- Reference Manager
- Simple TEXT file
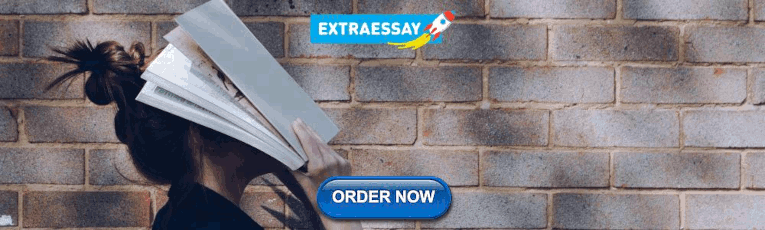
People also looked at
Perspective article, a perspective on future tiger shark research.
- 1 Hawaii Institute of Marine Biology, University of Hawaii at Manoa, Kaneohe, HI, United States
- 2 Department of Agriculture and Fisheries, Brisbane, QLD, Australia
This “Perspectives” paper identifies aspects of tiger shark ( Galeocerdo cuvier ) biology that are currently unknown or for which additional data are needed to improve interpretive power. Some of these data gaps may be regional. Technical or methodological approaches to acquiring these data are suggested. Some of these technologies already exist, some are in development and some exist in concept only. Reproductive biology and behavior, social interactions and the behavioral ecology of sub-adults are among the areas identified as deserving of future research effort.
Introduction
Tiger sharks ( Galeocerdo cuvier ) are large, iconic predators with a circumglobal distribution in warm waters ( Compagno, 1984 ). They play an important role in ecosystem function ( Heithaus and Dill, 2002 ; Ferreira et al., 2017 ; inter alia ) and are among the top three shark species identified in attacks on humans (International Shark Attack File 1 ). This paper identifies several aspects of tiger shark biology that are currently unknown or poorly understood and which we believe are critical to our understanding of this important predator. These data gaps may vary regionally. We focus on questions that are specific to tiger sharks and which are amenable to manipulative or empirical field experiments (as opposed to correlative analyses) There may be other fruitful areas for investigation that are not covered here. Tools for investigating the biology of vagile marine species are constantly and rapidly improving. These include increasingly sophisticated molecular techniques, portable analysis units (e.g., ultrasonic scanners) and multi-sensor electronic tags for tracking three-dimensional movements and sampling the environment surrounding the focal animal. We suggest technical solutions or methodological approaches for acquiring data to fill gaps in our knowledge of tiger shark biology. Some of these technologies already exist, some are in development and others currently exist in concept only.
Reproductive Biology and Behavior
Topic: pupping, mating and gestation locations.
Tiger sharks have low reproductive output ( Simpfendorfer, 1992 ; Whitney and Crow, 2007 ) making survival of juveniles (and protection of nursery areas) potentially critical to conservation strategies. Although many shark species utilize specific locations (e.g., coastal embayments or estuaries) as nursery areas (as defined by Heupel et al., 2007 ), tiger sharks apparently lack well-defined nursery areas ( Springer, 1967 ; Whitney and Crow, 2007 ; Driggers et al., 2008 ). For example, catch per unit effort (CPUE) surveys show tiger shark pups and juveniles are widely distributed at depths <100 m along the continental shelf of the southeastern United States with highest CPUE near the “Charleston Bump” ( Driggers et al., 2008 ). It is not known whether this broad distribution of pups results from widespread pupping by adult females or dispersal of pups from more specific parturition sites or an extended pupping season ( Driggers et al., 2008 ). The partial migration of adult females from remote Hawaiian atolls to the main Hawaiian Islands during pupping season ( Papastamatiou et al., 2013 ) suggests that there may be preferred tiger shark pupping habitats in Hawaii but this has not been confirmed. Similarly, if specific pupping sites do exist, it is not known if adult female sharks show repeated fidelity to those sites. Sulikowski et al. (2016) speculate there may be specific gestation grounds in the Bahamas but the wide ranging movements of pregnant sharks in other regions (e.g., Papastamatiou et al., 2013 ) indicate that, if such behavior exists, it may be regionally specific.
The seasonal timing of tiger shark mating is evident from mating scars on females (e.g., Whitney and Crow, 2007 ), but very little is known about the nature of mating behavior (see Meyer et al., 2018 ) or whether or not there are specific mating sites. Tiger sharks seem to be generally solitary, so finding a mate may be challenging unless there are specific locations and times when mating occurs. Within the main Hawaiian Islands, the probability of migration between islands for both adult male and female tiger sharks peaks during the winter mating season as does CPUE for adult males in shallow coastal habitats ( Papastamatiou et al., 2013 ; Meyer et al., 2018 ; A. Tester unpublished data). These observations, in conjunction with a single observation of a failed mating attempt by a camera-equipped male tiger shark in coral reef habitat ( Meyer et al., 2018 ), suggest shallow coastal areas are mating habitats for Hawaii tiger sharks. There are currently no similar data from other parts of the world.
Future Research
One strategy for addressing the nursery location/depth and gestation grounds questions would be to capture adult female tiger sharks during pupping season, scan them with portable ultrasound equipment to see if they are pregnant ( Sulikowski et al., 2016 ) and, if so, equip these sharks with cameras and accelerometry tags to detect pupping events. These sharks could also be equipped with satellite tags to provide insight into where pupping is occurring. These experiments should be geographically widespread.
A longer-term approach would be development of implantable devices that can detect key aspects of blood chemistry that indicate parturition. These physiological data would be transmitted to an externally mounted pop-off tag which would transmit these data via satellite. This dual internal/external tag technology has been successfully used to detect feeding behavior in several marine species including seals (e.g., Skinner et al., 2014 ). Similarly, equipping mature males with camera and depth and light level sensor packages during mating season (e.g., Meyer et al., 2018 ) would be the most direct path to elucidating the location and nature of mating behavior.
Topic: Reproductive Cycle
The reproductive cycle of tiger sharks remains one of the least understood aspects of its reproductive biology. Initial work in this area suggested a biennial reproductive cycle ( Rivera-López, 1970 ; Alves, 1977 ), and that mating appeared to occur before full-term females had pupped ( Branstetter et al., 1987 ). More recently, Castro (2009) surmised that North Atlantic tiger sharks probably have a gestation period of 12 months, based on the observation of tiger sharks carrying both unfertilized eggs and near-term young in May (boreal spring), indicating that females are possibly reproductively synchronous in this region. Conversely, Whitney and Crow (2007) inferred a triennial breeding cycle for Hawaiian tiger sharks, reporting that the proportion of captured sharks that were pregnant was lower than would be expected for a biennial reproductive cycle. The identification of potential differences in reproductive strategies between Atlantic and Indo-Pacific tiger sharks is critical to inform regional stock assessment and fishery management regimes.
Geographically diverse data on the reproductive state of large numbers of mature females is required. To avoid lethal sampling, portable ultrasound equipment could be used to assess pregnancy and in-utero pup sizes. Blood chemistry could be sampled from live sharks to determine temporal changes in hormone levels to deduce reproductive cycle status ( Sulikowski et al., 2016 ).
Topic: Sex Separation and Habitat Partitioning
Sexual segregation occurs among adults of many shark species ( Meyer et al., 2009 ; Papastamatiou et al., 2010 ) and existing evidence suggests this phenomenon may also occur in tiger sharks ( Meyer et al., 2014 ). Tiger shark sex ratio is reported to be close to 1:1 at birth in Hawaii ( Whitney and Crow, 2007 ), while significantly biased toward females off eastern Australia (1.26:1, Holmes, 2015 ). Concomitantly catches of adults tend to be heavily female-skewed in most coastal locations ( Simpfendorfer, 1992 ; Wintner and Dudley, 2000 ; Beerkircher et al., 2002 ; Meyer et al., 2014 ; inter alia ). This is thought to result from adult females occupying coastal areas (where most fishing effort occurs), while adult males occupy more offshore habitats for most of the year ( Papastamatiou et al., 2013 ). It is not known at what age this segregation occurs. Size-specific analyses of catch records off eastern Australia revealed catches of males on near-shore shark control gear declined when males reached >280 cm TL ( Holmes et al., 2012 ), potentially indicating greater offshore habitat selection with the onset of male sexual maturity (L 50 males = 297 cm TL, Holmes, 2015 ).
To distinguish gender-related habitat segregation from a population-wide disparity in sex ratio, simultaneous fishing should be conducted in inshore vs. offshore habitats at multiple locations around the world. Further size-specific analysis and movement data (e.g., Lea et al., 2018 ) might reveal the ontogeny of sexual segregation in other regions. Specifically targeting males for future tracking experiments would also elucidate differences in habitat selection.
Topic: Occurrence and Frequency of Multiple Paternity
Many carcharhinid species display multiple paternity which is a strategy to amplify the functional size and diversity of the reproductive population ( Daly-Engel et al., 2006 ). A single study of tiger sharks (using a small sample size) did not detect multiple maternity ( Holmes et al., 2018 ). Given its importance to conservation biology, multiple paternity should be more thoroughly investigated worldwide.
Opportunistic tissue sampling of tiger shark pups from known mothers should be conducted on a world-wide scale. Coupled with portable ultrasound equipment, it may be possible to use a biopsy needle to non-lethally sample pups from pregnant females.
Population Dynamics and Connectivity
Topic: population connectivity.
Whereas tiger shark populations seem to be distinct among the different oceans, there is still debate about within-ocean population structure and there appears to be a discrepancy between the within-basin population structure indicated by molecular genetics and the large-scale movements of tagged individuals in both the Atlantic and Indo-Pacific Oceans ( Holmes et al., 2014 ; Werry et al., 2014 ; Lea et al., 2015 ). A recent analysis using nuclear markers (microsatellites) indicated broad genetic differentiation between the Atlantic and the Indo-Pacific Ocean basins with considerable regional structure within the Atlantic and some structure within the Pacific – including a separate Hawaiian population ( Bernard et al., 2016 ). However, this distinction was not observed in a study using a larger sample size ( Holmes et al., 2017 ). Apparently, roaming individuals provide enough gene flow to maintain large, basin-wide populations. These different results indicate that further connectivity research is warranted globally.
Development of single nucleotide polymorphisms (SNP) for tiger sharks would complement microsatellite techniques and increase the power to detect genetic variation and metapopulations, even if sample sizes are small. Continued advances in tagging technology that allow for multi-year tracks of mature sharks (e.g., Lea et al., 2015 ), coupled with the advancement of molecular analyses, is likely to greatly improve our understanding of sex-biased dispersal, reproductive mixing and other spatiotemporal movement drivers that influence population structure and connectivity. Climate change should see a range extension through pole-ward movements in this species, which may increase the potential for greater trans -oceanic movements.
Behavioral Ecology
Topic: behavioral ecology of pups.
Virtually nothing is known about the behavior and habitat use of tiger shark pups during their first years of life including how quickly they disperse from their natal sites, what depths or types of habitat they select or how this changes with ontogeny. We do not know how early feeding experience impacts the feeding strategies of sharks later in their lives.
Active acoustic tracking (e.g., Holland et al., 1993 ) and passive acoustic monitoring using anchored receiver arrays (e.g., Heupel and Hueter, 2001 ) would greatly improve understanding of the behavior of neonate and juvenile tiger sharks. Young-of-the-year and juvenile tiger sharks are of sufficient body size to carry temperature-depth recorders and accelerometers to better understand three-dimensional spatial use and activity rates. Diet could be estimated using non-lethal techniques including gastric lavage ( Bangley et al., 2013 ) and stable isotope analyses ( Kinney et al., 2011 ). Respirometry experiments and feeding studies with captive juveniles would elucidate daily ration and energetics requirements ( Lowe, 2002 ).
Topic: Offshore Movements of Adults
Satellite telemetry ( Lea et al., 2015 ; Meyer et al., 2018 ) and catch records ( Polovina and Lau, 1993 ; Domingo et al., 2016 ) show tiger sharks make offshore migrations in both the Pacific and Atlantic. Adult tiger sharks in the Atlantic undertake repeated annual migrations which track latitudinal shifts in sea surface temperatures (SST) ( Lea et al., 2015 , 2018 ). Hammerschlag et al. (2012) correlated oceanic tiger shark movements in the mid-Atlantic with catch rates of pelagic fishes, although the causation is unknown. Intriguingly, some sharks in Hawaii (where there are no large seasonal temperature changes) also make extensive and repeated offshore looping excursions lasting several weeks and covering several hundred kilometers ( Meyer et al., 2018 ). Questions arise as to what are the drivers of these excursions or migrations and what (if anything) the animals are eating mid-ocean? Trophic position varies with location and oceanographic environment and as sharks move between habitats – including nearshore and pelagic realms ( Carlisle et al., 2012 , 2015 ; Ferreira et al., 2017 ) but what they are actually eating in mid-ocean remains largely unknown. It is possible that rafting seabirds are an important component. Other partial migrations may exist within tiger shark populations and may be related to ontogeny or nutritional status although there are no empirical data to support this hypothesis ( Gallagher et al., 2017 ).
In concept, sharks equipped with satellite-linked tags to detect feeding events ( Meyer and Holland, 2012 ) would reveal the depth and frequency of offshore feeding events. Animal-borne cameras would further elucidate the behavior. However, the problem comes with catching and identifying which sharks will undertake an offshore excursion after being tagged. Continued, long duration satellite telemetry in different regions will give further insight into temporal stability (or not) of feeding ecology. Identification of isotope or fatty acid signatures specific to seabirds (e.g., Cucherousset et al., 2012 ) would be a significant advance. The influence of nutritional status on movements is difficult to elucidate because nutritional status may change after a shark is tagged.
Topic: Feeding Ecology and Ecologic Services
Multiple diet studies of adult tiger sharks demonstrate them to be adaptable generalists that exploit a wide variety of prey which can vary both regionally and temporally ( Lowe et al., 1996 ; Simpfendorfer et al., 2001 ; Dicken et al., 2017 ; inter alia ). Recent stable isotope studies ( Hussey et al., 2015b ; Ferreira et al., 2017 ) confirm tiger sharks as apex predators but also that they are regionally and temporally adaptable and may function more as mesopredators in certain habitats (i.e., feeding on lower trophic level reef fishes, Ferreira et al., 2017 ). Additionally, there are observations of tiger sharks acting as facultative scavengers feeding at “bonanza” events such as whale carcasses ( Dudley et al., 2000 ; Clua et al., 2013 ) and also examples of individuals that apparently target specific prey such as fledgling albatross ( Lowe et al., 2006 ) and nesting sea turtles ( Fitzpatrick et al., 2012 ). Thus, there are several aspects of feeding ecology that remain unknown including questions about the degree of individual feeding specialization (or generalization; Matich et al., 2011 ) and how these originate (e.g., through experience during ontogeny). Are the various types of feeding strategy related to geographic location and do these lead to the disparate growth rates ( Branstetter et al., 1987 ; Meyer et al., 2014 ) that have been documented from different regions?
Neither the daily ration nor energetic requirements of adult tiger sharks have been directly measured ( Hammerschlag et al., 2013 ) so their impact on the supporting ecosystem is not well defined. In addition to direct predation, what is the role of tiger sharks in shaping an “ecology of fear” that influences the behavior of other species (e.g., Heithaus and Dill, 2002 ; Heithaus, 2005 ; Wirsing et al., 2007 ; inter alia ) and how does this relate to individual hunting strategies ( Towner et al., 2016 )?
Meta-analysis of existing diet studies should be conducted to look at regional structure in feeding ecology and there needs to be a broader geographic scope of diet studies, especially from open ocean areas. Extensive use of feeding tags, accelerometers and cameras, especially with longer deployment periods, would capture the daily feeding and energetic intake of these animals and provide insight into the direct and indirect effects they have on disparate habitats. Overcoming the logistical constraints of using swim-tunnel respirometry to measure metabolic rates of large sharks ( Payne et al., 2015 ) would allow empirical measurements of the energy requirements of adult tiger sharks.
Topic: Social Interactions of Adults
Virtually nothing is known regarding the social interactions of adult tiger sharks even though it is known that there can be multiple individuals using the same geographic habitat ( Hammerschlag et al., 2017 ; Meyer et al., 2018 ). What is the nature and frequency of intra-specific interactions? Is there any evidence of schooling? Do tiger sharks travel together or with other species?
Deploying “business card tags” ( Holland et al., 2009 ) on multiple sharks caught in well-defined areas could answer questions regarding the frequency and duration of intra-specific interactions – especially if tags were developed to download archived interaction data to moored receivers. Analyzing these data using network analysis techniques would undoubtedly be productive ( Stehfest et al., 2013 ). Deployment of shark-borne cameras would also be informative.
Topic: Extent of Lateral and Vertical Range and Impacts of Climate Change
Several studies demonstrate linkage between tiger shark distribution and water temperature ( Heithaus, 2001 ; Papastamatiou et al., 2013 ; Lea et al., 2015 , 2018 ; Payne et al., 2018 ; inter alia ). Tiger sharks occupy a broad thermal niche with a preference for temperatures between ∼17°C and ∼28°C ( Payne et al., 2018 ) and migrate in response to changing SSTs. However, the impacts of El Niño Southern Oscillation (ENSO) events are unknown. Overall tiger sharks may be climate change “winners”; they eat a wide variety of prey and are thus protected against climate-change driven demise of any one prey type. Warming SSTs may also extend their range toward the poles ( Last et al., 2010 ; Payne et al., 2018 ).
Electronic tagging studies – both acoustic and satellite-linked – have revealed distributions and aspects of habitat use for multiple species that are not obvious through catch statistics or mark/recapture studies (see Hussey et al., 2015a ). The extent of current range and any future changes in distribution may be detectable through the use of long-term acoustic transmitters and the establishment of collaborative acoustic arrays throughout the coastal margins of regions such as North America (Animal Telemetry Network; Block et al., 2016 ) and Australia (Integrated Marine Observing System Animal Tracking Facility; Hoenner et al., 2018 ) where they have access to a range of temperatures. The deployment of “oceanography” tags that can document in situ temperature, salinity and oxygen levels experienced by the sharks will help to define current physiological tolerance and habitat preferences and inform models of the impacts of climate change. Such data could be used to site future refugia. Satellite tagging of tiger sharks in the eastern Tropical Pacific where ENSO events are most extreme could shed light on their latitudinal range expansion along continental coasts with warming SSTs. A global review of historical and recent catch statistics might detect expansion of range (e.g., Last et al., 2010 ) and possible abandonment of areas that have become unsuitable (i.e., too hot; Payne et al., 2018 ).
Interactions with Humans
Topic: ecotourism.
Tiger sharks are components of ecotourism activities in multiple locations including Bahamas ( Hammerschlag et al., 2012 ), Fiji ( Brunnschweiler et al., 2014 ), South Africa ( Dicken and Hosking, 2009 ), and Hawaii ( Meyer et al., 2009 ) and shark tourism operations are increasing world-wide. In contrast to significant impacts of shark tourism on white shark ( Carcharadon carcharias ) biology ( Huveneers et al., 2018 ), two studies suggest a limited effect of shark-related ecotourism on tiger sharks ( Hammerschlag et al., 2012 , 2017 ). However, these were “indirect” measurements and from a single location. Thus, there is debate as to whether “provisioning” (i.e., chumming) exacerbates the influence (if any) of ecotourism activities on the biology of sharks associated with them.
Because the extent of provisioning varies from operation to operation and because they are sited in different environments, impact studies should be conducted on a case-by-case basis. Electronic tagging activities targeting sharks caught at ecotourism sites (e.g., Meyer et al., 2009 ) would elucidate impacts on their biology. Deploying tags that identify and quantify feeding events ( Meyer and Holland, 2012 ) would be especially useful in assessing the impact of provisioning on the feeding ecology of sharks associated with ecotourism sites.
Topic: Shark Behavior and Public Safety
Worldwide, tiger sharks are the second-most likely to be involved in attacks on humans (13%; International Shark Attack File 2 ) and, in some regions such as Hawaii (Division of Aquatic Resources, Hawaii Department of Land and Natural Resources 3 ), their impact is considerably greater. Given their generalist diet and the fact that tiger sharks are frequently close to humans it seems surprising that they do not bite humans more frequently. It is possible that there are fine scale (10 or 100 of meters) aspects of tiger shark behavior that reduce their interactions with recreational ocean users. Is there a “no-go” environment (e.g., depth or wave energy) that protects humans most of the time? These fine scale aspects of shark movement should be investigated.
Active acoustic tracking of adult tiger sharks ( Holland et al., 1999 ) in areas of high human-use would reveal fine-scale aspects of behavior that are not currently known. Double tagging focal animals with long-term transmitters detectable by acoustic receiver arrays would allow merging of fine-scale movement data with long-term habitat use data.
Topic: Sharks as Oceanographers
The use of animal-borne sensors is an emergent field in surveying the physical structure of the world’s oceans (e.g., Roquet et al., 2014 ) and is especially pertinent given the unprecedented rates of change and need for increased monitoring ( Abraham et al., 2013 ). The wide-ranging movements of tiger sharks (both laterally and vertically), their use of both coastal and offshore environments and their ability to tolerate capture and tagging ( Gallagher et al., 2014 ) make them ideal candidates for the role of “sharks as oceanographers”. In “proof of concept” experiments, tiger sharks equipped with “oceanography tags” are currently providing near-real time ocean profiles (pers. obs.).
Long-term programs that release tiger sharks equipped with “oceanography tags” in various regions could make a significant contribution to our understanding of the physical structure of the world’s tropical and semi-tropical oceans, coastal shelves and marginal seas while simultaneously revealing the habitat preferences of this important and iconic species.
Author Contributions
All authors listed have made a substantial, direct and intellectual contribution to the work, and approved it for publication.
Conflict of Interest Statement
The authors declare that the research was conducted in the absence of any commercial or financial relationships that could be construed as a potential conflict of interest.
- ^ http://www.floridamuseum.ufl.edu/shark-attacks/
- ^ http://dlnr.hawaii.gov/sharks/shark-incidents/incidents-list/
Abraham, J. P., Baringer, M., Bindoff, N. L., Boyer, T., Cheng, L. J., Church, J. A., et al. (2013). A review of global ocean temperature observations: implications for ocean heat content estimates and climate change. Rev. Geophys. 51, 450–483. doi: 10.1002/rog.20022
CrossRef Full Text | Google Scholar
Alves, M. I. M. (1977). Algumas considerações sobre a reprodução do cação jaguara, Galeocerdo cuvieri (Le Sueur, 1822) (Selachii: Carcharhinidae). Arquivos de Ciências do Mar 17, 121–125.
PubMed Abstract | Google Scholar
Bangley, C. W., Rulifson, R. A., and Overton, A. S. (2013). Evaluating the efficiency of flushed stomach-tube lavage for collecting stomach contents from dogfish sharks. Southeast. Nat. 12, 523–533. doi: 10.1656/058.012.0305
Beerkircher, L. R., Cortes, E., and Shivji, M. (2002). Characteristics of shark bycatch observed on pelagic longlines off the southeastern United States, 1992-2000. Mar. Fish. Rev. 64, 40–49.
Google Scholar
Bernard, A. M., Feldheim, K. A., Heithaus, M. R., Wintner, S. P., Wetherbee, B. M., and Shivji, M. S. (2016). Global population genetic dynamics of a highly migratory, apex predator shark. Mol. Ecol. 25, 5312–5329. doi: 10.1111/mec.13845
PubMed Abstract | CrossRef Full Text | Google Scholar
Block, B. A., Holbrook, C. M., Simmons, S. E., Holland, K. N., Ault, J. S., Costa, D. P., et al. (2016). Toward a national animal telemetry network for aquatic observations in the United States. Animal Biotelem. 4:6. doi: 10.1186/s40317-015-0092-1
Branstetter, S. T., Musick, J. A., and Colvocoresses, J. A. (1987). A comparison of the age and growth of the tiger shark, Galeocerdo cuvieri , from off virginia and from the northwestern gulf of Mexico. Fish. Bull. 85,269–279.
Brunnschweiler, J. M., Abrantes, K. G., and Barnett, A. (2014). Long-term changes in species composition and relative abundances of sharks at a provisioning site. PLoS One 9:e86682. doi: 10.1371/journal.pone.0086682
Carlisle, A. B., Goldman, K. J., Litvin, S. Y., Madigan, D. J., Bigman, J. S., Swithenbank, A. M., et al. (2015). Stable isotope analysis of vertebrae reveals ontogenetic changes in habitat in an endothermic pelagic shark. Proc. Biol. Sci. 282:20141446.
Carlisle, A. B., Kim, S. L., Semmens, B. X., Madigan, D. J., Jorgensen, S. J., Perle, C. R., et al. (2012). Using stable isotope analysis to understand the migration and trophic ecology of northeastern Pacific white sharks ( Carcharodon carcharias ). PLoS One 7:e30492. doi: 10.1371/journal.pone.0030492
Castro, J. I. (2009). Observations on the reproductive cycles of some viviparous North American sharks. Aqua 15, 205–222.
Clua, E., Chauvet, C., Read, T., Werry, J. M., and Lee, S. Y. (2013). Behavioural patterns of a tiger shark ( Galeocerdo cuvier ) feeding aggregation at a blue whale carcass in prony bay. New caledonia. Mar. Freshw. Behav. Physiol. 46, 1–20. doi: 10.1080/10236244.2013.773127
Compagno, L. J. V. (1984). FAO species catalogue. Volume 4. Sharks of the world. An annotated and illustrated catalogue of shark species known to date. Part 2. Carcharhiniformes. FAO Fish. Synop. No. 125 4(Pt 2), 251–655.
Cucherousset, J., Boulêtreau, S., Azémar, F., Compin, A., Guillaume, M., and Santoul, F. (2012). “Freshwater Killer Whales”: beaching behavior of an alien fish to hunt land birds. PLoS One 7:e50840. doi: 10.1371/journal.pone.0050840
Daly-Engel, T. S., Grubbs, R. D., Holland, K. N., Toonen, R. J., and Bowen, B. W. (2006). Assessment of multiple paternity in single litters from three species of carcharhinid sharks in hawaii. Environ. Biol. Fish. 76, 419–424. doi: 10.1007/s10641-006-9008-5
Dicken, M. L., and Hosking, S. G. (2009). Socio-economic aspects of the tiger shark diving industry within the aliwal shoal marine protected area, South africa. Afr. J. Mar. Sci. 31, 227–232. doi: 10.2989/AJMS.2009.31.2.10.882
Dicken, M. L., Hussey, N. E., Christiansen, H. M., Smale, M. J., Nkabi, N., Cliff, G., et al. (2017). Diet and trophic ecology of the tiger shark ( Galeocerdo cuvier ) from South african waters. PLoS One 12:e0177897. doi: 10.1371/journal.pone.0177897
Domingo, A., Coelho, R., Cortes, E., Garcia-Cortes, B., Mas, F., Mejuto, J., et al. (2016). Is the tiger shark Galeocerdo cuvier a coastal species? Expanding its distribution range in the atlantic ocean using at-sea observer data. J. Fish Biol. 88, 1223–1228. doi: 10.1111/jfb.12887
Driggers, W. B., Ingram, G. W., Grace, M. A., Gledhill, C. T., Henwood, T. A., Horton, C. N., et al. (2008). Pupping areas and mortality rates of young tiger sharks Galeocerdo cuvier in the western North atlantic ocean. Aquat. Biol. 2, 161–170. doi: 10.3354/ab00045
Dudley, S. F., Anderson-Reade, M. D., Thompson, G. S., and McMullen, P. B. (2000). Concurrent scavenging off a whale carcass by great white sharks, Carcharodon carcharias , and tiger sharks, Galeocerdo cuvier . Fish. Bull. 98, 646–649.
Ferreira, L. C., Thums, M., Heithaus, M. R., Barnett, A., Abrantes, K. G., Holmes, B. J., et al. (2017). The trophic role of a large marine predator, the tiger shark Galeocerdo cuvier . Sci. Rep. 7:7641. doi: 10.1038/s41598-017-07751-2
Fitzpatrick, R., Thums, M., Bell, I., Meekan, M. G., Stevens, J. D., and Barnett, A. (2012). A comparison of the seasonal movements of tiger sharks and green turtles provides insight into their predator-prey relationship. PLoS One 7:e51927. doi: 10.1371/journal.pone.0051927
Gallagher, A. J., Serafy, J. E., Cooke, S. J., and Hammerschlag, N. (2014). Physiological stress response, reflex impairment, and survival of five sympatric shark species following experimental capture and release. Mar. Ecol. Prog. Ser. 496, 207–218. doi: 10.3354/meps10490
Gallagher, A. J., Skubel, R. A., Pethybridge, H. R., and Hammerschlag, N. (2017). Energy metabolism in mobile, wild-sampled sharks inferred by plasma lipids. Conserv. physiol. 5:cox002. doi: 10.1093/conphys/cox002
Hammerschlag, N., Gallagher, A. J., and Carlson, J. K. (2013). A revised estimate of daily ration in the tiger shark with implication for assessing ecosystem impacts of apex predators. Funct. Ecol. 27, 1273–1274. doi: 10.1111/1365-2435.12157
Hammerschlag, N., Gallagher, A. J., Wester, J., Luo, J., and Ault, J. S. (2012). Don’t bite the hand that feeds: assessing ecological impacts of provisioning ecotourism on an apex marine predator. Funct. Ecol. 26, 567–576. doi: 10.1111/j.1365-2435.2012.01973.x
Hammerschlag, N., Gutowsky, L. F. G., Gallagher, A. J., Matich, P., and Cooke, S. J. (2017). Diel habitat use patterns of a marine apex predator (tiger shark, Galeocerdo cuvier ) at a high use area exposed to dive tourism. J. Exp. Mar. Biol. Ecol. 495, 24–34. doi: 10.1016/j.jembe.2017.05.010
Heithaus, M. R. (2001). The biology of tiger sharks, Galeocerdo cuvier , in shark bay, western australia: sex ratio, size distribution, diet, and seasonal changes in catch rates. Environ. Biol. Fish. 61, 25–36. doi: 10.1023/A:1011021210685
Heithaus, M. R. (2005). Habitat use and group size of pied cormorants ( Phalacrocorax varius ) in a seagrass ecosystem: possible effects of food abundance and predation risk. Mar. Biol. 147, 27–35. doi: 10.1007/s00227-004-1534-0
Heithaus, M. R., and Dill, L. M. (2002). Food availability and tiger shark predation risk influence bottlenose dolphin habitat use. Ecology 83, 480–491. doi: 10.1890/0012-9658 (2002)083[0480:FAATSP]2.0.CO;2
Heupel, M. R., Carlson, J. K., and Simpfendorfer, C. A. (2007). Shark nursery areas: concepts, definition, characterization and assumptions. Mar. Ecol. Prog. Ser. 337, 287–297. doi: 10.3354/meps337287
Heupel, M. R., and Hueter, R. E. (2001). “Use of an automated acoustic telemetry system to passively track juvenile blacktip shark movements,” in Electronic Tagging and Tracking in Marine Fisheries , eds J. R. Sibert and J. L. Nielsen (Dordrecht: Springer), 217–236.
Hoenner, X., Huveneers, C., Steckenreuter, A., Simpfendorfer, C., Tattersall, K., Jaine, F., et al. (2018). Australia’s continental-scale acoustic tracking database and its automated quality control process. Sci. Data 5:170206. doi: 10.1038/sdata.2017.206
Holland, K. N., Meyer, C. G., and Dagorn, L. C. (2009). Inter-animal telemetry: results from first deployment of acoustic ‘business card’tags. Endanger. Species Res. 10, 287–293. doi: 10.3354/esr00226
Holland, K. N., Wetherbee, B. M., Lowe, C. G., and Meyer, C. G. (1999). Movements of tiger sharks ( Galeocerdo cuvier ) in coastal hawaiian waters. Mar. Biol. 134, 665–673. doi: 10.1038/s41598-018-23006-0
Holland, K. N., Wetherbee, B. M., Peterson, J. D., and Lowe, C. G. (1993). Movements and distribution of hammerhead shark pups on their natal grounds. Copeia 1993, 495–502. doi: 10.2307/1447150
Holmes, B. J. (2015). The Biology and Ecology of the Tiger Shark (Galeocerdo cuvier) on the East Coast of Australia. Ph.D. Thesis, The University of Queensland, St Lucia, Brisbane, Australia.
Holmes, B. J., Pepperell, J. G., Griffiths, S. P., Jaine, F. R., Tibbetts, I. R., and Bennett, M. B. (2014). Tiger shark ( Galeocerdo cuvier ) movement patterns and habitat use determined by satellite tagging in eastern Australian waters. Mar. Biol. 161, 2645–2658. doi: 10.1007/s00227-014-2536-1
Holmes, B. J., Pope, L. C., Williams, S. M., Tibbetts, I. R., Bennett, M. B., and Ovenden, J. R. (2018). Lack of multiple paternity in the oceanodromous tiger shark ( Galeocerdo cuvier ). R. Soc. Open Sci. 5:171385. doi: 10.1098/rsos.171385
Holmes, B. J., Sumpton, W. D., Mayer, D. G., Tibbetts, I. R., Neil, D. T., and Bennett, M. B. (2012). Declining trends in annual catch rates of the tiger shark ( Galeocerdo cuvier ) in queensland, Australia. Fish. Res. 12, 38–45. doi: 10.1016/j.fishres.2012.06.005
Holmes, B. J., Williams, S. M., Otway, N. M., Nielsen, E. E., Maher, S. L., Bennett, M. B., et al. (2017). Population structure and connectivity of tiger sharks ( Galeocerdo cuvier ) across the Indo-pacific ocean basin. R. Soc. Open Sci. 4:170309. doi: 10.1098/rsos.170309
Hussey, N. E., Kessel, S. T., Aarestrup, K., Cooke, S. J., Cowley, P. D., Fisk, A. T., et al. (2015a). Aquatic animal telemetry: a panoramic window into the underwater world. Science 348:1255642. doi: 10.1126/science.1255642
Hussey, N. E., MacNeil, M. A., Siple, M. C., Popp, B. N., Dudley, S. F., and Fisk, A. T. (2015b). Expanded trophic complexity among large sharks. Food Webs 4, 1–7. doi: 10.1016/j.fooweb.2015.04.002
Huveneers, C., Watanabe, Y. Y., Payne, N. L., and Semmens, J. M. (2018). Interacting with wildlife tourism increases activity of white sharks. Conserv. physiol. 6, coy019. doi: 10.1093/conphys/coy019
Kinney, M. J., Hussey, N. E., Fisk, A. T., Tobin, A. J., and Simpfendorfer, C. A. (2011). Communal or competitive? Stable isotope analysis provides evidence of resource partitioning within a communal shark nursery. Mar. Ecol. Prog. Ser. 439, 263–276. doi: 10.3354/meps09327
Last, P., White, W., Gledhill, D., Hobday, A., Brown, R., Edgar, G., et al. (2010). Long-term shifts in abundance and distribution of a temperature fish fauna: a response to climate change and fishing practices. Glob. Ecol. Biogeogr. 20, 58–72. doi: 10.1111/j.1466-8238.2010.00575.x
Lea, J. S., Wetherbee, B. M., Queiroz, N., Burnie, N., Aming, C., Sousa, L. L., et al. (2015). Repeated, long-distance migrations by a philopatric predator targeting highly contrasting ecosystems. Sci. Rep. 5:11202. doi: 10.1038/srep11202
Lea, J. S., Wetherbee, B. M., Sousa, L. L., Aming, C., Burnie, N., Humphries, N. E., et al. (2018). Ontogenetic partial migration is associated with environmental drivers and influences fisheries interactions in a marine predator. ICES J. Mar. Sci. 75, 1383–1392. doi: 10.1093/icesjms/fsx238
Lowe, C. G. (2002). Bioenergetics of free-ranging juvenile scalloped hammerhead sharks ( Sphyrna lewini ) in Kâne’ohe bay, Ō’ahu, HI. J. Exp. Mar. Biol. Ecol. 278, 141–156. doi: 10.1016/S0022-0981(02)00331-3
Lowe, C. G., Wetherbee, B. M., Crow, G. L., and Tester, A. L. (1996). Ontogenetic dietary shifts and feeding behavior of the tiger shark, Galeocerdo cuvier , in Hawaiian waters. Environ. Biol. Fish. 47, 203–211. doi: 10.1007/BF00005044
Lowe, C. G., Wetherbee, B. M., and Meyer, C. G. (2006). Using acoustic telemetry monitoring techniques to quantify movement patterns and site fidelity of sharks and giant trevally around french frigate shoals and midway atoll. Atoll Res. Bull. 543, 281–303.
Matich, P., Heithaus, M. R., and Layman, C. A. (2011). Contrasting patterns of individual specialization and trophic coupling in two marine apex predators. J. Animal Ecol. 80, 294–305. doi: 10.1111/j.1365-2656.2010.01753.x
Meyer, C. G., Anderson, J. M., Coffey, D. M., Hutchinson, M. R., Royer, M. A., and Holland, K. N. (2018). Habitat geography around Hawaii’s oceanic islands influences tiger shark ( Galeocerdo cuvier ) spatial behaviour and shark bite risk at ocean recreation sites. Sci. Rep. 8:4945. doi: 10.1038/s41598-018-23006-0
Meyer, C. G., Dale, J. J., Papastamatiou, Y. P., Whitney, N. M., and Holland, K. N. (2009). Seasonal cycles and long term trends in abundance and species composition of sharks associated with cage diving ecotourism activities in Hawaii. Environ. Conserv. 36, 104–111. doi: 10.1017/S0376892909990038
Meyer, C. G., and Holland, K. N. (2012). Autonomous measurement of ingestion and digestion processes in free-swimming sharks. J. Exp. Biol. 215, 3681–3684. doi: 10.1242/jeb.075432
Meyer, C. G., O’Malley, J. M., Papastamatiou, Y. P., Dale, J. J., Hutchinson, M. R., Anderson, J. M., et al. (2014). Growth and maximum size of tiger sharks ( Galeocerdo cuvier ) in Hawaii. PLoS One 9:e84799. doi: 10.1371/journal.pone.0084799
Papastamatiou, Y. P., Itano, D. G., Dale, J. J., Meyer, C. G., and Holland, K. N. (2010). Site fidelity and movements of sharks associated with ocean-farming cages in hawaii. Mar. Freshw. Res. 61, 1366–1375. doi: 10.1071/MF10056
Papastamatiou, Y. P., Meyer, C. G., Carvalho, F., Dale, J. J., Hutchinson, M. R., and Holland, K. N. (2013). Telemetry and random walk models reveal complex patterns of partial migration in a large marine predator. Ecology 94, 2595–2606. doi: 10.1890/12-2014.1
Payne, N. L., Meyer, C. G., Smith, J. A., Houghton, J. D., Barnett, A., Holmes, B. J., et al. (2018). Combining abundance and performance data reveals how temperature regulates coastal occurrences and activity of a roaming apex predator. Glob. Chang. Biol. 24, 1884–1893. doi: 10.1111/gcb.14088
Payne, N. L., Snelling, E. P., Fitzpatrick, R., Seymour, J., Courtney, R., Barnett, A., et al. (2015). A new method for resolving uncertainty of energy requirements in large water breathers: the ‘mega-flume’seagoing swim-tunnel respirometer. Methods Ecol. Evol. 6, 668–677. doi: 10.1111/2041-210X.12358
Polovina, J. J., and Lau, B. B. (1993). Temporal and spatial distribution of catches of tiger sharks, Galeocerdo cuvier , in the pelagic longline fishery around the Hawaiian Islands. Mar. Fish. Rev. 55, 1–3.
Rivera-López, J. (1970). Studies on the Biology of the Nurse Shark, Ginglymostoma Cirratum Bonnaterre, and the Tiger Shark, Galeocerdo Cuvieri Péron and Le Sueur . MS Thesis. University of Puerto Rico, Mayaguez, PuertoRico.
Roquet, F., Williams, G., Hindell, M. A., Harcourt, R., McMahon, C., Guinet, C., et al. (2014). A Southern Indian ocean database of hydrographic profiles obtained with instrumented elephant seals. Sci. Data 1:140028. doi: 10.1038/sdata.2014.28
Simpfendorfer, C. (1992). Biology of tiger sharks ( Galeocerdo cuvier ) caught by the queensland shark meshing program off townsville, Australia. Mar. Freshw. Res. 43, 33–43. doi: 10.1071/MF9920033
Simpfendorfer, C. A., Goodreid, A. B., and McAuley, R. B. (2001). Size, sex and geographic variation in the diet of the tiger shark, Galeocerdo cuvier , from Western Australian waters. Environ. Biol. Fish. 61, 37–46. doi: 10.1023/A:1011021710183
Skinner, J. P., Mitani, Y., Burkanov, V. N., and Andrews, R. D. (2014). Proxies of food intake and energy expenditure for estimating the time–energy budgets of lactating northern fur seals Callorhinus ursinus . J. Exp. Mar. Biol. Ecol. 461, 107–115. doi: 10.1016/j.jembe.2014.08.002
Springer, S. (1967). “Social organization of shark populations,” in Sharks, Skates and Rays , eds P. W. Gilbert, R. W. Mathewson, and D. P. Rall (Baltimore, MD: John Hopkins Press), 149–174.
Stehfest, K. M., Patterson, T. A., Dagorn, L., Holland, K. N., Itano, D., and Semmens, J. M. (2013). Network 524 analysis of acoustic tracking data reveals the structure and stability of fish aggregations in the ocean. Animal Behav. 85, 839–848. doi: 10.1016/j.anbehav.2013.02.003
Sulikowski, J. A., Wheeler, C. R., Gallagher, A. J., Prohaska, B. K., Langan, J. A., and Hammerschlag, N. (2016). Seasonal and life-stage variation in the reproductive ecology of a marine apex predator, the tiger shark Galeocerdo cuvier, at a protected female-dominated site. Aquat. Biol. 24, 175–184. doi: 10.3354/ab00648
Towner, A. V., Leos-Barajas, V., Langrock, R., Schick, R. S., Smale, M. J., Kaschke, T., et al. (2016). Sex-specific and individual preferences for hunting strategies in white sharks. Funct. Ecol. 30, 1397–1407. doi: 10.1111/1365-2435.12613
Werry, J. M., Planes, S., Berumen, M. L., Lee, K. A., Braun, C. D., and Clua, E. (2014). Reef-fidelity and migration of tiger sharks, Galeocerdo cuvier , across the coral sea. PLoS One 9:e83249. doi: 10.1371/journal.pone.0083249
Whitney, N. M., and Crow, G. L. (2007). Reproductive biology of the tiger shark ( Galeocerdo cuvier ) in Hawaii. Mar. Biol. 151, 63–70. doi: 10.1007/s00227-006-0476-0
Wintner, S. P., and Dudley, S. F. (2000). Age and growth estimates for the tiger shark, Galeocerdo cuvier , from the east coast of South Africa. Mar. Freshw. Res. 51, 43–53. doi: 10.1071/MF99077
Wirsing, A. J., Heithaus, M. R., and Dill, L. M. (2007). Fear factor: do dugongs ( Dugong dugon ) trade food for safety from tiger sharks ( Galeocerdo cuvier )? Oecologia 153, 1031–1040.
Keywords : tiger sharks, behavior, research perspectives, tracking, ecology, population connectivity
Citation: Holland KN, Anderson JM, Coffey DM, Holmes BJ, Meyer CG and Royer MA (2019) A Perspective on Future Tiger Shark Research. Front. Mar. Sci. 6:37. doi: 10.3389/fmars.2019.00037
Received: 28 June 2018; Accepted: 23 January 2019; Published: 14 February 2019.
Reviewed by:
Copyright © 2019 Holland, Anderson, Coffey, Holmes, Meyer and Royer. This is an open-access article distributed under the terms of the Creative Commons Attribution License (CC BY) . The use, distribution or reproduction in other forums is permitted, provided the original author(s) and the copyright owner(s) are credited and that the original publication in this journal is cited, in accordance with accepted academic practice. No use, distribution or reproduction is permitted which does not comply with these terms.
*Correspondence: Kim N. Holland, [email protected]
This article is part of the Research Topic
Future Directions in Research on Marine Megafauna
Thank you for visiting nature.com. You are using a browser version with limited support for CSS. To obtain the best experience, we recommend you use a more up to date browser (or turn off compatibility mode in Internet Explorer). In the meantime, to ensure continued support, we are displaying the site without styles and JavaScript.
- View all journals
- My Account Login
- Explore content
- About the journal
- Publish with us
- Sign up for alerts
- Open access
- Published: 21 April 2022
Retrospective genomics highlights changes in genetic composition of tiger sharks ( Galeocerdo cuvier ) and potential loss of a south-eastern Australia population
- Alice Manuzzi ORCID: orcid.org/0000-0001-8116-9710 1 ,
- Belen Jiménez-Mena 1 ,
- Romina Henriques 1 ,
- Bonnie J. Holmes 2 ,
- Julian Pepperell 3 ,
- Janette Edson 4 ,
- Mike B. Bennett 5 ,
- Charlie Huveneers 6 ,
- Jennifer R. Ovenden 7 &
- Einar E. Nielsen 1 , 5
Scientific Reports volume 12 , Article number: 6582 ( 2022 ) Cite this article
2362 Accesses
2 Citations
127 Altmetric
Metrics details
- Marine biology
- Population genetics
Over the last century, many shark populations have declined, primarily due to overexploitation in commercial, artisanal and recreational fisheries. In addition, in some locations the use of shark control programs also has had an impact on shark numbers. Still, there is a general perception that populations of large ocean predators cover wide areas and therefore their diversity is less susceptible to local anthropogenic disturbance. Here we report on temporal genomic analyses of tiger shark ( Galeocerdo cuvier ) DNA samples that were collected from eastern Australia over the past century. Using Single Nucleotide Polymorphism (SNP) loci, we documented a significant change in genetic composition of tiger sharks born between ~1939 and 2015. The change was most likely due to a shift over time in the relative contribution of two well-differentiated, but hitherto cryptic populations. Our data strongly indicate a dramatic shift in the relative contribution of these two populations to the overall tiger shark abundance on the east coast of Australia, possibly associated with differences in direct or indirect exploitation rates.
Similar content being viewed by others
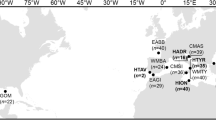
Ancient DNA SNP-panel data suggests stability in bluefin tuna genetic diversity despite centuries of fluctuating catches in the eastern Atlantic and Mediterranean
Adam J. Andrews, Gregory N. Puncher, … Alessia Cariani
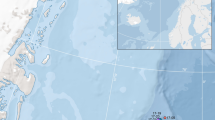
High genomic diversity in the endangered East Greenland Svalbard Barents Sea stock of bowhead whales (Balaena mysticetus)
José Cerca, Michael V. Westbury, … Lutz Bachmann
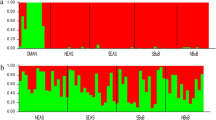
Genomic investigations provide insights into the mechanisms of resilience to heterogeneous habitats of the Indian Ocean in a pelagic fish
Wilson Sebastian, Sandhya Sukumaran, … A. Gopalakrishnan
Introduction
Intraspecific genetic diversity is essential for long-term population persistence to avoid the negative effects of inbreeding 1 , to buffer against environmental variation in time and space 2 , and to ensure adaptability to a changing environment 3 . High genetic diversity may also have positive ecosystem effects by promoting productivity, abundance, and stability of community structure 4 , 5 , 6 . Thus, species with many discrete populations with different genetic adaptations, including life history strategies, are assumed better at withstanding exploitation and environmental variation, than a single panmictic population. Intraspecific diversity protection is a specified objective of the Convention on Biological Diversity (CBD; www.cbd.int ), but is rarely an integral part of monitoring activities, in particular for species that are not critically endangered 7 , 8 . Indeed, the lack of dedicated sampling programs and the many species requiring monitoring makes it logistically impossible to oversee intra-specific diversity in most instances. In addition, the difficulties associated with the use of high-resolution genetic methods when sample sizes are low or when tissue samples vary in age, composition and provenance 9 also hinder our ability to assess intra-specific diversity.
The use of DNA extracted from museum specimens, in combination with modern molecular analytical tools, has revolutionized the ability to assess genetic changes at contemporary time scales 10 , 11 . Studies on processes such as intra-population loss of diversity, adaptive change caused by evolutionary drivers in the environment, as well as the movement, decline, or extirpation of populations through time and space can now be undertaken 5 , 12 . In the marine realm, there are numerous examples of local population reductions and extinctions, in particular for large sharks and rays 13 , 14 , but closely coupling these incidences with genetic and genomic data is challenging due to the lack of temporal genetic data for elasmobranch species. Spatiotemporal genetic samples (i.e. obtained across several locations and years 15 ) can be used to test for changes in distribution of elasmobranch populations including potential extirpations and replacements, as well as intra-population changes in levels of genetic variability, and putative adaptive genetic changes in the timeframe of recent environmental changes or exploitation. This is of significant interest with respect to populations of large sharks, where there is a strong need for identification of genetic populations and their historical trajectories, as they represent both the relevant unit for evolution and management 9 , 16 in order to contribute significantly to short-term sustainable exploitation as well as long-term protection of intra-specific biodiversity. Failing to identify and incorporate biologically-discrete populations in management may cause overharvesting of particular (cryptic) populations or population segments, resulting in extinction or reduction of genetic diversity within populations 4 , 7 .
The tiger shark ( Galeocerdo cuvier ) is one of the world’s largest sharks, with a circumglobal distribution in tropical and sub-tropical waters 17 , 18 . Throughout its range, the species is exploited by multiple users, from target and bycatch in commercial, artisanal, and recreational fisheries 19 , 20 , to shark control operations to improve bather safety 21 , 22 , which have been linked to on-going population decline and reduced average size of individuals 22 , 23 , 24 . The species is globally listed as Near Threatened on the International Union for the Conservation of Nature’s (IUCN) Red List of Threatened Species due to a suspected decline of ~ 30% over the past ~ 60 years 25 . This general decline covers variable regional population trends ranging from relatively stable abundances, e.g. North-western Atlantic 26 , to severe local depletions as observed in the Red Sea, Eritrea, Iranian Gulf, as well India and Pakistan 27 . In the Arabian Seas on-going local reported declines of 30–50% over three generations led the species to be locally assessed as Vulnerable 27 . Of greater concern, however, is the reported 74% decline in catch per unit effort (CPUE) for tiger sharks off Queensland (eastern Australia) over the past 25 years, which was accompanied by a 21% decline in the average size of individuals 24 . Thus, despite its cosmopolitan nature and dispersal potential, local depletions appear to be relatively common in the species, suggesting some degree of population isolation could occur. However, there is still a lack of adequate spatially resolved data to understand regional population trends of tiger sharks as they are commonly caught in unreported fisheries. Current assessments of the tiger shark population structure have been based on microsatellite and mitochondrial genetic markers, and have demonstrated a clear inter-basin genetic split between the Atlantic and Indo-Pacific Oceans, but a general lack of intra-basin population structuring 28 , 29 , 30 . Although this may be a true biological characteristic of the species, it could also reflect analytical challenges related to obtaining sufficient samples with good spatial coverage and the application of low-resolution genetic markers. Thus, in a recent review of research priorities, the development of high-resolution SNPs (Single Nucleotide Polymorphisms) to further resolve tiger shark meta-population structure was strongly recommended 31 . Moreover, temporal genetic information to assess the anthropogenic effects of exploitation is virtually absent. Such temporal genetic data are necessary to determine both intra-population changes in diversity by genetic drift, migration and selection, but also extent of population displacement or extirpation. Due to its Near Threatened status, potential for over-exploitation and risk to human lives, tiger shark management and conservation actions are high on the public agenda 32 . As large sharks are highly mobile, management has been primarily focused on international or regional policies, with limited value of enforcing strong local “domestic” regulations 16 . However, the detection of fine-scale population structure and the effects of local depletion or extirpation on intraspecific genetic diversity could lead to a general paradigm shift in large shark management from a global or regional perspective to a much stronger local focus.
Here, we investigated a possible link between the reported decline in tiger shark numbers and local genetic diversity off the east coast of Australia. While mating system can affect effective population size 33 , 34 and declines in genetic diversity can be moderated by gene flow 35 , genetic diversity has been shown to be linked to effective population size. Indeed, a reduction in population size (e.g. bottleneck) can lead to a rapid decline in heterozygosity and allele diversity 36 . Such relationship has also been reported in empirical studies (e.g. 37 , 38 ). We chose east coast Australia because of documented reductions in tiger shark CPUE in that region and because samples from this region are relatively abundant across both spatial and temporal scales; i.e. tiger sharks samples were available along the eastern Australian coastline and from as early as ~1939 to 2015. This provided an unparalleled opportunity to assess how the alleged population decline may have affected the genetic composition of the species over the past century. In order to collect genetic data over the widest spatial and temporal range, we extracted DNA from tissue samples taken from shark jaws archived in museums and private collections, mostly retained as trophies from fishing competitions, and performed retrospective genomic analyses 39 . As these trophies are listed with local game-fishing clubs, their size, catch date and location are well documented.
Tiger sharks in Australia are thought to be part of a large Indo-Pacific population 28 , and satellite-tag tracking studies have revealed extensive movements up to several thousand kilometres in the region 40 , 41 with some individuals seen moving as far as New Caledonia (maximum reported distances: of 1141 and 1800 km) 42 , 43 . In this context, we hypothesised that tiger sharks in eastern Australia, form a single panmictic population, from tropical Queensland to temperate Victoria 42 , 44 . Therefore, by employing a local spatiotemporal approach, our study aimed to assess if (i) tiger sharks in eastern Australia are indeed panmictic, (ii) reported declines are related to possible population structure in the species and if so, (iii) whether population distributions and relative abundances have remained stable over the last 100 years. This is the first study of its kind, not only for the region and tiger sharks, but also for elasmobranchs in general.
Bioinformatics pipeline and data filtering
Sequencing yielded an average of 2,833,138 reads per individual, with slightly lower numbers for historical jaw samples (median 1,973,493) than contemporary tissue samples (median 2,301,001). After running the bioinformatics pipeline, 78% of the original sequences went into transcriptome mapping. All samples showed a very low percentage of contaminants, confirming the validity of the capture strategy for sequencing enrichment with shark DNA. An average of 0.12% of the cleaned reads was of mitochondrial origin and excluded from further analysis, except for the few cases when it was used for species confirmation. Out of the 20,000 catshark derived baits, 4544 (22.72%) were post-hoc mapped back to the tiger shark transcriptome 45 covering 4137 scaffolds. Of these, 4143 had captured reads with a depth of coverage higher than non-target regions. Scaffolds with a bait had an average coverage of 68.5×, while scaffolds from off-target regions had an overall average depth of 40.7× (35.8× for historical and 43.4× for contemporary samples). Coverage was higher and less variable in contemporary than in historical samples. Single nucleotide polymorphisms (SNPs) were called from all transcriptomic sequences and we identified 35,061 raw SNP variants for 122 samples in 2978 reference scaffolds. After filtering, 4580 SNPs remained. SNPs with significant departures from Hardy–Weinberg Equilibrium (HWE) were removed to produce a final dataset consisting of 1840 SNP loci genotyped in 115 samples from G. cuvier specimens caught between ~1939 and 2015. In addition, we removed samples that had higher levels of missing data (below 70% call rate), had lack of length/weight information that did not allow to calculate the age, or had erroneous species labelling (identified as a different species), that could indicate a possible contamination with reads from other shark species. The final dataset thus contained 106 samples (Fig. 1 , Table S1 ). Very low levels of DNA damage was observed, confirming DNA was well preserved in jaws 39 , at least for the relatively short time period (~ 80 years) compared to true ancient DNA (aDNA) studies. Thus, it is highly unlikely that the final SNPs represent artefacts due to deamination or other DNA damage in the historical jaw samples.
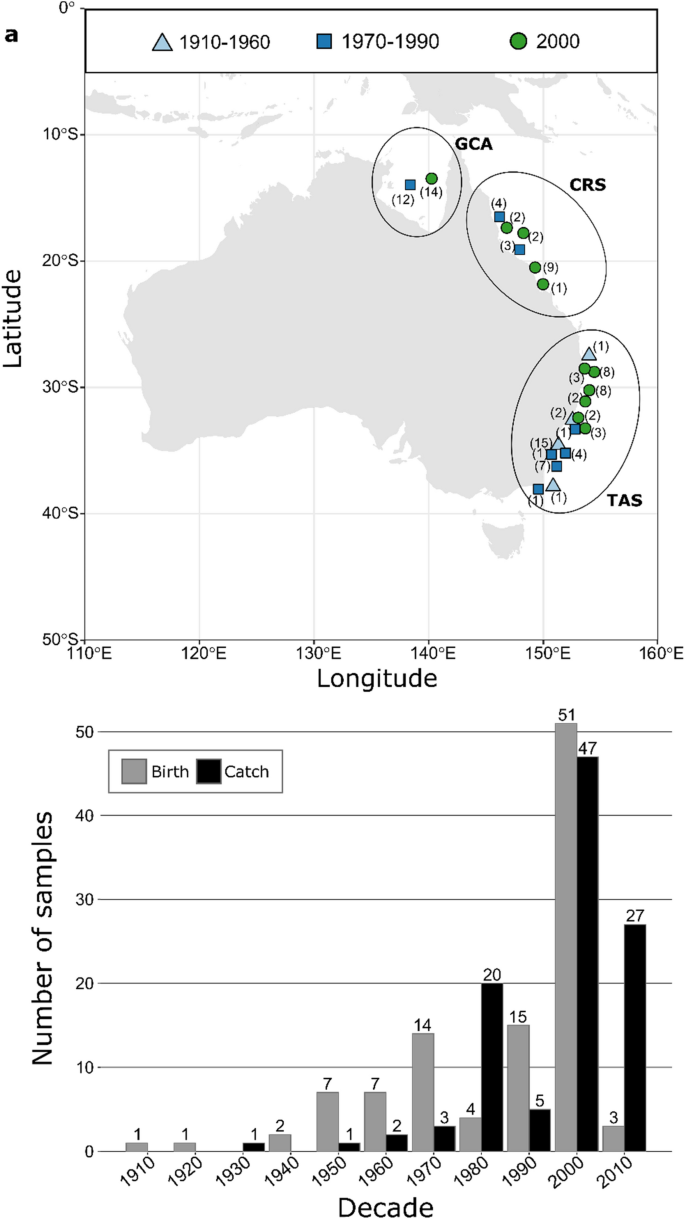
Sampling locations and distribution through time and space. ( a ) Samples distribution along the east coast of Australia. Samples are grouped by decades of birth (1910–1960, 1970–1990 and 2000) as explained in the text and the colours identify the three time-periods. Group names refer to the three major regions where samples were collected: Gulf of Carpentaria (GCA), Coral Sea (CRS) and Tasman Sea (TAS). ( b ) The histogram shows the difference between decade of catch and calculated decade of birth and associated sample numbers, as reported above each bar. Grey bars identify the calculated years of birth, while black numbers refer to the years of catch.
Data analysis for temporal and spatial genomic variability
To examine the stability of patterns over time, we used a temporal genetic analysis based on the back-calculated decade of birth of tiger shark individuals, which showed clear evidence of temporal genetic differentiation. This was apparent for the temporal dataset as a whole (Table 1 ), where pairwise F ST estimates increased with time. Temporal differentiation was also apparent for the Tasman Sea samples alone, where the majority of the oldest historical samples originated (Table S2 ). In contrast, contemporary tiger shark samples (2000 and 2010) from the Gulf of Carpentaria (GCA), Coral Sea (CRS) and Tasman Sea (TAS), showed little evidence of genetic structuring, with estimated non-significant pairwise F ST values of 0.006 between GCA and CRS, and 0.001 between CRS and TAS (Table S3 ). A Principal Coordinates Analysis (PCoA) of spatiotemporal F ST values (Fig. 2 ) showed a separation of samples along axis 1, explaining 88.7% of the variation. The 1910–1960 TAS samples were the most distinct, with the 1970–1990 TAS samples intermediate between the TAS oldest samples and a cluster of the remaining samples. The Principal Component Analysis (PCA) of all spatiotemporally collected individuals (Fig. 3 a) supported the genetic differentiation of historical samples as they clustered differently, with a proportion of the individuals forming a relatively distinct cluster from the contemporary samples. In contrast to Fig. 3 a, an individual based PCA of spatially-collected contemporary samples only (Fig. 3 b) did not display any apparent clustering of individuals according to location. The presence of a distinct group of individuals in the historical samples was supported by the results from the Discriminant Analysis of Principal Components (DAPC), where the Bayesian Information Criterion (BIC) analysis and the k-means algorithm identified K = 2 as the number of clusters that best explains the structure in the dataset (Fig. 4 ). Further analytical support for two populations was given by the results of the snmf algorithm (LEA package) that also identified K = 2 as the most likely number of clusters (Fig. S1 ). The DAPC analysis showed ~ 70% (13 of 19) of ‘cluster 2’ individuals in the 1910–1960 TAS samples, ~ 36% (5 of 14) in the 1970–1990 samples and a lack among the TAS samples from 2000. For the GCA samples, only a single ‘cluster 2’ individual (1 of 14) was found (2000). Likewise, only one ‘cluster 2’ individual (1 of 14) was found among the CRS 2000 samples. The Fisher exact test confirms that the change over time was statistically significant with all the comparisons between historical versus contemporary collections (TAS_1910–1960 vs TAS 1970–1990, TAS 1910–1960 vs TAS_2000, and TAS_1970–1990 vs TAS_2000) being significant (p-val = 0.041, 0.00001 and 0.003 respectively). Patterns of Site Frequency Spectra (SFS) and the pi and Watterson theta indices (Fig. S2 , Table S4 ) showed that older collections and contemporary collections behaved similarly with no evident bias (e.g. excess of singletons) in older samples. When pooling all putative ‘cluster 1’ and ‘cluster 2’ individuals across samples, the resulting mean F ST between the two population groups was 0.013, thus substantially higher than between any pair of spatiotemporal samples, further supporting a mixed populations hypothesis. The distribution of F ST values across loci showed that a high number of loci (Fig. S3 ) contributed to the differentiation, suggesting that genetic differences between the two groups were not caused by technical artefacts or contemporary evolution at one or a few loci. However, while BayeScan did not detect any outliers, pcadapt identified 14 possible outlier loci between the two clusters. Most of these loci (9 of 14) showed an overall higher allele frequency for ‘cluster 2’. The mean level of heterozygosity in the two groups was statistically different (Fig. 5 ), with 0.23 (± 0.01) for ‘cluster 1’ and 0.26 (± 0.01) for ‘cluster 2’. Average missing data was lower for ‘cluster 1’ than ‘cluster 2’ (0.35% and 1.14%, respectively), likely reflecting the average age of samples. However, there was no correlation between mean individual heterozygosity and proportion of missing data using a linear model ( p = 0.25; R 2 = 0.003).
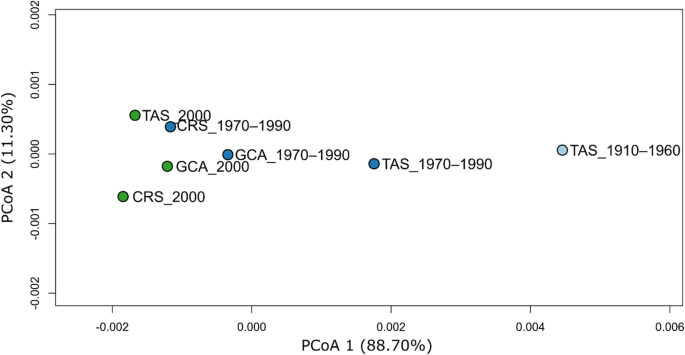
Principal Coordinates Analysis (PCoA) of mean pairwise FST’s between spatiotemporal tiger shark samples from eastern Australia. Groups are based on back-calculated ages and refer to: Gulf of Carpentaria (GCA), Coral Sea (CRS) and Tasman Sea (TAS). The axes report the percentage of variance explained.
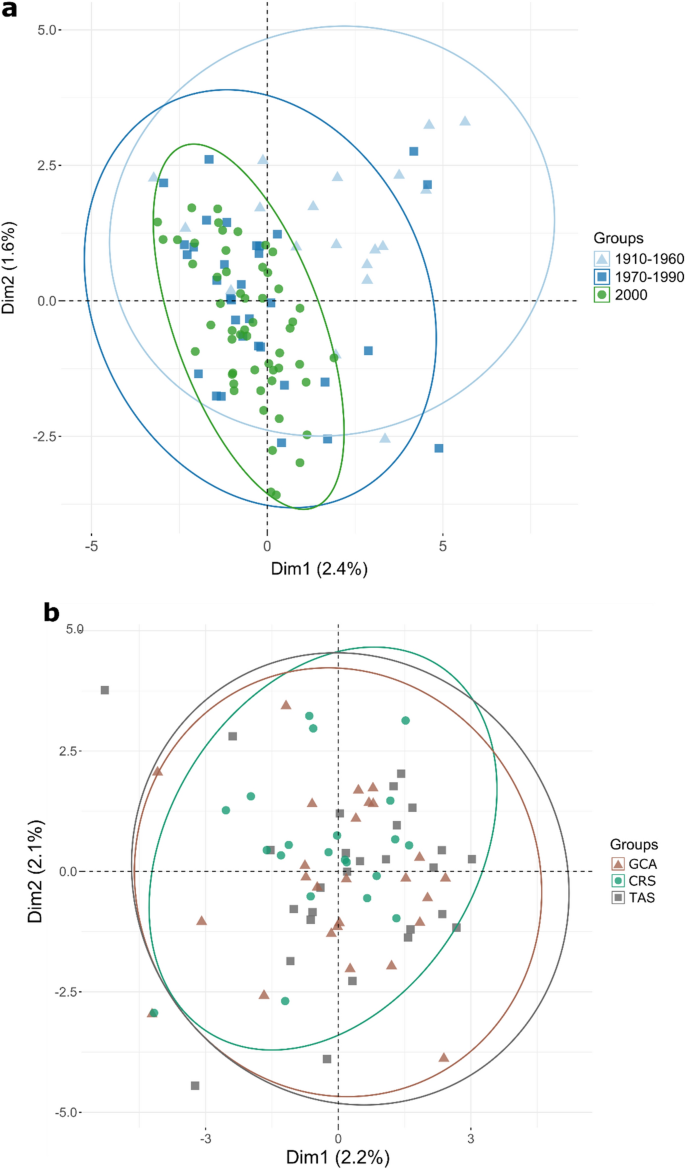
Principal Component Analysis (PCA) by time periods and locations. ( a ) PCA of all individual genotypes for the samples with back-calculated age of birth and ( b ) PCA of contemporary samples based on decade of catch (2000–2010) covering the Gulf of Carpentaria (GCA), Coral Sea (CRS) and Tasman Sea (TAS).
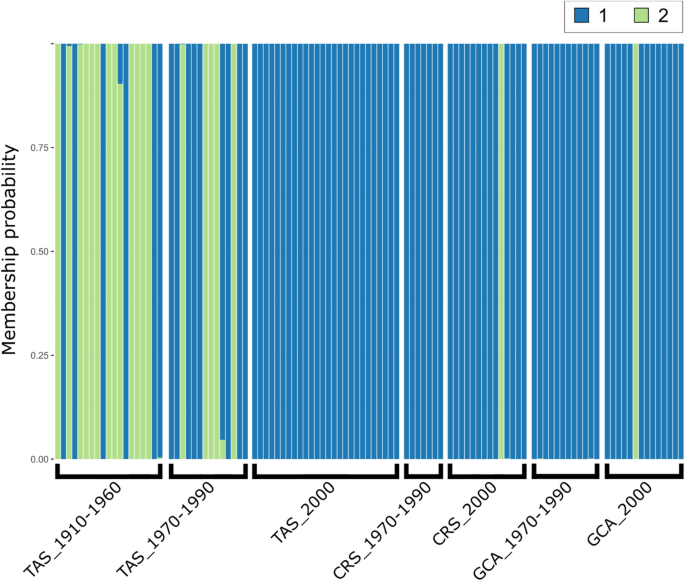
Discriminant Analysis of Principal Components (DAPC) for K = 2. The plot illustrates the spatiotemporal occurrence of individuals from the two hypothesized clusters in time and space. Samples grouped by time and space are labelled along the x-axis, only collections encompassing more than six samples were included. The y-axis reports the membership probability of each sample to belong of either clusters (‘1’ in blue and ‘2’ in green).
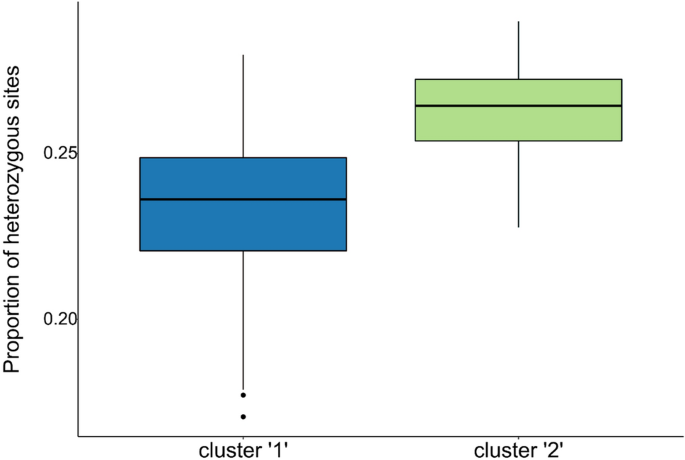
Boxplot of the average proportion of heterozygous SNPs loci for the two clusters. Average proportion of heterozygous SNP loci over total loci genotyped for the two identified clusters. Cluster 1 is composed of mainly contemporary and northern samples, while cluster 2 individuals are almost exclusively found in southern historical samples (see Fig. 4 for explanation).
This is the first study to demonstrate that archived samples in combination with modern genomic tools can reveal temporal changes in biodiversity in large sharks, which otherwise would have remained unnoticed. We identified genomic differences, consistent with two hitherto unidentified populations, at a relatively local scale (Eastern Australia) for a large migratory shark, the tiger shark. However, this pattern was only evident when historical samples were included, suggesting the displacement or extirpation of a local population. This conclusion is based on the findings that the genetic clustering of our spatiotemporal genotypes provided best statistical support for two population groups, with downstream analysis confirming that these units are genetically distinct and contain different levels of genetic diversity. One of the identified populations was most abundant in the oldest and southern-most samples (i.e. Tasman Sea), and almost completely absent from contemporary (including southern samples) and northern-most samples. We propose that the most parsimonious explanation for this absence is a shift in the relative population abundance likely associated with human fishing activities. Our results mirror the findings of Brown and Roff 46 that reported a major decline in abundance of tiger sharks over three generations off the Queensland coast, with greater declines detected at the southern sites. However, we recognise that a number of factors could have partially contributed to our observations and uncertainties around this apparent dramatic shift in abundance, which we discuss below.
Our findings are unlikely to be the result of technical issues associated with the use of historical DNA (hDNA). In general, capture sequencing of hDNA has a lower sequencing depth and coverage leading to fewer mapped reads and thus more missing data with increasing sample age 47 , both of which could affect downstream population genomic inferences 48 , 49 , 50 . Although our historical samples presented fewer reads than the contemporary samples, the level of missing data was generally low, even for the oldest samples (maximum level of missing data allowed was 5% per SNP). For example, in ‘cluster 2’ all but two individuals had less than 5% missing data (average of 1.14%, median 0.14%), while all but two individuals in ‘cluster 1’ had less than 1% missing data (average 0.35%, median 0.00%). ‘Cluster 2’ individuals generally showed a higher level of heterozygosity than individuals from ‘cluster 1’, which is the opposite of the expected pattern of reduced individual heterozygosity due to low coverage caused by allelic drop-out 42 . The opposite pattern of increased diversity due to a high occurrence of singletons caused by DNA degradation was also implausible due to the filtering, only including SNPs with a minimum allele count of three in the dataset. In addition, the genetic differentiation that we observed between clusters over time was not caused by a few spurious high-differentiated loci (which could be the result of a technical issue in the genomic pipeline), but was found to be spread across the transcriptome. Moreover, if ‘cluster 2’ genotypes were artefacts created by poor quality DNA, this would still fail to explain the complete absence of those genotypes in the historical northern samples with equal DNA quality (GCA). The observed pattern of differentiation is consistent with a scenario of genetic drift accumulated across the genome, at an evolutionary time scale in two semi-independent populations of tiger sharks, as clearly indicated by the genetic cluster analysis and the transcriptome-wide patterns of differentiation (i.e. differentiation was driven my multiple loci spread throughout the transcriptome). Further strength to this hypothesis come from the results obtained by the two independent clustering methods (DAPC and snmf function in LEA), representing different algorithm and population genetic models/assumptions. Both approaches consistently identified the most likely presence of a distinct cluster in the older southern samples, which was not detectable in the most recent/northern collections. Thus, the relatively large differentiation between the two putative population clusters, the differences in their levels of heterozygosity, and the finding that not all historical Tasman Sea samples clustered together, renders the alternative hypothesis of very strong short-term genetic drift in a single panmictic population distributed across the central Indo-Pacific less plausible . In summary, the reported temporal genetic differentiation is unlikely to be caused by artefacts in sequencing and in the bioinformatics pipeline, by historical genetic drift in a single panmictic population, or by strong temporal genetic signatures of intra-population environmental selection (which would imply differentiation to be driven by a few loci).
The use of material from historical collections together with genomic-scale analyses provided a relatively large sample size and sufficient statistical power for individual-based cluster analyses, presenting a unique window to explore population composition of tiger sharks in the past. The collection of historical and contemporary samples from the east coast of Australia comprised a huge logistic effort and, at least for the oldest specimens, represents the majority of high quality samples available and practically possible to sample in the community to date. Our samples thus provide relatively solid evidence of changes in population abundance, but still are unlikely to provide the full picture of genetic variation across space and time in tiger sharks off eastern Australia, in particular with respect to population mixing. Although location of catch was accurate, the sampling effort was mainly opportunistic, and thus variation in composition of samples with respect to age and sex, as well as season of sampling and method, may have influenced our results. Unfortunately, there is little information on sex for the historical samples and thus this assumption cannot be directly proven.
Previous tagging studies investigating tiger shark dispersal have shown complex movement patterns including large-scale migrations, seasonal residency, and sex- and size-based dispersal or partial migrations 42 , 51 , 52 , 53 , 54 , 55 , 56 , 57 , 58 . In eastern Australia, the movements of tiger shark are closely related to water temperature 42 , 58 . Prey densities associated with these gradients may also drive adult shark movements to regularly return to specific areas to feed, i.e. established turtle nesting sites 58 . As a species that undergoes ontogenetic diet shift to larger-bodied 45 prey items as they mature 59 , this could also partly explain a higher occurrence of the local (‘cluster 2’) population in the historical Tasman Sea samples, which were predominately large trophy specimens. However, little is still known about the use of space by tiger sharks, particularly those that are sexually mature, and there is some suggestion that reproductive cues associated with changes in thermal gradient may also drive movements of large females toward the insular regions of the Pacific Ocean 58 .
Sampling the same individual twice or kin sampling might skew the genetic diversity estimated 60 . However, these were not an issue in our study as, firstly, samples were obtained from dead individuals, and secondly, our kinship analysis did not show related individuals in our samples. Despite possible sampling-associated uncertainties, the observed patterns of temporal genetic divergence are clear. Firstly, our results based on contemporary samples are consistent with two recent studies that detected a single genetic population off the Australian east coast 28 , 29 and are also compatible with the present understanding of population structure in the Indo-Pacific 29 , 30 . Secondly, the presence of the two identified cryptic groups was not random in space and time. Individuals from ‘cluster 2’ were almost exclusively detected in the southern part of the species’ distribution, most abundant in the oldest samples, and almost absent from contemporary samples. Consequently, we hypothesize tiger sharks in east Australian waters consisted of at least two populations in the past, but likely comprise a single population now. This may sound counterintuitive in the light of satellite tag-tracking studies that have shown evidence of individuals migrating over 1000 km 23 , 43 , 61 . However, large migrations and local populations at fine geographical scales are not mutually exclusive since dispersal events may be related to foraging and not reproductive purpose hence contributing differently to a population genetic make-up. Thus, evidence of fine-scale structure could be linked to basic “triangle migrations 16 , 62 of fishes between parturition sites and juvenile and adult habitats 63 . Partial migration, ubiquitous among animal populations, and ontogenetic variation in dispersive behaviour, documented in tiger sharks 53 , 54 , 64 ), might have also affected estimate of genetic diversity. Indeed, pooling of life stages, i.e. across sizes, during data analysis can obscure estimates of stock structure and dispersal 65 . This is, however, unlikely to be an issue in our study because we were able to identify clear stock structure regardless of the majority of our samples being from large tiger sharks known to be the most dispersive 54 , 57 , 64 .
The observed pattern of population structure suggests a southern Australian distribution of one of the populations (‘cluster 2’), with the apparently more abundant population (‘cluster 1’) currently found throughout the entire distribution, with a previous limited intrusion to southern New South Wales (based on the small number of individuals in ‘cluster 1’ from the historical TAS samples). It is possible that the southerly population (‘cluster 2’) was a coastal and more resident ecotype and the other population is currently more widespread, more offshore and more migratory, as found in bony fishes such as Atlantic cod ( Gadus morhua ) 66 and European anchovy ( Engraulis encrasicolus ) 67 and in marine mammals like the bottlenose dolphin ( Tursiops truncatus ) 68 . Intraspecific differences in movement and residency patterns is also increasingly being reported in sharks 69 , 70 , 71 , including in large predatory species as tiger sharks 70 . Importantly, our findings suggest that the abundance of the putative southerly population has declined significantly compared to pre-1990s levels. The apparent local depletion of tiger sharks at the south eastern distribution of the species in Australia supports previous studies showing a reduction in the abundance and mean size of tiger sharks caught in this region 23 , 24 , 46 . Although the species is not commonly commercially targeted off eastern Australia, it is caught as bycatch, in Queensland and New South Wales’ shark control programs and in recreational fisheries off New South Wales. Off Queensland, tiger shark catch-per-unit-effort has dropped by 74% over the past 25 years, while the average size declined by 21% 24 , with most of the decline occurring in the southern part of the state 23 . Catch-per-unit-effort also declined in the NSW beach meshing program 22 . This reported strong reduction in tiger shark abundance, which coincides with the increase in lethal shark mitigation measures, is of concern and may imply that ongoing lethal mitigation measures as the possible drivers of the observed population declines in the region. Recent estimates of tiger shark bycatch obtained from commercial logbook records indicate between 5 and 10 t routinely caught off Queensland, and approximately 3 t per year off New South Wales. However, New South Wales also accounts for approximately 10 t of tiger shark recreational catches 72 , 73 . Further, since 1936 the game fishing in New South Wales has targeted large tiger sharks for capture point scores and continues to do so over several competitions annually 71 . Illegal foreign fishing vessels that target large sharks for their fins have been apprehended in the Tasman Sea region and north into the Coral Sea, with tiger shark found to comprise about 20% of the total biomass of sharks caught 74 . Overall, these activities may have selectively removed the southern population (‘cluster 2’), most likely through asserting a higher level of exploitation than on the more widespread northern population. Ongoing climate change can also contribute to shifting distributions and abundance of marine fish species and populations 63 , particularly in Australia 75 , 76 , since the region has been identified as one of the global hotspots for ocean warming 77 . Thus, it is possible that increasing sea temperatures could have negatively affected the putative southern population or contributed to the increase of the northern population in southern waters. This ‘tropicalization’ of southern Australian waters is well studied with several ‘northern’ species shifting their distribution ranges further south, and increasing in abundance in the Tasman Sea (e.g. 78 ). Nevertheless, in the light of the recent dramatic demographic changes in tiger sharks and other large shark populations in the region, it is likely that fishing has played a significant role in the reported changes in abundance of these two genetically differentiated populations. Still, genetic analysis of more contemporary samples from along the east coast of Australia and the Pacific Ocean 28 using e.g. a targeted genotyping approach focusing on the most informative SNPs 79 , could help to further elucidate the apparent change of abundance of the two populations of tiger sharks in eastern Australian waters.
The apparent occurrence (and loss) of localized cryptic populations of tiger sharks, at a finer geographical level than previously believed, raises a number of concerns regarding identification and monitoring of intraspecific biodiversity in large sharks. Our study suggests that localized populations may be more common than anticipated from recent genetic studies using markers with lower resolution 28 , 80 . Thus, reported local, in particular coastal, depletions of large sharks 14 could also be associated with severe reductions or losses of entire populations that has remained unnoticed due to replacement with individuals from other and possibly more wide-ranging populations. This eradication of semi-independent evolutionary lineages will not only have a local effect on population abundance, but also affect the evolutionary potential of the species as a whole and the ecosystem services they provide 4 . This highlights the importance of developing high-resolution genomic resources for elasmobranchs and other high gene flow marine organisms 81 , 82 which can provide information on a large number of variable sites in the genome (e.g. SNPs). By genotyping many individuals for a high number of SNPs, it may be possible to identify putative populations in species with general low levels of genetic differentiation such as sharks. More importantly, our work also points to significant challenges regarding the scale of current management and biodiversity protection schemes for large sharks. Sustainable management of local populations, through matching the scale of governance with population structure, is important for the protection of the evolutionary legacy of the species and the potential for adapting to future environmental changes 83 . It is also important for the maintenance of healthy marine ecosystems that could provide services to human society 84 . Accordingly, management focus will need to include localized protection measures, such as local seasonal closures or marine reserves to properly match the geographic scale of the population 85 . Specifically, for the east coast of Australia, we recommend to further investigate our findings, to elucidate the current abundance and distribution of the two populations and establish measures to protect the putative southern population component, which appears to have faced a significant historical decline, primarily driven by either direct and indirect exploitation. Overall, our work highlights the need to include historical samples in studies of population structure of large migratory marine species, as these harbour a treasure trove of information and can shed light on complex demographic patterns and aid in the development of accurate conservation actions at relevant geographical scales.
Sample collection
Tiger shark specimens were caught over a time-span of close to 80 years (~1939–2015). Samples originated from north-eastern and eastern Australia, extending from the Gulf of Carpentaria (GCA), through the Coral Sea (CRS) to the Tasman Sea (TAS) (Fig. 1 a). Contemporary tissue samples (2000–2015) were obtained as fin-clips from sharks caught in the Queensland Shark Control Program, the New South Wales Shark Meshing Program, commercial and recreational landings, and sharks caught for tagging and tracking research purposes 86 . Historical samples (~1939–1999) comprised dried tiger shark jaws and vertebrae obtained from museum collections, fishers, and other private or public collections. The initial dataset consisted of 115 unique sharks. As tiger sharks are long-lived and the sampled individuals were highly variable in age, we estimated the year of birth for each sample to allow for a more accurate temporal genetic comparison (Fig. 1 b). For example, a large shark sampled in 2010 could have been born the same year as a smaller shark sampled in 1990. Individual year of birth was estimated using a locally derived relationship between total length (L T ) and age. Growth rate estimation (t) was based on vertebral aging for both females and males using the Von Bertalanffy growth function (VBGF) ( 1 ) as 76 :
where L 0 and L ∞ represent the length-at-birth and theoretical asymptotic length, respectively, and k represents the growth coefficient. We assumed different parameters for males and females (males: L ∞ = 441.1 cm, k = 0.08, and L 0 = 123.4 cm; females: L ∞ = 379.9 cm, k = 0.06, and L 0 = 116.8 cm), and a combined set where information on sex was not available (L ∞ = 433.7 cm, k = 0.06, and L 0 = 121.5 cm). For individuals with only fork length (L F ) available, total length was calculated using the relationship L T = 22.607 + 1.096 L F 87 . For the 20 sharks without length information, total weight (W T ) was used to first obtain L F using a regression equation parameterized for tiger sharks in the north-western Atlantic 88 , the closest population to our target species from which there is available data (L F = ((W T /2.5281) × 10 –6 ) (1/3.2603) ).
DNA extraction and target capture
Historical tissue material was collected following the protocol described in Nielsen et al. 39 and involved the collection of “bio-swarf” produced when drilling a 3.5-mm hole in the calcified cartilage of jaws or vertebrae. Extraction of DNA from the bio-swarf and contemporary fin tissue was performed with the Bioline ISOLATE II Genomic DNA kit according to the manufacturer’s protocol, using 18–37 mg (average 27 mg) of tissue per extraction. For genomic library preparation, DNA from contemporary samples was sheared to an average fragment size of 200 bp with a M220 focused ultrasonicator (Covaris, USA). DNA from historical material was fragmented due to degradation over time and therefore used directly. Genomic-capture libraries were prepared using the KAPA Hyper Prep Kit (Kapa Biosystems, USA) according to manufacturer’s instructions. A total of 50 ng input DNA per sample was used with a one in five dilution of the TruSeq DNA HT dual-index adaptors (Illumina, USA). Ten PCR cycles for library amplification were used for the contemporary samples and twelve for the historical. Selected regions of genomic DNA were captured with a MyBaits (MYcroarray) target enrichment kit, consisting of 20.000 biotinylated RNA baits (120 bp each) developed from pancreas, liver and brain derived transcriptome sequences 89 of the small-spotted catshark ( Scyliorhinus canicula ). At the time of bait development this was the most taxonomically similar species from which a large genomic resource was available for bait design that would likely capture tiger shark transcribed regions. For more details about DNA extraction, library preparation, and bait design see Nielsen et al. 39 . All DNA samples were captured individually, using 135 ng of DNA library as input, which had previously been treated with a 1× AMPure XP beads clean-up. Hybridization capture of tiger shark DNA was conducted for 24 h at 60 °C in solution for subsequent paired-end (2 × 125 bp) sequencing on an Illumina HiSeq2000 v4. Prior to sequencing, the captured libraries were amplified using thirteen PCR cycles and purified using 0.8× AMPure XP beads. Quality Control (QC) steps were performed using a Bioanalyzer (Agilent Technologies, CA, USA), thus the final sequencing libraries could be pooled in equal nM concentrations. Samples were sequenced in two lanes. One “historical” lane consisting of 38 jaw samples and 5 fin samples, and a “contemporary” lane with 75 fin samples and 2 jaw samples. The difference in sample number per lane accounted for more variable template numbers among historical samples and thus secured a higher minimum number of sequences per individual. The lanes reciprocally included the same jaw samples that were sequenced in both lanes, similar to one of the contemporary tissue samples in the historical lane, allowing for estimation of “lane effects” and to evaluate reproducibility of multilocus genotypes through the molecular, bioinformatics and population genomics pipelines.
We customized a bioinformatics pipeline to ensure removal of potential contaminants, artefacts and low quality reads 90 before proceeding with the downstream analysis. Briefly, the de-multiplexed reads were controlled for quality using FastQC 91 . All adaptors were removed using AdapterRemoval 92 and reads were filtered by length and quality, with a minimum length of 30 bp and base quality of 28. Filtered reads were merged using FLASH 93 with default parameters, and checked for contaminants using Kraken2 94 . Both unpaired and concordantly merged reads were mapped against possible sources of contaminants (bacteria, fungi) using the Bowtie2 95 “sensitive” option. The cleaned reads were also mapped against the mitochondrial genome of tiger shark (NCBI Reference Sequence: NC_022193.1 96 ). Previous studies have shown that “off-target capture” is common, i.e. capture of genomic regions of both nuclear and mitochondrial origin not matching the baits. For example, in highly degraded samples target template DNA may not bind and amplify as well as in good quality samples and templates, resulting in more amplification of non-targeted regions of the genome 10 , 11 . Moreover, mtDNA sequences are commonly captured, or directly sequenced, due to the high copy number of mitochondrial DNA compared to nuclear DNA 82 , 97 . This phenomenon may even be desirable, as it allows assessment of mtDNA diversity. After removal of mitochondrial sequences, the reads were mapped against the transcriptome of tiger shark 45 , using the BWA-mem algorithm. This transcriptome includes 179,867 unique contigs greater than200 bp length (average 822 bp, maximum 15,892 bp). After mapping, PCR duplicates were removed using Picard-tools ( http://broadinstitute.github.io/picard/ ). We checked the patterns of DNA damage of the remaining reads, using Mapdamage2.0 98 . Coverage and depth of the target regions were estimated using Samtools 99 , and finally we called SNPs using Freebayes 100 with default parameters. The raw SNPs obtained were further filtered to keep only biallelic SNPs with quality above 30 and minimum allele count of three. Only SNPs with a maximum level of missing data of 20% were maintained. Additionally, we filtered for excess depth to reduce the possible presence of paralogs and multi-copy loci. Linkage disequilibrium (LD) between SNPs within bins of 800 bp (maximum length of the merged reads plus 150 bp each side) was estimated using the prune function in bcftools (SAMtools package), by calculating the square correlation between alleles of each pair of loci, r 2 101 and keeping only SNPs with an r 2 < 0.25. To test the reliability of our final SNPs, we compared genotypes for duplicate control samples and only maintained SNPs that matched more than 80% of the pairwise comparisons (to allow for missing data). Finally, we filtered for significant departure from HWE ( p < 0.05) to remove systematic genotyping errors. All filtering steps but the LD pruning were done using VCFtools 102 .
Back-calculated year of birth ranged between 1917 (the oldest) and 2012 (the youngest). For all downstream analysis, we grouped samples into four time periods based on their estimated decade of birth: 1910–1960, 1970–1990 and 2000, the latter comprising all contemporary samples (2000–2015). These date ranges were used as named time periods throughout the manuscript. The three periods were also associated with different catch rates in the study area, with the highest catch rate between 1960 and 1980, which significantly decreased after 2000 24 , especially in the southern part of Queensland 9 , 103 . For the temporal analysis we estimated temporal genetic differentiation using all samples (n = 106) including individuals belonging to different spatiotemporal sample groups, namely GCA_1970-1990 (n = 12), GCA_2000 (n = 14), CRS_1970-1990 (n = 7), CRS_2000 (n = 15), TAS_1910-1960 (n = 19), TAS_1970-1990 (n = 14) and TAS_2000 (n = 26). All estimations of pairwise F ST 104 between spatial and temporal samples were performed using the R package StAMPP 105 and their significance was assessed with 1,000 permutations over loci. For all FST comparisons the p-values were adjusted using a False Discovery Rate (FDR) method. A Principal Coordinates Analysis (PCoA) was applied to the pairwise F ST matrix to summarize and plot the differences reported in the table using the pcoa function in the ape 5.0 package 106 in R. We performed a Principal Component Analysis (PCA) to explore the spatial and temporal structure of individual genotypes using the R package adegenet 107 . An initial PCA of all samples revealed two identical genotypes (two types of archived tissue from the same individual) and one of them was subsequently removed. A PCA of the contemporary samples revealed two extreme outliers of which one of them was identified as another species (spinner shark; Carcharhinus brevipinna ) based on mtDNA sequences. Both samples were removed from further analysis. For the temporal estimates the dataset (n = 106) was divided into the three periods: 1910–1960 (n = 19), 1970–1990 (n = 33) and 2000 (n = 55). In order to assess current spatial genetic differentiation a subset of 74 contemporary individuals (based on year of catch) from the three different areas: GCA (n = 26), CRS (n = 21) and TAS (n = 27) were selected. In order to test for possible differences in individual heterozygosity among population groups, we used an ad-hoc R script, to calculate the proportion of heterozygous loci out of all genotyped loci for an individual, thereby accounting for differences in total number of genotyped loci (i.e. missing data) across individuals. The boxplot was realized using the ggplot2 package in R 108 to highlight the median of each cluster. The distribution of F ST across loci was plotted as a function of heterozygosity using ggplot2. Estimates of Weir and Cockerham 104 F ST and heterozygosity were obtained using VCFtools. A test for selection was performed using pcadapt 109 , with a qvalue of 0.1 as cut-off to identify putative selective outliers and bayescan 99 with parameters ‐n 5000 ‐thin 10 ‐nbp 20 ‐pilot 5000 ‐burn 50000 ‐pr_odds 100. To detect the presence of possible genetic clusters identified by the pairwise F ST analysis, a Discriminant Analysis of Principal Components (DAPC) was applied as it better detects variability and division among populations compared to other software for population structure analysis (e.g. STRUCTURE 110 ) as it is free of assumptions about any underlying population genetics model. For the DAPC we used the adegenet package in R. Since the results of a DAPC analysis can be highly affected by the number of discriminant functions used, we ran the xvalDapc function first to select the best number of discriminant functions to represent the data. The model was corrected for overfitting by using the a-score estimate, with 26 PCs retained representing the optimal trade-off between power of discrimination and over-fitting. The recommended number of PCs obtained using this approach (26) was used to run a Bayesian information criterion (BIC) analysis and the k-means algorithm to identify the most likely number of clusters that could explain the variability within our dataset. For comparison, the snmf function implemented in the LEA package 111 was also tested, since it represents a different model-based approach for clusters identification in large datasets. The value of K that best explain the data was selected using the cross-entropy criterion, with 100 repetitions for K value. A Fishers exact test was applied to pairwise comparisons between different temporal collections to determine whether any possible change in relative proportion among groups was statistically significant. Estimates of relatedness were performed using VCFtools to exclude kin sampling that could skew the estimates of genetic diversity. Finally, Site Frequency Spectrum per temporal and spatial collections were generated in ANGSD 112 to further investigate whether our results could be affected by technical biases (e.g. excess of singletons). Due to the lack of knowledge on the ancestral state, the folded version of the “realSFS” function was used to polarize the allele frequencies on the reference used to produce the vcf file (-anc -fold 1). In addition, Pi and Watterson theta were estimated for each temporal and spatial group using the “sfsr” function ( https://github.com/andrewparkermorgan/sfsr ).
Hoffman, J. I. et al. High-throughput sequencing reveals inbreeding depression in a natural population. Proc. Natl. Acad. Sci. U.S.A. 111 , 3775–3780 (2014).
Article ADS CAS PubMed PubMed Central Google Scholar
Schindler, D. E., Armstrong, J. B. & Reed, T. E. The portfolio concept in ecology and evolution. Front. Ecol. Environ. 13 , 257–263 (2015).
Article Google Scholar
Merilä, J. & Hendry, A. P. Climate change, adaptation, and phenotypic plasticity: The problem and the evidence. Evol. Appl. 7 , 1–14 (2014).
Article PubMed PubMed Central Google Scholar
Schindler, D. E. et al. Population diversity and the portfolio effect in an exploited species. Nature 465 , 609–612 (2010).
Article ADS CAS PubMed Google Scholar
Mimura, M. et al. Understanding and monitoring the consequences of human impacts on intraspecific variation. Evol. Appl. 10 , 121–139 (2017).
Article PubMed Google Scholar
Hughes, A. R., Inouye, B. D., Johnson, M. T. J., Underwood, N. & Vellend, M. Ecological consequences of genetic diversity. Ecol. Lett. 11 , 609–623 (2008).
Laikre, L. et al. Neglect of genetic diversity in implementation of the convention on biological diversity: Conservation in practice and policy. Conserv. Biol. 24 , 86–88 (2010).
Hoban, S. M. et al. Bringing genetic diversity to the forefront of conservation policy and management. Conserv. Genet. Resour. 5 , 593–598 (2013).
Dudgeon, C. L. et al. A review of the application of molecular genetics for fisheries management and conservation of sharks and rays. J. Fish Biol. 80 , 1789–1843 (2012).
Article CAS PubMed Google Scholar
Bi, K. et al. Unlocking the vault: Next-generation museum population genomics. Mol. Ecol. 22 , 6018–6032 (2013).
Article CAS PubMed PubMed Central Google Scholar
Burrell, A. S., Disotell, T. R. & Bergey, C. M. The use of museum specimens with high-throughput DNA sequencers. J. Hum. Evol. 79 , 35–44 (2015).
van der Valk, T., Díez-Del-Molino, D., Marques-Bonet, T., Guschanski, K. & Dalén, L. Historical genomes reveal the genomic consequences of recent population decline in Eastern Gorillas. Curr. Biol. 29 , 165–170 (2019).
Article PubMed CAS Google Scholar
Dulvy, N. K., Sadovy, Y. & Reynolds, J. D. Extinction vulnerability in marine populations. Fish Fish. 4 , 25–64 (2003).
Dulvy, N. K. et al. Extinction risk and conservation of the world’s sharks and rays. Elife 3 , e00590 (2014).
Fenderson, L. E., Kovach, A. I. & Llamas, B. Spatiotemporal landscape genetics: Investigating ecology and evolution through space and time. Mol. Ecol. 29 , 218–246 (2020).
Chapman, D. D., Feldheim, K. A., Papastamatiou, Y. P. & Hueter, R. E. There and back again: A review of residency and return migrations in sharks, with implications for population structure and management. Ann. Rev. Mar. Sci. 7 , 547–570 (2015).
Compagno, L. J. V. FAO species catalogue, Vol. 4. Sharks of the world. An annotated and illustrated catalogue of shark species known to date. Part 2. In Carcharhiniformes: FAO Fisheries Synopsis, 251–655 (1984).
Gaither, M. R., Bowen, B. W., Rocha, L. A. & Briggs, J. C. Fishes that rule the world: circumtropical distributions revisited. Fish Fish. 17 , 664–679 (2016).
Williams, L. E. Queensland’s Fisheries Resources: Current Condition and Recent Trends 1988–2000 (Department of Primary Industries, 2002).
Google Scholar
Macbeth, W. G., Geraghty, P. T., Peddemors, V. M. & Gray, C. A. Observer-Based Study of Targeted Commercial Fishing for Large Shark Species in Waters Off Northern New South Wales (2009).
Paterson, R. A. Effects of long-term anti-shark measures on target and non-target species in Queensland, Australia. Biol. Conserv. 52 , 147–159 (1990).
Reid, D. D., Robbins, W. D. & Peddemors, V. M. Decadal trends in shark catches and effort from the New South Wales, Australia, shark meshing program 1950–2010. Mar. Freshw. Res. 62 (62), 676–693 (2010).
Holmes, B. J. et al. Declining trends in annual catch rates of the tiger shark ( Galeocerdo cuvier ) in Queensland, Australia. Fish. Res. 129–130 , 38–45 (2012).
Roff, G., Brown, C. J., Priest, M. A. & Mumby, P. J. Decline of coastal apex shark populations over the past half century. Commun. Biol. 1 , 1–11 (2018).
C Ferreira, C. & Simpfendorfer, L. C. Tiger shark: Galeocerdo cuvier . The IUCN Red List of Threatened Species, Vol. 8235 (2019).
Baum, J. K. & Blanchard, W. Inferring shark population trends from generalized linear mixed models of pelagic longline catch and effort data. Fish. Res. 102 , 229–239 (2010).
Jabado, R. W. et al. Troubled waters: Threats and extinction risk of the sharks, rays and chimaeras of the Arabian Sea and adjacent waters. Fish Fish. 19 , 1043–1062 (2018).
Holmes, B. J. et al. Population structure and connectivity of tiger sharks ( Galeocerdo cuvier ) across the indo-pacific ocean basin. R. Soc. Open Sci. 4 , 170309 (2017).
Article ADS PubMed PubMed Central CAS Google Scholar
Pirog, A. et al. Genetic population structure and demography of an apex predator, the tiger shark Galeocerdo cuvier . Ecol. Evol. 9 , 5551–5571 (2019).
Sort, M. et al. Come together: Calibration of tiger shark ( Galeocerdo cuvier ) microsatellite databases for investigating global population structure and assignment of historical specimens. Conserv. Genet. 13 , 209 (2021).
Holland, K. N. et al. A perspective on future tiger shark research. Front. Mar. Sci. 6 , 37 (2019).
Muter, B. A., Gore, M. L., Gledhill, K. S., Lamont, C. & Huveneers, C. Australian and US news media portrayal of sharks and their conservation. Conserv. Biol. 27 , 187–196 (2013).
Kaeuffer, R., Pontier, D., Devillard, S. & Perrin, N. Effective size of two feral domestic cat populations ( Felis catus L.): Effect of the mating system. Mol. Ecol. 13 , 483–490 (2004).
Portnoy, D. S., McDowell, J. R., McCandless, C. T., Musick, J. A. & Graves, J. E. Effective size closely approximates the census size in the heavily exploited western Atlantic population of the sandbar shark, Carcharhinus plumbeus . Conserv. Genet. 10 , 1697 (2008).
Chevolot, M., Ellis, J. R., Rijnsdorp, A. D., Stam, W. T. & Olsen, J. L. Temporal changes in allele frequencies but stable genetic diversity over the past 40 years in the Irish Sea population of thornback ray, Raja clavata. Heredity 101 , 120–126 (2008).
Nei, M., Maruyama, T. & Chakraborty, R. The bottleneck effect and genetic variability in populations. Evolution 29 , 1–10 (1975).
Elizabeth Alter, S., Rynes, E. & Palumbi, S. R. DNA evidence for historic population size and past ecosystem impacts of gray whales. PNAS 104 , 15162–15167 (2007).
Article ADS PubMed CAS Google Scholar
Chehida, Y. B. et al. Harbor porpoise losing its edges: Genetic time series suggests a rapid population decline in Iberian waters over the last 30 years. BioRxiv 14 , 105. https://doi.org/10.1101/2021.08.19.456945 (2021).
Nielsen, E. E. et al. Extracting DNA from ‘jaws’: High yield and quality from archived tiger shark ( Galeocerdo cuvier ) skeletal material. Mol. Ecol. Resour. 17 , 431–442 (2017).
Ferreira, L. C. et al. Crossing latitudes—Long-distance tracking of an apex predator. PLoS ONE 10 , e0116916 (2015).
Article PubMed PubMed Central CAS Google Scholar
Heithaus, M. R., Wirsing, A. J., Dill, L. M. & Heithaus, L. I. Long-term movements of tiger sharks satellite-tagged in Shark Bay, Western Australia. Mar. Biol. 151 , 1455–1461 (2007).
Holmes, B. J. et al. Tiger shark ( Galeocerdo cuvier ) movement patterns and habitat use determined by satellite tagging in eastern Australian waters. Mar. Biol. 161 , 2645–2658 (2014).
Werry, J. M. et al. Reef-fidelity and migration of Tiger Sharks, Galeocerdo cuvier , across the Coral Sea. PLoS ONE 9 , e83249 (2014).
Stevens, J. D. & McLoughlin, K. J. Distribution, size and sex composition, reproductive biology and diet of sharks from Northern Australia. Mar. Freshw. Res. 42 , 151–199 (1991).
Swift, D. G. et al. Evidence of positive selection associated with placental loss in tiger sharks. BMC Evol. Biol. 16 , 126–126 (2016).
Brown, C. J. & Roff, G. Life-history traits inform population trends when assessing the conservation status of a declining tiger shark population. Biol. Conserv. 239 , 108230 (2019).
McGaughran, A. Effects of sample age on data quality from targeted sequencing of museum specimens: What are we capturing in time? BMC Genomics 21 , 188 (2020).
Parks, M. & Lambert, D. Impacts of low coverage depths and post-mortem DNA damage on variant calling: A simulation study. BMC Genomics 16 , 19 (2015).
Nielsen, R., Paul, J. S., Albrechtsen, A. & Song, Y. S. Genotype and SNP calling from next-generation sequencing data. Nat. Rev. Genet. 12 , 443–451 (2011).
Wang, J. Pedigree reconstruction from poor quality genotype data. Heredity 122 , 719–728 (2019).
Acuña-Marrero, D. et al. Residency and movement patterns of an apex predatory shark ( Galeocerdo cuvier ) at the galapagos marine reserve. PLoS ONE 12 , e0183669 (2017).
Meyer, C. G., Papastamatiou, Y. P. & Holland, K. N. A multiple instrument approach to quantifying the movement patterns and habitat use of tiger ( Galeocerdo cuvier ) and Galapagos sharks ( Carcharhinus galapagensis ) at French Frigate Shoals, Hawaii. Mar. Biol. 157 , 1857–1868 (2010).
Papastamatiou, Y. P. et al. Telemetry and random-walk models reveal complex patterns of partial migration in a large marine predator. Ecology 94 , 2595–2606 (2013).
Lea, J. S. E. et al. Repeated, long-distance migrations by a philopatric predator targeting highly contrasting ecosystems. Sci. Rep. 5 , 1–11 (2015).
Blaison, A. et al. Seasonal variability of bull and tiger shark presence on the west coast of Reunion Island, western Indian Ocean. Afr. J. Mar. Sci. 37 , 199–208 (2015).
Sulikowski, J. A. et al. Seasonal and life-stage variation in the reproductive ecology of a marine apex predator, the tiger shark Galeocerdo cuvier , at a protected female-dominated site. Aquat. Biol. 24 , 175–184 (2016).
Ajemian, M. J. et al. Movement patterns and habitat use of tiger sharks ( Galeocerdo cuvier ) across ontogeny in the Gulf of Mexico. PLoS ONE 15 , e0234868 (2020).
Niella, Y., Butcher, P., Holmes, B., Barnett, A. & Harcourt, R. Forecasting intraspecific changes in distribution of a wide-ranging marine predator under climate change. Oecologia 198 , 111–124 (2022).
Article ADS PubMed Google Scholar
Lowe, C. G., Wetherbee, B. M., Crow, G. L. & Tester, A. L. Ontogenetic dietary shifts and feeding behavior of the tiger shark, Galeocerdo cuvier , in Hawaiian waters. Environ. Biol. Fishes 47 , 203–211 (1996).
Als, T. D. et al. All roads lead to home: Panmixia of European eel in the Sargasso Sea. Mol. Ecol. 20 , 1333–1346 (2011).
Fitzpatrick, R. et al. A comparison of the seasonal movements of tiger sharks and green turtles provides insight into their predator-prey relationship. PLoS ONE 7 , e51927 (2012).
Jones, F. R. H. Fish Migration (Edward Arnold, 1968).
Krueck, N. C., Treml, E. A., Innes, D. J. & Ovenden, J. R. Ocean currents and the population genetic signature of fish migrations. Ecology 101 , e02967 (2020).
Lea, J. S. E. et al. Ontogenetic partial migration is associated with environmental drivers and influences fisheries interactions in a marine predator. ICES J. Mar. Sci. 75 , 1383–1392 (2018).
Phillips, N. M., Devloo-Delva, F., McCall, C. & Daly-Engel, T. S. Reviewing the genetic evidence for sex-biased dispersal in elasmobranchs. Rev. Fish. Biol. Fish. 31 , 821–841 (2021).
Hemmer-Hansen, J. et al. A genomic island linked to ecotype divergence in Atlantic cod. Mol. Ecol. 22 , 2653–2667 (2013).
Moan, A. L., Gagnaire, P.-A. & Bonhomme, F. Parallel genetic divergence among coastal-marine ecotype pairs of European anchovy explained by differential introgression after secondary contact. Mol. Ecol. 25 , 3187–3202 (2016).
Lowther-Thieleking, J. L., Archer, F. I., Lang, A. R. & Weller, D. W. Genetic differentiation among coastal and offshore common bottlenose dolphins, Tursiops truncatus , in the eastern North Pacific Ocean. Mar. Mamm. Sci. 31 , 1–20 (2015).
Bradford, R. et al. Evidence of diverse movement strategies and habitat use by white sharks, Carcharodon carcharias , off southern Australia. Mar. Biol. 167 , 96 (2020).
Vaudo, J. J. et al. Intraspecific variation in vertical habitat use by tiger sharks ( Galeocerdo cuvier ) in the western North Atlantic. Ecol. Evol. 4 , 1768–1786 (2014).
Stehfest, K. M., Patterson, T. A., Barnett, A. & Semmens, J. M. Intraspecific differences in movement, dive behavior and vertical habitat preferences of a key marine apex predator. Mar. Ecol. Prog. Ser. 495 , 249–262 (2014).
Article ADS Google Scholar
Department of Agriculture & Fisheries Queensland. QFish Online Database .
Rowling, K., Hegarty, A.-M. & Ives, M. Status of fisheries resources in NSW 2008/09. In Status of Fisheries Resources in NSW 2008/09 (2010).
Marshall, L. The Fin Blue Line: Quantifying Fishing Mortality Using Shark Fin Morphology (University of Tasmania, 2011).
Poloczanska, E. et al. Marine Climate Change in Australia: Impacts and Adaptation Responses. 2012 Report Card (CSIRO, 2012).
Poloczanska, E. S. et al. Climate change and Australian marine life. Oceanogr. Mar. Biol. 45 , 407 (2007).
Hobday, A. J. & Pecl, G. T. Identification of global marine hotspots: Sentinels for change and vanguards for adaptation action. Rev. Fish Biol. Fish. 24 , 415–425 (2014).
Gervais, C. R., Champion, C. & Pecl, G. T. Species on the move around the Australian coastline: A continental-scale review of climate-driven species redistribution in marine systems. Glob. Change Biol. 27 , 3200–3217 (2021).
Nielsen, E. E. et al. Gene-associated markers provide tools for tackling illegal fishing and false eco-certification. Nat. Commun. 3 , 1–7 (2012).
Article CAS Google Scholar
Bernard, A. M. et al. Global population genetic dynamics of a highly migratory, apex predator shark. Mol. Ecol. 25 , 5312–5329 (2016).
Ovenden, J. R., Dudgeon, C., Feutry, P., Feldheim, K. & Maes, G. E. Genetics and genomics for fundamental and applied research on Elasmobranchs. In Shark Research: Emerging Technologies and Applications for the Field and Laboratory (eds Carrier, J. C. et al. ) (CRC Press, 2019).
Johri, S., Doane, M., Allen, L. & Dinsdale, E. Taking advantage of the genomics revolution for monitoring and conservation of chondrichthyan populations. Diversity 11 , 49 (2019).
Hoffmann, A. et al. A framework for incorporating evolutionary genomics into biodiversity conservation and management. Clim. Change Responses. https://doi.org/10.1186/s40665-014-0009-x (2015).
Daily, G. C. et al. Ecosystem services: Benefits supplied to human societies by natural ecosystems. Issues Ecol. Numb. 2 , 1–16 (1997).
Ward-Paige, C. A., Keith, D. M., Worm, B. & Lotze, H. K. Recovery potential and conservation options for elasmobranchs. J. Fish Biol. 80 , 1844–1869 (2012).
Approved by the University of Queensland Animal Ethics Committee (CMS/300/08/DPI/SEAWORLD and CMS/326/11/DPI), the Department of Primary Industries and Fisheries (permit numbers 100541, 165491 and 56095) and the Department of Environment and Resource Mana.
Holmes, B. J. et al. Age and growth of the tiger shark Galeocerdo cuvier off the east coast of Australia. J. Fish Biol. 87 , 422–448 (2015).
Kneebone, J., Natanson, L. J., Andrews, A. H. & Howell, W. H. Using bomb radiocarbon analyses to validate age and growth estimates for the tiger shark, Galeocerdo cuvier , in the western North Atlantic. Mar. Biol. 154 , 423–434 (2008).
Mulley, J. F., Hargreaves, A. D., Hegarty, M. J., Heller, R. S. & Swain, M. T. Transcriptomic analysis of the lesser spotted catshark ( Scyliorhinus canicula ) pancreas, liver and brain reveals molecular level conservation of vertebrate pancreas function. BMC Genomics 15 , 1–18 (2014).
Therkildsen, N. O. & Palumbi, S. R. Practical low-coverage genomewide sequencing of hundreds of individually barcoded samples for population and evolutionary genomics in nonmodel species. Mol. Ecol. Resour. 17 , 194–208 (2017).
Andrews, S. FastQC: A Quality Control Tool for High Throughput Sequence Data (2010).
Schubert, M., Lindgreen, S. & Orlando, L. AdapterRemoval v2: Rapid adapter trimming, identification, and read merging. BMC. Res. Notes 9 , 88–88 (2016).
Magoc, T. & Salzberg, S. L. FLASH: Fast length adjustment of short reads to improve genome assemblies. Bioinformatics 27 , 2957–2963 (2011).
Wood, D. E., Lu, J. & Langmead, B. Improved metagenomic analysis with Kraken 2. Genome Biol. 20 , 257 (2019).
Langmead, B. & Salzberg, S. L. Fast gapped-read alignment with Bowtie 2. Nat. Methods 9 , 357–359 (2012).
Chen, X., Yu, J., Zhang, S., Ding, W. & Xiang, D. Complete mitochondrial genome of the tiger shark Galeocerdo cuvier (Carcharhiniformes: Carcharhinidae). Mitochondrial DNA 25 , 441–442 (2014).
Picardi, E. & Pesole, G. Mitochondrial genomes gleaned from human whole-exome sequencing. Nat. Methods 9 , 523–524 (2012).
Jónsson, H., Ginolhac, A., Schubert, M., Johnson, P. L. F. & Orlando, L. MapDamage2.0: Fast approximate Bayesian estimates of ancient DNA damage parameters. Bioinformatics 29 , 1682–1684 (2013).
Li, H. et al. The sequence alignment/map format and SAMtools. Bioinformatics 25 , 2078–2079 (2009).
Garrison, E. & Marth, G. Haplotype-Based Variant Detection from Short-Read Sequencing (2012).
Hill, W. G. & Robertson, A. Linkage disequilibrium in finite populations. Theor. Appl. Genet. 38 , 226–231 (1968).
Danecek, P. et al. The variant call format and VCFtools. Bioinformatics 27 , 2156–2158 (2011).
Simpfendorfer, C. A., de Jong, S. K. and Sumpton, W. Long-term trends in large shark populationsfrom inshore areas of the Great Barrier Reef World Heritage Area: Results from the Queensland SharkControl Program. In Marine and Tropical Sciences Research Facility Transition Program Report (2010).
Weir, B. S. & Cockerham, C. C. Estimating F-statistics for the analysis of population structure. Evolution 38 , 1358–1358 (1984).
CAS PubMed Google Scholar
Pembleton, L. W., Cogan, N. O. I. & Forster, J. W. StAMPP: An R package for calculation of genetic differentiation and structure of mixed-ploidy level populations. Mol. Ecol. Resour. 13 , 946–952 (2013).
Paradis, E. & Schliep, K. ape 5.0: An environment for modern phylogenetics and evolutionary analyses in R. Bioinformatics 35 , 526–528 (2019).
Jombart, T. adegenet: A R package for the multivariate analysis of genetic markers. Bioinformatics 24 , 1403–1405 (2008).
Wickham, H. et al. ggplot2: Elegant Graphics for Data Analysis. https://ggplot2.tidyverse.org (Springer-Verlag, New York, 2016).
Luu, K., Bazin, E. & Blum, M. G. B. pcadapt: An R package to perform genome scans for selection based on principal component analysis. Mol. Ecol. Resour. 17 , 67–77 (2017).
Jombart, T., Devillard, S. & Balloux, F. Discriminant analysis of principal components: A new method for the analysis of genetically structured populations. BMC Genet. 11 , 1–15 (2010).
Frichot, E. & François, O. LEA: An R package for landscape and ecological association studies. Methods Ecol. Evol. 6 , 925–929 (2015).
Korneliussen, T. S., Albrechtsen, A. & Nielsen, R. ANGSD: Analysis of next generation sequencing data. BMC Bioinform. 15 , 1–13 (2014).
Download references
Acknowledgements
The “Genojaws” project was part funded by the Danish Council for Independent Research Grant DFF—6108-00583 and Australian Research Grant DP170102043. We thank the representative of the following institutes and museums that assisted with the sample collections: John West (Taronga Zoo), Sophia Smiley (Waverley Library), Mark McGrouther (Australian Museum), Jeff Johnson (Queensland Museum) and Dennis Reid (formerly New South Wales Department of Primary Industries). We also wish to thank the recreational anglers that permitted us to sample their jaws, or helped us locate other samples: Cameron Baber, Mike Baber, Ivan Bennett, Mark Curtis, Brett Deeney, Neil Grieves, Mark Henrickson, Ken Hoff, Gary Hoff, Leanne Hoff, Gus Johnson, Ed Vander Kruk, David Litchfield, Stuart Lyall, John McIntyre, Mark Mikkelsen, Greg Miles, Brad Page, Nigel Rushworth, Peter Silcock, Mark Stevenson, Terry Titchener, Dave Tubman, Steve Viney, Jamie Ward, Grahame Williams, Karen Wright and David Yiend. Finally, we wish to thank Danielle Davenport and Camilla Christensen for valuable discussions and insights throughout the course of this study.
Author information
Authors and affiliations.
National Institute of Aquatic Resources, Technical University of Denmark, Vejlsøvej 39, 8600, Silkeborg, Denmark
Alice Manuzzi, Belen Jiménez-Mena, Romina Henriques & Einar E. Nielsen
School of Science, Technology & Engineering, University of the Sunshine Coast, Sippy Downs, QLD, 4556, Australia
Bonnie J. Holmes
Pepperell Research and Consulting, PO Box 1475, Noosaville DC, QLD, 4566, Australia
Julian Pepperell
Queensland Brain Institute, The University of Queensland, Brisbane, QLD, 4072, Australia
Janette Edson
School of Biomedical Sciences, The University of Queensland, Brisbane, QLD, 4072, Australia
Mike B. Bennett & Einar E. Nielsen
College of Science and Engineering, Flinders University, Adelaide, SA, 5001, Australia
Charlie Huveneers
Molecular Fisheries Laboratory, School of Biomedical Sciences, The University of Queensland, Brisbane, QLD, 4072, Australia
Jennifer R. Ovenden
You can also search for this author in PubMed Google Scholar
Contributions
E.E.N. and J.R.O. conceived of the study. E.E.N., J.P. and M.B. conducted the field work. A.M., B.J.M., R.H. and E.E.N. wrote the manuscript with input from all other authors. E.E.N., J.R.O. and J.E. conducted the lab work. A.M. conducted all bioinformatics and population genomics analyses with help from R.H. and B.J.M. Input regarding the biology of the species, fisheries data and estimates of length/age were provided by B.H., M.B. J.P. and C.H.
Corresponding author
Correspondence to Alice Manuzzi .
Ethics declarations
Competing interests.
The authors declare no competing interests.
Additional information
Publisher's note.
Springer Nature remains neutral with regard to jurisdictional claims in published maps and institutional affiliations.
Supplementary Information
Supplementary information., rights and permissions.
Open Access This article is licensed under a Creative Commons Attribution 4.0 International License, which permits use, sharing, adaptation, distribution and reproduction in any medium or format, as long as you give appropriate credit to the original author(s) and the source, provide a link to the Creative Commons licence, and indicate if changes were made. The images or other third party material in this article are included in the article's Creative Commons licence, unless indicated otherwise in a credit line to the material. If material is not included in the article's Creative Commons licence and your intended use is not permitted by statutory regulation or exceeds the permitted use, you will need to obtain permission directly from the copyright holder. To view a copy of this licence, visit http://creativecommons.org/licenses/by/4.0/ .
Reprints and permissions
About this article
Cite this article.
Manuzzi, A., Jiménez-Mena, B., Henriques, R. et al. Retrospective genomics highlights changes in genetic composition of tiger sharks ( Galeocerdo cuvier ) and potential loss of a south-eastern Australia population. Sci Rep 12 , 6582 (2022). https://doi.org/10.1038/s41598-022-10529-w
Download citation
Received : 16 March 2021
Accepted : 06 April 2022
Published : 21 April 2022
DOI : https://doi.org/10.1038/s41598-022-10529-w
Share this article
Anyone you share the following link with will be able to read this content:
Sorry, a shareable link is not currently available for this article.
Provided by the Springer Nature SharedIt content-sharing initiative
By submitting a comment you agree to abide by our Terms and Community Guidelines . If you find something abusive or that does not comply with our terms or guidelines please flag it as inappropriate.
Quick links
- Explore articles by subject
- Guide to authors
- Editorial policies
Sign up for the Nature Briefing newsletter — what matters in science, free to your inbox daily.

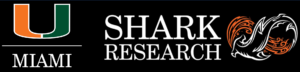
Peer-Reviewed Journal Publications
Gallagher, A.J., Brownscombe, J.W., Alsudairy, N.A., Casagrande, A.B., Fu, C., Harding, L., Harris, S.D., Hammerschlag, N., Howe, W., & Huertas, A.D., et al. (2022). Tiger sharks support the characterization of the world’s largest seagrass ecosystem. Nat Commun 13, 6328. https://doi.org/10.1038/s41467-022-33926-1; https://www.nature.com/articles/s41467-022-33926-1?fbclid=PAAaZFRW4hLDbC1OP-Lkitw3ld2labXK913Bd-sjMuYcdxHi4zG0SOiu78ajk
Bowlby HD, Hammerschlag N, Irion DT and Gennari E (2022) How continuing mortality affects recovery potential for prohibited sharks: The case of white sharks in South Africa. Frontiers in Conservation Science 3:988693. doi: 10.3389/fcosc.2022.988693; https://www.frontiersin.org/articles/10.3389/fcosc.2022.988693/full
Irschick DJ, Christiansen F, Hammerschlag N, Martin J, Madsen P, Wyneken J, Brooks A, Gleiss A, Fossette S, Siler C, Gamble T, et al. (2022) 3D Visualization Processes for Recreating and Studying Organismal Form. iScience ; 25(9): 104867; https://www.cell.com/iscience/fulltext/S2589-0042(22)01139-7
Andrzejaczek S, Lucas TC, Goodman MC, Hussey NE, Armstrong AJ, Carlisle A, Coffey DM, Gleiss AC, Huveneers C, Jacoby DM, Meekan MG…Hammerschlag N, et al. (2022) Diving into the vertical dimension of elasmobranch movement ecology. Science Advances ; 8(33):eabo1754. https://www.science.org/doi/full/10.1126/sciadv.abo1754
Lubitz N, Bradley M, Sheaves M, Hammerschlag N, Daly R, Barnett A. (2022). The role of context in elucidating drivers of animal movement. Ecology and Evolution , 12, e9128. https://doi.org/10.1002/ece3.9128
McClain MA, Hammerschlag N, Gallagher AJ, Drymon JM, Grubbs RD, Guttridge TL, Smukall MJ, Frazier BS, Daly-Engel TS (2022) Age-Dependent Dispersal and Relatedness in Tiger Sharks ( Galeocerdo cuvier ). Front. Mar. Sci. 9:900107. doi: 10.3389/fmars.2022.900107
Hammerschlag N, Gutowsky LFG, Rider MJ, Roemer R, Gallagher AJ (2022) Urban sharks: residency patterns of marine top predators in relation to a coastal metropolis. Marine Ecology Progress Series 691:1-17. https://doi.org/10.3354/meps14086
Griffin LP, Casselberry GA, Lowerre‐Barbieri SK, Acosta A, Adams AJ, Cooke SJ, Filous A, Friess C, Guttridge TL, Hammerschlag N, Morley D, Rider MJ, Skomal GB, Smukall MJ, Danylchuk AJ, Brownscombe JW. (2022) Predator–prey landscapes of large sharks and game fishes in the Florida Keys. Ecological Applications :e2584. https://esajournals.onlinelibrary.wiley.com/doi/abs/10.1002/eap.2584
Talwar BS, Bradley D, Berry C, Bond ME, Bouyoucos IA, Brooks AML, Fields KYA, Gallagher AJ, Guttridge TL, Guttridge AE, Hammerschlag N, et al. (2022) Estimated life-history traits and movements of the Caribbean reef shark ( Carcharhinus perezi ) in The Bahamas based on tag-recapture data. Marine Biology 169, 55: https://doi.org/10.1007/s00227-022-04044-9
Rangel BS, Hammerschlag N, Martinelli LA, Moreira RG. (2022) Effects of urbanization on the nutritional ecology of a highly active coastal shark: Preliminary insights from trophic markers and body condition. Science of The Total Environment . https://doi.org/10.1016/j.scitotenv.2022.154082
Jorgensen SJ, Micheli F, White TD, Van Houtan KS, Alfaro-Shigueto J, Andrzejaczek S, Arnoldi NS, Baum JK, Block B, Britten GL, Butner C, Cardeñosa D, Chapple TK, Clarke D, Cortés E, Dulvy NK, Fowler S, Gallagher AJ, Gilman E, Godley BJ, Graham RT, Hammerschlag N, et al. (2022) Emergent research and priorities for shark and ray conservation. Endangered Species Research . 47:171-203. https://www.int-res.com/abstracts/esr/v47/p171-203/
Verkamp H, Hammerschlag N, Quinlan J, Langan J, Sulikowski J. (2022) Preliminary investigation of reproductive hormone profiles in the blacktip shark ( Carcharhinus limbatus) , a placental viviparous species, in southern Florida. Marine and Freshwater Research . doi:10.1071/MF21235
Hammerschlag N, McDonnell LH, Rider MJ, Street GM, Hazen EL, Natanson LJ, McCandless CT, Boudreau MR, Gallagher A J, Pinsky ML, Kirtman B (2022). Ocean warming alters the distributional range, migratory timing, and spatial protections of an apex predator, the tiger shark ( Galeocerdo cuvier ). Global Change Biology , 00, 1–16. https://doi.org/10.1111/gcb.16045
Black C, Merly L, Hammerschlag N. (2021) Bacterial communities in multiple tissues across the body surface of three coastal shark species. Zoological Studies 60:69. doi:10.6620/ZS.2021.60-69 .
Williams LH, Anstett A, Bach Muñoz V, Chisholm J, Fallows C, Green JR, Higuera Rivas JE, Skomal G., Winton M, Hammerschlag N. (2021) Sharks as exfoliators: widespread chafing between marine organisms suggests an unexplored ecological role. Ecology, e03570. https://doi.org/10.1002/ecy.3570
Bates AE, Primack RB, Duarte CM, and PAN-Environment Working Group (including Hammerschlag N, Rider MJ, Albano PS) (2021). Global COVID-19 lockdown highlights humans as both threats and custodians of the environment. Biological Conservation , (263). 109175. https://doi.org/10.1016/j.biocon.2021.109175.
Rider MJ, Kirsebom OS, Gallagher AJ, Staaterman E, Ault JS, Sasso CR, Jackson T, Browder JA, Hammerschlag N. (2021) Space use patterns of sharks in relation to boat activity in an urbanized coastal waterway. Marine Environmental Research . 172, 105489 https://doi.org/10.1016/j.marenvres.2021.105489 .
Calich HJ, Rodríguez JP, Eguíluz VM, Hammerschlag N, Pattiaratchi C, Duarte CM, Sequeira AM. (2021) Comprehensive analytical approaches reveal species-specific search strategies in sympatric apex predatory sharks. Ecography https://doi.org/10.1111/ecog.05953
Jacoby DM, Fairbairn BS, Frazier BS, Gallagher AJ, Heithaus MR, Cooke SJ, Hammerschlag N. (2021) Social network analysis reveals the subtle impacts of tourist provisioning on the social behaviour of a generalist marine apex predator. Frontiers in Marine Science, 1202. https://doi.org/10.3389/fmars.2021.665726
Albano PS, Fallows C, Fallows M, Sedgwick O, Schuitema O, Bernard ATF, Hammerschlag N. (2021) Successful parks for for sharks: no-take marine reserve provides conservation benefits to endemic and threatened sharks off South Africa ; Biological Conservation; https://doi.org/10.1016/j.biocon.2021.109302
Lowerre‐Barbieri SK, Friess C, Griffin LP, Morley D, Skomal GB, Bickford JW, Hammerschlag N, Rider MJ, Smukall MJ, van Zinnicq Bergmann MP, Guttridge TL, t al. (2021) Movescapes and eco‐evolutionary movement strategies in marine fish: Assessing a connectivity hotspot. Fish and Fisheries; https://doi.org/10.1111/faf.12589
Rangel BS, Hammerschlag N, Sulikowski JA, Moreira RG. (2021) Physiological markers suggest energetic and nutritional adjustments in male sharks linked to reproduction. Oecologia. https://doi.org/10.1007/s00442-021-04999-4
Queiroz N, et al. (2021) Reply to: Caution over use of ecological big-data for conservation. Nature 595, E20-E28. https://www.nature.com/articles/s41586-021-03464-9
Queiroz N, et al. (2021) Reply to: Shark mortality cannot be assessed by fishery overlap alone. Nature 595, E8-E16. https://www.nature.com/articles/s41586-021-03397-3
Tinari AM, Hammerschlag N. (2021) An ecological assessment of large coastal shark communities in South Florida. Ocean & Coastal Management, 211(1):105772. https://doi.org/10.1016/j.ocecoaman.2021.105772 .
Gutowsky LF, Rider M, Roemer RP, Gallagher AJ, Heithaus MR, Cooke SJ, Hammerschlag N. (2021) Large sharks exhibit varying behavioral responses to major hurricanes. Estuarine, Coastal and Shelf Science, 256(9):107373. https://doi.org/10.1016/j.ecss.2021.107373
Sequeira AM, O’Toole M, Keates TR, McDonnell LH, Braun CD, Hoenner X, Jaine FR, Jonsen ID, Newman P, Pye J, Bograd SJ, et al. (2021) A standardisation framework for bio‐logging data to advance ecological research and conservation. Methods in Ecology and Evolution, 12(6):996-1007. https://doi.org/10.1111/2041-210X.13593
Wosnick N, Niella Y, Hammerschlag N, Chaves AP, Hauser-Davis RA, da Rocha RC, Jorge MB, de Oliveira RW, Nunes JL. (2021) Negative metal bioaccumulation impacts on systemic shark health and homeostatic balance. Marine Pollution Bulletin, 168(3):112398. https://doi.org/10.1016/j.marpolbul.2021.112398
Rangel BS, Hammerschlag N, Sulikowski JA, Moreira RG (2021) Dietary and reproductive biomarkers in a generalist apex predator reveal differences in nutritional ecology across life stages. Marine Ecology Progress Series, 664:149-163 . https://doi.org/10.3354/meps13640
Friess C, Lowerre-Barbieri SK, Poulakis GR, Hammerschlag N, et al. (2021) Regional-scale variability in the movement ecology of marine fishes revealed by an integrative acoustic tracking network. Friess et al. 2021_egional-scale variability in the movement ecology of marine fishes . Marine Ecology Progress Series, 663:157-177. https://doi.org/10.3354/meps13637
de Sousa Rangel B, Moreira RG, Niella YV, Sulikowski JA, Hammerschlag N. (2021) Metabolic and nutritional condition of juvenile tiger sharks exposed to regional differences in coastal urbanization . Science of The Total Environment, 780:146548. https://doi.org/10.1016/j.scitotenv.2021.146548
Rider MJ, McDonnell LH, Hammerschlag N. (2021) Multi-year movements of adult and subadult bull sharks (Carcharhinus leucas): philopatry, connectivity, and environmental influences. Aquatic Ecology, 55(3). https://doi.org/10.1007/s10452-021-09845-6
de Sousa Rangel B, Hammerschlag N, Moreira RG (2021) Urban living influences the nutritional quality of a juvenile shark species. Science of The Total Environment, 776:146025. https://doi.org/10.1016/j.scitotenv.2021.146025
Gallagher AJ, Shipley ON, van Zinnicq Bergmann MPM, Brownscombe JW, Dahlgren CP, Frisk MG, Griffin LP, Hammerschlag N, Kattan S, Papastamatiou YP, Shea BD, Kessel ST, Duarte CM (2021) Spatial Connectivity and Drivers of Shark Habitat Use Within a Large Marine Protected Area in the Caribbean, The Bahamas Shark Sanctuary. Frontiers in Marine Science, 7:608848. https://doi.org/10.3389/fmars.2020.608848
Shipley ON, Lee CS, Fisher NS, Sternlicht JK, Kattan S, Staaterman ER, Hammerschlag N, Gallagher AJ (2021) Metal concentrations in coastal sharks from The Bahamas with a focus on the Caribbean Reef shark. Scientific Reports, 11(218). https://doi.org/10.1038/s41598-020-79973-w
McDonnell LH, Jackson TL, Burgess GH, Phenix L, Gallagher AJ, Albertson H, Hammerschlag N, Browder JA. (2020) Saws and the city: smalltooth sawfish Pristis pectinata encounters, recovery potential, and research priorities in urbanized coastal waters off Miami, Florida, USA. Endangered Species Research, 43:543-53. https://doi.org/10.3354/esr01085
Moorhead SG, Gallagher AJ, Merly L, Hammerschlag N. (2020) Variation of body condition and plasma energy substrates with life stage, sex, and season in wild‐sampled nurse sharks Ginglymostoma cirratum. Journal of Fish Biology, 98(3):680-693. https://doi.org/10.1111/jfb.14612
Morgan A, Calich C, Sulikowski J, Hammerschlag N. (2020) Evaluating spatial management options for tiger shark (Galeocerdo cuvier) conservation in US Atlantic Waters. ICES Journal of Marine Science , 77(7-8):3095-3109. https://doi.org/10.1093/icesjms/fsaa193
Skubel RA, Wilson K, Papastamatiou YP, Verkamp HJ, Sulikowski JA, Benetti D, Hammerschlag N. (2020) A scalable, satellite-transmitted data product for monitoring high-activity events in mobile aquatic animals. Animal Biotelemetry, 8(1). https://doi.org/10.1186/s40317-020-00220-0
Ajemian MJ, Drymon JM, Hammerschlag N, Wells RJD, Street G, Falterman B, et al. (2020) Movement patterns and habitat use of tiger sharks ( Galeocerdo cuvier ) across ontogeny in the Gulf of Mexico. PLoS ONE, 15(7):e0234868. https://doi:10.1371/journal.pone.0234868
Cartolano MC, Berenshtein I, Heuer RM, Pasparakis C, Rider M, Hammerschlag N, Paris CB, Grosell M, McDonald MD. (2020) Impacts of a local music festival on fish stress hormone levels and the adjacent underwater soundscape. Environmental Pollution, 265(Pt A):114925. https://doi.org/10.1016/j.envpol.2020.114925
Cooke SJ, J, Bergman JN, Nyboer EA, Reid AJ, Gallagher AJ, Hammerschlag N, Van de Riet K, Vermaire JC (2020) Overcoming the concrete conquest of aquatic ecosystems. Biological Conservation, 247:108589. https://doi.org/10.1016/j.biocon.2020.108589
Gallagher AJ, Amon DJ, Bervoets T, Shipley ON, Hammerschlag N, Sims DW, (2020) The Caribbean needs big marine protected areas . Science, 367(6479):749.
AtallahBenson L, Merly L, Cray C, Hammerschlag N (2020) Serum protein analysis of nurse sharks. Journal of Aquatic Animal Health, 32(2):77-82. https://doi.org/10.1002/aah.10100
Bohaboy EC, Guttridge TL, Hammerschlag N, Van Zinnicq Bergmann MP, Patterson WF. (2019) Application of three-dimensional acoustic telemetry to assess the effects of rapid recompression on reef fish discard mortality. ICES Journal of Marine Science, 77(1):83-96. https://doi.org/10.1093/icesjms/fsz202
Flecker AS, Twining CW, Schmitz OJ, Cooke SJ, Hammerschlag N. (2019) Aquatic Predators Influence Micronutrients: Important but Understudied. Trends in Ecology & Evolution , 34(10):882-883. https://doi.org/10.1016/j.tree.2019.07.006
Shipley ON, Gallagher AJ, Shiffman DS, Kaufman L, Hammerschlag N. (2019) Diverse resource-use strategies in a large-bodied marine Predator guild: evidence from differential use of resource subsidies and intraspecific isotopic variation. Marine Ecology Progress Series , 623:71-83. https://doi.org/10.3354/meps12982
Queiroz N, et al. (2019) Global spatial risk assessment of sharks under the footprint of fisheries. Nature , 572:461-466. https://doi.org/10.1038/s41586-019-1444-4
Hammerschlag N (2019) Quantifying shark predation effects on prey: dietary data limitations and study approaches. Endangered Species Research, 38:147–151. https://doi.org/10.3354/esr00950
Shiffman DS, Heithaus MR, Kaufman L, Hammerschlag N (2019) Intraspecific differences in relative isotopic niche area and overlap of co-occurring sharks. Aquatic Ecology, 53(2):233-250. doi: 10.1007/s10452-019-09685-5
Martin KL, Abel DC, Crane D, Hammerschlag N, Burge EJ. (2019) Blacktip shark Carcharhinus limbatus presence at fishing piers in South Carolina: fidelity and environmental drivers. Journal of Fish Biology , 94(3):469-480. https://doi.org/10.1111/faf.12358
Merly L, Lange L, Meÿer M, Hewitt AM, Koen P, Fischer C, Muller J, Schilack V, Wentzel M, Hammerschlag N. (2019) Blood plasma levels of heavy metals and trace elements in white sharks (Carcharodon carcharias) and potential health consequences. Marine Pollution Bulletin , 142(4):85-92. https://doi.org/10.1016/j.marpolbul.2019.03.018
Hays GC, Bailey H, Bograd SJ, Bowen WD, Campagna C, Carmichael RH, Casale P, Chiaradia A, Costa DP, Cuevas E, Bruyn PJND, Dias MP, Duarte CM, Dunn DC, Dutton PH, Esteban N, Friedlaender A, Goetz KT, Godley BJ, Halpin PN, Hamann M, Hammerschlag N, et al. (2019) Translating Marine Animal Tracking Data into Conservation Policy and Management. Trends in Ecology & Evolution, 34(5):459-473. https://doi.org/10.1016/j.tree.2019.01.009
Hammerschlag N, Schmitz OJ, Flecker AJ, Lafferty KD, Sih A, Atwood T, Gallagher AJ, Irschick DJ, Skubel R, Cooke SJ. (2019) Ecosystem Function and Services of Aquatic Predators in the Anthropocene. Trends in Ecology & Evolution , 34(4):369-383. https://doi.org/10.1016/j.tree.2019.01.005
Hammerschlag N, Williams L, Fallows M, Fallows C. (2019) Disappearance of white sharks leads to the novel emergence of an allopatric apex predator, the sevengill shark. Scientific Reports, 9(1):1908. https://doi.org/10.1038/s41598-018-37576-6
Rooker JR, Dance MA, Wells RJD, Ajemian MJ, Block BA, Castelton MR, Drymon JM, Falterman BJ, Franks JS, Hammerschlag N, Hoffmaer ER, Kraus RT, McKinney JA, Secor DH, Stunz GW, Walter JF. (2019) Population connectivity of pelagic megafauna in the Cuba-Mexico-U.S. triangle. Scientific Reports, 9(1):1663. https://doi.org/10.1038/s41598-018-38144-8
Wosnick N, Niella YV, Navas CA, Monteiro-Fiho ELA, Freire CA, Hammerschlag N. (2019) Multispecies thermal dynamics of air-exposed ectothermic sharks and its implications for fisheries conservation. Journal of Experimental Marine Biology & Ecology , 513(1):1-9. https://doi.org/10.1016/j.jembe.2019.01.002
Enchelmaier AC, Babcock EA, Hammerschlag N. (2018) Survey of fishes within a restored mangrove habitat of a subtropical bay . Estuarine Coastal and Shelf Science, 244:106021. https://doi.org/10.1016/j.ecss.2018.11.009
Calich H, Estevanez M, Hammerschlag N. (2018) Overlap between highly suitable habitats and longline gear management areas reveals vulnerable and protected regions for highly migratory sharks. Marine Ecology Progress Series , 602:183-195. https://doi.org/10.3354/meps12671
Wosnick N, Navas CA, Niella YV, Monteiro-Filho ELA, Freire CA, Hammerschlag N. (2018) Thermal Imaging Reveals Changes in Body Surface Temperatures of Blacktip Sharks (Carcharhinus limbatus) during Air Exposure. Physiological and Biochemical Zoology , 91(5):1005-1012. https://doi.org/10.1086/699484
Glynn, P.W., Coffman, B., Primov, K.D., Moorhead, S.G., Vanderwoude, J., Barrales, R.N., Williams, M.K. and Roemer , R.P., (2018) Benthic ctenophores (Platyctenida: Coeloplanidae) in South Florida: predator–prey interactions. Invertebrate Biology .
Hammerschlag N, Skubel RA, Sulikowski J, Irschick DJ, Gallagher AJ. (2018) A Comparison of Reproductive and Energetic States in a Marine Apex Predator (the Tiger Shark, Galeocerdo cuvier). Physiological and Biochemical Zoology , 91(4):933-942. https://10.1086/698496
Sequeira AMM, Rodríguez JPP, Eguíluz VM, Harcourt R, Hindell M, Sims DW, Duarte CW, Costa DP, Fernández-Gracia J, Ferreira LC, Hays GC, Heupel MR, Meekan MG, Aven A, Bailleul F, Baylis AMM, Berumen ML, Braun CD, Burns J, Caley MJ, Campbell R, Carmichael RH, Clua E, Einoder LD, Friedlaender A, Goebel ME, Goldsworthy SD, Guinet C, Gunn J, Hamer D, Hammerschlag N, Hammill M, Hückstädt LA, Humphries NE, Lea MA, Lowther A, Mackay A, McHuron E, McKenzie J, McLeay L, McMahon CR, Mengersen K, Muelbert MMC, Pagano AM, Page B, Queiroz N, Robinson PW, Shaffer SA, Shivji M, Skomal GB, Thorrold SR, Villegas-Amtmann S, Weise M, Wells R, Wetherbee B, Wiebkin A, Wienecke B, Thums M. (2018). Convergence of marine megafauna movement patterns in coastal and open oceans. Proceedings of the National Academy of Sciences , 115(12). https://doi.org/10.1073/pnas.1716137115
Skubel RA, Kirtman BP, Fallows C, Hammerschlag N (2018) Patterns of long-term climate variability and predation rates by a marine apex predator, the white shark Carcharodon carcharias . Marine Ecology Progress Series , 587:129-139.
Hammerschlag N, Barley SC, Irschick DJ, Meeuwig JJ, Nelson ER, Meekan MG (2018) Predator declines and morphological changes in prey: evidence from coral reefs depleted of sharks. Marine Ecology Progress Series , 586:127-139. https://doi.org/10.3354/meps12426
Hammerschlag N, Meÿer M, Seakamela S M, Kirkman S, Fallows C, Creel S. (2017) Physiological stress responses to natural variation in predation risk: evidence from white sharks and seals. Ecology , 98(12):3199–3210. https://doi.org/10.1002/ecy.2049
Jerome JM, Gallagher AJ, Cooke SJ, Hammerschlag N. (2017) Integrating reflexes with physiological measures to evaluate coastal shark stress response to capture. ICES Journal of Marine Science , 75(2):796-804. https://doi.org/10.1093/icesjms/fsx191
Macdonald C, Gallagher AJ, Barnett A, Brunnschweiler J, Shiffman DS, Hammerschlag N. (2017) Conservation potential of apex predator tourism. Biological Conservation , 215: 132-141. https://doi.org/10.1016/j.biocon.2017.07.013
Klimley AP, Flagg M, Hammerschlag N, Hearn A. (2017) The value of using measurements of geomagnetic field in addition to irradiance and sea surface temperature to estimate geolocations of tagged aquatic animals. Animal Biotelemetry , 5:19. DOI: 10.1186/s40317-017-0134-y
Glynn PW, Coffman B, Fuller MPC, Moorhead SG, Williams MK, Primov KD, Fortson TN, Barrales RN, Glynn PJ. (2017) Benthic ctenophores (Platyctenida: Coeloplanidae) in south Florida: environmental conditions, habitats, abundances, and behaviors. Invertebrate Biology x(x): 1-15. https://doi.org/10.1111/ivb.12189
Acuña-Marrero D, Smith ANH, Hammerschlag N, Hearn A, Anderson MJ, Calich H, et al. (2017) Residency and movement patterns of an apex predatory shark (Galeocerdo cuvier) at the Galapagos Marine Reserve. PLoS ONE , 12(8). https://doi.org/10.1371/journal.pone.0183669
Irschick DJ, Fu A, Lauder G, Wilga C, Kuo C-Y, Hammerschlag N. (2017) A comparative morphological analysis of body and fin shape for eight shark species. Biological Journal of the Linnean Society , 122(3):1-16. https://doi.org/10.1093/biolinnean/blx088
Shiffman DS, Macdonald C, Ganz HY, Hammerschlag N. (2017) Fishing practices and representations of shark conservation issues among users of a land-based shark angling online forum. Fisheries Research , 196: 13-26. https://doi.org/10.1016/j.fishres.2017.07.031
Kough AS, Cronin H, Skubel R, Belak CA, Stoner AW. (2017) Efficacy of an established marine protected area at sustaining a queen conch Lobatus gigaspopulation during three decades of monitoring. Mar Ecol Prog Ser 573:177-189. https://doi.org/10.3354/meps12163
Hammerschlag N, Gutowsky LFG, Gallagher AJ, Matich P, Cooke SJ. (2017) Diel habitat use patterns of a marine apex predator (tiger shark, Galeocerdo cuvier) at a high use area exposed to dive tourism. Journal of Experimental Marine Biology and Ecology , 495(2): 24-34. https://doi.org/10.1016.j.jembe.2017.05.010
Hammerschlag N, Gallagher AJ. (2017) Extinction Risk and Conservation of the Earth’s National Animal Symbols. BioScience , 67(8): 744-749. https://doi.org/10.1093/biosci/bix054
Gallagher AJ, Shiffman DS, Byrnes EE, Hammerschlag-Peyer CM, Hammerschlag N. (2017) Patterns of resource use and isotopic niche overlap among three species of sharks occurring within a protected subtropical estuary. Aquatic Ecology , 51(1):435-448. DOI: 10.1007/s10452-017-9627-2
Cooke SJ, Gallagher AJ, Sopinka NM, Nguyen VM, Skubel RA, Hammerschlag N, Boon S, Young N, and Danylchuk AJ (2017) Considerations for effective science communication. FACETS , 2(1):233-248. https://doi.org/10.1139/facets-2016-0055
Gallagher AJ, Skubel RA, Pethybridge HR, Hammerschlag N. (2017) Energy metabolism in mobile, wild-sampled sharks inferred by plasma lipids. Conservation Physiology , 5(1). https://doi.org/10.1093/conphys/cox002
Matulik AG, Kerstetter DW, Hammerschlag N, Divoll T, Hammerschmidt CR, Evers DC. (2017) Bioaccumulation and biomagnification of mercury and methylmercury in four sympatric coastal sharks in a protected subtropical lagoon. Marine Pollution Bulletin , 116(1):357-364. https://doi.org/10.1016/j.marpolbul.2017.01.033
Hammerschlag N, Meyer C, Grace M, Kessel S, Sutton T, Harvey E, Paris C, Kerstetter D, Cooke SJ. (2017) Shining a Light on Fish at Night: an overview of patterns and processes operating in fish and fisheries at night and in the perpetual darkness of deep and polar seas. Bulletin of Marine Science -Miami- , 93(2): 253-284. https://doi.org/10.5345/bms.2016.1082
Hammerschlag N, Skubel R, Calich H, Nelson ER, Shiffman DS, Wester J, Macdonald C, Cain S, Jennings L, Enchaelmaier A, Gallagher AJ. (2017) Nocturnal and crepuscular behavior in elasmobranchs: a review of movement, habitat use, foraging, and reproduction in the dark. Bulletin of Marine Science -Miami- , 93(2):355-374.
Rangel BdS, Wosnick N, Hammerschlag N, Ciena AP, Kfoury Junior JR. Rici REG (2016) A preliminary investigation into the morphology of oral papillae and denticles of blue sharks (Prionace glauca) with inferences about its functional significance across life stages. Journal of Anatomy , 230(3):389-397. https://doi.org/10.1111/joa.12574
Gallagher AJ, Hammerschlag N, Danylchuk AJ, Cooke SJ. (2016) Shark recreational fisheries: Status, challenges, and research needs. Ambio; 1-14.
Gallagher AJ, Staaterman ER, Cooke SJ, Hammerschlag N. (2016) Behavioral responses to fisheries capture among various shark species reveal mechanisms of species-specific sensitivity. Canadian Journal of Fisheries and Aquatic Sciences; 74(1): 1-7.
Fallows C, Fallows M, Hammerschlag N. (2016) Effects of lunar phase on predator-prey interactions between white shark (Carcharodon carcharias) and Cape fur seals (Arctocephalus pusillus pusillus). Environmental Biology of Fishes; 99(11): 805-812.
Hammerschlag N, Davis DA, Mondo K, Seely MS, Murch SJ, Glover WB, Divoll T, Evers DC, Mash DC. (2016) Cyanobacterial Neurotoxin BMAA and Mercury in Sharks. Toxins 2016, 8, 238.
Hammerschlag N, Bell I, Fitzpatrick I, Gallagher AJ, Hawkes LA, Meekan MG, Stevens JD, Thums M, Witt MJ, Barnett A. (2016) Behavioral evidence suggests facultative scavenging by a marine apex predator during a food pulse. Behavioral Ecology and Sociobiology; 70(1): 1777-1788.
Roemer RP, Gallagher AJ, Hammerschlag N. (2016) Shallow water tidal flat use and associated specialized foraging behavior of the great hammerhead shark (Sphyrna mokarran). Marine and Freshwater Behaviour and Physiology; 49(4): 235-249.
Cooke SJ, Nguyen VM, Wilson AD, Donaldson MR, Gallagher A, Hammerschlag N, Haddaway NR. (2016) The need for speed in a crisis discipline: perspectives on peer review duration and implications for conservation science. Endangered Species Research 30: 11-19
Shiffman, D.S. and Hammerschlag, N. (2016) Shark Conservation and Management Policy: A Review and Primer for Non-Specialists. Animal Conservation; 19(5): 401-412.
Fu A, Lauder G, Wilga C, Kuo C, Hammerschlag N, Irschick DJ. (2016) Ontogeny of head and caudal fin shape of an apex marine predator: the tiger shark (Galeocerdo cuvier). Journal of Morphology; 277(5): 556-564.
Shiffman, DS and Hammerschlag, N. (2016) Preferred conservation policies of shark researchers. Conservation Biology; 30(4): 805-815.
Graham F, Rynne P, Estevanez M, Luo J, Ault JS, Hammerschlag N. (2016) Use of marine protected areas and exclusive economic zones in the subtropical western North Atlantic Ocean by large highly mobile sharks. Diversity and Distributions; 22(5): 534-546.
Queiroz N, Humphries N.E., Mucientes G., Hammerschlag N, Lima F.P, Scales K.L, Miller P.I., Sousa L.L., Seabra R., Sims D.W. (2016) Ocean-wide tracking of pelagic sharks reveals extent of overlap with longline fishing hotspots. Proceedings of the National Academy of Sciences; 113(6): 1582-1587.
Sulikowski J, Wheeler CR, Gallagher AJ, Prohaska BK, Langan JA, Hammerschlag N. (2016) Seasonal and life-stage variation in the reproductive ecology of a marine apex predator, the tiger shark Galeocerdo cuvier, at a protected female dominated site. Aquatic Biology, 24: 175-184
Creel S, Becker M, Christianson D, Dröge E, Hammerschlag N, Haward MW, Karanth U, Loveridge A, Macdonald DW, Wigganson M, M’soka J, Murray D, Rosenblatt E, Schuette P. (2015) Questionable policy for large carnivore hunting . Science, 350(6267): 1473-1475
Gallagher AJ, Cooke SJ, Hammerschlag N. (2015) Risk perceptions and conservation ethic among recreational anglers targeting threatened sharks in the subtropical Atlantic. Endangered Species Research, 29(1): 81-93.
Thaler, A.D.T. and Shiffman, D.S. (2015) Fish tales: Combating fake science in popular media. Ocean and Coastal Management
Nguyen VM, Haddaway NR, Gutowsky LFG, Wilson ADM, Gallagher AJ, Donaldson MR, Hammerschlag N, Cooke SJ. (2015) How Long Is Too Long in Contemporary Peer Review? Perspectives from Authors Publishing in Conservation Biology Journals. PLoS ONE 10(8): e0132557.
Gallagher AJ, Hammerschlag N, Cooke SJ, Costa DP, Irschick DJ (2015) One size does not always fit all: a reply to Stroud and Feeley. Trends in Ecology and Evolution; 30(6): 297-298.
Hammerschlag N, Broderick AC, Coker JW, Coyne MS, Dodd M, Frick MG, Godfrey MH, Godley BJ, Griffin DB, Hartog K, Murphy SR, Murphy TM, Nelson ER, Williams KL, Witt MJ, Hawkes LA (2015) Evaluating the landscape of fear between apex predatory sharks and mobile sea turtles across a large dynamic seascape. Ecology, 96(8): 2117-2126.
Gallagher AJ, Vianna GMS, Papastamatiou YP, Macdonald C, Guttridge TL, Hammerschlag N. (2015) Biological effects, conservation potential, and research priorities of shark diving tourism. Biological Conservation, 184: 365-379
Gallagher AJ, Hammerschlag N, Cooke SJ, Costa DP, Irschick DJ (2015) Evolutionary theory as a tool for predicting extinction risk. Trends in Ecology and Evolution, 30(2): 61-65
Fallows C, Benoit HP, Hammerschlag N. (2015) Intraguild predation and partial consumption of blue sharks (Prionace glauca) by Cape fur seals (Arctocephalus pusillus pusillus). African Journal of Marine Science; 37(1): 125-128.
D.S. Shiffman, A.J. Gallagher, J. Wester C.C. Macdonald, A.D. Thaler, S.J. Cooke, and N. Hammerschlag. (2015) A letter of clarification from the authors of “trophy fishing for species threatened with extinction”. Marine Policy 53, 213-214.
Ferry, L., and Shiffman, D.S. (2014) The Value of Taxon-focused Science: 30 Years of Elasmobranchs in Biological Research and Outreach. Copeia 743-746.
Hammerschlag N, Cooke SJ, Gallagher AJ, Godley BJ. (2013) Considering the fate of electronic tags: user responsibility and interactions when encountering tagged marine animals; Methods in Ecology and Evolution; 11(5): 1147-1153.
Shiffman DS, Hammerschlag N. (2014) An assessment of the scale, practices, and conservation implications of Florida’s charterboat-based recreational shark fishery. Fisheries, 39(9): 395-407
Irschick, DJ, Hammerschlag N. (2014) Morphological scaling of body form in four shark species differing in ecology and life-history. Biological Journal of the Linnean Society; 114(1): 126-135.
Shiffman, D.S, Gallagher AJ, Wester, Macdonald C, Thaler AD, Cooke SJ, Hammerschlag N (2014) Trophy fishing for species threatened with extinction: a way forward building on a history of conservation Marine Policy, 50: 318-322
Shiffman, D.S. (2014) Commentary: Keeping swimmers safe without killing sharks is a revolution in shark control. Animal Conservation DOI: 10.1111/acv.12155
Gallagher AJ, Orbesen ES, Hammerschlag N, Serafy JE (2014) Survival and vulnerability of pelagic shark species to pelagic longline bycatch. Global Ecology and Conservation; 1: 50-59.
Rumbold D, Wasno B, Hammerschlag N, Volety A. (2014) Mercury accumulation in sharks from the coastal waters of Southwest Florida. Archives of Environmental Contamination and Toxicology; 67(3): 402-412.
Irschick DI., Hammerschlag N. (2014) A new metric for measuring condition in large predatory sharks. Journal of Fish Biology; 85(3), 917-926.
Gallagher, AJ, Wagner, DN, Irschick, DJ, Hammerschlag, N. (2014) Body condition predicts energy stores in apex predatory sharks. Conservation Physiology; 2 (1): cou022
Shiffman, DS, Frazier, B, Kucklick, J, Abel, D, Brandes, J and Sancho, G. (2014) Feeding ecology of the sandbar shark (Carcharhinus plumbeus) in South Carolina estuaries revealed through δ13C and δ15N stable isotope analysis. Marine and Coastal Fisheries; 6(1): 156-169
Gallagher AJ, Hammerschlag N, Shiffman DS, Giery ST (2014) Evolved for extinction: the cost and conservation implications of extreme specialization in hammerhead sharks. BioScience; 64(7): 619-624.
Staaterman ER, Bhandiwad AA, Gravinese PM, Moeller PM, Reichenbach ZC, Shantz AA, Shiffman DS, Toth LT, Warneke AM, Gallagher AJ. (2014) Lights, camera, science: The utility and growing popularity of film festivals at scientific meetings. Ideas in Ecology and Evolution 7: 11-16.
Cooke SJ, Hogan ZS, Butcher PA, Stokesbury MJW, Raghavan R, Gallagher AJ, Hammerschlag N, Danylchuk AJ. (2014) Angling for endangered fish: Conservation problem or conservation action? Fish and Fisheries; 17(1): 249-265.
Gallagher AJ, Romeiro J, Canabal D, Canabal V, Hammerschlag N (2014) Novel social behaviors in a threatened apex marine predator, the oceanic whitetip shark Carcharhinus longimanus. Ethology Ecology & Evolution; 26(4), 413-417.
Gallagher, AJ, Serafy, JE, Cooke, SJ, Hammerschlag, N (2014) Physiological stress response, reflex impairment, and survival of five sympatric shark species following experimental capture and release. Marine Ecology Progress Series; 494: 207-218
ECM Parsons, DS Shiffman, ES Darling, N Spillman, and AJ Wright. (2013) How Twitter Literacy Can Benefit Conservation Scientists. Conservation Biology.
Darling E, Shiffman D, Drew J, and Cote Isabelle (2013) The role of twitter in the life cycle of a scientific publication. Ideas in Ecology and Evolution 6, 32-43.
Hammerschlag N, Gallagher AJ, Carlson JK. (2013) A revised estimate of daily ration in the tiger shark ( Galeocerdo cuvier ) with implications for assessing ecosystem impacts of apex predators. Functional Ecology 27 (5): 1273-1274.
Fallows C, Gallagher AJ, Hammerschlag N (2013) White Sharks (Carcharodon carcharias) Scavenging on Whales and Its Potential Role in Further Shaping the Ecology of an Apex Predator. PLoS ONE 8(4): e60797. doi:10.1371/journal.pone.0060797.
Carr, LA, Stier, AC, Fietz, K, Montero, I, Gallagher, AJ, Bruno, JF. (2013) Illegal shark fishing in the Galapagos Marine Reserve Marine Policy 39: 317-321.
Hammerschlag N, Luo J, Irschick DJ, Ault JS (2012) A Comparison of Spatial and Movement Patterns between Sympatric Predators: Bull Sharks ( Carcharhinus leucas ) and Atlantic Tarpon ( Megalops atlanticus ). PLoS ONE 7(9): e45958. doi:10.1371/journal.pone.0045958.
Shifman DS. (2012) Twitter as a tool for conservation education and outreach: what scientific conferences can do to promote live-tweeting. Journal of Environmental Studies and Sciences DOI 10.1007/s13412-012-0080-1.
Shiffman DS, Gallagher AJ, Boyle MD, Hammerschlag-Peyer CM, Hammerschlag N. (2012, Cover) Stable Isotope Analysis as a Tool for Elasmobranch Conservation Research: A Primer for Non-Specialists. Marine and Freshwater Research 63:635-643.
Hammerschlag N, Gallagher AJ, Wester J, Luo J, Ault JS. (2012, Cover) Don’t bite the hand that feeds: assessing ecological impacts of provisioning ecotourism on an apex marine predator. Functional Ecology 26(3): 567-576
Gallagher AJ, Kyne PM, Hammerschlag N. (2012) Ecological risk assessment and its application to elasmobranch conservation and management. Journal of Fish Biology 85(5): 1727-1748
Martin RA, Hammerschlag N. (2012) Marine predator—prey contests: Ambush and speed versus vigilance and agility . Marine Biology Research 8:1, 90-94.
Mondo K, Hammerschlag N, Basile M, Pablo J, Banack SA, Mash DC. (2012) Cyanobacterial Neurotoxin β-N-Methylamino-L-alanine (BMAA) in Shark Fins . Marine Drugs 10(2), 509-520; doi:10.3390/md10020509
Thaler AD, Zelnio KA, Freitag A, MacPherson R, Shiffman DS, Bik H, Goldstein MC, McClain C. (2012) Digital environmentalism: tools and strategies for the evolving online ecosystem. SAGE Reference — Environmental Leadership: A Reference Handbook D. Gallagher (Ed.).
Fallows C, Martin RA, Hammerschlag N. (2012) Comparisons between white shark-pinniped interactions at Seal Island (South Africa) with other sites in California (United States). Global Perspectives on the Biology and Life History of the Great White Shark, ed. Michael L. Domeier, CRC Press, Boca Raton, FL.
Hammerschlag N, Martin RA, Fallows C, Collier R, Lawrence R. (2012) Investigatory Behavior towards surface objects and Non-consumptive Strikes on Seabirds by White Sharks ( Carcharodon carcharias ) at Seal Island, South Africa. Global Perspectives on the Biology and Life History of the Great White Shark, ed. Michael L. Domeier, CRC Press, Boca Raton, FL: 91-103.
Hammerschlag N, Trussell G. (2011) Beyond the Body Count: Behavioral Downgrading of Planet Earth. Science. (E-Letter, 11 November 2011).
Gallagher AJ, Jackson T, Hammerschlag N. (2011) Evidence of tiger shark ( Galeocerdo cuvier ) scavenging on avian prey and its possible connection between large-scale bird die-offs in the Florida Keys. Florida Scientist, 74(4):264-269.
Staaterman ER, Clark CW, Gallagher AJ, deVries, MS, Claverie T, Patek SN. (2011) Rumbling in the benthos: the acoustic behavior of the California mantis shrimp and the presence anthropogenic noise. Aquatic Biology, 13(2):97–105.
Hammerschlag N, Sulikowski J. (2011) Killing for Conservation: The Need for Alternatives to Lethal Sampling of Apex Predatory Sharks. Endangered Species Research, 14(2):135–140.
Gallagher AJ, Hammerschlag N. (2011) Global Shark Currency: The Distribution, Frequency and Economic Value of Shark Eco-tourism. Current Issues in Tourism, 14(8):1–16. DOI: 10.1080/13683500.2011.585227.
Hammerschlag N, Gallagher AJ, Lazarre DM. (2011) A Review of Shark Satellite Tagging Studies. Journal of Experimental Marine Biology and Ecology, 398(1):1–8.
Hammerschlag N, Gallagher AJ, Lazarre DM, Slonim C. (2011) Range extension of the endangered great hammerhead shark Sphyrna mokarran in the Northwest Atlantic: Preliminary data and significance for conservation. Endangered Species Research, 13(2):111–116.
Serrano X, Grosell M, Serafy JE. (2011) Osmoregulatory capabilities of the gray snapper, Lutjanus griseus : salinity challenges and field observations. Marine and Freshwater Behaviour and Physiology , 44(3):1-12.
Brand LE, Pablo J, Compton A, Hammerschlag N, Mash DC. (2010) Cyanobacterial Blooms and the Occurrence of the neurotoxin beta-N-methylamino-L-alanine (BMAA) in South Florida Aquatic Food Webs. Harmful Algae , 9(6):620–635.
Hammerschlag N, Heithaus MR, Serafy JE. (2010) The influence of predation risk and food supply on nocturnal fish foraging distributions along a subtropical mangrove-seagrass ecotone. Marine Ecology Progress Series , 414:223-235.
Hammerschlag N, Ovando D, Serafy, JE. (2010) Seasonal diet and feeding habits of juvenile fishes foraging along a subtropical marine ecotone. Aquatic Biology, 9(3):279–290.
Hammerschlag N, Morgan A, Serafy JE. (2010) Relative predation risk for fishes along a subtropical mangrove-seagrass ecotone. Marine Ecology Progress Series, 401: 259–267.
Hammerschlag N, Serafy JE. (2010) Nocturnal fish utilization of a subtropical mangrove-seagrass ectone. Marine Ecology, 31(2):364–374.
Gallagher AJ, Lorenz FH, Bushnell PG, Brill RW, Mandelman JW. (2010) Blood Gas, Oxygen Saturation, pH, and Lactate Values in Elasmobranch Blood Measured with a Commercially Available Portable Clinical Analyzer and Standard Laboratory Instruments , Journal of Aquatic Animal Health, 22(4) 229–234.
Serrano X, Grosell M, Serafy JE. (2010) Salinity selection and preference of the grey snapper Lutjanus griseus : field and laboratory observations. Journal of Fish Biology, 76(7): 1592–1608.
Martin RA, Rossmo DK, Hammerschlag N. (2009) Hunting patterns and geographic profiling of white shark predation. Journal of Zoology, 279(2): 111–118.
Milano GR, Hammerschlag N, Barimo J, Serafy JE. (2007) Restoring essential fish habitat in southeast Florida: Mangrove and seagrass habitat design components and successful monitoring. Bulletin of Marine Science, 80(3): 928-929.
Hammerschlag N, Martin RA, Fallows C. (2006) Effects of environmental conditions on predator-prey interactions between white sharks ( Carcharodon carcharias ) and Cape fur seals ( Arctocephalus pusillus pusillus ) at Seal Island, South Africa. Environmental Biology of Fishes , 76(2): 341–350.
Hammerschlag N. (2006) Osmoregulation in Elasmobranchs: A review for fish biologists, behaviourists and ecologists. Marine and Freshwater Behaviour and Physiology, 39(3): 209–228.
Martin RA, Hammerschlag N, Collier R, Fallows C. (2005) Predatory Behaviour of White Sharks ( Carcharodon carcharias ) at Seal Island, South Africa. Journal of the Marine Biological Association of the UK , 85(5): 1121–1135.
Hammerschlag N, Fallows C. (2005) Galapagos sharks ( Carcharhinus galapagensis ) at the Bassas da India atoll: first record from the Mozambique Channel and possible significance as a nursery area. South African Journal of Science , 101: 375–377.
Hammerschlag N. (2004) A review of osmoregulation in freshwater and marine elasmobranchs. In: R.A. Martin and D. MacKinlay (ed.) Extended Abstract in Proceedings of the American Fisheries Society, fourth International Congress on the Biology of Fish, Manaus, Brazil: 35-41.
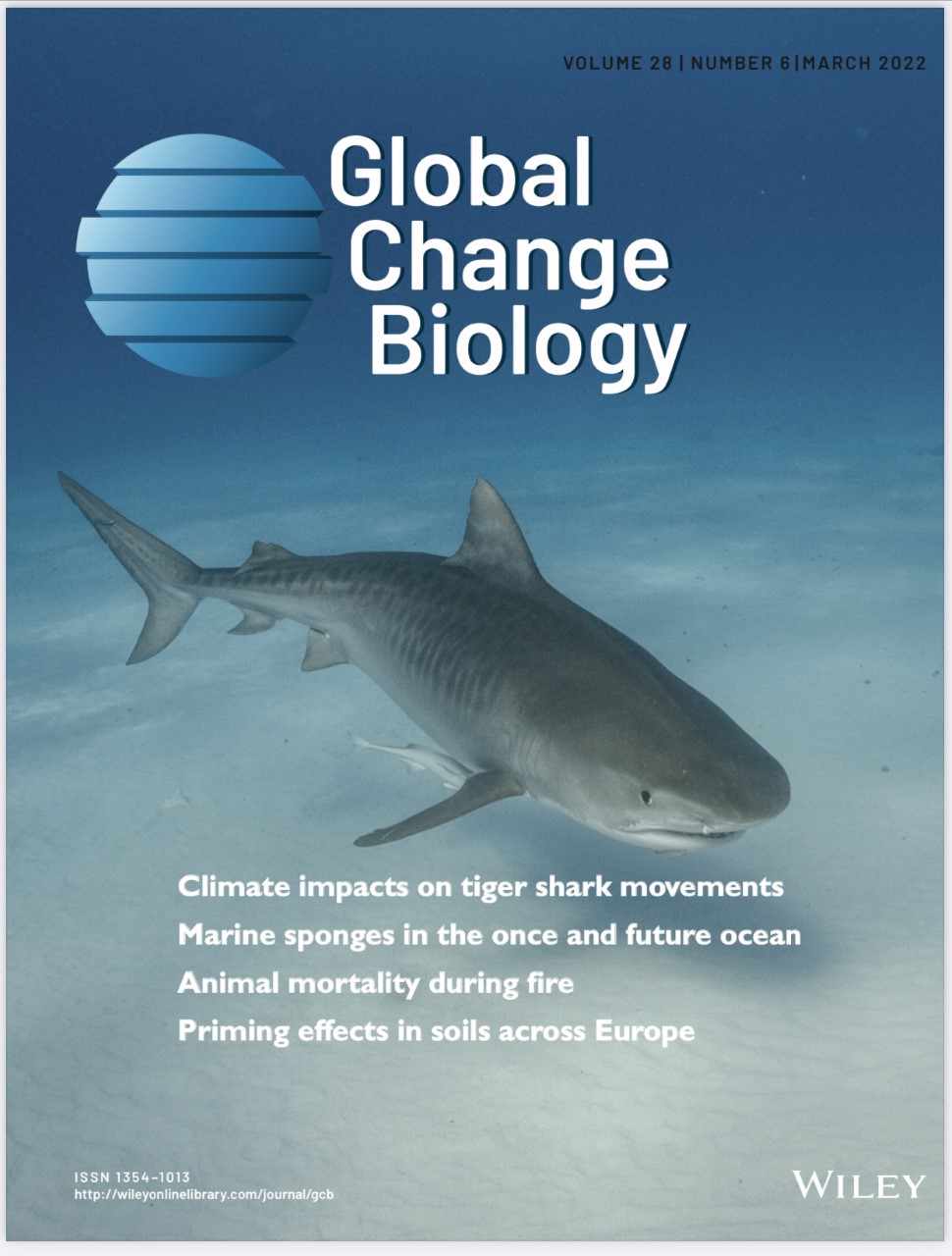
Privacy Statement and Legal Notices Copyright © 2018, University of Miami All rights reserved.
4600 Rickenbacker Causeway Miami, Fl 33149-1098 +1 305 421 4000
This site uses cookies. By continuing to browse the site, you are agreeing to our use of cookies.
Cookie and Privacy Settings
We may request cookies to be set on your device. We use cookies to let us know when you visit our websites, how you interact with us, to enrich your user experience, and to customize your relationship with our website.
Click on the different category headings to find out more. You can also change some of your preferences. Note that blocking some types of cookies may impact your experience on our websites and the services we are able to offer.
These cookies are strictly necessary to provide you with services available through our website and to use some of its features.
Because these cookies are strictly necessary to deliver the website, refuseing them will have impact how our site functions. You always can block or delete cookies by changing your browser settings and force blocking all cookies on this website. But this will always prompt you to accept/refuse cookies when revisiting our site.
We fully respect if you want to refuse cookies but to avoid asking you again and again kindly allow us to store a cookie for that. You are free to opt out any time or opt in for other cookies to get a better experience. If you refuse cookies we will remove all set cookies in our domain.
We provide you with a list of stored cookies on your computer in our domain so you can check what we stored. Due to security reasons we are not able to show or modify cookies from other domains. You can check these in your browser security settings.
We also use different external services like Google Webfonts, Google Maps, and external Video providers. Since these providers may collect personal data like your IP address we allow you to block them here. Please be aware that this might heavily reduce the functionality and appearance of our site. Changes will take effect once you reload the page.
Google Webfont Settings:
Google Map Settings:
Google reCaptcha Settings:
Vimeo and Youtube video embeds:
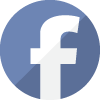

Tiger sharks have social preferences for one another
New research indicates that baited shark diving can influence tiger shark social behavior.
Scientists at the University of Miami Rosenstiel School of Marine and Atmospheric Science (UM) and the Institute of Zoology at the Zoological Society London (ZSL) found that tiger sharks, often considered a solitary nomadic species, are social creatures, having preferences for one another.
A first of its kind, the study also evaluated if exposure of the tiger shark to baited dive tourism impacted their social behavior. The study was conducted at a site named Tiger Beach, located off the north-west side of Little Bahama bank in the Bahamas. The area is known for hosting shark diving encounters, where the sharks are attracted with chum and often fed in front of dive tourists.
The research team tagged and tracked the movements of tiger sharks over the course of three years. They then applied a tool called Social Network Analysis to the tracking data to examine if tiger sharks exhibited social grouping behavior and if this social behavior differed at locations where sharks were exposed to baited shark dive tourism. The study not only found that tiger sharks formed social groups, but also discovered that at sites where tiger sharks were being fed by dive tourism operators, tiger sharks became more aggregated, but interactions between sharks became more random, suggesting a breakdown in social organization.
"Given that tiger sharks spend months at a time out in the open ocean as solitary predators, it's amazing to me that they show social preferences for one another when they are at the Tiger Beach area," said Neil Hammerschlag, senior author of the study and research associate professor at the UM Rosenstiel School. "For nearly two decades, I have spent countless hours diving at Tiger Beach, always wondering if these apex predators interacted socially. Now we know."
Baited shark dives are often conducted by dive tourism companies to attract the animals so that tourists may observe them. This approach has been known to cause mixed feelings among conservationists and shark experts, due to the possible long-term impacts on the predators, such as changes to their natural foraging behavior. This study found that tiger sharks aggregated at the dive sites, but social preferences between sharks were less prevalent as compared to areas outside of these dive sites. These results suggest that feeding sharks may disrupt their social organization, but only temporarily, as the study found that tiger sharks resumed their social groupings outside of the dive sites.
"The boundary between wildlife and people is becoming increasingly thin, so as well as observing a new social behavior for the first time in what was once thought of as a solitary shark, we also measured the impacts of human activity on these predators' interactions. They seem to show some resilience to the bait feeding," said David Jacoby, ZSL Honorary Research Associate and lead author of the study.
The social behavior of predators is an important area of study as it provides another tool to help scientists and wildlife managers build a picture of how they live, what drives them to form social groups, and the roles they play within the wider ecosystem.
Video: https://www.youtube.com/watch?v=NlgWTtPnMlg
- Wild Animals
- Coral Reefs
- Environmental Awareness
- Rainforests
Story Source:
Materials provided by University of Miami Rosenstiel School of Marine & Atmospheric Science . Original written by Diana Udel. Note: Content may be edited for style and length.
Journal Reference :
- David M. P. Jacoby, Bethany S. Fairbairn, Bryan S. Frazier, Austin J. Gallagher, Michael R. Heithaus, Steven J. Cooke, Neil Hammerschlag. Social Network Analysis Reveals the Subtle Impacts of Tourist Provisioning on the Social Behavior of a Generalist Marine Apex Predator . Frontiers in Marine Science , 2021; 8 DOI: 10.3389/fmars.2021.665726
Cite This Page :
Explore More
- Next-Gen Solar Cells: Perovskite Semiconductors
- When Faces Appear Distorted: Rare Condition
- End of Planet Formation
- Enormous Ice Loss from Greenland Glacier
- Signs of Life Detectable in Single Ice Grain
- Tudor Era Horse Cemetery
- When Do Babies Become Conscious?
- Secrets of the Van Allen Belt Revealed
- Robotic Prostheses, Exoskeletons
- Bronze Age Families: Clothing, Recipes, Pets
Trending Topics
Strange & offbeat.
Click through the PLOS taxonomy to find articles in your field.
For more information about PLOS Subject Areas, click here .
Loading metrics
Open Access
Peer-reviewed
Research Article
A Comparison of the Seasonal Movements of Tiger Sharks and Green Turtles Provides Insight into Their Predator-Prey Relationship
Affiliations School of Marine and Tropical Biology, James Cook University, Cairns, Queensland, Australia, Reef Channel, Cairns, Queensland, Australia
Affiliations School of Environmental Systems Engineering and the University of Western Australia Oceans Institute, The University of Western Australia, Crawley, Western Australia, Australia, Australian Institute of Marine Science, c/o University of Western Australia Oceans Institute, Crawley, Western Australia, Australia
Affiliation Queensland Department of Environment and Heritage Protection, Townsville Queensland, Australia
Affiliation Australian Institute of Marine Science, c/o University of Western Australia Oceans Institute, Crawley, Western Australia, Australia
Affiliation CSIRO Marine and Atmospheric Research, Hobart, Tasmania, Australia
* E-mail: [email protected]
Affiliations School of Life and Environmental Sciences, Deakin University, Melbourne, Victoria, Australia, Fisheries, Aquaculture and Coasts Centre, Institute for Marine and Antarctic Studies, University of Tasmania, Hobart, Tasmania, Australia, Reef Channel, Cairns, Queensland, Australia
- Richard Fitzpatrick,
- Michele Thums,
- Ian Bell,
- Mark G. Meekan,
- John D. Stevens,
- Adam Barnett
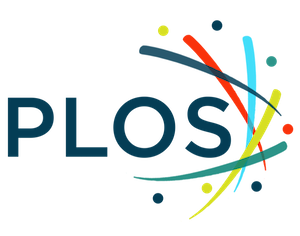
- Published: December 19, 2012
- https://doi.org/10.1371/journal.pone.0051927
- Reader Comments
During the reproductive season, sea turtles use a restricted area in the vicinity of their nesting beaches, making them vulnerable to predation. At Raine Island (Australia), the highest density green turtle Chelonia mydas rookery in the world, tiger sharks Galeocerdo cuvier have been observed to feed on green turtles, and it has been suggested that they may specialise on such air-breathing prey. However there is little information with which to examine this hypothesis. We compared the spatial and temporal components of movement behaviour of these two potentially interacting species in order to provide insight into the predator-prey relationship. Specifically, we tested the hypothesis that tiger shark movements are more concentrated at Raine Island during the green turtle nesting season than outside the turtle nesting season when turtles are not concentrated at Raine Island. Turtles showed area-restricted search behaviour around Raine Island for ∼3–4 months during the nesting period (November–February). This was followed by direct movement (transit) to putative foraging grounds mostly in the Torres Straight where they switched to area-restricted search mode again, and remained resident for the remainder of the deployment (53–304 days). In contrast, tiger sharks displayed high spatial and temporal variation in movement behaviour which was not closely linked to the movement behaviour of green turtles or recognised turtle foraging grounds. On average, tiger sharks were concentrated around Raine Island throughout the year. While information on diet is required to determine whether tiger sharks are turtle specialists our results support the hypothesis that they target this predictable and plentiful prey during turtle nesting season, but they might not focus on this less predictable food source outside the nesting season.
Citation: Fitzpatrick R, Thums M, Bell I, Meekan MG, Stevens JD, Barnett A (2012) A Comparison of the Seasonal Movements of Tiger Sharks and Green Turtles Provides Insight into Their Predator-Prey Relationship. PLoS ONE 7(12): e51927. https://doi.org/10.1371/journal.pone.0051927
Editor: Graeme Clive Hays, University of Wales Swansea, United Kingdom
Received: September 5, 2012; Accepted: November 7, 2012; Published: December 19, 2012
Copyright: © 2012 Fitzpatrick et al. This is an open-access article distributed under the terms of the Creative Commons Attribution License, which permits unrestricted use, distribution, and reproduction in any medium, provided the original author and source are credited.
Funding: Funding was supplied by Digital Dimensions, Australia, through the production of documentaries related to shark research. The funders had no role in study design, data collection and analysis, decision to publish, or preparation of the manuscript.
Competing interests: Funding for this project was from a commercial source, Digital Dimensions. RF and AB are affiliated with a commercial company The Reef Channel. This does not alter the authors’ adherence to all the PLOS ONE policies on sharing data and materials.
Introduction
Predators play important roles in ecosystems by influencing the distribution, behaviour and abundance of their prey and predator-prey interactions have long been recognised as important in ecosystem dynamics [1] , [2] , [3] . However predator-prey interactions can be difficult to observe and quantify. One way is to compare the spatial and temporal components of movement behaviour of potentially interacting species. By comparing movement patterns of predator and prey we can gain some understanding of when, where and how often predator and prey overlap spatially and therefore gauge the chance of interactions [4] , [5] , [6] , [7] . Such studies can vastly improve our understanding of large mobile predators’ spatial use in relation to their prey and shed light on their foraging strategies. For instance, is the predators’ foraging strategy to move directly between distinct habitats exploiting seasonally abundant prey, or do they concentrate on targeting a specific prey and therefore their movements are dictated by the prey’s movements?
The tiger shark, Galeocerdo cuvier, is the apex predator in tropical coral reef systems, yet we know relatively little about the ecosystem role of this species in these environments. The limited information available suggests that they utilise large (hundreds of km) home ranges, within which they appear to move continuously between distant foraging areas [8] , [9] , [10] , [11] . They can return to specific locations to take advantage of seasonally abundant prey like fledging albatross, Phoebastria spp. [11] , [12] . Studies in some of these locations suggest that they have both demographic and behavioural effects on prey species [13] , [14] .
Although the diet of tiger sharks is broad, there is some evidence to suggest that they specialise on hunting air-breathing animals, particularly turtles [15] , [16] , [17] and they are considered the biggest predation threat (excluding humans) to these marine reptiles [18] .
In the northern Great Barrier Reef, Australia, green turtles Chelonia mydas returning to natal rookeries during the austral summer are an abundant and predictable food source for tiger sharks. Raine Island and the surrounding sand cays in this location host the largest green turtle breeding population in the world [19] with turtle arrivals for nesting reaching a peak during the first week of December when an average of 5000 (range = 250–12000/night) turtles can attempt to nest in a single night [20] . These animals exhibit strong site-attachment to Raine Island for up to four months during reproduction (courtship, copulation and nesting), exposing them to the possibility of concentrated predation pressure from tiger sharks for an extended period [19] . Tiger sharks are frequently observed scavenging on floating turtle carcases and occasionally seen attacking live turtles [19] (pers. obs.). Outside the reproductive season, green turtles disperse widely to distant foraging grounds [19] , however the movements of tiger sharks in this region are unknown.
The coincidence of tiger sharks and this abundant food source of green turtles offered a unique opportunity to investigate the spatial relationship between this apex predator and its prey. We deployed satellite tags on tiger sharks and green turtles and analysed the movement behaviour of both species in order to: 1) determine whether tiger sharks concentrate their movements around Raine Island during the green turtle nesting season and 2) investigate the movement behaviour of green turtles and tiger sharks outside of the nesting season when turtles are more highly dispersed and not concentrated at Raine Island. We hypothesised that if tiger sharks were using the Raine Island area to focus their foraging effort on turtles (alive and dead) then their seasonal use of the waters near Raine Island should be highest during turtle nesting season and their movements might be less concentrated at Raine Island at the end of the nesting season when green turtles disperse widely to foraging grounds.
Ethics Statement
Research was approved and conducted under Australian Fisheries Management Authority Scientific Permit #901193 and Great Barrier Reef Marine Park Authority G11/33231.1.
Raine Island (11° 35′ S, 144° 02′ E) lies ∼80 km offshore from mainland Australia in the Far Northern Section of the Great Barrier Reef Marine Park. An elongate sand cay, approximately 830 m long and 430 m wide is located at the leeward end of an oval patch reef that is 3.5 km long and 0.75 km wide, with an area of 210 ha. The fringing reef of Raine Island slopes precipitously to meet the sea floor at depths of 200–300 m [19] .
We deployed eight satellite-linked transmitters on green turtles and ten on tiger sharks at Raine Island in late spring and summer during the turtle nesting season between 2002 and 2007 ( Table 1 ). The green turtles were all instrumented with SPOT transmitters (Wildlife computers, Redmond, Washington, USA). Shark deployments included one ST18 (Telonics, Mesa, Arizona, USA), four SPOT tags and five SPLASH tags (Wildlife computers, Redmond, Washington, USA). All tags relay position-only information via the ARGOS satellite network, except the SPLASH tags which also relay summaries of time at depth (±0.5 m) and time at temperature (±0.05°C) binned within 14 user defined data ranges over 6 h collection periods. One shark was tagged in consecutive summers. This individual was first tagged on 26 th November 2006 with SPLASH tag 72587, and then was recaptured 1 year later on 26 th November 2007. The SPLASH tag was removed and replaced with SPLASH tag 79975. The turtle tags were programmed to transmit from 04∶00–11∶00 h every day for December, January, February, March and April and the remaining months were duty cycled to transmit every 3 days. The rationale in selecting the transmit h was based on green turtles at Raine Island appearing to spend more time at the surface in the morning than in the afternoon when the wind speed increases (Bell pers. obs.). Therefore there would be a higher likelihood of obtaining uplinks from the tagged turtles basking in the calmest part of the day. The tiger sharks tags were programmed to transmit at all times.
- PPT PowerPoint slide
- PNG larger image
- TIFF original image
https://doi.org/10.1371/journal.pone.0051927.t001
Tiger sharks were attracted to a small boat using tuna heads threaded onto a buoyant rope, so the bait was floating on the surface and the rope attached to the boat. Once the shark had taken the bait, the boat was pulled along the bait line so that the boat was drawn close to the shark. A custom-designed tail clamp was then attached to the caudal peduncle with the use of a detachable 4 m pole. The clamp held a 5 m rope and large buoy, so once the clamp was attached it limited the swimming movement of the shark due to the drag of the buoy. The shark was then restrained using a harness at the back deck of the larger mothership vessel. The satellite transmitter was attached to the dorsal fin by two 5 mm diameter short, threaded nylon rods that passed through the fin and were secured on the other side by two washers and nuts, or a plastic plate and nuts [11] . The position of the transmitter on the fin was such that the antenna extended out of the water when the fin broke the surface. Transmitters were attached to green turtles that had successfully nested. The transmitter attachment procedure commenced immediately following oviposition or as the turtle was heading back to the sea. The SPOT tag was attached to the carapace using a fast drying epoxy resin (International Epiglass HT9000 Fast laminating resin). To avoid generating to much heat, we used less catalyst than the manufacturer’s instructions, so turtles were held in an enclosure for approximately 6 h allowing the epoxy sufficient time to fully set.
Data Analysis
Movement behavior..
The Bayesian state-space switching model (SSSM) developed by Jonsen et al. [21] , [22] was applied to each individual tiger shark and green turtle track. Satellite-derived locations using the Service Argos telemetry system are reported at irregular time intervals and can be prone to considerable error [23] . The SSSM allows for regular position estimates to be inferred from the Argos satellite positions by taking into account error from the Argos location class (B, A, 0, 1, 2, 3) and the dynamics of the animal’s movement; the mean turning angle and autocorrelation in speed and direction [24] . It also identifies two discrete behavioural modes from these data, nominally, transiting and area-restricted search (ARS) behaviour, assuming that while transiting, turn angles should be closer to 0 and autocorrelation should be higher than when in ARS [25] . While area-restricted search behaviour is associated with foraging [26] it can also be resting or breeding behaviour [27] , [28] .
The SSSMs were fitted using the freely available software, JAGS 3.2.0 [29] from R: A Language and Environment for Statistical Computing [30] using code developed by Ian Jonsen (bsam) [21] , [22] . We ran two Monte-Carlo Markov Chains (MCMC) for each model with 60 000 iterations following a 30 000 burn-in (thin = 10). We used a 12 h time step for tiger sharks and 6–12 h time step for the turtles depending on the temporal resolution of the ARGOS data ( Table 1 ). The SSSM classifies behaviour by using the means of the MCMC samples and delineating a cut-off at 1.5. Mean estimates below 1.5 were considered to represent transiting and estimates above 1.5 were considered to represent area-restricted search [24] and mean estimates of 1.5 were considered uncertain.
To assess whether shark movement was more concentrated at Raine Island during the green turtle nesting season we calculated the distance between each location and Raine Island for each shark using the sp package in R. We designated the period from November to February as the turtle nesting season based on the green turtle movement behaviour. We used linear mixed effects models to model the distance from Raine Island as a function of turtle season (nesting or not nesting). The random effect was the individual shark and we used the corAR1 function to account for the within-group correlation structure. We log-transformed the response to normalise its distribution and fitted the models in R using package nlme [31] . We used an information theoretic approach [32] to test the hypothesis by comparing the weight of Akaike’s information criterion corrected for small sample sizes ( w AIC c ) of the slope model (Distance from Raine ∼ season) to the intercept-only model (Distance to Raine ∼1) [32] .
To determine whether sharks showed variation in diving behaviour or thermal environments used between behavioural modes, we calculated the proportion of observations within each depth and temperature bin for each behavioural mode for each animal with diving data. All means presented in the text are accompanied by the standard deviation.
The number of locations obtained per day was 0.99±0.82 for tiger sharks and 2.94±1.77 for green turtles ( Table 1 ). For sharks, there were periods within each track with no recorded satellite locations. This resulted in the low mean number of positions calculated for each shark ( Table 1 ). The median length of these periods ranged from 1–4 days. The deployments provided data for 16–365 days for the sharks and 89–408 days for turtles ( Table 1 ). There was little uncertainty in the behavioural mode estimates with only 0.3% of state estimates at 1.5. The largest proportion of time was spent in area-restricted search mode for both turtles (0.95±0.03) and sharks (0.90±0.12).
From the deployment date, green turtles spent 76±24 days in area-restricted search mode around Raine Island ( Fig. 1 ). Apart from one turtle (88365) that switched to transit mode in early December, the turtles switched to transit mode from early February to mid March (Julian day range: 33–74), equating to a mean Julian day of 51±18 (20 th February). The switch to transit mode was accompanied with a largely northward migration ( Fig. 1 ). This switch probably relates to the transition between the nesting phase and the post-nesting migration to foraging grounds. Turtles spent 9.16±7.20 days in transit mode before a long period in area-restricted search mode (putative foraging) which lasted for the remainder of their deployments ( Table 1 ) predominantly in the Torres Strait ( Fig. 1 ). One turtle headed directly west of Raine to forage off the coast of Cape York Peninsula and another went south to the Howick group ( Fig. 1 ). When in the nesting phase (based on the time each turtle switched to transit mode) around Raine Island, turtles were 5.97±2.02 km from Raine Island. On their foraging grounds they were 272.03±72.51 km from Raine Island.
Turtle ID is indicated in the top right and duration of the deployment in the bottom left of each map. Each turtle’s path is coded by behavioural mode; red = area-restricted search and black = transit. Maps show Cape York Peninsula, Raine Island and the bottom of Papua New Guinea (PNG) (top).
https://doi.org/10.1371/journal.pone.0051927.g001
Tiger shark movement patterns were more variable than those of green turtles with approximately half the animals showing discrete periods of area-restricted search separated by transit movement ( Fig. 2 ). Sharks mainly displayed concentrated area-restricted search movements when they were in the vicinity of Raine Island. Sharks did not show concentrated area-restricted search in any other discrete location, except for shark 79975. This shark left Raine Island three days after tagging, spending 12 days in transit to the Torres Strait Islands ( Fig. 2 ), in a similar region to that of green turtle foraging areas where it proceeded to spend the rest of the time (105 days). These results are likely, in part, to be related to the shorter (on average) deployment lengths for sharks than turtles.
Shark ID is indicated in the top right and duration of the deployment in the bottom left of each map. Each shark’s path is coded by behavioural mode; red = area-restricted search and black = transit. Maps show Cape York Peninsula, Raine Island and the bottom of Papua New Guniea (PNG) (top).
https://doi.org/10.1371/journal.pone.0051927.g002
Tiger sharks largely remained in the vicinity of Raine Island ( Fig. 3a ). During the green turtle nesting phase (Nov – Feb) the sharks were 68.89±71.58 km from Raine Island and outside of the nesting phase they were 115.82±131.08 km from Raine ( Fig. 3b ). We found no evidence to suggest that shark distance from Raine Island was different in the nesting season compared to outside the nesting season with the intercept only model (null model) having much higher support ( w AIC c = 0.78) than the slope model ( w AIC c = 0.22) ( Figs. 3 and 4 ). However, some sharks did travel further afield. Sharks 79974 and 62849 moved into the Coral Sea, to the south-east of Papua New Guinea ( Figs. 2 and 5 ). These sharks also had two of the three longest data records (209 and 356 days) ( Table 1 ). Interestingly, these transits were of short duration and didn’t result in area-restricted search behaviour in the Coral Sea and both sharks returned to Raine Island outside of the green turtle nesting season ( Fig. 5 ). In contrast, shark 79972 which had location data for 231 days stayed relatively close to Raine Island, never leaving the northern Great Barrier Reef region and never showing transit movement ( Figs. 2 and 5 ). While there were no differences in proximity to Raine Island overall between nesting and non-nesting season, half of the shark deployments conformed with the prediction of being closer to Raine Island in the green turtle nesting season. For the tiger sharks with long enough deployments (62849, 79972 and 79974), two of these fit the prediction ( Fig. 5 ). During the 2010/11 summer expeditions to Raine Island two satellite tagged tiger sharks were also observed feeding on a dead turtle, but neither animal was recaptured.
Mean and standard error distance from Raine Island for each month for all tiger sharks and green turtles (a). Mean and standard error distance from Raine Island for tiger sharks during the green turtle nesting season (nesting) and outside the nesting season (not nesting) (b).
https://doi.org/10.1371/journal.pone.0051927.g003
Plots show green turtles (a) and tiger sharks (b) during the green turtle nesting season and green turtles (c) and tiger sharks (d) outside the nesting season. Black dots show shark locations. Warmer colours correspond to more points. Maps show Cape York Peninsula and Papua New Guinnea at the top left and right.
https://doi.org/10.1371/journal.pone.0051927.g004
Plots show the mean and standard error of monthly distance from Raine Island for each tiger shark. Dashed line shows the nesting period for which turtles were in area-restricted search mode around Raine Island prior to the switch to transit movement mode and migration to the foraging grounds. Numbers to the left of the dashed line refer to the mean and sd of distance from Raine Island during the green turtle nesting period and numbers to the right of the dashed line shows the mean and sd of distance from Raine Island outside the green turtle nesting period. Note that the y-axis is different for each shark to allow comparisons between months for each shark.
https://doi.org/10.1371/journal.pone.0051927.g005
The majority of tiger sharks with depth data spent most of their time in the 10, 20 and 50 m depth bins with smaller proportions of time in the depth bins up to 400 m ( Fig. 6 ). One of the sharks that travelled into the Coral Sea (79974) spent more time in these deeper depth bins than the other sharks. A similar pattern was seen with time at temperature with the majority of sharks inhabiting temperatures around 27–33°C ( Fig. 6 ). There was not a close correspondence with switches in one behavioural mode to the other and variation in time at depth and time at temperature ( Fig. 6 ).
Plots show the proportion of time spent in each depth (left plots) and temperature (right plots) bin for each behavioural mode for the tiger sharks with SPLASH tags. Note that the first three sharks did not have any transit behaviour.
https://doi.org/10.1371/journal.pone.0051927.g006
There is now growing recognition of the structuring role of top-order predators in ecosystems [33] , [34] and there is a need for behavioural studies that integrate information on the movements and interactions of apex predators and their prey [7] . Our study is one of the few to investigate the movements of a top-order marine predator and its air-breathing prey over a large (10–100 s km) spatial scale (for studies at scales of m–km see [4] , [14] ). On average, tiger sharks spent most of their time in the vicinity of Raine Island, where the majority of area-restricted search behaviour took place, irrespective of the green turtle season. When green turtles left Raine for their foraging grounds in the Torres Strait, tiger sharks did not conduct migrations to other discrete foraging grounds. Our results suggest that while tiger sharks might target green turtles when they are concentrated at Raine Island during the nesting season, they might not focus principally on this prey source outside the nesting season when turtles are widely dispersed. These observations further support the view that tiger sharks are generalist feeders [35] .
The tracks of tiger sharks were highly variable both spatially and temporally. The movement further afield by some tiger sharks in autumn occurred after the green turtles had migrated away from Raine Island in March. Some sharks (e.g. 54738, 54739 and 79975) had already moved over 150 km away from Raine Island prior to green turtles leaving and others were present at Raine Island and the surrounding areas outside of the peak green turtle nesting season. Although sharks 62849 and 79974 moved large distances away from Raine Island, they both returned and spent time at the island outside of the green turtle nesting season. The return of these sharks to Raine Island was not simply a consequence of their relatively long data records (356 and 209 d respectively), since the shark with the 2 nd longest data record (79972) spent 231 days in the Raine Island region and showed no transit movement. Such individual variability in movement behaviour and habitat use among individual tiger sharks appears typical of the species, since tagging studies in other localities have recorded similar patterns [9] , [11] , [36] . For example, at the French Frigate Shoals, tagging showed that some animals were present year round, whereas others visited the atoll in summer to forage on fledging albatross and then departed before returning in subsequent years [11] . In Shark Bay, Western Australia tiger shark numbers increase when dugongs are abundant, but as with the turtles in the current study the long-term movements of tiger sharks do not match those of dugongs [9] . Tiger sharks tagged in Shark Bay showed variable movement patterns outside the seasonal occurrence of their prey with some individuals remaining in the Shark Bay region while others made larger excursions that included offshore waters [9] . Meyer et al. [10] , [11] suggested that tiger shark movements presumably include some element of exploration, enabling them to discover new foraging locations and over time, build up detailed spatio-temporal maps of productive prey patches. Individual differences in movement behaviour and the use of key habitats have also been observed in broadnose sevengill sharks, Notorynchus cepedianus [37] , [38] , a species that fills an apex predator role in temperate coastal waters.
With the exception of shark 79975 that moved to the north of Cape York, a similar area that four tagged green turtles also migrated to, none of the tiger sharks moved to green turtle foraging grounds to the north of Raine Island. These results imply that either there is sufficient other types of prey in the region of Raine island or that other species of turtles are present in the area to provide food for the sharks. We would not expect that some green turtles remain to forage as the area around Raine Island is relatively deep with no known seagrass habitat and the closest recognised foraging grounds are ∼80 km away in the adjacent coastal region [19] , [20] . While there is seagrass habitat in the coastal region adjacent to Raine Island, the movement behaviour and the kernel density plots showed that tiger sharks still concentrated their movements at Raine Island both inside and outside the turtle nesting season. Even if turtles are present at Raine Island outside of the nesting season, they are much more widely dispersed at this time making this food source less predictable and searching over larger areas would be required for tiger sharks to continue to target them. Multi-season data are required to conclusively answer the question, specifically to determine whether tiger sharks arrive at Raine Island at the start of the nesting season. Our tagging subjects were all caught at Raine Island and the deployments therefore commenced during green turtle nesting season. In addition, we had a low sample size with which to examine the hypothesis as only half the sharks provided data outside the green turtle nesting season. However, our results suggest that movement of this predator and its turtle prey are not strongly linked throughout the entire year.
Three tiger sharks made forays into the open ocean beyond the edge of the continental shelf. These excursions only lasted between 6–8 days. Similarly, tiger sharks in Shark Bay, Western Australia moved offshore into waters with depths of ∼800 m, but did not remain there for protracted periods [9] . Other tagging studies in Hawaii and French Frigate Shoals recorded regular offshore movements of tiger sharks across deep waters [8] , [11] . Tiger sharks tagged at Raine Island spent most of their time above 100 m but made dives exceeding 600 m depth. This is very similar to the depth behaviour exhibited by tiger sharks at the French Frigate Shoals [11] . The reasons for the offshore movements in our study are unknown; clearly they had little to do with adult green turtles, since these animals migrated through shelf waters. It is possible that these movements are exploratory since they displayed transit type movements of short durations. Offshore movements of some tiger sharks in Hawaii were linked to patterns of oceanic productivity [11] and there are anecdotal reports of seasonal bursts of productivity in the Coral Sea that are related to spawning events of tunas and myctophid fishes.
Tag Performance on Sharks
The tiger sharks tracked for 209, 231 and 356 days are the longest satellite tag deployments on this species (but see [36] for a track of 297 d) and with the exception of salmon sharks, Lamna ditropis ( n = 68, 6–1335 days), they are also some of the longest satellite tracks for any shark [39] . However, the remaining deployment periods (16–119 days) were comparable to results of previous studies that attached satellite tags to dorsal fins of tiger sharks [9] , [11] , [36] . Suspected reasons for the premature cessation of data uplinks are the exhaustion of batteries, antenna breakage, animal mortality, damage to the tag, detachment of tags from the animals, and the biofouling of the saltwater switch, which may be particularly problematic in tropical waters [11] , [40] . The antenna of the tag recovered in our study was covered in algae causing the antenna to bend ( Fig. S1 ). However, the cessation of data uplinks after 42 days was probably due to battery failure as the level of fouling to bend the antenna could not occur in such a short time. Tags deployed on tiger sharks transmitted on average approximately one location per day. However, the raw locations were not regularly spaced through time with high daily variation and gaps in the data record. Even though the tiger shark tracks had periods with fewer raw satellite locations than the 12 h interval at which the SSSM locations were being estimated we do not think that the gaps were large enough to impact the accuracy of the SSSM location estimates, see [28] . This is quite common for tracking studies on marine vertebrates, due to limited and/or short duration surface intervals, biofouling or tag defects [40] . These problems influence the number of location fixes and suggests that Argos satellite tags may only provide limited movement information for this species. The newer models of satellite tags with Fastloc™ GPS are capable of acquiring the data required for a location fix in a much shorter period of time and with greater location accuracy. These tags will be beneficial in future work as short surface intervals will still result in high quality location data [41] . Also, combining satellite tag technology with other methods such as acoustic tracking and/or stable isotopes should provide more comprehensive information on movement patterns, habitat use and species interactions [11] , [37] , [38] , [42] . Approaches such as the SSSM used here are therefore essential in order to make the most out of the typically low spatial and temporal resolution data obtained from Argos tracking studies on marine vertebrates.
Individual tiger sharks displayed high spatial and temporal variation in movement behaviour which was not closely linked to the movement behaviour of green turtles. On average tiger shark movements were concentrated at Raine Island throughout the year. The concentrated spatial and temporal overlap of tiger sharks and green turtles during the green turtle nesting season on Raine Island suggest these predators could have a significant effect on green turtle behaviour and populations during the nesting season. Given that green turtles (and sea turtles generally) are widely dispersed outside the nesting season and that tiger sharks remained largely concentrated at Raine Island year round, we suggest that tiger sharks do not focus on this less predictable food source outside the green turtle nesting season. While our approach was based solely on movement data and cannot conclusively determine whether tiger sharks target green turtles, it has provided information on the spatial and temporal overlap between these two species. Closer examination of the predator-prey relationship between these two species requires spatial data at finer scales over longer time scales combined with information on tiger shark diet. Our approach has resulted in more accurate tracks, revealed changes in behaviour and provided insight into the predator-prey relationship between tiger sharks and green turtles.
Supporting Information
Satellite transmitter recovered from tiger shark. Picture shows algal growth on the recovered transmitter (A). Satellite transmitter still attached to shark showing how the algal growth bends the antenna (B).
https://doi.org/10.1371/journal.pone.0051927.s001
Acknowledgments
We thank the staff of Digital Dimensions, J. Rumney from Eye to Eye Marine Encounters and the crew of Undersea Explorer for help with data acquisition. We also thank Ian Jonsen for providing his SSSM code.
Author Contributions
Conceived and designed the experiments: RF AB JDS IB. Performed the experiments: RF AB IB. Analyzed the data: MT. Contributed reagents/materials/analysis tools: RF IB JDS. Wrote the paper: AB MT MM.
- View Article
- Google Scholar
- 3. Estes JA (1996) Predation and diversity; Levin S, editor. San Diego, CA.: Academic Press. 857–878 p.
- 20. Limpus CJ (2008) A biological review of marine turtles in Australia: Green turtle. Brisbane: Queensland Department of Environment. 96 p.
- 29. Plummer M. (2003) JAGS: A Program for Analysis of Bayesian Graphical Models Using Gibbs Sampling, Vienna, Austria.
- 30. R Development Core Team (2012). R: A language and environment for statistical computing, reference index version 2.15.1. R Foundation for Statistical Computing, Vienna, Austria. ISBN 3-900051-07-0, URL http://www.R-project.org .
- 31. Pinheiro J, Bates D, DebRoy S, Sarkar D, R Core team (2012) Linear and Nonlinear Mixed Effects Models, Package ‘nlme’.
- 32. Burnham KP, Anderson DR (2002) Model selection and multimodel Inference: a practical information-theoretic approach. New York: Springer-Verlag.
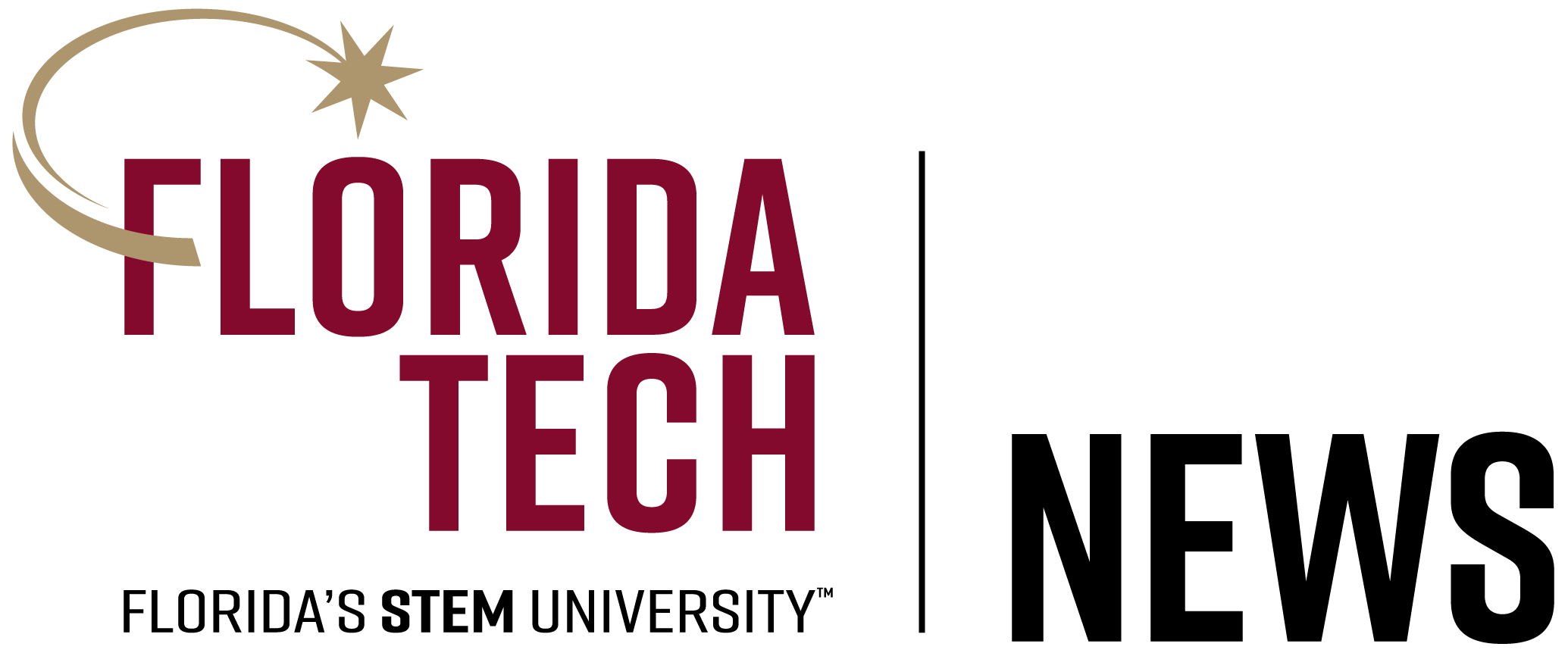
Tiger Sharks Return to Nurseries, New Study Finds
As conservationists learn better ways to preserve and protect marine life, new Florida Tech research investigating the movement patterns of tiger sharks may help safeguard the near-threatened species.
“Age-Dependent Dispersal and Relatedness in Tiger Sharks (Galeocerdo cuvier),” is a new publication featuring Florida Tech shark biologist Toby Daly-Engel , Margaret (Maggie) McClain, a MS spell out MS graduate from the University of West Florida (UWF) and a student of Daly-Engel’s when she taught at UWF, and researchers from the University of Miami, Florida State University, Mississippi State University, South Carolina Department of Natural Resources, Saving the Blue, Bimini Biological Field Station, Beneath the Waves and the Mississippi-Alabama Sea Grant Consortium. The paper was published in the July edition of Frontiers in Marine Sciences and the research looked at the northeastern Gulf of Mexico, Bahamas, Florida and South Carolina coasts to study the dispersal patterns of tiger sharks.
Researchers discovered that not only do female sharks return to nurseries for reproduction, but so do male sharks, with the goal of finding mates. They also discovered 8.9 percent of the tiger sharks studied were closely related to one another, showing that sharks returned repeatedly to the areas studied, but also that the species is declining in numbers, likely due to overfishing and environmental changes.
Sharks are what Daly-Engel and other researchers call “roving predators,” in that they don’t have a specific territory, making the discovery of their consistent return to specific areas even more noteworthy. The mother sharks will use shallow areas, such as river lagoons, as nurseries. This includes the Indian River Lagoon, a common nursery for bull sharks. Past research showed that a lot of female sharks use the same habitats where they were born to give birth to their own pups, but researchers assumed that since male sharks didn’t have to give birth, they didn’t return to the nursery habitats.
In a diverse shark population Daly-Engel referred to as “taking a bunch of watercolors and mixing them together,” McClain dug deeper into the data by taking out the largest sharks from the sample and reanalyzing it. When McClain got down to sharks that were under 8.5 feet, the team saw there were three unique genetic populations of sharks just in this small part of the world.
“It’s this repeated behavior that males are apparently doing as well,” Daly-Engel said. “What we were able to see in our data, the signal that they were looking at, we found it while looking at nuclear DNA, the DNA that an animal inherits from both its mom and its dad. Because that signal showed up nice and strong, we know that it must result from both male and female sharks, going back to the same areas year after year to reproduce over hundreds of thousands of years.”
Knowing the movement patterns of sharks can help with conservation. Currently, the World Conservation Union lists the tiger shark as “near threatened” throughout its range, as the species is targeted for its fins, flesh and oil and indirectly as bycatch in commercial and recreational fisheries worldwide. Having a better understanding of their movement patterns also re-emphasizes the importance of nursery habitats, like the Indian River Lagoon, which are facing environmental threats due to nutrient pollution and stormwater run-off leading to harmful algal blooms.
The tiger sharks may be known as roving predators, but this study shows they in fact stay close to specific area.
“It’s amazing that there are these separate gene pools,” Daly-Engel said. “Instead of one big world of tiger sharks, you have all these different countries, and there’s differences going back over thousands and even millions of years. You could say that they’re creatures of habit.”
Related Articles
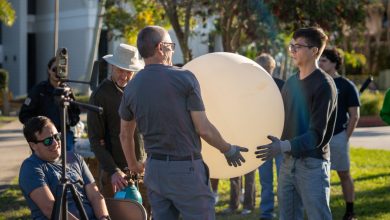
Meteorology Students, Faculty Deploy Weather Balloon from Crawford Green
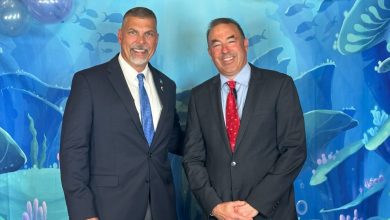
Florida Tech, East Coast Zoological Foundation Partner for Lagoon Research Group at New Aquarium
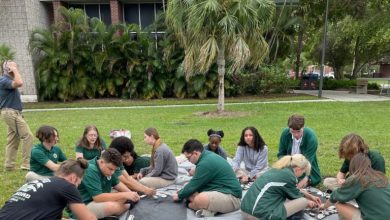
Indian River Lagoon Research Institute Hosts Workshop to Construct Oyster Mats
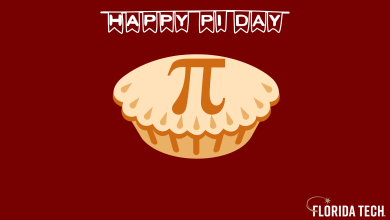
A Celebration of Pi
Academia.edu no longer supports Internet Explorer.
To browse Academia.edu and the wider internet faster and more securely, please take a few seconds to upgrade your browser .
Enter the email address you signed up with and we'll email you a reset link.
- We're Hiring!
- Help Center
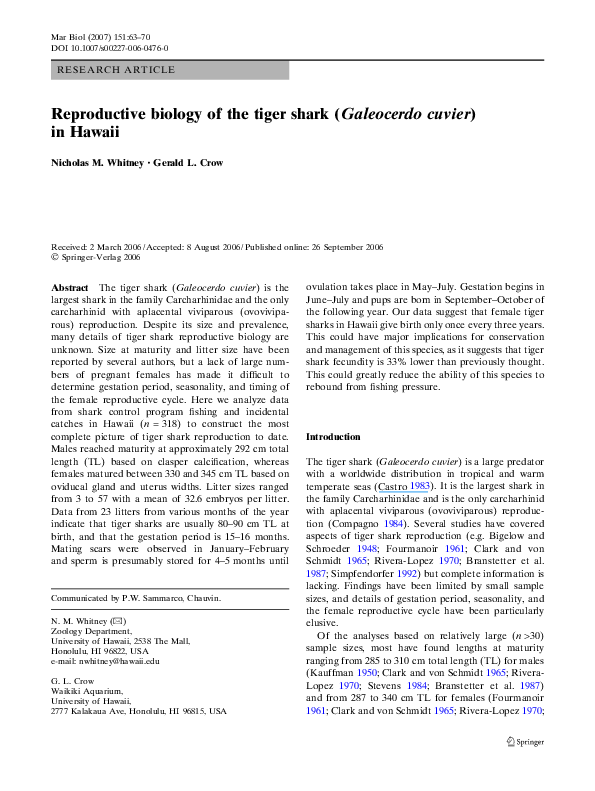
Reproductive biology of the tiger shark ( Galeocerdo cuvier ) in Hawaii

2007, Marine Biology
Related Papers
Neil Hammerschlag
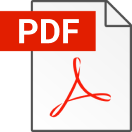
Journal of Animal Ecology
Sheldon Dudley
Eric Hoffmayer
Frontiers in Marine Science
Chris Buckner
Fundamental characteristics of the reproductive biology of female sand tiger sharks Carcharias taurus are needed to understand the periodicity, seasonality and environmental factors essential for reproduction in this iconic species. Animals in managed care, such as aquariums, provide the unique opportunity for longitudinal study in contrast to in situ sharks that are examined opportunistically, and at a single point in time. Additionally, comparison of reproductive observations from successfully reproducing in situ sharks and aquarium sharks may help elucidate reasons for lack of reproduction among aquarium sharks and aid the development of assisted reproductive techniques for managed populations. Reproductive status of in situ and aquarium female sharks was assessed using ultrasonography and plasma hormone (17β-estradiol, testosterone, and progesterone) monitoring. The reproductive cycle was divided into eight stages based on ovarian activity and uterine contents. In situ sharks we...
Marine and Coastal Fisheries
Zoo Biology
Marine and Freshwater Research
The reproductive biology of the blue shark (Prionace glauca) in the western North Pacific Ocean was investigated to contribute to future stock assessments because of limitations of recent studies and the lack of information about the reproductive cycle. Reproductive data were obtained from 490 males (precaudal length (PCL), 33.4–252.0cm) and 432 females (PCL, 33.4–243.3cm). Size at 50% maturity was estimated to be 160.9cm for males and 156.6cm PCL for females. Litter size varied from 15 to 112 (mean 35.5) and was positively correlated with maternal PCL. Parturition, ovulation and mating occurred sequentially from spring to summer. The gestation period was estimated to be 11 months. The ovarian follicles of pregnant females developed synchronously throughout the gestation period along with embryonic growth, indicating that females reproduce annually. Our results showed that the productivity of North Pacific blue sharks is higher than previously thought, based on larger fecundity and ...
Zoologia (Curitiba)
mARCIA SOUSA
Journal of Fish Biology
Thomas Poirout
Brazilian Journal of Oceanography
George Burgess
RELATED PAPERS
La canyamel dels ducs. Del trapig a la taula
Josep Antoni Gisbert Santonja
Peter Stetson
Mehdi Savaghebi
Fitria Usman
Abant İzzet Baysal Üniversitesi Eğitim Fakültesi Dergisi
ergün yurtbakan
Janka Slatinska
susu kambing
Dhaka University Journal of Science
Reazul Alam Refat
Juliana Kain
Chiara Bellio
Vanessa Soeiro
IEEE Transactions on Applied Superconductivity
Manfred Wanner
AL QUDS : Jurnal Studi Alquran dan Hadis
Irham Yuwana
Heloisa Szymanski
Fazir Mohamad
Journal of women's health (2002)
Cindy Amundsen
Experimental Dermatology
Agustín España
International Journal of Public Health Science
suprijandani suprijandani
Didasc@lia: Didáctica y Educación
MILTON PERALTA FONSECA
Synthesiology English edition
Koji Wakita
shaloo manocha
Bas Bruning
Vytautas Aukstakalnis
Cyril Perret
Werner R Murhadi
See More Documents Like This
RELATED TOPICS
- We're Hiring!
- Help Center
- Find new research papers in:
- Health Sciences
- Earth Sciences
- Cognitive Science
- Mathematics
- Computer Science
- Academia ©2024
A review of tonic immobility as an adaptive behavior in sharks
- Published: 27 April 2023
- Volume 106 , pages 1455–1462, ( 2023 )
Cite this article
- Abraham Miranda Páez ORCID: orcid.org/0000-0003-0985-1816 1 ,
- Edgar Mauricio Hoyos Padilla ORCID: orcid.org/0000-0002-4318-2655 2 , 3 &
- A. Peter Klimley ORCID: orcid.org/0000-0002-6097-0522 4 , 5
558 Accesses
13 Altmetric
Explore all metrics
Tonic immobility remains one of the least understood behaviors in nature. Despite this, the behavior has been described in a diversity of species across the animal kingdom. Tonic immobility has been observed in sharks and rays both in the laboratory and field. However, actual scientific studies of tonic immobility have been completed on only a few species of elasmobranchs. The behavior is frequently induced by handling an animal in a certain way rather than utilizing chemical anesthesia in order to assess body condition and implant electronic tracking devices. This behavior functions as (1) an innate defensive passive response against a predatory attack, (2) a component of courtship and copulation, and (3) a protective mechanism limiting the effect of overwhelming sensory stimulation. We present a review of the behavioral, physiological, and neurological processes that result in tonic immobility in sharks, and compare this information to the processes of tonic immobility that are better understood in mammals.
This is a preview of subscription content, log in via an institution to check access.
Access this article
Price includes VAT (Russian Federation)
Instant access to the full article PDF.
Rent this article via DeepDyve
Institutional subscriptions
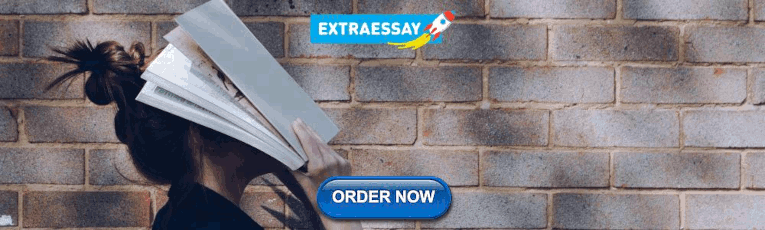
Similar content being viewed by others
Killer Whales: Behavior, Social Organization, and Ecology of the Oceans’ Apex Predators
Sea Otter Behavior: Morphologic, Physiologic, and Sensory Adaptations
The Function(s) of Sleep
Data availability.
Data sharing not applicable to this article as no datasets were generated or analyzed during the current study.
Code availability
Not applicable.
Bandler R, Carrive P, Depaulis A (1991) Emerging principles of organization in the midbrain periaqueductal gray matter. In: Depaulis A, Bandler R (eds) The midbrain periaqueductal gray matter: functional, anatomical and neurochemical organization. Plenum press, New York, pp 1–8
Google Scholar
Bandler R, Shipley MT (1994) Columnar organization in the midbrain periaqueductal gray: modules for emotional expression? Trends Neurosci 17:379–389
Article CAS PubMed Google Scholar
Bandler R, Keay KA (1996) Columnar organization in the midbrain periaqueductal gray and the integration of emotional expression. In: Holstege G, Bandler R, Saper CB (eds) The emotional motor system. Prog Brain Res 107. Elsevier Science BV, Amsterdam, pp 285–300
Bodznick D (1991) Elasmobranch vision: multimodal integration in the brain. J Exp Zool 5(Supp):108–116
Bonfil R, Meÿer M, Scholl MC, Johnson R, O´Brien S, Oosthuizen H. (2005) Transoceanic migration, spatial dynamics, and population linkages of white sharks. Science 310(5745):100–103. https://doi.org/10.1126/science.1114898
Bres M (1993) The behaviour of sharks. Rev Fish Biol Fish 3(2):133–159
Article Google Scholar
Brooks EJ, Sloman KA, Liss S, Hassan-Hassanein L, Danylchuk AJ, Cooke SJ, Mandelman JW, Skomal GB, Sims DW, Suski CD (2011) The stress physiology of extended duration tonic immobility in the juvenile lemon shark, Negaprion brevirostris (Poey, 1868). J Exp Mar Biol Ecol 409:351–360
Brunnschweiler JM, Pratt HL Jr (2008) Putative male–male agonistic behavior in free-living zebra sharks, Stegostoma fasciatum . Open Fish Sci J 1:23–27
Carli G, Lefebvre L, Silvano G, Vierucci S (1976) Suppression of accompanying reactions to prolonged noxious stimulation during animal hypnosis in the rabbit. Exp Neurol 53:1–11
Carrier JC, Pratt HL Jr (2004) Group reproductive behaviors in free-living nurse sharks, Ginglymostoma cirratum . Copeia 1994:646–656
Clayton L, Seeley KE (2019) Sharks and medicine. In: Miller RE, Lamberski N, Calle PP (eds) Fowler’s zoo and wild animal medicine current therapy. Elsevier Inc. Missouri.Vol 9 pp 338–344
Compagno LJV (1999) Checklist of living elasmobranches. In: Hamlet WC (ed) Sharks, skates, & rays. Johns Hopkins University Press, Baltimore MD, The biology of elasmobranch fishes, pp 471–498
Dannemann JP, White WJ, Marshall WK, Lang CM (1988) An evaluation of analgesia associated with the immobility response in laboratory rabbits. Lab Animal Sci 38:51–56
Davie PS, Franklin CE, Grigg GC (1993) Blood pressure and heart rate during tonic immobility in the black tipped reef shark. Carcharhinus Melanoptera Fish Physiol Biochem 12(2):95–100
De Swaef E, Vercauteren M, Pieraets L, Declercq AM (2020) Fight, flight or freeze. Tonic immobility in sharks. Vlaams Diergeneeskd Tijdschr 89:243–250
Fleischmann A, Urca G (1993) Tail-pinch induced analgesia and immobility: altered responses to noxious tail-pinch by prior pinch of the neck. Brain Res 601:28–33
Gallup GG Jr (1974) Animal hypnosis: factual status of a fictional concept. Psychol Bull 81:836–853
Article PubMed Google Scholar
Gallup GG Jr (1977) Tonic immobility: the role of fear and predation. Psychol Rec 1:41–61
Gallup GG Jr, Maser JD (1977) Tonic immobility: evolutionary underpinnings of human catalepsy and catatonia. In: Seligman MEP (ed) Psychopathology: experimental models. Freeman WH, New York, pp 334–457
Gruber SH, Keyes RS (1981) Keeping sharks for research. In: Hawkings AD (ed) Aquarium systems. Academic Press, London, pp 373–402
Henningsen AD (1994) Tonic immobility in 12 elasmobranchs: use as an aid in captive husbandry. Zoo Biol 13:325–332
Hofmann MH (1999) Nervous system. In: Hamlet WC (ed) Sharks, skates, & rays. Johns Hopkins University Press, Baltimore MD, The biology of elasmobranch fishes, pp 273–299
Holland KN, Wetherbee BM, Lowe CG, Meyer CG (1999) Movements of tiger sharks ( Galeocerdo cuvier ) in coastal Hawaiian waters. Mar Biol 134:665–673
Hoyos-Padilla EM, Klimley AP, Galván-Magaña F, Antoniou A (2016) Contrasts in the movements and habitat use of juvenile and adult white sharks ( Carcharodon carcharias ) at Guadalupe Island. Mexico Anim Biotelemetry 4:14. https://doi.org/10.1186/s40317-016-0106-7
Humphreys RK, Ruxton GD (2018) A review of thanatosis (death feigning) as an anti-predator behaviour. Behav Ecol Sociobiol 72:22. https://doi.org/10.1007/s00265-017-2436-8
Article PubMed PubMed Central Google Scholar
Jones RB (1986) The tonic immobility reaction of the domestic fowl: a review. Worlds Poult Sci J 42:82–96
Kessel ST, Hussey NE (2015) Tonic immobility as an anaesthetic for elasmobranch during surgical implantations procedures. Can J Fish Aquat Sci 72:1287–1291
Kingsbury MA, Kelly AM, Schrock SE, Goodson JL (2011) Mammal-like organization of the avian midbrain central gray and a reappraisal of the intercollicular nucleus. PLoS One 6(6):e20720
Article CAS PubMed PubMed Central Google Scholar
Kittelberger JM, Land BR, Bass AH (2006) Midbrain periaqueductal gray and vocal patterning in a teleost fish. J Neurophysiol 96(1):71–85
Klemm WR (2001) Behavioral arrest: in search of neural control system. Prog Neurobiol 65:453–471
Klimley AP (1980) Observations of courtship and copulation in the nurse shark, Ginglymostoma cirratum . Copeia 1980:878–882
Klimley AP (1985) Schooling in the large predator, Sphyrna lewini , a species with low risk of predation: a non-egalitarian state. Zeitschrift für Tierpsychologie (=Ethology) 70:297–319
Kreidl A (1916) Uber hypnose bei Fschen. Pfluger’s Arch Gen Physiol 164:441–444
Kozlowska K, Walker P, McLean L, Carrive P (2015) Fear and the defense cascade: clinical implications and management. Harvard Rev Psychiat 23(4):263287. https://doi.org/10.1097/HRP.0000000000000065
Leite-Panissi CR, Rodrigues CL, Brentegani MR, Menescal-De-Oliveira L (2001) Endogenous opiate analgesia induced by tonic immobility in guinea pigs. Brazil J Med Biol Res 34:245–250
Article CAS Google Scholar
Lissman HW (1946) The neurological basis of the locomotory rhythm in the spinal dogfish ( Scyallum canicula , Acanthias vulgaris ) I. Reflex Behavior. J Exp Zool 23:143–161
Mangold E (1920) Die tierische hypnose (Einschliesslich tonische tetanische und Totsell-reflex: Reactions- Akinese der Protisten). Ergelon Physiol 18:79–117
Miranda A, De La Cruz F, Zamudio SR (2006) Immobility response elicited by clamping the neck induces antinociception in a “tonic pain” test in mice. Life Sci 79:1108–1113
Miranda PA, Vázquez LP, Martínez ML, Sandoval HV, Villanueva BI, Zamudio HSR (2014) The effects of orexin A and orexin B on two forms of immobility responses and on analgesia. Adv Neuroim Biol 5:235–242. https://doi.org/10.3233/NIB-140092
Miranda PA, Zamudio SR, Vázquez LP, Campos RC, Ramírez SJE (2016) Involvement of opioid and GABA systems in the ventrolateral periaqueductal gray on analgesia associated with tonic immobility. Pharmacol Biochem Behav 142:72–78
Miyatake T, Nakayama S, Nishi Y, Nakajima S (2009) Tonically immobilized selfish prey can survive by sacrificing others. Proc R Soc 276:2763–2767
Monassi CR, Leite-Panissi CR, Menescal-de-Oliveira L (1999) Ventrolateral periaqueductal gray matter and the control of tonic immobility. Brain Res Bull 50:201–208
Morgan M, Whitney P, Gold M (1998) Immobility and flight associated with antinociception produced by activation of the ventral and lateral/dorsal regions of the rat periaqueductal gray. Brain Res 804:159–166
Morgan M, Clayton C (2005) Defensive behaviors evoked from the ventrolateral periaqueductal of the rat: comparison of opioid and GABA disinhibition. Behav Brain Res 164:61–66
Moskowitz AK (2004) “Scared stiff”: catatonia as evolutionary-based fear response. Psychol Rev 111(4):984–1002
Mukharror DA, Susiloningtyas D, Ichsan M (2019) Tonic immobility induction and duration on halmahera walking shark ( Hemischyllium halmahera ). IOP C Ser Earth Env 404:012080
Myrberg AA, Gruber SH (1974) The behavior of the bonnethead shark, Sphyrna lewini . Copeia 1974:358–374
Northcutt RG (1977) Elasmobranch central nervous system organization and its possible evolutionary significance. Am Zool 17:411–429
Northcutt RG (1978) Brain organization in the cartilaginous fishes. In: Hodgson ES, Mathewson RF (eds) Sensory biology of sharks, skates, and rays. Arlington VA: Office of Naval Research, pp 117–193
Pyle P, Schramm MJ, Keiper C, Anderson SD (1999) Predation on a white shark (Carcharodon carcharias) by a killer whale (Orcinus orca) and a possible case of competitive displacement. Mar Mam Sci 15(2):563–568
Ramos CM, Souza SLF, Menescal OL (2008) Modulation of tonic immobility in guinea pig PAG by homocysteic acid, a glutamate agonist. Physiol Behav 94:468–473
Ratner SC (1967) Comparative aspects of hypnosis. In: Gordon JE (ed) Handbook of clinical and experimental hypnosis. McMillan, New York
Reese WG, Angel C, Newton JEO (1984) Immobility reactions: a modified classification. Pay J Biol Sci Jul-Sept:137–143
Reynolds DV (1969) Surgery in the rat during electrical analgesia induced by focal brain stimulation. Science 164(3878):444–445
Schaefer JG (1921) Uber den lagerreflextonus von Raja clavata . Biol Zentrabl 41:291–295
Tricas TC, Deacon K, Last P, McCosker JE, Walker TI, Taylor L (1997) Sharks and rays. Taylor L (ed) Sydney, Reader's Digest
Vázquez LP, Mendoza RLG, Ramírez SJE, Chamorro CGA, Miranda PA (2017) Analgesic and anxiolytic effects of [Leu 31 , Pr o3 4]-neuropeptide Y microinjected into the periaqueductal gray in rats. Neuropeptides 66:81–89
Vázquez LP, Miranda PA, Valencia FK, Sánchez CA (2022) Defensive and emotional behavior modulation by serotonin in the periaqueductal gray. Cell Mol Neurobiol. https://doi.org/10.1007/s10571-022-01262-z
Watsky MA, Gruber SH (1990) Induction and duration of tonic immobility in the lemon shark Negaprion brevirostris . Fish Physiol Biochem 8:207–210
Wells RMG, McNeil H, McDonald JA (2005) Fish hypnosis: induction of an atonic immobility reflex. Marine Behav Physiol 38(1):71–78. https://doi.org/10.1080/10236240400029341
Whitman PA (1984) Tonic immobility in juvenile sandbar sharks, Carcharhinus plumbeus (Nardo, 1827) (Pisces, Carcharhinidae). M. S. thesis, Univ West Va. Morgantown WV, USA
Whitman PA, Marshal JA, Keller BC Jr (1986) Tonic immobility in the smooth dogfish shark, Mustelus canis (Pisces, Carcharinidae). Copeia 3:829–832
Williamson MJ, Dudgeon C, Slade R (2018) Tonic immobility in the zebra shark, Stegostoma fasciatum , and its use for capture methodology. Environ Biol Fish 101:741–748
Wilson AL (2004) Death feigning: an adaptive, antipredator response. Bio 755 # 1, pp 1–5. https://people.eku.edu/rithcisong/behavcol/awilson_feigning.pdf
Yopak KE, Lisney TJ, Collin SP, Montgomery JC (2007) Variation in brain organization and cerebellar foliation in chondrichthyans: sharks and holocephalans. Brain Behav Evol 69(4):280–300. https://doi.org/10.1159/000100037
Yopak KE, McMeans BC, Mull CG, Feindel KW, Kovacs KM, Lydersen C, Fisk AT, Collin SP (2019) Comparative brain morphology of the Greenland and Pacific sleeper sharks and its functional implications. Sci Rep 9:10022. https://doi.org/10.1038/s41598-019-462255
Yoshida M (2021) Immobility behaviors in fish: a comparison with other vertebrates. In: Sakai M (ed) Death-feigning in insects. Entomology Monographs. Springer, Singapore. https://doi.org/10.1007/978-981-33-6598-8_11
Download references
Acknowledgements
We are grateful for the funds provided by the Instituto Politécnico Nacional (SIP 20210587). EMHP and AMP are fellows of SNI (CONACYT) Mexico. Authors thanks to Dr. Priscila Vázquez-León for the supplied material, and the anonymous reviewers whose comments allow improve the manuscript.
Author information
Authors and affiliations.
Departamento de Fisiología, Escuela Nacional de Ciencias Biológicas, Instituto Politécnico Nacional, Wilfrido Massieu Esq. Manuel Stampa S/N Col. Nueva Industrial Vallejo, Alcaldía Gustavo A. Madero, 07738, Mexico City, Mexico
Abraham Miranda Páez
Pelagios Kakunjá A.C., La Paz, Baja California Sur, Mexico
Edgar Mauricio Hoyos Padilla
Fins Attached Marine Research and Conservation, Colorado Springs, CO, USA
Department of Wildlife, Fish, & Conservation Biology, University of California, Davis, CA, 95616, USA
A. Peter Klimley
Biotelemetric Consultants, 2870 Eastman Lane, Petaluma, CA, 94954, USA
You can also search for this author in PubMed Google Scholar
Contributions
All authors have been involved throughout the review and participated significantly to the writing. Specifically: conceived and designed the review and wrote the paper: AMP; corrected and edited the manuscript: MHP; wrote and edited extensively the manuscript: APK.
Corresponding author
Correspondence to A. Peter Klimley .
Ethics declarations
Ethical statement, consent to participate.
All the authors agree with the contents of the manuscript and give their consent to submit.
Consent for publication
This work is an original review carried out by the authors and all of us agree with its submission in the present form to the RFBF. The manuscript is not currently under consideration in another journal.
Conflict of interest
The authors declare no competing interests.
Additional information
Publisher's note.
Springer Nature remains neutral with regard to jurisdictional claims in published maps and institutional affiliations.
Rights and permissions
Springer Nature or its licensor (e.g. a society or other partner) holds exclusive rights to this article under a publishing agreement with the author(s) or other rightsholder(s); author self-archiving of the accepted manuscript version of this article is solely governed by the terms of such publishing agreement and applicable law.
Reprints and permissions
About this article
Páez, A.M., Padilla, E.M.H. & Klimley, A.P. A review of tonic immobility as an adaptive behavior in sharks. Environ Biol Fish 106 , 1455–1462 (2023). https://doi.org/10.1007/s10641-023-01413-1
Download citation
Received : 28 December 2022
Accepted : 03 April 2023
Published : 27 April 2023
Issue Date : June 2023
DOI : https://doi.org/10.1007/s10641-023-01413-1
Share this article
Anyone you share the following link with will be able to read this content:
Sorry, a shareable link is not currently available for this article.
Provided by the Springer Nature SharedIt content-sharing initiative
- Periaqueductal gray
- Find a journal
- Publish with us
- Track your research

An official website of the United States government
The .gov means it’s official. Federal government websites often end in .gov or .mil. Before sharing sensitive information, make sure you’re on a federal government site.
The site is secure. The https:// ensures that you are connecting to the official website and that any information you provide is encrypted and transmitted securely.
- Publications
- Account settings
Preview improvements coming to the PMC website in October 2024. Learn More or Try it out now .
- Advanced Search
- Journal List

Extinction risk and conservation of the world’s sharks and rays
Nicholas k dulvy.
IUCN Species Survival Commission Shark Specialist Group, Department of Biological Sciences, Simon Fraser University, Burnaby, Canada
Earth to Ocean Research Group, Department of Biological Sciences, Simon Fraser University, Burnaby, Canada
Sarah L Fowler
IUCN Species Survival Commission Shark Specialist Group, NatureBureau International, Newbury, United Kingdom
John A Musick
Virginia Institute of Marine Science, College of William and Mary, Gloucester Point, United States
Rachel D Cavanagh
British Antarctic Survey, Natural Environment Research Council, Cambridge, United Kingdom
Peter M Kyne
Research Institute for the Environment and Livelihoods, Charles Darwin University, Darwin, Australia
Lucy R Harrison
John k carlson.
Southeast Fisheries Science Center, NOAA/National Marine Fisheries Service, Panama City, United States
Lindsay NK Davidson
Sonja v fordham.
Shark Advocates International, The Ocean Foundation, Washington, DC, United States
Malcolm P Francis
National Institute of Water and Atmospheric Research, Wellington, New Zealand
Caroline M Pollock
Global Species Programme, International Union for the Conservation of Nature, Cambridge, United Kingdom
Colin A Simpfendorfer
Centre for Sustainable Tropical Fisheries and Aquaculture, James Cook University, Townsville, Australia
School of Earth and Environmental Sciences, James Cook University, Townsville, Australia
George H Burgess
Florida Program for Shark Research, Florida Museum of Natural History, University of Florida, Gainsville, United States
Kent E Carpenter
IUCN Species Programme Species Survival Commission, Old Dominion University, Norfolk, United States
Conservation International Global Marine Species Assessment, Old Dominion University, Norfolk, United States
Leonard JV Compagno
Shark Research Center, Iziko, South African Museum, Cape Town, South Africa
David A Ebert
Pacific Shark Research Center, Moss Landing Marine Laboratories, Moss Landing, United States
Claudine Gibson
Michelle r heupel.
School of Earth and Environmental Sciences, Australian Institute of Marine Science, Townsville, Australia
Suzanne R Livingstone
Global Marine Species Assessment, Biodiversity Assessment Unit, IUCN Species Programme, Conservation International, Arlington, United States
Jonnell C Sanciangco
John d stevens.
Marine and Atmospheric Research, Commonwealth Scientific and Industrial Research Organisation, Hobart, Australia
Sarah Valenti
William t white, associated data.
DOI: http://dx.doi.org/10.7554/eLife.00590.024
DOI: http://dx.doi.org/10.7554/eLife.00590.025
The rapid expansion of human activities threatens ocean-wide biodiversity. Numerous marine animal populations have declined, yet it remains unclear whether these trends are symptomatic of a chronic accumulation of global marine extinction risk. We present the first systematic analysis of threat for a globally distributed lineage of 1,041 chondrichthyan fishes—sharks, rays, and chimaeras. We estimate that one-quarter are threatened according to IUCN Red List criteria due to overfishing (targeted and incidental). Large-bodied, shallow-water species are at greatest risk and five out of the seven most threatened families are rays. Overall chondrichthyan extinction risk is substantially higher than for most other vertebrates, and only one-third of species are considered safe. Population depletion has occurred throughout the world’s ice-free waters, but is particularly prevalent in the Indo-Pacific Biodiversity Triangle and Mediterranean Sea. Improved management of fisheries and trade is urgently needed to avoid extinctions and promote population recovery.
DOI: http://dx.doi.org/10.7554/eLife.00590.001
eLife digest
Ocean ecosystems are under pressure from overfishing, climate change, habitat destruction and pollution. These pressures have led to documented declines of some fishes in some places, such as those living in coral reefs and on the high seas. However, it is not clear whether these population declines are isolated one-off examples or, instead, if they are sufficiently widespread to risk the extinction of large numbers of species.
Most fishes have a skeleton that is made of bone, but sharks and rays have a skeleton that is made of cartilage. A total of 1,041 species has such a skeleton and they are collectively known as the Chondrichthyes. To find out how well these fish are faring, Dulvy et al. worked with more than 300 scientists around the world to assess the conservation status of all 1,041 species.
Based on this, Dulvy et al. estimate that one in four of these species are threatened with extinction, mainly as a result of overfishing. Moreover, just 389 species (37.4% of the total) are considered to be safe, which is the lowest fraction of safe species among all vertebrate groups studied to date.
The largest sharks and rays are in the most peril, especially those living in shallow waters that are accessible to fisheries. A particular problem is the ‘fin trade’: the fins of sharks and shark-like rays are a delicacy in some Asian countries, and more than half of the chondrichthyans that enter the fin trade are under threat. Whether targeted or caught by boats fishing for other species, sharks and rays are used to supply a market that is largely unmonitored and unregulated. Habitat degradation and loss also pose considerable threats, particularly for freshwater sharks and rays.
Dulvy et al. identified three main hotspots where the biodiversity of sharks and rays was particularly seriously threatened—the Indo-Pacific Biodiversity Triangle, Red Sea, and the Mediterranean Sea—and argue that national and international action is needed to protect them from overfishing.
DOI: http://dx.doi.org/10.7554/eLife.00590.002
Introduction
Populations and species are the building blocks of the communities and ecosystems that sustain humanity through a wide range of services ( Mace et al., 2005 ; Díaz et al., 2006 ). There is increasing evidence that human impacts over the past 10 millennia have profoundly and permanently altered biodiversity on land, especially of vertebrates ( Schipper et al., 2008 ; Hoffmann et al., 2010 ). The oceans encompass some of the earth’s largest habitats and longest evolutionary history, and there is mounting concern for the increasing human influence on marine biodiversity that has occurred over the past 500 years ( Jackson, 2010 ). So far our knowledge of ocean biodiversity change is derived mainly from retrospective analyses usually limited to biased subsamples of diversity, such as: charismatic species, commercially-important fisheries, and coral reef ecosystems ( Carpenter et al., 2008 ; Collette et al., 2011 ; McClenachan et al., 2012 ; Ricard et al., 2012 ). Notwithstanding the limitations of these biased snapshots, the rapid expansion of fisheries and globalized trade are emerging as the principal drivers of coastal and ocean threat ( Polidoro et al., 2008 ; Anderson et al., 2011b ; McClenachan et al., 2012 ). The extent and degree of the global impact of fisheries upon marine biodiversity, however, remains poorly understood and highly contentious. Recent insights from ecosystem models and fisheries stock assessments of mainly data-rich northern hemisphere seas, suggest that the status of a few of the best-studied exploited species and ecosystems may be improving ( Worm et al., 2009 ). However, this view is based on only 295 populations of 147 fish species and hence is far from representative of the majority of the world’s fisheries and fished species, especially in the tropics for which there are few data and often less management ( Sadovy, 2005 ; Newton et al., 2007 ; Branch et al., 2011 ; Costello et al., 2012 ; Ricard et al., 2012 ).
Overfishing and habitat degradation have profoundly altered populations of marine animals ( Hutchings, 2000 ; Lotze et al., 2006 ; Polidoro et al., 2012 ), especially sharks and rays ( Stevens et al., 2000 ; Simpfendorfer et al., 2002 ; Dudley and Simpfendorfer, 2006 ; Ferretti et al., 2010 ). It is not clear, however, whether the population declines of globally distributed species are locally reversible or symptomatic of an erosion of resilience and chronic accumulation of global marine extinction risk ( Jackson, 2010 ; Neubauer et al., 2013 ). In response, we evaluate the scale and intensity of overfishing through a global systematic evaluation of the relative extinction risk for an entire lineage of exploited marine fishes—sharks, rays, and chimaeras (class Chondrichthyes)—using the Red List Categories and Criteria of the International Union for the Conservation of Nature (IUCN). We go on to identify, (i) the life history and ecological attributes of species (and taxonomic families) that render them prone to extinction, and (ii) the geographic locations with the greatest number of species of high conservation concern.
Chondrichthyans make up one of the oldest and most ecologically diverse vertebrate lineages: they arose at least 420 million years ago and rapidly radiated out to occupy the upper tiers of aquatic food webs ( Compagno, 1990 ; Kriwet et al., 2008 ). Today, this group is one of the most speciose lineages of predators on earth that play important functional roles in the top-down control of coastal and oceanic ecosystem structure and function ( Ferretti et al., 2010 ; Heithaus et al., 2012 ; Stevens et al., 2000 ). Sharks and their relatives include some of the latest maturing and slowest reproducing of all vertebrates, exhibiting the longest gestation periods and some of the highest levels of maternal investment in the animal kingdom ( Cortés, 2000 ). The extreme life histories of many chondrichthyans result in very low population growth rates and weak density-dependent compensation in juvenile survival, rendering them intrinsically sensitive to elevated fishing mortality ( Musick, 1999b ; Cortés, 2002 ; García et al., 2008 ; Dulvy and Forrest, 2010 ).
Chondrichthyans are often caught as incidental, but are often retained as valuable bycatch of fisheries that focus on more productive teleost fish species, such as tunas or groundfishes ( Stevens et al., 2005 ). In many cases, fishing pressure on chondrichthyans is increasing as teleost target species become less accessible (due to depletion or management restrictions) and because of the high, and in some cases rising, value of their meat, fins, livers, and/or gill rakers ( Fowler et al., 2002 ; Clarke et al., 2006 ; Lack and Sant, 2009 ). Fins, in particular, have become one of the most valuable seafood commodities: it is estimated that the fins of between 26 and 73 million individuals, worth US$400-550 million, are traded each year ( Clarke et al., 2007 ). The landings of sharks and rays, reported to the Food and Agriculture Organization of the United Nations (FAO), increased steadily to a peak in 2003 and have declined by 20% since ( Figure 1A ). True total catch, however, is likely to be 3–4 times greater than reported ( Clarke et al., 2006 ; Worm et al., 2013) . Most chondrichthyan catches are unregulated and often misidentified, unrecorded, aggregated, or discarded at sea, resulting in a lack of species-specific landings information ( Barker and Schluessel, 2005 ; Clarke et al., 2006 ; Iglésias et al., 2010 ; Bornatowski et al., 2013 ). Consequently, FAO could only be ‘hopeful’ that the catch decline is due to improved management rather than being symptomatic of worldwide overfishing ( FAO, 2010 ). The reported chondrichthyan catch has been increasingly dominated by rays, which have made up greater than half of reported taxonomically-differentiated landings for the past four decades ( Figure 1B ). Chondrichthyan landings were worth US$1 billion at the peak catch in 2003, since then the value has dropped to US$800 million as catch has declined ( Musick and Musick, 2011 ). A main driver of shark fishing is the globalized trade to meet Asian demand for shark fin soup, a traditional and usually expensive Chinese dish. This particularly lucrative trade in fins (not only from sharks, but also of shark-like rays such as wedgefishes and sawfishes) remains largely unregulated across the 86 countries and territories that exported >9,500 mt of fins to Hong Kong (a major fin trade hub) in 2010 ( Figure 1C ).

( A ) The landed catch of chondrichthyans reported to the Food and Agriculture Organization of the United Nations from 1950 to 2009 up to the peak in 2003 (black) and subsequent decline (red). ( B ) The rising contribution of rays to the taxonomically-differentiated global reported landed catch: shark landings (light gray), ray landings (black), log ratio [rays/sharks], (red). Log ratios >0 occur when more rays are landed than sharks. The peak catch of taxonomically-differentiated rays peaks at 289,353 tonnes in 2003. ( C ) The main shark and ray fishing nations are gray-shaded according to their percent share of the total average annual chondrichthyan landings reported to FAO from 1999 to 2009. The relative share of shark and ray fin trade exports to Hong Kong in 2010 are represented by fin size. The taxonomically-differentiated proportion excludes the ‘nei’ (not elsewhere included) and generic ‘sharks, rays, and chimaeras’ category.
DOI: http://dx.doi.org/10.7554/eLife.00590.003
Red List status of chondrichthyan species
Overall, we estimate that one-quarter of chondrichthyans are threatened worldwide, based on the observed threat level of assessed species combined with a modeled estimate of the number of Data Deficient species that are likely to be threatened. Of the 1,041 assessed species, 181 (17.4%) are classified as threatened: 25 (2.4%) are assessed as Critically Endangered (CR), 43 (4.1%) Endangered (EN), and 113 (10.9%) Vulnerable (VU) ( Table 1 ). A further 132 species (12.7%) are categorized as Near Threatened (NT). Chondrichthyans have the lowest percentage (23.2%, n = 241 species) of Least Concern (LC) species of all vertebrate groups, including the marine taxa assessed to date ( Hoffmann et al., 2010 ). Almost half (46.8%, n = 487) are Data Deficient (DD) meaning that information is insufficient to assess their status ( Table 1 ). DD chondrichthyans are found across all habitats, but particularly on continental shelves (38.4% of 482 species in this habitat) and deepwater slopes (57.6%, Table 2 ). Of the 487 DD species for which we had sufficient maximum body size (n = 396) and geographic distribution data (n = 378), we were able to predict that at least a further 68 DD species are likely to be threatened ( Table 3 , Supplementary file 1 ). Accounting for the uncertainty in threat levels due to the number of DD species, we estimate that more than half face some elevated risk: at least one-quarter (n = 249; 24%) of chondrichthyans are threatened and well over one-quarter are Near Threatened ( Table 1 ). Only 37% are predicted to be Least Concern ( Table 1 ).
Observed and predicted number and percent of chondrichthyan species in IUCN Red List categories
DOI: http://dx.doi.org/10.7554/eLife.00590.004
CR, Critically Endangered; EN, Endangered; VU, Vulnerable; NT, Near Threatened; LC, Least Concern; DD, Data Deficient. Number threatened is the sum total of the categories CR, EN and VU. Species number and number threatened are expressed as percentage of the taxon, whereas the percentage of each species in IUCN categories is expressed relative to the total number of species.
Number and percent of chondrichthyans in IUCN Red List categories by their main habitats
DOI: http://dx.doi.org/10.7554/eLife.00590.005
CR, Critically Endangered; EN, Endangered; VU, Vulnerable; NT, Near Threatened; LC, Least Concern; DD, Data Deficient.
Summary of predictive Generalized Linear Models for life history and ecological correlates of IUCN status
DOI: http://dx.doi.org/10.7554/eLife.00590.006
Species were scored as threatened (CR, EN, VU) = 1 or Least Concern (LC) = 0 for n = 367 marine species. AIC c is the Akaike information criterion corrected for small sample sizes and ΔAIC is the change in AIC c . The models are ordered by increasing complexity and decreasing AIC weight (largest ΔAIC to lowest), coefficient of determination ( R 2 ), and prediction accuracy (measured using Area Under the Curve, AUC).
Drivers of threat
The main threats to chondrichthyans are overexploitation through targeted fisheries and incidental catches (bycatch), followed by habitat loss, persecution, and climate change. While one-third of threatened sharks and rays are subject to targeted fishing, some of the most threatened species (including sawfishes and large-bodied skates) have declined due to incidental capture in fisheries targeting other species. Shark-like rays, especially sawfishes, wedgefishes and guitarfishes, have some of the most valuable fins and are highly threatened. Although the global fin trade is widely recognized as a major driver of shark and ray mortality, demand for meat, liver oil, and even gillrakers (of manta and other devil rays) also poses substantial threats. Half of the 69 high-volume or high-value sharks and rays in the global fin trade are threatened (53.6%, n = 37), while low-value fins often enter trade as well, even if meat demand is the main fishery driver ( Supplementary file 2A ). Coastal species are more exposed to the combined threats of fishing and habitat degradation than those offshore in pelagic and deepwater ecosystems. In coastal, estuarine, and riverine habitats, four principal processes of habitat degradation (residential and commercial development, mangrove destruction, river engineering, and pollution) jeopardize nearly one-third of threatened sharks and rays (29.8%, n = 54 of 181, Supplementary file 2B ). The combined effects of overexploitation and habitat degradation are most acute in freshwater, where over one-third (36.0%) of the 90 obligate and euryhaline freshwater chondrichthyans are threatened. Their plight is exacerbated by high habitat-specificity and restricted geographic ranges ( Stevens et al., 2005 ). Specifically, the degradation of coastal, estuarine and riverine habitats threatened 14% of sharks and rays: through residential and commercial development (22 species, including river sharks Glyphis spp.); mangrove destruction for shrimp farming in Southeast Asia (4 species, including Bleeker’s variegated stingray Himantura undulata ); dam construction and water control (8 species, including Mekong freshwater stingray Dasyatis laosensis ), and pollution (20 species). Many freshwater sharks and rays suffer multiple threats and have narrow geographic distributions, for example the Endangered Roughnose stingray (Pastinachus solocirostris) that is found only in Malaysian Borneo and Indonesia (Kalimantan, Sumatra and Java). Population control of sharks, in particular due to their perceived risk to people, fishing gear, and other fisheries has contributed to the threatened status of at least 12 species ( Supplementary file 2B ). Sharks and rays are also threatened due to capture in shark control nets (e.g. Dusky shark Carcharhinus obscurus ), and persecution to minimise: damage to fishing nets (e.g. Green sawfish Pristis zijsron ); their predation on aquacultured molluscs (e.g. Estuary stingray Dasyatis fluviorum ); interference with spearfishing activity (e.g. Grey nurse shark Carcharias taurus ), and the risk of shark attack (e.g. White shark Carcharodon carcharias ). So far the threatened status of only one species has been directly linked to climate change (New Caledonia catshark Aulohalaelurus kanakorum , Supplementary file 2B ). the climate-sensitivity of some sharks has been recognized ( Chin et al., 2010 ) and the status of shark and ray species will change rapidly in climate cul-de-sacs, such as the Mediterranean Sea ( Lasram et al., 2010 ).
Correlates and predictors of threat
Elevated extinction risk in sharks and rays is a function of exposure to fishing mortality coupled with their intrinsic life history and ecological sensitivity ( Figures 2–6 ). Most threatened chondrichthyan species are found in depths of less than 200 m, especially in the Atlantic and Indian Oceans, and the Western Central Pacific Ocean (79.6%, n = 144 of 181, Figure 2 ). Extinction risk is greater in larger-bodied species found in shallower waters with narrower depth distributions, after accounting for phylogenetic non-independence ( Figures 3 and 4 ). The traits with the greatest relative importance (>0.95) are maximum body size, minimum depth, and depth range. In comparison, geographic range (measured as Extent of Occurrence) has a much lower relative importance (0.79, Figure 3 ), and in the predictive models it improved the variance explained by 2% and the prediction accuracy by 1% ( Table 3 ). The probability that a species is threatened increases by 1.2% for each 10 cm increase in maximum body length, and decreases by 10.3% for each 50 m deepening in the minimum depth limit of species. After accounting for maximum body size and minimum depth, species with narrower depth ranges have a 1.2% greater threat risk per 100 m narrowing of depth range. There is no significant interaction between depth range and minimum depth limit. Geographic range, measured as the Extent of Occurrence, varies over six orders of magnitude, between 354 km 2 and 278 million km 2 and is positively correlated with body size (Spearman’s ρ = 0.58), and hence is only marginally positively related to extinction risk over and above the effect of body size. Accounting for the body size and depth effects, the threat risk increases by only 0.5% for each 1,000,000 km 2 increase in geographic range ( Table 4 ). The explanatory and predictive power of our life history and geographic distribution models increased with complexity, though geographic range size contributed relatively little additional explanatory power and a high degree of uncertainty in the parameter estimate ( Tables 3 and 4 ). The maximum variance explained was 69% ( Table 4 ) and the predictive models (without controlling for phylogeny) explained 30% of the variance and prediction accuracy was 77% ( Table 3 ).

Each vertical line represents the depth range (surface-ward minimum to the maximum reported depth) of each species and is colored according to threat status: CR (red), EN (orange), VU (yellow), NT (pale green), LC (green), and DD (gray). Species are ordered left to right by increasing median depth. The depth limit of the continental shelf is indicated by the horizontal gray line at 200 m. The Polar Seas include the following FAO Fishing Areas: Antarctic–Atlantic (Area 48), Indian (Area 58), Pacific (Area 88), and the Arctic Sea (Area 18).
DOI: http://dx.doi.org/10.7554/eLife.00590.007
Figure 2—figure supplement 1.

DOI: http://dx.doi.org/10.7554/eLife.00590.008

The data were standardized by subtracting the mean and dividing by one standard deviation to allow for comparison among parameters. The relative importance is calculated as the sum of the Akaike weights of the models containing each variable. Chondrichthyans were scored as threatened (CR, EN, VU) = 1 or Least Concern (LC) = 0 for n = 367 marine species. Threat status was modeled using General Linear Mixed-effects Models, with size, depth and geography treated as fixed effects and taxonomy hierarchy as a random effect to account for phylogenetic non-independence.
DOI: http://dx.doi.org/10.7554/eLife.00590.009

Probability that a species is threatened due to the combination of intrinsic life history sensitivity (maximum body size, cm total length, TL) and accessibility to fisheries which is represented as minimum depth limit, depth range, and geographic range size (Extent of Occurrence). The lines represent the variation in body size-dependent risk for the upper quartile, median, and lower quartile of each range metric. The examplar species are all of similar maximum body length and the difference in risk is largely due to differences in geographic distribution. Chondrichthyans were scored as threatened (CR, EN, VU) = 1 or Least Concern (LC) = 0 for n = 366 marine species. The lines are the best fits from General Linear Mixed-effects Models, with maximum body size and geographic distribution traits treated as fixed effects and taxonomy hierarchy as a random effect to account for phylogenetic non-independence. Each vertical line in each of the ‘rugs’ represents the maximum body size and Red List status of each species: threatened (red) and LC (green).
DOI: http://dx.doi.org/10.7554/eLife.00590.010

IUCN Red List status as a function of maximum body size (total length, TL cm) and accessibility to fisheries in marine chondrichthyans in three main habitats: coastal and continental shelf <200 m (‘Continental shelf’); neritic and oceanic pelagic <200 m (‘Pelagic’); and, deepwater >200 m (‘Deepwater’), n = 367 (threatened n = 148; Least Concern n = 219). The upper and lower ‘rug’ represents the maximum body size and Red List status of each species: threatened (upper rugs) and Least Concern (lower rugs). The lines are best fit using Generalized Linear Mixed-effects Models with 95% confidence intervals ( Table 9 ).
DOI: http://dx.doi.org/10.7554/eLife.00590.011

Threat among marine chondrichthyan families varies with life history sensitivity (maximum length) and exposure to fisheries (depth distribution). ( A ) Proportion of threatened data sufficient species and the richness of each taxonomic family. Colored bands indicate the significance levels of a one-tailed binomial test at p=0.05, 0.01, and 0.001. Those families with significantly greater (or lower) than expected threat levels at p<0.05 against a null expectation that extinction risk is equal across families (35.6%). ( B ) The most and least threatened taxonomic families. ( C ) Average life history sensitivity and accessibility to fisheries of 56 chondrichthyan families. Significantly greater (or lower) risk than expected is shown in red (green).
DOI: http://dx.doi.org/10.7554/eLife.00590.012
Figure 6—source data 1.
DOI: http://dx.doi.org/10.7554/eLife.00590.013
Summary of explanatory Generalized Linear Mixed-effect Models of the life history and geographic distributional correlates of IUCN status
DOI: http://dx.doi.org/10.7554/eLife.00590.014
Species were scored as threatened (CR, EN, VU) = 1 or Least Concern (LC) = 0 for n = 367 marine species. AIC c is the Akaike information criterion corrected for small sample sizes; ΔAIC is the change in AIC c . The models are ordered by increasing complexity and decreasing AIC weight (largest ΔAIC to lowest). R 2 GLMM( m ) is the marginal R 2 of the fixed effects only and R 2 GLMM( c ) is the conditional R 2 of the fixed and random effects.
By habitat, one-quarter of coastal and continental shelf chondrichthyans (26.3%, n = 127 of 482) and almost half of neritic and epipelagic species (43.6%, n = 17 of 39) are threatened. Coastal and continental shelf and pelagic species greater than 1 m total length have a more than 50% chance of being threatened, compared to ∼12% risk for a similar-sized deepwater species ( Figure 5 ). While deepwater chondrichthyans, due to their slow growth and lower productivity, are intrinsically more sensitive to overfishing than their shallow-water relatives ( García et al., 2008 ; Simpfendorfer and Kyne, 2009 ) for a given body size they are less threatened—largely because they are inaccessible to most fisheries ( Figure 5 ).
As a result of their high exposure to coastal shallow-water fisheries and their large body size, sawfishes (Pristidae) are the most threatened chondrichthyan family and arguably the most threatened family of marine fishes ( Figure 6 ). Other highly threatened families include predominantly coastal and continental shelf-dwelling rays (wedgefishes, sleeper rays, stingrays, and guitarfishes), as well as angel sharks and thresher sharks; five of the seven most threatened families are rays. Least threatened families are comprised of relatively small-bodied species occurring in mesopelagic and deepwater habitats (lanternsharks, catsharks, softnose skates, shortnose chimaeras, and kitefin sharks, Figure 6 , Figure 6—source data 1 ).
Geographic hotspots of threat and conservation priority by habitat
Local species richness is greatest in tropical coastal seas, particularly along the Atlantic and Western Pacific shelves ( Figure 7A ). The greatest uncertainty, where the number of DD species is highest, is centered on four areas: (1) Caribbean Sea and Western Central Atlantic Ocean, (2) Eastern Central Atlantic Ocean, (3) Southwest Indian Ocean, and (4) the China Seas ( Figure 7B ). The megadiverse China Seas face the triple jeopardy of high threat in shallow waters ( Figure 7CD ), high species richness ( Figure 7A ), and a large number of threatened endemic species ( Figure 8 ), combined with high risk due to high uncertainty in status (large number of DD species, Figure 7B ). Whereas the distribution of threat in coastal and continental shelf chondrichthyans is similar to the overall threat pattern across tropical and mid-latitudes, the spatial pattern of threat varies considerably for pelagic and deepwater species. Threatened neritic and epipelagic oceanic sharks are distributed throughout the world’s oceans, but there are also at least seven threat hotspots in coastal waters: (1) Gulf of California, (2) southeast US continental shelf, (3) Patagonian Shelf, (4) West Africa and the western Mediterranean Sea, (5) southeast South Africa, (6) Australia, and (7) the China Seas ( Figure 7D ). Hotspots of deepwater threatened chondrichthyans occur in three areas where fisheries penetrate deepest: (1) Southwest Atlantic Ocean (southeast coast of South America), (2) Eastern Atlantic Ocean, spanning from Norway to Namibia and into the Mediterranean Sea, and (3) southeast Australia ( Figure 7E ).

( A ) Total chondrichthyan richness, ( B ) the number of Data Deficient and threat by major habitat: ( C ) coastal and continental shelf (<200 m depth), ( D ) neritic and epipelagic (<200 m depth), and ( E ) deepwater slope and abyssal plain (>200 m) habitats. Numbers expressed as the total number of species in each 23,322 km 2 cell. The numbers are hotspots refereed to in the text.
DOI: http://dx.doi.org/10.7554/eLife.00590.015

Endemics were defined as species with an Extent of Occurrence of <500,000 km 2 (n = 66). Irreplaceable cells with the greatest number of small range species are shown in red, with blue cells showing areas of lower, but still significant irreplaceability. Irreplaceability is the sum of the inverse of the geographic range sizes of all threatened endemic species in the cell. A value of 0.1 means that on average a single cell represents one tenth of the global range of all the species present in the cell. The numbers are hotspots referred to in the text.
DOI: http://dx.doi.org/10.7554/eLife.00590.016
Hottest hotspots of threat and priority
Spatial conservation priority can be assigned using three criteria: (1) the greatest number of threatened species ( Figure 7A ), (2) greater than expected threat (residuals of the relationship between total number of species and total number of threatened species per cell, Figure 9 ), and (3) high irreplaceability—high numbers of threatened endemic species ( Figure 8 ). Most threatened marine chondrichthyans (n = 135 of 169) are distributed within, and are often endemic to (n = 73), at least seven distinct threat hotspots (e.g., for neritic and pelagic species Figure 7D ). With the notable exception of the US and Australia, threat hotspots occur in the waters of the most intensive shark and ray fishing and fin-trading nations ( Figure 1C ). Accordingly these regions should be afforded high scientific and conservation priority ( Table 5 ).

Residuals of the relationship between total number of data sufficient chondrichthyans and total number of threatened species per cell, where positive values (orange to red) represent cells with higher threat than expected for their richness alone.
DOI: http://dx.doi.org/10.7554/eLife.00590.017
Scientific and conservation priority according to threat, knowledge and endemicity by FAO Fishing Area
DOI: http://dx.doi.org/10.7554/eLife.00590.018
Endemics were defined as those species found only within a single FAO Fishing Area. FAO Fishing Areas were ranked according to greatest species richness, percent threatened species, percent Data Deficient species, number of endemic species and number of threatened endemic species.
The greatest number of threatened species coincides with the greatest richness ( Figure 7A vs 7C–E ); by controlling for species richness we can reveal the magnitude of threat in the pelagic ocean and two coastal hotspots that have a greater than expected level of threat: the Indo-Pacific Biodiversity Triangle and the Red Sea. Throughout much of the pelagic ocean, threat is greater than expected based on species richness alone, species richness is low (n = 30) and a high percentage (86%) are threatened (n = 16) or Near Threatened (n = 10). Only four are of Least Concern (Salmon shark Lamna ditropis, Goblin shark Mitsukurina owstoni, Longnose pygmy Shark Heteroscymnoides marleyi, and Largetooth cookiecutter shark Isistius plutodus ) ( Figure 9 ). The Indo-Pacific Biodiversity Triangle, particularly the Gulf of Thailand, and the islands of Sumatra, Java, Borneo, and Sulawesi, is a hotspot of greatest residual threat especially for coastal sharks and rays with 76 threatened species ( Figure 9 ). Indeed, the Gulf of Thailand large marine ecosystem has the highest threat density with 48 threatened chondrichthyans in an area of 0.36 million km 2 . The Red Sea residual threat hotspot has 29 threatened pelagic and coastal species ( Figure 9 ). There are 15 irreplaceable marine hotspots that harbor all 66 threatened endemic species ( Figure 8 ; Supplementary file 2C ).
In a world of limited funding, conservation priorities are often based on immediacy of extinction, the value of biodiversity and conservation opportunity ( Marris, 2007 ). In this study, we provide the first estimates of the threat status and hence risk of extinction of chondrichthyans. Our systematic global assessment of the status of this lineage that includes many iconic predators reveals a risky combination of high threat (17% observed and 23.9% estimated), low safety (Least Concern, 23% observed and >37% estimated), and high uncertainty in their threat status (Data Deficient, 46% observed and 8.7% estimated). Over half of species are predicted to be threatened or Near Threatened (n = 561, 53.9%, Table 1 ). While no species has been driven to global extinction—as far as we know—at least 28 populations of sawfishes, skates, and angel sharks are locally or regionally extinct ( Dulvy et al., 2003 ; Dulvy and Forrest, 2010 ). Several shark species have not been seen for many decades. The Critically Endangered Pondicherry shark ( Carcharhinus hemiodon ) is known only from 20 museum specimens that were captured in the heavily-fished inshore waters of Southeast Asia: it has not been seen since 1979 ( Cavanagh et al., 2003 ). The now ironically-named and Critically Endangered Common skate ( Dipturus batis ) and Common angel shark ( Squatina squatina ) are regionally extinct from much of their former geographic range in European waters ( Cavanagh and Gibson, 2007 ; Gibson et al., 2008 ; Iglésias et al., 2010 ). The Largetooth sawfish ( Pristis pristis ) and Smalltooth sawfish ( Pristis pectinata ) are possibly extinct throughout much of the Eastern Atlantic, particularly in West Africa ( Robillard and Séret, 2006 ; Harrison and Dulvy, 2014 ).
Our analysis provides an unprecedented understanding of how many chondrichthyan species are actually or likely to be threatened. A very high percentage of species are DD (46%, 487 species); that is one of the highest rates of Data Deficiency of any taxon to date ( Hoffmann et al., 2010 ). This high level of uncertainty in status further elevates risk and presents a key challenge for future assessment efforts. We outline a first step through our estimation that 68 DD species are likely to be threatened based on their life histories and distribution. Numerous studies have retrospectively explained extinction risk, but few have made a priori predictions of risk ( Dulvy and Reynolds, 2002 ; Davidson et al., 2012 ). Across many taxa, extinction risk has been shown to be a function of an extrinsic driver or threat ( Jennings et al., 1998 ; Davies et al., 2006 ) and the corresponding life history and ecological traits: large body size (low intrinsic rate of population increase, high trophic level), small geographic range size, and ecological specialization. Maximum body size is an essential predictor of threat status, we presume because of the close relationship between body size and the intrinsic rate of population increase in sharks and rays ( Smith et al., 1998 ; Frisk et al., 2001 ; Hutchings et al., 2012 ). Though we note that this proximate link may be mediated ultimately through the time-related traits of growth and mortality ( Barnett et al., 2013 ; Juan-Jordá et al., 2013 ). Our novel contribution is to show that depth-related geographic traits are more important for explaining risk than geographic range per se. The shallowness of species (minimum depth limit) and the narrowness of their depth range are important risk factors ( Figure 3 ). We hypothesize that this is so because shallower species are more accessible to fishing gears and those with narrower depth ranges have lower likelihood that a proportion of the species distribution remains beyond fishing activity. For example, the Endangered Barndoor skate ( Dipturus laevis ) was eliminated throughout much of its geographic range and depth distribution due to bycatch in trawl fisheries, yet may have rebounded because a previously unknown deepwater population component lay beyond the reach of most fisheries ( Dulvy, 2000 ; Kulka et al., 2002 ; COSEWIC, 2010 ). We find that geographic range (measured as Extent of Occurrence) is largely unrelated to extinction risk. This is in marked contrast to extinction risk patterns on land ( Jones et al., 2003 ; Cardillo et al., 2005 ; Anderson et al., 2011a ) and in the marine fossil record ( Harnik et al., 2012a , 2012b ), where small geographic range size is the principal correlate of extinction risk. We suggest that this is because fishing activity is now widespread throughout the world’s oceans ( Swartz et al., 2010 ), and even species with the largest ranges are exposed and often entirely encompassed by the footprint of fishing activity. By contrast, with a few exceptions (mainly eastern Atlantic slopes, Figure 7E ), fishing has a narrow depth penetration and hence species found at greater depths can still find refuge from exploitation ( Morato et al., 2006 ; Lam and Sadovy de Mitcheson, 2010 ).
The status of chondrichthyans is arguably among the worst reported for any major vertebrate lineage considered thus far, apart from amphibians ( Stuart et al., 2004 ; Hoffmann et al., 2010 ). The percentage and absolute number of threatened amphibians is high (>30% are threatened), but a greater percentage are Least Concern (38%), and uncertainty of status is lower (32% DD) than for chondrichthyans. Our discovery of the high level of threat in freshwater chondrichthyans (36%) is consistent with the emerging picture of the intense and unmanaged extinction risk faced by many freshwater and estuarine species ( Darwall et al., 2011 ).
Our threat estimate is comparable to other marine biodiversity status assessments, but our findings caution that ‘global’ fisheries assessments may be underestimating risk. The IUCN Global Marine Species Assessment is not yet complete, but reveals varying threat levels among taxa and regions ( Polidoro et al., 2008 , 2012 ). The only synoptic summary to-date focused on charismatic Indo-Pacific coral reef ecosystem species. Of the 1,568 IUCN-assessed marine vertebrates and invertebrates, 16% (range: 12–34% among families) were threatened ( McClenachan et al., 2012 ). This is a conservative estimate of marine threat level because although they may be more intrinsically sensitive to extinction drivers, charismatic species are more likely to garner awareness of their status and support for monitoring and conservation ( McClenachan et al., 2012 ). The predicted level of chondrichthyan threat (>24%) is distinctly greater than that provided by global fisheries risk assessments. These studies provide modeled estimates of the percentage of collapsed bony fish (teleost) stocks in both data-poor unassessed fisheries (18%, Costello et al., 2012 ) and data-rich fisheries (7–13%, Branch et al., 2011 ). This could be because teleosts are generally more resilient than elasmobranchs ( Hutchings et al., 2012 ), but in addition we caution that analyses of biased geographic and taxonomic samples may be underestimating risk of collapse in global fisheries, particularly for species with less-resilient life histories.
Our work relies on consensus assessments by more than 300 scientists. However, given the uncertainty in some of the underlying data that inform our understanding of threat status, such as fisheries catch landings data, it is worth considering whether these uncertainties mean our assessments are downplaying the true risk. While there are methods of propagating uncertainty through the IUCN Red List Assessments ( Akcakaya et al., 2000 ), in our experience this approach was uninformative for even the best-studied species, because it generated confidence intervals that spanned all IUCN Categories. Instead it is worth considering whether our estimates of threat are consistent with independent quantitative estimates of status. The Mediterranean Red List Assessment workshop in 2005 prompted subsequent quantitative analyses of catch landings, research trawl surveys, and sightings data. Quantitative trends could be estimated for five species suggesting they had declined by 96% to >99.9% relative to their former abundance suggesting they would meet the highest IUCN Threat category of Critically Endangered ( Ferretti et al., 2008 ). By comparison the earlier IUCN regional assessment for these species, while suggesting they were all threatened, was more conservative for two of the five species: Hammerhead sharks ( Sphyrna spp.)—Critically Endangered, Porbeagle shark ( Lamna nasus )—Critically Endangered, Shortfin mako ( Isurus oxyrinchus )—Critically Endangered, Blue shark ( Prionace glauca )—Vulnerable, and Thresher shark ( Alopias vulpinus )—Vulnerable.
We can also make a complementary comparison to a recent analysis of the status of 112 shark and ray fisheries ( Costello et al., 2012 ). The median biomass relative to the biomass at Maximum Sustainable Yield (B/B MSY ) of these 112 shark and ray fisheries was 0.37, making them the most overfished groups of any of the world’s unassessed fisheries. Assuming B MSY occurs at 0.3 to 0.5 of unexploited biomass then the median biomass of shark and ray fisheries had declined by between 81% and 89% by 2009. These biomass declines would be sufficient to qualify all of these 112 shark and ray fisheries for the Endangered IUCN category if they occurred within a three-generation time span. By comparison our results are considerably more conservative. Empirical analyses show that an IUCN threatened category listing is triggered only once teleost fishes (with far higher density-dependent compensation) have been fished down to below B MSY ( Dulvy et al., 2005 ; Porszt et al., 2012 ). Hence, our findings are consistent with only around one-quarter of chondrichthyan species having been fished down below the B MSY target reference point. While there may be concern that expert assessments may overstate declines and threat, it is more likely that our conservative consensus-based approach has understated declines and risk in sharks and rays.
For marine species, predicting absolute risk of extinction remains highly uncertain because, even with adequate evidence of severe decline, in many instances the absolute population size remains large ( Mace, 2004 ). There remains considerable uncertainty as to the relationship between census and effective population size ( Reynolds et al., 2005 ). Therefore, Red List categorization of chondrichthyans should be interpreted as a comparative measure of relative extinction risk, in recognition that unmanaged steep declines, even of large populations, may ultimately lead to ecosystem perturbations and eventually biological extinction. The Red List serves to raise red flags calling for conservation action, sooner rather than later, while there is a still chance of recovery and of forestalling permanent biodiversity loss.
Despite more than two decades of rising awareness of chondrichthyan population declines and collapses, there is still no global mechanism to ensure financing, implementation and enforcement of chondrichthyan fishery management plans that is likely to rebuild populations to levels where they would no longer be threatened ( Lack and Sant, 2009 ; Techera and Klein, 2011 ). This management shortfall is particularly problematic given the large geographic range of many species. Threat increased only slightly when geographic range is measured as the Extent of Occurrence; however, geographic range becomes increasingly important when it is measured as the number of countries (legal jurisdictions) spanned by each species. The proportion of species that are threatened increases markedly with geographic size measured by number of Exclusive Economic Zones (EEZs) spanned; one-quarter of threatened species span the EEZs of 18 or more countries ( Figure 10 ). Hence, their large geographic ranges do not confer safety, but instead exacerbates risk because sharks and rays require coherent, effective international management.

Frequency distribution of number of jurisdictions spanned by all chondrichthyans (black, n = 1,041) and threatened species only (red, n = 174), for ( A ) country EEZs, and ( B ) the overrepresentation of threatened species spanning a large number of country EEZs, shown by the log ratio of proportion of threatened species over the proportion of all species. The proportion of threatened species is greater than the proportion of all species where the log ratio = 0, which corresponds to range spans of 16 and more countries.
DOI: http://dx.doi.org/10.7554/eLife.00590.019
With a few exceptions (e.g., Australia and USA), many governments still lack the resources, expertise, and political will necessary to effectively conserve the vast majority of shark and rays, and indeed many other exploited organisms ( Veitch et al., 2012 ). More than 50 sharks are included in Annex I (Highly Migratory Species) of the 1982 Law of the Sea Convention, implemented on the high seas under the 1992 Fish Stocks Agreement, but currently only a handful enjoy species-specific protections under the world’s Regional Fishery Management Organizations ( Table 6 ), and many of these have yet to be implemented domestically. The Migratory Sharks Memorandum of Understanding (MoU) adopted by the Parties to the Convention on Migratory Species (CMS) so far only covers seven sharks, yet there may be more than 150 chondrichthyans that regularly migrate across national boundaries ( Fowler, 2012 ). To date, only one of the United Nations Environment Programme’s Regional Seas Conventions, the Barcelona Convention for the Conservation of the Mediterranean Sea, includes chondrichthyan fishes and only a few of its Parties have taken concrete domestic action to implement these listings. Despite two decades of effort, only ten sharks and rays had been listed by the Convention on International Trade in Endangered Species (CITES) up to 2013 ( Vincent et al., 2014 ). A further seven species of shark and ray were listed by CITES in 2013—the next challenge is to ensure effective implementation of these trade regulations ( Mundy-Taylor and Crook, 2013 ). OSPAR (the Convention for the Protection of the marine Environment of the North-East Atlantic) lists many threatened shark and ray species, but its remit excludes fisheries issues. Many chondrichthyans qualify for listing under CITES, CMS, and various regional seas conventions, and should be formally considered for such action as a complement to action by Regional Fisheries Management Organizations (RFMOs) ( Table 6 ).
Progress toward regional and international RFMO management measures for sharks and rays
DOI: http://dx.doi.org/10.7554/eLife.00590.020
Bans on ‘finning’ (slicing off a shark’s fins and discarding the body at sea) are the most widespread shark conservation measures. While these prohibitions, particularly those that require fins to remain attached through landing, can enhance monitoring and compliance, they have not significantly reduced shark mortality or risk to threatened species ( Clarke et al., 2013 ). Steep declines and the high threat levels in migratory oceanic pelagic sharks suggest raising the priority of improved management of catch and trade through concerted actions by national governments working through RFMOs as well as CITES, and CMS ( Table 7 ).
Management recommendations: the following actions would contribute to rebuilding threatened chondrichthyan populations and properly managing associated fisheries
DOI: http://dx.doi.org/10.7554/eLife.00590.021
A high proportion of catch landings come from nations with a large number of threatened chondrichthyans and less-than-comprehensive chondrichthyan fishery management plans. Future research is required to down-scale these global Red List assessments and analyses to provide country-by-country diagnoses of the link between specific fisheries and specific threats to populations of more broadly distributed species ( Wallace et al., 2010 ). Such information could be used to focus fisheries management and conservation interventions that are tailored to specific problems. There is no systematic global monitoring of shark and ray populations and the national fisheries catch landings statistics provide invaluable data for tracking fisheries trends in unmanaged fisheries ( Newton et al., 2007 ; Worm et al., 2013 ). However, the surveillance power of such data could be greatly improved if collected at greater taxonomic resolution. While there have been continual improvements, catches are under-reported ( Clarke et al., 2006 ), and for those that are reported only around one-third is reported at the species level ( Fischer et al., 2012 ). To complement improved catch landings data, we recommend the development of repeat regional assessments of the Red List Status of chondrichthyans to provide an early warning of adverse changes in status and to detect and monitor the success of management initiatives and interventions. Aggregate Red List Threat indices for chondrichthyans, like those available for mammals, birds, amphibians, and hard corals ( Carpenter et al., 2008 ) would provide one of the few global scale indicators of progress toward international biodiversity goals ( Walpole et al., 2009 ; Butchart et al., 2010 ).
Our global status assessment of sharks and rays reveals the principal causes and severity of global marine biodiversity loss, and the threat level they face exposes a serious shortfall in the conservation management of commercially-exploited aquatic species ( McClenachan et al., 2012 ). Chondrichthyans have slipped through the jurisdictional cracks of traditional national and international management authorities. Rather than accept that many chondrichthyans will inevitably be driven to economic, ecological, or biological extinction, we warn that dramatic changes in the enforcement and implementation of the conservation and management of threatened chondrichthyans are urgently needed to ensure a healthy future for these iconic fishes and the ecosystems they support.
IUCN Red List Assessment process and data collection
We applied the Red List Categories and Criteria developed by the International Union for Conservation of Nature (IUCN) ( IUCN, 2004 ) to 1,041 species at 17 workshops involving more than 300 experts who incorporated all available information on distribution, catch, abundance, population trends, habitat use, life histories, threats, and conservation measures.
Some 105 chondrichthyan fish species had been assessed and published in the 2000 Red List of Threatened Species prior to the initiation of the Global Shark Red List Assessment (GSRLA). These assessments were undertaken by correspondence and through discussions at four workshops (1996—London, UK, and Brisbane, Australia; 1997—Noumea, New Caledonia, and 1999—Pennsylvania, USA). These assessments applied earlier versions of the IUCN Red List Criteria and, where possible, were subsequently reviewed and updated according to version 3.1 Categories and Criteria during the GSRLA. The IUCN Shark Specialist Group (SSG) subsequently held a series of 13 regional and thematic Red List workshops in nine countries around the world ( Table 8 ). Prior to the workshops, each participant was asked to select species for assessment based on their expertise and research areas. Where possible, experts carried out research and preparatory work in advance, thus enabling more synthesis to be achieved during each workshop. SSG Red List-trained personnel facilitated discussion and consensus sessions, and coordinated the production of global Red List Assessments for species in each region. For species that had previously been assessed, participants provided updated information and assisted in revised assessments. Experts completed assessments for some wide-ranging, globally distributed species over the course of several workshops. In total, 302 national, regional, and international experts from 64 countries participated in the GSRLA workshops and the production of assessments. All Red List Assessments were based on the collective knowledge and pooled data from dedicated experts across the world, ensuring global consultation and consensus to achieve the best assessment for each species with the knowledge and resources available (‘Acknowledgements’). Any species assessments not completed during the workshops were finalized through subsequent correspondence among experts.
The locations, dates, number of participants and the number of countries represented at each of the SSG Red List workshops, along with unique totals
DOI: http://dx.doi.org/10.7554/eLife.00590.022
The SSG evaluated the status of all described chondrichthyan species that are considered to be taxonomically valid up to August 2011 (see “Systematics, missing species and species coverage” below). Experts compiled peer-reviewed Red List documentation for each species, including data on: systematics, population trends, geographic range, habitat preferences, ecology, life history, threats, and conservation measures. The SSG assessed all species using the IUCN Red List Categories and Criteria version 3.1 ( IUCN, 2001 ). The categories and their standard abbreviations are: Critically Endangered (CR), Endangered (EN), Vulnerable (VU), Near Threatened (NT), Least Concern (LC), and Data Deficient (DD). Experts further coded each species according to the IUCN Habitats, Threats and Conservation Actions Authority Files, enabling analysis of their habitat preferences, major threats and conservation action requirements. SSG Program staff entered all data into the main data fields in the IUCN Species Information Service Data Entry Module (SIS DEM) and subsequently transferred these data into the IUCN Species Information Service (SIS) in 2009.
Systematics, missing species and species coverage
The SSG collated data on order, family, genus, species, taxonomic authority, commonly-used synonyms, English common names, other common names, and taxonomic notes (where relevant). For taxonomic consistency throughout the species assessments, the SSG followed Leonard J V Compagno’s 2005 Global Checklist of Living Chondrichthyan Fishes ( Compagno, 2005 ), only deviating from this where there was extensive opposing consensus with a clear and justifiable alternative, as adjudicated by the IUCN SSG’s Vice Chairs of Taxonomy, David E Ebert and William T White.
Keeping pace with the total number of chondrichthyans is a challenging task, especially given the need to balance immediacy against taxonomic stability. One-third of all species have been described in the past thirty years. Scientists have described a new chondrichthyan species, on average, almost every 2–3 weeks since the 1970s ( Last, 2007 ; White and Last, 2012 ). Since Leonard V J Compagno completed the global checklist in 2005, scientists have recognized an additional ∼140 species (mostly new) living chondrichthyan species. This increase in the rate of chondrichthyan descriptions in recent years is primarily associated with the lead up to the publication of a revised treatment of the entire chondrichthyan fauna of Australia ( Last and Stevens, 2009 ), requiring formal descriptions of previously undescribed taxa. In particular, three CSIRO special publications published in 2008 included descriptions of 70 previously undescribed species worldwide ( Last et al., 2008a , 2008b , 2008c ). The number of new species described in 2006, 2007 and 2008 was 21, 23, and 81, respectively, with all but nine occurring in the Indo–West Pacific. Additional nominal species of chondrichthyans are also included following resurrection of previously unrecognized species such as the resurrection of Pastinachus atrus for the Indo–Australian region, previously considered a synonym of P. sephen ( Last and Stevens, 1994 ). Scientists excluded some nominal species of dubious taxonomic validity from this assessment. Thus, the total number of chondrichthyan species referred to in this paper (1,041) does not include all recent new or resurrected species, which require future work for their inclusion in the GSRLA.
Many more as yet undescribed chondrichthyan species exist. The chondrichthyan faunas in several parts of the world (e.g., the northern Indian Ocean) are poorly known and a large number of species are likely to represent complexes of several distinct species that require taxonomic resolution, for example some dogfishes, skates, eagle rays, and stingrays ( Iglésias et al., 2010 ; White and Last, 2012 ). Many areas of the Indian and Pacific Oceans are largely unexplored and, given the level of micro-endemism documented for a number of chondrichthyan groups, it is likely that many more species will be discovered in the future ( Last, 2007 ; Naylor et al., 2012 ). For example, recent surveys of Indonesian fish markets revealed more than 20 new species of sharks out of the approximately 130 recorded in total ( White et al., 2006 ; Last, 2007 ; Ward et al., 2008 ).
Distribution maps
SSG experts created a shapefile of the geographic distribution for each chondrichthyan species with GIS software using the standard mapping protocol for marine species devised by the IUCN GMSA team ( http://sci.odu.edu/gmsa/ ). The map shows the Extent of Occurrence of the species cut to one of several standardized basemaps depending on the ecology of the species (i.e., coastal and continental shelf, pelagic and deepwater). The distribution maps for sharks are based on original maps provided by the FAO and Leonard JV Compagno. Maps for some of the batoids were originally provided by John McEachran. New maps for recently described species were drafted where necessary. The original maps were updated, corrected, or verified by experts at the Red List workshops or out-of-session assessors and SSG staff and then sent to the GMSA team who modified the shapefiles and matched them to the distributional text within the assessment.
Occurrence and habitat preference
SSG assessors assigned countries of occurrence from the ‘geographic range’ section of the Red List documentation and classified species to the FAO Fishing Areas ( http://www.iucnredlist.org/technical-documents/data-organization ) in which they occur ( Figure 2—figure supplement 1 ). Each species was coded according to the IUCN Habitats Authority File ( http://www.iucnredlist.org/technical-documents/classification-schemes/habitats-classification-scheme-ver3 ). These categorizations are poorly developed and often irrelevant for coastal and offshore marine animals. For the purposes of analysis presented here we assigned chondrichthyans to five unique habitat-lifestyle combinations (coastal and continental shelf, pelagic, meso- and bathypelagic, deepwater, and freshwater) mainly according to depth distribution and, to a lesser degree, position in the water column. The pelagic group includes both neritic (pelagic on the continental shelf) and epipelagic oceanic (pelagic in the upper 200 m of water over open ocean) species. Species habitats were classified based on the findings from the workshops combined with a review of the primary literature, FAO fisheries guides and field guides ( Cavanagh et al., 2003 ; Cavanagh and Gibson, 2007 ; Cavanagh et al., 2008 ; Gibson et al., 2008 ; Camhi et al., 2009 ; Kyne et al., 2012 ). Species habitat classifications tended to be similar across families, but for some species the depth distributions often spanned more than one depth category and for these species habitat was assigned according to the predominant location of each species throughout the majority of its life cycle ( Compagno, 1990 ). This issue was mainly confined to coastal and continental shelf species that exhibited distributions extending down the continental slopes (e.g., some Dasyatis , Mustelus , Rhinobatos , Scyliorhinus , Squalus , and Squatina ). We caution that some of the heterogeneity in depth distribution or unusually large distributions may reflect taxonomic uncertainty and the existence of species complexes ( White and Last, 2012 ). We defined the deep sea as beyond the continental and insular shelf edge at depths greater than or equal to 200 m. Coastal and continental shelf includes predominantly demersal species (those spending most time dwelling on or near the seabed), and excluded neritic chondrichthyans. Pelagic species included macrooceanic and tachypelagic ocean-crossing epipelagic sharks with circumglobal distributions as well as sharks suspected of ocean-crossing because they exhibit circumglobal but disjunct distributions, for example Galapagos shark ( Carcharhinus galapagensis ).
Our classification resulted in a total of 33 obligate freshwater and 1,008 marine and euryhaline chondrichthyans of which 482 species were found predominantly in coastal and continental shelf, 39 in pelagic, 479 in deepwater, and eight in meso- and bathypelagic habitats. To evaluate whether the geographic patterns of threat are robust to alternate unique or multiple habitat classifications we considered two alternate classification schemes, one where species were classified into a single habitat and another where species were classified in one or more habitats. The alternate unique classification scheme yielded 42 pelagic ( Camhi et al., 2009 ), and 452 deepwater chondrichthyans ( Kyne and Simpfendorfer, 2007 ), leaving 517 coastal and continental shelf and 33 obligate freshwater species (totaling 1,044, based on an older taxonomic scheme). When species were classified in more than one habitat this resulted in 513 species in the coastal and continental shelf, 564 in deepwater, 54 in pelagic, and 13 meso- and bathypelagic habitats. We found the geographic pattern of threat was robust to the choice of habitat classification scheme, and we present only the unique classification (482 coastal and continental shelf, 39 pelagic, 479 deepwater habitat species).
Major threats
SSG assessors coded each species according to the IUCN Major threat Authority File ( http://www.iucnredlist.org/technical-documents/classification-schemes/habitats-classification-scheme-ver3 ). We coded threats that appear to have an important impact, but did not describe their relative importance for each species.
The term ‘bycatch’ and its usage in the IUCN Major threat Authority File do not capture the complexity and values of chondrichthyan fisheries. Some chondrichthyans termed ‘bycatch’ are actually caught as ‘incidental or secondary catch’ as they are used to a similar extent as the target species or are sometimes highly valued or at least welcome when the target species is absent. ‘Unwanted bycatch’ refers to cases where the chondrichthyans are not used and fishers would prefer to avoid catching them (Clarke, S personal communication, Sasama Consulting, Shizuoka, Japan). If the levels of unwanted bycatch are severe enough, chondrichthyans can be actively persecuted to avoid negative and costly gear interactions—such as caused the near extirpation of the British Columbian population of Basking shark ( Cetorhinus maximus ) ( Wallace and Gisborne, 2006 ).
Red List Assessment
We assigned a Red List Assessment category for each species based on the information above using the revised 2001 IUCN Red List Categories and Criteria (version 3.1; http://www.iucnredlist.org/technical-documents/categories-and-criteria ). We provided a rationale for each assessment justifying the classification along with a description of the relevant criteria used in the designation. Data fields also present the reason for any change in Red List categories from previous assessments (i.e., genuine change in status of species, new information on the species available, incorrect data used in previous assessments, change in taxonomy, or previously incorrect criteria assigned to species); the current population trend (i.e., increasing, decreasing, stable, unknown); date of assessment; names of assessors and evaluators (effectively the peer-reviewers); and any notes relevant to the Red List category. The Red List documentation for each species assessment is supported by references to the primary and secondary literature cited in the text.
Data entry, review, correction, and consistency checking
Draft regional Red List Assessments and supporting data were collated and peer-reviewed during the workshops and through subsequent correspondence to produce the global assessment for each species. At least one member of the SSG Red List team was present at each of the workshops to facilitate a consistent approach throughout the data collection, review and evaluation process. Once experts had produced draft assessments, SSG staff circulated summaries (comprised of rationales, Red List Categories and Criteria) to the entire SSG network for comment. As the workshops took place over a >10-year period, some species assessments were reviewed and updated at subsequent workshops or by correspondence. Each assessment received a minimum of two independent evaluations as a part of the peer-review process, either during or subsequent to the consensus sessions (a process involving 65 specialists and experts across 23 participating countries) prior to entry into the database and submission to the IUCN Red List Unit.
SSG Red List-trained personnel undertook further checks of all assessments to ensure consistent application of the Red List Categories and Criteria to each species, and the then SSG Co-chair Sarah L Fowler, thoroughly reviewed every assessment produced from 1996 to 2009. Following the data review and evaluation process, all species assessments were entered in the Species Information Service database and checked again by SSG Red List Unit staff. IUCN Red List Program staff made the final check prior to the acceptance of assessments in the Red List database and publication of assessments and data online ( http://www.iucnredlist.org/ ).
Subpopulation and regional assessments
We included only global species assessments in this analysis. In many cases, subpopulation and regional assessments were developed for species before a global assessment could be made. For very wide-ranging species, such as the oceanic pelagic sharks, a separate workshop was held to combine these subpopulation or regional assessments ( Table 8 ). A numerical value was assigned to each threat category in each region where the species was assessed, and where possible these values were then averaged to calculate a global threat category ( Gärdenfors et al., 2001 ). Hence, the Red List categories of some species may differ regionally; for example, porbeagle shark ( Lamna nasus ) is classified as VU globally, but CR in the Northeast Atlantic and Mediterranean Sea. Often population trends were not available across the full distribution of a species. In these cases, the degree to which the qualifying threshold was met was modified according to the degree of certainty with which the trend could be extrapolated across the full geographic range of a species. The calculation of the overall Red List category for globally distributed species is challenging, particularly when a combination of two or more of the following issues occurs: (1) trend data are available only for a part of the geographic range; (2) regional trend data or stock assessments are highly uncertain; (3) the species is data-poor in some other regions; (4) the species is subject to some form of management in other regions; and, (5) the species is moderately productive ( Dulvy et al., 2008 ). This situation is typified by the Blue shark ( Prionace glauca ) that faces all of these issues. The best abundance trend data come from the Atlantic Ocean, but the different time series available occasionally yield conflicting results; surveys of some parts of the Atlantic exhibit declines of 53–80% in less than three generations ( Dulvy et al., 2008 ; Gibson et al., 2008 ), while a 2008 stock assessment conducted for the International Commission for the Conservation of Atlantic Tuna (ICCAT) indicate, albeit with substantial uncertainty, that the North Atlantic Blue shark population biomass is still larger than that required to generate Maximum Sustainable Yield (B MSY ) ( Gibson et al., 2008 ). The Blue shark is one of the most productive of the oceanic pelagic sharks, maturing at 4–6 years of age with an annual rate of population increase of ∼28% per year and an approximate B MSY at ∼42% of virgin biomass, B 0 ( Cortés, 2008 ; Simpfendorfer et al., 2008 ). While the available data may support the regional listing of the Atlantic population of this species in a threatened category, the assessors could not extrapolate this to the global distribution because the species may be subject to lower fishing mortality in other regions. Hence the Blue shark was listed as NT globally. Further details on this issue and additional data requirements to improve the assessment and conservation of such species are considered elsewhere ( Gibson et al., 2008 ; Camhi et al., 2009 ).
Red Listing marine fishes
We assessed most threatened chondrichthyans (81%, n = 148 of 181) using the Red List population reduction over time Criterion A. Only one of the threatened species, the Skate ( Dipturus ) was assessed under the higher decline thresholds of the A1 criterion, where ‘population reduction in the past, where the causes are clearly reversible AND understood AND have ceased’. The remaining threatened species were assessed using the IUCN geographic range Criterion B (n = 29) or the small population size and decline Criterion C (n = 4: Borneo shark Carcharhinus borneensis , Colclough’s shark Brachaelurus colcloughi , Northern river shark Glyphis garricki , and Speartooth shark Glyphis glyphis ). The Criterion A decline assessments were based on statistical analyses and critical review of a tapestry of local catch per unit effort trajectories, fisheries landings trajectories (often at lower taxonomic resolution), combined with an understanding of fisheries selectivity and development trajectories.
We assessed most chondrichthyans using the Red List criterion based on population reduction over time (Criterion A). The original thresholds triggering a threatened categorization were Criterion A1: VU 20%; EN 50%; and CR 80% decline over the greater of the past (A1) or future (A2) 10 years or three generations (IUCN Categories and Criteria version 2.3). IUCN raised these thresholds in 2001 to VU, ≥30%; EN, ≥50%; and CR, ≥80% decline over the greater of 10 years or three generations in the past (A2), future (A3) and ongoing (A4), and changed A1 to a reduction over the past 10 yrs or 3 generations of VU ≥50%; EN ≥70%; CR ≥90%, where the causes of reduction are understood AND have ceased AND are reversible. This was in response to concerns that the original thresholds were too low for managed populations that are being deliberately fished down to MSY (typically assumed to be 50% of virgin biomass under Schaeffer logistic population growth) ( Reynolds et al., 2005 ). This revision was designed to improve consistency between fisheries limit reference points and IUCN thresholds reducing the likelihood of false alarms—where a sustainably exploited species incorrectly triggers a threat listing ( Dulvy et al., 2005 ; Porszt et al., 2012 ). Empirical testing shows that this has worked and demonstrates that a species exploited at fishing mortality rates consistent with achieving MSY ( F MSY ) would lead to decline rates that would be unlikely to be steep enough to trigger a threat categorization under these new thresholds ( Dulvy et al., 2005 ).
It is incontrovertible that a species that has declined by 80% over the qualifying time period is at a greater relative risk of extinction than another that declined by 40% (in the same period). Regardless, there may be a wide gap in the population decline trajectory between the point at which overfishing occurs and the point where the absolute risk of extinction becomes a real concern ( Musick, 1999a ). In addition, fisheries scientists have expressed concern that decline criteria designed for assessing the extinction risk of a highly productive species may be inappropriate for species with low productivity and less resilience ( Musick, 1999a ), although this was addressed with the use of generation times to rescale decline rates to make productivity comparable ( Reynolds et al., 2005 ; Mace et al., 2008 ). In response to concerns that IUCN decline thresholds are too low and risk false alarms, the American Fisheries Society (AFS) developed alternate decline criteria ( Musick, 1999a ) to classify North American marine fish populations ( Musick et al., 2000 ). This approach only categorizes species that have undergone declines of 70–99% over the greater of three generations or 10 years. Nonetheless, most of the species so listed by AFS also appear on the relevant IUCN Specialist Group lists and vice versa, although the risk categories are slightly different. The reason for the concordance is that in most instances the decline had far exceeded 50% over the appropriate timeframe long before it was detected. Consequently, SSG scientists generally agreed in assigning threat categories to species that had undergone large declines, but many were reluctant to assign a VU classification to species that were perceived to be at or near 50% virgin population levels and presumably near B MSY . In practice, the latter were usually classified as NT unless other circumstances (highly uncertain data, combined with widespread unregulated fisheries) dictated a higher level of threat according to the precautionary principle.
Statistical analysis
Modeling correlates of threat.
Vulnerability to population decline or extinction is a function of the combination of the degree to which intrinsic features of a species’ behavior, life history and ecology (sensitivity) may reduce the capacity of a species to withstand an extrinsic threat or pressure (exposure). We tested the degree to which intrinsic life histories and extrinsic fishing activity influenced the probability that a chondrichthyan species was threatened. Threat category was modeled as a binomial response variable; with LC species assigned a score of 0, and VU, EN & CR species assigned a 1. We used maximum body length (cm), geographic range size (Extent of Occurrence, km 2 ), and depth range (maximum–minimum depth, m) as indices of intrinsic sensitivity, and minimum depth (m) and mean depth (maximum–minimum depth/2) as a measure of exposure to fishing activity. All variables were standardized to z -scores by subtracting the mean and dividing by the standard deviation to minimize collinearity (variance inflation factors were less than 2). Mean depth was not included in model evaluation as it was computed from, and hence, correlated to minimum depth (Spearman’s ρ = 0.52). We fitted Generalized Linear Mixed-effect Models with binomial error and a logit link to model the probability of a species being threatened, using taxonomic structure as a nested random effect (e.g., order/family/genus) to account for phylogenetic non-independence. The probability of a species i being threatened was assumed to be binomially distributed with a mean p i , such that the linear predictor of p i was:
where β i,j and β i,k are the fitted coefficients for life history or geographic range traits j and k , and X i,j and X i,k are the trait values of j and k for species i ( Tables 4 and 9 ). The effect of a one standard deviation increase in the coefficient of interest was computed as:
following ( Gelman and Hill, 2006 ). Models were fitted using the lmer function in the R package lme4 ( Bates et al., 2011 ). The amount of variance explained by the fixed effects only and the combined fixed and random effects of the binomial GLMM models was calculated as the marginal R 2 GLMM( m ) and conditional R 2 GLMM( c ), respectively, using the methods described by Nagakawa and Schlielzeth (2012) .
Parameter estimates for General Linear Mixed-effects Models testing the probability that a species is threatened p(THR) given either categorical habitat class or continuous measure of depth distribution and maximum size
DOI: http://dx.doi.org/10.7554/eLife.00590.023
The improvement of model fit by inclusion of phylogenetic random effect was calculated as the difference in AIC (ΔAIC) between the GLMM (with phylogenetic random effect) and a GLM as ΔAIC = AIC(GLMM)-AIC(GLM). p (THR) was binomially distributed assuming species that were CR, EN or VU were threatened (1) and LC species were not (0). We present ΔAIC for two other threat classifications, assuming: THR also includes NT species, or THR was a continuous categorical variable ranging from LC = 0 to CR = 5.
Estimating the proportion of potentially threatened DD species
We predicted the number of Data Deficient species that are potentially threatened based on the maximum body size and geographic distribution traits ( Table 3 ; Supplementary file 1 ). Specifically, based on the explanatory models described above, all variables were log 10 transformed and we fitted generalized linear models of increasing complexity assuming a binomial error and logit link ( Equation 2 ; Table 3 ). Model performance was evaluated using Receiver Operating Characteristics by comparing the predicted probability that the species was threatened p (THR) against the true observed status (Least Concern = 0, and threatened [VU, EN & CR] = 1) ( Sing et al., 2005 ; Porszt et al., 2012 ). The prediction accuracy was calculated as the Area Under the Curve (AUC) of the relationship between false positive rates and true positive rates, where a false positive is a model prediction of ≥0.5 and true observed status is 0 (or <0.5 and 1) and a true positive is a prediction of ≥0.5 and true observed status is 1 (or <0.5 and 0). True and false positive rates, and accuracy (AUC) were calculated using the R package ROCR ( Sing et al., 2005 ). The probability that a DD species was threatened p(THR) DD was predicted based on the available life history and distributional traits. DD species with p(THR) DD ≥ 0.5 were classified as threatened and <0.5 as Least Concern. This optimum classification threshold was confirmed by comparing accuracy across the full range of possible thresholds (from 0 to 1). We fitted models using the gls function and calculated pseudo- R 2 using the package rms.
With these models we can estimate the number and proportion of species in each category ( Table 1 ). We estimated that 68 of 396 DD species are potentially threatened, and hence the remainder (396–68 = 328) is likely to be either Least Concern or Near Threatened. Assuming these species are distributed between these categories according to the observed ratio of NT:LC species of 0.5477 this results in a total of 312 (29.9%) Near Threatened species (132 known + 180 estimated) and 389 (37.4%) Least Concern species (241 known +148 estimated). After apportioning the DD species among threatened (68), NT (312), and LC (389), only 91 (8.7%; 487–396) are likely to be Data Deficient ( Table 1 ).
Spatial analysis
The SSG and the GMSA created ArcGIS distribution maps as polygons describing the geographical range of each chondrichthyan depending on the individual species’ point location and depth information. Pelagic species distribution maps were digitized by hand from the original map sources. For spatial analyses, we merged all species maps into a single shapefile. We mapped species using a hexagonal grid composed of individual units (cells) that retain their shape and area (∼23,322 km 2 ) throughout the globe. Specifically, we used the geodesic discrete global grid system, defined on an icosahedron and projected to the sphere using the inverse Icosahedral Snyder Equal Area (ISEA) ( Sahr et al., 2003 ). A row of cells near longitude 180°E/W was excluded, as these interfered with the spatial analyses ( Hoffmann et al., 2010 ). Because of the way the marine species range maps are buffered, the map polygons are likely to extrapolate beyond known distributions, especially for any shallow-water, coastal species, hence not only will range size itself likely be an overestimate, but so will the number of hexagons.
We excluded obligate freshwater species from the final analysis as their distribution maps have yet to be completed. The maps of the numbers of threatened species represent the sum of species that have been globally assessed as threatened, in IUCN Red List categories VU, EN or CR, existing in each ∼23,322 km 2 cell. We caution that this should not be interpreted to mean that species existing within that grid cell are necessarily threatened in this specific location, rather that this location included species that are threatened, on average, throughout their Extent of Occurrence. The number of threatened species was positively related to the species richness of cells ( F 1, 14,846 = 1.5 e 5 , p<0.001, r 2 = 0.91). To remove this first-order effect and reveal those cells with greater and lower than expected extinction risk, we calculated the residuals of a linear regression of the number of threatened species on the number of non-DD species (referred to as data sufficient species). Cells with positive residuals were mapped to show areas of greater than expected extinction risk compared to cells with equal or negative residuals. Hexagonal cell information was converted to point features and smoothed across neighboring cells using ordinary kriging using a spherical model in the Spatial Analyst package of ArcView. Such smoothing can occasionally lead to contouring artefacts, such as the yellow wedge west of southern Africa in Figure 7D , and we caution against over-interpreting marginal categorization changes.
We identified hotspots of threatened endemic chondrichthyans to guide conservation priorities. To describe the potential cost of losing unique chondrichthyan faunas, we calculated irreplaceability scores for each cell. Irreplaceability scores were calculated for each species as the reciprocal of its area of occupancy measured as the number of cells occupied. For example, for a species with an Extent of Occurrence spanning 100 hexagons, each hexagon in its range would have an irreplaceability 1/100 or 0.01 in each of the 100 hexagons of its Extent of Occurrence. The irreplaceability of each cell was calculated by averaging log 10 transformed irreplaceability scores of each species in each cell. Averaging irreplaceability scores controls for varying species richness across cells. We calculated irreplaceability both for all chondrichthyans and for threatened species only. Irreplaceability was also calculated using only endemic threatened species, whereby endemicity was defined as species having an Extent of Occurrence of <50,000, 100,000, 250,000 or 500,000 km 2 . Different definitions of endemicity gave similar patterns of irreplaceability and we present the results of only the largest-scale definition of endemicity. Hence the irreplaceability of threatened species and particularly the threatened endemic chondrichthyans represents those locations or ‘hotspots’ ( Myers et al., 2000 ) at greatest risk of losing the most unique chondrichthyan biodiversity.
Fisheries catch landings and shark fin exports to Hong Kong
We extracted chondrichthyan landings reported to FAO by 146 countries and territories from a total of 128 countries (as some chondrichthyan fishing nations are overseas territories, unincorporated territories, or British Crown Dependencies) from FishStat ( FAO, 2011 ). We categorized landings into 153 groupings, comprised of 128 species-specific categories (e.g., angular roughshark, piked dogfish, porbeagle, Patagonian skate, plownose chimaera , s mall-eyed ray , etc) and 25 broader nei (nei = not elsewhere included) groupings (e.g., such as various sharks nei, threshers sharks nei, ratfishes nei, raja rays nei ). For each country, all chondrichthyan landings in metric tonnes (t) were averaged over the decade 2000–2009. Landings reported as ‘<0.5’ were assigned a value of 0.5 t. Missing data reported as ‘.’ were assigned a zero. Total annual chondrichthyan landings are underestimated as data are not reported for 1,522 out of a total count of 13,990 entries in the dataset. Therefore, 11% of chondrichthyan landings reported to the FAO over the 10-year period are ‘data unavailable, unobtainable’. We mapped FAO chondrichthyan landings as the national percent share of the average total landings from 2000 to 2009.
For the analysis of landings over time we removed the aggregate category ‘sharks, rays, skates, etc’ and all nine of the FAO chimaera reporting categories. The ‘sharks, rays, skates, etc’ FAO reported category comprised 15,684,456 tonnes of the reported catch from all countries during 1950–2009, which is a total of 45% of the total reported catch for this time period. However, the proportion of catch in this category has declined from around 50% of global catch to around 35%, presumably due to better reporting of ray catch and as sharks have declined or come under stronger protection ( Figure 1 ). The nine chimaera categories make up a small fraction of the global catch, 249,404.5 tonnes from 1950 to 2009, representing 0.72% of the total catch.
Hong Kong has long served as one of the world’s largest entry ports for the global shark fin trade. While fins are increasingly being exported to Mainland China where species-specific trade data is more difficult to obtain, each year (from 1996 to 2001) Hong Kong handled around half of all fin imports ( Clarke et al., 2006 ). Data on shark fin exports to Hong Kong were requested directly from the Hong Kong Census and Statistics Department ( Hong Kong Special Administrative Region Government, 2011 ). We mapped exports to Hong Kong as the proportion of the summed total weight of the four categories of shark fin exported to Hong Kong in 2010: (1) shark fins (with or without skin), with cartilage, dried, whether or not salted but not smoked (trade code: 3055950), (2) shark fins (with or without skin), without cartilage, dried, whether or not salted but not smoked (3055930), (3) shark fins (with or without skin), without cartilage, salted or in brine, but not dried, or smoked (3056940), and (4) shark fins (with or without skin), with cartilage, salted or in brine, but not dried or smoked (3056930). We could not correct the difference in weight due to product type. To identify the threat classification of the chondrichthyan species in the fin trade, we included records of the most numerous species used in the Hong Kong fin trade as well as those species with the most-valued fins ( Clarke et al., 2006 , 2007 ; Clarke, 2008 ).
Acknowledgements
We thank the UN Food and Agriculture Organization and John McEachran for providing distribution maps. We thank all SSG staff, interns and volunteers for logistical and technical support: Sarah Ashworth, Gemma Couzens, Kendal Harrison, Adel Heenan, Catherine McCormack, Helen Meredith, Kim O’Connor, Rachel Kay, Charlotte Walters, Lindsay MacFarlane, Lincoln Tasker, Helen Bates and Rachel Walls. We thank Rowan Trebilco, Wendy Palen, Cheri McCarty, and Roger McManus for their comments on the manuscript, and Statzbeerz and Shinichi Nagakawa for statistical advice. Opinions expressed herein are of the authors only and do not imply endorsement by any agency or institution associated with the authors.
Assessing species for the IUCN Red List relies on the willingness of dedicated experts to contribute and pool their collective knowledge, thus allowing the most reliable judgments of a species’ status to be made. Without their enthusiastic commitment to species conservation, this work would not be possible. We therefore thank all of the SSG members, invited national, regional and international experts who have attended Regional, Generic and Expert Review SSG Red List workshops, and all experts who have contributed data and their expertise by correspondence. A total of 209 SSG members and invited experts participated in regional and thematic workshops and a total of 302 scientists and experts were involved in the process of assessing and evaluating the species assessments. We express our sincere thanks and gratitude to the following people who have contributed to the GSRLA since 1996. We ask forgiveness for any names that may have been inadvertently omitted or misspelled.
Acuña E, Adams W, Affronte M, Aidar A, Alava M, Ali A, Amorim A, Anderson C, Arauz R, Arfelli C, Baker J, Baker K, Baranes A, Barker A, Barnett L, Barratt P, Barwick M, Bates H, Batson P, Baum J, Bell J, Bennett M, Bertozzi M, Bethea D, Bianchi I, Biscoito M, Bishop S, Bizzarro J, Blackwell R, Blasdale T, Bonfil R, Bradaï MN, Brahim K, Branstetter S, Brash J, Bucal D, Cailliet G, Caldas JP, Camara L, Camhi M, Capadan P, Capuli E, Carlisle A, Carocci F, Casper B, Castillo-Geniz L, Castro A, Charvet P, Chiaramonte G, Chin A, Clark T, Clarke M, Clarke S, Cliff G, Clò S, Coelho R, Conrath C, Cook S, Cooke A, Correia J, Cortés E, Couzens G, Cronin E, Crozier P, Dagit D, Davis C, de Carvalho M, Delgery C, Denham J, Devine J, Dharmadi, Dicken M, Di Giácomo E, Diop M, Dipper F, Domingo A, Doumbouya F, Drioli M, Ducrocq M, Dudley S, Duffy C, Ellis J, Endicott M, Everett B, Fagundes L, Fahmi, Faria V, Fergusson I, Ferretti F, Flaherty A, Flammang B, Freitas M, Furtado M, Gaibor N, Gaudiano J, Gedamke T, Gerber L, Gledhill D, Góes de Araújo ML, Goldman K, Gonzalez M, Gordon I, Graham K, Graham R, Grubbs R, Gruber S, Guallart J, Ha D, Haas D, Haedrich R, Haka F, Hareide N-R, Haywood M, Heenan A, Hemida F, Henderson A, Herndon A, Hicham M, Hilton–Taylor C, Holtzhausen H, Horodysky A, Hozbor N, Hueter R, Human B, Huveneers C, Iglésias S, Irvine S, Ishihara H, Jacobsen I, Jawad L, Jeong C-H, Jiddawi N, Jolón M, Jones A, Jones L, Jorgensen S, Kohin S, Kotas J, Krose M, Kukuev E, Kulka D, Lamilla J, Lamónaca A, Last P, Lea R, Lemine Ould S, Leandro L, Lessa R, Licandeo R, Lisney T, Litvinov F, Luer C, Lyon W, Macias D, MacKenzie K, Mancini P, Mancusi C, Manjaji Matsumoto M, Marks M, Márquez-Farias J, Marshall A, Marshall L, Martínez Ortíz J, Martins P, Massa A, Mazzoleni R, McAuley R, McCord M, McCormack C, McEachran J, Medina E, Megalofonou P, Mejia-Falla P, Meliane I, Mendy A, Menni R, Minto C, Mitchell L, Mogensen C, Monor G, Monzini J, Moore A, Morales M.R.J, Morey G, Morgan A, Mouni A, Moura T, Mycock S, Myers R, Nader M, Nakano H, Nakaya K, Namora R, Navia A, Neer J, Nel R, Nolan C, Norman B, Notarbartolo di Sciara G, Oetinger M, Orlov A, Ormond C, Pasolini P, Paul L, Pegado A, Pek Khiok A.L, Pérez M, Pérez-Jiménez J.C, Pheeha S, Phillips D, Pierce S, Piercy A, Pillans R, Pinho M, Pinto de Almeida M, Pogonoski J, Pollard D, Pompert J, Quaranta K, Quijano S, Rasolonjatovo H, Reardon M, Rey J, Rincón G, Rivera F, Robertson R, Robinson L, J.R, Romero M, Rosa R, Ruίz C, Saine A, Salvador N, Samaniego B, San Martín J, Santana F, Santos Motta F, Sato K, Schaaf-DaSilva J, Schembri T, Seisay M, Semesi S, Serena F, Séret B, Sharp R, Shepherd T, Sherrill-Mix S, Siu S, Smale M, Smith M, Snelson, Jr, F, Soldo A, Soriano-Velásquez S, Sosa-Nishizaki O, Soto J, Stehmann M, Stenberg C, Stewart A, Sulikowski J, Sundström L, Tanaka S, Taniuchi T, Tinti F, Tous P, Trejo T, Treloar M, Trinnie F, Ungaro N, Vacchi M, van der Elst R, Vidthayanon C, Villavicencio-Garayzar C, Vooren C, Walker P, Walsh J, Wang Y, Williams S, Wintner S, Yahya S, Yano K, Zebrowski D & Zorzi G.
Funding Statement
The funders had no role in study design, data collection and interpretation, or the decision to submit the work for publication.
Funding Information
This paper was supported by the following grants:
- Conservation International to Sarah L Fowler.
- Packard Foundation to Sarah L Fowler.
- Save Our Seas Foundation to Nicholas K Dulvy.
- UK Department of Environment and Rural Affairs to Sarah L Fowler.
- US State Department to Nicholas K Dulvy, Sarah L Fowler.
- US Department of Commerce to Nicholas K Dulvy.
- Marine Conservation Biology Institute to Sarah L Fowler.
- Pew Marine Fellows Program to Sarah L Fowler.
- Mohamed bin Zayed Species Conservation Foundation to Nicholas K Dulvy.
- Zoological Society of London to Nicholas K Dulvy.
- Canada Research Chairs Program to Nicholas K Dulvy.
- Natural Environment Research Council, Canada to Nicholas K Dulvy.
- Tom Haas and the New Hampshire Charitable Foundation to Sarah L Fowler.
- Oak Foundation to Sarah L Fowler.
- Future of Marine Animal Populations, Census of Marine Life to Sarah L Fowler.
- IUCN Centre for Mediterranean Cooperation to Sarah L Fowler.
- UK Joint Nature Conservation Committee to Sarah L Fowler.
- National Marine Aquarium, Plymouth UK to Sarah L Fowler.
- New England Aquarium Marine Conservation Fund to Sarah L Fowler.
- The Deep, Hull, UK to Sarah L Fowler.
- Blue Planet Aquarium, UK to Sarah L Fowler.
- Chester Zoo, UK to Nicholas K Dulvy, Sarah L Fowler.
- Lenfest Ocean Program to Sarah L Fowler.
- WildCRU, Wildlife Conservation Research Unit, University of Oxford, UK to Sarah L Fowler.
- Institute for Ocean Conservation Science, University of Miami to Sarah L Fowler.
- Flying Sharks to Nicholas K Dulvy.
Additional information
The authors declare that no competing interests exist.
NKD, Conception and design, Acquisition of data, Analysis and interpretation of data, Drafting or revising the article, Contributed unpublished essential data or reagents.
SLF, Conception and design, Acquisition of data, Analysis and interpretation of data, Drafting or revising the article, Contributed unpublished essential data or reagents.
JAM, Acquisition of data, Drafting or revising the article.
CG, Acquisition of data, Drafting or revising the article.
RDC, Conception and design, Acquisition of data, Analysis and interpretation of data, Drafting or revising the article.
PMK, Acquisition of data, Analysis and interpretation of data, Drafting or revising the article.
LRH, Acquisition of data, Analysis and interpretation of data, Drafting or revising the article.
SV, Acquisition of data, Analysis and interpretation of data, Drafting or revising the article.
JKC, Analysis and interpretation of data, Drafting or revising the article.
LNKD, Analysis and interpretation of data, Drafting or revising the article.
MPF, Analysis and interpretation of data, Drafting or revising the article.
GHB, Analysis and interpretation of data, Drafting or revising the article.
SRL, Analysis and interpretation of data, Drafting or revising the article.
SVF, Analysis and interpretation of data, Drafting or revising the article, Contributed unpublished essential data or reagents.
JCS, Analysis and interpretation of data, Drafting or revising the article, Contributed unpublished essential data or reagents.
JDS, Analysis and interpretation of data, Drafting or revising the article, Contributed unpublished essential data or reagents.
CMP, Acquisition of data, Analysis and interpretation of data.
CAS, Conception and design, Analysis and interpretation of data, Drafting or revising the article.
KEC, Analysis and interpretation of data, Contributed unpublished essential data or reagents.
LJVC, Acquisition of data, Drafting or revising the article, Contributed unpublished essential data or reagents.
DAE, Drafting or revising the article, Contributed unpublished essential data or reagents.
MRH, Drafting or revising the article, Contributed unpublished essential data or reagents.
WTW, Drafting or revising the article, Contributed unpublished essential data or reagents.
Additional files
Supplementary file 1..
The Data Deficient chondrichthyan species that are potentially threatened.
Supplementary file 2.
( A ) IUCN Red List status of chondrichthyans in the fin trade, including (i) families with the most-valued fins, and (ii) the most prevalent species utilized in the Hong Kong fin trade. ( B ) Chondrichthyan species threatened by (i) control measures, and (ii) habitat destruction and degradation, pollution or climate change with the corresponding IUCN threat classification ( Salafsky et al., 2008 ). ( C ) Irreplaceable: the 66 threatened endemic sharks and rays ordered in decreasing irreplaceability.
Major datasets
The following dataset was generated:
The International Union for Conservation of Nature, 2013, The IUCN Red List of Threatened Species, http://www.iucnredlist.org/ , Publicly available.
- Akcakaya HR, Ferson S, Burgman MA, Keith DA, Mace GM, Todd CR. Making consistent IUCN classifications under uncertainty. Conservation Biology. 2000; 14 :1001–1013. doi: 10.1046/j.1523-1739.2000.99125.x. [ CrossRef ] [ Google Scholar ]
- Anderson SC, Farmer RG, Ferretti F, Houde ALS, Hutchings JA. Correlates of vertebrate extinction risk in Canada. Bioscience. 2011a; 61 :538–549. doi: 10.1525/bio.2011.61.7.8. [ CrossRef ] [ Google Scholar ]
- Anderson SC, Flemming JM, Watson R, Lotze HK. Serial exploitation of global sea cucumber fisheries. Fish and Fisheries. 2011b; 12 :317–339. doi: 10.1111/j.1467-2979.2010.00397.x. [ CrossRef ] [ Google Scholar ]
- Barker MJ, Schluessel V. Managing global shark fisheries: suggestions for prioritizing management strategies. Aquatic Conservation: Marine and Freshwater Ecosystems. 2005; 15 :325–347. doi: 10.1002/aqc.660. [ CrossRef ] [ Google Scholar ]
- Barnett LAK, Winton MV, Ainsley SM, Cailliet GM, Ebert DA. Comparative demography of skates: life-history correlates of productivity and implications for management. PLOS ONE. 2013; 8 :e65000. doi: 10.1371/journal.pone.0065000. [ PMC free article ] [ PubMed ] [ CrossRef ] [ Google Scholar ]
- Bates D, Maechler M, Bolker B. lme4: linear mixed-effects models using S4 classes. 2011 R package version 0.999999-2. [ Google Scholar ]
- Bornatowski H, Braga RR, Vitule JRS. Shark mislabeling threatens biodiversity. Science. 2013; 340 :923. doi: 10.1126/science.340.6135.923-a. [ PubMed ] [ CrossRef ] [ Google Scholar ]
- Branch TA, Jensen OP, Ricard D, Ye Y, Hilborn R. Contrasting global trends in marine fishery status obtained from catches and from stock assessments. Conservation Biology. 2011; 25 :777–786. doi: 10.1111/j.1523-1739.2011.01687.x. [ PubMed ] [ CrossRef ] [ Google Scholar ]
- Butchart SHM, Walpole M, Collen B, van Strien A, Scharlemann JPW, Almond REA, Baillie JEM, Bomhard B, Brown C, Bruno J, Carpenter KE, Carr GM, Chanson J, Chenery AM, Csirke J, Davidson NC, Dentener F, Foster M, Galli A, Galloway JN, Genovesi P, Gregory RD, Hockings M, Kapos V, Lamarque JF, Leverington F, Loh J, McGeoch MA, McRae L, Minasyan A, Morcillo MH, Oldfield TEE, Pauly D, Quader S, Revenga C, Sauer JR, Skolnik B, Spear D, Stanwell-Smith D, Stuart SN, Symes A, Tierney M, Tyrrell TD, Vie JC, Watson R. Global biodiversity: indicators of recent declines. Science. 2010; 328 :1164–1168. doi: 10.1126/science.1187512. [ PubMed ] [ CrossRef ] [ Google Scholar ]
- Camhi MD, Valenti SV, Fordham SV, Fowler SL, Gibson C. Newbury, UK: IUCN Species Survival Commission Shark Specialist Group; 2009. The conservation status of pelagic sharks and rays: Report of the IUCN shark specialist group pelagic shark red list workshop; p. 78. [ Google Scholar ]
- Cardillo M, Mace GM, Jones KE, Bielby J, Binida-Edmonds ORP, Sechrest W, Orme CDL, Purvis A. Multiple causes of high extinction risk in large mammal species. Science. 2005; 309 :1239–1241. doi: 10.1126/science.1116030. [ PubMed ] [ CrossRef ] [ Google Scholar ]
- Carpenter KE, Abrar M, Aeby G, Aronson RB, Banks S, Bruckner A, Chiriboga A, Cortes J, Delbeek JC, DeVantier L, Edgar GJ, Edwards AJ, Fenner D, Guzman HM, Hoeksema BW, Hodgson G, Johan O, Licuanan WY, Livingstone SR, Lovell ER, Moore JA, Obura DO, Ochavillo D, Polidoro BA, Precht WF, Quibilan MC, Reboton C, Richards ZT, Rogers AD, Sanciangco J, Sheppard A, Sheppard C, Smith J, Stuart S, Turak E, Veron JEN, Wallace C, Weil E, Wood E. One-third of reef-building corals face elevated extinction risk from climate change and local impacts. Science. 2008; 321 :560–563. doi: 10.1126/science.1159196. [ PubMed ] [ CrossRef ] [ Google Scholar ]
- Cavanagh RD, Fowler SL, Camhi MD. Pelagic sharks and the FAO international plan of action for the conservation and management of sharks. In: Camhi MD, Pikitch EK, Babcock EA, editors. Sharks of the open ocean. Biology, fisheries and conservation. Oxford: Blackwell; 2008. pp. 478–492. [ Google Scholar ]
- Cavanagh RD, Gibson C. Gland, Switzerland and Malaga, Spain: IUCN Species Survival Commission Shark Specialist Group; 2007. Overview of the conservation status of cartilaginous fishes (Chondrichthyans) in the Mediterranean Sea; p. 42. [ Google Scholar ]
- Cavanagh RD, Kyne PM, Fowler SL, Musick JA, Bennett MB. Brisbane, Australia: The University of Queensland, School of Biomedical Sciences; 2003. The conservation status of Australasian chondrichthyans: Report of the IUCN shark specialist group Australia and Oceania regional red list workshop; p. 170. [ Google Scholar ]
- CCMLR . Hobart, Tasmania: 2011. Conservation Measure 41-10 (2010) Limits on the exploratory fishery for Dissostichus spp. in Statistical Subarea 88.2 in the 2010/11 season. Commission on the Conservation of Antarctic Marine Living Resource; p. 3. [ Google Scholar ]
- Chin A, Kyne PM, Walker TI, McAuley RB. A new integrated risk assessment for climate change: analysing the vulnerability of sharks and rays on Australia’s Great Barrier Reef. Global Change Biology. 2010; 16 :1936–1953. doi: 10.1111/j.1365-2486.2009.02128.x. [ CrossRef ] [ Google Scholar ]
- Clarke SC. Use of shark fin trade data to estimate historic total shark removals in the Atlantic Ocean. Aquatic Living Resources. 2008; 21 :373–381. doi: 10.1051/alr:2008060. [ CrossRef ] [ Google Scholar ]
- Clarke SC, Harley SJ, Hoyle SD, Rice JS. Population trends in Pacific oceanic sharks and the utility of regulations on shark finning. Conservation Biology. 2013; 27 :197–209. doi: 10.1111/j.1523-1739.2012.01943.x. [ PubMed ] [ CrossRef ] [ Google Scholar ]
- Clarke SC, McAllister MK, Milner-Gulland EJ, Kirkwood GP, Michielsens CGJ, Agnew DJ, Pikitch EK, Nakano H, Shivji MS. Global estimates of shark catches using trade records from commercial markets. Ecology Letters. 2006; 9 :1115–1126. doi: 10.1111/j.1461-0248.2006.00968.x. [ PubMed ] [ CrossRef ] [ Google Scholar ]
- Clarke SC, Milner-Gulland EJ, Bjørndal T. Social, economic and regulatory drivers of the shark fin trade. Marine Resource Economics. 2007; 22 :305–327. [ Google Scholar ]
- CMS Memorandum of understanding on the conservation of migratory sharks. United nations environment programme, convention on migratory species. 2010 http://www.cms.int/pdf/en/summary_sheets/sharks.pdf
- Collette BB, Carpenter KE, Polidoro BA, J Jorda MJ, Boustany A, Die DJ, Elfes C, Fox W, Graves J, Harrison L, McManus R, Minte-Vera CV, Nelson RJ, Restrepo V, Schratwieser J, Sun C-L, Amorim A, Brick Peres M, Canales C, Cardenas G, Chang S-K, Chiang W-C, de Oliveira Leite JN, Harwell H, Lessa R, Fredou FL, Oxenford HA, Serra R, Shao K-T, Sumaila R, Wang S-P, Watson R, Yáñez E. High value and long-lived - double jeopardy for tunas and billfishes. Science. 2011; 333 :291–292. doi: 10.1126/science.1208730. [ PubMed ] [ CrossRef ] [ Google Scholar ]
- Compagno LJV. Alternative life-history styles of cartilaginous fishes in time and space. Environmental Biology of Fishes. 1990; 28 :33–75. doi: 10.1007/BF00751027. [ CrossRef ] [ Google Scholar ]
- Compagno LJV. Checklist of living chondrichthyan fishes. In: Fowler SL, Cavanagh R, Camhi M, Burgess GH, Caillet GM, Fordham S, Simpfendorfer CA, Musick JA, editors. Sharks, Rays and Chimaeras: the Status of the Chondrichthyan Fishes. Gland, Switzerland: IUCN/SSC Shark Specialist Group; 2005. pp. 401–423. [ Google Scholar ]
- Cortés E. Life history patterns and correlations in sharks. Reviews in Fisheries Science. 2000; 8 :299–344. [ Google Scholar ]
- Cortés E. Incorporating uncertainty into demographic modelling: application to shark populations and their conservation. Conservation Biology. 2002; 18 :1048–1062. doi: 10.1046/j.1523-1739.2002.00423.x. [ CrossRef ] [ Google Scholar ]
- Cortés E. Comparative life history and demography of pelagic sharks. In: Camhi MD, Pikitch EK, Babcock EA, editors. Sharks of the Open Ocean. Biology, Fisheries and Conservation. Oxford: Blackwell; 2008. pp. 309–322. [ Google Scholar ]
- COSEWIC . Ottawa, Canada: 2010. COSEWIC assessment and status report on the Barndoor skate (Dipturus laevis) in Canada. Committee on the Status of Endangered Wildlife in Canada. p. xiii + 71. [ Google Scholar ]
- Costello C, Ovando D, Hilborn R, Gaines SD, Deschenes O, Lester SE. Status and solutions for the world’s unassessed fisheries. Science. 2012; 338 :517–520. doi: 10.1126/science.1223389. [ PubMed ] [ CrossRef ] [ Google Scholar ]
- Darwall WRT, Holland RA, Smith KG, Allen D, Brooks EGE, Katarya V, Pollock CM, Shi YC, Clausnitzer V, Cumberlidge N, Cuttelod A, Dijkstra KDB, Diop MD, Garcia N, Seddon MB, Skelton PH, Snoeks J, Tweddle D, Vie JC. Implications of bias in conservation research and investment for freshwater species. Conservation Letters. 2011; 4 :474–482. doi: 10.1111/j.1755-263X.2011.00202.x. [ CrossRef ] [ Google Scholar ]
- Davidson AD, Boyer AG, Kim H, Pompa-Mansilla S, Hamilton MJ, Costa DP, Ceballos G, Brown JH. Drivers and hotspots of extinction risk in marine mammals. Proceedings Of the National Academy Of Sciences Of the United States Of America. 2012; 109 :3395–3400. doi: 10.1073/pnas.1121469109. [ PMC free article ] [ PubMed ] [ CrossRef ] [ Google Scholar ]
- Davies RG, Orme CDL, Olson V, Thomas GH, Ross SG, Ding TS, Rasmussen PC, Stattersfield AJ, Bennett PM, Blackburn TM, Owens IPF, Gaston KJ. Human impacts and the global distribution of extinction risk. Proceedings of the Royal Society B-Biological Sciences. 2006; 273 :2127–2133. doi: 10.1098/rspb.2006.3551. [ PMC free article ] [ PubMed ] [ CrossRef ] [ Google Scholar ]
- Díaz S, Fargione J, Chapin FS, III, Tilman D. Biodiversity loss threatens human well-being. PLOS Biology. 2006; 4 :1300–1305. doi: 10.1371/journal.pbio.0040277. [ PMC free article ] [ PubMed ] [ CrossRef ] [ Google Scholar ]
- Dudley SFJ, Simpfendorfer CA. Population status of 14 shark species caught in the protective gillnets off KwaZulu-Natal beaches, South Africa, 1978-2003. Marine and Freshwater Research. 2006; 57 :225–240. doi: 10.1071/MF05156. [ CrossRef ] [ Google Scholar ]
- Dulvy NK. Dipturus [Raja] laevis, Mitchill, 1818. IUCN, Gland, Switzerland. 2000 http://www.iucnredlist.org/details/39771/0
- Dulvy NK, Baum JK, Clarke S, Compagno LJV, Cortés E, Domingo A, Fordham S, Fowler S, Francis MP, Gibson C, Martínez J, Musick JA, Soldo A, Stevens JD, Valenti S. You can swim but you can’t hide: the global status and conservation of oceanic pelagic sharks. Aquatic Conservation: Marine and Freshwater Ecosystems. 2008; 18 :459–482. doi: 10.1002/aqc.975. [ CrossRef ] [ Google Scholar ]
- Dulvy NK, Forrest RE. In: Life histories, population dynamics, and extinction risks in chondrichthyans. Sharks and their relatives II: biodiversity, adaptive physiology, and conservation. Carrier JC, Musick JA, Heithaus MR, editors. Boca Raton: CRC Press; 2010. pp. 635–676. [ Google Scholar ]
- Dulvy NK, Jennings SJ, Goodwin NB, Grant A, Reynolds JD. Comparison of threat and exploitation status in Northeast Atlantic marine populations. Journal of Applied Ecology. 2005; 42 :883–891. doi: 10.1111/j.1365-2664.2005.01063.x. [ CrossRef ] [ Google Scholar ]
- Dulvy NK, Reynolds JD. Predicting extinction vulnerability in skates. Conservation Biology. 2002; 16 :440–450. doi: 10.1046/j.1523-1739.2002.00416.x. [ CrossRef ] [ Google Scholar ]
- Dulvy NK, Sadovy Y, Reynolds JD. Extinction vulnerability in marine populations. Fish and Fisheries. 2003; 4 :25–64. doi: 10.1046/j.1467-2979.2003.00105.x. [ CrossRef ] [ Google Scholar ]
- FAO . Rome: 2010. The state of world fisheries and Aquaculture 2010. Food and Agriculture organization of the United nations; p. 197. [ Google Scholar ]
- FAO . Rome: 2011. FishSTAT capture production (1950-2009) database. Food and Agriculture organization of the United nations. http://www.fao.org/fishery/statistics/software/fishstat/en [ Google Scholar ]
- Ferretti F, Myers RA, Serena F, Lotze HK. Loss of large predatory sharks from the Mediterranean sea. Conservation Biology. 2008; 22 :952–964. doi: 10.1111/j.1523-1739.2008.00938.x. [ PubMed ] [ CrossRef ] [ Google Scholar ]
- Ferretti F, Worm B, Britten GL, Heithaus MR, Lotze HK. Patterns and ecosystem consequences of shark declines in the ocean. Ecology Letters. 2010; 13 :1055–1071. doi: 10.1111/j.1461-0248.2010.01489.x. [ PubMed ] [ CrossRef ] [ Google Scholar ]
- Fischer J, Erikstein K, D‘Offay B, Barone M, Guggisberg S. C1076. Rome: 2012. Review of the implementation of the international plan of action for the conservation and management of sharks. Food and Agriculture organization of the United nations; p. 125. [ Google Scholar ]
- Fowler S, Séret B. Shark fins in Europe: Implications for reforming the EU finning ban. European Elasmobranch Association and IUCN Shark Specialist Group. 2010:27. [ Google Scholar ]
- Fowler SL. Bonn, Germany: 2012. Background paper on the conservation status of migratory sharks. Convention on migratory species; p. 22. [ Google Scholar ]
- Fowler SL, Reed TM, Dipper FA. Elasmobranch Biodiversity, Conservation and Management. Gland, Switzerland and Cambridge, UK: IUCN Species Survival Commission Shark Specialist Group; 2002. [ Google Scholar ]
- Frisk MG, Miller TJ, Fogarty MJ. Estimation and analysis of biological parameters in elasmobranch fishes: a comparative life history study. Canadian Journal of Fisheries and Aquatic Sciences. 2001; 58 :969–981. doi: 10.1139/f01-051. [ CrossRef ] [ Google Scholar ]
- García VB, Lucifora LO, Myers RA. The importance of habitat and life history to extinction risk in sharks, skates, rays and chimaeras. Proceedings of the Royal Society B-Biological Sciences. 2008; 275 :83–89. doi: 10.1098/rspb.2007.1295. [ PMC free article ] [ PubMed ] [ CrossRef ] [ Google Scholar ]
- Gärdenfors U, Hilton-Taylor C, Mace GM, Rodríguez JP. The application of IUCN Red List Criteria regional levels. Conservation Biology. 2001; 15 :1206–1212. doi: 10.1046/j.1523-1739.2001.00112.x. [ CrossRef ] [ Google Scholar ]
- Gelman A, Hill J. Data analysis using regression and multilevel/hierarchial models. Cambridge: Cambridge University Press; 2006. [ Google Scholar ]
- Gibson C, Valenti SV, Fowler SL, Fordham SV. Newbury, UK: IUCN species survival Commission shark specialist group; 2008. The conservation status of Northeast Atlantic chondrichthyans. Report of the IUCN shark specialist group Northeast Atlantic red list workshop; p. 76. [ Google Scholar ]
- Harnik PG, Lotze HK, Anderson SC, Finkel ZV, Finnegan S, Lindberg DR, Liow LH, Lockwood R, McClain CR, McGuire JL, O’Dea A, Pandolfi JM, Simpson C, Tittensor DP. Extinctions in ancient and modern seas. Trends in Ecology & Evolution. 2012a; 27 :608–617. doi: 10.1016/j.tree.2012.07.010. [ PubMed ] [ CrossRef ] [ Google Scholar ]
- Harnik PG, Simpson C, Payne JL. Long-term differences in extinction risk among the seven forms of rarity. Proceedings of the Royal Society B-Biological Sciences. 2012b; 279 :4969–4976. doi: 10.1098/rspb.2012.1902. [ PMC free article ] [ PubMed ] [ CrossRef ] [ Google Scholar ]
- Harrison LR, Dulvy NK. Vancouver, British Columbia, Canada: International Union for the Conservation of Nature Species Survival Commission’s Shark Specialist Group; 2014. Sawfish: a global Strategy for conservation; p. 112. ISBN: 978-0-9561063-3-9. [ Google Scholar ]
- Heithaus MR, Wirsing AJ, Dill LM. The ecological importance of intact top-predator populations: a synthesis of 15 years of research in a seagrass ecosystem. Marine and Freshwater Research. 2012; 63 :1039–1050. doi: 10.1071/MF12024. [ CrossRef ] [ Google Scholar ]
- Hoffmann M, Hilton-Taylor C, Angulo A, Bohm M, Brooks TM, Butchart SHM, Carpenter KE, Chanson J, Collen B, Cox NA, Darwall WRT, Dulvy NK, Harrison LR, Katariya V, Pollock CM, Quader S, Richman NI, Rodrigues ASL, Tognelli MF, Vie J-C, Aguiar JM, Allen DJ, Allen GR, Amori G, Ananjeva NB, Andreone F, Andrew P, Ortiz ALA, Baillie JEM, Baldi R, Bell BD, Biju SD, Bird JP, Black-Decima P, Blanc JJ, Bolanos F, Bolivar-G W, Burfield IJ, Burton JA, Capper DR, Castro F, Catullo G, Cavanagh RD, Channing A, Chao NL, Chenery AM, Chiozza F, Clausnitzer V, Collar NJ, Collett LC, Collette BB, Fernandez CFC, Craig MT, Crosby MJ, Cumberlidge N, Cuttelod A, Derocher AE, Diesmos AC, Donaldson JS, Duckworth JW, Dutson G, Dutta SK, Emslie RH, Farjon A, Fowler S, Freyhof J, Garshelis DL, Gerlach J, Gower DJ, Grant TD, Hammerson GA, Harris RB, Heaney LR, Hedges SB, Hero J-M, Hughes B, Hussain SA, Icochea MJ, Inger RF, Ishii N, Iskandar DT, Jenkins RKB, Kaneko Y, Kottelat M, Kovacs KM, Kuzmin SL, La Marca E, Lamoreux JF, Lau MWN, Lavilla EO, Leus K, Lewison RL, Lichtenstein G, Livingstone SR, Lukoschek V, Mallon DP, McGowan PJK, McIvor A, Moehlman PD, Molur S, Muñoz Alonso A, Musick JA, Nowell K, Nussbaum RA, Olech W, Orlov NL, Papenfuss TJ, Parra-Olea G, Perrin WF, Polidoro BA, Pourkazemi M, Racey PA, Ragle JS, Ram M, Rathbun G, Reynolds RP, Rhodin AG, Richards SJ, Rodríguez LO, Ron SR, Rondinini C, Rylands AB, Sadovy de Mitcheson Y, Sanciangco JC, Sanders KL, Santos-Barrera G, Schipper J, Self-Sullivan C, Shi Y, Shoemaker A, Short FT, Sillero-Zubiri C, Silvano DL, Smith KG, Smith AT, Snoeks J, Stattersfield AJ, Symes AJ, Taber AB, Talukdar BK, Temple HJ, Timmins R, Tobias JA, Tsytsulina K, Tweddle D, Ubeda C, Valenti SV, van Dijk PP, Veiga LM, Veloso A, Wege DC, Wilkinson M, Williamson EA, Xie F, Young BE, Akçakaya HR, Bennun L, Blackburn TM, Boitani L, Dublin HT, da Fonseca GA, Gascon C, Lacher TE, Jnr, Mace GM, Mainka SA, McNeely JA, Mittermeier RA, Reid GM, Rodriguez JP, Rosenberg AA, Samways MJ, Smart J, Stein BA, Stuart SN. The impact of conservation on the status of the world’s vertebrates. Science. 2010; 33 :1503–1509. doi: 10.1126/science.1194442. [ PubMed ] [ CrossRef ] [ Google Scholar ]
- Hong Kong Special Administrative Region Government . Hong Kong: 2011. Census and statistics Department. Hong Kong special Administrative region government. http://www.censtatd.gov.hk/home/index.jsp [ Google Scholar ]
- Hutchings JA. Collapse and recovery of marine fishes. Nature. 2000; 406 :882–885. doi: 10.1038/35022565. [ PubMed ] [ CrossRef ] [ Google Scholar ]
- Hutchings JA, Myers RA, Garcia VB, Lucifora LO, Kuparinen A. Life-history correlates of extinction risk and recovery potential. Ecological Applications. 2012; 22 :1061–1067. doi: 10.1890/11-1313.1. [ PubMed ] [ CrossRef ] [ Google Scholar ]
- IATTC . C-11-10. La Jolla, USA: 2011. Resolution on the conservation of oceanic whitetip sharks caught in association with fisheries in the Antigua convention area. Inter-American Tropical Tuna Commission (IATTC) p. 2. [ Google Scholar ]
- Iglésias SP, Toulhout L, Sellos DP. Taxonomic confusion and market mislabelling of threatened skates: Important consequences for their conservation status. Aquatic Conservation - Marine and Freshwater Ecosystems. 2010; 20 :319–333. doi: 10.1002/aqc.1083. [ CrossRef ] [ Google Scholar ]
- IOTC . Mahé, Seychelles: 2011. Report of the Fourteenth session of the IOTC scientific Committee. Indian ocean tuna Commission; p. 33. [ Google Scholar ]
- IUCN . Gland Switzerland and Cambridge, UK: 2001. IUCN red list categories and criteria: version 3.1. IUCN Species Survival Commission; p. 38. [ Google Scholar ]
- IUCN . Gland, Switzerland: 2004. Guidelines for using the IUCN red list categories and criteria. IUCN Species Survival Commission; p. 87. [ Google Scholar ]
- Jackson JBC. The future of the oceans past. Philosophical Transactions of the Royal Society B: Biological Sciences. 2010; 365 :3765–3778. doi: 10.1098/rstb.2010.0278. [ PMC free article ] [ PubMed ] [ CrossRef ] [ Google Scholar ]
- Jennings S, Reynolds JD, Mills SC. Life history correlates of responses to fisheries exploitation. Proceedings of the Royal Society B-Biological Sciences. 1998; 265 :333–339. doi: 10.1098/rspb.1998.0300. [ CrossRef ] [ Google Scholar ]
- Jones KE, Purvis A, Gittleman JL. Biological correlates of extinction risk in bats. American Naturalist. 2003; 161 :601–614. doi: 10.1086/368289. [ PubMed ] [ CrossRef ] [ Google Scholar ]
- Juan-Jordá MJ, Mosqueira I, Freire J, Dulvy NK. Life in 3-D: life history strategies of tunas, bonitos and mackerels. Reviews in Fish Biology and Fisheries. 2013; 23 :135–155. doi: 10.1007/s11160-012-9284-4. [ CrossRef ] [ Google Scholar ]
- Kriwet J, Witzmann F, Klug S, Heidtke UHJ. First direct evidence of a vertebrate three-level trophic chain in the fossil record. Proceedings of the Royal Society B-Biological Sciences. 2008; 275 :181–186. doi: 10.1098/rspb.2007.1170. [ PMC free article ] [ PubMed ] [ CrossRef ] [ Google Scholar ]
- Kulka DW, Frank KT, Simon JE. Barndoor skate in the northwest Atlantic off Canada: distribution in relation to temperature and depth based on commercial fisheries data. 2002:17. Department of Fisheries and Oceans, Canadian Science Advisory Secretariat Research Document, 2002/073. [ Google Scholar ]
- Kyne PM, Carlson JK, Ebert DA, Fordham SV, Bizzarro JJ, Graham RT, Kulka DW, Tewes EE, Harrison LR, Dulvy NK. Vancouver, Canada: 2012. The Conservation Status of North American, Central American, and Caribbean chondrichthyans. IUCN Species Survival Commission Shark Specialist Group; p. 148. [ Google Scholar ]
- Kyne PM, Simpfendorfer CA. Newbury, England: 2007. A collation and summarization of available data on deepwater chondrichthyans: biodiversity, life history and fisheries. IUCN Species Survival Commission Shark Specialist Group; p. 137. [ Google Scholar ]
- Lack M, Sant G. Cambridge: 2009. Trends in global shark catch and recent developments in management. TRAFFIC International; p. 33. [ Google Scholar ]
- Lam VYY, Sadovy de Mitcheson Y. The sharks of South East Asia – unknown, unmonitored and unmanaged. Fish and Fisheries. 2010; 12 :51–74. doi: 10.1111/j.1467-2979.2010.00383.x. [ CrossRef ] [ Google Scholar ]
- Lasram FBR, Guilhaumon FO, Albouy C, Somot S, Thuiller W, Mouillot D. The Mediterranean Sea as a ‘cul-de-sac’ for endemic fishes facing climate change. Global Change Biology. 2010; 16 :3233–3245. doi: 10.1111/j.1365-2486.2010.02224.x. [ CrossRef ] [ Google Scholar ]
- Last PR. The state of chondrichthyan taxonomy and systematics. Marine and Freshwater Research. 2007; 58 :7–9. doi: 10.1071/MF07003. [ CrossRef ] [ Google Scholar ]
- Last PR, Stevens JD. Hobart, Australia: 1994. Sharks and rays of Australia, CSIRO, Division of Fisheries. [ Google Scholar ]
- Last PR, Stevens JD. Hobart, Australia: 2009. Sharks and rays of Australia, CSIRO, Division of Fisheries. [ Google Scholar ]
- Last PR, White WT, Pogonoski J. Vol. 22. Hobart, Australia: 2008a. Descriptions of new Australian chondrichthyans. CSIRO Marine and Atmospheric Research Paper; p. 368. [ Google Scholar ]
- Last PR, White WT, Pogonoski J. Vol. 14. Hobart, Australia: 2008b. Descriptions of new dogfishes of the genus Squalus (Squaloidea: Squalidae). CSIRO Marine and Atmospheric Research Paper; p. 136. [ Google Scholar ]
- Last PR, White WT, Pogonoski J, Gledhill DC. Vol. 21. Hobart, Australia: 2008c. Descriptions of new Australian skates (Batoidea: Rajoidea). CSIRO Marine and Atmospheric Research Paper; p. 187. [ Google Scholar ]
- Lotze HK, Lenihan HS, Bourque BJ, Bradbury RH, Cooke RG, Kay MC, Kidwell SM, Kirby MX, Peterson CH, Jackson JBC. Depletion, degradation, and recovery potential of estuaries and coastal seas. Science. 2006; 312 :1806–1809. doi: 10.1126/science.1128035. [ PubMed ] [ CrossRef ] [ Google Scholar ]
- Mace G, Masundire H, Baillie J, Ricketts T, Brooks T. Biodiversity. In: Hassan R, Scholes R, Ash N, editors. Ecosystems and human well-being: current state and trends: findings of the condition and trends working group. Washington DC: Island Press; 2005. pp. 77–122. [ Google Scholar ]
- Mace GM, Collar NJ, Gaston KJ, Hilton-Taylor C, Akcakaya HR, Leader-Williams N, Milner-Gulland EJ, Stuart SN. Quantification of extinction risk: IUCN’s system for classifying threatened species. Conservation Biology. 2008; 22 :1424–1442. doi: 10.1111/j.1523-1739.2008.01044.x. [ PubMed ] [ CrossRef ] [ Google Scholar ]
- Mace PM. In defence of fisheries scientists, single species models and other scapegoats: confronting the real problems. Marine Ecology Progress Series. 2004; 274 :285–291. [ Google Scholar ]
- Marris E. Conservation priorities: what to let go. Nature. 2007; 450 :152–155. doi: 10.1038/450152a. [ PubMed ] [ CrossRef ] [ Google Scholar ]
- McClenachan L, Cooper AB, Carpenter KE, Dulvy NK. Extinction risk and bottlenecks in the conservation of charismatic marine species. Conservation Letters. 2012; 5 :73–80. doi: 10.1111/j.1755-263X.2011.00206.x. [ CrossRef ] [ Google Scholar ]
- Morato T, Watson R, Pitcher TJ, Pauly D. Fishing down the deep. Fish and Fisheries. 2006; 7 :24–34. doi: 10.1111/j.1467-2979.2006.00205.x. [ CrossRef ] [ Google Scholar ]
- Mundy-Taylor V, Crook V. Cambridge: 2013. Into the Deep: Implementing CITES measures for commercially-valuable sharks and manta rays. TRAFFIC; p. 106. [ Google Scholar ]
- Musick JA. Criteria to define extinction risk in marine fishes. Fisheries. 1999a; 24 :6–14. doi: 10.1577/1548-8446(1999)0242.0.CO;2. [ CrossRef ] [ Google Scholar ]
- Musick JA. Ecology and conservation of long-lived marine animals. In: Musick JA, editor. Life in the slow lane: ecology and conservation of long-lived marine animals. Bethesda, Maryland: American Fisheries Society Symposium; 1999b. pp. 1–10. [ Google Scholar ]
- Musick JA, Harbin MM, Berkeley SA, Burgess GH, Eklund AM, Findley L, Gilmore RG, Golden JT, Ha DS, Huntsman GR, McGovern JC, Parker SJ, Poss SG, Sala E, Schmidt TW, Sedberry GR, Weeks H, Wright SG. Marine, estuarine, and diadromous fish stocks at risk of extinction in North America (exclusive of Pacific salmonids) Fisheries. 2000; 25 :6–30. [ Google Scholar ]
- Musick JA, Musick S. Vol. 569. Rome, Italy: 2011. Sharks (Special Topics C3), in review of the state of the world Marine fishery resources. Food and Agriculture organization of the United nations; pp. 245–254. [ Google Scholar ]
- Myers N, Mittermeier RA, Mittermeier CG, da Fonseca GAB, Kent J. Biodiversity hotspots for conservation priorities. Nature. 2000; 403 :853–858. doi: 10.1038/35002501. [ PubMed ] [ CrossRef ] [ Google Scholar ]
- NAFO NAFO conservation and enforcement measures. North Atlantic fisheries Organisation. 2011 http://www.nafo.int/fisheries/frames/regulations.html
- Nakagawa S, Schielzeth H. A general and simple method for obtaining R 2 from generalized linear mixed-effects models. Methods in Ecology and Evolution. 2012; 4 :133–142. doi: 10.1111/j.2041-210x.2012.00261.x. [ CrossRef ] [ Google Scholar ]
- Naylor GJP, Caira JN, Jensen K, Rosana KAM, White WT, Last PR. A DNA sequence-based approach to the identification of shark and ray species and its implications for global elasmobranch diversity and parasitology. Bulletin of the American Museum of Natural History. 2012; 367 :1–262. doi: 10.1206/754.1. [ CrossRef ] [ Google Scholar ]
- NEAFC Fisheries (High seas fishing Notifications—North East Atlantic fisheries Commission) Notice 2009. 2009 http://www.legislation.govt.nz/regulation/public/2009/0138/latest/DLM3929500.html
- Neubauer P, Jensen OP, Hutchings JA, Baum JK. Resilience and recovery of overexploited marine populations. Science. 2013; 340 :347–349. doi: 10.1126/science.1230441. [ PubMed ] [ CrossRef ] [ Google Scholar ]
- Newton K, Côté IM, Pilling GM, Jennings S, Dulvy NK. Current and future sustainability of island coral reef fisheries. Current Biology. 2007; 17 :655–658. doi: 10.1016/j.cub.2007.02.054. [ PubMed ] [ CrossRef ] [ Google Scholar ]
- Polidoro BA, Brooks T, Carpenter KE, Edgar GJ, Henderson S, Sanciangco J, Robertson DR. Patterns of extinction risk and threat for marine vertebrates and habitat-forming species in the Tropical Eastern Pacific. Marine Ecology-Progress Series. 2012; 448 :93–104. doi: 10.3354/meps09545. [ CrossRef ] [ Google Scholar ]
- Polidoro BA, Livingstone SR, Carpenter KE, Hutchinson B, Mast RB, Pilcher NJ, Sadovy de Mitcheson Y, Valenti SV. Status of the world’s marine species. In: Vié J-C, Hilton-Taylor C, Stuart SN, editors. Wildlife in a changing world: an analysis of the 2008 IUCN Red List of threatened species. Gland, Switzerland: International Union for Conservation of Nature; 2008. pp. 55–65. [ Google Scholar ]
- Porszt E, Peterman RM, Dulvy NK, Cooper AB, Irvine JR. Reliability of indicators of declines in abundance. Conservation Biology. 2012; 26 :894–904. doi: 10.1111/j.1523-1739.2012.01882.x. [ PubMed ] [ CrossRef ] [ Google Scholar ]
- Reynolds JD, Dulvy NK, Goodwin NB, Hutchings JA. Biology of extinction risk in marine fishes. Proceedings of the Royal Society B-Biological Sciences. 2005; 272 :2337–2344. doi: 10.1098/rspb.2005.3281. [ PMC free article ] [ PubMed ] [ CrossRef ] [ Google Scholar ]
- Ricard D, Minto C, Jensen OP, Baum JK. Examining the knowledge base and status of commercially exploited marine species with the RAM Legacy Stock Assessment Database. Fish and Fisheries. 2012; 13 :380–398. doi: 10.1111/j.1467-2979.2011.00435.x. [ CrossRef ] [ Google Scholar ]
- Robillard M, Séret B. Cultural importance and decline of sawfish (Pristidae) populations in West Africa. Cybium. 2006; 30 :23–30. [ Google Scholar ]
- Sadovy Y. Trouble on the reef: the imperative for managing vulnerable and valuable fisheries. Fish and Fisheries. 2005; 6 :167–185. doi: 10.1111/j.1467-2979.2005.00186.x. [ CrossRef ] [ Google Scholar ]
- Sahr K, White D, Kimerling AJ. Geodesic discrete global grid systems. Cartography and Geographic Information Science. 2003; 30 :121–134. doi: 10.1559/152304003100011090. [ CrossRef ] [ Google Scholar ]
- Salafsky N, Salzer D, Stattersfield AJ, Hilton-Taylor C, Neugarten R, Butchart SHM, Collen B, Cox N, Master LL, O’Connor S, Wilkie D. A standard lexicon for biodiversity conservation: unified classifications of threats and actions. Conservation Biology. 2008; 22 :897–911. doi: 10.1111/j.1523-1739.2008.00937.x. [ PubMed ] [ CrossRef ] [ Google Scholar ]
- Schipper J, Chanson JS, Chiozza F, Cox NA, Hoffmann M, Katariya V, Lamoreux J, Rodrigues ASL, Stuart SN, Temple HJ, Baillie J, Boitani L, Lacher TE, Jnr, Mittermeier RA, Smith AT, Absolon D, Aguiar JM, Amori G, Bakkour N, Baldi R, Berridge RJ, Bielby J, Black PA, Blanc JJ, Brooks TM, Burton JA, Butynski TM, Catullo G, Chapman R, Cokeliss Z, Collen B, Conroy J, Cooke JG, da Fonseca GAB, Derocher AE, Dublin HT, Duckworth JW, Emmons L, Emslie RH, Festa-Bianchet M, Foster M, Foster S, Garshelis DL, Gates C, Gimenez-Dixon M, Gonzalez S, Gonzalez-Maya JF, Good TC, Hammerson G, Hammond PS, Happold D, Happold M, Hare J, Harris RB, Hawkins CE, Haywood M, Heaney LR, Hedges S, Helgen KM, Hilton-Taylor C, Hussain SA, Ishii N, Jefferson TA, Jenkins RKB, Johnston CH, Keith M, Kingdon J, Knox DH, Kovacs KM, Langhammer P, Leus K, Lewison R, Lichtenstein G, Lowry LF, Macavoy Z, Mace GM, Mallon DP, Masi M, McKnight MW, Medellin RA, Medici P, Mills G, Moehlman PD, Molur S, Mora A, Nowell K, Oates JF, Olech W, Oliver WRL, Oprea M, Patterson BD, Perrin WF, Polidoro BA, Pollock C, Powel A, Protas Y, Racey P, Ragle J, Ramani P, Rathbun G, Reeves RR, Reilly SB, Reynolds JE, III, Rondinini C, Rosell-Ambal RG, Rulli M, Rylands AB, Savini S, Schank CJ, Sechrest W, Self-Sullivan C, Shoemaker A, Sillero-Zubiri C, De Silva N, Smith DE, Srinivasulu C, Stephenson PJ, van Strien N, Talukdar BK, Taylor BL, Timmins R, Tirira DG, Tognelli MF, Tsytsulina K, Veiga LM, Vié JC, Williamson EA, Wyatt SA, Xie Y, Young BE. The status of the world’s land and marine mammals: diversity, threat, and knowledge. Science. 2008; 322 :225–230. doi: 10.1126/science.1165115. [ PubMed ] [ CrossRef ] [ Google Scholar ]
- Simpfendorfer CA, Cortés E, Heupel MR, Brookes E, Babcock EA, Baum JK, McAuley R, Dudley SFJ, Stevens JD, Fordham S, Soldo A. An integrated approach to determining the risk of over-exploitation for data-poor pelagic Atlantic sharks. International Commission for the Conservation of Atlantic Tunas, Madrid, 2008/138/140. 2008:1–14. [ Google Scholar ]
- Simpfendorfer CA, Hueter RE, Bergman U, Connett SMH. Results of a fishery-independent survey for pelagic sharks in the western North Atlantic, 1977-1994. Fisheries Research. 2002; 55 :175–192. doi: 10.1016/S0165-7836(01)00288-0. [ CrossRef ] [ Google Scholar ]
- Simpfendorfer CA, Kyne PM. Limited potential to recover from overfishing raises concerns for deep-sea sharks, rays and chimaeras. Environmental Conservation. 2009; 36 :97–103. doi: 10.1017/S0376892909990191. [ CrossRef ] [ Google Scholar ]
- Sing T, Sander O, Beerenwinkel N, Lengauer T. ROCR: visualizing classifier performance in R. Bioinformatics. 2005; 21 :3940–3941. doi: 10.1093/bioinformatics/bti623. [ PubMed ] [ CrossRef ] [ Google Scholar ]
- Smith SE, Au DW, Show C. Intrinsic rebound potentials of 26 species of Pacific sharks. Marine and Freshwater Research. 1998; 49 :663–678. doi: 10.1071/MF97135. [ CrossRef ] [ Google Scholar ]
- Stevens JD, Bonfil R, Dulvy NK, Walker P. The effects of fishing on sharks, rays and chimaeras (Chondrichthyans), and the implications for marine ecosystems. ICES Journal of Marine Science. 2000; 57 :476–494. doi: 10.1006/jmsc.2000.0724. [ CrossRef ] [ Google Scholar ]
- Stevens JD, Walker TI, Cook SF, Fordham S. Threats faced by chondrichthyan fishes. In: Fowler SL, Cavanagh R, Camhi M, Burgess GH, Caillet GM, Fordham S, Simpfendorfer CA, Musick JA, editors. Sharks, rays and chimaeras: the status of the Chondrichthyan fishes. Gland, Switzerland and Cambridge, UK: IUCN Species Survival Commission Shark Specialist Group; 2005. pp. 48–57. [ Google Scholar ]
- Stuart SN, Chanson JS, Cox NA, Young BE, Rodrigues ASL, Fischman DL, Waller RA. Status and trends of amphibian declines and extinctions worldwide. Science. 2004; 306 :1783–1786. doi: 10.1126/science.1103538. [ PubMed ] [ CrossRef ] [ Google Scholar ]
- Swartz W, Sala E, Tracey S, Watson R, Pauly D. The spatial expansion and ecological footprint of fisheries (1950 to Present) PLOS ONE. 2010; 5 :e15143. doi: 10.1371/journal.pone.0015143. [ PMC free article ] [ PubMed ] [ CrossRef ] [ Google Scholar ]
- Techera EJ, Klein N. Fragmented governance: reconciling legal strategies for shark conservation and management. Marine Policy. 2011; 35 :73–78. doi: 10.1016/j.marpol.2010.08.003. [ CrossRef ] [ Google Scholar ]
- Veitch L, Dulvy NK, Koldewey H, Lieberman S, Pauly D, Roberts CM, Rogers AD, Baillie JEM. Avoiding empty ocean commitments at Rio +20. Science. 2012; 336 :1383–1385. doi: 10.1126/science.1223009. [ PubMed ] [ CrossRef ] [ Google Scholar ]
- Vincent ACJ, Sadovy de Mitcheson Y, Fowler SL, Lieberman S. The role of CITES in the conservation of marine fishes subject to international trade. Fish and Fisheries. 2014 doi: 10.1111/faf.12035. (In Press) [ CrossRef ] [ Google Scholar ]
- Wallace BP, DiMatteo AD, Hurley BJ, Finkbeiner EM, Bolten AB, Chaloupka MY, Hutchinson BJ, Abreu-Grobois FA, Amorocho D, Bjorndal KA, Bourjea J, Bowen BW, Dueñas RB, Casale P, Choudhury BC, Costa A, Dutton PH, Fallabrino A, Girard A, Girondot M, Godfrey MH, Hamann M, López-Mendilaharsu M, Marcovaldi MA, Mortimer JA, Musick JA, Nel R, Pilcher NJ, Seminoff JA, Troëng S, Witherington B, Mast RB. Regional management units for marine turtles: a novel framework for prioritizing conservation and research across multiple scales. PLOS ONE. 2010; 5 :e15465. [ PMC free article ] [ PubMed ] [ Google Scholar ]
- Wallace S, Gisborne B. Basking sharks: the slaughter of BC’s gentle giants. Vancouver: New Star Books; 2006. [ Google Scholar ]
- Walpole M, Almond REA, Besancon C, Butchart SHM, Campbell-Lendrum D, Carr GM, Collen B, Collette L, Davidson NC, Dulloo E, Fazel AM, Galloway JN, Gill M, Goverse T, Hockings M, Leaman DJ, Morgan DHW, Revenga C, Rickwood CJ, Schutyser F, Simons S, Stattersfield AJ, Tyrrell TD, Vie JC, Zimsky M. Tracking progress toward the 2010 biodiversity target and beyond. Science. 2009; 325 :1503–1504. doi: 10.1126/science.1175466. [ PubMed ] [ CrossRef ] [ Google Scholar ]
- Ward RD, Holmes BH, White WT, Last PR. DNA barcoding Australasian chondrichthyans: results and potential uses in conservation. Marine and Freshwater Research. 2008; 59 :57–71. doi: 10.1071/MF07148. [ CrossRef ] [ Google Scholar ]
- White WT, Last PR. A review of the taxonomy of chondrichthyan fishes: a modern perspective. Journal of Fish Biology. 2012; 80 :901–917. doi: 10.1111/j.1095-8649.2011.03192.x. [ PubMed ] [ CrossRef ] [ Google Scholar ]
- White WT, Last PR, Stevens JD, Yearsley GK, Dharmadi F. Canberra: 2006. Economically important sharks and rays of Indonesia, Australian Centre for international Agricultural research. [ Google Scholar ]
- Worm B, Davis B, Kettemer L, Ward-Paige CA, Chapman D, Heithaus MR, Kessel ST, Gruber SH. Global catches, exploitation rates, and rebuilding options for sharks. Marine Policy. 2013; 40 :194–204. doi: 10.1016/j.marpol.2012.12.034. [ CrossRef ] [ Google Scholar ]
- Worm B, Hilborn R, Baum JK, Branch TA, Collie JS, Costello C, Fogarty MJ, Fulton EA, Hutchings JA, Jennings S, Jensen OP, Lotze HK, Mace PM, McClanahan TR, Minto C, Palumbi SR, Parma AM, Ricard D, Rosenberg AA, Watson R, Zeller D. Rebuilding global fisheries. Science. 2009; 325 :578–585. doi: 10.1126/science.1173146. [ PubMed ] [ CrossRef ] [ Google Scholar ]
- eLife. 2014; 3: e00590.
Decision letter
eLife posts the editorial decision letter and author response on a selection of the published articles (subject to the approval of the authors). An edited version of the letter sent to the authors after peer review is shown, indicating the substantive concerns or comments; minor concerns are not usually shown. Reviewers have the opportunity to discuss the decision before the letter is sent (see review process ). Similarly, the author response typically shows only responses to the major concerns raised by the reviewers.
Thank you for sending your work entitled “Global extinction risk of sharks and rays: drivers, hotspots, and conservation priorities” for consideration at eLife . Your article has been favorably evaluated by a Senior editor and 2 peer reviewers, and generated considerable discussion that has unfortunately contributed to a longer response time than usual.
The Senior editor and the two reviewers discussed their comments before we reached this decision, and the Senior editor has assembled the following comments to help you prepare a revised submission.
The manuscript by Dulvy and colleagues provides a global assessment of the status of sharks and rays. This assessment stems from an iterative series of workshops harvesting the judgement and analyses of experts using standardized protocols commonly used by the IUCN and affiliates. Based on this assessment of more than 1000 species, the authors explore the patterning of risk assessments as a function of geography (e.g., depth) and life history (e.g., maximum size) of the species. The resulting data are highly informative, providing a clear picture of the distributions and patterning of threat among this taxon. The value of this work is that it provides the most thorough and comprehensive assessment of global threats to sharks and rays ever produced. It synthesizes the expert judgment of more scientists than any other work and covers more species.
The authors extend beyond their data on assessment of distributions and patterning to explore some of the putative drivers of the patterns, including social, economic, and political factors. While we recognize that we have entered an era where threats to biodiversity and climate force policy makers to demand answers that the scientific community is not ready to produce if it relies on the traditional evidentiary standards of the scientific literature, eLife is a life sciences and biomedical journal and we feel uneasy with the amount of opinion expressed in the Results section. Hence, we would ask you to consider the following in preparing a revised submission.
While the distributional assessments are founded in natural scientific rigor, the social scientific assessments are anecdotal and potentially biased. The juxtaposition of scientific rigor and conservation vigor is striking but distracting, particularly when blended in the Results section. The manuscript suffers through this attempt to be all-inclusive and the current submission is disjointed. While there is every effort made to identify data for all species within the taxon, only a subset of the legislation specific to the taxon is reported. By mixing currencies and rigor, the authors are making the manuscript difficult to read, or worse, easy to dismiss.
We either suggest that the more subjective points are vetted from the Results and migrated to the Discussion section, or that a combined Results and Discussion section is created, taking particular care to separate subjective interpretation from strong inferences derived from objective data.
Either way, please give serious consideration to the structure of the manuscript. In its current format, the strength of the analysis is lost within the volume of opinions and prose. Consider, perhaps, a structure of rigorous statistical assessment of the distribution of these species as a function of threat. This alone would be a valuable scientific contribution. The reporting of socioeconomics could be reported elsewhere, perhaps in an accompanying article or within a policy journal.
[Editors' note: further clarifications were requested prior to acceptance, as described below.]
Thank you for resubmitting your work entitled “Extinction risk and conservation of the world's sharks and rays” for further consideration at eLife . Your revised article has been favorably evaluated by a Senior editor and two peer reviewers. The manuscript has been improved but there are some remaining issues that need to be addressed before acceptance, as outlined below:
We are pleased to have finally secured reviews of your revision and are ready to accept the manuscript for publication once the two issues detailed below are satisfactorily addressed, namely: 1) the problem about the transparency of the data adjustments used for the different data sets, and 2) the addition of a more explicit, preferably statistically supported consideration of the uncertainty of expert opinions. Thoroughly addressing these two points will neutralize the ease with which skeptical readers can dismiss the main take home message of your paper and additionally will highlight the very distinct possibility that your analysis may be underestimating the severity of the extinction risk.
1) First, there are several places where the presentation applies different adjustments to the data that can be interpreted as modifying data only when it makes the status look worse. These are easily addressed.
2) Second, and a far bigger concern, is the accuracy of estimates from expert opinion. These types of data can have large uncertainties and embedded biases. As the authors note, “Most Chondrichthyan catches are unregulated and often misidentified, unrecorded, aggregated or discarded at sea, resulting in a lack of species-specific landings information.” These same issues raise the critical concern of how much stock we can put into even the opinions of the world's experts. Aside from saying that the experts applied IUCN Red List criteria and used all available data, there is little to help the reader evaluate the level of uncertainty in any of these estimates. As a result, a skeptical reader can easily dismiss much of the findings because of the inherent limits of assessments based largely on expert opinion. There have certainly been evaluations of uncertainty associated with IUCN redlist criteria in other studies, but this issue is largely ignored in the paper except for a discussion related to issues of the category definitions deeply buried in the supplemental materials. This discussion only addresses a few issues affecting uncertainty in these estimates. Although it alludes to some disagreements among experts in terms of assessment status, there is no analysis of the level of uncertainty one should place on these estimates.
There is also the possibility for comparison of the results with other forms of quantitative objective assessments for at least a subset of the data. Without directly addressing this issue of uncertainty somewhere in this paper, the value of this work is compromised and the interpretation of the findings could be misleading. One possible comparison would be with the global fisheries assessments published by Costello et al. in 2012 (Science, 338:517-520). Although they do not have anywhere near as many sharks and cartilaginous species in their analyses (112), they do estimate B/Bmsy for a group of these species using an objective quantitative approach. The median B/Bmsy for previously unassessed species in this analysis was 0.37. If I do a back of the envelope comparison and assume that Bmsy is typically 0.5 * unfished biomass, this would mean that the Costello analysis would have the median shark fishery at about 19% of its unfished biomass. This is actually worse than the values presented in this paper. Here, the median fishery (ignoring the data deficient species) would be at the lower edge of the Near Threatened category since 51% of species with categories are either Least Concerned or Near Threatened. Although this estimate suggests that sharks and rays are better off than the Costello assessment, at least this kind of comparison could be used to make the case that these expert opinion assessments are actually likely to be conservative rather than over-estimating the threatened status of species. Whether is make sense to include such a comparison is up to the authors, but I think the value of this broad extension of assessments to a much larger number of species would gain more credibility if there is a clear consideration of the uncertainty in expert opinions. I suspect that many readers will assume such expert analyses overestimate the nature of the problem. Quite the opposite may be true.
Author response
We either suggest that the more subjective points are vetted from the Results and migrated to the Discussion section, or that a combined Results and Discussion section is created, taking particular care to separate subjective interpretation from strong inferences derived from objective data .
Either way, please give serious consideration to the structure of the manuscript. In its current format, the strength of the analysis is lost within the volume of opinions and prose. Consider, perhaps, a structure of rigorous statistical assessment of the distribution of these species as a function of threat. This alone would be a valuable scientific contribution. The reporting of socioeconomics could be reported elsewhere, perhaps in an accompanying article or within a policy journal .
Following the guidance of the Senior editor and both reviewers, we have chosen to remove our systematic analysis of social, economic, and political factors, and instead we focus only on the natural science of marine extinction risk. We will seek to publish the social, economic, and political elements of our work elsewhere. We note, however, that all social, economic, and political information was collected in a systematic manner. Hence the anecdotal nature and biased availability of such data reflects the widespread lack of effective management of sharks and rays rather than a lack of scientific rigor. We have compiled the global catch landings trajectory, the trend of increasing ray catch, and the map of catch share and shark fin export into a single figure, which we refer to in the Introduction. This new figure provides important context for our threat analysis. Only in the Discussion have we considered the social, economic, and political context that has resulted in the worldwide elevation of extinction risk faced by chondrichthyans, as reveal here for the first time.
As recommended by the Senior editor and reviewers, we have used this space to elaborate on the statistical modeling of the ecological and geographic correlates of risk. We extended our explanatory statistical modeling to develop predictive models ( Table 3 ). We use these predictive models to classify and identify those Data Deficient sharks and rays are likely to be threatened based on their body size and degree of accessibility to fisheries (Supplementary file 1). These models explain between 58 and 80% of the variance in species extinction risk ( Tables 3 and 4 ).
1) First, as discussed in minor comments, there are several places where the presentation applies different adjustments to the data that can be interpreted as modifying data only when it makes the status look worse. These are easily addressed .
We have addressed this issue in response to the substantive comment below.
2) Second, and a far bigger concern, is the accuracy of estimates from expert opinion. These types of data can have large uncertainties and embedded biases. As the authors note, “Most Chondrichthyan catches are unregulated and often misidentified, unrecorded, aggregated or discarded at sea, resulting in a lack of species-specific landings information.” These same issues raise the critical concern of how much stock we can put into even the opinions of the world's experts. Aside from saying that the experts applied IUCN Red List criteria and used all available data, there is little to help the reader evaluate the level of uncertainty in any of these estimates. As a result, a skeptical reader can easily dismiss much of the findings because of the inherent limits of assessments based largely on expert opinion. There have certainly been evaluations of uncertainty associated with IUCN redlist criteria in other studies, but this issue is largely ignored in the paper except for a discussion related to issues of the category definitions deeply buried in the supplemental materials. This discussion only addresses a few issues affecting uncertainty in these estimates. Although it alludes to some disagreements among experts in terms of assessment status, there is no analysis of the level of uncertainty one should place on these estimates .
There is also the possibility for comparison of the results with other forms of quantitative objective assessments for at least a subset of the data. Without directly addressing this issue of uncertainty somewhere in this paper, the value of this work is compromised and the interpretation of the findings could be misleading. One possible comparison would be with the global fisheries assessments published by Costello et al. in 2012 (Science, 338:517-520). Although they do not have anywhere near as many sharks and cartilaginous species in their analyses (112), they do estimate B/Bmsy for a group of these species using an objective quantitative approach. The median B/Bmsy for previously unassessed species in this analysis was 0.37. If I do a back of the envelope comparison and assume that Bmsy is typically 0.5 * unfished biomass, this would mean that the Costello analysis would have the median shark fishery at about 19% of its unfished biomass. This is actually worse than the values presented in this paper. Here, the median fishery (ignoring the data deficient species) would be at the lower edge of the Near Threatened category since 51% of species with categories are either Least Concerned or Near Threatened. Although this estimate suggests that sharks and rays are better off than the Costello assessment, at least this kind of comparison could be used to make the case that these expert opinion assessments are actually likely to be conservative rather than over-estimating the threatened status of species. Whether is make sense to include such a comparison is up to the authors, but I think the value of this broad extension of assessments to a much larger number of species would gain more credibility if there is a clear consideration of the uncertainty in expert opinions. I suspect that many readers will assume such expert analyses overestimate the nature of the problem. Quite the opposite may be true .
We agree with the referee and appreciate the efforts to bring our attention to new literature. While we were aware of the Costello et al. paper, we were not aware that it contained this vital and alarming estimate of the sustainability of 112 shark and ray fisheries. These data do indeed suggest our analyses maybe conservative, we have also found subsequent trend data from the Mediterranean Sea that also suggest that our Red List Assessments are conservative and err on the side of underestimating risk. We have added two new paragraphs comparing our findings to both the Costello et al. 2012 paper and the Ferretti et al. 2008 paper.
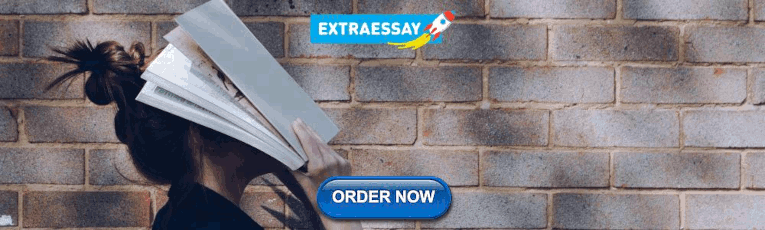
IMAGES
COMMENTS
The shark data used in this paper were collected as part of a multi-year, long-term research program evaluating the interannual behavior and physiology of large sharks throughout the coastal ...
This "Perspectives" paper identifies aspects of tiger shark (Galeocerdo cuvier) biology that are currently unknown or for which additional data are needed to improve interpretive power. Some of these data gaps may be regional. Technical or methodological approaches to acquiring these data are suggested. Some of these technologies already exist, some are in development and some exist in ...
The tiger shark ( Galeocerdo cuvier) has been relatively well assessed concerning biology and ecology aspects in both Atlantic and Pacific North America and in Caribbean waters. The amount of data in these regions has led to the species protection under capture quotas and with the creation of sanctuaries. The reality in developing countries ...
Abstract. This "Perspectives" paper identifies aspects of tiger shark (Galeocerdo cuvier) biology that are currently unknown or for which additional data are needed to improve interpretive ...
Tiger sharks (Galecerdo cuvier) are apex predators characterized by their broad diet, large size and rapid growth. Tiger shark maximum size is typically between 380 & 450 cm Total Length (TL), with a few individuals reaching 550 cm TL, but the maximum size of tiger sharks in Hawaii waters remains uncertain. A previous study suggested tiger sharks grow rather slowly in Hawaii compared to other ...
Tiger sharks were sampled from Ningaloo Reef and Shark Bay in Western Australia, the Great Barrier Reef ... Sample collection was a collaborative effort across multiple research programs in Australia and as a consequence, the number of samples for each location, sex, types of tissue collected and sampling methods varied among locations ...
Thus, in a recent review of research priorities, the development of high-resolution SNPs (Single Nucleotide Polymorphisms) to further resolve tiger shark meta-population structure was strongly ...
We used long-term observations of 55 individually identified tiger sharks (Galeocerdo cuvier) at a shark ecotourism site off Tahiti to estimate the prevalence, retention times and impacts of residual hooks and trailing line resulting from fisheries interactions.Thirty-eight percent of tiger sharks had at least one fisheries interaction resulting in retained hooks during the 8-year observation ...
(2013) A revised estimate of daily ration in the tiger shark (Galeocerdo cuvier) with implications for assessing ecosystem impacts of apex predators. Functional Ecology 27 (5): 1273-1274. Fallows C, Gallagher AJ, Hammerschlag N (2013) White Sharks (Carcharodon carcharias) Scavenging on Whales and Its Potential Role in Further Shaping the ...
New research indicates that baited shark diving can influence tiger shark social behavior. Scientists have found that tiger sharks, often considered a solitary nomadic species, are social ...
The research effort required to intercept and tag free-roaming tiger sharks—26 h of sampling over 10 days—was also considerably lower than the extensive time needed for human divers to perform photogrammetry transects (250 h over 30 days), for a much smaller surveyed area, demonstrating the time and cost efficiency of tiger shark surveying ...
During the reproductive season, sea turtles use a restricted area in the vicinity of their nesting beaches, making them vulnerable to predation. At Raine Island (Australia), the highest density green turtle Chelonia mydas rookery in the world, tiger sharks Galeocerdo cuvier have been observed to feed on green turtles, and it has been suggested that they may specialise on such air-breathing prey.
Like many elasmobranchs, tiger sharks comprise an important ecosystemic resource, structuring marine environments, maintaining food web stability and nutrient and energy cycling between trophic ...
But a recent paper that reviewed currently available tiger shark research papers, led by scientist Samuel Balanin, has reported several knowledge gaps that the scientists say should be the focus ...
Introduction. Tiger sharks (Galeocerdo cuvier) are wide-ranging - apex predators that consume a diverse array of vertebrate and invertebrate prey ,, while utilizing a broad variety of coastal and oceanic habitats -.They reproduce on a triennial cycle with average litter sizes of 30 to 50 pups ranging in size from 80 to 90 cm Total Length (TL) at birth .
Our current and ongoing tiger shark research grew out of an initial study (1993-1998) where we used a boat equipped with tracking equipment to follow individual tiger sharks for up to 50h. This study provided us with the first insights into the wide-ranging behavior shown by tiger sharks in Hawaii. Click here for further information on the ...
The paper was published in the July edition of Frontiers in Marine Sciences and the research looked at the ... as nurseries. This includes the Indian River Lagoon, a common nursery for bull sharks. Past research showed that a lot of female sharks use the same habitats where they were born to give birth to their own pups, but researchers assumed ...
Academia.edu is a platform for academics to share research papers. Reproductive biology of the tiger shark ( Galeocerdo cuvier ) in Hawaii ... One free-swimming shark captured in tiger sharks from the Hawaiian islands 100 1000 Pregnant (n= 4) Pregnant (n= 7) Non-pregnant (n= 36) Non-pregnant (n= 99) Oviducal Gland Width (mm) 80 Log uterus width ...
The hypotheses that tiger sharks using the island 1) are larger, on average, than tiger sharks using coastal waters off northeastern Brazil, and 2) move through wide distances across the equatorial pelagic environment are examined. The possible implications of tiger shark spatial distribution and behavior for species conservation are also ...
This "Perspectives" paper identifies aspects of tiger shark (Galeocerdo cuvier) biology that are currently unknown or for which additional data are needed to improve interpretive power. Some of these data gaps may be regional. Technical or methodological approaches to acquiring these data are suggested.
There is no current information that pain is alleviated during tonic immobility in sharks (Williamson et al. 2018).Tiger sharks (Galeocerdo cuvier) restrained upside-down at the side of the boat became immobile, and this made it easier to remove hooks from the mouth and the attachment or implantation of electronic transmitters on or in the body (Holland et al. 1999; Yoshida 2021).
Tiger Sharks Research Paper. 1182 Words5 Pages. Sharks are enormous mammals that live within the deep ocean waters all over the world. There are several different sharks in many parts of the world depending on temperature, seasons, food and more. Every shark is unique in their own way and every species is unique as well.
The landings of sharks and rays, reported to the Food and Agriculture Organization of the United Nations (FAO), increased steadily to a peak in 2003 and have declined by 20% since ( Figure 1A ). True total catch, however, is likely to be 3-4 times greater than reported ( Clarke et al., 2006; Worm et al., 2013).