
An official website of the United States government
The .gov means it’s official. Federal government websites often end in .gov or .mil. Before sharing sensitive information, make sure you’re on a federal government site.
The site is secure. The https:// ensures that you are connecting to the official website and that any information you provide is encrypted and transmitted securely.
- Publications
- Account settings
Preview improvements coming to the PMC website in October 2024. Learn More or Try it out now .
- Advanced Search
- Journal List
- Front Immunol
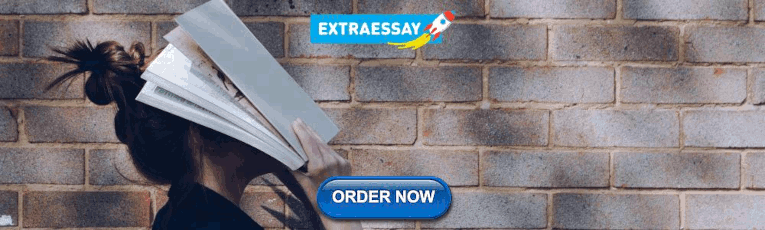
Brief Research Report: Anti-SARS-CoV-2 Immunity in Long Lasting Responders to Cancer Immunotherapy Through mRNA-Based COVID-19 Vaccination
Marta sisteré-oró.
1 Infection Biology Laboratory, Department of Medicine and Life Sciences, Universitat Pompeu Fabra (UPF), Barcelona, Spain
Diana D. J. Wortmann
Naína andrade, andres aguilar.
2 Instituto Oncologico Dr Rosell, Hospital Quiron-Dexeus Barcelona, Barcelona, Spain
Clara Mayo de las Casas
3 Laboratorio Oncología, Hospital Universitario Dexeus, Pangaea Oncology Lab, Barcelona, Spain
Florencia Garcia Casabal
Susana torres, eduardo bona salinas, laura raventos soler, andrea arcas, carlos esparre, beatriz garcia, joselyn valarezo, rafael rosell.
4 Laboratorio Oncología, Germans Trias i Pujol Health Science Insitute and Hospital (IGTP), Badalona, Spain
Roberto Güerri-Fernandez
5 Infectious Diseases Unit, Hospital del Mar, Universitat Autònoma de Barcelona, Barcelona, Spain
Maria Gonzalez-Cao
Andreas meyerhans.
6 Catalan Institution for Research and Advanced Studies (ICREA) Pg. Lluiís Companys 23, Barcelona, Spain
Associated Data
The raw data supporting the conclusions of this article will be made available by the authors, without undue reservation.
Cancer patients (CPs) have been identified as particularly vulnerable to SARS-CoV-2 infection, and therefore are a priority group for receiving COVID-19 vaccination. From the patients with advanced solid tumors, about 20% respond very efficiently to immunotherapy with anti-PD1/PD-L1 antibodies and achieve long lasting cancer responses. It is unclear whether an efficient cancer-specific immune response may also correlate with an efficient response upon COVID-19 vaccination. Here, we explored the antiviral immune response to the mRNA-based COVID-19 vaccine BNT162b2 in a group of 11 long-lasting cancer immunotherapy responders. We analysed the development of SARS-CoV-2-specific IgG serum antibodies, virus neutralizing capacities and T cell responses. Control groups included patients treated with adjuvant cancer immunotherapy (IMT, cohort B), CPs not treated with immunotherapy (no-IMT, cohort C) and healthy controls (cohort A). The median ELISA IgG titers significantly increased after the prime-boost COVID vaccine regimen in all cohorts (Cohort A: pre-vaccine = 900 (100-2700), 3 weeks (w) post-boost = 24300 (2700-72900); Cohort B: pre-vaccine = 300 (100-2700), 3 w post-boost = 8100 (300-72900); Cohort C: pre-vaccine = 500 (100-2700), 3 w post-boost = 24300 (300-72900)). However, at the 3 w post-prime time-point, only the healthy control group showed a statistically significant increase in antibody levels (Cohort A = 8100 (900-8100); Cohort B = 900 (300-8100); Cohort C = 900 (300-8100)) (P < 0.05). Strikingly, while all healthy controls generated high-level antibody responses after the complete prime-boost regimen (Cohort A = 15/15 (100%), not all CPs behaved alike [Cohort B= 12/14 (84'6%); Cohort C= 5/6 (83%)]. Their responses, including those of the long-lasting immunotherapy responders, were more variable (Cohort A: 3 w post-boost (median nAb titers = 95.32 (84.09-96.93), median Spike-specific IFN-γ response = 64 (24-150); Cohort B: 3 w post-boost (median nAb titers = 85.62 (8.22-97.19), median Spike-specific IFN-γ response (28 (1-372); Cohort C: 3 w post-boost (median nAb titers = 95.87 (11.8-97.3), median Spike-specific IFN-γ response = 67 (20-84)). Two long-lasting cancer responders did not respond properly to the prime-boost vaccination and did not generate S-specific IgGs, neutralizing antibodies or virus-specific T cells, although their cancer immune control persisted for years. Thus, although mRNA-based vaccines can induce both antibody and T cell responses in CPs, the immune response to COVID vaccination is independent of the capacity to develop an efficient anti-cancer immune response to anti PD-1/PD-L1 antibodies.
Introduction
The pandemic caused by the new severe acute respiratory syndrome coronavirus 2 (SARS-CoV-2) has a strong pathogenic impact on different subgroups of the general population ( 1 ). This generates the need to test efficacies of the rapidly developed COVID-19 vaccines in particularly vulnerable groups which have been excluded from the initial vaccine efficacy studies. Amongst these groups are immunocompromised individuals that are with active cancers, that receive immunosuppressive drugs i.e. after organ transplantation or that are infected with human immunodeficiency viruses ( 2 – 5 ). Due to their immunocompromised state, these individuals have a significantly higher mortality upon SARS-CoV-2 infection than non-compromised individuals and thus should be taken care of with high priority ( 6 , 7 ). However, there are now CPs that generate exceptional cancer responses after immunotherapy with anti-PD-1/PD-L1 antibodies and that achieve a long survival time ( 8 , 9 ). Such patients may possibly respond better to COVID-19 vaccination or may experience vaccine toxicity via an exaggerated immune response.
The mRNA-based COVID-19 vaccines from BioNTech/Pfizer and Moderna have been developed in record-speed and were given emergency approval in December 2020 after demonstrating to be safe and highly efficient ( 10 , 11 ). They are inducing virus-specific antibody and cellular immunity, and about a 95% protection from SARS-CoV-2-induced disease ( 12 , 13 ). However, vaccine immunogenicity in immunocompromised individuals is expected to be diminished to various degrees depending on the particular condition and state of immunosuppression ( 2 , 14 – 17 ). Here we report vaccination outcomes in a small cohort of solid CPs including a cohort of long survivors under immunotherapy (cohort B, subgroup B.1) with checkpoint inhibitors (mainly anti PD-1/PD-L1 antibodies) and relate them to COVID-19 vaccine studies of cancer patients that have been published until January 2022 ( 3 , 14 , 16 , 18 – 28 ).
Materials and Methods
Sample collection.
This is a post-authorization observational study that prospectively collected data and blood samples from CPs treated with anti PD-1/PD-L1 antibodies that were vaccinated with mRNA-based COVID-19 vaccines (cohort B, subgroups B.1 and B.2). Subgroup B.1 consists of long-lasting responders, which are CPs with advanced tumors that survive longer than the expected median survival achieved with non-immunological therapies. Subgroup B.2 consists of CPs under adjuvant therapy after receiving an initial primary therapy, either radiation or surgery. Adjuvant therapy is given to help prevent cancer relapse. The study included other cohorts as controls: one of CPs not treated with anti-PD-1 antibodies (cohort C) and a second cohort of healthy individuals vaccinated against COVID-19 (cohort A). From 14 patients treated with immunotherapy (cohort B), 11 were on active treatment (cohort B.1) and had received their last dose of anti PD-1/PD-L1 antibody within a median of 31 days (range 42 days to 21 days) before COVID-19 vaccination. Three patients (cohort B.2) had discontinued anti PD-1/PD-L1 treatment 8 months, 10 months and 14 months before COVID-19 vaccination due to immune-related adverse events that were finally resolved. These three patients maintained their cancer response in spite of not being under active anticancer treatment. From 6 CPs not treated with immunotherapy (cohort C), 4 were on active anticancer treatment: three of them with daily oral therapy (one with BRAF plus MEK (tyrosin-kinase inhibitors (TKIs)) and two with hormonal therapy), and 2 patients were on treatment with chemotherapy that received their last dose 14 days and 20 days before COVID-19 vaccination, respectively.
Participants received anti-COVID vaccinations following the standard schedule. The primary endpoint was to describe the specific IgG serum antibody response, the virus neutralizing capacity of these antibodies, and the T cell response. The study analyzed blood samples pre-vaccination, post-prime, and 3 weeks (w) and 9 w after the boost. The protocol of the study was approved by the institutional review board of “Grupo Hospitalario Quirón Salud-Catalunya”. Written informed consent was obtained from each patient.
Quantification of SARS-CoV-2 Spike-Specific IgG Antibody Responses and RBD Neutralization Capacity of Patient Sera
SARS-CoV-2-specific IgG antibodies towards the full-length SARS-CoV-2 S protein were assessed by an enzyme-linked immunosorbent assay (ELISA). Costar 96-well flat-bottom, high-binding plates (2240096, Bio-Rad) were coated with 2 μg/mL full-length SARS-CoV-2 S protein (40592-V08B1, Sino Biologicals) and blocked with 3% BSA (A4503, Sigma-Aldrich) in 1x PBS. Three-fold serial dilutions of serum samples were added in duplicates to the corresponding wells. Next, a detection step using an IgG HRP antibody (A18811, Life technologies) was performed and 3,3’,5,5’-Tetramethylbenzidine (TMB) substrate (T0440-100mL, Sigma-Aldrich) was dispensed and stopped with 1N H 2 SO 4 . Finally, absorbance was measured at 450 nm using a microplate reader (iMark™ Microplate Absorbance Reader #1681130, Bio-Rad). For each sample dilution duplicate, the mean absorbance was calculated. Endpoint-titres of S-specific binding antibodies were defined as the reciprocal of the last serum dilution that provided 3 times the mean optical density of the negative control (wells without sera). Endpoint-titres above a dilution of 1:2100 were scored positive.
Neutralising antibodies against the receptor binding domain (RBD) of the S protein were evaluated from patient sera using a commercial NeutraLISA assay (EI 2606-9601-4, Euroimmune). Sera were always analysed in duplicates and processed according to the manufacturer’s instructions (EI 2606-9601-4, Euroimmune). A microplate reader (iMark Microplate Reader, Bio-Rad) was used to measure the optical densities of the plates. Percentages of inhibition (% IH) against SARS-COV-2 were calculated as detailed by the assay’s manual: % IH = 100% - [(extinction sample x 100%)/average extinction blank]. Percentages of IH were interpreted as positive if ≥35%, as doubtful if ≥20 and <35, and as negative if <20.
Quantification of Spike-Specific T Cells From PBMCs
SARS-CoV-2-specific T cells were determined using an IFN-γ enzyme-linked immunospot (ELISpot) assay (3420-2H, Mabtech) according to the manufacturer’s instructions. Briefly, 250,000 PBMCs per well were incubated with SARS-CoV-2 spike peptides at a final concentration of 2 μg/ml for 16 - 24h (JPT-PM-WCPV-S, Sino Biologicals). A CEF peptide pool (JPT-PM-CEF-S1, Sino Biologicals, 2 ug/mL) and PMA (P8139, Sigma, 15ng/mL) plus ionomycin (I0634, Sigma, 250ng/mL) were used as positive controls. To quantify antigen-specific responses, mean spots of the RPMI control wells were subtracted from the positive wells, and the results were expressed as spot-forming units (SFU) per 10 6 PBMCs. T cell responses were considered positive if the results were ≥14 IFN-γ-secreting cells per 10 6 PBMCs.
The sample size for the study was not based on statistical hypothesis testing. The primary objective was to evaluate by descriptive summary statistics the immunogenicity at different times points in three cohorts of patients, including one cohort of long survivors treated with anti PD-1/PD-L1 antibodies. Statistical analyses and graphical representations of all data were performed using GraphPad PRISM7. For comparison of means and P-value determinations, the Mann-Whitney-U and the Kruskal Wallis test were conducted. P-values < 0.05 were considered significant.
Thirty-five persons participated in the study, including 20 CPs. Fourteen patients were on treatment with anti PD-1/PD-L1-based immunotherapy: three of them in the adjuvant setting (cohort B, subgroup B.2) and eleven patients were long lasting responders to immune-based therapies in advanced stage of cancer disease (cohort B, subgroup B.1). The study included two control cohorts: 6 CPs not treated with anti-PD-1/PD-1-L antibodies (cohort C) and 15 healthy persons (cohort A). All persons received after inclusion two doses of the BNT162b2 vaccine (prime at day 0 and boost after 21 days), except two persons from the healthy cohort that received ChAdOx1-S (boost after 90 days) and Ad26.COV2.S (one single dose), respectively. No previous infection by SARS-CoV-2 was recorded at inclusion in the study.
Noteworthy, all patients included in the immunotherapy cohort, were patients with a good control of the cancer disease, including 11 patients with long lasting responses to immunotherapy (six in complete response and five in partial response; median time on treatment was 36 months -range 12 months to 72 months-) (cohort B, subgroup B.1) and three patients with no active cancer disease and treated with immunotherapy in the adjuvant setting with anti-PD-1 antibodies (two as single agent and one patient in combination with bempegaldesleukin) (cohort B, subgroup B.2). From the cohort of CPs with non-immunologic treatments (cohort C), only one patient was in cancer progression at the time of being included into the current study. In cohort B, cancer treatment consisted of single anti-PD-1 or anti-PD-L1 antibodies in seven (63%) and three (27%) cases, respectively, and one (9%) patient received chemotherapy in combination with an anti-PD-1 antibody. From cohort C, two (33%) patients were under treatment with chemotherapy, one (17%) patient with targeted drugs against BRAF mutations and two (33%) patients were on treatment with hormonotherapy. Additional patient characteristics are included in Table 1 . There was no apparent increase in adverse events due to the BNT162b2 vaccine, as well as no increase in immune related adverse events due to cancer immunotherapy.
Table 1
Patient characteristics.
NA, Not applicable.
To assess SARS-CoV-2-specific humoral immune responses induced by the mRNA-based COVID-19 vaccine from Pfizer/BioNTech, ELISAs against the Spike protein (S) of the SARS-CoV-2 Wuhan isolate were carried out at 4 defined time-points, pre-vaccine, 3 w post prime, 3 w post boost and 9 w post boost. The median ELISA IgG titres significantly increased after the prime-boost vaccine regimen in all cohorts ( Figure 1A ) demonstrating that CPs were responsive to the vaccine and generated virus-specific antibodies. However, at the 3 w post-prime time-point, only cohort A (healthy control group) showed a statistically significant increase in antibody levels (P < 0.05). The respective increase in cohorts B and C was more variable and did not reach significance. Importantly, not all of the CPs generated high level antibody responses after the complete prime-boost regimen (Cohort B, 12/14=85,7%; Cohort C, 5/6 = 83%), while all individuals of the cohort A of healthy controls did (15/15=100%). Together this supports the strong immunogenicity of mRNA-based COVID-19 vaccines and reveals the immunocompromised state of some CPs, including also 2 cases from the cohort B of long lasting cancer immune responders.

SARS-CoV-2-specific immune responses after COVID-19 vaccination. Spike-specific IgG titers (A, B) , nAb titers (C, D) and spike-specific T cell responses (E, F) in healthy donors (Cohort A), CP under immunotherapy (anti-PD1/PD-L1) (IMT; Cohort B, subgroups B.1 and B.2) and CP not treated with immunotherapy (no-IMT; Cohort C) after two doses of mRNA-based COVID-19 vaccines. Individuals from subgroups B.1 are represented by circles and individuals from subgroup B.2 are represented by blue squares. Dotted lines indicate positive thresholds for Spike-specific IgG and T cells. In case of nAbs (C, D) , the dotted line determines the thresholds for negative, intermediate, and positive results according to manufacturer’s instructions. Patients with a supposedly previous asymptomatic SARS-CoV-2 infection are differently coloured (red circled symbols for CP19; blue circled symbols for CP7 and green circled symbols for CP16) and excluded from statistical analyses. Bars represent medians. Differences between the groups were calculated using Mann– Whitney test or Kruskal-Wallis test for comparison of two or three groups. Non-significant differences were indicated as “ns”. P-values below 0.05 were considered significant and wereindicated by asterisks: *p < 0.05; **p < 0.01; ***p < 0.001; ****p < 0.0001.
To further access vaccine-induced neutralising antibodies and virus-specific cellular immune responses, NeutraLISA and IFN-γ ELISpot assays were performed. The results were comparable to those of the ELISA assays. All cohorts experienced a significant increase in neutralising antibodies ( Figure 1C ) and cellular Th1 responses ( Figure 1E ) demonstrating the capacity of the mRNA-based vaccines to induce both antibody and T cell responses. Again, the response was more variable in cohorts B (B.1 and B.2) and C than in the healthy controls from cohort A, and not all cancer CPs responded to the vaccines (non-responders for the nAb: cohort B=28.6%, cohort C=17%; non-responders for the ELISPOT: cohort B=21.5%, cohort C=0%). A simple overview of all responses of the different groups is given in Figure S1 . To better visualize differences within the subgroups of cohort B, we plotted the responses of B.1 and B.2 in Figure S2 .
Noteworthy, there was one individual from cohort C with advanced breast cancer in complete response to hormonotherapy (CP19; red dots in Figures 1A, C, E ) that showed virus-specific IgG and Th1 responses, and a high neutralising antibody response before starting the vaccination scheme. This patient had been probably infected by SARS-CoV-2 previously. Nevertheless, before inclusion in the study, CP19 did neither have symptoms of a SARS-COV-2 infection nor was previously diagnosed of being infected. Interestingly, while vaccination increased the specific antibody response, the respective Th1 response was diminished. Patients CP7 (blue dots in Figures 1A, C, E ) (metastatic non-small cell lung cancer in complete response to anti-PD-L1 antibody; cohort B, subgroup B.1) and CP16 (green dots in Figures 1A, C, E ) (metastatic melanoma in progression after several lines of treatment, cohort C) were potentially SARS-CoV-2-infected before vaccination as they had clear positive S-specific IgG titres and borderline Th1 responses. Upon their first vaccination, both developed strong neutralising antibody responses and showed an increase in their specific T cells. However, as CP7 obtained anti-PDL1 antibodies just 1 week before the first vaccine dose, an immunotherapy-mediated enhancement of the response cannot be excluded. To avoid ambiguities, all 3 patients were not considered for the statistical evaluation.
Amongst the 20 CPs, there were three patients that did not respond properly to the prime-boost vaccination. These were CP1 and CP8 from the cohort B; subgroup B.1 and CP18 from cohort C. All these patients did not generate S-specific IgGs, neutralising antibodies or virus-specific T cells. An additional two patients, CP6 (stage III melanoma treated with adjuvant anti-PD-1 plus bempegaldesleukin, cohort B, subgroup B.2) and CP14 (metastatic non-small cell lung cancer in partial response to chemotherapy plus anti-EGFR antibody, cohort C), responded weakly with borderline responses in all three assays. The remaining 15 CPs showed a vaccine response that was comparable to the healthy control group. Together this demonstrates an important immune response heterogeneity in solid CPs and argues for the need of a careful diagnostic follow-up.
Comparison of the 3 w and 9 w post-boost responses revealed a rather stable immunity with no significant differences within the cohorts ( Figures 1B, D, F ). Only CP2 (metastatic non-small cell lung cancer in complete response to anti-PD-L1 antibody; cohort B, subgroup B.1) had a drop in the S-specific IgG titre by two serum dilutions. As this was not accompanied by a loss of neutralising antibodies or Th1 responses, it was not considered clinically relevant. Patients CP3 (metastatic colorectal cancer in complete response to anti-PD-1 antibody; cohort B, subgroup B.1) and CP11 (metastatic non-small cell lung cancer in complete response to anti-PD-L1 antibody, cohort B, subgroup B.1) showed a reduction of their neutralising antibodies which was for CP11 accompanied by an over 2-fold reduction in the Th1 response. However, most notably was the strong increase of all three immune response measures for CP6 (stage III melanoma treated with adjuvant anti-PD-1 plus bempegaldesleukin, cohort B, subgroup B.2). This patient was a weak responder to the vaccine itself but then had a huge increase in virus-specific IgG titre, neutralising antibodies and Th1 response ( Figures 1B, D, F ; highlighted in red). Thus, the combination of a checkpoint inhibitor with long lasting interleukin 2 may trigger superior vaccine-induced immunity in an otherwise low responder.
Our study demonstrates a significant albeit variable immunogenicity of mRNA-based COVID-19 vaccines for patients with solid tumours under anti-PD1/PDL1 inhibitor immunotherapy or chemotherapy. Long lasting responders to cancer immunotherapy did not have higher responses to COVID vaccines, although there was a relevant case with an exaggerated response during cancer treatment with the combination of anti-PD-1 antibody plus bempegdesleukin. There was no relevant toxicity due to vaccination nor cancer treatment. These data are in line with previous findings (summarised in Tables 2 , 3 ) and enable altogether the following general and clinically relevant conclusions. (1) mRNA-based COVID-19 vaccines are highly immunogenic and safe even in immunocompromised solid tumour patients under various therapy regimens. (2) Roughly up to 30% of solid tumour patients may not generate a proper vaccine response and thus require additional boosters. (3) The biology of the cancer type (i.e. haematological tumour vs solid tumour) dictates vaccine efficacy. (4) The therapy regimen may affect immune response induction and duration of immune memory.
Table 2
Summary of COVID-19 vaccination trials in cancer patients under diverse therapies.
Abbreviations: EC50, 50% effective concentration; CLL, chronic lymphocytic leukemia; CI,confidence interval; CP, Cancer patients; n, numbers; nAbs, neutralizing antibodies; MM, multiple myeloma; MPM, myeloproliferative malignancy; n.r., not reported; n.a., not applicable; Th1, T helper 1 cells; SC, solid cancer; HC, hematologic cancer; IQR,interquartile range; GMC, geometric mean concentration; CHT, chemotherapy; IMT, immunotherapy; TT, targeted therapy; anti-S, anti-spike protein; IFNγ,interferon-γ
*Total column values may not sum up to total as categories are not mutually exclusive (e.g. participants may have received CHT and TT)
Table 3
Summary of immune responses of COVID-19 vaccination trials in cancer patients under diverse therapies.
* Values obtained one month after the 3rd vaccination were: for patients under CHT 73% (22/30), for patients with TT 83% (5/6) and for IMT no patients were analyzed. 75% (27/36) of the CP seroconverted.
Our study included only 20 solid CPs under diverse therapies and thus is limited in its informative value. We therefore reviewed previously published work and included their results, as summarised in Tables 2 , 3 , to obtain the mentioned conclusions. In particular, the work of Thakkar et al., 2021 demonstrates the significant differences of the cancer type and therapy regimen on vaccination efficacy. Given that vaccines rely on the coordinated functioning of antigen-presenting cells and B and T lymphocytes, it is not surprising that haematological tumours and their therapies which affect these cell types, impede vaccine responses the most ( 23 ). Furthermore, since the induction of a vaccine response requires lymphocyte proliferation and differentiation, chemotherapies that target dividing cells are expected to influence this process negatively and thus reduce vaccine efficacies. Indeed, this is observed albeit the differences, for example, in SARS-CoV-2-S-specific antibody titres for CPs with or without chemotherapy were not statistically significant ( 23 ). However, the response level between the patients was very divergent and it may well be that the timing between cytotoxic drug intake and the vaccine schedule might have an important influence (29). Detailed studies on this are still lacking and clearly require further attention.
The three vaccine non-responders and the two low-responders represent together 25% of our patient group. They were low in both humoral and cellular immune responses. Importantly, one of them received bempegdesleukin that significantly boosted both types of responses suggesting that this low-responsive subgroup of patients has no inherent defect to respond and thus may benefit from booster vaccinations or combinations with other immune modulating drugs ( 29 ). This should particularly be true for vaccines, like the ones used here, that induce antibodies and cellular immunity because both antiviral effector mechanisms seem to cooperate in antiviral defence in a multiplicative, synergistic way ( 30 ).
Immunotherapy with checkpoint inhibitors is a common treatment option for patients with solid tumours. Respective therapeutic antibodies disrupt inhibitory signaling pathways in lymphocytes and thereby augment cellular immune responses. In the context of antiviral vaccines, this might have the immunological benefit of generating increased immune responses and thus better host protection against subsequent virus infections. However, our study revealed no significant differences in the vaccine responses between the immune cancer responders (cohort B) and the CPs not-treated with immunotherapy (cohort C), and both cohorts contained individuals that lacked an efficient response. Furthermore, there were no additional side effects compared to the healthy control group suggesting that the mRNA-based vaccine regimen is safe and well tolerated in solid CPs independent on the type of therapy, including those patients with excellent response to cancer immunotherapy. These findings are in line with those of others ( 2 , 14 , 23 , 27 , 29 , 31 ) and support the current recommendations to vaccinate CPs against COVID-19 independent of their therapy regimens ( 14 ).
In conclusion, our results here and the very recent findings of others consistently support COVID-19 vaccination of tumour patients to reduce their enhanced mortality risks upon SARS-CoV-2 infection. The existence of a significant portion of low-vaccine responders argues for diagnostic follow-up and booster vaccinations or novel combinations. Further studies that evaluate and optimise the timing between cancer therapies and vaccination schedules are warranted.
Data Availability Statement
Ethics statement.
The studies involving human participants were reviewed and approved by “Grupo Hospitalario Quirónsalud-Catalunya”. The patients/participants provided their written informed consent to participate in this study. Written informed consent was obtained from the individual(s) for the publication of any potentially identifiable images or data included in this article.
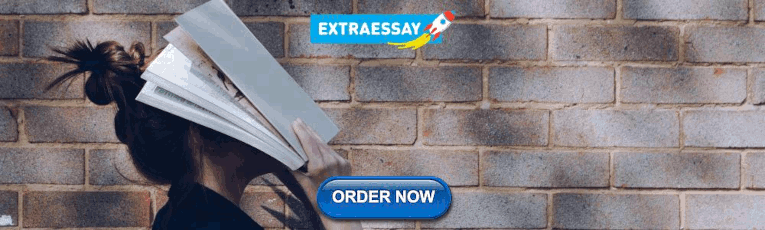
Author Contributions
Concept and funding acquisition: MG-C and AM. Experimental design: MS-O, MG-C and AM. Experiment performance: MS-O, DDJW and NA. Patient recruitment and handling: MG-C, RR, AAg and RG-F. Sample collection: AAg, CM, FGC, ST, EBS, LRS, AAr, CE, BG, JV, RR and RG-F. Figures and tables: MG-C, MS-O, DDJW and NA. Manuscript drafting: MS-O, DDJW and NA with corrections from AM, RR and MG-C. All authors contributed to the article and approved the submitted version.
The authors are supported by Spanish Melanoma Group Grant (III Beca GEM para Grupos Emergentes), the Spanish Ministry of Science and Innovation grant no. PID2019-106323RB-I00 AEI//10.13039/501100011033, the “Unidad de Excelencia María de Maeztu”, funded by the MCIN and the AEI (DOI: 10.13039/501100011033); Ref: CEX2018-000792-M.
Conflict of Interest
The authors declare that the research was conducted in the absence of any commercial or financial relationships that could be construed as a potential conflict of interest.
Publisher’s Note
All claims expressed in this article are solely those of the authors and do not necessarily represent those of their affiliated organizations, or those of the publisher, the editors and the reviewers. Any product that may be evaluated in this article, or claim that may be made by its manufacturer, is not guaranteed or endorsed by the publisher.
Supplementary Material
The Supplementary Material for this article can be found online at: https://www.frontiersin.org/articles/10.3389/fimmu.2022.908108/full#supplementary-material
Supplementary Figure 1
Percentages of responders from cohorts A, B.1, B.2 and C upon COVID-19 vaccination. The percentage of responders from Cohorts A (healthy donors), B.1 (CP under IMT, long lasting responders), B.2 (CP under IMT, adjuvant therapy) and C (CP not treated with IMT, no-IMT) in ELISA (A) , NeutraLISA (B) and ELISPOTs (C) after two doses of mRNA-based COVID-19 vaccines (3 w post boost time-point). Cohort A is represented by black bars, cohort B.1 by light blue bars, cohort B.2 by dark blue bars and cohort C by red bars. The ratio of responders to group size is given above each individual bar.
Supplementary Figure 2
SARS-CoV-2-specific immune responses from cohort B subgroups B.1 and B.2 after COVID-19 vaccination. Spike-specific IgG titers (A) , nAb titers (B) and Spike-specific T cell responses (C) in CPs under immunotherapy (anti-PD1/PD-L1) from subgroup B.1 (long lasting responders) and subgroup B.2 (adjuvant therapy) after two doses of mRNA-based COVID-19 vaccines. Dotted lines indicate positive thresholds for Spike-specific IgG and T cells. In case of nAbs (C, D) , the dotted line determines the thresholds for negative, intermediate, and positive results according to manufacturer’s instructions. Bars represent medians.
Thank you for visiting nature.com. You are using a browser version with limited support for CSS. To obtain the best experience, we recommend you use a more up to date browser (or turn off compatibility mode in Internet Explorer). In the meantime, to ensure continued support, we are displaying the site without styles and JavaScript.
- View all journals
- Explore content
- About the journal
- Publish with us
- Sign up for alerts
- Published: 28 August 2020
A special collection of reviews on frontiers in immunology
- Chenqi Xu ORCID: orcid.org/0000-0002-4968-6782 1 , 2 ,
- Hua-Bing Li 3 , 4 &
- Richard A. Flavell ORCID: orcid.org/0000-0003-4461-0778 5 , 6
Cell Research volume 30 , pages 827–828 ( 2020 ) Cite this article
3351 Accesses
Metrics details
- Cancer therapy
During this challenging period in human history when SARS-CoV-2 has infected more than 20 million people without any sign of an end in sight, we have never been so in need of a healthy immune system to shield ourselves against this highly infectious virus. The reality is that we are still lacking in immunological knowledge to quickly develop therapeutics, either a drug or a vaccine, to slow down the spread of the virus. However, past immunological research has successfully rewarded human society with various immunotherapies, such as the immune checkpoint blockade therapies that have treated numerous cancer patients. The immune system, which intersects with virtually every system in the human body, lies at the center of health and disease. The complexity of the human immune system reflects an ancient Chinese philosophy known as “Yin and Yang”. Immune function needs to be robust yet restrained to efficiently clean up external threats without causing self-damage. When there are “positive” types of immune cells, “negative” types of immune cells must exist simultaneously. At a molecular level, stimulatory and inhibitory molecules reciprocally interplay to elicit well-regulated immune responses. To understand the dynamic and complicated immune responses, immunologists have stepped out of their comfort zones to absorb knowledge from other fields and to apply the newest technologies in their research. Many new branches of immunology have emerged in recent years, including but not limited to, cancer immunology, immunometabolism, microbiota and immunity, neuroimmunology.
Inspired by the fast growth of the relatively “old” immunology field, we are honored to work together with world-renowned immunological experts to present a special review collection in Cell Research discussing our understanding and perspectives of immunological frontiers. Eleven review articles have been assembled to cover diverse topics including cancer immunology, immunometabolism, regulatory T cells (Treg), innate lymphoid cells (ILCs), microbiota/immune interplay, and the inflammasome.
Cancer immunotherapy can induce a durable response in patients, but the response rates are still low. The primary and secondary resistance is derived either from intrinsic tumor factors or from extrinsic microenvironment factors. Jedd Wolchok at Memorial Sloan Kettering Cancer Center and colleagues provide an overview of existing immunotherapies and discuss two categories of new approaches, i.e. direct (tumor) and indirect (microenvironment) modifiers, to improve the clinical outcome of immunotherapy. 1 One of the major clinical challenges of immune checkpoint blockade therapies is the development of autoimmune-like immune-related adverse events (irAEs). Vijay Kurchoo at Harvard Medical School and colleagues review the yin and yang of immune checkpoints in cancer and autoimmunity, with an intensive discussion of current approaches and future directions to reduce the risk of irAEs. 2 In addition to a blockade antibody preventing checkpoint-ligand interaction, other therapeutic reagents inhibiting checkpoint function are under rapid development. Chenqi Xu and a colleague at Shanghai Institute of Biochemistry and Cell Biology review current understandings of checkpoint signaling and discuss alternative paths to target immune checkpoints in cancer. 3 Innate immune response is an essential arm of antitumor immunity and its roles are less understood than the arm of adaptive immune response. Zhijian Chen at University of Texas Southwestern Medical Center and his colleagues review the antitumor roles of the cGAS-STING pathway and discuss how classic cancer therapies such as radiotherapy and chemotherapy elicit antitumor immune responses through cGAS activation. 4
Compared with immune signaling, immune metabolism is still at an early developmental stage. However, many other studies have justified the key role of immunometabolism in health and disease. From signaling aspect, immunometabolism is regulated by several serine/threonine kinase-mediated signaling networks, including the PI3K–AGC kinases, mTOR and LKB1–AMPK pathways. Hongbo Chi at St. Jude Children’s Hospital and colleagues review these key pathways and also provide perspectives about the pending questions and clinical directions. 5 Taking on a different angle, Will Bailis at Children’s Hospital of Philadelphia, Richard Flavell and colleagues at Yale University synthesize findings regarding the interplay between immune signaling and metabolism. 6 They discuss “top-down” regulation of metabolic protein expression and function by signal transduction and gene transcription as well as “bottom-up” regulations of the activity of signaling effectors and gene transcription by metabolites. Eva Pålsson-McDermott and Luke O’Neill at Trinity College Dublin discuss targeting immunometabolism as an anti-inflammation strategy. They first review metabolic therapies with anti-inflammatory effects, and then focus on glycolysis and the TCA cycle to prove the concept of targeting metabolic intermediates and enzymes in inflammation. 7 Besides immunometabolism, inflammasomes serve as key targets for controlling inflammation. Inflammasomes are a group of innate immune receptors sensing invading pathogens and cellular damage to induce production of inflammatory cytokines and pyroptotic cell death. Thirumala-Devi Kanneganti and colleagues at St. Jude Children’s Research Hospital review the transcriptional regulation of inflammasome components and related proteins, the post-translational mechanisms of inflammasome activation, and advances in the understanding of the structural basis of inflammasome activation. 8
In a specific organ, unique populations of immune cells are present to interplay with other tissue-resident cells and environmental factors like microbiota. Formally discovered 10 years ago, ILCs are tissue-resident innate immune cells that resemble transcriptional and functional diversity of the different classes of T cells. Lineage-specifying transcription factors are present to drive certain effector programs. Christoph S. N. Klose at Charité—Universitätsmedizin Berlin and David Artis at Cornell University provide a comprehensive summary and discussion of ILC development/heterogeneity and how ILCs impact innate and adaptive immunity. Moreover, influences of the nervous system, circadian rhythm, and developmental plasticity on ILC function have been reviewed. 9 Eran Elinav at the Weizmann Institute of Science and colleagues extensively review features of microbiome-immunity crosstalk in the intestine and extra-intestinal organs. They also highlight the importance of host immune-microbiome interactions in immune-mediated diseases and discuss how to translate current knowledge into future therapeutic interventions. 10
As a key part of the “yin” arm of the immune system, Treg cells suppress immune response to maintain self-tolerance and homeostasis. Functional abnormality of Treg cells are associated with autoimmunity, cancer and other diseases. Shimon Sakaguchi and a colleague at Osaka University review the transcriptional and epigenetic basis of Treg cell development and function. 11 In addition to the key transcriptional factor FoxP3, Treg-specific epigenome, such as DNA hypomethylation and histone modifications, is also required to induce stable and functional Treg. This review also summarizes and discusses relevant genetic anomalies or variations in autoimmune diseases as well as therapeutic applications of Treg cells.
In summary, the above review articles highlight the multifaceted organization of the immune system. Local and systematic immune responses provide a natural shield against pathogens and cancers but can also cause diseases if homeostasis is broken. There are more unknowns than knowns for us to explore. These articles are therefore made open access to readers to boost inter-discipline research to unravel the remaining secrets of immunity.
Murciano-Goroff, Y. R., Warner, A. B. & Wolchok, J. D. Cell Res. 30 , 507–519 (2020).
Article Google Scholar
Schnell, A., Bod, L., Madi, A. & Kuchroo, V. K. Cell Res. 30 , 285–299 (2020).
Article CAS Google Scholar
He, X. & Xu, C. Cell Res. 30 , 660–669 (2020).
Yum, S., Li, M. & Chen, Z. J. Cell Res. 30 , 639–648 (2020).
Saravia, J., Raynor, J. L., Chapman, N. M., Lim, S. A. & Chi, H. Cell Res. 30 , 328–342 (2020).
Shyer, J. A., Flavell, R. A. & Bailis, W. Cell Res. 30 , 649–659 (2020).
Palsson-McDermott, E. M. & O’Neill, L. A. J. Cell Res. 30 , 300–314 (2020).
Christgen, S., Place, D. E. & Kanneganti, T. D. Cell Res. 30 , 315–327 (2020).
Klose, C. S. N. & Artis, D. Cell Res. 30 , 475–491 (2020).
Zheng, D., Liwinski, T. & Elinav, E. Cell Res. 30 , 492–506 (2020).
Ohkura, N. & Sakaguchi, S. Cell Res. 30 , 465–474 (2020).
Download references
Author information
Authors and affiliations.
State Key Laboratory of Molecular Biology, CAS Center for Excellence in Molecular Cell Science, Shanghai Institute of Biochemistry and Cell Biology, Chinese Academy of Sciences, Shanghai, 200031, China
School of Life Science and Technology, ShanghaiTech University, Shanghai, 200031, China
Shanghai Institute of Immunology, Department of Liver Surgery, State Key Laboratory of Oncogenes and Related Genes, Ren Ji Hospital, Shanghai Jiao Tong University School of Medicine, Shanghai, 200025, China
Hua-Bing Li
Shanghai Jiao Tong University School of Medicine-Yale Institute for Immune Metabolism, Shanghai Jiao Tong University School of Medicine, Shanghai, 200025, China
Department of Immunobiology, Yale School of Medicine, New Haven, CT, USA
Richard A. Flavell
Howard Hughes Medical Institute, New Haven, CT, USA
You can also search for this author in PubMed Google Scholar
Corresponding authors
Correspondence to Chenqi Xu , Hua-Bing Li or Richard A. Flavell .
Rights and permissions
Reprints and permissions
About this article
Cite this article.
Xu, C., Li, HB. & Flavell, R.A. A special collection of reviews on frontiers in immunology. Cell Res 30 , 827–828 (2020). https://doi.org/10.1038/s41422-020-00403-7
Download citation
Published : 28 August 2020
Issue Date : October 2020
DOI : https://doi.org/10.1038/s41422-020-00403-7
Share this article
Anyone you share the following link with will be able to read this content:
Sorry, a shareable link is not currently available for this article.
Provided by the Springer Nature SharedIt content-sharing initiative
Quick links
- Explore articles by subject
- Guide to authors
- Editorial policies

Corrigendum: Brief Research Report: Virus-Specific Humoral Immunity at Admission Predicts the Development of Respiratory Failure in Unvaccinated SARS-CoV-2 Patients
Affiliations.
- 1 Intrahospital Infections Laboratory, National Centre for Microbiology, Instituto de Salud Carlos III (ISCIII), Madrid, Spain.
- 2 Unit of Infectious Diseases, Hospital Universitario "12 de Octubre", Instituto de Investigación Sanitaria Hospital "12 de Octubre" (imas12), Madrid, Spain.
- 3 Infecciones Víricas e Inmunidad en Enfermos Inmunodeprimidos, National Centre for Microbiology, Instituto de Salud Carlos III (ISCIII), Madrid, Spain.
- 4 Universidad Internacional de Valencia-VIU, Valencia, Spain.
- 5 Biología Viral, National Centre for Microbiology, Instituto de Salud Carlos III (ISCIII), Madrid, Spain.
- 6 Department of Internal Medicine, Hospital Universitario "12 de Octubre", Instituto de Investigación Sanitaria Hospital "12 de Octubre" (imas12), Madrid, Spain.
- 7 Centro de Investigación Biomédica en Red de Enfermedades Infecciosas (CIBERINFEC), Madrid, Spain.
- 8 Department of Medicine, Universidad Complutense, Madrid, Spain.
- PMID: 35990649
- PMCID: PMC9381374
- DOI: 10.3389/fimmu.2022.965809
[This corrects the article DOI: 10.3389/fimmu.2022.878812.].
Keywords: COVID disease severity; IgG; IgM 2; SARS-CoV-2; humoral response.
Copyright © 2022 Tajuelo, Carretero, García-Ríos, López-Siles, Cano, Vázquez, Más, Rodríguez-Goncer, Lalueza, López-Medrano, San Juan, Fernández-Ruiz, Aguado, McConnell and Pérez-Romero.
Publication types
- Published Erratum
- Journal Citations
- Research Topics
- Peer Review
- Community Support
- Young Minds
- Innovations

- Frontiers in Behavioral Neuroscience IF 3.104 Most cited journals in Neurosciences Journals Citations Most cited open-access journals in Neurosciences Journals Citations The Frontiers in Neuroscience journal series is the 1st most cited in Neurosciences. With an Impact Factor of 3.104, Frontiers in Behavioral Neuroscience is the 4th most cited open-access journal in Neurosciences. It is also the 5th most cited Behavioral Sciences journal in the World. Read the full Report for Frontiers in Behavioral Neuroscience here . The results are based on the 2016 Journal Citation Reports, released in 2017 by Clarivate Analytics (f.k.a.Thomson Reuters), for citations in 2016 of articles published in 2014-2015. The 2016 JCR Neurosciences category included 259 journals overall, and 37 open-access journals. The Behavioral Sciences category included 5,2 journals overall, and 3 open-access journals. Read the full analysis here .
- Frontiers in Cellular and Infection Microbiology IF 4.300 Most cited open-access journals in Microbiology Journals Citations With an Impact Factor of 4.300, Frontiers in Cellular and Infection Microbiology is the 5th most cited open-access journal in Microbiology. Read the full Report for Frontiers in Cellular and Infection Microbiology here . The results are based on the 2016 Journal Citation Reports, released in 2017 by Clarivate Analytics (f.k.a.Thomson Reuters), for citations in 2016 of articles published in 2014-2015. The 2016 JCR Microbiology category included 124 journals overall, and 19 open-access journals. Read the full analysis here .
- Frontiers in Cellular Neuroscience IF 4.555 Most cited journals in Neurosciences Journals Citations Most cited open-access journals in Neurosciences Journals Citations The Frontiers in Neuroscience journal series is the 1st most cited in Neurosciences. With an Impact Factor of 4.555, Frontiers in Cellular Neuroscience is the 2nd most cited open-access journal in Neurosciences. Read the full Report for Frontiers in Cellular Neuroscience here . The results are based on the 2016 Journal Citation Reports, released in 2017 by Clarivate Analytics (f.k.a.Thomson Reuters), for citations in 2016 of articles published in 2014-2015. The 2016 JCR Neurosciences category included 259 journals overall, and 37 open-access journals. Read the full analysis here .
- Frontiers in Chemistry IF 3.994 Read the full Report for Frontiers in Chemistry here .
- Frontiers in Computational Neuroscience IF 2.653 Read the full Report for Frontiers in Computational Neuroscience here .
- Frontiers in Endocrinology IF 3.675 Most cited open-access journals in Endocrinology & Metabolism Journals Citations With an Impact Factor of 3.675, Frontiers in Endocrinology is the 2nd most cited open-access journal in Endocrinology & Metabolism. Read the full Report for Frontiers in Endocrinology here . The results are based on the 2016 Journal Citation Reports, released in 2017 by Clarivate Analytics (f.k.a.Thomson Reuters), for citations in 2016 of articles published in 2014-2015. The 2016 JCR Endocrinology & Metabolism category included 138 journals overall, and 16 open-access journals. Read the full analysis here .
- Frontiers in Genetics IF 3.789 Read the full Report for Frontiers in Genetics here .
- Frontiers in Human Neuroscience IF 3.209 Most cited journals in Psychology Journals Citations Most cited journals in Neurosciences Journals Citations With an Impact Factor of 3.209, Frontiers in Human Neuroscience is the 1st most cited Psychology journal in the world. It is also the 8th most cited Neurosciences journal in the world. Read the full Report for Frontiers in Human Neuroscience here . The results are based on the 2016 Journal Citation Reports, released in 2017 by Clarivate Analytics (f.k.a.Thomson Reuters), for citations in 2016 of articles published in 2014-2015. The 2016 JCR Psychology category included 77 journals overall, and 2 open-access journals. The Neurosciences category included 259 journals overall, and 37 open-access journals. Read the full analysis here .
- Frontiers in Immunology IF 6.429 Most cited journals in Immunology Journals Citations Most cited open-access journals in Immunology Journals Citations With an Impact Factor of 6.429, Frontiers in Immunology is the 5th most cited Immunology journal in the world. It is also the 1st most cited open-access journal in Immunology. Read the full Report for Frontiers in Immunology here . The results are based on the 2016 Journal Citation Reports, released in 2017 by Clarivate Analytics (f.k.a.Thomson Reuters), for citations in 2016 of articles published in 2014-2015. The 2016 JCR Immunology category included 150 journals overall, and 18 open-access journals. Read the full analysis here .
- Frontiers in Microbiology IF 4.076 Most cited journals in Microbiology Journals Citations With an Impact Factor of 4.076, Frontiers in Microbiology is the 3rd most cited Microbiology journal in the world. Read the full Report for Frontiers in Microbiology here . The results are based on the 2016 Journal Citation Reports, released in 2017 by Clarivate Analytics (f.k.a.Thomson Reuters), for citations in 2016 of articles published in 2014-2015. The 2016 JCR Microbiology category included 124 journals overall, and 19 open-access journals. Read the full analysis here .
- Frontiers in Molecular Neuroscience IF 5.076 Read the full Report for Frontiers in Molecular Neuroscience here .
- Frontiers in Neural Circuits IF 3.879 Read the full Report for Frontiers in Neural Circuits here .
- Frontiers in Neuroanatomy IF 3.267 Most cited journals in Neurosciences Journals Citations Most cited open-access journals in Neurosciences Journals Citations The Frontiers in Neuroscience journal series is the 1st most cited in Neurosciences. With an Impact Factor of 3.267, Frontiers in Neuroanatomy is the 10th most cited open-acess journal in Neurosciences. It is also the 2nd most cited Anatomy & Morphology journal in the world. Read the full Report for Frontiers in Neuroanatomy here . The results are based on the 2016 Journal Citation Reports, released in 2017 by Clarivate Analytics (f.k.a.Thomson Reuters), for citations in 2016 of articles published in 2014-2015. The 2016 JCR Neurosciences category included 259 journals overall, and 37 open-access journals. The 2016 JCR Anatomy & Morphology category included 2,1 journals overall, and 1 open-access journals. Read the full analysis here .
- Frontiers in Neuroinformatics IF 3.870 Read the full Report for Frontiers in Neuroinformatics here .
- Frontiers in Neurology IF 3.552 Most cited open-access journals in Clinical Neurology Journals Citations With an Impact Factor of 3.552, Frontiers in Neurology is the 2nd most cited open-access journal in Clinical Neurology. Read the full Report for Frontiers in Neurology here . The results are based on the 2016 Journal Citation Reports, released in 2017 by Clarivate Analytics (f.k.a.Thomson Reuters), for citations in 2016 of articles published in 2014-2015. The 2016 JCR Clinical Neurology category included 194 journals overall, and 13 open-access journals. Read the full analysis here .
- Frontiers in Neurorobotics IF 2.486 Read the full Report for Frontiers in Neurorobotics here .
- Frontiers in Neuroscience IF 3.566 Most cited journals in Neurosciences Journals Citations Most cited open-access journals in Neurosciences Journals Citations The Frontiers in Neuroscience journal series is the 1st most cited in Neurosciences. With an Impact Factor of 3.566, Frontiers in Neuroscience is the 3rd most cited open-access journal in Neurosciences. Read the full Report for Frontiers in Neuroscience here . The results are based on the 2016 Journal Citation Reports, released in 2017 by Clarivate Analytics (f.k.a.Thomson Reuters), for citations in 2016 of articles published in 2014-2015. The 2016 JCR Neurosciences category included 259 journals overall, and 37 open-access journals. Read the full analysis here .
- Frontiers in Pediatrics IF 2.172 Read the full Report for Frontiers in Pediatrics here .
- Frontiers in Pharmacology IF 4.400 Most cited open-access journals in Pharmacology & Pharmacy Journals Citations With an Impact Factor of 4.400, Frontiers in Pharmacology is the 1st most cited open-access journal in Pharmacology & Pharmacy. Read the full Report for Frontiers in Pharmacology here . The results are based on the 2016 Journal Citation Reports, released in 2017 by Clarivate Analytics (f.k.a.Thomson Reuters), for citations in 2016 of articles published in 2014-2015. The 2016 JCR Pharmacology & Pharmacy category included 256 journals overall, and 20 open-access journals. Read the full analysis here .
- Frontiers in Physiology IF 4.134 Most cited journals in Physiology Journals Citations With an Impact Factor of 4.134, Frontiers in Physiology is the 2nd most cited Physiology journal in the world. Read the full Report for Frontiers in Physiology here . The results are based on the 2016 Journal Citation Reports, released in 2017 by Clarivate Analytics (f.k.a.Thomson Reuters), for citations in 2016 of articles published in 2014-2015. The 2016 JCR Physiology category included 84 journals overall, and 7 open-access journals. Read the full analysis here .
- Frontiers in Plant Science IF 4.298 Most cited journals in Plant Sciences Journals Citations With an Impact Factor of 4.298, Frontiers in Plant Science is the 1st most cited Plant Sciences journal in the world. Read the full Report for Frontiers in Plant Science here . The results are based on the 2016 Journal Citation Reports, released in 2017 by Clarivate Analytics (f.k.a.Thomson Reuters), for citations in 2016 of articles published in 2014-2015. The 2016 JCR Plant Sciences category included 211 journals overall, and 31 open-access journals. Read the full analysis here .
- Frontiers in Psychiatry IF 3.532 Read the full Report for Frontiers in Psychiatry here .
- Frontiers in Psychology IF 2.323 Most cited journals in Multidisciplinary Psychology Journals Citations With an Impact Factor of 2.323, Frontiers in Psychology is the 1st most cited Multidisciplinary Psychology journal in the world. Read the full Report for Frontiers in Psychology here . The results are based on the 2016 Journal Citation Reports, released in 2017 by Clarivate Analytics (f.k.a.Thomson Reuters), for citations in 2016 of articles published in 2014-2015. The 2016 JCR Multidisciplinary Psychology category included 128 journals overall, and 10 open-access journals. Read the full analysis here .

- Visit Frontiers
- @frontiersin
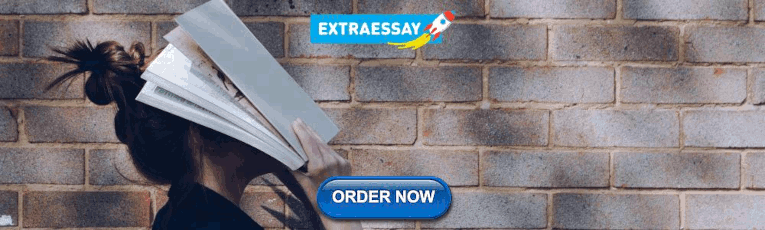
IMAGES
VIDEO
COMMENTS
Frontiers in Immunology. About us About us Who we are; Mission; Values; History; Leadership; Awards; Impact and progress; ... Horowitz JC, Rubinstein MP and Vlasova AN (2024) Brief research report: impact of vaccination on antibody responses and mortality from severe COVID-19. Front. Immunol. 15:1325243. doi: 10.3389/fimmu.2024.1325243 ...
luigi daniele notarangelo. Laboratory of Clinical Immunology and Microbiology, National Institute of Allergy and Infectious Diseases (NIH) Bethesda, United States. Field Chief Editor. Frontiers in Immunology.
Cancer patients (CPs) have been identified as particularly vulnerable to SARS-CoV-2 infection, and therefore are a priority group for receiving COVID-19 vaccination. From the patients with advanced solid tumors, about 20% respond very efficiently to immunotherapy with anti-PD1/PD-L1 antibodies and a …
Here we report vaccination outcomes in a small cohort of solid CPs including a cohort of long survivors under immunotherapy (cohort B, subgroup B.1) with checkpoint inhibitors (mainly anti PD-1/PD-L1 antibodies) and relate them to COVID-19 vaccine studies of cancer patients that have been published until January 2022 (3, 14, 16, 18-28).
Frontiers in Immunology is dedicated to propelling advancements in the field of Immunology by providing unrestricted access to articles and disseminating scientific knowledge to researchers and the public. ... Brief Research Report, Case Report, Classification, Clinical Trial, Conceptual Analysis, Correction, Curriculum, Instruction, and ...
Veronika Lukacs-Kornek. Frontiers in Immunology. doi 10.3389/fimmu.2024.1379225. The official journal of the International Union of Immunological Societies (IUIS) and the most cited in its field, leading the way for research across basic, translational and clinical immunology.
A special collection of reviews on frontiers in immunology. During this challenging period in human history when SARS-CoV-2 has infected more than 20 million people without any sign of an end in ...
Frontiers in Immunology. IF . Most cited journals in Immunology. Journals. Citations. ... Research Topics views & downloads (total) Contributing authors (total) Research Topics with eBooks (total) Visit the journal; Connect with us; For more information: Frontiers' Report. For a better visualisation in mobile or tablet, please turn it ...
A special collection of reviews on frontiers in immunology. Cell Research 30 , 827-828 ( 2020) Cite this article. During this challenging period in human history when SARS-CoV-2 has infected ...
Frontiers requires figures to be submitted individually, in the same order as they are referred to in the manuscript; the figures will then be automatically embedded at the end of the submitted manuscript. Kindly ensure that each figure is mentioned in the text and in numerical order.
Corrigendum: Brief Research Report: Virus-Specific Humoral Immunity at Admission Predicts the Development of Respiratory Failure in Unvaccinated SARS-CoV-2 Patients Front Immunol. 2022 Jun 30;13:965809. doi: 10.3389/fimmu.2022.965809. eCollection 2022. Authors Ana Tajuelo 1 ...
Read the full Report for Frontiers in Immunology here. The results are based on the 2016 Journal Citation Reports, released in 2017 by Clarivate Analytics (f.k.a.Thomson Reuters), for citations in 2016 of articles published in 2014-2015. ... Frontiers Research Network v2.0 . 2014. ALPSP Gold Award for Innovation in Publishing . 2013. Frontiers ...
We are now entering the third decade of the 21st Century, and, especially in the last years, the achievements made by scientists have been exceptional, leading to major advancements in the fast-growing field of Immunology. Frontiers has organized a series of Research Topics to highlight the latest advancements in research across the field of Immunology, with articles from the Associate Members ...
The Journal Impact Factor is the average number of citations received in the last year to articles published in the previous two years. It is measured each year by the Web of Science Group and reported in the Journal Citation Reports (JCR). The 2020 Journal Impact Factors are based on citations in 2020 to articles published in 2018 and 2019.
Keywords: HIV-infection, T-Lymphocytes, Immunological non-responders, Metabolism, Cell division, Cell death . Important Note: All contributions to this Research Topic must be within the scope of the section and journal to which they are submitted, as defined in their mission statements.Frontiers reserves the right to guide an out-of-scope manuscript to a more suitable section or journal at any ...