
An official website of the United States government
The .gov means it’s official. Federal government websites often end in .gov or .mil. Before sharing sensitive information, make sure you’re on a federal government site.
The site is secure. The https:// ensures that you are connecting to the official website and that any information you provide is encrypted and transmitted securely.
- Publications
- Account settings
Preview improvements coming to the PMC website in October 2024. Learn More or Try it out now .
- Advanced Search
- Journal List
- Proc Jpn Acad Ser B Phys Biol Sci
- v.89(7); 2013 Jul 25
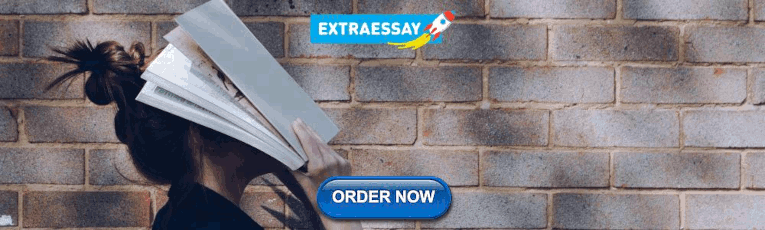
Sea-level rise caused by climate change and its implications for society
Nobuo mimura.
*1 Director, Institute for Global Change Adaptation Science (ICAS), Ibaraki University, Ibaraki, Japan.
Sea-level rise is a major effect of climate change. It has drawn international attention, because higher sea levels in the future would cause serious impacts in various parts of the world. There are questions associated with sea-level rise which science needs to answer. To what extent did climate change contribute to sea-level rise in the past? How much will global mean sea level increase in the future? How serious are the impacts of the anticipated sea-level rise likely to be, and can human society respond to them? This paper aims to answer these questions through a comprehensive review of the relevant literature. First, the present status of observed sea-level rise, analyses of its causes, and future projections are summarized. Then the impacts are examined along with other consequences of climate change, from both global and Japanese perspectives. Finally, responses to adverse impacts will be discussed in order to clarify the implications of the sea-level rise issue for human society.
1. Introduction
Sea-level rise is one of the most significant effects of climate change. High projected rates of future sea-level rise have captured the attention of the world. Particularly, countries which are located in low-lying areas as well as small islands are concerned that their land areas would be decreased due to inundation and coastal erosion and, at worst, a large proportion of their population may be forced to migrate to other countries. Therefore, this issue has resulted in heightened attention internationally, as the effects of climate change become apparent.
The level of the sea varies with time and space due to physical processes, such as tide and waves. Mean sea level at a given position is defined as the height of the sea surface averaged over a period of time, such as a month or a year, long enough that fluctuations caused by tide and waves are largely removed. 1 ) Mean sea level has also spatial distribution in the world. The mean sea level averaged over the global oceans is called global mean sea level (GMSL). The changes in local mean sea level usually differ from that of GMSL, because phenomena dominating in regional and local scales modify the global mean change. When we refer to sea-level change (rise) in this paper, it means the change (increase) in mean sea level, including both global and local, in a general sense. A specific word such as “global (local) sea-level rise” is used to denote the increase in global (local) mean sea level, where it is necessary to distinguish the two terms.
The causes of changes in sea level are not limited to those related to climate change. It is well known that the mean sea level has repeatedly had a large fluctuation due to the alternation of glacial and interglacial periods for the past several hundred thousand years in the Holocene. This fluctuation of mean sea level reached about 120 m. There are also much shorter-term fluctuations in sea level, such as tide, waves and tsunamis. Among such a wide range of fluctuations, climate change-related sea-level change has its own unique characteristics. Given its potential significant effects, there are many questions which scientific research is expected to answer. They include: To what extent did climate change contribute to GMSL rise in the past? How much will GMSL increase in the future due to climate change? How serious are the impacts of the expected sea-level rise, and can human society respond properly to them?
This paper aims to give answers to these questions, based on a review of recent research in the relevant areas. First, the present status of observed sea-level rise, analyses of its causes, and future projections are summarized. Then this paper will examine the impacts of sea-level rise along with other factors of climate change, from both global and Japanese perspectives. Finally, planned responses to the adverse impacts will be discussed.
The latest scientific understanding has been assessed in a systematic way by the Intergovernmental Panel on Climate Change (IPCC). The IPCC has published four major assessment reports since its establishment in 1988. The future sea-level rises by 2100 projected in the First to Fourth Assessment Reports are 31–110 cm (Business as usual scenario), 13–94 cm, 9–88 cm and 18–59 cm, respectively. 2 – 5 ) In each of the assessments, the IPCC used different climate models and scenarios for greenhouse gas (GHG) emissions. This is the major reason for the changes in the projected rise in mean sea level. In particular, the values given in the Fourth Assessment Report (AR4), published in 2007, are relatively low. This is because the assessment did not take account of the contribution of the Greenland Ice Sheet (GIS) and West Antarctica Ice Sheet (WAIS), as there was a lack of reliable understanding of the dynamics of these ice sheets, including collapse and outflow. As a result, some researchers considered that these estimates might be the low end of the future rise in sea level.
After the IPCC AR4 was published, progress has been made in various areas related to the sea-level rise issue. For the past changes in GMSL from 19th to 21st Century, by the improvement of analysis techniques applied to satellite altimeter data together with tide gauge data, more detailed and reliable sea-level change data became available (Cazenave and Llovel, 2010; Church and White, 2011; Ray and Douglas, 2011). 6 – 8 ) Increase in ocean heat content is caused by global warming, which in turn becomes a major factor of mean sea-level rise through thermal expansion of the sea water. Various techniques were also developed for correction of the past data observed by expendable bathythermograph (XBT) to produce a more reliable estimate of the changes in ocean heat content. Regarding the ice sheets in Greenland and Antarctica, although accurate quantitative assessments are still difficult, understanding of the mechanisms dominating the increase/reduction of the ice sheet mass has progressed. New projections of future sea-level rise, for both 21st century and a longer-term beyond this century have been made extensively based on the development of process-based models including atmospheric and oceanic general circulation models (AOGCMs). In parallel with the process-based models, semi-empirical models are developed for the projection by correlating the past data on global mean temperature and mean sea-level, although the empirical relation has no physical scientific basis. In the field of impact assessment, many have been made in order to understand the potential consequences of sea-level rise. In Japan, several projects for climate change impacts, including those for the coastal zone, have been implemented. Scientific papers and reports have been published from these projects, such as Project Team for Comprehensive Projection of Climate Change Impacts (2008, 2009). 9 , 10 ) The present paper provides an overview of the sea-level rise issue based on a review of these and other relevant publications.
2. Sea-level rise since the end of 19th century
We will first examine observed sea-level changes during the past 100 years. The observations have been made in two ways; one is tide gauge observation, and the other is by radar altimeters installed in satellites.
It is not easy to estimate the height of global mean sea level directly, because dense and uniform observations are needed for entire oceans. The data obtained by tide gauges has been compiled by the Permanent Service for Mean Sea Level (PSMSL) managed by Proudman Oceanographic Laboratory, Bidston Observatory in the UK. Today, data from about 1900 tide gauges in nearly 200 countries are sent to PSMSL. 11 ) Although the number is large, tide gauges are disproportionately located in the Northern Hemisphere. As uncorrected tide gauge data also reflect land movement at the observing location, selected tide gauges, which are located on stable ground, are used for the analysis for a long-term change in the global mean sea level. Further, the earth’s crust has been continuing a rebound (visco-elastic response) to the melt of large glaciers during the present interglacial period. This is known as Glacier Isostatic Adjustment (GIA, see 3.4 for further discussion). Therefore, correction of the GIA land movement is needed to estimate the mean sea-level. Lemke et al. (2007) 12 ) indicated that the GIA correction ranges from about 1 mm/year (or more) near to former ice sheets to a few tenths of a millimeter per year in the far field; the error in tide-gauge based GMSL change resulting from GIA is assessed as 0.15 mm/year.
Observations by satellite started with Topex/Poseidon, a satellite altimeter launched in 1992. This observation continues, using subsequent satellites such as Jason-1 and Jason-2. The satellite altimeter gives the distance between a fixed reference surface (typically a conventional reference ellipsoid) and the sea surface. Since the early 1990s satellite altimetry has become the main tool for precisely and continuously measuring sea level. This is due to its advantages over tide gauges, such as lack of influence of land movement, and a regular global coverage with frequent revisit cycles. At the beginning, the Topex/Poseidon altimeter had relatively large errors, but the precision of the estimate has greatly improved due to adjustment of the altimetry system and data processing.
There are similar studies to summarize the contemporary GMSL change such as Cazenave and Llovel (2010) 6 ) and Church and White (2011). 7 ) As they show close results, the result obtained by Church and White (2011) 7 ) is introduced here. Figure Figure1 1 is a summary of the long-term trend of GMSL from 1860 to 2010 obtained by superposing the two sources of observed data. From Fig. Fig.1 1 we can see that the rate of increase in GMSL was not large until 1930, but since then it has increased at an accelerating rate. Church and White (2011) 7 ) indicated that the increase of GMSL from 1880 to 2009 was 21 cm, and that the rate of increase almost doubled to 3.2 ± 0.4 mm/year for 1993 to 2009, relative to 1900 to 2009 when the rate was 1.7 ± 0.2 mm/year.

Global mean sea level from 1860 to 2009. 7 ) Blue line: estimated from coastal and island sea-level data with one standard deviation error (shading). Red line: estimated by Church and White (2006) 13 ) for 1870–2001 (solid line) with one standard deviation error (dashed lines). Black line: satellite altimeter data since 1993.
Figure Figure2 2 shows the change of GMSL for 1993 to 2008 obtained by satellite altimeters, 6 ) which corresponds to the latter part of Fig. Fig.1. 1 . This figure indicates a sudden increase in GMSL from 1997 to 1999, and a decrease in 2008. These irregular changes are considered to be related to an intense El Niño during 1997 to 1998, and La Niña in 2007. Cazenave and Llovel (2010) 6 ) also identified the influence of North Atlantic Oscillation (NAO) and Pacific Decadal Oscillation (PDO). These are marked longer-term fluctuations, with a period of 10 to 20 years. It is apparent that the fluctuation of the GMSL is a combination of anthropogenic climate change and such natural variations. It is also noted that since the start of the altimeter record in 1993, the rate of increase in GMSL was at the upper end of the sea level projections of the Third and Fourth Assessment Reports of IPCC.

Global mean sea level from satellite altimetry for 1993 to 2008. 6 ) Blue dots are raw 10-day data. The red line corresponds to a 90-day smoothing of the raw data. The −0.3 mm/year GIA correction has been applied.
3. Factors causing changes in sea level, and their contributions
3.1. factors causing changes in sea level..
The next question is what factors cause long-term changes in mean sea level. To answer this question we will examine the role of the various factors affecting mean sea level. The two basic factors are change of total volume of sea water, and movements of ground and ocean bottom that result in changes to the size and shape of the ocean basins. In addition to these, dynamic factors such as winds, atmospheric pressures, ocean currents, and waves, play a role. Thus there are many factors, with various temporal and spatial scales as shown below. 6 , 14 )
- • Thermal expansion of sea water
- • Growth/decay of land-based glaciers and ice caps
- • Growth/decay of ice sheets, such as Greenland Ice Sheet (GIS) and West Antarctica Ice Sheet (WAIS)
- • Terrestrial water storage, i.e. , dam reservoirs, lakes, etc, and depletion of groundwater
- • Isostatic adjustment of land mass, especially Glacier Isostatic Adjustment (GIA)
- • Tectonic movement including ground subsidence/uplift associated with earthquakes
- • Ground subsidence/uplift due to compaction of the ground, pumping up of ground water, etc
- • Sediment inflow from land
- • Changes in ocean currents
- • Changes in atmospheric pressure
- • Tide, tsunami, storm surges and waves
- • Natural inter-annual variations, such as the Pacific Decadal Oscillation (PDO)
Many of the factors listed above are individual factors directly connected with sea-level changes. Generally these factors cause sea-level changes as part of some geophysical phenomena or events. Among such phenomena, the most pronounced one to cause a change in sea level is the glacial-interglacial cycle. This took place six times during the past 500 thousand years. Estimated mean sea level and air temperature in Antarctica reveal similar temporal changes, as shown in Fig. Fig.3. 3 . In the last 140 thousand years, global mean sea-level was several meters higher than today during the last interglacial period, 130 to 120 thousand years B.P. The last glacial age started around 120 thousand years B.P., and the mean sea-level was about 120 m below the current level during the glacial maximum 20 thousand years B.P. At 15 thousand years B.P., the current interglacial period started, and sea level also started to rise as the ice sheets melted. Furthermore, during the warm period from 7000 to 6000 year B.P., sea level was 2 to 3 meters higher than the current level. The cause for this higher sea level, known as the Jomon Transgression in Japan, is visco-elastic response of the plate on which the Japan islands were located to the increased GMSL, In summary, these data show a large variation of GMSL on a geological time scale.

Sea level (blue, green: scale on the left) and Antarctic air temperature (orange, gray: scale on the right) over the last 550,000 years, from paleo-records (Left origin is the present-day). 15 ) The green symbols are coral and speleothem-based sea-level markers.
The focus of this paper is the change in mean sea level which took place from 100 years ago to the end of 21st century, a much shorter temporal scale compared with the alternation of glacial/interglacial periods. In the following sections, we will examine the latest scientific knowledge on sea-level change within a 100 year time scale, focusing on major direct factors.
3.2. Factors related to changes in volume of sea water contained in ocean basins.
Major factors that determine recent sea-level change are thermal expansion of sea water and melting of land-based ice. The latter includes melting and outflow of the ice sheets in Greenland and Antarctica.
When the increased concentration of GHGs in the atmosphere cause an increase in the air temperature, heat is transferred from the atmosphere to the ocean. Most of the heat uptake of the ocean takes place through the surface mixed layer, where water temperature and density are nearly uniform due to strong mixing of surface waters by the wind. Then the heat stored in the mixed layer is diffused to the deep layer through thermocline. As heat capacity of the ocean is about 1000 times larger than that of the atmosphere, it is estimated that the heat storage in the ocean accounts for about 90% of the heat which the earth absorbed for the past 40 years. This heat storage is 20 times larger than that of the atmosphere. Therefore, the increase of the heat content of the ocean is regarded as a major indicator of global warming. 16 ) Furthermore, it should be noted that it will take millennia for the ocean to reach a thermal equilibrium, because the convection and diffusion of heat in the ocean is a slow process. This means that the ocean heat uptake will continue for more than a thousand years, in contrast to the fact that the air temperature becomes stable in much shorter time.
Increased heat storage raises the water temperature, resulting in thermal expansion of sea water. Therefore, the ocean heat storage is a major factor for the mean sea-level rise related to global warming. In order to estimate the thermal expansion of the ocean, we need to know the spatial distribution of the sea water temperature. Expendable bathythermograph (XBT) and mechanical bathythermograph (MBT) have been used to observe the vertical distribution of the temperature. XBT observations started after the mid-1960s, while the data from MBT observations is available from after the World War II to around 1990. More recently, ARGO floats are frequently used for the observation of ocean conditions, including temperature and salinity. It has been suggested that significant positive temperature bias existed in the XBT and MBT data. For example, as an XBT falls in the ocean, the vertical positions are calculated using falling velocity formula for the XBT, which induced errors for the depth of temperature measurements. Intensive studies have been carried out for correction of the errors and statistical analyses for the existing XBT and MBT data, producing new estimates for the historical changes in the ocean heat content ( e.g. , Ishii and Kimoto, 2009; Levitus et al. , 2012). 17 , 18 )
Figure Figure4 4 shows a revised estimate for the ocean heat content (OHC) for two depth layers; 0–2000 m (red) and 700–2000 m (black). 18 ) The OHC of the 0–2000 m layer shows an overall increasing trend with a decrease during 1965 to 1970 and stagnation for 1980 to 1990. It is indicated that the global linear trend of OHC 0–2000 m is 0.43 × 10 22 J/year for 1955 to 2010, which corresponds to a total increase in heat content of 24.0 × 10 22 J, and a mean temperature increase of 0.09 ℃. From Fig. Fig.4, 4 , the 700–2000 m layer is responsible for about one third of the ocean warming of the 0–2000 m layer, indicating that major ocean warming has so far taken place in the relatively shallow ocean. Levitus et al. (2012) 18 ) also concludes that the heat storages for 0–2000 m and 0–700 m corresponds to a mean sea-level rise of 0.54 mm/year and 0.41 mm/year, respectively, for 1955 to 2010.

Time series for the World Ocean of ocean heat content for the 0–2000 m (red) and 700–2000 m (black) layers based on pendatal (five year) running mean analyses. 18 ) Reference period is 1955–2006. Each pentadal estimate is plotted at the midpoint of the 5-year period. The vertical bars represent ±2 × S.E. about the pentadal estimate for the 0–2000 m estimates and the grey-shaded area represent ±2 × S.E. about the pentadal estimate for the 0–700 m estimates. The blue bar chart at the bottom represents the percentage of one-degree square areas (globally) that have at least four pentadal one-degree square anomaly values used in their computation at 700 m depth. Blue line is the same as for the bar chart but for 2000 m depth.
Another major factor for sea-level rise is melting of land-based ice. This consists of two factors, namely melting of mountain glaciers and ice caps, and melting and outflow of the ice sheets in Greenland and Antarctica. There are many glaciers in the world, with more than 130 thousand of them being registered in the World Glacier Inventory prepared by the World Glacier Monitoring Service. 19 ) The water content stored in glaciers is considered to be equivalent to 0.5 m of GMSL rise. As glaciers and ice caps in high mountains are quite sensitive to the increased temperature, both accelerated melting and run-off have already occurred, causing glaciers to retreat and the formation of glacier lakes in regions such as the Himalayas.
On the other hand, the Greenland Ice Sheet (GIS) and West Antarctica Ice Sheet (WAIS) contain ice equivalent to about 7 m and 3–5 m sea-level rise, respectively. 6 ) Therefore behavior of these ice sheets has a major influence on future sea-level rise. After the publication of IPCC AR4 in 2007, many studies have focused on this point. The balance of the ice sheets is determined by surface melting/accumulation and outflow of ice to the peripheral coastal areas. Ice sheets increase their mass by snow accumulation, and lose it due to melting. Outflow from the coastal edges is another mechanism of loss. In Greenland, surface melting and loss of total mass are observed through observations of the gravity field using the Gravity Recovery and Climate Experiment (GRACE) satellite. Svendsen et al. (2013) 20 ) reported an acceleration of the GIS mass loss observed by GRACE. They indicate that acceleration of −8.8 to −18.6 Gt/year 2 are detected for different data for the period 2002–2010 (the former value is for 2003–2010). Based on such studies, Greenland is considered to contribute to the increase of global mean sea level. 12 )
A fact that draws further attention is the lubrication effect of melt water. During summer, melt water flows into crevasses, to reach the bottom layers between the glacier and base rock. This may exert a lubrication effect. If this phenomenon occurs on a large scale, the outflow of the ice sheet may accelerate. Another potential risk is the complete disintegration of the GIS in the long-term. This issue will be discussed in Section 4.2.
Next, we will consider the West Antarctica Ice Sheet (WAIS). Though there is the other ice sheet in East Antarctica, we will focus here on WAIS, because it is considered more vulnerable in terms of outflow. Formerly, as global warming increased the water vapor content of the atmosphere, and thus increased precipitation, the WAIS was considered to grow due to increased snow fall, 14 ) contributing to a fall in global mean sea level. However, it has been recognized recently that the outflow of the ice sheet is strongly related to the dynamics of the ice shelf stretching from the glacier edge to the Antarctic Ocean. As sea temperature recently increased, the ice shelf was warmed from below by sea water, resulting in enhanced melting. This may weaken the resistance against outflow of the ice sheet, and eventually contribute to faster outflow of the ice sheet. 12 ) Although many uncertainties remain, and more observations and studies are needed to reach a reliable understanding, long-term changes in the dynamics of the WAIS have a potential to accelerate the GMSL rise. Thus ice shelf behavior and outflow are areas of focus for estimation of future sea-level rise ( e.g. , Stocker et. al. , 2010). 21 )
Another factor is changes in water storage on land. This includes different forms of storage, such as reservoirs behind dams, ground water, wet lands, and soil moisture. If terrestrial water storage increases, the rate of sea-level rise will be reduced. As several hundreds of thousands of dams have been constructed in the world, dam reservoirs are considered to suppress sea-level rise. Although it is difficult to make a precise estimation of human activities such as dam reservoirs, irrigation, and extraction of ground water, Gornitz (2001) 22 ) estimated that the water stored behind dams was equivalent to −0.33– −0.27 mm/year of sea-level fall (here, a negative rate means sea-level fall). Pokhrel et al. , (2012) 23 ) suggested that ground water use, artificial reservoir water impoundment, climate-driven changes in terrestrial water storage and loss of water from closed basin have contributed a global sea-level rise of 0.77 mm/year from 1961 to 2003, about 42% of the sea-level rise which took place for the same period of time. On the other hand, other research shows that use of ground water, and extraction from large inland lakes such as the Aral Sea, has enhanced sea-level rise through increased freshwater use. Climate change will induce changes in both the natural hydrological cycle and the water demands/use by human beings. Therefore, it remains a challenge to evaluate and incorporate the effect of hydrological processes into projections of sea-level change.
3.3. Contribution of major factors.
As shown in the previous sections, sea-level change is caused by a combination of thermal expansion of sea water, melting and outflow of land-based ices, storage of water on land, etc. As it is important to analyze the contribution of these factors to estimate future changes in sea level, intensive studies have been performed. Comparison of the observed sea-level rise and its estimation is called the sea-level budget. Reducing the gap between these values has been a long challenge in the climate science. Table Table1 1 shows some of the summaries of the sea-level budget taken from Church et al. (2011) 16 ) and Cazenave and Llovel (2010). 6 )
Table 1.
Contributions of components to the budget of global mean sea-level rise (unit: mm/year)
Note: Uncertainty range of Cazenave & Llovel (2010)’s estimates is one standard deviation.
Table Table1 1 presents estimates for different periods from the late 20th century to 2008. For the period of 1972–2008, thermal expansion contributed about 40%, and the sum of thermal expansion and melting of glacier and ice caps explains approximately 75% of the observed rise. 16 ) For the period of 1993–2008 and nearly the same period of 1993–2007, two estimates from Church et al. (2011) 16 ) and Cazenave and Llovel (2010) 6 ) are shown in Table Table1. 1 . In both estimates, the observed rate of GMSL rise is almost the same, i.e. 3.22 mm/year and 3.3 mm/year, respectively. Although the estimated rates of GMSL rise do not agree with the observations, the two estimates give close values (2.54 mm/year and 2.85 mm/year). They also give similar values for the fractional contribution of each component: about 30% each for thermal expansion and glaciers and ice caps, and approximately 10% for the Greenland and Antarctica ice sheets. Comparisons between the two periods suggest that the proportion of thermal expansion decreases from 40% to 30% as ice contributions increase. It is also clear that both the Greenland and Antarctica ice sheets are an important component in closing the sea-level budget, particularly since 1993. 16 ) For the recent period of 2003–2007, only Cazenave and Llovel (2010) 6 ) data are available, which shows that the observed sea-level rise rate decreased for this period. As discussed in the previous section, the 2007 La Niña event might have caused a temporal decrease.
Reconstruction of the historical sea-level budget has improved considerably, as shown above, making the difference between observed and estimated sea-level rise smaller. This progress was made by re-analyses of ocean observation data and development of observations and modeling of the related phenomena. However, the budget of sea-level rise is not final, either. Producing more accurate and consistent estimates of the contributions are challenges for future studies.
3.4. Regional distribution and relative sea-level rise.
So far we have examined the global mean sea level averaged for entire oceans. However, in reality, the changes in sea level have a spatial distribution. 24 ) Figure Figure5 5 clearly shows the regional distribution of mean sea-level change. Such a distribution is attributed to local differences in density structure, ocean currents, frequency of low pressure systems, etc. Note that Fig. Fig.5 5 is for the average distribution during 1993 to 2001, and that the distribution will likely be different for other periods.

Spatial patterns in sea level trends over 1993–2001 observed by satellite altimetry. 24 )
Furthermore, actual changes in local mean sea level are a combination of those induced by changes in ocean volume, and by local crustal and land movement. Where the factors cause an increase in sea level it is called relative sea-level rise (RSLR). As this sea-level rise relative to land is the external force affecting coastal zones, it is necessary to estimate the RSLR for evaluating the impacts of sea-level rise.
A typical crustal movement is Glacier Isostatic Adjustment (GIA). This is a long-term rebound of the parts of the earth’s crust that were loaded with huge ice sheets during the glacial period. This movement is apparent in northern Europe, the northern part of North America, Siberia, and around Antarctica. Since GIA still continues today, it causes a sea-level fall relative to land instead of sea-level rise; for example, Stockholm in Sweden experienced −3.82 mm/year sea-level fall from 1880 to 2010, and Bergen in Norway also saw a sea-level fall of −0.54 ± 0.21 mm/year from 1883 to 2009. 25 ) The distribution of GIA is estimated by calculating the response of the crust to the disappearance of heavy ice sheets, assuming a viscoelastic body ( e.g. , Peltier, 2009). 26 )
On the other hand, land subsidence is a typical local phenomenon. Deltas formed by large rivers undergo subsidence due to consolidation, because they consist of a loose deposition of sediments. Human activities, such as extracting ground water for industrial use and irrigation, are another factor that causes land subsidence. For example, large scale subsidence took place in the Ganges delta, Bangladesh, and Chaophraya delta, Thailand (17 mm/year for 1960–1994). This increased the risk of floods and storm surges. Other than such factors, plate tectonics and uplift/subsidence due to earthquakes are also factors that cause local changes. The Great East Japan Earthquake in 2011 resulted in 1.2 m of subsidence in the Ojika Peninsula, Miyagi Prefecture. 27 )
Lastly, we will look at the sea-level changes around Japan. Figure Figure6 6 shows the changes around Japan for the past 100 years. 28 ) As the tectonic movement is considerable, tidal stations located on places with less active land movement were chosen to prepare Fig. Fig.6. 6 . A fluctuation with a period of nearly 20 years is apparent in the record, and the relative maximum appeared around 1950. After 1990 the mean sea level started to increase again. The speed of the recent increase is 4.8 mm/year, which is higher than the global average of 3.2 mm/year. There are possible reasons for the long-term fluctuations apparent in the long-term record shown in Fig. Fig.6, 6 , such as changes in water temperature and the routes of Kuroshio current around Japan. Although there have been some studies, 29 , 30 ) no definite reason for the fluctuation has yet been determined.

Sea-level change around Japan (1906–2011). 28 )
4. Projections of sea-level rise
4.1. projections for the 21st century..
Projections of sea-level rise are based on atmospheric and oceanic general circulation models (AOGCMs), as well as semi-empirical models. The former are called process-based models. These consist of models to simulate physical processes causing sea-level rise, such as the thermal expansion of the oceans, melting of land-based ice, and ice sheet changes. Generally, thermal expansion of the oceans is directly calculated by AOGCMs, while other factors, such as glacier melting and ice sheets changes, are calculated by models specific to each target phenomenon using output from AOGCMs as boundary conditions. Future projections using AOGCMs couple the physical processes of the climate system with future scenarios for GHG emissions. For this purpose, the IPCC’s Special Report on Emission Scenarios (SRES) 31 ) has been widely used. The SRES scenarios consist of six different GHG emission scenarios that were developed assuming various demographic, societal, economic, and technical change pathways (story lines).
Table Table2 2 summarizes projections for increases in global average temperature and GMSL made using the SRES scenarios presented in the IPCC AR4. 32 ) Global average temperature is projected to increase by 1.1–6.4 ℃ by the end of this century, relative to the period 1980–1990. The significant feature is that the magnitude of the temperature increase varies with the emission scenario, based on the socioeconomic development path set by the SRES scenario. However, even under the B1 scenario, which assumes the lowest emission path of GHGs, the temperature increase will reach 1.8 ℃ (a likely range of 1.1–2.9 ℃) by the end of the 21st century. For the most fossil fuel-intensive (A1FI) scenario, the temperature increase is projected to be 4.0 ℃, with a likely range upper limit of 6.4 ℃. If the global average temperature increases by such an amount, the Arctic and the midlands of large continents will show much higher increases, resulting in devastating effects over those regions.
Table 2.
Projected global average surface warming and sea level rise at the end of the 21st century (modified from IPCC, 2007b) 32 )
a) These estimates are assessed from a hierarchy of models that encompass a simple climate model, several Earth System Models of Intermediate Complexity and a large number of Atmosphere-Ocean General Circulation Models (AOGCMs).
Regarding sea-level rise, an 18–38 cm increase is projected for the B1 scenario, which gives the lowest temperature increase. The A1FI scenario, with the highest temperature rise, projects a 26–59 cm sea-level rise. The differences within the same scenario are due to different temperature changes projected by the various AOGCMs used for the sea-level rise projections. The IPCC AR4’s projection of sea-level rise is summarized as a 18–59 cm increase by the end of this century.
The projections shown in Table Table2 2 is based on process-based models. They are based only on thermal expansion and melting of land-based ice, and do not address the future dynamical behavior of the large polar ice sheets of Antarctica and Greenland in a changing climate. This is because the physical understanding of these process was not sufficient when the IPCC AR4 was published. Since IPCC AR4, progress has been made on the physical understanding and development of process-based models.
In parallel with the progress of the process-based models, higher estimates of rise in GMSL were published based on semi-empirical models. Rahmstorf (2007) 33 ) considers that large uncertainties exist even in the projection of thermal expansion, and estimates of the total volume of mountain glaciers and ice caps. Uncertainties associated with polar ice sheets are even larger. As pragmatic alternative to process-based models to estimate increase in the GMSL, a semi-empirical model has been developed based on an assumed linear relationship between the global mean temperature increase and the rate of sea-level rise. After calibrating this model using past data, Rahmstorf (2007) 33 ) applied the relationship to the projection of sea-level rise. The projected GMSL rise in 2100 is 0.5 to 1.4 m above the 1990 level, for the range of temperature increase of 1.4 to 5.8 ℃.
Figure Figure7, 7 , taken from Cazenave and Llovel (2010), 6 ) indicates the evolution of the global mean sea level between 1800 and 2100. Historical records and the observed trend are shown for 1800 to 2000. After 2000, the future projections of IPCC AR4 and those given by the empirical model by Rahmstorf (2007) 33 ) are superimposed. In Fig. Fig.7, 7 , a range of IPCC AR4’s projections is presented as a pink shaded band. However, this band covers only 20 to 50 cm rise in 2100, while IPCC AR4 gives 18–59 cm rise as the projection at 2100. Therefore, it should be noted that the pink shade does not cover the uppermost part of the IPCC AR4 projections and that the band should be overlapping with blue band for the projections by the semi-empirical model.

Evolution of global mean sea level between 1800 and 2100. 6 ) Thick black line: long-term sea level based on various observations for the 19th century. Red line: based on tide gauge data (from Church et al. 2004). 35 ) Green line: from satellite altimetry since 1993. Pink and light blue shaded region: projections from IPCC (2007a) 5 ) and from Rahmstorf (2007), 33 ) respectively. Note: The pink shaded band, which represents s range of projections from IPCC (2007a), 5 ) covers only 20 to 50 cm rise in 2100. However, IPCC (2007a) gives 18–59 cm rise as the projection at 2100. Therefore, it should be noted that the pink shade does not cover the uppermost part of the IPCC (2007a) projections and that the band should be overlapping with blue band.
There is a considerable difference in future projections for sea-level rise between process-based models and the semi-empirical model. A problem associated with the projections based on the semi-empirical model is assuming that sea-level change in the future will have the same relationship with the past, as the relationship is calibrated with the past record of the GMSL rise. However, it is not certain whether the past relationship will hold in the warmer world, because the climate system undergoes complex interactive changes, and rises in air temperature and GMSL may show a non-linear relationship. More effort is needed to resolve the reason for the large difference in the future projections between the two models. Nevertheless, a possibility cannot be excluded that the GMSL rise will exceed 1 m by the end of this century if climate change proceeds along a high temperature increase pathway.
Recently, a new set of scenarios, the Representative Concentration Pathways (RCPs), was proposed. 34 ) Considerable effort has been made to make new assessments for the future climate change using RCPs. New projections of GMSL rise incorporating the new scenarios and progress made after AR4 will be published as the IPCC Fifth Assessment Report in 2014.
4.2. Possibility of large sea-level rise.
A major concern is whether or not a huge sea-level rise of over several meters will take place. Each factor associated with sea-level change, as presented in Section 2, has its own time scale. For example, as the heat capacity of the ocean is very large compared with the atmosphere, it takes over 1000 years for the ocean to reach a thermal equilibrium after the atmosphere achieves its equilibrium. For this ocean warming, the deep ocean circulation plays a major role. Deep-ocean water is formed between Norway and Greenland, and near Antarctica. In these places, cold and dense water sinks from the surface to the deep sea, and is then transported to the wider ocean basins. As this deep ocean circulation plays an important role for transport of heat as well as other materials, it is called the “Conveyer Belt”. If there is still deep-water formation in the warmer world, the ocean will eventually warmed up fairly uniformly by the amount of the global surface temperature change. 36 ) Numerical experiments showed that sea level rose by 1 to 2 m after 2000 years for the doubled CO 2 (560 ppm) world. 14 ) This indicated that sea level rise will continue after the stabilization of global temperature is achieved.
A much larger sea-level rise is expected due to the melting and outflow of the Greenland Ice Sheet (GIS) and West Antarctica Ice Sheet (WAIS). The GIS has a potential to cause a 7 m of sea-level rise, which is considered to happen when a certain increased temperature (threshold temperature) continues to exist around Greenland. This threshold was pointed out to be around a 3 ℃ increase of the global mean temperature above pre-industrial level. 14 ) Furthermore, if the melting of GIS proceeds to reduce its height, resulting in acceleration of melting due to higher temperature in the lower elevations. Such a positive feedback would exist. Robinson et al. (2012) 37 ) suggest that the threshold is 1.6 ℃ (the uncertainty range is 0.8–3.2 ℃), and that the complete disintegration of the GIS needs this condition to continue more than 1000 years. Furthermore, if a higher temperature continues, an irreversible condition, where the GIS never recovers again, will be triggered even if the temperature returns to lower values after some time. 37 )
Albeit a far future, such a high sea-level would impose huge impacts on the whole world, if it happens. The only countermeasure against this phenomenon is to suppress the progress of climate change by major reductions in the emission of GHGs. Given its huge effects, a proactive policy is critically important for not triggering the collapse of the GIS and WAIS, although knowledge about the behavior of the GIS and WAIS is still limited.
In sumary, there are two categories in risks of sea-level rise, i.e. , impacts that occur within this century, and very long-term effects. We need to consider both time scales when planning responses.
5. Assessment of coastal impacts
What impacts will result from a further increase in mean sea level? This section will respond to this question by examining the results of relevant studies. Generally, the impacts of sea-level rise are identified as follows.
- 1) exacerbated inundation and flooding of low-lying coastal areas
- 2) increased coastal erosion
- 3) effects on coastal ecosystems such as salt marsh, mangroves and coral reefs
- 4) salt water intrusion into estuaries and aquifers
- 5) changes in sediment deposition along river channels
- 6) higher-order impacts on the natural environment and human society propagated from the above mentioned basic impacts
Many studies have been performed to assess these impacts, as summarized in the IPCC AR4. 38 ) In this section, the results of these studies will be introduced, focusing on major issues.
5.1. Impacts of coastal inundation and flooding.
(a) global assessment..
Exacerbation of inundation and flooding are among the most significant impacts of sea-level rise. If sea level does increase at an accelerated rate, and storm surges and high waves are superposed on it, areas of inundation are expected to expand significantly. To estimate the affected areas, and populations at risk due to such inundation on a global scale, many studies have been carried out ( e.g. , Nicholls et al. , 1999; Nicholls and Cazenave, 2010). 39 , 40 )
Among them, Maruyama and Mimura (2010) 41 ) estimated a global risk of inundation by combining the sea-level rise projection and 1/100 year storm surges calculated along world coastlines. In this study, sea-level rise and population change in each country were assumed based on four IPCC SRES scenarios (A1B, A2, B1, B2), and, in addition to them, coastal protection scenarios were set to evaluate the effect of coastal protection against sea-level rise and storm surges. The coastal protection scenarios consist of staged upgrade assumptions of coastal protection in a country, assuming that each country is able to introduce stronger coastal protection as it achieves higher economic development (index for this is GDP per capita). For example, as a country’s GDP per capita increases from a range of 801 to 2999 USD to over 20000 USD, the protected level for areas with over 5000 people/km 2 are upgraded from 1/1 year to 1/1000 year storm surges. Such an approach makes it possible to assess not only the intensity of hazards, such as sea-level rise and storm surge, but also the adaptive capacity of a country. Major results of this study are as follows.

Inundation areas in 2100 for SRES A1B scenario with coastal protection for 1/100 storm surges. 41 ) The dark color indicates inundation areas.
- • The present population at risk ( i.e. , people living in areas inundated by a 1/100 year storm surge) is estimated to be 270 million people worldwide. This number will increase to 670 and 450 million people by 2100 for the cases without and with coastal protection, respectively (A2 scenario). Note that the A2 scenario gives the largest number, and the numbers of people at risk vary with scenarios. For example, the people at risk in 2100 (with protection) are approximately 90, 90, and 240 million for A1B, B1 and B2, respectively.
- • About 80% of the people at risk live in Asia and the Pacific region today. This percentage would not change so much throughout the 21st century under the A1B scenario, because there are mega-deltas of large rivers, coastal mega-cities and small island countries in this region. This indicates that the Asia and Pacific region is particularly vulnerable to inundation and flooding.

Trend of affected people in the world and Asia for SRES A1B scenario. 41 )
Similarly, IPCC AR4 38 ) concluded that the population at risk is largest in the populous deltas in Asia and Africa, and that low, small islands, where places for safe migration are very much limited, are especially vulnerable as well.
(b) Impacts on Japan.
There are a number of studies for Japan. However, many studies estimated a inundation risk assuming no coastal protection, because data for coastal protection facilities were not easily available. Though these results gave an indicator for a potential risk of inundation, a more realistic assessment was needed. Recently, a study was undertaken to estimate the inundation risks in the three major bays (Tokyo, Osaka and Ise Bays) in the 2090s, using the heights of the present seawalls along these bays. 42 ) This study indicated the following results assuming that the typhoon (tropical cyclone) becomes 1.3 times stronger than the Ise Bay Typhoon in 1959; the inundation areas and people at risk will be 63–72 km 2 and 300–350 thousand, respectively, and economic damage will amount to 1.8–2.3 trillion JPY. The range of estimates comes from the three different scenarios for sea-level rise. This study also showed that, if sea-level rise and intensified storm surges are superposed, the current return period of 1/several hundred years for a high water level will be shortened to 1/several decades. The significance of this result is that the current design heights of seawalls might be insufficient for the higher sea level expected in the future.
As the superposition of sea-level rise and stronger typhoons cause higher risk, the future trend of typhoons is a major concern from the viewpoint of coastal protection. In this regard, Oouchi et al. (2006) 43 ) and Kitoh et al. (2009) 44 ) indicate that the number of stronger typhoons will increase, while the total number of typhoons may decrease.
However, as it is impossible to estimate the strength and trajectory of future typhoons precisely, a stochastic typhoon model has been developed. This stochastic typhoon model simulates the characteristics of typhoons for a given future climate condition, by giving probabilities of occurrence for typhoon characteristics ( i.e. , central pressure, movement speed and direction, etc.) in a calculation domain. Using this stochastic typhoon model, intensive studies have been carried out to estimate the changes in storm surges and high waves in the future ( e.g. , Hashimoto et al. , 2005; Yasuda et al. , 2009). 45 , 46 ) Furthermore, a new approach to use the output of AOGCMs for analysis of the storm surge risk was also implemented. 47 ) These studies indicate the possibility that unprecedentedly high storm surges that exceed the present design levels may occur in semi-enclosed bays and inland seas such as the Sea of Ariake. Importantly, these results have not yet been incorporated into the design codes for coastal structures. In order to improve the reliability of the estimates, more methodological development will be needed, including improved AOGCMs, and more collaboration between research communities in climate science and coastal engineering.
5.2. Impacts on coastal landforms and ecosystems.
(a) erosion of sandy beaches..
Among the impacts on coastal landforms, beach erosion has drawn attention for a long time. It is already a widespread problem all over the world. Many countries are suffering from beach erosion, which is attributed to various causes such as decrease of sediment supply from rivers, land subsidence, and interruption of longshore sediment transport by man-made structures. As sea level rises, causing waves to act on higher parts of the beach profile, erosion will be enhanced.
There are few studies that provide quantitative estimates of beach erosion due to sea-level rise. Mimura et al. (1994, 1996) 48 , 49 ) applied the Bruun Rule, which is based on the assumption of an equilibrium beach profile, to evaluate the erosion of sandy beaches in Japan. First, they confirmed that the Bruun Rule could macroscopically reproduce the beach erosion that took place on the Niigata Coast, by considering long-term land subsidence on the Niigata Coast (due to extraction of natural gas) as relative sea-level rise. Then, the total areas of eroded beach were estimated for a sea-level rise of 30, 65 and 100 cm. There were nearly 191 km 2 of sandy beaches in Japan in 1990s. These sandy beaches would be eroded by 57, 82 and 90% according to the assumed sea-level rise. These are surprisingly large impacts. Recently, another study reconfirmed these results; shorelines may retreat about 25 m on average by the end of the 21st century due to changes in sea level and wave heights (Sugawa et al. , 2011). 50 ) As the present average width of Japanese sandy beaches is about 30 m, this again suggests a possibility of large beach erosion.
These studies utilize the Bruun Rule, and concern has been expressed as to whether it is a valid approach for estimating beach erosion caused by sea-level rise ( e.g. , SCOR, 1991). 51 ) As sea-level rise is a global phenomenon, we need relevant ways to model the effects of sea-level rise on beach erosion. The Bruun Rule seems to be an important tool for this, if it is used with careful examination of applicability to avoid misuse such as application to coral and mud beaches. Another factor which makes national and global assessment difficult is the lack of data for the area, slope, sand diameter of beaches and incident waves. This is always a barrier to impact assessments.
Sandy beaches have multiple functions; they are not only places for recreation, but also work as a natural breakwater to protect inland areas. If sandy beaches disappear as a result of sea-level rise, waves and storm surges will impact higher areas along the coastline. This fact will force us to strengthen the existing seawalls and build new ones, such as along the Japanese coastlines. Japan has long suffered from beach erosion mainly due to loss of sediment sources and interruption of sediment transport by coastal structures such as jetties and breakwaters. As these factors vary spatially, such effects were not considered in assessments based on the Bruun Rule. Therefore, the erosion estimated above should be considered additional to the existing erosion trend, meaning that the future trend of beach erosion will be even more serious.
(b) Impacts on coastal ecosystems.
Coasts are well endowed with ecosystems such as salt marshes, tidal flats and mangroves. They are considered to experience the adverse effects of sea-level rise if they face lack of sediment supply from land, and lack of hinterland for migration. 38 , 52 ) Mangroves cannot survive in an environment with higher sea-level and salinity, because some mangrove species respire through aerial roots jetting above the sea surface. They will move to higher elevation or alter species as responses to the new environment. However, as the landward boundary is often already developed for human activities, they cannot migrate landward. As a result, the area of mangroves would decrease. A large scale retreat and decrease of mangrove areas is already happening in Thailand, for example, where the causes are land subsidence (relative sea-level rise).
Coral reefs are vulnerable to heat stress. This is not indicative of sea-level rise, but it is estimated that a 1–3 ℃ increase of sea water temperature will cause more frequent occurrence of coral bleaching, which will lead to death of the coral reefs. This is another major concern in terms of the ecological impacts of climate change.
5.3. Salt water intrusion.
Higher sea-level promotes sea water intrusion into river estuaries and aquifers, causing two further major issues; degradation of freshwater resources, and effects on bearing capacity of the ground.
Salt water intrusion has been widely recognized as a major impact of sea-level rise. 52 ) Although the real situation is complex, depending on the local conditions such as river discharge, tidal range, and geological setting, salt water intrusion is generally enhanced by sea-level rise. In fact, in large deltas such as Bangladesh, the salinity interface has advanced inland. 53 ) Furthermore, effects on water resources are also severe in small islands. There is a groundwater pool, called a freshwater lens, under small islands, which is an important water resource for coral islands, particularly low atolls. This freshwater lens is floating on sea water filled in porous coral islands. The volume of the lens changes depending on the area of an island. Therefore, if inundation and erosion due to sea-level rise reduce the island area, the freshwater lens under the island also shrinks. An estimate for Kiribati, an atoll country in the Pacific, indicated that the freshwater lens would shrink by more than 60%, if a 50 cm sea-level rise and 25% reduction of rainfall occurred simultaneously (World Bank, 2000). 54 ) Such a reduction of already scarce water resources is a critical issue for small islands.
Another major issue is the effect on bearing capacity of the ground. In many coastal zones, as the ground consists of loose sediment deposition, its bearing capacity is not high by its nature. In addition to this, sea-level rise raises the water table in the aquifers, increasing the risk of liquefaction due to earthquakes, and reducing bearing capacity of the ground. Reduction of stability of engineering structures, such as river dykes and seawalls, is also a problem. A case study for the coastal area in Tokyo Bay suggested an increase of liquefaction potential as a result of the combined effect of sea-level rise and higher river level. 55 ) Further, studies indicate a higher river surface level and salinity intrusion may bring about adverse effects on the stability of river dykes. 56 ) Such concerns have not attracted much attention so far. However, these are potentially important issues, especially for countries which have wide reclaimed lands in coastal zones and frequent earthquakes. In the future, we need to promote studies on the compound disasters of climate change and natural hazards such as earthquakes in order to better manage the social risks.
5.4. Effects on coastal structures and countermeasures.
There is a conglomeration of various facilities in coastal zones, including ports, fishery harbors, and natural disaster prevention facilities. These are designed based on the local mean sea level, design storm surges and waves. Therefore, when these conditions change, it is logical to revise the design standards to reflect this. As there are a huge number of such facilities in the world, these changes in design conditions could have enormous effects on both social safety and economic burden.
Isobe (2013) 57 ) summarizes the effects of sea-level rise on the design standards of facilities, including wave run-up, overtopping, weight of amour blocks, and stability of breakwaters. A primary effect of sea-level rise is an increase in the water depth in front of the structures, which, in turn, increases the wave height and makes the wave breaking point closer to the structures. These changes eventually cause higher wave run-up heights on seawall slopes. If a tropical cyclone (typhoon) becomes stronger, higher incident waves will occur, resulting in an even higher run-up. Isobe (2013) 57 ) shows a comparison of run-up heights on a model seawall in Tokyo Bay, calculated for the conditions with and without sea-level rise and stronger typhoons. As a model tropical cyclone, Ise Bay Typhoon in 1959 with a pressure depression of 70 hPa was taken, because it caused a historically high storm surge of 3.4 m in Japan. If a 60 cm sea-level rise and a stronger typhoon with 10% increase in the pressure depression are assumed, the difference in the maximum run-up height with and without the assumptions is more than three times larger than the sea-level rise of 60 cm. This means that, for the planning of a countermeasure, it is not enough to raise the seawalls by the amount of projected sea-level rise, but necessary to take into account the increased run-up height.
The following points were also mentioned. The amount of wave overtopping may increase six times even for a relatively small sea-level rise of 30 cm. As the design weight of amour blocks is proportional to the cube of wave height, it increases significantly even for a slight increase of wave height. Furthermore, sea-level rise reduces the stability of breakwaters by increasing buoyancy and wave forces acting on them.
There can be two countermeasures to this issue. One is to incorporate the future conditions induced by climate change into the design of new structures. This needs a higher initial construction cost, but the lifetime cost may be lower when the costs of later strengthening are taken into account. This concept is often called “climate proofing”, and is already partly realized in countries such as the UK and Canada.
The other countermeasure is stepwise strengthening (raising). As sea-level rise is a gradual and continuous phenomenon, this fact allows us to prepare countermeasures by monitoring the progress of sea-level rise and other changes in wave conditions. This is a kind of adaptive approach to the maintenance of costal structures. Although such an adaptive approach is not adopted in the Japan’s present guidelines, it seems necessary to formulate such an approach in the maintenance policy to respond to the changing external conditions.
6. Responses to adverse impacts of climate change and sea-level rise
Responses to climate change fall into two broad measures, mitigation and adaptation. Mitigation is to stabilize the climate system through the reduction of GHG emissions and sequestration of GHGs by forests, etc. As mitigation aims to keep climate change within the level to which human society and the ecosystem can adapt, it can be considered to avoid an “unmanageable situation” induced by a significant climate change. This unmanageable situation includes a large-scale irreversible change including disintegration of the GIS and WAIS.
The objective of adaptation, in turn, is to reduce the adverse impacts of climate change using a range of measures such as disaster risk reduction and increased resilience of food production and fresh water supplies. Even if mitigation succeeds in achieving the goal of stabilization, climate change will still proceed to a certain extent, resulting in some impacts on sectors and regions. Therefore, adaptation is considered a “measure to prepare for an unavoidable impact”. Planning for, and implementation of, adaptation is urgent, particularly for developing countries, for they will face larger impacts because of their inadequate infrastructure and low adaptive capacity. Given the uncertainty surrounding international agreements for mitigation, the importance of adaptation is increasing.
Countermeasures against coastal impacts are a part of adaptation. As coastal impacts are expected to be significant, from an early stage many studies have been carried out, focusing on coastal adaptation. The IPCC examined the impacts and responses in coastal zones by establishing the Coastal Zone Management Subgroup (CZMS) during its first assessment. 58 )
The IPCC CZMS proposed the three strategies of protection, accommodation, and retreat. Protection implies a strategy to defend inland areas using coastal structures such as seawalls and breakwaters. Accommodation is a concept to continue using coastal zones by changing their use, e.g. , raising houses on poles to prepare for possible inundation and converting rice paddies to fish ponds. The last strategy, retreat, is to regulate the land use of vulnerable areas, and relocate houses and economic activities to safer areas. After the IPCC First Assessment Report, these three strategies have evolved to propose concrete measures, as indicated in Fig. Fig.10 10 .

Evolution of planned coastal adaptation practices. 38 , 58 – 61 )
As retreat has the merit of conserving the coastal ecosystem, this strategy and ecosystem-based adaptation, such as enhancing coral reefs and mangroves, are often given priority. However, in the face of the tremendous damage of the Indian Ocean Tsunami in 2004, hurricane Katrina in 2006, and the Great East Japan Earthquake and Tsunami in 2011, people again recognized the important role of robust coastal protection structures as well as their limitation, especially for coastal mega cities and places with critical facilities such as large industries and nuclear power plants. It is projected that, in Asia, coastal megacities where more than 10 million inhabitants live, will increase as a result of population growth and economic development. Therefore, in the middle of the 21st century, climate change impacts will occur over and above such simultaneous increases in population and economic activity. It is therefore obvious that urban and infrastructure planning need to incorporate responses to climate change and sea-level rise.
In Japan, policies and facilities for coastal protection have been developed. However, as described in the previous sections, climate change would bring about storm surges, waves, and floods higher than the current protection standards. As forerunners have already occurred, we need to speed up the examination of how to incorporate these issues into strengthened policies and improved structural design standards for coastal protection.
At the same time, the coastal zone is characterized by the existence of diverse ecosystems and high primary productivity. There are also various human activities, such as coastal cities, transportation, fishery, tourism, and recreation. It has long been pointed out that, to ensure the co-existence and well-being of these activities and functions, integrated coastal management (ICM) is especially important. Therefore, it is most important that coastal adaptation be incorporated in the ICM. 38 )
7. Conclusions
In this paper we examine the present status of scientific understanding of the sea-level rise issue, and its environmental and social impacts. The major points discussed in this review can be summarized as follows.
- • Based on tidal observations since the end of 19th century and satellite observations from 1993, it is recognized that a global mean sea-level rise has occurred. Its speed was 1.7 ± 0.2 mm/year for a period of 1900 to 2009, but the rate has increased to 3.2 ± 0.4 mm/year since the end of 20th century.
- • Intensive studies have investigated the factors affecting contemporary sea-level rise. Their results are close to a quantitative explanation for the budget of the past sea-level rise. During the 20th century, thermal expansion and melting of mountain-based glacier and ice caps predominated. Although the roles of the Greenland Ice Sheet (GIS) and West Antarctic Ice Sheet (WAIS) were unknown previously, recent studies reveal that melting and outflow of these ice sheets have proceeded since the end of 20th century.
- • A projected sea-level rise of 18–59 cm by 2100, which was given by the IPCC Fourth Assessment report, can be understood as the lower limit of the possible increase. Given the roles of the GIS and WAIS, the projection may be revised upward. Although some studies based on semi-empirical models suggest that sea level would rise over 1 m by 2100, the absolute value needs further careful examination.
- • The sea-level rise projected for 2100 poses significant threats to coastal zones in the world. Particularly, when the intensification of tropical cyclones is superposed on sea-level rise, the population at risk from inundation is likely to amount to several hundred million. We should therefore consider that climate change is an issue for security on how to guarantee the safety of such a large number of people, including those in Asia and Africa.
- • Some studies suggest that tropical cyclones may be intensified due to climate change, although uncertainty still remains. To reduce the range of uncertainties for the risks of higher storm surge and waves, collaborative studies between climate science and coastal engineering should be promoted.
- • As sea-level rise is a global phenomenon, the total amount of its impacts could be huge, including beach erosion, degradation of coastal ecosystems and insufficient functions of coastal facilities. However, the global assessment of such impacts is still limited to a subset of the possible impacts, including inundation of land, population and wetlands.
- • The need for countermeasures against adverse impacts ( i.e. coastal adaptation) is inevitable. To this end, we need projections of impacts on a local scale, which is a major challenge to the research community. As a part of coastal adaptation, integrated coastal management is of special importance to ensure the co-existence and well-being of the natural environment and human activities in the coastal zone.
- • Asia and Africa, centers for population growth and economic development in the 21st century, as well as small islands, are particularly vulnerable to sea-level rise and climate change. In these areas, a combination of hard measures, i.e. , infrastructure development, and soft measures, i.e. , urban planning and disaster risk reduction should be enhanced to develop social capacity for adaptation.
- • The sea-level rise issue has two different dimensions; one is several tens cm of sea-level rise in the 21st century, and the other is a possibility of a large increase of over several meters in over 1000 years. There should be threshold conditions for the complete disintegration of the GIS and WAIS. This would cause a major sea-level rise. A key role of mitigation policy is not to trigger such a large and irreversible phenomenon by taking a proactive approach.
From this review, we can see that the sea-level rise issue includes an extremely wide range of disciplines from natural science, engineering and applied sciences to social sciences and humanities. As described in this paper, there has been progress in each area, motivated by high interest throughout the world. On the other hand, there remain many challenges to increase scientific understanding and accuracy of the estimates for bridging research results so as to develop effective countermeasures against the impacts of sea-level rise. This area is one which really needs inter-disciplinary collaboration, and the author hopes that efforts in that direction will be accelerated.
Acknowledgements
The author would like to express deep appreciation to Prof. Kiyoshi Horikawa, Professor Emeritus of the University of Tokyo and Member of The Japan Academy, and Prof. Taro Matsuno, Professor Emeritus of the University of Tokyo, and Member of The Japan Academy, for their scientific discussions. They are extremely effective to improve this paper. Appreciation is also extended to Prof. John Hay, The University of the South Pacific, for his helpful discussions and English proof reading.
Abbreviations
Nobuo Mimura was born in 1949 in Hiroshima. He graduated from the Faculty of Engineering at the University of Tokyo, specializing in urban engineering. He continued his study at the Graduate School of Engineering and obtained master’s and doctoral degrees in the field of urban engineering. He began his professional career as Research Associate in 1979 at the Prof. Kiyoshi Horikawa’s Laboratory at the University of Tokyo, where he started research in coastal engineering. Since then he has experienced Assistant Professor (1982 to 1983) and Associate Professor (1983 to 1984) at the University of Tokyo, and Associate Professor (1984 to 1995) and Professor (1995 to date) at Ibaraki University. He played a leading role in establishing the Institute for Global Change Adaptation Science (ICAS) at Ibaraki University in 2006, and has served as its director to the present. He has also served as assistant vice president of Ibaraki University since 2004. Dr. Mimura specializes in global environmental engineering, coastal engineering and adaptation policy to climate change. He is intensively engaged in studies on the impacts of climate change and sea-level rise in Japan, Asia and small island countries, leading several research projects in this field. He has also served as coordinating lead author of the Intergovernmental Panel on Climate Change (IPCC). As a result of his achievements, he was awarded Annual Research Award of the Japan Society of Civil Engineers in 1997, Grand Prix, Nikkei Global Environment Technology Award of the Japan Economic Newspaper Inc. in 1997, Environmental Protection Award of the Environment Agency of Japan in 1998, and Environmental Award of the Japan Society of Civil Engineers in 2006.
Thank you for visiting nature.com. You are using a browser version with limited support for CSS. To obtain the best experience, we recommend you use a more up to date browser (or turn off compatibility mode in Internet Explorer). In the meantime, to ensure continued support, we are displaying the site without styles and JavaScript.
- View all journals
- My Account Login
- Explore content
- About the journal
- Publish with us
- Sign up for alerts
- Open access
- Published: 15 February 2018
Ocean science research is key for a sustainable future
- Martin Visbeck 1 , 2
Nature Communications volume 9 , Article number: 690 ( 2018 ) Cite this article
37k Accesses
201 Citations
247 Altmetric
Metrics details
- Ocean sciences
- Sustainability
Human activity has already affected all parts of the ocean, with pollution increasing and fish-stocks plummeting. The UN’s recent announcement of a Decade of Ocean Science provides a glimmer of hope, but scientists will need to work closely with decision-makers and society at large to get the ocean back on track.
The ocean covers 71% of the Earth’s surface. It regulates our climate and holds vast and in some cases untouched resources. It provides us with basics such as food, materials, energy, and transportation, and we also enjoy the seascape for religious or recreational practices. Today, more than 40% of the global population lives in areas within 200 km of the ocean and 12 out of 15 mega cities are coastal. Doubling of the world population over the last 50 years, rapid industrial development, and growing human affluence are exerting increasing pressure on the ocean. Climate change, non-sustainable resource extraction, land-based pollution, and habitat degradation are threatening the productivity and health of the ocean (Fig. 1 ). It is in this context that over the last few years, scientists and societal actors have organized a bottom-up movement, which has ultimately led to the United Nations General Assembly proclaiming a Decade of Ocean Science for Sustainable Development (2021–2030). In the process, governments, industry, and scientists have raised awareness of the rapid degradation and over-use of the ocean. The final document from the Rio+20 summit, The future we want 1 , made extensive reference to the ocean, and the Global Ocean Commission articulated the need for more effective global ocean policies 2 . Moreover, the 2030 Agenda for Sustainable Development includes an explicit ocean goal (SDG14) 3 , 4 that led to the first-ever UN Ocean conference 5 to support its implementation. The ambition of the Decade of Ocean Science is to now use this gathering momentum to mobilize the scientific community, policy-makers, business, and civil society around a program of joint research and technological innovation 6 . I see reasons for optimism in four main areas. First, there is a tremendous opportunity to connect ocean sciences more directly with societal actors by promoting integrated ocean observation and solution-oriented research agendas (Fig. 2 ). Also, rich and poor nations are increasingly engaging in capacity development and resource sharing. And finally, the UN system and coastal states have a unique chance to seriously collaborate in multi-stakeholder processes to advance maritime spatial planning and effective ocean governance.
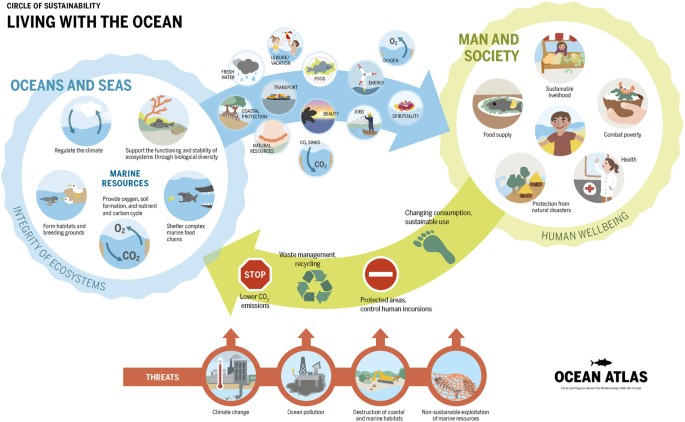
Human–ocean interactions highlighting ocean ecosystem services and their threads (taken from Ocean Atlas, 2017 12 )
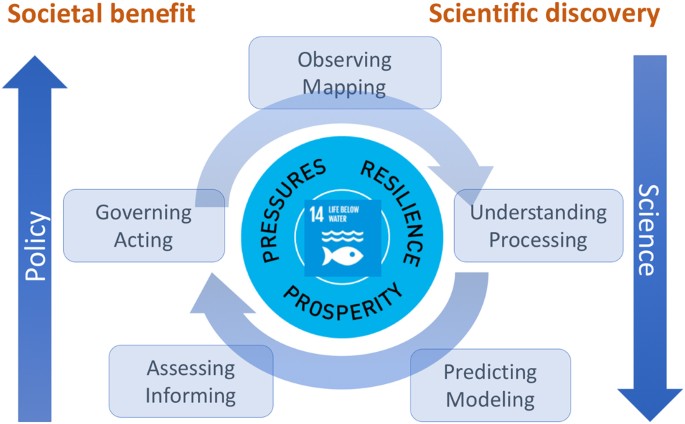
Increased need for ocean information to meet societal needs. Meeting a growing range of societal demands and achieving Sustainable Development Goal 14 (Conserve and sustainably use the oceans, seas, and marine resources) from the 2030 Agenda for Sustainable Development can only be fully realized if all elements of the ocean value chain are resourced adequately and more integrated science agendas are advanced. Figure adapted from ref. 9 . Sustainable Development Goal logo ©UNITED NATIONS. All rights reserved
A more integrated and sustainable ocean observing system
The Decade of Ocean Science will encourage actions towards a more integrated and sustainable ocean observing system to facilitate ocean discovery and environmental monitoring. The vast volume of the ocean and its complex coastlines are neither fully observed nor adequately understood. In particular the deep sea is a frontier of ocean sciences, where internationally coordinated research teams regularly discover new ocean phenomena of profound importance or new organisms and substances for potential future use. Sustained and systematic ocean observations are needed to document ocean change, initialize ocean system models and provide critical information for improved ocean understanding. Advances in ocean robotics and the combination of remote and in situ ocean observations offer new opportunities; and free and open data sharing and multi-stakeholder contributions by governments (rich and poor), the private sector and citizens are opening exciting new dimensions. International efforts, such as the Global Ocean Observing System, the Blue Planet initiative of the Group on Earth Observations and their Framework for Ocean Observing 7 provide a solid basis and an opportunity for growth. The upcoming decadal conference on ocean observations, OceanObs 19 8 will provide an excellent opportunity to advance our ocean observing ambitions.
A solution-oriented integrated ocean science agenda
The Decade of Ocean Science should address both deep disciplinary understanding of ocean processes and solution-oriented research to generate new knowledge. This will support societal actors in reducing ocean pressures, preserving and restoring ocean ecosystems and so safeguard ocean-related prosperity for generations to come. A solution-oriented integrated ocean science agenda can provide innovative ideas, improved assessments and fundamental knowledge in the context of sustainable development and improving human–ocean interactions 9 .
Our rapidly growing, affluent, and more technologically advanced societies are increasingly impacting their local and the global environment, leading to pollution by both chemical and physical wastes. Integrated research is needed to assess the human and environmental risks of ongoing and future types of ocean pollution, to generate new ideas to reduce the ocean pressures by promoting recycling, improved waste management and incentive and governance regimes to encourage more sustainable production and consumption. The most challenging ocean pollutants include: atmospheric carbon dioxide, which causes climate change, ocean warming, ocean acidification, and sea level rise; agricultural fertilizers, which lead to increased primary production but result in ocean deoxygenation; untreated waste water; invasive species; micro and macro plastics, the exponential increase of which has an environmental impact as yet only partially known.
Ocean hazards such as storm surges, harmful algal blooms, or coastline erosion can be devastating for coastal communities. Throughout human evolution civilization has developed strategies to increase our resilience to threats from the ocean. However, the rush for coastal recreation and access to the sea has produced newly built infrastructure that is increasingly vulnerable to ocean extreme events. Hard solutions, such as building walls and levees, could provide some mitigation. However, softer approaches, such as beach nourishment, restoration of mangroves and reef systems, would also provide natural protection and increase resilience to sea-level rise and storm surges. Marine protected areas, natural coastal defences, mining codes, or regulations to limit ocean pollution are all critical elements to safeguard ocean resilience.
Humans have always benefitted from the ocean and its diverse ecosystem services. We often speak of a healthy and productive ocean referring to the desire to maintain the ocean in a prosperous state. The ocean provides food for many, often poor, coastal communities; provides jobs, energy, and raw materials; and enables global trade and recreational and cultural services. The sustainability challenge is achieving long-term ocean prosperity for more affluent societies with a global population approaching 10 billion. Is there sufficient intergenerational will to sustain the overall long-term wealth and well-being of humans by safeguarding ocean resources and ecosystem productivity? What are the trade-offs and synergies between different strategies of marine food production and wild harvesting, different forms of energy harvesting and extraction of materials and ocean restoring zones? New research should develop and flesh out sustainable blue-green growth agendas and link it to efforts in ecosystem protection.
The Decade of Ocean Science should develop a new ocean narrative that can provide context and motivation to reduce ocean pressures, increase ocean resilience, and promote ocean prosperity for generations to come. At the same time, it can provide visibility to existing and new international ocean science programs, such as the new Future Earth Ocean Knowledge–Action network 10 that aims to connect academic and practical knowledge to address the pressing issues of ocean sustainability using the concept of co-design, co-production, and co-dissemination of ocean sustainability knowledge.
Global capacity building
The success of the Decade of Ocean Science will critically depend on global capacity building and resource-sharing between countries at different levels of wealth and development. The enormous need for more ocean information at the scientific, governmental, private sector, and public levels demands a step-change in ocean education at all levels. New technology to improve ocean observation, more sustainable ocean resource extraction, and the digital revolution are transforming the ocean sciences and information communities. How can we harness this opportunity? Perhaps new curricula at universities can provide the opportunity to engage a wide range of disciplines in the area of ocean sustainability. Global learning formats such as massive open online courses 11 , open access to ocean information and increased interactions between the academic and societal actor communities are all promising activities. In addition, partnerships between academic and civil society organizations can produce free ocean literacy material, such as the Ocean-Atlas 12 or the World Ocean Review 13 . However, more engagement at the primary and secondary school levels is urgently needed to promote ocean literacy. Training courses and exchange programs between south–south and north–south ocean actors, as well as courses for ocean professionals, hold tremendous potential to raise ocean awareness and promote better solutions.
Effective ocean governance
Finally, the Decade of Ocean Science, in conjunction with the 2030 Agenda for Sustainable Development and other international and regional ocean agendas, has shown the need for societal actors to reflect on effective ocean governance. From a regulatory perspective, coastal states can benefit from a systematic, multi-stakeholder assessment and spatial planning procedure. In many parts of the world, each cubic meter of ocean is expected to support several, often conflicting, demands. Spatial planning procedures that take the demands of neighboring countries and the global ocean system into account can help to find more sustainable and equitable regimes of ocean use and access. Science can help in this effort by reflecting on a range of human development scenarios and evaluating how best to sustain ocean prosperity while respecting planetary 14 and ocean boundaries. A good example of this is the ocean scenario team that is scoping out development pathways to reach SDG14 and ocean-related goals 15 by 2050 in the context of The World In 2050 project (TWI2050 16 ).
The increased awareness of the importance of the ocean to the future of humanity give grounds for cautious optimism and motivation for ambitious multilateral cooperation. The scientific community has been given a stage on which to shine during the Decade of Ocean Science for Sustainable Development. Let us come together, respect our disciplines and agendas but also be ready to embark on an exciting and transformative journey to realize the ocean we need for the future we want.
United Nations General Assembly. The future we want . Rio+20 conference outcome document A/RES/66/288. Available at https://sustainabledevelopment.un.org/futurewewant.html (United Nations, 2012).
Global Ocean Commission. The Future of Our Ocean: Next steps and priorities Report available at http://www.some.ox.ac.uk/research/global-ocean-commission (Global Ocean Commission, 2016).
Visbeck, M. et al. Securing blue wealth: the need for a special sustainable development goal for the ocean and coasts and for future ocean spatial planning. Mar. Policy 48 , 184–191 (2014).
Article Google Scholar
United Nations General Assembly. Transforming our world: the 2030 Agenda for Sustainable Development. A/RES/70/1 Available at http://www.un.org/sustainabledevelopment/development-agenda/ (United Nations, 2015).
United Nations. UN Ocean Conference 2017 . https://oceanconference.un.org/ (2017).
UNESCO. United Nations Decade of Ocean Science for Sustainable Development (2021-2030) UNESCO press release. https://en.unesco.org/ocean-decade (2017).
Task Team for an Integrated Framework for Sustained Ocean Observing. A Framework for Ocean Observing. IOC/INF-1284 (UNESCO, 2012).
OceanObs'19. OceanObs'19 Conference Announcement. https://www.oceanobs19.net (2018).
Visbeck, M. & Schneider, A. Future Earth’s Ocean in Global Change and Future Earth: The GeoScience Perspective (eds Beer, T., Li, J. & Alverson, K.) in press (Cambridge University Press, 2018).
Ocean-KAN development team. Future Earth Ocean Knowledge Action Network . http://www.futureearth.org/future-earth-ocean (2018).
Antia, A. & Visbeck, M. (eds) One Planet – One Ocean: From Science To Solutions, Massive Open Online Course . Available at https://www.oceanmooc.org (2017).
Visbeck, M. et al. Ocean-Atlas, Facts and Figures on the Threats to Our Marine Ecosystems. https://www.ocean-atlas.org (2017).
Lehmköster, J. (ed.) The World Ocean Review Series http://worldoceanreview.com/en/ (2010, 2013, 2014, 2015 & 2017).
Steffen, W. et al. Planetary boundaries: Guiding human development on a changing planet. Science 347 , 1259855 (2015).
Article PubMed Google Scholar
Schmidt S. et al. A Guide to SDG Interactions: from Science to Implementation. Ch.SDG14. Report available at https://www.icsu.org/publications/a-guide-to-sdg-interactions-from-science-to-implementation (2017).
TWI2050 project team. The World in 2050 . http://www.iiasa.ac.at/web/home/research/researchProjects/TWI2050.html (2017).
Download references
Author information
Authors and affiliations.
GEOMAR Helmholtz Centre for Ocean Research Kiel, Wischhofstr. 1-3, 24118, Kiel, Germany
Martin Visbeck
Kiel University, Christian-Albrechts-Platz 4, 24118, Kiel, Germany
You can also search for this author in PubMed Google Scholar
Contributions
M.V. wrote the manuscript.
Corresponding author
Correspondence to Martin Visbeck .
Ethics declarations
Competing interests.
The authors declare no competing financial interests.
Additional information
Publisher's note: Springer Nature remains neutral with regard to jurisdictional claims in published maps and institutional affiliations.
Rights and permissions
Open Access This article is licensed under a Creative Commons Attribution 4.0 International License, which permits use, sharing, adaptation, distribution and reproduction in any medium or format, as long as you give appropriate credit to the original author(s) and the source, provide a link to the Creative Commons license, and indicate if changes were made. The images or other third party material in this article are included in the article’s Creative Commons license, unless indicated otherwise in a credit line to the material. If material is not included in the article’s Creative Commons license and your intended use is not permitted by statutory regulation or exceeds the permitted use, you will need to obtain permission directly from the copyright holder. To view a copy of this license, visit http://creativecommons.org/licenses/by/4.0/ .
Reprints and permissions
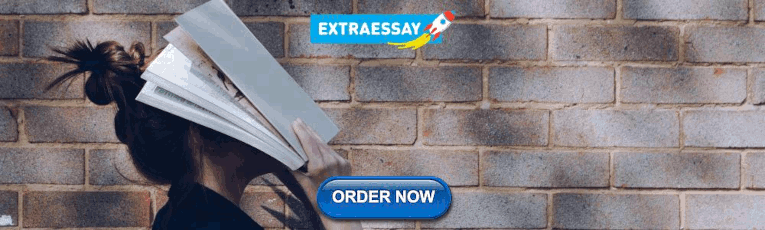
About this article
Cite this article.
Visbeck, M. Ocean science research is key for a sustainable future. Nat Commun 9 , 690 (2018). https://doi.org/10.1038/s41467-018-03158-3
Download citation
Received : 15 January 2018
Accepted : 24 January 2018
Published : 15 February 2018
DOI : https://doi.org/10.1038/s41467-018-03158-3
Share this article
Anyone you share the following link with will be able to read this content:
Sorry, a shareable link is not currently available for this article.
Provided by the Springer Nature SharedIt content-sharing initiative
This article is cited by
Isolation and screening of antimicrobial biosurfactants obtained from mangrove plant root–associated bacteria.
- Zainab Irfan
- Sayeed Mohammed Firdous
- Eswaramoorthy Thirumalaikumar
Naunyn-Schmiedeberg's Archives of Pharmacology (2024)
In search of value: the intricate impacts of benefit perception, knowledge, and emotion about climate change on marine protection support
- Minh-Hoang Nguyen
- Minh-Phuong Thi Duong
- Vuong-Quan Hoang
Journal of Environmental Studies and Sciences (2024)
Principles for transformative ocean governance
- Amanda T. Lombard
- Jai Clifford-Holmes
- Elisa Morgera
Nature Sustainability (2023)
A critical review of conventional and emerging wastewater treatment technologies
- Ravindra Sangamnere
- Tavishi Misra
- Rakesh Kumar
Sustainable Water Resources Management (2023)
Understanding and Adapting Ocean Decade Action
- Dhinakarasamy Inbakandan
Anthropocene Science (2023)
Quick links
- Explore articles by subject
- Guide to authors
- Editorial policies
Sign up for the Nature Briefing: Anthropocene newsletter — what matters in anthropocene research, free to your inbox weekly.

to submit an obituary
Please email [email protected] or call 530-896-7718. Please include your name, mailing address, and phone number along with the copy and photo.

Coastal Commission OKs seawater intake…
Share this:.
- Click to share on Twitter (Opens in new window)
- Click to share on Facebook (Opens in new window)
- Click to print (Opens in new window)
- Sponsored Content
Latest Headlines
Coastal commission oks seawater intake upgrades, benefiting proposed fish farm, research park.

The seawater intake systems upgrades would be capable of taking 11.8 million gallons of water per day out of the bay. The bolstered equipment would facilitate the operation of the proposed Nordic Aquafarms-run fish farm in Samoa on harbor district property.
“It’s not just about this aquaculture project, it’s also about marine research to be done on site. It’s about a whole host of other benefits that could come assuming this is something that happens,” Commissioner Mike Wilson – who also represents Humboldt County’s third district on the board of supervisors – said, referencing the National Marine Research and Innovation Park project.
The commission approved the improvements, but with several mitigation requirements: marsh restoration efforts, destroying invasive species and clearing wood treated with a toxic chemical from a Fields Landing dock.
Rob Holmlund, the harbor district’s development director, noted his agencies state mandate to implement conservation efforts and expressed willingness to work with coastal commission staff to monitor progress. As for the infrastructure upgrades, Holmlund detailed their specifications to the commission
“If you have a piece of paper in front of you, the diameter of the hole through which the water passes is smaller than the hole in a letter O on a piece of paper in front of you. So that is how small the screen size is,” Holmlund said, adding the velocity which water enters the system is relatively slow.
As Humboldt County weathers climate change, Wilson said the commission should consider increasing water volume in the Humboldt Bay to keep it cooler in the face of rising temperatures.
The project saw both support and opposition during public comment: Frank Egger, North Coast Rivers Alliance President, decried the proposed fish farm during public comment.
“The factory fish farm will cause significant adverse impacts on our fisheries. This application has been piecemealed through the California Environmental Quality Act process in direct indirect violation of CEQA. The entrainment issue is huge. Sea level rise and future tsunami impacts must be addressed. The California Pacific Ocean salmon season is shut down again the second year in the row,” Egger said.
The commission’s staff report detailed how the bolstered intake systems could suck in fish eggs and other aquatic life vital to the bay’s habitat, thus requiring the mitigation steps.
A Nordic Aquafarms spokesperson did not respond to a request for comment by the Times-Standard’s print deadline.
“It’s important to acknowledge the dire straits that the wild salmon fishery is in and kind of dramatic regulatory measures that the state of California and the federal government are playing are having to do considering the dire straits of that fishery, so we need to be extra careful that we’re not adding to the stress of that wild population.,” Commissioner Ann Notthoff said.
Jackson Guilfoil can be reached at 707-441-0506
More in News

SUBSCRIBER ONLY
Throwback thursday | commercial street paving spurred fight with railroad in 1924.

National Politics | Supreme Court orders Louisiana to use contested map for election

National Politics | US border arrests fall, bucking usual trend of spring spike

Arcata Planning Commission approves Gateway Area Plan, passes it to the city council
Suggestions or feedback?
MIT News | Massachusetts Institute of Technology
- Machine learning
- Social justice
- Black holes
- Classes and programs
Departments
- Aeronautics and Astronautics
- Brain and Cognitive Sciences
- Architecture
- Political Science
- Mechanical Engineering
Centers, Labs, & Programs
- Abdul Latif Jameel Poverty Action Lab (J-PAL)
- Picower Institute for Learning and Memory
- Lincoln Laboratory
- School of Architecture + Planning
- School of Engineering
- School of Humanities, Arts, and Social Sciences
- Sloan School of Management
- School of Science
- MIT Schwarzman College of Computing
Scientists develop an affordable sensor for lead contamination
Press contact :, media download.
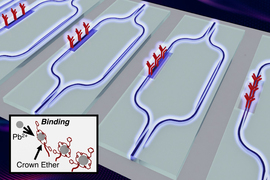
*Terms of Use:
Images for download on the MIT News office website are made available to non-commercial entities, press and the general public under a Creative Commons Attribution Non-Commercial No Derivatives license . You may not alter the images provided, other than to crop them to size. A credit line must be used when reproducing images; if one is not provided below, credit the images to "MIT."

Previous image Next image
Engineers at MIT, Nanyang Technological University, and several companies have developed a compact and inexpensive technology for detecting and measuring lead concentrations in water, potentially enabling a significant advance in tackling this persistent global health issue.
The World Health Organization estimates that 240 million people worldwide are exposed to drinking water that contains unsafe amounts of toxic lead, which can affect brain development in children, cause birth defects, and produce a variety of neurological, cardiac, and other damaging effects. In the United States alone, an estimated 10 million households still get drinking water delivered through lead pipes.
“It’s an unaddressed public health crisis that leads to over 1 million deaths annually,” says Jia Xu Brian Sia, an MIT postdoc and the senior author of the paper describing the new technology.
But testing for lead in water requires expensive, cumbersome equipment and typically requires days to get results. Or, it uses simple test strips that simply reveal a yes-or-no answer about the presence of lead but no information about its concentration. Current EPA regulations require drinking water to contain no more that 15 parts per billion of lead, a concentration so low it is difficult to detect.
The new system, which could be ready for commercial deployment within two or three years, could detect lead concentrations as low as 1 part per billion, with high accuracy, using a simple chip-based detector housed in a handheld device. The technology gives nearly instant quantitative measurements and requires just a droplet of water.
The findings are described in a paper appearing today in the journal Nature Communications , by Sia, MIT graduate student and lead author Luigi Ranno, Professor Juejun Hu, and 12 others at MIT and other institutions in academia and industry.
The team set out to find a simple detection method based on the use of photonic chips, which use light to perform measurements. The challenging part was finding a way to attach to the photonic chip surface certain ring-shaped molecules known as crown ethers, which can capture specific ions such as lead. After years of effort, they were able to achieve that attachment via a chemical process known as Fischer esterification. “That is one of the essential breakthroughs we have made in this technology,” Sia says.
In testing the new chip, the researchers showed that it can detect lead in water at concentrations as low as one part per billion. At much higher concentrations, which may be relevant for testing environmental contamination such as mine tailings, the accuracy is within 4 percent.
The device works in water with varying levels of acidity, ranging from pH values of 6 to 8, “which covers most environmental samples,” Sia says. They have tested the device with seawater as well as tap water, and verified the accuracy of the measurements.
In order to achieve such levels of accuracy, current testing requires a device called an inductive coupled plasma mass spectrometer. “These setups can be big and expensive,” Sia says. The sample processing can take days and requires experienced technical personnel.
While the new chip system they developed is “the core part of the innovation,” Ranno says, further work will be needed to develop this into an integrated, handheld device for practical use. “For making an actual product, you would need to package it into a usable form factor,” he explains. This would involve having a small chip-based laser coupled to the photonic chip. “It’s a matter of mechanical design, some optical design, some chemistry, and figuring out the supply chain,” he says. While that takes time, he says, the underlying concepts are straightforward.
The system can be adapted to detect other similar contaminants in water, including cadmium, copper, lithium, barium, cesium, and radium, Ranno says. The device could be used with simple cartridges that can be swapped out to detect different elements, each using slightly different crown ethers that can bind to a specific ion.
“There’s this problem that people don’t measure their water enough, especially in the developing countries,” Ranno says. “And that’s because they need to collect the water, prepare the sample, and bring it to these huge instruments that are extremely expensive.” Instead, “having this handheld device, something compact that even untrained personnel can just bring to the source for on-site monitoring, at low costs,” could make regular, ongoing widespread testing feasible.
Hu, who is the John F. Elliott Professor of Materials Science and Engineering, says, “I’m hoping this will be quickly implemented, so we can benefit human society. This is a good example of a technology coming from a lab innovation where it may actually make a very tangible impact on society, which is of course very fulfilling.”
“If this study can be extended to simultaneous detection of multiple metal elements, especially the presently concerning radioactive elements, its potential would be immense,” says Hou Wang, an associate professor of environmental science and engineering at Hunan University in China, who was not associated with this work.
Wang adds, “This research has engineered a sensor capable of instantaneously detecting lead concentration in water. This can be utilized in real-time to monitor the lead pollution concentration in wastewater discharged from industries such as battery manufacturing and lead smelting, facilitating the establishment of industrial wastewater monitoring systems. I think the innovative aspects and developmental potential of this research are quite commendable.”
Wang Qian, a principal research scientist at the Institute of Materials Research in Singapore, who also was not affiliated with this work, says, “The ability for the pervasive, portable, and quantitative detection of lead has proved to be challenging primarily due to cost concerns. This work demonstrates the potential to do so in a highly integrated form factor and is compatible with large-scale, low-cost manufacturing.”
The team included researchers at MIT, at Nanyang Technological University and Temasek Laboratories in Singapore, at the University of Southampton in the U.K., and at companies Fingate Technologies, in Singapore, and Vulcan Photonics, headquartered in Malaysia. The work used facilities at MIT.nano, the Harvard University Center for Nanoscale Systems, NTU’s Center for Micro- and Nano-Electronics, and the Nanyang Nanofabrication Center.
Share this news article on:
Related links.
- Jia Xu Brian Sia
- Luigi Ranno
- Juejun Hu Research Group
- Department of Materials Science and Engineering
Related Topics
- Public health
- Materials science and engineering
- Nanoscience and nanotechnology
Related Articles
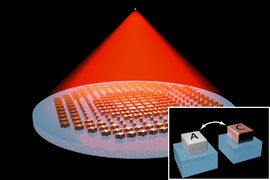
New “metalens” shifts focus without tilting or moving
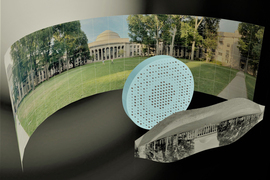
Engineers produce a fisheye lens that’s completely flat
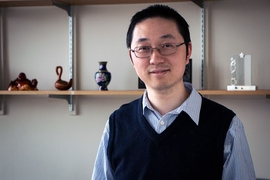
Exploring interactions of light and matter
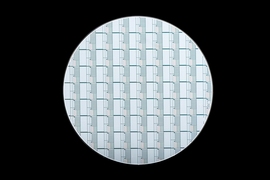
Researchers discover a new way to control infrared light
Previous item Next item
More MIT News
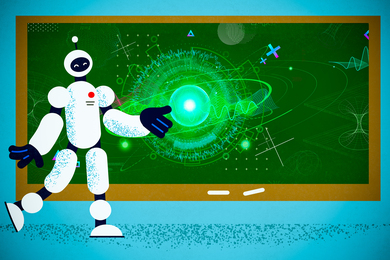
Scientists use generative AI to answer complex questions in physics
Read full story →
Elaine Liu: Charging ahead
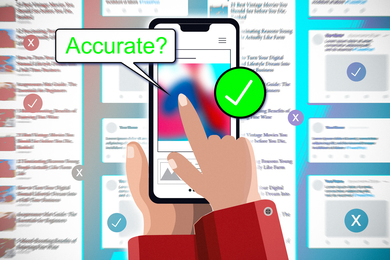
New tool empowers users to fight online misinformation
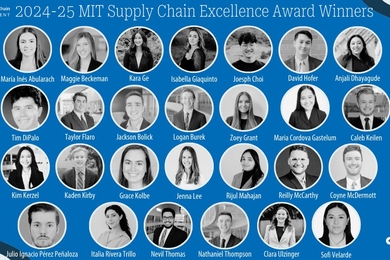
2024 MIT Supply Chain Excellence Awards given to 35 undergraduates
Faces of MIT: Reimi Hicks
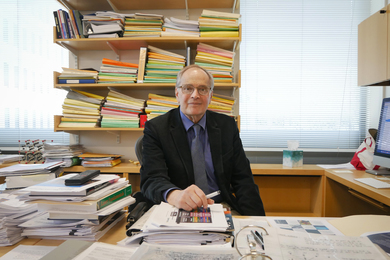
John Joannopoulos receives 2024-2025 Killian Award
- More news on MIT News homepage →
Massachusetts Institute of Technology 77 Massachusetts Avenue, Cambridge, MA, USA
- Map (opens in new window)
- Events (opens in new window)
- People (opens in new window)
- Careers (opens in new window)
- Accessibility
- Social Media Hub
- MIT on Facebook
- MIT on YouTube
- MIT on Instagram
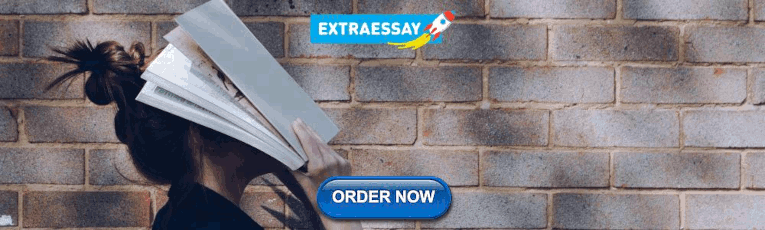
IMAGES
VIDEO
COMMENTS
In addition to industrial requirements, water supplies for many geographic locations are dwindling at an alarming rate. MENA region is home to over 6 % of the world population but has <2 % of the global freshwater resources [25].Access to fresh water is a perennial quest in Middle Eastern states; hence, water scarcity is considered a security risk for the entire region [26].
Desalinated water can be produced by using brackish water (salt content ≤ 10,000 mg/L) or seawater (salinity of 25,000 − 45,000 mg/L). Brackish water is defined as water that exist naturally in the environment (e.g. in estuaries and lakes) typically with a salt content of 1000 − 10,000 mg/L [2]. Given that seawater is practically ...
A portable seawater desalination system would be highly desirable to solve water challenges in rural areas and disaster situations. While many reverse osmosis-based portable desalination systems are already available commercially, they are not adequate for providing reliable drinking water in remote locations due to the requirement of high-pressure pumping and repeated maintenance. We ...
Her research explores high-performance sodium-ion battery materials, novel technologies for battery recycling, and water remediation. Lei Wang is a doctoral student under the supervision of Prof. Volker Presser at the Department of Materials Science and Engineering, Saarland University, and the INM - Leibniz Institute for New Materials in ...
Brine (concentrate) from desalination plants contains large quantities of minerals, enriched in concentration compared to sea water—thus the figures appearing in Table 2 could be multiplied by a ...
The globally and annually-averaged surface ocean pH T2000 in the Atlantic, Pacific, and Indian Oceans (60°N to 60°S) is 8.07 ± 0.02 (1σ uncertainty on gridded values, see Methods), 8.06 ± 0. ...
1 Introduction. Although fresh water is essential to maintain human life, the world is facing a global and domestic challenge to reliably supply its population with fresh and safe water, owing to shortages resulting from global population growth, 1-3 climate change, 4-7 contamination of clean water supplies, 8 and public policy. 9, 10 Water is also needed to produce hydrogen as a clean and ...
Key words: sea-water, solar power, desalination methods. Discover the world's research. 25+ million members; ... Sea-water Desalination in GCC Research Paper. July 2023. Abdelaziz Saafan;
Obtaining energy from renewable natural resources has attracted substantial attention owing to their abundance and sustainability. Seawater is a naturally available, abundant, and renewable resource that covers >70% of the Earth's surface. Reserve batteries may be activated by using seawater as a source of electrolytes. These batteries are very safe and offer a high power density, stable ...
Abstract and Figures. Sea water desalination is a process that separates saline water into two major components, the low dissolved salts concentration water stream (fresh or potable water) and the ...
a, c The sea level and T100 annual cycles, respectively, for ORA-S5 (black) averaged over the 1° ocean grid box nearest the Virginia Key tide gauge (purple; 25.7°N, 279.8°E). The seasonally ...
sea water that is able to generate el ectrical energy, where seawater is a compound o f NaCl, sea. water is a solution of electrolyte with bigg est solute. From the results of research cond ucted ...
The two basic factors are change of total volume of sea water, and movements of ground and ocean bottom that result in changes to the size and shape of the ocean basins. ... The focus of this paper is the change in mean sea level which took place from 100 years ago to the end of 21st century, a much shorter temporal scale compared with the ...
To date, one-step seawater electrolysis and two-step seawater electrolysis are the two main routes for the production of green hydrogen using seawater electrolysis (Figure 1).Some researchers believe that it is necessary to directly use seawater electrolysis to produce H 2, and the electrolyzer that can directly use seawater as a water source should be developed 8••, 9••, 10.
Prof. Jongyoon Han and research scientist Junghyo Yoon have developed a new portable desalination device that can deliver safe drinking water at the push of a button, reports Meghan Gunn and Kerri Anne Renzulli for Newsweek.The device "requires less power than a cell phone charger to run and produces clean drinking water that exceeds World Health Organization standards," writes Gunn and ...
The UN's recent announcement of a Decade of Ocean Science provides a glimmer of hope, but scientists will need to work closely with decision-makers and society at large to get the ocean back on ...
Explore the latest full-text research PDFs, articles, conference papers, preprints and more on SEAWATER ANALYSIS. Find methods information, sources, references or conduct a literature review on ...
The authors annually evaluated the reduction in the use of water (2.5 10 5 tons), nitrogen (5.6 NH 3 -N tons), and carbon emissions (134 tons) through the reuse of treated sewage. Advances in the management of solid waste and wastewater treatment include landfilling, the composting of organic matter, reductions in greenhouse gas emissions and ...
This paper presents the investigation of groundwater vulnerability to seawater intrusion of the aquifer system in the coastal area of Laspias River, NE Greece, for the year 2023, by applying the GALDIT, SITE and SIVI methods, in the context of the groundwater management of the area. The relevant research works include the collection and analysis of data and information regarding the geological ...
In this paper the effect of temperature, pressure, and salinity on the total attenuation coefficient for seawater containing a suspension of solid particles is investigated by employing suitable ...
The seawater intake systems upgrades would be capable of taking 11.8 million gallons of water per day out of the bay. The bolstered equipment would facilitate the operation of the proposed Nordic A…
Wang adds, "This research has engineered a sensor capable of instantaneously detecting lead concentration in water. This can be utilized in real-time to monitor the lead pollution concentration in wastewater discharged from industries such as battery manufacturing and lead smelting, facilitating the establishment of industrial wastewater ...
A water desalination system is a crucial technology designed to address water scarcity by converting seawater (SW) or brackish water (BW) into freshwater suitable for various uses, such as ...
Scientific collaborations with France and Spain dominate Moroccan water research with 59.5% of all the scientific output when there is a lack of co-operations between Moroccan institutions and ...