Thank you for visiting nature.com. You are using a browser version with limited support for CSS. To obtain the best experience, we recommend you use a more up to date browser (or turn off compatibility mode in Internet Explorer). In the meantime, to ensure continued support, we are displaying the site without styles and JavaScript.
- View all journals
- My Account Login
- Explore content
- About the journal
- Publish with us
- Sign up for alerts
- Open access
- Published: 07 August 2023
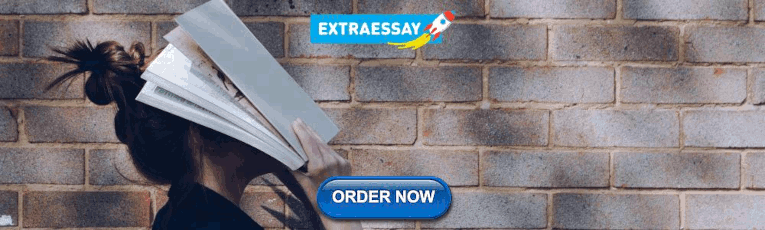
Mutational landscape of cancer-driver genes across human cancers
- Musalula Sinkala 1 , 2
Scientific Reports volume 13 , Article number: 12742 ( 2023 ) Cite this article
9658 Accesses
5 Citations
30 Altmetric
Metrics details
- Cancer genetics
- Cancer genomics
- Data integration
- Data mining
- Genome informatics
The genetic mutations that contribute to the transformation of healthy cells into cancerous cells have been the subject of extensive research. The molecular aberrations that lead to cancer development are often characterised by gain-of-function or loss-of-function mutations in a variety of oncogenes and tumour suppressor genes. In this study, we investigate the genomic sequences of 20,331 primary tumours representing 41 distinct human cancer types to identify and catalogue the driver mutations present in 727 known cancer genes. Our findings reveal significant variations in the frequency of cancer gene mutations across different cancer types and highlight the frequent involvement of tumour suppressor genes (94%), oncogenes (93%), transcription factors (72%), kinases (64%), cell surface receptors (63%), and phosphatases (22%), in cancer. Additionally, our analysis reveals that cancer gene mutations are predominantly co-occurring rather than exclusive in all types of cancer. Notably, we discover that patients with tumours displaying different combinations of gene mutation patterns tend to exhibit variable survival outcomes. These findings provide new insights into the genetic landscape of cancer and bring us closer to a comprehensive understanding of the underlying mechanisms driving the development of various forms of cancer.
Similar content being viewed by others
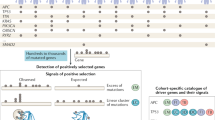
A compendium of mutational cancer driver genes
Francisco Martínez-Jiménez, Ferran Muiños, … Nuria Lopez-Bigas
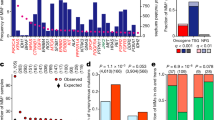
Landscape and function of multiple mutations within individual oncogenes
Yuki Saito, Junji Koya, … Keisuke Kataoka
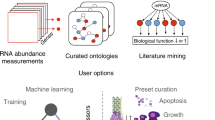
Interpreting pathways to discover cancer driver genes with Moonlight
Antonio Colaprico, Catharina Olsen, … Elena Papaleo
Introduction
Cancer is a complex disease characterised by mutations in genes that control various hallmarks of the disease, including escaping programmed cell death, promoting genome instability and mutations, and proliferative signalling 1 . Cancer genes include genes encoding cell surface receptors, oncogenes, tumour suppressor genes, kinases, phosphatases, and transcription factors 2 , 3 , 4 , 5 , 6 . Cancer genes of these classes transcribe mRNAs that encode proteins, which function in various oncogenic pathways that fuel oncogenesis by enabling various hallmarks of cancer 7 . The mutations in known cancer genes, unlike those in non-cancer driver genes 8 , 9 , 10 , qualitatively or quantitatively alter the function of genes and proteins and, consequently, alter the cellular processes in which these proteins participate. Moreover, oncogene mutations are linked with differences in patient survival, clinical outcomes, metastatic or recurrent tumours, and serve as predictors of tumour responsiveness to anti-cancer drugs 8 , 9 , 11 , 12 . There is, therefore, a need to understand the extent to which cancer genes are mutated in cancers of different tissues of origin.
Over the last few decades, our understanding of the genes, pathways, and their role in oncogenesis has grown significantly, leading to increased efforts to treat various cancer types 13 , 14 , 15 , 16 , 17 , 18 . Furthermore, many genetic aberrations have been identified in human cancers, and several of the proteins encoded by these genes are well-established drug targets, while others are promising drug targets 3 , 4 , 19 , 20 . However, despite this impressive list of known gene mutations, it covers only a few cancer types. To comprehend the extent and consequences of gene alterations affecting function, it is crucial to study the alteration of cancer genes across all human cancers and within each cancer category. Such knowledge has been successfully applied in the design of therapies explicitly targeting proteins altered by somatic and germline mutations in cancer genes 21 , 22 . However, we still do not completely understand the extent to which cancer genes and the classes thereof are altered in all human cancers.
Here, we utilise publicly available datasets generated by various cancer sequencing projects to understand the extent of cancer gene alterations in human cancers. Furthermore, we obtain information on known cancer genes compiled by the Catalog of Somatic Mutations in Cancer (COSMIC), Cancer Gene Consensus (CGC) database 23 , 24 . Then, we comprehensively analyse known cancer gene mutations across different cancer types by integrating information on tumour genetic alterations with known gene annotations. Our analysis provides novel biological insights into the mutational landscape of these cancer genes and shows the extent to which they co-occur or are exclusive in tumours of various tissues and their association with patient outcomes.
Mutational landscape of cancer genes in human cancers
Our study compiled a list of 727 known cancer genes based on information from the Catalogue of Somatic Mutations in Cancer (COSMIC) 24 and Cancer Gene Consensus (CGC) 23 database. This list includes genes that encode oncogenes (383), tumour suppressor genes (TSGs; 370), transcription factors (150), kinases (75), phosphatases (9), and cell surface receptor proteins (CSR; 63) (see Supplementary Fig. 1 , and Supplementary Data 1 ).
Next, we obtained whole-exome sequencing data from 138 cancer studies focusing on 41 different human cancer types and involving 20,331 samples (Supplementary Data 1 ). The number of samples in each study varied, with breast carcinoma representing the highest number of samples (2,585) and Small-Cell Lung Cancer the least (110), as shown in Fig. 1 a. We then calculated the somatic mutation frequencies in the 727 genes across the samples of the 41 cancer types (Supplementary Data 1 ) and found that the mutation frequency for different cancer genes ranged from 0% for the VHL (and other genes) in acute myeloid leukaemia to 94% for the TP53 gene in small cell lung carcinomas (Fig. 1 b,c). Other cancer genes with high mutation frequencies include KRAS (mutated in 84%) of pancreatic adenocarcinomas and PTEN (67%) of uterine corpus endometrial carcinomas, and JAK2 (75%) of myeloproliferative neoplasms (Fig. 1 c, also see Supplementary Data 1 ).
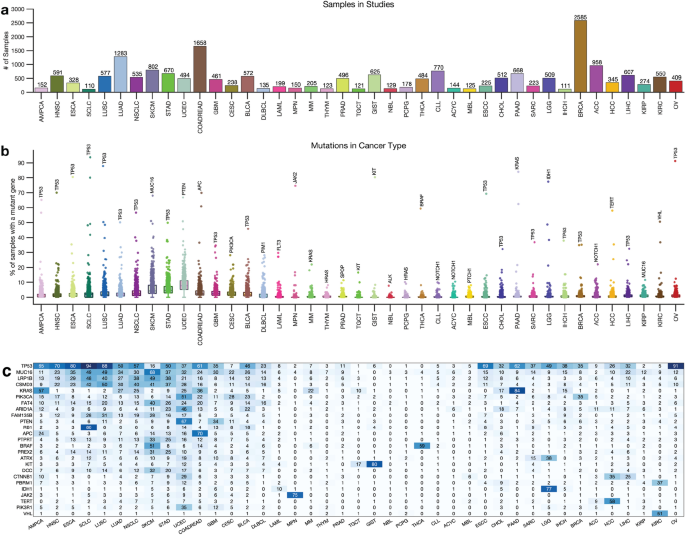
Distribution of cancer types and known cancer gene mutations in 20,066 tumours. ( a ) Distribution of 20,066 tumours across 43 human cancer types. The disease codes and abbreviations: UCEC, uterine corpus endometrial carcinoma; SKCM, skin cutaneous melanoma; BLCA, bladder urothelial carcinoma; UCS, uterine carcinosarcoma; OV, ovarian serous cystadenocarcinoma; LUSC, lung squamous cell carcinoma; STAD, stomach adenocarcinoma; LUAD, lung adenocarcinoma; ESCA, oesophageal adenocarcinoma; DLBC, diffuse large b-cell lymphoma; CESC, cervical squamous cell carcinoma; HNSC, head and neck squamous cell carcinoma; SARC, sarcoma; LIHC, liver hepatocellular carcinoma; BRCA, breast invasive carcinoma; COADREAD, colorectal adenocarcinoma; CHOL, cholangiocarcinoma; ACC, adrenocortical carcinoma; PAAD, pancreatic adenocarcinoma; PRAD, prostate adenocarcinoma; GBM, glioblastoma multiforme; KIRP, kidney renal papillary cell carcinoma; KIRC, kidney renal clear cell carcinoma; MESO, mesothelioma; LGG, brain lower grade glioma; UVM, uveal melanoma; PCPG, pheochromocytoma and paraganglioma; TGCT, testicular germ cell tumours; KICH, kidney chromophobe; THYM, thymoma; LAML, acute myeloid leukaemia; THCA, thyroid carcinoma. Panel ( b ) shows the mutation frequency of known cancer genes in each cancer type, and panel ( c ) is a clustered heatmap showing the most frequently mutated cancer genes across types, based on the percentage of tumours with altered genes. The heatmap was generated using unsupervised hierarchical clustering with the Cosine distance metric and complete linkage. The colour intensity increases to represent a higher percentage (see Supplementary Fig. 2 ).
Next, for each cancer type, we summarised the number of mutated genes in (1) none of the samples, (2) less than 5 per cent of the samples, and (3) more than 5% of the samples. Our analysis revealed that most cancer genes were not mutated cancer types, and a limited number of genes were found to be mutated in over 5% of the samples. For instance, only two known cancer genes were found to be mutated in over 5% of thymomas ( MUC16 and HRAS ), testicular germ cell tumours ( KRAS and KIT ), and thyroid carcinomas ( BRAF and NRAS ) (Fig. 1 c, Supplementary Fig. 2 , and Supplementary Data 1 ).
Furthermore, some cancer types had a significantly higher number of known cancer genes mutated in more than 5% of the samples, e.g., in uterine corpus endometrial carcinoma (568 known cancer genes mutated), stomach adenocarcinoma (330 genes), and skin cutaneous melanoma (314 genes). This finding shows that the extent to which the cancer genes are mutated across cancer types varies and that some cancer types have few mutations within the coding sequences of known cancer genes 25 , 26 .
Mutations of each cancer gene across all tumours
We calculated the mutation frequency of each cancer gene across 41 different human cancer types in all 20,331 samples. Our analysis revealed that 98.9% (719 out of 727) of cancer genes were mutated in at least one sample. Among the oncogenes, MUC16 (mutated in 18.9% of tumours), PIK3CA (12.4%), and KRAS (11.1%) were the most frequently mutated across the profiled samples (Fig. 2 a). Furthermore, among the TSGs, TP53 (36.6%), CSMD3 (13.7%), and LRP1B (13.5%) were the most frequently mutated (Fig. 2 b). PIK3CA (12.4%), BRAF (6.6%), and ATM (6.0%) were the top three mutated genes that encode protein kinases (Fig. 2 c). In addition, we found that PTPRT (6.5%) and PTEN (6.4%) were the most frequently mutated among the genes that encode protein phosphatases (Fig. 2 d), while TP53 (36.6%) and KMT2C (8.6%) were the top-two frequently mutated among the genes that encode transcription factors (Fig. 2 e). Furthermore, among the genes that encode cell surface receptors, we found that MUC16 (18.9%) and LRP1B (13.5%) were the most frequently mutated (Fig. 2 f). Overall, the top-five frequently mutated cancer genes across human cancers were TP53 (36.6%), MUC16 (18.9%), CSMD3 (13.7%), LRP1B (13.5%), and PIK3CA (12.4%) (Fig. 2 g).
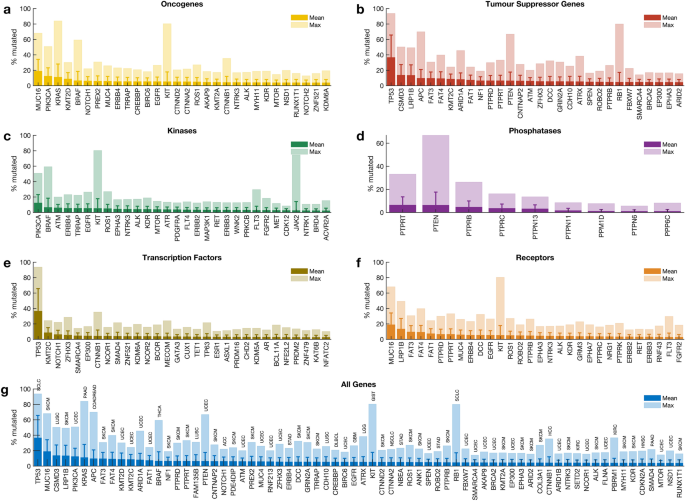
Frequency of cancer gene mutations in different gene classes. Frequency of known cancer gene mutations among: ( a ) oncogenes, ( b ) tumour suppressor genes, genes that encode ( c ) kinases, ( d ) phosphatases, ( e ) transcription factors, ( f ) cell surface receptor proteins, ( g ) all the genes.
The mutation frequencies we report here are reasonably consistent with previous reports, which indicated that TP53 (36.6% across all samples) is the most frequently altered gene, followed by PIK3CA (12.4%) 5 , 27 . Furthermore, we found that the extent to which the cancer genes are mutated in different cancer types varies significantly, a pattern likely to impact the treatment strategies that could be applied to cancers of different tissues 28 .
Mutations in categories of cancer genes
We were interested in evaluating the extent to which genes in particular categories of cancer genes (oncogenes, TSGs, transcription factors, kinases, phosphatases, and receptors) are mutated across human cancers. Here, we found mutations in the known cancer genes in all tumours. Of the six classes of cancer genes, the TSGs (91% of the tumours) and the oncogenes (89%) showed the highest frequency of mutations, followed by the transcription factors (72%), kinases (62%), receptors (60%), and the phosphatases (19%); (Supplementary Fig. 3 and Fig. 3 a). Overall, our analyses revealed that the mutational landscape of the six cancer gene classes was mainly consistent within cancer (Fig. 3 a). Therefore, we suggest that the observed correlation in mutation frequencies between cancer genes of different classes in a particular cancer type may indicate that gene mutations tend to co-occur (see Supplementary Fig. 4 ).
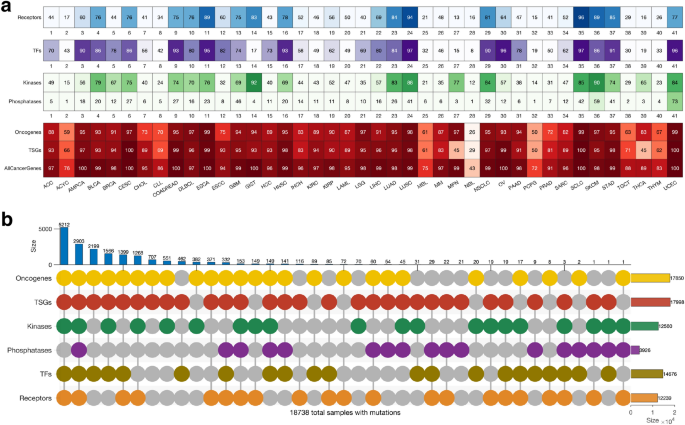
Gene alterations and mutational landscape in different cancer types. ( a ) The frequency of altered genes encoding cell surface receptors, transcription factors, kinases, phosphatases, oncogenes, tumour suppressor genes and all the cancer genes in different types of tumours. ( b ) A plot showing the mutual exclusivity and co-occurrence of mutations in the different classes of cancer genes, only considering mutations in tumours with mutations in genes that belong to more than one class. Refer to Supplementary Fig. 5 for the count of mutations exclusive to each class of genes.
Also, we found that 92% (18,738 samples) of all the tumours harboured mutations in genes involved in more than one of the six cancer gene classes (Fig. 3 b). Furthermore, we found 2,903 tumours harbouring mutations in all six classes of genes, and another 5,212 tumours harbouring mutations in all six classes of genes, except those that encode protein phosphatases (Fig. 3 b). Conversely, among all the cancer types, we found that 737 samples harboured mutations in only one class of the known cancer genes (Supplementary Fig. 5 ). The percentage of mutated cancer genes that are members of multiple cancer gene categories is shown in Supplementary Fig. 6 . Overall, our findings demonstrate that for most cancer types, the tumours tend to have mutations in the genes of at least five of the six classes of cancer genes.
Co-occurrence and exclusivity of mutations in cancer gene pairs
Given that we found a convolved pattern in the mutational landscape of the known cancer genes (Fig. 4 a and Supplementary Fig. 7 a and b), we were interested in determining the extent to which non-synonymous somatic gene mutations tend to be mutually exclusive or co-occur.
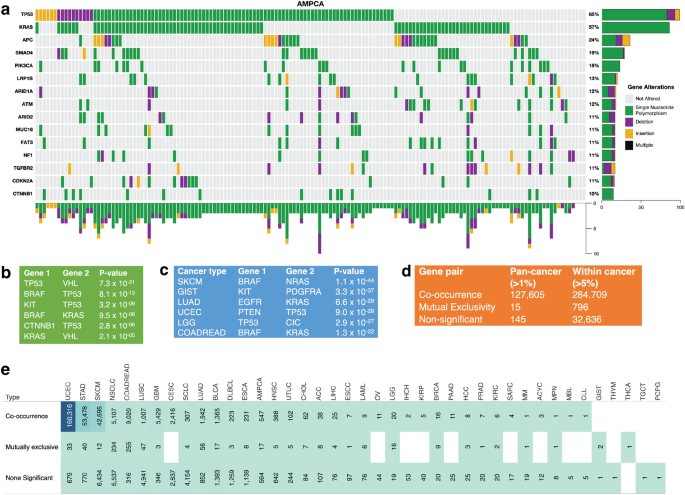
Analysis of co-occurrence and exclusivity of cancer gene mutations across cancer types. ( a ) A plot of non-synonymous somatic mutations in ampullary carcinoma, highlighting the co-occurrence and exclusivity of mutations in the 13 most frequently mutated genes. The plot columns represent samples, and the rows represent mutations. Also, refer to Supplementary Figs. 7 a and b for additional information. ( b ) Analysis of co-occurring and exclusive mutations in all cancer types and within each cancer. ( c ) The 7 gene pairs with the highest co-occurrence across pan-cancer studies. ( d ) The 7 gene pairs with the highest mutual exclusiveness within each cancer study. ( e ) Statistics on the number of cancer gene mutations that are co-occurring, mutually exclusive, or neither significantly co-occurring nor mutually exclusive.
First, we assessed mutations in 127,765 gene pairs, both present in at least 1% of 20,331 samples across 41 human cancer types (see the “Methods” section). Here, collectively across all the cancer types, our analysis revealed 127,605 gene pairs with significantly co-occurring mutations, 15 pairs with mutually exclusive mutations, and 145 pairs with non-statistically significant mutations (Fig. 4 b). Among the significantly mutually exclusive mutated gene pairs were VHL and TP53 ( p = 7.3 × 10 –21 ), TP53 and BRAF ( p = 8.0 × 10 –13 ), and BRAF and KRAS ( p = 9.5 × 10 –8 ), see Fig. 4 c and Supplementary Data 2 . Additionally, the 127,605 significantly co-occurring mutated gene pairs included PTEN and PIK3CA ( p = 2.1 × 10 –70 ), BRAF and ERBB4 ( p = 6.9 × 10 –63 ), and EGFR and ERBB4 ( p = 1.9 × 10 –59 ), see Supplementary Data 2 .
Second, we analysed the mutations in gene pairs that were found to be mutated in more than 5% of the tumours for each of the 41 cancer types. Our results indicate 284,709 gene pairs with significantly co-occurring mutations, 796 gene pairs with significantly mutually exclusive mutations, and 32,636 gene pairs that exhibit a non-statistically significant mutation pattern (see Supplementary Data 2 ). Furthermore, certain gene pairs were found to exhibit mutually exclusive or co-occurring mutations in specific cancer types. For example, among the top three exclusively mutated gene pairs are BRAF and NRAS in skin cutaneous melanoma ( p = 1.1 × 10 –44 ), KIT and PDGFRA in gastrointestinal stromal tumour ( p = 3.3 × 10 –37 ), and EGFR and KRAS in lung adenocarcinoma (p = 6.6 × 10 –29 ) (Fig. 4 c). Additionally, we identified specific cancer types in which certain gene pairs exhibit a significantly co-occurring mutation pattern, such as TP53 and ATRX in brain lower-grade glioma (p = 8.2 × 10 –59 ), TP53 and PTEN in uterine corpus endometrial carcinoma (p = 9.0 × 10 –29 ), FGFR1OP and MAP3K1 in colorectal adenocarcinoma (p = 2.7 × 10 –38 ) and uterine corpus endometrial carcinoma (p = 2.9 × 10 –05 ). The complete list of mutually exclusive and co-occurring mutated gene pairs in specific cancer types can be found in Supplementary Data 2 .
Our analysis of non-synonymous somatic gene mutations in known cancer genes revealed a convolved pattern of mutually exclusive and co-occurring mutations across different human cancer types. Notably, we found more exclusive and co-occurring gene pair mutations within cancer types (796 and 284,709 pairs) than across all types (15 and 127,605 pairs) (Fig. 4 d). This result suggests that there may be a selection for specific mutations in certain cancer gene pairs in specific cancer types 29 . Additionally, we propose that the exclusively mutated gene pairs identified in this study may disrupt divergent oncogenic pathways in specific cancer types, providing new insights into the genetic underpinnings of these diseases 30 , 31 .
Finally, we also analysed gene mutation patterns for each cancer type by aggregating the co-occurring, non-significant, and exclusive mutations per type. For example, as shown in Fig. 4 e, colorectal adenocarcinoma had the highest number of exclusively mutated gene pairs among the 41 cancer types, with 255 pairs identified. This was followed by non-small cell lung cancer (234 pairs) and lung adenocarcinoma (55 pairs). On the other hand, uterine corpus endometrial carcinoma exhibited the highest number of co-occurring mutations in gene pairs, with 160,320 pairs identified. This was followed by skin cutaneous melanoma (42,695 pairs) and stomach adenocarcinoma (53,478 pairs), as seen in Supplementary Data 2 . These results provide further insight into the distinct genetic profiles of different cancer types and the specific mutations that may drive their development.
Differences in gene pair co-occurrence and exclusivity among cancer types
We investigated the co-occurrence and exclusivity of mutations in the same cancer gene pairs across all cancer types. Interestingly, we found that certain gene pairs exhibit distinct mutation patterns in different cancer types. For instance, we observed that mutations in the TP53 and PIK3CA genes tend to be mutually exclusive in breast carcinoma, colorectal adenocarcinoma, and brain lower-grade glioma, but co-occur in non-small cell lung cancer (Fig. 5 a). Additionally, TP53 and KRAS mutations co-occur in lung adenocarcinoma and pancreatic ductal adenocarcinoma but are mutually exclusive in uterine corpus endometrial carcinoma and cholangiocarcinoma (Supplementary Data 2 ). These observations suggest that when gene pairs are co-mutated, they may work together to promote oncogenesis 32 , 33 , 34 , 35 , while when they are exclusively mutated, they may act independently and yield tumours with different phenotypic or molecular subtypes 30 , 31 , 36 .
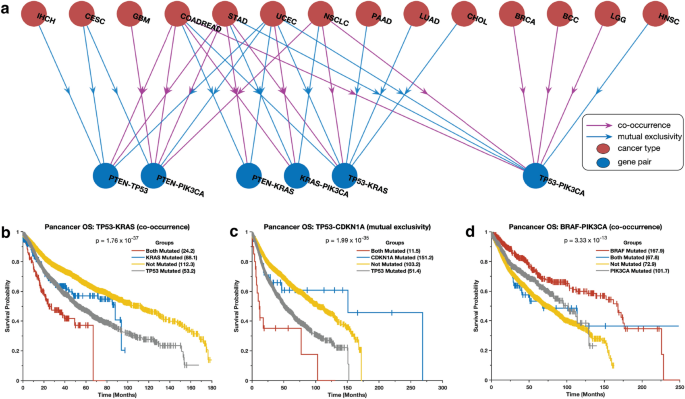
Combination of mutations associated with survival outcomes. ( a ) The correlation between the presence of specific mutations in the most widely studied cancer genes and patient survival rates for different types of cancer. The Kaplan–Meier curve displays the overall survival periods of patients with tumours that have ( b ) both TP53 and KRAS mutations, only TP53 mutations, only KRAS mutations, or no TP53 or KRAS mutations; ( c ) both TP53 and CDKN2A mutations, only TP53 mutations, only CDKN2A mutations, or no TP53 or CDKN2A mutations; ( d ) both PI3KCA and BRAF mutations, only PI3KCA mutations, only BRAF mutations, or no PI3KCA or BRAF mutations.
Previous research suggests that there may be a positive correlation between the number of exclusive mutations and co-occurring mutations in a given cancer type, potentially driven by the relationship between mutation burden 37 , 38 and the epistatic interaction between driver genes 39 , 40 . However, the results of the study showed that this was not the case, as there was no correlation between the number of exclusive mutations and co-occurring mutations, nor was there a correlation between the number of cancer-type samples and the number of exclusively mutated gene pairs observed (Supplementary Fig. 8 a and b). The lack of correlation was particularly pronounced in uterine corpus endometrial carcinoma, where 160,316 co-occurring gene pairs were found but only 33 exclusive gene pairs across 494 samples. The results suggest that the number of mutually exclusively mutated gene pairs may indicate the genomic complexity of a particular form of cancer and its link to alterations in different oncogenic pathways.
Disease outcomes of cancer patients are linked to the mutation patterns
We evaluated the impact of mutations in gene pairs on the overall survival of cancer patients by grouping patients into four groups based on the presence of mutations in a gene pair (see the “Methods” section). The groups were: (1) no mutations, (2) and (3) only one of the genes in the pair is mutated, and (4) both genes are mutated. Here, we found that mutations in gene pairs are associated with varied overall survival durations of patients afflicted. For example, in the case of some of the most studied genes in cancer 41 , 42 , we found that patients with tumours that harbour mutations in both KRAS and TP53 ( p = 1.76 × 10 –37 ) or CDKN1A and TP53 ( p = 1.99 × 10 –35 ) tended to exhibit worse survival outcomes than those with tumours in which one or none of these genes are mutated (Fig. 5 b and 5c, see Supplementary Data 3 ). Furthermore, we found that patients with tumours with mutations in PIK3CA and/or BRAF tended to exhibit better survival outcomes than those with mutations in TP53 , KRAS , and/or EGFR (Fig. 5 d and Supplementary Fig. 9 ). In addition, we found that the patients with tumours with mutations in PIK3CA , BRAF , CDH1 , and NRAS exhibit better survival outcomes than those without mutations in these genes (Supplementary Fig. 9 ; and Supplementary Data 3 ).
Our findings highlight the importance of understanding the impact of different combinations of gene mutations on cancer development and progression. Specifically, some cancer patients may exhibit significantly different disease outcomes due to the specific combination of mutations present in their tumours, as has been demonstrated in multiple studies 43 , 44 , 45 . For example, mutations in the KRAS that co-occur with STK11, KEAP1, and TP53 genes in lung cancer patients have been associated with poorer prognosis and reduced survival 44 .
Driver pathways of co-occurring and exclusively mutated genes
Our study sought to identify the driver pathways (gene combinations) for the top 10 most frequently mutated cancer genes in various cancer types. Using a detailed analysis of gene pairs, we found intriguing variations in patterns of co-occurring and mutually exclusive mutations across different cancer types (see “Methods” sections and Supplementary Data 4 ). In ampullary carcinoma, for example, we identified two sets of co-occurring mutated driver pathways (Fig. 6 a). The first set involves five genes ( TP53 , KRAS , APC , SMAD4 , and PIK3CA ) that exhibit a co-occurring mutation pattern, while the second set of five genes ( ARID1A , ATM , ARID3 , NF1 , and TGFBR2 ) are exclusively mutated. Similarly, in acute myeloid leukaemia, we found two gene sets; the first set includes six genes ( FLT3, DNMT3A, NPM1, IDH3, RUNX1, and IDH1 ) that exhibit a co-occurring mutation pattern, and the second set of four genes ( TET2, TP53, NRAS, and WT1 ), exhibit an exclusive mutation pattern (Fig. 6 b). In lung adenocarcinoma, we found two gene sets; the first set includes six genes ( TP53, EGFR, KRAS, KEAP1, STK11, and NF1 ) that exhibit a co-occurring mutation pattern, and the second set of four genes ( SMARCA4, ATM, RBM10, and APC ) (Fig. 6 c), exhibit an exclusive mutation pattern.
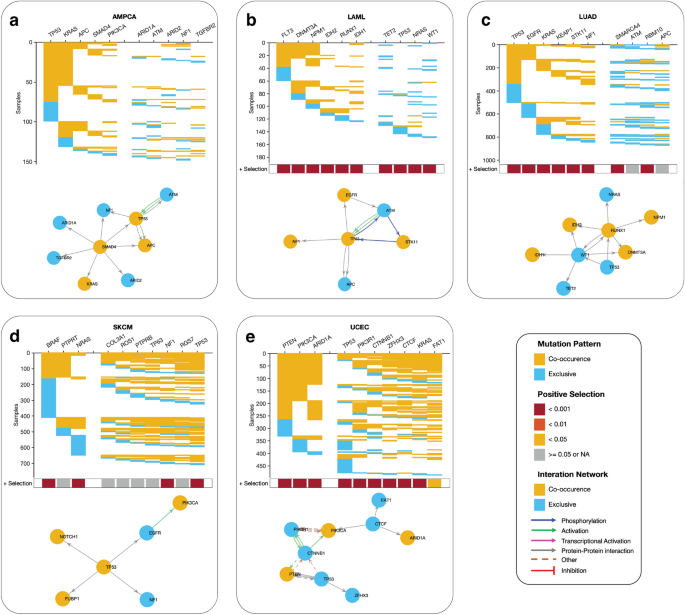
Predictive oncogenic pathways in different cancer types based on mutational landscapes. Two co-occurring pathways are predicted to drive oncogenesis based on the mutational landscape in ( a ) AMPCA, ( b ) LAML, ( c ) LUAD, ( d ) SKCM, and ( e ) ECEC. The coloured square marks at the bottom of each plot show a positive selection of mutations in each gene along each column (see the “Methods” section). The connectivity of network components within each panel was extracted from the KEA and ChEA databases and the UCSC super pathway.
However, our analysis revealed that driver gene mutations have a high co-occurrence in specific cancer types, including uterine corpus endometrial carcinoma, skin cutaneous melanoma, and basal cell carcinoma (Fig. 6 d,e, Supplementary Fig. 10 ). We also observed that the mutated genes of cancer driver pathways are significantly under positive selection across all cancer types, highlighting the potential importance of these genes in cancer development and progression.
Here, our findings suggest that different cancer types may exhibit distinct patterns of driver pathway mutations, and that further research is needed to fully understand the implications of these patterns for cancer subtypes and cancer treatment.
Cancer gene mutations in the context of cancer hallmarks
Owing to the importance of cancer hallmarks in designing better treatment strategies, we sought to determine the extent to which genes associated with each hallmark of cancer are altered across different types of human cancer (Fig. 7 and Supplementary Data 5 , see "Methods" section). Our analysis revealed that the highest number of mutated genes were found in the "escaping programmed cell death" hallmark (220 genes), followed by "invasion and metastasis" (213 genes), "proliferative signalling" (160 genes), and "genome instability and mutations" (129 genes), as shown in Fig. 7 . Notably, the most frequently mutated genes within these hallmarks were oncogenes and tumour suppressor genes that are not kinases, phosphatases, or cell surface receptors. This is of particular interest as current efforts in cancer research to identify drug targets primarily focus on kinases and cell surface receptors. Our findings suggest the potential for identifying a diverse range of drug targets among non-traditional cancer gene targets.
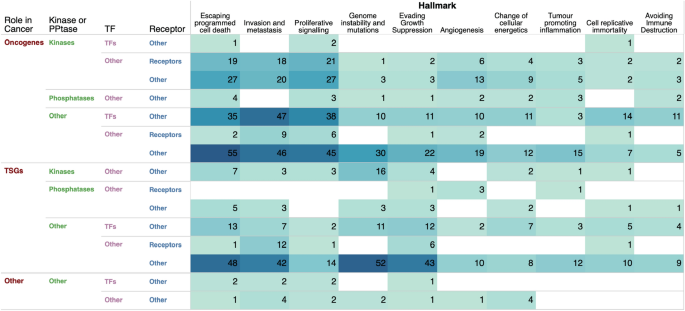
Alterations of cancer hallmark genes. The total number of cancer genes across each combination of cancer genes categories associated with the hallmarks of cancer.
In this study, we have conducted a systematic analysis of 727 cancer gene mutations across 41 human cancer types. Our results reveal the presence of non-synonymous mutations in known cancer genes in all samples examined, including mutations in oncogenes, TSGs, genes encoding transcription factors, kinases, phosphatases, and cell surface receptors. This suggests that various components of the cell signalling process are involved in oncogenesis. Furthermore, this finding demonstrates that various components of the cell signalling processes, including receptors that respond to stimuli, cytoplasmic enzymes, and nuclear proteins, are involved in oncogenesis. Interestingly, we found that not all samples of a particular cancer type harbour the same driver mutations, and the distribution of gene mutations within each cancer type varies significantly. These findings suggest that each patient may exhibit a different combination of mutations sufficient to perturb various oncogenic pathways, highlighting the importance of understanding the mutation profile of each patient's tumour for the optimization of personalised cancer treatments.
In recent years, there has been a growing understanding of the importance of identifying driver mutations in different cancer types 3 , 8 , 10 , 31 . Studies have identified specific driver mutations in various cancers, such as EGFR mutations in lung cancer 46 and BRAF mutations in melanoma 47 . These findings have led to the development of targeted therapies that specifically target the mutated genes or pathways, improving patient survival rates and quality of life 48 , 49 , 50 , 51 . For example, the discovery of EGFR mutations in lung cancer led to the development of targeted therapies such as gefitinib and erlotinib, which have demonstrated improved outcomes compared to traditional chemotherapy 52 , 53 . Similarly, the identification of BRAF mutations in melanoma led to the development of targeted therapies such as vemurafenib and dabrafenib, which have also improved patient outcomes 54 , 55 . Therefore, we suggest that a deeper understanding of the interplay between driver mutations in cancer can lead to more effective and personalised treatments for different cancer types and subtypes that reduce the burden of cancer and improve patient outcomes.
However, it is important to note that some tumours have fewer than 5% of their cancer genes mutated. For example, gene mutations are infrequent in thyroid carcinoma, testicular germ cell tumours, and thymomas, where only two cancer genes are mutated in more than 5% of the examined tumours. These exceptions reinforce the notion that multiple routes to oncogenesis may be independent of cancer gene mutations and involve alterations in other regulatory mechanisms, such as the epigenome 1 , 7 , 56 . Furthermore, these findings reveal that in cases where cancer gene mutations are infrequent, other genetic datasets, such as chromosomal changes, epigenetic changes, copy number variations, microsatellite instability, mRNA transcription abundance, and mutations in the non-coding and regulatory regions of the genome, should be considered to identify cancer genes 57 , 58 , 59 , 60 , 61 .
Despite the large amounts of genomic data this study analysed, we could not pinpoint commonly mutated cancer genes in samples of specific cancer types. This highlights the sparsity nature of gene mutations and the limited diversity of the presently available genome sequences, which impede the identification of commonly applicable drug targets and marker mutations within each cancer type 62 , 63 , 64 , 65 , 66 . Despite this, we discovered that in gene pairs specific to all types of cancer, the co-occurrence of mutations (284,709) is 378 times more likely than exclusive mutations (796). This demonstrates that genes involved in various cancer pathways have a greater tendency to co-mutate rather than mutate exclusively 40 , 67 . As a result, our findings suggest the presence of a limited number of context-dependent, co-occurring driver gene mutations, which could facilitate the identification of widely applicable drug targets and markers of aggressiveness across a multitude of patients' tumours.
Our findings also show that the co-occurrence and exclusive nature of cancer gene mutations significantly affect the disease outcome of patients with various forms of cancer. In addition, these results indicate that various gene alterations in specific gene pairs have a diverse impact on processes that drive disease aggressiveness 68 , 69 , 70 .
However, a limitation of our study is that we did not account for the distinction between COSMIC Tier 1 and Tier 2 cancer-driving genes in our analysis 23 . Tier 1 genes have well-documented activities relevant to cancer, while Tier 2 genes have strong indications but with emerging evidence 23 . By not distinguishing between these tiers, we may have overlooked some nuanced differences in the role these genes play in oncogenesis. This could potentially affect the interpretation of the data, especially in the context of clinical relevance. In future studies, a separate analysis of Tier 1 and Tier 2 genes might provide more detailed insights into their distinct roles and contributions to the development and progression of cancer.
In conclusion, to comprehensively evaluate the impact of different combinations of gene alterations on cancer development and treatment response, there is an urgent need for new molecular tools. While there is a vast amount of genomics data available, it is currently not feasible to study the impact of every possible combination of gene mutations due to the sheer size of the combinatorial space. It is important to note that the currently available genomics data is limited to a subset of patient tumours and cell lines, which only includes a fraction of the possible combinations of driver mutations that may exist. While databases 15 , 23 , 42 and computational tools can be used to predict the potential impact of certain gene mutations or their combinations 71 , 72 , 73 , the limitations of available data and the complexity of cancer genetics make it challenging to comprehensively evaluate the impact of every possible combination on cancer development and drug response. As such, future experiments that allow the altering of cancer genes in normal cells in different combinations will ultimately help to unlock the impact of a combination of cancer gene mutations on oncogenesis, disease aggressiveness, and the chemosensitivity of tumours. By developing new molecular tools and continuing to expand our knowledge of cancer genetics, we can move closer to achieving precision medicine in cancer treatment, where patients receive tailored therapies based on the genetic profile of their cancer.
In our study, we obtained a dataset of 366 cancer studies from the cBioPortal 74 version 5.2.5 ( http://www.cbioportal.org ) of deeply sequenced tumours. To ensure a high level of specificity, we excluded studies that employed targeted sequencing, datasets of paediatric tumours, and cancer cell lines, resulting in a final dataset of 206 cancer studies. Further, we filtered the dataset again to return only those from 138 cancer studies that also had clinical information for the profiled afflicted patients. The final datasets encompassed 20,331 patient-derived tumour samples representing 41 distinct human cancer types sequenced from the year 2012 to 2021 (see Supplementary Data 1 for details on the cancer studies). The elements of the data that we obtained from cBioPortal include somatic gene mutations (point mutations and small insertions/deletions) and comprehensively deidentified clinical data.
Compilation of cancer genes and their classes
In our study, we sourced data on cancer-driving genes the Catalogue of Somatic Mutations in Cancer 24 (COSMIC), specifically from the Consensus Cancer Gene Database 23 (version 95, released on November 24, 2021), which includes a total of 727 cancer-driving genes that have been rigorously vetted for evidence and manually curated, with 576 in Tier 1 and 151 in Tier 2. To further classify these known cancer genes, we divided them into five classes: oncogenes, tumour suppressor genes, kinases, phosphatases, cell surface proteins, and transcription factors. To achieve this, we obtained information on gene annotations from various databases, including the Sanger Consensus Cancer Gene Database 23 (699 oncogenes and TSGs), the UniProt Knowledgebase 75 (304 oncogenes and 741 TSGs), the TSGene database 76 (1,220 TSGs), the ChEA transcription factor database 77 (645 transcription factors), the TF2DNA database 78 (1,314 transcription factors), the Kinase Enrichment Analysis database 79 (428 kinases), the ONGene database 80 (725 oncogenes), and the Surfaceome database 81 (2,950 cell surface receptors). We then collated the cancer genes from all the above databases to obtain a list of 727 cancer genes (after removing overlapping genes), including 383 oncogenes, 370 tumour suppressor genes, 9 phosphatases, 75 kinases, 63 cell surface receptors, and 150 transcription factors (Supplementary Data 1 ).
Calculation of cancer gene mutations
We obtained the gene sequencing datasets of the samples for all the cancer genes. We then selected only the non-synonymous mutations that occurred within the genes. To evaluate the extent to which each cancer-driving gene is mutated in cancer, we calculated the somatic mutation frequency (including single nucleotide mutations, short indels, and insertions) for each gene across the 20,331 samples across each cancer type (Supplementary Data 1 ). Additionally, for each cancer type, we obtained summaries of the number of mutated genes in (1) none of the samples, (2) less than 5% of the samples, and (3) more than 5% of the samples.
Cancer gene mutations across gene classes
We aimed to determine the extent of mutations in cancer-associated genes within individual cancer types and across all human cancers. To achieve this, we calculated the non-synonymous somatic mutation frequency (including single nucleotide mutations, short indels, and insertions) for each of the 41 human cancer types represented among the 20,331 samples (Supplementary Data 1 ). Additionally, we calculated the frequency of non-synonymous somatic mutations for groups of genes, such as oncogenes, tumour suppressor genes, kinases, phosphatases, cell surface receptors, and transcription factors, across each of the 41 cancer types and the entire patient cohort (Supplementary Data 1 ). It should be noted that, for the calculations involving gene categories, we included genes that belong to each category and not only exclusively to one category.
Assessment of the co-occurrence and exclusivity of gene mutations
We used the hypergeometric Fisher test to evaluate the correlation in the mutation profile of cancer gene pairs. First, we obtained a list of mutated genes in more than 1% (550 cancer genes) of all tumours across all the samples. Next, we applied the Fisher test to each pair of the selected genes and utilised a cut-off p-value of 0.05 to identify statistically significant gene pair correlations. Furthermore, we used the magnitude of the odds ratio to identify gene pairs with co-occurring mutations (odds > 1 and p < 0.05) and gene pairs with mutually exclusive mutations (odds < 1 and p < 0.05). Additionally, we used the approach to identify cancer gene pairs within co-occurring or mutually exclusive mutation patterns within each of the 43 human cancer types (see Supplementary Data 2 ).
Correlation between mutations pattern and disease outcomes
We used the Kaplan–Meier 82 method to estimate the duration of overall survival and disease-free survival of patients with cancer who had (1) only one gene pair mutated, (2) the other gene pair mutated, (3) none of the gene pair mutated, and (4) both gene pairs mutated.
Identification of exclusive and co-occurring driver pathways
To identify the patterns of mutations associated with each cancer type and the corresponding cancer gene combinations, we applied the CoMDP algorithm 83 . This algorithm employs a mathematical programming method to identify de novo driver pathways in cancer from mutation profiles. Specifically, we aimed to identify pathways with mutated cancer genes that exhibit both high coverage (i.e., present in multiple samples) and high exclusivity, and show a statistically significant co-occurrence pattern.
To begin, we selected the top 10 mutated cancer genes for each cancer type as input for the CoMDP algorithm. These genes were selected from the significantly mutated genes identified using the MutSigCV2 algorithm 84 . In cases where MutSigCV2 identified fewer than 10 genes as significantly mutated, we included additional genes to bring the gene set size to 10. If a gene with very long coding regions or very long introns (including CSMD1, CSMD3, NRXN1, NRXN4, CNTNAP2, CNTNAP4, CNTNAP5, CNTN5, PARK2, LRP1B, PCLO, MUC16, MUC4, KMT2C, KMT2A, KMT2D, FAT1, FAT2, FAT3, and FAT4 ) were not identified as being significantly mutated using MutSigCV2 in a specific cancer type, it was excluded from the analysis for that cancer type 84 .
Next, we ran the CoMDP test for each cancer type with K = 10, where K equals the gene set size. The CoMDP analysis returned mutated driver pathways associated with the genes in each cancer type (Supplementary Data 4 ). Additionally, we obtained information on the selectivity of gene mutations in each cancer type from the supplementary data of Martincorena et al. 29 .
Association between cancer gene mutations and the cancer hallmarks
To investigate the relationship between the Hallmarks of Cancer 1 , 85 and the mutated gene sets, we accessed information on genes and proteins associated with various Hallmarks of Cancer from the COSMIC database 24 . We then calculated the number of mutated genes at a frequency of more than 1% in at least one cancer type within each class based on the various cancer hallmark gene sets (Supplementary Data 5 ).
Statistics and reproducibility
We used MATLAB 2022a 86 for all statistical analyses. We used two-sided statistical tests with a p -value < 0.05 to indicate statistical significance. To correct for multiple statistical testing, we applied the Benjamini & Hochberg procedure 87 , resulting in a q-value < 0.05 for each comparison.
Ethics approval
The study protocol was approved by The University of Cape Town; Health Sciences Research Ethics Committee IRB00001938. The publicly available datasets were collected by the cBioPortal.
Data availability
The data that support our results are available in this manuscript, the supplementary data, and from the following repositories: cBioPortal; https://www.cbioportal.org/ , and the COSMIC Consensus Cancer Genes; https://cancer.sanger.ac.uk/census .
Code availability
Custom code written in MATLAB for processing and analysing the presented data is freely available at https://github.com/smsinks/Analysis-of-Cancer-Gene-Mutations . In addition, the repository includes some pre-downloaded datasets and conversion files required for the analysis.
Hanahan, D. & Weinberg, R. A. Hallmarks of cancer: The next generation. Cell 144 , 646–674. https://doi.org/10.1016/j.cell.2011.02.013 (2011).
Article CAS PubMed Google Scholar
Kafita, D. et al. High ELF4 expression in human cancers is associated with worse disease outcomes and increased resistance to anticancer drugs. PLoS ONE 16 , e0248984. https://doi.org/10.1371/journal.pone.0248984 (2021).
Article CAS PubMed PubMed Central Google Scholar
Sinkala, M., Nkhoma, P., Mulder, N. & Martin, D. P. Integrated molecular characterisation of the MAPK pathways in human cancers reveals pharmacologically vulnerable mutations and gene dependencies. Commun. Biol. 4 , 9. https://doi.org/10.1038/s42003-020-01552-6 (2021).
Sinkala, M., Mulder, N. & Martin, D. P. Integrative landscape of dysregulated signaling pathways of clinically distinct pancreatic cancer subtypes. Oncotarget 9 , 29123–29139 (2018).
Article PubMed PubMed Central Google Scholar
Sanchez-Vega, F. et al. Oncogenic signaling pathways in the cancer genome atlas. Cell 173 , 321–337. https://doi.org/10.1016/j.cell.2018.03.035 (2018).
Liberzon, A. et al. The molecular signatures database (MSigDB) hallmark gene set collection. Cell Syst. 1 , 417–425. https://doi.org/10.1016/j.cels.2015.12.004 (2015).
Pavlova, N. N. & Thompson, C. B. The emerging hallmarks of cancer metabolism. Cell Metab. 23 , 27–47. https://doi.org/10.1016/j.cmet.2015.12.006 (2016).
Porta-Pardo, E., Valencia, A. & Godzik, A. Understanding oncogenicity of cancer driver genes and mutations in the cancer genomics era. FEBS Lett. 594 , 4233–4246. https://doi.org/10.1002/1873-3468.13781 (2020).
Scholl, C. & Frohling, S. Exploiting rare driver mutations for precision cancer medicine. Curr. Opin. Genet. Dev. 54 , 1–6. https://doi.org/10.1016/j.gde.2019.02.004 (2019).
Kishtagari, A., Levine, R. L. & Viny, A. D. Driver mutations in acute myeloid leukemia. Curr. Opin. Hematol. 27 , 49–57. https://doi.org/10.1097/MOH.0000000000000567 (2020).
Article PubMed Google Scholar
Sinkala, M., Mulder, N. & Martin, D. Machine learning and network analyses reveal disease subtypes of pancreatic cancer and their molecular characteristics. Sci. Rep. 10 , 1212. https://doi.org/10.1038/s41598-020-58290-2 (2020).
Article ADS CAS PubMed PubMed Central Google Scholar
Cramer, D. et al. Genetic interactions and tissue specificity modulate the association of mutations with drug response. Mol. Cancer Ther. 19 , 927–936. https://doi.org/10.1158/1535-7163.MCT-19-0045 (2020).
Schaub, F. X. et al. Pan-cancer alterations of the MYC oncogene and its proximal network across the cancer genome atlas. Cell Syst. 6 , 282–300. https://doi.org/10.1016/j.cels.2018.03.003 (2018).
Jn, W. et al. The cancer genome atlas pan-cancer analysis project. Nat. Genet. 45 , 1113–1120. https://doi.org/10.1038/ng.2764 (2013).
Article CAS Google Scholar
Korkut, A. et al. A pan-cancer analysis reveals high-frequency genetic alterations in mediators of signaling by the TGF-beta superfamily. Cell Syst 7 , 422–437. https://doi.org/10.1016/j.cels.2018.08.010 (2018).
Berger, A. C. et al. A comprehensive pan-cancer molecular study of gynecologic and breast cancers. Cancer Cell 33 , 690–705. https://doi.org/10.1016/j.ccell.2018.03.014 (2018).
Kafita, D., Nkhoma, P., Zulu, M. & Sinkala, M. Proteogenomic analysis of pancreatic cancer subtypes. PLoS ONE 16 , e0257084. https://doi.org/10.1371/journal.pone.0257084 (2021).
Dzobo, K. & Sinkala, M. Cancer stem cell marker CD44 plays multiple key roles in human cancers: Immune suppression/evasion, drug resistance, epithelial-mesenchymal transition, and metastasis. OMICS 25 , 313–332. https://doi.org/10.1089/omi.2021.0025 (2021).
Cancer Genome Atlas Network, Comprehensive molecular characterization of human colon and rectal cancer. Nature 487, 330–337 (2012). https://doi.org/10.1038/nature11252
Burotto, M., Chiou, V. L., Lee, J. M. & Kohn, E. C. The MAPK pathway across different malignancies: A new perspective. Cancer 120 , 3446–3456. https://doi.org/10.1002/cncr.28864 (2014).
Iorio, F. et al. A landscape of pharmacogenomic interactions in cancer. Cell 166 , 740–754. https://doi.org/10.1016/j.cell.2016.06.017 (2016).
Wang, L., Li, X., Zhang, L. & Gao, Q. Improved anticancer drug response prediction in cell lines using matrix factorization with similarity regularization. BMC Cancer 17 , 513. https://doi.org/10.1186/s12885-017-3500-5 (2017).
Sondka, Z. et al. The COSMIC cancer gene census: Describing genetic dysfunction across all human cancers. Nat. Rev. Cancer 18 , 696–705. https://doi.org/10.1038/s41568-018-0060-1 (2018).
Forbes, S. A. et al. COSMIC: Exploring the world’s knowledge of somatic mutations in human cancer. Nucleic Acids Res. 43 , D805-811. https://doi.org/10.1093/nar/gku1075 (2015).
Khurana, E. et al. Role of non-coding sequence variants in cancer. Nat. Rev. Genet. 17 , 93–108. https://doi.org/10.1038/nrg.2015.17 (2016).
Laumont, C. M. et al. Noncoding regions are the main source of targetable tumor-specific antigens. Sci. Transl. Med. https://doi.org/10.1126/scitranslmed.aau5516 (2018).
Sinkala, M., Mulder, N. & Patrick Martin, D. Metabolic gene alterations impact the clinical aggressiveness and drug responses of 32 human cancers. Commun. Biol. 2 , 414. https://doi.org/10.1038/s42003-019-0666-1 (2019).
Ben-David, U. et al. Genetic and transcriptional evolution alters cancer cell line drug response. Nature 560 , 325–330. https://doi.org/10.1038/s41586-018-0409-3 (2018).
Martincorena, I. et al. Universal patterns of selection in cancer and somatic tissues. Cell 173 , 1823. https://doi.org/10.1016/j.cell.2018.06.001 (2018).
Chiang, S. et al. IDH2 mutations define a unique subtype of breast cancer with altered nuclear polarity. Cancer Res 76 , 7118–7129. https://doi.org/10.1158/0008-5472.CAN-16-0298 (2016).
Watson, I. R., Takahashi, K., Futreal, P. A. & Chin, L. Emerging patterns of somatic mutations in cancer. Nat. Rev. Genet. 14 , 703–718. https://doi.org/10.1038/nrg3539 (2013).
Moro, M. et al. Metformin enhances cisplatin-induced apoptosis and prevents resistance to cisplatin in co-mutated KRAS/LKB1 NSCLC. J. Thorac. Oncol. 13 , 1692–1704. https://doi.org/10.1016/j.jtho.2018.07.102 (2018).
Richardson, D. R. et al. Genomic characteristics and prognostic significance of co-mutated ASXL1/SRSF2 acute myeloid leukemia. Am. J. Hematol. 96 , 462–470. https://doi.org/10.1002/ajh.26110 (2021).
Labbe, C. et al. Prognostic and predictive effects of TP53 co-mutation in patients with EGFR-mutated non-small cell lung cancer (NSCLC). Lung Cancer 111 , 23–29. https://doi.org/10.1016/j.lungcan.2017.06.014 (2017).
Hayashi, T. et al. RASA1 and NF1 are preferentially co-mutated and define a distinct genetic subset of smoking-associated non-small cell lung carcinomas sensitive to MEK inhibition. Clin. Cancer Res. 24 , 1436–1447. https://doi.org/10.1158/1078-0432.CCR-17-2343 (2018).
McConkey, D. J. & Choi, W. Molecular subtypes of bladder cancer. Curr. Oncol. Rep. 20 , 77. https://doi.org/10.1007/s11912-018-0727-5 (2018).
Sha, D. et al. Tumor mutational burden as a predictive biomarker in solid tumors. Cancer Discov. 10 , 1808–1825. https://doi.org/10.1158/2159-8290.CD-20-0522 (2020).
Skoulidis, F. & Heymach, J. V. Co-occurring genomic alterations in non-small-cell lung cancer biology and therapy. Nat. Rev. Cancer 19 , 495–509. https://doi.org/10.1038/s41568-019-0179-8 (2019).
Zhou, Y. et al. Integrated multi-omics data analyses for exploring the co-occurring and mutually exclusive gene alteration events in colorectal cancer. Hum. Mutat. 41 , 1588–1599. https://doi.org/10.1002/humu.24059 (2020).
El Tekle, G. et al. Co-occurrence and mutual exclusivity: What cross-cancer mutation patterns can tell us. Trends Cancer 7 , 823–836. https://doi.org/10.1016/j.trecan.2021.04.009 (2021).
Nguyen, D. T. et al. Pharos: Collating protein information to shed light on the druggable genome. Nucleic Acids Res. 45 , D995–D1002. https://doi.org/10.1093/nar/gkw1072 (2017).
Kelleher, K. J. et al. Pharos 2023: An integrated resource for the understudied human proteome. Nucleic Acids Res. 51 , D1405–D1416. https://doi.org/10.1093/nar/gkac1033 (2023).
Schmitz, R. et al. Genetics and pathogenesis of diffuse large B-cell lymphoma. N. Engl. J. Med. 378 , 1396–1407. https://doi.org/10.1056/NEJMoa1801445 (2018).
West, H. J. et al. Clinical efficacy of atezolizumab plus bevacizumab and chemotherapy in KRAS-mutated non-small cell lung cancer with STK11, KEAP1, or TP53 comutations: subgroup results from the phase III IMpower150 trial. J. Immunother. Cancer https://doi.org/10.1136/jitc-2021-003027 (2022).
Arbour, K. C. et al. Effects of co-occurring genomic alterations on outcomes in patients with KRAS-mutant non-small cell lung cancer. Clin. Cancer Res. 24 , 334–340. https://doi.org/10.1158/1078-0432.CCR-17-1841 (2018).
da Santos, C. G., Shepherd, F. A. & Tsao, M. S. EGFR mutations and lung cancer. Annu. Rev. Pathol. 6 , 49–69. https://doi.org/10.1146/annurev-pathol-011110-130206 (2011).
Ito, T. et al. BRAF heterogeneity in melanoma. Curr. Treat. Options Oncol. 22 , 20. https://doi.org/10.1007/s11864-021-00818-3 (2021).
Llovet, J. M., Montal, R., Sia, D. & Finn, R. S. Molecular therapies and precision medicine for hepatocellular carcinoma. Nat. Rev. Clin. Oncol. 15 , 599–616. https://doi.org/10.1038/s41571-018-0073-4 (2018).
Huang, R. & Zhou, P. K. DNA damage repair: Historical perspectives, mechanistic pathways and clinical translation for targeted cancer therapy. Signal Transduct. Target Ther. 6 , 254. https://doi.org/10.1038/s41392-021-00648-7 (2021).
Davies, H. et al. Mutations of the BRAF gene in human cancer. Nature 417 , 949–954. https://doi.org/10.1038/nature00766 (2002).
Article ADS CAS PubMed Google Scholar
Menzer, C. et al. Targeted therapy in advanced melanoma with rare BRAF mutations. J. Clin. Oncol. 37 , 3142–3151. https://doi.org/10.1200/JCO.19.00489 (2019).
Yang, J. J. et al. A phase III randomised controlled trial of erlotinib vs gefitinib in advanced non-small cell lung cancer with EGFR mutations. Br. J. Cancer 116 , 568–574. https://doi.org/10.1038/bjc.2016.456 (2017).
Koyama, N. & Uchida, Y. Clinical significance of erlotinib monotherapy for gefitinib-resistant non-small cell lung cancer with EGFR mutations. Anticancer Res. 33 , 5083–5089 (2013).
CAS PubMed Google Scholar
Halle, B. R. & Johnson, D. B. Defining and targeting BRAF mutations in solid tumors. Curr. Treat. Options Oncol. 22 , 30. https://doi.org/10.1007/s11864-021-00827-2 (2021).
Robert, C. et al. Improved overall survival in melanoma with combined dabrafenib and trametinib. N. Engl. J. Med. 372 , 30–39. https://doi.org/10.1056/NEJMoa1412690 (2015).
Singh, S. S. et al. Dual role of autophagy in hallmarks of cancer. Oncogene 37 , 1142–1158. https://doi.org/10.1038/s41388-017-0046-6 (2018).
Esteller, M. Cancer epigenomics: DNA methylomes and histone-modification maps. Nat. Rev. Genet. 8 , 286–298. https://doi.org/10.1038/nrg2005 (2007).
Zack, T. I. et al. Pan-cancer patterns of somatic copy number alteration. Nat. Genet. 45 , 1134–1140. https://doi.org/10.1038/ng.2760 (2013).
Geurts-Giele, W. R. et al. Somatic aberrations of mismatch repair genes as a cause of microsatellite-unstable cancers. J. Pathol. 234 , 548–559. https://doi.org/10.1002/path.4419 (2014).
Pfister, S. X. & Ashworth, A. Marked for death: targeting epigenetic changes in cancer. Nat. Rev. Drug Discov. 16 , 241–263. https://doi.org/10.1038/nrd.2016.256 (2017).
Zhao, X. et al. An integrated view of copy number and allelic alterations in the cancer genome using single nucleotide polymorphism arrays. Cancer Res. 64 , 3060–3071. https://doi.org/10.1158/0008-5472.can-03-3308 (2004).
Tamborero, D. et al. Comprehensive identification of mutational cancer driver genes across 12 tumor types. Sci. Rep. 3 , 2650. https://doi.org/10.1038/srep02650 (2013).
Chen, Y. et al. Identification of druggable cancer driver genes amplified across TCGA datasets. PLoS ONE 9 , e98293. https://doi.org/10.1371/journal.pone.0098293 (2014).
Liu, S. H. et al. DriverDBv3: A multi-omics database for cancer driver gene research. Nucleic Acids Res. 48 , D863–D870. https://doi.org/10.1093/nar/gkz964 (2020).
Vogelstein, B. et al. Cancer genome landscapes. Science 339 , 1546–1558. https://doi.org/10.1126/science.1235122 (2013).
Bailey, M. H. et al. Comprehensive characterization of cancer driver genes and mutations. Cell 173 , 371–385. https://doi.org/10.1016/j.cell.2018.02.060 (2018).
Dao, P. et al. BeWith: A between-within method to discover relationships between cancer modules via integrated analysis of mutual exclusivity, co-occurrence and functional interactions. PLoS Comput. Biol. 13 , e1005695. https://doi.org/10.1371/journal.pcbi.1005695 (2017).
Martin, I. et al. Negative impact on clinical outcome of the mutational co-occurrence of SF3B1 and DNMT3A in refractory anemia with ring sideroblasts (RARS). Leuk. Lymphoma 58 , 1686–1693. https://doi.org/10.1080/10428194.2016.1246725 (2017).
Iyer, G. et al. Prevalence and co-occurrence of actionable genomic alterations in high-grade bladder cancer. J. Clin. Oncol. 31 , 3133–3140. https://doi.org/10.1200/JCO.2012.46.5740 (2013).
Boddu, P. et al. Co-occurrence of FLT3-TKD and NPM1 mutations defines a highly favorable prognostic AML group. Blood Adv. 1 , 1546–1550. https://doi.org/10.1182/bloodadvances.2017009019 (2017).
Reva, B., Antipin, Y. & Sander, C. Predicting the functional impact of protein mutations: Application to cancer genomics. Nucleic Acids Res. 39 , e118. https://doi.org/10.1093/nar/gkr407 (2011).
Doss, G. P. C., Nagasundaram, N. & Tanwar, H. Predicting the impact of deleterious single point mutations in SMAD gene family using structural bioinformatics approach. Interdiscip. Sci. 4 , 103–115. https://doi.org/10.1007/s12539-012-0122-0 (2012).
Berber, I., Erten, C. & Kazan, H. Predator: Predicting the impact of cancer somatic mutations on protein-protein interactions. IEEE/ACM Trans. Comput. Biol. Bioinform. https://doi.org/10.1109/TCBB.2023.3262119 (2023).
Cerami, E. et al. The cBio cancer genomics portal: An open platform for exploring multidimensional cancer genomics data. Cancer Discov. 2 , 401–404. https://doi.org/10.1158/2159-8290.CD-12-0095 (2012).
UniProt, C. UniProt: The universal protein knowledgebase in 2021. Nucleic Acids Res. 49 , D480–D489. https://doi.org/10.1093/nar/gkaa1100 (2021).
Zhao, M., Kim, P., Mitra, R., Zhao, J. & Zhao, Z. TSGene 2.0: An updated literature-based knowledgebase for tumor suppressor genes. Nucleic Acids Res. 44 , D1023-1031. https://doi.org/10.1093/nar/gkv1268 (2016).
Lachmann, A. et al. ChEA: Transcription factor regulation inferred from integrating genome-wide ChIP-X experiments. Bioinformatics 26 , 2438–2444. https://doi.org/10.1093/bioinformatics/btq466 (2010).
Pujato, M., Kieken, F., Skiles, A. A., Tapinos, N. & Fiser, A. Prediction of DNA binding motifs from 3D models of transcription factors; identifying TLX3 regulated genes. Nucleic Acids Res. 42 , 13500–13512. https://doi.org/10.1093/nar/gku1228 (2014).
Lachmann, A. & Ma’ayan, A. KEA: Kinase enrichment analysis. Bioinformatics 25 , 684–686. https://doi.org/10.1093/bioinformatics/btp026 (2009).
Liu, Y., Sun, J. & Zhao, M. ONGene: A literature-based database for human oncogenes. J. Genet. Genom. 44 , 119–121. https://doi.org/10.1016/j.jgg.2016.12.004 (2017).
Article Google Scholar
Bausch-Fluck, D. et al. A mass spectrometric-derived cell surface protein atlas. PLoS ONE 10 , e0121314. https://doi.org/10.1371/journal.pone.0121314 (2015).
Rich, J. T. et al. A practical guide to understanding Kaplan-Meier curves. Otolaryngol. Head Neck Surg. 143 , 331–336. https://doi.org/10.1016/j.otohns.2010.05.007 (2010).
Zhang, J., Wu, L. Y., Zhang, X. S. & Zhang, S. Discovery of co-occurring driver pathways in cancer. BMC Bioinform. 15 , 271. https://doi.org/10.1186/1471-2105-15-271 (2014).
Lawrence, M. S. et al. Mutational heterogeneity in cancer and the search for new cancer-associated genes. Nature 499 , 214–218. https://doi.org/10.1038/nature12213 (2013).
Pavlova, N. N., Zhu, J. & Thompson, C. B. The hallmarks of cancer metabolism: Still emerging. Cell Metab. 34 , 355–377. https://doi.org/10.1016/j.cmet.2022.01.007 (2022).
MATLAB Version: 9.14.0.2137306 (R2023a) (Natick, Massachusetts, 2023).
Hochberg, Y. & Benjamini, Y. More powerful procedures for multiple significance testing. Stat. Med. 9 , 811–818. https://doi.org/10.1002/sim.4780090710 (1990).
Download references
Author information
Authors and affiliations.
Department of Biomedical Sciences, School of Health Sciences, University of Zambia, Lusaka, Zambia
Musalula Sinkala
Computational Biology Division, Faculty of Health Sciences, Institute of Infectious Disease and Molecular Medicine, University of Cape Town, Cape Town, South Africa
You can also search for this author in PubMed Google Scholar
Contributions
The study was conceptualized by M.S. The methodology was designed by M.S. and the data analysis performed M.S. M.S., drafted manuscript. Editing and reviewing of the manuscript was carried out by M.S. Data visualisations were produced by M.S.
Corresponding author
Correspondence to Musalula Sinkala .
Ethics declarations
Competing interests.
The authors declare no competing interests.
Additional information
Publisher's note.
Springer Nature remains neutral with regard to jurisdictional claims in published maps and institutional affiliations.
Supplementary Information
Supplementary legends., supplementary information 1., supplementary information 2., supplementary information 3., supplementary information 4., supplementary information 5., supplementary figures., rights and permissions.
Open Access This article is licensed under a Creative Commons Attribution 4.0 International License, which permits use, sharing, adaptation, distribution and reproduction in any medium or format, as long as you give appropriate credit to the original author(s) and the source, provide a link to the Creative Commons licence, and indicate if changes were made. The images or other third party material in this article are included in the article's Creative Commons licence, unless indicated otherwise in a credit line to the material. If material is not included in the article's Creative Commons licence and your intended use is not permitted by statutory regulation or exceeds the permitted use, you will need to obtain permission directly from the copyright holder. To view a copy of this licence, visit http://creativecommons.org/licenses/by/4.0/ .
Reprints and permissions
About this article
Cite this article.
Sinkala, M. Mutational landscape of cancer-driver genes across human cancers. Sci Rep 13 , 12742 (2023). https://doi.org/10.1038/s41598-023-39608-2
Download citation
Received : 26 September 2022
Accepted : 27 July 2023
Published : 07 August 2023
DOI : https://doi.org/10.1038/s41598-023-39608-2
Share this article
Anyone you share the following link with will be able to read this content:
Sorry, a shareable link is not currently available for this article.
Provided by the Springer Nature SharedIt content-sharing initiative
By submitting a comment you agree to abide by our Terms and Community Guidelines . If you find something abusive or that does not comply with our terms or guidelines please flag it as inappropriate.
Quick links
- Explore articles by subject
- Guide to authors
- Editorial policies
Sign up for the Nature Briefing: Cancer newsletter — what matters in cancer research, free to your inbox weekly.

Mutational landscape of cancer-driver genes across human cancers
Affiliations.
- 1 Department of Biomedical Sciences, School of Health Sciences, University of Zambia, Lusaka, Zambia. [email protected].
- 2 Computational Biology Division, Faculty of Health Sciences, Institute of Infectious Disease and Molecular Medicine, University of Cape Town, Cape Town, South Africa. [email protected].
- PMID: 37550388
- PMCID: PMC10406856
- DOI: 10.1038/s41598-023-39608-2
The genetic mutations that contribute to the transformation of healthy cells into cancerous cells have been the subject of extensive research. The molecular aberrations that lead to cancer development are often characterised by gain-of-function or loss-of-function mutations in a variety of oncogenes and tumour suppressor genes. In this study, we investigate the genomic sequences of 20,331 primary tumours representing 41 distinct human cancer types to identify and catalogue the driver mutations present in 727 known cancer genes. Our findings reveal significant variations in the frequency of cancer gene mutations across different cancer types and highlight the frequent involvement of tumour suppressor genes (94%), oncogenes (93%), transcription factors (72%), kinases (64%), cell surface receptors (63%), and phosphatases (22%), in cancer. Additionally, our analysis reveals that cancer gene mutations are predominantly co-occurring rather than exclusive in all types of cancer. Notably, we discover that patients with tumours displaying different combinations of gene mutation patterns tend to exhibit variable survival outcomes. These findings provide new insights into the genetic landscape of cancer and bring us closer to a comprehensive understanding of the underlying mechanisms driving the development of various forms of cancer.
© 2023. Springer Nature Limited.
- Neoplasms* / genetics
Featured Topics
Featured series.
A series of random questions answered by Harvard experts.
Explore the Gazette
Read the latest.
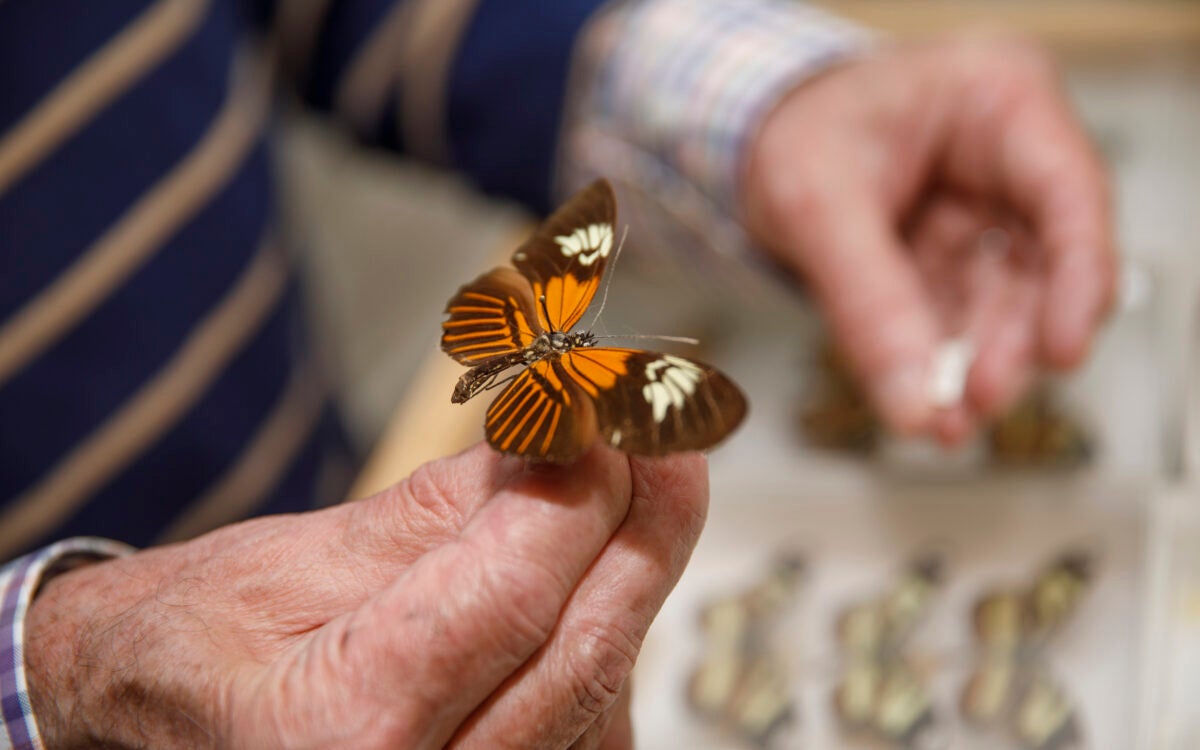
Amazon butterfly evolved from hybrids
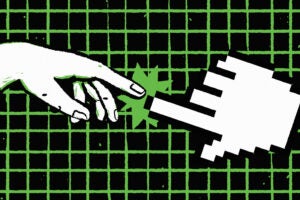
‘Harvard Thinking’: Is AI friend or foe? Wrong question.
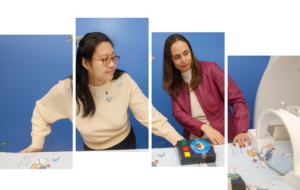
Getting ahead of dyslexia
CI Photos/iStock
Collaboration generates most complete cancer genome map
Stephanie McPherson
Broad Institute Communications
Pan-Cancer Project brings researchers closer to identifying all major cancer-causing genetic mutations
An international team has completed the most comprehensive study of whole cancer genomes, significantly improving the fundamental understanding of cancer and indicating new directions for developing diagnostics and treatments.
The discoveries, published today in 23 papers in Nature and its affiliated journals, are an important step toward a map of all major cancer-causing mutations in the genome.
The ICGC/TCGA Pan-Cancer Analysis of Whole Genomes Project (PCAWG, or the Pan-Cancer Project), a collaboration involving more than 1,300 scientists and clinicians from 37 countries, analyzed more than 2,600 whole genomes of 38 different tumor types — the largest publicly available whole-genome dataset in the cancer genomics field. Fifty-two members of the Broad Institute of MIT and Harvard contributed to this research throughout the six-year long project.
Using the collected data, 16 working groups examined multiple aspects of cancer development, causation, progression, and classification, confirming previous findings and generating new knowledge about cancer biology, including identifying a large diversity of molecular processes that generate cancer-causing mutations. The Pan-Cancer Project also improved and developed new methods for analyzing cancer genomes.
Previous cancer genome studies focused on the 1 percent of the genome that codes for proteins, known as the exome. The Pan-Cancer Project explored the remaining 99 percent of the genome, which includes regions that regulate the activity of genes.
“This large international effort shows the breadth of the types of research and new biological insight that are possible using whole cancer genome data,” said Gad Getz, an institute member and director of the Cancer Genome Computational Analysis Group at the Broad Institute who is also the director of bioinformatics at the Massachusetts General Hospital’s (MGH) Cancer Center and a professor of pathology at Harvard Medical School (HMS). Getz, a member of the PCAWG steering committee, is a co-senior author of three of the papers.
Other institutions represented on the project’s steering committee include the Ontario Institute for Cancer Research in Canada, the Wellcome Sanger Institute in the United Kingdom, the European Molecular Biology Laboratory in Germany and the University of California, Santa Cruz.
“It was heartening that this very large group was able to bring together disparate resources and work to come up with some groundbreaking findings,” said Rameen Beroukhim, an associate member of the Broad Institute, an associate professor at the Dana-Farber Cancer Institute, an associate professor of medicine at HMS, and a co-senior author of two of the papers.
Gad Getz, director of the Cancer Genome Computational Analysis Group at the Broad Institute (left), and Esther Rheinbay, an associate member of the institute, are part of an international consortium of scientists that analyzed more than 2,600 whole cancer genomes.
Credit: Bearwalk Cinema
Who’s driving?
Included in the suite of papers published today is an overview in Nature that describes how the collaborators collated and standardized existing genomic data from the consortium’s hundreds of research groups around the world. Moving these previously disparate datasets onto a common cloud computing platform was a major part of the project’s success. The paper also details some of the consortium’s most striking findings.
For example, the tumor genomes in the study each carry an average of four or five “driver mutations” — mutations that play a large role in driving the growth of cancer.
Prior to these studies, 30 percent of tumors had unexplained genetic causes, but by analyzing the entire tumor genome, the consortium scientists discovered more driver mutations, leaving only 5 percent of tumors with no known drivers.
Getz and the other PCAWG steering committee members are the senior authors of the overview paper.
Driving but not coding
Another paper, published in Nature , focused more closely on driver mutations in the regions of the genome that don’t code for proteins. The scientists were surprised to find so few of these non-coding drivers, given that 99 percent of the genome is non-coding. The team discovered that only 13 percent of drivers identified in this analysis were non-coding.
“When people started sequencing whole genomes, there was an expectation that we would find non-coding drivers on the same order as the protein-coding drivers. It was a bit surprising that we didn’t find as many as we would have expected,” said Esther Rheinbay, an associate member of the Broad Institute and co-first author of the non-coding drivers paper, who is also an assistant professor of medicine at HMS and the MGH Cancer Center. Jeremiah Wala and Ofer Shapira, former Beroukhim lab members, are also co-first authors, and Getz and Beroukhim are senior co-authors of this study.
“Using computational reconstruction methods, we were able to estimate the order and timing of genetic events that lead to cancer. We found that these genetic events often occur many years before the tumor is detected.” Ignaty Leshchiner, Broad Institute
While non-coding drivers may simply play a smaller role in cancer than previously assumed, they could also be relatively rare and more difficult to find, meaning that the search for these drivers is not over.
“One issue these studies highlight is that we still don’t have enough cancer genome data,” said Rheinbay. “We need many more whole cancer genome sequences — which will be coming in the near future because of decreasing DNA sequencing costs.”
Getz added that knowing where these non-coding drivers reside in the genome — mainly in the regulatory regions — could help focus future studies on these regions. “If we narrow the search enough, we might be able to generate cost-effective assays that would allow us to study a larger number of tumor samples at a lower cost,” he said.
Tumors over time
Another paper, in Nature, followed the development of specific tumors through time and tracked the progression of genetic changes. The scientists found that tumors of the same cancer type often shared the same cancer-initiating mutations. But as the tumors evolved, they acquired additional distinct driver mutations that were caused by different DNA-damaging processes, depending on the cancer type and the patient’s genetics and lifestyle.
“Using computational reconstruction methods, we were able to estimate the order and timing of genetic events that lead to cancer,” said Ignaty Leshchiner, a co-first author of the study, who is a group leader at the Broad Institute and a member of Getz’s lab. “We found that these genetic events often occur many years before the tumor is detected.”
This study suggests that since early-stage mutations are generally consistent within a cancer type, they could be targets for the prevention, early detection, and treatment of the disease. Getz and Beroukhim are co-authors of the paper, with Peter Van Loo of The Francis Crick Institute in the United Kingdom and Moritz Gerstung of European Bioinformatics Institute as corresponding authors.
A mutation’s source
In another study in Nature, researchers studied the molecular processes that cause cancer mutations, including those that damage DNA and others that, when broken, fail to properly repair DNA. These processes are known to generate distinct patterns, or signatures, of cancer mutations across the genome. By looking at these “mutational signatures,”scientists can identify the molecular events that caused these mutations.
The research team used mathematical models to analyze millions of mutations in thousands of cancer genomes in search of these signatures. The scientists discovered many more than were previously known, and found strong associations between the new signatures and specific DNA-damaging processes.
“This catalog can be used to understand the mechanisms that generate mutations and drive cancer in each patient.” Gad Getz, Broad Institute
“By analyzing the largest collection of whole cancer genomes studied thus far, we created the most comprehensive catalog of mutational signatures to date,” Getz said. “This catalog can be used to understand the mechanisms that generate mutations and drive cancer in each patient.”
Getz is a senior co-author of the study along with Steven Rozen of Duke-NUS Medical School in Singapore and Michael Stratton of the Wellcome Sanger Institute. Jaegil Kim and Nicholas Haradhvala from the Getz lab are co-first authors of the paper.
“Since every person is exposed to multiple sources of mutations throughout their lives, we need to use mathematical techniques to identify which mutations come from a particular biological source,” Kim said. “Having such a large dataset enabled us to map out these signatures with much greater precision.”
The authors also studied new types of mutation. “DNA can be mutated in a variety of ways, from changing single bases to removing entire sections of genetic code,” Haradhvala said. “This new dataset allowed us to analyze more types of mutation, expanding our understanding of the biological mechanisms of cancer.”
The results of the study will allow other researchers to identify the sources of cancer mutations in newly sequenced patient samples.
Looking ahead
The project has created and made available a comprehensive resource for cancer genomics researchers, including the raw genome sequencing data , software for cancer genome analysis, and multiple interactive websites exploring various aspects of the Pan-Cancer Project data.
“This global pan-cancer project demonstrates that we can learn a lot from studying whole cancer genomes,” Getz said. “We will continue learning about cancer biology and clinical applications from much larger genome datasets as they are generated in the future.”
He added that the data, findings, and methods reported in today’s 23 papers will help researchers and clinicians standardize cancer genome analysis, now that decreasing sequencing costs are making it possible to sequence more tumor genomes.
“We expect that this collection of papers will become the standard for analyzing whole cancer genomes,” Getz said.
The studies are also a major step toward personalized care for every cancer patient, by moving closer to a comprehensive list of cancer-causing mutations that oncologists could one day use to pinpoint the cause of a patient’s cancer.
“I want to be at a stage where, for every patient who comes to the doctor with cancer, we will be able to figure out what’s actually driving the tumor and how we can treat it,” Getz said.
PCAWG is a collaboration between the International Cancer Genome Consortium (ICGC), an umbrella organization that launches and coordinates cancer genomic research projects, and The Cancer Genome Atlas (TCGA), the cancer genomics program that was jointly managed by the National Cancer Institute and the National Human Genome Research Institute.
Share this article
You might like.
Genomic findings challenge thinking on what makes a species
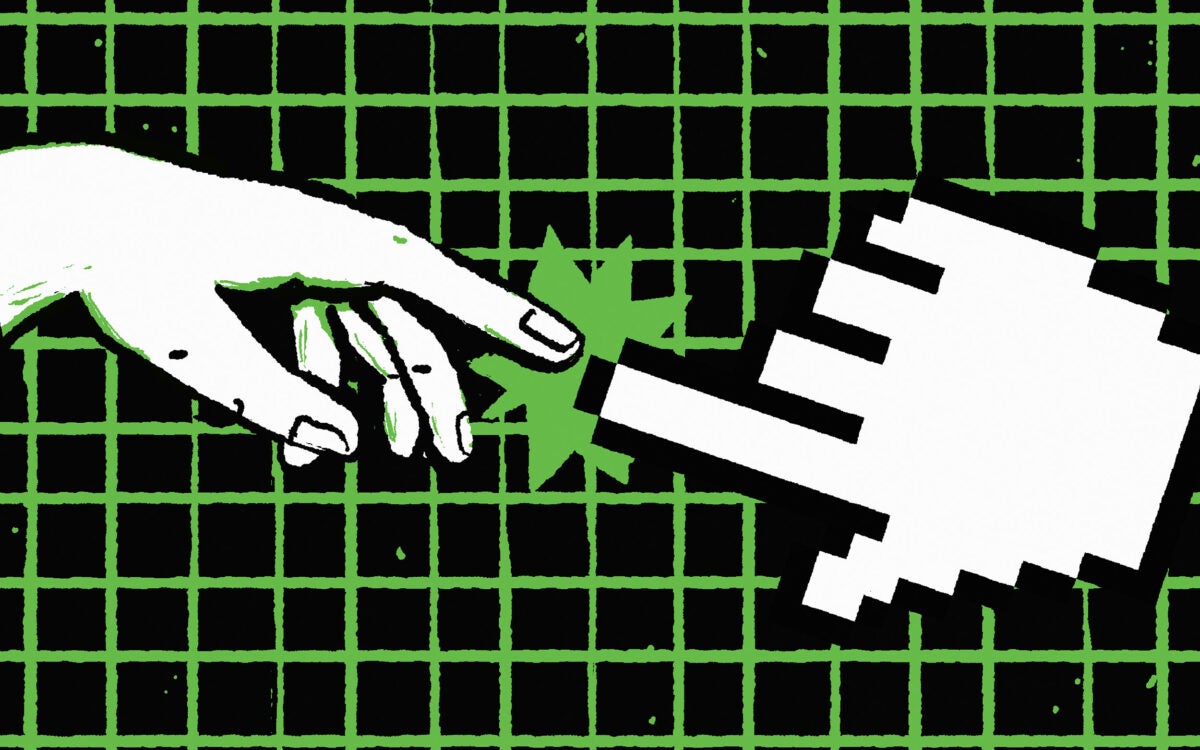
In podcast, a lawyer, computer scientist, and statistician debate ethics of artificial intelligence
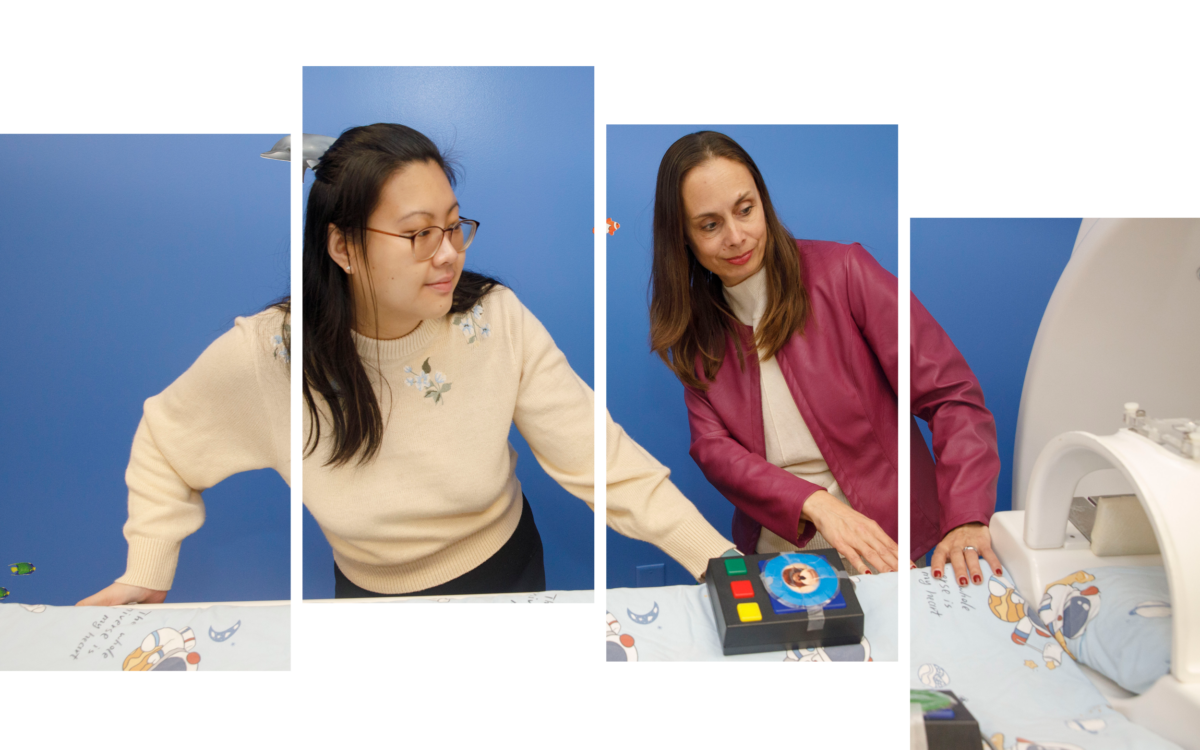
Harvard lab’s research suggests at-risk kids can be identified before they ever struggle in school
Harvard announces return to required testing
Leading researchers cite strong evidence that testing expands opportunity
When will patients see personalized cancer vaccines?
Sooner than you may think, says researcher who recently won Sjöberg Prize for pioneering work in field
For all the other Willie Jacks
‘Reservation Dogs’ star Paulina Alexis offers behind-the-scenes glimpse of hit show, details value of Native representation
Suggestions or feedback?
MIT News | Massachusetts Institute of Technology
- Machine learning
- Social justice
- Black holes
- Classes and programs
Departments
- Aeronautics and Astronautics
- Brain and Cognitive Sciences
- Architecture
- Political Science
- Mechanical Engineering
Centers, Labs, & Programs
- Abdul Latif Jameel Poverty Action Lab (J-PAL)
- Picower Institute for Learning and Memory
- Lincoln Laboratory
- School of Architecture + Planning
- School of Engineering
- School of Humanities, Arts, and Social Sciences
- Sloan School of Management
- School of Science
- MIT Schwarzman College of Computing
Scientists develop a rapid gene-editing screen to find effects of cancer mutations
Press contact :, media download.
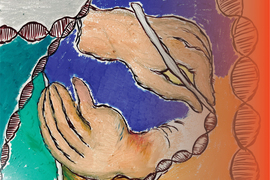
*Terms of Use:
Images for download on the MIT News office website are made available to non-commercial entities, press and the general public under a Creative Commons Attribution Non-Commercial No Derivatives license . You may not alter the images provided, other than to crop them to size. A credit line must be used when reproducing images; if one is not provided below, credit the images to "MIT."
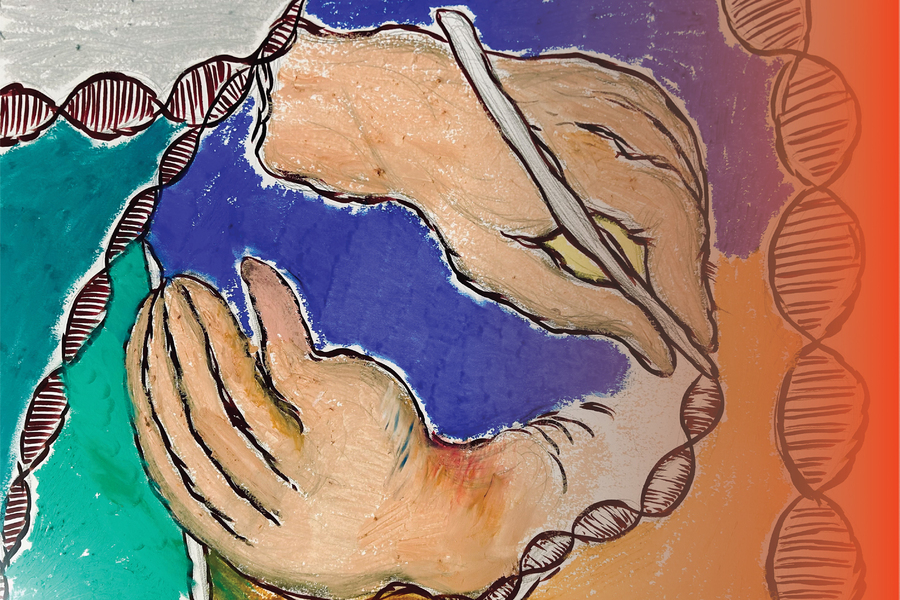
Previous image Next image
Tumors can carry mutations in hundreds of different genes, and each of those genes may be mutated in different ways — some mutations simply replace one DNA nucleotide with another, while others insert or delete larger sections of DNA.
Until now, there has been no way to quickly and easily screen each of those mutations in their natural setting to see what role they may play in the development, progression, and treatment response of a tumor. Using a variant of CRISPR genome-editing known as prime editing, MIT researchers have now come up with a way to screen those mutations much more easily.
The researchers demonstrated their technique by screening cells with more than 1,000 different mutations of the tumor suppressor gene p53, all of which have been seen in cancer patients. This method, which is easier and faster than any existing approach, and edits the genome rather than introducing an artificial version of the mutant gene, revealed that some p53 mutations are more harmful than previously thought.
This technique could also be applied to many other cancer genes, the researchers say, and could eventually be used for precision medicine, to determine how an individual patient’s tumor will respond to a particular treatment.
“In one experiment, you can generate thousands of genotypes that are seen in cancer patients, and immediately test whether one or more of those genotypes are sensitive or resistant to any type of therapy that you’re interested in using,” says Francisco Sanchez-Rivera, an MIT assistant professor of biology, a member of the Koch Institute for Integrative Cancer Research, and the senior author of the study.
MIT graduate student Samuel Gould is the lead author of the paper , which appears today in Nature Biotechnology .
Editing cells
The new technique builds on research that Sanchez-Rivera began 10 years ago as an MIT graduate student. At that time, working with Tyler Jacks, the David H. Koch Professor of Biology, and then-postdoc Thales Papagiannakopoulos, Sanchez-Rivera developed a way to use CRISPR genome-editing to introduce into mice genetic mutations linked to lung cancer.
In that study, the researchers showed that they could delete genes that are often lost in lung tumor cells, and the resulting tumors were similar to naturally arising tumors with those mutations. However, this technique did not allow for the creation of point mutations (substitutions of one nucleotide for another) or insertions.
“While some cancer patients have deletions in certain genes, the vast majority of mutations that cancer patients have in their tumors also include point mutations or small insertions,” Sanchez-Rivera says.
Since then, David Liu, a professor in the Harvard University Department of Chemistry and Chemical Biology and a core institute member of the Broad Institute, has developed new CRISPR-based genome editing technologies that can generate additional types of mutations more easily. With base editing, developed in 2016, researchers can engineer point mutations, but not all possible point mutations. In 2019, Liu, who is also an author of the Nature Biotechnology study, developed a technique called prime editing, which enables any kind of point mutation to be introduced, as well as insertions and deletions.
“Prime editing in theory solves one of the major challenges with earlier forms of CRISPR-based editing, which is that it allows you to engineer virtually any type of mutation,” Sanchez-Rivera says.
When they began working on this project, Sanchez-Rivera and Gould calculated that if performed successfully, prime editing could be used to generate more than 99 percent of all small mutations seen in cancer patients.
However, to achieve that, they needed to find a way to optimize the editing efficiency of the CRISPR-based system. The prime editing guide RNAs (pegRNAs) used to direct CRISPR enzymes to cut the genome in certain spots have varying levels of efficiency, which leads to “noise” in the data from pegRNAs that simply aren’t generating the correct target mutation. The MIT team devised a way to reduce that noise by using synthetic target sites to help them calculate how efficiently each guide RNA that they tested was working.
“We can design multiple prime-editing guide RNAs with different design properties, and then we get an empirical measurement of how efficient each of those pegRNAs is. It tells us what percentage of the time each pegRNA is actually introducing the correct edit,” Gould says.
Analyzing mutations
The researchers demonstrated their technique using p53, a gene that is mutated in more than half of all cancer patients. From a dataset that includes sequencing information from more than 40,000 patients, the researchers identified more than 1,000 different mutations that can occur in p53.
“We wanted to focus on p53 because it’s the most commonly mutated gene in human cancers, but only the most frequent variants in p53 have really been deeply studied. There are many variants in p53 that remain understudied,” Gould says.
Using their new method, the researchers introduced p53 mutations in human lung adenocarcinoma cells, then measured the survival rates of these cells, allowing them to determine each mutation’s effect on cell fitness.
Among their findings, they showed that some p53 mutations promoted cell growth more than had been previously thought. These mutations, which prevent the p53 protein from forming a tetramer — an assembly of four p53 proteins — had been studied before, using a technique that involves inserting artificial copies of a mutated p53 gene into a cell.
Those studies found that these mutations did not confer any survival advantage to cancer cells. However, when the MIT team introduced those same mutations using the new prime editing technique, they found that the mutation prevented the tetramer from forming, allowing the cells to survive. Based on the studies done using overexpression of artificial p53 DNA, those mutations would have been classified as benign, while the new work shows that under more natural circumstances, they are not.
“This is a case where you could only observe these variant-induced phenotypes if you're engineering the variants in their natural context and not with these more artificial systems,” Gould says. “This is just one example, but it speaks to a broader principle that we’re going to be able to access novel biology using these new genome-editing technologies.”
Because it is difficult to reactivate tumor suppressor genes, there are few drugs that target p53, but the researchers now plan to investigate mutations found in other cancer-linked genes, in hopes of discovering potential cancer therapies that could target those mutations. They also hope that the technique could one day enable personalized approaches to treating tumors.
“With the advent of sequencing technologies in the clinic, we'll be able to use this genetic information to tailor therapies for patients suffering from tumors that have a defined genetic makeup,” Sanchez-Rivera says. “This approach based on prime editing has the potential to change everything.”
The research was funded, in part, by the National Institute of General Medical Sciences, an MIT School of Science Fellowship in Cancer Research, a Howard Hughes Medical Institute Hanna Gray Fellowship, the V Foundation for Cancer Research, a National Cancer Institute Cancer Center Support Grant, the Ludwig Center at MIT, the Koch Institute Frontier Research Program via the Casey and Family Foundation Cancer Research Fund, Upstage Lung Cancer, and the Michael (1957) and Inara Erdei Cancer Research Fund, and the MIT Research Support Committee.
Share this news article on:
Related links.
- Francisco Sanchez-Rivera
- Koch Institute
- Department of Biology
Related Topics
- Broad Institute
Related Articles
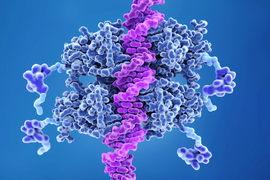
Gene-editing technique could speed up study of cancer mutations
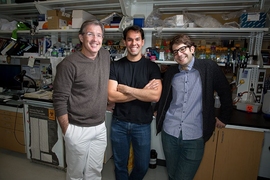
Fast modeling of cancer mutations
Previous item Next item
More MIT News
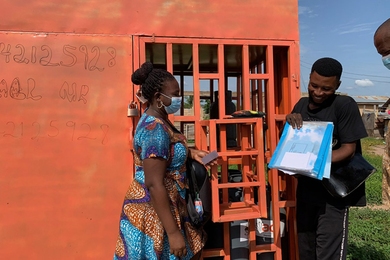
Q&A: Claire Walsh on how J-PAL’s King Climate Action Initiative tackles the twin climate and poverty crises
Read full story →
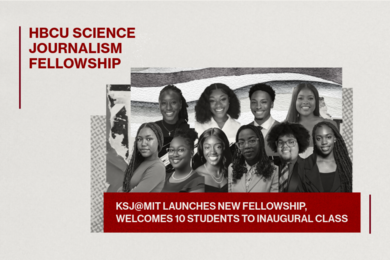
Knight Science Journalism Program launches HBCU Science Journalism Fellowship
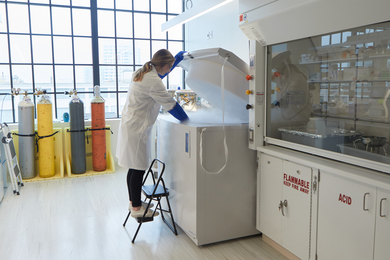
A home where world-changing innovations take flight
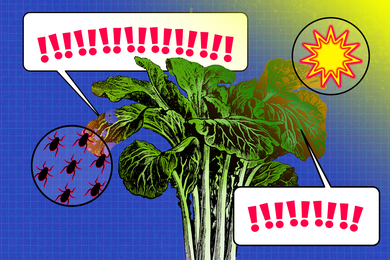
Plant sensors could act as an early warning system for farmers
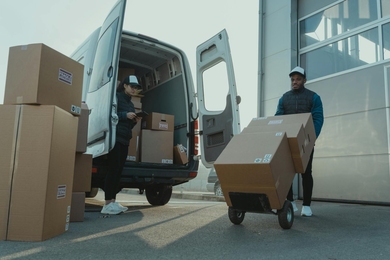
3 Questions: Enhancing last-mile logistics with machine learning
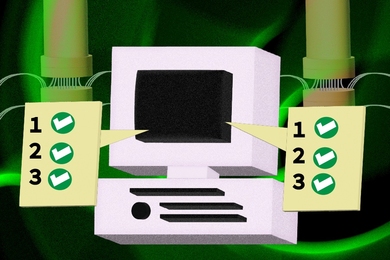
A blueprint for making quantum computers easier to program
- More news on MIT News homepage →
Massachusetts Institute of Technology 77 Massachusetts Avenue, Cambridge, MA, USA
- Map (opens in new window)
- Events (opens in new window)
- People (opens in new window)
- Careers (opens in new window)
- Accessibility
- Social Media Hub
- MIT on Facebook
- MIT on YouTube
- MIT on Instagram
Cancer Genomics Research
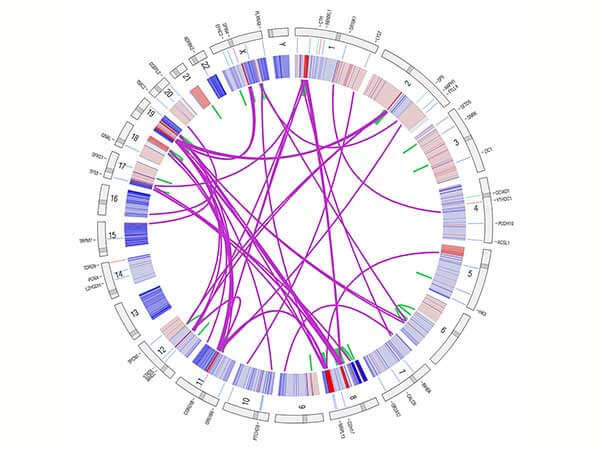
This Circos plot visualizes data from The Cancer Genome Atlas (TCGA) and allows scientists to explore the interrelationships among different data points.
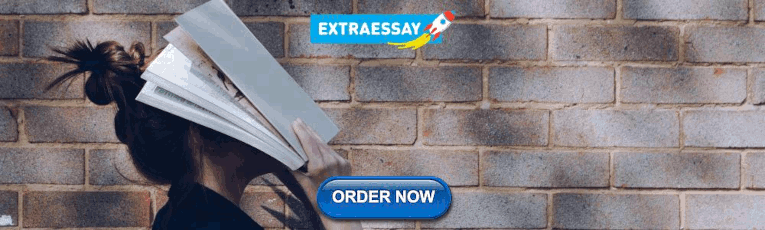
The Importance of Cancer Genomics Research
The study of cancer genomes (all the DNA in cancer cells) has revealed the mind-boggling complexity of genomic changes that drive cancer growth and survival. This knowledge has greatly expanded our understanding of how cancer develops and progresses. Ultimately, it has led to new ways of diagnosing and treating cancer, as well as new ways of identifying those at high risk of developing cancer. In short, genomics research has changed the way we see cancer.
Large-scale research projects such as The Cancer Genome Atlas (TCGA) , and its pediatric counterpart Therapeutically Applicable Research to Generate Effective Treatments (TARGET) , have surveyed and cataloged the genomic changes in multiple types of cancer. The discovery of novel cancer-associated genetic changes has led to an explosion of therapies that target specific changes and tests that identify patients whose cancers harbor those changes—an approach to cancer treatment known as precision medicine.
Genomics studies have also revealed unexpected genetic similarities across different types of tumors, shifting the way we define cancer. Treatments are typically given based on the tumor’s location in the body, such as the brain or lung. But a handful of new cancer drugs are used to treat tumors with specific genetic or molecular features, no matter where in the body the cancer started growing.
On the other hand, genomics research has also shown how different cancers can be , even when they started growing in the same organ. This finding has helped explain why some subtypes of tumors grow at different rates and why cancer treatments don’t work the same way in every patient.
NCI plays a key role in large-scale genomics research by facilitating collaborations between scientists of different disciplines, providing funding, and providing access to state-of-the-art technologies, data sets, and other resources .
In addition to genomics, cancer researchers are also creating large-scale catalogs of other kinds of molecular changes in cancer, including epigenomic, transcriptomic, proteomic, and metabolomic changes. These studies have further expanded our understanding of cancer and have led to new ideas for treating it.
The results of these projects illustrate the wide-ranging variation of genetic and molecular changes in cancer, and provide a better understanding of what causes cancer at the molecular level.
Selected NCI Activities in Cancer Genomics Research
Exploring the genetic and molecular foundations of cancer is a vital part of NCI’s research efforts. The following list highlights NCI-led or NCI-funded activities related to genomics and other “omics” research.
Building Genomics Data Sets
Large-scale research projects use omics technologies to catalog the molecular changes in multiple types of cancer.
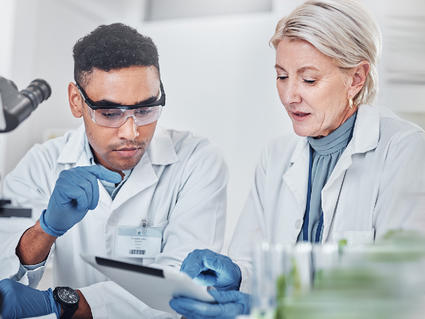
NCI Fiscal Year 2025 Professional Judgment Budget Proposal
Each year, NCI prepares a professional judgment budget to lead progress against cancer.
- The Human Tumor Atlas Network is constructing 3-dimensional atlases of human cancers as they change over time, including single-cell analyses of genomics, epigenomics, and transcriptomics. The network is generating adult and pediatric atlases and strives to include minority and underserved patients across cancer types and stages of disease.
- The Clinical Proteomic Tumor Analysis Consortium is using large-scale proteomic and genomic analyses, or proteogenomics, to characterize multiple types of cancer. The consortium is also using proteogenomics to answer questions about toxicity and resistance in clinical trials of new cancer drugs.
- NCI leads several projects to molecularly characterize large collections of cancers as well as collecting a rich profile of clinical features and outcomes. The Genomic Data Analysis Network develops cutting-edge computational tools and performs an initial analysis of the data from these projects, providing a launching pad for a broad community of researchers.
- The Participant Engagement and Cancer Genome Sequencing (PE-CGS) Research Network , a Cancer Moonshot initiative, is using direct participant engagement approaches to promote cancer genome sequencing programs for rare cancers, highly lethal cancers, cancers that occur at an early age, cancers that disproportionately affect certain populations, and cancers that are prevalent in understudied populations.
- The Childhood Cancer Data Initiative (CCDI) is gathering clinical care and research data—including genomics data—from children, teens, and young adults with cancer. CCDI has also created infrastructure to share this data with researchers to help them learn faster and on a larger scale than is possible for any single institution.
Connecting Genomics Data to Cancer Biology
NCI conducts and supports research to find out how genetic and other molecular changes in cancer cells affect cancer development, cancer progression, and treatment response.
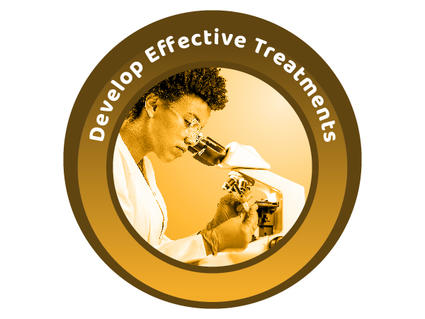
NCI Treatment Research and the National Cancer Plan
NCI supports a broad variety of research that aligns with the National Cancer Plan’s goal to develop effective treatments. Read about the plan and this goal.
- The Cancer Target Discovery and Development Program is a network of research centers devoted to studying how molecular changes found in cancers affect cancer cells and explore opportunities to target those changes with new therapies.
- The Epidemiology and Genomics Research Program supports research in human populations to understand genomic determinants of cancer occurrence and cancer outcomes, and translate findings to clinical and public health interventions.
- NCI researchers use molecular and genomic profiling to study the causes of cancer . This enables a better understanding of how normal cells transform into cancer cells, and to pinpoint internal processes and external exposures associated with specific molecular or genomic subtypes of cancer.
- The Cancer Systems Biology Consortium integrates and analyzes large omics data sets to get a birds-eye view of the molecular changes in cancer, how these changes interact with one another, and how they change over time and space.
Using Genomics in Clinical Trials
NCI clinical trials are among the first to use genomic testing to match patients to cancer treatments based on the genetic changes in their tumors.
- ComboMATCH is a group of clinical trials that are using genetic testing to find genetic changes in patients’ cancers and potentially match them to combinations of cancer treatments that target those genetic changes.
- ALCHEMIST is a set of precision medicine lung cancer trials designed to evaluate whether adding targeted therapy based on patients' tumor genetics can help prevent lung cancer from returning after surgery.
- NCI-COG Pediatric MATCH is an international cancer treatment clinical trial for children, teens, and young adults that is testing the use of precision medicine for childhood cancers.
Genomics Data Sharing
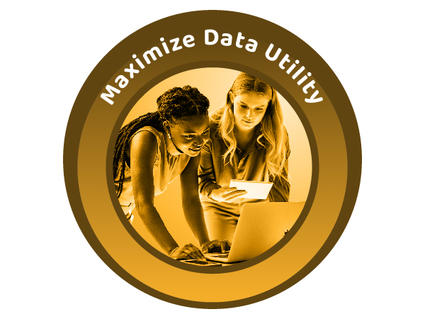
NCI, Data Sharing, and the National Cancer Plan
NCI is committed to providing access to research data, including genomic data, to accelerate progress against cancer. That commitment aligns with the National Cancer Plan’s goal to maximize data utility. Read about the plan and this goal.
NCI has spearheaded genomic data sharing practices since the first large-scale genomic characterization study, TCGA. NCI’s support for cancer omic data sharing continues by striving to make data as accessible as possible while protecting patient privacy.
The Cancer Research Data Commons is a cloud-based data science infrastructure that provides secure access to a large, comprehensive, and expanding collection of cancer research data, including data from TCGA, TARGET, and CCDI. Users can explore and use analytical and visualization tools for data analysis in the cloud.
Recent Research Findings in Cancer Genomics
- NCI’s ComboMATCH initiative will test new drug combinations guided by tumor biology
- New Way to Classify Meningioma Brain Tumors Suggests Potential Treatments
- NIH study illuminates origins of lung cancer in never smokers
- International study of rare childhood cancer finds genetic clues, potential for tailored therapy
- International research teams explore genetic effects of Chernobyl radiation
- NCI Genomic Data Commons: A Community Resource for Cancer Research
- Seeing Cancer Through New Lenses: A Broader Spectrum of Cancer Targets
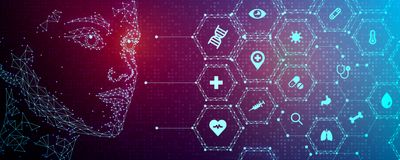
The Largest Whole-genome Sequencing Study in Cancer
Comprehensive genome sequencing of 13,880 tumors revealed somatic and germline mutations that could influence patient treatment and prognosis..
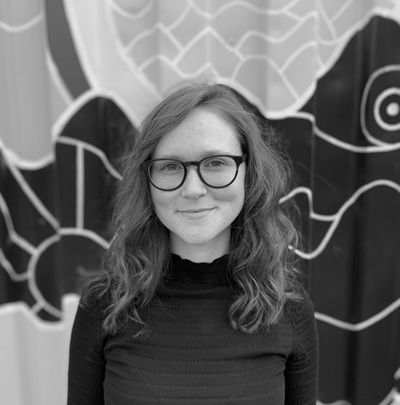
Danielle is an Assistant Editor at The Scientist. She has a background in neuroscience and molecular psychiatry. She has previously written for BioTechniques News, The Scientist, and Drug Discovery News.
View full profile.
Learn about our editorial policies.
ABOVE: Scientists developed a bioinformatics pipeline for integrating whole-sequencing data from 13,880 tumors with patient clinical data. They discovered genomic biomarkers of survival and treatment responsivity. ©iStock, ArtemisDiana
T here is no single genetic blueprint for cancer. Instead, each individual cancer draws on a collection of acquired mutations that endow the cells with a selective advantage and superior immune evasion and proliferation tactics. Thanks to next-generation sequencing technologies, many patients diagnosed with a particular cancer can discover whether their tumors harbor specific mutations that render them more susceptible to particular therapies. However, targeted approaches fail to capture the full suite of alterations and biomarkers nestled in the complex genetic architecture of a patient's tumor, potentially obscuring the best available treatment plan for an individual patient.
In a study published in Nature Medicine , researchers developed a bioinformatics pipeline for integrating whole-genome sequencing (WGS) data from 13,880 tumors with matching patient clinical data. 1 The large-scale study revealed somatic and germline DNA mutations that affect prognosis, highlighting the potential influence of comprehensive cancer genomics on patient outcomes.
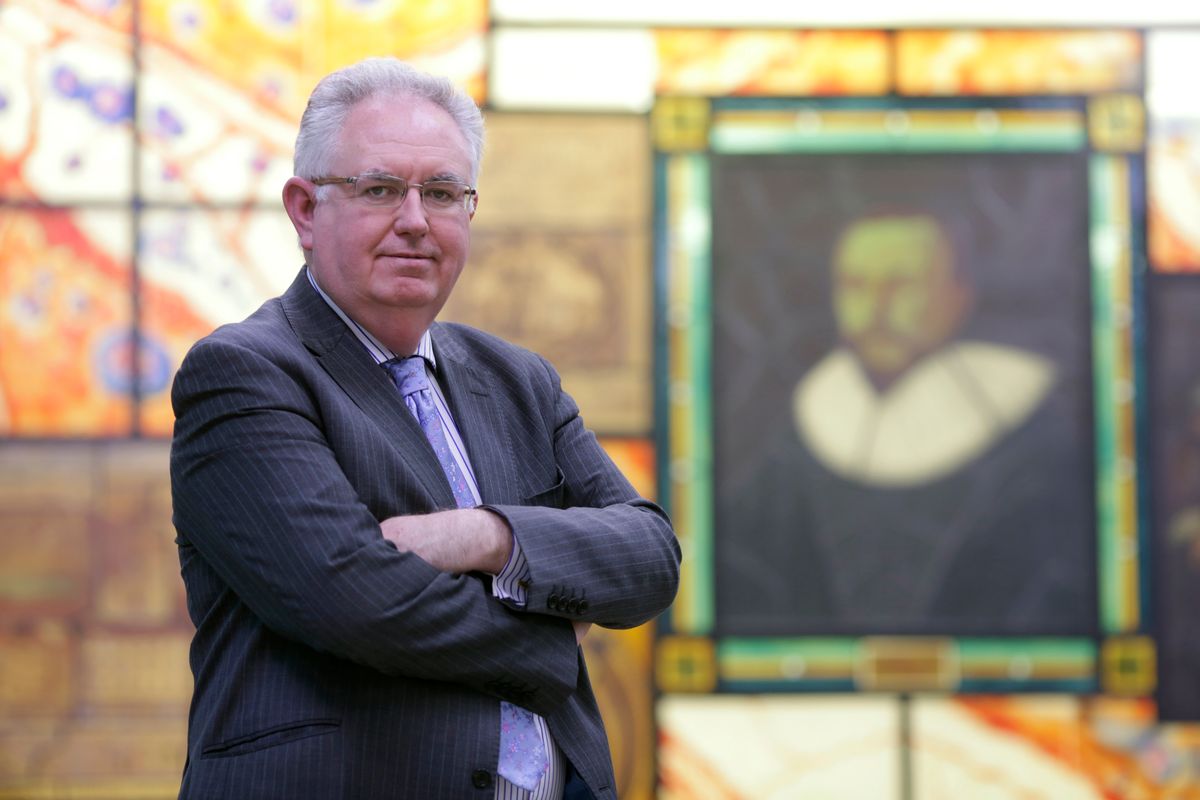
“Cancer is a disease of disordered genomes,” said Mark Caulfield , a genomic medicine researcher at Queen Mary University of London and a coauthor of the study. Many of the genetic mishaps that emerge are irrelevant to the cancer’s growth, but others carry information that would allow researchers to predict the tumor’s progression and vulnerabilities. Caulfield and his team set out to find these information-rich genetic fingerprints.
For many cancers, genomic testing to identify specific gene variants, like single-nucleotide polymorphisms, is already part of standard care. However, these targeted panels are limited in scope. “[A comprehensive approach] gives you the full picture of what’s happening in an individual patient’s tumor that you might miss if you just end up using targeted panels,” said Arul Chinnaiyan , a cancer researcher at the University of Michigan who was not involved in the study. “This study really brings together the importance of that.”
To explore the genes that shape health and disease, the UK government established the 100,000 Genomes Project in 2013. 2 Run by the government-owned company Genomics England, researchers set out to sequence the genomes of patients within the National Health Service (NHS), the UK’s publicly funded healthcare system. Within the Cancer Program of the initiative, researchers and clinicians teamed up to identify the genetic changes that drive cancer progression. In the present study, the researchers sequenced the patients’ tumors alongside their matched germline DNA. Analyzing a patient's normal, inherited DNA alongside tumor DNA can provide a bigger picture of susceptibility to other cancers or inform clinicians on which treatments are more appropriate for the patient given any genetic variants that affect drug metabolism. 3
The 13,880 samples covered 33 tumor types. In a single test, WGS can detect somatic small variants, including single nucleotide variants (SNV) and insertions and deletions, and copy number aberrations (CNA). By integrating longitudinal, real-world clinical data, the researchers could assess the role of these genomic factors in treatment response and survival.
“The entire scope of the study was quite impressive,” said Chinnaiyan.
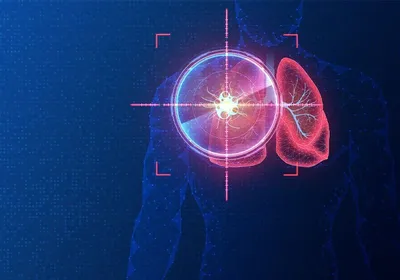
Precision Medicine: A New Era in Cancer Therapy
Caulfield and his team started by looking at the mutation rates for genes included in the most current version of the National Genomic Test Directory for Cancer (NGTDC), a list that specifies which genomic panels a patient is eligible for based on their specific cancer type. “The findings were quite striking,” said Caulfield. For example, they found that 94 percent of tumors from patients with glioblastoma multiforme, an aggressive form of brain cancer, had small variants in at least one gene, and 58 percent contained a CNA in at least one gene. Standard-of-care, targeted panels do not test for many of the observed mutations. “This complex genetic architecture led to the conclusion that to really characterize the genetic variation in glioma, a whole genome would be the best test,” said Caulfield.
The most frequently mutated gene across cancer types was tumor protein 53 ( TP53 ). However, within individual cancer types, the occurrence of each mutation was variable, highlighted by a high frequency of TP53 SNV in ovarian high grade serous carcinoma (90 percent) and a low frequency of the mutation in mesothelioma (seven percent).
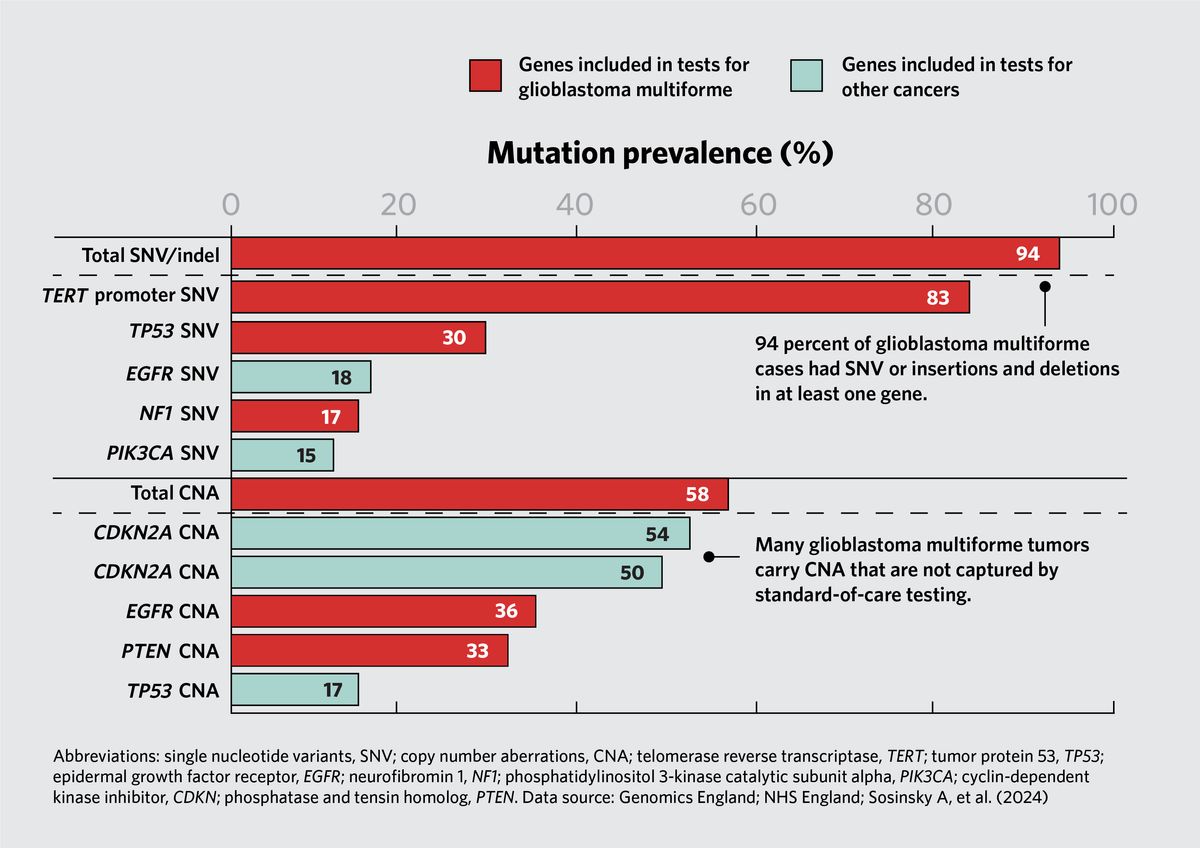
The research team also assessed mutation rates for genes that are not included in a cancer type’s standard-of-care testing protocol. For example, 54 percent of uterine corpus endometrial carcinomas and 49 percent of ovarian endometrioid adenocarcinomas carried a phosphatidylinositol 3-kinase catalytic subunit alpha ( PIK3CA ) mutation. However, in the UK healthcare system, this mutation is only tested in breast invasive carcinomas. These findings suggest that scientists could test the therapeutic potential of PIK3CA inhibitors in clinical trials for these cancers.
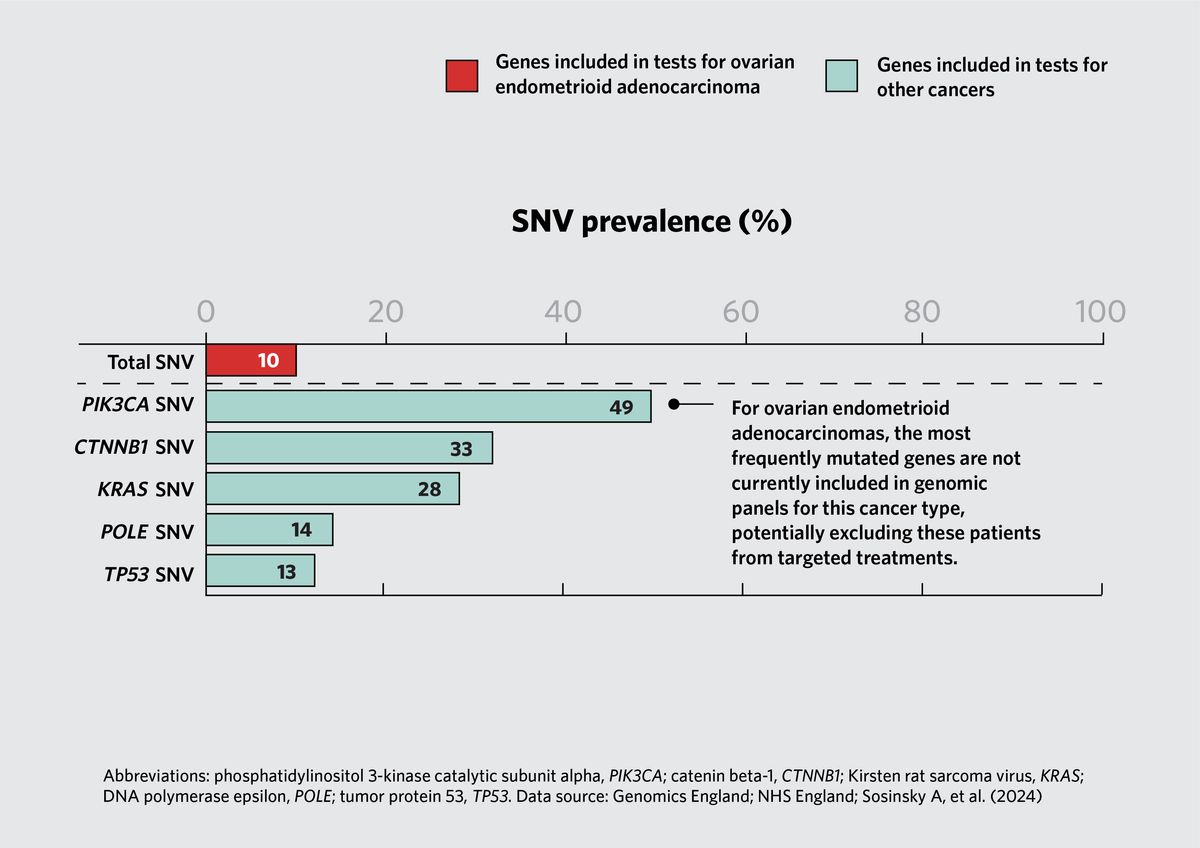
Homologous recombination deficiency (HRD) occurs when cells fail to repair double-stranded breaks in DNA via homologous recombination. Caulfield and his team found that 40 percent of ovarian high-grade serous carcinomas had genes with HRD. When they incorporated patient clinical data into their analysis, they found that patients whose tumors had high rates of HRD experienced better outcomes with platinum-based chemotherapies relative to patients with no genetic alterations in HR pathway genes; this was particularly true for patients with high-grade ovarian carcinomas.
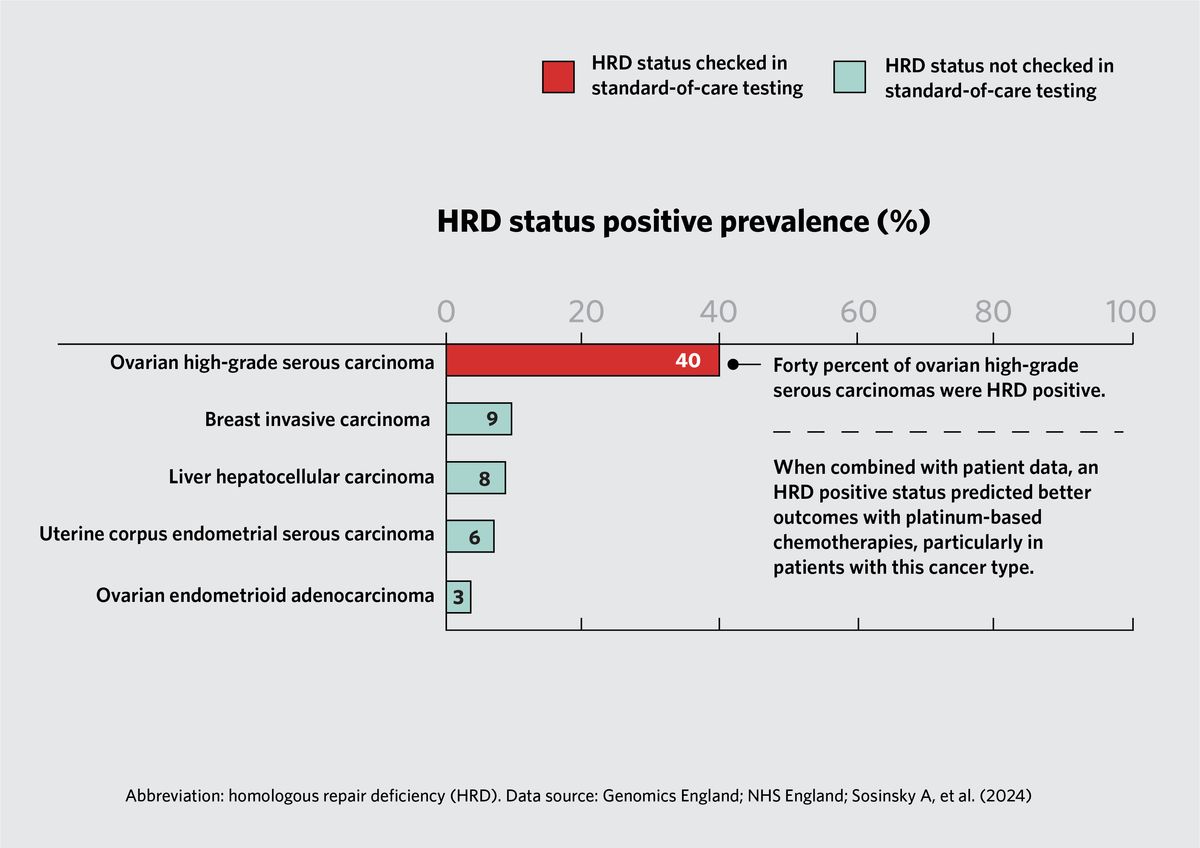
Because the research team used an integrated WGS approach, they could sequence patient tumor and non-tumor DNA in tandem in search of variants that carry information about patient predisposition to malignancy that can guide their clinical management. For example, 13 percent of patients with high-grade serous ovarian carcinoma had variants in BRCA1 and BRCA2 genes. Patients with this cancer type had an earlier cancer onset if they carried predisposing germline variants. “If we could harness that information in families where there is a strong cancer history, it may be that we'd be able to do a better job of screening and focusing screening on specific individuals,” said Caulfield.
The current study only included WGS data, and thus the pipeline only accounted for a patient’s genomic profile. “It would have been appealing to have included RNA sequencing or transcriptome sequencing because that gives you an epigenetic framework for the tumor microenvironment,” said Chinnaiyan. “They're sort of missing the functional aspect of the genome that RNA sequencing brings.”
Regardless, he noted that the genomic findings were impressive and intriguing. “[The study] is going to have an impact in terms of the cancer research community,” said Chinnaiyan, whose research group is already getting access to the data to support ongoing studies.
When the 100,000 Genomes Project started in 2013, genetic testing in cancer was limited. WGS has revolutionized cancer research, unraveling the complex genetic architecture of cancerous cells; however, the technology is largely restricted to research.
“The future of cancer care will almost certainly involve some degree of either genomic, or possibly multi-omics profiling, which will allow us to more correctly choose the therapies for patients,” said Caulfield. Chinnaiyan similarly sees WGS as playing a major role in cancer care of the future. “The longer-term vision for precision oncology is to carry out this comprehensive approach if the costs can be managed,” said Chinnaiyan. Although the cost to sequence an entire human genome has dropped substantially since the days of the Human Genome Project, it is still prohibitively expensive once processing, analysis, interpretation, and storage costs get factored in . 4,5
However, Caulfield hopes that day will come soon. He said, “Eventually, what will happen is that the price of a whole genome will fall down, and at that point, it's very likely that everyone will move towards whole-genome sequencing because it will be cost-effective. And what that will allow us to do is to detect unusual features in people's cancers that go beyond our current knowledge.”
Caulfield said that the next phase is to put WGS alongside clinical trials to understand whether they can identify biomarkers for treatment response. With the mutational architecture of a tumor and electronic clinical data in hand, researchers could follow a patient’s health journey beyond the end of a clinical trial.
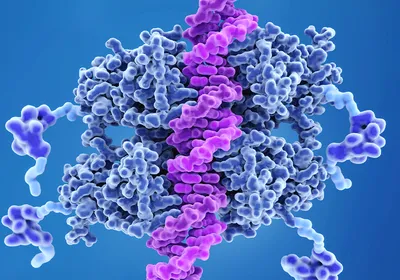
Viewing the Glioblastoma Tumor Microenvironment at Single Cell Resolution
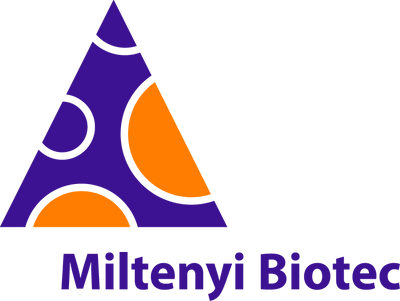
New Research Avenues Provide Hope for Metastatic Disease
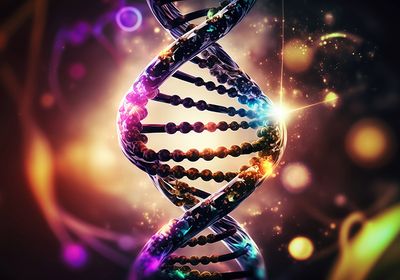
The Six-Base Genome Reveals Multimodal Data from a Single DNA Sample
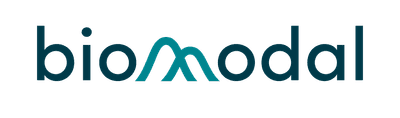
Advertisement
Modern therapies of nonsmall cell lung cancer
- Human Genetics • Review
- Open access
- Published: 12 September 2023
- Volume 64 , pages 695–711, ( 2023 )
Cite this article
You have full access to this open access article
- Andrzej Jachowski 1 ,
- Mikołaj Marcinkowski 1 ,
- Jakub Szydłowski 1 ,
- Oskar Grabarczyk 1 ,
- Zuzanna Nogaj 1 ,
- Łaz Marcin 1 ,
- Andrzej Pławski 2 ,
- Paweł Piotr Jagodziński 1 &
- Bartosz Kazimierz Słowikowski ORCID: orcid.org/0000-0002-6547-486X 1
2418 Accesses
3 Citations
Explore all metrics
Lung cancer (LC), particularly nonsmall cell lung cancer (NSCLC), is one of the most prevalent types of neoplasia worldwide, regardless of gender, with the highest mortality rates in oncology. Over the years, treatment for NSCLC has evolved from conventional surgery, chemotherapy, and radiotherapy to more tailored and minimally invasive approaches. The use of personalised therapies has increased the expected efficacy of treatment while simultaneously reducing the frequency of severe adverse effects (AEs). In this review, we discuss established modern approaches, including immunotherapy and targeted therapy, as well as experimental molecular methods like clustered regularly interspaced short palindromic repeat (CRISPR) and nanoparticles. These emerging methods offer promising outcomes and shorten the recovery time for various patients. Recent advances in the diagnostic field, including imaging and genetic profiling, have enabled the implementation of these methods. The versatility of these modern therapies allows for multiple treatment options, such as single-agent use, combination with existing conventional treatments, or incorporation into new regimens. As a result, patients can survive even in the advanced stages of NSCLC, leading to increased survival indicators such as overall survival (OS) and progression-free survival (PFS).
Similar content being viewed by others
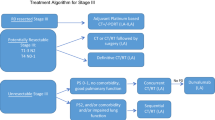
SEOM clinical guidelines for the treatment of non-small cell lung cancer (2018)
M. Majem, O. Juan, … M. Provencio
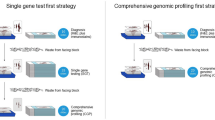
The Impact of Prior Single-Gene Testing on Comprehensive Genomic Profiling Results for Patients with Non-Small Cell Lung Cancer
Mary K. Nesline, Vivek Subbiah, … Shakti Ramkissoon
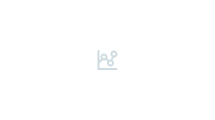
The Importance of Biomarker Testing in the Treatment of Advanced Non-Small Cell Lung Cancer: A Podcast
Fred R. Hirsch & Chul Kim
Avoid common mistakes on your manuscript.
Introduction
According to the latest data from GLOBOCAN, in 2020, more than 2.2 million people were diagnosed with lung cancer (LC), and almost 1.8 million deaths were noted worldwide. It made LC the most common cause of cancer-related death globally (Sung et al. 2021 ). Even with the increased smoking cessation and subsequent decrease in cases among men in developed countries, the overall statistics have grown since the last analysis in 2018 (Bray et al. 2018 ). National Cancer Institute’s Surveillance, Epidemiology, and End Results (SEER) program indicates that the 5-year survival rate in the USA (2010–2016) was 20.5%, which is a significant improvement compared to the rate from 1975, which was 11.5% (Thandra et al. 2021 ). This phenomenon can be attributed to the rapid development of diagnosis and treatment methods such as computed tomography (CT) and the introduction of immunotherapy and targeted therapies for LC. There are several risk factors responsible for LC development, such as age, gender, race/ethnicity, family history, tobacco smoking, cannabis smoking, environmental pollution, infections, inflammation, and chronic obstructive pulmonary disease (Thandra et al. 2021 ).
Tobacco smoking is the most critical risk factor in LC formation, as it accounts for over two-thirds of deaths and is a traceable cause in up to 80% of cases (Sung et al. 2021 ; Thandra et al. 2021 ). Combustion products contain various carcinogenic substances, such as polycyclic aromatic hydrocarbons and tobacco-specific N-nitrosamines. The presence of these carcinogens may lead to the activation of specific oncogenes (e.g. KRAS ) and inhibition of tumour suppressor genes (TSGs) (Dela Cruz et al. 2011 ; Akopyan and Bonavida 2006 ).
LC is divided into types and subtypes based on the histological features of the tumour cells. Small cell lung carcinoma (SCLC) and nonsmall cell lung carcinoma (NSCLC) are the two main types of LC, with NSCLC being much more common (85% of all LC cases) (Veronesi et al. 2017 ). NSCLC’s further subtypes include adenocarcinoma (LUAD), squamous cell carcinoma, and large cell carcinoma. In 2015, WHO introduced another subtype, neuroendocrine tumours, which consist of SCLC, large cell neuroendocrine carcinoma, and carcinoid. LUAD and squamous cell carcinoma are the most common types of lung tumours. Current diagnosis is more often based on immunochemistry than morphology, with biomarkers being the determining factor in situations of uncertainty (Veronesi et al. 2017 ). Genetic screening is becoming more common in LC with the development and cost reduction of next-generation sequencing (NGS). Specific mutations and gene alterations have been found to influence lung tumorigenesis. Those alterations are present in genes which encode proteins such as the epidermal growth factor receptor (EGFR), Kirsten rat sarcoma virus (KRAS), anaplastic lymphoma kinase (ALK), B-RAF, and ROS1.
Recognition of those mutations has facilitated the development of targeted therapy. Most defects affect signalling pathways within the cell due to tyrosine kinase (TK) activation. Monoclonal antibodies (mAbs) and tyrosine kinase inhibitors (TKIs) are used in selected patients to restrain the effects of mentioned alterations (Larsen et al. 2011 ). Epigenetic modifications have also been found to affect LC development. Hypermethylation of certain TSGs, due to increased levels of DNA methyltransferases, prevents them from being expressed and impacts lung tumorigenesis from its early stages (Langevin et al. 2015 ). There is also evidence of hypomethylation in the CpG sequences, which may lead to increased recombination during proliferation and chromosome instability. Some chromatin structural changes can be reversed, hence the potential for chromatin modifiers to be used in targeted therapy (Mehta et al. 2015 ). The main aim of this brief review is to collect and introduce the potential option of available, modern ways of battles with LC.
Conventional therapies
Due to the high mortality rate of NSCLC, there is a great need to develop new, more effective ways of curing patients (Siegel et al. 2014 ). New therapies begin to play a more significant role in NSCLC treatment. Nonetheless, classic approaches such as surgery, radiotherapy, and chemotherapy are still commonly used worldwide. Ongoing research on traditional therapies allows improving and using them more effectively. In this section, we want to outline the most common conventional treatment approaches briefly.
Surgical removal of a tumour has been the golden standard for patients in the early stage of NSCLC, but only if the tumour is resectable and the patient can survive the surgery (Zappa and Mousa 2016 ). The operation consists of removing the lobe, segment, or entire lung, depending on the tumour size, location, and level of the pulmonary reserve (Tandberg et al. 2018 ).
The oldest surgical intervention technique is open, posterolateral thoracotomy (conventional), and muscle-sparing thoracotomy (Wang et al. 2019 ). However, due to technological development, video-assisted thoracic surgery (VATS) has gained popularity. According to numerous studies, VATS appears significantly superior to thoracotomy, with fewer removed lymph nodes, less intraoperative bleeding and trauma, fewer postoperative complications, shorter hospitalisation, faster rehabilitation, and better 3- and 5-year survivals (Wang et al. 2019 ; Dziedzic et al. 2021 ). Usability limitations of VATS may arise from low experience in using this technique and higher costs. Nonetheless, available data shows that shorter hospitalisation and reduced postoperative healthcare requirements lower the costs of using VATS (Wang et al. 2019 ). In the past two decades, three-port VATS has been positively established and has become a conventional treatment for NSCLC. This relatively new variant of VATS involves making one 4-cm-long incision. It is a less invasive form of lung surgery proven to reduce trauma, decrease postoperative pain, and shorten the time needed for rehabilitation. However, it requires the extensive experience of the surgeon (Wang et al. 2017 ).
The latest and most challenging technique of surgical tumour removal in NSCLC is robot-assisted thoracic surgery (RATS). The biggest problems encountered in using RATS are related to the costs of this procedure and learning how to perform this technique (Lee et al. 2021 ). Robotic lobectomy may be recommended for operations requiring greater precision because robotic arms can provide more precise movements. The only systems currently available for RATS are da Vinci Systems (Veronesi 2015 ). According to most studies that compare RATS and VATS, there are no differences in mortality, overall survival (OS), disease-free survival, and intraoperative and postoperative parameters (Ma et al. 2021 ; Guo et al. 2019 ). However, a meta-analysis published in December 2020 suggests that RATS may result in better OS (Wu et al. 2021 ). This research implies that RATS is a safe and practicable technique in NSCLC treatment.
Radiotherapy
Radiotherapy is another important form of NSCLC treatment. It can be applied in every stage of NSCLC as adjuvant or neoadjuvant therapy (Uzel et al. 2019 ). Technological development and diagnostic imaging have made radiotherapy more precise and safer for patients. Research shows the superiority of three-dimensional conformal radiotherapy over two-dimensional therapy, which is characterised by poor control of tumour location (Chang et al. 2008 ). One of the latest techniques of this treatment is image-guided radiotherapy (IGRT). It can be used with four-dimensional CT or positron emission tomography, allowing tumour imaging before and during the procedure. IGRT maximised the precision of the radiation beam and reduced damage to healthy near-tumoural tissue (Kilburn 2016 ). One factor that can limit radiotherapy’s precision is respiratory movement during irradiation. Respiratory-gated conformal radiotherapy (RGRT) can be used to limit the impact of this factor. This technique involves sending the radiation beam toward the tumour during a predefined gate or blocking the patient’s breathing. Studies have shown that RGRT can reduce acute and late toxicity during chest irradiation. Nevertheless, this therapy is associated with higher financial costs (Giraud et al. 2011 ).
There are several techniques for radiotherapy: conventional with low doses of radiation and prolonged treatment time, stereotactic body radiation therapy (SBRT) with high doses but short treatment time, and intensity-modulated radiation therapy with modulated doses and longer treatment time. SBRT is a more complex technique, but many studies have shown that it is more convenient for patients due to shorter treatment times and lower morbidity, especially when used against peripheral tumours. Cancer foci located centrally are more challenging because of the increased risk of damage to the mediastinal structure (Tandberg et al. 2018 ; Donovan and Swaminath 2018 ; Kepka and Socha 2021 ).
Radiotherapy can be performed alone or with other types of NSCLC treatment, such as surgery, chemotherapy, immunotherapy, therapy with nanoparticles, targeted therapy, and CRISPR-Cas9 therapy.
Chemotherapy
Chemotherapy is commonly used in the IV stadium of NSCLC. Drugs used in chemotherapy, including LC, utilise the neoplastic cells’ capability of more intense mitosis compared to host cells, which makes these medicaments more toxic to the tumour. Platinum-based drugs are frequently used as they preferentially adduct with the N7 atom on guanine and adenosine. This bonding process impedes DNA replication and transcription, ultimately leading to apoptosis. Research has shown that the most effective outcomes are achieved through 4 rounds of chemotherapy that include platinum (Masters et al. 2015 ; Gridelli et al. 2004 ; Gerber and Schiller 2013 ).
The type of drugs administered to patients depends on their performance status (PS, scale 0–5), which describes their ability to function independently. According to The American Society of Clinical Oncology, the best combination of cytotoxic medicaments for patients with PS of 0 or 1 is cisplatin or carboplatin with paclitaxel , gemcitabine , docetaxel , vinorelbine , irinotecan , or pemetrexed (Masters et al. 2015 ). Patients treated this way can survive 8–10 months (Zappa and Mousa 2016 ). Nonetheless, a combination of these medicaments should be determined individually. Patients with a PS of 2 may be effectively treated only with nonplatinum drugs (Gridelli et al. 2004 ). Patients with a PS of 3 usually do not benefit from chemotherapy due to adverse effects (AE) and worse quality of life (Zappa and Mousa 2016 ).
Four of the most discussed forms of chemotherapy treatment are standard, sequential, alternating, and maintenance chemotherapy. All of them are effective in different groups of patients. However, it should be noted that only standard therapy is a validated and non-experimental method of chemotherapy (Grossi et al. 2007 ).
Sequential chemotherapy is based on applying non-cross-resistant medicaments in a specific sequence. It allows the application of more drugs with reduced toxicity by optimisation of dose of each medicament (Grossi et al. 2007 ). According to studies, sequential chemotherapy with a single agent followed by another can be promising for elderly feeble patients who do not qualify for platinum-based chemotherapy. For patients with good PS, an optimistic sequence of medicaments is a platinum-based doublet followed by a single, third-generation agent. The preliminary results of studies on this method have shown lower toxicity in this method with no differences in progression-free survival (PFS) and OS between this method and standard chemotherapy (Castro et al. 2002 ; Clark et al. 2001 ; Hosoe et al. 2003 ; Binder et al. 2007 ; Grossi et al. 2004 ; Edelman et al. 2004 ; Rinaldi et al. 2005 ; Chiappori et al. 2005 ).
Alternating chemotherapy is based on applying non-cross-resistant medicaments or their combination for a predetermined number of cycles or until progression. Optimal doses should be used as early as possible. Alternating administration of medicaments can reduce the toxicity of specific drugs (Grossi et al. 2007 ). Nonetheless, studies have shown that alternating chemotherapy is less effective for patients with good PS than standard therapy (Grossi et al. 2007 ). Despite this, several phase II trials have shown that alternating single-agent chemotherapy can be promising for elderly patients with bad PS (Mattson et al. 2000 ; Aguiar et al. 2005 ; Gridelli et al. 2007 ).
Maintenance chemotherapy is based on the administration of additional medicaments after planned in-advance chemotherapy. The drug used in this method can be one of those used in the standard cycle or a non-cross-resistant drug. The most effective outcome of maintenance chemotherapy is observed when the drug has been efficient during previous induction chemotherapy (Grossi et al. 2007 ). Studies have shown that maintenance chemotherapy can increase PFS, but OS is often not improved (Gerber and Schiller 2013 ). Two of the most promising drugs used in maintenance treatment are pemetrexed and bevacizumab . They present better OS; however, further research is required in this field (Gerber and Schiller 2013 ).
Chemotherapy can be combined with radiotherapy, immunotherapy, targeted therapy, surgery, and nanoparticle therapy. Currently, the most extensive research on cancer treatment has been on the combination of chemotherapy and surgical methods. The studies demonstrate that neo-adjuvant chemotherapy is primarily administered for the early stages of lung cancer. The procedure enables the reduction of tumour mass, making the operation feasible. Research shows that adjuvant chemotherapy is highly successful in decreasing mortality rates among patients diagnosed with stage IB cancer. There is a possibility that adjuvant chemotherapy, utilising platinum compounds, could become the standard for stage IB–IIIA of NSCL treatment.
- Immunotherapy
Immunotherapy is one of the latest forms of cancer treatment and focuses on the patient’s immune system. Neoplastic cells have developed numerous ways to avoid apoptosis and destruction by the immune system, so the immune system needs a specific boost provided by immunological treatment. There are several branches of immunotherapy, and the two focus on CD8 + T cells and factors that modify their function, i.e. cytokines—small proteins responsible for interactions between immune system cells. Interleukin-2 is the most effective cytokine regarding T cell activation, which can eventually lead to a reduction of tumour size (Rosenberg et al. 1984 , 1985 ; Rosenberg 2014 ; Taniguchi et al. 1983 ). Another option is using immune checkpoint inhibitors (ICIs). Their mechanism of action includes binding with receptor proteins located on the cytoplasmatic membrane of CD8 + T cells. These receptors enable cancer cells to avoid an immune system response by binding with cancer-produced proteins. Immunotherapeutic mAbs prevent from binding with these particles, increasing the capability of T cells to attack the tumour. The most commonly used antibodies in LC treatment are ipilimumab , pembrolizumab , and nivolumab (Steven et al. 2016 ). However, the tumour microenvironment may have highly immunosuppressive effects, which is the main obstacle in applying such medicaments. This area of research is being extensively investigated; learning about and eliminating these effects could be a promising possibility in helping the immune system destroy neoplastic cells (Steven et al. 2016 ).
Another successful immunotherapy method is adoptive cell therapy, based on genetic modification and proliferation of a patient’s T cells so they can effectively attack neoplastic cells. After modification, T cells are moved back to the patient. This approach has proven effective, but several potentially dangerous AEs currently limit the use of adoptive cell therapy (Dobosz and Dzieciątkowski 2019 ). Other promising immunotherapy options are oncolytic viruses and vaccines. The first one can be used to boost the immune system. Genetically modified viruses, which can penetrate only neoplastic cells, can cause their destruction. Molecules released during the disintegration of cancer cells stimulate the immune system to respond (Oiseth and Aziz 2017 ). Years ago, much hope arose among the scientific community that vaccines could also be used in preventing and treating cancers. Currently, there are only a few available and approved anticancer vaccines, but many of them, both allogeneic and autologous, are being investigated, and high hopes are placed on them (Dobosz and Dzieciątkowski 2019 ).
Immunotherapy appears to be extremely promising. Thanks to a large body of research conducted worldwide, it will be increasingly developed and may become a primary form of cancer treatment.
Immunotherapy in lung cancer
Immunotherapy and its incorporation of ICIs is the most promising branch of LC treatment. There have been attempts at developing alternative immunotherapeutic drugs, but none of them reached the efficacy achieved by ICIs (Steven et al. 2016 ).
Efficacy and safety
Compared to the standard NSCLC chemotherapy treatment approach, immunotherapy has proven to be more effective. Generally, ICIs may contribute to better OS and PFS values in previously treated NSCLC patients and be invaluable as first-line treatment (Reck et al. 2021 ; Zhou et al. 2021 ; Wang et al. 2020a ). In the clinical study of the metastatic LC, the use of ICIs alone with programmed death ligand-1 (PD-L1) expression ≥ 50% versus chemotherapy resulted in a doubling of the median OS and 5-year OS rate (Reck et al. 2021 ). Also, when combined with the cycles of standard chemotherapy, results have shown an increased OS and PFS (Reck et al. 2021 ). Additionally, clinical trials showed the overall relative safety of this approach compared to the conventional approach, even in cases of combining immunotherapies. However, this depends on the individual patient’s characteristics and draws particular attention to potential AEs (hui Jia et al. 2020 ; Zhao et al. 2021 ). To summarise the abovementioned results, many approaches combine treatment methods to achieve the best possible efficacy (Huang et al. 2021 ). Nevertheless, it is significant to consider the sex of a patient, as the analysis of clinical trials in cases of NSCLC and melanoma denotes that the particular ICI efficiency (i.e. pembrolizumab) decreases for women (Conforti et al. 2018 ).
Observed possible mild AEs include nausea, fatigue, dyspnea, and decreased appetite; however, given the antibody nature of immunotherapeutic drugs, AEs can escalate to severe conditions such as pneumonitis, hepatitis, colitis, psoriasis, transient thyroid malfunction, and diabetes mellitus (Zarogoulidis et al. 2017 ; Godwin et al. 2017 ; Iwama and Arima 2017 ). Furthermore, this implies that careful observation must continue long after termination of the treatment because of the hazard of developing long-term autoimmune conditions (Suresh et al. 2018 ; Puzanov et al. 2017 ).
The mechanism
The immune system destroys cancer cells through tumour-specific T cells, utilising the tumour-specific differentiation 8 T (CD8 T) cluster to distinguish hostile cells from the host cells. This response requires the presentation of tumour antigens by the antigen-presenting cells (Steven et al. 2016 ). In LC, several steps of this system may be disturbed, impairing the immune response, therefore allowing cancer cells to thrive. It may include an insufficient antigen expression on the surface of the tumour cells, a disturbance in the antigen-presenting process or local immunosuppressive conditions. To further suppress the immune response, cancer cells may exploit the ICI system by expressing specific ligands on their surface, such as the PD-L1 or B7 compound family, which bind to corresponding receptors on the T cells and inhibit their anti-tumour activity (Steven et al. 2016 ; Dobosz and Dzieciątkowski 2019 ; Oiseth and Aziz 2017 ).
Additionally, regulatory T cells may favour immunosuppression in the presence of specific cancer molecules. Furthermore, prolonged exposure to increased concentrations of hostile antigens or inflammatory signals (as in chronic infections or cancers) may lead to T cell exhaustion, making T cells lose their effector functions and express inhibitory signals (Wherry and Kurachi 2015 ). Both ICIs and T cell exhaustion can be reversed using immunotherapeutic drugs, allowing the reinduction of the immune cell response.
PD-1/PD-L1 axis
The most crucial immunological checkpoint exploit in LC involves the activation of a T cell programmed death receptor-1 (PD-1). PD-1 can be expressed in many haematopoietic and epithelial cells and the tumour microenvironment. Matching PD-L1 binds to the PD-1 receptor located on the surface of the T cell, downregulating its immune response (Fig. 1 A). In NSCLC, a matching PD-L1 may be expressed in approximately half of the cancer cells, making the tumour highly susceptible to immunotherapy (Steven et al. 2016 ). On the other hand, programmed death ligand-2 (PD-L2) is located on Th2 cells, helping to mediate the inflammation.
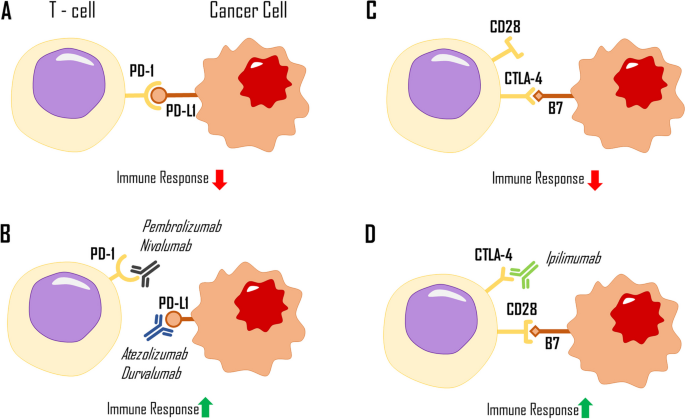
Interaction between immune checkpoint inhibitor ligands, their corresponding receptors ( A , C ), and most commonly used immunotherapeutic drugs ( B , D ). PD-1, programmed death receptor; PD-L1, programmed death ligand 1; CTLA4, cytotoxic T-lymphocyte associated protein 4; B7, B7 compound family molecules; CD28, cluster differentiation protein 28; immunoterapeutic drugs are portrayed as symbols of antibodies, visualising their immune checkpoint blocking capabilities
Depending on the specific drug, immunotherapeutic drugs in the form of mAbs adhere to either PD-L1 or its receptor. This binding prevents the immunosuppressive T cell signal by rendering them unable to bind together (Xia et al. 2019 ). The leading pharmaceuticals that use this axis are IgG-type antibodies such as atezolizumab and durvalumab , which target PD-L1, and nivolumab and pembrolizumab , which target the PD-1 receptor (Fig. 1 B). These drugs differ in their half-life, accompanying adverse effects, and possible immune-mediated adverse effects.
A crucial criterion of immunotherapy is the degree of PD-L1 expression on the surface of cancer cells, where PD-L1 ≥ 50% is the most favourable (Nasser et al. 2020 ). Individual predispositions, such as specific biomarkers and genetic landscapes, should be evaluated to ensure the best treatment option for a given patient (Rizvi et al. 2015 ). In the case of pembrolizumab , diagnostic trials include assessing tumour mutational burden, PD-L1 expression, and T cell response gene expression profile (Cristescu et al. 2018 ). Analysing these variables allows tailored therapy to a specific patient, advancing it into personalised precision therapy territory. The best-case scenario assumes treatment options with minimal use of chemotherapy or chemotherapy-free. However, knowing what is beneficial to a patient is most relevant, hence the importance of individual testing. Clinical trials, such as KEYNOTE ( pembrolizumab ), CHECKMATE ( nivolumab + ipilimumab ), and Impower ( atezolizumab ), confirm the efficacy of ICIs both as a monotherapy and in combination with standard chemotherapy such as cisplatin / carboplatin or paclitaxel , resulting in an overall increased overall survival median reaching 30 months. This makes it one of the most successful and currently best in use option for first-line treatments in NSCLC (Nasser et al. 2020 ).
CTLA-4 axis
Another important immune checkpoint involves the cytotoxic T-lymphocyte-associated protein-4 (CTLA-4) axis, which is one of the earliest and most studied ICIs. This receptor is located on the surface of T regulatory cells alongside CD28. These two compete for adherence to B7 compound family molecules, such as CD80 and CD86 (Fig. 1 C). The CTLA-4 receptor has a higher affinity for these ligands than CD28 and favours the downregulation of the immune response. Ipilimumab , a fully human kappa mAb used in treating melanoma, renal, and hepatic carcinoma, binds to the CTLA-4 receptor, making it unable to attach to its ligand, resulting in a more robust immune response due to the lack of prior-established inhibition (Fig. 1 D) (Nasser et al. 2020 ). This drug can be used in combination with PD-1/PD-L1 checkpoint inhibitors and standard chemotherapy while still producing beneficial results. In a clinical trial of CheckMate 9LA, after a 2-year update, treatment with nivolumab (PD-1), ipilimumab (CTLA-4), and chemotherapy resulted in higher overall survival (OS) and double the progression-free survival (PFS) compared to standard chemotherapy (Reck et al. 2021 ). Case studies have also observed a correlation between radiotherapy and CTLA-4 blockade immunotherapy, showing increased efficacy when both are combined in treatment (Formenti et al. 2018 ).
Other immunotherapies
There have been attempts at stimulating the immune system through Toll-like receptors located on the surface of B cells and plasmacytoid dendritic cells (Steven et al. 2016 ).
VEGF-targeted therapy
In NSCLC, cancer cells produce vascular endothelial growth factor (VEGF), particularly two forms of this molecule, VEGF-A and VEGF-B, which leads to intense angiogenesis and a better blood supply for tumour cells, enabling their growth and proliferation (Bonnesen et al. 2009 ; Holm et al. 1996 ). Targeting VEGF and its receptors can suppress angiogenesis and slow tumour progression by reducing the oxygen and nutrient supply to the tumour (Yamazaki and Morita 2006 ). VEGF is a glycoprotein produced by various cells, including macrophages, keratinocytes, endothelial cells, mesangial cells, platelets, and tumour cells (Sunderkotter et al. 1994 ; Rosen 2002 ; Duffy et al. 2013 ; Zhang et al. 2022 ). There are several forms of VEGF, including VEGF-A, VEGF-B, VEGF-C, VEGF-D, VEGF-E, and VEGF-F (Lohela et al. 2009 ). Hypoxia-induced factor (HIF) is a crucial factor for VEGF secretion, which is produced in response to oxygen deprivation. VEGF binds to the vascular endothelial growth factor receptor (VEGFR) to initiate the pathway. VEGFR is a tyrosine kinase receptor with three domains: intracellular (with TK activity), transmembrane, and extracellular (Ferrara et al. 2003 ). The binding of VEGF to the extracellular domain activates the intracellular domain, leading to the phosphorylation of signalling proteins essential for cell migration, proliferation, and survival (Kowanetz and Ferrara 2006 ; Neufeld et al. 1999 ).
VEGFR1 and VEGFR2 are the two most important receptors in the VEGFR group, expressed on the surface of various cells, including endothelial cells (Yamazaki and Morita 2006 ; Neufeld et al. 1999 ). Both receptors bind VEGF, but VEGFR1 shows a ten times higher affinity for VEGF than VEGFR2. However, VEGFR2 has stronger TK activity, and some studies suggest that VEGFR1 regulates VEGFR2 function (Ferrara 2004 ; Cébe-Suarez et al. 2006 ). There is also VEGFR3, which plays a role in lymphangiogenesis and is located on the surface of endothelial cells in lymphatic vessels (Hamrah et al. 2004 ).
During angiogenesis, VEGF initially induces vasodilation and increased vessel permeability. Next, endothelial cells proliferate and migrate to a site where a new capillary will be formed (Carmeliet 2000 ). Blood then flows through the preexisting vessel to the new vessel. Angiogenesis is a crucial process, not only important in wound healing or embryogenesis but also in carcinogenesis. Tumour cells require oxygen and nutrients to grow and proliferate, and new capillaries deliver these compounds. However, angiogenesis in tumours differs from that in healthy tissues. New vessels grow incoherently, often spiralling, and their permeability increases (Dudley 2012 ; Carmeliet 2005 ). This can lead to a weaker oxygen supply to the tumour cells, resulting in hypoxia. As a result, cells will produce more HIF, intensifying VEGF secretion and angiogenesis (Fig. 2 ) (Carmeliet 2005 ). Some monoclonal antibodies (mAbs), such as bevacizumab and ramucirumab , which can be used in LC treatment, operate by blocking angiogenesis.
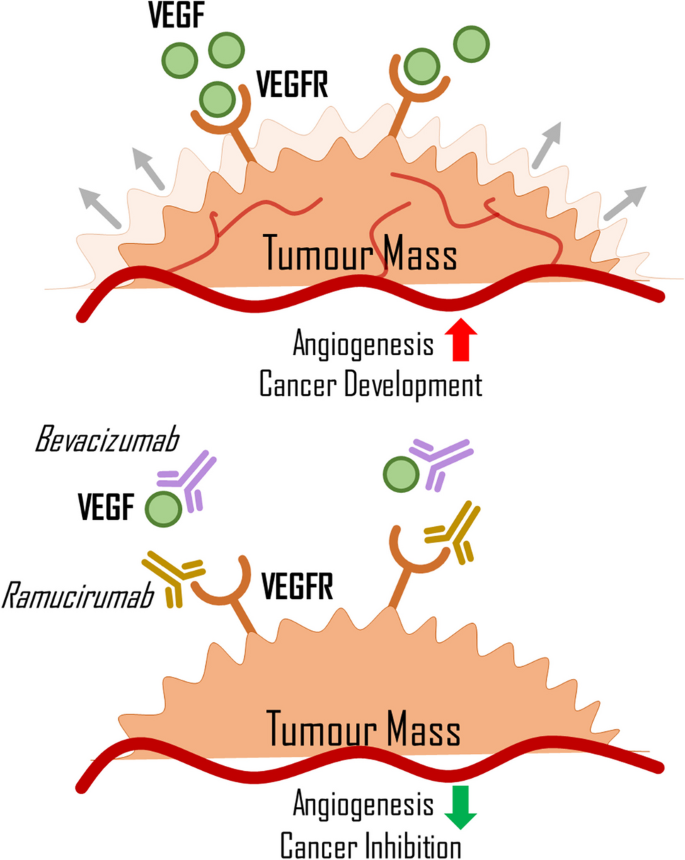
Interaction of VEGF and VEGFR with immunotherapeutic drugs. VEGF, vascular endothelial growth factor; VEGFR, vascular endothelial growth factor receptor; immunoterapeutic drugs are portrayed as symbols of antibodies, visualising their blocking capabilities. Red lines represent blood vessels; grey arrows visualize expanding tumour mass
Bevacizumab is a humanised monoclonal antibody that targets the VEGF-A molecule, preventing its attachment to its receptor and potentially inhibiting angiogenesis and tumour growth (Li et al. 2014 ; Garcia 2020 ). It is often used in combination with drugs like erlotinib or another monoclonal antibody, such as atezolizumab , in the treatment of NSCLC (Garcia 2020 ; Socinski et al. 2018 ; Cohen et al. 2005 ). While research shows that combination therapy with bevacizumab is generally safe, it can negatively affect the body, such as hypertension or pulmonary haemorrhage (Cohen et al. 2005 ; Piperdi et al. 2014 ). This is because bevacizumab reduces the level of VEGF, which impairs the remedial ability of the endothelium and weakens blood vessels, increasing the risk of rupture (Kamba and McDonald 2007 ; Johnson et al. 2004 ). Ramucirumab is another monoclonal antibody that has antiangiogenic properties. It binds to the extracellular domain of VEGFR2, preventing VEGF from binding to its receptor and blocking the formation of new blood vessels (Fig. 2 ) (Garon et al. 2014 ; Skobe et al. 1997 ). Recently approved in May 2020 for LC therapy, ramucirumab can be used with erlotinib when an activating mutation of EGFR is present in cancer cells (Nakagawa et al. 2019 ). As a newer drug in LC therapy, there is still some risk associated with its use .
Pathway targeted therapy
Targeted therapy for NSCLC focuses on identifying mutations in the EGFR and MAPK/ERK pathways, along with ALK and ROS1 gene fusions (Fig. 3 ). These mutations cause excessive stimulation of cell proliferation, differentiation, and growth, ultimately leading to carcinogenesis. Multiple studies demonstrate that these mutations can exist separately or concurrently. Molecular testing has confirmed that most forms of NSCLC, particularly LUADs, are positively correlated with mutations or alterations in EGFR , KRAS , ALK , ROS1 , and B-RAF , in decreasing frequency. Polymerase chain reaction and NGS, coupled with fluorescence in situ hybridisation (FISH), are widely employed for diagnosing these mutations in NSCLC patients (Dugay et al. 2017 ; Dogan et al. 2012 ).
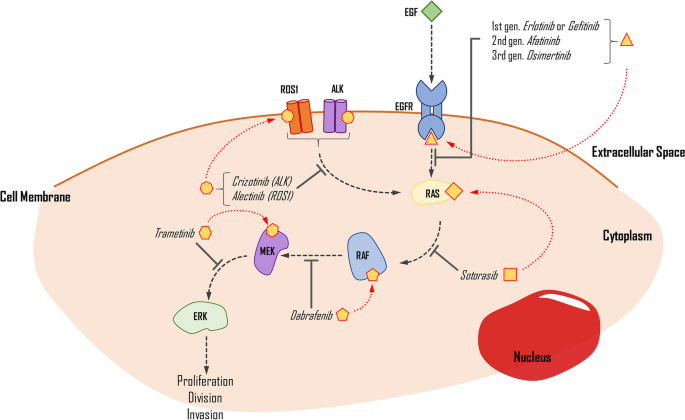
The simplified impact of selected drugs on the inhibition of the EGFR pathway. Medication is applied when proper mutations are detected in the genes that encode the pathway proteins. EGF, epidermal growth factor; EGFR, epidermal growth factor receptor; ROS1, proto-oncogene C-Ros 1; ALK, anaplastic lymphoma kinase; RAS, KRAS proto-oncogene; RAF, B-Raf proto-oncogene; MEK, mitogen-activated protein kinase kinase 1; ERK, mitogen-activated protein kinase kinase 3
EGFR is one of the most frequently overexpressed proteins in NSCLC, found in both wild-type and mutated variants, with prevalence rates of over 11% in Caucasian and about 50% in Asian populations. It is also more common in non-smokers than smokers (Dogan et al. 2012 ; Rude Voldborg et al. 1997 ). About 66% of patients with EGFR mutation have the in-frame deletion in exon 19, while less than 30% have a point mutation in L858R exon 21. Rare mutations make up the remainder (Castellanos et al. 2017 ).
Currently, there are several options for first-line treatment in advanced NSCLC, but different variants of mutations respond differently to the administered drugs, which are TKIs. These drugs work by preventing the phosphorylation process of compounds in the EGFR pathway, thus limiting cell division, causing cell cycle arrest, and initiating apoptosis (Abdelgalil et al. 2020 ). The first- and second-generation drugs work as reversible inhibitors of ATP binding to the TK domain, while third-generation drugs bind irreversibly (Gelatti et al. 2019 ). The most common variant, exon 19 deletion, responds better to first-line treatment (such as erlotinib or gefitinib ) than L858R. However, most EGFR-mutated cancers can develop resistance caused by a T790M mutation, which can be pre-existent or appear after implementing the TKIs (Castellanos et al. 2017 ). To address this resistance, a new approach has been taken that introduces an irreversibly binding drug called osimertinib . Initially, it was used as a second-line treatment in T790M-mutated NSCLC and significantly extended OS in patients. The genuine success of its implementation also transferred to first-line treatment. The FLAURA phase 3 trial compared osimertinib with first-generation TKIs, showing that the PFS was 18.9 and 10.2 months in osimertinib and standard TKIs (Soria et al. 2018 ). The findings imply that osimertinib treatment, irrespective of the presence of T790M mutation, improves overall survival (OS) and causes fewer adverse events (AEs) compared to standard treatment. Additionally, both approaches outperform platinum-based chemotherapy. However, the sequential approach gives more consistent results, with the osimertinib treatment implemented only after the gain of T790M mutation in EGFR -mutated NSCLC (Leonetti et al. 2019 ).
KRAS mutation occurs in 27.5% of NSCLC, mostly in LUAD (Judd et al. 2021 ). The most common variants are G12C (40%), G12V (19%), and G12D (15%). It is more often detected in women than men (Judd et al. 2021 ). Another study shows a correlation between smoking and the presence of KRAS mutations in 34% of patients diagnosed with NSCLC (Dogan et al. 2012 ). The protein product of this mutation, the consistently active form of KRAS, influences several pathways, with the most significant impact on the MAPK/ERK pathway, which causes overstimulation of cell proliferation and growth. KRAS mutated protein is hard to target, as its binding is not as tight as expected (Salgia et al. 2021 ). A recent trial of a new drug, sotorasib , a G12C KRAS protein irreversible inhibitor, shows promising effects in treatment. With a 37.1% response rate and 80.6% disease control, the PFS of patients who used this drug is 6.8 months, and the OS is 12.5 months, with 19.8% of grade 3 AES and 0.8% of grade 4 events. This drug binds to GDP-KRAS, the inactive form of the KRAS protein, and prevents it from changing into the GTP-KRAS form, which causes oncogenesis when the G12C mutation is present. The drug use is limited and is usually implemented as second-line treatment in advanced malignant NSCLC. Although superior to platinum-based chemotherapy, the outcome of the treatment varies depending on other mutations and the tolerability of the drug itself (Skoulidis et al. 2021 ).
The B-RAF proto-oncogene is commonly mutated in 2–4% of NSCLC cases, with most of these mutations occurring in LUADs (> 85%) and in current smokers. Different variants of B-RAF mutations determine the differentiation, with two main types identified—the V600E variant accounting for approximately 50% of all B-RAF mutations and non-V600E variants comprising the remainder. It has been reported that wild-type B-RAF patients have more prolonged OS than B-RAF mutated patients in those with EGFR mutations (O’Leary et al. 2019 ; Baik et al. 2017 ). B-RAF encodes a serine/threonine kinase, part of the MAPK/ERK pathway. Currently, two targets for inhibiting this pathway are available—B-RAF inhibitors (B-RAFi) or MEK inhibitors (MEKi). These drugs were previously used in metastatic melanoma and are now being used in NSCLC. Monotherapy with B-RAF inhibitors is not sufficient. Thus, combination therapy using both types of drugs is necessary to enhance cell cycle arrest and activate apoptosis. In clinical trials, treatment with dabrafenib and trametinib resulted in a 63% response rate and approximately 9.7 months of progression-free survival. The most common AEs reported in various trials were pyrexia, ALT increase, and vomiting. These findings suggest that BRAFi and MEKi therapy is effective and provides a promising treatment option for patients with B-RAF mutation (Baik et al. 2017 ; Planchard et al. 2017 ).
ALK/ROS1 gene rearrangement
The most common mutation of the ALK gene in NSCLC is the ALK-R gene rearrangement, which can be detected in up to 6% of patients. The ALK gene commonly fuses with EML4 , KIF5B , KLC1 , and TRP in cancers, with EMLA4 being the most frequent partner gene in NSCLC. These fusions increase the expression of an ALK protein—TK (Holla 2017 ; Du et al. 2018 ; Camidge et al. 2019 ). The ROS1 rearrangement is another common targetable mutation in NSCLC, present in 1–2% of patients. CD74-ROS1 and SLC34A2-ROS1 gene fusions are the most frequent variants, resulting in constant TK activity. Both fusions cause phosphorylation, which activates components in several pathways, such as MAPK/ERK, PI3K, and PLC-γ, leading to uncontrolled cell proliferation and inhibiting cell termination (Holla 2017 ; Mao and Wu 2017a , b ). The proteins resulting from the EML4-ALK and both ROS1 chromosomal rearrangements are target molecules for treating NSCLC ALK/ROS1 .
The first drug approved for ALK or ROS1 pathway signalling inhibition was crizotinib , which has an objective response rate (ORR) of 72% that is fast and durable. The median PFS was 19.3 months, and the median OS was 52.4 months when such mutations were present (Shaw et al. 2014 ; Shaw et al. 2019 ; Moro-Sibilot et al. 2019 ; Recondo et al. 2018 ). However, crizotinib lacks a durable response due to resistance gained toward it, resulting from the L1196M mutation. Alectinib is a drug introduced into clinical trials to respond to this mutation, with a 48–55% response rate in ALK -positive crizotinib-resistant NSCLCs. It has also been used in patients with no previous treatment, with a 93.5% ORR (Holla 2017 ). Another study showed that alectinib had significantly higher PFS (68.4% vs 48.7%) and ORR (82% vs 75.5%) over 12 months, in combination with lower toxicity (41% vs 50%) than crizotinib . These findings suggest that alectinib is solid choice to be used in first-line treatment for ALK-positive NSCLC patients (Camidge et al. 2019 ; McKeage 2015 ).
Brigatinib is a next-generation ALK inhibitor (since 2020 is approved by FDA as first-line treatment in NSCLC), and comparison with crizotinib favours the newer drug. The comparison shows a rate of PFS, 67% vs 43% (12-month period), a response rate of 71% vs 60%, and an intracranial response of 78% vs 29% (Camidge et al. 2018 ). Another study described a 24.0-month vs 11-month PFS in 24.9 months, with additional information about a delayed median time to worsening compared to crizotinib (Camidge et al. 2020 ).
CRISPR and nanotechnology
In this section, clustered regularly interspaced short palindromic repeat (CRISPR) and nanoparticles as the latest approaches in NSCLC treatment will be discussed separately and cooperatively. CRISPR is a genome-editing tool helpful in developing personalised therapies, whereas nanotechnology is mainly used to improve drug delivery systems. The latest research shows promising results of combining nanomedicine and CRISPR to improve delivery and activation for in vivo genome editing therapy in NSCLC (Taha et al. 2022 ).
CRISPR-CAS9/12A
CRISPR was initially discovered as part of an adaptive defence system in the Escherichia coli genome and was later adapted into an in vivo editing and targeting tool (Wiedenheft et al. 2012 ). What creates CRISPR is a small guide RNA that forms a complex with the inactivated DNA-binding protein Cas9 and together they attach to a precise region of the genome. With the production of RNA-guided nucleases (RGNs) like Cas9, allowing to obtain tailored specificities, many targets in the genomes of cells can be reached. The first step in genome editing involves creating a double-stranded DNA break at specific loci using the CRISPR-Cas system. The resulting break can be repaired by different pathways, including nonhomologous end-joining (NHEJ) and homology-directed repair (HDR), with HDR being a more precise mechanism (Sander and Joung 2014 ). The CRISPR system is typically combined with Cas9 nuclease, which is derived from the bacterial CRISPR-associated protein nine nucleases from Streptococcus pyogenes (Sander and Joung 2014 ). However, other systems that use Cas12 nucleases have shown promising results in the treatment of NSCLC (Feng et al. 2022 ). Bijoya et al. suggests that CRISPR-Cas12a can be used as a multiplex genome-editing tool and a system to promote HDR instead of NHEJ, which can lead to more precise DNA targeting (Paul and Montoya 2020 ). In 2020, Sreedurgalakshmi et al. comprehensively described the use of CRISPR-Cas in NSCLC treatment, including several issues that may lead to treatment resistance, such as mutations, molecular rearrangement, and conformational changes in ATP-binding domains (Sreedurgalakshmi et al. 2021 ). Therefore, CRISPR holds promise in different strategies, such as drug target validation and screening, generating mutant cell models, and discovering new targets for chemotherapy.
Today, one of the most common approaches in cancer research is investigating the roles of specific enzymes and domains in tumour growth to find new therapeutic targets to support chemotherapy. CRISPR is efficient in overcoming acquired drug resistance, as indicated by mutations in EGFR , KRAS , NRAS , B-RAF , and other genes. Therefore, it is necessary to investigate mutations responsible for modulating the sensitivity of these targets (Tsukumo et al. 2020 ; Terai et al. 2021 ; Floc’h et al. 2018 ; Park et al. 2021 ; Raoof et al. 2019 ; Gammelgaard et al. 2019 ; Wang et al. 2016 ; Caiola et al. 2020 ; Guerra et al. 2020 ).
Most patients diagnosed with advanced stages of NSCLC with EGFR mutations are treated with TKIs, but they often develop secondary resistance to drugs such as dasatinib , erlotinib , and osimertinib . Studies have shown the potential of the CRISPR system to overcome these issues or predict therapeutic outcomes by revealing genes and mediators, such as microRNA, responsible for drug sensitivity (Fig. 4 A). For example, the efficacy of dasatinib may be evaluated by revealing molecular alterations of YES1 (Garmendia et al. 2019 ). Erlotinib resistance might be caused by the presence of microRNA-214 (miR-214) or depletion of insulin-like growth factor 1 receptor (Liao et al. 2017 ; Hussmann et al. 2017 ). Acquired resistance to osimertinib might be overcome with ERK inhibition (Li et al. 2020 ). Enforced expression of miR-204 can downregulate caveolin-1 (CAV-1) expression and resensitise NSCLC cells for cisplatin , another commonly used drug that interferes with DNA replication in fast-proliferating cells.
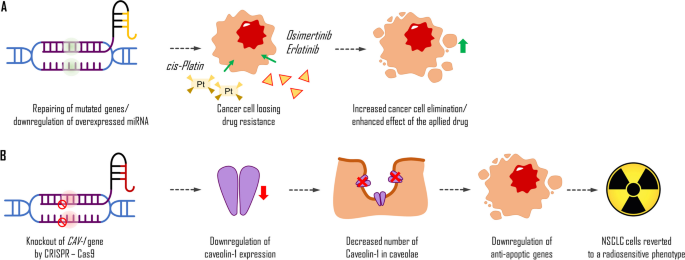
Potential application of Crisp-Cas system in the treatment of NSCLC. Increasing the possibilities of drugs used in the cancer treatment ( A ); caveolin-1 is a structural protein in caveolae. Its overexpression in cancer cells leads to the upregulation of anti-apoptotic genes. Thus, the knockout of the caveolin-1 (CAV-1) gene by CRISPR-Cas9-mediated gene editing leads to a decrease in its synthesis and a decrease in its number in caveolae. This process causes the downregulation of anti-apoptotic gene transcription in targeted NSCLC cells which reverts them to be sensitive to radiation ( B )
Moreover, the CRISPR-Cas system can also be used to improve more traditional treatment methods, such as radiotherapy, by targeting and suppressing factors responsible for radio-resistance, such as focal adhesion kinase in KRAS-mutant cells or gene knockout, which might revert NSCLC cells to a radiosensitive phenotype (Leiser et al. 2021 ; Moreno Roig et al. 2019 ; Tang et al. 2016 ). Recent research indicates that CAV-1 is highly expressed in some NSCLC cells, and the CRISPR-Cas9 knockout of that protein’s gene can revert such cells to a radiosensitive phenotype (Fig. 4 B) (Leiser et al. 2021 ).
Overall, CRISPR has demonstrated great potential in different strategies such as drug target validation and screening, generating mutant cell models, discovering new targets for chemotherapy, and improving traditional treatment methods like radiotherapy. Further research using the CRISPR system could provide valuable insights into the mechanisms of cancer and help develop more effective therapies for NSCLC. The abovementioned studies were all pre-clinical and conducted mostly in vitro using human cell models or in vivo on mouse models incorporating xenografts. Such mouse xenograft models involve the transplantation of cells, e.g. modified by CRISPR-Cas or human tumour cells into immunodeficient or humanised mice.
Recent research has shown the potential of CRISPR in improving diagnostic tools for NSCLC, such as next-generation sequencing (NGS). Wang et al. have demonstrated the benefits of using the CRISPR-Cas9 system in the early detection of circulating tumour DNA in NSCLC (Wang et al. 2020b ). This is crucial because late diagnosis is a significant challenge in treating this neoplasm. The ultimate treatment goal is to halt tumour metastasis, particularly to the brain, which is associated with a poor prognosis. The activated leukocyte cell adhesion molecule has been identified as a critical factor in brain metastasis formation, and its gene knockout using CRISPR has been suggested as a new therapeutic target (Justine et al. 2020 ). Other factors, such as myocardin, have been shown to promote pathways that enhance the epithelial-mesenchymal transition of NSCLC cells (Tong et al. 2020 ). Prior to the publication by Sreedurgalakshmi et al., only in vitro or in vivo studies using mouse models had been published. In 2020, the results of the first-in-human phase I clinical trial of CRISPR–Cas9 PD-1-edited T cells in patients with advanced NSCLC were reported (Lu et al. 2020 ). Twelve patients whose tumours were unresponsive to other available therapies were treated using this approach. The results showed that the treatment was generally safe and feasible, with a low frequency of off-target mutations (median 0.05%) (Lu et al. 2020 ).
To conclude, as indicated by the aforementioned reports, CRISPR-Cas9 technology holds great promise in the field of cancer research and treatment. It can contribute significantly to our understanding of cancer genomics by enabling the creation of genetically modified models of cancer within the laboratory setting. These models, in turn, aid researchers in comprehending the genetic mutations and molecular pathways underpinning various cancer types. This knowledge is pivotal in the development of targeted therapies. Furthermore, CRISPR-Cas9 can be employed to precisely target and edit the genes responsible for cancer initiation or progression. This capability has the potential to deactivate oncogenes or activate tumour suppressor genes, thus presenting an innovative approach to cancer treatment. Moreover, CRISPR technology can be effectively utilised to enhance immunotherapy by genetically modifying immune cells, such as T cells, to improve their efficiency in recognising and combating cancer cells. Another valuable application lies in addressing the significant challenge of drug resistance in cancer treatment. CRISPR technology plays a crucial role in elucidating how cancer cells develop resistance to chemotherapy or targeted therapies. This understanding can pave the way for the development of strategies to overcome drug resistance, ultimately enhancing the efficacy of cancer treatments. Additionally, this approach has the potential to usher in the era of personalised medicine. CRISPR-Cas9 could facilitate the development of personalised cancer treatments by analysing a patient’s tumour DNA and utilising CRISPR to target specific genetic mutations. This allows for the tailoring of treatments to align with an individual’s unique cancer profile. Lastly, in certain cases, genetic mutations directly contribute to cancer development. In such instances, CRISPR-Cas9 can be effectively applied in gene therapy approaches to correct these mutations or replace faulty genes, potentially leading to a genetic-level cure for cancer.
The convergence of cutting-edge technology and cancer research presents a promising future for more effective and personalised cancer treatments.
- Nanoparticles
Nanotechnology is rapidly becoming one of the most promising therapeutic strategies for treating widespread diseases such as diabetes, bacterial infections, cardiovascular diseases, and cancer (Mukherjee et al. 2019 ). Nanoparticles have been utilised for diagnosis and treatment, including active drug delivery and CRISPR-Cas systems (Mukherjee et al. 2019 ). Mukherjee et al. identified several nanomaterials, such as liposomal, polymeric, metal, and bio-nanoparticles, with promising theranostic effects in their 2019 study. These materials have been shown to improve drug delivery by increasing stability and site specificity, reducing the toxic effect on non-cancerous cells, and enabling a reduction in dosage frequency (Ngema et al. 2021 ). These benefits are significant for developing the CRISPR-Cas system for in vivo delivery and activation. When it comes to NSCLC treatment, albumin-based nanoparticles are currently being utilised across various stages of clinical trials to transport paclitaxel and cisplatin.
Three groups of carriers can be used for CRISPR-Cas delivery: biological, physical, and chemical. Among the chemical group, nanoparticles such as gold nanoparticles (AuNPs) and lipid nanoparticles (LNPs) have been demonstrated as a possible approach to the treatment of NSCLC (Taha et al. 2022 ). This strategy is expected to overcome the limitations of biological and physical methods, which can be immunogenic or toxic and mainly limited to ex vivo manipulations. AuNPs are bound with thiol-modified oligonucleotides, hybridised with a single-stranded donor oligonucleotide, complexed with Cas9 RNP, and coated with the endosomal disruptive polymer PAsp, which allows specific cell internalisation (Taha et al. 2022 ). However, AuNPs have a low level of HDR system induction.
Meanwhile, LNP technology is becoming increasingly popular due to its effectiveness in RNA-based vaccines against COVID-19. The method employs a combination of four types of lipids: cationizable (or ionisable) lipid, PEG lipid, helper phospholipid, and cholesterol. Its pH dependency and lack of proteins or peptides are thought to be responsible for its lower toxic or immunogenic level. Following the advancement of LNP technology, the first phase 1 clinical trial employing LNP-CRISPR took place in 2020 and a targeted organ was a human liver. Thus, such an approach holds promise for extending the scope of organs and genetic conditions amenable to targeting.
Given that most patients with NSCLC are diagnosed at advanced stages and develop secondary resistance to therapeutic methods, the development of both CRISPR and nanoparticle technology is essential for improving treatment outcomes.
Conventional therapies, including surgery, radiotherapy, and chemotherapy, are still the primary treatments for NSCLC. However, despite significant development and improvement in these treatments, OS and PFS remain unsatisfactory, especially for more advanced stages of cancer. Therefore, developing new and more advanced therapies and studying them is crucial.
Emerging experimental methods in medicine, such as CRISPR and nanotechnology, are essential for developing highly personalised therapies. The CRISPR-Cas9 and Cas12a systems provide a variety of approaches in both therapeutic and diagnostic processes. It is worth mentioning that CRISPR can be used for drug target validation, generating mutant cell models, discovering new targets for chemotherapy, and developing screening tests. It is significant that CRISPR-Cas9 already shows promising results in in-human clinical trials using modified T cells of patients. Nanomedicine focuses on improving drug delivery systems, but its application extends to the precise transport of the abovementioned genome-editing tools. However, more comprehensive studies are required to evaluate the safety and efficacy of these techniques.
Immunotherapy has gained popularity over the years and has become one of the most promising approaches to NSCLC treatment. It provides an increase in PFS and OS while still being relatively safe for most patients. Its nature incorporates a patient’s immune system instead of relying on aggressive cytotoxic drugs, allowing combination with other primary NSCLC therapies, such as chemotherapy.
Conventional therapies are based on resecting a tumour or using cytotoxic agents. In contrast, modern methodology targets the cause of tumorigenesis, resulting in a lower impact on surrounding non-cancerous tissue. Current technology and its continuous improvement allow for a diverse approach to LC care. Combining well-established and innovative forms of treatment enables oncologists to exploit individual variables, such as mutations or genetic landscapes, and increase a patient’s chance of survival. Future research should focus on integrating these forms of treatment and taking advantage of knowledge about another molecular pathway for challenging of lung tumour development.
Abbreviations
Adverse effect
Anaplastic lymphoma kinase
Gold nanoparticle
B-RAF inhibitors
Clustered regularly interspaced short palindromic repeat
Cytotoxic T-lymphocyte associated protein-4
Epidermal growth factor receptor
Homology-directed repair
Hypoxia-induced factor
Immune checkpoint inhibitor
Image-guided radiotherapy
Kirsten rat sarcoma virus
- Lung cancer
Lipid nanoparticle
Adenocarcinoma of the lung
Monoclonal antibody
MEK inhibitors
Next-generation sequencing
Non-homologous end-joining
Non-small cell lung cancer/carcinoma
Objective response rate
Overall survival
Programmed death receptor-1
Programmed death ligand-1
Programmed death ligand-2
Progression-free survival
Performance status
Robot-assisted thoracic surgery
Respiratory-gated radiotherapy
Stereotactic body radiation therapy
Tyrosine kinase
Tyrosine kinase inhibitor
Tumor suppressor gene
Video-assisted thoracic surgery
Vascular endothelial growth factor
Vascular endothelial growth factor receptor
Abdelgalil AA, Al-Kahtani HM, Al-Jenoobi FI (2020) Erlotinib. Profiles Drug Subst Excip Relat Methodol 45:93–117. https://doi.org/10.1016/BS.PODRM.2019.10.004
Article CAS PubMed Google Scholar
Aguiar D, Aguiar J, Bohn U (2005) Alternating weekly administration of paclitaxel and gemcitabine: a phase II study in patients with advanced non-small-cell lung cancer. Cancer Chemother Pharmacol 55(2):152–158. https://doi.org/10.1007/S00280-004-0897-8
Akopyan G, Bonavida B (2006) Understanding tobacco smoke carcinogen NNK and lung tumorigenesis (review). Int J Oncol 29(4):745–752. https://doi.org/10.3892/IJO.29.4.745/HTML
Baik CS, Myall NJ, Wakelee HA (2017) Targeting BRAF-mutant nonsmall cell lung cancer: from molecular profiling to rationally designed therapy. Oncologist 22(7):786–796. https://doi.org/10.1634/THEONCOLOGIST.2016-0458
Article PubMed PubMed Central Google Scholar
Binder D et al (2007) Docetaxel/gemcitabine or cisplatin/gemcitabine followed by docetaxel in the first-line treatment of patients with metastatic nonsmall cell lung cancer (NSCLC): results of a multicentre randomised phase II trial. Cancer Chemother Pharmacol 60(1):143–150. https://doi.org/10.1007/S00280-006-0358-7
Bonnesen B, Pappot H, Holmstav J, Skov BG (2009) Vascular endothelial growth factor A and vascular endothelial growth factor receptor 2 expression in nonsmall cell lung cancer patients: relation to prognosis. Lung Cancer 66(3):314–318. https://doi.org/10.1016/J.LUNGCAN.2009.02.013
Article PubMed Google Scholar
Bray F, Ferlay J, Soerjomataram I, Siegel RL, Torre LA, Jemal A (2018) Global cancer statistics 2018: GLOBOCAN estimates of incidence and mortality worldwide for 36 cancers in 185 countries. CA Cancer J Clin 68(6):394–424. https://doi.org/10.3322/CAAC.21492
Caiola E et al (2020) LKB1 deficiency renders NSCLC cells sensitive to ERK inhibitors. J Thorac Oncol 15(3):360–370. https://doi.org/10.1016/J.JTHO.2019.10.009
Camidge DR et al (2018) Brigatinib versus crizotinib in ALK-positive non-small-cell lung cancer. N Engl J Med 379(21):2027–2039. https://doi.org/10.1056/NEJMOA1810171
Camidge DR et al (2019) Updated efficacy and safety data and impact of the EML4-ALK fusion variant on the efficacy of alectinib in untreated ALK-positive advanced nonsmall cell lung cancer in the global phase III ALEX Study. J Thorac Oncol 14(7):1233–1243. https://doi.org/10.1016/j.jtho.2019.03.007
Camidge DR et al (2020) Brigatinib versus crizotinib in advanced ALK inhibitor-naive ALK-positive nonsmall cell lung cancer: second interim analysis of the phase III ALTA-1L trial. J Clin Oncol 38(31):3592–3603. https://doi.org/10.1200/JCO.20.00505
Article CAS PubMed PubMed Central Google Scholar
Carmeliet P (2000) Mechanisms of angiogenesis and arteriogenesis. Nat Med 6(4):389–395. https://doi.org/10.1038/74651
Carmeliet P (2005) VEGF as a key mediator of angiogenesis in cancer. Oncology 69 Suppl 3(SUPPL. 3):4–10. https://doi.org/10.1159/000088478
Castellanos E, Feld E, Horn L (2017) Driven by mutations: the predictive value of mutation subtype in EGFR-mutated non–small cell lung cancer. J Thorac Oncol 12(4):612–623. https://doi.org/10.1016/J.JTHO.2016.12.014
Cébe-Suarez S, Zehnder-Fjällman A, Ballmer-Hofer K (2006) The role of VEGF receptors in angiogenesis; complex partnerships. Cell Mol Life Sci 63(5):601–615. https://doi.org/10.1007/S00018-005-5426-3
Chang JY et al (2008) Image-guided radiation therapy for nonsmall cell lung cancer. J Thorac Oncol 3(2):177–186. https://doi.org/10.1097/JTO.0B013E3181622BDD
Chiappori A et al (2005) Phase II study of first-line sequential chemotherapy with gemcitabine-carboplatin followed by docetaxel in patients with advanced nonsmall cell lung cancer. Oncology 68(4–6):382–390. https://doi.org/10.1159/000086979
Clark JI et al (2001) Pilot study of sequential vinorelbine and cisplatin followed by docetaxel for selected IIIB and stage IV nonsmall cell lung cancer. Lung Cancer 34(2):271–277. https://doi.org/10.1016/S0169-5002(01)00251-3
Cohen MH, Johnson JR, Chen Y-F, Sridhara R, Pazdur R (2005) FDA drug approval summary: erlotinib (Tarceva) tablets. Oncologist 10(7):461–466. https://doi.org/10.1634/THEONCOLOGIST.10-7-461
Conforti F et al (2018) Cancer immunotherapy efficacy and patients’ sex: a systematic review and meta-analysis. Lancet Oncol 19(6):737–746. https://doi.org/10.1016/S1470-2045(18)30261-4
Cristescu R et al (2018) Pan-tumor genomic biomarkers for PD-1 checkpoint blockade-based immunotherapy. Science 362(6411). https://doi.org/10.1126/SCIENCE.AAR3593
De Castro J et al (2002) Sequential combination chemotherapy in patients with advanced nonsmall cell lung carcinoma. Carboplatin and gemcitabine followed by paclitaxel. Cancer 94(10):2797–2798. https://doi.org/10.1002/CNCR.10514
Dela Cruz CS, Tanoue LT, Matthay RA (2011) Lung cancer: epidemiology, etiology, and prevention. Clin Chest Med 32(4):605–644. https://doi.org/10.1016/j.ccm.2011.09.001
Dobosz P and Dzieciątkowski T (2019) The intriguing history of cancer immunotherapy. Front Immunol. https://doi.org/10.3389/FIMMU.2019.02965
Dogan S et al (2012) Molecular epidemiology of EGFR and KRAS mutations in 3026 lung adenocarcinomas: higher susceptibility of women to smoking-related KRAS-mutant cancers. Clin Cancer Res 18(22):6169. https://doi.org/10.1158/1078-0432.CCR-11-3265
Donovan EK, Swaminath A (2018) Stereotactic body radiation therapy (SBRT) in the management of non-small-cell lung cancer: clinical impact and patient perspectives. Lung Cancer (auckl) 9:13–23. https://doi.org/10.2147/LCTT.S129833
Du X, Shao Y, Qin HF, Tai YH, Gao HJ (2018) ALK-rearrangement in non-small-cell lung cancer (NSCLC). Thorac Cancer 9(4):423–430. https://doi.org/10.1111/1759-7714.12613
Dudley AC (2012) Tumor endothelial cells. Cold Spring Harb Perspect Med 2(3). https://doi.org/10.1101/CSHPERSPECT.A006536
Duffy AM, Bouchier-Hayes DJ, Harmey JH (2013) Vascular endothelial growth factor (VEGF) and its role in non-endothelial cells: autocrine signalling by VEGF. Accessed: Jun. 06, 2023. [Online]. Available: https://www.ncbi.nlm.nih.gov/books/NBK6482/
Dugay F et al (2017) Clinicopathological characteristics of ROS1- and RET-rearranged NSCLC in caucasian patients: data from a cohort of 713 non-squamous NSCLC lacking KRAS/EGFR/HER2/BRAF/PIK3CA/ALK alterations. Oncotarget 8(32):53336. https://doi.org/10.18632/ONCOTARGET.18408
Dziedzic DA, Zbytniewski M, Gryszko GM, Cackowski MM, Langfort R, Orlowski TM (2021) Video-assisted versus open thoracotomy lobectomy: comparison on lymphadenectomy and survival in early stage of lung cancer. J Thorac Dis 13(1):101–112. https://doi.org/10.21037/JTD-20-2251
Edelman MJ et al (2004) Randomised phase II trial of sequential chemotherapy in advanced nonsmall cell lung cancer (SWOG 9806): carboplatin/gemcitabine followed by paclitaxel or cisplatin/vinorelbine followed by docetaxel. Clin Cancer Res 10(15):5022–5026. https://doi.org/10.1158/1078-0432.CCR-04-0002
Feng C et al (2022) A simple and highly sensitive naked-eye analysis of EGFR 19del via CRISPR/Cas12a triggered no-nonspecific nucleic acid amplification. ACS Synth Biol 11(2):867–876. https://doi.org/10.1021/ACSSYNBIO.1C00521
Ferrara N (2004) Vascular endothelial growth factor: basic science and clinical progress. Endocr Rev 25(4):581–611. https://doi.org/10.1210/ER.2003-0027
Ferrara N, Gerber HP, LeCouter J (2003) The biology of VEGF and its receptors. Nat Med 9(6):669–676. https://doi.org/10.1038/NM0603-669
Floc’h N et al (2018) Antitumor activity of osimertinib, an irreversible mutant-selective EGFR tyrosine kinase inhibitor, in NSCLC harboring EGFR exon 20 insertions. Mol Cancer Ther 17(5):885–896. https://doi.org/10.1158/1535-7163.MCT-17-0758
Formenti SC et al (2018) Radiotherapy induces responses of lung cancer to CTLA-4 blockade. Nat Med 24(12):1845–1851. https://doi.org/10.1038/S41591-018-0232-2
Gammelgaard KR et al (2019) Up-regulated FGFR1 expression as a mediator of intrinsic TKI resistance in EGFR-mutated NSCLC. Transl Oncol 12(3):432–440. https://doi.org/10.1016/J.TRANON.2018.11.017
Garcia J et al (2020) Bevacizumab (Avastin®) in cancer treatment: a review of 15 years of clinical experience and future outlook. Cancer Treat Rev 86:102017. https://doi.org/10.1016/J.CTRV.2020.102017
Garmendia I et al (2019) YES1 drives lung cancer growth and progression and predicts sensitivity to dasatinib. Am J Respir Crit Care Med 200(7):888–899. https://doi.org/10.1164/RCCM.201807-1292OC
Garon EB et al (2014) Ramucirumab plus docetaxel versus placebo plus docetaxel for second-line treatment of stage IV non-small-cell lung cancer after disease progression on platinum-based therapy (REVEL): a multicentre, double-blind, randomised phase 3 trial. Lancet 384(9944):665–673. https://doi.org/10.1016/S0140-6736(14)60845-X
Gelatti ACZ, Drilon A, Santini FC (2019) Optimising the sequencing of tyrosine kinase inhibitors (TKIs) in epidermal growth factor receptor (EGFR) mutation-positive nonsmall cell lung cancer (NSCLC). Lung Cancer 137:113–122. https://doi.org/10.1016/J.LUNGCAN.2019.09.017
Gerber DE, Schiller JH (2013) Maintenance chemotherapy for advanced non-small-cell lung cancer: new life for an old idea. J Clin Oncol 31(8):1009–1020. https://doi.org/10.1200/JCO.2012.43.7459
Giraud P et al (2011) Respiratory gating techniques for optimisation of lung cancer radiotherapy. J Thorac Oncol 6(12):2058–2068. https://doi.org/10.1097/JTO.0B013E3182307EC2
Godwin JL et al (2017) Nivolumab-induced autoimmune diabetes mellitus presenting as diabetic ketoacidosis in a patient with metastatic lung cancer. J Immunother Cancer 5(1). https://doi.org/10.1186/S40425-017-0245-2
Gridelli C et al (2004) Treatment of advanced non-small-cell lung cancer patients with ECOG performance status 2: results of an European Experts Panel. Ann Oncol 15(3):419–426. https://doi.org/10.1093/ANNONC/MDH087
Gridelli C et al (2007) Single-agent pemetrexed or sequential pemetrexed/gemcitabine as front-line treatment of advanced nonsmall cell lung cancer in elderly patients or patients ineligible for platinum-based chemotherapy: a multicenter, randomised, phase II trial. J Thorac Oncol 2(3):221–229. https://doi.org/10.1097/JTO.0B013E318031CD62
Grossi F et al (2004) Sequential chemotherapy with paclitaxel plus cisplatin, followed by vinorelbine, followed by gemcitabine in advanced nonsmall cell lung cancer: an Alpe-Adria Thoracic Oncology Multidisciplinary group study (ATOM 001). Lung Cancer 46(1):99–106. https://doi.org/10.1016/j.lungcan.2004.03.003
Grossi F et al (2007) Sequential, alternating, and maintenance/consolidation chemotherapy in advanced nonsmall cell lung cancer: a review of the literature. Oncologist 12(4):451–464. https://doi.org/10.1634/THEONCOLOGIST.12-4-451
Guerra SL et al (2020) A deregulated HOX gene axis confers an epigenetic vulnerability in KRAS-mutant lung cancers. Cancer Cell 37(5):705-719.e6. https://doi.org/10.1016/J.CCELL.2020.03.004
Guo F, Ma D, Li S, Adamek M (2019) Compare the prognosis of Da Vinci robot-assisted thoracic surgery (RATS) with video-assisted thoracic surgery (VATS) for nonsmall cell lung cancer: A Meta-analysis. Medicine 98(39):e17089. https://doi.org/10.1097/MD.0000000000017089
Hamrah P, Chen L, Cursiefen C, Zhang Q, Joyce NC, Dana MR (2004) Expression of vascular endothelial growth factor receptor-3 (VEGFR-3) on monocytic bone marrow-derived cells in the conjunctiva. Exp Eye Res 79(4):553–561. https://doi.org/10.1016/J.EXER.2004.06.028
Holla VR et al (2017) ALK: a tyrosine kinase target for cancer therapy. Cold Spring Harb Mol Case Stud 3(1):a001115. https://doi.org/10.1101/MCS.A001115
Holm C, Gineitis D, McConville GS, Kazlauskas A (1996) Expression of PDGF, VEGF and their receptors in nonsmall cell lung tumor cell lines. Int J Oncol 9(5):1077–1086. https://doi.org/10.3892/IJO.9.5.1077
Hosoe S et al (2003) Gemcitabine and vinorelbine followed by docetaxel in patients with advanced non-small-cell lung cancer: a multi-institutional phase II trial of nonplatinum sequential triplet combination chemotherapy (JMTO LC00-02). Br J Cancer 88(3):342–347. https://doi.org/10.1038/SJ.BJC.6600723
Huang MY, Jiang XM, Wang BL, Sun Y, Lu JJ (2021) Combination therapy with PD-1/PD-L1 blockade in nonsmall cell lung cancer: strategies and mechanisms. Pharmacol Ther 219:107694. https://doi.org/10.1016/J.PHARMTHERA.2020.107694
huiJia X et al (2020) Efficacy and safety of neoadjuvant immunotherapy in resectable nonsmall cell lung cancer: a meta-analysis. Lung Cancer 147:143–153. https://doi.org/10.1016/J.LUNGCAN.2020.07.001
Article Google Scholar
Hussmann D, Madsen AT, Jakobsen KR, Luo Y, Sorensen BS, Nielsen AL (2017) IGF1R depletion facilitates MET-amplification as mechanism of acquired resistance to erlotinib in HCC827 NSCLC cells. Oncotarget 8(20):33300–33315. https://doi.org/10.18632/ONCOTARGET.16350
Iwama S, Arima H (2017) Clinical practice and mechanism of endocrinological adverse events associated with immune checkpoint inhibitors. Nihon Rinsho Meneki Gakkai Kaishi 40(2):90–94. https://doi.org/10.2177/JSCI.40.90
Johnson DH et al (2004) Randomised phase II trial comparing bevacizumab plus carboplatin and paclitaxel with carboplatin and paclitaxel alone in previously untreated locally advanced or metastatic non-small-cell lung cancer. J Clin Oncol 22(11):2184–2191. https://doi.org/10.1200/JCO.2004.11.022
Judd J et al (2021) Characterisation of KRAS mutation subtypes in nonsmall cell lung cancer. https://doi.org/10.1158/1535-7163.MCT-21-0201
Justine M et al (2020) ALCAM contributes to brain metastasis formation in non-small-cell lung cancer through interaction with the vascular endothelium. Neuro Oncol 22(7):955–966. https://doi.org/10.1093/NEUONC/NOAA028
Kamba T, McDonald DM (2007) Mechanisms of adverse effects of anti-VEGF therapy for cancer. Br J Cancer 96(12):1788–1795. https://doi.org/10.1038/SJ.BJC.6603813
Kepka L, Socha J (2021) Dose and fractionation schedules in radiotherapy for nonsmall cell lung cancer. Transl Lung Cancer Res 10(4):1969–1982. https://doi.org/10.21037/TLCR-20-253
Kilburn JM et al (2016) Image guided radiotherapy may result in improved local control in locally advanced lung cancer patients. Pract Radiat Oncol 6(3):e73. https://doi.org/10.1016/J.PRRO.2015.10.004
Kowanetz M, Ferrara N (2006) Vascular endothelial growth factor signaling pathways: therapeutic perspective. Clin Cancer Res 12(17):5018–5022. https://doi.org/10.1158/1078-0432.CCR-06-1520
Langevin SM, Kratzke RA, Kelsey KT (2015) Epigenetics of lung cancer. Transl Res 165(1):74–90. https://doi.org/10.1016/J.TRSL.2014.03.001
Larsen JE, Cascone T, Gerber DE, Heymach JV, Minna JD (2011) Targeted therapies for lung cancer: clinical experience and novel agents. Cancer J 17(6):512–527. https://doi.org/10.1097/PPO.0B013E31823E701A
Lee JH, Hong JI, Kim HK (2021) Robot-assisted thoracic surgery in nonsmall cell lung cancer. J Chest Surg 54(4):266. https://doi.org/10.5090/JCS.21.070
Leiser D et al (2021) Role of caveolin-1 as a biomarker for radiation resistance and tumor aggression in lung cancer. PLoS One 16(11):e0258951. https://doi.org/10.1371/JOURNAL.PONE.0258951
Leonetti A, Sharma S, Minari R, Perego P, Giovannetti E, Tiseo M (2019) Resistance mechanisms to osimertinib in EGFR-mutated nonsmall cell lung cancer. Br J Cancer 121(9):725. https://doi.org/10.1038/S41416-019-0573-8
Li H et al (2014) Addition of bevacizumab enhances antitumor activity of erlotinib against nonsmall cell lung cancer xenografts depending on VEGF expression. Cancer Chemother Pharmacol 74(6):1297–1305. https://doi.org/10.1007/S00280-014-2610-X
Li Y, Zang H, Qian G, Owonikoko TK, Ramalingam SR, Sun SY (2020) ERK inhibition effectively overcomes acquired resistance of epidermal growth factor receptor-mutant nonsmall cell lung cancer cells to osimertinib. Cancer 126(6):1339–1350. https://doi.org/10.1002/CNCR.32655
Liao J et al (2017) Down-regulation of miR-214 reverses erlotinib resistance in non-small-cell lung cancer through up-regulating LHX6 expression. Sci Rep 7(1). https://doi.org/10.1038/S41598-017-00901-6
Lohela M, Bry M, Tammela T, Alitalo K (2009) VEGFs and receptors involved in angiogenesis versus lymphangiogenesis. Curr Opin Cell Biol 21(2):154–165. https://doi.org/10.1016/J.CEB.2008.12.012
Lu Y et al (2020) Safety and feasibility of CRISPR-edited T cells in patients with refractory non-small-cell lung cancer. Nat Med 26(5):732–740. https://doi.org/10.1038/S41591-020-0840-5
Ma J, Li X, Zhao S, Wang J, Zhang W, Sun G (2021) Robot-assisted thoracic surgery versus video-assisted thoracic surgery for lung lobectomy or segmentectomy in patients with nonsmall cell lung cancer: a meta-analysis. BMC Cancer 21(1). https://doi.org/10.1186/S12885-021-08241-5
Mao Y, Wu S (2017a) ALK and ROS1 concurrent with EGFR mutation in patients with lung adenocarcinoma. Onco Targets Ther 10:3399. https://doi.org/10.2147/OTT.S133349
Mao Y, Wu S (2017b) OncoTargets and Therapy Dovepress ALK and ROS1 concurrent with EGFR mutation in patients with lung adenocarcinoma. Onco Targets Ther 10:3399. https://doi.org/10.2147/OTT.S133349
Masters GA et al (2015) Systemic therapy for stage IV non-small-cell lung cancer: American Society of Clinical Oncology Clinical Practice Guideline Update. J Clin Oncol 33(30):3488–3515. https://doi.org/10.1200/JCO.2015.62.1342
Mattson K et al (2000) Phase II study of docetaxel alternating with cisplatin in chemotherapy-naïve patients with advanced nonsmall cell lung cancer. Anticancer Drugs 11(1):7–13. https://doi.org/10.1097/00001813-200001000-00002
McKeage K (2015) Alectinib: a review of its use in advanced ALK-rearranged nonsmall cell lung cancer. Drugs 75(1):75–82. https://doi.org/10.1007/S40265-014-0329-Y
Mehta A, Dobersch S, Romero-Olmedo AJ, Barreto G (2015) Epigenetics in lung cancer diagnosis and therapy. Cancer Metastasis Rev 34(2):229–241. https://doi.org/10.1007/S10555-015-9563-3
Moreno Roig E et al (2019) HIF-1α and HIF-2α differently regulate the radiation sensitivity of NSCLC cells. Cells 8(1):45. https://doi.org/10.3390/CELLS8010045
Moro-Sibilot D et al (2019) Crizotinib in c-MET- or ROS1-positive NSCLC: results of the AcSé phase II trial. Ann Oncol 30(12):1985–1991. https://doi.org/10.1093/ANNONC/MDZ407
Mukherjee A, Paul M, Mukherjee S (2019) Recent progress in the theranostics application of nanomedicine in lung cancer. Cancers (Basel) 11(5):597. https://doi.org/10.3390/cancers11050597
Nakagawa K et al (2019) Ramucirumab plus erlotinib in patients with untreated, EGFR-mutated, advanced non-small-cell lung cancer (RELAY): a randomised, double-blind, placebo-controlled, phase 3 trial. Lancet Oncol 20(12):1655–1669. https://doi.org/10.1016/S1470-2045(19)30634-5
Nasser NJ, Gorenberg M, Agbarya A (2020) First line Immunotherapy for nonsmall cell lung cancer. Pharmaceuticals (Basel) 13(11):1–24. https://doi.org/10.3390/PH13110373
Neufeld G, Cohen T, Gengrinovitch S, Poltorak Z (1999) Vascular endothelial growth factor (VEGF) and its receptors. FASEB J 13(1):9–22. https://doi.org/10.1096/FASEBJ.13.1.9
Ngema LM, Adeyemi SA, Marimuthu T, Choonara YE (2021) A review on engineered magnetic nanoparticles in non-small-cell lung carcinoma targeted therapy. Int J Pharm 606:120870. https://doi.org/10.1016/j.ijpharm.2021.120870
O’Leary CG et al (2019) Targeting BRAF mutations in nonsmall cell lung cancer. Transl Lung Cancer Res 8(6):1119–1124. https://doi.org/10.21037/TLCR.2019.10.22
Oiseth SJ, Aziz MS (2017) Cancer immunotherapy: a brief review of the history, possibilities, and challenges ahead. J Cancer Metastasis Treat 3(10):250–261. https://doi.org/10.20517/2394-4722.2017.41
Article CAS Google Scholar
Park HR et al (2021) Acquired resistance to third-generation EGFR tyrosine kinase inhibitors in patients with de novo EGFR T790M-mutant NSCLC. J Thorac Oncol 16(11):1859–1871. https://doi.org/10.1016/J.JTHO.2021.06.013
Paul B, Montoya G (2020) CRISPR-Cas12a: functional overview and applications. Biomed J 43(1):8–17. https://doi.org/10.1016/J.BJ.2019.10.005
Piperdi B, Merla A, Perez-Soler R (2014) Targeting angiogenesis in squamous nonsmall cell lung canceR. Drugs 74(4):403. https://doi.org/10.1007/S40265-014-0182-Z
Planchard D et al (2017) Dabrafenib plus trametinib in patients with previously untreated BRAFV600E-mutant metastatic non-small-cell lung cancer: an open-label, phase 2 trial. Lancet Oncol 18(10):1307–1316. https://doi.org/10.1016/S1470-2045(17)30679-4
Puzanov I et al (2017) Managing toxicities associated with immune checkpoint inhibitors: consensus recommendations from the Society for Immunotherapy of Cancer (SITC) Toxicity Management Working Group. J Immunother Cancer 5(1). https://doi.org/10.1186/S40425-017-0300-Z
Raoof S et al (2019) Targeting FGFR overcomes EMT-mediated resistance in EGFR mutant nonsmall cell lung cancer. Oncogene 38(37):6399–6413. https://doi.org/10.1038/S41388-019-0887-2
Reck M et al (2021) Five-year outcomes with pembrolizumab versus chemotherapy for metastatic non-small-cell lung cancer with PD-L1 tumor proportion score ≥ 50. J Clin Oncol 39(21):2339–2349. https://doi.org/10.1200/JCO.21.00174
Reck M et al (2021) First-line nivolumab plus ipilimumab with two cycles of chemotherapy versus chemotherapy alone (four cycles) in advanced non-small-cell lung cancer: CheckMate 9LA 2-year update. ESMO Open 6(5). https://doi.org/10.1016/J.ESMOOP.2021.100273
Recondo G, Facchinetti F, Olaussen KA, Besse B, Friboulet L (2018) Making the first move in EGFR-driven or ALK-driven NSCLC: first-generation or next-generation TKI? Nat Rev Clin Oncol 15(11):694–708. https://doi.org/10.1038/S41571-018-0081-4
Rinaldi M et al (2005) Preliminary results of a multicenter randomised phase II trial of two different combinations of docetaxel (D) and gemcitabine (G) and of cisplatin/gemcitabine (CG) followed by docetaxel as first line therapy for locally advanced or metastatic non small cell lung cancer (NSCLC). https://doi.org/10.1200/jco.2005.23.16_suppl.7124 23(16_suppl):7124–7124. https://doi.org/10.1200/JCO.2005.23.16_SUPPL.7124
Rizvi NA et al (2015) Cancer immunology. Mutational landscape determines sensitivity to PD-1 blockade in nonsmall cell lung cancer. Science 348(6230):124–128. https://doi.org/10.1126/SCIENCE.AAA1348
Rosen LS (2002) Clinical experience with angiogenesis signaling inhibitors: focus on vascular endothelial growth factor (VEGF) blockers. Cancer Control 9(2 Suppl):36–44. https://doi.org/10.1177/107327480200902S05
Rosenberg SA (2014) IL-2: the first effective immunotherapy for human cancer. J Immunol 192(12):5451–5458. https://doi.org/10.4049/JIMMUNOL.1490019
Rosenberg SA et al (1984) Biological activity of recombinant human interleukin-2 produced in Escherichia coli. Science 223(4643):1412–1415. https://doi.org/10.1126/SCIENCE.6367046
Rosenberg SA et al (1985) Observations on the systemic administration of autologous lymphokine-activated killer cells and recombinant interleukin-2 to patients with metastatic cancer. N Engl J Med 313(23):1485–1492. https://doi.org/10.1056/NEJM198512053132327
Rude Voldborg B, Damstrup L, Spang-Thomsen M, Skovgaard Poulsen H (1997) Epidermal growth factor receptor (EGFR) and EGFR mutations, function and possible role in clinical trials. Ann Oncol 8(12):1197–1206. https://doi.org/10.1023/A:1008209720526
Salgia R, Pharaon R, Mambetsariev I, Nam A, Sattler M (2021) The improbable targeted therapy: KRAS as an emerging target in nonsmall cell lung cancer (NSCLC). Cell Rep Med 2(1):100186. https://doi.org/10.1016/J.XCRM.2020.100186
Sander JD, Joung JK (2014) CRISPR-Cas systems for editing, regulating and targeting genomes. Nat Biotechnol 32(4):347–355. https://doi.org/10.1038/nbt.2842
Shaw AT et al (2014) Crizotinib in ROS1-rearranged non–small-cell lung cancer. N Engl J Med 371(21):1963. https://doi.org/10.1056/NEJMOA1406766
Shaw AT et al (2019) Crizotinib in ROS1-rearranged advanced non-small-cell lung cancer (NSCLC): updated results, including overall survival, from PROFILE 1001. Ann Oncol 30(7):1121. https://doi.org/10.1093/ANNONC/MDZ131
Siegel R, Ma J, Zou Z, Jemal A (2014) Cancer statistics, 2014. CA Cancer J Clin 64(1):9–29. https://doi.org/10.3322/CAAC.21208
Skobe M, Rockwell P, Goldstein N, Vosseler S, Fusenig NE (1997) Halting angiogenesis suppresses carcinoma cell invasion. Nat Med 3(11):1222–1227. https://doi.org/10.1038/NM1197-1222
Skoulidis F et al (2021) Sotorasib for lung cancers with KRAS p. G12C mutation. N Engl J Med 384(25):2371–2381. https://doi.org/10.1056/NEJMOA2103695
Socinski MA et al (2018) Atezolizumab for first-line treatment of metastatic nonsquamous NSCLC. N Engl J Med 378(24):2288–2301. https://doi.org/10.1056/NEJMOA1716948
Soria J-C et al (2018) Osimertinib in untreated EGFR-mutated advanced non-small-cell lung cancer. N Engl J Med 378(2):113–125. https://doi.org/10.1056/NEJMOA1713137
Sreedurgalakshmi K, Srikar R, Rajkumari R (2021) CRISPR-Cas deployment in nonsmall cell lung cancer for target screening, validations, and discoveries. Cancer Gene Ther 28(6):566–580. https://doi.org/10.1038/S41417-020-00256-7
Steven A, Fisher SA, Robinson BW (2016) Immunotherapy for lung cancer. Respirology 21(5):821–833. Blackwell Publishing. https://doi.org/10.1111/resp.12789
Sunderkotter C, Steinbrink K, Goebeler M, Bhardwaj R, Sorg C (1994) Macrophages and angiogenesis. J Leukoc Biol 55(3):410–422. https://doi.org/10.1002/JLB.55.3.410
Sung H et al (2021) Global Cancer Statistics 2020: GLOBOCAN estimates of incidence and mortality worldwide for 36 cancers in 185 countries. CA Cancer J Clin 71(3):209–249. https://doi.org/10.3322/CAAC.21660
Suresh K, Naidoo J, Lin CT, Danoff S (2018) Immune checkpoint immunotherapy for nonsmall cell lung cancer: benefits and pulmonary toxicities. Chest 154(6):1416–1423. https://doi.org/10.1016/J.CHEST.2018.08.1048
Taha EA, Lee J, Hotta A (2022) Delivery of CRISPR-Cas tools for in vivo genome editing therapy: trends and challenges. J Control Release 342:345–361. https://doi.org/10.1016/J.JCONREL.2022.01.013
Tandberg DJ, Tong BC, Ackerson BG, Kelsey CR (2018) Surgery versus stereotactic body radiation therapy for stage I nonsmall cell lung cancer: a comprehensive review. Cancer 124(4):667–678. https://doi.org/10.1002/CNCR.31196
Tang KJ et al (2016) Focal adhesion kinase regulates the DNA damage response and its inhibition radiosensitizes mutant KRAS lung cancer. Clin Cancer Res 22(23):5851–5863. https://doi.org/10.1158/1078-0432.CCR-15-2603
Taniguchi T et al (1983) Structure and expression of a cloned cDNA for human interleukin-2. Nature 302(5906):305–310. https://doi.org/10.1038/302305A0
Terai H et al (2021) SHOC2 is a critical modulator of sensitivity to EGFR-TKIs in nonsmall cell lung cancer cells. Mol Cancer Res 19(2):317–328. https://doi.org/10.1158/1541-7786.MCR-20-0664
Thandra KC, Barsouk A, Saginala K, Aluru JS, Barsouk A (2021) Epidemiology of lung cancer. Wspolczesna Onkologia 25(1):45–52. https://doi.org/10.5114/WO.2021.103829
Tong X et al (2020) MYOCD and SMAD3/SMAD4 form a positive feedback loop and drive TGF-β-induced epithelial-mesenchymal transition in nonsmall cell lung cancer. Oncogene 39(14):2890–2904. https://doi.org/10.1038/S41388-020-1189-4
Tsukumo Y, Naito M, Suzuki T (2020) Influence of EGFR-activating mutations on sensitivity to tyrosine kinase inhibitors in a KRAS mutant nonsmall cell lung cancer cell line. PLoS One 15(3):e0229712. https://doi.org/10.1371/JOURNAL.PONE.0229712
Uzel EK, Figen M, Uzel Ö (2019) Radiotherapy in lung cancer: current and future role. Sisli Etfal Hastan Tıp Bul 53(4):353. https://doi.org/10.14744/SEMB.2019.25991
Veronesi G (2015) Robotic lobectomy and segmentectomy for lung cancer: results and operating technique. J Thorac Dis 7(Suppl 2):S122. https://doi.org/10.3978/J.ISSN.2072-1439.2015.04.34
Veronesi G, Bianchi F, Inamura K (2017) Article 193 Citation: Inamura K (2017) Lung cancer: understanding its molecular pathology and the 2015 WHO classification. Front Oncol 7:193. https://doi.org/10.3389/fonc.2017.00193
Wang H et al (2016) Tankyrase inhibitor sensitizes lung cancer cells to endothelial growth factor receptor (EGFR) inhibition via stabilizing angiomotins and inhibiting YAP signaling. J Biol Chem 291(29):15256–15266. https://doi.org/10.1074/JBC.M116.722967
Wang L, Liu D, Lu J, Zhang S, Yang X (2017) The feasibility and advantage of uniportal video-assisted thoracoscopic surgery (VATS) in pulmonary lobectomy. BMC Cancer 17(1):1–7. https://doi.org/10.1186/S12885-017-3069-Z/FIGURES/2
Wang C et al (2020a) The landscape of immune checkpoint inhibitor plus chemotherapy versus immunotherapy for advanced non-small-cell lung cancer: a systematic review and meta-analysis. J Cell Physiol 235(5):4913–4927. https://doi.org/10.1002/JCP.29371
Wang L et al (2020b) Improved EGFR mutation detection sensitivity after enrichment by Cas9/sgRNA digestion and PCR amplification. Acta Biochim Biophys Sin (shanghai) 52(12):1316–1324. https://doi.org/10.1093/ABBS/GMAA123
Wang Z et al (2019) Video-assisted thoracoscopic surgery versus muscle-sparing thoracotomy for nonsmall cell lung cancer: a systematic review and meta-analysis. BMC Surg 19(1). https://doi.org/10.1186/S12893-019-0618-1
Wherry EJ, Kurachi M (2015) Molecular and cellular insights into T cell exhaustion. Nat Rev Immunol 15(8):486–499. https://doi.org/10.1038/NRI3862
Wiedenheft B, Sternberg SH, Doudna JA (2012) RNA-guided genetic silencing systems in bacteria and archaea. Nature 482(7385):331–338. https://doi.org/10.1038/nature10886
Wu H, Jin R, Yang S, Park BJ, Li H (2021) Long-term and short-term outcomes of robot- versus video-assisted anatomic lung resection in lung cancer: a systematic review and meta-analysis. Eur J Cardiothorac Surg 59(4):732–740. https://doi.org/10.1093/EJCTS/EZAA426
Xia L, Liu Y, Wang Y (2019) PD-1/PD-L1 blockade therapy in advanced non-small-cell lung cancer: current status and future directions. Oncologist 24(S1):S31–S41. https://doi.org/10.1634/theoncologist.2019-IO-S1-s05
Yamazaki Y, Morita T (2006) Molecular and functional diversity of vascular endothelial growth factors. Mol Divers 10(4):515–527. https://doi.org/10.1007/S11030-006-9027-3
Zappa C, Mousa SA (2016) Nonsmall cell lung cancer: current treatment and future advances. Transl Lung Cancer Res 5(3):288–300. https://doi.org/10.21037/TLCR.2016.06.07
Zarogoulidis P et al (2017) Possible adverse effects of immunotherapy in nonsmall cell lung cancer; treatment and follow-up of three cases. Respir Med Case Rep 22:101–105. https://doi.org/10.1016/J.RMCR.2017.07.004
Zhang Y et al (2022) Platelet-derived PDGFB promotes recruitment of cancer-associated fibroblasts, deposition of extracellular matrix and Tgfβ signaling in the tumor microenvironment. Cancers (Basel) 14(8). https://doi.org/10.3390/CANCERS14081947/S1
Zhao Z, Gao Y, Xue Q, Gao S, He J (2021) Safety and efficacy of neoadjuvant immune checkpoint inhibitor therapy in patients with resectable non-small-cell lung cancer: a systematic review. Target Oncol 16(4):425–434. https://doi.org/10.1007/S11523-021-00818-1
Zhou C et al (2021) Camrelizumab plus carboplatin and pemetrexed versus chemotherapy alone in chemotherapy-naive patients with advanced non-squamous non-small-cell lung cancer (CameL): a randomised, open-label, multicentre, phase 3 trial. Lancet Respir Med 9(3):305–314. https://doi.org/10.1016/S2213-2600(20)30365-9
Download references
Author information
Authors and affiliations.
Department of Biochemistry and Molecular Biology, Poznań University of Medical Sciences, Święcickiego 6 Street, 60-781, Poznań, Poland
Andrzej Jachowski, Mikołaj Marcinkowski, Jakub Szydłowski, Oskar Grabarczyk, Zuzanna Nogaj, Łaz Marcin, Paweł Piotr Jagodziński & Bartosz Kazimierz Słowikowski
Institute of Human Genetics, Polish Academy of Sciences, Strzeszyńska 32 Street, 60-479, Poznań, Poland
Andrzej Pławski
You can also search for this author in PubMed Google Scholar
Contributions
Andrzej Jachowski: writing—original draft (“Introduction”), review and editing, literature searching; Mikołaj Marcinkowski: writing—original draft (“Immunotherapy”), review and editing, literature searching; Jakub Szydłowski: writing—original draft (“Pathway targeted therapy”), review and editing, literature searching; Oskar Grabarczyk: writing—original draft (“Introduction – conventional therapies”), literature searching; Zuzanna Nogaj: writing—original draft, conceptualization (“CRISPR-Cas”), literature searching; Marcin Łaz: writing—original draft (“Antiangiogenic therapy”), literature searching; Andrzej Pławski: writing—review and editing, supervision; Paweł Piotr Jagodziński: writing—review and editing, supervision; Bartosz Kazimierz Słowikowski: conceptualization, article idea, supervision, writing—review and editing, figure preparation.
Corresponding author
Correspondence to Bartosz Kazimierz Słowikowski .
Ethics declarations
Ethics approval.
This article does not contain any studies with human participants or animals performed by any of the authors.
Consent to participate
Not applicable/not required.
Consent for publication
Competing interests.
The authors declare no competing interests.
Additional information
Communicated by Ewa Ziętkiewicz
Publisher's note
Springer Nature remains neutral with regard to jurisdictional claims in published maps and institutional affiliations.
Rights and permissions
Open Access This article is licensed under a Creative Commons Attribution 4.0 International License, which permits use, sharing, adaptation, distribution and reproduction in any medium or format, as long as you give appropriate credit to the original author(s) and the source, provide a link to the Creative Commons licence, and indicate if changes were made. The images or other third party material in this article are included in the article's Creative Commons licence, unless indicated otherwise in a credit line to the material. If material is not included in the article's Creative Commons licence and your intended use is not permitted by statutory regulation or exceeds the permitted use, you will need to obtain permission directly from the copyright holder. To view a copy of this licence, visit http://creativecommons.org/licenses/by/4.0/ .
Reprints and permissions
About this article
Jachowski, A., Marcinkowski, M., Szydłowski, J. et al. Modern therapies of nonsmall cell lung cancer. J Appl Genetics 64 , 695–711 (2023). https://doi.org/10.1007/s13353-023-00786-4
Download citation
Received : 21 June 2023
Revised : 01 September 2023
Accepted : 01 September 2023
Published : 12 September 2023
Issue Date : December 2023
DOI : https://doi.org/10.1007/s13353-023-00786-4
Share this article
Anyone you share the following link with will be able to read this content:
Sorry, a shareable link is not currently available for this article.
Provided by the Springer Nature SharedIt content-sharing initiative
- Targeted therapy
- Find a journal
- Publish with us
- Track your research

Opening a new front against pancreatic cancer
A new type of investigational therapeutic in development for pancreatic cancer has shown unprecedented tumor-fighting abilities in preclinical models of the disease, suggesting it has the potential to offer novel treatment options for nearly all pancreatic tumors, a comprehensive study has found.
The inhibitors in this new class of oral medications, being developed by Revolution Medicines Inc., target the oncogenic or active cancer-causing form of RAS proteins (such as KRAS, NRAS, and HRAS). These RAS "oncoproteins" drive up to a third of all human cancers. The research findings -- conducted by a consortium of academic researchers led by Columbia scientists and the scientific team at Revolution Medicines -- were published in a paper appearing today in Nature .
Currently the third leading cause of death from cancer, pancreatic cancer kills about 50,000 people annually in the United States alone. Despite decades of research, the disease continues to stymie drug developers and oncologists. What's especially frustrating is that scientists know exactly what causes most cases at the cellular level. "For over four decades, we have known that there's one particular RAS protein, called KRAS, that's mutated and drives about 95% of all pancreatic ductal adenocarcinoma cases, and we've had no direct tools to attack it for most of that time," says Kenneth Olive, PhD, associate professor of medicine at Columbia University's Vagelos College of Physicians and Surgeons and Herbert Irving Comprehensive Cancer Center, one of the study's senior authors.
When the study's co-senior author, Mallika Singh, PhD, vice president for translational research at Revolution Medicines, told Olive the company had invented a class of inhibitors that had the potential to target all RAS mutations, he was incredulous. "My immediate reaction was skepticism," says Olive. "But I was curious, and we quickly established a collaboration."
Preclinical studies soon launched in the Olive lab at Columbia, led by Urszula Wasko, a PhD student in the molecular pharmacology graduate program. Early pilot experiments with RMC-7977 were remarkably effective. "We immediately knew we were working with something entirely different," says Olive. At the same time, Olive and Revolution Medicines worked to bring together pancreatic cancer experts from other academic institutions, including the University of Pennsylvania, Dana-Farber Cancer Institute, University of North Carolina at Chapel Hill, and Memorial Sloan Kettering. "Rather than compete against one another, we established a consortium and agreed to share data in real time. That was transformative," says Olive.
Pancreatic cancer researchers have developed many different preclinical models of the disease over the years, each with its own strengths and weaknesses. Rather than pick one, the expanded team tested RMC-7977 in all of them. "By unleashing a consortium of scientists on this problem, we were able to examine active RAS inhibition in every major class of model for pancreatic cancer, and this inhibitor performed really well in all," says Olive.
The preclinical tumor model Olive's lab has long favored is widely recognized for its broad resistance to treatment. "RMC-7977 as a single agent outperformed the best combination regimen that has ever been reported in the literature in that model system," he says, adding that it's the first time he's ever seen tumors routinely get smaller in those systems. Other models the consortium tested yielded similar results.
Because RMC-7977 also inhibits wild-type RAS proteins essential to the health of many normal cells, the scientists also carefully examined normal tissues in the treated animals. This work showed that tumor cells are uniquely sensitive to the inhibitor, while the impact in normal cells was minimal.
Though the initial responses in preclinical tumor models to the inhibitor were impressive, Olive hastens to point out that the tumors were not eliminated.
"In almost every case, the tumor came back," he says. In tissue culture, the investigators identified another oncogene, called MYC, that was altered in most of the resistant tumors, then developed a combination treatment that was effective against tumor cells that had developed resistance to the active RAS inhibitor. Those results suggest a combinatorial approach that is worth exploring in patients in the future.
In a field with a long history of failed drug development efforts, the new results are cause for optimism, Olive says. "I've been working on pancreatic cancer for almost 20 years, and I've never seen preclinical results like these. I think there is a real chance this approach will help change the standard of care for pancreatic cancer patients, but only clinical trials can determine that. I'm excited that Columbia is one of many institutions participating in the clinical development of these new agents."
- Pancreatic Cancer
- Brain Tumor
- Lung Cancer
- Breast Cancer
- Ovarian Cancer
- Colon Cancer
- Prostate Cancer
- Renal cell carcinoma
- Monoclonal antibody therapy
- Breast cancer
- Prostate cancer
- Drug discovery
- Tumor suppressor gene
Story Source:
Materials provided by Columbia University Irving Medical Center . Note: Content may be edited for style and length.
Journal Reference :
- Urszula N. Wasko, Jingjing Jiang, Tanner C. Dalton, Alvaro Curiel-Garcia, A. Cole Edwards, Yingyun Wang, Bianca Lee, Margo Orlen, Sha Tian, Clint A. Stalnecker, Kristina Drizyte-Miller, Marie Menard, Julien Dilly, Stephen A. Sastra, Carmine F. Palermo, Marie C. Hasselluhn, Amanda R. Decker-Farrell, Stephanie Chang, Lingyan Jiang, Xing Wei, Yu C. Yang, Ciara Helland, Haley Courtney, Yevgeniy Gindin, Karl Muonio, Ruiping Zhao, Samantha B. Kemp, Cynthia Clendenin, Rina Sor, William P. Vostrejs, Priya S. Hibshman, Amber M. Amparo, Connor Hennessey, Matthew G. Rees, Melissa M. Ronan, Jennifer A. Roth, Jens Brodbeck, Lorenzo Tomassoni, Basil Bakir, Nicholas D. Socci, Laura E. Herring, Natalie K. Barker, Junning Wang, James M. Cleary, Brian M. Wolpin, John A. Chabot, Michael D. Kluger, Gulam A. Manji, Kenneth Y. Tsai, Miroslav Sekulic, Stephen M. Lagana, Andrea Califano, Elsa Quintana, Zhengping Wang, Jacqueline A. M. Smith, Matthew Holderfield, David Wildes, Scott W. Lowe, Michael A. Badgley, Andrew J. Aguirre, Robert H. Vonderheide, Ben Z. Stanger, Timour Baslan, Channing J. Der, Mallika Singh, Kenneth P. Olive. Tumor-selective activity of RAS-GTP inhibition in pancreatic cancer . Nature , 2024; DOI: 10.1038/s41586-024-07379-z
Cite This Page :
Explore More
- Plastic Pollution Kills Ocean Embryos
- Most Massive Stellar Black Hole in Our Galaxy
- Coffee's Prehistoric Origin and It's Future
- Can Animals Count? New Rat Study
- A Single Atom Layer of Gold: Goldene
- Fool's Gold May Contain Valuable Lithium
- Exercise Cuts Stress-Related Brain Activity
- Microplastics Go from Gut to Other Organs
- Epilepsy Drug May Prevent Brain Tumors
- Evolution's Recipe Book
Trending Topics
Strange & offbeat.
The Straits Times
- International
- Print Edition
- news with benefits
- SPH Rewards
- STClassifieds
- Berita Harian
- Hardwarezone
- Shin Min Daily News
- Tamil Murasu
- The Business Times
- The New Paper
- Lianhe Zaobao
- Advertise with us
BRANDED CONTENT
Boosting cancer treatment accuracy: how gene testing helps, supported by philanthropic organisation tanoto foundation, pioneering genomic research by professor lim soon thye opens up new cancer treatment options.
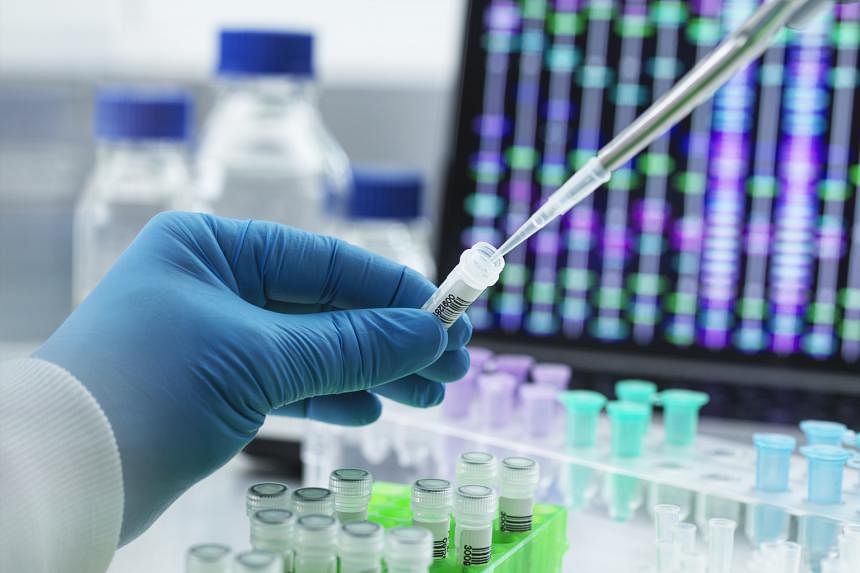
Singapore, like most modern, developed countries, has been grappling with a rising incidence of cancer. Today, one in four Singaporeans is likely to get some form of cancer during their lifetime, according to the Singapore Cancer Society .
The Singapore Cancer Registry Annual Report 2021 revealed that over 84,000 new cancer cases were reported between 2017 and 2021.
Lymphoma is among the different cancer types reported, with the aggressive form – n atural killer/T-cell lymphoma (NKTCL) – more prevalent in the Asian population. A rare blood cancer, it remains less studied and is poorly understood, according to the National Cancer Centre Singapore (NCCS).
As a result, treatment options are limited and survival rates are relatively low.
Professor Lim Soon Thye, chief executive officer at NCCS and professor at Duke-NUS Medical School, explains: “Lymphoma is a complex cancer which can manifest in various forms or exhibit different characteristics in different individuals. We need to better understand the disease and take a more targeted approach to treating individuals affected by it, so that we can improve treatment outcomes.”
His research is supported by the Tanoto Foundation Professorship in Medical Oncology at the SingHealth Duke-NUS Academic Medical Centre . The Professorship was established with the support of Tanoto Foundation in 2013 and has been a catalyst for several findings in the field since its inception.
Identifying cancer-causing genes
One major area of study conducted by Prof Lim and his NCCS team is genome sequencing and analysis.
In 2019, they collaborated with Singapore General Hospital (SGH), National University Cancer Institute, Singapore (NCIS) and Genome Institute of Singapore (GIS) under Agency for Science, Technology and Research (A*STAR) to study more than 21,000 participants from Asia and the United States. This has been described as the largest NKTCL genome-wide association study to date .
The study found that two genes, IL18RAP and HLA-DRB1, significantly increase NKTCL risk in patients.
Those with a risk allele (a variant form of a gene) are 39 per cent to 53 per cent more likely to develop NKTCL. Individuals with two identical risk alleles in IL18RAP, HLA-DRB1 and HLA-DPB1 are 18 times more predisposed to the disease.
“Our findings shed light on the need for genetic consultation to aid precision medicine for patients with NKTCL and point to the potential benefit of immunotherapies to tackle this deadly disease,” says Prof Lim.
Prof Lim also co-leads the Lymphoma Genomic Translational Research Laboratory at NCCS with leading oncologists, pathologists and scientists, taking a bench-to-bedside approach to lymphoma treatment research. The laboratory currently drives whole-genome sequencing of NKTCL in the International Cancer Genome Consortium (ICGC).
Personalised cancer treatment for lymphoma
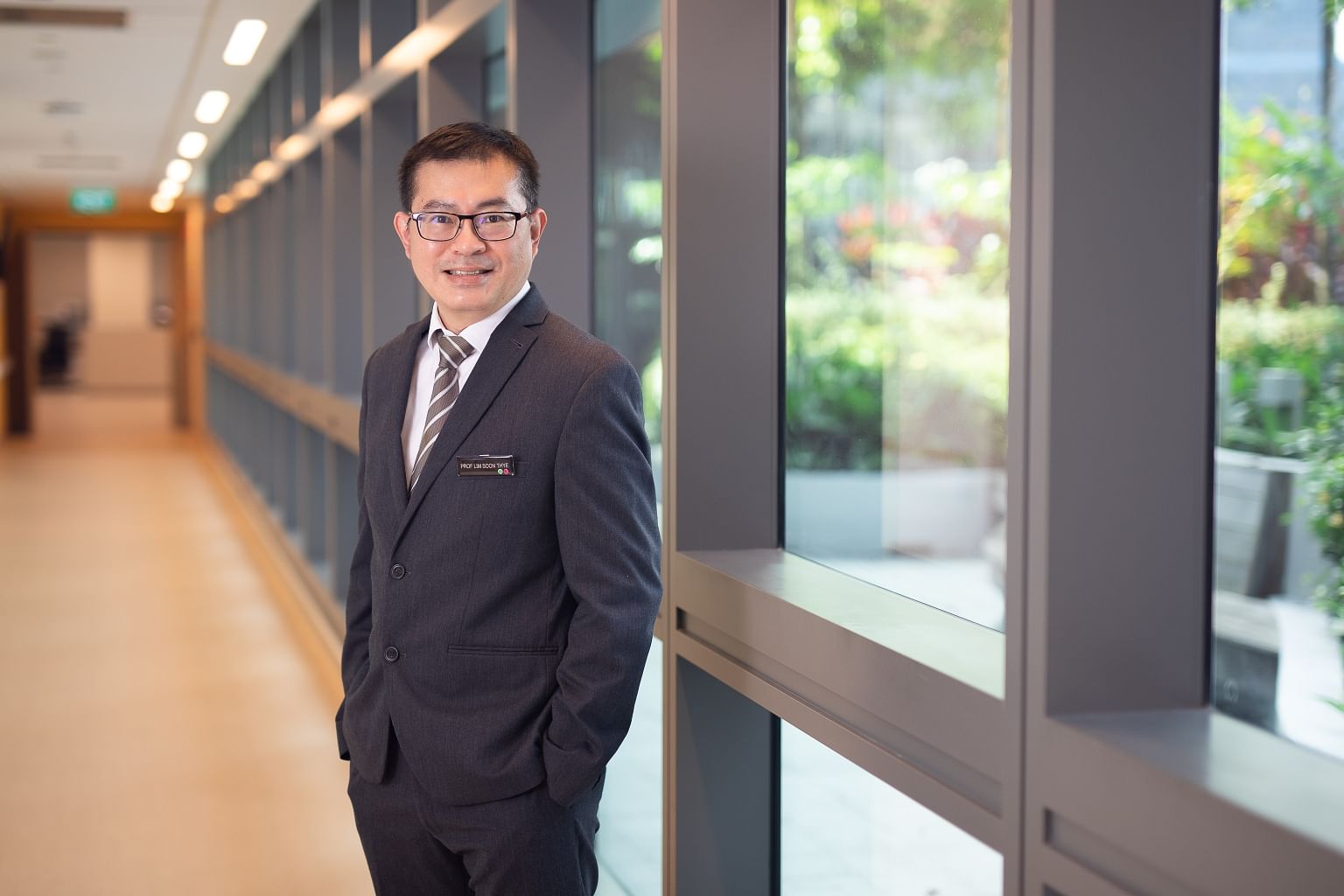
By 2030, a quarter of Singaporeans will be 65 or older. While medication, diet and healthy lifestyle can contribute to reducing cancer risk, genetic mutations inevitably occur in cells as individuals age.
The current standard treatment for NKCTL patients involves a combination of chemotherapy and radiotherapy, but patients often relapse and survival is not guaranteed beyond six months.
With Tanoto Foundation’s support, Prof Lim’s team performed whole-genome sequencing in 2020 and identified a mutation in the PD-L1 gene as a potential target in NKTCL patients .
While this gene regulates and prevents over-activation of the immune system, it also inadvertently prevents the immune system from attacking cancer cells. From the team’s findings, relapsed NKTCL patients with this gene mutation were more likely to show a positive response to anti-PD-1 therapy, a type of immunotherapy.
Immunotherapy treatments train the body’s immune system to fight cancer cells.
With this discovery, clinicians can identify patients who would benefit more from immunotherapy compared to standard cancer treatment options. This saves time and cost, and improves survival rates.
These findings have since been published in the journal Leukemia , and the mutation was integrated into a clinical-grade diagnostic panel to personalise treatment recommendations, which are now used in several countries.
Equitable access for all
Another key priority in Prof Lim’s clinical leadership role is to enhance and standardise the delivery of oncology care across SingHealth.
Cancer patients undergoing care and treatment at NCCS, Changi General Hospital, Sengkang General Hospital, SGH, and KK Women’s and Children’s Hospital now follow the same treatment pathways. This ensures equitable access to high-quality cancer support and resources – from screening, diagnostics, treatment, rehabilitation and palliative care to psychosocial support.
“Our research and clinical work must continue, as there is more that we need to understand about cancer to improve the quality of life and survivorship for individuals who are diagnosed with cancer,” says Prof Lim.
Tanoto Foundation’s support for Prof Lim is part of its wider commitment to medical research and development in Singapore, according to Ms Belinda Tanoto, a member of the Board of Trustees, Tanoto Foundation.
Since 2009, the foundation has contributed almost $21 million towards research and advocacy for treatments for major health issues, including oncology, cardiology and diabetes. The goal – to bring about an improvement in the overall well-being and lifespan of people in Asia and beyond.
In September 2023, Tanoto Foundation achieved a significant milestone in this area with the launch of its Medical Research Fund.
The fund will provide up to $5 million a year to support research into a range of diseases prevalent in Singapore, in the areas of cardiology, maternal and child health, diabetes, oncology and infectious diseases.
"We aim to support medical research to improve the health of Singaporeans and people throughout the region. We’re building an ecosystem of like-minded organisations to amplify the impact of meaningful research which will ultimately transform lives,” said Ms Tanoto.
Find out more about Tanoto Foundation here .
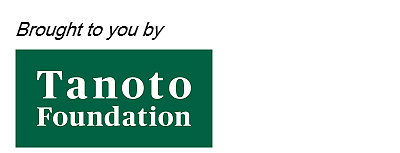
Join ST's WhatsApp Channel and get the latest news and must-reads.
- IMMUNOTHERAPY
- Branded content
- Gene therapy
Read 3 articles and stand to win rewards
Spin the wheel now
Together we are beating cancer
About cancer
Cancer types
- Breast cancer
- Bowel cancer
- Lung cancer
- Prostate cancer
Cancers in general
- Clinical trials
Causes of cancer
Coping with cancer
- Managing symptoms and side effects
- Mental health and cancer
- Money and travel
- Death and dying
- Cancer Chat forum
Health Professionals
- Cancer Statistics
- Cancer Screening
- Learning and Support
- NICE suspected cancer referral guidelines
Get involved
- Make a donation
By cancer type
- Leave a legacy gift
- Donate in Memory
Find an event
- Race for Life
- Charity runs
- Charity walks
- Search events
- Relay For Life
- Volunteer in our shops
- Help at an event
- Help us raise money
- Campaign for us
Do your own fundraising
- Fundraising ideas
- Get a fundraising pack
- Return fundraising money
- Fundraise by cancer type
- Set up a Cancer Research UK Giving Page
- Find a shop or superstore
- Become a partner
- Cancer Research UK for Children & Young People
- Our We Are campaign
Our research
- Brain tumours
- Skin cancer
- All cancer types
By cancer topic
- New treatments
- Cancer biology
- Cancer drugs
- All cancer subjects
- All locations
By Researcher
- Professor Duncan Baird
- Professor Fran Balkwill
- Professor Andrew Biankin
- See all researchers
- Our achievements timeline
- Our research strategy
- Involving animals in research
Funding for researchers
Research opportunities
- For discovery researchers
- For clinical researchers
- For population researchers
- In drug discovery & development
- In early detection & diagnosis
- For students & postdocs
Our funding schemes
- Career Development Fellowship
- Discovery Programme Awards
- Clinical Trial Award
- Biology to Prevention Award
- View all schemes and deadlines
Applying for funding
- Start your application online
- How to make a successful application
- Funding committees
- Successful applicant case studies
How we deliver research
- Our research infrastructure
- Events and conferences
- Our research partnerships
- Facts & figures about our funding
- Develop your research career
- Recently funded awards
- Manage your research grant
- Notify us of new publications
Find a shop
- Volunteer in a shop
- Donate goods to a shop
- Our superstores
Shop online
- Wedding favours
- Cancer Care
- Flower Shop
Our eBay store
- Shoes and boots
- Bags and purses
- We beat cancer
- We fundraise
- We develop policy
- Our global role
Our organisation
- Our strategy
- Our Trustees
- CEO and Executive Board
- How we spend your money
- Early careers
Cancer news
- Cancer News
- For Researchers
- For Supporters
- Press office
- Publications
- Update your contact preferences
ABOUT CANCER
GET INVOLVED
NEWS & RESOURCES
FUNDING & RESEARCH
You are here

What is cancer?
Cancer starts when cells change abnormally.

Cancer is when abnormal cells divide in an uncontrolled way. Some cancers may eventually spread into other tissues.
There are more than 200 different types of cancer.
1 in 2 people in the UK will get cancer in their lifetime.
Thanks to research many people are cured.
Cancer grows as cells multiply over and over
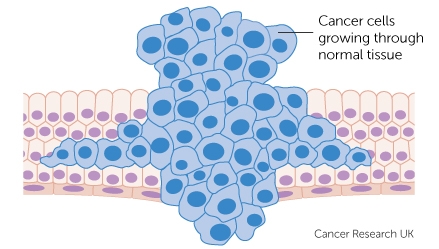
Cancer starts when gene changes make one cell or a few cells begin to grow and multiply too much. This may cause a growth called a tumour.
Some cancers can spread to other parts of the body
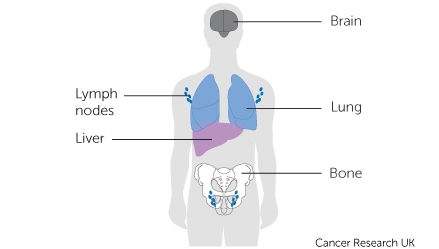
A primary tumour is the name for where a cancer starts.
Cancer can sometimes spread to other parts of the body – this is called a secondary tumour or a metastasis.
Cancer and its treatments can affect body systems, such as the blood circulation, lymphatic and immune systems, and the hormone system.
Is cancer genetic?
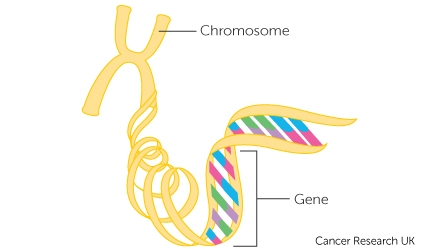
Sometimes cancers start due to inherited faulty genes passed down in families but this is rare.
Cancer can sometimes come back
Many cancers are cured. But in some people cancer can return.
Some cancers can’t be cured but treatment is often able to control them for some years.
There are 5 main cancer groups
Cancers are divided into groups according to the type of cell they start from. They include
Stages and grading of cancer
Staging and grading give an idea of how quickly a cancer may grow and which treatments may work best
The stage of a cancer means how big it is and whether it has spread.
Grading looks at how abnormal the cancer cells are .

Rate this page:

Prestigious cancer research institute has retracted 7 studies amid controversy over errors
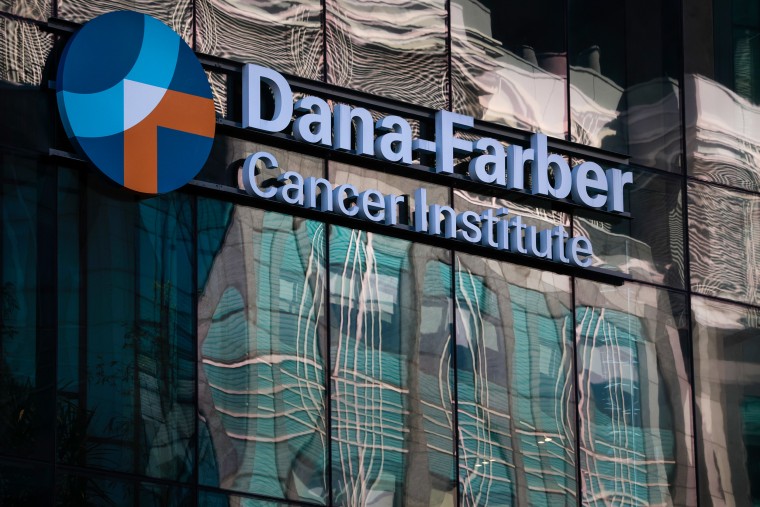
Seven studies from researchers at the prestigious Dana-Farber Cancer Institute have been retracted over the last two months after a scientist blogger alleged that images used in them had been manipulated or duplicated.
The retractions are the latest development in a monthslong controversy around research at the Boston-based institute, which is a teaching affiliate of Harvard Medical School.
The issue came to light after Sholto David, a microbiologist and volunteer science sleuth based in Wales, published a scathing post on his blog in January, alleging errors and manipulations of images across dozens of papers produced primarily by Dana-Farber researchers . The institute acknowledged errors and subsequently announced that it had requested six studies to be retracted and asked for corrections in 31 more papers. Dana-Farber also said, however, that a review process for errors had been underway before David’s post.
Now, at least one more study has been retracted than Dana-Farber initially indicated, and David said he has discovered an additional 30 studies from authors affiliated with the institute that he believes contain errors or image manipulations and therefore deserve scrutiny.
The episode has imperiled the reputation of a major cancer research institute and raised questions about one high-profile researcher there, Kenneth Anderson, who is a senior author on six of the seven retracted studies.
Anderson is a professor of medicine at Harvard Medical School and the director of the Jerome Lipper Multiple Myeloma Center at Dana-Farber. He did not respond to multiple emails or voicemails requesting comment.
The retractions and new allegations add to a larger, ongoing debate in science about how to protect scientific integrity and reduce the incentives that could lead to misconduct or unintentional mistakes in research.
The Dana-Farber Cancer Institute has moved relatively swiftly to seek retractions and corrections.
“Dana-Farber is deeply committed to a culture of accountability and integrity, and as an academic research and clinical care organization we also prioritize transparency,” Dr. Barrett Rollins, the institute’s integrity research officer, said in a statement. “However, we are bound by federal regulations that apply to all academic medical centers funded by the National Institutes of Health among other federal agencies. Therefore, we cannot share details of internal review processes and will not comment on personnel issues.”
The retracted studies were originally published in two journals: One in the Journal of Immunology and six in Cancer Research. Six of the seven focused on multiple myeloma, a form of cancer that develops in plasma cells. Retraction notices indicate that Anderson agreed to the retractions of the papers he authored.
Elisabeth Bik, a microbiologist and longtime image sleuth, reviewed several of the papers’ retraction statements and scientific images for NBC News and said the errors were serious.
“The ones I’m looking at all have duplicated elements in the photos, where the photo itself has been manipulated,” she said, adding that these elements were “signs of misconduct.”
Dr. John Chute, who directs the division of hematology and cellular therapy at Cedars-Sinai Medical Center and has contributed to studies about multiple myeloma, said the papers were produced by pioneers in the field, including Anderson.
“These are people I admire and respect,” he said. “Those were all high-impact papers, meaning they’re highly read and highly cited. By definition, they have had a broad impact on the field.”
Chute said he did not know the authors personally but had followed their work for a long time.
“Those investigators are some of the leading people in the field of myeloma research and they have paved the way in terms of understanding our biology of the disease,” he said. “The papers they publish lead to all kinds of additional work in that direction. People follow those leads and industry pays attention to that stuff and drug development follows.”
The retractions offer additional evidence for what some science sleuths have been saying for years: The more you look for errors or image manipulation, the more you might find, even at the top levels of science.
Scientific images in papers are typically used to present evidence of an experiment’s results. Commonly, they show cells or mice; other types of images show key findings like western blots — a laboratory method that identifies proteins — or bands of separated DNA molecules in gels.
Science sleuths sometimes examine these images for irregular patterns that could indicate errors, duplications or manipulations. Some artificial intelligence companies are training computers to spot these kinds of problems, as well.
Duplicated images could be a sign of sloppy lab work or data practices. Manipulated images — in which a researcher has modified an image heavily with photo editing tools — could indicate that images have been exaggerated, enhanced or altered in an unethical way that could change how other scientists interpret a study’s findings or scientific meaning.
Top scientists at big research institutions often run sprawling laboratories with lots of junior scientists. Critics of science research and publishing systems allege that a lack of opportunities for young scientists, limited oversight and pressure to publish splashy papers that can advance careers could incentivize misconduct.
These critics, along with many science sleuths, allege that errors or sloppiness are too common , that research organizations and authors often ignore concerns when they’re identified, and that the path from complaint to correction is sluggish.
“When you look at the amount of retractions and poor peer review in research today, the question is, what has happened to the quality standards we used to think existed in research?” said Nick Steneck, an emeritus professor at the University of Michigan and an expert on science integrity.
David told NBC News that he had shared some, but not all, of his concerns about additional image issues with Dana-Farber. He added that he had not identified any problems in four of the seven studies that have been retracted.
“It’s good they’ve picked up stuff that wasn’t in the list,” he said.
NBC News requested an updated tally of retractions and corrections, but Ellen Berlin, a spokeswoman for Dana-Farber, declined to provide a new list. She said that the numbers could shift and that the institute did not have control over the form, format or timing of corrections.
“Any tally we give you today might be different tomorrow and will likely be different a week from now or a month from now,” Berlin said. “The point of sharing numbers with the public weeks ago was to make clear to the public that Dana-Farber had taken swift and decisive action with regard to the articles for which a Dana-Farber faculty member was primary author.”
She added that Dana-Farber was encouraging journals to correct the scientific record as promptly as possible.
Bik said it was unusual to see a highly regarded U.S. institution have multiple papers retracted.
“I don’t think I’ve seen many of those,” she said. “In this case, there was a lot of public attention to it and it seems like they’re responding very quickly. It’s unusual, but how it should be.”
Evan Bush is a science reporter for NBC News. He can be reached at [email protected].

An official website of the United States government
The .gov means it’s official. Federal government websites often end in .gov or .mil. Before sharing sensitive information, make sure you’re on a federal government site.
The site is secure. The https:// ensures that you are connecting to the official website and that any information you provide is encrypted and transmitted securely.
- Publications
- Account settings
Preview improvements coming to the PMC website in October 2024. Learn More or Try it out now .
- Advanced Search
- Journal List
- Genes (Basel)

Breast Cancer Genetics: Diagnostics and Treatment
Carmen criscitiello.
1 Division of New Drugs and Early Drug Development for Innovative Therapies, European Institute of Oncology, IRCCS, 20141 Milan, Italy
2 Department of Oncology and Haematology (DIPO), University of Milan, 20122 Milan, Italy
Chiara Corti
Breast cancer (BC) genetics has become a fundamental aspect of BC management.
It influences screening, follow-up, prophylactic and therapeutic recommendations in women harboring a germinal BC susceptibility gene. In addition, it helps to identify patient subgroups with either a different prognosis or different response to treatment.
This Special Issue consists of one case report, two original research articles and five reviews, covering both diagnostic aspects and therapeutic implications of genetics in BC.
Pathogenic variants in the BC susceptibility genes represent the strongest hereditary risk factor for disease development, particularly in the context of early onset breast cancer (EOBC). Indeed, around 10–20% of EOBC cases are hereditary [ 1 ]. Consequently, individuals with a personal or family history of breast, ovarian, prostate or pancreatic cancer may benefit from hereditary risk evaluation to determine their own risk and family members’ risk for these and associated cancers. In this regard, Szczerba and colleagues examined 75 tumor samples from a cohort of Polish BC patients that had negative results for targeted breast cancer susceptibility genes 1 ( BRCA1 ) mutations (c.5266dupC, c.181T > G, c.4035delA, c.68_69delAG, c.3700_3704delGTAAA). All coding regions of the BRCA1/2 genes were sequenced with Next Generation Sequencing (NGS), with the detection of nine pathogenic variants and six variants of unknown significance (VUS). The authors also focused on methodological aspects of NGS, highlighting differences in variant calling files (VCF) obtained from the same FASTQ file, according to the variant calling algorithm used. The authors conclude that this observation could potentially affect the identification and interpretation of variants [ 2 ].
Moreover, recent studies have also shown germline BRCA1/2 status to be clinically relevant in the selection of therapy for patients already diagnosed with BC. Indeed, BRCA status predicts responsiveness to platinum-based chemotherapy as well as to inhibitors of poly(ADP-ribose) polymerase (PARP), highlighting the ability of these interventions to inhibit DNA repair pathways. From a surgical standpoint, surgical risk reduction remains a powerful tool in the therapeutic armamentarium for many women with genetic predisposing variants, as comprehensively highlighted by Berger and Golshan [ 3 ]. However, initial BC and contralateral BC risks should be clearly identified (i.e., highly penetrant genes compared to moderately penetrant genes), in order to fine tune risk reduction strategies and ideal timing, also in accordance with patient’s personal preferences [ 3 ]. While the survival benefit related to prophylactic bilateral mastectomy has been established, a growing body of evidence supports the oncological safety of nipple-sparing mastectomy as a risk-reducing procedure in BRCA -mutated patients, with low rates of new BCs, low rates of postoperative complications and high levels of satisfaction and postoperative quality of life, as reported by Rocco et al. [ 4 ]. However, larger multi-institutional studies with longer follow-up are needed to establish this procedure as the best surgical option in this setting.
Besides BRCA1/2 , pathogenic variants in other high- to moderate-risk genes such as tumor protein p53 ( TP53 ), partner and localizer of BRCA2 ( PALB2 ), phosphatase and tensin homolog ( PTEN ), checkpoint kinase 2 ( CHEK2 ) and ataxia-telangiectasia mutated ( ATM ) account for a smaller percentage of BC, and, in some cases, ovarian, prostate or pancreatic cancers [ 5 , 6 , 7 , 8 ].
In particular, ATM is involved in cell cycle control, apoptosis, oxidative stress and telomere maintenance, and its role as a risk factor for cancer development is well established [ 9 ]. Recent studies confirmed that some variants of ATM are associated with intermediate- and high-grade disease, a higher rate of lymph node metastatic involvement, HER2 positivity as well as the development of a contralateral breast tumor, as depicted by Stucci and colleagues [ 9 ]. Clinicopathologic characteristics of BC developed by ATM and checkpoint kinase 2 ( CHEK2 ) mutation were also explored by Toss and colleagues, who reviewed the archive of the local Family Cancer Clinic. Since 2018, 1185 multi-gene panel tests were performed. In total, 19 ATM and 17 CHEK2 mutation carriers affected by 46 different BCs were identified. A high rate of bilateral tumors was observed in ATM (26.3%) and CHEK2 mutation carriers (41.2%). While 64.3% of CHEK2 -mutant tumors were luminal A-like, 56.2% of ATM -mutant tumors were luminal B-like/HER2-negative. Moreover, 21.4% of CHEK2 -related invasive tumors showed a lobular histotype. About a quarter of all ATM -related BCs and a third of CHEK2 -related BCs were in situ carcinomas and more than half of ATM - and CHEK2 -related BCs were diagnosed at stage I-II. The biological and clinical characteristics of ATM - and CHEK2 -related tumors may help improve diagnosis, prognostication and targeted therapeutic approaches. Importantly, the authors advise the consideration and discussion of contralateral mastectomy for ATM and CHEK2 mutation carriers at the first diagnosis of BC.
This growing body of data regarding the identification of new ATM aberration as well as association with ancestry, prognosis and treatment outcomes could support clinicians in personalizing both treatments, as well as follow-up, in these patients [ 10 ]. Moreover, since mutations in ATM are involved in DNA repair mechanisms, ATM aberrations may sensitize cancer cells to platinum-derived drugs and PARPi, as BRCA1/2 mutations do. Some evidence suggests that ATM mutations could also be involved in the resistance to cyclin-dependent kinase 4 and 6 inhibitors (CDK4/6i) in luminal BC [ 10 ].
In this context, publicly available archives and case reports highlighting relationships among human gene variants and phenotypes are of particular importance. For example, Parenti et al. identified a new ATM deletion associated with a BRCA -negative patient who developed BC at the age of 34 [ 10 ]. Her mother had unilateral receptor-positive BC at the age of 45 with axillary lymph node involvement. The authors utilized SOPHiA Genetics Hereditary Cancer Solutions gene panel to detect a copy number variant (CNV), that was first validated by Multiplex Ligation-dependent Probe Amplification (MLPA). Afterward, long-range Polymerase Chain Reaction (PCR) and Sanger sequencing were used to characterize the breakpoint at DNA level (c.2838+2162_4110-292del) in proband and to also study segregation in the patient’s mother and sister. Further characterization at the RNA level on the proband’s mother and sister identified the presence of both the wild-type and the mutant allele in the mother’s sample. This abnormal ATM protein lacks the domain required for c-Abl protein interaction and mediation of cell cycle arrest in G1 phase. In addition, at least three other important domains are deleted from the ATM protein, such as the FAT (FRAP-ATM-TRRAP), PIKK (phosphatidylinositol 3-kinase-related kinase domain) and FATC (FAT C-terminal domain) domains, mediating most ATM functions.
Siddig et al. focused more broadly on the genetic landscape of EOBC, since 10–20% of these cancers are related to germline BC susceptibility genes. The authors provide an overview of somatic mutations, chromosome CNVs, single-nucleotide polymorphisms (SNPs), differential gene expression, microRNAs and gene methylation profile as well as of altered pathways resulting from those aberrations. Interestingly, the E-Cadherin/β-Catenin complex and the overall determinants of epithelial barrier integrity have been implicated in EOBC, with cell–cell adhesion genes such as CDH1 , GATA3 , CTNNB1 , MUC17 and FLG involved [ 1 , 11 ]. Eight stromal genes are differentially expressed in breast tumors from very young patients (≤35 years) compared to tumors from older age patients (≥50 years), with UQCRQ , ALDH1A3 , EGLN1 and IGF1 being overexpressed and FUT9 , IDI2 , PDHX and CCL18 being underexpressed. The TP53 gene typically shows a high mutational load in EOBC and plays an important pathogenic role by affecting cell cycle arrest mechanisms and the transcription of other genes, such as GAS7b , which regulates the cell structure and cell migration [ 12 ]. EOBC aggressive characteristics also appear to be linked to DNA methylations events [ 13 ]. EOBC displays several CNVs implicated in tumorigenesis (6q27, 6p32 and 7p21.1), advance-stage tumor progression (22q12.3 and 22q13.31), disease progression (19q13.32) and prognosis (CNV in BIRC5 gene). However, further studies that correlate the CNV profile with the gene and protein expression profile are needed. Finally, different SNPs may be linked to EOBC tumorigenesis, progression, resistance to chemotherapy and poor prognosis. Additionally, it is possible to discriminate BC arising in young women from that in older women using a microRNA profile [ 1 ].
In terms of future perspectives, even though several disease-causing mutations have been identified, therapy is often aimed at interfering with an aberrantly activated pathway, rather than rectifying the mutation in the DNA sequence. The Clustered Regularly Interspaced Short Palindromic Repeats (CRISPR)/Cas9 is a groundbreaking tool that is being utilized for the identification and validation of genomic targets bearing tumorigenic potential. CRISPR/Cas9 supersedes its gene editing predecessors through its unparalleled simplicity, efficiency and affordability. Ahmed and colleagues provide an overview of the CRISPR/Cas9 mechanism and discuss genes that were edited using this system for the treatment of BC. In addition, the authors shed light on the delivery methods, both viral and non-viral, that may be used to deliver the system, as well as on the main challenges associated with each method. However, despite great expectations, remarkable limitations related to ethics, off-target effects, mutagenesis and delivery necessitate further studies. For the conventional use of this system in the near future, both precise knowledge of pathogenic variants as well as the optimization of the system itself are essential.
In conclusion, the papers in this Special Issue cover various aspects of genetics in BC. Overall, they provide a summary of hereditary BC syndromes, personalized BC risk assessments, as well as historical and novel risk reduction approaches. They also offer a comprehensive overview regarding major advances in understanding the most frequent genetic aberrations, with potential implications for present and future treatment approaches.
Conflicts of Interest
The authors declare no conflict of interest.
Publisher’s Note: MDPI stays neutral with regard to jurisdictional claims in published maps and institutional affiliations.
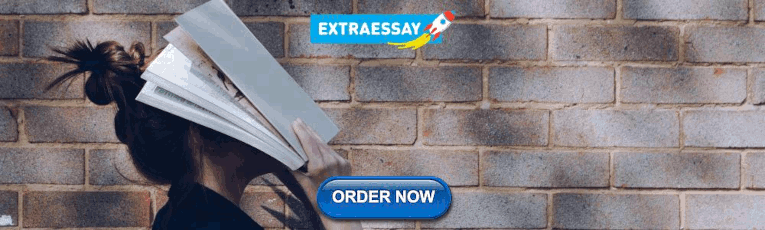
IMAGES
VIDEO
COMMENTS
Previous research suggests that there may be a positive correlation between the number of exclusive mutations and co-occurring mutations in a given cancer type, potentially driven by the ...
According to the International Agency for Research on Cancer (IARC), in 2020 there were approximately 19.3 million new cases of cancer, and 10 million deaths by this disease, 6 while 23.8 million cases and 13.0 million deaths are projected to occur by 2030. 73 In this regard, it is clear the increasing role that environmental factors ...
A comprehensive analysis of oncogenic driver genes and mutations in >9,000 tumors across 33 cancer types highlights the prevalence of clinically actionable cancer driver events in TCGA tumor samples. ... Institute for Research in Biomedicine (IRB Barcelona), The Barcelona Institute of Science and Technology, Baldiri Reixac, 10, 08028 Barcelona ...
P53 gene mutation is prevalent in several cancers. Therefore, Gendicine induces the expression of p53 restores its activity and destroys the tumor cells. Generally, Gendicine management showed 30-40% complete response and 50-60% partial response with a total response rate of 90%-96% in different therapeutic use.
Cancer is a genetic disease, and much cancer research is focused on identifying carcinogenic mutations and determining how they relate to disease progression. Three papers demonstrate how mutations are processed through networks of protein interactions to promote cancer (see the Perspective by Cheng and Jackson).
Abstract. A major goal of cancer research is to understand how mutations distributed across diverse genes affect common cellular systems, including multiprotein complexes and assemblies. Two challenges—how to comprehensively map such systems and how to identify which are under mutational selection—have hindered this understanding.
The molecular aberrations that lead to cancer development are often characterised by gain-of-function or loss-of-function mutations in a variety of oncogenes and tumour suppressor genes. In this study, we investigate the genomic sequences of 20,331 primary tumours representing 41 distinct human cancer types to identify and catalogue the driver ...
The result of this process is a control dataset, specific to a given cancer type, to be used for comparison concerning the observed cancer mutations. These shuffled mutation sets (synapseId: syn7187923) preserve mutational signatures associated with cancer while removing the local position-specific effects of cancer mutations within the range ...
Cancer Genetics is proud to be affiliated with the. The aim of Cancer Genetics is to publish high quality scientific papers on the cellular, genetic and molecular aspects of cancer, including cancer predisposition and clinical diagnostic applications. Specific areas of interest include descriptions of new chromosomal, molecular or epigenetic ….
Early NGS research initiatives, including The Cancer Genome Atlas, mitigated against these issues by restricting their focus to large, high-quality, ... NGS assays designed to explore the mutation status of cancer-associated genes beyond specific hotspot mutation loci reveal variants whose implications, either for the resulting protein function ...
The discoveries, published today in 23 papers in Nature and its affiliated journals, are an important step toward a map of all major cancer-causing mutations in the genome. The ICGC/TCGA Pan-Cancer Analysis of Whole Genomes Project (PCAWG, or the Pan-Cancer Project), a collaboration involving more than 1,300 scientists and clinicians from 37 ...
Caption: Using a variant of CRISPR genome-editing known as prime editing, MIT researchers have developed a method to screen cancer-associated genetic mutations much more easily and quickly than any existing approach. This illustration, by Samuel Gould's brother Owen Gould, is an artistic interpretation of the research and the idea of "rewriting the genome," explains Samuel.
The Cancer Target Discovery and Development Program is a network of research centers devoted to studying how molecular changes found in cancers affect cancer cells and explore opportunities to target those changes with new therapies. The Epidemiology and Genomics Research Program supports research in human populations to understand genomic determinants of cancer occurrence and cancer outcomes ...
The research team also assessed mutation rates for genes that are not included in a cancer type's standard-of-care testing protocol. For example, 54 percent of uterine corpus endometrial carcinomas and 49 percent of ovarian endometrioid adenocarcinomas carried a phosphatidylinositol 3-kinase catalytic subunit alpha ( PIK3CA ) mutation.
DICER1 syndrome is a rare genetic disorder that predisposes individuals to multiple cancer types. Through mutations of the gene encoding the endoribonuclease, Dicer, DICER1 syndrome disrupts the biogenesis and processing of miRNAs with subsequent disruption in control of gene expression. Since the first description of DICER1 syndrome, case reports have documented novel germline mutations of ...
Lung cancer (LC), particularly nonsmall cell lung cancer (NSCLC), is one of the most prevalent types of neoplasia worldwide, regardless of gender, with the highest mortality rates in oncology. Over the years, treatment for NSCLC has evolved from conventional surgery, chemotherapy, and radiotherapy to more tailored and minimally invasive approaches. The use of personalised therapies has ...
These mutations elicit a cancer phenotype similar to that of TET abnormalities. IDH1/IDH2 downregulation or mutation has been reported in chondrosarcoma , enchondroma [42,43], glioma , melanoma , and thyroid carcinoma [45,46]. Research has primarily focused on 5hmC as a candidate for a pathway to active DNA demethylation.
APA. Chicago. Columbia University Irving Medical Center. "Opening a new front against pancreatic cancer." ScienceDaily. ScienceDaily, 8 April 2024. <www.sciencedaily.com / releases / 2024 / 04 ...
The study found that two genes, IL18RAP and HLA-DRB1, significantly increase NKTCL risk in patients. Those with a risk allele (a variant form of a gene) are 39 per cent to 53 per cent more likely ...
Cancer is when abnormal cells divide in an uncontrolled way. Some cancers may eventually spread into other tissues. ... Cancer Research UK is a registered charity in England and Wales (1089464), Scotland (SC041666), the Isle of Man (1103) and Jersey (247). A company limited by guarantee. Registered company in England and Wales (4325234) and the ...
Poster, Presentation, Protocol or Paper. Deposit scholarly works such as posters, presentations, research protocols, conference papers or white papers. If you would like to deposit a peer-reviewed article or book chapter, use the "Scholarly Articles and Book Chapters" deposit option.
A large-scale sequencing study has shown that most somatic mutations in cancer cells are "passengers" that do not cause tumorigenesis, whereas 120 of the 518 genes screened (~23%) carry a "driver" mutation, which can function as cancer genes. ... In an update of the Hallmark paper, in 2011, Hanahan and Weinberg proposed four additional ...
April 9, 2024, 9:32 PM UTC. By Evan Bush. Seven studies from researchers at the prestigious Dana-Farber Cancer Institute have been retracted over the last two months after a scientist blogger ...
Breast cancer (BC) genetics has become a fundamental aspect of BC management. It influences screening, follow-up, prophylactic and therapeutic recommendations in women harboring a germinal BC susceptibility gene. In addition, it helps to identify patient subgroups with either a different prognosis or different response to treatment.
The Hub: (352) 294-0885. 747 SW 2nd Avenue Gainesville, FL 32601. Sid Martin Biotech: (386) 462-0880. 12085 Research Drive Alachua, FL 32615. A team led by neuroscientists at UF has discovered a key molecular player in fear conditioning, a specialized RNA with the acronym SLAMR.