Problem-Solving Theory: The Task-Centred Model
- Living reference work entry
- First Online: 12 April 2022
- Cite this living reference work entry
- Blanca M. Ramos 5 &
- Randall L. Stetson 6
Part of the book series: Social Work ((SOWO))
467 Accesses
This chapter examines the task-centred model to illustrate the application of problem-solving theory for social work intervention. First, it provides a brief description of the problem-solving model. Its historical development and key principles and concepts are presented. Next, the chapter offers a general overview of the crisis intervention model. The task-centred model and crisis intervention share principles and methods drawn from problem-solving theory. The remainder of the chapter focuses on the task-centred model. It reviews its historical background, viability as a framework for social work generalist practice, as well as its applicability with diverse client populations and across cultural settings. The structured steps that guide task-centred implementation throughout the helping process are described. A brief critical review of the model’s strengths and limitations is provided. The chapter concludes with a brief summary and some closing thoughts.
This is a preview of subscription content, log in via an institution to check access.
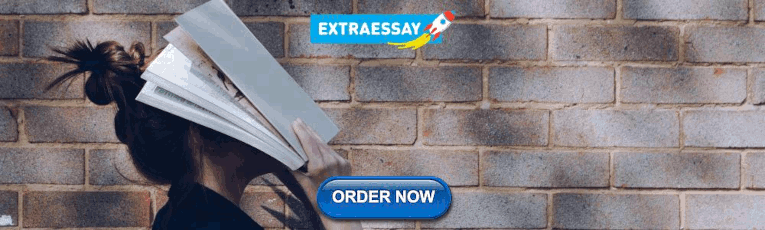
Access this chapter
Institutional subscriptions
Brieland D (1977) Historical overview. Soc Work 22(5):341–346. http://www.jstor.org.libezproxy2.syr.edu/stable/23712810
Google Scholar
Coady N, Lehmann P (2016) The problem-solving model: a framework for integrating the science and art of practice. In: Lehmann P, Coady N (eds) Theoretical perspectives for direct social work practice: a generalist-eclectic approach, 3rd edn. Springer
Chapter Google Scholar
D’Zurilla TJ, Goldfried MR (1971) Problem solving and behavior modification. J Abnorm Psychol 78(1):107–126. https://doi.org/10.1037/h0031360
Article Google Scholar
Dattilio F (1998) Cognitive behavioral therapy. In: Dattilio M (ed) Case studies in couple and family therapy: systems and cognitive perspectives. Guilford, New York, pp 62–82
Dohert W (1981) Cognitive processes in intimate conflicts: extending attribution theory. Am J Fam Ther 9:3–12
Duckword G (1967) A project in crisis intervention. Soc Casework 48(4):227–231
Fortune AE (2012) Development of the task-centered model. In: Rzepnicki TL, McCracken SG, Briggs HE (eds) From task-centered social work to evidence-based and integrative practice: reflections on history and implementation. Oxford University Press, pp 15–39
Fortune AE, Reid WJ (2011) Task-centered social work. In: Turner F (ed) Social work treatment: interlocking theoretical approaches, 6th edn. Oxford University Press, New York, pp 513–532
Fortune AE, McCallion P, Briar-Lawson K (Eds.) (2010) Social work practice research for the 21st century. New York: Columbia University Press
Fortune AE, Ramos BM, Reid WJ (2022) Task-Centered practice. In: Lisa Rapp-McCall, Kevin Corcoran & Albert R. Roberts, (eds.), Social workers’ desk reference, 4th edn Oxford University Press, New York
Fortune AE, Ramos BM, Reid WJ (2022) Task-Centered Practice. In: Lisa Rapp-McCall, Kevin Corcoran, Albert R Roberts, (Eds.). Social Workers’ Desk Reference, 4th edition. New York: Oxford University Press
Garfield SL (1994) Research on client variables in psychotherapy. In: Bergin A, Garfield S (eds) Handbook of psychotherapy and behavior change, 4th edn. Wiley, New York, pp 190–228
Golan N, Carey H, Hyttinnen E (1969) The emerging role of the social worker in the psychiatric emergency service. Community Ment Health J 5(1):55–61
Gorey KM, Thyer BA, Pawfuck DE (1998) Differential effectiveness of prevalent social work practice models: a meta-analysis. Soc Work 43:269–278
Hollis F (1970) The psychosocial approach to the practice of casework. In: Theories of social casework. University of Chicago Press, pp 33–75
Hoyt MF (2000) Some stories are better than others: doing what works in brief therapy and managed care. Brunner/Mazel, Philadelphia
Hubble M, Duncan B, Miller S (1999) Introduction. In: Hubble M, Duncan B, Miller S (eds) The heart and soul of change: what works in therapy. American Psychological Association, Washington, DC
Huh NS, Koh YS (2010) Task-centered practice in South Korea. In: Fortune AE, McCallion P, Briar-Lawson K (eds) Social work practice research for the 21st century. Columbia University Press, New York, pp 235–239
Jagt N, Jagt L (2010) Task-centered practice in the Netherlands. In: Fortune AE, McCallion P, Briar-Lawson K (eds) Social work practice research for the 21st century. Columbia University Press, New York, pp 208–212
Lo TW (2010) Task-centered practice in Hong Kong. In: Fortune AE, McCallion P, Briar-Lawson K (eds) Social work practice research for the 21st century. Columbia University Press, New York, pp 240–244
Malouff JM, Thorsteinsson EB, Schutte NS (2007) The efficacy of problem-solving therapy in reducing mental and physical health problems: a meta-analysis. Clin Psychol Rev 27(1):46–57
Marsh P (2010) Task-centered practice in Great Britain. In: Fortune AE, McCallion P, Briar-Lawson K (eds) Social work practice research for the 21st century. Columbia University Press, New York, pp 203–2007
Marsh P, Doel M (2005) The task-centred book. Routledge, Abingdon/New York
Book Google Scholar
Miley K, O’Melia M, DuBois (2017) Generalist social work practice: an empowering approach. Allyn & Bacon, Boston
Morris B (1968) Crisis intervention in a public welfare agency. Soc Casework 49(10):612–617
Naleppa M (2010) Task-centered practice in Germany. In: Fortune AE, McCallion P, Briar-Lawson K (eds) Social work practice research for the 21st century. Columbia University Press, New York, pp 213–216
Nezu AM, Nezu CM, D’Zurilla T (2012) Problem-solving therapy: a treatment manual. Springer
Nichols M, Schwartz R (2001) Family therapy. Allyn and Bacon, Needham Heights
Parad HJ (1958) Ego psychology and dynamic casework. Family Association of America, New York
Parad H (1965) Preventive casework: problems and implications. In: Parad H (ed) Crisis intervention: selected readings. Family Service Association of America, New York
Parad H (1966) The use of time-limited crisis interventions in community mental health programming. Soc Serv Rev 40(3):275–282
Parad H, Capland G (1960) A framework for studying families in crisis. Soc Work 5(3):3–15
Parad H, Parad G (1968) A study of crisis oriented planned short-term treatment. Soc Casework 49(6):346–355
Payne M (2014) Modern social work theory, 3rd edn. Palgrave Macmillan, Basingstoke
Perlman HH (1957) Social casework: a problem-solving process. University of Chicago Press, Chicago
Poal P (1990) Introduction to the theory and practice of crisis intervention. Quadernos Psicol 10:121–140
Ramos BM, Garvin C (2003) Task centered treatment with culturally diverse populations. In: Tolson E, Reid W, Garvin C (eds) Generalist practice: a task centered approach, pp. Columbia University Press, New York, pp 441–463
Ramos B, Tolson E (2016) The task-centered model. In: Lehmann P, Coady N (eds) Theoretical perspectives for direct social work practice: a generalist-eclectic approach, 3rd edn. Springer
Regehr C (2017) Crisis theory and social work treatment. In: Turner F (ed) Social work treatment: interlocking theoretical approaches. Oxford University Press
Reid WJ (1992) Task strategies: an empirical approach to social work practice. Columbia University Press, New York
Reid WJ, Epstein L (eds) (1972) Task-centered casework. Columbia University Press, New York
Reid W, Ramos B (2002) Intervención “Centrada en la Tarea”, un Modelo de Práctica de Trabajo Social. Rev Treball Soc 168:6–22
Reid WJ, Shyne AW (1969) Brief and extended casework. Columbia University Press, New York
Roberts A (2005) Bridging the past and present to the future of crisis intervention and case management. In: Roberts A (ed) Crisis intervention handbook: assessment, treatment, and research, 3rd edn. Oxford University Press
Rooney RH (2010) Task-centered practice in the United States. In: Fortune AE, McCallion P, Briar-Lawson K (eds) Social work practice research for the 21st century. Columbia University Press, New York, pp 195–202
Ruben D (1998) Social exchange theory: dynamics of a system governing the dysfunctional family and guide to assessment. J Contemp Psychother 8(3):307–325
Schatz MS, Jenkins LE, Sheafor BW (1990) Milford redefined: a model of initial and advanced generalist social work [Article]. J Soc Work Educ 26(3):217–231. https://doi.org/10.1080/10437797.1990.10672154
Strean HS (1968) Some reactions of case workers to the war on poverty. J Contemp Psychother 1:43–48
Strickler M (1965) Applying crisis theory in a community clinic. Soc Casework 46:150–154
Studt E (1968) Social work theory and implication for the practice of methods. Soc Work Educ Report 16:22–46
Tolson R, Reid W, Garvin C (2003) Generalist practice: a task-centered approach, 2nd edn. Columbia University Press, New York
Trotter C (2010) Task-centred practice in Australia. In Fortune AE, McCallion P, Briar-Lawson K (Eds.), Social work practice research for the 21st century, 235–239. New York: Columbia University Press
Watzlawick P, Bervin J, Jackson D (1967) Pragmatics of human communication. W.W. Norton, New York
Download references
Author information
Authors and affiliations.
State University of New York at Albany, Albany, NY, USA
Blanca M. Ramos
State University of New York at Oswego, Oswego, NY, USA
Randall L. Stetson
You can also search for this author in PubMed Google Scholar
Corresponding author
Correspondence to Blanca M. Ramos .
Editor information
Editors and affiliations.
School of Human Services and Social Work, Griffith University, Meadowbrook, QLD, Australia
Dorothee Hölscher
School of Social Sciences, UNSW Sydney, Sydney, NSW, Australia
Richard Hugman
Donna McAuliffe
Rights and permissions
Reprints and permissions
Copyright information
© 2022 Springer Nature Singapore Pte Ltd.
About this entry
Cite this entry.
Ramos, B.M., Stetson, R.L. (2022). Problem-Solving Theory: The Task-Centred Model. In: Hölscher, D., Hugman, R., McAuliffe, D. (eds) Social Work Theory and Ethics. Social Work. Springer, Singapore. https://doi.org/10.1007/978-981-16-3059-0_9-1
Download citation
DOI : https://doi.org/10.1007/978-981-16-3059-0_9-1
Received : 24 December 2021
Accepted : 25 January 2022
Published : 12 April 2022
Publisher Name : Springer, Singapore
Print ISBN : 978-981-16-3059-0
Online ISBN : 978-981-16-3059-0
eBook Packages : Social Sciences Reference Module Humanities and Social Sciences Reference Module Business, Economics and Social Sciences
- Publish with us
Policies and ethics
- Find a journal
- Track your research
Theories Used in Social Work
Social workers are employed throughout a variety of settings and guide people from all walks of life. Regardless of their context, social workers can leverage some core theories and practice models to help clients throughout the industry.
Common Theories and Practice Models in Social Work
In many ways, social work is a science. Social workers can guide their clients, but they don’t carry out their practice based on their own opinion and/or style. Instead, social workers study specific clinical theories that are grounded in research to inform how they implement clinical practice in a methodological manner. In fact, clinical social workers need to obtain both a bachelor’s and master’s degree in order to fully understand these theories and master therapeutic practice models.
At its core, social work focuses on “person-in-environment” (PIE) theory. This considers clients within their psychosocial contexts, and it connects to micro, mezzo, and macro levels of social work practice. This guide explores how each theory and practice model functions within the PIE theory.
Social workers learn about these theories during their education. You can learn more about these academic programs with these resources: a guide to social work bachelor’s degrees , master’s degrees , and online master’s degrees .
Why is Theory Important in Social Work?
As any social work professor can tell you, understanding clinical theories are an essential part of a social worker’s job. It allows social workers to explore certain origins of behavior with evidence-based approaches. Social workers also lean on these theories and practices to address client problems with research to back up their practice. This is especially important, as social workers need to avoid personal assumptions or biases from interfering with effective treatment plans.
Learning about these theories can also help social workers implement effective solutions rather than grasping at straws. If a certain therapeutic approach does not work, social workers can examine the reasons and use what they’ve learned to try a different approach.
Common Social Work Theories
Social workers can incorporate components of several different clinical theories in their work with clients. Some popular approaches for social workers include theories of systems, social learning, psychosocial development, psychodynamic, transpersonal, and rational choice.
Many of these theories have been developed within the past century, and several draw upon Sigmund Freud’s theories of psychoanalysis. Some of these theories encompass a broad outlook (such as systems theory), while others focus on specific conflicts (like psychosocial theory). Not every social worker uses every theory, while some social workers might use elements of each one. You can read more information about the most common social work theories below.
Systems theory assumes that human behavior is the result of a larger system comprised of several elements, including the relationships between these elements, as well as external factors like their environment. These factors could involve a person’s family, peers, school, work, or community. Sociologists have identified many different types of systems, including microsystems, mesosystems, exosystems, and macrosystems.
Social work professionals examine how the systems in which their clients live affect their behaviors. For instance, living in a system of poverty can have a significant impact on how a person makes decisions. Social workers can devise strategies based on these systems in order to provide a more concise treatment plan for their client.
Developed by psychologist Albert Bandura in the 1970s, social learning theory accounts for how the behavior of other people can affect somebody’s behavior. Bandura argued that individuals pick up behaviors by observing and imitating the people around them. Unlike behavioral theories, social learning theory proposes that people actively and mentally process other people’s behaviors before imitating them.
Social workers may take into account social learning theory when working with children who take on aggressive or violent behaviors, for example. The children may mimic their parents or other significant adults in their lives. When social workers are able to identify the origin of a child’s behaviors, they are able to effectively create a treatment approach.
Influenced by the seminal work of Freud, psychologist Erik Erikson proposes several stages of development relating to a person’s ego identity, personal identity, and social and cultural identity. Erikson’s theory argues that humans struggle with specific conflicts throughout different stages of their life. Those conflicts include:
- Trust vs. mistrust in infancy
- Autonomy vs. shame and doubt in early childhood
- Initiative vs. guilt in preschool age
- Industry vs. inferiority in school age
- Identity vs. role confusion in adolescence
- Intimacy vs. isolation in young adulthood
- Generativity vs. stagnation in middle adulthood
- Ego integrity vs. despair in maturity
Erikson’s theory suggests that if humans effectively navigate these tensions at each stage of their life, they can develop a healthy ego. Social workers may consider these conflicts when working with their clients. It is important to note that each stage correlates with an emotional stage which could also be in conflict with a developmental stage.
Introduced by Freud at the turn of the 20th century — and popularized by Carl Jung, Melanie Klein, and Anna Freud — psychodynamic theory argues that our personalities develop because of various internal forces. Freud wrote that our personalities are largely shaped during our early childhood, and our personality consists of three main parts: id (impulse), ego (decision-making), and superego (conscience). Psychodynamic theory also prioritizes a person’s unconscious thought process as the root of their behaviors.
Social workers may use psychodynamic theory to help clients examine the underlying causes of certain behaviors — often considering the clients’ childhood — to help explain why they act a certain way. Social workers may offer different types of therapies based on psychodynamic theory, including transference and dream analysis.
Transpersonal theory approaches humans with a holistic philosophy, and considers factors like spirituality, the relationship between the body and the mind, and consciousness. Psychologists generally do not consider transpersonal theory to be scientific, but many therapists or mental health professionals integrate elements of transpersonal theory into their practice. They might use meditation, mindfulness practices, or hypnotherapy on their patients.
Rational choice theory argues that people make decisions and carry out behaviors based on their own rational thought processes, especially if those decisions ultimately benefit the individual. This theory directly opposes some other clinical theories that suggest people make decisions on unconscious thought processes.
Although rational choice theory is often found within economic theory, social workers can also apply these principles to their job. To understand why clients make certain decisions, social workers can examine how those clients believed their choices would benefit them. Social workers also can develop solutions and suggest resources to assist clients with achieving their goals.
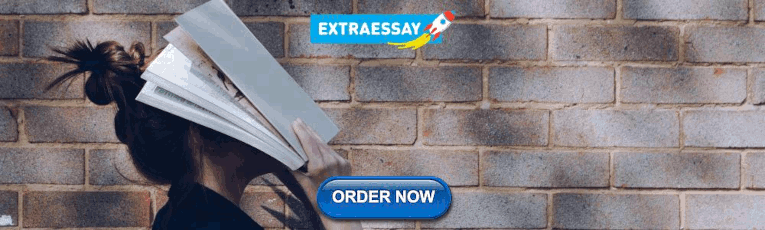
Common Practice Models in Social Work
While social workers integrate various clinical theories into their practice, they can also implement specific therapeutic models. The theories above may explain the causes of a person’s struggles; however, practice models allow social workers to carry out specific approaches to treat those struggles.
The section below outlines some of the most common practice models, including cognitive behavioral therapy, crisis intervention model, narrative therapy, problem-solving model, solution-focused therapy, and task-centered therapy. Some of these methods overlap or share characteristics with each other, but each serves a purpose for specific clients and circumstances.
Cognitive behavioral therapy (CBT) identifies unhealthy patterns of thinking and attempts to rewrite these patterns.
People often convince themselves that their frequently distorted thoughts are true. CBT forces individuals to question and confront these distortions. For instance, somebody might find themselves in fear of social situations, because they imagine a worst-case scenario that they will humiliate themselves. CBT pushes that person to examine these assumptions and instead consider new scenarios and outlooks.
People who struggle with anxiety and depression often find CBT helpful, and many clinical social workers incorporate CBT into their therapeutic practice.
Crisis intervention model is much what it sounds like: in times of acute psychological strain or distress, social workers and mental health professionals intervene before that crisis turns into harm. Albert Roberts and Allen Ottens propose seven steps to crisis intervention. These include conducting a safety assessment, establishing psychological contact, identifying the major problems, helping the patient explore their feelings, looking for new coping mechanisms, creating an action plan, and planning follow-ups.
Social workers can use the crisis intervention model for clients suffering from major trauma, post-traumatic stress disorder, or suicidal thoughts, among others. This model of crisis intervention works in a voluntary manner, which means that clients must be open to the process.
Narrative therapy is based on the theory that individuals turn their personal experiences into stories. In other words, they create narratives of their own lives. This type of therapy relies on four major principles: “objective truth” does not exist; reality is a social construct; language can influence how we view reality; and narratives help us organize our personal realities.
Narrative therapy encourages clients to distance themselves from their personal experiences by taking on the role of a narrator and rewriting the script. This can help them change harmful and disruptive thinking patterns, especially those shaped by trauma.
Helen Harris Perlman proposed the problem-solving model in the 1950s specifically for the field of social work. At the time, many social work theories and therapies relied on psychotherapy; Perlman instead argued that social workers could more effectively help clients by focusing on one problem at a time.
Focusing on smaller problems allows clients to develop and follow through with action plans to confront those issues in a manageable way. This method — also called “partializing” — would make therapies more manageable for social workers and clients alike, and professionals still use Perlman’s proposals.
Solution-focused therapy, or solution focused brief therapy, concentrates on an individual’s present and future situations. This therapy involves a departure from psychodynamic-influenced theories that focus on a person’s past and childhood.
Solution-focused therapy proposes immediate, manageable solutions that allow patients to better cope with their problems. A mental health professional or social worker employing this type of therapy might challenge a client to imagine their future life without their problem, or they might help individuals recognize and better harness copy mechanisms that they already use. Social workers might implement solution-focused therapy for adolescents with behavioral problems or families with conflicts, to name a couple of examples.
Task-centered practice shares many principles with the problem-solving model and solution-focused therapy, but it tends to follow an even more focused and quick approach. Task-centered practice usually only lasts 8-12 sessions, and clients concentrate on achieving measurable goals. Clients and social workers create action plans with specific tasks, and then clients carry out those tasks.
Social workers can integrate this type of therapy into many different types of settings. They might work with students with disruptive behavioral issues, soon-to-be-discharged hospital patients, or older clients at nursing homes.
Reviewed by:
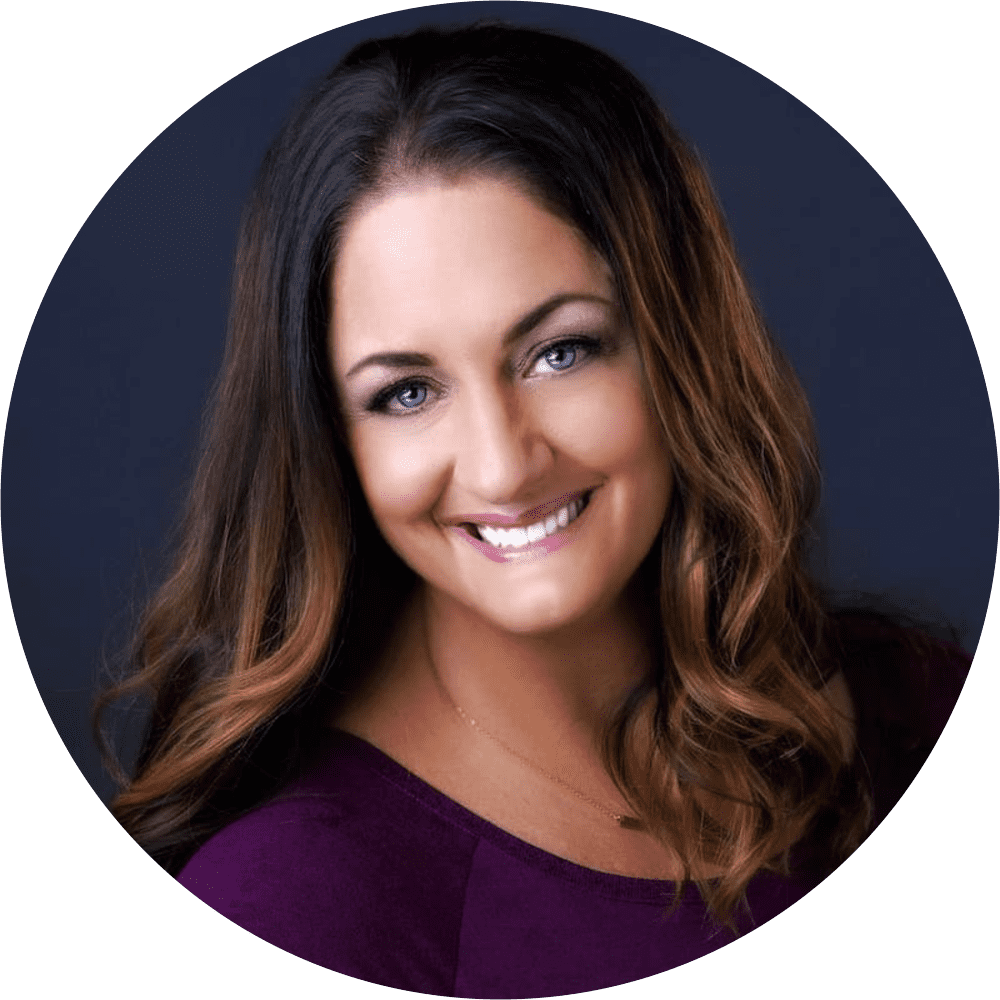
Melissa Russiano, LCSW, LISW
Melissa Russiano is a licensed clinical social worker in private practice that has organically developed into a specialty working with helping professionals. Russiano has a proven track record helping professionals avoid burnout in a unique way that holds clinicians accountable through laughter, tears, blunt (yet very supportive) feedback and quirky analogies that are grounded in solid theoretical research. Russiano practices solely in a virtual setting in the states of California, Florida, Ohio, Pennsylvania and Tennessee. Additionally, Russiano is a professor imparting her experiences and knowledge in the field to future social workers in a graduate program through Simmons University online.
Pursue your passion for helping others with a career in social work.
Discover schools with specialized programs that empower you to make a difference today.
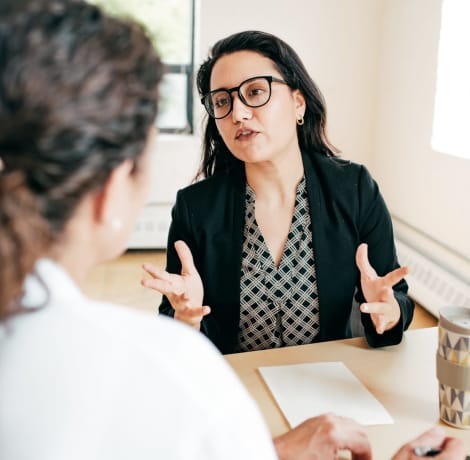
Problem-solving concepts and theories
Affiliation.
- 1 Mississippi StateUniversity, College of Veterinary Medicine, USA. [email protected]
- PMID: 14648495
- DOI: 10.3138/jvme.30.3.226
Many educators, especially those involved in professional curricula, are interested in problem solving and in how to support students' development into successful problem solvers. The following article serves as an overview of educational research on problem solving. Several concepts are defined and the transition from one theory to another is discussed. Educational theories describing problem solving in the context of behavioral, cognitive, and information-processing pedagogy are discussed. The final section of the article describes prior findings regarding expert-novice differences in problem solving of various kinds.
Publication types
- Education, Veterinary*
- Models, Educational*
- Problem Solving*
- Search Menu
- Advance articles
- Author Guidelines
- Submission Site
- Open Access Options
- Self-Archive Policy
- About Journal of Mechanics
- Editorial Board
- Advertising & Corporate Services
- Journals on Oxford Academic
- Books on Oxford Academic

Article Contents
1. introduction, 2. nonlocal elastic damage model, 3. analysis of the strain localization results, 4. selection of nonlocal kernel function, 5. conclusion, acknowledgments.
- < Previous
Determination of nonlocal kernels in nonlocal models for solving strain localization problems

- Article contents
- Figures & tables
- Supplementary Data
Delan Yang, Wei Chen, Yin Yao, Determination of nonlocal kernels in nonlocal models for solving strain localization problems, Journal of Mechanics , Volume 40, 2024, Pages 164–177, https://doi.org/10.1093/jom/ufae017
- Permissions Icon Permissions
The strain localization during the damage and failure of materials can be accurately predicted by nonlocal continuum theories. Yet, the results of theoretical predictions are directly affected by the nonlocal kernel formulation. In this study, the correlation between the nonlocal kernel formulation and the strain localization simulation results is revealed. First, based on the nonlocal damage theory, a novel free energy functional was proposed with strain and damage parameters as independent variables, and a constitutive relation considering nonlocality and elastic damage was derived within the framework of continuum thermodynamics. Then, one-dimensional governing equations were obtained through the dual-linearization method. Finally, strain localization predictions obtained with several nonlocal kernel formulations were presented, and the relationship between the nonlocal kernels and the solution was analyzed, thereby determining an appropriate nonlocal kernel function for the strain localization problem.
Under an external applied mechanical load, a finite-sized zone with highly concentrated strain is always formed in the material, namely a strain localization, which is responsible for material failure behaviors, e.g. the necking phenomenon. Strain localization has been a key issue in the research of the mechanical behaviors of materials. However, how to accurately characterize the strain localization behavior within the framework of continuum mechanics remains a challenge, and many existing theoretical results in this respect failed to reflect the physical reality [ 1 ]. This shortcoming is mainly due to the fact that the strain localization and material damage are determined by the microstructure evolution [ 2 ], which can not be characterized by classical continuum mechanics without any intrinsic length scale. To overcome this blemish, the nonlocal continuum theory considering the microstructural characteristics of materials has been applied to predict the damage and strain localization in materials, thus establishing a framework of nonlocal damage mechanics.
There have been 3 types of nonlocal damage models using different nonlocal variables, i.e. models using an equivalent strain as a nonlocal variable, models using a damage parameter as a nonlocal variable, and models using an energy release rate as a nonlocal variable [ 3 ], each of which has its own applications. Currently, the most commonly used nonlocal damage model can be referred to as the one proposed by Pijaudier-Cabot and Bazant [ 4 ], which uses a local measure of damage variables or the damage energy release rate based on classical continuum damage mechanics, thus ensuring a simple formulation of the stress–strain constitutive relation. However, Pijaudier-Cabot and Bazant's model may not be theoretically rigorous because the constitutive laws describing the damage evolution and the stress–strain correlation could not be derived within the framework of continuum thermodynamics. This is because in the Bazant model, only a dissipative variable is treated as a nonlocal variable by taking its weighted averaging or gradient forms [ 1 , 4 ], while the constitutive formulation of free energy density still shows a local form. Particularly, a Cauchy stress was always used as a stress measure, which is equivalent to a “contact force” interaction in the material, without considering the long-range effect due to the nonlocality, thus resulting in a contradictory with the concept of nonlocal continuum theory [ 5 ]. Subsequently, constitutive laws characterizing the damage evolution and the stress–strain relation with damage were proposed by Polizzoto et al. [ 6–9 ], but in which a gradient-enhanced form was used to characterize the nonlocality, rather than the integral-type constitutive equation that should be more appropriate to account for the effect of nonlocality [ 5 ]. In recent years, we have proposed a series of integral-type nonlocal damage constitutive models [ 11 , 12 ] based on the Cauchy stress boundary condition that consider nonlocal effects [ 10 ], and these models have successfully predicted the strain localization of elastic materials and elastic–plastic materials.
The determination of a universal nonlocal kernel function has also been a key research area in the field of nonlocal damage theories. In early studies, Kroner [ 13 ] and Kunin [ 14 ] obtained a nonlocal kernel function by fitting the lattice vibration dispersion curve, yet this nonlocal kernel was not suitable for damage and fracture problems. In 1972, Eringen [ 15 ] derived a complex nonlocal kernel by fitting the dispersion curve of aluminum, which, however, was not widely used in subsequent research. In 1998, Miller [ 16 ] used an atomic simulation method to obtain the discrete form of a nonlocal kernel, which was used to describe the nonlocal effect of the dislocation slip surface in the Peierls–Nabarro model. This nonlocal kernel was different from the Gaussian kernel function; it relied heavily on the slip system and thus was not unique. Eringen [ 17 ] argued that the nonlocal kernel function should be the Green function of certain linear differential operators, the physical meaning of which was difficult to explain. Then, Bazant and Jirasek [ 3 ] proposed a nonlocal kernel function for damage problems that satisfied a normalization condition in a limited area, yet it did not satisfy a symmetry condition and also lacked a reasonable physical explanation. Borino [ 18 ] and Polizzoto [ 19 ] proposed a nonlocal kernel function with symmetry that was complex and used indefinite parameters. Subsequently, Picu [ 20 ] carried out in-depth research on the expression of nonlocal nuclear functions based on the microstructure of the material and the atomic potential. In short, there is still a lack of a unified and widely acceptable standard for determining the form of nonlocal kernel functions.
Currently, many theoretical issues in nonlocal continuum mechanics that have not been fully solved or are still controversial involve the determination of the nonlocal kernel function, including the well-posedness of the nonlocal linear elastic initial boundary value problem that has attracted widespread attention. In the early 1980s, while studying crack problems in nonlocal elasticity, Atkinson first noticed that, for a certain type of nonlocal kernel, the solution to the mixed boundary value problem of linear nonlocal elasticity does not exist [ 21–23 ], and this attracted attention to the well-posedness of the boundary value problem in nonlocal elasticity theory. For isotropic and isotropic nonlocal initial-boundary value problems in nonlocal elasticity, Altan proved the uniqueness of the solution [ 24 ]. Yet, for mixed boundary value problems, a consensus is currently still lacking.
It is widely accepted that the well-posedness of nonlocal linear elastic mixed boundary value problems is related to the mathematical expression of the nonlocal kernel function, and thus, the expression of the nonlocal kernel needs to be modified [ 17 , 23 ], which involves the determination of the nonlocal kernel function. Recently, Huang et al. [ 10 ] introduced nonlocal surface residuals into stress boundary conditions and established a new nonlocal stress tensor, thereby converting the expression of nonlocal stress boundary conditions to the determination of nonlocal residuals. Based on this theory, a nonlocal damage model was established to analyze strain localization [ 11 , 12 ]. However, the effect of nonlocal kernel functions on the theoretical results was not analyzed. Hence, in conclusion, there is still a lack of comprehensive research and a widely accepted standard for the selection and determination of the nonlocal kernel formulation.
From the perspective of thermodynamics, the strain tensor and damage parameters in the free energy functional were regarded as nonlocal independent variables in the present study, and a theoretical model using integral-typed nonlocal constitutive descriptions of the damage evolution and stress–strain relation was established to simulate strain localization. First, the nonlocal damage constitutive equation and damage evolution equation in nonlocal elasticity were derived, and then an one-dimensional nonlocal damage model was presented to predict the strain localization of a brittle-elastic bar under a load and to study the stress–strain relationship. Finally, based on the nonlocal damage model, the strain localizations corresponding to different forms of nonlocal kernel functions were compared, thereby providing a reference for the selection of nonlocal kernel functions.
In this section, the integral-typed nonlocal constitutive descriptions of the damage evolution and stress–strain relation were derived within the framework of continuum thermodynamics. A one-dimensional linear nonlocal elastic model was established to describe the damage localization through double linearization, and the governing equations were obtained. On the basis of this model, the Gauss-type nonlocal kernel was used to simulate damage localization, and the results were compared with those of existing nonlocal damage models.
2.1 Damage constitutive equations and linearization treatment
According to the second law of thermodynamics [ 25 ], the entropy inequality is |$- \rho (\dot{\Psi } + \dot{\theta }\eta ) + {{\bf t}}:{{\bf d}} - {{\bf q}} \cdot (\nabla \theta )/\theta + \rho \overset{\frown}{\pi } \ge 0$| . Only small deformations are considered, the entropy is assumed to be locally closed, and there is no nonlocal entropy residual. Then, the Euler form of the nonlocal entropy inequality describing the irreversible process of elastic damage is [ 5 ]
where ϕ is the Helmholtz free energy density, |${{\bf \dot{\varepsilon }}}$| is the strain rate tensor, t is the nonlocal stress tensor conjugated with |${{\bf \dot{\varepsilon }}}$| , θ is the absolute temperature, q is the heat flow vector, η is the entropy density and |$\overset{\frown}{\pi }$| is the nonlocal energy residual. In thermodynamics problems with irreversible mechanisms, there is an internal variable in the energy functional that characterizes the dissipation mechanism [ 25 ], i.e. the damage variable. For the damage problem in this study, the strain, temperature and damage variables were selected as independent constitutive variables. According to the constitutive axiom [ 25 ], the constitutive variables involved in the entropy inequality can be expressed in the following nonlocal functional forms:
where x′ traverses all material points and Ω represents the damage variable. Eqs. ( 2 )–( 6 ) suggest that in nonlocal damage theory, due to the consideration of long-range interactions between material points, the constitutive variables are affected by the deformation field, temperature field and dissipation mechanism at all material points. According to previous studies [ 11 , 12 ], the free energy density Ψ is expressed by an additive functional. According to the Friedman–Katz representation theorem [ 26 ],
For the sake of simplicity, let ε ( x ) = ε, ε ( x′ ) = ε′ , θ( x ) = θ, θ( x′ ) = θ′, Ω( x ) = Ω, Ω( x′ ) = Ω′. Substituting Eq. ( 7 ) into Eq. ( 1 ) yields
In an independent thermodynamics process, |${{\bf \dot{\varepsilon }}}$| , |$\dot{\theta }$| and ∇θ can change arbitrarily, and from Eq. ( 2 ), it is known that ϕ, η, t and q are not related to |${{\bf \dot{\varepsilon }}}$| , |$\dot{\theta }$| and ∇θ. Thus,
Then, Eq. ( 11 ) can be rewritten as
Therefore, Y is the conjugate variable of Ω, which represents the damage energy release rate. Assuming that there is a convex dissipative potential |$\phi (\dot{\Omega })$| , in order to satisfy Eq. ( 13 ), the following can be obtained according to the orthogonality rule:
According to Legendre transformation, the dissipative residual potential ϕ*( Y ) is defined as follows:
By taking the derivative of Eq. ( 15 ), the general form of the damage evolution equation can be obtained:
Similar to the previous section, the effect of temperature on the damage constitutive is not taken into account, and it is assumed that the free energy density ψ has a quadratic form containing the damage variable:
By substituting Eq. ( 17 ) into Eq. ( 9 ) and simplifying the equation, the linear constitutive equation of elastic damage is obtained:
where K ( x ) = ∫ V C 1 ( x,x ′ ) dv ( x′ ).
Similarly, let φ* = λ Y 2 /2, where λ is a multiplier, which is related to the damage variable and material properties [ 29 ]. By substituting φ* = λ Y 2 /2 into Eq. ( 16 ) and from Eqs. ( 12 ) and ( 17 ), the elastic damage evolution equation is obtained:
2.2 One-dimensional nonlocal elastic damage model
In the above section, we used the laws of thermodynamics to derive the nonlocal elastic damage constitutive equation and the damage evolution equation, with the damage variable and strain as nonlocal independent variables. In this section, the equations are applied to a uniaxial tension problem. A one-dimensional linear nonlocal elastic damage model is established through a dual-linearization method.
As shown in Fig. 1 , the damage process of an isotropic elastic bar (length: L ) under quasi-static tension loads at both ends is analyzed. Without considering the thermal effects, the governing equations can be expressed as follows:
Balance equation:
Geometric equation:
Constitutive equation:
Damage evolution equation:
Boundary condition:
From Eqs. ( 22 ) and ( 23 ), it can be seen that the integral is in a limited range; hence, according to Bazant and Jirasek [ 3 ], the nonlocal kernel function is
This function satisfies the normalization condition ∫ L α( x, y ) dy = 1, and |$\bar{\alpha }(x,y)$| is a kernel function of any form. In the example below, this equation plays a significant role in simplifying the calculations.

A homogeneous elastic rod.
The coordinate system shown in Figure 1 was established. Since the length of the bar was set to a unit length, the value range of x / L was [−0.5, 0.5]. Symbol γ in the boundary conditions is referred to the surface tension caused by nonlocal effects at the boundary. Generally, γ is a function on the boundary domain. However, since in one-dimensional conditions, the boundaries are 2 points, γ was regarded as a constant in the calculation [ 10 ]. Since the rate of change of the damage variable reflected the loading history rather than a true time effect, it was assumed that |$\dot{\Omega } = d\Omega /d\varepsilon $| . Let |$\lambda = \frac{{1 - \Omega }}{s}$| , where s denotes the back stress [ 27 ]. By taking the integral of Eq. ( 23 ), the full form of the one-dimensional damage evolution equation can be obtained:
in which ε 0 denotes a critical strain at the transition from the elastic stage to the damage stage. The integral term in Eq. ( 26 ) can be transformed into
For the integral term in Eq. ( 27 ), the following linearization and simplification process is performed. The Taylor series expansion of ε( x ′) is obtained at x , and only the first-order gradient term of strain is preserved:
Substituting Eq. ( 28 ) into Eq. ( 27 ) yields
It can be obtained from the normalization condition that ∫ L α(| x ′ − x |) dx ′ = 1 [ 3 ]. Then, the first term is |$\frac{1}{2}\int_{{{{\varepsilon }_0}}}^{\varepsilon }{{E{{\varepsilon }^2}(x)d\varepsilon }} = \frac{1}{6}E[{{\varepsilon }^3}(x) - \varepsilon _0^3(x)]$| , and the second term can be transformed using d ε = |$\frac{{d\varepsilon }}{{dx}}dx$| . In this way, the square term of the first-order gradient appears in the equation. For the sake of simplicity, it is neglected. Then,
By substituting Eq. ( 30 ) into Eq. ( 26 ), the full form of the elastic damage evolution equation is obtained:
By substituting Eq. ( 31 ) into Eq. ( 22 ), performing a Taylor series expansion of ε 0 , and omitting the high-order (>2nd order) terms, the following linearized form can be obtained:
Hence, the simplified nonlocal damage constitutive and damage evolution equations were obtained through double linearization. From Eqs. ( 20 ) and ( 24 ), t ( x ) = p − γ, and from Eq. ( 32 ),
This equation is the second kind of Fredholm integral equation for the damage strain, whose solution exists and is unique. In the classic local damage model, the introduction of the damage variable can cause the equilibrium equation to have ill-posed characteristics. For example, the equilibrium equation changes from elliptical to hyperbolic in static problems, and vice versa in dynamic problems. This can affect the well-posedness of the solution [ 28 ]. Therefore, applying nonlocal theories to damage problems can standardize the governing equations, which is a very important feature of damage models with nonlocality.
The above-obtained nonlocal constitutive equations characterizing the damage evolution and stress–strain relation with damage are used in strain localization simulations. For the example of a one-dimensional bar under tension, the material properties are as follows: elastic modulus E = 1 × 10 5 MPa, back stress s = 1 × 10 −4 MPa and elastic limit strain ε 0 = 1 × 10 −3 . The Gaussian-type kernel function α( x, y ) is used:
where l is an intrinsic length. The intrinsic length is a length parameter related to the microstructural features in the material, e.g. atomic spacing, size of microvoids and microcracks, size of micro-inclusions, etc. Here, the intrinsic length can be considered to be on the same order of magnitude with the size of micro-defects, which was set to l = 0.08 L . In this way, Eq. ( 33 ) can be solved numerically. A force load was used, and the load step was 20 MPa. Since the damage model with nonlocality is only applicable after the onset of damage in the material, the initial load in the calculation was set to k 0 = 5. Figure 2 shows the strain distribution in the bar when the loading levels were at 10, 15 and 20 steps. It can be seen that there was evident damage localization in the bar during loading. Starting from both ends of the bar toward the midpoint, the strain changed slowly over a long distance, whereas in a narrow zone in the middle section, the deformation was highly concentrated, forming a localized strain peak. On both sides of the strain-localized area, the strain decreased rapidly, which was caused by unloading in the area due to the concentration of strain toward the central area. This phenomenon has not been described in previous nonlocal damage models. Since the strain changed drastically near the localized area, it was difficult to determine the accurate strain distribution in the area. Therefore, the presence of this phenomenon remains to be verified in future studies. In addition, as the load increased, the strain at each point in the bar increased; however, the increase was small outside the localized area and large within the localized area. This reflected that the deformation continued to concentrate toward the middle as the load increased.

Strain distribution along the bar under tensile loading.
Figure 3 shows the damage distribution in the bar at the final stage of loading. The damage distribution in the bar was similar to the strain distribution; that is, it was small over a long distance from the ends to the middle of the bar, meaning that the bar was basically in a damage-free state. However, the damage increased rapidly in the small area in the middle of the bar, indicating damage localization toward the center. Moreover, the length of the damage localization area was the same as the length of the strain localization area. Therefore, the strain localization can be called damage localization to a certain extent.

Damage distribution along the bar under tensile loading.
To validate the above results, the stress–strain curves of several typical points in the bar were analyzed. The strain was calculated by Eq. ( 33 ), and the stress was approximated using the local damage constitutive equation:
The damage variable was determined by Eq. ( 31 ). This facilitated a qualitative comparison with the results obtained by other nonlocal damage models because the stress–strain curves from existing models were all based on local stress measurements. For the convenience of description, the stress was normalized, i.e. stress/elastic modulus. Since the strains were distributed symmetrically to the center point x = 0, only the stress–strain curve on one side x ≤ 0 was calculated. Figure 4 shows the stress–strain curves of the points in the region with a small strain variation (−0.5 L ≤ x ≤ −0.12 L ) at load step 20.

Stress–strain curves at points in the nonlocalization region.
It can be seen from Figure 4 that in the region with a small strain variation (−0.5 L ≤ x ≤ −0.12 L ), the stress had a linear relationship with the strain. From Figs 2 and 3 , it can be seen that the strain in this region was much lower than that in the localized deformation region. The damage in the region during the entire loading process was minimal and almost negligible. Thus, in Eq. ( 35 ), 1 − Ω → 1, the stress and strain followed the linear elastic Hooke's law. In fact, according to damage mechanics, the stress included in the damage calculation in Eq. ( 35 ) was a macro-stress (also referred to as nominal stress). The ratio of the macro-stress to the effective stress is 1 − Ω, and the two are connected in material damage calculations. In regions with small strain variations and minimal damage, the macro-stress is approximately equal to the effective stress.
Figure 5 shows the stress–strain curves of several points in the strain attenuation area on both sides of the localized deformation region (−0.12 L ≤ x ≤ −0.04 L ). The strain in − 0.12 L ≤ x ≤ −0.04 L was smaller than that in − 0.5 L ≤ x ≤ −0.12 L . The damage was also negligible, and hence, the stress–strain curve was still linear. This reflected that the damage in this model was controlled by the strain. The smaller the strain was, the smaller the damage was.

Stress–strain curves at points in the strain attenuation area on both sides of the strain localization region.
The stress–strain curves of points in the localized strain region (−0.035 L ≤ x ≤ 0) are shown in Fig. 6 . As the strain increased rapidly and the damage grew in − 0.04 L ≤ x ≤ 0, the tangent stiffness of the stress–strain curve gradually decreased to zero, showing inelastic characteristics, and then became negative. The rate of increase of the stress gradually became smaller with the increase in the strain, and after reaching a certain limit, the stress began to attenuate with the increase in the strain, and closer to the midpoint, the decline was greater. The minimum was reached at the midpoint, showing a significant “strain softening” phenomenon. This phenomenon suggested that the deformation increased rapidly in the strain localization region, accompanied by increased micro-damage and micro-defects in the material, causing a decline in the material stiffness and its deformation resistance ability in this region. As a result, the deformation gradually lost control, which is reflected by the characteristics of “unstable” deformation in the macroscopic stress–strain curve. At this point, there was a significant difference between the macro-stress and the effective stress. The effective stress can always be regarded as the response of the nondamaged material to the external load, which characterizes the true resistance to deformation of the elastic material. The effective stress increased with the strain monotonically. However, the macro-stress reflected the degradation of the material stiffness and a decrease in the load-bearing capacity due to damage. As mentioned above, the effective stress was equal to the macro-stress in the region with small strain variations, but in the strain localization region, the macro-stress showed characteristics of stress degradation as a result of localized damage.

Stress–strain curves at points in the strain localization region.
In this section, using the one-dimensional nonlocal model in Section 1 , different nonlocal kernel functions were selected to predict the strain localization of a brittle elastic bar to study the effects of nonlocal kernel functions on the strain localization results. Additionally, taking Gaussian- and exponential-formed nonlocal kernels as examples, the correlation between the intrinsic length and the size of the strain localization region was further studied.
For the isotropic elastic bar in Fig. 1 , the governing equations, material constants and loading protocol remained unchanged, and the nonlocal kernel function was given by Eq. ( 25 ), which satisfied the normalization condition. With r = | x − y |, 7 common nonlocal kernel functions are listed below:
- Standard potential kernel function: $$\begin{eqnarray} \bar{\alpha }(r) &=& - 0.0009 \times \{ 1132.0 \\ && +\, \exp [10r] + \exp [ - 10r]\} .\end{eqnarray}$$ (36)
- Cosine kernel function: $$\begin{eqnarray} \bar{\alpha }(r) = 0.892 + 0.133\cos \{ \pi r[1 + 3\left| r \right| - 0.5]\} .\end{eqnarray}$$ (37)
Lennard–Jones kernel, i.e. the famous Lennard–Jones potential. When r = 0, the nonlocal kernel function has a singularity, disrupting the calculation. By studying the interactions and atomic potential of atoms in the microstructure of matter, Picu [ 20 ] proposed a new nonlocal kernel function that can approximate the Lennard–Jones potential as an exponential function, thereby avoiding singularities in calculations. The Picu kernel function is given below:
where Γ is the Gamma function, n = 1, m = 0.9, k 1 = 1 and k 2 = 2. In Eq. ( 32 ), with n = 1, m = 0.9, k 1 = 3 and k 2 = 4, the Lennard–Jones potential [ 20 ] can be obtained by the Picu kernel function. With n = 0.87, m = 1, k 1 = 2 and k 2 = 6, the Morse potential [ 20 ] can be obtained by the Picu kernel function.
- Triangular kernel function: $$\begin{eqnarray} \begin{array}{@{}*{1}{c}@{}} {\bar{\alpha }(r) = \displaystyle\frac{1}{l}\left[1 - \frac{r}{l}\right]\,\,,\,\,\,\,\,\,r \le l}\\ {\bar{\alpha }(r) = 0,\,\,\,\,\,\,\,\,\,\,\,\,\,\,\,\,\,\,\,\,\,\,\,\,\,\,r \ge l} \end{array}. \end{eqnarray}$$ (39)
- Gaussian kernel function: $$\begin{eqnarray} \bar{\alpha }(r) = \frac{1}{{2l\sqrt \pi }}\exp \left[ {-}\frac{{{{r}^2}}}{{4{{l}^2}}}\right].\end{eqnarray}$$ (40)
- Exponential kernel function: $$\begin{eqnarray} \bar{\alpha }(r) = \frac{1}{{2l}}\exp \left[ {-}\frac{r}{l}\right].\end{eqnarray}$$ (41)
The above nonlocal kernel functions were substituted into the nonlocal model in Section 1 to calculate the corresponding strain distributions at 10, 15 and 20 load steps. The results for kernel functions ( 1 )–( 5 ) are presented in Fig. 7 , and the 3 curves from bottom to top correspond to k = 10, 15 and 20, respectively.

Strain localization results obtained by using different nonlocal kernel functions: (a) standard potential kernel function, (b) cosine kernel function, (c) Picu kernel function, (d) Lennard–Jones potential simulated by Picu kernel function, (e) Morse potential simulated by Picu kernel function and (f) triangular kernel function.
It can be seen from Fig. 7(a)–(f) that the results for these nonlocal kernel functions showed a large inconsistency with the actual deformation localization. Specifically, the strain distributions calculated under the standard potential and triangular kernel functions were completely inconsistent with physical observations, indicating that these two kernel functions were not suitable for this model. The results of other kernel functions showed improvements to a certain extent. It can be seen that there was a strain concentration in the middle of the bar. However, the localization was not evident. Additionally, the strains at each point in the bar increased with the increase in the number of loading steps, and the amplitudes of the increase were the same. Hence, these kernel functions did not reflect the characteristics of strain localization, in which strains were gradually released and concentrated in the middle. Moreover, the results of certain kernel functions showed two strain concentration regions, which was also inconsistent with physical observations.
Figs 8 and 9 show the simulation results of the Gaussian and exponential kernel functions, calculated using different characteristic lengths. Figure 9 shows the strain localization predictions of the exponential nonlocal kernel function. Compared to Fig. 7 , the use of exponential kernel enables the theoretical prediction to better capture the strain distribution pattern in the localization zone. However, in contrast to the Gaussian kernel, the strain distribution in the bar fluctuated greatly for the exponential kernel, which was inconsistent with physical observations. In particular, when the characteristic length changed, the numerical results in the strain localization region fluctuated significantly, making it difficult to distinguish the localization and nonlocalization regions. Therefore, our results suggest that the Gaussian kernel had greater advantages than other kernel functions in simulating strain localization using the integral nonlocal damage model.

Strain localization results obtained by using the Gaussian kernel function with different characteristic lengths.

Strain localization results obtained by using the exponential kernel function with different characteristic lengths.
In order to further study the correlation between the nonlocal kernel and the strain localization result, the graphs of the selected kernel functions are shown in Fig. 10 . From Figs 7 to 10 , it can be seen that all kernel functions showed certain characteristics of a normal distribution, yet only the Gaussian and exponential kernel functions converged near the Y axis, which was similar to the strain distribution curve and was close to the ideal situation of strain localization, i.e. the shape of a Dirac function. By comparing the Gaussian and exponential kernel functions, it can be found that as the characteristic length decreased, the Gaussian kernel function gradually converged toward the Y -axis, and its crest gradually narrowed while the peak value increased. This was extremely consistent with the strain localization process. Additionally, according to Figs 8 and 9 , the strain localization results of the Gaussian kernel formulations with different intrinsic lengths showed similar characteristics, whereas this was not true for the exponential kernel formulations with different intrinsic lengths. Thus, the Gaussian kernel in the nonlocal damage model could achieve excellent results, and the formulation of the nonlocal kernel was closely related to the simulation results. In actual calculations, the closer the graph of the selected nonlocal kernel is to the ideal situation, the more accurate the simulation results can be. This finding provides a reliable basis for deriving reasonable forms of nonlocal kernels.

Shapes of different nonlocal kernel functions: (a) standard potential kernel function, (b) cosine kernel function, (c) Picu kernel function, (d) Lennard–Jones potential simulated by Picu kernel function, (e) Morse potential simulated by Picu kernel function, (f) triangular kernel function, (g) Gaussian kernel function ( l = 0.1 L , 0.08 L , 0.06 L ) and (h) exponential kernel function ( l = 0.1 L , 0.08 L , 0.06 L ).
Furthermore, when using the Gaussian nonlocal kernel function, the strain localization region corresponding to intrinsic lengths of l = 0.1 L , 0.08 L and 0.06 L were 0.08 L , 0.074 L and 0.052 L , respectively. As the characteristic length decreased, the strain localization region also decreased, and the two were of the same order of magnitude, which further verified the conclusion in Section 1 , that is, during the material damage process, there was a close correlation between the intrinsic length and the size of the strain localization region, and the former likely played a role in controlling the size of the latter. This finding reflects the impact of the microstructure on the strain localization process, which was consistent with our previous results.
In the present study, from the perspective of thermodynamics, the strain tensor and damage parameters in the free energy functional were regarded as nonlocal independent variables, and an integral nonlocal elastic damage model was established to simulate the strain localization phenomenon of brittle elastic materials. Since the nonlocal damage constitutive equations and damage evolution equations were derived based on the laws of thermodynamics, they were theoretically self-consistent. On this basis, a one-dimensional nonlocal elastic damage model was established to simulate the strain localization of an elastic bar under uniaxial loads. The simulation results accurately described the strain localization phenomenon of the elastic bar and the strain softening phenomenon in the damage concentration area. Compared with the results of some previous nonlocal elastic damage models, the strain in the bar was distributed on both sides of the strain localization region, with an evident unloading phenomenon, which caused the strain to be more concentrated near the midpoint. The macroscopic stress–strain curve in the strain localization region was smooth, which reflected softening characteristics, that is, the stress at each point in the strain localization region gradually decreased as the strain increased, and it reached the minimum at the midpoint. It is worth noting that the proposed model does not require a preset size of the strain localization area. Instead, the size of the strain localization region is controlled by the intrinsic length in the Gaussian nonlocal kernel function, thus reflecting the effect of the microstructure on the strain localization process.
Subsequently, based on the integral nonlocal elastic damage model established in this study, the effects of different nonlocal kernel functions on the strain localization results were analyzed. It was found that the results of the Gaussian kernel function were the most consistent with the physical observations, and the shapes of the Gaussian and exponential nonlocal kernel functions were close to their strain distribution results. When different intrinsic lengths were used in the Gaussian kernel function, the results could reflect the ideal characteristics of strain concentration toward the midpoint to varying extents, while the exponential kernel function did not have this characteristic. Therefore, the nonlocal kernel formulation was closely related to the strain localization results. When using nonlocal theory to solve strain localization problems, the closer the pattern of the nonlocal kernel formulation is to the ideal solution, the more accurate the simulation results will be. This finding provides a reference for the determination of a reasonable nonlocal kernel. Lastly, it was found that when different intrinsic lengths were used in the Gaussian nonlocal kernel function, the size of the strain localization region was of the same order of magnitude as the intrinsic length, thus verifying the correlation between the intrinsic length and the size of the strain localization region as well as the effect of the microstructure on the damage behavior. In conclusion, this study provides a reference for studying the evolution of the strain localization zone in the damage process.
The work reported here is supported by NSFC through grants (No. 12272043). The author also thanks LetPub ( www.letpub.com ) for its linguistic assistance during the preparation of this manuscript.
Bazant ZP , Pijaudier-Cabot G . Nonlocal continuum damage, localization, instability and convergence . ASME Journal of Applied Mechanics 1988 ; 55 ( 2 ): 287 – 293 .
Google Scholar
Bazant ZP . Why continuum damage is nonlocal: jusitification by quasiperiodic microcrack array . Mechanics Research Communications 1987 ; 14 ( 5 ): 407 – 419 .
Bazant ZP , Jirasek M . Nonlocal integral formulations of plasticity and damage: survey of progress . Journal of Engineering Mechanics 2002 ; 128 ( 11 ): 1119 – 1149 .
Pijaudier-Cabot G , Bazant ZP . Nonlocal damage theory . ASCE Journal of Engineering Mechanics 1987 ; 113 ( 10 ): 1512 – 1533 .
Eringen AC . Nonlocal Continuum Field Theories . New York : Springer , 2002 ;
Google Preview
Polizzotto C , Borino G . A thermodynamics-based formulation of gradient-dependent plasticity . European Journal of Mechanics A/Solids 1998 ; 17 ( 5 ): 741 – 761 .
Polizzotto C , Borino G , Fuschi P . A thermodynamically consistent formulation of nonlocal and gradient plasticity . Mechanics Research Communications 1998 ; 25 ( 1 ): 75 – 82 .
Peerlings RHJ , Borst de R , Brekelmans WAM , de Vree J . Gradient-enhanced damage for quasi-brittle materials . International Journal for Numerical Methods in Engineering 1996 ; 39 ( 19 ): 3391 – 3403 .
Peerlings RHJ , Geers MGD , Borst de R , Brekelmans W . A critical comparison of nonlocal and gradient-enhanced softening continua . International Journal of Solids and Structures 2001 ; 38 ( 44-45 ): 7723 – 7746 .
Huang ZX . Formulations of nonlocal continuum mechanics based on a new definition of stress tensor . Acta Mechanica 2006 ; 187 ( 11 ): 11 – 27 .
Huang LY , Yao Y . A thermodynamically nonlocal damage model using a surface-residual-based nonlocal stress . Journal of Mechanics 2021 ; 37 : 484 – 495 .
Chen W , Xia LZ , Yao Y . An investigation on the evolution of strain localization zone in metallic materials based on tensile tests and a 1-D nonlocal model . Journal of Mechanics 2023 ; 39 : 292 – 308 .
Kröner E . Elasticity theory of materials with long-range cohesive forces . International Journal of Solids and Structures 1967 ; 13 ( 5 ): 731 – 742 .
Kunin IA . The theory of elastic media with microstructure and the theory of dislocations . (In: Kroner E ). Mechanics of Generalized Continua , New York : Springer , 1968 ; 321 – 329 .
Eringen AC . Linear theory of nonlocal elasticity and dispersion of plane waves . International Journal of Engineering Science 1972 ; 10 ( 5 ): 425 – 435 .
Miller R , Phillips R , Beltz G , Ortiz M . A nonlocal formulation of the Peierls dislocation model . Journal of Mechanics and Physics of Solids 1998 ; 46 ( 10 ): 1845 – 1867 .
Eringen AC . On differential Eqs. of nonlocal elasticity and solutions of screw dislocation and surface waves . Journal of Applied Physics 1983 ; 54 ( 9 ): 4703 – 4710 .
Borino G , Failla B , Parrinello F . A symmetric formulation for nonlocal damage models . 2002 . Proceedings of 5th World Congress on Computational Mechanics (WCCM V) , Vienna University of Technology , Vienna, Austria , ISBN 3-9501554-0-6 .
Polizzotto C . Remarks on some aspects of nonlocal theories in solid mechanics . 2002 . Proceedings of 6th National Congress , SIMAI , Chia Laguna, Italy , CD-ROM .
Picu RC . On the functional form of nonlocal elasticity kernels . Journal of Mechanics and Physics of Solids 2002 ; 50 ( 9 ): 1923 – 1939 .
Atkinson C . On some recent crack tip stress calculations in nonlocal elasticity . Archives of Mechanics 1980 ; 32 ( 2 ): 317 – 328 .
Atkinson C . Crack problems in nonlocal elasticity . Archives of Mechanics 1980 ; 32 ( 4 ): 597 – 614 .
Atkinson C . Nonlocal elasticity and the line crack problem . Defects and Fracture . Proceedings of First International Symposium on Defects and Fracture, held at Tuczno, Poland, 1980. Dordrecht: Springer Netherlands , 1982 ; 121 – 128 .
Altan SB . Uniqueness of initial-boundary value problems in nonlocal elasticity . International Journal of Solids and Structures 1980 ; 25 ( 11 ): 1271 – 1278 .
Huang ZP . Fundamental of Continuum Mechanics . Beijing : Higher Education Press , 2012 .
Friedman , Katz M . A representation theorem for additive functionals . Archive for Rational Mechanics and Analysis 1966 ; 21 ( 1 ): 49 – 59 .
Lemaitre J . A Course on Damage Mechanics . Berlin : Springer-Verlag , 1992 .
Cheng JC . Mathematics Physics Eqs. and Their Approximation Methods . Beijing : Science Press , 2004 .
Lemaitre J . Coupled elasto-plasticity and damage constitutive Eqs. Computer Methods in Applied Mechanics and Engineering 1985 ; 51 ( 1-3 ): 31 – 49 .
Email alerts
Citing articles via.
- Advertising and Corporate Services
- Journals Career Network
Affiliations
- Online ISSN 1811-8216
- Copyright © 2024 Society of Theoretical and Applied Mechanics of the Republic of China, Taiwan
- About Oxford Academic
- Publish journals with us
- University press partners
- What we publish
- New features
- Open access
- Institutional account management
- Rights and permissions
- Get help with access
- Accessibility
- Advertising
- Media enquiries
- Oxford University Press
- Oxford Languages
- University of Oxford
Oxford University Press is a department of the University of Oxford. It furthers the University's objective of excellence in research, scholarship, and education by publishing worldwide
- Copyright © 2024 Oxford University Press
- Cookie settings
- Cookie policy
- Privacy policy
- Legal notice
This Feature Is Available To Subscribers Only
Sign In or Create an Account
This PDF is available to Subscribers Only
For full access to this pdf, sign in to an existing account, or purchase an annual subscription.
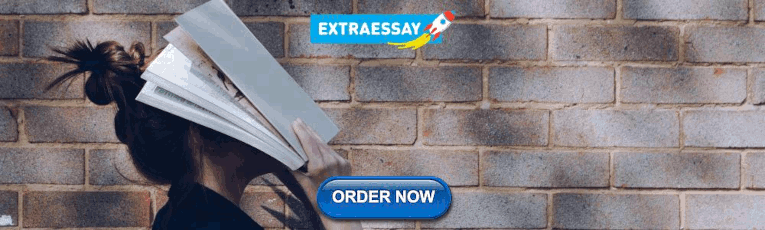
IMAGES
VIDEO
COMMENTS
Identify the people, information (data), and things needed to resolve the problem. Step. Description. Step 3: Select an Alternative. After you have evaluated each alternative, select the alternative that comes closest to solving the problem with the most advantages and fewest disadvantages.
Problem solving refers to cognitive processing directed at achieving a goal when the problem solver does not initially know a solution method. A problem exists when someone has a goal but does not know how to achieve it. Problems can be classified as routine or nonroutine, and as well defined or ill defined.
The problem solving is a personal and aimed process. That means that the activities done by an individual during the problem solving process are led to his/her personal aim (Mayer and Wittrock, 2006). An individual has to identify the problem first and then seek for possible solutions (Mayer and Wittrock, 2006).
This chapter focuses on the task-centred model (Reid and Epstein 1972) as a prime example of the major influence problem-solving theory has exerted in the practice of social work.First, as background for understanding the development of the task-centred model, the chapter offers a brief account of the historical development of the problem-solving model (Perlman 1957) and describes its key ...
inconsistency" of the situation; the problem solving consists of the removal of the conflict and the finding. of the desired object. b) a disorder in the objective situation or in the structure of ...
Problem solving is the process of articulating solutions to problems. Problems have two critical attributes. First, a problem is an unknown in some context. That is, there is a situation in which there is something that is unknown (the difference between a goal state and a current state). Those situations vary from algorithmic math problems to ...
personal resourcefulness influence problem-solving performance in both formal and informal contexts. In addition, these authors present a cycli-cal model of problem solving that identifies self-regulatory processes and sources of motivation that are central to successful problem solving in a wide range of situations.
Problem solving is the process of finding solutions to complex or challenging issues. It involves various skills, such as creativity, logic, analysis, and decision making. This article on Wikipedia provides an overview of different problem solving methods, models, techniques, and applications in various domains.
model, the IDEAL problem solver (Bransford & Stein, 1984), describes problem solving as a uni- form process of Identifying potential problems, Defining and representing the problem, Explor- ing possible strategies, Acting on those strate-. gies, and Looking back and evaluating the. effects of those activities.
Blanca M. Ramos and Randall L. Stetson. Abstract. This chapter examines the task-centred model to illustrate the application of problem-solving theory for social work intervention. First, it provides a brief description of the problem-solving model. Its historical development and key principles and concepts are presented.
A decision-making model is presented, which takes the problem-solving process through its latter stages. The theory is based on two axioms and three theorems pertaining to the process of decision making. These ... problem-solving theories vary widely in content and in the outcomes they seek to explain. Current problem-solving research
Most problem-solving models rely on data to inform decisions, which helps to maintain objectivity and fairness throughout the process. By using problem-solving methods to hear the opinions of everyone, you can eliminate bias when solving a problem. In addition, implementing problem-solving models can lead to more effective, thoughtful solutions.
2. Theory: Models of Problem Solving. During the past century, scholars proposed a wealth of models that aimed to capture how individuals solve problems ().Simon's (1947) foundational model unbundled problem solving into three phases (intelligence gathering, design, and choice). Many more models built on it, emphasizing different elements of problem solving.
In this chapter we (a) review the early development of the problem-solving model for social work practice; (b) discuss the later development of the problem solving model in terms of its extension to and further elaboration by generalist models of social work practice; (c) provide an overview of how the problem-solving model allows for the integration of the scientific and artistic elements of ...
In this chapter we describe the social problem-solving model that has generated most of the research and training programs presented in the remaining chapters of this volume. We also describe the major assessment methods and instruments that have been used to measure social problem-solving ability and performance in research as well as clinical practice.
Finding a suitable solution for issues can be accomplished by following the basic four-step problem-solving process and methodology outlined below. Step. Characteristics. 1. Define the problem. Differentiate fact from opinion. Specify underlying causes. Consult each faction involved for information. State the problem specifically.
The 4 Key Principles of a Problem-Based Curriculum. Through active learning. Learners can control their own learning, as well as submit and answer their own questions. Through integrated learning. Knowledge, understanding and skills go hand in hand, while classroom/book knowledge is linked to the real world, and the problem is the focus.
The term "problem" defines J. Linhart (1976, p. 385) as: . a) problem is an interactive relation between a subject and its surroundings, which incorporates the inner conflict that is solved by the subject by searching of transitions from initial condition to the final condition. b) the existence of a conflict causes the dynamics of an activity ...
Problem-Solving Model . Helen Harris Perlman proposed the problem-solving model in the 1950s specifically for the field of social work. At the time, many social work theories and therapies relied on psychotherapy; Perlman instead argued that social workers could more effectively help clients by focusing on one problem at a time.
Several concepts are defined and the transition from one theory to another is discussed. Educational theories describing problem solving in the context of behavioral, cognitive, and information-processing pedagogy are discussed. The final section of the article describes prior findings regarding expert-novice differences in problem solving of ...
At the end of this chapter, Appendixes A, B, and C, are three examples of problem-solving activities that we refer to as model-eliciting activities—so called because the products that students produce go beyond short answers to narrowly specified questions—which involve sharable, manipulatable, modifiable, and reusable conceptual tools (e.g., models) for constructing, describing ...
Some of the values upon which this theory is based include: learning to use naturally effective collaborative processes, critical-thinking and problem-solving skills, rich social contexts and multiple perspectives for learning, learning environments that are situated, learner-centered, integrated, and collaborative, authenticity, ownership, and ...
Delan Yang, Wei Chen, Yin Yao, Determination of nonlocal kernels in nonlocal models for solving strain localization problems, Journal of Mechanics, Volume 40, 2024, ... Therefore, applying nonlocal theories to damage problems can standardize the governing equations, which is a very important feature of damage models with nonlocality. ...