altmetric
Metrics details
- Biopolymers
- Polymer characterization
Soluble microbial products (SMPs) are of significant concern in the natural environment and in engineered systems. In this work, poly-γ-glutamic acid (γ-PGA), which is predominantly produced by Bacillus sp., was investigated in terms of pH-induced conformational changes and molecular interactions in aqueous solutions; accordingly, its sedimentation coefficient distribution and viscosity were also elucidated. Experimental results indicate that pH has a significant impact on the structure and molecular interactions of γ-PGA. The conformation of the γ-PGA acid form (γ-PGA-H) is rod-like while that of the γ-PGA sodium form (γ-PGA-Na) is sphere-like. The transformation from α-helix to random coil in the γ-PGA secondary structure is primarily responsible for this shape variation. The intramolecular hydrogen bonds in the γ-PGA-H structure decrease and intramolecular electrostatic repulsion increases as pH increases; however, the sedimentation coefficient distributions of γ-PGA are dependent on intermolecular interactions rather than intramolecular interactions. Concentration has a more substantial effect on intermolecular electrostatic repulsion and chain entanglement at higher pH values. Consequently, the sedimentation coefficient distributions of γ-PGA shift significantly at pH 8.9 from 0.1 to 1.0 g/L, and the viscosity of γ-PGA (5% w/v) significantly increases as pH increases from 2.3 to 6.0.
Similar content being viewed by others
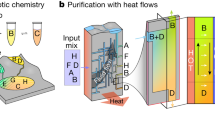
Heat flows enrich prebiotic building blocks and enhance their reactivity
Thomas Matreux, Paula Aikkila, … Christof B. Mast
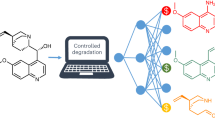
Computational synthesis design for controlled degradation and revalorization
Anna Żądło-Dobrowolska, Karol Molga, … Bartosz A. Grzybowski
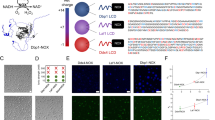
Local environment in biomolecular condensates modulates enzymatic activity across length scales
Marcos Gil-Garcia, Ana I. Benítez-Mateos, … Paolo Arosio
Introduction
Soluble microbial products (SMPs) secreted by microorganisms in the natural environment and in engineered systems are important and have been extensively studied in the fields of microbiology, geochemistry, biological wastewater treatment and biological fermentation 1 , 2 , 3 . They are constituents of natural organic matter, which is ubiquitous in water sources, and is a result of interactions between the hydrologic cycle, biosphere and geosphere 4 . In addition, SMPs are especially important in biological wastewater treatment systems because they often form a large portion of the soluble organic material in the effluent and cause membrane fouling 5 , 6 . Some SMPs, such as poly-γ-glutamic acid (γ-PGA), are commercially produced and used in many industries, including food, pharmaceuticals, healthcare, and water treatment 7 .
SMP characteristics can significantly affect their adsorption, stabilization/aggregation, dissolution and surface transformation in environmental processes and engineered systems 8 , 9 . Although clearly significant in the natural environment and engineered systems, better characterization of SMPs has proven to be challenging because the complexity of their constituents results in highly variable chemical structures, functional groups, molecular weight distribution, conformations, and surface charge 10 . Previous SMP studies have primarily focused on their origin, formation, and their various compounds, as well as their overall properties such as content, functional groups, molecular weight distribution, and hydrophobic and hydrophilic properties 10 , 11 . However, the relationships between their constituents and characteristics remain unclear. There is considerable more analytical work required to fully understand the characteristics of SMPs. Several studies have investigated various SMPs that have a regular composition and pattern, such as xanthan and γ-PGA, to reveal the relationship between their conformational changes and metal binding properties 12 , 13 .
γ-PGA is an SMP predominantly produced and secreted into the natural environment by water and soil bacteria belonging to the genus Bacillus sp., such as B. licheniformis , B. subtilis , B. megaterium , B. pumilis , B. mojavensis , B. amyloliquefaciens , and B. anthracis 13 . Some of the physiological functions carried out by γ-PGA include sequestration of toxic metal ions, and resistance to dewatering, nutrient shortage, and antibodies 7 , 13 . Additionally, γ-PGA has high water solubility, viscosity, biodegradability, biocompatibility, and is non-toxic towards human and the environment. Furthermore, this compound has potentially wide applications in the fields of medicine, cosmetics, foods, water treatment, and agriculture 14 , 15 .
Several investigations concerning the conformation of γ-PGA have been undertaken, mainly using circular dichroism (CD) measurements and infrared spectra 16 , 17 . Results have revealed that the unionized polymer takes on a helical conformation, while the ionized polymer behaves as a random coil. The intramolecular hydrogen bonds are considered the major force for the helical conformation; however, the conformational changes and molecular interactions, including intramolecular and intermolecular interactions of γ-PGA at different concentrations in aqueous solutions, have not been completely elucidated.
In dilute solutions, γ-PGA molecules are sequestered from each other and behave independently; the polymer chains interact primarily with the solvent molecules. Conversely, at concentrations, the polymer chains overlap and become entangled 18 ; thus, the intermolecular interactions are greatly increased when compared to the chains in dilute solutions. The highest yield of γ-PGA secreted into culture medium has been reported as an extremely high value of 73.0 g/L 19 . Thus, the effect of concentration on conformational changes and intramolecular and intermolecular interactions of γ-PGA could not be excluded in practical conditions, especially during the processes of fermentation, separation and purification of γ-PGA.
Therefore, the main objective of our study is to explore the properties of γ-PGA, an SMP produced by B. licheniformis , especially with respect to its conformations, as mediated by pH, and its intramolecular and intermolecular interactions in different concentrations. To that end, we interpreted the conformational-relevant properties (sedimentation coefficient distributions and viscosity) using a combination of Analytical Ultracentrifugation (AUC), Atomic Force Microscopy (AFM), Circular Dichroism (CD), Laser Light Scattering (LLS), and Fourier Transform Infrared Spectroscopy (FTIR) techniques. Applying these techniques can aid in a greater fundamental understanding of the characteristics and roles of γ-PGA (or structurally similar polymers) that are found in the natural environment and in the fermentation industry. Furthermore, this research can be applied in the fields of medicine, cosmetics, foods, water treatment, and agriculture.
Effect of pH on γ-PGA viscosity
The effect of pH on the viscosity of the γ-PGA solution and the γ-PGA − fraction was investigated (Fig. 1 ). The viscosity of the γ-PGA solution appeared to be highly related to the deprotonated degree of the COOH groups in the γ-PGA side chains. The viscosity increased significantly from 4.7 ± 0.1 to 14.8 ± 0.1 mPa·s as the pH increased from 2.3 to 6.0, after which the viscosity slightly increased from pH 6.0 to 8.0. Meanwhile, the acid form of γ-PGA-H rapidly ionized to form the mono-anionic γ-PGA − species as the pH increased from 3.0 to 6.0, while the viscosity of the γ-PGA − species slightly increased with an increase in pH from 6.0 to 8.0.
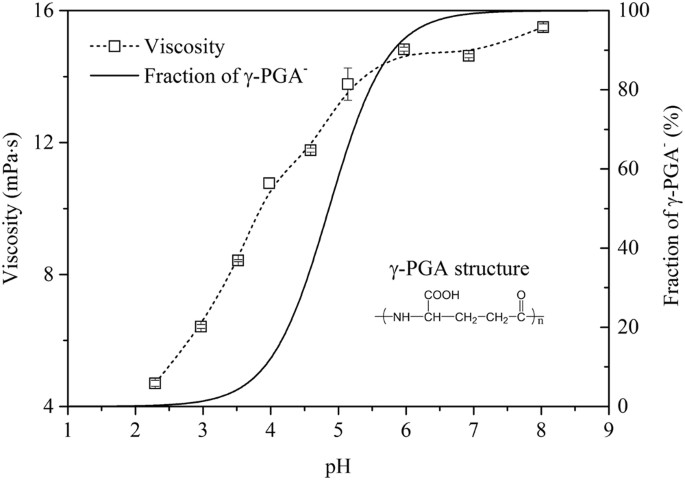
Effect of pH on the viscosity of a γ-PGA solution (5% w/v) and a γ-PGA − fraction.
Sedimentation coefficient distributions
The sedimentation coefficient distributions of γ-PGA at various concentrations (at pH 3.8, 5.9, and 8.9) were analyzed using the SEDFIT program. The continuous c ( s ) distribution model in the SEDFIT program can distinguish boundary spreading due to size heterogeneity from diffusion 20 . The sedimentation coefficient of dilute γ-PGA solutions (0.1 g/L) at pH 3.8, 5.9, and 8.9 are depicted in Fig. 2(a) . The naturally occurring γ-PGA (0.1 g/L) presented broad sedimentation coefficient distributions at pH 3.8, 5.9, and 8.9 (Fig. 2(a) ). The range of the sedimentation coefficient, s , which is commonly referred to in Svedberg (S) units and represents 10 −13 seconds, varied from approximately 1.3 to 4.0 S, with peaks at 2.5, 2.4, and 2.4 S at pH 3.8, 5.9, and 8.9, respectively.
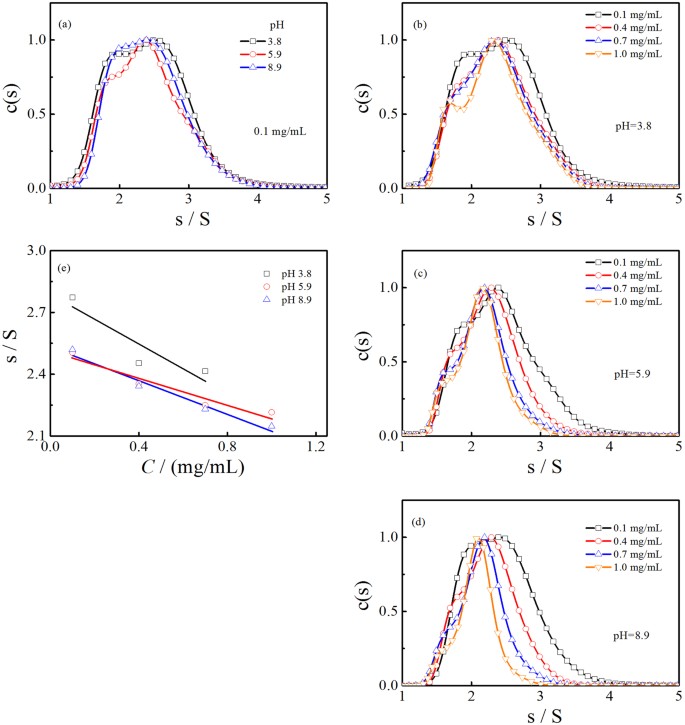
Sedimentation coefficient distributions of a 0.1 g/L γ-PGA at pH 3.8, 5.9, and 8.9 ( a ). Sedimentation coefficient distributions of γ-PGA from 0.1 to 1.0 g/L at pH 3.8 ( b ), pH 5.9 ( c ), and pH 8.9 ( d ). Concentration dependence of the γ-PGA sedimentation coefficient at different pH values ( e ). The c ( s ) distributions are normalized to the peak height and s represents Svedberg (S) units or 10 −13 sec.
The partial specific volume of the γ-PGA solution was determined to be 0.656, 0.715, and 0.730 mL/g at pH 3.8, 5.9, and 8.9, respectively. The variation of the partial specific volume with pH is significant and suggests that large differences in solvation is associated with pH. Two factors contribute to the partial specific volume; one is the intrinsic volume of the added solute and the other is the change in volume as a result of the interaction between the hydrated shell and the solute. As pH values increase, the conformational change and electrostatic interactions promote the binding of water molecules and ions to the carboxylated groups.
As the γ-PGA concentration increased, the peaks and distribution of the sedimentation coefficient varied at pH 3.8, 5.9, and 8.9. The effects of γ-PGA concentrations on the sedimentation coefficient distributions were more remarkable at higher pH values. At a lower pH (pH 3.8), the broad sedimentation coefficient distributions at 0.1 g/L γ-PGA were slightly narrow at 0.4, 0.7, and 1.0 g/L, with the peaks shifted to approximately 2.4, 2.3, and 2.3 S, respectively (Fig. 2(b) ). At pH 5.9, the width of the sedimentation coefficient distribution reduced gradually as the γ-PGA concentrations increased. The peaks for the 0.4, 0.7, and 1.0 g/L γ-PGA at pH 5.9 shifted to approximately 2.3, 2.2, and 2.1 S, respectively (Fig. 2(c) ). At a higher pH (pH 8.9), the areas of the sedimentation coefficient distributions were reduced as the concentration increased; the peaks at 0.4, 0.7, and 1.0 g/L γ-PGA shifted to approximately 2.3, 2.2, and 2.1 S, respectively (Fig. 2(d) ).
Multi-peak distributions can be seen under the various experimental conditions (Fig. 2 ), especially in low pH solutions, indicating that multiple species exist in the solution. As discussed below, γ-PGA appears as rod-like shapes in an acid solution. Furthermore, small and large particles coexist; however, when the rod-like γ-PGA turns to sphere-like shapes, the size difference becomes negligible. Consequently, the c ( s ) distribution becomes essentially unimodal with increasing pH.
Figure 2(e) demonstrates the concentration dependence of s . In dilute solutions, the weak interactions of the diffuse γ-PGA lead to a relatively large s . With increasing concentration, intermolecular interactions gradually increase as well. Thus, s generally decreases with increasing γ-PGA concentration. Furthermore, we can see that s is in direct proportion to the concentration of γ-PGA (Fig. 2(e) ). This is reasonable since the experimental concentration range is not high. The equation \(s={s}_{0}(1-{k}_{s}c)\) can be used here, where s 0 represents the sedimentation coefficient in infinite dilute solution and k s is the concentration coefficient.
Morphology of γ-PGA-H and γ-PGA-Na
The morphology of the acid form γ-PGA-H and sodium form γ-PGA-Na were observed using AFM (Fig. 3 ). The structure of γ-PGA-H (Fig. 3(a) ) was clearly distinct from that of γ-PGA-Na (Fig. 3(b) ). The γ-PGA structures presented in the AFM images should represent the shape of γ-PGA molecular and the size was considered as the rotating radius ( R R ), since the γ-PGA-H and γ-PGA-Na solutions used in AFM were more dilute (0.01 g/L) than in the AUC measurements.
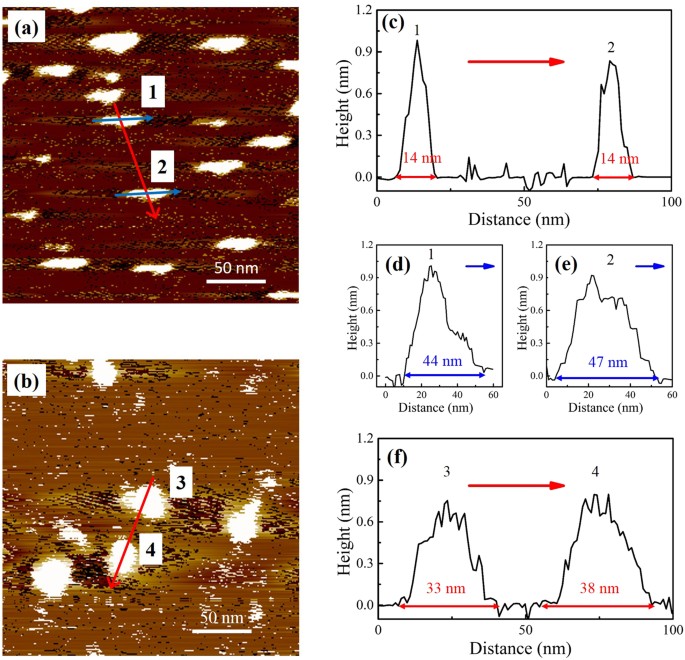
AFM images of the acid form γ-PGA-H ( a ) and sodium form γ-PGA-Na ( b ). Cross-sectional images of particles 1 and 2 (( c ), ( d ), ( e )). Cross-sectional images of particles 3 and 4 ( f ).
γ-PGA-H exhibited rod-like shapes and coexisted as both small and large particles (Fig. 3(a and b) ). For example, the widths of two typical particles were both 14 nm, while their lengths were 44 nm and 47 nm (Fig. 3(a) ). When a sodium ion replaced a hydrogen ion in the γ-PGA structure, the rod-like molecular structure changed to a sphere-like shape (Fig. 3(b) ). The diameters of two typical γ-PGA-Na spheres were approximately 33 nm and 38 nm. The heights in the cross-sectional images (Fig. 3(c–f) ) show that the particles collapsed upon drying in air and were present as flat structures on the mica surface. When compared to the γ-PGA-H structure, the γ-PGA-Na structure appeared to have a larger volume.
Conformation of γ-PGA at different pH values
CD spectroscopy and LLS were used to further explore the conformations of γ-PGA at different pH values. Here, the effects of pH on γ-PGA was investigated using CD spectroscopy. The secondary structure results were determined using the CD spectra deconvolution software CDNN 2.1 (Fig. 4 ). The secondary structure of the γ-PGA-H form (pH 2.5) consisted of 23% α-helix, 10% antiparallel, 12% parallel, 17% beta turn, and 38% random coil. With the decrease in γ-PGA-H percentage (i.e.: an increase of COO − groups in the γ-PGA side chains) there was a substantial decrease in the α-helix from 23% to 10% and a considerable increase in the antiparallel, parallel, and random coil from 10% to 13%, 12% to 16%, and 38% to 44%, respectively. These results indicate that the structure of the γ-PGA changes after ionization of the COOH groups in the γ-PGA side chains.
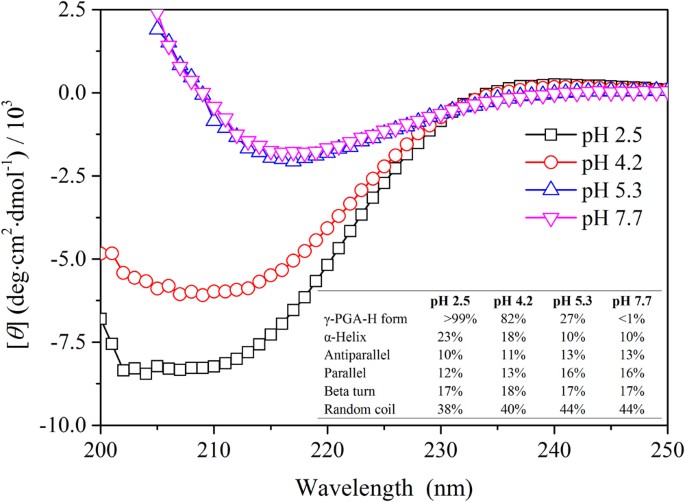
Effect of pH on γ-PGA CD spectroscopy; the inset represents the secondary structure results fitted by the CD spectra deconvolution software CDNN 2.1.
Sizes of γ-PGA, including < R g > and < R h >, and the apparent weight average molar mass < M w,app > at various pH values were determined using LLS measurements (Fig. 5 ). The < R g > of γ-PGA initially increased from pH 1.0 to 2.9, then steadied from pH 2.9 to 7.0, and then further increased at pH 8.8. These results were consistent with the AFM image data, thereby indicating that the γ-PGA-Na structure appeared to have a larger volume. Compared to the solely geometrically defined < R g >, < R h > is differently defined and independent. The much smaller value of < R h >, when compared to that of < R g >, suggests that the γ-PGA structure was deep drained by the water, so that the γ-PGA molecules collapsed and were present as flat structures on the mica surface in AFM images. The values of ρ ( ρ = < R g >/< R h >) were 2.1, 3.2, 2.3, 2.2, 2.4, 2.6 and 4.1 at pH 1.0, 2.9, 3.9, 5.0, 6.0, 7.0 and 8.8 respectively. The < R h > of γ-PGA only slightly increased from 5.31 nm to 6.83 nm with increasing pH, likely arising from the structural change from α-helix to random coil and increased ionic hydration. The ionic hydration γ-PGA may increase with the increasing degree of ionization and attraction of cationic counter ions at higher pH values. However, the < M w,app > of γ-PGA reduced gradually from 4.2 × 10 4 g/mol to 3.0 × 10 4 g/mol as the pH increased.
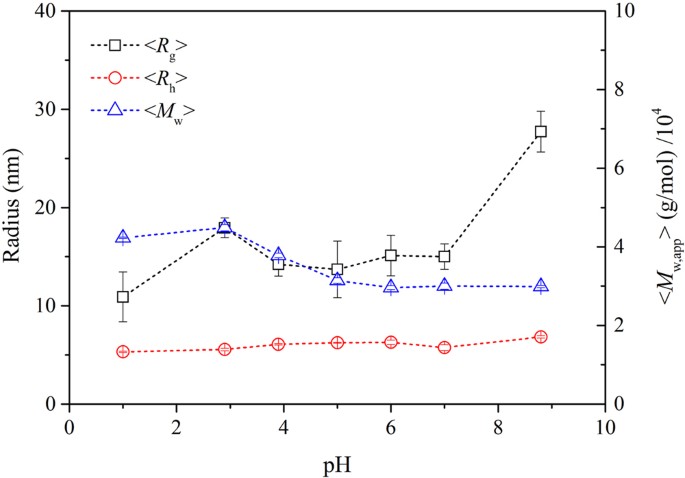
Effect of pH on < R g >, < R h >, and the apparent weight average molar mass, < M w,app >, of γ-PGA (3 g/L) as measured by LLS.
FTIR spectra of γ-PGA
At lower pH, it has been suggested that intramolecular hydrogen bonds are the dominant molecular force associated with γ-PGA 21 . FTIR spectra were used to confirm the existence of the hydrogen bonds in γ-PGA at pH 1.0 (99.9% γ-PGA-H form), 5.1 (36.5% γ-PGA-H form), and 9.5 (<0.1% γ-PGA-H form). In the γ-PGA-H form, the broad peak at 3310 cm −1 (Fig. 6 ) corresponded to hydrogen-bonded N–H stretching while the shoulder, which appears at around 3430 cm −1 , was attributable to non-hydrogen-bonded N–H 22 . With an increase in pH, the shoulder intensity increased due to the continuous dissociation of hydrogen bonds.
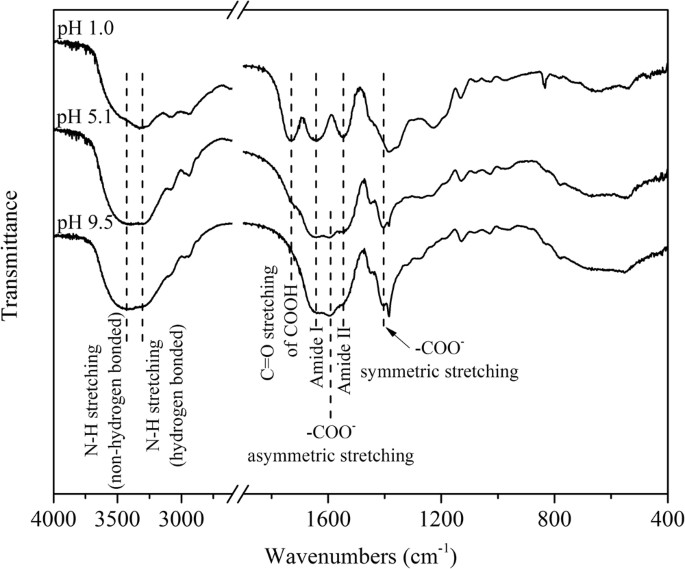
FTIR spectra of lyophilized γ-PGA at pH 1.0, 5.1, and 9.5.
A distinct peak around 1730 cm −1 at pH 1.0, which gradually disappeared at pH 5.1 and 9.5, could be assigned to the C=O stretching in COOH. With the deprotonation of COOH, the C=O in the zwitterionic form COO − shifted to about 1590 cm −1 (asymmetric stretching) and 1410 cm −1 (symmetric stretching) 23 . The intensity at 1590 cm −1 even overlapped the amide I and amide II bands at pH 5.1 and 9.5. The amide I band around 1643 cm −1 at pH 1.0 primarily originated from the C=O stretching vibration in the γ-PGA-H amide group because the amide I band represented 80% C=O stretching vibration of the amide group coupled to in-plane N–H bending and C–N stretching modes 24 . A small shift to 1653 cm −1 in the amide I band at pH 5.1 and 9.5 may arise from the dissociation of hydrogen bonds with the N–H.
Conventional viscosity studies of γ-PGA, and other SMPs, have focused on its relationship with concentration, molecular weight, and temperature 25 , 26 , 27 , 28 . However, the observation that the addition of acid to the medium effectively reduces the viscosity of the culture broth has not been reasonably explained; however, it has allowed for development of an efficient method to separate and recover γ-PGA from highly viscous culture broth, which minimizes purification and recovery costs for large-scale industrial appliations 29 . Therefore, in our study we focused on the relationship between viscosity and pH. We found that the observed results agree with previously published research 29 . At a defined concentration, molecular weight, and temperature, conformational changes and molecular interactions may be the primary cause for the significant increase in viscosity as pH increases.
The sizes determined by AFM in the dried state are different from those radii measured by LLS in the aqueous solution, presumably due to the different definition and sample preparation. AFM clearly demonstrates the distinct structures of the γ-PGA-H and γ-PGA-Na forms, thereby confirming conformational changes of γ-PGA. The decrease of α-helix and increase of random coil in the γ-PGA secondary structure, as analyzed by CD, are considered to be the main conformation responsible for the shape variation at different pH values. A similar helix to random coil structural change associated with pH variation has been substantiated for poly(glutamic acid) using CD studies 30 , 31 . The conformation of the poly(glutamic acid) was reported to change from random coil to α-helix as the solution pH decreased from 7.2 to 3.0 30 . Another study of γ-PGA, which was purified from B. licheniformis , used attenuated total reflectance FTIR (ATR-FTIR) to show that the exopolymer (0.1% w/v) is protonated and exhibits a helical conformation at a low pH, whereas a β-sheet-based conformation predominates at higher pH 13 .
Conformations of the γ-PGA-H acid form and γ-PGA-Na sodium form are schematically illustrated in Fig. 7(a) . The γ-PGA-H structure is stabilized by intramolecular hydrogen bonds. The un-ionized state of poly (γ-D-glutamic acid) was investigated using a combination of molecular dynamics and quantum mechanical calculations 21 . Theoretical models indicate that a left-handed helix with a 19-membered ring and hydrogen bonds set between the CO of the amide group i and the NH of amide group i + 3 is the most stable conformation. Weak intramolecular interactions between the side carboxylic oxygen and the NH of the backbone amide group are assumed to be responsible for the relatively high stability of the left-handed helix. More interestingly, hydrogen bonds in poly(γ-D-glutamic acid) would be three-centered after incubation at 65 °C for 48 h 32 . The α-helical conformation is metastable and transforms spontaneously into insoluble β-fibrils aggregates with the characteristic infrared trait. This has been attributed to the network of three-centered hydrogen bonds coupled to the side chain’s carboxyl and the main chain’s −NH groups 32 , 33 , 34 . The strong intramolecular hydrogen bonds between the CO and the NH of the amide group were confirmed by FTIR. However, the evidence of presumed hydrogen bonds between carboxylate groups of the side chains and NH of the amide group were not evident within the FTIR spectra. It is likely that these hydrogen bonds are weak intramolecular interactions.
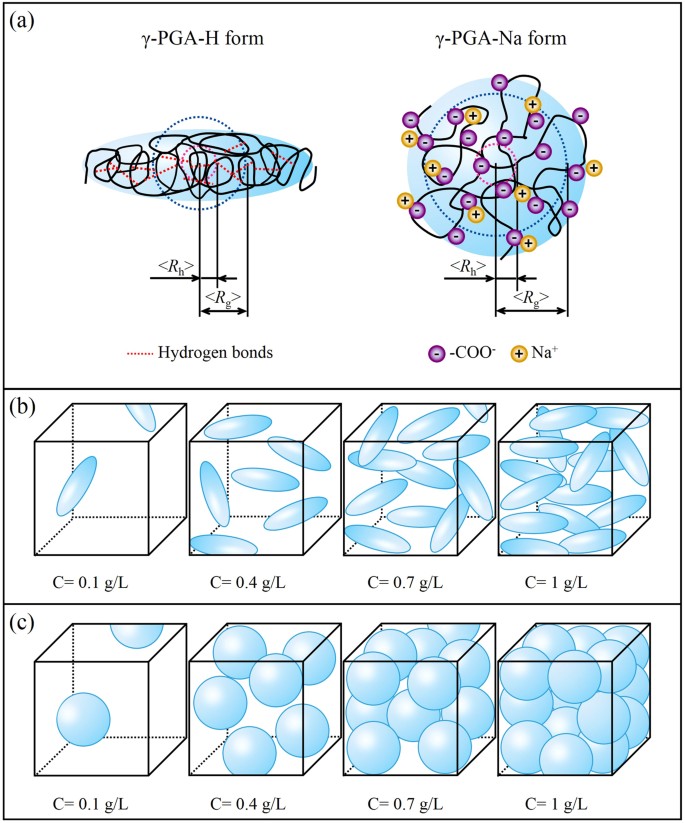
Schematic structural illustrations of the acid form γ-PGA-H and sodium form γ-PGA-Na ( a ), concentrations of γ-PGA-H ( b ) and γ-PGA-Na ( c ) in aqueous solutions at 0.1, 0.4, 0.7, and 1.0 g/L, used in AUC measurements. The side length of the cube is 100 nm in ( b ) and ( c ).
The random coil structure adopted by the γ-PGA-Na molecule may be attributed to two factors. Firstly, the deprotonated carboxylate groups destabilize and are therefore not available to maintain the hydrogen bonds between the side chains and the backbone. Secondly, each monomer of the γ-PGA molecule has one carboxylate group and this negatively charged carboxylate group has a strong electrostatic repulsion, which is resistant to the intramolecular interaction of hydrogen bonds between the CO and NH of the amide group.
Changes in molecular interactions of γ-PGA solutions at different pH were also confirmed by AUC measurements. Figure 7(b) and (c) illustrate the concentrations of γ-PGA-H and γ-PGA-Na in solutions at 0.1, 0.4, 0.7, and 1.0 g/L, which were used in AUC measurements. In a dilute solution (0.1 g/L), the molecules are separated from each other and behave independently. The polymer chain interacts primarily with the solvent molecules; therefore, the intermolecular interactions of γ-PGA in dilute solutions can be neglected. There were no significant differences in the sedimentation coefficient distributions for 0.1 g/L γ-PGA at pH 3.8, 5.9, and 8.9 (Fig. 2(a) ) despite the structures of γ-PGA being distinct at these three pH values. These results imply that the sedimentation coefficient distributions of γ-PGA are dependent on the intermolecular interaction, rather than the intramolecular interaction.
In contrast, as the γ-PGA concentration increased, the variation of structures associated with pH affected the peaks and distribution of the sedimentation coefficient (Fig. 2(c–e) ). According to the definition of sedimentation coefficient, s , a larger s represents a faster sedimentation velocity. The peaks and distribution of the sedimentation coefficient were shifted to slower sedimentation velocities as the pH increased with increasing concentrations from 0.1 to 1.0 g/L. These results indicate that the intermolecular interactions increase as the pH increases. Furthermore, the narrowed sedimentation coefficient distributions at higher pH values and increased concentrations suggest that the intermolecular interactions make the denser γ-PGA more homogeneous at higher concentrations.
Generally, the most probable intermolecular interactions in high concentration solutions of γ-PGA are electrostatic repulsion and chain entanglement. As expected, the negative charge derived from deprotonation of carboxylate groups increases with increasing pH, resulting in stronger intermolecular repulsion at a higher pH. The second virial coefficient, A 2 , of γ-PGA at pH 7.0 (phosphate buffer, 0.13 M ionic strength) was previously reported as a positive value ( A 2 = 1.44 × 10 −6 mol·L/g 2 ) 35 , which reflects repulsion between γ-PGA molecules at pH 7.0. Chain entanglement 18 is considerable when the γ-PGA molecules exist in a high concentration solution. Furthermore, the random coil structure of γ-PGA-Na likely promotes chain overlap and entanglement. Additionally, the stable conformation of γ-PGA at higher concentrations is probably due to the balance of intramolecular and intermolecular electrostatic repulsion and intermolecular chain entanglement.
Compared to the extracellular polymeric substances bounded to the microorganisms, the excreted SMPs are well dissolved in aqueous solutions and have much larger values of ρ 36 , 37 . The ρ value of bound extracellular polymeric substances of Bacillus megaterium TF10 with a negative A 2 ( A 2 = −2.9 × 10 −8 mol·L/g 2 ) at pH 7.0 and 0.1 M ionic strength was about 1.2 36 while the ρ value of its SMP was about 2.6 with a positive A 2 ( A 2 = 1.7 × 10 −6 mol·L/g 2 , similar to the positive A 2 of γ-PGA) at pH 7.0 and 0.75 M ionic strength 37 . The large values of ρ in aqueous solutions might confirmed that the γ-PGA is a well dissolved SMP.
γ-PGA purification
A γ-PGA solution (5% w/w), produced from B. licheniformis ATCC 9945 A, was supplied by Guangdong Demay biotechnology Co., China. The γ-PGA solution was concentrated and then washed repeatedly with deionized water by using a cross flow ultrafilter equipped with a Millipore Pellicon 2 Cassette (10 K Da molecular-weight cutoff polyethersulfone membrane) to remove ions and small molecules. The resulting γ-PGA solution was then filtered through a 0.22 μm hydrophilic PTFE filter and subsequently lyophilized. A portion of the lyophilized γ-PGA was further dissolved in distilled water and adjusted with excess HNO 3 or NaOH, and then dialyzed and lyophilized to obtain the acid form (γ-PGA-H) and sodium salt form (γ-PGA-Na) of γ-PGA for AFM images.
γ-PGA characterization
The purity of γ-PGA was determined by amino acid analysis using thin layer chromatography (TLC) after hydrolysis, according to Yokoi et al . 38 . Sole detection of glutamic acid in the TLC analysis confirmed the purity of γ-PGA (data not shown). The hydrolyzed γ-PGA product was further used to determine the glutamic acid content by colorimetric analysis, with glutamic acid as the standard. The glutamic acid content was determined as 7.02 ± 0.08 mmol/g. Metal ions were analyzed on an inductively coupled plasma mass spectrometer (ICP-MS, 7700X, Agilent Inc., USA). The most abundant metal ions were Na, Mg, K, and Ca, which were determined to be 29.69 ± 0.33 mg/g, 0.46 ± 0.01 mg/g, 0.74 ± 0.01 mg/g and 0.67 ± 0.03 mg/g, respectively. Based on the glutamic acid and metal ion results, the γ-PGA was determined to be primarily composed of the acid form (γ-PGA-H; 81.62%), with the remainder existing in the sodium salt form (γ-PGA-Na; 18.38%). The purity of γ-PGA was determined as 94 wt %. The pK a value of γ-PGA was determined to be 4.86, based on the previously reported acid-base titration method 39 using an automatic titrator (TIM865, Hach Co., USA).
Viscosity assay
The viscosity of γ-PGA at different pH values was analyzed on a BGD 155 Digital Viscometer (Biuged Laboratory Instruments, Guangzhou, China) at 20 °C. The concentration of γ-PGA used for viscosity analysis was 5% w/v and the pH was adjusted with HNO 3 or NaOH, with NaNO 3 added to control the ionic strength (I = 0.2 M).
Analytical ultracentrifugation (AUC) measurements
The analytical ultracentrifuge (AUC) technique was used to characterize the sedimentation coefficient based on sedimentation velocity (SV) analysis 40 , 41 . SV experiments were performed on a Proteomelab XL-A analytical ultracentrifuge (Beckman Coulter Instruments) coupled with a UV-vis absorption optics detector to monitor the time-dependent radial concentration of γ-PGA from 190 to 800 nm. Three cells equipped with a two-sector, charcoal-filled Epon centerpiece, quartz windows and a counterbalance were loaded into an An-60 Ti 4-hole rotor. γ-PGA solution (400 μL) and solvent (410 μL) were added as the sample and reference, respectively. Na 2 HPO 4 and Na 2 HPO 4 were used to adjust the pH of γ-PGA solution because HNO 3 and NaOH disrupt the signal from 190 to 260 nm. The ionic strength of the γ-PGA solution was kept at 0.2 M by addition of NaCl so that the net charges were completely screened. γ-PGA solution was thermostated at 20 °C and 0 rpm for at least 2 h before the angular velocity increased to the final rotational speed of 58,000 rpm. Approximately 200 scans of data for every sample were collected at a time interval of 3 min and were analyzed using the SEDFIT software program 42 , 43 . The Z-scores as well as the rmsd values of the fits in relation to that expected from the noise in the optical system are provided in Supplementary Information (Table S1 ). The Lamm equation describes the sedimentation and diffusion processes of molecules during centrifugation:
where c , t , r , D , ω and s are the concentration of the solute, sedimentation time, radial distance from the axis of rotation, diffusion coefficient, angular velocity and sedimentation coefficient, respectively. The sedimentation coefficient is defined as s = u / ω 2 r where u is the sedimentation velocity of the solute.
Partial specific volume ( v ), an important and essential parameter, is defined as the change in volume when a measured amount of solute is added to the solution. It can be calculated from the following equation:
where ρ 0 is the density of solvent, ρ is the density of solution, and c is the concentration of the solute. The density values obtained from the solutions at different concentrations were plotted against the concentration values, with \(\frac{\Delta \rho }{\Delta c}\) as the slope. The partial specific volume of the γ-PGA solution was measured using a DMA4500 densitometer (Anton Paar) and was determined to be 0.656, 0.715, and 0.730 mL/g at pH 3.8, 5.9, and 8.9, respectively.
Using the value of s and weight-average frictional ratio f/f 0 fitted from c ( s ) model and the parameters of partial-specific volume, density and viscosity of the solution, diffusion coefficient D can be calculated with the combination of Svedberg equation and Stokes-Einstein equation. Then, once the D is obtained, the hydrodynamic radius can be calculated from Stokes-Einstein equation.
Atomic force microscope (AFM) imaging
AFM (Park Systems, XE-100) images were obtained to characterize the morphology of the γ-PGA-H and γ-PGA-Na forms. Approximately 2 drops of the γ-PGA-H or γ-PGA-Na solution (0.01 g/L) were applied to a clean mica sheet and spin-coated (3000 rev/min) for 60 s in air. After the mica sheet dried, AFM images were acquired using a Park Systems microscope (XE-100) operating in non-contact mode at scanning rates of 0.3 or 0.5 with an image resolution of 256 by 256. The Silicon cantilever (PPP-NCHR, Nanosensors) used in the AFM was carried out with a spring constant of 42 N/m and resonance frequency of 330 kHz.
Circular dichroism (CD) measurements
CD measurements of γ-PGA solutions (0.1 g/L, in 0.1 mol/L NaF ionic medium) were performed on a Chirascan spectrometer (Applied Photophysics Co., UK) using a quartz cell of 1 cm path length. CD spectra were collected from 190 to 260 nm. Again, H 3 PO 4 and Na 2 HPO 4 were used to adjust the pH, as HNO 3 and NaOH would disrupt the signal of γ-PGA from 190 to 260 nm. The mean residue molar ellipticities were calculated according to the following equation 44 :
where [ θ ] obs is the observed ellipticity measured in degrees, MRW is the mean residue weight, l is the path length of the cell in centimeters, and c is the molar concentration of γ-PGA in g/L. CD spectra deconvolution software CDNN 2.1 (courtesy of Gerald Böhm, Martin-Luther-Universität Halle-Wittenberg, Germany) was used to analyze the secondary structure of γ-PGA.
Laser light scattering (LLS) measurements
LLS measurements were conducted according to our previous studies 36 , 39 on an ALV/DLS/SLS-5022F spectrometer equipped with a multi-τ digital time correlator (ALV5000) and a cylindrical 22 mW UNIPHASE He-Ne laser ( λ 0 = 632.8 nm) as the light source. The γ-PGA solutions were filtered using a 0.45 μm hydrophilic PTFE (Millex-LCR, Millipore Inc., USA) into a dust-free vial and tested at 20.0 ± 0.1 °C. The pH of the γ-PGA solution was adjusted with HNO 3 or NaOH, with NaNO 3 added to control the ionic strength (I = 0.2 M).
In static light scattering (SLS), the excess Rayleigh ratio, R ex , is related to the weight-averaged molecular weight ( M w ) and the z -average root-mean-square radius of gyration < R g 2 > z 1/2 (or simply < R g >) as described by 39 :
where K = 4 π 2 n 2 ( dn / dC ) 2 /( N A λ 0 4 ) with n , dn / dC , N A , and λ 0 representing the solvent refractive index, the specific refractive index increment, Avogadro’s number, and the wavelength of light in vacuum, respectively. q = (4 πn / λ 0 )sin( θ /2) with θ being the scattering angle. The value of dn / dC (specific refractive index increment) was determined with a precise differential refractometer at 20.0 °C and 633 nm. In SLS measurements, the intensity of scattered light at each angle was measured three times, indicating a variation of less than 5%. The relative errors of < R g > and M w,app were ±8% and ±5%.
In dynamic light scattering (DLS), the precisely measured intensity-intensity time correlation function G (2) ( q , τ ) was measured in the self-beating mode and the Laplace inversion of G (2) ( q , τ ) led to a line width distribution G ( Γ ). For a diffusive relaxation, Γ is directly related to the translational diffusion coefficient, D , which can be further converted to the hydrodynamic radius, < R h >, by using the Stokes-Einstein equation. The cumulant expansion analysis was used in the DLS data and the detailed theoretical analysis was provided in our previous study 36 . The G (2) ( q , τ ) at each pH value was measured four times and the standard deviation (StdDev) for each fitting was listed in Supplementary Information (Table S2 ).
FTIR spectroscopy analysis
FTIR spectra of the samples were recorded on an FTIR-7600 instrument (Lambda Scientific Pty Ltd., USA). The pH of the γ-PGA samples (10 mg/mL in deionized water) was adjusted to approximately 1.3, 7.0 and 10.0 using 0.2 M HCl or NaOH. The prepared samples were then lyophilized and directly mixed into FTIR grade KBr powder for testing.
Laspidou, C. S. & Rittmann, B. E. A unified theory for extracellular polymeric substances, soluble microbial products, and active and inert biomass. Water Res. 36 , 2711–2720 (2002).
Article CAS PubMed Google Scholar
More, T. T., Yadav, J. S. S., Yan, S., Tyagi, R. D. & Surampalli, R. Y. Extracellular polymeric substances of bacteria and their potential environmental applications. J. Environ. Manage. 144 , 1–25 (2014).
Tourney, J. & Ngwenya, B. T. The role of bacterial extracellular polymeric substances in geomicrobiology. Chem. Geol. 386 , 115–132 (2014).
Article ADS CAS Google Scholar
Bhatnagar, A. & Sillanpaa, M. Removal of natural organic matter (NOM) and its constituents from water by adsorption - a review. Chemosphere 166 , 497–510 (2017).
Ni, B. J. & Yu, H. Q. Microbial products of activated sludge in biological wastewater treatment systems: a critical review. Crit. Rev. Environ. Sci. Technol. 42 , 187–223 (2012).
Article Google Scholar
Yu, H. et al . Relationship between soluble microbial products (SMP) and effluent organic matter (EfOM): characterized by fluorescence excitation emission matrix coupled with parallel factor analysis. Chemosphere 121 , 101–109 (2015).
Bajaj, I. & Singhal, R. Poly (glutamic acid) - an emerging biopolymer of commercial interest. Bioresour. Technol. 102 , 5551–5561 (2011).
Matilainen, A., Vepsalainen, M. & Sillanpaa, M. Natural organic matter removal by coagulation during drinking water treatment: a review. Adv. Colloid Interface Sci. 159 , 189–197 (2010).
Wang, Z., Zhang, L., Zhao, J. & Xing, B. Environmental processes and toxicity of metallic nanoparticles in aquatic systems as affected by natural organic matter. Environ.-Sci. Nano 3 , 240–255 (2016).
Article CAS Google Scholar
Kunacheva, C. & Stuckey, D. C. Analytical methods for soluble microbial products (SMP) and extracellular polymers (ECP) in wastewater treatment systems: a review. Water Res. 61 , 1–18 (2014).
Ni, B. J., Rittmann, B. E. & Yu, H. Q. Soluble microbial products and their implications in mixed culture biotechnology. Trends Biotechnol. 29 , 454–463 (2011).
Causse, B. et al . Xanthan exopolysaccharide: Cu 2+ complexes affected from the pH-dependent conformational state; implications for environmentally relevant biopolymers. Environ. Sci. Technol. 50 , 3477–3485 (2016).
Article ADS CAS PubMed Google Scholar
He, L. M., Neu, M. P. & Vanderberg, L. A. Bacillus lichenformis gamma-glutamyl exopolymer: physicochemical characterization and U(VI) interaction. Environ. Sci. Technol. 34 , 1694–1701 (2000).
Buescher, J. M. & Margaritis, A. Microbial biosynthesis of polyglutamic acid biopolymer and applications in the biopharmaceutical, biomedical and food industries. Crit. Rev. Biotechnol. 27 , 1–19 (2007).
Ogunleye, A. et al . Poly-gamma-glutamic acid: production, properties and applications. Microbiology-(UK) 161 , 1–17 (2015).
Candela, T. & Fouet, A. Poly-gamma-glutamate in bacteria. Mol. Microbiol. 60 , 1091–1098 (2006).
Shih, I. L. & Van, Y. T. The production of poly-(gamma-glutamic acid) from microorganisms and its various applications. Bioresour. Technol. 79 , 207–225 (2001).
Teraoka, I. Models of polymer chains in Polymer solutions: an introduction to physical propertie s 64–65 (2002).
Tang, B. et al . Highly efficient rice straw utilization for poly-(gamma-glutamic acid) production by Bacillus subtilis NX-2. Bioresour. Technol. 193 , 370–376 (2015).
Wang, X. Y., Ye, X. D. & Zhang, G. Z. Investigation of pH-induced conformational change and hydration of poly(methacrylic acid) by analytical ultracentrifugation. Soft Matter 11 , 5381–5388 (2015).
Article ADS PubMed Google Scholar
Zanuy, D., Aleman, C. & Munoz-Guerra, S. On the helical conformation of un-ionized poly(gamma-D-glutamic acid). Int. J. Biol. Macromol. 23 , 175–184 (1998).
Appoh, F. E., Thomas, D. S. & Kraatz, H. B. Glutamic acid dendrimers attached to a central ferrocene core: synthesis and properties. Macromolecules 38 , 7562–7570 (2005).
Garcia-Alvarez, M. et al . Comb-like ionic complexes of cationic surfactants with bacterial poly(gamma-glutamic acid) of racemic composition. Macromol. Biosci. 5 , 30–38 (2005).
Stuart, B. H. Biological applications in Infrared spectroscopy: fundamentals and application s 142 (2004).
Irurzun, I. et al . Mark-Houwink parameters of biosynthetic poly(gamma-glutamic acid) in aqueous solution. Macromol. Chem. Phys. 202 , 3253–3256 (2001).
Richard, A. & Margaritis, A. Poly(glutamic acid) for biomedical applications. Crit. Rev. Biotechnol. 21 , 219–232 (2001).
Wu, Q., Xu, H., Liang, J. & Yao, J. Contribution of glycerol on production of poly(gamma-glutamic acid) in Bacillus subtilis NX-2. Appl. Biochem. Biotechnol. 160 , 386–392 (2010).
Zhu, F. et al . A novel approach for poly-gamma-glutamic acid production using xylose and corncob fibres hydrolysate in Bacillus subtillis HB-1. J. Chem. Technol. Biotechnol. 89 , 616–622 (2014).
Do, J. H., Chang, H. N. & Lee, S. Y. Efficient recovery of gamma-poly (glutamic acid) from highly viscous culture broth. Biotechnol. Bioeng. 76 , 219–223 (2001).
Agresti, C., Tu, Z., Ng, C., Yang, Y. & Liang, J. F. Specific interactions between diphenhydramine and alpha-helical poly(glutamic acid) - a new ion-pairing complex for taste masking and pH-controlled diphenhydramine release. Eur. J. Pharm. Biopharm. 70 , 226–233 (2008).
Gooding, E. A. et al . pH-dependent helix folding dynamics of poly-glutamic acid. Chem. Phys. 422 , 115–123 (2013).
Fulara, A. & Dzwolak, W. Bifurcated hydrogen bonds stabilize fibrils of poly(L-glutamic) acid. J. Phys. Chem. B 114 , 8278–8283 (2010).
Hernik-Magon, A. et al . Beware of cocktails: chain-length bidispersity triggers explosive self-assembly of poly-L-Glutamic acid beta(2)-fibrils. Biomacromolecules 17 , 1376–1382 (2016).
Yamaoki, Y. et al . An FT-IR study on packing defects in mixed beta-aggregates of poly(L-glutamic acid) and poly(D-glutamic acid): a high-pressure rescue from a kinetic trap. J. Phys. Chem. B 116 , 5172–5178 (2012).
de Cesaro, A., da Silva, S. B., da Silva, V. Z. & Zachia Ayub, M. A. Physico-chemical and rheological characterization of poly-gamma-glutamic acid produced by a new strain of Bacillus subtilis . Eur. Polym. J. 57 , 91–98 (2014).
Wang, L. L. et al . Spatial configuration of extracellular polymeric substances of Bacillus megaterium TF10 in aqueous solution. Water Res. 46 , 3490–3496 (2012).
Wang, L. L., Wang, L. F., Ye, X. D. & Yu, H. Q. Hydration interactions and stability of soluble microbial products in aqueous solutions. Water Res. 47 , 5921–5929 (2013).
Yokoi, H., Natsuda, O., Hirose, J., Hayashi, S. & Takasaki, Y. Characteristics of a biopolymer flocculant produced by Bacillus Sp. Py-90. J. Ferment. Bioeng. 79 , 378–380 (1995).
Wang, L. L. et al . pH dependence of structure and surface properties of microbial EPS. Environ. Sci. Technol. 46 , 737–744 (2012).
Laue, T. M. & Stafford, W. F. Modern applications of analytical ultracentrifugation. Annu. Rev. Biophys. Biomolec. Struct. 28 , 75–100 (1999).
Mächtle, W. & Börger, L. Sedimentation velocity In Analytical ultracentrifugation of polymers and nanoparticle s 62–64 (2006).
Lebowitz, J., Lewis, M. S. & Schuck, P. Modern analytical ultracentrifugation in protein science: a tutorial review. Protein Sci. 11 , 2067–2079 (2002).
Article CAS PubMed PubMed Central Google Scholar
Schuck, P. Size-distribution analysis of macromolecules by sedimentation velocity ultracentrifugation and Lamm equation modeling. Biophys. J. 78 , 1606–1619 (2000).
Article ADS CAS PubMed PubMed Central Google Scholar
Joyce, J. et al . Immunogenicity and protective efficacy of Bacillus anthracis poly-gamma-D-glutamic acid capsule covalently coupled to a protein carrier using a novel triazine-based conjugation strategy. J. Biol. Chem. 281 , 4831–4843 (2006).
Download references
Acknowledgements
This work was supported by the National Natural Science Foundation of China (No. 21607031) and the Science Project of Guangdong Province, China (No. 2014A010107023 and 2016A010103020).
Author information
Authors and affiliations.
State Key Laboratory of Applied Microbiology Southern China, Guangdong Provincial Key Laboratory of Microbial Culture Collection and Application, Guangdong Open Laboratory of Applied Microbiology, Guangdong Institute of Microbiology, Guangzhou, 510070, China
Ling-Ling Wang & Qing-Shan Shi
Faculty of Materials Science and Engineering, South China University of Technology, Guangzhou, 510640, China
Jian-Tao Chen & Guang-zhao Zhang
Department of Chemistry, University of Science and Technology of China, Hefei, 230026, China
Long-Fei Wang & Han-Qing Yu
Hefei National Laboratory for Physical Sciences at the Microscale, Department of Chemical Physics, University of Science and Technology of China, Hefei, 230026, China
Sha Wu & Xiao-dong Ye
You can also search for this author in PubMed Google Scholar
Contributions
L.L. Wang designed the study and wrote the main manuscript. L.L. Wang, J.T. Chen, L.F. Wang and S. Wu performed the experiments, analyzed the data and prepared the figures. G.Z. Zhang and X.D. Ye contributed to methodology development, assisted with analysis and discussed results. H.Q. Yu and Q.S. Shi critically reviewed the manuscript.
Corresponding author
Correspondence to Qing-Shan Shi .
Ethics declarations
Competing interests.
The authors declare that they have no competing interests.
Additional information
Publisher's note: Springer Nature remains neutral with regard to jurisdictional claims in published maps and institutional affiliations.
Electronic supplementary material
Supplementary information, rights and permissions.
Open Access This article is licensed under a Creative Commons Attribution 4.0 International License, which permits use, sharing, adaptation, distribution and reproduction in any medium or format, as long as you give appropriate credit to the original author(s) and the source, provide a link to the Creative Commons license, and indicate if changes were made. The images or other third party material in this article are included in the article’s Creative Commons license, unless indicated otherwise in a credit line to the material. If material is not included in the article’s Creative Commons license and your intended use is not permitted by statutory regulation or exceeds the permitted use, you will need to obtain permission directly from the copyright holder. To view a copy of this license, visit http://creativecommons.org/licenses/by/4.0/ .
Reprints and permissions
About this article
Cite this article.
Wang, LL., Chen, JT., Wang, LF. et al. Conformations and molecular interactions of poly-γ-glutamic acid as a soluble microbial product in aqueous solutions. Sci Rep 7 , 12787 (2017). https://doi.org/10.1038/s41598-017-13152-2
Download citation
Received : 06 April 2017
Accepted : 19 September 2017
Published : 06 October 2017
DOI : https://doi.org/10.1038/s41598-017-13152-2
Share this article
Anyone you share the following link with will be able to read this content:
Sorry, a shareable link is not currently available for this article.
Provided by the Springer Nature SharedIt content-sharing initiative
This article is cited by
Production of poly-gamma-glutamic acid (γ-pga) from sucrose by an osmotolerant bacillus paralicheniformis ncim 5769 and genome-based predictive biosynthetic pathway.
- Pranav G. Nair
- Emmanuel Joseph
- Mahesh S. Dharne
Biomass Conversion and Biorefinery (2023)
γ-PGA Fermentation by Bacillus subtilis PG-001 with Glucose Feedback Control pH-stat Strategy
- Jia-Qi Wang
- Jian-Ye Xia
Applied Biochemistry and Biotechnology (2022)
An anionic biopolymer γ-polyglutamate enhanced the microbially induced carbonate precipitation for soil improvement: mechanical behaviors and underlying mechanism
Acta Geotechnica (2022)
Biosorption of Cr(VI) by immobilized waste biomass from polyglutamic acid production
- Hui-Xue Ren
Scientific Reports (2020)
Assessment of cassava peels as renewable substrate for production of poly-γ-glutamic acid by Bacillus subtilis
- Thomas John
- Aliyu Salihu
- Elewechi Onyike
Environmental Sustainability (2020)
By submitting a comment you agree to abide by our Terms and Community Guidelines . If you find something abusive or that does not comply with our terms or guidelines please flag it as inappropriate.
Quick links
- Explore articles by subject
- Guide to authors
- Editorial policies
Sign up for the Nature Briefing newsletter — what matters in science, free to your inbox daily.


An official website of the United States government
The .gov means it’s official. Federal government websites often end in .gov or .mil. Before sharing sensitive information, make sure you’re on a federal government site.
The site is secure. The https:// ensures that you are connecting to the official website and that any information you provide is encrypted and transmitted securely.
- Publications
- Account settings
Preview improvements coming to the PMC website in October 2024. Learn More or Try it out now .
- Advanced Search
- Journal List
- Polymers (Basel)

Exploiting Potential Biotechnological Applications of Poly-γ-glutamic Acid Low Molecular Weight Fractions Obtained by Membrane-Based Ultra-Filtration
Odile francesca restaino.
1 Department of Experimental Medicine, Section of Biotechnology and Molecular Biology, University of Campania “Luigi Vanvitelli”, 80138 Naples, Italy; [email protected] (O.F.R.); [email protected] (E.C.); [email protected] (S.D.)
Sondos Hejazi
2 Department of Chemical Sciences, University of Naples “Federico II”, 80126 Naples, Italy; [email protected] (S.H.); ti.aninu@tafasoig (C.V.L.G.)
Domenico Zannini
3 Institute for Polymers, Composites and Biomaterials, National Council of Research, 80078 Pozzuoli, Italy; [email protected] (D.Z.); ti.rnc.bcpi@atagatnas (G.S.)
Concetta Valeria Lucia Giosafatto
Prospero di pierro.
4 Department of Agriculture, University of Naples “Federico II”, 80055 Naples, Italy; ti.aninu@orreipid
Elisabetta Cassese
Sergio d’ambrosio, gabriella santagata, chiara schiraldi, raffaele porta, associated data.
The data that support the findings of this study are available on request from the corresponding author.
Since the potentialities of applications of low molecular weight poly-γ-glutamic acid (γ-PGA) chains have been so far only partially explored, the separation of diverse molecular families of them, as well as their characterization for potential bioactivity and ability to form films, were investigated. Two different approaches based on organic solvent precipitation or on ultra- and nano-filtration membrane-based purification of inexpensive commercial material were employed to obtain size-specific γ-PGA fractions, further characterized by size exclusion chromatography equipped with a triple detector array and by ultra-high-performance liquid chromatography to assess their average molecular weight and their concentration. The γ-PGA low molecular weight fractions, purified by ultra-filtration, have been shown both to counteract the desiccation and the oxidative stress of keratinocyte monolayers. In addition, they were exploited to prepare novel hydrocolloid films by both solvent casting and thermal compression, in the presence of different concentrations of glycerol used as plasticizer. These biomaterials were characterized for their hydrophilicity, thermal and mechanical properties. The hot compression led to the attainment of less resistant but more extensible films. However, in all cases, an increase in elongation at break as a function of the glycerol content was observed. Besides, the thermal analyses of hot compressed materials demonstrated that thermal stability was increased with higher γ-PGA distribution po-lymer fractions. The obtained biomaterials might be potentially useful for applications in cosmetics and as vehicle of active molecules in the pharmaceutical field.
1. Introduction
Poly-γ-glutamic acid (γ-PGA) is a poly-amino acid produced in nature by Gram-positive bacteria of the Bacillus genus (e.g., Bacillus subtilis , B. subtilis subsp. natto , Bacillus licheniformis ), whose anionic polymeric structure is composed of D-glutamic acid and/or L-glutamic acid monomers linked by γ-amide bonds [ 1 , 2 ]. Nowadays, γ-PGA is considered a bio-homopolymer of high commercial interest, and it is already widely used in agriculture, environmental bioremediation, food industry, drug and cosmetic manufacturing, thanks to its biodegradable, edible, non-toxic, non-immunogenic and superabsorbent properties [ 2 , 3 ]. However, the different industrial applications of γ-PGA depend on its molecular weight (Mw), conformation, purity, solubility and pH conditions at which it is dissolved [ 2 ]. In water, at pH 7.0, γ-PGA solutions show high viscosity, whereas the homopolypeptide assumes an α-helix conformation in acidic conditions and a β-sheet-one at pH higher than 7.0 [ 4 ]. γ-PGA is also soluble in alcohols, but its solubility depends on the enantiomeric conformation. In fact, if the chains are composed of both D- and L-enantiomers, γ-PGA precipitates in ethanol, while if it contains only one type of enantiomer, it is soluble [ 3 ]. Furthermore, according to the microbial origin and to the culture conditions used in its fermentative production, the γ-PGA Mw ranges from less than 100 to 1000 kDa, and sometimes it can even reach 2000 kDa [ 1 , 5 ]. Ultra-high Mw γ-PGA chains (>1000–1500 kDa) can form colloids and could be used in the recycling of wastewater to remove heavy metals. Conversely, γ-PGA with a Mw > 700 kDa is widely used in cosmetics as skin moisturizing agent, being able to bind water in a proportion of 5000 times its weight, or in food industry as a thickener and cryoprotectant [ 1 , 5 ]. However, although both ultra-high and high Mw γ-PGA are quite exploited in industrial applications, their wider use is limited due to the high viscosity of their solutions at phy-siological pH values. Thus, in recent years, attention has been focused on the pre-paration of γ-PGA polymers of specific average sizes, mainly constituted from short Mw chains, that could be aimed at innovative commercial uses [ 2 ]. Oligomeric and low Mw (<100–200 kDa) γ-PGA have been used as drug carrier for tumor treatment [ 3 ] and, more recently, in the manufacturing of biodegradable films [ 6 ] potentially useful to produce materials with specific and tailored properties for applications in the pharmaceutical as well as cosmetic fields. Low Mw γ-PGA polymers are generally obtained by enzymatic digestion of those of high Mw chains of microbial origin, after their purification from the viscous fermentation broth.
The purification process has high costs and the recovery of the polymer can be hampered by the high viscosity of the fermentation broth [ 7 , 8 ]. The process generally includes centrifugation or sedimentation of the bacterial cells, γ-PGA recovery from the broth supernatant by alcohol precipitation (75–80% methanol or ethanol v / v ) or addition of divalent copper salts. Ethanol precipitation did not assure the removal of proteins that precipitate along with PGA, thus further purification steps are required as concentration by ultra-filtration membranes, dialysis/diafiltration or chromatography to remove small molecules, such as salts, exhaust nutrients and even glutamate monomers [ 7 , 8 , 9 , 10 ]. Then, the purified product is dried by freeze drying or spray drying [ 7 , 8 , 9 , 10 ]. Recently, new strategies for the recovery and the purification of γ-PGA from the broth supernatant were reported that, for example, include four-unit operations, such as acidification, plate and frame filtration, cyclic ultra-filtration and freeze drying, in order to avoid any inefficient precipitation step and to reduce the purification costs [ 7 ]. In another case, a hybrid reactor system that integrated cross-flow microfiltration modules in a fermentation vessel was employed to continuously produce γ-PGA and directly purify it by using two-stage membrane treatments [ 10 ]. This approach assured continuous, high-yield production and productivity of γ-PGA by recycling of the bacterial cells and an efficient downstream process up to high product purity by removing any inhibiting fermentation products [ 10 ]. As the fermentative conditions influence the titer of γ-PGA production, as well as the Mw and polydispersity index of the homopolypeptide, and because the downstream purification process is a complex and not efficient procedure in which the different γ-PGA chain length populations are not separated, the commercial samples sold on the market often contain not pure, highly dispersed γ-PGA multiple forms with different Mw. As the Mw is critical for the γ-PGA applications, new downstream purification approaches are needed, as the classical precipitation procedures are not good in obtaining specific, highly homogenous Mw fractions, but only a mixture of different size populations. Therefore, the use of low Mw γ-PGA fractions for diverse biotechnological applications has been so far poorly investigated, particularly in the field of film manufacturing. So far, low Mw γ-PGA has been used to obtain films by formulating, casting and drying different film-forming solutions (FFSs) [ 6 ], while other methods, such as thermal compression of the polymer, have not been investigated yet.
In the present study, selected and highly homogenous γ-PGA fractions of low Mw were obtained on a large scale by a membrane-based purification process of ultra-filtration (UF), dia-filtration (DF) and nano-filtration (NF) from an inexpensive commercial source of γ-PGA (COM-PGA) and compared to a homopolypeptide preparation obtained from the same source by methanol precipitation ( Figure 1 ). In order to quantify the γ-PGA concentration in all the samples, a new ultra-high-performance liquid chromatography (UHPLC) method was set up on the base of the L-glutamic acid monomer determination after acidic hydrolysis of the polymer. All the γ-PGA fractions were also characterized in terms of average Mw and poly-dispersity index by size exclusion chromatography coupled with a triple detector array (SEC-TDA) after determination, for the first time, of the γ-PGA dn·dc −1 value. Finally, the γ-PGA low Mw fractions obtained by UF, DF and NF and the homopolypeptide sample obtained by methanol precipitation (MET-PGA powder) were investigated for possible mammalian cell protection against dehydration and oxidative stress, as well as for the manufacturing of biomaterials potentially applicable in drug delivery systems and/or as dehydration masks in the cosmetic field. Manipulable γ-PGA films were produced not only by FFS casting but, for the first time, by hot compression of the powder samples and characterized in terms of mechanical, hydrophilic and thermal properties.

Flowchart of the processes for obtaining partially purified γ-PGA from COM-PGA by methanol precipitation (MET-PGA powder) or low Mw fractions of the homopolypeptide by membrane-based purification by micro-filtration (MF), ultra-(UF)/dia-filtration (DF) and nano-filtration (NF). The main γ-PGA fractions (MET-PGA powder, R1, R2 and R3), here reported in the green boxes, were then characterized and assayed for their properties.
2. Materials and Methods
2.1. materials.
COM-PGA of microbial origin was from Xi’an Fengzu Biological Technology Co., Ltd. (Xi’an, China). According to the manufacturer, this industrial bulk contained not purified γ-PGA obtained by microbial fermentation and suitable only for agriculture uses. Glycerol (GLY) (99.5%) was supplied from Carlo Erba Company (Milano, Italy), as well as methanol (99.9%) All other chemicals used for the buffer of SEC-TDA and UHPLC analyses and the standards were of analytical grade and from Sigma-Aldrich (St. Louis, MO, USA). For the biological assays, the Dulbecco’s modified eagle medium (DMEM), the heat-inactivated fetal bovine serum (FBS), the penicillin and the streptomycin, as well as the phosphate-buffered saline (PBS) and the trypsin, were all provided by Gibco Invitrogen (Milan, Italy).
2.2. Poly-γ-Glutamic Acid Purification by Organic Solvent Precipitation
Absolute methanol (1.2 L) was added to COM-PGA powder (300 g). The mixture was stirred by using a refrigerated incubator shaker (ISF-1-W, Kuhner, Birsfelden, Switzerland) for 12 h at 4 °C and then centrifuged at 10,000 rpm for 10 min (Avanti J-20 XP, Beckman Coulter, Brea, CA, USA). The supernatant was discarded, while the pellet was dried at 30 °C to remove the excess of methanol (left panel of Figure 1 ). The obtained powder (MET-PGA) was kept at 4 °C and used for film preparation and characterization.
2.3. Poly-γ-Glutamic Acid Fractionation by Ultra-Filtration and Nano-Filtration
COM-PGA (300 g) was dissolved overnight in 10 L of MilliQ water at room temperature under stirring conditions (600 rpm) and then microfiltered on a 0.65 μm polypropylene membrane (total filtering area of 0.05 m 2 , Sartopure PP2 MidiCaps, Sartorius Group, Göttingen, Germany) to remove undissolved materials by using a non-automatic Sartoflow Alpha system (Sartorius Group, Göttingen, Germany). As illustrated in the right part of Figure 1 , γ-PGA fractionation was performed in three steps by using two different UF membranes in sequence and then by using a NF membrane. The microfiltered solution was first treated on 100 kDa cut-off polyethersulfone cassette membranes (total filtering area of 0.1 m 2 , Sartorius Group, Germany) and concentrated by using an automatic tangential flow filtration system (Uniflux 10, UNICORN, GE Healthcare, Chicago, IL, USA), equipped with a 10 L sample reservoir, feeding pumps, level sensors, pH and conductivity meters and connected to a software able both to control the process parameters and to collect data and process parameters continuously [ 11 ]. After that, 95.0% of the sample was filtered and a continuous DF was performed by adding two volumes of MilliQ water (with respect to the concentrated volume) to the 100 kDa retentate (R1). The 100 kDa permeate (P1) was then ultra-filtered on 3 kDa cut-off polyethersulfone cassette membranes (0.5 m 2 of total filtering area, Sartorius Group, Germany) and concentrated by using a non-automatic Sartoflow Alpha system (Sartorius Group, Germany). The obtained 3 kDa retentate (R2) was then dia-filtered with two volumes of MilliQ water (with respect to the concentrated volume), while the 3 kDa permeate (P2) was then nano-filtered on 200 Da polyethersulfone spiral membranes (0.3 m 2 of total filtering area, Fluxa Filtri, Milan, Italy) by using a non-automatic Sartoflow Alpha system (Sartorius Group, Germany). Finally, the nano-filtration retentate (R3) was dia-filtered with two volumes of MilliQ water (with respect to the concentrated volume) and concentrated, while the obtained nano-filtration permeate (P3) was discarded. During all the processes, data of the pressure and volumes were collected, while conductivity and pH of the solutions were measured. The trans-membrane pressure (TMP) was calculated by using the following formula:
Fluxes were calculated as the volumes passed on a filtering area in 1 h (liter per square meter in 1 h, LMH). Samples were taken during each purification step to be freeze dried in order to determine their dry weight, the protein and inorganic material content and γ-PGA concentration. The selectivity of the membranes during the filtration processes was instead calculated on the base of the SEC-TDA analyses and on the dry weight of the samples according to the following formula:
2.4. Analytical Methods
2.4.1. determination of the dry weight, protein and water content of the samples.
Aliquots (10 mL) of samples of each step of the purification process, as well as the three collected retentate samples, were freeze dried (LIO 5P, 5Pascal, Milan, Italy) by using a previously reported method (18 h at −20 °C and 1.05 mbar and then 3 h at 20 °C and 0.04 mbar) [ 12 ] and weighted in order to determine their dry weight. The COM-PGA, the MET-PGA powder and the three retentate samples were also assayed to determine the percentage of total protein content with respect to the dry weight by using a colorimetric assay [ 13 ] and the bovine serum albumin (BSA) as standard (Bio-Rad Laboratories Inc., Hercules, CA, USA). The total protein percentage of the samples was calculated according to the following formula:
The water content of the COM-PGA, MET-PGA powder and of the three retentate samples was also investigated according to the method described by Shankar et al. [ 14 ].
2.4.2. γ-PGA Concentration Analyses by Ultra-High-Performance Liquid Chromatography
γ-PGA concentration analyses were performed by ultra-high-performance liquid chromatography (UHPLC) after setting up a new method that exploited a previously reported analytical procedure for organic acid determination [ 12 ] and an acidic hydrolysis protocol previously used to depolymerize different polysaccharide chains and lignin–carbohydrate complexes [ 15 , 16 , 17 ]. The COM-PGA, the MET-PGA powder and all the other freeze-dried samples of the purification process were hydrolyzed with 5 M HCl, at a concentration of 2.5 g·L −1 , for 6 h at 100 °C and 600 rpm (Thermomixer comfort, Eppendorf, Germany) to obtain L-glutamic acid monomers. Runs were performed by using an ultra-high-performance chromatography system (Ultimate 3000, Thermofisher-Dionex, Sunnyvale, CA, USA), by injecting 10 μL of the sample on a ion-exclusion column (Rezex ROA-organic acid H + , 300 × 78 mm, Phenomenex, Torrance, CA, USA) and by eluting in isocratic conditions with 0.1% H 2 SO 4 for 25 min at 0.8 mL·min −1 and at 40 °C with detection at 200 nm. A calibration curve of the γ-PGA standard after hydrolysis was built in the range from 0.025 to 0.100 g·L −1 on the base of the L-glutamic acid monomer area. The peak area values of this calibration curve were compared with the ones of the L-glutamic acid standard curve, built after hydrolysis in the same range, and the percentage of the γ-PGA recovery after reaction was calculated. The values of the γ-PGA concentrations, obtained by UHPLC analyses, were multiplied for the sample volumes to calculate the total amount (g) of the polymer in each sample of the purification process. The percentage of γ-PGA recovery in each sample of the purification process, with respect to the COM-PGA content, was calculated according to the following formula:
The percentage of γ-PGA content in the COM-PGA, in the MET-PGA powder and in the three retentate samples with respect to their dry weight was calculated according to the following formula:
The mass yield values were calculated as percentage ratio of the mass value of each sample on the mass value of the initial sample.
2.4.3. γ-PGA Molecular Weight Analyses by Size Exclusion Chromatography with Triple Detector Array
Mw analyses of the COM-PGA sample, of the MET-PGA powder and of the three retentate samples were performed by using a SEC-TDA instrument (Viscotek, Malvern, Italy) equipped with a triple detector array, including a refractive index (RI) detector, a four-bridge viscosimeter (VIS) and two laser detectors of right-angle (RALS) and low-angle light scattering (LALS). Runs were performed by using two gel-permeation columns put in series (TSK-GEL GMPWXL, 7.8 × 30.0 cm, Tosoh Bioscience, Turin, Italy), equipped with a guard column, at pH 7.0 and at 40 °C and by eluting with 0.1 M NaNO 3 at a flow rate of 0.6 mL·min −1 , according to a previously reported method [ 6 , 18 ]. The instrument was calibrated by using a polyethylene oxide (PEO) standard (22 kDa PolyCAL, Viscotek, Malvern, Italy). All the retentate samples were analyzed in duplicate to determine the γ-PGA average Mw, the poly-dispersity index (Mw/Mn) and the intrinsic viscosity (IV) on the base of the detector signals and on the base of the γ-PGA dn∙dc −1 value by applying the equations reported by the manufacturer:
where IV is the intrinsic viscosity (dL·g −1 ); C is the concentration (mg·mL −1 ); dn∙dc −1 is the refractive index increment (mL·g −1 ); and kRI, kVIS and kLALS are instrumental constants obtained by the universal calibration with PEO, performed according to the manufacturer’s procedure (Viscotek, information available from http://www.viscotek.com , accessed date 10 March 2022).
The γ-PGA dn∙dc −1 value was never reported in the literature so far, and it was experimentally determined after injection in triplicate solutions of the commercial γ-PGA standard in a concentration range from 0.1 to 0.5 g·L −1 , as previously described [ 18 ]. The commercial γ-PGA standard average Mw was 580 kDa ± 2 with a poly-dispersity index of 1.1 ± 0.1, as experimentally determined by using our instrument. By plotting the different γ-PGA concentrations versus the peak areas obtained from the RI signal, the γ-PGA dn∙dc −1 value was calculated according to the following formula:
where the injection volume was 0.1 mL; the RI of the solvent was 1.33; the constant kRI was 9.72 × 10 7 . The representative of each peak was calculated as the percentage ratio bet-ween the single peak RI area divided by the sum of the RI areas of all the peaks in each chromatogram [ 18 ]. In the retentate samples, the percentages of representativity of the Mw populations were also determined by dividing the populations in four different ranges in order to calculate the selectivity of the membranes, as reported above: Fraction A—between 150 and 50 kDa, Fraction B—between 50 and 20 kDa, Fraction C—between 20 and 3 kDa, and Fraction D—lower than 3 kDa.
2.5. Biological Activity Assay
2.5.1. dehydration assay.
COM-PGA, MET-PGA powder and the three retentate samples were tested for their ability to counteract dehydration in a cell-based assay. The dehydration assay was performed by using a spontaneously transformed, non-tumorigenic human keratinocyte cell line (HaCaT) provided by Istituto Zooprofilattico (Brescia, Italy). The cells were cultured in DMEM medium supplemented with 10% ( v / v ) FBS, 100 U·mL −1 of penicillin and 100 μg·mL −1 of streptomycin, in 24-multiwell plates (5 × 104/well) until a 70% cell confluence was reached (157,000 ± 10 cells/well). At this point, the culture medium was removed, a wash with PBS was performed, and the keratinocyte cell monolayers were treated for 2 h with solutions of the COM-PGA sample, MET-PGA powder and of the three retentate samples at a concentration of 1.5 g·L −1 in DMEM. All experiments were performed in triplicate. The negative control (−CTR), instead, was carried out in triplicate simply by diluting the culture medium in PBS without any treatment with γ-PGA samples. After 2 h treatment, the samples and the negative control were dehydrated by removal of the medium and incubated at 37 °C until a stress response, in terms of morphological changes detected by using an optical microscope (100-fold magnification) (Axiovert, Zeiss, Oberkochen, Germany), was observed. The positive control (+CTR) of the experiment was constituted by cell mono layers not subjected to dehydration and kept in the presence of the medium during the whole experiment. Cell viability was determined by assessing cell metabolism through the NAD(P)H-dependent cellular oxidoreductase enzyme activity by a colorimetric assay. Cells were incubated for 3 h with the 3-(4,5-dimethylthiazol-2-yl)-2,5-diphenyltetrazolium bromide (MTT) reagent (Cell proliferation Kit I, MTT, Merck, Rome, Italy) at 37 °C and then treated with 0.1 M HCl in isopropanol (Sigma-Aldrich, St. Louis, MO, USA) for 15 min at room temperature. The NAD(P)H oxidoreductase activity was evaluated by measuring the absorbance at 570 nm (PA800, Beckman Coulter, Brea, CA, USA), and the percentage cell viability in the γ-PGA treated samples was compared to the cell viability in the negative control.
2.5.2. Oxidative Stress Assay
COM-PGA, MET-PGA powder and the three retentate samples were compared for their ability to counteract the H 2 O 2 oxidative stress in a cell-mediated test (HaCaT) as rescuing agents. In the experiments 150,000 cell/well were seeded in 24-multiwell plates and cultivated for 24 h until a 40% cell confluence was reached (90,000 ± 10 cells/well). Then, the medium was removed, a wash with PBS was performed and 50 µM H 2 O 2 (Sigma-Aldrich, USA) was added. After 30 min, the samples were observed by using the optical microscope in order to evaluate the eventual morphological changes due to the oxidative stress. Then, the H 2 O 2 solution was removed, a wash with PBS was performed, and the keratinocyte mono layer cells were treated for 24 h in triplicate with solutions of COM-PGA, MET-PGA powder and of the three retentate samples at a concentration of 1.5 g·L −1 in DMEM (except for the CTR samples). The MTT viability assay was performed after 24 h, as previously described, and the percentage of cell viability of the samples was compared with the negative control. The negative control was simply prepared by diluting the culture medium in PBS and then performing the oxidative stress, while the positive control was not subjected to treatment with H 2 O 2 , and the cells were simply kept in the presence of the medium during the whole experiment.
2.6. Film Preparation and Characterization
2.6.1. preparation of films by casting.
Different FFSs (30 mL) containing 800 mg of either MET-PGA powder or retentates containing different γ-PGA fractions obtained by UF (R1, 55 kDa, R2, 18 kDa and R3, 6 kDa), were prepared by adjusting the pH to different values (2.0, 2.5, 3.0, 3.5, 4.0) by addition of 2.0 N HCl. All the FFSs were ultrasonicated (Bandelin SONOPULS ultrasonic homogenizers, Binder, Tuttlingen, Germany) for 20 min at 85% power by using 2 × 10% cycle. The FFSs were prepared in the absence or presence of different concentrations of GLY (1–10%, w / w γ-PGA). FFSs were poured into 60 × 15 mm polystyrene Petri dishes and left to dry in climatic chamber (25 °C and 45% relative humidity (RH) for 48 h). Dried films were conditioned at 25 °C and 50% RH for 2 h before the peeling and characterization.
2.6.2. Preparation of Films by Thermal Compression
Films of quite uniform thickness (≈500 μm) were prepared by compression molding in a Carver lab press (Carver Inc., No. 4122-12-12H, Wabash, IN, USA). Prior to film production, 2 g of γ-PGA powder samples (COM-PGA, MET-PGA, R1 and R2) were homogeneously mixed with 5% or 10% GLY ( w / w of γ-PGA) in a mortar by pestle. Each single dough obtained was finally shaped into small spherical pellets, then subjected to thermal compression. The samples were sandwiched between two flats of polytetrafluo-roethylene foils and heated between the plates of the press up to 150 °C for 2 min at 500 PSI. Then, the samples were removed from the hot press and cooled down to room temperature before the film peeling. Films were always conditioned at 25 °C and 50% RH for 2 h before their characterization.
2.6.3. Film Moisture Content and Solubility
For the determination of the moisture content (MC) of the films, small pieces of them (≈2 × 2 cm) were weighted, put in aluminum dishes and dried in an oven at 105 °C for 24 h. The weight before and after drying was recorded to calculate the MC by the following equation:
where Wi and Wd represent the weights of the initial and dried film, respectively.
Water solubility (WS) of the films was instead calculated by determining the initial weight (Wi) of the film samples after oven drying at 105 °C for 24 h and by immersing the dried specimens in 50 mL of distilled water continuously shaken for 24 h at 25 °C. Then, the insoluble film pieces were oven dried again at 105 °C to obtain the final dry weight (Wf) [ 19 ]. Film WS (%) was calculated according to equation
2.6.4. Film Mechanical Properties
All handleable films were cut into 1 cm × 8 cm strips and analyzed for their mechanical properties (tensile strength, TS; elongation at break, EB; Young’s module, YM) by means of a dynamometer Instron (Engineering Corp., Norwood, MA, USA), according to the procedure described in ASTM D882–10 [ 20 ]. Five specimens of each film sample were analyzed (5 cm gage length, 1 kN load and 20 mm/min speed) taking into consideration film thickness that was measured in five different points with a micrometer with a sensitivity of 0.001 mm (Electronic digital micrometer, DC-516, Alpa, Albiate Monza e Brianza, Italy).
2.6.5. Film Thermal Properties
Thermogravimetric analysis of MET-PGA-, R1- and R2-derived films was performed using a thermogravimetric analyzer TGA/DTG Perkin-Elmer PyrisDiamond, equipped with gas station. Samples (3–4 mg) were placed in an open ceramic crucible and heated from 25 °C to 600 °C at a speed rate of 10 °C·min −1 , under nitrogen flow of 30 mL·min −1 . Before testing, samples were conditioned for 24 h at 25 °C and 50% RH. Thermal properties of MET-PGA-, R1- and R2-derived films were investigated by using a Q2000 T zero differential scanning calorimeter (DSC), TA Instrument (New Castle, DE, USA), equipped with a liquid nitrogen accessory for fast cooling. The calorimeter was calibrated in temperature and energy using indium. Dry nitrogen was used as purge gas at a rate of 30 mL·min −1 . Samples (3–4 mg) were weighed and placed individually in aluminum pans; an empty pan was then used as reference. DSC measurements were performed in double heating run; the first one, occurring from 50 to 200 °C, at 10 C·min −1 , could reproduce the thermal history of the samples. After an isothermal step of one min, non-isothermal crystallization experiments were performed by cooling the specimens up to −50 °C, at a rate of 10 C·min −1 . Finally, a second heating ramp from −50 °C to 250 °C at 10 °C·min −1 was recorded. Before testing, the samples were conditioned for 24 h at 25 °C and 50% RH.
2.7. Statistical Analysis
For cell viability analyses, data were reported as averaged values of three replicates with their standard deviation values and were statistically analyzed by using Excel program (Microsoft, Redmond, WA, USA), using the Student’s t -test, considering statistically significant differences between data with p < 0.05. In terms of film characterization, in order to determine a significant difference between treatments, one-way analysis of variance (ANOVA) and Duncan’s multiple range tests ( p < 0.05) were performed using the Statistical Package for the Social Sciences (SPSS19, SPSS Inc., Chicago, IL, USA) software.
3.1. γ-PGA Quantitative Determination by UHPLC and Purification by Organic Solvent Precipitation
In order to determine the γ-PGA concentration in the commercial material and in all the purified samples, a new UHPLC method was set up on the base of the L-glutamic acid monomer determination after acidic hydrolysis of the homopolypeptide. The L-glutamic acid standard shows a peak at 5.5 min, as well as the γ-PGA standard after acidic hydrolysis (92.5% of the γ-PGA standard was recovered after reaction). Therefore, a calibration curve was built in the linearity range from 0.025 to 0.100 g·L −1 ( Figure S1 ). The obtained results indicate that the commercial dry sample contained only 51.5% of γ-PGA. Thus, 300 g of COM-PGA, containing around 154 g of γ-PGA, was mixed with methanol, and the obtained precipitated material (250 g) was dried overnight in the oven at 37 °C to eliminate methanol. Afterward, the dry material was grinded to obtain a powder (MET-PGA powder) that was further characterized and used for film preparation. At the end of this treatment, only 83% of the original powder was recovered (250 g) with a consequent γ-PGA content of 128.2 g. A similar percentage of recovery was previously reported by Manocha and Margaritis [ 21 ] by using ethanol to precipitate γ-PGA. The conductivity and the pH of the MET-PGA powder, when dissolved in water, were 13 mS·cm −1 and pH 6.4, respectively.
3.2. γ-PGA Purification by Using Filtration Membranes and Characterization
The COM-PGA was purified on different cut-off membranes, as illustrated in the right panel of Figure 1 , with the aim to recover fractions with the highest polymer concentration and of homogeneous averaged Mw size. The entire process is summarized in Table 1 and Figure 2 . An amount of 300 g of the COM-PGA was first solubilized in water (10 L), but 9.2% of the sample remained insoluble.

γ-PGA ultra-filtration (UF) and dia-filtration (DF) phases of 100 kDa ( A ), 3 kDa ( B ) membrane processes and the ultra-filtration phase of the nano-filtration purification ( C ), as indicated in the graphs. The curves of TMP, flux, conductivity and retentate volume during the processes are reported.
Summarized data of the γ-PGA filtration membrane-based purification process. (ND, not determined). Mass yield values were calculated as percentage ratio of the mass value of each sample on the mass value of the initial sample.
Thus, a micro-filtration step was performed to remove other insoluble material contained in the starting sample. A total of 85.7% of the initial γ-PGA was recovered in the microfiltered permeate that was initially concentrated and dia-filtered on 100 kDa membranes. The process parameters are reported in Figure 2 A.
The 100 kDa retentate (R1) had a concentration factor of 13.3, and it contained 15.5% of the initial material. The resulting permeate sample (P1) was then concentrated and dia-filtered on 3 kDa membranes ( Figure 2 B), achieving a conductivity decrease from 8.8 to 5.8 mS·cm −1 . The corresponding retentate (R2) was 11.6-fold concentrated, and it contained 12.3% of the initial COM-PGA. The resulting permeate (P2) was then treated on the nano-filtration membranes ( Figure 2 C), and during the process, conductivity decreased up to 4.0 mS∙cm −1 . The high TMP values reached did not permit a further dia-filtration step. The nano-filtration process allowed a recovery of 50.4% of the initial γ-PGA in the retentate (R3) (concentration factor of 4.4), while the remaining 3.3% was found in the nano-filtration permeate (P3). The selectivity values were 18.8%, 16.6%, 93.9% for the three steps of purification, respectively. In conclusion, the total γ-PGA recovery of the whole membrane process in the three retentate samples was 77.3%; the three retentate fractions were freeze dried, and their γ-PGA content resulted in an increase compared to the COM-PGA, since the γ-PGA content on the dry weight, also considering a water percentage of the freeze-dried samples between 6.0 and 10.0%, was 69.5%, 58.6% and 64.3%, respectively. The percentage protein concentrations on the recovered freeze-dried material of the three retentate samples was very low, between 0.3 and 3.2%.
3.3. γ-PGA Molecular Weight Analysis by SEC-TDA
To perform the SEC-TDA analyses of the samples, the dn∙dc −1 value of the γ-PGA standard was experimentally determined for the first time, and it emerged as 0.183 mL·g −1 ( Figure S1 ). COM-PGA, MET-PGA and the three obtained retentates were analyzed ( Figure 3 A). In Figure S2 , their complete chromatograms showed the diverse bio-polymer families that corresponded to the average Mw values reported and highlighted in Figure 3 B. Analyses showed the presence of three different populations of γ-PGA with low and ultra-low averaged Mw(between 78.3 and 5.6 kDa, with a poly-dispersity index between 1.07 and 2.73). The lowest Mw (5.6 kDa) fraction of γ-PGA constituted the main peak of the commercial sample with a representativity of 64.5%. The precipitation of the COM-PGA by methanol roughly halved the ultra-low Mw population, since the SEC-TDA analysis showed the presence of three peaks, with averaged Mw values like the values of the commercial sample (between 64.0 and 3.0 kDa) but with different representativity. Conversely, the representativity of the other two populations with higher Mw (64 and 20.6 kDa) increased by about 1.6- and 1.9-fold. The membrane-based purification process allowed for obtaining γ-PGA fraction samples that were more homogeneous in size. More specifically, R1 contained a homopolypeptide fraction with an average Mw of 54.7 kDa, a poly-dispersity index of 1.56 and a representativity of 98.5%. In R2, the main peak of 17.9 kDa with a representativity of 87.4% was found ( Figure 3 ), together with a higher Mw peak of 52.7 kDa, accounting for the 12.6% of γ-PGA. Finally, γ-PGA chains with Mw values lower than 10 kDa were collected only in R3. It is worthy to note that γ-PGA of average 3000 kDa Mw (between 2761 and 3254 kDa) was found in very small percentages (up to 1.5% of representativity) in all the analyzed samples.

Overlaid SEC-TDA chromatograms of the different γ-PGA samples: refractive index signals of the COM-PGA (red line), MET-PGA powder (blue line), as well as of the R1 (purple line), R2 (green line) and R3 (black line) fractions ( A ). Average Mw, poly-dispersity index (Mw/Mn), intrinsic viscosity (IV) and percentage representativity of each γ-PGA fraction analyzed by SEC-TDA ( B ).
3.4. Biological Activity: Dehydration and Oxidative Stress Assays
The biological activity of COM-PGA, MET-PGA powder and of the γ-PGA-containing R1, R2 and R3 fractions was investigated using HaCaT cells by an in vitro model for challenging both dehydration and oxidative stress. The dehydration test showed an 80% decrease in viability of the negative control, and a similar effect was observed in the presence of the COM-PGA sample ( Figure 4 A). Conversely, the addition of all the other γ-PGA samples was able to restore cell viability to various extents. In particular, R1 and R2 γ-PGA-containing fractions caused a marked improvement, with a recovery higher than 2.5-fold, whereas cell viability increased by less than 30% and 40% by employing R3 and MET-PGA powder (normalized to the positive control), respectively ( Figure 4 A).

Dehydration assay ( A ) and oxidative stress assay ( B ) on HaCat cells as rescue effect of COM-PGA, MET-PGA and of γ-PGA-containing R1, R2 and R3 fractions, normalized over the positive control and compared to the negative control. (Statistical analysis: * p < 0.05 and ** p < 0.01 compared to the negative control).
As far as the oxidative stress applied to HaCat monolayers is concerned, it reduced cell viability by about 70% compared to the positive control, while all γ-PGA-containing fractions were found to determine a significant rescue of the cell metabolism when added after the oxygen peroxide treatment ( Figure 4 B). In fact, while the COM-PGA seemed unable to improve cell viability, both MET-PGA powder and all the γ-PGA fractions obtained by ultra-filtration had a marked enhancing effect and, among them, the R3 fraction showed by far the best performance.
3.5. γ-PGA Film Preparation by FFS Casting/Drying and by Hot Compression
γ-PGA-based films were prepared by two different procedures, either by casting and drying of the FFSs containing 800 mg of the homopolypeptide and GLY at two different concentrations (5 and 10%, w / w of γ-PGA) or by hot compression for 2 min at 150 °C and 500 p.s.i. of the powders (2 g) of the different γ-PGA fractions, obtained by methanol precipitation or by ultra-filtration, after their mixing with GLY (5% w / w of γ-PGA). As it is possible to see from panel A of Figure 5 , all the films obtained by the FFS casting appeared brownish, and only the MET-PGA-based FFS containing 10% GLY and all the R3-based FFSs, prepared both in the absence or presence of GLY [ 22 ], did not allow for obtaining any manipulable films after casting and drying.

Films obtained by casting film forming solutions prepared with 800 mg of MET-PGA, R1, R2 and R3 γ-PGA fractions in the absence or presence of two different glycerol (GLY) concentrations; x, unhandleable sticky material ( A ). Films obtained by hot compression of 2 g of COM-PGA (γ-PGA), MET-PGA, R1 and R2 powders mixed with 5% GLY ( B ). Further experimental details are given in the text.
In contrast, all the films prepared with the R1 γ-PGA fraction (55 kDa), both in the absence and in the presence of the two different GLY concentrations, were easy to be peeled off from the plates and were very manipulable, even though they presented some spots on their surface. Finally, the R2 γ-PGA fraction (18 kDa) gave rise to brittle films when prepared either in the absence or presence of 5% GLY; conversely, brown and transparent films without any irregularities were obtained in the presence of 10% of GLY.
Panel B of Figure 5 shows the films obtained by hot compression of the γ-PGA powders (COM-PGA, MET-PGA, R1 and R2 γ-PGA fractions) previously mixed with 5% GLY, as described in Materials and Methods. These films showed a dark brown color and were handleable and quite homogenous, whereas no manipulable films were obtained by hot compression of the R3 γ-PGA powder, similarly to what occurred after casting and drying of the FFS containing R3 γ-PGA fraction.
3.6. Film Characterization for Mechanical and Hydrophylicity Properties
All the films prepared with the two different procedures were analyzed according to their mechanical (TS, EB and YM) and hydrophilicity (MC and WS) properties, and the detected results, reported in Table 2 , were compared. It was possible to analyze only the peelable and well-shaped materials, since unmanipulable films (either * sticky or ** brittle) were obtained by casting and drying the FFSs containing COM-PGA, with or without GLY, or MET-PGA and 10% GLY (both originating sticky materials) and R2 γ-PGA fraction prepared in the absence or presence of 5% GLY (originating brittle materials). Moreover, similar unmanipulable materials were obtained in the attempt to prepare γ-PGA films by hot compression from COM-PGA and 10% GLY or from MET-PGA in the absence of GLY, which gave rise to sticky and brittle materials, respectively. Finally, not even R3 γ-PGA fraction was able to produce manipulable films, both in the absence or presence of GLY, neither by casting and drying nor by hot compression of its powder. It is worthy to note that the thickness values of the films obtained by hot compression were markedly higher (2–4 times) as a consequence of the higher amount of the homopolypeptide used (2 g with respect to 0.8 g occurring in the cast FFSs) and that the effect of the presence of GLY in increasing film thickness was observed only when the films were prepared by casting and drying the γ-PGA FFSs.
Mechanical and hydrophilicity properties of the films obtained by either casting or hot compression of the different γ-PGA samples (ND, not detectable values because of the unmani-pulable (either * sticky or ** brittle) material obtained or because of *** the very low tensile strength (TS) values (<1.0 MPa) detected. Different small letters (a–g) indicate significant differences among the values reported in each column for each procedure ( p < 0.05). Further experimental details are given in the text.
The analyses of the mechanical properties showed a marked plasticizing effect of GLY in the films prepared by both procedures with all the different γ-PGA samples, as TS and YM always decreased, and EB values concurrently increased, as a function of GLY concentration enhancement. In particular, it deserves to be highlighted that both R1 and R2 γ-PGA films prepared in the presence of GLY (especially R2-derived film with 10% GLY) were endowed with a remarkably high extensibility. Conversely, the films obtained by hot compression of all the γ-PGA powder samples exhibited very low TS values (<1.0 MPa) and, as a consequence, undetectable YM values. Thus, it seems that the hot compression procedure led to production of less resistant but much more extensible films.
The films were also analyzed for their MC and WS after immersing the samples in distilled water for 24 h. As shown in Table 2 , the materials seemed to behave in a very similar way regardless of the method used for their manufacture. In fact, all the films showed a WS higher than 80%, except for R1-based films prepared by means of thermal compression in the absence or presence of 5% GLY, the WS of which was 62 and 71%, respectively. However, the hot compressed materials were found to possess a lower amount of water, as the measured MC was lower than that of the respective cast films prepared under the same conditions, with the only exception of the R2 γ-PGA films manufactured with 10% GLY.
3.7. Thermal Properties of γ-PGA Different Samples and of the Derived Films Manufactured by Hot Compression
The thermal properties of both MET-PGA and R1 and R2 powders, as well as of the derived films manufactured in the absence or presence of 10% GLY by hot compression, were evaluated by both thermogravimetric analysis and differential scanning calorimetry (DSC). However, it is worth considering that, in general, γ-PGA could exhibit different thermal characteristics depending on the microorganism used for fermentation and the fermentation conditions, which can in turn influence the Mw and, consequently, the physicochemical properties of the analyzed homopolypeptide sample [ 23 ].
3.7.1. Thermogravimetric Analysis
The thermogravimetric (TGA) and the differential thermogravimetric (DTG) curves of MET-PGA, R1 and R2 γ-PGA films, without and with glycerol, are reported in Figure 6 A,B and Figure 6 D,E, respectively. All curves were normalized with respect to the starting sample size.

Thermogravimetric ( A , D ), differential thermogravimetric ( B , E ) and differential scanning calorimetric (second heating ramp) ( C , F ) thermograms of MET-PGA, R1 γ-PGA and R2 γ-PGA films, without ( A – C ) and with ( D – F ) 10% glycerol (10GLY).
In order not to overburden the paper content, finalized to mainly emphasize the role of films, all the thermal analysis of powder-based samples, together with their interpretation and discussion, were postponed to the Supplementary Material file (Section S1, Figure S3A,B ). In Figure 6 A,B,D,E, the thermograms of neat and GLY-doped films are reported. Generally, from the analysis of weight loss curves ( Figure 6 A,D), different mass evolution steps could be detected, highlighting the complex pattern of film thermal degradation profiles. This outcome was better highlighted by analyzing the DTG curves of all the films. Specifically, for all neat samples ( Figure 6 B), at least three different regions of water evolutions could be found with their corresponding maxima peaks at around 50, 100 and 150 °C. In fact, as widely reported in the literature, the investigated region can be ascribed to free, bound and strongly bound water release, mostly if hydrophilic and hygroscopic polymers are concerned. It should be considered that γ-PGA-based samples are characterized by the presence of many polar groups, such as amino, carboxyl and hydroxyl residues, mostly involved in hydrogen bonding with water and, in any case, able to absorb and/or entrap water molecules in their three-dimensional networks. These findings, likewise well documented in the literature by some of the authors of the current paper [ 6 ], could also be explained by considering the thermal processing undergone by the films (compression molding), during which strong hydrogen bonds between water molecules and polymer polar residues occurred, responsible for water entrapment inside the polymer matrix network, releasable only at high temperature. Similar results were also found in the presence of GLY ( Figure 6 D,E); in these samples, the presence of two main regions of water evolution is well evident. The first one, up to 100 °C, was likely associated with the free and weakly bound water release, whereas the second temperature range between 100 and 200 °C was likely due to water evaporation coming from GLY-rich region, in which hydrogen bonding occurred. In fact, it is worthy to mention that water released at high temperature also came from the condensation reactions following polymer thermal degradation. Sabbah et al. [ 6 ] found that γ-PGA underwent the first depolymerization process at around 200 °C, consisting of an end-of-chain unzipping cyclodepolymerization reaction, leading to pyroglutamic acid. The short chains formed upon heating could depolymerize at around 250 °C, as shown by the small shoulder peak in both neat and doped films, whereas the main degradation process of high molecular weight polymers occurred in the wide range of 280–500 °C. From the comparison of DTG thermograms reported in Figure 6 B,E, it is worthy to note that in the presence of GLY, a clear splitting of the different γ-PGA Mw fractions degradation rate occurred [ 24 ]. Specifically, as shown in Table 3 and Figure 6 E, the lower molecular weight fractions, likely involved in hydrogen bonding with GLY, showed a maximum degradation rate peak at around 280 °C, about 20 °C lower with the analogous chain fractions of the neat polymers, degrading at 300 °C ( Figure 6 B) [ 25 ]. This result was ascribed to the increase in free volume and macromolecular mobility of the plasticized systems, as widely reported in the literature [ 26 ]. It was also likely that the thermal depolymerization of lower Mw macromolecular chains occurred simultaneously with GLYdegradation, thus explaining the higher peak intensity of this region. The third thermal peak, related to the higher γ-PGA molecular weight decomposition, occurred at 330 °C in all the samples, thus confirming both that the effect of the plasticizer was mainly marked in smaller Mw γ-PGA polymer residues and that a phase separation occurred during hot compression molding between regions of different molecular weights [ 27 , 28 ]. The final step of decomposition, observed by ana-lyzing the film samples in 450–550 °C range, was likely associated with the decarboxylation process leading to unsaturated chain fragments, non-volatile compounds in inert atmosphere (nitrogen).
Glass transition temperatures (Tg) and the starting endothermic peak (Tpeak) of all the films.
3.7.2. Differential Scanning Calorimetry
DSC thermograms, related to the second heating ramp of MET-PGA, R1 and R2 films, are reported in Figure 6 C,F. Apart from the expected chemical reaction, the first thermal heating of polymers by DSC analysis erases their thermal history ( Figure S3D–F ), while the second thermal run accounts for the real polymer properties at mole-cular level, evidencing all the involved transition phenomena [ 29 ]. In fact, due to the instrument limiting upper value of temperature, it was not possible to take a suitable guess related to the starting endothermic peaks visible for all samples at around 250 °C. In any event, from the differential thermal analysis (DTA) ( Figure S4A,B ), detectable by the same thermogravimetric analyzer used for TGA/DTG analysis in the range of 25–600 °C, and able to provide qualitative information on liable polymer transitions, no peaks could be found, apart from the ones related to polymer thermal degradation, better highlighted by DTG thermograms, as previously discussed. Thus, from DSC profiles, the starting endothermic peak found at 250 °C could likely be due to polymer degradation events. Nevertheless, from DSC analysis of the second heating run, it was possible to detect the glass transition temperature (Tg) of all the samples. The results, reported in Table 3 , demonstrated the plasticizing action of GLY, as the Tg decreased for all the tested films [ 30 ].
4. Discussion
Up to now, the use of different low Mw γ-PGA chains as natural and biodegradable sources for diverse biotechnological applications has been poorly investigated [ 6 ]. In particular, little exists in the literature on the specific application of low Mw fractions of the homopolypeptide as cosmeceutical agent or to produce biodegradable films. As the γ-PGA functions change according to the size, their homogeneous low Mw fractions might potentially exhibit different properties compared to both high or very high Mw γ-PGA or to the mixtures of different Mw chains of the homopolypeptide. Thus, a key point in assessing specific polymer properties and potential functions is the necessity to find new protocols to purify and prepare γ-PGA size-specific fractions and to characte-rize them in terms of concentration and Mw [ 2 ]. So far, the γ-PGA quantitative determination has been performed by spectrophotometric assays (e.g., colorimetric test of safradin-induced precipitation, or after cetyltrimethylammonium bromide precipitation, or UV absorbance) [ 31 , 32 ]. Although these methods are apparently rapid, most of them require extensive purification of the samples before the analyses to avoid interference of other components in the estimation of the real titer. Zeng et al. [ 32 ] used an HPLC method for the γ-PGA determination after a strong hydrolysis (i.e., 105 °C for 8 h). In the present study, a new UHPLC method was set up to precisely quantify γ-PGA, occurring even in raw samples, on the base of the L-glutamic acid amount after milder acidic hydrolysis of the samples and ion-exchange chromatography separation. This hydrolysis procedure, which already proved to be effective for the quantitative determination of other macromolecules [ 16 , 17 , 18 ], emerged as highly reliable (92% of recovery) also in evaluating the amount of γ-PGA. Besides, so far, γ-PGA molecular weight analyses have been performed by using methods such as SDS-PAGE (with methylene blue or alcian blue staining, as well as with densitometry band detection) and gel-permeation chromatography with RI detection [ 32 ]. In the present study, instead, we used SEC-TDA analyses that also involve the contemporary detection by light scattering signals and intrinsic viscosity, thus obtaining a more reliable determination of the γ-PGA Mw and poly-dispersity index. In fact, improving our previous results [ 6 ], the γ-PGA dn·dc −1 value was also experimentally determined for the first time, since in the literature, only the increment of the calculated, and not experimental, value of L-glutamic acid is reported [ 33 ]. Furthermore, improved procedures for γ-PGA purification are needed for a better characterization of the homopolypeptide. Alcohol precipitation is one of the main methods reported. However, it is not specific, and undesired polysaccharide and protein impurities contained in the fermentation broth generally remain in the γ-PGA samples and must then be removed with following acid or protease treatments [ 31 ]. Moreover, this method involves the consumption of high volumes of solvent, which raises environmental concerns, especially in large-scale production of the homopolypeptide [ 10 ]. Finally, alcohol precipitation does not fractionize γ-PGA in homogeneous Mw samples. In this respect, various methods, such as alkaline or enzymatic hydrolysis and ultrasonic irradiation, have been used to degrade the ultra-high and high Mw γ-PGA, obtained by fermentation, into low Mw homopolypeptide chains [ 31 ]. However, even these methods have limits, by degrading the polymer producing highly poly-dispersed molecular species. Size-specific γ-PGA samples (between 13 and 1300 kDa) have been previously obtained by using a hydrophilic silica-based anion exchange [ 31 ] or by gel-permeation chromatography [ 34 ]. However, these kinds of chromatographic methods are not applicable for large-scale γ-PGA preparations [ 34 ]. Therefore, membrane-based purification appeared more selective than alcohol-based precipitation and easy to scale up [ 11 ]. In the literature, ultra-filtration with polyethersulfone hollow fiber membranes (cut-off of 500 kDa, 300 kDa and 30 kDa) have been previously used to concentrate γ-PGA from a highly viscous culture broth and to reduce the amount of alcohol to be used in a second step of recovery [ 10 ]. An ultra-filtration process (with plate and frame cassettes of 10 and 100 kDa), coupled with nano-filtration was instead used in a previous work on lab scale to purify a small amount of γ-PGA (25 g). In this paper, we attempted a scale up of the previously developed tangential flow-based ultra-filtration process [ 6 ], to better recover some of the fractions with slight modifications of ultra-filtration membrane size in the second step, in order to purify a larger amount of COM-PGA (300 g) that contained a mixture of three different low Mw γ-PGA populations. The fractions were then compared with the sample obtained from the same raw material by methanol precipitation. This last procedure partially removed the very low Mw chains (<6.0 kDa) of the homopolypeptide from the COM-PGA, thus increasing the representativity of the other two macromolecular species and delivering high γ-PGA recovery percentages, but without an extensive purification of the commercial sample or selecting a precise size population of the homopolypeptide. The membrane-based process, instead, allowed for obtaining fractions with quite homogeneous sizes (54.7 and 17.9 kDa) and a higher purity grade. These fractions showed biological properties in terms of rescue effect against dehydration and oxidative stress. In fact, COM-PGA was not able to preserve the viability of the HaCaT cell monolayers, whereas the γ-PGA fractions with specific Mw sizes, such as R1 (54.7 kDa) and R2 (18.0 kDa), protected the cells from the dehydration stress and were rescuing the detrimental effect of oxygen peroxide treatment, with a significant outcome also by the R3 (6 KDa) γ-PGA fraction. The homopolypeptide size was shown to also affect the properties of the homopolymer-derived biomaterials. It is inte-resting to note that R3, containing very low Mw species of γ-PGA, was not able to produce any kind of films, neither in the absence nor presence of plasticizer, while COM-PGA, MET-PGA and the two retentate samples, R1 and R2, allowed for obtaining, under selected experimental conditions (i.e., pH and GLY concentration), manipulable films by casting/drying of FFSs or by hot compression of the sample powders. The mechanical properties of the manufactured γ-PGA-based materials demonstrated that GLY was able to promote a marked extensibility of these films, reducing contextually their TS and YM. These findings suggest a potential cosmetic application aimed at the preparation of hydration masks where the ability to stretch the skin is a prerequisite of paramount importance. In particular, an extraordinary extensibility (EB value of 785%) of the R2-based films when manufactured by hot compression and plasticized with 10% GLY was demonstrated. This behavior might be due to the fact that the thermal pressing at 150 °C of R2 powder mixed with 10% GLY led to an extensive esterification reaction between the carboxyl groups of the low Mw species of γ-PGA occurring in R2 sample and the hydroxyl groups of GLY that, in turn, improved the extensibility of the films as a consequence of the formation of a high number of covalent stable bonds between the plasticizer and the polymer matrix [ 35 ].
Finally, the thermal characterization of the materials obtained by hot compression showed an increase in their thermal stability corresponding to the enhancement of the Mw of γ-PGA molecular species. The thermogravimetric analyses of the different γ-PGA samples indicated at around 330 °C the peak of the thermal decomposition of the higher Mw species of γ-PGA (54.7 kDa) contained in R1 fraction, whereas the peak corresponding to the decomposition of the lower Mw species of γ-PGA (17.9 kDa) present in the R2 samples occurred just below 300 °C. In addition, from DSC analyses, it was possible to assess that R2 sample evidenced the lower glass transition temperature, as expected, given its lower molecular weight. Moreover, a worthy plasticizing effect of GLY was extended to all the films, thus confirming, at molecular level, the previous improvement of mechanical properties for all the GLY-doped samples. A recent study of Lee et al. [ 35 ] on the design of films from cellulose graft copolymers obtained by carver press demonstrated that the hot compression really led to an evident thermos-plasticizing effect. In conclusion, fractionated γ-PGA may be used for film formation with diverse possible uses, among which the bioactivity data here reported suggest some cosmeceutical applications. It is worth to mention that, for the first time, γ-PGA was used to prepare biomaterials by both casting and hot compression method. Although casting is the most successful technique for preparing homogeneous materials, it is less suitable for a large-scale process. In fact, the hot compression requires less time to produce materials and, therefore, it has been demonstrated to be a promising method for packaging ma-nufacture. Besides, the thermally obtained films ensure a superior processability and may also be proposed for packaging purposes. In the future, emphasis should be given to scale up experimentation on this hot compression method for the commercialization of γ-PGA-based biomaterials. However, further attempts are necessary in order to increase the very low TS found for the films prepared by means of this method ( Table 2 ).
5. Conclusions
The potential of γ-PGA as raw ingredient for ready-to-use market products has been extensively explored so far, even though the majority of the commercially inexpensive preparations of the homopolypeptide seem to result in low quality and purity. To better highlight the potential use of specific homogenous low molecular size populations of γ-PGA obtained by fermentation, a specific three-step membrane process was developed up to 300 g scale of the commercial product and compared to γ-PGA obtained from the same original source after precipitation by methanol. The retentate fractions, also cha-racterized by new analytical methods, permitted the obtaining of films by both casting and hot compression with good technological properties and diverse potential applications. Furthermore, since the results obtained by two skin damage experimental models showed that the low Mw forms of the homopolypeptide were able to counteract the desiccation and the oxidative stress of keratinocyte monolayers, all these data suggest possible applications of the γ-PGA-based films also as protective co-smeceutical devices.
Acknowledgments
We thank Rocco Di Girolamo (Department of Chemical Sciences, University of Naples Federico II) for his helpful technical assistance in preparing films by carver press.
Supplementary Materials
The following supporting information can be downloaded at: https://www.mdpi.com/article/10.3390/polym14061190/s1 , Section S1. Thermal analysis of MET-PGA, R1 and R2 powder based films Figure S1. UHPLC chromatogram of a standard (std) sample of γ-PGA (black line), analysed on the base of its L-glutamic acid content after acidic hydrolysis of the polymer, in comparison with the chromatogram of a representative γ-PGA sample (blue line) from the membrane purification process (A). RI chromatograms of SEC-TDA analyses of the different γ-PGA standards used to determine the dn·dc −1 value (B). Figure S2: Complete SEC-TDA chromatograms of the different γ-PGA samples with the signals of RI (red line), of the viscosimeter (blue line) and of the right angle (green) and low angle light scattering (black line): Commercial γ-PGA, MET-PGA powder, 100 kDa retentate (R1), 3 kDa retentate (R2) and of the nano-filtration retentate (R3). The dot blue lines indicate how were integrated the different species. Figure S3. Thermogravimetric TGA (A), differential thermogravimetric DTG (B) and differential scanning calorimetric analysis DSC (second heating run C) of powder samples. DSC first heating run of powders (D), neat films (E) and glycerol doped films (F). Figure S4. Derivative Thermogravimetry (DTG) and Differential Thermal Analysis (DTA) of MET-PGA, R1 and R2 neat films (A) and glycerol doped films (B).
Author Contributions
Conceptualization, C.S. and R.P.; methodology, O.F.R., S.H., C.V.L.G., E.C., S.D., G.S., D.Z. and P.D.P.; formal analysis, O.F.R., S.H., C.V.L.G., E.C., S.D., G.S., D.Z. and P.D.P.; investigation, O.F.R., S.H., C.V.L.G., E.C. and S.D.; writing—original draft preparation, O.F.R., S.H. and C.V.L.G.; writing—review and editing, C.S. and R.P.; funding acquisition, C.S. and R.P. All authors have read and agreed to the published version of the manuscript.
This research was funded by Contratto di sviluppo “Altergon Italia” (CdS000463), and by the grant PRIN: Progetti di Ricerca di Interesse Nazionale—Bando 2017—“CARDoon valorisation by InteGrAted biorefiNery (CARDIGAN)” of Italian Ministero dell’Università e della Ricerca (COD. 2017KBTK93). In addition, O.F.R. fellowship was granted by MUR PON01_2464.
Data Availability Statement
Conflicts of interest.
The authors declare no conflict of interest.
Publisher’s Note: MDPI stays neutral with regard to jurisdictional claims in published maps and institutional affiliations.
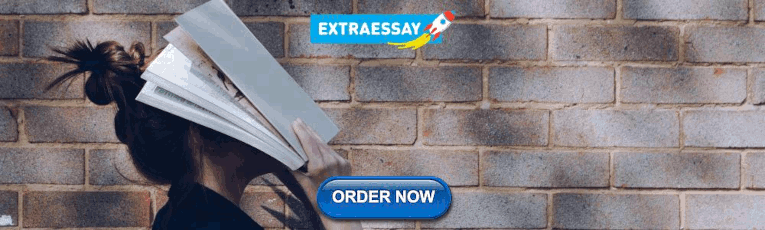
IMAGES
VIDEO
COMMENTS
What it is: The MD Formula Hydrating Serum is a lightweight serum designed to give the skin a deep hydrating effect. This advanced professional serum contains a powerful cocktail of 2 key ingredients Polyglutamic Acid at 1% and Vitamin C at 3% Polyglutamic Acid is significantly more hydrating than Hyaluronic Acid.
Hydrating Serum Polyglutamic Acid 1%, Vitamin C 3% 30ml. $89.00. Provides deep moisturization for hydrated skin. Enhances complexion with temporary brightness. Contains Polyglutamic Acid and Vitamin C for intense hydration and a radiant appearance. This formula is a skincare product that aims to provide deep moisturization and a temporary ...
MD Formula Hydrating Serum with Polyglutamic Acid 1% and Vitamin C 3% is a powerful and effective solution for dehydrated and dull skin. This serum is designed to provide intense hydration,...
POLYGLUTAMIC ACID. TYPE OF INGREDIENT: Hydrator. MAIN BENEFITS: Moisturizes skin, reduces the appearance of wrinkles and pores, replenishes cell moisture. WHO SHOULD USE IT: It is ideal for anyone with dry or dehydrated skin. Polyglutamic acid is safe for all skin types, however, if you have sensitive skin it is always best to patch test a new ...
Polyglutamic acid (PGA) is a polymer of the amino acid glutamic acid (GA). Depending on where the individual monomers connect, PGA can be gamma PGA (poly-γ-glutamic acid, γ-PGA), the form where the peptide bonds are between the amino group of GA and the carboxyl group at the end of the GA side chain, or alpha PGA, the form where the alpha-carboxyl is used to form the peptide bond.
Polyglutamic acid is a type of ingredient called a peptide and is made up of a chain of a particular type of amino acid called glutamic acid. Still, despite being a peptide, it doesn't work in the ...
Just like hyaluronic acid, Polyglutamic acid is a humectant. According to Elyse Love, MD, a board-certified dermatologist, that means the ingredient draws moisture into the skin when it is paired with a moisturizer. "This leads to increased skin hydration and improvement in the appearance of dullness and fine wrinkles," she explains. "It is a ...
Like hyaluronic acid, polyglutamic acid (PGA) is a moisturizing anti-aging ingredient that dermatologists say creates an instant glass skin complexion. ... MD, cosmetic dermatologist says. Like HA ...
Think of polyglutamic acid like cling wrap. "It forms a hydrogel film on the surface of the skin that prevents water from evaporating," says Dr. King. "Its occlusive property means that it helps ...
FaceGym, Hydro-Bound Hyaluronic Acid + Niacinamide Serum — $77.00. The larger, 30 mL bottle is back in stock for $77. The smaller, 15 mL bottle ($45) is currently on back-order. But trust me ...
Hydrating Serum Polyglutamic Acid 1%, Vitamin C 3% 30ml. £89.00 GBP. Provides deep moisturization for hydrated skin. Enhances complexion with temporary brightness. Contains Polyglutamic Acid and Vitamin C for intense hydration and a radiant appearance. This formula is a skincare product that aims to provide deep moisturization and a temporary ...
Poly(glutamic acid)s and their composites in medical applications4.1. Antimicrobial complexes. Bacterial biofilms formed on the surface of implantable devices pose a serious problem for human health. Additionally, when the skin is damaged, microorganisms can easily enter the body, resulting in an infection [80]. Therefore, it is important to ...
Karan Lal, MD, is a board-certified dermatologist at Schweiger Dermatology Group in Hillsborough, New Jersey. Quick Question: What Is Polyglutamic Acid? Despite its long name, you'll likely be hearing more and more about this powerhouse skincare ingredient. "Polyglutamic acid is the new humectant on the block," says Dr. Lal.
Buy MD Formula Hydrating Serum - Polyglutamic Acid 1%, Vitamin C 3% 30ml and other MD Formula products at www.feelunique.com. The MD Formula Hydrating Serum is a lightweight serum designed to give the skin a deep hydrating effect. Our advanced professional serum contains a powerful cocktail of 2 key ingredients Polyglutamic...
MD Formula (20) Price £GBP to £GBP. Availability. In stock only. Add to cart. Free Radical Defence Daily Moisturiser 50ml. Sale price £79.90 GBP. ... Hydrating Serum Polyglutamic Acid 1%, Vitamin C 3% 30ml. Sale price £89.00 GBP. Caviar LUX Nourish & Restore Night Moisturiser 50ml. Sale price £79.90 GBP.
Germantown, TN dermatologist Purvisha Patel, MD says that like HA, polyglutamic acid is a humectant with excellent water-binding capabilities. "It plumps surface skin cells and helps reduce the ...
Six types of γ-polyglutamic acid (γ-PGA)/cold water-soluble starch (St) composite film models were constructed using molecular dynamics simulation, and their properties were investigated and compared with the corresponding experimental values. The compatibility between the composite film components was analyzed using the radial distribution function and mean square displacement (MSD). The ...
1. Introduction. Poly-γ-glutamic acid (γ-PGA) is produced by a number of species of Bacillus.It is an unusual anionic polyamide that is made of d - and l-glutamic acid units connected by amide linkages between α-amino and γ-carboxylic groups.The γ-PGA is biodegradable, edible, water-soluble and non-toxic to humans and environment.
Introduction. Poly-γ-glutamic acid (γ-PGA) is an anionic, biodegradable, non-toxic polymer composed of D- and L-glutamic acid units linked by γ-glutamyl bonds. γ-PGA production with Bacillus subtilis is mainly known from B. subtilis (natto) in the production of natto, a traditional Japanese dish. But B. subtilis has also been considered as a promising organism for industrial production of ...
Poly-γ-glutamic acid (γ-PGA) is a natural, safe, non-immunogenic, biodegradable, and environmentally friendly glutamic biopolymer. γ-PGA has been regarded as a promising bio-based materials in the food field, medical field, even in environmental engineering field, and other industrial fields. Microbial synthesis is an economical and ...
The partial specific volume of the γ-PGA solution was determined to be 0.656, 0.715, and 0.730 mL/g at pH 3.8, 5.9, and 8.9, respectively. The variation of the partial specific volume with pH is ...
1. Introduction. Poly-γ-glutamic acid (γ-PGA) is a poly-amino acid produced in nature by Gram-positive bacteria of the Bacillus genus (e.g., Bacillus subtilis, B. subtilis subsp. natto, Bacillus licheniformis), whose anionic polymeric structure is composed of D-glutamic acid and/or L-glutamic acid monomers linked by γ-amide bonds [1,2].Nowadays, γ-PGA is considered a bio-homopolymer of ...