Modeling of Wind Power Plants and their Impact on Economic and Environmental Development
Contributed by the Advanced Energy Systems Division of ASME for publication in the J ournal of E nergy R esources T echnology .
- Split-Screen
- Article contents
- Figures & tables
- Supplementary Data
- Peer Review
- Open the PDF for in another window
- Cite Icon Cite
- Permissions
- Search Site
Suryani, E., Hendrawan, R., Muhandhis, I., Syafa'at, F., Mudjahidin, M., Handayani, F., Zahra, A., Chou, S., Dewabharata, A., and Karijadi, I. (April 29, 2024). "Modeling of Wind Power Plants and their Impact on Economic and Environmental Development." ASME. J. Energy Resour. Technol . doi: https://doi.org/10.1115/1.4065425
Download citation file:
- Ris (Zotero)
- Reference Manager
This research addresses the environmental dynamics of wind power plants and their impact on the economy and environment. A system dynamics framework is used as a tool for model development since it accommodates relationships between complex and nonlinear variables affecting the wind power plants and their impact on the economy and environment. The scientific contribution of this research is the creation of scenario modeling that describes the interrelationships of variables and parameters affecting wind power plants and their impact. Total wind energy depends on density, wind speed, blade cross-sectional area, and the efficiency of the Betz limit. The average fulfillment ratio after wind turbine installations is projected to increase from 0.047 to 0.117 due to the increase in wind energy production. Meanwhile, total CO 2 emissions are projected to decrease from an average of 67,306.48 tons to 36,830.57 tons due to the use of solid direct air capture.
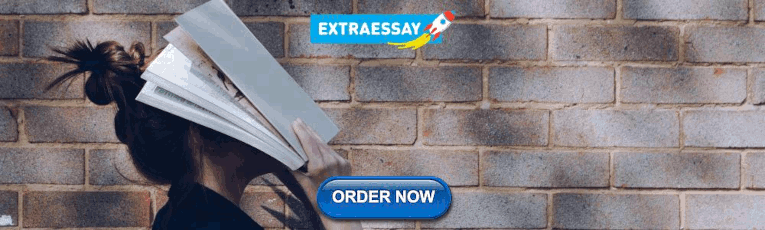
Get Email Alerts
Related articles, related proceedings papers, related chapters, affiliations.
- Accepted Manuscripts
- About the Journal
- Editorial Board
- Information for Authors
- Call for Papers
- Rights and Permission
- Online ISSN 1528-8994
- Print ISSN 0195-0738
ASME Journals
- About ASME Journals
- Submit a Paper
- Title History
ASME Conference Proceedings
- About ASME Conference Publications and Proceedings
- Conference Proceedings Author Guidelines
ASME eBooks
- About ASME eBooks
- ASME Press Advisory & Oversight Committee
- Book Proposal Guidelines
- Frequently Asked Questions
- Publication Permissions & Reprints
- ASME Membership
Opportunities
- Faculty Positions
- ASME Community
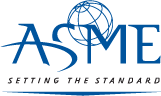
- Accessibility
- Privacy Statement
- Terms of Use
- Get Adobe Acrobat Reader
This Feature Is Available To Subscribers Only
Sign In or Create an Account
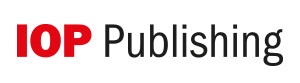
We apologize for the inconvenience...
To ensure we keep this website safe, please can you confirm you are a human by ticking the box below.
If you are unable to complete the above request please contact us using the below link, providing a screenshot of your experience.
https://ioppublishing.org/contacts/

An official website of the United States government
The .gov means it’s official. Federal government websites often end in .gov or .mil. Before sharing sensitive information, make sure you’re on a federal government site.
The site is secure. The https:// ensures that you are connecting to the official website and that any information you provide is encrypted and transmitted securely.
- Publications
- Account settings
Preview improvements coming to the PMC website in October 2024. Learn More or Try it out now .
- Advanced Search
- Journal List
- v.45(Suppl 1); 2016 Jan

Nuclear power in the 21st century: Challenges and possibilities
Akos horvath.
MTA Centre for Energy Research, KFKI Campus, P.O.B. 49, Budapest 114, 1525 Hungary
Elisabeth Rachlew
Department of Physics, Royal Institute of Technology, KTH, 10691 Stockholm, Sweden
The current situation and possible future developments for nuclear power—including fission and fusion processes—is presented. The fission nuclear power continues to be an essential part of the low-carbon electricity generation in the world for decades to come. There are breakthrough possibilities in the development of new generation nuclear reactors where the life-time of the nuclear waste can be reduced to some hundreds of years instead of the present time-scales of hundred thousand of years. Research on the fourth generation reactors is needed for the realisation of this development. For the fast nuclear reactors, a substantial research and development effort is required in many fields—from material sciences to safety demonstration—to attain the envisaged goals. Fusion provides a long-term vision for an efficient energy production. The fusion option for a nuclear reactor for efficient production of electricity has been set out in a focussed European programme including the international project of ITER after which a fusion electricity DEMO reactor is envisaged.
Introduction
All countries have a common interest in securing sustainable, low-cost energy supplies with minimal impact on the environment; therefore, many consider nuclear energy as part of their energy mix in fulfilling policy objectives. The discussion of the role of nuclear energy is especially topical for industrialised countries wishing to reduce carbon emissions below the current levels. The latest report from IPCC WGIII ( 2014 ) (see Box 1 for explanations of all acronyms in the article) says: “Nuclear energy is a mature low-GHG emission source of base load power, but its share of global electricity has been declining since 1993. Nuclear energy could make an increasing contribution to low-carbon energy supply, but a variety of barriers and risks exist ”.
Demand for electricity is likely to increase significantly in the future, as current fossil fuel uses are being substituted by processes using electricity. For example, the transport sector is likely to rely increasingly on electricity, whether in the form of fully electric or hybrid vehicles, either using battery power or synthetic hydrocarbon fuels. Here, nuclear power can also contribute, via generation of either electricity or process heat for the production of hydrogen or other fuels.
In Europe, in particular, the public opinion about safety and regulations with nuclear power has introduced much critical discussions about the continuation of nuclear power, and Germany has introduced the “Energiewende” with the goal to close all their nuclear power by 2022. The contribution of nuclear power to the electricity production in the different countries in Europe differs widely with some countries having zero contribution (e.g. Italy, Lithuania) and some with the major part comprising nuclear power (e.g. France, Hungary, Belgium, Slovakia, Sweden).
Current status
The use of nuclear energy for commercial electricity production began in the mid-1950s. In 2013, the world’s 392 GW of installed nuclear capacity accounted for 11 % of electricity generation produced by around 440 nuclear power plants situated in 30 countries (Fig. 1 ). This share has declined gradually since 1996, when it reached almost 18 %, as the rate of new nuclear additions (and generation) has been outpaced by the expansion of other technologies. After hydropower, nuclear is the world’s second-largest source of low-carbon electricity generation (IEA 2014 1 ).

Total number of operating nuclear reactors worldwide. The total number of reactors also include six in Taiwan (source: IAEA 2015) ( https://www.iaea.org/newscenter/focus/nuclear-power )
The Country Nuclear Power Profiles (CNPP 2 ) compiles background information on the status and development of nuclear power programmes in member states. The CNPP’s main objectives are to consolidate information about the nuclear power infrastructures in participating countries, and to present factors related to the effective planning, decision-making and implementation of nuclear power programmes that together lead to safe and economical operations of nuclear power plants.
Within the European Union, 27 % of electricity production (13 % of primary energy) is obtained from 132 nuclear power plants in January 2015 (Fig. 1 ). Across the world, 65 new reactors are under construction, mainly in Asia (China, South Korea, India), and also in Russia, Slovakia, France and Finland. Many other new reactors are in the planning stage, including for example, 12 in the UK.
Apart from one first Generation “Magnox” reactor still operating in the UK, the remainder of the operating fleet is of the second or third Generation type (Fig. 2 ). The predominant technology is the Light Water Reactor (LWR) developed originally in the United States by Westinghouse and then exploited massively by France and others in the 1970s as a response to the 1973 oil crisis. The UK followed a different path and pursued the Advanced Gas-cooled Reactor (AGR). Some countries (France, UK, Russia, Japan) built demonstration scale fast neutron reactors in the 1960s and 70s, but the only commercial reactor of this type currently operating is in Russia.

Nuclear reactor generations from the pioneering age to the next decade (reproduced with permission from Ricotti 2013 )
Future evolution
The fourth Generation reactors, offering the potential of much higher energy recovery and reduced volumes of radioactive waste, are under study in the framework of the “Generation IV International Forum” (GIF) 3 and the “International Project on Innovative Nuclear Reactors and Fuel Cycles” (INPRO). The European Commission in 2010 launched the European Sustainable Nuclear Industrial Initiative (ESNII), which will support three Generation IV fast reactor projects as part of the EU’s plan to promote low-carbon energy technologies. Other initiatives supporting biomass, wind, solar, electricity grids and carbon sequestration are in parallel. ESNII will take forward: the Astrid sodium-cooled fast reactor (SFR) proposed by France, the Allegro gas-cooled fast reactor (GFR) supported by central and eastern Europe and the MYRRHA lead- cooled fast reactor (LFR) technology pilot proposed by Belgium.
The generation of nuclear energy from uranium produces not only electricity but also spent fuel and high-level radioactive waste (HLW) as a by-product. For this HLW, a technical and socially acceptable solution is necessary. The time scale needed for the radiotoxicity of the spent fuel to drop to the level of natural uranium is very long (i.e. of the order of 200 000–300 000 years). The preferred solution for disposing of spent fuel or the HLW resulting from classical reprocessing is deep geological storage. Whilst there are no such geological repositories operating yet in the world, Sweden, Finland and France are on track to have such facilities ready by 2025 (Kautsky et al. 2013 ). In this context it should also be mentioned that it is only for a minor fraction of the HLW that recycling and transmutation is required since adequate separation techniques of the fuel can be recycled and again fed through the LWR system.
The “Strategic Energy Technology Plan” (SET-Plan) identifies fission energy as one of the contributors to the 2050 objectives of a low-carbon energy mix, relying on the Generation-3 reactors, closed fuel cycle and the start of implementation of Generation IV reactors making nuclear energy more sustainable. The EU Energy Roadmap 2050 provides decarbonisation scenarios with different assumptions from the nuclear perspective: two scenarios contemplate a nuclear phase-out by 2050, whilst three others consider that 15–20 % of electricity will be produced by nuclear energy. If by 2050 a generation capacity of 20 % nuclear electricity (140 GWe) is to be secured, 100–120 nuclear power units will have to be built between now and 2050, the precise number depending on the power rating (Garbil and Goethem 2013 ).
Despite the regional differences in the development plans, the main questions are of common interest to all countries, and require solutions in order to maintain nuclear power in the power mix of contributing to sustainable economic growth. The questions include (i) maintaining safe operation of the nuclear plants, (ii) securing the fuel supplies, (iii) a strategy for the management of radioactive waste and spent nuclear fuel.
Safety and non-proliferation risks are managed in accordance with the international rules issued both by IAEA and EURATOM in the EU. The nuclear countries have signed the corresponding agreements and the majority of them have created the necessary legal and regulatory structure (Nuclear Safety Authority). As regards radioactive wastes, particularly high-level wastes (HLW) and spent fuel (SF) most of the countries have long-term policies. The establishment of new nuclear units and the associated nuclear technology developments offer new perspectives, which may need reconsideration of fuel cycle policies and more active regional and global co-operation.
Open and closed fuel cycle
In the frame of the open fuel cycle, the spent fuel will be taken to final disposal without recycling. Deep geological repositories are the only available option for isolating the highly radioactive materials for a very long time from the biosphere. Long-term (80–100 years) near soil intermediate storages are realised in e.g. France and the Netherlands which will allow for permanent access and inspection. The main advantage of the open fuel cycle is its simplicity. The spent fuel assemblies are first stored in interim storage for several years or decades, then they will be placed in special containers and moved into deep underground storage facilities. The technology for producing such containers and for excavation of the underground system of tunnels exists today (Hózer et al. 2010 ; Kautsky et al. 2013 ).
The European Academies Science Advisory Board recently released the report on “Management of spent nuclear fuel and its waste” (EASAC 2014 ). The report discusses the challenges associated with different strategies to manage spent nuclear fuel, in respect of both open cycles and steps towards closing the nuclear fuel cycle. It integrates the conclusions on the issues raised on sustainability, safety, non-proliferation and security, economics, public involvement and on the decision-making process. Recently Vandenbosch et al. ( 2015 ) critically discussed the issue of confidence in the indefinite storage of nuclear waste. One complication of the nuclear waste storage problem is that the minor actinides represent a high activity (see Fig. 3 ) and pose non-proliferation issues to be handled safely in a civil used plant. This might be a difficult challenge if the storage is to be operated economically together with the fuel fabrication.

Radiotoxicity of radioactive waste
The open (or ‘once through’) cycle only uses part of the energy stored in the fuel, whilst effectively wasting substantial amounts of energy that could be recovered through recycling. The conventional closed fuel cycle strategy uses the reprocessing of the spent fuel following interim storage. The main components which can be further utilised (U and Pu) are recycled to fuel manufacturing (MOX (Mixed Oxide) fuel fabrication), whilst the smaller volume of residual waste in appropriately conditioned form—e.g. vitrified and encapsulated—is disposed of in deep geological repositories.
The advanced closed fuel cycle strategy is similar to the conventional one, but within this strategy the minor actinides are also removed during reprocessing. The separated isotopes are transmuted in combination with power generation and only the net reprocessing wastes and those conditioned wastes generated during transmutation will be, following appropriate encapsulation, disposed of in deep geological repositories. The main factor that determines the overall storage capacity of a long-term repository is the heat content of nuclear waste, not its volume. During the anticipated repository time, the specific heat generated during the decay of the stored HLW must always stay below a dedicated value prescribed by the storage concept and the geological host information. The waste that results from reprocessing spent fuel from thermal reactors has a lower heat content (after a period of cooling) than does the spent fuel itself. Thus, it can be stored more densely.
A modern light water reactor of 1 GWe capacity will typically discharge about 20–25 tonnes of irradiated fuel per year of operation. About 93–94 % of the mass of typical uranium oxide irradiated fuel comprises uranium (mostly 238 U), with about 4–5 % fission products and ~1 % plutonium. About 0.1–0.2 % of the mass comprises minor actinides (neptunium, americium and curium). These latter elements accumulate in nuclear fuel because of neutron capture, and they contribute significantly to decay heat loading and neutron output, as well as to the overall radiotoxic hazard of spent fuel. Although the total minor actinide mass is relatively small—20 to 25 kg per year from a 1 GWe LWR—it has a disproportionate impact on spent fuel disposal because of its long radioactive decay times (OECD Nuclear Energy Agency 2013 ).
Generation IV development
To address the issue of sustainability of nuclear energy, in particular the use of natural resources, fast neutron reactors (FNRs) must be developed, since they can typically multiply by over a factor 50 the energy production from a given amount of uranium fuel compared to current reactors. FNRs, just as today’s fleet, will be primarily dedicated to the generation of fossil-free base-load electricity. In the FNR the fuel conversion ratio (FCR) is optimised. Through hardening the spectrum a fast reactor can be designed to burn minor actinides giving a FCR larger than unity which allows breeding of fissile materials. FNRs have been operated in the past (especially the Sodium-cooled Fast Reactor in Europe), but today’s safety, operational and competitiveness standards require the design of a new generation of fast reactors. Important research and development is currently being coordinated at the international level through initiatives such as GIF.
In 2002, six reactor technologies were selected which GIF believe represent the future of nuclear energy. These were selected from the many various approaches being studied on the basis of being clean, safe and cost-effective means of meeting increased energy demands on a sustainable basis. Furthermore, they are considered being resistant to diversion of materials for weapons proliferation and secure from terrorist attacks. The continued research and development will focus on the chosen six reactor approaches. Most of the six systems employ a closed fuel cycle to maximise the resource base and minimise high-level wastes to be sent to a repository. Three of the six are fast neutron reactors (FNR) and one can be built as a fast reactor, one is described as epithermal, and only two operate with slow neutrons like today’s plants. Only one is cooled by light water, two are helium-cooled and the others have lead–bismuth, sodium or fluoride salt coolant. The latter three operate at low pressure, with significant safety advantage. The last has the uranium fuel dissolved in the circulating coolant. Temperatures range from 510 to 1000 °C, compared with less than 330 °C for today’s light water reactors, and this means that four of them can be used for thermochemical hydrogen production.
The sizes range from 150 to 1500 MWe, with the lead-cooled one optionally available as a 50–150 MWe “battery” with long core life (15–20 years without refuelling) as replaceable cassette or entire reactor module. This is designed for distributed generation or desalination. At least four of the systems have significant operating experience already in most respects of their design, which provides a good basis for further research and development and is likely to mean that they can be in commercial operation well before 2030. However, when addressing non-proliferation concerns it is significant that fast neutron reactors are not conventional fast breeders, i.e. they do not have a blanket assembly where plutonium-239 is produced. Instead, plutonium production happens to take place in the core, where burn-up is high and the proportion of plutonium isotopes other than Pu-239 remains high. In addition, new reprocessing technologies will enable the fuel to be recycled without separating the plutonium.
In January 2014, a new GIF Technology Roadmap Update was published. 4 It confirmed the choice of the six systems and focused on the most relevant developments of them so as to define the research and development goals for the next decade. It suggested that the Generation IV technologies most likely to be deployed first are the SFR, the lead-cooled fast reactor (LFR) and the very high temperature reactor technologies. The molten salt reactor and the GFR were shown as furthest from demonstration phase.
Europe, through sustainable nuclear energy technology platform (SNETP) and ESNII, has defined its own strategy and priorities for FNRs with the goal to demonstrate Generation IV reactor technologies that can close the nuclear fuel cycle, provide long-term waste management solutions and expand the applications of nuclear fission beyond electricity production to hydrogen production, industrial heat and desalination; The SFR as a proven concept, as well as the LFR as a short-medium term alternative and the GFR as a longer-term alternative technology. The French Commissariat à l’Energie Atomique (CEA) has chosen the development of the SFR technology. Astrid (Advanced Sodium Technological Reactor for Industrial Demonstration) is based on about 45 reactor-years of operational experience in France and will be rated 250 to 600 MWe. It is expected to be built at Marcoule from 2017, with the unit being connected to the grid in 2022.
Other countries like Belgium, Italy, Sweden and Romania are focussing their research and development effort on the LFR whereas Hungary, Czech Republic and Slovakia are investing in the research and development on GFR building upon the work initiated in France on GFR as an alternative technology to SFR. Allegro GFR is to be built in eastern Europe, and is more innovative. It is rated at 100 MWt and would lead to a larger industrial demonstration unit called GoFastR. The Czech Republic, Hungary and Slovakia are making a joint proposal to host the project, with French CEA support. Allegro is expected to begin construction in 2018 operate from 2025. The industrial demonstrator would follow it.
In mid-2013, four nuclear research institutes and engineering companies from central Europe’s Visegrád Group of Nations (V4) agreed to establish a centre for joint research, development and innovation in Generation IV nuclear reactors (the Czech Republic, Hungary, Poland and Slovakia) which is focused on gas-cooled fast reactors such as Allegro.
The MYRRHA (Multi-purpose hYbrid Research Reactor for High-tech Applications) 5 project proposed in Belgium by SCK•CEN could be an Experimental Technological Pilot Plant (ETPP) for the LFR technology. Later, it could become a European fast neutron technology pilot plant for lead and a multi-purpose research reactor. The unit is rated at 100 thermal MW and has started construction at SCK-CEN’s Mol site in 2014 planned to begin operation in 2023. A reduced-power model of Myrrha called Guinevere started up at Mol in March 2010. ESNII also includes an LFR technology demonstrator known as Alfred, also about 100 MWt, seen as a prelude to an industrial demonstration unit of about 600 MWe. Construction on Alfred could begin in 2017 and the unit could start operating in 2025.
Research and development topics to meet the top-level criteria established within the GIF forum in the context of simultaneously matching economics as well as stricter safety criteria set-up by the WENRA FNR demand substantial improvements with respect to the following issues:
- Primary system design simplification,
- Improved materials,
- Innovative heat exchangers and power conversion systems,
- Advanced instrumentation, in-service inspection systems,
- Enhanced safety,
and those for fuel cycle issues pertain to:
- Partitioning and transmutation,
- Innovative fuels (including minor actinide-bearing) and core performance,
- Advanced separation both via aqueous processes supplementing the PUREX process as well as pyroprocessing, which is mandatory for the reprocessing of the high MA-containing fuels,
- Develop a final depository.
Beyond the research and development, the demonstration projects mentioned above are planned in the frame of the SET-Plan ESNII for sustainable fission. In addition, supporting research infrastructures, irradiation facilities, experimental loops and fuel fabrication facilities, will need to be constructed.
Regarding transmutation, the accelerator-driven transmutation systems (ADS) technology must be compared to FNR technology from the point of view of feasibility, transmutation efficiency and cost efficiency. It is the objective of the MYRRHA project to be an experimental demonstrator of ADS technology. From the economical point of view, the ADS industrial solution should be assessed in terms of its contribution to closing the fuel cycle. One point of utmost importance for the ADS is its ability for burning larger amounts of minor actinides (the typical maximum in a critical FNR is about 2 %).
The concept of partitioning and transmutation (P&T) has three main goals: reduce the radiological hazard associated with spent fuel by reducing the inventory of minor actinides, reduce the time interval required to reach the radiotoxicity of natural uranium and reduce the heat load of the HLW packages to be stored in the geological disposal hence reducing the foot print of the geological disposal.
Advanced management of HLW through P&T consists in advanced separation of the minor actinides (americium, curium and neptunium) and some fission products with a long half-life present in the nuclear waste and their transmutation in dedicated burners to reduce the radiological and heat loads on the geological disposal. The time scale needed for the radiotoxicity of the waste to drop to the level of natural uranium will be reduced from a ‘geological’ value (300 000 years) to a value that is comparable to that of human activities (few hundreds of years) (OECD/NEA 2006 ; OECD 2012 ; PATEROS 2008 6 ). Transmutation of the minor actinides is achieved through fission reactions and therefore fast neutrons are preferred in dedicated burners.
At the European level, four building blocks strategy for Partitioning and Transmutation have been identified. Each block poses a serious challenge in terms of research & development to be done in order to reach industrial scale deployment. These blocks are:
- Demonstration of advanced reprocessing of spent nuclear fuel from LWRs, separating Uranium, Plutonium and Minor Actinides;
- Demonstration of the capability to fabricate at semi-industrial level dedicated transmuter fuel heavily loaded in minor actinides;
- Design and construct one or more dedicated transmuters;
- Fabrication of new transmuter fuel together with demonstration of advanced reprocessing of transmuter fuel.
MYRRHA will support this Roadmap by playing the role of an ADS prototype (at reasonable power level) and as a flexible irradiation facility providing fast neutrons for the qualification of materials and fuel for an industrial transmuter. MYRRHA will be not only capable of irradiating samples of such inert matrix fuels but also of housing fuel pins or even a limited number of fuel assemblies heavily loaded with MAs for irradiation and qualification purposes.
Options for nuclear fusion beyond 2050
Nuclear fusion research, on the basis of magnetic confinement, considered in this report, has been actively pursued in Europe from the mid-60s. Fusion research has the goal to achieve a clean and sustainable energy source for many generations to come. In parallel with basic high-temperature plasma research, the fusion technology programme is pursued as well as the economy of a future fusion reactor (Ward et al. 2005 ; Ward 2009 ; Bradshaw et al. 2011 ). The goal-oriented fusion research should be driven with an increased effort to be able to give the long searched answer to the open question, “will fusion energy be able to cover a major part of mankind’s electricity demand?”. ITER, the first fusion reactor to be built in France by the seven collaborating partners (Europe, USA, Russia, Japan, Korea, China, India) is hoped to answer most of the open physics and many of the remaining technology/material questions. ITER is expected to start operation of the first plasma around 2020 and D-T operation 2027.
The European fusion research has been successful through the organisation of EURATOM to which most countries in Europe belong (the fission programme is also included in EURATOM). EUROfusion, the European Consortium for the Development of Fusion Energy, manages European fusion research activities on behalf of EURATOM. The organisation of the research has resulted in a well-focused common fusion research programme. The members of the EUROfusion 7 consortium are 29 national fusion laboratories. EUROfusion funds all fusion research activities in accordance with the “EFDA Fusion electricity. Roadmap to the realisation of fusion energy” (EFDA 2012 , Fusion electricity). The Roadmap outlines the most efficient way to realise fusion electricity. It is the result of an analysis of the European Fusion Programme undertaken by all Research Units within EUROfusion’s predecessor agreement, the European Fusion Development Agreement, EFDA.
The most successful confinement concepts are toroidal ones like tokamaks and helical systems like stellarators (Wagner 2012 , 2013 ). To avoid drift losses, two magnetic field components are necessary for confinement and stability—the toroidal and the poloidal field component. Due to their superposition, the magnetic field winds helically around a system of nested toroids. In both cases, tokamak and stellarator, the toroidal field is produced by external coils; the poloidal field arises from a strong toroidal plasma current in tokamaks. In case of helical systems all necessary fields are produced externally by coils which have to be superconductive when steady-state operation is intended. Europe is constructing the most ambitious stellarator, Wendelstein 7-X in Germany. It is a fully optimised system with promising features. W7-X goes into operation in 2015. 8
Fusion research has now reached plasma parameters needed for a fusion reactor, even if not all parameters are reached simultaneously in a single plasma discharge (see Fig. 4 ). Plotted is the triple product n•τ E• T i composed of the density n, the confinement time τ E and the ion temperature T i . For ignition of a deuterium–tritium plasma, when the internal α-particle heating from the DT-reaction takes over and allows the external heating to be switched off, the triple product has to be about >6 × 10 21 m −3 s keV). The record parameters given as of today are shown together with the fusion experiment of its achievement in Fig. 4 . The achieved parameters and the missing factors to the ultimate goal of a fusion reactor are summarised below:
- Temperature: 40 keV achieved (JT-60U, Japan); the goal is surpassed by a factor of two
- Density n surpassed by factor 5 (C-mod,USA; LHD,Japan)
- Energy confinement time: a factor of 4 is missing (JET, Europe)
- Fusion triple product (see Fig. 4 : a factor of 6 is missing (JET, Europe)
- The first scientific goal is achieved: Q (fusion power/external heating power) ~1 (0,65) (JET, Europe)
- D-T operation without problems (TFTR (USA), JET, small tritium quantities have been used, however)
- Maximal fusion power for short pulse: 16 MW (JET)
- Divertor development (ASDEX, ASDEX-Upgrade, Germany)
- Design for the first experimental reactor complete (ITER, see below)
- The optimisation of stellarators (W7-AS, W7-X, Germany)

Progress in fusion parameters. Derived in 1955, the Lawson criterion specifies the conditions that must be met for fusion to produce a net energy output (1 keV × 12 million K). From this, a fusion “triple product” can be derived, which is defined as the product of the plasma ion density, ion temperature and energy confinement time. This product must be greater than about 6 × 10 21 keV m −3 s for a deuterium–tritium plasma to ignite. Due to the radioactivity associated with tritium, today’s research tokamaks generally operate with deuterium only ( solid dots ). The large tokamaks JET(EU) and TFTR(US), however, have used a deuterium–tritium mix ( open dots ). The rate of increase in tokamak performance has outstripped that of Moore’s law for the miniaturisation of silicon chips (Pitts et al. 2006 ). Many international projects (their names are given by acronyms in the figure) have contributed to the development of fusion plasma parameters and the progress in fusion research which serves as the basis for the ITER design
After 50 years of fusion research there is no evidence for a fundamental obstacle in the basic physics. But still many problems have to be overcome as detailed below:
Critical issues in fusion plasma physics based on magnetic confinement
- confine a plasma magnetically with 1000 m 3 volume,
- maintain the plasma stable at 2–4 bar pressure,
- achieve 15 MA current running in a fluid (in case of tokamaks, avoid instabilities leading to disruptions),
- find methods to maintain the plasma current in steady-state,
- tame plasma turbulence to get the necessary confinement time,
- develop an exhaust system (divertor) to control power and particle exhaust, specifically to remove the α-particle heat deposited into the plasma and to control He as the fusion ash.
Critical issues in fusion plasma technology
- build a system with 200 MKelvin in the plasma core and 4 Kelvin about 2 m away,
- build magnetic system at 6 Tesla (max field 12 Tesla) with 50 GJ energy,
- develop heating systems to heat the plasma to the fusion temperature and current drive systems to maintain steady-state conditions for the tokamak,
- handle neutron-fluxes of 2 MW/m 2 leading to 100 dpa in the surrounding material,
- develop low activation materials,
- develop tritium breeding technologies,
- provide high availability of a complex system using an appropriate remote handling system,
- develop the complete physics and engineering basis for system licensing.
The goals of ITER
The major goals of ITER (see Fig. 5 ) in physics are to confine a D-T plasma with α-particle self-heating dominating all other forms of plasma heating, to produce about ~500 MW of fusion power at a gain Q = fusion power/external heating power, of about 10, to explore plasma stability in the presence of energetic α-particles, and to demonstrate ash-exhaust and burn control.

Schematic layout of the ITER reactor experiment (from www.iter.org )
In the field of technology, ITER will demonstrate fundamental aspects of fusion as the self-heating of the plasma by alpha-particles, show the essentials to a fusion reactor in an integrated system, give the first test a breeding blanket and assess the technology and its efficiency, breed tritium from lithium utilising the D-T fusion neutron, develop scenarios and materials with low T-inventories. Thus ITER will provide strong indications for vital research and development efforts necessary in the view of a demonstration reactor (DEMO). ITER will be based on conventional steel as structural material. Its inner wall will be covered with beryllium to surround the plasma with low-Z metal with low inventory properties. The divertor will be mostly from tungsten to sustain the high α-particle heat fluxes directed onto target plates situated inside a divertor chamber. An important step in fusion reactor development is the achievement of licensing of the complete system.
The rewards from fusion research and the realisation of a fusion reactor can be described in the following points:
- fusion has a tremendous potential thanks to the availability of deuterium and lithium as primary fuels. But as a recommendation, the fusion development has to be accelerated,

Fusion time strategy towards the fusion reactor on the net (EFDA 2012 , Fusion electricity. A roadmap to the realisation of fusion energy)
In addition, there is the fusion technology programme and its material branch, which ultimately need a neutron source to study the interaction with 14 MeV neutrons. For this purpose, a spallation source IFMIF is presently under design. As a recommendation, ways have to be found to accelerate the fusion development. In general, with ITER, IFMIF and the DEMO, the programme will move away from plasma science more towards technology orientation. After the ITER physics and technology programme—if successful—fusion can be placed into national energy supply strategies. With fusion, future generations can have access to a clean, safe and (at least expected of today) economic power source.
The fission nuclear power continues to be an essential part of the low-carbon electricity generation in the world for decades to come. There are breakthrough possibilities in the development of new generation nuclear reactors where the life-time of the nuclear waste can be reduced to some hundreds of years instead of the present time-scales of hundred thousand of years. Research on the fourth generation reactors is needed for the realisation of this development. For the fast nuclear reactors a substantial research and development effort is required in many fields—from material sciences to safety demonstration—to attain the envisaged goals. Fusion provides a long-term vision for an efficient energy production. The fusion option for a nuclear reactor for efficient production of electricity should be vigorously pursued on the international arena as well as within the European energy roadmap to reach a decision point which allows to critically assess this energy option.
Box 1 Explanations of abbreviations used in this article
Biographies.
is Professor in Energy Research and Director of MTA Center for Energy Research, Budapest, Hungary. His research interests are in the development of new fission reactors, new structural materials, high temperature irradiation resistance, mechanical deformation.
is Professor of Applied Atomic and Molecular Physics at Royal Institute of Technology, (KTH), Stockholm, Sweden. Her research interests are in basic atomic and molecular processes studied with synchrotron radiation, development of diagnostic techniques for analysing the performance of fusion experiments in particular development of photon spectroscopic diagnostics.
1 http://www.iea.org/ .
2 https://cnpp.iaea.org/pages/index.htm .
3 GenIV International forum: ( http://www.gen-4.org/index.html ).
4 https://www.gen-4.org/gif/jcms/c_60729/technology-roadmap-update-2013 .
5 http://myrrha.sckcen.be/ .
6 www.sckcen.be/pateros/ .
7 https://www.euro-fusion.org/ .
8 https://www.ipp.mpg.de/ippcms/de/pr/forschung/w7x/index.html .
Contributor Information
Akos Horvath, Email: [email protected] .
Elisabeth Rachlew, Email: es.htk@kre .
- Bradshaw AM, Hamacher T, Fischer U. Is nuclear fusion a sustainable energy form? Fusion Engineering and Design. 2011; 86 :2770–2773. doi: 10.1016/j.fusengdes.2010.11.040. [ CrossRef ] [ Google Scholar ]
- EASAC. 2014. EASAC Report 23—Management of spent nuclear fuel and its waste. http://www.easac.eu/energy/reports-and-statements/detail-view/article/management-o.html .
- EFDA. 2012. Fusion electricity. A roadmap to the realization of fusion energy. https://www.euro-fusion.org/wpcms/wp-content/uploads/2013/01/JG12.356-web.pdf .
- Garbil, R., and G. Van Goethem. (ed.). 2013. Symposium on the “Benefits and limitations of nuclear fission for a low carbon economy”, European Commission, Brussels, ISBN 978-92.79.29833.2.
- Hózer, Z. S. Borovitskiy, G. Buday, B. Boullis, G. Cognet, S. A. Delichatsios, J. Gadó, A. Grishin, et al. 2010. Regional strategies concerning nuclear fuel cycle and HLRW in Central and Eastern European Countries. International conference on management of spent fuel from Nuclear Power Reactors, Vienna, Conference ID:38089 (CN-178).
- IEA (International Energy Authority). 2014. World Energy Outlook 2014. http://www.iea.org/ .
- IPCC. 2014. Summary for policymakers WGIII AR5, SPM.4.2.2 Energy supply.
- Kautsky, U., T. Lindborg, and J. Valentin (ed.). 2013. Humans and ecosystems over the coming millenia: A biosphere assessment of radioactive waste disposal in Sweden. Ambio 42(4): 381–526. [ PMC free article ] [ PubMed ]
- OECD. 2011–2012. Fact book: Economic, environmental and social statistics. Retrieved from http://www.oecd-ilibrary.org/economics/oecd-factbook-2011-2012_factbook-2011-en .
- OECD/NEA. 2006. Potential benefits and impacts of advanced nuclear fuel cycles with actinide partitioning and transmutation. ISBN: 978-92-64-99165-1, http://www.oecd-nea.org/science/reports/2011/6894-benefits-impacts-advanced-fuel.pdf .
- OECD Nuclear Energy Agency. 2013. Minor actinide burning in thermal reactors. A report by the Working Party on Scientific Issues of Reactor Systems, NEA #6997. http://www.oecd-nea.org/science/pubs/2013/6997-minor-actinide.pdf .
- Pitts R, Buttery R, Pinches S. Fusion: The way ahead. Physics World. 2006; 19 :20–26. doi: 10.1088/2058-7058/19/3/35. [ CrossRef ] [ Google Scholar ]
- Ricotti ME. Nuclear energy: Basics, present, future. EPJ Web of Conferences. 2013; 54 :01005. doi: 10.1051/epjconf/20135401005. [ CrossRef ] [ Google Scholar ]
- Vandenbosch R, Vandenbosch SE. Nuclear waste confidence: Is indefinite storage safe? APS Physics and Society. 2015; 44 :5–7. [ Google Scholar ]
- Wagner, F. 2012. Fusion energy by magnetic confinement. IPP 18/3, http://hdl.handle.net/11858/00-001M-0000-0026-E767-A .
- Wagner F. Physics of magnetic confinement fusion. EPJ Web of Conferences. 2013; 54 :01007. doi: 10.1051/epjconf/20135401007. [ CrossRef ] [ Google Scholar ]
- Ward DJ. The contribution of fusion to sustainable development. Fusion Engineering and Design. 2009; 82 :528–533. doi: 10.1016/j.fusengdes.2007.02.028. [ CrossRef ] [ Google Scholar ]
- Ward DJ, Cook I, Lechon Y, Saez R. The economic viability of fusion power. Engineering and Design. 2005; 75–79 :1221–1227. doi: 10.1016/j.fusengdes.2005.06.160. [ CrossRef ] [ Google Scholar ]
biomass power plants Recently Published Documents
Total documents.
- Latest Documents
- Most Cited Documents
- Contributed Authors
- Related Sources
- Related Keywords
Quantification and Environmental Assessment of Wood Ash from Biomass Power Plants: Case Study of Brittany Region in France
The increasing demand for energy is leading to the increasing use of renewable resources, such as biomass, resulting in the significant development of the wood energy sector in recent years. On the one hand, and to a certain extent, the sector has generated many benefits. On the other hand, the challenges related to wood ash (WA) management such as increasing tonnages, landfilling, restrictive regulations for reuse, etc., have been weighing more heavily in the debate related to the wood energy sector. However, all studies have assumed that no environmental impacts can be attributed to WA production. This study aims at discussing this assumption, whether the WA is a waste or a co-product of heat generation. In the first place, WA deposits were estimated using the biomass database and ash content from the literature regarding the collective, industrial and tertiary biomass power plants (BPP) in the French region of Brittany. Then, the impacts of the generated WA were estimated using the attributional life cycle assessment (LCA) method through two different impact allocation procedures (IAP), “from cradle to gate” (excluding the waste treatment). In Brittany, for the year 2017, an estimated amount of 2.8 to 8.9 kilotons of WA was generated, and this production should increase to 5 to 15.7 kilotons by 2050. The LCA conducted through this study gave an emission of 38.6 g CO2eq /kW h, with a major contribution from the production of the wood chips. Considering the environmental aspect, the IAP analysis indicated that energy and economic allocations were not relevant, and that, using the mass allocation, the environmental production of WA could represent 1.3% of the impacts of the combustion process in BPP. Therefore, WA, and especially the fly ash, can be considered as a waste from BPP heat production, without any environmental impact attributed to its generation.
Methods for the Determination of the Heat Transfer Coefficient in Air Cooled Condenser Used at Biomass Power Plants
In the present work, its show a summary of functional relationships developed for the application of dry condensation systems to Biomass Power Plants that present difficulties with access to water for condensation. The bibliographic review reveals the limitations of the analyzed works, in terms of the development of mathematical models and empirical correlations that allow evaluating the simultaneous effects of the surrounding meteorological variables on the average coefficient of heat transfer and the effect on the environment of the use of dry condensation. The analytical study is based on the weak solutions and their correlation with experimental quantities available in research already established in the area of action, a procedure is developed for the calculation of the average coefficients of heat transfer that includes the influence of local climatologically variables, the effect of the spatial distribution of the tubes package on the refrigerant and the confined confinement in inclined components, which increase the reliability of the thermo-hydraulic analysis and suppresses the need for the use of excess areas required by current methods. The proposed models and correlations allow the preparation of a procedure, by means of which all the possible operative variants are evaluated.
PENGUJIAN PERFORMA REAKTOR DOWNDRAFT BIOMASSA KOTORAN SAPI
Biomass power plants are electricity generators with alternative energy that utilize organicmaterials, in this case cow dung. The cow dung is then processed to produce syngas. Syngas is used as fuelto turn turbines. In previous studies, a cow manure gasification reactor was designed and manufactured.This reactor is part of a biomass power plant system (PLTBm) which is made separately. The power outputtarget of this PLTBm is 370 kW. The purpose of this study was to examine the performance of the downdraftreactor of cow dung biomass, namely discharge, temperature, and analyze the gas content released by thereactor so that the power that can be generated by the reactor can be obtained. The test results obtained acombustion chamber temperature of 580°C and a discharge of 0.285 m3/s. The composition of the outputgas is acetylene 58.16%, hexane 27.66%, butane 6.38%, and methane 7.8%. From the calculation results,the power generated by the reactor is 342 kW.
Community Capacity-Building Mobilization towards Energy Transitions in the Era of Thailand 4.0: A Case Study on Biomass Power Plants
In 2015, the National Energy Policy Council (NEPC) approved the latest Alternative Energy Development Plan (AEDP) 2015–2036, targeting electricity generation from biomass, biogas, and municipal solid waste by 2036 towards the Thailand 4.0 policy. The small biomass power plants are intensively promoted, contributing to many more public concerns. Therefore, this study provided new insight using the readiness and resilience in the communities near the biomass power plant generation in Southern Thailand. The community readiness model (CRM) and community health impact assessment (CHIA) were adopted using mixed methods during January–November 2019. A total of 999 respondents replied to the questionnaires, 153 informants were interviewed, and the panel was discussed and analyzed by descriptive statistics and content analysis. Findings illustrated that all stakeholder sectors strengthened community-driven development based on the average community readiness (3.01 ± 0.11) in a vague awareness stage, only with participation in information giving (75.38%) and having an impact pain point score of 7.64 ± 0.54, which was a highly intense level used to develop the public policy towards biomass power plants. Recent advanced community tools offered new insights for the first time about community strategic plans for sustainable biomass power generation, to achieve community security and values of democracy in Southern Thailand.
Using improved iterative gravity method to optimize the investment location of agricultural biomass power generation projects: a case study
Biomass power generation has characteristics of good quality of power generation, high reliability and mature technology. It plays significant aspects in maintaining the safety of energy, optimizing energy structure, alleviating environmental pollution and promoting the economic development in the rural areas. Analyzing the investment of biomass power generation in China systematically cannot only improve the scientificity of the investment process, but also guide the industry to develop rapidly and healthily. At present, the investment areas of agricultural biomass power generation projects are too concentrated and the fuel supply is difficult, which affect the normal operation of biomass power plants and lead to loss or on the verge of profit and loss of biomass power generation plants. This paper constructed the optimal model of investment location of agricultural biomass power generation projects using the iterative gravity algorithm based on the key factors analysis to affect the operation costs of agricultural biomass power plants. The model optimized the transportation lines and transportation distance, and gained the smallest transport costs of power generation materials after a few iterative calculations. This paper took Huantai County as an example, and determined the optimal investment location of agricultural biomass power project using the Region props toolbox of Matlab 7.4. The simulating calculation of Huantai County showed that the results given by this model are reliable, and this method to select the investment location of agricultural biomass power projects is feasible and effective.
Techno-Economic Analysis for the Optimal Design of a National Network of Agro-Energy Biomass Power Plants in Egypt
Extensive studies are conducted to investigate the potential and techno-economic feasibility of bioenergy routes in different countries. However, limited researches have been focused on the whole national agricultural bioenergy resources in Egypt. This research provides an assessment of the potential agricultural biomass resources for electric energy production in Egypt. It provides a strategic perspective for the design of a national network of biomass power plants to utilize the spatially available agricultural residues throughout a country. A comprehensive approach is presented and is applied to Egypt. First, the approach estimates the amount, type, and characteristics of the agricultural residues in each Egyptian governorate. Then, a techno-economic appraisal for locating a set of collection stations, and installing a direct combustion biomass power plant in each governorate is conducted. SAM simulation software is used for the technical and economic appraisals, and preliminary plant capacities are estimated assuming one plant in each governorate. Secondly, a new mixed integer linear programming (MILP) model is proposed and applied to optimally design a biomass supply chain national network to maximize the overall network profit. The network is composed of the collection stations, the potential biomass power plants, and the flow distribution of residues to supply the selected plants. Results indicate that the Egyptian agricultural residue resources can produce 10 million ton/year of dry residues, generate 11 TWh/year, an average levelized cost of electricity () of 6.77 ¢/kWh, and supply about 5.5% of Egypt’s current energy needs. Moreover, the optimization results reveal that a network of 5 biomass power plants with capacities of 460 MW each should be established in Egypt. This approach is thought to be particularly suitable to other developing countries whose energy demand depends on fossil fuels and poses a heavy economic burden, and whose residues are massive, wasted, and not industrialized. The obtained results may also enrich future comparative research that studies the impact and feasibility of implementing agro-residue based biomass electric energy generation.
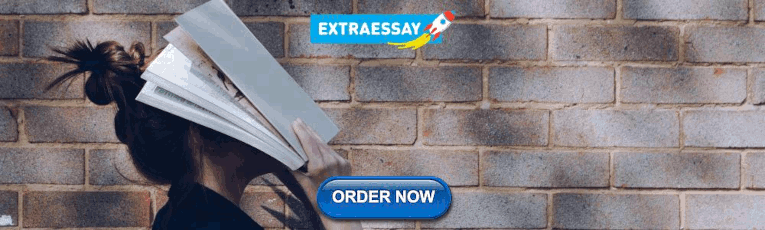
Latest advances on hybrid solar–biomass power plants
Understanding the potential of bio-carbon capture and storage from biomass power plant in indonesia.
Indonesia is currently experiencing a significant increase in population, industrialization and energy demand. As the energy demand increases, so does the production of climate-altering CO2 emission. Biomass power plants have emerged as a low carbon power generation alternative, utilizing agricultural and industrial waste. Biomass power plants have the potential of being a carbon-negative power generation technology in the near future by integrating carbon and capture storage (bio-CCS). The objective of this paper is to analyze and map potential CO2 emission in the processes of biomass power plants from gasification and firing or co-firing technology, then recommend suitable carbon capture technology based on the biomass power plant characteristics in Indonesia. The CO2 emission to be captured in the gasification process is 11-15% of the producer gas, while in co-firing it is 7-24% of the flue gas stream. Using biomass instead of coal in power plants reduces the electric efficiency and increases the plant’s in-house emission, but when analyzed in a wider boundary system it is apparent that the net GWP and CO2 emission of biomass power plants are way smaller than coal power plant, moreover when equipped with carbon capture unit. Biomass power plant that uses firing technology can reduce CO2 emission by 148% compared to typical coal power plant. Installing carbon capture unit in biomass firing power plants can further reduce the specific CO2 emission by 262%. If carbon capture technology is implemented to all existing biomass power plants in Indonesia, it could reduce the greenhouse gas emission up to 2.2 million tonnes CO2 equivalent annually. It is found that there are 3 significant designs for gasification technology: NREL design, Rhodes & Keith design and IGBCC+DeCO2 design. The first two designs are not suitable to be retrofitted into existing biomass power plants in Indonesia since they are based on a specific BCL/FERCO gasifier. While IGBCC+DeCO2 design still needs further study regarding its feasibility. While for firing, the most promising technology to be applied in the near future is solvent-based absorption because it is already on commercial scale for coal-based power plants and can be implemented for other source, e.g. biomass power plant. Bio-CCS in existing biomass power plant with firing technology is likely to be implemented in the near future compared to the gasification, because it applies the post combustion capture as an “end-of-pipe” technology which is generally seen as a more viable option to be retrofitted to existing power plants, resulting in potentially less expensive transition.
Assessment of Crop Residues for Power Generation and Optimal Sites for Biomass Power Plants Using NDVI and Landsat8 Bands in Punjab, India
Cost-effective flexibilisation of an 80 mw retrofitted biomass power plants: improved combustion control dynamics using virtual air flow sensors, export citation format, share document.
Thank you for visiting nature.com. You are using a browser version with limited support for CSS. To obtain the best experience, we recommend you use a more up to date browser (or turn off compatibility mode in Internet Explorer). In the meantime, to ensure continued support, we are displaying the site without styles and JavaScript.
- View all journals
- Explore content
- About the journal
- Publish with us
- Sign up for alerts
Latest science news, discoveries and analysis
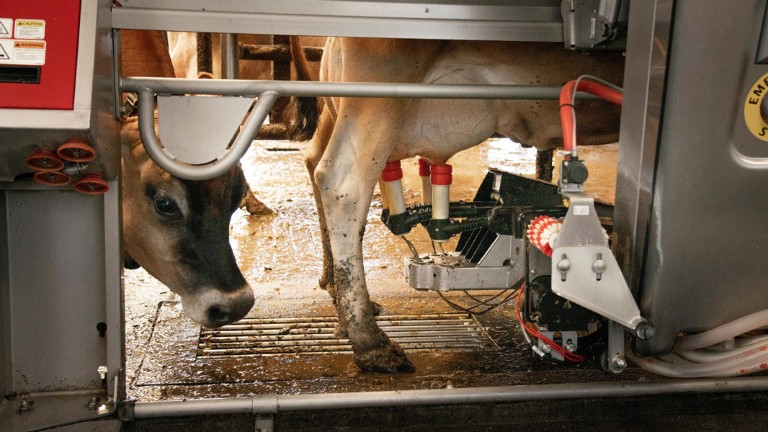
Bird flu virus has been spreading in US cows for months, RNA reveals
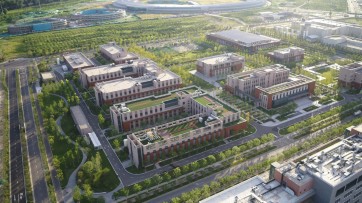
Superconductivity hunt gets boost from China's $220 million physics 'playground'
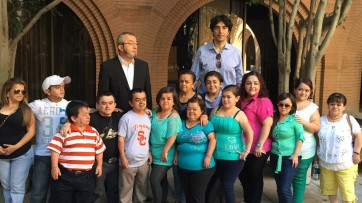
Could a rare mutation that causes dwarfism also slow ageing?
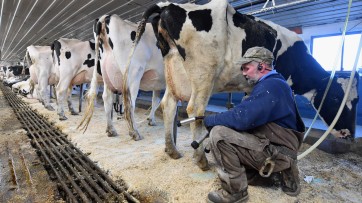
Bird flu in US cows: is the milk supply safe?
Future of humanity institute shuts: what's next for ‘deep future’ research, judge dismisses superconductivity physicist’s lawsuit against university, nih pay raise for postdocs and phd students could have us ripple effect, china's moon atlas is the most detailed ever made, ecologists: don’t lose touch with the joy of fieldwork chris mantegna.
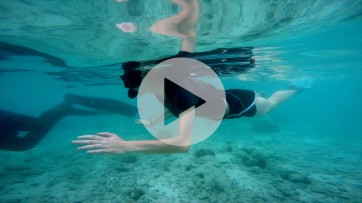
Should the Maldives be creating new land?
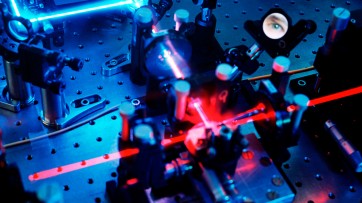
‘Shut up and calculate’: how Einstein lost the battle to explain quantum reality
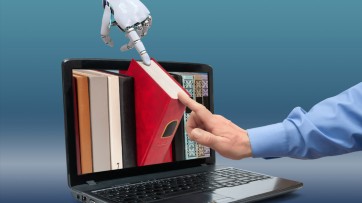
Algorithm ranks peer reviewers by reputation — but critics warn of bias
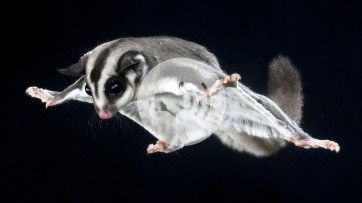
How gliding marsupials got their ‘wings’
First fetus-to-fetus transplant demonstrated in rats, what china’s mission to collect rocks from the far side could reveal about the moon, epic blazes threaten arctic permafrost. can fire-fighters save it, how reliable is this research tool flags papers discussed on pubpeer.
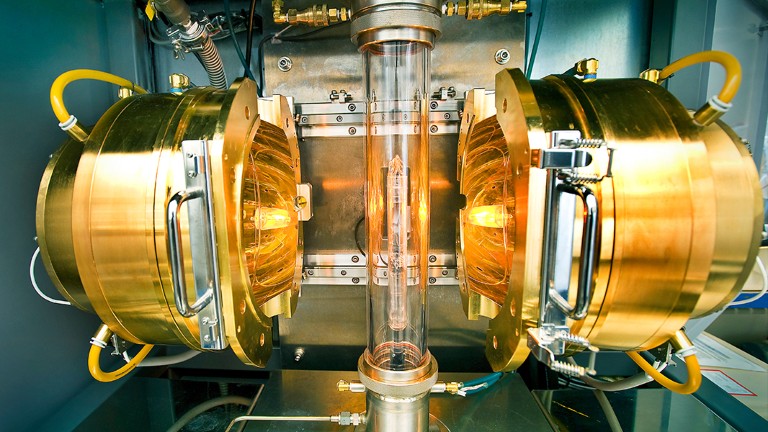
Retractions are part of science, but misconduct isn’t — lessons from a superconductivity lab
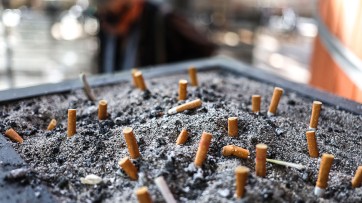
Any plan to make smoking obsolete is the right step
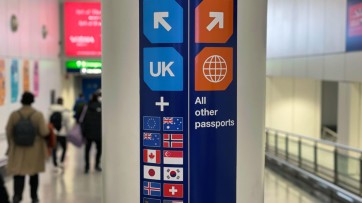
Citizenship privilege harms science
European ruling linking climate change to human rights could be a game changer — here’s how charlotte e. blattner, will ai accelerate or delay the race to net-zero emissions, current issue.
The Maldives is racing to create new land. Why are so many people concerned?
Surprise hybrid origins of a butterfly species, stripped-envelope supernova light curves argue for central engine activity, optical clocks at sea, research analysis.
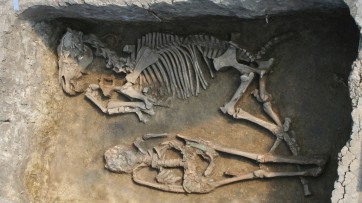
Ancient DNA traces family lines and political shifts in the Avar empire
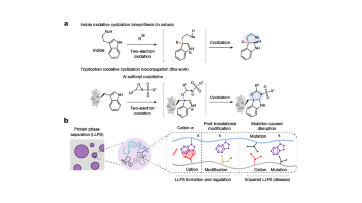
A chemical method for selective labelling of the key amino acid tryptophan
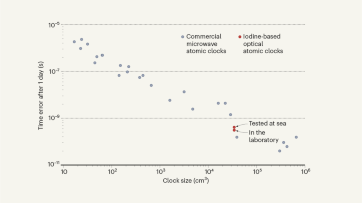
Robust optical clocks promise stable timing in a portable package
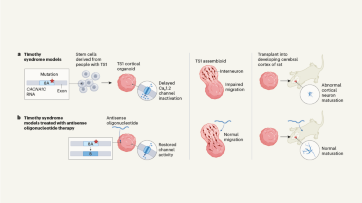
Targeting RNA opens therapeutic avenues for Timothy syndrome
Bioengineered ‘mini-colons’ shed light on cancer progression, galaxy found napping in the primordial universe, tumours form without genetic mutations, marsupial genomes reveal how a skin membrane for gliding evolved.
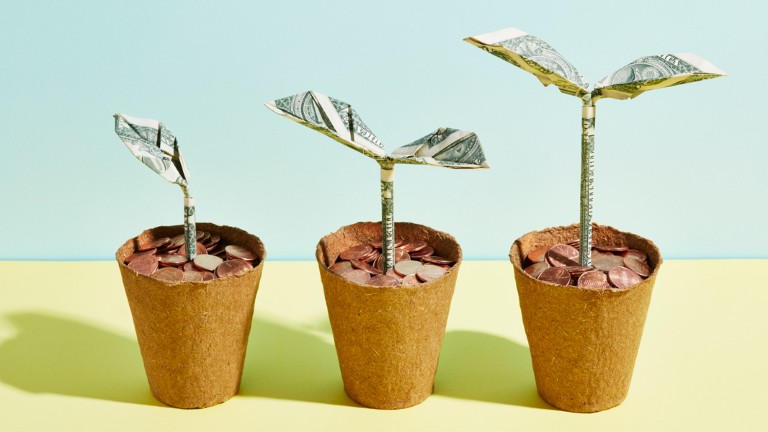
Scientists urged to collect royalties from the ‘magic money tree’
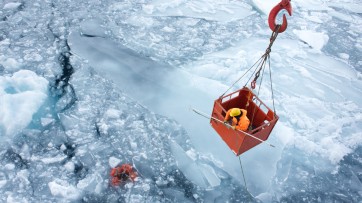
Breaking ice, and helicopter drops: winning photos of working scientists
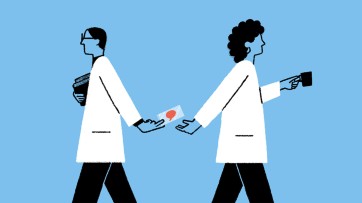
Shrouded in secrecy: how science is harmed by the bullying and harassment rumour mill
I strive to make the great barrier reef more resilient to heat stress, 85 million cells — and counting — at your fingertips, books & culture.
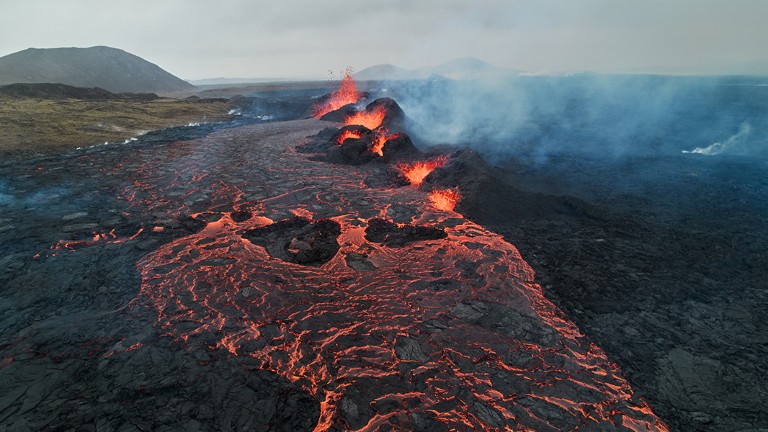
How volcanoes shaped our planet — and why we need to be ready for the next big eruption
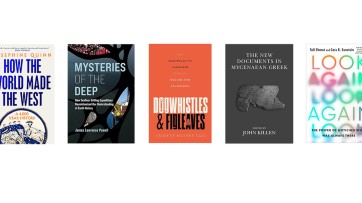
Dogwhistles, drilling and the roots of Western civilization: Books in brief
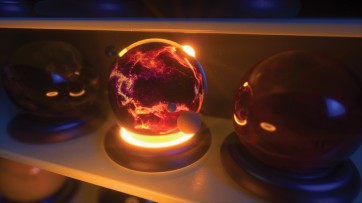
Cosmic rentals
Las borinqueñas remembers the forgotten puerto rican women who tested the first pill, dad always mows on summer saturday mornings, nature podcast.
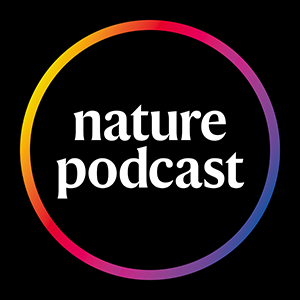
Latest videos
Nature briefing.
An essential round-up of science news, opinion and analysis, delivered to your inbox every weekday.

Quick links
- Explore articles by subject
- Guide to authors
- Editorial policies
- Share full article
Advertisement
Supported by
E.P.A. Severely Limits Pollution From Coal-Burning Power Plants
New regulations could spell the end for plants that burn coal, the fossil fuel that powered the country for more than a century.

By Lisa Friedman and Coral Davenport
The Biden administration on Thursday placed the final cornerstone of its plan to tackle climate change: a regulation that would force the nation’s coal-fired power plants to virtually eliminate the planet-warming pollution that they release into the air, or shut down.
The regulation from the Environmental Protection Agency requires coal plants in the United States to reduce 90 percent of their greenhouse pollution by 2039, one year earlier than the agency had initially proposed. The compressed timeline was welcomed by climate activists but condemned by coal executives who said the new standards would be impossible to meet.
The E.P.A. also imposed three additional regulations on coal-burning power plants, including stricter limits on emissions of mercury, a neurotoxin linked to developmental damage in children, from plants that burn lignite coal, the lowest grade of coal. The rules also more tightly restrict the seepage of toxic ash from coal plants into water supplies and limit the discharge of wastewater from coal plants.
Taken together, the regulations could deliver a death blow in the United States to coal, the fuel that powered the country for much of the last century but has caused global environmental damage. When burned, coal emits more carbon dioxide than any other fuel source.
The new rules regarding power plants come weeks after the administration’s other major climate regulations to limit emissions from cars and large trucks in a way that is designed to speed the adoption of electric vehicles. Transportation and electric power are the two largest sources in the United States of the carbon pollution that is driving climate change.
President Biden wants to cut that pollution by about 50 percent from 2005 levels by the end of this decade, and to eliminate emissions from the power sector by 2035.
The coal industry in the United States has been on a precipitous decline for over a decade, as environmental regulations and a boom in natural gas, wind and solar power have made it more expensive to burn coal, and power generation has shifted toward those cheaper, cleaner sources of electricity. In 2023, coal-fired power plants generated 16.2 percent of the nation’s electricity, according to the U.S. Energy Information Agency, down from a peak of 52 percent in 1990. There are about 200 coal-burning power plants still operating, with many concentrated in Pennsylvania, Texas and Indiana.
There are only a few ways to eliminate emissions from power plants that burn coal. The chief manner is carbon capture and sequestration, a process that traps emissions from a smokestack before they reach the atmosphere and then stores them. That technology is extremely expensive and not fully deployed at any American coal plant. Some researchers are exploring other technologies, such as converting coal plants to run on ammonia . But none of those have been widely implemented.
The limits on power plant emissions announced Thursday would also apply to future facilities that burn gas, requiring them to capture their emissions or to use a fuel that is nonpolluting. Gas-fired power plants that are currently in operation would be exempt.
“Today, E.P.A. is proud to make good on the Biden-Harris administration’s vision to tackle climate change and to protect all communities from pollution in our air, water, and in our neighborhoods,” Michael S. Regan, the E.P.A. administrator, said. “By developing these standards in a clear, transparent, inclusive manner, E.P.A. is cutting pollution while ensuring that power companies can make smart investments and continue to deliver reliable electricity for all Americans.”
Manish Bapna, the president of the Natural Resources Defense Council, an advocacy group, declared that “the age of unbridled climate pollution from power plants is finally over.”
The E.P.A. estimates that the rule controlling greenhouse gases from power plants would eliminate 1.38 billion metric tons of carbon dioxide between now and 2047, which is equivalent to preventing the annual emissions from 328 million gasoline-powered cars.
The agency estimates that the rule would cost industries $19 billion to comply between now and 2047, but says the economic benefits during the same period would be far greater. By stopping pollution from reaching the atmosphere, the regulation would help prevent $270 billion in damage to the economy from floods, wildfires, droughts, supply chain disruptions and increased commodity costs linked to climate change, the E.P.A. said.
The E.P.A. expects the regulation would also prevent other pollutants, such as soot, from escaping into the air, resulting in $120 billion in public health benefits between now and 2047. In 2035 alone, the agency projects that the rule will prevent up to 1,200 premature deaths, 870 hospital and emergency room visits, 360,000 asthma attacks, 48,000 school absence days and 57,000 lost workdays.
“Today is a good day for public health, particularly kids’ health,” said Harold Wimmer, president and chief executive of the American Lung Association.
Republicans, electric utilities and the coal industry are likely to challenge the regulations. They argue they would decimate jobs, increase blackouts and drive up electricity costs.
“We think it’s completely unrealistic,” said Michelle Bloodworth, the president and chief executive of America’s Power, a coal industry trade group.
There are about 42,000 jobs linked directly to coal mining today, down from about 73,000 a decade ago, according to the United States Bureau of Labor Statistics.
“This barrage of new E.P.A. rules ignores our nation’s ongoing electric reliability challenges and is the wrong approach at a critical time for our nation’s energy future,” said Jim Matheson, chief executive of the National Rural Electric Cooperative Association, which supplies electricity to many of the nation’s rural and suburban communities. “It undermines electric reliability and poses grave consequences for an already stressed electric grid.”
Former President Donald J. Trump, who is campaigning to return to the White House, has said he would overturn the regulation if he defeats Mr. Biden in November.
“I will cancel Biden’s power plant rule,” Mr. Trump said in a video address on his campaign website, adding that wind and solar energy “doesn’t work” because they are subsidized. That claim is false; fossil fuels receive billions of dollars annually in federal subsidies and wind and solar generate electricity more cheaply than oil, gas or coal even without extra financial help.
Under the plan, coal plants that are slated to operate through or beyond 2039 must reduce their greenhouse emissions 90 percent by 2032. Plants that are scheduled to close by 2039 would have to reduce their emissions 16 percent by 2030. Plants that retire before 2032 would not be subject to the rules.
The aging nature of the country’s existing coal plants means that many of the facilities could shut down before they would have to meet the most stringent limits. More than 200 coal plants have closed in the past decade, putting the average age of surviving plants at almost 50. The life span of an American coal plan t is about 60 years, according to the U.S. Energy Information Administration, and roughly one-quarter of the existing 200 plants are already slated to retire within the next five years.
The E.P.A. originally planned to also limit emissions limits from large gas plants that are currently operating. But the agency dropped that strategy after pushback from some moderate Democrats and the gas industry. Environmental justice groups also worried that a crackdown on large gas plants would cause utilities to more frequently run smaller gas units, known as peaker plants, that are frequently located in poor and minority communities that are already overburdened with pollution.
Under the new regulations, future natural gas plants that generate electricity at the rate of at least 40 percent of their maximum annual capacity would have to reduce their emissions 90 percent by 2032. New gas plants that generate electricity at less than 40 percent of their maximum annual capacity would be required to use low-polluting technology, such as energy-efficient turbines, but the standard would not be so stringent as to force those plants to install carbon capture and sequestration.
The E.P.A. cannot legally require that electric utilities use a specific technology or fuel. Instead, it can set limits on emissions that are so stringent that, in most cases, existing coal and new gas plants would have to install carbon capture technology or switch to a cleaner fuel.
Emily Grubert, an associate professor of sustainable energy policy at the University of Notre Dame, said it was possible but unlikely that coal plants would be able to continue operating under the new regulations.
A utility that installs expensive carbon capture technology in an aging coal plant would be unlikely to keep it running long enough to get a return on the investment, Ms. Grubert said. “Practically speaking, you’re talking about adding a billion dollars of capital investment to a plant that was at end of life anyway,” she said.
Minnkota, an electric cooperative based in Grand Forks, N.D., is planning what is expected to be the largest carbon capture project in the world at its coal-fired power station. Known as Project Tundra, it is projected to cost between $1.3 billion and $1.6 billion and is designed to cut the emissions from a 450 megawatt lignite coal unit by about 90 percent.
Mac McLennan, the chief executive of Minnkota, said that even with the carbon capture technology he was not fully certain his power plant would be able to keep operating under the new rules.
“It’s never captured a ton of CO2 yet. It’s not even constructed yet,” Mr. McLennan said, adding, “E.P.A. has made assumptions based on no real operating experience.”
Mr. McLennan said the utility started Project Tundra because it was preparing for a “carbon-constrained” world and that 42 percent of its generating capacity was already supplied by renewable energy. But he also said that when it gets seriously cold in North Dakota, coal is the most reliable fuel source to keep the heat on.
Meanwhile, Ms. Bloodworth said that the E.P.A. rules would exacerbate the challenges of a power grid that is already struggling to respond to surging demand for electricity.
The new regulations allow that in emergencies, such as responding to power outages in large storms, electric utilities could generate additional power from coal or gas plants without having to use carbon capture technology. And if a coal plant is scheduled to shut down by a certain date, but a state can demonstrate that its retirement would violate state rules on power reliability, the coal plant could be allowed to remain open for one additional year.
The crackdown on coal plants is nearly a decade in the making.
President Barack Obama tried to limit carbon pollution from coal-fired power plants, but his 2015 Clean Power Plan was blocked by the Supreme Court. The Trump administration then rolled back the rule and imposed its own plan to keep coal plants online longer.
In 2022, the Supreme Court found the E.P.A. had the authority to regulate emissions but could not force a nationwide transition away from the use of coal. Instead, it allowed the government to pursue only narrower policies that regulate how individual power plants operate.
Barbara Freese, the author of “Coal: A Human History,” noted the immense power the coal industry wielded for much of the last century. The industrial age, she wrote, “emerged literally in a haze of coal smoke.”
Ms. Freese, an environmental attorney and former assistant attorney general in Minnesota, said the industry’s decades-long campaign to question climate science and thwart regulation had delayed action until now to tackle greenhouse gases from coal plants.
“It has put us years behind schedule,” she said, adding, “It is infuriating we had to wait this long.”
Lisa Friedman is a Times reporter who writes about how governments are addressing climate change and the effects of those policies on communities. More about Lisa Friedman
Coral Davenport covers energy and environment policy, with a focus on climate change, for The Times. More about Coral Davenport
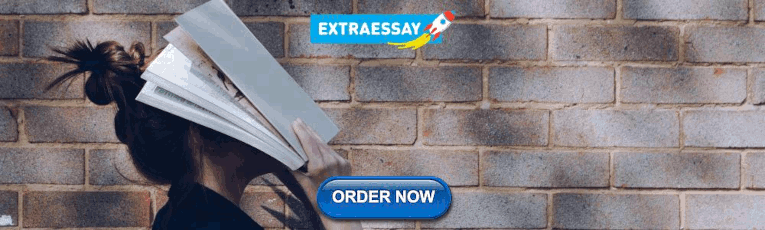
COMMENTS
Fig. 3 showed an increase of 2.9% in global energy demand in 2018. This is the strongest growth since the year 2010, almost doubling the 10-year average. There was a significant increase in the demand for all sources of energy; however, gas witnesses the strongest increase in demand, presenting an estimated demand of 168 million tons (about 43 % of the total increase in global demand).
The data of the power plant were obtained for a period of 8 years (2010-2017) from CEGCO annual reports 20,21.Throughout the studied period, several major malfunctions occurred to the power plant.
When the power ramp rate in the total power plant system is 4.5 % Pe 0 min −1, the power ramp rate in the coordinated control system is 3.9 % Pe 0 min −1 when load instruction is decomposed. In short, the decomposition of load instruction, i.e. Revised control III, accomplishes a scenario wherein the power ramp rate borne by the coordinated ...
Plant efficiency is calculated when these sophisticated controllers are used. NLC Tamilnadu Power Ltd (NTPL), a 500 MW capacity plant has operating efficiency of 37.2% is given in Table 2.From Table 2, Load variation is achieved in NTPL plant in the order of 5 MW.As per the plant design, time to achieve 5 MW change was found to be 60sec when the conventional controller was used.
Exergy efficiency varies from 60% to 90% for diff erent heat exchangers. The. analysis extends to other c omponents of the power plant, such as the condense r, deaerator, reheater, moisture ...
Energy Science & Engineering is a sustainable energy journal publishing high-impact fundamental and applied research that will help secure an ... thermal energy is harnessed employing concentrated solar power (CSP) plants such as Linear Fresnel collectors and parabolic trough collectors. In this paper, solar thermal technologies including soar ...
To compare the greenhouse gases emission of plants with the same capacity with RES, we have simulated the same power capacity hub for RES using thermal power plant as the base case via RETScreen ...
This review concentrated on a simple cycle GT power plant (GTPP) with a 2-shaft, regenerative, reheat, intercooler, complex cycle GT with effect intercooler, regenerative and reheat.
technology, hydropower provides 16,4% of global electricity production. [7] As to the energy. storage the storage hydropower (including pumpe d storage, PS) represents between 96 - 99%. of the ...
2 Research methodology. A review paper is a survey of existing literature on a topic that is used to explain the current state of the topic. ... built a forecast model of the hourly production of PV electricity for the next day at some power plants in mainland France by using binary regression tree, bagging, RF, gradient boosting (GB), and SVM ...
Virtual power plants represent the most immediate future of electricity generation, as they allow for intelligent consumption of energy in a distributed environment through the optimal management of demand and power generation. ... As a result, research papers incorporate this capacity to participate in the day-ahead electricity market in their ...
Application of machine learning to predict the thermal power plant process condition. Abstract This paper deals with the development of an algorithm for predicting thermal power plant process variables. The input data are described, and the data cleaning algorithm is presented along with the Python frameworks used.
It was found that the flash tank enhanced the net power by 0.72%. Further, as a resultant of using flash tank, the energy efficiency of the system increased from 31.68% to 31.91% while saving 25444.47 cubic meters water per year. In terms of exergy efficiency, a 0.72% increase was achieved.
Abstract. This research addresses the environmental dynamics of wind power plants and their impact on the economy and environment. A system dynamics framework is used as a tool for model development since it accommodates relationships between complex and nonlinear variables affecting the wind power plants and their impact on the economy and environment. The scientific contribution of this ...
Abstract. Hydroelectric energy has been in recent times placed as an important future source of renewable and clean energy. The advantage of hydropower as a renewable energy is that it produces negligible amounts of greenhouse gases, it stores large amounts of electricity at low cost and it can be adjusted to meet consumer demand.
Thermal Power Plant is converter of foss il fuel energy to. electricity in which during a cycle, steam is u sed to spin. a turbine driving electrical generator to p roduce. electricity. The first ...
Later, it could become a European fast neutron technology pilot plant for lead and a multi-purpose research reactor. The unit is rated at 100 thermal MW and has started construction at SCK-CEN's Mol site in 2014 planned to begin operation in 2023. A reduced-power model of Myrrha called Guinevere started up at Mol in March 2010.
Research papers. Comparative investigation on the thermodynamic performance of coal-fired power plant integrating with the molten salt thermal storage system. Author links open overlay panel Jing Xu a b c, Wenhao Liu a, Zhenpu Wang a, Suxia Ma a b, Guanjia Zhao a b, Yujiong Gu d. Show more.
a smart power plant that will be capable of autonomous operation. This paper will contemplate some of the ways to create a smarter power plant, knowing that the future holds countless possibilities. Creating the Smart Power Plant of the Future Mitsubishi Hitachi Power Systems As plants look to lower operating costs, respond to changing
In 2015, the National Energy Policy Council (NEPC) approved the latest Alternative Energy Development Plan (AEDP) 2015-2036, targeting electricity generation from biomass, biogas, and municipal solid waste by 2036 towards the Thailand 4.0 policy. The small biomass power plants are intensively promoted, contributing to many more public concerns.
Hydroelectric power. comes from flowing water winter and spring runoff from mountain s treams. and clear lakes. Water, whe n it is falling by the fo rce of g ravity, can be. used to tur n tur ...
A Virtual Power Plant (VPP) is a practical concept that aggregates various Renewable Energy Sources (RESs) to improve energy management efficiency and facilitate energy trading. ... After reviewing all the related studies, this paper determined the research gaps of the application of VPP for energy management, including optimal scheduling ...
Find breaking science news and analysis from the world's leading research journal.
At facilities called hydroelectric power plants, hydropower is generated. Some power plants are l ocated. on rivers, streams, and canals, but for a reliable water supply, dams are needed. Dams ...
The focus of this research is on energy output, with 1-year 100MW-QASP power plant data being examined for the EE. In this paper, it has been studied the different curves of power generation, irradiation curve and PR based on 4 quarterly datasheets of FY (2020-2021).
The E.P.A. also imposed three additional regulations on coal-burning power plants, including stricter limits on emissions of mercury, a neurotoxin linked to developmental damage in children, from ...
Cell efficiencies, market trends, cost of PV systems, and global research efforts over the last years are provided. Real monitored performances reveal a decrease of up to 10% of PV power output due to soiling effects. This paper discusses soiling mitigation approaches, a critical technical pathway to improve the power output of solar PV systems.