Academia.edu no longer supports Internet Explorer.
To browse Academia.edu and the wider internet faster and more securely, please take a few seconds to upgrade your browser .
Enter the email address you signed up with and we'll email you a reset link.
- We're Hiring!
- Help Center
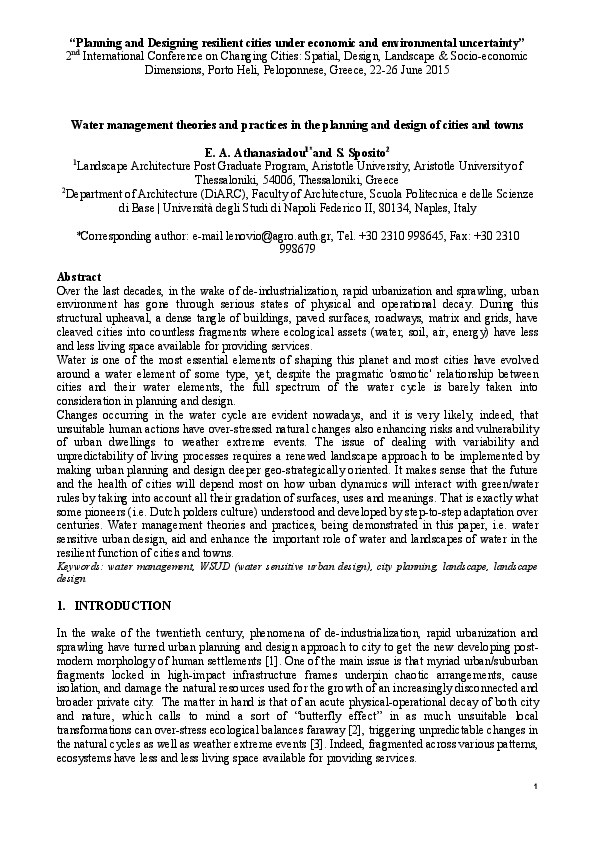
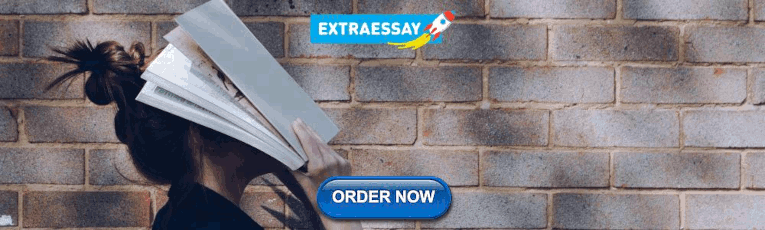
Water management theories and practices in the planning and design of cities and towns

Over the last decades, in the wake of de-industrialization, rapid urbanization and sprawling, urban environment has gone through serious states of physical and operational decay. During this structural upheaval, a dense tangle of buildings, paved surfaces, roadways, matrix and grids, have cleaved cities into countless fragments where ecological assets (water, soil, air, energy) have less and less living space available for providing services. Water is one of the most essential elements of shaping this planet and most cities have evolved around a water element of some type, yet, despite the pragmatic 'osmotic' relationship between cities and their water elements, the full spectrum of the water cycle is barely taken into consideration in planning and design. Changes occurring in the water cycle are evident nowadays, and it is very likely, indeed, that unsuitable human actions have over-stressed natural changes also enhancing risks and vulnerability of urban dwellings to weather extreme events. The issue of dealing with variability and unpredictability of living processes requires a renewed landscape approach to be implemented by making urban planning and design deeper geo-strategically oriented. It makes sense that the future and the health of cities will depend most on how urban dynamics will interact with green/water rules by taking into account all their gradation of surfaces, uses and meanings. That is exactly what some pioneers (i.e. Dutch polders culture) understood and developed by step-to-step adaptation over centuries. Water management theories and practices, being demonstrated in this paper, i.e. water sensitive urban design, aid and enhance the important role of water and landscapes of water in the resilient function of cities and towns. Keywords: water management, WSUD (water sensitive urban design), city planning, landscape, landscape design
Related Papers
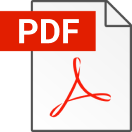
Jochen Eckart
Green Energy and Technology
Joan Marull , Carlos Smaniotto Costa
Sustainability Today
Jorge Prodanoff
SU RİSKİNİN ÖNLENMESİNDE KENTSEL PEYZAJIN ROLÜ
Sarıyya Rahımbaylı , Sümeyye KAHRAMAN
Flood and drought problems have an important place in Turkey as well as on a global scale. Drought and flooding have emerged over the past two decades as the two extremes of the most devastating hydrological spectrum of the climate crisis. These two extremes have caused approximately 600 billion dollars of damage since the beginning of the twenty-first century, affected 3.5 billion people, and caused material and moral losses on a large scale. According to the recently published reports of the Intergovernmental Panel on Climate Change (IPCC), changes in the hydrological cycle caused by climate change will be stronger, leading to longer-lasting droughts and floods. These problems also present the Anthropocene Age as the age when the most important decisions about our future will need to be taken. Detailed studies and meetings are held at global, national and local levels in order to manage disasters in the future. When viewed from a general perspective, natural disasters, which are considered as the main dynamics, and the issues that cause them (such as global urbanization, ecological crises, etc.) can be prevented by many interdisciplinary studies; scientific knowledge, ecological and holistic perspective, creation of flexible/resilient infrastructure, methods that can be adapted according to time and physical situation are all in the direction of minimizing the experienced crises and disaster effects. Landscape discipline also has an important place in the prevention of disasters. Landscape forms the layers of social, societal, ecological services and the transition between everyday experiences and wildlife. At the same time, it plays an effective role in the repair of the destruction and damaged ecosystem parts in cultural and natural areas where human lives are located. Urban landscape analyzes are effective in this repair, especially in the prevention of the destruction that occurs / will occur in the settlements where disasters intensify, or in the post-destructive renewal. There are important solutions to reduce the water risk, which is one of the biggest problems of the climate crisis; landscape designs in sponge cities, technological solutions positioned to prevent floods, permeable materials that can clean and absorb flood water, adoption of xeric landscape design in urban areas, etc. The aim of this study is to understand the role of urban landscape design examples used in preventing water risk in domestic and foreign literature, within the framework of modern technological analysis, and to understand their role in preventing water risk.
Journal of Urban Research
Nouran M O U S T A F A Mohamed
The 21st century is the century of City and Urbanization. As urban communities spread and populations grow, the impact on the natural environment escalates. The hydrological cycle is among the environmental aspects most impacted by urbanization. Consequently, this study suggests a “Water Sensitive Urban Design Tool” that comprises three dimensions: (i) determining the specific issues facing residential water demand; (ii) identifying the factors influencing these issues; and (iii) the relevant water sustainability objectives and criteria. The WSUD tool could be utilized by urban designers and planners to assess the water performance of residential urban areas. It could be employed to evaluate current conditions, suggested retrofitting options, or Greenfield developments. Thus, the tool not only measures the water sensitivity of residential areas but could also support decision-making and guide development. To evaluate the suggested WSUD tool and examine its suitability to the Egyptia...
Philipp Staufer
Since the beginning of industrialization urban areas have been rapidly growing. Recently, a paradigm shift has become noticeable, away from undamped economic and city growth towards more sustainable solutions. However, in the light of numerous negative trends of urban development there is still a bride gap between the ideal of sustainable development and reality. We suggest a far-reaching redefined approach of city planning predominantly focusing on water. By merging both modern urban water management and city planning we shaped a convincing idea which provides both a positive guiding principle and an operational framework including concrete measures which will considerably facilitate decision making processes. Thus, the suggested sector approach of water oriented city planning (WOCP) has the promising potential to be the key driver for an overall sustainable development of urban areas. WOCP includes a comprehensive bundle of different measures such as modern rainwater management, innovative urban surface design, new greening concepts, urban rainwater harvesting and water reuse, an urban hydraulic cross-linking system, and finally completely new types of housing and architecture. However, the described approach has to be sustained by an integrated and trans-disciplinary network of all relevant stakeholders as well as by further financial and legislative measures and instruments.
Matjaž Glavan
Lariyah Mohd Sidek
RELATED PAPERS
Revista de historiografía
Csaba Szabó
International Journal for Research in Applied Science & Engineering Technology (IJRASET)
IJRASET Publication
Uluslararası Sosyal Bilimler Dergisi
International Journal of Social Sciences
Universitas Philosophica
Alfonso Flórez
Journal of Gastroenterology
Syed Morshed
International Journal of Environmental Research and Public Health
Kath Peters
Connotations
Hannes Bergthaller
Journal of Clinical Psychology
Heike Gerger
Mikaail Kavanagh
Transactions of the Society of Instrument and Control Engineers
Hiroyuki Ukai
Journal of Economic Modeling Research
Economic Modeling Research
Századvég Publishing House
Arnis Vilks
Bonner Jahrbücher
Harald Floss
Physical Review B
Sotirios Baskoutas
Ibnu Maliki
Investigar la Comunicación desde Perspectivas, Teorías y Métodos Periféricos
Eugenia Isidro
Tidsskrift for Den norske lægeforening
Morten Vatn
Journal of Craniofacial Surgery
Jamie Perry
Arthritis & Rheumatism
vibeke strand
Mariam Saleem
Ahmed Faramawy
ffgfh jkjkj
RELATED TOPICS
- We're Hiring!
- Help Center
- Find new research papers in:
- Health Sciences
- Earth Sciences
- Cognitive Science
- Mathematics
- Computer Science
- Academia ©2024
- Tools and Resources
- Customer Services
- Agriculture and the Environment
- Case Studies
- Chemistry and Toxicology
- Environment and Human Health
- Environmental Biology
- Environmental Economics
- Environmental Engineering
- Environmental Ethics and Philosophy
- Environmental History
- Environmental Issues and Problems
- Environmental Processes and Systems
- Environmental Sociology and Psychology
- Environments
- Framing Concepts in Environmental Science
- Management and Planning
- Policy, Governance, and Law
- Quantitative Analysis and Tools
- Sustainability and Solutions
- Share This Facebook LinkedIn Twitter
Article contents
Water resource management: challenges and opportunities with game theory approaches.
- Kim Hang Pham Do Kim Hang Pham Do Massey University
- https://doi.org/10.1093/acrefore/9780199389414.013.763
- Published online: 24 February 2022
Water is essential to life and development in terms of both quantity and quality. Water resources continue to face various pressures brought about by climate change, growing population, and increased economic demand for water. Managing this unique and precious resource has become a global challenge. The conflicts over water issues often arise not only among stakeholders facing limited water resources but also from social and political aspects of the design, operation, and management of water supply projects. A fair and sustainable system of sharing water resources, therefore, is one of the greatest challenges we face in the 21st century. In the absence of negotiation and lack of clear property rights, water is a source for human conflicts.
Game theory as strategic analysis has provided powerful tools and been applied to many fields, including water resources management. The basic assumptions of game theory emphasize that rational players who pursue well-defined objectives and assume knowledge of others would accordingly form expectations of other decision makers’ behavior. Hence, game theory is used to predict agents’ behaviors toward fulfilling their own interests during the interactive decision-making process with other agents.
Since the 1950s, game theory has become an important tool for analyzing important aspects of water resource management. Yet despite the rapid increase in the application of game theoretical approaches to water resource management, many challenges remain. The challenges of the early 21st century, including resource constraints, financial instability, inequalities within and between countries, and environmental degradation, present opportunities to address and reach resolutions on how water is governed and managed to ensure that everyone has sufficient access to water.
- water resource management
- game theory models
- strategic analysis
- coalitional structures
- fair solutions
- sustainable development
You do not currently have access to this article
Please login to access the full content.
Access to the full content requires a subscription
Printed from Oxford Research Encyclopedias, Environmental Science. Under the terms of the licence agreement, an individual user may print out a single article for personal use (for details see Privacy Policy and Legal Notice).
date: 18 May 2024
- Cookie Policy
- Privacy Policy
- Legal Notice
- Accessibility
- [66.249.64.20|185.148.24.167]
- 185.148.24.167
Character limit 500 /500

An official website of the United States government
The .gov means it’s official. Federal government websites often end in .gov or .mil. Before sharing sensitive information, make sure you’re on a federal government site.
The site is secure. The https:// ensures that you are connecting to the official website and that any information you provide is encrypted and transmitted securely.
- Publications
- Account settings
Preview improvements coming to the PMC website in October 2024. Learn More or Try it out now .
- Advanced Search
- Journal List
- EPA Author Manuscripts

Reliability theory for microbial water quality and sustainability assessment
Allen teklitz.
a Department of Chemical and Environmental Engineering, University of Cincinnati, OH, USA
Christopher Nietch
b United States Environmental Protection Agency, Office of Research and Development, Cincinnati, OH, USA
M. Sadegh Riasi
Lilit yeghiazarian, associated data.
Microbial surface water contamination can disrupt critical ecosystem services such as recreation and drinking water supply. Prediction of water contamination and assessment of sustainability of water resources in the context of water quality are needed but are difficult to achieve – with challenges arising from the complexity of environmental systems, and stochastic variability of processes that drive contaminant fate and transport. In this paper we use reliability theory as a framework to address these issues. We define failure as exceedance of regulatory water contamination limits, and system components as reaches in the surface water network. We then methodically study the reliability of each component in the context of water quality, as well as the impact of individual components on overall water quality and sustainability. We obtain spatially distributed probability- and physics-based sustainability measures of reliability, vulnerability, resilience and the sustainability index. Finally, we use GIS as a platform to present these measures as geospatial products in an effort to foster public acceptance of probability-based methods in contaminant hydrology.
1. Introduction
As human impact on the environment increases, sustainable management of water resources and security of water quality has emerged as a top national priority. In the US, the leading cause of surface water impairment is fecal microbial contamination ( US EPA, 2008 ), which can potentially trigger massive outbreaks of gastrointestinal disease and thus presents a serious threat to public health ( Curriero et al., 2001 ). As a result, the imperative to understand processes governing microbial sources and dynamics in watersheds has emerged as part of the regulatory process both in the US and EU ( Kay et al., 2007 ). This knowledge is expected to translate into more accurate predictions of water contamination, and can be used as a foundation for development of physics-based sustainability metrics and, subsequently, water management strategies.
It is well known that the difficulties in understanding water contamination are rooted in the stochastic variability of contaminant fate and transport, and in the complexity of environmental systems ( Bertuzzo et al., 2007 ; Tyrrel and Quinton, 2003 ). The problem of stochastic variability that results from the inherent randomness of process parameters or drivers, is often addressed through Monte Carlo simulation of deterministic models, as commonly done in watershed modeling ( Mitsova-Boneva, 2007 ; Narasimhan et al., 2017 ; Sohrabi et al., 2003 ; Sparkman et al., 2017 ; Zheng and Han, 2016 ), or through fully stochastic approaches ( Lisle et al., 1998 ; Yeghiazarian and Samorodnitsky, 2013 ). To handle system complexity, these methods should be employed in systems frameworks ( Csete and Doyle, 2002 ; Harris and Heathwaite, 2012 ; Van Looy et al., 2015 ), that explicitly take into account the connectivity and interactions among individual components.
Similar challenges arise across multiple engineering fields, where reliability theory has emerged as a powerful instrument for component- and system-level analysis under uncertainty (see for example Abrishamchi et al., 2005 ; Franceschini and Tsai, 2008 ; Garrick et al., 1967 ; Hamed and El-Beshry, 2004 ; Jiang et al., 2015 ; Maier et al., 2001 ; Ng and Eheart, 2005 ; Park et al., 2008 ; Sahner et al., 1996 ; Sitar et al., 1987 ; Tartakovsky, 2013 ; 2007 ;; Thorndahl et al., 2008 ). System-level reliability theory addresses both the stochasticity of system processes, and the complexity and interconnectedness of system components. The former is achieved through modeling system processes as functions of random variables; and the latter – by analyzing the relationships and interactions among system components.
Reliability theory is used to analyze failure of complex, multi- component engineered systems, with failure typically defined as exceedance of safety thresholds ( Hoyland and Rausand, 1994 ). It can be employed both at the individual component and system levels, and aims to answer questions like “what is the probability of system failure?”, and “failure of which individual components is more likely to trigger system failure?”. In order to answer these questions, one must identify specific functions that a system, and any of its components, must perform. Failure at the component- or system-level is then defined as inability to perform these functions; and reliability theory provides a means for systematic assessment of failure.
Each function that a component is expected to perform is suitably described with its performance function . The performance function is used to compute the reliability of the component, and, if needed, its vulnerability and resilience. While failure in an engineered system is defined as inability to satisfy some performance criterion or function, in the context of water quality, failure can be defined as exceedance of regulatory thresholds of water contamination ( Jang et al., 1994 ; Park et al., 2008 ; Sitar et al., 1987 ); hence the respective performance function should comprise the mathematical model of contaminant fate and transport.
In environmental engineering problems, reliability theory has been applied to processes described by one performance function ( Abrishamchi et al., 2005 ; Franceschini and Tsai, 2008 ; Hamed and Bedient, 1997 ; Hamed and El-Beshry, 2004 ; Jang et al., 1994 ; Maier et al., 2001 ; Ng and Eheart, 2005 ; Park et al., 2008 ; Riasi et al., 2018 ; Sitar et al., 1987 ; Thorndahl et al., 2008 ; Wagner and Gorelick, 1987 ), essentially implementing a lumped, single component-level reliability analysis. In this paper, we address microbial contamination in surface water networks, which are geospatially distributed systems. Hence, we move away from the lumped approach towards a multi-component, distributed analysis. This allows for understanding of spatial distribution of risk and reliability. It also provides the ability to evaluate the impact of individual components on system failure.
In watersheds, streams and rivers are conduits for microbial activity and transport ( Campbell Grant et al., 2007 ), consequently stream reaches are formalized as the links of a watershed’s flow network. In reliability theory, each link can be represented as a single component with its own performance function. Individual component reliabilities can then be easily computed, as well as their impact on water quality at locations of strategic importance. Thus, moving from a lumped to a multicomponent, distributed representation of watersheds, in addition to general questions such as “what is the probability that water contamination will exceed a safety threshold?”, reliability theory can answer questions like “what are the specific areas in a watershed that will trigger the exceedance of contaminant safety threshold?”, “what is the spatial distribution of probability of failure in the entire watershed?”, and “which areas of the watershed would be most sensitive/responsive to implementation of management measures?”
Furthermore, translated into sustainability terms , reliability theory can answer questions like “what is the resilience of any particular area in the watershed, i.e. how quickly microbial concentration in the water at a given location will drop to safe levels”, and “how vulnerable is the system, i.e. how severe was water contamination at a given location and time?” ( Maier et al., 2001 ; Sarang et al., 2008 ). In essence, water resources sustainability defined as a function of reliability, vulnerability, and resilience ( Hashimoto et al., 1982 ; Loucks, 1997 ), can be analyzed using reliability theory.
Earlier studies on application of reliability concepts and reliability-based sustainability metrics to water quality problems have focused primarily on dissolved oxygen (DO) violations ( Maier et al., 2001 ; Sarang et al., 2008 ). More recently, reports have emerged on application of reliability, resilience and vulnerability concepts to sediments and chemical contaminants ( Hoque et al., 2016 ; 2012 ). To our knowledge, our previous study ( Park et al., 2008 ) and the present paper are the only applications of reliability theory and reliability-based sustainability analysis to microbial contamination of surface water. Along with the companion that focuses on water contamination with nutrients ( Teklitz et al., 2020 ), this paper presents a geospatial application of reliability theory to water resources management and sustainability. We call this tool STREAMS (STochastic Reliability-based Risk Evaluation And Mapping for watershed Systems and Sustainability).
Recent discussions on adoption of probability-based methods in contaminant hydrology highlighted the need to use conceptually simple modeling frameworks. Reliability theory is simple to explain and implement, and, for the sake of clarity, we have limited the physics of microbial fate and transport to a simple model (that can be later expanded as discussed in section 4 ). So, in this paper we have three main goals. First is to present reliability theory for distributed microbial surface water quality and sustainability at the watershed scale. This approach provides insight into the importance of individual components and their impact on water quality and sustainability at locations of strategic importance in the watershed, as well as the dependencies between physically distinct and distant watershed components. It also allows to obtain the spatial distribution of environmental sustainability metrics, such as reliability, resilience, vulnerability and sustainability index ( Hashimoto et al., 1982 ).
Our second goal is to derive these metrics from a stochastic, physics- based model of contaminant fate and transport. A stochastic physics-based approach serves two purposes: (1) it yields insight into the explicit relationship between governing processes and sustainability, and (2) it provides a foundation for reliability theory development and therefore for probability- and physics-based sustainability metrics.
The third goal is to facilitate watershed decision-making. To do so we have implemented the modeling framework in a Geographic Information System (GIS). The watershed is represented as a schematic network within ArcGIS. The ArcGIS Schematic Processor tool ( Whiteaker et al., 2006 ) is then used to model E. coli fate and transport in the flow network ( section 2.1 ). The Schematic Processor being part of the ArcGIS suite makes it a particularly attractive modeling platform, as the wealth of geospatial environmental information contained in ArcGIS becomes available to the modeler immediately, and also because its output is easily ingested in ArcGIS for visualization. We present results as visual geospatial products in the form of maps.
In what follows we apply reliability theory to water quality and sustainability in the context of microbial contamination, and demonstrate it in the case study of a watershed near Cincinnati, OH. We discuss further how systems reliability theory could be used to inform watershed management strategies, and propose additional lines of investigation to enhance its current capabilities. Reliability analysis is presented in section 2.2 , and sustainability metrics in section 2.3 . In each section, we consider relevant water quality and sustainability questions that can be answered in the framework of reliability theory. We demonstrate the application of STREAMS in a case study ( section 3 ). Discussion is presented in section 4 , and conclusion in section 5 .
2. Methodology
2.1. contaminant transport model in gis.
Watersheds can be characterized within GIS as a schematic network – a graphical representation of connectivity between features and landscape through a network of nodes and links. Each node represents a given feature such as a sub-watershed or catchment; links symbolize streams, channels or slopes, and represent, in other words, microbial transport routes ( Safwat et al., 2012 ; Whiteaker et al., 2006 ). Nodes are placed (i) at the centroid of every catchment, (ii) at junctions where reaches meet, and (iii) at the beginning and end of every reach. Then links are placed to connect the nodes. A node can also be placed to represent a point source contribution with a link connecting it to the network.
The ArcGIS Schematic Processor tool developed by Whiteaker et al. (2006) is based on the schematic network representation and can be used to model many watershed processes, including contaminant fate and transport ( Johnson et al., 2013 ; Tavakoly et al., 2016 ). This is achieved by assigning different “processing” behaviors to nodes and links that simulate hydrologic processes and contaminant transport in the network. Catchment nodes are used for generating contaminant loads based on catchment properties including area and land uses, and their associated links only pass the load to the subsequent stream node. Stream links are assigned the transport features such as decay. At junction nodes the processor adds up the upstream values.
Contaminants such as E. coli – the subject of this study, can be assumed to travel according to the first-order decay model ( Johnson et al., 2013 ):
where L is load defined as product of flow Q and concentration C ; λ is the first-order decay rate; t = S V is the travel time defined as stream length S divided by flow velocity V . Flow velocity is a function of flow, as described in section 3.2.5 ; L 0 is the initial input load:
where L NPS is the non-point source load, L PS is the point source load, and L u is the incoming upstream load.
To model non-point sources of contamination in the watershed, specific export coefficients are associated with each land use type as delineated by National Land Cover Database (NLCD 2011 – The Multi-Resolution Land Characteristics (MRLC) Consortium – www.mrlc.gov ). The input load from each catchment can then be calculated using:
where ϵ j is the export coefficient (load per area per year) for the respective land use of area A j within the catchment. Flow is calculated similarly: at each node the flows from upstream links are added and passed down to the downstream node. The streamflow contribution from each catchment is computed at the centroid node of the catchment and added at stream nodes.
Export coefficients, first-order decay rate, flows and travel times are random variables, therefore the output load is random as well. We performed Monte-Carlo (MC) simulations to produce realizations of contaminant loads in the case study watershed ( Section 3.2 ), which were then analyzed in the reliability framework as outlined in Section 3.3 .
2.2. Reliability analysis
In reliability theory, reliability is defined as the probability of successful performance of a given system under stressing load ( Haldar and Mahadevan, 2000 ). The maximum load under which the system is able to perform in a satisfactory manner, is called resistance, or capacity. Reliability analysis quantifies the likelihood of satisfactory system performance, and produces the probability that the total stressing load does not exceed resistance.
A reliability formulation usually involves a performance function g ( Y ) that describes the behavior of a certain process or a system and its relationship with resistance, where Y is the vector of relevant random variables. Failure in reliability problems is formulated in terms of exceeding the safety limit imposed by the system’s quality requirements. Mathematically, the system is in failure domain when g ( Y ) is negative, and in safe domain when g ( Y ) is positive. The failure, or the limit-state, surface defined as g ( Y ) = 0 can then be described as the boundary between the safe and failure domains of the system’s parameter space ( Haldar and Mahadevan, 2000 ; Sitar et al., 1987 ).
In the case of water contamination, the performance function can be written as
where L is the contaminant load calculated from equation (1) , and R is system resistance:
Here, Q is the flow and C c is the contaminant’s critical safety concentration limit, usually determined through the regulatory process. Then, the concept of failure in water quality problems can be formulated as contaminant load exceeding an established safety limit at the location of interest. The probability of failure is given as
where p f is the probability of failure. Reliability is defined as
Links in the schematic network can be thought of as system components. Here we analyze reliability of individual components, and seek answers to questions such as: “what is the spatial distribution of probability of exceeding contaminant standards in the watershed, or what are the failure probabilities of individual streams?” ( section 2.2.1 ), and “what are the areas that impact the probability of water contamination at strategic locations elsewhere in the watershed?” (conditional probabilities in section 2.2.2 ).
2.2.1. Component-level reliability
Let us say that each link i corresponds to component X i . Then the reliability of an individual component ( X i ) is calculated from the MC output as:
where X i S is the event of component X i functioning satisfactorily, or being in the safe domain, i.e. X i S ≡ g i ( Y ) > 0 , and P ( X i S ) is the corresponding probability. It is easy to compute the respective probabilities of failure, using equation (7) :
where X i F is the event that component X i fails, i.e. X i F ≡ g i ( Y ) < 0 , and P ( X i F ) is the probability of that event. Values of P ( X i F ) , computed for every link X i , can be mapped in ArcGIS to show the spatial distribution of probability of water contamination.
2.2.2. System-level conditional reliability
2.2.2.1. analysis conditioned on failing components..
The reservoir at the outlet of the watershed serves as a source of drinking water, and is a regional recreational resource. Therefore, in this case failure at the outlet can be defined as system failure. We are interested in the impact of upstream failures on the reservoir. This translates into the question “What is the probability that the outlet fails given failure of a specific link?”, or mathematically,
We compute this probability from the MC output. In practical terms, answering this question helps assess the importance of monitoring information from upstream areas of the watershed. For instance, if water samples collected at stream i show elevated levels of contaminants, then how likely is the outlet failure? In addition, one could determine which upstream streams should be monitored to potentially provide an early warning of the reservoir contamination.
2.2.2.2. Analysis conditioned on functioning components.
Similarly, we can ask the complementary question “What is the probability that the outlet fails if a given link has not failed?”, or mathematically,
In other words, if a monitoring station at stream i is measuring contaminants within the regulatory limits, what is the probability of having unsafe levels of contamination at the outlet?
Questions symbolized in equations ( 10 – 11 ) can be easily posed for any location in the watershed that is of interest due to its recreational or strategic value, such as proximity to uptake points for water treatment facilities, or various ecosystem functions.
2.3. Sustainability metrics
Results of reliability theory described in Section 2.2 form the foundation of sustainability analysis. We compute three metrics: reliability, vulnerability and resilience that provide measures of frequency, magnitude and duration of failure, i.e. water contamination. The sustainability index (SI) is a composite of these metrics.
2.3.1. Reliability
Reliability ( α ) is defined as the probability that the load on the system does not exceed its resistance, and is a complement of probability of failure. Reliability is computed from equation (7) , with the E. coli safety concentration limit C c of 126 CFU/100 ml for primary contact ( Ohio EPA et al., 2018 ), and represents the reliability of water quality in individual streams and at the watershed outlet.
2.3.2. Vulnerability
Vulnerability ( v ) is a measure of how likely a system is to experience a severe or critical failure. It is often of interest to examine the probability of a critical, or catastrophic, failure, which is typically determined by failure of a large magnitude or by severity of its impact on the system. As such, it can be specified by the watershed manager, or defined in terms of impact, e.g. a beach must be shut down due to water contamination. This is a very useful concept because it offers a way to compute reliability as a continuous, rather than dichotomous, measure.
This paper uses a critical failure level of 1030 CFU/100 ml, which is the EPA’s standard for secondary contact with E. Coli ( Ohio EPA et al., 2018 ). Vulnerability is then simply equals to the probability that the load exceeds the critical value ( Hashimoto et al., 1982 ; Maier et al., 2001 ). Vulnerability can be computed as:
where R iCritical is the critical load for component X i . Vulnerability ranges from zero to one, and the larger the value, the more vulnerable is the system to critical failure.
2.3.3. Resilience
Resilience is a measure of the system’s ability to recover from failure. In the classical interpretation of resilience in reliability theory, it is the probability that the system in failure domain at some point in time, will be in safe domain within a single time step ( Hashimoto et al., 1982 ). One interpretation of this definition for water quality is the probability that the level of water contamination becomes compliant within a single time step following an acute contamination event. Our focus in this paper is chronic contamination as opposed to single acute events; therefore, we take a slightly different interpretation. Best management practices (BMPs) are traditional means of reducing chronic water contamination. Hence we ask what is the probability that water quality will become compliant after application of BMPs, or, how much closer the system moves to compliance once in failure domain. Mathematically, it can be written as:
where γ i is the resilience of component X i . Resilience interpreted in this manner becomes a measure of the system’s ability to respond to human intervention, and highlights the areas of the watershed that would be most receptive to implementation of management measures. It can be shown that the system’s reliability after BMP implementation is
2.3.4. Sustainability index
The sustainability index (SI) is a composite measure that captures several sustainability metrics in a meaningful way, and that can be used to compare water bodies, guide water management and measure the improvement ( Loucks, 1997 ; Sandoval-Solis et al., 2011 ). The SI, in other words, is designed to help watershed managers make decisions that benefit the present and the future of the watershed through sustainable development. A classical definition of SI is ( Loucks, 1997 ; Sandoval-Solis et al., 2011 ):
In general, the SI can measure sustainability of different aspects in water resources management. Since in this study the sustainability metrics are defined in terms of water contamination, the SI represents sustainability of water resources in the context of water quality. As the values of sustainability metrics range from zero to one, and as the SI has a multiplicative form, the metric with the smaller value inherently has larger weight ( Sandoval-Solis et al., 2011 ). Hence, posing the measure of water quality sustainability in the form of equation (14) helps identify which primary metrics have the highest impact on the overall sustainability of the system.
3. Case study
3.1. study area.
This methodology is applied to a subwatershed within a mixed-use East Fork of the Little Miami River watershed in southwest Ohio. The selected area, called GRR, measures approximately 18 km 2 and drains downstream into a reservoir built in the 1970s for flood control. The reservoir serves as a source of drinking water, and is a regional recreational resource. Water quality was monitored for E. coli at four sites from May 2010 to May 2014. The annual geometric average of E. coli during this period is reported to be ~530 CFU/100 ml at the outlet of sub-watershed. In this study, we use this value to calibrate the schematic processor. GRR and its schematic network are shown in Fig. 1 .

(A) GRR subwatershed and land use coverage. (B) GRR schematic network in the ArcGIS Schematic Processor. (C) Schematic network of streams in GRR. Component IDs are shown in square brackets.
3.2. Model parameters
3.2.1. streamflow.
To estimate the streamflow in ungauged streams in GRR, streamflow contribution from each catchment ( i.e. drainage area) is assumed to be lognormally distributed throughout the year ( Granato, 2010 ) and its moments are estimated from regression equations developed by Koltun and Whitehead, (2002) :
where μ Q is mean annual flow; P 25, Q and P 75, Q are the 25th- and 75th-percentile annual streamflows for each catchment (in cubic feet per second); A is catchment area in square miles: Lat c and Long c are the latitude and longitude of the basin centroid in decimal degrees (39.1643°,84.0090°); P c is the mean annual precipitation at the basin centroid in inches (42.24 in , usclimatedata.com ); W is percentage of contributing basin area covered by water and wetlands (assumed to be zero); and V g is streamflow-variability index at streamflow gaging stations (assumed to be one). Using equations ( 16 – 17 ), standard deviation of flow can be estimated as σ Q = P 75 , Q − P 25 , Q 1.34898 ( Wan et al., 2014 ).
3.2.2. First-order decay rate
The first-order decay rate λ is assumed to be normally distributed with the mean μ λ and standard deviation σ λ , of (30.5 ± 6.4) × 10 −3 hr −1 ( Garcia-Armisen and Servais, 2009 ). These values were reported for free E. coli in river water and at temperature of 20° C and they are well within the range of reported values in the literature.
To capture the temperature dependency of decay rate, the following equation is used ( Blaustein et al., 2013 ):
where λ T is the first-order decay rate at temperature T , λ 20° C is the first-order decay rate at 20° C and Q 10 is the fitting parameter measuring the rate of change of λ as temperature increases by 10° C . The value of Q 10 for river water is determined to be ~1.520 ( Blaustein et al., 2013 ). The annual water temperature distribution is assumed to be a truncated normal distribution of 15 ± 8.5° C with the minimum and maximum of 0° C and 30° C , respectively. In the absence of water temperature measurements at GRR, the recorded values for water temperature for two closest USGS stations (USGS sites 03254520 and 03228039) during 2010–2014 were used to arrive at this distribution.
3.2.3. Export coefficients
Although there have been studies to determine export coefficients for E. coli and fecal coliforms within United States ( Line et al., 2008 ; Vidon et al., 2009 ), to the authors’ knowledge, a comprehensive study on the effect of land-use on export coefficients is missing. For this reason, in this study we use export coefficients reported in Kay et al., (2008) for fecal coliforms in the United Kingdom.
The export coefficient values are assumed to attain a lognormal distribution for each land use type; their geometric mean, μ g , ϵ , and standard deviation, σ g , ϵ , were obtained from overall ( i.e. combined base- and high-flow) values reported in Kay et al., (2008) . Geometric standard deviations were estimated from 95% confidence intervals (95% CIs) provided in Kay et al., (2008) using log 10 ( σ g , ϵ ) = N 3.92 ( log 10 C I 95 % , u p p e r − log 10 C I 95 % , l o w e r ) . Table 1 summarizes the export coefficients used in this study for each land use. Although these export coefficients are calculated based on the data collected in the United Kingdom, its detailed land-use classification facilitate the cross-referencing with standard NLCD land use types. These values are used as uncalibrated initial values for E. coli export coefficients.
Uncalibrated export coefficients.
3.2.4. Correlation among model variables
With the moments provided above, one can generate random distributions of flow Q , export coefficients ϵ, and first-order decay rate λ . Assuming similar decay rate at all streams, a single set of uncorrelated samples is generated for normally distributed first-order decay rates. These values are then modified using Equation (18) to capture the temperature dependency of decay rates.
Export coefficients and flows are correlated, as both are driven by rainfall over a small watershed. To capture this dependency, a Gaussian copula ( Bee, 2011 ; Joe, 1997 ; Sklar, 1959 ) is used with a Spearman correlation coefficient of 0.53 ( Bushon et al., 2017 ). Since GRR is a small watershed, the correlation factor among different export coefficients, and among flow values was assumed to be equal to 1.
3.2.5. Flow velocity
The flow-velocity relationship is derived by fitting a regression line in the form of V = aQ b to the recorded annual average streamflow and velocity of the GRR streams in the US National Hydrography Dataset (NHDPlus, Version 2). Given the nine streams in GRR, the mean squared error regression results in
where V is a set of realizations of the stream velocity in feet per second. Similar regressions have been used in Zoun (2010) and Gibson (2006) . Given the stream length and flow velocity, one can then obtain a set of random travel time values from t = S V .
3.2.6. Model calibration
To determine the number of iterations in Monte Carlo simulation, a convergence analysis was performed and a total number of 10,000 iterations were chosen for this study. Our analysis showed that both the geometric mean and coefficient of variation of E. coli concentration at the outlet of watershed undergo less than 1% change for simulation with more than 9000 iterations.
Model calibration was performed by multiplying the export coefficients by a calibration factor of 0.0246. This value was selected to allow for an exact match between the geometric mean of measured E. coli concentration of ~530 CFU/100 ml in GRR, and its corresponding value from Monte Carlo simulations at the outlet. The resulting export coefficients ranged from 1.8 × 10 7 CFU/km −2 hr −1 to 8 × 10 8 CFU/km −2 hr −1 that were well within the range of reported values for E. coli in the United States ( Vidon et al., 2009 ).
3.3. Results
3.3.1. independent component reliability.
The schematic stream network of GRR and the labeled stream network are shown in Fig. 1B – C . Independent component probabilities of failure are computed using equation (9) and mapped in Fig. 2A . As shown in this figure, all streams in GRR are highly prone to failure, which is consistent with the measurements at monitoring stations. Since the watershed is small, the microbial decay does not contribute significantly to water contamination at the outlet. Rather, the variation observed in the probabilities of failure can be linked to the variation in the land use and area in the corresponding catchments, and to the number of catchments draining into individual streams. For instance, the difference between the probability of failure of link 4 (0.79) and link 9 (0.91) is likely due to the higher forest coverage of the catchment draining into link 4. In general, the probability of failure of the main stem (links 7, 5, 3 and 1) is higher than that of incoming links.

(A) Map of component probabilities of failure. (B) Map of probabilities of failure at GRR outlet given failing (left), and functioning (right) components.
3.3.2. Conditional reliability
Conditional analysis can be used as a way to probe the sensitivity of the outlet to individual components in the system, or put differently, it determines the relative significance of individual components on system failure. Probabilities of failure at the outlet given failing and functioning components are computed with equations (10) and (11) , respectively, and mapped in Fig. 2B . Each value in the maps represents the probability that the outlet fails given failure/functioning of respective components. For example, the probability that the outlet fails given that component 2 is functioning is 0.13. Given that component 2 has failed, the probability of outlet failure is 0.87. These values are complementary and add to 1.
Values within each map are comparable. As GRR is small, and model parameters are not independent, it comes at no surprise that the state of the outlet is highly correlated with that of all streams within the system. In general, failure of any component leads to high probability of failure at the outlet, particularly the main stem components 3, 5 and 7. First-order streams, like 8 and 9 provide the same information as the main stem, but most, like components 2, 4 and 6, somewhat differ. For instance, there is a 14% chance that the outlet will fail when contamination levels at link 4 are below the safety threshold. Hence, in this system monitoring the main stem is sufficient to accurately predict the state at the outlet.
3.3.3. Sustainability analysis
Sustainability metrics of GRR are mapped in Fig. 3 . The reliability map shows probabilities that individual components are in the safe domain. Values of reliability are complementary to probabilities of failure at the component-level analysis ( Fig. 2A ). Generally, reliability is low throughout the watershed. The outlet and the main stem links are about 0.1, and the highest reliability of 0.21 is at link 4.

Maps of sustainability metrics and index. The red-green scale is flipped based on the map to show green for preferable numbers, and red for numbers that are less desirable. For vulnerability, numbers close to 0 are preferable. For reliability, resilience, and the sustainability index numbers closer to 1 are preferable. (For interpretation of the references to color in this figure legend, the reader is referred to the web version of this article.)
The vulnerability map shows the probability of a critical, or catastrophic, failure, in this paper defined as exceeding the safety limit for secondary contact. GRR exhibits overall low vulnerability to catastrophic failure, with highest vulnerability at 0.28 in link 9.
The resilience map shows probabilities that system components will recover from failure after implementation of BMPs. Here we simulated the effects of blanket BMP implementation across the watershed, resulting in a 15% reduction of contaminant loading into the streams. Our results show that, given this particular measure, all links have somewhat similar (very low) chances of recovering from their failure states, ranging from 0.04 to 0.07. This indicates that this particular BMP will not be effective.
The sustainability index SI combines the reliability, vulnerability and resilience metrics into a single composite measure ( equation (14) ). In agreement with sustainability metrics, the SI map shows that streams along the main stem have the lowest index score, and link 4 the highest.
To evaluate the impact of a wider range of BMPs, we calculated the resilience and sustainability index of all streams as a function of BMPs defined as percent reduction in contaminant loading ( Fig. 4 ). The curves in Fig. 4A can be fitted with a functional relationship
with a high coefficient of determination ( R 2 > 0.99). Resilience is bound between zero and one, with zero at zero contaminant reduction; and one at maximum reduction of 100%. Fig. 4A – B show that blanket contaminant reduction at the same level across the entire watershed will be more impactful as percent reduction increases. Fig. 4C , based on equation (13b) , shows the effect of percent reduction in contaminant loading on reliability. Overall the response is similar across the streams, as expected in a small watershed. Note that the sustainability index ( Fig. 4B ) only reaches the maximum of ~0.4–0.57 after 100% contaminant reduction. This is because the sustainability index is a lump parameter that is comprised of the pre- and post-BMP condition of the watershed streams. These values could be improved if the pre-BMP reliability and vulnerability values of the streams were better. This however remains a challenge in chronically contaminated waters. If water quality continuously improves, a re-evaluation of SI would eventually yield a higher value.

Effect of BMPs on (A) resilience, (B) sustainability index SI. (C) The reliability of individual streams as a result of BMP implementation.
4. Discussion and directions for future work
4.1. reliability theory as a framework for water quality and sustainability analysis.
Over the last 20 years the hydrological sciences have been undergoing a transformation as the research community focused on complex societal challenges stemming from the global scale of human impact on the environment. With the realization that sustainable management of water resources requires deep understanding and integration of hydro- geomorphological, ecological, social, economic and other aspects of water, cross-disciplinary approaches are becoming a norm in hydrologic research. Further, systems-oriented frameworks have emerged as the paradigm to understand the complexity of dynamics and interactions between system components and driving processes ( Kumar, 2015 ; Sidle et al., 2013 ; Wagener et al., 2010 ).
Reliability theory is a powerful tool for systems analysis, demonstrated in many applications across engineering disciplines. Its flexibility in interpretation makes it an attractive choice as a framework to conceptualize and analyze a wide range of diverse scientific problems. This flexibility starts with the definition of failure.
In the context of microbial water contamination – the focus of this paper, we defined failure as exceedance of E. coli concentration of regulatory standards for primary contact ( Ohio EPA et al., 2018 ). System components represent individual stream reaches in an agricultural watershed. Associated with each component is a limit-state surface that separates safe and failure domains. They are used to compute the spatial distribution of risk, reliability and related metrics; and gain insight into the impact of failure in individual streams on water quality at strategic locations of critical importance, such as a protected reservoir. It is not difficult to imagine how reliability theory could be expanded to other environmental analyses, such as for instance direct co-modeling of biogeochemical, ecological, and human sub-systems as they co-evolve with hydrology ( Troch et al., 2015 ), or in studies of ecological tipping points ( Sidle et al., 2013 ).
4.2. Probability-based sustainability measures as geospatial products
Reliability theory was developed to analyze performance of engineered systems under uncertainty. It is inherently probability-based, and hence well suited to produce probabilistic assessments of water contamination. The general public is already acclimated to some probability-based projections. The most notable example is, of course, weather forecasts, which have enforced the habit of every day decision-making under uncertainty. The ease of access to weather-related web applications with online stationary and dynamic radar maps available on every mobile device have facilitated public acceptance of randomness in natural phenomena, and of the resulting uncertainty in scientific predictions. The acceptance of stochastic models by the public and water practitioners has been much slower in contaminant hydrology ( Freeze, 2004 ; Rajaram, 2016 ). Could we take advantage of the momentum generated by climate studies to accustom the general public and watershed managers to probability-based decision-making in the context of water contamination?
There are many reasons for slow adoption of probability-based methods in contaminant hydrology among practitioners and general public. The participants of a recent debate in Water Resources Research on adoption of stochastic models by groundwater hydrology practitioners encourage conceptually simple, user-friendly modeling frameworks, direct use of data, and explicit modeling of uncertain and highly variable entities as random variables ( Cirpka and Valocchi, 2016 ; Fiori et al., 2016 ; Fogg and Zhang, 2016 ; Rajaram, 2016 ; Sanchez-Vila and Fernàndez-Garcia, 2016 ). To this list we would add geospatial visualization and accessibility. Developing models with GIS can materialize the former, and the latter by making these GIS-powered models accessible through web services and mobile apps.
Surface water quality modeling with GIS allows for immediate access to national hydrography (NHD Plus), land use (NLCD), soil (SSURGO) and other databases ( Johnson et al., 2013 ; Whiteaker et al., 2006 ). Because our modeling framework is implemented with GIS, all results are readily mapped and can be used immediately for decision-making. Each map pulls information from multiple national and local databases along with the modeling outcomes, and presents it in a visually compelling way with color-coded flow networks, as shown in Figs. 2 and and3. 3 . And, because the model contains random variables that directly represent random environmental parameters and driving processes, the output is inherently probabilistic and so the maps themselves display probabilities. We expect that full use of modern geospatial visualization capabilities as well as development of web services and mobile water contamination apps powered by GIS would enable the adoption of probability-based methods for decision making in water quality – much like the acceptance of NOAA’s weather maps.
Furthermore, the ongoing development of the National Water Model ( Maidment, 2016 ) facilitates direct access to real- or near-real time hydrologic data, hence enabling real-time hydrologic decision-making. Accessibility of water quality data of the same spatial and temporal resolution, especially microbial contamination monitoring data, lags behind due to cost and time requirements in sample processing. We expect that solutions to this challenge would eventually come from development of embedded and/or mobile sensing technologies.
In this study we produced several types of geospatial products to answer an array of probability-based inquiries to guide watershed management strategies. The component reliability map, for instance, shows the areas in the watershed that are at the highest risk for individual failure. Presented together on the same map, these probabilities reveal the spatial distribution of probability of exceeding contaminant standards in the entire watershed.
Conditional analysis maps help understand the impact that the state (failing or functioning) of individual streams has on strategic locations in the watershed, such as its outlet. These locations can be chosen based on the importance of ecological functions that they provide, such as inlets for water processing plants, recreational areas, or wildlife breeding grounds. Even in a watershed as small as GRR, with highly correlated parameters, the conditional analysis provided additional insight on streams with the greatest impact on the outlet. We expect that in larger and more complex systems the conditional analysis would be poised to serve as an early warning system and a guide to strategic placement of monitoring stations.
Three sustainability metrics, namely reliability, vulnerability and resilience, widely used in environmental science and engineering and related fields are a natural product of reliability theory. Several previous studies have applied reliability theory for calculation of these metrics from a component reliability perspective ( Maier et al., 2001 ; Park et al., 2008 ; Sarang et al., 2008 ). The analysis would then yield one number for each metric. In this paper we depart from the lumped approach and derive spatially distributed sustainability measures. This spatial distribution is then visualized in GIS as maps of reliability, vulnerability, resilience and sustainability index. Reliability is the complement of probability of failure computed for individual components. At values ranging from 0.083 to 0.21, reliability in GRR is low overall. However, vulnerability analysis indicates that probabilities of critically high water contamination levels, set for the secondary contact threshold, are not likely in this watershed.
The definition of resilience used in this paper has been tailored for chronic contamination problems. Traditionally, resilience is computed based on the amount of time it takes the system to move from the failure to the safe domain. While this definition works well for acute contamination events, it will likely not yield meaningful results when waters are chronically impaired. Hence, we defined resilience as the probability that the system will recover from its failure state after implementation of BMPs. Our resilience map therefore shows the measure of watershed’s response to BMPs. Further, this definition of resilience creates a conceptual connection between reliability theory and threshold behavior in complex systems, in particular with regard to positive tipping points that may occur when human innovation and interventions in degraded systems drive their recovery ( Sidle et al., 2013 ; Srinivasan and Kumar, 2015 ; Zehe and Sivapalan, 2009 ).
In our study we simulated BMPs across the entire watershed. However, while wide implementation of BMPs, especially with high levels of contaminant load reduction, will create significant gains in reliability, such a feat may not always be practical. The decision space would likely be constrained due to land ownership, accessibility and cost. Final decisions should be made based on the level of risk aversion that is appropriate or accepted given the ecosystem functions fulfilled by the watershed.
The composite SI reflects the current state of the watershed in regard to sustainable development. Among sustainability metrics, resilience has the lowest values, and therefore it has the highest impact on the SI. Since the resilience metric is shown to be responsive to BMPs in GRR, its overall sustainability can be improved through BMP implementation as well.
4.3. Physics-based sustainability measures
In this paper, sustainability metrics and a composite index are derived from a stochastic physics-based model of contaminant fate and transport. Therefore, the metrics inherently reflect measurable environmental parameters, and can potentially point to the processes and factors that need to be influenced in order to improve sustainability. The model used here for E. Coli transport is based on first-order decay of contaminants that enter the water from land in quantities determined by land use, but no other sources. While this simple model meets the needs of TMDL programs ( Johnson et al., 2013 ), the picture is likely to be very different if we consider other microbial species, as well as additional and more detailed mechanisms and processes in microbial fate and transport. For instance, emerging studies of microbial fate and transport highlight the importance of streambeds as microbial reservoirs ( Cho et al., 2010 ; Jamieson et al., 2005 ; Kim et al., 2010 ; Pandey et al., 2012 ; Yeghiazarian et al., 2006 ; Yeghiazarian and Samorodnitsky, 2013 ). This means that, moving forward, we should employ models that explicitly incorporate deposition and re-suspension of microorganisms from streambeds. Another critical factor in microbial transport is interactions with soil particles. In fact, attachment to eroded sediments makes erosion a major mode of microbial transport in surface water, especially from soils rich in clay such as in GRR ( Searcy et al., 2006 ; 2005 ; 2004;; Walker and Montemagno, 1999 ; Yeghiazarian et al., 2006 ). In a series of earlier papers we developed a stochastic physics-based model of Cryptosporidium transport that takes into account partitioning of microbes between solid and aqueous phases in water and on the soil surface, as well as deposition and re-suspension ( Yeghiazarian et al., 2009 ; 2006 ;; Yeghiazarian and Samorodnitsky, 2013 ). We then used this model as a foundation for a component-level reliability analysis of Cryptosporidium transport in overland flow over a single catchment ( Park et al., 2008 ). We are currently expanding this approach to implement system-level reliability analysis of entire watersheds. With integrated micro-scale processes, this framework could be viewed as a marriage of top-down and bottom-up modeling approaches.
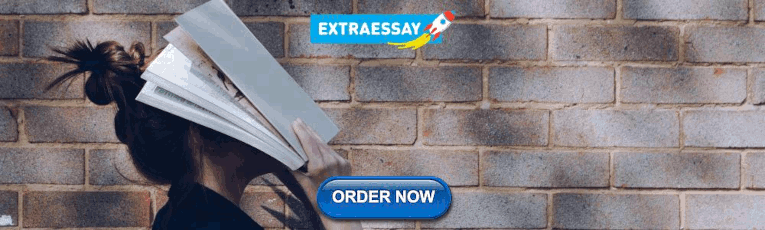
5. Conclusion
In this paper we use reliability theory as a framework for distributed water quality and sustainability analysis, with application to microbial contamination in surface water networks. Incorporation of a simple, stochastic physics-based model of microbial fate and transport enables derivation of physics-based sustainability metrics; and integration with ArcGIS makes a wealth of geospatial information available to the modeler. We expect that this work will help facilitate the adoption of probabilistic predictions of water quality security by water resources practitioners and the general public.
Supplementary Material
Grr_reliabilityanalysis_dataresults.
GRR_ReliabilityAnalysis_Data&Results.xlsx: This Excel sheet contains the list of nodes, links and hillslopes, the land cover distribution, the associated export coefficients, and the reliability analysis results.
ARCGIS Reliability Analysis
ArcGIS_InputFiles_GRR.zip: This zipped folder contains the ArcGIS files related to GRR subwatershed: Schematic network shapefiles, NLCD land cover raster, and NHDplus_V2 shapefile used to extract streamflow and stream velocity.
Acknowledgments
The authors gratefully acknowledge the National Science Foundation for supporting this research (CBET-1351361 grant). The U.S. Environmental Protection Agency (USEPA), through its Office of Research and Development, partially funded and collaborated in the research described herein. Any opinions expressed in this paper are those of the author(s) and do not reflect the views of the Agency, therefore, no official endorsement should be inferred. Any mention of trade names or commercial products does not constitute endorsement or recommendation for use by the USEPA.
Declaration of Competing Interest
The authors declare that they have no known competing financial interests or personal relationships that could have appeared to influence the work reported in this paper.
Appendix A. Supplementary data
Supplementary data to this article can be found online at https://doi.org/10.1016/j.jhydrol.2020.125711 .
- Abrishamchi A, Tajrishy M, Shafieian P, 2005. Uncertainty analysis in QUAL2E model of Zayandeh-Rood river . Water Environ. Res . 77 , 279–286. 10.2175/106143005×41861. [ PubMed ] [ CrossRef ] [ Google Scholar ]
- Bee M, 2011. Adaptive Importance Sampling for simulating copula-based distributions . Insur. Math. Econ . 48 , 237–245. 10.1016/j.insmatheco.2010.11.004. [ CrossRef ] [ Google Scholar ]
- Bertuzzo E, Maritan A, Gatto M, Rodriguez-Iturbe I, Rinaldo A, 2007. River networks and ecological corridors: Reactive transport on fractals, migration fronts, hydrochory . Water Resour. Res . 43. 10.1029/2006WR005533. [ CrossRef ] [ Google Scholar ]
- Blaustein RA, Pachepsky Y, Hill RL, Shelton DR, Whelan G, 2013. Escherichia coli survival in waters: Temperature dependence . Water Res . 47 , 569–578. 10.1016/j.watres.2012.10.027. [ PubMed ] [ CrossRef ] [ Google Scholar ]
- Bushon RN, Brady AMG, Christensen ED, Stelzer EA, 2017. Multi-year microbial source tracking study characterizing fecal contamination in an urban watershed . Water Environ. Res . 89 , 127–143. 10.2175/106143016X14798353399412. [ PubMed ] [ CrossRef ] [ Google Scholar ]
- Campbell Grant EH, Lowe WH, Fagan WF, 2007. Living in the branches: population dynamics and ecological processes in dendritic networks . Ecol. Lett , doi: 10.1111/j.1461-0248.2006.01007.x. [ PubMed ] [ CrossRef ] [ Google Scholar ]
- Cho KH, Pachepsky YA, Kim JH, Guber AK, Shelton DR, Rowland R, 2010. Release of Escherichia coli from the bottom sediment in a first-order creek: experiment and reach-specific modeling . J. Hydrol . 391 , 322–332. 10.1016/j.jhydrol.2010.07.033. [ CrossRef ] [ Google Scholar ]
- Cirpka OA, Valocchi AJ, 2016. Debates—Stochastic subsurface hydrology from theory to practice: does stochastic subsurface hydrology help solving practical problems of contaminant hydrogeology? Water Resour. Res . 52 , 9218–9227. 10.1002/2016wr019087. [ CrossRef ] [ Google Scholar ]
- Csete M, Doyle J, 2002. Reverse engineering of biological complexity . Science (80-.) 295 , 1664–1669. 10.1126/science.1069981. [ PubMed ] [ CrossRef ] [ Google Scholar ]
- Curriero FC, Patz JA, Rose JB, Lele S, 2001. The association between extreme precipitation and waterborne disease outbreaks in the United States, 1948–1994 . Am. J. Public Health 91 , 1194–1199. 10.2105/AJPH.91.8.1194. [ PMC free article ] [ PubMed ] [ CrossRef ] [ Google Scholar ]
- Fiori A, Cvetkovic V, Dagan G, Attinger S, Bellin A, Dietrich P, Zech A, Teutsch G, 2016. Debates—Stochastic subsurface hydrology from theory to practice: the relevance of stochastic subsurface hydrology to practical problems of contaminant transport and remediation. What is characterization and stochastic theory good for? Water Resour. Res . 52 , 9228–9234. 10.1002/2015wr017525. [ CrossRef ] [ Google Scholar ]
- Fogg GE, Zhang Y, 2016. Debates—Stochastic subsurface hydrology from theory to practice: a geologic perspective . Water Resour. Res . 52 , 9235–9245. 10.1002/2016wr019699. [ CrossRef ] [ Google Scholar ]
- Franceschini S, Tsai CW, 2008. Incorporating reliability into the definition of the margin of safety in total maximum daily load calculations . J. Water Resour. Plan. Manag . 134 , 34–44. 10.1061/(asce)0733-9496(2008)134:1(34). [ CrossRef ] [ Google Scholar ]
- Freeze RA, 2004. The role of stochastic hydrogeological modeling in real-world engineering applications . Stoch. Environ. Res. Risk Assess . 18 , 286–289. 10.1007/s00477-004-0194-4. [ CrossRef ] [ Google Scholar ]
- Garcia-Armisen T, Servais P, 2009. Partitioning and fate of particle-associated E. coli in river waters . Water Environ. Res . 81 , 21–28. 10.2175/106143008X304613. [ PubMed ] [ CrossRef ] [ Google Scholar ]
- Garrick BJ, Gekler WC, Goldfisher L, Karcher RH, Shimizu B, Wilson JH, 1967. Reliability Analysis Of Nuclear Power Plant Protective Systems , U.S. Atomic Energy Commission. Holmes and Narver, Inc., U.S. Atomic Energy Commission. doi: 10.2172/4568767. [ CrossRef ] [ Google Scholar ]
- Gibson CJ, 2006. Bacterial loadings Watershed Model in Copano Bay . University of Texas at Austin. [ Google Scholar ]
- Granato GE, 2010. Methods for development of planning-level estimates of stormflow at unmonitored sites in the conterminous United States , Federal Highway Administration. [ Google Scholar ]
- Haldar A, Mahadevan S, 2000. Probability, reliability and statistical methods in engineering design . J. Wiley & Sons, Incorporated. [ Google Scholar ]
- Hamed MM, Bedient PB, 1997. On the performance of computational methods for the assessment of risk from ground-water contamination . Ground Water 35 , 638–646. 10.1111/j.1745-6584.1997.tb00129.x. [ CrossRef ] [ Google Scholar ]
- Hamed MM, El-Beshry MZ, 2004. Uncertainty analysis in dissolved oxygen modeling in streams . Environ. Manage . 34 , 233–244. 10.1007/s00267-003-0244-6. [ PubMed ] [ CrossRef ] [ Google Scholar ]
- Harris GP, Heathwaite AL, 2012. Why is achieving good ecological outcomes in rivers so difficult? Freshw. Biol . 57 , 91–107. 10.1111/j.1365-2427.2011.02640.x. [ CrossRef ] [ Google Scholar ]
- Hashimoto T, Stedinger JR, Loucks DP, 1982. Reliability, resiliency, and vulnerability criteria for water resource system performance evaluation . Water Resour. Res . 18 , 14–20. 10.1029/WR018i001p00014. [ CrossRef ] [ Google Scholar ]
- Hoque YM, Tripathi S, Hantush MM, Govindaraju RS, 2016. Aggregate measures of watershed health from reconstructed water quality data with uncertainty . J. Environ. Qual . 45 , 709–719. 10.2134/jeq2015.10.0508. [ PubMed ] [ CrossRef ] [ Google Scholar ]
- Hoque YM, Tripathi S, Hantush MM, Govindaraju RS, 2012. Watershed reliability, resilience and vulnerability analysis under uncertainty using water quality data . J. Environ. Manage . 109 , 101–112. 10.1016/j.jenvman.2012.05.010. [ PubMed ] [ CrossRef ] [ Google Scholar ]
- Hoyland A, Rausand M, 1994. System reliability theory. Models and statistical methods., Wiley series in probability and mathematical statistics . John Wiley & Sons Inc, New York. [ Google Scholar ]
- Jamieson R, Joy DM, Lee H, Kostaschuk R, Gordon R, 2005. Transport and deposition of sediment-associated Escherichia coli in natural streams . Water Res . 39 , 2665–2675. 10.1016/j.watres.2005.04.040. [ PubMed ] [ CrossRef ] [ Google Scholar ]
- Jang Y-S, Sitar N, Der Kiureghian A, 1994. Reliability analysis of contaminant transport in saturated porous media . Water Resour. Res . 30 , 2435–2448. 10.1029/93WR03554. [ CrossRef ] [ Google Scholar ]
- Jiang S-H, Li D-Q, Cao Z-J, Zhou C-B, Phoon K-K, 2015. Efficient system reliability analysis of slope stability in spatially variable soils using Monte Carlo simulation . J. Geotech. Geoenviron. Eng . 141 , 04014096. 10.1061/(ASCE)GT.1943-5606.0001227. [ CrossRef ] [ Google Scholar ]
- Joe H, 1997. Multivariate Models and Multivariate Dependence Concepts . CRC Press. [ Google Scholar ]
- Johnson SL, Maidment DR, Kirisits MJ, 2013. TMDL balance: a model for coastal water pollutant loadings . J. Am. Water Resour. Assoc . 49 , 838–850. 10.1111/jawr.12044. [ CrossRef ] [ Google Scholar ]
- Kay D, Crowther J, Stapleton CM, Wyer MD, Fewtrell L, Anthony S, Bradford M, Edwards A, Francis CA, Hopkins M, Kay C, McDonald AT, Watkins J, Wilkinson J, 2008. Faecal indicator organism concentrations and catchment export coefficients in the UK . Water Res . 42 , 2649–2661. 10.1016/j.watres.2008.01.017. [ PubMed ] [ CrossRef ] [ Google Scholar ]
- Kay D, Edwards AC, Ferrier RC, Francis C, Kay C, Rushby L, Watkins J, McDonald AT, Wyer M, Crowther J, Wilkinson J, 2007. Catchment microbial dynamics: the emergence of a research agenda . Prog. Phys. Geogr . 31 , 59–76. 10.1177/0309133307073882. [ CrossRef ] [ Google Scholar ]
- Kim JW, Pachepsky YA, Shelton DR, Coppock C, 2010. Effect of streambed bacteria release on E. coli concentrations: Monitoring and modeling with the modified SWAT . Ecol. Modell . 221 , 1592–1604. 10.1016/j.ecolmodel.2010.03.005. [ CrossRef ] [ Google Scholar ]
- Koltun G, Whitehead M, 2002. Techniques for estimating selected streamflow characteristics of rural unregulated streams in Ohio . doi: 10.3133/wri024068. [ CrossRef ] [ Google Scholar ]
- Kumar P, 2015. Hydrocomplexity: addressing water security and emergent environmental risks . Water Resour. Res . 51 , 5827–5838. 10.1002/2015WR017342. [ CrossRef ] [ Google Scholar ]
- Line DE, White NM, Kirby-Smith WW, Potts JD, 2008. Fecal coliform export from four coastal North Carolina areas . J. Am. Water Resour. Assoc . 44 , 606–617. 10.1111/j.1752-1688.2008.00185.x. [ CrossRef ] [ Google Scholar ]
- Lisle IG, Rose CW, Hogarth WL, Hairsine PB, Sander GC, Parlange JY, 1998. Stochastic sediment transport in soil erosion . J. Hydrol . 204 , 217–230. 10.1016/S0022-1694(97)00123-6. [ CrossRef ] [ Google Scholar ]
- Loucks DP, 1997. Quantifying trends in system sustainability . Hydrol. Sci. J.-J. Des Sci. Hydrol . 42 , 513–530. 10.1080/02626669709492051. [ CrossRef ] [ Google Scholar ]
- Maidment DR, 2016. Open water data in space and time . JAWRA J. Am. Water Resour. Assoc . 52 , 816–824. 10.1111/1752-1688.12436. [ CrossRef ] [ Google Scholar ]
- Maier HR, Lence BJ, Tolson BA, Foschi RO, 2001. First-order reliability method for estimating reliability, vulnerability, and resilience . Water Resour. Res . 37 , 779–790. 10.1029/2000WR900329. [ CrossRef ] [ Google Scholar ]
- Mitsova-Boneva D, 2007. Exploring the variability in suspended sediment yield using BASINS-HSPF and probabilistic modeling: implications for land use planning . J. Environ. Informatics 9 , 29–40. 10.3808/jei.200700085. [ CrossRef ] [ Google Scholar ]
- Narasimhan B, Allen PM, Coffman SV, Arnold JG, Srinivasan R, 2017. Development and testing of a physically based model of streambank erosion for coupling with a basin-scale hydrologic model SWAT . JAWRA J. Am. Water Resour. Assoc . 53 , 344–364. 10.1111/1752-1688.12505. [ CrossRef ] [ Google Scholar ]
- Ng TL, Eheart JW, 2005. Effects of discharge permit trading on water quality reliability . J. Water Resour. Plan. Manag . 131 , 81–88. 10.1061/(Asce)0733-9496(2005)131:2(81). [ CrossRef ] [ Google Scholar ]
- Ohio EPA, Division of Surface Water, Standards & Technical Support Section, 2018. State of Ohio Water Quality Standards. Chapter 3745–1 of the ADMINISTRATIVE CODE , https://epa.ohio.gov/Portals/35/rules/01-all.pdf .
- Pandey PK, Soupir ML, Rehmann CR, 2012. A model for predicting resuspension of Escherichia coil from streambed sediments . Water Res . 46 , 115–126. 10.1016/j.watres.2011.10.019. [ PubMed ] [ CrossRef ] [ Google Scholar ]
- Park Y, Yeghiazarian L, Stedinger JR, Montemagno CD, 2008. Numerical approach to Cryptosporidium risk assessment using reliability method . Stoch. Environ. Res. Risk Assess . 22 , 169–183. 10.1007/s00477-007-0105-6. [ CrossRef ] [ Google Scholar ]
- Rajaram H, 2016. Debates—Stochastic subsurface hydrology from theory to practice: introduction . Water Resour. Res . 52 , 9215–9217. 10.1002/2016wr020066. [ CrossRef ] [ Google Scholar ]
- Riasi MS, Teklitz A, Shuster W, Nietch C, Yeghiazarian L, 2018. Reliability-based water quality assessment with load resistance factor design: application to TMDL . J. Hydrologic Eng . 23 , 1–10. 10.1061/(ASCE)HE.1943-5584.0001722. [ PMC free article ] [ PubMed ] [ CrossRef ] [ Google Scholar ]
- Safwat A, Whiteaker T, Teklitz A, Nietch C, Maidment D, Best EPH, Yeghiazarian L, 2012. Expanding water quality simulation capabilities of ArcGIS’ Schematic Processor tool , in: AWRA. New Orleans, LA. [ Google Scholar ]
- Sahner R, Trivedi KS, Puliafito A, 1996. Performance and Reliability Analysis of Computer Systems . Springer US, Boston, MA. doi: 10.1007/978-1-4615-2367-3. [ CrossRef ] [ Google Scholar ]
- Sanchez-Vila X, Fernandez-Garcia D, 2016. Debates—Stochastic subsurface hydrology from theory to practice: why stochastic modeling has not yet permeated into practitioners? Water Resour. Res . 52 , 9246–9258. 10.1002/2016wr019302. [ CrossRef ] [ Google Scholar ]
- Sandoval-Solis S, McKinney DC, Loucks DP, 2011. Sustainability index for water resources planning and management . J. Water Resour. Plan. Manag . 137 , 381–390. 10.1061/(asce)wr.1943-5452.0000134. [ CrossRef ] [ Google Scholar ]
- Sarang A, Vahedi A, Shamsai A, 2008. How to quantify sustainable development: a Risk-based approach to water quality management . Environ. Manage . 41 , 200–220. 10.1007/s00267-007-9047-5. [ PubMed ] [ CrossRef ] [ Google Scholar ]
- Searcy KE, Packman AI, Atwill ER, Harter T, 2005. Association of Cryptosporidium parvum with suspended particles: Impact on oocyst sedimentation . Appl. Environ. Microbiol . 71 , 1072–1078. 10.1128/aem.71.2.1072-1078.2005. [ PMC free article ] [ PubMed ] [ CrossRef ] [ Google Scholar ]
- Searcy KE, Packman AL, Atwill ER, Harter T, 2006. Deposition of Cryptosporidium oocysts in streambeds . Appl. Environ. Microbiol . 72 , 1810–1816. 10.1128/aem.72.3.1810-1816.2006. [ PMC free article ] [ PubMed ] [ CrossRef ] [ Google Scholar ]
- Sidle RC, Benson WH, Carriger JF, Kamai T, 2013. Broader perspective on ecosystem sustainability: consequences for decision making . Proc. Natl. Acad. Sci. U. S.A . 110 , 9201–9208. 10.1073/pnas.1302328110. [ PMC free article ] [ PubMed ] [ CrossRef ] [ Google Scholar ]
- Sitar N, Cawlfield JD, Der Kiureghian A, 1987. First-order reliability approach to stochastic analysis of subsurface flow and contaminant transport . Water Resour. Res . 23 , 794–804. 10.1029/WR023i005p00794. [ CrossRef ] [ Google Scholar ]
- Sklar M, 1959. Fonctions de répartition á n dimensions et leurs marges . Univ. Paris. [ Google Scholar ]
- Sohrabi TM, Shirmohammadi A, Chu TW, Montas H, Nejadhashemi AP, 2003. Uncertainty analysis of hydrologic and water quality predictions for a small watershed using SWAT2000 . Environ. Forensics 4 , 229–238. 10.1080/714044368. [ CrossRef ] [ Google Scholar ]
- Sparkman SA, Hogan DM, Hopkins KG, Loperfido JV, 2017. Modeling watershed-scale impacts of stormwater management with traditional versus low impact development design . JAWRA J. Am. Water Resour. Assoc . 53 , 1081–1094. 10.1111/1752-1688.12559. [ CrossRef ] [ Google Scholar ]
- Srinivasan V, Kumar P, 2015. Emergent and divergent resilience behavior in catastrophic shift systems . Ecol. Modell . 298 , 87–105. 10.1016/j.ecolmodel.2013.12.003. [ CrossRef ] [ Google Scholar ]
- Tartakovsky DM, 2013. Assessment and management of risk in subsurface hydrology: a review and perspective . Adv. Water Resour . 51 , 247–260. 10.1016/j.advwatres.2012.04.007. [ CrossRef ] [ Google Scholar ]
- Tartakovsky DM, 2007. Probabilistic risk analysis in subsurface hydrology . Geophys. Res. Lett . 34. 10.1029/2007GL029245. [ CrossRef ] [ Google Scholar ]
- Tavakoly AA, Maidment DR, McClelland JW, Whiteaker T, Yang Z-L, Griffin C, David CH, Meyer L, 2016. A GIS framework for regional modeling of riverine nitrogen transport: case study, San Antonio and Guadalupe Basins . JAWRA J. Am. Water Resour. Assoc . 52 , 1–15. 10.1111/1752-1688.12355. [ CrossRef ] [ Google Scholar ]
- Teklitz A, Nietch C, Whiteaker T, Riasi MS, Maidment D, Yeghiazarian L, 2020. STochastic Reliability-based Risk Evaluation And Mapping for watershed Systems and Sustainability (STREAMS) . J. Hydrol. Manuscript submitted for publication . [ PMC free article ] [ PubMed ] [ Google Scholar ]
- Thorndahl S, Schaarup-Jensen K, Jensen JB, 2008. Probabilistic modelling of combined sewer overflow using the First Order Reliability Method . Water Sci. Technol . 57 , 1337–1344. 10.2166/wst.2008.301. [ PubMed ] [ CrossRef ] [ Google Scholar ]
- Troch PA, Lahmers T, Meira A, Mukherjee R, Pedersen JW, Roy T, Valdes- Pineda R, 2015. Catchment coevolution: a useful framework for improving predictions of hydrological change? Water Resour. Res . 51 , 4903–4922. 10.1002/2015WR017032. [ CrossRef ] [ Google Scholar ]
- Tyrrel SF, Quinton JN, 2003. Overland flow transport of pathogens from agricultural land receiving faecal wastes . J. Appl. Microbiol . 94 , 87–93. 10.1046/j.1365-2672.94.s1.10.x. [ PubMed ] [ CrossRef ] [ Google Scholar ]
- Epa, U.S., 2008. Causes of Impairment for 303(d) Listed Waters . United States Environmental Protection Agency, Washington, D.C. [ Google Scholar ]
- Van Looy K, Piffady J, Tormos T, Villeneuve B, Valette L, Chandesris A, Souchon Y, 2015. Unravelling river system impairments in stream networks with an integrated risk approach . Environ. Manage . 55 , 1343–1353. 10.1007/s00267-015-0477-1. [ PubMed ] [ CrossRef ] [ Google Scholar ]
- Vidon P, Campbell M, Soyeux E, 2009. Eschrichia Coli and Fecal Coliform Export Rates in Two Agricultural Watersheds of the U.S. Midwest . Proc. Indiana Acad. Sci . 118 , 13–21. [ Google Scholar ]
- Wagener T, Sivapalan M, Troch PA, McGlynn BL, Harman CJ, Gupta HV, Kumar P, Rao PSC, Basu NB, Wilson JS, 2010. The future of hydrology: an evolving science for a changing world . Water Resour. Res . 46. 10.1029/2009wr008906. [ CrossRef ] [ Google Scholar ]
- Wagner BJ, Gorelick SM, 1987. Optimal groundwater quality management under parameter uncertainty . Water Resour. Res . 23 , 1162–1174. 10.1029/WR023i007p01162. [ CrossRef ] [ Google Scholar ]
- Walker MJ, Montemagno CD, 1999. Sorption of Cryptosporidium parvum oocysts in aqueous solution to metal oxide and hydrophobic substrates . Environ. Sci. Technol . 33 , 3134–3139. 10.1021/es990331f. [ CrossRef ] [ Google Scholar ]
- Wan X, Wang W, Liu J, Tong T, 2014. Estimating the sample mean and standard deviation from the sample size, median, range and/or interquartile range . BMC Med. Res. Methodol . 14 , 135. 10.1186/1471-2288-14-135. [ PMC free article ] [ PubMed ] [ CrossRef ] [ Google Scholar ]
- Whiteaker TL, Maidment DR, Goodall JL, Takamatsu M, 2006. Integrating arc hydro features with a schematic network . Trans. GIS 10 , 219–237. 10.1111/j.1467-9671.2006.00254.x. [ CrossRef ] [ Google Scholar ]
- Yeghiazarian L, Kalita P, Kuhlenschmidt MS, McLaughlin S, Montemagno CD, 2004. Field calibration and verification of a pathogen transport model . Water Environ. Res. Found . doi: 10.2166/9781780404271. [ CrossRef ] [ Google Scholar ]
- Yeghiazarian L, Samorodnitsky G, 2013. A fully stochastic approach bridging the microscopic behavior of individual microorganisms with macroscopic ensemble dynamics in surface flow networks . Water Resour. Res . 49 , 7820–7826. 10.1002/wrcr.20541. [ CrossRef ] [ Google Scholar ]
- Yeghiazarian L, Samorodnitsky G, Montemagno CD, 2009. A Poisson random field model of pathogen transport in surface water . Water Resour. Res . 45. 10.1029/2009WR007896. [ CrossRef ] [ Google Scholar ]
- Yeghiazarian L, Walker MJ, Binning P, Parlange J-Y, Montemagno CD, 2006. A combined micro- and macroscopic approach to modeling the transport of pathogenic microorganisms from non-point sources of pollution . Water Resour. Res . 42. 10.1029/2005WR004078. [ CrossRef ] [ Google Scholar ]
- Zehe E, Sivapalan M, 2009. Threshold behaviour in hydrological systems as (human) geo-ecosystems: manifestations, controls, implications . Hydrol. Earth Syst. Sci . 13 , 1273–1297. 10.5194/hess-13-1273-2009. [ CrossRef ] [ Google Scholar ]
- Zheng Y, Han F, 2016. Markov Chain Monte Carlo (MCMC) uncertainty analysis for watershed water quality modeling and management . Stoch. Environ. Res. Risk Assess . 30 , 293–308. 10.1007/s00477-015-1091-8. [ CrossRef ] [ Google Scholar ]
- Zoun R, 2010. Estimation of fecal coliform loadings to Galveston Bay . University of Texas at Austin. [ Google Scholar ]
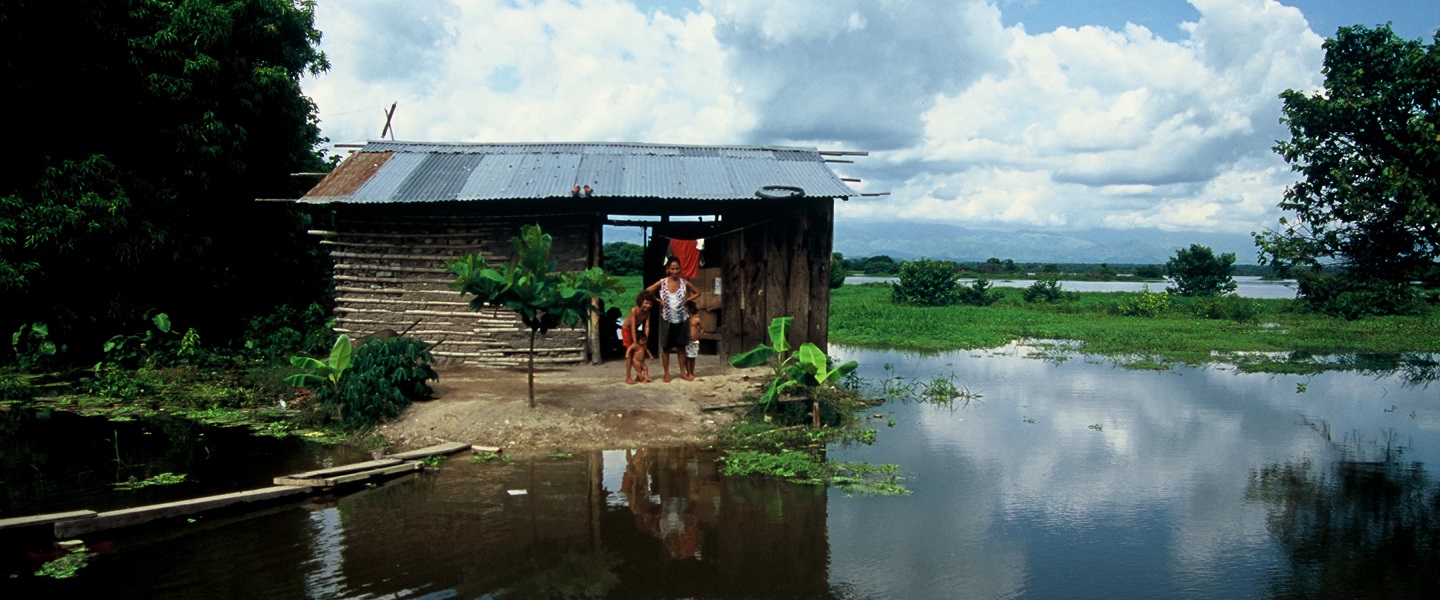
WATER RESOURCES MANAGEMENT
Water scarcity affects more than 40% of the global population. Water-related disasters account for 70% of all deaths related to natural disasters. The World Bank helps countries ensure sustainability of water use, build climate resilience and strengthen integrated management.
- Context & Challenges
- Results & Initiatives
Today, most countries are placing unprecedented pressure on water resources. The global population is growing fast, and estimates show that with current practices, the world will face a 40% shortfall between forecast demand and available supply of water by 2030. Furthermore, chronic water scarcity, hydrological uncertainty, and extreme weather events (floods and droughts) are perceived as some of the biggest threats to global prosperity and stability. Acknowledgment of the role that water scarcity and drought are playing in aggravating fragility and conflict is increasing.
View the Full Infographic>>
Feeding 10 billion people by 2050 will require a 50% increase in agricultural production , (which consumes 70% of the resource today), and a 15% increase in water withdrawals. Besides this increasing demand, the resource is already scarce in many parts of the world. Estimates indicate that over 40% of the world population live in water scarce areas, and approximately ¼ of world’s GDP is exposed to this challenge. By 2040, an estimated one in four children will live in areas with extreme water shortages . Water security is a major – and often growing –challenge for many countries today.
Climate change will worsen the situation by altering hydrological cycles, making water more unpredictable and increasing the frequency and intensity of floods and droughts. The roughly 1 billion people living in monsoonal basins and the 500 million people living in deltas are especially vulnerable. Flood damages are estimated around $120 billion per year (only from property damage), and droughts pose, among others, constraints to the rural poor, highly dependent on rainfall variability for subsistence.
The fragmentation of this resource also constrains water security. There are 276 transboundary basins, shared by 148 countries, which account for 60% of the global freshwater flow. Similarly, 300 aquifers systems are transboundary in nature, with 2.5 billion people worldwide are dependent on groundwater. The challenges of fragmentation are often replicated at the national scale, meaning cooperation is needed to achieve optimal water resources management and development solutions for all riparians. To deal with these complex and interlinked water challenges, countries will need to improve the way they manage their water resources and associated services.
To strengthen water security against this backdrop of increasing demand, water scarcity, growing uncertainty, greater extremes, and fragmentation challenges, clients will need to invest in institutional strengthening, information management, and (natural and man-made) infrastructure development. Institutional tools such as legal and regulatory frameworks, water pricing, and incentives are needed to better allocate, regulate, and conserve water resources. Information systems are needed for resource monitoring, decision making under uncertainty, systems analyses, and hydro-meteorological forecast and warning. Investments in innovative technologies for enhancing productivity, conserving and protecting resources, recycling storm water and wastewater, and developing non-conventional water sources should be explored in addition to seeking opportunities for enhanced water storage, including aquifer recharge and recovery. Ensuring the rapid dissemination and appropriate adaptation or application of these advances will be a key to strengthening global water security.
Last Updated: Oct 05, 2022
The World Bank is committed to assisting countries meet their economic growth and poverty reduction targets based on the Sustainable Development Goals ( SDGs ). Particularly, water resource management is tackled in SDG 6.5, but other SDGs and targets require water resource management for their achievement. Accordingly, the Bank has a major interest in helping countries achieve water security through sound and robust water resource management.
Water security is the goal of water resources management . For a rapidly growing and urbanizing global population, against a backdrop of increasing climatic and non-climatic uncertainties, it is not possible to "predict and plan" a single path to water security. To strengthen water security we need to build capacity, adaptability, and resilience for the future planning and management of water resources.
Water Resources Management (WRM) is the process of planning, developing, and managing water resources, in terms of both water quantity and quality, across all water uses. It includes the institutions, infrastructure, incentives, and information systems that support and guide water management. Water resources management seeks to harness the benefits of water by ensuring there is sufficient water of adequate quality for drinking water and sanitation services, food production, energy generation, inland water transport, and water-based recreational, as well as sustaining healthy water-dependent ecosystems and protecting the aesthetic and spiritual values of lakes, rivers, and estuaries. Water resource management also entails managing water-related risks, including floods, drought, and contamination. The complexity of relationships between water and households, economies, and ecosystems, requires integrated management that accounts for the synergies and tradeoffs of water's great number uses and values.
Water security is achieved when water's productive potential is leveraged and its destructive potential is managed . Water security differs from concepts of food security or energy security because the challenge is not only one of securing adequate resource provision – but also of mitigating the hazards that water presents where it is not well managed. Water security reflects the actions that can or have been taken to ensure sustainable water resource use, to deliver reliable water services, and to manage and mitigate water-related risks. Water security suggests a dynamic construct that goes beyond single-issue goals such as water scarcity, pollution, or access to water and sanitation, to think more broadly about societies' expectations, choices, and achievements with respect to water management. It is a dynamic policy goal, which changes as societies' values and economic well-being evolve, and as exposure to and societies' tolerance of water-related risks change. It must contend with issues of equity.
The Water Security and Integrated Water Resources Management Global Solutions Group (GSG) supports the Bank's analytical, advisory, and operational engagements to help clients achieve their goals of water security. Achieving water security in the context of growing water scarcity, greater unpredictability, degrading water quality and aquatic ecosystems, and more frequent droughts and floods, will require a more integrated and longer-term approach to water management. Key areas of focus will be ensuring sustainability of water resources, building climate resilience, and strengthening integrated management to achieve the Global Practice's (GP) goals and the SDGs. The GSG will work with a multiple GPs and Cross Cutting Solutions Areas (CCSAs) directly through water resources management or multi-sectoral projects and indirectly through agriculture, energy, environment, climate, or urban projects.
Robust water resource management solutions to complex water issues incorporate cutting-edge knowledge and innovation, which are integrated into water projects to strengthen their impact. New knowledge that draws on the World Bank Group’s global experiences, as well as partner expertise, are filling global knowledge gaps and transforming the design of water investment projects to deliver results. Multi-year, programmatic engagements in strategic areas are designed to make dramatic economic improvements in the long term and improve the livelihoods of millions of the world’s poorest people.
The Water Security Diagnostic Initiative is an analytical framework that can be used to examine the status and trends related to water resources, water services, and water-related risks, including climate change, transboundary waters, and virtual water trade. The framework helps countries determine if and to what extent water-related factors impact people, the economy, and the environment, and determine if and to what extent water-related factors provide opportunities for development and well-being.
The World Bank is proactively working to address new global challenges, by adapting its operations to reach those that most need it today. Working across sectors is ensuring that water considerations are addressed in energy, the environment, agriculture, urban and rural development, and within new global challenges. The Bank also supports transformational engagements and initiatives, which seek to optimize spatial, green, and co-benefits among water and other infrastructure sectors. A large proportion of World Bank-funded water resources management projects include institutional and policy components.
Recent initiatives include:
- Through the Federal Integrated Water Sector Project (INTERÁGUAS) , Brazil's federal government sought to integrate the water sector by improving coordination among and strengthening the capacity of the sector’s key federal institutions. In an ambitious innovation, the World Bank supported the government by helping to bring together the most important federal water sector agencies while supporting ongoing water reforms and institutional strengthening.
- The integration of nature-based solutions in the Bank’s water infrastructure projects has helped place a spotlight on the world’s growing infrastructure crisis, driven by climate change and growing populations. Embedding nature-based solutions into project designs can help deliver infrastructure services with greater impact and lower cost, all the while reducing risks from disaster, boosting water security and enhancing climate resilience.
- The publication of a National Framework for Integrated Urban Water Management in Indonesia , focuses on the potential for IUWM to address the severe and interrelated water security challenges faced by Indonesian cities.
- The Second Public Employment for Sustainable Agriculture and Water Management Project (PAMP II) supported the Government of Tajikistan in improving water resource management at local, basin and national levels, and in increasing crop yields through improved irrigation management. Key to improved irrigation was rehabilitation of irrigation and drainage infrastructure and support to Water Users Associations, which are community-based organizations linking farmers with irrigation service provider.
- The Water Management and Development Project in Uganda improved the integration of water resources planning, management and development, as well as access to water and sanitation services in priority urban areas. More than 1.01 million people received access to improved water sources, and 25,000 piped household water connections were rehabilitated from 2012-2018.
With 263 international rivers in the world, support for cooperative transboundary water management can make an important contribution towards improving the efficient and equitable management of water resources. The Bank supports transboundary waters through Multi-Donor Trust Funds (MDTF), knowledge pieces, and its lending portfolio:
- Central Asia Water & Energy Program ( CAWEP ) is a MDTF administered by the World Bank and financed by the European Commission, the Swiss State Secretariat for Economic Affairs, UK AID, and DFID. The MDTF is building energy and water security by leveraging the benefits of enhanced cooperation in Central Asia, including all five Central Asian countries plus Afghanistan.
- The Cooperation for International Waters in Africa (CIWA) is a MDTF administered by the World Bank and financed by Denmark, European Commission, the Netherlands, Norway, Sweden, and the United Kingdom. The trust fund finances upstream work in African International Rivers, 75% of which go to four priority basins – Nile, Niger, Volta, and Zambezi.
- The South Asia Water Initiative (SAWI) is a MDTF administered by the World Bank and financed by the governments of the United Kingdom, Australia, and Norway in South Asia. The trust fund provides recipient executed grants to initiatives in the major Himalayan River systems – the Indus, the Ganges, and the Brahmaputra.
- In the Mekong River Basin, the Bank is supporting riparian states such as Cambodia , the Lao People’s Democratic Republic , and Vietnam in strengthening their integrated water resource management and disaster risk management capacities, cooperating closely with the basin-wide Mekong River Commission.
- The Bank is also investing in knowledge pieces such as ROTI ( Retooling Operations with Transboundary Impacts ) to identify tools that promote riparian country coordination aimed at mitigating transboundary harm and leveraging benefits of investments in transboundary basins.
The Bank follows an integrated flood management agenda, which includes well-functioning early warning systems, infrastructure, and institutional arrangements for coordinated action to address increased variability and changes to runoff and flooding patterns. In addition, a new perspective, referred to as an "EPIC Response," is offered to better manage hydro-climatic risks: This perspective looks at floods and droughts not as independent events but rather as different ends of the same hydro-climatic spectrum that are inextricably linked. The EPIC response provides a comprehensive framework to help national governments lead a whole-of-society effort to manage these risks.
Water scarcity is also addressed in:
- The Water Scarce Cities Initiative , initially focusing on the Middle East and North Africa ( MENA ) region, seeking to bolster the adoption of integrated approaches to managing water resources and service delivery in water scarce cities as the basis for water security and climate resilience.
- Small Island States . The challenges and innovations of water management in small island states can be particularly vivid. These countries warrant particular attention not only because they are often neglected, but also because they provide an opportunity to focus on intensive reuse and non-conventional water resources development, which will be increasingly important knowledge for implementation in megacities and extremely water scarce settings. A scoping study is proposed on the state-of-the-art and the Bank’s portfolio.
Sustainable groundwater management is also a priority of the World Bank, and central to water security in many countries.
- Recognizing that groundwater is being depleted faster than it is replenished in many areas, the World Bank has collaborated with key global partners through years of consultations to develop a framework for groundwater governance. The 2030 Vision and Global Framework for Action represents a bold call for collectively responsible action among governments and the global community to ensure sustainable use of groundwater.
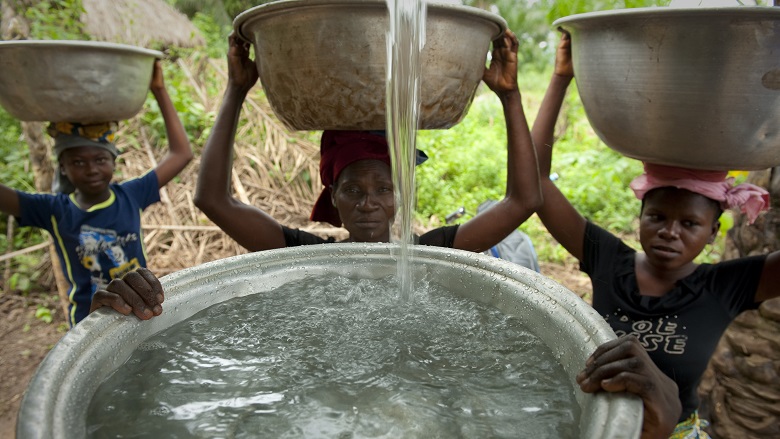
With or Without Water
Stay connected, additional resources.
This site uses cookies to optimize functionality and give you the best possible experience. If you continue to navigate this website beyond this page, cookies will be placed on your browser. To learn more about cookies, click here .
Pocosin Lakes National Wildlife Refuge Water Management Plan
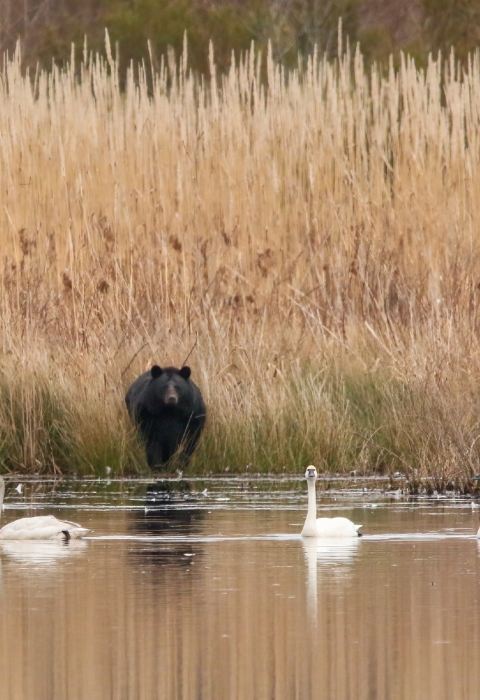
Columbia, N.C. — The U.S. Fish and Wildlife Service released the Pocosin Lakes National Wildlife Refuge Water Management Plan on Aug. 8, 2023, which provides a roadmap for refuge management in the coming years in a way that provides a clear understanding of the interconnectedness of water movement through the refuge and communities surrounding it.
The plan, which incorporates the diverse perspectives shared by members of the local community, includes strategies for expanding stakeholder engagement efforts and developing new educational tools. It identifies a series of complementary and supportive strategies aimed at broadening the understanding of water movement on the landscape across private lands, identifying barriers to water movement or other issues across the landscape.
As these barriers to water movement or other issues are identified, the plan also identifies opportunities for incentive programs and other possible solutions for addressing barriers and issues in the future. Focal areas described in the plan include water management infrastructure on the refuge, system functions and limitations, and management strategies to meet the goals and objectives outlined in the refuge’s Comprehensive Conservation Plan and protect Service investments in restoration.
“ Pocosin Lakes National Wildlife Refuge is an integral part of the fabric of the communities surrounding it. The plan, while informed by the unique environmental conditions at the refuge, was also informed by input from the community. Landowners and others shared their insights and perspectives during a public-comment period, and we look forward to continuing to be inclusive, open, and transparent in our ongoing conversations with adjacent landowners and others ,” said Rebekah Martin, project leader of the Coastal North Carolina National Wildlife Refuge Complex
Pocosin is a Native American term meaning “swamp on a hill.” These wetlands occur on peat domes and are at slightly higher elevations than the surrounding lands. Since the refuge ’s establishment in 1990, the Service has implemented several restoration projects to counteract the impacts of an extensive ditch system. Once drained, this system artificially dried out the peat soil, altering pocosin habitat and making the areas more susceptible to catastrophic wildfire. Pocosin wetlands on the refuge provide high quality habitat for wetland-dependent wildlife, reduce the chances of catastrophic wildfire, ease storm impacts, and repel the increasing threat of saltwater intrusion in northeastern North Carolina.
The refuge is located within a rainfall-driven system, meaning rainfall is the primary source of water in the system. During extreme weather conditions, the refuge can either experience too much or too little water. Too much rain leads to rapid surface runoff and may cause flooding issues. Too little water can lead to drought conditions, which adversely impacts the highly altered and artificially drained peatlands.
The drier peat soils make the landscape more susceptible to catastrophic, habitat-destroying wildfires which includes ground fire. Ground fires are very expensive to control and can result in severe elevation changes which affect drainage and destroys the native seedbank of native vegetation. Water management is critical for containing and controlling fire and perpetuating healthy pocosin habitats.
“Water management is essential for maintaining the integrity of these important pocosin wetlands,” Martin said “ The water management plan is based on the best science that we have now and incorporates nature-based solutions. It also gives us flexibility to adapt our strategies as we learn more from research and other regional hydrological studies. This approach will allow us to reconnect a healthy pocosin landscape and high-quality wildlife habitat.”
Funds from the Inflation Reduction Act of 2022 will be used to implement the water management plan at Pocosin Lakes National Wildlife Refuge. This funding allows the refuge to increase the resiliency of habitats and infrastructure to withstand severe and unanticipated weather events. The water management plan will bolster a resilient system of infrastructure already in place which retains and conserves rainwater to rewet the peat to mimic natural pocosin wetlands.
The Pocosin Lakes National Wildlife Refuge Water Management Plan can be found at : https://fws.gov/media/water-management-plan-and-environmental-assessment-pocosin-lakes-national-wildlife-refuge
For more information about the refuge go to: https://www.fws.gov/refuge/pocosin_lakes/ .
The mission of the U.S. Fish and Wildlife Service is working with others to conserve, protect, and enhance fish, wildlife, plants, and their habitats for the continuing benefit of the American people. For more information on our work and the people who can make it happen, visit fws.gov. Connect with the Service on Facebook , follow our tweets , watch the YouTube Channel and download photos from Flickr .
Media Contacts
Latest press releases.
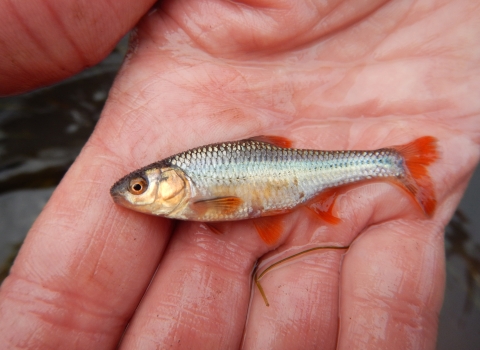
You are exiting the U.S. Fish and Wildlife Service website
You are being directed to
We do not guarantee that the websites we link to comply with Section 508 (Accessibility Requirements) of the Rehabilitation Act. Links also do not constitute endorsement, recommendation, or favoring by the U.S. Fish and Wildlife Service.
Hawaii residents disappointed as bill to safeguard water resources fails at sessions end
Hawaii residents are disappointed that the Aloha State’s lawmakers ended the legislative session without advancing a bill to strengthen the independence of the state’s Water Commission.
The bill’s demise comes at a critical time. The Hawaii Supreme Court recently determined that Attorney General Anne Lopez and the state Board of Land and Natural Resources (BLNR) made baseless allegations against a judge’s decision regarding East Maui streams.
Meanwhile, a citizens group known as the Red Hill Community Representative Initiative (CRI) has been advocating for the legislation after a significant water contamination affected roughly 93,000 residents in and around Joint Base Pearl Harbor-Hickam in 2021.
Marti Townsend, a resident and chair of the CRI, denounced the legislature for failing to advance the bill.
“From the ... decades-long foot-dragging in implementing legally required stream protections in East Maui, to the bald-faced lies about a lack of water for firefighting on Maui,” Townsend said. “Powerful special interests have pulled political strings to override the public interest for far too long,”
Prep for the polls: See who is running for president and compare where they stand on key issues in our Voter Guide
More: Hawaii legislature wraps up session - here's what passed and what didn't
On August 8, 2023, Lahaina, a historic town on Maui Island, fell victim to the deadliest wildfire in modern U.S. history. The blaze ravaged the community, reducing it to ashes, claiming the lives of over 100 individuals and displacing over 5,000 residents.
Last month, the Hawaii Supreme Court ruled that the Attorney General and BLNR attempted to exploit the aftermath of the Lahaina fire by submitting false accusations regarding a judge’s decision for East Maui streams.
The court determined that their submitted statements, aimed at increasing stream water diversion by Alexander & Baldwin, a former plantation now a real estate investment trust, were so evidently lacking in merit that they suggested bad faith.
“It seems that the BLNR tried to leverage the most horrific event in state history to advance its own interests,” according to the court’s decision.
Despite the submission of over 500 pages of written testimony in favor of the bill, Lopez and DLNR chair Dawn Chang stood as the sole voices who opposed it.
More: Hawaii is the first state to pass a Gaza ceasefire resolution. Here's what happens next
In November 2021, a major water contamination incident struck the facility catering to approximately 93,000 residents in and around Joint Base Pearl Harbor-Hickam.
In response to numerous reports of illnesses among local families who consumed the tainted water, the Pentagon announced plans in March 2022 to close Red Hill permanently.
Despite these actions, concerns persist among residents about water quality. Many continue to report symptoms akin to those experienced during the 2021 contamination, including rashes, headaches, heartburn, and fatigue.
A former Navy veteran, Lacey Quintero, told USA Today that she believes the Navy is aware that the Red Hill water is still contaminated and is misleading the public by claiming it is safe to drink.
What would the bill have done?
At its core, this bill aimed to minimize political interference in resource management decisions. It proposed empowering the Hawaiʻi Commission on Water Resource Management with the authority to select its own attorney and Chairperson, roles currently under gubernatorial control.
Additionally, it sought to protect Commission staff from arbitrary disciplinary actions, a concern brought to light by the departure of a former water deputy following the Maui wildfires.
The bill would have also established dedicated commission staff and a special fund to manage federal funding allocated for the cleanup of the Red Hill contamination.
Just before the sessions ended, State House and Senate Conferences agreed to defer the bill. In an interview with Spectrum News, Sen. Lorraine Inouye (D), chair of the Senate Committee on Water and Land, expressed her frustration.
“I am greatly disappointed in the outcome on (the bill) despite agreement between both legislative bodies that this bill is urgently needed and very important—not just for Red Hill but also for Lahaina,” Inouye said.
Jeremy Yurow is a politics reporting fellow based in Hawaii for the USA TODAY Network. You can reach him at [email protected] or on X, formerly Twitter @JeremyYurow

A .gov website belongs to an official government organization in the United States.
A lock ( ) or https:// means you've safely connected to the .gov website. Share sensitive information only on official, secure websites.
- Outbreaks of Vibrio Infections
- Clinical Overview
- Surveillance
- Publications
About Vibrio Infection
- Vibrio are bacteria that naturally live in coastal waters.
- About a dozen kinds of Vibrio can cause people to get an infection called vibriosis.
- People can get vibriosis after swallowing Vibrio or getting it in a wound.
- Vibrio infection can be serious. Know when to seek medical care.
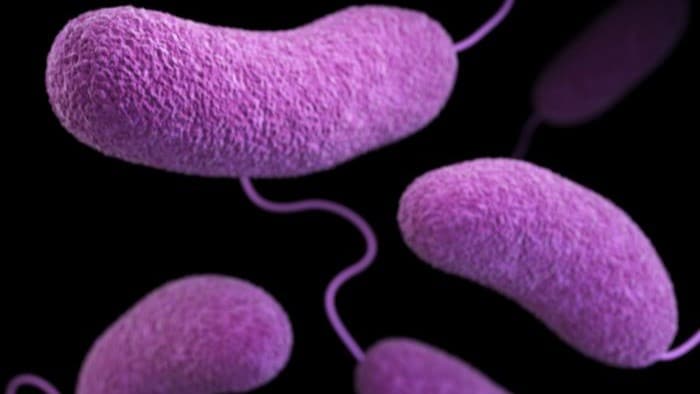
What are Vibrio ?
Vibrio are bacteria that naturally live in certain coastal waters. They are found in higher numbers in May through October, when water temperatures are warmer.
Coastal waters include salt water and brackish water. Brackish water is a mixture of salt water and fresh water. It is often found where rivers meet the ocean.
What is vibriosis?
About a dozen species (kinds) of Vibrio can cause a human illness called vibriosis. The most common species causing human illness in the United States are
- Vibrio parahaemolyticus
- Vibrio vulnificus
- Vibrio alginolyticus
Keep in mind
How do people get vibriosis.
Most people get vibriosis by eating raw or undercooked shellfish, particularly oysters .
Some people get vibriosis after an open wound comes in contact with coastal waters.
How common is vibriosis?
CDC estimates that:
- 80,000 cases of vibriosis happen each year in the United States.
- 52,000 cases of vibriosis are the result of eating contaminated food.
Common signs and symptoms of Vibrio infection
- Watery diarrhea
- Stomach cramps
Signs and symptoms of Vibrio bloodstream infection
- Dangerously low blood pressure
- Blistering skin lesions
Signs and symptoms of Vibrio wound infection
- Discoloration (turning a color other than normal)
- Discharge (leaking fluids)
Complications
Some Vibrio species, such as Vibrio vulnificus , can cause severe and life-threatening infections.
Some Vibrio infections lead to necrotizing fasciitis , a severe infection in which the flesh around an open wound dies. Some media reports call Vibrio vulnificus "flesh-eating bacteria." However, public health experts believe group A Streptococcus are the most common cause of necrotizing fasciitis in the United States.
Many people with Vibrio vulnificus infection can get seriously ill and need intensive care or limb amputation. About 1 in 5 people with this infection die, sometimes within a day or two of becoming ill.
When to seek emergency care
People at risk.
Anyone can get a Vibrio infection.
Some medical conditions and treatments can increase your risk for infection and severe complications. They include:
- Having liver disease, cancer, diabetes, HIV, or thalassemia
- Receiving immune-suppressing therapy for the treatment of disease
- Taking medicine to decrease stomach acid levels
- Having had recent stomach surgery
Some behaviors also can increase your risk of infection. These include:
- Eating raw seafood, particularly oysters
- Exposing an open wound to coastal waters
- Exposing an open wound to raw seafood or its drippings
Diagnosis and testing
Infection is diagnosed when Vibrio are found in the wound, blood, or stool (poop) of an ill person.
If you have signs and symptoms of infection, be sure to tell your healthcare provider if
- You recently ate raw or undercooked seafood, especially oysters
- Coastal water, including sale water or brackish water
- Raw or undercooked seafood or its drippings
Antibiotics are not recommended to treat mild Vibrio infections. People with diarrhea or vomiting should drink plenty of liquids to prevent dehydration. Dehydration is not having enough fluids in the body.
Antibiotics can be used to treat severe or prolonged Vibrio infections.
Vibrio wound infection is treated with antibiotics and surgery to remove dead or infected tissue. Surgery might include amputation.
Vibrio Infection
Some kinds of Vibrio cause an illness called vibriosis. Most people become infected by eating raw or undercooked shellfish, particularly oysters.
For Everyone
Health care providers, public health.
INTEGRATED WATER RESOURCES MANAGEMENT: THEORY AND PRACTICE
- Conference paper
- Cite this conference paper
- A. Dan Tarlock 4
Part of the book series: NATO Science Series ((NAIV,volume 77))
648 Accesses
1 Citations
Water resource planning and management are undergoing a paradigm shift. Historically, rivers have been viewed as communities to be exploited to the maximum extent possible for economic development. Water resource planning has primarily been an engineering exercise to achieve the optimum development of river basins for hydropower, flood control and consumptive use. Throughout the world, countries have constructed large-scale multiplepurpose dams and irrigation systems. Both international and domestic water law has supported optimum development by (1) creating semi-exclusive national rights to divert and store water and (2) and encouraging unilateral national water resources development.Water management meant the enforcement of existing entitlements and adherence to the original project purposes. The traditional vision of a river system of a commodity to be put to the optimum or maximum use remains the dominant paradigm in many parts of the world such as China, Central Asia, India and other areas as a matter of choice or necessity. However, the traditional paradigm is slowly being replaced by the alternative paradigm of ecologically sustainable development (ESD).
This is a preview of subscription content, log in via an institution to check access.
Access this chapter
- Available as PDF
- Read on any device
- Instant download
- Own it forever
- Compact, lightweight edition
- Dispatched in 3 to 5 business days
- Free shipping worldwide - see info
- Durable hardcover edition
Tax calculation will be finalised at checkout
Purchases are for personal use only
Institutional subscriptions
Unable to display preview. Download preview PDF.
Author information
Authors and affiliations.
Distinguished Professor of Law, Chicago-Kent College of Law, Chicago, IL, 60661-3691, USA
A. Dan Tarlock
You can also search for this author in PubMed Google Scholar
Editor information
Editors and affiliations.
University of Dundee, U.K.
Patricia Wouters
Scientific Information Center of Interstate Coordination, Water Commission Tashkent, Uzbekistan
Victor Dukhovny
Andrew Allan
Rights and permissions
Reprints and permissions
Copyright information
© 2007 Springer
About this paper
Cite this paper.
Tarlock, A.D. (2007). INTEGRATED WATER RESOURCES MANAGEMENT: THEORY AND PRACTICE. In: Wouters, P., Dukhovny, V., Allan, A. (eds) Implementing Integrated Water Resources Management in Central Asia. NATO Science Series, vol 77. Springer, Dordrecht. https://doi.org/10.1007/978-1-4020-5732-8_1
Download citation
DOI : https://doi.org/10.1007/978-1-4020-5732-8_1
Publisher Name : Springer, Dordrecht
Print ISBN : 978-1-4020-5730-4
Online ISBN : 978-1-4020-5732-8
eBook Packages : Earth and Environmental Science Earth and Environmental Science (R0)
Share this paper
Anyone you share the following link with will be able to read this content:
Sorry, a shareable link is not currently available for this article.
Provided by the Springer Nature SharedIt content-sharing initiative
- Publish with us
Policies and ethics
- Find a journal
- Track your research
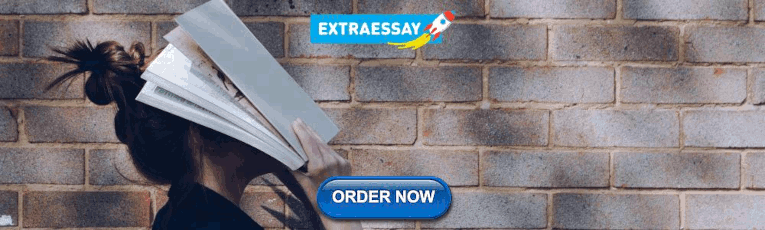
IMAGES
VIDEO
COMMENTS
Water management policies must also about ensuring sufficient water to produce the energy demanded by society. Humans and their economies and societies critically depend on reliable supplies of energy. Energy, as electricity and liquid and gaseous fuels, available when and where needed, requires water to produce, such as for cooling and ...
Water management theories and practices in the planning and design of cities and towns. ... but it necessitates landscape design to update traditional settlement arrangements in theory and practice. 3.1 Theories on water sensitive cities Cities have evolved around a river or a coastline bearing an osmotic relationship, yet although water is ...
Water scarcity and its geopolitical implications have been a cornerstone of scholarly discourse. However, literature often overlooks the nuanced relationship between human traits and water management. Addressing this oversight, this study synthesized data from 149 articles (1991-2023), revealing a substantial connection between human actions and water management dynamics. From this data, a ...
The answer to this question is equally open. This special feature on participatory water management attempts to propose responses to these three questions by outlining recent advances in theory, practice, and evaluation related to the implementation of participatory water management. The feature is largely based on an extensive range of case ...
Game theory approaches have been applied to deal with water resource management given that water is a common-pool resource subject to strategic behavior. As climate change is expected to result in water scarcity and the deterioration of water quality around the world, water quantity and quality are becoming key challenges for water managers and ...
2. Significance of water quality modeling. Water quality management is an essential component of overall integrated water resources management (UNESCO, Citation 2005).The output of the model for different pollution scenarios with water quality models is an imperative component of environmental impact assessment (Q. Wang et al., Citation 2013).Sound water quality is very limited in the world ...
Authors: Naim Haie. Provides a solid foundation for a comprehensive, systemic, and water-centric approach to water management. Integrates two performance principles essential for sustainable water use systems—equity and efficiency. Presents a transparent and autonomous structure for gathering water data. Part of the book series: Water ...
The papers included in our review of the empirical literature were selected based on the following criteria: 1. The paper assesses the impact of WCTs, including sprinkler or drip irrigation systems, laser leveling of fields, piped delivery systems, canal lining, and rehabilitation of irri- gation and delivery systems.
Hence, game theory is used to predict agents' behaviors toward fulfilling their own interests during the interactive decision-making process with other agents.Since the 1950s, game theory has become an important tool for analyzing important aspects of water resource management.
This paper provides an overview of the Special Issue on water supply and water scarcity. The papers selected for publication include review papers on water history, on water management issues under water scarcity regimes, on rainwater harvesting, on water quality and degradation, and on climatic variability impacts on water resources. Overall, the issue underscores the need for a revised water ...
Along with the companion that focuses on water contamination with nutrients (Teklitz et al., 2020), this paper presents a geospatial application of reliability theory to water resources management and sustainability. We call this tool STREAMS (STochastic Reliability-based Risk Evaluation And Mapping for watershed Systems and Sustainability).
1 Introduction. Water resource management must balance multiple priorities, needs and uses of water. The complexity of the human system (how people use water and how their actions impact water resources) coupled with the complexity of the natural system (how much water is available and how it responds to contamination and use) requires an integrated, holistic and interdisciplinary approach ...
Water quality is judged by water's chemical constituent concentrations and general quality parameters, like dissolved oxygen and suspended solids, against water quality criteria established for the intended use of the water, which can vary from water supply to industrial discharge conveyance. Water quality management is the control of ...
1. Introduction. Water pollution is a transboundary environmental problem manifesting across different political and institutional jurisdictions. The dynamics of water pollution emerges throughout scale, space, and time requiring a substantial amount of resources for management, which many local governments do not have the capacity or commitment to invest in. Water pollution cannot be ...
In this synthesis, we assess present research and anticipate future development needs in modeling water quality in watersheds. We first discuss areas of potential improvement in the representation of freshwater systems pertaining to water quality, including representation of environmental interfaces, in-stream water quality and process interactions, soil health and land management, and (peri ...
24 3 Integrated Water Resource Management: Principles and Applications. Development in Rio de Janeiro in 1992. Chapter 18 emphasized the need for an. integrated approach to manage water resources ...
Hypothesis 1: Compared to those supplied by other sources, well owners… : 1a: perceive their water quality as higher. : 1b: evaluate well water as higher on quality, reliability, health, and safety than water from other supplies (i.e., GWS and the public mains). : 1c: show stronger positive affective responses to well water. Hypothesis 2: Compared to those supplied by other sources, well ...
The present study applied a multi-criteria analysis to evaluate the best approach among six theoretical frameworks related to the integrated management of water-environmental resources, analyzing the frequency of multiple management criteria. The literature review covers the period from 1990 to 2015, with a notable presence of the theoretical frameworks of Integrated Water Resources ...
The Water Management and Development Project in Uganda improved the integration of water resources planning, management and development, as well as access to water and sanitation services in priority urban areas. More than 1.01 million people received access to improved water sources, and 25,000 piped household water connections were ...
Water-related issues in transboundary basins are generally complicated by the challenges of climate change, the historical evolution of the basin characteristics, and the different interests of the riparian countries. Therefore, dealing with water-sharing and water cooperation problems among basin countries needs to be based on multi-factor system analysis in the context of regional water ...
The continent of Africa is urbanizing rapidly and the demand for water in towns and cities is increasing. However, urban water management challenges have contributed to a crisis in demand and supply. The chapter assesses the study types, theories and empirical findings on water management in African cities. The chapter attempts to answer the ...
PID controller parameters proportional, integral and differential gain (Kp, Ki, Kd) are tuned with the help of Fuzzy Logic. The ranges of Kp, Ki and Kd are the fuzzy rules for the controller. Triangular geometric function-related type is used as fuzzy. The Simulink model for water level of boiler system controlled by Fuzzy Logic is developed.
Columbia, N.C. — The U.S. Fish and Wildlife Service released the Pocosin Lakes National Wildlife Refuge Water Management Plan on Aug. 8, 2023, which provides a roadmap for refuge management in the coming years in a way that provides a clear understanding of the interconnectedness of water movement through the refuge and communities surrounding it.
2025 Game Code - Recommended Changes. 2025 Game Code - Game Committee Recommendations. NJDEP| Fish & Wildlife | 2025 Game Code - Recommended Changes | Post Description.
Hawaii residents disappointed as bill to safeguard water resources fails at sessions end. Hawaii residents are disappointed that the Aloha State's lawmakers ended the legislative session without ...
The authors annually evaluated the reduction in the use of water (2.5 10 5 tons), nitrogen (5.6 NH 3 -N tons), and carbon emissions (134 tons) through the reuse of treated sewage. Advances in the management of solid waste and wastewater treatment include landfilling, the composting of organic matter, reductions in greenhouse gas emissions and ...
UK's water firm united Utilities forecasted higher 2024-2025 costs due to regulatory charges it might have to pay for overflows from high rainfall, even as it guided a 10% rise in revenues helped ...
Vibrio are bacteria that naturally live in certain coastal waters. They are found in higher numbers in May through October, when water temperatures are warmer. Coastal waters include salt water and brackish water. Brackish water is a mixture of salt water and fresh water. It is often found where rivers meet the ocean.
Both international and domestic water law has supported optimum development by (1) creating semi-exclusive national rights to divert and store water and (2) and encouraging unilateral national water resources development.Water management meant the enforcement of existing entitlements and adherence to the original project purposes.