
An official website of the United States government
The .gov means it’s official. Federal government websites often end in .gov or .mil. Before sharing sensitive information, make sure you’re on a federal government site.
The site is secure. The https:// ensures that you are connecting to the official website and that any information you provide is encrypted and transmitted securely.
- Publications
- Account settings
Preview improvements coming to the PMC website in October 2024. Learn More or Try it out now .
- Advanced Search
- Journal List
- CBE Life Sci Educ
- v.15(2); Summer 2016
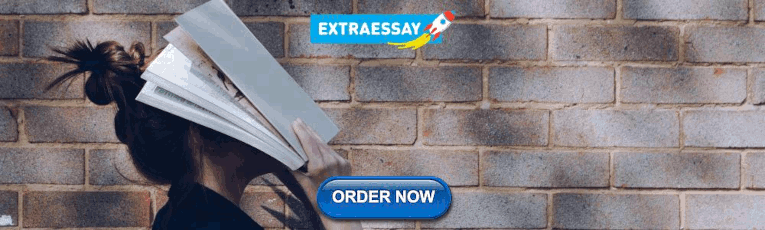
Insights from a Convocation: Integrating Discovery-Based Research into the Undergraduate Curriculum
Sarah c. r. elgin.
† Department of Biology, Washington University in St. Louis, St. Louis, MO 63130
Gita Bangera
‡ RISE Learning Institute, Bellevue College, Bellevue, WA 98007
Sean M. Decatur
§ Office of the President, Kenyon College, Gambier, OH 43022
Erin L. Dolan
‖ Texas Institute for Discovery Education in Science, University of Texas, Austin, TX 78712
Laura Guertin
¶ Department of Earth Science, Penn State Brandywine, Media, PA 19063
Wendy C. Newstetter
# College of Engineering, Georgia Institute of Technology, Atlanta, GA 30332
Elvyra F. San Juan
@ Office of the Chancellor, California State University System, Long Beach, CA 90802
Mary A. Smith
** Department of Biology, North Carolina A&T State University, Greensboro, NC 27411
Gabriela C. Weaver
†† Department of Chemistry, University of Massachusetts, Amherst, MA 01003
Susan R. Wessler
‡‡ Department of Botany and Plant Sciences, University of California, Riverside, Riverside, CA 92521
Kerry A. Brenner
§§ Board on Science Education, Washington, DC 20001
Jay B. Labov
‖‖ Board on Life Sciences, National Academies of Sciences, Engineering, and Medicine, Washington, DC 20001
The National Academies of Sciences, Engineering, and Medicine organized a convocation in 2015 to explore and elucidate opportunities, barriers, and realities of course-based undergraduate research experiences, known as CUREs, as a potentially integral component of undergraduate science, technology, engineering, and mathematics education. This paper summarizes the convocation and resulting report.
INTRODUCTION
Recommendation 2 of the February 2012 report Engage to Excel from the President’s Council of Advisors on Science and Technology ( PCAST, 2012 ) urges the science, technology, engineering, and mathematics (STEM) education community and funding agencies to “advocate and provide support for replacing standard laboratory courses with discovery-based research courses.” The report justifies this recommendation as follows:
Traditional introductory laboratory courses at the undergraduate level generally do not capture the creativity of STEM disciplines. They often involve repeating classical experiments to reproduce known results, rather than engaging students in experiments with the possibility of true discovery.… Engineering curricula in the first two years have long made use of design courses that engage student creativity. Recently, research courses in STEM subjects have been implemented at diverse institutions, including universities with large introductory course enrollments. These courses make individual ownership of projects and discovery feasible in a classroom setting, engaging students in authentic STEM experiences and enhancing learning and, therefore, they provide models for what should be more widely implemented. (pp. iv–v)
This recommendation has engendered wide discussion and motivated formation of a committee at the National Academies of Sciences, Engineering, and Medicine to organize a convocation to explore opportunities and challenges of developing, implementing, and sustaining course-based undergraduate research experiences (CUREs), one mechanism for reaching large numbers of undergraduates. This column reviews the considerations leading up to the May 2015 convocation and summarizes the report that emerged ( National Academies of Sciences, Engineering, and Medicine, 2015 ). 1
Emerging evidence (cited in the PCAST report and elsewhere) indicates that engaging students in research as early as possible during their undergraduate years is one of the best strategies for supporting and retaining undergraduates in STEM. Until recently, undergraduates have primarily participated in research through apprenticeships, wherein an individual faculty member (or one of their graduate students or postdoctoral fellows) supervises the work of one or several students. Apprenticeships can be beneficial and even life and career changing for many students, yet their one-on-one design inherently limits the number of students who can participate. Providing all beginning STEM students with an individualized mentored research experience in an apprentice-style program is not possible, given the large numbers of students. Faculty members (and other potential mentors from industry or elsewhere) have limited time, space, and resources to support undergraduates in their research activities. Most institutions have allocated only enough human and financial resources to involve a small fraction of their undergraduates in such experiences ( PCAST, 2012 ). Students who seek out such positions are generally those who are already interested in research. Competition for a limited number of slots excludes many students, including students who have little knowledge of science career structures or who may not have performed well in traditional academic studies but who are capable of engaging in a research experience. For all of these reasons, students from populations historically underrepresented in STEM fields may especially be precluded from gaining an apprentice-style research experience (e.g., National Research Council 2007 , 2011 ; Locks and Gregerman, 2008 ; National Academies of Sciences, Engineering, and Medicine, 2016 ). The PCAST report urges that access to a research experience during the undergraduate years should be seen as a pedagogical necessity for all students rather than a privilege for a small number of undergraduates.
Acting on this recommendation, many undergraduate STEM educators have been experimenting with different strategies for engaging more students in research experiences. There are now a number of different tested and emerging models available, and their successes suggest that this broad goal can be achieved through CUREs. 2 Traditional “cookbook” laboratories are being replaced with discovery-based research and related activities. These are occurring both in laboratories associated with lecture courses and in stand-alone laboratory courses, using on-campus, off-campus, and online resources. A CURE allows undergraduates to engage in research either collectively or individually as part of a regularly scheduled course. Recent efforts have been aided by the Web, which can provide access to large data sets in topics from genomics to environmental monitoring, remote access to research-grade instruments, access to the scientific literature, and the means to build project consortia and to link student and faculty researchers at dispersed sites.
If appropriately constructed, managed, and mentored, CURES may be able to provide students with many of the same benefits acquired from summer apprentice-style research experiences (e.g., Shaffer et al ., 2014 ). But CUREs also may provide additional advantages:
- By exposing more students to research and the nature of science earlier in their academic career, they can encourage students to explore the nature of various STEM topics and careers not otherwise considered.
- Course-based approaches may be a more effective and accessible starting point for many students, including minority, low-income, and first-generation college students ( Bangera and Brownell, 2014 ).
- Faculty members may be able to undertake research that would otherwise be difficult or impossible (e.g., Leung et al ., 2015 ; Pope et al ., 2015 ).
- CUREs can provide all participating students with a greater ability to use scientific thinking in other aspects of their lives.
However, large-scale undergraduate research, especially for first- or second-year students, is not yet the norm on most campuses, particularly at those institutions with more traditional views of classroom teaching and reward and incentive systems or those with little or no on-site research. To make this recommendation from PCAST a reality, faculty and administrators need to be convinced of the feasibility, efficacy, cost efficiency, student benefits, and overall value of the approach.
Because so many questions remain to be addressed regarding CUREs, the Board on Life Sciences, in collaboration with the Board on Science Education of the National Academies of Sciences, Engineering, and Medicine received support from three private foundations (see Acknowledgments ) to develop an initiative that could further explore and elucidate the opportunities, barriers, and realities of CUREs as a potentially integral component of undergraduate STEM education. In response, a two-day national convocation was organized by a committee appointed by the National Academies and was held in Washington, DC, from May 11 to 13, 2015. Participants explored the following questions:
- What models have been developed to engage larger numbers of undergraduates in research using an academic year course-based format? Is this general strategy viable for all STEM disciplines and all class levels, from freshman to senior? Are minority-serving institutions participating, and are these models effective in reaching underrepresented students?
- Is the evidence base currently robust enough to identify best practices for implementation, considering different goals and different approaches? What are the most important challenges?
- Can these best practices serve as drivers of institutional cultural change, tackling some of the present barriers to access, and are there examples where they have done so?
- Is it possible to scale up to all students, without losing essential elements of the research experience?
- How do we promote and insure access and equity for all students in such initiatives?
- Can we recommend best practices for dissemination, for “start-up” support? What are the most cost-effective strategies?
- Can a shared research agenda help resolve some of these questions?
A common theme—equity and access issues for all students, with an emphasis on students from those populations that historically have been underrepresented in STEM—was emphasized throughout the convocation.
The presentations and discussions that occurred at this convocation resulted in a summary report ( Figure 1 ). The organizing committee selected 12 existing CUREs, presented as case studies, and others were described during the panel discussions ( Table 1 ); these provide insights into the use of this strategy in a variety of settings, highlighting opportunities and challenges encountered. The committee also commissioned a paper from Dr. David Lopatto, Grinnell College, which focuses on assessment issues and is included as an appendix in the report. An extensive set of references is integrated into the convocation report. All participants also were invited to display posters of their work on course-based research opportunities, and the posters remained available throughout the event. 3 Thus, the convocation created a rich compendium of reference materials on CUREs brought together in the report and on the website, both of which are freely available.

Cover of the convocation report.
Case studies presented in the report by name, discipline, focus, and reach (individual faculty vs. national partnerships)
In this Feature , the authors describe activities at the National Academies aimed at understanding models and mechanisms for engaging undergraduates in research at scale and identifying the current state of knowledge and practice related to undergraduate research. We hope that the convocation report will stimulate broader conversations about the role of undergraduate research experiences for all students in undergraduate STEM education. Accordingly, the three foundations that supported the convocation also have provided financial support for follow-up dialogue through workshops at meetings of various scientific societies, including the American Society for Cell Biology and the Genetics Society of America. 4 The convocation, publication, and follow-up dialogue are all helping to inform a larger, more in-depth National Academies consensus study on undergraduate research experiences, including all mechanisms—CUREs, apprentice models, internships, and others. That study is being supported by the National Science Foundation’s Division of Undergraduate Education. The committee’s report should be available by Fall 2016. 5
OVERVIEW OF CONVOCATION THEMES
Multiple themes emerged during the convocation, including those laid out by the committee (see bullet points above) and others raised by other participants. An important topic throughout the convocation was the critical characteristics of CUREs. Dr. David Asai from the Howard Hughes Medical Institute proposed that students should know that they are engaged in working on a real scientific problem, that their work matters to the community, and how their discoveries are contributing to the field. According to Asai, CUREs should permit students to encounter and confront problems that are important and timely; their work should contribute to advancing or refining knowledge, rather than simply repeating or “rediscovering” something that is already known. How to structure CURES that can help students advance to this level of discovery, particularly students who enroll in CUREs early in their undergraduate careers and may have only a single exposure to this kind of experience, engendered a great deal of discussion.
In his address as the keynote speaker at the convocation, Dr. James Gates, professor of physics at the University of Maryland, College Park, a member of the National Academy of Sciences and cochair of the committee that authored Engage to Excel ( PCAST, 2012) , provided a historical perspective of the relationship of research in science and technology to the nation’s economy and well-being (chap. 2 of convocation report). During the latter half of the 19th century and most of the 20th century, average educational levels in the United States were higher than in other countries, which he argued fueled the economic engine of this country. Educational levels of people in the United States are now lower than those in most other developed countries, and median household income has fallen, especially during the past 30 yr. Given the increase in available information and changes in the nature of work, Gates argued that today’s workers will need to continually relearn and retool their skills over their working lifetimes. He emphasized and provided details about how emerging approaches to education, including efforts to allow larger numbers of students to engage in discovery-based research, can help meet the expanding need for workers trained in STEM fields. These points served as the basis for recommendation 2 in the PCAST report.
Assessment and Evaluation of CUREs
A plenary session focused on what emerging research indicates about the efficacy of CUREs on several levels. 6 Student and faculty enthusiasm for CUREs is, at present, largely based on student reports of learning gains and satisfaction with the experience ( Auchincloss et al ., 2014 ; Corwin et al ., 2015 ; Linn et al ., 2015 ). However, there are some well-documented studies showing that research experiences improve retention in the sciences (e.g., Locks and Gregerman, 2008 ; Estrada et al ., 2011 ; Schultz et al ., 2011 ; Eagan et al ., 2013 ; summarized in Corwin et al ., 2015 ), and several case studies presented at the convocation reported positive impacts. CURE assessments that use multiple indicators of student learning and program efficacy can provide greater insights concerning achievement of desired learning goals and affective behaviors of students and can offer guidance when starting new courses ( Corwin et al ., 2015 ; Linn et al ., 2015 ); more research of this type is needed.
Many CUREs are designed by individual faculty to align with their own research interests, an approach that has many benefits but results in assessments that are idiosyncratic and difficult to compare ( Lopatto, 2010 ; Linn et al ., 2015 ). In contrast, a group of coordinated national efforts ( Table 1 ) have attempted to address these issues by using common assessments, and some positive results have been reported ( Jordan et al ., 2014 ; Shaffer et al ., 2014 ). Speakers pointed out that collaborative projects and/or cooperatives of schools with common program goals and common sets of activities can develop a common set of metrics, providing unique opportunities for assessing their efforts. Moreover, speakers noted the potential for partnerships among state systems of higher education and public and private consortia for fostering the acceptance and institutionalization of research-based courses.
Leveraging Resources to Provide More Students with Opportunities for Research
A great deal of discussion at the convocation centered on issues of resources and costs of CUREs compared with more traditional teaching laboratories or apprentice-based models of research participation. There are surprisingly few data available on relative costs, and where available, most of the information is incomplete; for example, faculty are often not compensated for mentoring students under the apprenticeship model, so this cost is not recorded, but will be compensated for teaching a CURE. Benefits are also difficult to monetize. However, if CUREs can be demonstrated to increase retention of students, either in STEM or more broadly at the institution, the money from those students’ tuition can financially justify the strategy. Several presenters noted that research-based courses were powerful recruiting and retention tools for the departments or colleges that offer them. Several presenters reported that costs for running CUREs, even if slightly more expensive than traditional labs, could be borne by students through a small increase in lab fees. However, undergraduates who participated in the convocation pointed out that even a small increase in fees can be a much larger amount if included in high-interest student loans and could make a difference in their decision to enroll in a discovery-based lab course versus a more traditional one. Some people claimed that the CUREs they designed were less expensive; techniques such as DNA barcoding and synthetic biology open up many avenues at modest cost.
Several presenters emphasized that costs for CUREs can be reduced by taking advantage of local resources and partnerships. Specialized analytical instruments can be accessed remotely, often at low cost. Further, every campus needs to collect and analyze data on the operation of their physical plant, on the use of services, and so on, and students can be engaged in the effort. For example, California State University’s recent initiative, “The Campus as a Living Laboratory,” 7 and student research undertaken with the University of Wisconsin’s food services to better understand food use and waste by students 8 were both described. Some of the costs recovered can be directed back into the student research program (Cathy Middlecamp, University of Wisconsin–Madison, personal observation).
Opportunities and Challenges to Scaling CUREs
The convocation provided many examples of how to restructure a given course as a CURE, but we have little experience in “scaling up” these efforts. Expanding such efforts to all sections of a course or most courses within a department may seem desirable, but the logistics and infrastructure required to do so may seem prohibitive. A great deal of discussion during the convocation thus focused on issues of scaling up of CUREs. Because appropriate mentoring is hard to provide for large numbers, it was pointed out by David Shaffer (University of Wisconsin–Madison) that virtual internships (online challenges that prompt students to take action on a complex problem, reflect on their action, and develop ways of thinking about real-world practice) can enable many more students to participate, reaching more students than would be possible through internships in which students must be at the site of the internship to participate. However, several universities (e.g., University of Minnesota–Twin Cities, University of Texas–Austin; Table 1 ) are expanding wet-bench CUREs by organizing multiple research “streams,” among which a student can choose, using a hierarchical mentoring system. A parallel in engineering is the Vertically Integrated Projects program, described by Edward Coyle (Georgia Tech), in which senior students contribute to mentoring beginning students ( Table 1 ).
Several convocation speakers indicated that research-based courses can help a broad range of students decide whether they would like to pursue additional research opportunities, arguing that at least one such course should be mandatory for all students. Mandatory participation ensures that students who may lack the confidence to pursue such pathways on their own are able to do so ( Bangera and Brownell, 2014 ). However, requiring a research experience also means that some students may feel that they are being forced into something they do not want; the undergraduates present at the convocation noted that students who are working hard to maintain or increase their grade point average may be wary of a process in which the probability of failure may be high and course grades are not based on the usual criteria. Hence, for some students, an effort must be made to show them how a research experience will benefit them.
In a panel on institutional strategies, Goldie Byrd (North Carolina A&T State University) pointed out that department chairs, deans, and other administrators can support CUREs by actively promoting faculty professional development in teaching and mentoring and by supporting faculty time used to develop a CURE. The construction of new instructional spaces or the reconfiguration of existing spaces for CUREs also offers opportunities to change the culture of teaching and learning, as seen in the new Interdisciplinary Science Learning Laboratories at the University of Delaware and described by John Jungck. Efforts toward the establishment of endowments and special funds can send powerful signals to faculty, students, regents, and parents about the value of this kind of work. For those campuses undertaking or contemplating major curricular reforms, open consideration of investing in CUREs may provide opportunities to rethink the integration of research into undergraduate education and to retool the reward system for faculty, a major change strategy utilized by the City University of Hong Kong and described by Arthur Ellis.
Closing remarks stressed that the creation of new knowledge is a major function of universities. By welcoming students into this effort, we make them our partners and provide a sense of belonging in this field. As Robin Wright said in describing the freshman program at the University of Minnesota, “We’re talking to them as if they are emerging professional biologists, and we treat them as colleagues.” Teaching STEM by having students do research can be viewed as an active-learning strategy—and there is considerable evidence that active-learning strategies work (e.g., Freeman et al ., 2014 ). The convocation closed with enthusiasm for using CUREs to expand research opportunities to all students. Jim Gentile, now at the University of Arizona, concluded that “undergraduate research is quality education.” Collectively, the speakers and discussions engendered enthusiasm for several elements related to CUREs, and we hope that this report will be useful for those faculty and schools thinking about or planning to expand the use of CUREs in their curriculum.
Acknowledgments
The convocation and year of dialogue have been generously supported by the Alfred P. Sloan Foundation, the Howard Hughes Medical Institute, the Leona M. and Harry B. Helmsley Charitable Trust, and the President’s Committee of the National Academies of Sciences, Engineering, and Medicine.
1 The report is available for purchase or free download of a PDF at www.nap.edu/catalogue/21851 .
2 These kinds of research experiences are given different names and acronyms depending, e.g., on whether they are offered during the academic year or during the summer. In this paper, we have adopted the term “course-based research experiences” (CUREs) for consistency. The National Academies report provides additional information about differences in terminology.
3 Copies of posters, PowerPoint presentations, the meeting agenda, a list of participants, and a video of Lopatto’s discussion of his commissioned paper, as well as resources selected by the organizing committee and suggested by convocation participants, are available at www.dropbox.com/sh/lhxz8fokljbwe7i/AAAiwXqUmbshQurCxzCzIehga?dl=0 .
4 The list of meetings where these dialogues will occur as of the date of publication of this article and copies of presentations from sessions already completed are available at http://dels.nas.edu/global/bls/Year-of-Dialogue .
5 Additional information about this study is available at http://sites.nationalacademies.org/DBASSE/BOSE/CurrentProjects/DBASSE_090473 .
6 Given the limited amount of time to address many topics during the convocation, no topic was explored in detail. The consensus study now underway at the National Academies of Sciences, Engineering, and Medicine will address many of these issues more deeply. A primary charge to that committee is to examine the robustness of the research literature on assessment of CUREs and other types of undergraduate research experiences.
7 Additional information available at www.calstate.edu/cpdc/sustainability/liv-lab-grant .
8 Additional information available at http://nelson.wisc.edu/undergraduate/sustainability-certificate/syllabi/env_st_126-spring_2015_syllabus.pdf .
- Auchincloss LC, Laursen SL, Branchaw JL, Eagan K, Graham M, Hanauer DI, Lawrie G, McLinn CM, Pelaez N, Rowland S, et al. Assessment of course-based undergraduate research experiences: a meeting report. CBE Life Sci Educ. 2014; 13 :29–40. [ PMC free article ] [ PubMed ] [ Google Scholar ]
- Bangera G, Brownell SE. Course-based undergraduate research experiences can make scientific research more inclusive. CBE Life Sci Educ. 2014; 13 :602–606. [ PMC free article ] [ PubMed ] [ Google Scholar ]
- Corwin LA, Graham MJ, Dolan EL. Modeling course-based undergraduate research experiences: an agenda for future research and evaluation. CBE Life Sci Educ. 2015; 14 :es1. [ PMC free article ] [ PubMed ] [ Google Scholar ]
- Eagan JR, Hurtado S, Chang MJ, Garcia GA, Herrera FA, Garibay JC. Making a difference in science education the impact of undergraduate research programs. Am Educ Res J. 2013; 50 :683–713. [ PMC free article ] [ PubMed ] [ Google Scholar ]
- Estrada M, Woodcock A, Hernandez PR, Wesley P. Toward a model of social influence that explains minority student integration into the scientific community. J Educ Psychol. 2011; 103 :206–222. [ PMC free article ] [ PubMed ] [ Google Scholar ]
- Freeman S, Eddy SL, McDonough M, Smith MK, Okoroafor N, Jordt H, Wenderoth MP. Active learning increases student performance in science, engineering, and mathematics. Proc Natl Acad Sci USA. 2014; 111 :8410–8415. [ PMC free article ] [ PubMed ] [ Google Scholar ]
- Jordan TC, Burnett SH, Carson S, Caruso SM, Clase K, DeJong RJ, Dennehy JJ, Denver DR, Dunbar D, Elgin SR, et al. A broadly implementable research course for first year undergraduate students. mBio. 2014; 5 :1–13. [ PMC free article ] [ PubMed ] [ Google Scholar ]
- Leung W, Participating Students and Faculty of the Genomics Education Partnership Drosophila Muller F elements maintain a distinct set of genomic properties over 40 million years of evolution. G3: Genes Genomes Genetics. 2015; 5 :740. [ PMC free article ] [ PubMed ] [ Google Scholar ]
- Linn MC, Palmer E, Baranger A, Gerard E, Stone E. Undergraduate research experiences: impacts and opportunities. Science. 2015; 347 :627. [ PubMed ] [ Google Scholar ]
- Locks AM, Gregerman SR. Undergraduate research as an institutional retention strategy: the University of Michigan model. In: Taraban R, Blanton RL, editors. Creating Effective Undergraduate Research Programs in Science. New York: Teachers College Press; 2008. pp. 11–32. [ Google Scholar ]
- Lopatto D. Science in Solution: The Impact of Undergraduate Research on Student Learning. Washington, DC: Council on Undergraduate Research and Research Corporation for Science Advancement; 2010. [ Google Scholar ]
- National Academies of Sciences, Engineering, and Medicine. Integrating Discovery-Based Research into the Undergraduate Curriculum: Report of a Convocation. Washington, DC: National Academies Press; 2015. [ Google Scholar ]
- National Academies of Sciences, Engineering, and Medicine. Barriers and Opportunities for 2-Year and 4-Year STEM Degrees: Systemic Change to Support Students’ Diverse Pathways. Washington, DC: National Academies Press; 2016. [ PubMed ] [ Google Scholar ]
- National Research Council (NRC) Understanding Interventions That Encourage Minorities to Pursue Research Careers: Summary of a Workshop. Washington, DC: National Academies Press; 2007. [ Google Scholar ]
- NRC. Expanding Underrepresented Minority Participation: America’s Science and Technology Talent at the Crossroads. Washington, DC: National Academies Press; 2011. [ PubMed ] [ Google Scholar ]
- Pope WH, Bowman CA, Russell DA, Jacobs-Sera D, Asai DJ, Cresawn SG, Jacobs WR, Hendrix RW, Lawrence JG, Hatfull GF, et al. Whole genome comparison of a large collection of mycobacteriophages reveals a continuum of phage genetic diversity. Elife. 2015; 4 :e06416. [ PMC free article ] [ PubMed ] [ Google Scholar ]
- President’s Council of Advisors on Science and Technology. Engage to Excel: Producing One Million Additional College Graduates with Degrees in Science, Technology, Engineering and Mathematics. Washington, DC: U.S. Government Office of Science and Technology; 2012. [ Google Scholar ]
- Schultz PW, Hernandez PR, Woodcock A, Estrada M, Chance RC, Aguilar M, Serpe RT. Patching the pipeline: reducing educational disparities in the sciences through minority training programs. Educ Eval Policy Anal. 2011; 33 :95–114. [ PMC free article ] [ PubMed ] [ Google Scholar ]
- Shaffer CD, Alvarez CJ, Bednarski AE, Dunbar D, Goodman AL, Reinke C, Rosenwald AG, Wolyniak MJ, Bailey C, Barnard D, et al. A course-based research experience: how benefits change with increased investment in instructional time. CBE Life Sci Educ. 2014; 13 :111–130. [ PMC free article ] [ PubMed ] [ Google Scholar ]
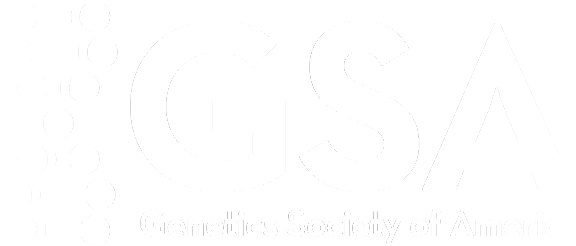
Genetics Society of America
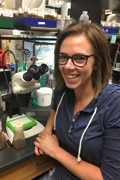
Using the tools of genetics and genomics, nearly 6,000 GSA members from more than 50 countries around the world investigate a wide variety of biological questions and applications.
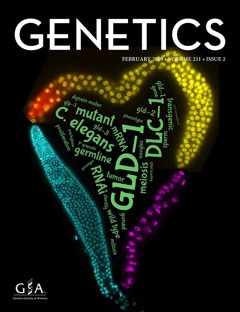
Learn more about why you should publish in the GSA Journals.
- Career Development
- Conferences
- Equity and Inclusion
- Online Events
- Partnerships
- Publications
- Join or Renew Membership
- Make a Donation
- Vote for Board Members
- Advance My Career
- Apply/Nominate for an Award
- Attend a Conference
- Read the Latest eNews
- Check Membership Status
- Find or Post a Job
- Join a Committee
- Submit a Manuscript
- Subscribe to GENETICS
Buy gear from the GSA Store
Genetics Research Project Laboratory: A Discovery-Based Undergraduate Research Course
Eugenia Villa-Cuesta and Lawrence Hobbie
Overview: This resource is an example of a discovery-based undergraduate research course in genetics, using the course-based undergraduate research experience (CURE) model. During the first part of the course students perform designed experiments with the goal of learning laboratory techniques, experimental design, and key features of the model system. During the second part of the course, students perform original research. Two example lab manuals from different offerings of the course are given, one using Drosophila mutants in mitochondrial function and one using Arabidopsis hormone mutants. Representative results of the experiments and students’ perceptions of learning are also included. Genetics Concept(s) Addressed:
Transmission/Patterns of Inheritance: How can one deduce information about genes, alleles, and gene functions from analysis of genetic crosses and patterns of inheritance?
Molecular Biology of Gene Function: How is genetic information expressed so it affects an organism’s structure and function?
Gene Expression and Regulation: How do genes and genomes control changes in an organism’s structure and function throughout its life cycle?
Genetics of Model Organisms: How do the results of molecular genetic studies in model organisms help us understand aspects of human genetics and genetic diseases?
Core Competencies Addressed:
Students should be able to locate, read, and comprehend primary literature research papers on genetics topics.
Students should be able to implement observational strategies to formulate a question. Students should be able to generate testable hypotheses.
Students should be able to design an experiment using appropriate controls and appropriate sample sizes.
Students should be able to gather and evaluate experimental evidence, including qualitative and quantitative data.
Students should be able to apply statistical methods when analyzing their data, and use patterns to construct a model.
Students should be able to generate and interpret graphs displaying experimental results.
Students should be able to communicate experimental results effectively, including writing research papers and giving presentations.
Students should be able to effectively explain genetics concepts to different audiences.
Intermediate undergraduate; biology/genetics majors Activity Type:
Discovery-based Laboratory Course Activity Length:
Full semester
Drosophila, Arabidopsis, discovery-based learning, Course-based undergraduate research experiece (CURE)
Villa-Cuesta, E. and Hobbie, L. (2016) Genetics Research Project Laboratory: A Discovery-Based Undergraduate Research Course. Genetics Society of America Peer-Reviewed Education Portal (GSA PREP) : 2016.003; doi: 10.1534/gsaprep.2016.003
Resource Justification and Instructor Guide
Drosophila Laboratory:
Drosophila Lab Manual
Drosophila Lab Instructor Guidelines
Arabidopsis Laboratory:
Arabidopsis Lab Manual
Arabidopsis Lab Instructor Guidelines
Donate to support our work.
Stay informed with the gsa e-news..
- Discovery research
Our mission is to transform understanding of life, health, and wellbeing through curiosity-driven research across a diverse range of perspectives and contexts.
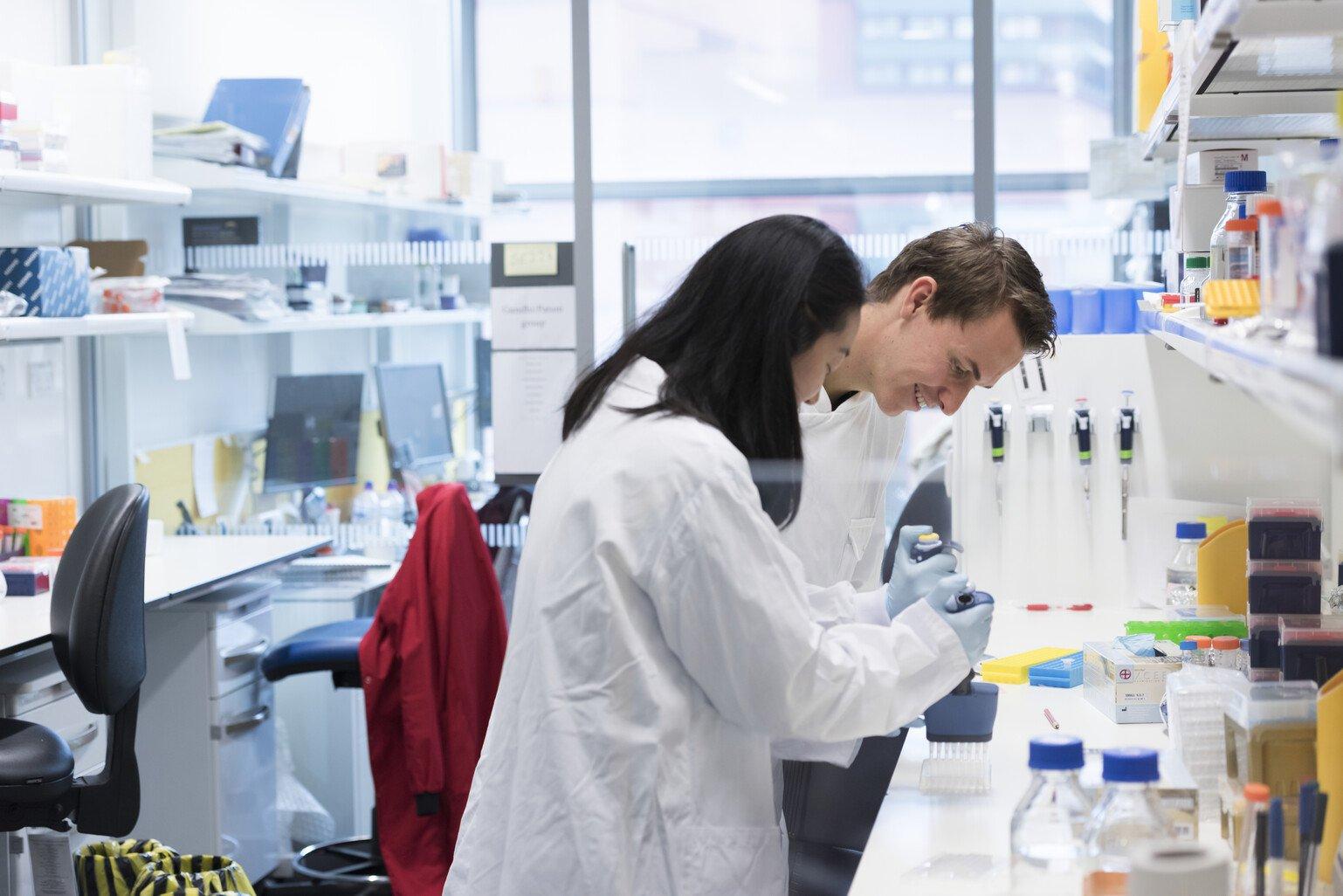
- Share with Facebook
- Share with X
- Share with LinkedIn
- Share with Email
The greatest advances in knowledge come from unexpected places, discovered by researchers who have the time and support they need to pursue new lines of investigation.
But 75% of researchers believe their creativity is being stifled , preventing them from making new breakthroughs.
We want to change this.
By funding ambitious, curiosity-driven research across a range of academic disciplines, we can give researchers from different backgrounds the resources they need to take on big questions and overcome barriers to progress.
What do we mean by ‘discovery research’?
Discovery research is our term for studies, across a breadth of disciplines, that lead to new knowledge and insights into life, health and wellbeing.
We have a long track record of funding curiosity-based ideas, which have led to important and unexpected advances.
For example, we:
- funded a project to discover which genes are active during early development in mice. The work led to the invention of single cell sequencing – now a technology that has made numerous biological questions answerable - and the creating of the Human Cell Atlas
- supported a study on gender and parenting by the Centre of Family Research in Cambridge that was used to successfully campaign for same-sex marriage in the United States.
In the search for these kinds of breakthroughs, we support a broad range of projects, from exploring the fundamental processes that underpin biology, to the development of new methodologies, clinical research, and the social, political, and cultural contexts of disease.
Why is discovery research a core focus for us?
Discovery research is expansive, only limited by the imaginations of researchers, and the resources available to them.
At a time when many funders are moving away from supporting curiosity-based discovery, and towards projects with a nearer-term impact, we have made a bold commitment to support discovery research as one of the best ways to achieve significant shifts in our understanding of health, life, and wellbeing.
Our independence means we can fund research over a long period of time, even when the link to health impact is not immediately clear. By doing this, we can support researchers to produce a bedrock of new knowledge and research capabilities that can be used to address the health challenges of today – and of tomorrow.
"By focusing on discovery research, we want to enable researchers to be ambitious, take on difficult problems, and adopt creative and innovative approaches to push the boundaries of our understanding of life, health and wellbeing."
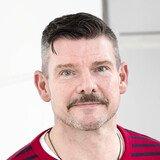
Michael Dunn
Director of Discovery Research
We fund transformative research across all fields and disciplines that generates new knowledge with the potential to improve health, life and wellbeing.
We support researchers with the resources, time and freedom to develop their skills and potential and investigate big questions.
Through our Discovery Research programme, we want to change the research ecosystem , optimising environments to provide the culture, tools, technology, methodologies and platforms needed for success.
We want to help break down silos, enabling collaborative and interdisciplinary research.
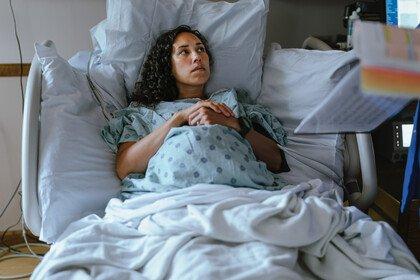
How do autistic people experience maternity care?
Dr Aimee Grant, Senior Lecturer in Public Health at Swansea University, tells us how her experience of autism and maternity care inspired her to research the topic, how it could improve autistic adults’ lives – and make healthcare more accessible.
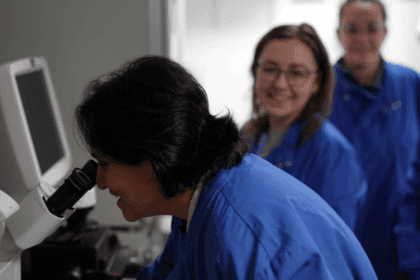
Human Cell Atlas: shaping the future of our health
The Human Cell Atlas aims to map all the cells in the human body. In what ways can these maps shape the future of our health? Learn more from Dr Sarah Teichmann and Prof Muzlifah Haniffa from the Human Cell Atlas Organising Committee.
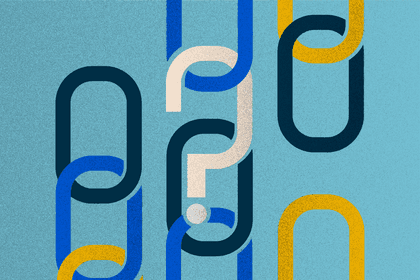
How directed funding is tackling barriers to progress in research
Christiane Hertz-Fowler, our Head of Directed Activity, outlines what we mean by directed funding, the types of initiatives it supports and how it can drive major advances in research.
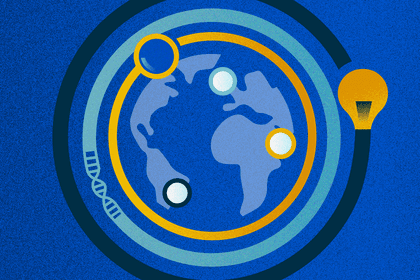
Supporting discovery research across the globe
Learn about discovery research Wellcome is funding across the globe and why it’s key to our strategy
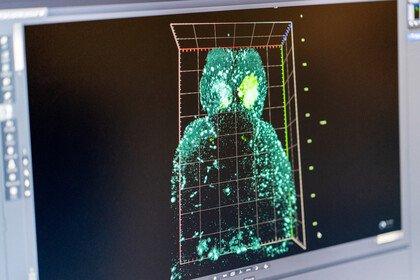
Unblocking barriers to progress in bioimaging
Bioimaging is vital to the future of health research, but there are barriers to access and progress in the field. Wellcome’s Marion Mercier and Luigi Martino explain how our new funding calls aim to change that.
What funding do we provide?
We prioritise research that has the potential to build and shape fields of enquiry, or open new ones, whether through a significant shift in understanding, or through the development of accessible new research tools, technologies, methodologies, and enabling platforms.
Pioneering discoveries are more likely to occur in creative environments between a diverse range of people, so we are working with partners and those we fund to support a thriving, inclusive research culture.
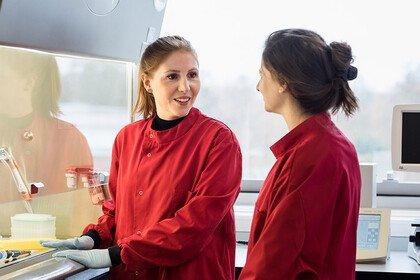
Research culture: let's reimagine how we work together
Current practices prioritise outputs over people’s wellbeing. We want to help build a better research culture – one that is creative, inclusive and honest.
Funding webinars for global researchers
Thank you to everyone who joined us online to learn how Wellcome Discovery Research funding can support research and researchers based in low- and middle-income countries around the world.
You can now watch the recordings of these sessions.
We encourage researchers to apply for our Discovery Research funding awards, which include dedicated schemes for early-career and mid-career researchers.
We also offer directed funding to tackle 'big questions' that require sizeable focus and make field-building investments in areas where open response activities are not sufficient to make progress.
Current specific discovery research funding opportunities
Discovery Research funding is available across three awards for researchers at various stages of their careers:
Wellcome Early-Career Awards
This award supports researchers to establish their independence and trajectory in discovery research.
Wellcome Career Development Awards
For mid-career researchers who are ready to lead a substantial, innovative research programme and achieve international standing.
Wellcome Discovery Awards
This award supports established researchers and teams.
Bioimaging Technology Development Awards
This award will fund teams of technology developers and users to create novel bioimaging tools that either answer ambitious biological questions or remove barriers to bioimaging in low-resource settings.
Does my project fit the criteria for funding?
For a more detailed description of what projects we will and won’t provide funding for, visit our remit page for Discovery Research.
We also invite organisations to apply for contract opportunities that support our mission .
How is Wellcome funded?
Wellcome is an independent charitable foundation. To find out where we get our grant funding from, visit our investments page .
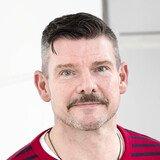
Christiane Hertz-Fowler
Head of Directed Activity
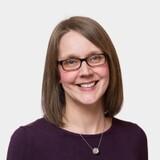
Morag Foreman
Head of Discovery Researchers
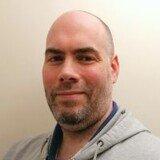
Head of Early Career and Career Development Researchers
Latest news and opinion
Latest report .
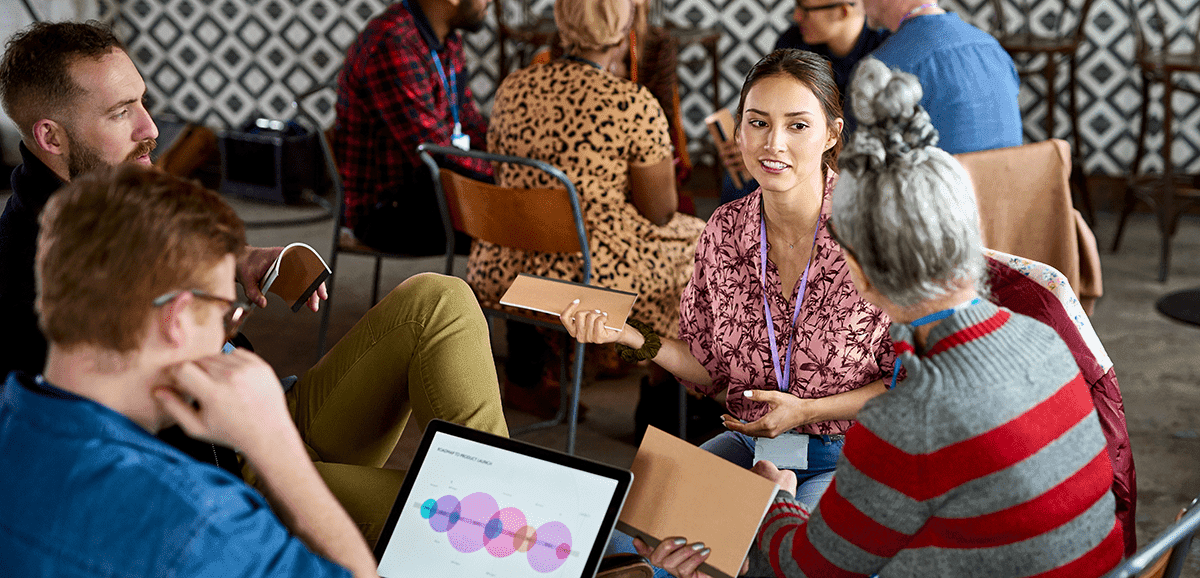
What researchers think about the culture they work in
Perspectives on and experiences of research culture, based on a survey of more than 4,000 researchers in the UK and globally.
- Research culture
Sign up to our research newsletter
Stay up to date with funding news, policy changes and events with our monthly email.
By clicking subscribe, you agree to receive this newsletter. You can unsubscribe any time. For information about how we handle your data, please read our privacy notice .
- Skip to primary navigation
- Skip to main content
- Skip to primary sidebar
- Skip to footer

Understanding Science
How science REALLY works...
- Understanding Science 101
- Scientific investigations may be inspired by many different factors.
- The early stages of an investigation often involve making observations, asking questions, sharing ideas and data, and learning what’s already been discovered about the topic.
- Science relies on the accumulated knowledge of the scientific community to move forward.
Exploration and discovery
The early stages of a scientific investigation often rely on making observations , asking questions, and initial experimentation — essentially poking around. But the routes to and from these stages are diverse. Intriguing observations sometimes arise in surprising ways, as in the discovery of radioactivity, which was inspired by the observation that photographic plates (an early version of camera film) stored next to uranium salts were unexpectedly exposed. Sometimes interesting observations (and the investigations that follow) are suddenly made possible by the development of a new technology . For example, the launch of the Hubble Space Telescope in 1990 allowed astronomers to make deeper and more focused observations of our universe than were ever before possible. These observations ultimately led to breakthroughs in areas as diverse as star and planet formation, the nature of black holes, and the expansion of the universe.
Sometimes, observations are clarified and questions arise through discussions with colleagues and reading the work of other scientists — as demonstrated by the discovery of the role of chlorofluorocarbons (CFCs) in ozone depletion…
EXPLORING AEROSOLS
In 1973, chemists had observed that CFCs were being released into the environment from aerosol cans, air conditioners, and other sources. But it was discussions with his colleague and advisor, Sherwood Rowland, that led Mario Molina to ask what their ultimate fate was. Since CFCs were rapidly accumulating in the atmosphere, the question was intriguing. But before he could tackle the issue (which would ultimately lead to a Nobel Prize and an explanation for the hole in the ozone layer), Molina needed more information. He had to learn more about other scientists’ studies of atmospheric chemistry, and what he learned pointed to the disturbing fate of CFCs.
- Take a sidetrip
Read Mario Molina’s whole story.
Furthermore, though observation and questioning are essential to the process of science, they are not enough to launch a scientific investigation on their own. Generally, scientists also need scientific background knowledge — all the information and understanding they’ve gained from their scientific training in school, supplemented by discussions with colleagues and reviews of the scientific literature . As in Mario Molina’s story, an understanding of what other scientists have already figured out about a particular topic is critical to the process. This background knowledge allows scientists to recognize revealing observations for what they are, to make connections between ideas and observations, and to figure out which questions can be fruitfully tackled with available tools. The importance of content knowledge to the process of science helps explain why science is often mischaracterized as a static set of facts contained in textbooks. Science is a process, but one that relies on accumulated knowledge to move forward.
THE SCIENTIFIC STATE OF MIND
Some scientific discoveries are chalked up to the serendipity of being in the right place at the right time to make a key observation — but rarely does serendipity alone lead to a new discovery. The people who turn lucky breaks into breakthroughs are generally those with the background knowledge and scientific ways of thinking needed to make sense of the lucky observation. For example, in 1896, Henri Becquerel made a surprising observation. He found that photographic plates stored next to uranium salts were spotted, as though they’d been exposed to light rays — even though they had been kept in a dark drawer. Someone else, with a less scientific state of mind and less background knowledge about physics, might have cursed their bad luck and thrown out the ruined plates.
But Becquerel was intrigued by the observation. He recognized it as something scientifically interesting, went on to perform follow-up experiments that traced the source of the exposure to the uranium, and in the process, discovered radioactivity. The key to this story of discovery lies partly in Becquerel’s instigating observation, but also in his way of thinking. Along with the relevant background knowledge, Becquerel had a scientific state of mind. Sure, he made some key observations — but then he dug into them further, inquiring why the plates were exposed and trying to eliminate different potential causes of the exposure to get to the physical explanation behind the happy accident.
Want to develop your own scientific state of mind? Visit Think science to get some handy tips.
- Teaching resources
Serendipity is often cited as a key factor in making scientific discoveries, but is that all there is — someone being lucky enough to get hit on the head by an apple? To learn more, explore The story of serendipity .
- Use our web interactive to help students document and reflect on the process of science.
- Learn strategies for building lessons and activities around the Science Flowchart: Grades 3-5 Grades 6-8 Grades 9-12 Grades 13-16
- Find lesson plans for introducing the Science Flowchart to your students in: Grades 3-5 Grades 6-8 Grades 9-16
- Get graphics and pdfs of the Science Flowchart to use in your classroom. Translations are available in Spanish, French, Japanese, and Swahili.
A blueprint for scientific investigations
Observation beyond our eyes
Subscribe to our newsletter
- The science flowchart
- Science stories
- Grade-level teaching guides
- Teaching resource database
- Journaling tool
- Misconceptions
- About University Overview Catholic, Marianist Education Points of Pride Mission and Identity History Partnerships Location Faculty and Staff Directory Social Media Directory We Soar
- Academics Academics Overview Program Listing Academic Calendar College of Arts and Sciences School of Business Administration School of Education and Health Sciences School of Engineering School of Law Professional and Continuing Education Intensive English Program University Libraries
- Admission Admission Overview Undergraduate Transfer UD Sinclair Academy International Graduate Law Professional and Continuing Education Campus Visit
- Financial Aid Affordability Overview Undergraduate Transfer International Graduate Law Consumer Information
- Diversity Diversity Overview Office of Diversity and Inclusion Equity Compliance Office
- Research Research Overview Momentum: Our Research UD Research Institute Office for Research Technology Transfer
- Life at Dayton Campus Overview Arts and Culture Campus Recreation City of Dayton Clubs and Organizations Housing and Dining Student Resources and Services
- Athletics Athletics Overview Dayton Flyers
- We Soar We Soar Overview Priorities Goals Impact Stories Volunteer Make a Gift
- Schedule a Visit
- Request Info
Explore More
- Academic Calendar
- Event Calendar
- College of Arts and Sciences Newsroom
- Blogs at UD
Discovery-Based Research
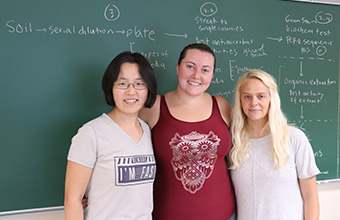
This fall, University of Dayton assistant professor Yvonne Sun will introduce students in her microbiology lab course to the potential rewards and pitfalls of real-world research through her new partnership with the Tiny Earth network.
Founded by University of Wisconsin-Madison professor Jo Handelsman, Tiny Earth is a network of instructors and students focused on crowdsourcing antibiotic discovery from the soil.
Tiny Earth’s goal is to encourage students to pursue careers in science through discovery-based field and laboratory research in introductory courses, with the potential for global impact. It also aims to address the world’s diminishing supply of effective antibiotics by having many student researchers concurrently tackle the same challenge by hunting for new antibiotic organisms in soil samples.
Sun, who joined the University of Dayton faculty in 2014, was one of 25 partner instructors who attended a five-day training program in July at the University of Connecticut. She joins a growing community of educators and researchers from 14 countries and 40 U.S. states.
Unlike standard laboratory courses, which often involve repeating classical experiments to reproduce known results, the discovery-based research model engages students in authentic research experiences in a classroom setting.
“Students should be excited, because I don’t know what the results are going to be,” Sun said. “It’s not like other lab classes where you know exactly what’s going to happen. I have no idea how many bacterial colonies are going to generate antibiotics or what obstacles the students will face.”
Nine juniors and seniors in one section of Sun’s general microbiology lab class will pilot the new course-based undergraduate research experience, or CURE. They will collect soil samples on the University of Dayton campus, grow bacteria colonies and then test them in the lab, in hopes of discovering new compounds.
The CURE approach allows Sun to expose a greater number of students to authentic research, despite limitations on her time, lab space and research funding. Students apply their newly learned skills to a real-world problem while developing their own approaches and ideas.
“I want students to think about: ‘If you want to find bacteria that can produce antibiotics, where would you want to go and why do you want to go there?’ So, the students are part of the research endeavor from the very beginning — they are part of the design,” Sun said.
Sun said the odds of finding bacteria that can produce antibiotics are high. Of the hundreds of students trained to date by the Tiny Earth network, only one had bacteria that failed to generate a colony that produced antibiotics.
“This is very common behavior for bacteria in soil, because the bacteria live in the soil together, so they are constantly fighting each other for nutrients,” Sun explained. “It is natural for them to produce antibiotics to kill off competition. Now, whether the antibiotics are novel and also nontoxic, that’s another issue.”
In addition to learning technical skills, students also discover that it’s OK when things don’t work, said Erica Rinehart, a University of Dayton biology doctoral student from North Lima, Ohio.
“You just have to troubleshoot and critically think about why it didn’t work and what you can do to make it better,” said Rinehart, who will serve as graduate teaching assistant and run the lab for the pilot class.
Sun hopes to incorporate discovery-based research into all of her general microbiology lab courses, or possibly develop a separate lab dedicated to this type of experiential learning.
Tiny Earth’s network of students and instructors share research findings and best practices. Headquartered at UW-Madison’s Wisconsin Institute for Discovery, the program is taking steps to build a database of compounds discovered by students and build a chemistry hub to centrally analyze samples. Tiny Earth also has partnerships with the Joint Genome Institute and the Illumina Corporation.
“Building this network is not just good for teaching, but also my own research program,” Sun said.
- - Dave Larsen, communication coordinator, College of Arts and Sciences
- Experiential Learning
- Faculty and Staff
In Memoriam: Don Vermillion
College faculty in the news: august 10, 2018.
Advertisement
Investigative Research Projects for Students in Science: The State of the Field and a Research Agenda
- Open access
- Published: 16 March 2023
- Volume 23 , pages 80–95, ( 2023 )
Cite this article
You have full access to this open access article
- Michael J. Reiss ORCID: orcid.org/0000-0003-1207-4229 1 ,
- Richard Sheldrake ORCID: orcid.org/0000-0002-2909-6478 1 &
- Wilton Lodge ORCID: orcid.org/0000-0002-9219-8880 1
3180 Accesses
2 Citations
6 Altmetric
Explore all metrics
One of the ways in which students can be taught science is by doing science, the intention being to help students understand the nature, processes, and methods of science. Investigative research projects may be used in an attempt to reflect some aspects of science more authentically than other teaching and learning approaches, such as confirmatory practical activities and teacher demonstrations. In this article, we are interested in the affordances of investigative research projects where students, either individually or collaboratively, undertake original research. We provide a critical rather than a systematic review of the field. We begin by examining the literature on the aims of science education, and how science is taught in schools, before specifically turning to investigative research projects. We examine how such projects are typically undertaken before reviewing their aims and, in more detail, the consequences for students of undertaking such projects. We conclude that we need social science research studies that make explicit the possible benefits of investigative research projects in science. Such studies should have adequate control groups that look at the long-term consequences of such projects not only by collecting delayed data from participants, but by following them longitudinally to see whether such projects make any difference to participants’ subsequent education and career destinations. We also conclude that there is too often a tendency for investigative research projects for students in science to ignore the reasons why scientists work in particular areas and to assume that once a written report of the research has been authored, the work is done. We therefore, while being positive about the potential for investigative research projects, make specific recommendations as to how greater authenticity might result from students undertaking such projects.
L’une des façons d’enseigner les sciences aux étudiants est de leur faire faire des activités scientifiques, l’objectif étant de les aider à comprendre la nature, les processus et les méthodes de la science. On peut avoir recours à des projets de recherche et d’enquête afin de refléter plus fidèlement certains éléments relevant de la science qu’en utilisant d’autres approches d’enseignement et d’apprentissage, telles que les activités pratiques de confirmation et les démonstrations faites par l’enseignant. Dans cet article, nous nous intéressons aux possibilités offertes par les projets de recherche dans lesquels les étudiants, individuellement ou en collaboration, entreprennent des recherches novatrices. Nous proposons un examen critique du domaine plutôt que d’y porter un regard systématique. Nous commençons par examiner la documentation portant sur les objectifs de l’enseignement des sciences et la manière dont les sciences sont enseignées dans les écoles, avant de nous intéresser plus particulièrement aux projets de recherche et d’enquête. Nous analysons la manière dont ces projets sont généralement menés avant d’examiner leurs buts et d’évaluer de façon plus approfondie quelles sont les conséquences pour les élèves de réaliser de tels projets. Nous constatons que nous avons besoin d’études de recherche en sciences sociales qui rendent explicites les avantages potentiels des projets de recherche et d’enquête scientifiques. Ces études devraient comporter des groupes de contrôle adéquats qui examinent les conséquences à long terme de ces projets, non seulement en recueillant des données différées auprès des participants, mais aussi en suivant ceux-ci de manière longitudinale de façon à voir si ces projets font une quelconque différence dans l’éducation subséquente et les destinations professionnelles ultérieures des participants. Nous concluons également que les projets de recherche et d’enquête des étudiants en sciences ont trop souvent tendance à ignorer les raisons pour lesquelles les scientifiques travaillent dans des domaines particuliers et à supposer qu’une fois que le rapport de recherche a été rédigé, le travail est terminé. Par conséquent, tout en demeurant optimistes quant au potentiel que représentent les projets de recherche et d’enquête, nous formulons des recommandations particulières en ce qui a trait à la manière dont une plus grande authenticité pourrait résulter de la réalisation de tels projets par les étudiants.
Similar content being viewed by others
Investigative School Research Projects in Biology: Effects on Students
Moving Research into the Classroom: Synergy in Collaboration
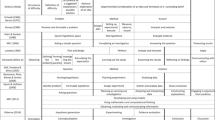
Reintroducing “the” Scientific Method to Introduce Scientific Inquiry in Schools?
Markus Emden
Avoid common mistakes on your manuscript.
Introduction
Many young people are interested in science but do not necessarily see themselves as able to become scientists (Archer & DeWitt, 2017 ; Archer et al., 2015 ). Others may not want to become scientists even though they may see themselves as succeeding in science (Gokpinar & Reiss, 2016 ). At the same time, in many countries, governments and industry want more young people to continue with science, primarily in the hope that they will go into science or science-related careers (including engineering and technology), but also because of the benefits to society that are presumed to flow from having a scientifically literate population. Making science more inclusive and accessible to everyone may need endeavours and support from across education, employers, and society (Royal Society, 2014 ; Institute of Physics, 2020 ).
However, getting more people to continue with science, once it is no longer compulsory, is only one purpose of school science (Mansfield & Reiss, 2020 ). Much of school science is focused on getting students to understand core content of science—things like the particulate theory of matter, and the causes of disease in humans and other organisms. Another strand in school science is on getting students to understand something of the practices of science, particularly through undertaking practical work. A further, recently emerging, position is that science education should help students to use their knowledge and critical understanding of the content and practices of science to strive for social and environmental justice (Sjöström & Eilks, 2018 ).
In this article, we are interested in the affordances of investigative research projects—discussed in more detail below but essentially pieces of work undertaken by students either individually or collaboratively in which they undertake original research. We provide a critical rather than a systematic review of the field and suggest how future research might be undertaken to explore in more detail the possible contribution of such projects. We begin by examining the literature on the aims of science education, and how science is taught in schools, before specifically turning to investigative research projects. We examine how such projects are typically undertaken before reviewing their aims and, in more detail, the consequences for students of undertaking such projects. We make recommendations as to how investigative research projects might more fruitfully be undertaken and conclude by proposing a research agenda.
Aims of Science Education
School science education typically aims to prepare some students to become scientists, while concurrently educating all students in science and about science (Claussen & Osborne, 2013 ; Hofstein & Lunetta, 2004 ; Osborne & Dillon, 2008 ). For example, in England, especially for older students, the current science National Curriculum for 5–16-year-olds is framed as providing a platform for future studies and careers in science for some students, and providing knowledge and skills so that all students can understand and engage with the natural world within their everyday lives (Department for Education, 2014 ). Accordingly, science education within the National Curriculum in England broadly aims to develop students’ scientific knowledge and conceptual understanding; develop students’ understanding of the nature, processes, and methods of science (aspects of ‘working scientifically’, including experimental, analytical, and other related skills); and ensure that students understand the relevance, uses, and implications of science within everyday life (Department for Education, 2014 ). Comparable aims are typically found in other countries (Coll & Taylor, 2012 ; Hollins & Reiss, 2016 ).
Science education often involves practical work, which is generally intended to help students gain conceptual understanding, practical and wider skills, and understanding of how science and scientists work (Abrahams & Reiss, 2017 ; Cukurova et al., 2015 ; Hodson, 1993 ; Millar, 1998 ). Essentially, the thinking behind much practical work is that students would learn about science by doing science. Practical work has often been orientated towards confirming and illustrating scientific knowledge, although it is increasingly orientated around reflecting the processes of investigation and inquiry used within the field of science, and providing understanding of the nature of science (Abrahams & Reiss, 2017 ; Hofstein & Lunetta, 2004 ).
In many countries, especially those with the resources to have school laboratories, practical work in science is undertaken at secondary level relatively frequently, although this is less the case with older students (Hamlyn et al., 2020 , 2017 ). Practical work is more frequent in schools within more advantaged regions (Hamlyn et al., 2020 ) and many students report that they would have preferred to do more practical work (Cerini et al., 2003 ; Hamlyn et al., 2020 ).
The impact of practical work remains less clear (Cukurova et al., 2015 ; Gatsby Charitable Foundation, 2017 ). Society broadly expects that students in any one country will experience practical work to similar extents, so it is unfeasible, for more than a handful of lessons (e.g. Shana & Abulibdeh, 2020 ), to apply experimental designs where some students undertake practical work while others do not. One study, where students were assigned to one of four different groups, concluded that while conventional practical work led to more student learning than did either watching videos or reading textbooks, it was no more effective than when students watched a teacher demonstration (Moore et al., 2020 ).
The study by Moore et al. ( 2020 ) illustrates an important point, namely, that students can acquire conceptual knowledge and theoretical understanding by ways other than engagement in practical work. Indeed, there are some countries where less practical work is undertaken than in others, yet students score well, on average, on international measures of attainment. Some, but relatively few, studies have focused on whether the extent of practical work, and/or whether practical work undertaken in particular ways, associates with any educational or other outcomes. There are some indications that more frequent practical work associates with benefits (Cukurova et al., 2015 ). For example, students in higher-performing secondary schools have reported that they undertake more frequent practical work than pupils in lower-performing schools, although this does not reflect the impact of practical work alone (Hamlyn et al., 2017 ). In a more recent study, Oliver et al. ( 2021a , b ), in their analysis of the science scores in the six Anglophone countries (Australia, Canada, Ireland, New Zealand, the UK, and the USA) that participated in PISA (Program for International Student Assessment) 2015, found that “Of particular note is that the highest level of student achievement is associated with doing practical work in some lessons (rather than all or most) and this patterning is consistent across all six countries” (p. 35).
Students often appreciate and enjoy practical work in science (Hamlyn et al., 2020 ; National Foundation for Educational Research, 2011 ). Nevertheless, students do not necessarily understand the purposes of practical work, some feel that practical work may not necessarily be the best way to understand some aspects of science, and some highlight that practical work does not necessarily give them what they need for examinations (Abrahams & Reiss, 2012 ; Sharpe & Abrahams, 2020 ). Teachers have also spoken about the challenges of devising and delivering practical work, and often value practical work for being motivational for students rather than for helping them to understand science concepts (Gatsby Charitable Foundation, 2017 ; National Foundation for Educational Research, 2011 ).
Teaching Approaches
Educational research has examined how teaching and learning could best be undertaken. Many teaching and learning approaches have been found to associate with students’ learning outcomes, such as their achievement (Bennett et al., 2007 ; Furtak et al., 2012 ; Hattie et al., 2020 ; Savelsbergh et al., 2016 ; Schroeder et al., 2007 ) and interest (e.g. Chachashvili-Bolotin et al., 2016 ; Swarat et al., 2012 ), both in science and more generally. However, considering different teaching and learning approaches is complicated by terminology (where the definitions of terms can vary and/or terms can be applied in various ways) and wider aspects of generalisation (where it can be difficult to determine trends across studies undertaken in diverse ways across diverse contexts).
Inquiry-based approaches to teaching and learning generally involve students having more initiative to direct and undertake activities to develop their understanding (although not necessarily without guidance and support from teachers), such as working scientifically to devise and undertake investigations. However, it is important to emphasise that inquiry-based approaches do not necessitate practical work. Indeed, there are many subjects where no practical work takes place and yet students can undertake inquiries. In science, examples of non-practical-based inquiries that could fruitfully be undertaken collaboratively or individually and using the internet and/or libraries include the sort of research that students might undertake to investigate a socio-scientific issue. An example of such research includes what the effects of reintroducing an extinct or endangered species might be on an ecosystem, such as the reintroduction of the Eurasian beaver ( Castor fiber ) into the UK, or the barn owl ( Tyto alba ) into Canada. Inquiry-based learning in school science has often been found to associate with greater achievement (Furtak et al., 2012 ; Savelsbergh et al., 2016 ; Schroeder et al., 2007 ), though too much time spent on inquiry can result in reduced achievement (Oliver et al., 2021a ).
Allied to inquiry-based approaches is project-based learning. Here, students take initiative, manifest autonomy, and exercise responsibility for addressing an issue (often attempting to solve a problem) that usually results in an end product (such as a report or model), with teachers as facilitators and guides. The project occurs over a relatively long duration of time (Helle et al., 2006 ), to allow time for planning, revising, undertaking, and writing up the study. Project-based learning tends to associate positively with achievement (Chen & Yang, 2019 ).
Context-based approaches to teaching and learning use specific contexts and applications as starting points for the development of scientific ideas, rather than more traditional approaches that typically cover scientific ideas before moving on to consider their applications and contexts (Bennett et al., 2007 ). Context-based approaches have been found to be broadly equivalent to other teaching and learning approaches in developing students’ understanding, with some evidence for helping foster positive attitudes to science to a greater extent than traditional approaches (Bennett et al., 2007 ). Specifically relating learning to students’ experiences or context (referred to as ‘enhanced context strategies’) often associates positively with achievement (Schroeder et al., 2007 ). The literature on context-based approaches overlaps with that on the use of socio-scientific issues in science education, where students develop their scientific knowledge and understanding by considering complicated issues where science plays a role but on its own is not sufficient to produce solutions (e.g. Dawson, 2015 ; Zeidler & Sadler, 2008 ). To date, the literature on context-based approaches and/or socio-scientific issues has remained distinct from that on investigative research projects but, as we will argue below, there might be benefit in considering their intersection.
Various other teaching and learning approaches have been found to be beneficial in science, including collaborative work, computer-based work, and the provision of extra-curricular activities (Savelsbergh et al., 2016 ). Similarly, but specifically focusing on chemistry, various teaching and learning practices have been found to associate positively with academic outcomes, including (most strongly) collaborative learning and problem-based learning (Rahman & Lewis, 2019 ).
Most attention has focused on achievement-related outcomes. Nevertheless, inquiry-based learning, context-based learning, computer-based learning, collaborative learning, and extra-curricular activities have often also been found to associate positively with students’ interests and aspirations towards science (Savelsbergh et al., 2016 ). While many teaching and learning approaches associate with benefits, it remains difficult definitively to establish whether any particular approach is optimal and/or whether particular approaches are better than others. Teaching and learning time are limited, so applying a particular approach may mean not applying another approach.
Investigative Research Projects
Science education has often (implicitly or explicitly) been orientated around students learning science by doing science, intending to help students understand the nature, processes, and methods of science. An early critique of pedagogical approaches that saw students as scientists was provided by Driver ( 1983 ) who, while not dismissing the value of the approach, cautioned against over-enthusiastic adoption on the grounds that, unsurprisingly, school students, compared to actual scientists, manifest a range of misconceptions about how scientific research is undertaken. Contemporary recommendations for practical work include schools delivering frequent and varied practical activities (in at least half of all science lessons), and students also having the opportunity to undertake open-ended and extended investigative projects (Gatsby Charitable Foundation, 2017 ).
Investigative research projects may be intended to reflect some aspects of science more accurately or authentically than other teaching and learning approaches, such as confirmatory practical activities and teacher demonstrations. Nevertheless, authenticity in science and science education can be approached and/or defined in various ways (Braund & Reiss, 2006 ), and the issue raises wider questions such as whether only (adult) scientists can authentically experience science, and who determines what science is and what authentic experiences of science are (Kapon et al., 2018 ; Martin et al., 1990 ).
Although too tight a definition can be unhelpful, investigative research projects in science typically involve students determining a research question (where the outcome is unknown) and approaches to answer it, undertaking the investigation, analysing the data, and reporting the findings. The project may be undertaken alone or in groups, with support from teachers and/or others such as scientists and researchers (Bennett et al., 2018 ; Gatsby Charitable Foundation, 2017 ). Students may have varying degrees of autonomy—but then that is true of scientists too.
Independent research projects in science for students have often been framed around providing students with authentic experiences of scientific research and with the potential for wider benefits around scientific knowledge and skills, attitudes, and motivations around science, and ultimately helping science to become more inclusive and accessible to everyone (Bennett et al., 2018 ; Milner-Bolotin, 2012 ). Considered in review across numerous studies, independent research projects for secondary school students (aged 11–19) have often (but not necessarily always) resulted in benefits, including the following:
Acquisition of science-related knowledge (Burgin et al., 2012 ; Charney et al., 2007 ; Dijkstra & Goedhart, 2011 ; Houseal et al., 2014 ; Sousa-Silva et al., 2018 ; Ward et al., 2016 );
Enhancement of knowledge and/or skills around aspects of research and working scientifically (Bulte et al., 2006 ; Charney et al., 2007 ; Ebenezer et al., 2011 ; Etkina et al., 2003 ; Hsu & Espinoza, 2018 ; Ward et al., 2016 );
Greater confidence in undertaking various aspects of science, including applying knowledge and skills (Abraham, 2002 ; Carsten Conner et al., 2021 ; Hsu & Espinoza, 2018 ; Stake & Mares, 2001 , 2005 );
Aspirations towards science-related studies and/or careers (Abraham, 2002 ; Stake & Mares, 2001 ), although students in other studies have reported unchanged and already high aspirations towards science-related studies and/or careers (Burgin et al., 2015 , 2012 );
Subsequently entering science-related careers (Roberts & Wassersug, 2009 );
Development of science and/or research identities and/or identification as a scientist or researcher (Carsten Conner et al., 2021 ; Deemer et al., 2021 );
Feelings and experiences of real science and doing science (Barab & Hay, 2001 ; Burgin et al., 2015 ; Chapman & Feldman, 2017 );
Wider awareness and/or understanding of science, scientists, and/or positive attitudes towards science (Abraham, 2002 ; Houseal et al., 2014 ; Stake & Mares, 2005 );
Benefits akin to induction into scientific or research communities of practice (Carsten Conner et al., 2018 );
Development of wider personal, studying, and/or social skills, including working with others and independent work (Abraham, 2002 ; Moote, 2019 ; Moote et al., 2013 ; Sousa-Silva et al., 2018 ).
Positive experiences of projects and programmes are often conveyed by students (Dijkstra & Goedhart, 2011 ; Rushton et al., 2019 ; Williams et al., 2018 ). For example, students have reported appreciating the greater freedom and independence to discover things, and that they felt they were undertaking real experiments with a purpose, and a greater sense of meaning (Bulte et al., 2006 ).
Nevertheless, it remains difficult to determine the extent of generalisation from diverse research studies undertaken in various ways and across various contexts: benefits have been observed across studies involving different foci (determining what was measured and/or reported), projects for students, and contexts and countries. Essentially, each individual research study did not cover and/or evidence the whole range of benefits. Many benefits have been self-reported, and only some studies have considered changes over time (Moote, 2019 ; Moote et al., 2013 ).
Investigative science research projects for students are delivered in various ways. For example, some projects are undertaken through formal programmes that provide introductions and induction, learning modules, equipment, and the opportunity to present findings (Ward et al., 2016 ). Some programmes put a particular emphasis on the presentation and dissemination of findings (Bell et al., 2003 ; Ebenezer et al., 2011 ; Stake & Mares, 2005 ). Some projects are undertaken through schools (Ebenezer et al., 2011 ; Ward et al., 2016 ); others entail students working at universities, sometimes undertaking and/or assisting with existing projects (Bell et al., 2003 ; Burgin et al., 2015 , 2012 ; Charney et al., 2007 ; Stake & Mares, 2001 , 2005 ) or in competitions (e.g. Liao et al., 2017 ). While many projects are undertaken in laboratory settings, some are undertaken outdoors, in the field (Carsten Conner et al., 2018 ; Houseal et al., 2014 ; Young et al., 2020 ).
Primary School
While much of the school literature on investigative research projects in science concentrates on secondary or university students, some such projects are undertaken with students in primary school. These projects are often perceived as enjoyable and considered to benefit scientific skills and knowledge and/or confidence in doing science (Forbes & Skamp, 2019 ; Liljeström et al., 2013 ; Maiorca et al., 2021 ; Tyler-Wood et al., 2012 ). Such projects often help students feel that they are scientists and doing science (Forbes & Skamp, 2019 ; Reveles et al., 2004 ).
For example, one programme for primary school students in Australia intended students to develop and apply skills in thinking and working scientifically with support by scientist mentors over 10 weeks. It involved the students identifying areas of interest and testable questions within a wider scientific theme, collaboratively investigating their area of interest through collecting and analysing data, and then presenting their findings. Data on the programme’s outcomes were obtained through interviews with students and by studying the reports that they wrote (Forbes & Skamp, 2016 , 2019 ). Participating students said that they appreciated the autonomy and practical aspects, and enjoyed the experiences. The students showed developments in thinking scientifically and around the nature of science, where science often became seen as something that could be interesting, enjoyable, student-led, collaborative, creative, challenging, and a way to understand how things work within the world (Forbes & Skamp, 2019 ). The experiences of thinking and working scientifically, and aspects such as collaborative working and learning from each other, were broadly considered to help develop students’ scientific identities and include them within a scientific community of practice. Some students felt that they were doing authentic (‘real’) science, in contrast to some of their earlier or other experiences of science at school, which had not involved an emphasis on working scientifically and/or specific activities within working scientifically, such as collecting and analysing data (Forbes & Skamp, 2019 ).
CREST Awards
CREST Awards are intended to give young people (aged 5–19) in the UK the opportunity to explore real STEM (science, technology, engineering, and mathematics) projects, providing the experience of ‘being a scientist’ (British Science Association, 2018 ). The scheme has been running since the 1980s and some 30,000 Awards are given each year. They exist at three levels (Bronze, Silver, and Gold), reflecting the necessary time commitment and level of independence and originality expected. The Awards are presented as offering the potential for participants to experience the process of engaging in a project, and developing investigation, problem-solving, and communication skills. They are also presented as something that can contribute to further awards (such as Duke of Edinburgh Awards) and/or competition entries (such as The Big Bang Competition). CREST Gold Awards can be used to enhance applications to university and employment. At Gold level, arranging for a STEM professional in a field related to the student’s work to act as a mentor is recommended, though not formally required. CREST Awards are assessed by teachers and/or assessors from industry or academia, depending on the Award level.
Classes of secondary school students in Scotland undertaking CREST Awards projects appeared to show some benefits around motivational and studying strategies, but less clearly than would be ideal (Moote, 2019 ; Moote et al, 2013 ). Students undertaking CREST Silver Awards between 2010 and 2013 gained better qualifications at age 16 and were more likely to study science subjects for 16–19-year-olds than other comparable students (matched on prior attainment and certain personal characteristics), although the students may have differed on unmeasured aspects, such as attitudes and motivations towards science and studying (Stock Jones et al., 2016 ). A subsequent randomised controlled trial found that year 9 students (aged 13–14) undertaking CREST Silver Awards and other comparable students ultimately showed similar science test scores, attitudes towards school work, confidence in undertaking various aspects of life (not covering school work), attitudes towards science careers (inaccurately referred to as self-efficacy), and aspirations towards science careers (Husain et al., 2019 ). Nevertheless, teachers and students perceived benefits, including students acquiring transferable skills such as time management, problem-solving, and team working, and that science topics were made more interesting and relevant for students (Husain et al., 2019 ). Overall, it remains difficult to form any definitive conclusions about impacts, given the diverse scope of CREST Awards but limited research. For example, whether and/or how CREST Awards projects are independent of or integrated with curricula areas may determine the extent of (curricula-based) knowledge gains.
Nuffield Research Placements
Nuffield Research Placements involve students in the UK undertaking STEM research placements during the summer between years 12 and 13, and presenting their findings at a celebration event (Nuffield Foundation, 2020 ). The scheme has been running since 1996 and a little over 1000 students participate each year. The programme is variously framed as an opportunity for students to undertake real research and develop scientific and other skills, and an initiative to enhance access/inclusion and assist the progression of students into STEM studies at university (Cilauro & Paull, 2019 ; Nuffield Foundation, 2020 ).
The application process is competitive, and requires a personal statement where students explain their interest in completing the placement. Students need to be studying at least one STEM subject in year 12, be in full-time education at a state school (i.e. not a private school that requires fees), and have reached a certain academic level at year 11. The scheme historically aimed to support and prioritise students from disadvantaged backgrounds, and is now only available for students from disadvantaged backgrounds based on family income, living or having lived in care, and/or being the first person in their immediate family who will study in higher education (Nuffield Foundation, 2020 ).
There have been indications that students who undertake Nuffield Research Placements are, on average, more likely to enrol on STEM subjects at top (Russell Group) UK universities and complete a higher number of STEM qualifications for 16–19-year-olds than other students (Cilauro & Paull, 2019 ). Nevertheless, it remains difficult to isolate independent impacts of the placements, given that (for example) students commence their 16–19 education prior to the placements.
Following their Nuffield Research Placements, students have reported increased understanding of what STEM researchers do in their daily work and unchanging (already high) enjoyment of STEM and interest in STEM job opportunities (Bowes et al., 2017 ; Cilauro & Paull, 2019 ). Wider benefits have been attributed to the placement, including skills in writing reports, working independently, confidence in their own abilities in general, and team working (Bowes et al., 2017 ). Students also often report that they feel they have contributed to an authentic research study in an area of STEM in which they are interested (Bowes et al., 2021 ).
Institute for Research in Schools Projects
The Institute for Research in Schools (IRIS) started in 2016 and has about 1000 or more participating students in the UK annually. It facilitates students to undertake a range of investigative research projects from a varied portfolio of options. For example, these projects have included CERN@School (Whyntie, 2016 ; Whyntie et al., 2015 , 2016 ), where students have been found to have positive experiences, developing research and data analysis skills, and developing wider skills such as collaboration and communication (Hatfield et al., 2019 ; Parker et al., 2019 ). Teachers who have facilitated projects for their students (Rushton & Reiss, 2019 ) report that the experiences produced personal and wider benefits around:
Appreciating the freedom to teach and engage in the research projects;
Connecting or reconnecting with science and research, including interest and enthusiasm (in science as well as teaching it) and with a role as a scientist, including being able to share past experiences or work as a scientist with students;
Collaborating with students and scientists, researchers, and others in different and/or new ways via doing research (including facilitating students and providing support);
Professional and skills development (refreshing/revitalising teaching and interest), including recognition by colleagues/others (strengthening recognition as a teacher/scientist, as having skills, as someone who provides opportunities/support for students).
The teachers felt that their students developed a range of specific and transferable benefits, including around research, communication, teamwork, planning, leadership, interest and enthusiasm, confidence, and awareness of the realities of science and science careers. Some benefits could follow and/or be enhanced by the topics that the students were studying, such as interest and enthusiasm linking with personal and wider/real-life relevance, for example, for topics like biodiversity (Rushton & Reiss, 2019 ).
Students in England who completed IRIS projects and presented their findings at conferences reported that the experiences were beneficial through developing skills (including communication, confidence, and managing anxiety); gaining awareness, knowledge, and understanding of the processes of research and careers in research; collaboration and sharing with students and teachers; developing networks and contacts; and doing something that may benefit their university applications (Rushton et al., 2019 ). Presenting and disseminating findings at conferences were considered to be inspirational and validating (including experiencing the impressive scientific and historical context of the conference venue), although also challenging, given limited time, competing demands, anxiety and nervousness, and uncertainty about how to engage with others and undertake networking (Rushton et al., 2019 ).
Although our principal interest is in investigative research projects in science at school, it is worth briefly surveying the literature on such projects at university level. This is because while such projects are rare at school level, normally resulting from special initiatives, there is a long tradition in a number of countries of investigative research projects in science being undertaken at university level, alongside other types of practical work.
Unsurprisingly, university science students typically report having little to no prior experience with authentic research, although they may have had laboratory or fieldwork experience on their pre-university courses (Cartrette & Melroe-Lehrman, 2012 ; John & Creighton, 2011 ). University students still perceive non-investigative-based laboratory work as meaningful experiences of scientific laboratory work, even if these might be less authentic experiences of (some aspects of) scientific research (Goodwin et al., 2021 ; Rowland et al., 2016 ).
Research experiences for university science students are often framed around providing students with authentic experiences of scientific research, with more explicit foci towards developing research skills and practices, developing conceptual understanding, conveying the nature of science, and fostering science identities (Linn et al., 2015 ). Considered in review across numerous studies, research experiences for university science students have often (but not necessarily always) resulted in benefits, including to research skills and practices and confidence in applying them, enhanced understanding of the reality of scientific research and careers, and higher likelihood of persisting or progressing within science education and/or careers (Linn et al., 2015 ).
For example, in one study, university students of science in England reported having no experience of ‘real’ research before undertaking a summer research placement programme (John & Creighton, 2011 ). After the programme, the majority of students agreed that they had discovered that they liked research and that they had gained an understanding of the everyday realities of research. Most of the students reported that their placement confirmed or increased their intentions towards postgraduate study and research careers (John & Creighton, 2011 ).
Implications and Future Directions
Investigative research projects in science have the potential for various benefits, given the findings from wider research into inquiry-based learning (Furtak et al., 2012 ; Savelsbergh et al., 2016 ; Schroeder et al., 2007 ), context-based learning (Bennett et al., 2007 ; Schroeder et al., 2007 ), and project-based learning (Chen & Yang, 2019 ). However, the potential for benefits involves broad generalisations, where inquiry-based learning (for example) covers a diverse range of approaches that may or may not be similar to those encountered within investigative research projects. Furthermore, we do not see investigative research projects as a universal panacea. It is, for example, unrealistic to expect that students can simultaneously learn scientific knowledge, learn about scientific practice, and engage skillfully and appropriately in aspects of scientific practice. Indeed, careful scaffolding from teachers is likely to be required for any, let alone all, of these benefits to result.
We are conscious that enabling students to undertake investigative research projects in science places particular burdens on teachers. Anecdotal evidence suggests that if teachers themselves have had a university education in which they undertook one or more such projects themselves (e.g. because they undertook a research masters or doctorate in science), they are more likely both to be enthused about the benefits of this way of working and to be able to help their students undertake research. It would be good to have this hypothesis investigated rigorously and, more importantly, to have data on effective professional development for teachers to help their students undertake investigative research projects in science. It is known that school teachers of science can benefit from undertaking small-scale research projects as professional development (e.g. Bevins et al., 2011 ; Koomen et al., 2014 ), but such studies do not seem rigorously to have followed individual teachers through into their subsequent day-to-day work with their students to determine the long-term consequences for the students.
Benefits accruing from investigative research projects are likely to be enhanced if there is an alignment between the form of the assessment and the intended outcomes of the investigative research project (cf. Molefe, 2011 ). The first author recalls how advanced level biology projects (for 16–18-year-olds) were assessed in England by one of the Examination Boards back in the 1980s. At the end of the course, each student who had submitted such a project had a 15-min viva with an external examiner. The mark scheme rewarded not only the sorts of things that any advanced level biology mark scheme would credit (use of literature, appropriate research design, care in data collection, thorough analysis, etc.) but originality too. There was therefore an emphasis on novel research. Indeed, occasionally students published sole- or co-authored accounts of their work in biology or biology education journals.
We mentioned above Driver’s ( 1983 ) caution about the extent to which it is realistic to envisage high school students undertaking investigative research projects that have more than superficial resemblance to those undertaken by actual scientists. Nevertheless, as the above review indicates, there is a strong strand within school science education of advocating the benefits of students designing and undertaking open-ended research projects (cf. Albone et al., 1995 ). Roth ( 1995 ) argued that for school science to be authentic, students need to:
(1) learn in contexts constituted in part by ill-defined problems; (2) experience uncertainties and ambiguities and the social nature of scientific work and knowledge; (3) learning is predicated on, and driven by, their current knowledge state; (4) experience themselves as parts of communities of inquiry in which knowledge, practices, resources and discourse are shared; (5) in these communities, members can draw on the expertise of more knowledgeable others whether they are peers, advisors or teachers. (p. 1)
Investigative research projects in science allow learners to learn about science by doing science, and therefore might help foster science identities. Science identities can involve someone recognising themselves and also being recognised by others as being a science person, and also with having various experiences, knowledge, and skills that are valued and recognised within the wider fields of science.
However, the evidence base, as indicated above and in the systematic review of practical independent research projects in high school science undertaken by Bennett et al. ( 2018 ), is still not robust. We need research studies that make explicit the putative benefits of investigative research projects in science, that have adequate control groups, and that look at the long-term consequences of such projects not only by collecting delayed data from participants (whether by surveys or interviews) but by following them longitudinally to see whether such projects make any difference to their subsequent education and career destinations. We also know very little about the significance of students’ home circumstances for their enthusiasm and capacity to undertake investigative research projects in science, though it seems likely that students with high science capital (DeWitt et al., 2016 ) are more likely to receive familial support in undertaking such projects (cf. Lissitsa & Chachashvili‐Bolotin, 2019 ).
We also need studies that consider more carefully what it is to engage in scientific practices. It is notable that the existing literature on investigative research projects for students in science makes no use of the literature on ethnographic studies of scientists at work—neither the foundational texts (e.g. Latour & Woolgar, 1979 ; Knorr-Cetina, 1983 ) nor more recent studies (e.g. Silvast et al., 2020 ). Too often there is a tendency for investigative research projects for students in science to ignore the reasons why scientists work in particular areas and to assume that once a written report of the research has been authored, the work is done. There can also be a somewhat simplistic belief that the sine qua non of an investigative research project is experimental science. Keen as we are on experimental science, there is more to being a scientist than undertaking experiments. For example, computer simulations (Winsberg, 2019 ) and other approaches that take advantage of advances in digital technologies are of increasing importance to the work of many scientists. It would be good to see such approaches reflected in more school student investigative projects (cf. Staacks et al., 2018 ).
More generally, greater authenticity would be likely to result if the following three issues were explicitly considered with students:
How should the particular focus of the research be identified? Students should be helped to realise that virtually all scientific research requires substantial funding. It may not be enough, therefore, for students to identify the focus for their work on the grounds of personal interest alone if they wish to understand how science is undertaken in reality. Here, such activities as participating in well-designed citizen science projects that still enable student autonomy (e.g. Curtis, 2018 ) can help.
Students should be encouraged, once their written report has been completed, to present it at a conference (as happens, for instance, with many IRIS projects) and to write it up for publication. Writing for publication is more feasible now that publication can be via blogs or on the internet, compared to the days when the only possible outlets were hard-copy journals or monographs.
What change in the world does the research wish to effect? Much student research in science seems implicitly to presume that science is neutral. The reality—back to funding again—is that most scientific research is undertaken with specific ends in mind (for instance, the development of medical treatments, the location of valuable mineral ores, the manufacture of new products for which desire can also be manufactured). It is not, of course, that we are calling for students unquestioningly to adopt the same values as those of professional scientists. Rather, we would encourage students to be enabled to reflect on such ends and values.
Abraham, L. (2002). What do high school science students gain from field-based research apprenticeship programs? The Clearing House, 75 (5), 229–232.
Article Google Scholar
Abrahams, I., & Reiss, M. (2012). Practical work: its effectiveness in primary and secondary schools in England. Journal of Research in Science Teaching, 49 (8), 1035–1055.
Abrahams, I., & Reiss, M. J. (Eds) (2017). Enhancing learning with effective practical science 11-16 . London: Bloomsbury.
Google Scholar
Albone, E., Collins, N., & Hill, T. (Eds) (1995). Scientific research in schools: a compendium of practical experience. Bristol: Clifton Scientific Trust.
Archer, L., Dawson, E., DeWitt, J., Seakins, A., & Wong, B. (2015). “Science capital”: a conceptual, methodological, and empirical argument for extending Bourdieusian notions of capital beyond the arts. Journal of Research in Science Teaching, 52 (7), 922–948.
Archer, L., & DeWitt, J. (2017). Understanding young people’s science aspirations: How students form ideas about ‘becoming a scientist’. Abingdon: Routledge.
Barab, S., & Hay, K. (2001). Doing science at the elbows of experts: issues related to the science apprenticeship camp. Journal of Research in Science Teaching, 38 (1), 70–102.
Bell, R., Blair, L., Crawford, B., & Lederman, N. (2003). Just do it? Impact of a science apprenticeship program on high school students’ understandings of the nature of science and scientific inquiry. Journal of Research in Science Teaching, 40 (5), 487–509.
Bennett, J., Dunlop, L., Knox, K., Reiss, M. J., & Torrance Jenkins, R. (2018). Practical independent research projects in science: a synthesis and evaluation of the evidence of impact on high school students. International Journal of Science Education, 40 (14), 1755–1773.
Bennett, J., Lubben, F., & Hogarth, S. (2007). Bringing science to life: a synthesis of the research evidence on the effects of context-based and STS approaches to science teaching. Science Education, 91 (3), 347–370.
Bevins, S., Jordan, J., & Perry, E. (2011). Reflecting on professional development. Educational Action Research, 19 (3), 399–411.
Bowes, L., Birkin, G., & Tazzyman, S. (2017). Nuffield research placements evaluation: final report on waves 1 to 3 of the longitudinal survey of 2016 applicants. Leicester: CFE Research.
Bowes, L., Tazzyman, S., Stutz, A., & Birkin, G. (2021). Evaluation of Nuffield future researchers. London: Nuffield Foundation.
Braund, M., & Reiss, M. (2006). Towards a more authentic science curriculum: the contribution of out-of-school learning. International Journal of Science Education , 28 , 1373–1388.
British Science Association. (2018). CREST Awards: getting started guide, primary. London: British Science Association.
Bulte, A., Westbroek, H., de Jong, O., & Pilot, A. (2006). A research approach to designing chemistry education using authentic practices as contexts. International Journal of Science Education, 28 (9), 1063–1086.
Burgin, S., McConnell, W., & Flowers, A. (2015). ‘I actually contributed to their research’: the influence of an abbreviated summer apprenticeship program in science and engineering for diverse high-school learners. International Journal of Science Education, 37 (3), 411–445.
Burgin, S., Sadler, T., & Koroly, M. J. (2012). High school student participation in scientific research apprenticeships: variation in and relationships among student experiences and outcomes. Research in Science Education, 42 , 439–467.
Carsten Conner, L., Oxtoby, L., & Perin, S. (2021). Power and positionality shape identity work during a science research apprenticeship for girls. International Journal of Science Education , 1–14.
Carsten Conner, L., Perin, S., & Pettit, E. (2018). Tacit knowledge and girls’ notions about a field science community of practice. International Journal of Science Education, Part B, 8 (2), 164–177.
Cartrette, D., & Melroe-Lehrman, B. (2012). Describing changes in undergraduate students’ preconceptions of research activities. Research in Science Education, 42 , 1073–1100.
Cerini, B., Murray, I., & Reiss, M. (2003). Student review of the science curriculum: major findings . London: Planet Science.
Chachashvili-Bolotin, S., Milner-Bolotin, M., & Lissitsa, S. (2016). Examination of factors predicting secondary students’ interest in tertiary STEM education. International Journal of Science Education , 38 (3), 366–390.
Chapman, A., & Feldman, A. (2017). Cultivation of science identity through authentic science in an urban high school classroom. Cultural Studies of Science Education, 12 , 469–491.
Charney, J., Hmelo-Silver, C., Sofer, W., Neigeborn, L., Coletta, S., & Nemeroff, M. (2007). Cognitive apprenticeship in science through immersion in laboratory practices. International Journal of Science Education, 29 (2), 195–213.
Chen, C.-H., & Yang, Y.-C. (2019). Revisiting the effects of project-based learning on students’ academic achievement: a meta-analysis investigating moderators. Educational Research Review, 26 , 71–81.
Cilauro, F., & Paull, G. (2019). Evaluation of Nuffield research placements: interim report. London: Nuffield Foundation.
Claussen, S., & Osborne, J. (2013). Bourdieu’s notion of cultural capital and its implications for the science curriculum. Science Education, 97 (1), 58–79.
Coll, R. K., & Taylor, N. (2012). An international perspective on science curriculum development and implementation. In B. J. Fraser, K. Tobin, & C. J. McRobbie (Eds), Second international handbook of science education (pp. 771–782). Springer, Dordrecht.
Chapter Google Scholar
Cukurova, M., Hanley, P., & Lewis, A. (2015). Rapid evidence review of good practical science. London: Gatsby Charitable Foundation.
Curtis, V. (2018). Online citizen science and the widening of academia: distributed engagement with research and knowledge production . Cham: Palgrave.
Dawson, V. (2015). Western Australian high school students’ understandings about the socioscientific issue of climate change. International Journal of Science Education , 37 (7), 1024–1043.
Deemer, E., Ogas, J., Barr, A., Bowdon, R., Hall, M., Paula, S., … Lim, S. (2021). Scientific research identity development need not wait until college: examining the motivational impact of a pre-college authentic research experience. Research in Science Education , 1–16. https://doi.org/10.1007/s11165-021-09994-6
Department for Education. (2014). The national curriculum in England: framework document. London: Department for Education. https://www.gov.uk/government/publications/national-curriculum-in-england-framework-for-key-stages-1-to-4 . Accessed 1 July 1 2017.
DeWitt, J., Archer, L., & Mau, A. (2016). Dimensions of science capital: exploring its potential for understanding students’ science participation. International Journal of Science Education, 38 , 2431–2449.
Dijkstra, E., & Goedhart, M. (2011). Evaluation of authentic science projects on climate change in secondary schools: a focus on gender differences. Research in Science & Technological Education, 29 (2), 131–146.
Driver, R. (1983). The pupil as scientist? Milton Keynes: Open University Press.
Ebenezer, J., Kaya, O. N., & Ebenezer, D. L. (2011). Engaging students in environmental research projects: perceptions of fluency with innovative technologies and levels of scientific inquiry abilities. Journal of Research in Science Teaching, 48 (1), 94–116.
Etkina, E., Matilsky, T., & Lawrence, M. (2003). Pushing to the edge: Rutgers Astrophysics Institute motivates talented high school students. Journal of Research in Science Teaching, 40 (10), 958–985.
Forbes, A., & Skamp, K. (2016). Secondary science teachers’ and students’ involvement in a primary school community of science practice: how it changed their practices and interest in science. Research in Science Education, 46 , 91–112.
Forbes, A., & Skamp, K. (2019). ‘You actually feel like you’re actually doing some science’: primary students’ perspectives of their involvement in the MyScience initiative. Research in Science Education, 49 , 465–498.
Furtak, E. M., Seidel, T., Iverson, H., & Briggs, D. (2012). Experimental and quasi-experimental studies of inquiry-based science teaching: a meta-analysis. Review of Educational Research, 82 (3), 300–329.
Gatsby Charitable Foundation. (2017). Good practical science. London: Gatsby Charitable Foundation.
Gokpinar, T., & Reiss, M. (2016). The role of outside-school factors in science education: a two-stage theoretical model linking Bourdieu and Sen, with a case study. International Journal of Science Education , 38 , 1278–1303.
Goodwin, E., Anokhin, V., Gray, M., Zajic, D., Podrabsky, J., & Shortlidge, E. (2021). Is this science? Students’ experiences of failure make a research-based course feel authentic. CBE-Life Sciences Education, 20 (1), 1–15.
Hamlyn, B., Hanson, T., Malam, S., Man, C., Smith, K., & Williams, L. (2020). Young people’s views on science education: science education tracker 2019: wave 2. London: Wellcome Trust.
Hamlyn, R., Matthews, P., & Shanahan, M. (2017). Young people’s views on science education: science education tracker research report February 2017. London: Wellcome Trust.
Hatfield, P., Furnell, W., Shenoy, A., Fox, E., Parker, B., Thomas, L., & Rushton, E. (2019). IRIS opens pupils’ eyes to real space research. Astronomy & Geophysics, 60 (1), 1.22–1.24.
Hattie, J., Bustamante, V., Almarode, J. T., Fisher, D., & Frey, N. (2020). Great teaching by design: from intention to implementation in the visible learning classroom. Thousand Oaks, CA: Corwin.
Helle, L., Tynjälä, P., & Olkinuora, E. (2006). Project-based learning in post-secondary education – theory, practice and rubber sling shots. Higher Education, 51 , 287–314.
Hodson, D. (1993). Re-thinking old ways: towards a more critical approach to practical work in school science. Studies in Science Education, 22 (1), 85–142.
Hofstein, A., & Lunetta, V. (2004). The laboratory in science education: foundations for the twenty-first century. Science Education, 88 (1), 28–54.
Hollins, M. & Reiss, M. J. (2016) A review of the school science curricula in eleven high achieving jurisdictions. The Curriculum Journal , 27 , 80-94.
Houseal, A., Abd-El-Khalick, F., & Destefano, L. (2014). Impact of a student-teacher-scientist partnership on students’ and teachers’ content knowledge, attitudes toward science, and pedagogical practices. Journal of Research in Science Teaching, 51 (1), 84–115.
Hsu, P.-L., & Espinoza, P. (2018). Cultivating constructivist science internships for high school students through a community of practice with cogenerative dialogues. Learning Environments Research, 21 , 267–283.
Husain, F., Wishart, R., Attygalle, K., Averill, P., Ilic, N., & Mayer, M. (2019). CREST Silver evaluation report. London: Education Endowment Foundation.
Institute of Physics. (2020). Limit Less: Support young people to change the world. London: Institute of Physics.
John, J., & Creighton, J. (2011). Researcher development: the impact of undergraduate research opportunity programmes on students in the UK. Studies in Higher Education, 36 (7), 781–797.
Kapon, S., Laherto, A., & Levrini, O. (2018). Disciplinary authenticity and personal relevance in school science. Science Education, 102 (5), 1077–1106.
Knorr-Cetina, K. D. (1983). New developments in science studies: the ethnographic challenge. The Canadian Journal of Sociology 8 (2), 153–177.
Koomen, M. H., Blair, R., Young-Isebrand, E., & Oberhauser, K. S. (2014). Science professional development with teachers: nurturing the scientist within. The Electronic Journal for Research in Science & Mathematics Education , 18 (6).
Latour, B. & Woolgar, S. (1979). Laboratory life: the social construction of scientific facts . Beverly Hills: Sage.
Liao, T., McKenna, J., & Milner-Bolotin, M. (2017). Four decades of High School Physics Olympics Competitions at the University of British Columbia. Physics in Canada , 73 (3), 127–129.
Liljeström, A., Enkenberg, J., & Pöllänen, S. (2013). Making learning whole: an instructional approach for mediating the practices of authentic science inquiries. Cultural Studies of Science Education, 8 , 51–86.
Linn, M., Palmer, E., Baranger, A., Gerard, E., & Stone, E. (2015). Undergraduate research experiences: impacts and opportunities. Science, 347 (6222), 1261757.
Lissitsa, S., & Chachashvili‐Bolotin, S. (2019). Enrolment in mathematics and physics at the advanced level in secondary school among two generations of highly skilled immigrants. International Migration , 57 (5), 216–234.
Maiorca, C., Roberts, T., Jackson, C., Bush, S., Delaney, A., Mohr-Schroeder, M., & Soledad, S. Y. (2021). Informal learning environments and impact on interest in STEM careers. International Journal of Science and Mathematics Education, 19 , 45–64.
Mansfield J., & Reiss M. J. (2020). The place of values in the aims of school science education. In D. Corrigan, C. Buntting, A. Fitzgerald, & A. Jones (Eds), Values in science education (pp. 191–209), Cham: Springer.
Martin, B., Kass, H., & Brouwer, W. (1990). Authentic science: a diversity of meanings. Science Education, 74 (5), 541–554.
Millar, R. (1998). Rhetoric and reality: what practical work in science is really for. In J. Wellington (Ed.), Practical work in school science. Which way now? (pp. 16–31). London: Routledge.
Milner-Bolotin, M. (2012). Increasing interactivity and authenticity of chemistry instruction through data acquisition systems and other technologies. Journal of Chemical Education , 89 (4), 477–481.
Molefe, M. L. (2011). A study of life sciences projects in science talent quest competitions in the Western Cape, South Africa, with special reference to scientific skills and knowledge . Unpublished PhD thesis.
Moore, A. M., Fairhurst, P., Correia, C. F., Harrison, C., & Bennett, J. M. (2020). Science practical work in a COVID-19 world: are teacher demonstrations, videos and textbooks effective replacements for hands-on practical activities? School Science Review , 102 (378), 7–12.
Moote, J. (2019). Investigating the longer-term impact of the CREST inquiry-based learning programme on student self-regulated processes and related motivations: views of students and teachers. Research in Science Education, 49 (1), 265–294.
Moote, J., Williams, J., & Sproule, J. (2013). When students take control: investigating the impact of the CREST inquiry-based learning program on self-regulated processes and related motivations in young science students. Journal of Cognitive Education and Psychology, 12 (2), 178–196.
National Foundation for Educational Research. (2011). Exploring young people’s views on science education. London: Wellcome Trust.
Nuffield Foundation. (2020). Nuffield research placements: guide for student applicants. London: Nuffield Foundation.
Oliver, M. C., Jerrim, J., & Adkins, M. J. (2021a). PISA: Engagement Attainment and interest in Science (PEAS) – Final Report. Available at https://www.nottingham.ac.uk/research/groups/lsri/documents/peas-report.pdf .
Oliver, M., McConney, A., & Woods-McConney, A. (2021b). The efficacy of inquiry-based instruction in science: a comparative analysis of six countries using PISA 2015. Research in Science Education , 51 , 595–616.
Osborne, J., & Dillon, J. (2008). Science education in Europe: critical reflections. London: The Nuffield Foundation.
Parker, B., Thomas, L., Rushton, E., & Hatfield, P. (2019). Transforming education with the Timepix detector – Ten years of CERN@school. Radiation Measurements, 127 (106090), 1–7.
Rahman, M. T., & Lewis, S. (2019). Evaluating the evidence base for evidence‐based instructional practices in chemistry through meta‐analysis. Journal of Research in Science Teaching , 1–29. https://doi.org/10.1002/tea.21610
Reveles, J., Cordova, R., & Kelly, G. (2004). Science literacy and academic identity formulation. Journal of Research in Science Teaching, 41 (10), 1111–1144.
Roberts, L., & Wassersug, R. (2009). Does doing scientific research in high school correlate with students staying in science? A half-century retrospective study. Research in Science Education, 39 , 251–256.
Roth, W.-M. (1995). Authentic school science knowing and learning in open-inquiry science laboratories. The Netherlands: Kluwer.
Rowland, S., Pedwell, R., Lawrie, G., Lovie-Toon, J., & Hung, Y. (2016). Do we need to design course-based undergraduate research experiences for authenticity? CBE-Life Sciences Education, 15 (4), 1–16.
Royal Society. (2014). Vision for science and mathematics education. London: The Royal Society.
Rushton, E., & Reiss, M. J. (2019). From science teacher to ‘teacher scientist’: exploring the experiences of research-active science teachers in the UK. International Journal of Science Education, 41 (11), 1541–1561.
Rushton, E., Charters, L., & Reiss, M. J. (2019). The experiences of active participation in academic conferences for high school science students. Research in Science & Technological Education , 1–19. https://doi.org/10.1080/02635143.2019.1657395
Savelsbergh, E., Prins, G., Rietbergen, C., Fechner, S., Vaessen, B., Draijer, J., & Bakker, A. (2016). Effects of innovative science and mathematics teaching on student attitudes and achievement: a meta-analytic study. Educational Research Review, 19 , 158–172.
Schroeder, C., Scott, T., Tolson, H., Huang, T.-Y., & Lee, Y.-H. (2007). A meta-analysis of national research: effects of teaching strategies on student achievement in science in the United States. Journal of Research in Science Teaching, 44 (10), 1436–1460.
Shana, Z., & Abulibdeh, E. S. (2020). Science practical work and its impact on high students’ academic achievement. Journal of Technology and Science Education , 10 (2), 199–215.
Sharpe, R., & Abrahams, I. (2020). Secondary school students’ attitudes to practical work in biology, chemistry and physics in England. Research in Science & Technological Education, 38 (1), 84–104.
Silvast, A., Laes, E., Abram, S., & Bombaerts, G. (2020). What do energy modellers know? An ethnography of epistemic values and knowledge models. Energy Research & Social Science , 66 , 101495.
Sjöström, J. & Eilks, I. (2018). Reconsidering different visions of scientific literacy and science education based on the concept of Bildung . In Y. J. Dori, Z. R. Mevarech, & D. R. Baker (Eds), Cognition, metacognition, and culture in STEM education (pp. 65–88). Cham: Springer.
Sousa-Silva, C., McKemmish, L., Chubb, K., Gorman, M., Baker, J., Barton, E., … Tennyson, J. (2018). Original Research by Young Twinkle Students (ORBYTS): when can students start performing original research? Physics Education, 53 (1), 1–12.
Staacks, S., Hütz, S., Heinke, H., & Stampfer, C. (2018). Advanced tools for smartphone-based experiments: phyphox. Physics Education , 53 (4), 045009.
Stake, J., & Mares, K. (2001). Science enrichment programs for gifted high school girls and boys: predictors of program impact on science confidence and motivation. Journal of Research in Science Teaching, 38 (10), 1065–1088.
Stake, J., & Mares, K. (2005). Evaluating the impact of science-enrichment programs on adolescents’ science motivation and confidence: the splashdown effect. Journal of Research in Science Teaching, 42 (4), 359–375.
Stock Jones, R., Annable, T., Billingham, Z., & MacDonald, C. (2016). Quantifying CREST: what impact does the Silver CREST Award have on science scores and STEM subject selection? London: British Science Association.
Swarat, S., Ortony, A., & Revelle, W. (2012). Activity matters: understanding student interest in school science. Journal of Research in Science Teaching , 49 (4), 515–537.
Tyler-Wood, T., Ellison, A., Lim, O., & Periathiruvadi, S. (2012). Bringing up girls in science (BUGS): the effectiveness of an afterschool environmental science program for increasing female students’ interest in science careers. Journal of Science Education and Technology, 21 , 46–55.
Ward, T., Delaloye, N., Adams, E. R., Ware, D., Vanek, D., Knuth, R., … Holian, A. (2016). Air toxics under the big sky: examining the effectiveness of authentic scientific research on high school students’ science skills and interest. International Journal of Science Education, 38 (6), 905–921.
Whyntie, T. (2016). CERN@School: forming nationwide collaborations for physics research in schools. Nuclear Physics News, 26 (1), 16–19.
Whyntie, T., Bithray, H., Cook, J., Coupe, A., Eddy, D., Fickling, R., … Shearer, N. (2015). CERN@school: demonstrating physics with the Timepix detector. Contemporary Physics, 56 (4), 451–467.
Whyntie, T., Cook, J., Coupe, A., Fickling, R., Parker, B., & Shearer, N. (2016). CERN@school: bringing CERN into the classroom. Nuclear and Particle Physics Proceedings, 273-275 , 1265–1270.
Williams, D., Brule, H., Kelley, S., & Skinner, E. (2018). Science in the learning gardens (SciLG): a study of students’ motivation, achievement, and science identity in low-income middle schools. International Journal of STEM Education, 5 (8), 1–14.
Winsberg, E. (2019). Computer simulations in science. In E. N. Zalta (Ed.), The Stanford Encyclopedia of Philosophy , https://plato.stanford.edu/archives/win2019/entries/simulations-science/ .
Young, J., Carsten Conner, L., & Pettit, E. (2020). ‘You really see it’: environmental identity shifts through interacting with a climate change-impacted glacier landscape. International Journal of Science Education, 42 (18), 3049–3070.
Zeidler, D. L., & Sadler, T. D. (2008). Social and ethical issues in science education: a prelude to action. Science & Education , 17 , 799–803.
Download references
Acknowledgements
We are very grateful to The Institute of Research in Schools for funding.
Author information
Authors and affiliations.
UCL Institute of Education, 20 Bedford Way, London, WC1H 0AL, UK
Michael J. Reiss, Richard Sheldrake & Wilton Lodge
You can also search for this author in PubMed Google Scholar
Corresponding author
Correspondence to Michael J. Reiss .
Additional information
Publisher's note.
Springer Nature remains neutral with regard to jurisdictional claims in published maps and institutional affiliations.
Rights and permissions
Open Access This article is licensed under a Creative Commons Attribution 4.0 International License, which permits use, sharing, adaptation, distribution and reproduction in any medium or format, as long as you give appropriate credit to the original author(s) and the source, provide a link to the Creative Commons licence, and indicate if changes were made. The images or other third party material in this article are included in the article's Creative Commons licence, unless indicated otherwise in a credit line to the material. If material is not included in the article's Creative Commons licence and your intended use is not permitted by statutory regulation or exceeds the permitted use, you will need to obtain permission directly from the copyright holder. To view a copy of this licence, visit http://creativecommons.org/licenses/by/4.0/ .
Reprints and permissions
About this article
Reiss, M.J., Sheldrake, R. & Lodge, W. Investigative Research Projects for Students in Science: The State of the Field and a Research Agenda. Can. J. Sci. Math. Techn. Educ. 23 , 80–95 (2023). https://doi.org/10.1007/s42330-023-00263-4
Download citation
Accepted : 01 February 2023
Published : 16 March 2023
Issue Date : March 2023
DOI : https://doi.org/10.1007/s42330-023-00263-4
Share this article
Anyone you share the following link with will be able to read this content:
Sorry, a shareable link is not currently available for this article.
Provided by the Springer Nature SharedIt content-sharing initiative
- Science investigative research projects
- Student autonomy
- Student engagement
- Authenticity
- Find a journal
- Publish with us
- Track your research
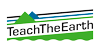
Teach the Earth the portal for Earth Education
From NAGT's On the Cutting Edge Collection

- Course Topics
- Atmospheric Science
- Biogeoscience
- Environmental Geology
- Environmental Science
- Geochemistry
- Geomorphology
- GIS/Remote Sensing
- Hydrology/Hydrogeology
- Oceanography
- Paleontology
- Planetary Science
- Sedimentary Geology
- Structural Geology
- Incorporating Societal Issues
- Climate Change
- Complex Systems
- Ethics and Environmental Justice
- Geology and Health
- Public Policy
- Sustainability
- Strengthening Your Department
- Career Development
- Strengthening Departments
- Student Recruitment
- Teacher Preparation
- Teaching Topics
- Biocomplexity
- Early Earth
- Earthquakes
- Hydraulic Fracturing
- Plate Tectonics
- Teaching Environments
- Intro Geoscience
- Online Teaching
- Teaching in the Field
- Two-Year Colleges
- Urban Students
- Enhancing your Teaching
- Affective Domain
- Course Design
- Data, Simulations, Models
- Geophotography
- Google Earth
- Metacognition
- Online Games
- Problem Solving
- Quantitative Skills
- Rates and Time
Service Learning
- Spatial Thinking
Teaching Methods
- Teaching with Video
- Undergrad Research
- Visualization
- Teaching Materials
- Two Year Colleges
- Departments
- Workshops and Webinars
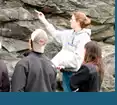
Undergraduate Research as Teaching Practice
- ⋮⋮⋮ ×
Inquiry and Discovery-based Projects Within Introductory Courses
Information compiled by Monica Bruckner, SERC
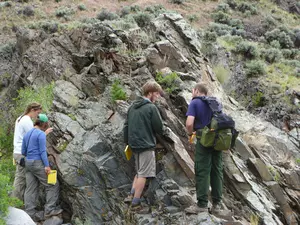
Inquiry and discovery-based projects, including field studies and interactive laboratory activities, can engage introductory students, majors and non-majors alike. These activities appeal to the affective domain and develop students' metacognitive skills . Hands-on, inquiry-based projects in the field allow students to observe their environment and to apply their classroom knowledge to the real world. When combined with data collection, students are able to take this a step further and take ownership of the data they collect and learn how to analyze that data to make sense of Earth processes. When fieldwork is not an option, classroom-based inquiry and discovery-based projects in the laboratory or virtual learning environments can still help students to apply their knowledge from various disciplines to solve problems and to relate what they learn in class to real-world situations.
Benefits of Inquiry and Discovery-based Projects
- Students learn concepts more deeply than in lecture-based classes because they have more direct experience.
- Students gain a better understanding of the processes of scientific inquiry because they do primary research.
- Students learn field and lab skills that are difficult to incorporate into traditionally-scheduled classes.
- Student resumes are filled with evidence of skills, report writing, publications and professional presentations.
- Students make direct connections between understanding Earth systems and managing the actions of human society.
How to Incorporate Inquiry and Problem-based Learning into the Classroom
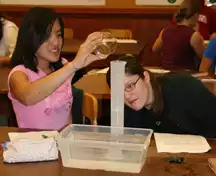
- Identify your learning objectives and goals and design your activity around these. This will help solidify students' conception of why they are doing the work. Learn more about learning objectives .
- Determine your project needs. This may include considering the time commitment you need to carry out the project, both in and out of class; what types of instruments you will need; travel considerations for off-campus field projects; and depending on the nature of the project, whether you need your department or college's permission to carry out the project.
- Set expectations for both you and your students and provide proper scaffolding for the activity. Remember that intro students may not have all the background knowledge and skills required to complete the project without your help and that they may struggle with the process of science when things don't turn out as they intend or expect them to. Informing students about the process of science may help alleviate students' frustrations when things go unexpectedly. In addition, developing a rubric for assessment may aid in aligning your expectations with those of the students.
- Consider how much and what type of support you will provide your students. Students learn a great deal from problem-solving on their own and through trial and error, but in some cases, it may be beneficial to provide students with guidance to alleviate their frustration when things don't go as expected or if they are struggling with the next steps.
- Develop your assessment prior to the activity and make sure students understand how they will be assessed. Developing a rubric is one example of a straight-forward, organized way to do this.
- Extend the experience. This can be done through a classroom discussion of what students learned through the project, whether it is knowledge gained or skills developed. Students could also develop their presentation skills by disseminating their findings to others via a department seminar, a presentation at a professional meeting, or if it is a service learning experience , students can disseminate their findings to the community through a newspaper article or by giving a presentation.
Guided Discovery Problems
Campus based learning, experience-based environmental projects, investigative case-based learning, undergraduate research, case studies.
« Previous Page Next Page »
Education During Coronavirus
A Smithsonian magazine special report
Science | June 15, 2020
Seventy-Five Scientific Research Projects You Can Contribute to Online
From astrophysicists to entomologists, many researchers need the help of citizen scientists to sift through immense data collections
:focal(300x157:301x158)/https://tf-cmsv2-smithsonianmag-media.s3.amazonaws.com/filer/e2/ca/e2ca665f-77b7-4ba2-8cd2-46f38cbf2b60/citizen_science_mobile.png)
Rachael Lallensack
Former Assistant Editor, Science and Innovation
If you find yourself tired of streaming services, reading the news or video-chatting with friends, maybe you should consider becoming a citizen scientist. Though it’s true that many field research projects are paused , hundreds of scientists need your help sifting through wildlife camera footage and images of galaxies far, far away, or reading through diaries and field notes from the past.
Plenty of these tools are free and easy enough for children to use. You can look around for projects yourself on Smithsonian Institution’s citizen science volunteer page , National Geographic ’s list of projects and CitizenScience.gov ’s catalog of options. Zooniverse is a platform for online-exclusive projects , and Scistarter allows you to restrict your search with parameters, including projects you can do “on a walk,” “at night” or “on a lunch break.”
To save you some time, Smithsonian magazine has compiled a collection of dozens of projects you can take part in from home.
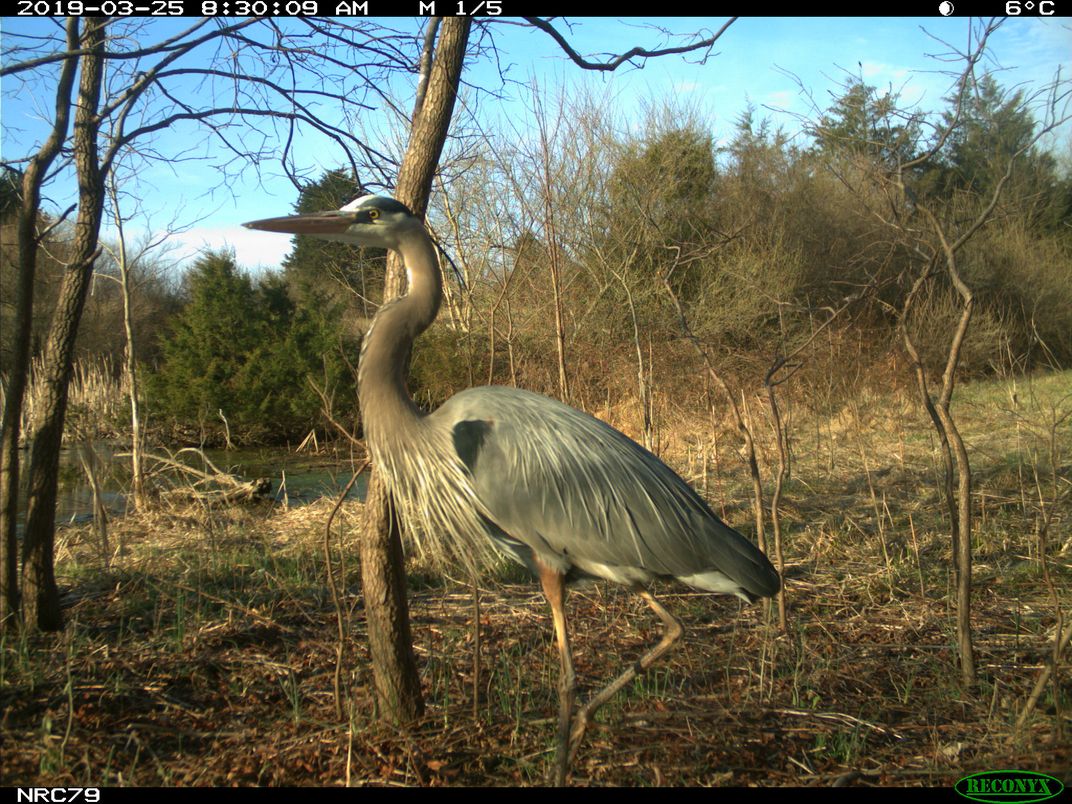
American Wildlife
If being home has given you more time to look at wildlife in your own backyard, whether you live in the city or the country, consider expanding your view, by helping scientists identify creatures photographed by camera traps. Improved battery life, motion sensors, high-resolution and small lenses have made camera traps indispensable tools for conservation.These cameras capture thousands of images that provide researchers with more data about ecosystems than ever before.
Smithsonian Conservation Biology Institute’s eMammal platform , for example, asks users to identify animals for conservation projects around the country. Currently, eMammal is being used by the Woodland Park Zoo ’s Seattle Urban Carnivore Project, which studies how coyotes, foxes, raccoons, bobcats and other animals coexist with people, and the Washington Wolverine Project, an effort to monitor wolverines in the face of climate change. Identify urban wildlife for the Chicago Wildlife Watch , or contribute to wilderness projects documenting North American biodiversity with The Wilds' Wildlife Watch in Ohio , Cedar Creek: Eyes on the Wild in Minnesota , Michigan ZoomIN , Western Montana Wildlife and Snapshot Wisconsin .
"Spend your time at home virtually exploring the Minnesota backwoods,” writes the lead researcher of the Cedar Creek: Eyes on the Wild project. “Help us understand deer dynamics, possum populations, bear behavior, and keep your eyes peeled for elusive wolves!"
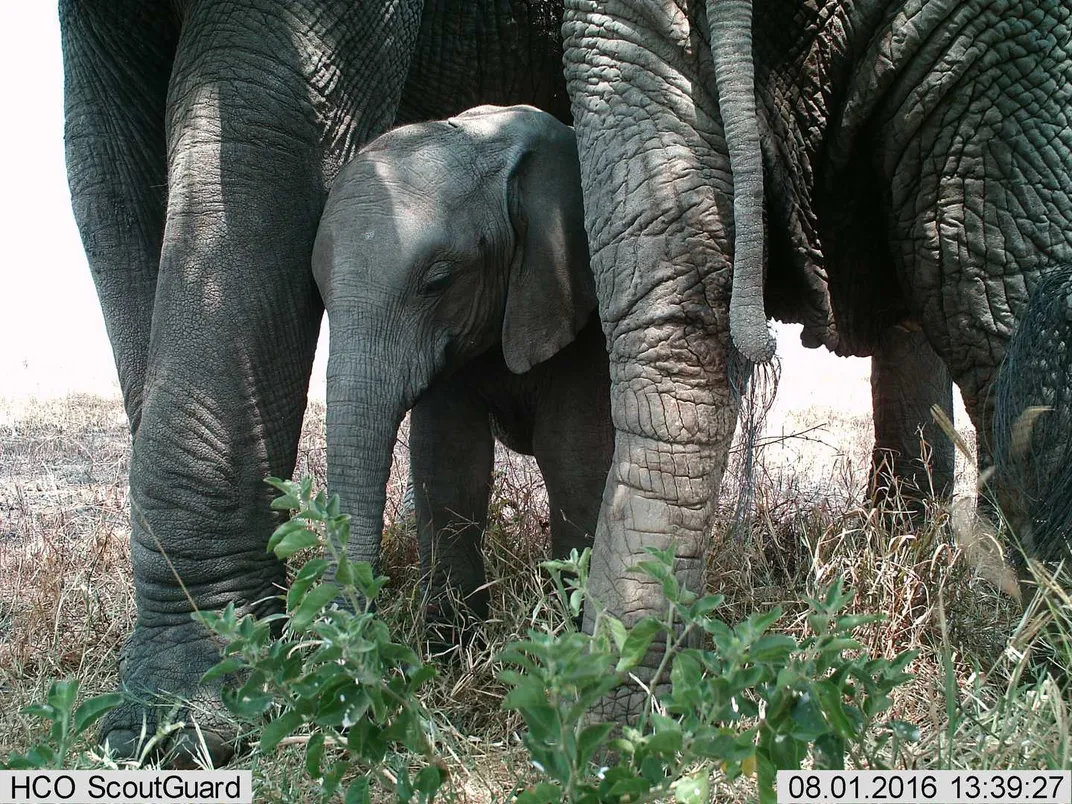
If being cooped up at home has you daydreaming about traveling, Snapshot Safari has six active animal identification projects. Try eyeing lions, leopards, cheetahs, wild dogs, elephants, giraffes, baobab trees and over 400 bird species from camera trap photos taken in South African nature reserves, including De Hoop Nature Reserve and Madikwe Game Reserve .
With South Sudan DiversityCam , researchers are using camera traps to study biodiversity in the dense tropical forests of southwestern South Sudan. Part of the Serenegeti Lion Project, Snapshot Serengeti needs the help of citizen scientists to classify millions of camera trap images of species traveling with the wildebeest migration.
Classify all kinds of monkeys with Chimp&See . Count, identify and track giraffes in northern Kenya . Watering holes host all kinds of wildlife, but that makes the locales hotspots for parasite transmission; Parasite Safari needs volunteers to help figure out which animals come in contact with each other and during what time of year.
Mount Taranaki in New Zealand is a volcanic peak rich in native vegetation, but native wildlife, like the North Island brown kiwi, whio/blue duck and seabirds, are now rare—driven out by introduced predators like wild goats, weasels, stoats, possums and rats. Estimate predator species compared to native wildlife with Taranaki Mounga by spotting species on camera trap images.
The Zoological Society of London’s (ZSL) Instant Wild app has a dozen projects showcasing live images and videos of wildlife around the world. Look for bears, wolves and lynx in Croatia ; wildcats in Costa Rica’s Osa Peninsula ; otters in Hampshire, England ; and both black and white rhinos in the Lewa-Borana landscape in Kenya.
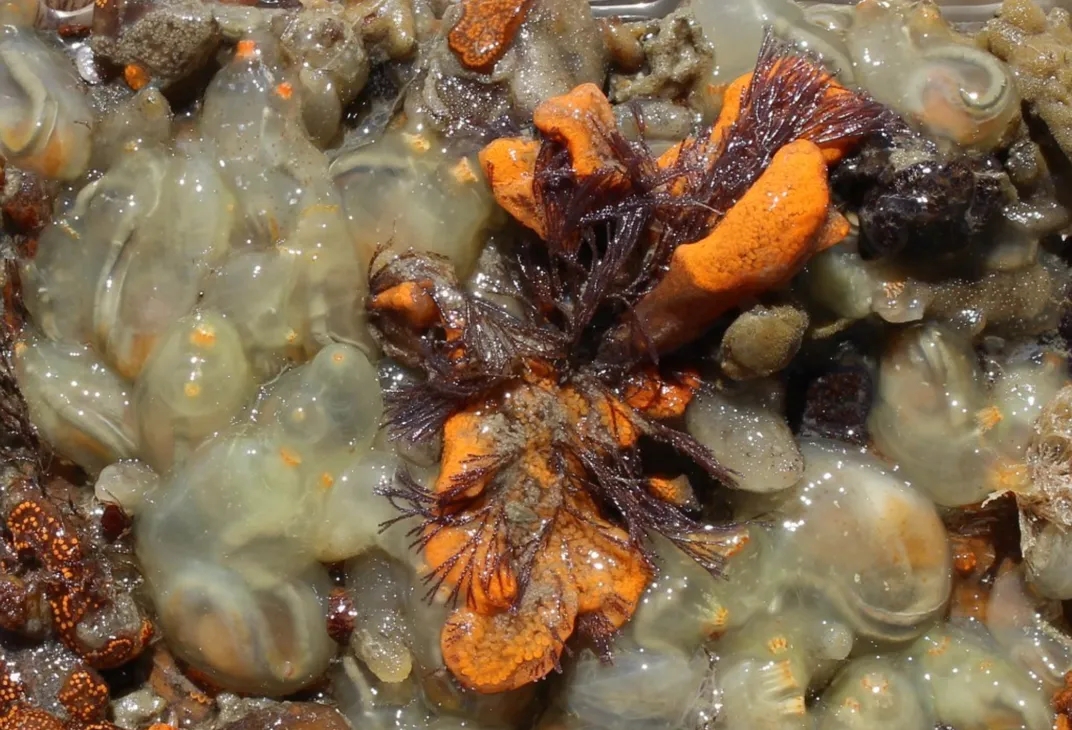
Under the Sea
Researchers use a variety of technologies to learn about marine life and inform conservation efforts. Take, for example, Beluga Bits , a research project focused on determining the sex, age and pod size of beluga whales visiting the Churchill River in northern Manitoba, Canada. With a bit of training, volunteers can learn how to differentiate between a calf, a subadult (grey) or an adult (white)—and even identify individuals using scars or unique pigmentation—in underwater videos and images. Beluga Bits uses a “ beluga boat ,” which travels around the Churchill River estuary with a camera underneath it, to capture the footage and collect GPS data about the whales’ locations.
Many of these online projects are visual, but Manatee Chat needs citizen scientists who can train their ear to decipher manatee vocalizations. Researchers are hoping to learn what calls the marine mammals make and when—with enough practice you might even be able to recognize the distinct calls of individual animals.
Several groups are using drone footage to monitor seal populations. Seals spend most of their time in the water, but come ashore to breed. One group, Seal Watch , is analyzing time-lapse photography and drone images of seals in the British territory of South Georgia in the South Atlantic. A team in Antarctica captured images of Weddell seals every ten minutes while the seals were on land in spring to have their pups. The Weddell Seal Count project aims to find out what threats—like fishing and climate change—the seals face by monitoring changes in their population size. Likewise, the Año Nuevo Island - Animal Count asks volunteers to count elephant seals, sea lions, cormorants and more species on a remote research island off the coast of California.
With Floating Forests , you’ll sift through 40 years of satellite images of the ocean surface identifying kelp forests, which are foundational for marine ecosystems, providing shelter for shrimp, fish and sea urchins. A project based in southwest England, Seagrass Explorer , is investigating the decline of seagrass beds. Researchers are using baited cameras to spot commercial fish in these habitats as well as looking out for algae to study the health of these threatened ecosystems. Search for large sponges, starfish and cold-water corals on the deep seafloor in Sweden’s first marine park with the Koster seafloor observatory project.
The Smithsonian Environmental Research Center needs your help spotting invasive species with Invader ID . Train your eye to spot groups of organisms, known as fouling communities, that live under docks and ship hulls, in an effort to clean up marine ecosystems.
If art history is more your speed, two Dutch art museums need volunteers to start “ fishing in the past ” by analyzing a collection of paintings dating from 1500 to 1700. Each painting features at least one fish, and an interdisciplinary research team of biologists and art historians wants you to identify the species of fish to make a clearer picture of the “role of ichthyology in the past.”
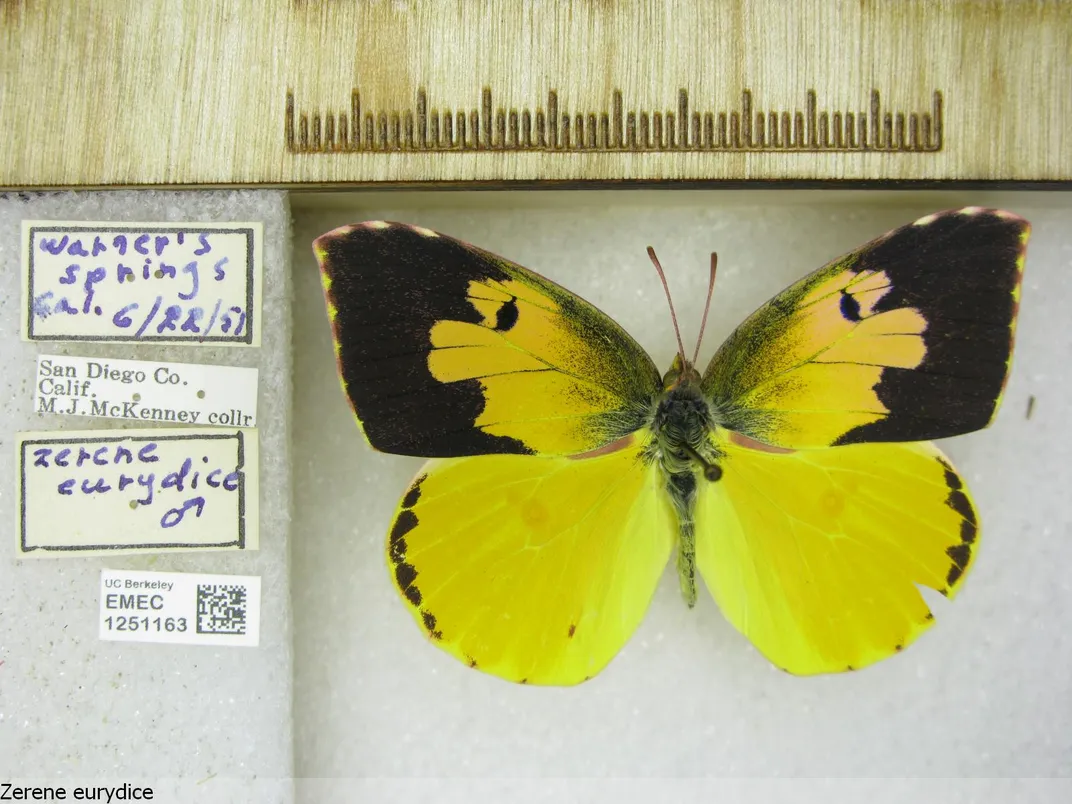
Interesting Insects
Notes from Nature is a digitization effort to make the vast resources in museums’ archives of plants and insects more accessible. Similarly, page through the University of California Berkeley’s butterfly collection on CalBug to help researchers classify these beautiful critters. The University of Michigan Museum of Zoology has already digitized about 300,000 records, but their collection exceeds 4 million bugs. You can hop in now and transcribe their grasshopper archives from the last century . Parasitic arthropods, like mosquitos and ticks, are known disease vectors; to better locate these critters, the Terrestrial Parasite Tracker project is working with 22 collections and institutions to digitize over 1.2 million specimens—and they’re 95 percent done . If you can tolerate mosquito buzzing for a prolonged period of time, the HumBug project needs volunteers to train its algorithm and develop real-time mosquito detection using acoustic monitoring devices. It’s for the greater good!
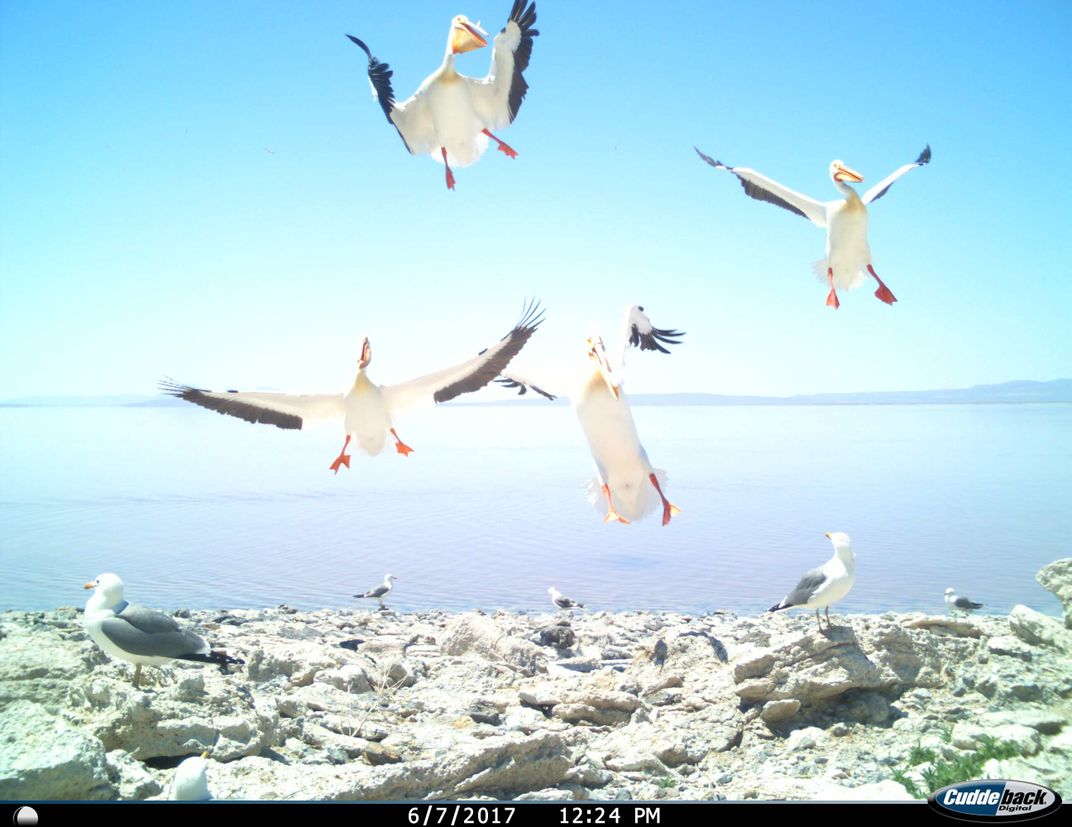
For the Birders
Birdwatching is one of the most common forms of citizen science . Seeing birds in the wilderness is certainly awe-inspiring, but you can birdwatch from your backyard or while walking down the sidewalk in big cities, too. With Cornell University’s eBird app , you can contribute to bird science at any time, anywhere. (Just be sure to remain a safe distance from wildlife—and other humans, while we social distance ). If you have safe access to outdoor space—a backyard, perhaps—Cornell also has a NestWatch program for people to report observations of bird nests. Smithsonian’s Migratory Bird Center has a similar Neighborhood Nest Watch program as well.
Birdwatching is easy enough to do from any window, if you’re sheltering at home, but in case you lack a clear view, consider these online-only projects. Nest Quest currently has a robin database that needs volunteer transcribers to digitize their nest record cards.
You can also pitch in on a variety of efforts to categorize wildlife camera images of burrowing owls , pelicans , penguins (new data coming soon!), and sea birds . Watch nest cam footage of the northern bald ibis or greylag geese on NestCams to help researchers learn about breeding behavior.
Or record the coloration of gorgeous feathers across bird species for researchers at London’s Natural History Museum with Project Plumage .
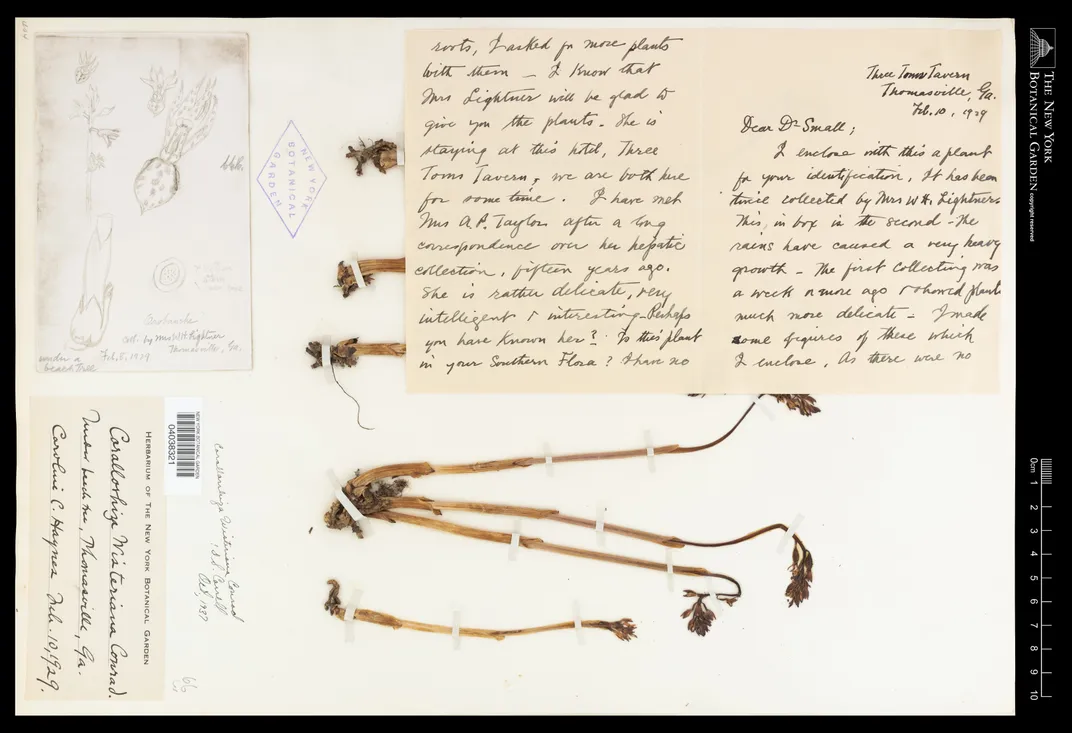
Pretty Plants
If you’re out on a walk wondering what kind of plants are around you, consider downloading Leafsnap , an electronic field guide app developed by Columbia University, the University of Maryland and the Smithsonian Institution. The app has several functions. First, it can be used to identify plants with its visual recognition software. Secondly, scientists can learn about the “ the ebb and flow of flora ” from geotagged images taken by app users.
What is older than the dinosaurs, survived three mass extinctions and still has a living relative today? Ginko trees! Researchers at Smithsonian’s National Museum of Natural History are studying ginko trees and fossils to understand millions of years of plant evolution and climate change with the Fossil Atmospheres project . Using Zooniverse, volunteers will be trained to identify and count stomata, which are holes on a leaf’s surface where carbon dioxide passes through. By counting these holes, or quantifying the stomatal index, scientists can learn how the plants adapted to changing levels of carbon dioxide. These results will inform a field experiment conducted on living trees in which a scientist is adjusting the level of carbon dioxide for different groups.
Help digitize and categorize millions of botanical specimens from natural history museums, research institutions and herbaria across the country with the Notes from Nature Project . Did you know North America is home to a variety of beautiful orchid species? Lend botanists a handby typing handwritten labels on pressed specimens or recording their geographic and historic origins for the New York Botanical Garden’s archives. Likewise, the Southeastern U.S. Biodiversity project needs assistance labeling pressed poppies, sedums, valerians, violets and more. Groups in California , Arkansas , Florida , Texas and Oklahoma all invite citizen scientists to partake in similar tasks.
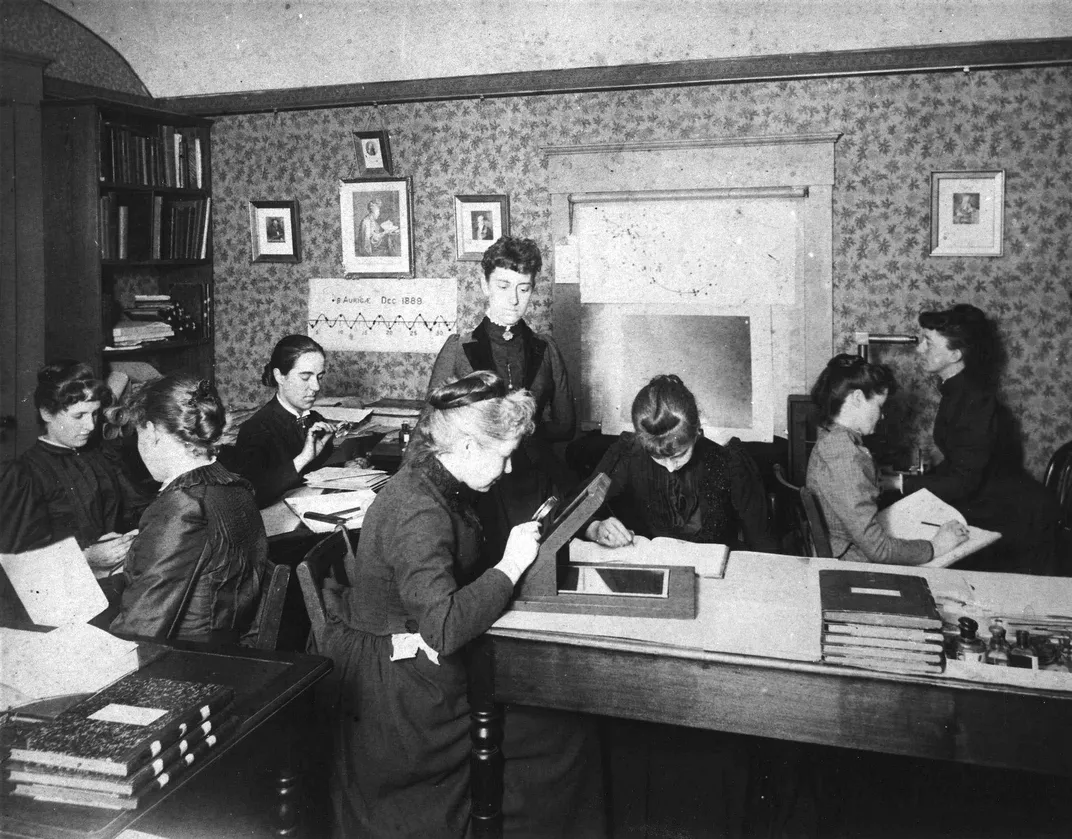
Historic Women in Astronomy
Become a transcriber for Project PHaEDRA and help researchers at the Harvard-Smithsonian Center for Astrophysics preserve the work of Harvard’s women “computers” who revolutionized astronomy in the 20th century. These women contributed more than 130 years of work documenting the night sky, cataloging stars, interpreting stellar spectra, counting galaxies, and measuring distances in space, according to the project description .
More than 2,500 notebooks need transcription on Project PhaEDRA - Star Notes . You could start with Annie Jump Cannon , for example. In 1901, Cannon designed a stellar classification system that astronomers still use today. Cecilia Payne discovered that stars are made primarily of hydrogen and helium and can be categorized by temperature. Two notebooks from Henrietta Swan Leavitt are currently in need of transcription. Leavitt, who was deaf, discovered the link between period and luminosity in Cepheid variables, or pulsating stars, which “led directly to the discovery that the Universe is expanding,” according to her bio on Star Notes .
Volunteers are also needed to transcribe some of these women computers’ notebooks that contain references to photographic glass plates . These plates were used to study space from the 1880s to the 1990s. For example, in 1890, Williamina Flemming discovered the Horsehead Nebula on one of these plates . With Star Notes, you can help bridge the gap between “modern scientific literature and 100 years of astronomical observations,” according to the project description . Star Notes also features the work of Cannon, Leavitt and Dorrit Hoffleit , who authored the fifth edition of the Bright Star Catalog, which features 9,110 of the brightest stars in the sky.
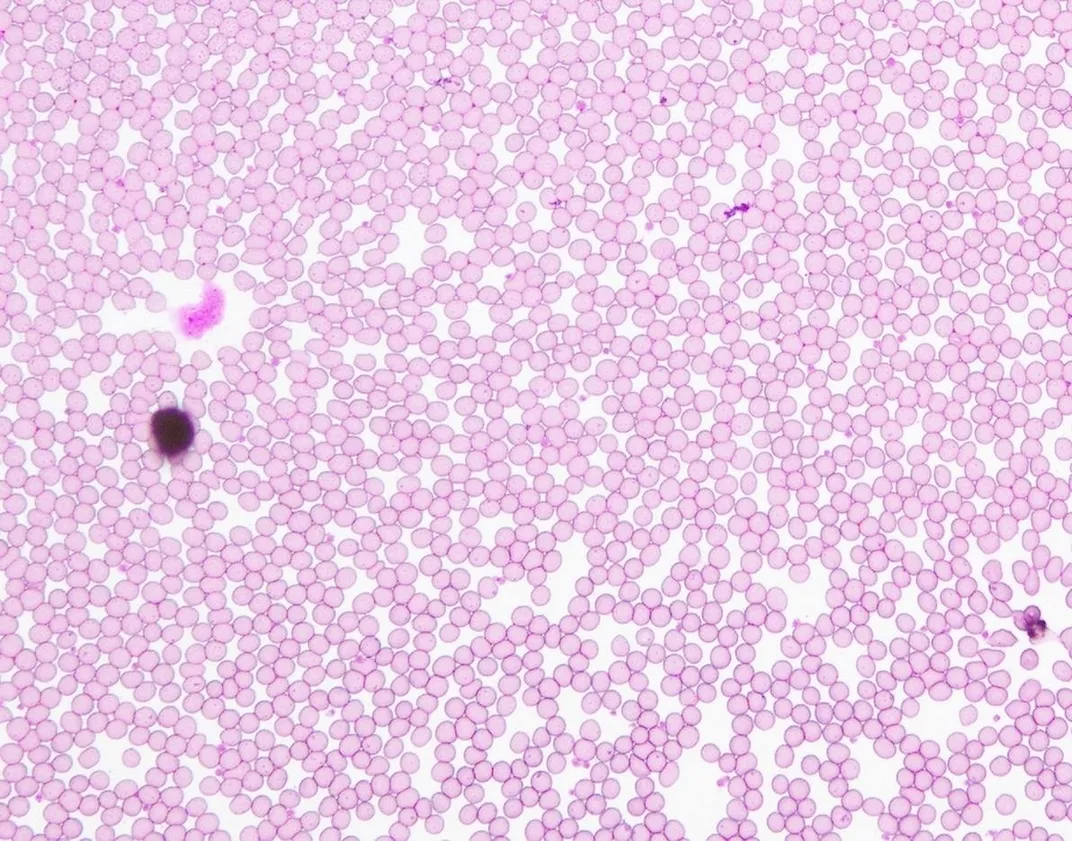
Microscopic Musings
Electron microscopes have super-high resolution and magnification powers—and now, many can process images automatically, allowing teams to collect an immense amount of data. Francis Crick Institute’s Etch A Cell - Powerhouse Hunt project trains volunteers to spot and trace each cell’s mitochondria, a process called manual segmentation. Manual segmentation is a major bottleneck to completing biological research because using computer systems to complete the work is still fraught with errors and, without enough volunteers, doing this work takes a really long time.
For the Monkey Health Explorer project, researchers studying the social behavior of rhesus monkeys on the tiny island Cayo Santiago off the southeastern coast of Puerto Rico need volunteers to analyze the monkeys’ blood samples. Doing so will help the team understand which monkeys are sick and which are healthy, and how the animals’ health influences behavioral changes.
Using the Zooniverse’s app on a phone or tablet, you can become a “ Science Scribbler ” and assist researchers studying how Huntington disease may change a cell’s organelles. The team at the United Kingdom's national synchrotron , which is essentially a giant microscope that harnesses the power of electrons, has taken highly detailed X-ray images of the cells of Huntington’s patients and needs help identifying organelles, in an effort to see how the disease changes their structure.
Oxford University’s Comprehensive Resistance Prediction for Tuberculosis: an International Consortium—or CRyPTIC Project , for short, is seeking the aid of citizen scientists to study over 20,000 TB infection samples from around the world. CRyPTIC’s citizen science platform is called Bash the Bug . On the platform, volunteers will be trained to evaluate the effectiveness of antibiotics on a given sample. Each evaluation will be checked by a scientist for accuracy and then used to train a computer program, which may one day make this process much faster and less labor intensive.
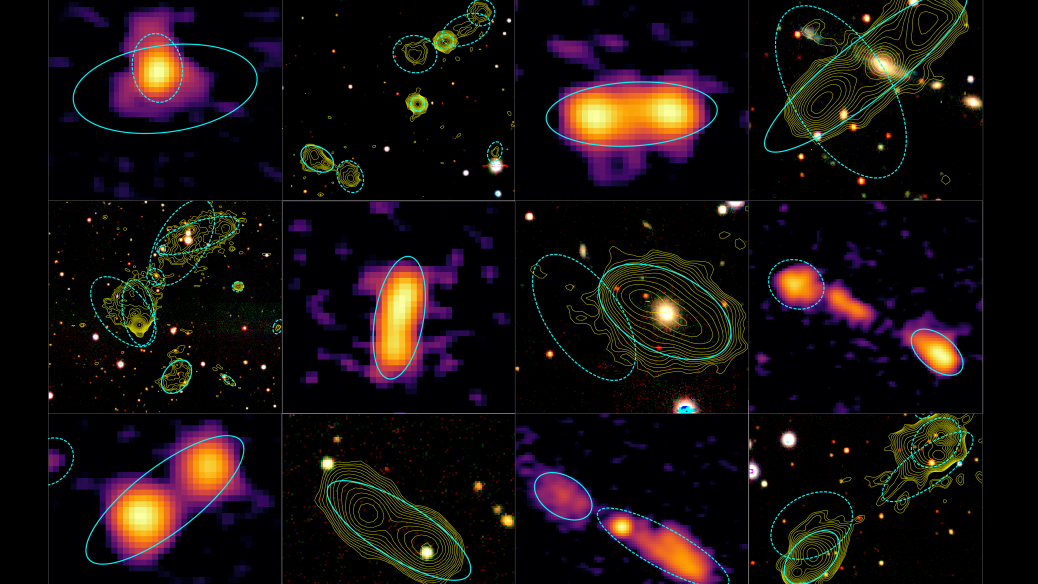
Out of This World
If you’re interested in contributing to astronomy research from the comfort and safety of your sidewalk or backyard, check out Globe at Night . The project monitors light pollution by asking users to try spotting constellations in the night sky at designated times of the year . (For example, Northern Hemisphere dwellers should look for the Bootes and Hercules constellations from June 13 through June 22 and record the visibility in Globe at Night’s app or desktop report page .)
For the amateur astrophysicists out there, the opportunities to contribute to science are vast. NASA's Wide-field Infrared Survey Explorer (WISE) mission is asking for volunteers to search for new objects at the edges of our solar system with the Backyard Worlds: Planet 9 project .
Galaxy Zoo on Zooniverse and its mobile app has operated online citizen science projects for the past decade. According to the project description, there are roughly one hundred billion galaxies in the observable universe. Surprisingly, identifying different types of galaxies by their shape is rather easy. “If you're quick, you may even be the first person to see the galaxies you're asked to classify,” the team writes.
With Radio Galaxy Zoo: LOFAR , volunteers can help identify supermassive blackholes and star-forming galaxies. Galaxy Zoo: Clump Scout asks users to look for young, “clumpy” looking galaxies, which help astronomers understand galaxy evolution.
If current events on Earth have you looking to Mars, perhaps you’d be interested in checking out Planet Four and Planet Four: Terrains —both of which task users with searching and categorizing landscape formations on Mars’ southern hemisphere. You’ll scroll through images of the Martian surface looking for terrain types informally called “spiders,” “baby spiders,” “channel networks” and “swiss cheese.”
Gravitational waves are telltale ripples in spacetime, but they are notoriously difficult to measure. With Gravity Spy , citizen scientists sift through data from Laser Interferometer Gravitational-Wave Observatory, or LIGO , detectors. When lasers beamed down 2.5-mile-long “arms” at these facilities in Livingston, Louisiana and Hanford, Washington are interrupted, a gravitational wave is detected. But the detectors are sensitive to “glitches” that, in models, look similar to the astrophysical signals scientists are looking for. Gravity Spy teaches citizen scientists how to identify fakes so researchers can get a better view of the real deal. This work will, in turn, train computer algorithms to do the same.
Similarly, the project Supernova Hunters needs volunteers to clear out the “bogus detections of supernovae,” allowing researchers to track the progression of actual supernovae. In Hubble Space Telescope images, you can search for asteroid tails with Hubble Asteroid Hunter . And with Planet Hunters TESS , which teaches users to identify planetary formations, you just “might be the first person to discover a planet around a nearby star in the Milky Way,” according to the project description.
Help astronomers refine prediction models for solar storms, which kick up dust that impacts spacecraft orbiting the sun, with Solar Stormwatch II. Thanks to the first iteration of the project, astronomers were able to publish seven papers with their findings.
With Mapping Historic Skies , identify constellations on gorgeous celestial maps of the sky covering a span of 600 years from the Adler Planetarium collection in Chicago. Similarly, help fill in the gaps of historic astronomy with Astronomy Rewind , a project that aims to “make a holistic map of images of the sky.”
Get the latest Science stories in your inbox.
/https://tf-cmsv2-smithsonianmag-media.s3.amazonaws.com/accounts/headshot/rachael.png)
Rachael Lallensack | READ MORE
Rachael Lallensack is the former assistant web editor for science and innovation at Smithsonian .
Science Fair Central
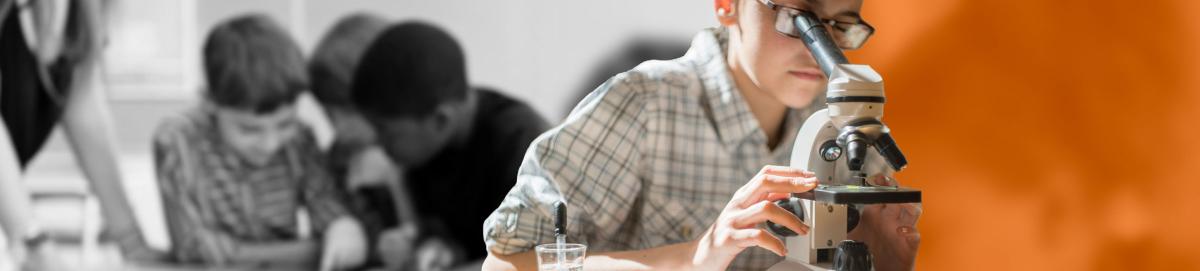
Investigation
The key to a good and manageable investigation is to choose a topic of interest, then ask what is called a “testable question.” Testable questions are those that can be answered through hands-on investigation by the student. The key difference between a general interest science question and a testable question is that testable questions are always about changing one thing to see what the effect is on another thing.
Select a Life Science, Earth Science or Physical Science category below to be presented with starter investigations.
Select a category and investigation.
Scientific Question A scientific question can be answered through an investigation.
What is tested? Scientists call this an independent variable. This is the one thing the investigator chooses to change.
What stays the same? A variable that is not changed, and stays the same, is called a constant.
Data collected: Data can be qualitative, describes physical properties, or quantitative, uses numbers and tools to collect data.
What factors affect seed germination?
What is tested? Factors (e.g. seeds in microwave, soaked seeds, etc.) What stays the same? Amount of water, light and soil nutrients Data collected: Amount of growth over time
What amounts of light promote algae growth in a fish tank?
What is tested? Hours of light in tank each day (e.g. 24 hours, 12 hrs, 6hrs, 2hrs, 0hrs.) What stays the same? Set up fish tank (e.g. gravel, plants, fish) Data collected: Amount of algae visible on sides of tank
What medium is best for seeds to sprout?
What is tested? Medium for planting (e.g. soil, cotton, rock wool, peat plugs, sand) What stays the same? Amount of light, water, plant type Data collected: Growth of plant over time
How does temperature affect the water uptake of celery plants?
What is tested? Temperature of water What stays the same? Size of celery plant, amount of water Data collected: Water uptake over time
Which parts of a bean seed are needed to grow a healthy bean plant?
What is tested? Seed parts (e.g. 1 cotyledon vs. 2 cotyledons, embryo, full seed) What stays the same? Soil, amount of light, temperature, location Data collected: Growth and health of bean plant over time
How are transpiration rates affected by the measured area of a leaf?
What is tested? Size area of leaves, type of leaf What stays the same? Number of leaves in transpiration collection bag, environmental conditions Data collected: Amount of transpiration
How does size affect the breathing rates of tropical fish?
What is tested? Size of fish What stays the same? Species of fish, time interval for observation Data collected: Average number of times different sized fish open and close their mouths over a given time period
How does temperature impact the activity of ants
What is tested? Temperature (eg. room temperature, refrigerator for 8 minutes) What stays the same? Number of ants in enclosed observatory Data collected: Pace and type of ant activity
How does time of day affect the problem solving ability of a hamster in a maze?
What is tested? Time of day (e.g. morning, noon, night) What stays the same? Maze, incentive Data collected: Time it takes to complete the maze
How does surface affect the travel rate of a caterpillar?
What is tested? Surfaces (e.g. bumpy, smooth, shiny, scratchy) What stays the same? Time, caterpillar Data collected: Distance traveled over time
What foods do worms prefer in a compost bin?
What is tested? Type of food (e.g. watermelon rind, coffee grounds, bread, corncobs) What stays the same? Number of and type of worm Data collected: Amount of food eaten over time
Which conditions attract the most insect pests (mosquitos, flies, gnats)?
What is tested? Conditions (e.g. dark or light clothing, sweet smelling or fragrance free plants, lactic acid/sweat) What stays the same? Time of day, length of time observed Data collected: Number of insect pests attracted to condition
What natural products are best at repelling insect pests (mosquitos, flies, gnats)?
What is tested? Natural products (e.g. citronella oil, eucalyptus oil, castor oil , peppermint oil, olive oil or vegetable cooking oil) What stays the same? Amount of oil, time of day, length of time observed Data collected: Number of insect pests attracted to product
What conditions affect the sleep of domesticated puppies?
What is tested? Conditions (e.g. ticking alarm clock, classical music, large stuffed dog, etc.) What stays the same? Species of puppy, age of puppy Data collected: Amount of time it takes puppy to fall asleep
How does temperature affect the development of a caterpillar?
What is tested? Temperature What stays the same? Age of caterpillar Data collected: Amount of time for metamorphosis
What type of food and feeder will attract the most cardinals?
What is tested? Bird food and feeder type What stays the same? Location, time of year Data collected: Number of cardinals attending each type of bird feeder with different foods at a specified time each day
How does moisture affect the tunneling ability of ants?
What is tested? Sand (dry and moist), Soil (dry and moist), Clay (dry and moist) What stays the same? Number of ants; type of closed observatory; temperature, light, food Data collected: Time it takes for ants to build a tunnel
How do laws/policies on deer affect deer populations?
What is tested? Laws/deer policies in different areas or states What stays the same? Time, data collection methods Data collected: Number of deer
How do the behaviors of domesticated cats compare to the behaviors of wild cats in zoo captivity?
What is tested? Type of domesticated cat (e.g. calico, Siamese, etc.), type of wild cat (e.g. lion, tiger, bobcat, etc.) What stays the same? Amount of time observed Data collected: Behaviors observed
Are natural sponges more absorbent than synthetic sponges?
What is tested? Type of natural sponge, type of synthetic sponge What stays the same? Amount of liquid absorbed, sponging method Data collected: Remaining liquid
Health / Human Body
What conditions impact productivity while doing homework.
What is tested? Conditions (e.g. tv, music, etc.) What stays the same? Time, age/skill level of participants Data collected: Amount of work completed
How does smell affect taste?
What is tested? Foods What stays the same? Nose plugs, time Data collected: Ability to identify foods while blindfolded
How do different style pencils or grips affect writing fatigue?
What is tested? Different style pencils or grips What stays the same? Writing phrase, age/skill of participants Data collected: Amount of time writing before fatigue
Which brand of skin moisturizer lasts the longest?
What is tested? Brand of body lotion What stays the same? Location, amount of lotion Data collected: Skin dryness over time
Do reflexes change with age?
What is tested? Age of participants What stays the same? Reflex test Data collected: Participant success at completing reflex task
How does photo-editing affect perception?
What is tested? Photo-editing techniques (e.g. lighting change, cropping, expanding a picture) What stays the same? Original picture Data collected: Participants perceptions of how a picture makes them feel
How do different liquids affect the health of a simulated tooth?
What is tested? Liquids (e.g. coffee, tea, juice, soda, vinegar, water, flavored water) What stays the same? Simulated tooth (e.g. chicken bone), amount of liquid, amount of time submersed in liquid Data collected: Characteristics of simulated tooth (e.g. firmness, brittleness, color, etc.)
How do different types of movement activity affect human heart rate?
What is tested? Activity (e.g. walking vs.running, etc.) What stays the same? Same person or people in each trial, starting heart rate, exercise route, temperature) Data collected: Heart rate of the person(s)
What conditions improve short term memory?
What is tested? Conditions (e.g. mnemonics, rehearsal, association, music) What stays the same? Test, age and initial skill of participants Data collected: Achievement on memory test
How does air moisture affect healing time of a stuffy nose?
What is tested? Type of moisture (e.g. cool mist, warm mist, no mist), hours of mist (e.g. 2 hrs., 8 hrs.) What stays the same? Age of participants, time onset of stuffy nose Data collected: Cleansing of nasal passage over time
Microorganisms
What is the best way to reduce odor in shoes.
What is tested? Odor reducing methods (e.g. open air, baking soda, etc.) What stays the same? Type of shoe, initial odor of shoes Data collected: Odor of shoe over time
At what temperature does a yogurt culture grow best?
What is tested? Temperature What stays the same? Type and amount of milk, yogurt bacteria, container size Data collected: Time it takes for the milk to turn to yogurt
How does the location of water samples affect the number of micro-organisms observed?
What is tested? Location What stays the same? Amount of water Data collected: Number and type of micro-organisms
What conditions keep bread mold from growing on bread?
What is tested? Conditions of containment, temperature, moisture What stays the same? Bread mold, type of bread Data collected: Spread of bread mold over time
How does the bacteria in the mouth of a human, dog, cat, or hamster compare?
What is tested? Animal (human, dog, cat, hamster) What stays the same? Number of scrapes from tongue, time for bacteria to grow Data collected: Amount of bacteria in culture after specified time
What conditions improve bread yeast growth?
What is tested? Water temperature, nutrient amount (sugar), nutrient type (type of sugar or other nutrient) What stays the same? Container size and shape, amount of water, type of yeast, amount of yeast Data collected: Height of foam in container
Environment
Does recycled paper decompose more quickly than non-recycled paper.
What is tested? Type of paper What stays the same? Time, location (e.g. depth buried in ground) Data collected: Condition of the paper
Which type of inside window covering is best for saving energy?
What is tested? Type of window treatment What stays the same? Amount of sunlight, outside temperature, time Data collected: Inside temperature
How does oil affect the growth of aquatic plants?
What is tested? Concentration or amount of oil What stays the same? Amount or water, number/type of aquatic plants Data collected: Condition of plant
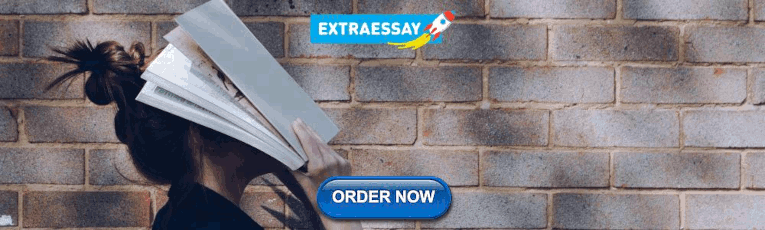
How does acid rain affect plant growth?
What is tested? Various concentrations of vinegar What stays the same? Type of plant, amount of light, soil nutrients Data collected: Condition of the plant over time
How does a surface affect the rate it is warmed?
What is tested? Surfaces (e.g. soil, water, sand) What stays the same? Amount and direction of heat, time observed Data collected: Temperature of surfaces at different time intervals
Does the sun heat saltwater and freshwater at the same rate?
What is tested? Salinity of water (grams of salt per liter) What stays the same? Container starting temperature Data collected: Temperature over time (1 hour)
How does topography affect wind speed?
What is tested? Topography/location (e.g. open field, city, forested area) What stays the same? Time tested Data collected: Wind speed
How does air temperature affect the rate of evaporation?
What is tested? Temperature What stays the same? Initial amount of water Data collected: Amount of water evaporated over time
How does weather affect mood?
What is tested? Weather (e.g. rainy, sunny, cloudy, cool, hot) What stays the same? Age of participants Data collected: Participants daily responses (3-4 weeks) to survey question: On a scale of 1-5 How do you feel today (Participants should complete survey prior to being told that it is about weather.)
Which method is best for melting icy pavement?
What is tested? Materials (e.g. types of salt, sand, kitty litter mixtures), methods: e.g amount of time material sits on ice, crushing ice before putting on ice melting material, etc.) What stays the same? Thickness of ice, temperature of air and ice Data collected: Amount of ice after 10 min and 20 min.
Which type of soil can hold the most water?
What is tested? Type of soil What stays the same? Amount of soil, amount of water, container Data collected: Amount of water loss
Which conditions stop erosion the best?
What is tested? Conditions of soil (e.g. plant cover, trench or terrace, no cover) What stays the same? Amount of water, slope, type of soil Data collected: Mass of filtered soil that eroded
How do different liquid solutions with varying pH levels affect rocks?
What is tested? Type of solution (e.g. vinegar, water, or mixture) What stays the same? Rocks tested (be sure to include chalk), amount of liquid Data collected: Effect on rock (e.g. bubbles or no bubbles)
What conditions are best for making the tallest sandcastle?
What is tested? Construction methods What stays the same? Amount of sand Data collected: Height of castle
What factors are best to grow sugar crystals?
What is tested? Temperature OR saturation (how much was dissolved in the water) What stays the same? Type of crystal, amount of water, temperature Data collected: Mass of crystals, number of crystals, length of time to form crystals
Do major earthquakes cause minor earthquakes nearby?
What is tested? Location of major earthquakes (e.g. check national geological service data) What stays the same? Distance from the major earthquake, time period, earthquake magnitude Data collected: Number of minor earthquakes that occur nearby during the time period
Which structure of model house is best at withstanding a model earthquake?
What is tested? Size of house, type of construction of house What stays the same? Amount/time shaken, shaking mechanism Data collected: Condition of shaken house
What is the best way to clean or remove deposits from a copper penny (or other precious metal)?
What is tested? Cleaning methods What stays the same? Initial color of penny, amount of copper in penny Data collected: Color of penny
How do fins on a rocket affect its flight?
What is tested? Size of fins What stays the same? Shape and number of fins, fin placement, type and size of rocket, propulsion system (amount of push), air movement, temperature, launch site Data collected: Distance the rocket flies or trajectory (how straight the rocket flies)
What conditions produce craters?
What is tested? Type of surface (e.g. sand, gravel, clay, silt or flour, mass of falling sphere What stays the same? Height from which sphere is dropped Data collected: Pattern of crater
How do fins on a straw rocket affect its flight?
What is tested? Size, shape and number of fins What stays the same? Type and size of rocket, propulsion system (amount of push), air movement, temperature, launch site Data collected: Distance the rocket flies or trajectory (how straight the rocket flies)
Which egg substitutes are best for different recipes?
What is tested? Type of egg substitute What stays the same? Recipe procedures except for egg Data collected: Taste, texture of food
How does temperature affect the rate of a simple reaction?
What is tested? Temperature What stays the same? Size of antacid tablet, amount of water Data collected: Amount of time for antacid tablet to dissolve
How does particle size affect the rate of a reaction?
What is tested? Particle size (whole, half, and powder) of an antacid tablet What stays the same? Amount of water, temperature of water Data collected: Amount of time for antacid tablet to dissolve
How does temperature affect the brewing of tea?
What is tested? Temperature What stays the same? Amount of water, type/size tea bag Data collected: Brew strength over time
Which detergent is best for removing stains?
What is tested? Detergent What stays the same? Size, type, and age of stain, fabric or carpet, physical process of stain removal Data collected: Color of fabric (e.g. stained, faded, completely disappeared)
What conditions are best to prevent soda from losing carbonation?
What is tested? Conditions [e.g. temperature- (refrigerated/room temp) and containers-glass, thermos, open cup, closed cup)] What stays the same? Brand of soda Data collected: Carbonation bubbles observed over time
Energy / Force
What type of paper makes the best paper airplane.
What is tested? Type of paper What stays the same? Design of plane, size of paper, thrust, air currents Data collected: Distance plane travels using the same amount of thrust
How does mass affect a pendulum swing?
What is tested? Mass (Number of washers/weights) What stays the same? Length of string, time tested Data collected: Number of swing periods
What is the best shock absorber for dropping an egg?
What is tested? Shock observers (e.g. feathers, foam, towels, etc.) What stays the same? Height of drop, size of egg Data collected: Wholeness of the egg (How much did the egg crack?)
How does the angle of impact affect patterns observed in falling water balloons?
What is tested? Angle (e.g. straight 90 degree vs. angled 45 degree) What stays the same? Size of water and procedures for making water balloon, force, height of drop Data collected: Pattern observed at impact site
What type of ball bounces the highest?
What is tested? Type of ball What stays the same? Size of ball, height from which ball is dropped Data collected: Bounce height
How does changing the height from which a ball is dropped impact its bounce?
What is tested? Drop height What stays the same? Ball Data collected: Bounce height
How does mass affect the distance of a catapulted object?
What is tested? Mass of object What stays the same? Design of catapult Data collected: Distance object travels
What is the best shape for a parachute?
What is tested? Shape of the parachute What stays the same? Drop height, wind, area of parachute Data collected: Time for parachute to complete descent
What is the best material to use for a parachute?
What is tested? Different materials What stays the same? Drop height, size and shape of parachute, wind Data collected: Time for parachute to complete descent
How does temperature affect a magnet’s strength?
What is tested? Temperature of magnet What stays the same? Type, shape, and size of magnet, type of thermometer, placement of thermometer on the magnet Data collected: Magnet’s ability to hold an object
What is the best insulator to keep ice from melting?
What is tested? Type of insulation in a container What stays the same? Amount of ice, starting temperature Data collected: Time for ice to completely melt
How does the size of a vibrating surface affect pitch?
What is tested? Size of object (e.g. length of a ruler) What stays the same? Method for vibrating the object Data collected: Pitch (e.g. high, medium, and low)
What materials conduct heat the best?
What is tested? Spoons made of different materials What stays the same? Amount of water, temperature of the water and air, amount of butter attaching a bead indicator to each spoon Data collected: Time it takes for heat to rise up the spoon causing the butter to melt and the bead indicator to detach from the spoon
How does the position of a fulcrum affect the ability of a lever to do work?
What is tested? Position of fulcrum What stays the same? Mass of load Data collected: Effort
Which conditions produce the best echo?
What is tested? Locations What stays the same? Sound making instrument Data collected: Loudness of echo
How do pulleys affect the amount of mass that can be lifted?
What is tested? Types of pulleys What stays the same? Mass Data collected: Ease of lifting mass
How does the surface area of a drum affect its pitch?
What is tested? Surface area of drum What stays the same? Material and construction of drum, shape of drum, drumstick, and force for hitting drum Data collected: Pitch
What materials are best for conducting electricity?
What is tested? Materials What stays the same? Battery, circuit arrangement Data collected: Ability of light bulb to light up
What conditions make for the fastest sailboat?
What is tested? Area of sail, material of sail, shape of boat, mass of boat What stays the same? Wind speed on fan Data collected: Distance traveled over time
What conditions make a top that spins the longest?
What is tested? Mass of the top, length of the axle What stays the same? Procedures for constructing the top Data collected: Spinning time
How do different surfaces affect friction?
What is tested? Different surfaces What stays the same? Slope of ramp, mass/shape/material of object slid on ramp Data collected: Time it takes object to slide down the ramp
How does the diameter of the hose affect the speed of water in a siphon?
What is tested? Diameter of water hose What stays the same? Siphoning procedure Data collected: Speed of water
Which paper towel absorbs the fastest?
What is tested? Brand of paper towel What stays the same? Amount of paper towel, amount of water Data collected: Time it takes for water to flow from one end of the paper towel to the other
How does temperature affect air pressure in a tire?
What is tested? Temperature What stays the same? Brand and type of tire Data collected: Air pressure
How does a container’s size and shape affect evaporation rates?
What is tested? Containers of varying size and shape (e.g. tall, short and wide, etc.) What stays the same? Initial amount of water, heat source Data collected: Amount of evaporation over time
How does exposure to sunlight affect the strength of a plastic bag?
What is tested? Plastic bags exposed to varying amounts of sunlight (e.g. 2 weeks, 1 week, 1 day, 1 hour etc.) What stays the same? Type of plastic bag, size of plastic bag Data collected: Amount of mass the bag can hold without breaking
Which types of paper have the least amount of fading in sunlight?
What is tested? Types of paper (e.g. construction paper, newspaper, advertisements, etc.) What stays the same? Area and thickness of paper Data collected: Fading over time
Which is the best liquid solvent for separating a marker stain?
What is tested? Solvents What stays the same? Marker stain, amount of solvent, time, filter paper Data collected: Number/colors observed on filter paper
Which type of microwave popcorn pops the best?
What is tested? Brand of microwave popcorn What stays the same? Time in microwave, microwave power level, container, position in microwave Data collected: Percentage of popcorn popped (number of popped kernels compared to number of unpopped kernels)
How does the type of liquid impact the time it takes for the liquid to freeze?
What is tested? Liquid type (e.g. water, syrup, detergent) What stays the same? Size of container, temperature Data collected: Time it takes to freeze
How does salinity affect th freezing point of liquids?
What is tested? Water with varying amounts of salt What stays the same? Temperature, size of container Data collected: Time it takes to freeze
What is the best way to remove wrinkles from fabric?
What is tested? Varying moisture amounts What stays the same? Type of fabric, amount of heat Data collected: Number of wrinkles observed
How does density affect the buoyancy of objects?
What is Tested? Objects of different mass and sizes What Stays the Same? Objects tested, liquid Data Collected: Level at which the object floats, is suspended or sinks.
Which brand of paper towels is the strongest when wet?
What is Tested? Brand of Paper Towels What Stays the Same? Amount of weight tested on each towel, size and position of paper towel Data Collected: Amount of weight it takes for the paper towel to break
How does temperature affect the drying time of paint?
What is Tested? Varying temperatures, type of paint What Stays the Same? Amount and surface of paint Data Collected: Dryness over time
Sample Scientific Investigation Projects
Kids workshops.
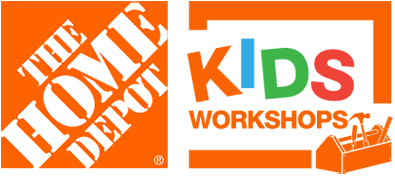
To learn more visit, homedepot.com/kids
Kids Workshops provide a mix of skill-building, creativity, and safety for future DIYers every month in Home Depot stores across the country. After registering for the next Workshop, download these exclusive extension activities from Discovery Education. Each extension provides opportunities to reimagine or use their Workshop creation in an unexpected new way.
Blooming Picture Frame
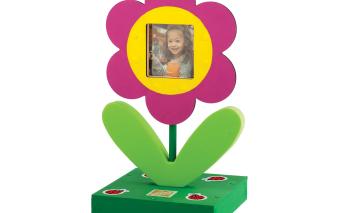
Use nature as inspiration for creative writing. Students will research a variety of flowers to create a poem for their Blooming Picture Frame.
Lattice Planter
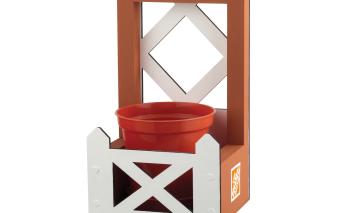
Explore the features of plants and consider how they impact our world. Students will select the type of plant they want to grow and map out a watering schedule to ensure it thrives in their Lattice Planter
Butterfly House
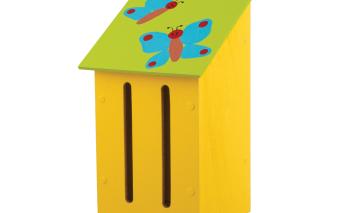
Do butterflies have a favorite spot to hang out? Students will create an observation chart, watch butterfly activity, and then analyze their results to select the ideal location for their Butterfly House.
- Learn center
- Design & UX
Discovery research is a UX essential — Here’s how to get started
Georgina Guthrie
April 13, 2022
Design is all about problem-solving. Often, these are quite big problems with complex answers — so designers break the process down into different stages to make it more manageable. These stages generally focus on research, design, and testing/development.
Today, we’ll take a closer look at how designers can move between the first two stages to gather information and test their ideas before fully launching into the development phase.
With this back-and-forth approach, it’s possible to analyze and make informed decisions about which findings to take forward. Discovery research ultimately leads to a final product that meets users’ needs— not just the designer’s assumptions .
What is discovery research?
Discovery research (also called generative, foundational, or exploratory research) is a process that helps designers understand user needs, behaviors, and motivations through different methods, such as interviews, surveys, and target market analysis.
Discovery research is related to product research but involves a broader analysis. Whereas the former deals with all kinds of research — for brands, innovations, products, and more — the latter is solely focused on the product.
How does discovery research help with design?
Discovery research helps designers understand user needs, behaviors, and motivations, which form the basis of key design decisions.
Conducting this early-stage analysis also ensures that designs are based on real user needs rather than the designer’s assumptions. This approach leads to products that feel more like tailor-made creations rather than a broad approximation of what users want.
Finally, it saves time and money by revealing potential problems before they become bigger (and more expensive) issues further down the line.
What are the main goals of discovery research?
- Understanding your users better : the first and most important goal of discovery research is to help you get under the skin of your users. By understanding user goals and pain points, you can design solutions that address their needs.
- Improving design decisions : the second goal of discovery research is to improve design decisions. Instead of simply creating a product the design team thinks is cool, you can develop a product roadmap based on relevant data.
- Save time and money : testing before leaping right into development means you can spot potential problems and work through them, investing time and resources wisely.
- Creating a shared vision : discovery research can create a shared vision for a project among the design team. Because the research provides a common understanding of user needs, design teams can more easily agree on what to prioritize.
What are the benefits of using both qualitative and quantitative research methods?
Qualitative research is based on open-ended questions and provides insights into people’s attitudes, opinions, and feelings. Typically, this research involves interviews, focus groups, or surveys. Quantitative research, on the other hand, uses closed-ended questions and focuses on hard data, including:
- Performance analytics : websites and apps contain a wealth of numerical data. Google Analytics can show you everything from the number of page views to time spent on a page.
- Target market analysis : demographic research looks at characteristics such as the age, gender, and location of your target market. It’s often collected through surveys and distributed via email.
The benefits of using qualitative and quantitative research methods are twofold.
Qualitative research is often viewed as ‘creative’ and exploratory, while quantitative research is considered more ‘scientific’ and focused. Both types of research reveal something different, each with its strengths and weaknesses.
Qualitative research is good for exploring new ideas and getting an in-depth understanding of user needs. However, it’s often less reliable than quantitative research and deals with smaller samples, which may not represent the wider population.
Quantitative research is good for obtaining hard data and measuring people’s feelings about specific topics or activities. The downside is it’s less nuanced than qualitative research and may provide a less multifaceted analysis of user needs.
Using both qualitative and quantitative research methods, designers can get a complete picture of user needs.
When should you run a discovery session?
Use a discovery session any time the design team needs to move forward in a design and/or when relying on guesswork or intuition is impossible or risky.
Here are some common real-life examples:
- New market opportunities : companies that want to enter a new market must understand user needs and identify opportunities to fill current gaps.
- Rebranding : before rebranding , organizations have to understand how users feel about the current brand, what they want from it, and what issues to avoid moving forward.
- Redesign : when redesigning a product, design teams need to understand what users like and dislike about the current product and how they can innovate in the future .
- Mergers : to ease the transition, merging companies need to understand how employees from both companies feel about the merger and design processes to meet their needs .
- New organizational strategy : when implementing a new strategy, organizations must consider how employees view the upcoming changes and communicate plans and expectations .
- Organizational problems : companies that are struggling with organizational problems must investigate the root cause of the problem to develop effective solutions.
How do you run a discovery research session?
The exact route you take will depend on your goals. Sometimes, you’ll want to use a mixture of methods (the more, the better). At other times, you’ll focus on one or two options. Here are some common discovery research methods.
User interviews
Interviews are a common qualitative research method. They involve sitting down with users and asking open-ended questions about their needs, behaviors, and motivations. Interviews are very useful for understanding user feelings and attitudes in their own words prior to any design work taking place.
Focus groups
Focus groups are a type of qualitative research that involves a group of people discussing a topic together. Not only does this help you find out how people feel about a design, but it also draws out deeper responses as participants build on each other’s comments.
Tips for running a focus group
- The ideal group size is around eight to ten people. To get started, you’ll need to define the topic of discussion and prepare some questions to spark conversation.
- When conducting the focus group, it’s important to moderate the discussion effectively. Keep things on track, offer up discussion points if the momentum slows, and ensure everyone can speak.
- Once the focus group is over, analyze the data you collected. Write a transcript of the discussion, or use diagramming software to help with the analysis.
Surveys are a quantitative research method that asks closed-ended questions about user needs. However, including a few open-ended questions is common to provide context for a user’s responses to closed-ended questions. This type of research is useful for obtaining hard data.
Decide what type of questions you want to ask: closed-ended or open-ended. Closed-ended questions have a ‘yes’ or ‘no’ answer, or participants can choose a specific response from a list of options. Open-ended questions can have a longer, freeform answer subject to various conditions.
Ethnographic user research
Ethnographic user research is a form of qualitative research in which you observe users in their natural environment. This type of research is useful for understanding user behaviors and needs.
Tips for conducting ethnographic user research
- Define the scope of your research, and decide on the observation methods prior to session kick-off.
- Choose one to three research methods that suit your resources and goals. Interviews, surveys, and user testing are all valid forms of observation.
- Once you collect user data, collate and analyze it. At this point, you’ll have dense information. Turning the raw data into business intelligence that makes sense for the wider team and stakeholders is important.
Diary studies
Diary studies are a qualitative research method asking participants to write down their thoughts and feelings about a given topic. Journaling gives a glimpse of a user’s thought processes, so you can better understand how they feel about a design or prototype.
Here are some questions you can ask to get the user thinking:
- What were your thoughts and feelings about the design/prototype?
- How easy was it to use the product?
- What did you like or dislike about it?
- Why did you feel that way?
- What problems did you encounter?
- How well did the design meet your needs?
Diary logging techniques you need to know
- Interval-contingent protocol : ask participants to record their thoughts and feelings at fixed intervals (e.g., every hour or every day). Use this type of diary study to understand how people feel over time.
- Event-contingent protocol : ask participants to record their thoughts and feelings after specific events, such as using a feature or carrying out a particular process. Choose this format to study how people react to specific events.
- Saturation sampling : ask participants to keep a diary until they have nothing new to say about the topic. Similar to interval methods, this diary study helps evaluate user feelings over time.
- Choice sampling : give participants a list of topics to choose from and ask them to record their thoughts and feelings about their chosen topic. This study helps you understand how people feel about different design aspects and what issues are most important to them.
Tips for conducting diary studies
- Make sure you store the data securely if the diaries contain personal or sensitive information.
- Define the study’s goals and the logic you’ll use to evaluate the data you receive. Diary studies can be time-consuming for both participants and researchers. As such, ensuring the study is well-designed and the results are worth the effort is crucial.
- Provide participants with an incentive to take part. Diary studies require time and energy, so it’s a good idea to compensate participants with a gift voucher or free product.
Sort cards are a type of qualitative research that involves asking participants to sort a set of cards into groups. The goal is to observe how people think about a particular topic and design intuitive products.
Where else can you find data?
Chatting with users is important, but don’t neglect the wealth of data already at your fingertips. Web analytics, social media, and customer support data can give you insights into how your users think and feel.
- Business data : if you’re working on an internal tool, you probably have access to a lot of data about how it’s used. This information is invaluable for understanding the steps users take to perform an action or solve a problem.
- Web analytics data : this data tells you how people are using your website or app. Use it to understand what pages are being visited, how much time users spend on a page, and what elements they interact with.
- Social media data : social media can be a great way to understand how people feel about your brand. Use social listening tools to track mentions of your brand and see what people are saying.
- Customer support data : if you offer customer support, the data can show you what problems people encounter when using your product.
- Competitor resources : it’s worth looking at competitor resources, such as websites, blog posts, and whitepapers, for ideas on improving or differentiating your product.
Analyzing and assessing discovery research
So, you’ve got all this data. Now what?
It’s time to assess it. Evaluating your discovery research involves looking at the numbers and determining how it fits together. You can write a report or create a diagram or graph to help you visualize it all.
When assessing qualitative data, it is important to consider the following factors:
- The quality and reliability of the data : bad data could send you in the wrong direction. If in doubt, chuck it out.
- The quantity of the data : too much could be a burden when turning it into reports. Too little might give you unreliable results.
- The context of the data : make sure you apply data to the relevant area, but at the same time, don’t look at it in isolation.
- The meaning of the data : only include responses that directly answer your questions. Don’t include irrelevant or unclear data.
- The validity of the data : data goes out of date. Disregard anything that’s no longer relevant.
Final thoughts
Data visualization features, like those in Cacoo , can help turn all those numbers into insight that makes sense.
Resources like persona templates , user story maps , and other research and design diagrams can help you see patterns and trends in the data and communicate your findings to others — including stakeholders who might not have a technical background.
Remember, your top priority is to make the data as understandable as possible for everyone on the team — whatever their background. After all, data is only useful if it’s used and understood!
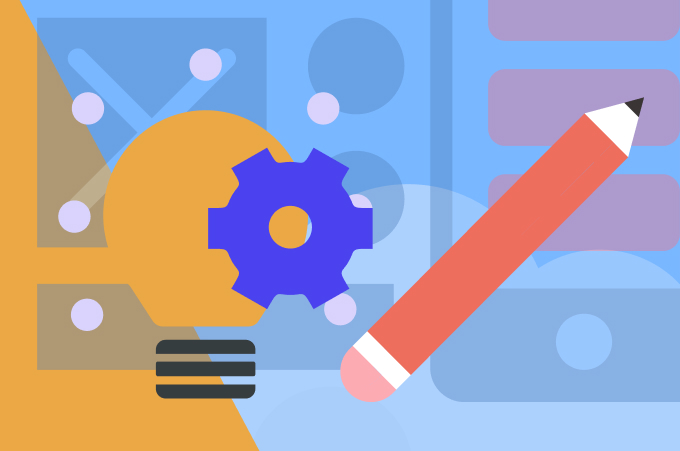
A detailed guide to the product design process
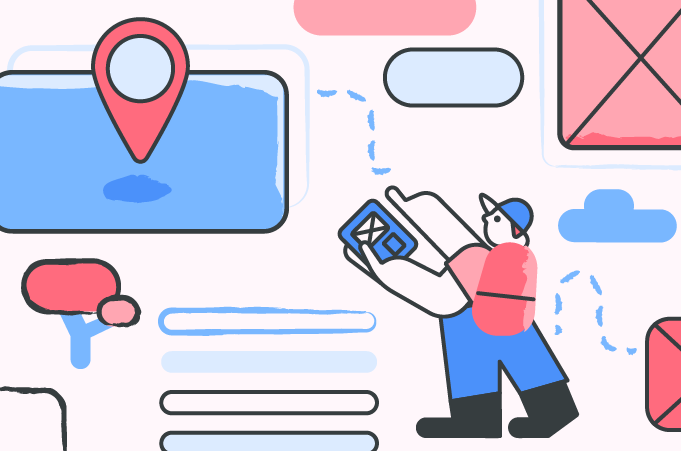
Here’s how to create a wireframe map (and why you should)
Subscribe to our newsletter.
Learn with Nulab to bring your best ideas to life
404 Not found
Your browser is not supported
Sorry but it looks as if your browser is out of date. To get the best experience using our site we recommend that you upgrade or switch browsers.
Find a solution
- Skip to main content
- Skip to navigation
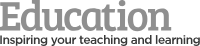
- Back to parent navigation item
- Find resources
Experiments and investigations
- Cross-curricular activities
- Meet the scientists
- Boost your knowledge
- Beyond the classroom
- Get funding
- About the RSC
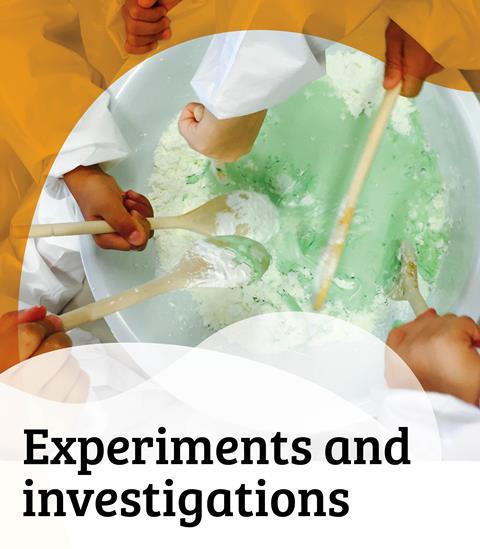
- More from navigation items
Inspiration and resources for experiments and investigations
Primary science investigations
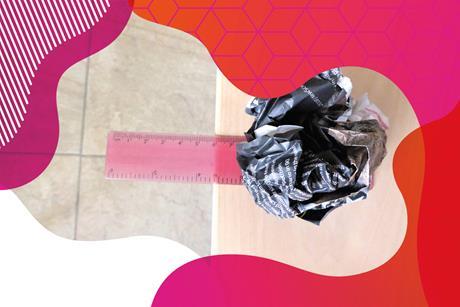
Air pressure, gases and the leaky bottle
Try this simple investigation to explore the effects of air pressure, with detailed teacher notes, classroom slides and a video demonstration.
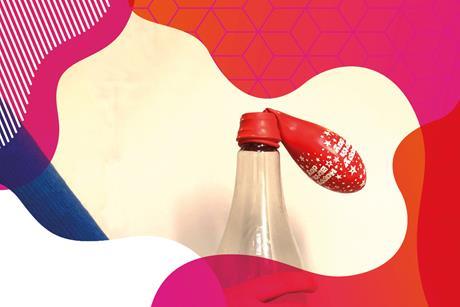
Properties of gases, air pressure and ‘sticky’ cups
Try this investigation to explore the effect of heat on gases, with detailed teacher notes, classroom slides and a video demonstration.
Dissolving, density and sugary drinks
Irreversible changes and the ‘freaky hand’, irreversible changes and the ‘fire extinguisher’, properties of solids and ‘biscuit bashing’, from kitchen to classroom.
Simple chemistry experiments using kitchen cupboard equipment.
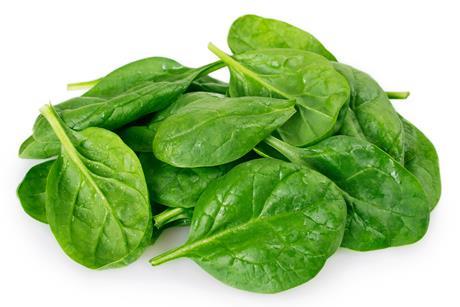
Chalky spinach
How does the food we eat affect our bodies? Discover the acid inside spinach, and why it can feel chalky
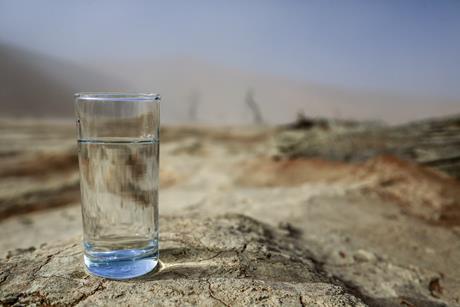
How to purify water
Explore the water cycle, and see how it can be used to purify your own water. A perfect experiment for the classroom, or at home, with kit list and safety instructions included.
Investigating surface tension with milk
Kitchen roll chromatography, how to make butter, demonstrations.
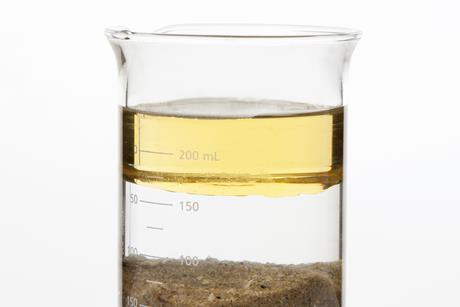
Separating mixtures | Primary science video demonstrations
Fun demonstrations of different mixtures, including racing liquids and rainbow colours.
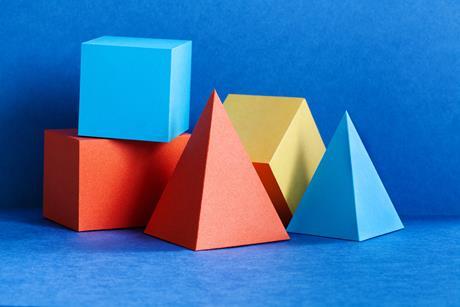
Solids | Primary science video demonstrations
Display some exciting solid properties to primary learners: including biscuit bashing, dissolving and heavy sugar.
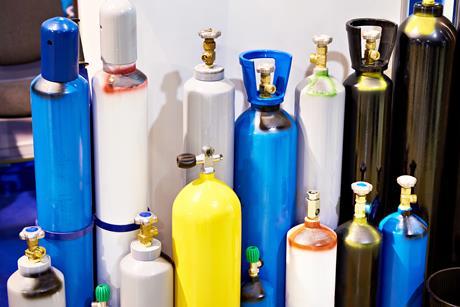
Gases | Primary science video demonstrations
Showing the properties of gases to primary learners, with examples from: the leaky bottle and the sticky cups
Developing scientific skills
Help your pupils develop their scientific skills with these experiments and resources
Asking scientific questions
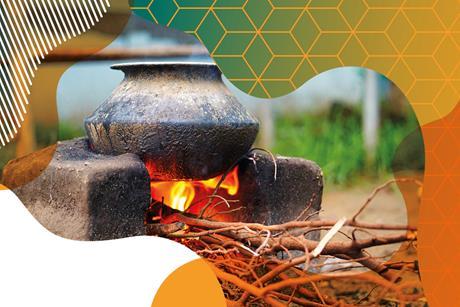
Pollutants produced by chemical changes | 9–11 years
Connect your curriculum teaching on chemical changes to engaging sustainability contexts. This topic web suggests classroom activities linked to plastic degradation and clean cooking.
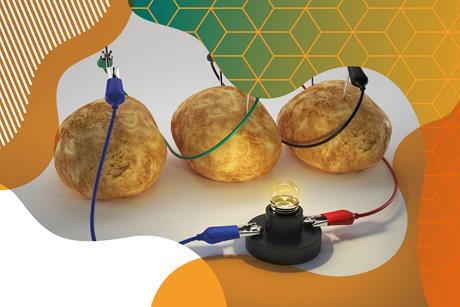
Electricity and batteries | 7–9 years
Connect your curriculum teaching on electricity to engaging sustainability contexts. This topic web suggests classroom activities linked to batteries and electric cars.
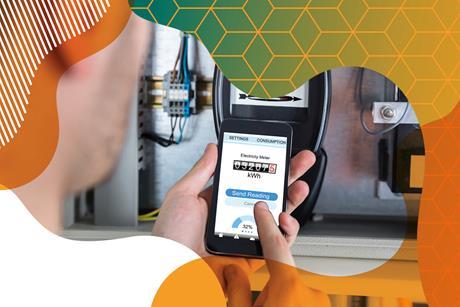
Electricity production and use | 9–11 years
Connect your curriculum teaching on electricity to engaging sustainability contexts. This topic web suggests classroom activities linked to sustainable sources of electricity and monitoring our electricity usage.
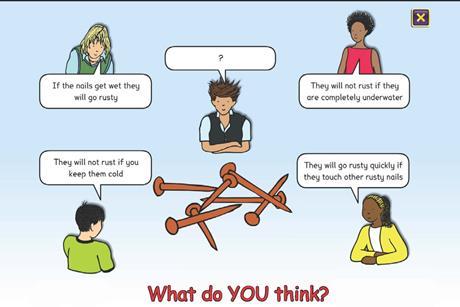
Science concept cartoons: rusting
In association with Millgate House Education
Use this conversation starter to get your pupils thinking about the process of rusting, and discussing different possibilities.
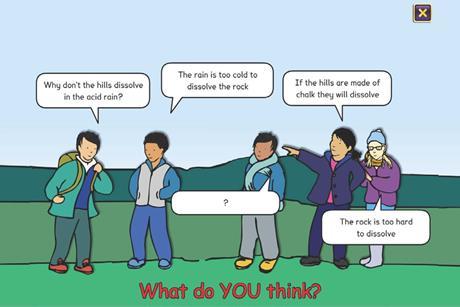
Science Concept Cartoons: Acid rain
Spark discussion and stimulate thinking about acid rain in a way that encourages pupils to share their ideas
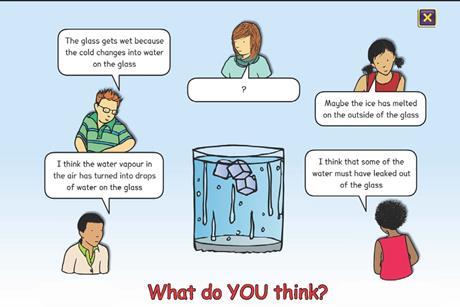
Science concept cartoons: condensation
Show this concept cartoon to your class to get them talking and thinking about condensation in new ways.
Observing and measuring
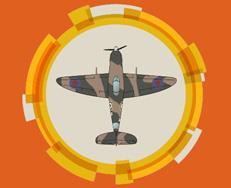
An Dàrna Cogadh: lìontan nam beachdan saidheans
Lìon nam beachdan mollaichte airson ceangal a dhèanamh eadar saidheans agus cuspair An Dàrna Cogadh. Faodaidh tu eachdraidh agus saidheans ionnsachadh còmhla le gnìomhachasan airson buidhnean de dh’aoisean diofraichte
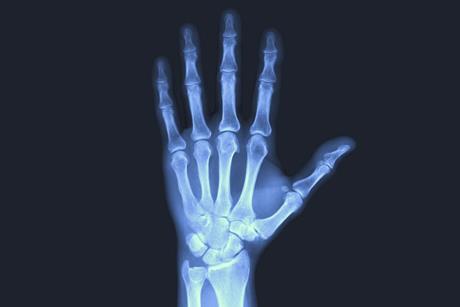
Bendy bones
What makes bones strong? Children can learn about the role that calcium plays in skeletons
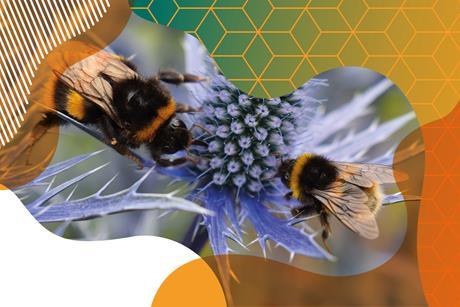
Biodiversity and habitats | 4–7 years
Connect your curriculum teaching on habitats to engaging sustainability contexts. This topic web suggests classroom activities linked to increasing biodiversity and protecting marine habitats.
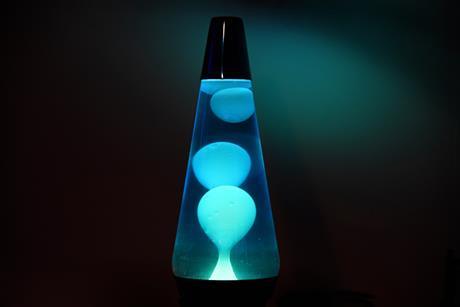
Changing materials | Primary science video demonstrations
Material changes are demonstrated using fun examples: bouncy custard, bath bombs, burning candles, fire extinguishers, lava lamps and many more.
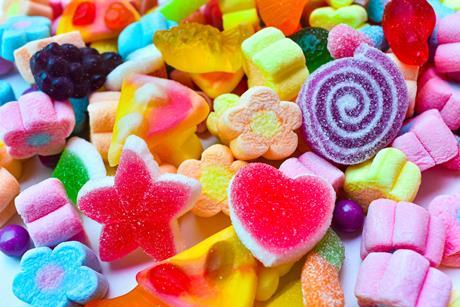
Colourful food: kitchen science podcasts
Introduce your students to colours in food with this short podcast.
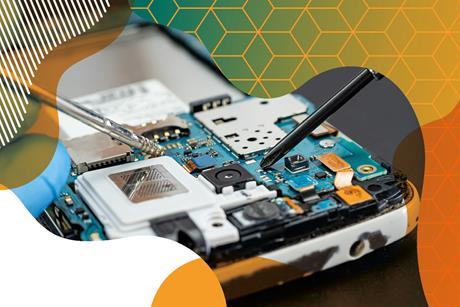
Decaying and materials in mobiles | 9–11 years
Connect your curriculum teaching on materials to engaging sustainability contexts. This topic web suggests classroom activities linked to the materials found in mobile phones and how different materials decay.
Making predictions
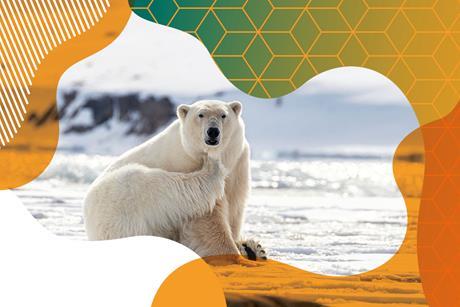
Protecting animals and their habitats | 7–9 years
Connect your curriculum teaching on habitats to engaging sustainability contexts. This topic web suggests classroom activities linked to protecting the habitats of orangutans and polar bears.
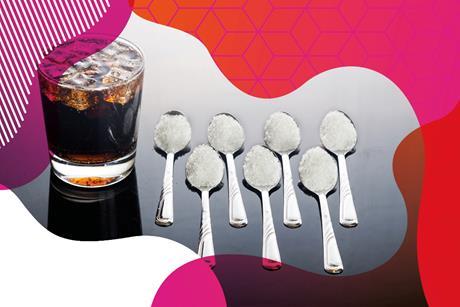
Try this investigation to get learners exploring the mass of sugar dissolved in their favourite drinks, with detailed teacher notes, classroom slides and a video demonstration.
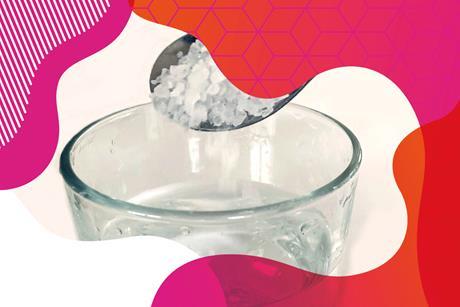
Freezing and the ‘intriguing ice’ experiment
Try this investigation to explore how materials change when they freeze, with detailed teacher notes, classroom slides and a video demonstration.
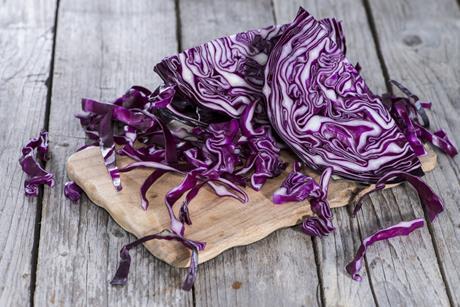
Red cabbage rainbows
In this activity, learners create rainbows using homemade red cabbage indicator paper. Includes video aimed at learners, kit list, instruction and explanation
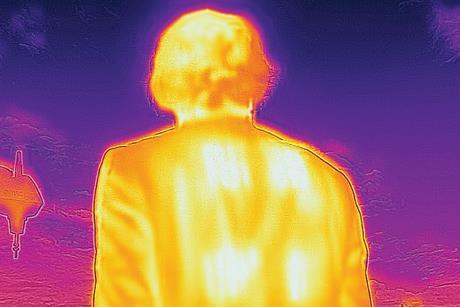
Insulation investigation
Which material makes the warmest jacket? Investigate the insulating properties of various materials with this activity for ages 7–14
Recording data
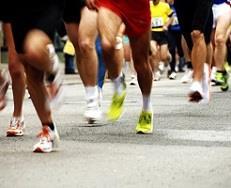
Chemistry in sport
Our very first global experiment compares the performance enhancement of student-made sports drinks vs water
Designing experiments
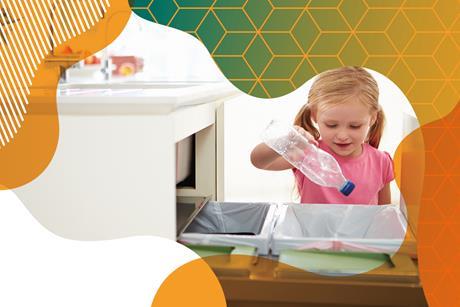
Materials, recycling and rubbish | 4–7 years
Connect your curriculum teaching on materials to engaging sustainability contexts. This topic web suggests classroom activities linked to recycling and how much rubbish is produced in your school.
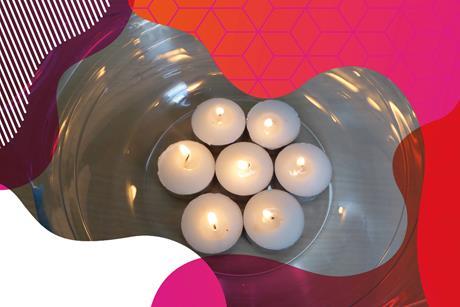
Try this investigation to explore what materials need to burn, with detailed teacher notes, classroom slides and a video demonstration.
Interpreting data
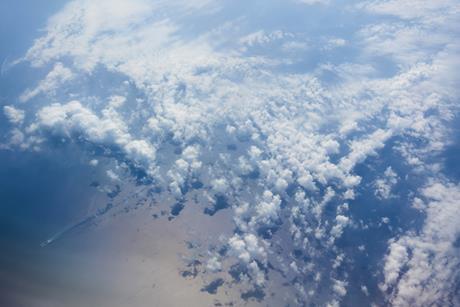
The life of water
Get hands on with H 2 O, changing states of matter and the water cycle. These experiments and investigations involve water in the context of space
Popular collections
Global experiments.
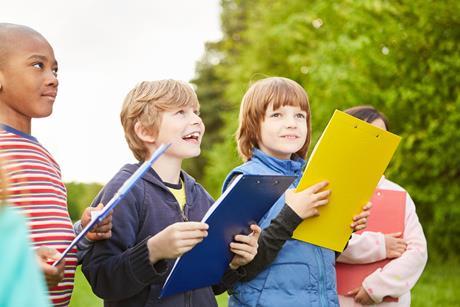
Engage your students with hands-on, fully-resourced, group activities that are adaptable for all ages
Edible experiments
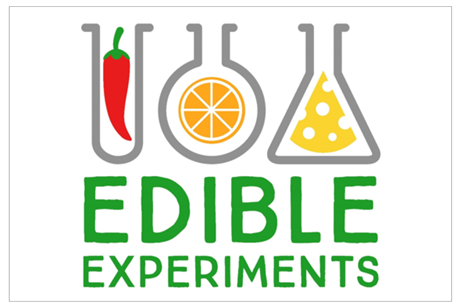
Discover the importance of chemistry in everyday eating experiences with this collection of edible experiments.
That's Chemistry!
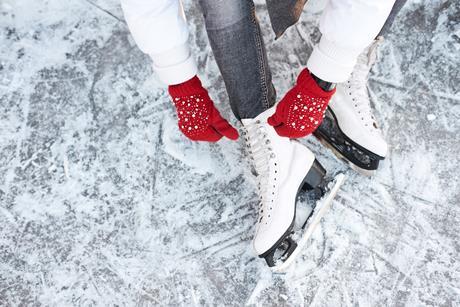
That’s Chemistry!
Discover the informative chapters of That’s Chemistry! Each chapter explains a chemistry concept and gives numerous ideas for activites to support students’ learning.
- Newsletters
- Find your local education coordinator
Site powered by Webvision Cloud
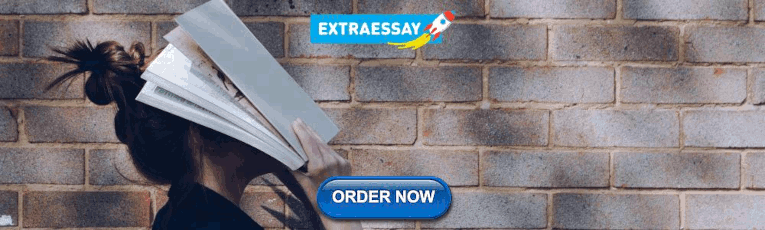
IMAGES
VIDEO
COMMENTS
For example, if three approaches were emitted during a reinforcement component and two instances of attention were scored, this would yield an integrity score of 67% for that component. However, if the same amount of each response was scored during an extinction component, this would yield a score of 33% for that component.
Integrating Discovery-Based Research into the Undergraduate Curriculum: Report of a Convocation The National Academy of Sciences was established in 1863 by an Act of Congress, signed by President Lincoln, as a private, nongovernmental institution to advise the nation on issues related
INTRODUCTION. Recommendation 2 of the February 2012 report Engage to Excel from the President's Council of Advisors on Science and Technology (PCAST, 2012) urges the science, technology, engineering, and mathematics (STEM) education community and funding agencies to "advocate and provide support for replacing standard laboratory courses with discovery-based research courses."
This resource is an example of a discovery-based undergraduate research course in genetics, using the course-based undergraduate research experience (CURE) model. During the first part of the course students perform designed experiments with the goal of learning laboratory techniques, experimental design, and key features of the model system.
Discovery research is expansive, only limited by the imaginations of researchers, and the resources available to them. At a time when many funders are moving away from supporting curiosity-based discovery, and towards projects with a nearer-term impact, we have made a bold commitment to support discovery research as one of the best ways to achieve significant shifts in our understanding of ...
A scaffold for scientific investigations The process of science involves many layers of complexity, but the key points of that process are straightforward:. There are many routes into the process, including serendipity (e.g., being hit on the head by the proverbial apple), concern over a practical problem (e.g., finding a new treatment for diabetes), and a technological development (e.g., the ...
The effects of variations in discovery instruction on learning have been reviewed in a combination of laboratory and field-based studies by Mayer (2004) by Kirschner, Sweller and Clark, (2006) and by Sweller, Kirschner and Clark, (2007). In his comprehensive review, Mayer (2004) discussed research reviews on the discovery of problem-solving rules
The early stages of a scientific investigation often rely on making observations, asking questions, and initial experimentation — essentially poking around. But the routes to and from these stages are diverse. Intriguing observations sometimes arise in surprising ways, as in the discovery of radioactivity, which was inspired by the observation that photographic plates (an early version of ...
Discovery science (also known as discovery-based science) is a scientific methodology which aims to find new patterns, correlations, and form hypotheses through the analysis of large-scale experimental data.The term "discovery science" encompasses various fields of study, including basic, translational, and computational science and research. ...
who each used two instructional simulations - one with a discovery-based design and the other with a tutorial-based design. Students' learning outcomes were assessed via pre-tests and post-tests of conceptual understanding. Students' interactions using the discovery-based program were recorded and coded as either systematic or unsystematic.
Discovery-Based Research. This fall, University of Dayton assistant professor Yvonne Sun will introduce students in her microbiology lab course to the potential rewards and pitfalls of real-world research through her new partnership with the Tiny Earth network. Founded by University of Wisconsin-Madison professor Jo Handelsman, Tiny Earth is a ...
One of the ways in which students can be taught science is by doing science, the intention being to help students understand the nature, processes, and methods of science. Investigative research projects may be used in an attempt to reflect some aspects of science more authentically than other teaching and learning approaches, such as confirmatory practical activities and teacher ...
Inquiry and discovery-based projects, including field studies and interactive laboratory activities, can engage introductory students, majors and non-majors alike. These activities appeal to the affective domain and develop students' metacognitive skills. Hands-on, inquiry-based projects in the field allow students to observe their environment ...
Take, for example, Beluga Bits, a research project focused on determining the sex, age and pod size of beluga whales visiting the Churchill River in northern Manitoba, Canada. With a bit of ...
This research used developmental research model as proposed by Plomp [5]. It consists of five phases; 1) Initial Investigation Phase, 2) Design Phase, 3) Realization Phase, 4) Test, Evaluation, and Revision Phase, and 5) Implementation Phase. Unfortunately, this research was conducted only until the fourth phase.
Course-based undergraduate research experiences (CUREs) provide a promising avenue to attract a larger and more diverse group of students into research careers. CUREs are thought to be distinctive in offering students opportunities to make discoveries, collaborate, engage in iterative work, and develop a sense of ownership of their lab course work.
The aim of this paper is to provide a preliminary insight into the use of a novel method of discovery instruction at degree level chemistry. The fundamental principle in instruction strategy was to provide minimal guidance to students with the intention of improving their autonomy. To do so, students only received feedback to their questions through online discussion board and they were not ...
Scientific Projects. Investigation. Scientific Steps. Presentation. The key to a good and manageable investigation is to choose a topic of interest, then ask what is called a "testable question.". Testable questions are those that can be answered through hands-on investigation by the student. The key difference between a general interest ...
Discovery research (also called generative, foundational, or exploratory research) is a process that helps designers understand user needs, behaviors, and motivations through different methods, such as interviews, surveys, and target market analysis. Discovery research is related to product research but involves a broader analysis.
8 Investigative projects. Students should have opportunities to do open-ended and extended investigative projects. There should be opportunities for students to do open-ended extended investigative projects in science. The school should have laboratory facilities such that all students who want to can carry out extended practical science ...
Inquiry-based learning is ampere learning real teaching strategy whereabouts students construct knowledge through adenine process off observations, investigation, and discovery. Examples of inquiry-based learning include observational field trips, science experiments, and hypothesis-based research projects. This
Try this investigation to explore the effect of heat on gases, with detailed teacher notes, classroom slides and a video demonstration. Dissolving, density and sugary drinks. Irreversible changes and the 'freaky hand'. Irreversible changes and the 'fire extinguisher'. Properties of solids and 'biscuit bashing'.