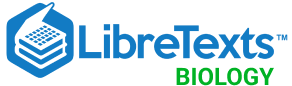
- school Campus Bookshelves
- menu_book Bookshelves
- perm_media Learning Objects
- login Login
- how_to_reg Request Instructor Account
- hub Instructor Commons
- Download Page (PDF)
- Download Full Book (PDF)
- Periodic Table
- Physics Constants
- Scientific Calculator
- Reference & Cite
- Tools expand_more
- Readability
selected template will load here
This action is not available.
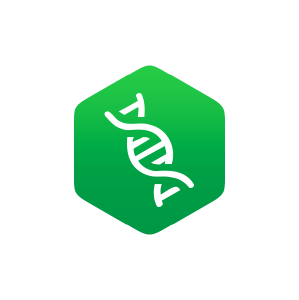
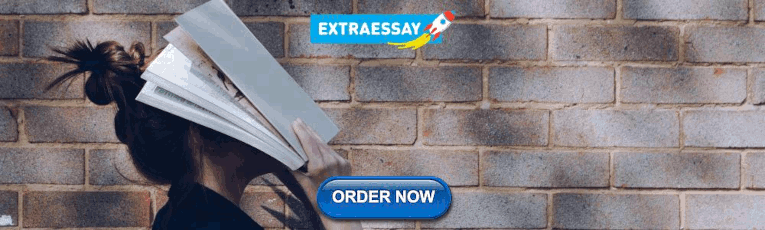
1.6: The law of independent assortment
- Last updated
- Save as PDF
- Page ID 73823
\( \newcommand{\vecs}[1]{\overset { \scriptstyle \rightharpoonup} {\mathbf{#1}} } \)
\( \newcommand{\vecd}[1]{\overset{-\!-\!\rightharpoonup}{\vphantom{a}\smash {#1}}} \)
\( \newcommand{\id}{\mathrm{id}}\) \( \newcommand{\Span}{\mathrm{span}}\)
( \newcommand{\kernel}{\mathrm{null}\,}\) \( \newcommand{\range}{\mathrm{range}\,}\)
\( \newcommand{\RealPart}{\mathrm{Re}}\) \( \newcommand{\ImaginaryPart}{\mathrm{Im}}\)
\( \newcommand{\Argument}{\mathrm{Arg}}\) \( \newcommand{\norm}[1]{\| #1 \|}\)
\( \newcommand{\inner}[2]{\langle #1, #2 \rangle}\)
\( \newcommand{\Span}{\mathrm{span}}\)
\( \newcommand{\id}{\mathrm{id}}\)
\( \newcommand{\kernel}{\mathrm{null}\,}\)
\( \newcommand{\range}{\mathrm{range}\,}\)
\( \newcommand{\RealPart}{\mathrm{Re}}\)
\( \newcommand{\ImaginaryPart}{\mathrm{Im}}\)
\( \newcommand{\Argument}{\mathrm{Arg}}\)
\( \newcommand{\norm}[1]{\| #1 \|}\)
\( \newcommand{\Span}{\mathrm{span}}\) \( \newcommand{\AA}{\unicode[.8,0]{x212B}}\)
\( \newcommand{\vectorA}[1]{\vec{#1}} % arrow\)
\( \newcommand{\vectorAt}[1]{\vec{\text{#1}}} % arrow\)
\( \newcommand{\vectorB}[1]{\overset { \scriptstyle \rightharpoonup} {\mathbf{#1}} } \)
\( \newcommand{\vectorC}[1]{\textbf{#1}} \)
\( \newcommand{\vectorD}[1]{\overrightarrow{#1}} \)
\( \newcommand{\vectorDt}[1]{\overrightarrow{\text{#1}}} \)
\( \newcommand{\vectE}[1]{\overset{-\!-\!\rightharpoonup}{\vphantom{a}\smash{\mathbf {#1}}}} \)
Introduction
The law of segregation lets us predict how a single feature associated with a single gene is inherited. In some cases, though, we might want to predict the inheritance of two characteristics associated with two different genes. How can we do this?
The law of segregation states that each gamete (sperm or egg cell) made by an organism will get just one of the two gene copies present in a parent organism, and that the gene copies are randomly allocated to the gametes. For instance, if an organism has a genotype of Aa , half of its gametes will contain an A allele, and the other half will contain an a allele.
You can use the link at the start of the paragraph to learn more about the law of segregation.
To make an accurate prediction, we need to know whether the two genes are inherited independently or not. That is, we need to know whether they "ignore" one another when they're sorted into gametes, or whether they "stick together" and get inherited as a unit.
When Gregor Mendel asked this question, he found that different genes were inherited independently of one another, following what's called the law of independent assortment . In this article, we'll take a closer look at the law of independent assortment and how it is used to make predictions. We'll also see when and why the law of independent assortment does (or doesn't!) hold true.
Note: If you are not yet familiar with how individual genes are inherited, you may want to check out the article on the law of segregation or the introduction to heredity video before you dive into this article.
What is the law of independent assortment?
Mendel's law of independent assortment states that the alleles of two (or more) different genes get sorted into gametes independently of one another. In other words, the allele a gamete receives for one gene does not influence the allele received for another gene.
Example: Pea color and pea shape genes
Let's look at a concrete example of the law of independent assortment. Imagine that we cross two pure-breeding pea plants: one with yellow, round seeds ( YYRR ) and one with green, wrinkled seeds ( yyrr ). Because each parent is homozygous, the law of segregation tells us that the gametes made by the wrinkled, green plant all are ry , and the gametes made by the round, yellow plant are all RY . That gives us F 1 offspring that are all RrYy .
The allele specifying yellow seed color is dominant to the allele specifying green seed color, and the allele specifying round shape is dominant to the allele specifying wrinkled shape, as shown by the capital and lower-case letters. This means that the F 1 plants are all yellow and round. Because they are heterozygous for two genes, the F 1 plants are called dihybrids ( di- = two, -hybrid = heterozygous).
A cross between two dihybrids (or, equivalently, self-fertilization of a dihybrid) is known as a dihybrid cross . When Mendel did this cross and looked at the offspring, he found that there were four different categories of pea seeds: yellow and round, yellow and wrinkled, green and round, and green and wrinkled. These phenotypic categories (categories defined by observable traits) appeared in a ratio of approximately 9:3:3:1.
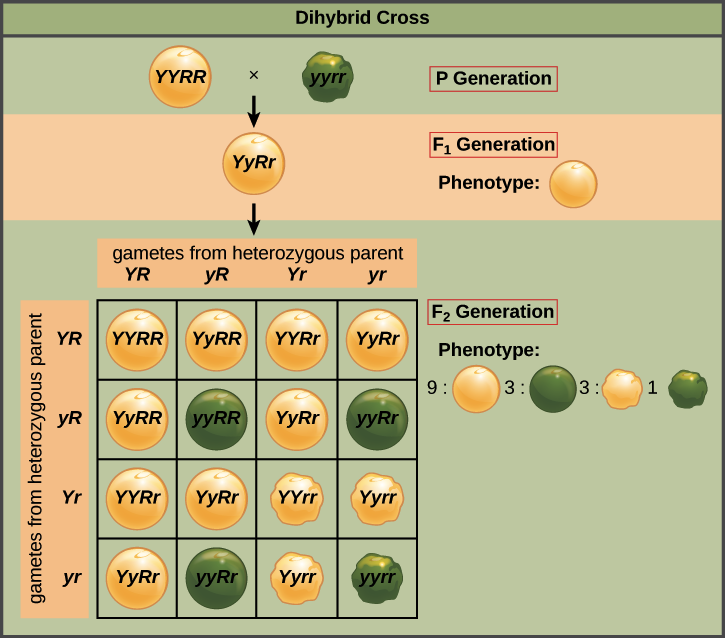
This ratio was the key clue that led Mendel to the law of independent assortment. That's because a 9:3:3:1 ratio is exactly what we'd expect to see if the F 1 plant made four types of gametes (sperm and eggs) with equal frequency: YR , Yr , yR , and yr . In other words, this is the result we'd predict if each gamete randomly got a Y or y allele, and, in a separate process, also randomly got an R or r allele (making four equally probable combinations).
We can confirm the link between the four types of gametes and the 9:3:3:1 ratio using the Punnett square above. To make the square, we first put the four equally probable gamete types along each axis. Then, we join gametes on the axes in the boxes of the chart, representing fertilization events. The 16 equal-probability fertilization events that can occur among the gametes are shown in the 16 boxes. The offspring genotypes in the boxes correspond to a 9:3:3:1 ratio of phenotypes, just as Mendel observed.
We can draw a Punnett square for a two-gene scenario by following the same basic rules as for a monohybrid cross, placing the gametes along the axes and combining them in the squares to represent fertilization events. However, since there are now more gamete types, there must also be more squares in the table: 4 possible types of maternal gametes x 4 possible types of paternal gametes = 16 squares total.
As with a single-gene Punnett square , we place all the possible types of gametes along the axes, then combine them in the squares where the columns and rows intersect to represent fertilization events (the formation of zygotes, or offspring).
To learn how you can use the rules of probability to predict the outcome of a dihybrid cross, see the probabilities in genetics article.
Independent assortment vs. linkage
The section above gives us Mendel's law of independent assortment in a nutshell, and lets us see how the law of independent assortment leads to a 9:3:3:1 ratio. But what was the alternative possibility? That is, what would happen if two genes didn't follow independent assortment?
In the extreme case, the genes for seed color and seed shape might have always been inherited as a pair. That is, the yellow and round alleles might always have stayed together, and so might the green and wrinkled alleles.
To see how this could work, imagine that the color and shape genes are physically stuck together and cannot be separated, as represented by the boxes around the alleles in the diagram below. For instance, this could happen if the two genes were located very, very close together on a chromosome (an idea we'll explore further at the end of the article).

Rather than giving a color allele and, separately, giving a shape allele to each gamete, the F 1 dihybrid plant would simply give one “combo unit” to each gamete: a YR allele pair or a yr allele pair.
We can use a Punnett square to predict the results of self-fertilization in this case, as shown above. If the seed color and seed shape genes were in fact always inherited as a unit, or completely linked , a dihybrid cross should produce just two types of offspring, yellow/round and green/wrinkled, in a 3:1 ratio. Mendel's actual results were quite different from this (the 9:3:3:1 ratio we saw earlier), telling him that the genes assorted independently.
The reason for independent assortment
To see why independent assortment happens, we need to fast-forward half a century and discover that genes are physically located on chromosomes. To be exact, the two copies of a gene carried by an organism (such as a Y and a y allele) are located at the same spot on the two chromosomes of a homologous pair . Homologous chromosomes are similar but non-identical, and an organism gets one member of the pair from each of its two parents.
The physical basis for the law of independent assortment lies in meiosis I of gamete formation, when homologous pairs line up in random orientations at the middle of the cell as they prepare to separate. We can get gametes with different combos of "mom" and "dad" homologues (and thus, the alleles on those homologues) because the orientation of each pair is random.
To see what this means, compare chromosome arrangement 1 (top) and chromosome arrangement 2 (bottom) at the stage of metaphase I in the diagram below. In one case, the red "mom" chromosomes go together, while in the other, they split up and mix with the blue "dad" chromosomes. If meiosis happens many times, as it does in a pea plant, we will get both arrangements—and thus RY , Ry , rY , and ry classes of gametes—with equal frequency.
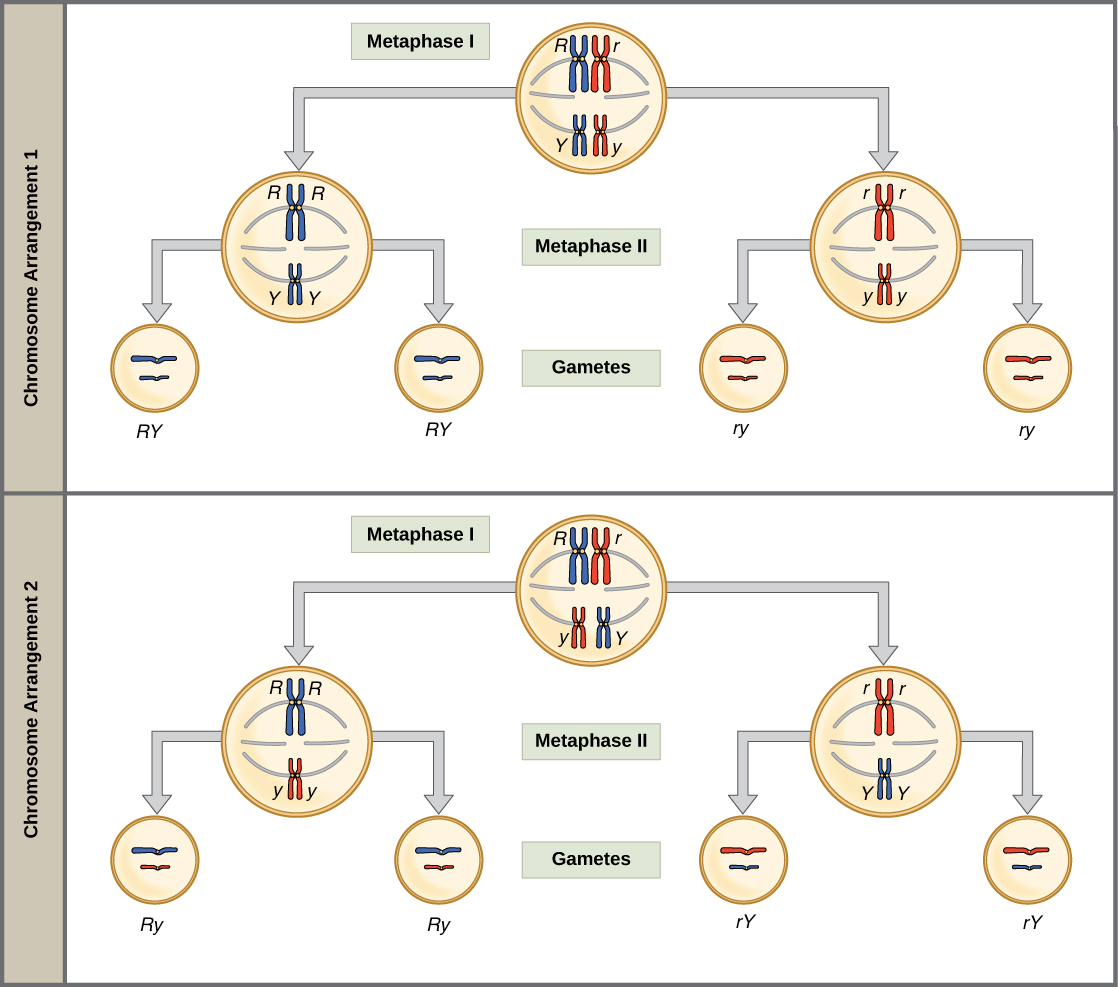
Genes that are on different chromosomes (like the Y and R genes) assort independently. The seed color and seed shape genes are on chromosomes 1 and 7 of the pea genome, respectively, in real life 1 . Genes that are far apart on the same chromosome also assort independently thanks to the crossing over, or exchange of homologous chromosome bits, that occurs early in meiosis I.
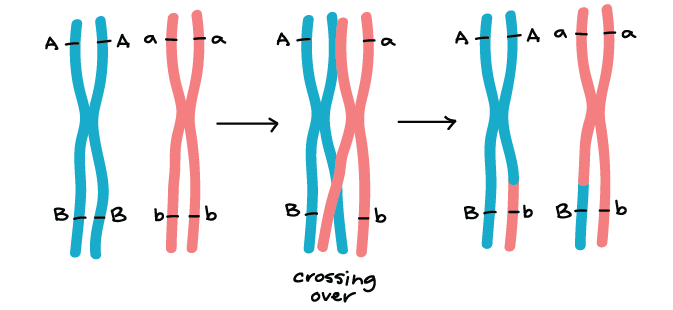
There are, however, gene pairs that do not assort independently. When genes are close together on a chromosome, the alleles on the same chromosome tend to be inherited as a unit more frequently than not. Such genes do not display independent assortment and are said to be linked . We'll take a closer look at genetic linkage in other articles and videos.
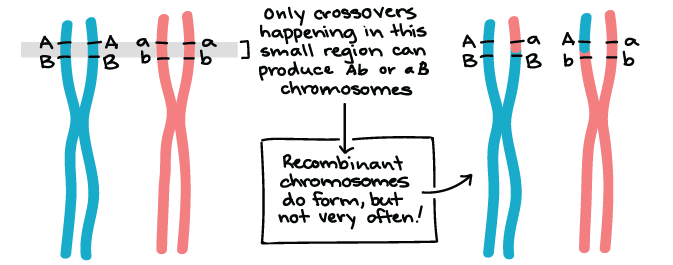
Check your understanding
Query \(\PageIndex{1}\)
Since all of the F 1 dogs are black and straight-furred, we know that black fur color and straight fur texture are dominant over yellow fur color and curly fur texture. If we call the color gene B/b and the texture gene C/c , and use capital letters for the dominant form of each gene and lowercase letters for the recessive form, we can assign the two parental dogs genotypes of BBcc (black and curly) and bbCC (yellow and straight-furred). When the parental dogs are crossed, they produce black, straight-furred F 1 dogs that are dihybrids: BbCc .
A cross between two F 1 dihybrid dogs results in the Punnett square shown below. The F 1 dogs can make four different types of gametes, which are represented along the two axes of the Punnett square. The squares of the table represent fertilization events in which the gametes on the axes combine. Since all of the gamete types are equally likely to be produced (because the genes assort independently, i.e., do not influence each other's inheritance), all the squares in the table represent equal-probability events, ones that occur 1/16 of the time.
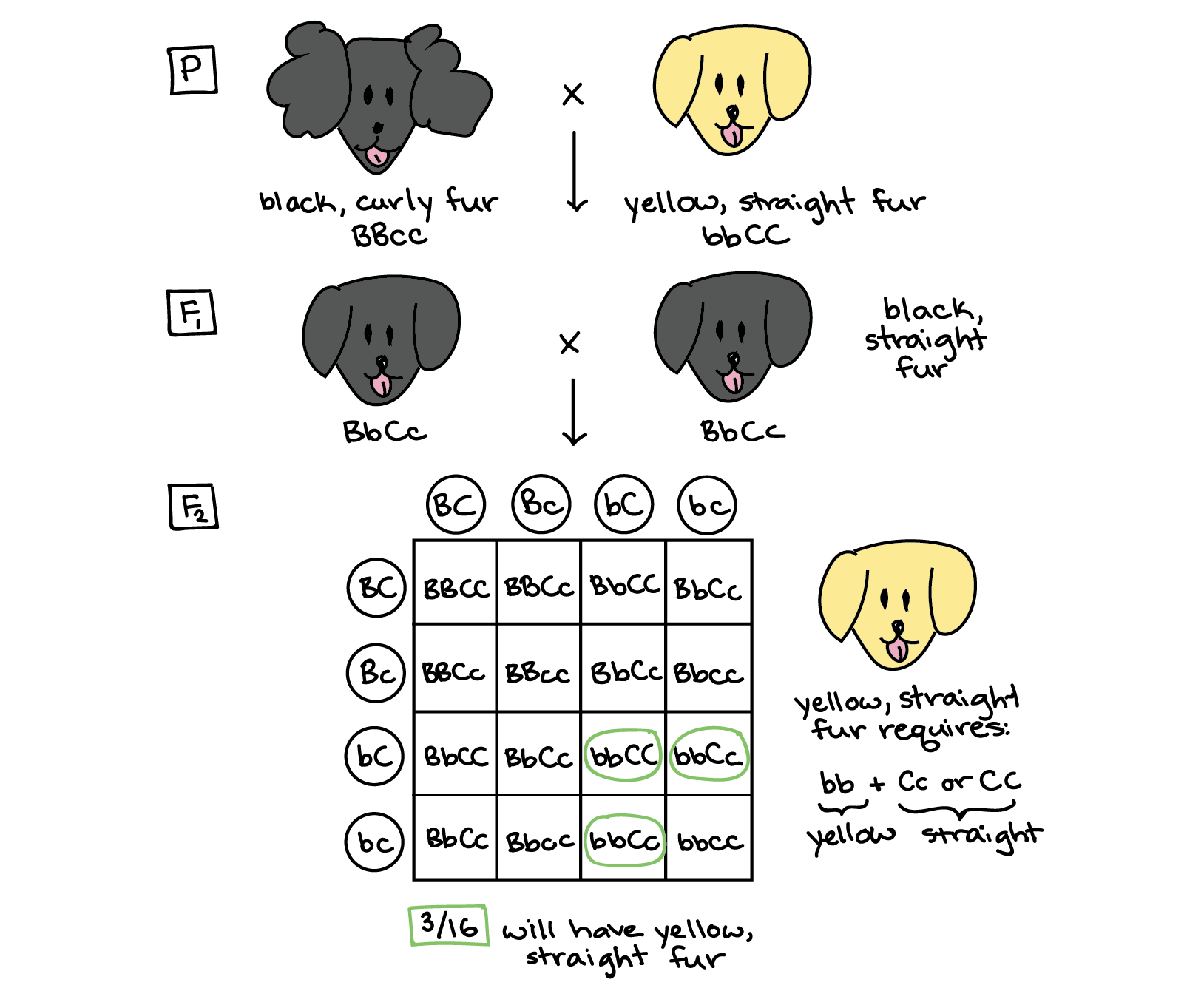
Now, we need to find the squares that correspond to the outcome we are interested in: a puppy with yellow, straight fur. To have yellow, straight fur, the puppy must get two recessive alleles for fur color ( bb genotype) and at least one dominant allele for fur texture ( Cc or CC genotype). If we go through the table and circle the genotypes that match these requirements, we'll find that 3 out of the 16 boxes correspond to yellow, straight-furred puppies. Thus, we would expect 3/16 of the F 2 puppies to have yellow, straight fur.
Answer: 3/16.
Contributors and Attributions
Khan Academy (CC BY-NC-SA 3.0; All Khan Academy content is available for free at www.khanacademy.org )
Attribution:
This article is a modified derivative of:
- “ Laws of inheritance ,” by OpenStax College, Biology ( CC BY 3.0 ). Download the original article for free at http://cnx.org/contents/[email protected] .
- " Laws of inheritance ," in Principles of Biology , by Robert Bear, David Rintoul, Bruce Snyder, Martha Smith-Caldas, Christopher Herren, and Eva Horne, OpenStax, ( CC BY 4.0 ). Download the original article for free at http://cnx.org/contents/[email protected] .
The modified article is licensed under a CC BY-NC-SA 4.0 license.
Works cited:
Reid, J. B., and Ross, J. J. (2011). Mendel's genes: Towards a full molecular characterization. Genetics 189 (1), 3-10. http://dx.doi.org/10.1534/genetics.111.132118 . Retrieved from www.ncbi.nlm.nih.gov/pmc/articles/PMC3176118/.
References:
Dihybrid. (2015). In The free dictionary . Retrieved from http://www.thefreedictionary.com/dihybrid .
Kimball, J. W. (2014, April 21). Genetic linkage and genetic maps. In Kimball's biology pages . Retrieved from https://www.biology-pages.info/L/Linkage.html
Purves, W. K., Sadava, D. E., Orians, G. H., and Heller, H.C. (2004). Genetics: Mendel and beyond. In Life: The science of biology (7th ed., pp. 187-212). Sunderland, MA: Sinauer Associates.
Raven, P. H., Johnson, G. B., Mason, K. A., Losos, J. B., and Singer, S. R. (2014). Patterns of inheritance. In Biology (10th ed., AP ed., pp. 221-238). New York, NY: McGraw-Hill.
Reece, J. B., Urry, L. A., Cain, M. L., Wasserman, S. A., Minorsky, P. V., and Jackson, R. B. (2011). Mendel and the gene idea. In Campbell Biology (10th ed., pp. 267-291). San Francisco, CA: Pearson.
The Adapa Project. (2014, August 13). What are the laws of segregation and independent assortment and why are they so important? In BioBook . Retrieved from https://adapaproject.org/bbk_temp/tiki-index.php?page=Leaf%3A+What+are+the+laws+of+segregation+and+independent+assortment+and+why+are+they+so+important%3F .
Introduction to Mendel's Law of Independent Assortment
Wikimedia Commons/CC BY-SA 3.0
- Cell Biology
- Weather & Climate
- B.A., Biology, Emory University
- A.S., Nursing, Chattahoochee Technical College
Independent assortment is a basic principle of genetics developed by a monk named Gregor Mendel in the 1860s. Mendel formulated this principle after discovering another principle known as Mendel's law of segregation, both of which govern heredity.
The law of independent assortment states that the alleles for a trait separate when gametes are formed. These allele pairs are then randomly united at fertilization. Mendel arrived at this conclusion by performing monohybrid crosses . These cross-pollination experiments were performed with pea plants that differed in one trait, such as the color of the pod.
Mendel began to wonder what would happen if he studied plants that were different with respect to two traits. Would both traits be transmitted to the offspring together or would one trait be transmitted independently of the other? It is from these questions and Mendel's experiments that he developed the law of independent assortment.
Mendel's Law of Segregation
Foundational to the law of independent assortment is the law of segregation . It was during earlier experiments that Mendel formulated this genetics principle.
The law of segregation is based on four main concepts:
- Genes exist in more than one form or allele.
- Organisms inherit two alleles (one from each parent) during sexual reproduction .
- These alleles separate during meiosis, leaving each gamete with one allele for a single trait.
- Heterozygous alleles exhibit complete dominance as one allele is dominant and the other recessive.
Mendel's Independent Assortment Experiment
Mendel performed dihybrid crosses in plants that were true-breeding for two traits. For example, a plant that had round seeds and yellow seed color was cross-pollinated with a plant that had wrinkled seeds and green seed color.
In this cross, the traits for round seed shape (RR) and yellow seed color (YY) are dominant. Wrinkled seed shape (rr) and green seed color (yy) are recessive.
The resulting offspring (or F1 generation ) were all heterozygous for round seed shape and yellow seeds (RrYy) . This means that the dominant traits of round seed shape and yellow color completely masked the recessive traits in the F1 generation.
Discovering the Law of Independent Assortment
The F2 Generation: After observing the results of the dihybrid cross, Mendel allowed all of the F1 plants to self-pollinate. He referred to these offspring as the F2 generation .
Mendel noticed a 9:3:3:1 ratio in the phenotypes . About 9/16 of the F2 plants had round, yellow seeds; 3/16 had round, green seeds; 3/16 had wrinkled, yellow seeds; and 1/16 had wrinkled, green seeds.
Mendel's Law of Independent Assortment: Mendel performed similar experiments focusing on several other traits such as pod color and seed shape; pod color and seed color; and flower position and stem length. He noticed the same ratios in each case.
From these experiments, Mendel formulated what is now known as Mendel's law of independent assortment. This law states that allele pairs separate independently during the formation of gametes . Therefore, traits are transmitted to offspring independently of one another.
How Traits Are Inherited
How genes and alleles determine traits.
Genes are segments of DNA that determine distinct traits. Each gene is located on a chromosome and can exist in more than one form. These different forms are called alleles, which are positioned at specific locations on specific chromosomes.
Alleles are transmitted from parents to offspring by sexual reproduction. They are separated during meiosis (process for the production of sex cells ) and united at random during fertilization .
Diploid organisms inherit two alleles per trait, one from each parent. Inherited allele combinations determine an organisms genotype (gene composition) and phenotype (expressed traits).
Genotype and Phenotype
In Mendel's experiment with seed shape and color, the genotype of the F1 plants was RrYy . Genotype determines which traits are expressed in the phenotype.
The phenotypes (observable physical traits) in the F1 plants were the dominant traits of round seed shape and yellow seed color. Self-pollination in the F1 plants resulted in a different phenotypic ratio in the F2 plants. The F2 generation pea plants expressed either round or wrinkled seed shape with either yellow or green seed color. The phenotypic ratio in the F2 plants was 9:3:3:1 . There were nine different genotypes in the F2 plants resulting from the dihybrid cross.
The specific combination of alleles that comprise the genotype determines which phenotype is observed. For example, plants with the genotype of (rryy) expressed the phenotype of wrinkled, green seeds.
Non-Mendelian Inheritance
Some patterns of inheritance do not exhibit regular Mendelian segregation patterns. In incomplete dominance, one allele does not completely dominate the other. This results in a third phenotype that is a mixture of the phenotypes observed in the parent alleles. For example, a red snapdragon plant that is cross-pollinated with a white snapdragon plant produces pink snapdragon offspring.
In co-dominance, both alleles are fully expressed. This results in a third phenotype that displays distinct characteristics of both alleles. For example, when red tulips are crossed with white tulips, the resulting offspring can have flowers that are both red and white.
While most genes contain two allele forms, some have multiple alleles for a trait. A common example of this in humans is ABO blood type . ABO blood types exist as three alleles, which are represented as (IA, IB, IO) .
Further, some traits are polygenic, meaning that they are controlled by more than one gene. These genes may have two or more alleles for a specific trait. Polygenic traits have many possible phenotypes and examples include traits such as skin and eye color.
- Dihybrid Cross in Genetics
- Mendel's Law of Independent Assortment
- Genes, Traits and Mendel's Law of Segregation
- What Is Mendel's Law of Segregation?
- True-Breeding Plants
- Heterozygous Traits
- Monohybrid Cross: A Genetics Definition
- Incomplete Dominance in Genetics
- What Does Homozygous Mean in Genetics?
- A Genetics Definition of Heterozygous
- What Is Genetic Dominance and How Does It Work?
- How Do Alleles Determine Traits in Genetics?
- Genes and Genetic Inheritance
- Probabilities for Dihybrid Crosses in Genetics
- Phenotype: How a Gene Is Expressed As a Physical Trait
- Law of Multiple Alleles
Independent Assortment
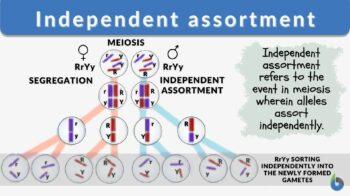
Independent assortment n., [ɪndɪˈpɛndənt əˈsɔːtmənt] Definition: random segregation and assortment of pairs of alleles during meiosis
Table of Contents
Independent Assortment Definition
Independent assortment refers to the alleles or genes that sort into the newly formed gametes independently of one another. This independent assortment is a result of the independent division of chromosomes into separate gametes. Take note that crossing over takes place where genes on each chromosome are rearranged. Thus, the principle of Independent Assortment by Gregor Mendel describes how different genes carrying different traits could occur in the new gametes after the full meiotic cell division. This principle is just one of the laws of Inheritance proposed by Gregor Mendel. Let us get to know more about Mendel’s laws below.
Law of Independent Assortment Definition
The Mendelian inheritance principles are derived from and named after the monk, Gregor Johann Mendel in the nineteenth century. The Mendelian principles were formulated based on certain experiments conducted by Mendel with pea plants in the monastery’s garden.
During gamete production , the normal diploid number of chromosomes is reduced to half the chromosome number during meiotic division to produce haploid gametes containing 23 chromosomes only. The normal human diploid cell contains 46 chromosomes; half the number of chromosomes is derived maternally, i.e., from the female gamete (the egg cell or ovum) and the other half is derived paternally, i.e., from the male gamete (the sperm cell ). During sexual reproduction , female and male gametes fuse together to produce a new organism or a diploid zygote .
Other Mendel’s laws
Mendel’s inheritance laws are the following:
- Law of Dominance and Uniformity states that alleles are either dominant or recessive and where a living organism with one or more dominant alleles will show the influence of this allele.
- Law of Segregation , where each gene alleles segregate from each other during the formation of the gamete to allow the gamete to carry one allele only for each gene. It is related to the Law of Independent Assortment .
The Law of Independent Assortment discusses the random genetic inheritance from both parents. As stated in the Law of Segregation, the so-called homologous chromosomes separate from each other during meiotic division . Therefore, the chromosomes of both maternal and paternal gametes are assorted independently; in other words, chromosomes found in one gamete do not necessarily end up in the same source after division. As a result, one gamete may eventually have all chromosomes from the maternal source while another gamete can end up having different mixtures of chromosomes from both maternal and paternal sources.
Got a question about homologous chromosome? Join us and participate in our Forum: Difference Between Homologous Chromosomes and Sister Chromatids .
Even though genes that are found on the same chromosome or linked genes (genetic linkage) are not randomly assorted, crossing over that takes place during meiosis allows such genes to rearrange. During this process, the exchange of homologous parts of chromosomes occurs in both maternal chromosomes and paternal chromosomes to ensure the independent assortment applies to linked genes as well. As a result, different gene combinations create great variety among individuals due to the production of gene mixtures that were not previously found.
Mendel’s law of independent assortment states that the resulting chromosomes are sorted randomly by mixing the maternal and paternal chromosomes. In the end, the zygote has a mix of chromosomes and not a defined set of specific traits from each parent. That’s why chromosomes are considered to be independently assorted so the zygote will eventually have a combination of different maternal and paternal chromosomes.
Since the number of chromosomes in each gamete is 23 and the number of gametes is 2; thus, the number of possible combinations is 2 23 , or 8,388,608 . This number of possibilities allows for great variability in progeny genes. This gene variation has a great effect on evolution and evolutionary processes.
Biology Definition:
The Law of Independent Assortment states that the process of random segregation and assortment of pairs of alleles during gamete formation will result in the production of gametes with all possible allele combinations in equal numbers. It is expressed in the inheritance of two or more different traits associated with different genes. It is one of the principles of inheritance of Gregor Mendel based on his work in garden peas. After the rediscovery of his work, they were accepted and referred to as the Mendelian Laws .
Principle of Independent Assortment
What does independent assortment mean? The law of independent assortment means that separate traits of different alleles are inherited independently from each other by the zygote. Where the random selection of one allele for a certain trait is not connected by any means to the selection of another allele for a different trait.
What is an independent assortment? Independent assortment states that the inheritance of various genes occurs independently of each other. In the law of independent assortment, the combination of genes and their probability is calculated and assumed by multiplying the probabilities of each gene. Moreover, the probability of having one gene does not influence the probability of having the other.
What stage of meiosis does independent assortment occur? Independent assortment in meiosis takes place in eukaryotes during metaphase I of meiotic division. It produces a gamete carrying mixed chromosomes. Gametes contain half the number of regular chromosomes in a diploid somatic cell. Thus, gametes are haploid cells that can undergo sexual reproduction at which two haploid gametes are fused together forming a diploid zygote having the complete set of chromosomes. The physical basis is the random distribution of chromosomes during the metaphase in relation to other chromosomes.
Why is independent assortment important? Independent assortment is responsible for the production of new genetic combinations in the organism along with crossing over. Thus, it contributes to genetic diversity among eukaryotes.
To define independent assortment, you should understand the law of segregation first. The law of segregation states that in meiosis, different gamete cells get two different independently assorted genes. On the other hand, the two maternal and paternal DNA are randomly separated allowing for more diversity in genes. The law of independent assortment is apparent during the random division of the maternal and paternal DNA sources. Due to random assortment, the gamete may get maternal genes, paternal genes, or a mixture of both. The genetic distribution is based on the initial stage of meiosis where these chromosomes are lined up randomly.
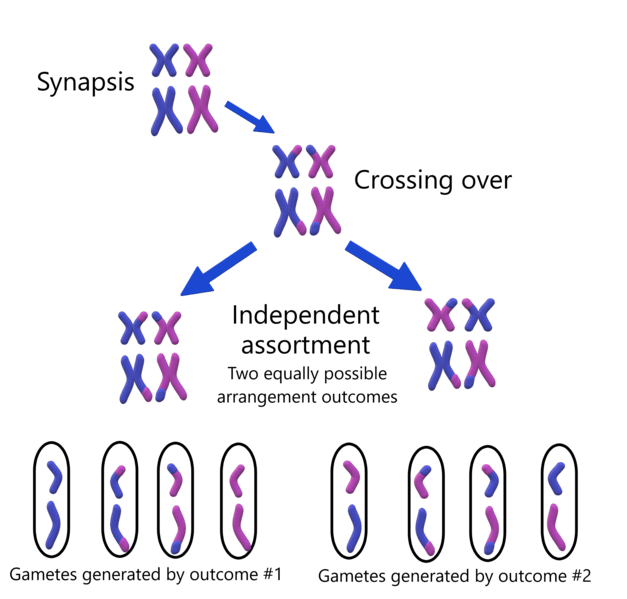
Independent Assortment Examples
Gregor Mandel carried out several experiments on pea plants. As a result, he was able to identify the way by which the units of heredity work, which are now known as genes after the discovery of DNA and genetic information.
How does independent assortment occur? Independent assortment occurs spontaneously when alleles of at least two genes are assorted independently into gametes. Consequently, the allele inherited by one gamete does not affect the allele inherited by other gametes.
Mendel noted that the transmission of different genes appeared to be independent events. In independent events, the probability of a particular combination of traits can be predicted by multiplying the individual probabilities of each trait. In independent events, the inheritance pattern of one trait will not affect the inheritance pattern of another.
For example, when Mendel crossed plants with round (R allele) yellow (Y allele) peas to plants with wrinkled green peas, all of the F1 peas expressed the dominant traits of round and yellow. In the F2, along with round yellow and wrinkled green peas, he observed round green and wrinkled yellow peas.
Each of the dominant traits was present in ¾ of the progeny and each of the recessive traits was present in ¼ of the progeny.
The four possible combinations of color and shape appeared in the phenotypic ratio of 9:3:3:1, which represents the independent assortment of the genes for the two pairs of traits in the gametes.
If you have ¾ yellow and ¾ round then independent events predict that ¾ x ¾ = 9/16 will be both yellow and round. The proportions of the other three combinations can be similarly calculated.
Mendel observed 9 yellow round: 3 yellow wrinkled: 3 green round: 1 green wrinkled peas.
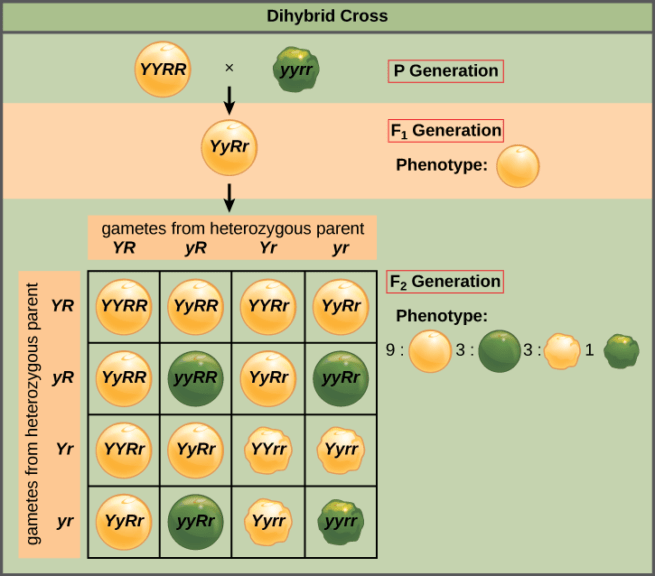
Later, after the discovery of chromosomes, and their behavior in meiosis , it was possible to explain independent assortment as a consequence of the independent movement of each pair of homologous chromosomes during meiosis. An independent assortment of genes is important to produce new genetic combinations that increase genetic variations within a population .
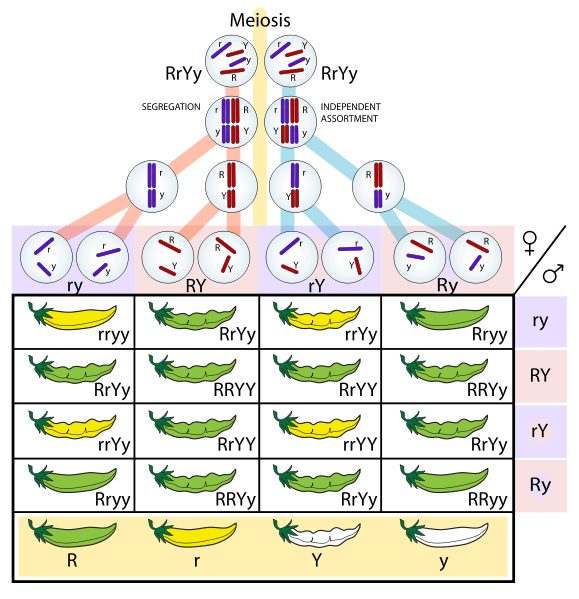
What is independent assortment as explained with a suitable example? Let’s take for example a random population of cats and track two traits: eye color (brown or green) and fur color (white or grey). The dominant allele for the eye color, for example, is brown eyes ( B ), and the recessive allele, green eye color ( b ). As for the color of the fur, let’s say that the white fur ( W ) allele is dominant over the gray fur allele ( w ). Heterozygous cats with dominant traits, brown eyes and white fur, will produce gametes at sexual maturity. During gamete production, the alleles for eye color will be sorted independent of the alleles for the fur color, if we are to base it on the law of segregation. The resulting gamete after meiosis will contain random alleles such that when two heterozygous cats are crossed, their offspring will likely have mixed traits. One of the kitten, for example, could have brown eye color ( BB or Bb ) and grey fur color ( ww ). Another kitten might have green eyes ( bb ) and grey fur ( ww ). Others still could have brown eyes and white fur (thus, possible genotypes could be BBWW, BBWw, BbWW, BbWw). Now, this is just an illustrative example. In nature, the eye and fur color traits are polygenic , meaning several alleles are involved in determining the phenotype of the offspring.
The independent assortment is now explained according to the behavior of chromosomes during meiosis and the random movement of each homologous pair of chromosomes during meiosis. Independent assortment is an important process for the production of new genetic combinations that contribute to the genetic diversity among individuals that reproduce sexually.
How do you know if a chromosome is homologous? Get insights here: Difference Between Homologous Chromosomes and Sister Chromatids . Join our Forum now!
Choose the best answer.
Send Your Results (Optional)
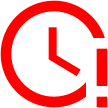
Further Reading
- Independent Assortment and Crossing Over – Biology Online Tutorial
- Mendel’s Law & Mendelian Genetics – Biology Online Tutorial
Explore more about homologous chromosome. Join our Forum: Difference Between Homologous Chromosomes and Sister Chromatids .
- Libretexts. (2020, December 1). 12.3D: Mendel’s Law of Independent Assortment. Biology LibreTexts. https://bio.libretexts.org/Bookshelves/Introductory_and_General_Biology/Book%3A_General_Biology_(Boundless)/12%3A_Mendel’s_Experiments_and_Heredity/12.3%3A_Laws_of_Inheritance/12.3D%3A_Mendels_Law_of_Independent_Assortment.
- Monaghan, F., & Corcos, A. (1984). On the origins of the Mendelian laws. Journal of Heredity, 75(1), 67-69.
- Ruch, D. G. (1998). A cookie model for the development of the concept of independent assortment. The American Biology Teacher, 60(9), 696-698.
- Semagn, K., Bjørnstad, Å., & Ndjiondjop, M. N. (2006). Principles, requirements and prospects of genetic mapping in plants. African Journal of Biotechnology, 5(25).
©BiologyOnline.com. Content provided and moderated by Biology Online Editors.
Last updated on September 20th, 2023
You will also like...
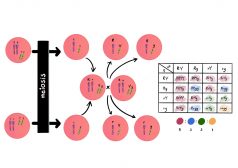
Independent Assortment and Crossing Over
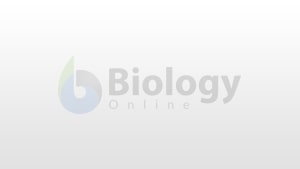
Genetics – Lesson Outline & Worksheets
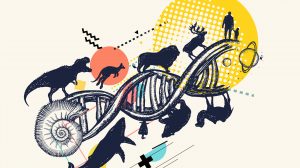
Genetics and Evolution
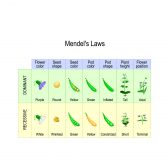
Mendel’s Law & Mendelian Genetics
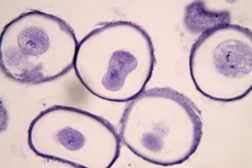
Meiosis – The Genetics of Reproduction
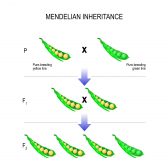
Inheritance and Probability
Related articles....
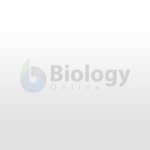
Mendel’s laws: definitions
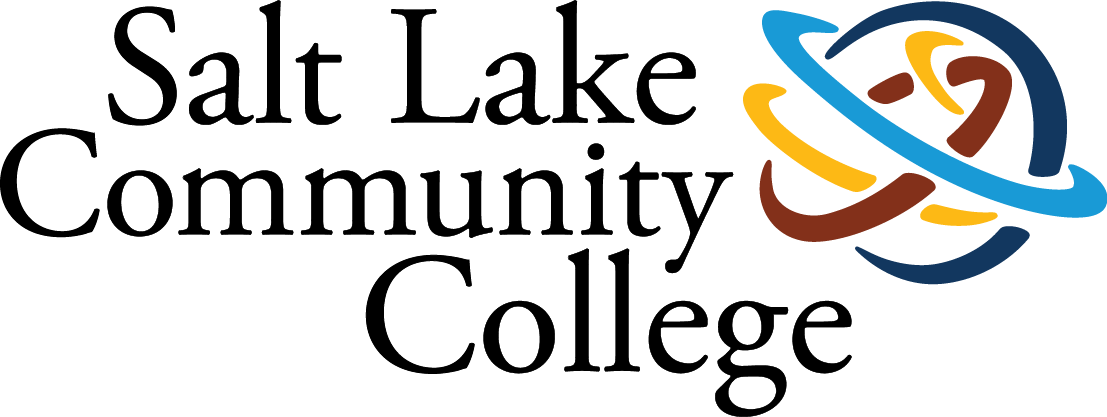
Want to create or adapt books like this? Learn more about how Pressbooks supports open publishing practices.
19.4 Independent Assortment
Mendel’s law of independent assortment states that genes do not influence each other with regard to the sorting of alleles into gametes, and every possible combination of alleles for every gene is equally likely to occur. The independent assortment of genes can be illustrated by the dihybrid cross, a cross between two true-breeding parents that express different traits for two characteristics.
Consider the characteristics of seed color and seed texture for two pea plants, one that has green, wrinkled seeds ( yyrr ) and another that has yellow, round seeds ( YYRR ). Because each parent is homozygous, the law of segregation indicates that the gametes for the green/wrinkled plant all are yr , and the gametes for the yellow/round plant are all YR . Therefore, the F 1 generation of offspring all are YyRr .
For the F 2 generation, each gamete receives either an R allele or an r allele and either a Y allele or a y allele. The law of independent assortment states that a gamete into which an r allele sorted would be equally likely to contain either a Y allele or a y allele. Thus, there are four equally likely gametes that can be formed when the YyRr heterozygote is self-crossed, as follows: YR , Yr , yR , and yr .
Arranging these gametes along the top and left of a 4 × 4 Punnett square gives us 16 equally likely genotypic combinations. From these genotypes, we see a phenotypic ratio of 9 round/yellow:3 round/green:3 wrinkled/yellow:1 wrinkled/green. These are the offspring ratios we would expect, assuming we performed the crosses with a large enough sample size.
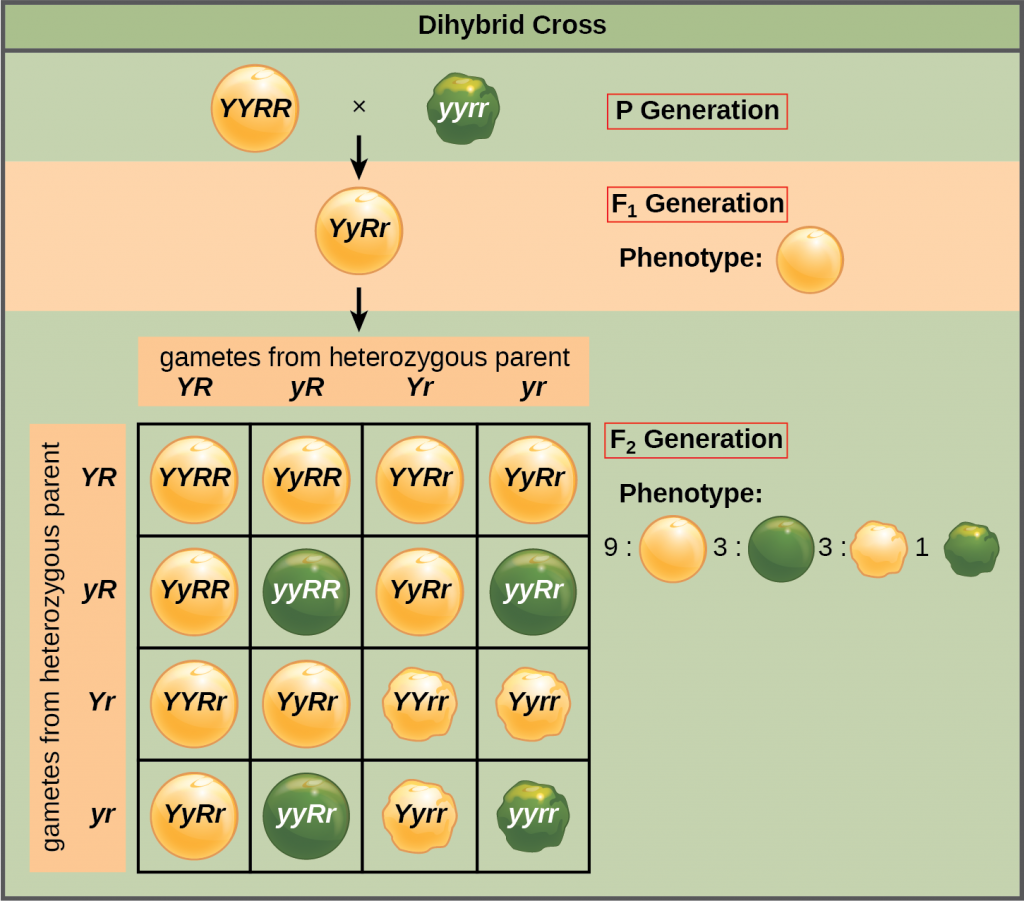
Because of independent assortment and dominance, the 9:3:3:1 dihybrid phenotypic ratio can be collapsed into two 3:1 ratios, characteristic of any monohybrid cross that follows a dominant and recessive pattern. Ignoring seed color and considering only seed texture in the above dihybrid cross, we would expect that three quarters of the F 2 generation offspring would be round, and one quarter would be wrinkled. Similarly, isolating only seed color, we would assume that three quarters of the F 2 offspring would be yellow and one quarter would be green. The sorting of alleles for texture and color are independent events, so we can apply the product rule.
Therefore, the proportion of round and yellow F 2 offspring is expected to be (3/4) × (3/4) = 9/16, and the proportion of wrinkled and green offspring is expected to be (1/4) × (1/4) = 1/16. These proportions are identical to those obtained using a Punnett square. Round, green and wrinkled, yellow offspring can also be calculated using the product rule, as each of these genotypes includes one dominant and one recessive phenotype. Therefore, the proportion of each is calculated as (3/4) × (1/4) = 3/16.
The law of independent assortment also indicates that a cross between yellow, wrinkled ( YYrr ) and green, round ( yyRR ) parents would yield the same F 1 and F 2 offspring as in the YYRR x yyrr cross.
The physical basis for the law of independent assortment also lies in meiosis I , in which the two members of each homologous chromosome pair line up in random orientation. Each gamete will contain one copy of each chromosome type (sometimes the maternal copy and sometimes the paternal copy); hence, many different gamete types are possible based on different combinations of paternal and maternal chromosomes (and therefore the genes on them).
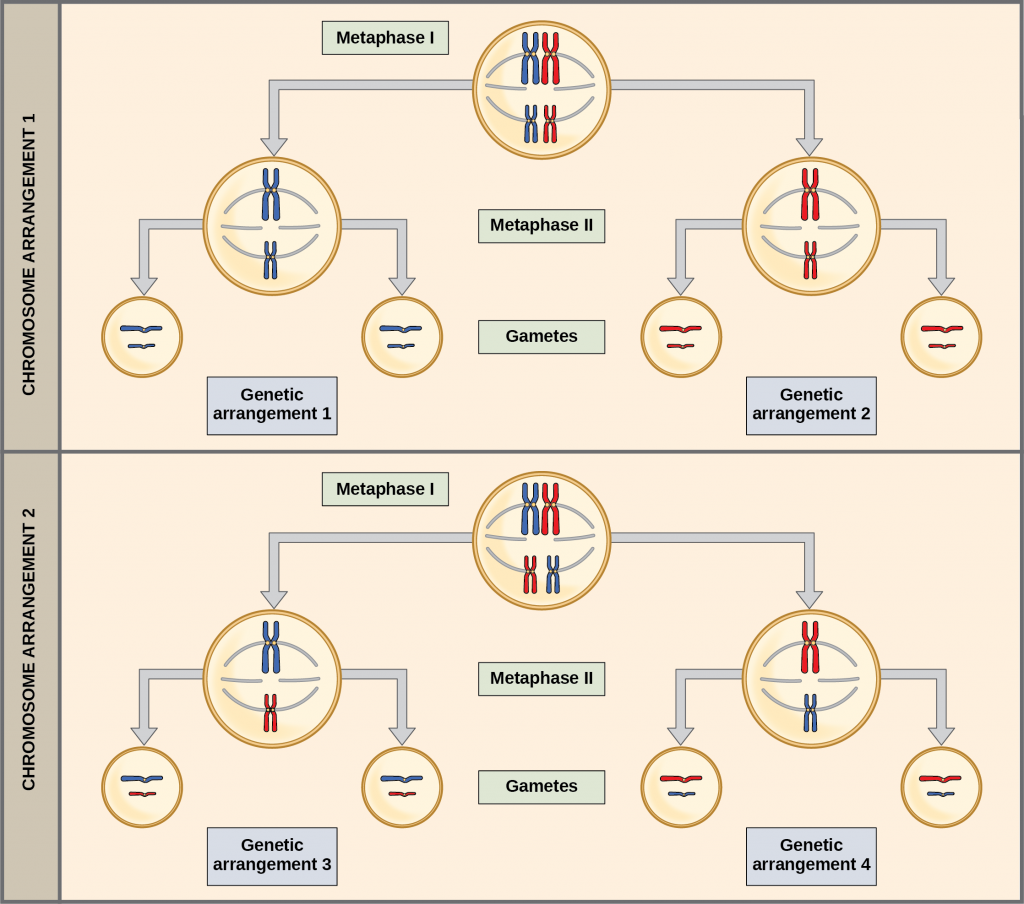
Identifying All Gamete Types Based on a Parental Genotype
To investigate the inheritance of traits, it is important to correctly predict all of the different gamete types that an individual of known genotype is expected to produce. Here, we need to remember that individuals are diploid and gametes are haploid. Hence individuals most often contain two copies of each gene (an exception is that human males only contain one copy of each X-linked gene) but gametes have only one copy of each gene. Because the segregation of alleles is random, half of the gametes are expected to contain one copy of the gene and the other half the other copy. When the assortment of genes is independent, either copy of gene 2 is equally likely to end up with either copy of gene 1.
What gamete types is an individual of genotype AabbCc expected to produce? Each gamete is expected to have one copy of gene A, one copy of gene B, and one copy of gene C. For gene A, each gamete would contain either the “A” or the “a” allele. Further, each gamete will contain a copy of “b” because that is the only allele for gene B in this individual. Since this individual is heterozygous for gene C, the same outcome is expected as for gene A: half of the gametes will contain the “C” allele and the other half will contain the “c” allele. An organized method to determine gamete types is to build a tree diagram in which each column represents a different gene.
Linked Genes
Although all of Mendel’s pea characteristics behaved according to the law of independent assortment, we now know that some allele combinations are not inherited independently of each other. Genes that are located on separate non-homologous chromosomes will always sort independently. However, genes on the same chromosome do not always sort independently. Linkage means that genes that are located physically close to each other on the same chromosome are more likely to be inherited together. However, because of the process of recombination, or “crossover,” it is possible for two genes on the same chromosome to behave independently, or as if they are not linked. To understand this, let’s consider the biological basis of gene linkage and recombination.
Homologous chromosomes possess the same genes in the same linear order. The alleles may differ on homologous chromosome pairs, but the genes to which they correspond do not. In preparation for the first division of meiosis, homologous chromosomes replicate and synapse. At this stage, segments of homologous chromosomes exchange linear segments of genetic material. This process is called recombination , or crossover , and it is a common genetic process. Because the genes are aligned during recombination, the gene order is not altered. Instead, the result of recombination is that maternal and paternal alleles are combined onto the same chromosome. Across a given chromosome, several recombination events may occur, causing extensive shuffling of alleles.
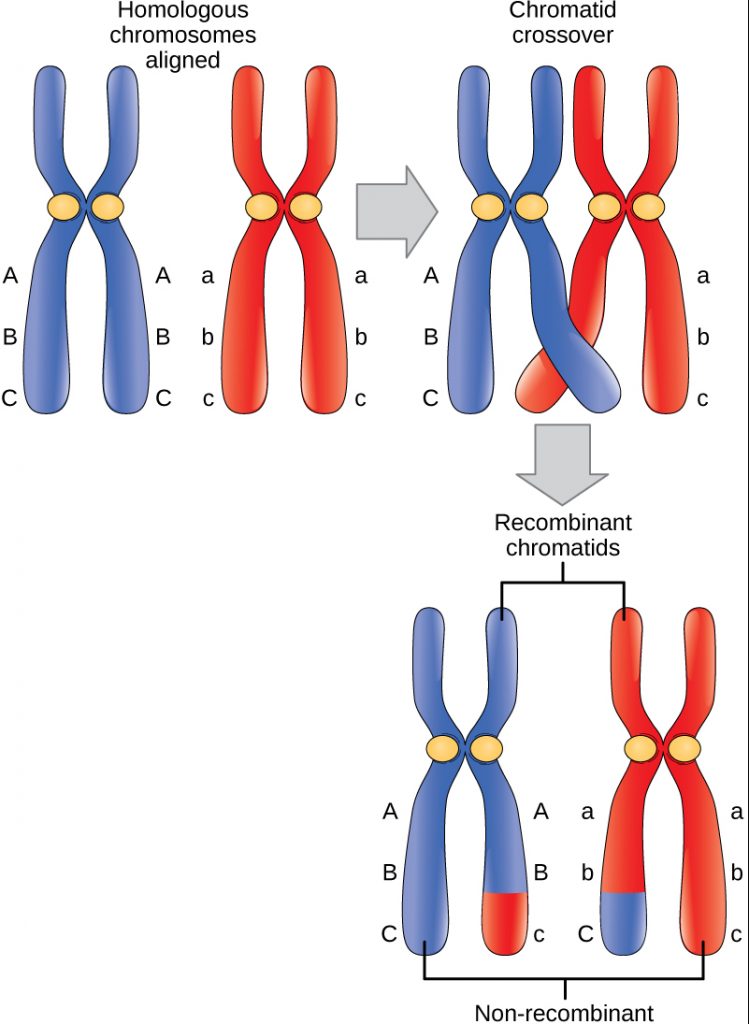
When two genes are located in close proximity on the same chromosome, they are considered linked, and their alleles, which are connected in the parental genome, tend to be transmitted through meiosis together.
On the diagram above representing a homologous chromosome pair, the “B” allele of gene B is linked to the “C” allele of gene C on one copy of the chromosome, and the “b” allele is linked to the “c” allele on the other copy. Gametes which retain this allelic arrangement are called parental or non-recombinant gametes (in this case BC and bc), and those in which the allelic arrangement is altered by crossover are called recombinant gametes (in this case Bc and bC). Because linked genes are more likely to be transmitted together, parental gametes are produced at a greater frequency than recombinant gametes. Thus, one can deduce which allele of gene C is linked to the “B” allele in a BbCc parent by identifying which two gamete types are produced at a higher frequency than the other two .
As the distance between two genes increases, the probability of one or more crossovers between them increases, and the genes behave more like they are on separate chromosomes. Geneticists have used the proportion of recombinant gametes (the ones unlike the parents) as a measure of how far apart genes are on a chromosome. Using this information, they have constructed elaborate maps of genes on chromosomes for well-studied organisms, including humans.
first round of meiotic cell division; referred to as reduction division because the daughter cells have half the number of chromosomes as the parent cell
phenomenon in which alleles that are located in close proximity to each other on the same chromosome are more likely to be inherited together
exchange of genetic material between homologous chromosomes resulting in chromosomes that incorporate genes from both parents of the organism
College Biology I Copyright © by Melissa Hardy is licensed under a Creative Commons Attribution-NonCommercial 4.0 International License , except where otherwise noted.
If you're seeing this message, it means we're having trouble loading external resources on our website.
If you're behind a web filter, please make sure that the domains *.kastatic.org and *.kasandbox.org are unblocked.
To log in and use all the features of Khan Academy, please enable JavaScript in your browser.
High school biology - NGSS
Course: high school biology - ngss > unit 6.
- Genes, traits, and the environment
- Mendelian inheritance and Punnett squares
Dihybrid cross and the law of independent assortment
- Creativity break: what are some new ways of thinking about problem solving?
- Understand: genes, traits, and the environment
- Apply: genes, traits, and the environment
Want to join the conversation?
- Upvote Button navigates to signup page
- Downvote Button navigates to signup page
- Flag Button navigates to signup page
Video transcript
12.4: Dihybrid Crosses
Chapter 1: scientific inquiry, chapter 2: chemistry of life, chapter 3: macromolecules, chapter 4: cell structure and function, chapter 5: membranes and cellular transport, chapter 6: cell signaling, chapter 7: metabolism, chapter 8: cellular respiration, chapter 9: photosynthesis, chapter 10: cell cycle and division, chapter 11: meiosis, chapter 12: classical and modern genetics, chapter 13: dna structure and function, chapter 14: gene expression, chapter 15: biotechnology, chapter 16: viruses, chapter 17: nutrition and digestion, chapter 18: nervous system, chapter 19: sensory systems, chapter 20: musculoskeletal system, chapter 21: endocrine system, chapter 22: circulatory and pulmonary systems, chapter 23: osmoregulation and excretion, chapter 24: immune system, chapter 25: reproduction and development, chapter 26: behavior, chapter 27: ecosystems, chapter 28: population and community ecology, chapter 29: biodiversity and conservation, chapter 30: speciation and diversity, chapter 31: natural selection, chapter 32: population genetics, chapter 33: evolutionary history, chapter 34: plant structure, growth, and nutrition, chapter 35: plant reproduction, chapter 36: plant responses to the environment.
The JoVE video player is compatible with HTML5 and Adobe Flash. Older browsers that do not support HTML5 and the H.264 video codec will still use a Flash-based video player. We recommend downloading the newest version of Flash here, but we support all versions 10 and above.

In pea plants Gregor Mendel also performed dihybrid crosses, breeding experiments between organisms that vary by two traits, like seed shape and seed color.
For example, if one parent is homozygous dominant for round and yellow seeds and the other is homozygous recessive for wrinkled and green seeds, all plants of the F1 generation area dihybrids, meaning that they're heterozygous for two traits.
The F1 plants display the dominant phenotype for both traits and have round and yellow seeds.
When they self fertilize, their progeny, the F2 generation, includes plants with round, yellow seeds, round green seeds, wrinkled yellow seeds, and wrinkled green seeds, in a nine to three to three to one ratio.
Importantly, the relationship of round to wrinkled seeded plants and yellow to green seeded, is three to one for both, indicating that these traits segregated independently, which demonstrates the principle of independent assortment.
To determine whether traits are inherited together or separately, Gregor Mendel crossed pea plants that differed in two traits. These parental plants were homozygous for both traits but displayed different phenotypes. The first generation of offspring were all dihybrids, heterozygotes exhibiting the two dominant phenotypes. When self-fertilized, the dihybrids consistently produced progeny with a 9:3:3:1 ratio of four possible phenotype combinations. This ratio suggested that inheriting one trait did not affect the likelihood of inheriting the other, establishing Mendel’s law of independent assortment.
Mendel’s Dihybrid Crosses Demonstrate the Principle of Independent Assortment
Gregor Mendel’s monohybrid crosses, between pea plants that differed in a single trait, demonstrated that (1) organisms randomly inherit one of two copies of each gene from each parent (Mendel’s first law, segregation), and (2) the dominant allele can mask the recessive allele’s effects on phenotype (the principle of uniformity).
To determine whether two traits were inherited separately or together, Mendel also performed crosses with pea plants that differed in two traits, such as pea color and pea shape. For these dihybrid crosses, Mendel first mated plants that were true breeding (i.e., homozygous) for different traits of the same two characteristics. For example, he crossed plants that bred true for round, yellow peas ( RRYY genotype) with those that bred true for wrinkled, green peas ( rryy genotype). This parental (P 0 ) generation produced offspring (F 1 generation) that were all heterozygous with dominant phenotypes. These dihybrids had RrYy genotypes and round, yellow peas.
Mendel then induced self-pollination in the F 1 dihybrids. Of the sixteen possible parental allele combinations, nine produce offspring with both dominant traits, yellow and round peas. Six fertilization events confer one dominant trait, with three producing yellow (dominant), wrinkled peas, and three creating green, round (dominant) peas. The one remaining possibility results in green, wrinkled peas, the two recessive phenotypes.
The proportion of phenotypes that Mendel observed in F 2 plants was consistently similar to this 9:3:3:1 ratio, which is expected only if each fertilization event is equally probable. Thus, observing this phenotypic ratio suggests that inheriting one of these traits (e.g., yellow or green pea color) does not influence the likelihood of inheriting one of the others (e.g., round or wrinkled peas). This finding is the crux of Mendel’s second law, the principle (or law) of independent assortment.
Linkage and Recombination Influence Trait Co-inheritance
Genes on separate, non-homologous chromosomes are independently assorted into gametes during meiosis. However, genes close to one another on the same chromosome are more likely to be distributed into the same gametes; a phenomenon called linkage. Thus, inheriting one trait can be linked to the likelihood of inheriting another. Mendel never reported linkage, although not all of the traits he studied are determined by loci on different chromosomes.
The alleles determining pod color and pea shape are on chromosomes 5 and 7, respectively, and are thus unlinked. For most of the other traits, the lack of linkage can be accounted for by recombination, which can cause the inheritance patterns of genes on the same chromosome to mimic independent assortment. During prophase I of meiosis, chromosome pairs line up, cross over, and swap homologous genetic segments, a process known as recombination. The closer two loci are to each other on a chromosome, the more likely they are to be on the same recombined segment and thus inherited together. Likewise, loci that are far apart are more likely to be inherited separately due to more recombination events dividing them.
Returning to Mendel’s traits, pea and flower color are determined by two chromosome 1 loci that are far apart. Similarly, the locus for flower position is far from the other chromosome 4 loci, pod shape and plant height. Due to recombination, it is unsurprising that linkage never manifested in these crosses. Loci for pod shape and plant height, however, are close enough to each other on chromosome 4 that some linkage is likely. Mendel never published the results of this particular crossing, so it is possible that he simply never carried out these experiments, making him one cross short of discovering linkage.
Clancy, Suzanne. 2008. “Genetic recombination.” Nature Education 1 (1): 40. [ Source ]
Lobo, Ingrid, and Kenna Shaw. 2008. “Discovery and Types of Genetic Linkage.” Nature Education 1 (1): 139. [ Source ]
Miko, Ilona. 2008. “Gregor Mendel and the principles of inheritance.” Nature Education 1 (1): 134. [ Source ]
Get cutting-edge science videos from J o VE sent straight to your inbox every month.
mktb-description
We use cookies to enhance your experience on our website.
By continuing to use our website or clicking “Continue”, you are agreeing to accept our cookies.

- Biology Article
- Law Independent Assortment
Law Of Independent Assortment
Mendel’s experimental study on the pattern of inheritance was a turning point in the history of genetics. Based on his investigation, Mendel proposed three laws of inheritance. Mendel’s Law of Inheritance is the fundamental principle of inheritance in genetics. Law of Dominance, Law of Segregation and Law of Independent Assortment are collectively known as Mendel’s Laws of Inheritance.
Explore more: Mendelian Genetics
Law of Inheritance
Mendel chose to perform a monohybrid cross of a pair of contrasting traits. The observations of the monohybrid cross led to the formulation of the Law of Segregation and Law of Dominance . Followed by this, Mendel performed a dihybrid cross taking two contradicting traits together for crossing.
Here we will discuss the Law of Independent Assortment.
Also Read: Mendel’s Laws Of Inheritance
Law of Independent Assortment
“The law of independent assortment states that the allels of different genes are inherited independently within the organisms that reproduce sexually.”
What is the Law of Independent Assortment?
According to the law of independent assortment, the alleles of two more genes get sorted into gametes independent of each other. The allele received for one gene does not influence the allele received for another gene.
Mendel’s experiment always portrayed that the combinations of traits of the progeny are always different from their parental traits. Based on this, he formulated the Law of Independent Assortment.
Also Read: Law Of Independent Assortment
Reasons for Independent Assortment
Independent assortment takes place during the process of meiosis. In this process, the chromosomes are halved and are known as haploid.
To understand the law of independent assortment, it is very important to understand the law of segregation. In this, two different genes are sorted into different gamete cells. On the other hand, the law of independent assortment occurs when the maternal and paternal genes are divided randomly.
Mendel’s Experiment on the Law of Independent Assortment
The Law of Independent Assortment states that during a dihybrid cross (crossing of two pairs of traits), an assortment of each pair of traits is independent of the other. In other words, during gamete formation, one pair of trait segregates from another pair of traits independently. This gives each pair of characters a chance of expression.
In the dihybrid cross, he chose round-yellow seed and wrinkled green seed and crossed them. He obtained only round yellow seeds in the F 1 generation. Later, self-pollination of F 1 progeny gave four different combinations of seeds in the F 2 generation. He obtained round-yellow, wrinkled-yellow, round green and wrinkled green seeds in the phenotypic ratio 9:3:3:1.
The phenotypic ratio 3:1 of yellow: green colour and the ratio 3:1 of the round: wrinkled seed shape during monohybrid cross was retained in the dihybrid cross as well. Thus, he concluded that characters are distributed independently and inherited independently. Based on this observation, he developed his third law – Law of Independent Assortment.
The dihybrid crosses between the parental genotype RRYY (round yellow seeds) and rryy (green wrinkled seeds) explains the law. Here the chances of formation of gametes with the gene R and the gene r are 50:50. Also, the chances of formation of gametes with the gene Y and the gene y are 50:50. Thus, each gamete should have either R or r and Y or y.
The Law of Independent Assortment states that the segregation of R and r is independent of the segregation of Y and y. This results in four types of gametes RY, Ry, rY, and ry. These combinations of alleles are different from their parental combination (RR, YY, rr and yy).
Explore more: Characteristics and Functions of Genes
Example of Law of Independent Assortment
Let us consider an example of rabbits with two visible traits:
- fur colour (black or white)
- eye colour (green or red)
Two-hybrid rabbits are crossed. Both the rabbits have a genotype BbGg. Before breeding each rabbit produced gametes. During this, the alleles are separated and the copy of each chromosome is assigned to different gamete. That means, regardless of the parental phenotype, the baby rabbits inherit different combinations of the traits. Alternatively, a baby rabbit can have a genotype Bbgg.
Also Read : Genetics
Learn more in detail about Law of Independent Assortment and other related topics at BYJU’S Biology .
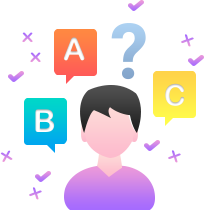
Put your understanding of this concept to test by answering a few MCQs. Click ‘Start Quiz’ to begin!
Select the correct answer and click on the “Finish” button Check your score and answers at the end of the quiz
Visit BYJU’S for all Biology related queries and study materials
Your result is as below
Request OTP on Voice Call
Leave a Comment Cancel reply
Your Mobile number and Email id will not be published. Required fields are marked *
Post My Comment

so perfect!thanks
Awesome n very helpful!

- Share Share
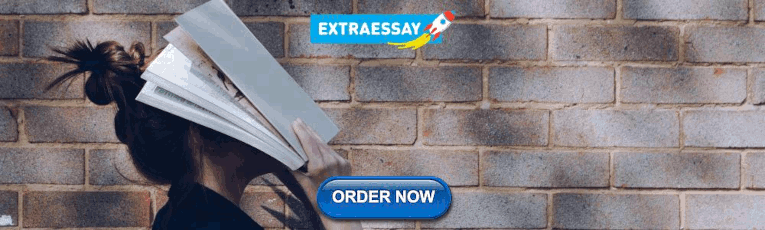
Register with BYJU'S & Download Free PDFs
Register with byju's & watch live videos.

Update image
- Principle of independent assortment
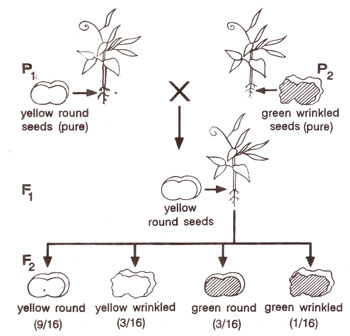
Support our developers
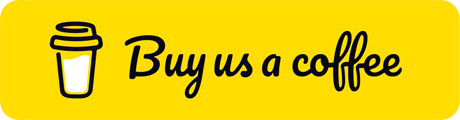
More in this section
- Mendel's Laws of Inheritance
- Gregor Mendel's life
- Pre-Mendelian experiments
- Mendel's experiments
- Symbols and terminology
- Principle of segregation (law of purity of gametes)
- Mendel's results, chromosome theory and linkage (present status)
- Molecular basis of Mendel's wrinkled seed character
- The rules of probability (product rule and sum rule)
- Mendelian genetics in humans
- Deviations from Mendel's finding
© 2012 - 2024 Biocyclopedia
Disclaimer Privacy Policy Feedback
Login to your account
Login / signup with.
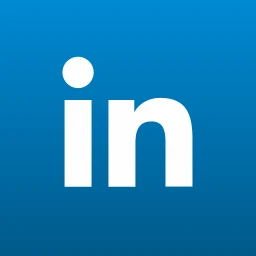
Meiosis and Mitosis and Mendel’s Law of Independent Assortment Essay
Meiosis and mitosis.
Meiosis and Mitosis entail the process of eukaryotic cell division, particularly of the nucleus. Both processes share a number of similarities but also differ on a range of factors. According to Gellert (2018), the key similarity between meiosis and mitosis is that they result in the formation of new cells. The processes also differ in various ways as discussed in the following sections.
Meiosis entails two nuclear divisions of two (diploid) cells resulting in the formation of four distinct nuclei, that is, two cells divide into four different cells. The resulting nuclei are genetically different and share a single chromosome set which is half the count of the original diploid cell. Meiosis is divided into meiosis I and meiosis II. During meiosis I, the homologous chromosomes divide to form dissimilar nuclei thereby reducing the ploidy level (Gellert, 2018). However, meiosis II, or second division takes place much more like a mitotic division.
Mitotic division or mitosis involves the nuclear division of a single cell resulting in the formation of two distinct but identical nuclei, that is, one cell divides into two different cells which are identical to the parent cell. The two nuclei formed out of mitosis share the same genetic identity as the parent cell. Mainly they have an equal number of groups of genetic material: diploid cells will have two chromosomes, and haploid cells will have one chromosome (Gellert, 2018). Therefore, the key difference between meiosis and mitosis is in the conduct of the chromosomes in the course of each process.
The cells formed out of a meiosis process especially in animals or diploid-dominant organisms only play a part in sexual reproduction hence the name ‘sexual reproduction’. Equally, the cells formed out of a mitosis process work in various parts of the body to help in growth or help to replace damaged or dead cells (Gellert, 2018). It is hence also referred to as ‘asexual reproduction’.
Mendel’s Law of Independent Assortment
Mendel’s law of independent assortment resulted from Mendel’s research on generational resemblance among families over long periods. His work focused on helping to explain why children inherited various features, called traits, from their parents. Mendel conducted his research using garden peas. He chose peas as the subject because the plants reproduce through sex just like human beings. The peas produce both female and male cells that are referred to as gametes (Mendel and Bateson, 2018). Mendel ensured that the peas and experiments were cautiously controlled by studying only one trait at a time.
The data collected was analyzed statistically. The results showed that each trait exhibited by the organisms was controlled by two factors. The factors are known as genes and they are found on chromosomes. Genes are in alternate forms, and the dissimilar gene forms are called alleles. Through his research studies, Mendel identified that different genes were hereditary autonomously of one another, hence the law of independent assortment. According to the law of independent assortment, every individual has two alleles of each gene and once the process of gamete production is completed, each gamete formed receives one of the alleles autonomously from one another. The law implies that each allele that goes to the gamete for one gene has no effect on the allele that goes to a different gene (Mendel and Bateson, 2018). The law is physically based on meiosis I which involves the process of gamete formation.
Gellert, A. (2018). Similarities of mitosis and meiosis. Sceiencing.com. Web.
Mendel, W. and Bateson, W. (2018). Mendel’s principles of heredity. Palala Press
- Chicago (A-D)
- Chicago (N-B)
IvyPanda. (2023, August 17). Meiosis and Mitosis and Mendel’s Law of Independent Assortment. https://ivypanda.com/essays/meiosis-and-mitosis-and-mendels-law-of-independent-assortment/
"Meiosis and Mitosis and Mendel’s Law of Independent Assortment." IvyPanda , 17 Aug. 2023, ivypanda.com/essays/meiosis-and-mitosis-and-mendels-law-of-independent-assortment/.
IvyPanda . (2023) 'Meiosis and Mitosis and Mendel’s Law of Independent Assortment'. 17 August.
IvyPanda . 2023. "Meiosis and Mitosis and Mendel’s Law of Independent Assortment." August 17, 2023. https://ivypanda.com/essays/meiosis-and-mitosis-and-mendels-law-of-independent-assortment/.
1. IvyPanda . "Meiosis and Mitosis and Mendel’s Law of Independent Assortment." August 17, 2023. https://ivypanda.com/essays/meiosis-and-mitosis-and-mendels-law-of-independent-assortment/.
Bibliography
IvyPanda . "Meiosis and Mitosis and Mendel’s Law of Independent Assortment." August 17, 2023. https://ivypanda.com/essays/meiosis-and-mitosis-and-mendels-law-of-independent-assortment/.
- The C-Fern Plant Laboratory Experiment
- Mitosis and Genetic Makeup of Different Species
- Structure of Deoxyribonucleic Acid
- Biology: Analysis of Egg Experiment
- Operant and Respondent Conditioning
- The Origin of Olives and Their Medicinal Uses
- Yersinia Pestis: Mechanism of Streptomycin Resistance
- Absorption and Digestion of Carbohydrates
Practice identifying cell parts by name or function. See this post for more information.
Biology Simulations
- Apr 9, 2019
Independent assortment, linked genes, and recombination
Updated: Jan 21
Mendel proposed the Law of Independent Assortment to explain his observations that the outcomes for one gene did not impact the outcomes for another gene. When he did crosses for multiple traits, new combinations occurred in the F2 generation that were not present in the P generation. For example, crossing true-breeding pea plants for yellow round seeds and green wrinkled seeds can result in an F2 generation that includes both yellow wrinkled seeds and green smooth seeds.
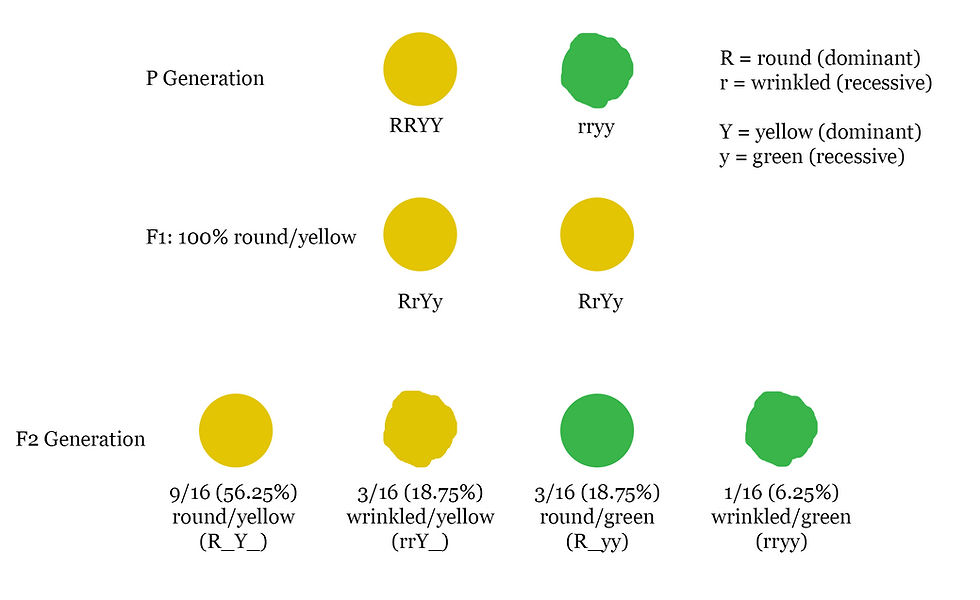
If genes are located on different chromosomes, a heterozygote has an equal chance of the dominant or recessive copy ending up in each gamete. This means that in a dihybrid cross there are four combinations that are equally likely to be passed on to the next generation.
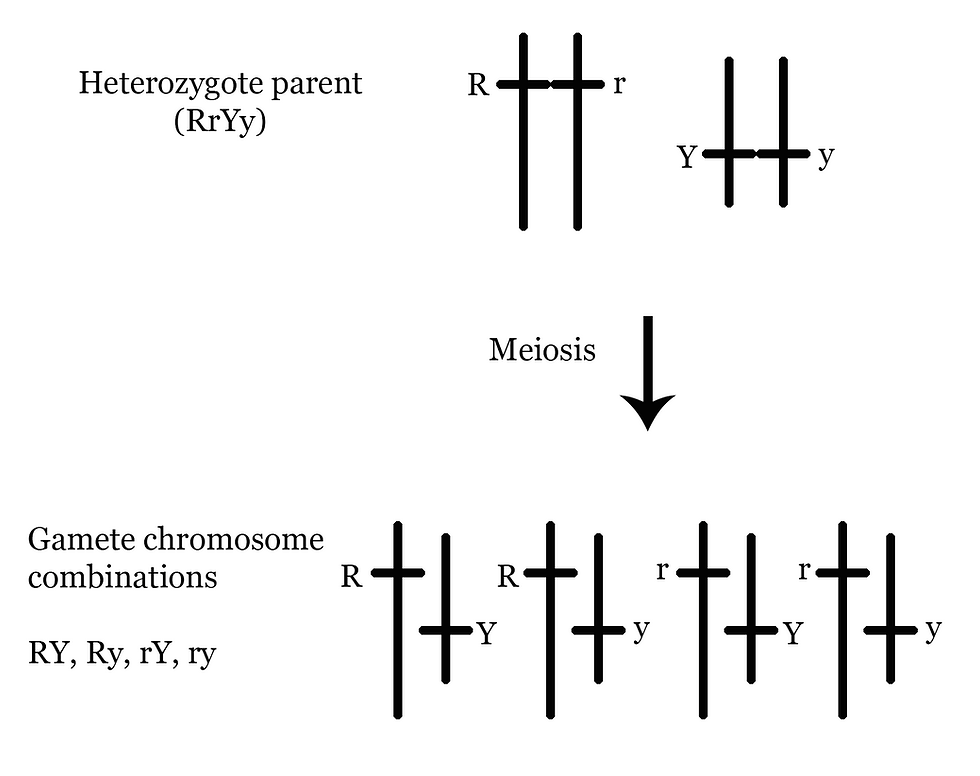
To find the expected outcomes for crossing two heterozygotes for both traits, you can make a sixteen-box Punnett square. Each parent has four different combinations that can be passed on that will be positioned along the top and side of the square. You can also skip the larger Punnett square and use math to find the combined probability. Multiply the chance of each trait occurring to get the probability that both traits appear together in the offspring.
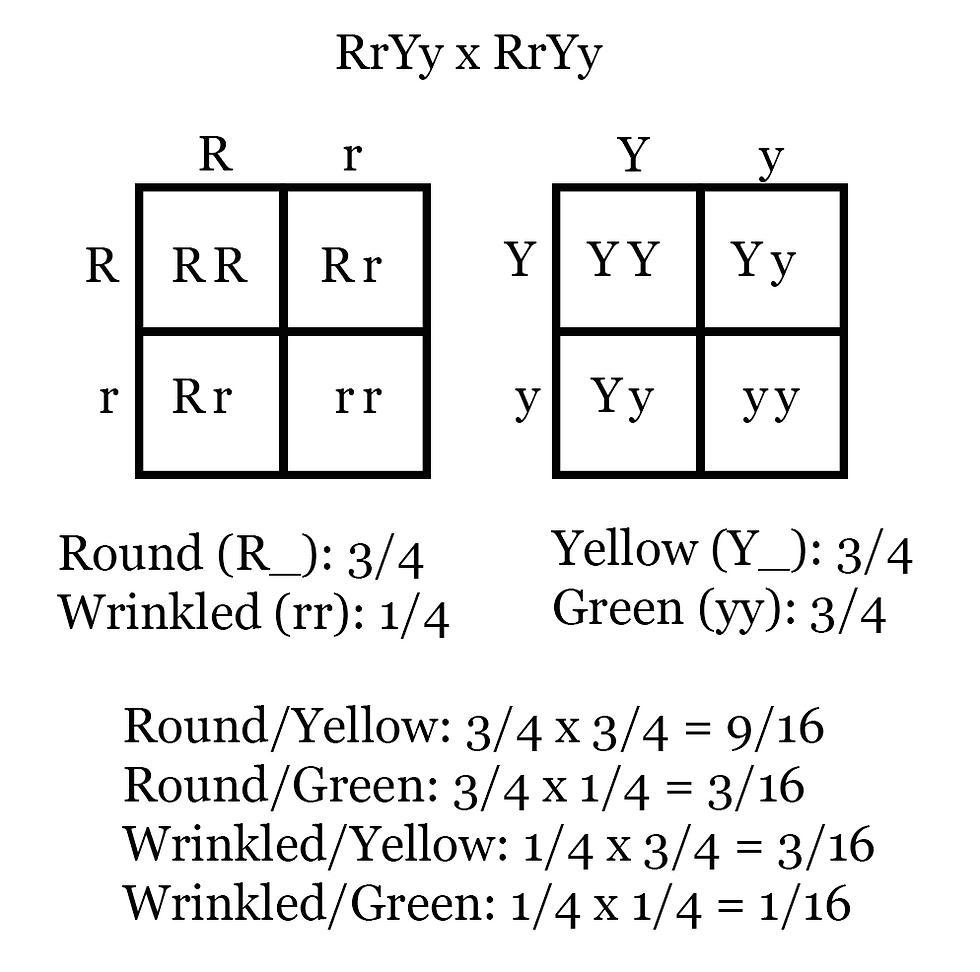
I prefer this method because it can be scaled up to three or more traits without becoming more difficult. For example, the chances of an individual having the dominant phenotype of three independently assorting traits is 27/64 (3/4 x 3/4 x 3/4). While you could make a 64 box Punnett square, the more boxes there are, the more chances of making a mistake.
Of course, all of this only works if the genes are unlinked. Alleles of genes close to each other on the same chromosome don't have an equal chance of being sorted into different gametes. Through crossing over, linked alleles may end up in different gametes, but at a lower rate than if they were on different chromosomes (or far apart on the same chromosome). The further apart linked genes are located on the chromosome, the more likely they are to be separated through crossing over. When this happens, the chromosome is called a recombinant. Offspring that show a phenotype combination that resulted from crossing over are also called recombinants.
Let's walk through an example of what will happen with linked genes when we cross a parent with both dominant traits to a parent with both recessive traits, using the fictional scenario in the Heredity IV simulation . In this case, the lack of pinna (external ear) and black eyes are dominant to the presence of pinna and white eyes. When our P cross is between black eye/no pinna and white eye/pinna parents (the genes are not sex-linked, so it doesn't matter if males or females have the dominant or recessive traits) the F1 generation results are 100% heterozygous black eye/no pinna. Because the P generation parents are homozygous this is the same result we get when doing a dihybrid cross for unlinked genes (see Heredity I simulation ).
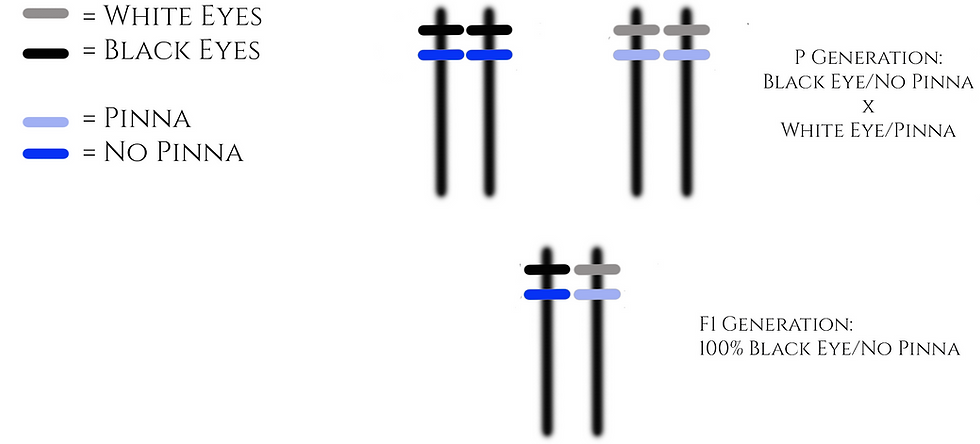
When we cross the F1 generation, though, the results will not be the 9:3:3:1 ratio that we expect from independently assorting genes.
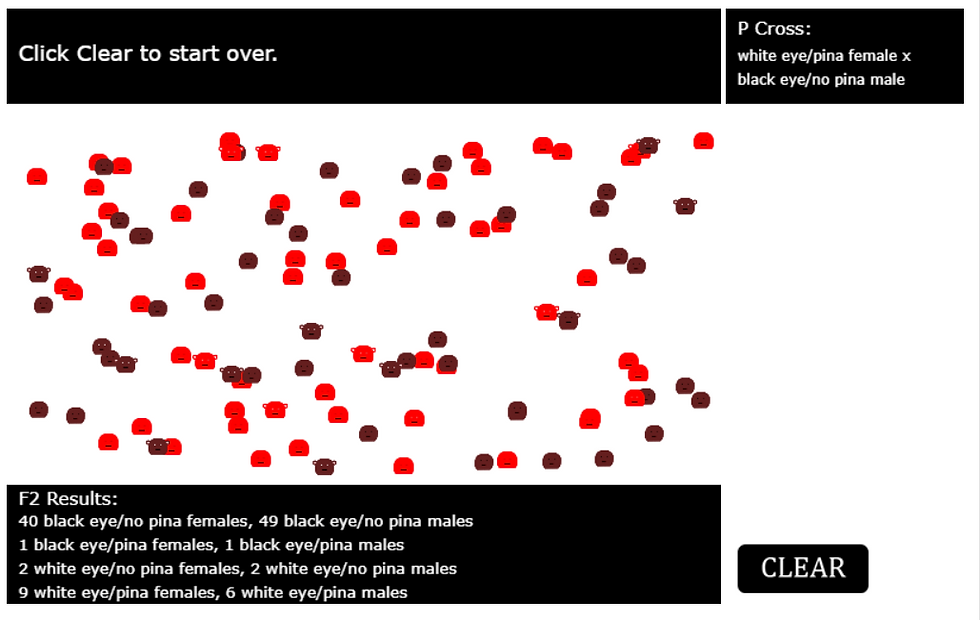
If no crossing over occurs during gamete formation in this F1 cross, 75% of the resulting offspring will have both dominant phenotypes and 25% will have both recessive phenotypes. When crossing over occurs, some gametes will have a different combination of alleles on the chromosome and may lead to offspring with one dominant and one recessive phenotype (black eye/pinna and white eyes/no pinna). These offspring are the recombinants. A lower than expected (3/16 + 3/16 = 3/8 or 37.5%) number of recombinant offspring indicates that genes are linked. In the sample data in the picture above, only 2% of the offspring are recombinants. However, in this case, it is difficult to estimate the rate of recombination because crossing over also results in offspring with the dominant phenotype (black eye/no pinna).
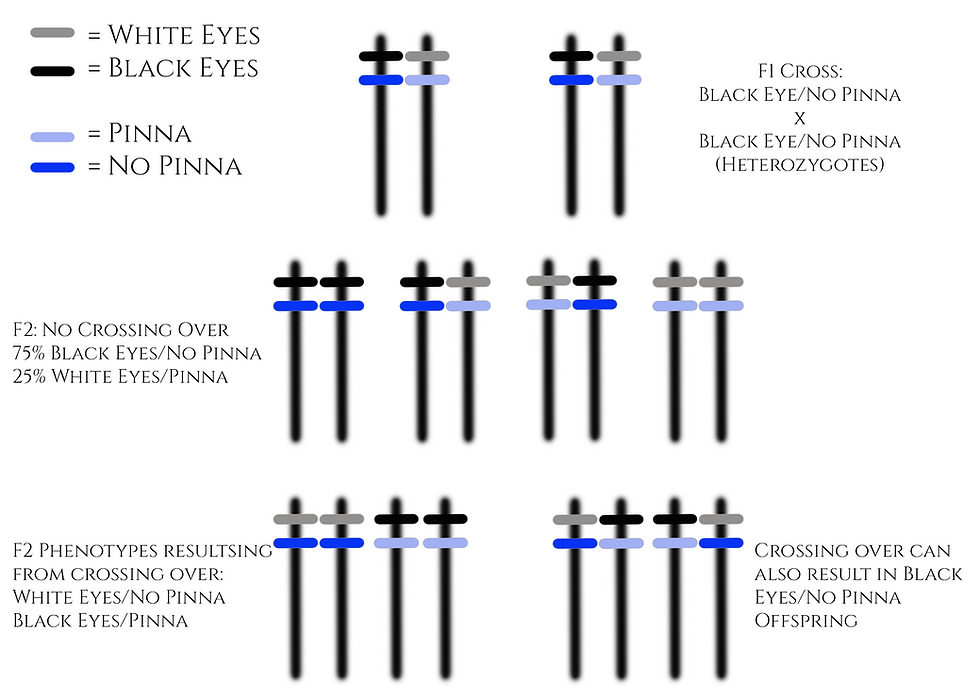
In an independently assorting dihybrid cross, a P generation in which one parent has the dominant version and the recessive version of the other and the other parent has the reverse, the F2 generation will have the same 9:3:3:1 expected results. When the two genes are linked, however, the results are different than what occurred when one parent was dominant for both traits and the other parent was recessive for both.
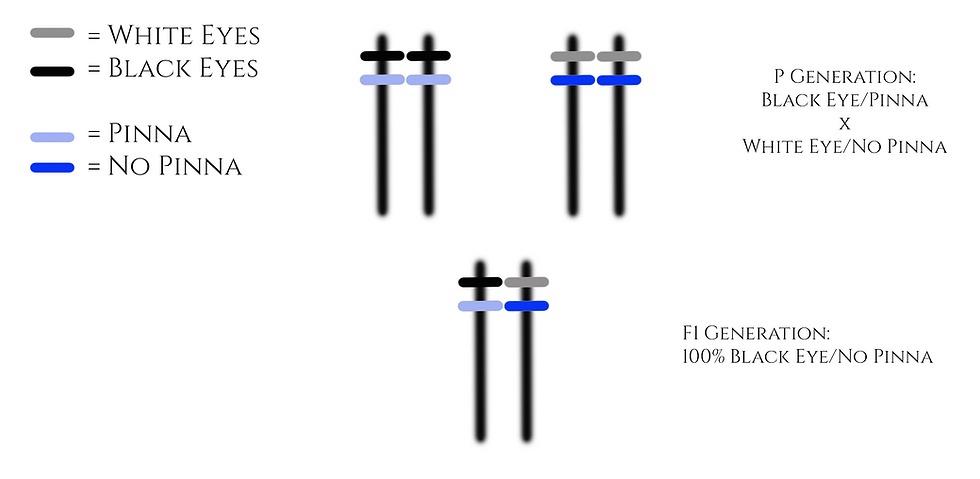
This example uses a P generation cross of black eye/pinna x white eye/no pinna. While the F1 is still 100% dominant phenotypes (black eye/no pinna), each chromosome is what would have been classified as recombinants in the last example.
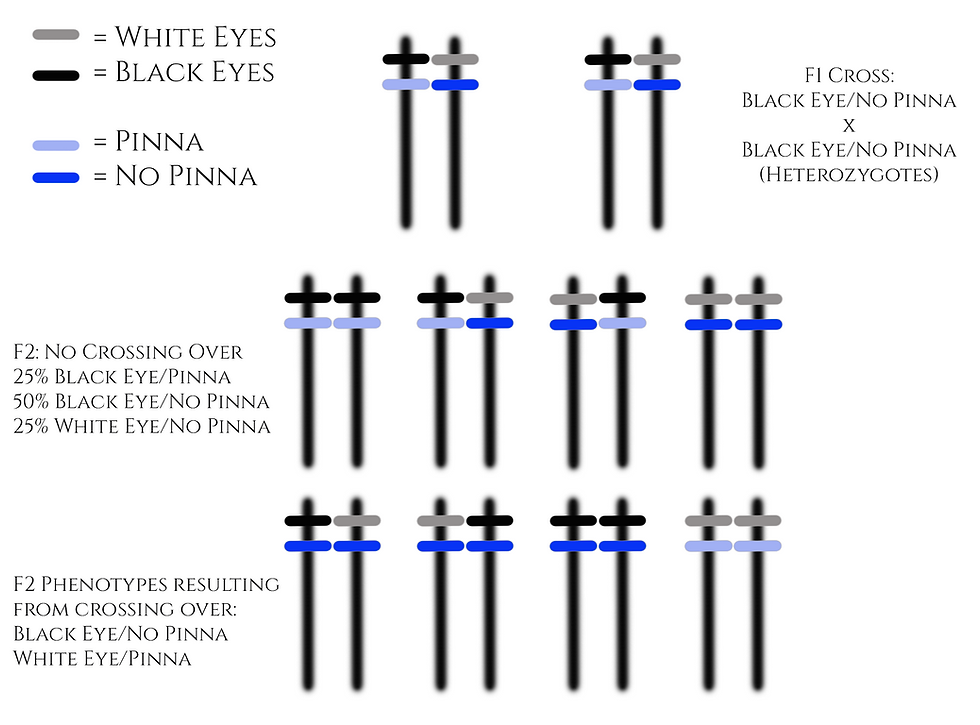
In this case, the rarest F2 phenotype will be both recessive traits (white eye/no pinna). In the sample data below, only 1% of the offspring have white eyes and the presence of pinna (both recessive traits). Because crossing over can lead to a phenotype that is also possible without crossing over (black eye/no pinna), this cross isn't ideal for estimating the rate of crossing over.
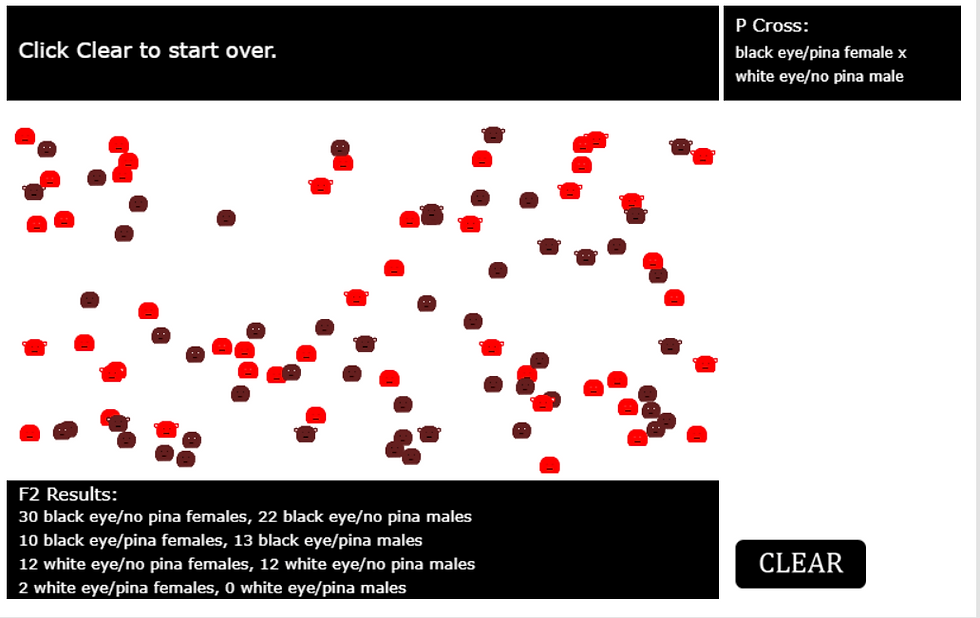
In order to estimate the recombination rate between the genes, we need a cross where there isn't overlap in the phenotypes resulting from crossing over and no crossing over. This can be done with a backcross. In a backcross, F1 heterozygotes are crossed to the parent with recessive phenotypes.

If we perform a backcross with unlinked genes, we should get a 1:1:1:1 ratio (see picture above, using pea seed traits). If the genes are linked there will be an over-representation of the parental phenotypes (dominant for both traits and recessive for both traits).
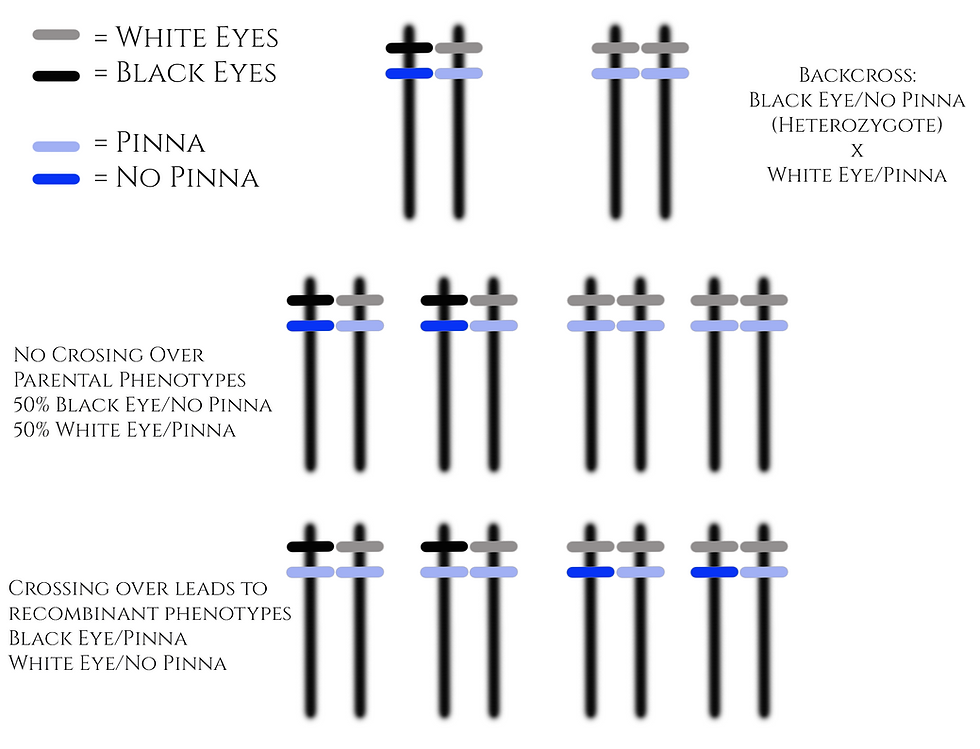
In this case, unlike the F1 cross examples discussed previously, a crossing over event between the two genes will lead to a recombinant phenotype (black eye/pinna and white eye/no pinna). For this reason, the backcross gives us a way of determining the rate of recombination, which is indicative of the distance between the genes.

The % recombination is equivalent to the map units/centiMorgans (cM) between the genes. This is a relative measure of distance on chromosomes and can be used to map the locations of genes. In the sample data in the picture above, 7.5% of the offspring are recombinants. This data suggests that the eye color and pinna genes are 7.5 cM apart. However, remember that it is best practice to base conclusions on an average of multiple trials.
If genes are located far enough apart on a chromosome, crossing over will occur often enough that the genes assort independently. At 50 cM the recombination rate is 50%, which means the expected 1:1:1:1 ratio for unlinked genes is observed.
For more information on the discovery of linked genes and the construction of the first fruit fly chromosome linkage maps, see this article from Nature Education.
- Instructional
Recent Posts
How to use chi-square to test an inheritance pattern
How to use chi-square to test for Hardy-Weinberg equilibrium
Join the email list for updates!
Thank you for using Biology Simulations! Please verify your email to confirm.
Law of Independent Assortment and Law of Segregation – A comprehensive Explanation
The world of genetics is a fascinating realm where the building blocks of life are unlocked, revealing the secrets of heredity and inheritance. Two fundamental principles, the Law of Segregation and the Law of Independent Assortment, play a crucial role in deciphering these mysteries. In this article, we will delve into these laws, demystifying the intricate processes governing the inheritance of traits from one generation to the next.
Table of Contents
The law of segregation: separation of alleles.
The Law of Segregation, proposed by Gregor Mendel in the mid-19th century, is one of the cornerstones of modern genetics. This law deals with the inheritance of single traits, such as eye color or seed shape in plants.
Key Points of the Law of Segregation:
- Alleles : Mendel’s law revolves around the concept of alleles, which are different versions of a gene. For instance, in humans, there are alleles for eye color, with brown and blue being common examples.
- Two Alleles : An organism inherits two alleles for each gene, one from each parent. These alleles can be either homozygous or heterozygous.
- Separation : The two alleles segregate or separate during the formation of gametes (sperm and egg cells). This means that only one allele from each gene passes into a gamete.
- Random Combination : When two gametes fuse during fertilization, they combine randomly, leading to diverse genetic outcomes in offspring.
The Law of Independent Assortment: Genes on Different Chromosomes
The Law of Independent Assortment, also formulated by Mendel, deals with the inheritance of traits located on different chromosomes. Chromosomes are structures in cells that contain genes.
Key Points of the Law of Independent Assortment:
- Multiple Traits : While the Law of Segregation deals with one trait at a time, the Law of Independent Assortment applies when considering two or more traits simultaneously. For instance, when looking at traits like hair color and height in humans.
- Different Chromosomes : Genes that govern different traits are located on separate chromosomes. Humans, for example, have 23 pairs of chromosomes, and genes for different traits are distributed among them.
- Random Arrangement : During the formation of gametes, chromosomes align randomly at the cell’s equator. This random arrangement ensures that maternal and paternal chromosomes for different traits assort independently.
- Variety in Offspring : Law of Independent assortment leads to a greater variety of genetic combinations in offspring, contributing to the diversity seen within species.
Mendel’s Pea Plant Experiments:
To illustrate these laws, Mendel conducted experiments with pea plants. One of his most famous experiments involved crossing pea plants with differing traits, such as flower color (purple or white) and seed shape (round or wrinkled).
In his experiments, Mendel observed that traits segregate independently. This means that the allele for purple flowers could be inherited with either the allele for round seeds or the allele for wrinkled seeds, creating various combinations of traits in the offspring.
Real-World Applications:
Understanding the Laws of Segregation and Independent Assortment has profound implications in various fields:
- Medicine : Knowledge of these laws helps geneticists predict the likelihood of certain traits or diseases in individuals based on their family history.
- Agriculture : Plant breeders use these principles to develop new varieties of crops with desired characteristics, such as disease resistance or higher yield.
- Evolution : These laws play a role in the creation of genetic diversity within species, which is essential for adaptation and evolution over time.
In conclusion, the Law of Segregation governs the inheritance of single traits, while the Law of Independent Assortment applies when considering multiple traits on different chromosomes. These laws, discovered by Gregor Mendel through his pioneering work with pea plants, remain fundamental in the field of genetics, providing insights into the inheritance of traits and the remarkable diversity of life on Earth.
Why Law of Independent Assortment Important?
The Law of Independent Assortment, proposed by Gregor Mendel, is essential in the field of genetics and biology for several critical reasons:
- Understanding Genetic Diversity : The law explains how different traits are inherited independently, leading to the creation of diverse genetic combinations in offspring. This genetic diversity is crucial for the adaptation and evolution of species over time. It allows for the introduction of new traits and the elimination of less favorable ones, contributing to a population’s fitness and survival.
- Plant and Animal Breeding : In agriculture and animal husbandry, the Law of Independent Assortment is applied to create new varieties or breeds with desirable characteristics. Breeders can selectively cross individuals with specific traits, knowing that the inheritance of one trait is not dependent on the inheritance of another. This principle has led to the development of crops with higher yields, disease resistance, and livestock with desired traits.
- Medical Genetics : In human genetics, understanding independent assortment is vital for predicting the likelihood of certain traits or diseases in individuals based on their family history. It allows genetic counselors and medical professionals to assess the inheritance of multiple genes simultaneously, which is essential for diagnosing genetic disorders, conducting pedigree analysis, and providing informed genetic counseling.
- Genetic Research : Independent assortment is a fundamental concept in genetic research. It underlies the principles of genetic mapping, linkage analysis, and genome-wide association studies. Researchers study how genes assort independently to identify genetic markers associated with specific diseases or traits.
- Evolutionary Biology : The law plays a significant role in evolutionary biology. It contributes to the generation of genetic diversity within populations, which is essential for natural selection and adaptation. Genetic diversity allows species to respond to changing environmental conditions and is a driving force behind evolutionary processes.
- Population Genetics : Population geneticists use the Law of Independent Assortment to study the genetic structure of populations. It helps analyze allele frequencies and genetic variation within and among populations, providing insights into evolutionary processes and population dynamics.
- Selective Breeding and Hybridization : The law is instrumental in creating hybrid plants and animals with desirable traits, such as drought resistance in crops or disease resistance in animals. This process has significant implications for food security and sustainable agriculture.
- Biotechnology : In biotechnology and genetic engineering, the principle of independent assortment is critical for the successful manipulation of genes. Scientists can introduce specific genes into organisms, knowing that these genes will segregate independently from the organism’s native genes.
In summary, the Law of Independent Assortment is essential because it elucidates the inheritance patterns of genes located on different chromosomes, leading to genetic diversity, informed breeding practices, medical genetics advancements, and a deeper understanding of evolutionary processes and population genetics. It has far-reaching applications across various scientific disciplines, agriculture, and medicine, making it a foundational concept in genetics and biology.
Why law of independent assortment is not universally applicable?
The Law of Independent Assortment, proposed by Gregor Mendel, describes the inheritance of different genes located on non-homologous chromosomes. According to this law, the alleles (gene variants) of one gene segregate independently from the alleles of another gene during gamete formation, leading to various genetic combinations in offspring. While this law is a fundamental concept in genetics, it is not universally applicable due to certain genetic and biological factors:
- Linked Genes : The law assumes that genes assort independently because they are located on different chromosomes or are far apart on the same chromosome. However, genes that are physically close to each other on the same chromosome tend to be inherited together, violating the principle of independent assortment. This phenomenon is called genetic linkage.
- Crossing Over : During meiosis (the process of gamete formation), homologous chromosomes exchange genetic material through a process called crossing over or recombination. This genetic exchange can break the linkage between alleles of genes on the same chromosome, promoting genetic diversity. However, it also means that genes on the same chromosome may not assort independently when crossing over occurs.
- Exceptions to Independent Assortment : Some genes do follow the Law of Independent Assortment because they are located on different chromosomes. However, many genes are not completely independent due to genetic linkage or other factors. For example, genes located on sex chromosomes (X and Y in humans) do not follow independent assortment because their inheritance depends on the sex of the offspring.
- Complex Traits : The Law of Independent Assortment simplifies genetic inheritance by considering one or a few genes at a time. In reality, many traits are controlled by multiple genes interacting with each other and influenced by environmental factors. This complexity can result in non-Mendelian patterns of inheritance, where genes do not assort independently.
- Epistasis : Epistasis is a genetic phenomenon where the expression of one gene masks or modifies the effects of another gene. In cases of epistasis, the alleles of genes interact, and their inheritance may not adhere to the principles of independent assortment.
- Environmental Influence : Environmental factors can also play a significant role in gene expression and trait development. Genes may interact with the environment in ways that affect their inheritance, making it challenging to predict genetic outcomes solely based on Mendelian genetics.
In summary, while the Law of Independent Assortment provides a foundational understanding of genetic inheritance, it is not universally applicable because genetic linkage, crossing over, exceptions to independent assortment, complex traits, epistasis, and environmental factors can all influence how genes are inherited and expressed. As a result, the inheritance of specific traits or genes may not always follow the principles outlined in Mendel’s law.
Why linked genes violate the law of independent assortment
Linked genes violate the Law of Independent Assortment because they are located on the same chromosome and tend to be inherited together, rather than assorting independently as Mendel’s law suggests. Here’s why linked genes do not follow independent assortment:
- Physical Proximity on Chromosomes : Linked genes are situated close to each other on the same chromosome. During meiosis, the process of gamete formation, homologous chromosomes align and segregate into different daughter cells. Because linked genes are physically close, they are less likely to be separated during this process.
- Crossing Over : While meiosis usually ensures the segregation of genes on different chromosomes (independent assortment), it can also lead to genetic recombination between homologous chromosomes. During crossing over, sections of homologous chromosomes are exchanged, breaking the linkage between alleles. However, when crossing over occurs between linked genes, it can result in new combinations of alleles on the same chromosome rather than the complete separation of alleles onto different chromosomes.
- Inheritance Patterns : When genes are linked, they tend to be inherited together in a manner that reflects their physical arrangement on the chromosome. This means that offspring are more likely to inherit a combination of alleles from one parent, rather than a random assortment of alleles from both parents.
To illustrate this, consider an example involving two genes, A and B, located on the same chromosome. If an individual carries the combination AB on one chromosome and ab on the other chromosome, due to physical linkage, they are more likely to pass on either AB or ab to their offspring, rather than assorting them independently. This can result in non-Mendelian inheritance patterns.
It’s important to note that while linked genes do not follow the Law of Independent Assortment, they can be used to map the relative positions of genes on a chromosome through genetic linkage analysis. By studying how frequently genes are inherited together, scientists can determine the physical distance between genes on a chromosome, providing valuable insights into genetics and gene mapping.
Difference between linkage and Law of independent assortment
Linkage and independent assortment are two contrasting principles that govern the inheritance of genes in organisms. Here are the key differences between linkage and independent assortment:
- Linkage : Linkage refers to the tendency of genes located on the same chromosome to be inherited together because they are physically close to each other.
- Independent Assortment : Independent assortment is a principle that describes how genes located on different chromosomes assort into gametes independently of each other, leading to various combinations of alleles.
- Linkage : Linked genes are those found on the same chromosome.
- Law of Independent Assortment : Law of Independent assortment pertains to genes located on different chromosomes.
- Linkage : Genes that are closely linked tend to stay together during meiosis, as they are less likely to undergo crossing over and separate into different gametes.
- Independent Assortment : Genes on different chromosomes assort independently during meiosis. They segregate into gametes without regard to the alleles at other gene loci.
- Linkage : Linked genes are usually inherited together, leading to non-Mendelian inheritance patterns where certain allele combinations are more common in offspring.
- Independent Assortment : Genes that assort independently produce a wide variety of allele combinations in offspring, adhering to Mendel’s principles of inheritance.
- Linkage : Linkage reduces the likelihood of crossing over between genes because they are physically close. When crossing over does occur, it can result in recombinant alleles, but linked genes are less likely to recombine.
- Independent Assortment : Crossing over predominantly occurs between non-sister chromatids of homologous chromosomes during meiosis, promoting genetic recombination and the creation of new allele combinations.
- Linkage : An example of linked genes is the ABO blood group system, where the A and B alleles are often inherited together because they are on the same chromosome.
- Independent Assortment : An example of independent assortment is the inheritance of genes for eye color (on one chromosome) and genes for hair color (on another chromosome) in humans.
- Linkage : Linked genes can be used for genetic mapping, as the frequency of recombination (crossing over) between them can indicate the distance between gene loci on a chromosome.
- Independent Assortment : Independent assortment is used to explain the variety of traits observed in offspring when considering genes located on different chromosomes.
In summary, linkage involves genes on the same chromosome that tend to be inherited together, while independent assortment involves genes on different chromosomes that assort into gametes independently, creating diverse allele combinations in offspring. These principles play a fundamental role in understanding inheritance patterns and genetic diversity.
Biotech Explorer
Why to buy yesmovies premium membership, how to get soap2day on roku tv - a comprehensive explanation | latest 2024, related articles.
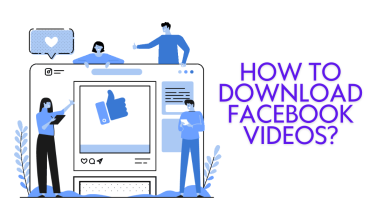
How to Download Videos from Facebook to iphone – Latest 2024
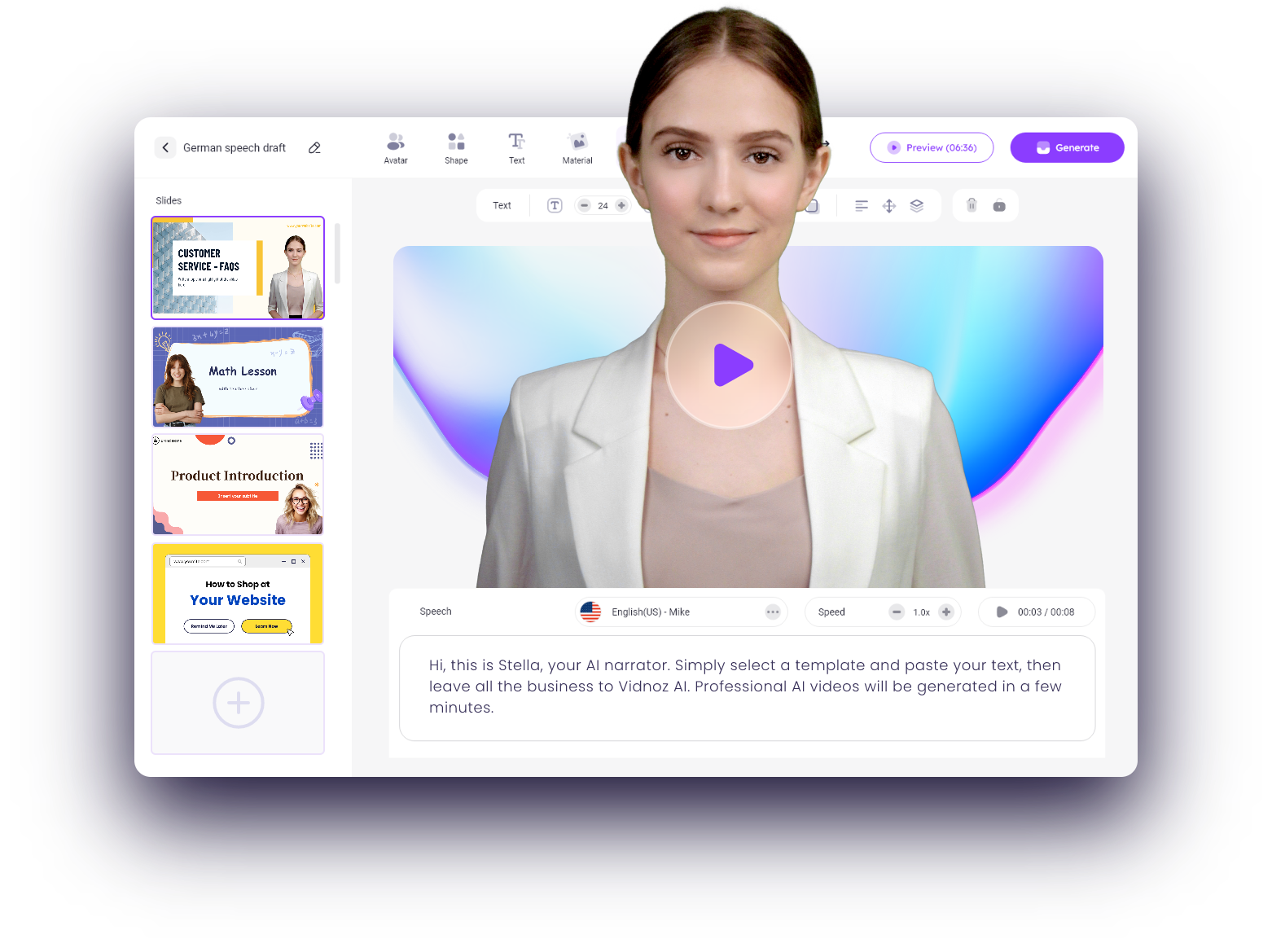
Ai video Editor & Free AI Video Generator – Latest 2024
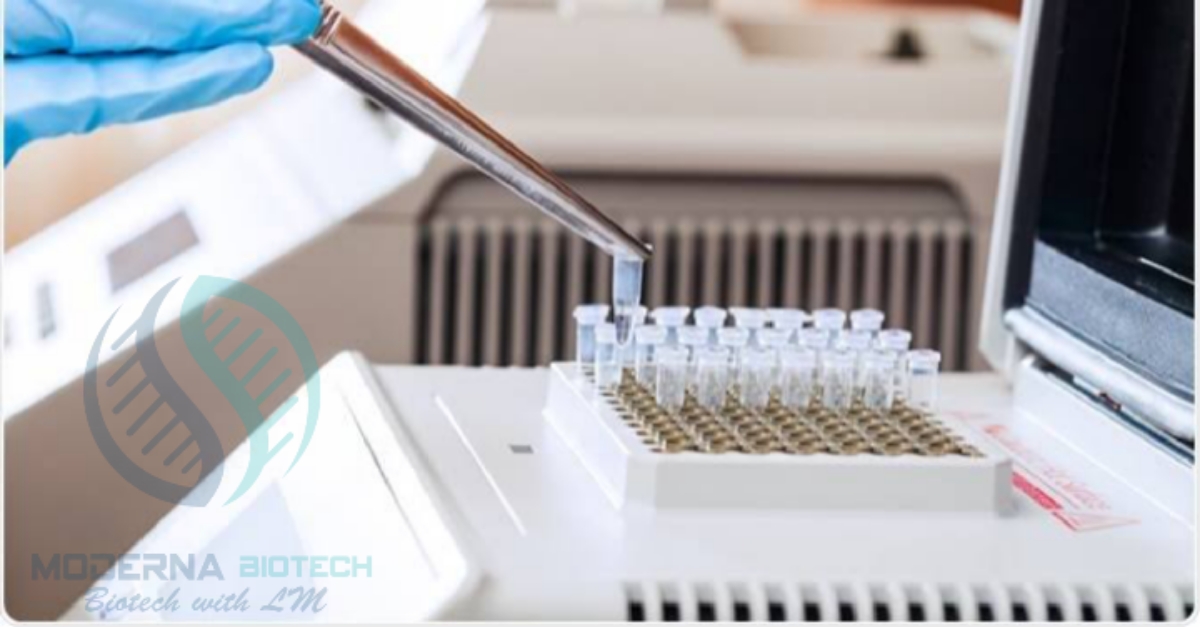
How does PCR work? | Polymerase chain reaction
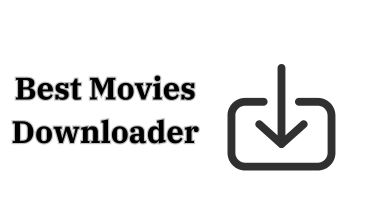
Soap2day Video Downloader – How to Download Movies from Soap2day
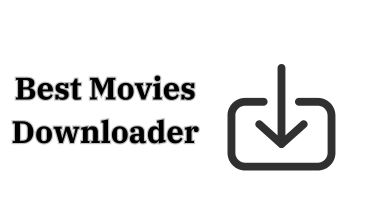
This page has been archived and is no longer updated
A classic Mendelian example of independent assortment: the 9:3:3:1 phenotypic ratio associated with a dihybrid cross (BbEe × BbEe).
Rate & certify.
- Flag Inappropriate
Delete Content

This image is linked to the following Scitable pages:

Some Genes Are Transmitted to Offspring in Groups via the Phenomenon of Gene Linkage
- Add Content to Group


- Gene Inheritance and Transmission
- Gene Expression and Regulation
- Nucleic Acid Structure and Function
- Chromosomes and Cytogenetics
- Evolutionary Genetics
- Population and Quantitative Genetics
- Genes and Disease
- Genetics and Society
- Cell Origins and Metabolism
- Proteins and Gene Expression
- Subcellular Compartments
- Cell Communication
- Cell Cycle and Cell Division
© 2014 Nature Education
- Press Room |
- Terms of Use |
- Privacy Notice |

Visual Browse
independent assortment Recently Published Documents
Total documents.
- Latest Documents
- Most Cited Documents
- Contributed Authors
- Related Sources
- Related Keywords
A rigorous measure of genome-wide genetic shuffling that takes into account crossover positions and Mendel’s second law
Comparative studies in evolutionary genetics rely critically on evaluation of the total amount of genetic shuffling that occurs during gamete production. Such studies have been hampered by the absence of a direct measure of this quantity. Existing measures consider crossing-over by simply counting the average number of crossovers per meiosis. This is qualitatively inadequate, because the positions of crossovers along a chromosome are also critical: a crossover toward the middle of a chromosome causes more shuffling than a crossover toward the tip. Moreover, traditional measures fail to consider shuffling from independent assortment of homologous chromosomes (Mendel’s second law). Here, we present a rigorous measure of genome-wide shuffling that does not suffer from these limitations. We define the parameter r¯ as the probability that the alleles at two randomly chosen loci are shuffled during gamete production. This measure can be decomposed into separate contributions from crossover number and position and from independent assortment. Intrinsic implications of this metric include the fact that r¯ is larger when crossovers are more evenly spaced, which suggests a selective advantage of crossover interference. Utilization of r¯ is enabled by powerful emergent methods for determining crossover positions either cytologically or by DNA sequencing. Application of our analysis to such data from human male and female reveals that (i) r¯ in humans is close to its maximum possible value of 1/2 and that (ii) this high level of shuffling is due almost entirely to independent assortment, the contribution of which is ∼30 times greater than that of crossovers.
Modeling Genetic Complexity in the Classroom
This classroom exercise aims to help students understand the three Ps of genetic complexity: polymorphic, polygenic, and pleiotropic. Using coin flips and dice rolls, students are able to generate the genotype and phenotype of a random individual. From there, students find a mate for this individual and determine the phenotype of their offspring. The randomness generated by the coin and dice mechanics illustrates the principles of independent assortment and segregation, variable gene expression, and environmental effects.
AbstractComparative studies in evolutionary genetics rely critically on evaluation of the total amount of genetic shuffling that occurs during gamete production. However, such studies have been ham-pered by the fact that there has been no direct measure of this quantity. Existing measures consider crossing over by simply counting the average number of crossovers per meiosis. This is qualitatively inadequate because the positions of crossovers along a chromosome are also critical: a crossover towards the middle of a chromosome causes more shuffling than a crossover towards the tip. More-over, traditional measures fail to consider shuffling from independent assortment of homologous chromosomes (Mendel’s second law). Here, we present a rigorous measure of genome-wide shuffling that does not suffer from these limitations. We define the parameter r̅ as the probability that the alleles at two randomly chosen loci will be shuffled in the production of a gamete. This measure can be decomposed into separate contributions from crossover number and position and from independent assortment. Intrinsic implications of this metric include the fact that r̅ is larger when crossovers are more evenly spaced, which suggests a novel selective advantage of crossover interference. Utilization of r̅ is enabled by powerful emergent methods for determining crossover positions, either cytologically or by DNA sequencing. Application of our analysis to such data from human male and female reveals that: (i) r̅ in humans is close to its maximum possible value of 1/2, (ii) this high level of shuffling is due almost entirely to independent assortment, whose contribution is ~30 times greater than that of crossovers.
Target-Site Resistances to ALS and PPO Inhibitors Are Linked in Waterhemp (Amaranthus tuberculatus)
It is generally expected that, in the case of multiple herbicide resistance, different resistance mechanisms within a weed will follow Mendel’s law of independent assortment. Research was conducted to investigate anecdotal observations suggesting that target site–based resistances to inhibitors of acetolactate synthase (ALS) and protoporphyrinogen oxidase (PPO) did not follow independent assortment in common waterhemp. Cosegregation of the two resistances was observed in backcross lines (population sensitive to both herbicides as recurrent parent). Specifically, whereas 52% of backcross plants were resistant to a PPO inhibitor, this percentage increased to 92% when the backcross plants were preselected for resistance to an ALS inhibitor. Molecular marker analysis confirmed that the corresponding genes (ALSandPPX2) were genetically linked. When data from all plants analyzed were pooled, the genetic distance between the two genes was calculated to be 7.5 cM. The two genes were found to be about 195 kb apart in the recently published grain amaranth genome, explaining the observed genetic linkage. There is likely enough recombination that occurs between the linked genes to prevent the linkage from having significant implications in terms of resistance evolution. Nevertheless, documentation of the happenstance linkage between target-site genes for resistance to ALS and PPO inhibitors in waterhemp is a reminder that one should not assume distinct resistance mechanism will independently assort.
On the independent loci assumption in phylogenomic studies
AbstractStudies using multi-locus coalescent methods to infer species trees or historical demographic parameters usually require the assumption that the gene tree for each locus (or SNP) is genealogically independent from the gene trees of other sampled loci. In practice, however, researchers have used two different criteria to delimit independent loci in phylogenomic studies. The first criterion, which directly addresses the condition of genealogical independence of sampled loci, considers the long-term effects of homologous recombination and effective population size on linkage between two loci. In contrast, the second criterion, which only considers the single-generation effects of recombination in the meioses of individuals, identifies sampled loci as being independent of each other if they undergo Mendelian independent assortment. Methods that use these criteria to estimate the number of independent loci per genome as well as intra-chromosomal “distance thresholds” that can be used to delimit independent loci in phylogenomic datasets are reviewed. To compare the efficacy of each criterion, they are applied to two species (an invertebrate and vertebrate) for which relevant genetic and genomic data are available. Although the independent assortment criterion is relatively easy to apply, the results of this study show that it is overly conservative and therefore its use would unfairly restrict the sizes of phylogenomic datasets. It is therefore recommended that researchers only refer to genealogically independent loci when discussing the independent loci assumption in phylogenomics and avoid using terms that may conflate this assumption with independent assortment. Moreover, whenever feasible, researchers should use methods for delimiting putatively independent loci that take into account both homologous recombination and effective population size (i.e., long-term effective recombination).
A powerful test of independent assortment that determines genome-wide significance quickly and accurately
Independent assortment, lack of host specificity leads to independent assortment of dipterocarps and ectomycorrhizal fungi across a soil fertility gradient, independent assortment of seed color and hairy leaf genes in brassica rapa l..
Rahman, M. 2014. Independent assortment of seed color and hairy leaf genes in Brassica rapa L. Can. J. Plant Sci. 94: 615–620. A genetic study of seed color and hairy leaf in Brassica rapa was conducted in progeny originating from the brown-seeded, hairy leaf B. rapa subsp. chinensis line and the Bangladeshi B. rapa var. trilocularis line. A joint segregation of both traits was also examined in the F2 and backcross populations. Seed color segregated into brown, yellow–brown, and yellow, which suggests that digenic control of brown or yellow–brown color was dominant over yellow seed color. Hairy leaves were found to be under monogenic control, and hairy leaf was dominant over non-hairy leaf. The data show that genes controlling seed color and hairy leaf are inherited independently.
Cootie Genetics
“Cootie Genetics” is a hands-on, inquiry-based activity that enables students to learn the Mendelian laws of inheritance and gain an understanding of genetics principles and terminology. The activity begins with two true-breeding Cooties of the same species that exhibit five observable trait differences. Students observe the retention or loss of traits among first-generation heterozygotes, hypothesize what happened to these traits, and design an experiment to test their hypotheses by mating the first-generation Cooties. With the second generation, Mendel’s principles of segregation and independent assortment of alleles are observed; dominant and recessive traits and tools students need to construct Punnett squares are apparent.
Export Citation Format
Share document.

Snapsolve any problem by taking a picture. Try it in the Numerade app?
UK Edition Change
- UK Politics
- News Videos
- Paris 2024 Olympics
- Rugby Union
- Sport Videos
- John Rentoul
- Mary Dejevsky
- Andrew Grice
- Sean O’Grady
- Photography
- Theatre & Dance
- Culture Videos
- Fitness & Wellbeing
- Food & Drink
- Health & Families
- Royal Family
- Electric Vehicles
- Car Insurance Deals
- Lifestyle Videos
- UK Hotel Reviews
- News & Advice
- Simon Calder
- Australia & New Zealand
- South America
- C. America & Caribbean
- Middle East
- Politics Explained
- News Analysis
- Today’s Edition
- Home & Garden
- Broadband deals
- Fashion & Beauty
- Travel & Outdoors
- Sports & Fitness
- Sustainable Living
- Climate Videos
- Solar Panels
- Behind The Headlines
- On The Ground
- Decomplicated
- You Ask The Questions
- Binge Watch
- Travel Smart
- Watch on your TV
- Crosswords & Puzzles
- Most Commented
- Newsletters
- Ask Me Anything
- Virtual Events
- Betting Sites
- Online Casinos
- Wine Offers
Thank you for registering
Please refresh the page or navigate to another page on the site to be automatically logged in Please refresh your browser to be logged in
Pupils warned of punishment if they search for exam papers on social media
Accounts claiming to sell exam papers are ‘almost always scams’, the regulator warned, article bookmarked.
Find your bookmarks in your Independent Premium section, under my profile
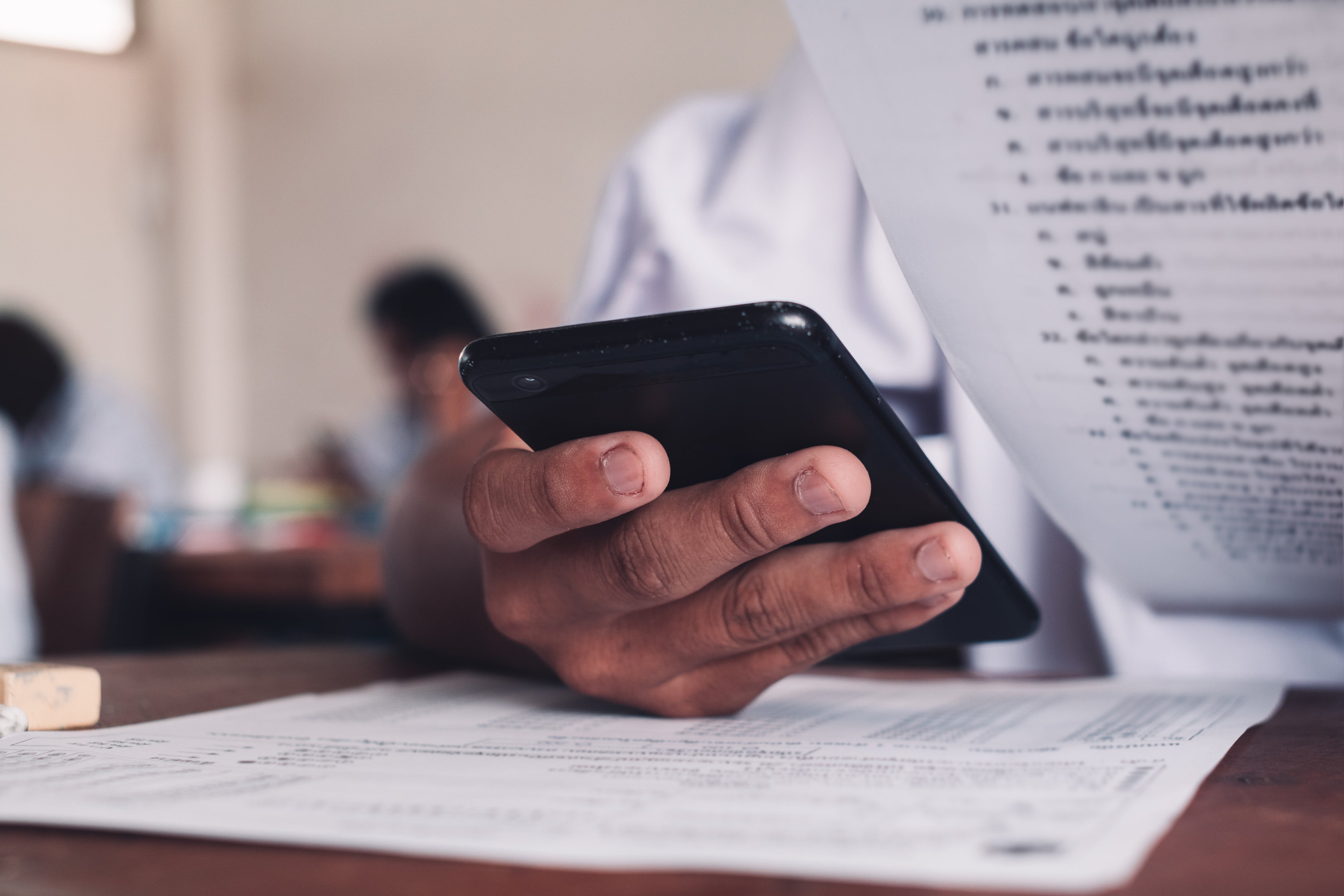
Sign up for a full digest of all the best opinions of the week in our Voices Dispatches email
Sign up to our free weekly voices newsletter, thanks for signing up to the voices dispatches email.
Pupils have been told they risk being disqualified if they look for exam papers on social media .
Teenagers who look for the exams or take their phones into an exam hall face the punishment, England’s exams regulator warned.
There were 2,180 cases with penalties for this type of offence in 2023, compared with 1,825 in 2022, according to figures from the exams regulator.
Students should report accounts claiming to sell this year’s exam papers – which are “almost always scams” – to teachers, the chief regulator has said.
Ahead of exam season, Ofqual has urged students to plan somewhere safe to leave their mobile device during exams after instances of students being found with mobile devices in exams have almost doubled since 2018.
Research for Ofqual last year suggested that most students do not intend to use their phones to cheat, but they do not want to be separated from them as they are worried about loss or damage.
Sir Ian Bauckham, chief regulator at Ofqual, said: “Students should also be aware of the risks of exam papers on social media.
“Accounts claiming to sell this year’s exam papers are almost always scams. Students should report these accounts to teachers.
“Students risk losing the qualification they’ve been studying for if they search for or communicate with social media accounts claiming to sell leaked exam papers.
“Sanctions can still apply even if the papers turn out to be fake. Buying papers is never worth the risk.”
Sir Ian added: “Students have been working hard to prepare for their exams, and nobody wants them to miss out on their grades and qualifications.
“Thankfully, most students are aware of the risks of malpractice and comply with the rules.
“It’s important that the rules are followed so that grades reflect what a student knows, understands and can do.”
Ofqual data, released in December last year, showed that there were 4,895 cases of malpractice during GCSEs, AS and A-level examinations which included students in 2023, up from 4,105 in 2022.
A spokesperson for the Joint Council for Qualifications (JCQ), which represents the UK’s major exam boards, said: “It is important students know to report fraudulent accounts claiming to sell exam papers on social media to their teachers.
“JCQ wishes all students well with their exams and assessments.”
Pepe Di’Iasio, general secretary of the Association of School and College Leaders (ASCL), said: “We are sure that the vast majority of students taking exams will stick to the rules, but there are always some who do not do so, and unfortunately the misuse of digital technology is a real headache.
“ Schools and colleges rigorously police exam rooms to ensure that devices are not brought in by candidates and they warn students not to try to find exam papers on social media.
“These are generally fake papers being circulated as a scam, but in the rare event of a genuine paper being leaked, any student accessing that paper risks disqualification.
“It is really important that students take heed of these warnings.”
Subscribe to Independent Premium to bookmark this article
Want to bookmark your favourite articles and stories to read or reference later? Start your Independent Premium subscription today.
New to The Independent?
Or if you would prefer:
Want an ad-free experience?
Hi {{indy.fullName}}
- My Independent Premium
- Account details
- Help centre
Support 110 years of independent journalism.
- The Weekend Essay
The rise of WhatsApp government
How the text message is transforming our democracies.
By Jonathan White
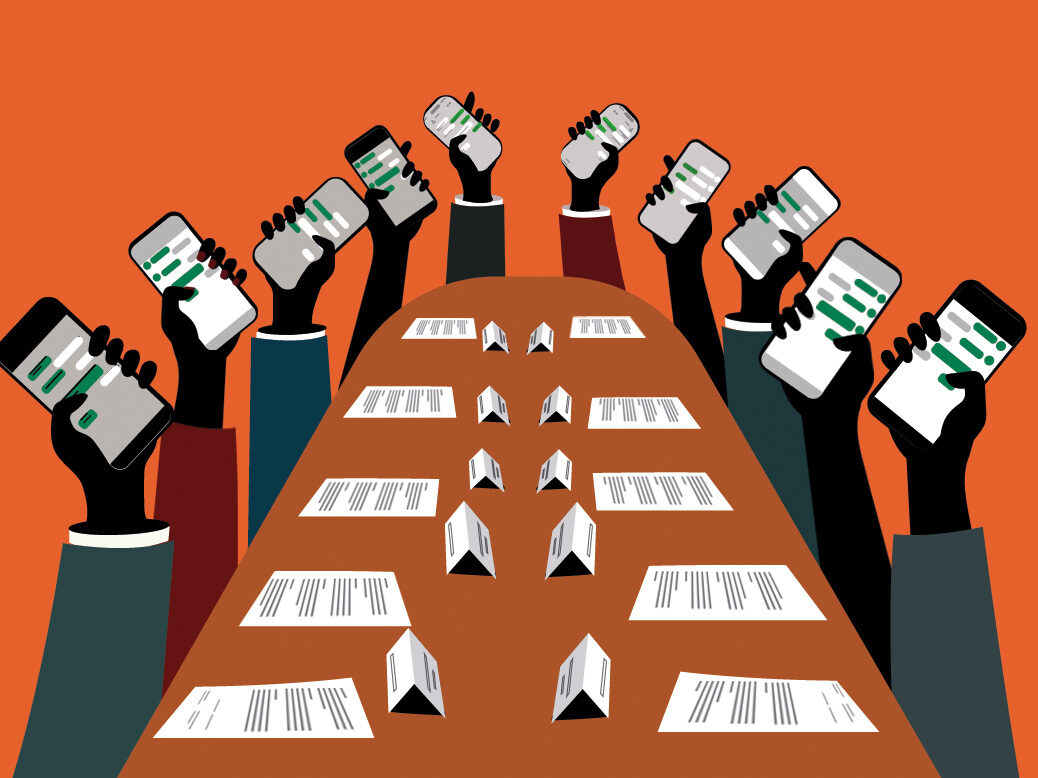
The exercise of public authority has always been shaped by the technologies available. From the printing press to the telegraph, radio to email, new inventions have left their mark on how decisions are taken and by whom. This pattern continues in the age of the smartphone, apparently indispensable to the professional lives of today’s officials and politicians. “You need one these days,” the then prime minister Boris Johnson noted during the Covid-19 pandemic, “I need to be in touch with people.” His style of communications would emerge when Dominic Cummings leaked his messages, including one describing the health secretary during the pandemic Matt Hancock as “totally f***ing hopeless”. Subsequent revelations from the period leave the distinct impression that the country is run by WhatsApp.
Britain is hardly alone in this. Concerns about text-message governance reach far and wide. In Brussels, the shadow of “Pfizergate” hangs over the European Commission president Ursula von der Leyen ahead of the European Parliament elections in June. The scandal dates to a New York Times piece in April 2021 which claimed the EU’s Covid vaccine deal with Pfizer had been negotiated by a series of messages and calls between her and the company’s chief executive. “That personal diplomacy played a big role in a deal,” said the newspaper. This suggestion of one-to-one negotiation on a high-profile matter prompted calls, notably from the European Ombudsman, for the messages to be made public. The Commission’s failure to do this led the ombudsman to conclude that this was a case of maladministration , and it is still facing lawsuits .
Critical discussion of government-by-text has tended to focus on access. Officials, it seems, do important business in a way the public can barely oversee. These concerns about transparency have been well made. That instant messaging services tend to be in the hands of large private companies is clearly another part of the problem. And there are the security issues that have been raised ever since President Barack Obama brought his Blackberry to the White House. But also at stake is how key decisions are taken in the moment. As discussions move from the meeting room to the virtual space of the chat group, they move into a world of heightened informality and fuzzy ethical boundaries.
Consider some features of the technology. Unlike a physical meeting, this is a form of interaction with no set beginning or end. Conversations begin at the initiative of one party, and depend on quick responses. A case in Spain at the height of the pandemic showed the risks. Madrid’s mayor José Luis Martínez-Almeida is said to have got agreement for medical supplies in a brief WhatsApp exchange around 1am on 24 March 2020. One councillor was excluded because he was not checking his phone, and others complained they were rushed into the agreement. Awkward details about the deliberation soon emerged: the deal involved a relative of the mayor, the supplies were overpriced and exorbitant commissions were charged, leading the contract to be denounced as a “scam” at the city’s expense. Almeida admitted it was a mistake but defended councillors’ actions at a time when it was so difficult to obtain scarce resources. An anti-corruption case against the suppliers was later investigated by a court.
The spontaneous nature of instant messaging means those involved are often being extracted from another activity or caught at an informal moment. This is a medium based on distraction and immediacy. Of course, much depends on how the technology is used. Not everyone is texting in their pyjamas or watching TV at the same time – but little ensures they are not.
The Saturday Read
Morning call.
- Administration / Office
- Arts and Culture
- Board Member
- Business / Corporate Services
- Client / Customer Services
- Communications
- Construction, Works, Engineering
- Education, Curriculum and Teaching
- Environment, Conservation and NRM
- Facility / Grounds Management and Maintenance
- Finance Management
- Health - Medical and Nursing Management
- HR, Training and Organisational Development
- Information and Communications Technology
- Information Services, Statistics, Records, Archives
- Infrastructure Management - Transport, Utilities
- Legal Officers and Practitioners
- Librarians and Library Management
- OH&S, Risk Management
- Operations Management
- Planning, Policy, Strategy
- Printing, Design, Publishing, Web
- Projects, Programs and Advisors
- Property, Assets and Fleet Management
- Public Relations and Media
- Purchasing and Procurement
- Quality Management
- Science and Technical Research and Development
- Security and Law Enforcement
- Service Delivery
- Sport and Recreation
- Travel, Accommodation, Tourism
- Wellbeing, Community / Social Services
There are questions of power at stake. Instant messaging allows leaders to detach themselves from the supporting officials and civil servants who might regulate their actions. Awkward individuals can be left out, and trusted advisers or favoured reporters brought in. Corporate interests can make themselves felt. This is a technology well-suited to bypassing hierarchies and creating shadow networks of influence, in a way that may not always be noticed. Those who might be stopped at the door in a physical setting can be “in the room” in a virtual one, while absences that would be notable in-person may be more easily overlooked.
The standard response of officials is to say that nothing of importance is decided this way. Replying to the European Ombudsman in summer 2022, the European Commission stated: “Due to their short-lived and ephemeral nature, text and instant messages in general do not contain important information relating to policies, activities and decisions of the Commission.” One can imagine a spectrum of uses to which messaging is put: if the making of decisions forms one pole, the other could be characterised as “harmless chitchat”, with a range of practices in the grey area in between (information sharing, deliberation, opinion formation, cultivation of contacts, private criticism of colleagues). But we have seen enough to know that not everything falls at the more innocuous end: that major decisions can be prepared and taken this way.
What such technologies encourage is the blurring of boundaries – between the formal and the informal, between different institutions, and between the business of government and the world beyond. Politics has always depended on rituals to reinforce the separation of offices and persons. Whether it is the crown of a monarch or the layout of a parliament, habits of dress and arrangements of space are intended to lend gravitas to a situation, shaping the self-understanding of participants as representatives of a wider interest – a social group, an institution, the public good. While some rituals can be replicated in mediatised communication, many cannot. Those who engage each other in this setting are deprived of the contextual cues intended elsewhere to depersonalise their encounter. Chumminess rules.
Government by instant messaging is emblematic of something wider – of a world in which key political decisions are taken informally, and power concentrated in the hands of key individuals and the networks they form. We tend to think of politics in terms of dry institutions and bureaucratic logic, but this picture can be quite misleading. From domestic to foreign policy, there is a wider story of the “ de-institutionalisation ” of power, as officials act with personal discretion and ties of trust override the formal definition of roles. This is what the making of a ruling elite looks like. Lockdowns may have given this trend a distinctive impetus, but the patterns are likely to endure. Whether it is policy on immigration, inflation or Israel , a key question will be which WhatsApp group leads it.
How much of this is really new? There have long been concerns in politics that the important conversations are had in the hallways, where no public record is taken. One can assume that instant messaging appeals in part because it allows those in authority to do what they are already inclined to do. So how much significance should we attach to the technology? Is it just the latest way of doing what has long been done and could be done without it?
One way it leaves a distinctive mark is by making these patterns of behaviour more traceable . Messages can be deleted, giving a sense of control in the moment, but no one can be certain that they have not meanwhile been copied or shared by their interlocutor(s). There is, as we have seen, ample potential for leaks – this way of operating can go badly wrong.
This makes the embrace of the technology more puzzling than it might seem. To be sure, it is attractively convenient. It may be socially and psychologically rewarding too, in so far as to be part of an “inner circle” is to have access and prestige, the chance to feel superior to those on the outside. Yet the traceability of these communications means they come with evident risks. For when they come to public attention – when newspapers can run stories on an official’s “personal diplomacy” – they cause discontent and reputational damage. These are foreseeable frictions. Von der Leyen had been caught in an earlier scandal in Germany to do with the transparency of her mobile-phone use while defence minister in Berlin. Though she was cleared of responsibility in that prior instance, she can hardly have been unaware of the pitfalls of messaging.
If the technology is embraced despite the risks, arguably this highlights something about the priorities of those in power. Informal methods appeal because those involved are far more interested in getting tangible “results” than living by the democratic rules. They feel it is enough to get things done, and are broadly contemptuous of the constraints on how. Scholars of politics distinguish between the legitimacy of good results and that of good methods – between output and procedural legitimacy. The prevalence of instant messaging reflects the dominance of the first over the second.
Output legitimacy has always been central to transnational institutions. It corresponds to the centrality of technocracy in settings such as the EU, where an instrumentalist, problem-solving outlook is central to public authority. Over time, however, it has also become central to authority in the national context. The weakening of parties, and of organised ideological politics in general, has meant that representatives define themselves less by normative commitments than by their capacity to act decisively. Aware that significant portions of their electorate are drawn to technocratic, populist or “ techno-populist ” styles of politics that seek to pursue the public good in an unmediated way, they too can become impatient with procedure and keen to demonstrate their problem-solving capacity. In the long run, that claim to legitimacy depends on getting good results, but in the first instance it simply depends on results – decisions that can be cited as signs of activity.
In this way instant messaging provides insight into the outlook of policymakers. For the very reason that it heightens the risks involved, it lays bare the extent of their attachment to irregular ways of governing in the service of getting things done.
This has implications for what it might mean to constrain the practice. Reformers tend to call for better regulation of the technology. The European Ombudsman has made various recommendations : that instant messages be recognised as EU documents, kept as records, and be available for inspection when requests for public access are made. Stronger rules on the use of communications technology exist in fields such as financial regulation, suggesting they can be drawn up where there is the political will.
But such moves neglect how the technology ties in with the wider appeal of irregular modes of rule. Make one technology transparent and others will be adopted to the extent they afford the same thing. Positive change would instead have to involve changing the wider conditions that make inappropriate uses of the technology attractive, rebalancing in particular the standards of legitimacy to which officials are held. In place of a technocratic emphasis on “outputs” alone, it would mean reviving a model of government in which wider participation and scrutiny are valued. It would mean strengthening constitutional mechanisms, and ensuring leaders are better tied to democratic institutions and organisations that can punish them for their transgressions.
Government by messaging makes the pathologies of contemporary politics more extreme and more traceable, but it is not the heart of the problem. It will not be deterred by rules of good conduct, nor by arbitrary or motivated leaks. Only structures that leave less discretionary power in the hands of individuals will do something to address the fundamentals.
[See also: Protest nation ]
Content from our partners
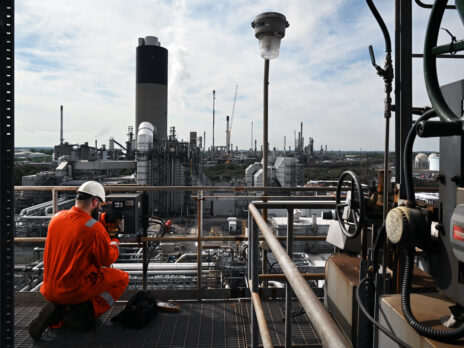
Solving the power puzzle
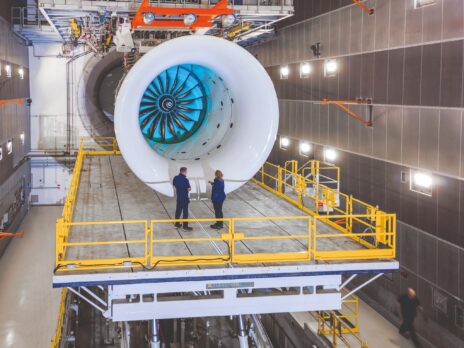
The UK can be a leader in pushing the aerospace sector to a sustainable future
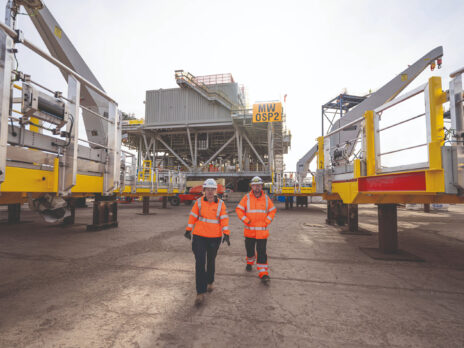
Harnessing Europe’s green power plant
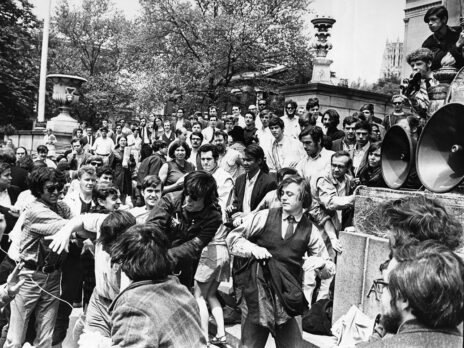
Student power
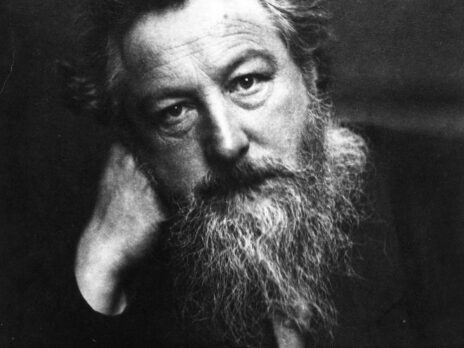
Labour’s moral purpose
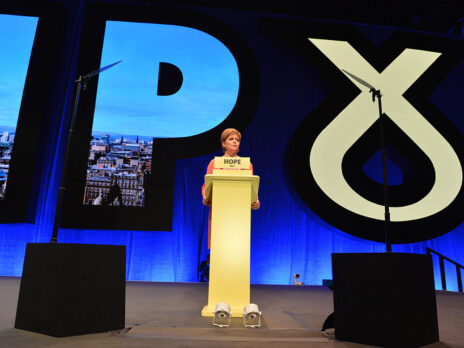
How the SNP lost itself in hyper-liberalism
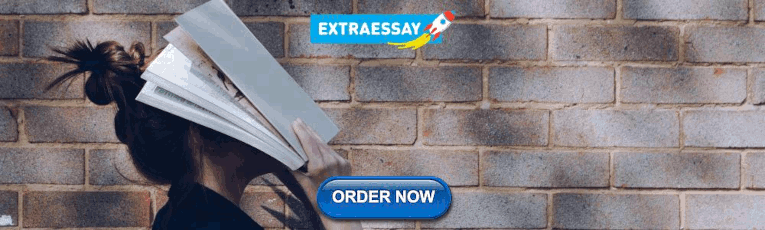
COMMENTS
Let's look at a concrete example of the law of independent assortment. Imagine that we cross two pure-breeding pea plants: one with yellow, round seeds (YYRR) and one with green, wrinkled seeds (yyrr).Because each parent is homozygous, the law of segregation tells us that the gametes made by the wrinkled, green plant all are ry, and the gametes made by the round, yellow plant are all RY.
Mendel provided his knowledge concerning reproduction mechanically during his period. The independent Law of assortment provides that off springs inherit traits from their parents. This is related to genes, DNA and chromosomes. In this context, genes of parents determine the traits of an off spring. These genes are located on the chromosomes ...
This ratio was the key clue that led Mendel to the law of independent assortment. That's because a 9:3:3:1 ratio is exactly what we'd expect to see if the F 1 plant made four types of gametes (sperm and eggs) with equal frequency: YR, Yr, yR, and yr. In other words, this is the result we'd predict if each gamete randomly got a Y or y allele ...
Updated on May 05, 2019. Independent assortment is a basic principle of genetics developed by a monk named Gregor Mendel in the 1860s. Mendel formulated this principle after discovering another principle known as Mendel's law of segregation, both of which govern heredity. The law of independent assortment states that the alleles for a trait ...
The Law of Independent Assortment states that different genes and their alleles are inherited independently within sexually reproducing organisms. During meiosis, chromosomes are separated into multiple gametes. Genes linked on a chromosome can rearrange themselves through the process of crossing-over.
Law of Independent Assortment Definition. The law of Independent Assortment is a Mendelian law stating that for every pair of unit factors, each of them would assort independently into the newly formed gametes. The Mendelian inheritance principles are derived from and named after the monk, Gregor Johann Mendel in the nineteenth century.
Independent assortment is a genetic term that refers to the variation of chromosomes, or genetic information, during sex cell division. This variation allows for genetic differentiation in offspring. Not surprisingly, the principle of independent assortment applies to the definition of independent assortment. It consists of two parts, the first ...
The Austrian monk Gregor Mendel conducted revolutionary experiments with pea plants in the early 1800s showing the existence of traits (he called them "factors") that offspring inherit from their parents. His work culminates in the three principles of Mendelian inheritance; the law of segregation, the law of independent assortment, and the law of dominance.
From these data, Mendel developed the third principle of inheritance: the principle of independent assortment. According to this principle, alleles at one locus segregate into gametes ...
19.4 Independent Assortment Mendel's law of independent assortment states that genes do not influence each other with regard to the sorting of alleles into gametes, and every possible combination of alleles for every gene is equally likely to occur. The independent assortment of genes can be illustrated by the dihybrid cross, a cross between two true-breeding parents that express different ...
Flag. Patrick Kang. 2 months ago. Dihybrid cross is just the name given to crossing of two identically heterozygous individuals, and it happens that it results in a 9:3:3:1 ratio. You can cross any two individuals by using a Punnet square, but they would not have the same 9:3:3:1 ratio. ( 1 vote) Upvote.
Mendel's Dihybrid Crosses Demonstrate the Principle of Independent Assortment. Gregor Mendel's monohybrid crosses, between pea plants that differed in a single trait, demonstrated that (1) organisms randomly inherit one of two copies of each gene from each parent (Mendel's first law, segregation), and (2) the dominant allele can mask the ...
The Law of Independent Assortment states that during a dihybrid cross (crossing of two pairs of traits), an assortment of each pair of traits is independent of the other. In other words, during gamete formation, one pair of trait segregates from another pair of traits independently. This gives each pair of characters a chance of expression.
Mendel's 2nd Law or the Law of Independent Assortment explains how two or more different traits separate from each other during sexual reproduction. It states that a heterozygous trait (Rr) will ...
By carrying out crosses between dihybrid plants, he was able to come up with his second law of inheritance, the Law of Independent Assortment of genes. This law states that individual genes encoding for individual traits are independently passed from parents to offspring. This occurs during gamete formation at anaphase I of the first meiotic ...
This principle is known as 'principle of independent assortment'. The independent assortment holds good for two or more than two pairs of characters. The following discussion will be devoted to the theoretical derivation of dihybrid and trihybrid ratios on the basis of independent assortment. Dihybrid cross. A dihybrid cross is represented in ...
Mendel's Law of Independent Assortment. Mendel's law of independent assortment resulted from Mendel's research on generational resemblance among families over long periods. His work focused on helping to explain why children inherited various features, called traits, from their parents. Mendel conducted his research using garden peas.
Mendel proposed the Law of Independent Assortment to explain his observations that the outcomes for one gene did not impact the outcomes for another gene. When he did crosses for multiple traits, new combinations occurred in the F2 generation that were not present in the P generation. For example, crossing true-breeding pea plants for yellow round seeds and green wrinkled seeds can result in ...
Evolution: These laws play a role in the creation of genetic diversity within species, which is essential for adaptation and evolution over time. In conclusion, the Law of Segregation governs the inheritance of single traits, while the Law of Independent Assortment applies when considering multiple traits on different chromosomes.
A classic Mendelian example of independent assortment: the 9:3:3:1 phenotypic ratio associated with a dihybrid cross (BbEe × BbEe). Some Genes Are Transmitted to Offspring in Groups via the ...
The independent assortment of alleles on non-homologous chromosomes allows for the random assortment of traits, further increasing genetic variation. ... Students looking for free, top-notch essay and term paper samples on various topics. Additional materials, such as the best quotations, synonyms and word definitions to make your writing ...
Independent Assortment . Human Male . Genome Wide . Gamete Production . High Level. Comparative studies in evolutionary genetics rely critically on evaluation of the total amount of genetic shuffling that occurs during gamete production. Such studies have been hampered by the absence of a direct measure of this quantity.
VIDEO ANSWER: Hello. Let's hear questions. Another question they were asking What causes independent assortment? That's the question. So you need to tell you answer for this question. So here independent assortment. So this independent assortment is
A student using their mobile phone during an exam (Getty Images/iStockphoto) Pupils have been told they risk being disqualified if they look for exam papers on social media. Teenagers who look for ...
From the printing press to the telegraph, radio to email, new inventions have left their mark on how decisions are taken and by whom. This pattern continues in the age of the smartphone, apparently indispensable to the professional lives of today's officials and politicians. "You need one these days," the then prime minister Boris Johnson ...