Cancer Genomics
Affiliations.
- 1 Instituto Nacional de Medicina Genómica, Ciudad de México, México; Laboratorio de Genómica del Cáncer, Instituto Nacional de Medicina Genómica, Ciudad de México, México.
- 2 Instituto Nacional de Medicina Genómica, Ciudad de México, México; Cátedras Consejo Nacional de Ciencia y Tecnología, Ciudad de México, México; Laboratorio de Genómica del Cáncer, Instituto Nacional de Medicina Genómica, Ciudad de México, México.
- 3 Instituto Nacional de Medicina Genómica, Ciudad de México, México.
- 4 Instituto Nacional de Medicina Genómica, Ciudad de México, México; Laboratorio de Genómica del Cáncer, Instituto Nacional de Medicina Genómica, Ciudad de México, México. Electronic address: [email protected].
- PMID: 36460546
- DOI: 10.1016/j.arcmed.2022.11.011
In the past decade, genomics has fundamentally changed our view of cancer biology, allowing comprehensive analyses of mutations, copy number alterations, structural variants, gene expression and DNA methylation profiles in large-scale studies across different cancer types. Efforts like The Cancer Genome Atlas (TCGA) and the International Cancer Genome Consortium (ICGC) have fostered international collaborations for cancer genomic analyses and have generated public databases that give scientists around the world access to thoroughly curated data, which have been extensively used as a tool for further hypothesis driven research on several aspects of cancer biology. In parallel, some of these findings are being translated into specific clinical benefits for cancer patients. In this review, we provide a brief historical description of the evolution of international public cancer genome projects and related databases, as well as we discuss about their impact on general cancer research.
Copyright © 2022. Published by Elsevier Inc.
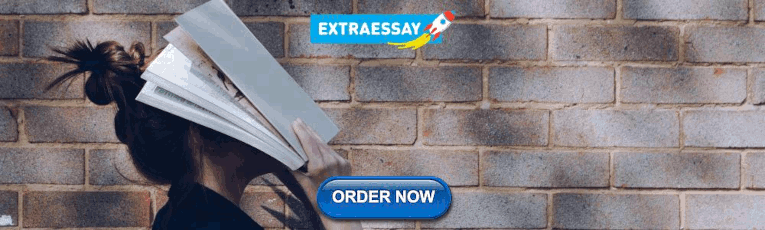
Publication types
- DNA Copy Number Variations
- Neoplasms* / genetics
- Introduction to Genomics
- Educational Resources
- Policy Issues in Genomics
- The Human Genome Project
- Funding Opportunities
- Funded Programs & Projects
- Division and Program Directors
- Scientific Program Analysts
- Contact by Research Area
- News & Events
- Research Areas
- Research investigators
- Research Projects
- Clinical Research
- Data Tools & Resources
- Genomics & Medicine
- Family Health History
- For Patients & Families
- For Health Professionals
- Jobs at NHGRI
- Training at NHGRI
- Funding for Research Training
- Professional Development Programs
- NHGRI Culture
- Social Media
- Broadcast Media
- Image Gallery
- Press Resources
- Organization
- NHGRI Director
- Mission & Vision
- Policies & Guidance
- Institute Advisors
- Strategic Vision
- Leadership Initiatives
- Diversity, Equity, and Inclusion
- Partner with NHGRI
- Staff Search
Cancer Genomics
Genomics is transforming how we study, diagnose and treat cancer.
Introduction
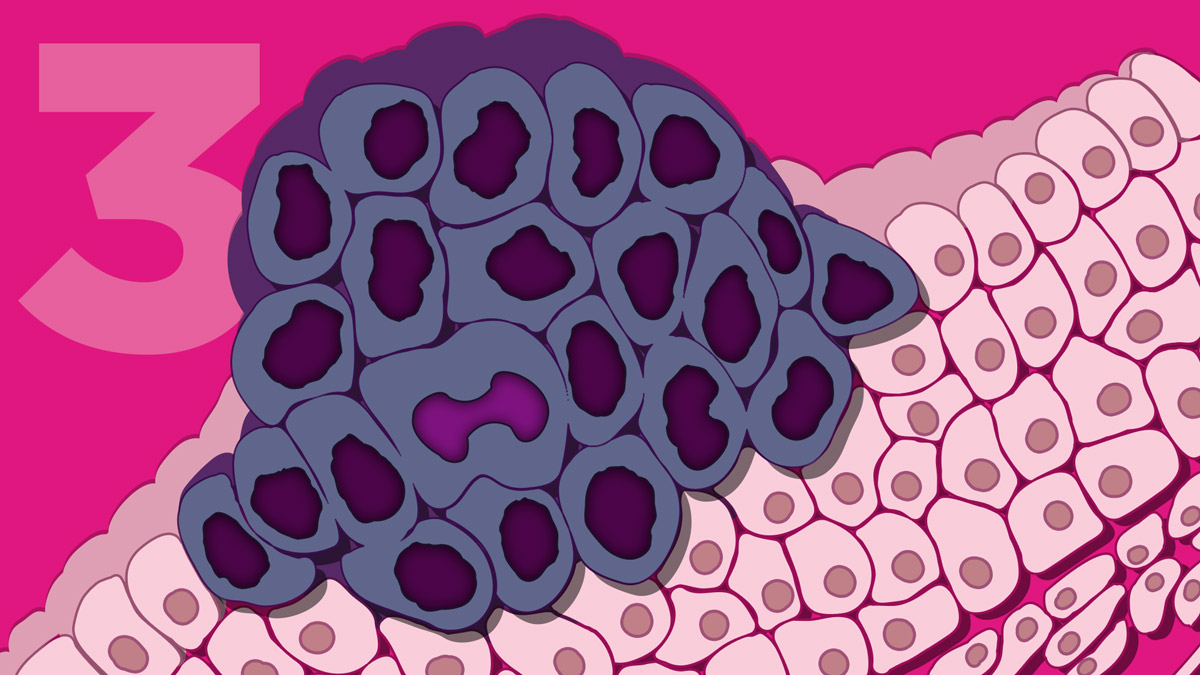
Did you know that we are increasingly able to detect cancers by testing just a blood sample? Or that we are moving toward treating cancers not by where they are found in the body, but by how their genomes have changed? Cancer is caused by changes in your genome, but advances in DNA sequencing technology are leading to a new understanding of cancer and new ways for diagnosing and treating many types of cancer.
Cancer is a group of genetic diseases that result from changes in the genome of cells in the body, leading them to grow uncontrollably. These changes involve DNA mutations in the genome. Our cells are constantly finding and fixing mutations that occur in our genome as the cells divide over and over again. But on rare occasions, some mutations slip through our cells' repair machinery, and those mutations can lead to cancer. The Human Genome Project has allowed us to establish what "normal" usually looks like for a human genome, so that we can now tell when changes in our genome have taken place that lead to cancer.
Large projects around the world, like The Cancer Genome Atlas in the United States and the Catalogue of Somatic Mutations (COSMIC) in the United Kingdom, have now determined the genome sequences of thousands of cancer samples of many cancer types. These projects have shown that some cancers have mutations in the same group of genes, even though they may have started in completely different tissues. Many of the mutations activate genes that normally promote cell growth or break genes that normally prevent cell growth. If we know more about the specific mutations that led to someone's cancer, no matter what tissue it was located in, then we can look for more specific and effective treatments for their cancer.
Using the Genome to Treat Cancer
Dr. Lukas Wartman , a physician and a researcher at Washington University, has both led cancer genomics efforts and experienced them himself. Lukas was diagnosed with a blood cancer called acute lymphoblastic leukemia (or ALL) in 2003, when he was in his fourth year of medical school. This led him to specialize in treating patients with leukemia, and studying the disease in the laboratory. While his university was helping pioneer cancer genome sequencing, Lukas suffered a relapse of his ALL with severe symptoms. So the laboratory, including Lukas's mentor, asked if they could study him.
The team compared the genome sequences of his normal cells and those of his cancerous blood cells. In his cancer's genome, they found mutations in a gene called FLT3 . The team then found that there was an existing medication that could be used for treating patients with mutations in this gene. Although it had originally been approved for treating other cancers, not blood cancer, they tried it anyway. Lukas started taking the medicine on a Friday, and his blood counts already showed better results by Monday. After a number of weeks, the leukemia cells were no longer detectable in his blood, meaning his cancer was in remission. He will still need more treatment, but Lukas would not have survived without the results of the genome sequencing that pointed him to a new therapy.
There are over 10,000 clinical trials for new therapies in cancer that are recruiting right now in the United States. In addition, cases like Lukas' have led to the resurgence of " n-of-1 " clinical trials, where doctors can gather enough information from just one patient to try a new therapy. Such trials are aided by patients who share their genomic data with researchers or even openly with other patients. Increasingly there is hope that as clinicians evaluate new cancer patients, they will be able to compare each new case with these large databases that might include positive responses to medications approved for a different cancer but with the same mutation(s), just like Lukas found for ALL.
Blood Tests to Detect Cancer
Unfortunately, some cancers are harder to evaluate because looking at their genomes would require difficult and painful biopsies or operations where tiny parts of the cancer tissue are removed for study. This also makes it harder for clinicians to monitor how treatment is working for some cancers because repeated biopsies are just not possible. Recent breakthroughs now allow the detection of circulating tumor DNA (or ctDNA ) in the blood of patients instead of directly sampling the tumor. As cancer cells grow very fast and die, they release some of their DNA into the bloodstream. We now have tests that are sensitive enough to detect and sequence these pieces of ctDNA in the bloodstream separately from the normal DNA of the patient - this is called a "liquid biopsy."
Although liquid biopsies are not yet in widespread use for cancer detection, improvements are being made all the time that move us closer to the routine use of ctDNA tests . One of the current approved uses for ctDNA is to test progression of non-small cell lung cancer by looking for specific mutations in the EGFR gene over time. Liquid biopsies can point to who will likely relapse after treatment, by detecting DNA with EGFR mutations that is circulating in the blood, sometimes better and more quickly than the now-used standard imaging techniques.
When Cancer Runs in Your Family
If you have a number of relatives who have developed cancer, you might be concerned about your own risk. By far, most cancers are not inherited, but there are a few examples of inherited cancers like Lynch syndrome (also known as hereditary non-polyposis colorectal cancer). This disorder is due to inherited mutations in any of six different genes , and leads to an increased risk of different types of cancers, most often in the colon. Breast cancer is another example; again most cases are not inherited, but men or women who have inherited mutations in the BRCA1 or BRCA2 genes have a much higher chance for developing breast cancer than other people. As we learn more about the genomic changes predisposing a person to cancer, we have been able to make screening tests available to many more people. The specific DNA sequences of the BRCA1 and BRCA2 genes were even the subject of a legal case that went all the way to the United States Supreme Court, who ruled that the sequences of your genes could not be patented. Before this ruling, only one company could provide BRCA1 or BRCA2 testing in the United States, but now there are a number of companies who can help if you'd like to have genomic testing for hereditary causes of breast cancer.
Science For Everyone
You can learn more about fighting cancer through the games at Re-Mission 2 . Cancer patients can learn more about current clinical trials through the NIH Clinical Trial Registry. If you have your own cancer genomic data that you'd like to share to help scientific discovery, you can talk to your oncologist and check out cBioPortal , Free the Data , or the Metastatic Breast Cancer Project .
Additional Resources:
- National Cancer Institute - Cancer Genomics Overview
- NHGRI - Circulating Tumor DNA: A New Generation of Cancer Biomarkers
- American Cancer Society - Cancer A-Z
- Cleveland's PBS Station IdeaStream - Be Well Cancer Research
Resources for Educators:
- Career Profile: Medical Geneticist | Video
- HHMI BioInteractive - Cancer Discovery Hands-On Activities
- HHMI BioInteractive - The Eukaryotic Cell Cycle and Cancer Interactive
- SciShow - Why Haven't We Cured Cancer?
- National Center for Case Study Teaching in Science - Colon Cancer: A Case of Genetic Bad Luck?
- The National Cancer Institute - The Genetics of Cancer
Resources for Healthcare Providers:
- National Cancer Institute - PDQ Cancer Information Summaries
- City of Hope - Cancer Genomics Education Program
- The Jackson Laboratory - Genetic Testing for Breast Cancer Course
- NHGRI - My Family Health Portrait: Family Tree to Review for Inherited Health Conditions
- The American College of Clinical Pharmacy and the American Society of Health-System Pharmacists - Oncology Pharmacy Preparatory Review and Recertification Course
- American Society of Human Genetics - Cancer Genomics Infographic
Last updated: April 9, 2018
We've updated our Privacy Policy to make it clearer how we use your personal data. We use cookies to provide you with a better experience. You can read our Cookie Policy here.
Informatics
Stay up to date on the topics that matter to you
Scientific Journal Publishes Paper With AI-Generated Introduction
A high-profile journal has published a paper featuring an ai-generated introductory sentence..

Complete the form below to unlock access to ALL audio articles.
A peer-reviewed scientific paper has gathered significant attention on the social media platform X (formerly Twitter), albeit for unfavorable reasons.
Published in Elsevier’s Surfaces and Interfaces journal, the first line of said paper’s introduction reads: “ Certainly, here is a possible introduction for your topic” .
If you’ve had any interaction with ChatGPT or other large language models (LLMs), you’re likely well acquainted with this phrasing.
It suggests that AI-assisted technologies have been utilized in writing the manuscript, titled: The three-dimensional porous mesh structure of Cu-based metal-organic-framework - aramid cellulose separator enhances the electrochemical performance of lithium metal anode batteries .
Though Elsevier does not condemn the adoption of AI and AI-assisted technologies in manuscript writing, its policies do require that authors disclose this information.
“We ask authors who have used AI or AI-assisted tools to insert a statement at the end of their manuscript immediately above the references or bibliography entitled ‘Declaration of AI and AI-assisted technologies in the writing process’. In that statement, we ask authors to specify the tool that was used and the reason for using the tool,” the journal’s website reads .
Despite the clear-cut evidence that LLMs have been used, the authors – Zhang et al. – failed to include such statement, which begs the question: how has this paper survived the processes preceding peer-review, peer-review itself and, finally, publication?
Want more breaking news?
Subscribe to Technology Networks ’ daily newsletter, delivering breaking science news straight to your inbox every day.
Elsevier has responded via X, stating, “Our policies are clear that LLMs can be used in the drafting of papers as long as it is declared by the authors on submission. We are investigating this paper and are in discussion with editorial team and the authors.” The journal has not yet released any further information.
The viral X thread features members of the scientific community expressing their frustration and disappointment with the situation. It also highlights other instances where LLM-generated text features in peer-reviewed journals, some of which are several months old and appear to have not yet been addressed.
AI’s future in scientific publishing
The controversial Elsevier paper comes at a time when the value, risks and ethical implications of using AI in scientific publishing are under constant evaluation.
While some tout the benefits of AI-assisted plagiarism detection, text optimization and the tailoring of articles, others heed caution at the technologies’ potential to introduce factual inaccuracies, debates surrounding authorship and biases into the literature.
At Technology Networks, we want to hear from you – what do you think about the use of AI and AI-assisted tools in scientific publication?
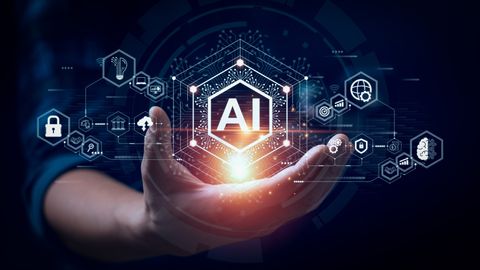
The Ethical Implications of AI in Scientific Publishing

The Cancer Genome Atlas Program (TCGA)
The Cancer Genome Atlas (TCGA), a landmark cancer genomics program, molecularly characterized over 20,000 primary cancer and matched normal samples spanning 33 cancer types. This joint effort between NCI and the National Human Genome Research Institute began in 2006, bringing together researchers from diverse disciplines and multiple institutions.
Over the next dozen years, TCGA generated over 2.5 petabytes of genomic, epigenomic, transcriptomic, and proteomic data. The data, which has already led to improvements in our ability to diagnose, treat, and prevent cancer, will remain publicly available for anyone in the research community to use.
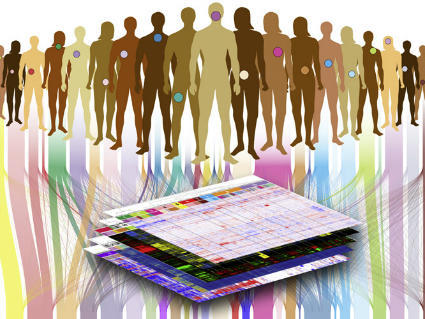
TCGA Outcomes & Impact
TCGA has changed our understanding of cancer, how research is conducted, how the disease is treated in the clinic, and more.
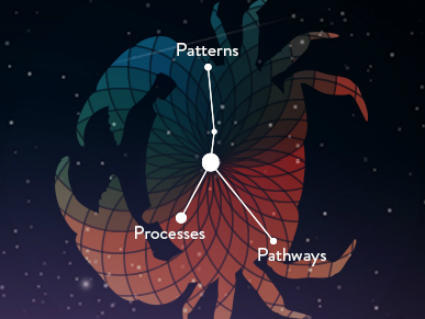
TCGA's Pan-Cancer Atlas
A collection of cross-cancer analyses delving into overarching themes on cancer, including cell-of-origin patterns, oncogenic processes, and signaling pathways. Published in 2018 at the program's close
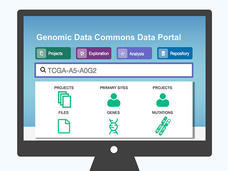
Access TCGA Data
Access TCGA data through the Genomic Data Commons Data Portal, along with web-based analysis and visualization tools.
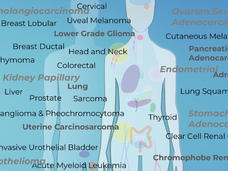
TCGA Cancers Selected for Study
An overview of the 33 cancers selected for molecular characterization and the criteria TCGA used to select them.
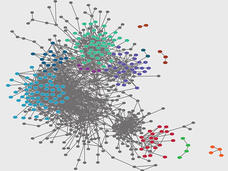
TCGA Computational Tools
Some of the data processing, visualization, and other computational tools developed by TCGA network researchers and collaborators.
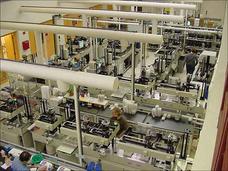
TCGA Molecular Characterization Platforms
Descriptions and supporting materials for each of the sequencing platforms and other technologies used to generate the TCGA data set.
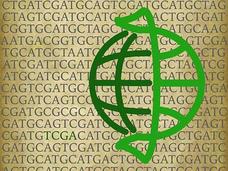
TCGA Timeline and Milestones
The events leading up to TCGA's inception in 2006 and major milestones in the program's history.
Thank you for visiting nature.com. You are using a browser version with limited support for CSS. To obtain the best experience, we recommend you use a more up to date browser (or turn off compatibility mode in Internet Explorer). In the meantime, to ensure continued support, we are displaying the site without styles and JavaScript.
- View all journals
- Explore content
- About the journal
- Publish with us
- Sign up for alerts
Series 20 March 2020
Clinical Cancer Genomics
Advances in sequencing and genomic technologies are rapidly expanding our understanding of cancer and in turn the role of genomics in clinical oncology. Nature Cancer presents a Series of commissioned Reviews covering the current impact of genomics in guiding cancer diagnosis and treatment, the potential role of emerging technologies, and efforts toward translating new discoveries to the clinic. This collection also includes related primary research articles and News and Views on the topic published by Nature Cancer.
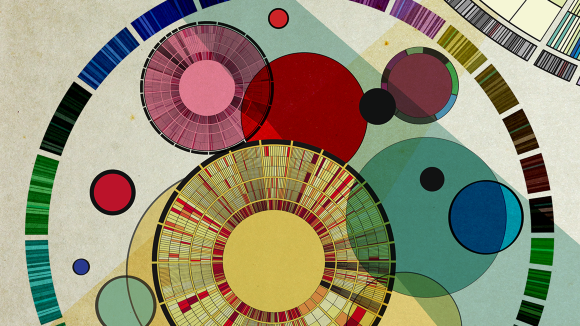
Spotlight on cancer genomics
The field of cancer genomics has been advancing at a rapid pace, opening up a wealth of possibilities for translational applications. In this issue, we are excited to launch a Series of commissioned articles that explore the role of genomics in cancer research and oncology, from current achievements to future directions.
Nature Cancer Reviews & Perspectives
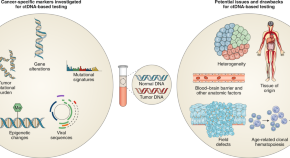
Circulating tumor DNA and liquid biopsy in oncology
Evaluation of circulating tumor DNA in blood has emerged as a powerful technology for oncology research. Lillian Siu and colleagues review the potential applications of liquid biopsy, highlighting clinical-trial designs to establish its clinical utility.
- David W. Cescon
- Scott V. Bratman
- Lillian L. Siu
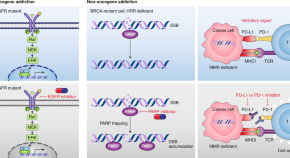
Genomics-guided pre-clinical development of cancer therapies
Garnett and colleagues review principles that underpin the pre-clinical development of genomics-guided cancer medicines, challenges that limit their impact, and new opportunities, such as CRISPR-based screening, for refining and extending their use.
- Hayley E. Francies
- Ultan McDermott
- Mathew J. Garnett
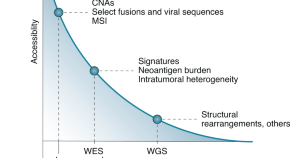
Discovery through clinical sequencing in oncology
Molecular characterization of tumors informs clinical cancer care. Here, Taylor and colleagues review the progress, opportunities, and challenges of scientific and translational discovery that leverages prospective data from clinical genomic screening.
- Mark T. A. Donoghue
- Alison M. Schram
- Barry S. Taylor
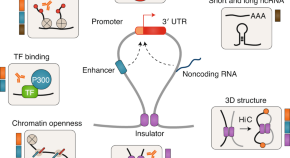
Illuminating the noncoding genome in cancer
Zhang and Meyerson review exciting advances in methodologies, models and datasets to study noncoding alterations in cancer, new insights into their roles in disease and potential translational implications.
- Xiaoyang Zhang
- Matthew Meyerson
Related Nature Cancer Comment & Opinion
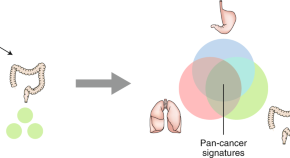
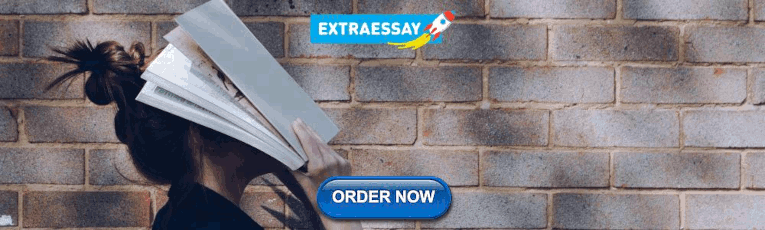
One size does not fit all for mutational signatures
Discerning and analyzing the mutational patterns that arise in the cancer genome can provide essential information on the process of tumorigenesis. An analytical framework and web-based tool now aim to aid in mutational signature assignment for improved tumor stratification.
- Giovanni Ciriello
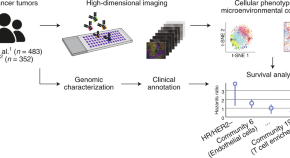
Mapping cell phenotypes in breast cancer
Tumor heterogeneity remains an obstacle to effective clinical management of breast cancer. Two new studies use high-dimensional imaging of single-cell protein expression in situ in clinical samples to link genomic alterations to multi-cellular features of the tumor microenvironment and reveal breast-cancer phenotypes associated with clinical outcome.
- Leeat Keren
- Michael Angelo
Prospecting whole cancer genomes
A new suite of studies from the Pan Cancer Analysis of Whole Genomes (PCAWG) Consortium provides the most detailed resolution of cancer genomes to date, extending our knowledge of driver genes, mutational features, structural alterations and more. Kreisberg, Ideker, Mills and Meric-Bernstam discuss the foundational and translational insights gained from this project.
- Jason F. Kreisberg
- Trey Ideker
- Gordon Mills
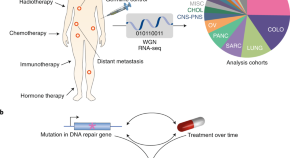
The genomic landscape of advanced cancer
Despite recent advances in cancer treatment, metastasis and therapy resistance remain among the major causes of cancer-related deaths worldwide. A recent pan-cancer study provides a comprehensive molecular profile of advanced and post-therapy tumors, integrating whole-genome and transcriptomic analysis with clinical outcomes.
- Esther Rheinbay
Related Nature Cancer Research Papers
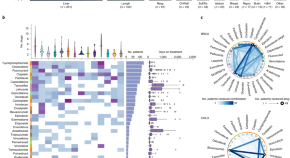
Pan-cancer analysis of advanced patient tumors reveals interactions between therapy and genomic landscapes
Marra and colleagues describe POG570, a pan-cancer, whole-genome, transcriptome and clinical dataset stressing the molecular interactions in advanced and post-therapy cancer patients.
- Erin Pleasance
- Emma Titmuss
- Marco A. Marra
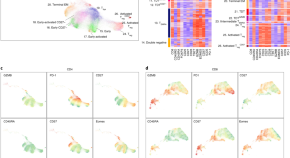
The T cell differentiation landscape is shaped by tumour mutations in lung cancer
Ghorani et al. use a multiomics approach to characterize the effect of tumour mutational burden on the differentiation of CD4 and CD8 T cell subpopulations in non-small cell lung cancer.
- Ehsan Ghorani
- James L. Reading
- Sergio A. Quezada
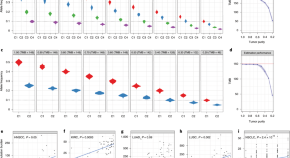
Multimodal genomic features predict outcome of immune checkpoint blockade in non-small-cell lung cancer
Anagnostou et al. present an improved predictor of response to immune checkpoint blockade that integrates estimates of tumor mutational burden corrected for tumor purity, RTK genomic alterations, a smoking-related mutational signature and HLA status.
- Valsamo Anagnostou
- Noushin Niknafs
- Victor E. Velculescu
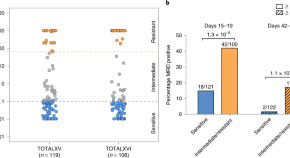
Integrative genomic analyses reveal mechanisms of glucocorticoid resistance in acute lymphoblastic leukemia
Autry et al. combine genome-wide genomic, epigenetic and transcriptomic analyses in an integrated polygenomic approach to identify mechanisms of glucocorticoid resistance in acute lymphoblastic leukemia.
- Robert J. Autry
- Steven W. Paugh
- William E. Evans
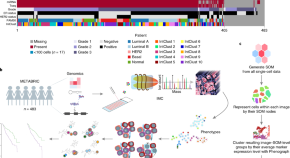
Imaging mass cytometry and multiplatform genomics define the phenogenomic landscape of breast cancer
Bodenmiller and colleagues pair imaging mass cytometry with data from the METABRIC cohort to define single-cell phenotypic and genomic features of breast cancer with spatial context, finding associations with breast cancer subtypes and prognosis.
- H. Raza Ali
- Hartland W. Jackson
- Bernd Bodenmiller
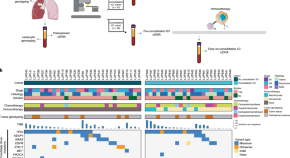
Circulating tumor DNA dynamics predict benefit from consolidation immunotherapy in locally advanced non-small-cell lung cancer
Diehn and colleagues report that assaying circulating DNA in patients receiving chemoradiation therapy for non-small-cell lung cancer could identify the patients most likely to benefit from consolidation immunotherapy.
- Everett J. Moding
- Maximilian Diehn
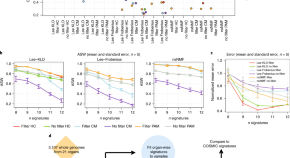
A practical framework and online tool for mutational signature analyses show intertissue variation and driver dependencies
Degasperi et al. introduce a practical framework and Signal, an online tool, to analyze mutational signatures. They find evidence of tissue-specific variability in mutational signatures, which may impact tumor classification and clinical application.
- Andrea Degasperi
- Tauanne Dias Amarante
- Serena Nik-Zainal
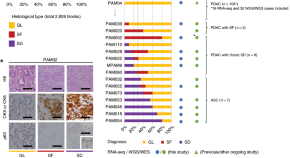
A unifying paradigm for transcriptional heterogeneity and squamous features in pancreatic ductal adenocarcinoma
Iacobuzio-Donahue and colleagues use integrated transcriptomic, histologic and mutational data to analyze squamous features of pancreatic ductal adenocarcinoma (PDAC), further refining the understanding of heterogeneity and evolution in PDAC.
- Akimasa Hayashi
- Christine A. Iacobuzio-Donahue
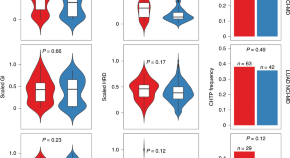
Higher prevalence of homologous recombination deficiency in tumors from African Americans versus European Americans
Ryan and colleagues analyze genomic features in tumors from African Americans and European Americans and find that homologous recombination deficiency is more prevalent in African Americans.
- Sanju Sinha
- Khadijah A. Mitchell
- Bríd M. Ryan
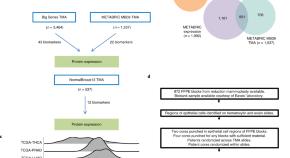
Age-correlated protein and transcript expression in breast cancer and normal breast tissues is dominated by host endocrine effects
Aparicio and colleagues identify gene expression changes in breast cancer datasets putatively associated with age-related endocrine effects, suggesting that patient age may influence the prognostic potential of certain biomarkers.
- Samuel Aparicio
Quick links
- Explore articles by subject
- Guide to authors
- Editorial policies


An official website of the United States government
The .gov means it’s official. Federal government websites often end in .gov or .mil. Before sharing sensitive information, make sure you’re on a federal government site.
The site is secure. The https:// ensures that you are connecting to the official website and that any information you provide is encrypted and transmitted securely.
- Publications
- Account settings
Preview improvements coming to the PMC website in October 2024. Learn More or Try it out now .
- Advanced Search
- Journal List
- Front Oncol
A Review of Cancer Genetics and Genomics Studies in Africa
Solomon o. rotimi.
1 Department of Translational Genomics, Keck School of Medicine, University of Southern California, Los Angeles, CA, United States
2 Department of Biochemistry, Covenant University, Ota, Nigeria
Oluwakemi A. Rotimi
Bodour salhia.
3 Norris Comprehensive Cancer Centre, University of Southern California, Los Angeles, CA, United States
Cancer is the second leading cause of death globally and is projected to overtake infectious disease as the leading cause of mortality in Africa within the next two decades. Cancer is a group of genomic diseases that presents with intra- and inter-population unique phenotypes, with Black populations having the burden of morbidity and mortality for most types. At large, the prevention and treatment of cancers have been propelled by the understanding of the genetic make-up of the disease of mostly non-African populations. By the same token, there is a wide knowledge gap in understanding the underlying genetic causes of, and genomic alterations associated with, cancer among black Africans. Accordingly, we performed a review of the literature to survey existing studies on cancer genetics/genomics and curated findings pertaining to publications across multiple cancer types conducted on African populations. We used PubMed MeSH terms to retrieve the relevant publications from 1990 to December 2019. The metadata of these publications were extracted using R text mining packages: RISmed and Pubmed.mineR. The data showed that only 0.329% of cancer publications globally were on Africa, and only 0.016% were on cancer genetics/genomics from Africa. Although the most prevalent cancers in Africa are cancers of the breast, cervix, uterus, and prostate, publications representing breast, colorectal, liver, and blood cancers were the most frequent in our review. The most frequently reported cancer genes were BRCA1 , BRCA2 , and TP53 . Next, the genes reported in the reviewed publications’ abstracts were extracted and annotated into three gene ontology classes. Genes in the cellular component class were mostly associated with cell part and organelle part , while those in biological process and molecular function classes were mainly associated with cell process, biological regulation, and binding, and catalytic activity, respectively. Overall, this review highlights the paucity of research on cancer genomics on African populations, identified gaps, and discussed the need for concerted efforts to encourage more research on cancer genomics in Africa.
Introduction
Cancer is the second leading cause of death globally ( 1 ). In Africa, cancer incidence and mortality continue to grow rapidly. According to the 2018 Globocan data, new cancer cases and cancer deaths in Africa were estimated at 1,049,800 and 700,800, respectively ( 2 ). In 2018, women in East Africa had the highest cumulative risk of dying from cancer globally. The burden of cancer in Africa is increasing, and this burden is expected to increase by 60% by the year 2030. To lower this projected increase in cancer burden, population-relevant biological studies and the identification of innate risk factors among African populations are needed ( 3 – 5 ).
As cancer is a genetic disease, scientific studies investigating its causes, diagnosis, and treatment in developing countries need to focus more on genetics and genomics. The African or Black population is not a homogenous group and, as such, necessitates the need for genomic/genetic studies to reflect the diverse African populations. The population history of Africa shows that the people of Africa are the most genetically and phenotypically diverse population ( 6 , 7 ). The peopling history of Africa has been described by Campbell et al. and Tucci & Akey ( 8 , 9 ), and their reviews showed that African ethnic groups and tribes are genetically heterogeneous. Hence, there is likely a critical contribution of the underlying within-group genetic differences to the disparity in cancer prognosis seen among Blacks ( 10 ). Therefore, cancer genetics/genomics studies are expected to significantly impact the understanding of the risk, susceptibility, diagnosis, and treatment of this disease.
The genomic heterogeneity of human populations was driven by ancient migration and heterogeneous adaptive pressures on the human genome, particularly on the African Continent ( 11 , 12 ). These evolutionary events resulted in the split of human populations into five distinct groups: southern Khoe-San, northern Khoe-San, central African hunter-gatherers, West Africans, and East Africans, out of which a subset migrated out of Africa and is now recognized as the out-of-Africa population ( 11 , 12 ). Therefore, the African continent could be considered to harbor the repository of human genomic diversity and serves as the resource reference for understanding the role of genomics in human health equity. This repository is further deepened by the present-day North African populations enriched with the genetic pool of the out-of-Africa’s Euro-Asian populations. Still, Africa’s contribution to global genetic and genomics information is grossly disproportionate to its population’s diversity and size. For example, very few African populations were included in the HapMap and 1000Genome projects ( 13 ). This is a serious shortcoming for a group of people that represent over 90% of human genomic diversity. A recent review of genome-wide association studies (GWAS) showed that Africans (including African Americans) only represent 2.4% of individuals included in all GWAS studies ( 14 ).
The proper understanding of genetics and genomics among African populations will expectantly improve prevention, diagnosis, and treatment outcomes of cancer. Although recent evidence shows that the burden of cancer is in Africa, there remains a huge deficit in requisite skills and infrastructure required to carry out the necessary research studies to alleviate this knowledge gap, requiring still non-African nations to fill this gap ( 15 ).
Accordingly, in this review, we discuss both genetics and genomics study findings across multiple cancer types in African populations. The goal here is to demonstrate the existing knowledge and to crucially identify the gaps that should be filled in order to address the cancer burden across Africa.
The peer-reviewed publications included in this review were extracted from PubMed and covered the period between January 1990 and December 2019, as shown in the flow chart in Figure 1 . Since PubMed Medical Subject Heading (MeSH) terms involve synonym control, it yields more precise and inclusive search results ( 16 ). Our literature search approach, therefore, utilized an integration of MeSH terms that incorporated “the disease” (neoplasm), 54 African countries, and combinations of study parameters (‘gene or protein or molecular biology or mutation or genetics or genomics’). After extracting African cancer papers, we next filtered those to include only papers pertaining to cancer molecular biology (protein or nucleic acid). Cancer molecular biology papers were then further filtered using “genetic* OR genomic* OR mutation*[MeSH Terms].” The final criteria were that the studies must utilize biospecimens of African origin. Two authors (SOR and OAR) manually verified these publications to ensure the accuracy of terms.

Flow diagram of the literature search strategy.
For the purpose of data extraction, the metadata and abstract of each publication returned from our search were collected in a single corpus and subjected to text-mining using the R packages RISmed ( 17 ) and Pubmed.mineR ( 18 ). The publications returned were analyzed in R to identify the cancer types/sites associated with each publication, as described by Acharya et al. ( 19 ). Furthermore, the R package “PubmedmineR” was used for obtaining the names and frequency of occurrence of genes denoted in “Human Genome Nomenclature Committee” (HGNC) symbols ( 20 ). For this purpose, we considered the genes reported in the abstract as the genes associated with the most prominent findings of the publications. Next, these genes were pulled and subjected to gene ontology functional profiling for three gene ontology classes (“molecular function”, “biological process”, and “cellular component”) using “goProfiles” ( 21 ).
The total numbers of publications returned by our search on the topics of cancer globally, as well as cancer, cancer molecular biology, and cancer genetics/genomics within Africa between 1990 and December 2019, are shown in Figure 1 . Out of nearly two and half million publications on cancer globally, only 7,697 (0.329%) papers were returned by our search on cancer in Africa, with only 1,456 (0.061%) related to molecular biology (protein or nucleic acid). Of these publications, only 375 articles were found using the search terms “genetic, genomics, mutations”.
Among all cancer publications pertaining to Africa, the cancer sites with the highest number of published studies represented cancers of the cervix, breast, liver, head/neck, and colorectal while, lung, brain, bladder, ovarian, and uterine cancers were the least frequently reported on ( Figure 2A ). For publications related to cancer molecular biology in Africa, breast, liver, colorectal, blood, and prostate cancer were the most frequent. In contrast, cancers of the brain, stomach, lung, skin, and uterine cancer had the fewest publications ( Figure 2B ). Most papers reporting cancer genetics or genomics reported on breast, colorectal, liver, blood, and ovarian cancer, with the fewest cancer genetics or genomics studies on the brain, stomach, lung, skin, and uterine cancers ( Figure 2C ).

The proportion of the number of publications on each cancer type. (A) Cancer in Africa (n=7,697) (B) Cancer Molecular Biology in Africa (n=1,456), and (C) Cancer Genetics/Genomics in Africa (n=375). *The total values presented in the pie charts are greater than the sum of publications in each category due to the multiplicity of cancer sites for some publications as exemplified by studies on breast/ovary and blood/lymph.
There were also disparities in the publications by country, as illustrated in Figures 3A–C . Nigeria had the most papers on cancer overall, followed by South Africa, Egypt, Tunisia, Morocco, and Kenya ( Figure 3A ). For cancer molecular biology papers, Egypt took the lead, followed by Tunisia, South Africa, and then Nigeria ( Figure 3B ). Tunisia, however, returned the most search results for cancer genetics/genomics papers followed by Egypt, South Africa, and Morocco ( Figure 3C ). Overall, only seven African countries contributed at least 10 cancer genetics/genomics publications, while 22 African countries returned no search results on cancer genetics/genomics studies. The search results show clear evidence of regional differences in publishing capacity, with North Africa and South Africa leading in cancer research.

Heat map showing the number of publications retrieved for (A) all cancer publications per African country; (B) cancer molecular biology publications per African country and (C) cancer genetics/genomics publications per African country. Countries without any publication in each category are shaded in white.
Next, we focused specifically on the list of 375 genetics/genomics publications for gene curation and review. We did this to identify the functional contributions of these studies to the understanding of biological processes associated with carcinogenesis, using functional correlations comparison ( 22 ). A total of 152 genes in the abstracts of 375 publications on cancer genomics were extracted and further annotated into the following gene ontology classes: cellular component, biological process, and molecular function ( Figures 4A–C ). In the cellular component class, the genes studied were mostly associated with cell part, organelle, organelle part, and cell membrane. In contrast, the genes in the biological process were mainly associated with cell process, biological regulation, response to stimulus, and positive regulation of the biological process. The molecular function ontology genes were mostly associated with binding, catalytic activity, molecular function regulator, molecular function transducer activity, and transcription regulation in the molecular function class, which are dysregulated in cancer. The most studied genes in the publications were BRCA1 , BRCA2 , TP53 , EGFR , and MLH1 ( Table 1 ), indicating a dearth of data on the plethora of other critical cancer-associated genes. Next, we reviewed some of the key findings reported across the 375 genomics papers for each of the major and most frequently published cancer types below.

Gene ontology of the genes reported in the abstracts of publications on cancer genomics in Africa. (A) Cellular component ontology, (B) Biological process ontology, and (C) Molecular function ontology.
List of top 20 genes reported in the abstracts of publications on cancer genetics and genomics in Africa.
Breast/Ovarian Cancer
Breast cancer has continued to be the leading cause of cancer morbidity and mortality in Africa, with an incidence and mortality rate of 37.9 and 17.2 per 100000, respectively, according to GLOBOCAN 2018 data ( 2 ). Breast cancer’s prominence in Africa dates back to around 3000BC in the ancient Egyptian medical text - the Edwin Smith Papyrus, the oldest cancer record ( 23 , 24 ). Not surprisingly, breast cancer had the highest number (n=82, 19%) of peer-reviewed cancer genetics/genomics publications in Africa. With the current understanding of cancer as a genomic disease and the unique phenotype that breast cancer presents in the people of African ancestry, attempts to address its burden require rigorous genomics investigations.
Together with cancer of the ovary, breast cancer risk is greatly increased in women with inherited mutation(s) in tumor suppressor genes ( 25 ). Not surprisingly, the earliest publications on breast and ovarian cancers in African populations focused on understanding the contribution of variations in the tumor suppressor genes BRCA1/2 and TP53 , particularly in North African populations of Morocco, Tunisia, Egypt, and Sudan ( 26 – 40 ). While these findings hold immense benefits for those populations, their BRCA variants are not dissimilar to those present in the out-of-Africa populations. This, therefore, limits the translational impact of such findings to controlling breast/ovarian cancer in the Sub-Saharan African populations.
Furthermore, the major epidemiological implication of BRCA mutations lies in identifying specific founder mutation(s) within each population, with the view of using it as a predictive molecular risk marker and treatment recommendation. For instance, advances in understanding the role of BRCA proteins in tumorigenesis have now led to improved therapeutic choices with the availability of PARP inhibitors for breast cancer patients with germline mutations ( 41 ). Also, the identification of founder BRCA gene mutations in populations like Ashkenazi-Jewish (Hungarian and Russian), Polish, Norwegian and Icelandic people has resulted in improved low-cost genetic testing and the determination of high-risk individuals for breast and ovarian cancers ( 42 , 43 ). Therefore, these have made it imperative for the founder mutations of the BRCA gene within Africa populations to be identified and included in breast cancer screening, diagnosis, and treatment.
In an attempt to consider BRCA contributions to breast cancer in Africa, Rebbeck et al. ( 44 ) published a global distribution of BRCA1 and BRCA2 germline mutations by including women from Nigeria and South Africa. However, the extent to which their subjects represent the ethnic and genetic diversity in these countries is unclear. They did note that the mutations observed in African American families were of African origin because they are unlike the mutations seen in out-of-African ethnic groups ( 44 – 46 ). This study of Rebbeck et al. ( 44 ) was part of the Consortium of Investigators of Modifiers of BRCA1/2 investigations, which only included the nation of South Africa ( http://cimba.ccge.medschl.cam.ac.uk/cimba-groups/study-groups/ ). A more detailed study of Zheng et al. ( 47 ) on Nigerian women established that up to 20% of inherited invasive breast cancer cases in Nigeria are associated with inherited mutations in BRCA1 , BRCA2 , PALB2 , or TP53 . Their findings on BRCA1 and BRCA2 built on the earlier report of Fackenthal et al. ( 48 ) that Nigerian breast cancer patients have a very high frequency of BRCA1 and BRCA2 mutations. These mutations were reported by Pitt et al. ( 49 ) to be associated with greater structural variation and aggressive biology in Nigerian women with HR + /HER2 − tumors. Similar findings were reported by Pegoraro et al. ( 50 ) in Black South Africans with ovarian epithelial malignancies.
Recently, Mahfoudh et al. ( 51 ) showed that the 5382insC BRCA1 mutation contributes to the development of triple-negative breast cancer (TNBC) in Tunisia. The higher mortality of breast cancer in women of West African ancestry is due in part to higher levels of TNBC (compared to whites), which is associated with the poorest prognosis of all breast cancer subtypes. Hence, BRCA screening in Africa could help identify women who can benefit from PARP inhibitors leading to improved clinical outcomes. In South Africa, Reeves et al. ( 52 ) characterized BRCA1 mutations in breast and/or ovarian cancer to identify founder mutations in Afrikaner families. However, this population is also of European ancestry, and the mutations that were identified were similar to those reported in the Netherlands and in Ashkenazi Jews ( 53 ). They also reported that variants of PALB2 , a partner and localizer of BRCA2 was also associated with the early onset of breast cancer in some South African patients ( 53 ). PALB2 functions as a scaffold between BRCA1 and BRCA2 . Similar PALB2 mutations have previously been identified in women of European ancestry but not in women with Nigerian ancestry, as reported by Sluiter et al. ( 54 ).
The first publication on BRCA mutations in the indigenous Sub-Saharan African population was by Zhang et al. ( 55 ), who identified an ancient BRCA1 mutation (Y101X) in Yoruba (Nigeria, West Africa) breast cancer patients. The team further reported a non-pathogenic novel exon 21 deletion of BRCA1 (c. 5277 + 480_5332+672del) in Nigeria in addition to a novel deleterious BRCA1 mutation (c. 1949_1950delTA) in a woman from Senegal (West Africa) ( 56 ). Another novel founder, BRCA2 mutation, was identified by var der Merwe et al. ( 53 ) in the Bantu-speaking Xhosa population (South Africa). Other studies have identified new BRCA mutations and their contribution to early-onset and sporadic breast and/or ovarian cancer in Arabic speaking countries ( 57 ) of Egypt ( 58 ), Tunisia ( 51 , 59 – 66 ), Algeria ( 67 – 69 ), Morocco ( 70 – 72 ), and Sudan ( 73 , 74 ), in addition to Senegal ( 75 ), Mauritius ( 76 ) and South Africa ( 50 , 77 – 79 ) in the Sub-Saharan region.
Additional studies on BRCA genes have expanded to identifying the population-based mutation frequency and screening/genetic testing in the Democratic Republic of the Congo ( 80 ), Morocco ( 81 ), Tunisia ( 82 , 83 ), Algeria ( 84 , 85 ), familial studies in Morocco ( 86 , 87 ), and large genomic rearrangement in Egypt ( 88 , 89 ). Of these, the contribution of BRCA mutations to male breast cancer was reported only in the Moroccan study by Guaoua et al. ( 86 ). A mutation in the TP53 gene often accompanies BRCA mutations in breast and ovarian cancers, making the mutations in these DNA repair genes relevant in therapeutic interventions ( 90 , 91 ). The publications on TP53 mutation have focused on its expression in breast cancer and the contribution of its polymorphism, particularly codon 72 to breast cancer ( 28 , 31 , 33 , 36 , 92 – 94 ), as well as to its interaction with MDM2 344T>A polymorphism in response to chemotherapy of breast cancer in Tunisia ( 95 ). Other DNA repair genes that have been studied in Africa include XRCC1 and XPD in Egypt ( 96 , 97 ). Overall, even though it is one of the most studied genes in African cancer research, there remains a very small number of publications on BRCA mutations in the indigenous African population, clearly showing a knowledge gap on a hereditary gene critical in managing incidence and clinical outcomes in breast cancer.
Exogenous factors that drive DNA damage include viruses and xenobiotics. The presence of these agents and genetic alterations that mediate the ensuing host-response can promote carcinogenesis. The first reports of virus-associated breast cancer in Africa were by Levine et al. ( 98 ) and Hachana et al. ( 99 ), who reported the presence of a human breast carcinoma virus (a virus similar to mouse mammary tumor virus) in 74% of tumors in Tunisia. These were the only two studies that reported this virus in Africa. Studies have also shown an association of the hepatitis C virus in Egypt ( 100 ) and Human papillomavirus (HPV) in Rwanda ( 101 ) to breast cancer progression. However, the most reported virus linked to breast cancer in Africa is the Epstein-Barr virus (EBV), with studies published in Algeria ( 102 ), Eritrea ( 103 ), Egypt ( 104 ), and Sudan ( 105 ). EBV was the first identified human oncogenic virus that was detected in Uganda in 1964 by Denis Parsons Burkitt ( 106 – 108 ) and its molecular pathogenesis has been reviewed by Lawson et al. ( 109 ). The virus is responsible for many cancers across the continent, and the host genomic factors that facilitate tumorigenesis are described below.
The detoxification of carcinogenic chemical entities is primarily catalyzed by cytochrome P450 (phase I) and a host of phase II xenobiotic-metabolizing enzymes. Polymorphisms in these genes dictate, in large part, the effect of xenobiotics on the biological system. Such polymorphisms have been reported in CYP1A1 and CYP1B1 in Nigeria and Egypt ( 110 , 111 ), CYP2D6 in South Africa ( 112 ), and CYP1A2 in Tunisia ( 113 ). Furthermore, as hormone-responsive cancers, these cytochrome P450 genes play critical roles in estrogen metabolism and the response of the tumor to endocrine therapy. For genes coding for phase II xenobiotics metabolizing enzymes, the deletion of GSTT1 and GSTM1 were reported by Khedhaier ( 114 ) to predict the early onset and prognosis of breast cancer among Tunisian women. The number of TA repeats in the promoter of low activity UGT1A1 was reported to be protective against breast cancer in pre-menopausal Nigerian women ( 115 , 116 ). Similarly, the association of polymorphisms in paraoxonase, cyclooxygenase, glyoxalase, and glutathione peroxidase genes with breast cancer were reported in Egypt and Rwanda ( 117 – 120 ).
Inflammation is a major hallmark of cancer, and it is known to contribute to aggressive tumor biology. This makes understanding the variations in immuno-oncogenic genes important in understanding the population biology of cancer in Africa. Mestiri et al. ( 121 , 122 ) reported that polymorphisms in TNF-α and TNFRII increase the susceptibility to breast cancer in Tunisian women, with TNFRII -196R prevalent in premenopausal women. Conversely, FASL (rs763110) was associated with a good prognosis in the same population ( 123 ). However, HLA-DQB1 and HLA-G +3142C>G (rs1063320) polymorphisms were related to increased breast cancer susceptibility ( 124 , 125 ). Pathogenic polymorphisms of other inflammatory genes like NRF2 , IL1α , IL1β , IL6 , IL8 , and CXCR2 have also been identified in Tunisian and Egyptian breast cancer patients ( 126 – 130 ).
Recent evidence suggests that inflammation-driven cancer in Blacks is influenced by vitamin D levels ( 131 , 132 ). To establish the association of vitamin D variants and related genes with breast cancer, El-Shorbagy et al. ( 133 ), Abd-Elsala et al. ( 134 ) and Shaker & Senousy ( 135 ) showed that polymorphisms in the vitamin D receptor ( VDR ) increases the risk of breast cancer in Egyptian women who carry the ATT haplotype. The risk of developing breast cancer due to these mutations was elevated in women who also carry RANKL (rs9533156), OPG (rs2073617), and CHI3L1 (rs4950928) ( 135 ). Similar studies have also reported the risk allele in Ethiopian women as VDR rs2228570 (FokI) ( 136 ) but the study of Wang et al. ( 137 ) did not identify variants in vitamin D related genes as risk factors for breast cancer in Nigerian women that were used as the ancestral population for African American women. This genome-wide association study, however, identified TYRP1 (rs41302073), a melanin synthesis regulatory gene, as a significant risk allele for breast cancer in their dataset that included African American and Barbadian women. Furthermore, the authors also used the same dataset to identify WWCI as an important susceptibility locus in the Hippo pathway for breast cancer ( 138 ).
Polymorphisms in the angiogenesis-associated genes have also been identified in breast cancer in African populations and include the LEP , LEPR , VEGF , and MMP2 . Leptin and LEPR Q223R (rs1137101) were identified as risk factors for breast cancer in Egyptian and Nigerian women ( 139 – 141 ) while leptin alone was notably reported as a key driver of breast cancer progression through the induction of JAK/STAT3 , ERK1/2 , and estrogen pathways in obese Egyptian women ( 142 ). Furthermore, variants of VEGF and MMPs , which induce the upregulation of these proteins, were reported as risk factors in North African countries of Morocco, Egypt, and Tunisia ( 143 – 148 ). Other overexpressed angiogenic proteins reported are EGFR in Tunisia ( 149 ) and IGFBP2 and IGFBP5 in Nigerian women ( 150 ). The authors proposed these angiogenic proteins as druggable targets in breast cancer treatment. Another therapeutic pathway that has been studied is the PIK3/AKT pathway. Jouali et al. ( 151 ) reported PIK3CA hotspot mutations in 13% of triple-negative breast cancer cases in Morocco. They suggested that this pathway could be of therapeutic importance for triple-negative breast cancer in Morocco.
Cancer is a polygenic disease, and scientific investigation to understand breast cancer’s population biology, therefore, cannot be simplified to a single genetic variant. Hence, techniques to investigate multiple genes at a time such as with next generation sequencing are now being utilized to understand the genetic risk factors of breast cancer in Africa. To that effect, genome-wide studies (GWAS) published primarily on breast cancer in African populations include GWAS in Tunisia and South Africa ( 152 – 154 ) and whole-exome sequencing in Tunisia and Egypt ( 155 – 157 ). In the Tunisian population, Shan et al. ( 154 ) and Hamdi et al. ( 152 ) identified rs1219648, rs2981582, rs8051542, rs889312, and rs889312 as breast cancer susceptibility single nucleotide polymorphisms (SNPs), with rs9911630 as the SNP with the strongest effect on the expression of BRCA1 and two long non-coding RNAs (NBR2 and LINC008854). The genome-wide copy number alteration analysis of breast cancer in South African women ( 153 ) identified the amplification in Xp22.3 and 6p21-p25, and other regions that affect known cancer genes like CCND1 , CDKN1A , MDM2 , TP53 , and SMAD2 . Meanwhile, the whole-exome sequencing study by Hamdi et al. ( 152 ) and Riahi et al. ( 156 ) linked breast cancer in Tunisian women to alterations in MMS19 , DNAH3 , POLK , KATβ6 , and RCC1 in BRCA1/2 mutation-negative patients with familial breast cancer. A similar study in Egypt also found other novel genetic variants responsible for familial breast cancer. These genetic variants are different from those linked to DNA damage repair (like BRCA1 and BRCA2 ) but are linked to other functional genes like NBPF10 , ZNF750 , CHTI5 , NPIPB11 , and PHIP , that are involved in RNA binding, transcriptional regulation, extracellular matrix, a structural protein, and signal transduction, respectively.
The contribution of epigenetic factors to risk and prognosis of breast cancer reported in Africa included the roles of tissue microRNA, circulating free mRNA, circulating long non-coding RNA ( 158 – 163 ) as well as DNA methylation status of breast cancer susceptibility genes like APC , ERα , RASSFIA , UCHL1 , COX-2 , and FHIT ( 161 , 164 – 167 ) in breast tumor across Africa.
Prostate Cancer
Prostate cancer continues to be the leading cause of cancer morbidity and mortality among African men ( 168 , 169 ). Although genetics is a major risk factor for this disease, there are only a few publications on prostate cancer genomics in Africa. In this subsection, we review 14 papers that were relevant to prostate cancer out of the list of 375 papers extracted. Prostate cancer presents with an aggressive phenotype among men of African descent, and like breast cancer, it is a hormone-responsive tumor. Consequently, early studies on this disease identified androgen’s influence in the control of normal prostate growth and, in its transformation into adenocarcinoma, a phenomenon called the “androgen hypothesis” ( 170 , 171 ). Therefore, peer-reviewed publications on prostate cancer genetics in African populations have reported genetic variants that contribute to elevated circulating androgens, including androgen reduced clearance and upregulated activity of androgen receptor. These include the polymorphisms in cytochrome P450 genes like CYP3A4 , CYP3A5 , CYP1A1 , CYP17 in Morocco, Tunisia, Nigeria, South Africa, and Senegal ( 172 – 176 ). Besides, alterations in CAG and GGN repeats in the androgen receptor gene have been reported as risk factors in North Africa, Ivory Coast, and Nigeria ( 177 , 178 ). Unlike the North African populations, prostate cancer in Sub-Saharan African populations and North African Berbers were associated with high frequencies of low size alleles (CAG under 18 repeats, and GGC under 15 repeats) ( 178 ). Other reported genetic variations that increase African populations’ susceptibility to prostate cancer include GSTM1 , GSTT1 , UDP-glucuronosyltransferase, and sulfotransferase in Tunisia and Algeria ( 179 – 182 ).
A deeper understanding of the disease’s polygenic risk was elucidated by four studies that have investigated the genome-wide genetic variations in prostate cancer across Africa. These included GWAS of prostate cancer in Tunisia, Ghana, and Uganda ( 183 – 185 ), as well as a whole-genome sequencing of six individuals in South Africa ( 186 ). It is interesting to note that these four studies did not identify any common high-risk prostate cancer variants. The Tunisian study identified three regions (on chromosomes 9, 17, and 22) containing 14 significant SNPs, three of which are shared with Caucasian populations ( 185 ). The Ghanaian study of Cook et al. ( 184 ) identified 30 most significant SNPs distributed across chromosomes 1, 2, 3, 5, 6, 7, 8, 9, 10, 13, and 20.
Meanwhile, the Ugandan study identified risk alleles on chromosomes 1, 6, 11, 13, 14, and 17 ( 183 ). Although the Ugandan and Ghanaian populations shared cytoband 6p21.32 in common, the nucleotide positions and risk alleles were still different. This chromosome position codes for HLA-DQB1 , which has been reported to be important for the adaptation of African ancestral populations to the African rainforest environment. These studies further add to the existing evidence of the heterogeneity of African populations ( 12 ) and that cancers in these populations may have a different biology. These findings provide further evidence for the need to disaggregate the Black population by genetic lineage in studying the contributions of genomics to racial disparities of diseases like prostate cancer. Importantly, it is yet to be revealed whether these differences influence the disease phenotype and disparity in outcome.
The most commonly reported genomic alteration that drives prostate tumorigenesis is TMRPSS2-ERG fusion, and this androgen-upregulating fusion is known to correlate with higher grades of the disease. Although men of African ancestry are known to present with higher disease grade, only three studies have examined the TMRPSS2-ERG fusion on the Continent ( 187 – 189 ). This fusion often results from either a chromosomal translocation or an interstitial deletion, and these studies reported rates that were less than 20% in Ghanaian and Black South African patients ( 187 – 189 ).
Liver Cancer
According to the 2018 GLOBOCAN data, hepatocellular carcinoma accounted for 8.4 cases per 100,000 and 8.3 deaths per 100,000 globally ( 2 ) and it is the 4 th most common cancer in Africa. We retrieved 46 publications that studied liver cancer genetics/genomics in Africa. Several of these studies investigated the contribution of the hepatitis virus and mycotoxins to this malignancy. These biotic and abiotic agents represent the major causes of this disease on the continent ( 190 , 191 ). Hence, a preponderance of publications on liver cancer in Africa focused on understanding the contribution of mutation and expression of TP53 , and other tumor suppressors like TP73 , RB , KLF6 , and CTNNB1 , to liver carcinogenesis ( 190 , 192 – 207 ), particularly in Senegal, Gambia, Nigeria, South Africa, Egypt, and Morocco. These studies identified the mutation in codon 249 of TP53 as a genetic risk factor for developing hepatocellular carcinoma following exposure to either the hepatitis virus or mycotoxins (see Lin et al. ( 208 ) for detailed mechanism). In Morocco, MDM2 309 T>G was associated with liver cancer ( 209 , 210 ). These mutations are known to upregulate this oncogene’s expression, which in turn binds p53 and prevents its tumor suppression function ( 209 ) resulting in increased genomic instability as demonstrated by loss of heterozygosity in chromosome 4-q13 in Black South Africans ( 211 ).
The development of hepatocellular carcinoma is often preceded by chronic inflammation of the liver. In Africa, hepatic inflammation is exacerbated by high-prevent co-morbid conditions like non-alcoholic fatty liver disease (NAFLD), non-alcoholic steatohepatitis (NASH), and liver cirrhosiss. For instance, the prevalence of NAFLD in Nigeria, Ethiopia, and South Africa has been reported to be 68.8%, 73%, and 87%, respectively ( 212 ).
Despite the pervasiveness of liver cancer across Africa, only the Egyptian and Tunisian populations have been studied for the contribution of variation in inflammation-related genes to this disease. These studies reported mutations in IL3R , IL17A , IL8 , IL1 , IL16 , IL12 , IL27 , and TNF-α as risk factors for hepatitis and hepatocellular carcinoma ( 213 – 219 ).
Other authors have focused on the development of biomarkers for liver cancer, using epigenetic factors like microRNAs. These include serum Mir-224, Mir-215, Mir-143, Mir-122, Mir-199a, and Mir-16 ( 220 , 221 ). Specifically, Mir-122 and Mir-222 levels were reported by Motawi et al. ( 222 ) as a discriminating biomarker for distinguishing liver injury from liver cancer. This group further reported that LncRNA HULC rs7763881 and MALAT rs619586 were associated with decreased susceptibility of Egyptian hepatitis virus-persistent carriers to liver cancer ( 223 ).
Mycotoxicosis, with concomitant early-life protein malnutrition, is an important driver of liver cancer in Africa ( 224 – 226 ). One group of enzymes that are involved in detoxifying mycotoxins are the glutathione-S-transferases (μ, θ, π, α, σ). Hence, individuals who do not express all the enzymes due to homozygous deletion are more susceptible to myco-carcinogens ( 227 ). Overall, two studies have identified the deletion of GSTM1 and GSTT1 haplotypes as risk factors for aflatoxin-associated hepatocellular carcinoma ( 228 , 229 ) in Africa.
The last group of genes that have been studied on hepatocellular carcinoma in Africa are those involved in angiogenesis, including VEGF , MMP , RASSF1A , and RECK . In Egypt, Samamoudy et al. ( 230 ) reported that patients with MMP9 (rs3918242) are at high risk of developing liver cancer while RECK (rs12814325) ( 231 ) could account for the disease progression and metastasis.
Cervical Cancer
Cervical cancer continues to be responsible for the highest cancer mortality in Africa, accounting for 2,000,000 deaths in 2018 ( 2 ), and its incidence rates continue to increase in most Sub-Saharan African countries ( 232 ). However, studies on cervical cancer genetics/genomics only represented 3% of the publications we retrieved. Similar to liver cancer, cervical cancer is viral-related and primarily caused by Human Papillomavirus (HPV). Several reviews have discussed the burden, distribution, and contribution of HPV serotypes to cervical cancer in Africa ( 233 – 235 ). Despite the burden of HPV in Africa, only a small proportion of women that are infected develop cervical cancer ( 236 , 237 ). It is, therefore, essential to understand the genetic factors that contribute to the risk of progression from HPV infection to cervical cancer across Africa.
One of such genetic factors that increase susceptibility to HPV-associated cervical carcinogenesis is the TP53 R72P mutation ( 238 ), which was reported in Gabon, Senegal, Sudan, Morocco, and South Africa; and this risk increases when combined with the chromosomal allelic loss of RB or with aberrant methylation of DAPK1 , RARB , TWIST1 , and CDH13 ( 79 , 239 – 244 ). Furthermore, aberrant methylation of these genes was proposed by Feng et al. ( 245 ) to be useful in Senegal for the screening of cervical cancer, either alone or in combination with cytology. The importance of this homozygous arginine polymorphism at codon 72 of TP53 in determining genetic susceptibility of a population has been shown in Israeli Jewish women who have been reported to have reduced susceptibility to HPV-associated cervical cancer ( 246 ).
The variations in genes involved in inflammatory and apoptotic response pathways have also been reported to increase African women’s susceptibility to cervical cancer ( 247 ). The reported polymorphisms in Africa include those of TLR 2/3/4/9 and IL1/10/15 genes in Tunisia and -308 promoter polymorphism of TNF-α in South Africa ( 248 – 250 ). Meanwhile, polymorphisms in FASR -670A and CASP8 -652 were associated with a reduced risk of developing cervical cancer in South African women ( 251 ).
Colorectal Cancer
Colorectal cancer is the 5 th most common cancer in Africa and accounted for 550,000 deaths in 2018 ( 2 ). We retrieved 56 publications on colorectal cancer genetics/genomics from Nigeria, Ghana, South Africa, Algeria, Tunisia, Morocco, and Egypt. The findings in these publications included: (1) the identification of I130K APC polymorphism in the indigenous Black population in South Africa and Tunisia to development of familial adenomatous polyposis coli ( 252 – 255 ), (2) the presence of mutations in the MUTYH , MLH1 , and MSH2 gene in patients with colorectal cancer and attenuated polyposis in Algeria, Egypt, Morocco, Tunisia, and South Africa ( 256 – 266 ), (3) the burden of KRAS and BRAF mutations in colorectal cases in Morroco, Nigeria, Ghana, Egypt and Tunisia ( 267 – 275 ) and (4) the level of microsatellite instability in South African, Nigerian, Ghanian, Tunisian, and Moroccan colorectal cancer patients ( 259 , 271 , 274 , 276 – 281 ). Other studies have also explored the contribution of epigenetic changes to colorectal cancer carcinogenesis in Africa ( 278 , 282 – 285 ). For example, the methylation of UCH1 and p14ARF genes were reported to drive colorectal cancer in the presence of TP53 mutation in Tunisia ( 282 , 283 , 286 , 287 ). Other studies on the North African populations reported the influence of polymorphisms in telomere and mitochondrial D-loop region on the clinicopathological characteristics of the colorectal cancers among their patients ( 288 , 289 ). Hence, the dearth of data on the genomics of this disease makes it difficult to explain the increase in the level of sporadic colorectal cancers reported in African countries, despite the difference in lifestyle and dietary habits. Profiling of these genes, including the use of targeted next-generation sequencing, in the screening and clinical management of this disease is essential in reducing its burden ( 255 , 290 ).
Lung Cancer
Across Africa, lung cancer ranks 6 th, with about 550,000 cases in 2018 ( 2 ). However, the burden of this disease is on the North African countries and South Africa ( 2 ). This burden reflects the pattern of tobacco smoking reported through national surveys ( 291 ). Lung cancer genetics/genomics studies have also largely been conducted on the North African populations of Tunisia and Egypt. These studies investigated the role of angiogenic pathway genes like EGFR and MMP-3 in lung carcinogenesis ( 292 – 297 ). The expression of EGFR was associated with poor prognosis, and the frequency of the mutations observed in Tunisian and Moroccan patients was similar to those of Europeans ( 294 , 296 , 298 ). However, Dhieh et al. ( 292 ) found that abnormal p53 expression in these patient populations was more frequent than in Europeans. Similarly, a nonsense mutation (Arg-196-Term) in exon 6 of TP53 was identified in the small cell lung cancer from gold miners in South Africa ( 299 ).
Cigarette and air pollution are major sources of lung carcinogens; hence, studies have reported polymorphisms in CYP1A1 , CYP1A2 , CYP2F1 , CYP2A6*2 , and CYP2A6*9 ( 300 – 305 ) in lung cancer patients in North Africa. These polymorphisms alter the detoxification rate of toxicants, and individuals who carry the slow metabolizer variants have an increased risk of lung cancer ( 300 ). For example, Hussein et al. ( 302 ) concluded that Egyptian smokers with CYP1A1 m1 (rs4646903) and CYP1A1 m2 (rs1048943) are more likely to develop squamous cell carcinoma. Furthermore, lung carcinogens are highly inflammatory and studies in Tunisia, for example, identified alterations in inflammatory genes- TNF-α , IL8 , IL17A , IL17F , CCR2 , and VDR Fok1 (rs2228570) and ApaI (rs7975232) that predispose to lung cancer ( 306 – 310 ).
There were additional studies that used epigenetic techniques to develop diagnostic or prognostic markers for non-small cell lung cancer in Egypt. These included the study of Haroun et al. ( 311 ) that identified FHIT methylation and that of Hetta et al. ( 312 ) which reported circulating microRNA-17 and microRNA-22 as potential biomarkers for early detection of lung cancer.
Bladder Cancer
Chronic inflammation with attendant oxidative stress induced by Schistosomia haematobium infection remains a major cause of bladder cancer in Africa ( 313 – 315 ), with squamous cell carcinoma being the most common ( 316 , 317 ). Schistosomiasis (or bilharzia) is a neglected tropical disease that is widespread across Africa ( 318 ). This cancer is the 10 th most prevalent cancer in Africa and accounted for 240,000 death in 2018. Studies on its genetics/genomics represented about 3% of the publications that we reviewed.
Its pathogenesis involves the bladder infection by S. haemotobium , which induces the formation of carcinogenic N-nitrosamine that contributes to squamous cell carcinogenesis ( 319 ), particularly in individuals with TP53 mutation ( 320 ). In addition, mutations in genes associated with inflammation and detoxification of carcinogenesis are critical risk factors. One of which is the polymorphisms in CYP2D6 and CYP1A1 that have been studied in Egypt and Tunisia ( 321 – 323 ) and that of CYP2D*1A , which was found to increase the risk and clinicopathological outcome of both transitional and squamous cell carcinomas in Egypt ( 322 ). Similar findings were reported in the same North African countries for individuals with GST null genotypes and NAT*5 (341T>C) ( 324 – 331 ).
The neoplastic transformation and progression of bladder cancer are enhanced through oxidative stress-induced genomic instability and chromosomal aberrations, which particularly involve the loss of heterozygosity on chromosomes 8 and 9 ( 332 – 338 ). These aberrations, coupled with p53 and p16 loss, have been reported in both bilharzial and non-bilharzial bladder cancer in Egypt and Tunisia ( 36 , 332 , 339 – 343 ).
The pattern of CpG island hypermethylation was studied by Gustierrez et al. ( 344 ) and they showed that the Schistosoma-associated tumors in Egyptian patients had higher hypermethylation of genes like E-cadherin, DAP-kinase, TP14 , TP15 , TP16 , APC , GSTP1 , and TP73 . Other authors have further proposed using these unique epigenetic modifications for the early diagnosis of bladder cancer by utilizing plasma circulating microRNA and urinary DNA methylation profile ( 345 , 346 ).
It is important to note that pesticides have also been implicated in bladder tumorigenesis ( 347 , 348 ) through oxidative stress and KRAS mutation in Egyptian occupationally-exposed individuals ( 347 ).
Other Solid Tumors
Studies in South Africa, Egypt, Sudan, and Tunisia identified the EBV as the major cause of head and neck cancer ( 349 – 354 ). The genetic risk factors that have been reported include TP53 mutations in Sudan and Egypt ( 355 – 357 ), XRCC1 , TNF-α , IL10 promoter, CYP1A1 , CYP2D6 , and NAT2 polymorphisms in Tunisia ( 358 – 361 ) as well as genome-wide aberrations associated with chromosomes 2p, 3p, 5q, and 18q and microsatelite instabilities ( 362 – 364 ) and mutations in the mitochondrial D-Loop region and Cytochrome b gene ( 365 ).
The genomic studies on the cancer of the brain, kidney, pancreas, and other organs are still emerging with very limited publications ( 366 – 378 ). The emphasis of these publications on the polymorphisms of genes associated with inflammatory response is an indication of the importance of this biological process to the neoplastic transformation of normal tissue and the progression of the malignancy. In addition, studies on retinoblastoma concentrated on identifying the constitutional mutations in RB within the North African populations ( 379 – 382 ) while publications on esophageal and gastric cancers focused on identifying the role of RAS genes mutations as drivers of genomic instability ( 383 – 386 ).
Lymph and Hematological Malignancies
The most prevalent lymphoma in Africa is Burkitt lymphoma. Its pattern and geographical spread are similar to that of malaria and ancient human migration on the continent ( 387 – 392 ). This aggressive pediatric B-cell non-Hodgkin lymphoma is caused by the EBV, which induces genomic instability in the B-cell that results in hyperproliferation ( 393 , 394 ) and it is associated with unique TP53 mutations that are clustered between codons 213 to 248 ( 395 – 397 ).
Other studies on lymphoma include: (1) the role of TP73 and FOXP3 in the pathogenesis of reactive lymphoid hyperplasia and diffuse B-cell lymphoma, as well as the contribution of HLA-G polymorphism to non-Hodgkin lymphoma in Egypt ( 398 – 400 ), (2) susceptibility of individuals with A/A genotype of TNF promoter (-308A/G) to non-Hodgkins lymphoma in Tunisia ( 401 ) and Egypt ( 402 ) and the identification of HLA-B*18 , DRB1*03 , DRB1*07 , and DQB1*02 as lymphoma susceptibility loci in Algerian children ( 403 ).
Studies from Egypt, Tunisia, and Morocco have identified the susceptibility or prognostic implications of mutations in FLT3-ITD , NPM-1 , KIT , NPM1 , HFE , DNMT3A , TERT , and NRAS in hematological malignancies ( 404 – 410 ). NRAS G12D and NRAS G13C mutations were reported in Nigerian leukemia patients Anyanwu et al. ( 411 ).
In order to provide an overview of research progress in African cancer genomics with the view of identifying the critical gaps, we searched and reviewed publications on cancer genetics and genomics in Africa. The 375 publications on cancer genetics/genomics retrieved on PubMed represented only 0.016% of total publications on cancer globally.
According to the 2018 GLOBOCAN data on cancer in Africa, the most frequently diagnosed cancers were breast, cervix, prostate, liver, and colorectum, while the leading causes of cancer deaths were from cancers of the cervix, breast, prostate, liver, and colorectum ( 2 ). However, of the top ten frequently diagnosed cancers and the leading cause of cancer deaths in Africa, only breast, colorectal, liver, and ovarian cancers were proportionately represented in cancer genetics/genomics studies returned from search terms.
Overall, Africans are grossly underrepresented in cancer genomics and molecular biology research globally. For example, research on prostate cancer in African men or breast cancer in African women, both leading causes of death in Africa, are still understudied compared to cancers in their non-Black and white counterparts ( 412 ).
Although Africa seems to be on the right track in terms of focusing on some of the top cancers, researchers and funding agencies, need to elevate and prioritize genetics and genomics research on cancers that remain hugely underrepresented or unrepresented in the literature for which there is a significant burden in Africa. These include cancers of the lung, ovary, stomach, bladder, prostate, and non-Hodgkin lymphoma, which are among the leading ten causes of death but remain understudied in the literature. Filling this research gap is essential to improving awareness, prevention, diagnosis, and treatment outcomes for people affected by cancer across the continent.
It is also worth noting that most studies on cancer in Africa are clustered to a few regions, mainly North Africa, Nigeria, Ghana, and South Africa. Most of the continent lacks any appreciable data, is often excluded from research efforts, and is devoid of the infrastructure and resources needed to contribute to cancer genomics/genetics discoveries.
It is important to reiterate that this review was based on publications that were indexed in Pubmed only. This is because Pubmed is considered as the most reputable index for biomedical publications, and the data we have retrieved are a good representation of the spectrum and scope of this review. It is also possible that our search did not retrieve some studies that included African populations, and this could be because those studies were not focused on African countries or groups but have used them for comparative purposes, thereby making the data obscure and less prominent in their findings. The use of MeSH terms ensured that relevant publications were extracted from Pubmed.
Conclusion and Future Directions
As presented in this review, the preponderance of the peer-reviewed publications on cancer genomics in Africa was on the North Africa populations. Hence, there is a need for a concerted effort to address the gaps in the contribution of genomic variance and alterations to cancer in Sub-Saharan African populations. Recently, Durvasula and Sankararaman ( 413 ) reported the presence of ghost archaic introgression into the genome of Sub-Saharan Africa populations, and some of this introgression included regions involved in carcinogenesis. This and the details presented in this review lay credence to the inadequacy of the use of predominantly Caucasian genomics data for cancer control in Africa. The use of personalized medicine and targeted therapy in cancer management rely on understanding the genomics of the population. Hence, there is a need to step up cancer genomics studies for Africa to benefit from medical advances. Also, because Africa is the root of humanity, understanding the genetic basis of this disease in Africans will contribute to improving cancer health equity globally.
In addition, scientific investigations on cancer racial disparity have largely considered the Black race as a homogenous group. However, the evidence is now emerging that there are within-group differences in cancer risk among Blacks ( 414 ). This review also clearly demonstrated the need to disaggregate Africa in cancer studies. To reduce cancer disparity and achieve equity in treatment outcomes, cancer genetics and genomics studies in African should endeavor to stratify populations by their ancestry roots, tribes, or languages rather than countries. This is imperative to identifying population-relevant genetic variants since African countries are geopolitical constructs that bear no relationship with the biological relatedness of the people that are clustered together in those countries.
Furthermore, every genomic study requires a reference to make an appropriate inference, but African populations are presently inadequately represented in the current reference genomes. To address this unmet need, Shermanet et al. ( 415 ) recently published a pan-African reference genome. The African Pan Genome sequences they assembled revealed that up to 10% of the genome will be missed by any efforts relying only on GRCh38 to study human variation. Yet, it is important to note that their study only included representative samples (5%) from Ibadan, Nigeria, and may not be a true “Pan African Genome” and may best represent the West African human population, which the Yoruba people belong to. Further research efforts are, therefore, needed to assemble more African reference genomes, which should be based on the genetic divergence of human populations in Africa.
Author Contributions
BS conceived, designed, and supervised the review. SR and OR collected and analyzed the data. BS, SR, and OR wrote the manuscript. All authors contributed to the article and approved the submitted version.
Fulbright Visiting Scholar Fellowship (Grant ID: E0604356) awarded to SR.
Conflict of Interest
The authors declare that the research was conducted in the absence of any commercial or financial relationships that could be construed as a potential conflict of interest.
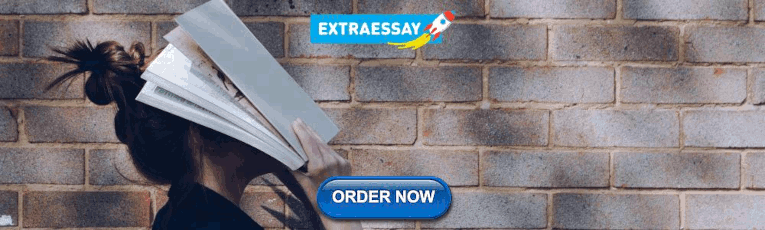
IMAGES
VIDEO
COMMENTS
Cancer genomics is the study of the totality of DNA sequence and gene expression differences between tumour cells and normal host cells. ... Research Highlights 09 Feb 2024 Nature Reviews Cancer ...
Challenges and considerations. The application of NGS technologies to the characterization of human tumours has provided unprecedented opportunities to understand the biological basis of different cancer types, develop targeted therapies and interventions, discover genomic biomarkers of drug response and resistance, and to guide clinical decision-making regarding the treatment of patients 1,2.
One of the biggest impacts that cancer genomics is having is on treatment choices. ... The NCI also supports the Cancer Target Discovery and Development Network — a group of 12 cancer research ...
Advances in cancer genomics research are enabling a more nuanced understanding of causal factors and informing opportunities to reduce the risk of certain cancers in some individuals. These ...
Immunohistochemistry research in cancer was presented in papers by Anastasiya Snezhkina and colleagues [22,23] in the journal issues of the "Medical Genetics, Genomics and Bioinformatics" series. The authors studied succinate dehydrogenase genes in neuroendocrine tumor—carotid paragangliomas [ 22 ].
In 1986, Renato Dulbecco's perspective paper "A turning point in cancer research", made clear that understanding of cancer's daunting complexity, required to focus on the sequence of the whole cellular genome. ... ML approaches have been recently incorporated on cancer genomics research and are achieving promising results, which potentially ...
The Biology of Cancer. Cancer is a disease that begins with genetic and epigenetic alterations occurring in specific cells, some of which can spread and migrate to other tissues. 4 Although the biological processes affected in carcinogenesis and the evolution of neoplasms are many and widely different, we will focus on 4 aspects that are particularly relevant in tumor biology: genomic and ...
The Cancer Target Discovery and Development Program is a network of research centers devoted to studying how molecular changes found in cancers affect cancer cells and explore opportunities to target those changes with new therapies. The Epidemiology and Genomics Research Program supports research in human populations to understand genomic determinants of cancer occurrence and cancer outcomes ...
Abstract. In the past decade, genomics has fundamentally changed our view of cancer biology, allowing comprehensive analyses of mutations, copy number alterations, structural variants, gene expression and DNA methylation profiles in large-scale studies across different cancer types. Efforts like The Cancer Genome Atlas (TCGA) and the ...
The development of cancer is an evolutionary process involving the sequential acquisition of genetic alterations that disrupt normal biological processes, enabling tumor cells to rapidly proliferate and eventually invade and metastasize to other tissues. We investigated the genomic evolution of prostate cancer through the application of three ...
Credit: National Cancer Institute. Genomic information about cancer is leading to better diagnoses and treatment strategies that are tailored to patients' tumors, an approach called precision medicine. As a result of research into the genomic changes associated with cancer, drugs have been developed to fight the disease in several ways:
In 2020, the Pan-Cancer Analysis of Whole Genomes Consortium published a series of papers that characterize over 2,600 cancer whole-genome sequences with unprecedented scope and sensitivity.
An up-to-date list of publications by The Cancer Genome Atlas Research Network, including analyses performed after the original cancer type-specific studies were completed. Skip to main content ... A comprehensive list of publications by The Cancer Genome Atlas program. This list is updated as the TCGA Analysis Network continues to study and ...
The aim of Cancer Genetics is to publish high quality scientific papers on the cellular, ... View full aims & scope. Elsevier's Oncology Journal Network. Cancer Genomics Consortium $3150. Article publishing charge for open access. 8 days. Time to first decision. 144 days. Review time. 164 days. Submission to acceptance. ... Research article ...
Cancer is a multifaceted disease arising from numerous genomic aberrations that have been identified as a result of advancements in sequencing technologies. While next-generation sequencing (NGS), which uses short reads, has transformed cancer research and diagnostics, it is limited by read length. Third-generation sequencing (TGS), led by the Pacific Biosciences and Oxford Nanopore ...
Explore Research Programs. Using a collaborative team science approach, the Center for Cancer Genomics engages in three complementary subdisciplines within cancer genomics: genome sequencing, functional genomics, and computational genomics. Cancer Target Discovery and Development (CTD²) Network. Human Cancer Models Initiative (HCMI)
In this paper, we will provide an overview of different cancer proteomics approaches. ... In addition, the proteomics data in CPTAC has been integrated with cBioportal, a useful information site for cancer genomics research, to facilitate the easy exploration and integrated analysis of proteomics data sets with clinical and genomic data . The ...
Lim, B., Lin, Y. & Navin, N. Advancing cancer research and medicine with single-cell genomics. Cancer Cell 37 , 456-470 (2020). Article CAS PubMed PubMed Central Google Scholar
Cancer is a group of genetic diseases that result from changes in the genome of cells in the body, leading them to grow uncontrollably. These changes involve DNA mutations in the genome. Our cells are constantly finding and fixing mutations that occur in our genome as the cells divide over and over again. But on rare occasions, some mutations ...
Results. We identified the 100 most-cited articles on cancer epigenetics, which collectively had been cited 147,083 times at the time of this writing. The top-cited article was cited 7,124 times, with an average of 375 citations per year since publication. In the period 1980-2018, the most prolific years were the years 2006 and 2010, producing ...
Read time: 1 minute. A peer-reviewed scientific paper has gathered significant attention on the social media platform X (formerly Twitter), albeit for unfavorable reasons. Published in Elsevier's Surfaces and Interfaces journal, the first line of said paper's introduction reads: " Certainly, here is a possible introduction for your topic".
The Cancer Genome Atlas (TCGA), a landmark cancer genomics program, molecularly characterized over 20,000 primary cancer and matched normal samples spanning 33 cancer types. This joint effort between NCI and the National Human Genome Research Institute began in 2006, bringing together researchers from diverse disciplines and multiple ...
Genomics-guided pre-clinical development of cancer therapies. Garnett and colleagues review principles that underpin the pre-clinical development of genomics-guided cancer medicines, challenges ...
The total numbers of publications returned by our search on the topics of cancer globally, as well as cancer, cancer molecular biology, and cancer genetics/genomics within Africa between 1990 and December 2019, are shown in Figure 1. Out of nearly two and half million publications on cancer globally, only 7,697 (0.329%) papers were returned by ...