Origins of the universe, explained
The most popular theory of our universe's origin centers on a cosmic cataclysm unmatched in all of history—the big bang.
The best-supported theory of our universe's origin centers on an event known as the big bang. This theory was born of the observation that other galaxies are moving away from our own at great speed in all directions, as if they had all been propelled by an ancient explosive force.
A Belgian priest named Georges Lemaître first suggested the big bang theory in the 1920s, when he theorized that the universe began from a single primordial atom. The idea received major boosts from Edwin Hubble's observations that galaxies are speeding away from us in all directions, as well as from the 1960s discovery of cosmic microwave radiation—interpreted as echoes of the big bang—by Arno Penzias and Robert Wilson.
Further work has helped clarify the big bang's tempo. Here’s the theory: In the first 10^-43 seconds of its existence, the universe was very compact, less than a million billion billionth the size of a single atom. It's thought that at such an incomprehensibly dense, energetic state, the four fundamental forces—gravity, electromagnetism, and the strong and weak nuclear forces—were forged into a single force, but our current theories haven't yet figured out how a single, unified force would work. To pull this off, we'd need to know how gravity works on the subatomic scale, but we currently don't.
It's also thought that the extremely close quarters allowed the universe's very first particles to mix, mingle, and settle into roughly the same temperature. Then, in an unimaginably small fraction of a second, all that matter and energy expanded outward more or less evenly, with tiny variations provided by fluctuations on the quantum scale. That model of breakneck expansion, called inflation, may explain why the universe has such an even temperature and distribution of matter.
After inflation, the universe continued to expand but at a much slower rate. It's still unclear what exactly powered inflation.
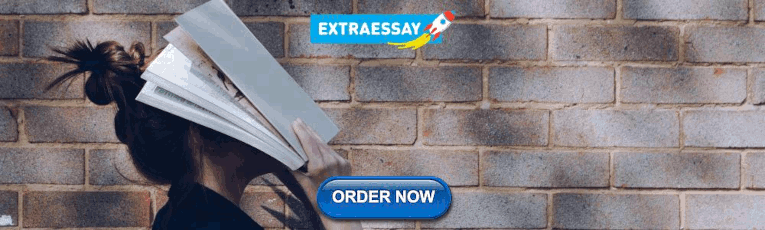
Aftermath of cosmic inflation
As time passed and matter cooled, more diverse kinds of particles began to form, and they eventually condensed into the stars and galaxies of our present universe.
For Hungry Minds
By the time the universe was a billionth of a second old, the universe had cooled down enough for the four fundamental forces to separate from one another. The universe's fundamental particles also formed. It was still so hot, though, that these particles hadn't yet assembled into many of the subatomic particles we have today, such as the proton. As the universe kept expanding, this piping-hot primordial soup—called the quark-gluon plasma—continued to cool. Some particle colliders, such as CERN's Large Hadron Collider , are powerful enough to re-create the quark-gluon plasma.
Radiation in the early universe was so intense that colliding photons could form pairs of particles made of matter and antimatter, which is like regular matter in every way except with the opposite electrical charge. It's thought that the early universe contained equal amounts of matter and antimatter. But as the universe cooled, photons no longer packed enough punch to make matter-antimatter pairs. So like an extreme game of musical chairs, many particles of matter and antimatter paired off and annihilated one another.
You May Also Like
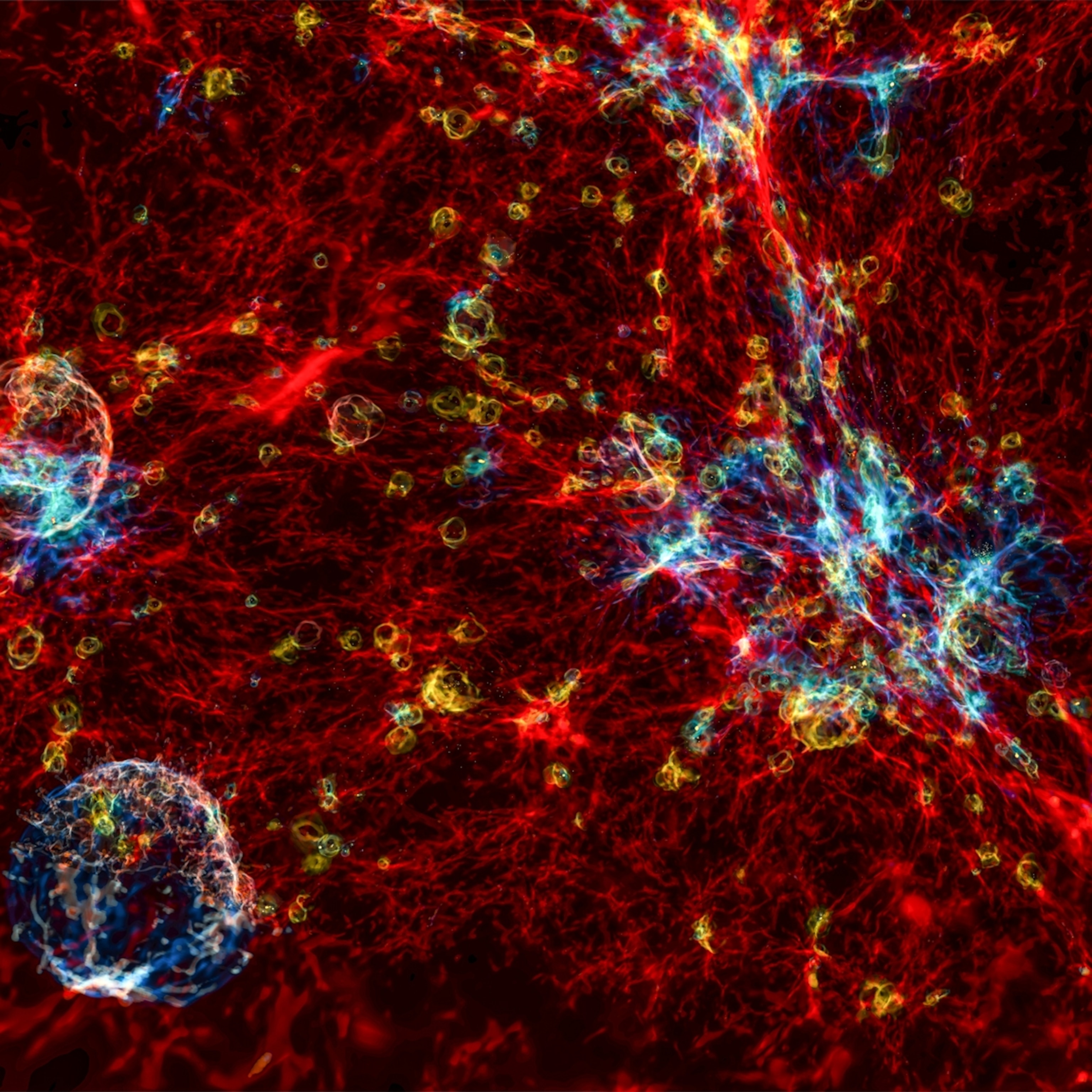
The world’s most powerful telescope is rewriting the story of space and time
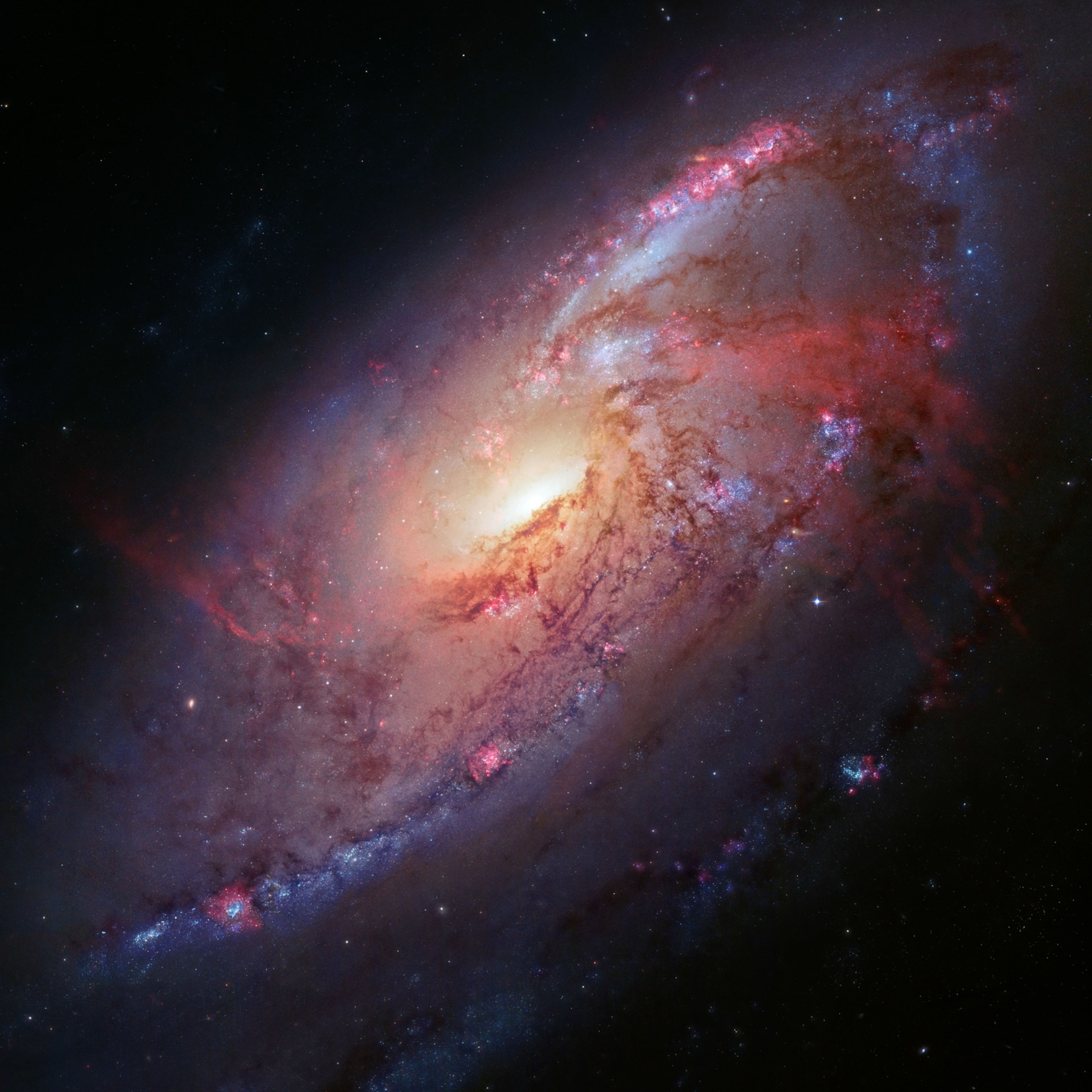
How fast is the universe really expanding? The mystery deepens.
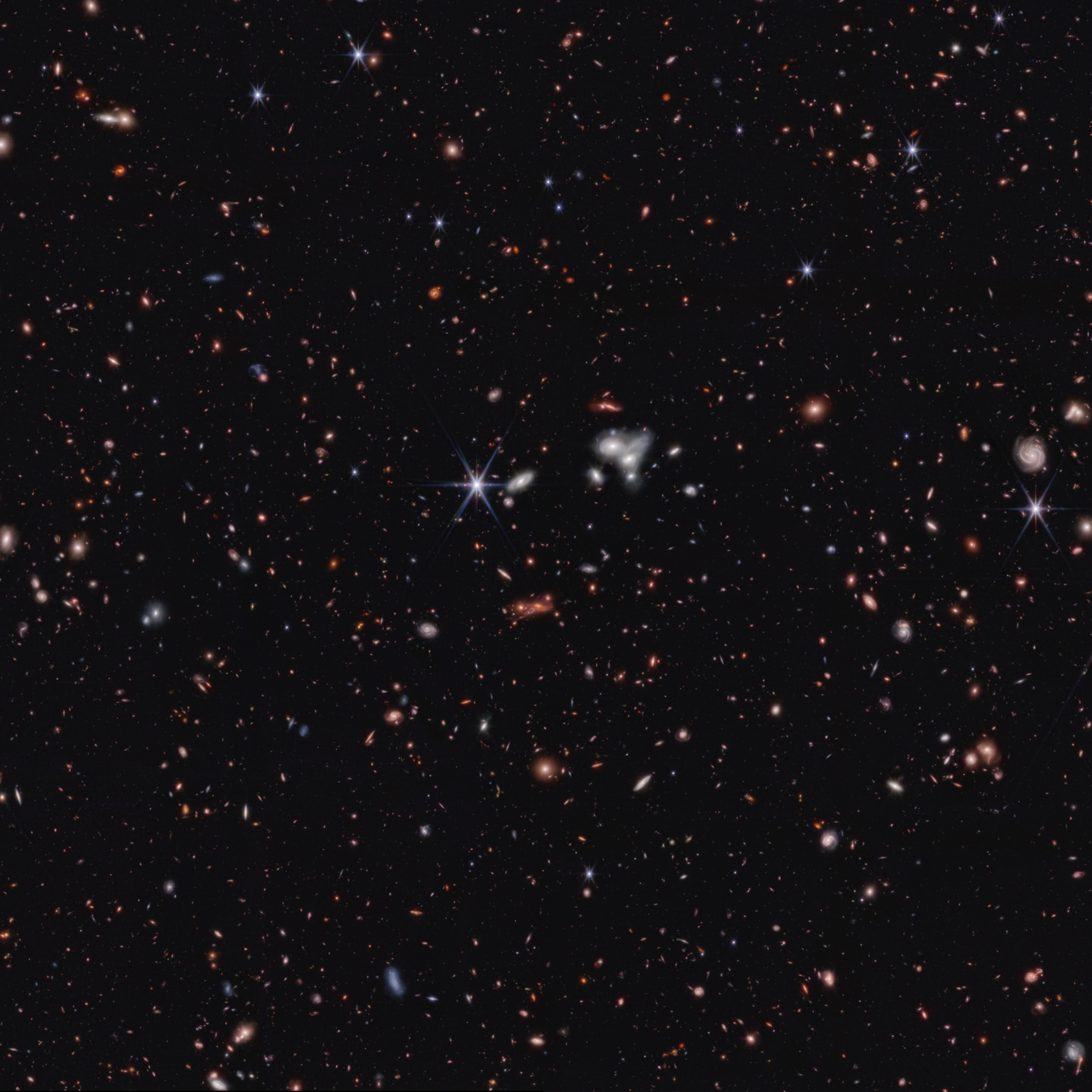
This supermassive black hole was formed when the universe was a toddler
Somehow, some excess matter survived—and it's now the stuff that people, planets, and galaxies are made of. Our existence is a clear sign that the laws of nature treat matter and antimatter slightly differently. Researchers have experimentally observed this rule imbalance, called CP violation , in action. Physicists are still trying to figure out exactly how matter won out in the early universe.
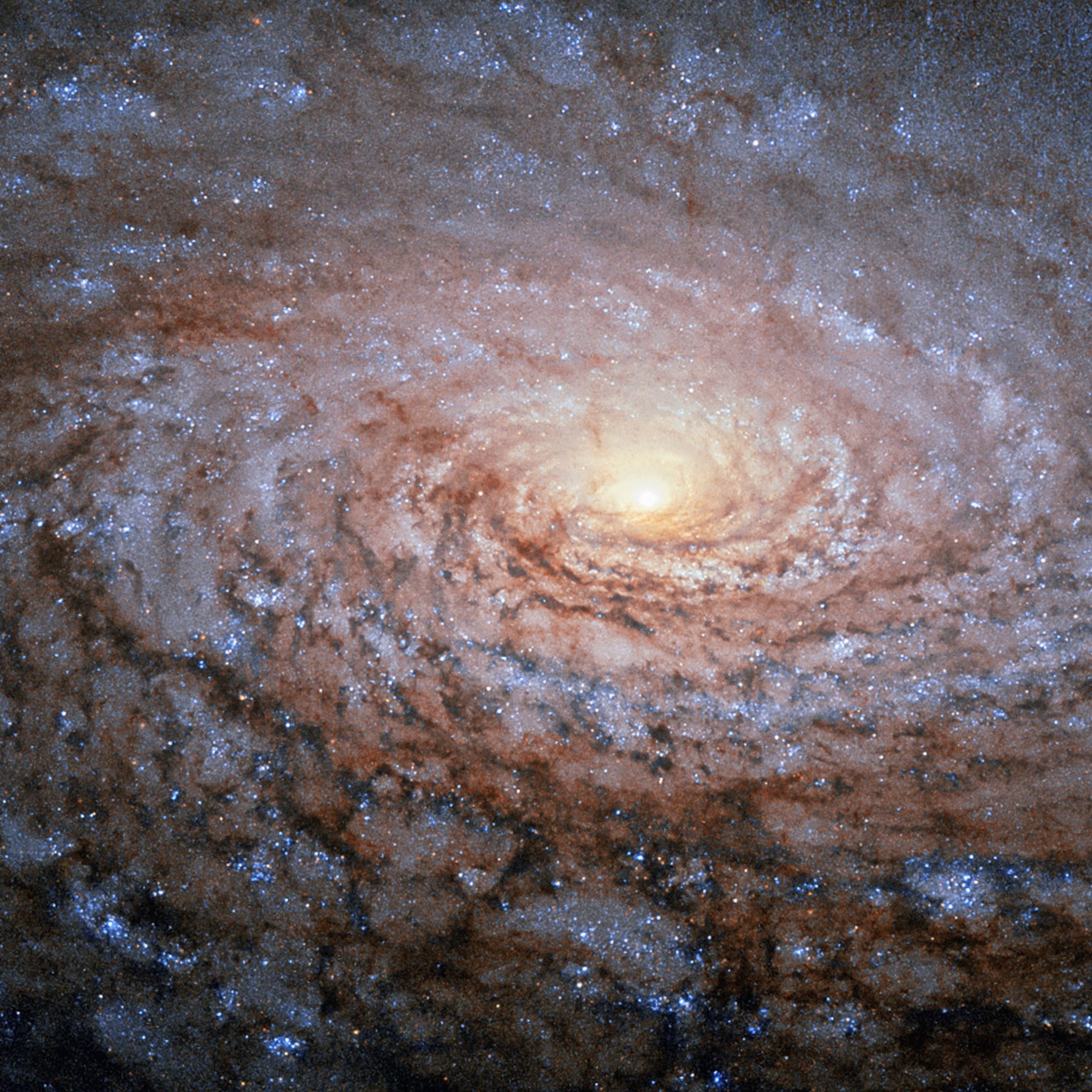
Building atoms
Within the universe's first second, it was cool enough for the remaining matter to coalesce into protons and neutrons, the familiar particles that make up atoms' nuclei. And after the first three minutes, the protons and neutrons had assembled into hydrogen and helium nuclei. By mass, hydrogen was 75 percent of the early universe's matter, and helium was 25 percent. The abundance of helium is a key prediction of big bang theory, and it's been confirmed by scientific observations.
Despite having atomic nuclei, the young universe was still too hot for electrons to settle in around them to form stable atoms. The universe's matter remained an electrically charged fog that was so dense, light had a hard time bouncing its way through. It would take another 380,000 years or so for the universe to cool down enough for neutral atoms to form—a pivotal moment called recombination. The cooler universe made it transparent for the first time, which let the photons rattling around within it finally zip through unimpeded.
We still see this primordial afterglow today as cosmic microwave background radiation , which is found throughout the universe. The radiation is similar to that used to transmit TV signals via antennae. But it is the oldest radiation known and may hold many secrets about the universe's earliest moments.
From the first stars to today
There wasn't a single star in the universe until about 180 million years after the big bang. It took that long for gravity to gather clouds of hydrogen and forge them into stars. Many physicists think that vast clouds of dark matter , a still-unknown material that outweighs visible matter by more than five to one, provided a gravitational scaffold for the first galaxies and stars.
Once the universe's first stars ignited , the light they unleashed packed enough punch to once again strip electrons from neutral atoms, a key chapter of the universe called reionization. In February 2018, an Australian team announced that they may have detected signs of this “cosmic dawn.” By 400 million years after the big bang , the first galaxies were born. In the billions of years since, stars, galaxies, and clusters of galaxies have formed and re-formed—eventually yielding our home galaxy, the Milky Way, and our cosmic home, the solar system.
Even now the universe is expanding , and to astronomers' surprise, the pace of expansion is accelerating. It's thought that this acceleration is driven by a force that repels gravity called dark energy . We still don't know what dark energy is, but it’s thought that it makes up 68 percent of the universe's total matter and energy. Dark matter makes up another 27 percent. In essence, all the matter you've ever seen—from your first love to the stars overhead—makes up less than five percent of the universe.
Related Topics
- BIG BANG THEORY
- SCIENCE AND TECHNOLOGY
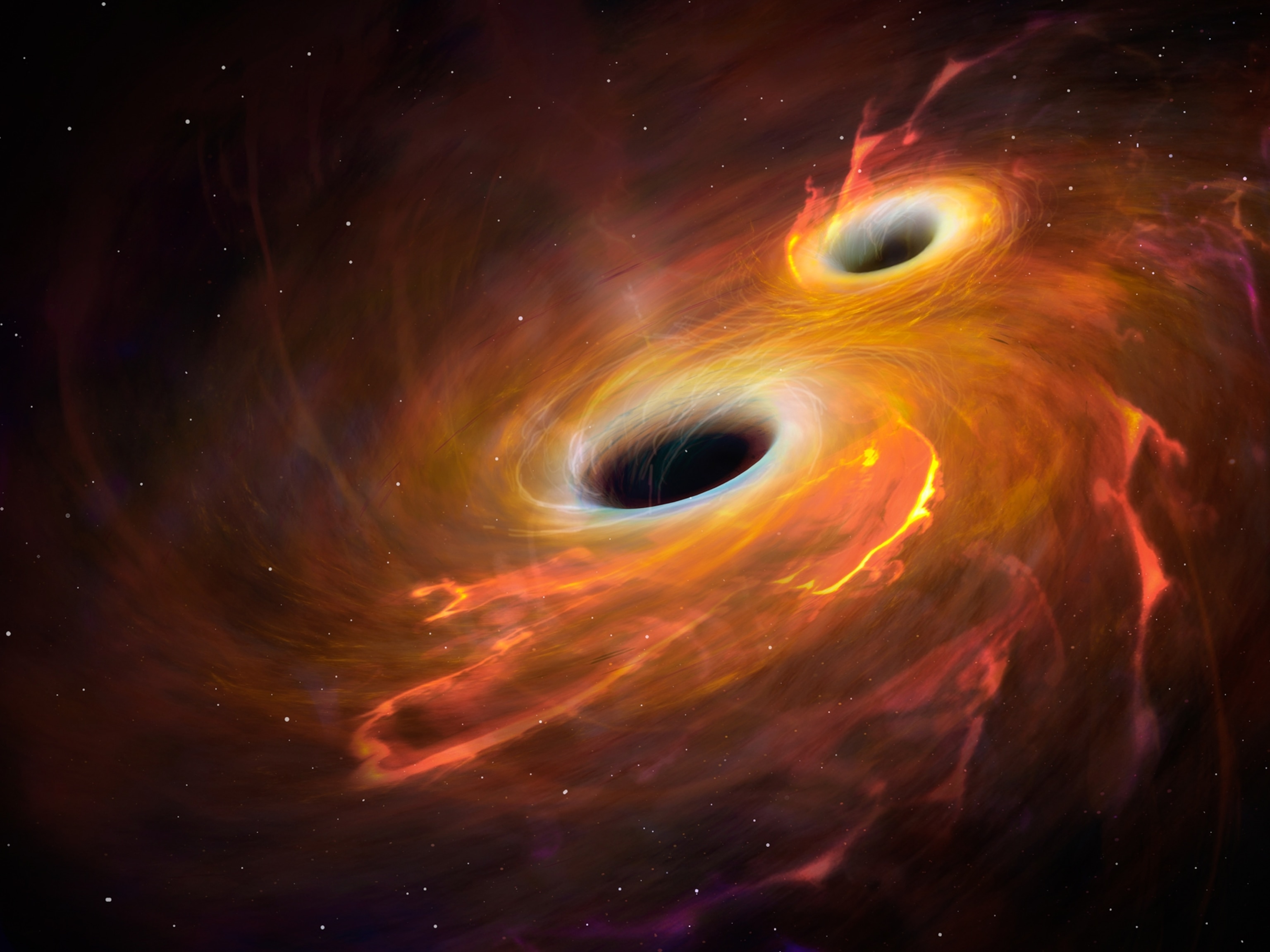
The 11 most astonishing scientific discoveries of 2023
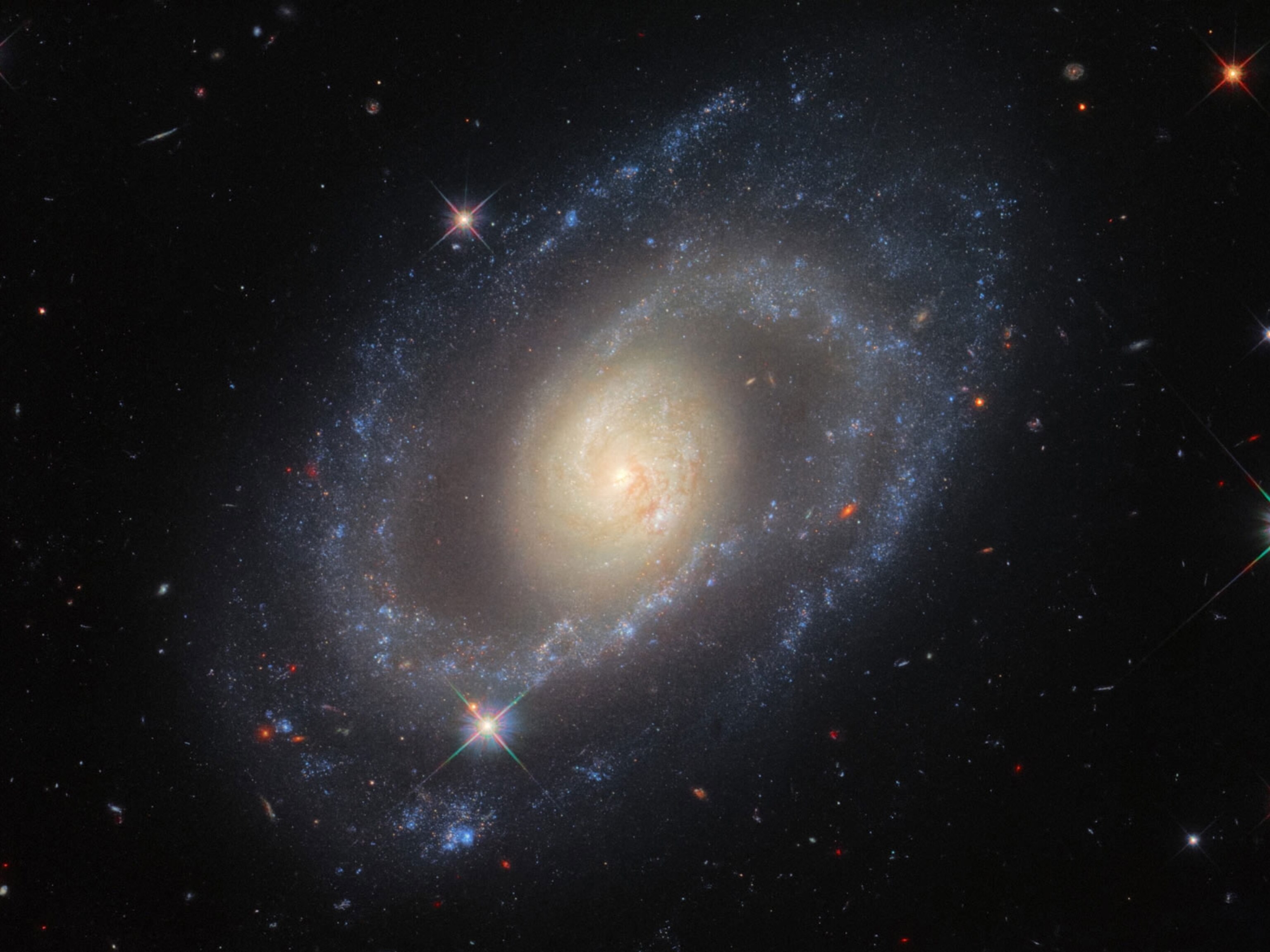
The universe is expanding faster than it should be
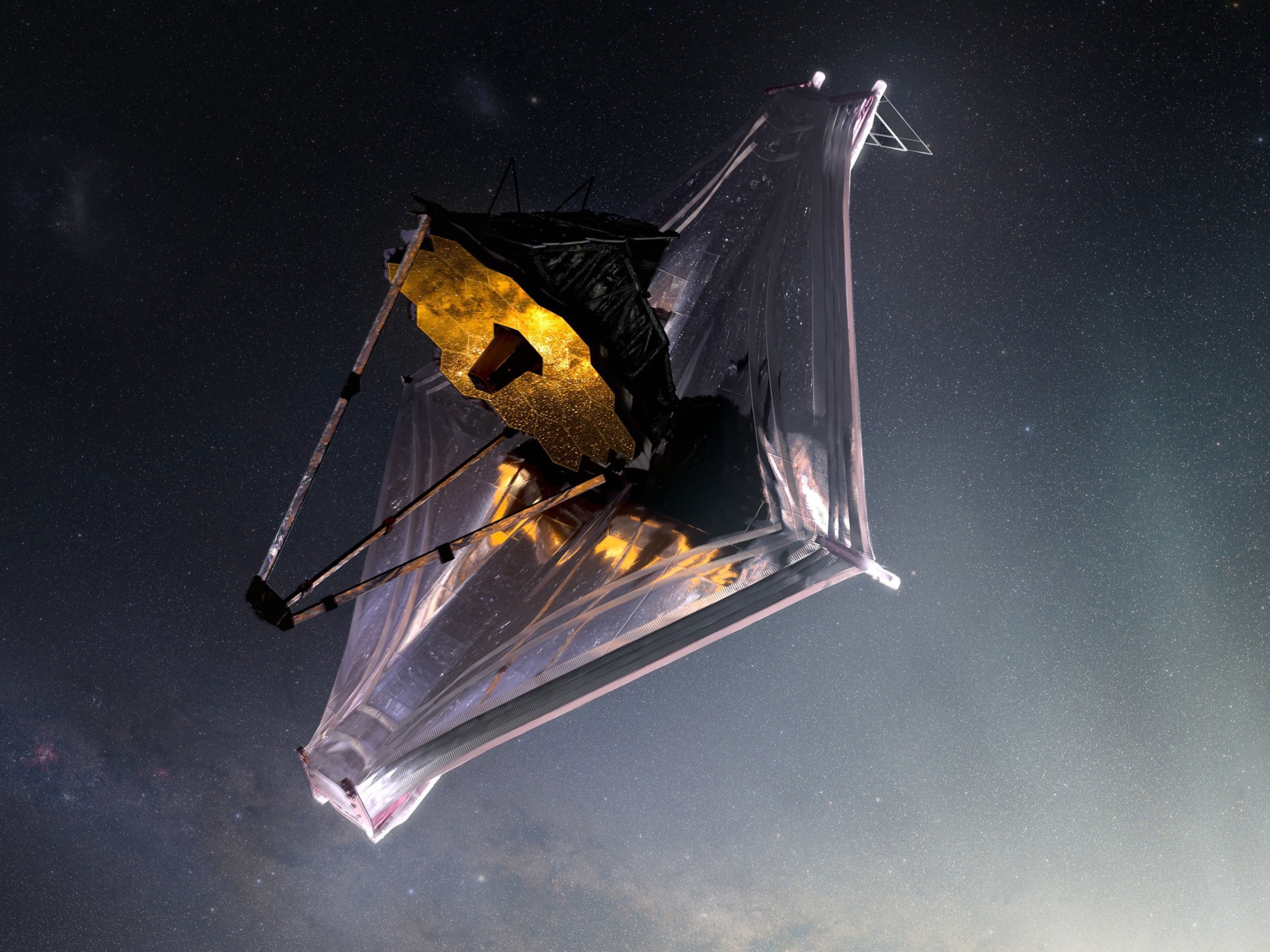
The world's most powerful space telescope has launched at last
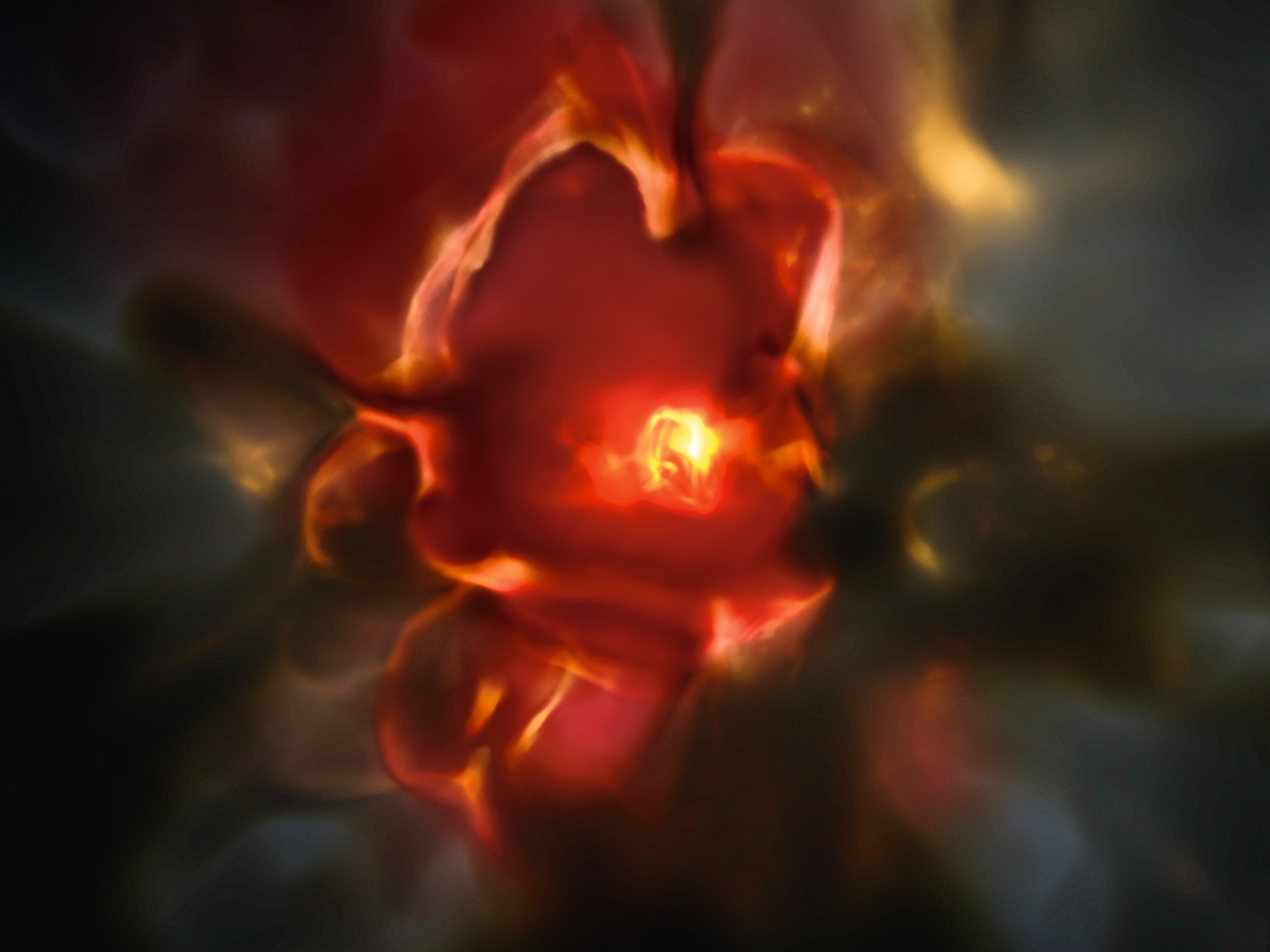
A First Glimpse of the Hidden Cosmos
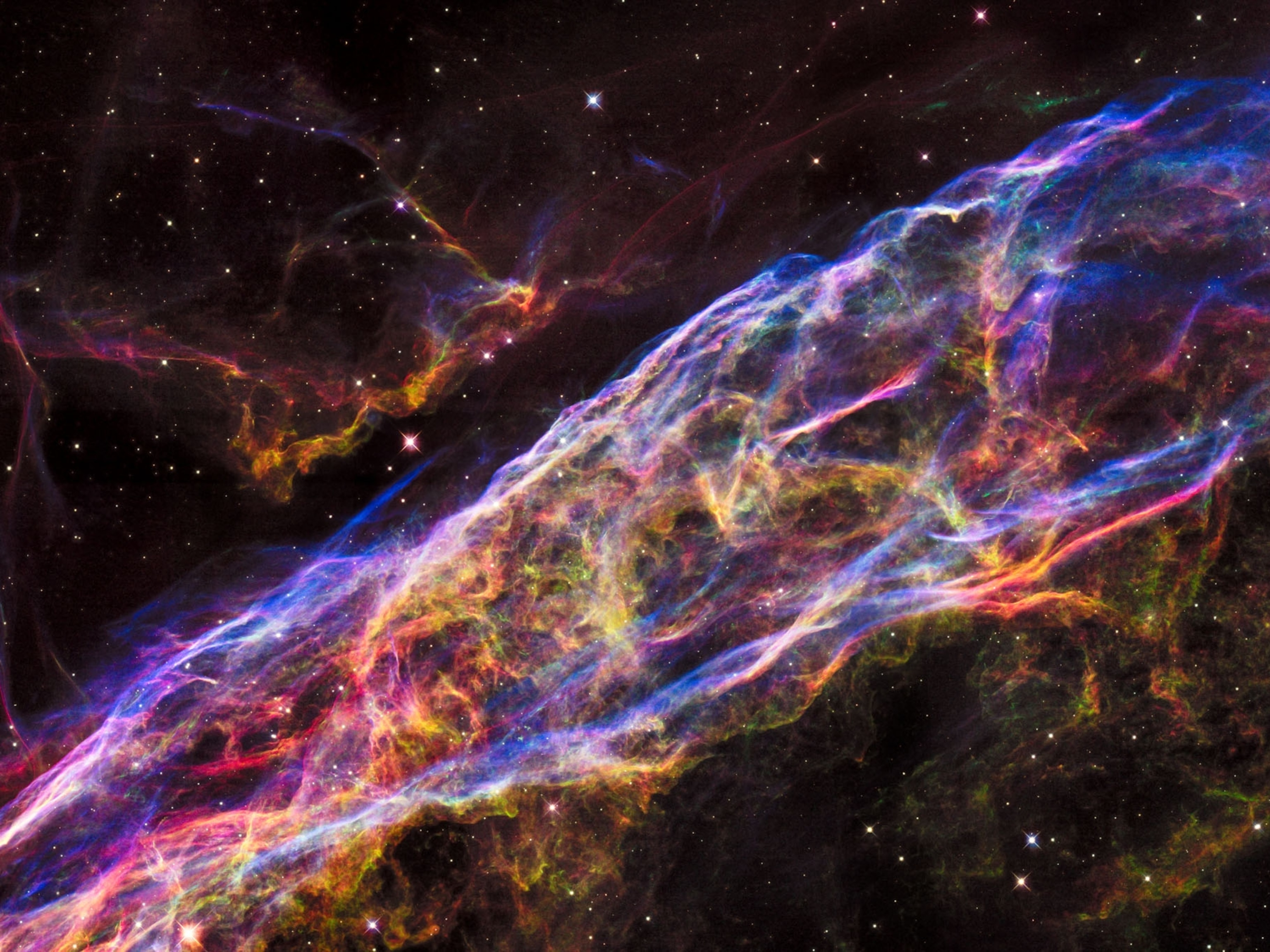
After 30 years, Hubble is still revealing new mysteries of the universe
- Environment
History & Culture
- History & Culture
- Mind, Body, Wonder
- Paid Content
- Terms of Use
- Privacy Policy
- Your US State Privacy Rights
- Children's Online Privacy Policy
- Interest-Based Ads
- About Nielsen Measurement
- Do Not Sell or Share My Personal Information
- Nat Geo Home
- Attend a Live Event
- Book a Trip
- Inspire Your Kids
- Shop Nat Geo
- Visit the D.C. Museum
- Learn About Our Impact
- Support Our Mission
- Advertise With Us
- Customer Service
- Renew Subscription
- Manage Your Subscription
- Work at Nat Geo
- Sign Up for Our Newsletters
- Contribute to Protect the Planet
Copyright © 1996-2015 National Geographic Society Copyright © 2015-2024 National Geographic Partners, LLC. All rights reserved
The Universe
Formation of the universe.
Before Hubble, most astronomers thought that the universe didn’t change. But if the universe is expanding, what does that say about where it was in the past? If the universe is expanding, the next logical thought is that in the past it had to have been smaller.
The Big Bang Theory
The Big Bang theory is the most widely accepted cosmological explanation of how the universe formed. If we start at the present and go back into the past, the universe is contracting, getting smaller and smaller. What is the end result of a contracting universe? According to the Big Bang theory, the universe began about 13.7 billion years ago. Everything that is now in the universe was squeezed into a very small volume. Imagine all of the known universe in a single, hot, chaotic mass. An enormous explosion—a big bang—caused the universe to start expanding rapidly. All the matter and energy in the universe, and even space itself, came out of this explosion. What came before the Big Bang? There is no way for scientists to know since there is no remaining evidence.
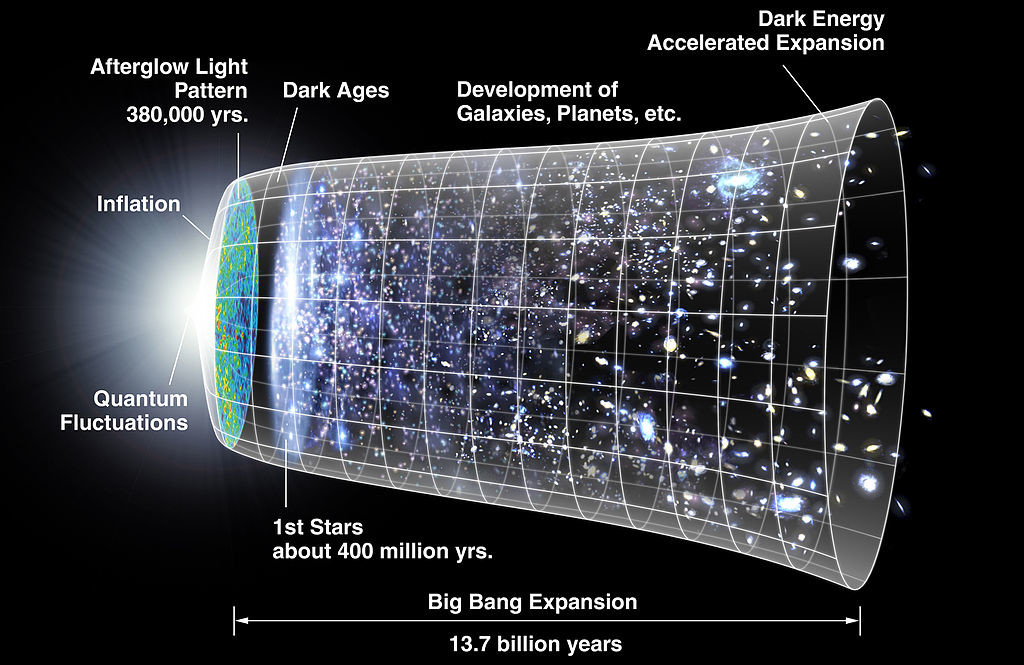
After the Big Bang
In the first few moments after the Big Bang, the universe was unimaginably hot and dense. As the universe expanded, it became less dense and began to cool. After only a few seconds, protons, neutrons, and electrons could form. After a few minutes, those subatomic particles came together to create hydrogen. Energy in the universe was great enough to initiate nuclear fusion and hydrogen nuclei were fused into helium nuclei. The first neutral atoms that included electrons did not form until about 380,000 years later.The matter in the early universe was not smoothly distributed across space. Dense clumps of matter held close together by gravity were spread around. Eventually, these clumps formed countless trillions of stars, billions of galaxies, and other structures that now form most of the visible mass of the universe. If you look at an image of galaxies at the far edge of what we can see, you are looking at great distances. But you are also looking across a different type of distance. What do those far away galaxies represent? Because it takes so long for light from so far away to reach us, you are also looking back in time.
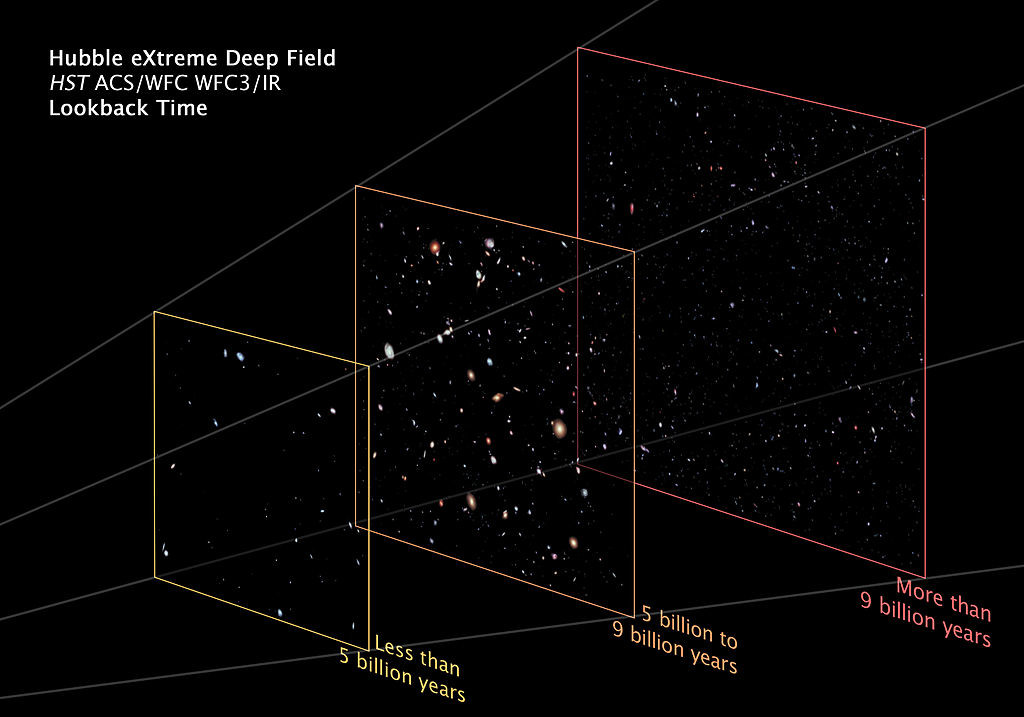
- Dynamic Earth: Introduction to Physical Geography. Authored by : R. Adam Dastrup. Located at : http://www.opengeography.org/physical-geography.html . Project : Open Geography Education. License : CC BY-SA: Attribution-ShareAlike
- Stephen Hawking - The Big Bang. Authored by : The Cosmos Is Also Within Us. Located at : https://youtu.be/gs-yWMuBNr4 . License : All Rights Reserved . License Terms : Standard YouTube License
- CERN's supercollider. Authored by : Brian Cox. Provided by : TED-Ed. Located at : https://youtu.be/u8C2Un6Gjhk . License : All Rights Reserved . License Terms : Standard YouTube License
- Why is our universe fine-tuned for life?. Authored by : Brian Greene. Provided by : TED. Located at : https://youtu.be/bf7BXwVeyWw . License : All Rights Reserved . License Terms : Standard YouTube License
- CMB Timeline300 no WMAP. Authored by : Ryan Kaldari. Provided by : NASA. Located at : https://commons.wikimedia.org/wiki/File:CMB_Timeline300_no_WMAP.jpg . License : Public Domain: No Known Copyright
- XDF-separated. Authored by : Z. Levay, F. Summers. Provided by : NASA and ESA. Located at : https://commons.wikimedia.org/wiki/File:XDF-separated.jpg . License : Public Domain: No Known Copyright
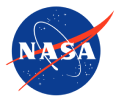
Cosmic History
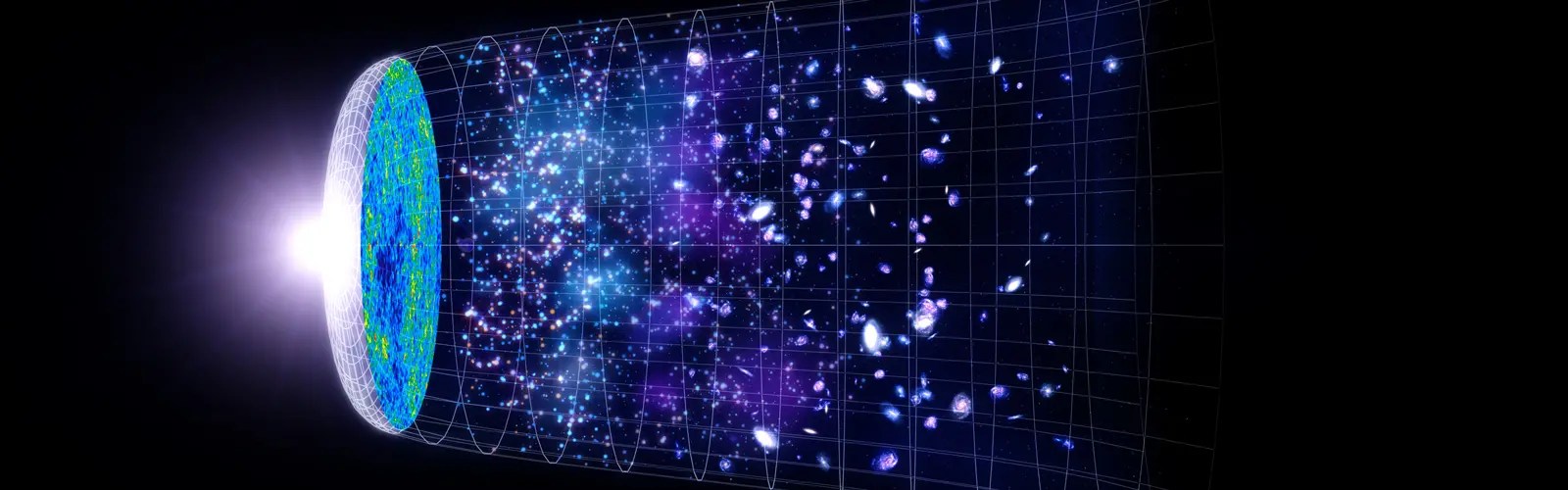
The Universe’s History
The origin, evolution, and nature of the universe have fascinated and confounded humankind for centuries. New ideas and major discoveries made during the 20th century transformed cosmology – the term for the way we conceptualize and study the universe – although much remains unknown. Here is the history of the universe according to cosmologists’ current theories.
Cosmic Inflation
Around 13.8 billion years ago, the universe expanded faster than the speed of light for a fraction of a second, a period called cosmic inflation. Scientists aren’t sure what came before inflation or what powered it. It’s possible that energy during this period was just part of the fabric of space-time. Cosmologists think inflation explains many aspects of the universe we observe today, like its flatness, or lack of curvature, on the largest scales. Inflation may have also magnified density differences that naturally occur on space’s smallest, quantum-level scales, which eventually helped form the universe’s large-scale structures.
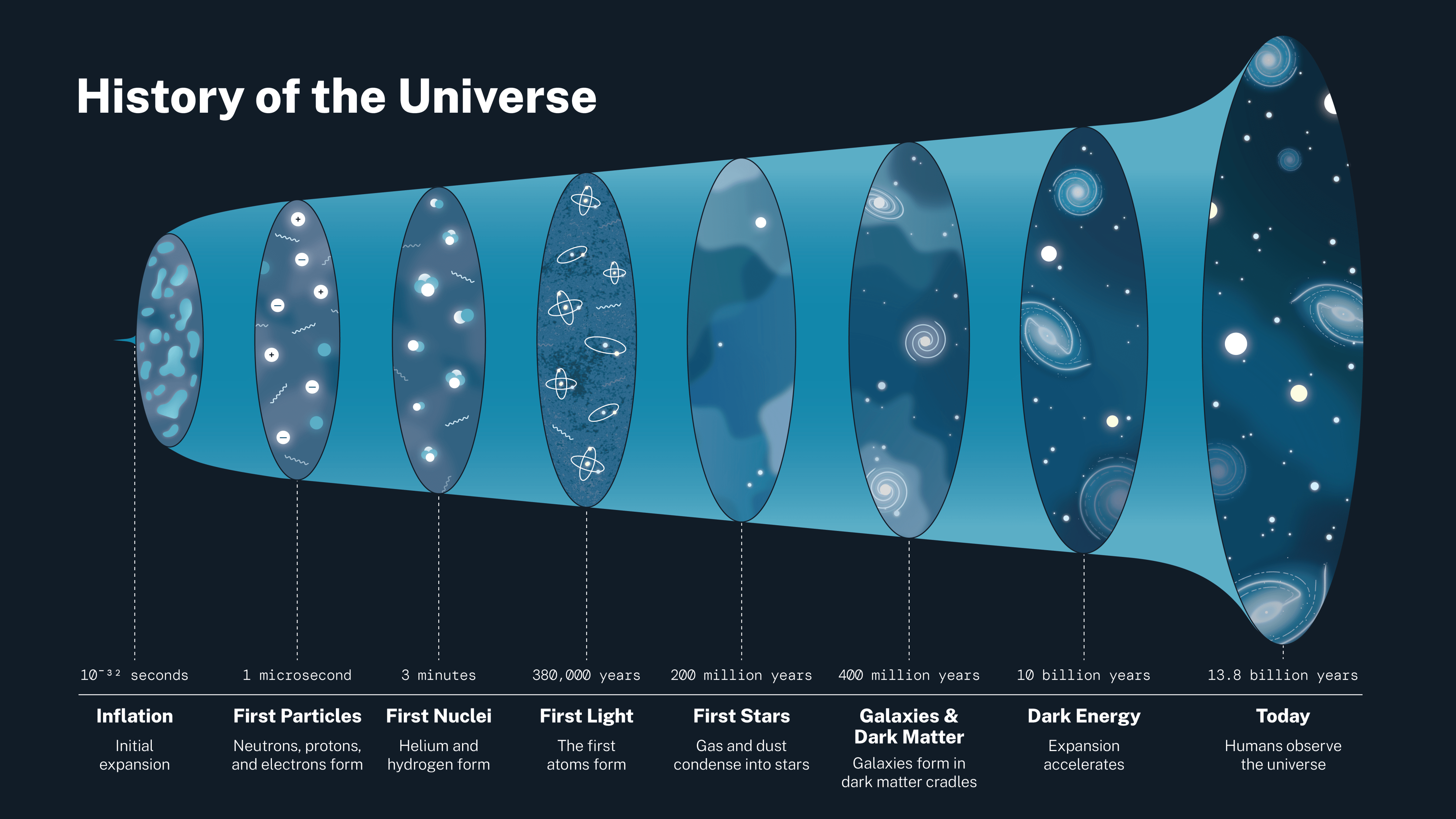
Big Bang and Nucleosynthesis
When cosmic inflation stopped, the energy driving it transferred to matter and light – the big bang. One second after the big bang, the universe consisted of an extremely hot (18 billion degrees Fahrenheit or 10 billion degrees Celsius) primordial soup of light and particles. In the following minutes, an era called nucleosynthesis, protons and neutrons collided and produced the earliest elements – hydrogen, helium, and traces of lithium and beryllium. After five minutes, most of today’s helium had formed, and the universe had expanded and cooled enough that further element formation stopped. At this point, though, the universe was still too hot for the atomic nuclei of these elements to catch electrons and form complete atoms. The cosmos was opaque because a vast number of electrons created a sort of fog that scattered light.
Big Bang News
What is Dark Energy? Inside our accelerating, expanding Universe
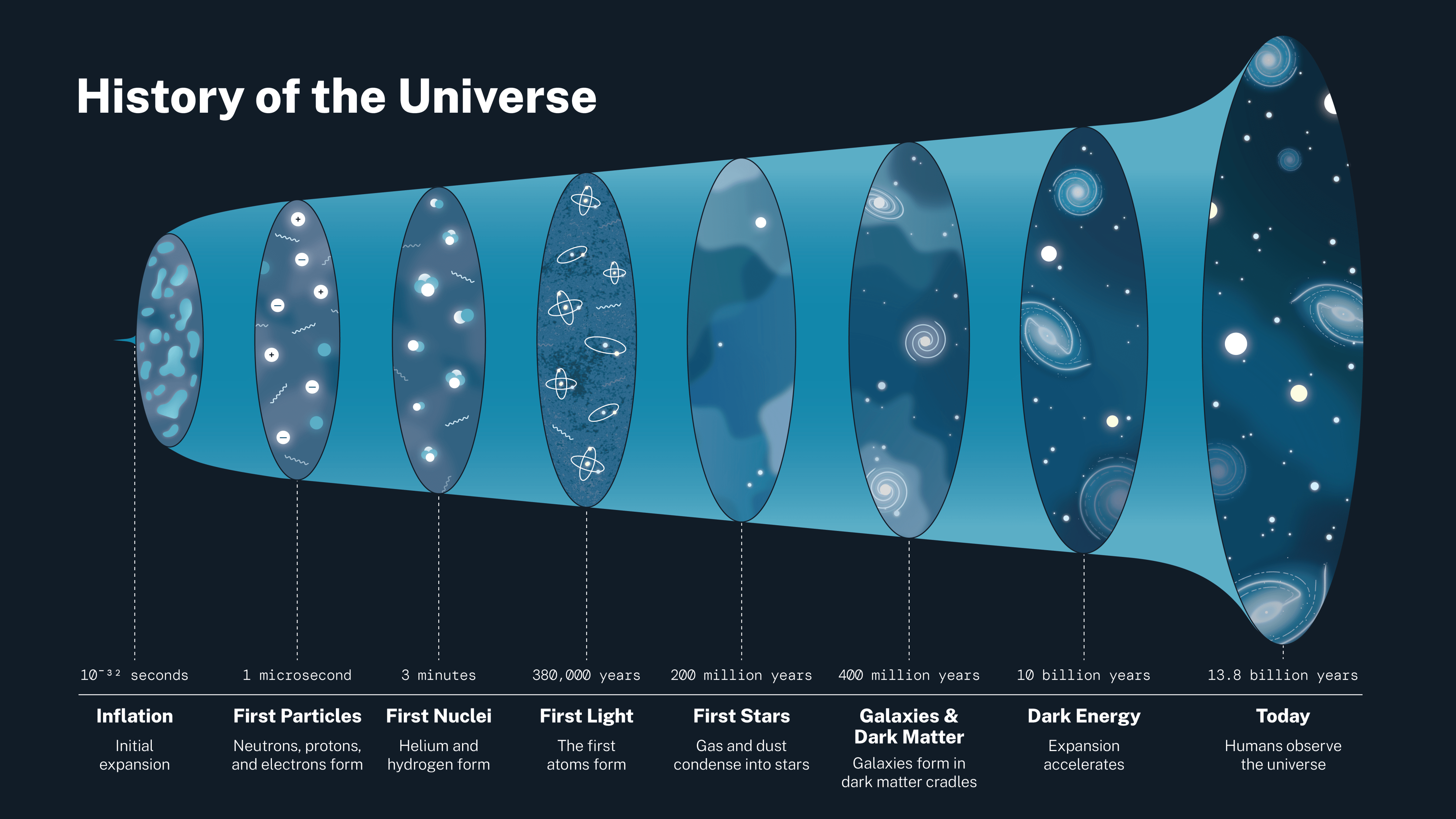
NASA’s Webb Identifies the Earliest Strands of the Cosmic Web
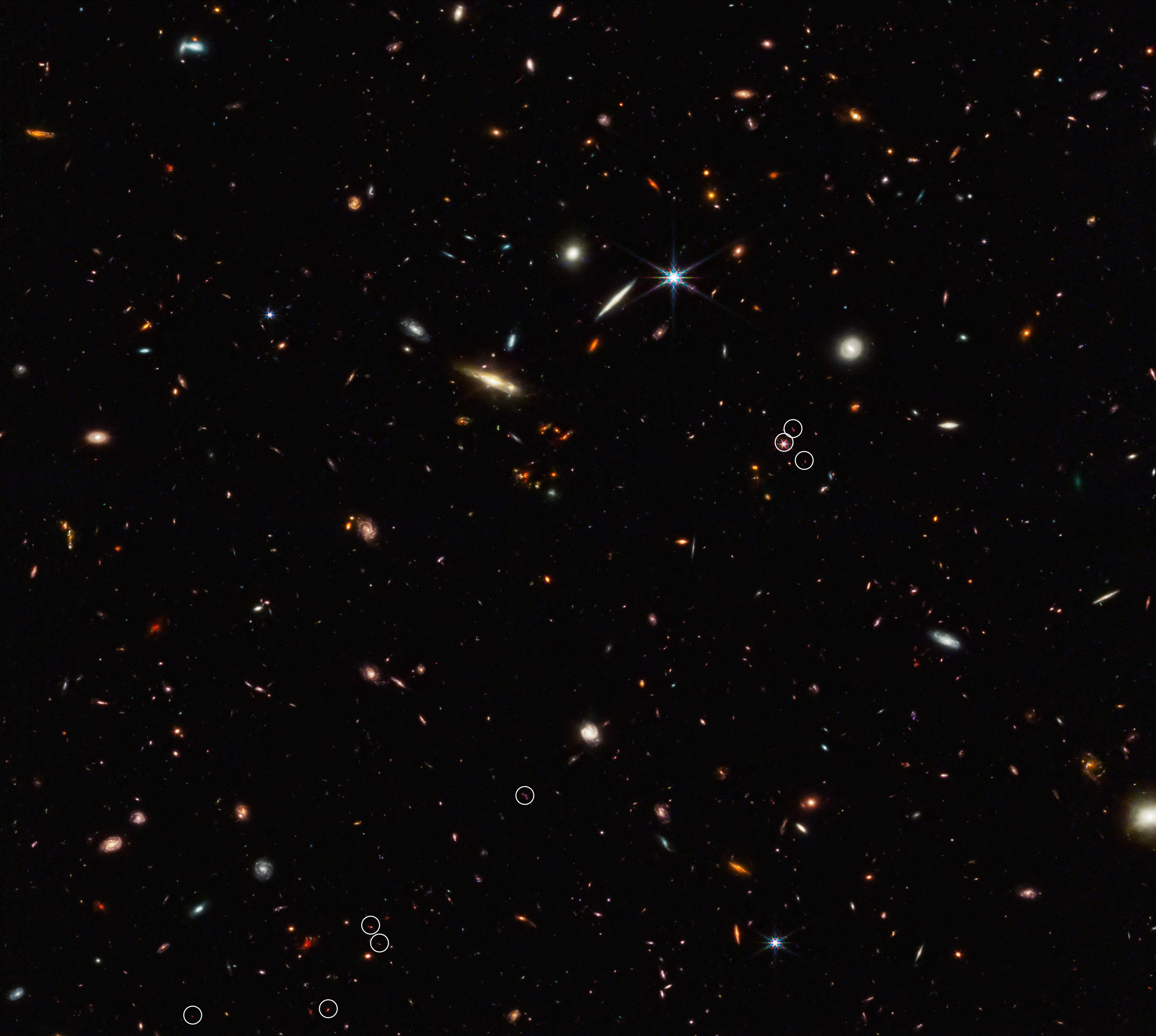
Lunar Crater Radio Telescope: Illuminating the Cosmic Dark Ages
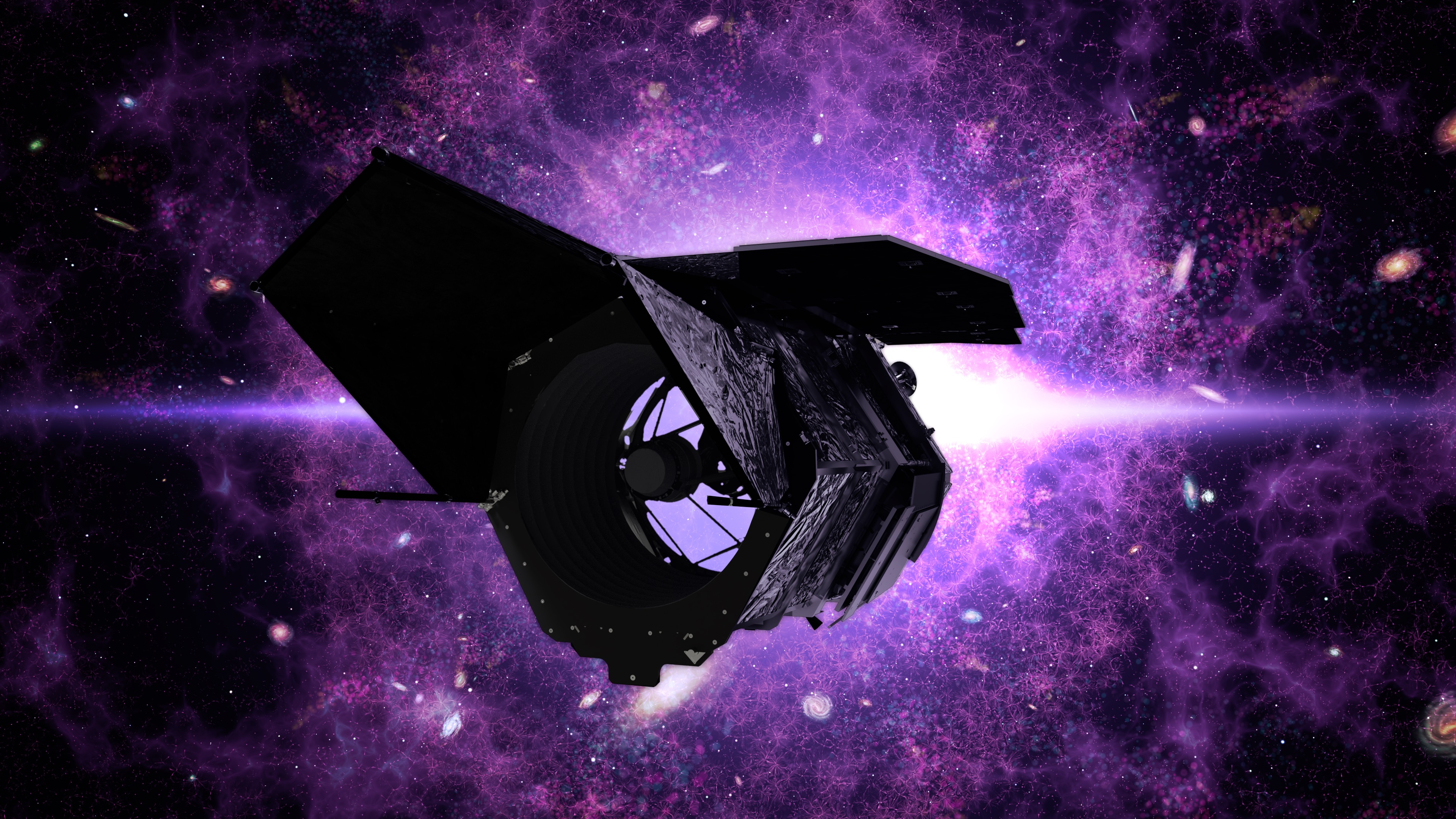
Roman Space Telescope Could Image 100 Hubble Ultra Deep Fields at Once
NASA’s Roman Space Telescope to Uncover Echoes of the Universe’s Creation
Recombination
Around 380,000 years after the big bang, the universe had cooled enough that atomic nuclei could capture electrons, a period astronomers call the epoch of recombination. This had two major effects on the cosmos. First, with most electrons now bound into atoms, there were no longer enough free ones to completely scatter light, and the cosmic fog cleared. The universe became transparent, and for the first time, light could freely travel over great distances. Second, the formation of these first atoms produced its own light. This glow, still detectable today, is called the cosmic microwave background. It is the oldest light we can observe in the universe.
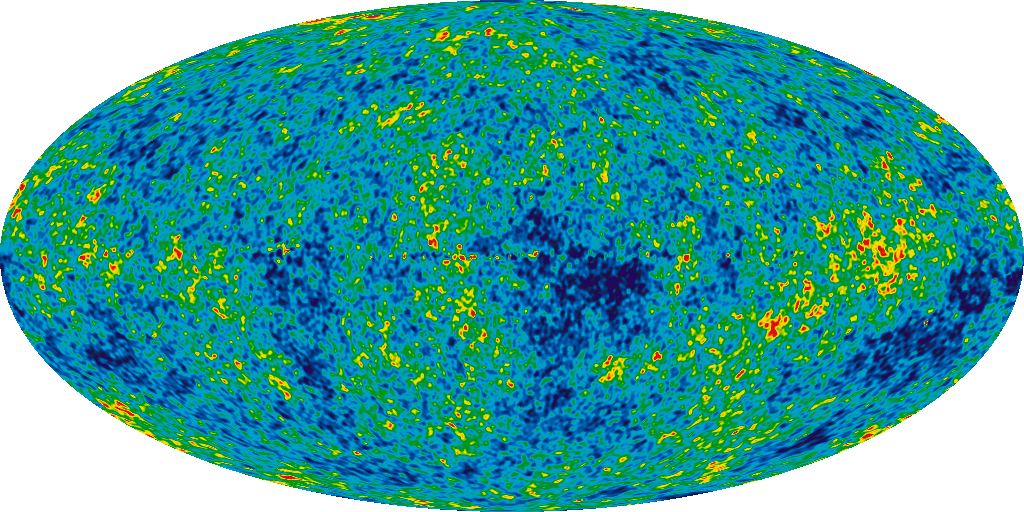
After the cosmic microwave background, the universe again became opaque at shorter wavelengths due to the absorbing effects of all those hydrogen atoms. For the next 200 million years the universe remained dark. There were no stars to shine. The cosmos at this point consisted of a sea of hydrogen atoms, helium, and trace amounts of heavier elements.
First Stars
Gas was not uniformly distributed throughout the universe. Cooler areas of space were lumpier, with denser clouds of gas. As these clumps grew more massive, their gravity attracted additional matter. As they became denser, and more compact, the centers of these clumps became hotter – hot enough eventually that nuclear fusion occurred in their centers. These were the first stars. They were 30 to 300 times more massive than our Sun and millions of times brighter. Over several hundred million years, the first stars collected into the first galaxies.
Reionization
At first, starlight couldn’t travel far because it was scattered by the relatively dense gas surrounding the first stars. Gradually, the ultraviolet light emitted by these stars broke down, or ionized, hydrogen atoms in the gas into their constituent electrons and protons. As this reionization progressed, starlight traveled farther, breaking up more and more hydrogen atoms. By the time the universe was 1 billion years old, stars and galaxies had transformed nearly all this gas, making the universe transparent to light as we see it today.
For many years, scientists thought the universe’s current expansion was slowing down. But in fact, cosmic expansion is speeding up. In 1998, astronomers found that certain supernovae, bright stellar explosions, were fainter than expected. They concluded this could only happen if the supernovae had moved farther away, at a faster rate than predicted.
Scientists suspect a mysterious substance they call dark energy is accelerating expansion. Future research may yield new surprises, but cosmologists suggest it’s likely the universe will continue to expand forever.
Discover More Topics From NASA
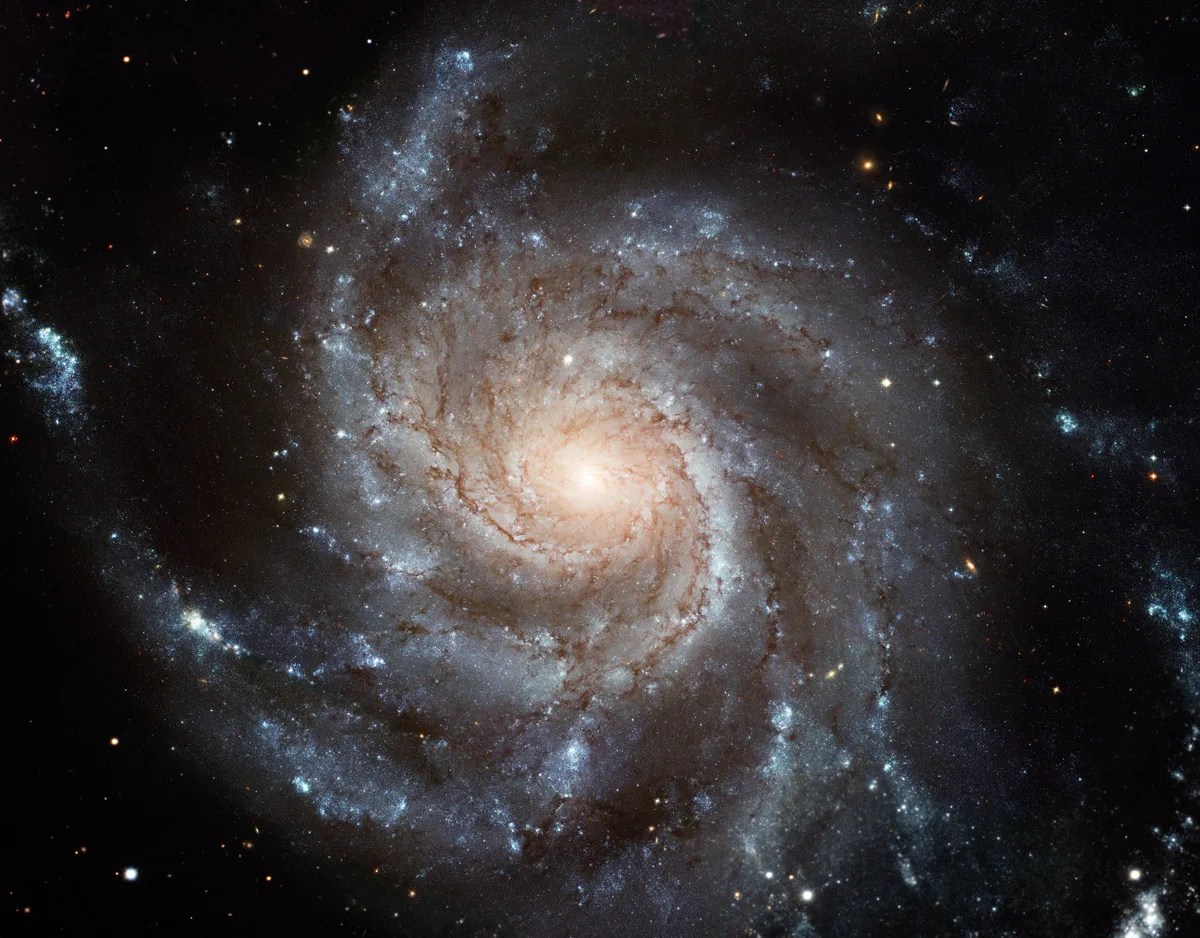
Black Holes
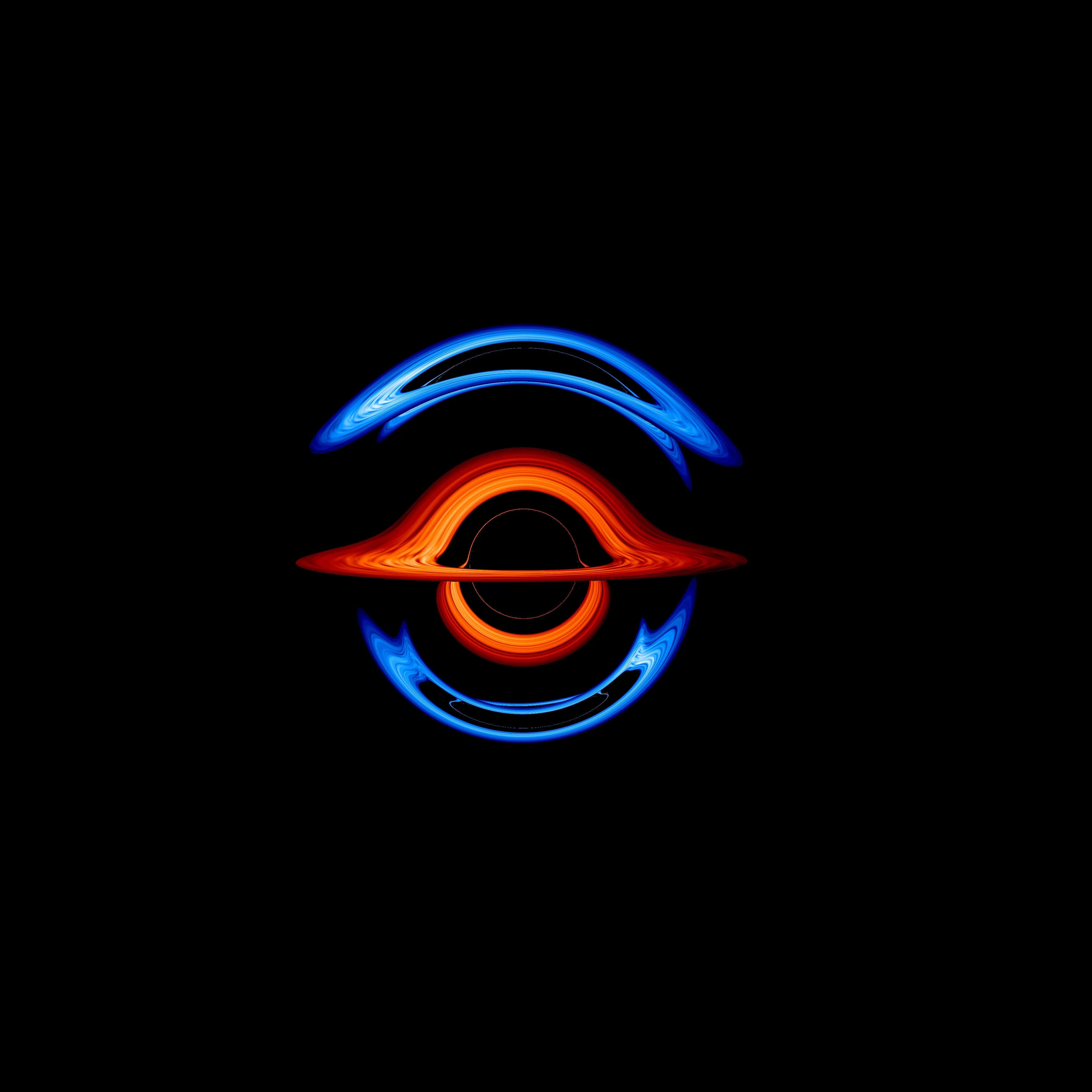
October 1, 1994
17 min read
The Evolution of the Universe
Some 15 billion years ago the universe emerged from a hot, dense sea of matter and energy. As the cosmos expanded and cooled, it spawned galaxies, stars, planets and life
By P. James E. Peebles , David N. Schramm , Edwin L. Turner & Richard G. Kron
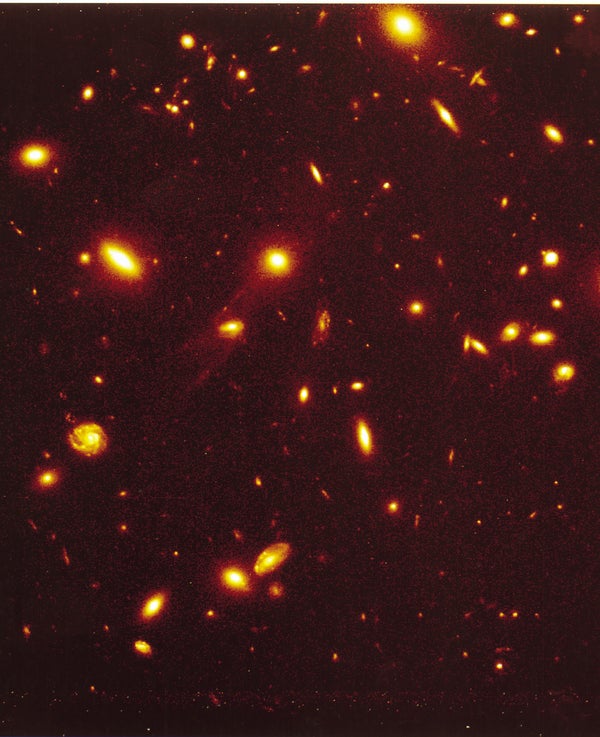
GALAXY CLUSTER is representative of what the universe looked like when it was 60 percent of its present age. The Hubble Space Telescope captured the image by focusing on the cluster as it completed 10 orbits. This image is one of the longest and clearest exposures ever produced. Several pairs of galaxies appear to be caught in one another’s gravitational field. Such interactions are rarely found in nearby clusters and are evidence that the universe is evolving.
Editor’s Note (10/8/19): Cosmologist James Peebles won a 2019 Nobel Prize in Physics for his contributions to theories of how our universe began and evolved. He describes these ideas in this article, which he co-wrote for Scientific American in 1994.
At a particular instant roughly 15 billion years ago, all the matter and energy we can observe, concentrated in a region smaller than a dime, began to expand and cool at an incredibly rapid rate. By the time the temperature had dropped to 100 million times that of the sun’s core, the forces of nature assumed their present properties, and the elementary particles known as quarks roamed freely in a sea of energy. When the universe had expanded an additional 1,000 times, all the matter we can measure filled a region the size of the solar system.
At that time, the free quarks became confined in neutrons and protons. After the universe had grown by another factor of 1,000, protons and neutrons combined to form atomic nuclei, including most of the helium and deuterium present today. All of this occurred within the first minute of the expansion. Conditions were still too hot, however, for atomic nuclei to capture electrons. Neutral atoms appeared in abundance only after the expansion had continued for 300,000 years and the universe was 1,000 times smaller than it is now. The neutral atoms then began to coalesce into gas clouds, which later evolved into stars. By the time the universe had expanded to one fifth its present size, the stars had formed groups recognizable as young galaxies.
On supporting science journalism
If you're enjoying this article, consider supporting our award-winning journalism by subscribing . By purchasing a subscription you are helping to ensure the future of impactful stories about the discoveries and ideas shaping our world today.
When the universe was half its present size, nuclear reactions in stars had produced most of the heavy elements from which terrestrial planets were made. Our solar system is relatively young: it formed five billion years ago, when the universe was two thirds its present size. Over time the formation of stars has consumed the supply of gas in galaxies, and hence the population of stars is waning. Fifteen billion years from now stars like our sun will be relatively rare, making the universe a far less hospitable place for observers like us.
Our understanding of the genesis and evolution of the universe is one of the great achievements of 20th-century science. This knowledge comes from decades of innovative experiments and theories. Modern telescopes on the ground and in space detect the light from galaxies billions of light-years away, showing us what the universe looked like when it was young. Particle accelerators probe the basic physics of the high-energy environment of the early universe. Satellites detect the cosmic background radiation left over from the early stages of expansion, providing an image of the universe on the largest scales we can observe.
Our best efforts to explain this wealth of data are embodied in a theory known as the standard cosmological model or the big bang cosmology. The major claim of the theory is that in the largescale average the universe is expanding in a nearly homogeneous way from a dense early state. At present, there are no fundamental challenges to the big bang theory, although there are certainly unresolved issues within the theory itself. Astronomers are not sure, for example, how the galaxies were formed, but there is no reason to think the process did not occur within the framework of the big bang. Indeed, the predictions of the theory have survived all tests to date.
Yet the big bang model goes only so far, and many fundamental mysteries remain. What was the universe like before it was expanding? (No observation we have made allows us to look back beyond the moment at which the expansion began.) What will happen in the distant future, when the last of the stars exhaust the supply of nuclear fuel? No one knows the answers yet.
Our universe may be viewed in many lights—by mystics, theologians, philosophers or scientists. In science we adopt the plodding route: we accept only what is tested by experiment or observation. Albert Einstein gave us the now well-tested and accepted Theory of General Relativity, which establishes the relations between mass, energy, space and time. Einstein showed that a homogeneous distribution of matter in space fits nicely with his theory. He assumed without discussion that the universe is static, unchanging in the large-scale average [see “How Cosmology Became a Science,” by Stephen G. Brush; SCIENTIFIC AMERICAN, August 1992].
In 1922 the Russian theorist Alexander A. Friedmann realized that Einstein’s universe is unstable; the slightest perturbation would cause it to expand or contract. At that time, Vesto M. Slipher of Lowell Observatory was collecting the first evidence that galaxies are actually moving apart. Then, in 1929, the eminent astronomer Edwin P. Hubble showed that the rate a galaxy is moving away from us is roughly proportional to its distance from us.
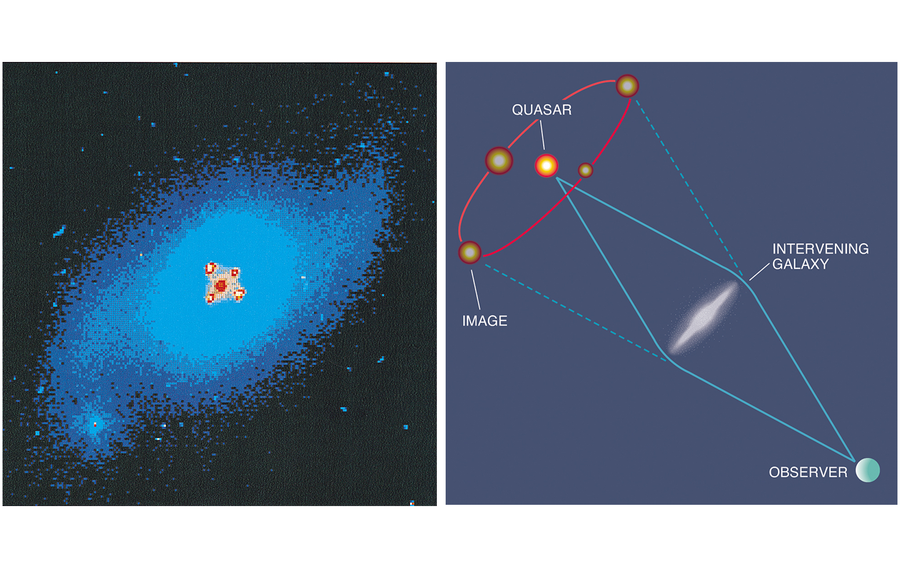
MULTIPLE IMAGES of a distant quasar ( left ) are the result of an effect known as gravitational lensing. The effect occurs when light from a distant object is bent by the gravitational field of an intervening galaxy. In this case, the galaxy, which is visible in the center, produces four images of the quasar. The photograph was produced using the Hubble telescope.
The existence of an expanding universe implies that the cosmos has evolved from a dense concentration of matter into the present broadly spread distribution of galaxies. Fred Hoyle, an English cosmologist, was the first to call this process the big bang. Hoyle intended to disparage the theory, but the name was so catchy it gained popularity. It is somewhat misleading, however, to describe the expansion as some type of explosion of matter away from some particular point in space.
That is not the picture at all: in Einstein’s universe the concept of space and the distribution of matter are intimately linked; the observed expansion of the system of galaxies reveals the unfolding of space itself. An essential feature of the theory is that the average density in space declines as the universe expands; the distribution of matter forms no observable edge. In an explosion the fastest particles move out into empty space, but in the big bang cosmology, particles uniformly fill all space. The expansion of the universe has had little influence on the size of galaxies or even clusters of galaxies that are bound by gravity; space is simply opening up between them. In this sense, the expansion is similar to a rising loaf of raisin bread. The dough is analogous to space, and the raisins, to clusters of galaxies. As the dough expands, the raisins move apart. Moreover, the speed with which any two raisins move apart is directly and positively related to the amount of dough separating them.
The evidence for the expansion of the universe has been accumulating for some 60 years. The first important clue is the redshift. A galaxy emits or absorbs some wavelengths of light more strongly than others. If the galaxy is moving away from us, these emission and absorption features are shifted to longer wavelengths—that is, they become redder as the recession velocity increases. This phenomenon is known as the redshift.
Hubble’s measurements indicated that the redshift of a distant galaxy is greater than that of one closer to the earth. This relation, now known as Hubble’s law, is just what one would expect in a uniformly expanding universe. Hubble’s law says the recession velocity of a galaxy is equal to its distance multiplied by a quantity called Hubble’s constant. The redshift effect in nearby galaxies is relatively subtle, requiring good instrumentation to detect it. In contrast, the redshift of very distant objects—radio galaxies and quasars—is an awesome phenomenon; some appear to be moving away at greater than 90 percent of the speed of light.
Hubble contributed to another crucial part of the picture. He counted the number of visible galaxies in different directions in the sky and found that they appear to be rather uniformly distributed. The value of Hubble’s constant seemed to be the same in all directions, a necessary consequence of uniform expansion. Modern surveys confirm the fundamental tenet that the universe is homogeneous on large scales. Although maps of the distribution of the nearby galaxies display clumpiness, deeper surveys reveal considerable uniformity.
The Milky Way, for instance, resides in a knot of two dozen galaxies; these in turn are part of a complex of galaxies that protrudes from the so-called local supercluster. The hierarchy of clustering has been traced up to dimensions of about 500 million light-years. The fluctuations in the average density of matter diminish as the scale of the structure being investigated increases. In maps that cover distances that reach close to the observable limit, the average density of matter changes by less than a tenth of a percent.
To test Hubble’s law, astronomers need to measure distances to galaxies. One method for gauging distance is to observe the apparent brightness of a galaxy. If one galaxy is four times fainter in the night sky than an otherwise comparable galaxy, then it can be estimated to be twice as far away. This expectation has now been tested over the whole of the visible range of distances.
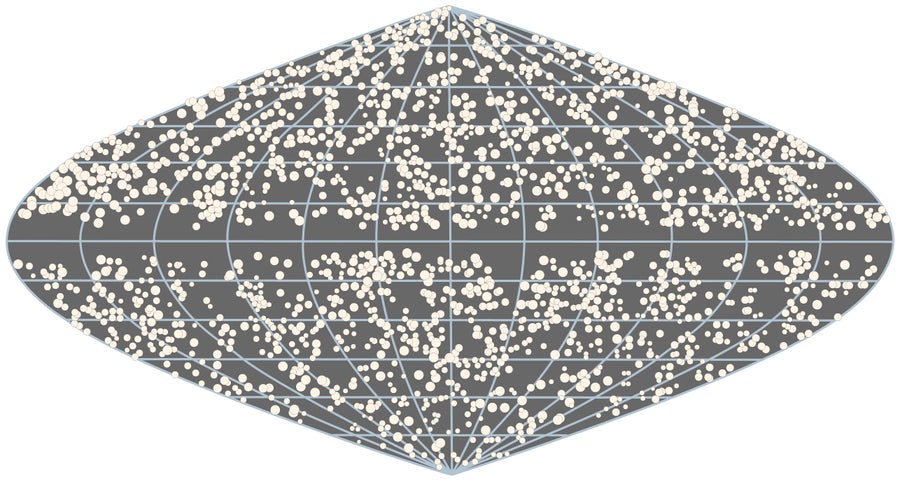
HOMOGENEOUS DISTRIBUTION of galaxies is apparent in a map that includes objects from 300 to 1,000 million light-years away. The only inhomogeneity, a gap near the center line, occurs because part of the sky is obscured by the Milky Way. Michael Strauss of the Institute for Advanced Study in Princeton, N.J., created the map using data from NASA’s Infrared Astronomical Satellite .
Some critics of the theory have pointed out that a galaxy that appears to be smaller and fainter might not actually be more distant. Fortunately, there is a direct indication that objects whose redshifts are larger really are more distant. The evidence comes from observations of an effect known as gravitational lensing. An object as massive and compact as a galaxy can act as a crude lens, producing a distorted, magnified image (or even many images) of any background radiation source that lies behind it. Such an object does so by bending the paths of light rays and other electromagnetic radiation. So if a galaxy sits in the line of sight between the earth and some distant object, it will bend the light rays from the object so that they are observable [see “Gravitational Lenses,” by Edwin L. Turner; SCIENTIFIC AMERICAN, July 1988]. During the past decade, astronomers have discovered more than a dozen gravitational lenses. The object behind the lens is always found to have a higher redshift than the lens itself, confirming the qualitative prediction of Hubble’s law.
Hubble’s law has great significance not only because it describes the expansion of the universe but also because it can be used to calculate the age of the cosmos. To be precise, the time elapsed since the big bang is a function of the present value of Hubble’s constant and its rate of change. Astronomers have determined the approximate rate of the expansion, but no one has yet been able to measure the second value precisely.
Still, one can estimate this quantity from knowledge of the universe’s average density. One expects that because gravity exerts a force that opposes expansion, galaxies would tend to move apart more slowly now than they did in the past. The rate of change in expansion is therefore related to the gravitational pull of the universe set by its average density. If the density is that of just the visible material in and around galaxies, the age of the universe probably lies between 12 and 20 billion years. (The range allows for the uncertainty in the rate of expansion.)
Yet many researchers believe the density is greater than this minimum value. So-called dark matter would make up the difference. A strongly defended argument holds that the universe is just dense enough that in the remote future the expansion will slow almost to zero. Under this assumption, the age of the universe decreases to the range of seven to 13 billion years.
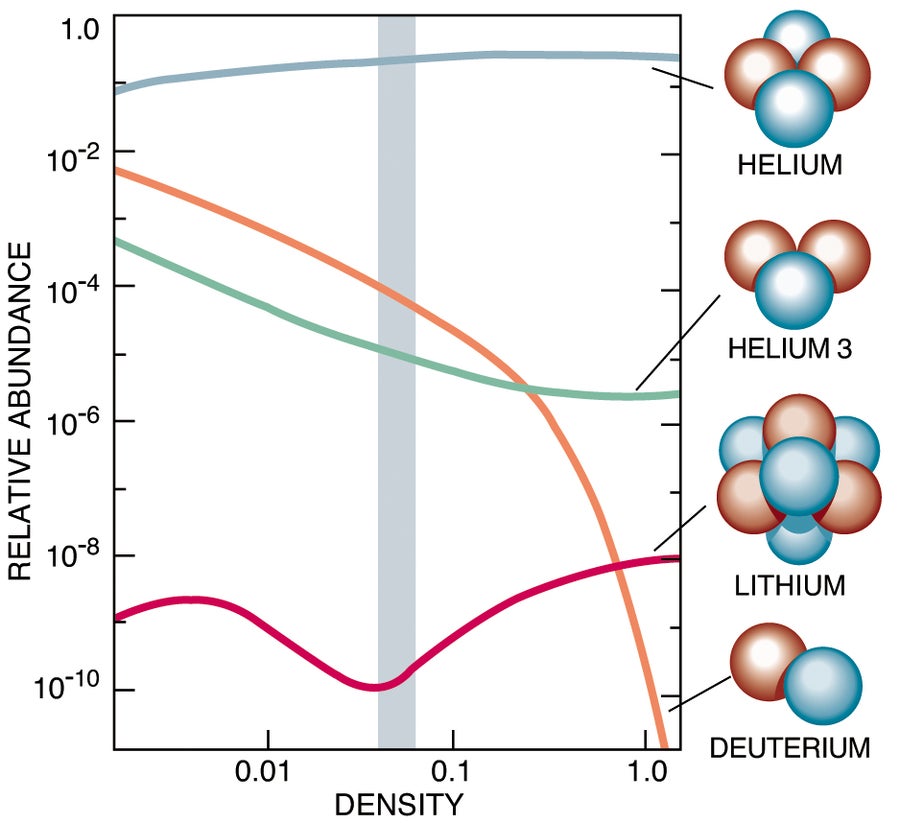
DENSITY of neutrons and protons in the universe determined the abundances of certain elements. For a higher density universe, the computed helium abundance is little different, and the computed abundance of deuterium is considerably lower. The shaded region is consistent with the observations, ranging from an abundance of 24 percent for helium to one part in 1010 for the lithium isotope. This quantitative agreement is a prime success of the big bang cosmology.
To improve these estimates, many astronomers are involved in intensive research to measure both the distances to galaxies and the density of the universe. Estimates of the expansion time provide an important test for the big bang model of the universe. If the theory is correct, everything in the visible universe should be younger than the expansion time computed from Hubble’s law.
These two timescales do appear to be in at least rough concordance. For example, the oldest stars in the disk of the Milky Way galaxy are about nine billion years old—an estimate derived from the rate of cooling of white dwarf stars. The stars in the halo of the Milky Way are somewhat older, about 15 billion years—a value derived from the rate of nuclear fuel consumption in the cores of these stars. The ages of the oldest known chemical elements are also approximately 15 billion years—a number that comes from radioactive dating techniques. Workers in laboratories have derived these age estimates from atomic and nuclear physics. It is noteworthy that their results agree, at least approximately, with the age that astronomers have derived by measuring cosmic expansion.
Another theory, the steady state theory, also succeeds in accounting for the expansion and homogeneity of the universe. In 1946 three physicists in England—Hoyle, Hermann Bondi and Thomas Gold—proposed such a cosmology. In their theory the universe is forever expanding, and matter is created spontaneously to fill the voids. As this material accumulates, they suggested, it forms new stars to replace the old. This steady state hypothesis predicts that ensembles of galaxies close to us should look statistically the same as those far away. The big bang cosmology makes a different prediction: if galaxies were all formed long ago, distant galaxies should look younger than those nearby because light from them requires a longer time to reach us. Such galaxies should contain more shortlived stars and more gas out of which future generations of stars will form.
The test is simple conceptually, but it took decades for astronomers to develop detectors sensitive enough to study distant galaxies in detail. When astronomers examine nearby galaxies that are powerful emitters of radio wavelengths, they see, at optical wavelengths, relatively round systems of stars. Distant radio galaxies, on the other hand, appear to have elongated and sometimes irregular structures. Moreover, in most distant radio galaxies, unlike the ones nearby, the distribution of light tends to be aligned with the pattern of the radio emission.
Likewise, when astronomers study the population of massive, dense clusters of galaxies, they find differences between those that are close and those far away. Distant clusters contain bluish galaxies that show evidence of ongoing star formation. Similar clusters that are nearby contain reddish galaxies in which active star formation ceased long ago. Observations made with the Hubble Space Telescope confirm that at least some of the enhanced star formation in these younger clusters may be the result of collisions between their member galaxies, a process that is much rarer in the present epoch.

DISTANT GALAXIES differ greatly from those nearby—an observation that shows that galaxies evolved from earlier, more irregular forms. Among galaxies that are bright at both optical ( blue ) and radio ( red ) wavelengths, the nearby galaxies tend to have smooth elliptical shapes at optical wavelengths and very elongated radio images. As redshift, and therefore distance, increases, galaxies have more irregular elongated forms that appear aligned at optical and radio wavelengths. The galaxy at the far right is seen as it was at 10 percent of the present age of the universe. The images were assembled by Pat McCarthy of the Carnegie Institute.
So if galaxies are all moving away from one another and are evolving from earlier forms, it seems logical that they were once crowded together in some dense sea of matter and energy. Indeed, in 1927, before much was known about distant galaxies, a Belgian cosmologist and priest, Georges Lemaître, proposed that the expansion of the universe might be traced to an exceedingly dense state he called the primeval “super-atom.” It might even be possible, he thought, to detect remnant radiation from the primeval atom. But what would this radiation signature look like?
When the universe was very young and hot, radiation could not travel very far without being absorbed and emitted by some particle. This continuous exchange of energy maintained a state of thermal equilibrium; any particular region was unlikely to be much hotter or cooler than the average. When matter and energy settle to such a state, the result is a so-called thermal spectrum, where the intensity of radiation at each wavelength is a definite function of the temperature. Hence, radiation originating in the hot big bang is recognizable by its spectrum.
In fact, this thermal cosmic background radiation has been detected. While working on the development of radar in the 1940s, Robert H. Dicke, then at the Massachusetts Institute of Technology, invented the microwave radiometer—a device capable of detecting low levels of radiation. In the 1960s Bell Laboratories used a radiometer in a telescope that would track the early communications satellites Echo-1 and Telstar. The engineer who built this instrument found that it was detecting unexpected radiation. Arno A. Penzias and Robert W. Wilson identified the signal as the cosmic background radiation. It is interesting that Penzias and Wilson were led to this idea by the news that Dicke had suggested that one ought to use a radiometer to search for the cosmic background.
Astronomers have studied this radiation in great detail using the Cosmic Background Explorer (COBE) satellite and a number of rocket-launched, balloon-borne and ground-based experiments. The cosmic background radiation has two distinctive properties. First, it is nearly the same in all directions. (As George F. Smoot of Lawrence Berkeley Laboratory and his team discovered in 1992, the variation is just one part per 100,000.) The interpretation is that the radiation uniformly fills space, as predicted in the big bang cosmology. Second, the spectrum is very close to that of an object in thermal equilibrium at 2.726 kelvins above absolute zero. To be sure, the cosmic background radiation was produced when the universe was far hotter than 2.726 degrees, yet researchers anticipated correctly that the apparent temperature of the radiation would be low. In the 1930s Richard C. Tolman of the California Institute of Technology showed that the temperature of the cosmic background would diminish because of the universe’s expansion.
The cosmic background radiation provides direct evidence that the universe did expand from a dense, hot state, for this is the condition needed to produce the radiation. In the dense, hot early universe thermonuclear reactions produced elements heavier than hydrogen, including deuterium, helium and lithium. It is striking that the computed mix of the light elements agrees with the observed abundances. That is, all evidence indicates that the light elements were produced in the hot, young universe, whereas the heavier elements appeared later, as products of the thermonuclear reactions that power stars.
The theory for the origin of the light elements emerged from the burst of research that followed the end of World War II. George Gamow and graduate student Ralph A. Alpher of George Washington University and Robert Herman of the Johns Hopkins University Applied Physics Laboratory and others used nuclear physics data from the war e›ort to predict what kind of nuclear processes might have occurred in the early universe and what elements might have been produced. Alpher and Herman also realized that a remnant of the original expansion would still be detectable in the existing universe.
Despite the fact that significant details of this pioneering work were in error, it forged a link between nuclear physics and cosmology. The workers demonstrated that the early universe could be viewed as a type of thermonuclear reactor. As a result, physicists have now precisely calculated the abundances of light elements produced in the big bang and how those quantities have changed because of subsequent events in the interstellar medium and nuclear processes in stars.
Our grasp of the conditions that prevailed in the early universe does not translate into a full understanding of how galaxies formed. Nevertheless, we do have quite a few pieces of the puzzle. Gravity causes the growth of density fluctuations in the distribution of matter, because it more strongly slows the expansion of denser regions, making them grow still denser. This process is observed in the growth of nearby clusters of galaxies, and the galaxies themselves were probably assembled by the same process on a smaller scale.
The growth of structure in the early universe was prevented by radiation pressure, but that changed when the universe had expanded to about 0.1 percent of its present size. At that point, the temperature was about 3,000 kelvins, cool enough to allow the ions and electrons to combine to form neutral hydrogen and helium. The neutral matter was able to slip through the radiation and to form gas clouds that could collapse to star clusters. Observations show that by the time the universe was one fifth its present size, matter had gathered into gas clouds large enough to be called young galaxies.
A pressing challenge now is to reconcile the apparent uniformity of the early universe with the lumpy distribution of galaxies in the present universe. Astronomers know that the density of the early universe did not vary by much, because they observe only slight irregularities in the cosmic background radiation. So far it has been easy to develop theories that are consistent with the available measurements, but more critical tests are in progress. In particular, different theories for galaxy formation predict quite different fluctuations in the cosmic background radiation on angular scales less than about one degree. Measurements of such tiny fluctuations have not yet been done, but they might be accomplished in the generation of experiments now under way. It will be exciting to learn whether any of the theories of galaxy formation now under consideration survive these tests.
The present-day universe has provided ample opportunity for the development of life as we know it—there are some 100 billion billion stars similar to the sun in the part of the universe we can observe. The big bang cosmology implies, however, that life is possible only for a bounded span of time: the universe was too hot in the distant past, and it has limited resources for the future. Most galaxies are still producing new stars, but many others have already exhausted their supply of gas. Thirty billion years from now, galaxies will be much darker and filled with dead or dying stars, so there will be far fewer planets capable of supporting life as it now exists.
The universe may expand forever, in which case all the galaxies and stars will eventually grow dark and cold. The alternative to this big chill is a big crunch. If the mass of the universe is large enough, gravity will eventually reverse the expansion, and all matter and energy will be reunited. During the next decade, as researchers improve techniques for measuring the mass of the universe, we may learn whether the present expansion is headed toward a big chill or a big crunch.
In the near future, we expect new experiments to provide a better understanding of the big bang. As we improve measurements of the expansion rate and the ages of stars, we may be able to confirm that the stars are indeed younger than the expanding universe. The larger telescopes recently completed or under construction may allow us to see how the mass of the universe affects the curvature of spacetime, which in turn influences our observations of distant galaxies.
We will also continue to study issues that the big bang cosmology does not address. We do not know why there was a big bang or what may have existed before. We do not know whether our universe has siblings—other expanding regions well removed from what we can observe. We do not understand why the fundamental constants of nature have the values they do. Advances in particle physics suggest some interesting ways these questions might be answered; the challenge is to find experimental tests of the ideas.
In following the debate on such matters of cosmology, one should bear in mind that all physical theories are approximations of reality that can fail if pushed too far. Physical science advances by incorporating earlier theories that are experimentally supported into larger, more encompassing frameworks. The big bang theory is supported by a wealth of evidence: it explains the cosmic background radiation, the abundances of light elements and the Hubble expansion. Thus, any new cosmology surely will include the big bang picture. Whatever developments the coming decades may bring, cosmology has moved from a branch of philosophy to a physical science where hypotheses meet the test of observation and experiment.
- Skip to main content
- Keyboard shortcuts for audio player
13.7 Cosmos & Culture
The origin of the universe: from nothing everything.
Marcelo Gleiser

A computer simulation of the formation of large-scale structures in the Universe, showing a patch of 100 million light-years and the resulting coherent motions of galaxies flowing towards the highest mass concentration in the centre. The snapshot refers to an epoch about 10 billion years back in time. Klaus Dolag/VIMOS-VLT Deep Survey/ESO hide caption
A computer simulation of the formation of large-scale structures in the Universe, showing a patch of 100 million light-years and the resulting coherent motions of galaxies flowing towards the highest mass concentration in the centre. The snapshot refers to an epoch about 10 billion years back in time.
Last week, I started a discussion of what I call "The Three Origins," focusing first on the origin of life. Although we are far from knowing how non-living matter became living organisms on primitive Earth some 3.5 billion years ago (or more), or how to repeat the feat in the laboratory, I consider this the "easiest" of the three questions.
Contrary to the origin of the Universe and the origin of mind, the origin of life is something we can study from the outside in, where we can have an external and objective view of what is going on. Even if, as I have argued, it seems impossible to know exactly how life originated on Earth (unless it could be proven that there is only one pathway from nonlife to life), we can still investigate the biochemical pathways leading to what we may call a living organism. In the case of the cosmos or the mind, things are subtler.
From what we know, all cultures have a creation narrative describing the origin of the world, of how everything came from nothing. As I explored in The Dancing Universe: From Creation Myths to the Big Bang , there are only a small number of possible answers to the origin of the cosmos. All creation myths presuppose the existence of some kind of divine or absolute power capable of creating the world. In the vast majority of cases, the Bible being one example, this absolute power is embodied in God, or a group of gods. In others, the Universe is eternal, without a starting moment in the distant past: it has existed forever and will exist forever. In others still, the cosmos emerges without any divine interference from a primal Nothingness, from an innate tendency to exist. This Nothing can be complete emptiness, a primal egg, or even a struggle between chaos and order. Not every creation myth uses divine intervention or supposes that time started in some moment in the past.
According to modern science, the origin of the universe is part of cosmology. In trying to describe a creation process through scientific language we encounter a serious challenge: if every effect results from a cause, we can follow the chain of causation backwards in time until we arrive at the First Cause. But what caused this cause? Aristotle, for one, used some kind of divine entity to solve this conundrum, the Unmoved Mover, the one that can cause without having been caused. Very convenient, but not scientifically satisfying.
As current astronomical observations resolutely point to a Universe with a beginning in the distant past (according to the latest measurements from the Planck satellite related here last week , at about 13.8 billion years ago), scientific models of the origin of the Universe must face the challenge of explaining or doing away with the problem of the First Cause.
The fundamental question is this: even if a scientific explanation exists, is it an acceptable answer to the question of the origin of the Universe? Defenders of scientism might argue that this is the best that we can do, that it is the only reasonable thing that we can do. Fair enough, if you believe that science should provide an answer to this question and if you are happy with the answers given.
The best answer we have at this point is that the Universe emerged spontaneously from a random quantum fluctuation in some sort of primordial quantum vacuum, the scientific equivalent of "nothing." However, this quantum vacuum is a very loaded nothing: it assumes the whole machinery of quantum field theory, the modern description of how elementary particles of matter interact with one another, was already in operation.
In the quantum realm, even the lowest energy state, the "vacuum," is not empty. Even if the energy of a quantum system is zero, it is never really zero due to the inherent quantum fluctuations about this state. A zero energy quantum state is as impossible as a perfectly still lake, with absolutely no disturbances on its surface. This quantum jitteriness amounts to fluctuations on the value of the energy; if one of these fluctuations is unstable it may grow big, like a soap bubble that blows itself up. The energy remains zero on average because of a clever interplay between the positive energy of matter and the negative energy of attractive gravity. This is the result that physicists like Stephen Hawking, Lawrence Krauss, Mikio Kaku and others speak of when they state that the "universe came out of quantum nothingness," or something to that extent.
The essential question, though, is whether this is indeed a satisfactory explanation to the question of cosmic origins, or simply part of one. The philosopher David Albert raised similar points in a recent review of Lawrence Krauss's book. Here is Krauss's response.
It is obvious that this quantum nothingness is very different from an absolute nothingness. Physicists may shrug this away stating that concepts like absolute nothingness are not scientific and hence have no explanatory value. It is indeed true that there is no such thing as absolute nothingness in science, since the vacuum is pregnant with all sorts of stuff. Any scientific explanation presupposes a whole conceptual structure that is absolutely essential for science to function: energy, space, time, the equations we use, the laws of Nature. Science can't exist without this scaffolding. So, a scientific explanation of the origin of the universe needs to use such concepts to make sense. It necessarily starts from something, which is the best that science can ever hope to do.
Even if we move on to the multiverse, things still need to be formulated in terms of fields, energy, spacetime, derivatives, etc. Furthermore, scientific hypotheses need to be testable and falsifiable, and we don't yet know how to do this with a quantum fluctuation that generates a universe. We can't set this experiment in the laboratory and examine the right conditions for universes to emerge from the quantum vacuum. Contrary to the origin of life question, we can't step out of the Universe to examine it from the outside in. At best, and this should be quite enough for a scientific explanation of cosmic origins, a model for the quantum origin of the Universe should lead to a cosmos compatible with current observations. Stepping out into the abstract multiverse may provide us with different plausible cosmoids and help us understand why our own Universe is so special. But unless there is a very clear selection principle that doesn't predicate our existence, the question as to why this Universe and not another will remain open.
And this is not at all bad. The fact that science answers so many questions doesn't mean it should answer all; or that some questions should only be answered through science. Before I am accused of advocating obscurantism, let me be clear. What I mean is that a scientific explanation to the origin of the Universe, at least one based in the current way we do science, cannot be self-contained. Sometimes we must have the humility to accept that our modes of explanation have limits and make peace with what we can do; and marvel at how much we can do without the pretense of knowing how to do everything.
You can keep up with more of what Marcelo is thinking on Facebook and Twitter: @mgleiser
- the big bang
CERN Accelerating science
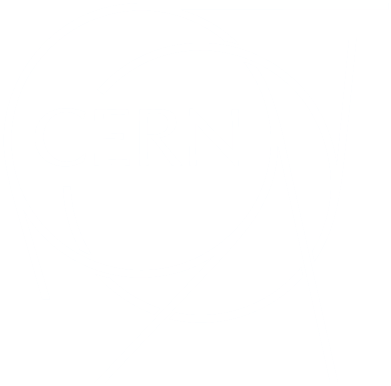
The early universe
All matter in the universe was formed in one explosive event 13.7 billion years ago – the Big Bang
The Big Bang
In 1929 the American astronomer Edwin Hubble discovered that the distances to far-away galaxies were proportional to their redshifts. Redshift occurs when a light source moves away from its observer: the light's apparent wavelength is stretched via the Doppler effect towards the red part of the spectrum. Hubble’s observation implied that distant galaxies were moving away from us, as the furthest galaxies had the fastest apparent velocities. If galaxies are moving away from us, reasoned Hubble, then at some time in the past, they must have been clustered close together.
Hubble’s discovery was the first observational support for Georges Lemaître’s Big Bang theory of the universe, proposed in 1927. Lemaître proposed that the universe expanded explosively from an extremely dense and hot state, and continues to expand today. Subsequent calculations have dated this Big Bang to approximately 13.7 billion years ago. In 1998 two teams of astronomers working independently at Berkeley, California observed that supernovae – exploding stars – were moving away from Earth at an accelerating rate. This earned them the Nobel prize in physics in 2011 . Physicists had assumed that matter in the universe would slow its rate of expansion; gravity would eventually cause the universe to fall back on its centre. Though the Big Bang theory cannot describe what the conditions were at the very beginning of the universe, it can help physicists describe the earliest moments after the start of the expansion.
In the first moments after the Big Bang, the universe was extremely hot and dense. As the universe cooled, conditions became just right to give rise to the building blocks of matter – the quarks and electrons of which we are all made. A few millionths of a second later, quarks aggregated to produce protons and neutrons. Within minutes, these protons and neutrons combined into nuclei. As the universe continued to expand and cool, things began to happen more slowly. It took 380,000 years for electrons to be trapped in orbits around nuclei, forming the first atoms. These were mainly helium and hydrogen, which are still by far the most abundant elements in the universe. Present observations suggest that the first stars formed from clouds of gas around 150–200 million years after the Big Bang. Heavier atoms such as carbon, oxygen and iron, have since been continuously produced in the hearts of stars and catapulted throughout the universe in spectacular stellar explosions called supernovae.
But stars and galaxies do not tell the whole story. Astronomical and physical calculations suggest that the visible universe is only a tiny amount (4%) of what the universe is actually made of. A very large fraction of the universe, in fact 26%, is made of an unknown type of matter called " dark matter ". Unlike stars and galaxies, dark matter does not emit any light or electromagnetic radiation of any kind, so that we can detect it only through its gravitational effects.
An even more mysterious form of energy called “dark energy” accounts for about 70% of the mass-energy content of the universe. Even less is known about it than dark matter. This idea stems from the observation that all galaxies seems to be receding from each other at an accelerating pace, implying that some invisible extra energy is at work.
Home — Essay Samples — Science — Big Bang Theory — The Origin of the Universe

The Origin of The Universe
- Categories: Big Bang Theory Universe
About this sample

Words: 445 |
Published: Mar 1, 2019
Words: 445 | Page: 1 | 3 min read
Works Cited
- Alpher, R. A., Bethe, H. A., & Gamow, G. (1948). The Origin of Chemical Elements. Physical Review, 73(7), 803-804.
- Hawking, S. (1988). A Brief History of Time. Bantam.
- Hubble, E. (1929). A Relation between Distance and Radial Velocity among Extra-Galactic Nebulae. Proceedings of the National Academy of Sciences of the United States of America, 15(3), 168–173.
- Liddle, A. R. (2003). An Introduction to Modern Cosmology. Wiley.
- Penrose, R. (1965). Gravitational collapse and space-time singularities. Physical Review Letters, 14(3), 57–59.
- Planck Collaboration, Ade, P. A. R., Aghanim, N., Armitage-Caplan, C., Arnaud, M., Ashdown, M., ... & Zonca, A. (2015). Planck 2015 results—XIII. Cosmological parameters. Astronomy & Astrophysics, 594, A13.
- Rees, M. J. (2003). Our Cosmic Habitat. Princeton University Press.
- Riess, A. G., Filippenko, A. V., Challis, P., Clocchiatti, A., Diercks, A., Garnavich, P. M., ... & Tonry, J. (1998). Observational evidence from supernovae for an accelerating universe and a cosmological constant. The Astronomical Journal, 116(3), 1009-1038.
- Silk, J. (2001). The Big Bang. W. H. Freeman.
- Weinberg, S. (1972). Gravitation and cosmology: principles and applications of the general theory of relativity. Wiley.
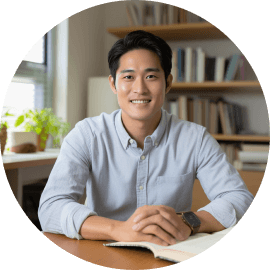
Cite this Essay
Let us write you an essay from scratch
- 450+ experts on 30 subjects ready to help
- Custom essay delivered in as few as 3 hours
Get high-quality help

Dr Jacklynne
Verified writer
- Expert in: Science
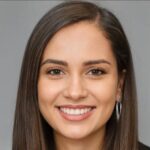
+ 120 experts online
By clicking “Check Writers’ Offers”, you agree to our terms of service and privacy policy . We’ll occasionally send you promo and account related email
No need to pay just yet!
Related Essays
3 pages / 1437 words
1 pages / 552 words
1 pages / 534 words
2 pages / 944 words
Remember! This is just a sample.
You can get your custom paper by one of our expert writers.
121 writers online
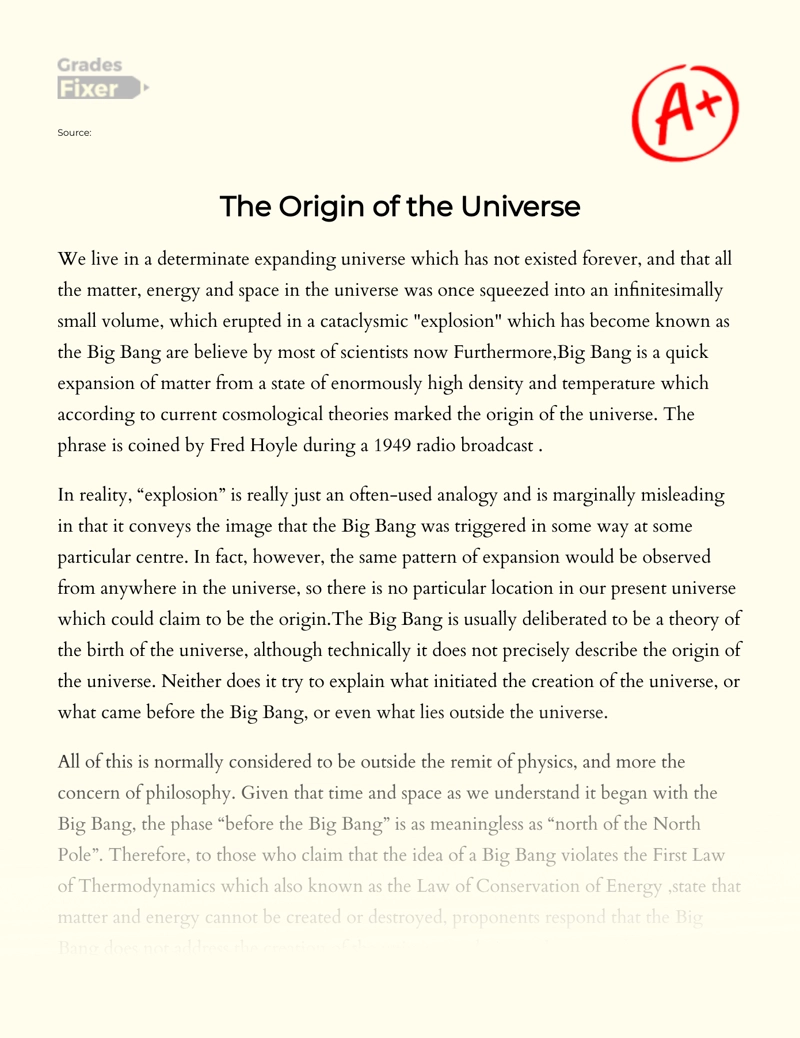
Still can’t find what you need?
Browse our vast selection of original essay samples, each expertly formatted and styled
Scientists believe the Universe began in a hot ‘big bang’ about 13,600 million years ago. The Universe continues to expand today. The evidence for the Big Bang theory includes the existence of a microwave background radiation, [...]
Billion years ago, there was an extra-ordinary event without which nothing would exist. It was the beginning of the universe. It was the time when a large amount of energy in an infinitely small space violently expanded and led [...]
In 2022, SpaceX intends to land humans on Mars using its new rocket, BFR, and interplanetary spaceship, BFS. In order to prepare for this mission, an unmanned BFS will fly to Mars in 2020, validating life support, EDL, and [...]
Dynamics related to the study of forces and torques and their effect on motion. It is the branch of physics (specifically classical mechanics). It is the opposite of kinematics. Kinematics studies the motion of objects without [...]
Cosmology is the scientific study of the large-scale properties of the universe as a whole. It endeavors the use of scientific method to understand the origin, evolution and ultimate fate of the universe. Cosmology involves the [...]
A line that stood out most to me in The Man Who Stole the Sun was when a government official remarked on how an individual does not need an atomic bomb -- that nations are what need it. There's a lot that can be unpacked in this [...]
Related Topics
By clicking “Send”, you agree to our Terms of service and Privacy statement . We will occasionally send you account related emails.
Where do you want us to send this sample?
By clicking “Continue”, you agree to our terms of service and privacy policy.
Be careful. This essay is not unique
This essay was donated by a student and is likely to have been used and submitted before
Download this Sample
Free samples may contain mistakes and not unique parts
Sorry, we could not paraphrase this essay. Our professional writers can rewrite it and get you a unique paper.
Please check your inbox.
We can write you a custom essay that will follow your exact instructions and meet the deadlines. Let's fix your grades together!
Get Your Personalized Essay in 3 Hours or Less!
We use cookies to personalyze your web-site experience. By continuing we’ll assume you board with our cookie policy .
- Instructions Followed To The Letter
- Deadlines Met At Every Stage
- Unique And Plagiarism Free
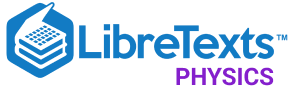
- school Campus Bookshelves
- menu_book Bookshelves
- perm_media Learning Objects
- login Login
- how_to_reg Request Instructor Account
- hub Instructor Commons
- Download Page (PDF)
- Download Full Book (PDF)
- Periodic Table
- Physics Constants
- Scientific Calculator
- Reference & Cite
- Tools expand_more
- Readability
selected template will load here
This action is not available.
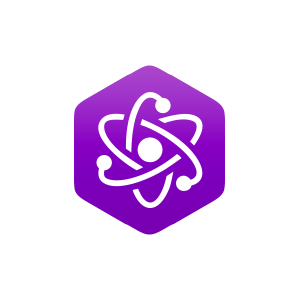
11.8: Evolution of the Early Universe
- Last updated
- Save as PDF
- Page ID 4956
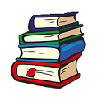
Learning Objectives
By the end of this section, you will be able to:
- Describe the evolution of the early universe in terms of the four fundamental forces
- Use the concept of gravitational lensing to explain astronomical phenomena
- Provide evidence of the Big Bang in terms of cosmic background radiation
- Distinguish between dark matter and dark energy
In the previous section, we discussed the structure and dynamics of universe. In particular, the universe appears to be expanding and even accelerating. But what was the universe like at the beginning of time? In this section, we discuss what evidence scientists have been able to gather about the early universe and its evolution to present time.
The Early Universe
Before the short period of cosmic inflation, cosmologists believe that all matter in the universe was squeezed into a space much smaller than an atom. Cosmologists further believe that the universe was extremely dense and hot, and interactions between particles were governed by a single force. In other words, the four fundamental forces (strong nuclear, electromagnetic, weak nuclear, and gravitational) merge into one at these energies (Figure \(\PageIndex{1}\)). How and why this “unity” breaks down at lower energies is an important unsolved problem in physics.
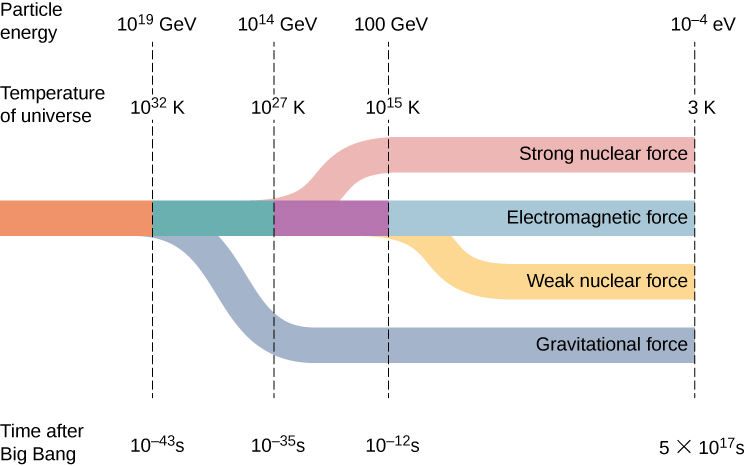
Scientific models of the early universe are highly speculative. Figure \(\PageIndex{2}\) shows a sketch of one possible timeline of events.
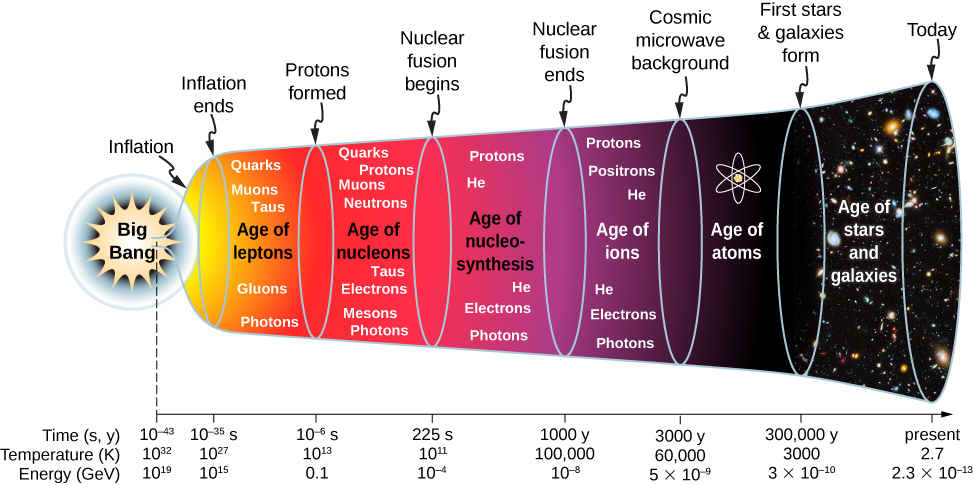
- Big Bang \((t < 10^{-43} s)\): The current laws of physics break down. At the end of the initial Big Bang event, the temperature of the universe is approximately \(T = 10^{32}K\).
- Inflationary phase \((t = 10^{-43} \, to \, 10^{-35})\): The universe expands exponentially, and gravity separates from the other forces. The universe cools to approximately \(T = 10^{27}K\).
- Age of leptons \((t = 10^{-35} \, to \, 10^{-6} s)\): As the universe continues to expand, the strong nuclear force separates from the electromagnetic and weak nuclear forces (or electroweak force). Soon after, the weak nuclear force separates from the electromagnetic force. The universe is a hot soup of quarks, leptons, photons, and other particles.
- Age of nucleons \((t = 10^{-6} \, to 225 \, s)\): The universe consists of leptons and hadrons (such as protons, neutrons, and mesons) in thermal equilibrium. Pair production and pair annihilation occurs with equal ease, so photons remain in thermal equilibrium: \[\gamma + \gamma \rightleftharpoons e^- + e^+ \nonumber \] \[\gamma + \gamma \rightleftharpoons p + \overline{p} \nonumber \] \[\gamma + \gamma \rightleftharpoons n + \overline{n}. \nonumber \] The number of protons is approximately equal to the number of neutrons through interactions with neutrinos: \[\nu_e + n \rightleftharpoons e^- + p \nonumber \] \[\overline{\nu}_e + p \rightleftharpoons e^+ + n. \nonumber \] The temperature of the universe settles to approximately \(10^{11} K\) —much too cool for the continued production of nucleon-antinucleon pairs. The numbers of protons and neutrons begin to dominate over their anti-particles, so proton-antiproton \((p\overline{p})\) and neutron-antineutron \((n\overline{n})\) annihilations decline. Deuterons (proton-neutron pairs) begin to form.
- Age of nucleosynthesis (\(t = 225 s\) to 1000 years): As the universe continues to expand, deuterons react with protons and neutrons to form larger nuclei; these larger nuclei react with protons and neutrons to form still larger nuclei. At the end of this period, about 1/4 of the mass of the universe is helium. (This explains the current amount of helium in the universe.) Photons lack the energy to continue electron-positron production, so electrons and positrons annihilate each other to photons only.
- Age of ions \((t = 1000\) to 3000 years): The universe is hot enough to ionize any atoms formed. The universe consists of electrons, positrons, protons, light nuclei, and photons.
- Age of atoms \((3000\) to 300,000 years): The universe cools below \(10^5\, K\) and atoms form. Photons do not interact strongly with neutral atoms, so they “decouple” (separate) from atoms. These photons constitute the cosmic microwave background radiation to be discussed later.
- Age of stars and galaxies \((t = 300,000\) years to present): The atoms and particles are pulled together by gravity and form large lumps. The atoms and particles in stars undergo nuclear fusion reaction.
Watch this video to learn more about Big Bang cosmology.
To describe the conditions of the early universe quantitatively, recall the relationship between the average thermal energy of particle ( E ) in a system of interacting particles and equilibrium temperature ( T ) of that system: \[E = k_BT, \nonumber \] where \(k_B\) is Boltzmann’s constant. In the hot conditions of the early universe, particle energies were unimaginably large.
Example \(\PageIndex{1}\): What Was the Average Thermal Energy of a Particle just after the Big Bang?
The average thermal energy of a particle in a system of interacting particles depends on the equilibrium temperature of that system. We are given this approximate temperature in the above timeline.
Cosmologists think the temperature of the universe just after the Big Bang was approximately \(T = 10^{32}K\). Therefore, the average thermal energy of a particle would have been
\[k_BT \approx (10^{-4}eV/K)(10^{32}K) = 10^{28} eV = 10^{19} GeV. \nonumber \]
Significance
This energy is many orders of magnitude larger than particle energies produced by human-made particle accelerators. Currently, these accelerators operate at energies less than \(10^4 \, GeV\).
Exercise \(\PageIndex{1}\)
Compare the abundance of helium by mass 10,000 years after the Big Bang and now.
about the same
Nucleons form at energies approximately equal to the rest mass of a proton, or 1000 MeV. The temperature corresponding to this energy is therefore
\[T = \dfrac{1000 \, MeV}{8.62 \times 10^{11} MeV \cdot K^{-1}} = 1.2 \times 10^{13}K. \nonumber \]
Temperatures of this value or higher existed within the first second of the early universe. A similar analysis can be done for atoms. Atoms form at an energy equal to the ionization energy of ground-state hydrogen (13 eV). The effective temperature for atom formation is therefore
\[T = \dfrac{13 \, eV}{8.62 \times 10^5 \, eV \cdot K_{-1}} = 1.6 \times 10^5 K. \nonumber \]
This occurs well after the four fundamental forces have separated, including forces necessary to bind the protons and neutrons in the nucleus (strong nuclear force), and bind electrons to the nucleus (electromagnetic force).
Nucleosynthesis of Light Elements
The relative abundances of the light elements hydrogen, helium, lithium, and beryllium in the universe provide key evidence for the Big Bang. The data suggest that much of the helium in the universe is primordial. For instance, it turns out that that 25% of the matter in the universe is helium, which is too high an abundance and cannot be explained based on the production of helium in stars.
How much of the elements in the universe were created in the Big Bang? If you run the clock backward, the universe becomes more and more compressed, and hotter and hotter. Eventually, temperatures are reached that permit nucleosynthesis , the period of formation of nuclei, similar to what occurs at the core of the Sun. Big Bang nucleosynthesis is believed to have occurred within a few hundred seconds of the Big Bang.
How did Big Bang nucleosynthesis occur? At first, protons and neutrons combined to form deuterons, \(^2H\). The deuteron captured a neutron to form triton, \(^3H\) - the nucleus of the radioactive hydrogen called tritium. Deuterons also captured protons to make helium \(^3He\). When \(^3H\) captures a proton or \(^3He\) captures a neutron, helium \(^4He\) results. At this stage in the Big Bang, the ratio of protons to neutrons was about 7:1. Thus, the process of conversion to \(^4He\) used up almost all neutrons. The process lasted about 3 minutes and almost \(25\%\) of all the matter turned into \(^4He\), along with small percentages of \(^2H\), \(^3H\) and \(^3He\). Tiny amounts of \(^7Li\) and \(^7Be\) were also formed. The expansion during this time cooled the universe enough that the nuclear reactions stopped. The abundances of the light nuclei \(^2H\), \(^4He\), and \(^7Li\) created after the Big Bang are very dependent on the matter density.
The predicted abundances of the elements in the universe provide a stringent test of the Big Bang and the Big Bang nucleosynthesis. Recent experimental estimates of the matter density from the Wilkinson Microwave Anisotropy Probe (WMAP) agree with model predictions. This agreement provides convincing evidence of the Big Bang model.
Cosmic Microwave Background Radiation
According to cosmological models, the Big Bang event should have left behind thermal radiation called the cosmic microwave background radiation (CMBR). The intensity of this radiation should follow the blackbody radiation curve ( Photons and Matter Waves ). Wien’s law states that the wavelength of the radiation at peak intensity is
\[\lambda_{max} = \dfrac{2.898 \times 10^{-3} \, m \cdot K}{T}, \nonumber \]
where T is temperature in kelvins. Scientists expected the expansion of the universe to “stretch the light,” and the temperature to be very low, so cosmic background radiation should be long-wavelength and low energy.
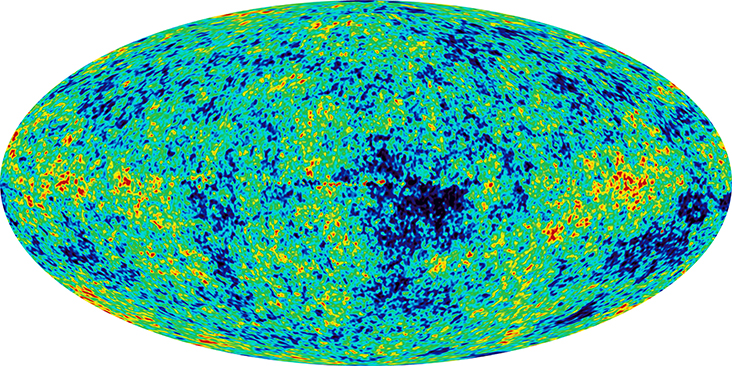
In the 1960s, Arno Penzias and Robert Wilson of Bell Laboratories noticed that no matter what they did, they could not get rid of a faint background noise in their satellite communication system. The noise was due to radiation with wavelengths in the centimeter range (the microwave region). Later, this noise was associated with the cosmic background radiation . An intensity map of the cosmic background radiation appears in Figure \(\PageIndex{3}\). The thermal spectrum is modeled well by a blackbody curve that corresponds to a temperature \(T = 2.7 \, K\) (Figure \(\PageIndex{4}\)).
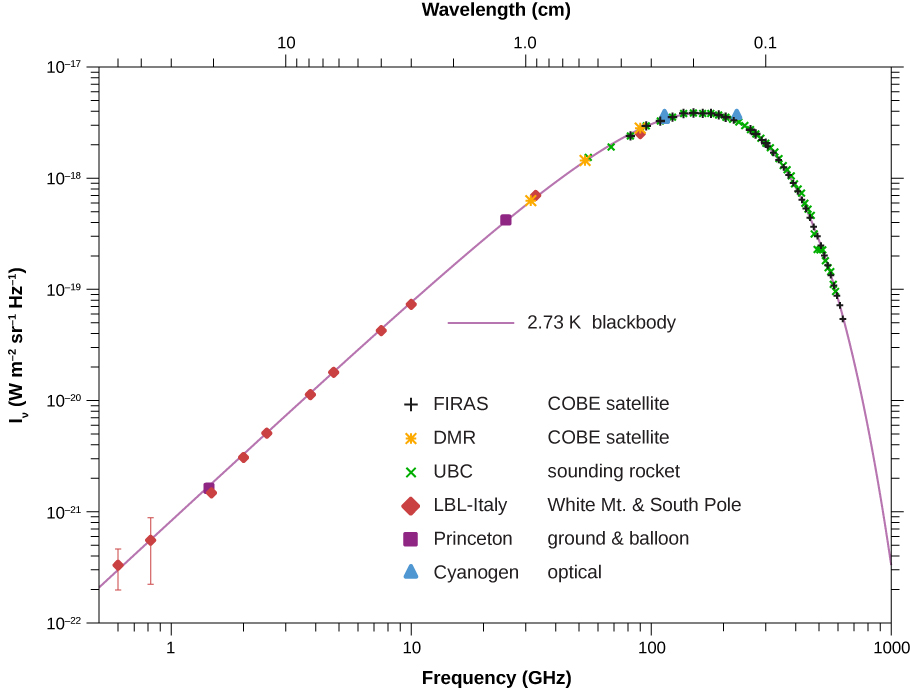
The formation of atoms in the early universe makes these atoms less likely to interact with light. Therefore, photons that belong to the cosmic background radiation must have separated from matter at a temperature T associated with 1 eV (the approximate ionization energy of an atom) . The temperature of the universe at this point was
\[k_BT \sim 1 \, eV \Rightarrow T = \dfrac{1 \, eV}{8.617 \times 10^5 eV/K} \sim 10^4 \, K. \nonumber \]
According to cosmological models, the time when photons last scattered off charged particles was approximately 380,000 years after the Big Bang. Before that time, matter in the universe was in the plasma form and the photons were “thermalized.”
Antimatter and Matter
We know from direct observation that antimatter is rare. Earth and the solar system are nearly pure matter, and most of the universe also seems dominated by matter. This is proven by the lack of annihilation radiation coming to us from space, particularly the relative absence of 0.511-MeV \(\gamma\) rays created by the mutual annihilation of electrons and positrons. (Antimatter in nature is created in particle collisions and in \(\beta^+\) decays, but only in small amounts that quickly annihilate, leaving almost pure matter surviving.)
Despite the observed dominance of matter over antimatter in the universe, the Standard Model of particle interactions and experimental measurement suggests only small differences in the ways that matter and antimatter interact. For example, neutral kaon decays produce only slightly more matter than antimatter. Yet, if through such decay, slightly more matter than antimatter was produced in the early universe, the rest could annihilate pair by pair, leaving mostly ordinary matter to form the stars and galaxies. In this way, the vast number of stars we observe may be only a tiny remnant of the original matter created in the Big Bang.
Dark Matter and Dark Energy
In the last two decades, new and more powerful techniques have revealed that the universe is filled with dark matter . This type of matter is interesting and important because, currently, scientists do not know what it is! However, we can infer its existence by the deflection of distant starlight. For example, if light from a distant galaxy is bent by the gravitational field of a clump of dark matter between us and the galaxy, it is possible that two images of the same galaxy can be produced (Figure \(\PageIndex{5}\)). The bending of light by the gravitational field of matter is called gravitational lensing . In some cases, the starlight travels to an observer by multiple paths around the galaxy, producing a ring (Figure \(\PageIndex{6}\)).
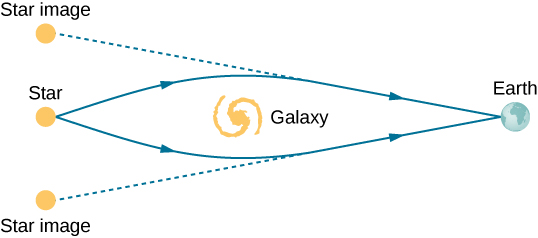
Based on current research, scientist know only that dark matter is cold, slow moving, and interacts weakly with ordinary matter. Dark matter candidates include neutralinos (partners of Z bosons, photons, and Higgs bosons in “supersymmetry theory”) and particles that circulate in tiny rings set up by extra spatial dimensions.
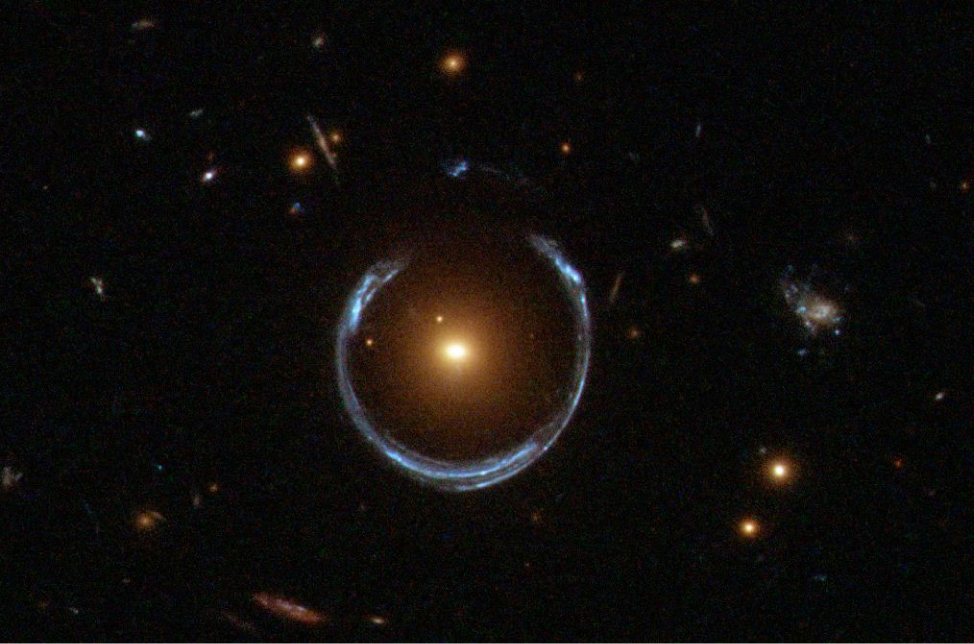
Increasingly precise astronomical measurements of the expanding universe also reveal the presence of a new form of energy called dark energy . This energy is thought to explain larger-than-expected values for the observed galactic redshifts for distant galaxies. These redshifts suggest that the universe is not only expanding, but expanding at an increasing rate. Virtually nothing is known about the nature and properties of dark energy. Together, dark energy and dark matter represent two of the most interesting and unsolved puzzles of modern physics. Scientists attribute \(68.3 \%\) of the energy of the universe to dark energy, \(26.8\%\) to dark matter, and just \(4.9\%\) to the mass-energy of ordinary particles (Figure \(\PageIndex{7}\)).
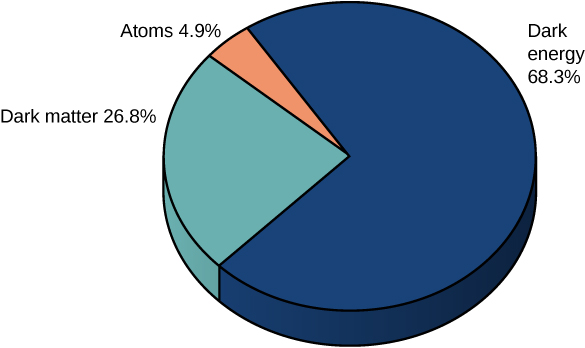
Given the current great mystery over the nature of dark matter and dark energy, Isaac Newton’s humble words are as true now as they were centuries ago: “I do not know what I may appear to the world, but to myself I seem to have been only like a boy playing on the sea-shore, and diverting myself in now and then finding a smoother pebble or a prettier shell than ordinary, whilst the great ocean of truth lay all undiscovered before me.”
The history of the universe: Big Bang to now in 10 easy steps
Take a trip through time to discover the history of the universe.
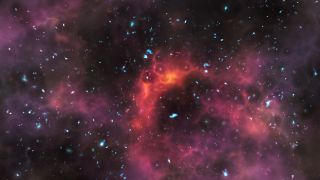
Step 1: How it all started
Step 2: the universe's first growth spurt, step 3: too hot to shine, step 4: let there be light, step 5: emerging from the cosmic dark ages, step 6: more stars and more galaxies, step 7: birth of our solar system, step 8: the invisible stuff in the universe, step 9: the expanding and accelerating universe, step 10: we still need to know more, additional resources, bibliography.
The history of the universe and how it evolved is broadly accepted as the Big Bang model, which states that the universe began as an incredibly hot, dense point roughly 13.7 billion years ago. So, how did the universe go from being fractions of an inch (a few millimeters) across to what it is today? Here is a breakdown of the Big Bang to now in 10 easy-to-understand steps.
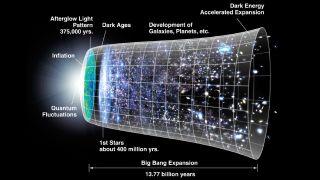
The Big Bang was not an explosion in space, as the theory's name might suggest. Instead, it was the appearance of space everywhere in the universe, researchers have said. According to the Big Bang theory, the universe was born as a very hot, very dense, single point in space. Cosmologists are unsure what happened before this moment, but with sophisticated space missions, ground-based telescopes and complicated calculations, scientists have been working to paint a clearer picture of the early universe and its formation. A key part of this comes from observations of the cosmic microwave background , which contains the afterglow of light and radiation left over from the Big Bang. This relic of the Big Bang pervades the universe and is visible to microwave detectors, which allows scientists to piece together clues of the early universe. In 2001, NASA launched the Wilkinson Microwave Anisotropy Probe (WMAP) mission to study the conditions as they existed in the early universe by measuring radiation from the cosmic microwave background. Among other discoveries, WMAP was able to determine the age of the universe — about 13.7 billion years old.
When the universe was very young — something like a hundredth of a billionth of a trillionth of a trillionth of a second (whew!) — it underwent an incredible growth spurt. During this burst of expansion, which is known as inflation, the universe grew exponentially and doubled in size at least 90 times.
"The universe was expanding, and as it expanded, it got cooler and less dense," David Spergel, a theoretical astrophysicist at Princeton University in Princeton, N.J., told SPACE.com. After inflation, the universe continued to grow, but at a slower rate.
As space expanded, the universe cooled and matter formed.
Light chemical elements were created within the first three minutes of the universe's formation. As the universe expanded, temperatures cooled and protons and neutrons collided to make deuterium, which is an isotope of hydrogen. Much of this deuterium combined to make helium.
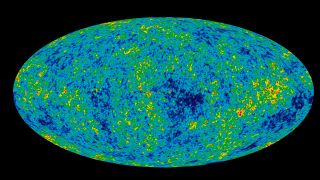
For the first 380,000 years after the Big Bang, however, the intense heat from the universe's creation made it essentially too hot for light to shine. Atoms crashed together with enough force to break up into a dense, opaque plasma of protons, neutrons and electrons that scattered light like fog.
About 380,000 years after the Big Bang, matter cooled enough for electrons to combine with nuclei to form neutral atoms. This phase is known as "recombination," and the absorption of free electrons caused the universe to become transparent. The light that was unleashed at this time is detectable today in the form of radiation from the cosmic microwave background. Yet, the era of recombination was followed by a period of darkness before stars and other bright objects were formed.
Roughly 400 million years after the Big Bang, the universe began to come out of its dark ages. This period in the universe's evolution is called the age of re-ionization. This dynamic phase was thought to have lasted more than a half-billion years, but based on new observations, scientists think re-ionization may have occurred more rapidly than previously thought. During this time, clumps of gas collapsed enough to form the very first stars and galaxies. The emitted ultraviolet light from these energetic events cleared out and destroyed most of the surrounding neutral hydrogen gas. The process of re-ionization, plus the clearing of foggy hydrogen gas, caused the universe to become transparent to ultraviolet light for the first time.
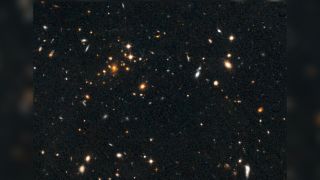
Astronomers comb the universe looking for the most far-flung and oldest galaxies to help them understand the properties of the early universe. Similarly, by studying the cosmic microwave background, astronomers can work backwards to piece together the events that came before. Data from older missions like WMAP and the Cosmic Background Explorer (COBE), which launched in 1989, and missions still in operation, like the Hubble Space Telescope, which launched in 1990, all help scientists try to solve the most enduring mysteries and answer the most debated questions in cosmology.
Our solar system is estimated to have been born a little after 9 billion years after the Big Bang, making it about 4.6 billion years old. According to current estimates, the sun is one of more than 100 billion stars in our Milky Way galaxy alone, and orbits roughly 25,000 light-years from the galactic core.
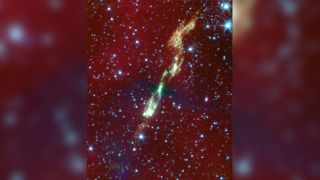
Many scientists think the sun and the rest of our solar system was formed from a giant, rotating cloud of gas and dust known as the solar nebula. As gravity caused the nebula to collapse, it spun faster and flattened into a disk. During this phase, most of the material was pulled toward the center to form the sun.
In the 1960s and 1970s, astronomers began thinking that there might be more mass in the universe than what is visible. Vera Rubin , an astronomer at the Carnegie Institution of Washington, observed the speeds of stars at various locations in galaxies. Basic Newtonian physics implies that stars on the outskirts of a galaxy would orbit more slowly than stars at the center, but Rubin found no difference in the velocities of stars farther out. In fact, she found that all stars in a galaxy seem to circle the center at more or less the same speed. This mysterious and invisible mass became known as dark matter . Dark matter is inferred because of the gravitational pull it exerts on regular matter. One hypothesis states the mysterious stuff could be formed by exotic particles that don't interact with light or regular matter, which is why it has been so difficult to detect.
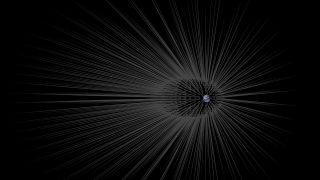
In the 1920s, astronomer Edwin Hubble made a revolutionary discovery about the universe. Using a newly constructed telescope at the Mount Wilson Observatory in Los Angeles, Hubble observed that the universe is not static, but rather is expanding. Decades later, in 1998, the prolific space telescope named after the famous astronomer, the Hubble Space Telescope , studied very distant supernovas and found that, a long time ago, the universe was expanding more slowly than it is today. This discovery was surprising because it was long thought that the gravity of matter in the universe would slow its expansion, or even cause it to contract.
– How big is the universe?
– What is the coldest place in the universe?
– How many black holes are there in the universe?
– What color is the universe?
Dark energy is thought to be the strange force that is pulling the cosmos apart at ever-increasing speeds, but it remains undetected and shrouded in mystery. The existence of this elusive energy, which is thought to make up 80% of the universe, is one of the most hotly debated topics in cosmology.
While much has been discovered about the creation and evolution of the universe, there are enduring questions that remain unanswered. Dark matter and dark energy remain two of the biggest mysteries, but cosmologists continue to probe the universe in hopes of better understanding how it all began.
The James Webb Space Telescope (JWST), launched in 2021, will continue the hunt for the elusive dark matter, as well as peering back to the beginning of time and the evolution of the universe using its infrared instruments.
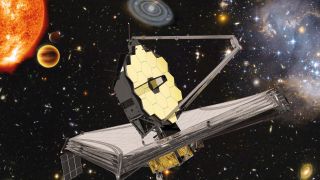
For more information about the evolution of the universe check out, " The History of the Universe " by David H. Lyth or " A Brief History of Time " by Stephen Hawking. You can also keep up to date with the discoveries of JWST, visit NASA's dedicated webpage or the European Space Agency's dedicated webpage .
Scientific American, " The Evolution of the Universe ", October 1994.
Walter Perry, " Origin and Evolution of the Universe ", Journal of Modern Physics, Volume 12, November 2021.
Bharat Ratra and Michael S. Vogeley, " The Beginning and Evolution of the Universe ", Publications of the Astronomical Society of the Pacific, Volume 120, March 2008,
NASA, " Brief History of the Universe ", December 2006.
Join our Space Forums to keep talking space on the latest missions, night sky and more! And if you have a news tip, correction or comment, let us know at: [email protected].
Get the Space.com Newsletter
Breaking space news, the latest updates on rocket launches, skywatching events and more!
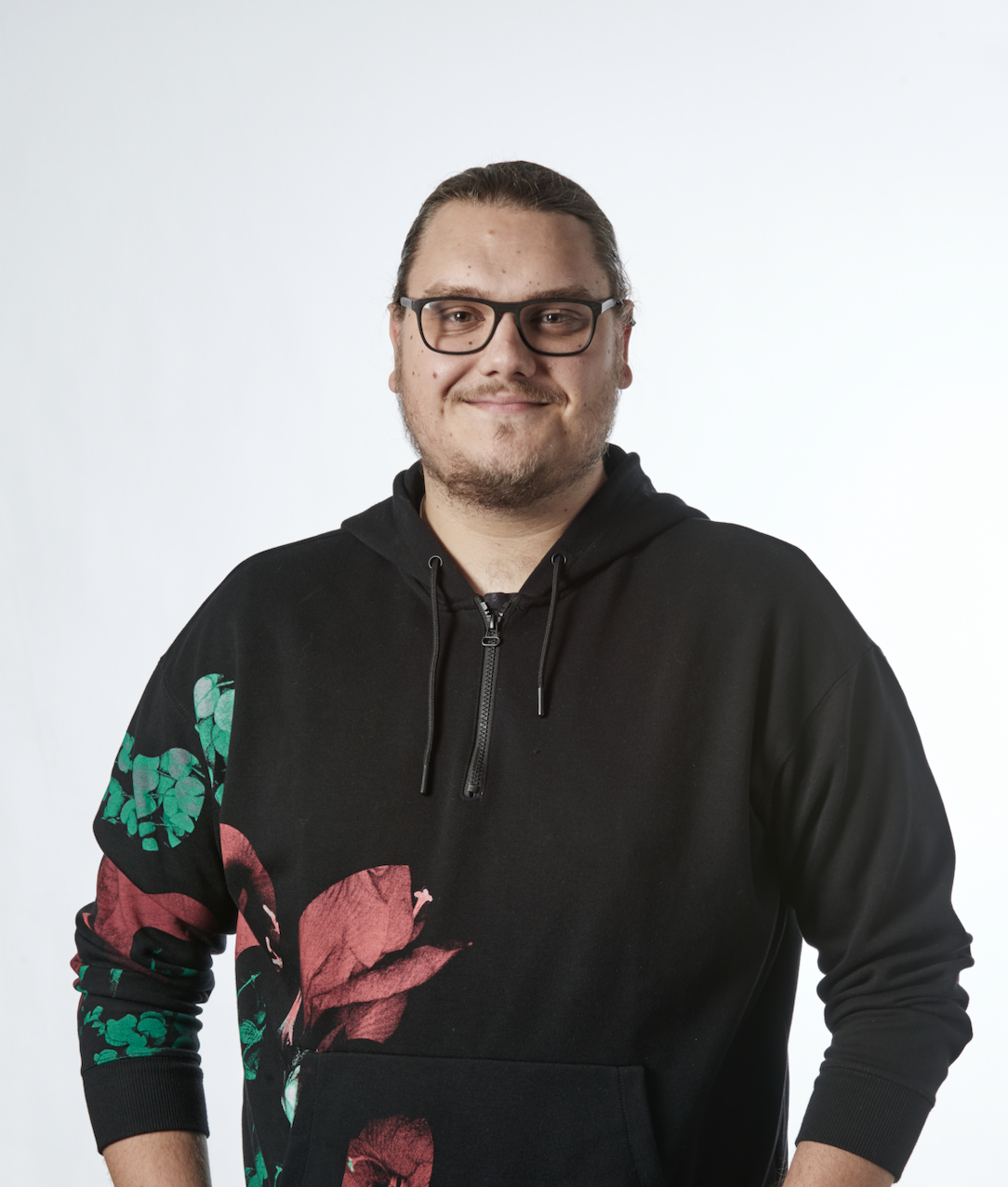
Scott is a staff writer for How It Works magazine and has previously written for other science and knowledge outlets, including BBC Wildlife magazine, World of Animals magazine, Space.com and All About History magazine . Scott has a masters in science and environmental journalism and a bachelor's degree in conservation biology degree from the University of Lincoln in the U.K. During his academic and professional career, Scott has participated in several animal conservation projects, including English bird surveys, wolf monitoring in Germany and leopard tracking in South Africa.
China's Chang'e 6 mission to collect samples of the far side of the moon enters lunar orbit (video)
China launches Chang'e 6 sample-return mission to moon's far side (video)
Boeing's Starliner rolled off launch pad to replace 'buzzing' rocket valve (photo)
Most Popular
- 2 A failed star and an ammonia trail could reveal how some giant exoplanets form
- 3 World's largest visible light telescope spies a galaxy cluster warping spacetime
- 4 SpaceX launching 20 satellites from California tonight on 2nd leg of Starlink doubleheader
- 5 Star Wars makes Darth Jar Jar official in 'Lego Star Wars: Rebuild the Galaxy' mini-series (video)
The Big Bang Theory: How the Universe Began
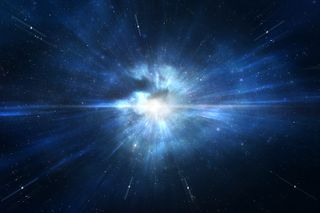
The Big Bang theory represents cosmologists ' best attempts to reconstruct the 14 billion year story of the universe based on the sliver of existence visible today.
Different people use the term "Big Bang" in different ways. Most generally, it illustrates the arc of the observable universe as it thinned out and cooled down from an initially dense, hot state. This description boils down to the idea that the cosmos is expanding, a broad principle analogous to survival of the fittest in biology that few would consider debatable.
More specifically, the Big Bang can also refer to the birth of the observable universe itself — the moment something changed, kickstarting the events that led to today. Cosmologists have argued for decades about the details of that fraction of a second, and the discussion continues today. [ From Big Bang to Present: Snapshots of Our Universe Through Time ]
The classic Big Bang theory
For most of human history, observers of the sky assumed it eternal and unchanging. Edwin Hubble dealt this story an experimental blow in the 1920s when his observations showed both that galaxies outside the Milky Way existed, and that their light appeared stretched — a sign that they were rushing away from Earth .
George Lemaître, a contemporary Belgian physicist, interpreted data from Hubble and others as evidence of an expanding universe, a possibility permitted by Einstein's recently published field equations of general relativity . Thinking backwards, Lemaître inferred that today's separating galaxies must have started out together in what he called the "primeval atom."
The first public use of the modern term for Lemaître's idea actually came from a critic — English astronomer Fred Hoyle. On March 28, 1949, Hoyle coined the phrase during a defense of his preferred theory of an eternal universe that created matter to cancel out the dilution of expansion. Hoyle said the notion that "all matter of the universe was created in one big bang at a particular time in the remote past," was irrational. In later interviews, Hoyle denied intentionally inventing a slanderous name , but the moniker stuck, much to the frustration of some.
"The Big Bang is a really bad term," said Paul Steinhardt, a cosmologist at Princeton. "The Big Stretch would capture the right idea." The mental image of an explosion causes all kinds of confusion, according to Steinhardt. It implies a central point, an expanding frontier, and a scene where light shrapnel flies faster than heavier chunks. But an expanding universe looks nothing like that, he said. There's no center, no edge, and galaxies large and small all slide apart in the same way (although more distant galaxies move away faster under the cosmologically recent influence of dark energy).
Regardless of its name, the Big Bang theory found widespread acceptance for its unparalleled ability to explain what we see. The balance of light with particles like protons and neutrons during the first 3 minutes, for instance, let early elements form at a rate predicting the current amounts of helium and other light atoms.
"There was a small window in time where it was possible for nuclei to form," said Glennys Farrar, a cosmologist at New York University. "After that, the universe kept expanding and they couldn't find each other, and before [the window] it was too hot."
A cloudy plasma filled the universe for the next 378,000 years, until further cooling let electrons and protons form neutral hydrogen atoms, and the fog cleared. The light emitted during this process, which has since stretched into microwaves, is the earliest known object researchers can study directly. Known as the cosmic microwave background (CMB) radiation, many researchers consider it the strongest evidence for the Big Bang.
An explosive update
But as cosmologists pushed farther back into the universe's first moments, the story unraveled. General relativity's equations suggested an initial speck of unlimited heat and density — a singularity. In addition to not making much physical sense, a singular origin didn't match the smooth, flat CMB. Fluctuations in the speck's formidable temperature and density would have produced swaths of sky with different properties , but the CMB's temperature varies by just a fraction of a degree. The curvature of space-time also looks quite flat, which implies an initially near-perfect balance of matter and curvature that most cosmologists find improbable.
Alan Guth proposed a new picture of the first fraction of a second in the 1980s, suggesting that the universe spent its earliest moments growing exponentially faster than it does today. At some point this process stopped, and putting on the brakes produced a dense and hot (but not infinitely so) mess of particles that takes the place of the singularity. "In my own mind I think of that as the Big Bang, when the universe got hot," Farrar said.
The inflation theory, as it's called, now has a plethora of competing models. Although no one knew much about what made the universe expand so rapidly, the theory has grown popular for its ability to explain the seemingly improbable featureless CMB: Inflation preserved minor fluctuations (which developed into today's galaxy clusters), while flattening the major ones. "It's a very sweet story," Steinhardt said, who helped develop the theory. "It's the one we tell our kids."
Beyond inflation
Recent research has introduced two wrinkles into the inflation theory's cosmic narrative. Work by Steinhardt and others suggests that inflation would have stopped in some regions (such as our observable universe) but continued in others, producing an array of separate territories with "every conceivable set of cosmological properties," as Steinhardt puts it. Many physicists find this " multiverse " picture distasteful, because it makes an infinite number of untestable predictions.
On the experimental front, cosmologists expect that inflation should have produced galaxy-spanning gravitational waves in the CMB just as it produced slight temperature and density variations. Current experiments should be sensitive enough to find them, but the primordial space-time ripples haven't shown up (despite one false alarm in 2014 ).
Many researchers await more precise CMB measurements that could kill, or validate , the many inflation models that still stand. Other physicists, however, don't see the cosmos's smoothness as a problem at all — it started off uniform and needs no explanation .
While experimentalists strive for new levels of precision, some theorists have turned away from inflation to seek other ways to squash the universe flat. Steinhardt, for instance, is working on a "big bounce" model, which pushes the starting clock back even further, to an earlier period of contraction that smoothed space-time and set the stage for an explosive expansion. He hopes that before too long, new signatures, in addition to problems like the lack of primordial gravitational waves, will set cosmologists up with a new creation story to tell. "Are there any other observable features to look for?" Steinhardt said, "Ask me again in a few years and I hope to have an answer."
Additional resources :
- Fermilab's Don Lincoln explains exactly what the Big Bang theory does, and doesn't say.
- Read about why some think it's odd how flat the universe is .
- PBS's SpaceTime explains why inflation has proved such an attractive idea .
Sign up for the Live Science daily newsletter now
Get the world’s most fascinating discoveries delivered straight to your inbox.
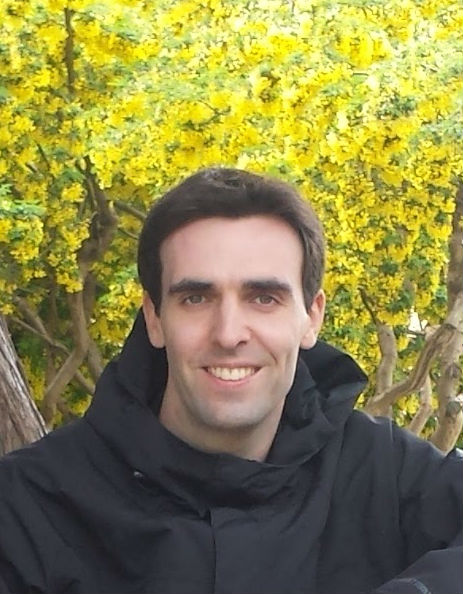
Charlie Wood is a staff writer at Quanta Magazine, where he covers physics both on and off the planet. In addition to Live Science, his work has also appeared in Popular Science, Scientific American, The Christian Science Monitor, and other publications. Previously, he taught physics and English in Mozambique and Japan, and he holds an undergraduate degree in physics from Brown University.
Researchers solve mystery of inexplicably dense galaxy at the heart of perfect 'Einstein ring' snapped by James Webb telescope
Gravitational waves reveal 1st-of-its-kind merger between neutron star and mystery object
Hoard of 17th-century coins hidden during English Civil War unearthed during kitchen renovation
Most Popular
- 2 Asteroid that exploded over Berlin was fastest-spinning space rock ever recorded
- 3 Antarctic ice hole the size of Switzerland keeps cracking open. Now scientists finally know why.
- 4 Why do dogs sniff each other's butts?
- 5 Earth from space: Mysterious wave ripples across 'galaxy' of icebergs in Arctic fjord
- 2 Japan captures 1st image of space debris from orbit, and it's spookily stunning
- 3 Siberia's 'gateway to the underworld' is growing a staggering amount each year
- 4 1,900-year-old Roman legionary fortress unearthed next to UK cathedral
Big Bang Theory and the Origin of Our Universe Essay
The introduction: some basic assumptions of the universe development, the body: the most widespread attempts to explain the big bang theory, the conclusion: the most reliable theories on bbt, works cited:.
Generally, the Big Bang theory is recognized to be one of the ways to explain the appearance of the Universe. They say that our Universe expanded from hot structure. The structure existed more than thirteen billion years ago; so, they say that the Big Bang took place exactly at that time. The theory is based on the observations of cosmology. On the other hand, many astronomers rely on the so-called Hubble’s law. According to scientific data taken from the popular website Cornell.
External galaxies are receding in such a way that their recessional speeds are proportional to the distance they are away from us. This observation is explained well by a uniform expansion of the universe. If the universe is expanding, it must have started out very small some time far in the past. It is this point which has been called the beginning of the universe or the Big Bang (1).
On the other hand, one is to keep in mind the CMB radiation, which also explains the way the Universe appeared. Thus, according to radiation investigations, some scientists say that billion years ago the Universe was hotter. For this reason, one may affirm that cosmic microwave background radiation represents the first photons. The CMB radiation was predicted in the late forties; however, the phenomenon was observed only in the mid of the sixties. Arno Penzias and Robert Wilson opened the CMB radiation.
Another important point, which is to be considered, is a large scale structure of the Universe. Thus, analyzing the structure, the scientists can study numerous aspects cosmology includes. Moreover, there is an opportunity to know more about the structure formation of our Universe.
The Thesis Statement
Generally, the Big Bang theory studies the process of cosmic evolution. The most important point is that the type of theory is recognized to be totally independent of other forms of evolution. The theory doesn’t disclose the origin of the Universe; it studies the way the Universe started.
Björn Feuerbacher and Ryan Scranton say that No similar consensus has been reached on ideas about the ultimate origin of the universe. This remains an area of active research. That said, BBT is nevertheless about origins – the origin of matter, the origin of the elements, the origin of large scale structure, the origin of the Cosmic Microwave Background Radiation, etc (1).
There are numerous misconceptions, which exist in persons’ mind about the BBT. The theory is not so easy to explain; however, the simplest affirmation is that when the Universe was expanded, it became cooler . The issue of the Universe expansion should be related to a space expansion. Anyway, there are many contradictions concerning the development of the Universe. Some scientists suppose that there was pre-existing volume, and the Universe had to reach the corresponding size. On the other hand, there are no facts, which could confirm the idea. Background knowledge about volume and geometry is at variance with the position. The BBT is also related to Einstein’s theory of General Relativity, and thus Björn Feuerbacher and Ryan Scranton state, “Energy determines the geometry and changes in the geometry of the universe, and, in turn, the geometry determines the movement of energy” (1). It is one more opinion, which is based on simple explanations. It is the so-called cosmological principle, which combines Einstein’s GR and BBT. Thus, there are two basic distributions of energy. The major assumptions are recognized to be homogeneous and isotropic distributions. The issues of matter, radiation and energy are rather important. One more important component of BBT is considered to be a dark energy. The basic parameters of BBT are the curvature of space, the scale factor, the Hubble Parameter, Deceleration Parameter, component densities, and Dark Energy Equation of State.
The basic problems with the Big Bang Theory are also to be taken into account. So, the central problems are the horizon problem, the flatness problem, and the monopole problem. According to the website. This general problem is called the horizon problem , because the inability to have received a signal from some distant source because of the finite speed of light is termed a horizon in cosmology. Thus, in the standard big bang theory we must simply assume the required level of uniformity (1). The main assumption of the flatness problem is that the development of the Universe on the basis of BBT is impossible due to its low geometrical curvature.
They say that there are only Grand Unified Theories (GUTs) which explain the issue of BBT. The theories are related to electromagnetic forces and their impact. The website UTK.edu explains, “Our current understanding of elementary particle physics indicates that such theories should produce very massive particles called magnetic monopoles , and that there should be many such monopoles in the Universe today” (1). Although the most important point is that the monopoles were not found.
Yuki D. Takahashi states, “The most conclusive evidence for the big bang arises from the observation of the cosmic background radiation” (1).
Cornell. edu. Cosmology and the Big Bang , 2011. Web.
Feuerbacher, B., Scranton, R. Evidence for Big Bang, 2006. Web.
Takahashi, Y. Big Bang: How Did the Universe Begin? 2000. Web.
UTK.edu. Problems with the Big Bang, 2011. Web.
- Chicago (A-D)
- Chicago (N-B)
IvyPanda. (2024, January 10). Big Bang Theory and the Origin of Our Universe. https://ivypanda.com/essays/big-bang-theory-and-the-origin-of-our-universe/
"Big Bang Theory and the Origin of Our Universe." IvyPanda , 10 Jan. 2024, ivypanda.com/essays/big-bang-theory-and-the-origin-of-our-universe/.
IvyPanda . (2024) 'Big Bang Theory and the Origin of Our Universe'. 10 January.
IvyPanda . 2024. "Big Bang Theory and the Origin of Our Universe." January 10, 2024. https://ivypanda.com/essays/big-bang-theory-and-the-origin-of-our-universe/.
1. IvyPanda . "Big Bang Theory and the Origin of Our Universe." January 10, 2024. https://ivypanda.com/essays/big-bang-theory-and-the-origin-of-our-universe/.
Bibliography
IvyPanda . "Big Bang Theory and the Origin of Our Universe." January 10, 2024. https://ivypanda.com/essays/big-bang-theory-and-the-origin-of-our-universe/.
- Curriculum-Based Measurement of Mathematics Skills
- Large-scale universe to Milky Way
- Photon Lifecycle in Technological Applications
- The Kepler Space Observatory
- Safety Role in Space Tourism
- Space Tourism and Safety
- Comparison of Mercury Planet and a Speck of Sand
- "Walking in the City" by Michel de Certeau
If you're seeing this message, it means we're having trouble loading external resources on our website.
If you're behind a web filter, please make sure that the domains *.kastatic.org and *.kasandbox.org are unblocked.
To log in and use all the features of Khan Academy, please enable JavaScript in your browser.
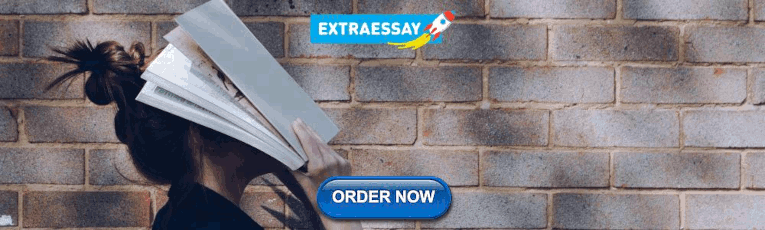
Big History Project
Course: big history project > unit 4.
- ACTIVITY: Planet Card Sort
- WATCH: Unit 4 Overview
- ACTIVITY: Unit 4 Vocab Tracking
- WATCH: Threshold 4 — Earth & Solar System
- ACTIVITY: Threshold Card —Threshold 4 Earth & the Solar System
- WATCH: How Did Earth and the Solar System Form?
READ: How Our Solar System Formed
- READ: The Rocket Scientist - Mary Golda Ross: Graphic Biography
- READ: Gallery — Earth & Solar System
- Quiz: Earth & the Formation of Our Solar System
How Our Solar System Formed
The birth of the sun, the birth of the planets, conditions on earth, earth’s moon, pluto and beyond, for further discussion.
- What were the factors working against life forming on the early Earth?
- Should we be surprised that life formed here at all?
Want to join the conversation?
- Upvote Button navigates to signup page
- Downvote Button navigates to signup page
- Flag Button navigates to signup page

What is the universe made of?
Matter and energy are the two basic components of the entire Universe. An enormous challenge for scientists is that most of the matter in the Universe is invisible and the source of most of the energy is not understood. How can we study the Universe if we can’t see most of it?
As our tools for observation grow more sophisticated, scientists at Center for Astrophysics | Harvard & Smithsonian will continue to be at the forefront of dark matter and dark energy research.
NASA’s Chandra X-ray Observatory and optical telescopes help map the distribution of dark matter in colliding galaxy clusters, like the Bullet Cluster. X-ray observations show a heated shock front where the gas from the clusters collided and slowed down, but gravitational lensing measurements show that dark matter was unaffected by the collision and separate from the normal matter.
It is theorized that when some dark matter particles collide, they annihilate and disappear in a flash of high-energy radiation. The Very Energetic Radiation Imaging Telescope Array System (VERITAS) in Arizona, which can detect gamma-ray radiation, is looking for the signature of dark matter annihilation.
The South Pole Telescope in Antarctica and Chandra are placing limits on dark energy by looking for its effects on galaxy cluster evolution throughout the history of the Universe. By comparing observations of galaxy clusters with experimental models, researchers are studying how dark energy competed with gravity throughout the history of the Universe.
Scientists at CfA have led the Baryon Oscillation Spectroscopic Survey (BOSS), analyzing millions of galaxies and charting their distribution in the Universe. The distribution has been shown to trace sound waves from the early Universe, like ripples in a pond, where some regions have higher numbers of galaxies, and others have less. Looking at these distributions, we can more accurately measure the distance to galaxies and map the effects of dark energy.
On the horizon, the Dark Energy Spectroscopic Instrument (DESI) will create a 3D map of the Universe, containing millions of galaxies out to 10 billion light years. This map will measure dark energy’s effect on the expansion of the Universe. And the Large Synoptic Survey Telescope (LSST) will observe billions of galaxies and discover unprecedented numbers of supernovae, constraining the properties of dark matter and dark energy.
Dark Matter and Dark Energy
Astronomer Fritz Zwicky was the first to notice the discrepancy between the amount of visible matter in a cluster of galaxies and the motions of the galaxies themselves. He suggested that there may be invisible matter, or what he called “dark matter”, interacting gravitationally with the visible matter. Later, astronomers noticed similar incongruities when observing nearby spiral galaxies. The outer edges of the galaxies rotated much faster than expected, suggesting “dark matter” existed throughout and extended beyond the visible galaxy.
Today, we can estimate the amount of dark matter in a galaxy based on how it causes light from a background source to bend. Using this “gravitational lensing” technique, we can measure the severity of that bend to get an idea of the galaxy’s mass. When the mass we calculate from the bend and the mass we can observe directly don’t agree, we know dark matter must be present.
Modern calculations say dark matter comprises about 27% of the Universe. We don’t yet know what it is, but we are searching for answers.
We have known that the Universe is expanding since the early 20th century. But recent observations of distant supernovae and other observations show that the Universe is not only expanding, but the expansion is accelerating. This astonishing discovery came as a complete surprise because the expansion of the Universe should slow down with time because of the gravitational attraction between galaxies and clusters of galaxies. The unseen repellant force required to explain this observation has been labelled “dark energy,” and current models say it makes up about 68% of the Universe.
That leaves only 5% of the Universe that is visible to us.
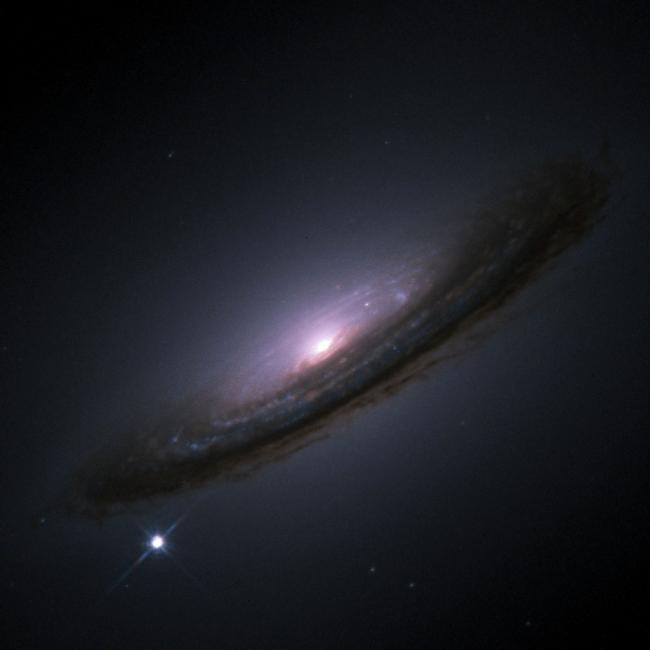
Supernova 1994D in this image from NASA's Hubble Space Telescope might look like a star, but it's the explosion of a white dwarf that nearly outshone an entire galaxy. Such supernovas — known as type Ia — are extremely similar to each other, allowing astronomers to use them to measure the rate of the expansion of the universe.
What We Know and What We Think
While we can’t see dark matter, we know it’s there. And we can investigate some of dark matter’s properties using gravitational lensing. This technique measures the gravitational pull galaxies exert on light from more distant sources. The warping and magnification of this light gives us insight into the amount, density, and distribution of dark matter in any given lensing galaxy. Theoretically, the current best explanation we have for dark matter is the existence of WIMPs, or Weakly Interacting Massive Particles. These theoretical particles should have certain predictable behaviors, but directly observing them and their byproducts so far has proved elusive.
As for dark energy, Einstein had assumed the Universe was static, neither expanding nor collapsing. However, his Theory of General Relativity predicted that the Universe was not static, and so he added a “cosmological constant,” to oppose gravity. He later called it the “biggest blunder” of his life after Hubble demonstrated that the Universe was expanding.
The discovery that the expansion of the Universe is accelerating revived the idea of the cosmological constant. The simplest interpretation of this constant is that it represents the energy of empty space. This “vacuum energy” is constant throughout space and time.
Another interpretation is that dark energy might be an energy field that varies over time and space. Or, perhaps we do not fully understand gravity. For example, maybe it acts differently on enormous scales. Astronomers are currently testing modifications to General Relativity to see if they can explain the Universe’s accelerating expansion.
- Moons and Satellites
- Neutron Stars and White Dwarfs
- Planetary Geology
- Planetary Nebulas
- Spectroscopy
- Star Clusters
- Star Formation
- Starburst Galaxies
- Stellar Structure and Evolution
- Supernovas & Remnants
- Variable Stars and Binaries
- Astrochemistry
- Atomic & Molecular Data
- Cosmic Microwave Background
- Dark Energy and Dark Matter
- Elemental Abundances
- Laboratory Astrophysics
- Stellar Astronomy
- Atomic and Molecular Physics
- Optical and Infrared Astronomy
- Solar, Stellar, and Planetary Sciences
- Theoretical Astrophysics
Related News
The giant magellan telescope’s final mirror fabrication begins, billions of celestial objects revealed in gargantuan survey of the milky way, the most precise accounting yet of dark energy and dark matter, dozens of newly discovered gravitational lenses could reveal ancient galaxies and the nature of dark matter, mars as a base for asteroid exploration and mining, astrophysicists reveal largest-ever suite of universe simulations, planets form in organic soups with different ingredients, interstellar comets like borisov may not be all that rare, center for astrophysics celebrates class of 2021 graduates, to map the universe, astrophysicists launch largest sky survey yet, abacussummit, dark energy spectroscopic instrument (desi), hitran and hitemp database, physics of the primordial universe, sloan digital sky survey (sdss), cfa redshift catalog, the star formation reference survey, telescopes and instruments, 1.5-meter tillinghast (60-inch) telescope, giant magellan telescope, magellan telescopes, pan-starrs-1 science consortium, south pole telescope, antarctica, submillimeter wave astronomy satellite.

Want to create or adapt books like this? Learn more about how Pressbooks supports open publishing practices.
17 Origin of the Universe and Our Solar System
Learning Objectives
By the end of this chapter, students should be able to:
- Explain the formation of the universe and how we observe it.
- Understand the origin of our solar system .
- Describe how the objects in our solar system are identified, explored, and characterized.
- Describe the types of small bodies in our solar system, their locations, and how they formed.
- Describe the characteristics of the giant planets , terrestrial planets , and small bodies in the solar system.
- Explain what influences the temperature of a planet’s surface.
- Explain why there is geological activity on some planets and not on others.
- Describe different methods for dating planets and the age of the solar system.
- Describe how the characteristics of extrasolar systems help us to model our own solar system.
The universe began 13.77 billion years ago when energy, matter, and space expanded from a single point. Evidence for the big bang is the cosmic “afterglow” from when the universe was still very dense, and red-shifted light from distant galaxies, which tell us the universe is still expanding.
The big bang produced hydrogen, helium, and lithium, but heavier elements come from nuclear fusion reactions in stars. Large stars make elements such as silicon, iron, and magnesium, which are important in forming terrestrial planets. Large stars explode as supernovae and scatter the elements into space.
Planetary systems begin with the collapse of a cloud of gas and dust. Material drawn to the center forms a star, and the remainder forms a disk around the star. Material within the disk clumps together to form planets. In our solar system , rocky planets are closer to the Sun, and ice and gas giants are farther away. This is because temperatures near the Sun were too high for ice to form, but silicate minerals and metals could solidify.
Early Earth was heated by radioactive decay, collisions with bodies from space, and gravitational compression. Heating melted Earth, causing molten metal to sink to Earth’s center and form a core, and silicate melt to float to the surface and form the mantle and crust. A collision with a planet the size of Mars knocked debris into orbit around Earth, and the debris coalesced into the moon. Earth’s atmosphere is the result of volcanic degassing, contributions by comets and meteorites, and photosynthesis.
The search for exoplanets has identified 12 planets that are similar in size to Earth and within the habitable zone of their stars. These are thought to be rocky worlds like Earth, but the compositions of these planets are not known for certain.
17.1 The Big Bang
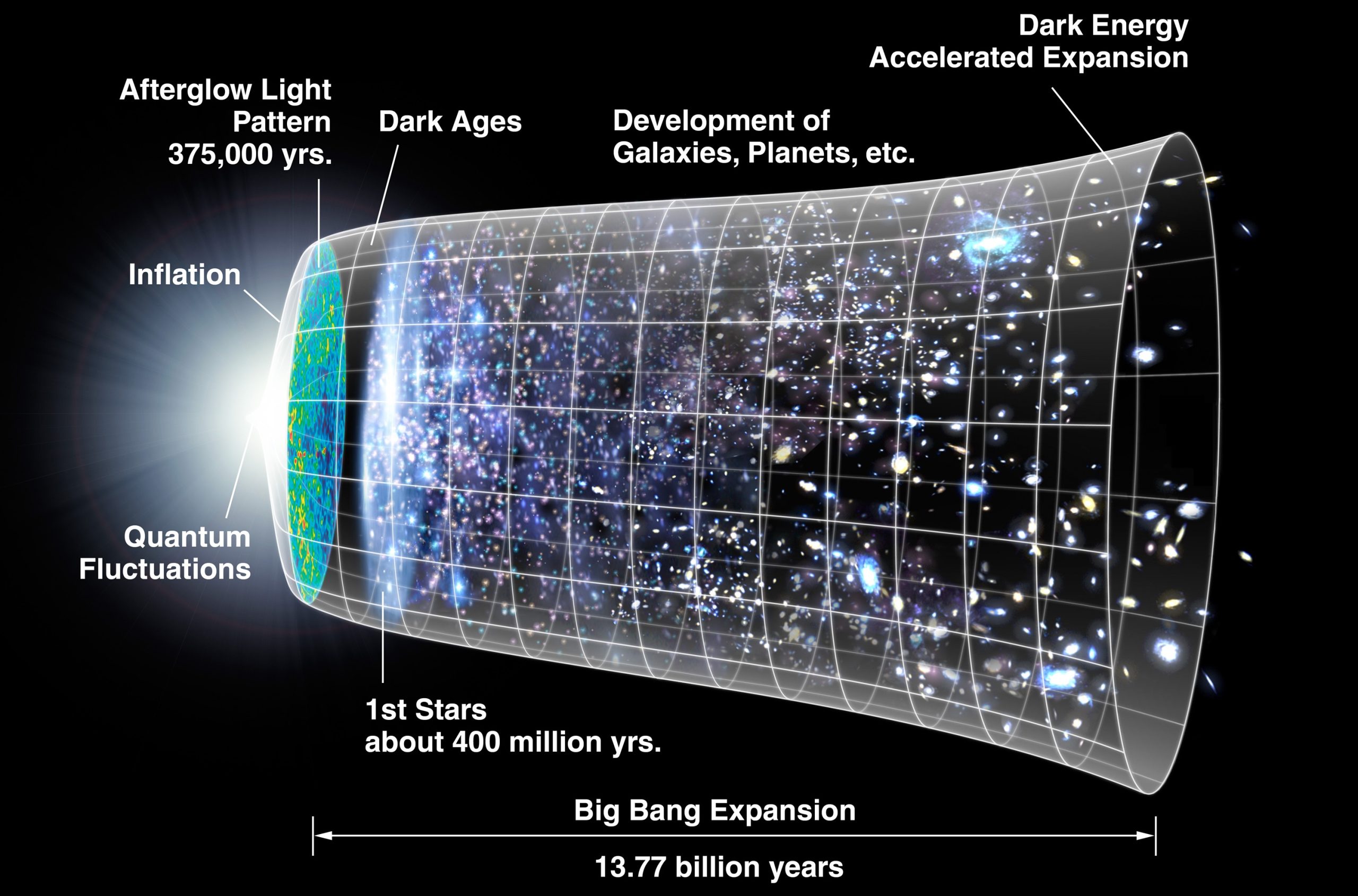
According to the big bang theory , the universe blinked violently into existence 13.77 billion years ago. The big bang is often described as an explosion, but imagining it as an enormous fireball isn’t accurate. The big bang involved a sudden expansion of matter, energy, and space from a single point. The kind of Hollywood explosion that might come to mind involves expansion of matter and energy within space, but during the big bang, space itself was created.
At the start of the big bang, the universe was too hot and dense to be anything but a sizzle of particles smaller than atoms, but as it expanded, it also cooled. Eventually some of the particles collided and stuck together. Those collisions produced hydrogen and helium, the most common elements in the universe, along with a small amount of lithium.
You may wonder how a universe can be created out of nothing, or how we can know that the big bang happened at all. Creating a universe out of nothing is mostly beyond the scope of this chapter, but there is a way to think about it. The particles that make up the universe have opposites that cancel each other out, similar to the way that we can add the numbers 1 and −1 to get zero (also known as “nothing”). As far as the math goes, having zero is exactly the same as having a 1 and a −1. It is also exactly the same as having a 2 and a −2, a 3 and a −3, two −1s and a 2, and so on. In other words, nothing is really the potential for something if you divide it into its opposite parts. As for how we can know that the big bang happened at all, there are very good reasons to accept that it is indeed how our universe came to be.
17.1.1 Looking Back to the Early Stages of the Big Bang
The notion of seeing the past is often used metaphorically when we talk about ancient events, but in this case it is meant literally. In our everyday experience, when we watch an event take place, we perceive that we are watching it as it unfolds in real time. In fact, this isn’t true. To see the event, light from that event must travel to our eyes. Light travels very rapidly, but it does not travel instantly. If we were watching a digital clock 1 m away from us change from 11:59 a.m. to 12:00 p.m., we would actually see it turn to 12:00 p.m. three billionths of a second after it happened. This isn’t enough of a delay to cause us to be late for an appointment, but the universe is a very big place, and the “digital clock” in question is often much, much farther away. In fact, the universe is so big that it is convenient to describe distances in terms of light years , or the distance light travels in one year. What this means is that light from distant objects takes so long to get to us that we see those objects as they were at some considerable time in the past. For example, the star Proxima Centauri is 4.24 light years from the sun. If you viewed Proxima Centauri from Earth on January 1, 2015, you would actually see it as it appeared in early October 2010.
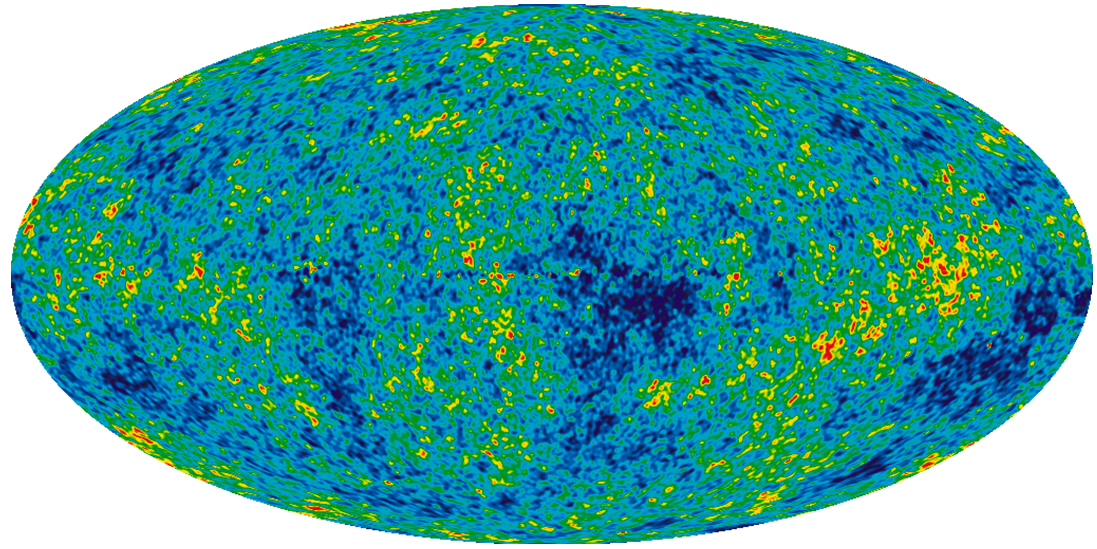
We now have tools that are powerful enough to look deep into space and see the arrival of light from early in the universe’s history. Astronomers can detect light from approximately 375,000 years after the big bang is thought to have occurred. Physicists tell us that if the big bang happened, then particles within the universe would still be very close together at this time. They would be so close that light wouldn’t be able to travel far without bumping into another particle and getting scattered in another direction. The effect would be to fill the sky with glowing fog, the “afterglow” from the formation of the universe.
In fact, this is exactly what we see when we look at light from 375,000 years after the big bang. The fog is referred to as the cosmic microwave background (or CMB), and it has been carefully mapped throughout the sky. The map displays the cosmic microwave background as temperature variations, but these variations translate to differences in the density of matter in the early universe. The red patches are the highest density regions and the blue patches are the lowest density. Higher density regions represent the eventual beginnings of stars and planets. The map has been likened to a baby picture of the universe.
17.1.2 The Big Bang is Still Happening, and We Can See the Universe Expanding
The expansion that started with the big bang never stopped. It continues today, and we can see it happening by observing galaxies that large clusters of billions of stars, called galaxies, are moving away from us. (The exception is the Andromeda galaxy with which we are on a collision course.) The astronomer Edwin Hubble came to this conclusion when he observed that the light from other galaxies was red-shifted. The red shift is a consequence of the Doppler effect. This refers to how we see waves when the object that is creating the waves is moving toward us or away from us.
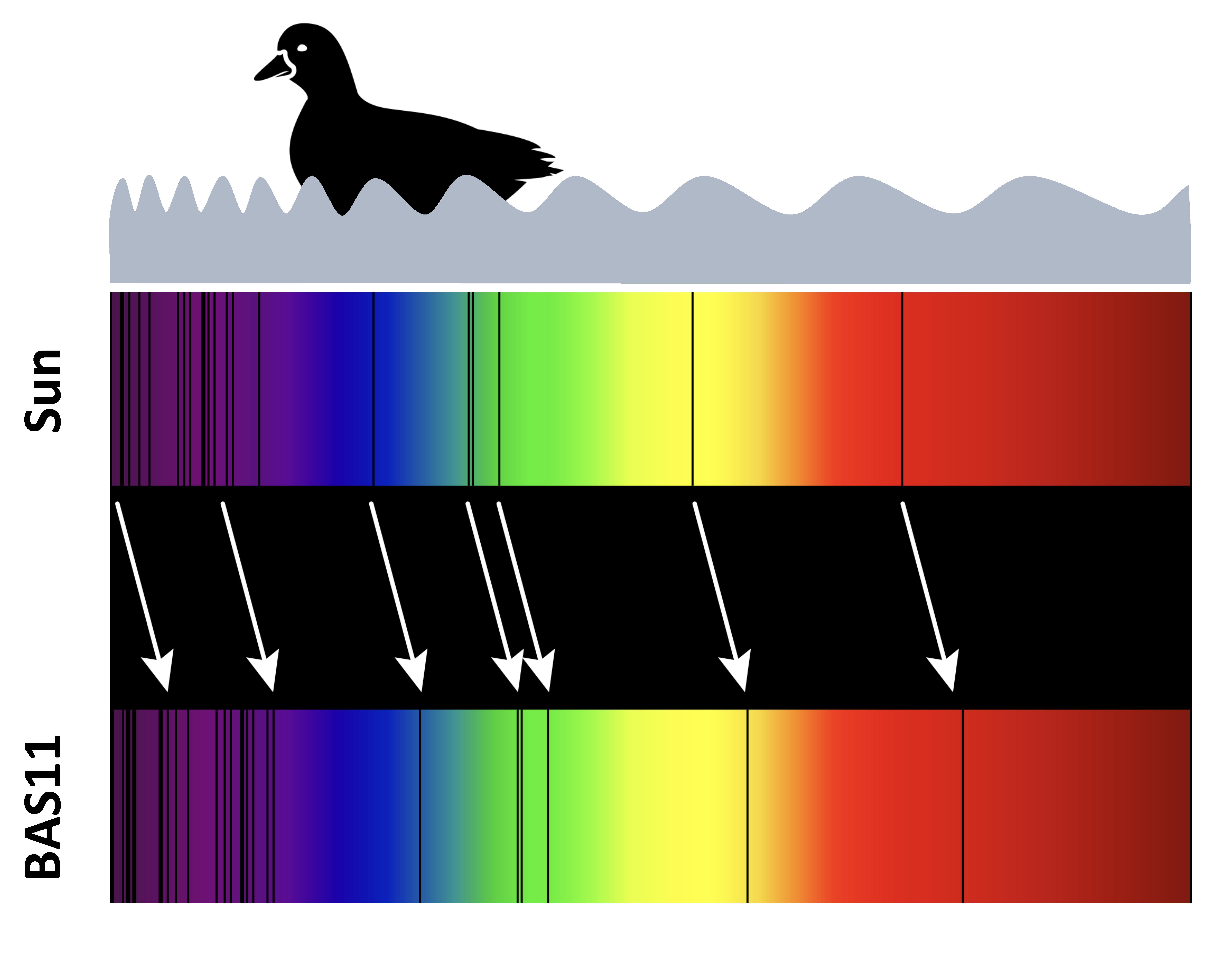
Before we get to the Doppler effect as it pertains to the red shift, let’s see how it works on something more tangible. The swimming duckling is generating waves as it moves through the water. It is generating waves that move forward as well as back, but notice that the ripples ahead of the duckling are closer to each other than the ripples behind the duckling. The distance from one ripple to the next is called the wavelength . The wavelength is shorter in the direction that the duckling is moving, and longer as the duckling moves away.
When waves are in air as sound waves rather than in water as ripples, the different wavelengths manifest as sounds with different pitches—the short wavelengths have a higher pitch, and the long wavelengths have a lower pitch. This is why the pitch of a car’s engine changes as the car races past you.
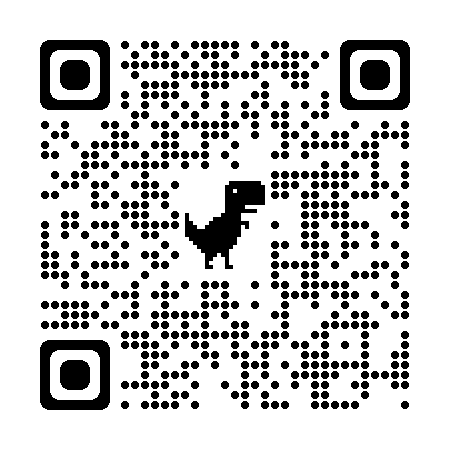
If you are using an offline version of this text, access the quiz for section 17.1 via the QR code.
17.2 Overview of Our Planetary System [1]
The solar system consists of the Sun and many smaller objects: the planets, their moons and rings, and such “debris” as asteroids , comets , and dust. Decades of observation and spacecraft exploration have revealed that most of these objects formed together with the Sun about 4.5 billion years ago. They represent clumps of material that condensed from an enormous cloud of gas and dust. The central part of this cloud became the Sun, and a small fraction of the material in the outer parts eventually formed the other objects.
During the past 50 years, we have learned more about the solar system than anyone imagined before the space age. In addition to gathering information with powerful new telescopes, we have sent spacecraft directly to many members of the planetary system . (Planetary astronomy is the only branch of our science in which we can, at least vicariously, travel to the objects we want to study.) With evocative names such as Voyager , Pioneer , Curiosity , and Pathfinder , our robot explorers have flown past, orbited, or landed on every planet, returning images and data that have dazzled both astronomers and the public. In the process, we have also investigated two dwarf planets , hundreds of fascinating moons, four ring systems, a dozen asteroids, and several comets (smaller members of our solar system that we will discuss later).
Our probes have penetrated the atmosphere of Jupiter and landed on the surfaces of Venus, Mars, our Moon , Saturn’s moon Titan, the asteroids Eros, Itokawa, Ryugu, and Bennu, and the Comet Churyumov-Gerasimenko (usually referred to as 67P). Humans have set foot on the Moon and returned samples of its surface soil for laboratory analysis. We have flown a helicopter drone on Mars. We have even discovered other places in our solar system that might be able to support some kind of life.
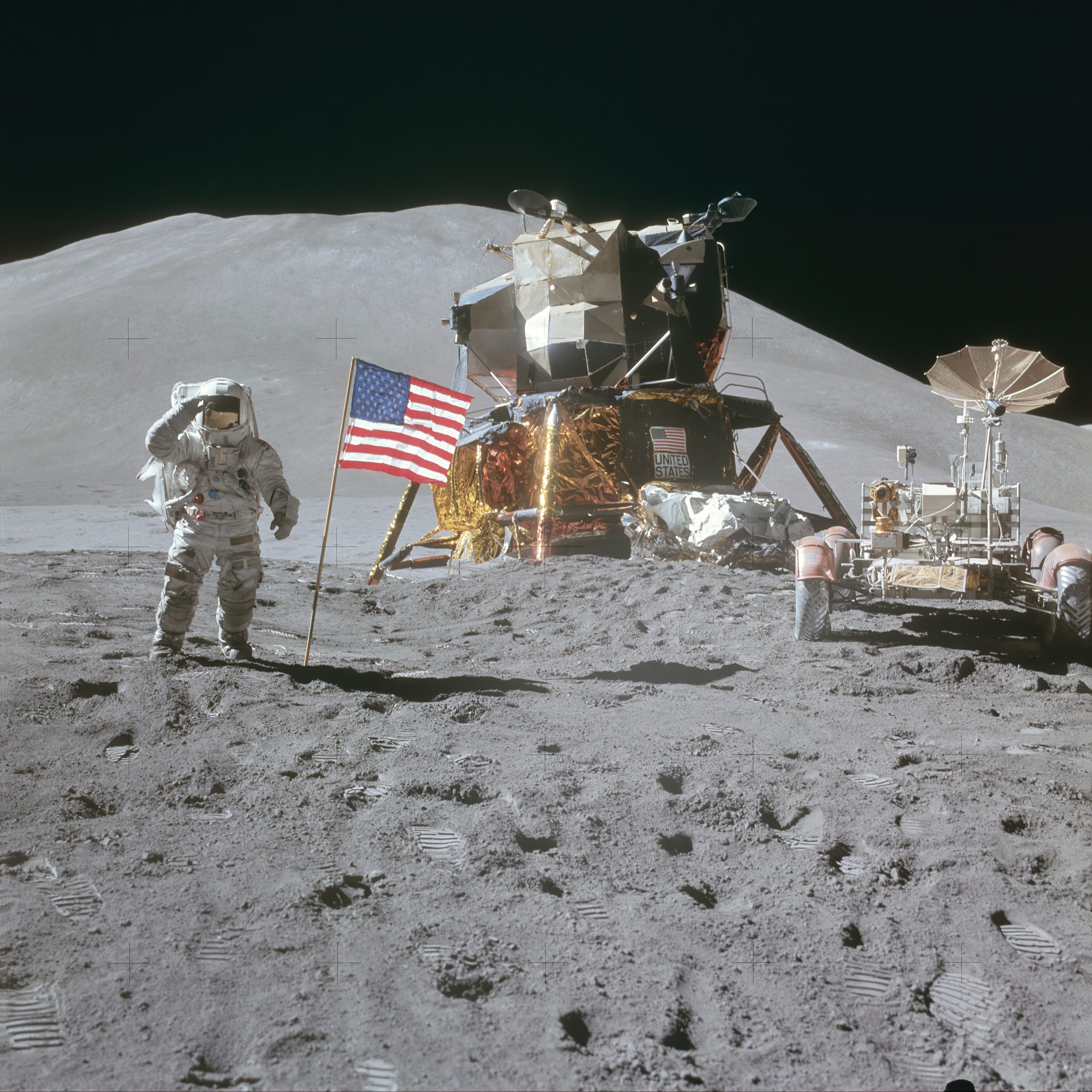
17.2.1 An Inventory
The Sun, a star that is brighter than about 80% of the stars in the Galaxy, is by far the most massive member of the solar system. It is an enormous ball about 1.4 million kilometers in diameter, with surface layers of incandescent gas and an interior temperature of millions of degrees. The Sun will be discussed in later chapters as our first, and best-studied, example of a star.
Table 17.1: Mass of members of the solar system. Note that the Sun is by far the most massive member of the solar system.
Most of the material of the planets in the solar system is actually concentrated in the largest one, Jupiter , which is more massive than all the rest of the planets combined. Astronomers were able to determine the masses of the planets centuries ago using Kepler’s laws of planetary motion and Newton’s law of gravity to measure the planets’ gravitational effects on one another or on moons that orbit them. Today, we make even more precise measurements of their masses by tracking their gravitational effects on the motion of spacecraft that pass near them.
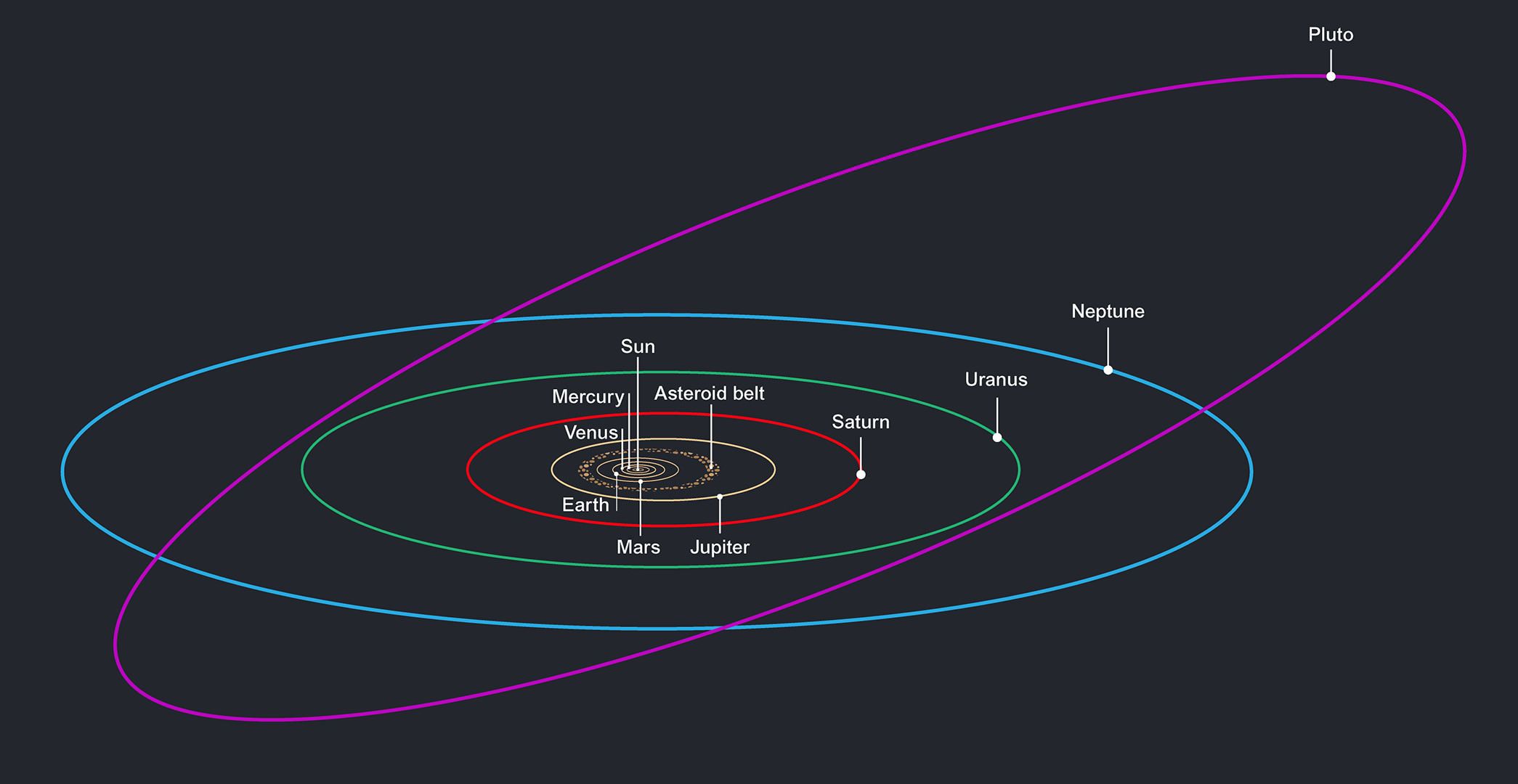
Beside Earth, five other planets were known to the ancients—Mercury, Venus, Mars, Jupiter, and Saturn—and two were discovered after the invention of the telescope: Uranus and Neptune. The eight planets all revolve in the same direction around the Sun. They orbit in approximately the same plane, like cars traveling on concentric tracks on a giant, flat racecourse. Each planet stays in its own “traffic lane,” following a nearly circular orbit about the Sun and obeying the “traffic” laws discovered by Galileo, Kepler, and Newton. Besides these planets, we have also been discovering smaller worlds beyond Neptune that are called trans-Neptunian object s or TNOs. The first to be found, in 1930, was Pluto , but others have been discovered during the twenty-first century. One of them, Eris , is about the same size as Pluto and has at least one moon (Pluto has five known moons). The largest TNOs are also classed as dwarf planets , as is the largest asteroid, Ceres . To date, more than 2600 of these TNOs have been discovered, and one, called Arrokoth, was explored by the New Horizons spacecraft.
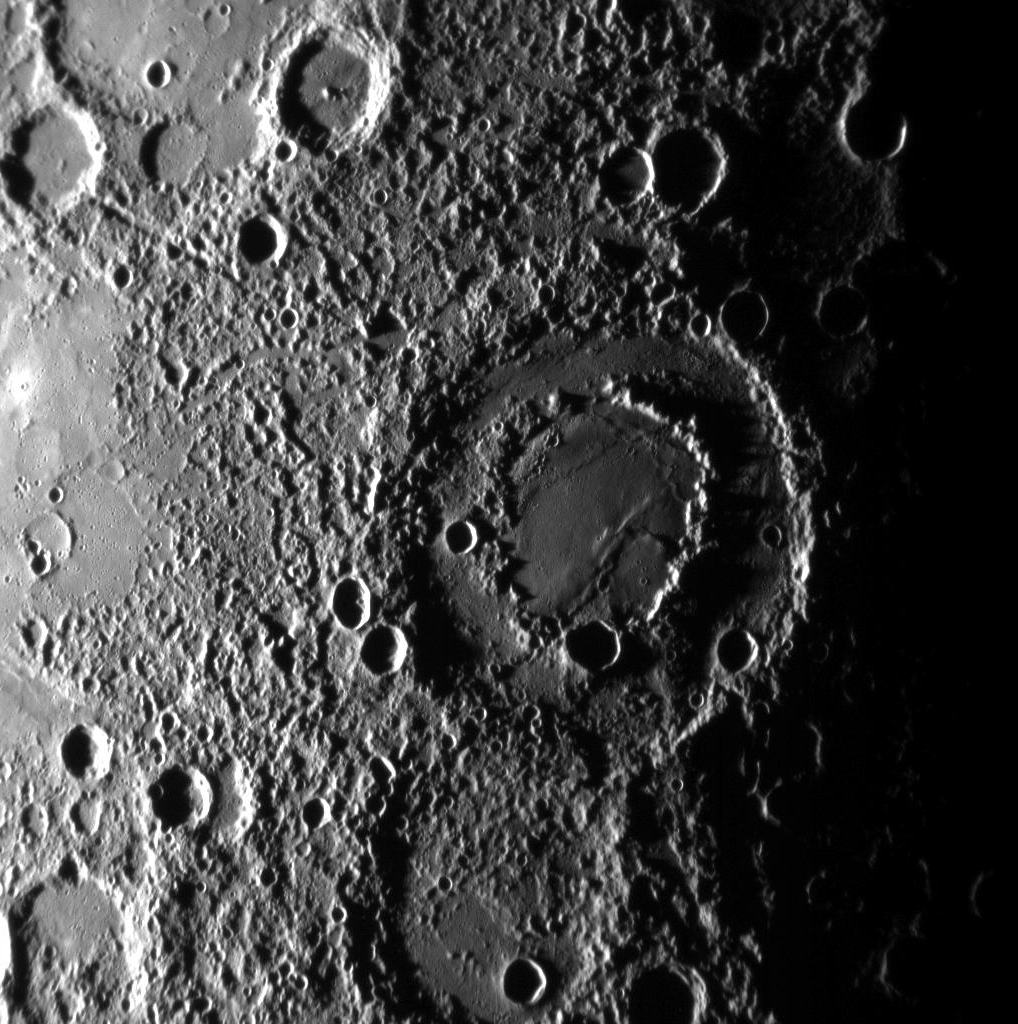
Each of the planets and dwarf planets also rotates (spins) about an axis running through it, and in most cases the direction of rotation is the same as the direction of revolution about the Sun. The exceptions are Venus , which rotates backward very slowly (that is, in a retrograde direction), and Uranus and Pluto , which also have strange rotations, each spinning about an axis tipped nearly on its side. We do not yet know the spin orientations of Eris, Haumea, and Makemake.
The four planets closest to the Sun (Mercury through Mars) are called the inner or terrestrial planets . Often, the Moon is also discussed as a part of this group, bringing the total of terrestrial objects to five (we generally call Earth’s satellite “the Moon,” with a capital M, and the other satellites “moons,” with lowercase m’s). The terrestrial planets are relatively small worlds, composed primarily of rock and metal. All of them have solid surfaces that bear the records of their geological history in the forms of craters , mountains , and volcanoes .
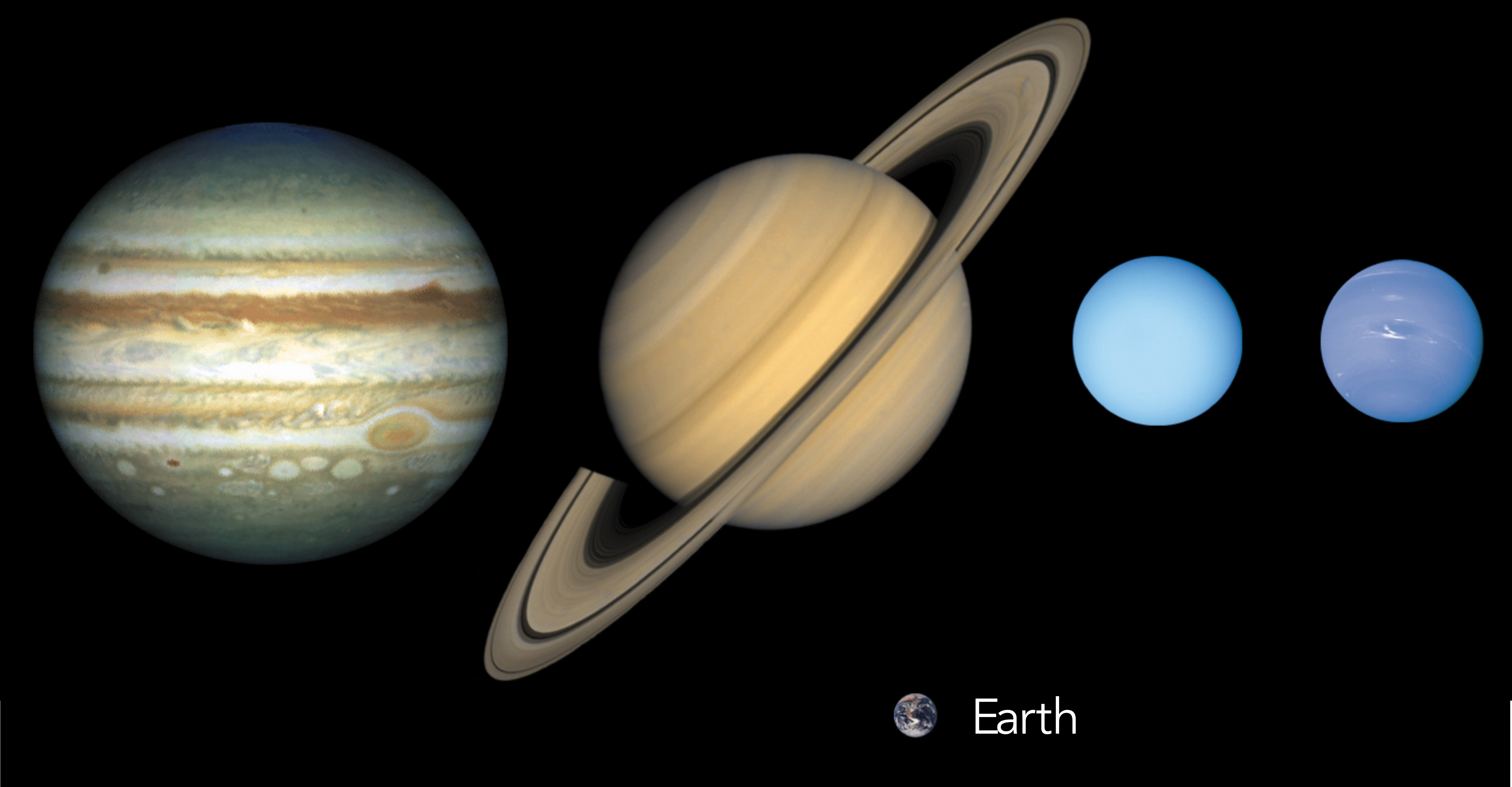
The next four planets (Jupiter through Neptune) are much larger and are composed primarily of lighter ices, liquids, and gases. We call these four the Jovian planets (after “Jove,” another name for Jupiter in mythology) or giant planets —a name they richly deserve. About 1,300 Earths could fit inside Jupiter, for example. These planets do not have solid surfaces on which future explorers might land. They are more like vast, spherical oceans with much smaller, dense cores.
Near the outer edge of the system lies Pluto , which was the first of the distant icy worlds to be discovered beyond Neptune (Pluto was visited by a spacecraft, the NASA New Horizons mission, in 2015).
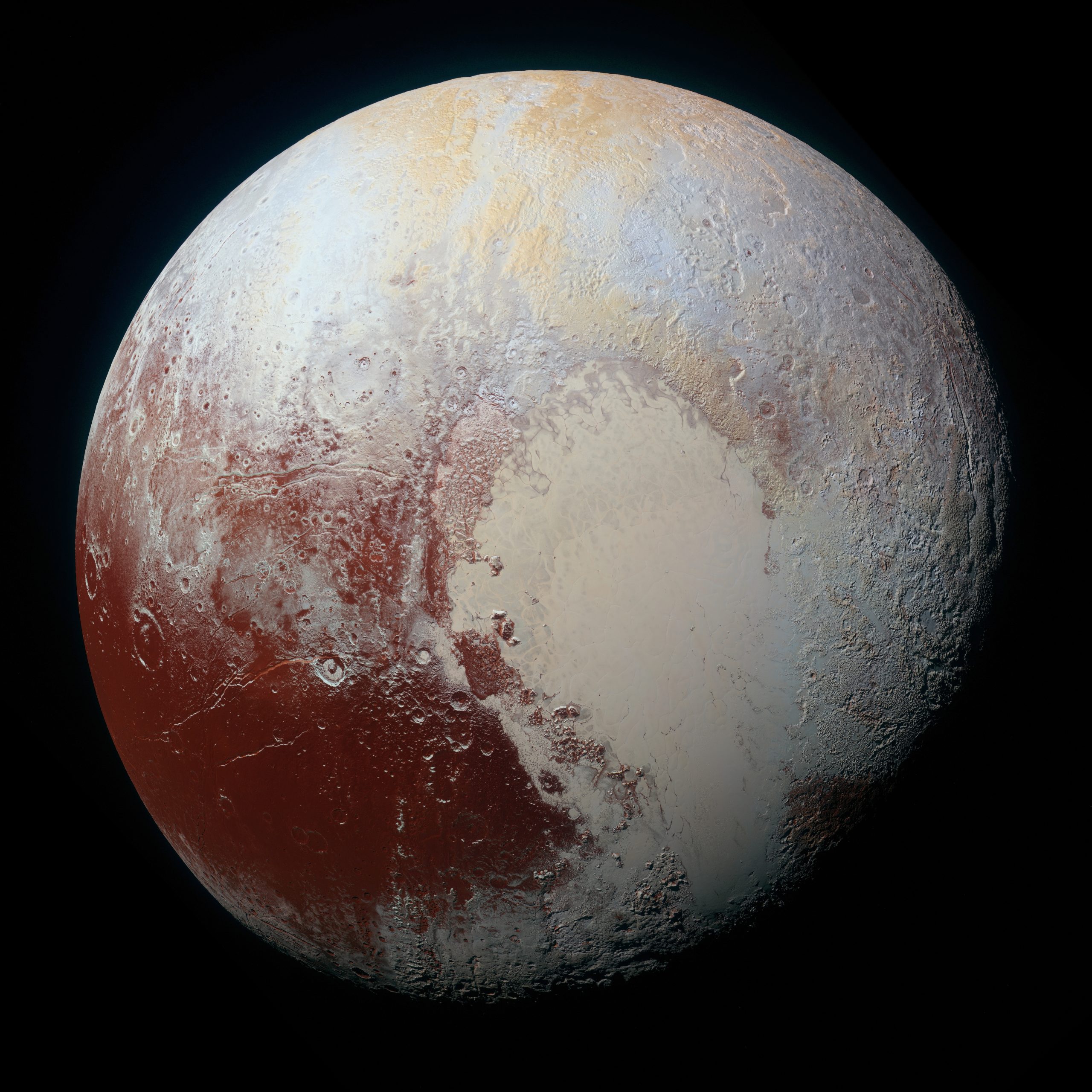
Table 17.2: The planets.
The outermost part of the solar system is known as the Kuiper belt , which is a scattering of rocky and icy bodies. Beyond that is the Oort cloud , a zone filled with small and dispersed ice traces. These two locations are where most comets form and continue to orbit, and objects found here have relatively irregular orbits compared to the rest of the solar system. Pluto, formerly the ninth planet, is located in this region of space. The XXVIth General Assembly of the International Astronomical Union (IAU) stripped Pluto of planetary status in 2006 because scientists discovered an object more massive than Pluto, which they named Eris. The IAU decided against including Eris as a planet, and therefore, excluded Pluto as well.
The IAU narrowed the definition of a planet to three criteria: 1) it must orbit a star (in our cosmic neighborhood, the Sun), 2) it must be big enough to have enough gravity to force it into a spherical shape, and 3) it must be big enough that its gravity cleared away any other objects of a similar size near its orbit around the Sun. Pluto passed the first two parts of the definition, but not the third. Pluto and Eris are currently classified as dwarf planets.
17.2.2 Smaller Members of the Solar System
Most of the planets are accompanied by one or more moons ; only Mercury and Venus move through space alone. There are more than 210 known moons orbiting planets and dwarf planets, and undoubtedly many other small ones remain undiscovered. The largest of the moons are as big as small planets and just as interesting. In addition to our Moon, they include the four largest moons of Jupiter (called the Galilean moons, after their discoverer) and the largest moons of Saturn and Neptune (confusingly named Titan and Triton).
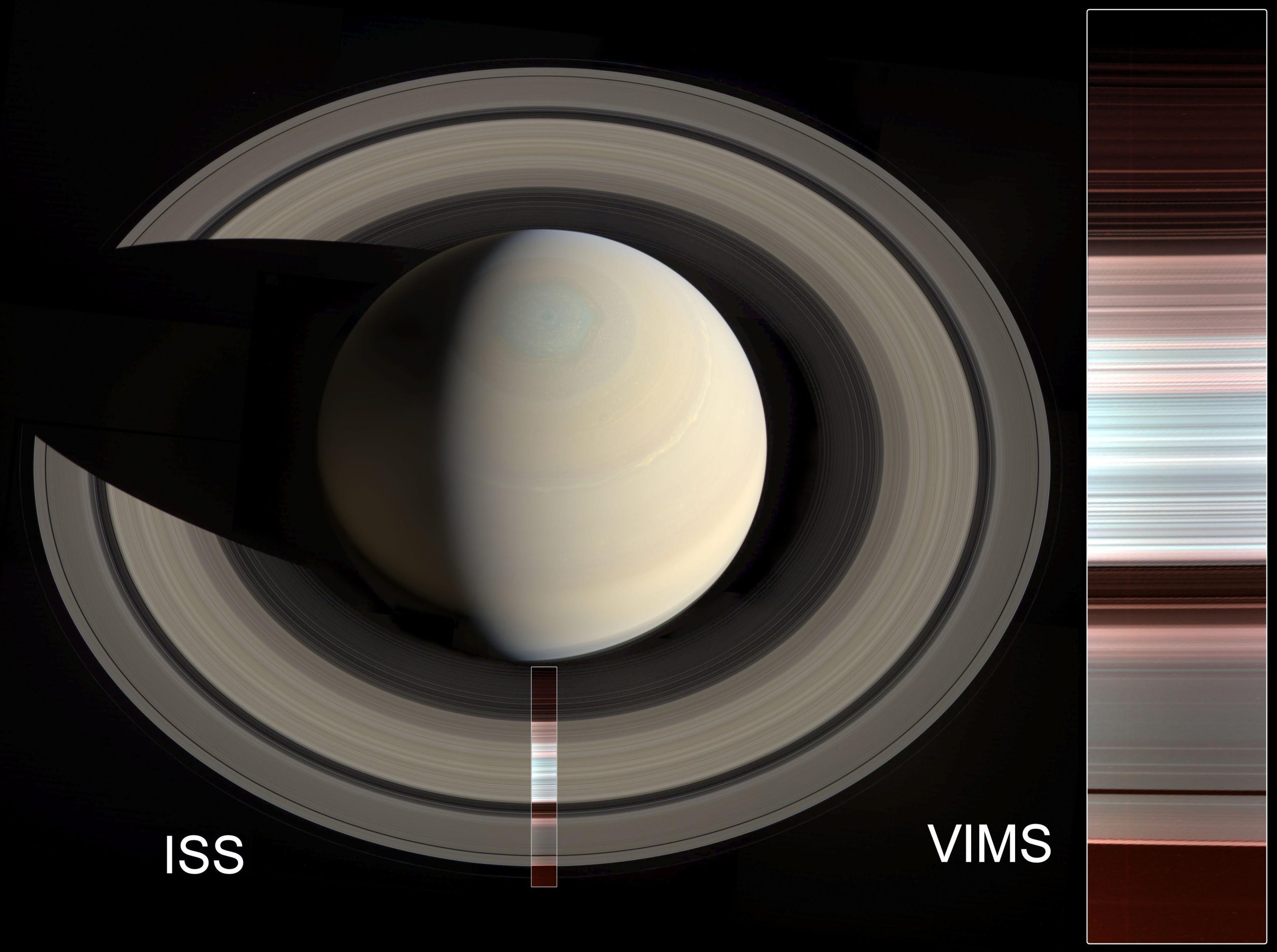
Each of the giant planets also has rings made up of countless small bodies ranging in size from mountains to mere grains of dust, all in orbit about the equator of the planet. The bright rings of Saturn are, by far, the easiest to see. They are among the most beautiful sights in the solar system. But, all four ring systems are interesting to scientists because of their complicated forms, influenced by the pull of the moons that also orbit these giant planets.
The solar system has many other less-conspicuous members. Another group is the asteroids , rocky bodies that orbit the Sun like miniature planets, mostly in the space between Mars and Jupiter (although some do cross the orbits of planets like Earth). Most asteroids are remnants of the initial population of the solar system that existed before the planets themselves formed. Some of the smallest moons of the planets, such as the moons of Mars, are very likely captured asteroids.
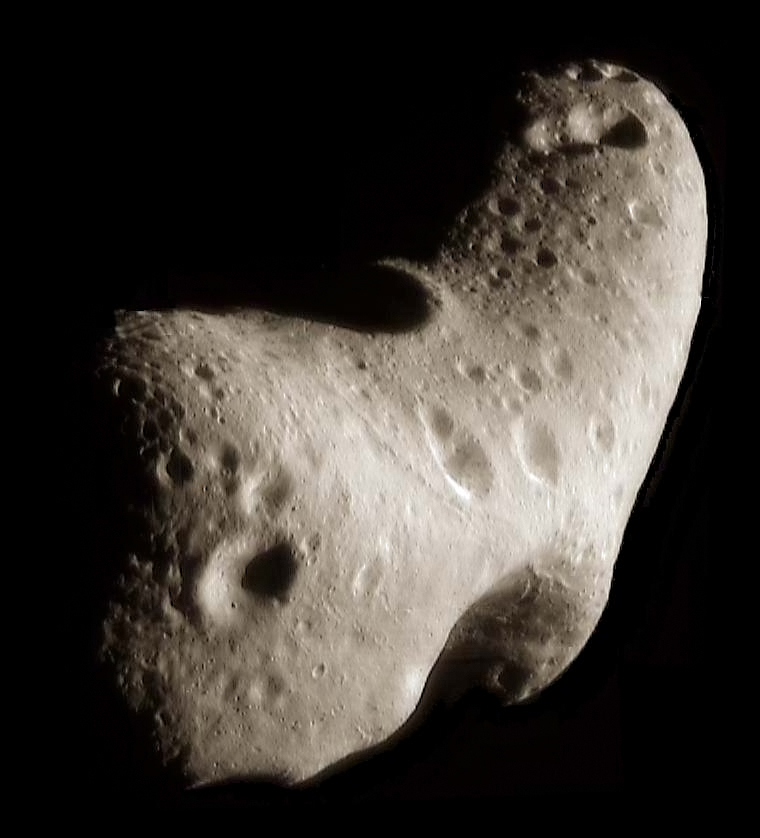
Another class of small bodies is composed mostly of ice, made of frozen gases such as water, carbon dioxide, and carbon monoxide; these objects are called comets . Comets also are remnants from the formation of the solar system, but they were formed and continue (with rare exceptions) to orbit the Sun in distant, cooler regions—stored in a sort of cosmic deep freeze. This is also the realm of the larger icy worlds, called dwarf planets.
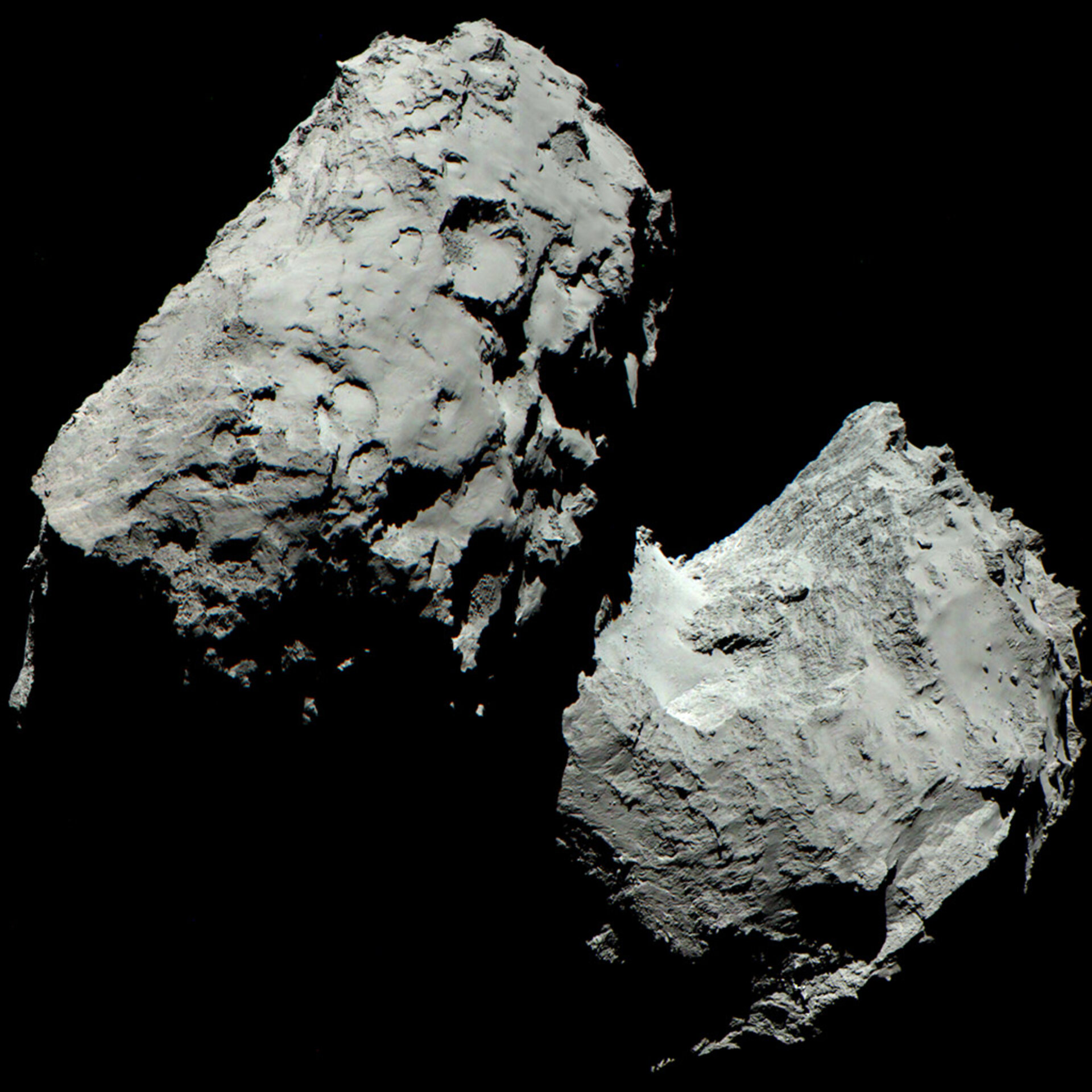
Finally, there are countless grains of broken rock, which we call cosmic dust, scattered throughout the solar system. When these particles enter Earth’s atmosphere (as millions do each day), they burn up, producing a brief flash of light in the night sky known as a meteor (meteors are often referred to as shooting stars). Occasionally, some larger chunk of rocky or metallic material survives its passage through the atmosphere and lands on Earth. Any piece that strikes the ground is known as a meteorite . You can see meteorites on display in many natural history museums and can sometimes even purchase pieces of them from gem and mineral dealers.
17.2.3 A Scale Model of the Solar System
Astronomy often deals with dimensions and distances that far exceed our ordinary experience. What does 1.4 billion kilometers—the distance from the Sun to Saturn—really mean to anyone? It can be helpful to visualize such large systems in terms of a scale model.
In our imaginations, let us build a scale model of the solar system, adopting a scale factor of 1 billion (10 9 )—that is, reducing the actual solar system by dividing every dimension by a factor of 10 9 . Earth, then, has a diameter of 1.3 centimeters, about the size of a grape. The Moon is a pea orbiting this at a distance of 40 centimeters, or a little more than a foot away. The Earth-Moon system fits into a standard backpack.
In this model, the Sun is nearly 1.5 meters in diameter, about the average height of an adult, and our Earth is at a distance of 150 meters—about one city block—from the Sun. Jupiter is five blocks away from the Sun, and its diameter is 15 centimeters, about the size of a very large grapefruit. Saturn is 10 blocks from the Sun; Uranus, 20 blocks; and Neptune, 30 blocks. Pluto, with a distance that varies quite a bit during its 249-year orbit, is currently just beyond 30 blocks and getting farther with time. Most of the moons of the outer solar system are the sizes of various kinds of seeds orbiting the grapefruit, oranges, and lemons that represent the outer planets.
In our scale model, a human is reduced to the dimensions of a single atom, and cars and spacecraft to the size of molecules. Sending the Voyager spacecraft to Neptune involves navigating a single molecule from the Earth–grape toward a lemon 5 kilometers away with an accuracy equivalent to the width of a thread in a spider’s web.
If that model represents the solar system, where would the nearest stars be? If we keep the same scale, the closest stars would be tens of thousands of kilometers away. If you built this scale model in the city where you live, you would have to place the representations of these stars on the other side of Earth or beyond.
By the way, model solar systems like the one we just presented have been built in cities throughout the world. In Sweden, for example, Stockholm’s huge Globe Arena has become a model for the Sun, and Pluto is represented by a 12-centimeter sculpture in the small town of Delsbo, 300 kilometers away. Another model solar system is in Washington on the Mall between the White House and Congress (perhaps proving they are worlds apart?).
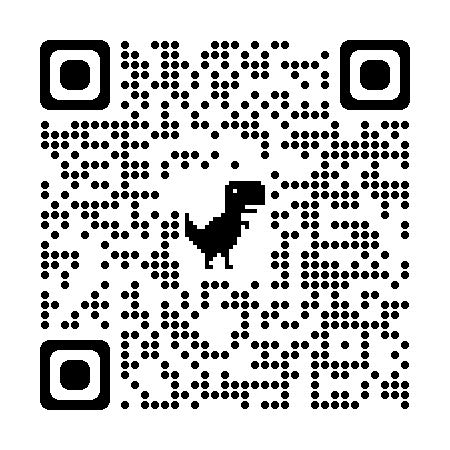
If you are using an offline version of this text, access the quiz for section 17.2 via the QR code.
17.3 Composition and Structure of Planets [2]
The fact that there are two distinct kinds of planets—the rocky terrestrial planets and the gas-rich Jovian planets—leads us to believe that they formed under different conditions. Certainly their compositions are dominated by different elements . Let us look at each type in more detail.
17.3.1 The Giant Planets
The two largest planets, Jupiter and Saturn , have nearly the same chemical makeup as the Sun; they are composed primarily of the two elements hydrogen and helium, with 75% of their mass being hydrogen and 25% helium. On Earth, both hydrogen and helium are gases, so Jupiter and Saturn are sometimes called gas planets. But, this name is misleading. Jupiter and Saturn are so large that the gas is compressed in their interior until the hydrogen becomes a liquid. Because the bulk of both planets consists of compressed, liquefied hydrogen, we should really call them liquid planets.
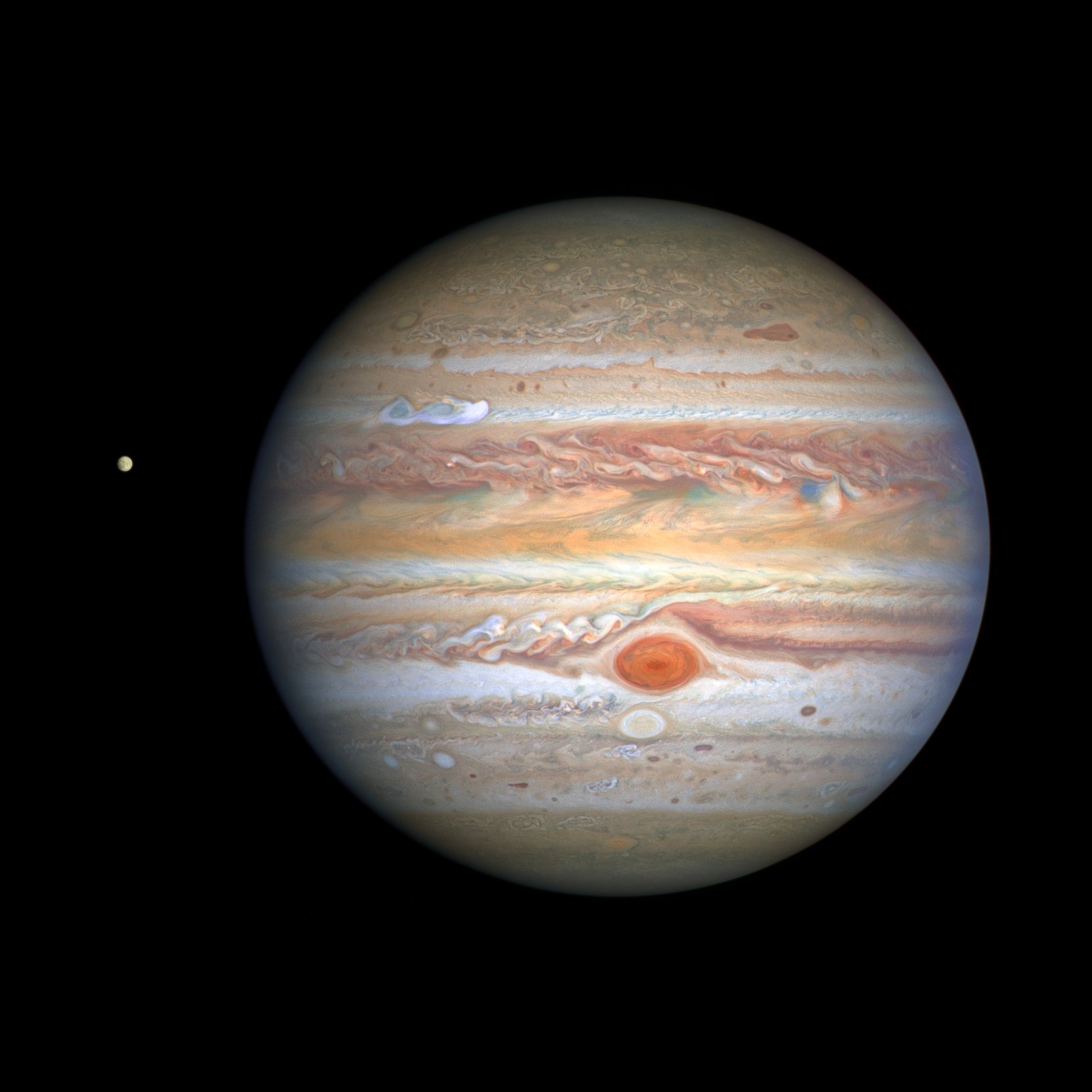
Under the force of gravity, the heavier elements sink toward the inner parts of a liquid or gaseous planet. Both Jupiter and Saturn, therefore, have cores composed of heavier rock, metal, and ice, but we cannot see these regions directly. In fact, when we look down from above, all we see is the atmosphere with its swirling clouds. We must infer the existence of the denser core inside these planets from studies of each planet’s gravity.
Uranus and Neptune are much smaller than Jupiter and Saturn, but each also has a core of rock, metal, and ice. Uranus and Neptune were less efficient at attracting hydrogen and helium gas, so they have much smaller atmospheres in proportion to their cores.
Chemically, each giant planet is dominated by hydrogen and its many compounds. Nearly all the oxygen present is combined chemically with hydrogen to form water (H 2 O). Chemists call such a hydrogen-dominated composition reduced . Throughout the outer solar system, we find abundant water (mostly in the form of ice) and reducing chemistry.
17.3.2 The Terrestrial Planets
The terrestrial planets are quite different from the giants. In addition to being much smaller, they are composed primarily of rocks and metals. These, in turn, are made of elements that are less common in the universe as a whole. The most abundant rocks, called silicates , are made of silicon and oxygen, and the most common metal is iron. We can tell from their densities that Mercury has the greatest proportion of metals (which are denser) and the Moon has the lowest. Earth , Venus , and Mars all have roughly similar bulk compositions: about one third of their mass consists of iron-nickel or iron-sulfur combinations; two thirds is made of silicates. Because these planets are largely composed of oxygen compounds (such as the silicate minerals of their crusts), their chemistry is said to be oxidized .
When we look at the internal structure of each of the terrestrial planets, we find that the densest metals are in a central core, with the lighter silicates near the surface. If these planets were liquid, like the giant planets, we could understand this effect as the result the sinking of heavier elements due to the pull of gravity. This leads us to conclude that, although the terrestrial planets are solid today, at one time they must have been hot enough to melt.
Differentiation is the process by which gravity helps separate a planet’s interior into layers of different compositions and densities. The heavier metals sink to form a core, while the lightest minerals float to the surface to form a crust. Later, when the planet cools, this layered structure is preserved. In order for a rocky planet to differentiate, it must be heated to the melting point of rocks, which is typically more than 1300 K.
17.3.3 Moons, Asteroids, and Comets
Chemically and structurally, Earth’s Moon is like the terrestrial planets, but most moons are in the outer solar system, and they have compositions similar to the cores of the giant planets around which they orbit. The three largest moons—Ganymede and Callisto in the Jovian system, and Titan in the Saturnian system—are composed half of frozen water, and half of rocks and metals. Most of these moons differentiated during formation, and today they have cores of rock and metal, with upper layers and crusts of very cold and—thus very hard—ice.
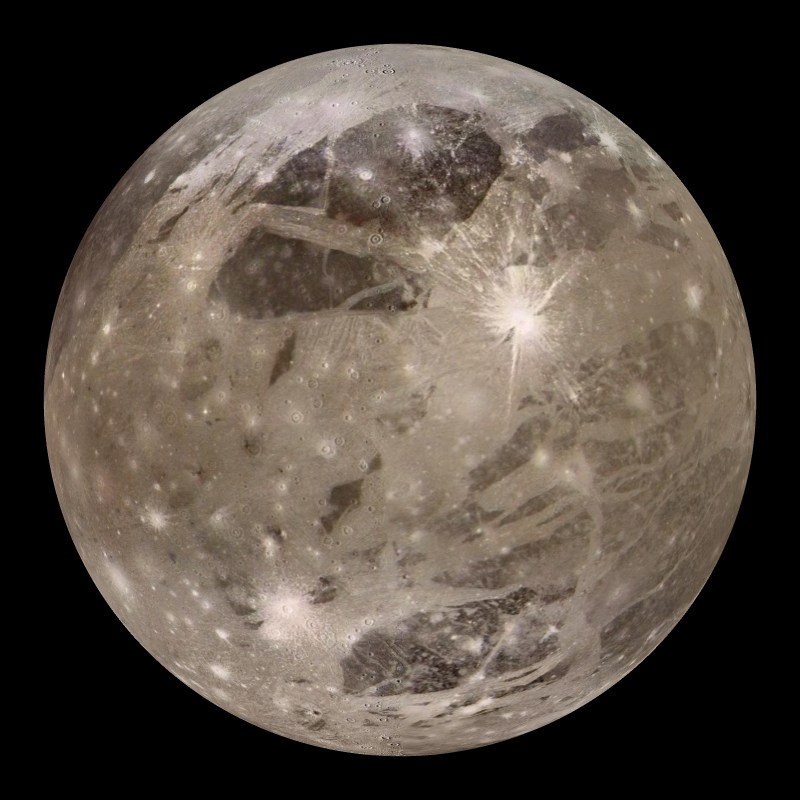
Most of the asteroids and comets , as well as the smallest moons , were probably never heated to the melting point. However, some of the largest asteroids, such as Vesta , appear to be differentiated; others are fragments from differentiated bodies. Many of the smaller objects seem to be fragments or rubble piles that are the result of collisions. Because most asteroids and comets retain their original composition, they represent relatively unmodified material dating back to the time of the formation of the solar system. In a sense, they act as chemical fossils, helping us to learn about a time long ago whose traces have been erased on larger worlds.
17.3.4 Temperatures: Going to Extremes
Generally speaking, the farther a planet or moon is from the Sun, the cooler its surface. The planets are heated by the radiant energy of the Sun, which gets weaker with the square of the distance. You know how rapidly the heating effect of a fireplace or an outdoor radiant heater diminishes as you walk away from it; the same effect applies to the Sun. Mercury , the closest planet to the Sun, has a blistering surface temperature that ranges from 280–430 °C on its sunlit side, whereas the surface temperature on Pluto is only about –220 °C, colder than liquid air.
Mathematically, the temperatures decrease approximately in proportion to the square root of the distance from the Sun. Pluto is about 30 AU at its closest to the Sun (or 100 times the distance of Mercury) and about 49 AU at its farthest from the Sun. Thus, Pluto’s temperature is less than that of Mercury by the square root of 100, or a factor of 10: from 500 K to 50 K.
In addition to its distance from the Sun, the surface temperature of a planet can be influenced strongly by its atmosphere . Without our atmospheric insulation (the greenhouse effect, which keeps the heat in), the oceans of Earth would be permanently frozen. Conversely, if Mars once had a larger atmosphere in the past, it could have supported a more temperate climate than it has today. Venus is an even more extreme example, where its thick atmosphere of carbon dioxide acts as insulation, reducing the escape of heat built up at the surface, resulting in temperatures greater than those on Mercury. Today, Earth is the only planet where surface temperatures generally lie between the freezing and boiling points of water. As far as we know, Earth is the only planet to support life.
17.3.5 Dating Planetary Surfaces [3]
How do we know the age of the surfaces we see on planets and moons? If a world has a surface (as opposed to being mostly gas and liquid), astronomers have developed some techniques for estimating how long ago that surface solidified. Note that the age of these surfaces is not necessarily the age of the planet as a whole. On geologically active objects (including Earth), vast outpourings of molten rock or the erosive effects of water and ice, which we call planet weathering , have erased evidence of earlier epochs and present us with only a relatively young surface for investigation.
One way to estimate the age of a surface is by counting the number of impact craters . This technique works because the rate at which impacts have occurred in the solar system has been roughly constant for several billion years. Thus, in the absence of forces to eliminate craters, the number of craters is simply proportional to the length of time the surface has been exposed. This technique has been applied successfully to many solid planets and moons .
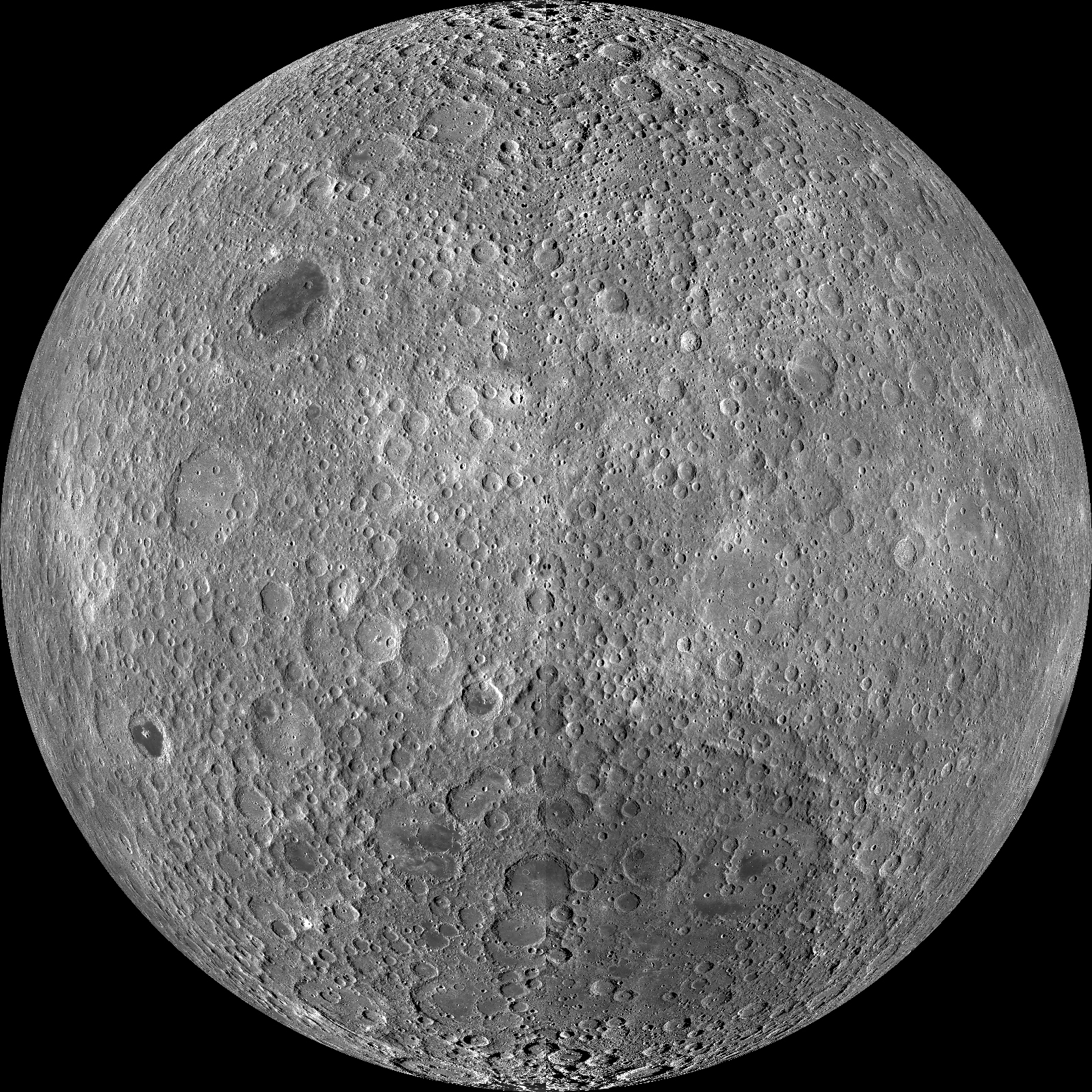
Bear in mind that crater counts can tell us only the time since the surface experienced a major change that could modify or erase preexisting craters. Estimating ages from crater counts is a little like walking along a sidewalk in a snowstorm after the snow has been falling steadily for a day or more. You may notice that in front of one house the snow is deep, while next door the sidewalk may be almost clear. Do you conclude that less snow has fallen in front of Ms. Jones’ house than Mr. Smith’s? More likely, you conclude that Jones has recently swept the walk clean and Smith has not. Similarly, the numbers of craters indicate how long it has been since a planetary surface was last “swept clean” by ongoing lava flows or by molten materials ejected when a large impact happened nearby.
Still, astronomers can use the numbers of craters on different parts of the same world to provide important clues about how regions on that world evolved. On a given planet or moon, the more heavily cratered terrain will generally be older (that is, more time will have elapsed there since something swept the region clean).
17.3.6 Radioactive Rocks
Another way to trace the history of a solid world is to measure the age of individual rocks. After samples were brought back from the Moon by Apollo astronauts, the techniques that had been developed to date rocks on Earth were applied to rock samples from the Moon to establish a geological chronology for the Moon. Furthermore, a few samples of material from the Moon, Mars, and the large asteroid Vesta have fallen to Earth as meteorites and can be examined directly.
Scientists measure the age of rocks using the properties of natural radioactivity . Around the beginning of the twentieth century, physicists began to understand that some atomic nuclei are not stable but can split apart (decay) spontaneously into smaller nuclei. The process of radioactive decay involves the emission of particles such as electrons , or of radiation in the form of gamma rays .
For any one radioactive nucleus, it is not possible to predict when the decay process will happen. Such decay is random in nature, like the throw of dice: as gamblers have found all too often, it is impossible to say just when the dice will come up 7 or 11. But, for a very large number of dice tosses, we can calculate the odds that 7 or 11 will come up. Similarly, if we have a very large number of radioactive atoms of one type (say, uranium), there is a specific time period, called its half-life , during which the chances are fifty-fifty that decay will occur for any of the nuclei.
A particular nucleus may last a shorter or longer time than its half-life, but in a large sample, almost exactly half of the nuclei will have decayed after a time equal to one half-life. Half of the remaining nuclei will have decayed after two half-lives pass, leaving only one half of a half—or one quarter—of the original sample.
If you had 1 gram of pure radioactive nuclei with a half-life of 100 years, then after 100 years you would have 1/2 gram; after 200 years, 1/4 gram; after 300 years, only 1/8 gram; and so forth. However, the material does not disappear. Instead, the radioactive atoms are replaced with their decay products. Sometimes the radioactive atoms are called parents and the decay products are called daughter elements.
In this way, radioactive elements with half-lives we have determined can provide accurate nuclear clocks. By comparing how much of a radioactive parent element is left in a rock to how much of its daughter products have accumulated, we can learn how long the decay process has been going on and hence how long ago the rock formed. The following table summarizes the decay reactions used most often to date lunar and terrestrial rocks.
Table 17.3: Radioactive decay reaction used to date rocks. The number after each element is its atomic weight, equal to the number of protons plus neutrons in its nucleus. This specifies the isotope of the element, different isotopes of the same element differ in the number of neutrons.
When astronauts first flew to the Moon, one of their most important tasks was to bring back lunar rocks for radioactive age-dating. Until then, astronomers and geologists had no reliable way to measure the age of the lunar surface. Counting craters had let us calculate relative ages (for example, the heavily cratered lunar highlands were older than the dark lava plains), but scientists could not measure the actual age in years. Some thought that the ages were as young as those of Earth’s surface, which has been resurfaced by many geological events. For the Moon’s surface to be so young would imply active geology on our satellite. Only in 1969, when the first Apollo samples were dated, did we learn that the Moon is an ancient, geologically dead world. Using such dating techniques, we have been able to determine the ages of both Earth and the Moon: each was formed about 4.5 billion years ago (although, as we shall see, Earth probably formed earlier than the Moon).
We should also note that the decay of radioactive nuclei generally releases energy in the form of heat. Although the energy from a single nucleus is not very large (in human terms), the enormous numbers of radioactive nuclei in a planet or moon (especially early in its existence) can be a significant source of internal energy for that world. Geologists estimate that about half of Earth’s current internal heat budget comes from the decay of radioactive isotopes in its interior.
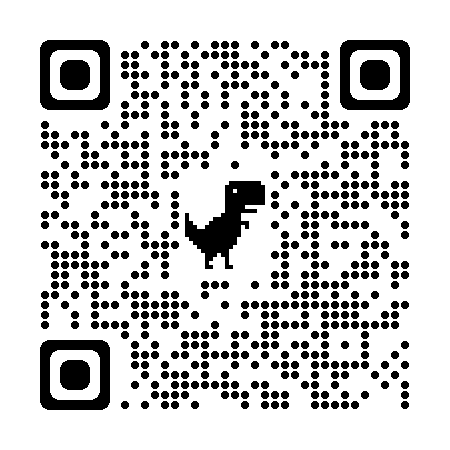
If you are using an offline version of this text, access the quiz for section 17.3 via the QR code.
17.4 Origin of the Solar System [4]
Much of astronomy is motivated by a desire to understand the origin of things: to find at least partial answers to age-old questions of where the universe , the Sun, Earth, and we ourselves came from. Each planet and moon is a fascinating place that may stimulate our imagination as we try to picture what it would be like to visit. Taken together, the members of the solar system preserve patterns that can tell us about the formation of the entire system. As we begin our exploration of the planets, we want to introduce our modern picture of how the solar system formed.
The recent discovery of thousands of planets in orbit around other stars has shown astronomers that many exoplanetary systems can be quite different from our own solar system. For example, it is common for these systems to include planets intermediate in size between our terrestrial and giant planets. These are often called superearths . Some exoplanet systems even have giant planets close to the star, reversing the order we see in our system.
17.4.1 Looking for Patterns
One way to approach our question of origin is to look for regularities among the planets. We found, for example, that all the planets lie in nearly the same plane and revolve in the same direction around the Sun. The Sun also spins in the same direction about its own axis. Astronomers interpret this pattern as evidence that the Sun and planets formed together from a spinning cloud of gas and dust that we call the solar nebula .
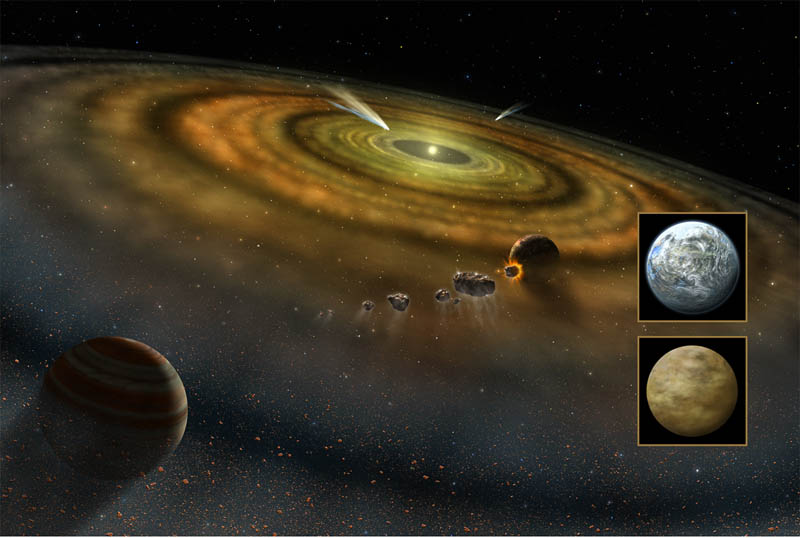
The composition of the planets gives another clue about origins. Spectroscopic analysis allows us to determine which elements are present in the Sun and the planets. The Sun has the same hydrogen-dominated composition as Jupiter and Saturn, and therefore appears to have been formed from the same reservoir of material. In comparison, the terrestrial planets and our Moon are relatively deficient in the light gases and the various ices that form from the common elements oxygen, carbon, and nitrogen. Instead, on Earth and its neighbors, we see mostly the rarer heavy elements such as iron and silicon. This pattern suggests that the processes that led to planet formation in the inner solar system must somehow have excluded much of the lighter materials that are common elsewhere. These lighter materials must have escaped, leaving a residue of heavy stuff.
The reason for this is not hard to guess, bearing in mind the heat of the Sun. The inner planets and most of the asteroids are made of rock and metal, which can survive heat, but they contain very little ice or gas, which evaporate when temperatures are high (to see what we mean, just compare how long a rock and an ice cube survive when they are placed in the sunlight). In the outer solar system, where it has always been cooler, the planets and their moons, as well as icy dwarf planets and comets , are composed mostly of ice and gas.
17.4.2 The Evidence from Far Away
A second approach to understanding the origins of the solar system is to look outward for evidence that other systems of planets are forming elsewhere. We cannot look back in time to the formation of our own system, but many stars in space are much younger than the Sun. In these systems, the processes of planet formation might still be accessible to direct observation. We observe that there are many other “ solar nebulas ” or circumstellar disks —flattened, spinning clouds of gas and dust surrounding young stars. These disks resemble our own solar system’s initial stages of formation billions of years ago.
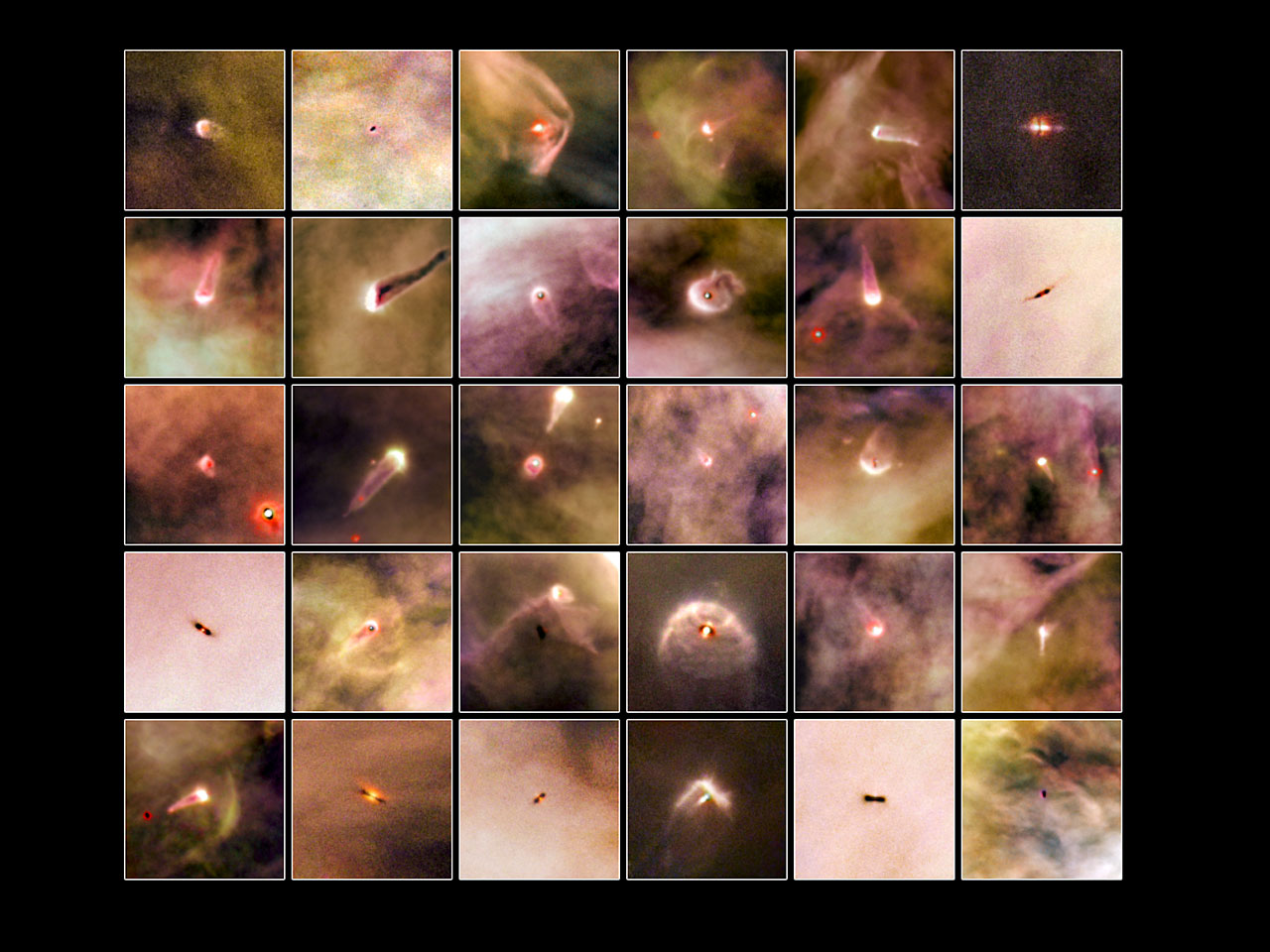
17.4.3 Building Planets
Circumstellar disks are a common occurrence around very young stars, suggesting that disks and stars form together. Astronomers can use theoretical calculations to see how solid bodies might form from the gas and dust in these disks as they cool. These models show that material begins to coalesce first by forming smaller objects, precursors of the planets, which we call planetesimals .
Today’s fast computers can simulate the way millions of planetesimals, probably no larger than 100 kilometers in diameter, might gather together under their mutual gravity to form the planets we see today. We are beginning to understand that this process was a violent one, with planetesimals crashing into each other and sometimes even disrupting the growing planets themselves. As a consequence of those violent impacts (and the heat from radioactive elements in them), all the planets were heated until they were liquid and gas, and therefore differentiated, which helps explain their present internal structures.
The process of impacts and collisions in the early solar system was complex and, apparently, often random. The solar nebula model can explain many of the regularities we find in the solar system, but the random collisions of massive collections of planetesimals could be the reason for some exceptions to the “rules” of solar system behavior. For example, why do the planets Uranus and Pluto spin on their sides? Why does Venus spin slowly and in the opposite direction from the other planets? Why does the composition of the Moon resemble Earth in many ways and yet exhibit substantial differences? The answers to such questions probably lie in enormous collisions that took place in the solar system long before life on Earth began.
Today, some 4.5 billion years after its origin, the solar system is—thank goodness—a much less violent place. However, some planetesimals have continued to interact and collide, and their fragments move about the solar system as roving “transients” that can make trouble for the established members of the Sun’s family, such as our own Earth.
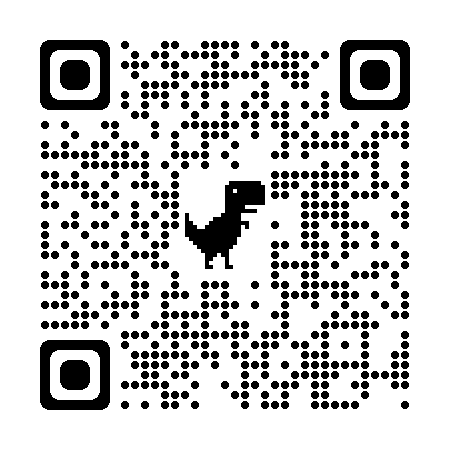
If you are using an offline version of this text, access the quiz for section 17.4 via the QR code.
Our solar system currently consists of the Sun, eight planets, five dwarf planets, nearly 200 known moons , and a host of smaller objects. The planets can be divided into two groups: the inner terrestrial planets and the outer giant planets. Smaller members of the solar system include asteroids (including the dwarf planet Ceres), which are rocky and metallic objects found mostly between Mars and Jupiter; comets , which are made mostly of frozen gases and generally orbit far from the Sun; and countless smaller grains of cosmic dust. When a meteor survives its passage through our atmosphere and falls to Earth, we call it a meteorite .
The ages of the surfaces of objects in the solar system can be estimated by counting craters: on a given world, a more heavily cratered region will generally be older than one that is less cratered. We can also use samples of rocks with radioactive elements in them to obtain the time since the layer in which the rock formed last solidified. The half-life of a radioactive element is the time it takes for half the sample to decay; we determine how many half-lives have passed by how much of a sample remains the radioactive element and how much has become the decay product. In this way, we have estimated the age of the Moon and Earth to be roughly 4.5 billion years.
Regularities among the planets have led astronomers to hypothesize that the Sun and the planets formed together in a giant, spinning cloud of gas and dust called the solar nebula . Astronomical observations show tantalizingly similar circumstellar disks around other stars. Within the solar nebula, material first coalesced into planetesimals ; many of these gathered together to make the planets and moons. The remainder can still be seen as comets and asteroids. Probably all planetary systems have formed in similar ways, but many exoplanet systems have evolved along quite different paths.
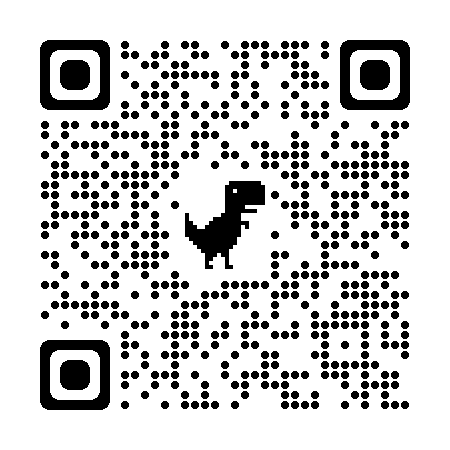
If you are using an offline version of this text, access the quiz for chapter 17 via the QR code.
Text References
Parts of this chapter are from OpenStax’s Astronomy (chapter 7) . 2016. CC BY 4.0 .
Chapter 17 Origin of Earth and the Solar System ( CC BY 4.0) by Karla Panchuk was added from Earle, Steven (2019) Physical Geology, 2nd edition. BC Campus https://opentextbc.ca/physicalgeology2ed/chapter/22-1-starting-with-a-big-bang
Figure References
Figure 17.1: The big bang. NASA/WMAP Science Team. 2006. Public domain. https://en.wikipedia.org/wiki/File:CMB_Timeline300_no_WMAP.jpg
Figure 17.2: Cosmic microwave background (CMB) map of the sky, a baby picture of the universe. NASA / WMAP Science Team. 2012. Public domain. https://commons.wikimedia.org/wiki/File:Ilc_9yr_moll4096.png
Figure 17.3: Doppler effect. Charly Whisky. 2007. CC BY-SA 3.0 . https://commons.wikimedia.org/wiki/File%3ADopplerfrequenz.gif
Figure 17.4: Red shift in light from the supercluster BAS11 compared to the sun’s light. Kindred Grey. 2022. CC BY 4.0 . Includes Duck by parkjisun from Noun Project ( Noun Project license ).
Figure 17.5: Astronauts on the Moon. NASA Johnson Space Center; Restored by Bammesk. 1971. Public domain. https://en.wikipedia.org/wiki/File:AS15-88-11866_-_Apollo_15_flag,_rover,_LM,_Irwin_-_restoration1.jpg
Figure 17.6: Orbits of the planets. Arabik4892. 2022. CC BY-SA 4.0 . https://commons.wikimedia.org/wiki/File:Planet_Orbits.jpg
Figure 17.7: Surface of Mercury. NASA/Johns Hopkins University Applied Physics Laboratory/Carnegie Institution of Washington. 2009. Public domain. https://commons.wikimedia.org/wiki/File:Mercury_Double-Ring_Impact_Basin.png
Figure 17.8: The four giant planets. Solar System Exploration, NASA. 2008. Public domain. https://commons.wikimedia.org/wiki/File:Gas_planet_size_comparisons.jpg
Figure 17.9: This intriguing image from the New Horizons spacecraft, taken when it flew by the dwarf planet Pluto in July 2015, shows some of its complex surface features. NASA / Johns Hopkins University Applied Physics Laboratory / Southwest Research Institute. 2015. Public domain. https://en.wikipedia.org/wiki/File:Pluto-01_Stern_03_Pluto_Color_TXT.jpg
Figure 17.10: Saturn and its A, B, and C rings in visible and (inset) infrared light. NASA/JPL-Caltech/Space Science Institute/G. Ugarkovic (ISS), NASA/JPL-Caltech/University of Arizona/CNRS/LPG-Nantes (VIMS). 2019. Public domain. https://commons.wikimedia.org/wiki/File:PIA23170-Saturn-Rings-IR-Map-20190613.jpg
Figure 17.11: Asteroid Eros. NASA/JPL/JHUAPL. 2000. Public domain. https://commons.wikimedia.org/wiki/File:Eros_-_PIA02923_(color).jpg
Figure 17.12: Comet Churyumov-Gerasimenko (67P). ESA/Rosetta/MPS for OSIRIS Team MPS/UPD/LAM/IAA/SSO/INTA/UPM/DASP/IDA. 2014. CC BY-SA 4.0 . https://commons.wikimedia.org/wiki/File:Comet_67P_True_color.jpg
Figure 17.13: Jupiter with its moon Europa on the left. NASA, ESA, STScI, A. Simon (Goddard Space Flight Center), and M.H. Wong (University of California, Berkeley) and the OPAL team. 2020. Public domain. https://commons.wikimedia.org/wiki/File:Jupiter_and_Europa_2020.tiff
Figure 17.14: Jupiter’s moon Ganymede. NOAA. 2009. Public domain. https://commons.wikimedia.org/wiki/File:Moon_Ganymede_by_NOAA.jpg
Figure 17.15: Our cratered Moon. NASA/Goddard/Arizona State University. 2011. Public domain. https://www.nasa.gov/mission_pages/LRO/news/lro-farside.html
Figure 17.16: Radioactive decay. Andrew Fraknoi, David Morrison, and Sidney Wolff. 2015. CC BY 4.0 . https://en.wikipedia.org/wiki/File:OSC_Astro_07_03_Decay_(1).jpg
Figure 17.17: NASA artist’s conception of various planet formation processes, including exocomets and other planetesimals, around Beta Pictoris, a very young type A V star. NASA/FUSE/Lynette Cook. 2007. Public domain. https://commons.wikimedia.org/wiki/File:NASA-ExocometsAroundBetaPictoris-ArtistView.jpg
Figure 17.18: Atlas of Planetary Nurseries. NASA/ESA and L. Ricci (ESO). 2009. CC BY 4.0 . https://esahubble.org/copyright/
- Source: OpenStax Astronomy (CC BY). Access for free at https://openstax.org/books/astronomy-2e/pages/7-1-overview-of-our-planetary-system ↵
- Source: OpenStax Astronomy (CC BY). Access for free at https://openstax.org/books/astronomy/pages/7-2-composition-and-structure-of-planets ↵
- Source: OpenStax Astronomy (CC BY). Access for free at https://openstax.org/books/astronomy/pages/7-3-dating-planetary-surfaces ↵
- Source: OpenStax Astronomy (CC BY). Access for free at https://openstax.org/books/astronomy/pages/7-4-origin-of-the-solar-system ↵
All of space and time and their contents, including planets, stars, galaxies, and all other forms of matter and energy.
The generic term for a group of planets and other bodies circling a star is planetary system. Our planetary system is the only one officially called “solar system,” because our Sun is sometimes called Sol.
A large astronomical body that is neither a star nor a stellar remnant.
The measure of the vibrational (kinetic) energy of a substance.
Originating or existing outside the solar system.
The theory that the Universe started with a expansive explosion. Shortly after, elements were created (mostly hydrogen) and galaxies started to form.
A process inside stars where smaller atoms combine and form larger atoms.
The generic term for a group of planets and other bodies circling a star.
The process of atoms breaking down randomly and spontaneously.
Any planet beyond our solar system.
A group of all atoms with a specific number of protons, having specific, universal, and unique properties.
The distance that light can travel through space in a year. One light year is 9.4607 × 10^12 km.
Radiation left over from the an early stage in the development of the universe at the time when protons and neutrons were recombining to form atoms.
A gravitationally-bound system of stars and interstellar matter.
The increase in wavelength of light resulting from the fact that the source of the light is moving away from the observer.
The distance between any two repeating portions of a wave (e.g., two successive wave crests).
to move in a circular or curving course or orbit. Not to be confused with rotate, when something spins on an axis
An object that orbits a planet or something else that is not a star. Besides planets, moons can circle dwarf planets, large asteroids, and other bodies.
A small rocky body orbiting the sun.
a celestial object consisting of a nucleus of ice and dust and, when near the Sun, a “tail” of gas and dust particles pointing away from the Sun
A small planetary-mass object that is in direct orbit of the Sun – something smaller than any of the eight classical planets, but still a world in its own right.
The layers of gases surrounding a planet or other celestial body.
To move in a circular or curving course or orbit. Not to be confused with rotate, when something spins on an axis.
To spin on an axis. Not to be confused with revolve, when something moves in a circular or curving course or orbit.
A bowl-shaped depression, or hollowed-out area, produced by the impact of a meteorite, volcanic activity, or an explosion.
A landform that rises above its surrounding area.
Place where lava is erupted at the surface.
A circumstellar disc in the outer Solar System, extending from the orbit of Neptune at 30 astronomical units (AU) to approximately 50 AU from the Sun.
A spherical layer of icy objects surrounding our Sun; likely occupies space at a distance between about 2,000 and 100,000 astronomical units (AU) from the Sun.
The gases that are part of the Earth, which are mainly nitrogen and oxygen.
A small body of matter from outer space that enters the Earth's atmosphere, becoming incandescent as a result of friction and appearing as a streak of light.
A stoney and/or metallic object from our solar system which was never incorporated into a planet and has fallen onto Earth. Meteorite is used for the rock on Earth, meteoroid for the object in space, and meteor as the object travels in Earth's atmosphere.
The branch of science which deals with celestial objects, space, and the physical universe as a whole.
A straight line passing from side to side through the center of a body or figure, especially a circle or sphere.
Reduction involves a half-reaction in which a chemical species decreases its oxidation number, usually by gaining electrons.
Mineral group in which the silica tetrahedra, SiO4-4, is the building block.
A solid material that is typically hard, shiny, malleable, fusible, and ductile, with good electrical and thermal conductivity.
Oxidation is the loss of electrons or an increase in the oxidation state of a chemical or atoms within it.
In planetary science, differentiation is the process of separating out the different components within a planetary body as a consequence of their physical or chemical behavior (e.g. density and chemical affinities).
An AU (or astronomical unit) is the average distance from Earth to the Sun.
The kelvin, symbol K, is the SI base unit of temperature. Absolute zero is 0 K, the equivalent of −273.15°C.
Breaking down rocks into small pieces by chemical or mechanical means.
A stable subatomic particle with a charge of negative electricity, found in all atoms and acting as the primary carrier of electricity in solids.
A penetrating form of electromagnetic radiation arising from the radioactive decay of atomic nuclei. It consists of the shortest wavelength electromagnetic waves, typically shorter than those of X-rays.
A radioactive atom that can and will decay.
The atom that is made after a radioactive decay.
Of or pertaining to an exoplanet, a planet outside the solar system.
Rotating, flattened disk of gas and dust from which the solar system originated.
Turn from liquid into vapor.
A body that could or did come together with many others under gravitation to form a planet.
The calculated amount of time that half of the mass of an original (parent) radioactive isotope breaks down into a new (daughter) isotope.
Introduction to Earth Science Copyright © 2023 by Laura Neser is licensed under a Creative Commons Attribution-NonCommercial-ShareAlike 4.0 International License , except where otherwise noted.
Share This Book
- The Universe
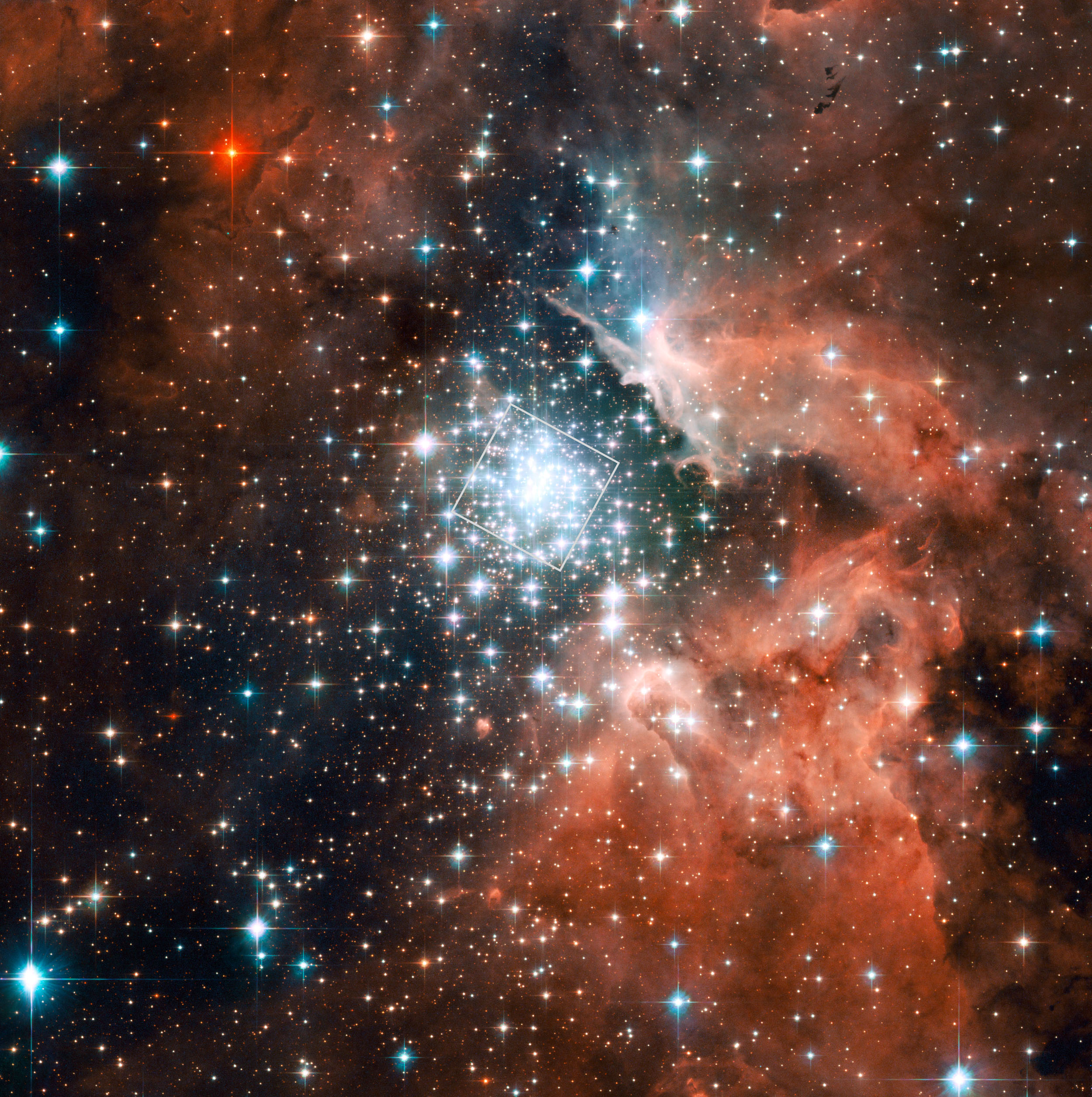
The Universe is everything we can touch, feel, sense, measure or detect. It includes living things, planets, stars, galaxies, dust clouds, light, and even time. Before the birth of the Universe, time, space and matter did not exist.
The Universe contains billions of galaxies, each containing millions or billions of stars. The space between the stars and galaxies is largely empty. However, even places far from stars and planets contain scattered particles of dust or a few hydrogen atoms per cubic centimeter. Space is also filled with radiation (e.g. light and heat), magnetic fields and high energy particles (e.g. cosmic rays).
The Universe is incredibly huge. It would take a modern jet fighter more than a million years to reach the nearest star to the Sun. Travelling at the speed of light (300,000 km per second), it would take 100,000 years to cross our Milky Way galaxy alone.
No one knows the exact size of the Universe, because we cannot see the edge – if there is one. All we do know is that the visible Universe is at least 93 billion light years across. (A light year is the distance light travels in one year – about 9 trillion km.)
The Universe has not always been the same size. Scientists believe it began in a Big Bang, which took place nearly 14 billion years ago. Since then, the Universe has been expanding outward at very high speed. So the area of space we now see is billions of times bigger than it was when the Universe was very young. The galaxies are also moving further apart as the space between them expands.
Story of the Universe
- Extreme life
- In the beginning
- The Big Bang
- The birth of galaxies
- What is space?
- Black Holes
- The mystery of the dark Universe
- Cosmic distances
Formation of Earth
Our planet began as part of a cloud of dust and gas. It has evolved into our home, which has an abundance of rocky landscapes, an atmosphere that supports life, and oceans filled with mysteries.
Chemistry, Earth Science, Astronomy, Geology
Manicouagan Crater
Asteroids were not only important in Earth's early formation, but have continued to shape our planet. A five-kilometer (three-mile) diameter asteroid is theorized to have formed the Manicouagan Crater about 215.5 million years ago.
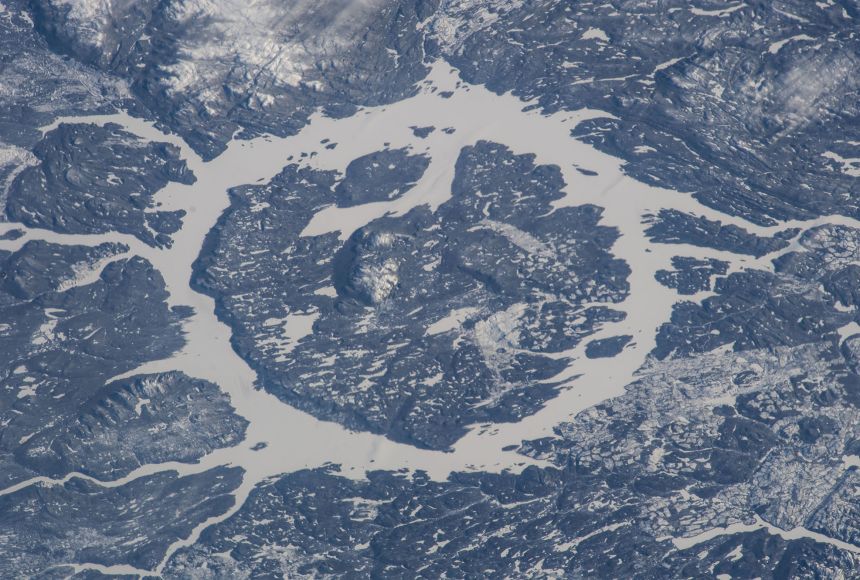
We live on Earth’s hard, rocky surface, breathe the air that surrounds the planet , drink the water that falls from the sky, and eat the food that grows in the soil. But Earth did not always exist within this expansive universe, and it was not always a hospitable haven for life. Billions of years ago, Earth, along with the rest of our solar system, was entirely unrecognizable, existing only as an enormous cloud of dust and gas. Eventually, a mysterious occurrence—one that even the world’s foremost scientists have yet been unable to determine—created a disturbance in that dust cloud, setting forth a string of events that would lead to the formation of life as we know it. One common belief among scientists is that a distant star collapsed, creating a supernova explosion, which disrupted the dust cloud and caused it to pull together. This formed a spinning disc of gas and dust, known as a solar nebula . The faster the cloud spun, the more the dust and gas became concentrated at the center, further fueling the speed of the nebula . Over time, the gravity at the center of the cloud became so intense that hydrogen atoms began to move more rapidly and violently. The hydrogen protons began fusing, forming helium and releasing massive amounts of energy. This led to the formation of the star that is the center point of our solar system—the sun—roughly 4.6 billion years ago. Planet Formation The formation of the sun consumed more than 99 percent of the matter in the nebula . The remaining material began to coalesce into various masses. The cloud was still spinning, and clumps of matter continued to collide with others. Eventually, some of those clusters of matter grew large enough to maintain their own gravitational pull, which shaped them into the planets and dwarf planets that make up our solar system today. Earth is one of the four inner, terrestrial planets in our solar system. Just like the other inner planets —Mercury, Venus, and Mars—it is relatively small and rocky. Early in the history of the solar system, rocky material was the only substance that could exist so close to the Sun and withstand its heat. In Earth's Beginning At its beginning, Earth was unrecognizable from its modern form. At first, it was extremely hot, to the point that the planet likely consisted almost entirely of molten magma . Over the course of a few hundred million years, the planet began to cool and oceans of liquid water formed. Heavy elements began sinking past the oceans and magma toward the center of the planet . As this occurred, Earth became differentiated into layers, with the outermost layer being a solid covering of relatively lighter material while the denser, molten material sunk to the center. Scientists believe that Earth, like the other inner planets , came to its current state in three different stages. The first stage, described above, is known as accretion, or the formation of a planet from the existing particles within the solar system as they collided with each other to form larger and larger bodies. Scientists believe the next stage involved the collision of a proto planet with a very young planet Earth. This is thought to have occurred more than 4.5 billion years ago and may have resulted in the formation of Earth’s moon. The final stage of development saw the bombardment of the planet with asteroids . Earth’s early atmosphere was most likely composed of hydrogen and helium . As the planet changed, and the crust began to form, volcanic eruptions occurred frequently. These volcanoes pumped water vapor, ammonia, and carbon dioxide into the atmosphere around Earth. Slowly, the oceans began to take shape, and eventually, primitive life evolved in those oceans. Contributions from Asteroids Other events were occurring on our young planet at this time as well. It is believed that during the early formation of Earth, asteroids were continuously bombarding the planet , and could have been carrying with them an important source of water. Scientists believe the asteroids that slammed into Earth, the moon, and other inner planets contained a significant amount of water in their minerals, needed for the creation of life. It seems the asteroids , when they hit the surface of Earth at a great speed, shattered, leaving behind fragments of rock. Some suggest that nearly 30 percent of the water contained initially in the asteroids would have remained in the fragmented sections of rock on Earth, even after impact. A few hundred million years after this process—around 2.2 billion to 2.7 billion years ago—photosynthesizing bacteria evolved . They released oxygen into the atmosphere via photosynthesis and, in a few hundred million years, were able to change the composition of the atmosphere into what we have today. Our modern atmosphere is comprised of 78 percent nitrogen and 21 percent oxygen, among other gases, which enables it to support the many lives residing within it.
Media Credits
The audio, illustrations, photos, and videos are credited beneath the media asset, except for promotional images, which generally link to another page that contains the media credit. The Rights Holder for media is the person or group credited.
Production Managers
Program specialists, last updated.
October 19, 2023
User Permissions
For information on user permissions, please read our Terms of Service. If you have questions about how to cite anything on our website in your project or classroom presentation, please contact your teacher. They will best know the preferred format. When you reach out to them, you will need the page title, URL, and the date you accessed the resource.
If a media asset is downloadable, a download button appears in the corner of the media viewer. If no button appears, you cannot download or save the media.
Text on this page is printable and can be used according to our Terms of Service .
Interactives
Any interactives on this page can only be played while you are visiting our website. You cannot download interactives.
Related Resources
- iai Academy
- iai Podcast
- iai Festivals
- About the iai
- Join the Team
- Debates and Talks
- Support the iai
- iai Education
- iai Speakers

Welcome to the IAI.
As a new reader of IAI News, your first article is completely free!
To discover 3000+ more articles and videos, start a free trial today.
New theory of gravity solves accelerating universe
Massive gravity and the end of dark energy.
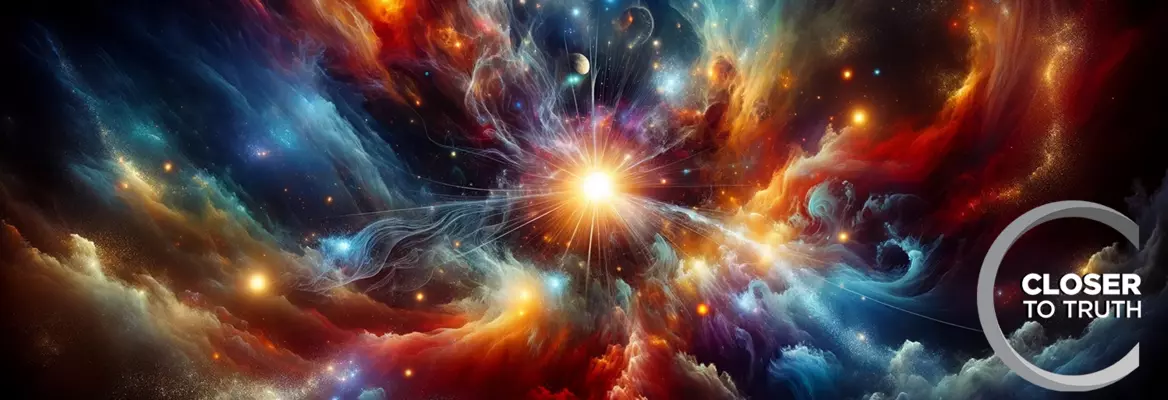
Claudia de Rham
The universe is expanding at an accelerating rate but Einstein’s theory of General Relativity and our knowledge of particle physics predict that this shouldn’t be happening. Most cosmologists pin their hopes on Dark Energy to solve the problem. But, as Claudia de Rham argues, Einstein’s theory of gravity is incorrect over cosmic scales, her new theory of Massive Gravity limits gravity’s force in this regime, explains why acceleration is happening, and eliminates the need for Dark Energy.
You can see Claudia de Rham live, debating in ‘Dark Energy and The Universe’ alongside Priya Natarajan and Chris Lintott and ‘Faster Than Light’ with Tim Maudlin and J oão Magueijo at the upcoming HowTheLightGetsIn Festival , on May 24th-27th.
This article is presented in partnership with Closer To Truth, an esteemed partner for the 2024 HowtheLightGetsIn Hay Festival. Closer To Truth explores some of humanity's deepest questions through discussions with the world's leading scientists, philosophers, and creative thinkers. Dive deeper into the profound questions of the universe with thousands of video interviews, essays, and full episodes of the long-running TV show at their website: www.closertotruth.com .
The beauty of cosmology is that it often connects the infinitely small with the infinitely big – but within this beauty lies the biggest embarrassment in the history of physics.
According to Einstein’s theory of General Relativity and our knowledge of particle physics, the accumulated effect of all infinitely small quantum fluctuations in the cosmos should be so dramatic that the Universe itself should be smaller than the distance between the Earth and the Moon. But as we all know, our Universe spans over tens of billions of light years: it clearly stretches well beyond the moon.
This is the “Cosmological Constant Problem”. Far from being only a small technical annoyance, this problem is the biggest discrepancy in the whole history of physics. The theory of Massive Gravity, developed by my colleagues and I, seeks to address this problem.
For this, one must do two things. First, we need to explain what leads to this cosmic acceleration; second, we need to explain why it leads to the observed rate of acceleration – no more no less. Nowadays it is quite popular to address the first point by postulating a new kind of Dark Energy fluid to drive the cosmic acceleration of the Universe. As for the second point, a popular explanation is the anthropic principle: if the Universe was accelerating at a different rate, we wouldn’t be here to ask ourselves the questions.
To my mind, both these solutions are unsatisfactory. In Massive Gravity, we don’t address the first point by postulating a new form of as yet undiscovered Dark Energy but rather by relying on what we do know to exist: the quantum nature of all the fundamental particles we are made out of, and the consequent vacuum energy, which eliminates the need for dark energy. This is a natural resolution for the first point and would have been adopted by scientists a long time ago if it wasn’t for the second point: how can we ensure that the immense levels of vacuum energy that fill the Universe don’t lead to too fast an acceleration? We can address this by effectively changing the laws of gravity on cosmological scales and by constraining the effect of vacuum energy.
The Higgs Boson and the Nature of Nothingness
At first sight, our Universe seems to be filled with a multitude of stars within galaxies. These galaxies are gathered in clusters surrounded by puffy “clouds” of dark matter. But is that it? Is there anything in between these clusters of galaxies plugged in filaments of dark matter? Peeking directly through our instruments, most of our Universe appears to be completely empty, with empty cosmic voids stretching between clusters of galaxies. There are no galaxies, nor gas, nor dark matter nor anything else really tangible we can detect within these cosmic voids. But are they completely empty and denuded of energy? To get a better picture of what makes up “empty space,” it is useful to connect with the fundamental particles that we are made of.
The discovery of the Higgs boson and its mechanism reveals fundamental insights into our understanding of nothingness
I still vividly remember watching the announcement of the discovery of the Higgs boson in 2012. By now most people have heard of this renowned particle and how it plays an important role in our knowledge of particle physics, as well as how it is responsible for giving other particles mass. However, what’s even more remarkable is that the discovery of the Higgs boson and its mechanism reveals fundamental insights into our understanding of nothingness .
To put it another way, consider “empty space" as an area of space where everything has been wiped away down to the last particle. The discovery of the Higgs boson indicates that even such an ideal vacuum is never entirely empty: it is constantly bursting with quantum fluctuations of all known particles, notably that of the Higgs.
This collection of quantum fluctuations I'll refer to as the “Higgs bath.” The Higgs bath works as a medium, influencing other particles swimming in it. Light or massless particles, such as photons, don’t care very much about the bath and remain unaffected. Other particles, such as the W and Z bosons that mediate the Weak Force , interact intensely with the Higgs bath and inherit a significant mass. As a result of their mass the Weak Force they mediate is fittingly weakened.
Accelerating Expansion
When zooming out to the limits of our observable Universe we have evidence that the Universe is expanding at an accelerating speed, a discovery that led to the 2011 Nobel Prize in Physics . This is contrary to what we would have expected if most of the energy in the Universe was localized around the habitable regions of the Universe we are used to, like clusters of galaxies within the filaments of Dark Matter. In this scenario, we would expect the gravitational attraction pulling between these masses to lead to a decelerating expansion.
So what might explain our observations of acceleration? We seem to need something which fights back against gravity but isn’t strong enough to tear galaxies apart, which exists everywhere evenly and isn't diluted by the expansion of the cosmos. This “something” has been called Dark Energy.
A different option is vacuum energy . We’ve long known that the sea of quantum fluctuations has dramatic effects on other particles, as with the Higgs Bath, so it’s natural to ask about its effect on our Universe. In fact, scientists have been examining the effect of this vacuum energy for more than a century, and long ago realized that its effects on cosmological scales should lead to an accelerated expansion of the Universe, even before we had observations that indicated that acceleration was actually happening. Now that we know the Universe is in fact accelerating, it is natural to go back to this vacuum energy and estimate the expected rate of cosmic acceleration it leads to.
The bad news is that the rate of this acceleration would be way too fast. The estimated acceleration rate would be wrong by at least twenty-eight orders of magnitude! This is the “Cosmological Constant Problem” and is also referred to as the “Vacuum Catastrophe.”
Is our understanding of the fundamental particles incorrect? Or are we using Einstein’s theory of General Relativity in a situation where it does not apply?
General Relativity may not be the correct description of gravity at large cosmological scales where gravity remains untested
The Theory of Massive Gravity
Very few possibilities have been suggested. The one I would like to consider is that General Relativity may not be the correct description of gravity at large cosmological scales where gravity remains untested.
In Einstein’s theory of gravity, the graviton like the photon is massless and gravity has an infinite reach. This means objects separated by cosmological scales, all the way up to the size of the Universe, are still under the gravitational influence of each other and of the vacuum energy that fills the cosmos between them. Even though locally the effect of this vacuum energy is small, when you consider its effect accumulated over the whole history and volume of the Universe, its impact is gargantuan, bigger than everything else we can imagine, so that the cosmos would be dominated by its overall effect. Since there is a lot of vacuum energy to take into account, this leads to a very large acceleration, much larger than what we see, which again is the Cosmological Constant Problem. The solution my colleagues and I have suggested is that perhaps we don’t need to account for all this vacuum energy. If we only account for a small fraction of it, then it would still lead to a cosmic acceleration but with a much smaller rate, compatible with the Universe in which we live. Could it be that the gravitational connection that we share with the Earth, with the rest of the Galaxy and our local cluster only occurs because we are sufficiently close to one another? Could it be that we do not share that same gravitational connection with very distant objects, for instance with distant stars located on the other side of the Universe some 10 thousand million trillion km away? If that were the case, there would be far less vacuum energy to consider and this would lead to a smaller cosmic acceleration, resolving the problem.
Just as Einstein himself tried to include a Cosmological Constant in his equations, what we need to do is add another term which acts as the mass of the graviton
In practice, what we need to do is understand how to weaken the range of gravity. But that’s easy: nature has already showed us how to do that. We know that the Weak Force is weak and has a finite range distance because the W and Z bosons that carry it are massive particles. So, in principle, all we have to do is simply to give a mass to the graviton. Just as Einstein himself tried to include a Cosmological Constant in his equations, what we need to do is add another term which acts as the mass of the graviton, dampening the dynamics of gravitational waves and limiting the range of gravity. By making the graviton massive we now have ourselves a theory of “massive gravity.” Easy! The possibility that gravity could have a finite range is not a question for science-fiction. In fact Newton, Laplace and many other incredible scientists after them contemplated the possibility. Even following our development of quantum mechanics and the Standard Model, many including Pauli and Salam considered the possibility of gravitons with mass. But that possibility was always entirely refuted! Not because it potentially contradicts observations – quite the opposite, it could solve the vacuum catastrophe and explain why our Universe’s expansion is accelerating – but rather because models of massive gravity appeared to be haunted by “ ghosts .” Ghosts are particles with negative energies that would cause everything we know, including you, me, the whole Universe, and possibly the structure of space and time to decay instantaneously. So if you want a theory of massive gravity you need to either find a way to get rid of these ghosts or to “ trap ” them.
For decades, preventing these supernatural occurrences seemed inconceivable. That’s until Gregory Gabadadze, Andrew Tolley and I found a way to engineer a special kind of “ghost trap” that allowed us to trick the ghost to live in a constrained space and do no harm. One can think of this like an infinite loop-Escherian impossible staircase in which the Ghosts may exist and move but ultimately end up nowhere.
Even in science, there are many cultures and mathematical languages or scientific arguments that individuals prefer.
Coming up with a new trick was one thing, but convincing the scientific community required even more ingenuity and mental flexibility. Even in science, there are many cultures and mathematical languages or scientific arguments that individuals prefer. So, throughout the years, whenever a new colleague had a different point of view, we were bound to learn their language, translate, and adjust our reasoning to their way of thinking. We had to repeat the process for years until no stone remained unturned. Overcoming that process was never the goal, though: it was only the start of the journey that would allow us to test our new theory of gravity.
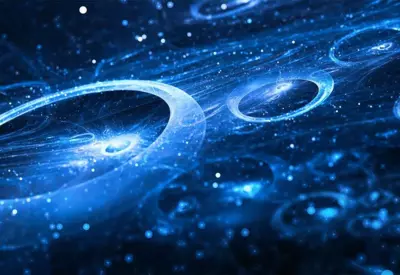
If gravitons have a mass, this mass should be tiny, smaller than the mass of all the other massive particles, even lighter than the neutrino. Consequently, detecting it may not be straightforward. Nevertheless, different features will appear which may make it possible to measure it.
The most promising way to test for massive gravity involves observations of gravitational waves . If gravitons are massive, then we’d expect that low-frequency gravitational waves will travel ever so slightly slower than high-frequency ones. Unfortunately, this difference would be too slight to measure with current ground-based observatories. However, we should have better luck with future observatories. Missions like the Pulsar Timing Array , LISA and the Simons Observatory will detect gravitational waves with smaller and smaller frequencies, making possible the observations we need. Whether the massive gravity theory developed by my collaborators and I will survive future tests is of course presently unknown, but the possibility is now open. After all, even if the outcome isn’t certain, when it comes to challenging the biggest discrepancy of the whole history of science, addressing the Cosmological Constant Problem, eliminating the need for dark energy, and reconciling the effect of vacuum energy with the evolution of the Universe, some risks may be worth taking.
Claudia de Rham has recently published her first book The Beauty of Falling: A Life in Pursuit of Gravity with Princeton University Press.
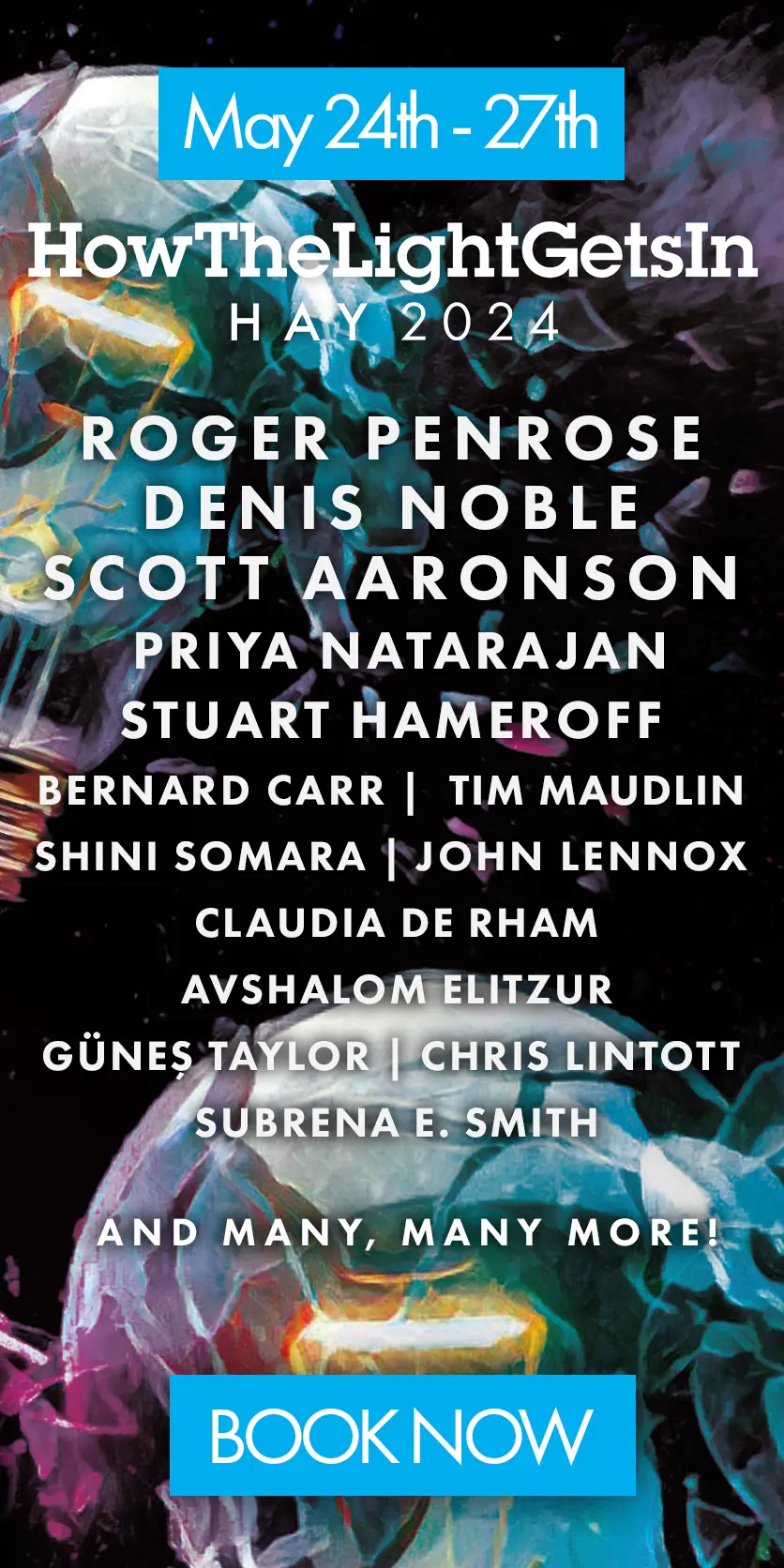
Love the iai?
Sign up to get exclusive access.
Related Posts:
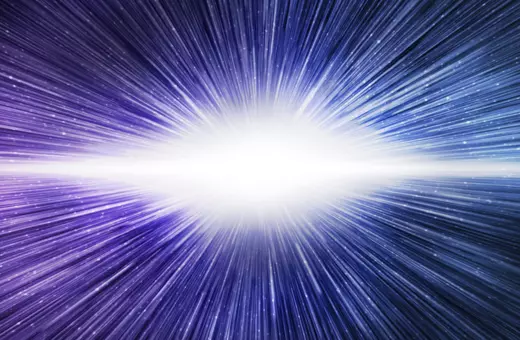
Was the Big Bang a white hole?
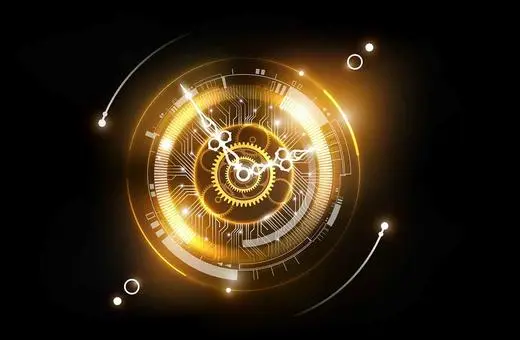
Ethics, death and the block universe
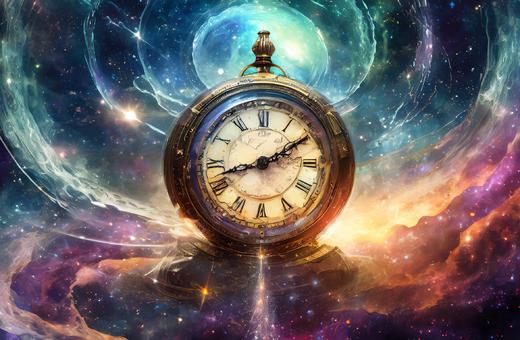
The mistake at the heart of the physics of time
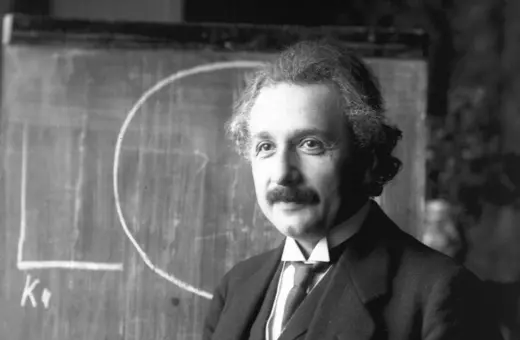
Einstein’s failed magic trick
Related videos:.
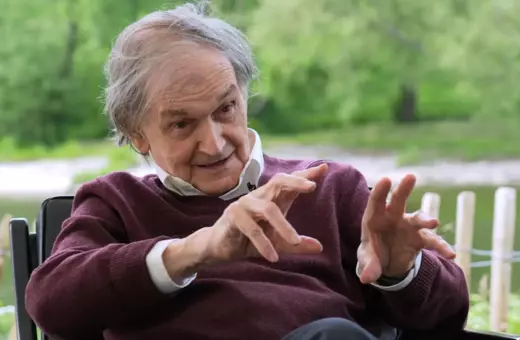
Roger Penrose | Interview
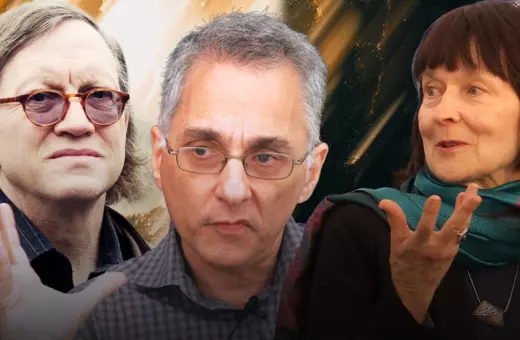
Fragments and reality
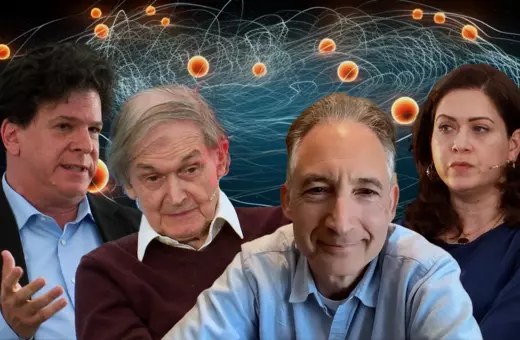
The trouble with string theory
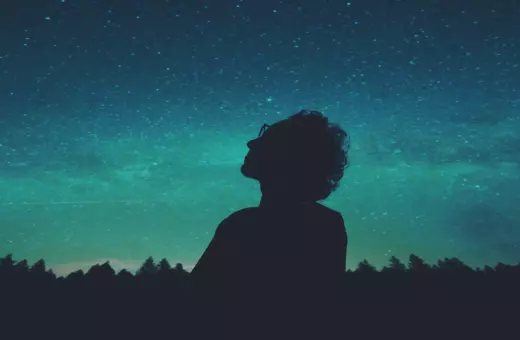
Consciousness and material reality
Continue reading.
Enjoy unlimited access to the world's leading thinkers.
Start by exploring our subscription options or joining our mailing list today.
Start Free Trial
Already a subscriber? Log in
Related Videos
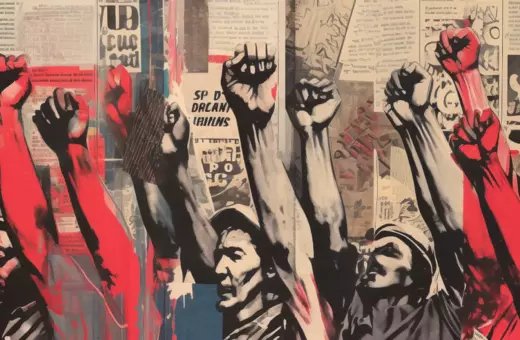
Inside anarchy
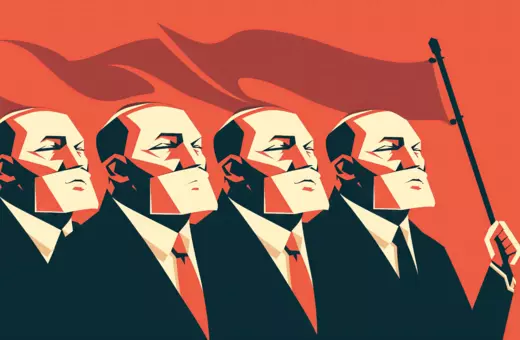
The challenge to optimism
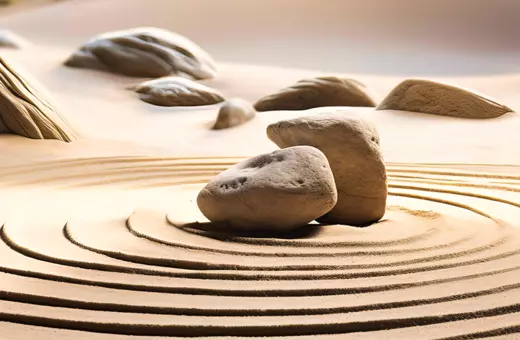
Consciousness came before life
Join the conversation.
Sign in to post comments or join now (only takes a moment) . Don't have an account? Sign in with Facebook, Twitter or Google to get started: -->
Advertisement
Supported by
Critic’s Pick
‘Kingdom of the Planet of the Apes’ Review: Hail, Caesar
The latest installment in an excellent series finds mythology turning into power.
- Share full article

By Alissa Wilkinson
For a series with a goofy premise — what if talking apes overthrew humanity — the “Planet of the Apes” universe is uncommonly thoughtful, even insightful. If science fiction situates us in a universe that’s just different enough to slip daring questions past our mental barriers, then the “Apes” movies are among the best examples. That very premise, launched with talking actors in ape costumes in the 1968 film, has given storytellers a lot to chew on, contemplating racism, authoritarianism, police brutality and, in later installments, the upending of human society by a brutal, fast-moving virus. (Oops.)
Those later virus-ridden installments, a trilogy released between 2011 and 2017, are among the series’ best, and well worth revisiting. The newest film, “Kingdom of the Planet of the Apes,” picks up exactly where that trilogy left off: with the death of Caesar, the ultrasmart chimpanzee who has led the apes away from what’s left of humanity and into a paradise. (The scene was a direct quotation of the story of Moses leading the Israelites to the Promised Land, but dying before he could set foot there.) The apes honor his memory and vow to keep his teachings, especially the first dictum — “ape not kill ape.” Caesar preached a gospel of peacefulness, loyalty, generosity, nonaggression and care for the earth; unlike the humans, they intend to live in harmony.
The teachings of peaceful prophets, however, tend to be twisted by power-seekers, and apparently this isn’t just a human problem. “Kingdom of the Planet of the Apes,” directed by Wes Ball from a screenplay by Josh Friedman, leaps forward almost immediately by “many generations” (years matter less in this post-human world), and the inevitable has happened. The apes have fractured into tribes, while Caesar has passed from historical figure to mythic one, a figure venerated by some and forgotten by most.
That there even was a Caesar is unknown to Noa (Owen Teague), a young chimpanzee whose father, Koro (Neil Sandilands) is leader of his clan and an avid breeder of birds. That clan has its own laws, mostly having to do with how to treat birds’ nests, and that’s all that Noa and his friends Anaya (Travis Jeffery) and Soona (Lydia Peckham) have known.
But then one day tragedy strikes, in the form of an attack on the clan by the soldiers of Proximus Caesar (Kevin Durand), the leader of a clan of coastal apes. Noa finds himself alone, searching for his clan, who have been carted away. On his journey Noa meets a human (Freya Allen) who, like the other humans, doesn’t speak.
At this point in the evolution of the virus, mutations have rendered any surviving humanity speechless and dull-witted, living in roving bands and running from predators; to the apes it’s as preposterous to imagine a talking human as a talking ape is to us. But he also meets Raka (Peter Macon), who believes himself to be the last of the faithful followers of Caesar’s peaceful teachings, even wearing Caesar’s diamond-shaped symbol around his neck. (Eagle-eyed viewers will recall that the symbol echoes the shape of the window in the room in which Caesar was raised as a baby.) Noa learns from Raka. And when he finds what he’s looking for, he realizes he has an important job to do.
“Kingdom of the Planet of the Apes” is not quite as transporting as the previous trilogy, perhaps because the apes now act so much like humans that the fruitful dissonance in our minds has mostly been mitigated. It’s simpler to imagine the apes as just stand-in humans when they’re all talking, and thus easier to just imagine you’re watching, say, “The Lion King” or something.
But there’s still a tremendous amount to mull over here, like Proximus Caesar, who borrows the idea of Caesar to prop up his own version of leadership. The real Caesar was undoubtedly strong and brave, but Proximus Caesar has mutated this into swagger and shows of force, an aggression designed to keep his apes in line. He is not brutal, exactly; He is simply insistently powerful and more than a bit of a fascist. Every morning, he greets his subjects by proclaiming that it is a “wonderful day,” and that he is Caesar’s rightful heir, and that they must all work together as one to build their civilization ever stronger.
Visual cues indicate that Proximus Caesar’s kingdom is modeled partly on the Roman Empire, with its colonizing influence and its intention to sweep the riches of the ancient human world — its history, its labor, its technology — into its own coffers. By telling his version of Caesar’s legacy, Proximus Caesar makes the apes believe they are part of some mighty, unstoppable force of history.
But of course, history has a habit of repeating itself, whether it’s ancient Rome or Egypt, and in Proximus Caesar’s proclamations one detects a bit of Ozymandias : Look on his works, ye mighty, and despair! “Kingdom of the Planet of the Apes” is set in the future, but like a lot of science fiction — “Dune,” for instance, or “Battlestar Galactica,” or Walter Miller’s “A Canticle for Leibowitz” — there’s a knowing sense that all this has happened before, and all this will happen again.
That’s what makes “Kingdom of the Planet of the Apes” powerful, in the end. It probes how the act of co-opting idealisms and converting them to dogmas has occurred many times over. What’s more, it points directly at the immense danger of romanticizing the past, imagining that if we could only reclaim and reframe and resurrect history, our present problems would be solved. Golden ages were rarely actually golden, but history is littered with leaders who tried to make people believe they were anyhow. It’s a great way to make people do their bidding.
There are some hints near the end of “Kingdom of the Planet of the Apes” of what might be next for the franchise, should it be fated to continue. But the uneasy fun of the series is we already know what happens, eventually; it was right there in the first movie, and the warning it poses remains bleak.
At the start of the 1968 film, the star Charlton Heston explains, “I can’t help thinking somewhere in the universe there has to be something better than man.” You might have expected, from a movie like this, that “better” species would be these apes. But it turns out we might have to keep looking.
Kingdom of the Planet of the Apes Rated PG-13, for scenes of peril and woe and a couple of funny, mild swear words. Running time: 2 hours 25 minutes. In theaters.
Alissa Wilkinson is a Times movie critic. She’s been writing about movies since 2005. More about Alissa Wilkinson
Explore More in TV and Movies
Not sure what to watch next we can help..
The Netflix stalker series “ Baby Reindeer ” combines the appeal of a twisty thriller with a deep sense of empathy. The ending illustrates why it’s become such a hit .
We have entered the golden age of Mid TV, where we have a profusion of well-cast, sleekly produced competence, our critic writes .
The writer-director Alex Garland has made it clear that “Civil War” should be a warning. Instead, the ugliness of war comes across as comforting thrills .
Studios obsessively focused on PG-13 franchises and animation in recent years, but movies like “Challengers” and “Saltburn” show that Hollywood is embracing sex again .
If you are overwhelmed by the endless options, don’t despair — we put together the best offerings on Netflix , Max , Disney+ , Amazon Prime and Hulu to make choosing your next binge a little easier.
Sign up for our Watching newsletter to get recommendations on the best films and TV shows to stream and watch, delivered to your inbox.
I was laid off from my job at a fancy convenience store chain when it suddenly shuttered. My final shift felt like 'The Purge.'
- Foxtrot, an upscale convenience-store chain, shuttered its 33 locations on April 23.
- Declan Rhodes, 25, was one of hundreds of employees laid off while on shift.
- He said the last day was like "The Purge," as workers kicked out customers and locked up for good.

This as-told-to essay is based on a conversation with Declan Rhodes, a 25-year-old former employee of Foxtrot , an upscale convenience store chain that was based in Chicago. On April 23, the company announced it had laid off its entire staff and shuttered operations across its 30-plus locations in Chicago, Austin, Dallas, and Washington DC. Business Insider verified Rhodes' employment history. The following has been edited for length and clarity.
In August 2022, I moved from Springfield, Missouri, where I got a degree in musical theater, to pursue artistic endeavors in Chicago .
I've worked all the "survival jobs" in the customer service and food industries. Little Caesars was my first job. But I landed a job at Foxtrot in January 2023. I was like, "OK, I'll do this. This will be a nice little survival job while I'm auditioning."
Foxtrot was a convenience store and coffee shop, similar to an upscale 7-Eleven. We sold and made different drinks as well as grab-and-go food, a lot of healthy alternatives, and gluten-free snacks.
I worked at the biggest location in Chicago. We had a wide range of clients — from older clientele, who I could smell old money on, to younger clientele, who either would come and study in the morning or work remotely.
I was brought in as a shift lead, a step below the assistant manager. It was not a salaried position, but I was doing full-time hours.
The silver lining was the queer-friendly environment and accepting atmosphere that I had with my coworkers.
But there was a disconnect from the corporate side.
A great example of this: We had a wonderful coworker who was in his 60s, maybe early 70s, one of the sweetest humans I've ever met. He was our main receiver. He would receive all of the products we would get. We used to have a working elevator that made it easier to load stuff onto a cart. Eventually, that elevator ended up breaking down. It was never fixed, never made a priority. We had several people young strapping people who would help him out. But eventually, this wonderful human ended up leaving.
The next day, one of the corporate people comes in and says, "Oh, I guess we've really got to get that elevator fixed now."
They had a facade of caring for their workers, but it felt like a lot of us were expendable.
There were red flags before the company went bust
Looking back, the biggest red flag was an eviction notice we'd gotten a week before the company closed.
It was explained by my assistant manager, who was told by his district manager, that there was some turnover at the corporate level, and it would be taken care of.
Related stories
We had also gotten a message in our work group chat from my general manager the weekend before saying that we were having supply-chain issues.
On April 23, I got up at my usual time, 4:45 a.m., to get there at 5:30 a.m. We opened the store as normal and started making lattes. At 8 a.m., my manager instructed us to stop selling gift cards. At 9 a.m., my manager attended an emergency company meeting. Every manager across every Foxtrot was on this Zoom call, I believe. They didn't know what it was about.
After the fact, I was informed by my manager that the phone call quite literally lasted 10 minutes. It was just: "Kick everyone out of the store, take out the trash, lock doors, and close the store officially." There was no "good luck" or "sorry" or well wishes in the next chapter. They were just as blindsided as we were.
At about 10:10 a.m., on a Google Hangout organized by my manager, we were instructed to close the store and leave the premises with all our personal belongings by 12 p.m. Not everyone who worked at my store was on that call. It's possible people did not realize they were losing their jobs until the companywide email was officially sent out at 11:38 a.m.
We were not given any clear direction. I'd probably say we had 20, 30 people in the store. It was pretty busy. Two guys looked like they were having a business meeting. My manager said, "Sorry, guys, we are closing forever."
It felt like "The Purge." My manager ended up taping two notices to our glass doors that said something along the lines of, "Thank you for letting us serve you. We are closing our doors for the final time."
A mob of people started collecting outside. I could see them taking videos and pictures of the sign.
Foxtrot employees are trying to hold the company to account
If I could talk directly to the CEO, I would say, "First and foremost, thank you for instilling an environment that felt accepting and tended to be warm and welcoming to new workers. On the flip side, a caveat: Shame on you for the way that you handled this, and absolutely shame on every single person who had an inkling that this was coming and did nothing."
The next day, my former coworkers and I went to my manager's boyfriend's restaurant. One of my other former coworkers is a teacher, but works on the weekends as a barista. He put his teacher cap on and printed out full documents with the WARN Act .
It is Illinois state law that for mass layoffs, you are to provide 30 to 60 days' notice of termination. Clearly, they didn't do that. We are full force taking part in a class-action lawsuit . It's surreal.
My next move after Foxtrot
It's back to square one in terms of finding a survival job.
I was trying to manifest — not the closure of the entire company, but a career shift in that I could be making an income doing fully creative projects. But the universe works in mysterious ways.
I have comfortable-enough savings. I should be OK for at least a few months. I might pick up some side hustles or gigs.
I'll donate plasma twice a week, and that's a nice little check there.
I tend to be a pretty optimistic person. I feel I will find another job. I'm just really trying to adapt and shift direction.
Watch: Nearly 50,000 tech workers have been laid off — but there's a hack to avoid layoffs
- Main content
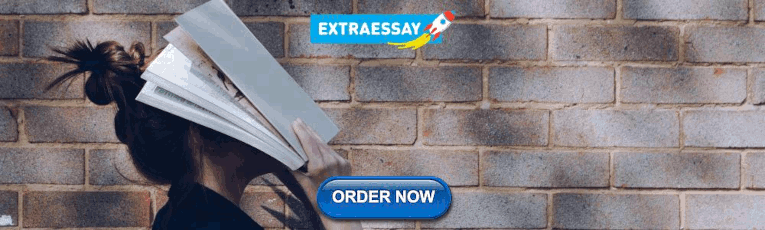
IMAGES
VIDEO
COMMENTS
A Belgian priest named Georges Lemaître first suggested the big bang theory in the 1920s, when he theorized that the universe began from a single primordial atom. The idea received major boosts ...
According to the Big Bang theory, the universe began about 13.7 billion years ago. Everything that is now in the universe was squeezed into a very small volume. Imagine all of the known universe in a single, hot, chaotic mass. An enormous explosion—a big bang—caused the universe to start expanding rapidly.
The Universe's History The origin, evolution, and nature of the universe have fascinated and confounded humankind for centuries. New ideas and major discoveries made during the 20th century transformed cosmology - the term for the way we conceptualize and study the universe - although much remains unknown. Here is the history of the universe according […]
Origin of the Universe. Cosmologists are closing in on the ultimate processes that created and shaped the universe. The universe is big in both space and time and, for much of humankind's history ...
Some 15 billion years ago the universe emerged from a hot, dense sea of matter and energy. As the cosmos expanded and cooled, it spawned galaxies, stars, planets and life. By P. James E. Peebles ...
Inflation projected infinitesimal quantum fluctuations in the young Universe into cosmic scales, leaving some patches with a little more or a little less matter. These variations became the scaffolding for the structure of the Universe. As the Universe expanded, the seething plasma of subatomic particles cooled to form hydrogen, the first atoms.
A computer simulation of the formation of large-scale structures in the Universe, showing a patch of 100 million light-years and the resulting coherent motions of galaxies flowing towards the ...
An even more mysterious form of energy called "dark energy" accounts for about 70% of the mass-energy content of the universe. Even less is known about it than dark matter. This idea stems from the observation that all galaxies seems to be receding from each other at an accelerating pace, implying that some invisible extra energy is at work.
The Universe: Big Bang to Now in 10 Easy Steps The widely accepted theory for the origin and evolution of the universe is the Big Bang model, which states that the universe began as an incredibly ...
Get custom essay. According to the standard theory, our universe sprang into existence as "singularity" around 13.7 billion years ago. "Singularity"a point or region of infinite mass density at which space and time are infinitely distorted by gravitational forces and which is held to be the final state of matter falling into a black hole.
The formation of atoms in the early universe makes these atoms less likely to interact with light. Therefore, photons that belong to the cosmic background radiation must have separated from matter at a temperature T associated with 1 eV (the approximate ionization energy of an atom) . The temperature of the universe at this point was
Step 2: The universe's first growth spurt. Step 3: Too hot to shine. Step 4: Let there be light. Step 5: Emerging from the cosmic dark ages. Step 6: More stars and more galaxies. Step 7: Birth of ...
By Charlie Wood. published 12 June 2019. The universe began with a bang. Cosmologists have predicted that stars didn't form for another 180 million years.(Image credit: Shutterstock) The Big Bang ...
The Introduction: Some Basic Assumptions Of The Universe Development. Generally, the Big Bang theory is recognized to be one of the ways to explain the appearance of the Universe. They say that our Universe expanded from hot structure. The structure existed more than thirteen billion years ago; so, they say that the Big Bang took place exactly ...
The Solar System that we live in consists of a medium-size star (the Sun) with eight planets orbiting it. The planets are of two different types. The four inner planets, those closest to the Sun, are Mercury, Venus, Earth, and Mars. They are smaller and composed mainly of metals and rocks. The four outer planets — Jupiter, Saturn, Uranus, and ...
The universe is all of space and time and their contents. It comprises all of existence, any fundamental interaction, physical process and physical constant, and therefore all forms of energy and matter, and the structures they form, from sub-atomic particles to entire galaxies.Space and time, according to the prevailing cosmological theory of the Big Bang, emerged together 13.787 ± 0.020 ...
Big Questions >. What is the universe made of? Matter and energy are the two basic components of the entire Universe. An enormous challenge for scientists is that most of the matter in the Universe is invisible and the source of most of the energy is not understood. How can we study the Universe if we can't see most of it?
The universe began 13.77 billion years ago when energy, matter, and space expanded from a single point. Evidence for the big bang is the cosmic "afterglow" from when the universe was still very dense, and red-shifted light from distant galaxies, which tell us the universe is still expanding.. The big bang produced hydrogen, helium, and lithium, but heavier elements come from nuclear fusion ...
2. The key process that allows astronomers to build large 3-dimensional galaxy surveys of the local universe is the cosmic expansion that was discovered about 100 years ago. Between 1912 and 1923, Slipher showed that the light from galaxies is redshifted due to the Doppler effect.
The Universe. The Universe is everything we can touch, feel, sense, measure or detect. It includes living things, planets, stars, galaxies, dust clouds, light, and even time. Before the birth of the Universe, time, space and matter did not exist. The Universe contains billions of galaxies, each containing millions or billions of stars.
Universe consists, constellation, in which Sun exists, is so big that from the core of constellation, light takes around 27 thousand years to reach up to sun. The solar system which is part of Milky Way galaxy is in disc-shaped spiral form. Essay # 1. Sun: Sun rotates round its axis from West to East.
noun. an opening in the Earth's crust, through which lava, ash, and gases erupt, and also the cone built by eruptions. Our planet began as part of a cloud of dust and gas. It has evolved into our home, which has an abundance of rocky landscapes, an atmosphere that supports life, and oceans filled with mysteries.
The universe is expanding at an accelerating rate but Einstein's theory of General Relativity and our knowledge of particle physics predict that this shouldn't be happening. ... essays, and full episodes of the long-running TV show at their website: www.closertotruth.com . The beauty of cosmology is that it often connects the infinitely ...
For a series with a goofy premise — what if talking apes overthrew humanity — the "Planet of the Apes" universe is uncommonly thoughtful, even insightful.
This as-told-to essay is based on a conversation with Declan Rhodes, a 25-year-old former employee of Foxtrot, an upscale convenience store chain that was based in Chicago.On April 23, the company ...