- Skip To Main Content
- Skip To Navigation
- Screen Reader Access

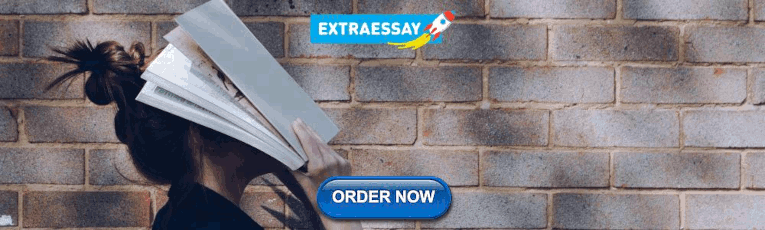
Search form
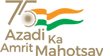
- Agricultural Sciences
- Astronomy & Space Sciences
- Chemical Sciences
- Cognitive Sciences and Psychology
- Computer Sciences and IT
- COVID-19 Research
- Earth, Atmosphere & Environment Sciences
- Energy Sciences
- Engineering Sciences
- Life Sciences & Biotechnology
- Mathematical Sciences
- Material Sciences
- Medical Sciences
- Pharmaceutical Sciences
- Physical Sciences
- Traditional Knowledge
- Other Areas
- Institutional
- International
- Grants for Seminar and Conferences
- Startup Grants
Ministries & Departments
- Centres of Excellence
- Thematic Centres
- Centres of Higher Learning
- National Academies
- Statewise S&T Organisations
- Industry Related Associations
- Laboratories
- International Organisations
- Civil Societies
- Science Centres & Planetaria
- All Programmes & Schemes
- Research and Development
- Human Resource and Development
- Women Schemes
- International Programmes
- Societal Development
- Academia Industry Partnerships
- School Students
- Graduate Students
- Post Graduate Students
- PhD Scholars
- Post Doctoral Fellowships
- Scholarships for Women
- Faculty and Scientists
- National Fellows
- Grassroot Innovations
- Industrial Innovations
- COVID-19 Technology
- Earth, Atmosphere & Env. Sciences
- Rural Technologies
Organisations sidebar menu
- Office of the PSA
- Ministries & Departments
- Geospatial Institutions
- Environmental Institutions
- Central Universities
- State Universities
- Indian Institute of Science (IISc)
- Indian Institute of Technology (IITs)
- Indian Institute of Science Education & Research (IISERs)
- All India Institute of Medical Sciences (AIIMS)
- National Institute of Pharmaceutical Education and Research (NIPERs)
- National Institutes of Technology (NITs)
- Indian Institutes of Information Technology (IIITs)
- List of S&T Organisations
- Statewise S&T Organisations
- State S&T Councils
- All Science Centres & Planetaria
- Regional Science Centres - Assam
- Regional Science Centres - Gujarat
ICAR-National Research Centre on Plant Biotechnology
National Institute For Plant Biotechnology (NIPB) is a premier research institution of the Indian Council of Agricultural Research (ICAR). The institute was founded in 1985 as the ‘Biotechnology Centre’ of Indian Agricultural Research Institute (IARI) for molecular biology and biotechnology research in crop plants. National Institute For Plant Biotechnology has been entrusted with the responsibility of developing new tools and techniques and delivering a breakthrough in biotechnology for crop improvement.
Research Areas: Agricultural Sciences, Life Sciences & Biotechnology
Contact name: dr ajit kumar shasany, director, contact address: pusa campus, new delhi, contact phone: 011-25848783, 011-25841787/25842789, email: [email protected], [email protected], fax: 91 +11 25843984, state: delhi, related links.

- Login as Faculty
- Login as Nodal Officer
- Registration
Faculty / Scientist
- Professor 1
- Principal Scientist 24
- Scientist 10
Scholarly Resources
- Journal Articles 665
- Conference / In Proceedings 8
- Books / Chapters 54
Resources Impact

Departments
- (9) Biochemistry
- (1) Bioinformatics
- (2) Biotechnology
- (1) Computer Application
- (11) Genetics and Plant Breeding
- (2) Plant Biotechnology
- (10) Plant Molecular Biology
Top ten Profiles
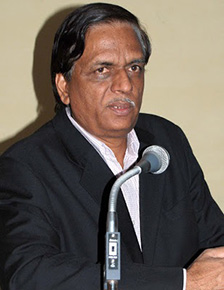
- 186 Publications

- 98 Publications
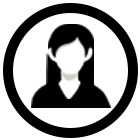
- 38 Publications
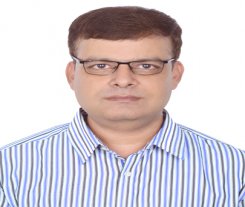
- 80 Publications
- 58 Publications
- 33 Publications
- 69 Publications
- 102 Publications
- 16 Publications
- 31 Publications
- 26 Publications
Publications
Author: Matsumoto T.;Wu J.;Kanamori H.;Katayose Y.;Fujisawa M.;Namiki N.;Mizuno H.;Yamamoto K.;Antonio B.A.;Baba T.;Sakata K.;Nagamura Y.;Aoki H.;Arikawa K.;Arita K.;Bito T.;Chiden Y.;Fujitsuka N.;Fukunaka R.;Hamada M.;Harada C.;Hayashi A.;Hijishita S.;Honda M.;Hosokawa S.;Ichikawa Y.;Idonuma A.;Iijima M.;Ikeda M.;Ikeno M.;Ito K.;Ito S.;Ito T.;Ito Y.;Ito Y.;Iwabuchi A.;Kamiya K.;Karasawa W.;Kurita K.;Katagiri S.;Kikuta A.;Kobayashi H.;Kobayashi N.;MacHita K.;Maehara T.;Masukawa M.;Mizubayashi T.;Mukai Y.;Nagasaki H.;Nagata Y.;Naito S.;Nakashima M.;Nakama Y.;Nakamichi Y.;Nakamura M.;Meguro A.;Negishi M.;Ohta I.;Ohta T.;Okamoto M.;Ono N.;Saji S.;Sakaguchi M.;Sakai K.;Shibata M.;Shimokawa T.;Song J.;Takazaki Y.;Terasawa K.;Tsugane M.;Tsuji K.;Ueda S.;Waki K.;Yamagata H.;Yamamoto M.;Yamamoto S.;Yamane H.;Yoshiki S.;Yoshihara R.;Yukawa K.;Zhong H.;Yano M.;Sasaki T.;Yuan Q.;Ouyang S.;Liu J.;Jones K.M.;Gansberger K.;Moffat K.;Hill J.;Bera J.;Fadrosh D.;Jin S.;Johri S.;Kim M.;Overton L.;Reardon M.;Tsitrin T.;Vuong H.;Weaver B.
- https://www.nature.com/articles/nature03895.pdf -->
Author: Sato S.;Tabata S.;Hirakawa H.;Asamizu E.;Shirasawa K.;Isobe S.;Kaneko T.;Nakamura Y.;Shibata D.;Aoki K.;Egholm M.;Knight J.;Bogden R.;Li C.;Shuang Y.;Xu X.;Pan S.;Cheng S.;Liu X.;Ren Y.;Wang J.;Albiero A.;Dal Pero F.;Todesco S.;Van Eck J.;Buels R.M.;Bombarely A.;Gosselin J.R.;Huang M.;Leto J.A.;Menda N.;Strickler S.;Mao L.;Gao S.;Tecle I.Y.;York T.;Zheng Y.;Vrebalov J.T.;Lee J.;Zhong S.;Mueller L.A.;Stiekema W.J.;Ribeca P.;Alioto T.;Yang W.;Huang S.;Du Y.;Zhang Z.;Gao J.;Guo Y.;Wang X.;Li Y.;He J.;Li C.;Cheng Z.;Zuo J.;Ren J.;Zhao J.;Yan L.;Jiang H.;Wang B.;Li H.;Li Z.;Fu F.;Chen B.;Han B.;Feng Q.;Fan D.;Wang Y.;Ling H.;Xue Y.;Ware D.;Richard McCombie W.;Lippman Z.B.;Chia J.M.;Jiang K.;Pasternak S.;Gelley L.;Kramer M.;Anderson L.K.;Chang S.B.;Royer S.M.;Shearer L.A.;Stack S.M.;Rose J.K.C.;Xu Y.;Eannetta N.;Matas A.J.;McQuinn R.;Tanksley S.D.;Camara F.;Guigó R.;Rombauts S.;Fawcett J.;Van De Peer Y.;Zamir D.;Liang C.;Spannagl M.;Gundlach H.;Bruggmann R.
- https://www.nature.com/articles/nature11119.pdf -->
Author: Appels R.;Eversole K.;Feuillet C.;Keller B.;Rogers J.;Stein N.;Pozniak C.J.;Choulet F.;Distelfeld A.;Poland J.;Ronen G.;Barad O.;Baruch K.;Keeble-Gagnère G.;Mascher M.;Ben-Zvi G.;Josselin A.A.;Himmelbach A.;Balfourier F.;Gutierrez-Gonzalez J.;Hayden M.;Koh C.S.;Muehlbauer G.;Pasam R.K.;Paux E.;Rigault P.;Tibbits J.;Tiwari V.;Spannagl M.;Lang D.;Gundlach H.;Haberer G.;Mayer K.F.X.;Ormanbekova D.;Prade V.;Wicker T.;Swarbreck D.;Rimbert H.;Felder M.;Guilhot N.;Kaithakottil G.;Keilwagen J.;Leroy P.;Lux T.;Twardziok S.;Venturini L.;Juhasz A.;Abrouk M.;Fischer I.;Uauy C.;Borrill P.;Ramirez-Gonzalez R.H.;Arnaud D.;Chalabi S.;Chalhoub B.;Cory A.;Datla R.;Davey M.W.;Jacobs J.;Robinson S.J.;Steuernagel B.;Van Ex F.;Wulff B.B.H.;Benhamed M.;Bendahmane A.;Concia L.;Latrasse D.;Alaux M.;Bartoš J.;Bellec A.;Berges H.;Doležel J.;Frenkel Z.;Gill B.;Korol A.;Letellier T.;Olsen O.A.;Šimková H.;Singh K.;Valárik M.;Van Der Vossen E.;Vautrin S.;Weining S.;Fahima T.;Glikson V.;Raats D.;Toegelová H.;Vrána J.;Sourdille P.;Darrier B.;Barabaschi D.;Cattivelli L.;Hernandez P.;Galvez S.;Budak H.;Jones J.D.G.;Witek K.;Yu G.;Small I.;Melonek J.
- https://science.sciencemag.org/content/sci/361/6403/eaar7191.full.pdf -->
Author: Takuji Sasaki .,
Author: Lukaszewski A.J.;Alberti A.;Sharpe A.;Kilian A.;Stanca A.M.;Keller B.;Clavijo B.J.;Friebe B.;Gill B.;Wulff B.;Chapman B.;Steuernagel B.;Feuillet C.;Viseux C.;Pozniak C.;Rokhsar D.S.;Klassen D.;Edwards D.;Akhunov E.;Paux E.;Alfama F.;Choulet F.;Kobayashi F.;Muehlbauer G.J.;Quesneville H.;Šimková H.;Rimbert H.;Gundlach H.;Budak H.;Sakai H.;Handa H.;Kanamori H.;Batley J.;Vrána J.;Rogers J.;Číhalíková J.;Doležel J.;Chapman J.;Poland J.A.;Wu J.;Khurana J.;Wright J.;Bader K.C.;Eversole K.;Barry K.;McLay K.;Mayer K.F.X.;Singh K.;Clissold L.;Pingault L.;Couderc L.;Cattivelli L.;Spannagl M.;Kubaláková M.;Caccamo M.;Mascher M.;Bellgard M.;Pfeifer M.;Zytnicki M.;Febrer M.;Alaux M.;Martis M.M.;Loaec M.;Colaiacovo M.;Singh N.K.;Glover N.;Guilhot N.;Stein N.;Olsen O.A.;Maclachlan P.R.;Chhuneja P.;Wincker P.;Sourdille P.;Faccioli P.;Ramirez-Gonzalez R.H.;Waugh R.;Šperková R.;Knox R.;Appels R.;Sharma S.;Ayling S.;Praud S.;Wang S.;Lien S.;Sandve S.R.;Matsumoto T.;Endo T.R.;Itoh T.;Nussbaumer T.;Wicker T.;Tanaka T.;Scholz U.;Barbe V.;Jamilloux V.;Ogihara Y.;Dubská Z.
Author: Ramanjulu Sunkar ., Thomas Girke ., Pradeep Kumar Jain ., Jian-Kang Zhu .,
Author: Mingsheng Chen ., Gernot Presting ., W. Brad Barbazuk ., Jose Luis Goicoechea ., Barbara Blackmon ., Guangchen Fang ., Hyeran Kim ., David Frisch ., Yeisoo Yu ., Shouhong Sun ., Stephanie Higingbottom ., John Phimphilai ., Dao Phimphilai ., Scheen Thurmond ., Brian Gaudette ., Ping Li ., Jingdong Liu ., Jamie Hatfield ., Dorrie Main ., Kasey Farrar ., Caroline Henderson ., Laura Barnett ., Ravi Costa ., Brian Williams ., Suzanne Walser ., Michael Atkins ., Caroline Hall ., Muhammad A. Budiman ., Jeffery P. Tomkins ., Meizhong Luo ., Ian Bancroft ., Jerome Salse ., Farid Regad ., Trilochan Mohapatra ., Nagendra K. Singh ., Akhilesh K. Tyagi ., Carol Soderlund ., Ralph A. Dean ., Rod A. Wing .,
Author: M. Farooq ., N. Gogoi ., S. Barthakur ., B. Baroowa ., N. Bharadwaj ., S. S. Alghamdi ., K. H. M. Siddique .,
Author: Zhiwen Wang ., Neil Hobson ., Leonardo Galindo ., Shilin Zhu ., Daihu Shi ., Joshua McDill ., Linfeng Yang ., Simon Hawkins ., Godfrey Neutelings ., Raju Datla ., Georgina Lambert ., David W. Galbraith ., Christopher J. Grassa ., Armando Geraldes ., Quentin C. Cronk ., Christopher Cullis ., Prasanta K. Dash ., Polumetla A. Kumar ., Sylvie Cloutier ., Andrew G. Sharpe ., Gane K.‐S. Wong ., Jun Wang ., Michael K. Deyholos .,
Author: Bhat J.A.;Shivaraj S.M.;Singh P.;Navadagi D.B.;Tripathi D.K.;Dash P.K.;Solanke A.U.;Sonah H.;Deshmukh R.
- https://www.mdpi.com/2223-7747/8/3/71/pdf?version=1553671589 -->


ICAR-National Institute for Plant Biotechnology
National research center, established : default.
Regional Station
KVK Farmer's & Outreach
Major awards.
Publication
Soil Health Card
Mera Gao Mera Gaurav
Swatch Bharat Abhiyan
IPR/Patent/Techonology Developed
Institute Technology Commercialized
Institute as Partner - AICRP's/Network Project/CRP's
Institute as corrdinationg centre - aicrp's/network project/crp's.

AICRP's/Network Project/CRP's

Search research topics, case studies, institutions and more:
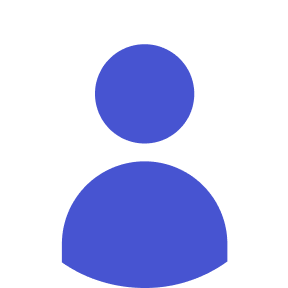
- Log in/sign up
National Research Centre on Plant Biotechnology
Specialised research institution.
(06/08/2021- Excerpt from Institution Website )
National Institute For Plant Biotechnology (NIPB) is a premiere research institution of the Indian Council of Agricultural Research (ICAR). The institute was founded in 1985 as the ‘Biotechnology Centre’ of Indian Agricultural Research Institute (IARI) for molecular biology and biotechnology research in crop plants. The prescience of the role of biotechnology in agriculture led to a bigger responsibility for this centre and it was elevated as National Research Centre on Plant Biotechnology in the year 1993 (Now NIPB).
National Institute For Plant Biotechnology has been entrusted with the responsibility of developing new tools and techniques and to deliver breakthrough in biotechnology for crop improvement. With a humble beginning and a few dedicated scientists, the centre could successfully deliver varieties such as Pusa Jai Kisan, which is one of the top three mustard varieties released by the ICAR till date. Besides, the centre has released a rice variety Improved Pusa Basmati-1 resistant to bacterial leaf blight using marker assisted selection (MAS) in collaboration with the Division of Genetics, IARI. Moricandia based CMS system developed at NIPB has contributed to the commercial production of mustard hybrids namely NRC Sankar Sarson (DRMR, Bharatpur) and Coral 432 (Advanta India). The rice blast resistance gene Pi54 identified, mapped, cloned and characterized at NIPB has been transferred in mega varieties of rice like Pusa Basmati and BPT 5204 and in many other varieties by the rice breeders using MAS. The centre has matched steps with the changing time and conducted research in basic and applied research for crop improvement resulting in many publications in high impact factor journals, patents and public private partnerships. The state-of-the-art infrastructure and expertise of the scientists have enabled the successful execution of International (rice, tomato and wheat) and National ( Pigeonpea, Mango, Mesorhizobium, Puccinia and Magnaporthe ) genome sequencing projects.
NIPB is also engaged in teaching postgraduate students with regular PhD and MSc degree programmes in Molecular Biology and Biotechnology.
The centre takes lead and has contributed substantially towards human resource development by developing strong inter-and intra-institutional linkages and organizing training programmes, summer/winter schools sponsored by Education Division of ICAR as well as other major National funding agencies.
Other National Research Centre on Plant Biotechnology links
Want to contribute or amend this page.
Found an inaccuracy, something out of date, or want to add something you think is important to understanding National Research Centre on Plant Biotechnology?
Add your knowledge by contacting us and requesting a page update.

Most Searched
- Birth Certificate
- Driving Licence
Website of National Research Centre on Plant Biotechnology

National Research Centre on Plant Biotechnology (NRCPB) is a premiere research institution of the Indian Council of Agricultural Research (ICAR). The institute was founded in 1985 as the 'Biotechnology Centre' of Indian Agricultural Research Institute (IARI) for molecular biology and biotechnology research in crop plants.
Related Links
Farm machinery online.
Farm Machinery Online is a web based application or Online Monitoring System by Ministry of Agriculture for faster and reliable information. Users can access reports related to MPR testing activities, detailed machine status, capital equipment, training, finance, revenue, etc.
Telephone Directory of Ministry of Agriculture & Farmers Welfare
Get contact details of employees of the Ministry of Agriculture & Farmers Welfare. Users can access information about name of the employee, designation, office address, room number, phone number, extension number, residence address, etc.
Website of Ministry of Agriculture & Farmers Welfare
E-book of indian council of agricultural research.
The Indian Council of Agricultural Research (ICAR) is an autonomous organisation under the Department of Agricultural Research and Education (DARE), Ministry of Agriculture and Farmers Welfare , Government of India. Formerly known as Imperial Council of Agricultural Research.
Website of National Agricultural Higher Education Project
Website of national agriculture infra financing facility.
The role of infrastructure is crucial for agriculture development and for taking the production dynamics to the next level. It is only through the development of infrastructure, especially at the post harvest stage that the produce can be optimally utilized with opportunity for value addition and fair deal for the farmers.
Website of Mission for Integrated Development of Horticulture (MIDH)
Mission for Integrated Development of Horticulture (MIDH) is a Centrally Sponsored Scheme for the holistic development of horticulture in the country during XII plan. User can find the details of schemes, operational guidelines and contact details of MIDH officials.
Agri Stack Portal
Website of agri-clinics and agri-business centres, website of upag - unified portal for agriculture statistics.
Its provides comprehensive information on crop estimates, secondary datasets, news and updates, resources, and more. Whether you're a farmer, researcher, or policymaker, we have everything you need to make informed decisions and stay up-to-date with the latest developments in the field.
Website of Agriculture Marketing Information System Network (Agmarknet)
The objective is to collect, analyses and disseminate market information to the farmers, traders, Policy makers and other stakeholders. More than 3200 markets are covered under the scheme and more than 2700 markets are reporting data at Agmarknet portal.
Website of Kisan Suvidha
You can get detailed information about the Kisan Suvidha. Its provides online access to the latest information at one platform from multiple IT systems/initiatives in the agricultural sector.
Website of Coconut Development Board
Get information about the Coconut Development Board and various activities it undertakes for Coconut sector. Users can also get information related to major schemes.
Website of Participatory Guarantee System of India
PGS-India (Participatory Guarantee System of India) is a quality assurance initiative that is locally relevant, emphasize the participation of stakeholders, including producers and consumers and operate outside the frame of third party certification. National Project on Organic Farming (NPOF) is a continuing central sector scheme since 10th Five Year Plan.
Direct Benefit Transfer (DBT) Portal for Agriculture Schemes
Get information about the Direct Benefit Transfer (DBT) Portal for Agriculture Schemes and various activities it undertakes for agriculture sector.
- Old website
- Employee Corner
- Skip to Main Content
- Screen Reader Access
- A - A+ A A- --> A+ A A- A A
- English Eng Hin

भारतीय कृषि अनुसंधान परिषद indian council of agricultural research Ministry of agriculture and Farmers Welfare

भारतीय कृषि अनुसंधान परिषद indian council of agricultural research Ministry of Agriculture and Farmers Welfare

National Research Centres
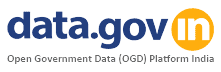
- Privacy Policy
- Web Information Manager
- Copyright Policy
- Hyperlinking Policy
- Accessibility Statement
@ Content Owned by Indian Council of Agricultural Research
@ Content Owned by ICAR - Directorate of Knowledge Management in Agriculture
Copyrights © 2022 All Rights Reserved By Indian Council of Agricultural Research Krishi Bhavan
- Total Visitors: 22001821
Like on Facebook
Subscribe on Youtube
Follow on Twitter
Like on instagram
Genomic Designing of Climate-Smart Oilseed Crops
- © 2019
- Chittaranjan Kole 0
ICAR-National Research Center on Plant Biotechnology, New Delhi, India
You can also search for this editor in PubMed Google Scholar
- Highlights the latest advances in plant molecular mapping and genome sequencing
- Demonstrates how advances in plant molecular breeding and genomics-assisted breeding can help produce oilseed crops that can adapt to climate change
- Written by leading experts in the field
5392 Accesses
85 Citations
2 Altmetric
This is a preview of subscription content, log in via an institution to check access.
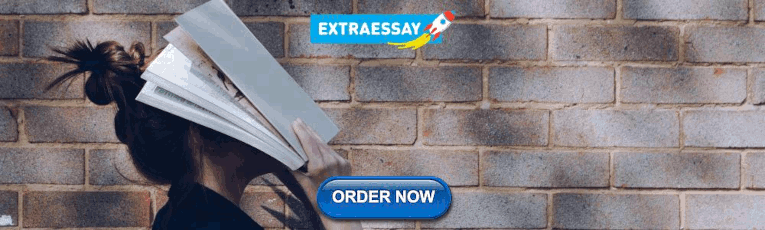
Access this book
- Available as EPUB and PDF
- Read on any device
- Instant download
- Own it forever
- Durable hardcover edition
- Dispatched in 3 to 5 business days
- Free shipping worldwide - see info
Tax calculation will be finalised at checkout
Other ways to access
Licence this eBook for your library
Institutional subscriptions
Table of contents (8 chapters)
Front matter, approaches, applicability, and challenges for development of climate-smart soybean.
- Juhi Chaudhary, SM Shivaraj, Praveen Khatri, Heng Ye, Lijuan Zhou, Mariola Klepadlo et al.
Genetic Solutions to Improve Resilience of Canola to Climate Change
- Harsh Raman, Rajneet Kaur Uppal, Rosy Raman
Climate-Smart Groundnuts for Achieving High Productivity and Improved Quality: Current Status, Challenges, and Opportunities
- Sunil S. Gangurde, Rakesh Kumar, Arun K. Pandey, Mark Burow, Haydee E. Laza, Spurthi N. Nayak et al.
Sunflower and Climate Change: Possibilities of Adaptation Through Breeding and Genomic Selection
- Dragana Miladinović, Nada Hladni, Aleksandra Radanović, Siniša Jocić, Sandra Cvejić
Progress Toward Development of Climate-Smart Flax: A Perspective on Omics-Assisted Breeding
- S. M. Shivaraj, Priyanka Dhakate, Humira Sonah, Tri Vuong, Henry T. Nguyen, Rupesh Deshmukh
Breeding Brassica juncea and B. rapa for Sustainable Oilseed Production in the Changing Climate: Progress and Prospects
- Priya Panjabi, Satish Kumar Yadava, Nitin Kumar, Rajkumar Bangkim, Nirala Ramchiary
Potential for Adaptation to Climate Change Through Genomic Breeding in Sesame
- Haiyang Zhang, Hongmei Miao, Ming Ju
Breeding for Climate Resilience in Castor: Current Status, Challenges, and Opportunities
- Xuegui Yin, Jiannong Lu, Kwadwo Gyapong Agyenim-Boateng, Shuai Liu
- global warming
- climate-smart genes
- abiotic stress
- genomics-assisted breeding
- molecular breeding
- allele mining
- oilseed rape
- castor bean
- climate change
About this book
This book highlights modern strategies and methods to improve oilseed crops in the era of climate change, presenting the latest advances in plant molecular breeding and genomics-driven breeding.
Spectacular achievements in the fields of molecular breeding, transgenics and genomics in the last three decades have facilitated revolutionary changes in oilseed- crop-improvement strategies and techniques. Since the genome sequencing of rice, as the first crop plant, in 2002, the genomes of about one dozen oilseed crops have been sequenced and more are to follow. This has made it possible to decipher the exact nucleotide sequence and chromosomal positions of agroeconomic genes. Most importantly, comparative genomics and genotyping-by-sequencing have opened up new vistas for exploring available biodiversity, particularly of wild crop relatives, for identifying useful donor genes.
Editors and Affiliations
Chittaranjan Kole
About the editor
Bibliographic information.
Book Title : Genomic Designing of Climate-Smart Oilseed Crops
Editors : Chittaranjan Kole
DOI : https://doi.org/10.1007/978-3-319-93536-2
Publisher : Springer Cham
eBook Packages : Biomedical and Life Sciences , Biomedical and Life Sciences (R0)
Copyright Information : Springer Nature Switzerland AG 2019
Hardcover ISBN : 978-3-319-93535-5 Published: 01 March 2019
eBook ISBN : 978-3-319-93536-2 Published: 15 February 2019
Edition Number : 1
Number of Pages : XXV, 498
Number of Illustrations : 12 b/w illustrations, 23 illustrations in colour
Topics : Plant Breeding/Biotechnology , Climate Change , Agriculture , Plant Genetics and Genomics
- Publish with us
Policies and ethics
- Find a journal
- Track your research
Ectopic Expression of Rice PYL3 Enhances Cold and Drought Tolerance in Arabidopsis thaliana
Affiliations.
- 1 ICAR-National Research Centre on Plant Biotechnology, Indian Agricultural Research Institute, New Delhi, 110012, India.
- 2 TERI-Deakin NanoBiotechnology Centre, The Energy and Resources Institute, New Delhi, 110003, India.
- 3 ICAR-Central Tuber Crops Research Institute, Thiruvananthapuram, 695017, India.
- 4 Division of Plant Physiology, ICAR-Indian Agricultural Research Institute, New Delhi, 110012, India.
- 5 ICAR-National Research Centre on Plant Biotechnology, Indian Agricultural Research Institute, New Delhi, 110012, India. [email protected].
- 6 TERI-Deakin NanoBiotechnology Centre, The Energy and Resources Institute, New Delhi, 110003, India. [email protected].
- PMID: 29574592
- DOI: 10.1007/s12033-018-0076-5
Abscisic acid (ABA) plays an important role in plant development and adaptation to abiotic stresses. The pyrabactin resistance-like (PYL) gene family has been characterized as intracellular ABA receptors in Arabidopsis. We describe here the functional characterization of PYL3 ABA receptor from a drought-tolerant rice landrace Nagina 22 (N22). The induced expression level of the PYL3 transcript was observed in the N22 under different stress treatments, including cold, drought, high temperature, salt and ABA. In contrast, the expression of PYL3 was down-regulated in drought-susceptible rice cv. IR64 in response to above stresses. C-terminal GFP translational fusion of OsPYL3 was localized to both cytosol and nucleus explaining in part functional conservation of PYL protein as ABA receptor. Arabidopsis transgenic lines overexpressing OsPYL3 were hypersensitive to ABA suggesting ABA signaling pathway-dependent molecular response of the OsPYL3. Further, constitutive overexpression of OsPYL3 in Arabidopsis led to improved cold and drought stress tolerance. Thus, OsPYL3 identified in this study could be a good candidate for genetic improvement of cold and drought stress tolerance of rice and other crop plants.
Keywords: ABA receptor; Abiotic stress; Cold; Drought; PYL; Rice.
- Abscisic Acid / pharmacology
- Arabidopsis / genetics
- Arabidopsis / growth & development*
- Cold Temperature
- Gene Expression Regulation, Plant
- Oryza / genetics
- Oryza / metabolism*
- Plant Proteins / genetics
- Plant Proteins / metabolism
- Plants, Genetically Modified / growth & development
- Receptors, Cell Surface / genetics*
- Receptors, Cell Surface / metabolism
- Recombinant Proteins / metabolism
- Stress, Physiological*
- Plant Proteins
- Receptors, Cell Surface
- Recombinant Proteins
- Abscisic Acid
Grants and funding
- NPTC/Indian Council of Agricultural Research
- NFBSFARA/Phen 2015/Indian Council of Agricultural Research
- JRF/Council of Scientific and Industrial Research
- SRF/Council of Scientific and Industrial Research
- INSPIRE/Department of Science and Technology, Ministry of Science and Technology
- Open supplemental data
- Reference Manager
- Simple TEXT file
People also looked at
Original research article, enhancing nutrient uptake and grapefruit ( citrus paradisi ) growth through soil application of beneficial bacteria ( bacillus spp.).
- 1 Soil, Water and Ecosystem Sciences Department, Citrus Research and Education Center, University of Florida, Lake Alfred, FL, United States
- 2 Microbiology and Cell Sciences Department, Citrus Research and Education Center, University of Florida, Lake Alfred, FL, United States
- 3 Soil, Water and Ecosystem Sciences Department, Southwest Florida Research and Education Center, University of Florida, Immokalee, FL, United States
The term plant growth-promoting rhizobacteria (PGPR) refers to a root-associated bacteria that possesses several benefits for soil and the plant. The increasing demand for sustainable food production necessitates a shift towards agricultural practices that mitigate adverse environmental impacts. Excessive use of chemical fertilizers and pesticides has raised concerns, prompting a surge of interest in harnessing the potential of beneficial soil microorganisms, particularly plant growth-promoting rhizobacteria. This study explores the impact of soil inoculation with PGPR treatments on grapefruit seedlings, including a commercial strain of Bacillus velezensis , a fresh inoculum of Bacillus amyloliquefaciens , a mixture of three selected citrus endophytic Bacillus spp. isolates, inorganic fertilizers, and combinations thereof. The aim was to test their ability to enhance growth and nutrient uptake reducing the input of chemical fertilization. Results indicated that the combination of Bacillus velezensis and inorganic fertilization significantly improved soil nutrient availability and enhanced plant growth, surpassing both negative (water) and positive (inorganic fertilization) controls. Shoot and root system biomass showed significant increases from Bacillus velezensis plus inorganic fertilization compared to other treatments. These findings provide insights into management methods that can reduce chemical inputs while promoting plant productivity. The demonstrated benefits of PGPR on grapefruit seedlings highlight a promising approach for future research and applications in sustainable citrus cultivation.
Introduction
Developing methods to enhance agricultural sustainability is necessary for ensuring not only an adequate food supply but also for upholding global environmental protection for future generations ( Nwachukwu et al., 2021 ; Wang et al., 2022 ). The activity of plant growth-promoting rhizobacteria (PGPR) has been documented in a wide range of strains across several different genera, including Azotobacter , Arthrobacter , Bacillus , Clostridium , Hydrogenophaga , Enterobacter , Pseudomonas , Serratia , Klebsiella, Microbacterium, Paenibacillus , and Azospirillum ( Grobelak et al., 2015 ; Kumar Meena et al., 2015 ; Kumar et al., 2018 ; Numan et al., 2018 ; Barnawal et al., 2019 ). Root colonization by microbes is active ( Compant et al., 2019 ) but it will be influenced by the root exudates from different plant species ( Elsharkawy et al., 2023 ) reported by Jamil et al. (2022) , and Antoszewski et al. (2022) . Among the rhizobacteria that can colonize plants, we can find Bacillus amyloliquefaciens ( Fan et al., 2015 ; Gamez et al., 2019 ), Bacillus subtilis ( Blake et al., 2021 ; Hu et al., 2023 ), Bacillus velezensis ( Shi et al., 2022 ; Sun et al., 2022 ) and some commercial strains of these species serve as biofertilizers and biocontrol agents. The ability of these strains to form resilient endospores can assist in ensuring their viability for the production of microbial inoculants ( Tsotetsi et al., 2022 ) when added to the soil. As a result, its utilization in agricultural practices is rapidly expanding ( Shi et al., 2022 ) as biostimulants for growth promotion ( Senger et al., 2022 ), biofertilization ( Bueno et al., 2022 ), and biocontrol agents ( Chen et al., 2020 ).
A microbial inoculant (MI) is a category of agricultural amendments that uses live microorganisms to provide fertilizer benefits for crops ( Gong et al., 2018 ). In numerous instances, utilizing microbial inoculants containing beneficial microorganisms instead of synthetic chemicals has raised attention for their ability to help plant growth, and offer enhanced nutrient availability while simultaneously fostering environmental benefits and augmenting soil productivity ( Luo et al., 2022 ; Mawarda et al., 2022 ; Etesami et al., 2023 ). Global markets for PGPR are growing, especially products involving multiple live bacterial species in “consortia”. When multiple PGPRs are used together, they appear to provide additive benefits to plants compared to a single PGPR ( Rosier et al., 2021 ).
Essentially, microbe-based biofertilizers can enhance both the correct development of plants as well as yield ( Rosier et al., 2021 ; Shi et al., 2022 ) in several agricultural plants such as wheat ( Triticum aestivum L.) ( Zahra et al., 2023 ), sweet corn ( Zea mays subsp. mays L.) ( Akinrinlola et al., 2018 ), and tomato ( Lycopersicon esculentum Mill.) ( Mengistie and Awlachew, 2022 ; Patani et al., 2023 ). They achieved this by augmenting the soil availability of mineral nutrients, solubilizing phosphorus, and synthesizing phytohormones, all of which contribute significantly to the overall health of plants ( Kumar et al., 2011 ; Gohil et al., 2022 ).
Citrus production in Florida has been adversely affected since the first detection of the Huanglongbing disease (HLB; Candidatus Liberibacter asiaticus), which is incurable and leads to an eventual tree death ( Dala-Paula et al., 2019 ). Therefore, the need for vigorous citrus rootstock seedlings is a key component for the good development of trees at young stages, to provide tolerance of biotic and abiotic challenges as well as enhance their overall growth and yield ( Vashisth et al., 2020 ). On the other hand, water and fertilizer play vital roles in the growth and fruit yield of citrus. The quantity of fertilizer used in citrus management is linked to both production costs and environmental concerns ( Ma et al., 2022 ), thus nutrient management has been implemented to promote citrus trees’ health in Florida where soils are poorly drained and have low fertility ( Hallman et al., 2022 ). Based on the current disease and nutrient problems the citrus industry in Florida is facing, the objective of this study is to evaluate the role of Bacillus spp. soil inoculations in citriculture, particularly in its ability to stimulate and enhance plant growth and improve nutrient uptake of grapefruit seedlings.
Materials and methods
The experimental site and grapefruit seedling establishment.
The experiment was conducted in a greenhouse located at the Citrus Research and Education Center (CREC), Lake Alfred, Florida (28.1026° N, 81.7120° W). ‘Duncan’ grapefruit ( Citrus Paradisi ) seedlings were propagated from disease-free seeds collected directly from the fruit grown in the CREC CUPS (Citrus Under Protective Screens; Schumann et al., 2023 ) facility. Seeds were rinsed three times with distilled water to remove any juice vesicles, and then soaked in distilled water to discard floating seeds. The selected seeds were dried with paper towels and the seed coats were carefully removed with a previously sanitized blade (GEM 62-0161 carbon steel extra sharp single-edge blade). Two seeds were planted into each growth media plug in germination trays of the Double Bio Dome Seed-Starting System (Park Seed, Greenwood, SC) and allowed to germinate in the greenhouse. After three weeks of completed germination, the tallest vigorous seedlings with true leaves were transferred individually into 1.67-L plastic pots filled with unsterilized substrate media of 50% Canadian peat moss with the addition of pine bark, perlite, and vermiculite. All plants were fertilized every week until the start of the experiment 18 weeks after transplanting, then fertilization was stopped and only treatments based on fertilizer (T1) and B. velezensis plus fertilizer (T6) continued receiving reduced fertilization every four weeks (25% of the full rate) ( Table 1 ). Monitoring and treating for any potential pest incidence were assessed weekly.
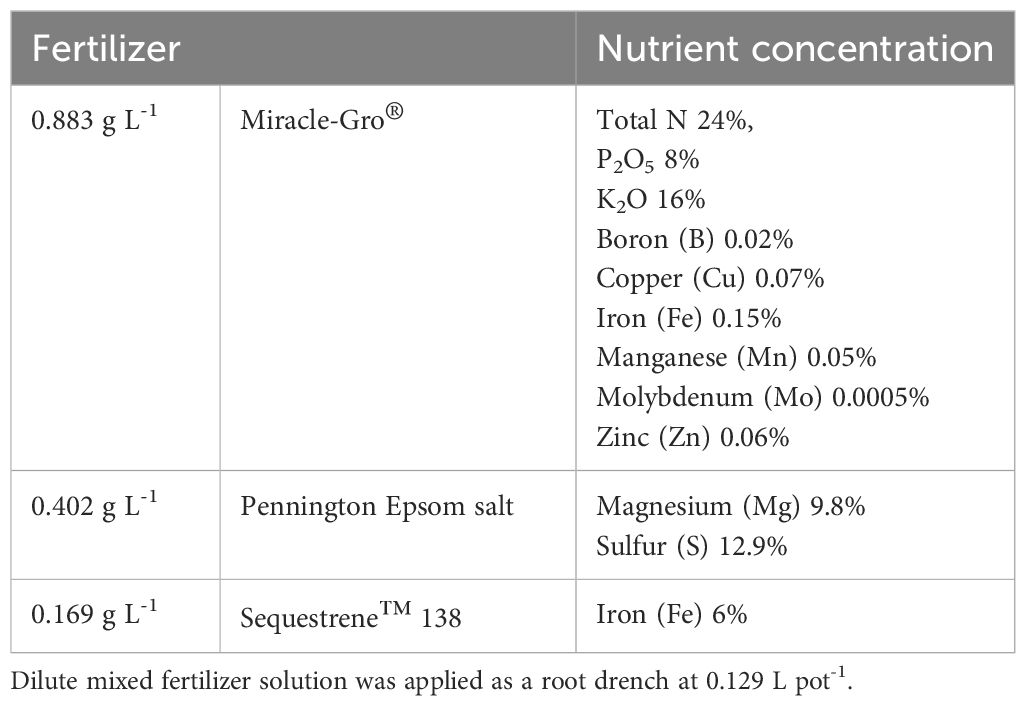
Table 1 Fertilizer description and nutrient concentration.
Experimental design and treatments
A randomized complete block design with seven treatments and five replications was used, with each plot (experimental unit) composed of four potted grapefruit seedlings. The treatments ( Table 2 ) were applied as a 0.129 L pot -1 root drench and correspond to: (1) fertilization ( Table 1 ), (2) B. velezensis (LALRISE VITA ® ; Lallemand Plant Care, Montreal, Canada), (3) fresh inoculum of B. amyloliquefaciens (commercial product Double Nickel 55 ® ; Certis, Columbia, MD) where dormant spores were activated in a bioreactor 24 hours before application, (4) fresh inoculum of a consortium of B. subtilis, B. megaterium , and Bacillus sp. (citrus endophytic isolates) ( Li et al., 2021 ), (5) a combination of fresh inoculum of B. amyloliquefaciens and the consortium of three B. subtilis isolates to test for additional synergy with bacterial combinations, (6) B. velezensis with the addition of fertilization and (7) water (control) with no additional application. The fertilizer treatment consisted of three different sources described in Table 1 . The fresh inoculum of Bacillus spp. consortia was prepared by culturing the bacterium in a nutrient broth medium with agitation at 30°C for 24 hours and adjusted to 10 9 cells per milliliter with water.
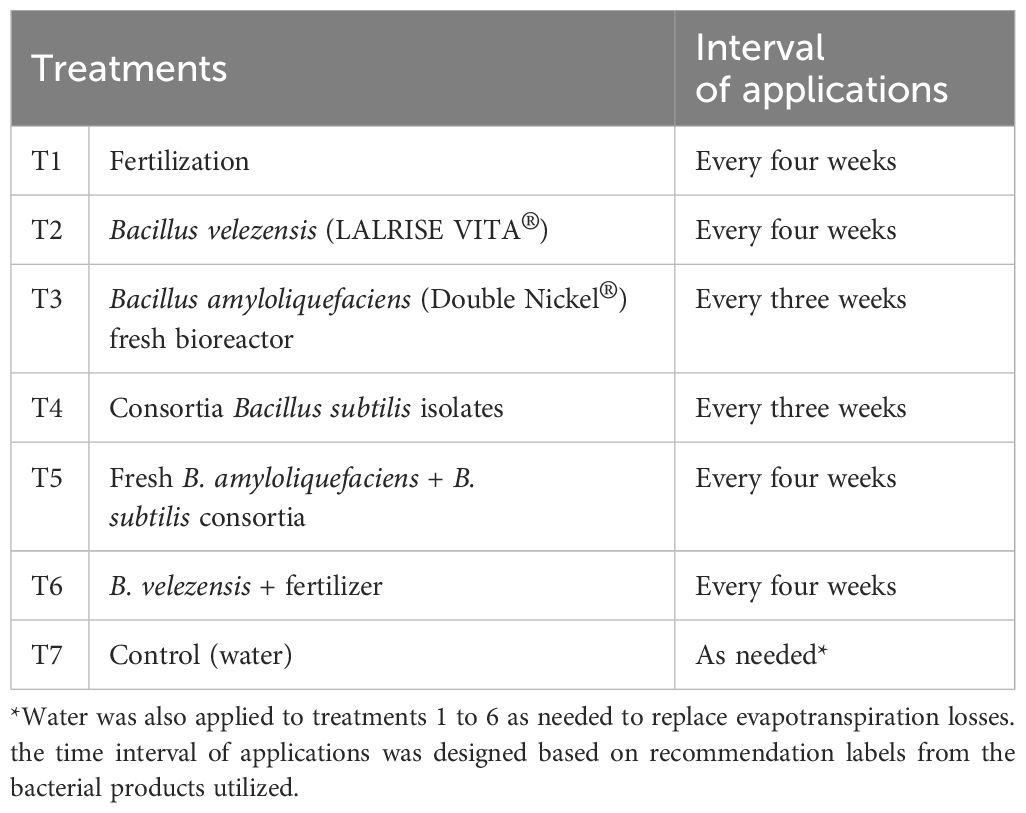
Table 2 Description of treatments applied as a root drench to the pots (0.129 L pot -1 ).
Plant growth measurements
Tree seedling heights and diameters in two perpendicular directions were measured every month to obtain canopy volume based on the formula of Thorne et al. (2002) , as well as trunk diameter measured 5 cm above the potting media. SPAD chlorophyll index (Konika Minolta, Ramsey, NJ) was collected monthly from three leaves per tree and averaged for each 4-tree plot. At the end of the experiment (three months after treatments began), every plot of four trees was photographed and four leaves per tree were collected and sent for N, P, K, Ca, Mg, S, Zn, B, Mn, Fe, and Cu concentration analysis at the Waters Agricultural Laboratory, Camilla, GA. Tree above-ground biomass and root biomass were obtained by separating leaves, shoots, and roots for each 4-tree plot. Bagged samples were placed in a drying oven (Fisher Scientific, Pittsburgh, PA) for 48 hours at 70°C, and were then weighed to obtain dry mass per plot.
Media sampling and estimation of microbial respiration
We hypothesized that microbial respiration from the most effective rhizobacterial treatments would increase compared to the controls. Growing media samples in the rhizosphere were obtained from the excavated root systems by gentle shaking into a plastic tray, to collect samples, mix and transfer them into plastic Ziploc bags. A mixed media sample from 4 trees was collected, representing the treatment plot and processed for microbial respiration in the lab by implementing the substrate-induced respiration method ( Anderson and Domsch, 1978 ). Empty Mason jars were weighed, then 50 grams of media from the samples was weighed into the jars. To measure the CO 2 respired, a 20 mL plastic scintillation vial was placed inside the jar with 4 mL of 1 M NaOH. After 10 days of incubation in a growth chamber in dark- conditions at 35 degrees Celsius, vials were removed from the jars and capped. Jars were placed in the drying oven at 105 degrees Celsius for 48 hours to dry the media. Dry media was then weighed to calculate the percentage of gravimetric water content. The determination of CO 2 produced by microbial respiration processes was based on the titration of 1 M NaOH in vials with standardized 0.1 M HCl and the use of phenolphthalein as a color indicator to determine the endpoint. An additional blank jar with a vial inside and no media was included in the incubation to calculate the titration amount with zero respiration.
Calculations
To obtain shoot biomass we summed the leaf and stem biomass and then conducted an ANOVA to find the significance among treatments. Similarly, the shoot-to-root ratio was calculated by dividing shoot biomass by root biomass. Finally, the N content in leaves was obtained by multiplying the leaf N concentration and the leaf biomass. In order to test the N fertilizer equivalent saved as a result of using PGPRs, a linear regression line of mg N applied per pot versus mg N/pot accumulated in leaves was calculated for treatments T1 (fertilizer) and T7 (control). The regression equation was then used to interpolate the mg N fertilizer equivalent contributed by each PGPR treatment according to its N accumulation in the leaf biomass.
To identify significant differences between treatments, Analysis of Variance (ANOVA) was performed using Rstudio (Rstudio Team (2020). Rstudio: Integrated Development for R. Rstudio, PBC, Boston, MA URL http://www.rstudio.com/ .) and conducting Post hoc comparisons with the Tukey HSD test to find statistical differences between means using the ‘agricolae’ package ( De Mendiburu, 2022 ). The data was tested to be normally and independently distributed, from the seven treatment combinations with 5 replications. Differences were considered statistically significant when P ≤ 0.05.
Results and discussion
Grapefruit seedling growth.
Results of grapefruit seedling growth measurements included height, canopy volume, trunk diameter, root, stem, and leaf biomass, and SPAD chlorophyll index. Tree height, canopy volume, trunk diameter, shoot biomass, root biomass, and chlorophyll index were significantly greater for plants inoculated with B. velezensis that also received fertilizer ( Figures 1 – 6 ). The B. subtilis + B. amyloliquefaciens consortium treatment (T5) had a significantly higher shoot biomass than the untreated negative control (T7), despite the lack of any added fertilizer. The growth promotion ability may have an effect on roots ( Shen et al., 2023 ) and can lead to an increase in water and nutrient uptake for the overall plant growth ( Wang et al., 2020 ). In our results, Supplementary Figure 3 shows a very highly significant correlation (p<0.001) between leaf N accumulated and root biomass per tree to illustrate the linkage between enhanced root biomass and N uptake. The B. velezensis inoculation + fertilizer treatment (T6) produced significantly more shoot biomass than any other treatment, even including T1, the fertilized positive control ( Figure 4 ), and the B. velezensis inoculation (T2), suggesting synergy between the B. velezensis and fertilizer. The ratio of shoot/root biomass is an estimate of response to physical, chemical, and biological factors related to above-ground architecture ( Lopez et al., 2023 ). Supplementary Figure 1 shows no significant differences among treatments suggesting that the relative changes in root and shoot growth according to treatments were approximately proportional. The B. subtilis + B. amyloliquefaciens treatment produced a significantly higher (p<0.001) SPAD chlorophyll index than the control ( Figure 6 ), but the other B. subtilis and B. amyloliquefaciens treatments did not show evidence of promoting tree growth ( Figures 1 – 6 ).
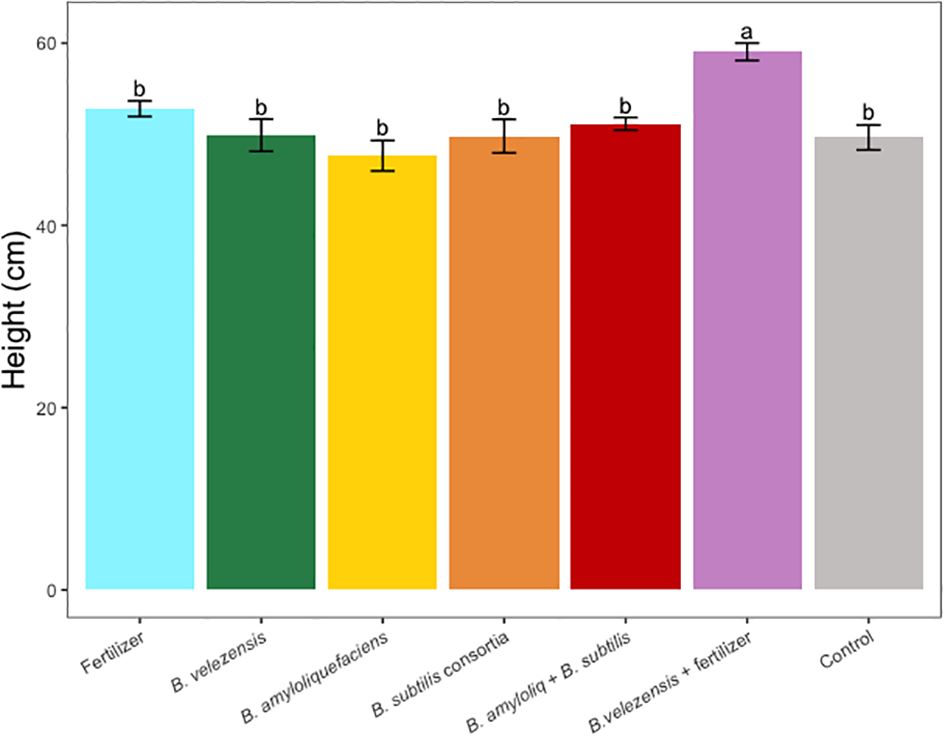
Figure 1 Average tree height per tree for treatments. Mean values and standard deviations were employed for representation. Different letters indicate significant differences between treatments (P < 0.05).
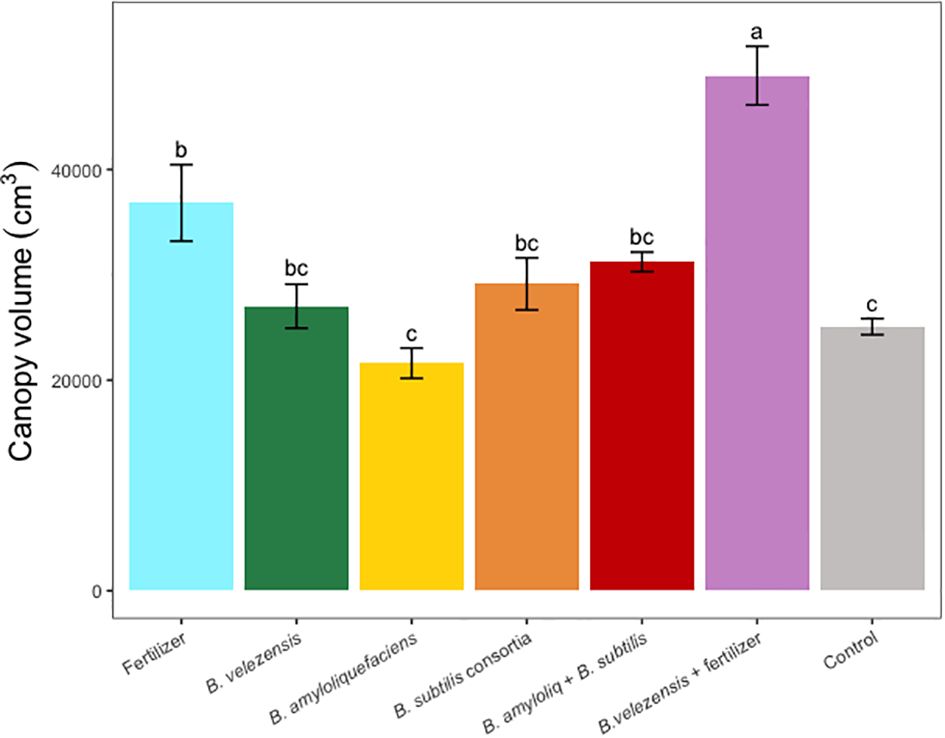
Figure 2 Average canopy volume per tree for treatments. Mean values and standard deviations were employed for representation. Different letters indicate significant differences between treatments (P < 0.05).
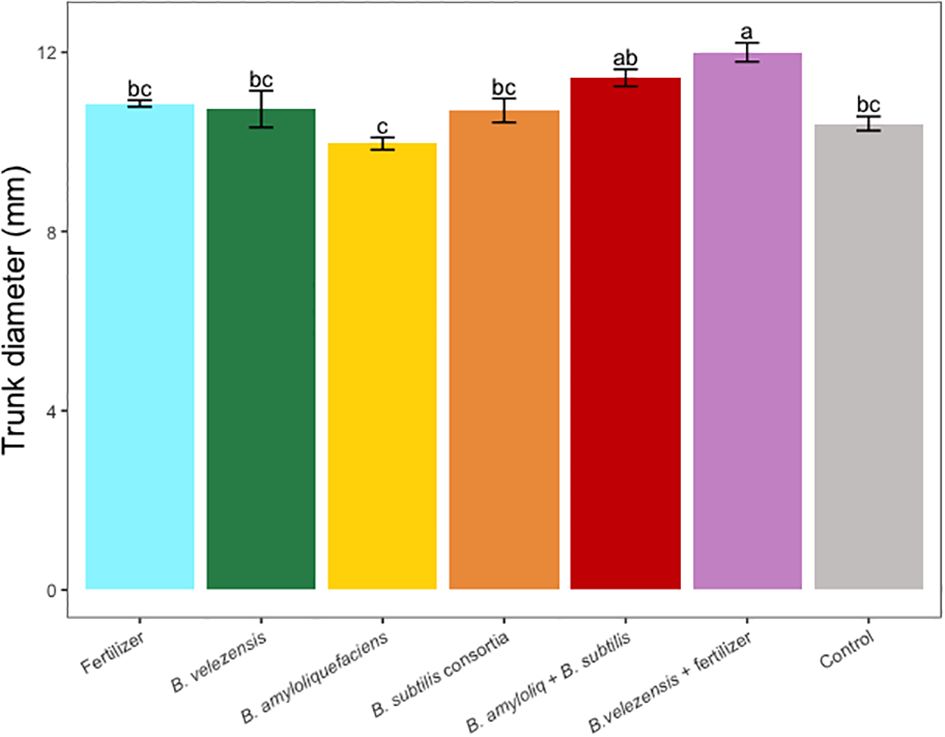
Figure 3 Average trunk diameter per tree for treatments. Mean values and standard deviations were employed for representation. Different letters indicate significant differences between treatments (P < 0.05).
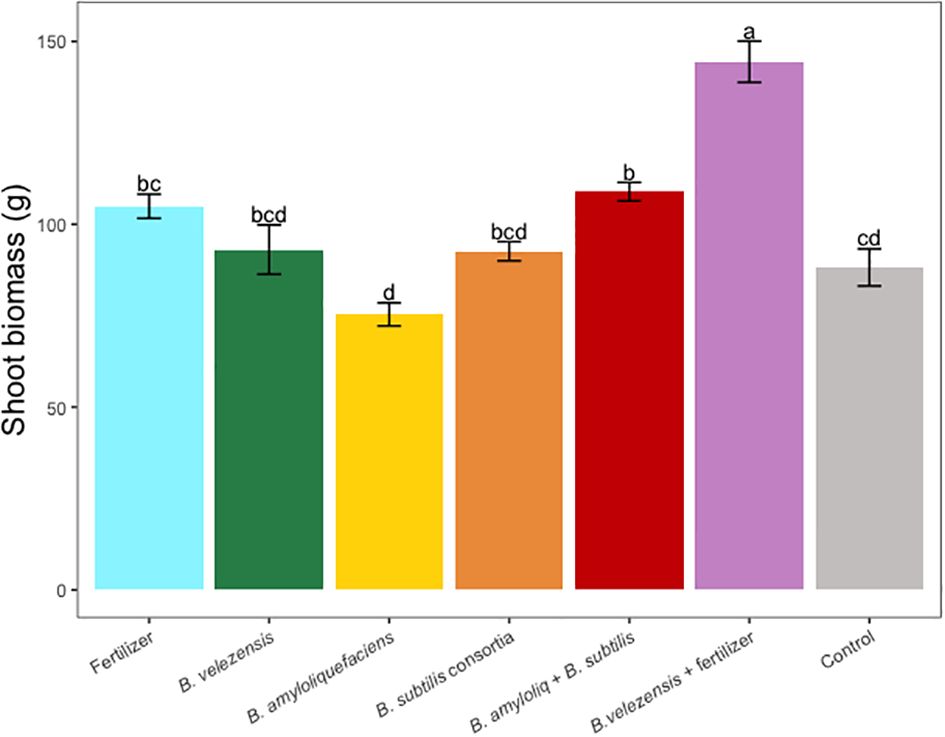
Figure 4 Average shoot (leaf + stem) dry biomass per tree for treatments. Mean values and standard deviations were employed for representation. Different letters indicate significant differences between treatments (P < 0.05).
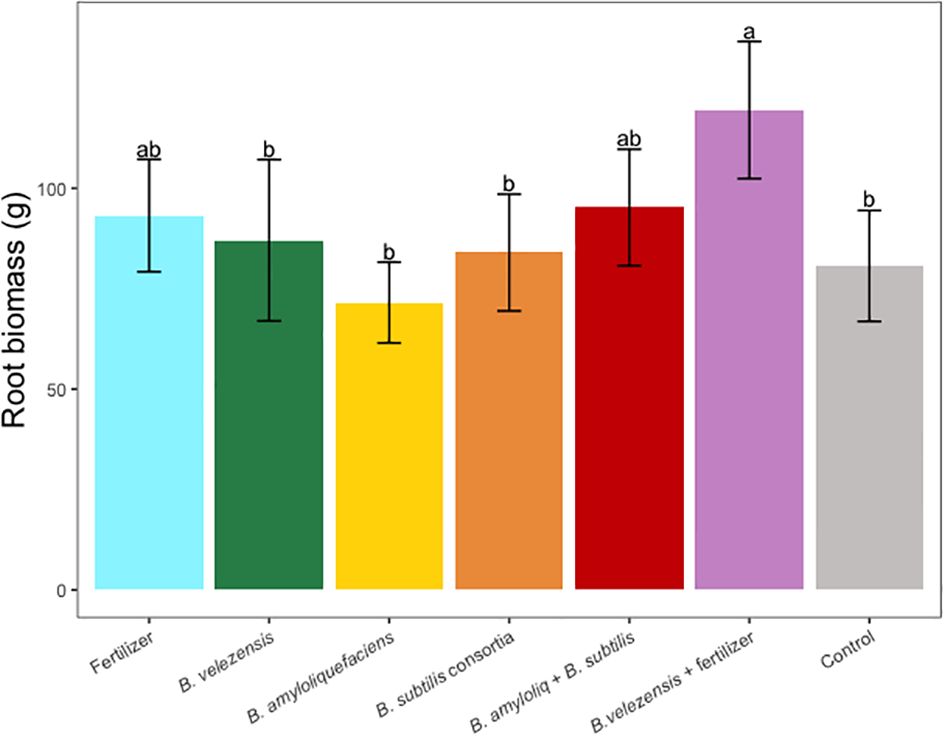
Figure 5 Average root dry biomass per tree for treatments. Mean values and standard deviations were employed for representation. Different letters indicate significant differences between treatments (P < 0.05).
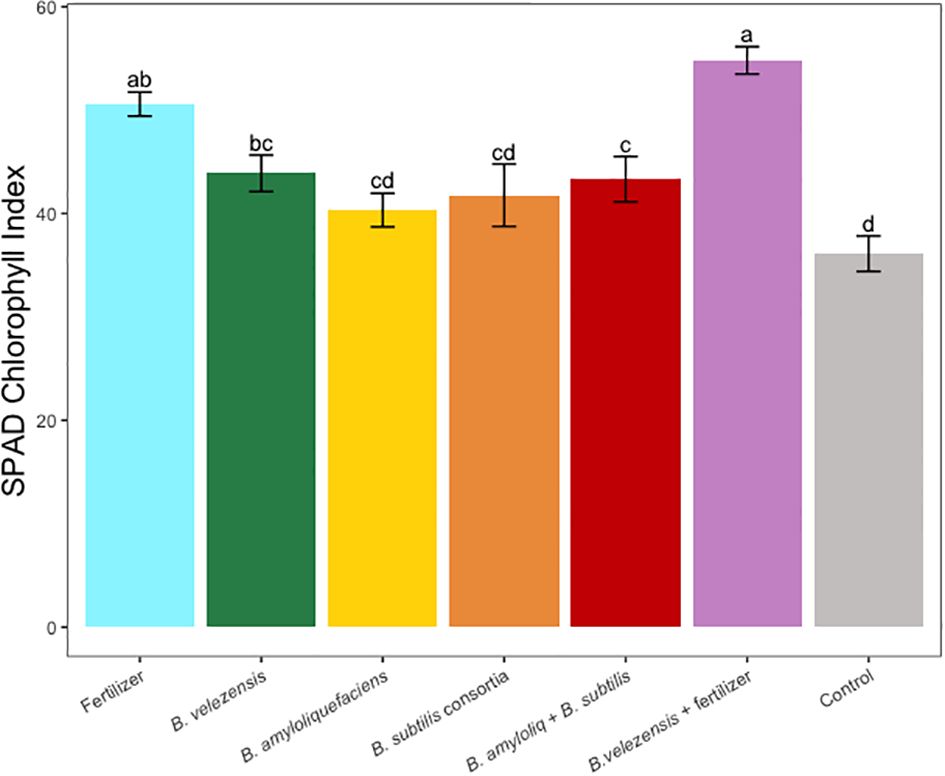
Figure 6 Average SPAD chlorophyll index for leaves sampled in treatments. Mean values and standard deviations were employed for representation. Different letters indicate significant differences between treatments (P < 0.05).
Soil inoculation with bacteria that promote plant growth is a promising technique for sustainable agricultural management and an effort to increase the efficiency of crops to uptake nutrient via symbiosis with microbes. In the present study, we anticipated that the applied bacteria would successfully establish around the rhizosphere in the potting media, as demonstrated in this study. However, when conducting microbial inoculation in open field soil conditions, the response of soil bacterial communities may be less consistent due to environmental variability such as tillage ( Sergaki et al., 2018 ), pesticides, fertilizers, and sewage sludge amendments applied in raw form ( Omotayo and Babalola, 2021 ). These environmental factors could disrupt the establishment and proliferation of the bacteria. Bacillus species are well known for their plant growth-promoting potential ( Gohil et al., 2022 ), and based on our results, we could see a marked increase in above and below-ground plant biomass with PGPR inoculation. Although the specific mechanisms by which PGPR promotes plant growth are not fully understood ( Backer et al., 2018 ), they are thought to include auxin secretion by B. velezensis , systemic induced resistance, phosphate solubilization ( Wang et al., 2020 ; Wang et al., 2022 ) pathogen control, and secondary metabolites ( Grobelak et al., 2015 ; Ghazy & El-Nahrawy, 2021 ) to protect and promote plant growth ( Wang et al., 2020 ).
Microbial respiration assay
We hypothesized that microbial respiration from the most effective bacterial treatments would vary compared to the controls, however there were no significant differences among the microbial respiration of the treatments ( Supplementary Figure 2 ). The positive and negative controls (untreated and fertilized) showed respiratory activity even though they were not inoculated with bacteria, and this indicates that unsterilized potting media can harbor a wide variety of microorganisms, whether they are beneficial ( Huang et al., 2012 ; Al-Mazroui and Al-Sadi, 2015 ) or pathogenic species ( Al-Sa’di et al., 2008 ; Al-Sadi et al., 2011 ). The fact that in the present work all the treatments showed microbial respiration activity suggests that it could have masked the activity of added PGPR treatments. From previous studies, we can identify the lack of analysis for bacterial communities’ characterization in potting media to assess changes in the activity of inoculated soil microbial communities ( Al-Sadi et al., 2016 ; Mawarda et al., 2022 ).
Leaf nutrient analyses
Inoculation with B. velezensis together with the addition of a low (25%) fertilizer rate significantly increased leaf nitrogen (N), magnesium (Mg), and sulfur (S) concentrations compared to the other treatments of Bacillus species along with the positive and negative control ( Table 3 ). Salvagiotti et al. (2009) reported a synergism in the uptake of N and S by plants, and a synergism of P and Mg uptake by plants was reported by Weih et al., 2021 . However, in our study P concentration differences were not significant ( Table 3 ) but increased levels of P uptake were observed from treatments inoculated with B. velezensis , B. amyloliquefaciens , and B. subtilis . Leaf concentrations of K, Ca ( Table 3 ), Mn, and Cu ( Table 4 ) were also not significantly different among treatments.
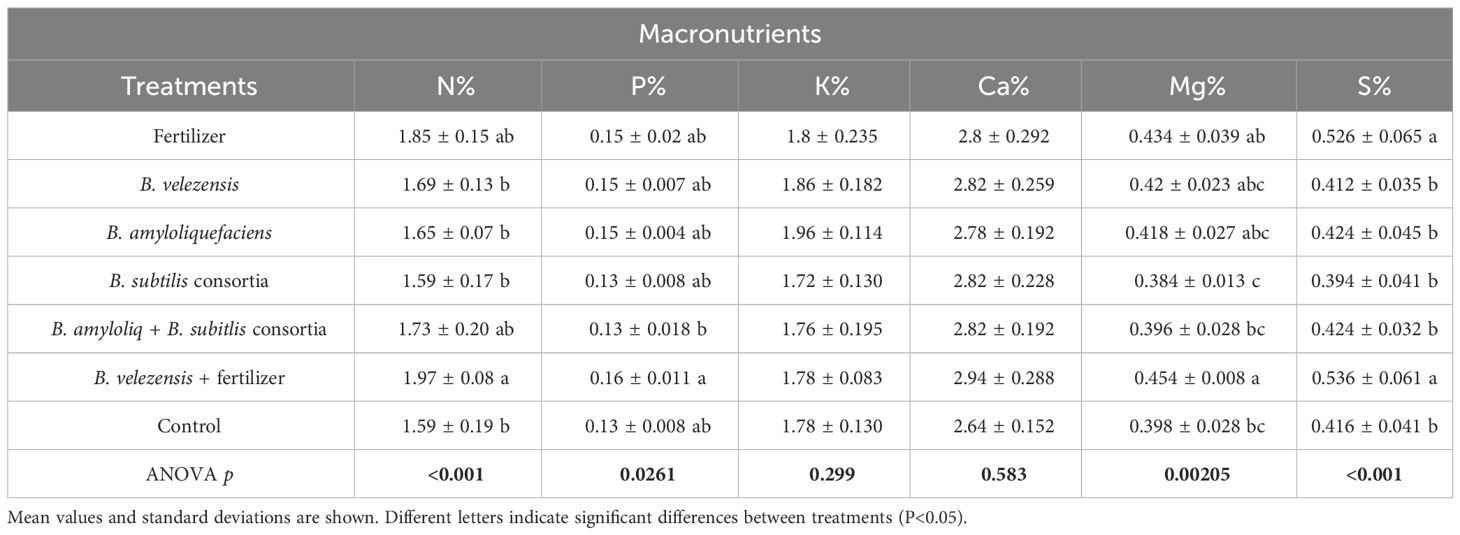
Table 3 The concentration of macronutrients in leaves for the different treatments.
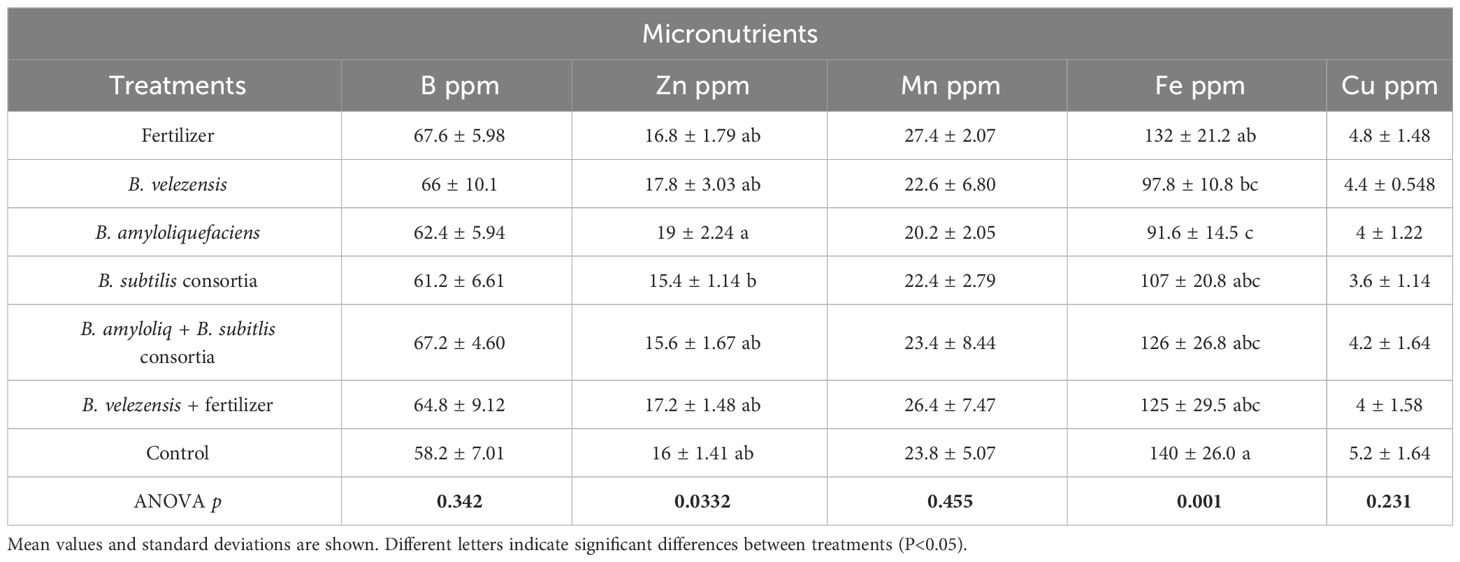
Table 4 The concentration of micronutrients in leaves for the different treatments.
Nitrogen is one of the essential nutrients for crop growth and has been widely studied ( Wang et al., 2014 ). In our study, due to a low rate of fertilization, leaf N concentration for all treatments was below the optimum rate required for citrus crops ( Obreza et al., 2020 ), however, leaf N concentration for the B. velezensis plus fertilizer treatment, significantly outperformed the rest of the treatments ( Table 3 ). A similar pattern was observed in the SPAD chlorophyll index ( Figure 6 ), where fertilizer treatment B. velezensis plus fertilizer had the highest average chlorophyll content correlated to N status in the leaf.
In the absence of fertilizer, there were non-significant differences in leaf nutrient concentrations among treatments inoculated only with bacteria ( Tables 3 , 4 ), emphasizing the importance of synergistically including fertilizer with PGPR inoculation as some previous studies found, increasing yield in tomato ( Solanum lycopersicum L.) ( Clagnan et al., 2023 ) and cowpea ( Vigna unguiculata L. Walp) ( Stamford et al., 2020 ). Surprisingly, leaf iron (Fe) concentrations were higher for the control treatment (T7; Table 4 ), although Fe concentration was at the optimum level for all the treatments. For the negative control (T7), it is possible that other nutrients were more limiting than Fe, resulting in a lack of biomass growth and a relative concentration of Fe in the leaves when compared to the more thrifty treatments.
Stamford et al. (2020) ; Clagnan et al. (2023) and Angulo et al. (2020) have examined the potential beneficial effects of microbial-based fertilizers on the rhizosphere in a competitive nutrient-limited environment. After soil inoculation, these microorganisms contribute to nitrogen fixation, solubilization/mobilization of soil nutrients, and secretion of substances that enhance plant growth ( Shahwar et al., 2023 ). The B. amyloliquefaciens + B subtilis consortia (T5) treatment accumulated the most leaf N per tree for any unfertilized treatment, and significantly more than B. amyloliquefaciens (T3; Supplementary Figure 4 ). PGPRs play a key role when unavailable nutrients are present in the soil or nearby the rhizosphere ( Prasad et al., 2019 ). From the regression line of N fertilizer applied versus leaf N accumulated (y = 0.4015x - 169.19), we estimated the amount of N contributed by PGPRs that was not derived by chemical N fertilizer. T6 was the highest (105 mg N/pot), followed by T5 (47 mg N/pot), T2 (16 mg N/pot), T4 (14 mg N/pot), and T3 (-23 mg N/pot). The negative value for T3 suggests microbial N immobilization. For T6, the amount of fertilizer N applied (82 mg N/pot) was subtracted from the interpolated result, leaving only the equivalent portion derived from PGPR activity (105 mg N/pot). The highest N fertilizer equivalent provided only by PGPRs was T5 ( B. amyloliquefaciens + triple B. subtilis consortia), which was 57% of the amount of chemical N fertilizer supplied in treatments 1 and 6. Gohil et al., 2022 reported that Bacillus sp. PG-8 was able to solubilize insoluble nutrients to help the plant growth. Phosphate solubilization is most frequently attributed to Bacillus isolates by 80% compared to Azotobacter , Pseudomonas , and least by Mesorhizobium ( Ahmad et al., 2008 ). The promotion of plant growth can be ascribed to various mechanisms, including the synthesis of plant growth-promoting hormones within the rhizosphere and the involvement in other plant growth-promoting activities ( Ahmad et al., 2008 ). In recent years, numerous strains of B. velezensis have been discovered and extensively examined for their impact on plant growth, disease, and pest resistance, and the induction of systemic plant defenses, while some strains have proven to be pivotal in enhancing crop yield.
Conclusions
This study demonstrated that PGPR inoculation using B. velezensis plus fertilizer as a root drench significantly increased root biomass, trunk diameter, tree height, canopy volume, and SPAD chlorophyll index of grapefruit seedlings. The combination of these factors can contribute to healthier and more robust plants. The increase in canopy volume is indicative of greater foliage density, which can have a direct influence on photosynthesis, light interception, and overall productivity. In the same context, the study also shows that the chlorophyll index, an indicator of photosynthetic activity, was significantly enhanced by the B. velezensis inoculation and fertilizer. Furthermore, the study reveals that leaf nutrient concentrations, particularly nitrogen (N), sulfur (S), and magnesium (Mg), were significantly increased in plants treated with PGPR and fertilizer compared to other treatments.
Another key finding of this research was the necessity of sufficient fertilizer in the root zone in order to obtain the desired benefits from PGPR inoculation. Future studies designed to establish the critical nutrient levels required for optimizing the function and benefits of PGPR would help refine recommendations for their widespread use in citrus cultivation. Nursery trees grown in artificial media and drenched with PGPR could reach marketable size sooner, with less resources. The increased root biomass of PGPR-grown nursery trees would be especially valuable during the transplanting and establishment of trees in the field.
Data availability statement
The raw data supporting the conclusions of this article will be made available by the authors, without undue reservation.
Author contributions
LC: Conceptualization, Data curation, Formal analysis, Investigation, Methodology, Software, Validation, Visualization, Writing – original draft, Writing – review & editing. JL: Resources, Writing – review & editing. NW: Resources, Writing – review & editing. SS: Writing – review & editing. AS: Conceptualization, Funding acquisition, Investigation, Methodology, Project administration, Resources, Software, Supervision, Writing – review & editing.
The author(s) declare financial support was received for the research, authorship, and/or publication of this article. This material is based upon work that is supported by the National Institute of Food and Agriculture, U.S. Department of Agriculture, under award number 2018-70016-27387.
Acknowledgments
We would like to recognize the assistance received from Napoleon Mariner, Perseverança Mungofa, Laura Waldo, and Timothy Ebert for their valuable guidance and experience.
Conflict of interest
The authors declare that the research was conducted in the absence of any commercial or financial relationships that could be construed as a potential conflict of interest.
Publisher’s note
All claims expressed in this article are solely those of the authors and do not necessarily represent those of their affiliated organizations, or those of the publisher, the editors and the reviewers. Any product that may be evaluated in this article, or claim that may be made by its manufacturer, is not guaranteed or endorsed by the publisher.
Supplementary material
The Supplementary Material for this article can be found online at: https://www.frontiersin.org/articles/10.3389/fhort.2024.1383013/full#supplementary-material
Supplementary Figure 1 | Average shoot/root dry biomass ratio for treatments. Mean values and standard deviations were employed for representation. Different letters indicate significant differences between treatments (P < 0.05).
Supplementary Figure 2 | Microbial respiration obtained from media samples represented as milligrams of CO 2 -C per gram of soil media respired by microbes. Mean values and standard deviations were employed for representation. Different letters indicate significant differences between treatments (P < 0.05).
Supplementary Figure 3 | Correlation relationship between leaf N accumulation per tree and root biomass per tree.
Supplementary Figure 4 | Leaf N accumulation per tree according to treatment.
Ahmad F., Ahmad I., Khan M. S. (2008). Screening of free-living rhizospheric bacteria for their multiple plant growth promoting activities. Microbiol. Res. 163, 173–181. doi: 10.1016/j.micres.2006.04.001
PubMed Abstract | CrossRef Full Text | Google Scholar
Akinrinlola R. J., Yuen G. Y., Drijber R. A., Adesemoye A. O. (2018). Evaluation of Bacillus strains for plant growth promotion and predictability of efficacy by in vitro physiological traits. Int. J. Microbiol. 2018, 11. doi: 10.1155/2018/5686874
CrossRef Full Text | Google Scholar
Al-Mazroui S. S., Al-Sadi A. M. (2015). Highly variable fungal diversity and the potential occurrence of plant pathogenic fungi in potting media, organic fertilizers and composts originating from 14 countries. J. Plant Pathol. 97, 6.
Google Scholar
Al-Sadi A. M., Al-Said F. A., Al-Jabri A. H., Al-Mahmooli I. H., Al-Hinai A. H., De Cock A. W. A. M. (2011). Occurrence and characterization of fungi and oomycetes transmitted via potting mixtures and organic manures. Crop Prot. 30, 38–44. doi: 10.1016/j.cropro.2010.09.015
Al-Sadi A. M., Al-Zakwani H. A., Nasehi A., Al-Mazroui S. S., Al-Mahmooli I. H. (2016). Analysis of bacterial communities associated with potting media. Springerplus 5, 1–5. doi: 10.1186/s40064-016-1729-0
Al-Sa’di A. M., Drenth A., Deadman M. L., Al-Said F. A., Khan I., Aitken E. A. B. (2008). Potential sources of Pythium inoculum into greenhouse soils with no previous history of cultivation. J. Phytopathol. 156, 502–505. doi: 10.1111/j.1439-0434.2008.01396.x
Anderson J. P. E., Domsch K. H. (1978). A physiological method for the quantitative measurement of microbial biomass in soil. Soil Biol. Biochem. 11, 215–221. doi: 10.1016/0038-0717(78)90099-8
Angulo J., Martínez-Salgado M. M., Ortega-Blu R., Fincheira P. (2020). Combined effects of chemical fertilization and microbial inoculant on nutrient use efficiency and soil quality indicators. Scientia Agropecuaria 11, 375–380. doi: 10.17268/sci.agropecu.2020.03.09
Antoszewski M., Mierek-Adamska A., Dąbrowska G. B. (2022). The importance of microorganisms for sustainable agriculture—a review. Metabolites 12, 1100. doi: 10.3390/metabo12111100
Backer R., Rokem J. S., Ilangumaran G., Lamont J., Praslickova D., Ricci E., et al. (2018). Plant growth-promoting rhizobacteria: Context, mechanisms of action, and roadmap to commercialization of biostimulants for sustainable agriculture. Front. Plant Sci. 9, 1473. doi: 10.3389/fpls.2018.01473
Barnawal D., Singh R., Singh R. P. (2019). “Role of plant growth promoting rhizobacteria in drought tolerance: regulating growth hormones and osmolytes,” in PGPR amelioration in sustainable agriculture (Cambridge, United Kingdom: Woodhead Publishing), 107–128. doi: 10.1016/B978-0-12-815879-1.00006-9
Blake C., Christensen M. N., Kovacs A. T. (2021). Molecular aspects of plant growth promotion and protection by bacillus subtilis. Mol. Plant-Microbe Interact. 34, 15–25. doi: 10.1094/MPMI-08-20-0225-CR
Bueno C. B., Dos Santos R. M., de Souza Buzo F., de Andrade da Silva M. S. R., Rigobelo E. C. (2022). Effects of chemical fertilization and microbial inoculum on Bacillus subtilis colonization in soybean and maize plants. Front. Microbiol. 13, 901157. doi: 10.3389/fmicb.2022.901157
Chen K., Tian Z., He H., Long C. A., Jiang F. (2020). Bacillus species as potential biocontrol agents against citrus diseases. Biol. control 151, 104419. doi: 10.1016/j.biocontrol.2020.104419
Clagnan E., Cucina M., De Nisi P., Dell’Orto M., D’Imporzano G., Kron-Morelli R., et al. (2023). Effects of the application of microbiologically activated bio-based fertilizers derived from manures on tomato plants and their rhizospheric communities. Sci. Rep. 13, 22478. doi: 10.1038/s41598-023-50166-5
Compant S., Samad A., Faist H., Sessitsch A. (2019). A review on the plant microbiome: Ecology, functions, and emerging trends in microbial application. J. Adv. Res. 19, 29–37. doi: 10.1016/j.jare.2019.03.004
Dala-Paula B. M., Plotto A., Bai J., Manthey J. A., Baldwin E. A., Ferrarezi R. S., et al. (2019). Effect of huanglongbing or greening disease on orange juice quality, a review. Front. Plant Sci. 9, 1976. doi: 10.3389/fpls.2018.01976
De Mendiburu F. (2022) agricolae: Statistical Procedures for Agricultural Research. R package version x.y.z. Available online at: https://CRAN.R-project.org/package=agricolae .
Elsharkawy M. M., Khedr A. A., Mehiar F., El-Kady E. M., Alwutayd K. M., Behiry S. I. (2023). Rhizobacterial colonization and management of bacterial speck pathogen in tomato by pseudomonas spp. Microorganisms 11, 1103. doi: 10.3390/microorganisms11051103
Etesami H., Jeong B. R., Glick B. R. (2023). Potential use of Bacillus spp. as an effective biostimulant against abiotic stresses in crops—A review. Curr. Res. Biotechnol. 5, 100128.
Fan B., Li L., Chao Y., Förstner K., Vogel J., Borriss R., et al. (2015). DRNA-seq reveals genomewide TSSs and noncoding RNAs of plant beneficial rhizobacterium Bacillus amyloliquefaciens FZB42. PloS One 10, e0142002. doi: 10.1371/journal.pone.0142002
Gamez R., Cardinale M., Montes M., Ramirez S., Schnell S., Rodriguez F. (2019). Screening, plant growth promotion and root colonization pattern of two rhizobacteria (Pseudomonas fluorescens Ps006 and Bacillus amyloliquefaciens Bs006) on banana cv. Williams (Musa acuminata Colla). Microbiol. Res. 220, 12–20. doi: 10.1016/j.micres.2018.11.006
Ghazy N., El-Nahrawy S. (2021). Siderophore production by Bacillus subtilis MF497446 and Pseudomonas koreensis MG209738 and their efficacy in controlling Cephalosporium maydis in maize plant. Arch. Microbiol. 203, 1195–1209. doi: 10.1007/s00203-020-02113-5
Gohil R. B., Raval V. H., Panchal R. R., Rajput K. N. (2022). Plant Growth-Promoting Activity of Bacillus sp. PG-8 Isolated From Fermented Panchagavya and Its Effect on the Growth of Arachis hypogea. Front. Agron. 4, 805454.
Gong H., Li J., Ma J., Li F., Ouyang Z., Gu C. (2018). Effects of tillage practices and microbial agent applications on dry matter accumulation, yield and the soil microbial index of winter wheat in North China. Soil Tillage Res. 184, 235–242. doi: 10.1016/j.still.2018.07.002
Grobelak A., Napora A., Kacprzak M. (2015). Using plant growth-promoting rhizobacteria (PGPR) to improve plant growth. Ecol. Eng. 84, 22–28. doi: 10.1016/j.ecoleng.2015.07.019
Hallman L., Kadyampakeni D., Ferrarezi R., Wright A., Ritenour M., Johnson E., et al. (2022). Impact of ground applied micronutrients on root growth and fruit yield of severely Huanglongbing-affected grapefruit trees. Horticulturae 8, 763. doi: 10.3390/horticulturae8090763
Hu G., Wang Y., Blake C., Nordgaard M., Liu X., Wang B., et al. (2023). Parallel genetic adaptation of Bacillus subtilis to different plant species. bioRxiv . 9, p001064. doi: 10.1099/mgen.0.001064
Huang T. P., Tzeng D. D. S., Wong A. C., Chen C. H., Lu K. M., Lee Y. H., et al. (2012). DNA polymorphisms and biocontrol of Bacillus antagonistic to citrus bacterial canker with indication of the interference of phyllosphere biofilms. PLoS ONE 7 (7), e42124. doi: 10.1371/journal.pone.0042124
Jamil F., Mukhtar H., Fouillaud M., Dufossé L. (2022). Rhizosphere signaling: Insights into plant–rhizomicrobiome interactions for sustainable agronomy. Microorganisms 10, 899. doi: 10.3390/microorganisms10050899
Kumar A., Prakash A., Johri B. N. (2011). “Bacillus as PGPR in crop ecosystem,” in Bacteria in agrobiology: crop ecosystems (Heidelberg, Germany: Springer Berlin Heidelberg), 37–59.
Kumar A., Singh V. K., Tripathi V., Singh P. P., Singh A. K. (2018). “Plant growth-promoting rhizobacteria (PGPR): perspective in agriculture under biotic and abiotic stress,” in New and future developments in microbial biotechnology and bioengineering: crop improvement through microbial biotechnology (Amsterdam, The Netherlands: Elsevier), 333–342. doi: 10.1016/B978-0-444-63987-5.00016-5
Kumar Meena R., Kumar Singh R., Pal Singh N., Kumari Meena S., Singh Meena V. (2015). Isolation of low temperature surviving plant growth - promoting rhizobacteria (PGPR) from pea (Pisum sativum L.) and documentation of their plant growth promoting traits. Biocatalysis Agric. Biotechnol. 4, 806–811. doi: 10.1016/j.bcab.2015.08.006
Li J., Pang Z., Lee D., Wang N. (2021). Biological control of citrus bacterial canker by native endophytic Bacillus spp. isolates. (Abstr.) Phytopathol. 111, S2.1. doi: 10.1094/PHYTO-111-10-S2.1
Lopez G., Ahmadi S. H., Amelung W., Athmann M., Ewert F., Gaiser T., et al. (2023). Nutrient deficiency effects on root architecture and root-to-shoot ratio in arable crops. Front. Plant Sci. 13, 5385. doi: 10.3389/fpls.2022.1067498
Luo L., Zhao C., Wang E., Raza A., Yin C. (2022). Bacillus amyloliquefaciens as an excellent agent for biofertilizer and biocontrol in agriculture: An overview for its mechanisms. Microbiol. Res. 259, 127016. doi: 10.1016/j.micres.2022.127016
Ma X., Li F., Chen Y., Chang Y., Lian X., Li Y., et al. (2022). Effects of fertilization approaches on plant development and fertilizer use of citrus. Plants 11, 2547. doi: 10.3390/plants11192547
Mawarda P. C., Lakke S. L., Dirk van Elsas J., Salles J. F. (2022). Temporal dynamics of the soil bacterial community following Bacillus invasion. IScience 25, 529–534. doi: 10.1016/j.isci.2022.104185
Mengistie G. Y., Awlachew Z. T. (2022). Evaluation of the plant growth promotion effect of Bacillus species on different varieties of tomato (Solanum lycopersicum L.) seedlings. Adv. Agric. 2022, 6. doi: 10.1155/2022/1771147
Numan M., Bashir S., Khan Y., Mumtaz R., Shinwari Z. K., Khan A. L., et al. (2018). Plant growth promoting bacteria as an alternative strategy for salt tolerance in plants: A review. Microbiol. Res. 209, 21–32. doi: 10.1016/j.micres.2018.02.003
Nwachukwu B. C., Ayangbenro A. S., Babalola O. O. (2021). Elucidating the rhizosphere associated bacteria for environmental sustainability. Agric. (Switzerland) 11, 1–18. doi: 10.3390/agriculture11010075
Obreza T., Zekri M., Hanlon E. (2020). Nutrition of Florida Citrus Trees, 3rd Edition: Chapter 4. Soil and Leaf Tissue Testing. EDIS . doi: 10.32473/edis-ss671-2020
Omotayo O. P., Babalola O. O. (2021). Resident rhizosphere microbiome’s ecological dynamics and conservation: Towards achieving the envisioned Sustainable Development Goals, a review. Int. Soil Water Conserv. Res. 9, 127–142. doi: 10.1016/j.iswcr.2020.08.002
Patani A., Prajapati D., Ali D., Kalasariya H., Yadav V. K., Tank J., et al. (2023). Evaluation of the growth-inducing efficacy of various Bacillus species on the salt-stressed tomato (Lycopersicon esculentum Mill.). Front. Plant Sci. 14, 1168155. doi: 10.3389/fpls.2023.1168155
Prasad M., Srinivasan R., Chaudhary M., Choudhary M., Jat L. K. (2019). Plant growth promoting rhizobacteria (PGPR) for sustainable agriculture. PGPR Amelioration Sustain. Agric. , 129–157. doi: 10.1016/B978-0-12-815879-1.00007-0
Rosier A., Beauregard P. B., Bais H. P. (2021). Quorum Quenching Activity of the PGPR Bacillus subtilis UD1022 Alters Nodulation Efficiency of Sinorhizobium meliloti on Medicago truncatula. Front. Microbiol. 11, 596299. doi: 10.3389/fmicb.2020.596299
Salvagiotti F., Castellarín J. M., Miralles D. J., Pedrol H. M. (2009). Sulfur fertilization improves nitrogen use efficiency in wheat by increasing nitrogen uptake. Field Crops Res. 113, 170–177. doi: 10.1016/j.fcr.2009.05.003
Schumann A., Singerman A., Ritenour M., Qureshi J., Alferez F. (2023). 2023–2024 Florida Citrus Production Guide: Citrus under Protective Screen (CUPS) Production Systems: CPG ch. 21, HS1304/CMG19, rev. 5/2023 (University of Florida, USA: EDIS). doi: 10.32473/edis-hs1304-2023
Senger M., Moresco E., Dalbosco M., Santin R., Inderbitzin P., Barrocas E. N. (2022). Methods to quantify Bacillus simplex-based inoculant and its effect as a seed treatment on field-grown corn and soybean in Brazil. J. Seed Sci. 44, 13. doi: 10.1590/2317-1545v44263329
Sergaki C., Lagunas B., Lidbury I., Gifford M. L., Schäfer P. (2018). Challenges and approaches in microbiome research: from fundamental to applied. Front. Plant Sci. 9, 1205. doi: 10.3389/fpls.2018.01205
Shahwar D., Mushtaq Z., Mushtaq H., Alqarawi A. A., Park Y., Alshahrani T. S., et al. (2023). Role of microbial inoculants as biofertilizers for improving crop productivity: a review. Heliyon 9 (2), e16134. doi: 10.1016/j.heliyon.2023.e16134
Shen Y., Yang H., Lin Z., Chu L., Pan X., Wang Y., et al. (2023). Screening of compound-formulated Bacillus and its effect on plant growth promotion. Front. Plant Sci. 14, 1174583. doi: 10.3389/fpls.2023.1174583
Shi J. W., Lu L. X., Shi H. M., Ye J. R. (2022). Effects of plant growth-promoting rhizobacteria on the growth and soil microbial community of Carya illinoinensis. Curr. Microbiol. 79, 352. doi: 10.1007/s00284-022-03027-9
Shi H., Lu L., Ye J., Shi L. (2022). Effects of two bacillus velezensis microbial inoculants on the growth and rhizosphere soil environment of prunus davidiana. Int. J. Mol. Sci. 23, 13639. doi: 10.3390/ijms232113639
Stamford N. P., da Silva E. V. N., da Silva Oliveira W., dos Santos Martins M., Moraes A. S., de Barros J. A., et al. (2020). Benefits of microbial fertilizer in interspecific interaction with textile sludges on cowpea in a Brazilian Ultisol and on wastes toxicity. Environ. Technol. Innovation 18, 100756. doi: 10.1016/j.eti.2020.100756
Sun X., Xu Z., Xie J., Hesselberg-Thomsen V., Tan T., Zheng D., et al. (2022). Bacillus velezensis stimulates resident rhizosphere Pseudomonas stutzeri for plant health through metabolic interactions. ISME J. 16, 774–787. doi: 10.1038/s41396-021-01125-3
Thorne M. S., Skinner Q. D., Smith M. A., Rodgers J. D., Laycock W. A., Cerekci S. A. (2002). Evaluation of a technique for measuring canopy volume of shrubs. Rangeland Ecol. Management/Journal Range Manage. Arch. 55, 235–241.
Tsotetsi T., Nephali L., Malebe M., Tugizimana F. (2022). Bacillus for plant growth promotion and stress resilience: What have we learned? Plants 11, 2482. doi: 10.3390/plants11192482
Vashisth T., Chun C., Ozores Hampton M. (2020). Florida citrus nursery trends and strategies to enhance production of field-transplant ready citrus plants. Horticulturae 6, 8. doi: 10.3390/horticulturae6010008
Wang J., Qu F., Liang J., Yang M., Hu X. (2022). Bacillus velezensis SX13 promoted cucumber growth and production by accelerating the absorption of nutrients and increasing plant photosynthetic metabolism. Scientia Hortic. 301, 111151. doi: 10.1016/j.scienta.2022.111151
Wang Y., Wang D., Shi P., Omasa K. (2014). Estimating rice chlorophyll content and leaf nitrogen concentration with a digital still color camera under natural light. Plant Methods 10, 1–11. doi: 10.1186/1746-4811-10-36
Wang C., Zhao D., Qi G., Mao Z., Hu X., Du B., et al. (2020). Effects of Bacillus velezensis FKM10 for Promoting the Growth of Malus hupehensis Rehd. Inhibiting Fusarium verticillioides. Front. Microbiol. 10, 2889. doi: 10.3389/fmicb.2019.02889
Wang H. W., Zhu Y. X., Xu M., Cai X. Y., Tian F. (2022). Co-application of spent mushroom substrate and PGPR alleviates tomato continuous cropping obstacle by regulating soil microbial properties. Rhizosphere 23, 100563. doi: 10.1016/j.rhisph.2022.100563
Weih M., Liu H., Colombi T., Keller T., Jäck O., Vallenback P., et al. (2021). Evidence for magnesium-phosphorus synergism and co-limitation of grain yield in wheat agriculture. Sci. Rep. 11, 9012. doi: 10.1038/s41598-021-88588-8
Zahra S. T., Tariq M., Abdullah M., Azeem F., Ashraf M. A. (2023). Dominance of Bacillus species in the wheat (Triticum aestivum L.) rhizosphere and their plant growth promoting potential under salt stress conditions. PeerJ 11, e14621.
PubMed Abstract | Google Scholar
Keywords: PGPR, beneficial bacteria, nutrient uptake, growth promotion, sustainable production, biofertilizer
Citation: Cano-Castro L, Li J, Wang N, Strauss SL and Schumann AW (2024) Enhancing nutrient uptake and grapefruit ( Citrus paradisi ) growth through soil application of beneficial bacteria ( Bacillus spp.). Front. Hortic. 3:1383013. doi: 10.3389/fhort.2024.1383013
Received: 06 February 2024; Accepted: 22 April 2024; Published: 03 May 2024.
Reviewed by:
Copyright © 2024 Cano-Castro, Li, Wang, Strauss and Schumann. This is an open-access article distributed under the terms of the Creative Commons Attribution License (CC BY) . The use, distribution or reproduction in other forums is permitted, provided the original author(s) and the copyright owner(s) are credited and that the original publication in this journal is cited, in accordance with accepted academic practice. No use, distribution or reproduction is permitted which does not comply with these terms.
*Correspondence: Arnold W. Schumann, [email protected]
This article is part of the Research Topic
Citriculture: Sustaining Quality Production
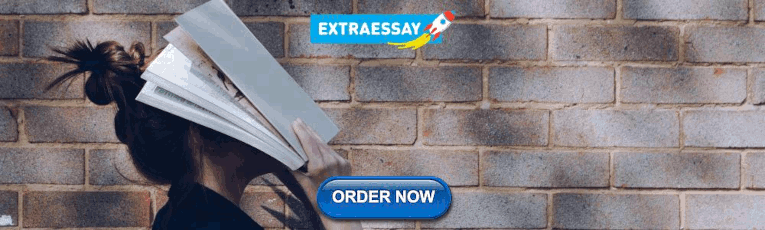
IMAGES
VIDEO
COMMENTS
National Institute For Plant Biotechnology (NIPB) is a premiere research institution of the Indian Council of Agricultural Research (ICAR). The institute was founded in 1985 as the 'Biotechnology Centre' of Indian Agricultural Research Institute (IARI) for molecular biology and biotechnology research in crop plants.
National Institute For Plant Biotechnology (NIPB) is a premier research institution of the Indian Council of Agricultural Research (ICAR). The institute was founded in 1985 as the 'Biotechnology Centre' of Indian Agricultural Research Institute (IARI) for molecular biology and biotechnology research in crop plants.
Top ten Profiles. Dr N K Singh Professor. 186 Publications. Projects. 15299. 11565. View Profile. Dr Kishor Gaikwad Principal Scientist. 98 Publications.
Institute Address National Reserach Center on Plant Biotechnology , Pusa Campus , New Delhi , New Delhi , 110012 Phone : 91-011-25848783 | Fax : 91-011-25843984 Email : [email protected] Website : www.nrcpb.org
National Institute for Plant Biotechnology (NIPB) LBS Centre, Pusa Campus, New Delhi - 110012, INDIA. ... This website belongs to National Institute for Plant Biotechnology, Indian Council of Agricultural Research, an autonomous organization under the Department of Agricultural Research and Education, Ministry of Agriculture, Government of ...
Overview. (06/08/2021- Excerpt from Institution Website) National Institute For Plant Biotechnology (NIPB) is a premiere research institution of the Indian Council of Agricultural Research (ICAR). The institute was founded in 1985 as the 'Biotechnology Centre' of Indian Agricultural Research Institute (IARI) for molecular biology and ...
National Research Centre for Plant Biotechnology, Hisar ( CPB ), a collaborator of ICAR, is responsible for the research, genetic diversity analysis, propagation of tissue culture technology, training and mass propagation of planting material of newly developed fruits, crop varieties and rare medicinal, horticultural, forest, ornamental and ...
ICAR-National Institute for Plant Biotechnology, New Delhi organized a two-day workshop for school students (IX-XII) on "Plant Biotechnology: Science, Applications, and Opportunities" during November 10-11, 2022. The workshop was sponsored by the Society for Plant Biochemistry & Biotechnology, New Delhi. The Chief guest of the valedictory ...
Share This. National Research Centre on Plant Biotechnology (NRCPB) is a premiere research institution of the Indian Council of Agricultural Research (ICAR). The institute was founded in 1985 as the 'Biotechnology Centre' of Indian Agricultural Research Institute (IARI) for molecular biology and biotechnology research in crop plants.
ICAR-National Research Centre on Mithun, Medziphema, Nagaland: 9. ICAR-National Research Centre on Orchids, Pakyong, Sikkim: 10. ICAR-National Research Centre on Pig, Guwahati: 11. ICAR-National Research Centre on Plant Biotechnology, New Delhi: 12. ICAR-National Research Centre on Seed Spices, Ajmer: 13. ICAR-National Research Centre on Yak ...
He served National Research Centre on Plant Biotechnology, IARI, New Delhi as researcher and teacher for about 20 years. He is a scientist of global repute working in the area of molecular genetics and genomics. Dr. Mohapatra has over 145 research papers in national and international journals of repute and several book chapters.
Affiliations 1 ICAR-National Research Centre on Plant Biotechnology, ICAR-Indian Agricultural Research Institute, New Delhi, 110012, India.; 2 Division of Crop Improvement, ICAR-Central Tuber Crops Research Institute, Thiruvananthapuram, 695017, India.; 3 TERI-Deakin Nanobiotechnology Centre, Gurgaon, 122 001, India.; 4 ICMR-All India Institute of Medical Science, Ansari Nagar, New Delhi ...
ICAR-National Research Center on Plant Biotechnology, New Delhi, India. View editor publications. You can also search for this editor in PubMed Google Scholar. Highlights the latest advances in plant molecular mapping and genome sequencing ... ICAR-National Research Center on Plant Biotechnology, New Delhi, India
ICAR-National Institute for Plant Biotechnology Lal Bahadur Shastri Centre, Pusa Campus New Delhi - 110012 www.nipb.icar.gov.in AnnuAl RepoRt 2020. ii Published by ... ICAR-NIPB Research Achievements 1. Search and Deployment of Genes for Stress Tolerance and Grain Quality in Rice 1
1 ICAR-National Research Centre on Plant Biotechnology, Indian Agricultural Research Institute, New Delhi, 110012, India. 2 TERI-Deakin NanoBiotechnology Centre, The Energy and Resources Institute, New Delhi, 110003, India. 3 ICAR-Central Tuber Crops Research Institute, Thiruvananthapuram, 695017, India.
ICAR-Indian Institute of Wheat and Barley Research, Regional Station, Flowerdale, Shimla Current institution ICAR-national research centre on plant biotechnology, New Delhi
sponsored by ICAR; 1-21 February, 2016, ICAR-National Research Centre on Plant Biotechnology, New Delhi, India. 125 pp Practical Manual Compiled and Edited by Dr. Prasanta K Dash, Senior Scientist & Course Director ICAR-National Research Centre on Plant Biotechnology, New Delhi-110012, India Course co-ordinators Dr. Rhitu Rai, Senior Scientist
National Institute for Plant Biotechnology (NIPB) LBS Centre, Pusa Campus, New Delhi - 110012, INDIA. ... This website belongs to National Institute for Plant Biotechnology, Indian Council of Agricultural Research, an autonomous organization under the Department of Agricultural Research and Education, Ministry of Agriculture, Government of ...
ICAR-National Institute for Plant Biotechnology (earlier National Research Centre on Plant Biotechnology) Pusa Delhi INDIA Pham Thi Thu Ha High Agricultural Technology Research Institute for ...
The term plant growth-promoting rhizobacteria (PGPR) refers to a root-associated bacteria that possesses several benefits for soil and the plant. The increasing demand for sustainable food production necessitates a shift towards agricultural practices that mitigate adverse environmental impacts. Excessive use of chemical fertilizers and pesticides has raised concerns, prompting a surge of ...
National Institute for Plant Biotechnology (NIPB) LBS Centre, Pusa Campus, New Delhi - 110012, INDIA. ... This website belongs to National Institute for Plant Biotechnology, Indian Council of Agricultural Research, an autonomous organization under the Department of Agricultural Research and Education, Ministry of Agriculture, Government of ...
biotechnology research in crop plants. The prescience of the role of biotechnology in agriculture led to a bigger responsibility for this centre and it was elevated as National Research Centre on Plant Biotechnology (NRCPB) in the year 1993 (Now NIPB). ICAR-National Institute for Plant Biotechnology
Student Internship Position in Biotechnology/ Computational Biology Applications are invited for one position for Student internship as part of Social Scientific Responsibility under Science and Engineering Research Board (SERB) funded project at National Institute for Plant Biotechnology (NIPB), New Delhi.