Click through the PLOS taxonomy to find articles in your field.
For more information about PLOS Subject Areas, click here .
Loading metrics
Open Access
Peer-reviewed
Research Article
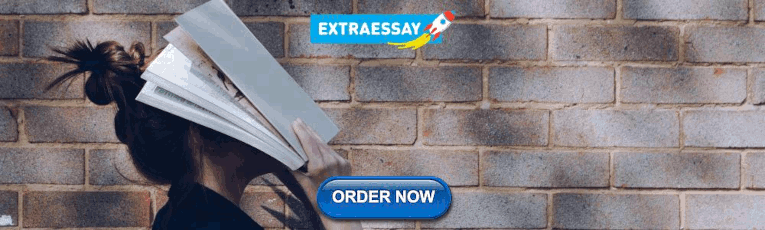
The Last Frontier: Catch Records of White Sharks ( Carcharodon carcharias ) in the Northwest Pacific Ocean
* E-mail: [email protected]
Affiliation Great Lakes Institute for Environmental Research, University of Windsor, Windsor, Ontario, Canada
Affiliation Independent Researcher, Taipei, Taiwan
Affiliation School of Marine Science and Technology, Tokai University, Shimizu, Shizuoka, Japan
Affiliation Division of Marine and Freshwater Biological Resources, Sakhalin Research Institute of Fisheries & Oceanography, Yuzhno-Sakhalinsk, Russia
Affiliations Moss Landing Marine Laboratories, Moss Landing, California, United States of America, Monterey Bay Aquarium, Monterey, California, United States of America
Affiliations KwaZulu-Natal Sharks Board, Umhlanga Rocks, South Africa, Biomedical Resource Unit, University of KwaZulu-Natal, Durban, South Africa
Affiliation Shark Advocates International (a project of The Ocean Foundation), Washington, DC, United States of America
- Heather M. Christiansen,
- Victor Lin,
- Sho Tanaka,
- Anatoly Velikanov,
- Henry F. Mollet,
- Sabine P. Wintner,
- Sonja V. Fordham,
- Aaron T. Fisk,
- Nigel E. Hussey
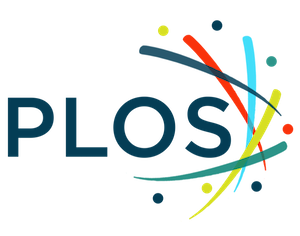
- Published: April 16, 2014
- https://doi.org/10.1371/journal.pone.0094407
- Reader Comments
White sharks are highly migratory apex predators, globally distributed in temperate, sub-tropical, and tropical waters. Knowledge of white shark biology and ecology has increased recently based on research at known aggregation sites in the Indian, Atlantic, and Northeast Pacific Oceans; however, few data are available for the Northwest Pacific Ocean. This study provides a meta-analysis of 240 observations of white sharks from the Northwest Pacific Ocean between 1951 and 2012. Records comprise reports of bycatch in commercial fisheries, media accounts, personal communications, and documentation of shark-human interactions from Russia (n = 8), Republic of Korea (22), Japan (129), China (32), Taiwan (45), Philippines (1) and Vietnam (3). Observations occurred in all months, excluding October-January in the north (Russia and Republic of Korea) and July-August in the south (China, Taiwan, Philippines, and Vietnam). Population trend analysis indicated that the relative abundance of white sharks in the region has remained relatively stable, but parameterization of a 75% increase in observer effort found evidence of a minor decline since 2002. Reliably measured sharks ranged from 126–602 cm total length (TL) and 16–2530 kg total weight. The largest shark in this study (602 cm TL) represents the largest measured shark on record worldwide. For all countries combined the sex ratio was non-significantly biased towards females (1∶1.1; n = 113). Of 60 females examined, 11 were confirmed pregnant ranging from the beginning stages of pregnancy (egg cases) to near term (140 cm TL embryos). On average, 6.0±2.2 embryos were found per litter (maximum of 10) and gestation period was estimated to be 20 months. These observations confirm that white sharks are present in the Northwest Pacific Ocean year-round. While acknowledging the difficulties of studying little known populations of a naturally low abundance species, these results highlight the need for dedicated research to inform regional conservation and management planning.
Citation: Christiansen HM, Lin V, Tanaka S, Velikanov A, Mollet HF, Wintner SP, et al. (2014) The Last Frontier: Catch Records of White Sharks ( Carcharodon carcharias ) in the Northwest Pacific Ocean. PLoS ONE 9(4): e94407. https://doi.org/10.1371/journal.pone.0094407
Editor: William Hughes, University of Sussex, United Kingdom
Received: November 20, 2013; Accepted: March 11, 2014; Published: April 16, 2014
Copyright: © 2014 Christiansen et al. This is an open-access article distributed under the terms of the Creative Commons Attribution License , which permits unrestricted use, distribution, and reproduction in any medium, provided the original author and source are credited.
Funding: Support for this project was provided in part by Canada Research Chair program NSERC Ocean Tracking Network to ATF. HMC was supported in part by scholarships and graduate assistantships from the University of Windsor. The funders had no role in study design, data collection and analysis, decision to publish, or preparation of the manuscript.
Competing interests: The authors have declared that no competing interests exist.
Introduction
Details on the population status and spatial/temporal distribution of threatened species are critical to focus conservation efforts [1] , [2] . Typically, species that have globally distributed populations are exposed to a range of region-specific threats and pressures and consequently management actions require data for each region [3] – [5] . Regional declines of large marine predators including elasmobranchs have been documented [6] , [7] , with potential cascading effects on marine food webs [8] . For many large elasmobranch species, data on spatial and temporal distributions are limited due to their migratory behavior, the relative rarity of sightings, and the nature of the environment they inhabit [9] , [10] .
The white shark ( Carcharodon carcharias ) is a large (maximum size 6 m, [11] , [12] ) marine apex predator with a global distribution, occurring in temperate, sub-tropical, and tropical waters [13] – [16] . The life history characteristics of white sharks (e.g., natural low abundance, slow growth, late maturity, low fecundity) make them vulnerable to exploitation [17] , [18] . Population estimates conducted in California [19] , South Africa [20] , [21] , and Australia [22] indicate relatively low regional population sizes with genetic diversity constricted by philopatric behavior [23] . White sharks are classified as threatened (globally Vulnerable) by the International Union for Conservation of Nature (IUCN), listed on the Convention on International Trade of Endangered Species of Wild Fauna and Flora (CITES) Appendix II, listed on both Appendix I and II of the Convention on Migratory Species (CMS), and are protected by national legislation in Australia, Canada (Atlantic Ocean), Croatia, European Union, Maldives, Malta, Mexico, Namibia, New Zealand, South Africa, and in all United States waters (except in the Western Pacific) [24] , [25] . For the countries of the Northwest Pacific Ocean, participation in CITES and CMS is variable, while none appear to impose complete national white shark protection. Specifically, the only domestic, white shark-specific conservation measure identified in the region applies in China, but permits with unclear conditions may allow for some take ( Table 1 ).
- PPT PowerPoint slide
- PNG larger image
- TIFF original image
https://doi.org/10.1371/journal.pone.0094407.t001
The white shark is a charismatic species and consequently one of the most studied and protected shark species [26] ; however, there is still much to learn about their basic biology and ecology. The majority of recent research on white shark movement and feeding ecology has focused on regional population hotspots or aggregation sites in the Northeast Pacific, South Africa, and surrounding Australia (including New Zealand and New Caledonia), with only a few studies examining the lesser-known populations of the Mediterranean [27] – [29] and the Northwest Atlantic [30] ( Fig. 1 ). For the Northwest Pacific region, there is a paucity of available data on white sharks with only a few reports of incidental captures and white shark-human interactions (bites) from Japan [31] – [36] and Russia [37] , [38] . This disparity in research focus is so large that the Proceedings book of the last international white shark symposium [39] contained no data for the Northwest Pacific Ocean [26] . Currently, the frequency of occurrence and geographical extent of the population of white sharks in this region is unknown. Based on repeated long distance migrations recorded in South Africa and the Northeast Pacific [13] , [16] , [40] , [41] , it is possible that white sharks transit to the Northwest Pacific from other regional populations, such as Australia, however, evidence suggests that white sharks caught off Japan form a genetically distinct population that has a vastly different growth rate from the Northeast Pacific and South African populations [36] . It is therefore likely that there is a separate resident population of white sharks inhabiting the Northwest Pacific region.
Known global white shark aggregation sites. Question marks indicate suitable latitudinal ranges where white sharks may occur, but little is known about current population trends.
https://doi.org/10.1371/journal.pone.0094407.g001
Considering the conservation concern for white sharks, the lack of protective measures in the Northwest Pacific region and the limited data available for the associated population, the aims of this study were to; i) provide a comprehensive record of the distribution of white shark catches and sightings in the Northwest Pacific Ocean, ii) describe spatio-temporal patterns in white shark occurrence, iii) examine relative population trends over the past 60 years, iv) document size and sex based trends, and v) provide details on the reproductive biology of females. These data provide a baseline to stimulate further research and to inform regional management for this little known population.
The study region of the Northwest Pacific Ocean was defined as the waters surrounding Russia, the Republic of Korea, Japan, China, Taiwan, the Philippines and Vietnam. A literature search was conducted using the ISI Web of Science. Search terms included: “white shark”, “ Carcharodon carcharias ” and “Russia or Republic of Korea or Japan or China or Taiwan or Philippines or Vietnam”. This resulted in 12 studies, six of which contained no relevant data for the Northwest Pacific Ocean and were therefore excluded. Regional observations of white sharks were defined as any records of fishery landings/discards, interactions with humans (bites), or museum specimens. To document white shark observations from media reports and websites, a search was conducted using Google. Personal communications and observations by the authors were also included. In Japan, the Ibaraki Prefectural Oarai Aquarium works directly with local fishermen, including those involved in set net fisheries, to document white shark catches.
Data Analysis
Data were filtered to remove duplicate observations. The amount of information recorded for each white shark observation ranged from basic data that included only the country of capture to detailed records that included date of capture, city landed, method of capture, size (cm total length - TL), weight (kg), sex, and if the individual was pregnant. In most instances, the locations of white sharks were based on landing sites rather than actual catch locations (see Table S1 ). To examine population trends over time, a generalized linear model was fit to the observation data period to examine the magnitude of change in population relative to a set of reference dates following the approach developed by McPherson and Myers [42] . Values equal to one or less suggest a stable or increasing population, while values larger than one are indicative of a decline in relative abundance. This model enables testing of sensitivity to changes in observer effort over the reference period of 1951–2011. Consequently, +75%, 0% and −75% changes in observer effort were selected to incorporate a wide range of potential observer variation.
We documented whether length and weight were measured by scientifically trained staff or estimated by laypeople/untrained fishermen, from photos, or by jaw size. When both an estimate from a fishermen and calculated TL from jaws or photos were available, the TL from the latter was used. When multiple weight estimates were available the lowest estimate was retained. The length-weight relationship was calculated using the equation logW = log a + b *log TL where W is weight (kg), TL is total length (cm) and a and b are constants (where log a is the intercept and b is the slope). Significant differences by sex were tested using an analysis of covariance (ANCOVA) on log-transformed data. Since estimated weights were used for several individuals ( Table S1 ), the validity of the data was tested using an ANCOVA with length-weight data obtained from white sharks caught in beach protection nets off KwaZulu-Natal, South Africa (KwaZulu-Natal Sharks Board, unpublished data), as well as global length-weight data collected by trained scientists [17] , [27] , [43] (see references therein). Pregnant females were excluded from this analysis.
Due to the number of observations where sex data were available (113, 47%), the sex ratio for each country was determined, but further statistical analysis, e.g. segregation by size and sex or temporal differences by sex, were not conducted. Embryos of pregnant individuals were categorized by size as early term (<40 cm TL), mid-term (between 40 and 100 cm TL) or full term (>100 cm TL) [17] , [35] , [44] . For individuals where embryo size was described by stage, this description was maintained. To estimate gestation length, data from embryos and free-swimming juveniles from the Northern and Southern Hemispheres ( Table S2 ) were combined on one time scale, where January equals month 0 in the Northern Hemisphere and July equals month 0 in the Southern Hemisphere. Linear regression was performed on the embryo size versus month of capture data and gestation length was determined using the slope of the regression, and the size at birth (we used 126 cm TL, the smallest free-swimming individual in the Northwest Pacific).
A total of 248 white shark observations were documented; after the removal of duplicates 240 reliable observations remained ( Table S1 ). Records of white sharks were found in seven countries ranging from the most northerly in temperate waters off Russia to the most southerly in tropical waters off Vietnam ( Fig. 2A , 3 ), spanning a straight line latitudinal distance of 4,300 km. Location of landing was recorded for 169 observations, while the remaining reports only included the country of landing ( Fig. 2A, 2B ). Observations of white sharks occurred between 1951 and 2012; the year of capture was recorded for 212 animals. The number of observations increased over time, with two peaks occurring in 1992 and 2009 and no observations documented between 1960 and 1974 ( Fig. 4A ). Correct species identification for the 240 white sharks was confirmed through records from trained scientists, personal observations, preserved remains, photographs or media reports ( Fig. 5 ; Table S1 ). The method of capture was documented for 79 observations with 56 animals caught in set nets, 12 by set lines, 3 each by either gillnet, seine or trawls and one each by harpoon and crab basket ( Table S1 ). The majority of these captures occurred in Japan (76) with 25 occurring in the set net fishery off Ibaraki and 11 in the set line fishery surrounding Okinawa. Of these captures, most sharks were caught in spring (April–June) (38), followed by winter (January–March) (18), summer (July–September) (12), and autumn (October–December) (10).
Color legend for country of observation occurrence applies to entire figure. A) Approximate location of observation or landing for individual white sharks. Circles on land indicate observations that only reported country of landing. Annual average sea surface temperature is indicated by color gradient. B) Percentage of white shark observations by country landed. C) Size of white shark observed by country landed (top two panels) and as a percent of all observations (bottom panel). Note–the Philippines observations did not have an associated animal size.
https://doi.org/10.1371/journal.pone.0094407.g002
A) Male captured on February 17, 2009 in Taitung, Taiwan measuring 500 cm total length and weighing 1020 kg. Photo obtained from: http://tw.myblog.yahoo.com/jw!duL4dwaTBB7FYwm6Q0vtIm8d/gallery?cfid=144&act=&fid=144&nfid=&yuid=jw!duL4dwaTBB7FYwm6Q0vtIm8d&page=1&.crumb=8mdZj9IAdn6 B) Male captured on July 19, 2007 in Aniva Bay Sakhalin, Russia measuring 504 cm total length and weighing 1111 kg.
https://doi.org/10.1371/journal.pone.0094407.g003
A) White shark observations by year. Black circles indicate data included in population trend analysis. Gray circles indicate data from focused monitoring in Japan that was excluded prior to undertaking the analysis. B) Estimates of changes in relative abundance for any reference year between 1951–2011 under different assumptions of trends in observation effort.
https://doi.org/10.1371/journal.pone.0094407.g004
Data are shown as a percentage; Media Report - from newspapers or online news sources; Photo - confirmed by pictures of the individual shark (either through personal communications or online but not through news source); Preserved - individuals or remains of an individual, i.e. jaws that are held in a personal or museum collection; Scientific Confirmation - observations that have been previously reported in the scientific literature; Author Observations - personal observations or communications by the authors.
https://doi.org/10.1371/journal.pone.0094407.g005
When considering all regional observations, month of capture was reported for 146 animals ( Fig. 6 ). White sharks were reported across all months and seasonal trends mirrored those of documented fishery captures in Japan with spring having the highest number of observations (59), followed by winter (39), summer (24), and autumn (24). In the more northern countries (Russia and the Republic of Korea) white sharks were not observed in autumn and early winter from October–January ( Fig. 6A ). For Japan, white sharks were observed across all months of the year with a peak between April–May ( Fig. 6B ). In the most southerly countries (China, Taiwan, the Philippines, and Vietnam) white sharks were observed in all months except during summer (July–August) ( Fig. 6C ).
A) Republic of Korea (blue bars) and Russia (black bars) B) Japan (gray bars) C) China (yellow bars), Taiwan (light blue bars), Vietnam (dark blue bars), and the Philippines (orange bars), D) All observations combined. Winter and summer months are shaded gray.
https://doi.org/10.1371/journal.pone.0094407.g006
There were two time periods where focused white shark monitoring occurred in Japan (1990–1994 and 2005–2009). To avoid any biases caused by increased observation effort these data points were removed prior to population trend analysis. When accounting for no change in observer effort over time, the population trend estimate indicated that the relative abundance of white sharks has been stable or increasing in the region until recently (2007) ( Fig. 4B ). Parameterization of a 75% decrease in observer effort indicated the relative abundance of white sharks has remained stable or increased throughout the reference period, while a 75% increase in observer effort indicated a minor decline in relative abundance since 2002.
Size of animal (TL) was recorded for 175 observations ( Fig. 2C ; Table S1 ). For this study, sharks whose weight and total length were estimated are identified in Table S1 . The smallest recorded shark measured 126 cm TL and was caught in Primorye, Russia, while the largest shark was landed in Seven Star Lake, Taiwan and was estimated to be 670–700 cm TL ( Fig. 2C ). The largest accurately measured shark was caught in the East China Sea and measured 602 cm TL. White sharks <200 cm TL were observed in Russia (n = 1)(September), the Republic of Korea (1), Japan (2) (July) and Vietnam (1) (June). Weight was measured or estimated for 162 white shark observations ( Table S1 ). The lowest weight recorded was 16 kg (for the 126 cm TL specimen-Primorye, Russia), while the largest estimated weight was 3000 kg for a 520 cm TL shark caught in Hikari City, Japan. The most reliable weight for the heaviest animal documented was 2530 kg, a 555 cm TL shark that was recorded by a fishery wholesaler in NingPuo, China. There was no significant difference in the slope of the length-weight regression between females and males, so data were combined (interaction of length and sex: F = 0.3 and P = 0.59). The intercept for females was higher than males indicating that females grow larger than males. When testing between combined sex length-weight data for the Northwest Pacific and global data, no significant difference between slopes was detected (interaction of length and region: F = 0.06 and P = 0.81). The relationship between length and weight was expressed as: Northwest Pacific W = 1.04e −5 TL 3.008 ( r 2 = 0.72), global data W = 5.86e −7 TL 3.476 ( r 2 = 0.84). A regression for all data was calculated as W = 1.61e −6 TL 3.309 ( r 2 = 0.85) ( Fig. 7 ).
Regressions were fit to white shark data for the Northwest Pacific (black circles/line), global data recorded by trained scientists (orange circles/line) and all data combined (dashed black line). Pregnant females from the Northwest Pacific (black stars) were not included in the analysis.
https://doi.org/10.1371/journal.pone.0094407.g007
Sex was recorded on 113 occasions. A total of 53 males and 60 females were documented ( Fig. 8 ; Table S1 ) of which 11 (18.3%) were pregnant and one individual was suspected to be pregnant ( Fig. 9 ; Table 2 ). The observed sex ratio was not significantly different from 1∶1 for all countries combined (1∶1.1, Χ 2 = 0.43, p = 0.51) or individually China (1∶2.8, Χ 2 = 3.3, p = 0.07), Japan (1∶0.7, Χ 2 = 2.3, p = 0.13) and Republic of Korea (1∶3, Χ 2 = 1.0, p = 0.32), while it was significantly biased towards females for Taiwan (1∶4, Χ 2 = 7.2, p<0.01). Sex was reported for one shark in Russia (male) and for none in Vietnam and the Philippines.
The occurrence of male, female and pregnant female white sharks in the Northwest Pacific. Circles on land underneath country label indicate observations that did not report a specific landing location. For pregnant females, black squares indicate early term, black triangles indicate mid term and black circles indicate full term for individuals where embryo size was reported.
https://doi.org/10.1371/journal.pone.0094407.g008
A) Pregnant female captured on November 7, 2008 in Southern Bay Dock, East Taiwan, measuring 542 cm total length and weighing 1930 kg B) Ovary C) Egg cases measuring 10 cm. Photos obtained from: http://tw.m.wretch.yahoo.com/album/redo0905/12 .
https://doi.org/10.1371/journal.pone.0094407.g009
https://doi.org/10.1371/journal.pone.0094407.t002
Pregnant females ranged in size from 450–600 cm TL and contained an average of six embryos (ranging from egg cases to 10 embryos) ( Table 2 ). Egg cases were found in females captured in early autumn, mid-term embryos were present in winter, and full-term embryos (130–150 cm TL) were present in spring ( Table 2 ). The relationship between embryo size and month of capture predicted a gestation period of 20 months (n = 9, slope = 6.4 cm/month, SE = 1.0 cm/month, p<0.01, r 2 = 0.84) ( Fig. 10 ). Pregnant white sharks were geographically separated during the various stages of gestation; egg cases and early term embryos were present in individuals from the southern locations of Taiwan and Okinawa, whereas full term embryos were found in individuals mostly captured near mainland Japan.
Data are for white shark observations in this study and globally. Month zero is January in Northern Hemisphere and July in Southern Hemisphere. For unborn animals, black circles indicate embryos from this study (Northwest Pacific; n = 9); orange circles indicate embryos from the Northern Hemisphere (n = 4), while gray circles indicate embryos from the Southern Hemisphere (n = 5). For free-swimming juvenile sharks, orange triangles indicate individuals from the Northern Hemisphere (n = 18), and gray triangles indicate individuals from the Southern Hemisphere (n = 17). The solid black line indicates the linear regression for embryos from this study, while the dashed black lines indicate the confidence intervals. The red dashed line indicates size at birth.
https://doi.org/10.1371/journal.pone.0094407.g010
Determining the spatial and temporal distribution of threatened species is a critical first step for effective conservation measures. This is the first study to collate and present observations of white sharks in the Northwest Pacific Ocean in order to define the geographic extent of the population. While we do not assume that we obtained all records in the region over the past 60 years, these observations provide important baseline data for this little known population, providing a benchmark to instigate further research for population-specific conservation and management efforts.
The observations of white sharks in the Northwest Pacific increased over time and showed a bimodal trend that coincided with focused monitoring of catches (1990–1994; [33] ) and sampling of white sharks by Ibaraki Prefectural Oarai Aquarium staff (2005–2009; [36] ) in Japan, and consequently represent a bias in observed trends. Aside from these two periods, there was no formal monitoring program of white shark catches and actual observations may be higher due to fishermen releasing individuals at sea and/or not reporting catches [33] . The number of observers reporting large white sharks in this region have likely increased over time due to a combination of improved fishing gear/effort and increasing media coverage due to technological advances and heightened public interest in large marine predators. However, smaller individuals likely do not elicit the same media response and catches may go unreported or may be misidentified with other species in the family Lamnidae, resulting in lower observation numbers for this size class [45] . Additionally, during the study period there was no requirement to report catches of white sharks, which may have affected observer effort. When accounting for an increase, no change, and decrease in observer effort over the study period within the generalized linear model, minimal effects of observer effort on model results were observed for all scenarios. Overall the population trend analysis, which identifies changes in relative abundance and not absolute population estimates, found that the Northwest Pacific population was relatively stable. Evidence for a recent declining trend in relative abundance of sharks with 75% increase in observer effort, identifies that it is important to enact ongoing monitoring to detect if this decline continues. Previously this analysis has documented an increase in white shark abundance off the coast of Massachusetts [30] , and steep declines in relative abundance of white shark in the eastern Adriatic and eastern Canada [42] .
There are no directed fisheries for the relatively rare white shark; however, bycatch in artisanal and commercial fisheries has been reported from the coast of California [46] , Mexico [47] , Australia [48] , and in beach protection programs off Australia [48] and South Africa [49] . In the current study, the majority of the animals categorized as bycatch were between 300–580 TL, which contrasts with data from the above regions where most animals were young-of-the-year or juveniles (<300 cm TL) [46] – [48] . It has been proposed that larger white sharks (>300 cm TL) are less susceptible to entanglement in fishing gear [46] , [49] , but this finding is not supported by our data for the Northwest Pacific. Given that in this study the largest numbers of white sharks documented as fisheries bycatch were caught in Japan, specifically the set net fishery off Ibaraki, further research including biological sampling, could shed light on this discrepancy and other related questions of regional population size and distribution.
Temperatures in this region vary both seasonally and geographically, ranging from 0°C at northern latitudes off Russia to 28°C off Vietnam [50] , [51] . White sharks were not observed during autumn and early winter in the most northerly latitudes, similar to the absence of white sharks in the northern latitudes of the Northeast Pacific [52] . Similarly, sharks were absent from the most southern latitudes during July-August, suggesting they have a preferred temperature niche. White sharks have been documented in water temperatures from 3–27°C [13] – [15] , [40] , [52] , [53] ; but typically spend most of their time in a narrow range of water temperatures, from 16.4–24.7°C [47] , [54] – [57] . As the SST off Japan encompasses these temperature ranges [58] , [59] , it is possible that sharks move along the Japanese coastline concurrent with the seasonal change in SST. Alternatively, white sharks may inhabit these temperatures due to prey availability [57] . While there are documented seal rookeries along the Japanese coastline [60] , [61] further research is required to determine the extent white sharks utilize them as a food source. Regardless of the cause of habitat preference (temperature or prey availability) these data suggest that Japan is an important aggregation site for the regional population similar to Central California and Guadalupe Island in the Northeast Pacific [15] , [62] .
Prior to this study, the largest white shark on record worldwide was reported to be 600 cm TL and was caught in Western Australia [12] . One shark in this study was estimated to be 670–700 cm TL; however, examination of the jaw dimensions revealed it to be smaller (approximately 600 cm TL; Author Observation). The longest reliably measured shark in this study now represents the largest white shark on record worldwide (602 cm TL); this shark was measured by a fish factory owner following instructions from one of the authors (see Fig. S1 ). Fishermen commonly exaggerate when estimating the size of large fish [12] , [33] , which may result in a bias in this and other observation data; however, the length-weight data from the Northwest Pacific Ocean (including estimated lengths and weights) agreed with global data recorded by trained scientists providing confidence in the quality of the data. This study reports some of the heaviest white sharks on record and consequently adds important data to the upper end of the length-weight relationship for this species (KwaZulu-Natal Sharks Board, unpublished data) [17] , [27] , [43] .
Single regional nursery grounds for white sharks have long been hypothesized [15] , [55] , but recent genetic and tracking data indicates the presence of multiple nursery grounds off Eastern Australia [63] . Similarly, individuals <200 cm TL in this study were geographically widespread. Assuming that these small juveniles have relatively restricted home ranges [64] , our data suggests the occurrence of multiple nursery grounds in the Northwestern Pacific with associated implications for management. White sharks of all sizes were observed throughout the region, suggesting either a lack of size segregation or regionally structured populations. In South Africa, sub-adults and small adults aggregate at pinniped colonies and make offshore migrations [13] , [55] , while larger adults, including pregnant females, are typically found in the tropical waters of the Western Indian Ocean [65] . Similarly in the Northeast Pacific, size segregation occurs and adult sharks aggregate to one of two locations (Central California or Guadalupe Island) and make defined offshore migrations [15] . White sharks have also been documented to use specific habitats by size within aggregation sites [66] . Accepting that observation data provides only a snap shot of a species’ distribution and movement, it is likely, that size segregation occurs in the region but the coarseness of the observation data is unable to determine this.
Conclusions about sexual segregation by season or location could not be made due to under-reporting of sex. Similar to size segregation, it is possible that fine-scale sexual segregation may be occurring within the Northwest Pacific white shark population, but tracking data is required to confirm this. Sexual segregation in white sharks has been described in the Northeast Pacific Ocean, whereby males make annual migrations and large likely pregnant females migrate biannually, remaining in offshore waters for a prolonged period of time [15] , [40] . In the Neptune Islands, South Australia, females are only observed from autumn to mid-winter while males are present year round [64] . Conversely in False Bay, South Africa, females are present year-round while males are only present during autumn and winter [67] . Because fishing pressure can affect one sex unequally in sexually segregated populations [68] , it is important to determine the extent of sexual segregation occurring in the region.
Of the 11 pregnant females documented from the Northwest Pacific, four were described previously [32] , [33] , [35] and there was one additional suspected pregnant female ( Table 1 ). The geographic segregation of these pregnant individuals by gestation stage may suggest that pregnant females have variable habitat preferences during this period. Previous opportunistic sampling of white sharks has allowed the description of the reproductive system in mature males [69] and pregnant females [32] , [33] , [35] ; however, little is known about the migration patterns of pregnant females. For the Northeast Pacific, it is thought that females remain offshore during gestation and return to inshore nurseries to pup [15] , [70] . This hypothesis is supported by data from other regions, with near-term females being captured nearshore in Kenya [65] and Tunisia [71] . The estimated 20-month gestation period for sharks in the Northwest Pacific is slightly longer than the previously suggested 18-month gestation period [15] , [44] , [70] . The spring (April–June) parturition in the Northwest Pacific, is slightly before the late spring – mid-summer (May–August) parturition found in the Northeastern Pacific [15] , and earlier (assuming offset by 6 months) from the southern hemisphere South Australia parturition, which is purported to occur in summer – mid-autumn (December–May) [48] .
Conclusions and Recommendations
White sharks are protected globally by a variety of measures ( Table 1 ); however, participation in these agreements varies in the Northwest Pacific. Regional management units may provide a mechanism to preserve genetic diversity and protect distinct population segments [4] . This highlights the need for improved regional protection of white sharks. International cooperation among countries throughout the Northwest Pacific is required to establish a management agenda, initiate systematic monitoring and biological sampling programs, and to reach agreement over commitments to international conventions [72] . Regional workshops that involve various stakeholders (i.e. policy makers, scientists, and local fisherman) should be conducted to determine the best practices to accommodate local customs and requirements [73] . Formal monitoring programs such as the recently enacted regulation in Taiwan requiring fishermen to report catches of white sharks of all size classes [74] , if properly implemented and enforced, would allow for a more accurate assessment of population trends in the region and help to determine aggregation sites.
There are several broad areas of scientific research that should be conducted to promote conservation [1] . Satellite tagging studies can provide details on migration (by size and sex) [40] , [41] , aggregation sites, and potential nursery grounds [16] , [22] , while acoustic tagging can give fine-scale details on localized movements during residency periods [62] , [67] . Identifying aggregation sites will provide locations to conduct biological sampling to better understand the role of white sharks in regional food webs and continue population assessments. Chemical tracer techniques can be successfully used to infer the diet and trophic ecology of these large predators [75] , [76] and hormone analysis, for example, can elucidate information on reproductive status [77] . Combining photographic identification with mark recapture or acoustic tracking data can be used to quantitatively investigate population trends [21] , [78] . In addition, identification of aggregation sites can facilitate economically profitable shark-based ecotourism [79] , [80] . While not without concern over possible harmful effects on ecosystems, white shark cage diving operations at aggregation sites in Australia, Mexico, New Zealand, South Africa, and the US yield financial benefits for operators and associated communities as well as opportunities for education and research [79] .
Mitochondrial DNA analyses of Japanese white sharks indicate these individuals form a monophyletic clade separate from other geographic regions including the Northeast Pacific [36] . Furthermore, of more than 200 white sharks satellite tagged in the Northeast Pacific [14] , [40] , [70] , [81] – [85] , no animals have yet undertaken trans-oceanic migrations to the Northwest Pacific region. This, coupled with the large number of observations in this study across the entire Northwest Pacific and the relatively stable abundance of animals over the past 60 years, support the occurrence of a distinct sub-population of white sharks, which is widely dispersed from northern temperate to southern tropical latitudes.
Supporting Information
White shark total length measuring protocol.
https://doi.org/10.1371/journal.pone.0094407.s001
Records of White Shark Observations in the Northwest Pacific Ocean 1951–2012.
https://doi.org/10.1371/journal.pone.0094407.s002
Global records of white shark embryos and free-swimming juveniles.
https://doi.org/10.1371/journal.pone.0094407.s003
Acknowledgments
We wish to thank the Ibaraki Prefectural Oarai Aquarium staff for providing details on observation records in Japan and J. McPherson for assisting with data analysis. We would also like to thank the editor and two anonymous reviewers for their thoughtful comments and suggestions.
Author Contributions
Conceived and designed the experiments: HMC NEH. Performed the experiments: HMC. Analyzed the data: HMC NEH. Contributed reagents/materials/analysis tools: VL ST AV HFM SPW SVF. Wrote the paper: HMC NEH. Editoral input: VL ST AV HFM SPW SVF ATF.
- View Article
- Google Scholar
- 11. Mollet HF, Cailliet GM, Klimley AP, Ebert DA, Testi AD, et al. (1996) A review of length validation methods and protocols to measure large white sharks. In: Klimley AP, Ainley DG, editors. Great White Sharks: The Biology of Carcharodon carcharias . San Diego: Academic Press. pp. 91–108.
- 12. Castro JI (2012) A summary of observations on the maximum size attained by the white shark, Carcharodon carcharias . In: Domeier ML, editor. Global Perspectives on the Biology and Life History of the White Shark. Boca RatonxcBoca Raton: CRC Press. pp. 85–90.
- 15. Domeier ML (2012) A new life-history hypothesis for white sharks, Carcharodon carcharias , in the Northeastern Pacific. In: Domeier ML, editor. Global Perspectives on the Biology and Life History of the White Shark. Boca Raton: CRC Press. pp. 199–223.
- 16. Duffy CAJ, Francis MP, Manning MJ, Bonfil R (2012) Regional population connectivity, oceanic habitat, and return migration revealed by satellite tagging of white sharks, Carcharodon carcharias , at New Zealand aggregation sites. In: Domeier ML, editor. Global Perspectives on the Biology and Life History of the White Shark. Boca Raton: CRC Press. pp. 301–318.
- 17. Francis MP (1996) Observations on a pregnant white shark with a review of reproductive biology. In: Klimley AP, Ainley DG, editors. Great White Sharks: The Biology of Carcharodon carcharias . San Diego: Academic Press. pp. 157–172.
- 19. Dewar H, Eguchi T, Hyde J, Kinzey D, Kohin S, et al. (2013) Status review of the Northeastern Pacific population of white sharks ( Carcharodon carcharias ) under the Endangered Species Act. National Oceanic and Atmospheric Administration, National Marine Fisheries Service, Southwest Fisheries Science Center.
- 20. Cliff G, Dudley SFJ, Jury MR (1996) Catches of white sharks in KwaZulu-Natal, South Africa and environmental influences. In: Klimley AP, Ainley DG, editors. Great White Sharks: The Biology of Carcharodon carcharias . San Diego: Academic Press. pp. 351–362.
- 22. Strong Jr WR, Bruce BD, Murphy RC, Nelson DR (1996) Population dynamics of great white sharks, Carcharodon carcharias , in the Spencer Gulf region, South Australia. In: Klimley AP, Ainley DG, editors. Great White Sharks: The Biology of Carcharodon carcharias . San Diego: Academic Press. pp. 401–414.
- 24. Camhi MD, Valenti SV, Fordham SV, Fowler SL, Gibson C (2009) The conservation status of pelagic sharks and rays: Report of the IUCN Shark Specialist Group Pelagic Shark Red List Workshop. Newbury: IUCN Species Survival Commission Shark Specialist Group. 78 p.
- 25. Kyne PM, Carlson JK, Ebert DA, Fordham SV, Bizzarro JJ, et al. editors (2012) The Conservation Status of North American, Central American, and Caribbean Chondrichthyans. IUCN Species Survival Commission Shark Specialist Group, Vancouver, Canada. 148 p.
- 26. Domeier ML (2012) Global Perspectives on the Biology and Life History of the White Shark. Boca Raton: CRC Press. 543 p.
- 27. Fergusson IK (1996) Distribution and autecology of the white shark in the eastern North Atlantic Ocean and the Mediterranean Sea. In: Klimley AP, Ainley DG, editors. Great White Sharks: The Biology of Carcharodon carcharias . San Diego: Academic Press. pp. 321–345.
- 29. De Maddalena A, Heim W (2012) Mediterranean Great White Sharks: A Comprehensive Study Including All Recorded Sightings. Jefferson: McFarland & Company Inc. 254 p.
- 30. Skomal GB, Chisholm J, Correia SJ (2012) Implications of increasing pinniped populations on the diet and abundance of white sharks off the coast of Massachusetts. In: Domeier ML, editor. Global Perspectives on the Biology and Life History of the White Shark. Boca Raton: CRC Press. pp. 405–417.
- 35. Uchida S, Todo M, Teshima K, Yano K (1996) Pregnant white sharks and full-term embryos from Japan. In: Klimley AP, Ainley DG, editors. Great White Sharks: The Biology of Carcharodon carcharias . San Diego: Academic Press. pp. 139–155.
- 39. International White Shark Symposium February 2–6, 2010. Honolulu, Hawaii.
- 40. Domeier ML, Nasby-Lucas N (2012) Sex-specific migration patterns and sexual segregation of adult white sharks, Carcharodon carcharias , in the Northeastern Pacific. In: Domeier ML, editor. Global Perspectives on the Biology and Life History of the White Shark. Boca Raton: CRC Press. pp. 133–146.
- 43. Ezcurra JM, Lowe CG, Mollet HF, Ferry LA, O'Sullivan JB (2012) Captive feeding and growth of young-of-the-year white sharks, Carcharodon carcharias , at the Monterey Bay Aquarium. In: Domeier ML, editor. Global Perspectives on the Biology and Life History of the White Shark. Boca Raton: CRC Press. pp. 3–15.
- 45. Weng K, Honebrink R (2013) Occurrence of White Sharks ( Carcharodon carcharias ) in Hawaiian Waters. J Mar Biol doi: https://doi.org/10.1155/2013/598745 .
- 46. Lowe CG, Blasius ME, Jarvis ET, Mason TJ, Goodmanlowe GD, et al. (2012) Historic fishery interactions with white sharks in the Southern California Bight. In: Domeier ML, editor. Global Perspectives on the Biology and Life History of the White Shark. Boca Raton CRC Press. pp. 405–417.
- 47. Santana-Morales O, Sosa-Nishizaki O, Escobedo-Olvera MA, Oñate-González EC, O’Sullivan JB, et al. (2012) Incidental catch and ecological observations of juvenile white sharks, Carcharodon carcharias , in Western Baja California, Mexico, conservation implications. In: Domeier ML, editor. Global Perspectives on the Biology and Life History of the White Shark. Boca Raton: CRC Press. pp. 187–198.
- 48. Malcolm H, Bruce BD, Stevens JD (2001) A review of the biology and status of white sharks in Australian waters. In: Report to Environment Australia, Marine Species Protection Program. Hobart: CSIRO Marine Research.
- 51. Locarnini RA, Mishonov AV, Antonov JI, Boyer TP, Garcia HE, et al. (2010) World Ocean Atlas 2009. In: Levitus S, editor. Volume 1 : Temperature. Washington DC: NOAA Atlas NESDIS 68, U.S. Government Printing Office.184 p.
- 53. Francis MP, Duffy CAJ, Bonfil R, Manning MJ (2012) The third dimension vertical habitat use by white sharks, Carcharodon carcharias , in New Zealand and in Oceanic and tropical waters of the Southwest Pacific Ocean. In: Domeier ML, editor. Global Perspectives on the Biology and Life History of the White Shark. Boca Raton: CRC Press. pp. 319–342.
- 55. Dudley SFJ (2012) A review of research on the white shark, Carcharodon carcharias , in Southern Africa. In: Domeier ML, editor. Global Perspectives on the Biology and Life History of the White Shark. Boca Raton: CRC Press. pp. 511–533.
- 58. Moriyasu S (1972) The Tsushima Current. In: Stommel H, Yoshida K, editors. Kuroshio, University of Washington Press, U.S.A. pp. 353–369.
- 62. Jorgensen SJ, Chapple TK, Anderson S, Hoyos M, Reeb C, et al. (2012) Connectivity among white shark coastal aggregation areas in the Northeastern Pacific. In: Domeier ML, editor. Global Perspectives on the Biology and Life History of the White Shark. Boca Raton: CRC Press. pp. 159–167.
- 63. Bruce BD, Bradford RW (2012) Habitat use and spatial dynamics of juvenile white sharks, Carcharodon carcharias , in Eastern Australia. In: Domeier ML, editor. Global Perspectives on the Biology and Life History of the White Shark. Boca Raton: CRC Press Boca Raton. pp. 225–253.
- 66. Robbins RL, Booth DJ (2012) Seasonal sexual and size segregation of white sharks, Carcharodon carcharias , in Eastern Australia. In: Domeier ML, editor. Global Perspectives on the Biology and Life History of the Great White Shark. Boca Raton: CRC Press. pp. 287–299.
- 69. Pratt Jr HL (1996) Reproduction in the male white shark. In Klimley AP, Ainley DG, editors. Great white sharks: The biology of Carcharodon carcharias . San Diego: Academic Press. pp. 131–138.
- 74. Lee IC (2013) Fisheries Agency’s new rule aims to help shark research. Available: http://www.taipeitimes.com/News/taiwan/archives/2013/03/26/2003558045 . Accessed 2013 Apr 25.
- 76. Hussey NE, McCann HM, Cliff G, Dudley SFJ, Wintner SP, et al. (2012) Size-based analysis of diet and trophic position of the white shark, Carcharodon carcharias , in South African waters. In: Domeier ML, editor. Global Perspectives on the Biology and Life History of the Great White Shark. Boca Raton: CRC Press. pp. 27–49.
- 78. Nasby-Lucas N, Domeier ML(2012) Use of photo identification to describe a white shark aggregation at Guadalupe Island, Mexico. In: Domeier ML, editor. Global Perspectives on the Biology and Life History of the Great White Shark. Boca Raton: CRC Press. pp. 381–392.
- 88. Norman JR, Fraser FC (1937) Giant Fishes, Whales and Dolphins. Putnam, London. 376 p.
- 89. Ellis R, McCosker JE (1991) Great white shark. Stanford: Standford University Press. 270 p.

Search form
- Find Stories
- For Journalists
Scientists take a deep dive into how sharks use the ocean
Researchers compiled the largest set of biologging data revealing how 38 species of sharks, rays, and skates move vertically in oceans around the world.
Using sophisticated electronic tags, scientists have assembled a large biologging dataset to garner comparative insights on how sharks, rays, and skates – also known as “elasmobranchs” – use the ocean depths. While some species spend their entire lives in shallow waters close to our shores on the continental shelf, others plunge hundreds of meters or more off the slope waters into the twilight zone, beyond where sunlight penetrates. This new understanding of how elasmobranchs use the ocean will enable policymakers and resource managers the opportunity to examine the threats these animals face, and guide future management and conservation plans.

Samantha Andrzejaczek, a postdoctoral research fellow at Stanford’s Hopkins Marine Station, (second from right) tagging a tiger shark. (Image credit: Alex Kydd)
A study published Aug. 19 in Science Advances , led by Stanford University and ZSL (Zoological Society of London) researchers, is the largest global investigation of where and when a diverse group of elasmobranchs move vertically. A team of 171 researchers from 135 institutions across 25 countries brought together two decades of data from satellite and archival tags that remotely tracked the movements and behaviors of 38 species in oceans across the globe.
“For the first time, we have a standardized, global database that we used to fill important knowledge gaps about the diving behaviors of sharks and rays,” said Samantha Andrzejaczek, co-lead author of the study and a postdoctoral research fellow at the Hopkins Marine Station of Stanford University. “This will enable better understanding of what fisheries interact with elasmobranchs and how to improve management of many of these long-lived animals.”
Movement in three dimensions
Scientists already have a wealth of movement data about many marine species that inhabit the near-surface spaces of the coastal ocean. Drones, scuba divers, and other methods, for example, survey marine communities and populations to a depth of about 50 meters, but animal movement in three dimensions, especially in the deeper, vertical spaces of the ocean, is far less understood.
“Sharks and rays are iconic but threatened ocean species. Key to their effective management is an understanding of their basic ecology,” said David Curnick, head of the Ocean Predator Lab at ZSL and co-lead author of the paper. “Yet, for many species, we know relatively little about their fundamental behavior, and what we do know is often restricted to what we can observe in surface waters.”
Over the past 20 years, a variety of electronic tags have come of age that provide the opportunity to tag numerous elasmobranch species. Scientists at Stanford have been at the forefront of biologging tag development and applying these technologies on sharks and rays.
One of the common vertical movements among elasmobranchs appears to match up with the ocean’s diel (twice a day) vertical migration. At daybreak, tiny fish and invertebrates – followed by the animals that prey on them – begin migrating from the bright, uppermost ocean layer to the relative safety of darker, deeper water. At night, they return to the surface to feed.
“We think that sharks and rays in their diel migrations are following food resources up and down the water column,” said Andrzejaczek .
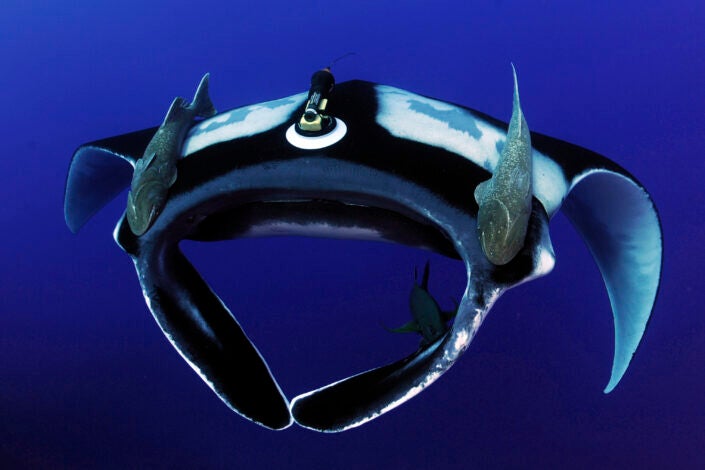
An oceanic manta ray (Mobula birostris) at a dive site called the Boiler near San Benedicto Island in Mexico. (Image credit: Guy Stevens/Manta Trust 2015)
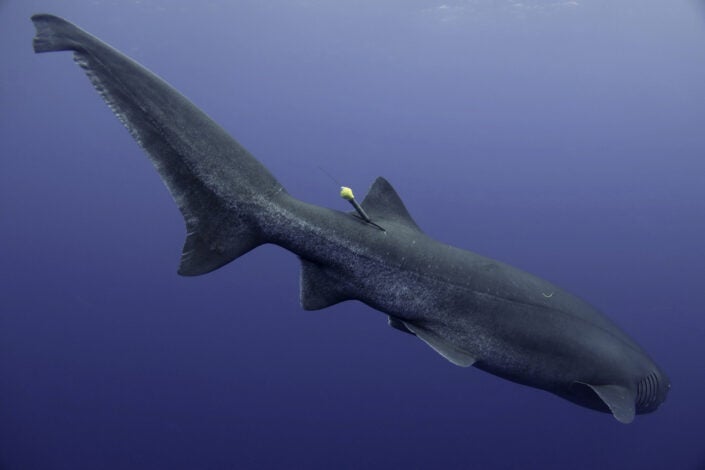
A tagged sixgill shark descending to deeper depths. (Image credit: Mark Royer)
The study found that about one-third of species frequently dive to depths where the water is cold, often low on oxygen due to biological activity related to productivity, and visibility is limited. Sensor records show that white sharks ( Carcharodon carcharias ) dive deeper than 1200 meters while whale sharks ( Rhincodon typus ) have reached 1896 meters, which is near the pressure limit of 2000 meters for today’s sensors.
“Deep divers might be looking for food in deeper water or avoiding hunters themselves as potential prey,” said Andrzejaczek. “Some sharks and rays are small, and some of the biggest sharks and rays will feed on them. We found that 13 species had individuals that dive to more than 1000 meters, which is extremely deep.” Some may require cooling-off periods while at depth. “When large sharks spend too long in the warm surface waters, they may have to dive to cool down, a form of behavioral thermoregulation,” she added.
The researchers also identified overlaps among species in the same vertical spaces. Whale sharks, tiger sharks, and oceanic manta rays showed strikingly similar vertical distributions, although they have very different evolutionary histories. Predator-and-prey relationships likely drive this proximity. “The oceanic manta ray and whale shark both feed on plankton, and the tiger shark has been known to predate on both those species,” said Andrzejaczek.
A foundation for future management
The photic zone or epipelagic – the ocean region receiving sunlight – stretches from the surface to about 200 meters and can potentially be a dangerous area for elasmobranchs. That is where they are most likely to be exposed to fishing gear as either target species or as bycatch. Of the 38 species in the study, researchers found that 26 spent more than 95% of their time in the top 250 meters of the water column.

Samantha Andrzejaczek with some of the Block lab’s satellite tags. (Image credit: Andrew Brodhead)
More than one-third of all sharks and rays are threatened with extinction, according to the IUCN Red List of Threatened Species.
“These data provide the foundation for future management of global elasmobranch resources, and it has taken a team of scientists thousands of hours tagging and tracking the sharks with global satellite and biologging systems to make this possibility happen,” said Barbara Block , the Prothro Professor of Marine Sciences at Stanford, whose tagging programs such as TOPP, contributed 25% of the data set.
Understanding how elasmobranchs use vertical habitats is crucial to understanding their current and future ecological roles in the ocean and their risks to various threats. Scientists could utilize this and future databases to investigate how changing ocean temperature and oxygen levels may influence species’ distributions and create new conditions and threats.
“Human beings are unaccustomed to thinking of habitat in the vertical dimension,” said Andrzejaczek. “We hope this study can make people realize that we need management strategies that incorporate this overlooked dimension of elasmobranch behavior. For example, we could use these data to better understand how sharks and human fisheries interact.”
This three-year study brought together data from increasingly sophisticated and more accurate tags with sensitive sensors that can withstand the rigors of the environment while riding along on a shark or ray and function in deep water, as well as improved analytical tools to incorporate different types of movement data. The key ingredient has been cooperation among biologging scientists from around the world.
“Large-scale scientific studies like this one are not possible without a monumental collaborative effort,” said Curnick. “We reconcile the collective knowledge and expertise of researchers from around the world. The result is far greater than any one researcher or institution could achieve on their own.”
This work was led by Stanford University and the Zoological Society of London. Additional Stanford co-authors include graduate student Maurice Goodman; research data analyst Mike Castleton; research scientist Jonathan Dale; and researcher Robert Schallert. Former Stanford graduate students and postdoctoral scholars George Shillinger, Andre Boustany, Kevin Weng, Taylor Chapple, and Sal Jorgensen are also co-authors. Block is also a professor of biology and a senior fellow at the Stanford Woods Institute for the Environment .
This research was funded by the Bertarelli Foundation, the Moore Foundation, the Sloan Foundation, Instituto Politecnico Nacional, the Darwin Initiative, the Georgia Aquarium, the Rolex Awards for Enterprise, and the Whitley Fund for Nature.
Internet Explorer lacks support for the features of this website. For the best experience, please use a modern browser such as Chrome, Firefox, or Edge.

Shark Conservation Starts with Shark Science
August 30, 2023
NOAA Fisheries invests millions of dollars into shark research to base management decisions on the best available science.
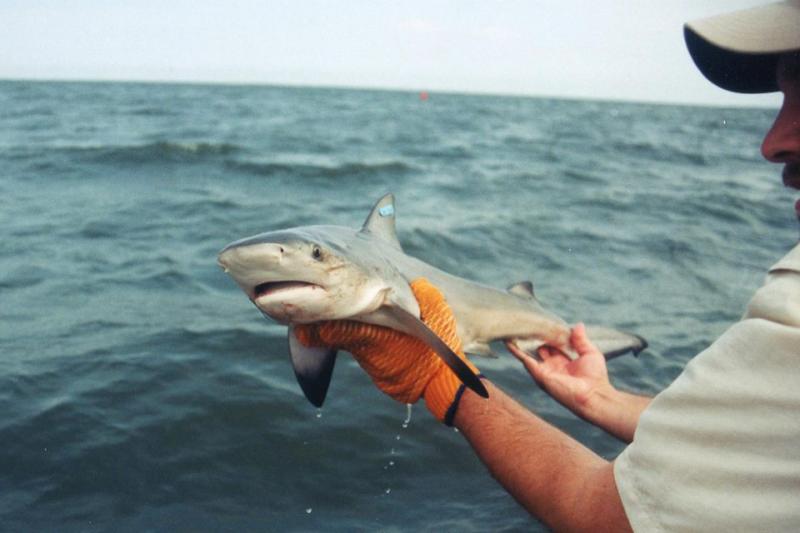
NOAA Fisheries invests millions of dollars in shark (and skate and ray) research so that management decisions can be based on the best available science. In fact, we are one of the largest providers of funding for shark science in the world. This includes programs conducted by NOAA Fisheries scientists as well as grants for states, academic institutions, and other organizations.
Regional Research, Shared Globally
Sharks are found throughout all U.S. waters, so we study them in every region. Each NOAA Fisheries Science Center conducts shark research to meet the needs of regional fishery managers.
For example, the Northeast Fisheries Science Center conducts surveys, sampling, and tagging programs for sharks, spiny dogfish, and skates along the east coast.The Southeast Fisheries Science Center does surveys , research, and stock assessments . These form the foundation for shark management in U.S. waters and international fisheries in the Atlantic Ocean.
Our shark research is peer-reviewed to ensure the science is high-caliber and suitable for management purposes. Since 2018, NOAA Fisheries scientists have contributed to more than 100 shark research publications on dozens of species. The research is submitted to journals or published in reports that are available to everyone through the NOAA Central Library .
Driving Research with Funding
Since 2018, NOAA and NOAA Fisheries have awarded more than $7 million to outside organizations for shark-focused research projects. We do this through grant programs such as the Saltonstall-Kennedy Grant Program , Bycatch Reduction Engineering Program , Sea Grant , and Cooperative Research Program . The region-specific Species Recovery Grant Program supports research for sharks and rays listed as threatened or endangered under the Endangered Species Act. These investments in shark science allow us to continually improve our shark management.
We fund and conduct research, assess stocks, work with U.S. fishermen, and implement appropriate management measures on shark harvests. Through these efforts, we have made significant progress toward ending overfishing and rebuilding overfished shark stocks for long-term sustainability.
More Information
- Celebrating 30 Years of Atlantic Shark Management
- Podcast: Atlantic Sharks: 30 Years of Successes and Lessons
- Atlantic Blacktip Sharks: A Management Success Story
- Sharpen Your Shark Facts
Recent News
Louisiana dolphin shot dead.
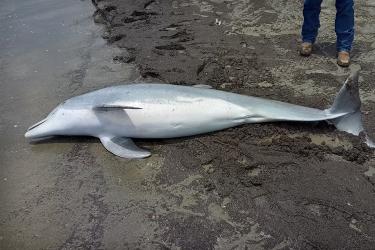
Reaching a Major Oyster Restoration Milestone in Virginia’s York River
Unwelcome Catch: Fishermen's Stewardship Role Reeling in Marine Debris
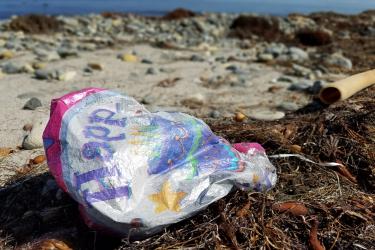
Last updated by Office of Sustainable Fisheries on September 01, 2023

Oceanic Whitetip Sharks: A Plan for Recovery
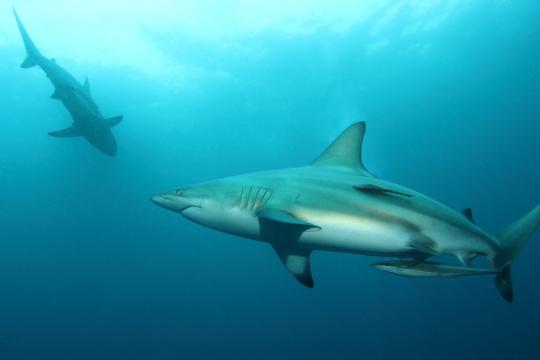
Atlantic Sharks: 30 Years of Successes and Lessons
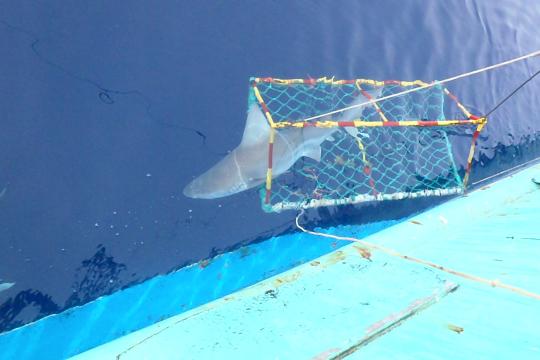
Story Map: Atlantic Shark Surveys

General Category Bluefin Fishery to Reopen for Four Days, December 20-23, 2021
Thank you for visiting nature.com. You are using a browser version with limited support for CSS. To obtain the best experience, we recommend you use a more up to date browser (or turn off compatibility mode in Internet Explorer). In the meantime, to ensure continued support, we are displaying the site without styles and JavaScript.
- View all journals
- My Account Login
- Explore content
- About the journal
- Publish with us
- Sign up for alerts
- Open access
- Published: 22 July 2020
Investigating the presence of microplastics in demersal sharks of the North-East Atlantic
- Kristian J. Parton 1 ,
- Brendan J. Godley 1 ,
- David Santillo 2 ,
- Muhammad Tausif 3 ,
- Lucy C. M. Omeyer 1 &
- Tamara S. Galloway 4
Scientific Reports volume 10 , Article number: 12204 ( 2020 ) Cite this article
10k Accesses
47 Citations
486 Altmetric
Metrics details
- Environmental impact
- Ichthyology
- Marine biology
- Ocean sciences
Microplastic pollution is ubiquitous in the marine environment and is ingested by numerous marine species. Sharks are an understudied group regarding their susceptibility to microplastic ingestion. Here, we provide evidence of ingestion of microplastic and other anthropogenic fibres in four demersal sharks species found in the waters of the United Kingdom and investigate whether body burdens of contamination vary according to species, sex or size. Sharks were collected from the North-East Atlantic. Stomachs and digestive tracts of 46 sharks of 4 species were examined and 67% of samples contained at least one contaminant particle. Although we acknowledge modest sample size, estimated particle burden increased with body size but did not vary systematically with sex or species. A total of 379 particles were identified, leading to median estimates ranging from 2 to 7.5 ingested contaminants per animal for the 4 species. The majority were fibrous in nature (95%) and blue (88%) or black (9%) in colour. A subsample of contaminants (N = 62) were subject to FT-IR spectroscopy and polymers identified as: synthetic cellulose (33.3%), polypropylene (25%), polyacrylamides (10%) and polyester (8.3%). The level of risk posed to shark species by this level of contamination is unknown. Nevertheless, this study presents the first empirical evidence and an important baseline for ingestion of microplastics and other anthropogenic fibres in native UK shark species and highlights the pervasive nature of these pollutants.
Similar content being viewed by others
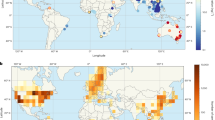
Underestimated burden of per- and polyfluoroalkyl substances in global surface waters and groundwaters
Diana Ackerman Grunfeld, Daniel Gilbert, … Denis M. O’Carroll
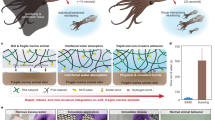
Bioadhesive interface for marine sensors on diverse soft fragile species
Camilo Duque Londono, Seth F. Cones, … Xuanhe Zhao
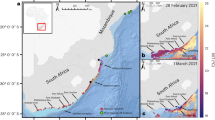
Climate change-driven cooling can kill marine megafauna at their distributional limits
Nicolas Lubitz, Ryan Daly, … Adam Barnett
Introduction
Plastics in the marine environment.
Research on plastic in the marine environment has accelerated rapidly in the last decade, with numerous publications describing its impact on ecosystems and marine taxa 1 , 2 , 3 , 4 , 5 , 6 , 7 . It is estimated that between 4.8 and 12.7 million tonnes of plastic enter the oceans every year from a variety of sources 6 . Plastic is a popular material due to its durability, low production cost and efficiency in its uses 8 . It is these properties, alongside its often disposable nature that leads to its prevalence in the environment for many years 9 .
Microplastics (defined as plastic particles < 5 mm) 10 are ubiquitous in the marine environment 11 , 12 , 13 . Despite this knowledge, quantitative assessments of their abundance are still fairly limited 14 , although some estimates place their abundance at 5.25 trillion particles globally, weighing in at over 250,000 tonnes 5 . Microplastics, in the form of fibres, fragments or beads/spheres, assimilate in the marine ecosystem via multiple avenues. Larger pieces of plastic can disintegrate over time due to UV radiation exposure, wave action and physical abrasion, eventually fragmenting into microscopic particles 15 . Microplastics are also found in many everyday items used by humans including cosmetic products and can be produced by clothing wear 16 , 17 , 18 , 19 . These can then reach the oceans via wastewater treatment plants 20 .
Ingestion of microplastics in marine species
Ingestion of microplastics is reported in many marine species including turtles, marine mammals and fish 1 , 21 , 22 , 23 , 24 , 25 . Alongside these larger species, microplastics have been reported in invertebrates such as zooplankton and crustaceans 26 , 27 , 28 . Our understanding of the impacts of microplastic ingestion is better understood in the latter group, with reports suggesting dose-dependent detrimental effects on feeding behaviour, development, reproduction and lifespan 29 , 30 , 31 .
Microplastic ingestion in elasmobranchs
Elasmobranchs are relatively understudied in regards to threats from plastic pollution 32 , 33 , nonetheless their susceptibility to microplastic ingestion has been reported in a handful of scientific publications 22 , 34 , 35 , 36 , 37 , 38 , 39 . It is thought that some species of elasmobranch may be at higher risk of microplastic ingestion based on their feeding strategies or habitat use 35 . Filter feeding species (such as whale sharks and basking sharks) that occupy habitats which overlap areas with high densities of plastic pollution have been suggested to be at higher risk of microplastic ingestion 35 , 40 , 41 . Many shark species, however, are non-filter feeders, instead feeding on a range of larger organisms such as fish, crustaceans, marine turtles and marine mammals, all of which have records of microplastic ingestion 22 , 23 , 24 , 27 .
North-East Atlantic demersal elasmobranchs
The North-East Atlantic is home to numerous shark and ray species, including small to medium sized demersal sharks. These species can be found at varying depths from 5 to 900 m 42 , 43 , most often residing in benthic habitats 44 , 45 . They feed on a wide range of small teleost fishes, crustaceans and cephalopods 44 , 46 . Due to their habitat choice they are often caught in demersal fisheries as bycatch, however targeted fisheries for these species also exist 47 , 48 . The exposure of microplastics to demersal shark species globally, is currently poorly investigated, with only a few reports of plastic ingestion, mostly situated in and around the Mediterranean Sea 22 , 36 , 37 , 38 , 49 , 50 . There have, however, been multiple studies of plastic ingestion in bony fish in the region, with ingestion rates varying from 1 to 47% across the species 22 , 51 , 52 , 53 , 54 .
Here we carry out the first detailed comparative study of microplastic ingestion in four shark species in the North-East Atlantic (small-spotted catshark; Scyliorhinus canicula , starry smooth-hound; Mustelus asterias , spiny dogfish; Squalus acanthias and bull huss; Scyliorhinus stellaris ). These species were chosen due to their availability as bycatch in local fisheries. Alongside this, all four species are primarily demersal in their habitat choice, therefore studying microplastic ingestion within them may provide insights into contaminant levels for this marine biome and as a result indicate whether these species would be suitable bio-indicators for marine pollution. Given interspecific differences in habitat niche, ontogenetic shifts in diet and sex variation in life history strategies, we hypothesized that there would be differences in contaminant load among species, between sex and among size classes.
Materials and methods
Collection and dissection of shark samples.
The study was conducted in Cornwall, UK using sharks caught as bycatch in a demersal hake fishery, fishing in and around the North-East Atlantic and Celtic Sea (ICES rectangles: VIIg, VIIh and VIIf). Four species of sharks were investigated (Total N = 46), including: small-spotted catshark ( Scyliorhinus canicula ) (n = 12), spiny dogfish ( Squalus acanthias ) (n = 12), starry smooth-hound ( Mustelus asterias) (n = 12) and bull huss ( Scyliorhinus stellaris ) (n = 10). Standard shark morphometric measurements were taken for each species (for full details see Supplementary Materials).
Necropsy and analysis
Upon dissection, the entire gastrointestinal tracts were removed (stomach and intestines) and 10 ml (20–50% of total volume depending on species) of their contents were removed for analysis and visual inspection of gut contents (see Supplementary Fig. S1 ). Samples were treated with 20% potassium hydroxide (KOH) as recent studies have highlighted its efficacy at digesting fish ingesta 53 , 55 , 56 , 57 and heated for 48 h at 60 °C to aid digestion of biological materials. Digested samples were filtered and subsequently analysed under a digital stereo microscope (Leica M165C) and classified by type (fibre, fragment or bead) and colour, as well as measured (mm). A subsample of the contaminants identified (including potential fragments and fibres) underwent Fourier Transform Infrared spectroscopy (FT-IR) to gain insights into their polymer make-up and possible origins. Substantial measures were taken to reduce and control for contamination of samples throughout laboratory work, including the running of procedural blanks and air-borne contamination blanks at every stage of the necropsy and subsequent analysis (for full details, including quality control and contamination control measures see Supplementary Materials). All methods were carried out in accordance with relevant guidelines and regulations.
All statistical analyses were conducted on raw data. A negative binomial generalised linear model (GLM) was used to investigate the influence of species, sex and individual length on the estimated number of ingested fibres, using the MASS package 58 in R v3.5.1. 59 All combinations of terms were examined and ranked by Akaike’s Information Criteria (AIC) using subset selection of the maximal model using the MuMIn package v1.42.1. 60 Top ranked models were defined as models ΔAIC ≤ 2 units of the best supported model, after excluding further models where a simpler model attained stronger weighting 61 .
Particle terminology
Throughout the manuscript a range of terms are used to describe the various identified contaminants. The following terms are hereby explained. Microplastics and/or microplastic fibres refer to traditional petrochemical-derived polymer compounds. Anthropogenic fibres encompass compounds that are naturally occurring, however have been repurposed for human use, this includes the likes of synthetic regenerated cellulose, viscose, rayon and cotton. Contaminants/contaminant particles, in this context, refers to both microplastics and anthropogenic fibres as an umbrella term for compounds not-naturally occurring within these sharks.
Descriptive statistics
In total, 46 individual sharks were analysed, of which 56.5% were male, although proportion varied across species (Proportion male for individual species: small-spotted catshark 66.6%, starry smooth-hound 25%, spiny dogfish 83.3%, bull huss 50%). Overall, 67.4% of sharks were classified as adults although again, the proportion differed among species (Proportion adult for individual species: small-spotted catshark 75%, starry smooth-hound 66.6%, spiny dogfish 58.3%, bull huss 58.3%).
Almost all particles identified in sharks were classified as fibres, with only two fragments identified, and no beads/spheres found. Of the 46 sharks analysed in this study, samples from 67% (31/46) contained at least one contaminant particle and incidence was relatively consistent across species (small-spotted catshark 66.6%, starry smooth-hound 75%, spiny dogfish 58%, bull huss 70%). Estimated number of fibres varied across the four shark species: estimated median fibres (IQ range; range): Overall: 4(0–9; 0–770) (IQ range; range), starry smooth-hound (7.5(3.8–28.75; 0–735), small spotted catshark (2(0–4; 0–6), spiny dogfish (4(0–4; 0–12), bull huss (5(1.3–13.8; 0–770).
Fibres ranged in length from 0.3 mm to 14.4 mm and had an average length of 2.7 mm ± 2.6 SD (see Fig. 1 ). The vast majority of fibres were blue (88.0%) or black (8.8%) in colour, with the remaining colours including: red, yellow and other (clear, green and white) each making up 3.8% (see Fig. 2 A-D). The two fragments identified were blue and white in colour. Fibres larger than 5 mm (n = 50) were considered here as macroplastics and were excluded from the analysis, although can be found grouped together in the ≥ 5 mm category on Fig. 1 .
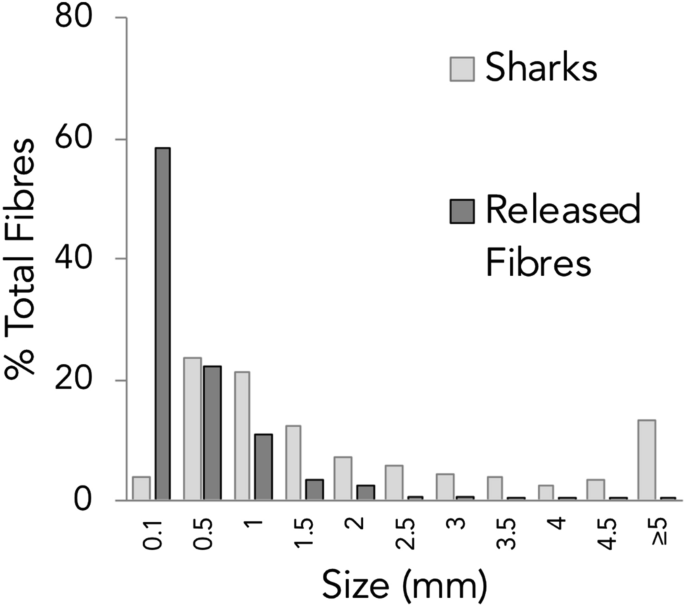
Fibre length distribution. Fibre lengths as a proportion of total fibres for fibres found in shark species (light grey) and fibres released in laboratory conditions after washing of various cotton and polyethylene terephthalate textiles. Palacios Marin AV, (2019) Release of microfibres from comparative common textile structures during laundering (Unpublished Masters dissertation). University of Leeds, UK.
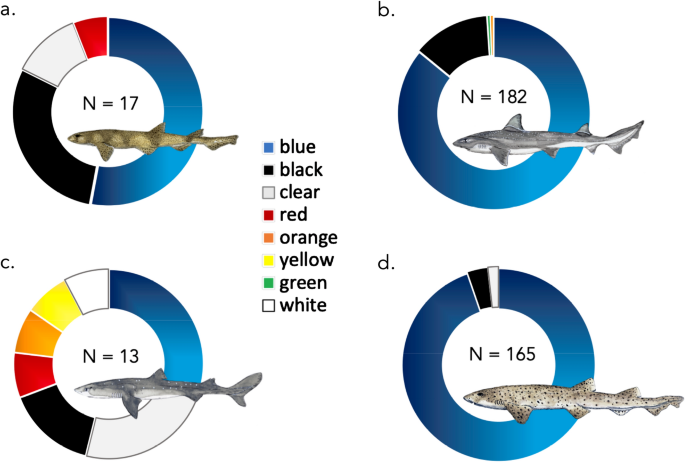
Composition of colours of ingested fibres, found across both the stomachs and intestines of four species of north-east Atlantic demersal sharks: ( a) small-spotted catshark ( Scyliorhinus canicula ), ( b ) starry smooth-hound ( Mustelus asterias ), ( c ) spiny dogfish ( Squalus acanthias ) and ( d ) bull huss ( Scyliorhinus stellaris ). Total N of coloured fibres identified annotated within figure. Elasmobranch drawings by Lucie Jones.
Differences between species, sex and body size
The estimated number of ingested microfibres was positively influenced by individual shark body length, however it did not differ between species or sex (See Fig. 3 A,B, Supplementary Table S2 and Supplementary Fig. S5 ). It should be noted two individuals in this study (one starry smooth-hound and one bull huss) had much higher levels, with the sample from the former individual containing 147 fibres and the sample from the latter containing 154 fibres. Upon visual examination, these fibres appeared to be strands of blue rope, subsequently confirmed as olefin polypropylene. (Supplementary Figures S4 – S8 have been created with these outliers removed/added for comparison).
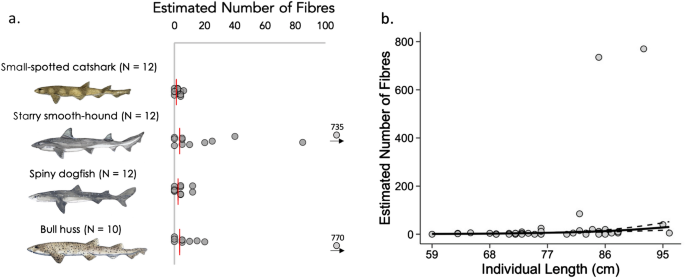
Estimated fibre ingestion and relationship with total length (cm). ( a ) Expected number of fibres based on extrapolation from full stomach/GI tract volumes. Medians marked by red line. N = annotated. Elasmobranch drawings by Lucie Jones. ( b ) Relationship between the estimated number of ingested fibres and individual length. Lines denote predictions from the top ranked model presented in Supplementary Table S2 . Standard errors are shown by the dashed lines.
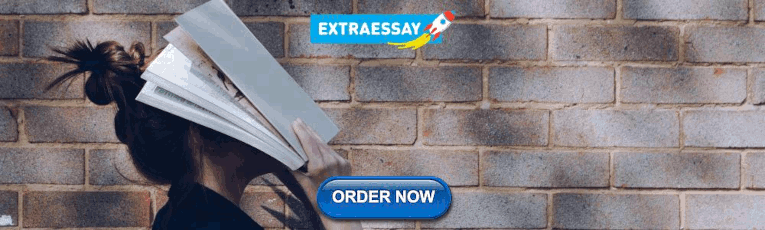
Polymer identification
A subsample of contaminant particles (n = 60 fibres, n = 2 fragments) were subject to FT-IR analysis (16% of total contaminants identified). However, when we consider the sample set without the two outliers mentioned above which were olefin polypropylene fibres, the subsample of contaminants that underwent FT-IR spectroscopy equalled 79% of all particles isolated.
Our analysis revealed 33.3% of fibres (n = 20) were cellulose derivatives (Alpha & Ecteola modified), however further analysis by light microscopy revealed these cellulose fibres were anthropogenic in nature due to their uniform diameter distribution across the fibre length and observation of convoluted structure of the fibre; a characteristic of cotton fibres (see Supplementary Fig. S2 ). Polyacrylamides made up 10% of fibres (n = 6), 8.3% of fibres were polyesters (n = 5) and 1.7% were cellophane (n = 1). Another 25% (n = 15) registered as Olefin polypropylene. Combined with the aforementioned microplastic contaminants (polyester and polyacrylamide), this results in a total of 43.3% of particles being true microplastics.
The remaining 21.6% of fibres (n = 13) were either unidentifiable due to low spectral match scores (n = 7) or returned as biological in nature (n = 6). Biological returns were excluded from broader statistical analysis. See Fig. 4 & Supplementary Table S1 .
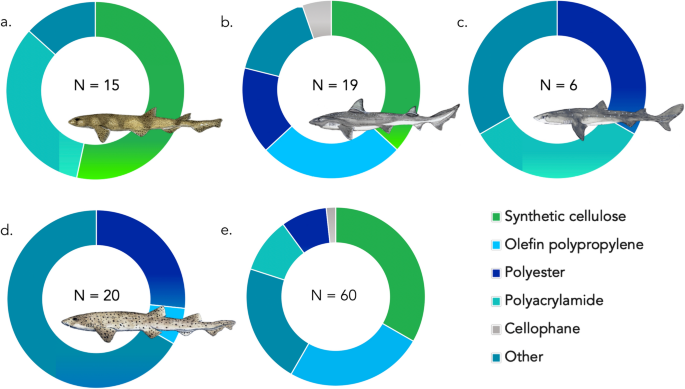
Composition of polymer make up of fibres between shark species. N of polymers identified in each species annotated on figure. ( a ) small-spotted catshark. ( b ) starry smooth-hound. ( c ) Spiny dogfish. ( d ) bull huss. ( e ) Total polymer percentages for all four species. Other = Biological materials and/or low spectral match scores. Elasmobranch drawings by Lucie Jones.
The two fragments identified returned as polyethylene and polypropylene (see Supplementary Fig. S3 ).
Our study is the first to demonstrate the presence of microplastic and anthropogenic particle contaminants in resident UK shark species in the North-East Atlantic. Despite there being no substantial differences in microplastic uptake among the shark species studied here, the research provides an important empirical baseline for future work investigating contaminant levels in UK sharks. Greater levels of contamination might be expected in animals that inhabit other parts of the UK with lower water quality. Although we have not demonstrated any health impacts on the sharks, the presence of these particle contaminants indicates their pervasiveness in the marine environment. With increasing global plastic production and its prevalence in every day products, the abundance of such marine pollutants is likely set to increase.
Contaminant particle ingestion by species, sex and size
Nearly 70% of all sharks sampled in our study contained at least one contaminant particle in their digestive tracts. Although this is likely to be a conservative estimate of incidence, this number is significantly higher than many other reports for similar shark species around the world 22 , 36 , 39 , 50 , 62 , 63 see Table 1 . Studies by Alomar and Deudero 38 and Smith 36 revealed ingestion rates of microplastics at 16.8% in blackmouth catsharks sampled in the Mediterranean, and 15% in small-spotted catsharks from the North sea, respectively 36 , 63 . Interestingly, the Mediterranean is considered by some to be one of the worst affected oceans with regards to plastic pollution 5 , 64 , 65 , therefore ingestion of contaminant particles may have been expected to have been lower in North-East Atlantic. The only other study to have been conducted on similar species and within a similar ocean area is that of Neves et al. 22 , which found microplastic ingestion rates of 20% in small-spotted catsharks collected from the North-East Atlantic coast of Portugal, with microplastics being mostly fibrous in nature.
The contaminants found within our sharks is consistent with other studies investigating the presence of pollutants in the marine environment 23 , 66 , 67 , 68 , and their colours 23 , 66 , 68 , 69 . Fibres are quickly becoming the most ubiquitous contaminant type in many compartments of marine ecosystems, as well as in the gut contents of numerous marine species including turtles, seals and cetaceans 23 , 24 , 70 , 71 . Fibres have a number of potential sources, including break-off from fishing and maritime equipment such as nets and ropes 72 , fibre shedding from automotive tyre wear and the washing of synthetic fabrics in clothing, as well as breakage and release from other textiles 16 , 19 , 73 , 74 , 75 .
We hypothesised that there would be differences in estimated contaminant load among species, between sexes and across size classes. The expected number of ingested fibres was only influenced by individual length (TL cm) with more found in larger sharks. As we were unable to control for location/habitat in this study, this remains to be explored in further detail. While diet could be an additional influencing factor for these shark species, with the current presented data and relatively small sample size we can only speculate as to the factors influencing contaminant burden in these demersal sharks.
Ingestion pathways
There are at least two potential ingestion pathways for contaminant particles by demersal shark species. Firstly, via the presence of contaminants directly in their food source. Microplastics and other anthropogenic materials have been reported in several prey species for these sharks, including crustaceans and molluscs 28 , 51 , 52 , 76 , 77 . Some of these prey items have also been to shown to take-up and translocate microplastics around their bodies in laboratory conditions 30 , 78 , as well as transfer microplastics up the food-web 79 . The species in this study show some variation in their published dietary strategies with starry smooth-hounds and spiny dogfish having fairly specialist diets, compared to small-spotted catsharks and bull huss which are more generalist 44 , 46 , 80 , 81 . We may have expected the generalist feeding sharks to have more contaminants due to feeding on a wider range of prey items, however this was not evident.
The second pathway for exposure could be through direct engulfment alongside target prey species. Habitat use has been identified as a potential driver of plastic ingestion for other elasmobranch species, including whale sharks and manta rays 41 , as well as bony fish species 82 . The sharks analysed in this study all display similar strategies while feeding in their demersal habitat, in that to swallow their prey, they engulf it whole using suction feeding 83 , 84 . In doing so, many of these species will ingest large quantities of sediment alongside their prey. Although the majority of this is immediately expelled from the mouth, some makes its way to the gut 85 , 86 . Numerous studies have revealed that microplastics eventually sink to the seafloor and rest in the sediment 87 , 88 , 89 , 90 . Naturally occurring sediment particles occur quite regularly in the guts of these shark species and it is highly likely that many of the ingested microfibres will be excreted alongside natural sediment particles. The potential for either plastic or natural particles to cause internal damage before excretion remains to be tested.
Existing studies have attempted to analyse environmental microplastic contamination in the North-East Atlantic, both on the sea surface and the sediment 68 , 90 . Lusher et al. 68 found that 94% of samples from surface waters in the North-East Atlantic contained what they believed to be potential microplastics, although after further analysis 63% of these appeared to be matt black anthropogenic fibres and not true microplastics. These matt black fibres are similar in description to many of the fibres found in our current study. When analysed under Raman FT-IR, Lusher et al. 68 found they were matched closely with cellulose and rayon, again similar to the cellulose fibres found in this study. In a separate study, Maes et al. 90 identified microplastic particles in 89% of sediment samples from the North Sea and English channel, with most of the plastics considered spheres (microbeads) and fibres 90 , however these authors do not allude to regenerated cellulose fibres in their samples, which may have been present, but not recorded. Given these environmental levels, it should, therefore, be no surprise that approximately 70% of the sharks in this study contained at least 1 contaminant particle.
Analysing the polymer make-up of marine plastics can reveal potential sources, fate and causes for ingestion 23 , 24 . The use of FT-IR spectrometry to analyse environmental samples is a reliable method of determining their polymer make-up 91 , 92 , 93 and should be fundamental to any future study. The polymers we identified largely reflect the results of similar studies in the marine environment 23 , 94 , 95 , 96 and are also similar to polymer diversity of microplastics globally 23 , 66 , 97 , with polypropylene being one of the most widely abundant polymers identified worldwide 23 .
Synthetic cellulose fibres are being recorded in environmental samples across multiple studies 23 , 66 , 88 , 98 , although currently their diverse origins remains somewhat understudied. These anthropogenic fibres made up a third of the analysed contaminants and were identified as regenerated cellulose, such as viscose and rayon, as well as lyocell and cotton, with the likely source of such fibres being textiles or personal hygiene items 19 , 73 , 74 . Spectral libraries for FT-IR set-ups must continue to expand moving forwards, in order to develop reliable databases that are capable of accurately identifying regenerated cellulose fibres within environmental samples.
Estimates show that an average clothes wash of 6 kg can release more than 700,000 fibres into waste water facilities and although some will be retained by these facilities, many will inevitably make their way into the marine environment, often via river systems 19 . These released fibres such as polyester and cotton are globally in-demand between 24–46 million tonnes per year 99 . Interestingly, the fibre lengths identified in the digestive tracts of sharks were similar to that of fibres released upon washing of various textiles under laboratory conditions (see Fig. 1 ), highlighting the washing of clothes as a major route for fibres to enter the environment.
Potential implications
As we only tested a sub-sample of gut content for each animal (20 ml), the proportional incidence of anthropogenic contaminants we report is a conservative estimate. Due to the microscopic size of these synthetic fibres, direct internal organ damage is unlikely, when compared to ingestion of larger macro-plastics, although the ability of small fibres to cause inflammatory damage is acknowledged in other contexts 100 , 101 , 102 . Translocation of relatively large (150 µm) particles can occur across the vertebrate gut via persorption (the passage of particles through the epithelial layers of the gastro-intestinal tract), whilst smaller particles are taken up through normal digestive processes such as pinocytosis and phagocytosis, circulating through the blood and lymph vessels. Thus, there is the opportunity for such circulating particles to enter cells and induce inflammatory damage before being excreted 103 . Fibres of 100–1,000 µm will most likely pass straight through the digestive tract and be excreted with other waste products 23 .
Future research could aim to assess whether certain fibres present exposure risks of associated contaminants and/or persistent organic pollutants 23 , 24 . Certain textiles may contain toxic chemicals such as BPA (bisphenol A) and BPS (bisphenol S) 104 , with both chemicals capable of causing disruption to reproductive and endocrine systems as well as growth suppression in marine taxa, at relatively low doses 105 , 106 , 107 , 108 . Other studies have shown different associated contaminants can present inherent biological risks to various species, including elasmobranchs 34 , 35 , 40 , 109 .
Research has revealed that spiny dogfish and small-spotted catshark are regularly sold in fish and chip shops under pseudonyms such as “Rock”, “Rock salmon” and “Murgey” 110 . If contaminants are able to pass from the digestive tract to the muscle tissue of these shark species, then humans may inadvertently be consuming these pollutants. Although currently there is no conclusive evidence to suggest these pollutants present inherent health risks to humans, we recommend further research to investigate the presence or absence of these contaminant particles in the muscle tissues of these shark species and other fish consumed across the world.
Conclusions
This study presents the first evidence of microplastics and anthropogenic fibre contaminants in a range of native UK demersal shark species. Although not occurring in as high levels as in other marine megafauna, the presence of anthropogenic particles in these marine species highlights their ubiquitous nature. Although highly unlikely, neither individual nor population level effects of this level of contamination are known. Due to these low levels of ingestion, these species are perhaps not ideal candidates to be used as bio-indicators for marine pollution in demersal habitats when compared to other bony fish species. Nonetheless, if inorganic pollutants can attach to these microfibres, alongside a future increase in their prevalence throughout the marine environment, biological side-effects may occur. Further research on the sources and pathways of anthropogenic fibres may inform policy to reduce their overall prevalence in the environment. By limiting their production in everyday products (through supporting reduction, reuse and replacement of fibre-generating materials from the resource flow) and implementing strategies to prevent their initial entry into the oceans there lies the potential to dramatically reduce the occurrence of microfibres in the marine environment and across food webs.
Data availability
Data is available from the British Oceanographic Centre (Marine).
Denuncio, P. et al. Plastic ingestion in Franciscana dolphins, Pontoporia blainvillei (Gervais and d’Orbigny, 1844), from Argentina. Mar. Pollut. Bull. 62 , 1836–1841 (2011).
CAS PubMed Google Scholar
Ryan, P. G., Shomura, R. S. & Godfrey, M. L. The marine plastic debris problem of Southern Africa: types of debris, their environmental effects, and control measures. Proc. Second Int. Conf. Mar. debris 623–634 (1989). doi:NOAA-TM-NMFS-SWFSC-15
Cole, M., Lindeque, P., Halsband, C. & Galloway, T. S. Microplastics as contaminants in the marine environment: A review. Mar. Pollut. Bull. 62 , 2588–2597 (2011).
Galloway, T. S. & Lewis, C. N. Marine microplastics spell big problems for future generations. Proc. Natl. Acad. Sci. 113 , 2331–2333 (2016).
ADS CAS PubMed Google Scholar
Eriksen, M. et al. Plastic Pollution in the World’s Oceans: More than 5 Trillion Plastic Pieces Weighing over 250,000 Tons Afloat at Sea. PLoS ONE 9 , e111913 (2014).
ADS PubMed PubMed Central Google Scholar
Jambeck, J. R. et al. Marine pollution. Plastic waste inputs from land into the ocean. Science 347 , 768–71 (2015).
Bucci, K., Tulio, M. & Rochman, C. What is known and unknown about the effects of plastic pollution: a meta-analysis and systematic review. Ecol. Appl. https://doi.org/10.1002/eap.2044 (2019).
Article Google Scholar
Ryan, P. G., Moore, C. J., Van Franeker, J. A. & Moloney, C. L. Monitoring the abundance of plastic debris in the marine environment. Philos. Trans. R. Soc. B Biol. Sci. 364 , 1999–2012 (2009).
CAS Google Scholar
Barnes, D. K. A., Galgani, F., Thompson, R. C. & Barlaz, M. Accumulation and fragmentation of plastic debris in global environments. Philos. Trans. R. Soc. B Biol. Sci. 364 , 1985–1998 (2009).
Arthur, C., Baker, J. E. & Bamford, H. A. Proceedings of the International Research Workshop on the Occurrence, Effects, and Fate of Microplastic Marine Debris, September 9–11, 2008, University of Washington Tacoma, Tacoma, WA, USA. in (2009).
Sussarellu, R. et al. Oyster reproduction is affected by exposure to polystyrene microplastics. Proc. Natl. Acad. Sci. USA 113 , 2430–2435 (2016).
Galloway, T. S., Cole, M. & Lewis, C. Interactions of microplastic debris throughout the marine ecosystem. Nat. Ecol. Evol. 1 , 0116 (2017).
Google Scholar
Koelmans, A. A., Besseling, E. & Foekema, E. M. Leaching of plastic additives to marine organisms. Environ. Pollut. 187 , 49–54 (2014).
Avio, C. G., Gorbi, S. & Regoli, F. Plastics and microplastics in the oceans: From emerging pollutants to emerged threat. Mar. Environ. Res. 128 , 2–11 (2017).
Browne, M. A., Galloway, T. & Thompson, R. Microplastic-an emerging contaminant of potential concern?. Integr. Environ. Assess. Manag. 3 , 559–561 (2007).
PubMed Google Scholar
De Falco, F. et al. Evaluation of microplastic release caused by textile washing processes of synthetic fabrics. Environ. Pollut. 236 , 916–925 (2018).
Carney Almroth, B. M. et al. Quantifying shedding of synthetic fibers from textiles; a source of microplastics released into the environment. Environ. Sci. Pollut. Res. 25 , 1191–1199 (2018).
Hernandez, E., Nowack, B. & Mitrano, D. M. Polyester textiles as a source of microplastics from households: a mechanistic study to understand microfiber release during washing. Environ. Sci. Technol. 51 , 7036–7046 (2017).
Napper, I. E. & Thompson, R. C. Release of synthetic microplastic plastic fibres from domestic washing machines: Effects of fabric type and washing conditions. Mar. Pollut. Bull. 112 , 39–45 (2016).
Murphy, F., Ewins, C., Carbonnier, F. & Quinn, B. Wastewater treatment works (WwTW) as a source of microplastics in the aquatic environment. Environ. Sci. Technol. 70 , 1–5 (2016).
Nadal, M. A., Alomar, C. & Deudero, S. High levels of microplastic ingestion by the semipelagic fish bogue Boops boops (L.) around the Balearic Islands. Environ. Pollut. 214 , 517–523 (2016).
Neves, D., Sobral, P., Ferreira, J. L. & Pereira, T. Ingestion of microplastics by commercial fish off the Portuguese coast. Mar. Pollut. Bull. 101 , 119–126 (2015).
Duncan, E. M. et al. Microplastic ingestion ubiquitous in marine turtles. Glob. Chang. Biol. 25 , 744–752 (2018).
Nelms, S. E., Galloway, T. S., Godley, B. J., Jarvis, D. S. & Lindeque, P. K. Investigating microplastic trophic transfer in marine top predators. Environ. Pollut. 238 , 999–1007 (2018).
Schuyler, Q., Hardesty, B. D., Wilcox, C. & Townsend, K. Global analysis of anthropogenic debris ingestion by sea turtles. Conserv. Biol. 28 , 129–139 (2014).
Setälä, O., Fleming-Lehtinen, V. & Lehtiniemi, M. Ingestion and transfer of microplastics in the planktonic food web. Environ. Pollut. 185 , 77–83 (2014).
Murray, F. & Cowie, P. R. Plastic contamination in the decapod crustacean Nephrops norvegicus (Linnaeus, 1758). Mar. Pollut. Bull. 62 , 1207–1217 (2011).
Devriese, L. I. et al. Microplastic contamination in brown shrimp ( Crangon crangon , Linnaeus 1758) from coastal waters of the Southern North Sea and Channel area. Mar. Pollut. Bull. 98 , 179–187 (2015).
Botterell, Z. L. R. et al. Bioavailability and effects of microplastics on marine zooplankton: a review. Environ. Pollut. 245 , 98–110 (2019).
Gandara e Silva, P. P., Nobre, C. R., Resaffe, P., Pereira, C. D. S. & Gusmão, F. Leachate from microplastics impairs larval development in brown mussels. Water Res. 106 , 364–370 (2016).
Cole, M., Lindeque, P., Fileman, E., Halsband, C. & Galloway, T. S. The impact of polystyrene microplastics on feeding, function and fecundity in the marine copepod Calanus helgolandicus . Environ. Sci. Technol. 49 , 1130–1137 (2015).
Stelfox, M., Hudgins, J. & Sweet, M. A review of ghost gear entanglement amongst marine mammals, reptiles and elasmobranchs. Mar. Pollut. Bull. 111 , 6–17 (2016).
Parton, K., Galloway, T. & Godley, B. Global review of shark and ray entanglement in anthropogenic marine debris. Endanger. Species Res. 39 , 173–190 (2019).
Fossi, M. C. et al. Are whale sharks exposed to persistent organic pollutants and plastic pollution in the Gulf of California (Mexico)? First ecotoxicological investigation using skin biopsies. Comp. Biochem. Physiol. Part C Toxicol. Pharmacol. 199 , 48–58 (2017).
Germanov, E. S., Marshall, A. D., Bejder, L., Fossi, M. C. & Loneragan, N. R. Microplastics: no small problem for filter-feeding megafauna. Trends Ecol. Evol. 33 , 227–232 (2018).
Smith, L. E. Plastic ingestion by Scyliorhinus canicula trawl captured in the North Sea. Mar. Pollut. Bull. 130 , 6–7 (2018).
Valente, T. et al. Exploring microplastic ingestion by three deep-water elasmobranch species: a case study from the Tyrrhenian Sea. Environ. Pollut. 253 , 342–350 (2019).
Alomar, C. & Deudero, S. Evidence of microplastic ingestion in the shark Galeus melastomus Rafinesque, 1810 in the continental shelf off the western Mediterranean Sea. Environ. Pollut. 223 , 223–229 (2017).
Bernardini, I., Garibaldi, F., Canesi, L., Fossi, M. C. & Baini, M. First data on plastic ingestion by blue sharks ( Prionace glauca ) from the Ligurian Sea (North-Western Mediterranean Sea). Mar. Pollut. Bull. 135 , 303–310 (2018).
Fossi, M. C. et al. Large filter feeding marine organisms as indicators of microplastic in the pelagic environment: the case studies of the Mediterranean basking shark ( Cetorhinus maximus ) and fin whale ( Balaenoptera physalus ). Mar. Environ. Res. 100 , 17–24 (2014).
Germanov, E. S. et al. Microplastics on the menu: plastics pollute indonesian manta ray and whale shark feeding grounds. Front. Mar. Sci. 6 , 679 (2019).
Sulikowski, J. et al. Use of satellite tags to reveal the movements of spiny dogfish Squalus acanthias in the western North Atlantic Ocean. Mar. Ecol. Prog. Ser. 418 , 249–254 (2010).
ADS Google Scholar
Sims, D., Nash, J. & Morritt, D. Movements and activity of male and female dogfish in a tidal sea lough: alternative behavioural strategies and apparent sexual segregation. Mar. Biol. 139 , 1165–1175 (2001).
Ellis, J. R., Pawson, M. G. & Shackley, S. E. The comparative feeding ecology of six species of shark and four species of ray (Elasmobranchii) In The North-East Atlantic. J. Mar. Biol. Assoc. UK 76 , 89 (2009).
Fordham, S., Fowler, S. L., Coelho, R. P., Goldman, K. & Francis, M. P. Squalus acanthias. The IUCN Red List of Threatened Species 2016 (2016). Available at: https://www.iucnredlist.org/species/91209505/2898271 . (Accessed: 2nd December 2019)
Domi, N., Bouquegneau, J. M. & Das, K. Feeding ecology of five commercial shark species of the Celtic Sea through stable isotope and trace metal analysis. Mar. Environ. Res. 60 , 551–569 (2005).
Hammond, T. R. & Ellis, J. R. Bayesian assessment of northeast atlantic spurdog using a stock production model & with prior for intrinsic population growth rate set by demographic methods. J. Northw. Atl. Fish. Sci 35 , 299–308 (2004).
Revill, A. S., Dulvy, N. K. & Holst, R. The survival of discarded lesser-spotted dogfish ( Scyliorhinus canicula ) in the Western English Channel beam trawl fishery. Fish. Res. 71 , 121–124 (2005).
Bellas, J., Martínez-Armental, J., Martínez-Cámara, A., Besada, V. & Martínez-Gómez, C. Ingestion of microplastics by demersal fish from the Spanish Atlantic and Mediterranean coasts. Mar. Pollut. Bull. 109 , 55–60 (2016).
Anastasopoulou, A., Mytilineou, C., Smith, C. J. & Papadopoulou, K. N. Plastic debris ingested by deep-water fish of the Ionian Sea (Eastern Mediterranean). Deep Sea Res. Part I Oceanogr. Res. Pap. 74 , 11–13 (2013).
Murphy, F., Russell, M., Ewins, C. & Quinn, B. The uptake of macroplastic & microplastic by demersal & pelagic fish in the Northeast Atlantic around Scotland. Mar. Pollut. Bull. 122 , 353–359 (2017).
Rummel, C. D. et al. Plastic ingestion by pelagic and demersal fish from the North Sea and Baltic Sea. Mar. Pollut. Bull. 102 , 134–141 (2016).
Foekema, E. M. et al. Plastic in north sea fish. Environ. Sci. Technol. 47 , 8818–8824 (2013).
Lusher, A. L., O’Donnell, C., Officer, R. & O’Connor, I. Microplastic interactions with North Atlantic mesopelagic fish. ICES J. Mar. Sci. J. du Cons. 73 , 1214–1225 (2016).
Dehaut, A. et al. Microplastics in seafood: Benchmark protocol for their extraction and characterization. Environ. Pollut. 215 , 223–233 (2016).
Kühn, S. et al. The use of potassium hydroxide (KOH) solution as a suitable approach to isolate plastics ingested by marine organisms. Mar. Pollut. Bull. 115 , 86–90 (2017).
Bessa, F. et al. Occurrence of microplastics in commercial fish from a natural estuarine environment. Mar. Pollut. Bull. 128 , 575–584 (2018).
Venables, W. & Ripley, B. Modern applied statistics with S 4th edn. (Springer, Berlin, 2002).
MATH Google Scholar
R Core Team. R: A language and environment for statistical computing. (2018).
Barton, K. MuMIn: Multi-model inference. (2015).
Richards, S. A., Whittingham, M. J. & Stephens, P. A. Model selection and model averaging in behavioural ecology: the utility of the IT-AIC framework. Behav. Ecol. Sociobiol. 65 , 77–89 (2011).
Cliff, G., Dudley, S. F. J., Ryan, P. G. & Singletonc, N. Large sharks and plastic debris in KwaZulu-Natal, South Africa. Mar. Freshw. Res. 53 , 575–581 (2002).
Deudero, S. & Alomar, C. Mediterranean marine biodiversity under threat: Reviewing influence of marine litter on species. Mar. Pollut. Bull. 98 , 58–68 (2015).
Cózar, A. et al. Plastic accumulation in the mediterranean sea. PLoS ONE 10 , 1–12 (2015).
Suaria, G. et al. The Mediterranean Plastic Soup: synthetic polymers in Mediterranean surface waters. Sci. Rep. 6 , 37551 (2016).
ADS CAS PubMed PubMed Central Google Scholar
Gago, J., Carretero, O., Filgueiras, A. V. & Viñas, L. Synthetic microfibers in the marine environment: A review on their occurrence in seawater and sediments. Mar. Pollut. Bull. 127 , 365–376 (2018).
Compa, M., Ventero, A., Iglesias, M. & Deudero, S. Ingestion of microplastics and natural fibres in Sardina pilchardus (Walbaum, 1792) and Engraulis encrasicolus (Linnaeus, 1758) along the Spanish Mediterranean coast. Mar. Pollut. Bull. 128 , 89–96 (2018).
Lusher, A. L., Burke, A., O’Connor, I. & Officer, R. Microplastic pollution in the Northeast Atlantic Ocean: Validated and opportunistic sampling. Mar. Pollut. Bull. 88 , 325–333 (2014).
Steer, M., Cole, M., Thompson, R. C. & Lindeque, P. K. Microplastic ingestion in fish larvae in the western English Channel. Environ. Pollut. 226 , 250–259 (2017).
Lusher, A. Microplastics in the Marine Environment: Distribution, Interactions and Effects. in Marine Anthropogenic Litter 245–307 (Springer International Publishing, 2015). https://doi.org/10.1007/978-3-319-16510-3_10 .
Zhu, J. et al. Cetaceans and microplastics: First report of microplastic ingestion by a coastal delphinid Sousa chinensis . Sci. Total Environ. 659 , 649–654 (2019).
Welden, N. A. & Cowie, P. R. Degradation of common polymer ropes in a sublittoral marine environment. Mar. Pollut. Bull. 118 , 248–253 (2017).
Hartline, N. L. et al. Microfiber Masses Recovered from Conventional Machine Washing of New or Aged Garments. Environ. Sci. Technol. 50 , 11532–11538 (2016).
Salvador Cesa, F., Turra, A. & Baruque-Ramos, J. Synthetic fibers as microplastics in the marine environment: a review from textile perspective with a focus on domestic washings. Sci. Total Environ. 598 , 1116–1129 (2017).
Wagner, S. et al. Tire wear particles in the aquatic environment—a review on generation, analysis, occurrence, fate and effects. Water Res. 139 , 83–100 (2018).
Van Cauwenberghe, L. & Janssen, C. R. Microplastics in bivalves cultured for human consumption. Environ. Pollut. 193 , 65–70 (2014).
Watts, A. J. R. et al. Uptake and retention of microplastics by the shore crab Carcinus maenas . Environ. Sci. Technol. 48 , 8823–8830 (2014).
Browne, M. A., Dissanayake, A., Galloway, T. S., Lowe, D. M. & Thompson, R. C. Ingested Microscopic Plastic Translocates to the Circulatory System of the Mussel, Mytilus edulis (L.). Environ. Sci. Technol. 42 , 5026–5031 (2008).
Farrell, P. & Nelson, K. Trophic level transfer of microplastic: Mytilus edulis (L.) to Carcinus maenas (L.). Environ. Pollut. 177 , 1–3 (2013).
Saldanha, L., Almeida, A. J., Andrade, F. & Guerreiro, J. Observations on the Diet of Some Slope Dwelling Fishes of Southern Portugal. Int. Rev. der gesamten Hydrobiol. und Hydrogr. 80 , 217–234 (1995).
Martinho, F., Sá, C., Falcão, J., Cabral, H. N. & Pardal, M. Â. Comparative feeding ecology of two elasmobranch species, Squalus blainville and Scyliorhinus canicula, off the coast of Portugal. Fish. Bull. 110 , 71–84 (2012).
Ogonowski, M. et al. Microplastic Intake, Its Biotic Drivers, and Hydrophobic Organic Contaminant Levels in the Baltic Herring. Front. Environ. Sci. 7 , 134 (2019).
Wilga, C. & Motta, P. Conservation and variation in the feeding mechanism of the spiny dogfish squalus acanthias. J. Exp. Biol. 201 , (1998).
Huber, D. R. & Motta, P. J. Comparative analysis of methods for determining bite force in the spiny dogfishSqualus acanthias. J. Exp. Zool. 301A , 26–37 (2004).
Kalmijn, A. J. The Electric Sense of Sharks and Rays. J. Exp. Biol. 55 , (1971).
Smith, J. W. & Merriner, J. V. Food habits and feeding behavior of the Cownose Ray, Rhinoptera bonasus , in Lower Chesapeake Bay. Estuaries 8 , 305 (1985).
Martin, J., Lusher, A., Thompson, R. C. & Morley, A. The deposition and accumulation of microplastics in marine sediments and bottom water from the Irish continental shelf. Sci. Rep. 7 , 10772 (2017).
Woodall, L. C. et al. The deep sea is a major sink for microplastic debris. R. Soc. Open Sci. 1 , 140317 (2014).
Ling, S. D., Sinclair, M., Levi, C. J., Reeves, S. E. & Edgar, G. J. Ubiquity of microplastics in coastal seafloor sediments. Mar. Pollut. Bull. 121 , 104–110 (2017).
Maes, T. et al. Microplastics baseline surveys at the water surface and in sediments of the North-East Atlantic. Front. Mar. Sci. 4 , 135 (2017).
Shim, W. J., Hong, S. H. & Eo, S. E. Identification methods in microplastic analysis: a review. Anal. Methods 9 , 1384–1391 (2017).
Jung, M. R. et al. Validation of ATR FT-IR to identify polymers of plastic marine debris, including those ingested by marine organisms. Mar. Pollut. Bull. 127 , 704–716 (2018).
Hidalgo-Ruz, V., Gutow, L., Thompson, R. C. & Thiel, M. Microplastics in the marine environment: a review of the methods used for identification and quantification. Environ. Sci. Technol. 46 , 3060–3075 (2012).
Remy, F. et al. When microplastic is not plastic: the ingestion of artificial cellulose fibers by macrofauna living in seagrass macrophytodetritus. Environ. Sci. Technol. 49 , 11158–11166 (2015).
Cai, L. et al. Characteristic of microplastics in the atmospheric fallout from Dongguan city, China: preliminary research and first evidence. Environ. Sci. Pollut. Res. 24 , 24928–24935 (2017).
Comnea-Stancu, I. R., Wieland, K., Ramer, G., Schwaighofer, A. & Lendl, B. On the identification of rayon/viscose as a major fraction of microplastics in the marine environment: discrimination between natural and manmade cellulosic fibers using fourier transform infrared spectroscopy. Appl. Spectrosc. 71 , 939–950 (2017).
White, E. M. et al. Ingested micronizing plastic particle compositions and size distributions within stranded post-hatchling sea turtles. Environ. Sci. Technol. 52 , 10307–10316 (2018).
Lusher, A. L., McHugh, M. & Thompson, R. C. Occurrence of microplastics in the gastrointestinal tract of pelagic and demersal fish from the English Channel. Mar. Pollut. Bull. 67 , 94–99 (2013).
Ladewig, S. M., Bao, S. & Chow, A. T. Natural fibers: a missing link to chemical pollution dispersion in aquatic environments. Environ. Sci. Technol. 49 , 12609–12610 (2015).
Pham, C. K. et al. Plastic ingestion in oceanic-stage loggerhead sea turtles ( Caretta caretta ) off the North Atlantic subtropical gyre. Mar. Pollut. Bull. 121 , 222–229 (2017).
Ivar do Sul, J. A. & Costa, M. F. The present and future of microplastic pollution in the marine environment. Environ. Pollut. 185 , 352–364 (2014).
Ryan, P. G. et al. Impacts of plastic ingestion on post-hatchling loggerhead turtles off South Africa. Mar. Pollut. Bull. 107 , 155–160 (2016).
Galloway, T. S. Micro- and Nano-plastics and Human Health. in Marine Anthropogenic Litter 343–366 (Springer International Publishing, 2015). https://doi.org/10.1007/978-3-319-16510-3_13
Xue, J., Liu, W. & Kannan, K. Bisphenols, benzophenones, and bisphenol a diglycidyl ethers in textiles and infant clothing. Environ. Sci. Technol. 51 , 5279–5286 (2017).
Park, S., Hong, Y., Lee, J., Kho, Y. & Ji, K. Chronic effects of bisphenol S and bisphenol SIP on freshwater waterflea and ecological risk assessment. Ecotoxicol. Environ. Saf. 185 , 109694 (2019).
Huang, Q. et al. Embryonic exposure to low concentration of bisphenol A affects the development of Oryzias melastigma larvae. Environ. Sci. Pollut. Res. 19 , 2506–2514 (2012).
Huang, Q. et al. New insights into the metabolism and toxicity of bisphenol A on marine fish under long-term exposure. Environ. Pollut. 242 , 914–921 (2018).
Aluru, N., Leatherland, J. F. & Vijayan, M. M. Bisphenol A in oocytes leads to growth suppression and altered stress performance in juvenile rainbow trout. PLoS ONE 5 , e10741 (2010).
Rochman, C. M., Kurobe, T., Flores, I. & Teh, S. J. Early warning signs of endocrine disruption in adult fish from the ingestion of polyethylene with and without sorbed chemical pollutants from the marine environment. Sci. Total Environ. 493 , 656–661 (2014).
Hobbs, C. A. D., Potts, R. W. A., Bjerregaard Walsh, M., Usher, J. & Griffiths, A. M. Using DNA Barcoding to Investigate Patterns of Species Utilisation in UK Shark Products Reveals Threatened Species on Sale. Sci. Rep. 9 , 1028 (2019).
Download references
Acknowledgements
The authors thank Dr Sarah Nelms and Dr Emily Duncan for their help with laboratory analysis and general advice, illustrator Lucie Jones for her elasmobranch drawings and the anonymous reviewers and the editor, whose comments greatly improved the manuscript. This work was undertaken with approval of the University of Exeter Animal Ethics Committee. NERC Grant NE/S003975/1.
Author information
Authors and affiliations.
Centre for Ecology and Conservation, College of Life and Environmental Sciences, University of Exeter, Penryn Campus, Penryn, TR10 9EZ, UK
Kristian J. Parton, Brendan J. Godley & Lucy C. M. Omeyer
Greenpeace Research Laboratories, School of Biosciences, Innovation Centre Phase 2, University of Exeter, Exeter, UK
David Santillo
Textile Technology Research Group, School of Design, University of Leeds, Leeds, LS2 9JT, UK
Muhammad Tausif
Biosciences, College of Life and Environmental Sciences, Geoffrey Pope Building, University of Exeter, Stocker Road, Exeter, Devon, EX4 4QD, UK
Tamara S. Galloway
You can also search for this author in PubMed Google Scholar
Contributions
T.G. oversaw training of K.P. in lab skills required for analysis. K.P. conducted processing of samples and led data collection, D.S. helped conduct analysis of samples using FT-IR, M.T. analysed fibres to elucidate their origins and helped create Fig. 1 . B.G. oversaw creation of figures and tables. L.O. conducted statistical analysis. K.P. B.G., T.G. and M.T. wrote the main manuscript text. All authors reviewed the manuscript.
Corresponding author
Correspondence to Kristian J. Parton .
Ethics declarations
Competing interests.
The authors declare no competing interests.
Additional information
Publisher's note.
Springer Nature remains neutral with regard to jurisdictional claims in published maps and institutional affiliations.
Supplementary information
Supplementary figure s1., supplementary figure s2., supplementary figure s3., supplementary figure s4., supplementary figure s5., supplementary figure s6., supplementary figure s7., supplementary figure s8., supplementary materials., supplementary table s1., supplementary table s2., rights and permissions.
Open Access This article is licensed under a Creative Commons Attribution 4.0 International License, which permits use, sharing, adaptation, distribution and reproduction in any medium or format, as long as you give appropriate credit to the original author(s) and the source, provide a link to the Creative Commons license, and indicate if changes were made. The images or other third party material in this article are included in the article’s Creative Commons license, unless indicated otherwise in a credit line to the material. If material is not included in the article’s Creative Commons license and your intended use is not permitted by statutory regulation or exceeds the permitted use, you will need to obtain permission directly from the copyright holder. To view a copy of this license, visit http://creativecommons.org/licenses/by/4.0/ .
Reprints and permissions
About this article
Cite this article.
Parton, K.J., Godley, B.J., Santillo, D. et al. Investigating the presence of microplastics in demersal sharks of the North-East Atlantic. Sci Rep 10 , 12204 (2020). https://doi.org/10.1038/s41598-020-68680-1
Download citation
Received : 13 December 2019
Accepted : 26 June 2020
Published : 22 July 2020
DOI : https://doi.org/10.1038/s41598-020-68680-1
Share this article
Anyone you share the following link with will be able to read this content:
Sorry, a shareable link is not currently available for this article.
Provided by the Springer Nature SharedIt content-sharing initiative
This article is cited by
Understanding microplastic pollution of marine ecosystem: a review.
- Swati Sharma
- Aprajita Bhardwaj
- Anita Saini
Environmental Science and Pollution Research (2023)
Overview of microplastics in the environment: type, source, potential effects and removal strategies
- Risky Ayu Kristanti
- Tony Hadibarata
- Ariani Hatmanti
Bioprocess and Biosystems Engineering (2023)
Impact of waste of COVID-19 protective equipment on the environment, animals and human health: a review
- Yanping Cheng
Environmental Chemistry Letters (2022)
Understanding of environmental pollution and its anthropogenic impacts on biological resources during the COVID-19 period
- Jiban Kumar Behera
- Pabitra Mishra
- Bhaskar Behera
Environmental Science and Pollution Research (2022)
Effect of microplastics in water and aquatic systems
- Merlin N Issac
- Balasubramanian Kandasubramanian
Environmental Science and Pollution Research (2021)
By submitting a comment you agree to abide by our Terms and Community Guidelines . If you find something abusive or that does not comply with our terms or guidelines please flag it as inappropriate.
Quick links
- Explore articles by subject
- Guide to authors
- Editorial policies
Sign up for the Nature Briefing: Anthropocene newsletter — what matters in anthropocene research, free to your inbox weekly.


An official website of the United States government
The .gov means it’s official. Federal government websites often end in .gov or .mil. Before sharing sensitive information, make sure you’re on a federal government site.
The site is secure. The https:// ensures that you are connecting to the official website and that any information you provide is encrypted and transmitted securely.
- Publications
- Account settings
Preview improvements coming to the PMC website in October 2024. Learn More or Try it out now .
- Advanced Search
- Journal List
- PMC10276984

Habitat-use of the vulnerable Atlantic Nurse Shark: a review
Vanessa brito bettcher.
1 Theoretical and Applied Ichthyology Lab (LICTA), Federal University of the State of Rio de Janeiro, Rio de Janeiro, RJ, Brazil
2 Postgraduate Program in Ecology and Evolution (PPGEE), Rio de Janeiro State University (UERJ), Rio de Janeiro, RJ, Brazil
Ana Clara Sampaio Franco
3 Postgraduate Program in Neotropical Biodiversity (PPGBIO), Universidade Federal do Estado do Rio de Janeiro (UNIRIO), Rio de Janeiro, RJ, Brazil
Luciano Neves dos Santos
Associated data.
The following information was supplied regarding data availability:
The lists of the studies obtained through a literature review of the distribution and habitat association of the Atlantic Nurse Shark Ginglymostoma cirratum are available in the Supplemental Files .
Human activities have led to the loss of critical habitats for aquatic species at such an accelerated rate that habitat modification is considered a leading threat to biodiversity. Sharks and rays are considered the second most threatened group of vertebrates that have also suffered from habitat loss, especially in nursery grounds and reef-associated species. In this sense, actions toward the conservation of critical grounds for species survival are urgently needed, especially for those threatened with extinction. This study aimed to gather and provide information on the worldwide distribution and habitat association of the ‘vulnerable’ Atlantic Nurse Shark Ginglymostoma cirratum through a literature review performed at the Dimensions research database. A total of 30 studies published between 1950 and 2021 were retained since they defined at least the type of habitat in which G. cirratum was associated. Most studies covered the Floridian ecoregion, where G. cirratum is more common and abundant. Reefs, seagrass, sandy, rocky, mangrove, and macroalgae accounted for the majority of habitat associations, with a higher diversity of habitats detected within marine protected areas (MPAs). Ginglymostoma cirratum was recorded at a maximum depth of 75 m, temperatures ranging from 25 °C to 34 °C, and salinities between 31 and 38 ppt. Neonates were associated with shallower habitats (<20 m), mostly reefs, rocks, macroalgae, sandy shores, and seagrass, in an average temperature of 26 °C and salinity of 36 ppt. Breeding events and habitats were reported by 11 studies, 72.7% of them in shallow waters, mostly inside MPAs (90.9%). Our findings highlighted the key role played by MPAs in protecting essential grounds for threatened species, such as the Atlantic Nurse Shark. Major ecoregions ( e.g ., the Eastern Atlantic) are still underrepresented in the scientific literature as long as studies aim specifically to assess G. cirratum habitat association. Thus, further insights into the essential habitats needed to conserve the Atlantic Nurse Shark can still emerge from future studies. Considering the recent IUCN extinction risk status change in G. cirratum ( i.e ., Data Deficient to ‘Vulnerable’ ), new conservation measures that integrate habitat protection and management are urgently needed and should consider the data collected herein.
Introduction
Species occurrence is an expression of the “fundamental niche” which reflects the physiological limits imposed for a given species by climatic and chemical parameters ( Soberón & Peterson, 2005 ). Within favorable conditions, species select essential habitats based on their evolutionary history, but also in pursuit of prey abundance, predator risk, and refuge availability ( Soberón & Peterson, 2005 ). The assessment of species-habitat associations enables modeling species’ potential distribution, especially for endangered species, investigating possible causes of decline, or even predicting the effects of future habitat alterations on species distributions, supporting the creation of conservation plans that are more accurate under a future of global changes ( Redhead et al., 2016 ). Thus, defining the physical conditions that enable species occurrence is essential to understanding the nature of those associations. Unlike in terrestrial systems, knowledge of marine habitat alteration effects is moving forward very slowly and remains barely known for most species, despite these systems being constantly subjected to several human impacts ( e.g ., fisheries, pollution, and acidification) ( Airoldi, Balata & Beck, 2008 ).
Habitat is also a mediating factor underlying species co-occurrence, which reflects in increased reef fish abundance and richness in the presence of more rich and complex habitats ( Chittaro, 2004 ). However, many human activities ( e.g ., urbanization, fisheries, and pollution) have been interfering with the quality, diversity, availability, and complexity of habitats in aquatic ecosystems. Consequently, these human activities also interfere with the composition and structure of fish assemblages, by potentially disrupting competitive and mutualistic fish relationships. Many of these complex interactions are the same which support ecosystem services, such as fisheries, erosion control, flooding, food production, and tourism, among others ( Dulvy, Sadovy & Reynolds, 2003 ; Worm et al., 2006 ; Capitani et al., 2021 ). Elasmobranchs, mainly medium and large-size species, are commonly known for their role in structuring and connecting food chains and ecosystems such as through nutrient cycling, habitat modification, and biocontrol of weak and sick individuals, and invasive species by reef-associated mesopredators ( Estes et al., 2016 ; Roff et al., 2016 ; Jorgensen et al., 2022 ). However, sharks and rays are among the most threatened vertebrates today, with 387 species listed as threatened in the IUCN Red List ( Bascompte, Melian & Sala, 2005 ; Desbiens et al., 2021 ; Dulvy et al., 2021 ). In addition to fishing, which currently threatens 100% of those species, the group is threatened by habitat loss (31.2% of the threatened chondrichthyans), climate change, and pollution ( Dulvy et al., 2021 ). Threats to elasmobranch habitat are represented by several categories in the IUCN classification. Still, they have potential synergistic effects, such as climate change that not only influence species’ thermal affinity and, consequently, their distribution but also threaten habitat conservation, leading to habitat degradation through coral bleaching ( Dulvy et al., 2021 ).
Recently listed as ‘vulnerable’ (VU) on the IUCN Red List, the Atlantic Nurse Shark, Ginglymostoma cirratum , was included in this category due to threats mainly imposed by fishing and continuous habitat decline, both in extension and quality ( Carlson et al., 2021 ; Ward-Paige et al., 2010 ). Therefore, the protection of essential areas is one of the conservation actions indicated for the protection of this species ( Carlson et al., 2021 ), which frequently is achieved through the implementation of protected areas and no-take zones. Marine protected areas (MPAs) are often used as a strategy for elasmobranch conservation, especially to reduce the impact of fisheries, but also to preserve essential ecosystems for species at their different life stages ( MacKeracher, Diedrich & Simpfendorfer, 2019 ). The urgency to identify and protect essential habitats for the species has been evidenced. The Shark Special Protection Zone in the Dry Tortugas’ courtship and the mating ground is one example of an MPA proposed by Carrier & Pratt (1998) that is closed for marine boat traffic during the breeding season of the Atlantic Nurse Shark. The success is evidenced by the mating site fidelity of some individuals ( Pratt et al., 2022 ). Other evidence of MPAs’ importance to protect the Atlantic Nurse Shark is the recent discovery of their occurrence in its southernmost distribution, in a mosaic of no-take and sustainable use MPAs of São Paulo, Brazil, after being considered locally extinct ( Rosa et al., 2006 ; Santos et al., 2023 ). Even though they have been proven to be essential for species protection, these areas are often fewer and smaller than necessary to cover the home range of certain species, including the Atlantic Nurse Shark ( Ward-Paige, 2017 ; Dwyer et al., 2020 ), and are subjected to several political limitations, which may undermine their potential to protect threatened species. Dwyer et al. (2020) showed that 50 km long MPAs would be necessary to protect 50% of all Atlantic Nurse Shark local individuals from fishing. This is because contrary to what has been believed that this is a sedentary species, the Atlantic Nurse Shark showed mean weekly dispersal distances of 8.02 ± 5.03 km ( Dwyer et al., 2020 ). Other records have also evidenced long-distance migrations of the species (541 km, Kohler & Turner, 2001 ; 335 km, Pratt et al., 2018 ) highlighting the need for a regional perspective on the protection of the Atlantic Nurse Shark.
The Atlantic Nurse Shark occurs from North Carolina (USA) to southern Brazil, colonizing several coastal ecosystems, such as sandy flats, coral reefs, seagrasses, and mangroves. However, the specific role played by each habitat during the life cycle of the species ( i.e ., mating, feeding, and recruitment) is essential to understand the distribution patterns and advising conservation agencies towards protection measures for this species. This is particularly crucial since the same habitats that are essential to G. cirratum are those that have been threatened by urbanization and ocean warming ( Carlson et al., 2021 ; Capitani et al., 2021 ; Garzon et al., 2021 ), posing an even greater threat to this ‘vulnerable’ species. Considering (a) the challenge to obtain primary data on habitat use of such a largely distributed species, (b) habitat modification as one of the major threats to biodiversity and ecosystem functioning, (c) the conservation actions indicated for G. cirratum , and (d) the current demand for scientific data on ecosystem assessment, a literature review was carried out to provide information on ecological factors associated with the occurrence of G. cirratum populations and to point out and discuss priority areas for species conservation.
Survey methodology
A literature review was performed at the Dimensions research database to assess the geographical distribution and essential habitats associated with the Atlantic Nurse Shark G. cirratum . The Dimensions research database is a recent alternative to perform reviews of the scientific literature more comprehensive than Web of Science and Scopus and less general than Google Scholar ( Hook, Porter & Herzog, 2018 ; Martín-Martín et al., 2021 ; Singh et al., 2021 ). We used secondary data to address two questions: (1) the geographical distribution of the Atlantic Nurse Sharks; and (2) the type of habitats associated with the populations of the Atlantic Nurse Sharks. To achieve the first goal, a literature review was performed on 26th January 2021 using as keywords the scientific name of the species and its synonyms according to Froese & Pauly (2021) (see Appendix S1 for further details on keywords). We retained only articles that provided the exact location where the Atlantic Nurse Sharks were sampled or observed. To assess the association of the G. cirratum with habitats we performed another search on the 10th of August 2021 (see Appendix S2 for further details on keywords). We retained all the studies that described the habitat with which the Atlantic Nurse Shark was associated. The studies listed in the supporting information (SI) of the review performed by Osgood & Baum (2015) were also screened. Studies that did not describe the habitat of the study site where the sharks were collected/observed and those that we could not access were excluded from our database.
The habitat types were divided into six categories: Macroalgae (algae, benthic algae, seaweed, brown algae, and green algae); Mangrove (mangrove lined barrier islands, mangrove forests, mangrove-fringed bay, mangrove barrier, and mangrove islands); Reefs (reefs, flat reef, reef canyon, reef front, ocean reefs, fringing reefs, corals, coral bottom, corals and sponges, amidst colonies of octocorals, hard bottom, and calcareous algae); Rocky (rocky substrate, rocky shores, under rocks, boulders, cliffs, and channels); Sandy (oolitic sand banks, sandy shelf, sandy shoal, sand bottom, sandy substratum, and lagoon); and Seagrass (mud and patchy seagrass, and seagrass beds). We also recorded, from each retained study, the following information: study site, geographic coordinates, and whenever available, the sex ratio and total length of Atlantic Nurse Sharks, and water temperature, depth, and salinity. Sharks smaller than 31.5 cm TL were considered neonates, while individuals larger than 215.0 cm TL were classified as adults ( Castro, 2000 ), regardless of sex (considering that most of the studies did not provide individual sizes per sex).
Publications were classified into their field of research according to the Australian and New Zealand Standard Research Classification (ANZSRC) used by Dimensions. We used Google Earth to estimate the geographical coordinates of the occurrences when the authors provided only the name of the sampling site. The occurrences of the Atlantic Nurse Shark were assigned to ecoregions and provinces following the Marine Ecoregions of the World (MEOW) as proposed by Spalding et al. (2007) using the shapefile in Google Earth. Maps with the geographical distribution of G. cirratum were built using a shapefile of the MEOWs (downloaded from: https://www.worldwildlife.org/publications/marine-ecoregions-of-the-world-a-bioregionalization-of-coastal-and-shelf-areas ) in Quantum GIS 3.16.1 ( QGIS, 2022 ).
We also evaluated whether the study sites coincided with the limits of MPAs by overlaying a layer with the geographic coordinates of all study sites with the World Database on Protected Areas ( UNEP-WCMC & IUCN, 2021 ). The Bahamian Shark Sanctuary was also considered a MPA. This evaluation was not performed in large-scale studies that did not provide the exact coordinate of the capture/observation, since we could not evaluate whether the sharks were sampled in or out of the MPAs limits. All habitat types and locations in the database were considered, whenever the article provided information on single or multiple locations with more than one habitat type.
The first literature review yielded 194 articles and 109 were retained to depict the geographic distribution of the Atlantic Nurse Shark ( Appendix S1 ). Publications from the Southwestern Atlantic dated from 1967 to 2021 ( N = 16) and from the Northwestern Atlantic from 1964 to 2021 ( N = 93) ( Fig. 1 ). Studies in the field of biological sciences accounted for most publications (62.4%), peaking in recent years. Most studies were performed in the Northwestern Atlantic (87.3%), particularly in the Floridian ecoregion (39.2%). Only two studies covered the Eastern Atlantic distribution of the Atlantic Nurse Shark ( Trape, 2008 ; Karl, Castro & Garla, 2012 ) ( Fig. 2 ).

Colors indicate the field of research according to the Australian and New Zealand standard research classification (ANZSRC).

The second search yielded 31 studies (29 articles and two book chapters) but excluded one article that referred to Ginglymostoma unami (Pacific nurse shark) and eight to Carcharias taurus (grey nurse shark). Six additional studies were included in our database due to previous knowledge of the authors that they dealt with Atlantic Nurse Shark habitats. Out of the 13 articles in the SI from Osgood & Baum (2015) , three were already present in our database. We were unable to find five articles, and out of those, five did not address the Atlantic Nurse shark habitat. One article was excluded because it relied on secondary data, and as a result, we added Gilmore et al. (1977) to the database. The final database was composed of 30 articles. Studies on the habitat colonized by Atlantic Nurse Sharks were published between 1950 and 2021 ( Appendix S2 ) and followed a similar pattern to the distribution studies. Most data were obtained from the Floridian ecoregion ( N = 13; Gilmore et al., 1977 ; Smith & Herrkind, 1992 ; Carrier & Pratt, 1998 ; Castro, 2000 ; Wiley & Simpfendorfer, 2007 ; Pratt & Carrier, 2007 ; Adams & Paperno, 2007 ; Ward-Paige et al., 2010 ; Hannan et al., 2012 ; Pratt et al., 2018 ; Rangel, Hammerschlag & Moreira, 2021 ; Gutowsky et al., 2021 ; Tinari & Hammerschlag, 2021 ), followed by surrounding ecoregions, the Bahamian ( N = 5; Hansell et al., 2018 ; Shipley et al., 2018 ; Ward-Paige et al., 2010 ; Bruns & Henderson, 2020 ; Lennon & Sealey, 2021 ), and Western Caribbean ( N = 4; Eggleston & Lipcius, 1992 ; Chapman et al., 2005 ; Pikitch et al., 2005 ; Ward-Paige et al., 2010 ). There was also data from Northeastern Brazil ( N = 3; Ferreira et al., 2013 ; Afonso, Andrade & Hazin, 2014 ; Niella, Hazin & Afonso, 2017 ), Fernando de Noronha and Atoll das Rocas ( N = 3; Garla et al., 2009 ; Garla, Garrone-Neto & Gadig, 2014 ; Afonso et al., 2016 ), Eastern Caribbean ( N = 3; Smith-Vaniz, Jelks & Rocha, 2006 ; DeAngelis et al., 2008 ; Stoffers et al., 2021 ), and Guianan ( N = 1; Springer, 1950 ) ecoregions ( Fig. 3A ). A greater variety of habitats was detected at the Tropical Northwestern Atlantic province, where reefs (32.9%), sandy bottom (21.1%), and seagrass (19.7%) were the dominant habitats, and smaller contributions of rocky bottom, mangrove, and macroalgae were also found. The South Atlantic province had a lower variety of habitats associated with the Nurse Sharks, being composed of reefs and sandy and rocky bottoms (28.6% for each type) ( Fig. 3B ). Studies performed within MPAs had a greater abundance and diversity of habitat types than in locations outside these borders ( Fig. 4 ).

The most reported abiotic variable in the retained studies ( N = 30) was depth (73.3%), followed by temperature (23.3%), and salinity (13.3%). Populational data available in the studies ( N = 30) were size (53.3%), description of breeding events (36.6%), and sex ratio (28.6%). The systems where the Atlantic Nurse Sharks were observed had a maximum depth of 75 m, temperatures ranging from 25 °C to 34 °C, and salinities varying from 31 to 38 ppt.
The habitat of neonates was addressed in only two studies ( Pratt & Carrier, 2007 ; Garla et al., 2009 ; Garla, Garrone-Neto & Gadig, 2014 ), with occurrences in reefs, rocky, macroalgae, sandy, and seagrass at a maximum depth of 20 m, with an average temperature of 26 °C and a salinity of 36 ppt. Adult sharks ranged from 215 to 380 TL cm and were recorded in all habitat categories ( Castro, 2000 ; Chapman et al., 2005 ; Pratt & Carrier, 2007 ; DeAngelis et al., 2008 ; Hannan et al., 2012 ; Afonso, Andrade & Hazin, 2014 ; Shipley et al., 2018 ; Hansell et al., 2018 ; Tinari & Hammerschlag, 2021 ; Stoffers et al., 2021 ) ( Fig. 5 ). The majority of studies did not discriminate variation in size and sex per type of habitat.

A total of 11 (36.6%) studies provided information on breeding events in the habitats described, 72.7% in shallow waters. Mating grounds were 90.9% of those locations and 63.4% were characterized as nursery grounds, most of them inside MPAs (90.9%). Neonates and small juveniles were associated with all six habitat types. Mating events were related only to seagrass, sandy bottoms, and/or macroalgae flats. No mention of the habitats’ relation with reproductive events was detected for Northeastern Brazil and Guianan ecoregions.
Studies that provided sex ratio or abundance of females and males were divided into three groups to address possible sex segregation of habitat use. The one female: one male ratio group occurred in all habitat types (1F:1.077−1.02M; Ferreira et al., 2013 ; Afonso, Andrade & Hazin, 2014 ; Hannan et al., 2012 ; Rangel, Hammerschlag & Moreira, 2021 ). The group 1:0.5 had higher occurrences in sandy and seagrass types but also occurred in reefs and rocky habitats (1F:0.43−0.56 M; Chapman et al., 2005 ; DeAngelis et al., 2008 ; Pratt et al., 2018 ). The third group of one female: two males occurred in mangroves, reefs, and seagrass (1F:1:2.05M; Wiley & Simpfendorfer, 2007 ) ( Fig.5 ).
Our study consolidates information on the essential habitats associated with the occurrence of the Atlantic Nurse Shark populations. Our results can assist in the strategic (not opportunistic) creation of MPAs to protect this species which is necessary for the conservation of sharks, as highlighted by Baldi et al. (2017) . Additionally, we emphasize herein the lack of studies on the habitat structural complexity that mediates the interaction of the Atlantic Nurse Shark with other species and with abiotic variables (beyond temperature and salinity). These are also key information for modeling studies that can allow us to foresee and identify critical regions for the conservation of this ‘‘Vulnerable’’ species, and to manage habitats that could collapse with Atlantic Nurse Sharks’ disappearance. We also provide a set of gaps that may serve as a road map for future studies.
Although research on the Atlantic Nurse Shark has increased in the last decade, knowledge of its ecology in the Eastern Atlantic and its habitat use patterns are still lacking in the scientific literature. Recently, evidence of a nursery area, mostly sandy substrate, was described for the Atlantic Nurse Shark and other shark species in Cabo Verde (Sal Rei Bay, Boa Vista Island), with sizes ranging from 43 to 140 cm ( Rosa et al., 2023 ). Despite the sharks sampled in temperate realms on the first search, most studies (96.8%) were performed in Tropical Atlantic Waters. This may be related to the warmer temperatures found associated with Atlantic Nurse Shark abundance. The higher number of Atlantic Nurse Shark-habitat associations and diversity of habitats occurred in the Northwestern Atlantic, which comprehends the greatest expenditure in shark tourism along the Atlantic Nurse Shark distribution ( Cisneros-Montemayor et al., 2013 ). These places are usually chosen precisely for the abundance of sharks ( Gallagher et al., 2015 ) and, thus, enable higher research efforts. Therefore, it is important to highlight that our findings reflect the habitats associated with the Atlantic Nurse Sharks that were evaluated, not necessarily covering the entire range and diversity of habitats for the species, as studies focusing on its habitat use are still scarce. However, these findings are indicative of the habitats that harbor individuals and populations of the Atlantic Nurse Sharks and may be an indication for future studies to analyze habitat selection and correlate it with sex and ontogeny. Thus, it is possible that habitats of Atlantic Nurse Sharks that do not have the same visibility as tourist sites may be under greater impact but with less investment in research.
Sharks with larger sizes and occurrences in medium depths are more likely to be threatened due to lower population growth rates and refugees from fisheries ( Dulvy et al., 2021 ). The Atlantic Nurse Shark is an example of a shallow-water shark that reaches up to 380 cm ( Tinari & Hammerschlag, 2021 ). Thus, it is likely subjected to numerous coastal human impacts throughout its entire life, worsened by a lower ability to replace individuals removed from overfishing in populations, and a higher exposition to fisheries in shallow habitats ( Dulvy et al., 2021 ). Considering that fishing pressure is an economic activity that already poses a great threat to chondrichthyans ( Dulvy et al., 2021 ), our findings suggest that habitat loss could also be influencing the vulnerability of coastal populations. In addition, as most of the habitats used by G. cirratum are inserted in zones of high presence of touristic activities, it can be expected an even greater impact on the populations subjected to both habitat modification and behavior/movement influence. Considering the species’ recent change in the classification of extinction risk (from ‘ Data Deficient’ to ‘Vulnerable’ ), new conservation plans should focus on mitigating the impacts of fishing and preserving the critical species’ habitat based on the data collected by this study to prevent further increase in extinction risk.
Besides the relationship between the Atlantic Nurse Shark populations and their essential habitats of occurrence, our findings could also provide insights into the ecological requirements of this species regarding water conditions. Populations were commonly found associated with shallow, coastal, warm, and saline waters in the studies retained herein. Even though these variables are important and may be an indicator of the species requirements, many other variables can be relevant in determining chondrichthyans’ occurrence. Visibility was not provided by the studies in this search, even though most of the study sites are recognized as high visibility areas ( e.g ., Glover’s Atoll, Bahamas, Florida Keys, Fernando de Noronha, Turks and Caicos, US Virgin Islands). Since Afonso, Andrade & Hazin, (2014) and Niella, Hazin & Afonso, (2017) related higher abundances with lower transparencies in Recife, Brazil, whether the Atlantic Nurse shark is dependent or not upon high transparency is yet to be revealed. Therefore, studies investigating the influence of visibility on the Atlantic Nurse Shark occurrence and other variables such as dissolved oxygen, hydrodynamics, and site isolation and connectivity, are still needed.
The IUCN Red List assessment of the Atlantic Nurse Shark indicates its occurrence in rocky, reefs, sandy, mangroves, and seagrasses habitats ( Carlson et al., 2021 ), but our findings indicate reefs as the most important habitat for Atlantic Nurse Sharks. They represent at least 25% of the habitats encountered for all life stages, at both Northern and Southern Atlantic within and out of MPAs and were also described as nursery grounds. These habitats are known for providing refuge during the resting behavior on caves and crevices ( Compagno, 2001 ) and are also the habitats where their main prey can be found in higher abundances ( i.e ., macroinvertebrates and small fish from the family Haemulidae; Castro, 2000 ). According to Carlson et al. (2021) , the loss of coral reefs in Central America and the Southwestern Atlantic could be related to a decline in Atlantic Nurse Sharks’ observations. Coral reefs are known for their high diversity of species and structural complexity, supporting fishing stocks all over the world ( Magel et al., 2019 ). Although Bascompte, Melian & Sala (2005) predicted top-down structuring of ecosystems with the Atlantic Nurse Shark as one of the top predators, studies have shown that reef-associated sharks occupy a mesopredator function ( Roff et al., 2016 ; Desbiens et al., 2021 ). The destabilization generated by reef habitat degradation has effects that may scale up to the ecosystem level, leading to the loss of filtering services, oxygen depletion, and the extinction of commercially important fish and invertebrates ( Worm et al., 2006 ). The effect of altered habitats and the relevance of habitat in maintaining species’ healthy has already been evidenced by Rangel, Hammerschlag & Moreira (2021) , which showed lower nutritional quality in urban Atlantic Nurse Sharks compared to non-urban sharks. Thus, further studies should focus on the effects of the loss or degradation of specific habitats on the populational attributes of the Atlantic Nurse Sharks which are key to unveiling the patterns described in this study and can subsidize conservation measures with context-dependent information.
Although highly threatened by urbanization, deforestation, and grounding, mangroves are another example of a habitat that is important for several marine organisms, often acting as reproduction and recruitment zones. Neonates and small juveniles of Northwestern Atlantic populations of G. cirratum have been described to utilize mangroves as shelter during resting periods ( Castro, 2000 ; Pratt & Carrier, 2007 ). However, no published data have investigated the use of this habitat by the Atlantic Nurse Sharks in the South Atlantic, even though this region harbors the world’s most extensive range of continuous mangroves, the Amazon Macrotidal Mangrove Coast ( Souza-Filho, 2005 ). Seagrasses were also not mentioned in habitat studies in the Southern Atlantic for any life stages although associated with reproduction events in the Northwestern Atlantic ( Carrier & Pratt, 1998 ; Wiley & Simpfendorfer, 2007 ; Pratt et al., 2018 ; Tinari & Hammerschlag, 2021 ; Pratt et al., 2022 ). The high fluvial discharge and consequent lower visibility may be one of the reasons seagrasses are not reported on the Northern coast of Brazil ( Copertino, Creed & Lanari, 2016 ). However, there are hotspots for seagrass meadows on the Brazilian Northeast coast and sporadic occurrences on the southern distribution of Atlantic Nurse Sharks ( Copertino, Creed & Lanari, 2016 ). Does this species not use these habitat types in Southern Waters or is there a gap in knowledge? Further studies would be required to address this question.
The occurrence of mainly females in sandy and seagrass habitats and the mating events described in these habitats might be related as some studies describe its use during breeding season as an avoidance strategy of males’ attempts to copulate or associated with thermoregulation during gestation ( Pratt & Carrier, 2007 ; Afonso et al., 2016 ; Pratt et al., 2022 ). While males have been described by Pratt & Carrier (2007) to use deeper surrounding reefs as resting and staging areas. Studies in the Dry Tortugas have also described sex segregation in the use of space throughout the year, where males stay annually from May to July and females arrive biennially or triennially with peaks in June (when mating occurs) and September/August ( Pratt & Carrier, 2007 ; Pratt et al., 2018 ; Pratt et al., 2022 ). Contrarily, in Recife, Northeastern Brazil, no pregnant female was captured, and the CPUE was lower from May to August with a seasonal pattern for males during the first quarter of the year ( Ferreira et al., 2013 ). Further studies describing sex and size segregation in the use of habitat should be replicated enabling a complex understanding of possible habitat fidelity for some groups, philopatry, and allowing large-scale mapping of Atlantic Nurse Sharks’ movement and reproductive habitat use. Populational and genetic studies are also essential to assist in the definition of habitats to be protected considering their use as steppingstones in corridors between populations exchange. Until conducting more comprehensive and integrated research, we will remain unaware of the species’ dependence on specific habitats and their relationship to the site, as well as the possible influence of life stages that may trigger the periodic migration of individuals, such as reproduction in certain areas, and even refuge strategies that can increase the survival rate of neonates.
The Florida Keys, Dry Tortugas and Bahamas, USA, and Fernando de Noronha, Brazil are some localities known as breeding sites for the Atlantic Nurse Sharks ( Fowler, 1906 ; Castro, 2000 ; Brooks et al., 2012 ; Afonso et al., 2016 ; Pratt et al., 2018 ). In agreement with Pratt et al. (2022) , the Dry Tortugas is the breeding ground described in more detail for the Atlantic Nurse Shark. Although the authors describe characteristics shared with Fernando de Noronha and the Bahamas, such as the sand and seagrass flats, low-lying islands, high temperatures, and shallow waters near deep waters, it is still not well documented why this site is so important for the species’ reproduction ( Pratt et al., 2022 ). Despite the increase in the number of studies in the last 14 years, it is still not possible to address the question proposed by Pratt & Carrier (2007) : “What defines optimal habitat for Atlantic Nurse Shark’ neonates?”. Neonates were recorded in most habitats categorized here; however, all these data refer to only two localities (Fernando de Noronha, Brazil, and Dry Tortugas, USA) made by studies not directly focused on species-habitat relations. Thus, it is not possible to understand if the neonates actively select habitats that increase shelter against predation and not even if breeding events have taken place on these sites. Although the term “nursery grounds” was used in the studies to define areas with the presence of neonates and juveniles in consecutive years ( Pratt & Carrier, 2007 ; Garla et al., 2009 ; Garla, Garrone-Neto & Gadig, 2014 ; Pratt et al., 2018 ), to define an area as a nursery, the place must meet the criteria proposed by Heupel, Carlson & Simpfendorfer (2007) which includes (1) sharks more commonly encountered in the area than other areas; (2) sharks with a tendency to remain or return for extended periods; and (3) the area or habitat is repeatedly used across years. Considering Heupel, Carlson & Simpfendorfer (2007) definition, Pratt et al. (2022) suggest that not only the Dry Tortugas is not used as a nursery but also that the Atlantic Nurse Shark does not use nurseries at all since the ovulation takes between 2–3 weeks ( Castro, 2013 ) and consequently the release of fully developed pups could occur in different areas.
Overall, data on Atlantic Nurse Shark’s occurrence and habitat use, species interaction, and their interaction with abiotic conditions are still scarce throughout its entire distribution, but especially in the South Atlantic where the species is most threatened. Such information is critical while evaluating potential occurrence areas, as it can help identify satellite patches of specific habitats surrounding the study area that certain groups, like juveniles and pregnant females, might use. By incorporating this patchy perspective into their evaluations of the species and, potentially, protected areas, researchers and conservation agencies can gain a more comprehensive understanding of this species. Critical habitats for neonates and breeding sites are yet to be identified in Northeastern Brazil, and Guianan ecoregions. As highlighted by Pratt et al. (2022) , breeding grounds are known to occur in remote areas, suggesting that anthropogenic development may have impacted coastal potential grounds. Considering that females seem to prefer shallow water during breeding, making them more vulnerable to fishing and other anthropogenic stressors ( e.g ., tourism, boat traffic, mooring, pollution, loss of mangroves ecosystems, and acoustic pollution; Carrier & Pratt, 1998 ; Arthington et al., 2016 ; Dulvy et al., 2021 ; Wosnick et al., 2021 ). The only known reproduction site for this species in the entire Southwestern Atlantic are the oceanic islands Fernando de Noronha and Rocas Atoll ( Afonso et al., 2016 ). The study made by Karl, Castro & Garla (2012) showed through microsatellite analysis that Atlantic Nurse Shark populations from these islands were separated from the Brazilian coast highlighting the knowledge gaps on the reproductive events for a wide distribution of the species. In addition, studies are also needed to understand if the differences found in our study ( i.e ., no record for G. cirratum use of mangroves and seagrass in the South Atlantic) are due to a habitat selectivity of this species in the North and South Atlantic or due to poor sampling efforts in these regions.
The creation of new MPAs as long as the revision of the limits of the existing ones might both benefit the Atlantic Nurse Sharks and also be benefited from the increased knowledge of their requirements, considering their importance in ecosystem processes. Even though this process depends on the conservation status of some species, but also on politics and societal awareness, MPAs deliver the main conservation strategy to elasmobranchs through monitoring or prohibition of fishing ( i.e ., the main threat to this group) ( Carlson et al., 2021 ; Dulvy et al., 2021 ). Our findings highlighted a role played by MPAs in protecting essential habitats since the greater diversity of habitats was found within their limits. According to Albano et al. (2021) , MPAs are more effective for elasmobranchs when they include species-specific habitats of shark species, thus novel MPAs should consider our findings which highlight the importance of protecting shallow sandy and seagrass habitats mainly during the reproductive period and reef habitats for all life stages. The recent discovery of the Atlantic Nurse Shark occurrence in São Paulo, Brazil shows that it is still possible to find essential habitats where they occur and has been poorly explored by researchers ( Santos et al., 2023 ). Currently, 7.9% of the Atlantic Nurse Shark occurrence area overlaps with MPAs, and the need for more numerous and larger MPAs (from tens to hundreds of km) encompassing a greater variety and connectivity between habitats are being evidenced ( Chapman et al., 2005 ; Albano et al., 2021 ; Dwyer et al., 2020 ). A significant reduction in the Atlantic Nurse Shark demography at Rocas Atoll, Brazil, a no-take MPA, was predicted for the Intergovernmental Panel on Climate Change scenarios until 2100 (IPCC; Pörtner et al., 2019 , Capitani et al., 2021 ). However, since the knowledge of the habitats required along the entire distribution of the Atlantic Nurse Sharks and their association with other water variables are still scarce, predictions for the future of climate change can underestimate the impacts on this species. Thus, understanding the ecological requirements, and the habitat structural complexity used by the different sex and size classes of the Atlantic Nurse Sharks is critical for defining optimal drivers for the species but also for trophic interactions associated with the habitats used.
Conclusions
Conservation measures essential for the protection of the ‘Vulnerable’ Atlantic Nurse Shark include the creation of protected areas, fishing restriction measures, and closing access during the reproductive period. However, these measures rely on accurate scientific information that is still lacking for many populations of this species, thus it is crucial that a greater effort be undertaken in these regions so we may delineate efficient conservation measures. Otherwise, we would be spending valuable and limited resources in regions that do not encompass the living area of the target species making it difficult to protect G. cirratum , reduce mortality or restore its depleted populations ( Dwyer et al., 2020 ). Further studies incorporating modeling techniques would also be useful to assist in the decision-making regarding this species and should incorporate habitat availability for the occurrence of Atlantic Nurse Sharks. Furthermore, studies that consider the future scenarios of tropicalization (increasing abundance of warm-affinity species) are also needed to understand new distribution ranges for the Atlantic Nurse Shark ( McLean et al., 2021 ).
Supplemental Information
Supplemental information 1, supplemental information 2, acknowledgments.
We thank Dr. Natascha Wosnick for the helpful comments.
Funding Statement
This study was funded by the Coordenação de Aperfeiçoamento de Pessoal de Nível Superior–Brasil (CAPES)–(doctorate scholarship to Vanessa Bettcher B, 88887.370376/2019-00, Code 001); Fundação Carlos Chagas Filho de Amparo à Pesquisa do Estado do Rio de Janeiro, Brazil (postdoctoral fellowship to Ana Clara S. Franco, E-26/202.423/2019; and research grants to Luciano N. Santos, E-26/200.489/2023), and National Council for Scientific and Technological Development (CNPq), Brazil (research grant to Luciano N. Santos, ref. 315020/2021-0). There was no additional external funding received for this study. The funders had no role in study design, data collection and analysis, decision to publish, or preparation of the manuscript.
Additional Information and Declarations
The authors have no conflicts of interest to declare.
Vanessa Brito Bettcher conceived and designed the experiments, performed the experiments, analyzed the data, prepared figures and/or tables, authored or reviewed drafts of the article, and approved the final draft.
Ana Clara Sampaio Franco conceived and designed the experiments, performed the experiments, analyzed the data, prepared figures and/or tables, authored or reviewed drafts of the article, and approved the final draft.
Luciano Neves dos Santos conceived and designed the experiments, authored or reviewed drafts of the article, and approved the final draft.
Research Papers

A novel categorisation system to organize a large photo identification database for white sharks, Carcharodon carcharias

New insights into the evolutionary history of white sharks, Carcharodon carcharias

An integrated mark-recapture and genetic approach to estimate the population size of white sharks in South Africa

Semi-automated software for dorsal fin photographic identification of marine species: application to Carcharodon carcharias

The effect of kelp density on white shark movements in the Dyer Island Nature Reserve, South Africa

Effective number of white shark (Carcharodon carcharias, Linnaeus) breeders is stable over four successive years in the population adjacent to eastern Australia and New Zealand. Ecology and Evolution.

The role of controlled human-animal interactions in changing the negative perceptions towards white sharks, in a sample of white shark cage diving tours participants
Publications, masters and phd projects in preparation.
Hessa A.P., Pindral N., Rowlinson M., Capper A., Andreotti S. (2021-submitted) “Preliminary indications of a trophic change in the Dyer Island Nature Reserve Complex, following the decline in [...]
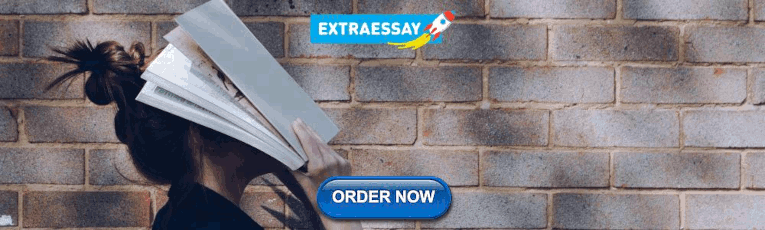
IMAGES
COMMENTS
SRI South Africa. Shark Research Institute (SRI) conducts and sponsors rigorous, peer-reviewed field research about sharks and uses science-based information to educate and advocate for shark conservation policies and protections by the world's governing bodies, including CITES.
A plurality of respondents (45.8%) agreed with the statement that the science concerning the sustainability of shark fisheries is currently uncertain, and 15.2% of respondents agreed with the ...
Declining abundance index. We find that, globally, the abundance of oceanic sharks declined by 71.1% (95% credible interval, 63.2-78.4%) (Fig. 1) from 1970 to 2018, at a steady rate averaging 18 ...
The landings of sharks and rays, reported to the Food and Agriculture Organization of the United Nations (FAO), increased steadily to a peak in 2003 and have declined by 20% since ( Figure 1A ). True total catch, however, is likely to be 3-4 times greater than reported ( Clarke et al., 2006; Worm et al., 2013).
Global demand for shark products, such as fins and meat, as well as high levels of bycatch, have caused widespread declines in shark populations globally 1, 2, 3, with the potential to affect the ...
Open in viewer. From 2017 to 2019, national waters accounted for 95% of shark fishing mortality by number of individuals and 71% of catch by tonnage (tables S14 and S15). Global fishing mortality increased from 76 million sharks in 2012 to more than 80 million in 2017, averaging 79 million from 2017 to 2019 (table S15).
Abstract. Shark depredation is a complex social-ecological issue that affects a range of fisheries worldwide. Increasing concern about the impacts of shark depredation, and how it intersects with the broader context of fisheries management, has driven recent research in this area, especially in Australia and the United States.
In this paper, we recognize that ... In part, this results from the exponential increase in shark research, 83 but also a concerted effort to improve fisheries stock assessments 21, 32 and the development of new analysis techniques. 33, 34 Unlocking these data was made possible by working closely with local researchers in regional assessment ...
I have read papers that show that certain shark fisheries can be sustainable and some currently are: More research needs to be done I believe, but there is sufficient science to suggest these fisheries can and do exist: I am engaged in fisheries management, keep up on status reports, and find that scientists usually present facts
Each year, global landings of sharks and other fisheries resource species are reported by fishing states to the FAO (Fig. 1).Since 1950, Chondrichthyes (sharks, rays, skates and chimaeras) have comprised between 1% and 2% of the total landings (Fig. 1 A, average proportion of 1.2%). Sharks made up about half of the total Chondrichthyes landings over that time frame (Fig. 1 B).
Abstract. Despite over 70 years of research on shark repellents, few practical and reliable solutions to prevent shark attacks on humans or reduce shark bycatch and depredation in commercial fisheries have been developed. In large part, this deficiency stems from a lack of fundamental knowledge of the sensory cues that drive predatory behavior ...
White sharks are highly migratory apex predators, globally distributed in temperate, sub-tropical, and tropical waters. Knowledge of white shark biology and ecology has increased recently based on research at known aggregation sites in the Indian, Atlantic, and Northeast Pacific Oceans; however, few data are available for the Northwest Pacific Ocean. This study provides a meta-analysis of 240 ...
The importance of research and public opinion to conservation management of sharks and rays: a synthesis. Marine and Freshwater Research 62 , 518 (2011). Article CAS Google Scholar
Sensor records show that white sharks ( Carcharodon carcharias) dive deeper than 1200 meters while whale sharks ( Rhincodon typus) have reached 1896 meters, which is near the pressure limit of ...
These form the foundation for shark management in U.S. waters and international fisheries in the Atlantic Ocean. Our shark research is peer-reviewed to ensure the science is high-caliber and suitable for management purposes. Since 2018, NOAA Fisheries scientists have contributed to more than 100 shark research publications on dozens of species.
Shark movement varied according to shark size, as in Hoyos‐Padilla et al. , with larger sharks swimming in open and deeper waters during the day and shallower during the night. Meanwhile, juveniles occupied shallower and coastal waters than adults throughout the observed time periods. ... Marine Biology Research, 8 (1), 90-94. 10.1080 ...
Sharks have an important and major role in maintaining the ocean ecosystem and also serving as an. indicator of the ocean health. They control the populations of prey species by eliminating the ...
The North-East Atlantic is home to numerous shark and ray species, including small to medium sized demersal sharks. These species can be found at varying depths from 5 to 900 m 42 , 43 , most ...
Elasmobranchs (sharks, rays, and skates) are caught throughout fisheries globally, leading to over one-third of species being threatened with extinction 1.Oceanic shark populations have undergone an average 71% decline over the last half century, owing to an 18-fold increase in relative fishing pressure 2.Incidental capture or 'bycatch' is a primary driver of population declines, and poses ...
Survey methodology. A literature review was performed at the Dimensions research database to assess the geographical distribution and essential habitats associated with the Atlantic Nurse Shark G. cirratum.The Dimensions research database is a recent alternative to perform reviews of the scientific literature more comprehensive than Web of Science and Scopus and less general than Google ...
Dr Eugene Gudger collected reports of whale sharks from all over the world and published 47 papers on these sharks in 40 years. Dr Fay Wolfson also documented whale shark records from all over the world and published a bibliography on the species as well as a paper summarising all the known (320) occurrences from published records and verified ...
Research Papers: The following findings were published to date in peer-reviewed scientific journals as a result of the ongoing partnership between the Shark & Marine Research Institute, Stellenbosch University, and the Department of Environment, Forestry, and Fishery (Branch Oceans and Coasts) (IF - impact factor indicated in bold).