(502) 895-9500 | [email protected]
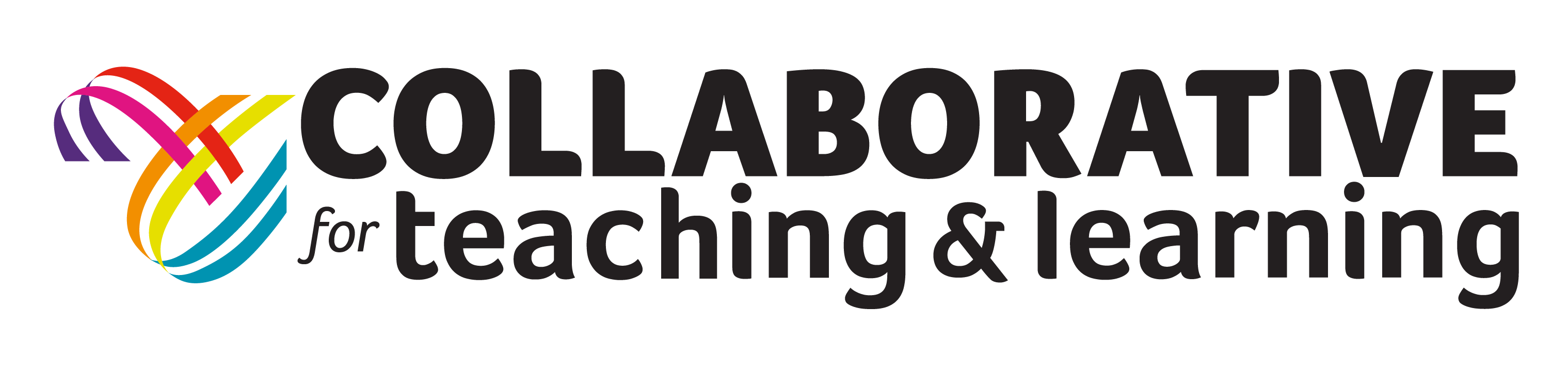
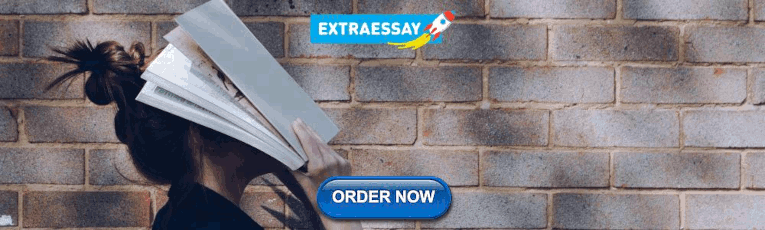
Multiple Representations – What is it and do you have any suggestions?
Written by jmosier.
The Principles and Standards for School Mathematics, National Council of Teachers of Mathematics (NCTM) [1] have six principles for guiding teachers and schools in providing sound research-based mathematics education. Those principles are: equity, curriculum, teaching, learning, assessment, and technology. The ten Standards, however, are specific to what mathematics instruction should enable a student to know and do: content – Number and Operations, Geometry, Measurement, and Data Analysis and Probability and process – problem solving, reasoning and proof, communication, and representations.
Recent conversations with teachers have to do with the teaching process standard of representations and more specifically, providing opportunities for students to show what they know in multiple ways and what would be the benefit to their mathematical understanding?
What does it mean to represent what you know in multiple ways?
For purposes of this discussion, investigation into Curriculum and Evaluation Standards for School Mathematics, NCTM [2] for grades K – 12, state that connections should be such that students can:
- Relate representations of concepts or procedures to one another;
- Describe results using graphical, numerical, physical, algebraic, and verbal models or representations;
- See mathematics as an integrated whole;
- Recognize equivalent representations of the same concept.
It is through these representations that students can link various models of numbers, solve problems in multiple ways, and bridge from the concrete to the abstract.
What are some strategies for incorporating multiple representations instructionally?
- Connecting the various ways for solving equations with the Numeric Values (Tabular), Algebraic Solution, Graphic Representation, Sentence (explanation of the solution) or NAGS
The following is the description of group work that provides the opportunity for students to show what they know about solving various equations (linear with variables on each side, linear systems, and other function forms) and how each of these solutions are linked and equilvalent.
1) Using one of the following approaches, work in pairs to solve the (linear system):
Tabular values
In order to be able to share the results and process used with another group, each person is to take notes and record results. Then one representative from each of these groups (jigsaw method) forms yet another group for synthesizing the results.
2) One person from each of the three groups (Algebra, Graphing, and Tabular Values) form another group to discuss the following:
- share the results;
- check for consistency in solutions and make any necessary edits;
- record results in the graphic organizer;
- explain what the solution of the (linear system) means and reflect on the process.
3) The groups then provide multiple representations of the solution(s) of the problem posed using NAGS: Number (Tabular Values), Algebraic Solution, Graphing Solution, Sentence – explanation of what the solution provides.
4) Results are to be posted and explained to the entire group.
EXIT SLIP: At the end of class, have students complete the following reflection, “ How can representing solutions in different ways/forms help you understand the mathematics in a rigorous, more complete way?”
Various graphic organizers can be designed as a note-taking device so that students can track their understanding.
Connecting the use of a concrete model to the abstraction using algebra tiles
This model links the use of a concrete model (algebra tiles) for solving linear equations to verbalizing what the steps are using words, and then to the abstraction of the algebraic symbols. These experiences allow the student to mentally synchronize the visual, verbal, and written forms for solving linear equations; and to kinesthetically engage in with the mathematics.

Connecting the various ways to represent Rational Numbers
As students begin to develop number sense and connect equivalent forms of ways that number can be represented, multiple models of that number can be introduced. By doing so, some operations with Rational Numbers can be connected and justified rather than purely approaching the operation from an algorithmic stance.
An example might be:

The connected and equivalent Rational Number forms are fractions, decimal fractions, percents, placement on the number line, circle model, and a set model. Using a graphic organizer where one of the representations is completed and students have to complete the other models provides opportunities for students to gain flexibility of toggling amongst the various Rational Number forms.

[1] Principles and Standards for School Mathematics, National Council of Teachers of Mathematics,2000
[2] Curriculum and Evaluation Standards for School Mathematics, NCTM, 1989, pp 32, 84, and 146
Subscribe for Updates
Get the latest posts, and stay up to date with the latest strategies to keep kids reading at a higher level.
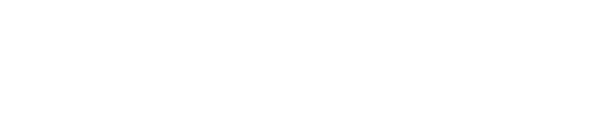
Or search by topic
Number and algebra
- The Number System and Place Value
- Calculations and Numerical Methods
- Fractions, Decimals, Percentages, Ratio and Proportion
- Properties of Numbers
- Patterns, Sequences and Structure
- Algebraic expressions, equations and formulae
- Coordinates, Functions and Graphs
Geometry and measure
- Angles, Polygons, and Geometrical Proof
- 3D Geometry, Shape and Space
- Measuring and calculating with units
- Transformations and constructions
- Pythagoras and Trigonometry
- Vectors and Matrices
Probability and statistics
- Handling, Processing and Representing Data
- Probability
Working mathematically
- Thinking mathematically
- Mathematical mindsets
- Cross-curricular contexts
- Physical and digital manipulatives
For younger learners
- Early Years Foundation Stage
Advanced mathematics
- Decision Mathematics and Combinatorics
- Advanced Probability and Statistics
- Multiple Representations
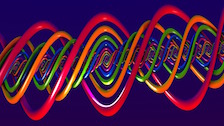
Catrina's Cards Age 5 to 7 Challenge Level
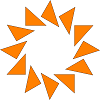
Triangle or No Triangle? Age 5 to 7 Challenge Level
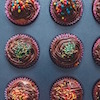
Birthday Sharing Age 5 to 7 Challenge Level
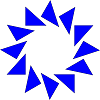
Name That Triangle! Age 7 to 11 Challenge Level
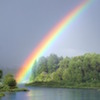
The Remainders Game Age 7 to 14 Challenge Level
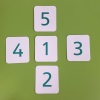
Magic Crosses Age 7 to 14 Challenge Level
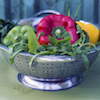
Remainders Age 7 to 14 Challenge Level
- More Multiple Representations

- For Parents
- For Teachers
- Teaching Topics
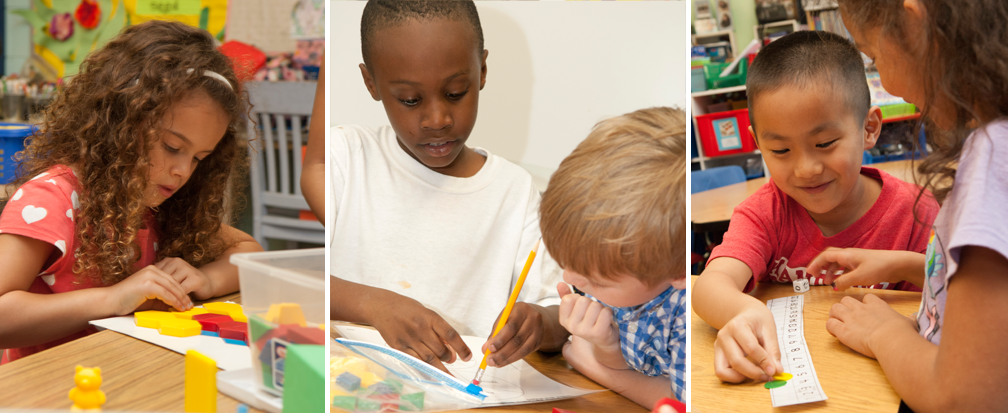
- EM Quickfacts
- Developing Conceptual Understanding
- Repeated Exposure for Long-Term Learning
- Frequent Practice of Basic Computation Skills
- Building Proficiency Through Multiple Methods
- EM History and Development
- Research and Results
- Understanding EM
- About UCSMP/UChicago STEM Education
- About the Publisher
- How to Order
Building Conceptual Understanding through Concrete, Real-Life Examples
If children are introduced to abstract concepts before they have a solid basis for understanding those concepts, they tend to resort to memorization and rote learning, which is not a solid foundation for further learning. Everyday Mathematics focuses on first developing student’s understanding of concepts through:
- Real world examples and concrete objects (manipulatives)
- Pictorial representations
- Discussion of ideas and methods
The use of multiple representations is carefully built into the Everyday Mathematics curriculum to ensure that students truly understand the concepts they are learning.
In Grade 2 students use manipulatives, other real objects, and pictures to explore division of whole numbers:

Related Links
Teaching everyday mathematics.
Access guides to assessment , computation , differentiation , pacing , and other aspects of Everyday Mathematics instruction.
Everyday Mathematics Virtual Learning Community
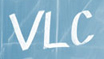
Join the Virtual Learning Community to access EM lesson videos from real classrooms, share EM resources, discuss EM topics with other educators, and more.
Professional Development
The UChicago STEM Education offers strategic planning services for schools that want to strengthen their Pre-K–6 mathematics programs.
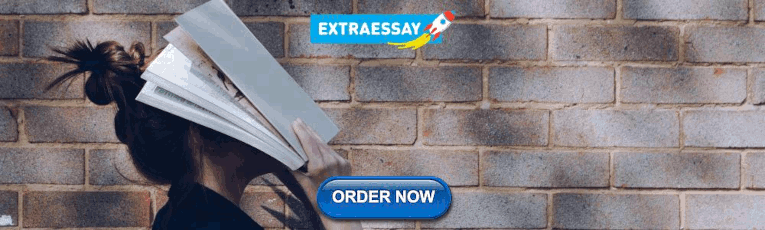
On the Publisher's Site
McGraw-Hill Education's website features supplemental materials, games, assessment and planning tools, technical support, and more.
About the Authors
Find out more information about the creators of Everyday Mathematics .

Advertisement
Why Use Multiple Representations in the Mathematics Classroom? Views of English and German Preservice Teachers
- Published: 06 March 2015
- Volume 14 , pages 363–382, ( 2016 )
Cite this article
- Anika Dreher 1 ,
- Sebastian Kuntze 1 &
- Stephen Lerman 2
2472 Accesses
25 Citations
14 Altmetric
Explore all metrics
Dealing with multiple representations and their connections plays a key role for learners to build up conceptual knowledge in the mathematics classroom. Hence, professional knowledge and views of mathematics teachers regarding the use of multiple representations certainly merit attention. In particular, investigating such views of preservice teachers affords identifying corresponding needs for teacher education. However, specific empirical research is scarce. Taking into account the possible role of culture, this study consequently focuses on views about using multiple representations held by more than 100 English and more than 200 German preservice teachers. The results indicate that there are culture-dependent aspects of preservice teachers’ views, but also that there are common needs for professional development.
This is a preview of subscription content, log in via an institution to check access.
Access this article
Price includes VAT (Russian Federation)
Instant access to the full article PDF.
Rent this article via DeepDyve
Institutional subscriptions
Similar content being viewed by others
Transforming Professional Practice in Numeracy Teaching
Multiple Intelligences Theory—Howard Gardner
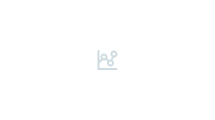
Research on early childhood mathematics teaching and learning
Camilla Björklund, Marja van den Heuvel-Panhuizen & Angelika Kullberg
Acevedo Nistal, A., van Dooren, W., Clarebout, G., Elen, J. & Verschaffel, L. (2009). Conceptualising, investigating and stimulating representational flexibility in mathematical problem solving and learning: A critical review. ZDM The International Journal on Mathematics Education, 41 (5), 627–636.
Article Google Scholar
Ainsworth, S. (2006). A conceptual framework for considering learning with multiple representations. Learning and Instruction, 16 , 183–198.
Arbuckle, J. L. (2012). IBM SPSS Amos 19 user’s guide . Chicago, IL: IBM.
Google Scholar
Ball, D. L. (1993). Halves, pieces, and twoths: Constructing representational contexts in teaching fractions. In T. Carpenter, E. Fennema & T. Romberg (Eds.), Rational numbers: An integration of research (pp. 157–196). Hillsdale, NJ: Erlbaum.
Ball, D.L. & Hill, H.C. (2008). Mathematical knowledge for teaching (MKT) measures. Mathematics released items 2008 . Retrieved from http://sitemaker.umich.edu/lmt/files/LMT_sample_items.pdf .
Ball, D. L., Thames, M. H. & Phelps, G. (2008). Content knowledge for teaching: What makes it special? Journal of Teacher Education, 59 (5), 389–407.
Bodemer, D. & Faust, U. (2006). External and mental referencing of multiple representations. Computers in Human Behavior, 22 , 27–42.
Bossé, M. J., Adu-Gyamfi, K. & Cheetham, M. (2011). Translations among mathematical representations: Teacher beliefs and practices. International Journal of Mathematics Teaching and Learning . Retrieved from http://www.cimt.plymouth.ac.uk/journal/bosse4.pdf .
Brenner, M., Herman, S., Ho, H. & Zimmer, J. (1999). Cross-national comparison of representational competence. Journal for Research in Mathematics Education, 30 (5), 541–557.
Charalambous, C. Y. & Pitta-Pantazi, D. (2007). Drawing on a theoretical model to study students’ understandings of fractions. Educational Studies in Mathematics, 64 , 293–316.
Doerr, H. & Lerman, S. (2009). The procedural and the conceptual in mathematics pedagogy: What teachers learn from their teaching. In M. Tzekaki, M. Kaldrimidou & H. Sakonidis (Eds.), Proceedings of 33th Conference of the International Group for the Psychology of Mathematics Education (Vol. 2, pp. 433–440). Thessaloniki, Greece: PME.
Dreher, A. (2012). Vorstellungen von Lehramtsstudierenden zum Nutzen vielfältiger Darstellungen im Mathematikunterricht [Preservice teachers' views on using multiple representations in the mathematics classroom]. In M. Ludwig & M. Small (Eds.), Beiträge zum Mathematikunterricht 2012 (pp. 205–208). Münster: WTM-Verlag.
Dreher, A. & Kuntze, S. (2015). Teachers’ professional knowledge and noticing: The case of multiple representations in the mathematics classroom. Educational Studies in Mathematics, 88 (1), 89–114.
Duval, R. (2006). A cognitive analysis of problems of comprehension in a learning of mathematics. Educational Studies in Mathematics, 61 , 103–131.
Elia, I., Panaoura, A., Eracleous, A. & Gagatsis, A. (2007). Relations between secondary pupils’ conceptions about functions and problem solving in different representations. Journal of Science and Mathematics Education, 5 (3), 533–556.
Even, R. (1990). Subject matter knowledge for teaching and the case of functions. Educational Studies in Mathematics, 21 (6), 521–544.
Goldin, G. & Shteingold, N. (2001). Systems of representation and the development of mathematical concepts. In A. A. Cuoco & F. R. Curcio (Eds.), The role of representation in school mathematics (pp. 1–23). Boston, Virginia: NCTM.
Graham, A. T., Pfannkuch, M. & Thomas, M. (2009). Versatile thinking and the learning of statistical concepts. ZDM The International Journal on Mathematics Education, 41 (5), 681–695.
Gravemeijer, K., Lehrer, R., van Oers, B. & Verschaffel, L. (2002). Symbolizing, modeling and tool use in mathematics education . Dordrecht, The Netherland: Kluwer.
Hill, H. C., Schilling, S. G. & Ball, D. L. (2004). Developing measures of teachers’ mathematics knowledge for teaching. Elementary School Journal, 105 , 11–30.
Jöreskog, K. G. (1971). Statistical analysis of sets of cogeneric tests. Psychometrica, 36 (2), 109–133.
Kaiser, G. (2002). Educational philosophies and their influence on mathematics education – An ethnographic study in English and German mathematics classrooms. Zentralblatt für Didaktik der Mathematik 34 ,(6), 241–257.
Kline, R. B. (2005). Principles and practice of structural equation modeling (2nd ed.). New York, NY: Guilford.
Kultusministerkonferenz (KMK). (2003). Bildungsstandards im Fach Mathematik für den Mittleren Schulabschluss [Education standards in mathematics for an intermediate school-leaving certificate]. Retrieved from http://www.kmk.org/fileadmin/veroeffentlichungen_beschluesse/2003/2003_12_04-Bildungsstandards-Mathe-Mittleren-SA.pdf .
Kunter, M., Baumert, J., Blum, W., Klusmann, U., Krauss, S. & Neubrand, M. (2011). Professionelle Kompetenz von Lehrkräften. Ergebnisse des Forschungsprogramms COACTIV [Professional competence of teachers. Results from the COACTIV Projekt]. Münster: Waxmann.
Kuntze, S. (2012). Pedagogical content beliefs: global, content domain-related and situation-specific components. Educational Studies in Mathematics, 79 (2), 273–292.
Kuntze, S., & Dreher, A. (2014). PCK and the awareness of affective aspects reflected in teachers’ views about learning opportunities – a conflict? In B. Pepin & B. Rösken-Winter (Eds.), From beliefs to dynamic affect systems in mathematics education: Exploring a mosaic of relationships and interactions -Advances in Mathematics Education series (pp. 295–318). New York, NY: Springer.
Kuntze, S., Lerman, S., Murphy, B., Kurz-Milcke, E., Siller, H.-S. & Winbourne, P. (2011). Development of pre-service teachers’ knowledge related to big ideas in mathematics. In B. Ubuz (Ed.), Proceedings of the 35th Conference of the International Group for the Psychology of Mathematics Education (Vol. 3, pp. 105-112). Ankara, Turkey: PME.
Leikin, R., Leikin, M., Waisman, I. & Shaul, S. (2013). Effect of the presence of external representations on accuracy and reaction time in solving mathematical double-choice problems by students of different levels of instruction. International Journal of Science and Mathematics Education, 11 (5), 1049–1066.
Lerman, S. (2001). A review of research perspectives on mathematics teacher education. In F.-L. Lin & T. J. Cooney (Eds.), Making sense of mathematics teacher education (pp. 33–52). Doderecht, The Netherland: Kluwer.
Malle, G. (2004). Grundvorstellungen zu Bruchzahlen [Basic ideas “Grundvorstellungen” of rational numbers]. Mathematik Lehren, 123 , 4–8.
McLeod, D. B. & McLeod, S. H. (2002). Synthesis—beliefs and mathematics education: Implications for learning, teaching and research. In G. C. Leder, E. Pehkonen & G. Torner (Eds.), Beliefs: A hidden variable in mathematics education (pp. 115–123). Dordrecht, The Netherland: Kluwer.
Meira, L. (1998). Making sense of instructional devices: The emergence of transparency in mathematical activity. Journal for Research in Mathematics Education, 29 (2), 121–142.
National Council of Teachers in Mathematics. (2000). Principles and standards for school mathematics . Reston, VA: NCTM.
Pajares, F. M. (1992). Teachers’ beliefs and educational research: Cleaning up a messy construct. Review of Educational Research, 62 (3), 307–332.
Pehkonen, E. & Pietilä, A. (2003). On relationships between beliefs and knowledge in mathematics education . Retrieved from http://www.dm.unipi.it/~didattica/CERME3/proceedings/Groups/TG2/TG2_pehkonen_cerme3.pdf .
Pepin, B. (1999). Epistemologies, beliefs and conceptions of mathematics teaching and learning: The theory, and what is manifested in mathematics teachers’ practices in England, France and Germany. In B. Hudson, F. Buchberger, P. Kansanen & H. Seel (Eds.), Didaktik/Fachdidaktik as science(s) of the teaching profession (pp. 127–146). Umeå: TNTEE.
Qualifications and Curriculum Authority (2007). textit{ Mathematics – Programme of study for key stages 3 and attainment targets }. Retrieved from http://webarchive.nationalarchives.gov.uk/20101221004558/http:/curriculum.qcda.gov.uk/uploads/QCA-07-3338-p_Maths_3_tcm8-403.pdf .
Rau, M. A., Aleven, V. & Rummel, N. (2009). Intelligent tutoring systems with multiple representations and self-explanation prompts support learning of fractions. In V. Dimitrova, R. Mizoguchi & B. du Boulay (Eds.), Proceedings of the 14th International Conference on Artificial Intelligence in Education (pp. 441–448). Amsterdam: Ios Press.
Renkl, A., Berthold, K., Große, C. S. & Schwonke, R. (2013). Making better use of multiple representations: How fostering metacognition can help. In R. Azevedo & V. Aleven (Eds.), International handbook of metacognition and learning technologies (pp. 397–408). New York, NY: Springer.
Schnotz, W. & Bannert, M. (2003). Construction and interference in learning from multiple representation. Learning and Instruction, 13 , 141–156.
Shulman, L. S. (1986). Those who understand: Knowledge growth in teaching. Educational Researcher, 15 (2), 4–14.
Siegler, R. S., Carpenter, T., Fennell, F., Geary, D., Lewis, J., Okamoto, Y., Thompson, L. & Wray, J. (2010). Developing effective fractions instruction: A practice guide . Washington, DC: National Center for Education Evaluation and Regional Assistance.
Staub, F. & Stern, E. (2002). The nature of teachers’ pedagogical content beliefs matter for students’ achievement gains: Quasi-experimental evidence from elementary mathematics. Journal of Educational Psychology, 94 (2), 344–355.
Steinbring, H. (2000). Mathematische Bedeutung als eine soziale Konstruktion – Grundzüge der epistemologisch orientierten mathematischen Interaktionsforschung [Mathematical meaning as a social construction – Main features of the epistemology oriented mathematical interaction research]. Journal für Mathematik-Didaktik, 21 (1), 28–49.
Tall, D. (1988). Concept image and concept definition. In J. de Lange & M. Doorman (Eds.), Senior secondary mathematics education (pp. 37–41). Utrecht: OW & OC.
Törner, G. (2002). Mathematical beliefs—A search for a common ground. In G. Leder, E. Pehkonen & G. Törner (Eds.), Beliefs: A hidden variable in mathematics education? (pp. 73–94). Dordrecht, The Netherlands: Kluwer.
Tripathi, P. (2008). Developing mathematical understanding through multiple representations. Mathematics Teaching in the Middle School, 13 (8), 438–445.
van der Meij, J. & de Jong, T. (2006). Supporting students’ learning with multiple representations in a dynamic simulation-based learning environment. Learning and Instruction, 16 , 199–212.
Download references
Acknowledgments
The data gathering phase of this study has been supported in the framework of the project ABCmaths which was funded with support from the European Commission (503215-LLP-1-2009-1-DE-COMENIUS-CMP). This publication reflects the views only of the authors, and the Commission cannot be held responsible for any use which may be made of the information contained therein.
Author information
Authors and affiliations.
Ludwigsburg University of Education, Ludwigsburg, Germany
Anika Dreher & Sebastian Kuntze
Department of Education, Centre for Mathematics Education, London South Bank University, 103 Borough Road, SE1 0AA, London, UK
Stephen Lerman
You can also search for this author in PubMed Google Scholar
Corresponding author
Correspondence to Anika Dreher .
Rights and permissions
Reprints and permissions
About this article
Dreher, A., Kuntze, S. & Lerman, S. Why Use Multiple Representations in the Mathematics Classroom? Views of English and German Preservice Teachers. Int J of Sci and Math Educ 14 (Suppl 2), 363–382 (2016). https://doi.org/10.1007/s10763-015-9633-6
Download citation
Received : 01 September 2014
Accepted : 08 February 2015
Published : 06 March 2015
Issue Date : July 2016
DOI : https://doi.org/10.1007/s10763-015-9633-6
Share this article
Anyone you share the following link with will be able to read this content:
Sorry, a shareable link is not currently available for this article.
Provided by the Springer Nature SharedIt content-sharing initiative
- Multiple representations
- Preservice teachers
- Transnational design
- Find a journal
- Publish with us
- Track your research
principle Provide multiple means of Representation
Learners differ in the ways that they perceive and comprehend information that is presented to them. For example, those with sensory disabilities (e.g., blindness or deafness); learning disabilities (e.g., dyslexia); language or cultural differences, and so forth may all require different ways of approaching content. Others may simply grasp information quicker or more efficiently through visual or auditory means rather than printed text. Also learning, and transfer of learning, occurs when multiple representations are used, because they allow students to make connections within, as well as between, concepts. In short, there is not one means of representation that will be optimal for all learners ; providing options for representation is essential.
Representation | Guidelines & Checkpoints
Guideline 1 perception.
Interact with flexible content that doesn't depend on a single sense like sight, hearing, movement, or touch.
checkpoint 1.1 Offer ways of customizing the display of information
Checkpoint 1.2 offer alternatives for auditory information, checkpoint 1.3 offer alternatives for visual information, guideline 2 language & symbols.
Communicate through languages that create a shared understanding.
checkpoint 2.1 Clarify vocabulary and symbols
Checkpoint 2.2 clarify syntax and structure, checkpoint 2.3 support decoding of text, mathematical notation, and symbols, checkpoint 2.4 promote understanding across languages, checkpoint 2.5 illustrate through multiple media, guideline 3 comprehension.
Construct meaning and generate new understandings.
checkpoint 3.1 Activate or supply background knowledge
Checkpoint 3.2 highlight patterns, critical features, big ideas, and relationships, checkpoint 3.3 guide information processing and visualization, checkpoint 3.4 maximize transfer and generalization, udl guidelines, provide multiple means of engagement, provide options for recruiting interest ( guideline 7), optimize individual choice and autonomy ( checkpoint 7.1), optimize relevance, value, and authenticity ( checkpoint 7.2), minimize threats and distractions ( checkpoint 7.3), provide options for sustaining effort & persistence ( guideline 8), heighten salience of goals and objectives ( checkpoint 8.1), vary demands and resources to optimize challenge ( checkpoint 8.2), foster collaboration and community ( checkpoint 8.3), increase mastery-oriented feedback ( checkpoint 8.4), provide options for self regulation ( guideline 9), promote expectations and beliefs that optimize motivation ( checkpoint 9.1), facilitate personal coping skills and strategies ( checkpoint 9.2), develop self-assessment and reflection ( checkpoint 9.3), provide multiple means of representation, provide options for perception ( guideline 1), offer ways of customizing the display of information ( checkpoint 1.1), offer alternatives for auditory information ( checkpoint 1.2), offer alternatives for visual information ( checkpoint 1.3), provide options for language & symbols ( guideline 2), clarify vocabulary and symbols ( checkpoint 2.1), clarify syntax and structure ( checkpoint 2.2), support decoding of text, mathematical notation, and symbols ( checkpoint 2.3), promote understanding across languages ( checkpoint 2.4), illustrate through multiple media ( checkpoint 2.5), provide options for comprehension ( guideline 3), activate or supply background knowledge ( checkpoint 3.1), highlight patterns, critical features, big ideas, and relationships ( checkpoint 3.2), guide information processing and visualization ( checkpoint 3.3), maximize transfer and generalization ( checkpoint 3.4), provide multiple means of action & expression, provide options for physical action ( guideline 4), vary the methods for response and navigation ( checkpoint 4.1), optimize access to tools and assistive technologies ( checkpoint 4.2), provide options for expression & communication ( guideline 5), use multiple media for communication ( checkpoint 5.1), use multiple tools for construction and composition ( checkpoint 5.2), build fluencies with graduated levels of support for practice and performance ( checkpoint 5.3), provide options for executive functions ( guideline 6), guide appropriate goal-setting ( checkpoint 6.1), support planning and strategy development ( checkpoint 6.2), facilitate managing information and resources ( checkpoint 6.3), enhance capacity for monitoring progress ( checkpoint 6.4), expert learners who are…, purposeful & motivated, resourceful & knowledgeable, strategic & goal-directed.
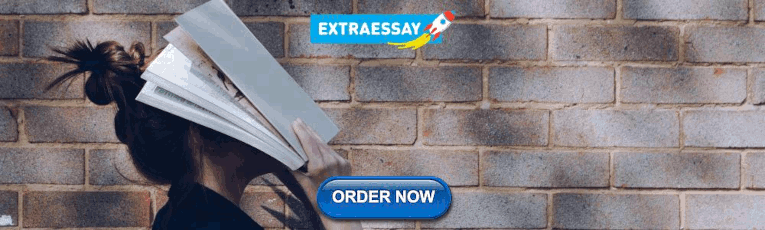
COMMENTS
The Principles and Standards for School Mathematics, National Council of Teachers of Mathematics (NCTM)[1] have six principles for guiding teachers and schools in providing sound research-based mathematics education. Those principles are: equity, curriculum, teaching, learning, assessment, and technology. The ten Standards, however, are specific to what mathematics instruction should enable a ...
Multiple representations (mathematics education) In mathematics education, a representation is a way of encoding an idea or a relationship, and can be both internal (e.g., mental construct) and external (e.g., graph). Thus multiple representations are ways to symbolize, to describe and to refer to the same mathematical entity.
Definition of multiple representations. Multiple representations (MRs) is externalizing internal mathematical concepts and ideas by employing a combination of more than one representation tools such as images, graphs, symbols, drawings, and writings (Huinker & Bill, 2017; Jonassen, 2000; Morrow-Leong, Moore, & Gojak, 2020).
The use of multiple representations in the teaching and learning of mathematics is a major topic in mathematics education that has gained significant importance in recent decades (Ozgun-Koca, 1998). ... definition and meaning of representation, (2) types of representation, (3) modes of representation, (4) translation
Definition. A representation consists of symbols that take the place of something else (from the real world). ... Multiple representations can better cover the complete domain and elicit a wider range of learning processes. This is also what educational practice does; most educational material houses a multitude of representational formats ...
In other words, multiple representations are powerful tools but like all powerful tools they need careful handling if learners are to use them successfully. In this chapter, I will review the evidence that suggests that multiple representations serve a number of important roles in science education.
Brenner ME and Moseley B. Preparing students for algebra: The role of multiple representations in problem solving. Proceedings of the Sixteenth Annual Conference for the Psychology of Mathematics Education 1994 Baton Rouge Louisiana State University 138-144. Google Scholar.
Research findings showed that students did not always understand the role of multiple representations despite the efforts of a science teacher (Treagust et al. 2003).For instance, an argument offered in physics education for enhanced student understanding is to present physical concepts or problems using multiple representations in the form of words, diagrams, graphs, tables, or bar charts ...
Multiple representations can be used as a psychometric tool to successfully measure elementary school pre-service teachers' creative thinking abilities in mathematics. ... Definition of multiple representations. Multiple representations (MRs) is externalizing internal mathematical concepts and ideas by employing a combination of more than one ...
Multiple Representations Mathematicians use many different representations when solving problems, such as tables, graphs, diagrams and algebra. These tasks encourage students to use a variety of different representations to gain valuable insights into mathematical situations.
problems) and symbolic representations (e.g., equations) can enhance students' learning. Because no single visual representation perfectly depicts the complexity of mathematical concepts, instructors often use multiple visual representations, where the different representations emphasize complementary conceptual aspects.
The use of multiple representations enhances mathematical discourse by providing different ways that students can communicate their ideas. In multiplicative contexts, the representations are diverse, but connected. For example, equal-sized groups are often illustrated with discrete objects, such as cookies, balloons, or animals. ...
Multiple Representations. When children are curious about why something works, multiple ways of representing the same concept or idea can offer insights and deepen understanding. The problems below will give your learners opportunities to consider different representations, whether they are created by the children themselves or by someone else.
In the last three decades many research studies focused on the topic of multiple representations and their role for learning mathematics. As a result, there is a broad consensus in the scientific ...
Everyday Mathematics focuses on first developing student's understanding of concepts through: Real world examples and concrete objects (manipulatives) Pictorial representations. Discussion of ideas and methods. The use of multiple representations is carefully built into the Everyday Mathematics curriculum to ensure that students truly ...
Multiple representations mean that a concept is repeatedly represented by different types of representations, such as verbal, graphical, mathematical representations, and that students are exposed to the same concept several times (Prain & Waldrip, 2006, p.1844).
Dealing with multiple representations and their connections plays a key role for learners to build up conceptual knowledge in the mathematics classroom. Hence, professional knowledge and views of ...
Dealing with multiple representations and their connections plays a key role for learners to build up conceptual knowledge in the mathematics classroom. Hence, professional knowledge and views of mathematics teachers regarding the use of multiple representations certainly merit attention. In particular, investigating such views of preservice teachers affords identifying corresponding needs for ...
different representations, for example by symbols or in graphical representation registers (Duval, 2006), stand for a mathematical object. Indeed, teachers' support for learners is important when dealing with multiple representations, as conversions between representation registers are complex for students and have shown to be
He has extensive experience in working with college academic support services as an instructor of mathematics, physics, chemistry and biology. Many mathematical concepts can be represented in ...
Representation. Learners differ in the ways that they perceive and comprehend information that is presented to them. For example, those with sensory disabilities (e.g., blindness or deafness); learning disabilities (e.g., dyslexia); language or cultural differences, and so forth may all require different ways of approaching content. Others may ...
addition, the definition of multiple representations also refers to the involvement of various media in the learning process (Ainsworth, 2006). Although there is a definition of multiple representations that refers to the use of various media, in chemistry learning, these different media are often integrated with macroscopic, submicroscopic ...
Multiple representations and multi-media can support learning in many di•erent ways. In this paper, it is claimed that by identifying the functions that they can serve, many of the conflicting findings arising out of the existing evaluations of multi-representational learning environments can be explained. This